- Key Laboratory of Bio-Resources and Eco-Environment of Ministry of Education, State Key Laboratory of Hydraulics and Mountain River Engineering, College of Life Sciences, Sichuan University, Chengdu, China
Actin depolymerization factors (ADFs), as actin-binding proteins, act a crucial role in plant development and growth, as well as in response to abiotic and biotic stresses. Here, we found that CARK3 plays a role in regulating hypocotyl development and links a cross-talk between actin filament and drought stress through interaction with ADF4. By using bimolecular fluorescence complementation (BiFC) and GST pull-down, we confirmed that CARK3 interacts with ADF4 in vivo and in vitro. Next, we generated and characterized double mutant adf4cark3-4 and OE-ADF4:cark3-4. The hypocotyl elongation assay indicated that the cark3-4 mutant seedlings were slightly longer hypocotyls when compared with the wild type plants (WT), while CARK3 overexpressing seedlings had no difference with WT. In addition, overexpression of ADF4 significantly inhibited long hypocotyls of cark3-4 mutants. Surprisingly, we found that overexpression of ADF4 markedly enhance drought resistance in soil when compared with WT. On the other hand, drought tolerance analysis showed that overexpression of CARK3 could rescue adf4 drought susceptibility. Taken together, our results suggest that CARK3 acts as a regulator in hypocotyl elongation and drought tolerance likely via regulating ADF4 phosphorylation.
Introduction
Actin filaments (F-actin), as a scaffold in cells, play a fundamental role in numerous cellular processes, including morphogenesis, motility and cell elongation. In addition, the studies have shown that F-actin also functions in response to abiotic and biotic stresses in planta (Lin et al., 2014). The dynamic of F-actin is regulated by actin-binding proteins (ABPs), including actin depolymerization factors (ADFs), formin family proteins and adenylyl cyclase-associated proteins (Liu et al., 2018; Jiang et al., 2019).
ABPs are involved in regulating a vast array of fundamental biological processes, such as the normal organism development (Ketelaar et al., 2004). For example, the actin-binding protein Rice Morphology Determinant (RMD) in rice (Oryza sativa) promotes statolith mobility in gravisensing endodermal cells, and for proper auxin distribution in light-grown shoots through reorganization of the actin cytoskeleton (Song et al., 2019). ADF7 and ADF10 directly modulate pollen tube elongation in Arabidopsis (Zheng et al., 2013; Jiang et al., 2017; Jiang et al., 2022). UV-B inhibits Arabidopsis hypocotyl elongation due to the fact that actin filaments shift from bundles to a loose arrangement (Du et al., 2020b). PRF4 and PRF5, as actin-binding proteins, could regulate vesicle trafficking and polarity establishment during pollen germination by enhancing the interaction between AtFH5 (Arabidopsis formin homology 5) and actin filaments (Liu et al., 2021). In the dark, ADF4 mutants have longer hypocotyl and epidermal cells when compared with wild-type seedlings (Henty et al., 2011; Yao et al., 2022). However, the molecular mechanism of ADF4 is not uncovered in regulating the hypocotyl elongation.
In addition, ABPs have been implicated in integration of cellular responses to extracellular and intracellular signals during biotic and abiotic stresses. GhVLN4 from cotton, as one type of major ABPs responsible for microfilament bundling, overexpression of which shows resistance to Verticilium dahliae, salt and drought stresses in Arabidopsis. (Ge et al., 2021). Actin-depolymerizing factor 1 (ADF) is repressed by MYB73, which is known as a negative regulator in salt tolerance, and then regulates actin filaments organization (Wang et al., 2021). Additionally, ADF4 is mediated by CPK3 to control pathogen-induced actin reorganization during immune signaling, and phosphorylated by CKL2 to regulate actin filaments in stomata in response to dehydration (Guo et al., 2016; Lu et al., 2020). However, the recent studies have revealed that ADF5 has evolved F-actin-bundling activity, thus positively regulates drought stress (Qian et al., 2019). DaADF3 from Deschampsia antarctica is induced by drought stress (Byun et al., 2021). Knockdown of TaADF3 from wheat leads to Puccinia striiformis susceptibility, which is accompanied by increased ROS production (Tang et al., 2015). ADFs regulate the dynamics of actin filaments in response to environmental cues.
Our previous studies have shown that CAK3 kinase protein could form dimer to phosphorylate ABA (abscisic acid) receptors, and subsequently trigger ABA signaling pathway (Li et al., 2022). Here, we observed that CARK3 T-DNA mutants exhibited long hypocotyl compared with the wild-type plants. Further, we found that CARK3 phosphorylates ADF4, and the association cooperatively mediates the hypocotyl growth in the dark. Moreover, the interaction between CARK3 and ADF4 led us to investigate a role of CARK3 during drought stress. The evaluation of drought tolerance reveals that overexpression of ADF4 could resume cark3-4 drought sensitivity in Arabidopsis.
Materials and methods
Plant materials and growth conditions
Plant materials and growth conditions Arabidopsis plants used in this study were all in the Columbia (Col-0) background. The cark3-4 and adf4 T-DNA insertion mutants, OE-ADF4 #10, #17, #18 and CARK3-OE 2 overexpression plants were previously described (Wang et al., 2020; Yao et al., 2022). Plants were grown on soil-vermiculite mixtures at 22°C under 60% relative humidity with cycles of 16 h light and 8 h dark. For plate experiment, seeds were stratified in distilled water for 3 days at 4°C in dark. The imbibed seeds were surface sterilized with a 20% bleach solution for 15 min and then washed 5 times with sterilized water, and then sown on Murashige and Skoog (MS) medium containing 2% sucrose and 1.0% agar, pH 5.8.
Bimolecular fluorescence complementation (BiFC) assay
The ADF4-YFPN and CARK3-YFPC constructs were previously described (Wang et al., 2020; Yao et al., 2022). The constructs were transformed into Agrobacteria GV3101 stain. And then the indicated YFPN/YFPC combination were co-expressed in 4-week old tobacco leaves. After 2 days, the signals of YFP were observed with a Leica confocal laser scanning microscope (DM4 B). 3 leaves were observed each time and three biological repeats were performed.
GST pull-down assay
To test the interaction of CARK3 with ADF4 in vitro, GST-CARK3, GST and His-ADF4 were purified as previously described (Li et al., 2022; Yao et al., 2022). 5 μg GST-CARK3 or GST bound glutathione-agarose beads were incubated with His-ADF4 in binding buffer (50 mM Tris-HCl, pH 8.0, 200 mM NaCl, 10% glycerol, 0.1% Tween 20) at room temperature for 30 min and washed 10 times with binding buffer. The pulled-down compounds were eluted with 2× SDS sample buffer (24 mM Tris-Cl, pH 6.8, 10% glycerol, 0.8% SDS, and 2% 2-mercaptoethanol) and boiled at 98°C for 10 min. Next, proteins were analyzed by immunoblotting with GST and His antibodies.
In vitro kinase assay
To investigate CARK3-mediated phosphorylation of ADF4, the purified ADF4 was incubated with His-CARK3-KD (Kinase domain) in kinase buffer (20 mM Tris, pH 7.5, 1 mM MgCl2, 100 mM NaCl, and 1 mM DTT, 5 mM ATP), which was previously described (Wang et al., 2020). After incubation at 30°C for 30 min, the reaction mixture was terminated by adding an equal volume of 2 × SDS-PAGE (Sodium Dodecyl Sulfate, Polyacrylamide Gel Electrophoresis) loading buffer, and boiled at 98°C for 5 min. The proteins were separated by SDS–PAGE and analyzed with Anti-Phosphoserine/threonine Rabbit Polyclonal (Cell Signaling Technology).
Physiological analysis
To test the interaction of CARK3 with ADF4 in response to soil drought stress, we obtained the double mutant adf4cark3-4 and OE-ADF4:cark3-4 by crosses. For drought tolerance experiment, 7 d seedlings indicated genotypes were grown under the same conditions and then subjected to drought stress treatment by withholding water for 12 or 13 days. Then, 2 days after rehydration, the morphological changes of plants were recorded, including survival rate and fresh weight (FW).
