- School of Pharmaceutical Sciences, Changchun University of Chinese Medicine, Changchun, China
In the planting of crops, especially medicinal plants, formula fertilization is important for improving the utilization rate of elements, soil quality, crop yield, and quality. Therefore, it is important to study targeted fertilizer application schemes for sustainable agricultural development and environmental protection. In this study, an L9(34) orthogonal design was used to conduct a field experiment to study the effects of NPK combined application on the growth and pharmacodynamic component biosynthesis of Atractylodes chinensis (DC.) Koidz. Results showed that after applying a base fertilizer at the seedling stage (late May), topdressing at the vegetative stage (late June) and fruit stage (late August) was beneficial to the growth and development of A. chinensis. The high concentrations of phosphorus were conducive to the accumulation of yield and effective components, and the best harvest time was after late October. Principal component analysis (PCA) showed that the comprehensive score of T6 treatment was the highest, indicating that the optimal fertilization scheme for the high yield and high quality of A. chinensis was (N2P3K1): N 180, P2O5 225, and K2O 105 kg⋅ha–1. A signaling response analysis showed that during the growth and development of A. chinensis, the T6 fertilization scheme had clear effects on the activity and gene expression of the key enzymes acetyl-CoA carboxylase (ACC) and farnesyl pyrophosphate synthase (FPPS). Under the T4 [(N2P1K2): N 180, P2O5 75, and K2O 210 kg⋅ha–1] fertilization scheme, the activity and gene expression of the key enzyme 3-hydroxy-3-methylglutaryl-CoA reductase (HMGR) were higher. Moreover, ACC was closely related to the synthesis of the polyacetylene component atractylodin, and FPPS played an important regulatory role in the synthesis of sesquiterpene components atractylenolide II, β-eudesmol, and atractylon. In summary, the high phosphorus fertilization scheme T6 could notably increase the yield of A. chinensis, and promote the accumulation of polyacetylene and sesquiterpene volatile oils by increasing the expression of ACC and FPPS. Therefore, we postulate that the precise application of nutrients (NPK) plays a vital role in the yield formation and quality regulation of A. chinensis.
Introduction
Atractylodes chinensis (DC.) Koidz., belongs to the Asteraceae family; it is mainly distributed in East Asia, where it is known as “Cangzhu” in China and “Sojutsu” in Japan (Toshinari et al., 2020; Wang L.Q. et al., 2020). The rhizomes of A. chinensis are used as medicine, mainly for the treatment of humoral imbalances and digestive diseases (Hossen et al., 2018). The medicinal history of Atractylodis Rhizoma can be traced back to the Han Dynasty (206BC-220AD) when the first Chinese Pharmacopeia, “Shen-nong-ben-cao-jing,” called it “Zhu.” Later, the medicinal materials were divided into A. chinensis and Atractylodes lancea (Thunb.) DC. (Cho et al., 2016).
The main components of the rhizomes of A. chinensis are volatile oils, such as polyacetylenes and sesquiterpenes, the polyacetylene component is mainly atractylodin. Acetyl-CoA carboxylase (ACC) is an important key enzyme in its biosynthetic pathway, and the expression of ACC is easily induced by external factors; e.g., jasmonic acid and fungi can increase or decrease its expression (Figueroa-Balderas et al., 2006; Romero-Munar et al., 2017). The components of sesquiterpenes include atractylon, β-eudesmol, and atractylenolide II, which are mainly synthesized in plants through the mevalonate (MVA) pathway, while 3-hydroxy-3-methylglutaryl-CoA reductase (HMGR) mainly catalyzes the formation of MVA (Aarthy et al., 2018). Farnesyl diphosphate (FPP) is the precursor of all sesquiterpenes, and farnesyl pyrophosphate synthase (FPPS) catalyzes the synthesis of FPP (Wen and Jia, 2021). Therefore, HMGR and FPPS are essential in the biosynthesis of sesquiterpenes (Zhou et al., 2017).
With the sharp increase in demand for medicinal drugs, the wild resources of A. chinensis have been exploited indiscriminately and led to a sharp decline in the reserves of wild resources of A. chinensis in North China and Northeast China. Therefore, a standardized cultivation protocol for A. chinensis is urgently needed. Studies have shown that the synthesis of effective substances in medicinal plants is affected by various soil factors, such as macro elements N, P, K, and trace elements, such as Fe, Zn, and Mn (Singh and Misra, 2000; Aruna et al., 2020; Shiponi and Bernstein, 2021). Soil content of NPK can significantly affect the plant growth and development and the accumulation of medicinal substances; the effect of a mixed application is better than that of a single application (Bernstein et al., 2019). At the same time, the application of organic fertilizer, inorganic fertilizer, and compound fertilizer can ensure the supply of soil nutrients and improve the soil that can greatly increase the yield and quality of crops per unit area (Ferreira et al., 2019; Al-Amri, 2021). However, different crops have different demand ratios for NPK, and the addition of a single fertilizer at too high or too low level will affect growth and development (Bai et al., 2022). Therefore, the study of the effects of NPK on the growth and development of A. chinensis and the formation of pharmacodynamic components is needed to deliver a standardized plan for planting. The clarification of the molecular mechanism of bioactive substance biosynthesis in A. chinensis is key to improve the quality of medicinal materials and solving the shortage of resources.
In summary, this study used field experiments and the L9(34) orthogonal experiment design to reveal the changes in physiological and ecological indicators, such as growth indexes (plant height, stem and leaf fresh weight, fibrous root fresh weight, and rhizome fresh weight) and pharmacodynamic components (atractylenolide II, β-eudesmol, atractylodin, and atractylon) of A. chinensis under different NPK applications. At the same time, by measuring the activity and gene expression of the key biosynthetic enzymes ACC, HMGR, and FPPS, to explore the molecular regulatory mechanisms of the synthesis of polyacetylenes and sesquiterpenes in the rhizomes of A. chinensis under different NPK applications. We aimed to lay a theoretical foundation for further improving the yield and quality of A. chinensis, and provide a basis for the reasonable application of fertilizers at planting.
Materials and Methods
Experimental Site
The test site was located in Changnong A. chinensis planting base, Ankou Town, Liuhe County, Jilin Province, China (42°12′37.65″N, 125°36′4.94″E, and altitude 399 m). The experiment was conducted on private land with the permission of the landowner and did not involve endangered or protected species. The basic physical and chemical properties of the soil were: pH 5.65, organic matter content 51.73 g⋅kg–1, available nitrogen content 41.89 mg⋅kg–1, available phosphorus content 94.40 mg⋅kg–1, and available potassium content 143.54 mg⋅kg–1.
Experimental Design and Field Management
The experiment used annual A. chinensis seedlings with similar growth, no damage on the surface, and no pests or diseases; they were purchased from Changnong Industrial Group Co., Ltd. (Jilin, China). The L9(34) orthogonal fertilization scheme was adopted, with 3 factors of NPK at 4 levels. The specific fertilization amounts are shown in Table 1. A total of 10 different fertilization treatments (3 replicates) were set, with a total area of 150 m2 and a total of 30 plots. Each plot was 3 m2 (1.5 m × 2.0 m), and the row spacing was 15 cm × 20 cm. A 0.5 m wide walkway was set between the plots, and a 40 cm deep plastic film was buried on both sides of the walkway to block the water and nutrient flow. Transplantation took place in May 2019, except for fertilizer application, other treatments (such as, irrigation, weeding, and protection measures) were consistent with base management. In late May (seedling period), an application of base fertilizer with N: 60.0%, P: 100.0%, and K: 33.3% was applied. Topdressing was conducted in late June (vegetative period) and late August (fruit period), with N: 20.0% and K: 33.3%. Fertilizer formations were: urea (N, total nitrogen ≥ 46%), calcium superphosphate (P2O5, total phosphorus ≥ 46%), and potassium sulfate (K2O, total potassium ≥ 50%). The fertilization method involved uniform spraying on the soil surface of different treatment combinations of NPK dissolved in the same volume of water.
Sample Collection and Growth Indexes Determination
At 30, 60, 90, 120, and 150 days post-transplantation (dpt), 6 plants in each parallel plot were randomly sampled. After measuring the growth indexes (plant height, stem and leaf fresh weight, fibrous root fresh weight, and rhizome fresh weight), the non-medicinal parts were removed and cleaned. Some parts were stored in a refrigerator at −80°C, and the remaining parts were dried in shade for further use.
Determination of Pharmacodynamic Components
The dried rhizomes of A. chinensis were ground into fine powder and passed through a 50 mesh (300 μm) sieve. Methanol (50 ml) was added to 300 mg of the accurately weighed sample. The solution was thoroughly vortexed for 1 min and sonicated (power 250 W and frequency 40 kHz) for 60 min at room temperature. The extract was left to cool, and then weighed. The lost weight was made up with methanol, the mixture was shaken well and filtered through 0.2 μm polytetrafluoroethylene (PTFE) membrane filters for further high-performance liquid chromatography (HPLC)–UV analysis.
An Agilent Eclipse XDB-C18 reversed-phase chromatographic column (4.6 mm × 250 mm and 5 μm particle size) was used to determine the contents of atractylenolide II, β-eudesmol, atractylodin, and atractylon at a temperature of 32°C. The mobile phase was acetonitrile (A) and 0.2% phosphoric acid aqueous solution (B), and the flow rate was 1.0 ml/min. Gradient elution was performed as follows: 0–3 min, 55% solvent A; 3–20 min, 55%→60% solvent A; 20–35 min, 60%→65% solvent A; 35–55 min, 65%→85% solvent A; 55–60 min, 85%→95% solvent A; and holding at 100% solvent A for 10 min. UV detection wavelength was 0–28 min, 208 nm (atractylenolide II, β-eudesmol), 28–45 min, 340 nm (atractylodin), 45–60 min, 220 nm (atractylon) and the injection volume was 15 μl. As shown in Figure 1, the method was accurate, stable, and reproducible.
