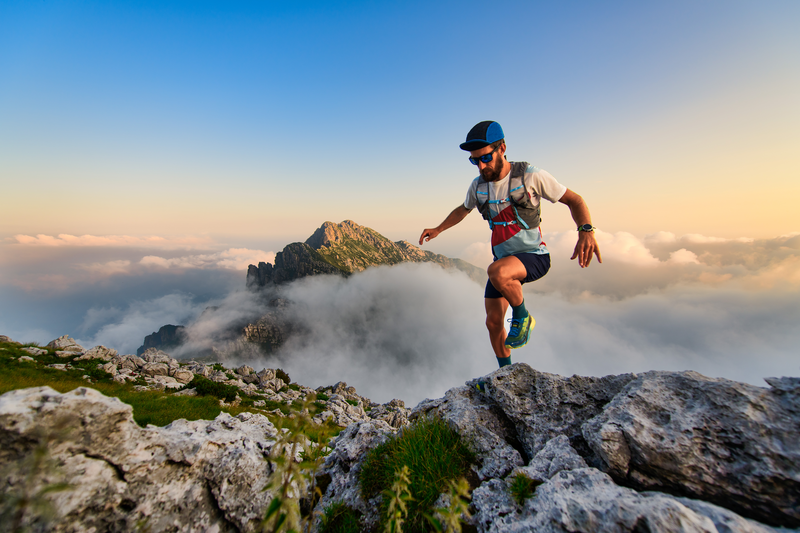
94% of researchers rate our articles as excellent or good
Learn more about the work of our research integrity team to safeguard the quality of each article we publish.
Find out more
ORIGINAL RESEARCH article
Front. Plant Sci. , 09 January 2023
Sec. Plant Pathogen Interactions
Volume 13 - 2022 | https://doi.org/10.3389/fpls.2022.1065627
This article is part of the Research Topic Advances in Integrated Disease Management (IDM) For Soil-Borne Plant Pathogens: Innovative Approaches and Underlying Action Mechanism at Molecular Level View all 13 articles
Rabproteins are the largest members of the small G protein family and are widely distributed in eukaryotes. It comprises eight subfamilies and is responsible for regulating vesicle transport, plant growth and development, and biotic and abiotic stress responses. In this study, the small G protein gene StRab5b was cloned from potato, and its biological information, expression profile and induced expression level, overexpression and gene silencing were examined on regulating potato resistance to Phytophthora infestans using PCR, qPCR and Virus-induced gene silencing (VIGS). Our results indicate that the amino acid of StRab5b shows the highest and lowest homology with NbRab5b in N. benthamiana and StRab in potato respectively. StRab5b expression varied among different potato tissues and varieties, and was induced by P. infestans infection. Transiently ectopic expression of StRab5b in N. benthamiana enhanced its resistance to P. infestans, whereas, silencing of StRab5b and its homologous gene facilitated pathogen infection in potato and N. benthamiana respectively. Furthermore, stable expression of the StRab5b gene in potatoes enhanced its redox-stress response capacity, as manifested by the accumulation of H2O2 in infected leaves and subsequent increase in the activity and expression of ROS scavenging enzymes, thereby attenuating the development of P. infestans and ultimately reducing the lesions on infected potato leaves. In addition, the LOX gene transcripts and JA level were upregulated rapidly after inoculation with P. infestans. Collectively, our results suggest that StRab5b positively regulates the resistance against potato late blight (PLB) via JA-mediated defense signaling pathway.
The potato (Solanum tuberosum L.) is the most important non-gramineous crop worldwide. However, its tuber yield and quality are devastatingly affected by PLB caused by P. infestans. In China, in the early 1950s and the 1960s, PLB caused yield losses amounting to approximately 1 billion dollars (Li et al., 1997). Furthermore, the frequent variation in dominant races of P. infestans in potatoes in recent years has been considerable, resulting in a loss of disease resistance (Saville et al., 2016). At present, it is extremely difficult to control PLB, and an effective way is urgently required to excavate novel functional genes for investigating their resistance functions.
Plants have developed sophisticated pathogen-surveillance systems and can mount defense responses against microbial attack. Microbial parasites in the environment constantly challenge plants, which in turn, defend themselves through the innate immune system. Specifically, plants detect potential invaders, including bacteria, fungi, and oomycetes, mainly through a two-layered immunity system. First, when the host plants defend pathogen infection, the pattern recognition receptors (PRRs) located on the cell membrane recognize the pathogen associated molecular patterns (PAMPs) of Phytophthora pathogens, and elicit the first layer of immune system (PAMP-triggered immunity, PTI) (Naveed et al., 2020). Microbe-associated molecular patterns (MAMPs) are motifs of microbes which can be recognized by plants in response to infection (Robinson and Bostock, 2015). Then, MAMPs triggered PTI defense responses that can effectively counteract potential microbes. Second, pathogens secrete and deliver effectors into the host cells, that neutralize the functions of immune regulators (Saville et al., 2016). Resistance proteins (R) recognize pathogen effectors, activating a rapid immune response known as effector-triggered immunity (ETI), which results in host cell death, a phenomenon known as the hypersensitive response (HR). These pathogen effectors can evolve new functions through mutations to suppress immunity and promote disease progression (Wang et al., 2019).
During the establishment of HR, Salicylic acid (SA), JA, and ethylene (ET) biosynthesis are activated through PTI, ETI, and systemic acquired resistance (SAR). The accumulation of SA, JA and ET leads to enhanced resistance to pathogens in plants (Li et al., 2004). Particularly, recent studies have reported that the transcription factor StbZIP61 is involved in the SA signaling pathway that regulates the defense response of potato against P. infestans (Zhou et al., 2018) and enhances potato resistance to salt stress (Efimova et al., 2019). Similarly, activating JA and ET signaling, instead of SA signaling by eliciting β-cryptogein, triggers resistance to P. infestans (Starý et al., 2019).
In addition, when plants are subjected to biotic or abiotic stress, rapid accumulation of reactive oxygen species (ROS) occurs, leading to programmed cell death (PCD) and HR. However, cells have evolved a set of ROS-scavenging enzymes, including superoxide dismutase (SOD), peroxidase (POD), catalase (CAT), and ascorbate peroxidase (APX) to balance cellular ROS levels (Beers and Sizer, 1952). Recent evidence suggests that the higher activities of POD and SOD play key roles in scavenging excessive ROS in late plant-pathogen interaction. Higher levels of H2O2 were also represented in potato transgenic plants after inoculation with P. infestans (Zhang et al., 2014). Zhang et al. (2020) found that ROS can be scavenged by SOD, POD, and CAT activities. In sweet potato, SOD and APX activities of transgenic plants were increased under salt stress (Yan et al., 2016). In conclusion, ROS and antioxidant enzymes are involved in the signal pathway of plant-pathogen interaction and stress response.
