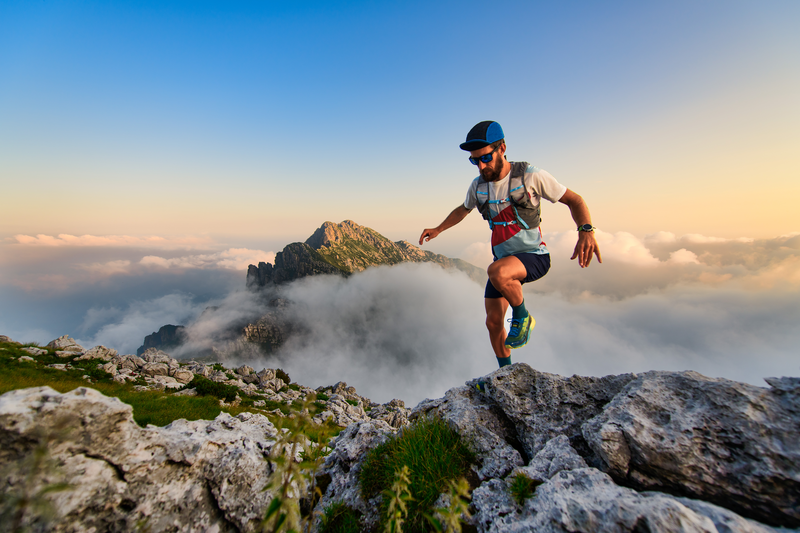
94% of researchers rate our articles as excellent or good
Learn more about the work of our research integrity team to safeguard the quality of each article we publish.
Find out more
REVIEW article
Front. Plant Sci. , 04 January 2023
Sec. Plant Abiotic Stress
Volume 13 - 2022 | https://doi.org/10.3389/fpls.2022.1083960
This article is part of the Research Topic Transcriptome & Metabolic Profiling: An Insight Into the Abiotic Stress Response Crosstalk in Plants View all 13 articles
Environmental stresses such as drought, high salinity, and low temperature can adversely modulate the field crop’s ability by altering the morphological, physiological, and biochemical processes of the plants. It is estimated that about 50% + of the productivity of several crops is limited due to various types of abiotic stresses either presence alone or in combination (s). However, there are two ways plants can survive against these abiotic stresses; a) through management practices and b) through adaptive mechanisms to tolerate plants. These adaptive mechanisms of tolerant plants are mostly linked to their signalling transduction pathway, triggering the action of plant transcription factors and controlling the expression of various stress-regulated genes. In recent times, several studies found that Zn-finger motifs have a significant function during abiotic stress response in plants. In the first report, a wide range of Zn-binding motifs has been recognized and termed Zn-fingers. Since the zinc finger motifs regulate the function of stress-responsive genes. The Zn-finger was first reported as a repeated Zn-binding motif, comprising conserved cysteine (Cys) and histidine (His) ligands, in Xenopus laevis oocytes as a transcription factor (TF) IIIA (or TFIIIA). In the proteins where Zn2+ is mainly attached to amino acid residues and thus espousing a tetrahedral coordination geometry. The physical nature of Zn-proteins, defining the attraction of Zn-proteins for Zn2+, is crucial for having an in-depth knowledge of how a Zn2+ facilitates their characteristic function and how proteins control its mobility (intra and intercellular) as well as cellular availability. The current review summarized the concept, importance and mechanisms of Zn-finger motifs during abiotic stress response in plants.
Crops usually encounter a wide range of hostile climatic fluctuations during their life cycles. Such abnormal environmental fluctuations are covered by stressors of both biotic origins, including infection by pathogens (virus, bacteria, fungi, etc.), attack by weeds, and insects, as well as by abiotic components also (Moulick et al., 2018d; Ghosh et al., 2020a; Ghosh et al., 2020b; Ghosh et al., 2021; Ghosh et al., 2022a; Ghosh et al., 2022b; Mateos Fernández et al., 2022; Tonnang et al., 2022; Jain et al., 2022a; Jain et al., 2022b). Among the abiotic stress including heat and chilling stress (Aslam et al., 2022; Haider et al., 2022; Ullah et al., 2022; Verma et al., 2022), limitation of water (drought), limitation of nutrients, elevated levels of salt, and hazardous/toxic metals and metalloids in the soil (Hernandez et al., 2000; Moulick et al., 2019; Saha et al., 2019; Sahoo et al., 2019; Moulick et al., 2021; Choudhury et al., 2022a; Choudhury et al., 2022b; Choudhury and Moulick, 2022; Mazumder et al., 2022).
Environmental stress can adversely modulate the field crop’s ability to maintain its yield potential, i.e., their determining yield despite satisfactory inputs and other factors. A field crop/plant’s vulnerability to adverse deviations from (maximum yield) yield potential is usually calculated by measuring the respective crop’s yield stability (Bhadra et al., 2021). The difference between the actual yield and yield potential of a particular site (agro-environment) is regarded as the yield gap (Mueller et al., 2012). Crops seldom touch their real yield potential in most broad-acre agri-environmental systems due to experiencing stress (s) during crop’s life cycle. Stress-affected plants exhibited three primary response segments: first is the alarm or apprehension phase, sometimes also called the initiation of stress; the next phase, i.e., the second phase is characterized by resistance or fight phase through the initiation of defence systems; and the last or third phase is the exhaustion phase or collapse stage where loss due to stress is evident (Larcher, 2003; Moulick et al., 2019; Sahoo et al., 2019).
Among the stresses, salinity (high Na+) is the critical agro-environmental factor that limits growth and yield/productivity. Under salinity stress, crops usually modulate their physiological processes by endorsing water acquisition and retention, and shifting the ion homeostasis management process (Parida and Das, 2005; Barman et al., 2022; Roy et al., 2022). At the same time, scarcity of available water to plants or drought stress results in decreased survival of plants, growth, and development due to metabolic imbalances. Drought is often linked with a lack of accessibility of groundwater in the land/soil but can also be worsened by greater evapotranspiration (Jaleel et al., 2009; Choudhury et al., 2022c; Jadhav et al., 2022). Such stress (drought) may occur under dry/humid conditions and with elevated air temperatures. The disparity in the water loss due to evapotranspiration flux and water uptake from soil may attribute to the key reason behind imposing drought stress (Lipiec et al., 2013; Dash et al., 2022; Sagar et al., 2022). On the other hand, toxic heavy metals and metalloids are also posing a serious threat to achieving agricultural sustainability in crop production with significant accumulation in the edible parts imposing a menace to the food chain (Moulick et al., 2016a; Moulick et al., 2016b; Moulick et al., 2018a; Moulick et al., 2018b; Moulick et al., 2018c; Saha et al., 2019; Chowardhara et al., 2019a; Chowardhara et al., 2019b; Saha et al., 2022).
From a genetic point of view, stress is a set of certain environmental conditions that prevent a crop from experiencing its complete genetic expression. An abiotic component-induced stress, not only due to the exchanges (mainly with signalling) with other organisms and that can negatively impact particular organisms in an agro-environment, is regarded as abiotic stress. The effect of abiotic stresses on the agro-environmental sector is a crucial threat presently intensified by anthropogenic activities and global warming (Kang et al., 2017; Mukherjee and Hazra, 2022).
Reports indicate that abiotic stresses not only impose adverse on a crop’s anatomy, physiology, biochemistry and but subsequently limit essential metabolic processes like respiration, photosynthesis, and growth when lingered for a long time often inducing death also (Hirayama and Shinozaki, 2010; Lima et al., 2015; Basu et al., 2016). Plants are equipped with mechanisms (physiological and metabolic) that may be crucial in lightening/mitigating agro-environmental stresses. These stress-induced shuffling crops’ metabolic machinery is controlled using the initiation of genetic networks or pathways. The outcome of this genetic alternation is imparting greater/better tolerance or resistance to certain stress (s) (Claeys and Inzéand Inzé, 2013; Thorpe et al., 2013). Upon exposure to environmental stresses, dangerous by-products to crop health were found to be instrumental to plants’ normal health and wellbeing. H2O2 (hydrogen peroxide), superoxide radicals, OH-radicals (hydroxyl radicals), are regarded as reactive oxygen species (ROS), generated as a result of leakage of electrons that occurs during the photorespiration and photosynthesis process (Thorpe et al., 2013; Kao, 2017; Moulick et al., 2021). Within the plant cells, the proper antioxidant defense machinery and ROS build-up sustain a steady-state balance (Hasanuzzaman et al., 2012). Keeping a satisfactory level of ROS within the cell permits adequate operation of redox biology (metabolic reactions) and the management of numerous phycio-biochemical processes vital for plant’s growth and development. This kind of harmony among ROS formation and ROS quenching is an example of intermediate level of ROS management/homeostasis (Hasanuzzaman et al., 2019).
These reduced oxygen radicals or ROS were reported to adversely influence key components of a crop’s metabolic cycle resulting in significant damage to cellular processes and death (Nouman et al., 2014). In order to lessen the excessive ROS production and subsequent oxidative stress, plants/crops have well-maintained anti-oxidative machinery that consists of non-enzymatic as well as an enzymatic unit that can bring equilibrium among ROS generation and quenching and protection of cellular damage even PCD or programed cell death also (Raja et al., 2017; Duan et al., 2012; Das and Roychoudhury, 2014; Choudhury et al., 2021a; Hossain et al., 2021).
The magnitude of ROS induced damage to biomolecules subjected to many factors like the concentration of target biomolecule(s), site of the particular biomolecule(s) in relation to the ROS production site, the rate constant of the reaction among biomolecules and ROS, efficacy ROS quenching components are to name a few (Davies, 2005). Increasing the antioxidant level within the plant cells can be achieved by spontaneously through use of genetic engineering or by supplementing can boost up the defense system of the plant and saving them from the adverse impact of ROS generated as a result of environmental stress (Mishra et al., 2006; Gupta et al., 2009; Kaur et al., 2022; Upadhyay et al., 2022). SOD (superoxide dismutase), CAT (catalase), and POX or peroxides are well-recognized enzymes involved in antioxidant systems that adjust the ROS homeostasis by reducing OH• into H2O2 of crops (Gill and Tuteja, 2010; Nouman et al., 2016). Whereas, in coordination, with enzymatic components, the non-enzymatic units of the antioxidant system (glutathione, flavanoid, lipids carotenoids, etc.) work on H2O2 through various means (Duan et al., 2012; Yin et al., 2020; Choudhury et al., 2022c).
At present, the main concern for the researchers working on developing suitable strategies to mitigate abiotic stress in crops, the main challenge is the complexities regarding stressor (s), i.e., abiotic components and the responses by crops towards the stressors. Apart from agronomic practices, irrigation management comparatively newer avenues like seed priming technology (Moulick et al., 2016a; Moulick et al., 2017; Moulick et al., 2018a; Moulick et al., 2018b; Moulick et al., 2018c), potentials of wild relatives (Hossain et al., 2022) potentials of metabolomics and next generation sequences are to name a few (Choudhury et al., 2021b; Hossain et al., 2021).In order to properly understand how stressors and crops interact at the molecular level, i.e. replication, transcription and translation. One such exciting topic of how the information imposed upon exposure to a stressor communicates at the cellular level is to illustrate the contributions made by transcription factors or Tfs. Among the well-known TFs, Zn-Fingers are of prime interest.
The Zn-finger was first reported as a repeated Zn-binding motif, comprising conserved cysteine (Cys) and histidine (His) ligands, in Xenopus laevis oocytes as a transcription factor (TF) IIIA (TFIIIA) (Miller et al., 1985). Since its first report, a wide range of Zn-binding motifs has been recognized and termed Zn-fingers. To meet the cellular demand, many proteins employ non-protein (often metallic ions) as cofactors. Among the metallic ions considered cofactors, transition metal ions are the most important due to their significant influence on modulating a wide range of cellular activities. From the periodic table’s perspective, d-block elements are found to be more actively involved as cofactors. Zn is one cofactor that can influence as much as >10% of human proteins with a pronounced impact on structural and catalytic activities (Andreini et al., 2006; Maret and Li, 2009; Kochańczyk et al., 2015). In the proteins where Zn2+ is mostly attached to amino acid residues and thus espousing a tetrahedral coordination geometry. The physical nature of Zn-proteins, defining the attraction of Zn-proteins for Zn2+is crucial for knowing how a Zn2+ facilitates their characteristic function and how proteins control its mobility (intra and intercellular) as well as cellular availability. In the mammalian genomes, encoded Zn-finger proteins dominate in number and are known as TF-regulators. A specific number and variety within Zn-finger or Zn-containing domains contribute to different cellular processes like regulation of transcription, binding to nucleic acid, and folding of proteins. Intermolecular attachment spots are the most significant and sort after when compared with similar intermolecular bindings. Due to having structural complexities (peptide chain composition, orientation, etc.), the intermolecular attachment of ligands and their respective targets poses serious challenges to analysis analyze (Maret et al., 2004; Kochańczyk et al., 2016). Findings have shown that an optimum level of Zn2+ concentration is essential to maintaining the stability and folding of protein subunits and for satisfactory performance of the catalytic activity of a particular enzyme (Keilin and Mann, 1940; Vallee and Neurath, 1954; Parraga et al., 1988). This innovative concept of small Zn2+-stabilized domains was further supported by the in-depth analysis of the TFIIIA sequence, which exhibited that a continuous stretch of nine tandemly repeated 30 amino acid residues (13 to 276) having two invariant pairs of Cys along with His residues coordinating one Zn2+. This particular pattern was later coined as ZF/zinc finger (Miller et al., 1985; Fairall et al., 1986; Rhodes and Klug, 1986).
In this review, we are going to assess the contributions made by ZFs in elaborating and imparting abiotic stress tolerance in field crops with unique references to salinity, water stress, thermos-stress, heavy metals, irradiation and elevated CO2 levels.
Zinc Finger Proteins (ZFPs) contain a highly conserved signature domain consisting of 20–30 amino acid residues having the consensus sequence of CX2–4CX3FX5LX2HX3–5H (X denotes any amino acid). Structural differences in different ZFPs are basically due to the differences in positions and numbers of cysteine (Cys) and histidine (His) residues that interacts and bind to the zinc ion and are constituted of several sub-groups which are designated as Cys4/C4 (GATA-1), Cys6/C6 (GAL4), Cys8/C8, Cys2HisCys/C2HC (Retroviral nucleocapsid), Cys2His2/C2H2 (TFIIIA), Cys2HisCys5/C2HC5 (LIM domain), Cys3His/C3H/CCCH, Cys3HisCys4/C3HC4 (RING finger) and Cys4HisCys3/C4HC3 (Requium), DnaJ-like zinc finger protein and many others (Lyu and Cao, 2018; Han et al., 2020; Yuce and Ozkan, 2020; Ali et al., 2022). As ZFPs are basically the members of the most prominent transcription factor family having a DNA binding domain, they are generally localized to the nucleus after translation. However, the zinc finger domain, an important structural motif, is also reported to be involved in other cellular activities such as RNA binding, membrane association and protein-protein recognitions and interaction (Han et al., 2020). Thus, different types of ZFPs are localized to cellular compartments as per their specific role in cellular activities. Protein sorting in a cell can also offer clues about the functions of these proteins (Wang et al., 2020).
Among all the sub-groups of ZFPs, the C2H2 (TFIIIA) has been extensively studied in plants. It represents the large proportions of ZFPs in plants where 189 members in rice (Agarwal et al., 2007), 321 in Soybean (Yuan et al., 2018), 122 in Cucumis sativus (Yin et al., 2020) and many more members in other crops have been reported. Its DNA-binding motif is one of the best-characterized motifs having two residues each of Cys and His amino acids tetrahedrally combined into a zinc ion (Xie et al., 2019). Focussing on plant-specific C2H2 type ZFPs (Q-type C2H2-ZFPs), it is reported to have different lengths of long spacer between two Zn finger motifs as compared to other eukaryotic organisms. Apart from the Zn finger motif, the core sequence KXKRSKRXR, which is present in the N-terminal of protein sequences, acts as an NLS for sorting of C2H2 type ZFPs to the nucleus (Xie et al., 2019) and the QALGGH motif present in the helical region is required for binding to DNA (Kielbowicz-Matuk, 2012). Having multiple functions in the nucleus, most of the reported C2H2-type ZFPs in plants were found to be localized in the nucleus. Several experiments were conducted with the C2H2 type ZFP genes to confirm the nuclear localization, such as FEMU2 of Chlamydomonas reinhardtii, fused with the β-glucuronidase (GUS) reporter gene was bombarded into onion epidermal cell and found that FEMU2 protein was in fact localized to the nucleus. A similar experiment was conducted with JcZFP8 gene of Jatropha curcas fused with the GFP reporter gene under the control of the CaMV35S promoter. The 35Sp : JcZFP8:GFP gene construct was injected into tobacco protoplast for transient expression, and the fluorescence signal results clearly showed the localization of 35Sp : JcZFP8:GFP fusion protein into the nucleus of the tobacco cells (Shi et al., 2018). WRKYs with its conserved consensus sequence of WRKYGQK along with zinc-finger-like motifs of C2H2 and C2HC type ZFPs also have a NLS for their localization to the nucleus (Bakshi and Oelmüller, 2014). In the nucleus, it acts as a transcription factor and binds to the TTGAC(C/T) W-box cis-element in the promoter of their target genes (Bakshi and Oelmüller, 2014; Chen W et al., 2019) and transcriptionally regulates the expression of target genes (Cheng et al., 2017).
Similarly, another extensively studied sub-class of plant ZFPs is Cys3His/C3H/CCCH type. Plant genome encodes large numbers of CCCH type ZFPs and has been identified and characterized in crop plants such as Chickpea (Pradhan et al., 2017), Brassica rapa (Pi et al., 2018) and many other plants. The CCCH-type ZFPs of plants have conserved CCCH motifs ranging from one to six copies, with the most prevalent consensus sequence of the C-X7–8-C-X5-C-X3-H motif in the middle of the protein sequence (Pi et al., 2018; Chen et al., 2020). Although C-X7–8-C-X5-C-X3-H is the most common motif in CCCH-type ZFPs in plants, other structural variations in the consensus sequences suggest different cellular localisation patterns and roles in cellular activities (Han et al., 2020). Many of the CCCH-type ZFPs are localized to the nucleus, such as AtZFP1, KHZ1 and KHZ2 of Arabidopsis (Han et al., 2014), SAW1 and OsC3H10 of rice (Wang et al., 2020; Seong et al., 2020). CCCH-type ZFPs such as AtTZF2/3, ZFP36L3 and ZC3H12a are localized to the cytoplasm [84-86), where some of the CCCH-type ZFPs, namely OsC3H10, AtTZF4-6, etc. gets co-localized with stress granules (SGs) and processing bodies (PBs) (Seong et al., 2020). Likewise, some CCCH-type ZFPs are localized to plasma membranes such as AtOZF1and AtOZF2 (Oxidation-related Zinc Finger 1) of Arabidopsis and PeC3H74 of Moso bamboo (Huang TL et al., 2012; Chen et al., 2020) where some of the members are involved in secondary wall synthesis in response to biotic and abiotic stresses (Zhang et al., 2018; Chen et al., 2020), while several other members of CCCH type ZFPs such as OsLIC of rice, AtTZF of Arabidopsis shuttle between the nucleus and the cytoplasm (Wang et al., 2008; Bogamuwa and Jang, 2013). Shuttling of CCCH type ZFPs between cytoplasm and nucleus is due to the presence of leucine-rich NESs (Nuclear Export Signals) and NLSs (Nuclear Localization Signals) which are mainly present in their N- or C- terminal of the protein sequence (Han et al., 2020). These shuttle signals are current in several CCCH-type ZFPs across the crop species indicating their potential role in stress responses and signal transduction (Wang et al., 2008; Chai et al., 2012). Sub-cellular localization to cytoplasm and membrane was observed in another class of ZFPs called RING ZFPs. Arabidopsis RING ZFPs, AtRZFP fused with GFP under the control of 35S promoter was transformed into onion epidermal cells, and the signal of AtRZFP-GFP was clearly observed in the cytoplasm and plasma membrane (Zang et al., 2016). Some of the RING ZFPs located in the plasma membrane and cytoplasm include AtAIRP1, RHA2a, AtATL78 of Arabidopsis, OsRDCP1, OsSIRH2-14, OsRFPv6 of rice, LjCZF1 of Lotus japonicas, VpRH2 of grape are located to the plasma membrane (Han et al., 2021) and ZmXerico2 of maize (Gao et al., 2012), OsSIRH2-14 and OsSIRP1 of rice (Hwang et al., 2016), AtAIRP4, EMR of Arabidopsis (Park et al., 2018), CaDSR1, CaASRF1 of pepper (Lim et al., 2018; Joo et al., 2019) are located in the cytoplasm. Other studies suggested that other than plasma membrane and cytoplasm, RING ZFPs are also localized to nucleus and other cellular compartments. For instance, CaASRF1, CaAIRF1, CaDSR1 of pepper (Lim et al., 2017), AtHOS1, AtATRF1 of Arabidopsis (Tian et al., 2015; Kim et al., 2017; Qin et al., 2017) and OsSADR1 of rice (Park et al., 2018) were located to nucleus, whereas, OsSIRH2-14 of rice was not only located in the cytoplasm and plasma membrane but was also found to be localized to Golgi bodies (Park et al., 2019). Likewise, wheat TaDIS1 was also reported to be localized to Golgi bodies (Lv et al., 2020). Rice RING-H2 zinc finger proteins OsHCI-1 and OsMAR-1 were found to be localized to the cytoskeleton particularly in microtubules (Lim et al., 2013; Park et al., 2018) and RING-H2 ZFPs of wild tomato SpRING was found to be localized to the endoplasmic reticulum.
