- State Key Laboratory of Crop Biology, College of Agronomy, Shandong Agricultural University, Tai’an, China
The wheat dough quality is of great significance for the end-use of flour. Some genes have been cloned for controlling the protein fractions, grain protein content, starch synthase, grain hardness, etc. Using a unigene map of the recombinant inbred lines (RILs) for “TN 18 × LM 6,” we mapped a quantitative trait locus (QTL) for dough stability time (ST) and SDS-sedimentation values (SV) on chromosome 6A (QSt/Sv-6A-2851). The peak position of the QTL covered two candidate unigenes, and we speculated that TraesCS6A02G077000 (a xylanase inhibitor protein) was the primary candidate gene (named the TaXip gene). The target loci containing the three homologous genes TaXip-6A, TaXip-6B, and TaXip-6D were edited in the variety “Fielder” by clustered regularly interspaced short palindromic repeats–associated protein 9 (CRISPR/Cas9). Two mutant types in the T2:3 generation were obtained (aaBBDD and AAbbdd) with about 120 plants per type. The SVs of aaBBDD, AAbbdd, and WT were 31.77, 27.30, and 20.08 ml, respectively. The SVs of the aaBBDD and AAbbdd were all significantly higher than those of the wild type (WT), and the aaBBDD was significantly higher than the AAbbdd. The STs of aaBBDD, AAbbdd, and WT were 2.60, 2.24, and 2.25 min, respectively. The ST for the aaBBDD was significantly higher than that for WT and was not significantly different between WT and AAbbdd. The above results indicated that XIP in vivo can significantly affect wheat dough quality. The selection of TaXip gene should be a new strategy for developing high-quality varieties in wheat breeding programs.
Introduction
Wheat (Triticum aestivum. L) is the main source of nutrition and feeds more than 30% of the world’s population (Rasheed et al., 2018; Wang et al., 2019). The demand for wheat with high-quality attributes has increased globally due to the growing population and the rising living standards in countries worldwide (Peña et al., 2008; Kumar et al., 2019). The presence of wheat gluten gives the dough viscoelasticity and ductility, and it can be processed into a variety of foods to meet people’s needs (Veraverbeke and Delcour, 2002).
The stability time (ST) and SDS-sedimentation value (SV) are the key quality traits for wheat and play a critical role in determining end-use products. ST is the main parameter of the farinograph, which determines the final quality of bread, steamed bread, noodles, and other wheat foods (Tsilo et al., 2011). The length of the ST reflects the resistance of the dough to kneading, and doughs with high ST values have good flexibility and high gluten strength (Veraverbeke and Delcour, 2002; Dangi et al., 2019). The SV can be used as an essential indicator for detecting the quality of gluten, which positively correlates with dough rheological properties (Kaur et al., 2013). In general, the strong flour demands that the ST and SV are greater than 8 min and 40 ml, respectively (Ma et al., 2021). Moreover, because the mensuration of SV is simple and convenient and uses less flour, it is usually used as an indicator of early generation selection in wheat quality breeding programs (Peña et al., 2008).
The ST and SV are parameters highly influenced by the protein compositions, i.e., glutenins and gliadins, their fractions, the ratio between them as well as the total protein content (Payne et al., 1988; Deng et al., 2006; Rogers et al., 2006; Caballero et al., 2008; Dhaka and Khatkar, 2015). Some genes have been cloned in controlling the protein compositions, high molecular weight glutenin subunits (HMW-GS; Thompson et al., 1985; Anderson and Greene, 1989), low molecular weight glutenin subunits (LMW-GS) (D’Ovidio et al., 1992; Ikeda et al., 2002), and gliadin (Rafalski et al., 1984; Sugiyama et al., 1986). The genes regulated other wheat quality traits, such as grain protein content (Uauy et al., 2006; Hagenblad et al., 2012) and grain hardness (Gautier et al., 1994; Chen et al., 2006), were also cloned. Some transcription factors, such as TaNAC019, directly activated the expression of HMW-GS genes by binding to a specific motif in their promoters and interacting with the TaGlu-1 regulator TaGAM (Gao et al., 2021).
Cereals contain proteinaceous inhibitors of endo-xylanases (McLauchlan et al., 1999). The inhibitors of xylanase in wheat are grouped into three classes: TAXI (Triticum aestivum xylanase inhibitor; Debyser et al., 1997, 1999), XIP (xylanase inhibitory protein; McLauchlan et al., 1999; Elliott et al., 2003), and TLXI (thaumatin-like xylanase inhibitor; McLauchlan et al., 1999). The XIP-type xylanase inhibitor genes are responsible for plant defense (Takahashi-Ando et al., 2007). The effect of the XIP in vivo on the grain quality of wheat has not been reported.
Gene editing is an important tool to study gene function. In the past few years, clustered regularly interspersed short palindromic repeats (CRISPR) have achieved the ability to control the specific introduction of directed sequence variation (Gilbert et al., 2013; Shan et al., 2013; Soda et al., 2018). This technology has been applied to the genetic studies of wheat quality. Zhang S. J. et al. (2018) used CRISPR/Cas9 to silence HWM-GS in wheat, which significantly reduced dough strength and bread-baking quality. Sánchez-León et al. (2018) found that CRISPR/Cas9 could be used to produce low-gluten foodstuff and serve as source material to introgress this trait into elite wheat varieties. Li et al. (2020) edited TaSBEIIa in both winter and spring wheat varieties by CRISPR/Cas9 modification of starch composition, structure, and properties.
In this study, we found a quantitative trait locus (QTL) under multi-environments for the ST and SV, QSt/Sv-6A-2851, on chromosome 6A using TL-recombinant inbred lines (RIL) population. This locus contains the xylanase inhibitor protein (Xip) gene. We performed functional validation of the TaXip gene using the CRISPR/Cas9 mutagenesis system.
Materials and Methods
RIL Population, Field Trials, and Quality Trait Measurements
The RIL population of 184 lines used in the study was derived by single-seed descent (SSD) methods from a cross of “TN18 × LM6” (TL-RIL, F11 in 2015; Zhang et al., 2019). TN18 is a cultivated variety developed by our research group and LM6 is an excellent line developed by the Linyi Academy of Agricultural Sciences. The field trials were conducted by Guo et al. (2020) at the experimental farm of Shandong Agricultural University in Tai’an for three growing seasons with two replications: 2011–2012 (E1), 2012–2013 (E2), and 2013–2014 (E3).
The seed samples for the TL-RIL population obtained from the harvested grains were stored at room temperature for approximately three months and then milled using a Bühler experimental mill (Bühler mill, Bühler-Miag Company, Braunschweig, Germany; Guo et al., 2020). The flour was used to test the SV and farinograph parameters including ST. The SV was determined with a sedimentation volume instrument (BAU-A type) and the farinograph parameters were determined by a farinograph (Brabender GmbH and CoKG).
