- 1Hubei Key Laboratory of Food Crop Germplasm and Genetic Improvement, Food Crops Institute, Hubei Academy of Agricultural Sciences, Wuhan, China
- 2College of Agronomy, Qingdao Agricultural University, Qingdao, China
- 3Food Futures Institute and College of Science, Health, Engineering and Education, Murdoch University, Perth, WA, Australia
Elucidation of the composition, functional characteristics, and formation mechanism of wheat quality is critical for the sustainable development of wheat industry. It is well documented that wheat processing quality is largely determined by its seed storage proteins including glutenins and gliadins, which confer wheat dough with unique rheological properties, making it possible to produce a series of foods for human consumption. The proportion of different gluten components has become an important target for wheat quality improvement. In many cases, the processing quality of wheat is closely associated with the nutritional value and healthy effect of the end-products. The components of wheat seed storage proteins can greatly influence wheat quality and some can even cause intestinal inflammatory diseases or allergy in humans. Genetic and environmental factors have great impacts on seed storage protein synthesis and accumulation, and fertilization and irrigation strategies also greatly affect the seed storage protein content and composition, which together determine the final end-use quality of wheat. This review summarizes the recent progress in research on the composition, function, biosynthesis, and regulatory mechanism of wheat storage proteins and their impacts on wheat end-product quality.
Introduction
Wheat (Triticum aestivum) is one of the largest grain crops in the world, and its quality mainly comprises processing and nutritional quality. The term “wheat quality” usually refers to the processing quality, which is mainly dependent on the content and characteristics of storage proteins in wheat grains (Shewry and Halford, 2002; Ma et al., 2019) and directly determines wheat’s market price and end-use value. Since storage proteins contain some components that can cause human intestinal inflammatory diseases or allergy, the concept of wheat “protein quality” is often used to cover the scope of the processing and nutritional quality (Scherf et al., 2016a).
Wheat processing quality is represented by the physical and chemical characteristics of the dough, which make it possible to process wheat into a variety of food products (Payne, 1987; He et al., 2005; Don et al., 2006; Zhang et al., 2007, 2014b, 2021; Li et al., 2015; Gao et al., 2016b; Chen et al., 2019; Jiang et al., 2019). Dough properties are mainly determined by gluten proteins, glutenins, and gliadins (Shewry and Halford, 2002). Glutenins can be subdivided into high molecular weight glutenin (HMW-GS) and low molecular weight glutenin (LMW-GS; Shewry et al., 2002; Veraverbeke and Delcour, 2002). HMW-GS is the main factor determining gluten elasticity, which is encoded by the Glu-1 genes including Glu-A1, Glu-B1, and Glu-D1 loci on the long arm of chromosomes 1A, 1B, and 1D, respectively. Each locus comprises two linked genes encoding two different types (X type and Y type) of HMW-GS subunits (McIntosh et al., 1991; Liu et al., 2003; Sun et al., 2006; Zheng et al., 2011; Peng et al., 2015; Yu et al., 2019). Gliadins are mainly monomer proteins, including ω-, α/β-, and γ-gliadins (Kasarda et al., 1984; Zhou et al., 2022). According to the Chinese National Standard (Wang et al., 2013), wheat can be divided into four categories based on the usage and gluten strength: (1) Strong gluten wheat: the endosperm is hard and the wheat flour produces very strong gluten, which is suitable for baking bread; (2) Medium strong gluten wheat: the endosperm is hard and the gluten is rather strong and is suitable for making instant noodles, dumplings, steamed bread, noodles, and other foods; (3) Medium gluten wheat: the endosperm is hard and the gluten strength is moderate and is suitable for making noodles, dumplings, steamed bread, and other foods; and (4) Weak gluten wheat: the endosperm is soft and the gluten is weak and is suitable for making cake, biscuit, and other foods. Strong gluten dough has high ductility resistance and can maintain stability (Ma et al., 2019). The dough can retain the gas produced during fermentation in discrete cells evenly distributed in the dough (Don et al., 2006). A lower gluten strength can cause the excessive expansion of gas cells during baking, resulting in the collapse of cell walls and aggregation of cells, and thereby a rough bread texture (Don et al., 2006). Therefore, strong gluten wheat has always been an important goal of wheat breeding programs.
Generally, the protein content in wheat grains ranges from 10 to 18% (Qi et al., 2012; Liu et al., 2018). To some extent, the protein content is positively correlated with wheat processing quality, particularly dough strength. However, the protein content and grain yield are usually negatively correlated with each other (Kibite and Evans, 1984). In real production, a large amount of nitrogen fertilizer is often applied in order to promote wheat yield and protein content, which tends to reduce the nitrogen use efficiency and cause negative impacts on the environment (Justes et al., 1994). In recent years, multiple methods have been developed with the aim to simultaneously improve wheat yield and protein content, such as the utilization of new genes and optimization of water and fertilization regimes (Alhabbar et al., 2018; Balotf et al., 2018; Roy et al., 2018, 2020, 2021; Yang et al., 2018; Yu et al., 2018a,b; Li et al., 2021a). However, the effect of protein content on wheat quality is rather complex due to the presence of gliadin components in the storage protein. Gliadins have an odd number of cysteine residues and a negative effect on wheat processing quality (Lindsay and Skerritt, 1999; Wieser, 2007; Rasheed et al., 2014). Therefore, high-quality wheat should be characterized by a higher content of glutenins and a lower content of gliadins, and wheat processing quality is not necessarily related to the grain protein content.
In Australia, researchers have been pursuing the breeding goal of wheat varieties with low-protein content but high quality since 2000, targeting at the improvement of wheat quality by optimizing the gluten composition, namely, a higher glu/gli ratio (Roy et al., 2018, 2020, 2021). In this approach, the protein content is no longer a target. Since there is a negative correlation between the grain protein content and yield, a low-protein content naturally means a higher yield without sacrificing the quality. However, considering the nutritional value of protein, the breeding goal of low-protein and high-quality wheat is not suitable for some developing countries. Therefore, “three-high wheat” (high quality, high yield, and high protein) should be the breeding goal for most countries.
Genetics and Applications in Relation to Wheat Quality Breeding
Wheat quality can be improved by manipulation of the main storage protein genes. As a matter of fact, many effective genes have been efficiently utilized for decades, such as GluD1 (5 + 10) and GluB1 (17 + 18; Payne et al., 1981, 1987; Payne, 1987; Altpeter et al., 2004; Mohan and Gupta, 2015). The common HMW-GS alleles have been assigned with quality scores to facilitate their application in breeding (Payne et al., 1987). Although there are six HMW-GS genes in the wheat genome, most hexaploid wheat varieties only have three to five HMW glutenin subunits due to the silencing of some genes (Ma et al., 2003), such as the genes encoding the Ay subunit (Yu et al., 2019). Roy et al. (2018, 2020, 2021) found that the expression of Ay subunit has positive effects on grain protein content, grain yield, and quality. A new storage protein family consisting of the avenin-like proteins has also been identified to have great breeding value for the improvement of wheat quality (Chen et al., 2016). Since the genetic control of wheat quality has been comprehensively reviewed (Shewry and Tatham, 1997; Vasila and Anderson, 1997; Gras et al., 2001; She et al., 2011; Ortolan and Steel, 2017; Ma et al., 2019; Sharma et al., 2020; Wang et al., 2020), this review will not focus on this aspect.
Manipulation of Fertilization and Watering Regimes
Seed storage proteins can account for 40–60% of wheat processing quality (Békés et al., 2006), and those unaccounted quality variations can be attributed to environmental factors. In wheat production, fertilization and watering strategies are also often considered for quality improvement (Li et al., 2018, 2019b; Yu et al., 2018a, 2021). As nitrogen (N) is one of the most important and essential elements for wheat, N fertilizer is usually the most efficient input for simultaneously increasing grain protein content and grain yield in wheat production (Zebarth et al., 2007; Malik et al., 2013; Zhen et al., 2018, 2020; Zhong et al., 2018, 2020; Ding et al., 2020; Xia et al., 2020; Hermans et al., 2021; Landolfi et al., 2021; Lyu et al., 2021; Dong et al., 2022; Liu et al., 2022; Ma et al., 2022). Kichey et al. (2007) demonstrated that 50–95% of nitrogen in mature grains is derived from the remobilization of nitrogen stored in the tissues before anthesis. However, nitrogen applied later in the growth period, namely, at anthesis or during grain filling, often increases grain protein content (Gooding and Davies, 1992; Sultana et al., 2017; Zhong et al., 2018, 2020). Li et al. (2018, 2019b) reported that nitrogen application during the grain filling period in winter wheat can significantly increase the uptake and accumulation of nitrogen. Yu et al. (2018a) reported that apart from the influence of genotype, grain yield and protein content have similar responses to nitrogen availability, with the former being slightly more sensitive than the latter. Furthermore, Yu et al. (2018a) proposed an N-mediated mechanism for protein polymerization in wheat grains: N promotes PPIase SUMOylation by interacting with SUMO1, facilitating the transport of PPIase into cytoplasm to support the formation of protein polymers (Yu et al., 2018a; Figure 1). Zhong et al. (2018, 2020) reported that at the same N application rate (240 kg ha−1), N topdressing can better promote the protein content and quality of wheat grains at the emergence of the top first leaf than at the emergence of the top third leaf of the main stem. The timing of N topdressing can significantly regulate γ-gliadins and HMW-GSs, while has almost no effect on the LMW-GS level, leading to a higher HMW-GS/LMW-GS ratio (Zhong et al., 2018). Furthermore, a delay of N topdressing was found to alter the grain hardness and flour allergenicity (Zhong et al., 2019). Ding et al. (2020) found that an increase in total N provision (210–270 kg ha−1) in the Yangtze River basin of China could enhance wheat grain yield, grain protein content, and nitrogen efficiency, with the appropriate topdressing timing and N application dose depending on the environment. Moreover, the biotic and abiotic stresses during wheat growth also significantly affect the quality of wheat (Duan et al., 2020). Among various stresses, drought has been identified to have a severe negative impact on wheat quality, particularly at the early grain filling stage (Gu et al., 2015). Usually, drought can cause stomatal closure, inhibit photosynthesis, increase respiration, and ultimately reduce starch biosynthesis, thereby leading to low yield of plants (Deng et al., 2018; Zhu et al., 2020). However, on the other hand, drought can enhance the content of wheat storage proteins to contribute to improved baking quality (Dong et al., 2012; Gu et al., 2015; Zhou et al., 2018). Different watering conditions were found to result in significant differences in the phosphorylation level of corresponding phosphoproteins in wheat grains (Zhang et al., 2014a). The changes in protein and starch synthesis during drought may be ascribed to post-translational protein modifications such as phosphorylation (Zhang et al., 2014a; Xia et al., 2018).
