- 1Crop Science Group, Institute of Crop Science and Resource Conservation, University of Bonn, Bonn, Germany
- 2Agroecology and Organic Farming Group, Institute of Crop Science and Resource Conservation, University of Bonn, Bonn, Germany
- 3Department of Ecological Plant Protection, Faculty of Organic Agricultural Sciences, University of Kassel, Witzenhausen, Germany
- 4Crop Systems Analysis Group, Wageningen University, Wageningen, Netherlands
- 5Université Paris-Saclay, INRAE, CNRS, AgroParisTech, GQE-Le Moulon, Gif-sur-Yvette, France
Cropping system diversification through annual intercropping provides a pathway for agricultural production with reduced inputs of fertilizer and pesticides. While several studies have shown that intercrop performance depends on the genotypes used, the available evidence has not been synthesized in an overarching analysis. Here, we review the effects of genotypes in cereal/legume intercropping systems, showing how genotype choice affects mixture performance. Furthermore, we discuss the mechanisms underlying the interactions between genotype and cropping system (i.e., sole cropping vs. intercropping). Data from 69 articles fulfilling inclusion criteria were analyzed, out of which 35 articles reported land equivalent ratio (LER), yielding 262 LER data points to be extracted. The mean and median LER were 1.26 and 1.24, respectively. The extracted genotype × cropping system interaction effects on yield were reported in 71% out of 69 publications. Out of this, genotype × cropping system interaction effects were significant in 75%, of the studies, whereas 25% reported non-significant interactions. The remaining studies did not report the effects of genotype × cropping system. Phenological and morphological traits, such as differences in days to maturity, plant height, or growth habit, explained variations in the performance of mixtures with different genotypes. However, the relevant genotype traits were not described sufficiently in most of the studies to allow for a detailed analysis. A tendency toward higher intercropping performance with short cereal genotypes was observed. The results show the importance of genotype selection for better in cereal/legume intercropping. This study highlights the hitherto unrevealed aspects of genotype evaluation for intercropping systems that need to be tackled. Future research on genotype effects in intercropping should consider phenology, root growth, and soil nutrient and water acquisition timing, as well as the effects of weeds and diseases, to improve our understanding of how genotype combination and breeding may help to optimize intercropping systems.
Introduction
In the past few decades, agricultural intensification has resulted in increased yields of pure line crops (Blomqvist et al., 2020); this has been accompanied by the simplification and homogenization of production systems and concentration on very few species as human diet staples (Khoury et al., 2014). Genetic uniformity and loss of diversity in the agricultural landscape (Hazell and Wood, 2008; Gregory and George, 2011) are characteristics of intensive agriculture, increasing vulnerability to climate change (Lin et al., 2008), and pathogen invasions (Anderson et al., 2004; Savary et al., 2019). Diversifying crop production systems is a promising pathway to tackle such vulnerabilities (Renard and Tilman, 2019; Hufnagel et al., 2020; Tscharntke et al., 2021). Diversification approaches can be classified into two categories: (1) integration of underutilized crops into the system; and (2) diversification of the production system through crop rotation, mixed cropping, and/or catch crops (Mustafa et al., 2019). More efficient utilization of resources with beneficial effects on the environment could also be gained by the integration of livestock with temporal and spatial crop diversification, such as forage legume intercropping with grain cereals (Danso-Abbeam et al., 2021). Crop diversification includes practices that significantly improve crop productivity, especially benefiting rural smallholders (Makate et al., 2016), and enhance overall ecosystem services without compromising crop yield (Tamburini et al., 2020; Beillouin et al., 2021; Ditzler et al., 2021). Annual intercropping is one form of cropping system diversification, which allows high productivity and reduction of fertilizer and pesticide input (Bedoussac et al., 2015; Li et al., 2020b) thereby substantially minimizing the negative environmental impacts of agriculture. Furthermore, crop diversification provides insurance against crop failure for farmers (Lithourgidis et al., 2011; Gaba et al., 2015).
Mixing crop species may be done with annual crops or perennial crops on a gradient of complexity from two to several species (Malézieux et al., 2009; Finckh and Wolfe, 2015). Cereal/legume intercropping systems are widely used across the world, particularly by smallholders, producing high-quality cereal and legume grains in an economically sustainable, environmentally friendly, and efficient way. Using legume crops in a mixture with cereals may significantly mitigate N2O fluxes derived from fertilizer, hence providing an effective way to reduce greenhouse gas emissions from cropping systems (Senbayram et al., 2015). Furthermore, intercropping was found to produce higher cereal protein concentration (Bedoussac and Justes, 2010; Timaeus et al., 2021b), higher grain yields (Yu et al., 2016), higher yield stability (Raseduzzaman and Jensen, 2017), and better abiotic and biotic stress resistance (Bedoussac et al., 2015; Timaeus et al., 2021a) than sole crops.
Intercropping performance is often measured by the land equivalent ratio (LER), an index measuring the relative land area required to produce the same yields (or any other services, such as biomass) in sole crops as obtained from a unit area of intercrop. An LER greater than one indicates that intercropping uses the land more efficiently than pure stands to produce the desired outputs (Mead and Willey, 1980).
Several studies have shown that the general performance of intercropping systems depends on the genotypes used in the mixture (e.g., Hauggaard-Nielsen and Jensen, 2001) and that the performance in a mixed stand can be poorly correlated to performance in a pure stand (Annicchiarico et al., 2019). Different genotypes of legumes may have different responses in terms of phenology and morphology (Annicchiarico and Filippi, 2007) when compared in sole crops vs. mixtures. Hence, a specific selection of genotypes for intercropping is important (Giles et al., 2017), and intercrop yield advantage could be achieved by selecting specific traits of both species (Berghuijs et al., 2020). Therefore, it has been suggested that specific breeding of genotypes for intercropping is needed to improve complementarity of the intercropping partners (Annicchiarico et al., 2019; Haug et al., 2021).
Cereal/legume mixtures could include systems where both species have similar phenology but contrasting morphology, or, alternatively, contrasting phenology and morphology, resulting in temporal and/or spatial niche complementarity (Gaudio et al., 2019). The ecological niche separation concept posits that the different species involved may have different resource requirements at different times, as well as for different sources of nutrition (Malézieux et al., 2009). In addition to niche complementarity, intercrop performance can also be due to additional ecological mechanisms (Loreau and Hector, 2001). Facilitation effects may exist between mixed species, such as synergy in the use of phosphorus (Hinsinger et al., 2011; Li et al., 2020). The species complementarity effect, which measures the overall shift of relative yields in mixtures vs. sole crop, has a higher effect on yield gain than the selection effect, which defines how these shifts in relative yields are correlated to sole crop yields (Li et al., 2020a). Complementarity is a paramount feature in cereal/legume intercrops grown under low-nitrogen (N) conditions, in which biological N fixation by the legume and strong competition for soil-N by the cereal may synergize to enhance yield and grain quality.
Choosing plant genotypes for specific intercropping systems is, however, laborious and costly, if only because assessing intercropping performance also requires the inclusion of sole crops in field experiments for comparison and estimation of the benefits of mixing. Testing genotypes in mixtures easily results in a curse of dimensionality. For instance, with five genotypes of a cereal and five genotypes of a mixture, 25 mixtures should be tested along with 10 pure stands. Optimal species traits likely depend on the companion species, such that all possible combinations are preferably tested. Note that incomplete designs have been proposed to deal with this challenge of dimensionality (Hinsinger et al., 2011), and shown to be efficient to estimate mixing abilities (Haug et al., 2021). Testers and reciprocal breeding schemes have been proposed to co-breed species (Sampoux et al., 2020). Recent technologies, such as genomic selection strategies, could help select traits for breeding for intercropping accurately (Bančič et al., 2021). However, better knowledge on genotypes and their associated trait effects in intercropping is needed to make selection more targeted.
General and specific mixing ability of genotypes of single species has been studied to determine contrasting traits in sole cropping and in mixtures, and the theoretical background has been discussed with respect to species mixtures (Wright, 1985). Historically, multiple studies have evaluated different crop genotypes for complementarity in intercropping (Francis et al., 1976; Smith, 1985; Smith and Zobel, 1991; Davis and Woolley, 1993). Abundant research has been conducted, but the knowledge on genotype effects in intercropping is fragmented and has not been compiled to deliver necessary knowledge for designing optimized intercropping systems. Here, we aim to provide a current update by linking recent advances through a review. In particular, we address the knowledge gap concerning the mechanisms involved in genotype × cropping system interaction. This review is intended to answer the following questions: (i) How do different genotypes and/or traits of a species in cereal/legume intercropping systems affect the performance of the mixture? (ii) What are the mechanisms underlying the interaction of the genotypes in the intercropping system? and (iii) What are the current knowledge gaps in genotype evaluation for intercropping systems?
Materials and Methods
Literature Search and Publication Screening
We conducted a systematic map, using the science databases Web of Science, Scopus, Science Direct, and Google Scholar. Keywords used for searching suitable publications were “genotype interaction in inter/mixed cropping system” OR “cultivars interaction in inter/mixed cropping system” OR “varieties interaction in inter/mixed cropping system” OR “cereals in inter/mixed cropping system” and scientific names (genus and species name) and common names of cereals species with intercropping and mixed cropping. The slash (/) was not used in a search; here, it is used for simplified expression of search terms (i.e., intercropping OR mixed cropping). A full list of the search terms is given in the Supplementary Table 1. In addition, secondary literature cited in selected articles were also looked up and included if relevant. The latest search was conducted on 12 April 2021.
To select the relevant articles, we used the following inclusion criteria: (i) studies from cereal/legume intercropping with both grain and forage legumes, (ii) studies evaluated at least two genotypes of at least one of the mixed species, (iii) peer-reviewed full-length articles published in English, (iv) studies reporting original research data, and (v) only field experiments, excluding greenhouse or pot experiments. No restriction was made against the type of mixture design, e.g., with respect to plant density, such as additive, replacement (substitution), or intermediate design. The information extracted from the original research articles was categorized in a digital database and analyzed following the Preferred Reporting Items for Systematic Review and Meta-Analyses (PRISMA) guidelines (Moher et al., 2009).
Variables and Data Extraction
Data on genotype performance originated from different management and different zones, resulting in large differences in yield. Hence, an index was necessary to characterize the performance of genotypes in intercropping in relation to their respective pure stands (Mead and Willey, 1980). We used the LER (Equation 1) as a key metric to measure intercrop yield advantage (or disadvantage) by reference to the pure crop yields of mixed genotypes. We also retrieved the results of any ANOVA analyzing genotype and cropping system main effects and their interaction. Furthermore, individual studies were scrutinized by assessing conclusions and interpretations about the effects of different traits (phenology and morphology) of species in mixtures to identify the general mechanisms responsible for cereal/legume intercropping yield advantage.
Different variables were extracted from each study (Table 1) in the core set of publications. Information, like intercropping design (design of the mixing system, i.e., substitutive or additive or intermediate), country of the experiment, number of genotypes, and other related variables, was extracted from each publication. Significance (or non-significance) of “genotype” effect, “cropping system” effect (pure vs. mixed stand), and “genotype” × “cropping system” interaction effect on yield data was extracted from ANOVA tables of the articles. This was done by extracting results from the ANOVA of each article; any differences among articles regarding the structure of statistical analysis (e.g., fixed vs. random effects) were disregarded. The mechanisms of intercropping performance were extracted from the description of results, and the full article was consulted if needed. Some studies reported various types of mixtures, from different species of either cereals or legumes. In addition, in these cases, data were extracted from all combinations in which at least two genotypes of at least one of the partners were evaluated.
The LER (Equation 1) of each genotype combination was extracted from the subset of articles reporting them, either directly when represented numerically, or in figures. Data from figures were digitalized using a web-based plot digitizer (Rohatgi, 2020), an online system used to extract data from images efficiently and accurately (Burda et al., 2017; Cramond et al., 2019). LER was reported in figures only in five articles (Rao and Willey, 1983; Odo, 1991; Watiki et al., 1993; Kontturi et al., 2011; Pappa et al., 2012; Barillot et al., 2014). The majority of the studies reported mean LER per genotype combination across multiple environments. However, in some cases, the studies reported data individually from each environment. If the mean LER across different environments was not reported, this mean was computed for each genotype combination of the species in the intercrop from the individual environments. When a study reported only the partial land equivalent ratio (PLER), the total LER was calculated for each genotype combination of the species in intercropping by summing the PLERs:
where LERc+l is the LER of the cereal genotype c with the legume genotype l; and PLERc/l is the partial LER of genotype c in mixture with legume genotype l (and reciprocally for PLERl/c). This genotype combination-specific LER was used in further analysis. If neither LER nor PLER was reported, LER for each genotype in a given cereal–legume combination was calculated from yields in mono-cropping and intercropping.
When other treatments were applied (such as different row spacing, and sowing density or proportion), LERs were extracted or calculated from only one treatment. If different levels of N were used, data for each level of fertilizer were considered and averages computed for each genotype combination. In one study, results from two species of cereals or legumes were reported. Thus, data were recorded from each genotype combination from each species and analyzed. Therefore, at least 2 data points from each article (depending on number of genotypes of cereals and legumes) were extracted. In this way, we obtained 262 LER data points.
Since only few (10%) LER data points were reported from forage legume species combinations with cereals (2 articles with oats, 1 article with finger millet, and 2 articles with maize) all data from forage and grain legumes were combined and analyzed together.
