- 1College of Forestry, Guizhou University, Guiyang, China
- 2Guizhou Academy of Forestry, Guiyang, China
- 3Guizhou Province Institute of Mountain Resources, Guiyang, China
- 4College of Pharmacy, Guizhou University of Traditional Chinese Medicine, Guiyang, China
Seed dormancy is a complex adaptive trait of plants that are influenced by several physiological and environmental factors. The endangered plant Cinnamomum migao is also known to exhibit seed dormancy and low germination, which may influence its regeneration; however, these characteristics remain unexplored. To our knowledge, this study is the first to examine the type of dormancy and improve the germination percentage of C. migao seeds. We evaluated the structure and characteristics of the embryo and endocarp of C. migao seeds as well as the effects of endogenous inhibitors. Furthermore, we assessed the effects of light, stratification, alternating temperature, and gibberellic acid 3 (GA3) on the dormancy release of these seeds. The embryo was well developed the endocarp was water-permeable, and no obvious mechanical hindrance to germination was observed. However, the endocarp and embryo contained phenols and other germination inhibitors. The seed extracts of C. migao delayed the germination of cabbage and ryegrass seeds, which indicates the presence of endogenous inhibitors. These findings suggest that C. migao seeds exhibit physiological dormancy. Light and an alternating temperature (15/20°C) did not influence germination. However, GA3 pretreatment, alternating temperatures, and warm stratification relieved dormancy. GA3 pretreatment combined with the 15°C stratification treatment was most effective in rapidly releasing the C. migao seed dormancy. Our findings may facilitate the storage and conservation of this endangered plant, which is currently underrepresented in ex situ collections.
Introduction
Seed dormancy describes the phenomenon in which seeds with vigor and integrity fail to germinate within a certain period even when the environmental conditions (e.g., water, light, temperature, and oxygen) are suitable (Finch-Savage and Leubner-Metzger, 2006; Yan and Chen, 2020). Seed dormancy is a physiological and ecological adaptation that enables plants to survive in complex environments. Dormancy also aids wild plant seeds in maintaining their vitality under harsh environmental conditions, thus contributing to plant survival, species continuation, and evolution (Stevens et al., 2020). Fresh seeds that do not germinate for 4–6 weeks under suitable conditions are considered dormant (Ensslin et al., 2018). Previous studies have estimated that 50–90% of wild plant seeds exhibit dormancy (Tang et al., 2004). According to the widely recognized classification system of Baskin and Baskin (2004), seed dormancy can be categorized into five classes: physiological dormancy (PD), morphological dormancy (MD), physical dormancy (PY), morphophysiological dormancy (MPD), and combinational dormancy (PY + PD). These classes are further categorized into subclasses, levels, and types (Baskin and Baskin, 2004).
Dormancy can prevent the seeds from germinating in unsuitable seasons, thus reducing species competition and ensuring population reproduction under adverse conditions (Rubio de Casas et al., 2012; Nonogaki, 2019). However, this trait poses a challenge when large numbers of seedlings are required for horticultural purposes, particularly for the conservation and rapid recovery of endangered plant populations (Cho et al., 2018; Gao et al., 2021). For the restoration of endangered populations in the wild, it may take several seasons for the seeds to become nondormant, which may result in low final germination percentages. Moreover, seeds are often lost during the dormancy period because of animal foraging, microbial attack, and soil erosion; this hinders the recovery of plant populations (Kildisheva et al., 2020; Ma et al., 2020; Zida et al., 2020).
Cinnamomum migao H. W. Li—an evergreen arbor belonging to Lauraceae—is a rare endemic species in China. Its distribution is limited to the area surrounding the dry hot valley formed by a small watershed in the Guizhou, Yunnan, and Guangxi Provinces in Southwest China (Li et al., 2013). C. migao has been classified as near-threatened in the Red List of Biodiversity in China: Volume of Higher Plants (Ministry of Ecology and Environment, P.R.C., 2011). It produces high-quality wood that is used for making high-grade furniture and wood carvings (Shi et al., 2003). The fruit is rich in sugars, amino acids, and volatile oils and is widely used as a spice and traditional medicine in Southwest China, particularly as a substitute for Lauraceae spices such as Litsea lancilimba and Litsea cubeba (Qiu and Du, 2010; Zhou et al., 2010; Wang et al., 2016). However, the wild population of C. migao has been reported to be endangered and its natural regeneration faces various obstacles. The population of this arbor comprises adult trees aged >50 years and seedlings are extremely scarce. Previous evidence has confirmed seed dormancy in C. migao (Zhou et al., 2010; Huang et al., 2019); seed germination is very slow even in suitable environment, and the natural germination percentage is <36% (Gao et al., 2015). Our group further confirmed that seedlings germinated from C. migao seeds are scare in the wild (Li, 2017; Huang et al., 2019). The low germination percentage may result from seed dormancy, which prevents the supplementation C. migao seedlings. This is a primary barrier of natural regeneration of C. migao, ultimately making it an endangered species (Shi et al., 2003; Huang et al., 2021).
However, to our knowledge, the factors responsible for the low seed dormancy of C. migao and effective methods for dormancy release are unknown. This study, therefore, aimed to uncover the primary factors responsible for seed dormancy, such as anatomy, morphology, and physiology. The secondary objective was to explore the most effective method for C. migao seed dormancy release using alternating temperature, light/dark phase, gibberellic acid 3 (GA3), and clod/warm stratification. Our ultimate goal was to determine the type of seed dormancy and find a highly effective method to release seed dormancy of this arbor. Our findings may be a point of reference for future interventions aiming at raising seedlings ex situ and reintroducing them into the wild.
Materials and Methods
Fresh Seed Collection
Cinnamomum migao fruits were collected from Luodian County, Guizhou Province, China (25°26′40″N, 106°31′51″; 667 m altitude) in October–November 2017. The ripened fruits were transported to the laboratory immediately after collection. The fruits were then peeled, washed under running water, and placed in a ventilated dark room. The seeds enclosed by the endocarp were stored in the dark at 20 ± 2°C and approximately 60% relative humidity for 1 week before use.
Morphology of Seeds and Embryos
The transverse axis diameter, longitudinal axis diameter, and thickness of C. migao seeds were measured using a vernier caliper (0.01 mm). The caliper was slowly clamped along the seed ridge to separate the embryo from the endocarp, and embryo development was observed and photographed under a fluorescence microscope (Leica M205 FA). Three replicates of 30 seeds were measured.
Determination of Seed and Endocarp Characteristics
Seed Weight, Moisture Content, and Viability
In the beginning of December 2017, C. migao seeds were crushed and sieved through a No. 20 mesh screen, weighed accurately to 50 g, and oven-dried at 103°C for 17 h and then weighed again. Water content was determined based on the difference between fresh and dry weights. To test seed viability, embryos were placed in a 0.50% 2,3,5-triphenyltetrazolium chloride solution and then incubated in the dark at 25°C for 12 h. Embryos that had been kept in boiling water for 20 min for inactivation served as the control. Seed viability was determined based on the coloring position and depth of the embryos (Souza et al., 2010). Three replicates of 30 seeds each were used (Supplementary Figure S1: viability test results).
Imbibition Rate and Fruit Endocarp Characteristics
The seeds were categorized into three groups: first (control), seeds with the endocarp; second, seeds with partial endocarp (one-fifth of the endocarp was cut off); and third, seeds without the endocarp (Supplementary Figure S2). Seeds belonging to these three groups (50 seeds each) were placed in separate beakers with 200 ml of distilled water. They were then placed in a 25°C incubator and weighed using an electronic balance (0.0001 g) every 2 h until the weights stabilized. Before weighing, the water on the surface of the seeds was soaked using absorbent paper. Water absorption was calculated as the percentage of initial weight increase. For ultrastructural observation, the endocarps were fixed with 2.5% glutaraldehyde solution, washed with 0.1 M phosphate buffer, fixed with 1% osmic acid in 0.1 M phosphate buffer (pH 7.4) at room temperature (20°C) for 1–2 h, rinsed with 0.1 M phosphate buffer (pH 7.4), and then dehydrated using an ethanol gradient. The samples were dried in a critical point dryer (quorum K850) and sprayed with 30% gold using an ion-sputtering instrument (IXRF MSP-2S) for approximately 30 s. The endocarp ultramicrostructure was observed under a scanning electron microscope (Hitachi su8100).
Effects of H2O2, NaOH, and H2SO4 Treatments on Seed Germination
Toward the end of November, whole seeds were soaked in 15, 20, and 40% H2O2; 10, 20, and 40% NaOH; and 30, 50, and 98% H2SO4 for 10 min. Whole germination units (seed enclosed by endocarp, hereafter indicated as “seeds”) were soaked in distilled water. Subsequently, the seeds were washed under running water for 2 h, disinfected with 1% NaClO solution for 20 min at room temperature, and washed with sterile distilled water for the germination test (Chien and Lin, 1994). All seeds were disinfected after treatments to avoid seed contamination from the previous operation steps. Seeds were incubated at 25°C in dark and light (22.4 μmol m−2 s−1) at 40 ± 5% humidity; the treatment temperature was 25°C. Three replicates of 50 seeds each were used for the treatments.
Inhibitory Effects of C. migao Seed Extract on Germination
Extraction Method
The endocarps and embryos were separated from the C. migao seeds in the end of November; these parts were then separated and frozen using liquid nitrogen. Next, 50 g of the endocarps and embryos were weighed and extracted using the continuous reflux method. On the basis of the polarity gradient of solvents—petroleum ether (30–60°C, polarity: 0.01) <acetic acid (polarity: 4.3) <acetone (polarity: 5.4) <methanol (polarity: 6.6) <water (polarity: 10.2; 50°C extraction was used in this layer), 200 ml of each extraction solvent was added. After 12 h, the extract was concentrated to approximately 1 ml using a rotary evaporator (RE-6000A). It was then fully dried in an evaporation dish inside a water bath at 20°C; the residue was dissolved in 1 ml of ethyl acetate, and distilled water was added to increase the volume to 50 ml (containing 2% ethyl acetate). This served as a stock solution of 1 g tissue (g ml−1) and was stored at 4°C for <5 days (Shao et al., 2016).
Evaluation of Germination Inhibition
During the end of November, the 1-g tissue (g ml−1) extracts of endocarps and embryos were diluted to 5, 10, 15, and 20% with distilled water. Next, 10 ml of extract or distilled water was added into sterile glass petri dishes (diameter, 9 cm; 3 filter paper were placed at the bottom). Distilled water containing 2% ethyl acetate served as the control. A total of 50 Chinese cabbage (Brassica rapa var. glabra) seeds (Variety Fengkang 90, Guanhe zhuangyuan Co., Ltd., China) were added to each plate replicate, and seeds were incubated at 25°C in the dark. Germination was monitored for 3 days until no further germination was noted; at this time, the length of the seedling hypocotyls and roots were measured using a vernier caliper. After selecting the extract with the strongest inhibitory effect, ryegrass (Lolium perenne) seeds (Big boss, Royal Barenbrug Group, Netherlands) were used to confirm the inhibitory effect of this extract following the same method. Three replicates of 50 seeds were used per treatment.
