- 1Tropical Crops Genetic Resources Institute, Chinese Academy of Tropical Agricultural Sciences, Haikou, Hainan, China
- 2Hainan Yazhou Bay Seed Lab, Hainan, China
- 3Key Laboratory of Tropical Crops Germplasm Resources Genetic Improvement and Innovation of Hainan Province, Hainan, China
- 4Haikou Experimental Station, Chinese Academy of Tropical Agricultural Sciences, Haikou, Hainan, China
Although a few studies have elucidated the creation of bitter gourd mutants, the suitable concentration and duration of ethyl methanesulfonate (EMS) mutagenesis have not been determined. In this study, mutant collection was conducted to create new germplasms and widen genetic diversity. By employing the seeds of the inbred line Y52 as the mutagenic material, EMS as the mutagen, and the suitable mutagenic conditions for bitter gourd seeds (EMS concentration 0.2%, mutagenic time 10 h), we mutated 10,000 seeds and acquired 3223 independent M1 lines. For the randomly selected 1000 M2 lines, 199 M2 lines with visible phenotypes were found, and 167 M2 lines were mutants of fruit shape, size, and tubercles. Furthermore, fourteen dwarf, eleven leaf color, five leaf shape, and eight meristem defect mutants were discovered in this mutant collection. In addition, three lines of 1253, 2284, and 3269 represented recessive mutants crossed with Y52. Furthermore, the yellow leaf lines of 2284 and 3269 were not mutated at the same gene locus. This study constructed a mutant collection through innovative new germplasms and provided valuable resources for bitter gourd breeding and functional gene research.
Introduction
Bitter gourd (Momordica charantia L., 2n = 22), also named balsam pear, bitter melon, bitter cucumber, or African cucumber, belongs to the family Cucurbitaceae, and is cultivated in Asia and Africa. As an important vegetable, it has many other pharmacological benefits for human immunodeficiency virus (HIV) infection and diabetes and plays a vital role in our life (Behera et al., 2010). Owing to land shortages and the worsening environment, breeding is focused on promoting crop yield. Bitter gourd is facing the problem of using less land to obtain higher yields, and biotechnology can solve this problem. For a long time, the basic research of bitter gourd was concentrated on establishing recombinant inbred lines and constructing genetic linkage maps using molecular markers (Cui et al., 2017; Cui et al., 2018; Rao et al., 2018; Kaur et al., 2021; Yang et al., 2022; Zhong et al., 2022).
Creating mutant materials to conduct the functional study of related genes in genetic research is necessary when the ratio of spontaneous variation is very low. Consequently, an efficient method for creating mutants is urgently required. Recently, gene editing technology with the CRISPR-Cas system has been widely used in rice, wheat, and tomato (Li et al., 2018; Lin et al., 2020; Wang et al., 2022), but it is coupled with an efficient transformation rate. Nevertheless, the transformation system has not been established in bitter gourd, resulting in the impossibility of gene editing in bitter gourd.
In bitter gourd, the functional study of genetics is considerably behind other Cucurbitaceae crops like watermelon and cucumber because of the lack of mutants (Deng et al., 2022; Zhang et al., 2022). A long-recognized large-scale method for mutant acquisition is mutagenization using chemical or physical mutagens. Physical mutagens such as fast neutrons, gamma rays, and x-rays mainly cause nucleotide deletion, and their mutation frequency is low (Li et al., 2017). As a chemical mutagen, EMS mutagenesis was successfully used in crop breeding and functional genomics research. EMS mutagenesis technology is feasible in bitter gourd because it is appropriate to most plants rather than their transformation rate (Lian et al., 2020).
EMS is an effective mutagen that can introduce random base pair changes (mainly G:C/AT) in the genome after replication, bringing less damage to plants (Greene et al., 2003). For example, in Arabidopsis thaliana, approximately 700 mutations in each EMS mutant line and 50000 M1 lines are sufficient to make a 95% chance of mutation in any G:C base pair in the genome (Jander et al., 2003). Besides, the mutants at M2 or more advanced generations of recessive homozygous are crossed with the world-type and generate second filial generation (F2) progeny that can be easily discovered by the mutated genes using MutMap (Abe et al., 2012; Qu and Qin, 2014).
Because EMS mutagenesis is easy to control, exhibits a high mutation rate, and causes less harm to plants, it was widely used in rice (Henry et al., 2014), maize (Lu et al., 2018), soybean (Li et al., 2017), pepper (Arisha et al., 2015), cabbage (Sun et al., 2022), watermelon (Deng et al., 2022), cucumber (Zhang et al., 2022) and Gossypium hirsutum L (Lian et al., 2020). EMS concentrations differ from one crop to another, and a suitable mutagen concentration can be used to achieve the desired effect. Generally, the optimal lethality among the M1 generation is approximately 50% (Arisha et al., 2015; Lian et al., 2020; Dutta et al., 2021). However, the optimal and specific protocol of EMS treatment conditions has not been determined because of limited studies on EMS treatment experiments in bitter gourd. Therefore, there is an urgent need to conduct an EMS mutagenesis study and determine the suitable concentration and duration time of EMS for bitter gourd seeds.
There are few studies on the creation of bitter gourd mutants. Some mutants related to vine length, fertility, and nutrient contents were generated by gamma rays (Co60 source) on a small scale (Dutta et al., 2021); however, they did not significantly improve functional genomic research and breeding. Therefore, this study focused on establishing an optimal EMS technique and guidance for other scientists in bitter gourd research. Furthermore, the mutant collection successfully created by EMS mutagenesis will contribute new germplasms to bitter gourd breeding and accelerate the process of gene functional analysis.
Materials and methods
Plant material
The cultivated inbred line Y52 was used in this study, and artificial self-pollinated seeds were supplied by the Institute of Tropical Crop Genetic Resources in Hainan province, China.