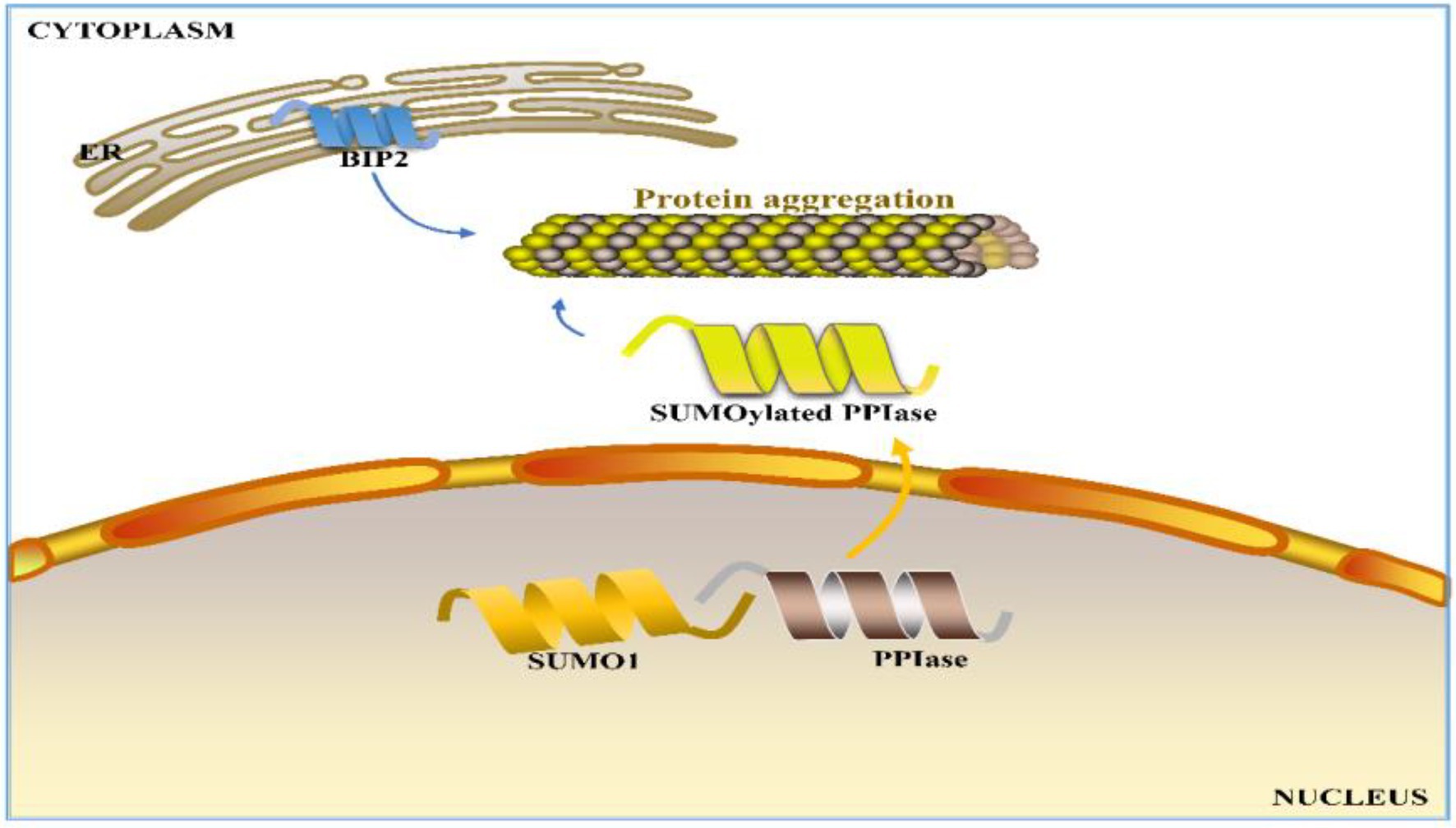
Figure 1. Proposed N-regulated mechanism for wheat grain protein polymerization in the cytoplasm. ER: endoplasmic reticulum, BIP2: luminal-binding protein 2 precursor, SUMO1: small ubiquitin-related modifier 1, and PPIase: peptidyl-prolyl cis-trans isomerase.
To reduce the yield loss caused by drought, moderate to high amounts of nitrogen fertilizer is often applied during wheat growth. A recent study showed that high-nitrogen fertilization under drought can increase the enzymatic protein synthesis for nitrogen and carbohydrate metabolism (Duan et al., 2020). Liu et al. (2022) reported that high-nitrogen treatments under drought conditions can either independently or coordinately facilitate the accumulation of wheat storage protein and gluten macropolymer, as well as improve lipid accumulation and protein secondary structure. The content of random coils and β-sheets of gluten proteins was also increased (Liu et al., 2022). These changes can contribute to the improvement of baking quality. Moreover, irrigation strategies under drought conditions have great impacts on crop yield and quality (Flagella et al., 2010; Xu et al., 2018b; Jha et al., 2019; Li et al., 2019b, 2021a). Li et al. (2021a) proposed an irrigation method that integrates micro-sprinkling irrigation and fertilizer, which could synergistically improve the grain yield and protein content of winter wheat. Compared with conventional irrigation, this method can reduce the total amount of water use and provide water and nitrogen at later growth stages, making more water and nitrogen available to wheat plants after flowering, which can reduce the canopy temperature and significantly delay leaf senescence and finally enhance the grain yield and protein content simultaneously.
Also, studies of glutamine synthetase activity in wheat developing grains and flag leaves have demonstrated that high-nitrogen availability facilitates the participation of glutamine in biological processes (Yu et al., 2018a, 2021; Zhong et al., 2018, 2020). A number of studies have revealed that application of sulfur fertilizer can significantly improve wheat quality (Zhao et al., 1999a,b; Luo et al., 2000; Yu et al., 2021). Based on the differences in the distribution of cysteine residues among wheat gluten subunits, wheat storage proteins can be categorized into three types of subunits, including sulfur-poor subunits (ω-gliadins), sulfur-medium subunits (HMW-GS and α/β-gliadins), and sulfur-rich subunits (LMW-GS and γ-gliadins; Shewry et al., 1995). It is worth noting that this classification is based on the number of cysteine residues within each subunit instead of the total sulfur amount (Lindsay and Skerritt, 1999; Wieser, 2007; note: apart from cysteine, methionine is another sulfur containing amino acid). Since the disulfide bond is believed to be the foundation of gluten rheological properties, for a long time, it has been generally believed that sulfur’s positive effects on wheat quality are implemented through mediating the gluten component ratios based on their sulfur or cysteine contents (Ma et al., 2019). However, Yu et al. (2021) recently proposed a different regulatory mechanism through proteomics, transcriptomics, metabolomics, and field experiments (Figure 2). It clearly demonstrated that sulfur does not mediate the gluten component ratios based on their sulfur or cysteine contents (Yu et al., 2021). Their study showed that the application of sulfur enhances the accumulation of free glycine at the beginning of grain filling and then promotes the participation of glycine in glutenin biosynthesis. Glycine belongs to aspartate acid family, and its content disparity between gliadins (1.75%) and glutenins (13.33%) marks the main difference of the two gluten components (Yu et al., 2021). A higher content of free glycine under sulfur fertilizer treatment can more significantly promote the biosynthesis of glutenins than that of gliadins, resulting in a high glu/gli ratio (Yu et al., 2021). The gene network regulating the biosynthesis and accumulation of glutenin components is mediated by S-adenosylmethionine (SAM; Yu et al., 2021). In addition, a high concentration of SAM indicates that more secondary metabolites are involved in the final development of grains. Chen et al. (2014) found that the downregulation of SAM decarboxylase genes would reduce the rice grain length, pollen viability, seed setting rate, grain yield per plant, and abiotic stress (salinity, drought, and chilling) tolerance, indicating a positive effect of SAM on rice yield.
