- 1School of Life Science, Changchun SCI-TECH University, Changchun, Jilin, China
- 2School of Architecture and Urban Planning, Changchun University of Architecture and Civil Engineering, Changchun, Jilin, China
Recent changing patterns of global climate have turned out to be a severe hazard to the horticulture crops production. A wide range of biotic and abiotic stresses often affect plants due to their sessile nature. Horticultural crop losses are mainly caused by abiotic factors such as drought, salt, heat, cold, floods, and ultraviolet radiation. For coping up with these adversities, well-developed mechanisms have been evolved in plants, which play a role in perceiving stress signals and enabling optimal growth responses. Interestingly, the use of phytohormones for suppressing the impact of abiotic stress has gained much attention in recent decades. For circumvention of stress at various levels, including physiological, molecular, as well as biochemical, a sophisticated mechanism is reported to be provided by the phytohormones, thus labeling these phytohormones a significant role in plant growth and development. Phytohormones can improves tolerance against abiotic stresses by increasing seed germination, seedling growth, leaf photosynthesis, root growth, and antioxidant enzymes and reducing the accumulation of reactive oxygen species, malonaldehyde, and electrolyte leakage. Recent discoveries highlight the significant role of a variety of phytohormones including melatonin (MEL), Gamma-aminobutyric acid (GABA), jasmonic acid (JA), salicylic acid (SA), brassinosteroids (BRs), and strigolactones (SLs) in abiotic stress tolerance enhancement of horticultural plants. Thus, current review is aimed to summarize the developmental concepts regarding role of phytohormones in abiotic-stress mitigation, mainly in horticultural crops, along with the description of recent studies which identified the role of different phytohormones in stressed environments. Hence, such a review will help in paving the path for sustainable agriculture growth via involvement of phytohormones in enhancement of abiotic stress tolerance of horticultural crops.
Introduction
With recent changes in global climate, along with the elevations in world population, an increment in the agricultural productivity is a need of time. The estimated agricultural output by the mid-century must be 70 percent more than the current output, for fulfilling the requirements of world growing population (Francini and Sebastiani, 2019). Moreover, climate variabilities also significantly affect production of horticultural crops. Various abiotic and biotic stresses are important factors, limiting the agricultural yield and productivity (Mangal et al., 2022). The response of plants towards varying environmental stimuli is one of the most critical questions, both for agronomists and plant biologists. Amid different environmental stresses affecting growth and development of plants, salt, drought and heat stress are the most important, as well as common ones (Wani et al., 2016). Due to the complex characteristics associated with stress tolerance, traditional breeding techniques show low efficacy, thus needing advancements for filling the gap between world food supply and demand. Development of new and effective methods is a necessity in this area. One of the viable alternatives and realistic option for growing highly productive climate-resilient crops is the phytohormones. Recently, phytohormones are emerged as highly eco-friendly alternative approach, which help to enhance abiotic stress tolerance, particularly in horticultural plants. Phytohormones are the plants’ released chemical regulators targeting the regulation of plant responses, growth, and development under environmental stresses (Kohli et al., 2013; Verma et al., 2016). The important role of phytohormones under abiotic stresses is through coordination of differential signal transduction pathways (Saini et al., 2021; Salvi et al., 2021). They also get involve in regulation of different stimuli, both internal and external, thus bringing key changes in development of plants (Ku et al., 2018). Hitherto, the role of phytohormones as signaling molecules in abiotic stress resistance has been studied in horticultural plants (Wu et al., 2018; Sytar et al., 2019), and these phytohormones also play significant role in crop production of horticultural plants (Figure 1) (Ciura and Kruk, 2018). In recent studies on abiotic stress tolerance, the regulatory role of phytohormones in various plant processes including physiological, molecular, and biochemical, has been highlighted (Arif et al., 2020). In the light of above background, the key focus of current review is to highlight the conceptual improvements in abiotic stress tolerance of horticultural plants through different phytohormones’ functioning, including brassinosteroids (BRs), melatonin (MEL), salicylic acid (SA), jasmonates (JAs), strigolactones (SLs), and gamma-aminobutyric acid (GABA). Figure 1 depicts the illustration of role of these phytohormones.
Effects of abiotic stress on horticultural crop performance
Starting from seed germination and through the whole life cycles, plants face multiple stresses (Figure 2). Various abiotic stressors cause crop losses via affecting quality and yield of crop products, including salinity, heat, drought, and nutrient deficiency stresses (Andreotti, 2020). Abiotic stresses impact is not only on yield but also on the product quality, causing morphological, physiological, and biochemical alterations (Rao et al., 2016). Recent changing climatic events also pose multiple abiotic stresses to horticultural crops. Changing climate is also labeled as an eminent challenge that agriculture sector must suffer in the future (Francini and Sebastiani, 2019; Shahid et al., 2021; Gao et al., 2022). A wide range of stress responses are reported in plants, including decline in photosynthetic machinery yield, leaf water potential, membrane integrity, photosynthetic pigments, plant growth, and yield (Ullah et al., 2018). Further, single and/or multiple stress conditions affecting 90 percent of agricultural lands. The horticulture sector is therefore actively seeking for new agronomic tools that are able to contrast the adversities of environmental factors, while maintaining the overall sustainability as well as quality of the production. In this regard, the horticultural plants are protected through various plant hormones against abiotic stressors (Table 1).
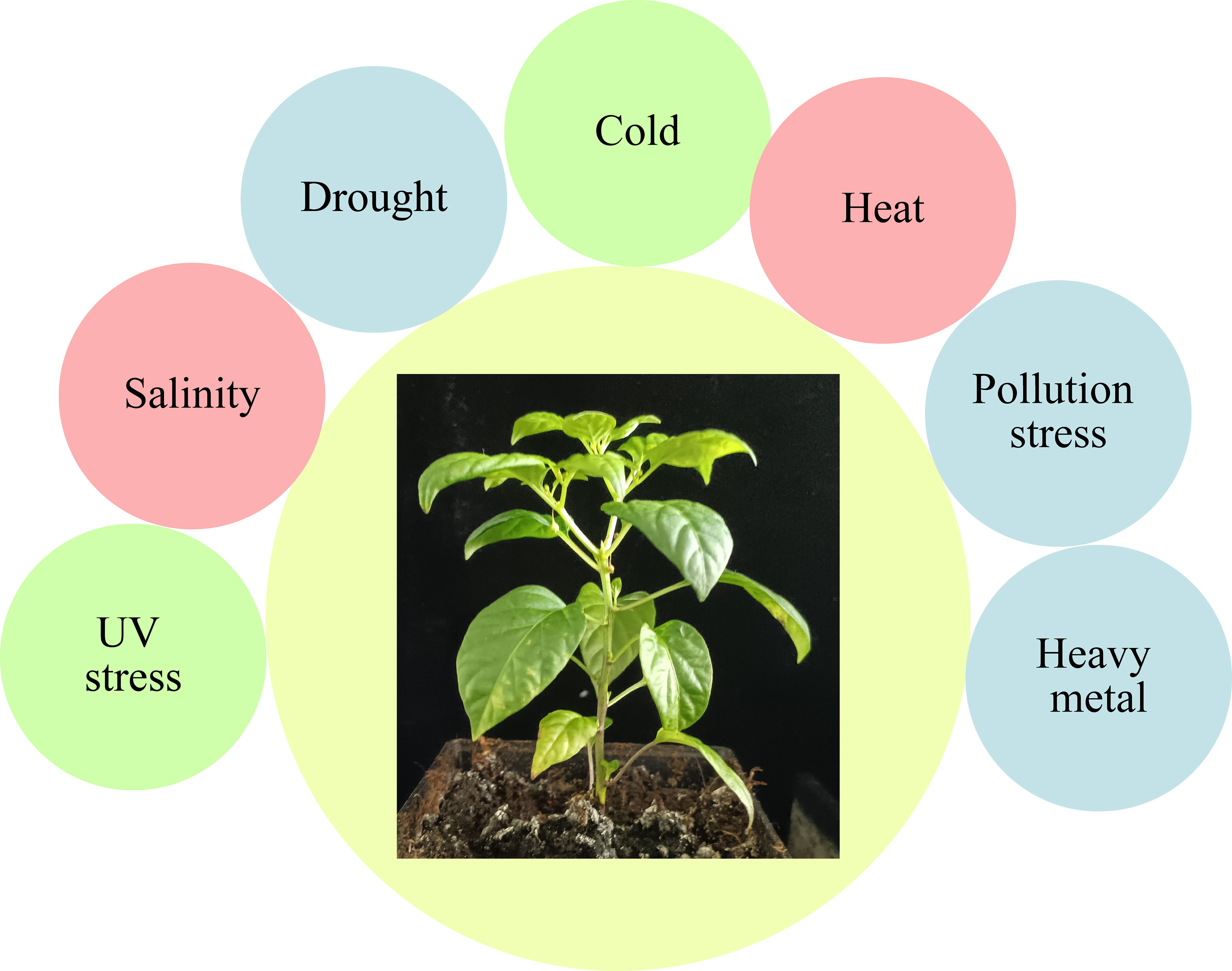
Figure 2 Illustration of several stress factors that impede horticulture plants growth and development.
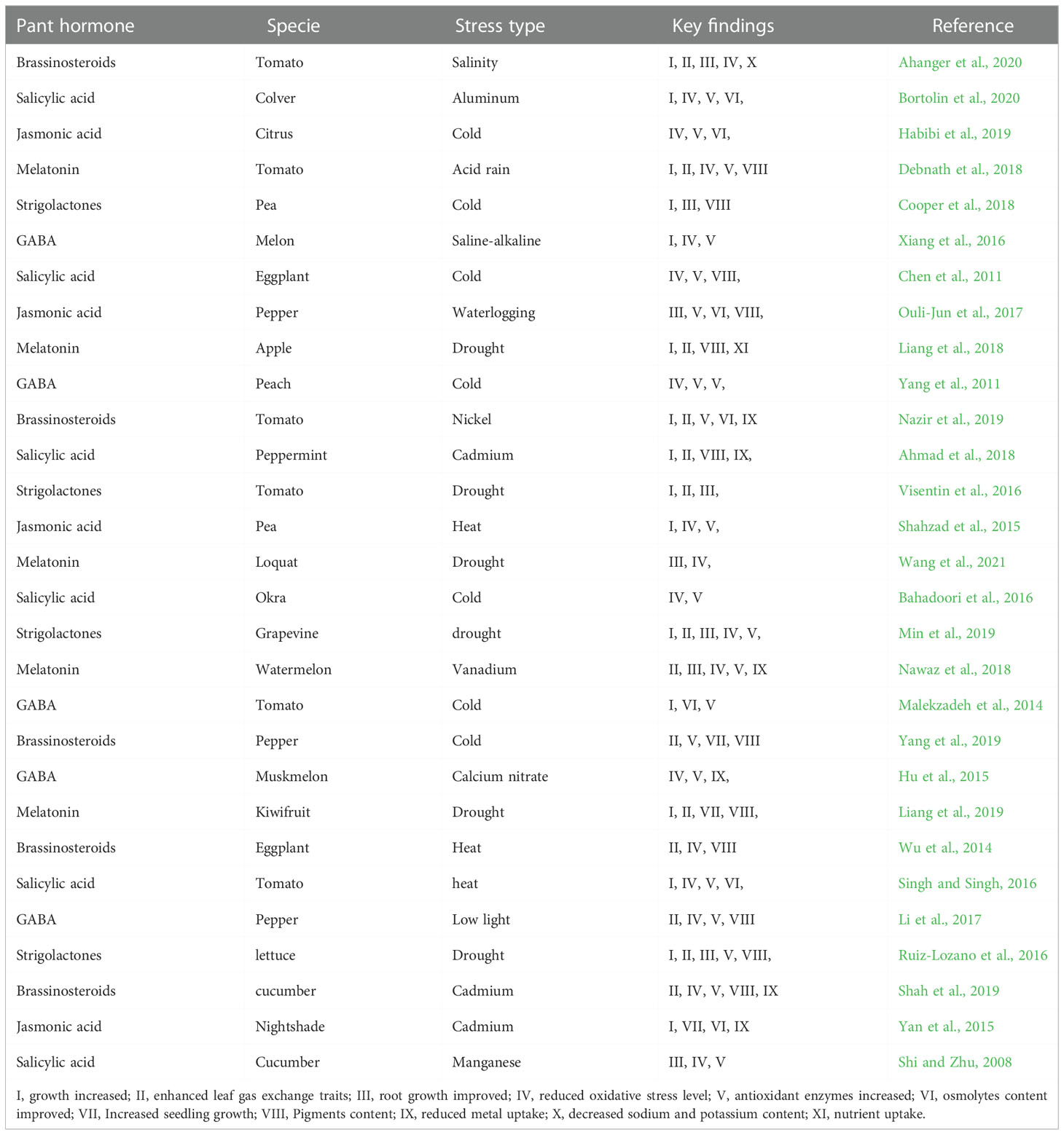
Table 1 Phytohormone-mediated regulation of abiotic stress-impacts in horticultural crops: a summary of representative research.