GATA-1 which is one of the sub-group members of ZFPs conserved families of transcription factors regulating the expression genes involved in cellular processes (Zhang et al., 2015). With the consensus sequence of CX2CX17−20CX2C along with DNA binding domain, it binds to the WGATAR (W = T/A, R = G/A) sequence in the promoter region of the target genes (Behringer and Schwechheimer, 2015; Gupta et al., 2017). As a transcription factor family, it has to be localized to the nucleus for its activities and to confirm it a study was conducted using the GATA gene of Poplar (P. deltoids) where Arabidopsis plant was transformed with PdGNC-GFP gene fusion under the control of CaMV35S promoter. Its nuclear localization was confirmed as the 35S: PdGNC-GFP fusion protein was detected in the nucleus (An et al., 2014).
Likewise, Brachpodium distachyon BdGATA13-eGFP gene fusion under the control of 35S promoter which was used for the transformation of tobacco leaves was found to be localized into the nucleus (Guo et al., 2021). However, reports on FIP (FtsH5 Interacting Protein), which is a type of GATA-1 ZFPs highlighted its localization to plastid as well specifically to thylakoid membrane in response to abiotic stress signals (Lopes et al., 2018). Similarly, DnaJ-like ZFPs with their characteristic C-terminal tandem 4× repeats of the CxxCxxxG are reported to have roles in the accumulation of carotenoids in plastids of non-pigmented tissues (Osorio, 2019) and inducing chromoplast biogenesis and simultaneously repressing the chloroplast biogenesis and chlorophyll biosynthesis in the nucleus of de-etiolating cotyledons cells (Sun et al., 2019). With its functions specific to the nucleus and chloroplast, DnaJ-like ZFPs are localized to both the nucleus and chloroplast. Chloroplast localization of DnaJ like ZFPs is due to the presence of N-terminal chloroplast transit peptide (cTP) along with C-terminal zinc finger domain (ZF) which is separated by two trans-membrane domains (TMs) (Chen et al., 2021a). For nuclear localization, ubiquitination of lysine58 in ORANGE/OR (a type of DnaJ like ZFPs) by UBC19 was reported to be essential to generate truncated OR ZFPs proteins (Chen et al., 2021a). Sub-cellular localization studies of another class of ZFPs called as FCS-like ZFPs (FLZs) were conducted as it was found to be active both in cytoplasm and nucleus. FCS-like ZFPs are reported to act as scaffold proteins for plant-specific SnRK1 complex which is involved in various stress responses and are reported to be localized to both cytoplasm and the nucleus in plants like Arabidopsis and Maize (Jamsheer et al., 2018a; Chen et al., 2021b). The distribution of ZFPs to different compartments of plant cell clearly indicates that this class of proteins are involved in several cellular processes either at both transcriptional and post-transcriptional level.
Many ZFPs acts as critical transcriptional regulators that correlate with their localization into the nucleus. However, it also interacts with RNA and other proteins to regulate the post- transcriptional expression of the target genes at RNA and protein levels, respectively (Han et al., 2020). As a transcription factor, it binds to the cis-acting element and subsequently activates or represses the expression of downstream target genes. The conserved zinc finger structure helps it bind to DNA double helix at specific sites to act as a transcription factor (Han et al., 2021). In plants like Arabidopsis, durum wheat and rice, a highly conserved sequence QALGGH of Q-type C2H2-ZFPs provide the ability for ZFPs to recognize the target sites and to further regulate the expression of downstream genes through activator or repressor domain (Lyu and Cao, 2018; Xie et al., 2019). However, the QALGGH sequence is not the only key and ubiquitous sequence for binding to target genes (Liu et al., 2022), the C2H2 type ZFPs may bind to the target site of the genes through DNA binding domain which is present in long spacers between the two adjacent zinc finger motifs (Sakamoto et al., 2004).
Apart from binding to DNA as a transcription factor, C2H2 ZFPs can also bind to RNA based on their bases and folding backbones, which recognize variants of phosphoric acid skeletons in RNA (Lin and Lin, 2018; Han et al., 2020). It is found that amino acid residues of C2H2 zinc finger proteins positioned at -1 and +2 of the a-helix play a vital role in its binding to RNA (Han et al., 2020). Upon binding to RNA, some of the members of ZFPs such as cleavage and polyadenylation specificity factor 30 (CPSF30) belonging to CCCH/Cys3His/C3H type ZFPs are involved in the polyadenylation step of pre-mRNA processing after forming a complex which is collectively called as CPSF (Shimberg et al., 2016). The RNA binding AtCPSF30 known for involvement in the polyadenylation step of pre-mRNA also interacts with other molecules like calmodulin, however, its RNA binding activity gets reduced in presence of its other interacting molecules like calmodulin (Lee et al., 2012). For interacting with other proteins including other zinc finger proteins, ZFPs like the C2H2 type utilize domains such as L-box motif and EAR motif for interaction resulting in binding/prevention of binding of the target protein to DNA which in turn regulates the expression of downstream genes (Gamsjaeger et al., 2007; Brayer and Segal, 2008). Among the two motifs, the EAR motif which is the smallest known repressor domain in plants is reported to be essential for the inhibition of transcriptional activities (Hiratsu et al., 2004).
Correspondingly, WRKY proteins which are the type of Cys(2)-His(2) (C2H2) or Cys(2)-HisCys (C2HC) ZFPs, have two highly conserved domains with WRKYGQK sequences of WRKY domain and C-terminal zinc finger domain (Rushton et al., 2010). The target DNA binding site generally recognizes W-box cis-elements in the promoter region of target genes. For instance, a WRKY protein of Hylocereus polyrhizus HpWRKY44 was found to be directly binding to the W-box element present in the promoter of HpCytP450-like1 and transcriptionally activated the HpCytP450-like1, resulting into induced betalain biosynthesis in pitaya fruit (Cheng et al., 2017). However, some WRKY proteins bind to the promoter region other than W-box cis-elements (Chen et al., 2019). The selective binding of different WRKY TF members to W-box cis-element is based on neighboring DNA sequences outside of the W-box core motif (Bakshi and Oelmüller, 2014). Several experiments highlighted the role of the WRKY gene family to be associated with the regulation of transcriptional reprogramming in response to environmental stresses. For example, PROPER genes of Arabidopsis which encodes for small peptides which act as molecular patterns associated with injury or damage are perceived by PEPR1 and PEPR2 (leucine-rich repeat receptor kinases) and amplify the defense responses. Upon receiving the stress signal WRKY TF binds to the promoter of these two kinases and regulates their expression (Logemann et al., 2013).
Advancement in molecular techniques has helped to characterize various TF and regulatory element functions on a genome-wide scale, such as the utilization of the DAP-seq technique to discover the binding sites of TFs in DNA (O’Malley et al., 2016). Similarly, transcriptional role and binding sites of ZFPs like AtWRKY33 under biotic were successfully identified using the techniques like ChIP-seq and revealed that AtWRKY33 negatively regulates the NCED3 and NCED5 (ABA biosynthesis genes) to impart resistance against biotic stress like necrotrophic fungus (Liu et al., 2015). Some C2H2 type ZFPs are reported to transcriptionally regulate the expression of genes involved in programme cell death of the plants, thereby inducing PCD of plant cells (Yin et al., 2020).
Similar to C2H2 type ZFPs, many C3H/CCCH type ZFPs are keys to regulating transcriptional activities with the presence of its conserved activator or repressor domains. For instance, rice OsLIC and Ehd4 protein have a conserved EELR domain in its C terminal, which acts as the key component for transcriptional activation of the target genes (Wang et al., 2008). CCCH type ZFPs such as OsLIC and Ehd4 of rice, AtTZF1 and AtTZF6 (PEI1) of Arabidopsis can bind to the promoter region of target genes (Wang et al., 2008; Bogamuwa and Jang, 2013; Wang et al., 2020). However, in some of the CCCH-type ZFPs, the transcriptional activator domain, called EELR-like, was found to be in N-terminal. This EELR-like domain of ZFPs such as AtC3H17 and AtZFP1were found to play an essential role in transcriptional activation of downstream salt-responsive genes like AtP5CS1, AtGSTU5, SOS1 and ABA-dependent responsive genes RD22, COR15A and RAB18 (Seok et al., 2016; Seok et al., 2018). In addition to transcriptional activators, some other proteins are transcriptional repressors. For negatively regulating the transcription of target genes, CCCH ZFPs like GLUB-1-BINDING ZINC FINGER 1 (OsGZF1) and ILA1-interacting protein 4 (IIP4) of rice have repressor motifs. After binding of ZFPs in the promoter region of target genes like MYB61, CESAs and GluB-1, it negatively regulates the expression of CESAs and MYB61 for secondary wall synthesis and GluB-1 for accumulation of gluten (Chen et al., 2014; Zhang et al., 2018). In addition to its involvement in transcriptional activities, CCCH ZFPs like AtTZF1, KHZ1 and KHZ2 of Arabidopsis also has been reported to be involved in post-transcriptional regulation of gene expression by binding to mRNA through its RNA binding motif (Yan et al., 2017; Pi et al., 2018). It is reported that with the help of the RNA binding domain, ZFPs namely, OsTZF1 bind to mRNA at the 3’ un-translated region specifically at AU-rich elements (AREs) (Jan et al., 2013). In plants like Arabidopsis, ZFPs like HUA1 with its RNA binding ability regulates flower development through pre-mRNA processing of AGAMOUS (Rodriguezcazorla et al., 2018) and FRIGIDA-ESSENTIAL 1 (FES1) promotes the winter annual growth habits in a FRIGIDA-dependent manner by regulating mRNA levels of FLOWERING LOCUS C (FLC) (Schmitz et al., 2005). A recent study has found that the splicing efficiency of FLC pre-mRNA can be inhibited by KHZ1 and KHZ2 (RNA binding ZFPs) and promotes flowering in Arabidopsis through other independent pathways (Yan et al., 2020). Likewise, it has been reported that zinc finger homeodomain proteins (ZF-HD) another class of ZFPs are essential for the induction of flowers in plants like Arabidopsis. As the induction of flowers is affected by environmental stresses, ZF-HD1 proteins over-expresses during such stress condition and helps in coping with stress through transcriptional activation of genes like ERD1 (Shalmani et al., 2019).
In plants, through in vitro studies, KTEL (V) residue at the N terminus of ZFPs was observed in each zinc finger motif proving to be a key interface for RNA binding (Wang et al., 2008) and plant-specific TZF motif (RR-TZF) & RR sequence in AtTZF1 were found to be the essential motifs for binding to AREs of RNA leading to mRNA degradation (Qu et al., 2014). More recently two putative mRNA binding domains namely RRM and OST-HTH/LOTUS were identified in CCCH type ZFP (AtC3H18L) sequence (Xu et al., 2020).
Some ZFPs are co-localized to PBs and SGs. These PBs and SGs have essential roles in the post-transcriptional regulation of several genes, more importantly, during plant tolerance against environmental stresses (Bogamuwa and Jang, 2016). Some ZFPs, namely AtTZF1, AtTZF4, AtTZF5, and AtTZF6 of Arabidopsis, with their cytoplasmic shuttling characteristics, work in association with these PBs and SGs for post-transcriptional regulation of target proteins (Jang, 2016; Seong et al., 2020). Different from the rest of the ZFPs, most of the RING zinc finger proteins have E3 ubiquitin ligase activity and it is involved in post-transcriptional activities of the ubiquitin-proteasome pathway. In this pathway, E3 RING ZFPs help in the recognition of the substrate proteins and degrades or change the activity of target proteins through ubiquitination (Swatek et al., 2019). For instance, AtATL78, StRFP2, AtRZF1, DRIP1, DRIP2, OsDIS1 and HOS1 post-transcriptionally regulate the target proteins such as DREB2A, OsNek6, OsSKIPa, AtERF53, ICE1, OsARK4, OsHRK1, AtRma1 and CaRma1H1, etc. through ubiquitination (Han et al., 2021) of which many of the target proteins like CaRma1H1 and AtRma1 are involved in the transport of the aquaporin PIP2;1 from the ER. It is also reported to have roles in transcriptional and post-transcriptional regulations of different genes involved in ABA-dependent pathways, ROS and Ca2+ signalling pathways (Han et al., 2021). Transcriptionally it is involved in the activation of genes encoding enzymes of ABA pathways such as ABA aldolase, NCED, ZEP and short-chain dehydrogenase/reductase (Vaičiukynė et al., 2019) and post-transcriptionally it is involved in forming a complex with other proteins such as PP2Cs and SnRK2s for ubiquitination and phosphorylation of other proteins and transcription factors like protein phosphatase 1 (CaADIP1), CaATBZ1, AtAIRP3, bZIP, AtKPNB1, TaSTP, GDU1 and RD21 which further activates or represses the transcription of ABA-responsive genes (Sah et al., 2016; Joo et al., 2020; Lv et al., 2020; Oh et al., 2020). In the MAPK signalling pathway, zinc finger proteins such as RGLG1 and RGLG2 have been involved in post-transcriptional modification of MAPKKK18 in response to environmental stress like drought. In ROS and Ca2+ signalling pathways, it is involved in transcriptional activation of genes encoding antioxidant enzymes like SOD and POD in response to environmental stresses (Zang et al., 2016). The ORANGE or OR proteins which are the type of DnaJ type ZFPs with their dual sub-cellular localization abilities, perform transcriptional and post-transcriptional activities in the nucleus and plastids. In the nucleus, it interacts with eRF1-2 (eukaryotic release factor 1-2) for transcriptional regulation of the downstream gene involved in cell elongation of petiole and plastids. It plays a role in post-transcriptional regulation by interacting with phytoene synthase to induce biogenesis of chromoplast and accumulation of carotenoid in non-pigmented tissues, simultaneously interacting with TCP14, a type of TFs to repress ELIPs (Early Light Induced Proteins) and biogenesis of chloroplast in de-etiolating cotyledons (Sun et al., 2019; Chen et al., 2021b). Whereas, some reports suggest that DnaJ ZFPs function as molecular chaperones in post-transcriptional or rather post-translational maintenance of structures and functions of its interacting proteins (Ali et al., 2022). The FCS-like zinc finger (FLZ) proteins which are reported to be localized to the cytoplasm and nucleus extensively interact with the kinase subunit of the SnRK1 complex and act as an adaptor to facilitate the interactions of effector proteins with the SnRK1 complex. In plants, it was found to be acting as a transcriptional activator by mediating the interactions of SnRK1 and effector proteins under various environmental stresses, however many of them are reported as negative regulators of SnRK1 signalling (Jamsheer et al., 2018a and Jamsheer et al., 2018b).
Zinc finger proteins play an extensive role in plant tolerance to various abiotic stress, such as drought, high salt, cold, high light, and osmotic and oxidative stresses (Wang et al., 2019; Han et al., 2020). In the process of external stress resistance, plants have evolved a set of complex and effective defence mechanisms, including signal perception, signal transduction, transcriptional regulation and response, to reduce or avoid damage to plants and ensure their average growth (Figures 1, 2) (Noman et al., 2019; Liu et al., 2022).
Figure 1 C2H2 zinc finger proteins are involved in plant stress responses. Source: (Liu et al., 2022).
Figure 2 Some important Zinc Finger Proteins (ZNPs) involved in abiotic stress factors of the plants (1st order of the hierarchy is the name of the crop/plant, 2nd order is the type of the protein and 3rd order is the name of the ZNPs) (Source: Modified after Noman et al. (2019) with the permission from the Elsevier Rights Links (https://s100.copyright.com/), Licence No.: 5371200779212, Dated 17th August 2022).
Several studies found that Zn-finger motifs significantly function during abiotic stress response in plants. In the first report, a wide range of Zn-binding motifs has been recognized and termed as Zn-fingers. Since the zinc finger motifs regulate the function of stress-adaptation genes. The Zn-finger was first reported as a repeated Zn-binding motif, comprising conserved cysteine (Cys) and histidine (His) ligands, in Xenopus laevis oocytes as a transcription factor (TF) IIIA (TFIIIA) (Laity et al., 2001; Ciftci-Yilmaz and Mittler, 2008; Kielbowicz-Matuk, 2012; Lyu and Cao, 2018). In the proteins where Zn2+ is mainly attached to amino acid residues and thus espousing a tetrahedral coordination geometry. The physical nature of Zn-proteins, defining the attraction of Zn-proteins for Zn2+, is crucial for having an in-depth knowledge of how a Zn2+ facilitates their characteristic function and how proteins control its mobility (intra and intercellular) as well as cellular availability.
Many C2H2-type zinc finger proteins involved in the abiotic stress signalling pathway were identified based on stress induction, mutant, compliment, and ectopic expression analysis. Phytohormones are responsible for abiotic stress resistance and participate in the process of response to various stresses via C2H2-type zinc finger proteins, especially ABA (Abscisic acid) (Roychoudhury et al., 2013; Ku et al., 2018; Takahashi et al., 2018; Chong et al., 2020). ABA, acting as a pivotal regulator of abiotic stress responses in plants, induces the expression of stress-related genes and triggers a range of adaptive physiological responses under abiotic stress conditions in the plant (Ku et al., 2018; Takahashi et al., 2018; Chong et al., 2020). C2H2 zinc finger proteins regulate plants in response to abiotic stresses through two ABA-mediated signal pathways: ABA-dependent and ABA-independent signal pathways (Smekalova et al., 2014; Ku et al., 2018; Takahashi et al., 2018; Chong et al., 2020). In addition to the ABA signal pathway, C2H2 zinc finger proteins enhance abiotic stress resistance by the MAPK (mitogen-activated protein kinase) signaling pathway (Smekalova et al., 2014; Ku et al., 2018; Takahashi et al., 2018; Chong et al., 2020; He et al., 2020; Lin et al., 2021). MAPKs, as highly conserved signaling transduction modules, play an essential role in regulating responses to adverse environmental stresses (Lin et al., 2021). Typically, a MAPK module is composed of at least three protein kinases, including MAPK (MPK), MAPK kinase (MAPKK/MAP2K/MKK/MEK) and MAPK kinase (MAPKKK/MAP3K/MEKK). The MAPK cascade amplifies and conveys stress signals from signaling receptors to downstream stress response factors through a sequential phosphorylation manner (De Zelicourt et al., 2016; He et al., 2020; Lin et al., 2021). Thus, C2H2 zinc finger proteins regulate abiotic stress responses via both the ABA signaling pathway and MAPK signaling transduction pathway and constitute a certain degree of crosstalk and a complex regulatory network (Figure 3) (Liu et al., 2022).