The RAS superfamily of small G proteins are divided into five subfamilies: RHO, RAB, RAS, RAN and ARF. RAB proteins belong to the largest subfamily of RAS superfamily. RAB GTPase generally regulates its activity through two regulatory proteins: Guanine nucleotide exchange factors (GEFs) are used to dissociate the GDP to form an active GTP-RAB-effector, and GTPase activating proteins (GAPs) are used to decompose GTP into GDP. GDP-RAB combines with GDI to form inactive GDP-RAB-GDI, thus entering the next regulation cycle (Ebine and Ueda, 2009). RAB proteins are widely distributed in plants, animals, and microorganisms. To date, 52 Rab genes have been cloned from rice (Oryza sativa L.) and 57 from Arabidopsis thaliana (Rutherford and Moore, 2002; Du et al., 2013). In potato, the Rab gene StRab5b is located on the 11th chromosome of the genome (https://solgenomics.net/locus/82544/view). Previous studies have shown that Rab proteins regulate both abiotic and biotic stress responses in plants, plant hormone-mediated signal transduction, plant growth and development, and vesicular trafficking (Yamaguchi-Shinozaki et al., 1990; Moshkov and Novikova, 2008; Szumlanski and Nielsen, 2009; Falchi et al., 2010; Gao et al., 2012). Research of plant bacterial diseases showed that the overexpression of OsRab11 in rice resulted in elevated resistance to the pathogen Pseudomonas syringae through induced JA response genes (Hong et al., 2013). Small G protein, VmRab7, regulates the vegetative growth and pathogenicity of Valsa mali, and knockout of VmRab7 blocks autophagy (Zhang et al., 2021). In previous studies on plant fungal diseases, Rab2 was significantly expressed in the early stage of infection by wheat leaf rust (Puccinia triticina). The active OsRac1 caused HR responses and substantially reduced disease lesions against rice blast fungus (Ono et al., 2001). In Arabidopsis, RabG3bOX-transgenic plants displayed unrestricted hypersensitive PCD against a fungal toxin and a fungal pathogen Alternaria brassicicola (Kwon et al., 2009). Additionally, higher expression of TaRab7 implied that it was related with defense to wheat-stripe rust fungus (Liu et al., 2012). Moreover, the overexpression of the small G protein StRab caused small lesions on potato leaves after inoculation with P. infestans (Gao et al., 2012), indicating that the Rab genes and their associated proteins show potential to influence disease progression. However, limited research has been done on the resistance of small G protein to PLB.
In this study, we aimed to elucidate the role of the small G protein StRab5b in the regulation of potato resistance against late blight. For this purpose, the StRab5b gene was cloned, gene sequences and expression profile were analyzed; transient expression and gene silencing were performed in N. benthamiana and potato. Stable expression of StRab5b in potatoes was obtained and used to confirm its role in regulating potato resistance against P. infestans. These results lay a stone for resistant breeding against potato late blight.
Potato cultivar ‘Desiree’ was used for gene amplification and genetic transformation. Plants were grown in vitro on Murashige and Skoog (MS) medium, under a luminous flux intensity of 3,000–4,000 Lx, 80% relative humidity, and a photoperiod of 16 h/8 h light/dark, at 25°C. The P. infestans strain (14-3) was kindly provided by Prof. Francine Gover, Wageningen University and Research (WUR), Netherlands. Tubers of six potato varieties ‘Zihuabai’ (ZHB), ‘Desiree’ (DXR), ‘Longshu No.7’ (L7), ‘Zhongshu No.4’ (ZS4), ‘Atlantic’ (DXY) and ‘Shepody’ (XPD), were planted in a nutrient pot and cultivated in a chamber under a luminous flux density of 18,000 Lx, 75% relative humidity, and a photoperiod of 16 h/8 h light/dark, at 25°C. These plants were used for expression profile analysis. Desiree seedlings cultivated in pots for 20 d were selected for induced expression.
Primers were designed using Primer 5.0 software and synthesized by the Beijing Hooseen Biotechnology Company, China. The primer sequences used in this study are listed in Supplementary Table 1.
Luria-Bertani liquid medium (10 g tryptone, 5 g yeast extract, and 5 g NaCl) was prepared, and H2O was added to bring the volume to 1,000 mL. YEB liquid medium (5 g beef extracts, 5 g tryptone, 5 g sucrose, 1 g yeast extract, MgSO4·H2O 0.5 g) with pH 7.0 was prepared, and H2O was added to bring the volume to 1,000 mL. Agar powder (15 g) was added to each mixture media to prepare the corresponding solid medium. P. infestans was induced to produce zoospores at 4°C for 4 h. Zoospore suspensions were adjusted to a concentration of 5 × 105 mL-1 sporangia using a hemocytometer (Neubauer-improved, Germany). Approximately 10−15 μL of spore suspension was inoculated on plant leaves.
Relative expression quantitation was performed using Quantity one software for semi-quantitative RT-PCR (St Rab5b and PDS), and GAPDH was used as a reference gene. Q-PCR was performed on a LightCycler (96, Roche, Basel, Switzerland) using the SYBR Premix Ex Taq II Kit (TaKaRa Bio, Shiga, Japan). We analyzed the expression levels of ACS, LOX, NPR1, SOD, APX1, POD, CAT2, and PIO and used Actin as a reference gene.
Total RNA was extracted from potato plantlets grown for 30 d using the RNAiso reagent (TaKaRa Bio), and genomic DNA was extracted using the DNAiso reagent (TaKaRa). The quality of RNA and DNA was determined by performing gel electrophoresis on 1% agarose gels. The first cDNA chain was synthesized according to manufacturer instructions of a HiScript II reverse transcription Kit (Nanjing Vazyme Biotechnology Co., Ltd).
The PCR-amplification mixture (50 μL) included 10 μL of 5X Prime Star Buffer (Mg2+ plus), 4 μL of dNTP Mix (2.5 mM), 1 μL of Primer Rab5bF/Rab5bR, 1 μL of cDNA template, 0.5 μL of Prime STAR HS DNA Polymerase, and 32.5 μL of ddH2O. Target fragments were purified using the EasyPure Quick Gel Extraction Kit (Beijing TransGen Biotech Company, China) according to manufacturer’s instructions. Plasmid extraction was performed according to the manufacturer’s instructions of the TIANprep Mini Plasmid Kit II (Beijing TIANGEN Biotech Company). PLG-Rop and pBIA1300-221 were provided by Prof. Yan Zhao, from the Institute of Genetics and Development, Chinese Academy of Sciences, Beijing, China. WUR kindly provided the P. infestans strains, pTRV1 and pTRV2 vectors. Fastdigest endonucleases KpnI, BglII, XbaI, and SacI were purchased from Thermo Fisher Scientific, (Waltham, MA, USA).
Phylogenetic analyses were performed with DNAMAN software. The Rab or Rab5b sequences of Oryza sativa (AF323991), Nicotiana benthamiana (DQ335217), Solanum tuberosum (ABK96799 and AK323357), Hevea brasiliensis (KC577146), Physcomitrella patens (AB379973), Phoenix dactylifera (AK287492), Nicotiana sylvestris (X63875), Mangifera indica (KF768563), Athaliana (D89824), Selaginella moellendorffii (KF516567), Medicago sativa (X79278), Triticum aestivum (X59133), Lotus japonicus (Z73939), and Medicago truncatula (BT147767) were retrieved from Genbank separately.
The StRab5b gene was linked to the pEASY-Blunt Simple cloning vector and transferred into DH5α cells (TransGen Biotech Company, Beijing, China). Positive DH5α colonies were screened and sequenced. Target gene purification was performed using the EasyPure Quick Gel Extraction Kit (TransGen Biotech Company). Recombinant vectors PLG-Rop and pEASY-Blunt Simple-StRab5b were digested with BglII and KpnI to obtain the PLG-StRab5b vector. Recombinant plasmid PLG-StRab5b and vector pBIA1300-221 were digested with XbaI and SacI to obtain the recombinant plasmid pBIA1300-StRab5b. Plasmid pBIA1300-StRab5b was transferred into Agrobacterium tumefaciens GV3101 for preservation.
The length and width of the leaf lesions formed on the plant leaves were measured using an electronic caliper (DL91150, China). Each lesion was measured three times (Vleeshouwers et al., 1999). The lesion area (A1) was calculated using the formula: A1 = 1/4π × length × width.