For hypocotyl elongation assay, genotypes were grown in the dark for 4 d after germination and then representative seedlings were photographed. At least 20 seedlings per genotype were measured as one biological replicate using ImageJ software (http://imagej.nih.gov/ij/).
Stomatal aperture was carried out as previously described (Li et al., 2022). 3-week old leaves were peeled and then incubated in opening buffer (50 mM KCl, 10 μM CaCl2, 10 mM MES pH 6.15) for 3 h to induce stomatal opening. For ABA treatment, 5 and 20 μM ABA was added to the opening buffer for 30 min or 2 h. Images were taken using Leica TCS SP5 II HCS confocal microscope.
For nitroblue tetrazolium (NBT) staining to test superoxides, 3-week-old leaves were treated with or without 50 μM ABA for 1 days, and then incubated in NBT buffer for overnight. Chlorophyll was removed with 95% ethanol.
Statistical analysis
In this study, statistical data were analyzed using GraphPad Prism version 8 software (GraphPad Software, La Jolla, CA, USA). Data were collected in triplicates and subjected to analysis of variance (ANOVA). Post hoc means separation was done with Tukey’s test at P < 0.05.
Results
CARK3 is involved in hypocotyl elongation
In this study, we found that cark3-4 T-DNA mutants and RNAi plants exhibited longer length of hypocotyl than WT (Figure 1). In addition, the overexpression of CARK3 lines had markedly shorter hypocotyl when compared with the wild-type plants (Figure 1). These results suggest that CARK3 modulates hypocotyl growth in Arabidopsis.
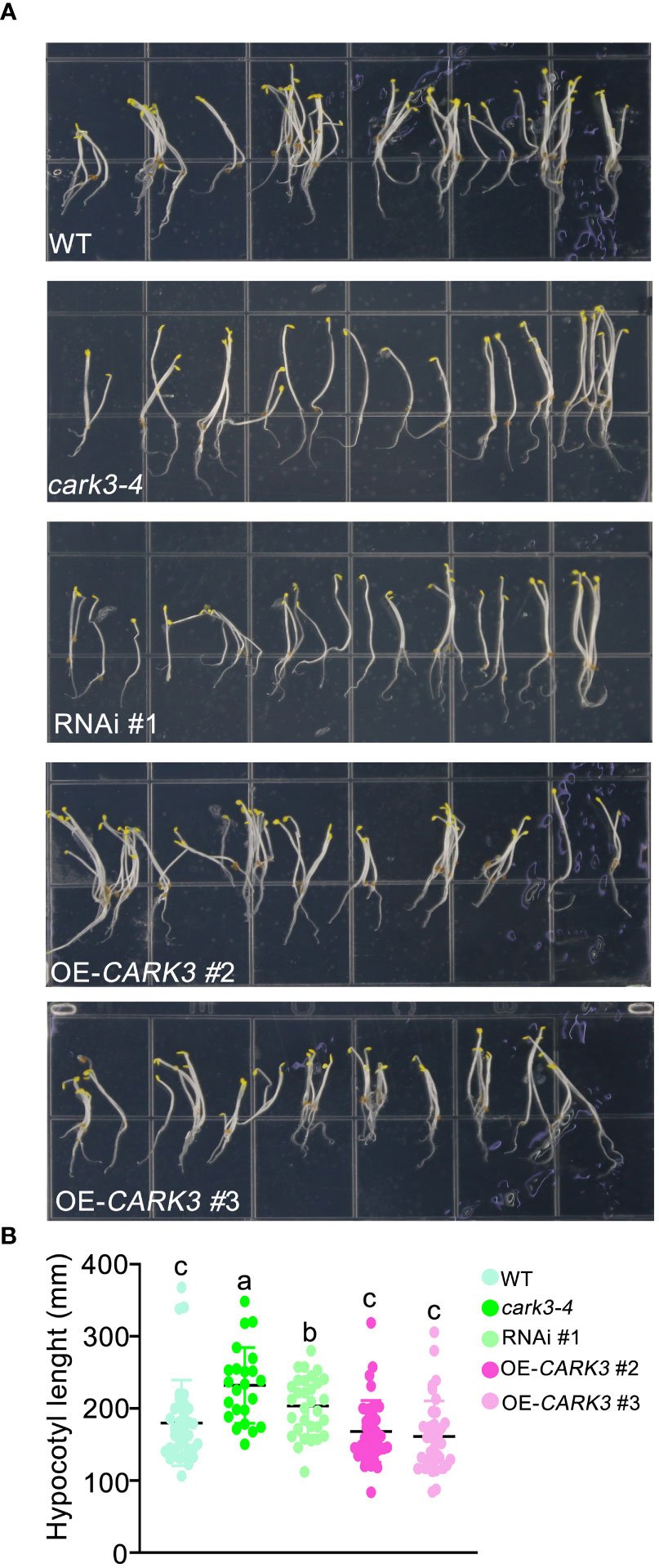
Figure 1 CARK3 is involved in hypocotyl elongation. (A) Seedlings from wild-type plants, CARK3 T-DNA insertion mutant (cark3-4), RNAi #1 plant and CARK3-overexpressing lines (CARK3-OE 2/3) grown on 1/2 MS growth medium in the dark for 4 d. (B) The graph shows the average hypocotyl length measured from a minimum of 20 seedlings. Each point represents an individual seedling. Lines and error bars represent the mean and 95% confidence interval for each genotype, respectively. Bars with different alphabets indicate significant difference by Tukey test at P < 0.05, while those with a common alphabet indicate no significant difference (P > 0.05).
CARK3 phosphorylates ADF4
In our previous study, we reported that 14-3-3κ coordinates ADF4 to regulate hypocotyl elongation (Yao et al., 2022). The 14-3-3 proteins usually interact with kinase proteins to mediate signal transduction by altering their activity, or affinity to other proteins (Paul et al., 2012). Thus, we proposed that CARK3 would interact with 14-3-3κ. However, the bimolecular fluorescence complementation (BiFC) assay showed that CARK3 could not interact with 14-3-3κ in vivo. Next, we tested the association of CARK3 with ADF4 using the BiFC assay. The yellow fluorescent signals were observed in tobacco cells when co-expression of ADF4-YFPN and CARK3-YFPC. However, no signal was found, when co-expression of ADF4-YFPN and YFPC or YFPN and CARK3-YFPC (Figure 2A). These results indicate that CARK3 interact with ADF4 in vivo.