Phytohormones: Key mediators of plant responses to abiotic stresses
Other than playing roles in developmental processes, endogenous phytohormones are significantly involved in abiotic stress tolerance, and are mentioned as key mediators of responses in plants under stress conditions. Plant hormones are small and signaling molecules, acting virtually to some extent, in every aspect of growth and development of plants (Ullah et al., 2018). The acting mechanism behind various processes can vary with different hormones. Thus, a single hormone is sometimes observed to regulate a wide range of processes, both cellular and developmental, whereas, simultaneously, a single process might get regulated by multiple hormones (Ciura and Kruk, 2018). Phytohormones which are crucial for growth and development of plants include BRs, MEL, SA, JA, GABA, and SLs (Figure 3), providing support and management to plants against biotic and abiotic stressors (Sytar et al., 2019). Hence, the application of phytohormones is carried out for enhancing the future crop stress management research.
Brassinosteroids
Brassinosteroids (BRs) are steroidal plant compounds that are non-toxic, multifunctional, and poly-hydroxylated (Yasir and Wasaya, 2021). 28-homobrassinolide (28-HBL), Brassinolide (BL) and 24-epibrassinolide (24-EBL) are the most effective BRs which are commonly used in plant molecular and physiological research. Seed germination, cell division, senescence, stomatal opening, seedling development, root growth, and vascular differentiation are all aided by BRs (Ullah et al., 2018; Chaudhuri et al., 2022). Different morphological and physiological processes in horticultural crops are effectively controlled by BRs (Mumtaz et al., 2022). Currently, it is clarified that BRs may confer significant tolerance in plants towards many abiotic stressors such as salt, water logging, drought, metals, and high and low temperatures (Ahammed et al., 2020; Kour et al., 2021). The stress protection and growth regulatory ability of BRs nexuses strongly with the protein and nucleic acid biosynthesis, and photosynthesis related metabolic processes (Upreti and Sharma, 2016). Ding et al. (2012) reported that BRs supplementation significantly enhanced growth and antioxidant enzymes pool and reduced electrolyte leakage (EL), malonaldehyde (MDA), sodium and chloride content in eggplant under salt stress. Under salt stress, BRs application efficiently improved strawberry growth characteristics, ion homeostasis, activity of antioxidant enzymes, leaf photosynthesis, and cellular membrane integrity (Karlidag et al., 2011). Furthermore, 24-EBL treatment increased photosynthesis-related characteristics in pepper under drought stress (Hu et al., 2013). Mahesh et al. (2013) discovered that 24-EBL and 28-HBL supplementation boosted osmolyte content, antioxidant enzymes, and reduced levels of MDA under drought stress in radish crop. Kapoor et al. (2014) revealed that 24-EBL application enhanced radish photosynthetic pigments content, level of secondary metabolites under metal (cadmium and mercury) toxicity. Moreover, 24-EBL significantly modulates the ascorbate-glutathione (AsA-GSH) pool.
Exogenous BRs have been proven to alleviate the deleterious effects of abiotic stress on a number of horticultural plants. In Cucumis sativus L., Solanum lycopersicum L., Prunus persica L., and Raphanus sativus L., BRs boosted antioxidant enzymes (SOD, CAT, POD, APX), chlorophyll content, secondary metabolites, and gas exchange components (Ge et al., 2014; Choudhary et al., 2012). Furthermore, the use of 24-EBR significantly enhanced secondary metabolite, leaf photosynthetic parameters, and chlorophyll content in cucumber (Yu et al., 2004). Choudhary et al. (2011) discovered that supplementation with 24-EBR effectively improved growth parameters, secondary metabolites, antioxidant enzyme activity, and decreased oxidative stress biomarkers in Raphanus sativus L. under copper stress. Under metal toxicity, 24-EBL efficiently enhanced root architectural system, chlorophyll content, antioxidant metabolites, and AsA-GSH pool and lowered oxidative damage in grape (Zhou et al., 2018). Under nickel toxicity, 24-EPR supplementation effectively improved metabolic function of tomato (Soares et al., 2016). Under cadmium stress, Hayat et al. (2012) discovered that BRs treatment boosted growth status, yield attributes, photosynthetic pigments content, and antioxidant system in Solanum lycopersicum. In another study, when grapevine seedlings are exposed to chilling stress, 24-EBL application balances the antioxidant machinery, and improves osmolyte content (Xi et al., 2013). The positive regulation of growth and development of sweet pepper plants by BR application has been reported through suppressing the adversities of different abiotic stress factors. (Hegazi et al., 2017), pea (Shahid et al., 2011), cucumber (Hu et al., 2010), and fenugreek (Swamy et al., 2014), and radish (Alper and Sharma, 2013) by overcoming the negative effect of multiple abiotic stresses. Though the variability in BRs’ physiological responses is due to complex actions of molecular mechanisms, the plants’ stress response management is highly dependent on their potential of improving tolerance towards abiotic stresses through BRs. Deep investigations are required in future for effective gains and enhanced scope of BRs research, mainly through studying the BRs stress tolerance mechanisms.
Melatonin
Melatonin is a potential antioxidant with a low molecular weight (Nawaz et al., 2016). Previous research demonstrated that MEL is involved in a variety of plant activities during the vegetative and reproductive stages of plants, including seed germination, seedling growth, root architecture, blooming, plant growth, and leaf senescence (Jahan et al., 2021a; Wu et al., 2021). It has been reported hitherto that plant growth status is maintained normally due to positive effects of MEL under abiotic stresses (Tiwari et al., 2020; Heshmati et al., 2021). The molecule was detected in horticultural plants in 1993, in morning glory and tomato, according to Van Tassel (1997). In plants most common way, MEL controls stress via increasing the antioxidative defense mechanisms, as well as reactive oxygen species (ROS) scavenging. Nawaz et al. (2018) revealed the surprisingly increased growth traits, activity of antioxidant enzymes, root morphology, chlorophyll content, and suppressed oxidative damage in MEL-pretreated watermelon seedlings in vanadium toxicity. Foliar application of melatonin dramatically reduced ROS-induced damage and enhanced growth, metabolites level, and activity of antioxidant enzymes in strawberry seedling under cadmium stress (Wu et al., 2021). Sharma and Zheng (2019) revealed the protective role of MEL in horticultural plants through prevention of damages to antioxidative defense system, photosynthetic apparatus, by regulation of oxidative stress and other defense mechanisms. Various positive effects of exogenously applied MEL are present in literature, including prevention of photosynthetic damages to chloroplast, and improvement of intact spongy tissues, leaves water content, and turgor pressures under drought stress (Tiwari et al., 2020).
The ROS scavenging efficiency gets enhanced and antioxidant defense system of plants gets triggered by the application of MEL. In horticultural plants, the related phenomenal mechanisms for scavenging of H2O2 and regulating superoxide anions are well studied in the form of ascorbate-glutathione cycle’ regulation (Vielma et al., 2014; Ahammed et al., 2019). The photosynthetic damage induced by abiotic stress has been minimized with exogenous treatment of MEL in tomato (Jahan et al., 2021b), watermelon (Li et al., 2017a), peach (Cao et al., 2018), cucumber (Zhang et al., 2020), and pepper (Korkmaz et al., 2017). Furthermore, in Malus hupehensis, the inhibitory effect of UV-B radiation efficiently mitigated by MEL (1 µM) supplementation. MEL controlled photosynthetic properties and decreased damages to leaf membrane. Furthermore, MEL treatment resulted in a considerable decrease in H2O2 levels as well as increased antioxidant machinery (Wei et al., 2019). MEL application significantly improved growth status, photosynthesis, anti-oxidant activity of enzymes, and depletion of ROS generation in ozone-stressed grape leaves (Liu et al., 2021). Jahan et al. (2020) observed that MEL application significantly increased micro and macro-nutrient absorption under nickel toxicity in tomato roots and leaves, whereas MEL treatment significantly lowered nickel deposition in both root and shoot system. Exogenous MEL application significantly reduced ROS production and chlorophyll degradation in leafy vegetables like fenugreek, whereas MEL application significantly enhanced antioxidant enzymes, proline content, and photosynthetic pigments under drought condition (Zamani et al., 2019). Additionally, in pepper plants, the H2O2, and MDA contents and EL level were reduced, and antioxidant enzymes, leaf mineral content, gas exchange elements, leaf area, and seedling biomass were enhanced by the application of MEL under cold stress (Korkmaz et al., 2021). These results suggest the signaling function of MEL, which help to enhance growth and defense mechanisms of horticultural plants under abiotic stresses.