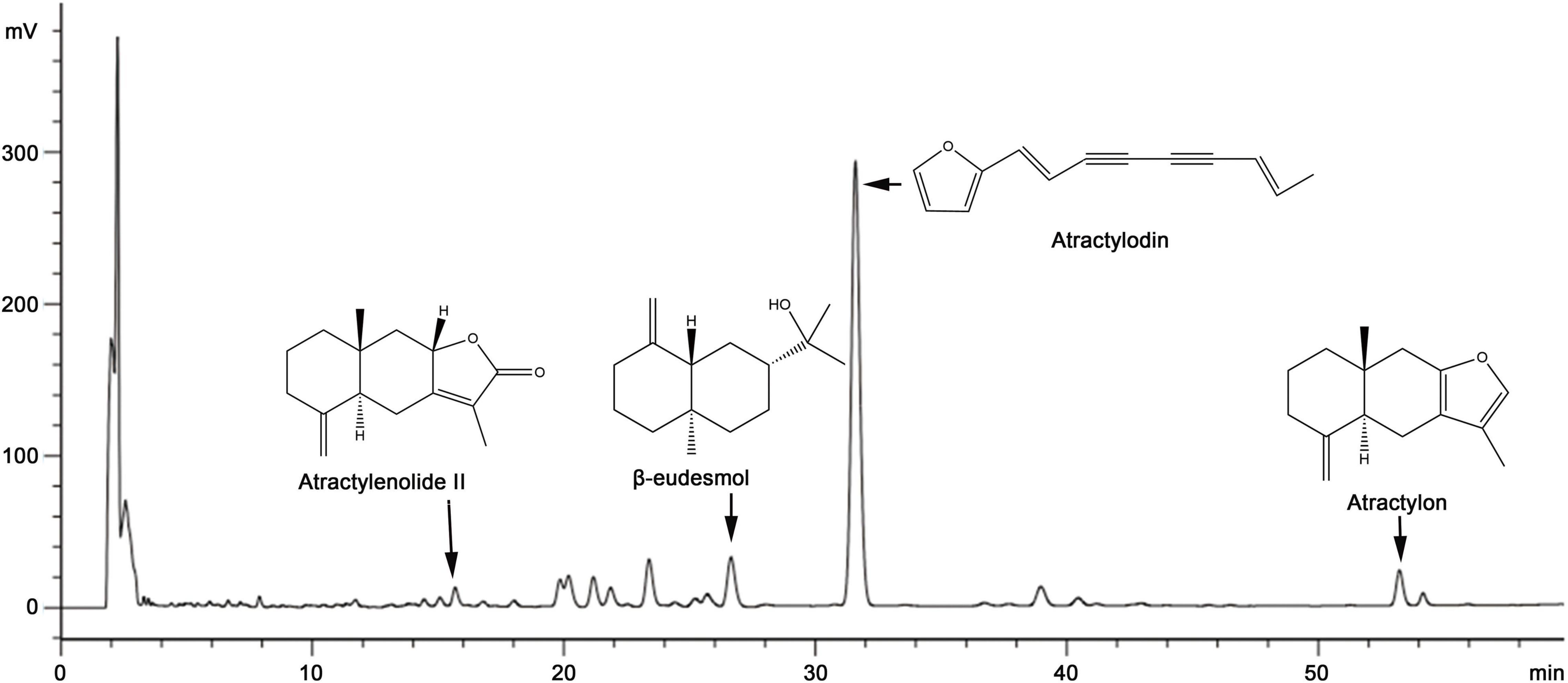
Figure 1. A typical high-performance liquid chromatography (HPLC)–UV chromatogram of several classical volatile oils presents in Atractylodes chinensis.
Determination of Key Enzyme Activity
Enzyme activity was assayed according to the method of Kim et al. (2014), with slight modifications. The rhizome samples of A. chinensis were weighed (0.2 g), added to 1.8 ml precooled 0.01 mol⋅L–1 phosphate buffer saline (PBS) (pH 7.4, NaCl 8 g, Na2HPO4 1.44 g, and KH2PO4 0.24 g), and ground into a homogenate with liquid nitrogen. The slurry was centrifuged at 10,000 r⋅min–1 at 4°C for 20 min to remove the cell debris; then the supernatant was taken to determine the activities of ACC, HMGR, and FPPS using an enzyme-linked immunosorbent assay (ELISA) kit. The specific operation was as follows: take the supernatant (50 μl), add the corresponding enzyme labeled reagent (100 μl); leave at 37°C for 1 h, discard the solution, wash; color, using spectrophotometry at 450 nm wavelength absorbance; and calculate the activity of the sample.
Quantitative RT-PCR Determination of Key Enzyme Genes
According to the instructions of the Takara plant total RNA extraction kit, total RNA was extracted from A. chinensis rhizomes. The concentration and purity of RNA were determined by a Multiskan Sky full wavelength enzyme labeling instrument, and it was reverse transcribed into cDNA. Using cDNA as the template and EF-1α as the internal reference gene (Sang et al., 2017), according to a 20 μl reaction system (SYBR® Premix Ex Taq™ 10.4 μl, 10 μmol⋅L–1 Forward Primer 0.8 μl, 10 μmol⋅L–1 Reverse Primer 0.8 μl, cDNA template 2 μl, ddH2O 6 μl) and reaction program [95°C pre-denaturation for 30 s; 40 cycles (95°C denaturation for 5 s, and 64°C annealing for 1 min)]. The key enzyme genes ACC, HMGR, and FPPS were quantitatively analyzed for the different fertilizer combinations, and each sample had 3 biological replicates. Gene expression was calculated using the comparative threshold cycle (Ct) method. The levels of gene expression in all groups were expressed as a ratio to the control (seedling) group value. The primer sequences are listed in Table 2.
Statistical Analysis
For each variable, SPSS 21.0, GraphPad prism 8.0, and Origin 2019 were used for the statistical analysis and graph plotting. All the data were analyzed by variance analysis. Student–Newman–Keuls post-special test was used to detect the multiple comparisons between treatments, p < 0.05. Principal component analysis (PCA) was used to analyze the selected pharmacodynamic components and physiological parameters of A. chinensis.
Results
Effects of Different NPK Combinations on the Growth Indexes of Atractylodes chinensis
Growth indexes reflect the vitality of plants, which is one important indicator for evaluating the adaptability of plants to soil environments. Changes in each index under different fertilization schemes are shown in Table 3. The results showed that: (i) the plant height of A. chinensis increased quickly 30–60 dpt. At 60-120 dpt, the plant height increased slowly. At 150 dpt, the average plant height under T3 scheme was the tallest (18.34 cm), which was 1.42-fold that of the control group. (ii) The variation trend of fresh weight of stem and leaf of A. chinensis at 30–120 dpt was similar to that of the plant height. Under different fertilization schemes, the stem and leaf fresh weight of T3 and T6 were relatively large. At 150 dpt, the plant entered the wilting stage, and fresh weight of the stem and leaf decreased. (iii) The fresh weight of fibrous roots of A. chinensis increased gradually during the growth period. At 30–90 dpt, there was little difference in the fresh weight of the fibrous roots under the different fertilizer combinations. At 150 dpt, the difference in the fibrous root fresh weight of each fertilization treatment was notable, and the T6 scheme was the largest, which was 1.41-fold of the control group. Similarly, (iv) rhizome fresh weight showed a gradual upward trend in the growth period. At 150 dpt, the fresh weight of rhizomes in each experimental group reached a maximum, and the average weight was 2.35–4.90 g. The fresh weight of rhizomes in the T6 scheme was the largest, and there was no notable difference between T3 and T6. It can be seen from the above that basal fertilizer application at the seedling stage (0 days), and topdressing at the vegetative growth stage (30 days) and fruit stage (90 days) were conducive to the growth and development of A. chinensis. Moreover, A. chinensis had good adaptability under the T3 and T6 fertilization schemes, and the plants showed good growth and vitality.
Effects of NPK Combined Application on the Concentration of Pharmacodynamic Components in Atractylodes chinensis
The concentrations of the four main volatile oils in A. chinensis during the whole growth and development period were analyzed (Figure 2). The concentration of atractylenolide II in rhizomes changed in a “V”-shaped pattern during 30–150 dpt under the different NPK fertilization treatments (Figure 2A). The concentration of atractylenolide II showed a downward trend throughout 30–120 dpt, but the concentration was high under T6 scheme at each stage. At 150 dpt, the concentration of atractylenolide II increased, and the T7 scheme was the highest, which was 1.61-fold that of the control group. There were no significant differences between the T2, T3, T6, and T7 schemes (p > 0.05). Combined with the changes in rhizome fresh weight, it can be seen that the T6 fertilization scheme effectively promoted the synthesis and accumulation of atractylenolide II.