Data Analysis
The main effects of genotype and intercropping and their interaction effects were assessed by counting and calculating the proportion of articles that reported significant or non-significant effects on yields. In addition to the analysis of LER, a fixed-effects ANOVA model was used to test the effect of cereal species, design, and interaction effect on LER across cereal species by categorizing the dataset by cereal species. Because the number of data points of wheat was low (n = 5), and data records from barley and rice were only from replacement design, we excluded these three from the analysis. The number of data records per cereal species varied from 25 (finger millet) to 131 (maize). Similarly, a fixed-effects ANOVA model was used to test the effect of legume species, design, and interaction on LER across legume species by categorizing the dataset by legume species. However, faba bean, grass pea, guar and hairy vetch, berseem clover, and bitter vetch were excluded because the number of data points (two to four) was low. The mean comparison was done by Tukey’s honestly significant difference (HSD) test.
To assess the potential of genotype choice for optimizing LER, we calculated three indices using the extracted data from the articles (averages across the site years); to obtain these indices, we first calculated the maximum, median, and minimum LER across different genotype combinations for each article. Then (i) the difference between maximum and median LER was used as a measure for the potential of combined genotype choice to improve LER in comparison to a random choice; similarly, (ii) the difference between minimum and median LER was taken as a measure for the risk to choose an inappropriate genotype combination in comparison to a random choice; and (iii) the range, i.e., the difference between maximum and minimum LER from an article was used to characterize the maximum genotype combination effect within a study. The median used to calculate all three statistics were calculated from each individual article. The three statistics are equivalent when only two genotypes were evaluated. Because of sampling effects, it is expected that all three differences would tend to increase (in absolute terms) with increasing number of genotype combinations tested within a study (Schwarz, 2011); therefore, we plotted the indices against the number of genotype combinations. The extracted LER data were subjected to descriptive statistics; all analyses were conducted with R (R CoreTeam, 2020), and figures were produced using the R package ggplot2 (Wickham, 2016).
Results
Geographical Distribution and Characteristics of Studies
From about 4,000 search hits using all search terms, only 69 articles fulfilled the inclusion criteria (Table 2). The reported research studies were conducted in 28 different countries (Supplementary Table 2). The majority of data came from Africa (37%) followed by Europe (24%) and Asia (18%). The included studies considered different contrasting characteristics of genotypes of cereals and legumes evaluated.
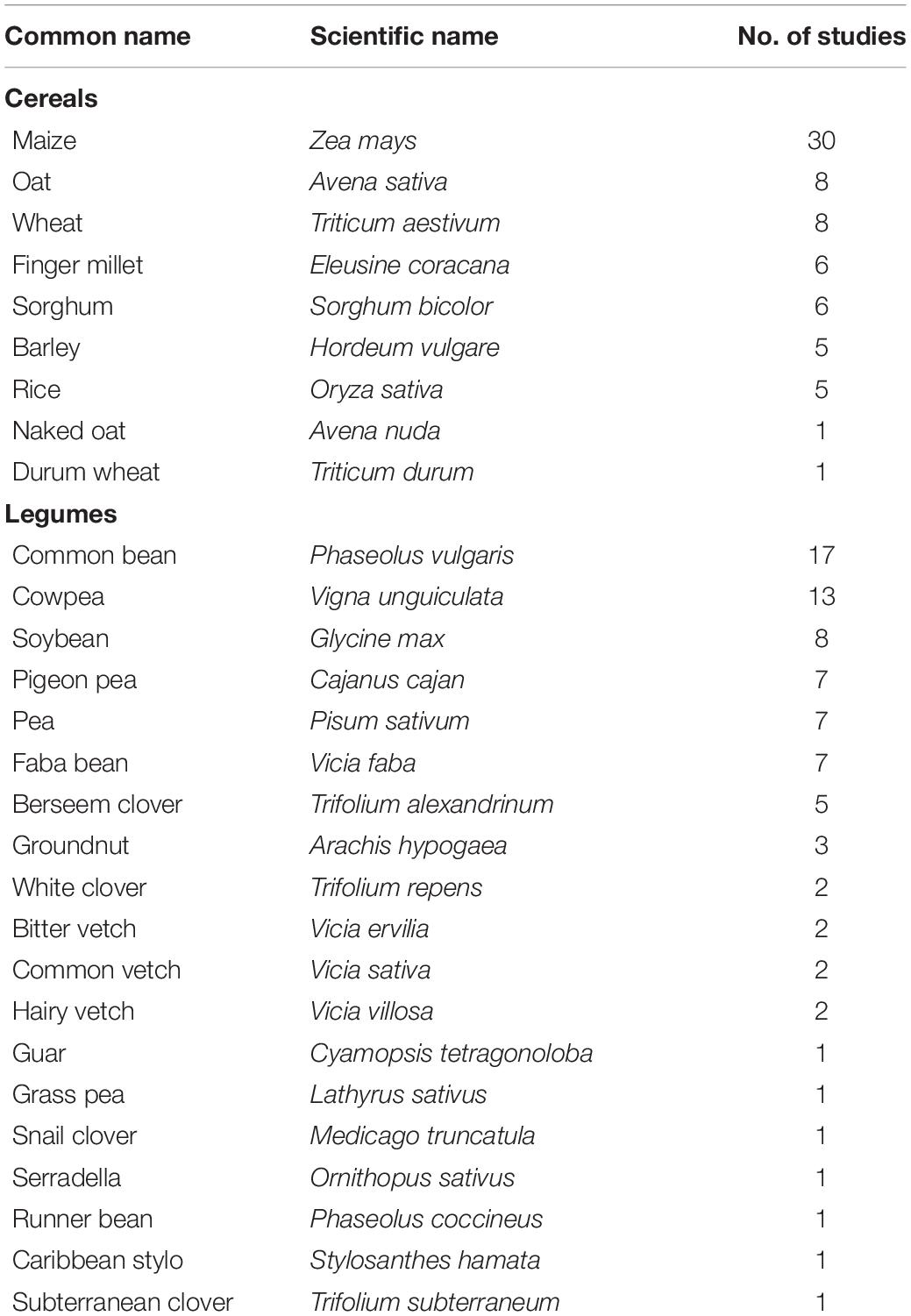
Table 2. List of cereal and legume species in the 69 selected studies investigating genotype effects in intercropping; because some studies tested more than two species, the sum of studies across all crop species (152) is greater than 2 × 69 = 138.
Overall, 9 cereal crop species and 19 legume species were evaluated in 69 publications with maize as the most frequently evaluated cereal species followed by oat and wheat. Common bean was the most frequently evaluated legume followed by cowpea and soybean. In the considered studies, common bean was only intercropped with maize. A single genotype was used in 62% of the studies for one of the partner species, i.e., in these studies, genotypic variation was only investigated in the other partner. On average, 4 cereal genotypes or 3 legume genotypes were compared per study, when excluding the single genotype studies (Figure 1). The most diverse comparison included 8 genotypes of cereal (Avena sativa) and 7 genotypes of legume species (Trifolium alexandrinum), in a total of 56 cereal–clover combinations.
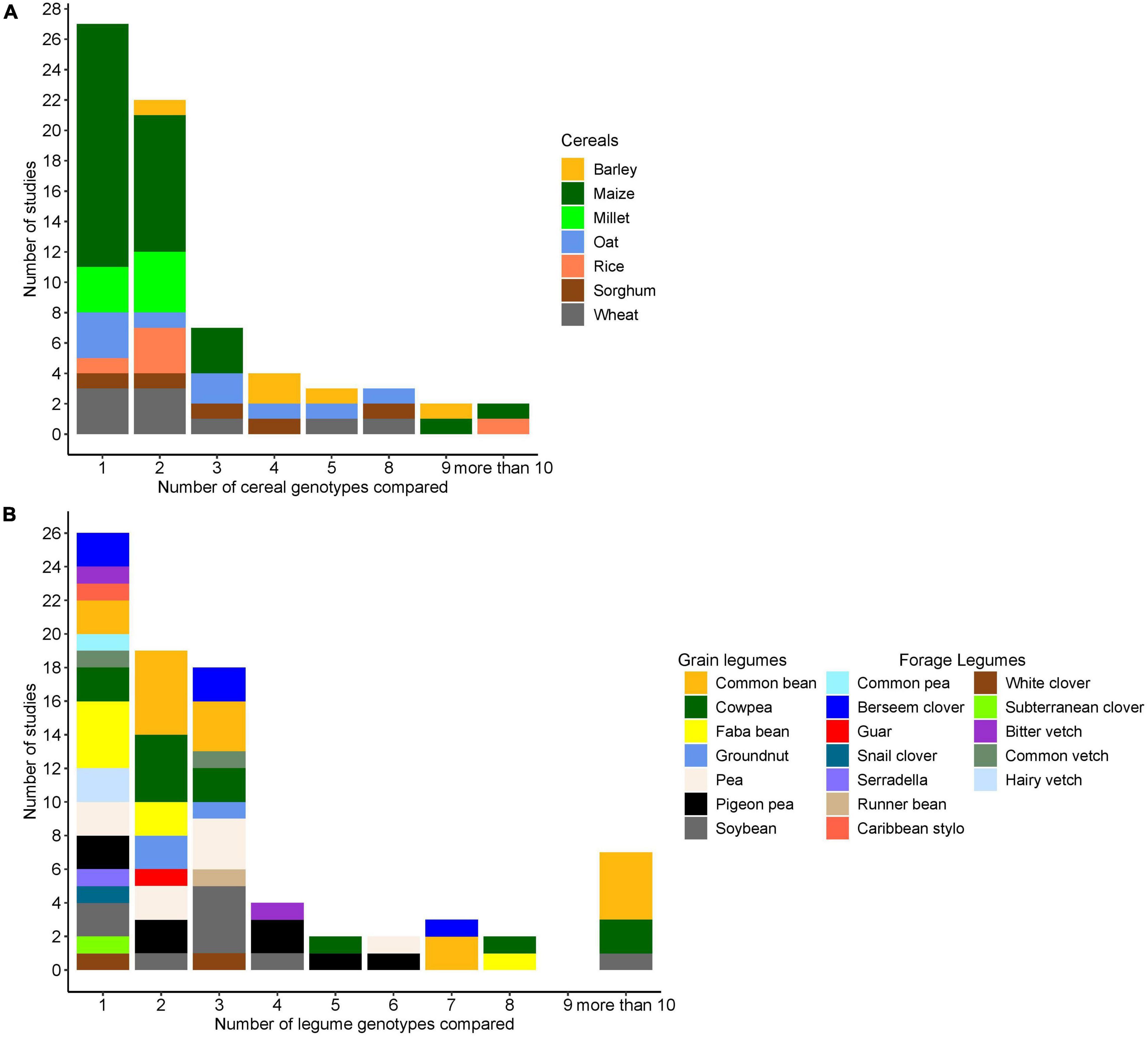
Figure 1. (A) Number of cereal genotypes evaluated in combination with legume species (each combination was categorized based on the cereal species). (B) Number of legume genotypes evaluated in combination with cereal species (each combination was categorized based on the legumes species). In both cases, if one genotype of one partner is evaluated, the other partner had at least 2 genotypes.
The majority of studies (55) evaluated grain legumes, whereas eight studies evaluated forage legumes, and a small proportion (6) of studies evaluated both forage and grain legumes together. The number of genotypes used in the studies varied, with similar numbers of studies reporting on (i) combinations of two or more cereal genotypes with two or more legume genotypes, (ii) one cereal genotype combined with two or more legume genotypes; or (iii) one legume genotype combined with two or more cereal genotypes (Table 3).
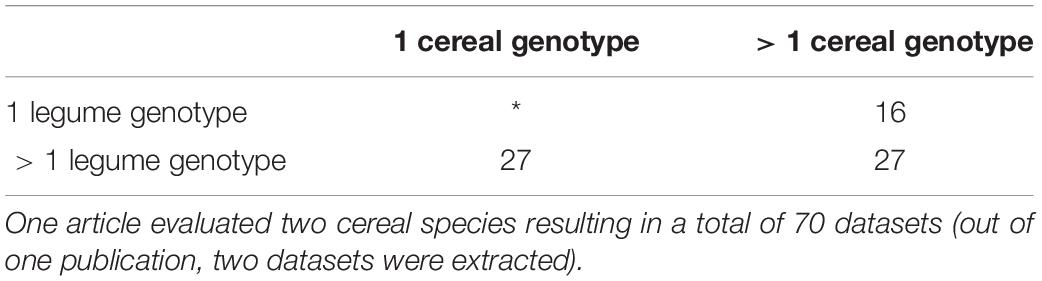
Table 3. Number of studies with one or more than one genotype of cereal and/or legume (*not included in this review) from 69 studies.
Effect of Cropping System and Genotypes of Cereal/Legume on Intercropping Performance
Genotype × Cropping System Interaction
The extracted genotype × cropping system interaction effects on yield were reported in 49 (71%) studies out of 69 publications. Out of this, genotype × cropping system interaction effects were significant in 37 (75%) of the studies, whereas 12 (25%) of the studies reported non-significant interactions. The remaining studies did not report the effects of genotype × cropping system. In addition, intercropping main effects were reported in 38 (55%) studies. Out of this, the effect was significant in 27 (71%) and non-significant in 11 (29%) of the publications. Genotype main effects were reported in 37 (53%) studies; out of this, the genotype effect was significant in 25 (67%) and non-significant in 12 (33%) of the publications. The remaining studies did not mention the effects of cropping system and genotype effects.
