- C.A. Biosystems Engineering, Autonomous University of Querétaro, Querétaro, Mexico
Blackberry fruits are appreciated as a source of nutrients and compounds related to benefit human health. However, they are highly perishable and very susceptible to decay factors. Current methods to improve and maintain blackberry quality are limited in use because of the fruit's fragile physical properties. Regarding these properties, it has been reported that the activities of certain enzymes are linked to senescence and fruit softening processes. This study was aimed to assess the effect of salicylic acid (SA) and chitosan (COS) as preharvest treatments on the physiology related to improving fruit conservation and preserving the marketability index of blackberry fruit. The preharvest treatments were foliar sprayed on blackberry plants at different concentrations. The activities of enzymes superoxide dismutase (SOD), catalase (CAT), phenylalanine ammonia-lyase (PAL), and polygalacturonase (PG) were measured. Total soluble solids (TSS), titratable acidity (TA), TSS/TA ratio, and marketability index (MI) were analyzed after 144 h of storage. The application of 3 mM of SA and 0.25% of COS treatments preserved the MI of blackberries by reducing leakage, red drupelet reversion (RDR), and mycelium presence in the fruit. SA application increased SOD, CAT, and PAL activities. Our results also showed that SA and COS preharvest treatments modified the activity of the cell wall degrading enzyme PG, which might play a role in improving the shelf life and resistance to decay factors of blackberry fruit without any significant effects on physicochemical properties like TSS, TA, and the TSS/TA ratio.
1. Introduction
Blackberries (Rubus sp.) are aggregate fruits formed by multiple arranged drupelets (Hummer, 2017). In recent decades, blackberries have become highly popular worldwide, mainly due to their distinctive taste, nutritional properties, and high content of compounds that provide beneficial effects to human health (Złotek et al., 2014). However, their thin skin cause leakage and accelerated decay and also make them susceptible to mechanical damage, which can reduce their shelf life (Junqueira-Gonçalves et al., 2016). In addition to their fragile nature, blackberries are susceptible to the occurrence of red drupelets after harvest, this condition is referred to as red drupelet reversion (RDR). This change of color could be attributed to the exposure of pigments after the breaking or collapse of the fruit, which can be aggravated by vibration during transportation and affects their integrity (Clark et al., 2007; Edgley et al., 2019). The main reasons for the rejection of blackberries during commercialization are RDR, leakage of the fruit, and visible decay symptoms. At this moment, cold storage is the main method to extend the blackberry shelf life. Freshly harvested blackberries have been reported to last up to 14 days at 0°C with relative humidity above 90%, although the average shelf life for blackberry fruits is 2–5 days (Perkins-Veazie, 2016). On the other hand, blackberries are non-climacteric fruits, so, harvesting of blackberries close to a fully ripened stage is recommended (Benichou et al., 2018). Numerous studies report improvement in berry's shelf life (Huynh et al., 2019) through diverse treatments such as coatings (Baldwin, 2016), modified packaging (Junqueira-Gonçalves et al., 2016), controlled atmosphere storage (Yahia et al., 2019), sanitation (Horvitz, 2017), and irradiation (Butot et al., 2018). However, most of the research regarding this topic has not been developed under commercial production conditions. These methods require preconditioning of the samples and are not easily available; also some of them are not fully compatible with the current blackberry production model. As the fruits ripen, the alteration in the metabolism leads to changes in the cell wall composition that could result in weakened cell walls and ultimately compromised cell wall integrity (Muro-Villanueva et al., 2019). Moreover, the fruit softening process is related to the activation of certain degrading enzymes including polygalacturonase (PG), responsible for pectin degradation, one of the main components for cellular adhesion (Wakabayashi et al., 2000). The reduction or slowing down in cell wall degradation enzyme activity could enhance physical fruit properties related to decay (Lo'ay, 2017). For example, SA and COS, two commonly used elicitors, have shown positive effects reducing softening of fruits (Lo'ay and El-Boray, 2018; Mannozzi et al., 2018). Additionally, SA and COS have exhibited the potential to prevent fruit decay by activating plant defensive responses (Lucini et al., 2018; Shi et al., 2018; El-Mogy et al., 2019; He et al., 2019; Hidangmayum et al., 2019). These responses can include the phenylpropanoid metabolism activation, which is related to defense activities such as cell wall reinforcement and antimicrobial effects (Taiz and Zeiger, 2006; Li et al., 2012). These enzymatic responses can be activated through the antioxidant systems in plants, which include enzymes like superoxide dismutase (SOD) and catalase (CAT). SOD catalyzes the conversion of superoxide to hydrogen peroxide and molecular oxygen (Saibi and Brini, 2018); moreover, CAT is an indispensable enzyme that directly converts hydrogen peroxide into water and molecular oxygen in reactive oxygen species (ROS) detoxification during stress conditions (Garg and Manchanda, 2009). The high presence of ROS can be detrimental to fruit quality by causing the oxidation of several components of the cell wall affecting its integrity (Halliwell and Gutteridge, 2015). The formation of specialized compounds can also occur via enzymatic synthesis through the activation of phenylalanine ammonia-lyase (PAL), which is a key enzyme in the synthesis of phenolic compounds that has been related to defense activities such as cell wall reinforcement, repellent, and antimicrobial activities (Kessmann et al., 1994; Taiz and Zeiger, 2006). Most of the methods reported to date for the conservation of blackberry fruits require postharvest intervention, which can be detrimental to blackberry quality and are limited by its fragile biomechanical properties. Also, preharvest treatments could be more suitable for very fragile fruits such as blackberries. Based on previous reports on other fruits, it was hypothesized that preharvest treatments of SA and COS could improve the blackberry shelf life by modifying the metabolism related to senescence processes. There are no previous reports regarding the effect of preharvest treatments of blackberry plants and fruits with SA and COS, to evaluate the effects on its shelf life, enzymatic activity, and physiochemical properties, which was the purpose of the present study.
2. Materials and Methods
2.1. Location and Plant Material
The experiment (September 2020) was conducted in a commercial crop field of “Tupi” blackberry plants (4 years old) in compliance with the USDA National Organic Program (NOP), located in Senegal de las Palomas community, Querétaro, Mexico (20°26′10.1″N, 100°05′06.6″W). The climatic conditions were as follows: a sub-humid climate, with a mean annual temperature of 16.5 °C and mean accumulated rainfall of 572 mm. Pruning was carried out according to standard cultural practices for blackberry (late May). Fertilization consisted of organic microbial fertilizers with the addition of organic liquid humic acids (late May and early June, respectively).