Salicylic acid
Salicylic acid is a versatile natural phenolic compound and an important signaling molecule (Prakash et al., 2021). SA has been demonstrated to be important in the regulation of plant physiological processes such as seedling growth, root growth, leaf photosynthesis, ion homeostasis, secondary metabolite production, fruit ripening, and antioxidant enzymes system (Hernández et al., 2017; Rajeshwari and Bhuvaneshwari, 2017). Miao et al. (2020) revealed that SA supplementation considerably enhanced cucumbers growth characteristics, photosynthetic capability, and root architecture system under salt stress. Under high temperature stress, SA application considerably boosted leaf water potential, metabolites, leaf gas exchange components, antioxidant enzyme system, and decreased ROS-induced damage in Solanum lycopersicum L. (Jahan et al., 2019). SA significantly improved potato growth status, antioxidant enzyme activity, proline content, chlorophyll content, and decreased oxidative damage and reduced cadmium accumulation under cadmium toxicity (Li et al., 2019). Hormonal priming with SA in cucumber seed improves germination of seeds, development of seedling and yield of crop (Rehman et al., 2011). Under water scarcity environments, SA treatment enhanced plant fresh weight, leaf water potential, photosynthetic apparatus, antioxidant enzymes system, anatomical response, and decreased cell damage in tomato (Lobato et al., 2021). Under heat stress, SA increased thermotolerance, chlorophyll concentration, leaf water content, and antioxidant enzyme activity in pepper (Zhang et al., 2019). Moreover, SA improved the photosynthesis of pepper (Kaya, 2021), peach (Zhao et al., 2021), cucumber (Shi et al., 2006), and melon (Zhang et al., 2015). According to Chen et al. (2011), SA application can prevent cold stress-induced oxidative damage in eggplant seedlings by enhancing the activity of antioxidant enzyme and upregulating gene expression. Under high temperature conditions, SA supplementation lowered H2O2 concentration and controlled the system of antioxidant enzymes in banana plants (Kang et al., 2003). Plants subjected to ozone stress, SA treatment significantly increased seed germination, nitrogen absorption, and root properties (Santisree et al., 2020). Spraying of SA improved antioxidant enzyme activity in pepper leaves subjected to UV-B exposure (Mahdavian et al., 2008).
The extant literature highlights the mitigating effects of exogenous SA in horticultural crops under abiotic stress, including spinach (Shin et al., 2018), tobacco (Dat et al., 2000), rosemary (El-Esawi et al., 2017), pea (Embiale et al., 2016), and strawberry (Ergin et al., 2016). The most prevalent plant responses mediated by SA in horticultural plants are enhanced chlorophyll content, secondary metabolites, proline level, and antioxidant enzyme activity. Conversely, other plant responses to abiotic stress conditions include reduced oxidative damage (strawberry, tobacco, and rosemary) and improved growth characteristics, and yield. The synergistic and antagonistic interactions of SA with nutrients, under both favorable and stressed environments, helps to modulate the growth and development of plants. For example, exogenous application of SA significantly reduced salt (Na+) absorption while increasing absorption of mineral nutrients under salt stress in cucumber (Yildirim et al., 2008). Significant increments in antioxidant enzymes (AsA-GSH pathway) and reductions in oxidative damage, along with relevant gene expressions, are exhibited in eggplant seedlings under cold stress (Chen et al., 2011). SA application successfully decreased oxidative damage and boosted antioxidant defense system in okra during cold stress (Bahadoori et al., 2016). SA has the ability to mitigate the harmful environmental effects on horticultural crops.
Jasmonates
Jasmonic acid (JA) and methyl jasmonate (MeJA) belong to a group of multifunctional compounds called Jasmonates (JAs) (Ullah et al., 2018). They are key plant signaling molecules that regulate plant responses to environmental stress and play a variety of role in plants growth and development (Eyidogan et al., 2012). Furthermore, Photosynthesis, root elongation, stomatal development, leaf senescence, chlorophyll breakdown, and nutritional balance are all regulated by JA (Siva et al., 2015). The crucial role of JA in stress tolerance and adaptability of plants is well documented. Interestingly, the resistance of plants towards environmental stress factors in increased by JA (Tables 1, 2). Exogenous MeJA increased cold stress tolerance in peaches via ROS-mediated oxidative damage maintenance and enhanced antioxidant defense mechanism (Jin et al., 2009). Under heat stress, JA application increased antioxidant defense system of grapes seedling (Chen et al., 2006). Furthermore, JA significantly enhanced sugar beet production, antioxidant enzyme activity, and water scarcity tolerance (Ghafari et al., 2020). According to Sayyari et al. (2011), MeJA supplementation significantly increased secondary metabolite content, antioxidant enzyme activity, and mitigated chilling injury in Punica granatum L. In another study, loquat fruit were treatment with MeJA under cold stress. MeJA treatment effectively reduced harmful effect of chilling injury and enhanced antioxidant enzymes system (Jin et al., 2014). Under salinity stress, JA effectively improved growth characteristics, proline, metabolite content, and reduced oxidative damage in bitter melon (Alisof et al., 2020). Under cadmium toxicity, low MeJA significantly lowered MDA levels while increasing root development, antioxidant enzyme defense system, and chlorophyll content in Capsicum frutescens L. (Yan et al., 2013). Faghih et al. (2017) reported considerable increments in antioxidant activity by pretreatment of MeJA under salt stress in strawberry seedlings.
Previous literature revealed under abiotic stress environment, JA improved growth status in Solanum nigrum (Yan et al., 2015), increased seed germination in okra (Azooz et al., 2015), enhanced pigments content in strawberries (Yosefi et al., 2020), enhanced leaf gas exchange parameters (Choudhary and Agrawal, 2014) increased antioxidant defense system in cauliflower (Wu et al., 2012), increased osmolytes content in tomato (Bali et al., 2018), reduced ROS production in Malus crabapple (Qiu et al., 2019), and reduced heavy metal accumulation in horticultural plants (Dar et al., 2015; Zhao et al., 2016). Amid jasmonates, JA is found notably well known, best characterized and most abundant one. The defense mechanisms of horticultural crops are well-regulated by JA, when exposed to abiotic stresses including drought (Ghafari et al., 2020), salinity (Abouelsaad and Renault, 2018), cold (Zhao et al., 2013), alkalinity (Ge et al., 2010), heat (Chen et al., 2006), and metal stress (Bali et al., 2019). Thus, the JA is capable of reducing various environmental stress vulnerabilities (Raza et al., 2021). Furthermore, exogenous MeJA helped to increase endogenous levels of JA in pea crop (Shahzad et al., 2015). Meng et al. (2009) indicated that MeJA treatment reduced phenolic content while increasing antioxidant enzyme activity due to chilling damage index. In citrus, JA treatment significantly boosted proline content and antioxidant enzyme activity while decreasing ROS-induced oxidative damage (Habibi et al., 2019). Ouli-Jun et al. (2017) reported that the metabolism, root respiration, antioxidant enzymes activity, and osmolytes content were significantly enhanced, whereas, the hydroxyl free radical’s accumulation, MDA and EL content were declined through the exogenous application of MeJA under waterlogging stress in pepper plants. Hence, the adaption of horticultural crops in abiotic stress conditions is positively regulated by JAs.
Strigolactones
Strigolactones (SLs) are newly discovered multifunctional carotenoid derivative compounds of the plant hormone (Raza et al., 2021). SLs positively regulates seedling growth, photosynthetic efficiency, leaf senescence, blooming, and ion homeostasis (Banerjee and Roychoudhury, 2018). Exogenous supplementation of SLs significantly increased growth, relative water content, anti-oxidant enzyme activity, gas exchange parameters, chlorophyll fluorescence elements and contents in Vitis vinifera under drought conditions, while drastically lowering the oxidative injury, stomatal opening, and EL level (Min et al., 2019). Furthermore, the application of SLs activates the transcription of genes related to SLs production. SLs production promotes root architecture and arbuscular mycorrhizal fungus symbiosis, which boosts nutrient intake (Bhoi et al., 2021). Exogenous SLs modified root growth pattern of tomato (Santoro et al., 2020). Similarly, SL applied to apple plants exogenously exhibited significant increases in chlorophyll content and seedlings’ net photosynthetic rate, when exposed to potassium chloride (KCL) toxicity (Zheng et al., 2021). Furthermore, under KCL toxicity, SLs application enhanced POD and SOD activity while decreasing oxidative stress by increasing accumulation of proline, sustaining absorption of mineral nutrient and osmotic equilibrium. The SLs applied exogenously under salt stress significantly increased cucumbers seedlings’ leaf photosynthetic capability, AsA-GSH pool, and decreased oxidative damage (Zhang et al., 2015). Under drought, SLs treatment considerably improved stomata’s sensitivity in tomato (Visentin et al., 2016).
Additionally, the stress tolerance of various horticultural crops was enhanced under abiotic stress conditions by endogenous SLs, which label them as endogenous growth regulators (Ha et al., 2014; Sytar et al., 2019). Banerjee et al. (2017) stated that SLs have received a significant attention during recent years due to their crucial roles in regulation of multiple processes, both physiological and molecular, throughout the responses of plants towards abiotic stresses. SLs primarily functions as a second messenger in shoot branching by dampening auxin transport in the stem and so suppressing axillary bud development (Saeed et al., 2017). Furthermore, in low-light conditions, SLs foliar spray improved tomato growth traits, chlorophyll fluorescence parameters, pigment molecule and photosynthetic assimilation. Furthermore, SLs application increased antioxidant enzyme gene expression while decreasing H2O2 and MDA levels in tomato under low light-stress (Lu et al., 2019). Exogenous application of SLs in peas significantly increased photosynthetic pigment and shoot branching under cold stress (Cooper et al., 2018). Exogenous SLs improved salt stress tolerance in Solanum lycopersicum L. by boosting antioxidant defense mechanism, chlorophyll and carotenoids content, and endogenous SLs synthesis (Liu et al., 2022). Seed priming with SLs in Lupine resulted in improved germination of seeds and growth of seedlings, and helped to enhance proline content and decline MDA content. Additionally, the antioxidant enzymes activity and glyoxalase system of lupine seedlings showed improvements after application of SLs (Omoarelojie et al., 2020). SLs supplementation regulating antioxidant defense system, increasing nutrient uptake, and reduced MDA accumulation in cucumber under salt stress (Zhang et al., 2022). Under cadmium toxicity, SLs significantly reduced MDA accumulation and enhanced root vigor, activity of antioxidant enzymes, and flavonoid biosynthesis by modulating there encoding gene in melon seedlings (Chen et al., 2022). Overall, SLs shows a vital function in the abiotic stress physiology of horticultural crops in response to various environmental stresses.
GABA
One of the non- proteinogenic amino acid in plants is the Gamma-aminobutyric acid (GABA), which acts as a signaling molecule, having well-recognized ubiquitous status and multifaceted roles (Ansari et al., 2021). In horticultural crops, GABA is known to mediate various physiological function such as regulation of seed germination (Kumar et al., 2019), osmolyte accumulation (Li et al., 2021), balanced photosynthetic capacity (Wu et al., 2020), root architecture (Seifkalhor et al., 2019), plant yield regulation (Sita and Kumar, 2020), and ion homeostasis (Fait et al., 2008), redox homeostasis (Ansari et al., 2021), and antioxidant defense system (Hasan et al., 2021). The morphological and physiological functions of plants including production of proline, soluble sugar, and polyamine metabolism, as well as photosynthetic efficiency, are reported to be significantly improved by the application of GABA under abiotic stress (Jalil and Ansari, 2020). Chen et al. (2018) stated that this molecule is also known for mitigating excess ROS formation under stress conditions, mainly through activation of antioxidant defense mechanism. Furthermore, exogenous GABA supplementation markedly enhanced leaf photosynthesis traits, modulate stomatal opening, improved root growth, balanced ion homeostasis, enhanced osmolytes accumulation, and stress related protein in black pepper under PEG-induced stress (Vijayakumari and Puthur, 2016). Malekzadeh et al. (2013) revealed that foliar application of GABA efficiently improved seedling growth status, proline content, leaf water potential, stomatal opening, and antioxidant enzymes activity in tomato seedling under cold stress. According to Su et al. (2019), GABA shunt components are essential for ion homoeostasis. Under cold stress, GABA treatment significantly increased biomass yield, chlorophyll content, antioxidant enzymes, and lowered ROS production and cell membranes integrity in peaches (Shang et al., 2011). GABA is the primary mediator of induced leaf senesces under oxidative stress. Under short/low light stress, GABA increased chilies stress resistance through activation of antioxidant defense system, and increasing photochemical efficiency. Furthermore, Li et al. (2017b) described that GABA treatment increased gas exchange traits, chlorophyll content and fluorescence characteristics, SOD and CAT activity, and decreased levels of MDA content in chilies.
