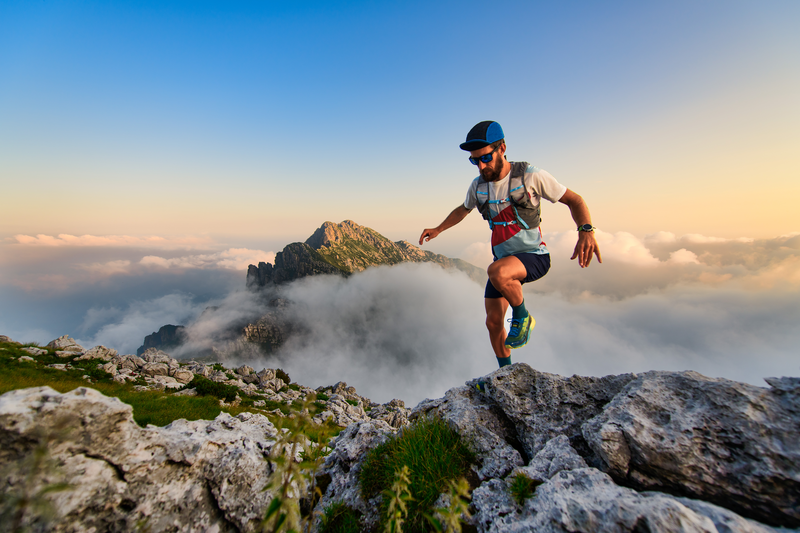
94% of researchers rate our articles as excellent or good
Learn more about the work of our research integrity team to safeguard the quality of each article we publish.
Find out more
ORIGINAL RESEARCH article
Front. Plant Sci. , 13 December 2022
Sec. Functional Plant Ecology
Volume 13 - 2022 | https://doi.org/10.3389/fpls.2022.1064504
Shrubs play a major role in maintaining ecosystem stability in the arid deserts of Central Asia. During the long-term adaptation to extreme arid environments, shrubs have developed special assimilative branches that replace leaves for photosynthesis. In this study, four dominant shrubs with assimilative branches, namely Haloxylon ammodendron, Haloxylon persicum, Calligonum mongolicum, and Ephedra przewalskii, were selected as the research objects, and the dry mass, total length, node number, and basal diameter of their assimilative branches and the average length of the first three nodes were carefully measured, and the allometric relationships among five traits of four species were systematically compared. The results indicated that: (1) Four desert shrubs have different assimilative branches traits. Compared with H. persicum and H. ammodendron, C. mongolicum and E. przewalskii have longer internodes and fewer nodes. The dry mass of H. ammodendron and the basal diameter of H. persicum were the smallest; (2) Significant allometric scaling relationships were found between dry mass, total length, basal diameter, and each trait of assimilative branches, all of which were significantly less than 1; (3) The scaling exponents of the allometric relationship between four traits and the dry mass of assimilative branches of H. persicum were greater or significantly greater than those of H. ammodendron. The scaling exponents of the relationships between the basal diameter, dry mass, and total length of E. przewalskii were higher than those of the other three shrubs. Therefore, although different species have adapted to drought and high temperatures by convergence, there was great variability in morphological characteristics of assimilative branches, as well as in the scaling exponents of relationships among traits. The results of this study will provide valuable insights into the ecological functions of assimilative branches and survival strategies of these shrubs to cope with aridity and drought in desert environments.
The environment profoundly affects the creation and variation of plant morphological structure. The environmental conditions in the arid region in China, Central Asia are harsh, and plants have developed various adaptation strategies under adverse conditions such as high temperature, drought, and soil nutrient deficiency for a long time (Gonzalez-Paleo and Ravetta, 2018; Li et al., 2018; Brodribb et al., 2020; Peguero-Pina et al., 2020; Yao et al., 2021; Zhou et al., 2022). As the organs with plasticity in the process of plant evolution, photosynthetic systems (e.g., leaves) are very sensitive to changes in environmental factors such as water, temperature, light, and CO2 concentration (Gratani et al., 2006; Bradshaw, 2006), and their traits are important indicators of plant adaptation strategies in response to environmental changes (Wei et al., 2020; Bergholz et al., 2021; Zhou et al., 2022).
The shrubs with assimilative branches, e.g., Haloxylon ammodendron, Haloxylon persicum, Calligonum mongolicum, and Ephedra przewalskii, are typical xerophyte species, which are widely distributed in desert regions of Central Asia (Orlovsky and Birnbaum, 2002; Hu et al., 2021). During the long-term adaptation to the adverse conditions of frequent drought and high temperature (Zhou et al., 2022), the leaves of the four shrubs degenerated into membrane scales and fleshy specialization or completely degenerated and shrunk to thin rods, and the annual woodless branches replaced the leaves for photosynthesis, completing the life history of plants (Pyankov et al., 1999; Li et al., 2011; Liu et al., 2016). They can resist various stresses, such as sterility, drought, high temperature, salt, and sand burial, and play an important role in maintaining the structure and function of desert ecosystems, reducing wind speed, improving desert microclimate, and promoting the establishment and growth of other desert plants (Wang et al., 2011; Buras et al., 2012; Fan et al., 2016; Hu et al., 2021; Wu et al., 2021).
Composed of multiple nodes and internodes and situated at the front of the shrub branch, assimilative branches are the most vigorous parts of the four shrub branching systems (Li and Li, 1981; Zhou et al., 2022). As a consequence of long-term adaptation to arid conditions, assimilative branches help the plants reduce heat load and water loss, and their vertical growth directly reduces the amount of radiation absorbed (Ehleringer and Cooper, 1992; Li et al., 2014; Ávila-Lovera et al., 2020). In addition, when the assimilative branches experience extreme drought, their surface area can be reduced by curling, wilting, or falling off to reduce water loss (Yu et al., 2012). The formation of assimilative branches is the culmination of the evolution of vegetation under drought conditions (Lyshede, 1979). Studies have shown that plants with assimilative branches are better able to adapt to drought conditions than other plants (Delzon et al., 2010; Li et al., 2014; Zhou et al., 2022). Assimilative branches are situated between leaves and stems and have unique structural characteristics, and their traits affect the carbon sequestration, competitive ability, and mechanical stability of the plant (Li and Li, 1981; Shuang et al., 2009; Li ZB et al., 2013; Yue et al., 2013; Zhou et al., 2022).