The Genetic Map of Unigenes and Quantitative Trait Locus Analysis for the TL-RILs
By RNA sequencing each line of the TL-RIL population, a genetic map of unigenes based on the physical position in reference genome RefSeq v1.1 (IWGSC, 2018) was previously constructed by our group (Zhang, 2019). The map included 27,452 sites; 28,811 unigenes; 31,445 sub-unigenes; and 117,758 SNP/InDels. Using the unigene map and phenotypic data (Guo et al., 2020), we mapped QTLs by IciMapping4.1 and MapQTL5.0 software. For IciMapping4.1, inclusive composite interval mapping (ICIM) was carried out with a step size of 0.5 cm. The parameter for handling missing phenotypic data was “Deletion.” For MapQTL 5.0, the multiple-QTL model (MQM) package with a mapping step size of 0.5 cm was used to map QTLs. The LOD threshold for declaring a significant QTL in both the software was a LOD > 2.5.
DNA and RNA Extraction and Primer Design
For the variety “Fielder” and the progenies of gene editing, total DNA was extracted using a DNA extraction kit (Tiangen, Beijing, China). The quality and concentration of the total DNA were determined using a NanoDrop 2000c spectrophotometer (Thermo Fisher Scientific, Wilmington, DE, United States). Total RNA was extracted using an AG21019 RNA extraction kit (Accurate, Changsha, China), digested with DNase to remove residual DNA, and reverse transcribed into cDNA using a Prime Script TMRT-PCR kit AG11711 (Accurate, Changsha, China). The quality and concentration of the total RNA were determined using 1% agarose gel electrophoresis and a NanoDrop 2000c spectrophotometer (Thermo, Wilmington, DE, United States). Genomic DNA was extracted using the Tiangen DNA quick Plant System (Tiangen, Beijing, China) for deep sequencing.
Amplification of the TaXip Genes
Total DNA and cDNA were used to amplify TaXip-6A, TaXip-6B, and TaXip-6D. Sequence amplification was performed with FastPfu high-fidelity enzyme (TransGen Biotech, Beijing, China). The primers were TAXIP6A-F: ccttaggattcactcctgcg; TAXIP6A-R: gttccgagtggtgatcagc; TAXIP6B-F: gcgctagagcagag gatcctaac; TAXIP6B-R: ggcttgtggaagcatagctcc; TAXIP6B-R: gtc ggatacgaattggcg; and TAXIP6D-R: aactgtgcgaccaatctgttc). PCR was run at 95°C for 5 min, followed by 33 cycles of denaturing at 94°C, annealing for 30 s, and extension at 72 C for 60 s, with a final extension at 72°C for 5 min on an ABI PCR system 2400. The PCR products were separated by electrophoresis, recovered, and ligated to a pEASY®-Blunt Cloning Kit (TransGen Biotech, Beijing, China). They were transformed into E. coli competent cells and selected on LB plates containing 50 μg/ml ampicillin. Positive clones were sequenced. Complete multiple alignments of sequences (Supplementary Figure 1) and translations of nucleotides into amino acid sequences were performed by DNAMAN Version 5.0 (Gong et al., 2013).
Acquisition and Culture of Gene Editing Plants
The sequences of the TaXip genes were obtained from the cloned sequence from “Fielder” and used to design sgRNA target sequences in CRISPR-direct1 and CRISPOR.2 The sgRNA2 was 5′-GTCCAACCGCTCCGCGCTCG-3′ from 374 to 393 bp (start at ATG) with protospacer adjacent motif (PAM) site CCC, and sgRNA1 was 5′-ACAACATCCGCGGCGGCCCG-3′ from 550 to 569 bp with PAM site GGG.
Using the designed primer (MT1T2-XF0: gacaacatccgcggcg gcccggttttagagctagaaatagc; MT1T2-XR0: gtccaaccgctccgcgctcgcgc ttcttggtgcc; MT1T2-XF: aataatggtctcaggcgacaacatccgcggcggcccg; and MT1T2-XR: attattggtctctaaacgtccaaccgctccgcgctcg) and MT1T2 vector as templates, PCR amplification was conducted to obtain the intermediate vector fragment containing two sgRNAs (964 bp). The sgRNAs were connected to the pBUE411 vector by an enzymatic ligation reaction. The schematic map of the binary vector is shown in Supplementary Figure 2. The RB/LB represent the left and right borders of the vector; TaU3P was the wheat U3 gene promoter and used to drive sgRNAs; sgRNA site refers to the guide RNA clone site; sgRNA SC was the sgRNA scaffold; PUbi was the ubiquitin gene promoter and used to drive zCas9; zCas9 was the maize codon-optimized Cas9; Tnos was the Nos terminator; and P35S was the 35S promoter to drive bar (Xing et al., 2014). After sequencing the target sites, the binary vector was transformed into the wheat variety “Fielder” by Agrobacterium tumefaciens-mediated transformation (Zhang S. J. et al., 2018). A total of 29 T0 plants were yielded in August 2019. In the greenhouse of Shandong Agricultural University, T0 generation seeds of different genotypes were selected and T1 plants were planted. After identifying the mutant types of T2 plants, the seeds of T2 mutant plants were sown in flowerpots with 4 plants in each flowerpot and 30 flowerpots for each edited type (T2:3 lines). The seeds harvested in the T2 generation were the T2:3 lines that were used for ST and SV measurements. All plants were grown in the greenhouse with 16 h of light and 8 h of darkness at 25°C. The grains from WT plants were similarly harvested and served as controls in the experiments.
Genomic DNA was extracted from the leaves of the genome-edited plants. To identify mutation types of T0, T1, and T2 generations, specific primers (g6aF: ggagtgagtac ggtgtgcGTTGGCGGCTACGGCACC; g6aR: gagttggatgctggatg gCACCGGACCGTCGCCGT; g6bF: ggagtgagtacggtgtgcATCG GCGGCTACGGCACC; g6bR: gagttggatgctggatggCACCGGAC CGTCGCCGTT; g6dF: ggagtgagtacggtgtgcCATCGGCGGCTAC GGCG; g6dR: gagttggatgctggatggCGGACCGTCGCCGTCAG GT) were designed to sequence the target regions of the A, B, and D genomes. Then, the PCR products were amplified and sequenced by Hi-Tom (Liu et al., 2019). Next-generation sequencing technology was used to detect the mutation sites. Li et al. (2021) reported that the lowest average ratio for plants with albino phenotype was over 80% after the TaPDS gene was edited. This indicated an editing threshold for displaying loss of function phenotype. We used this strategy to classify different genotypes.