Land Equivalent Ratio as Metric to Gauge Yield Advantage of Genotypes in Intercropping
From the 69 studies used for data extraction, 35 studies yielded 36 datasets (one study used two cereal species) and either reported the LERs directly or allowed calculation from the reported yield data. From these 36 datasets, 262 data points (cereal/legume genotype combinations) were extracted, based on a total of 85 cereal and 126 legume genotypes, with a number of cereal/legume combinations (LER) ranging from 2 to 22 per study.
The calculated mean and the median LER were 1.26 and 1.24, respectively (Figure 2), and LER was greater than 1.0 in 85% of the single cases. Although the number of data points for some cereals, especially wheat, may not be sufficient to compare the median LER with other cereals, the overall outcome was robustly > 1 with the highest median LER of 1.38 (n = 25) found in finger millet. The strikingly high variation in maize is in part due to the number of studies. In barley-based cropping systems, all of the LER data were greater than 1 (n = 22, range 1.05–1.48) (Figure 3).
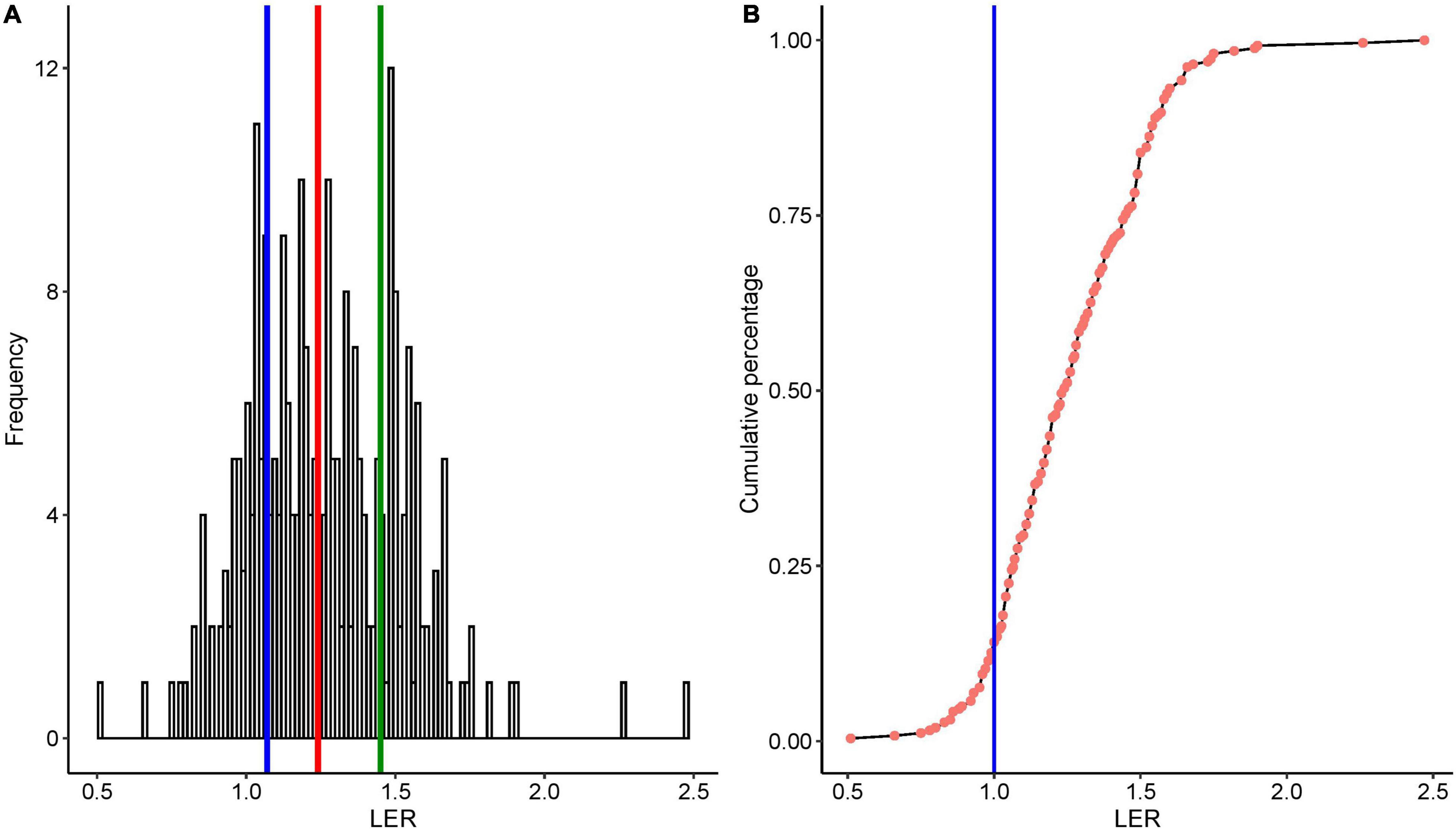
Figure 2. (A) Frequency distribution of LER from 35 studies, quartiles marked by blue and green; median marked by red-colored vertical lines; (B) cumulative percentage distribution of LER from 35 studies, 36 (datasets); the vertical blue line in panel (B) shows LER = 1.
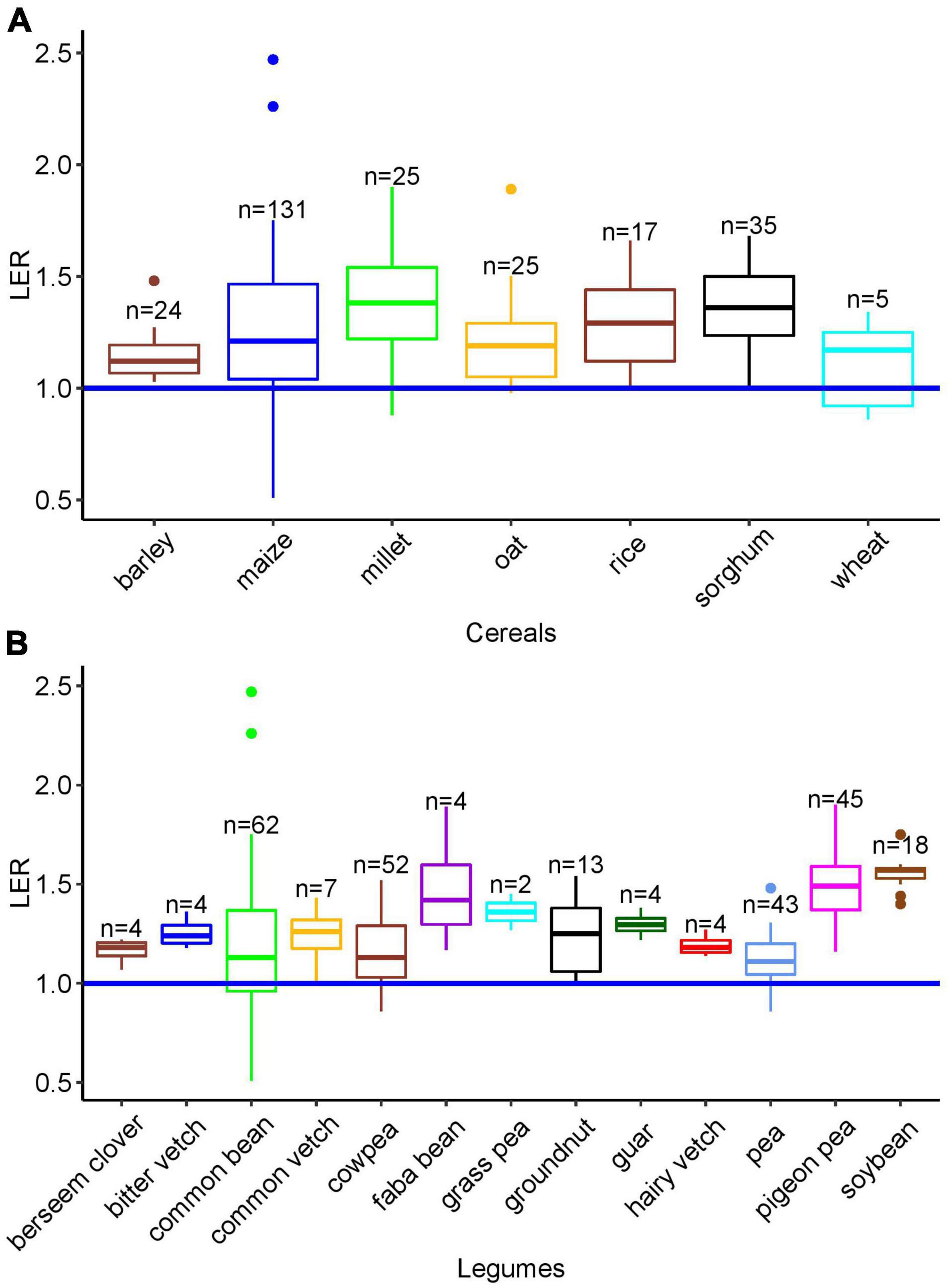
Figure 3. (A) LER of intercropping systems with different cereal components. (B) LER of intercropping systems with different legume components. Extracted from 35 studies with median (horizontal line), upper and lower quartiles (boxes), and 1.5 interquartile range (IQR) (whiskers). The horizontal blue line was drawn at LER = 1; n: number of data points. Although wheat, faba bean, berseem clover, bitter vetch, hairy vetch, and guar data were excluded from the ANOVA (n < 5), the data are shown in this graph for comparison.
The ANOVA resulted in highly significant differences across cereal species and design (p < 0.01). In addition, the interaction effect was significant (p < 0.05). The pairwise means comparison revealed that finger millet reached higher LERs in additive designs as compared to replacement designs, whereas no effect of design was found in maize and sorghum (see Supplementary Table 3 for ANOVA and Figure 4A). The ANOVA, across legume species and design, resulted in highly significant differences across legume species with pigeon pea and soybean exceeding other species but non-significant effects of design and interaction effect (p > 0.05) (see Supplementary Table 4 for ANOVA) (Figure 4B).
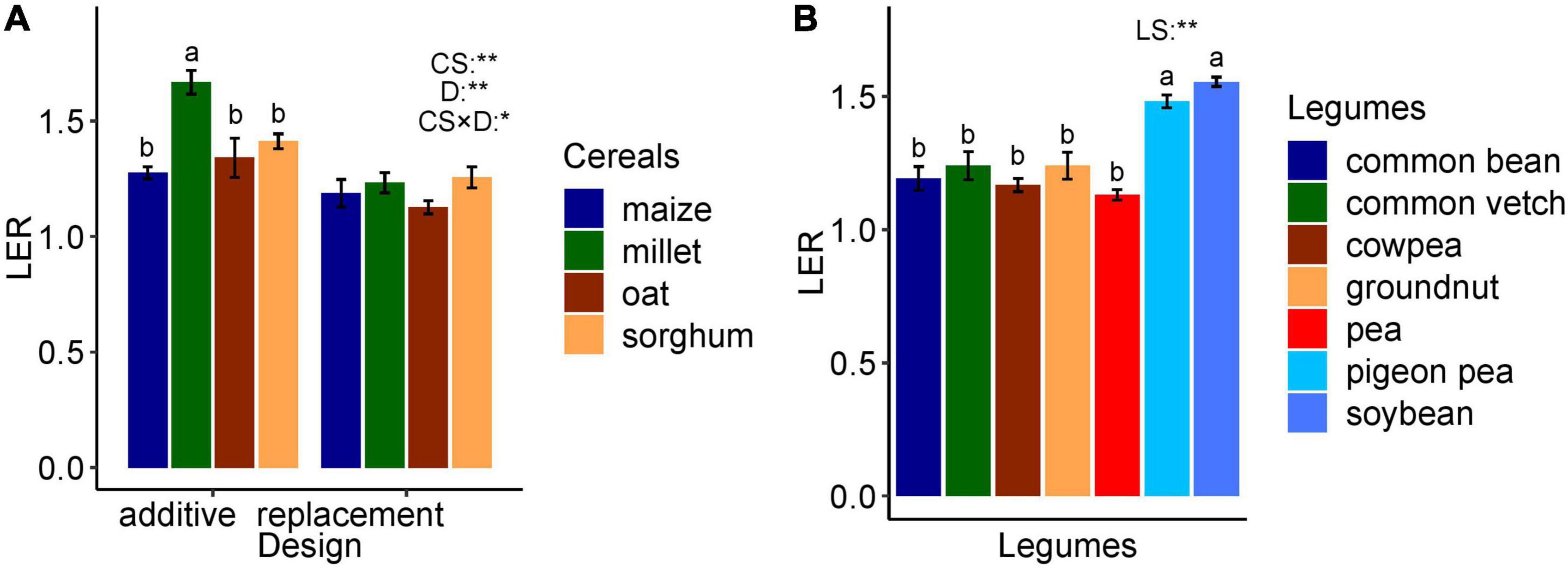
Figure 4. (A) Effect of cereal species and design on LER. (B) Effect of legume species on LER. The letters show the statistical differences between species. CS, cereals species; D, design; CS × D, species interaction with design; LS, legumes species; *significant (p < 0.05), **highly significant (p < 0.01), and the error bar is the standard error of the mean. The two designs (additive and replacement) are not represented for legumes because the effect of design is not significant.
