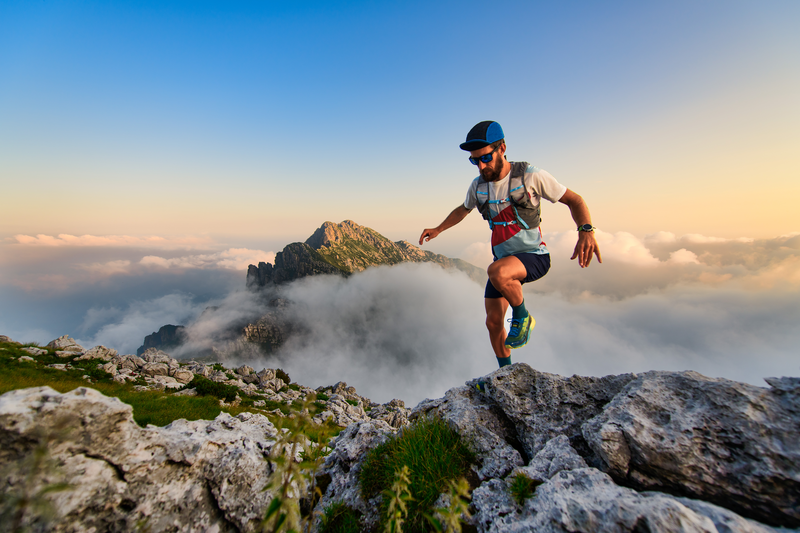
94% of researchers rate our articles as excellent or good
Learn more about the work of our research integrity team to safeguard the quality of each article we publish.
Find out more
REVIEW article
Front. Plant Sci. , 16 September 2022
Sec. Plant Pathogen Interactions
Volume 13 - 2022 | https://doi.org/10.3389/fpls.2022.823233
This article is part of the Research Topic Lipid Metabolism and Membrane Structure in Plant Biotic Interactions View all 6 articles
Lipids are central at various stages of host–pathogen interactions in determining virulence and modulating plant defense. Free fatty acids may act as substrates for oxidizing enzymes [e.g., lipoxygenases (LOXs) and dioxygenases (DOXs)] that synthesize oxylipins. Fatty acids and oxylipins function as modulators of several pathways in cell-to-cell communication; their structural similarity among plant, fungal, and bacterial taxa suggests potential in cross-kingdom communication. We provide a prospect of the known role of fatty acids and oxylipins in fungi and bacteria during plant–pathogen interactions. In the pathogens, oxylipin-mediated signaling pathways are crucial both in development and host infection. Here, we report on case studies suggesting that oxylipins derived from oleic, linoleic, and linolenic acids are crucial in modulating the pathogenic lifestyle in the host plant. Intriguingly, overlapping (fungi-plant/bacteria-plant) results suggest that different inter-kingdom pathosystems use similar lipid signals to reshape the lifestyle of the contenders and occasionally determine the outcome of the challenge.
Host–pathogen recognition relies upon an elaborate multi-molecular communication. In this context, oxylipins—oxidized fatty acids (FAs)—produced in several phyla can regulate numerous events associated with physiological and pathological processes (Blée, 2002; Andreou et al., 2009; Mosblech et al., 2009; Ambaw et al., 2021; Liu et al., 2021; Fernandes and Ghag, 2022). The term oxylipin (Gerwick et al., 1993) refers to a broad family of secondary metabolites originated from the oxidation of polyunsaturated and/or monounsaturated FAs (PUFAs and/or MUFAs) (Mosblech et al., 2009), which comprises a complex array of products: alcohols, aldehydes, ketones, acids, and hydrocarbon gases, generated via enzymatic and non-enzymatic processes. Oxylipins share significant structural and functional similarities across different mammal, plant, fungal, and bacterial taxa, including part of their biosynthetic pathway, structure, function, and modifications.
In plant, oxylipins are signaling molecules involved in the regulation of development and immunity. Jasmonates are the better-characterized oxylipins in plants and include jasmonic acid (JA), its precursor 12-oxo-phytodienoic acid (12-OPDA), and JA derivatives such as methyl jasmonate and JA-isoleucine. JA and its derivatives act as defense regulators, influencing the reproductive and pathogenetic processes during the interaction with both beneficial and pathogenic microorganisms (Andreou et al., 2009; Deboever et al., 2020); pathogen infection and pest wounding are the best-studied environmental triggers, in this context (Farmer et al., 2003; Block et al., 2005; Gorman et al., 2021; Shaban et al., 2021). The well-characterized JA pathway activation starts with conjugated lipids in the chloroplast membranes (e.g., monogalactosyldiacylglycerol). Lipase A1 mediates the release of α-linolenic acid, and LOX enzyme catalyzes PUFA dioxygenation. Oxygenation may happen at several positions along the carbon chain. For JA, important PUFA precursors are α-linolenic acid (Wasternack and Strnad, 2018) or, alternatively, the hexadecatrienoic acid (Chini et al., 2018). Local defenses and systemic acquired resistances (e.g., SAR, ISR) require JA involvement (Ryan and Moura, 2002; Yu et al., 2022) to express a distinct set of defense-related genes (Okada et al., 2015). Biosynthesis of other plant oxylipins is initiated by the 9 and 13-LOX and α-dioxygenase (α-DOX), or monooxygenases, which all catalyze the oxygenation of linoleic acid and linolenic acid (mainly) into reactive hydroperoxides, followed by a secondary modification by cytochrome P450 enzymes or peroxygenases (Blée, 2002; Hamberg et al., 2003; Andreou and Feussner, 2009). Oxylipins can also be produced nonenzymatically through free radical-mediated oxygenation (Oenel et al., 2017). Plant (or phyto-) oxylipins are produced under abiotic and biotic stress, as well as during beneficial interactions (Blée, 2002; Feussner and Wasternack, 2002; Prost et al., 2005). Their role spans from antimicrobial agents to signaling molecules. Recently, the employment of oxylipins as effective and commercial antimicrobial agents has been discussed (Deboever et al., 2020). Plants produce diversified oxylipins for different purposes, and oxylipins can act directly against the pathogen (such as Fusarium spp.) but also as attractors of biocontrol agents (such as Trichoderma spp.) (Gol et al., 2018; Lombardi et al., 2018; Wang et al., 2020). As signaling molecules, they have been implicated in several pathways, including morphogenesis, and as determinants of the hypersensitive response (HR) in incompatible plant–pathogen interactions (Mehta et al., 2021).
In fungi and bacteria, oxylipins control lifestyle and quorum sensing inter alia (Su et al., 1995; Tsitsigiannis et al., 2005b). The “oxylipin regulation system” in fungi and bacteria can employ host oxylipins to improve virulence (e.g., enhancing toxin production or switching to biofilm stage) (Burow et al., 2000; Brodhagen et al., 2008; Martínez et al., 2019) and affect reproduction rate within host tissues by increasing the sporulation (Scarpari et al., 2014). Bacterial oxylipins impact the plant defense mechanisms, as in the case of coronatine production in Pseudomonas syringae, a toxin that mimics the plant hormone JA-isoleucine with the aim to induce the opening of stomata to enable bacterial ingress (Zheng et al., 2012). These features support the hypothesis that oxylipins may act as “words” in the lipid common language in host–pathogen communication with a paracrine activity, as suggested by Niu and colleagues (Tsitsigiannis and Keller, 2007; Christensen and Kolomiets, 2011; Niu et al., 2020).
Discriminating which oxylipins are produced by the host and which by the pathogen is not trivial, since the two actors will produce the same molecules at the same time. Oxylipins play a relevant ecological role for the producers (i.e., plants, fungi, and bacteria) and their interspecific interactions (Siebers et al., 2016; Beccaccioli et al., 2021b). In plant–pathogen interactions, plants (the host) produce oxylipins to systemically signal pathogen attack, mounting an efficient defense system and interfering with pathogen growth and reproduction (Burow and Nesbitt, 1997; Brodhagen et al., 2008; Andreou et al., 2009; Scarpari et al., 2014).
Numerous evidence shows that oxylipins mediate interspecies signaling among eukaryotes (Pohl and Kock, 2014). Trichoderma virens stimulates symbiont-induced systemic resistance in maize by promoting the release of 12-OPDA and α-ketol of octadecadienoic in the xylem sap (Wang et al., 2020). In plants, 9-HPODE and 13-HPODE alter the secondary metabolism and conidiation in Aspergillus flavus (Calvo et al., 1999), Colletotrichum graminicola (Gorman et al., 2021), Verticillium dahliae (Shaban et al., 2021), Fusarium oxysporum (Fernandes and Ghag, 2022), and inter alia. Fungal oxylipins are involved in the control of sexual and asexual structures formation, secondary metabolism, density-dependent growth, and in the interaction with hosts (Reverberi et al., 2010; Brodhun and Feussner, 2011; Christensen and Kolomiets, 2011). In bacteria, very recent studies in Pseudomonas aeruginosa and Xylella fastidiosa suggest a role in mediating autocrine or paracrine signals in the communication with their hosts or vectors (Martínez et al., 2019; Niu et al., 2020; Scala et al., 2020).