Studies have shown that plants eventually develop a combination of traits to adapt to the environment through the internal adaptation of different functional traits, and there is a certain correlation among the different trait combinations (Xue and Cao, 2010; Reich and Cornelissen, 2014). Allometric scaling is a phenomenon that reflects the link between different relative growth rates and both the two traits of an organism. Since first proposed, the proportional relationship among plant functional traits has been proved to be a valid theory that is also widely used (West et al., 1997; Niklas and Enquist, 2001; Niklas, 2004; Han and Fang, 2008; Wu et al., 2021). Studies have shown that the scaling exponents of the relationships between leaf dry mass, twig mass, and leaf area are isometric (Liu et al., 2008). At the whole plant level, the proportionate allocation of biomass in leaves versus stems, supporting the leaves scale, is in accordance with the 3/4 power law expected for the metabolic theory (Huang et al., 2016). Desert plants have internal coordination among different functional traits to cope with heat and drought stresses by making morphological adjustment, which is the most intuitive strategy (Li and Xu, 2008), and a series of morphological traits of assimilative branches are critical for plant survival in arid environments. Researchers discovered significant allometric relationships between H. ammodendron twig mass, dry mass fraction of assimilative branches, and stem mass, as well as the surface area of assimilative branches and stem cross-sectional area (Li ZB et al., 2013). For decades, the morphological and anatomical structures, as well as the photosynthetic physiological characteristics, of green assimilative branches have been investigated (Li and Li, 1981; Su et al., 2005; Yan et al., 2008; Liu et al., 2016; Zhang et al., 2018; Wang et al., 2021; Zhang et al., 2022). However, very limited information is available on the convergence or divergence of morphological traits of assimilative branches of various desert shrubs.
In the present study, four dominant desert shrubs, including H. ammodendron, H. persicum, C. mongolicum, and E. Przewalskii in the Gurbantunggut Desert, northwestern China, were selected as the research objects, and the total length of assimilative branches, the total number of nodes, the basal diameter, the dry mass, and other traits were determined. The purpose of this study was to explore the differences in morphological traits of assimilative branches among different shrubs and the allometric relationships between these traits. Although different species have adapted to drought and high temperature by convergence, according to existing studies, we hypothesize that different shrub species have different functional traits and trait associations of assimilative branches. The results of this study will provide valuable insights into the ecological functions of assimilative branches and the survival strategies of these shrubs to adapt to arid desert environments.
The study site is located in the southeast part (44.34° N, 87.85° E, altitude of 515 m) of the Gurbantunggut Desert (34°09′–49°08′N, 73°25′–96°24′E), northern Xinjiang, China. This desert (4.88 × 104 km2) is the second largest desert in China and also a typical temperate inland desert in Central Asia. It is characterized as an area with low precipitation of 50–200 mm, mean relative humidity of < 45%, high evaporation rate (> 2000 mm), long winter, short spring, sufficient sunshine, and wide daily and annual temperature ranges (Zhang and Chen, 2002; Wang et al., 2003; Tao et al., 2013). The Gurbantunggut Desert is China’s only fixed semi-fixed desert that is affected by cold and humid air currents in the Atlantic Ocean (Qian and Wu, 2010). As a result, the climate, vegetation composition, and richness in the Gurbantunggut Desert vary similarly to that of any other desert in China. (Zhang and Chen, 2002). The desert vegetation is mainly composed of H. persicum, H. ammodendron, and small shrubs such as E. przewalskii and Artemisia songarica, as well as ephemeral and ephemeroid plants that do not exist in other temperate deserts in China (Mao and Zhang, 1994; Wang et al., 2003; Yuan and Tang, 2010). In addition, biological soil crusts are widely distributed, and they are important biological factors that maintain the stability of the surface of deserts (Mao and Zhang, 1994; Zhang and Chen, 2002; Zhang and Wang, 2008).
The assimilative branches of H. persicum, H. ammodendron, C. mongolicum, and E. przewalskii (Figure 1) were collected from a long-term monitoring plot (Beishawo Plot) in later July 2019. Referring to the manual of methodological standards of Cornelissen et al., 2003, six well-grown individuals of H. persicum, H. ammodendron, and C. mongolicum species with similar height and crown size were chosen from a 100 m × 100 m sampling plot, and 10 green, mature, and fully-developed intact current-year assimilative branches from the mid and upper parts of an individual plant canopy were randomly collected in various directions for different species. For E. przewalskii, with a small body size, 30 plants of similar height and crown size were selected, and two intact current-year assimilative branches were collected from each individual. All plant samples (n = 60 for each species) were labeled and quickly placed into foam boxes with ice blocks before transferring them to the lab for analysis.
Figure 1 The morphology of assimilative branches of four shrubs (EP: E. przewalskii; CM: C. mongolicum; HA: H. ammodendron; and HP: H. persicum) in the Gurbantunggut Desert.
In the laboratory, the number of internodes of assimilative branches (NI) was counted, and the length of assimilative branches (LAB, cm) and also the average length of the first three internodes (i.e., located at the base, not the top) of assimilative branches (AL, cm) were measured with a ruler with the precision of 1 mm. The basal diameter of assimilative branches (BD, mm) was measured three times with a vernier caliper with the precision of 0.01 mm, and then the average BD was used. Finally, the assimilative branches were oven-dried at 70°C for 48 h to reach the constant weight, and then their dry mass (DM, g) was recorded with the scale with an accuracy of 0.1 mg. The parameters were expressed as mean ± standard error (mean ± SE).