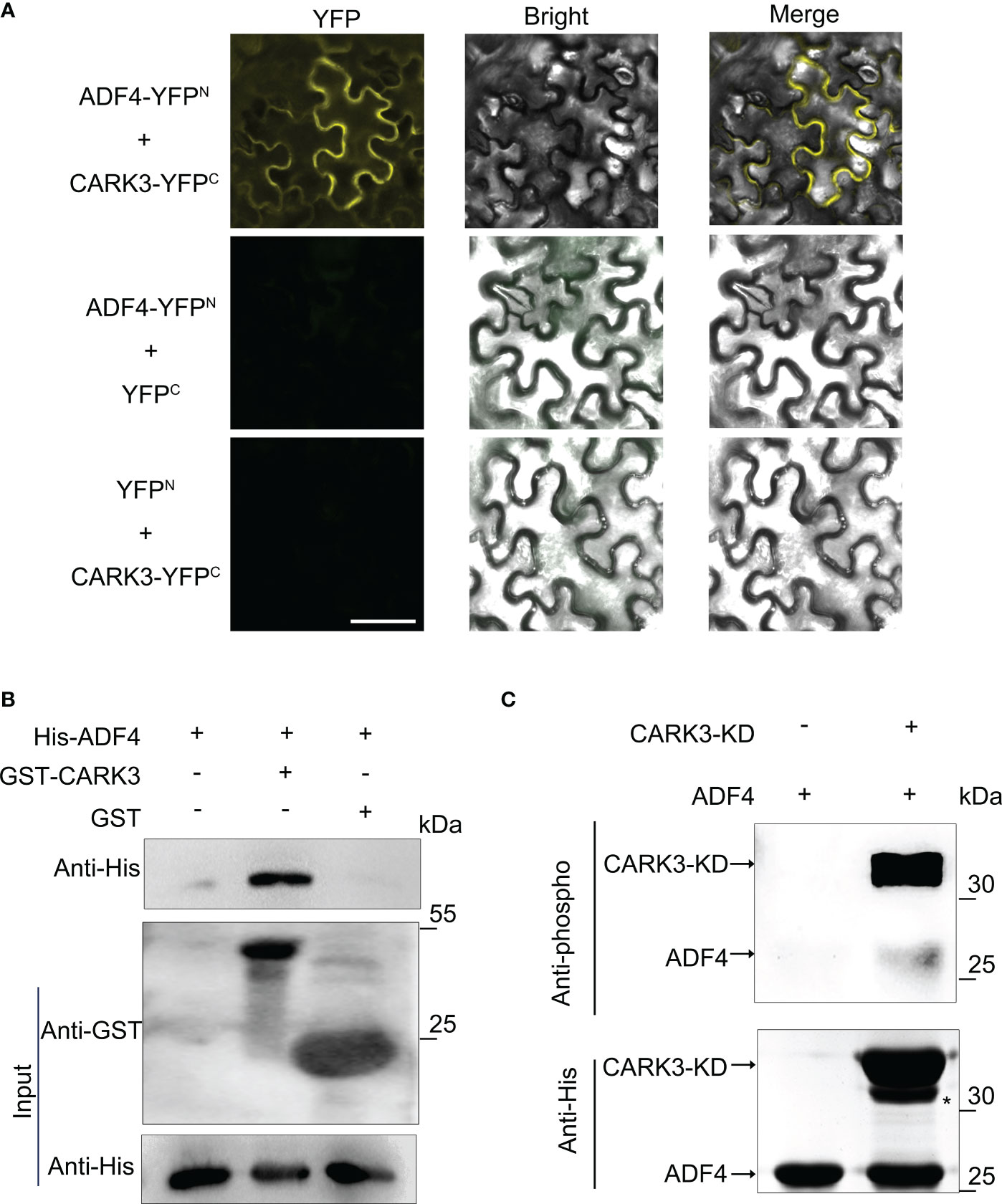
Figure 2 CARK3 interacts with ADF4. (A) BiFC assays showing interaction between CARK3 and ADF4 in tobacco leaves. Negative controls used were ADF4-YFPN +YFPC and YFPN +CARK3-YFPC. Scale bars = 50 μm. (B) Pull-down assays showing that GST-CARK3 interacts with His-ADF4. His-ADF4 pulled down by GST-CARK3 was detected by anti-His antibody. GST was used as a negative control and did not interact with His-ADF4. (C) In vitro phosphorylation of ADF4 by CARK3. Western blot assay with anti-phosphoserine/threonine antibody. Anti-His antibody was used to test loading.
To further confirm the physical interaction of CARK3 and ADF4, we then performed an in vitro pull-down assay using bacterially expressed purified proteins. GST and His-ADF4, GST-CARK3 and His-ADF4 were co-incubated with GST resin, respectively. The bound proteins were tested with anti-GST and anti-His antibodies, respectively. As indicated in Figure 2B, GST-CARK3 could pull-downed His-ADF4, while GST could not, indicating that CARK3 physically interacts with ADF4 in vitro.
CARK3 has been shown to phosphorylate ABA receptors to mediate ABA signaling pathway (Li et al., 2022). ADF4 has revealed to be phosphorylated by CKL2 (Arabidopsis thaliana casein kinase 1-like protein 2) and CPK3 (Arabidopsis calcium-dependent protein kinase 3), showing multiple potential Ser/Thr phosphorylation sites (Guo et al., 2016; Lu et al., 2020). Thus, ADF4 could be a substrate of CARK3. To address this, an in vitro kinase assay was conducted. The phosphorylation band was observed, when ADF4 was incubated with CARK3. However, no band was detected in the gel without CARK3 (Figure 2C). The results suggest that CARK3 phosphorylates ADF4 in vitro.
CARK3-mediated ADF4 regulates hypocotyl elongation
To investigate ADF4 phosphorylated by CARK3 in regulation of hypocotyl elongation, we generated adf4cark3-4 double mutant and OE-ADF4:cark3-4 by crossing cark3-4 with adf4 and OE-ADF4, respectively (Yao et al., 2022). Next, sterilized seeds from WT, adf4, cark3-4, adf4cark3-4 and OE-ADF4:cark3-4 were plated on 1/2 MS plates grown vertically in the dark. After 4 d, we observed that adf4cark3-4 double mutants displayed longer hypocotyls when compared with the cark3-4 plants, and no significant difference with adf4 or cark3-4 (Figures 3A, B). On the other hand, the hypocotyl length in the cark3-4 mutant was shorter than that of the OE-ADF4:cark3-4 seedlings, which had longer hypocotyl length than OE-ADF4 transgenic plants (Figures 3C, D). The hypocotyl length of the adf4 seedlings exhibited longer than that of WT, which is consistent with the previous report (Henty et al., 2011; Yao et al., 2022). Considering that CARKs are involved in ABA signaling, we also investigated ABA-mediated hypocotyl growth in darkness. The results showed that cark3-4 seedlings were insensitive to ABA (Figure S1), which may due to the fact that cark3-4 plants have higher germination late that WT under ABA treatment (Wang et al., 2020). These results suggest that the phosphorylation of ADF4 by CARK3 mediates hypocotyl elongation in Arabidopsis.