Sedimentation Values and Stability Time Determination and Statistical Analysis for Mutant Lines and “Fielder”
For the variety “Fielder” (wild type, WT) and the T2:3 mutant lines, about 500 g grains were obtained from mixed samples of 120 individual plants, respectively. The flour was milled by a small experimental mill of Quadrumat Junior (Brabender GmbH and CoKG, Germany) with no xylanases added and then passed through a 80-mesh sieve. The separate flour samples were used to test the SV and ST. The SV was tested based on Peña et al. (1990) and corrected to 14% flour moisture content. The ST is a farinograph parameter which was determined using Automatic Farinograph-AT (Brabender GmbH and CoKG, Germany) referred to Tian (2006).
SPSS 17.0 software (SPSS, Chicago, IL, United States) was used for statistical analysis of the ST and SV. Multiple comparisons using LSD to identify where the differences lay. P-value less than 0.05 or 0.01was considered as significant or extremely significant.
Results
Acquisition of the Candidate Gene of the Stability Time and Sedimentation Values
Using the TL-RIL population and their genetic map of unigenes based on RNA-Seq technology (Zhang, 2019), we mapped the QTLs for quality traits. Of these, a QTL for ST and SV, QSt/Sv-6A-2851, was detected under multi-environments with peak positions ranging from 2850.3 to 2860.4 cM by IciMapping 4.1 and MapQTL 5.0 software (Figure 1A and Supplementary Table 1). The peak position of QSt/Sv-6A-2851 covered STRG.003769 and two annotated unigenes in RefSeq v1.1 (IWGSC, 2018), TraesCS6A02G076900 (46974751–46979670 bp) and TraesCS6A02G077000 (47104969–47106326 bp). The STRG.003769 is a transcript with no complete gene structure. The TraesCS6A02G076900 was annotated as an ABC-2-type transporter family protein. The TraesCS6A02G077000 was annotated as a xylanase inhibitor protein (named the TaXip gene). Sequence analysis revealed that TraesCS6A02G076900 had three SNPs in the 5′UTR, two SNPs in the intron region, and one SNP in the 3′UTR without amino acid changes. But the TraesCS6A02G077000 had one SNP at the 390 bp (start at ATG) in the exon region that led to amino acid changes (cysteine to tryptophan). Additionally, our group found a QTL cluster that contained SV and ST on chromosome 6A between TraesCS6A02G070900 and TraesCS6A02G125200 using the same TL-RIL population and its molecular marker map (Guo et al., 2020). Xylanases are widely used as additives in the baking industry to improve processing and product quality (Goesaert et al., 2005). The xylanase inhibitor protein is likely to inhibit the decomposition of xylanase in vivo (Simpson et al., 2003). Therefore, we speculated that TraesCS6A02G077000 was the candidate gene of the QTL QSt/Sv-6A-2851.
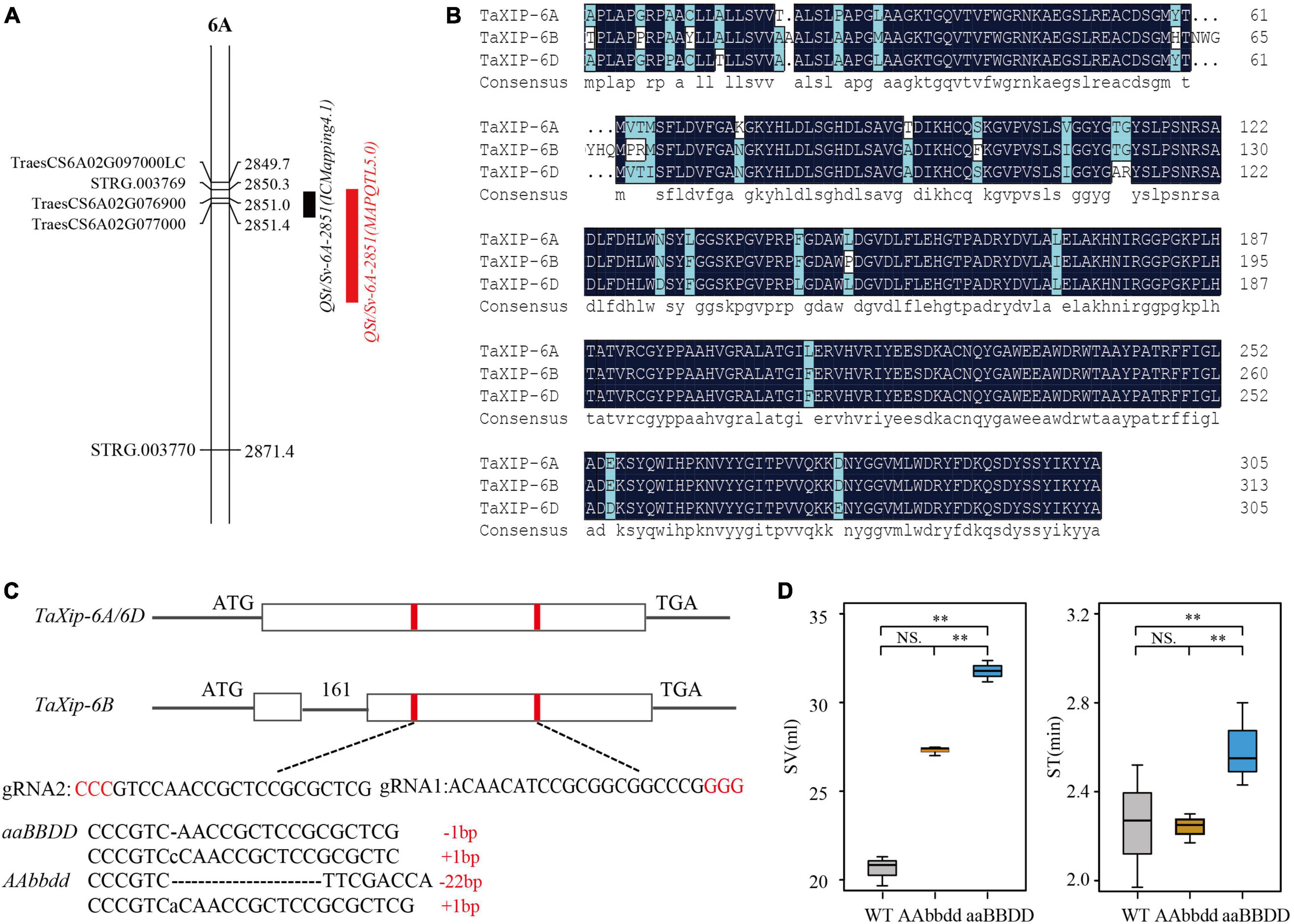
Figure 1. Mapping of QSt/Sv-6A-2851 and development of TaXIP mutants by CRISPR/Cas9-mediated genome editing. (A) QTL mapping for QSt/Sv-6A-2851 using IciMapping 4.1 (black bar), MapQTL5.0 (red bar) software. (B) Amino acid sequence alignment for three homologous genes TaXip-6A, TaXip-6B, and TaXip-6D in “Fielder.” (C) Schematic map of the sgRNAs target site selection in the TaXip genes and the T2:3 mutant types (aaBBDD and AAbbdd) induced by CRISPR/Cas9. The vertical bar is exon and the horizontal line is intron, the protospacer-adjacent motif (PAM) is highlighted in red. (D) Difference for SV and ST between T2:3 mutant lines and WT (Fielder). “**”represents extremely significant difference.