Based on the decomposition of Type I sums of squares, the nested ANOVA was used to separately analyze the effects of different species, individuals, and assimilative branches on all traits (Nested Procedure, SAS version 8.0; SAS Institute Inc., Cary, NC, USA) (Liu et al., 2010). One-way ANOVA was used to compare the significance of differences in morphological traits of assimilative branches of the four desert plants. Levene’s test was used to test the equality of variances, and Tukey’s HSD test was used for multiple comparisons when group variances were homogeneous; Tamhane’s T2 test was used for multiple comparisons of means with unequal variances (Búrquez et al., 2010). All parameters were expressed as mean ± standard error (mean ± Er). SPSS 19.0 (SPSS Inc. Chicago, Illinois, USA) was used for data analysis.
Model II regression analysis was employed to estimate the correlation between the traits of assimilative branches. The relationship between any two traits is represented by Y = β·Xα, where X and Y denote two parameter values, and α is the scaling exponent (West et al., 1997; Niklas, 2004; Niklas, 2005; Warton et al., 2006; Han and Fang, 2008; Smith, 2009). α = 1 indicates an isometric relationship, i.e., the dependent variable and the independent variable change uniformly or in the same proportion; α ≠ 1 represents an allometric relationship (Zhu et al., 2011). When determining allometric parameters, the power function is generally converted into the equation as follows: logY = logβ + α· logX; the reduced major axis (RMA) linear regression (i.e., Model Type II) was conducted to estimate the scaling slope, a 95% confidence interval (95% CI), and the coefficient of determination (R2). Allometric scaling analysis was performed using the SMATR package (Falster, 2003). Origin 2019 (Originlab Corporation, Northampton, MA, USA) was used for the graphical representation of data.
The results of the hierarchical variation component analysis of each variable (Figure 2) revealed that NI and AL had the most variation among species, accounting for 83.64% and 86.25% of the total variation, respectively, and little variation among individual species and assimilative branches, all of which accounted for less than 10% of the total variation. The variation among LAB and DM species was very small, accounting for less than 10% of the total variation, whereas the variation among individual species and assimilative branches was large and similar, accounting for 42.8-50.1% of the total variation. BD revealed significant variation between species, individuals, and assimilative branches, accounting for 32.2%, 39.6% and 28.2% of total variation, respectively.
Figure 2 The hierarchical variation component analysis of each variable (sums of squares of type I ANOVA); LAB: Length of assimilative branches, NI: Number of internodes, DM: Dry mass of assimilative branches, BD: Average basal diameter of assimilative branches, and AL: Average length of the first three internodes of assimilating branches.
C. mongolicum had a higher LAB (15.53 cm ± 2.26) than the other three species (13.46 cm ± 0.63, 12.66 cm ± 1.40, and 11.94 cm ± 0.95) (P > 0.05). (Figure 3A). Among the four species, H. ammodendron and H. persicum had the highest NI (19.8 ± 0.93 and 18.4 ± 1.14, respectively), while C. mongolicum and E. przewalskii had much lower NIs (6.8 ± 1.07 and 5.4 ± 0.20, respectively), and E. przewalskii had the lowest value (5.4 ± 0.20). (Figure 3B). The DM of H. ammodendron (0.0437 g ± 0.006) was significantly lower than that of E. przewalskii, C. mongolicum, and H. persicum (0.0766 g ± 0.006, 0.0680 g ± 0.013, and 0.0659 g ± 0.009, respectively), and no significant difference was found between the latter three species (Figure 3C). E. przewalskii, H. ammodendron, and C. mongolicum had the highest BD (1.39 mm ± 0.029, 1.36 mm ± 0.055, and 1.28 mm ± 0.038, respectively), with no significant difference between the three species; however, H. persicum had the lowest BD (1.13 mm ± 0.047). (Figure 3D). C. mongolicum and E. przewalskii had higher ALs (2.54 cm ± 0.19 and 2.64 cm ± 0.06, respectively) than the two Haloxylon species (1.06 cm ± 0.06 and 0.81 cm ± 0.02). (Figure 3E). In short, the assimilative branch traits of four desert shrubs differed.
Figure 3 Five traits of assimilative branches of four desert shrubs; Species: HP = Haloxylon persicum, HA = Haloxylon ammodendron, CM = Calligonum mongolicum, and EP = Ephedra przewalskii; Functional traits: (A) LAB = Length of assimilative branches, (B) NI = Number of internodes, (C) DM = Dry mass of assimilative branches, (D) BD = Average basal diameter of assimilative branches, and (E) AL = Average length of the first three internodes of assimilative branches. Different lowercase letters represent significant differences (P < 0.05). Mean ± SE (n = 6 and 30).
There were significant positive correlations between LAB, NI, BD, and DM (P < 0.001), AL and DM (P < 0.05), and all scaling exponents were significantly less than one P < 0.001 (Figure 4; Supplementary Table S1), indicating that the rate of linear increase in LAB, NI, BD, and AL was lower than in DM. The scaling exponent for LAB and DM of C. mongolicum had the largest value (0.832), while that of H. ammodendron had the smallest value (0.597); the scaling exponent of H. persicum and E. przewalskii exhibited moderate values, and both these species significantly differed from the other two species (Figure 4A; Supplementary Table S1). Furthermore, for LAB vs. DM, H. persicum had a higher mean Y-intercept than E. przewalskii, indicating that H. persicum had a larger LAB than E. przewalskii at given SM. The scaling exponent between NI and DM of C. mongolicum showed the largest value (0.772), followed by that of E. przewalskii (0.556), and those of H. persicum and H. ammodendron had the smallest values with no significant difference. The Y-intercept of H. ammodendron was significantly higher than that of H. persicum (Figure 4B; Supplementary Table S1), i.e., the former species produced higher NI than the latter at a given DM. The scaling exponents between BD and DM of E. przewalskii and H. persicum (0.267 and 0.258, respectively) had considerably higher values than those of C. mongolicum (0.219) and H. ammodendron (0.151), and H. ammodendron had the lowest value of scaling exponent (Figure 4C; Supplementary Table S1). In addition, the Y-intercept for BD vs. DM of E. przewalskii was higher than that of H. persicum. For AL vs. DM, the scaling exponent of C. mongolicum and H. persicum had markedly higher values (0.419 and 0.347, respectively) than that of H. ammodendron (0.273), while E. przewalskii showed no significant difference in scaling slope with the other three species (Figure 4D; Supplementary Table S1).