2.2. Treatments
Salicylic acid (SA, J.T. Baker®, USA) and low molecular weight chitosan (COS, deacetylation degree ≥90%, 50,000–190,000 Da. Alzor® Biotechnologies, Mexico) treatments were prepared at different concentrations (SA 1, SA 2, SA 3 mM, COS 0.25%, COS 0.5% and COS 1%). For our study, plants and fruit were sprayed until a dripping point using a hand-held sprayer between 7:00 and 8:00 am. Plants and fruits sprayed with distilled water were used as controls (9-10 plants per treatment with three replicates). After 5 h of treatment application, fruits were harvested based on commercial criteria. Black and brilliant blackberries with fully developed drupelets located in similar parts of the plant with no evidence of mechanical damage, discoloration, or infection were harvested for the study.
2.3. Marketability Index (MI)
The shelf life of the blackberry fruit was estimated by the calculation of an MI. The fruit was collected in commercial 6 oz PET clamshells and put into thermal isolation storage. The samples were kept at 0–1 °C and 90–95% relative humidity for 12 h, then at the ambient temperature of 22–24 °C and 90–95% relative humidity for 132 h. Four clamshells with 12–14 blackberries each were monitored per treatment. Each blackberry was carefully removed from the clamshell container for evaluation. The RDR, considered as the occurrence of two or more red drupelets within the same blackberry after harvest, leakage, considered as drupelet liquid spillage, and mycelium presence considered as presence or absence of visible growth of mycelium in the fruit surface (Figure 1) were registered as percentages every 24 h throughout 144 h. The MI was calculated as follows: MI (%) = 100− [(% RDR + % Leakage + % Mycelium)/3], a minimum value of 85% was considered for a clamshell to be acceptable for commercialization (Clark and Perkins-Veazie, 2011).
Samples from the clamshells were collected after 144 h and stored at −70 °C. Two treatments, COS 0.25% and SA 3 mM, from the MI test were selected for physicochemical properties, enzymatic activity, and total anthocyanin content assays.
2.4. Physicochemical Properties
Five blackberry fruits from each clamshell (15 blackberries total per treatment), were selected and homogenized by mixing in a blender. Total Soluble Solids (TSS) were measured as °Brix using a digital refractometer (H196801, Hanna Instruments, USA). TA was measured according to AOAC 942.15 (AOAC, 2000). Titratable acidity was expressed as a percent of citric acid. The TSS/TA ratio was calculated as proposed by Mikulic-Petkovsek et al. (2012). All determinations were assayed in triplicates.
2.5. Enzymatic Activity
Polygalacturonase (EC 3.2.1.15) activity was determined as viscosity reduction percentage according to Abeles and Biles (1991) and Brummell et al. (2004) with slight modifications. About 500 mg of frozen fruit were grounded with 5 ml of ice-cold 12% polyethylene glycol. Samples were centrifuged at 13,000 g for 10 min at 4 °C. The pellet was incubated for 2 h at 4 °C with 50 mM NaCl/50 mM acetate buffer (pH = 4.4). The samples were centrifuged at 13,000 g for 10 min at 4 °C. The supernatant was recovered as crude enzyme extract. About 1 ml of extract and 4 ml of 2% pectin solution in acetate buffer of 50 mM were mixed. The viscosity was estimated by determining the time required for the solution to pass from the 0.4 to the 0.9 ml mark of a 1 ml pipette and activity was expressed as relative PG activity and registered at five different times at ambient temperature. The lowest relative viscosity at the first measurement was considered as 100%. SOD (EC 1.15.1.1), CAT (EC 1.11.1.6), and PAL (EC 4.3.1.24), activities were determined in a similar way to Beauchamp and Fridovich (1971), Aebi (1984), and Dickerson et al. (1984), respectively. Briefly, 500 mg of sample previously obtained and homogenized were weighted and frozen grounded with 1 to 4 ml of phosphate extraction buffer (50 mM). The sample was centrifuged for 20 min at 10,000 rpm and 4 °C. The supernatant was collected as enzyme extract for SOD, CAT, and PAL determinations. For SOD activity, 50 μl of enzyme extract were added to a 2 ml reaction mix containing phosphate buffer (50 mM), nitro blue tetrazolium chloride (NBT 98%, Sigma-Aldrich), methionine (Sigma-Aldrich), riboflavin (Sigma-Aldrich), then the mix was exposed to uniform light for 20 min. Absorbance was read at 560 nm. One SOD unit was defined as the amount of enzyme that inhibits the rate of NBT reduction by 50% under the above assay conditions. For CAT activity, 30 μl of enzyme extract were mixed with 100 μl of hydrogen peroxide 100 mM in 1.9 ml phosphate buffer (50 mM). The decrease in absorbance was measured at 240 nm for 1 min. One unit of CAT activity was equal to 1 μmol of H2O2 degraded per minute. For PAL activity, 100 μl of enzyme extract were mixed with 1.15 ml of borate 0.1 M/10 mM L-Phenylalanine (pH = 8.8) buffer. Samples were incubated for 1 h at 40 °C, 0.25 ml of HCl 1 N were added to stop the reaction. Samples were rested for 10 min at ambient temperature then absorbance was measured at 290 nm and compared to a trans-cinnamic acid calibration curve. Total protein was determined as Bradford (1976) by Bradford reagent method. Enzyme activity is expressed as U/mg of protein for SOD, CAT, and PAL. COS of 0.25% and SA of 3 mM treatments were selected for enzymatic activity assays. All determinations were assayed by triplicate.
2.6. Total Anthocyanin Content (TAC)
Total anthocyanin content was determined in a similar way to Lee et al. (2016) and Paunović et al. (2017) by the pH differential method, with slight modifications. The crude extract was obtained by adding 5 ml of HCl/MetOH of 1% (v/v) to 0.5 g of sample previously grounded and homogenized. The samples were vortexed for 30 s and then sonicated at 45 Hz for 10 min at ambient temperature. The samples were then centrifuged at 4,000 rpm and 20 °C for 5 min, the supernatant was collected as a crude extract. About 1.90 ml of 0.025 M potassium chloride buffer of pH = 1 was added to 0.05 ml of extract, concurrently 1.90 ml of 0.4 M sodium acetate buffer pH = 4.5 was added to another 0.05 ml of extract. Samples were rested for 15 min and the absorbance was measured at 515 nm and 700. Total anthocyanin content was expressed as mg of cyanidin-3-glucoside (C3G) per 100 g of fresh weight.
