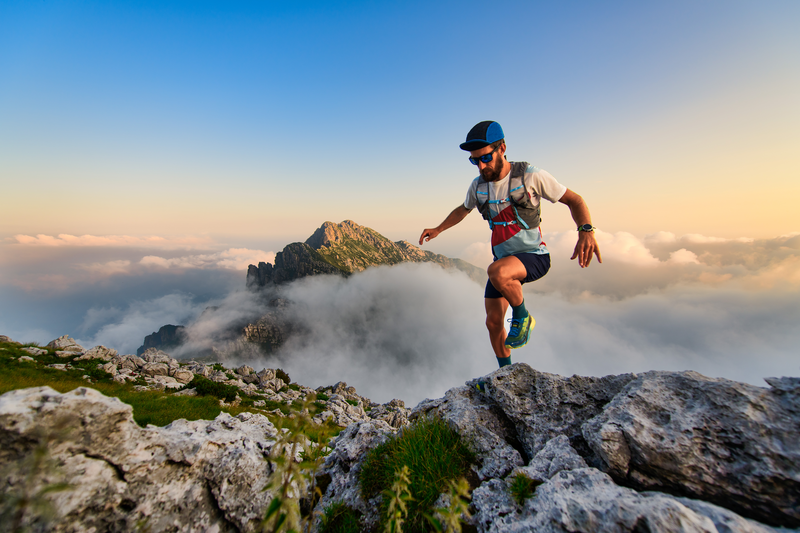
95% of researchers rate our articles as excellent or good
Learn more about the work of our research integrity team to safeguard the quality of each article we publish.
Find out more
REVIEW article
Front. Plant Sci. , 21 March 2022
Sec. Plant Pathogen Interactions
Volume 13 - 2022 | https://doi.org/10.3389/fpls.2022.847199
This article is part of the Research Topic Disease and Pest Resistance in Rice View all 6 articles
Bacterial blight (BB) of rice caused by Xanthomonas oryzae pv. oryzae (Xoo) is one of the most serious bacterial diseases that hinder the normal growth and production of rice, which greatly reduces the quality and yield of rice. The effect of traditional methods such as chemical control is often not ideal. A series of production practices have shown that among the numerous methods for BB controlling, breeding and using resistant varieties are the most economical, effective, and environmentally friendly, and the important basis for BB resistance breeding is the exploration of resistance genes and their functional research. So far, 44 rice BB resistance genes have been identified and confirmed by international registration or reported in journals, of which 15 have been successfully cloned and characterized. In this paper, research progress in recent years is reviewed mainly on the identification, map-based cloning, molecular resistance mechanism, and application in rice breeding of these BB resistance genes, and the future influence and direction of the remained research for rice BB resistance breeding are also prospected.
As a food crop closely related to human beings, rice is related to the food needs of many people in China and the world. Relevant data show that the annual yield of rice accounts for about 43% of China’s total grain output, and it is an important food crop in China. Therefore, stable yield and yield increase in rice are important measures to ensure food security. However, in the growth process of rice, many diseases inevitably occur. On the one hand, the occurrence of these diseases seriously reduces the yield and quality of rice, and on the other hand, it also restricts the development of social economy.
Bacterial blight (BB) of rice caused by Gram-negative bacteria Xanthomonas oryzae pv. oryzae (Xoo) is one of the most serious bacterial diseases that hinder the normal growth and production of rice and is a global bacterial disease of rice (Gnanamanickam et al., 1999). Rice BB is a kind of bacterial disease. Although it is different from fungal spores or rice planthoppers and other pests by means of atmospheric circulation remote transmission, it can be transmitted to other countries with wind, rain, or diseased rice varieties. Since its first discovery in Japan in 1884, the disease has spread to major rice-producing countries such as East Asia and Southeast Asia and has now occurred to varying degrees in Africa, the Americas, South Europe, and Australia in addition to in Asia (Gonzalez et al., 2007). Related studies have shown that the yield reduction rate of 20–30% by moderate infection of BB in the field can be as high as 50% in severe cases, or even absolute yield.
Rice BB is a typical disease in line with the “gene-to-gene” hypothesis. Due to the continuous differentiation of Xoo physiological races and the emergence of new virulent pathogen populations, some rice germplasm resources with resistance genes will lose their resistance after planting for a period of time (Waikhom et al., 2015). In the past century, scientists from all over the world have done a lot of research on the disease control of rice BB. However, both biological control and chemical control have failed to achieve the ideal control effect. A series of practical breeding practices have also shown that among the numerous means of BB control, the research and cultivation of resistant rice varieties are the most economical, effective, and environmentally friendly, and the foundation and key to the success of disease resistance breeding are the excavation of disease resistance genes.
It is a long process from identification to mapping of rice resistance genes. As the smallest species in Gramineae, the whole genome sequencing of rice has been completed, which provides great convenience for the mapping of BB resistance genes (Yu et al., 2002).
Up to now, there are 44 rice BB resistance genes confirmed by international registration and reported in journals. Among them, 28 were dominant disease resistance genes (Xa2 and Xa31 belonged to the same, and Xa3 and Xa26 belonged to the same), 16 were recessive disease resistance genes [xa5, xa8, xa13, xa15, xa19, xa20, xa24, xa25, xa28, xa32, xa33, xa34, xa41, xa42, xa42 (2), and xa44], 37 had been mapped, and 15 had been cloned (Xa1, Xa2/Xa31, Xa3/Xa26, Xa4, xa5, Xa7, Xa10, xa13, Xa14, Xa21, Xa23, xa25, Xa27, xa41, and Xa45).
According to the location of the genes that have been mapped at present, there are different numbers of BB resistance genes on 10 chromosomes except rice chromosomes 9 and 10 (Figure 1). Most resistance genes were distributed on chromosome 11, nearly 1/3. In addition, more resistance genes were distributed on chromosomes 4 and 6. This indicated that rice BB resistance genes were clustered on chromosome. The location of these resistance genes on chromosomes laid a foundation for the deep cloning of genes and their application in rice disease resistance breeding.
Up to now, the resistance genes to BB in rice that have not been mapped are derived from mutation and local varieties, with a total of seven genes, namely xa15, Xa16, Xa17, Xa18, xa19, xa20, and xa28. xa15, a recessive gene resistant to BB of rice, is derived from the mutant of variety M41 and has resistance to Japanese races I, II, III, and IV (Nakai et al., 2008). Xa16, a dominant gene resistant to BB of rice, is derived from the variety Tetep and has resistance to Japanese race V (Ogawa, 1993). Xa17, a dominant gene resistant to BB of rice, is derived from the variety Asominori and has resistance to Japanese race II (Ogawa, 1993). Xa18, a dominant gene resistant to BB of rice, was derived from Toyonishiki, Milyang 23, IR24, and was resistant to Myanmar strain H8584 (Ogawa, 1993). xa19, a recessive gene resistant to BB of rice, is derived from the mutant XM5 of variety IR24 and has resistance to six Filipino races (Satoru et al., 2008). xa20, a recessive gene resistant to BB of rice, is derived from the mutant XM6 of variety IR24 and has resistance to six Filipino races (Taura et al., 1992b). xa28, a recessive gene resistant to BB of rice, is derived from the variety Lota Sail and resistant to race 2 of the Philippines (Lee et al., 2003).
Two resistance genes were located on rice chromosome 1: Xa29 and xa34.
Xa29, a dominant gene resistant to BB of rice, was derived from the penetration line B5 of medicinal wild rice and was resistant to the Philippine race PXO61. The gene was located between RFLP markers C904 and R596 on chromosome 1, and the genetic distance was 1.3 cM (Tan et al., 2004).
xa34, a recessive gene resistant to rice BB, derived from Sri Lanka variety BG1222, is resistant to Chinese race 5226. The gene was located between the markers RM10929 and BGID25 on chromosome 1, with a genetic distance of 0.2 cM and a physical distance of about 204 kb, and was co-segregated with markers BGID34 and BGID36 (Shen et al., 2011). The F2 genetic segregation population was constructed using xa34 vector variety BG1222 as the resistance donor and JG30 as the susceptible variety. The disease resistance was identified by screening the recombinants and developing the recombinant F3 family, which was finely located on chromosome 1 of rice and located in the 56.47 kb region between the molecular markers RM10926 and InDelnv7 (Shen et al., 2011).
Only one resistance gene was located on rice chromosome 2: xa24.
This gene is a recessive gene against rice BB. This gene was identified in DV86 and then confirmed as a new gene. The gene was resistant to Filipino races 4, 6, 10, and Chinese strains Zhe173, JL691, and KS-1-21 during the whole growth period and was mapped between SSR markers RM14222 and RM14226 with a genetic distance of 0.14 cM and a physical distance of 71 kb (Khush and Angeles, 1999; Wu et al., 2008a).
Two resistance genes were mapped on rice chromosome 3: Xa11 and xa42.
Xa11, a dominant gene resistant to rice BB, is derived from IRBB11 (in the genetic background of IR24), resistant to Japanese races IB, II, IIIA, and V. The gene was located between markers RM347 and KUX11 at the short arm of chromosome 3, and the genetic distances from the two markers were 2.0 and 1.0 cM, respectively (Goto et al., 2012).
xa42, a recessive gene resistant to BB of rice, was derived from the mutant XM14 of IR24 which was resistant to six Japanese races. The gene was identified by linkage analysis around the chromosome 3 centromere region between DNA markers KGC3_16.1 and RM15189 (Constantine et al., 2016). After that, the gene was further narrowed to the 57 kb chromosome region between DNA markers KGC3_16.341 and KGC3_16.399 (Busungu et al., 2018). The gene was co-segregated with two DNA markers KGC3_16.370 and KGC3_16.371.
Seven resistance genes were located on chromosome 4 of rice: Xa1, Xa2/Xa31, Xa12, Xa14, Xa25, Xa38, and Xa45.
Xa1 is resistant to rice BB dominant gene, resistant to Japanese strains X-17, T7174, etc. The gene was identified from cultivated rice Kogyoku and Java14 and was located on chromosome 4 (Sakaguchi, 1967). The gene was subsequently found to be closely linked to three RFLP markers XNpb235, XNpb264, and C600 (Yoshimura et al., 1996).
Xa2 is resistant to rice BB dominant gene, resistant to Japanese strains X-17, X-14, etc. The gene was located on chromosome 4 with a linkage rate of 2–16% with Xa1. The gene was fine mapped in the 190 kb region between markers HZR950-5 and HZR970-4 by SSR (He et al., 2006). Xa31 is located in the 0.2 cM interval between G235 and C600 at the long arm end of chromosome 4 in rice, and the physical region of this interval in Minghui 63 is about 100 kb (Ogawa et al., 1978). Xa2 and Xa31 have the same sequence, which is actually the same gene.
Xa12, a dominant gene resistant to BB of rice, is derived from rice variety Java14 and resistant to Indonesian race V (Bao et al., 2006). This gene is located at the long arm end of chromosome 4 (Taura et al., 1992a). Xa1 is closely linked to Xa12.
Xa14, a dominant gene resistant to rice BB, is resistant to race 4 in the Philippines. This gene is preliminarily located on chromosome 4 through rice trisomic technology (Tan et al., 1998). The F2 population was constructed by using susceptible pearl dwarf and near-isogenic line CBB14 containing Xa14 as parents, and then, Xa14 was located in the 0.21 cM interval between RG620 and G282 by RFLP analysis. By using IR24 × IRBB14 hybrid F2 to isolate 599 individuals, Xa14 was finally finely mapped between molecular markers 52940 and RM5473 (Wang et al., 2009).
Xa25, a dominant gene resistant to rice BB, is derived from a somatic mutant HX3 and is resistant to Philippine races 1, 3, and 4. The gene was mapped between two SSR markers RM6748 and RM1153 at the end of the long arm of chromosome 4 by linkage analysis, and the linkage distances were 93 and 30 cM, respectively (Ying et al., 2002).
Xa38, a dominant gene resistant to BB of rice, was identified from Oryza nivara IRGC81825 and was resistant to all seven prevalent pathogenic Xoos in northern India (Cheema et al., 2008). The gene was mapped within the genetic distance of 35 cM at the long arm of chromosome 4 through 191 polymorphic SSR markers and located between markers RM317 and RM562. Through further screening, the gene was finally located between marker RM17499 and STS markers based on annotated genes LOC_Os04g53060 and LOC_Os04g53120, about 38.4 kb distance.
Xa45, a dominant gene resistant to rice BB, is resistant to AXO1947 and T7174. The gene is an allele of Xa1, which is distributed on chromosome 4 and also encodes NLR-like proteins, and was successfully cloned recently (Biaoming et al., 2020).
Only one resistance gene was located on chromosome 5 of rice: xa5.
This gene is a recessive gene for resistance to BB of rice. Three varieties DV85, DV86, and DZ78 from Bangladesh are resistant to PXO61 and PXO86. The gene was located between SNPS marker RS7 at the short arm end and SSR marker RM611, with a genetic distance of 0.5 cM and a physical distance of 70 kb (Blair et al., 2003).
