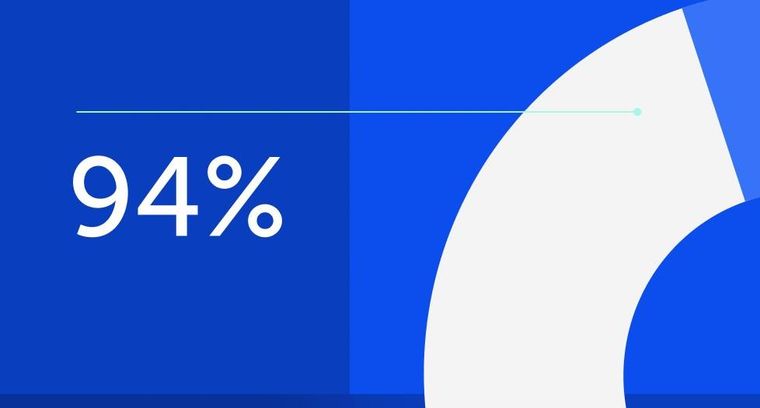
94% of researchers rate our articles as excellent or good
Learn more about the work of our research integrity team to safeguard the quality of each article we publish.
Find out more
ORIGINAL RESEARCH article
Front. Plant Sci., 12 December 2022
Sec. Sustainable and Intelligent Phytoprotection
Volume 13 - 2022 | https://doi.org/10.3389/fpls.2022.1083848
This article is part of the Research TopicAphids as Plant Pests: From Biology to Green Control TechnologyView all 11 articles
Banker plant systems increase biological pest control by supporting populations of natural enemies, i.e., using non-pest arthropod species as alternative prey. However, the presence of alternative prey does not always result in improved control of the target pest species owing to the complexity of biotic interactions. To increase the effectiveness of banker plants in IPM programs, a fine understanding of the indirect interactions between target aphid and alternative prey mediated by biocontrol agents is necessary. In this study, we first established a banker plant system, banker plant (Vicia faba)–alternative prey (Megoura japonica)–predator (Harmonia axyridis), to control the target pest (Myzus persicae) on pepper. We found that M. japonica strongly preferred faba bean as a host plant and posed no risk to Solanaceous crops. Harmonia axyridis adults had no significant predation preference for the alternative prey. In the short term, the interaction direction of the two aphid species depended on the relative initial density and the timescale. Harmonia axyridis showed a stronger negative effect on M. persicae than that on M. japonica. In the long term, the presence of alternative prey, M. japonica, enhanced the control effect of H. axyridis to M. persicae with initial density of 100–500 aphids per plant. The presence of the alternative prey could proliferate the population of H. axyridis, with from 0.2- to 2.1-fold increase of H. axyridis eggs. Overall, we put forward a strategy for setting the initial density of alternative prey of the banker plant system to target the high and low density of aphids, which highlighted the importance of indirect interactions in designing a proper banker plant system.
Conservation biological control (CBC) could enhance the survival, longevity, and fecundity of natural enemies by habitat management to increase their effectiveness in pest control (Gurr et al., 2017). The key to an effective CBC is an early colonization and establishment of natural enemies in crops, when pest populations are still at low densities (Symondson et al., 2002). Different types of functional plants have been proposed to support natural enemies, increasing the efficiency and sustainability of biological control of pests (Xie et al., 2012; Zhao et al., 2017; Hatt et al., 2018; Hatt et al., 2019b; Damien et al., 2020). As an important form of CBC, banker plant system could preserve populations of beneficial arthropods in crops by providing alternative prey/hosts and could provide an on-farm refuge for spontaneous populations for sustainably effective pest control (Frank, 2010; Huang et al., 2011; Li et al., 2015). The use of banker plant systems has been increasingly investigated and developed for greenhouse and field crops (Zheng et al., 2017; Xu et al., 2020; Chen et al., 2022). However, research studies mainly focus on the establishment of the banker plant system (Wang et al., 2020; Wang et al., 2021), and further research is needed to understand the quantitative relationship among trophies when the banker plant system applied to pest control (Li et al., 2020).
The population dynamic of species in ecosystem not only depends on the direct feeding behavior in food web (Symondson et al., 2002) but also on the indirect interaction among species (Montoya et al., 2009; Zhang et al., 2019). In agroecosystem, preys without direct resource competition could have indirect interaction mediated by shared natural enemies (van Veen et al., 2006; Frost et al., 2016; Holt and Bonsall, 2017). These interactions affect the predator’s predation in the short term (Desneux and O’Neil, 2008; Jaworski et al., 2013) and even direct the predator and prey population dynamics in the long term (e.g., apparent competition, Holt, 1977; Jaworski et al., 2015; Desneux et al., 2019). Understanding how pests, alternative prey, and natural enemy interact in complex managed environments is essential to the pest management in agriculture (Chailleux et al., 2014). When the banker plant system is used to control the target pests in the field, there is no direct resource competition between the alternative prey and the target pests. Whether the indirect interaction mediated by the shared natural enemies affects the population dynamics is still uncovered.
Depending on the temporal or spatial scale, the behavior of prey, and the quality and density of prey, the natural enemy–mediated indirect interactions can take different forms, such as apparent competition, mutualism, amensalism, and commensalism (Chaneton and Bonsall, 2000; Chailleux et al., 2014). Natural enemy–mediated indirect interactions contribute to pest population dynamics, and insufficient efforts have been made to generate predictions that would facilitate the use of indirect interactions in biological control in different spatial scales (Chailleux et al., 2014). On a large scale, maintaining a high level of biodiversity has been proved to promote the growth of natural enemies of pests and improve the control of target pests (Karp et al., 2018). Small-scale experiments show that natural enemies benefit from mixed food by alternative prey (Liu et al., 2006; Messelink et al., 2008). Many studies have also shown that alternative prey/food can help natural enemy populations establish in crops before pest arrival (Gurr et al., 2016; Jaworski et al., 2019; Sanchez et al., 2021). Whereas alternative prey could induce a decrease in pest densities in crops through indirect interactions with a shared natural enemy (i.e., apparent competition) (Liu et al., 2006; Desneux and O’Neil, 2008), generalist predators are widely used as biocontrol agents to regulate populations of pest in agriculture (Symondson et al., 2002; van Lenteren, 2012). When the alternative prey is applied to pest biological control programs, it is necessary to clarify the indirect interactions induced by the generalist predators (Chailleux et al., 2014; Costa and Anjos, 2020).