During environment stress such as salt stress, drought, heavy metals, low and high temperature stresses (Sita and Kumar, 2020; Ansari et al., 2021; Hasan et al., 2021), the production of GABA is escalated with such an intensity that exceeds the cellular levels of this non-proteinogenic amino acid than those amino acids having role in protein synthesis (Srivastava et al., 2021). Notably, the production of high amounts of GABA were observed under abiotic stress conditions, and there found a nexus between its metabolism and other factors including defense against oxidative stress, antioxidant enzymes’ upregulation, osmolytes regulation, and balance ion homeostasis (Khan et al., 2021). The abiotic stress resistance of plants has been successfully improved through GABA priming. The growth of muskmelon was also significantly increased with application of GABA under calcium nitrate stress. Furthermore, GABA treatment significantly increased ADC, PAO, OCD, DAO and SMDC activity. Exogenous GABA treatment successfully increased spermidine and spermine levels while decreasing level of putrescine in leaves, enhancing polyamine biosynthetic concentration (Hu et al., 2015). Furthermore, exogenous application in carrots, tomato, and peach increases endogenous GABA levels, resulting in increased enzymatic activity, pigment content, and ultimately resistance of plants against abiotic stress (Yang et al., 2011; Koike et al., 2013; Bashir et al., 2021). The oxidative stress recovery is also linked with GABA application, which helps to scavenge the excess ROS production through a disturbance in intracellular redox
Future outlook
The adversities linked with abiotic stress factors are surging day-by-day, gaining attention of scientific research from plant biologists so as to avoid threats to sustainable agricultural production I future. Phytohormones have emerged as a viable technique in current stress management because they protect plants from numerous abiotic stressors by boosting antioxidant enzyme activity, lowering oxidative damage, and promoting plant development (Figure 3). Therefore, in flood-, drought-, and saline-prone areas worldwide, the sustainability of crops production can be maintained through utilization of phytohormones, which are proved to better the abiotic stress resistance of horticultural crops. Along with improving the stress resistance in abiotic stresses, the application of phytohormones is also known for ensuring the harmonization of germination process, mainly by increasing the viability and breaking the seed dormancy. Current review is an attempt to provide useful insights into the exogenous application of phytohormones and the role played by these in developing and enhancing the plants’ defense mechanisms. In recent two decades, the identification and characterization of metabolizing enzymes related to phytohormones has been focused by the researchers. Further, for better understanding of growth-regulation mechanisms induced by phytohormones and bringing more clarity to elusive interactive events, intensive executions of phytohormones crosstalk research is being made recently. Finally, in future research, the aim of modernizing agricultural production with the engineering of abiotic stress-resistant crops can be aligned with the manipulation of phytohormones level and their subsequent action at pertinent developmental stage in appropriate tissue/organ.
Author contributions
YZ and YH: Conceptualization, Literature survey, Writing major original draft, Review structure. XW and XC: Literature survey, Writing- review and editing, Figure designing. KW: Literature survey, Writing- review and editing. YW: Reviewing and editing, References collection. YH: Supervision. All authors contributed to the article and approved the submitted version.
Conflict of interest
The authors declare that the research was conducted in the absence of any commercial or financial relationships that could be construed as a potential conflict of interest.
Publisher’s note
All claims expressed in this article are solely those of the authors and do not necessarily represent those of their affiliated organizations, or those of the publisher, the editors and the reviewers. Any product that may be evaluated in this article, or claim that may be made by its manufacturer, is not guaranteed or endorsed by the publisher.
References
Abouelsaad, I., Renault, S. (2018). Enhanced oxidative stress in the jasmonic acid-deficient tomato mutant def-1 exposed to NaCl stress. J. Plant Physiol. 226, 136–144. doi: 10.1016/j.jplph.2018.04.009
Ahammed, G. J., Li, X., Liu, A., Chen, S. (2020). Brassinosteroids in plant tolerance to abiotic stress. J. Plant Growth Regul. 39, 1451–1464. doi: 10.1007/s00344-020-10098-0
Ahammed, G. J., Xu, W., Liu, A., Chen, S. (2019). Endogenous melatonin deficiency aggravates high temperature-induced oxidative stress in solanum lycopersicum l. Environ. Exp. Bot. 161, 303–311. doi: 10.1016/j.envexpbot.2018.06.006
Ahanger, M. A., Mir, R. A., Alyemeni, M. N., Ahmad, P. (2020). Combined effects of brassinosteroid and kinetin mitigates salinity stress in tomato through the modulation of antioxidant and osmolyte metabolism. Plant Physiol. Biochem. 147, 31–42. doi: 10.1016/j.plaphy.2019.12.007
Ahmad, B., Jaleel, H., Sadiq, Y., Khan, M. M. A., Shabbir, A. (2018). Response of exogenous salicylic acid on cadmium induced photosynthetic damage, antioxidant metabolism and essential oil production in peppermint. Plant Growth Regul. 86, 273–286. doi: 10.1007/s10725-018-0427-z
Alisof, S., Einali, A., Sangtarash, M. H. (2020). Jasmonic acid-induced metabolic responses in bitter melon (Momordica charantia) seedlings under salt stress. J. Hortic. Sci. Biotechnol. 95, 247–259. doi: 10.1080/14620316.2019.1663135
Alper, S. L., Sharma, A. K. (2013). ). the SLC26 gene family of anion transporters and channels. Mol. Asp Med. 34, 494–515. doi: 10.1016/j.mam.2012.07.009
Andreotti, C. (2020). Management of abiotic stress in horticultural crops: Spotlight on biostimulants. Agronomy 10, 1514. doi: 10.3390/agronomy10101514
Ansari, M. I., Jalil, S. U., Ansari, S. A., Hasanuzzaman, M. (2021). GABA shunt: A key-player in mitigation of ROS during stress. Plant Growth Regul. 94, 131–149. doi: 10.1007/s10725-021-00710-y
Arif, Y., Sami, F., Siddiqui, H., Bajguz, A., Hayat, S. (2020). Salicylic acid in relation to other phytohormones in plant: A study towards physiology and signal transduction under challenging environment. Environ. Exp. Bot. 175, 104040. doi: 10.1016/j.envexpbot.2020.104040
Azooz, M. M., Metwally, A., Abou-Elhamd, M. F. (2015). Jasmonate-induced tolerance of hassawi okra seedlings to salinity in brackish water. Acta Physiol. Plantar. 37, 77. doi: 10.1007/s11738-015-1828-5
Bahadoori, S., Behrooz, E., Mokhtar, H., Surur, K., Mosadegh, P. S., Hassankeloo, N. T., et al. (2016). Effects of seed priming with salicylic acid and polyamines on physiological and biochemical characteristics of okra (Abelmoschus esculentus) under low temperature stress. J. Plant Process Funct. 5, 145–156.
Bali, S., Jamwal, V. L., Kaur, P., Kohli, S. K., Ohri, P., Gandhi, S. G., et al. (2019). Role of p-type ATPase metal transporters and plant immunity induced by jasmonic acid against lead (Pb) toxicity in tomato. Ecotoxicol. Environ. Saf. 174, 283–294. doi: 10.1016/j.ecoenv.2019.02.084
Bali, S., Kaur, P., Kohli, S. K., Ohri, P., Thukral, A. K., Bhardwaj, R., et al. (2018). Jasmonic acid induced changes in physio-biochemical attributes and ascorbate-glutathione pathway in lycopersicon esculentum under lead stress at different growth stages. Sci. Total Environ. 645, 1344–1360. doi: 10.1016/j.scitotenv.2018.07.164
Banerjee, A., Roychoudhury, A. (2018). Strigolactones: multi-level regulation of biosynthesis and diverse responses in plant abiotic stresses. Acta physiologiae plantarum 40, 1–10. doi: 10.1007/s11738-018-2660-5
Banerjee, A., Wani, S. H., Roychoudhury, A. (2017). Epigenetic control of plant cold responses. Front. Plant Sci. 8. doi: 10.3389/fpls.2017.01643
Bashir, R., Riaz, H. N., Anwar, S. (2021). Morpho-physiological changes in carrots by foliar γ-aminobutyric acid under drought stress. Braz. J. Bot. 44, 57–68. doi: 10.1007/s40415-020-00676-7
Bhoi, A., Yadu, B., Chandra, J., Keshavkant, S. (2021). Contribution of strigolactone in plant physiology, hormonal interaction and abiotic stresses. Planta 254, 1–21. doi: 10.1007/s00425-021-03678-1
Bortolin, G. S., Teixeira, S. B., de Mesquita, P. R., Ávila, G. E., Carlos, F. S., da Silva Pedroso, C. E., et al. (2020). Seed priming with salicylic acid minimizes oxidative effects of aluminum on trifolium seedlings. J. Soil Sci. Plant Nutr. 20, 2502–2511. doi: 10.1007/s42729-020-00316-9
Cao, S. F., Shao, J. R., Shi, L. Y., Xu, L. W., Shen, Z. M., Chen, W., et al. (2018). Melatonin increases chilling tolerance in postharvest peach fruit by alleviating oxidative damage. Sci. Rep. 8, 806. doi: 10.1038/s41598-018-19363-5
Chaudhuri, A., Halder, K., Abdin, M. Z., Majee, M., Datta, A. (2022). Abiotic stress tolerance in plants: Brassinosteroids navigate competently. Int. J. Mol. Sci. 23, 14577. doi: 10.3390/ijms232314577
Chen, H., Liu, T., Xiang, L., Hu, L., Hu, X. (2018). GABA enhances muskmelon chloroplast antioxidants to defense salinity- alkalinity stress. Russ. J. Plant Physiol. 654, 674–679.
Chen, X., Shi, X., Ai, Q., Han, J., Wang, H., Fu, Q. (2022). Transcriptomic and metabolomic analyses reveal that exogenous strigolactones alleviate the response of melon root to cadmium stress. Hortic. Plant J. 8, 637–649. doi: 10.1016/j.hpj.2022.07.001
Chen, P. Q., Yu, S. L., Zhan, Y. N., Kang, X. L. (2006). Effects of jasmonate acid on thermotolerance of grape seedlings. J. Shihezi Univ. 24, 87–91.