Four resistance genes were located on chromosome 6 of rice: Xa7, Xa27, xa33, and xa42 (2).
Xa7, the dominant gene for resistance to BB of rice, was derived from three varieties DV85, DV86, and DZ78 from Bangladesh. It was resistant to PXO61, PXO86, PXO79, and SCB4-1 and was the dominant gene for resistance at booting stage. The gene was located between markers GDSSR02 and RM20593, and the genetic distances from markers were 0.07 and 0.14 cM, respectively. The gene was further locked in a range of 28 kb through map cloning and radiation mutagenesis (Chen et al., 2021).
Xa27, a dominant gene resistant to BB of rice, was derived from tetraploid small-grain wild rice and resistant to race 2, 5 of the Philippines. The gene was located in the range of 0.052 cM between RFLP markers M964 and M1197 and was co-segregated with RFLP markers M631, M1230, and M449. RGP markers C12560S and S12715 were of RFLP markers M964 and M1197, and the genetic distance between them was 0.9 cM (Gu et al., 2004).
xa33, a recessive gene resistant to BB of rice, was derived from the variety Ba7 and resistant to the Thai race TXO16. The gene was located between RM30 and RM400 by SSR marker-assisted selection. Xa33 and Xa7 are closely linked to SSR markers RM20590, but the resistance characteristics are significantly different from Xa7 and Xa27 (Kumar et al., 2012).
xa42 (2), a recessive gene resistant to rice BB, was derived from a self-bred variety Baixiangzhan (BXZ). xa42 (2) showed broad-spectrum resistance to five Xoo pathogenic types in China, including the epidemic high-toxic Xoo pathogenic Chinese Race V (CV). The gene was mapped on chromosome 6 by linkage analysis and restricted to 3.9 cM region by genetic mapping, flanked by RM20558/RM20547 and RM20580 (Liang et al., 2017). The other seven markers were developed from this interval for high resolution mapping, and xa42 (2) was reduced to a 34.8 kb fragment defined by RM20572 and DT46.
Two resistance genes were located on rice chromosome 7: xa8 and Xa33.
xa8 is a recessive gene resistant to rice BB, PI231129 from the United States, a strain resistant to northwestern India, with a genetic distance of 19.9 cM to marker RM214 (Sidhu et al., 1978). With the emergence of new SSR markers in the region, the gene was located between two continuous SSR markers, 7.0 and 9.9 cM, RM21044 and RM21045, respectively (Vikal et al., 2014).
Xa33, a dominant gene resistant to rice BB, was derived from Oryza nivara IRGC105710. The material showed broad-spectrum high resistance to BB and resistance to Indian race I-VII. The gene was initially mapped within 36.3 cM distance between RM5711 and RM6728 on chromosome 7 by 72 SSR markers. Subsequently, through the 2011 individuals of BC2F2 population, the gene was finally finely mapped within the genetic distance of 2.1 cM between RMWR7.1 and RMWR7.6 (Natraj et al., 2012).
Only one resistance gene located on rice chromosome 8: xa13.
This gene is a recessive gene for resistance to BB of rice, derived from varieties BJ1 and DV85, and has specific resistance to race 6 of the Philippines (Ogawa, 1993). The gene was further mapped to a 9.2 kb fragment by recombination and a newly developed CAPS marker ST12 (Chu et al., 2006).
Fifteen resistance genes were located on chromosome 11 of rice: Xa3/Xa26, Xa4, Xa10, Xa21, Xa22, Xa23, Xa30, Xa32, Xa35, Xa36, Xa39, Xa40, xa41, Xa43, and xa44.
Xa3/Xa26, Xa22, and Xa4 were dominant genes resistant to rice BB and were located on M3H8 subclones at the end of the long arm of chromosome 11, between markers RM224 and RM114. The genetic distances between Xa3/Xa26, Xa22, and Xa4 and RFLP marker R1056 were 0, 0.4, and 0.5 cM, respectively. Marker R1506 was located at 116.2 cM on chromosome clone OSJBa0047M04 (Chuntai et al., 2003; Yang et al., 2003). By analyzing the sequence of BAC clone 3H8, RKb gene was identified as Xa26 gene (Sun et al., 2004). Xa3/Xa26 was finely mapped on a 20 kb chromosome fragment of BAC clone M3H8 through the utilization of five double-exchange resistant plants of “Zhenshan 97”/“Minghui 63” recombinant inbred lines and finally cloned successfully in a BAC clone M3H8 of MH63 (Yi et al., 2006).
Xa10, a dominant gene resistant to rice BB, derived from cultivar Cas209, has specific resistance to four races in the Philippines (Yoshimura et al., 1983). The gene was located on chromosome 11, with a map distance of 5.3 cM from RAPD molecular marker O072000. Through linkage analysis of RFLP markers and F2 mapping population, Xa10 was mapped to the proximal side of E1981S with a genetic distance of 0.93 cM (Gu et al., 2008). Based on five new RFLP markers developed from Nipponbare genome sequence, Xa10 was fine mapped between the proximal marker M491 with a genetic distance of 0.28 cM and the distal marker M419 with a physical distance of 74 kb. Finally, Xa10 was successfully map cloned (Dongsheng et al., 2014).
Xa21, a dominant gene resistant to rice BB, was derived from long-drug wild rice, resistant to races 1–6 in the Philippines. Xa21 gene was located in the 8.5 cM interval on chromosome 11 (Ronald et al., 1992). At the same time, Xa21 gene was co-segregated with molecular markers such as RG103, RAPD818, and RAPD248. By using the molecular marker RG103 as a probe to screen the 16 overlapping clones, Xa21 gene was identified in a 9.6 kb fragment (Song et al., 1995).
Xa23 is a dominant gene resistant to rice BB. Xa23 gene has a wide spectrum of resistance. There are 20 domestic and foreign BB identification strains resistant to Philippine race 1–10, Chinese pathogenic race 1–7, and Japanese race 1–3. Xa23 was identified in 1998 and then transferred to “Jingang 30” to develop a near-isogenic line CBB23 (Li et al., 2006). By using the F2 generation of hybrid between “Jingang 30” and CBB23 as the mapping population, molecular markers closely linked to Xa23 were searched, BAC library and Shotgun library were constructed, and the corresponding sequencing and genetic transformation were carried out. Finally, Xa23 gene was successfully cloned (Chunlian, 2006). The gene is located between markers C189 and RM206, and the genetic distances are 0.8 and 1.9 cM, respectively. The genetic distance from the RFLP marker C1003A on the same side of C189 is 0.4 cM. The markers C1003A and RM206 cover five clones: OSJNBa0072L08, OSJNBa006K21, P0480H08, OSJNBa0029K08, and B1356F10, and the genetic position is 84.6–88.4 cM.
Xa30, a dominant gene resistant to rice BB, is derived from common wild rice variety Y238 and is resistant to PXO99 (Philippine race 6). Four markers RM1341, V88, C189, and STS03 that reveal resistance and susceptibility polymorphism were screened from 343 molecular markers and then used for molecular detection and linkage analysis of 303 individuals in BC6F2 population. The results showed that the above four markers were all located in the long arm of chromosome 11 of rice, and the genetic distances with Xa30 were 11.4, 11.4, 4.4, and 2.0 cM, respectively, and they were located on the same side of Xa30 gene (Jin et al., 2007). The genetic distance between Xa30 and marker STS03 was 2.0 cM, closer to the end of long arm than Xa23, and the genetic distance between RM206 and RM224 was more than 10 cM. Therefore, the position of Xa30 on chromosome was closer to centromere than that of Xa3/Xa26.
Xa32 (Zheng et al., 2009), Xa35 (Guo et al., 2010), and Xa36 (Miao et al., 2010) were all dominant genes for resistance to BB of rice. Xa32 comes from C4064, resistant to Pr I, IV-IX, and KXO85. Xa35 is derived from Acc. No.101133, resistant to PXO61, PXO112, and PXO339. Xa36 comes from C4059, resistant to PXO99. The three were all located at the long arm end of chromosome 11. The genetic distances between Xa4 and Xa36 and the marker RM224 were 1.1 and 1.3 cM, respectively. It was speculated that Xa36 was closer to the long arm end than Xa4. Xa35 was co-segregated with the marker RM114, closest to the long arm end. The genetic distances between Xa32 and Xa36 and the marker RM5926 were 2.6 and 3.8 cM, respectively. It was speculated that Xa32 was between Xa36 and Xa35. Five pairs of polymorphic molecular markers were newly developed between resistant material Q143 and susceptible parent JG30, which were K58, K57, Y72FF, Y77, and Y99, respectively (Zheng et al., 2009). These five polymorphic markers were closely linked to the Xa32 gene, and Xa32 was located within 0.16 cM between the end marker Y72FF of the long arm of chromosome 11 and the marker Y77 of rice. Then, the physical map of Xa32 gene locus was constructed, and Xa32 gene was located within the range of about 70 kb at the long arm end of rice chromosome 11.
Xa39, a dominant gene resistant to BB of rice, was derived from a rice introgression line FF329, which was screened from BC1F4, a backcross progeny of PSBRC66 (P66) and Huang Huazhan (HHZ). Xa39 was located at a distance of 97.4 kb between RM26985 and DM13 on chromosome 11 (Zhang et al., 2015).
Xa40, a dominant resistance gene to rice BB, is a new resistance gene identified from indica rice IR65482-7-216-1-2, showing high level resistance to all Korean Xoo races, including the new Xoo race K3a (Kim et al., 2015). The gene was mapped to an 80 kb region between RM27320 and ID55.WA18-5 on chromosome 11, which contains eight predicted candidate genes.
xa41, a recessive gene resistant to rice BB, derived from Oryza barthii and Oryza glaberrima, is resistant to BAI3. After screening the polymorphism of 169 rice germplasms in the promoter of the susceptible gene OsSWEET14 for BB, the gene was found (Hutin et al., 2016). The deletion of OsSWEET14 gene promoter region18 bp represents a new resistance allele, namely xa41.
Xa43, a dominant gene resistant to rice BB, derived from P8, is resistant to Korean race HP01009. The target region was dissected by QTL analysis and fine mapping, and the mapping region of this gene was narrowed to 27.83–27.95 Mbp of PCR-based DNA markers IBb27os11_14 and S_BB11. ssr_9, with a physical interval of about 119 kb (Kim et al., 2019).
xa44, a recessive gene resistant to rice BB, derived from P6, is resistant to the Korean race HB1009. Due to the additional development based on PCR markers in the target region, the target region of SNP flanking (28.00–28.12 Mbp) was further narrowed. Through fine mapping of 520 BC1F2 individuals, R gene xa44 was identified in a fragment of about 120 kb, with DNA markers # 46. g0689400 and RM27318 on both sides (Kim, 2018).
Two resistance genes were located on rice chromosome 12: xa25 and xa32.
xa25, a recessive gene resistant to BB of rice, is derived from Minghui 63 and has transformation resistance to Philippine race 9 (PXO339) at seedling and adult stages. This gene is located at 9.5 cM between RFLP markers R887 and G1314 on chromosome 12 of rice (Chen et al., 2002).
xa32, a recessive gene resistant to BB of rice, is a new germplasm Y76 derived from the somatic hybridization between wild wart grain rice and cultivated rice in Xishuangbanna, Yunnan. It is resistant to PXO99 (race 6 of the Philippines), and the genetic distance from the marker RM20A is 1.7 cM (Ruan et al., 2008).
In summary, the basic information of 44 rice BB resistance genes that have been mapped can be found in Table 1.
After breaking through the first line of defense PTI, pathogenic microorganisms evolved effector proteins to inhibit the immune response caused by pathogen associated molecule patterns (PAMPs) in order to successfully infect plants. At the same time, plants have evolved R genes to recognize effectors and cause hypersensitive reaction of cells. Only when the avirulence genes of the pathogen and the R genes of the host cell exist at the same time, the plant disease resistance gene can play its own disease resistance function (Figure 2). Therefore, after gene mapping, people continue to clone new rice R genes to resist the infection of new avirulence genes. Next, the cloning and function of rice BB resistance gene will be described in detail.
Xa1 gene is the first reported NLR resistance genes in BB resistance gene. The gene was located in a YAC clone by DNA hybridization and recombination events and then isolated by map cloning (Yoshimura et al., 1996). But unlike the strategy of cloning Xa21, Xa1 was cloned by cDNA (Yoshimura et al., 1998). The gene contains four exons and three introns, one of which has no coding sequence. The gene encoding product is a cytoplasmic protein composed of 1,802 amino acids. Its amino end contains the NBS domain, and the carboxyl end contains the LRR functional domain, that is, the NBS-LRR-type resistance protein to BB (Yoshimura et al., 1996).