Determination of Seed Extract Components
Gas chromatography (GC)-mass spectrometry (MS) was performed using HP6890/5975c GC–MS (Agilent, United States). For sample determination, 2 μl of the extract [ethyl acetate and methanol extracts of the endocarp, 1 g tissue (g ml−1)] was injected with a microsyringe. The chromatographic conditions were as follows: capillary column, hp-5 ms (60 m × 0.25 mm × 0.25 μm); initial temperature, 70°C (hold for 2 min), which was increased to 270°C at a rate of 5°C min−1 and then to 310°C at a rate of 8°C min−1 (hold for 9 min) for a total operation time of 56 min; vaporization chamber temperature, 250°C; carrier gas, high purity He (99.999%); pressure, 7.65 psi; carrier gas flow rate, 1.0 ml min−1; split ratio, 10:1; and solvent delay time, 6.0 min. The mass spectrum conditions were as follows: EI ion source with 230°C ion source temperature, 150°C quadrupole temperature, 70-EV electron energy, 34.6 μA emission current, 1,894 v multiplier voltage, 280°C interface temperature, and 29–450 amu mass range (Chen et al., 2010). Peaks in the total ion flow diagram were retrieved via the MS computer data system and were compared with the National Institute of Standards and Technology 2014 and Wiley 275 standard mass spectra to identify the volatile chemical components. The relative mass fraction of each chemical component was determined using the peak area normalization method.
Seed Germination Test
Effects of Light and Temperature Treatments on Seed Germination
The in situ underground relative constant temperature, surface variable temperature, and light conditions were simulated. The light conditions were as follows: incubation in the dark (24 h) and dark/light (8/16 h), with 22.5 μmol m−2 s−1 light intensity and 40 ± 5% humidity. The constant treatment temperatures were 10°C, 20°C, 25°C, and 30°C. The alternating temperatures were 10/20°C, 20/25°C, and 25/30°C; the period of temperature change was 8/16 h. For each treatment, three replicates of 50 fresh seeds were used [fresh seeds were germinated in a sterile seed germination box (length 30 cm × width 20 cm × height 10 cm; 3 layers of filter paper were placed at the bottom) at 40 ± 5% humidity]. The seeds were observed every day, and the criterion for germination was radicle emergence (>2 mm). The experiment began in the early days of December 2017, and the treatment period was 240 days.
Effects of Hormone Treatment on Seed Germination
Fresh, intact seeds were soaked in GA3 aqueous solutions at concentrations of 100 mg L−1, 200 mg L−1, 400 mg L−1, 600 mg L−1, and 800 mg L−1 for 8 h. Afterward, the seeds were disinfected with 1% NaClO solution for 20 min at room temperature and then washed with sterile distilled water. Three replicates of 50 seeds were used per treatment. The seeds were incubated in germination boxes at 25°C in the dark for 30 days (length 30 cm × width 20 cm × height 10 cm); the conditions were dark incubation and 40 ± 5% humidity, and the treatment temperature was 25°C. The experiment began in the early days of December 2017, and the treatment period was 240 days.
Effects of Stratification Treatment on Seed Germination
The seeds were categorized into two groups of 50 seeds each and soaked in distilled water and 200 mg L−1 GA3 solution for 8 h. After disinfection with 1% NaClO solution for 20 min at room temperature, the seeds were washed with sterile distilled water. They were then mixed with wet sand (2 mm diameter; 30% humidity) in a ratio of 1:5 and divided into two equal parts, which were incubated at 4°C and 15°C for 240 days. Every 30 days, 150 seeds from each treatment (three replicates of 50 seeds) were assessed for germination in the dark at. The experiment began in the early days of December 2017, and the treatment period was 240 days.
Data Analysis
Final germination percentage, germination energy, and germination index (GI) were calculated using the following formulas:
where Gt is the number of germinated seeds per day and Dt is that of germination time (d).
The SPSS 21.0 statistical software package (IBM, Chicago, IL, United States) was used for data processing. One-way ANOVA and Duncan’s multiple comparison analysis were used to compare the treatment outcomes. All data were expressed as means ± standard deviations (SD). The data were inverse sine-transformed using the asin() function; data were expressed as means ± SDs and were analyzed using the SPSS 17.0 software (IBM, United States). Statistical differences among the treatments were analyzed using one-way ANOVA followed by Duncan’s post-hoc test. Statistical significance was set at p < 0.05. Origin 2019b (Origin Lab, Northampton, Ma, United States) and Adobe Illustrator 17.0 (California, ADBE, United States) software were used for drawing and processing.
Results
Characteristics of Seeds
The mature C. migao fruits (Figure 1A) were baccate, 1.2–1.3 cm in diameter, and spherical with a slightly flat top (Figure 1B); these grew on the top of a goblet-shaped receptacle (Supplementary Figures S1A–D). The fresh seeds (encapsulated by the endocarp) were black and had a unique aroma. The seeds dried in the dark were brown and pea-shaped (Figure 1C).
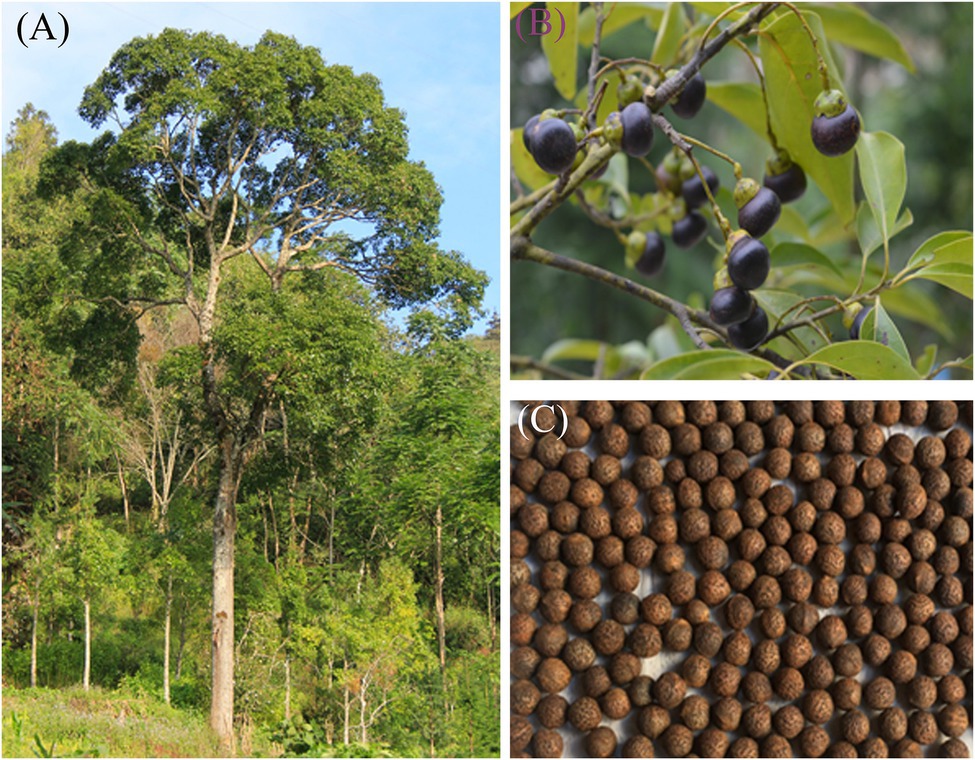
Figure 1. Morphology of Cinnamomum migao mature fruits and seeds. Mature C. migao tree (A), fruits (B), and dried seeds encapsulated by the endocarp (C).
The transverse and longitudinal axis diameters of the seeds were 10.73 ± 0.19 mm and 9.73 ± 0.09 mm, respectively, and the seed thickness was 9.31 ± 0.20 mm. The thousand-grain weight of the dry seeds was 421.34 ± 5.32 g, moisture content was 12.19 ± 1.21%, and viability was 98.67 ± 1.15%.
Characteristics of the Endocarp and Embryo and Evaluation of Seed Imbibition
The endocarp was ossified and closely adhered to the testa, resulting in the separation of the testa from the embryo (Figure 2A). Inside the endocarp were two thick cotyledons (Figure 2B). The radicle, hypocotyl, and germ were small and cone-shaped, connecting the two cotyledons at the apex (Figure 2C). The obvious differentiation of the radicle, hypocotyl, and each part of the embryo indicates that these parts develop completely as the seeds mature (Figure 2D). The endocarp had a layer of stone cells (Figures 2E–H).
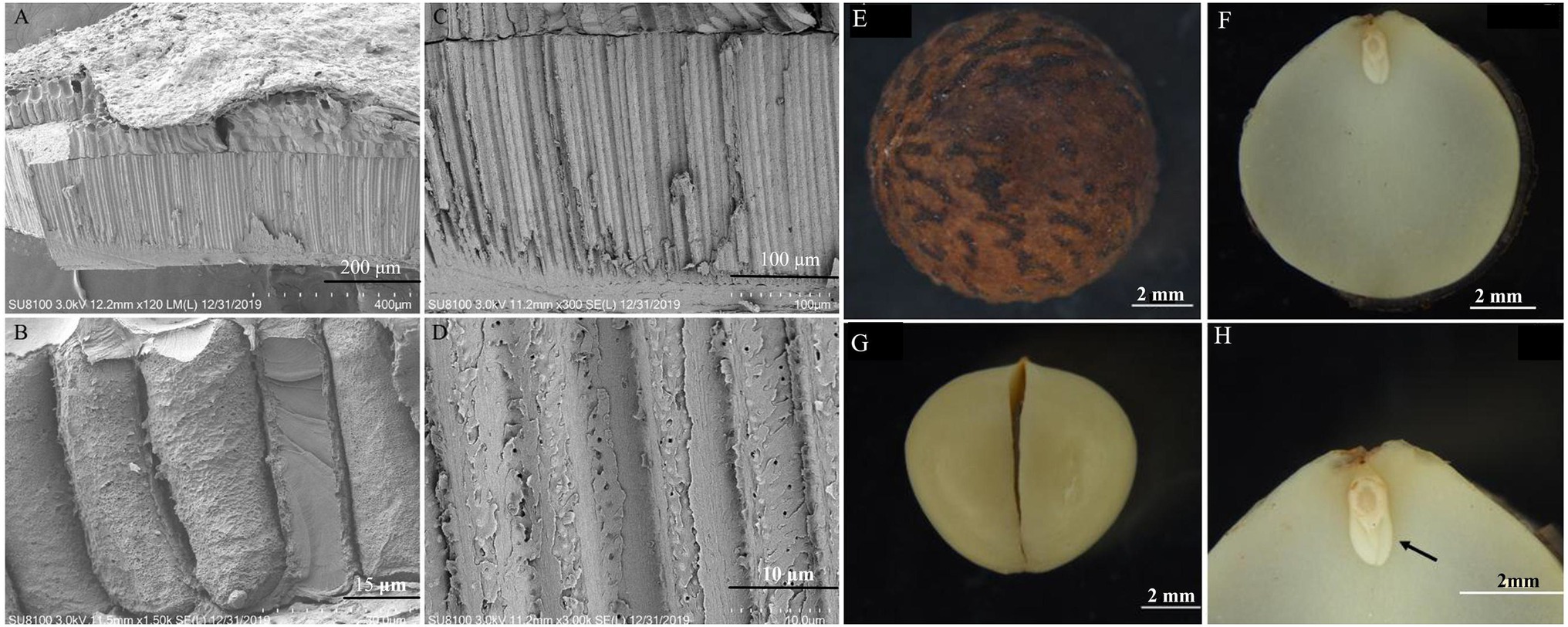
Figure 2. Morphology and anatomy of the Cinnamomum migao seed embryo and endocarp. Scanning electron microscope images of the endocarp (A–D), scale bar: 2 mm. Light microscope images of the dry seed (E), embryo (F), cotyledon (G), plumule (arrow), hypocotyl, and radicle (H).