Figure 3 The signalling pathways of zinc finger proteins during abiotic stress response in plants. Note: The lines marked as solid indicate regulation, and the dashed lines indicate putatively. The C2H2 zinc finger proteins are SCOF-1, ZFP245, ZFP179, AZF1/2/3, ZFP36, IbZFP1, OsZFP213, ZAT4, DST, ZAT10/STZ, ZAT12, ZAT7 and ZAT6. Source: (Liu et al., 2022).
In many regions of the globe, soil salinity is significant abiotic stress that inhibits plant production. Salinity stress creates nutrient imbalances, is a source of harmful ions, and alters the osmotic condition of plants (Yaghoubian et al., 2021). It has been reported that more than 108 × 108 km2 of land throughout the world are affected by salinity (Riaz et al., 2019). Due to salinity and problematic soils, millions of hectares in the humid areas of South and Southeast Asia that are technically suitable for various field crops, particularly rice cultivation, are left uncultivated or are cultivated with extremely poor yields. (Mainuddin et al., 2019; Mainuddin et al., 2020). In addition, mineral shortages and toxicity often exacerbate the issue of salinity since it seldom occurs alone. These soil stresses change in magnitude and interactions throughout time and space, making long-term adaptation dependent on its degree of tolerance to all environmental stresses (Sarkar et al., 2022). Moreover, the ill response of soil and water salinity in the different crops varied significantly.
As transcription factors, zinc finger proteins (ZNPs) play an essential part in a wide variety of cellular processes, including RNA binding, transcription control, stress tolerance, and plant growth and development in response to phytohormones) (Noman et al., 2019). Scientists have identified a large number of zinc finger proteins in higher plants that can regulate the various environmental cues (Yu et al., 2015). Around 189 stress-induced zinc finger proteins especially for indica rice and maize have been classified and amongst them, Cys2/His2- and CCCH-types have received greater attention (Agarwal et al., 2007) Multiple C2H2-type zinc finger proteins in rice, including ZFP36, ZFP179, ZFP182, ZFP245, and ZFP252, have been implicated in salt, drought, and oxidative stress responses (Xu et al., 2008; Wang et al., 2015; Wang et al., 2022a). It has been found that drought and salt tolerance in rice are improved by overexpressing the ZFP252 zinc finger protein gene by elevated synthesis of free proline and soluble sugars (Xu et al., 2008) and increased ROS scavenging activity (Huang et al., 2009). Huang et al. (2007) found that the production of ZFP182 in transgenic tobacco or overexpression in rice plants boosted their salt tolerance, ZFP182 may have a curative function in salt tolerance in plants. Wang et al. (2022a) reported that some C2H2 type Zinc finger protein plays a significant role in salinity tolerance in rice seedlings by enhancing ABA catabolism. They also stated that overexpression of OsZFP15-like Zinc finger protein increased the production of reactive oxygen species (ROS) and decreased tolerance to oxidative stress, resulting in increased salinity stress tolerance in rice seedlings. The A20/AN1-type (ZmZnF1) and ring-binding type (ZmZnF2) zinc finger proteins found in maize kernels are reported to induce by ABA, mannitol and NaCl stresses. Similarly, simultaneous overexpression of these zinc finger proteins in transgenic lines of rice significantly increased the Na induced stress tolerance (Yu et al., 2015). It has been observed that transgenic Arabidopsis plants that constitutively produce the Cys2/His2 zinc finger protein Zat7 exhibit slower growth and development and a higher degree of tolerance for the effects of salt stress and the ability to tolerate high salinity is lost when the EAR motif of Zat7 has a mutation or deletion (Ciftci-Yilmaz et al., 2007). Wang et al. (2022b) found that the overexpression of C2H2-type zinc finger protein ‘MdZAT17’ in transgenic apple and Arabidopsis reduces the sensitivity to abscisic acid (ABA) and regulates salt tolerance positively. They also reported that the growth of both wild-type and transgenic Arabidopsis seedlings was inhibited under salt stress, but the growth shyness of transgenic plants was pointedly lower than that of wild-type seedlings. In another study (Xu et al., 2022) has been identified 36 B-box (BBX) family of proteins in maize consists of zinc-finger transcription factors that have a significant role in the regulation of different abiotic stresses, including drought and salinity.
Water is one of the fundamental inputs for the growth and yield of any arable crop. Still, both excess and deficit water supply in different growth stages can significantly reduce crop production. In the present era of climate change, aberrant weather conditions have increased the excess and deficit supply of soil moisture in most growing regions worldwide. Crop physiological and biological adaptability with the excess and deficiency supply of soil moisture has emerged as a potential research question (Osakabe et al., 2014). Multiple Zinc finger proteins have been identified from various crops has a definite role concerning drought and excess moisture stress of the field crops. Numbers of ZFPs have been identified in transgenic rice that has significant functions in enhancing drought, and excess moisture tolerance (Huang et al., 2009; Li et al., 2013; Wang et al., 2022a). Huang et al. (2009) reported that ZFP245 type ZNPs have significantly enhanced the cold and drought tolerance in rice by augmenting free proline and antioxidant concentration in transgenic rice plants. They further suggested that ZFP245 transgenic rice plants under 14 days drought stressed condition has survived at 70-80% with 7 days recovery period. It has been postulated that under cold or drought stress, ZFPs boost the SOD and POD enzymatic activities in transgenic rice seedlings and thereby help to enhance the abiotic stress tolerance by triggering the ROS-scavenging mechanism (Rizhsky et al., 2004). In harmony to the previous findings, (Sakamoto et al., 2004) found that two Cys-2/His-2-type ZNPs viz. AZP2 and STZ in transgenic Arabidopsis have significantly overexpressed under drought-stressed conditions and facilitated the plant to tolerate drought stress. (Gao et al., 2012) reported that ‘CgZFP1’, a Cys2/His2 type ZFP isolated from Chrysanthemum has a significant role in regulating drought and salinity stress in transgenic Arabidopsis. In severer drought conditions, abscisic acid plays an important role in enhancing the plant’s leaf senescence rate, reducing the yield. It has been found that a Cys-2/His-2-type ZNP, ´MdZAT10´, reduced the sensitivity to abscisic acid in apples and in addition to that, MdZAT10 (overexpressed in Arabidopsis) has a beneficial effect on seed germination and seedling growth (Yang et al., 2021). In another study, Mukhopadhyay et al. (2004) isolated a ZNP from rice viz. “OSISAP1” (induced by abscisic acid) overexpressed in transgenic tobacco increased tolerance to cold, dehydration, and salt stress at early growing stages. Giri et al. (2011) reported that Stress-associated ZNP isolated from rice viz. A20-AN1 increased the abiotic stress tolerance in transgenic Arabidopsis plants.
Most of the study suggested that ZNPs isolated from various plant sources involved in regulating abiotic stress factors has manually controlled the drought and salinity stress simultaneously (Huang et al., 2009; Lawrence et al., 2022). But application or overexpression of ZNPs in different transgenic crops is precise in nature.
Temperature stress due to both cold and high temperatures is considered a vital abiotic stress a plant faces during its growth and development (Liu et al., 2018). Thus, a clear-cut concept about the bio-physical-chemical impacts of temperature on a plant and its subsequent response mechanism is crucial to breeding improved stress-tolerant cultivars (Liu et al., 2018). Many Zn-finger proteins are responsible for mitigating the different temperature-related stresses in plants. CCCH Zn-finger proteins can control the expression of cold-induced genes; these proteins can improve cold tolerance in plants. Lin et al. (2011) found that the expressions of the cold-temperature genes viz. COR15A, RD29A, KIN1 etc. were upregulated in the cold-stress tolerant different Arabidopsis lines. Enhancement of the cold-stress tolerance in the plants can also be explained through signalling pathways of ABA, which are controlled by CCCH Zn-finger proteins (Xie et al., 2019). Transgenic switchgrass lines with induced Zn-finger protein, PvC3H72, survived even at –5°C temperature, while the lines with the low Zn-finger proteins could not survive (Xie et al., 2019). Zn-finger protein, DgC3H1, can improve the proline and soluble sugar levels in Chrysanthemum plants along with increased-level of SOD and POD, which ultimately make the plants able to survive under cold stress (Bai et al., 2021). C2H2 Zn-finger proteins also play a pivotal part in mitigating cold stress by regulating the ABA pathways. Kim et al. (2001) reported that the overexpression of C2H2 Zn-finger proteins results in cold-stress tolerance in soybean and tobacco by controlling ABA-response elements which facilitate COR gene-expression responsible for developing the plant cold-tolerance. The ABA-independent pathway for cold tolerance through C2H2 Zn-finger proteins is represented in Figure 4. In addition to the above two Zn-finger proteins, the AZF: Arabidopsis Zn-finger protein and STZ: salt-tolerance Zn-finger proteins are also responsible for cold-stress tolerance in Arabidopsis (Kodaira et al., 2011). The AZF genes, AZF, AZF2, AZF3, and STZ gene regulate the ABA-dependant pathway of Arabidopsis by regulating the ATPase gene, Na+, and Li+ outflows in plants (Lippuner et al., 1996).
Figure 4 ABA-independent pathways for cold-tolerance by C2H2 Zn-finger proteins. Note: Zn-finger proteins also regulate the several pathways of plants under heat-induced stresses. Huang et al. (2008) found A20/AN1-type Zn-finger proteins in japonica rice regulating the heat-induced stresses in the plants. Moreover, they reported that Zn-finger protein, ZFP177 was responsive to heat stress tolerance in plants. Overexpression of Zn-finger protein, ZFP177, is also responsible for the heat tolerance in tobacco plants (Mukhopadhyay et al., 2004).
For any HM stress, the plant has a three-tier strategy including absorption or isolation of HM inside the plant, HM removal through a series of chelating mechanisms and ROS removal which are accumulated during HM stress. Other than this a few subsidiary events of the HM-tolerance mechanism include an impedance of HM transport within the plant through HM binding to the cytoderm (composed of cellulosic material) (Chen et al., 2019). Few HM ions bind to the active groups (-COOH, -OH) of cellulose (inside cytoderm) which reduces the quantity of HM that enters the protoplasm resulting in damage alleviation caused by HM (Nedelkoska and Doran, 2000; Clemens et al., 2013). Three types of zinc finger transcription factors (ZF-TFs) (GATA-type, CCCH-type, and C2H2-type) are expressed differentially and reported to be up-regulated under cadmium stress in cotton roots, some of which are shown to be associated with secondary cell wall biosynthesis (Chen et al., 2019). Up-regulation in the homologs of cellulose synthase genes during cadmium stress reveals the involvement of ZF-TF in cellular layer modification as one of the possible modes of action for imparting HM-stress tolerance in cotton plants.
In Arabidopsis thaliana, upon exposure to HMs exhibited some interesting findings. With the help of yeast-two-hybrid model when interaction among the different members of the HIPP family and the related zinc finger TF or transcription factors, have borne a particular interaction pattern of ATHB29 and HIPP proteins (of cluster III). Thus, a purposeful connection among ATHB29 and HIPP26 is also shown by experiments conducted with HIPP26 (mutants), displayed reformed expression pattern levels of such genes earlier known to be controlled by ATHB29 (Barth et al., 2009). Chakrabarty et al. (2009) while investigating genome‐wide expression pattern of Zn-finger proteins in plants exposed to As3+ and As5+ reported a contrasting scenario. Proteins of the zinc‐finger family were found to be downregulated under As5+ stressed situation whereas, Zinc‐finger C3HC4‐type proteins were found to exhibited both up and down regulated expression profile upon exposed to As3+ stressed situation. Authors like Abercrombie et al. (2008); Chakrabarty et al. (2009); Tripathi et al. (2012) have presented the expression profile of Oryza sativa (monocot) and Arabidopsis thaliana (dicot). Under As stressed condition both exhibited a downregulated Zn-finger protein expression profile. Shah et al. (2022) observed that spermine (Spm), a polyamine compound upon supplanting in common bean (P. vulgaris) exposed to As stress have enhanced the expression of PvC3H24, PvC3H25, PvC3H26 and PvC3H27 (Zn-finger proteins). The authors concluded that, Spm confer tolerance to As induced phyto-toxicity by modulating polyamine metabolism, antioxidant defense system along with facilitating (as enhancer) for zinc-finger proteins related genes expressions. Huang et al. (2012) reported some interesting findings while analysing the transcriptomic profile of rice roots exposed to As stress. among the 231 TFs, the zinc-finger protein (expressed particularly in inflorescence meristem or ZIM) had a share of only 3.46%. The authors have concluded that under As stress ZIM-TF were enhanced in a noteworthy fashion.
HM homeostasis strategies mainly involve the phytochelatin, metallothionein and metallochaperones (responsible for safe transport of metal ions inside the cell) induction (Harrison et al., 2000; de Abreu-Neto et al., 2013; Zhang et al., 2014) as well as vacuolar sequestration mediated by phytochelatin binding (Yang and Chuand Chu, 2011). Barth et al. (2009) found a functional association between a ZF-homeodomain (ZF-HD) TF (known as ATHB29) and arrays of HIPP26 (a type of metallochaperone protein) in A. thaliana under HM stress by double checking mutant line assay (mutated for HIPP26 functional loss) for the expression of stress-responsive genes which showed that the genes up-regulated under the influence of ATHB29 are inhibited in the absence of functional interaction between HIPP26 and ZF-HD.
Plant stress-associated proteins (SAPs) contain ZF domains (either [Cys2-Cys2]n finger motifs or [Cys and His]n residues; ‘n’ represents multiple numbers of motif repeat) at the N- or C-terminal (Jin et al., 2007). These SAPs can be a key player in stress signaling through protein-protein interactions (PPIs) via their ZF-domain (Opipari et al., 1990; Kanneganti and Gupta, 2008). Additionally, SAP10 of A. thaliana was found to be coding for a nuclear or cytoplasmic protein that might act early in the signal transduction of the HM-stress tolerance response (Ströher et al., 2009; Dixit and Dhankher, 2011). Many of the HM defense responses shown by the plants are due to the major contribution of cellular receptors involved in signaling cascades like MAPK (three-tier phosphorylation module) (Rodriguez et al., 2010; Sinha et al., 2011; Jalmi and Sinha, 2015) which modulates downstream WRKY and ZAT TFs (containing ZF motifs) (Ogawa et al., 2009; Opdenakker et al., 2012). PPI (protein-protein interaction) study performed by Nguyen et al. (2012) between ZAT-TF (ZAT10; a ZF-containing TF) and Arabidopsis MAPK (MPK3, MPK6) under HM stress has paved a conceptual understanding that MAPKs are involved in HM stress signaling either through ZF-TFs or transcription factors containing ZF-motifs. Major plant stress tolerance responsive signaling factors includes EF-Tu receptor, ETR1/ETR2, SIT1, ER etc. Ca signaling is important in heavy metal stress hormone signaling, as is MAPK signaling, which uses Calmodulin, a Calcineurin B-like protein, and Ca dependent kinase (Steinhorst and Kudla, 2014). Among these, MAPK signalling is one of the major signalling pathways involved in alleviating heavy metal stress. Two transcription factors, viz., MPK3 and MPK6, get activated under high Cd stress mediated by ROS signalling (Liu et al., 2010). Calcium- and cadmium-responsive mitogen activated protein kinase (MAPKKK) in Arabidopsis remains a major signal transduction protein component (Suzuki et al., 2001).
HM stress always generates oxidative stress and causes destabilization in the balance between ROS and the antioxidant system (Zhang et al., 2013; Jin et al., 2016; Yin et al., 2016; Xu et al., 2019). Different transcription factors (TFs) families including zinc finger-TFs are involved in such ROS-mediated stress responses (Singh et al., 2019). HM accumulation ROS mediated functional disruption of biomolecules among several other damages (Stohs and Bagchi, 1995; Cuypers et al., 2009; Haider et al., 2021). Under HM-stress SlRING1-ZFP overexpression in tomatoes led to more chlorophyll content and photosynthetic rate. Moreover, the maximal photochemical efficiency of photosystem II was evidently improved by SlRING1-ZFP mediated minimization of ROS levels and electrolyte leakage (Ahammed et al., 2020). Soybean ZFP (GmRZFP1) and Arabidopsis ZFP (AtOHRP) are reported to be involved in oxidative stress (activity induced by ROS) in plants thereby promoting ROS scavenging enzyme systems (Wu et al., 2010; Li et al., 2015). Putting together, zinc finger protein (ZFP) is involved in HM stress alleviation and detoxification of ROS as two underlined strategies of HM tolerance. Abscisic acid enhances plant stress response mechanisms under drought stress. Ring-zinc finger protein overexpression enhances genes like AtNECD3, which codes for a key enzyme in ABA biosynthesis (Ko et al., 2006). Zinc finger protein also enhances major genes’ expression (ABA3, ABI5, etc.) responsible for ABA biosynthesis in rice (Zeng et al., 2015). Zinc finger protein ZFP-185 regulates ABA and GA synthesis modulation, which enhances stress response (Zhang et al., 2016). Flowering and gibberellin biosynthesis are also controlled by ZFP in Chrysanthemum (Yang et al., 2014). The effect of different Zinc finger protein in mitigating various abiotic stresses has been presented in Table 1.
Under abiotic stress, including HM, many functional proteins undergo the ubiquitin proteosome-mediated degradation process due to the altered quaternary structure of the protein (resulting in misfolded design) and hence no longer required for average growth and development (Blasiak et al., 2019). This way of aberrant protein identification and removal ensures better survival of plants under stress conditions (Stone, 2019). Most RING zinc finger proteins have E3 ubiquitin ligase activity (Joazeiro and Weissman, 2000; Ahammed et al., 2020) similar to the last enzyme of the ubiquitin-proteasome pathway (which plays a specific role in recognising target substrates and then degrades the target protein or changes the activity of the target protein). Hence RING-ZFP can be engaged in ubiquitin proteosome-mediated degradation of the misfolded or altered protein (Swatek et al., 2019) as a stress tolerance mechanism.
Further bioinformatics analysis on RING-ZFP (possess intra- and extra-cellular domains) has already revealed research findings on extracellular domain binding (negatively charged) of RING-ZFP to the positively charged extracellular harmful metal ions suggesting a sensor-like activity of ZFP upon HM accumulation. Additionally, the intracellular domain of RING-ZFP interacts with and promotes ROS-mediated ABA signalling (Suh et al., 2016).
A GSH-dependent pathway and phytochelatin (polymerized GSH encoded by PCS)-conjugated vacuolar sequestration are two established mechanisms contributing to HM-tolerance (Flores-Cáceres et al., 2015; Hernández et al., 2015; Jozefczak et al., 2015). Cd-tolerant Arabidopsis phenotypes resulted from ZAT6 over-expression, when undergoing BSO (an irreversible inhibitor of GSH biosynthesis; γ-glutamylcysteine synthetase; Reliene and Schiestl (2006) treatment, completely loses its HM-tolerance ability which implies that the ZAT6-mediated enhanced Cd tolerance is GSH-dependent (Chen et al., 2016). Furthermore, Chen and his co-workers have also found GSH1 (encoding γ-glutamylcysteine synthetase) as the transcriptional target of ZAT6 through qRT-PCR (outcome: positive regulation of GSH1 by ZAT6) and transient expression analysis (outcome: activation of GSH1 promoter activity by ZAT6). Putting together, it is clear that GSH1 which regulates HM tolerance in Arabidopsis is under transcriptional control of ZAT6, a ZF-TF. Phytochelatin synthesis was found to be under ZAT6 (acts as TF for gene encoding PCS1 and PCS2) transcriptional regulation too under HM-stress (Chen et al., 2016). Phytochelatin synthesis additionally augments the effect of GSH towards Cd-tolerance by accumulating Cd followed by phytochelatin-conjugated vacuolar sequestration (Wawrzynski et al., 2006; Lin and Aarts, 2012). Saad et al. (2018) demonstrated that LmSAP overexpression in tobacco seedlings enhanced the expression of several genes encoding metallothionein proteins (thiol group of cysteine amino acid residue binds with metal ions and/or ROS, (Yuan et al., 2008; Hassinen et al., 2009).