Chen, S., Zimei, L., Cui, J., Jiangang, D., Xia, X., Liu, D., et al. (2011). Alleviation of chilling-induced oxidative damage by salicylic acid pretreatment and related gene expression in eggplant seedlings. Plant Growth Regul. 65, 101–108. doi: 10.1007/s10725-011-9579-9
Choudhary, K. K., Agrawal, S. B. (2014). Ultraviolet-b induced changes in morphological, physiological, and biochemical parameters of two cultivars of pea (Pisum sativum l.). Ecotox. Environ. Safe. 100, 178–187. doi: 10.1016/j.ecoenv.2013.10.032
Choudhary, S. P., Bhardwaj, R., Gupta, B. D., Dutt, P., Gupta, R. K., Kanwar, M., et al. (2011). Enhancing effects of 24-epibrassinolide and putrescine on the antioxidant capacity and free radical scavenging activity of raphanus sativus seedlings under Cu ion stress. Acta Physiol. Plantar. 33, 1319–1333. doi: 10.1007/s11738-010-0665-9
Choudhary, S. P., Kanwar., M., Bhardwaj, R., Yu, J. Q., Tran, L. S. P. (2012). Chromium stress mitigation by polyamine-brassinosteroid application involves phytohormonal and physiological strategies in raphanus sativus l. PloS One 7, e33210. doi: 10.1371/journal.pone.0033210
Ciura, J., Kruk, J. (2018). Phytohormones as targets for improving plant productivity and stress tolerance. J. Plant Physiol. 229, 32–40. doi: 10.1016/j.jplph.2018.06.013
Cooper, J. W., Hu, Y., Beyyoudh, L., Yildiz Dasgan, H., Kunert, K., Beveridge, C. A., et al. (2018). Strigolactones positively regulate chilling tolerance in pea and in arabidopsis. Plant Cell Environ. 41, 1298–1310. doi: 10.1111/pce.13147
Dar, T. A., Uddin, M., Khan, M. M. A., Hakeem, K. R., Jaleel, H. (2015). Jasmonates counter plant stress: a review. Environ. Exp. Bot. 115, 49–57. doi: 10.1016/j.envexpbot.2015.02.010
Dat, J. F., Lopez-Delgado, H., Foyer, C. H., Scott, I. M. (2000). Effects of salicylic acid on oxidative stress and thermotolerance in tobacco. J. Plant Physiol. 156, 659–665. doi: 10.1016/s0176-1617(00)80228-x
Debnath, B., Hussain, M., Irshad, M., Mitra, S., Li, M., Liu, S., et al. (2018). Exogenous melatonin mitigates acid rain stress to tomato plants through modulation of leaf ultrastructure, photosynthesis and antioxidant potential. Molecules 23, 388. doi: 10.3390/molecules23020388
Debnath, B., Li, M., Liu, S., Pan, T., Ma, C., Qiu, D. (2020). Melatonin-mediate acid rain stress tolerance mechanism through alteration of transcriptional factors and secondary metabolites gene expression in tomato. Ecotoxicol. Environ. Saf. 200, 110720. doi: 10.1016/j.ecoenv.2020.110720
Ding, H. D., Zhu, X. H., Zhu, Z. W., Yang, S. J., Zha, D. S., Wu, X. X. (2012). Amelioration of salt-induced oxidative stress in eggplant by application of 24-epibrassinolide. Biol. Plant 56, 767–770. doi: 10.1007/s10535-012-0108-0
Djennane, S., Hibrand-Saint Oyant, L., Kawamura, K., Lalanne, D., Laffaire, M., Thouroude, T., et al. (2014). Impacts of light and temperature on shoot branching gradient and expression of strigolactone synthesis and signalling genes in rose. Plant Cell Environ. 37, 742–757. doi: 10.1111/pce.12191
El-Esawi, M. A., Elansary, H. O., El-Shanhorey, N. A., Abdel-Hamid, A. M., Ali, H. M., Elshikh, M. S. (2017). Salicylic acid-regulated antioxidant mechanisms and gene expression enhance rosemary performance under saline conditions. Front. Physiol. 8. doi: 10.3389/fphys.2017.00716
Embiale, A., Hussein, A., Husen, A., Sahile, S., Mohammed, K. (2016). Differential sensitivity of pisum sativum l. cultivars to water-defcit stress: changes in growth, water status, chlorophyll fuorescence and gas exchange attributes. J. Agron. 15, 45–57. doi: 10.3923/ja.2016.45.57
Ergin, S., Gülen, H., Kesici, M., Turhan, E., Ipek, A., Köksal, N. (2016). Effects of high temperature stress on enzymatic and nonenzymatic antioxidants and proteins in strawberry plants. Turk J. Agric. For. 40, 908–917. doi: 10.3906/tar-1606-144
Eyidogan, F., Oz, M. T., Yucel, M., Oktem, H. A. (2012). “Signal transduction of phytohormones under abiotic stresses,” in Phytohormones and abiotic stress tolerance in plants (Berlin, Heidelberg: Springer), (pp. 1–(pp48). doi: 10.1007/978-3-642-25829-9_1
Faghih, S., Ghobadi, C., Zarei, A. (2017). Response of strawberry plant cv. ‘Camarosa’ to salicylic acid and methyl jasmonate application under salt stress condition. J. Plant Growth Regul. 36, 651–659. doi: 10.1007/s00344-017-9666-x
Fait, A., Fromm, H., Walter, D., Galili, G., Fernie, A. R. (2008). Highway or byway: The metabolic role of the GABA shunt in plants. Trends Plant Sci. 13, 14–19. doi: 10.1016/j.tplants.2007.10.005
Francini, A., Sebastiani, L. (2019). Abiotic stress effects on performance of horticultural crops. Horticulturae 5, 67. doi: 10.3390/books978-3-03921-751-9
Gao, J., Shahid, R., Ji, X., Li, S. (2022). Climate change resilience and sustainable tropical agriculture: Farmers’ perceptions, reactive adaptations and determinants of reactive adaptations in hainan, China. Atmosphere 13 (6), 955. doi: 10.3390/atmos13060955
Ge, Y., Li, C., Tang, R., Sun, R., Li, J. (2014). “Effects of postharvest brassinolide dipping on quality parameters and antioxidant activity in peach fruit,” in Proceedings of III international symposium on postharvest pathology: using science to increase food availability. 377–384. doi: 10.17660/actahortic.2016.1144.56
Ge, Y. X., Zhang, L. J., Li, F. H., Chen, Z. B., Wang, C., Yao, Y. C., et al. (2010). Relationship between jasmonic acid accumulation and senescence in drought-stress. Afr. J. Agric. Res. 5, 1978–1983. doi: 10.5897/AJAR10.207
Ghafari, H., Tadayon, M. R., Nadeem, M., Razmjoo, J., Cheema, M. (2020). Foliage applications of jasmonic acid modulate the antioxidant defense under water defcit growth in sugar beet. Span. J. Agric. Res. 17, e0805. doi: 10.5424/sjar/2019174-15380
Habibi, F., Ramezanian, A., Rahemi, M., Eshghi, S., Guillén, F., Serrano, M., et al. (2019). Postharvest treatments with γ-aminobutyric acid, methyl jasmonate, or methyl salicylate enhance chilling tolerance of blood orange fruit at prolonged cold storage. J Sci. Food Agric. 99, 6408–6417. doi: 10.1002/jsfa.9920
Ha, C. V., Leyva-Gonzalez, M. A., Osakabe, Y., Tran, U. T., Nishiyama, R., Watanabe, Y., et al. (2014). Positive regulatory role of strigolactone in plant responses to drought and salt stress. Proc. Natl. Acad. Sci. U.S.A. 111, 851–856. doi: 10.1073/pnas.1322135111
Hasan, M. M., Alabdallah, N. M., Alharbi, B. M., Waseem, M., Yao, G., Liu, X. D., et al. (2021). GABA: A key player in drought stress resistance in plants. Int. J. Mol. Sci. 22, 10136. doi: 10.3390/ijms221810136
Hayat, S., Alyemeni, M. N., Hasan, S. A. (2012). Foliar spray of brassinosteroids enhances yield and quality of solanum lycopersicum under cadmium stress. Saudi. J. Bio. Sci. 19, 325–335. doi: 10.1016/j.sjbs.2012.03.005
Hegazi, A. M., El-Shraiy, A. M., Ghoname, A. (2017). Mitigation of salt stress negative effects on sweet pepper using arbuscular mycorrhizal fungi (AMF), bacillus megaterium and brassinosteroids (BRs). Gesunde Pflanzen. 69, 91–102. doi: 10.1007/s10343-017-0393-9
Hernández, J. A., Diaz-Vivancos, P., Barba-Espín, G., Clemente-Moreno, M. J. (2017). “On the role of salicylic acid in plant responses to environmental stresses,” in Salicylic acid: a multifaceted hormone (Singapore: Springer), (pp. 17–34). doi: 10.1007/978-981-10-6068-7_2
Heshmati, S., Dehaghi, M. A., Farooq, M., Wojtyla, Ł., Maleki, K., Heshmati, S. (2021). Role of melatonin seed priming on antioxidant enzymes and biochemical responses of carthamus tinctorius l. under drought stress conditions. Plant Stress 2, 100023. doi: 10.1016/j.stress.2021.100023
Hu, W., Wu, Y., Zeng, J., He, L., Zeng, Q. (20102010). Chill-induced inhibition of photosynthesis was alleviated by 24-epibrassinolide pretreatment in cucumber during chilling and subsequent recovery. Photosynthetica 48, 537–544. doi: 10.1007/s11099-010-0071-y
Hu, X., Xu, Z., Xu, W., Li, J., Zhao, N., Zhou, Y. (2015). Application of γ-aminobutyric acid demonstrates a protective role of polyamine and GABA metabolism in muskmelon seedlings under Ca (NO3) 2 stress. Plant Physiol. Biochem. 92, 1–10. doi: 10.1016/j.plaphy.2015.04.006
Hu, W. H., Yan, X. H., Xiao, Y. A., Zeng, J. J., Qi, H. J., Ogweno, J. O. (2013). 24-epibrassinosteroid alleviate drought-induced inhibition of photosynthesis in capsicum annuum l. Sci. Hortic. 150, 232–237. doi: 10.1016/j.scienta.2012.11.012
Jahan, M. S., Guo, S., Baloch, A. R., Sun, J., Shu, S., Wang, Y. (2020). Melatonin alleviates nickel phytotoxicity by improving photosynthesis, secondary metabolism and oxidative stress tolerance in tomato seedlings. Ecotox. Environ. Saf. 197, 110593. doi: 10.1016/j.ecoenv.2020.110593
Jahan, M. S., Guo, S., Sun, J., Shu, S., Wang, Y., Abou El-Yazied, A., et al. (2021b). Melatonin-mediated photosynthetic performance of tomato seedlings under high-temperature stress. Plant Physiol. Biochem. 167, 309–320. doi: 10.1016/j.plaphy.2021.08.002
Jahan, M. S., Shu, S., Wang, Y., Hasan, M., El-Yazied, A. A., Alabdallah, N. M. (2021a). Melatonin pretreatment confers heat tolerance and repression of heat-induced senescence in tomato through the modulation of ABA- and GA-mediated pathways. Front. Plant Sci. 12. doi: 10.3389/fpls.2021.650955
Jahan, M. S., Wang, Y., Shu, S., Zhong, M., Chen, Z., Wu, J., et al. (2019). Exogenous salicylic acid increases the heat tolerance in tomato (Solanum lycopersicum l.) by enhancing photosynthesis efficiency and improving antioxidant defense system through scavenging of reactive oxygen species. Sci. Hortic. 247, 421–429. doi: 10.1016/j.scienta.2018.12.047
Jalil, S. U., Ansari, M. I. (2020). “Physiological role of gamma-aminobutyric acid in salt stress tolerance,” in Salt and drought stress tolerance in plants (Cham: Springer), (pp. 337–350). doi: 10.1007/978-3-030-40277-8_13
Jin, P., Duan, Y., Wang, L., Wang, J., Zheng, Y. (2014). Reducing chilling injury of loquat fruit by combined treatment with hot air and methyl jasmonate. food bio. Technology 7, 2259–2266. doi: 10.1007/s11947-013-1232-3
Jin, P., Zheng, Y., Tang, S., Rui, H., Wang, C. Y. (2009). A combination of hot air and methyl jasmonate vapor treatment alleviates chilling injury of peach fruit. Postharvest Biol. Technol. 52, 24–29. doi: 10.1016/j.postharvbio.2008.09.011
Kang, G. Z., Wang, C. H., Sun, G. C., Wang, Z. X. (2003). Salicylic acid changes activities of H2O2-metabolizing enzymes and increases the chilling tolerance of banana seedlings. Environ. Exp. Bot. 50, 9–15. doi: 10.1016/s0098-8472(02)00109-0
Kapoor, D., Rattan, A., Gautam, V., Kapoor, N., Bhardwaj, R. (2014). 24-epibrassinolide mediated changes in photosynthetic pigments and antioxidative defence system of radish seedlings under cadmium and mercury stress. J. Stress Physiol. Biochem. 10, 110–121.