During infection, host–pathogen communication determines the fate of the interaction. Here, we show how oxylipins represent a common language shared among plant and pathogens, both fungal and bacterial. Some case studies related to oxylipin-mediated plant–pathogen interaction regarding interaction between host plants and filamentous fungi or bacterial pathogens will be presented.
From a chemical point of view, oxylipins are products of oxygenation of MUFAs or PUFAs. FAs are the main components of several complex lipids (acylglycerols, glycerophospholipids, glycolipids, sphingolipids, and sterol) from where they may be cleaved. Free FAs are diversified by length and degree of unsaturation. PUFA availability is crucial for the cell because, based on the degree of saturation, they alter cell membrane fluidity, the arrangement and availability of receptors, influencing signal transduction regulation. In this regard, fatty acids may act directly on the receptors (de Angelis et al., 2016).
Oxidation of FAs may be spontaneous in the presence of reactive oxygen species (ROS) or a consequence of enzymatic activity. The study of oxylipins in fungi followed the discovery of the role of lipid hydroperoxides in Aspergillus parasiticus (Fabbri et al., 1983) and of the psi factors (precocious sexual inducers), a series of fatty acid-derived oxylipins involved in the regulation of the development of spores and conidia (Champe et al., 1987). Different studies have elucidated the biosynthetic pathways of these molecules. Phospholipids and acylglycerides provide the substrate to oxylipin synthesis: phospholipases release oleic, linoleic, linolenic, and arachidonic acids, central elements for the formation of fungal oxylipins (Sakuradani et al., 2009; Beccaccioli et al., 2019).
The first evidence of enzymatic formation of oxylipins in fungi was found in Gaeumannomyces graminis, the causal agent of root and crown rot diseases. G. graminis produces oxylipins via FA oxidation with LOXs, DOXs [including the transformation of linoleic acid into dihydroxy-linoleate by 8-DOX (also named linoleate diol synthase or LDS)], and cyclooxygenase (COX) (Su et al., 2000). More recently, fatty acid dioxygenase-cytochrome P450 fusion enzymes have been identified in several pathogenic fungi, along with the implication of several oxylipins such as 8-, 9-, or 10-hydroperoxy metabolite biosynthesis (Oliw, 2021). Each fatty acid may generate different oxylipins based on enzymatic activity and spontaneous reactions available in the oxidant environment. We list known fungal oxylipins (Figure 1) and present examples of their function in relation to their chemical origin based on the class of oxygenase (LOX, DOX or LDS, and COX) (Figure 2), with a focus on the role in plant–fungus interaction.
Figure 1. Fungal oxylipins. Oleic acid (18:1) can be oxidized by LDS enzyme and converted in hydroperoxyoctanoic acid (HPOMEs); other enzymes (e.g. isomerases) convert HPOME in hydroxyoctanoic acid (HOME), and di-hydroxyoctanoic acid (diHOME). Linoleic acid (18:2) can be oxidized by LOX enzyme and converted in hydroperoxyoctadecadienoic acid (HPODE); other enzymes (e.g. reductases) convert HPODE in hydroxyoctadecadienoic acid (HODE), and di-hydroxyoctadecadienoic acid (diHODE). Linoleic acid (18:2) is also the substrate of LDS that catalyzes the conversion in hydroperoxyoctadecadienoic acid (HPODE); other enzymes (e.g. epoxidases) convert HPODE in hydroxyoctadecadienoic acid (HODE), di-hydroxyoctadecadienoic acid (diHODE), and epoxyoctadecenoic acids (EPOME). LOX enzyme acts upon α/δ-Linolenic acid (18:3) to generate hydroperoxyoctadecatrienoic acid (HPOTE); HPOTE is the substrate of other enzymes to generate hydroxyoctadecatrienoic acid (HOTE) and di-hydroxyoctadecatrienoic acid (diHOTE). Arachidonic acid (20:4) can be oxidized by LOX and converted in hydroperoxyeicosatetraenoic acid (HPETE); HPETE is the substrate of hydroxyeicosatetraenoic acid (HETE), di-hydroxyeicosatetraenoic acid (diHETE). COX enzyme convert the arachidonic acid (20:4) in prostaglandins (PGs).
Figure 2. Oxylipin functions. Linoleic acid-derived oxylipins are described for Aspergillus spp., Fusarium spp., and maize. For Aspergillus spp., the main signaling process mediated by lipoxygenases (LOX) and linoleate diol synthases (LDS) is reported, for Fusarium spp. is described the LDS involvement, and for maize the LOX-mediated functions. Linolenic acid oxylipins derived from LOX activity shared the same functions in Aspergillus spp. and Fusarium spp.
LOX-mediated oxygenation may occur on linoleic or linolenic FAs. Early evidence was reported in Aspergillus nidulans; activity on linoleic acid generates oxylipins 9-HODE and 9-HPODE that are involved in sporulation control (Calvo et al., 1999). LOX activity on linoleic acid may also form 13-HODE and 13-HPODE, inhibiting mycotoxin production in Aspergillus spp. (Burow and Nesbitt, 1997) and promoting sporulation (Calvo et al., 1999). Both 9-LOX and 13-LOX, which differ in the position at which they cause oxygenation, stimulate cAMP production by fungal G protein-coupled receptor (GPCR) signaling (Affeldt et al., 2012), suggesting its activation to transduce autocrine signaling. In the mycoparasite Trichoderma atroviride, LOX1 is indispensable for 6-pentyl-2H-pyran-2-one production and to parasitize and antagonize host fungi, for conidiation in darkness, response to injury (i.e., 9-HODE, 13-HODE, 9-oxoOTrE, and 13-HOTrE), and production of volatile organic compounds. LOX1 in T. atroviride is required during the interaction with the host plant, particularly in Arabidopsis thaliana, to induce systemic resistance against the plant-pathogenic fungus Botrytis cinerea (Speckbacher et al., 2020). Fungal oxylipins derived from linolenic acid by LOX-oxidation may generate several JA analogs borrowed by the plant and act as precursors for JA, methyl-JA, and 12-OH-JA formation. These compounds were first identified in Fusarium oxysporum and Aspergillus niger (Miersch et al., 1999a,b). In plants, 12-OH-JA represents the inactive form of JA. It is likely to prevent the inhibitive effects of accumulation of JA on plant development and growth. In Ganoderma lucidum, the presence of 12-OH-JA also regulates host colonization by inhibiting methyl-JA formation, important for plant defense (Patkar et al., 2015). More recently, research has shown that Magnaporthe oryzae, the causal agent of the rice blast, can autonomously produce analogs of JA to control its pathogenic development (Liu et al., 2021).
Several fungi possess genes encoding for DOX which is involved in oxylipin biosynthesis. DOX enzymes contain a catalytic domain often fused to a functional cytochrome P450 at the C-terminal end. Therefore, they constitute a peculiar group within the peroxidase-cyclooxygenase superfamily. Their oxidizing activity toward linoleic acid produces five different hydroperoxides. The CYP450 domain can then transform these hydroperoxides into diols, epoxy alcohols, and allene oxides which all support sporulation, hyphal branching, and development in filamentous fungi. Fungal pathogens with biotrophic [e.g., Blumeria graminis (Rupasinghe et al., 2006), Ustilago maydis (Huber et al., 2002)], hemibiotrophic [e.g., Fusarium verticillioides (Scala et al., 2014)], and necrotrophic [e.g., Botrytis cinerea (Niu et al., 2020)] phenotypes express these “fusion enzymes” during pathogenesis to support virulence.