EMS experiment
Ten thousand seeds with a small opening at the seed coat of Y52 were soaked in ddH2O overnight and then embedded in 0.2% EMS at room temperature with gentle shaking for 10 h. The treated seeds were detoxified with 0.1 mol/L sodium thiosulfate five times. Afterward, the seeds were washed 15 times with ddH2O for 5 min each time. Finally, the seeds were wrapped with a wet cloth and transferred to an incubator at 37 °C in a dark condition until germination.
The germinated seeds were planted into small pots 3–5 days later and placed in a greenhouse until 4–6 true leaves appeared. Then, the seedlings were sown into the soil in an open field. By artificial self-pollination, 3223 M1 plants generated M2 mature seeds, and 10–60 M2 seeds were harvested, labeled, and saved. In addition, 10–20 M2 seeds from each individual M1 plant were randomly selected to sow into the soil, and their morphological phenotype was detected.
Results
EMS mutagen experiment condition
Because high concentrations of EMS and prolonged treatment can damage seeds and cause germination problems, we first detected the suitable conditions for bitter gourd mutagenesis. To increase the efficiency of mutagenesis, we created an opening at the embryo of each seed coat. Then, the preliminary assay of EMS treatment was completed, and we counted the germination seeds, and statistical analysis was done 15 days later (Table 1).
In our preliminary assay, the control group seeds’ germination rate was more than 90%. However, the germination rate with 0.5% EMS treatment in 4 h or 10 h were all lower than 50%, indicating this dose of EMS was not suitable for bitter gourd seeds. In contrast, when the seeds were treated with 0.2% EMS for 10 h, the germination rate was around 50%–60%, which was close to the half-lethal dose, indicating 0.2% EMS for 10 h treatment is the right condition for bitter gourd seeds. Besides, we also used 0.2% EMS for 4 h treatment and found that the mutagenesis effect is poor for bitter gourd.
M1 Population
Y52 is a cultivated species with a length of 30–40 cm and a weight of about 0.5 kg. Therefore, it was selected as the basic material for mutagenesis. Nearly 10,000 seeds were treated with 0.2% EMS for 10 h, and 5319 seeds were germinated, then all the germinated seedlings were planted in the soil (Table 2). After one month, we found that 4356 plants survived and 783 plants died, which may have been caused by the injury of the EMS mutagen.
M2 Population
Bitter gourd is a monoecious plant with staminate and pistillate flowers born on different internodes. Self-pollination is required for bitter gourd mutant propagation. However, some plants failed to pollinate because female and male flowers did not bloom simultaneously, resulting in no seeds. Finally, only 3223 M1 plants were successfully harvested (Table 2), and we constructed a bitter gourd mutant collection containing 3223 mutants.
Because bitter gourd needs artificial pollination and has a large workload, we randomly selected 1000 mutants for planting, 10–20 seeds for each, and approximately 10,000–20,000 seedlings were planted. As a result, the phenotypic variation of M2 generation was very abundant, approximately 19.9% (Table 3), including plant height, leaf color, leaf shape, fruit shape, and meristem defect.
Dwarf phenotype
Approximately fourteen mutants were not higher than 60 cm or had short internodes, leading to low plant height (Figure 1). Among fourteen mutants, nine lines of 454, 931, 1313, 1372, 2078, 2981, 3070, 3080, and 3114 had arrested growth and were no more than 30 cm tall. Moreover, there were 3–4 plants with dwarf phenotype in lines 931, 2981, and 3070 with a segregation rate close to 4:1 (Supplemental Table 1), consistent with Mendel’s classical genetic law. In contrast, there were only one or two mutants in other lines, which was inconsistent with Mendelian inheritance law. The possible reason is that the number of family plants is insufficient and does not have a statistical effect. Additionally, lines 681, 942, and 2194 are not only nanoid but also have small leaves, and lines 1833 and 3210 have short internodes (Figure 1).
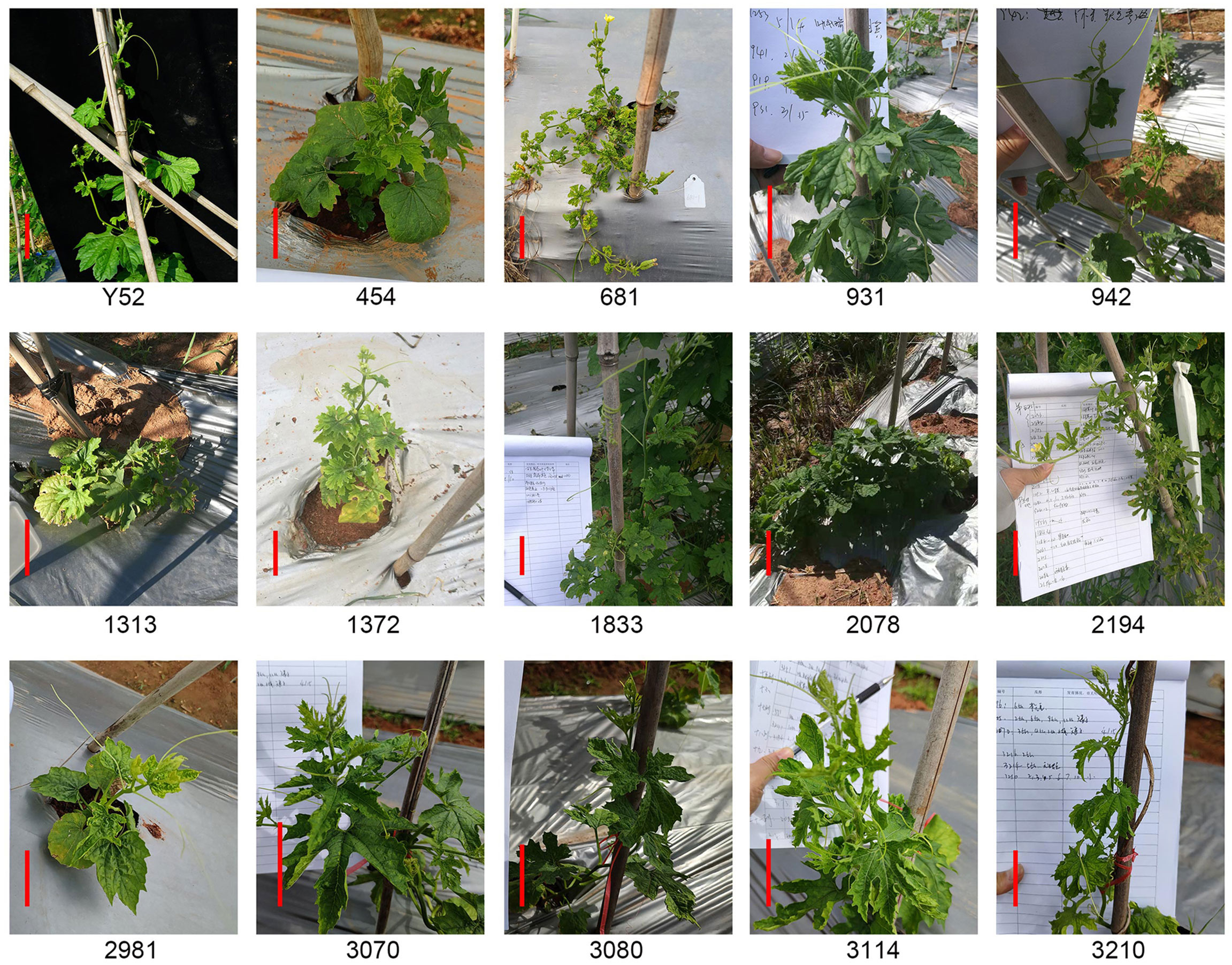
Figure 1 Different dwarf mutants in the M2 generation compared to Y52. Scale bar in red color = 5 cm.