Xa1 is highly resistant to type I physiological race of BB in Japan and has specific resistance. Different from other resistance genes, the expression of Xa1 is induced by pathogen infection and trauma rather than constitutive expression. Studies have shown that the expression of Xa1 is induced by both compatible physiological races and incompatible physiological races, indicating that the damage caused by pathogen infection may induce the expression of Xa1 gene, and the increase in Xa1 gene expression may improve the efficiency of Xa1 interacting with an avirulence gene, thereby activating the Xa1-mediated disease resistance signaling pathway, so Xa1 plays a major role in the identification of pathogens (Ji et al., 2020). Recently, Xa2, Xa14, Xa31, and Xa45 have also been successfully cloned. These four genes, as alleles of Xa1, also encode NLR proteins (Ji et al., 2020; Zhang et al., 2020).
Xa3 was located in IRBB13, and Xa26 gene was identified in rice cultivar “Minghui 63.” After DNA fingerprinting analysis, fine mapping, phenotypic comparison after inoculation with Xoo and sequence analysis of candidate genes, these two genes were found to be the same gene and renamed as Xa3/Xa26 (Yoshimura et al., 1995; Peng et al., 1998; Yi et al., 2006). The Xa3/Xa26 gene was constitutively expressed. The coding region was 3,309 bp in length, with two exons and a 105 bp intron located on the cell membrane. The gene encodes a LRR receptor protein kinase composed of 1,103 amino acids, which belongs to the same type as Xa21. The structural characteristics of the two genes are very similar, including an amino-terminal signal peptide composed of 30 amino acids, an extracellular LRR domain composed of 26 incomplete LRRs, a single transmembrane region (TM), and an intracellular protein serine/threonine kinase domain (Sun et al., 2004). The amino acid of 88 ~ 771 encodes 26 incomplete leucine-rich repeats (LLRs), which are conserved sequences encoded by these genes. The main function is to identify specific pathogens and then stimulate disease resistance through signal transduction (Song et al., 1995).
Xa3/Xa26 belongs to LRR-STK resistance type and is a multi-gene family, including four members: RKa, RKb, RKc, and RKd. The gene is clustered and arranged on chromosome 11 with high conservation (Wang, 2015). It was found that the Xa3/Xa26 LRR domain may be involved in the identification of different Xoo races (Jing et al., 2009). Xa3/Xa26 is only specifically expressed in vascular bundles and surrounding cells and is low-level constitutive expression (Cao et al., 2007). The infection of pathogens had no obvious effect on the transcriptional expression intensity (Sun et al., 2004). The resistance of Xa3/Xa26 was affected by the growth period of rice. The rice containing Xa3/Xa26 was susceptible to PXO61 at seedling stage and resistant to PXO61 at adult stage. This was due to the dose effect of Xa3/Xa26. The expression level of Xa3/Xa26 was very low at the two-leaf stage and reached the highest at the tillering stage. The overexpression of Xa3/Xa26 at the seedling stage showed resistance to BB of rice. The resistance of Xa3/Xa26 is also affected by genetic background. Studies have shown that the broad and narrow resistance spectrum and the strength of resistance of Xa3 are significantly different under different genetic backgrounds, and even there is a phenomenon of recessive transformation, which is also the reason for the diversification of Xa3 gene naming (Xa4b, Xa6, and xa9). The resistance is different in different indica rice and japonica rice, but in general, the resistance of Xa3/Xa26 in japonica rice is stronger than that in indica rice (Cao et al., 2007). The Xa3/Xa26 gene family reflects the competition and co-evolution of rice BB resistance genes and pathogens. Some genes have been resistant to BB and then lost resistance. However, in the later evolution process, they have regained the function of disease resistance by point mutation (Cao et al., 2007).
Xa4 was located on the M3H8 subclone at the end of the long arm of chromosome 11 and located between markers RM224 and RM114 (Lee et al., 2003). The genetic distance between Xa4 and RFLP marker R1056 was 0.5 cM, and the marker R1506 was located at 116.2 cM on the chromosome clone OSJBa0047M04. Through recombinant test and sequence analysis, the Xa4 gene was fine mapped within 47Kb (Sun et al., 2003) and then successfully cloned. Xa4 encodes a wall-associated kinase (WAK) consisting of 707 amino acids, containing a predicted galacturonic acid binding domain, a calcium-binding epidermal growth factor domain, a transmembrane helix, and a STK domain. The protein encoded by recessive xa4 has an amino acid variation (D152E) after the galacturonic acid binding domain (Keming et al., 2017).
Xa4 is a dominant gene, which was identified in cultivated rice varieties IR20, IR22, IR72, and TKM6. Xa4 is mainly resistant to Philippine races 1, 5, 7, and 8 and also has strong resistance to seven BB races in China (Amante-Bordeos et al., 1992). The cell WAK encoded by Xa4 can accelerate the synthesis of cell cellulose, thereby inhibiting cell wall porosity, strengthening plant cell wall, and effectively preventing the invasion of pathogens. It is a natural barrier for plant protection. In addition, Xa4 can achieve excellent improvement of plant height without affecting the yield of grain crops. By reducing the height of the plant and improving the mechanical strength of the plant, the plant is not easy to break under harsh natural conditions, which further improves the lodging resistance of rice plants and is conducive to practical production and utilization. Due to the simultaneous improvement of multiple key agronomic traits, Xa4 has been widely used in the global rice breeding program (Keming et al., 2017).
xa5 was derived from three Bangladesh varieties DV85, DV86, and DZ78 and then was initially located on chromosome 5 (Iyer and Mccouch, 2004). By using near-isogenic lines IRBB5 and IR24 as parents, xa5 was finely mapped between SNPS marker RS7 and SSR marker RM611 at the short arm end of chromosome 5, with genetic distance of 0.5 cM and physical distance of 70 kb (Blair et al., 2003). Different from the above functional complementation verification methods of cloned genes, the confirmation of candidate genes of xa5 was performed by mutant analysis, and then cloned successfully (Iyer and Mccouch, 2004). Chinese researchers have continued to complete the functional complementation test of xa5 candidate genes, which further confirmed the previous results (Jiang et al., 2006).
xa5 is an incomplete recessive gene for resistance during the whole growth period, which is constitutive expression like its allelic dominant gene Xa5 (Jiang et al., 2006). The coding product of this gene is the γ subunit of transcription factor TFIIA, which is composed of 106 amino acids. The corresponding DNA sequence contains four exons (one of which has no coding sequence) and three introns. TFIIAγ is a transcription factor of eukaryotes. It has 50% homology with TFIIAγ of Drosophila and human, and 85–93% homology with Arabidopsis, maize, barley, sugarcane, and wheat. Most plants and rice have the same TFIIAγ susceptibility alleles.
xa5 is resistant to races 1, 2, 3, and 5 of the Philippines (Li et al., 1999). xa5 can inhibit the transfer of Xoo invading rice, thereby resisting BB of rice (Iyer-Pascuzzi et al., 2008). In addition, this gene has a typical structure of disease resistance gene, and glutamic acid is the 39th amino acid of its coding product. If the xa5 coding sequence is mutated here, the expression product cannot interact with non-toxic effectors, and rice also loses its disease resistance function (Jiang et al., 2006). Therefore, the disease resistance of this gene is due to the change of two bases, which makes the encoded hydrophobic valine become hydrophilic glutamic acid. There are two pathogenic variants of Xoo, which are effectors (TALEs) of Xoo and Alternaria solani. They can directly interact with TFIIAγ5 and activate the expression of susceptible genes in the host. The mutation of recessive gene xa5 destroys its combination with TALE, thus triggering plant defense responses (Yuan et al., 2016).
Xa7, a dominant gene resistant to rice BB, is a dominant gene resistant to rice BB at booting stage in three varieties DV85, DV86, and DZ78 from Bangladesh (Sidhu et al., 1978). It is located between markers GDSSR02 and RM20593, with genetic distances of 0.07 and 0.14 cM, respectively. In addition, the variety Zhenhui 084 of Xa7 was also bred. After identification, Xa7 has resistance to multiple physiological races of the Philippines in the whole growth period, which is of great significance for rice resistance to BB (Porter et al., 2003). Xa7 was initially located at 107.5 cM on the long arm of chromosome 6 in rice, and then, the genetic map constructed by molecular markers was finely mapped. Through a series of polymorphic molecular markers such as AFLP, STS, and SSR, Xa7 was mapped between M1 and M3 with a genetic distance of 2.7 cM (Porter et al., 2003). Under the utilization of more susceptible F2 plants of IR24 × IRBB7, Xa7 was located in the region of 118.5 kb (Nipponbare genome; Shen et al., 2008). But the gene was not cloned for a long time. Recently, the cloning of Xa7 gene has made breakthrough progress. At the same time, Xa7 was mutated by radiation. Nine lines with high susceptibility to BB were screened from more than 20,000 mutant lines over 5 years. One mutant line happened to have chromosome deletion at the location of Xa7 gene. The size of the deletion fragment was determined to be 107 kb by high-throughput sequencing. By comparing the overlapping regions between the gene localization region and the chromosome deletion region, Xa7 was further locked in the 28 kb region. Through a large number of verification of transgenic functions of rice, Xa7 was finally cloned successfully (Chen et al., 2021).
Studies have shown that Xoo’s two important TALEs (AvrXa7 and PthXo3) have overlapping regions in Xa7 promoter effector binding elements (EBEs). Xa7 is induced by PXO86 (avrXa7) and PXO61 (pthXo3) at the same time. AvrXa7 and PthXo3 can trigger Xa7 expression, which makes rice materials containing the gene have broad-spectrum characteristics (Chen et al., 2021). In addition, by using IRBB7, IRBB4, and IRBB21 as donors, the polymeric resistance restorer lines with Xa4 + Xa7 double genes and Xa4 + Xa7 + Xa21 triple genes were obtained, which provided the possibility for breeding new resistant varieties.
It was found that after more than 10 years of field planting, the resistance of Xa7 gene could be maintained for more than 10 years (Cruz et al., 2000). Related studies have shown that the resistance of Xa7 to BB is stronger and more durable than that of genes such as Xa4 and Xa10 (Webb et al., 2010). In general, high temperature is conducive to bacterial growth and promotion of infection. However, under the environmental conditions of high-temperature stress, the resistance pathway mediated by disease resistance gene Xa7 can more effectively limit the growth and reproduction of Beauveria bassiana compared with genes such as Xa3, Xa4, Xa5, and Xa10 (Cohen et al., 2017). RNA-Seq analysis showed that in susceptible plants, high temperature upregulated genes in plant hormone abscisic acid biosynthesis and response pathways, while in plants with Xa7-mediated resistance, high temperature inhibited the expression of these genes. Moreover, salicylic acid is an important plant disease resistance hormone in plant disease resistance response. Under high-temperature stress, the response genes of both resistant and susceptible plants to salicylic acid have been downregulated, indicating that the resistance enhancement mediated by Xa7 under high temperature may not depend on salicylic acid signal transduction. This may be the reason why the resistance of resistance gene Xa7 is more durable (Cohen et al., 2017). Other studies have shown that Xa7 is induced by the secretion effector (TALEs) AvrXa7 of Xoo to produce defense responses to prevent the invasion of Xanthomonas oryzae. This induction is faster and stronger at high temperatures, and Xa7 is more resistant.
Xa10 was originally found in Cas209 and has race-specific resistance to BB of rice (Yoshimura et al., 1983). Xa10 was initially located on the long arm of chromosome 11 of rice, in the 0.28 cM interval between markers M491 and M419, and was co-segregated with S723 and M604 (Gu et al., 2008). Then, the gene was cloned by map. Xa10 encodes a small molecule protein composed of 126 amino acid residues, and it is predicted that the gene contains four potential transmembrane helixes and an ED domain at the C-terminal. The promoter region of Xa10 has elements that bind to AvrXa10, and its transcription is specifically activated by AvrXa10 (Dongsheng et al., 2014).
The protein encoded by Xa10 is located on the endoplasmic reticulum membrane in the form of hexaploid. It causes the hypersensitivity of host cells by affecting the balance of endoplasmic reticulum and intracellular calcium ions, leading to cell death, thereby limiting the continued reproduction and infection of pathogens, thereby producing BB resistance. The expression of Xa10 in rice, tobacco, and salad cells leads to programmed cell death. It is speculated that Xa10 is at the end of a signal pathway that can be induced to trigger programmed cell death, and this is achieved through a conservative system that maintains endoplasmic reticulum and Ca2+ homeostasis. In the F2 population of IR24 × IRBB10, it was found that Xa10-mediated resistance to PXO99A did not follow Mendelian genetic law; that is, the segregation ratio was not 3:1 (Gu et al., 2008). The new near-isogenic line IRBB10A of Xa10 was developed under the genetic background of IR24. Its expression was induced by physiological race PXO99A, but it was not induced by uninoculated or inoculated with PXO99A without avrXa10, indicating that AvrXa10 could specifically recognize EBEAvrXa10 and initiate the expression of Xa10.
xa13 was located on the long arm of chromosome 8, and the genetic distance was less than 0.4 cM by using the F2 line of the hybrid offspring of IR24 × IRBB13 and the F2 line of the hybrid offspring of IRBB13 × IR65598-112 (Sanchez et al., 1999). Subsequently, xa13 was successfully cloned through fine mapping and candidate target gene analysis. xa13 is a recessive disease resistance gene, which has specific resistance to PXO99. xa13 is composed of five exons, encoding SWEET protein containing 307 amino acids, which is located on the plasma membrane (Vikal et al., 2014).