Amplification of the TaXip Homologous Genes
Three homologs of TaXip were identified by BLAST analysis in the IWGSC database3 : TaXip-6A (TraesCS6A02G077000), TaXip-6B (TraesCS6B02G103900), and TaXip-6D (TraesCSU02G026500). These homologous genes were amplified separately in the parents TN18 and LM6 of the TL-RILs and the variety “Fielder” (used in gene editing). For TN18 and LM6, an SNP site at the 390 bp was found in the TaXip-6A gene. It was base C in TN18 and G in LM6, encoding cysteine and tryptophan, respectively, which is in accordance with the RNA-Seq result. The exon sequences of TaXip-6B and TaXip-6D were not different between the parents. In “Fielder,” TaXip-6A and TaXip-6D have only one exon with 915 bp open reading frames and encode 305 amino acids. In contrast, TaXip-6B has one intron and two exons, 939 bp open reading frames, and encodes 313 amino acids (Figure 1B). Based on the amino acid sequences and domains, paired comparison results showed that the identities between TaXip-6A and TaXip-6D, between TaXip-6A and TaXip-6B, and between TaXip-6B and TaXip-6D were 94.75, 91.69, and 91.37%, respectively.
Obtaining of Gene Editing Plants by CRISPR/Cas9
The sgRNA targets for TaXip-6A, TaXip-6B, and TaXip-6D were designed based on the conserved domains in all the three sub-genomes. Two sgRNAs (sgRNA1 and sgRNA2) were designed to target the first exon on TaXip-6A and TaXip-6D, the second exon on TaXip-6B (Figure 1C). The pBUE411 vector was designed to create In/Del in the fourth base after PAM. In the T0 generation, a total of 29 mutated plants were identified with 7, 7, 5, and 10 mutant plants that were edited for A, D, AB, and ABD sub-genome(s), respectively. In the T2:3 generation, two genotypes of mutants were obtained (Figure 1C): the aaBBDD genotype with an editing ratio greater than 80% for subgenome A and less than 20% for B and D, and the AAbbdd genotype with an editing ratio greater than 80% for subgenomes B and D and less than 20% for A. In the aaBBDD, 1 bp was deleted or 1 bp was inserted for 6A and in the AAbbdd 22 bp was deleted for 6B and 1 bp was inserted for 6D (Figure 1C). After protein prediction,4 we found that all these mutations would lead to frameshift mutations and make the termination codon appear in advance, leading to protein functional inactivation (Supplementary Figure 3).
Changes for the SV and ST Between Wild and Mutant Genotypes
The SVs of the two mutant genotypes, aaBBDD and AAbbdd, and WT were 31.77, 27.30, and 20.08 ml, respectively (Supplementary Table 2). The SVs of aaBBDD and AAbbdd were significantly higher than that of the WT (Figure 1D); the SV of aaBBDD was significantly larger than that of AAbbdd and WT; and the SV of AAbbdd was significantly larger than that of WT. These results indicated that TaXip-6A, TaXip-6B, and TaXip-6D significantly influenced the SV, but the effect of TaXip-6A was greater than those of TaXip-6B and TaXip-6D. The STs of aaBBDD, AAbbdd, and WT were 2.60, 2.24, and 2.25 min, respectively (Supplementary Table 3). The ST of the mutant genotype aaBBDD was significantly higher than that of WT and AAbbdd, but was not significantly different between WT and AAbbdd (Figure 1D). This indicates that TaXip-6A significantly affected the ST, while TaXip-6B and TaXip-6D have little effect on the ST.
Discussion
For the genes of XIP class, Xip-I, Xip-III, Xip-R1, Xip-R2, Xip-II, and xip-9023, xip-366 (Elliott et al., 2002, 2009; Igawa et al., 2005; Takahashi-Ando et al., 2007; Liu et al., 2017) have been cloned in wheat with the functions of plant defense by binding glycoside hydrolase families 10 (GH10) and 11 (GH11; Payan et al., 2004). TaXIP-6A was most similar to wheat XIP-III, XIP-I, and rice acidic class III chitinase (Park et al., 2002) with a protein sequence identity of 98.69, 87.25, and 36.59%, respectively. The sequence alignment of TaXIP-6A, TaXIP-6B, and TaXIP-6D conservation of the two Arg residues (Figure 2; red boxed) proved to be engaged in salt bridges with the catalytic Glu residue (Figure 2; blue bold and red boxed) in XIP-I. This feature suggests that TaXIP-6A, TaXIP-6B, and TaXIP-6D lack chitinolytic activity, as demonstrated in the case of XIP-I (Payan et al., 2003). It indicated that TaXIP functions as an inhibitor of xylanase. The TaXip-6A gene was expressed notably in the grain at the later stage of grain development.5 In this study, we indicated that the knock-out XIP in vivo significantly affected the SV and ST. That is to say, the endogenous XIP affected dough quality. The addition of fungal xylanase in vitro can improve the processing quality of dough and end-product quality of bread (Wang et al., 2005; Ghoshal et al., 2013, 2017; Ahmad et al., 2014; Leys et al., 2019). So we speculated that the SV and ST, as important indicators for dough quality, may be affected by XIP through xylanase. But what is the mechanism of XIP and xylanase interaction, and how the xylanase improves the dough quality needs to be further studied.