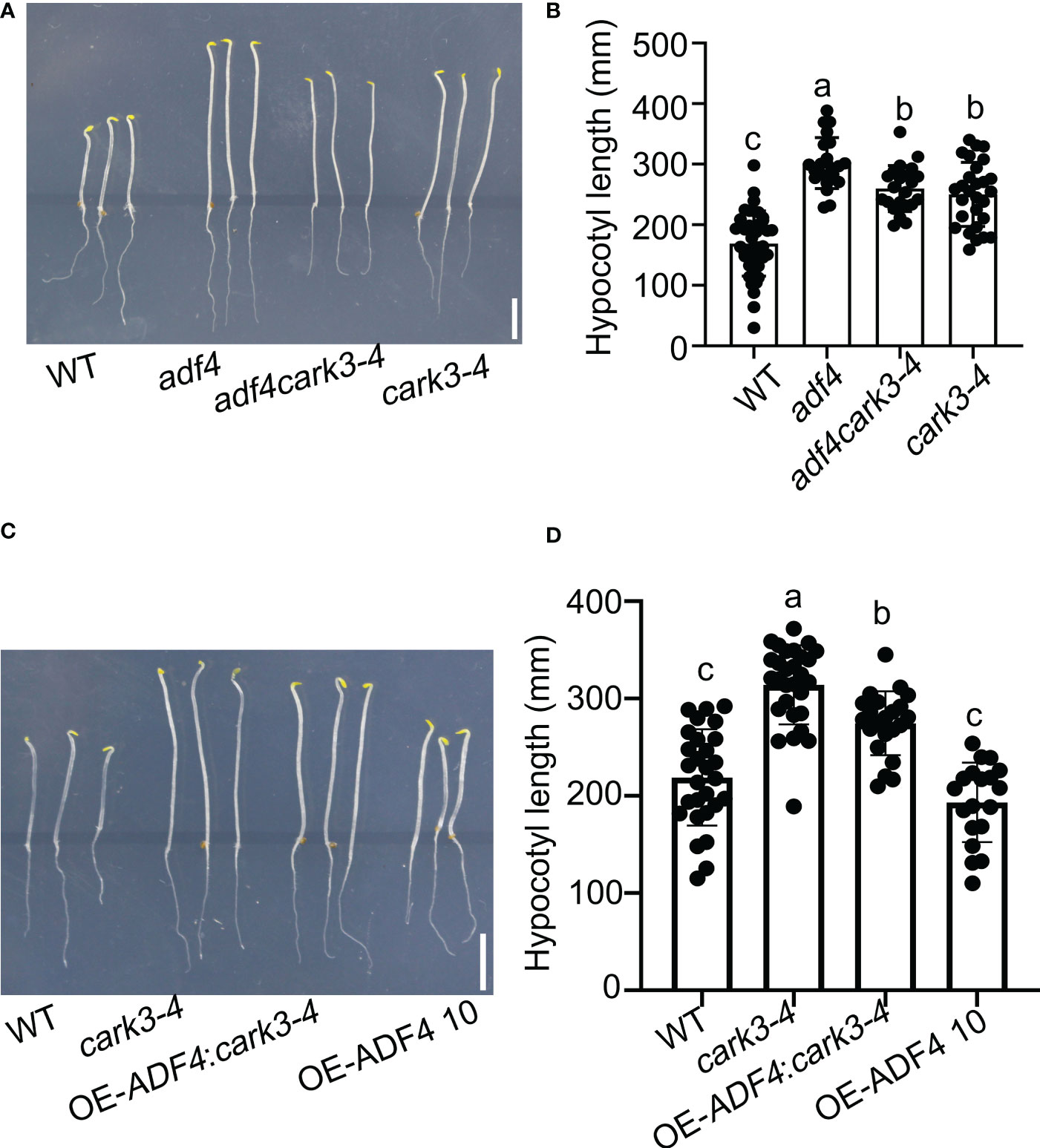
Figure 3 Overexpression of ADF4 partially inhibited hypocotyl growth of cark3-4 mutants. (A) adf4cark3-4 seedlings developed shorter hypocotyls when compared with adf4 mutants. (C) OE-ADF4: cark3-4 seedlings developed shorter hypocotyls than cark3-4 mutants. (B, D) The graphs shows the average hypocotyl length measured from a minimum of 20 seedlings in (A) and (C), respectively. Each point represents an individual seedling. Lines and error bars represent the mean and 95% confidence interval for each genotype, respectively. Bars with different alphabets indicate significant difference by Tukey test at P < 0.05, while those with a common alphabet indicate no significant difference (P > 0.05).
Overexpression of ADF4 enhances long-term drought tolerance
The previous studies have shown that CARK3 phosphorylates ABA receptors, triggers ABA binding, resulting in plant resistance to drought stress (Wang et al., 2020). In addition, ADF4 is also involved in stomatal closure through reorganization actin filaments (Guo et al., 2016). Thus, we proposed that CARK3 participates in drought tolerance, through regulation of ADF4 activity. To test this, we performed the drought tolerance assay in soil. The results showed that CARK3-OE plants had higher survival rate, while cark3-4 mutants had lower when compared with the wild-type (WT) plants (Figures 4A, B). We further examined stomatal closure in response to ABA. The data showed that the cark3-4 mutant was insensitive to ABA-induced stomatal closure under low (5 μM) and high (20 μM) concentration of ABA treatment (Figure 4C). Interestingly, we found that OE-ADF4 lines were similar to WT, with 100% survival rate (Figures 5A, B), while the percent survival of adf4 was significantly lower than those of WT after drought treatment. The results of fresh weight also confirmed the phenotype analysis (Figure 5C). Additionally, the adf4 mutant was sensitive to the low concentration of exogenous ABA (Figure 5D), which is consistent with the previous report (Guo et al., 2016). However, we here found that the adf4 mutant was insensitive to ABA-induced stomatal closure, the plants overexpressing ADF4 were sensitive under the high concentration of exogenous ABA treatment (Figure 5D). These data reveal that CARK3 and ADF4 are positive regulators in response to soil drought stress in Arabidopsis.
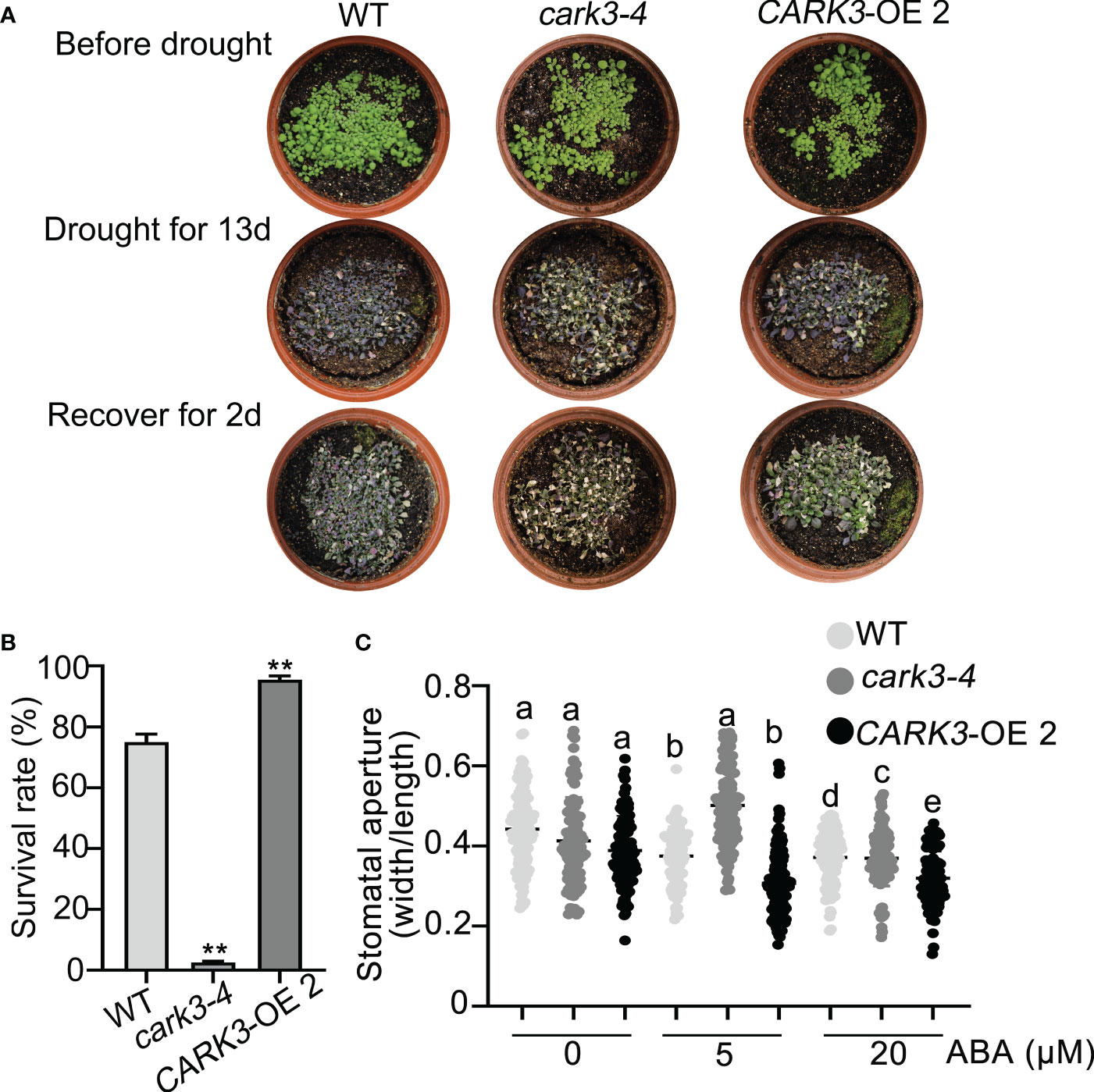
Figure 4 Overexpression of CARK3 enhance Arabidopsis drought tolerance. (A) The representative images of the plants from WT, cark3-4 and CARK3-OE that were grown for 7 days under normal conditions, then treated for 13 days with drought, and rewatered for 2 days. (B) Quantitative analysis of survival rates in (A). Mean ± SEM, n = 3. **P < 0.05, Student’s t-test. The experiment was repeated three times, at least five pots at a time. (C) ABA-induced stomatal closure was measured with or without ABA treatment for 30 min. Low (5 μM) or high (20 μM) concentrations of ABA were added to the opening buffer. Each point represents some individual stomata, at least 50 stomata per genotype were measured. Bars with different alphabets indicate significant difference by Tukey test at P < 0.05, while those with a common alphabet indicate no significant difference (P > 0.05).