Different promoter regions of xa13 lead to differences in protein products encoded by its dominant allele Xa13 gene (Ginny et al., 2010). xa13 was hardly induced by pathogens and injuries, and its dominant allele Xa13 could be strongly induced by PXO99 but not by mechanical injuries. It is believed that xa13 is different from other disease-resistant genes, and its disease-resistant function is caused by the loss of the power of induced transcription caused by natural selection (Yuan et al., 2009). Both xa13 and its dominant allele Xa13 were expressed at low levels in rice leaves, but at high levels in inflorescence and pollen sac of rice. Studies have found that inhibiting the expression of xa13 and Xa13 at the same time will improve the resistance of plants, but will lead to male sterility in rice, indicating that xa13 gene controls the disease resistance of rice and the growth and development of pollen (Li et al., 2012).
Copper is not only an important trace element in plants but also an important component of some pesticides. It can inhibit the growth of BB, and BB strain PXO99 is more sensitive to copper than other strains, which is also the reason why xa13 has specific resistance to PXO99. The corresponding dominant gene Xa13 of xa13 is a susceptible gene, which is basically consistent with the coding region of xa13, and is different from the mutation of 18 bases in its promoter region. Because of the mutation of the cis-acting element of the promoter, PthXo1 secreted by the pathogen cannot bind to its promoter. PthXo1 cannot bind to the promoter of xa13 gene and thus cannot induce the expression of xa13 gene. Thus, plant host cells can inhibit the growth of PXO99A by maintaining the balance of copper ion concentration in vascular bundle ducts, thereby making plants resistant. In rice containing Xa13, copper transporters can play a role in the xylem of plants by binding to the coding product of Xa13, removing copper ions that can inhibit the pathogenic function of Xoo, leading to the rapid growth and reproduction of Xoo in xylem, and rice is susceptible (Yuan et al., 2010; Yuan and Wang, 2013).
Xa21 is the first resistance gene isolated by map-based cloning, showing excellent resistance to physiological races 1–6 of BB in the Philippines (Song et al., 1995). This gene has been transferred into near-isogenic lines with IR24 as the background and has been widely used in rice genetic breeding. It was also found that Xa21 gene was co-segregated with molecular markers such as RG103, RAPD818, and RAPD248. The Xa21 gene was located and cloned by map-based cloning, but the homologous sequence method was also used in the study. The efficiency of cloning gene was greatly improved by using this method (Wang, 1996).
The gene contains an 843 bp intron that encodes a protein composed of 1,025 amino acids, and the encoded protein belongs to the receptor-like kinase (RLK; Song et al., 1995). The protein structure is divided into nine regions, including signal peptide region, unknown function region, LRRs, a charged region, TM, a charged region, perimembrane region (JM), serine–threonine kinase domain (STK), and carboxy-terminal tail region. The resistance of Xa21 is related to the LRRs and STK regions. The resistance mediated by Xa21 depends on the activity of these two regions. The former is composed of 23 incomplete LRRs, which are involved in protein–protein interactions and are related to pathogen identification (Wang et al., 1998). The latter is a typical signal molecule for early defense immune response of plants (Park et al., 2010).
It was found that the downstream signal transduction was determined by the kinase region and was transcribed throughout the whole process without the influence of Xoo infection. However, its disease resistance was affected by various stages of rice growth and development. With the growth and development of rice, its disease resistance gradually increased, and it was completely resistant at tillering stage (Zhang et al., 2010). The disease resistance of Xa21 is similar to that of Xa3/Xa26 and is also affected by genetic background (Jing et al., 2009). Under the background of “IR24” or “Minghui 63,” the resistance of Xa21 and Xa3/Xa26 to both compatible and non-compatible races gradually increased from seedling stage to adult stage, and the resistance change was the most obvious during the four-leaf stage to the peak tillering stage. However, under the background of “Mudanjiang 8,” Xa21 and Xa3/Xa26 resistance was not affected by growth period.
With the deepening of research, more and more Xa21 binding proteins (XB) have been found to be involved in Xa21-mediated disease resistance (Pruitt et al., 2015). For example, XB3 is a positive regulator of XA21-mediated immune response. The combination of XA21 and XB3 will promote the phosphorylation of XB3 and stimulate disease resistance (Xu et al., 2006). XB10 is a negative regulator of XA21-mediated immune response, which can inhibit the expression of defense genes by binding to XA21 (Park et al., 2017). XB15 is a PP2C protein phosphatase that can negatively regulate cell death and negatively regulate XA21-mediated immune response (Wang et al., 2007). XB24 is an ATP enzyme that can promote the phosphorylation of XA21 (Ying et al., 2010). The autophosphorylation of Ser686, Thr688, and Ser689 can stabilize XA21 to resist development-controlled protease activity (Peng et al., 2008).
The avirulence gene AvrXa21 of XA21 is named RaxX. When the pathogen invades, the LRR region of XA21 protein recognizes and binds to the effector RaxX protein of the pathogen, thereby initiating the PTI process (Park et al., 2008). The specific interaction process between Xa21 and RaxX is that endoplasmic reticulum molecular chaperones and auxiliary molecular chaperones participate in the biosynthesis of Xa21. After the synthesis of Xa21 polypeptide, it needs to be processed by endoplasmic reticulum and transported to plasma membrane before it can play its biological function. When XB24 is combined with the intracellular near membrane region of XA21, XA21 is in the inactive state of self-phosphorylation. When RaxX binds to the extracellular LRRs domain of XA21, XB24 separates from Xa21 and activates the kinase domain of XA21. The self-phosphorylated Thr705 transfers the phosphoryl group to another residue of XA21, which activates XA21. Then, the XA21 kinase domain is segmented and transported to the cell core, which binds to the transcription factor (TF) WRKY62 and further triggers the expression of defense genes (Chen et al., 2010).
A new gene resistant to BB from common wild rice was identified by resistance spectrum evaluation, resistance-type comparison, and genetic analysis, which was named Xa23 (Li et al., 2006). Xa23 gene was transferred to “Jingang 30” and cultivated into near-isogenic line CBB23. The F2 generation of “Jingang 30” and CBB23 hybrids was used as mapping population, and then, the molecular markers closely linked to Xa23 were found through various ways. BAC library and Shotgun library were constructed for sequencing and genetic transformation. Finally, Xa23 gene was successfully cloned (Chunlian, 2006). Xa23 was located between markers C189 and RM206, with a genetic distance of 0.8 and 1.9 cM, respectively. The genetic distance of RFLP marker C1003A from the same side of C189 was 0.4 cM. Markers C1003A and RM206 covered five clones: OSJNBa0072L08, OSJNBa006K21, P0480H08, OSJNBa0029K08, and B1356F10, with a genetic position of 84.6–88.4 cM.
Xa23 gene has a broad spectrum of resistance, resistance to Philippine races 1 ~ 10, Chinese pathogenic races 1 ~ 7, and Japanese races 1 ~ 3, a total of 20 domestic and foreign BB identification strains, and completely dominant and whole growth resistance (Wang et al., 2014b). Through the identification of Xa23 transgenic plants resistance to BB, it was found that when the Xa23 gene insertion site was different, the degree of disease resistance was significantly different, and the degree of disease resistance of T0 plants can be accurately and stably inherited to T1 and T2 generations (Zhou et al., 2011). Xa23 encodes a protein consisting of 113 amino acids, which has 50% homology with the known Executor protein XA10. The transmembrane helix structure of Xa23 protein is also partially overlapped with that of Xa10 protein. Unlike Xa10, Xa23 has broad-spectrum resistance and can be activated by TALE effector AvrXa23 (Wang et al., 2015). Xa23 transcription is specifically induced by AvrXa23. The susceptible xa23 allele has the same open reading frame region (ORF113) as the dominant gene Xa23 for disease resistance. The reason for the polymorphism between the two genes is that the promoter region is different. The susceptible xa23 lacks the TALE binding element (EBE) that can specifically bind to AvrXa23. Under normal conditions, the ORF113 of Xa23 had a very low transcription level in resistant and susceptible varieties, but it was induced by pathogenic bacteria in CBB23 and transgenic plants. This induced expression was not detected in susceptible varieties JG30, Nipponbare, and MDJ8. ORF113-RNAi plants were susceptible to PXO99A, indicating that Xa23-mediated resistance needs to increase the expression of ORF113. There is a 7 bp polymorphism in the promoter region of resistant Xa23 in susceptible xa23 and CBB23 in JG30, which is related to the effector binding element of AvrXa23 (Wang et al., 2015). These results suggest that Xa23 plays a role in disease resistance by identifying TALEs in pathogens.
xa25 is derived from the cultivated rice “Minghui 63.” The gene is located between RFLP markers R887 and G1314 on chromosome 12 of rice, with the map distances of 3.0 and 7.3 cM, respectively (Chen et al., 2002), and then isolated through map cloning (Liu et al., 2011). xa25 has specific resistance to physiological race PXO339 and belongs to the sugar transporter (SWEET) family gene (Rmer et al., 2010). xa25 encodes a MtN3/saliva family protein containing 296 amino acids, which is ubiquitous in eukaryotes. Its allelic dominant susceptibility gene Xa25 also encodes a member of the MtN3 gene family, and the encoded product is a protein composed of 293 amino acids. The resistance mediated by Xa25 is different from that of most other R genes. It shows recessive resistance at seedling stage and dominant resistance at adult stage (Liu et al., 2011). Not only the number of amino acids is different, but also the encoded protein has eight amino acids.
xa25 showed unique resistance to PXO339 by inhibiting the growth of Xoo at seedling stage and mature stage, respectively. The transfer of Xa25 to resistant rice carrying xa25 could lead to the weakening of resistance to PXO339. Only PXO339 in different strains could rapidly induce the expression of Xa25, but did not induce the expression of xa25. It was speculated that Xa25 and Xa13 were also induced by specific TAL effectors in pathogens, thus causing host susceptibility. To confirm this hypothesis, promoter structure analysis showed that xa25 from resistant varieties and Xa25 from susceptible varieties had multiple sites in the promoter region, which may be the cause of different resistance (Liu et al., 2011).
The cis-acting element of dominant Xa25 gene promoter contains PthXo2 binding site, which can be recognized and induced by effectors. Xa25 is a sucrose transporter gene. Its expression can leak sucrose, glucose, and other photosynthetic products to the intercellular space of rice. These substances are used by pathogenic bacteria, which are beneficial to the infection and reproduction of pathogenic bacteria, and then make plants susceptible. However, there is a mutation at this site on the cis-acting element of the recessive xa25 gene promoter, so that it could not be recognized by the pathogen effector PthXo2, and thus, the plant showed resistance to diseases (Zhou et al., 2015). Therefore, compared with Xa25, the characteristics of xa25-encoded protein and its expression pattern in the interaction between rice and Xoo indicate that the resistance mechanism mediated by xa25 is different from most R proteins (Hutin et al., 2016).
Xa27, derived from Oryzae minuta, has broad-spectrum resistance to Xoo. Xa27 was introduced into the susceptible variety “IR24” by hybridization and backcross, and the near isogenic line IRBB27 was constructed, and Xa27 was isolated by map cloning strategy (Gu et al., 2004). Xa27 and avrXa27 are the first combinations of resistance genes and corresponding nontoxic genes cloned from rice. Xa27 has no intron and only one exon, which is a non-intragenic gene. Induced by the coding product of the non-toxic gene avrXa27 interacting with it, the gene encodes a protein consisting of 113 amino acids and containing a α-helix domain. The resistance and susceptibility alleles of Xa27 also encode the same protein (Gu et al., 2005). The difference between the two genes is that the xa27 promoter has a 10 bp sequence upstream of the promoter ATG, and 25 bases upstream of the TATA frame.
Although the resistance allele and susceptible allele of Xa27 encoded the same protein, Xa27 was only expressed in the adjacent cell tissues of rice infection under the infection of X. oryzae containing avrXa27. This indicated that the induced expression of Xa27 was not induced by the system, but by the pathogen, and was only induced by the non-affinity strain containing avrXa27. This mechanism acts as a local defense and belongs to non-systematic defense. In addition, the Xa27 infiltration system could resist the BB strain that originally infected IRBB27, and the promoter replacement experiment proved that the expression difference of Xa27 in the resistant and susceptible parents was controlled by the promoter (Gu et al., 2005). Xa27 gel block test showed that the affinity between Xa27 promoter and AvrXa27 was at least 100 times that between xa27 promoter and AvrXa27. When Xa27 was driven by the PR1 gene promoter, the plants were resistant to both compatible and non-compatible pathogens, and the secondary cell wall of vascular bundle was thickened at the infection site, suggesting that Xa27 might resist the invasion of pathogens by increasing the thickness of the secondary cell wall of vascular bundle. In order to verify the accuracy of the speculation, further subcellular localization found that XA27 may be transported to the vascular bundle cavity and the xylem parenchyma cell wall by cell exocytosis and accumulate more XA27 in vascular tissue. When the amino acid anchor signal sequence of XA27 was mutated, XA27 could not be subcellularly located and rice plants lost the ability to resist disease (Wu et al., 2008b).