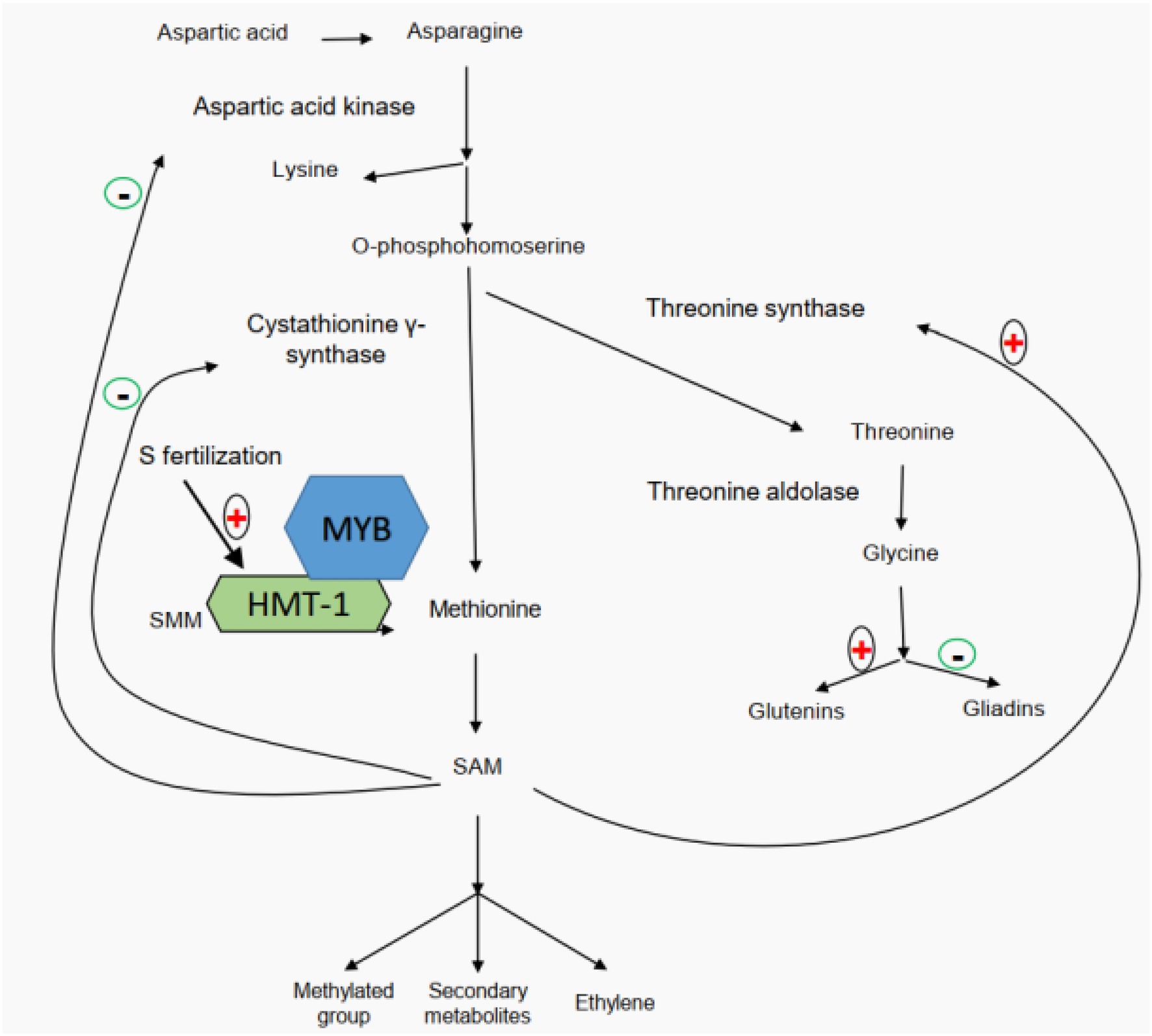
Figure 2. Sulfur-mediated regulation network of wheat gluten component biosynthesis (modified from Yu et al., 2021).
Gene Networks Regulating Storage Protein Biosynthesis
The wheat storage protein genes have spatiotemporal specific expression, and generally function at the middle and late stage of seed development (Diaz et al., 2002; Dong et al., 2007; Gao et al., 2021). Although wheat storage protein synthesis is regulated by many factors, it is mainly regulated at the transcriptional level (Gao et al., 2021). In recent years, important progress has been achieved in research on the regulation of wheat storage protein synthesis (Table 1). A series of conserved cis-elements in the promoter region of wheat seed storage protein genes have been identified, including the bZIP binding sites (GCN4-like motifs, ATGAG/CTCAT and G-box motif, TTACGTGG), DNA binding with one finger (DOF) binding sites (PB-box, TGTAAAG), R2R3MYB-binding sites (AACAAC), RY repeat sites (RY-box, CATGCA), and other basal promoter elements (Aryan et al., 1991; Juhasz et al., 2011; Ravel et al., 2014; Guo et al., 2015; Makai et al., 2015). Thirty conserved motifs and three conserved cis-regulatory modules (CCRMs) were found within the 1-kb region upstream of the start codon of Glu-1: CCRM3 (−950 to −750), CCRM 2 (−650 to −400), and CCRM 1 (−300 to −101; Li et al., 2019a). All three CCRMs can regulate the expression of wheat storage proteins and the 300 bp promoter (−300 to −1) can ensure the precise initiation of Glu-1 gene expression in the endosperm at 7 days after flowing and maintain its expression pattern during seed development. Further analysis revealed that CCRM1-1 (−208 to −101) is the core region for maintaining the endosperm-specific expression of Glu-1 genes (Li et al., 2019a). In addition, various transcription factors (TFs) involved in gluten gene regulation have been identified, such as bZIP, DOF, MYB (myeloblastosis), and B3. A bZIP transcription factor SPA (storage protein activator) can bind to the GCN4-like motif (GLM and ATGAG/CTCAT) in the promoters of HMW-GS genes to enhance their expression in common wheat (Albani et al., 1997; Conlan et al., 1999; Ravel et al., 2014). Averagely, the expression intensity of SPA-B is 10- and 7-fold that of SPA-A and SPA-D, respectively (Ravel et al., 2009). SPA markers are associated with dough viscoelasticity such as dough strength, extensibility, and tenacity (Ravel et al., 2009). As a bZIP transcription factor, the SPA Heterodimerizing Protein (SHP) prevents the binding of SPA to the cis-motifs and represses the synthesis of both LMW-GS and HMW-GS (Boudet et al., 2019). Thus, the glu/gli ratio is decreased in common wheat (Boudet et al., 2019). Wheat prolamin-box binding factor (WPBF), a DOF transcription factor, was first identified from wheat as a homolog of barley prolamin-box binding factor (BPBF; Dong et al., 2007). WPBF binds the prolamin box of the gliadin promoter region and interacts with TaQM (cloned from the wheat root cDNA library; QM, initially found as a putative tumor suppressor gene) to activate the expression of LMW-GS and gliadin genes during wheat grain development (Dowdy et al., 1991; Mena et al., 1998; Dong et al., 2007). TaPBF-D, another DOF transcription factor, binds in vitro the prolamin box of Glu-1By8 and Glu-1Dx2 promoters and decreases their C-methylation level, and its overexpression was found to enhance HMW-GS accumulation in wheat grains (Zhu et al., 2018). TaGAMyb, a TF of the R2R3MYB family, binds to a C/TAACAAA/CC-like motif in the HMW-GS gene promoter, recruits the histone acetyltransferase TaGCN5, and activates the expression of the Glu-1Dy by facilitating the acetylation of histones H3K9 and H3K14 (Diaz et al., 2002; Guo et al., 2015). TaFUSCA3 is a wheat B3-superfamily TF specifically binding to the RY motif of the Glu-1Bx7 promoter region to activate the Glu-1Bx7 expression (Sun et al., 2017). TF interactions between TaSPA and TaFUSCA3 were discovered (Sun et al., 2017). It is well known that NAM-ATAF-CUC (NAC) TFs participate in a series of biological processes, including abiotic and biotic stress responses and organ development (Uauy et al., 2006; Xue et al., 2011; Liang et al., 2014; Borrill et al., 2017; Guerin et al., 2019). Recently, some NAC TFs (TaNAC019, TaNAC100, and TaSPR) in wheat have been identified to regulate grain storage protein synthesis (Gao et al., 2021; Li et al., 2021b; Shen et al., 2021). TaNAC019, a wheat endosperm-specific NAC TF, binds to the motif ([AT]NNNNNN[ATC][CG]A[CA]GN[ACT]A) in the promoter region of Glu-1 genes. In coordination with TaGAMyb, it directly activates the expression of HMW-GS genes and indirectly modulates that of TaSPA (Gao et al., 2021). In a wheat natural population, two allelic variations of TaNAC019-B, TaNAC019-BI, and TaNAC019-BII were identified (Gao et al., 2021). TaNAC019-BI can improve flour processing quality and is an important candidate gene for wheat quality improvement (Gao et al., 2021). However, two recent studies demonstrated that both TaNAC100 and TaSPR function as repressors of seed storage protein expression in common wheat, indicating that further research is needed for better utilization of such TFs in breeding (Li et al., 2021b; Shen et al., 2021). The TaDME (wheat DEMETER) gene encoding 5-methylcytosine DNA glycosylase on the long arm of group 5 chromosomes suppresses the LMW-GS and gliadin gene expression by activating the demethylation of their promoters in the endosperm (Wen et al., 2012). It is worth noting that these studies have been mainly focused on the molecular regulatory mechanism of HMW-GS, LMW-GS, gliadins, or the total seed storage protein, and future research should be targeted at the regulatory mechanism for each subtype of gluten components, including different LMW-GSs (i-, m-, s-,α-, ω-, and γ-types) and gliadin components (α/β-, ω-, and γ-gliadins), so as to fine-tune wheat processing quality and improve the quality of wheat products for human consumption (Rasheed et al., 2014; Ma et al., 2019).