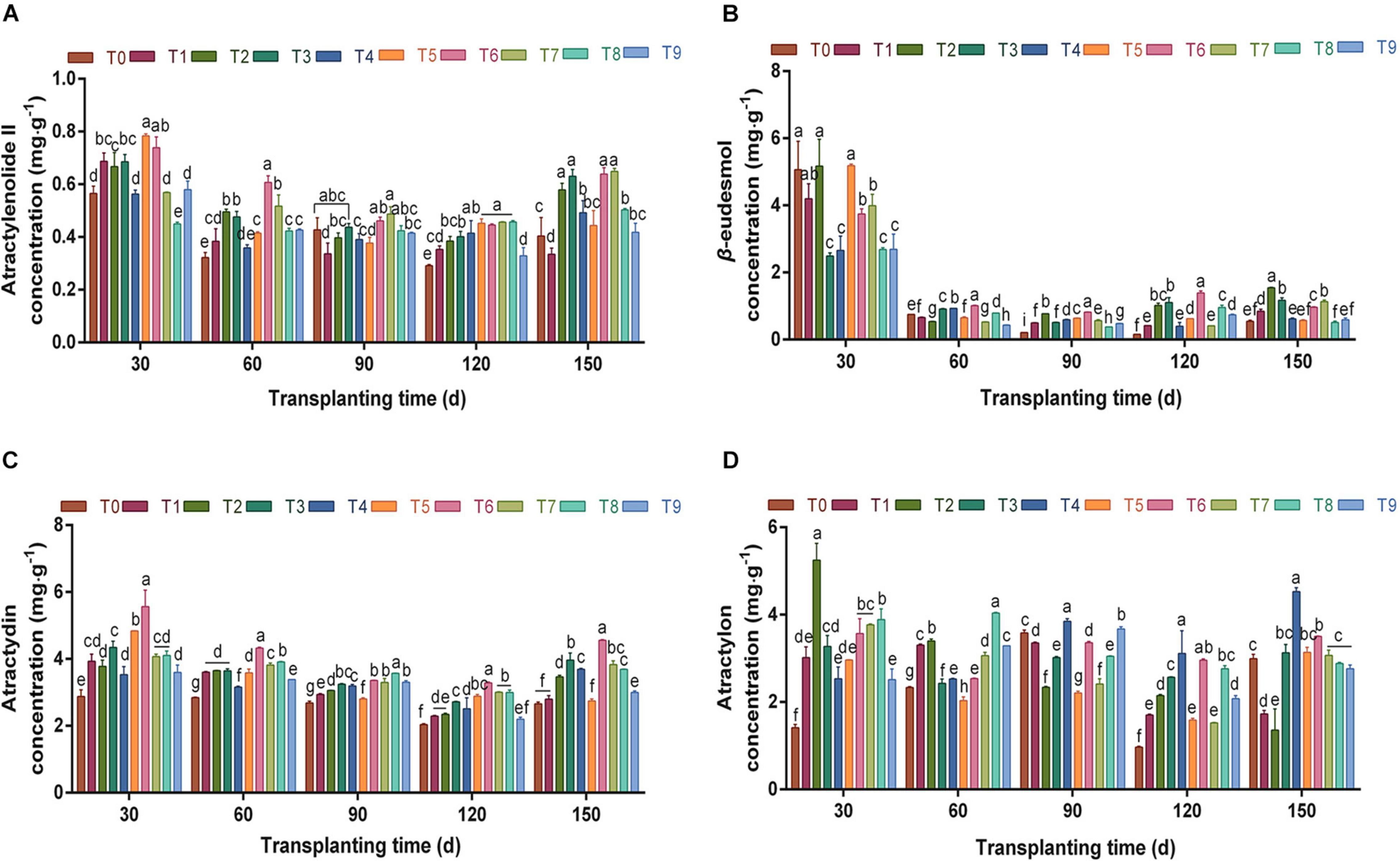
Figure 2. The effects of different NPK combinations on atractylenolide II (A), β-eudesmol (B), atractylodin (C), and atractylon concentration (D) in rhizomes of A. chinensis (n = 3). (a) Initial effective component concentration of A. chinensis seedlings: atractylenolide II 0.2757 ± 0.0017 mg⋅g –1; β-eudesmol 2.9488 ± 0.0958 mg⋅g–1; atractylodin 2.2449 ± 0.0132 mg⋅g–1; and atractylon 1.8074 ± 0.0421 mg⋅g–1. (b) Different lowercase letters indicate that the effects of different NPK combinations on A. chinensis were significantly different in the same period (p < 0.05). (c) The composition of fertilization treatments: T0, N0P0K0; T1, N1P1K1; T2, N1P2K2; T3, N1P3K3; T4, N2P1K2; T5, N2P2K3; T6, N2P3K1; T7, N3P1K3; T8, N3P2K1; and T9, N3P3K2.
Under different NPK fertilization treatments, the concentration of β-eudesmol in the rhizome was the highest at 30 dpt, and was notably decreased between 60 and 150 days compared with 30 days (Figure 2B). At 120 dpt, the concentration of β-eudesmol was between 0.1588 and 1.3881 mg⋅g–1, and the β-eudesmol concentration was highest under the T6 fertilization scheme; furthermore, each fertilization group was significantly higher than that of the control group (p < 0.05). At 150 dpt, the concentration of β-eudesmol in T2 was the highest, which was increased by 51.92% compared with the previous period. Thus, exploring the variation law of yield and the content of medicinal components in different periods under each fertilization scheme, and clarifying the optimal harvest period of medicinal materials, are important measures for reducing agricultural production costs and improving economic benefits.
It can be seen from Figure 2C that during the whole growth and development period, the variation of atractylodin concentration in the rhizome of A. chinensis was similar to that of atractylenolide II. Under different fertilizer combinations in each period, the atractylodin concentration in the T6 scheme was significantly higher than that in the control group (p < 0.05). At 150 dpt, the concentration of atractylodin was 2.6588–4.5589 mg⋅g–1, and the T6 fertilization scheme was the highest, which was 1.71-fold that of the control group. It can be seen from the above that the T6 fertilization scheme significantly promoted the formation of sesquiterpene and polyacetylene volatile oils in the rhizomes of A. chinensis.
During the whole growth and development period, atractylon concentration in the rhizomes showed a “V”-shaped trend (Figure 2D). Between 90 and 150 dpt, the atractylon concentration under T4 was significantly higher than that under other fertilization treatments (p < 0.05). In the harvest period (150 days), the concentration of atractylon was 4.5252 mg⋅g–1, which was 1.51-fold that of the control group T0. It can be seen that a specific ratio of NPK can be targeted to improve the content of one or some components in crops, and provide a basis for promoting agricultural precision production through fertilizer application.
Effects of NPK Combined Application on the Accumulation of Pharmacodynamic Components in Atractylodes chinensis
The proportion and total amount of effective components are important economic characteristics of Daodi herbs (Peng et al., 2021). Under different NPK combinations, the overall variation trend of the total concentrations of 4 volatile oils in rhizomes of A. chinensis was similar to that of single component concentration; however, the proportion of individual volatile oil concentration to the total concentration of all 4 volatile oils was different (Figure 3A). At 150 dpt, the total volatile oil concentration was 8.1594–16.5610 mg⋅g–1, and the concentration of the T6 scheme was the highest, which was increased by 46.36% compared with the control group. The accumulation of active ingredients determines the quality of medicinal materials. The contents of the 4 volatile oils were calculated by analyzing the concentrations of 4 effective components and the average rhizome weight. The biomass of A. chinensis increased with increased transplantation time (Table 3), and the total volatile oil content under different fertilization treatments showed an upward trend (Figure 3B). During the whole growth and development period, T3 and T6 schemes maintained high volatile oil content. At 150 dpt, the total volatile oil content of T3, T4, and T6 treatments increased most notably compared with the control group, and the total volatile oil content of T6 was the highest followed by T3 treatment. There was no significant difference between T4 and T3, indicating that different fertilizer ratios may also play a similar role in promotion, providing a basis for reducing fertilizer consumption and reducing agricultural production costs.
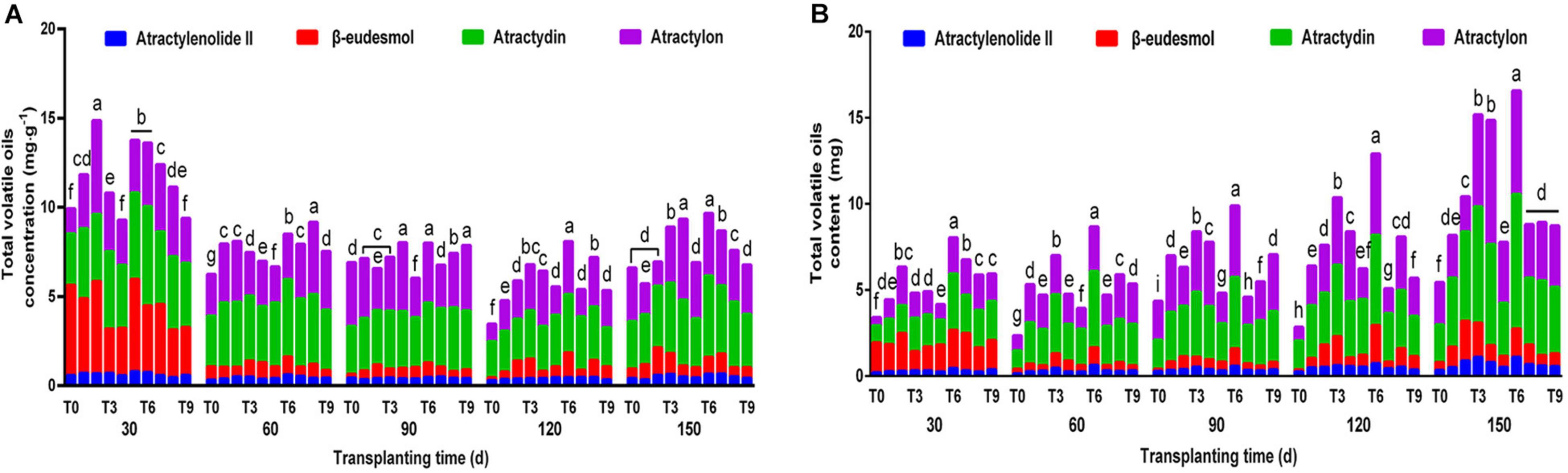
Figure 3. The effects of NPK combined application on total volatile oil concentration (A) and content (B) of A. chinensis (n = 3). Note: (a) The total concentration and content of volatile oil in A. chinensis seedlings were 7.2768 ± 0.1528 mg⋅g–1 and 1.8338 ± 0.0385 mg, respectively. (b) Different lowercase letters indicate that the effects of different NPK combinations on A. chinensis were significantly different in the same period (p < 0.05). (c) The composition of fertilization treatments: T0, N0P0K0; T1, N1P1K1; T2, N1P2K2; T3, N1P3K3; T4, N2P1K2; T5, N2P2K3; T6, N2P3K1; T7, N3P1K3; T8, N3P2K1; and T9, N3P3K2.