Figure 4 Allometric relationships among LAB, NI, BD, AL, and DM of four desert shrubs. (A) The relationship between LAB and DM, (B) The relationship between NI and DM, (C) The relationship between BD and DM, (D) The relationship between AL and DM. Species: HP = Haloxylon persicum, HA = Haloxylon ammodendron, CM = Calligonum mongolicum, and EP = Ephedra przewalskii; Functional traits: LAB = Length of assimilative branches, NI = Number of internodes, DM = Dry mass of assimilative branches, BD = Average basal diameter of assimilative branches, and AL = Average length of the first three internodes of assimilative branches; Different lowercase letters in the upper right-hand corner of the plot of scaling exponents (α) represent significant differences (P < 0.05).
Similarly, significant positive correlations between NI, BD, AL, and LAB of the four shrubs (P < 0.001) were identified (Figure 5; Supplementary Table S2). The scaling slope between NI and LAB of C. mongolicum (0.867) and E. przewalskii (0.836) did not significantly differ, but the Y-intercept of C. mongolicum was higher than that of E. przewalskii; The scaling exponents between NI and LAB of H. persicum and H. ammodendron had markedly lower values (0.661 and 0.593, respectively) than those of the other two species, and their Y-intercepts also differed (Figure 5A; Supplementary Table S2). The scaling exponents between BD and LAB of E. przewalskii, H. persicum, and H. ammodendron exhibited significantly higher values (0.401, 0.369, and 0.366, respectively) than that of C. mongolicum (0.181), and there was no significant difference in Y-intercepts between H. ammodendron and E. przewalskii, which were greater than that of H. persicum (Figure 5B; Supplementary Table S2). No significant differences in values of the scaling exponent between AL and LAB of the four shrubs were observed, but the Y-intercepts had significant differences among these shrubs (Figure 5C; Supplementary Table S2).
Figure 5 Allometric relationships among NI, BD, AL, and LAB of four desert shrubs. (A) The relationship between NI and LAB, (B) The relationship between BD and LAB, (C) The relationship between AL and LAB. Species: HP = Haloxylon persicum, HA = Haloxylon ammodendron, CM = Calligonum mongolicum, and EP = Ephedra przewalskii; Functional traits: LAB = Length of assimilative branches, NI = Number of internodes, BD = Average basal diameter of assimilative branches, AL = Average length of the first three internodes of assimilative branches; Different lowercase letters in the upper right-hand corner of the plot of scaling exponents (α) represent significant differences (P < 0.05).
The scaling exponents between NI and BD of the four shrubs had significantly greater values than 1 (P < 0.001). C. mongolicum had the highest scaling slope (4.784), followed by E. przewalskii (2.084), whereas H. persicum had the lowest scaling slope (1.606). There was no significant difference in scaling exponents among E. przewalskii, H. ammodendron, and H. persicum. The Y-intercept of H. persicum was significantly higher than that of H. ammodendron, which was also significantly higher than that of E. przewalskii (Figure 6; Supplementary Table S3). Consequently, the allometric relationships among multiple assimilative branch traits also showed strong inconsistency.
Figure 6 The allometric relationship between NI and BD of four desert shrubs; Species: HP = Haloxylon persicum, HA = Haloxylon ammodendron, CM = Calligonum mongolicum, and EP = Ephedra przewalskii; NI = Number of internodes, BD = Average basal diameter of assimilative branches. Different lowercase letters in the upper right-hand corner of the plot of scaling exponents (α) represent significant differences (P < 0.05).
Leaf morphological traits clearly reflected the response and adaptation of plants to the environment and affected the material and energy exchanges between plants and the surrounding environment, as well as the viability, development, and change in the growth direction of plants (Smith and Geller, 1980; Lambers et al., 2008; Vogel, 2009; Li et al., 2012; Jin et al., 2019; Ren, 2022). Some studies have reported that after long-term drought stress, shrubs have evolved assimilative branches to adapt to the desert environment (Li et al., 2014; Ávila-Lovera et al., 2020). The nested ANOVAs of the present study demonstrated that the variation of NI and AL was the greatest among species, whereas the variation of LAB, BD and DM was primarily affected by the plants in the sample plot and relevant to assimilative branch collection, rather than the species.
Although H. ammodendron and H. persicum are members of the same genus, the traits of their assimilative branches differed. Figure 3 shows that H. ammodendron developed assimilative branches with shorter internodes, larger basal diameter, a greater number of nodes, and a lower dry mass than H. persicum. This morphological difference could indicate that H. ammodendron and H. persicum have evolved distinct strategies for maintaining effective photosynthesis, reducing water evaporation, and even reproducing offspring. Our findings are consistent with Zhang’s findings on the individual morphology of H. ammodendron and H. persicum (Zhang, 2002). Plants can spatially arrange their canopy structure by changing the length, diameter, and distribution of their branches, according to studies. H. ammodendron was larger and had greater branching ability and a denser canopy than H. persicum (Li et al., 2009; Wang et al., 2011; Xu et al., 2012). Therefore, we speculate that the morphological characteristics of assimilative branches of two Haloxylon species are related to the size and configuration of individual plants. The shorter the branch lengths of H. ammodendron, the more assimilative the branches, confirming Corner (1949) second rule that there was a trade-off between branch size and number (Westoby and Wright, 2003).