Inoculation method of P. infestans for analysis of the expression profile of StRab5b was described as follows. The seedlings of different potato varieties were cultured in a pot (10 cm height * 10 diameter) for 50 d. The abaxial surface of potato leaves was scratched with sterile toothpicks and inoculated with 10 uL zoospore suspension (5 × 105 mL-1) of P. infestans on one side; the other side was inoculated with water as control. The inoculated leaves were placed in a plastic box with wet filter paper on the bottom and kept in an incubator (day and night 16 h/8 h, 25/18°C).
Induction of StRab5b after inoculation with P. infestans: Potato seedlings (‘Desiree’) were planted in a pot (10 cm height * 10 diameter) for 20 d, then inoculated with 10 μL of sporulation of P. infestans (5 × 105 mL-1). Then, inoculated leaves were collected at 0, 12, 24, 48, 72, 96, and 108 h post-inoculation (hpi). Using potato GAPDH as a reference gene, RT-PCR was performed to detect the relative expression levels of StRab5b, and the results were analyzed using Quantity one software.
A. tumefaciens containing pBIA1300-StRab5b and pBIA1300 were inoculated in 10 mL YEB liquid medium (100 μg/mL rifampicin and 50 μg/mL kanamycin). The oscillating culture was incubated overnight at 180 rpm, followed by centrifugation for 2 min at 7,104×g to collect the bacteria; then, the culture was resuspended in a liquid mixture containing 150 μM acetyleugenone, 10 mM MgCl2, and 10 mM MES. The bacterial solution was suitable for use when the OD600 value reached 0.5–0.6. The bacterial liquid (15–20 μL) was drawn using a needle-free syringe, injected into the right side of the abaxial surface of the N. benthamiana leaf, and incubated for 24 h (night and day alternate 16 h/8 h) at 21°C. The N. benthamiana leaves were inoculated with P. infestans spores and incubated for 4 h at 4°C before being transferred to a 21-23°C incubator with 80% humidity. The lesion area was measured at different inoculation time points.
Recombinant vectors pTRV2 and pEASY-Blunt Simple-StRab5b were digested with XbaI and SacI to construct the silence-expression vector pTRV2-Rab5b (98% amino acid identity with Rab5b of N. benthamiana). The Agrobacterium tumefaciens vector pTRV1 was provided by WUR. Plasmid pTRV2-Rab5b was then transferred to A. tumefaciens GV3101. A. tumefaciens plasmids were resuspended with VIGS mixture containing 20 mM MgCl2, 100 mM MES, and 20 mM acetosyringone. The photobleaching phenotype was obtained by inhibiting the expression of endogenous phytoene desaturase gene (PDS). A 536 bp fragment of StPDS (95% amino acid identity with PDS of N. benthamiana) was linked with pTRV2 and transformed into A. tumefaciens GV3101 (pTRV2-PDS). Thereafter, A. tumefaciens containing pTRV1 was mixed with pTRV2-PDS or pTRV2-StRab5b at a 1:1 ratio after the OD600 value reached 0.5−0.6. A needle-free syringe was used to draw 20 μL of the bacterial suspension to inject into N. benthamiana and potato leaves. Three to four leaves of each plant were inoculated. After photobleaching, leaf samples were collected to identify the relative expression levels of PDS, StRab5b and StRab5b homologous gene using RT-PCR, after 25 d (N. benthamiana) and 60 d (potato). GAPDH was used as a reference.
Potato stems (2 cm long) were placed in the suspended Agrobacterium solution, soaked for 10 min, and dried with sterile filter paper. Stems were then transferred to the co-culture medium (MS + 1.0 mg/L IAA + 0.2 mg/L GA3 + 2.0 mg/L ZT + 0.5 mg/L 6-BA, pH 5.8) and cultured in darkness for 2–3 d. Thereafter, they were transferred to the bud inductive-differentiation medium (MS + 1.0 mg/L IAA + 0.2 mg/L GA3 + 2.0 mg/L ZT + 0.5 mg/L 6-BA + 50 mg/L Kan + 300 mg/L TMT, pH 5.8), and incubated at 25°C under a light intensity of 4,000 μmol/m2·s, and a photoperiod of 16 h/8 h. The medium was replaced at 2-week intervals. When adventitious buds grew to 2.0 cm, they were transferred to the rooting medium (MS + 50 mg/L Kan, pH 5.8). When plantlets were formed, total leaf RNA was extracted, and positive transgenic plants were identified using PCR and q-PCR separately.
Leaves infected with P. infestans were decolorized in anhydrous ethanol by boiling for 2 to 2.5 h. Decolorized leaves were placed in 0.5% trypan blue solution (0.5 g trypan blue dissolved in 100 mL distilled H2O), stained for 10–15 min, washed twice with distilled water, and then treated with lactophenol water (lactic acid, phenol, and dd H2O mixed in a ratio of 1:1:1).
Leaves infected with P. infestans were soaked overnight in 1 mg/mL 3,3′-Diaminobenzidine (DAB) dyeing solution (0.1 g DAB dissolved in 100 mL distilled water, pH 3.8). The leaves were decolorized by boiling for 2 h in absolute ethanol, then treated with lactophenol water (lactic acid, phenol, and water were mixed in a ratio of 1:1:1), and photographed. The H2O2 content of P. infestans-inoculated transgenic plants was determined as described by Zhang et al. (2014).
Activities of APX CAT, POD, and SOD enzymes were determined according to manufacturer’s instructions using the corresponding kits (APX-1-W, CAT-1-W, POD-1-Y and SOD-1-W), purchased from Suzhou Keming Biotechnology Co., Ltd., (Suzhou, China). The infected leaf samples were collected from all treated plants. The data were analyzed using SPSS 16.0.
0.1 g smples collected from potato leaves were cut into pieces, and grinded into powders in liquid nitrogen. The content of JA in potato was determined by plant JA ELISA Kit (Jiangsu Jingmei Biological Technology Co., Ltd) (Yi et al., 2020).
The potato StRab5b gene was amplified using Prime STAR HS DNA Polymerase (Takara). The fragment identified via agarose gel electrophoresis and the amplicon size was approximately 607 bp. To analyze the conserved domains of StRab5b, Rab 5b proteins isolated from rice, N. benthamiana, lotus, and Physcomitrella patens were retrieved from Genbank and used for multi-sequence analysis. The results suggested that StRab5b contained the basic general characteristics of Rab proteins, including RabF2 (83−87), RabF4 (YYRGA, 102−106) and RabF5 (131−135) domains, which are the conserved domains of the Rab proteins. Additionally, it also contained the GTP/GDP binding domain, phosphate binding loop G1 (39−46), G2 (79−80), and the coordination of GTP β and γ phosphate motifs G3 (88−91) and G5 (176−178), binding motifs NKAD (146−149) and ETSA (174−177) (Figure 1A). Therefore, the potato StRab5b protein belongs to Rab protein family.
Figure 1 Conservative domain and phylogenetic tree analysis of StRab5b (A) The conserved domains of StRab5b. (B) Phylogenetic tree of StRab5b. The phylogenetic tree was constructed with DNAMAN v. 6.0. The accession number of each protein is as follows: Oryza sativa (AF323991), Nicotiana benthamiana (DQ335217), Solanum tuberosum (ABK96799 and AK323357), Hevea brasiliensis (KC577146), Physcomitrella patens (AB379973), Phoenix dactylifera (AK287492), Nicotiana sylvestris (X63875), Mangifera indica (KF768563), Arabidopsis thaliana (D89824), Selaginella moellendorffii (KF516567), Medicago sativa (X79278), Triticum aestivum (X59133), Lotus japonicus (Z73939), and Medicago truncatula (BT147767). The symbol * represents the middle value between two sequence numbers.