LDS-derived oxylipins have different roles across fungal species. In F. verticillioides, linoleic acid is the substrate of LDS from which 8-HPODE and 8,13-diHODE are derived. These oxylipins can influence fungal growth and mycotoxin production (Scala et al., 2013, 2014). LDS oxylipins also promote pathogenesis and modulate the expression of maize oxylipins which, in turn, produce 9-LOX oxylipins to trigger JA-mediated defense (Battilani et al., 2018). In A. nidulans, 5,8-diHODE, produced from linoleic acid, regulates sexual development and the conidiation/sporulation ratio (Champe et al., 1987; Mazur et al., 1990; EbrahimáEl-Zayat, 1991; Tsitsigiannis and Keller, 2007; Brown et al., 2008). In Aspergillus fumigatus and Aspergillus flavus, 5,8-diHODE and 7,8-diHODE induce cell differentiation and lateral branching through GPCRs. Psi-producing oxidase (essentially a group of oxygenases such as LDS) is largely conserved among filamentous fungi; the synthesis of diol-containing oxylipins, primarily 5,8- and 7,8-diHODE, might represent a crucial step in the organization of fungal “morphology” (namely hyphal branching and polarity) and even “escape” from antifungals (Niu et al., 2020). For instance, in the pathogenic ascomycete Magnaporthe grisea, 5,8-diHODE accumulates during appressoria formation (Niu et al., 2020) paving the way for host infection. Apparently, these species of diol-oxylipins generate a signaling network in the mycelial mat to tightly adapt hyphal morphology to the environment.
COX produces active mediators of inflammatory response from arachidonic acid oxygenation. The main findings on fungal development focus on oxylipins deriving from linoleic acid involved in sporulation and mycotoxin biosynthesis in A. flavus, A. parasiticus (Calvo et al., 1999), and F. verticillioides (Gao et al., 2007). Recently, research has also focused on fungal production of jasmonate analogs (Eng et al., 2021). Information is more scarce on oxylipins generated from arachidonic acid, a fatty acid mainly present in extremophile fungi such as Mortierella alpina (Kikukawa et al., 2018). In A. fumigatus, arachidonic acid is oxygenated by COX-like enzymes to produce prostaglandins to enhance virulence (Tsitsigiannis et al., 2005a). In Cryptococcus neoformans and Candida albicans, several studies show that fungal prostaglandins promote colonization and infection (Noverr et al., 2001, 2003).
Mechanisms of oxylipin biosynthesis and signaling are similar in plants and fungi. Here, we report examples on how plant and fungus exploit the oxylipins to exchange messages involved in the outcome of the interaction.
The structural similarity between plant and fungal oxylipins can be exploited to reciprocally manipulate each other's signaling. In A. flavus on maize, thanks to this structural similarity, the pathogen's oxylipins can act on the plant's oxylipinogenic pathways, and vice versa. This cross talk among host and pathogen was demonstrated in different experimental settings, as reported below. In Aspergillus cultures, the exogenous application of plant oxylipins (i.e., 9-HPODE naturally produced by maize) increases sporulation and mycotoxin production. In Aspergillus deletion mutants for the DOX ppoA and ppoC, the wild-type phenotype can be restored through the insertion of maize lipoxygenase, suggesting a complementing activity. Inoculation of peanut seeds with Aspergillus ppo-mutants reduces LOX expression in seed, suggesting that fungal oxylipins are related to the plant LOX expression (Brodhagen et al., 2008).
The hydroperoxide 9-HODE generated by LOX activity offers an example of a shared signaling molecule. In plants, it induces programmed cell death, as observed in tomato protoplasts (Knight et al., 2001); in fungi, it induces sporulation and the cAMP-G protein-coupled pathway-mediated signaling (Calvo et al., 1999; Affeldt et al., 2012). Furthermore, during the interaction between maize and specific fungal pathogens, the disruption of 9-LOX from maize promotes increased resistance to the fungal pathogen and decreased mycotoxin contamination (Gao et al., 2007). 9-HODE seems to be very important for the progression of infection.
The F. verticillioides-maize pathosystem provides numerous examples of oxylipins as mediators of fungus–plant interaction. A close correlation exists between the fumonisin B1 accumulation and oxylipin signaling. When fumonisin is produced, maize increases the release of 9-HODE, suggesting an active role in infection development (Dall'Asta et al., 2014; Beccaccioli et al., 2021a). Further evidence shows that, in maize, the 9-LOX (ZmLOX3) deletion alters F. verticillioides fitness, decreasing conidiation and fumonisin B1 synthesis (Gao et al., 2007). In addition, when FvLDS1 is deleted in the pathogen and ZmLOX3 is mutagenized in the host, resistance to the infection increased, ZmLOX4, ZmLOX5, and ZmLOX12 expression was upregulated, and the fungus showed decreased fumonisin production. The ZmLOX4 and ZmLOX5 mutants were more susceptible to F. verticillioides and showed reduced levels of JA, suggesting the relevance of JA-mediated defense signaling. Intriguingly, F. verticillioides infecting the ZmLOX3 mutant is less effective at accumulating oxylipins from linoleate diol synthase and properly infecting maize kernels; this suggests that the oxylipins derived by ZmLOX3 activity are central to fungal virulence (Battilani et al., 2018).
The coexistence of a common language among hosts and pathogens supports the idea that a common receptor may also exist, and it could be represented by the GPCRs (Brown et al., 2018). Oxylipin perception has been explored only partially. In mammals, oxylipins are perceived by GPCRs (Funk, 2001; Noverr et al., 2003) in the plasma membrane (Funk, 2001). G2A is a GPCR receptor, characterized in the lymphoid tissues, and is able to recognize oxylipins derived from linoleic and arachidonic acid (Obinata et al., 2005). GPCR promotes root growth and ROS scavenging probably in the context of oxylipins pathway perception of abiotic stress in Arabidopsis and cotton (Lu et al., 2019). In fungi, several research studies showed the GPCR involvement in numerous functions among which oxylipin sensing (Affeldt et al., 2012). G proteins have a crucial role in sensing external ligands such as nutrients, hormones, proteins, pheromones and other peptides, ions, hydrophobic surfaces, and light (Kochman, 2014). Several works suggest that fungal GPCR-mediated signaling is linked to pathogenesis and could be considered a target for disease control (Brown et al., 2018). Secondary metabolism seems to be related to the G protein pathway as well through the activity of transcription factors related to toxin biosynthesis (Gao et al., 2021).
The “story” of oxylipins in bacteria is far more recent than in fungi. Only recently, this class of lipid compounds was found to be significant in regulating different aspects of the bacterial lifestyle. The molecules from which oxylipins originate, that is, lipids, have a quite vast background. Many lipid molecules in bacterial cells, such as hopanoids and ornitholipids, are absent in eukaryotes, while others are shared (e.g., phospholipids). Lipids play important roles in bacterial cell-to-cell communication by regulating quorum sensing (QS) and in the interactions with the host and the vector (Siebers et al., 2016). In phytopathogenic bacteria, different types of lipids can determine compatibility with the host. Numerous research papers describe the role of FAs as diffusible signal factors (DSF) acting as modulators of different pathways in cell-to-cell communication to modulate QS and virulence (Wang and Qian, 2019). In phytopathogenic bacteria such as X. fastidiosa responsive of olive quick decline syndrome (OQDS), DSF-based QS model promotes biofilm formation and stickiness, determining (a) degradation of pit membranes to enable cross-vessel diffusion in the xylem; (b) twitching motility of bacterial cells; and (c) adhesion to the xylem surface and the switch from the planktonic endophytic lifestyle to the sessile insect-acquisition stage (Chatterjee et al., 2008; Beaulieu et al., 2013; Ionescu et al., 2016). The QS regulation is based on a delicate balance of several DSFs [e.g., MUFAs: lauroleic acid (C12:1), myristoleic acid (14:1), palmitoleic acid (16:1), oleic acid (18:1)] (Lindow et al., 2014; Ionescu et al., 2016).
As reported in Figure 1, oleic acid is the major substrate for the DOX-mediated synthesis of 10-HPOME and 7,10-diHOME (Martínez and Campos-Gómez, 2016). The role of oxylipins in mediating autocrine or paracrine signaling in communication is reported in several recent papers (Martínez et al., 2019; Niu et al., 2020; Scala et al., 2020). Despite their importance in eukaryotes and in plant–fungi interactions, the role of oxylipins is overlooked in phytopathogenic bacteria. Only recently, Martinez and colleagues demonstrate that unsaturated FAs can act as substrates for oxidizing enzymes (e.g., LOX and DOX) to form oxylipins that, in the opportunistic bacterial pathogen Pseudomonas aeruginosa, may transform into mono- and di-hydroxylated derivatives during the interaction with the host (e.g., Drosophila, lettuce) (Martínez and Campos-Gómez, 2016).
Lipoxygenase LOXA, which was first identified in the human parasite P. aeruginosa, was the first prokaryotic lipoxygenase ever to be characterized. LOXA transforms arachidonic acid into 15-hydroxyheicosatetraenoic acid (15-HETE) (Vance et al., 2004). LOXA is secreted by P. aeruginosa in the lungs and oxidizes the PUFAs. Several biological roles, including interference with the host lipid signaling, and modulation of bacterial invasion have been hypothesized (Morello et al., 2019).