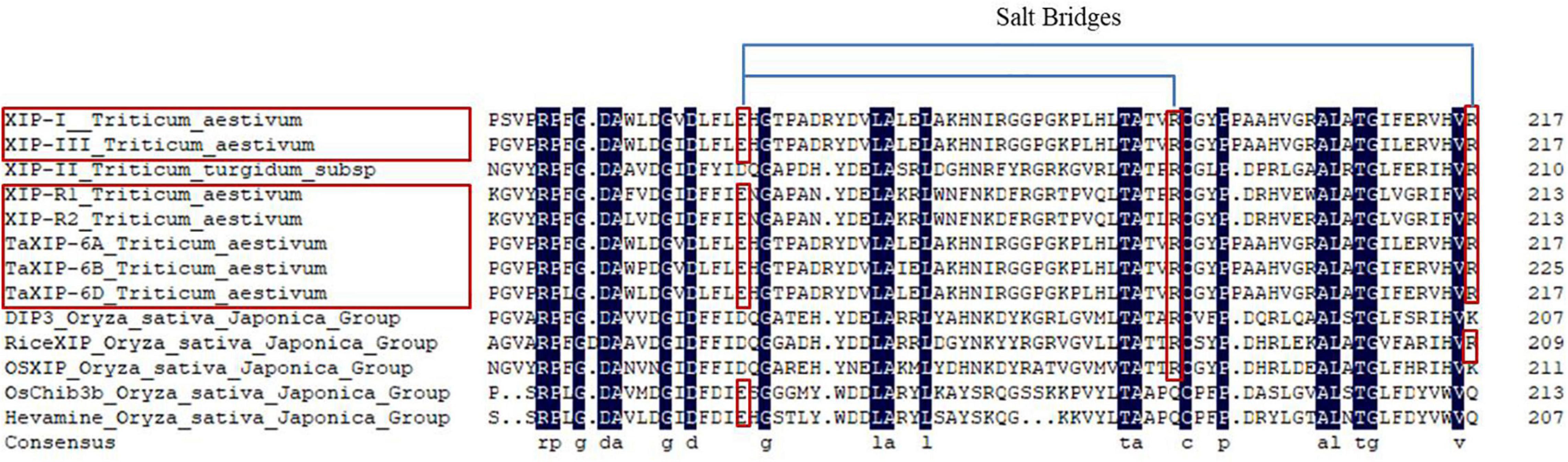
Figure 2. Comparison of the amino acid residues surrounding the chitinase “active site” region of XIPs and chitinase. Conserved amino acid residues are shaded. The two Arg residues (red boxed) and the catalytic Glu residue are joined by blue lines. The names of the sequences with salt bridges are boxed in red. Genbank accession numbers are as follows: XIP-I: CAD19479.1; XIP-II: CAC87260.1; XIP-III: BAD99103.1; XIP-R1: BAF74363.1; XIP-R2: BAF74364.1; RiceXIP: BAG89082.1; DIP3: AFM95334.1; OSXIP: AXF92897.1; OsChib3b: BAA22266.1.
The factors affecting wheat quality have mainly been focused on protein (Sharma et al., 2020), starch (Rakszegi et al., 2006), and fat (Bonnand-Ducasse et al., 2010). HMW-GS is one of the most important factors affecting dough rheological properties and bread-making quality. Payne et al. (1980, 1987) established a standard of Glu-1 quality score based on SV that was widely used to evaluate the quality of wheat varieties (Weegels et al., 1996; Gianibelli et al., 2001; Esmaeilzadeh Moghaddam et al., 2011). Ram et al. (2015) found that Glu-B1i and Glu-D1d showed a highly significant positive effect (P < 0.001) on SV and also had additive effects. The allelic genes of Glu-1 caused the SV variations of several or more than 10 units (ml) (Wang et al., 2018; Chen et al., 2019). The SV decreased by about 22 ml by silencing the five homologous Glu-1 genes (1Ax2*, 1Bx7, 1By9, 1Dx5, and 1Dy10) (Zhang Y. J. et al., 2018). In this study, the SVs of aaBBDD and AAbbdd were 11.69 and 7.22 ml higher than WT, respectively. The allelic gene selection of XIP should be a new strategy for developing high-quality varieties of wheat.
Data Availability Statement
The original contributions presented in the study are included in the article/Supplementary Material, further inquiries can be directed to the corresponding authors.
Author Contributions
SL contributed to the conception of the study. ZS performed the experiment and the data analyses. MZ and BG performed the unigene map construction. ZS and YG contributed to the quality mensuration. ZS, SL, and YA wrote the manuscript. XH, GL, and YZ assisted in gene editing and plant culture. All authors read and approved the manuscript.
Funding
This work was supported by the National Key Research and Development Program of China (2021YFD1200600) and the Agricultural Variety Program of Shandong Province, China (2019LZGC001).
Conflict of Interest
The authors declare that the research was conducted in the absence of any commercial or financial relationships that could be construed as a potential conflict of interest.
Publisher’s Note
All claims expressed in this article are solely those of the authors and do not necessarily represent those of their affiliated organizations, or those of the publisher, the editors and the reviewers. Any product that may be evaluated in this article, or claim that may be made by its manufacturer, is not guaranteed or endorsed by the publisher.
Acknowledgments
We thank Genying Li and Shujuan Zhang (Shandong Academy of Agricultural Sciences) for their help with gene-editing.
Supplementary Material
The Supplementary Material for this article can be found online at: https://www.frontiersin.org/articles/10.3389/fpls.2022.811668/full#supplementary-material
Footnotes
- ^ http://crispr.dbcls.jp/
- ^ http://crispor.tefor.net/
- ^ http://plants.ensembl.org/Triticum_aestivum/Info/Index
- ^ http://www.detaibio.com/sms2/translate.html
- ^ http://www.wheat-expression.com/
References
Ahmad, Z., Butt, M. S., Ahmed, A., Riaz, M., Sabir, S. M., Farooq, U., et al. (2014). Effect of aspergillus niger xylanase on dough characteristics and bread quality attributes. J. Food Sci. Technol. 51, 2445–2453. doi: 10.1007/s13197-012-0734-8
Anderson, O. D., and Greene, F. C. (1989). The characterization and comparative analysis of high-molecular-weight glutenin genes from genomes A and B of a hexaploid bread wheat. Theor. Appl. Genet. 77, 689–700. doi: 10.1007/bf00261246
Bonnand-Ducasse, M., Valle, G. D., Lefebvre, J., and Saulnier, L. (2010). Effect of wheat dietary fibres on bread dough development and rheological properties. J. Cereal Sci. 52, 200–206. doi: 10.1016/j.jcs.2010.05.006
Caballero, L., Martin, L. M., and Alvarez, J. B. (2008). Relationships between the HMW- glutenin and LMW-glutenin subunits and sds-sedimentation volume in Spanish hulled wheat lines. Czech J. Genet. Plant Breed. 44, 114–117. doi: 10.17221/8/2008-cjgpb
Chen, F., He, Z. H., Xia, X. C., Xia, L. Q., Zhang, X. Y., Lillemo, M., et al. (2006). Molecular and biochemical characterization of puroindoline a and b alleles in Chinese landraces and historical cultivars. Theor. Appl. Genet. 112, 400–409. doi: 10.1007/s00122-005-0095-z
Chen, Q., Zhang, W. J., Gao, Y. J., Yang, C. F., Gao, X., Peng, H. R., et al. (2019). High molecular weight glutenin subunits 1Bx7 and 1By9 encoded by Glu-B1 locus affect wheat dough properties and sponge cake quality. J. Agric. Food Chem. 67, 11796–11804. doi: 10.1021/acs.jafc.9b05030
Dangi, P., Chaudhary, N., and Khatkar, B. S. (2019). Rheological and microstructural characteristics of low molecular weight glutenin subunits of commercial wheats. Food Chem. 297:124989. doi: 10.1016/j.foodchem.2019.124989