2.7. Statistical Analysis
Data were analyzed by one-way ANOVA. Mean separations were performed by Tukey-Kramer HSD test at α = 0.05. Percentages comparison of RDR, leakage, and decay were performed. A percentage comparison for PG activity was performed using a transformation function. The analyses were performed using IBM® SPSS Statistics v 25 (SPSS Inc., Chicago, IL, USA) and JMP® v 12.1.0 (SAS Institute Inc., North Carolina, USA).
3. Results
3.1. MI and Individual Decay Factors
In order to estimate the blackberry shelf life, the MI was integrated by three decay factors considered relevant for blackberry commercialization, which was also analyzed individually as shown in Table 1. SA of 3 mM and COS of 0.25% treatments were selected for further discussion since 0.25% of COS presented no statistical difference with the higher COS concentration treatments and SA of 3 mM maintained the MI above the minimum 85% required for the study. The MI of the control group dropped to 68.63% after 144 h, SA of 3 mM and COS of 0.25% significantly preserved the MI showing 92.67 and 93.15%, respectively. The fruit treated with SA of 3 mM and COS of 0.25% showed no incidence of RDR while the control group presented 25% of the fruit affected with RDR. The leakage percentage and mycelium presence in the fruit were also lower with both 3 mM of SA and 0.25% of COS treatments.
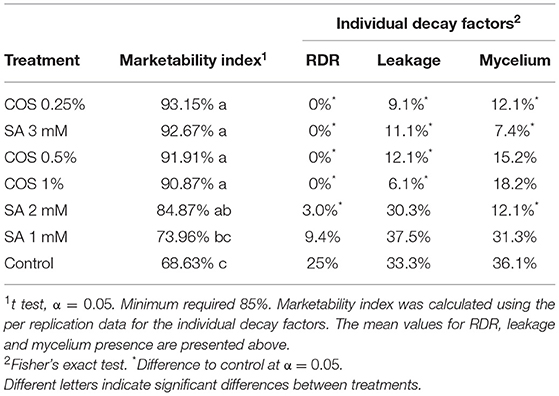
Table 1. Marketability index (MI) and percentage of individual decay factors present on blackberry fruit after 144 h of storage.
We documented the changes in the blackberry fruit every 24 h and selected one representative picture for each treatment at 48, 96, and 144 h after storage for a visual comparison. As shown in Figure 2, as the storage time increases, the leakage and mycelium presence in the fruit also tends to increase, especially in the control group (Figure 2C).
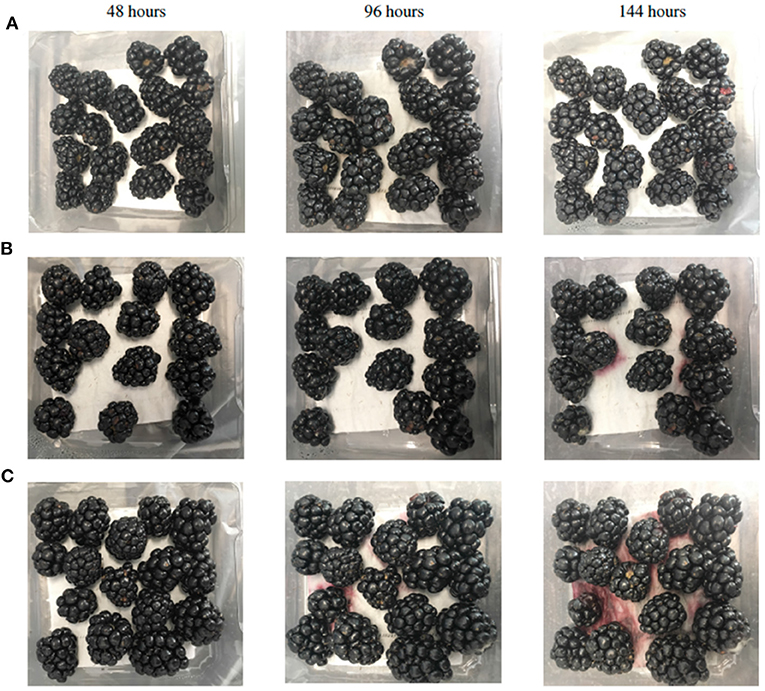
Figure 2. Visual comparison of blackberry fruit at different times of storage, (A) COS of 0.25%, (B) SA of 3 mM, and (C) Control. One representative picture for each treatment is presented.
3.2. Physicochemical Properties
Treatments did not show a significant difference in fruit physicochemical traits evaluated on TSS, TA, and TSS/TA ratio. TSS values were 7.37, 7.47, and 7.46 °Brix. TA had values of 1.90, 1.78, and 1.88%. TSS/TA ratios were 3.88, 4.22, and 4.0 for 3 mM of SA, 0.25% of COS, and control, respectively. In previous reports for blackberries, TSS/TA ratios range from 1.94 up to 14.39 and are highly dependent on the cultivar (Mikulic-Petkovsek et al., 2012; Złotek et al., 2014). Our results showed that the preharvest COS of 0.25% and SA of 3 mM treatments did not affect negatively the organoleptic properties of the blackberry fruit under experimental conditions conducted.
3.3. Enzymatic Activity and TAC
There were significant differences in the activity of key enzymes involved in fruit senescence and decay (Table 2). The PG activity, a key enzyme related to fruit softening, was reduced with both COS of 0.25% and SA of 3 mM treatments, showing 73.03 and 80.67%, respectively, compared to 85.33% in the control group. PAL activity, a key enzyme related to defense responses and the phenylpropanoid metabolism, which includes anthocyanin synthesis, increased with both COS of 0.25% and SA of 3 mM treatments. Even though PAL activation was observed with both treatments, only the COS of 0.25% showed a higher anthocyanin content compared to the control. For SOD activity, a reduction was observed with COS of 0.25%, and the activity was significantly higher on SA of 3 mM treated fruit. CAT activity presented no significant difference between the COS of 0.25% treatment and SA of 3 mM treatments, although the CAT activity for the control group was lower than the SA of 3 mM treatment. The 0.25% of COS treatment did not seem to have an effect in the activation of the blackberry enzymatic antioxidant system under the conditions assayed. All together, these results suggest that the 3 mM of SA and 0.25% of COS treatments effectively promoted the activation of the phenylpropanoid mechanism while reducing the activity of cell wall degrading enzymes such as PG.