Health Effects of Wheat Grains and the Underlying Regulatory Mechanism
Gluten can cause human diseases related to digestion of wheat flour products, such as celiac disease, non-celiac gluten sensitivity, and gluten allergy (Scherf et al., 2016a). The intake of too much proline-rich gluten can reduce pepsin activity in the gastrointestinal tract, resulting in the accumulation of flour polypeptides rich in Pro and Gln in the small intestine (Scherf et al., 2016a). Previous studies have demonstrated that gliadins are the most toxic wheat protein components related to celiac disease, and glutenins are classified as non-toxic or weakly toxic (Barone and Zimmer, 2016; Scherf et al., 2016a,b). In order to reduce the toxicity of wheat gluten, a variety of flour treatment methods have been developed, including chemical, physical, and enzymatic methods (Buddrick et al., 2015; Scherf et al., 2018; Xue et al., 2019; Abedi and Pourmohammadi, 2021). In addition, some genetic methods have also been used to knock out or silence gliadin coding genes. Generally, RNAi can reduce the content of total gliadin in wheat gluten by 60–80% (Gil-Humanes et al., 2010). However, some negative effects on the processing quality were observed in RNAi wheat lines (Gil-Humanes et al., 2010, 2014). For instance, CRISPR-Cas9 editing was applied to silence the α-gliadin gene to reduce immune reactivity by 85%, but the treatment also greatly reduced the gluten content by 85% and led to an obvious decline in processing quality (Sánchez-León et al., 2018). At present, the greatest challenge is to find a technical solution to reduce wheat gliadin and increase gluten content with high yield and high total protein.
Yu et al. (2021) showed that sulfur treatment can reduce sulfur-poor ω-gliadins (the most abundant among all gliadin subtypes) by up to 31.4% in the total gluten, particularly the ω5-gliadin known to cause WDEIA (wheat-dependent exercise-induced anaphylaxis disease), which could be reduced by 83.9%. The α/β-gliadins, ω1,2-gliadins, and γ-gliadins, which are known to cause celiac disease, were also reduced by up to 25.9% under sulfur treatment. Carcinogen acrylamide is a processing contaminant usually formed from free asparagine and reducing sugars through the Maillard reaction (Mottram et al., 2002; Stadler et al., 2002; Zyzak et al., 2003). It has been discovered in a range of baked, fried, roasted, and toasted foods, including bread, pies, cakes, biscuits, batter, and breakfast cereals (Raffan and Halford, 2019). Since free asparagine is the major precursor for the formation of acrylamide during food processing especially high temperature baking, its accumulation mechanism in wheat grains has emerged as a hot research topic (Mottram et al., 2002; Stadler et al., 2002; Raffan et al., 2021). In living cells, aspartate is the substrate of asparagine, which is formed through enzymes that catalyze the ATP-dependent transfer of an amino group from glutamine (Gaufichon et al., 2010). Five asparagine synthetase genes have been found in the wheat genome, including TaASN1, TaASN2, TaASN3.1, TaASN3.2, and TaASN4 (Xu et al., 2018a; Raffan and Halford, 2021). Among these genes, TaASN2 is seed-specific with the highest expression in the embryo (Gao et al., 2016a; Curtis et al., 2019). It has been revealed that free asparagine is commonly present in wheat even under normal growth conditions (Curtis et al., 2018). Both environmental factors and agricultural practice can affect its accumulation (Zhong et al., 2018, 2020). In addition, adverse growing conditions such as sulfur deficiency and pathogen infection can increase asparagine concentration (Raffan and Halford, 2019). World Health Organization1 has stated that acrylamide in the diet has potential cancer-causing effects. The food industry is in demand of available raw materials with lower acrylamide-forming potential. So far, numerous studies have been carried out to reduce acrylamide in wheat products, mainly by reducing the free asparagine concentration in wheat grains. For example, Muttucumaru et al. (2006) reported that sulfur application can reduce the asparagine accumulation in mature wheat grains, making the wheat products healthier for human daily consumption. More recently, Raffan et al. (2021) successfully reduced the asparagine concentration through CRISPR-Cas9 approach to knock out the six alleles of TaASN2, a seed-specific asparagine synthetase gene in wheat.
Conclusion
The formation mechanism of wheat processing quality has been extensively studied via a broad range of biological approaches. Sulfur deficiency in soil has been reported as a global issue, which has negative impacts on wheat quality. An adequate level of sulfur fertilization is highly recommended in modern wheat farming to gain high processing quality as well as desirable nutritional value and healthy effect of the wheat end-products. Nitrogen fertilization after flowering should be considered for better processing quality. In the predicted drought season, low-protein content wheat cultivars may be selected for cultivation so that the grain starch can be allocated with more biosynthesis capacity to reduce yield loss. Molecular biological research has been mostly focused on the regulatory mechanism of the biosynthesis of various gluten components, which has led to the discovery of some key TFs that influence the quality. In future, TFs regulating specific HMW-GS subunits, LMW-GS types, and particularly the gliadin subtypes should be focused so that the relevant molecular markers can be used in breeding to meet a broad range of consumer needs.
Author Contributions
YP, YZ, ZY, JZ, and DX prepared the first draft. JD and WM critically reviewed and revised the manuscript. All authors contributed to the article and approved the submitted version.
Funding
This work is supported by National Natural Science Foundation of China (31701506), Youth Science Fund of Hubei Academy of Agricultural Sciences (2020NKYJJ03), and Fund of Hubei Key Laboratory of Food Crop Germplasm and Genetic Improvement (2020LZJJ04).
Conflict of Interest
The authors declare that the research was conducted in the absence of any commercial or financial relationships that could be construed as a potential conflict of interest.
Publisher’s Note
All claims expressed in this article are solely those of the authors and do not necessarily represent those of their affiliated organizations, or those of the publisher, the editors and the reviewers. Any product that may be evaluated in this article, or claim that may be made by its manufacturer, is not guaranteed or endorsed by the publisher.
Acknowledgments
We are grateful to Jiangsheng Wu (College of Plant Science and Technology, Huazhong Agricultural University, Wuhan, China) for his critical revision of the manuscript.
Footnotes
References
Abedi, E., and Pourmohammadi, K. (2021). Chemical modifications and their effects on gluten protein: An extensive review. Food Chem. 343:128398. doi: 10.1016/j.foodchem.2020.128398
Albani, D., HammondKosack, M. C. U., Smith, C., Conlan, S., Colot, V., Holdsworth, M., et al. (1997). The wheat transcriptional activator SPA: A seed-specific bZIP protein that recognizes the GCN4-like motif in the bifactorial endosperm box of prolamin genes. Plant Cell 9, 171–184. doi: 10.1105/tpc.9.2.171
Alhabbar, Z., Yang, R., Juhasz, A., Xin, H., She, M., Anwar, M., et al. (2018). NAM gene allelic composition and its relation to grain-filling duration and nitrogen utilisation efficiency of Australian wheat. PLoS One 13:e0205448. doi: 10.1371/journal.pone.0205448
Altpeter, F., Popelka, J. C., and Wieser, H. (2004). Stable expression of 1Dx5 and 1Dy10 high-molecular-weight glutenin subunit genes in transgenic rye drastically increases the polymeric glutelin fraction in rye flour. Plant Mol. Biol. 54, 783–792. doi: 10.1007/s11103-004-0122-5