Debyser, W., Derdelinckx, G., and Delcour, J. A. (1997). Arabinoxylan solubilization and inhibition of the barley malt xylanolytic system by wheat during mashing with wheat wholemeal adjunct: evidence for a new class of enzyme inhibitors in wheat. J. Am. Soc. Brew. Chem. 55, 153–156. doi: 10.1094/asbcj-55-0153
Debyser, W., Peumans, W. J., Van Damme, E. J. M., and Delcour, J. A. (1999). Triticum aestivum Xylanase Inhibitor (TAXI), a new class of enzyme inhibitor affecting breadmaking performance. J. Cereal Sci. 30, 39–43. doi: 10.1006/jcrs.1999.0272
Deng, Z. Y., Tian, J. C., and Hu, R. B. (2006). The accumulation of high molecular weight glutenin subunits (HMW-GS) and their relation to dough rheological quality in Chinese winter wheat. Aust. J. Agric. Res. 57, 41–46. doi: 10.1071/AR05080
Dhaka, V., and Khatkar, B. S. (2015). Effects of gliadin/glutenin and HMW-gs/LMW-gs ratio on dough rheological properties and bread–making potential of wheat varieties. J. Food Qual. 38, 71–82. doi: 10.1111/jfq.12122
D’Ovidio, R., Tanzarella, O. A., and Porceddu, E. (1992). Nucleotide sequence of a low-molecular-weight glutenin from Triticum durum. Plant Mol. Biol. 18, 781–784. doi: 10.1007/BF00020020
Elliott, G., Durand, A., Hughes, R. K., Kroon, P. A., D’Ovidio, R., and Juge, N. (2009). Isolation and characterisation of a xylanase inhibitor Xip-II gene from durum wheat. J. Cereal Sci. 50, 324–331. doi: 10.1016/j.jcs.2009.06.013
Elliott, G. O., Hughes, R. K., Juge, N., Kroon, P. A., and Williamson, G. (2002). Functional identification of the cDNA coding for a wheat endo-1,4-beta-D-xylanase inhibitor. FEBS Lett. 519, 66–70. doi: 10.1016/S0014-5793(02)02710-2
Elliott, G. O., Mclauchlan, W. R., Williamson, G., and Kroon, P. A. (2003). A wheat xylanase inhibitor protein (XIP-I) accumulates in the grain and has homologues in other cereals. J. Cereal Sci. 37, 187–194. doi: 10.1006/jcrs.2002.0493
Esmaeilzadeh Moghaddam, M., Jalal Kamali, M. R., Kazemi, S., Amini, A., Bozorgipour, R., Najafian, G., et al. (2011). Assessment of high molecular weight glutenin sub-units and baking quality related traits in some of the Iranian bread wheat (Triticum aestivum L.) landraces. Crop Breed. J. 1, 29–40.
Gao, Y. J., An, K. X., Guo, W. W., Chen, Y. M., Zhang, R. J., Zhang, X., et al. (2021). The endosperm-specific transcription factor TaNAC019 regulates glutenin and starch accumulation and its elite allele improves wheat grain quality. Plant Cell 33, 603–622. doi: 10.1093/plcell/koaa040
Gautier, M. F., Aleman, M. E., Guirao, A., Marion, D., and Joudrier, P. (1994). Triticum aestivum puroindolines, two basic cystine-rich seed proteins: cDNA sequence analysis and developmental gene expression. Plant Mol. Biol. 25, 43–57. doi: 10.1007/BF00024197
Ghoshal, G., Shivhare, U. S., and Banerjee, U. C. (2013). Effect of xylanase on quality attributes of whole-wheat bread. J. Food Qual. 36, 172–180. doi: 10.1111/jfq.12034
Ghoshal, G., Shivhare, U. S., and Banerjee, U. C. (2017). Rheological properties and microstructure of xylanase containing whole wheat bread dough. J. Food Sci. Technol. 54, 1928–1937. doi: 10.1007/s13197-017-2627-3
Gianibelli, M. C., Larroque, O. R., MacRitchie, F., and Wrigley, C. W. (2001). Biochemical, genetic, and molecular characterization of wheat glutenin and its compo-nent subunits. Cereal Chem. 78, 635–646. doi: 10.1094/CCHEM.2001.78.6.635
Gilbert, L. A., Larson, M. H., Morsut, L., Liu, Z., Brar, G. A., Torres, S. E., et al. (2013). CRISPR-mediated modular RNA-guided regulation of transcription in eukaryotes. Cell 154, 442–451. doi: 10.1016/j.cell.2013.06.044
Goesaert, H., Brijs, K., Veraverbeke, W. S., Courtin, C. M., Gebruers, K., and Delcour, J. A. (2005). Wheat flour constituents: how they impact bread quality, and how to impact their functionality. Trends Food Sci. Technol. 16, 12–30. doi: 10.1016/j.tifs.2004.02.011
Gong, C. Y., Cao, S. H., Fan, R. C., Bo, W., Chen, G. P., Wang, X. P., et al. (2013). Identification and phylogenetic analysis of a CC-NBS-LRR encoding gene assigned on chromosome 7B of wheat. Int. J. Mol. Sci. 14, 15330–15347. doi: 10.3390/ijms140815330
Guo, Y., Zhang, G. Z., Guo, B. J., Qu, C. Y., Zhang, M. X., Kong, F. M., et al. (2020). QTL mapping for quality traits using a high-density genetic map of wheat. PLoS One 15:e0230601. doi: 10.1371/journal.pone.0230601
Hagenblad, J., Asplund, L., Balfourier, F., Ravel, C., and Leino, M. W. (2012). Strong presence of the high grain protein content allele of NAM-B1 in Fennoscandian wheat Theor. Appl. Genet. 125, 1677–1686. doi: 10.1007/s00122-012-1943-2
Igawa, T., Tokai, T., Kudo, T., Yamaguchi, I., and Kimura, M. (2005). A wheat xylanase inhibitor gene, Xip-I, but not Taxi-I, is significantly induced by biotic and abiotic signals that trigger plant defense. Biosci. Biotechnol. Biochem. 69, 1058–1063. doi: 10.1271/bbb.69.1058
Ikeda, T. M., Nagamine, T., Fukuoka, H., and Yano, H. (2002). Identification of new low-molecular-weight glutenin subunit genes in wheat. Theor. Appl. Genet. 104, 680–687. doi: 10.1007/s001220100756
IWGSC (2018). Shifting the limits in wheat research and breeding using a fully annotated reference genome. Science 361:eaar7191. doi: 10.1126/science.aar7191
Kaur, A., Singh, N., Ahlawat, A. K., Kaur, S., Singh, A. M., Chauhan, H., et al. (2013). Diversity in grain, flour, dough and gluten properties amongst Indian wheat cultivars varying in high molecular weight subunits (HMW-GS). Food Res. Int. 53, 63–72. doi: 10.1016/j.foodres.2013.03.009