There was no significant difference in BD and LAB between H. ammodendron and E. przewalskii in this study, but H. ammodendron had significantly lower DM than E. przewalskii. Therefore, the findings, E. przewalskii had a lower specific leaf area (SLA) than H. ammodendron, implying that evergreen plants (E. przewalskii) have lower SLA and photosynthetic efficiency, as well as a longer leaf lifespan (Liu and Liang, 2016). The DM of H. ammodendron was lower than that of the other three shrubs, which could be attributed to the fine structure of the assimilative branches. Previous research has shown that the water storage tissues in H. ammodendron assimilative branches are well developed, accounting for more than 50% of their radius, and that the assimilative branches of this succulent plant have a very high moisture content, possibly due to a large amount of water loss after drying, resulting in a significant reduction in DM (Tan et al., 2011; Zan and Zhuang, 2017; Wei et al., 2020). E. przewalskii is an evergreen plant that has been subjected to more environmental stress than the other three species. According to studies, the dry mass of evergreens is greater than that of other deciduous plants, implying that the leaf construction cost of evergreens is frequently greater than that of deciduous plants (Niklas, 1999; Cornelissen et al., 2003; Li et al., 2008; Zhu et al., 2011).
The NI of H. ammodendron and H. persicum was significantly higher than that of C. mongolicum and E. przewalskii, as shown in Figure 3. This difference was consistent with the individual size differences observed in four shrubs previously studied. As a result, we hypothesized that there might be a link between NI and plant individual size. Furthermore, studies have shown that the structural characteristics of leaves change with plant size, though some changes are not significant (Price et al., 2014; Huang et al., 2016; Chang et al., 2021). For leaves that initially grow between the internodes, there is a larger number of nodes per assimilative branch, which indicates that the shrub originally had more full-grown leaves. In addition, we found that the AL of H. ammodendron and H. persicum had significantly smaller values than that of C. mongolicum and E. przewalskii, and LAB and DM of H. ammodendron and H. persicum were slightly smaller than those of C. mongolicum and E. przewalskii at later growth stages. Therefore, we speculated that the length of assimilative branches developed at the earlier stages of plant growth may affect the total length of all assimilative branches, which may be related to the potential configuration of plants. For C. mongolicum and H. ammodendron with similar heights, studies have shown that the latter has the fractal dimension of the branches twice that of the former and also a more complex branch structure (Guo et al., 2015). From a biomechanical perspective, internode length, dry mass, and basal diameter may potentially play critical roles in plant resistance to bending and also in structural stability (Yang et al., 2016; Stubbs et al., 2022). Tall plants with complex branch structures not only bear greater water loss through transpiration and mechanical damage but also are more susceptible to strong wind interference. Therefore, by adjusting the length, dry mass, and basal diameter of assimilative branches, their ability to resist bending can be enhanced to some extent (Li et al., 2021).
In addition, it is undeniable that the differences in all traits of assimilative branches should not be ignored to a certain extent for the other appendages carried by assimilative branches (Li et al., 2009). Moreover, assimilative branches are not only the most important photosynthetic organ but also the basis of sexual reproduction. Since the construction and maintenance of tips, stipules, and other appendages undoubtedly require biomass allocation, the existence of these structures may affect the existence of assimilative branches and cause changes in them, just as the fruit structure affects the cross-species variation in seed yield (Henery and Westoby, 2001). However, it must be pointed out that the four shrubs can grow and develop normally in the arid environment due to their multiple channels and successful adaptation to drought, and any adaptation mode has its value (Zhang, 2002).
The allometric relationship among different functional traits is an ecological strategy for plants to promote resource utilization which reflects the ability of plants to coordinately adapt to environmental changes (Wu et al., 2021). The results of a goodness-of-fit test for all morphological traits of assimilative branches showed a good fit, and all traits showed a non-proportional increasing trend, indicating that the allocation ratio of resources among assimilating branch traits was different. Since DM, NI, and LAB of assimilative branches are the material basis of plant growth and reproduction, as well as the key factor for morphogenesis of photosynthetic organs, resources are more likely to be allocated to them, similar to the law of “diminishing returns” of investment supported by Niklas (Niklas et al., 2007). The allometric relationship among morphological traits of assimilative branches had specificity, indicating that different species have adapted to environmental conditions in different types of the morphological structure of their leaves (Yang et al., 2004; Guerra and Scremin-Dias, 2018). It might be due to differences in fine structures (palisade tissue, stomatal structure, etc.) of assimilative branches.
We found that the scaling exponent between all traits and the DM of assimilative branches of H. persicum also were higher or significantly higher than those of H. ammodendron, and there were no significant differences in the scaling exponent between NI, BD, and AL and between LAB, NI, and BD. The habitat heterogeneity and different ecological adaptation strategies of plants lead to differences in investment trade-off mechanisms, which directly or indirectly affect the growth, development, and reproduction of plants (Xu et al., 2014; Wu et al., 2021).
The scaling exponents between LAB, NI, AL, and DM, as well as between NI and LAB and NI and BD in assimilative branches of C. mongolicum were higher than those of the other three shrubs, while the scaling exponent between BD, DM, and LAB was the smallest. The results indicated that compared with the other three shrubs in C. mongolicum, more resources were allocated for the length and number of nodes of assimilative branches but less for their diameter. This may be related to the clonal growth of C. mongolicum (Li SY et al., 2013; Fan et al., 2016). Studies have reported that the assimilative branches of C. mongolicum show high phenotypic plasticity when exposed to heterogeneous environmental conditions such as wind erosion and sand burial (Balestri and Lardicci, 2013; Fan et al., 2016; Fan et al., 2018a; Fan et al., 2018b). Clonal plants acquire resources in the micro-environment through morphological changes and biomass allocation; for example, to escape from unfavorable habitat conditions as soon as possible and reduce their losses, they mainly establish the simplified structure and slow down their growth by developing thinner stolons, increasing the length of internodes, and reducing the number of branches (D’Hertefeldt and van der Putten, 1998; Zhang et al., 2022).
In addition, the allometric relationships between some traits of E. przewalskii were not significantly different from those of the other three plants. Among them, the assimilative branches of E. przewalskii and H. ammodendron had the same allometric relationship between their BD and LAB, which indicated that although there are quite phylogenetic and individual differences between them, they have experienced the same geological and historical events and the arid and seasonal temperate desert climate during their evolution (Xie et al., 2012) and the basal diameter and total length of their assimilative branches have a relatively consistent resource allocation rate.