Aryan, A. P., An, G., and Okita, T. W. (1991). Structural and functional analysis of promoter from gliadin, an endosperm-specific storage protein gene of Triticum aestivum L. Mol. Gen. Genet. 225, 65–71. doi: 10.1007/BF00282643
Balotf, S., Islam, S., Kavoosi, G., Kholdebarin, B., Juhasz, A., and Ma, W. (2018). How exogenous nitric oxide regulates nitrogen assimilation in wheat seedlings under different nitrogen sources and levels. PLoS One 13:e0190269. doi: 10.1371/journal.pone.0190269
Barone, M. V., and Zimmer, K. P. (2016). Endocytosis and transcytosis of gliadin peptides. Mol. Cell Pediatr. 3:8. doi: 10.1186/s40348-015-0029-z
Békés, F., Kemény, S., and Morell, M. (2006). An integrated approach to predicting end-product quality of wheat. Eur. J. Agron. 25, 155–162. doi: 10.1016/j.eja.2006.04.008
Borrill, P., Harrington, S. A., and Uauy, C. (2017). Genome-wide sequence and expression analysis of the NAC transcription factor family in Polyploid wheat. G three 7, 3019–3029. doi: 10.1534/g3.117.043679
Boudet, J., Merlino, M., Plessis, A., Gaudin, J. C., Dardevet, M., Perrochon, S., et al. (2019). The bZIP transcription factor SPA Heterodimerizing protein represses glutenin synthesis in Triticum aestivum. Plant J. 97, 858–871. doi: 10.1111/tpj.14163
Buddrick, O., Cornell, H., and Small, D. (2015). Reduction of toxic gliadin content of wholegrain bread by the enzyme caricain. Food Chem. 170, 343–347. doi: 10.1016/j.foodchem.2014.08.030
Chen, X. Y., Cao, X. Y., Zhang, Y. J., Islam, S., Zhang, J. J., Yang, R. C., et al. (2016). Genetic characterization of cysteine-rich type-b avenin-like protein coding genes in common wheat. Sci. Rep. 6:30692. doi: 10.1038/srep30692
Chen, M., Chen, J. J., Fang, J. Y., Guo, Z. F., and Lu, S. Y. (2014). Down-regulation of S-adenosylmethionine decarboxylase genes results in reduced plant length, pollen viability, and abiotic stress tolerance. Plant Cell Tissue Organ Cult. 116, 311–322. doi: 10.1007/s11240-013-0405-0
Chen, Q., Zhang, W. J., Gao, Y. J., Yang, C. F., Gao, X., Peng, H. R., et al. (2019). High molecular weight glutenin subunits 1Bx7 and 1By9 encoded by Glu-B1 locus affect wheat dough properties and sponge cake quality. J. Agric. Food Chem. 67, 11796–11804. doi: 10.1021/acs.jafc.9b05030
Conlan, R. S., Hammond-Kosack, M., and Bevan, M. (1999). Transcription activation mediated by the bZIP factor SPA on the endosperm box is modulated by ESBF-1 in vitro. Plant J. 19, 173–181. doi: 10.1046/j.1365-313x.1999.00522.x
Curtis, T. Y., Powers, S. J., Wang, R., and Halford, N. G. (2018). Effects of variety, year of cultivation and Sulphur supply on the accumulation of free asparagine in the grain of commercial wheat varieties. Food Chem. 239, 304–313. doi: 10.1016/j.foodchem.2017.06.113
Curtis, T. Y., Raffan, S., Wan, Y., King, R., Gonzalez-Uriarte, A., and Halford, N. G. (2019). Contrasting gene expression patterns in grain of high and low asparagine wheat genotypes in response to Sulphur supply. BMC Genomics 20:628. doi: 10.1186/s12864-019-5991-8
Deng, X., Liu, Y., Xu, X. X., Liu, D. M., Zhu, G. R., Yan, X., et al. (2018). Comparative proteome analysis of wheat flag leaves and developing grains under water deficit. Front. Plant Sci. 9:425. doi: 10.3389/fpls.2018.00425
Diaz, I., Vicente-Carbajosa, J., Abraham, Z., Martinez, M., Isabel-La Moneda, I., and Carbonero, P. (2002). The GAMYB protein from barley interacts with the DOF transcription factor BPBF and activates endosperm-specific genes during seed development. Plant J. 29, 453–464. doi: 10.1046/j.0960-7412.2001.01230.x
Ding, J., Li, F., Le, T., Wu, P., Zhu, M., Li, C., et al. (2020). Nitrogen management strategies of tillage and no-tillage wheat following rice in the Yangtze river basin, China: grain yield, grain protein, nitrogen efficiency, and economics. Agronomy 10:155. doi: 10.3390/agronomy10020155
Don, C., Mann, G., Bekes, F., and Hamer, R. J. (2006). HMW-GS affect the properties of glutenin particles in GMP and thus flour quality. J. Cereal Sci. 44, 127–136. doi: 10.1016/j.jcs.2006.02.005
Dong, F., Feng, L., Ya, J., Feng, Y., Qiu, Y., Jin, L., et al. (2022). Effect of nitrogen topdressing rate on yield and quality of black-grained wheat varieties (strains). J. Nuc. Agric. Sci. 36, 435–444. doi: 10.11869/j.issn.100-8551.2022.02.0435
Dong, K., Ge, P., Ma, C. Y., Wang, K., Yan, X., Gao, L. Y., et al. (2012). Albumin and globulin dynamics during grain development of elite Chinese wheat cultivar Xiaoyan 6. J. Cereal Sci. 56, 615–622. doi: 10.1016/j.jcs.2012.08.016
Dong, G. Q., Ni, Z. F., Yao, Y. Y., Nie, X. L., and Sun, Q. X. (2007). Wheat Dof transcription factor WPBF interacts with TaQM and activates transcription of an alpha-gliadin gene during wheat seed development. Plant Mol. Biol. 63, 73–84. doi: 10.1007/s11103-006-9073-3
Dowdy, S. F., Lai, K. M., Weissman, B. E., Matsui, Y., Hogan, B. L., and Stanbridge, E. J. (1991). The isolation and characterization of a novel cDNA demonstrating an altered mRNA level in nontumorigenic Wilms' microcell hybrid cells. Nucleic Acids Res. 19, 5763–5769. doi: 10.1093/nar/19.20.5763
Duan, W. J., Zhu, G. R., Zhu, D., and Yan, Y. M. (2020). Dynamic proteome changes of wheat developing grains in response to water deficit and high-nitrogen fertilizer conditions. Plant Physiol. Biochem. 156, 471–483. doi: 10.1016/j.plaphy.2020.08.022
Flagella, Z., Giuliani, M. M., Giuzio, L., Volpi, C., and Masci, S. (2010). Influence of water deficit on durum wheat storage protein composition and technological quality. Eur. J. Agron. 33, 197–207. doi: 10.1016/j.eja.2010.05.006
Gao, Y. J., An, K. X., Guo, W. W., Chen, Y. M., Zhang, R. J., Zhang, X., et al. (2021). The endosperm-specific transcription factor TaNAC019 regulates glutenin and starch accumulation and its elite allele improves wheat grain quality. Plant Cell 33, 603–622. doi: 10.1093/plcell/koaa040
Gao, R., Curtis, T. Y., Powers, S. J., Xu, H., Huang, J., and Halford, N. G. (2016a). Food safety: structure and expression of the asparagine synthetase gene family of wheat. J. Cereal Sci. 68, 122–131. doi: 10.1016/j.jcs.2016.01.010
Gao, X., Liu, T., Yu, J., Li, L., Feng, Y., and Li, X. (2016b). Influence of high-molecular-weight glutenin subunit composition at Glu-B1 locus on secondary and micro structures of gluten in wheat (Triticum aestivum L.). Food Chem. 197, 1184–1190. doi: 10.1016/j.foodchem.2015.11.085
Gaufichon, L., Reisdorf-Cren, M., Rothstein, S. J., Chardon, F., and Suzuki, A. (2010). Biological functions of asparagine synthetase in plants. Plant Sci. 179, 141–153. doi: 10.1016/j.plantsci.2010.04.010
Gil-Humanes, J., Piston, F., Barro, F., and Rosell, C. M. (2014). The shutdown of celiac disease-related gliadin epitopes in bread wheat by RNAi provides flours with increased stability and better tolerance to over-mixing. PLoS One 9:e91931. doi: 10.1371/journal.pone.0091931
Gil-Humanes, J., Piston, F., Tollefsen, S., Sollid, L. M., and Barro, F. (2010). Effective shutdown in the expression of celiac disease-related wheat gliadin T-cell epitopes by RNA interference. Proc. Natl. Acad. Sci. U. S. A. 107, 17023–17028. doi: 10.1073/pnas.1007773107
Gooding, M. J., and Davies, W. P. (1992). Foliar urea fertilization of cereals - a review. Fert. Res. 32, 209–222. doi: 10.1007/Bf01048783
Gras, P. W., Anderssen, R. S., Keentok, M., Békés, F., and Appels, R. (2001). Gluten protein functionality in wheat flour processing: a review. Aust. J. Agric. Res. 52, 1311–1323. doi: 10.1071/AR01068
Gu, A. Q., Hao, P. C., Lv, D. W., Zhen, S. M., Bian, Y. W., Ma, C. Y., et al. (2015). Integrated proteome analysis of the wheat embryo and endosperm reveals central metabolic changes involved in the water deficit response during grain development. J. Agric. Food Chem. 63, 8478–8487. doi: 10.1021/acs.jafc.5b00575