In liquid culture, X. fastidiosa accumulates different oxylipins, in particular, 10-HPOME, 10-HOME, and epoxyoctadecamonoenoic acids (EpOMEs), with different intra/extra-cellular distribution. LOX enzyme-derived oxylipins (i.e., 13-HODE, 9-HODE; 8,13-diHODE, 13HOTrE, and methyl jasmonic acid) are less represented (Christensen and Kolomiets, 2011). The plant stress hormone methyl jasmonate is secreted by X. fastidiosa in vitro and, overall, in large amounts in artificially infected plant tissues (Nomura et al., 2005). These results indicate that X. fastidiosa can synthesize and secrete oxylipins suggesting that, although oxygenation may occur inside the cell, oxylipins are transported through the outer membrane and accumulate in the medium (Martínez and Campos-Gómez, 2016; Scala et al., 2018). Recent in vitro studies on X. fastidiosa subsp. pauca demonstrate that the 9-LOX-derived oxylipins promote biofilming, whereas DOX-derived oxylipins stimulate planktonic growth and inhibit biofilm formation (Scala et al., 2020).
In P. aeruginosa, DOXs including diol synthase catalyze the stereospecific oxygenation of oleic acid (Martínez and Campos-Gómez, 2016) to synthesize 10-HOME and 7,10-diHOME. These oleic acid-derived oxylipins are involved in regulating motility, biofilm formation, and virulence. In the QS regulating system, they inhibit type III pili-induced-motility by stimulating the expression of type IV pili, thus promoting twitching and aggregation in micro-colonies and biofilm formation, in vitro. These oxylipins are promoters of virulence on Drosophila melanogaster and lettuce (Martínez and Campos-Gómez, 2016). P. aeruginosa can co-opt host oxylipins to let them operate as environment-specific QS signals. A recent study (Martínez et al., 2019) highlighted a new oxylipin-dependent quorum sensing system (ODS) and the role of oxylipins produced from the host's oleic acid as auto-inducers of lifestyle switch in P. aeruginosa (Martínez et al., 2019).
Research on X. fastidiosa highlights similar trends (Scala et al., 2018, 2020), suggesting that oxylipins' role in autocrine bacterial cell communication (Niu et al., 2020) could be common across bacterial families (Martínez et al., 2019).
Although studies on oxylipins in plant–bacteria interactions are few and primarily focused plant oxylipins, nevertheless, a pivotal study by Martínez and Campos-Gómez (2016) suggests that oxylipins may also be crucial in bacterial communication. This section focuses on several recent studies that suggest that bacterial oxylipins may also be important signals in the intricate signaling network between plant hosts and pathogenic bacteria.
The example of Arabidopsis thaliana inoculated with Pseudomonas syringae pv. tomato (Pto) represents one of the first studies based on the cross talk mediated by the oxylipins during plant–bacteria interaction. The pre-treatment of plant with 9-LOX- and α-DOX-oxylipins from linoleic acid activated SAR and protected plant tissues against infection through a JA-independent signaling pathway (Hamberg et al., 2003; Prost et al., 2005; Truman et al., 2007; Vellosillo et al., 2007; Jung et al., 2009; Xia et al., 2009; Chanda et al., 2011; Vincent et al., 2012). In plant, the presence of 9-LOX-derived oxylipins induces brassinosteroid synthesis (hormones important for plant development and growth), SAR, and cell wall-based defense such as callose deposition (Hamberg et al., 2003; Vellosillo et al., 2007; Marcos et al., 2015). Among 9-oxylipins, 9-ketooctadecatrienoic acid pre-treatment modifies hormone homeostasis during infection and interferes with the hormonal changes caused by bacterial effectors (Vellosillo et al., 2007). JA, generated within 13-LOX pathway of linolenic acid, participates in the establishment of SAR after infection by biotrophic bacteria (Truman et al., 2007). 13-LOX derivatives participate in plant defense as regulators of gene expression, cell death, and antimicrobials (Stintzi, 2000; Vollenweider et al., 2000; Montillet et al., 2004; Prost et al., 2005).
Details of the oxylipin-mediated communication between plant and phytopathogenic bacteria were provided by several studies on X. fastidiosa. The infection caused by X. fastidiosa was evaluated in plant model systems. A. thaliana induces the expression of ethylene/JA pathway to limit oxidative damage and represses the salicylic acid pathway (Rogers, 2012; Pereira et al., 2019); Nicotiana tabacum leads to a differential accumulation of specific lipid entities, including the oxylipins (Scala et al., 2018). Oxylipins emerged as hallmarks of pathogenic invasion in host tissues: Plants infected with X. fastidiosa accumulate oleic acid- and linoleic acid-derived oxylipins (e.g., 7,10-diHOME and 13-HODE). The study of oxylipin metabolism in the X. fastidiosa pathosystem was investigated also in Olea europaea. Symptomatic trees of the susceptible olive cultivar Ogliarola salentina accumulate ten lipid compounds that should be defined as hallmarks of OQDS (Scala et al., 2019). Identified hallmarks include 10-HOME (DOX-oxylipins); oleic and linoleic acid; LOX-derived 9- and 13-oxylipins (9-HODE, 9-OXODE, 9-HOTRE, 13-HODE, 13-OXODE, and 13-HOTRE). All the identified compounds were tested in vitro to unveil their effect on planktonic or biofilming state of X. fastidiosa subsp. pauca. The 7,10-diHOME, a DOX-derived oxylipin, downregulates biofilm formation, while LOX oxylipins from linoleic acid stimulate it. Recently, combined mass spectrometry/machine learning approach demonstrated that 13-HODE is a hallmark of OQDS and a susceptibility factor for olive tree toward X. fastidiosa (Scala et al., 2022). Considering the lipidome profile of the bacteria alone, the host–pathogen pathosystem, and available literature (Chatterjee et al., 2008; Rapicavoli et al., 2018; Roper et al., 2019; Scala et al., 2020), we argue that in plant tissue infected by X. fastidiosa, oxylipin involvement occurs from the early stage of infection just before host–pathogen recognition. At this stage, the pathogen modulates the planktonic-biofilm through DFS-QS and ODS; DOX-oxylipins are mainly accumulated for extensive vessel colonization, leading the planktonic state. At a later stage of infection, the host identifies the pathogen, activates the defense response, and triggers plant LOX-oxylipins. The accumulation of LOX oxylipins leads to a switch to the “acquisition phase” in the pathogen, a step of the infection cycle that culminates with the bacteria acquisition from the xylem sap to the insect vector (Figure 3). LOX accumulation is induced by the bacterium–host recognition and stimulates biofilm formation, vector acquisition, and extensive vascular blockage in plants, in accordance with the results obtained in the A. thaliana-Pto interaction. The activation of the LOX oxylipin pathway, in response to the plant–pathogen interaction, seems to be an adaptive strategy for the bacteria to cope with harsh environmental conditions and establish pathogenic insult with the host (Mosblech et al., 2009; Wasternack, 2014). A reversible cycle is de facto in place among the dual state of bacteria up to the host immune-system activation. Arguably, the pathogen regulates its behavior through FAs (as the DSFs) and oxylipins to trigger systemic invasion, limiting biofilm formation and acquisition by insect vectors.
Figure 3. Hypothetical model involving oxylipins as signals in X. fastidiosa–host interaction. At the begin of host–bacteria interaction, the host does not recognize the presence of the pathogen, the bacterium modulates the planktonic-biofilm by itself, thought DFS-QS and ODS, and accumulates mainly DOX-oxylipins. Later, the plant recognizes the pathogen, activates the defense response, and triggers plant LOX-oxylipins. The bacterial pathogen switches in the “acquisition phase” by DSF-QS and ODS, accumulating mainly LOX-oxylipins. The bacterium–host interaction stimulates the bacteria biofilming, vector acquisition, and extensive vascular blockage plants, favored by a LOX oxylipins pathway in response to as an adaptive strategy to cope with harsh environmental conditions and to establish pathogenic insult with their host.
These studies suggest that oxylipins have a significant role in determining the fate of the interactions: Resistance vs. susceptibility and that plant on one side and bacteria on the other try to adapt to the oxylipin signature formed during their interaction to exploit the weaknesses of the opponent.
Lipid-mediated signal communication is an issue ongoing with numerous implications in plant–fungi and plant–bacteria disease control. This review focused primarily on oxidized lipids, the oxylipins, implicated in host–pathogen interactions, notably when the plant is exposed to fungal or bacterial disease. In plants, the oxylipins—jasmonates apart—are mainly involved in the defense against pathogens; in pathogens, a dual role emerges: oxylipins are produced as autocrine signals to mediate the development and differentiation (i.e., sexual sporulation in fungi; phase transition in bacteria), and paracrine signals to entertain a communication during plant diseases. The LOX pathway is the best characterized in plants and seems to be crucial for communicating with both fungi and bacteria, probably antagonizing and modulating the pathogen's response. LDS pathway seems to be crucial for the pathogen invasion (i.e., fungi and bacteria). The identification of oxylipins common or structurally similar in plants, fungi, and bacteria consolidates the theory of cross-kingdom communication. We can suggest that the ability to “recognize and react” to specific oxylipins may drive the fate of the interaction for the host: susceptibility vs. resistance.