Leaf color
Besides dwarf, leaf color is another easily observed trait. A total of eleven lines had color changes owing to chlorophyll synthesis defects (Abe et al., 2012), mainly leaf yellowing and leaf albinism (Figure 2). The leaves of the whole plant line 239, 3135, 3186, and 3206 were light yellow, and five lines of 1091, 1668, 2078, 3217, and 3269 had yellow leaves at the top of the plants while the leaves of line 2824 near the ground were yellow. Furthermore, we also found an albino mutant line 2401 with partial leaves is albinism. Of all these mutant lines, 1668, 2401, 2824, and 3269 had a segregation of 4:1 (Supplemental Table 2).
Leaf shape
We selected five mutants with prominent variations in leaf shape, namely 372, 681, 1253, 2002, and 3064 (Figure 3). The leaf blades of lines 372 and 2002 were upward curling, and 2002 is more serious. Line 681 has a small leaf size with some blades bulging upward. Unlike 681, the leaf edge of 3064 is blunt. The mutant of 1253 has severe defect with withered leaves, but they can grow and bloom with fewer female flowers. Unfortunately, the five mutants have the same sterility problem and can only be conservated by heterozygotes which increases the difficulty of gene localization. Moreover, the segregation ratio of 372, 1253, and 3064 are close to 4:1 except the line 681and 2002 (Supplemental Table 3).
Shoot apical meristem defect
The shoot apical meristem defect directly affected the yield of bitter gourd. Eight lines with shoot apical meristem defects were obtained by mutant collection screening (Figure 4). Three lines of 345, 1372, and 3211 have flat and wide shoot apical meristem, and the apical meristem of lines 1084, 1479, 1519, and 2837 develops into petiole, while the apical meristem of line 1319 was dead or withered. In addition, the segregation of all the lines was inconsistent with the Mendelian genetic law due to insufficient plants (Supplemental Table 4).
Fruit shape mutants
In our study, the variation of fruit shape was the most abundant phenotype, including fruit length, fruit transverse diameter, the pattern of fruit bibbing and warts, the shape of the blossom end, and the shape of the base of the fruit. From the 1000 M2 lines, we screened 167 mutants with various fruit shapes (Supplemental Table 5). Among the 167 mutants, eighteen mutants (Figure 5A) with a nearly round phenotype at the blossom end and thirty-eight mutants (Figure 5B) with a truncated phenotype at the base of the fruit were found compared with Y52.