Karlidag, H., Yildirim, E., Turan, M. (2011). Role of 24-epibrassinolide in mitigating the adverse effects of salt stress on stomatal conductance, membrane permeability, and leaf water content, ionic composition in salt stressed strawberry (Fragaria x ananassa). Sci. Hortic. 130, 133–140. doi: 10.1016/j.scienta.2011.06.025
Kaya, C. (2021). Nitrate reductase is required for salicylic acid-induced water stress tolerance of pepper by upraising the AsA-GSH pathway and glyoxalase system. Physiol. Plantar. 172, 351–370. doi: 10.1111/ppl.13153
Khan, M. I. R., Jalil, S. U., Chopra, P., Chhillar, H., Ferrante, A., Khan, N. A. (2021). Role of GABA in plant growth, development and senescence. Plant Gene. 26, 100283. doi: 10.1016/j.plgene.2021.100283
Kohli, A., Sreenivasulu, N., Lakshmanan, P., Kumar, P. P. (2013). The phytohormone crosstalk paradigm takes center stage in understanding how plants respond to abiotic stresses. Plant Cell Rep. 32, 945–957. doi: 10.1007/s00299-013-1461-y
Koike, S., Matsukura, C., Takayama, M., Asamizu, E., Ezura, H. (2013). Suppression of γ-aminobutyric acid (GABA) transaminases induces prominent GABA accumulation, dwarfism and infertility in the tomato (Solanum lycopersicum l.). Plant Cell Physiol. 54, 793–807. doi: 10.1093/pcp/pct035
Korkmaz, A., Değer, Ö., Szafrańska, K., Köklü, Ş., Karaca, A., Yakupoğlu, G., et al. (2021). Melatonin effects in enhancing chilling stress tolerance of pepper. Sci. Hortic. 289, 110434. doi: 10.1016/j.scienta.2021.110434
Korkmaz, A., Karaca, A., Kocacinar, F., Cuci, Y. (2017). The effects of seed treatment with melatonin on germination and emergence performance of pepper seeds under chilling stress. J. Agric. Sci. 23, 167–176.
Kour, J., Kohli, S., Khanna, K., Bakshi, P., Sharma, P., Singh, A. D., et al. (2021). Brassinosteroid signaling, crosstalk and physiological functions in plants under heavy metal stress. Front. Plant Sci. 12. doi: 10.3389/fpls.2021.608061
Kumar, V., Khare, T., Srivastav, A., Surekha, C., Shriram, V., Wani, S. H. (2019). Oxidative stress and leaf senescence: important insights. senescence signal. Cont. Plants., 139–163. doi: 10.1016/b978-0-12-813187-9.00009-3
Ku, Y. S., Sintaha, M., Cheung, M. Y., Lam, H. M. (2018). Plant hormone signaling cross talks between biotic and abiotic stress responses. Int. J. Mol. Sci. 19, 3206. doi: 10.3390/ijms19103206
Liang, B., Ma, C., Zhang, Z., Wei, Z., Gao, T., Zhao, Q. (2018). Long term exogenous application of melatonin improves nutrient uptake fuxes in apple plants under moderate drought stress. Environ. Exp. Bot. 155, 650–661. doi: 10.1016/j.envexpbot.2018.08.016
Liang, D., Ni, Z., Xia, H., Xie, Y., Lv, X., Wang, J., et al. (2019). Exogenous melatonin promotes biomass accumulation and photosynthesis of kiwifruit seedlings under drought stress. Sci. Hortic. 246, 34–43. doi: 10.1016/j.scienta.2018.10.058
Li, H., Chang, J., Chen, H., Wang, Z., Gu, X., Wei, C., et al. (2017a). Exogenous melatonin confers salt stress tolerance to watermelon by improving photosynthesis and redox homeostasis. Front. Plant Sci. 8. doi: 10.3389/fpls.2017.00295
Li, Y., Fan, Y., Ma, Y., Zhang, Z., Yue, H., Wang, L., et al. (2017b). Effects of exogenous γ-aminobutyric acid (GABA) on photosynthesis and antioxidant system in pepper (Capsicum annuum l.) seedlings under low light stress. J. Plant Growth Regul. 36, 436–449. doi: 10.1007/s00344-016-9652-8
Liu, C., Kang, H., Wang, Y., Yao, Y., Gao, Z., Du, Y. (2021). Melatonin relieves ozone stress in grape leaves by inhibiting ethylene biosynthesis. Front. Plant Sci. 12. doi: 10.3389/fpls.2021.702874
Liu, H., Li, C., Yan, M., Zhao, Z., Huang, P., Wei, L., et al. (2022). Strigolactone is involved in nitric oxide-enhanced the salt resistance in tomato seedlings. J. Plant Res. 135, 337–350. doi: 10.1007/s10265-022-01371-2
Li, Q., Wang, G., Wang, Y., Yang, D., Guan, C., Ji, J. (2019). Foliar application of salicylic acid alleviates the cadmium toxicity by modulation the reactive oxygen species in potato. Ecotox. Environ. Saf. 172, 317–325. doi: 10.1016/j.ecoenv.2019.01.078
Li, Z. G., Xiang, R. H., Wang, J. Q. (2021). Hydrogen sulfide–phytohormone interaction in plants under physiological and stress conditions. J. Plant Growth Regul. 40, 2476–2484. doi: 10.1007/s00344-021-10350-1
Lobato, A. K. D. S., Barbosa, M. A. M., Alsahli, A. A., Lima, E. J. A., Silva, B. R. S. D. (2021). Exogenous salicylic acid alleviates the negative impacts on production components, biomass and gas exchange in tomato plants under water deficit improving redox status and anatomical responses. Physiol. Plantar. 172, 869–884. doi: 10.1111/ppl.13329
Lu, T., Yu, H., Li, Q., Chai, L., Jiang, W. (2019). Improving plant growth and alleviating photosynthetic inhibition and oxidative stress from low-light stress with exogenous GR24 in tomato (Solanum lycopersicum l.) seedlings. Front. Plant Sci. 10. doi: 10.3389/fpls.2019.00490
Mahdavian, K., Ghorbanli, M., Kalantari, K. M. (2008). Role of salicylic acid in regulating ultraviolet radiation-induced oxidative stress in pepper leaves. Rus. J. Plant Physiol. 55, 560–563. doi: 10.1134/s1021443708040195
Mahesh, B., Parshavaneni, B., Ramakrishna, B., Rao, S. S. R. (2013). Effect of brassinosteroids on germination and seedling growth of radish (Raphanus sativus l.) under PEG-6000 induced water stress. Am. J. Plant Sci. 4, 2305–2313. doi: 10.4236/ajps.2013.412285
Malekzadeh, P., Khara, J., Heydari, R. (2013). Alleviating effects of exogenous gamma-aminobutyric acid on tomato seedling under chilling stress. Physiol. Mol. Biol. Plants. 20, 133–137. doi: 10.1007/s12298-013-0203-5
Malekzadeh, P., Khara, J., Heydari, R. (2014). Alleviating effects of exogenous gamma amino butyric acid on tomato seedling under chilling stress. Physiol. Mol. Biol. Plants. 20, 133–137. doi: 10.1007/s12298-013-0203-5
Mangal, V., Lal, M. K., Tiwari, R. K., Altaf, M. A., Sood, S., Kumar, D., et al. (2022). Molecular insights into the role of reactive oxygen, nitrogen and sulphur species in conferring salinity stress tolerance in plants. J. Plant Growth Regul. doi: 10.1007/s00344-022-10591-8
Meng, X., Han, J., Wang, Q., Tian, S. (2009). Changes in physiology and quality of peach fruits treated by methyl jasmonate under low temperature stress. Food Chem. 114, 1028–1035. doi: 10.1016/j.foodchem.2008.09.109
Miao, Y., Luo, X., Gao, X., Wang, W., Li, B., Hou, L. (2020). Exogenous salicylic acid alleviates salt stress by improving leaf photosynthesis and root system architecture in cucumber seedlings. Sci. Hortic. 272, 109577. doi: 10.1016/j.scienta.2020.109577
Min, Z., Li, R., Chen, L., Zhang, Y., Li, Z., Liu, M., et al. (2019). Alleviation of drought stress in grapevine by foliar-applied strigolactones. Plant Physiol. Biochem. 135, 99–110. doi: 10.1016/j.plaphy.2018.11.037
Mumtaz, M. A., Hao, Y., Mehmood, S., Shu, H., Zhou, Y., Jin, W., et al. (2022). Physiological and transcriptomic analysis provide molecular insight into 24-epibrassinolide mediated cr (VI)-toxicity tolerance in pepper plants. Environ. Poll 306, 119375. doi: 10.1016/j.envpol.2022.119375
Nawaz, M. A., Huang, Y., Bie, Z., Ahmed, W., Reiter, R. J., Niu, M., et al. (2016). Melatonin: current status and future perspectives in plant science. Front. Plant Sci. 6. doi: 10.3389/fpls.2015.01230
Nawaz, M. A., Jiao, Y., Chen, C., Shireen, F., Zheng, Z., Imtiaz, M. (2018). Melatonin pretreatment improves vanadium stress tolerance of watermelon seedlings by reducing vanadium concentration in the leaves and regulating melatonin biosynthesis and antioxidant trelated gene expression. J. Plant Physiol. 220, 115–127. doi: 10.1016/j.jplph.2017.11.003
Nazir, F., Hussain, A., Fariduddin, Q. (2019). Interactive role of epibrassinolide and hydrogen peroxide in regulating stomatal physiology, root morphology, photosynthetic and growth traits in solanum lycopersicum l under nickel stress. Environ. Exp. Bot. 162, 479–495. doi: 10.1016/j.envexpbot.2019.03.021
Omoarelojie, L. O., Kulkarni, M. G., Finnie, J. F., Pospíšil, T., Strnad, M., Van Staden, J. (2020). Synthetic strigolactone (rac-GR24) alleviates the adverse effects of heat stress on seed germination and photosystem II function in lupine seedlings. Plant Physiol. Biochem. 155, 965–979. doi: 10.1016/j.plaphy.2020.07.043
Ouli-Jun, Z. C. H., Zhou-Bin, L., Ge, W., Bo-Zhi, Y., Xue-Xiao, Z. (2017). Mitigation of waterlogging-induced damages to pepper by exogenous MeJA. Pak. J. Bot. 49, 1127–1135.