Despite numerous findings regarding the specific oxylipins that are exchanged during the interactions, many questions remain open. One of these concerns the understanding of the receptor and transduction system of oxylipins that remains still unclear.
MB, MR, and VS: conceptualization. MSa, MB, and NP: methodology. MR, MSc, and SL: investigation and funding acquisition. MB and VS: writing—original draft. MB, VS, BM, MZ, MSc, NP, and SL: writing—reviewing and editing. MB: visualization. MR and VS: supervision. All authors contributed to the article and approved the submitted version.
This study was funded by MIPAAFT, Project Oli.Di.X.I.It (Olive growing and defense against Xylella fastidiosa and vector insects in Italy), D.M. 23773 of 6/09/2017, Project SALVAOLIVI (Safeguard and enhancement of the Italian olive-growing heritage with research actions in the phytosanitary defense sector), D.M.33437 of 12/21/20. MIUR National Operational Program Research and Innovation 2014-2020 (PON RI 2014-2020) Development of Nutraceuticals from Natural Sources - ARS01_01166. PUT National Operational Program “Enterprises and Competitiveness” 2014–2020 ERDF, UR - Development of new technologies in precision agriculture for the sustainable production of potato genotypes with high nutritional qualities (Acronym SOS TATA), no. F / 200088 / 01-03 / X45.
The authors declare that the research was conducted in the absence of any commercial or financial relationships that could be construed as a potential conflict of interest.
All claims expressed in this article are solely those of the authors and do not necessarily represent those of their affiliated organizations, or those of the publisher, the editors and the reviewers. Any product that may be evaluated in this article, or claim that may be made by its manufacturer, is not guaranteed or endorsed by the publisher.
12-OH-JA, 12-hydroxy jasmonic acid; COX, cyclooxygenase; CYP450, cytochrome P450; DOX, dioxygenase; FA, fatty acid; GPCR, G protein-coupled receptors; HR, hypersensitive response; ISR, induced systemic resistance; JA, jasmonic acid; LDS, linoleate diol synthase; LOX, lipoxygenase; MUFA, monounsaturated fatty acid; ODS, oxylipin-dependent quorum sensing system; OQDS, olive quick decline syndrome; PTO, Pseudomonas syringae pathovar tomato; PUFA, polyunsaturated fatty acid; QS, quorum sensing; ROS, reactive oxygen species; SAR, systemic acquired resistance.
Affeldt, K. J., Brodhagen, M., and Keller, N. P. (2012). Aspergillus oxylipin signaling and quorum sensing pathways depend on G protein-coupled receptors. Toxins 4, 695–717. doi: 10.3390/toxins4090695
Ambaw, Y. A., Pagac, M. P., Irudayaswamy, A. S., Raida, M., Bendt, A. K., Torta, F. T., et al. (2021). Host/malassezia interaction: a quantitative, non-invasive method profiling oxylipin production associates human skin eicosanoids with Malassezia. Metabolites 11, 700. doi: 10.3390/metabo11100700
Andreou, A., Brodhun, F., and Feussner, I. (2009). Biosynthesis of oxylipins in non-mammals. Prog. Lipid Res. 48, 148–170. doi: 10.1016/j.plipres.2009.02.002
Andreou, A., and Feussner, I. (2009). Lipoxygenases - structure and reaction mechanism. Phytochemistry 70, 1504–1510. doi: 10.1016/j.phytochem.2009.05.008
Battilani, P., Lanubile, A., Scala, V., Reverberi, M., Gregori, R., Falavigna, C., et al. (2018). Oxylipins from both pathogen and host antagonize jasmonic acid-mediated defence via the 9-lipoxygenase pathway in Fusarium verticillioides infection of maize. Mol. Plant Pathol. 19, 2162–2176. doi: 10.1111/mpp.12690
Beaulieu, E. D., Ionescu, M., Chatterjee, S., Yokota, K., Trauner, D., and Lindow, S. (2013). Characterization of a diffusible signaling factor from Xylella fastidiosa. MBio. 4, e00539–12. doi: 10.1128/mBio.00539-12
Beccaccioli, M., Reverberi, M., and Scala, V. (2019). Fungal lipids: biosynthesis and signalling during plant-pathogen interaction. Front. Biosci. 24, 172–185. doi: 10.2741/4712
Beccaccioli, M., Salustri, M., Scala, V., Ludovici, M., Cacciotti, A., D'Angeli, S., et al. (2021a). The effect of fusarium verticillioides fumonisins on fatty acids, sphingolipids, and oxylipins in maize germlings. Int. J. Mol. Sci. 22, 2435. doi: 10.3390/ijms22052435
Beccaccioli, M., Scala, V., and Massimo, R. (2021b). “Communication with plants,” in Encyclopedia of Mycology (Amsterdam: Oliver Walter), 114–122.
Blée, E. (2002). Impact of phyto-oxylipins in plant defense. Trends Plant Sci. 7, 315–321. doi: 10.1016/S1360-1385(02)02290-2
Block, A., Schmelz, E., Jones, J. B., and Klee, H. J. (2005). Coronatine and salicylic acid: the battle between Arabidopsis and Pseudomonas for phytohormone control. Mol. Plant Pathol. 6, 79–83. doi: 10.1111/j.1364-3703.2004.00265.x
Brodhagen, M., Tsitsigiannis, D. I., Hornung, E., Goebel, C., Feussner, I., and Keller, N. P. (2008). Reciprocal oxylipin-mediated cross-talk in the Aspergillus–seed pathosystem. Mol. Microbiol. 67, 378–391. doi: 10.1111/j.1365-2958.2007.06045.x
Brodhun, F., and Feussner, I. (2011). Oxylipins in fungi. FEBS J. 278, 1047–1063. doi: 10.1111/j.1742-4658.2011.08027.x
Brown, N. A., Schrevens, S., Van Dijck, P., and Goldman, G. H. (2018). Fungal G-protein-coupled receptors: mediators of pathogenesis and targets for disease control. Nat. Microbiol. 3, 402–414. doi: 10.1038/s41564-018-0127-5
Brown, S. H., Zarnowski, R., Sharpee, W. C., and Keller, N. P. (2008). Morphological transitions governed by density dependence and lipoxygenase activity in Aspergillus flavus. Appl. Environ. Microbiol. 74, 5674–5685. doi: 10.1128/AEM.00565-08
Burow, G. B., Gardner, H. W., and Keller, N. P. (2000). A peanut seed lipoxygenase responsive to Aspergillus colonization. Plant Mol. Biol. 42, 689–701. doi: 10.1023/A:1006361305703
Burow, G. B., and Nesbitt, T. C. (1997). Seed lipoxygenase products modulate Aspergillus mycotoxin biosynthesis. Mol. Plant Microbe Interact. 10, 380–387. doi: 10.1094/MPMI.1997.10.3.380
Calvo, A. M., Hinze, L. L., Gardner, H. W., and Keller, N. P. (1999). Sporogenic effect of polyunsaturated fatty acids on development of Aspergillus spp. Appl. Environ. Microbiol. 65, 3668–3673. doi: 10.1128/AEM.65.8.3668-3673.1999
Champe, S. P., Rao, P., and Chang, A. (1987). An endogenous inducer of sexual development in Aspergillus nidulans. Microbiology 133, 1383–1387. doi: 10.1099/00221287-133-5-1383
Chanda, B., Xia, Y. E., Mandal, M. K., Yu, K., Sekine, K., Gao, Q., et al. (2011). Glycerol-3-phosphate is a critical mobile inducer of systemic immunity in plants. Nat. Genet. 43, 421–427. doi: 10.1038/ng.798
Chatterjee, S., Wistrom, C., and Lindow, S. E. (2008). A cell–cell signaling sensor is required for virulence and insect transmission of Xylella fastidiosa. Proc. Natl. Acad. Sci. 105, 2670–2675. doi: 10.1073/pnas.0712236105
Chini, A., Monte, I., Zamarreño, A. M., Hamberg, M., Lassueur, S., Reymond, P., et al. (2018). An OPR3-independent pathway uses 4, 5-didehydrojasmonate for jasmonate synthesis. Nat. Chem. Biol. 14, 171. doi: 10.1038/nchembio.2540