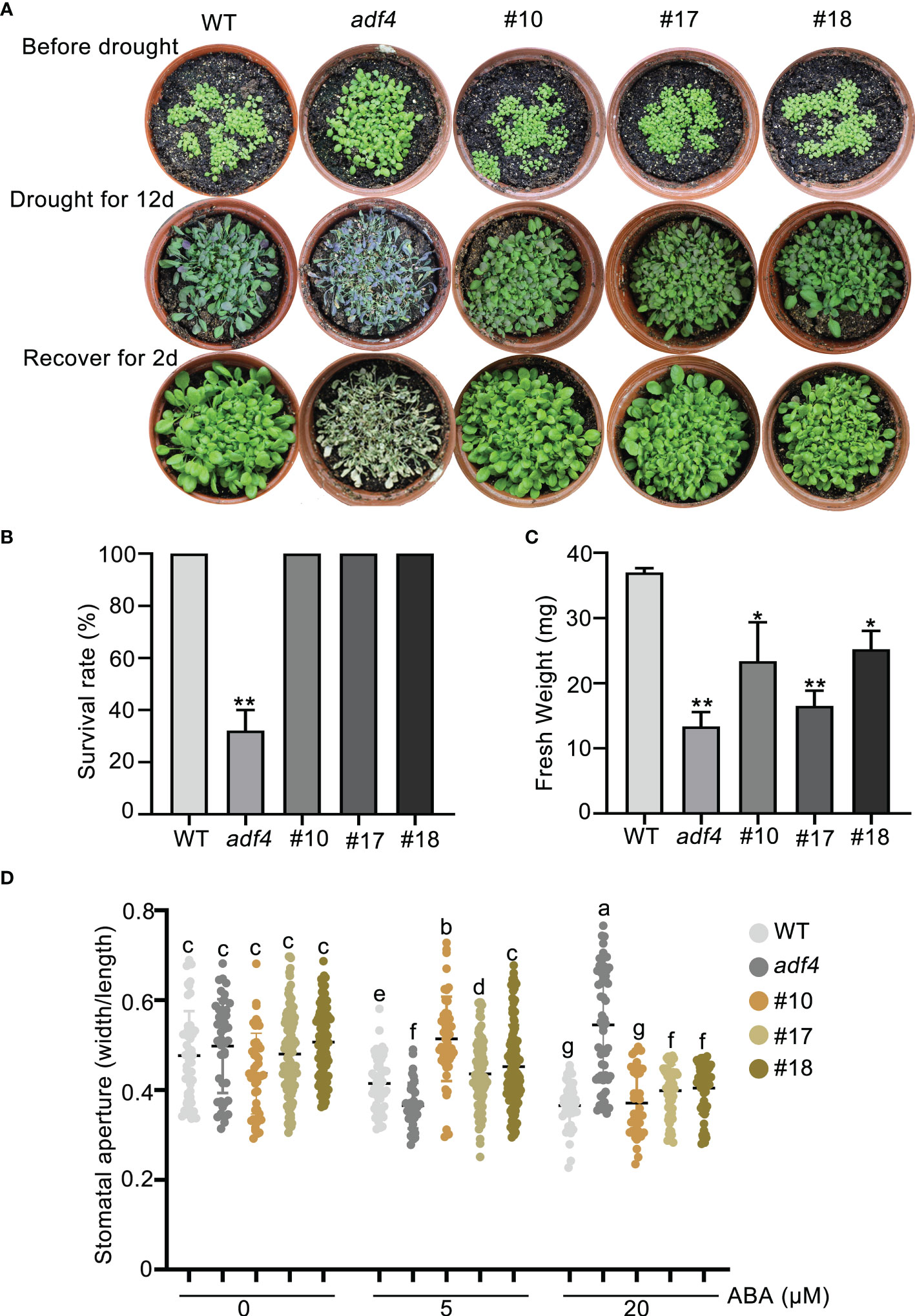
Figure 5 Overexpression of ADF4 enhance Arabidopsis drought tolerance. (A) Images of plants before and after drought and after rewatering, including WT, adf4 and OE-ADF4 lines. The plants were grown for 7 days under normal conditions, then treated for 12 days without watering and rewatered for 2 days. (B, C) Quantities of survival rates and fresh weight of per pot from genotypes after rewatering in (A). The error bars indicate the SEM from three replicates (n = 3). *P < 0.05, **P < 0.01, Student’s t-test. The experiment was repeated three times, at least three pots at a time. (D) Stomatal aperture of WT, adf4 and overexpression of ADF4 plants. Four-week-old Arabidopsis leaves were treated with or without low (5 μM) and high (20 μM) concentrations of ABA for 2 h, respectively. Each point represents some individual stomata, at least 50 stomata per genotype were measured (the ratio of width to length). Bars with different alphabets indicate significant difference by Tukey test at P < 0.05, while those with a common alphabet indicate no significant difference (P > 0.05). #10, #17 and #18 represent the overexpression of ADF4 lines in wild-type plants.
Drought resistance by CARK3 is dependent on ADF4
To determine the genetic relation of CARK3 with ADF4 in drought resistance, we performed drought tolerance experiment. The results showed that the adf4cark3-4 double mutant had lower level of survival rate than WT, which had 100% survival rate and higher level than that of adf4 or cark3-4 genotype (Figures 6A, B). Similarly, WT plants had highest fresh weight after rewatered following 12-day dehydration (Figure 6C). ABA-induced stomatal closure assay also confirmed that the loss of ADF4 rescued the ABA insensitivity of cark3-4 mutant under both low and high levels of exogenous ABA treatment (Figure 6D). Next, we analyzed OE-ADF4:cark3-4 plants in drought tolerance compared with OE-ADF4, cark3-4 and WT, respectively. The results showed that the percent survival rate of WT, OE-ADF4 and OE-ADF4:cark3-4 plants were indistinguishable (Figure 7A). However, OE-ADF4:cark3-4 showed better and had higher level of fresh weight when compared WT OE-ADF4 plants (Figures 7B, C). For ABA-induced stomatal aperture assay, we observed that the stomatal sensitivity of OE-ADF4:cark3-4 plants to ABA recovered to that of the WT plants after low concentration of ABA treatment (Figure 7D). ABA induced-stomatal closure under drought stress also contributes to the production of hydrogen peroxide (H2O2) (An et al., 2019; Pei et al., 2022). In this study, we found that H2O2 accumulation in adf4 mutants was higher than that in WT, while the ADF4-overexpressing line (#17) produced less H2O2 after ABA treatment (50 μM) (Figure S2). These phenotypic data indicate overexpression of ADF4 mediates long-term drought tolerance through the its phosphorylation by CARK3.