Out of twenty-three sequences of sorghum gene homologs identified by BLASTP searches of the Xtxp270-QTL genomic region on chromosome 10, two gene homologs encoding ZFPs (SbZFP17 and SbZFP346) were found to be up-regulated under HM stress (Abou-Elwafa et al., 2019). Another HM-tolerance study on Arabidopsis reported decreased HM-tolerance upon mutation in the gene encoding a ZFP (ZAT6) (Chen et al., 2016).
Over-expression of a gene encoding an HM (Si) transporter in rice was found to promote strong cell membrane structure and activate regulators (ZF protein viz. Lsd1 and dof, protein kinase domain) of the UV-B tolerance signal transduction pathway (Fang et al., 2011), suggesting the possible involvement of zinc finger motif in irradiance-induced signalling.
The B‐box (BBX) proteins are a family of zinc finger TFs containing one or two BBX motifs, which has already elucidated its role in PPI (Gangappa and Botto, 2014; Zhang et al., 2017). Fang et al. (2019) monitored the transcript levels of eight BBX genes in the apple, which were significantly induced by UV‐B radiation. Further, MdBBX20 overexpression in apple callus promoted the expression of structural genes encoding anthocyanin pigments and their subsequent accumulation under UV‐B radiation, possibly by its transcriptional coactivator role (promoter modulation of several proteins) that coordinates with MdHY5 (Fang et al., 2019). In fact, the direct relationship between the expression profile of rhl41 (encode for a ZFP) and collective accumulation of anthocyanin and chlorophyll under UV irradiation suggests the disguised role of zinc finger in photo-protection and increased level of photosynthetic efficiency (both are evolved as strategies of light and radiation tolerance in plants) respectively (Iida et al., 2000).
Exposure of plants to light intensities that exceed the electron utilization capacity of the chloroplastic photosystem (PS) and light-harvesting complex (LHC) dramatically impacts nuclear gene expression. In this regard, the interaction and genetically association (binding component unknown to date) between GATA-type zinc finger-TF (ZML) and CryR1 (cryptochrome involved in growth and regulation) induce the formation of ZML heterodimer regulating the expression of photo-protective genes (Shaikhali et al., 2012).
Protective role (as a shield of LHC of PS-I, II and as an antioxidant; quenching of excited electrons during an imbalanced state of excitation transfer at the LHC) of flavonoid (anthocyanin) class of metabolites are very prominent under light and irradiance stress (Hughes et al., 2014; Tattini et al., 2014). HY5 (a type of Leucine zipper TF) positively regulates light‐responsive gene expression (Nawkar et al., 2017) through interaction (via phytochrome interacting factor) with BBX-ZFP resulting in anthocyanin accumulation under light and/or radiation stress (Wei et al., 2016; Zhang et al., 2017). MdBBX20 responds to UV‐B signaling and forms an active heterodimer with HY5 (one of the key regulatory factors for UV-B response; facilitating the transcriptional activity of HY5 (An et al., 2019; Fang et al., 2019).
Modulation in antenna size of LHC is another approach adopted by the plant to tolerate incoming high light and radiation stress (Rossel et al., 2002; Kimura et al., 2003; Mittler et al., 2004). Genetic alteration of ZAT10 (ZF-TF) has a modulatory impact on high light-induced transcriptome (Rossel et al., 2002) whose products are targeted to chloroplast suggesting the involvement of ZF-TF (zinc finger motif) in plants’ manipulation of chloroplastic apparatus.
The experiment setup (95% CO2+1% O2 treatment; Artificial high-CO2 atmosphere) designed by Jamil et al. (2019) reported abundant levels of C2H2-type ZFP (DkZF1-5) transcripts. Even though there are no direct in-vivo reports on ZF-motif-mediated stress tolerance under the influence of elevated CO2 to date but still, the existing elucidated protein-protein interactions among the expressed product of DkZF1-5 transcripts might have some synergistic regulatory role under CO2 stress. Notably, comparative transcriptome analysis had already unveiled up-regulation in stress-related TFs (WRKY; domain containing C2HC ZF motif) (Rushton et al., 2010) in immature California grapes under CO2 gaseous treatment (Rosales et al., 2016; Romero et al., 2019). ABA phytohormone maintenance (balance between anabolism and catabolism) and their optimal levels are influenced by elevated CO2 (linear relationship between CO2 concentration and ABA synthesis) (Seo et al., 2000; Iuchi et al., 2001; Xiong et al., 2001; Xiong et al., 2002; Xiong and Zhu, 2003; Zou et al., 2007). Both of the above concepts indicate the possible involvement of WRKY with ABA-inducible and -repressible genes (under the influence of HVA22 promoter; Zou et al. (2004) under elevated CO2 suggesting ABA-mediated stomatal response to CO2 stress in plants. The present interpretation and previously made elucidations suggest the involvement of WRKY-TF as a functional node integrating stress signaling. Non-fluctuating concentrations of existing intracellular monosaccharides and disaccharides (soluble sugars like glucose and sucrose) (Katny et al., 2005; Zou et al., 2007)and increased accumulation of storage polysaccharides (starch) (Poorter et al., 1997) under elevated CO2 remain a mystery which can be taken up as future research to establish the link between ABA-signaling and intracellular starch proportion of the cell under elevated CO2 situation.
From the discussion of the current overview of the recent study, it can be concluded that abiotic stresses, particularly drought, high salinity, heavy metal, photo-stress, and high and low temperatures, are the major hindrances that limit crop productivity. After overviewing various earlier studies, the current study revealed that Zn-finger motifs have a significant role in the better understanding of abiotic stress. The study also recognized that a wide range of Zn-binding motifs, termed Zn-fingers’ proteins, had been identified. However, the function of stress-adaptation Zn-finger motifs is fully controlled by various genes. Speaking of abiotic stress and illustration the role of ZFP in wide range of plants, drought, salinity, temperatures etc. seems to be predominated. However, the involvements of ZFP in heavy metal stress are comparatively less than the other stresses. Moreover, the consequences of radio-nucleotides exposure to plants and the behaviour of ZFs will be an area of interest in the near future, as there is hardly any article addressing this issue. On the other hand, there is an ample scope to work on the effect of HM on the yield of crops as associated with various zinc finger protein. Another area of interest will be the documentation of the role of ZFP when plants were exposed to multiple stresses in different magnitudes. The information on the concept, importance, and mechanisms of Zn-finger motifs during abiotic stress response in plants will be helpful for the sustainability of crop production in the modern era of the changing climate.
Conceptualization, DM, KB, BP, SM, TS, MS, UM, MB, and AH; writing—original draft preparation, DM, UM, SS, AR, KB, BP, SM, TS, MS, MB, VB, and AH; writing—review and editing, MB, MS, VB, SM, SH, and AH; Funding, MB, VB, MS, SM, and AH; All authors have read and agreed to submit the manuscript in Front. Plant Sci.
This is a collaborative write-up to prove information on abiotic stress response in plants. This publication was supported by the Slovak University of Agriculture, Nitra, Tr. A. Hlinku 2, 949 01 Nitra, Slovak Republic, under the projects ‘APVV-20-0071 and EPPN2020-OPVaI-VA-ITMS313011T813’.
We gratefully acknowledge to the funded project ‘APVV-20-0071 and EPPN2020-OPVaI-VA-ITMS313011T813′.
The authors declare that the research was conducted in the absence of any commercial or financial relationships that could be construed as a potential conflict of interest.
All claims expressed in this article are solely those of the authors and do not necessarily represent those of their affiliated organizations, or those of the publisher, the editors and the reviewers. Any product that may be evaluated in this article, or claim that may be made by its manufacturer, is not guaranteed or endorsed by the publisher.
ABA, abscisic acid; APX, ascorbate peroxidase; AtERF5, a class I ERF protein; CAT, catalase; COR, cold-regulated genes; DREB/CBF, C-repeat binding factors; EIN3, ethylene-induced 3; ENA1, Na+- exporting P-type ATPase gene; FIT, fer-like iron deficiency-induced transcription factor; FSD1, iron superoxide dismutase 1; GR, glutathione reductase; GSH1/2, glutathione 1/2; LP2, plasma membrane receptor-like kinase leaf panicle 2; MAPK, mitogen-activated protein kinase; MDA, malonaldehyde; NCED, 9-cis-epoxy-carotenoid dioxygenase; OsDREB2A, dehydration-responsive element-binding 2A; OsP5CS, pyrroline 5 carboxylate synthetase; OsProT, proline transporter; PCS1/2, phytochelatin synthases 1/2; PMR2, Ca2+ ATPase gene; POD, peroxidase; PRXZ4, peroxidase 24 precursor; RbohD, respiratory burst oxidase homologue; RD29A, a classical stress-response gene; ROS, reactive oxygen species; SOD, superoxide dismutase.
Abercrombie, J. M., Halfhill, M. D., Ranjan, P., Rao, M. R., Saxton, A. M., Yuan, J. S., et al. (2008). Transcriptional responses of arabidopsis thaliana plants to as (V) stress. BMC Plant Biol. 8, 87. doi: 10.1186/1471-2229-8-87
Abou-Elwafa, S. F., Amin, A. E. E. A. Z., Shehzad, T. (2019). Genetic mapping and transcriptional profiling of phytoremediation and heavy metals responsive genes in sorghum. Ecotoxicol. Environ. Safety. 173, 366–372. doi: 10.1016/j.ecoenv.2019.02.022
Agarwal, P., Arora, R., Ray, S., Singh, A. K., Singh, V. P., Takatsuji, H., et al. (2007). Genome-wide identification of C2H2 zinc-finger gene family in rice and their phylogeny and expression analysis. Plant Mol. Biol. 65, 467–485. doi: 10.1007/S11103-007-9199-Y
Ahammed, G. J., Li, C. X., Li, X., Liu, A., Chen, S., Zhou, J. (2020). Overexpression of tomato RING E3 ubiquitin ligase gene SlRING1 confers cadmium tolerance by attenuating cadmium accumulation and oxidative stress. Physiol. Plant 173, 449–459. doi: 10.1111/ppl.13294
Ali, F., Wang, Q., Fazal, A., Wang, L. J., Song, S., Kong, M. J., et al. (2022). The DnaJ-like zinc finger protein ORANGE promotes proline biosynthesis in drought-stressed arabidopsis seedlings. Int. J. Mol. Sci. 23 (7), 3907. doi: 10.3390/ijms23073907
Andreini, C., Banci, L., Bertini, I., Rosato, A. (2006). Counting the zinc-proteins encoded in the human genome. J. Proteome Res. 5 (1), 196–201. doi: 10.1021/pr050361j
An, Y., Han, X., Tang, S., Xia, X., Yin, W. (2014). Poplar GATA transcription factor PdGNC is capable of regulating chloroplast ultrastructure, photosynthesis, and vegetative growth in arabidopsis under varying nitrogen levels. Plant Cell Tissue Organ Culture. 119 (2), 313–327. doi: 10.1007/s11240-014-0536-y
An, J. P., Zhang, X. W., Bi, S. Q., You, C. X., Wang, X. F., Hao, Y. J. (2019). MdbHLH93, an apple activator regulating leaf senescence, is regulated by ABA and MdBT2 in antagonistic ways. New Phytol. 222, 735–751. doi: 10.1111/nph.15628
Aslam, M., Fakher, B., Ashraf, M. A., Cheng, Y., Wang, B., Qin, Y. (2022). Plant low-temperature stress: Signaling and response. Agron. 12 (3), 702. doi: 10.3390/agronomy12030702
Bai, H., Lin, P., Li, X., Liao, X., Wan, L., Yang, X., et al. (2021). DgC3H1, a CCCH zinc finger protein gene, confers cold tolerance in transgenic chrysanthemum. Sci. Hortic. 281, 109901. doi: 10.1016/j.scienta.2021.109901
Bakshi, M., Oelmüller, R. (2014). WRKY transcription factors: Jack of many trades in plants. Plant Signal Behav. 9 (2), e27700. doi: 10.4161/psb.27700
Barman, D., Sathee, L., Padhan, B. K., Watts, A. (2022). “Compatible solutes engineering to balance salt (Na+) and ROS-induced changes in potassium homeostasis”. In Response of field crops to abiotic stress: Current status and future prospects Choudhury, Eds. S, Moulick, D (Boca Raton, USA: CRC Press), 139–152.
Barth, O., Vogt, S., Uhlemann, R., Zschiesche, W., Humbeck, K. (2009). Stress induced and nuclear localized HIPP26 from arabidopsis thaliana interacts via its heavy metal associated domain with the drought stress related zinc finger transcription factor ATHB29. Plant Mol. Biol. 69 (1–2), 213–226. doi: 10.1007/s11103-008-9419-0
Basu, S., Ramegowda, V., Kumar, A., Pereira, A. (2016). Plant adaptation to drought stress. F1000 Res. 5, 1554. doi: 10.12688/f1000research.7678.1
Behringer, C., Schwechheimer, C. (2015). B-GATA transcription factors – insights into their structure, regulation, and role in plant development. Front. Plant Sci. 6. doi: 10.3389/fpls.2015.00090
Bhadra, P., Maitra, S., Shankar, T., Hossain, A., Praharaj, S., Aftab, T. (2012). “Climate change impact on plants: Plant responses and adaptations,” in Plant perspectives to global climate changes. Eds. Aftab, T., Roychoudhury, A. (Elsevier Inc., Academic Press), 1–24. doi: 10.1016/B978-0-323-85665-2.00004-2
Blasiak, J., Pawlowska, E., Szczepanska, J., Kaarniranta, K. (2019). Interplay between autophagy and the ubiquitin-proteasome system and its role in the pathogenesis of age-related macular degeneration. Int. J. Mol. Sci. 20, 210. doi: 10.3390/ijms20010210
Bogamuwa, S., Jang, J. (2013). The arabidopsis tandem CCCH zinc finger proteins AtTZF4, 5 and 6 are involved in light-, abscisic acid and gibberellic acid-mediated regulation of seed germination. Plant Cell Environ. 36, 1507–1519. doi: 10.1111/pce.12084
Bogamuwa, S., Jang, J. (2016). Plant tandem CCCH zinc finger proteins interact with ABA, drought, and stress response regulators in processing-bodies and stress granules. PloS One 11, e0151574. doi: 10.1371/journal.pone.0151574
Brayer, K. J., Segal, D. J. (2008). Keep your fingers off my DNA: Protein-protein interactions mediated by C2H2 zinc finger domains. Cell Biochem. Biophys. 50, 111–131. doi: 10.1007/s12013-008-9008-5
Chai, G., Hu, R., Zhang, D., Qi, G., Zuo, R., Cao, Y., et al. (2012). Comprehensive analysis of CCCH zinc finger family in poplar (Populus trichocarpa). BMC Genom. 13, 253. doi: 10.1186/1471-2164-13-253
Chakrabarty, D., Trivedi, P. K., Misra, P., Tiwari, M., Shri, M., Shukla, D., et al. (2009). Comparative transcriptome analysis of arsenate and arsenite stresses in rice seedlings. Chemosphere 74 (5), 688–702. doi: 10.1016/j.chemosphere.2008.09.082
Cheng, M. N., Huang, Z. J., Hua, Q. Z., Shan, W., Kuang, J. F., Lu, W. J., et al. (2017). The WRKY transcription factor HpWRKY44 regulates CytP450-like1 expression in red pitaya fruit (Hylocereus polyrhizus). Hortic. Res. 4, 17039. doi: 10.1038/hortres.2017.39
Chen, W. F., Wei, X. B., Rety, S., Huang, L. Y., Liu, N. N., Dou, S. X., et al. (2019). Structural analysis reveals a “molecular calipers” mechanism for a LATERAL ORGAN BOUNDARIES DOMAIN transcription factor protein from wheat. Journal of Biological Chemistry 294 (1), 142–156.
Chen, F., Liu, H. L., Wang, K., Gao, Y. M., Wu, M., Xiang, Y. (2020). Identification of CCCH zinc finger proteins family in moso bamboo (Phyllostachys edulis), and PeC3H74 confers drought tolerance to transgenic plants. Front.Plant Sci. 11, 579255. doi: 10.3389/fpls.2020.579255
Chen, S., Li, X., Yang, C., Yan, W., Liu, C., Tang, X., et al. (2021a). Genome-wide identification and characterization of FCS-like zinc finger (FLZ) family genes in maize (Zea mays) and functional analysis of ZmFLZ25 in plant abscisic acid response. Int. J. Mol. Sci. 22 (7), 3529. doi: 10.3390/ijms22073529
Chen, Y., Sun, A., Wang, M., Zhu, Z., Ouwerkerk, P. B. F. (2014). Functions of the CCCH type zinc finger protein OsGZF1 in regulation of the seed storage protein GluB-1 from rice. Plant Mol. Biol. 84, 621–634. doi: 10.1007/s11103-013-0158-5
Chen, W. C., Wang, Q., Cao, T. J., Lu, S. (2021b). UBC19 is a new interacting protein of ORANGE for its nuclear localization in arabidopsis thaliana. Plant Signal Behav. 16 (11), 1964847. doi: 10.1080/15592324.2021.1964847
Chen, J., Yang, L., Yan, X., Liu, Y., Wang, R., Fan, T., et al. (2016). Zinc-finger transcription factor ZAT6 positively regulates cadmium tolerance through the glutathione-dependent pathway in arabidopsis. Plant Physiol. 171, 707–719. doi: 10.1104/pp.15.01882
Chong, L., Guo, P., Zhuand Zhu, Y. (2020). Mediator complex: A pivotal regulator of ABA signaling pathway and abiotic stress response in plants. Int. J. Mol. Sci. 21, 7755. doi: 10.3390/ijms21207755
Choudhury, S., Mazumder, M. K., Moulick, D., Sharma, P., Tata, S. K., Ghosh, D., et al. (2022a). Computational study of the role of secondary metabolites for mitigation of acid soil stress in cereals using dehydroascorbate and mono-dehydroascorbate reductases. Antioxidant. 11 (3), 458. doi: 10.3390/antiox11030458
Choudhury, S., Moulick, D. (2022). Response of field crops to abiotic stress: Current status and future prospects. (Boca Raton, USA: CRC Press), 1–332 doi: 10.1201/9781003258063
Choudhury, S., Moulick, D., Ghosh, D., Soliman, M., Alkhedaide, A., Gaber, A., et al. (2022b). Drought-induced oxidative stress in pearl millet (Cenchrus americanus l.) at seedling stage: Survival mechanisms through alteration of morphophysiological and antioxidants activity. Life. 12 (8), 1171. doi: 10.3390/life12081171
Choudhury, S., Moulick, D., Mazumder, M. K., Pattnaik, B. K., Ghosh, D., Vemireddy, L. R., et al. (2022c). An In vitro and in silico perspective study of seed priming with zinc on the phytotoxicity and accumulation pattern of arsenic in rice seedlings. Antioxidants 11 (8), 1500. doi: 10.3390/antiox11081500
Choudhury, S., Moulick, D., Mazumder, M. K. (2021a). Secondary metabolites protect against metal and metalloid stress in rice: An in silico investigation using dehydroascorbate reductase. Acta Physiol. Plantarum. 43 (1), 1–10. doi: 10.1007/s11738-020-03173-2
Choudhury, S., Sharma, P., Moulick, D., Mazumder, M. K. (2021b). Unrevealing metabolomics for abiotic stress adaptation and tolerance in plants. J.Crop Sci. Biotechnol. 24, 5479–5493. doi: 10.1007/s12892-021-00102-8
Chowardhara, B., Borgohain, P., Saha, B., Awasthi, J. P., Moulick, D., Panda, S. K. (2019a). Phytotoxicity of cd and zn on three popular Indian mustard varieties during germination and early seedling growth. Biocatalysis Agric. Biotechnol. 21, 101349. doi: 10.1016/j.bcab.2019.101349
Chowardhara, B., Borgohain, P., Saha, B., Prakash, J., Awasthi, D. M., Panda, S. K. (2019b). Assessment of phytotoxicity of zinc on Indian mustard (Brassica juncea) varieties during germination and early seedling growth. Ann. Plant Soil Res. 21 (3), 239–244. doi: 10.1016/j.bcab.2019.101349
Ciftci-Yilmaz, S., Mittler, R. (2008). The zinc finger network of plants. Cell Mol. Life Sci. 65, 1150–1160. doi: 10.1007/s00018-007-7473-4
Ciftci-Yilmaz, S., Morsy, M. R., Song, L., Coutu, A., Krizek, B. A., Lewis, M. W., et al. (2007). The EAR-motif of the Cys2/His2-type zinc finger protein Zat7 plays a key role in the defense response of arabidopsis to salinity stress. J.Biol. Chem. 282 (12), 9260–9268. doi: 10.1074/jbc.M611093200
Claeys, H., Inzéand Inzé, D. (2013). The agony of choice: How plants balance growth and survival under water-limiting conditions. Plant Physiol. 162, 1768–1779. doi: 10.1104/pp.113.220921
Clemens, S., Aarts, M. G. M., Thomine, S., Verbruggen, N. (2013). Plant science: the key to preventing slow cadmium poisoning. Trend. Plant Sci. 18, 92–99. doi: 10.1016/j.tplants.2012.08.003
Cuypers, A., Smeets, K., Vangronsveld, J. (2009). “Heavy metal stress in plants,” in Plant stress biology: From genomics to systems biology. Ed. Hirt, H. (Weinheim: Wiley-VCH Verlag GmbH & Co. KGaA), 161–178.