Analysis of the Best Fertilization Plan for Atractylodes chinensis With Different NPK
Principal component analysis was used to comprehensively evaluate the yield and quality of A. chinensis under different NPK fertilization formulas during the harvest period (150 days). The component scores of the PCA are shown in Figure 4 for the case of PC1 and PC2 which contributed 96.1% of the variance. PC1 contributed up to 80.2% of total variance and PC2 contributed up to 15.9% of total variance. The differences among the treatments were mainly controlled by PC1. The treatments with an increase in NPK composition were found to be displaced toward the right along PC1 compared with the control treatment. The current research results demonstrated that under the soil fertility status represented by the experimental area, the optimal fertilization plan for the high yield and high quality of A. chinensis was N 180, P2O5 225, and K2O 105 kg⋅ha–1. In other planting areas, this may need to be adjusted and optimized according to local soil conditions to achieve high-quality and high-yields.
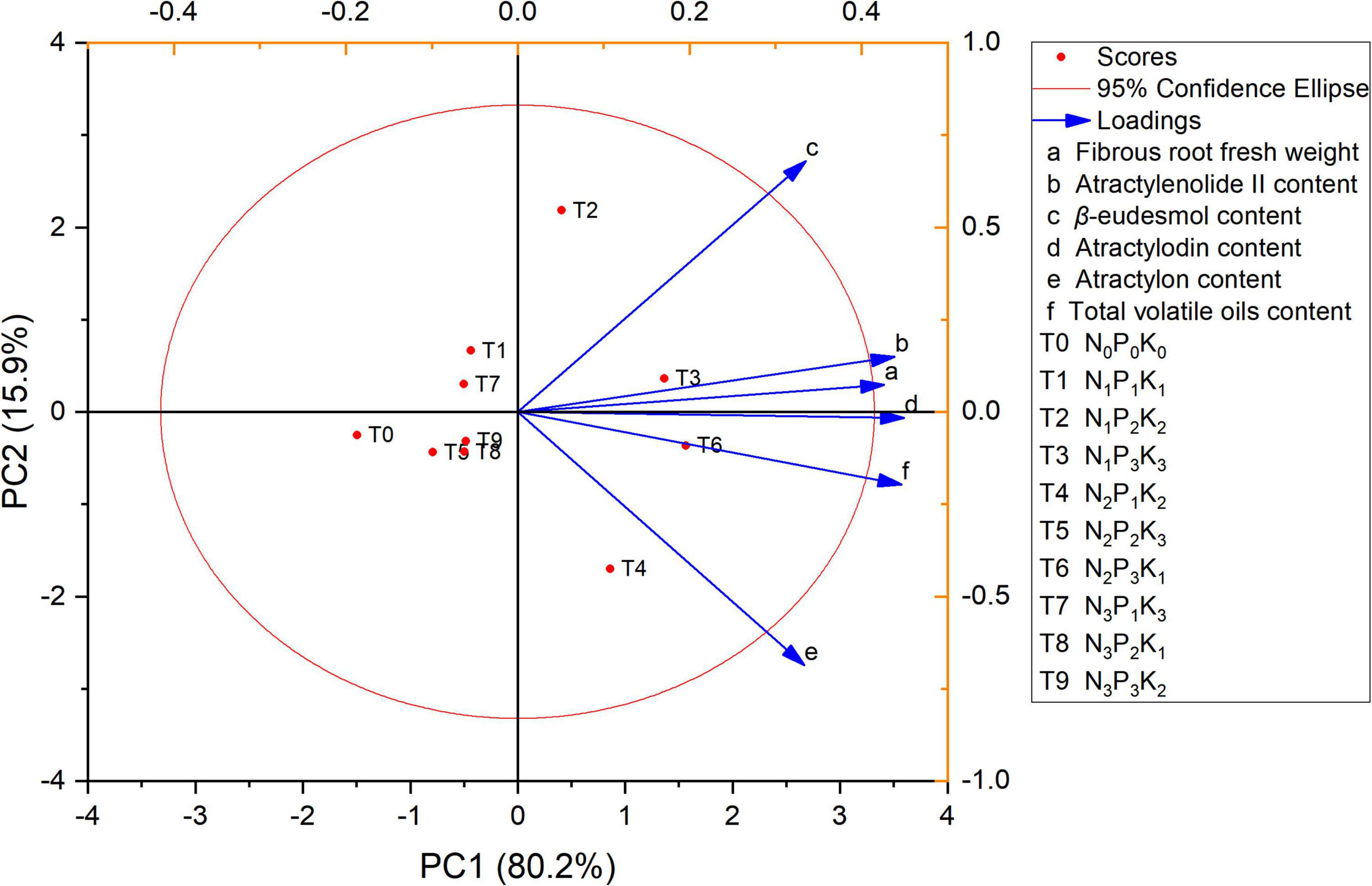
Figure 4. Multi-index principal component analysis (PCA) score map of A. chinensis under different NPK combined application.
Effects of NPK Combined Application on Key Enzymes of Atractylodes chinensis
The key enzymes of volatile oil biosynthesis in A. chinensis were investigated (Figure 5). According to Figure 5A, compared with the control group T0, the ACC activity in rhizomes under different N, P, and K fertilization treatments changed significantly from 30 days to 150 dpt (p < 0.05), and the overall trend was “V”-shaped. The ACC activity decreased gradually from 30 days to 120 dpt. During this growth period, T3 and T6 schemes had a greater effect on the ACC activity; e.g., the ACC activity at 120 dpt was significantly higher than that of other fertilization groups (p < 0.05), which were 1.20- and 1.16-fold of the control group T0, respectively. At 150 dpt, ACC activity increased slightly compared with the previous period, and the range was 209.24–245.44 U⋅L–1.
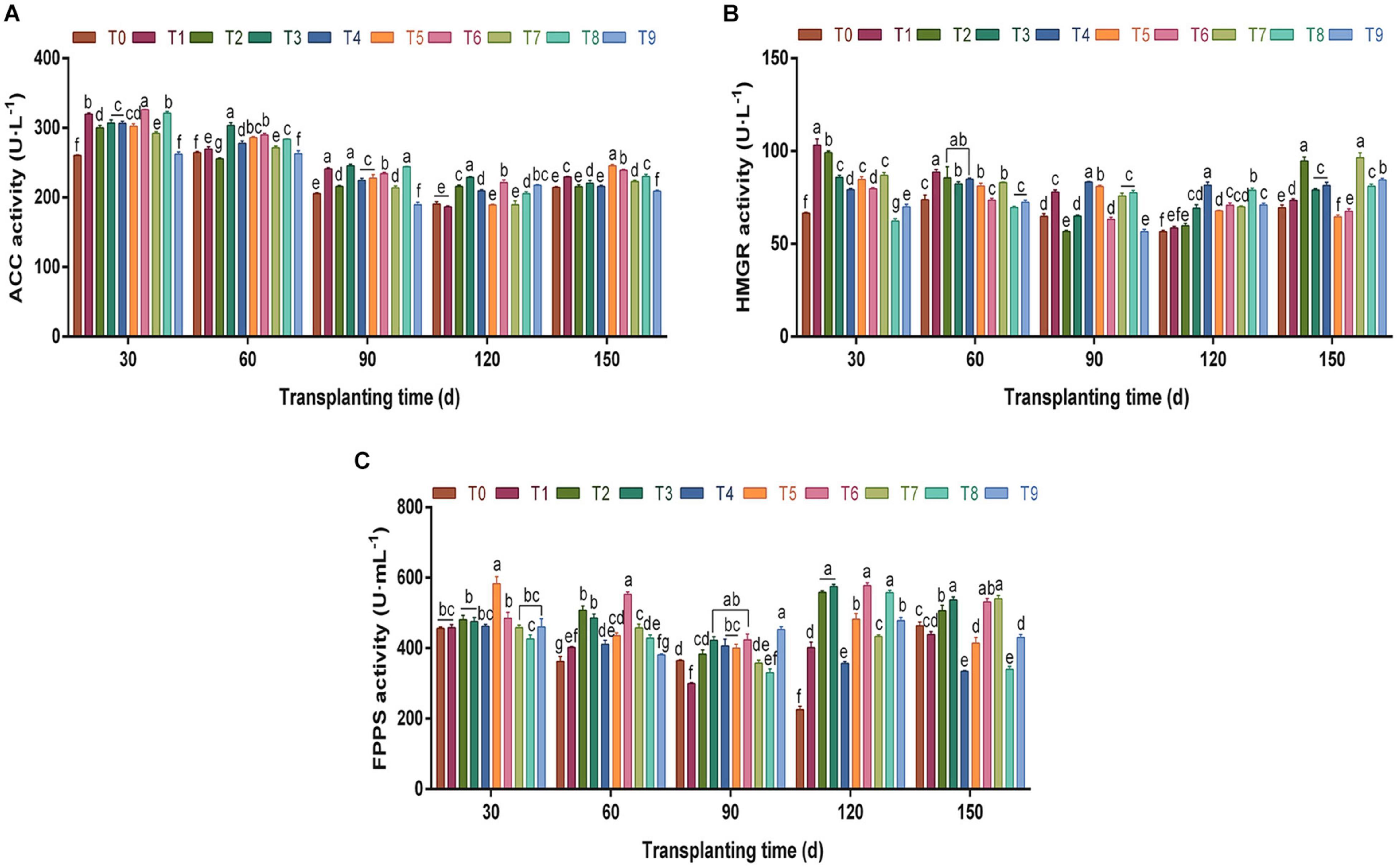
Figure 5. The effects of different NPK combinations on the activities of key enzymes acetyl-CoA carboxylase (ACC) (A), 3-hydroxy-3-methylglutaryl-CoA reductase (HMGR) (B), and farnesyl pyrophosphate synthase (FPPS) (C) in the rhizomes of A. chinensis (n = 3). (a) Initial key enzyme activities of A. chinensis seedlings: ACC 207.76 ± 1.85 U⋅L–1; HMGR 63.38 ± 1.19 U⋅L–1; and FPPS 326.67 ± 8.90 U⋅ml–1. (b) Different lowercase letters indicate that the effects of different NPK combinations on A. chinensis were significantly different in the same period (p < 0.05). (c) The composition of fertilization treatments: T0, N0P0K0; T1, N1P1K1; T2, N1P2K2; T3, N1P3K3; T4, N2P1K2; T5, N2P2K3; T6, N2P3K1; T7, N3P1K3; T8, N3P2K1; and T9, N3P3K2.