Furthermore, the phylogenetic tree was constructed with StRab5b and the other 15 Rab proteins, derived from Oryza sativa, Nicotiana benthamiana, Solanum tuberosum, Hevea brasiliensis, Physcomitrella patens, Phoenix dactylifera, Nicotiana sylvestris, Mangifera indica, Arabidopsis thaliana, Selaginella moellendorffii, Medicago sativa, Triticum aestivum, Lotus japonicus, and Medicago truncatula, showed that StRab5b exhibited the highest similarity (95%) with NbRab5b, whereas, it showed the lowest similarity (44%) with TaRab (Figure 1B).
The relative expression levels of StRab5b in ‘ZHB’, ‘XPD’, ‘L7’, ‘DXR’, ‘ZS4’, and ‘DXY’ showed a decreasing tendency (Figure 2A). The expression profiles indicated that the expression levels of StRab5b differed substantially among different organs; the highest expression was in young leaves, followed by expression in stems, old leaves, and roots (Figure 2B). The tendency of symptoms in ‘Desiree’ leaves at different time points increased firstly at 12 hpi, followed by reaching the highest point 72 hpi, then dropping to basal level at 108 hpi, indicating the induction of StRab5b by P. infestans (Figures 2C, D).
Figure 2 Expression pattern and inducible expression of the StRab5b gene in potato (A) Relative expression levels of StRab5b in six different potato varieties. (B) Relative expression levels of StRab5b in different tissues in cultivar ‘Desiree’. (C) Phenotype of potato leaves inoculated with Phytophthora infestans and the relative expression levels of StRab5b in cultivar ‘Desiree’ at 0–108 hpi. Results were obtained from three biological replicates. (D) Quantification of relative expression of StRab5b in cultivar ‘Desiree’ at 0–108 hpi. Different lower case letters show significant differences at p< 0.05. Error bars represent the standard deviation (SD) of three biological replicates.
Transient expression of StRab5b in N. benthamiana leaves was performed via infiltration, followed by inoculation with P. infestans. The lesions on the control leaves gradually increased after inoculation, and reached the largest size (102.1 mm2) at 108 hpi; it is much bigger than the lesion size on leave which transiently expressed StRab5b, indicating that transiently ectopic expression of StRab5b could enhance N. benthamiana resistance to P. infestans (Figure 3A).
Figure 3 The function of the StRab5b gene was verified by both transient expression and VIGS in Nicotiana benthamiana and potato (A) The differences of lesion in Nicotiana benthamiana inoculated after transient expression of StRab5b homologous gene at 0–108 hpi. (B) The Establishment of VIGS system and identification of StRab5b homologous gene silencing efficiency in N. benthamiana. Results were obtained from three biological replicates. Photobleaching occurs after 25 dpi. Lanes 1-3 represents the plants injected with pTRV1:pTRV2-Rab5b, and lanes 4-6 represents the plants injected with pTRV1:pTRV2 (C)The leaf symptoms and the lesion area size of silenced StRab5b homologous gene in Nicotiana benthamiana at different times. (D) The formation of VIGS system in potato and determination of StRab5b gene silencing efficiency. Results were obtained from three biological replicates. Photobleaching occurs after 60 dpi. Lanes 1-3 represents the plants injected with pTRV1:pTRV2-Rab5b, and lanes 4-6 represents the plants injected with pTRV1:pTRV2. (E)The leaf symptoms and the lesion area size of silenced StRab5b gene in potato at different times. Error bars represent the standard deviation (SD) of three biological replicates. Different lower case letters indicate significant differences at p< 0.05.
To verify the function of StRab5b on regulating the resistance to P. infestans, VIGS was used to silence StRab5b and NbRab5b in potato and N. benthamiana respectively. PDS gene was taken alone as an indicator. When photobleaching was observed on N. benthamiana and potato leaves at 25 and 60 dpi, respectively, the expression of StRab5b, NbRab5b and PDS genes was quantified using qRT-PCR and the average silencing efficiencies of StRab5b, NbRab5b and PDS were 59%, 65%, and 100%, respectively (Figures 3B, D). The leaf lesion was recorded upon inoculation with P. infestans. The lesion area on the infiltrated leaves was 259.9, 253.4, 284, and 152.8 mm2 at different time points, which were significantly larger than that on control leaves at the same time point. The average increase in the rate of the lesion area was 3.1 mm2/h (Figure 3C). However, the leaf lesion areas of potato were 67.4, 83.7, 63.6, and 40.1 mm2 at 96 hpi, respectively (Figure 3E).
Positive transgenic lines were obtained via Agrobacteria-mediated transformation of StRab5b in potato cultivar ‘Desiree’ (Figure 4A). The relative expression levels of StRab5b among the nine transgenic lines were variable, but significantly higher than its expression levels in control plants. After 96 hpi, the leaf lesions among three transgenic lines (2, 3, and 8) did not differ extensively (5.1, 5.4, and 5.6 mm2, respectively) (Figure 4C). Meanwhile, the lesions on control leaves increased gradually and reached 96.9 mm2 at the same inoculation point (Figure 4B). The dead cells on potato leaves were monitored with trypan blue staining; much less blue color was observed on transgenic leaves, indicating a lower number of dead cells on transgenic leaves compared with those in control leaves (Figure 4D). However, Quantitative analyze the amount of colonization of P. infestans showed that there were significant differences among the three transgenic lines and EV. In addition, the phenotypic characteristics of transgenic plants showed that the stems, leaves and tubers of transgenic plants presented as red, while the control remained green (Supplementary Figures 1A, B). The anthocyanin accumulation in leaves and tubers of transgenic lines were greatly higher than that in the EV control (Supplementary Figure 1C).
Figure 4 Identification of resistance to Phytophthora infestans in transgenic potato plants (A) The relative expression levels of StRab5b gene in 9 transgenic lines. Different lower case letters indicate significant differences at p< 0.05. Error bars represent the standard deviation (SD) of three biological replicates. (B) The differences of lesion area on transgenic plants inoculated with P. infestans at 0−96 h Results were obtained from five biological replicates. (C) Quantification of the lesion area on transgenic plants inoculated with P. infestans at 0−96 h Different lower case letters indicate significant differences at p< 0.05. Error bars represent the standard deviation (SD). (D) Trypan blue staining and the amount of colonization after inoculated with P. infestans in transgenic leaves. Results were obtained from three biological replicates. PIO gene is an indicator of P. infestans and Actin is a reference gene.
DAB staining was performed to detect whether H2O2 was involved in the resistance of StRab5b to late blight. A brownish color was observed in the StRab5b transgenic leaves at 24 hpi and the color deepened at 48 and 96 hpi. However, no signal was observed in control leaves at 24 hpi, and brownish color on control leaves was lighter than that on the transgenic leaves (Figure 5A). The quantification of H2O2 accumulation is shown in Figure 5B. The accumulation of H2O2 increased after inoculation, peaked at 72 hpi, then decreased in three transgenic and control plants. Compared with the H2O2 accumulation level in control (0.097 μmol/g), the highest H2O2 level of 0.156 μmol/g was detected in transgenic line 2 at 72 hpi, followed by other two transgenic lines 3 and 8 with values of 0.136 and 0.132 μmol/g, respectively. The value of H2O2 was significantly higher than that in control plants after inoculation. These results suggested that StRab5b positively regulates potato resistance through H2O2 accumulation.
Figure 5 Qualitative and quantitative detection of H2O2 accumulation after inoculation with Phytophthora infestans (A) DAB staining to detect H2O2 accumulation on transgenic plants. Leaves of transgenic plants were inoculated with Phytophthora infestans on the right side, while water was used on the left side as control treatment. Three biological replicates were prepared. Lesion expansion was observed at 0, 24, 48, 72, and 96 hpi. (B) Accumulation of H2O2 on transgenic plants. Different lower case letters indicate significant differences at p< 0.05. Error bars represent the standard deviation (SD) of three biological replicates.