Dash, G. K., Barik, M., Parida, S., Baig, M. J., Swain, P., Sahoo, S. K., et al. (2022). “Drought and high-temperature stress tolerance in field crops”, in Response of field crops to abiotic stress: Current status and future prospects. Eds. Choudhury, S., Moulick, D.. (Boca Raton, USA: CRC Press) 103-109.
Das, K., Roychoudhury, A. (2014). Reactive oxygen species (ROS) and response of antioxidants as ROS-scavengers during environmental stress in plants. Front. Environ. Sci. 2, 53. doi: 10.3389/fenvs.2014.00053
Davies, M. J. (2005). The oxidative environment and protein damage. Biochim. Biophys. Acta 1703 (2), 93–109. doi: 10.1016/j.bbapap.2004.08.007
de Abreu-Neto, J. B., Turchetto-Zolet, A. C., de Oliveira, L. F. V., Bodanese Zanettini, M. H., Margis Pinheiro, M. (2013). Heavy metal-associated isoprenylated plant protein (HIPP): characterization of a family of proteins exclusive to plants. FEBS J. 280 (7), 1604–1616. doi: 10.1111/febs.12159
De Zelicourt, A., Colcombet, J., Hirtand Hirt, H. (2016). The role of MAPK modules and ABA during abiotic stress signaling. trends. Plant Sci. 21, 677–685. doi: 10.1016/j.tplants.2016.04.004
Dixit, A. R., Dhankher, O. P. (2011). A novel stress-associated protein ‘AtSAP10’ from arabidopsis thaliana confers tolerance to nickel, manganese, zinc, and high temperature stress. PloS One 6 (6), e20921. doi: 10.1371/journal.pone.0020921
Duan, J., Zhang, M., Zhang, H., Xiong, H., Liu, P., Ali, J., et al. (2012). OsMIOX, a myo-inositol oxygenase gene, improves drought tolerance through scavenging of reactive oxygen species in rice (Oryza sativa l.). Plant Sci. 196, 143–151. doi: 10.1016/j.plantsci.2012.08.003
Fairall, L., Rhodes, D., Klug, A. (1986). Mapping of the sites of protection on a 5 s RNA gene by the xenopus transcription factor IIIA: A model for the interaction. J. Mol. Biol. 192 (3), 577–591. doi: 10.1016/0022-2836(86)90278-0
Fan, Y., Zhang, Y., Rui, C., et al (2021). Zinc finger transcription factor ZAT family genes confer multi-tolerances in gossypium hirsutum l. J. Cotton Res. 4, 24. doi: 10.1186/s42397-021-00098-0
Fang, H., Dong, Y., Yue, X., Hu, J., Jiang, S., Xu, H., et al. (2019). The b-box zinc finger protein MdBBX20 integrates anthocyanin accumulation in response to ultraviolet radiation and low temperature. Plant Cell Environ. 42 (7), 2090–2104. doi: 10.1111/pce.13552
Fang, C. X., Wang, Q. S., Yu, Y., Li, Q. M., Zhang, H. L., Wu, X. C., et al. (2011). Suppression and overexpression of Lsi1 induce differential gene expression in rice under ultraviolet radiation. Plant Growth Reg. 65 (1), 1–10. doi: 10.1007/s10725-011-9569-y
Flores-Cáceres, M. L., Hattab, S., Hattab, S., Boussetta, H., Banni, M., Hernández, L. E. (2015). Specific mechanisms of tolerance to copper and cadmium are compromised by a limited concentration of glutathione in alfalfa plants. Plant SciPlant Sci. 233, 165–173. doi: 10.1016/j.plantsci.2015.01.013
Gamsjaeger, R., Liew, C. K., Loughlin, F. E., Crossley, M., Mackay, J. P. (2007). Sticky fingers: Zinc-fingers as protein-recognition motifs. Trends Biochem. Sci. 32, 63–70. doi: 10.1016/j.tibs.2006.12.007
Gangappa, S. N., Botto, J. F. (2014). The BBX family of plant transcription factors. Trends Plant Sci. 19 (7), 460–470. doi: 10.1016/j.tplants.2014.01.010
Gao, H., Song, A., Zhu, X., Chen, F., Jiang, J., Chen, Y., et al. (2012). The heterologous expression in arabidopsis of a chrysanthemum Cys2/His2 zinc finger protein gene confers salinity and drought tolerance. Plant. 235 (5), 979–993. doi: 10.1007/s00425-011-1558-x
Ghosh, D., Brahmachari, K., Brestic, M., Ondrisik, P., Hossain, A., Skalicky, M., et al. (2020a). Integrated weed and nutrient management improve yield, nutrient uptake and economics of maize in the rice-maize cropping system of Eastern India. Agron 10 (12), 1906. doi: 10.3390/agronomy10121906
Ghosh, D., Brahmachari, K., Das, A., Hassan, M. M., Mukherjee, P. K., Sarkar, S., et al. (2021). Assessment of energy budgeting and its indicator for sustainable nutrient and weed management in a rice-Maize-Green gram cropping system. Agron. 11 (1), 166. doi: 10.3390/agronomy11010166
Ghosh, D., Brahmachari, K., Sarkar, S., Dinda, N. K., Das, A., Moulick, D. (2022a). Impact of nutrient management in rice-maize-greengram cropping system and integrated weed management treatments on summer greengram productivity. Ind. J. Weed Sci. 54 (1), 25–30. doi: 10.5958/0974-8164.2022.00004.1
Ghosh, D., Brahmachari, K., Skalicky, M., Hossain, A., Sarkar, S., Dinda, N. K., et al. (2020b). Nutrients supplementation through organic manures influence the growth of weeds and maize productivity. Molecules 25 (21), 4924. doi: 10.3390/molecules25214924
Ghosh, D., Brahmachari, K., Skalický, M., Roy, D., Das, A., Sarkar, S., et al. (2022b). The combination of organic and inorganic fertilizers influence the weed growth, productivity and soil fertility of monsoon rice. PloS One 17 (1), e0262586. doi: 10.1371/journal.pone.0262586
Gill, S. S., Tuteja, N. (2010). Reactive oxygen species and antioxidant machinery in abiotic stress tolerance in crop plants. Plant Physiol. Biochem. 48, 909–930. doi: 10.1016/j.plaphy.2010.08.016
Giri, J., Vij, S., Dansana, P. K., Tyagi, A. K. (2011). Rice A20/AN1 zinc-finger containing stress-associated proteins (SAP1/11) and a receptor-like cytoplasmic kinase (OsRLCK253) interact via A20 zinc-finger and confer abiotic stress tolerance in transgenic arabidopsis plants. New Phytol. 191 (3), 721–732. doi: 10.1111/j.1469-8137.2011.03740.x
Guo, J., Bai, X., Dai, K., Yuan, X., Guo, P., Zhou, M., et al. (2021). Identification of GATA transcription factors in Brachypodium distachyon and functional characterization of BdGATA13 in drought tolerance and response to gibberellins. Front. Plant Sci. 11, 2386. doi: 10.3389/fpls.2021.763665
Gupta, D. K., Nicoloso, F. T., Schetinger, M. R. C., Rossato, L. V., Pereira, L. B., Castro, G. Y., et al. (2009). Antioxidant defense mechanism in hydroponically grown zea mays seedlings under moderate lead stress. J. Hazardous Materials 172 (1), 479–484. doi: 10.1016/j.jhazmat.2009.06.141
Gupta, P., Nutan, K. K., Singla-Pareek, S. L., Pareek, A. (2017). Abiotic stresses cause differential regulation of alternative splice forms of GATA transcription factor in rice. Front. Plant Sci. 8, 1944. doi: 10.3389/fpls.2017.01944
Haider, F. U., Liqun, C., Coulter, J. A., Cheema, S. A., Wu, J., Zhang, R., et al. (2021). Cadmium toxicity in plants: Impacts and remediation strategies. Ecotoxicol. Environ. Safety. 211, 111887. doi: 10.1016/j.ecoenv.2020.111887
Haider, S., Raza, A., Iqbal, J., Shaukat, M., Mahmood, T. (2022). Analyzing the regulatory role of heat shock transcription factors in plant heat stress tolerance: A brief appraisal. Mol. Biol. Rep. ;49 (6), 1–15. doi: 10.1007/s11033-022-07190-x
Han, G., Lu, C., Guo, J., Qiao, Z., Sui, N., Qiu, N., et al. (2020). C2H2 zinc finger proteins: Master regulators of abiotic stress responses in plants. Front. Plant Sci. 11, 115. doi: 10.3389/fpls.2020.00115
Han, G., Qiao, Z., Li, Y., Wang, C., Wang, B. (2021). The roles of CCCH zinc-finger proteins in plant abiotic stress tolerance. Int. J. Mol. Sci. 22 (15), 8327. doi: 10.3390/ijms22158327
Han, G., Wang, M., Yuan, F., Sui, N., Song, J., Wang, B. (2014). The CCCH zinc finger protein gene AtZFP1 improves salt resistance in arabidopsis thaliana. Plant Mol. Biol. 86, 237–253. doi: 10.1007/s11103-014-0226-5
Harrison, M. D., Jones, C. E., Solioz, M., Dameron, C. T. (2000). Intracellular copper routing: the role of chaperones. Trends Biochem. Sci. 25, 29–32. doi: 10.1016/S0968-0004(99)01492-9
Hasanuzzaman, M., Bhuyan, M. H. M. B., Anee, T. I., Parvin, K., Nahar, K., Mahmud, J. A., et al. (2019). Regulation of ascorbate-glutathione pathway in mitigating oxidative damage in plants under abiotic stress. Antioxidants 8 (9), 384. doi: 10.3390/antiox8090384
Hasanuzzaman, M., Hossain, M. A., Silva, J. A., Fujita, M. (2012). “Plant response and tolerance to abiotic oxidative stress: Antioxidant defense is a key factor,” in Crop stress and its management: Perspectives and strategies (Singapore: Springer), 261–315.
Hassinen, V., Vallinkoski, V.-M., Issakainen, S., Tervahauta, A., Kärenlampi, S., Servomaa, K. (2009). Correlation of foliar MT2b expression with cd and zn concentrations in hybrid aspen (Populus tremula×tremuloides) grown in contaminated soil. Environ. pollut. 157, 922–930. doi: 10.1016/j.envpol.2008.10.023
Hernandez, J. A., Jimenez, A., Mullineaux, P. M., Sevilla, F. (2000). Tolerance of pea (Pisum sativum l.) to long term salt stress is associated with induction of antioxidant defenses. Plant Cell Environ. 23, 853–862. doi: 10.1046/j.1365-3040.2000.00602.x
Hernández, L. E., Sobrino-Plata, J., Montero-Palmero, M. B., Carrasco-Gil, S., Flores-Cáceres, M. L., Ortega-Villasante, C., et al. (2015). Contribution of glutathione to the control of cellular redox homeostasis under toxic metal and metalloid stress. J. Exp. Bot. 66, 2901–2911. doi: 10.1093/jxb/erv063
He, X., Wang, C., Wang, H., Li, L., Wang, C. (2020). The function of MAPK cascades in response to various stresses in horticultural plants. Front. Plant Sci. 11, 952. doi: 10.3389/fpls.2020.00952
Hiratsu, K., Mitsuda, N., Matsui, K., Ohme-Takagi, M. (2004). Identification of the minimal repression domain of SUPERMAN shows that the DLELRL hexapeptide is both necessary and sufficient for repression of transcription in arabidopsis. Biochem. Biophys. Res. Commun. 321, 172–178. doi: 10.1016/j.bbrc.2004.06.115
Hirayama, T., Shinozaki, K. (2010). Research on plant abiotic stress responses in the post-genome era: past, present and future. Plant J. 61, 1041–1052. doi: 10.1111/j.1365-313X.2010.04124.x
Hossain, A., Maitra, S., Pramanick, B., Bhutia, K. L., Ahmad, Z., Moulik, D., et al. (2022). “Wild relatives of plants as sources for the development of abiotic stress tolerance in plants,” in Plant perspectives to global climate changes (Springer, Singapore: Academic Press), 471–518. doi: 10.1016/B978-0-323-85665-2.00011-X
Hossain, A., Pramanick, B., Bhutia, K. L., Ahmad, Z., Moulick, D., Maitra, S., et al. (2021). “Emerging roles of osmoprotectant glycine betaine against salt-induced oxidative stress in plants: A major outlook of maize (Zea mays l.),” in Frontiers in plant-soil interaction (Springer, Singapore: Academic Press), 567–587. doi: 10.1016/B978-0-323-90943-3.00015-8
Huang, P., Ju, H., Min, J., Zhang, X., Chung, J., Cheong, H., et al. (2012). Molecular and physiological characterization of the arabidopsis thaliana oxidation-related zinc finger 2, a plasma membrane protein involved in ABA and salt stress response tThrough the ABI2-mediated signaling pathway. Plant Cell Physiol. 53, 193–203. doi: 10.1093/pcp/pcr162
Huang, T. L., Nguyen, Q. T. T., Fu, S. F., Lin, C. Y., Chen, Y. C., Huang, H. J. (2012). Transcriptomic changes and signalling pathways induced by arsenic stress in rice roots. Plant Mol. Biol. 80 (6), 587–608. doi: 10.1007/s11103-012-9969-z
Huang, J., Sun, S. J., Xu, D. Q., Yang, X., Bao, Y. M., Wang, Z. F., et al. (2009). Increased tolerance of rice to cold, drought and oxidative stresses mediated by the overexpression of a gene that encodes the zinc finger protein ZFP245. biochem. Biophy. Res. Commun. 389 (3), 556–561. doi: 10.1016/j.bbrc.2009.09.032
Huang, J., Wang, M., Jiang, Y., Bao, Y., Huang, X., Sun, H., et al. (2008). Expression analysis of rice A20/AN1-type zinc finger genes and characterization of ZFP177 that contributes to temperature stress tolerance. Gene. 420, 135–144. doi: 10.1016/j.gene.2008.05.019
Huang, J., Yang, X., Wang, M. M., Tang, H. J., Ding, L. Y., Shen, Y., et al. (2007). A novel rice C2H2-type zinc finger protein lacking DLN-box/EAR-motif plays a role in salt tolerance. Biochim. Biophys. Acta 1769, 220–227. doi: 10.1016/j.bbaexp.2007.02.006
Hughes, N. M., Carpenter, K. L., Keidel, T. S., Miller, C. N., Waters, M. N., Smith, W. K. (2014). Photosynthetic costs and benefits of abaxial versus adaxial anthocyanins in colocasia esculenta ‘Mojito’. Plant. 240 (5), 971–981. doi: 10.1007/s00425-014-2090-6
Hwang, S. G., Kim, J. J., Lim, S. D., Park, Y. C., Moon, J. C., Jang, C. S. (2016). Molecular dissection of Oryza sativa salt-induced RING finger protein 1 (OsSIRP1): possible involvement in the sensitivity response to salinity stress. Physiol. Plant 158 (2), 168–179. doi: 10.1111/ppl.12459
Iida, A., Kazuoka, T., Torikai, S., Kikuchi, H., Oeda, K. (2000). A zinc finger protein RHL41 mediates the light acclimation response in arabidopsis. Plant J. 24, 191–203. doi: 10.1046/j.1365-313x.2000.00864.x
Iuchi, S., Kobayashi, M., Taji, T., Naramoto, M., Seki, M., Kato, T., et al. (2001). Regulation of drought tolerance by gene manipulation of 9-cis-epoxycarotenoid dioxygenase, a key enzyme in abscisic acid biosynthesis in arabidopsis. Plant J. 27 (4), 325–333. doi: 10.1046/j.1365-313x.2001.01096.x
Jadhav, S. S., Kumari, R., Mahtha, S. K., Purama, R. K., Lamba, V., Yadav, G. (2022). “Metabolomics and molecular physiology perspective for drought and salinity stress tolerance. response of field crops to abiotic stress: Current status and future prospects”, in Response of field crops to abiotic stress: Current status and future prospects. Eds. Choudhury, S, Moulick, D (Boca Raton, USA: CRC Press), 153–166.
Jain, R. G., Fletcher, S. J., Manzie, N., Robinson, K. E., Li, P., Lu, E., et al. (2022b). Foliar application of clay-delivered RNA interference for whitefly control. Nat. Plant 8 (5), 535–548. doi: 10.1038/s41477-022-01152-8
Jain, R., Fletcher, S., Mitter, N. (2022a). Effective RNAi-mediated control of the crop pest whitefly. Nat. Plant 8 (5), 461–462. doi: 10.1038/s41477-022-01160-8
Jaleel, C. A., Manivannan, P., Wahid, A., Farooq, M., Al-Juburi, H. J., Somasundaram, R. (2009). Drought stress in plants: A review on morphological characteristics and pigments composition. Int. J. Agric. Biol. 11, 100–105.