Different from ACC, T3 and T6 with high phosphorus fertilization did not perform well in improving HMGR activity (Figure 5B). On the contrary, the T4 fertilization scheme greatly promoted HMGR activity in the middle and late growth stages of A. chinensis. For example, after 120 dpt, HMGR activity under T4 was significantly higher than that in other fertilization groups (p < 0.05), reaching 81.58 U⋅L–1, 1.44-fold that of the control group.
Under different NPK fertilization treatments, the overall changing trend of FPPS activity (Figure 5C) was similar to that of ACC. Between 60 and 150 dpt, the FPPS activities under T3 and T6 were significantly higher than those in the control group (p < 0.05). During the harvest period (150 days), the FPPS activities under T3 and T6 were 537.0833 U⋅ and 531.3889 U⋅ml–1, respectively, which were 15.88 and 14.65% higher than those in the control group. It can be seen from the above that the high phosphorus fertilization schemes T3 and T6 produced a great improvement in the activities of ACC and FPPS in the growth and development of A. chinensis, while T4 specifically improved the activity of HMGR.
Effects of NPK Combined Application on Key Enzyme Genes of Atractylodes chinensis
During growth and development, the relative expression of key enzyme gene ACC showed a “V”-shaped trend (Figure 6A). Under different fertilization schemes, the relative expression trend of the ACC gene was similar to that of enzyme activity, and the relative expression of ACC was high under T3 and T6 at each stage. Particularly, at 60 dpt, the schemes T3 and T6 were significantly higher than those of other fertilization groups (p < 0.05), which were 167.40 and 105.94% higher than those of the control group, respectively.
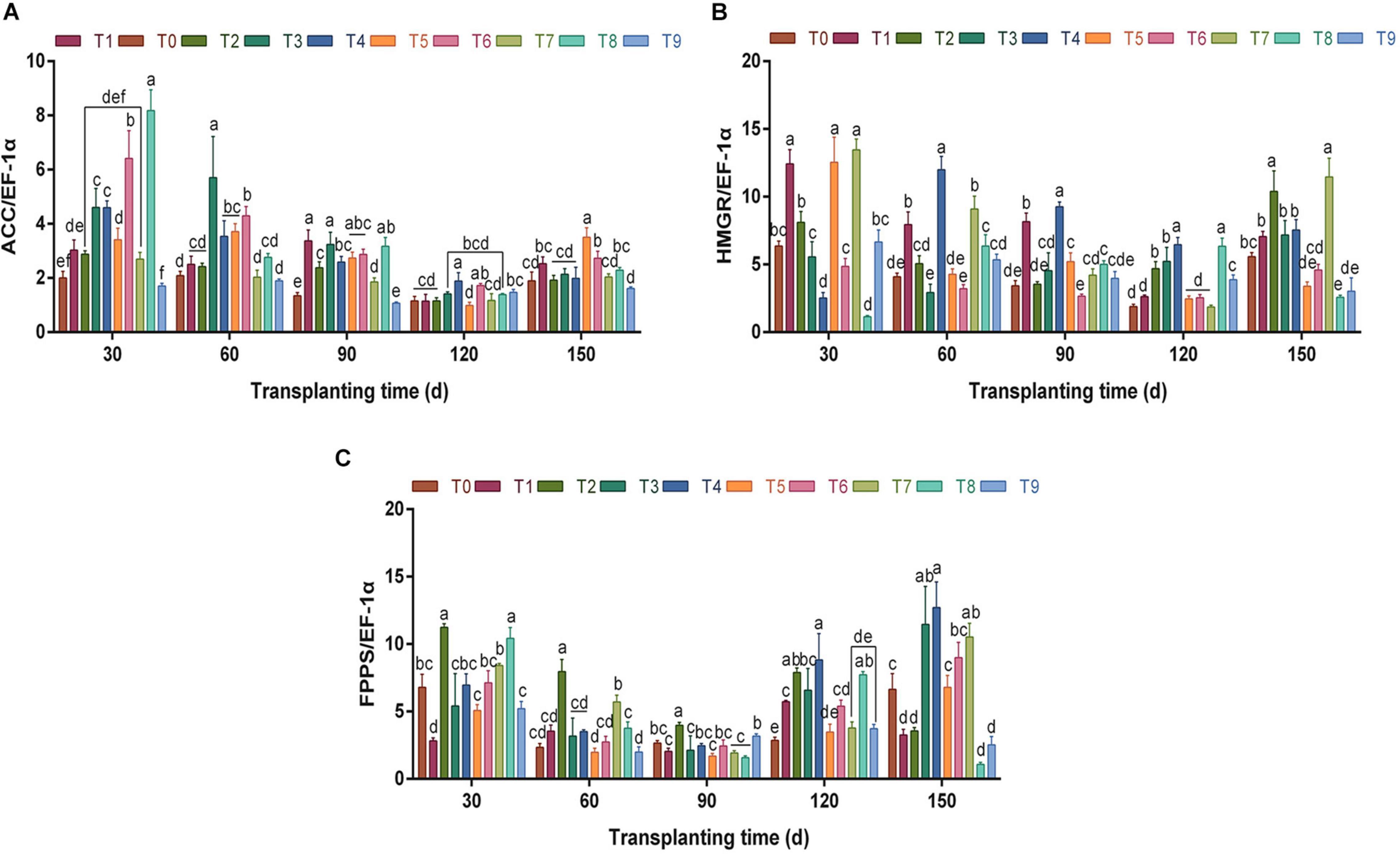
Figure 6. The effects of different NPK combinations on the relative expression levels of key enzyme genes ACC (A), HMGR (B), and FPPS (C) in the rhizomes of A. chinensis (n = 3). (a) The relative gene expression level is calculated based on the expression level of A. chinensis seedlings. (b) Different lowercase letters indicate that the effects of different NPK combinations on A. chinensis were significantly different in the same period (p < 0.05). (c) The composition of fertilization treatments: T0, N0P0K0; T1, N1P1K1; T2, N1P2K2; T3, N1P3K3; T4, N2P1K2; T5, N2P2K3; T6, N2P3K1; T7, N3P1K3; T8, N3P2K1; and T9, N3P3K2.
The relative expression of HMGR was similar to that of ACC (Figure 6B). At 30–120 dpt, the relative expression levels of HMGR showed a downward trend as a whole, and the expression levels under T4 were the highest between 60 and 120 dpt, which were 2.93-, 2.70-, and 3.43-fold that of the control group, respectively. At 150 dpt, the relative expression level of HMGR showed an upward trend compared with the previous period, and the relative expression level was between 2.57 and 11.46.
Different from the key enzyme genes ACC and HMGR, the relative expression level of FPPS (Figure 6C) was the lowest at 90 dpt. At this time, with the exception of the T2 scheme, there were no significant differences between other fertilization treatments and the control group (p > 0.05). Between 90 and 150 dpt, the relative expression of FPPS showed an overall upward trend. At 150 dpt, the relative expression of FPPS in T3, T4, T6, and T7 was significantly higher than that in the control group (p < 0.05). In summary, the changes of gene expression levels of the 3 key enzymes were similar to enzyme activities, which proved that precise fertilizer application could promote the synthesis and accumulation of metabolites by regulating the expression of enzymes and genes.
Correlation Analysis of Pharmacodynamic Components and Key Enzyme Activity and Gene Expression of Atractylodes chinensis Under Different NPK Combined Applications
Addressing the changes of pharmacodynamic components, key enzyme activities, and gene expression levels during the growth and development of A. chinensis, Spearman’s correlation analysis was performed on the concentrations of atractylenolide II, β-eudesmol, atractylodin, and atractylon, the activities of the key enzymes ACC, HMGR, FPPS, and the relative expression of key enzyme genes ACC, HMGR, and FPPS. The results are shown in Figure 7.