The xa41 gene is a recessive resistance gene identified in African wild rice. It was found by analyzing the promoter polymorphism of OsSWEET14, the main susceptible gene of BB in 169 rice germplasm resources (Hutin et al., 2016). The 18 bp deletion in the promoter region of OsSWEET14 gene represents a new resistance allele, named xa41. The recessive gene xa41 and its allelic dominant gene Xa41 also encode MtN3/saliva family proteins. The promoter of Xa41 contains four binding sites of TALE (AvrXa7, Tal5, PthXo3, and TalC), which can be induced to express, so that the plant is susceptible (Yu et al., 2011). xa41 is the 18 bp deletion allele of Xa41 at the promoter, and the 18 bp deletion overlaps with the recognition sites of AvrXa7 and Tal5, thus producing resistance to Xoo strains containing these two TALEs (Streubel et al., 2013).
The SWEET susceptible gene families Xa13, Xa25, and Xa41 were induced to express due to the presence of TALE binding elements in the promoter, which were identified by the pathogen. This part of the susceptible genes effectively obtained breeding materials with broad-spectrum disease resistance through gene editing technology (Oliva et al., 2019; Xu et al., 2019). In the future, such susceptible genes can be directly edited in high-yield and high-quality varieties or super hybrid rice parents to create new varieties with high yield and high resistance in large scale.
Previously, several major resistance genes such as Xa2, Xa14, and Xa31 were located near the cloned Xa1 gene, but the cloning of these genes has not been completed. Recently, Xa2, Xa14, Xa31, and Xa45 were successfully cloned (Ji et al., 2020). These four genes, as alleles of Xa1, are all distributed on chromosome 4 and also encode NLR proteins, indicating the existence of gene duplication and functional evolution. Xa2 and Xa31 have the same sequence, which is actually the same gene. These genes are highly conserved and structurally similar at nucleotide and predicted amino acid levels. The main difference is that the repeat numbers of 93 amino acid residues at the C-terminal of protein are different. For example, the repeat numbers of XA14 and XA45 are 4 and 7, respectively.
Xa1 gene is a member of NBS-LRR plant disease resistance genes (Yoshimura et al., 1998). The analysis of disease resistance genes near Xa1 gene on the long arm of chromosome 4 reveals that the region is rich in NBS-LRR sequences (Freddy and Nishimura, 2018). Xa1 and its alleles Xa2, Xa14, Xa31, and Xa45 are a class of NLR genes, and the mediation of its disease resistance mechanism is also related to TALE. The NLR protein encoded by Xa1 had specific resistance to BB. XA1 stimulated resistance to BB by identifying several TALEs including PthXo1, Tal4, and Tal9d. Further studies have found that truncated interference TALE (iTALE) lacking transcriptional activation domain can interfere with the resistance given by XA1 (Ji et al., 2016). The resistance spectrum of Xa1 alleles was also inhibited to some extent by iTALEs, and the resistance spectrum of Xa2, Xa31, and Xa14 to Xoo was different from that of Xa1, but also inhibited by iTALEs. This is the first report that the resistance genes inhibited by iTALE can mediate different resistance. These genes encode NBS-LRR proteins, and the key to determining the main difference is the functional domain of LRR at the carboxyl end (Biaoming et al., 2020).
Up to now, more than 40 rice BB resistance genes have been discovered, but most of the resistance genes are inevitably controlled by recessive genes, the control spectrum is narrow and the resistance is weak. Therefore, there are few resistance genes that can be put into practical production. In the actual breeding practice, the widely used BB resistance genes include xa5, xa13, and Xa23. In recent years, single-gene rice varieties have been planted in different degrees throughout the country. In the long run, the genetic structure of local rice pathogens will be changed, and mutant new races will be produced to infect the original resistance source, resulting in the disappearance of the resistance of the original varieties and bringing serious economic losses to the local crops. It has been reported that the application of single antigen will produce new Xoo strains in some areas. For example, Chinese race VIII that can cause Xa21 disease was found in Guangdong, China (Zhang, 2005).
Therefore, it is not limited to the use of one resistance gene, but the comprehensive utilization of multiple resistance genes has become an effective means of breeding and production. Different rice BB resistance genes are polymerized into the same variety through reasonable comprehensive utilization, rotation, and mixed utilization, so that the target crops can obtain better resistance (Dai et al., 2010). The common forms of gene polymerization are two-gene polymerization, three-gene polymerization, and four-gene polymerization. The common forms of gene combination are double dominant gene combination and one recessive gene combination. The common gene binding forms in China are: Xa4 + Xa21, Xa21 + Xa23, Xa7 + Xa21, Xa4 + Xa21 + Xa23, and Xa4 + xa5 + xa13 + Xa21. For example, the Xa4 + xa5 polymerization system was developed by MAS technology (Cao et al., 2007). The xa5 + xa13 + Xa21 polyline bred by molecular marker-assisted backcross breeding strategy showed high resistance (Pradhan et al., 2015). The resistance genes Xa21 and Pi54 to BB and blast were polymerized into the restorer line RPHR-1005, and the progeny materials with high resistance to BB and blast were obtained by marker-assisted backcross breeding (Kumar et al., 2016). These genes showed high resistance and broad-spectrum characteristics by polymerization or combination.
In summary, the existing breeding practices have proved that the polymerization of different resistance genes into the same variety can enhance the resistance of varieties to varying degrees, broaden the resistance spectrum of varieties, and delay the decline of resistance of varieties through the interaction between resistance genes.
The resistance of rice and the pathogenicity of BB is a process of co-evolution and long-term competition. The change of any party will cause the change of the other party. The mutation of the physiological race of BB will cause the variation of rice, and the external pressure on rice will also cause the mutation of the physiological race of BB. In order to effectively control the mutation and infection of rice BB, it is necessary to continuously explore new resistant resources (Lee et al., 2011).
Xa1 gene was isolated and cloned by Japanese researchers in 1998 (Yoshimura et al., 1998). It is reported that many full-length TALEs can trigger Xa1-mediated resistance. However, interfering iTALE in pathogen PXO99A interferes with Xa1-mediated resistance. iTALE exists in many Xoo strains, resulting in the narrowing of the resistance of Xa1, so the application of Xa1 is not much (Ji et al., 2016). The genes such as Xa4 and xa5 excavated later are more resistant and broad-spectrum than Xa1 and have been widely used in practical production (Li et al., 1999). The main rice varieties in China have also introduced resistance genes such as Xa3, Xa21, and Xa23, which once effectively controlled BB and made the incidence of BB lighter and lighter. However, with the gradual warming of the global environment and the continuous variation of BB, new pathogenic varieties have emerged, leading to the gradual loss of resistance of main rice varieties. However, under the environmental conditions of high-temperature stress, the disease resistance pathway mediated by the disease resistance gene Xa7 can more effectively limit the growth and reproduction of BB compared with genes such as Xa3, Xa4, Xa5, and Xa10 (Cohen et al., 2017). Xa7 has been successfully cloned recently, and under the condition of global warming, Xa7 reflects the stronger breeding value of its own genes (Chen et al., 2021).
It can be seen that although significant progress has been made in molecular breeding for BB resistance in China, the variation of pathogen itself and the stress of adverse environmental conditions still threaten the effectiveness of existing resistance genes. The disease resistance of rice is essentially the result of the interaction between the target crop rice and pathogenic bacteria under specific conditions and the two evolve and compete with each other. In the long-term interaction process, due to the continuous emergence of new pathogenic strains and the evolution of pathogenic physiological races, the effectiveness of existing rice resistance genes continues to decrease. Therefore, continuous cloning of new genes with broad-spectrum and long-lasting disease resistance from rice to resist the infection of rice BB is the basis and key to the success of disease resistance breeding.
Due to the unique climatic conditions and geographical location, wild rice and local rice resources in China are extremely rich, and many excellent genetic traits have been retained in the long-term self-evolution for many years. Particularly, wild rice resources not only show the characteristics of high resistance to BB, but also are partially immune.
At present, there are many effective genes for resistance to BB cloned from wild rice resources. For example, Xa21 is the first cloned gene for resistance to BB in rice, which is from wild rice with long stamens (Park et al., 2012). Xa23 is a broad-spectrum resistance gene to BB found in long-acting wild rice (Zhou et al., 2015). Xa27 is derived from tetraploid small-grain wild rice (Bimolata et al., 2013). xa41 is identified on wild rice in Africa. These resistance genes excavated from wild rice germplasm resources have broad-spectrum resistance to Xoo. For example, Xa21, Xa23, Xa27, and other resistance genes excavated from wild rice varieties have been widely used in production practice and achieved ideal resistance effect. In addition, there are also some resistance genes from wild rice: xa32 in the new germplasm Y76 obtained by somatic hybridization between wild rice verrucous and cultivated rice from Xishuangbanna, Yunnan (Ruan et al., 2008); Xa36 from Australian wild rice and cultivated rice hybrids (Miao et al., 2010); and Xa38 from annual common wild rice (Ellur et al., 2016), etc. These genes have been mapped but not cloned and have broad application prospects.
The wild rice resources that have been explored and utilized and other resistance genes that have not been found are valuable breeding resources for rice resistance to BB. By revealing the resistance mechanism of wild rice and local rice, it is of great significance to enrich the resistance gene pool of rice and further clarify the genetic basis of disease resistance in rice cultivation.
With the continuous warming of global climate, the continuous growth of population and the continuous reduction of cultivated land, the traditional agricultural production methods, and methods cannot meet the needs of the development of modern society. Therefore, there is an urgent need for scientific and technological innovation to improve crop varieties and traits. Through gene editing, specific target genes can be targeted modified, which can greatly make up for the deficiency of natural mutation and achieve the specific purpose required for production (Hassan et al., 2021).
The development of gene editing technology has experienced a long process, from the initial zinc finger nuclei (ZFNs), transcription activator-like effector nucleases (TALENs) to CRISPR/cas9. The editing efficiency and operability of gene editing technology have been continuously improved, which has attracted extensive attention. It is worth noting that by knocking out the promoter and other key elements of host susceptibility genes, the expression of crop susceptibility genes by pathogenic microorganisms can be regulated and disease-resistant germplasm can be created. For example, knocking out the EBE region of the promoters of susceptibility genes OSSWEET11, OSSWEET 14, and OSSWEET 13 in rice leads to the inability of TaL effector protein to activate the expression of relevant susceptibility genes, resulting in extensive resistance of rice to BB (Xu et al., 2019).
The breakthrough of gene editing technology represented by CRISPR/cas9 makes the current gene operation more mobile and feasible. CRISPR/cas9 gene editing technology is simpler and cheaper than traditional breeding methods (Wang et al., 2014a). The research shows that CRISPR/cas9 gene editing technology has greatly improved the gene editing efficiency of tomato, wheat, rice, soybean, and other crops. The second generation CRISPR/cas9 gene editing technology gradually formed in the later stage is easier to realize single-base editing. Single-base editing can realize base substitution from cytosine (c) to thymine (T) and adenine (a) to guanine (g; Komor et al., 2016). For example, by fusing RNA adenylate deaminase with cas9 notch enzyme, the conversion of target base a to G can be realized (Gaudelli et al., 2018). The translation of genes can be terminated in advance by converting the codon encoded by amino acids into a stop codon (Kuscu et al., 2017). These new single-base editing strategies greatly expand the application scope of gene editing technology and lay a foundation for obtaining new disease resistant materials.
Although the low editing efficiency and high miss rate still exist in gene editing (Qin et al., 2020), which limits the large-scale application of this technology, the rapid development of gene editing technology in the past two decades can now create genetic variations with high accuracy and specificity. Therefore, gene editing technology is also widely used in polyploid breeding, germplasm resource innovation, and molecular design breeding. It is believed that with the maturity of this technology, it will play a greater role in crop breeding.
YY, YuhZ, JS, WL, and JC contributed to conceptualization. YY, YuhZ, JS, WL, XC, XW, JZho, CYu, JW, SW, XY, YujZ, JZhu, and CYa were involved in collecting and reading the literature. YY, YuhZ, JS, WL, XC, XW, JZho, CYu, JW, SW, and BZ contributed to writing—original draft preparation. YY, YuhZ, JS, WL, and JC were involved in writing—review and editing. All authors contributed to the article and approved the submitted version.
This research was funded by Zhejiang Provincial Key Research and Development Plan (2019C02006), the National Natural Science Foundation of China for young scholar (31101208), the Key Program of Zhejiang Provincial Foundation for Natural Science (LZ16C130002), Zhejiang Fundamental Public Welfare Research Program (LGN19C140008), and State Key Laboratory for Managing Biotic and Chemical Threats to the Quality and Safety of Agro-products (2010DS700124-ZZ1907).