This study aims to identify the quantitative relationship among trophies when the banker plant system applied to pest control. Targeting the widely distributed and important pest of protected vegetables, Myzus persicae (Aparicio et al., 2020; Wang et al., 2022), we explored the interactions when the Harmonia axyridis banker plant system was applied to M. persicae control. We hypothesized that (1) Harmonia axyridis adults do not show predation preference for the two aphid species; (2) the direction, sign, and strength of the indirect interactions mediated by shared predator between the two aphid species depends on the relative initial density and time; (3) the alternative prey, M. japonica, preserve populations of H. axyridis and provide sustainably effective pest control in caged study.
Initial colonies of the green peach aphid (Myzus persicae), the bean aphid (Megoura japonica), and the harlequin ladybird (Harmonia axyridis) were collected from Beijing Noah Organic Farm (116°59′E, 40°6′N), Beijing, China. The aphids, M. persicae, was reared on Capsicum annuum var. Zhongjiao 105 (Institute of Vegetables and Flowers, CAAS), and M. japonica was reared on Vicia faba var. Kexi (Sichuan Kexi Seed Co., Ltd.). The ladybeetle H. axyridis was reared in cages (35 × 35 × 55 cm) made of nylon mesh net and aluminum alloy frames. They were fed ad libitum with M. japonica reared on faba bean plants. All colonies were kept at 25 ± 2°C, 60 ± 5% relative humidity (RH), 16-hour light: 8-h dark (L16:D8-h) photoperiod in the insectary of the Institute of Plant Protection, Beijing Academy of Agriculture and Forestry Sciences (BAAFS). The culture environment was regulated using an automatic environmental management system (LT-100, Suntech, Beijing, China).
The tomato, eggplant, and pepper seedlings were grown in plastic trays (54 × 28 × 6 cm, 21 plants per tray) and transplanted individually in plastic pots once they reached 15 cm in height. All plants were cultured individually in plastic pots (H = 25 cm, D = 15 cm) with commercial substrate (Pindstrup®) at 25 ± 2°C, 60 ± 5% RH, and L16:D8-h photoperiod. The tomato, eggplant, and pepper plants reaching 30–35 cm in height with five to seven fully expanded true leaves, and faba bean plants with five to seven fully expanded true leaves were used for experiments.
Newborn nymph (<12 h) of Megoura japonica were placed individually on the back of faba bean leaves in plastic Petri dish (H = 1.5 cm, D = 5 cm). The leaves bases were covered with absorbent cotton to moisturize. Dishes were kept in the laboratory at 25 ± 2°C, 60 ± 5% RH, and L16:D8 h. The leaves were replaced every 2 days. Development and survival data were recorded daily. The presence of an exuvium was used as the criterion for molting to the next developmental stage. After the emergence of adults, the number of newborn nymphs was recorded and the nymphs were removed from the dishes daily until the death of the adult. One hundred newborn nymphs were used for the life table study (n = 100).
A choice experiment was conducted to evaluate the host specificity of M. japonica to the faba bean and three Solanaceous vegetables (tomato, eggplant, and pepper). One 2.5-cm (in diameter) leaf disc of each plant was placed on the wet filter paper with an equal distance between them to form a square in a plastic Petri dish (H = 1.5 cm, D = 9 cm). Thirty third-instar nymphs of M. japonica were released onto the center of the filter paper. Dishes were kept in the laboratory at 25 ± 2°C, 60 ± 5% RH, and L16:D8 h. The number of M. japonica on each leaf disc (treatment) was counted at 3, 6, 12, and 24 h after the release of aphids into the Petri dish. The position of different leaf discs was determined randomly and rotated in each replication. The experiment was a randomized complete block design and replicated 16 times (n = 16).
A survival experiment was conducted to determine survival or development of M. japonica on different host plants (faba bean, tomato, eggplant, and pepper). Ten fourth-instar nymphs of M. japonica were introduced to the head of the potted plant with five to seven fully expanded true leaves. Each plant was placed randomly at an interval of 3 m in a greenhouse. The greenhouse was maintained at 26 ± 2°C, 70 ± 10% RH, and L16:D8 h. The number of M. japonica that survived on each plant of different host plants was recorded at 24, 48, 72, and 96 h. Each treatment (host plant) was replicated 10 times (n = 10).
On the basis of the daily predation of H. axyridis adults on M. persicae and M. japonica, we set five levels of prey complex. The total number of preys per dish remained constant at 180. The ratios tested of M. persicae and M. japonica were 180:0, 120:60, 90:90, 60:120, and 0:180. In each experimental unit, third-instar nymphs of M. persicae and M. japonica were randomly chosen from the stock colony reared in the laboratory and carefully transferred onto a Petri dish (9 cm in diameter) using a fine brush for 1 h of acclimatization, after which one newly hatched (< 24 h) adult of H. axyridis was added to the center of the dish. Male and female H. axyridis were tested separately. All adults suffered starving for 24 h before being used in preference experiments. The experiments were conducted in the laboratory (25 ± 1°C, 65 ± 5% RH, and L16:D8 h). After 24 h, each prey type consumed by the predator was counted. Ten replicates were prepared for each prey complex (n = 10).
In greenhouse, we studied the dynamic relationship, interaction direction, sign, and strength of the predator (H. axyridis), alternative prey (M. japonica), and target pest (M. persicae) when banker plant system applied to M. persicae control using a 2 x 2 factorial design (Figure 1). The first three-level treatment varied the alternative prey density (0, 200, and 400 per plant). The second three-level treatment varied the target pest density (100, 300, and 500 per plant) in the microcosms. One potted pepper and faba bean were set oppositely in a microcosm (35 × 35 × 55 cm). Third-instar nymphs of M. persicae and M. japonica were randomly chosen from the colonies reared in the laboratory and released on the pepper and faba bean plant, respectively. After 1 day of acclimatization, one pair newly hatched (<24 h) adults of H. axyridis was released. Population dynamics of the three insect species were monitored 39 days following the introduction of predators. Adults and nymphs of H. axyridis, M. persicae, and M. japonica were counted on all leaves every 3 days. Plants were fertilized and watered to avoid any abiotic stress. Each treatment was repeated 15 times (n = 15).
Figure 1 The scheme of the interaction of the predator (Harmonia axyridis), alternative prey (Megoura japonica), and target pest (Myzus persicae) when the banker plant system applied to biological aphid control on pepper.