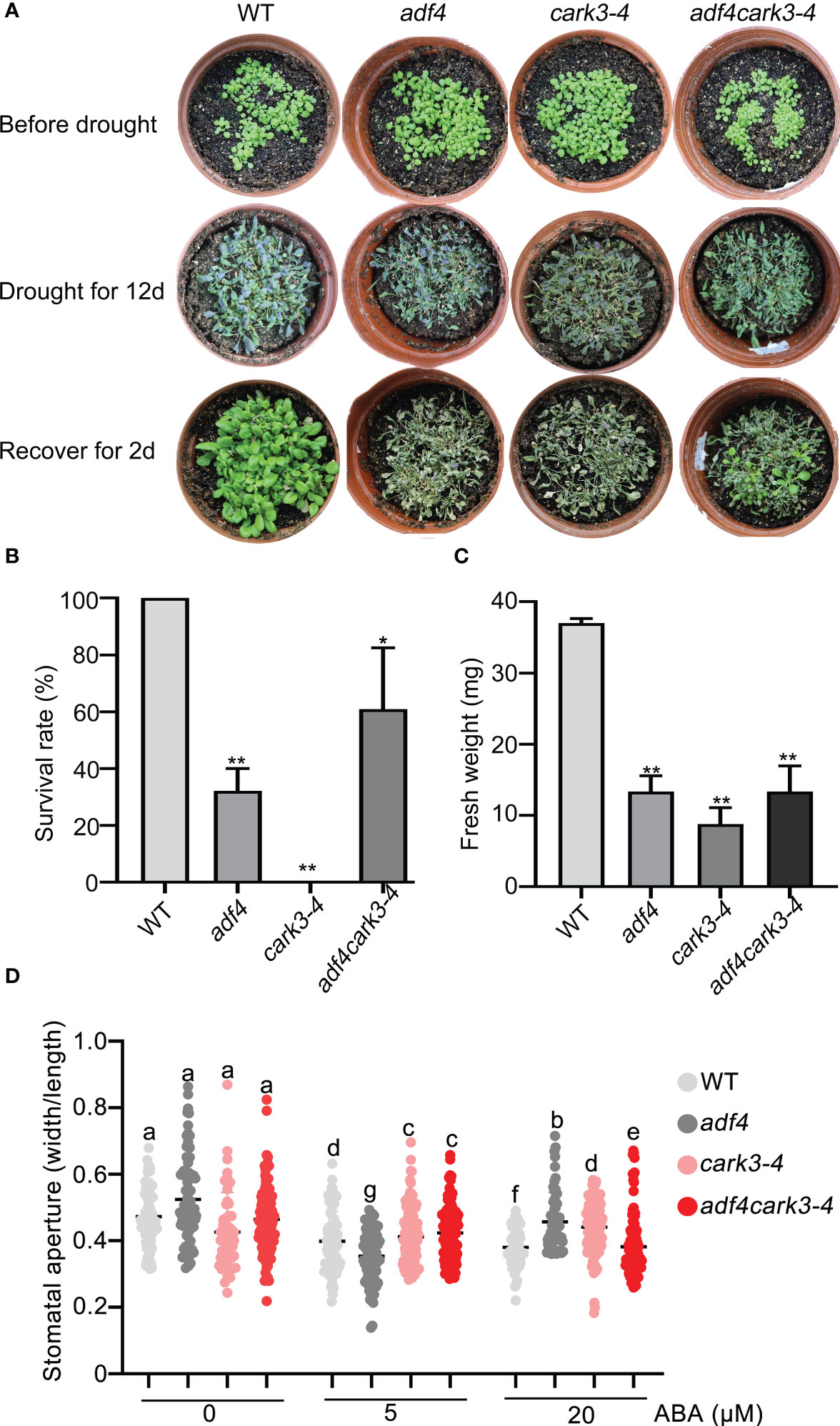
Figure 6 adf4 partially resumes cark3 in response to soil drought stress. (A) Phenotypic comparison of WT, cark3-4, adf4 and adf4cark3-4 plants (upper) grown in soil after water was withheld for 12 days (middle) and the plants were then rewatered for 2 day (bottom). Three independent experiments were performed that yielded similar results; 5 pots per genotype for each time. (B, C) The survival rates and fresh weight were assessed in three replicates (n = 3). *P < 0.05, **P < 0.05, Student’s t-test. (D) Stomatal closure (the ratio of stomatal width/length) was measured in WT, cark3-4, adf4 and adf4cark3-4 plants in response to 5 μM and 20 μM exogenous ABA. Each point represents some individual stomata, at least 50 stomata per genotype were measured. Bars with different alphabets indicate significant difference by Tukey test at P < 0.05, while those with a common alphabet indicate no significant difference (P > 0.05).
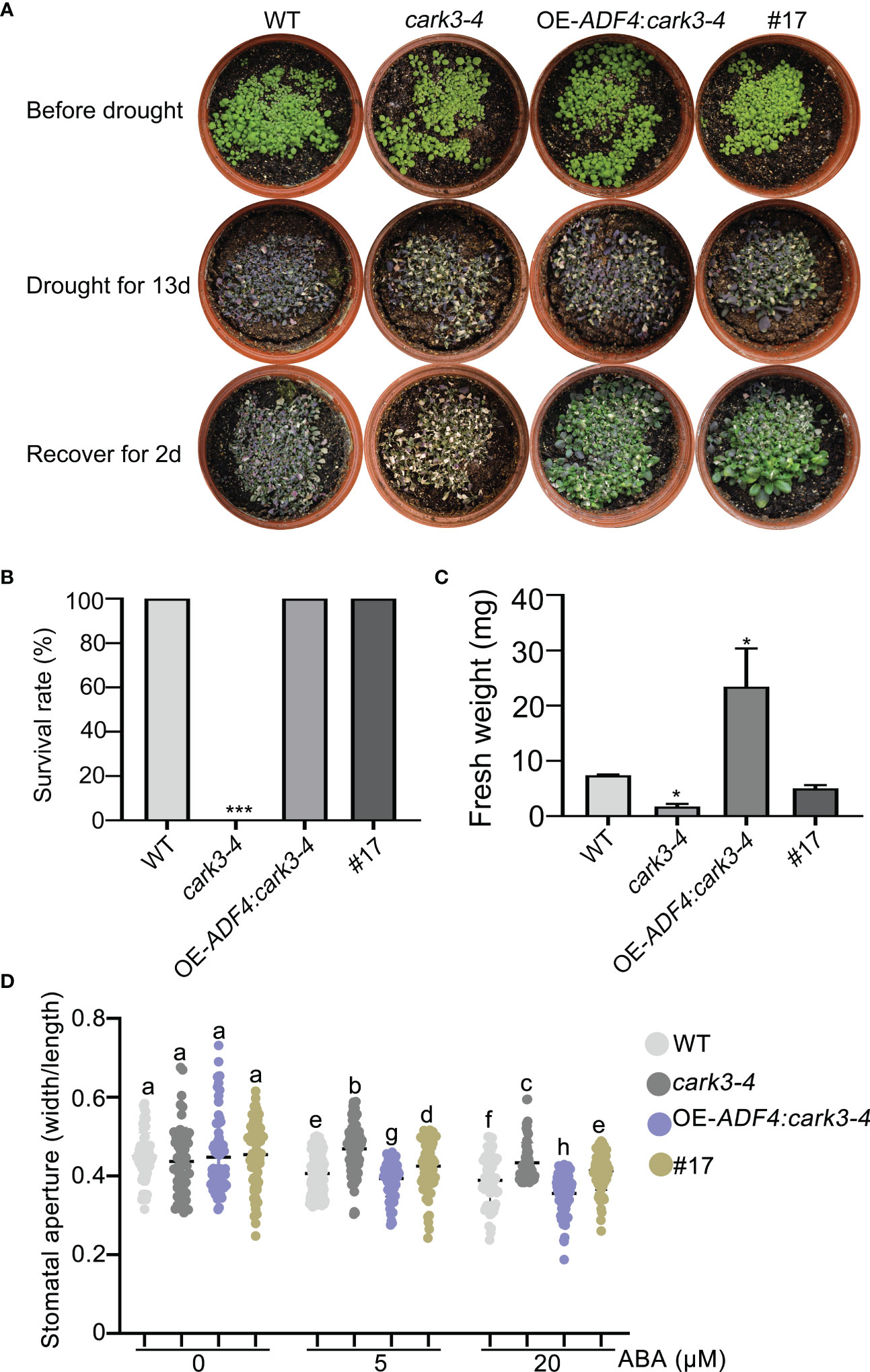
Figure 7 Overexpression of CARK3 rescues adf4 drought sensitivity. (A) Images of plants before and after drought and after rewatering, including WT, cark3-4 and OE-ADF4: cark3-4 genotypes. The plants were grown for 7 days under normal conditions, then treated for 13 days with drought, and rewatered for 2 days. (B, C) The survival rates and fresh weight were assessed in three replicates (n = 3). *P < 0.05, ***P < 0.001, Student’s t-test. (D) ABA-induced stomatal closure was measured in each plant indicated under low (5 μM) and high (20 μM) concentrations of ABA treatment for 2 h. Each point represents some individual stomata, at least 50 stomata per genotype were measured. Bars with different alphabets indicate significant difference by Tukey test at P < 0.05, while those with a common alphabet indicate no significant difference (P > 0.05).