The Potential of Genotype Choice for Intercropping
The distribution of the LERs within the studies around the median (Figure 5) indicates that genotype-specific effects play a role in the performance of mixtures in comparison to sole crops. Overall, the range (i.e., difference between maximum LER and minimum LER within a study) varied between 0 and 1.98, showing the potential of large genotype effects in intercropping. Conversely, there was a risk to obtain low LERs by non-appropriate genotype choice (i.e., as indicated by the difference of minimum LER and median LER, red points in Figure 5); the difference between minimum and median ranged from − 0.55 to 0. The largest LER range (1.96) was found in a study with 20 different genotypes combinations (10 bean and two maize genotypes) (Santalla et al., 2001); in the only other study with 20 genotypes combination (Hauggaard-Nielsen and Jensen, 2001), the range was 0.27, i.e., quite moderate (Table 5).
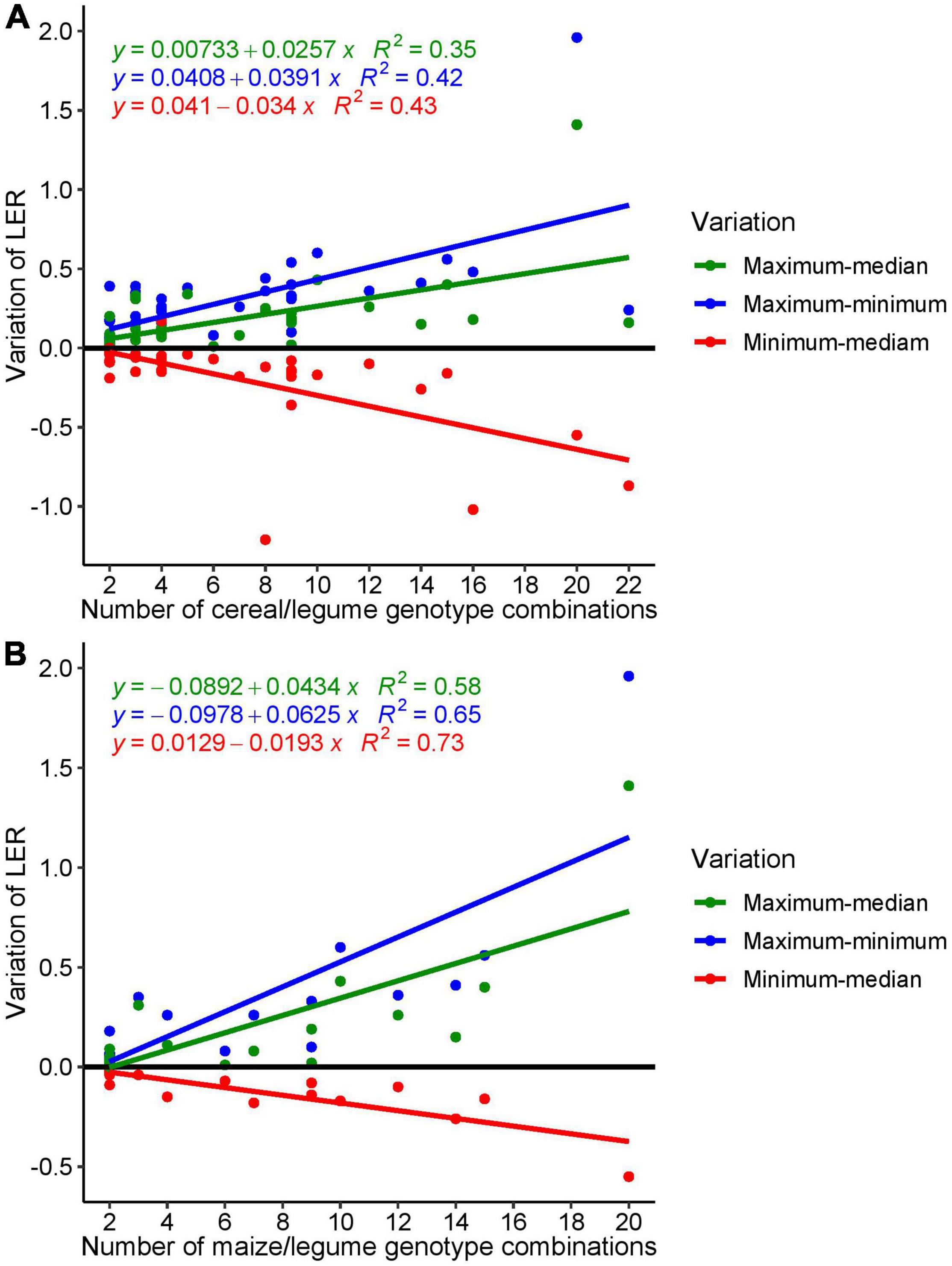
Figure 5. Variation of extracted LER: (A) all data points from cereal/legume intercropping extracted from 35 studies and (B) LER variation from maize-based intercropping extracted from 16 studies.
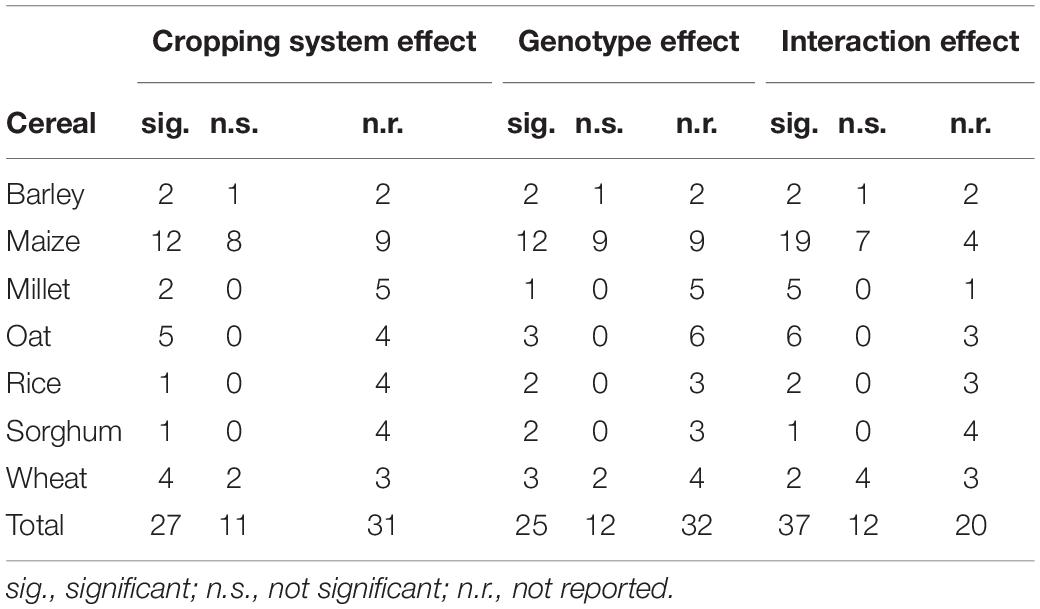
Table 4. Number of studies reporting significant and non-significant genotype, cropping system, and interaction effects, categorized by cereals.
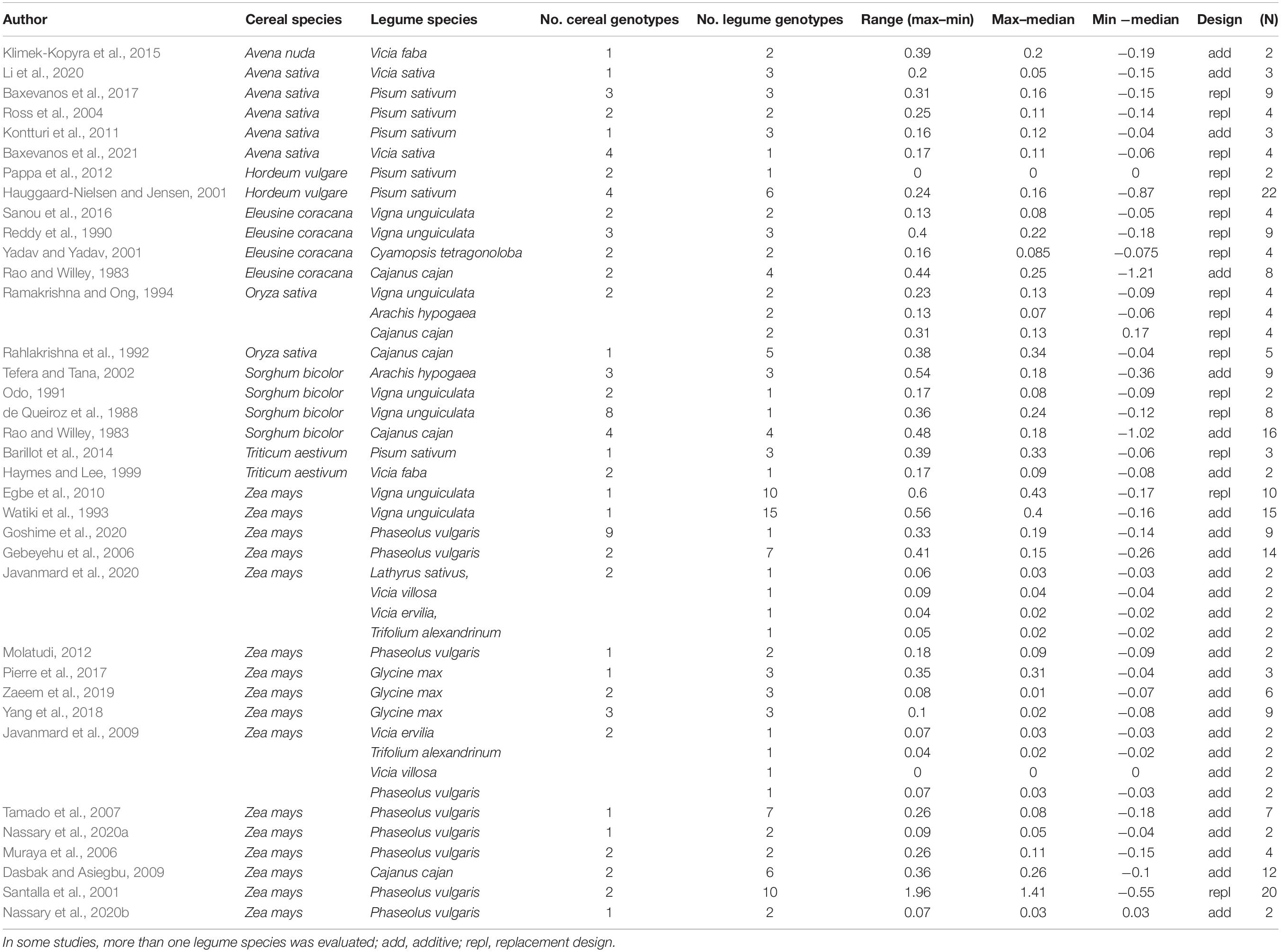
Table 5. Deviations of LER from the median in cereal/legume intercropping extracted from 35 studies including between 2 and 20 mixtures, i.e., different genotype combinations (N).
To elaborate the effect of genotypes on intercropping performance in terms of LER, the studies from maize-based were analyzed in detail. A total of 16 studies reported LER in maize-intercropping system and yielded 138 LER data records. The analysis shows that with the increasing number of maize genotypes included in the study, the LER range (maximum–minimum) increased significantly with an R2 of 0.58 (p = 0.00063) and 0.47 (p = 0.0046) in the regression of LER against number of genotype combinations, when the study of Santalla et al. (2001) that represents an outlier in terms of the number of genotypes combination tested (20 compared to 2–15) was included or excluded, respectively (Figure 5B).
Mechanisms Underlying the Interactions Between Genotypes and Cropping System
In 20 out of the 69 studies, contrasting phenological or architectural characteristics of cereal and/or legume genotypes were highlighted, suggesting that the temporal and spatial differences among genotypes contributed to intercrop performance. These traits were broadly categorized into phenological and morphological traits (Table 6).