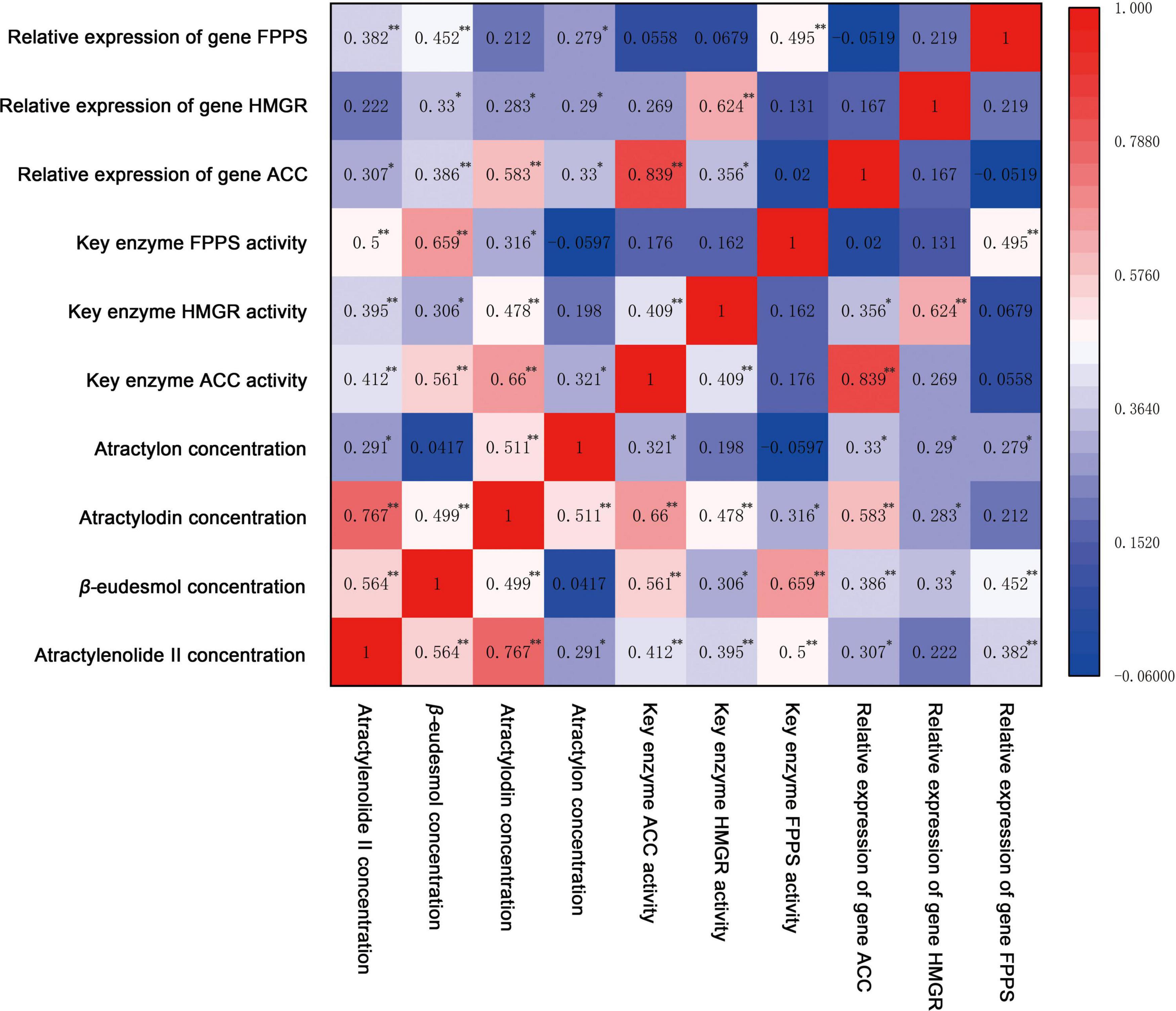
Figure 7. The correlation analysis of pharmacodynamic components with key enzyme activity and gene relative expression of A. chinensis under different NPK combination applications. * indicates significant correlation at p < 0.05 (bilateral), ** indicates significant correlation at p < 0.01 (bilateral).
The correlation results (Figure 7) showed that there was a highly significant positive correlation between the concentration of polyacetylene component atractylodin and sesquiterpene components atractylenolide II, β-eudesmol, and atractylon (p < 0.01). The correlation between atractylodin and atractylenolide II was the highest (0.767), and the correlation between atractylodin and β-eudesmol and atractylon was slightly lower (0.499, 0.511). At the same time, atractylenolide II was significantly positively correlated with β-eudesmol (p < 0.01) and atractylone (p < 0.05). Among the activities of key enzymes ACC, HMGR, and FPPS, there was no correlation except that ACC was highly positively correlated with HMGR (0.409). Similarly, there was no correlation between the relative expression of key enzyme genes ACC, HMGR, and FPPS, indicating that the expression of 3 genes was relatively independent. However, there was a highly significant positive correlation between the corresponding genes and enzymes (p < 0.01). Among them, ACC activity had the highest correlation with the relative expression of the ACC gene (0.839), while HMGR and FPPS activities had a slightly lower correlation with the relative expression of the corresponding genes (0.624, 0.495).
The correlation analysis showed that atractylodin was positively correlated with the activities of key enzymes ACC, HMGR, and FPPS, and the relative expression of key enzyme genes ACC and HMGR. Among them, atractylodin was significantly positively correlated with the ACC enzyme and gene in the polyacetylene biosynthesis pathway, and the correlation coefficient was large (0.660, 0.583). Meanwhile the correlation coefficient with enzymes HMGR and FPPS was small (0.478, 0.316), indicating that polyacetylene atractylodin was mainly regulated by ACC and its genes, and was also affected by HMGR and FPPS. Atractylenolide II was significantly positively correlated with the activities of ACC, HMGR, and FPPS (p < 0.01); the correlation coefficients were 0.412, 0.395, and 0.500, indicating that the biosynthesis of atractylenolide II was mainly regulated by FPPS and ACC. β-eudesmol was positively correlated with the 3 key enzymes and genes to varying degrees, and was extremely significantly positively correlated with the activities of FPPS, ACC, and gene expression (p < 0.01). Among them, the correlation coefficient of FPPS was the largest (0.659), indicating that the biosynthesis of β-eudesmol in rhizomes was mainly regulated by FPPS. Atractylon was significantly correlated with the expression of key enzyme genes ACC, HMGR, and FPPS (p < 0.05), and had a similar correlation coefficient, indicating that the synthesis of atractylon in rhizomes was affected by 3 genes. In summary, the synthesis of volatile oil in the rhizomes of A. chinensis is a complex process involving multiple enzymes and genes. ACC and FPPS play important regulatory roles in the biosynthesis of polyacetylenes and sesquiterpenes, respectively.
Discussion
Insights Into the Impact of NPK on Growth Indexes
Formula fertilization is an advanced agricultural technology for the scientific application of large amounts of the elements and trace elements according to the laws of crop fertilizer demand, soil fertilizer supply performance, and fertilizer effect (Mary Isabella Sonali et al., 2022). This technology can not only improve the yield of cash crops, but also improve their quality, which greatly promotes research regarding plant-soil fertilizer systems (Habibur et al., 2021). This study clearly indicated that the availability of nutrients (NPK) had a significant impact on A. chinensis productivity. Initially, we found that the growth of A. chinensis plant height and stem leaf fresh weight showed better adaptability and responsiveness to the T3 scheme, which highlighted that the higher levels of phosphorus and potassium content in soil could make the plant stems robust and leaves lush. Phosphorus is involved in energy metabolism and photosynthesis during plant growth (Yan et al., 2021), and potassium plays an important role in carbohydrate and protein metabolism (Hassanein et al., 2021), indicating that a reasonable proportion of nutrition (NPK) factors directly promote the absorption and assimilation of plants, thereby affecting their growth and development (Yildirim et al., 2011). Subsequently, we analyzed the fresh weight of fibrous roots and the rhizomes of A. chinensis, because these two important attributes directly determine the yield of crops. The results showed that under high phosphorus fertilization schemes (T3, T6) both were significantly greater than in other treatment groups. Therefore, we speculated that the higher levels of soil phosphorus would significantly promote the root development of A. chinensis. Excitingly, other researchers have observed similar effects of phosphorus content in soil on crop roots, which provided an important basis for the study of an A. chinensis fertilization formula (Pelá et al., 2018).
Insights Into the Impact of NPK on Pharmacodynamic Components
The scientific and precise application of NPK is conducive for increasing crop yields (Jabborova et al., 2021). The planting of Chinese medicinal materials should not only consider yield, but also pay attention to the quality (Ostadi et al., 2020). These 2 jointly determine the cost and benefit of subsequent processing, which is of great importance in production. In this study, we used different ratios of NPK to regulate soil nutrient composition (Table 1). When plants can obtain plentiful essential nutrients, the production of secondary metabolites will be greatly promoted (Wang J. et al., 2020). However, the effects of different NPK ratios on metabolic pathways are different. We found that the metabolic pathways of polyacetylenes and sesquiterpenes showed a good response to the T6 fertilization treatment, which was mainly manifested by a notable increase in the concentrations of atractylodin, β-eudesmol, and atractylenolide II. Interestingly, T4 fertilization treatment could specifically increase the concentration of the sesquiterpene atractylon, which stimulated ideas for the development of precision agriculture. The comprehensive analysis of the 4 effective components (Figure 3) showed that T6 fertilization notably increased the accumulation of total volatile oil in A. chinensis, and the harvest content was the highest after late October. Therefore, the current research results demonstrated that the greater application of phosphorus fertilizer can allow A. chinensis to achieve the higher yields of volatile oil. Similarly, Meysam et al. (2017) found that the application of phosphate fertilizer could increase the total volatile oil production of German Chamomile. We speculated that this may be closely related to the participation of phosphorus in the composition of key molecules, such as nucleic acids and ATP (Campos et al., 2018).
In addition, a single analysis may not be sufficient to determine the relationship between physiological performance and soil nutrient factors in soil-plant systems, which should be combined with other analytical procedures. The biological laws of soil nutrient factors on plants need to be further explored and analyzed. PCA uses variable rotation to reduce variable dimensions and create one or more new variables (Sun et al., 2012). In this study, PCA was used to evaluate the correlation between nutritional factors and physiological characteristics (Figure 4). PCA study showed that the T6 (N2P3K1) fertilization scheme had the best effect; this was the optimal and most favorable fertilizer composition for improving the yield and quality of A. chinensis. It should be noted that optimizing NPK composition to achieve efficient and sustainable agricultural production seems to involve complex processes (Roy et al., 2020a,b, 2021a,b), which would totally depend on the harmonious behavior of plant-soil systems (Wang J. et al., 2020). However, for the long-term effect of NPK fertilizer, such optimization is necessary. The current research offers new insights for sustainable agricultural research and crop fertilization formulation, suggesting that accurately adding NPK in certain proportions or compositions according to plant characteristics, will be reliable and cost-effective.