According to Özgökçe et al. (2018), the life table raw data were analyzed using the computer program TWOSEX-MSChart (Chi, 2017b) based on the age-stage, two-sex life table theory (Chi and Liu, 1985; Chi, 1988). The variances and standard errors of the developmental time, fecundity, longevity, and population parameters were estimated using the bootstrap technique (Efron and Tibshirani, 1993) with 100,000 resampling. The bootstrap routine is embedded in the TWOSEX program. The difference between treatments was examined by using paired bootstrap test (Efron and Tibshirani, 1993).
The data obtained from the host specificity experiments did not follow normal distribution (Shapiro–Wilk test, P< 0.05) and/or homoscedasticity (Bartlett’s test, P< 0.05), and Friedman test and Kruskal–Wallis test was used to compare the differences among treatments for the choice rate of M. japonica on different plants in laboratory choice experiments and number of M. japonica colonizing on different plants in greenhouse, respectively.
Manly’s model was used to estimate the predation preference of H. axyridis to M. japonica and M. persicae at different prey ratios. Manly (1973) equation:
Ai was the number of individuals of a given prey type i available for predation by H. axyridis (= total number of prey available for predation) and ri was the number of a prey type i that has not been attacked. The number of prey types was n = 2 and βj = 1/n when prey was chosen randomly.
The Wilcoxon signed-rank test of two related samples in non-parametric test was used to compare the predation preference of H. axyridis, and the Mann–Whitney U-test of two independent samples was used to compare the difference in predation preference between male and female adults (P< 0.05).
To differentiate long-term from short-term interactions between preys, the data were divided into two time periods. Short-term interactions were assessed on days 0–9 and long-term interactions on days until the end of experiments according to the predation pressure in the system.
Log response ratios (RRs) were used separately for each aphid species as a measure of the strength of the negative impact of predation, and mean ratios of the log abundance of the two aphid species were used as a measure of the symmetry of the impact of predation on each aphid species (Gelman and Hill, 2007). The impacts of predation at each treatment level were compared with the control (no alternative prey, M. japonica). Log RRs was also used to estimate the log-proportional difference between the mean of a particular treatment level and that of a control (Hedges et al., 1999). For each experiment, the mean ratios of log abundance at each treatment level of different sampling days were tested for significant differences from the control without alternative prey using t-test.
A delta correction (RRΔ) and its variance [var (RRΔ)] were used on the basis of the standard deviation (SD), sample size (N), and mean (X) of the treatment (T) and control (C) following Lajeunesse (2015):
As the ladybird abundance data did not follow normal distribution (Shapiro–Wilk test, P< 0.05) and/or homoscedasticity (Bartlett’s test, P< 0.05), Kruskal–Wallis test was used to compare the differences among treatments. All statistical analyses were performed using SPSS 25.0 (SPSS Inc., Chicago, IL, USA).
The developmental times, longevity, reproductive periods, and fecundity of M. japonica on faba bean are shown in Table 1. The developmental time periods of the pre-adult and adult stage were 5.63 ± 0.08 days and 15.03 ± 0.95 days, respectively. The longevity and reproductive periods were 19.02 ± 0.99 days and 9.14 ± 0.53 days, respectively. The fecundity was 44.09 ± 3.34 offspring (Table 1).
Megoura japonica significantly preferred faba bean leaf disc in the laboratory choice experiments (3H: X2 = 39.146, P< 0.001; 6H: X2 = 33.064, P< 0.001; 12H: X2 = 39.992, P< 0.001; 24H: X2 = 30.157, P< 0.001). The number of M. japonica chose faba bean increased over time, whereas the number of M. japonica on eggplant, pepper, and tomato decreased rapidly over time (Figure 2A). Megoura japonica developed better on the faba bean plants than that on the Solanaceae crops. The population size of the bean aphid on faba bean plants reached the 3.5 times of the initial value after 96 h, whereas the population size on eggplant, pepper, and tomato decreased significantly (24H: X2 = 29.431, P< 0.001; 48H: X2 = 33.056, P< 0.001; 72H: X2 = 37.981, P< 0.001; 96H: X2 = 37.956, P< 0.001). Although the number of M. japonica on tomato plants was significantly higher than that on eggplant and pepper at 24 h, they almost went extinction on eggplant, pepper, and tomato at 72 h, indicating that those plants were unsuitable hosts for M. japonica (Figure 2B).
Figure 2 The choice rate of Megoura japonica on different plants over time in laboratory choice experiments (A) and mean (± SE) of Megoura japonica colonizing on different plants in greenhouse (B). Means capped with different letters in the same sampling time are significantly different (P < 0.05; Friedman test for choice rate and Kruskal–Wallis test for mean of M. japonica) among treatments.
Predation preference of H. axyridis strongly depended on prey relative abundance with a disproportionately high predation on the most abundant prey (Figures 3A, C). On the basis of the analyses of Manly’s Beta values (βj), predation preference did not change across various M. persicae and M. japonica relative prey ratios (Figures 3B, D). Female H. axyridis consumed a slightly higher number of prey than male, but Manly’s Beta values were no significant difference between genders (60:120 M. persicae:M. japonica, P = 0.560; 90:90 M. persicae:M. japonica, P = 0.845; 120:60 M. persicae:M. japonica, P = 0.46).
Figure 3 Mean (± SE) of consumed Myzus persicae and Megoura japonica, predation preference of female (A, B) and male (C, D) Harmonia axyridis in various prey complex. Wilcoxon signed-rank test of two related samples in non-parametric test was used to compare the predation preference of H. axyrdis.
When the initial density of M. persicae is low (100 aphids per plant) (i.e., the initial density of M. persicae is less than that of M. japonica.), the density of M. persicae is significantly different at the same time (X2 = 22.182, P< 0.001) but no interaction between time (X2 = 2.431, P = 0.051). The density of M. persicae was significantly higher than that of the control on the sixth and ninth days (6 days: X2 = 25.068, P< 0.001; 9 days: X2 = 24.227, P< 0.001; Figure 4A). When the initial density of M. persicae is medium (300 aphids per plant), the density of M. persicae among treatments is significantly different at the same time (X2 = 6.357, P = 0.002). In addition, the density of M. persicae was significantly higher than that of the control on the ninth day (3 days: X2 = 2.638, P = 0.267; 6 days: X2 = 5.439, P = 0.066; 9 days: X2 = 6.145, P = 0.046; Figure 4B). With higher initial density of M. persicae (500 aphids per plant), no significant difference was found among treatments (X2 = 0.392, P = 0.676) and sampling times (3 days: X2 = 0.383, P = 0.826; 6 days: X2 = 0.127, P = 0.939; 9 days: X2 = 1.127, P = 0.569; Figure 4C).