However, E. przewalskii, an evergreen shrub, is better able to cope with environmental stress than the other three deciduous plants and prefers to allocate limited photosynthetic products for leaf thickening to resist harsh environments during the non-growing seasons and improve its stress resistance (Cornelissen et al., 2003; Wei et al., 2020). In addition, several reports have shown that evergreen plants take a long time to establish the supporting structures (e. g., basal diameter), which may decrease their photosynthetic efficiency so that they accumulate photosynthetic products by extending the lifespan of their leaves and increasing the length of time during which photosynthesis occurs in leaves (Westoby and Wright, 2003; Wright et al., 2004; Li et al., 2008; Choat et al., 2012).
Under the restriction of resources, natural selection develops the final geometry and structure of plant leaves in the most economical way. Only by constantly adjusting the proportion of distributed internal resources and ultimately establishing a morphological structure conducive to their growth can plants enhance their adaptation to adversity (Yang et al., 2004). As the important organ of the desert shrub, the complexity of morphology and internal structure of assimilative branches is closely related to the environment. During the long-term evolution, under the influence of systematic classification, genetic development, and environmental factors, each species has its unique biological characteristics and evolutionary adaptation process, and there are differences in the response and adaptation to the external environment; thus, different species have the same or different allometric relationships among their various traits (Liu et al., 2008). However, there are many similar adaptation strategies. Data obtained on the morphological and structural parameters of assimilating branches of four shrubs are limited, and therefore, more data need to be collected to verify the specific reasons for these similarities and differences.
In conclusion, we discovered that the variation of NI and AL was greatest among species, whereas the variation of LAB, BD and DM was greater among individuals and assimilative branches. The characteristics of four desert shrubs’ assimilative branches differed. C. mongolicum and E. przewalskii have longer internodes and fewer nodes than H. persicum and H. ammodendron. H. ammodendron had the smallest dry mass and H. persicum had the smallest basal diameter. The reason could be that different plants have different functional requirements for different leaf forms. The scaling exponents between dry mass, length, basal diameter, and each assimilative branch trait differed between four shrub species, all of which were significantly less than one, indicating that the resource allocation ratio among assimilative branch traits varied. The allometric relationship between each trait-pair of assimilative branches differed significantly between four shrub species, which could be related to plant characteristics such as individual size and species specificity formed as a result of long-term adaptation to the desert environment. From the standpoint of the allometric growth relationship between traits, the findings of our study provide an in-depth understanding of the survival strategies and ecological functions of shrubs with assimilative branches that have adapted to the arid desert environment with high temperatures.
The original contributions presented in the study are included in the article/Supplementary Material. Further inquiries can be directed to the corresponding authors.
YT and D-QZ: conceptualization, investigation, writing – review and editing, and preparation. H-HM: data analysis, writing – original draft. YT, B-FY, Y-GL and X-BZ: investigation, formal analysis, and methodology. Y-MZ and YT: conceptualization, resources, supervision, funding acquisition, project administration, and writing – review and editing. All authors contributed to the article and approved the submitted version.
This study was supported by the Xinjiang Natural Science Foundation (Title: Stoichiometric Variation and Influencing Factors of Assimilative Branches of Shrubs in the Junggar Desert), the National Natural Science Foundation of China (nos. U2003214, 42171070, and 41977099), and the CAS Youth Innovation Promotion Association (grant number Y201976).
We thank Yuan-Yuan Zhang, Jing-Ming Yan, and Zhi-Li Han for their invaluable help with fieldwork.
The authors declare that the research was conducted in the absence of any commercial or financial relationships that could be construed as a potential conflict of interest.
All claims expressed in this article are solely those of the authors and do not necessarily represent those of their affiliated organizations, or those of the publisher, the editors and the reviewers. Any product that may be evaluated in this article, or claim that may be made by its manufacturer, is not guaranteed or endorsed by the publisher.
The Supplementary Material for this article can be found online at: https://www.frontiersin.org/articles/10.3389/fpls.2022.1064504/full#supplementary-material
Ávila-Lovera, E., Garcillán, P., Silva-Bejarano, C., Santiago, L. S. (2020). Functional traits of leaves and photosynthetic stems of species from a sarcocaulescent scrub in the southern Baja California peninsula. Am. J. Bot. 107, 1410–1422. doi: 10.1002/ajb2.1546
Balestri, E., Lardicci, C. (2013). The impact of physical disturbance and increased sand burial on clonal growth and spatial colonization of sporobolus virginicus in a coastal dune system. PloS One 8, e72598. doi: 10.1371/journal.pone.0072598
Bergholz, K., Kober, K., Jeltsch, F., Schmidt, K., Weiss, L. (2021). Trait means or variance–what determines plant species’ local and regional occurrence in fragmented dry grasslands? Ecol. Evol. 11, 3357–3365. doi: 10.1002/ece3.7287
Bradshaw, A. D. (2006). Unravelling phenotypic plasticity-why should we bother? New Phytol. 170, 644–648. doi: 10.1111/j.1469-8137.2006.01761.x
Brodribb, T. J., Powers, J., Cochard, H., Choat, B. (2020). Hanging by a thread? forests and drought. Science 368, 261–266. doi: 10.1126/science.aat7631
Buras, A., Wucherer, W., Zerbe, S., Noviskiy, Z., Muchitdinov, N., Shimshikov, B., et al. (2012). Allometric variability of haloxylon species in central Asia. For. Ecol. Manage. 274, 1–9. doi: 10.1016/j.foreco.2012.02.023
Búrquez, A., Martínez-Yrízar, A., Núñez, S., Quintero, T., Aparicio, A. (2010). Aboveground biomass in three sonoran desert communities: Variability within and among sites using replicated plot harvesting. J. Arid Environ. 74, 1240–1247. doi: 10.1016/j.jaridenv.2010.04.004
Chang, Y. N., Xu, C. B., Yang, H., Zhou, J. X., Hua, W. P., Zhang, S. H., et al. (2021). Leaf structural traits were variant with plant size in even-aged stands of sapindus mukorossi. Front. Plant Sci. 12. doi: 10.3389/fpls.2021.692484
Choat, B., Jansen, S., Brodribb, T. J., Cochard, H., Delzon, S., Bhaskar, R., et al. (2012). Global convergence in the vulnerability of forests to drought. Nature 491, 752–755. doi: 10.1038/nature11688
Cornelissen, J. H. C., Lavorel, S., Garnier, E., Díaz, S., Buchmann, N., Gurvich, D. E., et al. (2003). A handbook of protocols for standardised and easy measurement of plant functional traits worldwide. Aust. J. Bot. 51, 335–380. doi: 10.1071/bt02124
Corner, E. J. H. (1949). The durian theory or the origin of the modern tree. Ann. Bot. 13, 367–414. doi: 10.1093/oxfordjournals.aob.a083225
Delzon, S., Douthe, C., Sala, A., Cochard, H. (2010). Mechanism of water stress induced cavitation in conifers: Bordered pit structure and function support the hypothesis of seal capillary-seeding. Plant Cell Environ. 33, 2101–2111. doi: 10.1111/j.1365-3040.2010.02208.x
D’Hertefeldt, T., van der Putten, W. H. (1998). Physiological integation of the clonal plant carex arenaria and its response to soil-bome pathogens. Oikos 81, 229–237. doi: 10.2307/3547044
Ehleringer, J. R., Cooper, T. A. (1992). On the role of orientation in reducing photoinhibitory damage in photosynthetic-twig desert shrubs. Plant Cell Environ. 15, 301–306. doi: 10.1111/j.1365-3040.1992.tb00977.x
Falster, D., Warton, D. I., Wright, I. J. (2003). (S)MATR: Standardised major axis tests and routines. (version 1.0) [EB/OL]. http://www.bio.mq.edu.au/ecology/SMATR/.