The imbibition data of the C. migao seeds with the endocarp, with the partial endocarp, and without the endocarp are shown in Supplementary Figure S3. The seed weigh rapidly increased in the first 6 h. After 2 h of imbibition, the mass increases were 10.51, 12.38, and 21.65% for the seeds with the endocarp, with the partial endocarp, and without the endocarp, respectively; after 6 h, these were 21.47, 23.58, and 32.29%, respectively (Figure 3). Mass increase began to plateau after 12 h. The seeds with the endocarp, with the partial endocarp, and without the endocarp attained saturation after 72, 60, and 48 h of imbibition, respectively. Final mass increases were 40.64, 41.21, and 41.76%, respectively, with no significant differences across treatments (p > 0.05; Figure 3).
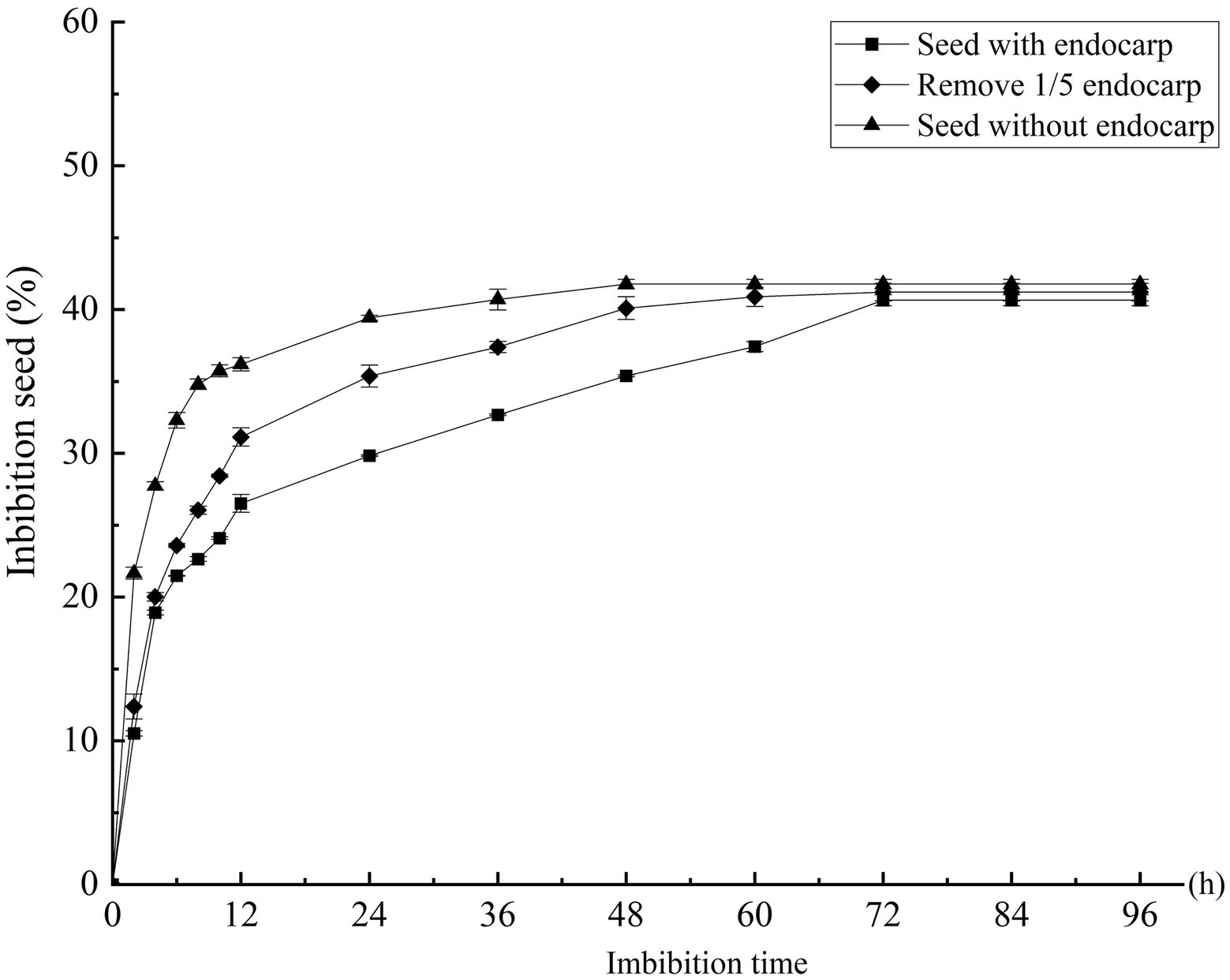
Figure 3. Effect of different treatments on the water absorption rate of Cinnamomum migao seeds. The x-axis denotes the water imbibition time of the seeds, and the y-axis denotes the water absorption percentage. Data are presented as means ± standard deviations for three replicates. Three replicates of 30 seeds each were used for each treatment.
Effects of Oxidant Treatment on Seed Dormancy and Germination
To further investigate how the endocarp influences germination, we treated the seeds with NaOH, H2SO4, and H2O2 before the germination test. Owing to corrosion or oxidation by chemical solvents, the endocarp thinned or the outer structure carbonized, blackened, and peeled off. The final germination percentage of seeds pretreated with 20% H2O2 was the highest at 34% (Figure 4A), which was significantly greater than that of the unstratified seeds (p < 0.05). The final germination percentages did not differ significantly (p > 0.05) in seeds pretreated with NaOH (Figure 4B). In seeds pretreated with H2SO4, the final germination percentage was 31.33% after pretreatment with 50% H2SO4 (Figure 4C), which was significantly higher than that of the unstratified seeds (p < 0.05). However, pretreatment with 98% H2SO4 significantly (p < 0.05) reduced the final germination percentage of the seeds.
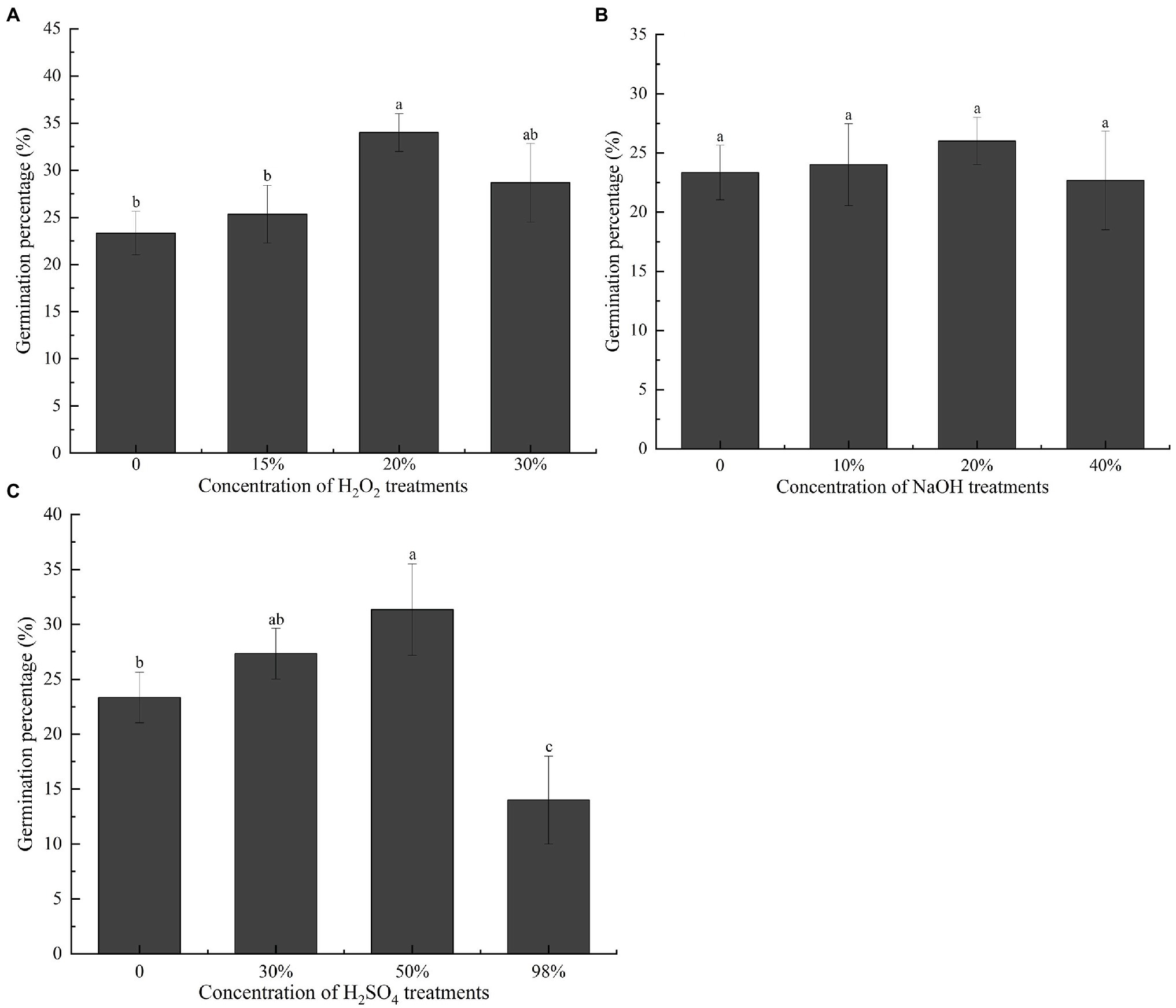
Figure 4. Effect of different chemical treatments on the final germination percentage of Cinnamomum migao seeds. Data are presented as means ± standard deviations (n = 3), and the values marked with different letters in various treatments vary significantly at p < 0.05. Three replicates of 50 seeds each were used for each treatment. (A) Different concentration of H2O2 treatment germination percentage of C. migao seed, (B) different concentration of NaOH treatment germination percentage of C. migao seed, (C) different concentration of H2SO4 treatment germination percentage of C. migao seed.