Prakash, V., Singh, V. P., Tripathi, D. K., Sharma, S., Corpas, F. J. (2021). Nitric oxide (NO) and salicylic acid (SA): A framework for their relationship in plant development under abiotic stress. Plant Bio. 23, 39–49. doi: 10.1111/plb.13246
Qiu, Y., An, K., Sun, J., Chen, X., Gong, X., Ma, L., et al. (2019). Investigating the effect of methyl jasmonate and melatonin on resistance of malus crabapple ‘Hong jiu’ to ozone stress. Environ. Sci. Poll Res. 26, 27761–27768. doi: 10.1007/s11356-019-05946-w
Rajeshwari, V., Bhuvaneshwari, V. (2017). Salicylic acid induced salt stress tolerance in plants. Int. J. Plant Biol. Res. 5, 1067.
Rao, N. S., Shivashankara, K. S., Laxman, R. H. (2016). Abiotic stress physiology of horticultural crops, vol 311 (India: Springer). doi: 10.1007/978-81-322-2725-0
Raza, A., Javed, R., Zahid, Z., Sharif, R., Hafeez, M. B., Ghouri, M. H., et al. (2021). “Strigolactones for sustainable plant growth and production under adverse environmental conditions,” in Plant performance under environmental stress (Cham: Springer), (pp. 129–166). doi: 10.1007/978-3-030-78521-5_6
Rehman, H., Farooq, M., Basra, S. M., Afzal, I. (2011). Hormonal priming with salicylic acid improves the emergence and early seedling growth in cucumber. J. Agric. Soc Sci. 7, 109–113.
Ruiz-Lozano, J. M., Aroca, R., Zamarreño, Á.M., Molina, S., Andreo-Jiménez, B., Porcel, R., et al. (2016). Arbuscular mycorrhizal symbiosis induces strigolactone biosynthesis under drought and improves drought tolerance in lettuce and tomato. Plant Cell Environ. 39, 441–452. doi: 10.1111/pce.12631
Saeed, W., Naseem, S., Ali, Z. (2017). Strigolactones biosynthesis and their role in abiotic stress resilience in plants: a critical review. Front. Plant Sci. 8. doi: 10.3389/fpls.2017.01487
Saini, S., Kaur, N., Pati, P. K. (2021). Phytohormones: key players in the modulation of heavy metal stress tolerance in plants. Ecotox. Environ. Saf. 223, 112578. doi: 10.1016/j.ecoenv.2021.112578
Salvi, P., Manna, M., Kaur, H., Thakur, T., Gandass, N., Bhatt, D., et al. (2021). Phytohormone signaling and crosstalk in regulating drought stress response in plants. Plant Cell Rep. 40, 1305–1329. doi: 10.1007/s00299-021-02683-8
Santisree, P., Jalli, L. C. L., Bhatnagar-Mathur, P., Sharma, K. K. (2020). “Emerging roles of salicylic acid and jasmonates in plant abiotic stress responses,” in Protective chemical agents in the amelioration of plant abiotic stress: biochemical molecular perspectives (Hoboken: Wiley), (pp–342-373). doi: 10.1002/9781119552154.ch17
Santoro, V., Schiavon, M., Gresta, F., Ertani, A., Cardinale, F., Sturrock, C. J., et al. (2020). Strigolactones control root system architecture and tip anatomy in solanum lycopersicum l. plants under p starvation. Plants 9, 612. doi: 10.3390/plants9050612
Sayyari, M., Babalar, M., Kalantari, S., Martínez-Romero, D., Guillén, F., Serrano, M., et al. (2011). Vapour treatments with methyl salicylate or methyl jasmonate alleviated chilling injury and enhanced antioxidant potential during postharvest storage of pomegranates. Food. Chem. 124, 964–970. doi: 10.1016/j.foodchem.2010.07.036
Seifkalhor, M., Aliniaeifard, S., Hassani, B. (2019). Diverse role of γ-aminobutyric acid in dynamic plant cell responses. Plant Cell. Rep. 38, 847–867. doi: 10.1007/s00299-019-02396-z
Serna, M., Coll, Y., Zapata, P. J., Botella, M.Á, Pretel, M. T., Amorós, A. (2015). A brassinosteroid analogue prevented the effect of salt stress on ethylene synthesis and polyamines in lettuce plants. Sci. Hortic. 185, 105–112. doi: 10.1016/j.scienta.2015.01.005
Shah, A. A., Ahmed, S., Yasin, N. A. (2019). 24-epibrassinolide triggers cadmium stress mitigation in cucumis sativus through intonation of antioxidant system. South Afr. J. Bot. 127, 349–360. doi: 10.1016/j.sajb.2019.11.003
Shahid, M. A., Pervez, M. A., Balal, R. M. (2011). Brassinosteroid (24-epibrassinolide) enhances growth and alleviates the deleterious effects induced by salt stress in pea (Pisum sativum l.). Aust. J. Crop Sci. 5, 500.
Shahid, R., Shijie, L., Shahid, S., Altaf, M. A., Shahid, H. (2021). Determinants of reactive adaptations to climate change in semi-arid region of Pakistan. J. Arid Environments 193, 104580. doi: 10.1016/j.jaridenv.2021.104580
Shahzad, R., Waqas, M., Khan, A. L., Hamayun, M., Kang, S. M., Lee, I. J. (2015). Foliar application of methyl jasmonate induced physio hormonal changes in pisum sativum under diverse temperature regimes. Plant Physiol. Biochem. 96, 406–416. doi: 10.1016/j.plaphy.2015.08.020
Shang, H., Cao, S., Yang, Z., Cai, Y., Zheng, Y. (2011). Effect of exogenous γ-aminobutyric acid treatment on proline accumulation and chilling injury in peach fruit after long term cold storage. J. Agric. Food Chem. 59, 1264–1268. doi: 10.1021/jf104424z
Sharma, A., Zheng, B. (2019). Melatonin mediated regulation of drought stress: physiological and molecular aspects. Plants 8, 190. doi: 10.3390/plants8070190
Shi, Q., Bao, Z., Zhu, Z., Ying, Q., Qian, Q. (2006). Effects of different treatments of salicylic acid on heat tolerance, chlorophyll fluorescence, and antioxidant enzyme activity in seedlings of cucumis sativa l. Plant Growth Regul. 48, 127–135. doi: 10.1007/s10725-005-5482-6
Shin, H., Min, K., Arora, R. (2018). Exogenous salicylic acid improves freezing tolerance of spinach (Spinacia oleracea l.) leaves. Cryobiology 81, 192–200. doi: 10.1016/j.cryobiol.2017.10.006
Shi, Q., Zhu, Z. (2008). Effects of exogenous salicylic acid on manganese toxicity, element contents and anti-oxidative system in cucumber. Environ. Exp. Bot. 63, 317–326. doi: 10.1016/j.envexpbot.2007.11.003
Singh, S. K., Singh, P. K. (20162016). Effect of seed priming of tomato with salicylic acid on growth, flowering, yield and fruit quality under high temperature stress conditions. Int. J. Adv. Res. 4, 723–727.
Sita, K., Kumar, V. (2020). Role of gamma amino butyric acid (GABA) against abiotic stress tolerance in legumes: a review. Plant Physiol. Rep. 25, 654–663. doi: 10.1007/s40502-020-00553-1
Siva, G., Sivakumar, S., Kumar, G. P., Vigneswaran, M., Vinoth, S., Selvan, A. M., et al. (2015). Optimization of elicitation condition with jasmonic acid, characterization and antimicrobial activity of psoralen from direct regenerated plants of psoralea corylifolia l. Biocatal Agric. Biotechnol. 4, 624–631. doi: 10.1016/j.bcab.2015.10.012
Soares, C., de Sousa, A., Pinto, A., Azenha, M., Teixeira, J., Azevedo, R. A. (2016). Effect of 24-epibrassinolide on ROS content, antioxidant system, lipid peroxidation and Ni uptake in solanum nigrum l. under Ni stress. Environ. Exp. Bot. 122, 115–125. doi: 10.1016/j.envexpbot.2015.09.010
Srivastava, V., Mishra, S., Chowdhary, A. A., Lhamo, S., Mehrotra, S. (2021). The γ-aminobutyric acid (GABA) towards abiotic stress tolerance. compatible solutes engineering for crop plants facing climate change (Cham: Springer), 171–187. doi: 10.1007/978-3-030-80674-3_7
Su, N., Wu, Q., Chen, J., Shabala, L., Mithofer, A., Wang, H., et al. (2019). GABA operates upstream of h+-ATPase and improves salinity tolerance in arabidopsis by enabling cytosolic k+ retention and na+ exclusion. J. Exp. Bot. 70, 6349–6361. doi: 10.1093/jxb/erz367
Swamy, K. N., Vardhini, B. V., Ramakrishna, B., Anuradha, S., Siddulu, N., Rao, S. S. R. (2014). Role of 28-homobrassinolide on growth biochemical parameters of trigonella foenum-graecum l. plants subjected to lead toxicity. Int. J. Multi Curr. Res. 2, 317–332.
Sytar, O., Kumari, P., Yadav, S., Brestic, M., Rastogi, A. (2019). Phytohormone priming: regulator for heavy metal stress in plants. J. Plant Growth Regul. 38, 739–752. doi: 10.1007/s00344-018-9886-8
Tiwari, R. K., Lal, M. K., Naga, K. C., Kumar, R., Subhash, C. K. N. (2020). Emerging roles of melatonin in mitigating abiotic and biotic stresses of horticultural crops. Sci. Hortic. 272, 109592. doi: 10.1016/j.scienta.2020.109592
Ullah, A., Sun, H., Yang, X., Zhang, X. (2018). A novel cotton WRKY gene, GhWRKY6-like, improves salt tolerance by activating the ABA signalling pathway and scavenging of reactive oxygen species. Physiol. Plant 162, 439–454. doi: 10.1111/ppl.12651
Upreti, K. K., Sharma, M. (2016). “Role of plant growth regulators in abiotic stress tolerance,” in Abiotic stress physiology of horticultural crops (New Delhi: Springer), (pp. 19–46). doi: 10.1007/978-81-322-2725-0_2
Van Tassel, D. L. (1997). Identification and quantification of melatonin in higher plants (Davis: University of California).