Four antioxidant enzyme activities (APX, CAT, POD, and SOD) were monitored after inoculation. The results suggested that APX, CAT, POD, and SOD activities in StRab5b-transgenic leaves were substantially higher than those in control plants at different inoculation time points (Figure 6). Particularly, SOD, POD, and APX showed a decreasing to- increasing pattern after inoculation, whereas, CAT showed an increasing to- decreasing pattern. Maximum activities of APX and SOD appeared at 72 hpi (54408.5 nmol/min·g and 2180.2 U/g), maximum activities of POD were 566.8 U/g at 96 hpi, except CAT, which had 2 peaks at 48 hpi and 96 hpi (353.1 and 376.8 nmol/min·g), respectively. The expression levels of these four genes (APX1, CAT2, POD, and SOD) that encode ROS scavenge enzymes were examined using qPCR after inoculation. The relative expression levels of four genes are consistent with the trend of enzyme activities. The maximum relative expression levels of APX1, CAT2, POD, and SOD genes were 5.3, 4.2, 6.2, and 7.5-fold, respectively.
Figure 6 Antioxidant enzyme activities and relative expression levels of genes in transgenic plants after inoculation with Phytophthora infestans (A) The differences of Ascorbate peroxidase activity and expression levels of APX1 in transgenic plants at 0−96 hpi. (B) The differences of Catalase activity and expression levels of CAT2 in transgenic plants at 0−96 hpi. activity (0−96 h). (C) The differences of Peroxidase activity and expression levels of POD in transgenic plants at 0−96 hpi. (D) The differences of Superoxide dismutase activity and expression levels of SOD in transgenic plants at 0−96 hpi. Different lower case letters indicate significant differences at p< 0.05. Error bars represent the standard deviation (SD) of three biological replicates.
Signaling pathways associated with SA, JA, and ET are directly involved in plant resistance to pathogens. To determine whether JA, SA, or ET are involved in StRab5b-mediated resistance to P. infestans, several marker genes related to different signaling pathways were analyzed at different time points. The results suggested that the expression levels of LOX and JA content in three transgenic lines (2, 3, and 8) were induced at 24 hpi and subsequently dropped at 48 hpi, followed by reaching the highest level at 72 hpi (158.2, 140.2, and 124.5-fold expression levels in the LOX gene, and 33.2, 24.4, and 20.7 ng/g JA content, respectively); moreover, JA content in transgenic plants was significantly higher than that in EV. Whereas, both NPR1 and ACS showed a different induction pattern compared with the LOX gene. They reached the peak at 24 hpi, then decreasing gradually. However, if we compared to the expression of the three marker genes, the induction of LOX was much more dramatic than ACS or NPR1. The induction rate was 67.5-fold at 24 hpi and 141.0-fold at 72 hpi separately, indicating that JA was the dominant molecular signal, which was involved in StRab5b-mediated resistance against P. infestans (Figure 7).
Figure 7 Transcriptional levels of jasmonic acid JA-, salicylic acid SA- and ethylene ET-related genes in transgenic plants after inoculation with Phytophthora infestans. (A) Relative expression levels of ACS. ACS gene expression levels were examined by qPCR at 0–96 hpi. (B) Relative expression levels of NPR1. NPR1 gene expression levels were examined by qPCR at 0–96 hpi. (C) Relative expression levels of LOX. LOX gene expression levels were examined by qPCR at 0–96 hpi. (D) Determination of JA content in transgenic potato lines inoculated with late blight. The JA content was examined using ELISA at 0–96 hpi. Different lowercase letters show significant differences at p< 0.05. Error bars represent the standard deviation (SD) of three biological replicates.
Protein StRab5b is an important member of the small G protein Rab family that differs from previously characterized StRab proteins in amino acid structure and domains. The conserved domain motif RabF2 (YYRGA) of the StRab5b was analyzed using multiple sequence alignment. The guanine-binding motif NKAD and the guanine-binding and dissociation motif ETSA were consistent with the expression of TaRab5b protein (Chen et al., 2005). The specific motifs of G1, G3, G5, RabF4, and RabF5 were consistent with those of Dunaliella salina and potato StRab proteins. Although the homology of potato StRab and StRab5b was only 46% at amino acid level, StRab5b showed a structural and functional similarity to the Rab protein (Gao et al., 2012; Yu et al., 2013). Therefore, we replicated the StRab5b gene, analyzed its expression profile, and induced expression.
In this study, the relative expression levels of StRab5b were variable among different tissues. The highest expression level was detected in young leaves, followed by stems, old leaves and roots. This is in line with the order of the relative expression levels of another small G protein, StRac (Zhang et al., 2020). Additionally, the relative expression levels of StRab5b among different varieties is also variable, the order from higher to lower is ZHB, DXR, L7, ZS4, DXY, and XPD. It is also partially confirmed by the results that the relative expression of StBAG3 was also highest in ‘Zihuabai’ (Lu et al., 2017). Such different expression profiles are likely genotype-dependent. The clear induction pattern of StRab5b was also confirmed in ‘Desiree’, indicating the response of StRab5b to P. infestans infection.
Virus-induced gene silencing (VIGS) is an important method for studying gene function and has been used routinely in N. benthamiana, Lycopersicum esculentum, and A. thaliana (Ratcliff et al., 2001; Brigneti et al., 2004; Lacomme and Chapman, 2008; Senthil-Kumar and Mysore, 2014). In the present study, the StRab5b silenced leaves were more susceptible to P. infestans than control leaves (Figure 3). Similar studies have been reported in N. benthamiana and potato. Specifically, small GTPase NbRanBP1-1-silencing reduced resistance to P. infestans in plants of N. benthamiana (Mizuno et al., 2019). Consistently, when silencing EIN2, which was involved in pre-invasion phase, facilitated the penetration rate of P. infestans (Rin et al., 2017). However, the silencing of Matrix metalloprotease 1 made N. benthamiana more susceptible to P. infestans, but enhanced Nep1-like protein induced cell death (Ha et al., 2017). Silencing five candidate genes TRV:12 (sodium dicarboxylate cotransporter), TRV:17 (UDP-arabinose 4-epimerase), TRV:21 (2-oxoglutarate dehydrogenase), TRV:22 (Lipoxygenase), and TRV:48 (suberization-associated anionic peroxidase) from N. benthamiana facilitated the infection of P. infestans (Du et al., 2013). Conversely, silencing StERF3 in potatoes not only enhanced foliage resistance to P. infestans, but also promoted plant tolerance to salt stress (Tian et al., 2015).
Currently, small G proteins reportedly respond to abiotic stress factors and regulate plant defense or stress-related gene expression (Miao et al., 2018). The H2O2 was previously recognized as a toxic metabolite in plant cells. However, it has been known that H2O2 also mediates stimulus responses to plant cells as a signaling factor (Bailey-Serres and Mittler, 2006). According to previous research, small G proteins of the RAB family play important roles in the establishment of plant defense responses (Lamb and Dixon, 1997). In this study, the results confirmed that H2O2 accumulation in the transgenic lines might be caused by StRab5b expression on the membrane. Previous studies on rice disease resistance have shown that small GTPase OsRac1 induces hydrogen peroxide products, and enhances resistance to rice blast disease in transgenic plants (Ono et al., 2001). Overexpression of NtRop activated H2O2 products in Arabidopsis transgenic lines (Cao et al., 2008). In potato, stable expression DN-AtRop1 led to H2O2 accumulation associated smaller lesions on leaves after inoculation with P. infestans (Zhang et al., 2014). Among Rab proteins, overexpression of StRab in potato reduced the leaf lesion area and increased H2O2 production after inoculation with P. infestans (Gao et al., 2012). However, less H2O2 was observed in miR172a transgenic tomato plants after inoculation with P. infestans than in the wild type (Luan et al., 2018). These findings indicate that the involvement of H2O2 in disease resistance is a complex phenomenon, and that H2O2 accumulation in plant tissues experiences early and late stages. Furthermore, when plants lose the ability for regulation in the late stage, H2O2 may be induced in the early or late stages after inoculation, and may thus increase in concentration, leading to the cell death.