Fan, B. L., Ma, Q. L., Guo, S. J., Zhang, J. H., Zhang, X. J., Zhang, W. X., et al. (2016). Ecophysiological responses of mother and daughter ramets in response to wind erosion and sand burial in the clonal shrub plant calligonum mongolicum. Acta Botanica Boreali-Occidental. Sin. 36, 2491–2497. doi: 10.7606/j.issn.1000-4025.2016.12.2491
Fan, B. L., Zhao, C. M., Sun, K. (2018a). Impacts of sand burial and wind erosion on regeneration and growth of a desert clonal shrub. Front. Plant Sci. 9. doi: 10.3389/fpls.2018.01696
Fan, B. L., Zhou, Y. F., Ma, Q. L., Yu, Q. S., Zhao, C. M., Sun, K. (2018b). The bet-hedging strategies for seedling emergence of calligonum mongolicum to adapt to the extreme desert environments in northwestern China. Front. Plant Sci. 9. doi: 10.3389/fpls.2018.01167
Gonzalez-Paleo, L., Ravetta, D. A. (2018). Relationship between photosynthetic rate, water use and leaf structure in desert annual and perennial forbs differing in their growth. Photosynthetica 56, 1177–1187. doi: 10.1007/s11099-018-0810-z
Gratani, L., Covone, F., Larcher, W. (2006). Leaf plasticity in response to light of three evergreen species of the Mediterranean maquis. Trees 20, 549–558. doi: 10.1007/s00468-006-0070-6
Guerra, A., Scremin-Dias, E. (2018). Leaf traits, sclerophylly and growth habits in plant species of a semiarid environment. Braz. J. Bot. 41, 131–144. doi: 10.1007/s40415-017-0416-x
Guo, C. X., Yuan, H. B., Xu, X. Y., Liu, H. J., Wang, D. Z., Li, X. M., et al. (2015). A comparison on architecture of 7 psammophyte shrubs at lower reaches of shiyang river basin. Acta Botanica Boreali-Occidental. Sin. 35, 1031–1036. doi: 10.7606/j.issn.1000-4025.2015.05.1031
Han, W. X., Fang, J. Y. (2008). Review on the mechanism models of allometric scaling laws: 3/4 vs. 2/3 power. Chin. J. Plant Ecol. 32, 951–960. doi: 10.3773/j.issn.1005-264x.2008.04.025
Henery, M. L., Westoby, M. (2001). Seed mass and seed nutrient content as predictors of seed output variation between species. Oikos 92, 479–490. doi: 10.1034/j.1600-0706.2001.920309.x
Huang, Y. X., Lechowicz, M. J., Price, C. A., Li, L., Wang, Y., Zhou, Q. W. (2016). The underlying basis for the trade-off between leaf size and leafing intensity. Funct. Ecol. 30, 199–205. doi: 10.1111/1365-2435.12491
Hu, D., Lv, G. H., Qie, Y. D., Wang, H. F., Yang, F., Jiang, L. M. (2021). Response of morphological characters and photosynthetic characteristics of haloxylon ammodendron to water and salt stress. Sustainability 13, 388. doi: 10.3390/su13010388
Jin, S., Yan, S. J., Huang, L. J., Cheng, Y., Ma, W. W., Wang, Y. X., et al. (2019). Research progress in trade-offs among leaf functional traits. J. Sichuan Forestry Sci. Technol. 40, 96–103. doi: 10.16779/j.cnki.1003-5508.2019.05.020
Lambers, H., Chapin, F. S., III, Pons, T. L. (2008). Plant physiological ecology (New York: Springer-Verlag).
Li, T., Deng, J. M., Wang, G. X., Cheng, D. L., Yu, Z. L. (2009). Isometric scaling relationship between leaf number and size within current-year shoots of woody species across contrasting habitats. Polish J. Ecol. 57, 659–667. doi: 10.1017/S0032247408007912
Li, Z. L., Li, R. A. (1981). Anatomical observation of assimilating branches of nine xerophytes in GanSu. Acta Botan. Sin. 23, 181–185.