Christensen, S. A., and Kolomiets, M. V. (2011). The lipid language of plant-fungal interactions. Fungal Genet. Biol. 48, 4–14. doi: 10.1016/j.fgb.2010.05.005
Dall'Asta, C., Giorni, P., Cirlini, M., Reverberi, M., Gregori, R., Ludovici, M., et al. (2014). Maize lipids play a pivotal role in the fumonisin accumulation. World Mycotoxin J. 8, 87–97. doi: 10.3920/WMJ2014.1754
de Angelis, L., Rinaldi, T., Cirigliano, A., Bello, C., Reverberi, M., Amaretti, A., et al. (2016). Functional roles of the fatty acid desaturases encoded by KLOLE1, FAD2 and FAD3 in the yeast Kluyveromyces lactis. Microbiology 162, 1435–1445. doi: 10.1099/mic.0.000315
Deboever, E., Deleu, M., Mongrand, S., Lins, L., and Fauconnier, M.-L. (2020). Plant–pathogen interactions: underestimated roles of phyto-oxylipins. Trends Plant Sci. 25, 22–34. doi: 10.1016/j.tplants.2019.09.009
EbrahimáEl-Zayat, A. A. (1991). Structure and synthesis of sporogenic psi factors from Aspergillus nidulans. J. Chem. Soc. Chem. Commun. 1486–87. doi: 10.1039/c39910001486
Eng, F., Marin, J. E., Zienkiewicz, K., Gutiérrez-Rojas, M., Favela-Torres, E., and Feussner, I. (2021). Jasmonic acid biosynthesis by fungi: derivatives, first evidence on biochemical pathways and culture conditions for production. PeerJ 9, e10873. doi: 10.7717/peerj.10873
Fabbri, A. A, Fanelli, C., Panfili, G., Passi, S., and Fasella, P. (1983). Lipoperoxidation and aflatoxin biosynthesis by Aspergillus parasiticus and Aspergillus falvus. Microbiology 129, 3447–3452. doi: 10.1099/00221287-129-11-3447
Farmer, E. E., Alméras, E., and Krishnamurthy, V. (2003). Jasmonates and related oxylipins in plant responses to pathogenesis and herbivory. Curr. Opin. Plant Biol. 6, 372–378. doi: 10.1016/S1369-5266(03)00045-1
Fernandes, L. B., and Ghag, S. B. (2022). Molecular insights into the jasmonate signaling and associated defense responses against wilt caused by Fusarium oxysporum. Plant Physiol. Biochem. 174, 22–34. doi: 10.1016/j.plaphy.2022.01.032
Feussner, I., and Wasternack, C. (2002). The lipoxygenase pathway. Annu. Rev. Plant Biol. 53, 275–297. doi: 10.1146/annurev.arplant.53.100301.135248
Funk, C. D. (2001). Prostaglandins and leukotrienes: advances in eicosanoid biology. Science 294, 1871–1875. doi: 10.1126/science.294.5548.1871
Gao, J., Xu, X., Huang, K., and Liang, Z. (2021). Fungal G-protein-coupled receptors: a promising mediator of the impact of extracellular signals on biosynthesis of ochratoxin A. Front. Microbiol. 12, 193. doi: 10.3389/fmicb.2021.631392
Gao, X., Shim, W.-B., Göbel, C., Kunze, S., Feussner, I., Meeley, R., et al. (2007). Disruption of a maize 9-lipoxygenase results in increased resistance to fungal pathogens and reduced levels of contamination with mycotoxin fumonisin. Mol. Plant Microbe Interact. 20, 922–933. doi: 10.1094/MPMI-20-8-0922
Gerwick, W. H., Proteau, P. J., Nagle, D. G., Wise, M. L., Jiang, Z. D., Bernart, M. W., et al. (1993). Biologically active oxylipins from seaweeds. Hydrobiologia 260, 653–665. doi: 10.1007/BF00049085
Gol, S., Pena, R. N., Rothschild, M. F., Tor, M., and Estany, J. (2018). A polymorphism in the fatty acid desaturase-2 gene is associated with the arachidonic acid metabolism in pigs. Sci. Rep. 8, 1–9. doi: 10.1038/s41598-018-32710-w
Gorman, Z., Tolley, J. P., Koiwa, H., and Kolomiets, M. V. (2021). The synthesis of pentyl leaf volatiles and their role in resistance to anthracnose leaf blight. Front. Plant Sci. 1760. doi: 10.3389/fpls.2021.719587
Hamberg, M., Sanz, A., Rodriguez, M. J., Calvo, A. P., and Castresana, C. (2003). Activation of the fatty acid α-dioxygenase pathway during bacterial infection of tobacco leaves: formation of oxylipins protecting against cell death. J. Biol. Chem. 278, 51796–51805. doi: 10.1074/jbc.M310514200
Huber, S., Lottspeich, F., and Kämper, J. (2002). A gene that encodes a product with similarity to dioxygenases is highly expressed in teliospores of Ustilago maydis. Mol. Genet. Genomics 267, 757–771. doi: 10.1007/s00438-002-0717-y
Ionescu, M., Yokota, K., Antonova, E., Garcia, A., Beaulieu, E., Hayes, T., et al. (2016). Promiscuous diffusible signal factor production and responsiveness of the Xylella fastidiosa Rpf system. MBio 7, e01054–e01016. doi: 10.1128/mBio.01054-16
Jung, H. W., Tschaplinski, T. J., Wang, L., Glazebrook, J., and Greenberg, J. T. (2009). Priming in systemic plant immunity. Science 324, 89–91. doi: 10.1126/science.1170025
Kikukawa, H., Sakuradani, E., Ando, A., Shimizu, S., and Ogawa, J. (2018). Arachidonic acid production by the oleaginous fungus Mortierella alpina 1S-4: A review. J. Adv. Res. 11, 15–22. doi: 10.1016/j.jare.2018.02.003
Knight, V. I., Wang, H., Lincoln, J. E., Lulai, E. C., Gilchrist, D. G., and Bostock, R. M. (2001). Hydroperoxides of fatty acids induce programmed cell death in tomato protoplasts. Physiol. Mol. Plant Pathol. 59, 277–286. doi: 10.1006/pmpp.2001.0366
Kochman, K. (2014). Superfamily of G-protein coupled receptors (GPCRs)-extraordinary and outstanding success of evolution. Postepy Hig Med. Dosw. 68, 1225–1237. doi: 10.5604/17322693.1127326
Lindow, S., Newman, K., Chatterjee, S., Baccari, C., Iavarone, A. T., and Ionescu, M. (2014). Production of Xylella fastidiosa diffusible signal factor in transgenic grape causes pathogen confusion and reduction in severity of Pierce's disease. Mol. Plant Microbe Interact. 27, 244–254. doi: 10.1094/MPMI-07-13-0197-FI
Liu, Y., Pagac, M., Yang, F., Patkar, R. N., and Naqvi, N. I. (2021). Fungal jasmonate as a novel morphogenetic signal for pathogenesis. J. Fungi 7, 693. doi: 10.3390/jof7090693
Lombardi, N., Vitale, S., Turr,à, D., Reverberi, M., Fanelli, C., Vinale, F., et al. (2018). Root exudates of stressed plants stimulate and attract Trichoderma soil fungi. Mol. Plant Microbe Interact. 31, 982–994. doi: 10.1094/MPMI-12-17-0310-R
Lu, P., Magwanga, R. O., Kirungu, J. N., Dong, Q., Cai, X., Zhou, Z., et al. (2019). Genome-wide analysis of the cotton G-coupled receptor proteins (GPCR) and functional analysis of GTOM1, a novel cotton GPCR gene under drought and cold stress. BMC Genom. 20, 1–17. doi: 10.1186/s12864-019-5972-y
Marcos, R., Izquierdo, Y., Vellosillo, T., Kulasekaran, S., Cascón, T., Hamberg, M., et al. (2015). 9-Lipoxygenase-derived oxylipins activate brassinosteroid signaling to promote cell wall-based defense and limit pathogen infection. Plant Physiol. 169, 2324–2334. doi: 10.1104/pp.15.00992
Martínez, E., and Campos-Gómez, J. (2016). Oxylipins produced by Pseudomonas aeruginosa promote biofilm formation and virulence. Nat. Commun. 7, 13823. doi: 10.1038/ncomms13823
Martínez, E., Cosnahan, R. K., Wu, M., Gadila, S. K., Quick, E. B., Mobley, J. A., et al. (2019). Oxylipins mediate cell-to-cell communication in Pseudomonas aeruginosa. Commun. Biol. 2, 1–10. doi: 10.1038/s42003-019-0310-0
Mazur, P., Meyers, H. V., Nakanishi, K., and Champe, S. P. (1990). Structural elucidation of sporogenic fatty acid metabolites from Aspergillus nidulans. Tetrahedron Lett. 31, 3837–3840. doi: 10.1016/S0040-4039(00)97482-3