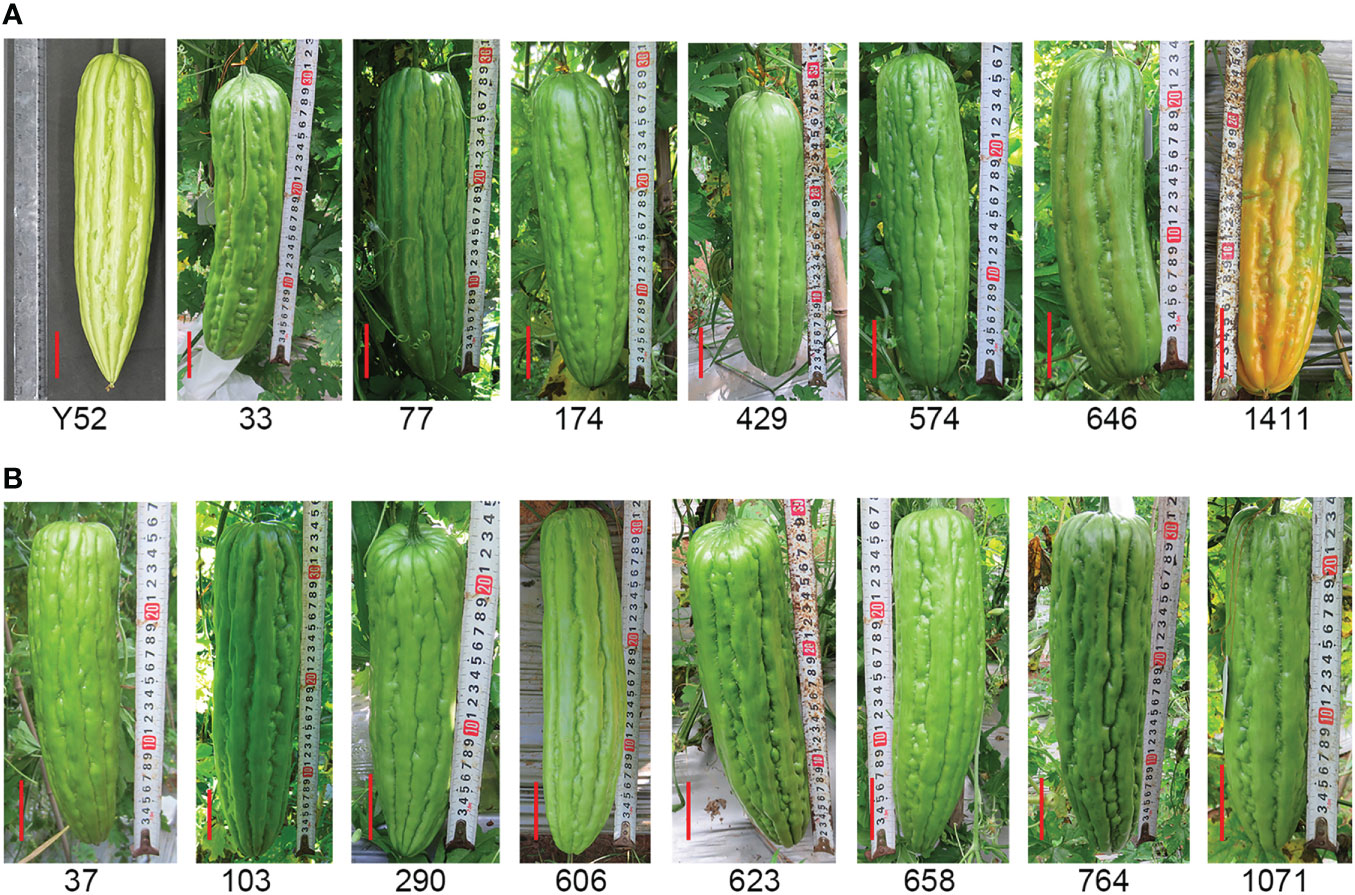
Figure 5 Mutation for fruit shape. (A) Mutants with nearly round phenotype at the blossom end. (B) Mutants with a truncated phenotype at the base of the fruit compared with Y52. Scale bar in red color = 5 cm.
Besides, compared with Y52 (Figure 6A) there were three kinds of mutation related to the pattern of fruit bibbing and warts: flat, grain, and stripe warts (Figures 6B–D) of three, twenty-eight, and nine mutants, respectively. Second, we found fifteen slender fruit mutants with a small transverse diameter of 3–6 cm (Figure 6E). Additionally, there were twenty-six mutants with fruit lengths less than 20 cm, while the fruit length of Y52 was between 30–40 cm (Figure 6F). Bending fruit is another prominent phenotype, and twenty-seven mutants were found (Figure 6G).
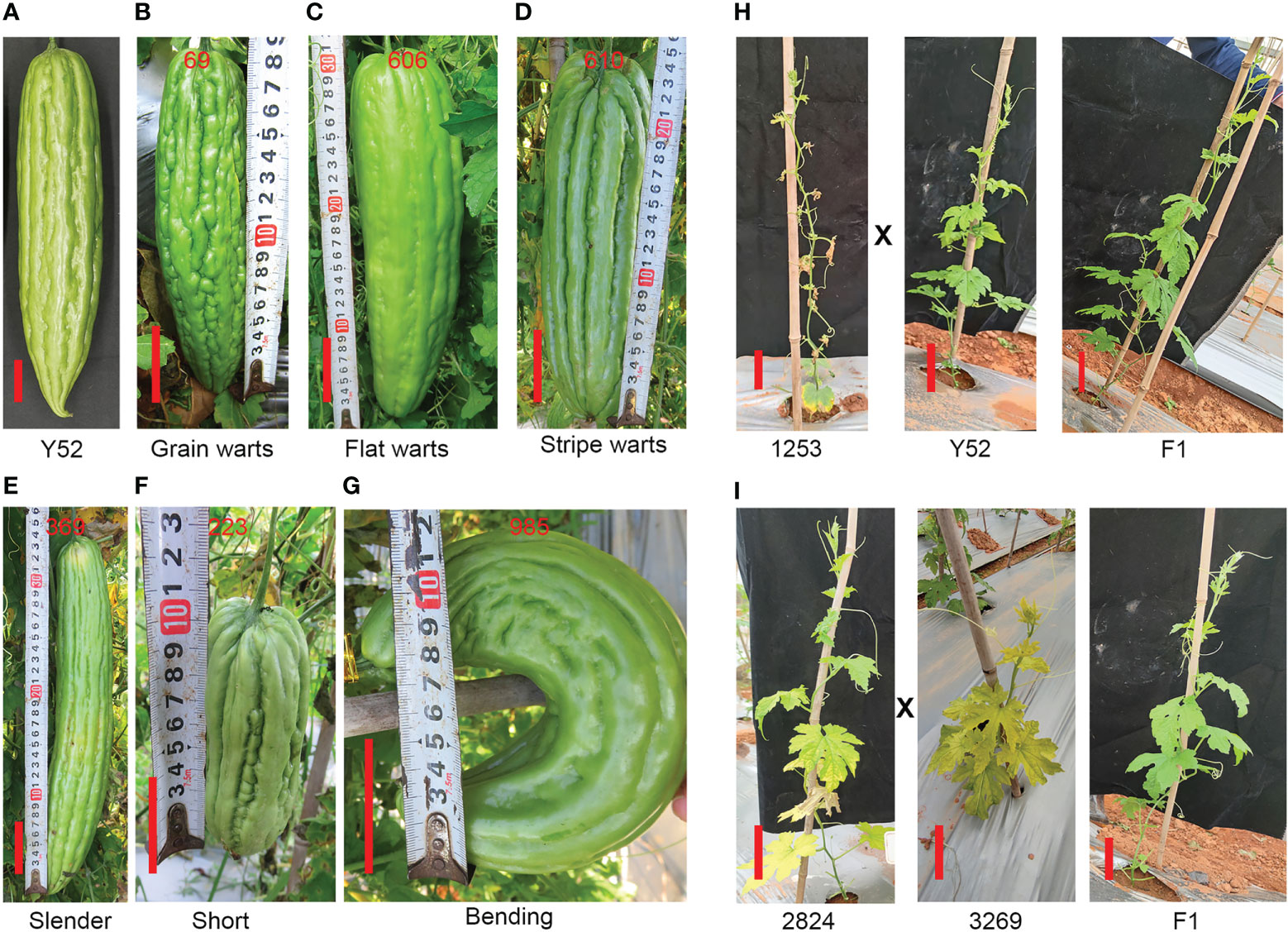
Figure 6 Different kinds of phenotype of fruit (A–G) and the phenotype of the F1 generation (H, I). (A) Fruit of Y52. (B–D) The phenotype of fruit with grain, flat and stripe warts. (E–G) Slender, short and bending fruit. The phenotype of the F1 generation generated by Y52 crossed with 1253 (H), and 2824 crossed with 3269 (I) compared to Y52, respectively. Scale bar in red color = 5 cm.