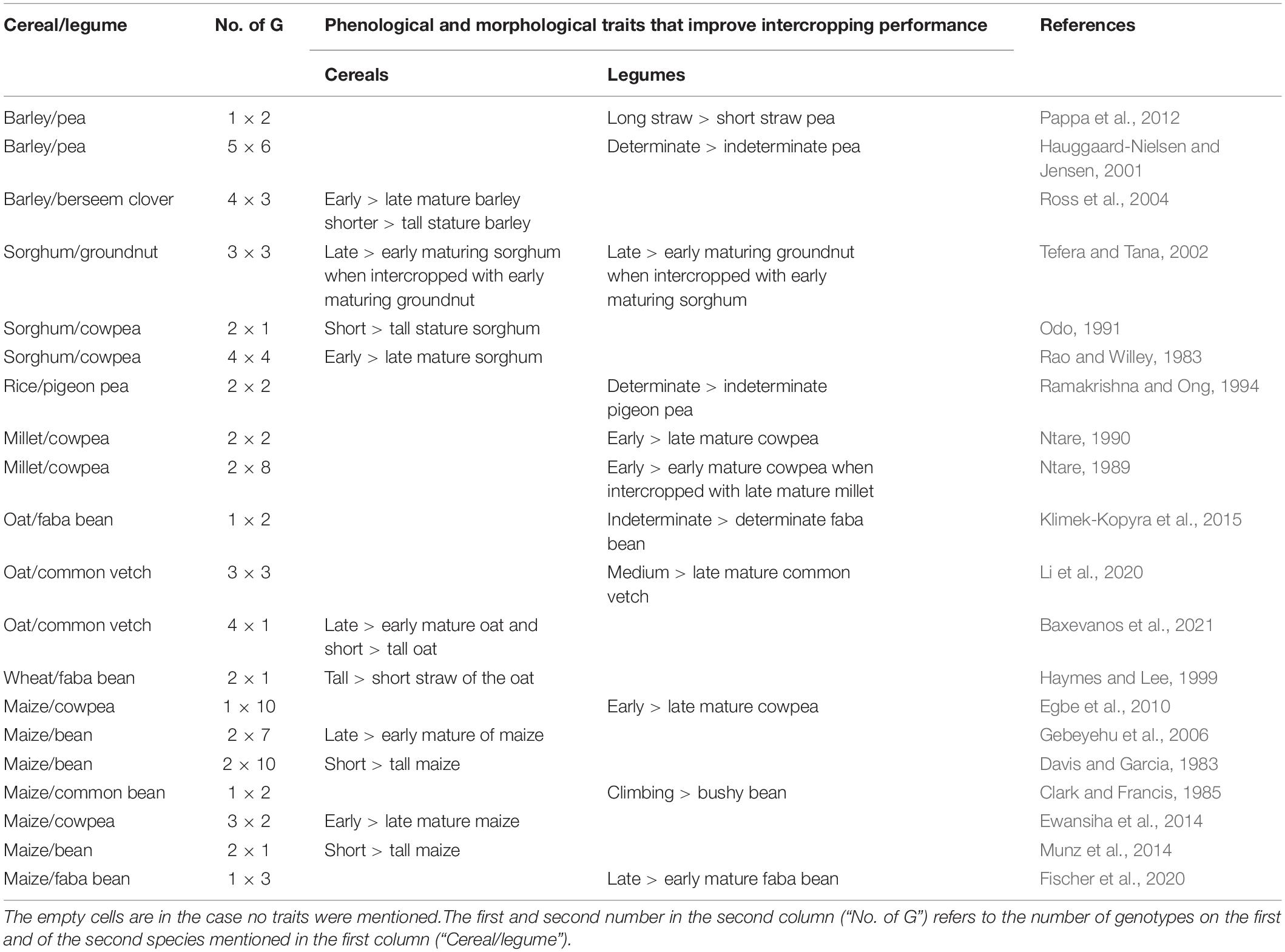
Table 6. Mechanisms of genotypes (G) complementarity in cereal/legume intercropping as mentioned in the consulted literature.
The phenological traits include growth duration (days to maturity, days required from emergence to flowering, and harvesting time), whereas morphological traits include shoot architecture (plant height) and growth habit (determinate/indeterminate growth) of the genotypes of each species. The reported phenological legume traits that affect intercropping, growth habit, and growth duration were reported more often than the morphological traits (long/short straw and climbing/bushy beans). However, no trend can be extracted from the provided information. In case of the cereals, only the phenological trait growth duration and the morphological trait plant height were reported. Three studies reported a better intercropping performance for early maturing cereals (maize, barley, and sorghum), whereas three others for late-maturing cereals (sorghum, oat, and maize). In case of plant height, five out of six studies reported improved intercropping performance for shorter cereal genotypes. Thus, besides a tendency for higher intercropping performance in case of short cereal genotypes, no conclusion can be drawn.
Discussion
Evaluation of the Performance of Different Cereal/Legume Species and Genotypes
The systematic assessment of LER from 35 independent studies showed the mean and median values of 1.26 and 1.24 (Figure 2A). This result is not far from the previously published meta-analysis result median values of 1.17 (Yu et al., 2015),1.16 (Yu et al., 2016), and 1.3 (Martin-Guay et al., 2018). These studies focus on the yield performance of crop species mixtures regardless of genotype. The median LER of 1.24 across 16 maize-based studies in our study is in line with a meta-analysis from 43 studies of maize/soybean of intercropping that reported an LER of 1.32 (Xu et al., 2020). Although the mean and median varied among different cereals, median LER was above one in all cereals.
The species and design effects were highly significant (p < 0.01) (Figure 4A), with a significant interaction (p < 0.05), mainly due to the higher LER of finger millet (1.66) compared to other species in additive designs. However, in replacement designs, no differences were observed among species. The overall LER was higher in additive designs compared to replacement designs. In an additive design, the planting density of both species in the mixture may be equivalent or somewhat reduced compared to their sole stand resulting in planting densities leading to density equivalent ratios > 1 and up to 2. For example, pea–oat mixtures may be composed of 100% peas and 20% oats compared to the pure stand densities (Gronle et al., 2015) or wheat–winter pea mixtures of 70% wheat with 50% pea (Timaeus et al., 2022). In replacement designs, the density of one sole crop species is proportionally (based on sole crop densities) replaced by the other species resulting in a density equivalent ratio of 1. For example, they may be composed of 50% barley and 50% pea compared to pure stand densities (Pappa et al., 2012). Although the planting proportion has an effect on LER, the range of effects depends on the species in the mixture because tillering in the case of cereals can compensate variable sowing densities (e.g., Finckh and Mundt, 1992; Finckh et al., 1999).
Compared to other cereal crops, millet was intercropped with short legumes, such as cowpea and pigeon pea. Intercropping the tall millet and sorghum cereals with shorter legumes permits better radiation use efficiency (Marshall and Willey, 1983; Matthews et al., 1991). Due to less resource competition by spatial segregation, yield in mixture and mono-cropping is comparable for both species which increased LER in additive compared to replacement designs. Nevertheless, a meta-analysis by Raseduzzaman and Jensen (2017) reported that in intercropping, replacement designs lead to higher yield stability compared to additive designs. The ANOVA across legume species (excluding faba bean, grass pea, guar and hairy vetch, berseem clover, and bitter vetch with n < 4 data points) resulted in significant differences. However, the effects of design and interaction were not significant (p > 0.05) (see Supplementary Table 5) with greater LER for pigeon pea and soybean compared to other legume species (see Supplementary Table 6). These two legume species are frequently intercropped with C4 cereals, such as maize, millet, and sorghum, which may increase the LER due to temporal niche differentiation (Yu et al., 2015; Xu et al., 2020).
The interaction between different cereal and legume genotypes and different cropping systems was significant in 75% of the studies that reported interaction effects of genotype × cropping system. This implies that in many studies, genotypes behave differently in sole vs. intercropping, often resulting in changes in the performance ranking of varieties between the sole crop and mixture (Woolley and Rodriguez, 1987; Baxevanos et al., 2017). The analyses of variation of different genotypes of cereal/legume intercrops within each selected study (Figure 5) revealed that the choice of the specific genotype combination could result in positive or negative yield effects compared to the median of all genotype combinations within each study. The largest LER range was found in a study with 20 different genotypes combinations (10 bean and two maize genotypes) (Santalla et al., 2001). This indicates the potential for high LER in case of appropriate genotype choice and highlights the potential for genotype or trait combination to optimize intercropping systems. However, this finding also emphasizes the need to develop a more general understanding of the mechanisms underlying these differences.
Concept of Cereal/Legume Intercropping Niche Complementarity
Out of 20 studies assessing the mechanisms underlying the intercropping performance, 10 studies reported that intercropping performance was improved by cereal genotype, whereas the remaining 10 studies reported that the improvement was by legumes genotype. In some studies, however, a relatively high number of genotypes did not affect the intercropping performance. For instance, in the study of Hauggaard-Nielsen and Jensen (2001), none of the five barley genotypes affected LER, whereas pea genotype affected intercropping performance in terms of LER (Table 6).
In an intercropping system with annual species, the niche differentiation is a general mechanism underlying the yield advantage and better resource use efficiencies (Lithourgidis et al., 2011). Niche differentiation improves the use of resources according to species complementarity for light interception and the use of both soil mineral N and atmospheric N (Bedoussac et al., 2015). The selection of cereal and legume genotypes for better complementarity is important because the traits required for intercropping are those which enhance the complementary effects between the partners (Davis and Woolley, 1993). Niche differentiation among plant species occurs for the various environmental resources, such as light, water, and nutrient availability. It is driven by plant phenology and morphology that allows for partitioning of resources over time and space that facilitates coexistence (Silvertown, 2004). The trait differences in genotypes of cereals and legumes result in differences in phenology and morphology of the plants. Therefore, in cereal/legume mixtures, both species could have similar phenology but contrasting morphology or contrasting phenology and morphology, resulting in temporal and/or spatial niche complementarity (Gaudio et al., 2019). The contrasting characteristics of the genotypes play an important role in the complementarity of the species in intercropping (Hauggaard-Nielsen and Jensen, 2001; Gebeyehu et al., 2006).
The ecological niche separation concept describes the fact that different species involved may have different resource requirements at different times, as well as different sources of nutrition, e.g., root exploitation of top subsoil layers by one component vs. deeper exploitation by the other component, different growth patterns, or different affinities for the same nutrient (Malézieux et al., 2009). The temporal and spatial segregation of species in intercropping is useful in two ways: better resource capture, hence utilization of more resources, and enhanced resource use efficiency in a given unit of resource (Willey, 1990). The maturity rate and the growth habit of cereal and legumes define either the domination or suppression of one of the species in the mixture (Baxevanos et al., 2021). However, besides niche separation, additional mechanisms, such as mutual beneficial interactions via the soil microbiome, including biological N fixation, have to be considered (Hauggaard-Nielsen et al., 2009). Thus, in cereal legume mixtures, the contribution of biological N fixation through the leguminous partner is affected by the mineral N-supply level with strong effects on the competitive interactions and overall biological N fixation by the legume (Wang et al., 2015; Li et al., 2021).
Temporal Niche Complementarity of Cereal/Legume Intercropping
A trend for enhanced intercrop performance due to a specific trait related to phenology or temporal combination cannot be identified from the evaluated studies. Days required for maturity is one of the important factors for complementarity of species in intercropping. In this review, out of 20 studies reported that phenological and morphological traits affected intercropping performance with 12 studies indicated that the difference of days of maturity of different genotypes of cereals and/or legumes had an effect on the intercropping performance. However, it also varies in some cases, with a late-maturing genotype of either of the species meeting better the aim of cultivation compared to an early maturing genotype. In contrast, early genotypes could also be better compared to late maturing genotypes of one of the species (Table 6). In the study of Ntare (1989), intercropping an early maturing cowpea genotype with a relatively late-maturing millet genotype performed better by reducing the co-growth period to escape moisture scarcity and minimizing all components not affected equally in drought-prone areas. Another example of temporal complementarity is the combination of determinate field peas with a cereal where peas started maturing and releasing N from the roots around the time when the cereal flowers and requires increased N to fill its grains (Jensen et al., 2020; Timaeus et al., 2021b). The rate of development and time between sowing and harvesting of the components in intercropping provide the opportunity of temporally complementary use of incident radiation, thereby improving intercropping performance (Keating and Carberry, 1993). Tefera and Tana (2002) reported that the temporal niche complementarity of different genotypes in sorghum/groundnut intercropping influences the general performance of intercropping: partners that have a lower co-growth period produced higher yields compared to genotypes that have equal or higher co-growth period. Similar temporal niche complementarity was reported for millet/cowpea (Ntare, 1990), maize/cowpea systems (Egbe et al., 2010), and bean/maize systems (Gebeyehu et al., 2006). Depending on the aim of cultivation, the selection of cereal and legume genotypes with contrasting maturity periods will increase the intercropping yield advantage (Ross et al., 2004).
Spatial Niche Complementarity of Cereal/Legume Genotypes
Spatial niche complementarity can be exploited by the spatial arrangement of one component to maintain its full population, whereas allowing more space (and thus more resources) for another component (Willey, 1990). The spatial arrangement for better resource use efficiency could be classified as above-ground (canopy structure of both components) and below-ground (root system) (Gaudio et al., 2019). Canopy structure has considerable implications for intercropping systems. The erect open canopy of one component allows more transmission of radiation to shorter crops and enables more radiation use efficiency (Willey, 1990). The use of abiotic resources is improved according to species complementarity for light interception and the use of both soil mineral and atmospheric N.
In this review, 11 studies reported morphological differences of the genotype of either cereal or legumes to be involved in intercropping complementarity (Table 6). In most of these articles (7), plant height was observed. Whether the taller or the shorter genotype performed better varied. However, a tendency toward higher intercropping performance was observed with short cereal genotypes. Plant height and branching of long cycle pea genotypes varied between the sole and mixed cropping systems. This reveals the importance of the pea genotype choice in terms of morphology for intercropping systems (Barillot et al., 2014). The study by Hauggaard-Nielsen and Jensen (2001) revealed that pea genotypes with determinate growth absorbed more radiation under the barley canopy, which enhanced the intercropping performance compared to intercropping systems with indeterminate pea genotypes.
The growth habit of different genotypes of one species significantly affects the performance of other species, and thereby intercropping performance mainly by affecting radiation interception. Ramakrishna and Ong (1994) reported that the indeterminate pigeon pea genotype with indeterminate growth habit reduces the yield of rice by half due to the competitive advantage for radiation. In the barley/pea intercropping system, spatial complementarity due to pea genotypes has resulted in better N use efficiency of barley. An indeterminate pea genotype resulted in a greater proportion of peas in the intercrop yield due to high competitiveness, whereas a determinate pea genotype with normal leaves caused the highest degree of complimentary use of N sources by allowing barley to exploit the soil N sources efficiently, and they contribute with fixed N. However, indeterminate pea genotypes caused a reduced N uptake and yield of barley (Hauggaard-Nielsen and Jensen, 2001; Pappa et al., 2012). Based on the analyzed studies, we cannot draw a conclusion. In two articles, the intercropping performance was higher in case the growth of the legume partner was determinate, whereas in one study, it was higher for the indeterminate genotype.