Mehta, S., Chakraborty, A., Roy, A., Singh, I. K., and Singh, A. (2021). Fight hard or die trying: current status of lipid signaling during plant–pathogen interaction. Plants 10, 1098. doi: 10.3390/plants10061098
Miersch, O., Bohlmann, H., and Wasternack, C. (1999a). Jasmonates and related compounds from Fusarium oxysporum. Phytochemistry 50, 517–523. doi: 10.1016/S0031-9422(98)00596-2
Miersch, O., Porzel, A., and Wasternack, C. (1999b). Microbial conversion of jasmonates-hydroxylations by Aspergillus niger. Phytochemistry 50, 1147–1152. doi: 10.1016/S0031-9422(98)00698-0
Montillet, J., Cacas, J., Garnier, L., Montané, M., Douki, T., Bessoule, J., et al. (2004). The upstream oxylipin profile of Arabidopsis thaliana: a tool to scan for oxidative stresses. Plant J. 40, 439–451. doi: 10.1111/j.1365-313X.2004.02223.x
Morello, E., Pérez-Berezo, T., Boisseau, C., Baranek, T., Guillon, A., Bréa, D., et al. (2019). Pseudomonas aeruginosa lipoxygenase LoxA contributes to lung infection by altering the host immune lipid signaling. Front. Microbiol. 10, 1826. doi: 10.3389/fmicb.2019.01826
Mosblech, A., Feussner, I., and Heilmann, I. (2009). Oxylipins: structurally diverse metabolites from fatty acid oxidation. Plant Physiol. Biochem. 47, 511–517. doi: 10.1016/j.plaphy.2008.12.011
Niu, M., Steffan, B. N., Fischer, G. J., Venkatesh, N., Raffa, N. L., Wettstein, M. A., et al. (2020). Fungal oxylipins direct programmed developmental switches in filamentous fungi. Nat. Commun. 11, 1–13. doi: 10.1038/s41467-020-18999-0
Nomura, K., Melotto, M., and He, S.-Y. (2005). Suppression of host defense in compatible plant–Pseudomonas syringae interactions. Curr. Opin. Plant Biol. 8, 361–368. doi: 10.1016/j.pbi.2005.05.005
Noverr, M. C., Erb-Downward, J. R., and Huffnagle, G. B. (2003). Production of eicosanoids and other oxylipins by pathogenic eukaryotic microbes. Clin. Microbiol. Rev. 16, 517–533. doi: 10.1128/CMR.16.3.517-533.2003
Noverr, M. C., Phare, S. M., Toews, G. B., Coffey, M. J., and Huffnagle, G. B. (2001). Pathogenic yeasts Cryptococcus neoformans and Candida albicans produce immunomodulatory prostaglandins. Infect. Immun. 69, 2957–2963. doi: 10.1128/IAI.69.5.2957-2963.2001
Obinata, H., Hattori, T., Nakane, S., Tatei, K., and Izumi, T. (2005). Identification of 9-hydroxyoctadecadienoic acid and other oxidized free fatty acids as ligands of the G protein-coupled receptor G2A. J. Biol. Chem. 280, 40676–40683. doi: 10.1074/jbc.M507787200
Oenel, A., Fekete, A., Krischke, M., Faul, S. C., Gresser, G., Havaux, M., et al. (2017). Enzymatic and non-enzymatic mechanisms contribute to lipid oxidation during seed aging. Plant Cell Physiol. 58, 925–933. doi: 10.1093/pcp/pcx036
Okada, K., Abe, H., and Arimura, G. (2015). Jasmonates induce both defense responses and communication in monocotyledonous and dicotyledonous plants. Plant Cell Physiol. 56, 16–27. doi: 10.1093/pcp/pcu158
Oliw, E. H. (2021). Fatty acid dioxygenase-cytochrome P450 fusion enzymes of filamentous fungal pathogens. Fungal Genet. Biol. 157, 103623. doi: 10.1016/j.fgb.2021.103623
Patkar, R. N., Benke, P. I., Qu, Z., Chen, Y. Y. C., Yang, F., Swarup, S., et al. (2015). A fungal monooxygenase-derived jasmonate attenuates host innate immunity. Nat. Chem. Biol. 11, 733–740. doi: 10.1038/nchembio.1885
Pereira, W. E. L., Ferreira, C. B., Caserta, R., Melotto, M., and de Souza, A. A. (2019). Xylella fastidiosa subsp. pauca and fastidiosa colonize arabidopsis systemically and induce anthocyanin accumulation in infected leaves. Phytopathology 109, 225–232. doi: 10.1094/PHYTO-05-18-0155-FI
Pohl, C. H., and Kock, J. L. F. (2014). Oxidized fatty acids as inter-kingdom signaling molecules. Molecules 19, 1273–1285. doi: 10.3390/molecules19011273
Prost, I., Dhondt, S., Rothe, G., Vicente, J., Rodriguez, M. J., Kift, N., et al. (2005). Evaluation of the antimicrobial activities of plant oxylipins supports their involvement in defense against pathogens. Plant Physiol. 139, 1902–1913. doi: 10.1104/pp.105.066274
Rapicavoli, J. N., Blanco-Ulate, B., Muszyński, A., Figueroa-Balderas, R., Morales-Cruz, A., Azadi, P., et al. (2018). Lipopolysaccharide O-antigen delays plant innate immune recognition of Xylella fastidiosa. Nat. Commun. 9, 1–12. doi: 10.1038/s41467-018-02861-5
Reverberi, M., Punelli, F., Scarpari, M., Camera, E., Zjalic, S., Ricelli, A., et al. (2010). Lipoperoxidation affects ochratoxin A biosynthesis in Aspergillus ochraceus and its interaction with wheat seeds. Appl. Microbiol. Biotechnol. 85, 1935–1946. doi: 10.1007/s00253-009-2220-4
Rogers, E. E. (2012). Evaluation of arabidopsis thaliana as a model host for Xylella fastidiosa. Mol. Plant Microbe Interact. 25, 747–754. doi: 10.1094/MPMI-11-10-0270
Roper, C., Castro, C., and Ingel, B. (2019). Xylella fastidiosa: bacterial parasitism with hallmarks of commensalism. Curr. Opin. Plant Biol. 50, 140–147. doi: 10.1016/j.pbi.2019.05.005
Rupasinghe, S., Schuler, M. A., Kagawa, N., Yuan, H., Lei, L., Zhao, B., et al. (2006). The cytochrome P450 gene family CYP157 does not contain EXXR in the K-helix reducing the absolute conserved P450 residues to a single cysteine. FEBS Lett. 580, 6338–6342. doi: 10.1016/j.febslet.2006.10.043
Ryan, C. A., and Moura, D. S. (2002). Systemic wound signaling in plants: a new perception. Proc. Natl. Acad. Sci. 99, 6519–6520. doi: 10.1073/pnas.112196499
Sakuradani, E., Ando, A., Ogawa, J., and Shimizu, S. (2009). Improved production of various polyunsaturated fatty acids through filamentous fungus Mortierella alpina breeding. Appl. Microbiol. Biotechnol. 84, 1–10. doi: 10.1007/s00253-009-2076-7
Scala, V., Camera, E., Ludovici, M., Dall'Asta, C., Cirlini, M., Giorni, P., et al. (2013). Fusarium verticillioides and maize interaction in vitro: relationship between oxylipin cross-talk and fumonisin synthesis. World Mycotoxin J. 6, 343–351. doi: 10.3920/WMJ2012.1527
Scala, V., Giorni, P., Cirlini, M., Ludovici, M., Visentin, I., Cardinale, F., et al. (2014). LDS1-produced oxylipins are negative regulators of growth, conidiation and fumonisin synthesis in the fungal maize pathogen Fusarium verticillioides. Front. Microbiol. 5, 669. doi: 10.3389/fmicb.2014.00669
Scala, V., Pucci, N., Salustri, M., Modesti, V., L'Aurora, A., Scortichini, M., et al. (2019). Bacterial and plant produced lipids can exacerbate the olive quick decline syndrome caused by xylella. bioRxiv. 867523. doi: 10.1101/867523
Scala, V., Pucci, N., Salustri, M., Modesti, V., L'Aurora, A., Scortichini, M., et al. (2020). Xylella fastidiosa subsp. pauca and olive produced lipids moderate the switch adhesive versus non-adhesive state and viceversa. PLoS ONE 15, e0233013. doi: 10.1371/journal.pone.0233013
Scala, V., Reverberi, M., Salustri, M., Pucci, N., Modesti, V., Lucchesi, S., et al. (2018). Lipid profile of Xylella fastidiosa Subsp. pauca associated with the olive quick decline syndrome. Front. Microbiol. 9, 1839. doi: 10.3389/fmicb.2018.01839