Figure 4 Population abundance of Myzus persicae (Mp) in different treatments with the initial density of M. persicae is 100 (A), 300 (B), and 500 (C). Mj, Megoura japonica. Different letters next to the curves indicate significant differences among treatments (P<0.05).
On the basis of the density of M. persicae in control without alternative prey, the effect of H. axyridis on M. persicae varied from positive to negative with the increase of the initial density of M. persicae, and the positive effect increased as the initial density of the alternative prey, M. japonica (Figure 5). The negative impact of predation for M. persicae weakened with time at low initial density (Figure 5A). At intermediate and higher initial density, the negative impact of predation on the target prey M. persicae weakened with time and initial density of alternative prey (Figures 5B, C).
Figure 5 Relationships among the relative abundance of Myzus persicae (Mp) and Megoura japonica (Mj) in different treatments for log response ratios with the initial density of M. persicae is 100 (A), 300 (B), and 500 (C), and sampling after 3 (D), 6 (E), and 9 (F) days.
The log abundance of M. persicae and M. japonica was significantly different among treatments (Figures 5D–F). On day 3, the mean ratio of treatments with higher initial density of M. persicae (500:200 M. persicae:M. japonica and 500:400 M. persicae:M. japonica) were marginally greater than 1 (Figures 5D–F). Whereas the treatments with higher initial density of M. persicae on day 3 were significantly greater than that of the control (100:200 M. persicae:M. japonica, P< 0.000; 100:400 M. persicae:M. japonica, P< 0.001; Figure 5D). With the increase of time, the mean ratio of the log abundance of M. persicae and M. japonica was lower than 1 (Figures 5D–F). This indicated that different initial density of alternative prey had a more significant negative impact on M. persicae than that on M. japonica. Moreover, the treatments with lower initial density of M. persicae on days 6 and 9 were significantly greater than that of the control (Figures 5D–F). The mean ratio of treatment (300:200 M. persicae:M. japonica, 0.73 ± 0.01) on day 9 was significantly lower than that of the control (0.98 ± 0.02, t = −2.45, P = 0.01).
Overall, a higher number of M. persicae were registered throughout the duration of the experiment with than that without alternative prey (Figure 6). At low initial density, the population of M. persicae in control decreased and subsided on the 18th day. Whereas the population of M. persicae in treatments with alternative prey increased and then decreased, reaching the peak on the ninth day, which was 1.7 times of the initial density (Figure 6A). The population of M. persicae in control at intermediate and higher initial density decreased and subsided before the treatments with M. japonica (Figures 6B, C).
Figure 6 Population abundance of Myzus persicae (Mp) in different treatments through the whole term with the initial density of M. persicae is 100 (A), 300 (B), and 500 (C). Mj, Megoura japonica.
Harmonia axyridis was sustained by the alternative prey and varied with different initial density in the system (Figure S1). The predator egg abundance was positively related to the total initial density of aphids (F= 42.893, df =11,133, P< 0.001; Figure S2A). In addition, the total initial density of aphids positively affected the hatching rate and net reproductive rate of H. axyridis egg (F = 7.673, df =11,133, P=0.006; Figure S2B; F= 31.824, df =11,133, P< 0.001; Figure S2C).
The banker plant system, integrating the essence of augmentative and CBC, has been widely used to aid establishment, development, and dispersal of beneficials employed in pest biological control (Parolin et al., 2012; Miller et al., 2018; Wang et al., 2021; Ardanuy et al., 2022). However, the size of the founder population of the banker plant system has received little attention despite their importance in biological control efficacy and adoption (Sanchez et al., 2021). In this study, we explored the dynamic relationship and interaction degree of the predator (H. axyridis), alternative prey (M. japonica), and target pest (M. persicae). We found that the interaction direction of the two aphids depends on the relative initial density and time. Harmonia axyridis showed a stronger negative effect on M. persicae than that on M. japonica. Megoura japonica with different initial densities had different effects on the proliferation of H. axyridis. During the whole experimental period, the presence of alternative prey, M. japonica, enhanced the control effect of H. axyridis to M. persicae with initial density of 100–500 aphids per plant.
Alternative prey generally has been shown to enhance predator densities and to have a negative effect on the population of another prey species, resulting in increased biocontrol services on target pests in agroecosystems (Desneux and O’Neil, 2008; Eubanks and Finke, 2014; Ramirez and Eubanks, 2016; Wang et al., 2021). When alternative prey and target pests co-existed, the spatial location of the two prey species may reduce the efficiency of indirect interactions, especially likely when the alternative prey is provided by using mulch or banker plants (Chailleux et al., 2014). Therefore, understanding how alternative prey and beneficials interact when banker plant was used is essential for pest management programs. On the timescale of this study, the interaction direction of the two aphids is related to the relative initial density and time. Harmonia axyridis showed a stronger negative effect on M. persicae than that on M. japonica. However, the highest hatching rate of H. axyridis eggs is 26.12%, which indicates that intraguild predation (IGP) may occur in the system. Previous experience of cannibalism did not increase further cannibalism frequency of H. axyridis (Ovchinnikov et al., 2019). Some studies have shown that intraspecific predation would negatively affect the predator numerical response, resulting in different forms indirect interactions (Holt and Lawton, 1994; Liu et al., 2006). Conversely, supplementing non-prey food resources, such as floral resources (e.g., marigold), could reduce IGP of H. axyridis in agroecosystems (Liang et al., 2022).
Predators could affect the colonization and abundance of a single prey by direct predation (i.e., consumptive effects) and by altering prey activity, behavior, and development (i.e., non-consumptive effects) (Orrock et al., 2008). Moreover, predator’s habit (e.g., predation preference) could also modify the strength, the direction, and the symmetry of indirect interactions among preys (Holt and Bonsall, 2017). If the predator has no preference, then the indirect interaction mediated by shared predator among preys would change from negative to positive due to the predator satisfaction or prey switching (Abrams and Matsuda, 1996; Jaworski et al., 2015). As predator preference leads to apparent competition, a critical first step was to establish if there was predator preference between the two prey species (Jaworski et al., 2013; Emery and Mills, 2020). Our results indicated that H. axyridis adults show no preference for the two aphid species when their densities varied in a short period (i.e., 24 h).