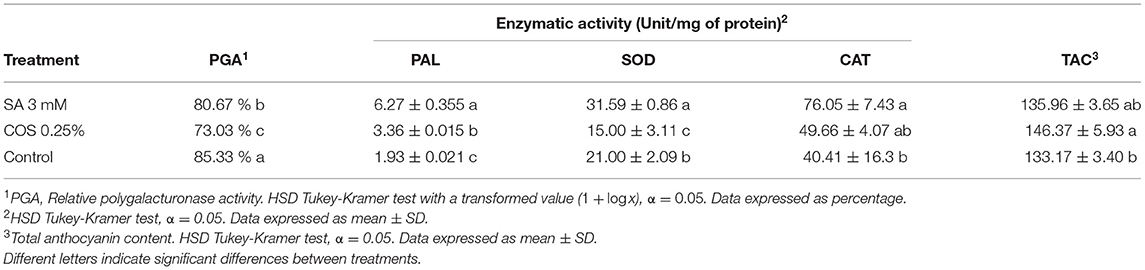
Table 2. Enzymatic activity and total anthocyanin content (TAC) in blackberry fruit after 144 h of storage.
4. Discussion
Blackberry fruits are very fragile so the leakage of the fruit content is quite common without the proper handling. The occurrence in RDR is a very specific disorder that happens in blackberries, even though it is still unclear if it affects the fruit properties at a physicochemical level, the change of color exhibited by blackberries affected with RDR is perceived as a sign of bad quality by the consumers. The presence of mycelium is another unmistakable factor for rejection during blackberry commercialization. In general, blackberries tend to be very prone to damage during harvesting and transportation causing the fruit to present some or all of these undesirable conditions. Also, cell wall degradation or mechanical damage can promote pathogen invasion in the fruit skin since most of the pathogens rely on some physical or physiological disorder to invade plant tissue (Kader, 2002). For our study, the presence of leakage and RDR in the control group showed consistency with previously reported observations of higher electrolyte leakage and the presence of RDR (Edgley et al., 2019). The fruits treated with the COS of 0.25% and SA of 3 mM treatments presented lower percentage of leakage compared to control (Figure 2). In this regard, it has been reported that the mechanical damage in cells can promote some pigments to be exposed and change color (Clark et al., 2007). Our results suggested that the reduction in leakage might be associated with increased blackberry resistance to decay factors, specifically pathogen invasion, and RDR; they also showed that SA of 3 mM and COS of 0.25% treatments preserved the fruit for a longer period of time under the assayed conditions. Although different techniques and conditions were used, we found our results consistent with previously reported studies regarding the use of SA and COS on blackberries and similar fruits (Moreno et al., 2015; El-Mogy et al., 2019; Rodŕıguez et al., 2020; Vilaplana et al., 2020).
In general, blackberry fruits soften rapidly once harvested. The application of SA of 3 mM and COS of 0.25% resulted in lower activity of PG in our study (Table 2). The softening process in fruits have been associated with the activation of a range of cell wall-modifying hydrolases which lead to the cell wall disassembly, particularly, PG that has been related to the pectin degradation and cell wall dissolution (Wakabayashi et al., 2000; Brummell et al., 2004; Payasi et al., 2009). The decrease in PG activity observed in our study was similar with previous reports regarding cell wall degradation using SA and COS. Although postharvest treatments were applied, COS and SA could reduce the loss of firmness in fruits by delaying or suppressing the expression and activity of cell wall degradation enzymes like PG (El-Mogy et al., 2019; He et al., 2019; Zhang et al., 2019). Our results suggested that the preharvest COS of 0.25% and SA of 3 mM treatments might reduce or delay the cell wall degrading process and ultimately enhance blackberry shelf life by delaying cell wall decomposition and possibly delaying pathogen invasion.
Anthocyanins are phenolic compounds that have strong antioxidant properties and also provide blackberries with their distinctive color (Paredes-López et al., 2010). The anthocyanin content in our study increased with the COS of 0.25% treatment in comparison to control (Table 2). Our results suggest that COS of 0.25% had a similar effect as reported by Tezotto-Uliana et al. (2014), Barikloo and Ahmadi (2018), and Grande-Tovar et al. (2018) in other fruits. In addition to COS's properties as an activator for the metabolic synthesis of phenolic compounds, it has been reported that COS promotes fruit preservation for longer periods of time (Saavedra et al., 2016; Mannozzi et al., 2018). However, the anthocyanin content with the COS of 0.25% treatment presented no statistical difference in comparison with the SA of 0.3 mM treatment. In our study, the TAC was determined only one time at the end of the storage period; therefore, there is no conclusive information at the moment to determine if COS of 0.25% promoted anthocyanin synthesis, or alternately, it presented a protective effect against anthocyanin degradation in blackberry fruit. It is relevant to mention that the presence of RDR has been related to decreased anthocyanin contents, structural and physicochemical changes in blackberry fruits (Edgley et al., 2019; Kim et al., 2019). This information suggests that the conditions in specific drupelets of the blackberries affected by RDR could lead to the degradation of pigments, including anthocyanins, as well as cell wall components (Clark et al., 2007; Edgley et al., 2019). To our knowledge, there is no consensus to this date between the influence of RDR on anthocyanin content in blackberry fruit, as contrastive results have been reported (Pérez-Pérez et al., 2016; Kim et al., 2019; Flores-Sosa et al., 2021).
It has been reported that ripening fruits have a higher conversion ROS rate due to oxidative processes (Wang et al., 2009). The imbalance between antioxidant enzymes and ROS can cause oxidative stress if there are high radical levels present in the environment or as plant metabolism by-products. The high presence of ROS can be detrimental to fruit quality by causing the oxidation of several components of the cell wall affecting its integrity (Halliwell and Gutteridge, 2015). Some undesirable conditions in fruits like browning, elevated content of hydrogen peroxide, oxidative stress, and fruit ripening are frequently related to SOD and CAT activities (Mondal et al., 2004; Asghari and Aghdam, 2010; Saba and Moradi, 2016). The higher activity in SOD and CAT with the SA of 3 mM treatment might indicate higher protection against oxidative processes within the blackberry fruit. On the other hand, the COS of 0.25% treatment did not seem to have an effect in the activation of the blackberry enzymatic antioxidant system under the conditions assayed.