Discussion
Plants need to respond to photomorphogenesis when grown in the light, and also undergo skotomorphogenesis in the dark, exhibiting long hypocotyls, as well as survive in response to multiple developmental and environmental cues. Hypocotyl length is usually controlled by cell elongation which is regulated by the dynamics of actin filaments (Fan et al., 2013; Zhao et al., 2015; Du et al., 2020b). In this study, we demonstrated that the phosphorylation of ADF4 mediated by CARK3 controls hypocotyl cell elongation and modulates soil dehydration in Arabidopsis.
Previous studies have revealed cross-talk between auxin and ABA signaling transduction (Emenecker et al., 2021). Auxin could induce reorganization of actin filaments (Du et al., 2020a). Loss of AUX1 (auxin transporter AUXIN RESISTANT 1) leads to failure of actin reorganization in response to IAA treatment (Arieti and Staiger, 2020). ADF4 modulates auxin distribution and transport through regulating the dynamics of actin filaments, which is required for cell expansion (Deeks and Hussey, 2009; Jiang et al., 2020). Additionally, ABA receptors PYL8 (RCAR3) and PYL9 (RCAR1) could interact with the transcription factor PIF3 to regulate hypocotyl elongation (Qi et al., 2020). Our previous study shows that CARKs phosphorylate ABA receptors (Zhang et al., 2018). Thus, the interaction of ADF4 and CARK3 offers exciting new insights into the cross-talk between ABA signaling and actin filament in hypocotyl elongation.
The previous studies have revealed that adf4 mutants have smaller stomatal aperture than the wild-type plants under 2 μM ABA treatment, indicating that ADF4 acts a negative regulator in ABA-mediated drought tolerance (Guo et al., 2016). However, adf4 mutants displayed lower survival rate compared with WT, when genotypes were subjected to long-term drought stress (Figure 5). We inferred that the inhibition of ADF4 activity by CARK3, leading to fail actin filament disassembly during stomatal closure. However, CKL2 inhibits ADF4 activity on severing actin filaments, leading to actin arrays being reorganized into highly bundled long cables and maintain stomatal closure (Guo et al., 2016). The clade A PP2C phosphatase would inhibit CKL2 kinase activity, when water is supplied (Shi et al., 2021). Thus, overexpression of ADF4 triggers F-actin in guard cell from cortical filaments to randomly distributed (Hwang and Lee, 2001), then CKL2 and CARK3 inhibit ADF4 activity, and then actin filaments changes to longitudinal direction, which stabilizes guard cells in closed stomata. Similarly, CPK3-mediated ADF4 governs actin filaments organization and activate pattern- and effector-triggered immunity (Lu et al., 2020). In summary, ADF4 is mediated by upstream regulators during plant development and in response to abiotic and biotic stresses.
Data availability statement
The original contributions presented in the study are included in the article/Supplementary Material. Further inquiries can be directed to the corresponding author.
Author contributions
XYL and YY conceived and designed the experiment. LP and JH conducted the BiFC analysis. HY and QY preformed Arabidopsis crossing. KL, YH, and LC conducted drought tolerance experiments. XFL, YY, and XYL analyzed the results and wrote the paper. All authors contributed to the article and approved the submitted version.
Funding
This study was funded by the Science & Technology Department of Sichuan Province (2022NSFSC0162) and the Fundamental Research Funds for Central Universities (10822041A2018).
Conflict of interest
The authors declare that the research was conducted in the absence of any commercial or financial relationships that could be construed as a potential conflict of interest.
Publisher’s note
All claims expressed in this article are solely those of the authors and do not necessarily represent those of their affiliated organizations, or those of the publisher, the editors and the reviewers. Any product that may be evaluated in this article, or claim that may be made by its manufacturer, is not guaranteed or endorsed by the publisher.
Supplementary material
The Supplementary Material for this article can be found online at: https://www.frontiersin.org/articles/10.3389/fpls.2022.1065677/full#supplementary-material
Supplementary Figure 1 | Statistical analysis of hypocotyl length of seedlings in darkness. Seeds were sown on plants containing 0.1 μM ABA. Over 20 seedlings per genotype were measured after 4 d. Violin plot showing the average stomatal aperture (width/length). Student’s t-test, *P < 0.05.
Supplementary Figure 2 | ABA-induced ROS accumulation. NBT staining indicates different levels of ABA-induced H2O2 production in leaves of the plants indicated. To detect O2-, 4-week-old plant leaves were staining with NBT after 2 days with or without 50 μM ABA.
References
An, J., Li, Q., Yang, J., Zhang, G., Zhao, Z., Wu, Y., et al. (2019). Wheat f-box protein TaFBA1 positively regulates plant drought tolerance but negatively regulates stomatal closure. Front. Plant Sci. 10. doi: 10.3389/fpls.2019.01242
Arieti, R. S., Staiger, C. J. (2020). Auxin-induced actin cytoskeleton rearrangements require AUX1. New Phytol. 226, 441–459. doi: 10.1111/nph.16382
Byun, M. Y., Cui, L. H., Lee, A., Oh, H. G., Yoo, Y. H., Lee, J., et al. (2021). Abiotic stress-induced actin-depolymerizing factor 3 from deschampsia antarctica enhanced cold tolerance when constitutively expressed in rice. Front. Plant Sci. 12. doi: 10.3389/fpls.2021.734500
Du, M., Spalding, E. P., Gray, W. M. (2020a). Rapid auxin-mediated cell expansion. Annu. Rev. Plant Biol. 71, 379–402. doi: 10.1146/annurev-arplant-073019-025907
Du, M., Wang, Y., Chen, H., Han, R. (2020b). Actin filaments mediated root growth inhibition by changing their distribution under UV-b and hydrogen peroxide exposure in arabidopsis. Biol. Res. 53, 54. doi: 10.1186/s40659-020-00321-3
Emenecker, R. J., Cammarata, J., Yuan, I., Strader, L. C. (2021). Abscisic acid modulates auxin-responsive hypocotyl elongation. Preprint. doi: 10.1101/2021.04.25.441358
Fan, T., Zhai, H., Shi, W., Wang, J., Jia, H., Xiang, Y., et al. (2013). Overexpression of profilin 3 affects cell elongation and f-actin organization in arabidopsis thaliana. Plant Cell Rep. 32, 149–160. doi: 10.1007/s00299-012-1349-2
Ge, D., Pan, T., Zhang, P., Wang, L., Zhang, J., Zhang, Z., et al. (2021). GhVLN4 is involved in multiple stress responses and required for resistance to verticillium wilt. Plant Sci. 302, 110629. doi: 10.1016/j.plantsci.2020.110629
Guo, Y., Zhao, S., Jiang, Y., Zhao, Y., Huang, S., Yuan, M., et al. (2016). Casein Kinase1-like Protein2 regulates actin filament stability and stomatal closure via phosphorylation of actin depolymerizing factor. Plant Cell 28, 1422–1439. doi: 10.1105/tpc.16.00078