Inhibitory Effects of C. migao Seed Extract on Cabbage and Ryegrass Seed Germination
Cinnamomum migao endocarp and embryo extracts inhibited the germination of Chinese cabbage seeds (Supplementary Figure S3). Ethyl acetate endocarp extract, when applied at concentrations of 5, 10, 15, and 20%, significantly reduced the seed germination percentage of Chinese cabbage by 8.16, 17.69, 22.45, and 33.33%, respectively, compared with the control (Figure 5A). At the 5% concentration, acetone, methanol, and water extracts significantly (p < 0.05) inhibited the germination of cabbage seeds (Figure 5B). The methanol extract had the strongest inhibitory effect; concentrations of 5, 10, 15, and 20% reduced the final germination percentages of cabbage seeds by 10.20, 21.09, 29.93, and 44.90%, respectively, compared with the control (Figure 5B). Ryegrass seeds were treated with the C. migao extract showing the strongest inhibitory effects (endocarp: ethyl acetate extract; embryo: methanol extract) to confirm the inhibitory effects. The ryegrass seeds were significantly inhibited as the extract concentration increased (Figure 5C), and even the lowest concentration (5%) significantly reduced the final germination percentages. In addition, the growth of the cabbage and ryegrass seedlings was influenced by the C. migao seed extracts (Supplementary Tables S1–S3), with the ethyl acetate and methanol extracts of the endocarp and embryo, respectively, displaying the strongest inhibitory effects on the growth of cabbage seedlings.
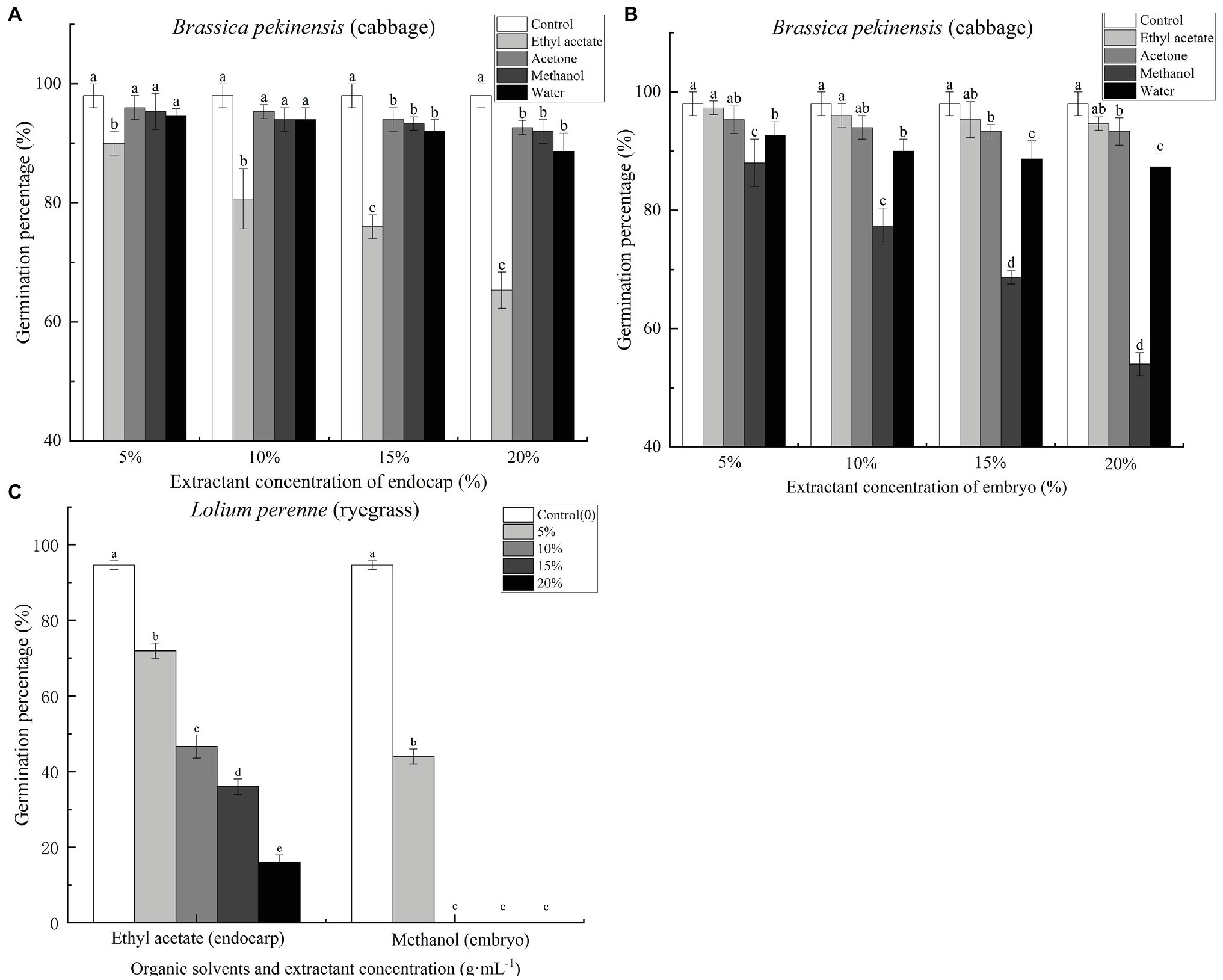
Figure 5. Inhibitory effects of different extracts of Cinnamomum migao seeds on the germination of cabbage and ryegrass seeds. (A) Endocarp extracts and (B) Embryo extracts. (C) Effect of endocarp and embryo extracts on ryegrass seed germination. The x-axis denotes the concentration of the extracts, and the y-axis denotes the germination rate. Data are presented as means ± standard deviations (n = 3). The values marked with different letters indicate the significance levels among the various extract treatments at the same concentration (p < 0.05). Three replicates of 50 seeds each were used for each treatment.
Evaluation of Endogenous Inhibitors in Seed Extract
The ethyl acetate and methanol extracts of C. migao seed endocarps were analyzed using GC-MS (Supplementary Figure S4). We identified 58 endogenous chemical compounds, including 25 in the ethyl acetate extract and 33 in the methanol extract. The primary components were as follows: 4 phenolic compounds, 11 aldehydes, 2 ethers, 8 ketones, 6 alcohols, 9 fatty acids, 11 lipids, 1 benzene, 1 pyrazine, 1 anhydride, and 1 alkene (Figure 6).
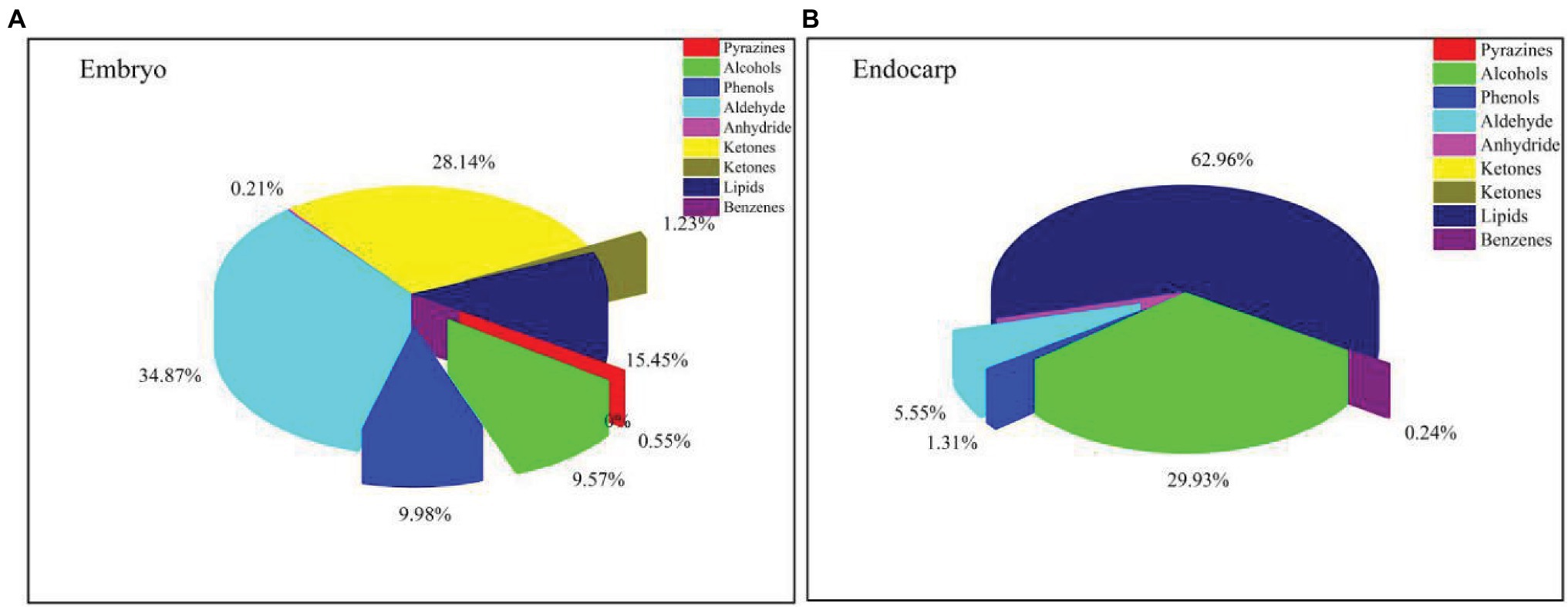
Figure 6. Types and relative percentages of the identified compounds in Cinnamomum migao fruits. (A), Methanol extract of the C. migao embryo, concentration: 1 g tissue (g ml−1). (B), Ethyl acetate extract of the C. migao endocarp, concentration: 1 g tissue (g ml−1).
Effects of Light and Temperature on Seed Germination
The temperature and light treatments influenced the initial seed germination and resulted in significant differences (p < 0.05) in the final germination percentage, germination vigor, and GI of C. migao seeds (Figure 7A). Seeds incubated at 30°C did not germinate until day 46, and the final germination percentage was the lowest at 4.0% (Figure 7B). ANOVA results (Supplementary Table S5) demonstrated that light (F = 0.706, p = 0.408) or the interaction between light and temperature (F = 0.157, p = 0.986) had no significant influence on germination. Nonetheless, the effect of temperature on the final germination percentage was significant (F = 55.33, p < 0.001). Thus, temperature is the primary factor in seed germination.