The RRs could quantify the effect size of predation prey individually in proportion to their abundance in the controls. This also served as an initial indicator of potential asymmetrical effects between the two aphid species (Desneux et al., 2019; Emery and Mills, 2020). We found that the interaction direction and intensity of H. axyridis predation on the two aphids in the system were related to the relative initial density of the two aphid species and timescales. The negative effect of H. axyridis on M. persicae was stronger than that of M. japonica in the short term (9 days). In addition, consistent with the results of the study by Abrams and Matsuda (1996), we found that the interaction direction between the two aphid species changed with different initial density ratio. The t-test of the mean ratios of the log abundance of two preys could be used to test for asymmetry in comparison with the mean ratios of the control treatments (Emery and Mills, 2020). Nevertheless, we used the t-test with control without alternative prey and found that the effect of H. axyridis on M. persicae changed from positive to negative with the increase of the initial density of M. persicae, and the positive effect increased with the increase of the initial density of M. japonica.
The asymmetric interaction between the two aphids mediated by H. axyridis may be related to the predation rate on different aphids or the asymmetric non-consumption effect of prey (Emery and Mills, 2020). We found that, although the initial density of M. persicae is high, the alternative prey generally trended to upward and the M. persicae shows a downward trend in the long term. Although H. axyridis adults did not show predation preference in Petri dishes, we do not take in consideration the effect of living plants on predation preference. Studies have shown that plant morphology impacts the activity and prey availability of predatory ladybugs (Grevstad and Klepetka, 1992). According to the experimental observation, Myzus persicae is the apical meristem scattered in pepper plants, whereas M. japonica are evenly distributed in faba bean plants, which may lead to differences in predation on different aphids, causing the asymmetric effect of H. axyridis impact on two aphids. Although the literature on short-term interaction has received less attention, it may have negative effects on biological control (Bompard et al., 2013; Chailleux et al., 2014; Blubaugh et al., 2018; Emery and Mills, 2020). Our study confirmed that the existence of alternative prey did not affect the control effect of H. axyridis on M. persicae.
Studies on indirect interaction mediated by shared natural enemies mainly focused on two pests on one plant (Liu et al., 2006; Bompard et al., 2013; Jaworski et al., 2015), leading to a hard discrimination between indirect interactions and resource competition (Bompard et al., 2013). Noonburg and Byers (2005) showed that the negative competitive impact of invasive species on native species is more likely to be apparent competition than resource competition. Meanwhile, Jones et al. (2009) stated that the effect of resource competition is higher than that of apparent competition in ecosystem. In our research, two preys apparently do not experience resource competition, but only indirect interaction mediated by shared predators. If natural enemies can quickly gather in habitat patches containing two preys with high density, then short-term apparent competition will also appear, and its mechanism is controlled by spatial effects (Holt and Lawton, 1994). However, we failed to test spatial effects in this study. In addition, the environmental factors (e.g., fertilizer application and climate warming) could also modulate natural enemy-mediated indirect interactions between pests, which could trigger multiple indirect bottom-up effects and increase both interspecific competition and overall biocontrol service (Han et al., 2019; Han et al., 2020; Han et al., 2022).
The establishment of biological control agents in the banker plant system is a probabilistic event that depends on the size of the founder population and the banker plant species (Sanchez et al., 2021). Compared with other studies in which mix-stage predators were introduced (Messelink et al., 2008; Bompard et al., 2013; Jaworski et al., 2015; Han et al., 2020), only one pair of H. axyridis adults was released. Allee effects had been suggested, which led to the decline of the suitability of predator population in the banker plant system (Sanchez et al., 2021). Therefore, the possible Allee effects may account for the differences in the effect of banker the plant system on H. axyridis abundance. Moreover, the timely replacement of banker plant may enhance the establishment of biological control agents in the banker plant system. Studies have proved that early planting and timely replanting of functional plants (e.g., repellent plants) in crops would ensure a more effective and suitable pest control (Hatt et al., 2019a; Wang et al., 2022).
Studies have shown that it is difficult to distinguish the negative effects of experiments from the operational problems related to complex experimental design, and it is difficult to detect the strongly apparent competition effect through experiments (Chaneton and Bonsall, 2000). Consequently, apparent competition and mutualism can be methodologically dissected in this food web when the banker plant system was applied to aphid control. This work adds to the previous literature on indirect food web interactions and the potentially indirect benefits of the banker plant system in pest management programs (Chailleux et al., 2014). On the basis of the co-effects of different initial density of alternative prey on target pest and the proliferation of predator, H. axyridis, we put forward two strategies for pest biological control. When the density of target prey is low, higher density of alternative prey in the banker plant system could enhance population buildup of beneficials without affecting the control effect on pest. When the density of target prey is high, lower density of alternative prey could directly support population of beneficials and facilitate indirectly the potential negative effect (i.e., apparent competition) on target pest in the ecosystem. Overall, we put forward a strategy for setting the initial density of alternative prey of the banker plant system to target the high and low density of aphids, which highlighted the importance of indirect interactions in designing a proper banker plant system.
The raw data supporting the conclusions of this article will be made available by the authors, without undue reservation.
SW, SL, and JW conceived the research. JW, SL, and YY performed the experiments. JW, SL, and SW analyzed the data. JW, SL, YY, YL, ZJ, ND, PH, and SW wrote the manuscript. All authors contributed to the article and approved the submitted version.
This study was sponsored by projects of the Youth Program of National Natural Science Foundation of China (32202372), the Beijing Key Laboratory of Environment Friendly Management on Fruit Diseases and Pests in North China, the National Natural Science Foundation of China (32001904), the Technical Innovation Program of Beijing Academy of Agriculture and Forestry Sciences (KJCX20200110), the Major Science and Technology Program of China Tobacco Corporation (11020210104LS-06), and the Beijing Technology Program (Z201100008020014).
The authors acknowledge technical assistance related to analyzing the data from Dr. Huang Ningxing from the Institute of Plant Protection, Beijing Academy of Agriculture and Forestry Sciences, and capturing theory form Dr. Jaworski Coline C. from the Department of Zoology, University of Cambridge.
The authors declare that the research was conducted in the absence of any commercial or financial relationships that could be construed as a potential conflict of interest.
All claims expressed in this article are solely those of the authors and do not necessarily represent those of their affiliated organizations, or those of the publisher, the editors and the reviewers. Any product that may be evaluated in this article, or claim that may be made by its manufacturer, is not guaranteed or endorsed by the publisher.