Henty, J. L., Bledsoe, S. W., Khurana, P., Meagher, R. B., Day, B., Blanchoin, L., et al. (2011). Arabidopsis actin depolymerizing factor4 modulates the stochastic dynamic behavior of actin filaments in the cortical array of epidermal cells. Plant Cell 23, 3711–3726. doi: 10.1105/tpc.111.090670
Hwang, J. U., Lee, Y. (2001). Abscisic acid-induced actin reorganization in guard cells of dayflower is mediated by cytosolic calcium levels and by protein kinase and protein phosphatase activities. Plant Physiol. 125, 2120–2128. doi: 10.1104/pp.125.4.2120
Jiang, Y., Chang, M., Lan, Y., Huang, S. (2019). Mechanism of CAP1-mediated apical actin polymerization in pollen tubes. Proc. Natl. Acad. Sci. U.S.A. 116, 12084–12093. doi: 10.1073/pnas.1821639116
Jiang, Y., Lu, Q., Huang, S. (2022). Functional non-equivalence of pollen ADF isovariants in arabidopsis. Plant J. 110, 1068–1081. doi: 10.1111/tpj.15723
Jiang, H., Shui, Z., Xu, L., Yang, Y., Li, Y., Yuan, X., et al. (2020). Gibberellins modulate shade-induced soybean hypocotyl elongation downstream of the mutual promotion of auxin and brassinosteroids. Plant Physiol. Biochem. 150, 209–221. doi: 10.1016/j.plaphy.2020.02.042
Jiang, Y., Wang, J., Xie, Y., Chen, N., Huang, S. (2017). ADF10 shapes the overall organization of apical actin filaments by promoting their turnover and ordering in pollen tubes. J. Cell Sci. 130, 3988–4001. doi: 10.1242/jcs.207738
Ketelaar, T., Allwood, E. G., Anthony, R., Voigt, B., Menzel, D., Hussey, P. J. (2004). The actin-interacting protein AIP1 is essential for actin organization and plant development. Curr. Biol. 14, 145–149. doi: 10.1016/j.cub.2004.01.004
Lin, F., Qu, Y., Zhang, Q. (2014). Phospholipids: molecules regulating cytoskeletal organization in plant abiotic stress tolerance. Plant Signal Behav. 9, e28337. doi: 10.4161/psb.28337
Liu, C., Zhang, Y., Ren, H. (2018). Actin polymerization mediated by AtFH5 directs the polarity establishment and vesicle trafficking for pollen germination in arabidopsis. Mol. Plant 11, 1389–1399. doi: 10.1016/j.molp.2018.09.004
Liu, C., Zhang, Y., Ren, H. (2021). Profi liu promotes formin-mediated actin filament assembly and vesicle transport during polarity formation in pollen. Plant Cell 33, 1252–1267. doi: 10.1093/plcell/koab027
Li, X., Xie, Y., Zhang, Q., Hua, X., Peng, L., Li, K., et al. (2022). Monomerization of abscisic acid receptors through CARKs-mediated phosphorylation. New Phytol. 235, 533–549. doi: 10.1111/nph.18149
Lu, Y. J., Li, P., Shimono, M., Corrion, A., Higaki, T., He, S. Y., et al. (2020). Arabidopsis calcium-dependent protein kinase 3 regulates actin cytoskeleton organization and immunity. Nat. Commun. 11, 6234. doi: 10.1038/s41467-020-20007-4
Paul, A. L., Denison, F. C., Schultz, E. R., Zupanska, A. K., Ferl, R. J. (2012). 14-3-3 phosphoprotein interaction networks - does isoform diversity present functional interaction specification? Front. Plant Sci. 3. doi: 10.3389/fpls.2012.00190
Pei, D., Hua, D., Deng, J., Wang, Z., Song, C., Wang, Y., et al. (2022). Phosphorylation of the plasma membrane h+-ATPase AHA2 by BAK1 is required for ABA-induced stomatal closure in arabidopsis. Plant Cell 34, 2708–2729. doi: 10.1093/plcell/koac106
Qian, D., Zhang, Z., He, J., Zhang, P., Ou, X., Li, T., et al. (2019). Arabidopsis ADF5 promotes stomatal closure by regulating actin cytoskeleton remodeling in response to ABA and drought stress. J. Exp. Bot. 70, 435–446. doi: 10.1093/jxb/ery385
Qi, L., Liu, S., Li, C., Fu, J., Jing, Y., Cheng, J., et al. (2020). PHYTOCHROME-INTERACTING FACTORS interact with the ABA receptors PYL8 and PYL9 to orchestrate ABA signaling in darkness. Mol. Plant 13, 414–430. doi: 10.1016/j.molp.2020.02.001
Shi, Y., Liu, X., Zhao, S., Guo, Y. (2021). The PYR-PP2C-CKL2 module regulates ABA-mediated actin reorganization during stomatal closure. New Phytol. 233, 2168–2184. doi: 10.1111/nph.17933
Song, Y., Li, G., Nowak, J., Zhang, X., Xu, D., Yang, X., et al. (2019). The rice actin-binding protein RMD regulates light-dependent shoot gravitropism. Plant Physiol. 181, 630–644. doi: 10.1104/pp.19.00497
Tang, C., Deng, L., Chang, D., Chen, S., Wang, X., Kang, Z. (2015). TaADF3, an actin-depolymerizing factor, negatively modulates wheat resistance against puccinia striiformis. Front. Plant Sci. 6. doi: 10.3389/fpls.2015.01214
Wang, L., Qiu, T., Yue, J., Guo, N., He, Y., Han, X., et al. (2021). Arabidopsis ADF1 is regulated by MYB73 and is involved in response to salt stress affecting actin filament organization. Plant Cell Physiol. 62, 1387–1395. doi: 10.1093/pcp/pcab081
Wang, H.-H., Qiu, Y., Yu, Q., Zhang, Q., Li, X., Wang, J., et al. (2020). Close arrangement of CARK3 and PMEIL affects ABA-mediated pollen sterility in arabidopsis thaliana. Plant. Cell Environ. 43, 2699–2711. doi: 10.1111/pce.13871
Yao, H., Li, X., Peng, L., Hua, X., Zhang, Q., Li, K., et al. (2022). Binding of 14-3-3κ to ADF4 is involved in the regulation of hypocotyl growth and response to osmotic stress in arabidopsis. Plant Sci. 320. doi: 10.1016/j.plantsci.2022.111261
Zhang, L., Li, X., Li, D., Sun, Y., Li, Y., Luo, Q., et al. (2018). CARK1 mediates ABA signaling by phosphorylation of ABA receptors. Cell Discovery 4, 30. doi: 10.1038/s41421-018-0029-y
Zhao, S., Zhao, Y., Guo, Y. (2015). 14-3-3 lambda protein interacts with ADF1 to regulate actin cytoskeleton dynamics in arabidopsis. Sci. China Life Sci. 58, 1142–1150. doi: 10.1007/s11427-015-4897-1
Keywords: hypocotyl elongation, drought tolerance, actin depolymerization factor, CARK3, phosphorylation
Citation: Peng L, He J, Yao H, Yu Q, Zhang Q, Li K, Huang Y, Chen L, Li X, Yang Y and Li X (2022) CARK3-mediated ADF4 regulates hypocotyl elongation and soil drought stress in Arabidopsis. Front. Plant Sci. 13:1065677. doi: 10.3389/fpls.2022.1065677
Received: 10 October 2022; Accepted: 08 December 2022;
Published: 21 December 2022.
Edited by:
Mahmoud Magdy, Ain Shams University, EgyptReviewed by:
Chun-Hai Dong, Qingdao Agricultural University, ChinaBenjamin Karikari, University for Development Studies, Ghana
Xiaoyun Li, South China Normal University, China
Copyright © 2022 Peng, He, Yao, Yu, Zhang, Li, Huang, Chen, Li, Yang and Li. This is an open-access article distributed under the terms of the Creative Commons Attribution License (CC BY). The use, distribution or reproduction in other forums is permitted, provided the original author(s) and the copyright owner(s) are credited and that the original publication in this journal is cited, in accordance with accepted academic practice. No use, distribution or reproduction is permitted which does not comply with these terms.
*Correspondence: Xiaoyi Li, bGl4aWFveWkyMDJAc2N1LmVkdS5jbg==
†These authors have contributed equally to this work
‡ORCID: Xiaoyi Li, orcid.org/0000-0002-4607-0819