The phenylpropanoid metabolism which is largely modulated by PAL, has a key role in the resistance against stress factors. The phenolic acids produced via PAL have shown antifungal as well as antioxidant activities (Muro-Villanueva et al., 2019). Our results showed an increase in PAL activity, which might have an effect on the synthesis of compounds related to cell reinforcement in blackberry. The activation of the phenylpropanoid pathway is a common defensive response in plants and can promote cell wall reinforcement, repellent, and antimicrobial activities (Haslam, 1988; Taiz and Zeiger, 2006; Muro-Villanueva et al., 2019). It has been observed a significantly positive correlation between anti-fungal activity and total phenolic compound content in plants (Mohamed et al., 2017; Shen and Yang, 2017; Xu et al., 2018). As stated by Muro-Villanueva et al. (2019), down-regulation or block in phenylpropanoid metabolism could lead to accumulation of growth-inhibitory compounds, a reduction in lignin content could result in weak xylem vessel cell walls, vascular collapse, and ultimately compromised cell wall integrity. On the other hand, activation of phenylpropanoid metabolism could result in enhancing the cell wall integrity through the production of additional phenolics (Cocuron et al., 2019). This activation of the PAL enzyme could be ultimately related to the extended shelf life observed in the blackberries treated with COS of 0.25% and SA of 3 mM.
Based on our results, the preharvest application of COS exhibited an overall decrease in the decay incidence in the blackberries, which can be attributed to the inhibition of the PG enzyme and consequently, a reduction in the degradation of cell structures, and the overall antifungal properties of COS (Nia et al., 2021; Wu et al., 2021). Similarly, the impact of preharvest applications of SA on maintaining the MI of the blackberry fruit could be attributed to the reduction in the fruit softening processes and the delay of the ripening by the antagonist effect of SA with senescence-related phytohormones (Garćıa-Pastor et al., 2020b; Gomes et al., 2021; Wang et al., 2021). Even COS and SA showed similar results on the blackberry MI preservation, different mechanisms could be in action for each case. The antimicrobial effects of COS on fruits have been attributed to the polycationic structure by directly interacting and changing the microbial cell wall properties and ultimately inactivating them (Xing et al., 2016; Rajestary et al., 2021). By contrast, the effects of SA on fruits could be related to the effects on the biosynthetic pathways and overall physiological responses in plants (Asghari and Aghdam, 2010; Garćıa-Pastor et al., 2020a).
Blackberry fruit is highly perishable, and it is known to be fragile, which is a limitation to the techniques and methods that can be applied to maintain its quality throughout its commercialization. From our observations, the reduction in RDR incidence and the longer shelf life of the blackberries treated with SA of 3 mM and COS of 0.25% could be attributed mainly to the PAL enzyme activation and the decrease in PG activity. Also, studies are reporting that blackberry handling largely increases the possibility of developing physiological disorders compared with not handled fruit (Edgley et al., 2020). Therefore, to preserve blackberry integrity and shelf life, minimal handling is desired. As mentioned before, most physical and chemical methods reported to date regarding the improvement of blackberry shelf life rely on postharvest interventions. Our results suggested that the application of preharvest treatments focused on modifying blackberry physiology could have positive implications in its shelf life blackberry and make it less prone to decay factors by reducing cell wall degrading enzyme activity, promoting antioxidant enzyme activity, and preventing quality detrimental processes, such as cellular damage, pigment degradation, or oxidative damage.
5. Conclusion
In this study, we presented two preharvest treatments for improving blackberry shelf life. Our results suggested that SA of 3 mM and CH of 0.25% preharvest applications could have promising results as a complementary method to improve blackberry shelf life by maintaining fruit physicochemical properties, slowing down the decay processes and activating PAL activity. Preharvest treatments are more suitable for blackberry production by avoiding additional operations after harvesting the fruit. It is important to consider the integration of SA and COS as preharvest treatments as they could represent an economic and technical advantage for blackberry production as a complementary, affordable, and minimal handling method.
Data Availability Statement
The raw data supporting the conclusions of this article will be made available by the authors, without undue reservation.
Author Contributions
JM-C: contributed to initial conceptualization for the research, field and lab works, data generation, writing the original draft, and funding acquisition. IT-P: supervised project administration, he also contributed with funding acquisition, field work, and data analysis. RG-G: provided lab facilities, materials, and contributed to reviewing and editing. ER-G: contributed to conceptualization and provided some facilities. ET-P: performed some lab work and provided some materials. All authors contributed to the article and approved the submitted version.
Funding
This study was partially funded by CONACYT (Ref: 500818).
Conflict of Interest
The authors declare that the research was conducted in the absence of any commercial or financial relationships that could be construed as a potential conflict of interest.
Publisher's Note
All claims expressed in this article are solely those of the authors and do not necessarily represent those of their affiliated organizations, or those of the publisher, the editors and the reviewers. Any product that may be evaluated in this article, or claim that may be made by its manufacturer, is not guaranteed or endorsed by the publisher.
Acknowledgments
The authors would like to express their gratitude to Mr. Alex A, Mr. Resti AM, and their team for facilitating plant material in favor of the conducted research. JM-C would also like to acknowledge the late Dr. Ramón Alvar Martínez Peniche for his support and outstanding contributions to this field.
References
Abeles, F. B., and Biles, C. L. (1991). Cellulase activity in developing apple fruits. Scientia Horticulturae 47, 77–87.
Aebi, H. (1984). [13] catalase in vitro, in Methods in enzymology, Vol. 105 (New York, NY: Elsevier), 121–126.
AOAC (2000). Acidity titratable of fruit products (aoac official method 942.15), in Official Methods of Analysis of AOAC International (Rockville, MD:AOAC International).
Asghari, M., and Aghdam, M. S. (2010). Impact of salicylic acid on post-harvest physiology of horticultural crops. Trends Food Sci. Technol. 21, 502–509. doi: 10.1016/j.tifs.2010.07.009
Baldwin, E. A. (2016). The commercial storage of fruits, vegetables, and florist and nursery stocks, in Agriculture Handbook Number 66. The Commercial Storage of Fruits, Vegetables, and Florist and Nursery Stocks (Washington, DC: USDA), 133.
Barikloo, H., and Ahmadi, E. (2018). Shelf life extension of strawberry by temperatures conditioning, chitosan coating, modified atmosphere, and clay and silica nanocomposite packaging. Scientia Horticulturae 240, 496–508. doi: 10.1016/j.scienta.2018.06.012
Beauchamp, C., and Fridovich, I. (1971). Superoxide dismutase: improved assays and an assay applicable to acrylamide gels. Anal. Biochem. 44, 276–287.