The Supplementary Material for this article can be found online at: https://www.frontiersin.org/articles/10.3389/fpls.2022.1083848/full#supplementary-material
Abrams, P. A., Matsuda, H. (1996). Positive indirect effects between prey species that share predators. Ecology 77 (2), 610–616. doi: 10.2307/2265634
Aparicio, Y., Gabarra, R., Arno, J. (2020). Interactions among Myzus persicae, predators and parasitoids may hamper biological control in Mediterranean peach orchards. Entomol. Gen. 40 (3), 217–228. doi: 10.1127/entomologia/2020/0946
Ardanuy, A., Figueras, M., Matas, M., Arnó, J., Agustí, N., Alomar, Ò., et al. (2022). Banker plants and landscape composition influence colonisation precocity of tomato greenhouses by mirid predators. J. Pest Sci. 95, 447–459. doi: 10.1007/s10340-021-01387-y
Blubaugh, C. K., Asplund, J. S., Eigenbrode, S. D., Morra, M. J., Philips, C. R., Popova, I. E., et al. (2018). Dual-guild herbivory disrupts predator-prey interactions in the field. Ecology 99 (5), 1089–1098. doi: 10.1002/ecy.2192
Bompard, A., Jaworski, C. C., Bearez, P., Desneux, N. (2013). Sharing a predator: can an invasive alien pest affect the predation on a local pest? Popul. Ecol. 55 (3), 433–440. doi: 10.1007/s10144-013-0371-8
Chailleux, A., Mohl, E. K., Teixeira Alves, M., Messelink, G. J., Desneux, N. (2014). Natural enemy-mediated indirect interactions among prey species: Potential for enhancing biocontrol services in agroecosystems. Pest Manage. Sci. 70 (12), 1769–1779. doi: 10.1002/ps.3916
Chaneton, E. J., Bonsall, M. B. (2000). Enemy-mediated apparent competition: empirical patterns and the evidence. Oikos 88 (2), 380–394. doi: 10.1034/j.1600-0706.2000.880217.x
Chen, X., Jaworski, C. C., Dai, H., Liang, Y., Guo, X., Wang, S., et al. (2022). Combining banker plants to achieve long-term pest control in multi-pest and multi-natural enemy cropping systems. J. Pest Sci. 95, 685–697. doi: 10.1007/s10340-021-01428-6
Chi, H. (1917). TWOSEX-MSChart: A computer program for the age-stage, two-sex life table analysis. (Taichung: National Chung Hsing University). Available at: http://140.120.197.173/Ecology/Download/Twosex-MSChart.rar.
Chi, H. (1988). Life-table analysis incorporating both sexes and variable development rates among individuals. Environ. Entomol. 17 (1), 26–34. doi: 10.1093/ee/17.1.26
Chi, H., Liu, H. (1985). Two new methods for the study of insect population ecology. Bull. Inst. Zool. Acad. Sin. 24 (2), 225–240.
Chi, H., You, M., Atlıhan, R., Smith, C. L., Kavousi, A., Özgökçe, M. S., et al (2020). Age-stage, two-sex life table: an introduction to theory, data analysis, and application. Entomol. Gen. 40 (2), 103–24. doi: 10.1127/entomologia/2020/0936
Chi, H., Güncan, A., Kavousi, A., Gharakhani, G., Atlihan, R., Özgökçe, MS., et al (2022). TWOSEX-MSChart: the key tool for life table research and education. Entomol. Gen. in press. doi: 10.1127/entomologia/2022/1851
Costa, M. I. S., Anjos, L. (2020). The occurrence of apparent competition and apparent mutualism in a modeled greenhouse system with two non-competing pests and a shared biocontrol agent. Neotrop. Entomol. 49 (6), 874–881. doi: 10.1007/s13744-020-00820-8
Damien, M., Llopis, S., Desneux, N., van Baaren, J., Le Lann, C. (2020). How does floral nectar quality affect life history strategies in parasitic wasps? Entomol. Gen. 40 (2), 147–156. doi: 10.1127/entomologia/2020/0906
Desneux, N., Kaplan, I., Yoo, H. J. S., Wang, S., O’Neil, R. J. (2019). Temporal synchrony mediates the outcome of indirect effects between prey via a shared predator. Entomol. Gen. 39 (2), 127–136. doi: 10.1127/entomologia/2019/0824
Desneux, N., O’Neil, R. J. (2008). Potential of an alternative prey to disrupt predation of the generalist predator, Orius insidiosus, on the pest aphid, Aphis glycines, via short-term indirect interactions. Bull. Entomol. Res. 98 (6), 631–639. doi: 10.1017/S0007485308006238
Efron, B., Tibshirani, R. J. (1993). An Introduction to the Bootstrap (New York, USA: Chapman & Hall Inc).
Emery, S. E., Mills, N. J. (2020). Effects of predation pressure and prey density on short-term indirect interactions between two prey species that share a common predator. Ecol. Entomol. 45 (4), 821–830. doi: 10.1111/een.12855
Eubanks, M. D., Finke, D. L. (2014). Interaction webs in agroecosystems: beyond who eats whom. Curr. Opin. Insect Sci. 2, 1–6. doi: 10.1016/j.cois.2014.06.005
Frank, S. D. (2010). Biological control of arthropod pests using banker plant systems: past progress and future directions. Biol. Control 52 (1), 8–16. doi: 10.1016/j.biocontrol.2009.09.011
Frost, C. M., Peralta, G., Rand, T. A., Didham, R. K., Varsani, A., Tylianakis, J. M. (2016). Apparent competition drives community-wide parasitism rates and changes in host abundance across ecosystem boundaries. Nat. Commun. 7, 12644. doi: 10.1038/ncomms12644
Gelman, A., Hill, J. (2007). Data analysis using regression and multilevel/hierarchical models (Cambridge, U. K: Cambridge University Press).