Guerin, C., Roche, J., Allard, V., Ravel, C., Mouzeyar, S., and Bouzidi, M. F. (2019). Genome-wide analysis, expansion and expression of the NAC family under drought and heat stresses in bread wheat (T. aestivum L.). PLoS One 14:e0213390. doi: 10.1371/journal.pone.0213390
Guo, W. W., Yang, H., Liu, Y. Q., Gao, Y. J., Ni, Z. F., Peng, H. R., et al. (2015). The wheat transcription factor TaGAMyb recruits histone acetyltransferase and activates the expression of a high-molecular-weight glutenin subunit gene. Plant J. 84, 347–359. doi: 10.1111/tpj.13003
He, Z. H., Liu, L., Xia, X. C., Liu, J. J., and Pena, R. J. (2005). Composition of HMW and LMW glutenin subunits and their effects on dough properties, pan bread, and noodle quality of Chinese bread wheats. Cereal Chem. 82, 345–350. doi: 10.1094/Cc-82-0345
Hermans, W., Mutlu, S., Michalski, A., Langenaeken, N. A., and Courtin, C. M. (2021). The contribution of sub-aleurone cells to wheat endosperm orotein content and gradient is dependent on cultivar and N-fertilization level. J. Agric. Food Chem. 69, 6444–6454. doi: 10.1021/acs.jafc.1c01279
Jha, S. K., Ramatshaba, T. S., Wang, G. S., Liang, Y. P., Liu, H., Gao, Y., et al. (2019). Response of growth, yield and water use efficiency of winter wheat to different irrigation methods and scheduling in North China plain. Agric. Water Manag. 217, 292–302. doi: 10.1016/j.agwat.2019.03.011
Jiang, P., Xue, J., Duan, L., Gu, Y., Mu, J., Han, S., et al. (2019). Effects of high-molecular-weight glutenin subunit combination in common wheat on the quality of crumb structure. J. Sci. Food Agric. 99, 1501–1508. doi: 10.1002/jsfa.9323
Juhasz, A., Makai, S., Sebestyen, E., Tamas, L., and Balazs, E. (2011). Role of conserved non-coding regulatory elements in LMW glutenin gene expression. PLoS One 6:e29501. doi: 10.1371/journal.pone.0029501
Justes, E., Mary, B., Meynard, J. M., Machet, J. M., and Thelierhuche, L. (1994). Determination of a critical nitrogen dilution curve for winter-wheat crops. Ann. Bot. 74, 397–407. doi: 10.1006/anbo.1994.1133
Kasarda, D. D., Okita, T. W., Bernardin, J. E., Baecker, P. A., Nimmo, C. C., Lew, E. J., et al. (1984). Nucleic acid (cDNA) and amino acid sequences of alpha-type gliadins from wheat (Triticum aestivum). Proc. Natl. Acad. Sci. U. S. A. 81, 4712–4716. doi: 10.1073/pnas.81.15.4712
Kibite, S., and Evans, L. E. (1984). Causes of negative correlations between grain-yield and grain protein-concentration in common wheat. Euphytica 33, 801–810. doi: 10.1007/Bf00021906
Kichey, T., Hirel, B., Heumez, E., Dubois, F., and Le Gouis, J. (2007). In winter wheat (Triticum aestivum L.), post-anthesis nitrogen uptake and remobilisation to the grain correlates with agronomic traits and nitrogen physiological markers. Field Crops Res. 102, 22–32. doi: 10.1016/j.fcr.2007.01.002
Landolfi, V., Visioli, G., and Blandino, M. (2021). Effect of nitrogen fertilization and fungicide application at heading on the gluten protein composition and rheological quality of wheat. Agronomy 11:1687. doi: 10.3390/agronomy11091687
Li, Y. W., An, X. L., Yang, R., Guo, X. M., Yue, G. D., Fan, R. C., et al. (2015). Dissecting and enhancing the contributions of high-molecular-weight glutenin subunits to dough functionality and bread quality. Mol. Plant 8, 332–334. doi: 10.1016/j.molp.2014.10.002
Li, J. H., Lina, X., Xie, L., Tian, X., Liu, S. Y., Jin, H., et al. (2021b). TaNAC100 acts as an integrator of seed protein and starch synthesis conferring pleiotropic effects on agronomic traits in wheat. Plant J. 108, 829–840. doi: 10.1111/tpj.15485
Li, J. H., Wang, K., Li, G. Y., Li, Y. L., Zhang, Y., Liu, Z. Y., et al. (2019a). Dissecting conserved cis-regulatory modules of Glu-1 promoters which confer the highly active endosperm-specific expression via stable wheat transformation. Crop J. 7, 8–18. doi: 10.1016/j.cj.2018.08.003
Li, J., Wang, Z., Yao, C., Zhang, Z., Liu, Y., and Zhang, Y. (2021a). Micro-sprinkling irrigation simultaneously improves grain yield and protein concentration of winter wheat in the North China plain. Crop J. 9, 1397–1407. doi: 10.1016/j.cj.2020.12.009
Li, J. P., Wang, Y. Q., Zhang, M., Liu, Y., Xu, X. X., Lin, G., et al. (2019b). Optimized micro-sprinkling irrigation scheduling improves grain yield by increasing the uptake and utilization of water and nitrogen during grain filling in winter wheat. Agric. Water Manag. 211, 59–69. doi: 10.1016/j.agwat.2018.09.047
Li, J. P., Xu, X. X., Lin, G., Wang, Y. Q., Liu, Y., Zhang, M., et al. (2018). Micro-irrigation improves grain yield and resource use efficiency by co-locating the roots and N-fertilizer distribution of winter wheat in the North China plain. Sci. Total Environ. 643, 367–377. doi: 10.1016/j.scitotenv.2018.06.157
Liang, C. Z., Wang, Y. Q., Zhu, Y. N., Tang, J. Y., Hu, B., Liu, L. C., et al. (2014). OsNAP connects abscisic acid and leaf senescence by fine-tuning abscisic acid biosynthesis and directly targeting senescence-associated genes in rice. Proc. Natl. Acad. Sci. U. S. A. 111, 10013–10018. doi: 10.1073/pnas.1321568111
Lindsay, M. P., and Skerritt, J. H. (1999). The glutenin macropolymer of wheat flour doughs: structure-function perspectives. Trends Food Sci. Technol. 10, 247–253. doi: 10.1016/S0924-2244(00)00004-2
Liu, J., Feng, B., Xu, Z., Fan, X., Jiang, F., Jin, X., et al. (2018). A genome-wide association study of wheat yield and quality-related traits in Southwest China. Mol. Breeding 38, 1–11. doi: 10.1007/s11032-017-0759-9
Liu, Z., Yan, Z., Wan, Y., Liu, K., Zheng, Y., and Wang, D. (2003). Analysis of HMW glutenin subunits and their coding sequences in two diploid Aegilops species. Theor. Appl. Genet. 106, 1368–1378. doi: 10.1007/s00122-002-1175-y
Liu, J., Zhang, J., Zhu, G., Zhu, D., and Yan, Y. (2022). Effects of water deficit and high N fertilization on wheat storage protein synthesis, gluten secondary structure, and breadmaking quality. Crop J. 10, 216–223. doi: 10.1016/j.cj.2021.04.006
Luo, C., Branlard, G., Griffin, W. B., and McNeil, D. L. (2000). The effect of nitrogen and Sulphur fertilisation and their interaction with genotype on wheat glutenins and quality parameters. J. Cereal Sci. 31, 185–194. doi: 10.1006/jcrs.1999.0298
Lyu, X., Liu, Y., Li, N., Ku, L., Hou, Y., and Wen, X. (2021). Foliar applications of various nitrogen (N) forms to winter wheat affect grain protein accumulation and quality via N metabolism and remobilization. Crop J. doi: 10.1016/j.cj.2021.10.009 (in press)
Ma, Q., Tao, R., Ding, Y., Zhang, X., Li, F., Zhu, M., et al. (2022). Can split application of slow-release fertilizer improve wheat yield, nitrogen efficiency and their stability in different ecological regions? Agronomy 12:407. doi: 10.3390/agronomy12020407
Ma, W. J., Yu, Z. T., She, M. Y., Zhao, Y., and Islam, S. (2019). Wheat gluten protein and its impacts on wheat processing quality. Front. Agr. Sci. Eng. 6, 279–287. doi: 10.15302/J-FASE-2019267
Ma, W., Zhang, W., and Gale, K. R. (2003). Multiplex-PCR typing of high molecular weight glutenin alleles in wheat. Euphytica 134, 51–60. doi: 10.1023/A:1026191918704
Makai, S., Eva, C., Tamas, L., and Juhasz, A. (2015). Multiple elements controlling the expression of wheat high molecular weight glutenin paralogs. Funct. Integr. Genomics 15, 661–672. doi: 10.1007/s10142-015-0441-4
Malik, A. H., Kuktaite, R., and Johansson, E. (2013). Combined effect of genetic and environmental factors on the accumulation of proteins in the wheat grain and their relationship to bread-making quality. J. Cereal Sci. 57, 170–174. doi: 10.1016/j.jcs.2012.09.017
McIntosh, R. A., Hart, G. E., and Gale, M. D. (1991). Catalog of gene symbols for wheat - 1991 supplement. Cereal Res. Commun. 19, 491–508.