In plants, H2O2 is mainly produced through NADPH oxidase and polyamine oxidase (PAO) pathways. The production of H2O2 in plants generally suffer abiotic stress through the POD pathway. The extracellular PH value temporarily increases, which can activate the peroxidase of cell wall, and reduces O2 to H2O2 (Khokon et al., 2010). The activities of SOD and POD were higher in miR172 transgenic tomato plants after inoculation with P. infestans, accompanied by less H2O2 (Luan et al., 2018). As shown in Figure 6, the inoculated StRab5b-transgenic plants had higher APX, SOD, POD, CAT activities and expression levels of ROS related-genes, and increased H2O2 accumulation. These results suggested that StRab5b in potato may modulate antioxidants to eliminate redundant H2O2 and prevent cellular membrane injury after P. infestans infection. Similar results have been found in tomato, and the amounts of H2O2 and O2− were increased with the continuous salt stress in both roots and leaves. Compared to control, the plants had increased activities of SOD, CAT, POD, and APX after 6 or 12 days of salt stress (Raziq et al., 2022). In tomato, the melatonin-induced H2O2 generation through RBOH was attributed to activate some genes expression (CDPK1, MAPK1 and ERD15). Conversely, the inhibition of RBOH reduced stress defense response and antioxidant enzyme activity (SOD, CAT and APX) (Gong et al., 2017). In addition, a previous study reported that APX1 and SOD genes showed higher levels in SA- or CdCl2-treated potato plants, when compared to CK plants (Li et al., 2019). Brassinosteroid (BR) activated the expression of the StPOD gene, and the expression level was partially increased in potato tubers at the end of wound healing (Han et al., 2022). Some researchers believe that the highly active antioxidant enzymes are used to eliminate active oxygen, and the concentration of H2O2 is reduced.
Plant defense responses refer to the resistance of plants to external infection, including the synthesis of pathogenesis-related (PR) proteins. Plant hormones SA, JA, and ET play major roles in the plant immune system (Verma et al., 2016). There are many studies regarding plant resistance and JA signaling pathway. For example, JA may be involved in resistance regulated by AtRop1 (Zhang et al., 2014). Overexpression of OsRab11 in Arabidopsis enhances plant resistance to Pseudomonas syringae by inducing the expression of JA-responsive genes, rather than SA-responsive genes (Hong et al., 2013). These results are consistent with those obtained in the present study. Thus, the key enzyme-encoding gene in the JA signaling pathway, i.e., LOX and JA content were dramatically induced in StRab5b transgenic lines at different inoculation time points, indicating the involvement of JA signaling pathway on potato resistance manipulated by StRab5b. This is in contrast with the enhanced late blight resistance observed by the overexpression of LecRK-I.9 in Arabidopsis by reducing SA-responsive gene expression (Bouwmeester et al., 2014). SA and ROS were confirmed as the primary signaling molecules that mediated potato resistance to P. infestans (Lamb and Dixon, 1997; Na et al., 2012), and the present study clearly highlights the involvement of the JA pathway in potato resistance to P. infestans. However, the cross talk of JA and SA signaling pathways were confirmed via hormone-responsive transcription factors WRKY70 and PDF1.2, which regulate plant defense responses (Petersen et al., 2000; Li et al., 2004). In other words, the mechanism underlying the involvement of small G proteins on manipulating potato resistance against late blight resistance is rather complex, and different signaling pathways interact with each other through key transcription factors to improve durable resistance in solanaceous crops. Whether the same mechanism occurs in small G protein-mediated establishment of potato resistance remains to be explored. Further experiments are needed to delineate the signaling transduction pathway mediated by small G proteins in potato.
The original contributions presented in the study are included in the article/Supplementary Material, further inquiries can be directed to the corresponding author/s.
ZT and ZZ conceived the experiments. JuZ designed and guided the experiments scheme. ZT, ZZ, LK and ML completed the experiments. ZT, XG, JY, JiZ and YF provided consumable materials/reagents/software and analyzed the data. ZT wrote the paper. All authors contributed to the article and approved the submitted version.
The study was funded by Key Natural Science Foundation of Inner Mongolia Autonomous Region (2022ZD10); Natural Science Foundation of China (31860495); Potato Innovation Team in Modern Agricultural Industry Technology System of Hebei Province (HBCT2018080201); The project of Hebei province education department (QN2018111) and Inner Mongolia natural science foundation (2019MS03057).
We thank Wageningen University and Research (WUR), Netherlands for providing the P. infestans strains.
The authors declare that the research was conducted in the absence of any commercial or financial relationships that could be construed as a potential conflict of interest.
All claims expressed in this article are solely those of the authors and do not necessarily represent those of their affiliated organizations, or those of the publisher, the editors and the reviewers. Any product that may be evaluated in this article, or claim that may be made by its manufacturer, is not guaranteed or endorsed by the publisher.