Grevstad, F. S., Klepetka, B. W. (1992). The influence of plant architecture on the foraging efficiencies of a suite of ladybird beetles feeding on aphids. Oecologia 92 (3), 399–404. doi: 10.1007/BF00317466
Gurr, G. M., Lu, Z., Zheng, X., Xu, H., Zhu, P., Chen, G., et al. (2016). Multi-country evidence that crop diversification promotes ecological intensification of agriculture. Nat. Plants 2, 16014. doi: 10.1038/nplants.2016.14
Gurr, G. M., Wratten, S. D., Landis, D. A., You, M. (2017). Habitat management to suppress pest populations: progress and prospects. Annu. Rev. Entomol. 62, 91–109. doi: 10.1146/annurev-ento-031616-035050
Han, P., Becker, C., Le Bot, J., Larbat, R., Lavoir, A. V., Desneux, N. (2020). Plant nutrient supply alters the magnitude of indirect interactions between insect herbivores: From foliar chemistry to community dynamics. J. Ecol. 108 (4), 1497–1510. doi: 10.1111/1365-2745.13342
Han, P., Lavoir, A. V., Rodriguez-Saona, C., Desneux, N. (2022). Bottom-up forces in agroecosystems and their potential impact on arthropod pest management. Annu. Rev. Entomol. 67, 239–259. doi: 10.1146/annurev-ento-060121-060505
Han, Z., Tan, X., Wang, Y., Xu, Q., Zhang, Y., Harwood, J. D., et al. (2019). Effects of simulated climate warming on the population dynamics of Sitobion avenae (Fabricius) and its parasitoids in wheat fields. Pest. Manage. Sci. 75 (12), 3252–3259. doi: 10.1002/ps.5447
Hatt, S., Uyttenbroeck, R., Lopes, T., Chen, J., Piqueray, J., Monty, A., et al. (2018). Effect of flower traits and hosts on the abundance of parasitoids in perennial multiple species wildflower strips sown within oilseed rape (Brassica napus) crops. Arthropod. Plant Interact. 12, 787–797. doi: 10.1007/s11829-017-9567-8
Hatt, S., Xu, Q., Francis, F., Chen, J. (2019a). Intercropping oilseed rape with wheat and releasing Harmonia axyridis sex pheromone in northern China failed to attract and support natural enemies of aphids. Biotechnol. Agron. Soc Environ. 23 (3), 147–152. doi: 10.25518/1780-4507.17921
Hatt, S., Xu, Q., Francis, F., Osawa, N. (2019b). Aromatic plants of East Asia to enhance natural enemies towards biological control of insect pests. A review. Entomol. Gen. 38 (4), 275–315. doi: 10.1127/entomologia/2019/0625
Hedges, L. V., Gurevitch, J., Curtis, P. S. (1999). The meta-analysis of response ratios in experimental ecology. Ecology 80 (4), 1150–1156. doi: 10.1890/0012-9658(1999)080[1150:TMAORR]2.0.CO;2
Holt, R. D. (1977). Predation, apparent competition, and the structure of prey communities. Theor. Popul. Biol. 12 (2), 197–129. doi: 10.1016/0040-5809(77)90042-9
Holt, R. D., Bonsall, M. B. (2017). Apparent competition. Annu. Rev. Ecol. Evol. Syst. 48, 447–471. doi: 10.1146/annurev-ecolsys-110316022628
Holt, R. D., Lawton, J. H. (1994). The ecological consequences of shared natural enemies. Annu. Rev. Ecol. Syst. 25, 495–520. doi: 10.1146/annurev.es.25.110194.002431
Huang, N., Enkegaard, A., Osborne, L. S., Ramakers, P. M. J., Messelink, G. J., Pijnakker, J., et al. (2011). The banker plant method in biological control. Crit. Rev. Plant Sci. 30 (3), 259–278. doi: 10.1080/07352689.2011.572055
Jaworski, C. C., Bompard, A., Genies, L., Amiens-Desneux, E., Desneux, N. (2013). Preference and prey switching in a generalist predator attacking local and invasive alien pests. PloS One 8 (12), e82231. doi: 10.1371/journal.pone.0082231
Jaworski, C. C., Chailleux, A., Bearez, P., Desneux, N. (2015). Apparent competition between major pests reduces pest population densities on tomato crop, but not yield loss. J. Pest Sci. 88 (4), 793–803. doi: 10.1007/s10340-015-0698-3
Jaworski, C. C., Xiao, D., Xu, Q., Ramirez-Romero, R., Guo, X., Wang, S., et al. (2019). Varying the spatial arrangement of synthetic herbivore-induced plant volatiles and companion plants to improve conservation biological control. J. Appl. Ecol. 56 (5), 1176–1188. doi: 10.1111/1365-2664.13353
Jones, T. S., Godfray, H. C., van Veen, F. J. (2009). Resource competition and shared natural enemies in experimental insect communities. Oecologia 159 (3), 627–635. doi: 10.1007/s00442-008-1247-z
Karp, D. S., Chaplin-Kramer, R., Meehan, T. D., Martin, E. A., DeClerck, F., Grab, H., et al. (2018). Crop pests and predators exhibit inconsistent responses to surrounding landscape composition. Proc. Natl. Acad. Sci. U.S.A. 115 (33), 7863–7870. doi: 10.1073/pnas.1800042115
Lajeunesse, M. J. (2015). Bias and correction for the log response ratio in ecological meta-analysis. Ecology 96 (8), 2056–2063. doi: 10.1890/14-2402.1
Liang, Y., Chen, X., Dai, H., Wang, J., Guo, X., Wang, S., et al. (2022). Flower provision reduces intraguild predation between predators and increases aphid biocontrol in tomato. J. Pest Sci. 95, 461–472. doi: 10.1007/s10340-021-01396-x
Li, S., Tan, X., Desneux, N., Benelli, G., Zhao, J., Li, X., et al. (2015). Innate positive chemotaxis to pollen from crops and banker plants in predaceous biological control agents: Towards new field lures? Sci. Rep. 5, 12729. doi: 10.1038/srep12729
Liu, C., Yan, L., Li, H., Wang, G. (2006). Effects of predator-mediated apparent competition on the population dynamics of Tetranychus urticae on apples. BioControl 51 (4), 453–463. doi: 10.1007/s10526-005-4363-z
Li, S., Wang, J., Huang, N., Jin, Z., Wang, S., Zhang, F. (2020). Research progress and prospect on banker plant systems of predators for biological control. Sci. Agric. Sin. 53 (19), 3975–3987. doi: 10.3864/j.issn.0578-1752.2020.19.011
Manly, B. F. J. (1973). A linear model for frequency-dependent selection by predators. Res. Popul. Ecol. 14, 137–150. doi: 10.1007/BF02518839
Messelink, G. J., van Maanen, R., van Steenpaal, S. E. F., Janssen, A. (2008). Biological control of thrips and whiteflies by a shared predator: Two pests are better than one. Biol. Control 44 (3), 372–379. doi: 10.1016/j.biocontrol.2007.10.017
Miller, P., Tracey, L., Rebek, E. J. (2018). Banker plants for aphid biological control in greenhouses. J. Integr. Pest Manage. 9, 1–8. doi: 10.1093/jipm/pmy002