Jalmi, S. K., Sinha, A. K. (2015). ROS mediated MAPK signaling in abiotic and biotic stress-striking similarities and differences. Front. Plant Sci. Plant Sci. 6. doi: 10.3389/fpls.2015.00769
Jamil, W., Wu, W., Gong, H., Huang, J. W., Ahmad, M., Zhu, Q. G., et al. (2019). C2H2-type zinc finger proteins (DkZF1/2) synergistically control persimmon fruit deastringency. Int. J. Mol. Sci. 20 (22), 5611. doi: 10.3390/ijms20225611
Jamsheer, K. M., Sharma, M., Singh, D., Mannully, C. T., Jindal, S., Shukla, B. N., et al. (2018a). FCS-like zinc finger 6 and 10 repress SnRK1 signalling in arabidopsis. Plant J. 94 (2), 232–245. doi: 10.1111/tpj.13854
Jamsheer, K. M., Shukla, B. N., Jindal, S., Gopan, N., Mannully, C. T., Laxmi, A. (2018b). The FCS-like zinc finger scaffold of the kinase SnRK1 is formed by the coordinated actions of the FLZ domain and intrinsically disordered regions. J. Biol. Chem. 293 (34), 13134–13150. doi: 10.1074/jbc.RA118.002073
Jang, J. (2016). Arginine-rich motif-tandem CCCH zinc finger proteins in plant stress responses and post-transcriptional regulation of gene expression. Plant Sci. 252, 118–124. doi: 10.1016/j.plantsci.2016.06.014
Jan, A., Maruyama, K., Todaka, D., Kidokoro, S., Abo, M., Yoshimura, E., et al. (2013). OsTZF1, a CCCH-tandem zinc finger protein, confers delayed senescence and stress tolerance in rice by regulating stress-related genes. Plant Physiol. 161, 1202–1216. doi: 10.1104/pp.112.205385
Jiang, A.-L., Xu, Z.-S., Zhao, G.-Y., Cui, X.-Y., Chen, M., Li, L.-C., et al (2014). Genome-wide analysis of the C3H zinc finger transcription factor family and drought responses of members in aegilops tauschii. Plant Mol. Biol. Report 32, 1241–1256.
Jin, Y., Liu, L., Zhang, S., He, R., Wu, Y., Chen, G., et al. (2016). Cadmium exposure to murine macrophages decreases their inflammatory responses and increases their oxidative stress. Chemosphere. 144, 168–175. doi: 10.1016/j.chemosphere.2015.08.084
Jin, Y., Wang, M., Fu, J., Xuan, N., Zhu, Y., Lian, Y., et al. (2007). Phylogenetic and expression analysis of ZnF-AN1 genes in plants. Genomics. 90 (2), 265–275. doi: 10.1016/j.ygeno.2007.03.019
Joazeiro, C. A., Weissman, A. M. (2000). RING finger proteins: Mediators of ubiquitin ligase activity. Cell 102, 549–552. doi: 10.1016/s0092-8674(00)00077-5
Jonak, C., Nakagami, H., Hirt, H. (2004). Heavy metal stress. activation of distinct mitogen-activated protein kinase pathways by copper and cadmium. Plant Physiol. 136 (2), 3276–3283.
Joo, H., Lim, C. W., Lee, S. C. (2019). Roles of pepper bZIP transcription factor CaATBZ1 and its interacting partner RING-type E3 ligase CaASRF1 in modulation of ABA signalling and drought tolerance. Plant J. 100, 399–410. doi: 10.1111/tpj.14451
Joo, H., Lim, C. W., Lee, S. C. (2020). The pepper RING-type E3 ligase, CaATIR1, positively regulates abscisic acid signalling and drought response by modulating the stability of CaATBZ1. Plant Cell Environ. Cell Environ. 43, 1911–1924. doi: 10.1111/pce.13789
Jozefczak, M., Bohler, S., Schat, H., Horemans, N., Guisez, Y., Remans, T., et al. (2015). Both the concentration and redox state of glutathione and ascorbate influence the sensitivity of arabidopsis to cadmium. Ann. Bot. 116, 601–612. doi: 10.1093/aob/mcv075
Kang, J. S., Singh, H., Singh, G., Kang, H., Kalra, V. P., Kaur, J. (2017). Abiotic stress and its amelioration in cereals and pulses: A review. Int. J. Curr. Microbiol. Appl. Sci. 6, 1019–1045. doi: 10.20546/ijcmas.2017.603.120
Kanneganti, V., Gupta, A. K. (2008). Overexpression of OsiSAP8, a member of stress associated protein (SAP) gene family of rice confers tolerance to salt, drought and cold stress in transgenic tobacco and rice. Plant Mol. Biol. 66 (5), 445–462. doi: 10.1007/s11103-007-9284-2
Kao, C. H. (2017). Mechanisms of salt tolerance in rice plants: Cell wall-related genes and expansins. J. Taiwan Agric. Res. 66, 87–93. doi: 10.6156/JTAR/2017.06602.01
Katny, M. A. C., Hoffmann-Thoma, G., Schrier, A. A., Fangmeier, A., Jäger, H. J., van Bel, A. J. (2005). Increase of photosynthesis and starch in potato under elevated CO2 is dependent on leaf age. J. Plant Physiol. 162 (4), 429–438. doi: 10.1016/j.jplph.2004.07.005
Kaur, H., Kaur, H., Kaur, H., Srivastava, S. (2022). The beneficial roles of trace and ultratrace elements in plants. Plant Growth Regul., 1–18. doi: 10.1007/s10725-022-00837-6
Keilin, D., Mann, T. (1940). Carbonic anhydrase. purification and nature of the enzyme. Biochem.J. 34 (8-9), 1163. doi: 10.1042/bj0341163
Khan, M., Samrana, S., Zhang, Y., Malik, Z., Khan, M. D., Zhu, S. (2020). Reduced glutathione protects subcellular compartments from Pb-induced ROS injury in leaves and roots of upland cotton (Gossypium hirsutum l.). Front. Plant Sci. 11. doi: 10.3389/fpls.2020.00412
Kielbowicz-Matuk, A. (2012). Involvement of plant C(2)H(2)-type zinc finger transcription factors in stress responses. Plant Sci. 185, 78–85. doi: 10.1016/j.plantsci.2011.11.015
Kim, J. C., Lee, S. H., Cheong, Y. H., Yoo, C. M., Lee, S. I., Chun, H. J., et al. (2001). A novel cold-inducible zinc finger protein from soybean, SCOF-1, enhances cold tolerance in transgenic plants. Plant J. 25, 247–259. doi: 10.1046/j.1365-313x.2001.00947.x
Kim, J. H., Lee, H. J., Park, C. M. (2017). HOS1 acts as a key modulator of hypocotyl photo morphogenesis. Plant Signal. Behav. 12, e1315497. doi: 10.1080/15592324.2017.1315497
Kimura, M., Yamamoto, Y. Y., Seki, M., Sakurai, T., Sato, M., Abe, T., et al. (2003). Identification of arabidopsis genes regulated by high light stress using cDNA microarray. Photochem. Photobiol. 77, 226–233. doi: 10.1562/0031-8655(2003)077<0226:ioagrb>2.0.co;2
Kochańczyk, T., Drozd, A., Krężel, A. (2015). Relationship between the architecture of zinc coordination and zinc binding affinity in proteins–insights into zinc regulation. Metallomics 7 (2), 244–257. doi: 10.1039/C4MT00094C
Kochańczyk, T., Nowakowski, M., Wojewska, D., Kocyła, A., Ejchart, A., Koźmiński, W., et al. (2016). Metal-coupled folding as the driving force for the extreme stability of Rad50 zinc hook dimer assembly. Sci. Rep. 6 (1), 1–12. doi: 10.1039/c4mt00094c
Kodaira, K., Qin, F., Tran, L. P., Maruyama, K., Kidokoro, S., Fujita, Y., et al. (2011). Arabidopsis Cys2/His2 zinc-finger proteins AZF1 and AZF2 negatively regulate abscisic acid-repressive and auxin-inducible genes under abiotic stress conditions. Plant Physiol. 157, 742–756. doi: 10.1104/pp.111.182683
Ko, J. H., Yang, S. H., Han, K. H. (2006). Upregulation of an arabidopsis RING-H2 gene, XERICO, confers drought tolerance through increased abscisic acid biosynthesis. Plant Journal: For Cell Mol. Biol. 47 (3), 343–355. doi: 10.1111/j.1365-313X.2006.02782.x
Kovtun, Y., Chiu, W. L., Tena, G., Sheen, J. (2000). Functional analysis of oxidative stress-activatedmitogenactivated protein kinase cascade in plants. PANS 97, 2940–2945. doi: 10.1073/pnas.97.6.2940
Ku, Y. S., Sintaha, M., Cheung, M. Y., Lam, H. M. (2018). Plant hormone signaling crosstalks between biotic and abiotic stress responses. Int. J. Mol. Sci. 19, 3206. doi: 10.3390/ijms19103206
Laity, J. H., Lee, B. M., Wright, P. E. (2001). Zinc finger proteins: New insights into structural and functional diversity. Curr. Opin. Struct. Biol. 11, 39–46. doi: 10.1016/S0959-440X(00)00167-6
Larcher, W. (2003). Physiological plant ecology: Ecophysiology and stress physiology of functional groups (Berlin/Heidelberg, Germany: Springer), 345–437.
Lawrence, S. D., Blackburn, M. B., Shao, J., Novak, N. G. (2022). The response to cabbage looper infestation in arabidopsis is altered by lowering levels of Zat18 a q-type C2H2 zinc finger protein. J. Plant Interaction. 17 (1), 198–205. doi: 10.1080/17429145.2021.2024285
Lee, S. J., Jung, H. J., Kang, H., Kim, S. Y. (2012). Arabidopsis zinc finger proteins AtC3H49/AtTZF3 and AtC3H20/AtTZF2 are involved in ABA and JA responses. Plant Cell Physiol. 53, 673–686. doi: 10.1093/pcp/pcs023
Lima, J. M., Nath, M., Dokku, P., Raman, K., Kulkarni, K., Vishwakarma, C., et al. (2015). Physiological, anatomical and transcriptional alterations in a rice mutant leading to enhanced water stress tolerance. AoB Plant 7, plv023. doi: 10.1093/aobpla/plv023
Lim, C. W., Baek, W., Lee, S. C. (2017). The pepper RING-type E3 ligase CaAIRF1 regulates ABA and drought signaling via CaADIP1 protein phosphatase degradation. Plant Physiol. 173, 2323–2339. doi: 10.1104/pp.16.01817
Lim, C. W., Baek, W., Lee, S. C. (2018). Roles of pepper bZIP protein Ca DILZ 1 and its interacting partner RING-type E3 ligase Ca DSR 1 in modulation of drought tolerance. Plant J. 96, 452–467. doi: 10.1111/tpj.14046
Lim, S. D., Cho, H. Y., Park, Y. C., Ham, D. J., Lee, J. K., Jang, C. S. (2013). The rice RING finger E3 ligase, OsHCI1, drives nuclear export of multiple substrate proteins and its heterogeneous overexpression enhances acquired thermo tolerance. J. Exp. Bot. 64, 2899–2914. doi: 10.1093/jxb/ert143
Lin, Y. F., Aarts, M. G. (2012). The molecular mechanism of zinc and cadmium stress response in plants. Cell Mol. Life Sci. 69, 3187–3206. doi: 10.1007/s00018-012-1089-z
Lin, C. Y., Lin, L. Y. (2018). The conserved basic residues and the charged amino acid residues at the alpha-helix of the zinc finger motif regulate the nuclear transport activity of triple C2H2 zinc finger proteins. PloS One 13, e0191971. doi: 10.1371/journal.pone.0191971
Lin, P., Pomeranz, M., Jikumaru, Y., Kang, S. G., Hah, C., Fujioka, S., et al. (2011). The arabidopsis tandem zinc finger protein AtTZF1 affects ABA- and GA-mediated growth, stress and gene expression responses. Plant J. 65, 253–268. doi: 10.1111/j.1365-313X.2010.04419.x
Lin, L., Wu, J., Jiang, M., Wang, Y. (2021). Plant mitogen-activated protein kinase cascades in environmental stresses. Int. J. Mol. Sci. 22, 1543. doi: 10.3390/ijms22041543
Lipiec, J., Doussan, C., Nosalewicz, A., Kondracka, K. (2013). Effect of drought and heat stresses on plant growth and yield: A review. Int. Agrophys. 27, 463–477. doi: 10.2478/intag-2013-0017
Lippuner, V., Cyert, M., Gasser, C. (1996). Two classes of plant cDNA clones differentially complement yeast calcineurin mutants and increase s alt tolerance of wild-type yeast. J. Biol. Chem. 271 (22), 12859–12866. doi: 10.1074/jbc.271.22.12859
Liu, Y., Khan, A. R., Gan, Y. (2022). C2H2 zinc finger proteins response to abiotic stress in plants. Int. J. Mol. Sci. 23, 2730. doi: 10.3390/ijms23052730
Liu, X. M., Kim, K. E., Kim, K. C., Nguyen, X. C., Han, H. J., Jung, M. S., et al. (2010). Cadmium activates arabidopsis MPK3 and MPK6 via accumulation of reactive oxygen species. Phytochemistry 71 (5–6), 614–618. doi: 10.1016/j.phytochem.2010.01.005
Liu, S., Kracher, B., Ziegler, J., Birkenbihl, R. P., Somssich, I. E. (2015). Negative regulation of ABA signaling by WRKY33 is critical for arabidopsis immunity towards botrytis cinerea 2100. Life 4, e07295. doi: 10.7554/eLife.07295.033
Liu, Y., Xu, C., Zhu, Y., Zhang, L., Chen, T., Zhou, F., et al. (2018). The calcium-dependent kinase OsCPK24 functions in cold stress responses in rice. J. Integr. Plant Biol. 60, 173–188. doi: 10.1111/jipb.12614
Li, D., Xu, X., Hu, X., Liu, Q., Wang, Z., Zhang, H., et al. (2015). Genome-wide analysis and heavy metal-induced expression profiling of the HMA gene family in Populus trichocarpa. Front. Plant Sci. 6, 1149. doi: 10.3389/fpls.2015.01149
Li, S., Zhao, B., Yuan, D., Duan, M., Qian, Q., Tang, L., et al. (2013). Rice zinc finger protein DST enhances grain production through controlling Gn1a/OsCKX2 expression. Proceed. Natl. Acad. Sci. United States Amer. 110 (8), 3167–3172. doi: 10.1073/pnas.1300359110
Logemann, E., Birkenbihl, R. P., Rawat, V., Schneeberger, K., Schmelzer, E., Somssich, I. E. (2013). Functional dissection of the PROPEP2 and PROPEP3 promoters reveals the importance of WRKY factors in mediating microbe-associated molecular pattern-induced expression. New Phytol. 198, 1165–1177. doi: 10.1111/nph.12233
Lopes, K. L., Rodrigues, R. A., Silva, M. C., Braga, W. G., Silva-Filho, M. C. (2018). The zinc-finger thylakoid-membrane protein FIP is involved with abiotic stress response in arabidopsis thaliana. Front. Plant Sci. 9, 504. doi: 10.3389/fpls.2018.00504
Luo, X., Bai, X., Zhu, D., Li, Y., Ji, W., Cai, H., et al. (2012). GsZFP1, a new Cys2/His2-type zinc-finger protein, is a positive regulator of plant tolerance to cold and drought stress. Planta 235 (6), 1141–1155. doi: 10.1007/s00425-011-1563-0
Lv, Q., Zhang, L., Zan, T., Li, L., Li, X. (2020). Wheat RING E3 ubiquitin ligase TaDIS1 degrade TaSTP via the 26S proteasome pathway. Plant Sci. 296, 110494. doi: 10.1016/j.plantsci.2020.110494
Lyu, T., Cao, J. (2018). Cys(2)/His(2) zinc-finger proteins in transcriptional regulation of flower development. Int. J. Mol. Sci. 19, 2589. doi: 10.3390/ijms19092589
Mainuddin, M., Bell, R. W., Gaydon, D. S., Kirby, J. M., Glover, M., Akanda, M. A. R., et al. (2019). An overview of the Ganges coastal Zone: Climate, hydrology, land use and vulnerability. J. Ind. Soc Coast. Agric. Res. 37 (2), 1–11.
Mainuddin, M., Maniruzzaman, M., Gaydon, D. S., Sarkar, S., Rahman, M. A., Sarangi, S. K., et al. (2020). A water and salt balance model for the polders and islands in the Ganges delta. J. Hydrol 587, 125008. doi: 10.1016/j.jhydrol.2020.125008
Maret, W., Albrecht Messerschmidt, W. B., Cygler, M. (2004). Handbook of metalloproteins (Boca Raton, USA: CRC Press).
Maret, W., Li, Y. (2009). Coordination dynamics of zinc in proteins. Chem. Rev. 109 (10), 4682–4707. doi: 10.1021/cr800556u
Martin, R. C., Glover-Cutter, K., Baldwin, J. C., Dombrowski, J. E. (2012). Identification and characterization of a salt stress-inducible zinc finger protein from festuca arundinacea. BMC Res. Notes 5, 66. doi: 10.1186/1756-0500-5-66
Mateos Fernández, R., Petek, M., Gerasymenko, I., Juteršek, M., Baebler, Š., Kallam, K., et al. (2022). Insect pest management in the age of synthetic biology. Plant Biotechnol. J. 20 (1), 25–36. doi: 10.1111/pbi.13685
Mazumder, M. K., Moulick, D., Choudhury, S. (2022). Iron (Fe3+)-mediated redox responses and amelioration of oxidative stress in cadmium (Cd2+) stressed mung bean seedlings: A biochemical and computational analysis. J. Plant Biochem. Biotechnol. 31 (1), 49–60. doi: 10.1007/s13562-021-00654-4
Miller, J., McLachlan, A. D., Klug, A. (1985). Repetitive zinc-binding domains in the protein transcription factor IIIA from xenopus oocytes. EMBO J. 4 (6), 1609–1614. doi: 10.1002/j.1460-2075.1985.tb03825.x
Mishra, S., Srivastava, S., Tripathi, R. D., Govindarajan, R., Kuriakose, S. V., Prasad, M. N. V. (2006). Phytochelatin synthesis and response of antioxidants during cadmium stress in bacopa monnieri l. Plant Physiol. Biochem. 44 (1), 25–37. doi: 10.1016/j.plaphy.2006.01.007
Mittler, R., Vanderauwera, S., Gollery, M., Van Breusegem, F. (2004). Reactive oxygen gene network of plants. Trend. Plant Sci. 9, 490–498. doi: 10.1016/j.tplants.2004.08.009
Mostofa, M. G., Seraj, Z. I., Fujita, M. (2015). Interactive effects of nitric oxide and glutathione in mitigating copper toxicity of rice (Oryza sativa l.) seedlings. Plant Signaling Behav. 10 (3), e991570. doi: 10.4161/15592324.2014.991570
Moulick, D., Chowardhara, B., Panda, S. K. (2019). “Agroeco-toxicological aspect of arsenic (As) and cadmium (Cd) on field crops and its mitigation: current status and future prospect,” in Plant-metal interactions (Cham: Springer), 217–246. doi: 10.1007/978-3-030-20732-8_11
Moulick, D., Ghosh, D., Santra, S. C. (2016a). An assessment of some physicochemical properties and cooking characteristics of milled rice and associated health risk in two rice varieties of arsenic contaminated areas of West Bengal, India. Int. J. Res. Agric. Food Sci. 6, 44–55.