Mutant analysis
The mutant of 1253 has seventeen plants in the M2 generation and five plants with withered leaves, and the segregation ratio was close to 4:1, which means that it is a recessive mutation. To check the recessive or dominant mutation, we hybridized 1253 with Y52 and found that the phenotype of the F1 generation was similar to Y52, which indicated that 1253 was a recessive mutant (Figure 6H). Furthermore, we selected two mutants of 2824 (segregation ratio 4:1) and 3269 (segregation ratio 4:1) with yellow leaves to establish whether the two mutants mutated at the same gene. We hybridized 2824 with 3269 and found that the phenotype of the F1 generation was similar to Y52, indicating that the two mutants had different gene mutations (Figure 6I).
Specific mutants
Three mutants of 620, 681, and 1152 were selected as specific mutants. Some shoots, leaves, and fruits of 620 were albino, though the seed coat was black; the next generation of seedlings was albino and did not survive (Figure 7A). The mutant of 681 has two plants with a dwarf phenotype and thumb-sized fruits without seeds (Figure 7B), and it was used as a male parent and hybridized with Y52 to keep it. The mutant of 1152 was another interesting mutant with a yellow ovary and premature senescence of leaves attached to charcoal maggot disease (Figure 7C). Unfortunately, the segregation rate of the three mutants was inconsistent with Mendel’s classical genetic law, and the difficulty of gene mapping increased.
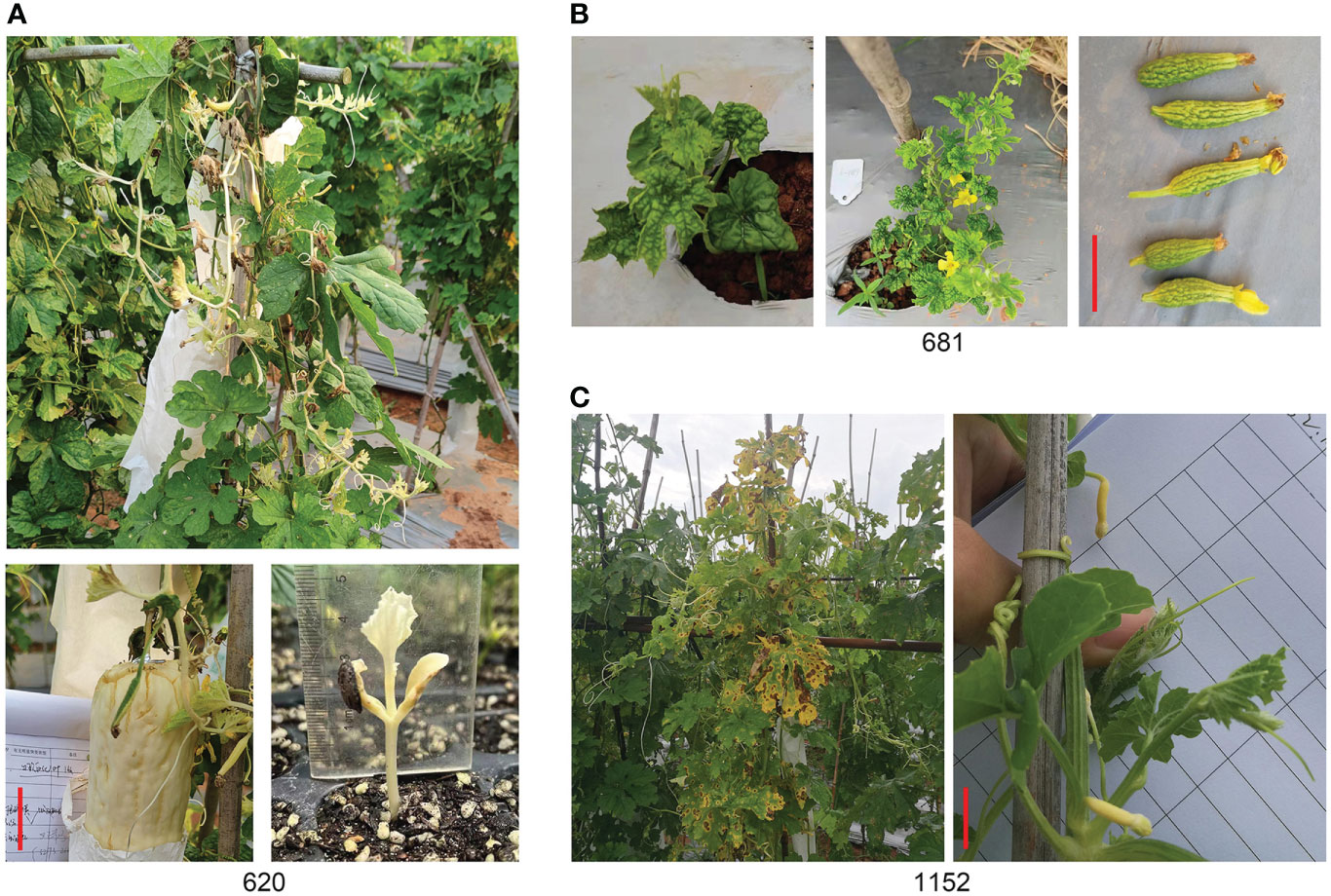
Figure 7 Specific mutants. (A) Albino mutant of line 620. (B) Thumb fruit of line 681. (C) Yellow fruit of line 1152. Scale bar in red color = 5 cm.