Montoya, J. M., Woodward, G., Emmerson, M. C., Sole, R. V. (2009). Press perturbations and indirect effects in real food webs. Ecology 90 (9), 2426–2433. doi: 10.1890/08-0657.1
Noonburg, E. G., Byers, J. E. (2005). More harm than good: when invader vulnerability to predators enhances impact on native species. Ecology 86 (10), 2555–2560. doi: 10.1890/05-0143
Orrock, J. L., Grabowski, J. H., Pantel, J. H., Peacor, S. D., Peckarsky, B. L., Sih, A., et al. (2008). Consumptive and nonconsumptive effects of predators on metacommunities of competing prey. Ecology 89 (9), 2426–2435. doi: 10.1890/07-1024.1
Ovchinnikov, A. N., Belyakova, N. A., Ovchinnikova, A. A., Reznik, S. Y. (2019). Factors determining larval cannibalistic behavior in invasive and native populations of the multicolored Asian ladybird, Harmonia axyridis. Entomol. Gen. 38 (2), 243–254. doi: 10.1127/entomologia/2019/0702
Özgökçe, M. S., Chi, H., Atlıhan, R., Kara, H. (2018). Demography and population projection of Myzus persicae (Sulz.) (Hemiptera: Aphididae) on five pepper (Capsicum annuum L.) cultivars. Phytoparasitica 46 (2), 169–169. doi: 10.1007/s12600-018-0651-0
Parolin, P., Bresch, C., Poncet, C., Desneux, N. (2012). Functional characteristics of secondary plants for increased pest management. Int. J. Pest Manage. 58, 369–377. doi: 10.1080/09670874.2012.734869
Ramirez, R. A., Eubanks, M. D. (2016). Herbivore density mediates the indirect effect of herbivores on plants via induced resistance and apparent competition. Ecosphere 7 (2), e01218. doi: 10.1002/ecs2.1218
Sanchez, J. A., López-Gallego, E., Pérez-Marcos, M., Perera-Fernández, L. (2021). The effect of banker plants and pre-plant release on the establishment and pest control of Macrolophus pygmaeus in tomato greenhouses. J. Pest Sci. 94 (2), 297–307. doi: 10.1007/s10340-020-01257-z
Symondson, W. O., Sunderland, K. D., Greenstone, M. H. (2002). Can generalist predators be effective biocontrol agents? Annu. Rev. Entomol. 47, 561–594. doi: 10.1146/annurev.ento.47.091201.145240
van Lenteren, J. C. (2012). The state of commercial augmentative biological control: plenty of natural enemies, but a frustrating lack of uptake. BioControl 57, 1–20. doi: 10.1007/s10526-011-9395-1
van Veen, F. J. F., Morris, R. J., Godfray, H. C. (2006). Apparent competition, quantitative food webs, and the structure of phytophagous insect communities. Annu. Rev. Entomol. 51, 187–208. doi: 10.1146/annurev.ento.51.110104.151120
Wang, J., Li, S., Fang, Y., Zhang, F., Jin, Z., Desneux, N., et al. (2022). Enhanced and sustainable control of Myzus persicae by repellent plants in organic pepper and eggplant greenhouses. Pest Manage. Sci. 78 (2), 428–437. doi: 10.1002/ps.6681
Wang, J., Li, S., Yang, J., Guo, M., Dai, H., Ramirez-Romero, R., et al. (2021). The fitness of mass rearing food on the establishment of Chrysopa pallens in a banker plant system under fluctuating temperature conditions. Insects 12 (11), 1014 doi: 10.3390/insects12111014
Wang, Y., Yao, F., Soares, M. A., Basiri, S. E., Amiens-Desneux, E., Campos, M. R., et al. (2020). Effects of four non-crop plants on life history traits of the lady beetle Harmonia axyridis. Entomol. Gen. 40 (3), 243–252. doi: 10.1127/entomologia/2020/0933
Xie, H., Chen, J., Cheng, D., Zhou, H., Sun, J., Liu, Y., et al. (2012). Impact of wheat-mung bean intercropping on English grain aphid (Hemiptera: Aphididae) populations and its natural enemy. J. Econ. Entomol. 105 (3), 854–859. doi: 10.1603/ec11214
Xu, Q., Wang, S., Li, S., Hatt, S. (2020). “Conservation biological control in organic greenhouse vegetables,” in Integrative biological control progress in biological control. Eds. Gao, Y., Hokkanen, H. M., Menzler-Hokkanen, I. (Cham, Switzerland: Springer). doi: 10.1007/978-3-030-44838-7_8
Zhang, Y., Fan, J., Fu, Y. (2019). Plant-mediated interactions between two cereal aphid species: promotion of aphid performance and attraction of more parasitoids by infestation of wheat with phytotoxic aphid Schizaphis graminum. J. Agric. Food Chem. 67 (10), 2763–2773. doi: 10.1021/acs.jafc.8b06150
Zhao, J., Guo, X., Tan, X., Desneux, N., Lucia, Z., Zhang, F., et al. (2017). Using Calendula officinalis as floral resource to enhance aphid and thrips suppression by the flower bug Orius sauteri (Hemiptera: Anthocoridae). Pest Manage. Sci. 73, 515–520. doi: 10.1002/ps.4474
Keywords: aphid, banker plants, IPM, indirect interaction, population quantitative relationship
Citation: Wang J, Yang Y, Li Y, Jin Z, Desneux N, Han P, Wang S and Li S (2022) Direct and indirect effects of banker plants on population establishment of Harmonia axyridis and aphid control on pepper crop. Front. Plant Sci. 13:1083848. doi: 10.3389/fpls.2022.1083848
Received: 29 October 2022; Accepted: 21 November 2022;
Published: 12 December 2022.
Edited by:
Julian Chen, Institute of Plant Protection (CAAS), ChinaReviewed by:
Javad Karimi, Ferdowsi University of Mashhad, IranCopyright © 2022 Wang, Yang, Li, Jin, Desneux, Han, Wang and Li. This is an open-access article distributed under the terms of the Creative Commons Attribution License (CC BY). The use, distribution or reproduction in other forums is permitted, provided the original author(s) and the copyright owner(s) are credited and that the original publication in this journal is cited, in accordance with accepted academic practice. No use, distribution or reproduction is permitted which does not comply with these terms.
*Correspondence: Su Wang, d2FuZ3N1QGlwZXBiYWFmcy5jbg==; Shu Li, bGlzaHVAaXBlcGJhYWZzLmNu
Disclaimer: All claims expressed in this article are solely those of the authors and do not necessarily represent those of their affiliated organizations, or those of the publisher, the editors and the reviewers. Any product that may be evaluated in this article or claim that may be made by its manufacturer is not guaranteed or endorsed by the publisher.
Research integrity at Frontiers
Learn more about the work of our research integrity team to safeguard the quality of each article we publish.