Gaps of Genotype and Trait Evaluation in Cereal/Legume Intercropping
Even though ample research reported on cereal legume intercropping, the number of publications that evaluated cereal/legume genotypes for complementarity in intercropping systems was very limited. Among the studies analyzed (69), only 20 (29%) articles indicate the contrasting traits of genotypes that contribute to intercropping performance. From those, the general mechanisms underlying the genotype cropping system were broadly classified as phenological and morphological heterogeneity of cereal and/or legume genotypes. However, in most of the studies, the contrasting characteristics of genotypes of either cereal or legumes and/or both of the species were not described well. The phenology of the crops has an impact on resource use over time (Gaudio et al., 2019). Consequently, cultivating genotypes with different phenological characteristics results in different temporal niche complementarity. The latter can increase the land use efficiencies, especially if N is released after grain filling of the legumes benefiting the cereals. Nevertheless, in most of the studies, sufficient information on phenology was not provided, and none of the studies reported the differences in the phenological stages of the genotypes.
Root growth and thus water and nutrient uptake are some of the most important factors in temporal and spatial heterogeneity (Hauggaard-Nielsen and Jen Yin et al., 2020). Root system distribution in time and space can partly explain competition. For instance, barley roots grow faster than pea roots (Hauggaard-Nielsen et al., 2001) and start nutrient acquisition earlier. Different genotypes of either the cereals or the legumes could have different root characteristics, which influence the competitive ability of the species. Streit et al. (2019) reported that mixtures of winter faba bean and winter wheat over yielded more below- than above-ground. The authors concluded that genotype differences in root biomass and over-yielding indicate the breeding potential of winter faba bean cultivars for mixed cropping. Legumes provide N to the agroecosystem through their exclusive capability to fix atmospheric N in a symbiotic relationship with soil rhizobia, but different genotypes of a legume species might have different capabilities in nodulation (Rodiño et al., 2011). Only a very limited number of studies considered the nutrient acquisition of different genotypes of cereals and legumes in intercropping. Different species have temporal niche differentiation in nutrient acquisition (Zhang et al., 2017). The symbiotic association of different legume genotypes and their rhizobia could also differ. The spatial complementarity of the genotypes in the nutrient acquisition is therefore important to increase the performance of intercropping. Hence, future research needs to address how different genotypes respond to nutrient competition, with a particular focus on below-ground traits.
Pest and disease resistance is one of the most important advantages of intercropping (Finckh et al., 2021). However, there are only a limited number of studies, which have considered genotype differences concerning pest and disease resistance in cereal/legume intercropping. Recent work has highlighted the importance of plant–plant interactions, either direct by mechanical, physical, or chemical cues, or mediated through soil/air microbiota, and the way they can affect plant immune system or other functions (Subrahmaniam et al., 2018; Khashi u Rahman et al., 2019; Zhu and Morel, 2019; Pélissier et al., 2021). Life cycle assessment (LCA) is a convenient, effective, and rarely used [but see Naudin et al. (2014)] approach for analyzing the environmental impact of cereal/legume intercropping, especially on the N cycle.
There are only a few studies considering the socio-economic importance of genotypes of both cereals and legumes species. Goshime et al. (2020) involved the farmers in the evaluation of genotypes. Different quality parameters of the genotypes not included in most of the articles hence could affect the acceptance of intercropping by farmers. The forage quality differences of legume genotypes were mostly ignored, and the number of studies on this topic is very limited. The consumer and market preference of different genotypes of cereals and/or legumes is also important in the selection of genotypes for intercropping. Therefore, in addition to morphological and phenological traits, other traits (roots, water and nutrient acquisition, and quality) and advantages in pest and weed suppression deserve attention to understand the mixing ability of different genotypes. Future research should consider pedigree analysis, functional genes, or key traits when selecting varieties tested in intercropping.
Summary and Conclusion
We evaluated the observations of studies that included at least two genotypes of one species in cereal/legume intercropping. While the number of studies is inadequate for obtaining a comprehensive and reliable insight, our results point to the potential of genotype selection in intercropping, and future research should therefore emphasize genotype × cropping system interaction in cereal/legume intercropping. In total, the majority of the studies reported that there was a significant genotype–cropping system interaction revealing the importance of genotype selection for intercropping for more land productivity. Among the 69 analyzed studies, only 35 studies reported LER values. We determined a median LER of 1.24, which indicated that a combination of specific genotype cereals and legumes improves the land productivity by 24% on average. In addition, 85% of the LER data points of cereal/legume intercropping were greater than 1. On the other hand, 15% of the specific cereal/legume genotype combinations resulted in LER < 1 revealing that judicious choice of genotype combination in cereal/legume is indispensable.
Furthermore, the ANOVA across cereal species and design indicated that different species have different land-use efficiency in the different design types with finger millet having higher land-use efficiency than other crops in additive designs, whereas no difference was observed between the species in replacement designs. The number of studies, which report LER from different wheat genotypes, was very limited [but see Timaeus et al. (2022)]; because of the high importance of wheat for global food security, we suggest that more research is needed to investigate the performance of different wheat genotypes in intercropping. Conversely, the effect of design on land use efficiency in legumes is not significant, whereas species effect is significant. Temporal and spatial heterogeneity between the genotypes of the cereals and those of the legumes was mentioned in the selected studies as the main mechanism enhancing the overall performance of cereal–legume intercropping. However, the spatiotemporal heterogeneity of genotypes was not described sufficiently in most of the studies to allow a detailed analysis. Hence, future research studies should consider and report the genotypes’ traits more comprehensively, including root growth, soil nutrient and water acquisition, and diseases, among others. In most studies, only some agronomic traits of genotypes were emphasized ignoring other genotypic functional traits. Furthermore, we recommend that future research needs to evaluate a higher number of genotypes and their traits on various sites and under different climate and management conditions. It is impossible to test all possible combinations (genotype × genotype × environment × management) of intercropping in field trials. The complex interactions in intercropping can be disentangled by process-based agroecological models, which can help to identify the relevant influencing factors of intercrop performance. However, the prerequisite is an understanding of the basic mechanisms.
Author Contributions
All authors listed have made a substantial, direct, and intellectual contribution to the work, and approved it for publication.
Funding
The presented study has been funded by the Deutsche Forschungsgemeinschaft (DFG, German Research Foundation) under Germany’s Excellence Strategy-EXC 2070-390732324 (PhenoRob), by the German Federal Ministry of Education and Research (BMBF) in the framework of the funding measure “Soil as a Sustainable Resource for the Bio-economy- BonaRes,” project BonaRes (Module A): BonaRes Center for Soil Research, subproject “Sustainable Subsoil Management—Soil3” (Grant 031B0151A), and it was also a part of the project ReMIX “Redesigning European cropping systems based on species MIXtures” funded by the EU’s Horizon 2020 Research and Innovation Program (Grant Agreement No. 727217).
Conflict of Interest
The authors declare that the research was conducted in the absence of any commercial or financial relationships that could be construed as a potential conflict of interest.
Publisher’s Note
All claims expressed in this article are solely those of the authors and do not necessarily represent those of their affiliated organizations, or those of the publisher, the editors and the reviewers. Any product that may be evaluated in this article, or claim that may be made by its manufacturer, is not guaranteed or endorsed by the publisher.
Supplementary Material
The Supplementary Material for this article can be found online at: https://www.frontiersin.org/articles/10.3389/fpls.2022.846720/full#supplementary-material
References
Anderson, P. K., Cunningham, A. A., Patel, N. G., Morales, F. J., Epstein, P. R., and Daszak, P. (2004). Emerging infectious diseases of plants: pathogen pollution, climate change and agrotechnology drivers. Trends Ecol. Evol. 19, 535–544. doi: 10.1016/j.tree.2004.07.021
Annicchiarico, P., Collins, R. P., De Ron, A. M., Firmat, C., Litrico, I., and Hauggaard-Nielsen, H. (2019). Do we need specific breeding for legume-based mixtures? Adv. Agron. 157, 141–215. doi: 10.1016/bs.agron.2019.04.001
Annicchiarico, P., and Filippi, L. (2007). A field pea ideotype for organic systems of northern Italy. J. Crop Improv. 20, 193–203. doi: 10.1300/J411v20n01_11
Bančič, J., Werner, C. R., Gaynor, R. C., Gorjanc, G., Odeny, D. A., Ojulong, H. F., et al. (2021). Modeling Illustrates That Genomic Selection Provides New Opportunities for Intercrop Breeding. Front. Plant Sci. 12:605172. doi: 10.3389/fpls.2021.605172
Barillot, R., Combes, D., Pineau, S., Huynh, P., and Escobar-Gutiérrez, A. J. (2014). Comparison of the morphogenesis of three genotypes of pea (Pisum sativum) grown in pure stands and wheat-based intercrops. AoB Plants 6:plu006. doi: 10.1093/aobpla/plu006
Baxevanos, D., Tsialtas, I. T., Vlachostergios, D., Hadjigeorgiou, I., Dordas, C., and Lithourgidis, A. (2017). Cultivar competitiveness in pea-oat intercrops under Mediterranean conditions. Field Crops Res. 214, 94–103. doi: 10.1016/j.fcr.2017.08.024
Baxevanos, D., Tsialtas, I. T., Voulgari, O., Pankou, C. I., Vlachostergios, D., and Lithourgidis, A. S. (2021). Oat genotypic requirement for intercropping with vetch under Mediterranean conditions. J. Agric. Sci. 158, 695–706. doi: 10.1017/S0021859621000071
Bedoussac, L., Journet, E. P., Hauggaard-Nielsen, H., Naudin, C., Corre-Hellou, G., and Jensen, E. S. (2015). Ecological principles underlying the increase of productivity achieved by cereal-grain legume intercrops in organic farming. A review. Agron. Sustain. Dev. 35, 911–935. doi: 10.1007/s13593-014-0277-7
Bedoussac, L., and Justes, E. (2010). The efficiency of a durum wheat-winter pea intercrop to improve yield and wheat grain protein concentration depends on N availability during early growth. Plant Soil 330, 19–35. doi: 10.1007/s11104-009-0082-2
Beillouin, D., Ben-Ari, T., Malézieux, E., Seufert, V., and Makowski, D. (2021). Positive but variable effects of crop diversification on biodiversity and ecosystem services. Glob. Chang. Biol. 27, 4697–4710. doi: 10.1111/gcb.15747
Berghuijs, H. N. C., Wang, Z., Stomph, T. J., Weih, M., Van der Werf, W., and Vico, G. (2020). Identification of species traits enhancing yield in wheat-faba bean intercropping: development and sensitivity analysis of a minimalist mixture model. Plant Soil 455, 203–226. doi: 10.1007/s11104-020-04668-0
Blomqvist, L., Yates, L., and Brook, B. W. (2020). Drivers of increasing global crop production: a decomposition analysis. Environ. Res. Lett. 15:0940b6. doi: 10.1088/1748-9326/ab9e9c
Burda, B. U., O’Connor, E. A., Webber, E. M., Redmond, N., and Perdue, L. A. (2017). Estimating data from figures with a Web-based program: considerations for a systematic review. Res. Synth. Methods 8, 258–262. doi: 10.1002/jrsm.1232
Clark, E. A., and Francis, C. A. (1985). Bean-maize intercrops: a comparison of bush and climbing bean growth habits. Field Crops Res. 10, 151–166. doi: 10.1016/0378-4290(85)90023-1
Cramond, F., O’mara-Eves, A., Doran-Constant, L., Rice, A. S. C., Macleod, M., and Thomas, J. (2019). The development and evaluation of an online application to assist in the extraction of data from graphs for use in systematic reviews. Wellcome Open Res. 3:157. doi: 10.12688/wellcomeopenres.14738.2
Danso-Abbeam, G., Dagunga, G., Ehiakpor, D. S., Ogundeji, A. A., Setsoafia, E. D., and Awuni, J. A. (2021). Crop–livestock diversification in the mixed farming systems: implication on food security in Northern Ghana. Agric. Food Secur. 10:35. doi: 10.1186/s40066-021-00319-4
Dasbak, M. A. D., and Asiegbu, J. E. (2009). Performance of pigeon pea genotypes intercropped with maize under humid tropical ultisol conditions. J. Anim. Plant Sci. 4, 329–340.
Davis, J. H. C., and Garcia, S. (1983). Competitive ability and growth habit of indeterminate beans and maize for intercropping. Field Crops Res. 6, 59–75. doi: 10.1016/0378-4290(83)90048-5
Davis, J. H. C., and Woolley, J. N. (1993). Genotypic requirement for intercropping. Field Crops Res. 34, 407–430. doi: 10.1016/0378-4290(93)90124-6
de Queiroz, M. A., Willey, R. W., and Galwey, N. W. (1988). The effect of intercropping with cowpea on genotype x environment interaction in sorghum. Euphytica 39, 175–184. doi: 10.1007/BF00039871
Ditzler, L., Van Apeldoorn, D. F., Schulte, R. P. O., Tittonell, P., and Rossing, W. A. H. (2021). Redefining the field to mobilize three-dimensional diversity and ecosystem services on the arable farm. Eur. J. Agron. 122:126197. doi: 10.1016/j.eja.2020.126197
Egbe, O., Alibo, S., and Nwueze, I. (2010). Evaluation of some extra-early- and early-maturing cowpea varieties for intercropping with maize in southern Guinea Savanna of Nigeria. Agric. Biol. J. North Am. 1, 845–858. doi: 10.5251/abjna.2010.1.5.845.858
Ewansiha, S. U., Kamara, A. Y., and Onyibe, J. E. (2014). Performance of cowpea cultivars when grown as an intercrop with maize of contrasting maturities. Arch. Agron. Soil Sci. 60, 597–608. doi: 10.1080/03650340.2013.829565
Finckh, M. R., Gacek, E. S., Czembor, H. J., and Wolfe, M. S. (1999). Host frequency and density effects on powdery mildew and yield in mixtures of barley cultivars. Plant Pathol. 48, 807–816. doi: 10.1046/j.1365-3059.1999.00398.x
Finckh, M. R., Junge, S. M., Schmidt, J. H., Sisic, A., and Weedon, O. D. (2021). “Intra- and interspecific diversity: the cornerstones of agroecological crop health management,” in Intercropping for Sustainability: Research Developments and Their Application, eds J.-N. Aubertot, I. Bertelsen, C. Bickler, R. Carlton, A. J. Karley, B. Keilor, et al. (Wellesbourne: Warwick Enterprise Park), 193–206.