Discussion
Unlike Arabidopsis thaliana, rice, wheat, and soybean, the bitter gourd seed covered with a hard coat hindered the full contact between seed and mutagen, leading to decreased mutagenesis efficiency. To solve the problem and improve the mutagenesis efficiency, we used tweezers to clamp a small opening at the seed coat to enable the EMS mutagen to come into full contact with the seeds. Simultaneously, we added 0.1% Tween 20 to enable the seeds to encounter the mutagen evenly and easily clean. In this study, different EMS concentrations and mutagenesis times were evaluated. The germination rate of bitter gourd seeds treated with 0.2% EMS for 10 h was the closest to 50% (Arisha et al., 2015; Lian et al., 2020). Therefore, this condition was selected for subsequent experiments.
Because most of the M1 mutants acquired by the EMS mutagenesis were heterozygotes, abundant phenotypic variation was observed in the M2 generation. Similar to our results, leaf color mutation was easy to find, and we had already discovered eleven mutants with yellow or yellow-green leaves, and the chlorophyll synthesis deficiency mainly caused the yellow leaf mutants found in previous studies (Abe et al., 2012). Although the leaf color mutants survived but had smaller plants and fruits. Identifying mutation sites that lead to chlorophyll synthesis deficiency is crucial to breeders. Consistent with our results, many gene mutations can cause leaf color deficiency (Ma et al., 2017; Rong et al., 2019), the F1 generation produced by 2824 and 3269 did not have yellow leaves (Figure 6I), and they have different gene mutations.
Leaf shape, including size, margin, and rolling. Two specific mutants, 372 and 2002, with curly leaves were found in the M2 generation. Moderate leaf rolling can help to construct the ideal plant architecture and promote photosynthetic efficiency (Rong et al., 2019). Many genes that control leaf rolling in rice have been cloned, such as CFL2, SRL1, ZHD1, REL1/2, and SLL2 (Zhang et al., 2020). In the family of Cucurbitaceae, the CsPHB gene was regulated by miRNA165/166 to control leaf curly in cucumber but was never reported in bitter gourd (Rong et al., 2019). Thus, the two leaf curly mutants were valuable germplasms in bitter gourd and will decipher the gene function of the interest phenotype.
Dwarf mutants are crucial to understanding the regulatory mechanisms for plant height, development, and productivity (Bae et al., 2021) because they can be planted at high density and are resistant to lodging (Zhu et al., 2019; Sun et al., 2020). In addition, a class of plant hormones such as GA, BR, and cytokinin were reported to regulate cell elongation and division that could cause the dwarf phenotype when the level of hormones was abnormal, and many dwarf mutants have been found in various crops such as rice sd1, wheat Rht-B1b/D1b and cucumber Csdw (Wei et al., 2019). Fourteen dwarf mutants with short internodes and small leaf sizes were explored in this study. Previous studies reported that plant height-related genes are associated with leaf sizes such as OsNAL1, OsNAL7, and OsDNL-4 (Bae et al., 2021). Similar to recent studies, six mutants of line 681, 942, 1372, 1833, 2194, and 3210 have short plant heights and small leaf sizes.
Fruit phenotype, including fruit shape, size, color, and surface texture possibly caused by long-term domestication, artificial selection, natural selection, or environmental factors, are crucial characteristics for different markets and consumers, and the variety of fruit gives rise to uncovering the molecular mechanism and genetic basis (Snouffer et al., 2020). For example, in bitter gourd, the fruit of wild type with tubercles is small, round, or spindle, while the cultivated species are larger, longer, and do not taper at both ends (Behera et al., 2010). In the mutant collection, we found small, slender, and bending fruits (Figures 6E–G). Moreover, we also found some mutants with different tubercles like flat, grain, and stripe warts on the skin (Figures 6B–D). Some studies have proved vital genes or QTLs that control fruit shapes, such as CsFUL and CsTRM5 in cucumber, CmOFP1a in melon, and ClSUN25-26-27a in watermelon (Pan et al., 2020; Snouffer et al., 2020; Boualem et al., 2022). Furthermore, some mutants with a good fruit shape, that do not taper at both ends, and meet our breeding objectives were kept for future study. Therefore, further molecular analysis is needed and important to discover these genes controlling related fruit traits.
Conclusion
In this study, we confirmed the optimal EMS mutagenesis conditions for bitter gourd seeds of Y52 (EMS concentration 0.2% and mutagenic time 10 h). Furthermore, a mutant collection including 3223 mutants was constructed. We classified them according to the detection of phenotype characters into leaf shape mutation, leaf color mutation, dwarfing mutation, apical meristem mutation, fruit size, and shape mutation. These mutants not only bring new germplasms for breeding but can also accelerate gene functional study in bitter gourd.
Data availability statement
The original contributions presented in the study are included in the article/Supplementary Material. Further inquiries can be directed to the corresponding author.
Author contributions
RY, YY, and XW conceived and designed the project. RY and XW conducted the EMS mutation experiment, RY and YN collected most of the phenotype. KY, XH, ZL and ZQ participated in some mutant phenotype collected. RY and XW wrote the manuscript, and YY revised the manuscript. All authors contributed to the article and approved the submitted version.