Mena, M., Vicente-Carbajosa, J., Schmidt, R. J., and Carbonero, P. (1998). An endosperm-specific DOF protein from barley, highly conserved in wheat, binds to and activates transcription from the prolamin-box of a native B-hordein promoter in barley endosperm. Plant J. 16, 53–62. doi: 10.1046/j.1365-313x.1998.00275.x
Mohan, D., and Gupta, R. K. (2015). Gluten characteristics imparting bread quality in wheats differing for high molecular weight glutenin subunits at Glu D1 locus. Physiol. Mol. Biol. Plants 21, 447–451. doi: 10.1007/s12298-015-0298-y
Mottram, D. S., Wedzicha, B. L., and Dodson, A. T. (2002). Acrylamide is formed in the Maillard reaction. Nature 419, 448–449. doi: 10.1038/419448a
Muttucumaru, N., Halford, N. G., Elmore, J. S., Dodson, A. T., Parry, M., Shewry, P. R., et al. (2006). Formation of high levels of acrylamide during the processing of flour derived from sulfate-deprived wheat. J. Agric. Food Chem. 54, 8951–8955. doi: 10.1021/jf0623081
Ortolan, F., and Steel, C. J. (2017). Protein characteristics that affect the quality of vital wheat gluten to be used in baking: a review. Compr. Rev. Food Sci. Food Saf. 16, 369–381. doi: 10.1111/1541-4337.12259
Payne, P. I. (1987). Genetics of wheat storage proteins and the effect of allelic variation on bread-making quality. Annu. Rev. Plant Physiol. Plant Mol. Biol. 38, 141–153. doi: 10.1146/annurev.pp.38.060187.001041
Payne, P. I., Holt, L. M., and Law, C. N. (1981). Structural and genetical studies on the high-molecular-weight subunits of wheat glutenin: part 1: allelic variation in subunits amongst varieties of wheat (Triticum aestivum). Theor. Appl. Genet. 60, 229–236. doi: 10.1007/BF02342544
Payne, P. I., Nightingale, M. A., Krattiger, A. F., and Holt, L. M. (1987). The relationship between hmw glutenin subunit composition and the bread-making quality of british-grown wheat-varieties. J. Sci. Food Agric. 40, 51–65. doi: 10.1002/jsfa.2740400108
Peng, Y., Yu, K., Zhang, Y., Islam, S., Sun, D., and Ma, W. (2015). Two novel y-type high molecular weight glutenin genes in chinese wheat landraces of the yangtze-river region. PLoS One 10:e0142348. doi: 10.1371/journal.pone.0142348
Qi, L. J., Hu, X. X., Zhou, G. Y., Wang, S., Li, J. M., Lu, W., et al. (2012). Analysis of wheat protein quality in main wheat producing areas of China from 2004 to 2011. Sci. Agric. Sin. 45, 4242–4251. doi: 10.3864/j.issn.0578-1752.2012.20.014
Raffan, S., and Halford, N. G. (2019). Acrylamide in food: Progress in and prospects for genetic and agronomic solutions. Ann. Appl. Biol. 175, 259–281. doi: 10.1111/aab.12536
Raffan, S., and Halford, N. G. (2021). Cereal asparagine synthetase genes. Ann. Appl. Biol. 178, 6–22. doi: 10.1111/aab.12632
Raffan, S., Sparks, C., Huttly, A., Hyde, L., Martignago, D., Mead, A., et al. (2021). Wheat with greatly reduced accumulation of free asparagine in the grain, produced by CRISPR/Cas9 editing of asparagine synthetase gene TaASN2. Plant Biotechnol. J. 19, 1602–1613. doi: 10.1111/pbi.13573
Rasheed, A., Xia, X. C., Yan, Y. M., Appels, R., Mahmood, T., and He, Z. H. (2014). Wheat seed storage proteins: advances in molecular genetics, diversity and breeding applications. J. Cereal Sci. 60, 11–24. doi: 10.1016/j.jcs.2014.01.020
Ravel, C., Fiquet, S., Boudet, J., Dardevet, M., Vincent, J., Merlino, M., et al. (2014). Conserved cis-regulatory modules in promoters of genes encoding wheat high-molecular-weight glutenin subunits. Front. Plant Sci. 5:621. doi: 10.3389/fpls.2014.00621
Ravel, C., Martre, P., Romeuf, I., Dardevet, M., El-Malki, R., Bordes, J., et al. (2009). Nucleotide polymorphism in the wheat transcriptional activator spa influences its pattern of expression and has pleiotropic effects on grain protein composition, dough viscoelasticity, and grain hardness. Plant Physiol. 151, 2133–2144. doi: 10.1104/pp.109.146076
Roy, N., Islam, S., Al-Habbar, Z., Yu, Z., Liu, H., Lafiandra, D., et al. (2021). Contribution to breadmaking performance of two different HMW glutenin 1Ay alleles expressed in hexaploid wheat. J. Agr. Food Chem. 69, 36–44. doi: 10.1021/acs.jafc.0c03880
Roy, N., Islam, S., Ma, J. H., Lu, M. Q., Torok, K., Tomoskozi, S., et al. (2018). Expressed ay HMW glutenin subunit in Australian wheat cultivars indicates a positive effect on wheat quality. J. Cereal Sci. 79, 494–500. doi: 10.1016/j.jcs.2017.12.009
Roy, N., Islam, S., Yu, Z. T., Lu, M. Q., Lafiandra, D., Zhao, Y., et al. (2020). Introgression of an expressed HMW 1Ay glutenin subunit allele into bread wheat cv. Lincoln increases grain protein content and breadmaking quality without yield penalty. Theor. Appl. Genet. 133, 517–528. doi: 10.1007/s00122-019-03483-1
Sánchez-León, S., Gil-Humanes, J., Ozuna, C. V., Gimenez, M. J., Sousa, C., Voytas, D. F., et al. (2018). Low-gluten, nontransgenic wheat engineered with CRISPR/Cas9. Plant Biotechnol. J. 16, 902–910. doi: 10.1111/pbi.12837
Scherf, K. A., Ciccocioppo, R., Pohanka, M., Rimarova, K., Opatrilova, R., Rodrigo, L., et al. (2016a). Biosensors for the diagnosis of celiac disease: current status and future perspectives. Mol. Biotechnol. 58, 381–392. doi: 10.1007/s12033-016-9940-3
Scherf, K. A., Koehler, P., and Wieser, H. (2016b). Gluten and wheat sensitivities - An overview. J. Cereal Sci. 67, 2–11. doi: 10.1016/j.jcs.2015.07.008
Scherf, K. A., Wieser, H., and Koehler, P. (2018). Novel approaches for enzymatic gluten degradation to create high-quality gluten-free products. Food Res. Int. 110, 62–72. doi: 10.1016/j.foodres.2016.11.021
Sharma, A., Garg, S., Sheikh, I., Vyas, P., and Dhaliwal, H. S. (2020). Effect of wheat grain protein composition on end-use quality. J. Food Sci. Technol. 57, 2771–2785. doi: 10.1007/s13197-019-04222-6
She, M., Ye, X., Yan, Y., Howit, C., Belgard, M., and Ma, W. (2011). Gene networks in the synthesis and deposition of protein polymers during grain development of wheat. Funct. Integr. Genomics 11, 23–35. doi: 10.1007/s10142-010-0196-x
Shen, L. S., Luo, G. B., Song, Y. H., Xu, J. Y., Ji, J. J., Zhang, C., et al. (2021). A novel NAC family transcription factor SPR suppresses seed storage protein synthesis in wheat. Plant Biotechnol. J. 19, 992–1007. doi: 10.1111/pbi.13524
Shewry, P. R., and Halford, N. G. (2002). Cereal seed storage proteins: structures, properties and role in grain utilization. J. Exp. Bot. 53, 947–958. doi: 10.1093/jexbot/53.370.947
Shewry, P. R., Halford, N. G., Belton, P. S., and Tatham, A. S. (2002). The structure and properties of gluten: an elastic protein from wheat grain. Philos. Trans. R. Soc. Lond. Ser. B Biol. Sci. 357, 133–142. doi: 10.1098/rstb.2001.1024
Shewry, P. R., Napier, J. A., and Tatham, A. S. (1995). Seed storage proteins: structures and biosynthesis. Plant Cell 7, 945–956. doi: 10.1105/tpc.7.7.945
Shewry, P. R., and Tatham, A. S. (1997). Disulphide bonds in wheat gluten proteins. J. Cereal Sci. 25, 207–227. doi: 10.1006/jcrs.1996.0100
Stadler, R. H., Blank, I., Varga, N., Robert, F., Hau, J., Guy, P. A., et al. (2002). Acrylamide from maillard reaction products. Nature 419, 449–450. doi: 10.1038/419449a
Sultana, H., Armstrong, R., Suter, H., Chen, D. L., and Nicolas, M. E. (2017). A short-term study of wheat grain protein response to post-anthesis foliar nitrogen application under elevated CO2 and supplementary irrigation. J. Cereal Sci. 75, 135–137. doi: 10.1016/j.jcs.2017.03.031
Sun, X., Hu, S., Liu, X., Qian, W., Hao, S., Zhang, A., et al. (2006). Characterization of the HMW glutenin subunits from Aegilops searsii and identification of a novel variant HMW glutenin subunit. Theor. Appl. Genet. 113, 631–641. doi: 10.1007/s00122-006-0327-x
Sun, F. S., Liu, X. Y., Wei, Q. H., Liu, J. N., Yang, T. X., Jia, L. Y., et al. (2017). Functional characterization of TaFUSCA3, a B3-superfamily transcription factor gene in the wheat. Front. Plant Sci. 8:1133. doi: 10.3389/fpls.2017.01133
Uauy, C., Distelfeld, A., Fahima, T., Blechl, A., and Dubcovsky, J. (2006). A NAC gene regulating senescence improves grain protein, zinc, and iron content in wheat. Science 314, 1298–1301. doi: 10.1126/science.1133649
Vasila, I. K., and Anderson, O. D. (1997). Genetic engineering of wheat gluten. Trends Plant Sci. 2, 292–297. doi: 10.1016/S1360-1385(97)89950-5
Veraverbeke, W. S., and Delcour, J. A. (2002). Wheat protein composition and properties of wheat glutenin in relation to breadmaking functionality. Crit. Rev. Food Sci. Nutr. 42, 179–208. doi: 10.1080/10408690290825510
Wang, L., Chen, A., Lin, Z., Zhao, R., Lan, J., and Dai, C (2013). GB/T 17320–2013: Quality Classification of Wheat Varieties; General Administration of Quality Supervision, Inspection and Quarantine of P.R.C: Beijing, China.