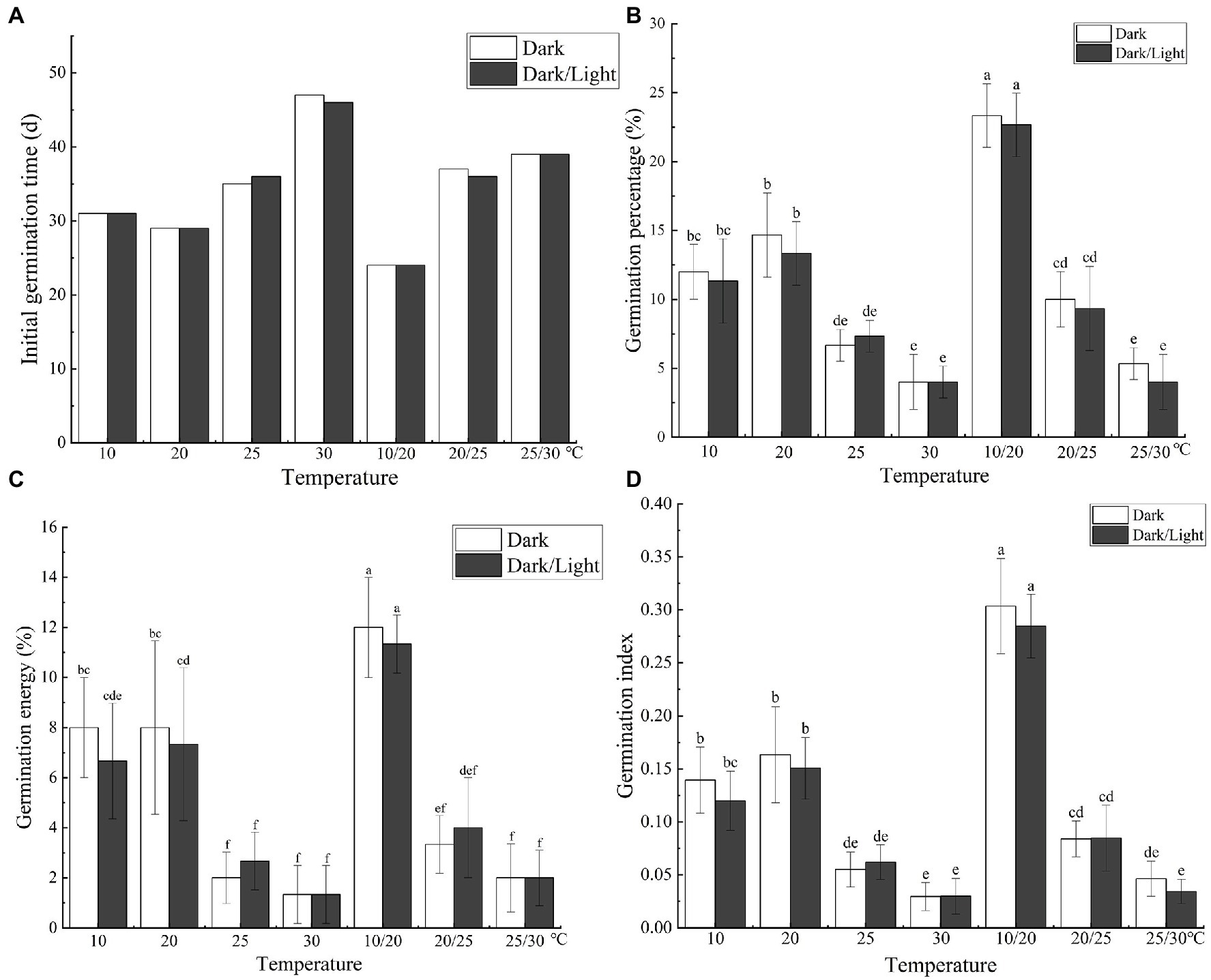
Figure 7. Effects of temperature and light treatments on the germination characteristics of Cinnamomum migao seeds. Data are presented as means ± standard deviations (n = 3), and the values marked with different letters in different treatments vary significantly (p < 0.05). Three replicates of 50 seeds were used in the germination test. (A) Initial germination time, (B) germination percentage, (C) germination energy, (D) germination index.
Effects of Exogenous Hormone Treatment on Seed Germination
Pretreatment with GA3—a dormancy-releasing plant hormone—can effectively reduce the initial germination time of C. migao seeds. The final germination percentage, germination vigor, and GI improved with an increase in the GA3 concentration, reaching a peak at 200 mg L−1 and then decreasing (Figure 8). After the treatment with 200 mg L−1 GA3, the initial germination time was reduced to 19 days, which was 5 days less than that of the control seeds. The final seed germination percentage, germination vigor, and GI were 44.67 ± 3.06%, 23.33 ± 1.15%, and 0.64 ± 0.05 (representing growths of 91.47, 84.14, and 113.33%), respectively, compared with the control group (p < 0.05).
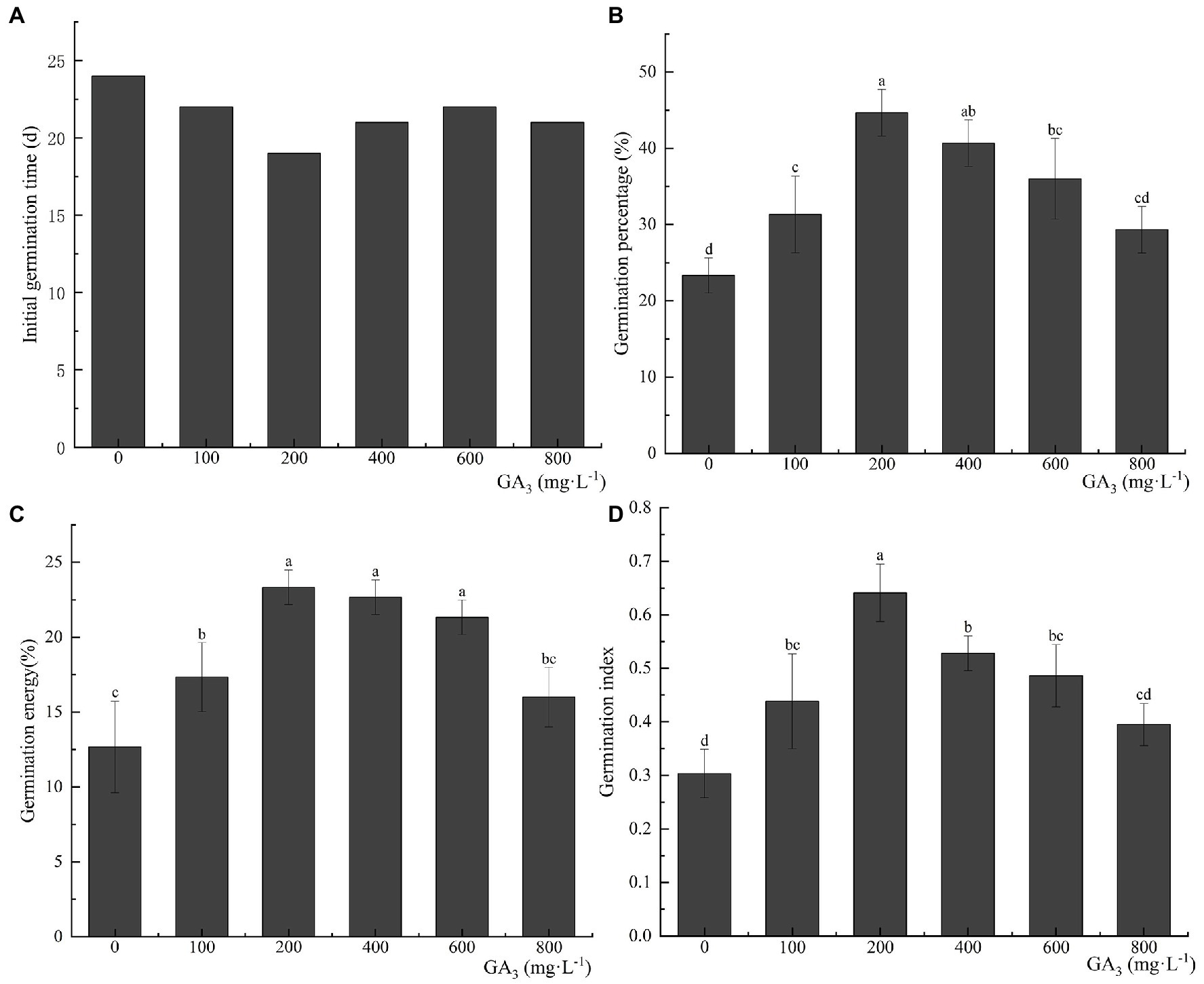
Figure 8. Effects of exogenous GA3 treatment on the germination characteristics of Cinnamomum migao seeds. Data are presented as means ± standard deviations (n = 3), and the values marked with different letters in different treatments vary significantly (p < 0.05). Three replicates of 50 seeds each were used in the germination test. (A) Initial germination time, (B) germination percentage, (C) germination energy, (D) germination index.
Effects of Stratification Period on Seed Germination
The results of the stratification experiment revealed that the final germination percentage of C. migao seeds was higher under the cold/warm stratification + GA3 treatment than that under the stratification treatment alone (Figure 9). In addition, the germination percentage was higher in the warm-temperature stratification treatment (15°C) than that in the cold-temperature treatment (4°C). The germination ability of the seeds treated at 15 and 4°C with GA3 peaked at 60 (13 days for initial germination) and 90 (12 days for initial germination) days of stratification, respectively, which was significantly less than that of the unstratified seeds (Figure 9A). The final germination percentage, germination vigor, and GI were significantly higher (p < 0.05) in the 15°C + GA3- and 4°C + GA3-treatment groups than in the unstratified seeds by 73.11, 89.96, and 128.13% as well as 57.12, 82.90, and 123.44%, respectively (Figures 9B–D). The germination ability of seeds treated at 15 and 4°C peaked after 120 and 150 days of stratification, respectively. The initial germination time in both treatments was 15 days, which was 9 days less than that of the unstratified seeds. When 15°C + GA3 and 4°C + GA3 treatment compared with treatment in the absence of GA3, the final germination percentage, germination vigor, and GI of the unstratified seeds increased significantly by 188.60, 216.67, and 333.33% and 174.32, 194.63, and 306.67%, respectively (p < 0.05). After reaching the peak, the germination of the seeds under different treatments exhibited a downward trend with prolonged stratification time. The final germination percentage and vigor of seeds in 120–180 days declined in a nonsignificant manner (p > 0.05). In the stratification of 180–240 days, the seeds in each treatment group showed different degrees of reduction in the germination. Seeds in the 15°C + GA3 and 4°C + GA3 treatment groups had significantly reduced final germination percentages than those in the same temperature treatment groups but without GA3. Overall, stratification can effectively increase the final germination percentage of C. migao seeds, and GA3 treatment can significantly shorten the stratification period and can thus reduce the initial germination time. The warm-temperature (15°C) treatment was more effective in releasing the dormancy of the seeds than the low-temperature (4°C) treatment, and adding GA3 (15°C + GA3) to the warm-temperature stratification treatment further increased the final germination percentage.