Benichou, M., Ayour, J., Sagar, M., Alahyane, A., Elateri, I., and Aitoubahou, A. (2018). Postharvest technologies for shelf life enhancement of temperate fruits, in Postharvest Biology and Technology of Temperate Fruits (Marrakech: Springer), 77–100.
Bradford, M. M. (1976). A rapid and sensitive method for the quantitation of microgram quantities of protein utilizing the principle of protein-dye binding. Anal. Biochem. 72, 248–254.
Brummell, D. A., Dal Cin, V., Crisosto, C. H., and Labavitch, J. M. (2004). Cell wall metabolism during maturation, ripening and senescence of peach fruit. J. Exp. Bot. 55, 2029–2039. doi: 10.1093/jxb/erh227
Butot, S., Cantergiani, F., Moser, M., Jean, J., Lima, A., Michot, L., et al. (2018). Uv-c inactivation of foodborne bacterial and viral pathogens and surrogates on fresh and frozen berries. Int. J. Food Microbiol. 275, 8–16. doi: 10.1016/j.ijfoodmicro.2018.03.016
Clark, J. R., and Perkins-Veazie, P. (2011). “apf-45”primocane-fruiting blackberry. HortScience 46, 670–673. doi: 10.21273/HORTSCI.46.4.670
Clark, J. R., Stafne, E. T., Hall, H. K., and Finn, C. E. (2007). Blackberry breeding and genetics. Plant Breeding Rev. 29, 19. doi: 10.1002/9780470168035.ch2
Cocuron, J.-C., Casas, M. I., Yang, F., Grotewold, E., and Alonso, A. P. (2019). Beyond the wall: high-throughput quantification of plant soluble and cell-wall bound phenolics by liquid chromatography tandem mass spectrometry. J. Chromatography A 1589, 93–104. doi: 10.1016/j.chroma.2018.12.059
Dickerson, D., Pascholati, S., Hagerman, A. E., Butler, L., and Nicholson, R. (1984). Phenylalanine ammonia-lyase and hydroxycinnamate: Coa ligase in maize mesocotyls inoculated with helminthosporium maydis or helminthosporium carbonum. Physiol. Plant Pathol. 25, 111–123.
Edgley, M., Close, D., and Measham, P. (2020). Red drupelet reversion in blackberries: a complex of genetic and environmental factors. Scientia Horticulturae 272, 109555. doi: 10.1016/j.scienta.2020.109555
Edgley, M., Close, D., Measham, P., and Nichols, D. (2019). Physiochemistry of blackberries (rubus l. subgenus rubus watson) affected by red drupelet reversion. Postharvest Biol. Technol. 153, 183–190. doi: 10.1016/J.POSTHARVBIO.2019.04.012
El-Mogy, M. M., Ali, M. R., Darwish, O. S., and Rogers, H. J. (2019). Impact of salicylic acid, abscisic acid, and methyl jasmonate on postharvest quality and bioactive compounds of cultivated strawberry fruit. J. Berry Res. 9, 333–348. doi: 10.3233/JBR-180349
Flores-Sosa, A. R., Soto-Magaña, D., Gonzalez-de la Vara, L. E., Sanchez-Segura, L., Bah, M., Rivera-Pastrana, D. M., et al. (2021). Red drupelet reversion in blackberries caused by mechanical damage is not linked to a reduction in anthocyanin content. Postharvest Biol. Technol. 180, 111618. doi: 10.1016/j.postharvbio.2021.111618
García-Pastor, M. E., Zapata, P. J., Castillo, S., Martínez-Romero, D., Guillén, F., Valero, D., et al. (2020a). The effects of salicylic acid and its derivatives on increasing pomegranate fruit quality and bioactive compounds at harvest and during storage. Front. Plant Sci. 11, 668. doi: 10.3389/fpls.2020.00668
García-Pastor, M. E., Zapata, P. J., Castillo, S., Martínez-Romero, D., Valero, D., Serrano, M., et al. (2020b). Preharvest salicylate treatments enhance antioxidant compounds, color and crop yield in low pigmented-table grape cultivars and preserve quality traits during storage. Antioxidants 9, 832. doi: 10.3390/antiox9090832
Garg, N., and Manchanda, G. (2009). Ros generation in plants: boon or bane? Plant Biosyst. 143, 81–96. doi: 10.1080/11263500802633626
Gomes, E. P., Borges, C. V., Monteiro, G. C., Belin, M. A. F., Minatel, I. O., Junior, A. P., et al. (2021). Preharvest salicylic acid treatments improve phenolic compounds and biogenic amines in ‘niagara rosada' table grape. Postharvest Biol. Technol. 176, 111505. doi: 10.1016/j.postharvbio.2021.111505
Grande-Tovar, C. D., Chaves-Lopez, C., Serio, A., Rossi, C., and Paparella, A. (2018). Chitosan coatings enriched with essential oils: effects on fungi involved in fruit decay and mechanisms of action. Trends Food Sci. Technol. 78, 61–71. doi: 10.1016/j.tifs.2018.05.019
Halliwell, B., and Gutteridge, J. M. (2015). Free Radicals in Biology and Medicine. Oxford: Oxford University Press.
Haslam, E. (1988). Plant polyphenols (syn. vegetable tannins) and chemical defense—a reappraisal. J. Chem. Ecol. 14, 1789–1805.
He, Y., Bose, S. K., Wang, M., Liu, T., Wang, W., Lu, H., et al. (2019). Effects of chitosan oligosaccharides postharvest treatment on the quality and ripening related gene expression of cultivated strawberry fruits. J. Berry Res. 9, 11–25. doi: 10.3233/JBR-180307
Hidangmayum, A., Dwivedi, P., Katiyar, D., and Hemantaranjan, A. (2019). Application of chitosan on plant responses with special reference to abiotic stress. Physiol. Mol. Biol. Plants 25, 313–326. doi: 10.1007/s12298-018-0633-1
Horvitz, S. (2017). Postharvest handling of berries, in Postharvest Handling (Rijeka: Intech Open), 107–123.
Hummer, K. (2017). Blackberries: an introduction, in Blackberries and Their Hybrids. Crop Production Science in Horticulture, Vol. 27 (Boston, MA: CABI). 1.