NPK, Pharmacodynamic Components, Key Enzymes, and Genes Interactions
The accumulation mode of secondary metabolites in plants is multicomponent and multi-active (Chen et al., 2007), and the purpose of regulating plant secondary metabolism is to promote the accumulation of some active compounds (Zhou et al., 2018). However, plant secondary metabolites are regulated by many enzymes and genes owing to their diversity and complex synthesis mechanisms (Pickett, 2014). Therefore, the in-depth exploration of metabolic pathways is key to clarifying the molecular mechanism of the biosynthesis of medicinal substances, improving the quality of medicinal materials, and solving the shortage of medicinal plant resources (Zhang et al., 2021). This study found that fertilizer application can induce changes in enzymes and gene levels in plants, promote the accumulation of secondary metabolites, and thus enhance plant growth and competitive advantage in the natural environment (Krausfeldt et al., 2019). Among them, the promotion effects of T3 and T6 fertilization schemes on the activities and gene expression of key enzymes ACC and FPPS were notable. The T4 fertilization treatment was the superior scheme for HMGR activity and gene overexpression. This well corresponds to the accumulation level of total volatile oil in the rhizomes of A. chinensis (Figure 3), fully indicating that precision fertilizer application can effectively regulate the expression of key enzymes and genes in synthesis pathways, and thus effectively promote the accumulation of metabolites (Ali et al., 2021). In addition, we found a significant correlation between key enzyme activity and gene expression and the concentration of active ingredients (Figure 7). These results clearly show that the upregulation of ACC enzymes and genes are key factors in the synthesis of atractylodin, a polyacetylene component. The expression of FPPS enzyme and gene is essential for the synthesis of sesquiterpene components atractylenolide II, β-eudesmol, and atractylon. HMGR enzymes and genes regulate the synthesis of β-eudesmol, atractylodin, and atractylon to a certain extent. Therefore, we speculated that the high phosphorus fertilization scheme T6 could not only increase the accumulation of medicinal parts of A. chinensis through primary metabolism, but also further activate secondary metabolism to promote the synthesis and accumulation of volatile oil components by increasing the expression of ACC and FPPS, which is similar to the findings of a new study on medical cannabis (Shiponi and Bernstein, 2021).
In summary, we believe that exploring the effects of nutrition (NPK) factors on the traits and qualities of different medicinal plants and developing specific and targeted fertilizer application schemes are of great import for promoting agricultural precision production, reducing agricultural production costs, and improving the quality of medicinal materials. At the same time, we recognize that the interaction between fertilizer application and environmental factors (light, temperature, and water conditions) should be strengthened in a future study.
Conclusion
The purpose of this study was to investigate the effects of nutrients (NPK) on the growth indexes (plant height, stem and leaf fresh weight, fibrous root fresh weight, and rhizome fresh weight), pharmacodynamic components (atractylenolide II, β-eudesmol, atractylodin, and atractylon), key enzymes (ACC, HMGR, and FPPS), and the genes of A. chinensis. Under different NPK combinations, the T6 (N2P3K1) scheme notably promoted the yield and volatile oil content of A. chinensis. Therefore, we believe that the process of planting A. chinensis involves, “heavy application of P fertilizer, and appropriate application of N and K fertilizer.” In addition, we noticed that the synthesis of polyacetylene atractylodin was closely related to ACC; furthermore, FPPS had an important regulatory effect on the synthesis of sesquiterpenes atractylenolide II, β-eudesmol, and atractylon. At the same time, the T6 fertilization program notably improved the key enzymes ACC and FPPS activity and gene expression. In summary, reasonable NPK administration can both effectively promote the growth of the medicinal parts of A. chinensis, and also promote the accumulation of medicinal ingredients by regulating the expression of key enzymes and genes in the synthesis pathway.
Data Availability Statement
The datasets presented in this study can be found in online repositories. The names of the repository/repositories and accession number(s) can be found in the article/supplementary material.
Author Contributions
JS: data curation, formal analysis, software, and writing-original draft. HL: investigation and methodology. YJ: sample collection and data curation. LjW: methodology. CX: conceptualization, project administration, funding acquisition, and writing-review & editing. LlW: conceptualization, funding acquisition, and supervision. All authors contributed to the article and approved the submitted version.
Funding
This work was supported by the National Nature Science Foundations of China [81803649], and the Science and Technology Project of Jilin Provincial Department of Science and Technology [20191102035YY].
Conflict of Interest
The authors declare that the research was conducted in the absence of any commercial or financial relationships that could be construed as a potential conflict of interest.
Publisher’s Note
All claims expressed in this article are solely those of the authors and do not necessarily represent those of their affiliated organizations, or those of the publisher, the editors and the reviewers. Any product that may be evaluated in this article, or claim that may be made by its manufacturer, is not guaranteed or endorsed by the publisher.
References
Aarthy, T., Mulani, F. A., Pandreka, A., Kumar, A., Nandikol, S. S., Haldar, S., et al. (2018). Tracing the biosynthetic origin of limonoids and their functional groups through stable isotope labeling and inhibition in neem tree (Azadirachta indica) cell suspension. BMC Plant Biol. 18:230. doi: 10.1186/s12870-018-1447-6
Al-Amri, S. M. (2021). Response of growth, essential oil composition, endogenous hormones and microbial activity of Mentha piperita to some organic and biofertilizers agents. Saudi J. Biol. Sci. 28, 5435–5441. doi: 10.1016/J.SJBS.2021.06.094
Ali, I., Ullah, S., Iqbal, A., Quan, Z., Liang, H., Ahmad, S., et al. (2021). Combined application of biochar and nitrogen fertilizer promotes the activity of starch metabolism enzymes and the expression of related genes in rice in a dual cropping system. BMC Plant Biol. 21:600. doi: 10.1186/s12870-021-03384-w
Aruna, O. A., Wutem, S. E., Adeniyi, O., Oluwagbenga, D., Christopher, M. A., Charity, A., et al. (2020). Different organic manure sources and NPK fertilizer on soil chemical properties, growth, yield and quality of okra. Sci. Rep. 10:16083. doi: 10.1038/s41598-020-73291-x
Bai, S. H., Omidvar, N., Gallart, M., Kämper, W., Tahmasbian, I., Farrar, M. B., et al. (2022). Combined effects of biochar and fertilizer applications on yield: a review and meta-analysis. Sci. Total Environ. 808:152073. doi: 10.1016/J.SCITOTENV.2021.152073
Bernstein, N., Gorelick, J., Zerahia, R., and Koch, S. (2019). Impact of N, P, K, and humic acid supplementation on the chemical profile of medical Cannabis (Cannabis sativa L). Front. Plant Sci. 10:736. doi: 10.3389/fpls.2019.00736
Campos, P., Borie, F., Cornejo, P., López-Ráez, J. A., López-García, Á, and Seguel, A. (2018). Phosphorus acquisition efficiency related to root traits: is Mycorrhizal symbiosis a key factor to wheat and barley cropping? Front. Plant Sci. 9:752. doi: 10.3389/fpls.2018.00752
Chen, A. X., Lou, Y. G., Mao, Y. B., Lu, S., Wang, L. J., and Chen, X. Y. (2007). Plant terpenoids: biosynthesis and ecological functions. J. Integr. Plant Biol. 49, 179–186. doi: 10.1111/j.1744-7909.2007.00395.x
Cho, H. D., Unyong, K., Joon, H. S., Han, Y. E., Junghyun, K., Seul, G. L., et al. (2016). Classification of the medicinal plants of the genus Atractylodes using high-performance liquid chromatography with diode array and tandem mass spectrometry detection combined with multivariate statistical analysis. J. Sep. Sci. 39, 1286–1294. doi: 10.1002/jssc.201501279
Ferreira, C. M. H., López-Rayo, S., Lucena, J. J., Soares, E. V., and Soares, H. M. V. M. (2019). Evaluation of the efficacy of two new biotechnological-based Freezedried fertilizers for sustainable fe deficiency correction of soybean plants grown in calcareous soils. Front. Plant Sci. 10:1335. doi: 10.3389/fpls.2019.01335
Figueroa-Balderas, R. E., Garcia-Ponce, B., and Rocha-Sosa, M. (2006). Hormonal and stress induction of the gene encoding common bean acetyl-coenzyme a carboxylase. Plant Physiol. 142, 609–619. doi: 10.1104/PP.106.085597