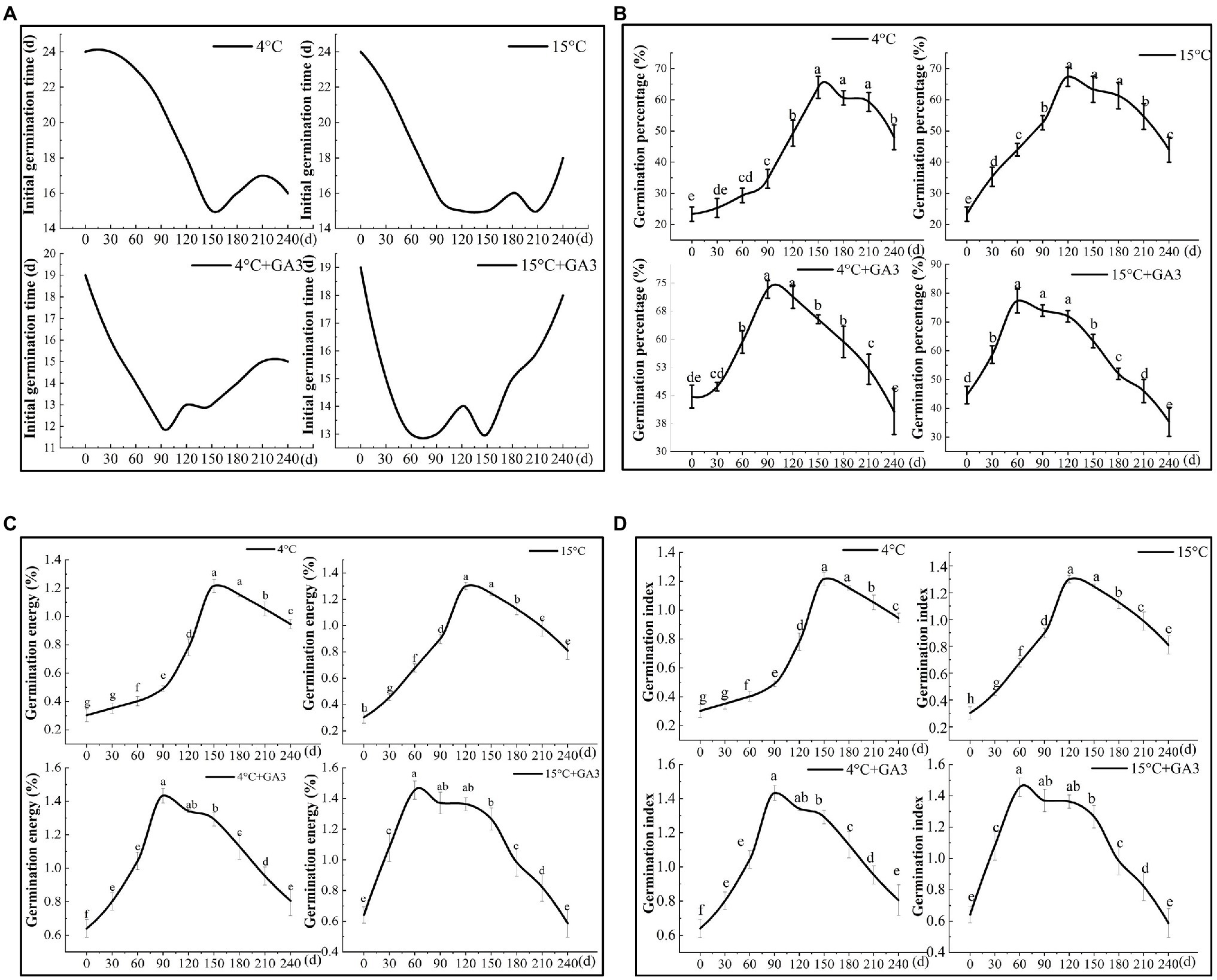
Figure 9. Effects of stratification on the germination characteristics of Cinnamomum migao seeds. Data are presented as means ± standard deviations (n = 3), and the values marked with different letters in different treatments vary significantly (p < 0.05). Three replicates of 50 seeds were used in the germination test. (A) Initial germination time, (B) germination percentage, (C) germination energy, (D) germination index.
Discussion
Dormancy Class and Potential Factors
The complex and diverse factors responsible for seed dormancy are associated with changes in the pericarp, seed coat, and embryo (Meyer, 2008). Therefore, we systematically investigated the possible causes of C. migao seed dormancy from the aspects of morphology, anatomy, and physiology.
MD refers to the dormancy due to the small embryo size, incomplete development, or lack of differentiation after seed maturation. The morphology of the seed embryo is generally spatulate, rudimentary, or linear; in some species, it occupies ≤1% of the seed volume (Jaganathan, 2020; Liu et al., 2020). For example, the Lamprocapnos spectabilis seed endosperm comprises the entire internal tissue of the seed, and the embryo is almost invisible (Cho et al., 2020). Similarly, the Acer yangjuechi seed embryo exhibits poor development and low vigor, which lead to a germination failure under suitable conditions (Chen et al., 2017). However, our results show that the mature embryos of C. migao seeds are fully developed, indicating the absence of MD (Figure 2; Supplementary Figure S1).
The endocarp of C. migao is water-permeable, and thus they do not have PY (Figure 2). Furthermore, no significant difference in the final percentages of imbibition was detected among the seeds with the endocarp, with the partial endocarp, and without the endocarp (Figure 3). The imbibition of C. migao seeds was similar to that of Phoebe bournei seeds belonging to the same family, in which the pericarp does not hinder imbibition (Li and Min, 2020). C. migao seeds with the partial endocarp absorbed water faster than those with the intact endocarp; seeds without the endocarp absorbed water even faster than those with the partial endocarp. This finding is similar the results obtained for the endocarp of Prunus mahaleb (Murphy et al., 2010) and Phillyrea angustifolia (Mira et al., 2015). However, in the present study, the intact seeds were capable of absorbing the same amount of water as that of the seeds without the endocarp, although the imbibition was slower. Hence, the endocarp was not impermeable, which eliminated the PY of C. migao (Figure 3).
To assess PD, we examined whether the C. migao endocarp and embryo extracts contain compounds that inhibit the germination of cabbage and ryegrass seeds. Specifically, the ethyl acetate extract of the endocarp and the methanol extract of the embryo showed significant inhibitory effects. The methanol extract completely inhibited ryegrass germination at concentrations of >0.05 g ml−1 (Figure 5B). The primary components of the extracts with the strongest inhibitory effects were lipids, fatty acids, ketones, aldehydes, and phenolic compounds. Previous studies have demonstrated that inhibitory compounds in the endocarp or the hard middle layer of the testa contribute to low and unstable seed germination (Hamilton and Carpenter, 1976; Richmond and Ghisalberti, 1994; Wu and Shen, 2021). Phenolic and lipid compounds present in the seeds have been widely shown to induce seed dormancy and prevent germination (Graeber et al., 2012; Inácio et al., 2013; Smýkal et al., 2014). Inhibitory compounds present in the seeds can cause physiological inhibition by hindering the growth potential or breakthrough ability of the embryo; as a result, it fails to break the mechanical resistance of the endocarp, thereby negatively influencing seed germination (Baskin and Baskin, 2014). Similarly, previous studies have reported that phenolic compounds present in Lycopersicon esculentum (tomato), Fagus sylvatica, and Pinus laricio in the seeds inhibit the metabolism of key enzymes involved in seed germination (Muscolo et al., 2001; El-Araby et al., 2006). Therefore, according to the classification system of Baskin and Baskin (2004), we determined that C. migao seed dormancy is physiological in nature and is due to endogenous inhibitors and low growth potential of the embryo.
Effects of Various Treatments on C. migao Seed Dormancy
For seeds with obvious dormancy, its release involves changes in the external environment and the physiology and biochemistry of the seed. The release of seed dormancy primarily occurs through the regulation of light, temperature, hormones, and stratification (Cosmas et al., 2019; Hou et al., 2019; Yang et al., 2019). Therefore, we evaluated the effects of light, temperature, an exogenous hormone, and stratification on C. migao seed dormancy and germination.
No significant differences in dormancy release were observed in the seeds under the same temperature but different light treatments. Light neither reduced the initial germination time nor influence the final germination percentage, germination vigor, or GI (Supplementary Table S5). Light-requiring seeds are generally small (Koutsovoulou et al., 2014; Yang et al., 2020), which allows the external light to be perceived by the seed pigments, integrating the metabolism and signal transduction of abscisic acid and GA to control seed dormancy and germination (Bentsink and Koornneef, 2008; Shu et al., 2016). However, C. migao seeds are relatively large and are encapsulated by the firm endocarp (Figures 1, 2), which prevents the internal pigments from perceiving external light (Liu and Lin, 1995).
The low constant temperature and alternating temperatures reduced the initial germination time and significantly increased the final germination percentage, germination vigor, and GI, with the treatment effect being the strongest at 10/20°C. Compared with the interaction of light and temperature, temperature alone significantly promoted the release of dormancy (Figure 7). The natural distribution of C. migao is limited to dry and hot valleys, and the 10/20°C alternating temperature condition is similar to the habitat temperature in spring (Tian et al., 2015). The changing temperature stimulates enzymatic activities in these seeds, which in turn promotes the transformation of the stored materials and releases the dormancy. The 10/20°C alternating temperature treatment may be in line with the natural temperature change in the environment (Chahtane et al., 2016; Kildisheva et al., 2019).