Huynh, N. K., Wilson, M. D., Eyles, A., and Stanley, R. A. (2019). Recent advances in postharvest technologies to extend the shelf life of blueberries (Vaccinium sp.), raspberries (Rubus idaeus L.) and blackberries (Rubus sp.). J. Berry Res. 9, 687–707. doi: 10.3233/JBR-190421
Junqueira-Gonçalves, M. P., Alarcón, E., and Niranjan, K. (2016). The efficacy of potassium sorbate-coated packaging to control postharvest gray mold in raspberries, blackberries and blueberries. Postharvest Biol. Technol. 111, 205–208. doi: 10.1016/j.postharvbio.2015.09.014
Kader, A. A. (2002). Postharvest Technology of Horticultural Crops, Vol. 3311. (Washington, DC: University of California Agriculture and Natural Resources).
Kessmann, H., Staub, T., Ligon, J., Oostendorp, M., and Ryals, J. (1994). Activation of systemic acquired disease resistance in plants. Eur. J. Plant Pathol. 100, 359–369.
Kim, M. J., Lee, M. Y., Shon, J. C., Kwon, Y. S., Liu, K.-H., Lee, C. H., et al. (2019). Untargeted and targeted metabolomics analyses of blackberries–understanding postharvest red drupelet disorder. Food Chem. 300, 125169. doi: 10.1016/j.foodchem.2019.125169
Lee, S. G., Vance, T. M., Nam, T.-G., Kim, D.-O., Koo, S. I., and Chun, O. K. (2016). Evaluation of ph differential and hplc methods expressed as cyanidin-3-glucoside equivalent for measuring the total anthocyanin contents of berries. J. Food Meas. Characterization 10, 562–568. doi: 10.1007/s11694-016-9337-9
Li, M., Yu, M., Zhang, Z., Liu, Z., and Pan, Y. (2012). Control of black spot disease caused by alternaria alternata on jujube (ziziphus jujuba mill. cv. dongzao) using harpinxoo protein. J. Horticult. Sci. Biotechnol. 87, 250–254. doi: 10.1080/14620316.2012.11512860
Lo'ay, A. (2017). Preharvest salicylic acid and delay ripening of ‘superior seedless' grapes. Egyptian J. Basic Appl. Sci. 4, 227–230. doi: 10.1016/j.ejbas.2017.04.006
Lo'ay, A., and El-Boray, M. (2018). Improving fruit cluster quality attributes of ‘flame seedless' grapes using preharvest application of ascorbic and salicylic acid. Scientia Horticulturae 233, 339–348. doi: 10.1016/j.scienta.2018.02.010
Lucini, L., Baccolo, G., Rouphael, Y., Colla, G., Bavaresco, L., and Trevisan, M. (2018). Chitosan treatment elicited defence mechanisms, pentacyclic triterpenoids and stilbene accumulation in grape (vitis vinifera l.) bunches. Phytochemistry 156, 1–8. doi: 10.1016/j.phytochem.2018.08.011
Mannozzi, C., Tylewicz, U., Chinnici, F., Siroli, L., Rocculi, P., Dalla Rosa, M., et al. (2018). Effects of chitosan based coatings enriched with procyanidin by-product on quality of fresh blueberries during storage. Food Chem. 251, 18–24. doi: 10.1016/j.foodchem.2018.01.015
Mikulic-Petkovsek, M., Schmitzer, V., Slatnar, A., Stampar, F., and Veberic, R. (2012). Composition of sugars, organic acids, and total phenolics in 25 wild or cultivated berry species. J. Food Sci. 77, C1064–C1070. doi: 10.1111/j.1750-3841.2012.02896.x
Mohamed, M. S., Saleh, A. M., Abdel-Farid, I. B., and El-Naggar, S. A. (2017). Growth, hydrolases and ultrastructure of fusarium oxysporum as affected by phenolic rich extracts from several xerophytic plants. Pesticide Biochem. Physiol. 141, 57–64. doi: 10.1016/j.pestbp.2016.11.007
Mondal, K., Sharma, N., Malhotra, S., Dhawan, K., and Singh, R. (2004). Antioxidant systems in ripening tomato fruits. Biologia Plantarum 48, 49–53.
Moreno, B. M., Rizzolo, R. G., de Moraes Fagundes, C., Bender, A., and Antunes, L. E. C. (2015). Efeito do ácido salicílico na pré–colheita de amora preta cv. tupy. Revista Iberoamericana de Tecnología Postcosecha 16, 234–239. Available online at: https://www.redalyc.org/articulo.oa?id=81343176013
Muro-Villanueva, F., Mao, X., and Chapple, C. (2019). Linking phenylpropanoid metabolism, lignin deposition, and plant growth inhibition. Curr. Opin. Biotechnol. 56, 202–208. doi: 10.1016/j.copbio.2018.12.008
Nia, A. E., Taghipour, S., and Siahmansour, S. (2021). Pre-harvest application of chitosan and postharvest aloe vera gel coating enhances quality of table grape (vitis vinifera l. cv.‘yaghouti') during postharvest period. Food Chem. 347, 129012. doi: 10.1016/j.foodchem.2021.129012
Paredes-López, O., Cervantes-Ceja, M. L., Vigna-Pérez, M., and Hernández-Pérez, T. (2010). Berries: improving human health and healthy aging, and promoting quality life—a review. Plant Foods Hum. Nutrit. 65, 299–308. doi: 10.1007/s11130-010-0177-1
Paunović, S. M., Mašković, P., Nikolić, M., and Miletić, R. (2017). Bioactive compounds and antimicrobial activity of black currant (ribes nigrum l.) berries and leaves extract obtained by different soil management system. Scientia Horticulturae 222, 69–75. doi: 10.1016/j.scienta.2017.05.015
Payasi, A., Mishra, N. N., Chaves, A. L. S., and Singh, R. (2009). Biochemistry of fruit softening: an overview. Physiol. Mol. Biol. Plants 15, 103–113.
Pérez-Pérez, G., Fabela-Gallegos, M., Vázquez-Barrios, M., Rivera-Pastrana, D., Palma-Tirado, L., Mercado-Silva, E., et al. (2016). Effect of the transport vibration on the generation of the color reversion in blackberry fruit, in VIII International Postharvest Symposium: Enhancing Supply Chain and Consumer Benefits-Ethical and Technological Issues 1194 (Cartagena), 1329–1336.
Perkins-Veazie, P. (2016). The commercial storage of fruits, vegetables, and florist and nursery stocks. in Agriculture Handbook Number 66. The Commercial Storage of Fruits, Vegetables, and Florist and Nursery Stocks (Washington, DC: USDA), 237.