Scala, V., Salustri, M., Loreti, S., Pucci, N., Cacciotti, A., Tatulli, G., et al. (2022). Mass spectrometry-based targeted lipidomics and supervised machine learning algorithms in detecting disease, cultivar and treatment biomarkers in Xylella fastidiosa subsp. pauca-Infected Olive Trees. Front. Plant Sci. 13, 833245. doi: 10.3389/fpls.2022.833245
Scarpari, M., Punelli, M., Scala, V., Zaccaria, M., Nobili, C., Ludovici, M., et al. (2014). Lipids in Aspergillus flavus-maize interaction. Front. Microbiol. 5, 74. doi: 10.3389/fmicb.2014.00074
Shaban, M., Khan, A. H., Noor, E., Malik, W., Ali, H. M. W., Shehzad, M., et al. (2021). A 13-Lipoxygenase, GhLOX2, positively regulates cotton tolerance against Verticillium dahliae through JA-mediated pathway. Gene 796, 145797. doi: 10.1016/j.gene.2021.145797
Siebers, M., Brands, M., Wewer, V., Duan, Y., Hölzl, G., and Dörmann, P. (2016). Lipids in plant–microbe interactions. Biochim. Biophys. Acta Mol. Cell Biol. Lipids 1861, 1379–1395. doi: 10.1016/j.bbalip.2016.02.021
Speckbacher, V., Ruzsanyi, V., Martinez-Medina, A., Hinterdobler, W., Doppler, M., Schreiner, U., et al. (2020). The lipoxygenase lox1 is involved in light-and injury-response, conidiation, and volatile organic compound biosynthesis in the mycoparasitic fungus trichoderma atroviride. Front. Microbiol. 11, 2004. doi: 10.3389/fmicb.2020.02004
Stintzi, A. (2000). The Arabidopsis male-sterile mutant, opr3, lacks the 12-oxophytodienoic acid reductase required for jasmonate synthesis. Proc. Natl. Acad. Sci. 97, 10625–10630. doi: 10.1073/pnas.190264497
Su, C., Brodowsky, I. D., and Oliw, E. H. (1995). Studies on linoleic acid 8R-dioxygenase and hydroperoxide isomerase of the fungus Gaeumannomyces graminis. Lipids 30, 43–50. doi: 10.1007/BF02537040
Su, C., Sahlin, M., and Oliw, E. H. (2000). Kinetics of manganese lipoxygenase with a catalytic mononuclear redox center. J. Biol. Chem. 275, 18830–18835. doi: 10.1074/jbc.M001408200
Truman, W., Bennett, M. H., Kubigsteltig, I., Turnbull, C., and Grant, M. (2007). Arabidopsis systemic immunity uses conserved defense signaling pathways and is mediated by jasmonates. Proc. Natl. Acad. Sci. 104, 1075–1080. doi: 10.1073/pnas.0605423104
Tsitsigiannis, D. I., Bok, J. W., Andes, D., Nielsen, K. F., Frisvad, J. C., and Keller, N. P. (2005a). Aspergillus cyclooxygenase-like enzymes are associated with prostaglandin production and virulence. Infect. Immun. 73, 4548–4559. doi: 10.1128/IAI.73.8.4548-4559.2005
Tsitsigiannis, D. I., and Keller, N. P. (2007). Oxylipins as developmental and host-fungal communication signals. Trends Microbiol. 15, 109–118. doi: 10.1016/j.tim.2007.01.005
Tsitsigiannis, D. I., Kowieski, T. M., Zarnowski, R., and Keller, N. P. (2005b). Three putative oxylipin biosynthetic genes integrate sexual and asexual development in Aspergillus nidulans. Microbiology 151, 1809–1821. doi: 10.1099/mic.0.27880-0
Vance, R. E., Hong, S., Gronert, K., Serhan, C. N., and Mekalanos, J. J. (2004). The opportunistic pathogen Pseudomonas aeruginosa carries a secretable arachidonate 15-lipoxygenase. Proc. Natl. Acad. Sci. 101, 2135–2139. doi: 10.1073/pnas.0307308101
Vellosillo, T., Martínez, M., López, M. A., Vicente, J., Cascón, T., Dolan, L., et al. (2007). Oxylipins produced by the 9-lipoxygenase pathway in Arabidopsis regulate lateral root development and defense responses through a specific signaling cascade. Plant Cell 19, 831–846. doi: 10.1105/tpc.106.046052
Vincent, D., Du Fall, L. A., Livk, A., Mathesius, U., Lipscombe, R. J., Oliver, R. P., et al. (2012). A functional genomics approach to dissect the mode of action of the Stagonospora nodorum effector protein SnToxA in wheat. Mol. Plant Pathol. 13, 467–482. doi: 10.1111/j.1364-3703.2011.00763.x
Vollenweider, S., Weber, H., Stolz, S., Chételat, A., and Farmer, E. E. (2000). Fatty acid ketodienes and fatty acid ketotrienes: michael addition acceptors that accumulate in wounded and diseased Arabidopsis leaves. Plant J. 24, 467–476. doi: 10.1046/j.1365-313x.2000.00897.x
Wang, F.-F., and Qian, W. (2019). The roles of histidine kinases in sensing host plant and cell–cell communication signal in a phytopathogenic bacterium. Philos. Trans. R. Soc. B 374, 20180311. doi: 10.1098/rstb.2018.0311
Wang, K.-D., Borrego, E. J., Kenerley, C. M., and Kolomiets, M. V. (2020). Oxylipins other than jasmonic acid are xylem-resident signals regulating systemic resistance induced by Trichoderma virens in maize. Plant Cell 32, 166–185. doi: 10.1105/tpc.19.00487
Wasternack, C. (2014). Action of jasmonates in plant stress responses and development–applied aspects. Biotechnol. Adv. 32, 31–39. doi: 10.1016/j.biotechadv.2013.09.009
Wasternack, C., and Strnad, M. (2018). Jasmonates: news on occurrence, biosynthesis, metabolism and action of an ancient group of signaling compounds. Int. J. Mol. Sci. 19, 2539. doi: 10.3390/ijms19092539
Xia, X.-J., Huang, L.-F., Zhou, Y.-H., Mao, W.-H., Shi, K., Wu, J.-X., et al. (2009). Brassinosteroids promote photosynthesis and growth by enhancing activation of Rubisco and expression of photosynthetic genes in Cucumis sativus. Planta 230, 1185–1196. doi: 10.1007/s00425-009-1016-1
Yu, Y., Gui, Y., Li, Z., Jiang, C., Guo, J., and Niu, D. (2022). Induced systemic resistance for improving plant immunity by beneficial microbes. Plants 11, 386. doi: 10.3390/plants11030386
Keywords: lipids, oxylipins, Xylella fastidiosa, Fusarium spp, Aspergillus spp, Olea europaea L., Zea mays (L)
Citation: Beccaccioli M, Pucci N, Salustri M, Scortichini M, Zaccaria M, Momeni B, Loreti S, Reverberi M and Scala V (2022) Fungal and bacterial oxylipins are signals for intra- and inter-cellular communication within plant disease. Front. Plant Sci. 13:823233. doi: 10.3389/fpls.2022.823233
Received: 26 November 2021; Accepted: 24 August 2022;
Published: 16 September 2022.
Edited by:
Fiona L. Goggin, University of Arkansas, United StatesReviewed by:
Sotiris Tjamos, Agricultural University of Athens, GreeceCopyright © 2022 Beccaccioli, Pucci, Salustri, Scortichini, Zaccaria, Momeni, Loreti, Reverberi and Scala. This is an open-access article distributed under the terms of the Creative Commons Attribution License (CC BY). The use, distribution or reproduction in other forums is permitted, provided the original author(s) and the copyright owner(s) are credited and that the original publication in this journal is cited, in accordance with accepted academic practice. No use, distribution or reproduction is permitted which does not comply with these terms.
*Correspondence: Massimo Reverberi, bWFzc2ltby5yZXZlcmJlcmlAdW5pcm9tYTEuaXQ=
Disclaimer: All claims expressed in this article are solely those of the authors and do not necessarily represent those of their affiliated organizations, or those of the publisher, the editors and the reviewers. Any product that may be evaluated in this article or claim that may be made by its manufacturer is not guaranteed or endorsed by the publisher.
Research integrity at Frontiers
Learn more about the work of our research integrity team to safeguard the quality of each article we publish.