The Supplementary Material for this article can be found online at: https://www.frontiersin.org/articles/10.3389/fpls.2022.1065627/full#supplementary-material
Bailey-Serres, J., Mittler, R. (2006). The role of reactive oxygen species in plant cells. Plant Phyiol. 141, 311. doi: 10.1104/pp.104.900191
Beers, R. F., Sizer, I. W. (1952). A spectrophotometric method for measuring the breakdown of hydrogen peroxide by catalase. J. Biol. Chem. 195, 133–140. doi: 10.1016/S0021-9258(19)50881-X
Bouwmeester, K., Han, M., Blanco-Portales, R., Song, W., Weide, R., Guo, L. Y., et al. (2014). The arabidopsis lectin receptor kinase LecRK-I.9 enhances resistance to phytophthora infestans in solanaceous plants. Plant Biotechnol. J. 12, 10–16. doi: 10.1111/pbi.12111
Brigneti, G., Martín-Hernández, A. M., Jin, H., Chen, J., Baulcombe, D. C., Baker, B., et al. (2004). Virus-induced gene silencing in solanum species. Plant J. 39, 264–272. doi: 10.1111/j.1365-313X.2004.02122.x
Cao, Y. R., Li, Z. G., Chen, T., Zhang, Z. G., Zhang, J. S., Chen, S. Y. (2008). Overexpression of a tobacco small G protein gene NtRop1 causes salt sensitivity and hydrogen peroxide production in transgenic plants. Sci. China. 5, 383–390. doi: 10.1007/s11427-008-0060-6
Chen, X., Zhou, R., Jia, J. (2005). Isolation, primary expression analysis of a full-length cDNA clone encoding small GTP-binding protein gene TaRab5B in wheat. Acta Agron. Sin. 7, 897–901. doi: 10.3321/j.issn:0496-3490.2005.07.013
Du, J., Tian, Z. D., Liu, J., Vleeshouwers, V. G., Shi, X., Xie, C. (2013). Functional analysis of potato genes involved in quantitative resistance to phytophthora infestans. Mol. Biol. Rep. 40, 957–967. doi: 10.1007/s11033-012-2137-3
Ebine, K., Ueda, T. (2009). Unique mechanism of plant endocytic/vacuolar transport pathways. J. Plant Res. 122, 21–30. doi: 10.1007/s10265-008-0200-x
Efimova, M. V., Mukhamatdinova, E. A., Kovtun, I. S., Kabil, F. F., Medvedeva, Y. V., Kuznetsov, V. V. (2019). Jasmonic acid enhances the potato plant resistance to the salt stress in vitro. dokl. Biol. Sci. 488, 149–152. doi: 10.1134/S0012496619050077
Falchi, R., Cipriani, G., Marrazzo, T., Nonis, A., Vizzotto, G., Ruperti, B. (2010). Identification and differential expression dynamics of peach small GTPases encoding genes during fruit development and ripening. J. Exp. Bot. 61, 2829–2842. doi: 10.1093/jxb/erq116
Gao, W., Tian, Z. D., Liu, J., Lin, Y., Xie, C. H. (2012). Isolation, characterization and functional analysis of StRab, a cDNA clone from potato encoding a small GTP-binding protein. Sci. Hortic. 135, 80–86. doi: 10.1016/j.scienta.2011.12.019
Gong, B., Yan, Y. Y., Wen, D., Shi, Q. H. (2017). Hydrogen peroxide produced by NADPH oxidase: a novel downstream signaling pathway in melatonin-induced stress tolerance in solanum lycopersicum. Physiol. Plant 160 (4), 396–409. doi: 10.1111/ppl.12581
Ha, J. H., Jang, H. A., Moon, K. B., Baek, K. H., Choi, G. J., Choi, D., et al. (2017). Nicotiana benthamiana matrix metalloprotease 1 (NMMP1) gene confers disease resistance to phytophthora infestans in tobacco and potato plants. J. Plant Physiol. 218, 189–195. doi: 10.1016/j.jplph.2017.08.010
Han, Y., Yang, R., Zhang, X., Wang, Q., Wang, B., Zheng, X., et al. (2022). Brassinosteroid accelerates wound healing of potato tubers by activation of reactive oxygen metabolism and phenylpropanoid metabolism. Foods. 11, 906. doi: 10.3390/foods11070906
Hong, M. J., Lee, Y. M., Son, Y. S., Im, C. H., Yi, Y. B., Rim, Y. G., et al. (2013). Rice Rab11 is required for JA-mediated defense signaling. Son. Biochem. Biophys. Res. Commun. 434, 797–802. doi: 10.1016/j.bbrc.2013.04.014
Khokon, M. A. R., Uraji, M., Munemasa, S., Okuma, E., Nakamura, Y., Mori, I., et al. (2010). Chitosan-induced stomatal closure accompanied by peroxidase-mediated reactive oxygen species production in arabidopsis. Biosci. Biotechnol. Biochem. 11, 2313–2315. doi: 10.1271/bbb.100340
Kwon, S. I., Hong, J. C., Bae, K., Jin, H. J., Jin, H. C., Park, O. K. (2009). Role of an arabidopsis rab GTPase RabG3b in pathogen response and leaf senescence. J. Plant Biol. 52, 79–87. doi: 10.1007/s12374-009-9011-4
Lacomme, C., Chapman, S. (2008). Use of potato virus X (PVX)-based vectors for gene expression and virus-induced gene silencing (VIGS). Curr. Protoc. Microbiol. 2. doi: 10.1002/9780471729259.mc16i01s8
Lamb, C., Dixon, R. A. (1997). The oxidative burst in plant disease resistance. Annu. Rev. Plant Physiol. Plant Mol. Biol. 48, 251–275. doi: 10.1146/annurev.arplant.48.1.251
Li, J., Brader, G., Palva, E. T. (2004). The WRKY70 transcription factor: a node of convergence for jasmonate-mediated and salicylate-mediated signals in plant defense. Plant Cell. 16, 319–331. doi: 10.1105/tpc.016980
Liu, F. R., Guo, J., Bai, P. F., Duan, Y. H., Wang, X. D., Chen, Y. L., et al. (2012). Wheat TaRab7 GTPase is part of the signaling pathway in responses to stripe rust and abiotic stimuli. PloS One 7, e37146. doi: 10.1371/journal.pone.0037146
Li, Q., Wang, G., Wang, Y. R., Yang, D., Guan, C. F., Ji, J. (2019). Foliar application of salicylic acid alleviate the cadmium toxicity by modulation the reactive oxygen species in potato. Ecotoxicol. Environ. Saf. 172, 317–325. doi: 10.1016/j.ecoenv.2019.01.078
Li, R. G., Wu, N. F., Fan, Y. L. (1997). Research progress of potato resistance to late blight. J. Potato. 1997, 243–250.
Luan, Y., Cui, J., Li, J., Jiang, N., Liu, P., Meng, J. (2018). Effective enhancement of resistance to phytophthora infestans by overexpression of miR172a and b in solanum lycopersicum. Planta 247, 127–138. doi: 10.1007/s00425-017-2773-x
Lu, X. G., Zhang, G., Tian, Z. M., Hou, D. Y., Zhao, J., Zhang, Z. W., et al. (2017). Cloning of cDNA StBAG3 and induced express by pathogen of potato late blight. Acta Agric. Boreali-Occidentalis Sin. 32, 142–148. doi: 10.7668/hbnxb.2017.05.022
Miao, H. X., Sun, P. G., Liu, J. H., Wang, J. Y., Xu, B. Y., Jin, Z. Y. (2018). Overexpression of a novel ROP gene from the banana (MaROP5g) confers increased salt stress tolerance. Int. J. Mol. Sci. 19, 3108. doi: 10.3390/ijms19103108
Mizuno, Y., Ohtsu, M., Shibata, Y., Tanaka, A., Camagna, M., Ojika, M., et al. (2019). Nicotiana benthamiana RanBP1-1 is involved in the induction of disease resistance via regulation of nuclear-cytoplasmic transport of small GTPase ran. Front. Plant Sci. 10. doi: 10.3389/fpls.2019.00222
Moshkov, I. E., Novikova, G. V. (2008). Superfamily of plant monomeric GTP-binding proteins: 2. rab proteins are the regulators of vesicles trafficking and plant responses to stresses. Russ. J. Plant Physiol. 55, 119–129. doi: 10.1134/S1021443708010159
Naveed, Z. A., Wei, X. Y., Chen, J. J., Mubeen, H., Ali, G. S. (2020). The PTI to ETI continuum in Phytophthora-plant interactions. Front. Plant Sci. 11. doi: 10.3389/fpls.2020.593905
Na, R., Zhang, Z., Yu, X., Zhang, X., Yang, F., Zhao, J. (2012). ROS and salicylic acid (SA) play roles on the resistance establishment of the potato cultivar zihuabai to Phytophthora infestans. J. Plant Dis. Prot. 119, 191–199. doi: 10.1007/BF03356441