Kumar, A., Kapoor, P., Chunduri, V., Sharma, S., and Garg, M. (2019). Potential of aegilops sp. for improvement of grain processing and nutritional quality in wheat (Triticum aestivum L.). Front. Plant Sci. 10:308. doi: 10.3389/fpls.2019.00308
Leys, S., De Bondt, Y., Schreurs, L., and Courtin, C. M. (2019). Sensitivity of the bacillus subtilis xyn a xylanase and its mutants to different xylanase inhibitors determines their activity profile and functionality during bread making. J. Agric. Food Chem. 67, 11198–11209. doi: 10.1021/acs.jafc.9b04712
Li, J. H., Zhang, S. J., Zhang, R. Z., Gao, J., Qi, Y. P., Song, G. Q., et al. (2021). Efficient multiplex genome editing by CRISPR/Cas9 in common wheat. Plant Biotechnol. J. 19, 427–429. doi: 10.1111/PBI.13508
Li, J. Y., Jiao, G. A., Sun, Y. W., Chen, J., Zhong, Y. X., Yan, L., et al. (2020). Modification of starch composition, structure and properties through editing of TaSBEIIa in both winter and spring wheat varieties by CRISPR/Cas9. Plant Biotechnol. J. 19, 937–951. doi: 10.1111/pbi.13519
Liu, Q., Wang, C., Jiao, X. Z., Zhang, H. W., Song, L. L., Li, Y. X., et al. (2019). Hi-TOM: a platform for high-throughput tracking of mutations induced by CRISPR/Cas systems. China Life Sci. 62, 1–7. doi: 10.1007/s11427-018-9402-9
Liu, X. Y., Zhang, Y. K., Wei, Z. H., Chen, H. G., and Jia, X. C. (2017). Molecular cloning and characterizations of xylanase inhibitor protein from wheat (Triticum Aestivum). J. Food Sci. 82, 1582–1587. doi: 10.1111/1750-3841.13773
Ma, M. M., Li, Y. C., Xue, C., Xiong, W., Peng, Z. P., Han, X., et al. (2021). Current situation and key parameters for improving wheat quality in china. Front. Plant Sci. 12:638525. doi: 10.3389/fpls.2021.638525
McLauchlan, W. R., Garcia-Conesa, M. T., Williamson, G., Roza, M., Ravestein, P., and Maat, J. (1999). A novel class of protein from wheat which inhibits xylanases. Biochem. J. 338, 441–446. doi: 10.1042/bj3380441
Park, S. M., Kim, D. H., Truong, N. H., and Itoh, Y. (2002). Heterologous expression and characterization of classIII chitinases from rice (Oryza sativa L.). Enzyme Microb. Technol. 30, 697–702. doi: 10.1016/s0141-0229(02)00042-x
Payan, F., Flatman, R., Porciero, S., Williamson, G., Juge, N., and Roussel, A. (2003). Structural analysis of xylanase in hibitor protein I (XIP-I), a protein aceous xylanase inhibitor from wheat (Triticum aestivum, var.Soisson). Biochem. J. 372, 399–405. doi: 10.1042/BJ20021802
Payan, F., Leone, P., Porciero, S., Furniss, C., Tahir, T., Williamson, G., et al. (2004). The dual nature of the wheat xylanase protein inhibitor XIP- I: structural basis for the inhibition of family 10 and family 11 xylanases. J. Biol. Chem. 279, 36029–36037. doi: 10.1074/jbc.M404225200
Payne, P. I., Holt, L. M., Krattiger, A. F., and Carrillo, J. M. (1988). Relationships between seed quality characteristics and HMW glutenin subunit composition determined using wheats grown in Spain. J. Cereal Sci. 7, 229–235. doi: 10.1016/S0733-5210(88)80004-3
Payne, P. I., Law, C. N., and Mudd, E. E. (1980). Control by homologous group 1 chomosomes of the high-molecular-weight subunits of glutenin, a major protein of wheat endosperm. Theor. Appl. Genet. 58, 113–120. doi: 10.1007/BF00263101
Payne, P. I., Nightingale, M. A., Krattiger, A. F., and Holt, L. M. (1987). The relationship between HMW glutenin subunit composition and the bread-making quality of British-grown wheat varieties. J. Sci. Food Agric. 40, 51–65. doi: 10.1002/jsfa.2740400108
Peña, R. J., Amaya, A., Rajaram, S., and Mujeeb-Kazi, A. (1990). Variation in quality characteristics associated with some spring 1B/1R translocation wheats. J. Cereal Sci. 12, 105–112. doi: 10.1016/S0733-5210(09)80092-1
Peña, R. J., Trethowan, R., Pfeiffer, W. H., and Ginkel, M. V. (2008). Quality (end-use) improvement in wheat. J. Crop Prod. 5, 1–37. doi: 10.1300/J144v05n01_02
Rafalski, J. A., Scheets, K., Metzler, M., Peterson, M., Hedgcoth, C., and Söll, D. G. (1984). Developmentally regulated plant genes: the nucleotide sequence of a wheat gliadin genomic clone. EMBO J. 3, 1409–1415. doi: 10.1002/j.1460-2075.1984.tb01985.x
Rakszegi, M., Lang, L., and Bedo, Z. (2006). Importance of starch properties in quality oriented wheat breeding. Cereal Res. Commun. 34, 637–640. doi: 10.1556/crc.34.2006.1.159
Ram, S., Sharma, S., and Sharma, I. (2015). Allelic diversity of HMW and lmw glutenins in indian wheats and their relationship with sedimentation volume and mixograph parameters. Cereal Res. Commun. 43, 492–503. doi: 10.1556/0806.43.2015.001
Rasheed, A., Mujeeb-Kazi, A., Ogbonnaya, F. C., He, Z. H., and Rajaram, S. (2018). Wheat genetic resources in the post-genomics era: promise and challenges. Ann. Bot. 121, 603–616. doi: 10.1093/aob/mcx148
Rogers, W. J., Payne, P. I., and Harinder, K. (2006). The HMW glutenin subunit and gliadin compositions of german-grown wheat varieties and their relationship with bread-making quality. Plant Breed. 103, 89–100. doi: 10.1111/j.1439-0523.1989.tb00356.x
Sánchez-León, S., Gil-Humanes, J., Ozuna, C. V., Giménez, M. J., Sousa, C., Voytas, D. F., et al. (2018). Low-gluten, nontransgenic wheat engineered with CRISPR/Cas9. Plant Biotechnol. J. 16, 902–910. doi: 10.1111/pbi.12837