Moulick, D., Ghosh, D., Santra, S. C. (2016b). Evaluation of effectiveness of seed priming with selenium in rice during germination under arsenic stress. Plant Physiol. Biochem. 109, 571–578. doi: 10.1016/j.plaphy.2016.11.004
Moulick, D., Ghosh, D., Skalicky, M., Gharde, Y., Mazumder, M. K., Choudhury, S., et al. (2022). Interrelationship among rice grain arsenic, micronutrients content and grain quality attributes: An investigation from genotype× environment perspective. Front. Environ. Sci. 25 (27). doi: 10.3389/fenvs.2022.857629
Moulick, D., Samanta, S., Sarkar, S., Mukherjee, A., Pattnaik, B. K., Saha, S., et al. (2021). Arsenic contamination, impact and mitigation strategies in rice agro-environment: An inclusive insight. Sci. Total Environ. 800, 149477. doi: 10.1016/j.scitotenv
Moulick, D., Santra, S. C., Ghosh, D. (2017). Seed priming with Se alleviate as induced phytotoxicity during germination and seedling growth by restricting as translocation in rice (Oryza sativa l cv IET-4094). Ecotoxicol Environ. Safety. 145, 449–456. doi: 10.1016/j.ecoenv.2017.07.060
Moulick, D., Santra, S. C., Ghosh, D. (2018a). Effect of selenium induced seed priming on arsenic accumulation in rice plant and subsequent transmission in human food chain. Ecotoxicol Environ. Safety. 152, 67–77. doi: 10.1016/j.ecoenv.2018.01.037
Moulick, D., Santra, S. C., Ghosh, D. (2018b). Rice seed priming with se: a novel approach to mitigate as induced adverse consequences on growth, yield and as load in brown rice. J. Hazardous Material. 355, 187–196. doi: 10.1016/j.jhazmat.2018.05.017
Moulick, D., Santra, S. C., Ghosh, D. (2018c). Seed priming with Se mitigates as-induced phytotoxicity in rice seedlings by enhancing essential micronutrient uptake and translocation and reducing as translocation. Environ. Sci. Poll. Res. 25 (27), 26978–26991. doi: 10.1007/s11356-018-2711-x
Moulick, D., Santra, S. C., Ghosh, D. (2018d). “Consequences of paddy cultivation in arsenic-contaminated paddy fields of lower Indo-Gangetic plain on arsenic accumulation pattern and selected grain quality traits: A preliminary assessment”, in Mech. of Arsenic Toxic. and Tolerance in Plants. Ed. Hasanuzzaman, M. (Singapore: Springer), 49–78. doi: 10.1007/978-981-13-1292-2_3
Mueller, N. D., Gerber, J. S., Johnston, M., Ray, D. K., Ramankutty, N., Foley, J. A. (2012). Closing yield gaps through nutrient and water management. Nat. 490, 254–257. doi: 10.1038/nature11420
Mukherjee, A., Hazra, S. (2022). “Impact of elevated CO2 and O3 on field crops and adaptive strategies through agro-technology,” in Response of field crops to abiotic stress (Boca Raton, USA: CRC Press), 177–190.
Mukhopadhyay, A., Vij, S. ,. A., Tyagi, A. K. (2004). Overexpression of a zinc-finger protein gene from rice confers tolerance to cold, dehydration, and salt stress in transgenic tobacco. Proceed. Natl. Acad. Sci. United States Amer 101 (16), 6309–6314. doi: 10.1073/pnas.0401572101
Nawkar, G. M., Kang, C. H., Maibam, P., Park, J. H., Jung, Y. J., Chae, H. B., et al. (2017). HY5, a positive regulator of light signaling, negatively controls the unfolded protein response in arabidopsis. Proc. Nat. Acad. Sci. 21, 14(8):2084–2089. doi: 10.1073/pnas.1609844114
Nedelkoska, T. V., Doran, P. M. (2000). Hyper accumulation of cadmium by hairy roots of thlaspi caerulescens. Biotechnol. Bioeng. 67, 607–615. doi: 10.1002/(SICI)1097-0290(20000305)67:5<607::AID-BIT11>3.0.CO;2-3
Nguyen, T. P., Ho, T. B. (2012). Detecting disease genes based on semi-supervised learning and protein–protein interaction networks. Artif. Intell. Med. 54 (1), 63–71.
Noman, A., Aqeel, M., Khalid, N., Islam, W., Sanaullah, T., Anwar, M., et al. (2019). Zinc finger protein transcription factors: Integrated line of action for plant antimicrobial activity. Microbial. Pathogen. 132, 141–149. doi: 10.1016/j.micpath.2019.04.042
Nouman, W., Anwar, F., Gull, T., Newton, A., Rosa, E., Domínguez-Perles, R. (2016). Profiling of polyphenolics, nutrients and antioxidant potential of germplasm’s leaves from seven cultivars of Moringa oleifera lam. Ind. Crop Prod. 83, 166–176. doi: 10.1016/j.indcrop.2015.12.032
Nouman, W., Basra, S. M. A., Yasmeen, A., Gull, T., Hussain, S. B., Zubair, M., et al. (2014). Seed priming improves the emergence potential, growth and antioxidant system of moringa oleifera under saline conditions. Plant Growth Regul. 73, 267–278. doi: 10.1007/s10725-014-9887-y
Ogawa, I., Nakanishi, H., Mori, S., Nishizawa, N. K. (2009). Time course analysis of gene regulation under cadmium stress in rice. Plant Soil. 325, 97. doi: 10.1007/s11104-009-0116-9
Oh, T. R., Yu, S. G., Yang, H. W., Kim, J. H., Kim, W. T. (2020). AtKPNB1, AtKPNB1, an arabidopsis importin-b protein, is downstream of the RING E3 ubiquitin ligase AtAIRP1 in the ABA-mediated drought stress response. Plant. 252, 93. doi: 10.1007/s00425-020-03500-4
O’Malley, R. C., Huang, S. C., Lewsey, M. G., Bartlett, A., Nery, J. R., Galli, M., et al. (2016). Cistrome and epicistrome features shape the regulatory DNA landscape. Cell. 165, 1280–1292. doi: 10.1016/j.cell.2016.04.038
Opdenakker, K., Remans, T., Vangronsveld, J., Cuypers, A. (2012). Mitogen activated protein (MAP) kinases in plant metal stress: Regulation and responses in comparison to other biotic and abiotic stresses. Int. J.Mol. Sci. 13, 7828–7853. doi: 10.3390/ijms13067828
Opipari, A. W., Boguski, M. S., Dixit, V. M. (1990). The A20 cDNA induced by tumor necrosis factor alpha encodes a novel type of zinc finger protein. J. Biol. Chem. 265 (25), 14705–14708. doi: 10.1016/S0021-9258(18)77165-2
Osakabe, Y., Osakabe, K., Shinozaki, K., Tran, L. S. P. (2014). Response of plants to water stress. Front. Plant Sci. 5. doi: 10.3389/fpls.2014.00086
Osorio, C. E. (2019). The role of orange gene in carotenoid accumulation: Manipulating chromoplasts toward a colored future. Front. Plant Sci. 10. doi: 10.3389/fpls.2019.01235
Parida, A. K., Das, A. B. (2005). Salt tolerance and salinity effects on plants: A review. Ecotoxic. Environ. Saf. 60, 324–349. doi: 10.1016/j.ecoenv.2004.06.010
Park, Y. C., Chapagain, S., Jang, C. S. (2018). The microtubule-associated RING finger protein 1 (OsMAR1) acts as a negative regulator for salt-stress response through the regulation of OCPI2 (O. sativa chymotrypsin protease inhibitor 2). Planta 247 (4), 875–886. doi: 10.1007/s00425-017-2834-1
Park, Y. C., Lim, S. D., Moon, J. C., Jangand Jang, C. S. (2019). A rice really interesting new gene h 2-type e 3 ligase, OsSIRH2-14, enhances salinity tolerance via ubiquitin/26 s proteasome-mediated degradation of salt-related proteins. Plant Cell Environ. 42 (11), 3061–3076. doi: 10.1111/pce.13619
Parraga, G., Horvath, S. J., Eisen, A., Taylor, W. E., Hood, L., Young, E. T., et al. (1988). Zinc-dependent structure of a single-finger domain of yeast ADR1. Science 241 (4872), 1489–1492. doi: 10.1126/science.3047872
Pi, B., He, X., Ruan, Y., Jang, J. C., Huang, Y. (2018). Genome-wide analysis and stress-responsive expression of CCCH zinc finger family genes in Brassica rapa. BMC Plant Biol. 18 (1), 1–15. doi: 10.1186/s12870-018-1608-7
Poorter, H., Van Berkel, Y., Baxter, R., Den Hertog, J., Dijkstra, P., Gifford, R. M., et al. (1997). The effect of elevated CO2 on the chemical composition and construction costs of leaves of 27 C3 species. Plant Cell Environ. 20 (4), 472–482. doi: 10.1046/j.1365-3040.1997.d01-84.x
Pradhan, S., Kant, C., Verma, S., Bhatia, S. (2017). Genome-wide analysis of the CCCH zinc finger family identifies tissue specific andstress responsive candidates in chickpea (Cicer arietinum l.). PloS One 12, e0180469. doi: 10.1371/journal.pone.0180469
Qin, X., Huang, S., Liu, Y., Bian, M., Shi, W., Zuo, Z., et al. (2017). Overexpression of a RING finger ubiquitin ligase gene AtATRF1 enhances aluminium tolerance in arabidopsis thaliana. J. Plant Biol. 60, 66–74. doi: 10.1007/s12374-016-0903-9
Qu, J., Kang, S. G., Wang, W., Musier-Forsyth, K., Jang, J. C. (2014). The arabidopsis thaliana tandem zinc finger 1 (AtTZF1) protein in RNA binding and decay. Plant J. 78, 452–467. doi: 10.1111/tpj.12485
Raja, V., Majeed, U., Kang, H., Andrabi, K. I., John, R. (2017). Abiotic stress: Interplay between ROS, hormones and MAPKs. Environ. Exp. Bot. 137, 142–157. doi: 10.1016/j.envexpbot.2017.02.010
Reliene, R., Schiestl, R. H. (2006). Glutathione depletion by buthionine sulfoximine induces DNA deletions in mice. Carcinogenesis 27 (2), 240–244. doi: 10.1093/carcin/bgi222
Rhodes, D., Klug, A. (1986). An underlying repeat in some transcriptional control sequences corresponding to half a double helical turn of DNA. Cell 46 (1), 123–132. doi: 10.1016/0092-8674(86)90866-4
Riaz, M., Arif, M. S., Ashraf, M. A., Mahmood, R., Yasmeen, T., Shakoor, M. B., et al. (2019). A comprehensive review on rice responses and tolerance to salt stress. Adv.Rice Res. Abiotic Stress. Tolerance (Amsterdam: Elsevier), 133–158. doi: 10.1016/b978-0-12-814332-2.00007-1
Rizhsky, L., Davletova, S., Liang, H., Mittler, R. (2004). The zinc finger protein Zat12 is required for cytosolic ascorbate peroxidase 1 expression during oxidative stress in arabidopsis. J. Biol. Chem. 279 (12), 11736–11743. doi: 10.1074/JBC.M313350200
Rodriguezcazorla, E., Ortunomiquel, S., Candela, H., Baileysteinitz, L. J., Yanofsky, M. F., Martinezlaborda, A., et al. (2018). Ovule identity mediated by pre-mRNA processing in arabidopsis. PloS Genet. 14, e1007182. doi: 10.1371/journal.pgen.1007182
Rodriguez, M. C., Petersen, M., Mundyand Mundy, J. (2010). Mitogen-activated protein kinase signaling in plants. Annu. Rev. Plant Biol. 61, 621–649. doi: 10.1146/annurev-arplant-042809-112252
Romero, I., Alegria-Carrasco, E., de Pradena, A. G., Vazquez-Hernandez, M., Escribano, M. I., Merodio, C., et al. (2019). WRKY transcription factors in the response of table grapes (cv. Autumn royal) to high CO2 levels and low temperature. Postharvest Biol. Technol. 150, 42–51. doi: 10.1016/j.postharvbio.2018.12.011
Rosales, R., Romero, I., Fernandez-Caballero, C., Escribano, M. I., Merodio, C., Sanchez- Ballesta, M. T. (2016). Low temperature and short-term high-CO2 treatment in postharvest storage of table grapes at two maturity stages: effects on transcriptome profiling. Front. Plant Sci. 7, e1020. doi: 10.3389/fpls.2016.01020
Rossel, J. B., Wilson, I. W., Pogson, B. J. (2002). Global changes in gene expression in response to high light in arabidopsis. Plant Physiol. 130, 1109–1120. doi: 10.1104/pp.005595
Roychoudhury, A., Paul, S., Basu, S. (2013). Cross-talk between abscisic acid-dependent and abscisic acid-independent pathways during abiotic stress. Plant Cell Rep. 32, 985–1006. doi: 10.1007/s00299-013-1414-5
Roy, D., Vishwanath, P. D., Sreekanth, D., Mahawar, H., Ghosh, D. (2022). “Salinity and osmotic stress in field crops: Effects and way out,” in Response of field crops to abiotic stress: Current status and future prospects. Eds. Choudhury, S., Moulick, D. (Boca Raton, USA: CRC Press), 123. doi: 10.1201/9781003258063-11
Rushton, P. J., Somssich, I. E., Ringler, P., Shen, Q. J. (2010). WRKY transcription factors. Trend. Plant Sci. 15, 247–258. doi: 10.1016/j.tplants.2010.02.006
Saad, R. B., Hsouna, A. B., Saibi, W., Hamed, K. B., Brini, F., Ghneim-Herrera, T. (2018). A stress-associated protein, LmSAP, from the halophyte Lobularia maritima provides tolerance to heavy metals in tobacco through increased ROS scavenging and metal detoxification processes. J. Plant Physiol. 231, 234–243. doi: 10.1016/j.jplph.2018.09.019
Sagar, L., Praharaj, S., Singh, S., Attri, M., Pramanick, B., Maitra, S., et al. (2022). Drought and heat stress tolerance in field crops: Consequences and adaptation strategies. response of field crops to abiotic stress: Current status and future prospects. In Response of field crops to abiotic stress: Current status and future prospects. Eds. Choudhury, S, Moulick, D (Boca Raton, USA: CRC Press), 91–102.
Saha, B., Chowardhara, B., Chowra, U., Panda, C. K. (2022). Aluminum toxicity and ionic homeostasis in plants. response of field crops to abiotic stress: Current status and future prospects. In Response of field crops to abiotic stress: Current status and future prospects. Eds. Choudhury, S, Moulick, D (Boca Raton, USA: CRC Press), 79–90.
Saha, B., Chowardhara, B., Kar, S., Devi, S. S., Awasthi, J. P., Moulick, D., et al. (2019). “Advances in heavy metal-induced stress alleviation with respect to exogenous amendments in crop plants,” in Priming and pretreatment of seeds and seedlings (Singapore: Springer), 313–332. doi: 10.1007/978-981-13-8625-1_15
Sahoo, S., Borgohain, P., Saha, B., Moulick, D., Tanti, B., Panda, S. K. (2019). “Seed priming and seedling pre-treatment induced tolerance to drought and salt stress: recent advances,” in Priming and pretreatment of seeds and seedlings (Singapore: Springer), pp.253–pp.263. doi: 10.1007/978-981-13-8625-1_12
Sah, S. K., Reddy, K. R., Li, J. (2016). Abscisic acid and abiotic stress tolerance in crop plants. Front. Plant Sci. 7. doi: 10.3389/fpls.2016.00571
Sakamoto, H., Maruyama, K., Sakuma, Y., Meshi, T., Iwabuchi, M., Shinozaki, K., et al. (2004). Arabidopsis Cys2/His2-type zinc-finger proteins function as transcription repressors under drought, cold, and high-salinity stress conditions. Plant Physiol. 136, 2734–2746. doi: 10.1104/pp.104.046599
Sarkar, S., Gaydon, D. S., Brahmachari, K., Poulton, P. L., Chaki, A. K., Ray, K., et al. (2022). Testing APSIM in a complex saline coastal cropping environment. Environ. Model. Software 147, 105239. doi: 10.1016/j.envsoft.2021.105239
Schmitz, R. J., Hong, L., Michaels, S., Amasino, R. M. (2005). FRIGIDA-ESSENTIAL 1 interacts genetically with FRIGIDA and FRIGIDALIKE 1 to promote the winter-annual habit of arabidopsis thaliana. Develop. 132, 5471–5478. doi: 10.1242/dev.02170
Seok, H., Nguyen, L. V., Park, H., Tarte, V. N., Ha, J., Lee, S., et al. (2018). Arabidopsis non-TZF gene AtC3H17 functions as a positive regulator in salt stress response. Biochem. Biophys. Res. Commun. 498, 954–959. doi: 10.1016/j.bbrc.2018.03.088
Seok, H. Y., Woo, D. H., Park, H. Y., Lee, S. Y., Tran, H. T., Lee, E. H., et al. (2016). AtC3H17, a non-tandem CCCH zinc finger protein, functions as a nuclear transcriptional activator and has pleiotropic effects on vegetative development, flowering and seed development in arabidopsis. Plant Cell Physiol. 57, 603–615. doi: 10.1093/pcp/pcw013
Seong, S. Y., Shim, J. S., Bang, S. W., Kim, J. K. (2020). Overexpression of OsC3H10, a CCCH-zinc finger, improves drought tolerance inRice by regulating stress-related genes. Plant. 9, 1298. doi: 10.3390/plants9101298
Seo, M., Peeters, A. J., Koiwai, H., Oritani, T., Marion-Poll, A., Zeevaart, J. A., et al. (2000). The arabidopsis aldehyde oxidase 3 (AAO3) gene product catalyzes the final step in abscisic acid biosynthesis in leaves. Proceed. Natl. Acad. Sci. 97 (23), 12908–12913. doi: 10.1073/pnas.220426197
Shah, A. A., Riaz, L., Siddiqui, M. H., Nazar, R., Ahmed, S., Yasin, N. A., et al. (2022). Spermine-mediated polyamine metabolism enhances arsenic-stress tolerance in phaseolus vulgaris by expression of zinc-finger proteins related genes and modulation of mineral nutrient homeostasis and antioxidative system. Environ. pollut. 300, 118941. doi: 10.1016/j.envpol.2022.118941
Shaikhali, J., de Dios Barajas-Lopéz, J., Ötvös, K., Kremnev, D., Garcia, A. S., Srivastava, V., et al. (2012). The CRYPTOCHROME1-dependent response to excess light is mediated through the transcriptional activators zinc finger protein expressed in inflorescence meristem LIKE1 and ZML2 in arabidopsis. Plant Cell. 24 (7), 3009–3025. doi: 10.1105/tpc.112.100099
Shalmani, A., Muhammad, I., Sharif, R., Zhao, C., Ullah, U., Zhang, D., et al. (2019). Zinc finger-homeodomain genes: Evolution, functional differentiation, and expression profiling under flowering-related treatments and abiotic stresses in plants. Evol. Bioinform. 15, 1176934319867930. doi: 10.1177/1176934319867930
Shimberg, G. D., Michalek, J. L., Oluyadi, A. A., Rodrigues, A. V., Zucconi, B. E., Neu, H. M., et al. (2016). Cleavage and polyadenylation specificity factor 30: An RNA-binding zinc-finger protein with an unexpected 2Fe–2S cluster. Proceed. Nation Acad. Sci. 113 (17), 4700–4705. doi: 10.1073/pnas.1517620113
Shi, X., Wu, Y., Dai, T., Gu, Y., Wang, L., Qin, X., et al. (2018). JcZFP8, a C2H2 zinc finger protein gene from Jatrophacurcas, influences plant development in transgenic tobacco. Electronic. J. Biotechnol. 34, 76–82. doi: 10.1016/j.ejbt.2018.05.008
Singh, A., Kumar, A., Yadav, S., Singh, I. K. (2019). Reactive oxygen species-mediated signaling during abiotic stress. Plant Gene. 18, 100173. doi: 10.1016/j.plgene.2019.100173