Habibur, R., Abdus, S., Razzab, A., Tanjila, N. T., and Mohammed, H. S. (2021). Effects of the selected combination of boron and zinc in presence of different doses of NPK fertilizers on yield and quality of okra seed. Am. J. Biol. Environ. Stat. 7, 19–28. doi: 10.11648/J.AJBES.20210701.13
Hassanein, R. A., Hussein, O. S., Abdelkader, A. F., Farag, A. I, Hassan, Y. E., and Ibrahim, M. (2021). Metabolic activities and molecular investigations of the ameliorative impact of some growth biostimulators on chilling-stressed coriander (Coriandrum sativum L.) plant. BMC Plant Biol. 21:361. doi: 10.1186/s12870-021-03021-6
Hossen, M. J., Chou, J. Y., Li, S. M., Fu, X. Q., Yin, C. L., Guo, H., et al. (2018). An ethanol extract of the rhizome of Atractylodes chinensis exerts anti-gastritis activities and inhibits Akt/NF-κB signaling. J. Ethnopharmacol. 228, 18–25. doi: 10.1016/j.jep.2018.09.015
Jabborova, D., Sayyed, R. Z., Azimov, A., Jabbarov, Z., Matchanov, A., Enakiev, Y., et al. (2021). Impact of mineral fertilizers on mineral nutrients in the ginger rhizome and on soil enzymes activities and soil properties. Saudi J. Biol. Sci. 28, 5268–5274. doi: 10.1016/J.SJBS.2021.05.037
Kim, Y. J., Lee, O. R., Oh, J. Y., Jang, M. G., and Yang, D. C. (2014). Functional analysis of 3-hydroxy-3-methylglutaryl coenzyme a reductase encoding genes in triterpene saponin-producing ginseng. Plant Physiol. 165, 373–387. doi: 10.1104/pp.113.222596
Krausfeldt, L. E., Farmer, A. T., Castro Gonzalez, H. F., Zepernick, B. N., Campagna, S. R., and Wilhelm, S. W. (2019). Urea is both a carbon and nitrogen source for Microcystis aeruginosa: tracking 13C incorporation at bloom pH conditions. Front. Microbiol. 10:1064. doi: 10.3389/fmicb.2019.01064
Mary Isabella Sonali, J., Kavitha, R., Kumar, P. S., Rajagopal, R., Gayathri, K. V., Ghfar, A. A., et al. (2022). Application of a novel nanocomposite containing micro-nutrient solubilizing bacterial strains and CeO2 nanocomposite as bio-fertilizer. Chemosphere 286:131800. doi: 10.1016/j.chemosphere.2021.13180
Meysam, G. J., Mohsen, M., Issa, K., and Maryam, R. (2017). The changes of yield and essential oil components of German Chamomile (Matricaria recutita L.) under application of phosphorus and zinc fertilizers and drought stress conditions. J. Saudi Soc. Agric. Sci. 16, 60–65. doi: 10.1016/j.jssas.2015.02.003
Ostadi, A., Javanmard, A., Machiani, M. A., Morshedloo, M. R., Nouraein, M., Rasouli, F., et al. (2020). Effect of different fertilizer sources and harvesting time on the growth characteristics, nutrient uptakes, essential oil productivity and composition of Mentha x piperita L. Ind. Crops Prod. 148:112290. doi: 10.1016/j.indcrop.2020.112290
Pelá, A., Ribeiro, M. A., Bento, R. U., Cirino, L. H. B., and Reis Júnior, R. A. (2018). Enhanced-efficiency phosphorus fertilizer: promising technology for carrot crop. Hortic. Bras. 4, 492–497. doi: 10.1590/s0102-053620180411
Peng, Z., Zhang, Y., Yan, B., Zhan, Z., Chi, X., Xu, Y., et al. (2021). Diverse intercropping patterns enhance the productivity and volatile oil yield of Atractylodes lancea (Thunb.) DC. Front. Plant Sci. 12:663730. doi: 10.3389/fpls.2021.663730
Pickett, J. A. (2014). Genetic engineering of secondary metabolism for plant protection. New Biotechnol. 31:S55. doi: 10.1016/j.nbt.2014.05.1737
Romero-Munar, A., Del-Saz, N. F., Ribas-Carbó, M., Flexas, J., Baraza, E., Florez-Sarasa, I., et al. (2017). Arbuscular mycorrhizal symbiosis with Arundo donax decreases root respiration and increases both photosynthesis and plant biomass accumulation. Plant Cell Environ. 40, 1115–1126. doi: 10.1111/pce.12902
Roy, R., Mostofa, M. G., Wang, J., Fornara, D., Sarker, T., and Zhang, R. (2021a). Revegetation intervention of drought-prone coal-mined spoils using Caragana korshinskii under variable water and nitrogen-phosphorus resources. Agric. Water Manag. 246:106712. doi: 10.1016/J.AGWAT.2020.106712
Roy, R., Mostofa, M. G., Wang, J., Sikdar, A., and Sarker, T. (2020a). Improvement of growth performance of Amorpha fruticosa under contrasting regime of water and fertilizer in coal-contaminated spoils using response surface methodology. BMC Plant Biol. 20:181. doi: 10.1186/s12870-020-02397-1
Roy, R., Wang, J., Mostofa, M. G., and Fornara, D. (2021b). Optimal water and fertilizer applications improve growth of Tamarix chinensis in a coal mine degraded area under arid conditions. Physiol. Plant. 172, 371–390. doi: 10.1111/ppl.13147
Roy, R., Wang, J., Mostofa, M. G., Fornara, D., Sikdar, A., Sarker, T., et al. (2020b). Fine-tuning of soil water and nutrient fertilizer levels for the ecological restoration of coal-mined spoils using Elaeagnus angustifolia. J. Environ. Manage. 270:110855. doi: 10.1016/j.jenvman.2020.110855
Sang, X. H., Gu, W., Chao, J. G., Liu, Q. Z., Jiang, L., Han, Y., et al. (2017). Selection of reference genes of Atractylodes lancea and its application in bio-synthesis of active ingredients. Plant Physiol. J. 53, 1680–1686. doi: 10.13592/j.cnki.ppj.2017.0065
Shiponi, S., and Bernstein, N. (2021). The highs and lows of p supply in medical cannabis: effects on cannabinoids, the Ionome, and Morpho-physiology. Front. Plant Sci. 12:657323. doi: 10.3389/fpls.2021.657323
Singh, P., and Misra, A. (2000). Influence of graded level of iron on growth and essential oil production in Mentha spicata L. J. Med. Aromat. Plant Sci. 22, 557–562.
Sun, W., Zhou, Z., Li, Y., Xu, Z., Xia, W., and Zhong, F. (2012). Differentiation of flue-cured tobacco leaves in different positions based on neutral volatiles with principal component analysis (PCA). Eur. Food Res. Technol. 235, 745–752. doi: 10.1007/s00217-012-1799-3
Toshinari, I., Tetsuya, O., Nao, N., Yuto, N., Tadayoshi, O., Masaki, K., et al. (2020). Correction to: antiinflammatory constituents of Atractylodes chinensis rhizome improve glomerular lesions in immunoglobulin A nephropathy model mice. J. Nat. Med. 74:616. doi: 10.1007/s11418-020-01405-w
Wang, J., Chen, X., Chi, Y., Chu, S., Hayat, K., Zhi, Y., et al. (2020). Optimization of NPK fertilization combined with phytoremediation of cadmium contaminated soil by orthogonal experimentl. Ecotoxicol. Environ. Saf. 189:109997. doi: 10.1016/j.ecoenv.2019.109997
Wang, L. Q., Zhang, H., Wu, X., Wang, Z. Y., Fang, W. W., Jiang, M., et al. (2020). Phylogenetic relationships of Atractylodes lancea, A. chinensis and A. macrocephala, revealed by complete plastome and nuclear gene sequences. PLoS One 15:e0227610. doi: 10.1371/journal.pone.0227610
Wen, L. Z., and Jia, C. Z. (2021). Advances in biotechnological production of santalenes and santalols. Chin. Herbal Med. 13, 90–97. doi: 10.1016/j.chmed.2020.11.002
Yan, L., Sunoj, V. S. J., Short, A. W., Lambers, H., Elsheery, N. I., Kajita, T., et al. (2021). Correlations between allocation to foliar phosphorus fractions and maintenance of photosynthetic integrity in six mangrove populations as affected by chilling. New Phytol. 232, 2267–2282. doi: 10.1111/nph.17770
Yildirim, E., Karlidag, H., Turan, M., Dursun, A., and Goktepe, F. (2011). Growth, nutrient uptake, and yield promotion of broccoli by plant growth promoting Rhizobacteria with manure. Hortscience 46, 932–936. doi: 10.21273/HORTSCI.46.6.932
Zhang, R., Tan, S. Q., Zhang, B. L., Hu, P. C., and Li, L. (2021). Cerium-promoted ginsenosides accumulation by regulating endogenous methyl Jasmonate biosynthesis in hairy roots of Panax ginseng. Molecules 26:5623. doi: 10.3390/MOLECULES26185623
Zhou, J. Y., Sun, K., Chen, F., Yuan, J., Li, X., and Dai, C. C. (2018). Endophytic Pseudomonas induces metabolic flux changes that enhance medicinal sesquiterpenoid accumulation in Atractylodes lancea. Plant Physiol. Biochem. 130, 473–481. doi: 10.1016/j.plaphy.2018.07.016
Keywords: Atractylodes chinensis, NPK fertilizers, polyacetylenes, sesquiterpenes, key enzymes, gene expression
Citation: Sun J, Luo H, Jiang Y, Wang L, Xiao C and Weng L (2022) Influence of Nutrient (NPK) Factors on Growth, and Pharmacodynamic Component Biosynthesis of Atractylodes chinensis: An Insight on Acetyl-CoA Carboxylase (ACC), 3-Hydroxy-3-Methylglutaryl-CoA Reductase (HMGR), and Farnesyl Pyrophosphate Synthase (FPPS) Signaling Responses. Front. Plant Sci. 13:799201. doi: 10.3389/fpls.2022.799201
Received: 21 October 2021; Accepted: 16 February 2022;
Published: 18 March 2022.
Edited by:
Antonio Lupini, Mediterranea University of Reggio Calabria, ItalyReviewed by:
Rana Roy, Sylhet Agricultural University, BangladeshYanbo Hu, Northeast Forestry University, China
Copyright © 2022 Sun, Luo, Jiang, Wang, Xiao and Weng. This is an open-access article distributed under the terms of the Creative Commons Attribution License (CC BY). The use, distribution or reproduction in other forums is permitted, provided the original author(s) and the copyright owner(s) are credited and that the original publication in this journal is cited, in accordance with accepted academic practice. No use, distribution or reproduction is permitted which does not comply with these terms.
*Correspondence: Chunping Xiao, YnR4bndAMTYzLmNvbQ==; Lili Weng, NzM1MTEwNDYyQHFxLmNvbQ==