Rajestary, R., Landi, L., and Romanazzi, G. (2021). Chitosan and postharvest decay of fresh fruit: meta-analysis of disease control and antimicrobial and eliciting activities. Comprehensive Rev. Food Sci. Food Safety 20, 563–582. doi: 10.1111/1541-4337.12672
Rodríguez, M. C., Yépez, C. V., González, J. H. G., and Ortega-Toro, R. (2020). Effect of a multifunctional edible coating based on cassava starch on the shelf life of andean blackberry. Heliyon 6, e03974. doi: 10.1016/j.heliyon.2020.e03974
Saavedra, G. M., Figueroa, N. E., Poblete, L. A., Cherian, S., and Figueroa, C. R. (2016). Effects of preharvest applications of methyl jasmonate and chitosan on postharvest decay, quality and chemical attributes of fragaria chiloensis fruit. Food Chem. 190, 448–453. doi: 10.1016/j.foodchem.2015.05.107
Saba, M. K., and Moradi, S. (2016). Internal browning disorder of eight pear cultivars affected by bioactive constituents and enzyme activity. Food Chem. 205, 257–263. doi: 10.1016/j.foodchem.2016.03.022
Saibi, W., and Brini, F. (2018). Superoxide dismutase (sod) and abiotic stress tolerance in plants: An overview. Superoxide Dismutase: Structure, Synthesis and Applications, ed Magliozzi, S., (Sfax: Nova Science Publishers), 101–142.
Shen, Y., and Yang, H. (2017). Effect of preharvest chitosan-g-salicylic acid treatment on postharvest table grape quality, shelf life, and resistance to botrytis cinerea-induced spoilage. Scientia Horticulturae 224, 367–373. doi: 10.1016/j.scienta.2017.06.046
Shi, Z., Wang, F., Lu, Y., and Deng, J. (2018). Combination of chitosan and salicylic acid to control postharvest green mold caused by penicillium digitatum in grapefruit fruit. Scientia Horticulturae 233, 54–60. doi: 10.1016/j.scienta.2018.01.039
Tezotto-Uliana, J. V., Fargoni, G. P., Geerdink, G. M., and Kluge, R. A. (2014). Chitosan applications pre-or postharvest prolong raspberry shelf-life quality. Postharvest Biol. Technol. 91, 72–77.
Vilaplana, R., Guerrero, K., Guevara, J., and Valencia-Chamorro, S. (2020). Chitosan coatings to control soft mold on fresh blackberries (rubus glaucus benth.) during postharvest period. Scientia Horticulturae 262, 109049.
Wakabayashi, K., Chun, J.-P., and Huber, D. J. (2000). Extensive solubilization and depolymerization of cell wall polysaccharides during avocado (perseaamericana) ripening involves concerted action of polygalacturonase and pectinmethylesterase. Physiologia Plantarum 108, 345–352.
Wang, B., Wang, J., Feng, X., Lin, L., Zhao, Y., and Jiang, W. (2009). Effects of 1-mcp and exogenous ethylene on fruit ripening and antioxidants in stored mango. Plant Growth Regul. 57, 185. doi: 10.1007/s10725-008-9335-y
Wang, P., Lu, S., Zhang, X., Hyden, B., Qin, L., Liu, L., et al. (2021). Double nced isozymes control aba biosynthesis for ripening and senescent regulation in peach fruits. Plant Sci. 304, 10739. doi: 10.1016/j.plantsci.2020.110739
Wu, P., Xin, F., Xu, H., Chu, Y., Du, Y., Tian, H., et al. (2021). Chitosan inhibits postharvest berry abscission of ‘kyoho' table grapes by affecting the structure of abscission zone, cell wall degrading enzymes and so2 permeation. Postharvest Biol. Technol. 176, 111507. doi: 10.1016/j.postharvbio.2021.111507
Xing, Y., Xu, Q., Li, X., Chen, C., Ma, L., Li, S., et al. (2016). Chitosan-based coating with antimicrobial agents: preparation, property, mechanism, and application effectiveness on fruits and vegetables. Int. J. Polym. Sci. 2016, 4851730. doi: 10.1155/2016/4851730
Xu, D., Deng, Y., Han, T., Jiang, L., Xi, P., Wang, Q., et al. (2018). In vitro and in vivo effectiveness of phenolic compounds for the control of postharvest gray mold of table grapes. Postharvest Biol. Technol. 139, 106–114. doi: 10.1016/j.postharvbio.2017.08.019
Yahia, E. M., Fadanelli, L., Mattè, P., and Brecht, J. K. (2019). Chapter 13—controlled atmosphere storage, in Postharvest Technology of Perishable Horticultural Commodities, ed Yahia, E. M., (Duxford: Woodhead Publishing), 439–479.
Zhang, C., Xiong, Z., Yang, H., and Wu, W. (2019). Changes in pericarp morphology, physiology and cell wall composition account for flesh firmness during the ripening of blackberry (rubus spp.) fruit. Scientia Horticulturae 250, 59–68. doi: 10.1016/j.scienta.2019.02.015
Keywords: elicitor, shelf-life, chitosan, salicylic acid, Rubus sp.
Citation: Martínez-Camacho JE, Guevara-González RG, Rico-García E, Tovar-Pérez EG and Torres-Pacheco I (2022) Delayed Senescence and Marketability Index Preservation of Blackberry Fruit by Preharvest Application of Chitosan and Salicylic Acid. Front. Plant Sci. 13:796393. doi: 10.3389/fpls.2022.796393
Received: 22 October 2021; Accepted: 27 January 2022;
Published: 04 March 2022.
Edited by:
María Serrano, Miguel Hernández University of Elche, SpainReviewed by:
Kalyan Barman, Banaras Hindu University, IndiaMohamed A. Taher, Mansoura University, Egypt
Alexander Prosekov, Kemerovo State University, Russia
Copyright © 2022 Martínez-Camacho, Guevara-González, Rico-García, Tovar-Pérez and Torres-Pacheco. This is an open-access article distributed under the terms of the Creative Commons Attribution License (CC BY). The use, distribution or reproduction in other forums is permitted, provided the original author(s) and the copyright owner(s) are credited and that the original publication in this journal is cited, in accordance with accepted academic practice. No use, distribution or reproduction is permitted which does not comply with these terms.
*Correspondence: Irineo Torres-Pacheco, irineo.torres@uaq.mx