Sinha, A. K., Jaggi, M., Raghuram, B., Tutejaand Tuteja, N. (2011). Mitogen-activated protein kinase signaling in plants under abiotic stress. Plant Signal. Behav. 6, 196–203. doi: 10.4161/psb.6.2.14701
Smekalova, V., Doskocilova, A., Komis, G., Samaj, J. (2014). Crosstalk between secondary messengers, hormones and MAPK modules during abiotic stress signalling in plants. Biotechnol. Adv. 32, 2–11. doi: 10.1016/j.biotechadv.2013.07.009
Steinhorst, L., Kudla, J. (2014). Signaling in cells and organisms – calcium holds the line. Curr. Opin. Plant Biol. 22, 14–21. doi: 10.1016/j.pbi.2014.08.003
Stohs, S. J., Bagchi, D. (1995). Oxidative mechanisms in the toxicity of metal ions. Free Radic. Biol. Med. 18, 321–336. doi: 10.1016/0891-5849(94)00159-H
Stone, S. L. (2019). Role of the ubiquitin proteasome system in plant response to abiotic stress. Int. Rev. Cell Mol. Biol. 343, 65–110. doi: 10.1016/bs.ircmb.2018.05.012
Ströher, E., Wang, X., Roloff, N., Klein, P., Husemann, A., Dietz, K. (2009). Redox-dependent regulation of the stress-induced zinc-finger protein SAP12 in arabidopsis thaliana. Mol. Plant 2 (2), 357–367. doi: 10.1093/mp/ssn084
Suh, J. Y., Kim, S. J., Oh, T. R., Cho, S. K., Yang, S. W., Kim, W. T. (2016). Arabidopsis tóxicos en levadura 78 (AtATL78) mediates ABA-dependent ROS signaling in response to drought stress. Biochem. Biophys. Res. Commun. 469, 8–14. doi: 10.1016/j.bbrc.2015.11.061
Sun, T., Zhou, F., Huang, X. Q., Chen, W. C., Kong, M. J., Zhou, C. F., et al. (2019). ORANGE represses chloroplast biogenesis in etiolated arabidopsis cotyledons via interaction with TCP14. Plant Cell. 31, 2996–3014. doi: 10.1105/tpc.18.00290
Suzuki, N., Koizumi, N., Sano, H. (2001). Screening of cadmium-responsive genes in arabidopsis thaliana: Screening of cadmium-responsive genes in arabidopsis thaliana. Plant Cell Environ. 24 (11), 1177–1188. doi: 10.1046/j.1365-3040.2001.00773.x
Swatek, K. N., Usher, J. L., Kueck, A. F., Gladkova, C., Mevissen, T. E., Pruneda, J. N., et al. (2019). Insights into ubiquitin chain architecture using ub-clipping. Nat. 572, 533–537. doi: 10.1038/s41586-019-1482-y
Takahashi, F., Kuromori, T., Sato, H., Shinozaki, K. (2018). Regulatory gene networks in drought stress responses and resistance in plants. Adv. Exp. Med. Biol. 1081, 189–214. doi: 10.1007/978-981-13-1244-1_11
Tattini, M., Landi, M., Brunetti, C., Giordano, C., Remorini, D., Gould, K. S., et al. (2014). Epidermal coumaroyl anthocyanins protect sweet basil against excess light stress: Multiple consequences of light attenuation. Physiol. Plant 152 (3), 585–598. doi: 10.1111/ppl.12201
Thorpe, G. W., Reodica, M., Davies, M. J., Heeren, G., Jarolim, S., Pillay, B., et al. (2013). Superoxide radicals have a protective role during H2O2 stress. Mol. Biol. Cell. 24, 2876–2884. doi: 10.1091/mbc.e13-01-0052
Tian, M., Lou, L., Liu, L., Yu, F., Zhao, Q., Zhang, H., et al. (2015). The RING finger E3 ligase STRF1 is involved in membrane trafficking and modulates salt-stress response in arabidopsis thaliana. Plant J. 82, 81–92. doi: 10.1111/tpj.12797
Tonnang, H. E., Sokame, B. M., Abdel-Rahman, E. M., Dubois, T. (2022). Measuring and modelling crop yield losses due to invasive insect pests under climate change. curr. opin. Insect Sci. 50, 100873. doi: 10.1016/j.cois.2022.100873
Tognetti, V. B., Van Aken, O., Morreel, K., Vandenbroucke, K., van de Cotte, B., De Clercq, I., et al. (2010). Perturbation of indole-3-butyric acid homeostasis by the UDPglucosyltransferase UGT74E2 modulates arabidopsis architecture and water stress tolerance. Plant Cell 22, 2660–2679. doi: 10.1105/tpc.109.071316
Tripathi, R. D., Tripathi, P., Dwivedi, S., Dubey, S., Chatterjee, S., Chakrabarty, D., et al. (2012). Arsenomics: Omics of arsenic metabolism in plants. Front. Physiol. 3. doi: 10.3389/fphys.2012.00275
Tsai, T. M., Huang, H. J. (2006). Effects of iron excess on cell viability and mitogen-activated protein kinase activation in rice roots. Physiol. Plant 127, 583–592. doi: 10.1111/j.1399-3054.2006.00696.x
Ullah, A., Nadeem, F., Nawaz, A., Siddique, K. H., Farooq, M. (2022). Heat stress effects on the reproductive physiology and yield of wheat. J. Agron. Crop Sci. 208 (1), 1–17. doi: 10.1111/jac.12572
Upadhyay, M. K., Majumdar, A., Srivastava, A. K., Bose, S., Suprasanna, P., Srivastava, S. (2022). Antioxidant enzymes and transporter genes mediate arsenic stress reduction in rice (Oryza sativa l.) upon thiourea supplementation. Chemosphere 292, 133482. doi: 10.1016/j.chemosphere.2021.133482
Vaičiukynė, M., Žiauka, J., Žūkienė, R., Vertelkaitė, L., Kuusienė, S. (2019). Abscisic acid promotes root system development in birch tissue culture: A comparison to aspen culture and conventional rooting-related growth regulators. Physiol. Plant 165 (1), 114–122. doi: 10.1111/ppl.12860
Vallee, B. L., Neurath, H. (1954). Carboxypeptidase, a zinc metalloprotein. J.Amer.Chem. Soc 76 (19), 5006–5007. doi: 10.1021/ja01648a088
Verma, N., Giri, S. K., Singh, G., Gill, R., Kumar, A. (2022). Epigenetic regulation of heat and cold stress responses in crop plants. Plant Gene. 29, 100351. doi: 10.1016/j.plgene.2022.100351
Wang, F., Tong, W., Zhu, H., Kong, W., Peng, R., Liu, Q., et al. (2016). A novel Cys2/His2 zinc finger protein gene from sweetpotato, IbZFP1, is involved in salt and drought tolerance in transgenic arabidopsis. Planta 243 (3), 783–797. doi: 10.1007/s00425-015-2443-9
Wang, K., Ding, Y., Cai, C., Chen, Z., Zhu, C. (2019). The role of C2H2 zinc finger proteins in plant responses to abiotic stresses. Physiol. Plant 165, 690–700. doi: 10.1111/ppl.12728
Wang, B., Fang, R., Chen, F., Han, J., Liu, Y., Chen, L., et al. (2020). A novel CCCH-type zinc-finger protein SAW1 activates OsGA20ox3to regulate gibberellin homeostasis and anther development in rice. J. Integr. Plant Biol. 62, 1594–1606. doi: 10.1111/jipb.12924
Wang, Y., Liao, Y., Quan, C., Li, Y., Yang, S., Ma, C., et al. (2022a). C2H2-type zinc finger OsZFP15 accelerates seed germination and confers salinity and drought tolerance of rice seedling through ABA catabolism. Environ. Expe. Bot. 199, 104873. doi: 10.1016/j.envexpbot.2022.104873
Wang, W., Liu, B., Xu, M., Jamil, M., Wang, G. (2015). ABA-induced CCCH tandem zinc finger protein OsC3H47 decreases ABA sensitivity and promotes drought tolerance in oryza sativa. Biochem. Biophy. Res. Commun. 464 (1), 33–37. doi: 10.1016/j.bbrc.2015.05.087
Wang, L., Xu, Y., Zhang, C., Ma, Q., Joo, S., Kim, S., et al. (2008). OsLIC, a novel CCCH-type zinc finger protein with transcription activation, mediates rice architecture via brassinosteroids signaling. PloS One 3, e3521. doi: 10.1371/journal.pone.0003521
Wang, D. R., Yang, K., Wang, X., You, C. X. (2022b). A C2H2-type zinc finger transcription factor, MdZAT17, acts as a positive regulator in response to salt stress. J. Plant Physiol. 275, 153737. doi: 10.1016/j.jplph.2022.153737
Wawrzynski, A., Kopera, E., Wawrzynska, A., Kaminska, J., Bal, W., Sirko, A. (2006). Effects of simultaneous expression of heterologous genes involved in phytochelatin biosynthesis on thiol content and cadmium accumulation in tobacco plants. J. Exp. Bot. 57, 2173–2182. doi: 10.1093/jxb/erj176
Wei, C. Q., Chien, C. W., Ai, L. F., Zhao, J., Zhang, Z., Li, K. H., et al. (2016). The arabidopsis b-box protein BZS1/BBX20 interacts with HY5 and mediates strigolactone regulation of photo morphogenesis. J. Genet. Genomics 43, 555–563. doi: 10.1016/j.jgg.2016.05.007
Wu, X. C., Cao, X. Y., Chen, M., Zhang, X. K., Liu, Y. N., Xu, Z. S., et al. (2010). Isolation and expression pattern assay of a C3HC4-type RING zinc finger protein gene GmRZFP1 in Glycine max (L.). J. Plant Genet. Res. 11, 343–348.
Xie, M., Sun, J., Gong, D., Kong, Y. (2019). The roles of arabidopsis C1-2i subclass of C2H2-type zinc-finger transcription factors. Genes 10, 653. doi: 10.3390/genes10090653
Xiong, L., Gong, Z., Rock, C. D., Subramanian, S., Guo, Y., Xu, W., et al. (2001). Modulation of abscisic acid signal transduction and biosynthesis by an Sm-like protein in arabidopsis. Dev. Cell. 1 (6), 771–781. doi: 10.1016/S1534-5807(01)00087-9
Xiong, L., Lee, H., Ishitani, M., Zhu, J. K. (2002). Regulation of osmotic stress-responsive gene expression by thelos6/aba1 locus inarabidopsis. J. Biol. Chem. 277 (10), 8588–8596. doi: 10.1074/jbc.M109275200
Xiong, L., Zhu, J. K. (2003). Regulation of abscisic acid biosynthesis. Plant Physiol. 133 (1), 29–36. doi: 10.1104/pp.103.025395
Xu, Z., Dong, M., Peng, X., Ku, W., Zhao, Y., Yang, G. (2019). New insight into the molecular basis of cadmium stress responses of wild paper mulberry plant by transcriptome analysis. Ecotoxicol. Environ. Safety. 171, 301–312. doi: 10.1016/j.ecoenv.2018.12.084
Xu, D. Q., Huang, J., Guo, S. Q., Yang, X., Bao, Y. M., Tang, H. J., et al. (2008). Overexpression of a TFIIIA-type zinc finger protein gene ZFP252 enhances drought and salt tolerance in rice (Oryza sativa l.). FEBS Lett. 582 (7), 1037–1043. doi: 10.1016/j.febslet.2008.02.052
Xu, L., Liu, T., Xiong, X., Liu, W., Yu, Y., Cao, J. (2020). AtC3H18L is a stop-codon read-through gene and encodes a novel non-tandem CCCH zinc-finger protein that can form cytoplasmic foci similar to mRNP granules. Biochem. Biophys. Res. Commun. 528, 140–145. doi: 10.1016/j.bbrc.2020.05.081
Xu, X., Li, W., Yang, S., Zhu, X., Sun, H., Li, F., et al. (2022). Identification, evolution, expression and protein interaction analysis of genes encoding b-box zinc-finger proteins in maize. J. Integr. Agric. 8, 91. doi: 10.1016/j.jia.2022.08.091
Yaghoubian, I., Ghassemi, S., Nazari, M., Raei, Y., Smith, D. L. (2021). Response of physiological traits, antioxidant enzymes and nutrient uptake of soybean to azotobacter chroococcum and zinc sulfate under salinity. South Afr. J. Bot. 143, 42–51. doi: 10.1016/j.sajb.2021.07.037
Yang, Y., Ma, C., Xu, Y., Wei, Q., Imtiaz, M., Lan, H., et al. (2014). A zinc finger protein regulates flowering time and abiotic stress tolerance in chrysanthemum by modulating gibberellin biosynthesis. Plant Cell 26 (5), 2038–2054.
Yang, Z., Chuand Chu, C. (2011). “Towards understanding plant response to heavy metal stress,” in Abiotic stress in plants - mechanisms and adaptations (InTech.), 59–78. doi: 10.5772/24204
Yang, K., Li, C. Y., An, J. P., Wang, D. R., Wang, X., Wang, C. K., et al. (2021). The C2H2-type zinc finger transcription factor MdZAT10 negatively regulates drought tolerance in apple. Plant Physiol. Biochem. 167, 390–399. doi: 10.1016/j.plaphy.2021.08.014
Yan, Z., Shi, H., Liu, Y., Jing, M., Han, Y. (2020). KHZ1 and KHZ2, novel members of the autonomous pathway, repress the splicing efficiency of FLC pre-mRNA in arabidopsis. J. Exp. Bot. 71 (4), 1375–1386. doi: 10.1093/jxb/erz499
Yin, K., Lv, M., Wang, Q., Wu, Y., Liao, C., Zhang, W., et al. (2016). Simultaneous bioremediation and biodetectionbio-detection of mercury ion through surface display of carboxylesterase E2 from pseudomonas aeruginosa PA1. Water Res. 103, 383–390. doi: 10.1016/j.watres.2016.07.053
Yin, J., Wang, L., Zhao, J., Li, Y., Huang, R., Jiang, X., et al. (2020). Genome-wide characterization of the C2H2 zinc-finger genes in Cucumis sativus and functional analyses of four CsZFPs in response to stresses. BMC Plant Biol. 20 (1), 1–22. doi: 10.1186/s12870-020-02575-1
Yu, G.-H., Jiang, L.-L., Ma, X.-F., Xu, Z.-S., Liu, M.-M., Shan, S.-G., et al. (2014). A soybean C2H2-type zinc finger gene GmZF1 enhanced cold tolerance in transgenic arabidopsis. PloS One 9 (10), e109399. doi: 10.1371/journal.pone.0109399
Yuan, J., Chen, D., Ren, Y., Zhang, X., Zhao, J. (2008). Characteristic and expression analysis of ACCEPTED MANUSCRIPT a metallothionein gene, OsMT2b, down-regulated by cytokinin suggests functions in root development and seed embryo germination of rice. Plant Physiol. 146, 1637–1650. doi: 10.1104/pp.107.110304
Yuan, S., Li, X., Li, R., Wang, L., Zhang, C., Chen, L., et al. (2018). Genome-wide identification and classification of soybean C2H2 zinc finger proteins and their expression analysis in legume-rhizobium symbiosis. Front. Microbiol. 9, 126. doi: 10.3389/fmicb.2018.00126
Yuce, K., Ozkan, A. I. (2020). “Cys2His2 zinc finger proteins boost survival ability of plants against stress conditions,” in Plant stress physiology (London: Intech Open). doi: 10.5772/intechopen.92590
Yu, L. X., Shen, X., Setter, T. (2015). L. molecular and functional characterization of two drought-induced zinc finger proteins, ZmZnF1 and ZmZnF2 from maize kernels. Environ. Expe. Bot. 111, 13–20. doi: 10.1016/j.envexpbot.2014.10.004
Zang, D., Li, H., Xu, H., Zhang, W., Zhang, Y., Shi, X., et al. (2016). An arabidopsis zinc finger protein increases abiotic stress tolerance by regulating sodium and potassium homeostasis, reactive oxygen species scavenging and osmotic potential. Front. Plant Sci. 7. doi: 10.3389/fpls.2016.01272
Zeng, D.-E., Hou, P., Xiao, F., Liu, Y. (2015). Overexpression of arabidopsis XERICO gene confers enhanced drought and salt stress tolerance in rice (Oryza sativa l.). J. Plant Biochem. Biotechnol. 24 (1), 56–64. doi: 10.1007/s13562-013-0236-4
Zhang, C., Hou, Y., Hao, Q., Chen, H., Chen, L., Yuan, S., et al. (2015). Genome-wide survey of the soybean GATA transcription factor gene family and expression analysis under low nitrogen stress. PloS One 10, e0125174. doi: 10.1371/journal.pone.0125174
Zhang, Y., Lan, H., Shao, Q., Wang, R., Chen, H., Tang, H., et al. (2016). An A20/AN1-type zinc finger protein modulates gibberellins and abscisic acid contents and increases sensitivity to abiotic stress in rice (Oryza sativa). J. Exp. Bot. 67 (1, January), 315–326. doi: 10.1093/jxb/erv464
Zhang, X., Shang, F., Huai, J., Xu, G., Tang, W., Jing, Y., et al. (2017). A PIF1/PIF3-HY5-BBX23 transcription factor cascade affects photo morphogenesis. Plant Physiol. 174 (4), 2487–2500. doi: 10.1104/pp.17.00418
Zhang, T., Wang, X., Lu, Y., Cai, X., Ye, Z., Zhang, J. (2014). Genome-wide analysis of the cyclin gene family in tomato. Int. J. Mol. Sci. 15 (1), 120–140. doi: 10.3390/ijms15010120
Zhang, D., Xu, Z., Cao, S., Chen, K., Li, S., Liu, X., et al. (2018). An uncanonical CCCH-tandem zinc-finger protein represses secondary wall synthesis and controls mechanical strength in rice. Mol. Plant 11, 163–174. doi: 10.1016/j.molp.2017.11.004
Zhang, W., Yin, K., Li, B., Chen, L. (2013). A glutathione s-transferase from Proteus mirabilis involved in heavy metal resistance and its potential application in removal of Hg2+. J. Hazard Mater. 261, 646–652. doi: 10.1016/j.jhazmat.2013.08.023
Zhang, H., Sun, Z., Feng, S., Zhang, J., Zhang, F., Wang, W., et al. (2022). The C2H2-type zinc finger protein PhZFP1 regulates cold stress tolerance by modulating galactinol synthesis in petunia hybrida. J. Exp. Bot. 73 (18), 6434–6448. doi: 10.1093/jxb/erac274
Zou, X., Seemann, J. R., Neuman, D., Shen, Q. J. (2004). A WRKY gene from creosote bush encodes an activator of the abscisic acid signaling pathway. J. Biol. Chem. 279 (53), 55770–55779. doi: 10.1074/jbc.M408536200
Keywords: abiotic stresses, Zn-finger proteins, mechanisms, plants, gene signalling ABA - abscisic acid
Citation: Moulick D, Bhutia KL, Sarkar S, Roy A, Mishra UN, Pramanick B, Maitra S, Shankar T, Hazra S, Skalicky M, Brestic M, Barek V and Hossain A (2023) The intertwining of Zn-finger motifs and abiotic stress tolerance in plants: Current status and future prospects. Front. Plant Sci. 13:1083960. doi: 10.3389/fpls.2022.1083960
Received: 29 October 2022; Accepted: 22 November 2022;
Published: 04 January 2023.
Edited by:
Poonam Yadav, Institute of Environment and Sustainable Development, Banaras Hindu University, IndiaReviewed by:
Munish Kumar Upadhyay, Indian Institute of Technology Kanpur, IndiaCopyright © 2023 Moulick, Bhutia, Sarkar, Roy, Mishra, Pramanick, Maitra, Shankar, Hazra, Skalicky, Brestic, Barek and Hossain. This is an open-access article distributed under the terms of the Creative Commons Attribution License (CC BY). The use, distribution or reproduction in other forums is permitted, provided the original author(s) and the copyright owner(s) are credited and that the original publication in this journal is cited, in accordance with accepted academic practice. No use, distribution or reproduction is permitted which does not comply with these terms.
*Correspondence: Viliam Barek, dmlsaWFtLmJhcmVrQHVuaWFnLnNr; Akbar Hossain, YWtiYXIuaG9zc2FpbkBid21yaS5nb3YuYmQ=
Disclaimer: All claims expressed in this article are solely those of the authors and do not necessarily represent those of their affiliated organizations, or those of the publisher, the editors and the reviewers. Any product that may be evaluated in this article or claim that may be made by its manufacturer is not guaranteed or endorsed by the publisher.
Research integrity at Frontiers
Learn more about the work of our research integrity team to safeguard the quality of each article we publish.