Funding
This research was supported by grants from the Hainan Province Science and Technology Special Fund (ZDYF2020063), The Major Science and Technology plan of Hainan Province (ZDKJ2021010), Hainan Yazhou Bay Seed Lab (B21HJ0305), and Hainan Province Science and Technology Special Fund (ZDYF2020059).
Conflict of interest
The authors declare that the research was conducted in the absence of any commercial or financial relationships that could be construed as a potential conflict of interest.
Publisher’s note
All claims expressed in this article are solely those of the authors and do not necessarily represent those of their affiliated organizations, or those of the publisher, the editors and the reviewers. Any product that may be evaluated in this article, or claim that may be made by its manufacturer, is not guaranteed or endorsed by the publisher.
Supplementary material
The Supplementary Material for this article can be found online at: https://www.frontiersin.org/articles/10.3389/fpls.2022.1069750/full#supplementary-material
References
Abe, A., Kosugi, S., Yoshida, K., Natsume, S., Takagi, H., Kanzaki, H., et al. (2012). Genome sequencing reveals agronomically important loci in rice using MutMap. Nat. Biotechnol. 30 (2), 174–178. doi: 10.1038/nbt.2095
Arisha, M. H., Shah, S. N., Gong, Z. H., Jing, H., Li, C., Zhang, H. X. (2015). Ethyl methane sulfonate induced mutations in M2 generation and physiological variations in M1 generation of peppers (Capsicum annuum l.). Front. Plant Sci. 6. doi: 10.3389/fpls.2015.00399
Bae, K. D., Um, T. Y., Yang, W. T., Park, T. H., Hong, S. Y., Kim, K. M., et al. (2021). Characterization of dwarf and narrow leaf (dnl-4) mutant in rice. Plant Signal Behav. 16 (2), 1849490. doi: 10.1080/15592324.2020.1849490
Behera, T. K., Behera, S., Bharathi, L. K.. (2010). Bitter gourd: botany, horticulture, breeding. Hortic. Rev. 101–141. doi: 10.1002/9780470543672.ch2
Boualem, A., Berthet, S., Devani, R. S., Camps, C., Fleurier, S., Morin, H., et al. (2022). Ethylene plays a dual role in sex determination and fruit shape in cucurbits. Curr. Biol. 32 (11), 2390–2401.e2394. doi: 10.1016/j.cub.2022.04.031
Cui, J., Cheng, J., Nong, D., Peng, J., Hu, Y., He, W., et al. (2017). Genome-wide analysis of simple sequence repeats in bitter gourd (Momordica charantia). Front. Plant Sci. 8. doi: 10.3389/fpls.2017.01103
Cui, J., Luo, S., Niu, Y., Huang, R., Wen, Q., Su, J., et al. (2018). A RAD-based genetic map for anchoring scaffold sequences and identifying QTLs in bitter gourd (Momordica charantia). Front. Plant Sci. 9. doi: 10.3389/fpls.2018.00477
Deng, Y., Liu, S., Zhang, Y., Tan, J., Li, X., Chu, X., et al. (2022). A telomere-to-telomere gap-free reference genome of watermelon and its mutation library provide important resources for gene discovery and breeding. Mol. Plant 15 (8), 1268–1284. doi: 10.1016/j.molp.2022.06.010
Dutta, S., Hazra, P., Saha, S., Acharya, B., Bhattacharjee, T., Kumar Maurya, P., et al. (2021). Applied mutagenesis could improve economically important traits in bitter gourd (Momordica charantia l.). J. Genet. 100, 34282734. doi: 10.1007/s12041-021-01283-5
Greene, E. A., Codomo, C. A., Taylor, N. E., Henikoff, J. G., Till, B. J., Reynolds, S. H., et al. (2003). Spectrum of chemically induced mutations from a large-scale reverse-genetic screen in arabidopsis. Genetics 164 (2), 731–740. doi: 10.1093/genetics/164.2.731
Henry, I. M., Nagalakshmi, U., Lieberman, M. C. (2014). Efficient genome-wide detection and cataloging of EMS-induced mutations using exome capture and next-generation sequencing. Plant Cell 26 (4), 1382–1397. doi: 10.1105/tpc.113.121590
Jander, G., Baerson, S. R., Hudak, J. A., Gonzalez, K. A., Gruys, K. J., Last, R. L. (2003). Ethylmethanesulfonate saturation mutagenesis in arabidopsis to determine frequency of herbicide resistance. Plant Physiol. 131 (1), 139–146. doi: 10.1104/pp.102.010397
Kaur, G., Pathak, M., Singla, D., Chhabra, G., Chhuneja, P., Kaur Sarao, N. (2021). Quantitative trait loci mapping for earliness, fruit, and seed related traits using high density genotyping-by-Sequencing-Based genetic map in bitter gourd (Momordica charantia l.). Front. Plant Sci. 12. doi: 10.3389/fpls.2021.799932
Lian, X., Liu, Y., Guo, H., Fan, Y., Wu, J., Guo, H., et al. (2020). Ethyl methanesulfonate mutant library construction in gossypium hirsutum l. for allotetraploid functional genomics and germplasm innovation. Plant J. 103 (2), 858–868. doi: 10.1111/tpj.14755
Li, Z., Jiang, L., Ma, Y., Wei, Z., Hong, H., Liu, Z., et al. (2017). Development and utilization of a new chemically-induced soybean library with a high mutation density. J Integr. Plant Biol. 59 (1), 60–74. doi: 10.1111/jipb.12505
Lin, Q., Zong, Y., Xue, C., Wang, S., Jin, S., Zhu, Z., et al. (2020). Prime genome editing in rice and wheat. Nat. Biotechnol. 38 (5), 582–585. doi: 10.1038/s41587-020-0455-x