Shan, Q. W., Wang, Y. P., Li, J., Zhang, Y., Chen, K. L., Liang, Z., et al. (2013). Targeted genome modification of crop plants using a CRISPR-Cas system. Nat. Biotechnol. 31, 686–688. doi: 10.1038/nbt.2650
Sharma, A., Garg, S., Sheikh, I., Vyas, P., and Dhaliwal, H. S. (2020). Effect of wheat grain protein composition on end-use quality. J. Food Sci. Technol. 57, 2771–2785. doi: 10.1007/s13197-019-04222-6
Simpson, D. J., Fincher, G. B., Huang, A. H. C., and Cameron-Mills, V. (2003). Structure and function of cereal and related higher plant (1-4)-β-xylan endohydrolases. J. Cereal Sci. 37, 111–127. doi: 10.1006/jcrs.2002.0488
Soda, N., Verma, L., and Giri, J. (2018). CRISPR-Cas9 based plant genome editing: significance, opportunities and recent advances. Plant Physiol. Biochem. 131, 2–11. doi: 10.1016/j.plaphy.2017.10.024
Sugiyama, T., Rafalski, A., and Soll, D. (1986). The nucleotide sequence of a wheat gamma-gliadin genomic clone. Plant Sci. 44, 205–209. doi: 10.1016/0168-9452(86)90092-0
Takahashi-Ando, N., Inaba, M., Ohsato, S., Igawa, T., Usami, R., and Kimura, M. (2007). Identification of multiple highly similar XIP-type xylanase inhibitor genes in hexaploid wheat. Biochem. Biophys. Res. Commun. 360, 880–884. doi: 10.1016/j.bbrc.2007.06.151
Thompson, R. D., Bartels, D., and Harberd, N. P. (1985). Nucleotide sequence of a gene from chromosome 1D of wheat encoding a HMW-glutenin subunit. Nucleic Acids Res. 13, 6833–6846. doi: 10.1093/nar/13.19.6833
Tsilo, T. J., Simsek, S., Ohm, J. B., Hareland, G. A., Chao, S., and Anderson, J. A. (2011). Quantitative trait loci influencing endosperm texture, dough-mixing strength, and bread-making properties of the hard red spring wheat breeding lines. Genome 54, 460–470. doi: 10.1139/g11-012
Uauy, C., Distelfeld, A., Fahima, T., Blechl, A., and Dubcovsky, J. (2006). A NAC gene regulating senescence improves grain protein, zinc, and iron content in wheat. Science 314, 1298–1301. doi: 10.1126/science.1133649
Veraverbeke, W. S., and Delcour, J. A. (2002). Wheat protein composition and properties of wheat glutenin in relation to breadmaking functionality. Crit. Rev. Food Sci. Nutr. 42, 179–208. doi: 10.1080/10408690290825510
Wang, M. W., Vlieta, T. V., and Hamer, R. J. (2005). Interaction of water unextractable solids and xylanasewith gluten protein: effect of wheat cultivar. J. Cereal Sci. 41, 251–258. doi: 10.1016/j.jcs.2004.07.007
Wang, Y. Q., Li, M., Guan, Y. B., Li, L., Sun, F. S., Han, J. P., et al. (2019). Effects of additional cysteine residue of avenin-like b protein by site-directed mutagenesis on dough properties in wheat (Triticum aestivum L.). J. Agric. Food Chem. 67, 8559–8572. doi: 10.1021/acs.jafc.9b02814
Wang, Z. Z., Huang, L., Wu, B. H., Hu, J. L., Jiang, Z. L., Qi, P. F., et al. (2018). Characterization of an integrated active Glu-1Ay allele in common wheat from wild emmer and its potential role in flour improvement. Int. J. Mol. Sci. 19:923. doi: 10.3390/ijms19040923
Weegels, P. L., Hamer, R. J., and Schofield, J. D. (1996). Functional properties of wheat glutenin. J. Cereal. Sci. 23, 1–17. doi: 10.1006/jcrs.1996.0001
Xing, H. L., Dong, L., Wang, Z. P., Zhang, H. Y., Han, C. Y., Liu, B., et al. (2014). A CRISPR/Cas9 toolkit for multiplex genome editing in plants. BMC Plant Biol. 14:327. doi: 10.1186/s12870-014-0327-y
Zhang, M. X. (2019). Construction of Genetic Map for Unigenes And QTL Mapping for Nitrogen Use Efficiency Related Traits in Wheat. Ph.D. thesis. Tai’an: Shandong Agricultural University.
Zhang, M. X., Gao, M. G., Zheng, H. H., Yuan, Y. Y., Zhou, X. W., Guo, Y., et al. (2019). QTL mapping for nitrogen use efficiency and agronomic traits at the seedling and maturity stages in wheat. Mol. Breed. 39, 71–88. doi: 10.1007/s11032-019-0965-8
Zhang, S. J., Zhang, R. Z., Song, G. Q., Gao, J., Li, W., Han, X. D., et al. (2018). Targeted mutagenesis using the Agrobacterium tumefaciens-mediated CRISPR-Cas9 system in common wheat. BMC Plant Biol. 18:302. doi: 10.1186/s12870-018-1496-x
Keywords: wheat, dough quality, xylanase inhibitor protein (XIP), CRISPR/Cas9, SDS-sedimentation values (SV), stability time (ST)
Citation: Sun Z, Zhang M, An Y, Han X, Guo B, Lv G, Zhao Y, Guo Y and Li S (2022) CRISPR/Cas9-Mediated Disruption of Xylanase inhibitor protein (XIP) Gene Improved the Dough Quality of Common Wheat. Front. Plant Sci. 13:811668. doi: 10.3389/fpls.2022.811668
Received: 09 November 2021; Accepted: 02 March 2022;
Published: 05 April 2022.
Edited by:
Maria Raffaella Ercolano, University of Naples Federico II, ItalyReviewed by:
Kurniawan Rudi Trijatmiko, International Rice Research Institute (IRRI), PhilippinesAwais Rasheed, Quaid-i-Azam University, Pakistan
Copyright © 2022 Sun, Zhang, An, Han, Guo, Lv, Zhao, Guo and Li. This is an open-access article distributed under the terms of the Creative Commons Attribution License (CC BY). The use, distribution or reproduction in other forums is permitted, provided the original author(s) and the copyright owner(s) are credited and that the original publication in this journal is cited, in accordance with accepted academic practice. No use, distribution or reproduction is permitted which does not comply with these terms.
*Correspondence: Ying Guo, guoying@sdau.edu.cn; Sishen Li, ssli@sdau.edu.cn