Verma, V., Ravindran, P., Kumar, P. P. (2016). Plant hormone-mediated regulation of stress responses. BMC Plant Biol. 16, 86. doi: 10.1186/s12870-016-0771-y
Vielma, J. R., Bonilla, E., Chacín-Bonilla, L., Mora, M., Medina-Leendertz, S., Bravo, Y. (2014). Effects of melatonin on oxidative stress, and resistance to bacterial, parasitic, and viral infections: a review. Acta Trop. 137, 31–38. doi: 10.1016/j.actatropica.2014.04.021
Vijayakumari, K., Puthur, J. T. (2016). γ-aminobutyric acid (GABA) priming enhances the osmotic stress tolerance in piper nigrum linn. plants subjected to PEG-induced stress. Plant Growth Regul. 78, 57–67. doi: 10.1007/s10725-015-0074-6
Visentin, I., Vitali, M., Ferrero, M., Zhang, Y., Ruyter-Spira, C., Novák, O., et al. (2016). Low levels of strigolactones in roots as a component of the systemic signal of drought stress in tomato. New Phytol. 212, 954–963. doi: 10.1111/nph.14190
Wang, D., Chen, Q., Chen, W., Guo, Q., Xia, Y., Wang, S. (2021). Physiological and transcription analyses reveal the regulatory mechanism of melatonin in inducing drought resistance in loquat (Eriobotrya japonica Lind l.) seedlings. Environ. Exp. Bot. 181, 104291. doi: 10.1016/j.envexpbot.2020.104291
Wani, S. H., Kumar, V., Shriram, V., Sah, S. K. (2016). Phytohormones and their metabolic engineering for abiotic stress tolerance in crop plants. Crop J. 4, 162–176. doi: 10.1016/j.cj.2016.01.010
Wei, L., Deng, X. G., Zhu, T., Zheng, T., Li, P. X., Wu, J. Q., et al. (2015). Ethylene is involved in brassinosteroids induced alternative respiratory pathway in cucumber (Cucumis sativus l.) seedlings response to abiotic stress. Front. Plant Sci. 6. doi: 10.3389/fpls.2015.00982
Wei, Z., Li, C., Gao, T., Zhang, Z., Liang, B., Lv, Z., et al. (2019). Melatonin increases the performance of malus hupehensis after UV-b exposure. Plant Physiol. Biochem. 139, 630–641. doi: 10.1016/j.plaphy.2019.04.026
Wu, X., Jia, Q., Ji, S., Gong, B., Li, J., Lü, G. (2020). Gamma-aminobutyric acid (GABA) alleviates salt damage in tomato by modulating na+ uptake, the GAD gene, amino acid synthesis and reactive oxygen species metabolism. BMC Plant Biol. 20, 1–21. doi: 10.21203/rs.3.rs-33418/v2
Wu, J., Shu, S., Li, C., Sun, J., Guo, S. (2018). Spermidine-mediated hydrogen peroxide signaling enhances the antioxidant capacity of salt stressed cucumber roots. Plant Physiol. Biochem. 128, 152–162. doi: 10.1016/j.plaphy.2018.05.002
Wu, S., Wang, Y., Zhang, J., Gong, X., Zhang, Z., Sun, J., et al. (2021). Exogenous melatonin improves physiological characteristics and promotes growth of strawberry seedlings under cadmium stress. Hortic. Plant J. 7, 13–22. doi: 10.1016/j.hpj.2020.06.002
Wu, X., Yao, X., Chen, J., Zhu, Z., Zhang, H., Zha, D. (2014). Brassinosteroids protect photosynthesis and antioxidant system of eggplant seedlings from high-temperature stress. Acta Physiol. Plant 36, 251–261. doi: 10.1007/s11738-013-1406-7
Wu, Y., Zhang, D., Chu, J. Y., Boyle, P., Wang, Y., Brindle, I. D., et al. (2012). The arabidopsis NPR1 protein is a receptor for the plant defense hormone salicylic acid. Plant Cell Rep. 1, 639–647. doi: 10.1016/j.celrep.2012.05.008
Xiang, L., Hu, L., Xu, W., Zhen, A., Zhang, L., Hu, X. (2016). Exogenous γ-aminobutyric acid improves the structure and function of photosystem ii in muskmelon seedlings exposed to salinity-alkalinity stress. PloS One 11, e0164847. doi: 10.1371/journal.pone.0164847
Xi, Z., Wang, Z., Fang, Y., Hu, Z., Hu, Y., Deng, M., et al. (2013). Effects of 24-epibrassinolide on antioxidation defense and osmoregulation systems of young grapevines (Vitis vinifera l.) under chilling stress. Plant Growth Regul. 71, 57–65. doi: 10.1007/s10725-013-9809-4
Yan, Z., Chen, J., Li, X. (2013). Methyl jasmonate as modulator of cd toxicity in capsicum frutescens var fasciculatum seedlings. Ecotoxicol Environ. Saf. 98, 203–209. doi: 10.1016/j.ecoenv.2013.08.019
Yang, A., Cao, S., Yang, Z. (2011). γ-aminobutyric acid treatment reduces chilling injury and activates the defence response of peach fruit. Food Chem. 129, 1619–1622. doi: 10.1016/j.foodchem.2011.06.018
Yang, P., Wang, Y., Li, J., Bian, Z. (2019). Effects of brassinosteroids on photosynthetic performance and nitrogen metabolism in pepper seedlings under chilling stress. Agronomy 9, 839. doi: 10.3390/agronomy9120839
Yan, Z., Zhang, W., Chen, J., Li, X. (2015). Methyl jasmonate alleviates cadmium toxicity in solanum nigrum, by regulating metal uptake and antioxidative capacity. Biol. Plant 59, 373–381. doi: 10.1007/s10535-015-0491-4
Yasir, T. A., Wasaya, A. (2021). Brassinosteroids signaling pathways in plant defense and adaptation to stress. Plant Growth Regul., 197–206. doi: 10.1007/978-3-030-61153-8_9
Yildirim, E., Turan, M., Guvenc, I. (2008). Effect of foliar salicylic acid applications on growth, chlorophyll, and mineral content of cucumber grown under salt stress. J. Plant Nutr. 31, 593–612. doi: 10.1080/01904160801895118
Yosefi, A., Mozafari, A. A., Javadi, T. (2020). Jasmonic acid improved in vitro strawberry (Fragaria × ananassa duch.) resistance to PEG-induced water stress. Plant Cell Tiss Org Cult 142, 549–558. doi: 10.1007/s11240-020-01880-9
Yu, J. Q., Huang, L. F., Hu, W. H., Zhou, Y. H., Mao, W. H., Ye, S. F., et al. (2004). A role for brassinosteroids in the regulation of photosynthesis in cucumis sativus l. J. Exp. Bot. 55, 1135–1143. doi: 10.1093/jxb/erh124
Zamani, Z., Amiri, H., Ismaili, A. (2019). Improving drought stress tolerance in fenugreek (Trigonella foenum-graecum) by exogenous melatonin. Plant Biosyst. Int. J. Deal Aspec Plant Biol. 154, 643–655. doi: 10.1080/11263504.2019.1674398
Zhang, Z., Lan, M., Han, X., Wu, J., Wang-Pruski, G. (2019). Response of ornamental pepper to high temperature stress and role of exogenous salicylic acid in mitigating high temperature. J. Plant Growth Regul. 39, 133–146. doi: 10.1007/s00344-019-09969-y
Zhang, T., Shi, Z., Zhang, X., Zheng, S., Wang, J., Mo, J. (2020). Alleviating effects of exogenous melatonin on salt stress in cucumber. Sci. Hortic. 262, 109070. doi: 10.1016/j.scienta.2019.109070
Zhang, Y., Xu, S., Yang, S., Chen, Y. (2015). Salicylic acid alleviates cadmium-induced inhibition of growth and photosynthesis through upregulating antioxidant defense system in two melon cultivars (Cucumis melo l.). Protoplasma 252, 911–924. doi: 10.1007/s00709-014-0732-y
Zhao, S., Ma, Q., Xu, X., Li, G., Hao, L. (2016). Tomato jasmonic acid deficient mutant spr2 seedling response to cadmium stress. J. Plant Growth Regul. 35, 603–610. doi: 10.1007/s00344-015-9563-0
Zhao, Y., Song, C., Brummell, D. A., Qi, S., Lin, Q., Duan, Y. (2021). Jasmonic acid treatment alleviates chilling injury in peach fruit by promoting sugar and ethylene metabolism. Food Chem. 338, 128005. doi: 10.1016/j.foodchem.2020.128005
Zhao, M. L., Wang, J. N., Shan, W., Fan, J. G., Kuang, J. F., Wu, K. Q. (2013). Induction of jasmonate signalling regulators MaMYC2s and their physical interactions with MaICE1 in methyl jasmonate-induced chilling tolerance in banana fruit. Plant Cell Environ. 36, 30–51. doi: 10.1111/j.1365-3040.2012.02551.x
Zhao, H., Zhang, K., Zhou, X., Xi, L., Wang, Y., Xu, H. (2017). Melatonin alleviates chilling stress in cucumber seedlings by up-regulation of cszat12 and modulation of polyamine and abscisic acid metabolism. Sci. Rep. 7, 4998. doi: 10.1038/s41598-017-05267-3
Zheng, X., Li, Y., Xi, X., Ma, C., Sun, Z., Yang, X., et al. (2021). Exogenous strigolactones alleviate KCl stress by regulating photosynthesis, ROS migration and ion transport in malus hupehensis rehd. Plant Physiol. Biochem. 159, 113–122. doi: 10.1016/j.plaphy.2020.12.015
Keywords: environmental stresses, horticultural plant growth, plant hormones, plant physiology, photosynthesis
Citation: Zheng Y, Wang X, Cui X, Wang K, Wang Y and He Y (2023) Phytohormones regulate the abiotic stress: An overview of physiological, biochemical, and molecular responses in horticultural crops. Front. Plant Sci. 13:1095363. doi: 10.3389/fpls.2022.1095363
Received: 11 November 2022; Accepted: 05 December 2022;
Published: 06 January 2023.
Edited by:
Muhammad Ahsan Altaf, Hainan University, ChinaReviewed by:
Rana Roy, Sylhet Agricultural University, BangladeshMuhammad Anwar, Shenzhen University, China
Noshin Ilyas, PMAS Arid Agriculture University, Pakistan
Copyright © 2023 Zheng, Wang, Cui, Wang, Wang and He. This is an open-access article distributed under the terms of the Creative Commons Attribution License (CC BY). The use, distribution or reproduction in other forums is permitted, provided the original author(s) and the copyright owner(s) are credited and that the original publication in this journal is cited, in accordance with accepted academic practice. No use, distribution or reproduction is permitted which does not comply with these terms.
*Correspondence: Yuhui He, eWhlMjYwMkBnbWFpbC5jb20=; Zmx5dWh1aTAwOUBzaW5hLmNvbQ==