Wang, D., Li, F., Cao, S., and Zhang, K. (2020). Genomic and functional genomics analyses of gluten proteins and prospect for simultaneous improvement of end-use and health-related traits in wheat. Theor. Appl. Genet. 133, 1521–1539. doi: 10.1007/s00122-020-03557-5
Wen, S. S., Wen, N. A., Pang, J. S., Langen, G., Brew-Appiah, R. A. T., Mejias, J. H., et al. (2012). Structural genes of wheat and barley 5-methylcytosine DNA glycosylases and their potential applications for human health. Proc. Natl. Acad. Sci. U. S. A. 109, 20543–20548. doi: 10.1073/pnas.1217927109
Wieser, H. (2007). Chemistry of gluten proteins. Food Microbiol. 24, 115–119. doi: 10.1016/j.fm.2006.07.004
Xia, J., Zhu, D., Chang, H., Yan, X., and Yan, Y. (2020). Effects of water-deficit and high-nitrogen treatments on wheat resistant starch crystalline structure and physicochemical properties. Carbohydr. Polym. 234:115905. doi: 10.1016/j.carbpol.2020.115905
Xia, J., Zhu, D., Wang, R. M., Cui, Y., and Yan, Y. M. (2018). Crop resistant starch and genetic improvement: a review of recent advances. Theor. Appl. Genet. 131, 2495–2511. doi: 10.1007/s00122-018-3221-4
Xu, H. W., Curtis, T. Y., Powers, S. J., Raffan, S., Gao, R. H., Huang, J. H., et al. (2018a). Genomic, biochemical, and modeling analyses of asparagine synthetases from wheat. Front. Plant Sci. 8:2237. doi: 10.3389/fpls.2017.02237
Xu, X. X., Zhang, M., Li, J. P., Liu, Z. Q., Zhao, Z. G., Zhang, Y. H., et al. (2018b). Improving water use efficiency and grain yield of winter wheat by optimizing irrigations in the North China plain. Field Crops Res. 221, 219–227. doi: 10.1016/j.fcr.2018.02.011
Xue, L., Li, Y., Li, T., Pan, H., Liu, J., Fan, M., et al. (2019). Phosphorylation and enzymatic hydrolysis with alcalase and papain effectively reduce allergic reactions to gliadins in normal mice. J. Agric. Food Chem. 67, 6313–6323. doi: 10.1021/acs.jafc.9b00569
Xue, G. P., Way, H. M., Richardson, T., Drenth, J., Joyce, P. A., and McIntyre, C. L. (2011). Overexpression of TaNAC69 leads to enhanced transcript levels of stress up-regulated genes and dehydration tolerance in bread wheat. Mol. Plant 4, 697–712. doi: 10.1093/mp/ssr013
Yang, R. C., Juhasz, A., Zhang, Y. J., Chen, X. Y., Zhang, Y. J., She, M. Y., et al. (2018). Molecular characterisation of the NAM-1 genes in bread wheat in Australia. Crop Pasture Sci. 69, 1173–1181. doi: 10.1071/Cp18273
Yu, Z., Islam, S., She, M., Diepeveen, D., Zhang, Y., Tang, G., et al. (2018a). Wheat grain protein accumulation and polymerization mechanisms driven by nitrogen fertilization. Plant J. 96, 1160–1177. doi: 10.1111/tpj.14096
Yu, Z., Juhasz, A., Islam, S., Diepeveen, D., Zhang, J., Wang, P., et al. (2018b). Impact of mid-season Sulphur deficiency on wheat nitrogen metabolism and biosynthesis of grain protein. Sci. Rep. 8:2499. doi: 10.1038/s41598-018-20935-8
Yu, Z. T., Peng, Y. C., Islam, M. S., She, M. Y., Lu, M. Q., Lafiandra, D., et al. (2019). Molecular characterization and phylogenetic analysis of active y-type high molecular weight glutenin subunit genes at Glu-A1 locus in wheat. J. Cereal Sci. 86, 9–14. doi: 10.1016/j.jcs.2019.01.003
Yu, Z., She, M., Zheng, T., Diepeveen, D., Islam, S., Zhao, Y., et al. (2021). Impact and mechanism of Sulphur-deficiency on modern wheat farming nitrogen-related sustainability and gliadin content. Commun. Biol. 4:945. doi: 10.1038/s42003-021-02458-7
Zebarth, B. J., Botha, E. J., and Rees, H. (2007). Rate and time of fertilizer nitrogen application on yield, protein and apparent efficiency of fertilizer nitrogen use of spring wheat. Can. J. Plant Sci. 87, 709–718. doi: 10.4141/Cjps06001
Zhang, P. P., He, Z. H., Chen, D. S., Zhang, Y., Larroque, O. R., and Xia, X. C. (2007). Contribution of common wheat protein fractions to dough properties and quality of northern-style Chinese steamed bread. J. Cereal Sci. 46, 1–10. doi: 10.1016/j.jcs.2006.10.007
Zhang, P. P., Jondiko, T. O., Tilley, M., and Awika, J. M. (2014b). Effect of high molecular weight glutenin subunit composition in common wheat on dough properties and steamed bread quality. J. Sci. Food Agric. 94, 2801–2806. doi: 10.1002/jsfa.6635
Zhang, M., Ma, C. Y., Lv, D. W., Zhen, S. M., Li, X. H., and Yan, Y. M. (2014a). Comparative phosphoproteome analysis of the developing grains in bread wheat (Triticum aestivum L.) under well-watered and water-deficit conditions. J. Proteome Res. 13, 4281–4297. doi: 10.1021/pr500400t
Zhang, F. L., Wang, C. M., and Li, D. (2021). Study on the quality evaluation of steamed bread and the physical and chemical properties of wheat. Agricul. Biotechnol. 10, 125–127. doi: 10.19759/j.cnki.2164-4993.2021.02.029
Zhao, F., Hawkesford, M., and McGrath, S. (1999a). Sulphur assimilation and effects on yield and quality of wheat. J. Cereal Sci. 30, 1–17. doi: 10.1006/jcrs.1998.0241
Zhao, F. J., Salmon, S. E., Withers, P. J. A., Monaghan, J. M., Evans, E. J., Shewry, P. R., et al. (1999b). Variation in the breadmaking quality and rheological properties of wheat in relation to Sulphur nutrition under field conditions. J. Cereal Sci. 30, 19–31. doi: 10.1006/jcrs.1998.0244
Zhen, S. M., Deng, X., Li, M. F., Zhu, D., and Yan, Y. M. (2018). 2D-DIGE comparative proteomic analysis of developing wheat grains under high-nitrogen fertilization revealed key differentially accumulated proteins that promote storage protein and starch biosyntheses. Anal. Bioanal. Chem. 410, 6219–6235. doi: 10.1007/s00216-018-1230-4
Zhen, S. M., Deng, X., Xu, X. X., Liu, N. N., Zhu, D., Wang, Z. M., et al. (2020). Effect of high-nitrogen fertilizer on gliadin and glutenin subproteomes during kernel development in wheat (Triticum aestivum L.). Crop J. 8, 38–52. doi: 10.1016/j.cj.2019.06.002
Zheng, W., Peng, Y. C., Ma, J. H., Appels, R., Sun, D. F., and Ma, W. J. (2011). High frequency of abnormal high molecular weight glutenin alleles in Chinese wheat landraces of the Yangtze-River region. J. Cereal Sci. 54, 401–408. doi: 10.1016/j.jcs.2011.08.004
Zhong, Y., Vidkjær, N. H., Massange-Sanchez, J. A., Laursen, B. B., Gislum, R., Borg, S., et al. (2020). Changes in spatiotemporal protein and amino acid gradients in wheat caryopsis after N-topdressing. Plant Sci. 291:110336. doi: 10.1016/j.plantsci.2019.110336
Zhong, Y., Wang, W., Huang, X., Liu, M., Hebelstrup, K., Yang, D., et al. (2019). Nitrogen topdressing timing modifies the gluten quality and grain hardness related protein levels as revealed by iTRAQ. Food Chem. 277, 135–144. doi: 10.1016/j.foodchem.2018.10.071
Zhong, Y., Xu, D., Hebelstrup, K. H., Yang, D., Cai, J., Wang, X., et al. (2018). Nitrogen topdressing timing modifies free amino acids profiles and storage protein gene expression in wheat grain. BMC Plant Biol. 18:353. doi: 10.1186/s12870-018-1563-3.doi:10.1186/s12870-018-1563-3
Zhou, J. X., Liu, D. M., Deng, X., Zhen, S. M., Wang, Z. M., and Yan, Y. M. (2018). Effects of water deficit on breadmaking quality and storage protein compositions in bread wheat (Triticum aestivum L.). J. Sci. Food Agric. 98, 4357–4368. doi: 10.1002/jsfa.8968
Zhou, Z., Liu, C., Qin, M., Li, W., Hou, J., Shi, X., et al. (2022). Promoter DNA hypermethylation of TaGli-γ-2.1 positively regulates gluten strength in bread wheat. J. Adv. Res. 36, 163–173. doi: 10.1016/j.jare.2021.06.021
Zhu, J. T., Fang, L. L., Yu, J. Q., Zhao, Y., Chen, F. G., and Xia, G. M. (2018). 5-Azacytidine treatment and TaPBF-D over-expression increases glutenin accumulation within the wheat grain by hypomethylating the Glu-1 promoters. Theor. Appl. Genet. 131, 735–746. doi: 10.1007/s00122-017-3032-z
Zhu, D., Zhu, G. R., Zhang, Z., Wang, Z. M., Yan, X., and Yan, Y. M. (2020). Effects of independent and combined water-deficit and high-nitrogen treatments on flag leaf proteomes during wheat grain development. Int. J. Mol. Sci. 21:2098. doi: 10.3390/ijms21062098
Keywords: wheat quality, fertilization, watering regime, regulatory mechanism, sulfur deficiency
Citation: Peng Y, Zhao Y, Yu Z, Zeng J, Xu D, Dong J and Ma W (2022) Wheat Quality Formation and Its Regulatory Mechanism. Front. Plant Sci. 13:834654. doi: 10.3389/fpls.2022.834654
Edited by:
Vincenzo Rossi, Council for Agricultural and Economics Research (CREA), ItalyReviewed by:
Patricia Giraldo, Polytechnic University of Madrid, SpainGrazia Maria Borrelli, Council for Agricultural and Economics Research (CREA), Italy
Yueming Yan, Capital Normal University, China
Fei Xiong, Yangzhou University, China
Copyright © 2022 Peng, Zhao, Yu, Zeng, Xu, Dong and Ma. This is an open-access article distributed under the terms of the Creative Commons Attribution License (CC BY). The use, distribution or reproduction in other forums is permitted, provided the original author(s) and the copyright owner(s) are credited and that the original publication in this journal is cited, in accordance with accepted academic practice. No use, distribution or reproduction is permitted which does not comply with these terms.
*Correspondence: Wujun Ma, dy5tYUBtdXJkb2NoLmVkdS5hdQ==