Li, T., Yang, X., Yu, Y., Si, X., Zhai, X., Zhang, H., et al. (2018). Domestication of wild tomato is accelerated by genome editing. Nat. Biotechnol. 10, 30272626. doi: 10.1038/nbt.4273
Lu, X., Liu, J., Ren, W., Yang, Q., Chai, Z., Chen, R., et al. (2018). Gene-indexed mutations in maize. Mol. Plant 11 (3), 496–504. doi: 10.1016/j.molp.2017.11.013
Ma, X., Sun, X., Li, C., Huan, R., Sun, C., Wang, Y., et al. (2017). Map-based cloning and characterization of the novel yellow-green leaf gene ys83 in rice (Oryza sativa). Plant Physiol. Biochem. 111, 1–9. doi: 10.1016/j.plaphy.2016.11.007
Pan, Y., Wang, Y., McGregor, C., Liu, S., Luan, F., Gao, M., et al. (2020). Genetic architecture of fruit size and shape variation in cucurbits: a comparative perspective. Theor. Appl. Genet. 133 (1), 1–21. doi: 10.1007/s00122-019-03481-3
Qu, L. J., Qin, G. (2014). Generation and identification of arabidopsis EMS mutants. Methods Mol. Biol. 1062, 225–239. doi: 10.1007/978-1-62703-580-4_12
Rao, P. G., Behera, T. K., Gaikwad, A. B., Munshi, A. D., Boopalakrishnan, G. (2018). Mapping and QTL analysis of gynoecy and earliness in bitter gourd (Momordica charantia l.) using genotyping-by-Sequencing (GBS) technology. Front. Plant Sci. 9. doi: 10.3389/fpls.2018.01555
Rong, F., Chen, F., Huang, L., Zhang, J., Zhang, C., Hou, D., et al. (2019). A mutation in class III homeodomain-leucine zipper (HD-ZIP III) transcription factor results in curly leaf (cul) in cucumber (Cucumis sativus l.). Theor. Appl. Genet. 132 (1), 113–123. doi: 10.1007/s00122-018-3198-z
Snouffer, A., Kraus, C., van der Knaap, E. (2020). The shape of things to come: ovate family proteins regulate plant organ shape. Curr. Opin. Plant Biol. 53, 98–105. doi: 10.1016/j.pbi.2019.10.005
Sun, X., Li, X., Lu, Y., Wang, S., Zhang, X., Zhang, K., et al. (2022). Construction of a high-density mutant population of Chinese cabbage facilitates the genetic dissection of agronomic traits. Mol. Plant 15 (5), 913–924. doi: 10.1016/j.molp.2022.02.006
Sun, Y., Zhang, H., Fan, M., He, Y., Guo, P. (2020). A mutation in the intron splice acceptor site of a GA3ox gene confers dwarf architecture in watermelon (Citrullus lanatus l.). Sci. Rep. 10 (1), 14915. doi: 10.1038/s41598-020-71861-7
Wang, K., Shi, L., Liang, X., Zhao, P., Wang, W., Liu, J., et al. (2022). The gene TaWOX5 overcomes genotype dependency in wheat genetic transformation. Nat. Plants 8 (2), 110–117. doi: 10.1038/s41477-021-01085-8
Wei, C., Zhu, C., Yang, L., Zhao, W., Ma, R., Li, H., et al. (2019). A point mutation resulting in a 13 bp deletion in the coding sequence of cldf leads to a GA-deficient dwarf phenotype in watermelon. Hortic. Res. 6, 132. doi: 10.1038/s41438-019-0213-8
Yang, J., Weng, Y., Li, H., Kong, Q. (2022). Epidermal patterning factor 2-like (McEPFL2): A putative candidate for the continuous ridge (cr) fruit skin locus in bitter gourd (Momordica charantia l.). Genes (Basel) 13 (7), 35885929. doi: 10.3390/genes13071148
Zhang, M., Song, M., Davoudi, M., Cheng, F., Yin, J., Zha, G., et al. (2022). The mutation of c-24 reductase, a key enzyme involved in brassinolide biosynthesis, confers a novel compact plant architecture phenotype to cucumber. Theor. Appl. Genet. 135 (8), 2711–2723. doi: 10.1007/s00122-022-04144-6
Zhang, X., Wang, Y., Zhu, X., Wang, X., Zhu, Z., Li, Y., et al. (2020). Curled flag leaf 2, encoding a cytochrome P450 protein, regulated by the transcription factor Roc5, influences flag leaf development in rice. Front. Plant Sci. 11. doi: 10.3389/fpls.2020.616977
Zhong, J., Cheng, J., Cui, J., Hu, F., Dong, J., Liu, J., et al. (2022). MC03g0810, an important candidate gene controlling black seed coat color in bitter gourd (Momordica spp.). Front. Plant Sci. 13. doi: 10.3389/fpls.2022.875631
Keywords: bitter gourd, EMS, mutant collection, phenotype, germplasm
Citation: Yu R, Niu Y, Wang X, Yang K, Han X, Liu Z, Qi Z and Yang Y (2022) Construction of a density mutant collection in bitter gourd via new germplasms innovation and gene functional study. Front. Plant Sci. 13:1069750. doi: 10.3389/fpls.2022.1069750
Received: 14 October 2022; Accepted: 25 October 2022;
Published: 22 November 2022.
Edited by:
Qiusheng Kong, Huazhong Agricultural University, ChinaReviewed by:
Libo Han, Fujian Agriculture and Forestry University, ChinaXiaoqin Liu, Peking University, China
Copyright © 2022 Yu, Niu, Wang, Yang, Han, Liu, Qi and Yang. This is an open-access article distributed under the terms of the Creative Commons Attribution License (CC BY). The use, distribution or reproduction in other forums is permitted, provided the original author(s) and the copyright owner(s) are credited and that the original publication in this journal is cited, in accordance with accepted academic practice. No use, distribution or reproduction is permitted which does not comply with these terms.
*Correspondence: Yan Yang, eXppcWlAMTI2LmNvbQ==
†These authors have contributed equally to this work