Finckh, M. R., and Mundt, C. C. (1992). Plant competition and disease in genetically diverse wheat populations. Oecologia 91, 82–92. doi: 10.1007/BF00317245
Finckh, M. R., and Wolfe, M. S. (2015). “Biodiversity enhancement,” in Plant Diseases and Their Management in Organic Agriculture, eds M. R. Finckh, A. H. C. van Bruggen, and L. Tamm (Minnesota: The American Phytopathological Society), 153–174.
Fischer, J., Böhm, H., and Heβ, J. (2020). Maize-bean intercropping yields in Northern Germany are comparable to those of pure silage maize. Eur. J. Agron. 112:125947. doi: 10.1016/j.eja.2019.125947
Francis, C. A., Flor, C. A., and Temple, S. R. (1976). “Adapting varieties for intercropping systems in the tropics,” in Multiple Cropping, eds R. I. Papendick, P. A. Sánchez, and G. B. Triplett (Wisconsin: American Society of Agronomy), 235–253. doi: 10.2134/asaspecpub27.c12
Gaba, S., Lescourret, F., Boudsocq, S., Enjalbert, J., Hinsinger, P., Journet, E. P., et al. (2015). Multiple cropping systems as drivers for providing multiple ecosystem services: from concepts to design. Agron. Sustain. Dev. 35, 607–623. doi: 10.1007/s13593-014-0272-z
Gaudio, N., Escobar-Gutiérrez, A. J., Casadebaig, P., Evers, J. B., Gérard, F., Louarn, G., et al. (2019). Current knowledge and future research opportunities for modeling annual crop mixtures. A review. Agron. Sustain. Dev. 39:20. doi: 10.1007/s13593-019-0562-6
Gebeyehu, S., Simane, B., and Kirkby, R. (2006). Genotype × cropping system interaction in climbing beans (Phaseolus vulgaris L.) grown as sole crop and in association with maize (Zea mays L.). Eur. J. Agron. 24, 396–403. doi: 10.1016/j.eja.2006.01.005
Giles, C. D., Brown, L. K., Adu, M. O., Mezeli, M. M., Sandral, G. A., Simpson, R. J., et al. (2017). Response-based selection of barley cultivars and legume species for complementarity: root morphology and exudation in relation to nutrient source. Plant Sci. 255, 12–28. doi: 10.1016/j.plantsci.2016.11.002
Goshime, M. M., Solomon, A. S., and Alemayehu, Z. L. (2020). Performance evaluation and selection of new maize hybrids under sole and inter crop production systems. J. Plant Breed. Crop Sci. 12, 219–227. doi: 10.5897/jpbcs2020.0898
Gregory, P. J., and George, T. S. (2011). Feeding nine billion: the challenge to sustainable crop production. J. Exp. Bot. 62, 5233–5239. doi: 10.1093/jxb/err232
Gronle, A., Heß, J., and Böhm, H. (2015). Weed suppressive ability in sole and intercrops of pea and oat and its interaction with ploughing depth and crop interference in organic farming. Org. Agric. 5, 39–51.
Haug, B., Messmer, M. M., Enjalbert, J., Goldringer, I., Forst, E., Flutre, T., et al. (2021). Advances in Breeding for Mixed Cropping – Incomplete Factorials and the Producer / Associate Concept. Front. Plant Sci. 11:620400. doi: 10.3389/fpls.2020.620400
Hauggaard-Nielsen, H., Ambus, P., and Jensen, E. S. (2001). Temporal and spatial distribution of roots and competition for nitrogen in pea-barley intercrops - A field study employing 32p technique. Plant Soil 236, 63–74. doi: 10.1023/A:1011909414400
Hauggaard-Nielsen, H., Gooding, M., Ambus, P., Corre-Hellou, G., Crozat, Y., Dahlmann, C., et al. (2009). Pea-barley intercropping for efficient symbiotic N2-fixation, soil N acquisition and use of other nutrients in European organic cropping systems. Field Crops Res. 113, 64–71. doi: 10.1016/j.fcr.2009.04.009
Hauggaard-Nielsen, H., and Jensen, E. S. (2001). Evaluating pea and barley cultivars for complementarity in intercropping at different levels of soil N availability. Field Crops Res. 72, 185–196. doi: 10.1016/S0378-4290(01)00176-9
Haymes, R., and Lee, H. C. (1999). Competition between autumn and spring planted grain intercrops of wheat (Triticum aestivum) and field bean (Vicia faba). Field Crops Res. 62, 167–176. doi: 10.1016/S0378-4290(99)00016-7
Hazell, P., and Wood, S. (2008). Drivers of change in global agriculture. Philos. Trans. R. Soc. B Biol. Sci. 363, 495–515. doi: 10.1098/rstb.2007.2166
Hinsinger, P., Betencourt, E., Bernard, L., Brauman, A., Plassard, C., Shen, J., et al. (2011). P for two, sharing a scarce resource: soil phosphorus acquisition in the rhizosphere of intercropped species. Plant Physiol. 156, 1078–1086. doi: 10.1104/pp.111.175331
Hufnagel, J., Reckling, M., and Ewert, F. (2020). Diverse approaches to crop diversification in agricultural research. A review. Agron. Sustain. Dev. 40:14. doi: 10.1007/s13593-020-00617-4
Javanmard, A., Amani Machiani, M., Lithourgidis, A., Morshedloo, M. R., and Ostadi, A. (2020). Intercropping of maize with legumes: a cleaner strategy for improving the quantity and quality of forage. Clean. Eng. Technol. 1:100003. doi: 10.1016/j.clet.2020.100003
Javanmard, A., Nasab, A. D. M., Javanshir, A., Moghaddam, M., and Janmohammadi, H. (2009). Forage yield and quality in intercropping of maize with different legumes as double-cropped. J. Food Agric. Environ. 7, 163–166.
Jensen, E. S., Carlsson, G., and Hauggaard-Nielsen, H. (2020). Intercropping of grain legumes and cereals improves the use of soil N resources and reduces the requirement for synthetic fertilizer N: a global-scale analysis. Agron. Sustain. Dev. 40:5. doi: 10.1007/s13593-020-0607-x
Keating, B. A., and Carberry, P. S. (1993). Resource capture and use in intercropping: solar radiation. Field Crops Res. 34, 273–301. doi: 10.1016/0378-4290(93)90118-7
Khashi u Rahman, M., Zhou, X., and Wu, F. (2019). The role of root exudates, CMNs, and VOCs in plant–plant interaction. J. Plant Interact. 14, 630–636. doi: 10.1080/17429145.2019.1689581
Khoury, C. K., Bjorkman, A. D., Dempewolf, H., Ramirez-Villegas, J., Guarino, L., Jarvis, A., et al. (2014). Increasing homogeneity in global food supplies and the implications for food security. Proc. Natl. Acad. Sci. U. S. A. 111, 4001–4006. doi: 10.1073/pnas.1313490111
Klimek-Kopyra, A., Kulig, B., Oleksy, A., and Zając, T. (2015). Agronomic performance of naked oat (Avena nuda L.) and faba bean intercropping. Chil. J. Agric. Res. 75, 168–173. doi: 10.4067/S0718-58392015000200005
Kontturi, M., Laine, A., Niskanen, M., Hurme, T., Hyövelä, M., and Peltonen-Sainio, P. (2011). Pea oat intercrops to sustain lodging resistance and yield formation in northern European conditions. Acta Agric. Scand. B Soil Plant Sci. 61, 612–621. doi: 10.1080/09064710.2010.536780
Li, C., Hoffland, E., Kuyper, T. W., Yu, Y., Li, H., Zhang, C., et al. (2020a). Yield gain, complementarity and competitive dominance in intercropping in China: a meta-analysis of drivers of yield gain using additive partitioning. Eur. J. Agron. 113:125987. doi: 10.1016/j.eja.2019.125987
Li, C., Hoffland, E., Kuyper, T. W., Yu, Y., Zhang, C., Li, H., et al. (2020b). Syndromes of production in intercropping impact yield gains. Nat. Plants 6, 653–660. doi: 10.1038/s41477-020-0680-9
Li, R., Zhang, Z., Tang, W., Huang, Y., Coulter, J. A., and Nan, Z. (2020). Common vetch cultivars improve yield of oat row intercropping on the Qinghai-Tibetan plateau by optimizing photosynthetic performance. Eur. J. Agron. 117:126088. doi: 10.1016/j.eja.2020.126088
Li, X.-F., Wang, Z.-G., Bao, X.-G., Sun, J.-H., Yang, S.-C., Wang, P., et al. (2021). Long-term increased grain yield and soil fertility from intercropping. Nat. Sustain. 4, 943–950. doi: 10.1038/s41893-021-00767-7
Lin, B. B., Perfecto, I., and Vandermeer, J. (2008). Synergies between agricultural intensification and climate change could create surprising vulnerabilities for crops. Bioscience 58, 847–854. doi: 10.1641/B580911
Lithourgidis, A. S., Dordas, C. A., Damalas, C. A., and Vlachostergios, D. N. (2011). Annual intercrops: an alternative pathway for sustainable agriculture. Aust. J. Crop Sci. 5, 396–410.
Loreau, M., and Hector, A. (2001). Partitioning selection and complementarity in biodiversity experiments. Nature 412, 72–76. doi: 10.1038/35083573
Makate, C., Wang, R., Makate, M., and Mango, N. (2016). Crop diversification and livelihoods of smallholder farmers in Zimbabwe: adaptive management for environmental change. Springerplus 5:1135. doi: 10.1186/s40064-016-2802-4
Malézieux, E., Crozat, Y., Dupraz, C., Laurans, M., Makowski, D., Ozier-Lafontaine, H., et al. (2009). Mixing plant species in cropping systems: concepts, tools and models. A review. Agron. Sustain. Dev. 29, 43–62. doi: 10.1051/agro:2007057
Marshall, B., and Willey, R. W. (1983). Radiation interception and growth in an intercrop of pearl millet/groundnut. Field Crops Res. 7, 141–160. doi: 10.1016/0378-4290(83)90018-7
Martin-Guay, M. O., Paquette, A., Dupras, J., and Rivest, D. (2018). The new Green Revolution: sustainable intensification of agriculture by intercropping. Sci. Total Environ. 615, 767–772. doi: 10.1016/j.scitotenv.2017.10.024
Matthews, R. B., Azam-Ali, S. N., Saffell, R. A., Peacock, J. M., and Williams, J. H. (1991). Plant growth and development in relation to the microclimate of a sorghum/groundnut intercrop. Agric. For. Meteorol. 53, 285–301. doi: 10.1016/0168-1923(91)90048-U
Mead, R., and Willey, R. W. (1980). The concept of LER and advantages in yields. Expl. Agric. 16, 217–228.