The role of the exogenous hormone GA3 in releasing seed dormancy and increasing the final germination percentage has been extensively studied (Linkies and Leubner-Metzger, 2012). GA3 treatment has been demonstrated to effectively enhance the final germination percentage of Buglossoides arvensis and Cyclocarya paliurus seeds (Fang et al., 2006; de Las Mercedes et al., 2021). GA3 improves α-amylase hydrolysis and glyoxylate cycle enzymatic activities in seeds, thereby activating energy metabolism and enhancing the growth potential of the embryo (Song et al., 2019). In addition, the cold/warm stratification treatment has been shown to release PY (Wolkis et al., 2020). Similar to physical polishing, stratification increases the growth potential of the embryo, thus allowing it to overcome the mechanical resistance to its growth (Baskin and Baskin, 2014). Our results showed that seed treatment with 200 mg L−1 pure exogenous GA3 significantly reduces the initial germination time and the final germination percentage (Figure 8). The 15°C stratification was significantly better than the 4°C stratification, and stratification after GA3 pretreatment resulted in earlier germination peaks than those observed with stratification alone. The combination of 15°C + GA3 was particularly effective, inducing initial germination 6 days earlier than the control seeds. The final germination percentage was as high as 77.33 ± 4.16%, which is significantly higher than those noted in the alternating temperature, light, single hormone, and single stratification treatments (Figure 9). Therefore, combining GA3 pretreatment with stratification was most effective in the rapid release of C. migao seed dormancy (Figure 9B). The hormone treatment activates and accelerates the metabolic activities of the seeds, promoting cell division and radicle growth (Yang et al., 2019), and the stratification treatment influences the hormone contents and metabolic processes. The combination of exogenous hormones and stratification treatment thus accelerated the physiological and biochemical processes.
Release of Seed Dormancy and Natural Regeneration of the C. migao Population
Temperature and cold stratification treatment were effective in releasing the dormancy of C. migao seeds (Figure 9); however, light and dark treatments had no significant effect on dormancy release (Figure 7). The habitats of C. migao—the forests—are heavily impacted by human activities, including fruit harvesting as well as understorey vegetation and leaf litter removal. Therefore, although a large number of fruits fall on the ground after ripening, these are not covered by litter (Zhou, 2003). Owing to the relatively dry surface soil conditions in winter, C. migao seeds may be unable to absorb sufficient water for effective alternating temperature stratification. This phenomenon has been observed in several species. Exposure to the soil surface increases the risk of animal (e.g., rodents) foraging in winter (Gao et al., 2021). In fact, we noted several C. migao seeds that were apparently chewed by rodents. Therefore, the lack of an effective stratification treatment because of human disturbance may significantly hinder the release of dormancy and lead to an extreme shortage of C. migao seedlings. The results provide important insights into the successful regeneration of the C. migao population. For example, covering the seeds under the litter to ensure effective stratification in winter will facilitate seed germination. On the other hand, collecting seeds to artificially release seed dormancy can prevent animal foraging (Chen et al., 2020). Furthermore, the reintroduction of C. migao seedlings into wild population should be considered an effective method to increase the population of this arbor (Wright et al., 2009).
Conclusion
Cinnamomum migao seeds absorb water easily, and the embryo is well developed; no obvious mechanical hindrance to the germination process was identified. However, the seed embryo and endocarp were rich in phenols, aldehydes, which reduced the final germination percentages of cabbage and ryegrass seeds. These compounds may inhibit the germination of fresh C. migao seeds and thus induce/enhance dormancy. The abovementioned results suggest that C. migao seeds exhibit PD. Our findings demonstrated that seed dormancy release was not influenced by light; however, an exogenous hormone, temperature, and stratification treatments influenced seed dormancy release. Overall, GA3 pretreatment followed by a 15°C stratification treatment for 60 days was most effective in relieving C. migao seed dormancy.
Data Availability Statement
The raw data supporting the conclusions of this article will be made available by the authors, without undue reservation.
Author Contributions
X-lH and X-fL: conceptualization and data curation. J-zC: writing–original draft preparation. Q-wS and J-mL: methodology. X-fX, LP, and LZ: writing–review and editing. All authors have read and approved the final version of the manuscript.
Funding
This work was supported by the National Natural Science Foundation of Unite Project, China (grant number: U1812403-2) and the Guizhou Science and Technology Program [Qiankehe (2019) 2774].
Conflict of Interest
The authors declare that the research was conducted in the absence of any commercial or financial relationships that could be construed as a potential conflict of interest.
Publisher’s Note
All claims expressed in this article are solely those of the authors and do not necessarily represent those of their affiliated organizations, or those of the publisher, the editors and the reviewers. Any product that may be evaluated in this article, or claim that may be made by its manufacturer, is not guaranteed or endorsed by the publisher.
Acknowledgments
We express our sincere gratitude toward the three reviewers for their insightful comments that greatly improved the quality of our manuscript.
Supplementary Material
The Supplementary Material for this article can be found online at: https://www.frontiersin.org/articles/10.3389/fpls.2022.770940/full#supplementary-material
References
Baskin, J. M., and Baskin, C. C. (2004). A classification system for seed dormancy. Seed Sci. Res. 14, 1–16. doi: 10.1079/SSR2003150
Baskin, C. C., and Baskin, J. M. (2014). Seeds: Ecology, Biogeography, and Evolution of Dormancy and Germination. San Diego, CA: Elsevier.
Bentsink, L., and Koornneef, M. (2008). Seed dormancy and germination. Arabidopsis Book 6:e0119. doi: 10.1199/tab.0119
Chahtane, H., Kim, W., and Lopez-Molina, L. (2016). Primary seed dormancy: a temporally multilayered riddle waiting to be unlocked. J. Exp. Bot. 68, erw377–erw869. doi: 10.1093/jxb/erw377
Chen, X., Liu, Y., Zhao, M., and Tu, S. (2017). Embryo development and dormancy releasing of Acer yangjuechi, the extremely endangered plant. Sci. Silvae Sin. 53, 65–73. doi: 10.11707/j.1001-7488.20170408
Chen, D. L., Luo, X. P., Yuan, Z., Bai, M. J., and Hu, X. W. (2020). Seed dormancy release of Halenia elliptica in response to stratification temperature, duration and soil moisture content. BMC Plant Biol. 20:352. doi: 10.1186/s12870-020-02560-8
Chen, M. L., Zhou, T., Jiang, W. K., Jin, Y. L., and Yang, Z. N. (2010). Researches on relationship between genetic differentiation and chemical variation of Cinnamomum migao. China J. Chin. Mater. Med. 36, 1409–1415. doi: 10.4268/cjcmm20111101
Chien, C. T., and Lin, T. P. (1994). Mechanism of hydrogen peroxide in improving the germination of Cinnamomum camphora seed. Seed Sci. Technol. 35, 85–86.
Cho, J. S., Jang, B. K., and Lee, C. H. (2018). Seed dormancy and germination characteristics of the endangered species Cicuta virosa L. in South Korea. Hortic. Environ. Biotechnol. 59, 473–481. doi: 10.1007/s13580-018-0062-7
Cho, J. S., Jang, B. K., Lee, S. M., Lee, I. J., and Lee, C. H. (2020). Factors affecting the dormancy and germination of bleeding heart [Lamprocapnos spectabilis (L.) Fukuhara] seeds. Plant Biol. 22, 514–521. doi: 10.1111/plb.13089
Cosmas, P., Agathar, K., Ronald, M., John, C. T., and Simon, M. (2019). Preliminary evaluation of different seed dormancy breaking methods in wild tuber cowpea (Vigna vexillata). Can. J. Agric. Crops 4, 33–40. doi: 10.20448/803.4.1.33.40
de Las Mercedes, L. M., Ricardo, S. M., Diego, B., and Rubén, C. G. (2021). Effect of contrasting maternal nitrogen environments on Buglossoides arvensis seed germination response to gibberellic and abscisic acids. Weed Res. 61, 221–230. doi: 10.1111/WRE.12475
El-Araby, M. M., Moustafa, S., Ismail, A. I., and Hegazi, A. (2006). Hormone and phenol levels during germination and osmopriming of tomato seeds, and associated variations in protein patterns and anatomical seed features. Acta Agron. Hung. 54, 441–457. doi: 10.1556/AAgr.54.2006.4.7
Ensslin, A., Van de Vyver, A., Vanderborght, T., and Godefroid, S. (2018). Ex situ cultivation entails high risk of seed dormancy loss on short-lived wild plant species. J. Appl. Ecol. 55, 1145–1154. doi: 10.1111/1365-2664.13057
Fang, S., Wang, J., Wei, Z., and Zhu, Z. (2006). Methods to break seed dormancy in Cyclocarya paliurus (Batal) Iljinskaja. Sci. Hortic. 110, 305–309. doi: 10.1016/j.scienta.2006.06.031
Finch-Savage, W. E., and Leubner-Metzger, G. (2006). Seed dormancy and the control of germination. New Phytol. 171, 501–523. doi: 10.1111/j.1469-8137.2006.01787.x
Gao, R., Hou, J., Zhao, R., Yang, X., and Walck, J. L. (2021). Seed dormancy and germination of a critically endangered plant, Elaeagnus mollis, on the loess plateau of China. Eur. J. For. Res. 140(Suppl. 1), 1–11. doi: 10.1007/s10342-020-01342-z
Gao, P., Liu, J. M., Li, X. L., Luo, C., and Wang, J. C. (2015). Biological characteristics of Cinnamomum migao seed. Guangdong Agric. Sci. 2015:22. doi: 10.16768/j.issn.1004-874x.2015.22.005
Graeber, K., Nakabayashi, K., Miatton, E., Leubner-Metzger, G. L., and Soppe, W. J. J. (2012). Molecular mechanisms of seed dormancy. Plant Cell Environ. 35, 1769–1786. doi: 10.1111/j.1365-3040.2012.02542.x
Hamilton, D. F., and Carpenter, P. L. (1976). Regulation of seed dormancy in Elaeagnus angustifolia by endogenous growth substances. Can. J. Bot. 54, 1068–1073. doi: 10.1139/b76-113
Hou, L., Wang, M., Wang, H., Zhang, W. H., and Mao, P. (2019). Physiological and proteomic analyses for seed dormancy and release in the perennial grass of Leymus chinensis. Environ. Exp. Bot. 162, 95–102. doi: 10.1016/j.envexpbot.2019.02.002
Huang, X. L., Chen, J. Z., Liu, J. M., Li, J., Wu, M., and Tong, B. (2019). Autotoxicity hinders the natural regeneration of Cinnamomum migao H.W. Li in Southwest China. Forests 10:919. doi: 10.3390/f10100919
Huang, X. L., Tian, T., Chen, J. Z., Wang, D., Tong, B., and Liu, J. (2021). Transcriptome analysis of Cinnamomum migao seed germination in medicinal plants of Southwest China. BMC Plant Biol. 21:270. doi: 10.1186/s12870-021-03020-7
Inácio, M. C., Moraes, R. M., Mendonça, P. C., Morel, L. J. F., and França, S. C. (2013). Phenolic compounds influence seed dormancy of Palicourea rigida H.B.K. (Rubiaceae), a medicinal plant of the Brazilian savannah. Am. J. Plant Sci. 4, 129–133. doi: 10.4236/ajps.2013.41017
Jaganathan, G. K. (2020). Defining correct dormancy class matters: morphological and morphophysiological dormancy in Arecaceae. Ann. For. Sci. 77:100. doi: 10.1007/s13595-020-01010-7
Kildisheva, O. A., Dixon, K. W., Silveira, F. A. O., Chapman, T., Di Sacco, A., Mondoni, A., et al. (2020). Dormancy and germination: making every seed count in restoration. Restor. Ecol. 28(Suppl. 3), S256–S265. doi: 10.1111/rec.13140
Kildisheva, O. A., Erickson, T. E., Kramer, A. T., Zeldin, J., and Merritt, D. J. (2019). Optimizing physiological dormancy break of understudied cold desert perennials to improve seed-based restoration. J. Arid. Environ. 170:104001. doi: 10.1016/j.jaridenv.2019.104001
Koutsovoulou, K., Daws, M. I., and Thanos, C. A. (2014). Campanulaceae: a family with small seeds that require light for germination. Ann. Bot. 113, 135–143. doi: 10.1093/aob/mct250
Li, L. X. (2017). Study on Cinnamomum migao population characteristics in Guizhou. Guizhou University.