The authors declare that the research was conducted in the absence of any commercial or financial relationships that could be construed as a potential conflict of interest.
All claims expressed in this article are solely those of the authors and do not necessarily represent those of their affiliated organizations, or those of the publisher, the editors and the reviewers. Any product that may be evaluated in this article, or claim that may be made by its manufacturer, is not guaranteed or endorsed by the publisher.
Amante-Bordeos, A., Sitch, L. A., Nelson, R., Dalmacio, R. D., Oliva, N. P., and Leung, H. A. (1992). Transfer of bacterial blight and blast resistance from the tetraploid wild rice Oryza minuta to cultivated rice, Oryza sativa. Theor. Appl. Genet. 84–84, 345–354. doi: 10.1007/BF00229493
Bao, S. Y., Tan, M. P., and Lin, X. H. (2006). Genetic mapping of the region including a bacterial blight resistance gene Xa12 in rice. Subtrop. Plant Sci. 35, 1–4.
Biaoming, Z., Haitao, Z., Fang, L., Yidan, O., Meng, Y., Xianghua, L., et al. (2020). Multiple alleles encoding atypical NLRs with unique central tandem repeats in rice confer resistance to Xanthomonas oryzae pv. oryzae. Plant Commun. 1:100088. doi: 10.1016/j.xplc.2020.100088
Bimolata, W., Kumar, A., Sundaram, R. M., Laha, G. S., Qureshi, I. A., Reddy, G. A., et al. (2013). Analysis of nucleotide diversity among alleles of the major bacterial blight resistance gene Xa27 in cultivars of rice (Oryza sativa) and its wild relatives. Planta 238, 293–305. doi: 10.1007/s00425-013-1891-3
Blair, M. W., Garris, A. J., Iyer, A. S., Chapman, B., Kresovich, S., and McCouch, S. R. (2003). High resolution genetic mapping and candidate gene identification at the xa5 locus for bacterial blight resistance in rice (Oryza sativa L.). Theor. Appl. Genet. 107, 62–73. doi: 10.1007/s00122-003-1231-2
Busungu, C., Taura, S., Jun-Ichi, S., Anai, T., and Ichitani, K. (2018). High-resolution mapping and characterization of xa42, a resistance gene against multiple Xanthomonas oryzae pv. oryzae races in rice (Oryza sativa L.). Breed. Sci. 68, 188–199. doi: 10.1270/jsbbs.17094
Cao, Y., Liu, D., Li, H., Sun, X., Yu, Z., Xu, C., et al. (2007). Functional analysis of Xa3/Xa26 family members in rice resistance to Xanthomonas oryzae pv. oryzae. Theor. Appl. Genet. 115, 887–895. doi: 10.1007/s00122-007-0615-0
Cheema, K. K., Grewal, N. K., Vikal, Y., Sharma, R., Lore, J., Das, A., et al. (2008). A novel bacterial blight resistance gene from Oryza nivara mapped to 38 kb region on chromosome 4L and transferred to Oryza sativa L. Genet. Res. 90, 397–407. doi: 10.1017/S0016672308009786
Chen, X., Chern, M., Canlas, P. E., Ruan, D., Jiang, C., et al. (2010). An ATPase promotes autophosphorylation of the pattern recognition receptor XA21 and inhibits XA21-mediated immunity. Proc. Natl. Acad. Sci. U. S. A. 107, 8029–8034. doi: 10.1073/pnas.0912311107
Chen, X., Liu, P., Mei, L., He, X., Chen, L., Liu, H., et al. (2021). Xa7, a new executor R gene that confers durable and broad-spectrum resistance to bacteria-blight disease in rice. Plant Commun. 2:100143. doi: 10.1016/j.xplc.2021.100143
Chen, H., Wang, S., and Zhang, Q. (2002). New gene for bacterial blight resistance in rice located on chromosome 12 identified from Minghui 63, an elite restorer line. Phytopathology 92, 750–754. doi: 10.1094/PHYTO.2002.92.7.750
Chu, Z., Yuan, M., Yao, J., Ge, X., Yuan, B., Xu, C., et al. (2006). Promoter mutations of an essential gene for pollen development result in disease resistance in rice. Genes Dev. 20, 1250–1255. doi: 10.1101/gad.1416306
Chuntai, W., Mingpu, T., Xin, X., Guosong, W., Duanpin, Z., and Xinghua, L. (2003). Localizing the bacterial blight resistance gene, Xa22(t), to a 100-kilobase bacterial artificial chromosome. Phytopathology 93, 1258–1262. doi: 10.1094/PHYTO.2003.93.10.1258
Cohen, S. P., Liu, H., Argueso, C. T., Pereira, A., and Leach, J. E. (2017). RNA-Seq analysis reveals insight into enhanced rice Xa7-mediated bacterial blight resistance at high temperature. PLoS One 12:e0187625. doi: 10.1371/journal.pone.0187625
Constantine, B., Satoru, T., Jun-Ichi, S., and Katsuyuki, I. (2016). Identification and linkage analysis of a new rice bacterial blight resistance gene from XM14, a mutant line from IR24. Breed. Sci. 66, 636–645. doi: 10.1270/jsbbs.16062
Cruz, C. V., Bai, J., Ona, I., Leung, H., Nelson, R. J., Mew, T. W., et al. (2000). Predicting durability of a disease resistance gene based on an assessment of the fitness loss and epidemiological consequences of avirulence gene mutation. Proc. Natl. Acad. Sci. U. S. A. 97, 13500–13505. doi: 10.1073/pnas.250271997
Dai, L. Y., Liu, X. L., Xiao, Y. H., and Wang, G. L. (2010). Recent advances in cloning and characterization of disease resistance genes in Rice. J. Integ. Plant Biol. 49, 112–119. doi: 10.1111/j.1744-7909.2006.00413.x
Dongsheng, T., Junxia, W., Xuan, Z., Keyu, G., Chengxiang, Q., Xiaobei, Y., et al. (2014). The rice TAL effector-dependent resistance protein XA10 triggers cell death and calcium depletion in the endoplasmic reticulum. Plant Cell 26, 497–515. doi: 10.1105/tpc.113.119255
Ellur, R. K., Khanna, A., Gopala, K. S., Bhowmick, P. K., and Singh, A. K. (2016). Marker-aided incorporation of Xa38, a novel bacterial blight resistance gene, in PB1121 and comparison of its resistance spectrum with xa13+Xa21. Sci. Rep. 6:29188. doi: 10.1038/srep29188
Freddy, M., and Nishimura, M. T. (2018). Structural, functional, and genomic diversity of plant NLR proteins: an evolved resource for rational engineering of plant immunity. Annu. Rev. Phytopathol. 56, 243–267. doi: 10.1146/annurev-phyto-080417-045817
Gaudelli, N. M., Komor, A. C., Rees, H. A., Packer, M. S., Badran, A. H., Bryson, D. I., et al. (2018). Programmable base editing of AT to GC in genomic DNA without DNA cleavage. Nature 559:E8. doi: 10.1038/s41586-018-0070-x
Ginny, A., Junhui, Z., Sheng, H., Ting, L., Bo, L., Frank, W., et al. (2010). Rice xa13 recessive resistance to bacterial blight is defeated by induction of the disease susceptibility gene Os-11N3. Plant Cell 22, 3864–3876. doi: 10.1105/tpc.110.078964
Gnanamanickam, S. S., Priyadarisini, V. B., Narayanan, N. N., Vasudevan, P., and Kavitha, S. (1999). An overview of bacterial blight disease of rice and strategies for its management. Curr. Sci. 77, 1435–1444.
Gonzalez, C., Szurek, B., Manceau, C., Mathieu, T., Séré, Y., and Verdier, V. (2007). Molecular and pathotypic characterization of new Xanthomonas oryzae strains from West Africa. Mol. Plant-Microbe Interact. 20, 534–546. doi: 10.1094/MPMI-20-5-0534
Goto, T., Matsumoto, T., Furuya, N., Tsuchiya, K., and Yoshimura, A. (2012). Mapping of bacterial blight resistance gene Xa11 on rice chromosome 3. Jpn. Agric. Res. Q. 43, 221–225. doi: 10.6090/jarq.43.221
Gu, K., Sangha, J. S., Li, Y., and Yin, Z. (2008). High-resolution genetic mapping of bacterial blight resistance gene Xa10. Theor. Appl. Genet. 116, 155–163. doi: 10.1007/s00122-007-0655-5
Gu, K., Tian, D., Yang, F., Wu, L., Sreekala, C., Wang, D., et al. (2004). High-resolution genetic mapping of Xa27(t), a new bacterial blight resistance gene in rice, Oryza sativa L. Theor. Appl. Genet. 108, 800–807. doi: 10.1007/s00122-003-1491-x
Gu, K., Yang, B., Tian, D., Wu, L., Wang, D., Sreekala, C., et al. (2005). R gene expression induced by a type-III effector triggers disease resistance in rice. Nature 435, 1122–1125. doi: 10.1038/nature03630
Guo, S. B., Zhang, D. P., and Lin, X. H. (2010). Identification and mapping of a novel bacterial blight resistance gene Xa35(t) originated from Oryza minuta. Entia Agric. Sin. 43, 2611–2618.
Hassan, M. M., Zhang, Y., Yuan, G., De, K., Chen, J. G., Muchero, W., et al. (2021). Construct design for CRISPR/Cas-based genome editing in plants. Trends Plant Sci. 26, 1133–1152. doi: 10.1016/j.tplants.2021.06.015
He, Q., Li, D., Zhu, Y., Tan, M., Zhang, D., and Lin, X. (2006). Fine mapping of Xa2, a bacterial blight resistance gene in rice. Mol. Breed. 17, 1–6. doi: 10.1007/s11032-005-8698-2
Hutin, M., Sabot, F., Ghesquière, A., Koebnik, R., and Szurek, B. A. (2016). Knowledge-based molecular screen uncovers a broad-spectrum OsSWEET14 resistance allele to bacterial blight from wild rice. Plant J. 84, 694–703. doi: 10.1111/tpj.13042
Iyer, A. S., and Mccouch, S. R. (2004). The rice bacterial blight resistance gene xa5 encodes a novel form of disease resistance. Mol. Plant-Microbe Interact. 17, 1348–1354. doi: 10.1094/MPMI.2004.17.12.1348
Iyer-Pascuzzi, A. S., Jiang, H., Huang, L., and McCouch, S. R. (2008). Genetic and functional characterization of the rice bacterial blight disease resistance gene xa5. Phytopathology 98, 289–295. doi: 10.1094/PHYTO-98-3-0289
Ji, C., Ji, Z., Liu, B., Cheng, H., and Chen, G. (2020). Xa1 allelic R genes activate rice blight resistance suppressed by interfering TAL effectors. Plant Commun. 1:100087. doi: 10.1016/j.xplc.2020.100087
Ji, Z., Ji, C., Liu, B., Zou, L., Chen, G., and Yang, B. (2016). Interfering TAL effectors of Xanthomonas oryzae neutralize R-gene-mediated plant disease resistance. Nat. Commun. 7:13435. doi: 10.1038/ncomms13435
Jiang, G. H., Xia, Z. H., Zhou, Y. L., Wan, J., Li, D. Y., Chen, R. S., et al. (2006). Testifying the rice bacterial blight resistance gene xa5 by genetic complementation and further analyzing xa5 (Xa5) in comparison with its homolog TFIIAγ1. Mol. Gen. Genomics. 275, 354–366. doi: 10.1007/s00438-005-0091-7
Jin, X. W., Wang, C. L., Yang, Q., Jiang, Q. X., Fan, Y. L., Liu, G. C., et al. (2007). Breeding of near-isogenic line CBB30 and molecular mapping of Xa30(t), a new resistance gene to bacterial blight in rice. Sci. Agric. Sin. 40, 1094–1100. doi: 10.3321/j.issn:0578-1752.2007.06.002
Jing, Z., Jing, F., Li, X., Xu, C., and Wang, S. (2009). Dissection of the factors affecting development-controlled and race-specific disease resistance conferred by leucine-rich repeat receptor kinase-type R genes in rice. Theor. Appl. Genet. 119, 231–239. doi: 10.1007/s00122-009-1032-3
Keming, H., Jianbo, C., Jie, Z., Xia, F., Ke, Y., Zhang, H., et al. (2017). Improvement of multiple agronomic traits by a disease resistance gene via cell wall reinforcement. Nat. Plants 3:17009. doi: 10.1038/nplants.2017.9
Khush, G. S., and Angeles, E. R. (1999). A new gene for resistance to race 6 of bacterial blight in rice, Oryza sativa L. Rice Genet. Newsl. 16, 92–93.