Moher, D., Liberati, A., Tetzlaff, J., and Altman, D. G. (2009). Preferred reporting items for systematic reviews and meta-analyses: the PRISMA statement. BMJ 339, 332–336. doi: 10.1136/bmj.b2535
Molatudi, R. L. (2012). Grain yield and biomass response of a maize/dry bean intercrop to maize density and dry bean variety. Afr. J. Agric. Res. 7, 3139–3146. doi: 10.5897/ajar10.170
Munz, S., Feike, T., Chen, Q., Claupein, W., and Graeff-Hönninger, S. (2014). Understanding interactions between cropping pattern, maize cultivar and the local environment in strip-intercropping systems. Agric. For. Meteorol. 19, 152–164. doi: 10.1016/j.agrformet.2014.05.009
Muraya, M. M., Omolo, E. O., and Ndirangu, C. M. (2006). Development of high yielding synthetic maize (Zea mays L.) varieties suitable for intercropping with common bean (Phaseolus vulgaris L.). Asian J. Plant Sci. 5, 163–169. doi: 10.3923/ajps.2006.163.169
Mustafa, M. A., Mayes, S., and Massawe, F. (2019). “Crop diversification through a wider use of underutilised crops: A strategy to ensure food and nutrition security in the face of climate change,” in Sustainable Solutions for Food Security: Combating Climate Change by Adaptation, eds A. Sarkar, S. Sensarma, and G. vanLoon (Cham: Springer), 125–149. doi: 10.1007/978-3-319-77878-5_7
Nassary, E. K., Baijukya, F., and Ndakidemi, P. A. (2020a). Assessing the productivity of common bean in intercrop with maize across agro-ecological zones of smallholder farms in the Northern highlands of Tanzania. Agriculture 10:117. doi: 10.3390/agriculture10040117
Nassary, E. K., Baijukya, F., and Ndakidemi, P. A. (2020b). Productivity of intercropping with maize and common bean over five cropping seasons on smallholder farms of Tanzania. Eur. J. Agron. 113:125964. doi: 10.1016/j.eja.2019.125964
Naudin, C., Van Der Werf, H. M. G., Jeuffroy, M. H., and Corre-Hellou, G. (2014). Life cycle assessment applied to pea-wheat intercrops: a new method for handling the impacts of co-products. J. Clean. Prod. 73, 80–87. doi: 10.1016/j.jclepro.2013.12.029
Ntare, B. R. (1989). Evaluation of cowpea cultivars for intercropping with pearl millet in the Sahelian zone of West Africa. Field Crops Res. 20, 31–40. doi: 10.1016/0378-4290(89)90021-X
Ntare, B. R. (1990). Intercropping morphologically different cowpeas with pearl millet in a short season environment in the sahel. Exp. Agric. 26, 41–47. doi: 10.1017/S0014479700015386
Odo, P. E. (1991). Evaluation of short and tall sorghum varieties in mixtures with cowpea in the sudan savanna of nigeria: land equivalent ratio, grain yield and system productivity index. Exp. Agric. 27, 435–441. doi: 10.1017/S0014479700019426
Pappa, V. A., Rees, R. M., Walker, R. L., Baddeley, J. A., and Watson, C. A. (2012). Legumes intercropped with spring barley contribute to increased biomass production and carry-over effects. J. Agric. Sci. 150, 584–594. doi: 10.1017/S0021859611000918
Pélissier, R., Violle, C., and Morel, J. B. (2021). Plant immunity: good fences make good neighbors? Curr. Opin. Plant Biol. 62:102045. doi: 10.1016/j.pbi.2021.102045
Pierre, H., Kinama, J., Olubayo, F., Wanderi, S., Muthomi, J., and Nzuve, F. (2017). Effect of Intercropping Maize and Promiscuous Soybean on Growth and Yield. J. Exp. Agric. Int. 18, 1–21. doi: 10.9734/jeai/2017/36923
R CoreTeam (2020). R: A Language and Environment for Statistical Computing. Vienna: R Foundation for Statistical Computing.
Rahlakrishna, A., On, C. K., and Rliidy, N. (1992). Canopy duration and structure of pigeon pea intercropped with upland rice. Exp. Agric. 28, 295–307.
Ramakrishna, A., and Ong, C. K. (1994). Productivity and light interception in upland rice-legume intercropping systems. Trop. Agric. 71, 5–11.
Rao, M. R., and Willey, R. W. (1983). Effects of Genotype in Cereal/Pigeonpea Intercropping on the Alfisols of the Semi-Arid Tropics of India. Exp. Agric. 19, 67–78. doi: 10.1017/S0014479700010528
Raseduzzaman, M., and Jensen, E. S. (2017). Does intercropping enhance yield stability in arable crop production? A meta-analysis. Eur. J. Agron. 91, 25–33. doi: 10.1016/j.eja.2017.09.009
Reddy, K. C., Van Der Ploeg, J., and Maga, I. (1990). Genotype effects in millet / cowpea intercropping in the semi-arid tropics of Niger. Exp. Agric. 26, 387–396. doi: 10.1017/S0014479700001319
Renard, D., and Tilman, D. (2019). National food production stabilized by crop diversity. Nature 571, 257–260. doi: 10.1038/s41586-019-1316-y
Rodiño, A. P., De La Fuente, M., De Ron, A. M., Lema, M. J., Drevon, J. J., and Santalla, M. (2011). Variation for nodulation and plant yield of common bean genotypes and environmental effects on the genotype expression. Plant Soil 346, 349–361. doi: 10.1007/s11104-011-0823-x
Rohatgi, A. (2020). WebPlotDigitizer. Available online at: https://automeris.io/WebPlotDigitizer (accessed May 31, 2021).
Ross, S. M., King, J. R., O’Donovan, J. T., and Spaner, D. (2004). Intercropping berseem clover with barley and oat cultivars for forage. Agron. J. 96, 1719–1729. doi: 10.2134/agronj2004.1719
Sampoux, J. P., Giraud, H., and Litrico, I. (2020). Which recurrent selection scheme to improve mixtures of crop species? Theoretical expectations. G3 10, 89–107. doi: 10.1534/g3.119.400809
Sanou, J., Bationo, B. A., Barry, S., Nabie, L. D., Bayala, J., and Zougmore, R. (2016). Combining soil fertilization, cropping systems and improved varieties to minimize climate risks on farming productivity in northern region of Burkina faso. Agric. Food Secur. 5:20. doi: 10.1186/s40066-016-0067-3
Santalla, M., Rodio, A. P., Casquero, P. A., and De Ron, A. M. (2001). Interactions of bush bean intercropped with field and sweet maize. Eur. J. Agron. 15, 185–196. doi: 10.1016/S1161-0301(01)00104-6
Savary, S., Willocquet, L., Pethybridge, S. J., Esker, P., McRoberts, N., and Nelson, A. (2019). The global burden of pathogens and pests on major food crops. Nat. Ecol. Evol. 3, 430–439. doi: 10.1038/s41559-018-0793-y
Schwarz, C. R. (2011). “Statistics of range of a set of normally distributed numbers,”in W CORS and OPUS for Engineers, ed. T. Soler, (Reston: American Society of Civil Engineers), 41–45. doi: 10.1061/9780784411643.ch06
Senbayram, M., Wenthe, C., Lingner, A., Isselstein, J., Steinmann, H., Kaya, C., et al. (2015). Legume-based mixed intercropping systems may lower agricultural born N2O emissions. Energy Sustain. Soc. 6:2. doi: 10.1186/s13705-015-0067-3
Silvertown, J. (2004). Plant coexistence and the niche. Trends Ecol. Evol. 19, 605–611. doi: 10.1016/j.tree.2004.09.003
Smith, M. E. (1985). Variety development for multiple cropping systems. CRC Crit. Rev. Plant Sci. 3, 133–168. doi: 10.1080/07352688509382207
Smith, M. E., and Zobel, R. W. (1991). “Plant Genetic Interactions in Alternative Cropping Systems: Considerations for Breeding Methods,” in Plant Breeding and Sustainable Agriculture: Considerations for Objectives and Methods: Proceedings of a Symposium, eds D. A. Sleper, T. C. Barker, and P. J. Bramel-Cox (Massachusetts: Crop Science Society of America), 57–81. doi: 10.2135/cssaspecpub18.c4
Streit, J., Meinen, C., Nelson, W. C. D., Siebrecht-Schöll, D. J., and Rauber, R. (2019). Above- and belowground biomass in a mixed cropping system with eight novel winter faba bean genotypes and winter wheat using FTIR spectroscopy for root species discrimination. Plant Soil 436, 141–158. doi: 10.1007/s11104-018-03904-y
Subrahmaniam, H. J., Libourel, C., Journet, E. P., Morel, J. B., Muños, S., Niebel, A., et al. (2018). The genetics underlying natural variation of plant–plant interactions, a beloved but forgotten member of the family of biotic interactions. Plant J. 93, 747–770. doi: 10.1111/tpj.13799
Tamado, T., Fininsa, C., and Worku, W. (2007). Agronomic Performance and Productivity of Common Bean (Phaseolus vulgaris L.) Varieties in Double Intercropping with Maize (Zea mays L.) in Eastern Ethiopia. Asian J. Plant. Sci. 6, 749–756. doi: 10.3923/ajps.2007.749.756
Tamburini, G., Bommarco, R., Wanger, T. C., Kremen, C., van der Heijden, M. G. A., Liebman, M., et al. (2020). Agricultural diversification promotes multiple ecosystem services without compromising yield. Sci. Adv. 6:eaba1715. doi: 10.1126/SCIADV.ABA1715
Tefera, T., and Tana, T. (2002). Agronomic performance of sorghum and groundnut cultivars in sole and intercrop cultivation under semiarid conditions. J. Agron. Crop Sci. 188, 212–218. doi: 10.1046/j.1439-037X.2002.00553.x
Timaeus, J., Weedon, O., Backes, G., and Finckh, M. (2021a). “Plant traits, plasticity and growth dynamics in wheat-pea species mixtures: Evaluation of contrasting wheat genotypes,” in Conference: Intercropping for sustainability: Research developments and their application, (Reading: Association of Applied Biologists).
Timaeus, J., Weedon, O., and Finckh, M. (2021b). “Wheat-pea species mixtures as resource efficient and high-performance food cropping systems: Evaluation of contrasting wheat genotypes,” in Proceedings of the Intercropping for sustainability: Research developments and their application, (Reading: Association of Applied Biologists).
Timaeus, J., Weedon, O. D., and Finckh, M. R. (2022). Harnessing the potential of wheat-pea species mixtures: evaluation of multifunctional performance and wheat diversity. Front. Plant Sci. doi: 10.3389/fpls.2022.846237
Tscharntke, T., Grass, I., Wanger, T. C., Westphal, C., and Batáry, P. (2021). Beyond organic farming – harnessing biodiversity-friendly landscapes. Trends Ecol. Evol. 36, 919–930. doi: 10.1016/j.tree.2021.06.010
Wang, Z., Bao, X., Li, X., Jin, X., Zhao, J., Sun, J., et al. (2015). Intercropping maintains soil fertility in terms of chemical properties and enzyme activities on a timescale of one decade. Plant Soil 391, 265–282. doi: 10.1007/s11104-015-2428-2
Watiki, J. M., Fukai, S., Banda, J. A., and Keating, B. A. (1993). Radiation interception and growth of maize/cowpea intercrop as affected by maize plant density and cowpea cultivar. Field Crop. Res. 35, 123–133. doi: 10.1016/0378-4290(93)90145-D
Wickham, H. (2016). ggplot2: Elegant Graphics for Data Analysis. Berlin: Springer Science & Business Media.
Willey, R. W. (1990). Resource use in intercropping systems. Agric. Water Manag. 17, 215–231. doi: 10.1016/0378-3774(90)90069-B
Woolley, J. N., and Rodriguez, W. (1987). Cultivar X Cropping System Interactions in Relay. Exp. Agric. 23, 181–192. doi: 10.1017/S0014479700016975
Wright, A. J. (1985). Selection for improved yield in inter-specific mixtures or intercrops. Theor. Appl. Genet. 69, 399–407. doi: 10.1007/BF00570909
Xu, Z., Li, C., Zhang, C., Yu, Y., van der Werf, W., and Zhang, F. (2020). Intercropping maize and soybean increases efficiency of land and fertilizer nitrogen use; A meta-analysis. Field Crops Res. 246:107661. doi: 10.1016/j.fcr.2019.107661
Yadav, R. S., and Yadav, O. P. (2001). The performance of cultivars of pearl millet and clusterbean under sole cropping and intercropping systems in arid zone conditions in India. Exp. Agric. 37, 231–240. doi: 10.1017/S0014479701002046
Yang, S., Chen, W., Chen, W., Tian, C., Sui, X., and Chen, W. (2018). Influence of rhizobial inoculation and crop variety on dry matter accumulation of crops in maize-soybean intercropping system. Int. J. Adv. Agric. Res. 6, 101–115.
Yin, W., Chai, Q., Zhao, C., Yu, A., Fan, Z., Hu, F., et al. (2020). Water utilization in intercropping: a review. Agric. Water Manag. 241:106335. doi: 10.1016/j.agwat.2020.106335
Yu, Y., Stomph, T. J., Makowski, D., and van der Werf, W. (2015). Temporal niche differentiation increases the land equivalent ratio of annual intercrops: a meta-analysis. Field Crops Res. 184, 133–144. doi: 10.1016/j.fcr.2015.09.010
Yu, Y., Stomph, T. J., Makowski, D., Zhang, L., and van der Werf, W. (2016). A meta-analysis of relative crop yields in cereal/legume mixtures suggests options for management. Field Crops Res. 198, 269–279. doi: 10.1016/j.fcr.2016.08.001
Zaeem, M., Nadeem, M., Pham, T. H., Ashiq, W., Ali, W., Gilani, S. S. M., et al. (2019). The potential of corn-soybean intercropping to improve the soil health status and biomass production in cool climate boreal ecosystems. Sci. Rep. 9:13148. doi: 10.1038/s41598-019-49558-3
Zhang, W. P., Liu, G. C., Sun, J. H., Fornara, D., Zhang, L. Z., Zhang, F. F., et al. (2017). Temporal dynamics of nutrient uptake by neighbouring plant species: evidence from intercropping. Funct. Ecol. 31, 469–479. doi: 10.1111/1365-2435.12732
Keywords: cultivar combination, intercropping performance, mixture, mixing ability, trait combination
Citation: Demie DT, Döring TF, Finckh MR, van der Werf W, Enjalbert J and Seidel SJ (2022) Mixture × Genotype Effects in Cereal/Legume Intercropping. Front. Plant Sci. 13:846720. doi: 10.3389/fpls.2022.846720
Received: 31 December 2021; Accepted: 24 February 2022;
Published: 01 April 2022.
Edited by:
Sean Mayes, University of Nottingham, United KingdomCopyright © 2022 Demie, Döring, Finckh, van der Werf, Enjalbert and Seidel. This is an open-access article distributed under the terms of the Creative Commons Attribution License (CC BY). The use, distribution or reproduction in other forums is permitted, provided the original author(s) and the copyright owner(s) are credited and that the original publication in this journal is cited, in accordance with accepted academic practice. No use, distribution or reproduction is permitted which does not comply with these terms.
*Correspondence: Dereje T. Demie, ZGVyZWplLmRlbWllQHVuaS1ib25uLmRl
†These authors have contributed equally to this work