Li, X. W., Li, J., and Henk, W. (2013). Flora of China. Beijing: Science publishing house Missouri botanical garden publishing house. Available at: http://www.iplant.cn/info/Cinnamomum%20migao?t=foc
Li, T., and Min, X. H. (2020). Dormancy characteristics and germination requirements of Phoebe bournei seed. Sci. Hortic. 260:108903. doi: 10.1016/j.scienta.2019.108903
Linkies, A., and Leubner-Metzger, G. (2012). Beyond gibberellins and abscisic acid: how ethylene and jasmonates control seed germination. Plant Cell Rep. 31, 253–270. doi: 10.1007/s00299-011-1180-1
Liu, N., and Lin, T. (1995). Micromorphological characteristics of endocarp and testa of four Cinnamomum medicinal plants in Guizhou. J. Guizhou Nor. Univ. 3, 22–26.
Liu, H. N., Zhang, K. L., Zhao, D. Q., Sun, J., Meng, J. S., and Tao, J. (2020). Advances in studies of seed dormancy and germination. Mol. Plant Breed. 18, 621–627. doi: 10.13271/j.mpb.018.000621
Ma, M., Collins, S. L., and Du, G. (2020). Direct and indirect effects of temperature and precipitation on alpine seed banks in the Tibetan plateau. Ecol. Appl. 30:e02096. doi: 10.1002/eap.2096
Meyer, S. E. (2008). “Rosa L.: Rose, briar,” in The Woody Plant Seed Manual, Agriculture Handbook No. 727. eds. F. T. Bonner and R. P. Karrfalt (Washington, DC: US Department of Agriculture, Forest Service), 974–980.
Ministry of Ecology and Environment, P.R.C (2011). The list of red species of biodiversity in China: higher plants volume.
Mira, S., Veiga-Barbosa, L., and Pérez-García, F. (2015). Seed germination characteristics of Phillyrea angustifolia L. and P. latifolia L. (Oleaceae), two Mediterranean shrub species having lignified endocarp. Ann. For. Res. 58, 27–37. doi: 10.15287/afr.2015.304
Murphy, K., Bradley, J., and James, H. E. (2010). Effect of endocarp removal, gibberellin, stratification and sulfuric acid on germination of mahaleb (Prunus mahaleb L.) seeds. Am. Eurasian J. Agric. Environ. Sci. 49, 27–33. doi: 10.1128/JCM.01311-10
Muscolo, A., Panuccio, M., and Sidari, M. (2001). The effect of phenols on respiratory enzymes in seed germination-respiratory enzyme activities during germination of pinus laricio seeds treated with phenols extracted from different forest soils. Plant Growth Regul. 35, 31–35. doi: 10.1023/A:1013897321852
Nonogaki, H. (2019). Seed germination and dormancy: the classic story, new puzzles, and evolution. J. Integr. Plant Biol. 61, 541–563. doi: 10.1111/jipb.12762
Qiu, D. W., and Du, M. D. (2010). Research and industrialization of the subsystem of Guizhou specialty medicinal Cinnamomum migao of fruit. Guiyang Coll. J. Tradit. Chin. Med. 25, 48–51. doi: 10.16588/j.cnki.issn1002-1108.2003.01.030
Richmond, G. S., and Ghisalberti, E. L. (1994). Seed dormancy and germination mechanisms in Eremophila (Myoporaceae). Aust. J. Bot. 42:705. doi: 10.1071/BT9940705
Rubio de Casas, R. R., Kovach, K., Dittmar, E., Barua, D., Barco, B., and Donohue, K. (2012). Seed after-ripening and dormancy determine adult life history independently of germination timing. New Phytol. 194, 868–879. doi: 10.1111/j.1469-8137.2012.04097.x
Shao, C., Liu, J. Y., Zhang, S. N., and Zhang, Y. Y. (2016). Bioassay of endogenous germination inhibitors in Trillium kamtschaticum seed. Seed Sci. Technol. 44, 224–232. doi: 10.15258/sst.2016.44.1.16
Shi, H., Shi, Q., Wang, T., Qin, J., and Wang, Y. (2003). “Cinnamomum migao, a endemic the miao nationality plant.” in Special Edition of National Miao Medicine Symposium in China. China.
Shu, K., Liu, X. D., Xie, Q., and He, Z. H. (2016). Two faces of one seed: hormonal regulation of dormancy and germination. Mol. Plant 9, 34–45. doi: 10.1016/j.molp.2015.08.010
Smýkal, P., Vernoud, V., Blair, M. W., Soukup, A., and Thompson, R. D. (2014). The role of the testa during development and in establishment of dormancy of the legume seed. Front. Plant Sci. 5:351. doi: 10.3389/fpls.2014.00351
Song, Q., Cheng, S., Chen, Z., Nie, G., Xu, F., Zhang, J., et al. (2019). Comparative transcriptome analysis revealing the potential mechanism of seed germination stimulated by exogenous gibberellin in Fraxinus hupehensis. BMC Plant Biol. 19:199. doi: 10.1186/s12870-019-1801-3
Souza, V. C., Pollyanna, F. M. A., Leonaldo, A. A., Irenice, G. O., and Lamartine, S. O. (2010). Germination of seeds of the invasive plant Sesbania virgata (Cav.) Pers. under effects of light, temperature, and dormancy overcoming. Semina Ciências Agrárias 31, 889–894. doi: 10.5433/1679-0359.2010v31n4p889
Stevens, A. V., Nicotra, A. B., Godfree, R. C., and Guja, L. K. (2020). Polyploidy affects the seed, dormancy and seedling characteristics of a perennial grass, conferring an advantage in stressful climates. Plant Biol. 22, 500–513. doi: 10.1111/plb.13094
Tang, A. J., Long, C. L., and Dao, Z. L. (2004). Review on development of seed dormancy mechanisms. Acta Bot. Yunnanica 26, 241–251. doi: 10.1088/1009-0630/6/5/011
Tian, Y. C., Liang, M. Z., and Hu, B. Q. (2015). Analysis on characteristics of extre-me temperature changes in red river basin from 1961 to 2011. Res. Devel. Mar. 31, 312–315. doi: 10.3969/j.issn.1005-8141.2015.03.014
Wang, J. H., Zhang, N., Wang, J., and Wu, K. Y. (2016). Main nutritional components in Cinnamomum migao seed. Guizhou Agric. Sci. 44, 117–119.
Wolkis, D., Blackwell, B., and Villanueva, S. K. (2020). Conservation seed physiology of the ciénega endemic, Eryngium sparganophyllum (Apiaceae). Conserv. Physiol. 8:coaa017. doi: 10.1093/conphys/coaa017
Wright, B. R., and Clarke, P. J. (2009). Fire, aridity and seed banks. What does seed bank composition reveal about community processes in fire-prone desert. J. Vegetation Sci. 20, 663–674. doi: 10.1111/j.1654-1103.2009.01051.x
Wu, Y., and Shen, Y. B. (2021). The structural and chemical characteristics of the pericarp are important in Tilia miqueliana seed dormancy. New For. 52, 875–888. doi: 10.1007/s11056-020-09820-3
Yan, A., and Chen, Z. (2020). The control of seed dormancy and germination by temperature, light and nitrate. Bot. Rev. 86, 39–75. doi: 10.1007/s12229-020-09220-4
Yang, B., Cheng, J., Wang, J., Cheng, Y., He, Y., Zhang, H., et al. (2019). Physiological characteristics of cold stratification on seed dormancy release in rice. Plant Growth Regul. 89, 131–141. doi: 10.1007/s10725-019-00516-z
Yang, L., Liu, S., and Lin, R. (2020). The role of light in regulating seed dormancy and germination. J. Integr. Plant Biol. 62, 1310–1326. doi: 10.1111/jipb.13001
Zhou, T. (2003). Study on molecular Pharmacognosy of Cinnamomum migao. Guiyang College of Traditional Chinese Medicine. H.W. Li of Miao minority nationality herbs and its related species.
Zhou, T., Yang, Z. N., Jiang, W. K., Ai, Q., and Guo, L. P. (2010). Variation and regularity of volatile oil constituents in fruits of national medicine Cinnamomum migao. China J. Chin. Mat. Med. 35, 852–856. doi: 10.4268/cjcmm20100710
Keywords: regeneration, Cinnamomum migao, dormancy and germination trait, endogenous inhibitor, seed stratification
Citation: Chen J-z, Huang X-l, Xiao X-f, Liu J-m, Liao X-f, Sun Q-w, Peng L and Zhang L (2022) Seed Dormancy Release and Germination Requirements of Cinnamomum migao, an Endangered and Rare Woody Plant in Southwest China. Front. Plant Sci. 13:770940. doi: 10.3389/fpls.2022.770940
Edited by:
Xiaowen Hu, Lanzhou University, ChinaReviewed by:
Hongyuan Ma, Northeast Institute of Geography and Agroecology, Chinese Academy of Science (CAS), ChinaCarol C. Baskin, University of Kentucky, United States
Copyright © 2022 Chen, Huang, Xiao, Liu, Liao, Sun, Peng and Zhang. This is an open-access article distributed under the terms of the Creative Commons Attribution License (CC BY). The use, distribution or reproduction in other forums is permitted, provided the original author(s) and the copyright owner(s) are credited and that the original publication in this journal is cited, in accordance with accepted academic practice. No use, distribution or reproduction is permitted which does not comply with these terms.
*Correspondence: Ji-ming Liu, Z3VpZGFoMzY1QDEyNi5jb20=; Xiao-long Huang, a2Fyc3QwNjIzQDE2My5jb20=