Kim, S. M. (2018). Identification of novel recessive gene xa44(t) conferring resistance to bacterial blight races in rice by QTL linkage analysis using an SNP chip. Theor. Appl. Genet. 131, 2733–2743. doi: 10.1007/s00122-018-3187-2
Kim, S. M., Reinke, R. F., and Sundaram, R. M. (2019). A novel resistance gene for bacterial blight in rice, Xa43(t) identified by GWAS, confirmed by QTL mapping using a bi-parental population. PLoS One 14:e0211775. doi: 10.1371/journal.pone.0211775
Kim, S.-M., Suh, J.-P., Qin, Y., Noh, T.-H., Reinke, R. F., and Jena, K. K. (2015). Identification and fine-mapping of a new resistance gene, Xa40, conferring resistance to bacterial blight races in rice (Oryza sativa L.). Theor. Appl. Genet. 128, 1933–1943. doi: 10.1007/s00122-015-2557-2
Komor, A. C., Kim, Y. B., Packer, M. S., Zuris, J. A., and Liu, D. R. (2016). Programmable editing of a target base in genomic DNA without double-stranded DNA cleavage. Nature 533, 420–424. doi: 10.1038/nature17946
Kumar, V. A., Balachiranjeevi, C. H., Naik, S. B., Rambabu, R., Rekha, G., Madhavi, K. R., et al. (2016). Marker-assisted introgression of the major bacterial blight resistance gene, Xa21 and blast resistance gene, Pi54 into RPHR-1005, the restorer line of the popular rice hybrid, DRRH3. J. Plant Biochem. Biotechnol. 25, 400–409. doi: 10.1007/s13562-016-0352-z
Kumar, P. N., Sujatha, K., Laha, G. S., Rao, K. S., and Sundaram, R. M. (2012). Identification and fine-mapping of Xa33, a novel gene for resistance to Xanthomonas oryzae pv. oryzae. Phytopathology 102, 222–228. doi: 10.1094/PHYTO-03-11-0075
Kuscu, C., Parlak, M., Tufan, T., Yang, J., Szlachta, K., Wei, X., et al. (2017). CRISPR-STOP: gene silencing through base-editing-induced nonsense mutations. Nat. Methods 14, 710–712. doi: 10.1038/nmeth.4327
Lee, S. W., Han, M., Park, C. J., Seo, Y. S., and Jeon, J. S. (2011). The molecular mechanisms of rice resistance to the bacterial blight pathogen, Xanthomonas oryzae pathovar oryzae. Adv. Botani. Res. 60, 51–87. doi: 10.1016/B978-0-12-385851-1.00002-0
Lee, K. S., Rasabandith, S., Angeles, E. R., and Khush, G. S. (2003). Inheritance of resistance to bacterial blight in 21 cultivars of rice. Phytopathology 93, 147–152. doi: 10.1094/PHYTO.2003.93.2.147
Li, Z. K., Luo, L. J., Mei, H. W., Paterson, A. H., Zhao, X. H., Zhong, D. B., et al. (1999). A “defeated” rice resistance gene acts as a QTL against a virulent strain of Xanthomonas oryzae pv. oryzae. Mol. Gen. Genet. 261, 58–63. doi: 10.1007/s004380050941
Li, J. B., Wang, C. L., Xia, M. Y., Zhao, K. J., and Lu, X. G. (2006). Enhancing bacterial blight resistance of hybrid rice restorer lines through marker-assisted selection of Xa23 gene. Acta Agron. Sin. 32, 1423–1429. doi: 10.3321/j.issn:0496-3490.2006.10.001
Li, C., Wei, J., Lin, Y., and Chen, H. (2012). Gene silencing using the recessive rice bacterial blight resistance gene xa13 as a new paradigm in plant breeding. Plant Cell Rep. 31, 851–862. doi: 10.1007/s00299-011-1206-8
Liang, L. Q., Wang, C. Y., Zeng, L. X., Wang, W. J., Feng, J. Q., Bing, C., et al. (2017). The rice cultivar Baixiangzhan harbours a recessive gene xa42 (t) determining resistance against Xanthomonas oryzae pv. oryzae. Plant Breed. 136, 603–609. doi: 10.1111/pbr.12493
Liu, Q., Yuan, M., Zhou, Y., Li, X., Xiao, J., and Wang, S. (2011). A paralog of the MtN3/saliva family recessively confers race-specific resistance to Xanthomonas oryzae in rice. Plant Cell Environ. 34, 1958–1969. doi: 10.1111/j.1365-3040.2011.02391.x
Miao, L., Wang, C., Zheng, C., Che, J., Gao, Y., Wen, Y., et al. (2010). Preliminary mapping of new genes resistant to bacterial blight in rice. Sci. Agric. Sin. 43, 3051–3058. doi: 10.3864/j.issn.0578-1752.2010.15.001
Nakai, H., Kuwahara, S., and Senga, S. (2008). Genetic analysis of an induced mutant of rice for a quantitative resistance to bacterial leaf blight. Jpn. J. Breed. 40, 397–409. doi: 10.1270/jsbbs1951.40.397
Natraj, K. P., Sujatha, K., Laha, G. S., Srinivasa Rao, K., Mishra, B., Viraktamath, B. C., et al. (2012). Identification and fine-mapping of Xa33, a novel gene for resistance to Xanthomonas oryzae pv. oryzae. Phytopathology 102, 222–228. doi: 10.1094/PHYTO-03-11-0075
Ogawa, T. (1993). Methods and strategy for monitoring race distribution and identification of resistance genes to bacterial leaf blight (Xanthomonas campestris pv. oryzae) in rice. Jpn. Agric. Res. Q. 27, 71–80.
Ogawa, T., Morinaka, T., Fujii, K., and Kimura, T. (1978). Inheritance of resistance of rice varieties Kogyoku and Java 14 to bacterial group V of Xanthomonas oryzae. Jpn. J. Phytopathol. 44, 137–141. doi: 10.3186/jjphytopath.44.137
Oliva, R., Ji, C., Atienza-Grande, G., Huguet-Tapia, J. C., and Yang, B. (2019). Broad-spectrum resistance to bacterial blight in rice using genome editing. Nat. Biotechnol. 37, 1344–1350. doi: 10.1038/s41587-019-0267-z
Park, C. J., Canlas, P. E., and Ronald, P. C. (2012). Establishment of glucocorticoid-mediated transcriptional induction of the rice XA21 pattern recognition receptor. J. Plant Biol. 55, 43–49. doi: 10.1007/s12374-011-9188-1
Park, C. J., Lee, S. W., Chern, M., Sharma, R., Canlas, P. E., Song, M. Y., et al. (2010). Ectopic expression of rice Xa21 overcomes developmentally controlled resistance to Xanthomonas oryzae pv. oryzae. Plant Sci. 179, 466–471. doi: 10.1016/j.plantsci.2010.07.008
Park, C. J., Peng, Y., Chen, X., Dardick, C., and Ronald, P. C. (2008). Rice XB15, a protein phosphatase 2C, negatively regulates cell death and XA21-mediated innate immunity. PLoS Biol. 6:e282. doi: 10.1371/journal.pbio.0060231
Park, C. J., Wei, T., Sharma, R., and Ronald, P. C. (2017). Overexpression of rice auxilin-Like protein, XB21, induces necrotic lesions, up-regulates endocytosis-related genes, and confers enhanced resistance to Xanthomonas oryzae pv. oryzae. Rice 10:27. doi: 10.1186/s12284-017-0166-1
Peng, Y., Bartley, L. E., Chen, X., Dardick, C., Chern, M., Ruan, R., et al. (2008). OsWRKY62 is a negative regulator of basal and Xa21-mediated defense against Xanthomonas oryzae pv. oryzae in rice. Mol. Plant 1, 446–458. doi: 10.1093/mp/ssn024
Peng, K. M., Zhang, H. B., and Zhang, Q. F. (1998). A BAC library constructed to the rice cultivar "Minghui 63" for cloning genes of agronomic importance. Acta Bot. Sin. 40, 1108–1114.
Porter, B. W., Chittoor, J. M., Yano, M., Sasaki, T., and White, F. F. (2003). Development and mapping of markers linked to the rice bacterial blight resistance gene Xa7. Crop Sci. 43, 1484–1492. doi: 10.2135/cropsci2003.1484
Pradhan, S. K., Nayak, D. K., Mohanty, S., Behera, L., Barik, S. R., Pandit, E., et al. (2015). Pyramiding of three bacterial blight resistance genes for broad-spectrum resistance in deepwater rice variety, Jalmagna. Rice 8:51. doi: 10.1186/s12284-015-0051-8
Pruitt, R. N., Schwessinger, B., Joe, A., Thomas, N., Liu, F., Albert, M., et al. (2015). The rice immune receptor XA21 recognizes a tyrosine-sulfated protein from a gram-negative bacterium. Sci. Adv. 1:e1500245. doi: 10.1126/sciadv.1500245
Qin, R., Li, J., Liu, X., Xu, R., and Wei, P. (2020). SpCas9-NG self-targets the sgRNA sequence in plant genome editing. Nat. Plants 6, 197–201. doi: 10.1038/s41477-020-0603-9
Rmer, P., Recht, S., Strauss, T., Elsaesser, J., and Lahaye, T. (2010). Promoter elements of rice susceptibility genes are bound and activated by specific TAL effectors from the bacterial blight pathogen, Xanthomonas oryzae pv. oryzae. New Phytol. 187, 1048–1057. doi: 10.1111/j.1469-8137.2010.03217.x
Ronald, P. C., Albano, B., Tabien, R., Abenes, L., Wu, K.-S., McCouch, S., et al. (1992). Genetic and physical analysis of the rice bacterial blight disease resistance locus, Xa21. Mol. Gen. Genet. 236, 113–120. doi: 10.1007/BF00279649
Ruan, H.-H., Yan, C.-Q., An, D.-R., Liu, R.-H., and Chen, J.-P (2008). Identifying and Mapping New Gene xa32 (t) for Resistance to Bacterial Blight (Xanthomonas oryzae pv. oryzae, Xoo) from Orza meyeriana L. Airiti Library. 17, 170–174.
Sakaguchi, S. (1967). Linkage studies on the resistance to bacterial leaf blight, Xanthomonas oryzae (Uyeda et Ishiyama) Dowson, in rice. Bull. Nat. Inst. Agric. Sci. 16, 1–18.
Sanchez, A. C., Ilag, L. L., Yang, D., Brar, D. S., Ausubel, F., Khush, G. S., et al. (1999). Genetic and physical mapping of xa13, a recessive bacterial blight resistance gene in rice. Theor. Appl. Genet. 98, 1022–1028. doi: 10.1007/s001220051163
Satoru, T., Tsugufumi, O., Atsushi, Y., Ryoichi, I., and Takeshi, O. (2008). Identification of a recessive resistance gene in induced mutant line XM5 of rice to rice bacterial blight. Jpn. J. Breed. 41, 427–432.
Shen, C., Huang, Z., Zeng, L., Yang, J., and Zhu, X. (2008). High-resolution mapping and gene prediction of Xanthomonas Oryzae pv. oryzae resistance gene Xa7. Mol. Breed. 22, 433–441. doi: 10.1007/s11032-008-9187-1
Shen, C., Liu, X., Zeng, L., Ouyang, D., Yang, J., and Zhu, X. (2011). Genetic analysis and molecular mapping of a novel recessive gene xa34(t) for resistance against Xanthomonas oryzae pv. oryzae. Theor. Appl. Genet. 122, 1331–1338. doi: 10.1007/s00122-011-1534-7
Sidhu, G. S., Khush, G. S., and Mew, T. W. (1978). Genetic analysis of bacterial blight resistance in seventy-four cultivars of rice, Oryza sativa L. Theor. Appl. Genet. 53, 105–111. doi: 10.1007/BF00272687
Song, W.-Y., Wang, G.-L., Chen, L.-L., Kim, H.-S., Pi, L.-Y., Holsten, T., et al. (1995). A receptor kinase-like protein encoded by the rice disease resistance gene, Xa21. Science 270, 1804–1806. doi: 10.1126/science.270.5243.1804
Streubel, J., Pesce, C., Hutin, M., Koebnik, R., Boch, J., and Szurek, B. (2013). Five phylogenetically close rice SWEET genes confer TAL effector-mediated susceptibility to Xanthomonas oryzae pv. oryzae. New Phytol. 200, 808–819. doi: 10.1111/nph.12411
Sun, X., Cao, Y., Yang, Z., Xu, C., Li, X., Wang, S., et al. (2004). Xa26, a gene conferring resistance to Xanthomonas oryzae pv. oryzae in rice, encodes an LRR receptor kinase-like protein. Plant J. 37, 517–527. doi: 10.1046/j.1365-313X.2003.01976.x
Sun, X., Yang, Z., Wang, S., and Zhang, Q. (2003). Identification of a 47-kb DNA fragment containing Xa4, a locus for bacterial blight resistance in rice. Theor. Appl. Genet. 106, 683–687. doi: 10.1007/s00122-002-1117-8
Tan, G. X., Xiang, R., Weng, Q. M., Shi, Z. Y., and He, G. C. (2004). Mapping of a new resistance gene to bacterial blight in rice line introgressed from Oryza officinalis. Acta Genet. Sin. 31, 724–729.