Ono, E., Wong, H., Kawasaki, T., Hasegawa, M., Kodama, O., Shimamoto, K., et al. (2001). Essential role of the small GTPase rac in disease resistance of rice. P. Nat. Acad. Sci. U.S.A. 98, 759–764. doi: 10.1073/pnas.021273498
Petersen, M., Brodersen, P., Naested, H., Andreasson, E., Lindhart, U., Johansen, B., et al. (2000). Arabidopsis, MAP kinase 4 negatively regulates systemic acquired resistance. Cell 103, 1111–1120. doi: 10.1016/S0092-8674(00)00213-0
Ratcliff, F., Martin-Hernandez, A. M., Baulcombe, D. C. (2001). Technical advance. tobacco rattle virus as a vector for analysis of gene function by silencing. Plant J. 25, 237–245. doi: 10.1046/j.0960-7412.2000.00942.x
Raziq, A., Wang, Y., Din, A. M. U., Sun, J., Shu, S., Guo, S. R. (2022). A comprehensive evaluation of salt tolerance in tomato (Var. ailsa craig): Responses of physiological and transcriptional changes in RBOH’s and ABA biosynthesis and signalling genes. Int. J. Mol. Sci. 23, 1603. doi: 10.3390/ijms23031603
Rin, S., Mizuno, Y., Shibata, Y., Fushimi, M., Katou, S., Sato, I., et al. (2017). EIN2-mediated signaling is involved in preinvasion defense in Nicotiana benthamiana against potato late blight pathogen, Phytophthora infestans. Plant Signal. Behav. 12, e1300733. doi: 10.1080/15592324.2017.1300733
Robinson, S. M., Bostock, R. M. (2015). β-glucans and eicosapolyenoic acids as MAMPs in plant–oomycete interactions: past and present. Front. Plant Sci. 5. doi: 10.3389/fpls.2014.00797
Rutherford, S., Moore, I. (2002). The arabidopsis rab GTPase family: another enigma variation. Curr. Opin. Plant Biol. 5, 518–528. doi: 10.1016/s1369-5266(02)00307-2
Saville, A. C., Martin, M. D., Ristaino, J. B., Ristaino, J. B. (2016). Historic late blight outbreaks caused by a widespread dominant lineage of Phytophthora infestans (Mont.) de bary. PloS One 11, e0168381. doi: 10.1371/journal.pone.0168381
Senthil-Kumar, M., Mysore, K. S. (2014). Tobacco rattle virus-based virus-induced gene silencing in nicotiana benthamiana. Nat. Protoc. 9, 1549–1562. doi: 10.1038/nprot.2014.092
Starý, T., Satková, P., Piterková, J., Mieslerová, B., Luhová, L., Mikulík, J., et al. (2019). The elicitin β-cryptogein’s activity in tomato is mediated by jasmonic acid and ethylene signalling pathways independently of elicitin-sterol interactions. Planta. 249, 739–749. doi: 10.1007/s00425-018-3036-1
Szumlanski, A. L., Nielsen, E. (2009). The rab GTPase RabA4d regulates pollen tube tip growth in Arabidopsis thaliana. Plant Cell. 21, 526–544. doi: 10.1105/tpc.108.060277
Tian, Z., He, Q., Wang, H., Liu, Y., Zhang, Y., Shao, F., et al. (2015). The potato ERF transcription factor StERF3 negatively regulates resistance to Phytophthora infestans and salt tolerance in potato. Plant Cell Physiol. 56, 992–1005. doi: 10.1093/pcp/pcv025
Verma, V., Ravindran, P., Kumar, P. P. (2016). Plant hormone-mediated regulation of stress responses. BMC Plant Biol. 16, 86. doi: 10.1186/s12870-016-0771-y
Vleeshouwers, V. G. A. A., van Dooijeweert, W., Paul Keizer, L. C., Sijpkes, L., Govers, F., Colon, L. T. (1999). A laboratory assay for phytophthora infestans resistance in various solanum species reflects the field situation. Eur. J. Plant Pathol. 105, 241–250. doi: 10.1023/A:1008710700363
Wang, J., Gao, C., Li, L., Cao, W., Dong, R., Ding, X., et al (2019). Ttransgenic rxlr effector pitg_15718.2 suppresses immunity and reduces vegetative growth in potato. Int. J. Mol. Sci. 20, 3031. doi: 10.3390/ijms20123031
Yamaguchi-Shinozaki, K., Mundy, J., Chua, N. H. (1990). Four tightly linked rab genes are differentially expressed in rice. Plant Mol. Biol. 14, 29–39. doi: 10.1007/BF00015652
Yan, H., Li, Q., Park, S. C., Wang, X., Liu, Y. J., Zhang, Y. G., et al. (2016). Overexpression of CuZnSOD and APX enhance salt stress tolerance in sweet potato. Plant Physiol. Biochem. 109, 20–27. doi: 10.1016/j.plaphy.2016.09.003
Yi, M., Zhao, L., Wu, K., Liu, C., Deng, D., Zhao, K., et al. (2020). Simultaneous detection of plant growth regulators jasmonic acid and methyl jasmonate in plant samples by a monoclonal antibody-based ELISA. Analyst. 145 (11), 4004–4011. doi: 10.1039/D0AN00203H
Yu, Z., Chai, X., Zhang, X., Zhang, T., Xue, F. (2013). Cloning and expression analysis of small GTP-binding protein gene from dunaliellasalina (DsRab) under salt stress. Biotechnol. Bull. 29, 77–83. doi: 10.13560j.cnki.biotech.bull.1985.2013.09.009
Zhang, Y. N., Jin, Y. L., Cui, H. X., Yan, X. Y., Fan, K. L. (2020). Nanozyme-based catalytic theranostics. RSC Advances. 10, 10–20. doi: 10.1039/c9ra09021e
Zhang, X. L., Wu, Q., Tain, R. Z., Xu, L. S., Huang, L. L. (2021). Functional analysis of small G protein VmRab7 in Visa mali. J. Nor. For. Uni. 36, 158–163. doi: 10.3969/j.issn.1001–7461.2021.02.23
Zhang, Z., Yang, F., Na, R., Zhang, X., Yang, S., Gao, J., et al. (2014). AtROP1 negatively regulates potato resistance to Phytophthora infestans via NADPH oxidase-mediated accumulation of H2O2. BMC Plant Biol. 14, 392. doi: 10.1186/s12870-014-0392-2
Zhang, Z., Zhang, X., Na, R., Yang, S., Tian, Z., Zhao, Y., et al. (2020). StRac1 plays an important role in potato resistance against phytophthora infestans via regulating H2O2 production. J. Plant Physiol. 253, 153249. doi: 10.1016/j.jplph.2020.153249
Keywords: Small G proteins, potato late blight, antioxidant enzyme activities, plant disease resistance, gene expression, hormone signaling pathways
Citation: Tian Z, Zhang Z, Kang L, Li M, Zhang J, Feng Y, Yin J, Gong X and Zhao J (2023) Small G Protein StRab5b positively regulates potato resistance to Phytophthora infestans. Front. Plant Sci. 13:1065627. doi: 10.3389/fpls.2022.1065627
Received: 10 October 2022; Accepted: 16 December 2022;
Published: 09 January 2023.
Edited by:
Dr. Raja Asad Ali Khan, Hainan University, ChinaReviewed by:
Erik Andreasson, Swedish University of Agricultural Sciences, SwedenCopyright © 2023 Tian, Zhang, Kang, Li, Zhang, Feng, Yin, Gong and Zhao. This is an open-access article distributed under the terms of the Creative Commons Attribution License (CC BY). The use, distribution or reproduction in other forums is permitted, provided the original author(s) and the copyright owner(s) are credited and that the original publication in this journal is cited, in accordance with accepted academic practice. No use, distribution or reproduction is permitted which does not comply with these terms.
*Correspondence: Jun Zhao, emhhb2p1bkBpbWF1LmVkdS5jbg==; Xuechen Gong, bmt4Z3hjQDE2My5jb20=
†These authors have contributed equally to this work
Disclaimer: All claims expressed in this article are solely those of the authors and do not necessarily represent those of their affiliated organizations, or those of the publisher, the editors and the reviewers. Any product that may be evaluated in this article or claim that may be made by its manufacturer is not guaranteed or endorsed by the publisher.
Research integrity at Frontiers
Learn more about the work of our research integrity team to safeguard the quality of each article we publish.