Tan, Z. B., Zhang, Q., Zhu, L. H., and Wang, C. L. (1998). RFLP mapping of a rice bacterial blight resistance gene Xa-14. Hereditas 20, 30–33.
Taura, S., Ogawa, T., Tabien Re, S., Khush, G., Yoshimura, A., and Omura, T. (1992a). Resistance gene of rice cultivar, Taichung native 1 to Philippine races of bacterial blight pathogens. Jpn. Soc. Breed. 42, 195–201. doi: 10.1270/jsbbs1951.42.195
Taura, S., Ogawa, T., Yoshimura, A., Ikeda, R., and Lwata, N. (1992b). Identification of a recessive resistance gene to rice bacterial blight of mutant line XM6, Oryza sativa L. Jpn. J. Breed. 42, 7–13. doi: 10.1270/jsbbs1951.42.7
Vikal, Y., Chawla, H., Sharma, R., Lore, J. S., and Singh, K. (2014). Mapping of bacterial blight resistance gene xa8 in rice (Oryza sativa L.). Indian J. Genet. Plant Breed. 74:589. doi: 10.5958/0975-6906.2014.00895.5
Waikhom, B., Anirudh, K., Reddy, M., Meenakshi, S. R., Sankar, L. G., Ahmed, Q. I., et al. (2015). Nucleotide diversity analysis of three major bacterial blight resistance genes in rice. PLoS One 10:e0120186. doi: 10.1371/journal.pone.0120186
Wang, G. L. (1996). The cloned gene, Xa21, confers resistance to multiple Xanthomonas oryzae pv. oryzae isolates in transgenic plants. Mol. Plant-Microbe Interact. 9, 850–855. doi: 10.1094/MPMI-9-0850
Wang, S. P. (2015). Ortholog alleles at Xa3/Xa26 locus confer conserved race-specific resistance against Xanthomonas oryzae in rice. Mol. Plant 5, 281–290.
Wang, Y., Cheng, X., Shan, Q., Zhang, Y., Liu, J., Gao, C., et al. (2014a). Simultaneous editing of three homoeoalleles in hexaploid bread wheat confers heritable resistance to powdery mildew. Nat. Biotechnol. 32, 947–951. doi: 10.1038/nbt.2969
Wang, C., Fan, Y., Zheng, C., Qin, T., Zhang, X., and Zhao, K. (2014b). High-resolution genetic mapping of rice bacterial blight resistance gene Xa23. Mol. Genet. Genom. 289, 745–753. doi: 10.1007/s00438-014-0848-y
Wang, Y. S., Pi, L. Y., Chen, X., Chakrabarty, P. K., Jiang, J., Leon, A. D., et al. (2007). Rice XA21 binding protein 3 is a ubiquitin ligase required for full Xa21-mediated disease resistance. Plant Cell 18, 3635–3646. doi: 10.1105/tpc.106.046730
Wang, G. L., Ruan, D. L., Song, W. Y., Sideris, S., Chen, L. L., Pi, L. Y., et al. (1998). Xa21D encodes a receptor-like molecule with a leucine-rich repeat domain that determines race-specific recognition and is subject to adaptive evolution. Plant Cell 10, 765–779. doi: 10.1105/tpc.10.5.765
Wang, C., Wen, G., Lin, X., Liu, X., and Zhang, D. (2009). Identification and fine mapping of the new bacterial blight resistance gene, Xa31(t), in rice. Eur. J. Plant Pathol. 123, 235–240. doi: 10.1007/s10658-008-9356-4
Wang, C., Zhang, X., Fan, Y., Ying, G., Zhu, Q., Zheng, C., et al. (2015). XA23 is an executor R protein and confers broad-spectrum disease resistance in rice. Mol. Plant 8, 290–302. doi: 10.1016/j.molp.2014.10.010
Webb, K. M., Oña, I., Bai, J., Garrett, K. A., Mew, T., Cruz, C. M. V., et al. (2010). A benefit of high temperature: increased effectiveness of a rice bacterial blight disease resistance gene. New Phytol. 185, 568–576. doi: 10.1111/j.1469-8137.2009.03076.x
Wu, X., Li, X., Xu, C., and Wang, S. (2008a). Fine genetic mapping of xa24, a recessive gene for resistance against Xanthomonas oryzae pv. oryzae in rice. Theor. Appl. Genet. 118, 185–191. doi: 10.1007/s00122-008-0888-y
Wu, L., Mei, L. G., and Yin, S. Z. (2008b). XA27 depends on an amino-terminal signal-anchor-like sequence to localize to the apoplast for resistance to Xanthomonas oryzae pv oryzae. Plant Physiol. 148, 1497–1509. doi: 10.1104/pp.108.123356
Xu, W. H., Wang, Y. S., Liu, G. Z., Chen, X., Tinjuangjun, P., Pi, L. Y., et al. (2006). The autophosphorylated Ser686, Thr688, and Ser689 residues in the intracellular juxtamembrane domain of XA21 are implicated in stability control of rice receptor-like kinase. Plant J. 45, 740–751. doi: 10.1111/j.1365-313X.2005.02638.x
Xu, Z., Xu, X., Gong, Q., Li, Z., Li, Y., Wang, S., et al. (2019). Engineering broad-spectrum bacterial blight resistance by simultaneously disrupting variable TALE-binding elements of multiple susceptibility genes in rice. Mol. Plant 12, 1434–1446. doi: 10.1016/j.molp.2019.08.006
Yang, Z., Sun, X., Wang, S., and Zhang, Q. (2003). Genetic and physical mapping of a new gene for bacterial blight resistance in rice. Theor. Appl. Genet. 106, 1467–1472. doi: 10.1007/s00122-003-1205-4
Yi, X., Cao, Y., Xu, C., Li, X., and Wang, S. (2006). Xa3, conferring resistance for rice bacterial blight and encoding a receptor kinase-like protein, is the same as Xa26. Theor. Appl. Genet. 113, 1347–1355. doi: 10.1007/s00122-006-0388-x
Ying, P., Bartley, L. E., Canlas, P., and Ronald, P. C. (2010). OsWRKY IIa transcription factors modulate rice innate immunity. Rice 3, 36–42. doi: 10.1007/s12284-010-9039-6
Ying, G. D., Hai, X. Y., Hua, S. L., and Mei, L. Z. O. (2002). RAPD analysis of a new resistance gene Xa-25(t) to rice bacterial blight. Chin. J. Rice Sci. 16, 179–181.
Yoshimura, A., Mew, T. W., Khush, G. S., and Omura, T. (1983). Inheritance of resistance to bacterial blight in rice cultivar CAS 209. Phytopathology 73, 1409–1412. doi: 10.1094/Phyto-73-1409
Yoshimura, S., Umehara, Y., Kurata, N., Nagamura, Y., Sasaki, T., Minobe, Y., et al. (1996). Identification of a YAC clone carrying the Xa-1 allele, a bacterial blight resistance gene in rice. Theor. Appl. Genet. 93, 117–122. doi: 10.1007/BF00225736
Yoshimura, S., Yamanouchi, U., Katayose, Y., Toki, S., Wang, Z. X., Kono, I., et al. (1998). Expression of Xa1, a bacterial blight-resistance gene in rice, is induced by bacterial inoculation. Proc. Natl. Acad. Sci. U. S. A. 95, 1663–1668. doi: 10.1073/pnas.95.4.1663
Yoshimura, S., Yoshimura, A., Iwata, N., Mccouch, S. R., and Nelson, R. J. (1995). Tagging and combining bacterial blight resistance genes in rice using RAPD and RFLP markers. Mol. Breed. 1, 375–387. doi: 10.1007/BF01248415
Yu, J., Hu, S., Wang, J., Wong, G., Li, S., Liu, B., et al. (2002). A draft sequence of the rice genome (Oryza sativa L. ssp. indica). Science 296, 79–92. doi: 10.1126/science.1068037
Yu, Y., Streubel, J., Balzergue, S., Champion, A., and Szurek, B. (2011). Colonization of rice leaf blades by an African strain of Xanthomonas oryzae pv. oryzae depends on a new TAL effector that induces the rice nodulin-3 Os11N3 gene. Mol. Plant-Microbe Interact. 24, 1102–1113. doi: 10.1094/MPMI-11-10-0254
Yuan, M., Chu, Z., Li, X., Xu, C., and Wang, S. (2010). The bacterial pathogen Xanthomonas oryzae overcomes rice defenses by regulating host copper redistribution. Plant Cell 22, 3164–3176. doi: 10.1105/tpc.110.078022
Yuan, Z. C. M., Li, X., Caiguo, X., and Wang, S. (2009). Pathogen-induced expressional loss of function is the key factor in race-specific bacterial resistance conferred by a recessive R gene xa13 in rice. Plant Cell Physiol. 50, 947–955. doi: 10.1093/pcp/pcp046
Yuan, M., and Wang, S. (2013). Rice MtN3/saliva/SWEET family genes and their homologs in cellular organisms. Mol. Plant 6, 665–674. doi: 10.1093/mp/sst035
Yuan, M., Wang, S., Li, X., Xiao, J., Chu, Z., Yang, Z., et al. (2016). A host basal transcription factor is a key component for infection of rice by TALE-carrying bacteria. elife 5:e19605. doi: 10.7554/eLife.19605
Zhang, Q. (2005). Highlights in identification and application of resistance genes to bacterial blight. Zhongguo Shuidao Kexue. 19, 453–459. doi: 10.1360/aps040074
Zhang, J., Li, X., Jiang, G., Xu, Y., and He, Y. (2010). Pyramiding of Xa7 and Xa21 for the improvement of disease resistance to bacterial blight in hybrid rice. Plant Breed. 125, 600–605. doi: 10.1111/j.1439-0523.2006.01281.x
Zhang, B., Zhang, H., Li, F., Ouyang, Y., and Wang, S. (2020). Multiple alleles encoding atypical NLRs with unique central tandem repeats (CTRs) in rice confer resistance to Xanthomonas oryzae pv. oryzae. Plant Commun. 1:100088. doi: 10.1016/j.xplc.2020.100088
Zhang, F., Zhuo, D. L., Huang, L. Y., Wang, W. S., and Zhou, Y. L. (2015). Xa39, a novel dominant gene conferring broad-spectrum resistance to Xanthomonas oryzae pv. oryzae in rice. Plant Pathol. 64, 568–575. doi: 10.1111/ppa.12283
Zheng, C. K., Wang, C. L., Yuan-Jie, Y. U., Liang, Y. T., and Zhao, K. J. (2009). Identification and molecular mapping of Xa32(t), a novel resistance gene for bacterial blight (Xanthomonas oryzae pv. oryzae) in rice. Acta Agron. Sin. 35, 1173–1180. doi: 10.1016/S1875-2780(08)60089-9
Zhou, J., Peng, Z., Long, J., Sosso, D., Liu, B., Eom, J. S., et al. (2015). Gene targeting by the TAL effector PthXo2 reveals cryptic resistance gene for bacterial blight of rice. Plant J. 82, 632–643. doi: 10.1111/tpj.12838
Keywords: rice bacterial blight, resistance genes, gene mapping, map-based cloning, resistance mechanism, resistance breeding
Citation: Yang Y, Zhou Y, Sun J, Liang W, Chen X, Wang X, Zhou J, Yu C, Wang J, Wu S, Yao X, Zhou Y, Zhu J, Yan C, Zheng B and Chen J (2022) Research Progress on Cloning and Function of Xa Genes Against Rice Bacterial Blight. Front. Plant Sci. 13:847199. doi: 10.3389/fpls.2022.847199
Received: 04 February 2022; Accepted: 24 February 2022;
Published: 21 March 2022.
Edited by:
Zuhua He, Center for Excellence in Molecular Plant Sciences (CAS),ChinaReviewed by:
Yuanhu Xuan, Shenyang Agricultural University, ChinaCopyright © 2022 Yang, Zhou, Sun, Liang, Chen, Wang, Zhou, Yu, Wang, Wu, Yao, Zhou, Zhu, Yan, Zheng and Chen. This is an open-access article distributed under the terms of the Creative Commons Attribution License (CC BY). The use, distribution or reproduction in other forums is permitted, provided the original author(s) and the copyright owner(s) are credited and that the original publication in this journal is cited, in accordance with accepted academic practice. No use, distribution or reproduction is permitted which does not comply with these terms.
*Correspondence: Jianping Chen, amlhbnBpbmdjaGVuQG5idS5lZHUuY24=; Yong Yang, eWFuZ3lvbmdAemFhcy5hYy5jbg==
†These authors have contributed equally to this work and share first authorship
Disclaimer: All claims expressed in this article are solely those of the authors and do not necessarily represent those of their affiliated organizations, or those of the publisher, the editors and the reviewers. Any product that may be evaluated in this article or claim that may be made by its manufacturer is not guaranteed or endorsed by the publisher.
Research integrity at Frontiers
Learn more about the work of our research integrity team to safeguard the quality of each article we publish.