Li, Z. B., Li, P. H., Wang, Q., Xu, L. (2013). Allometric characteristics of terminal twigs of tamarix ramosissima and haloxylon ammodendron. Bull. Botan. Res. 33, 274–281. doi: 10.7525/j.issn.1673-5102.2013.03.005
Li, Y. H., Li, Z., Xin, Z. M., Liu, M. H., Li, Y. L., Hao, Y. G. (2018). Effect of morphology on blade surface temperature. J. Plant Ecol. 42, 202–208. doi: 10.17521/cjpe.2017.0127
Li, L., Li, X. Y., Xu, X. W., Lin, S. L., Chen, F. L. (2014). Photosystem II activity in the leaves and assimilative branches of alhagi sparsifolia shap. under brief elevated temperature. Acta Physiol. Plant 36, 1919–1926. doi: 10.1007/s11738-014-1567-z
Li, Y. H., Lu, Q., Wu, B., Zhu, Y. J., Liu, D. J., Zhang, J. X., et al. (2012). A review of leaf morphology plasticity linked to plant response and adaptation characteristics in arid ecosystems. Chin. J. Plant Ecol. 36, 88–98. doi: 10.3724/SP.J.1258.2012.00088
Li, H., Ma, R. Y., Qiang, B., He, C., Han, L., Wang, H. Z. (2021). Effect of current-year twig stem configuration on the leaf display efficiency of populus euphratica. Chin. J. Plant Ecol. 45, 1251–1262. doi: 10.17521/cjpe.2020.0425
Liu, Z. G., Cai, Y. L., Li, K. (2008). Studies on the leaf size-twig size spectrum of subtropical evergreen board-leaved woody species. Chin. J. Plant Ecol. 32, 363–369. doi: 10.3773/j.issn.1005-264x.2008.02.013
Liu, G. F., Freschet, G. T., Pan, X., Cornelissen, J. H. C., Li, Y., Dong, M. (2010). Coordinated variation in leaf and root traits across multiple spatial scales in Chinese semi-arid and arid ecosystems. New Phytol. 188, 543–553. doi: 10.1111/j.1469-8137.2010.03388.x
Liu, M. X., Liang, G. L. (2016). Research progress on leaf mass per area. Chin. J. Plant Ecol. 40, 847–860. doi: 10.17521/cjpe.2015.0428
Liu, Y. B., Li, X. R., Li, M. M., Liu, D., Zhang, W. L. (2016). Leaf (or assimilation branch) epidermal micromorphology of desert plant in arid and semi-arid areas of China. Chin. J. Plant Ecol. 40, 1189–1207. doi: 10.17521/cjpe.2016.0129
Li, Y., Xu, H. (2008). Water and carbon balances of haloxylon ammodendron: intergated study at physiological, plant abd community level. Arid Land Geogr. 31, 313–323. doi: 10.13826/j.cnki.cn65-1103/x.2008.03.001
Li, S. Y., Xu, X. W., Lei, J. Q., Zhou, H. W., Li, Y. G., Jin, Z. Z., et al. (2013). Response and adaptive strategy of photosynthetic organ growth of calligonum caput-medusae schrenk to wind erosion in the central taklimakan desert. Chin. J. Eco-Agricul. 21, 860–866. doi: 10.3724/SP.J.1011.2013.00860
Li, G. Y., Yang, D. M., Sun, S. C. (2008). Allometric relationships between lamina area, lamina mass and petiole mass of 93 temperate woody species vary with leaf habit, leaf form and altitude. Funct. Ecol. 22, 557–564. doi: 10.1111/j.1365-2435.2008.01407.x
Li, S., Zheng, X. J., Tang, L. S., Li, Y. (2011). Morphological investigation of desert shrubs of china’s junggar basin based on allometric theory. Chin. J. Plant Ecol. 35, 471–479. doi: 10.3724/SP.J.1258.2011.00471
Lyshede, O. B. (1979). Xeromorphic features of three stem assimilants in relation to their ecology. Bot. J. Linn. Soc 78, 85–98. doi: 10.1111/j.1095-8339.1979.tb02187.x
Mao, Z. M., Zhang, T. M. (1994). The conspectus of ephemeral flora in northern xinjiang. Arid Zone Res. 3, 1–26.
Niklas, K. J. (1999). A mechanical perspective on foliage leaf form and function. New Phytol. 143, 19–31. doi: 10.1046/j.1469-8137.1999.00441.x
Niklas, K. J. (2004). Plant allometry: Is there a grand unifying theory? Biol. Rev. 79, 871–889. doi: 10.1017/s1464793104006499
Niklas, K. J. (2005). Modelling below-and above-ground biomass for nonwoody and woody plants. Ann. Bot. 95, 315–321. doi: 10.1093/aob/mci028
Niklas, K. J., Cobb, E. D., Niinemets, U., Peter, R., Sellin, A., Shipley, B., et al. (2007). “ diminishing returns “ in the scaling of functional leaf traits across and within species groups. Proc. Natl. Acad. Sci. U. S. A. 104, 8891–8896. doi: 10.1073/pnas.0701135104
Niklas, K. J., Enquist, B. J. (2001). Invariant scaling relationships for interspecific plant biomass production rates and body size. Proc. Natl. Acad. Sci. U. S. A. 98, 2922–2927. doi: 10.1073/pnas.041590298
Orlovsky, N., Birnbaum, E. (2002). The role of haloxylon species for combating desertification in central Asia. Plant Biosyst. 136, 233–240. doi: 10.1080/11263500212331351139
Peguero-Pina, J. J., Vilagrosa, A., Alonso-Forn, D., Ferrio, J. P., Sancho-Knapik, D., Gil-Pelegrín, E. (2020). Living in drylands: Functional adaptations of trees and shrubs to cope with high temperatures and water scarcity. Forests 11, 1011–1028. doi: 10.3390/f11101028
Price, C. A., Wright, I. J., Ackerly, D. D., Niinemets, Ü., Reich, P. B., Veneklaas, E. J. (2014). Are leaf functional traits “ invariant “ with plant size, and what is “ invariance “ anyway? Funct. Ecol. 28, 1330–1343. doi: 10.1111/1365-2435.12298
Pyankov, V. I., Black, C. C., Artyusheva, E. G., Voznesenskaya, E. V., Ku, M. S. B., Edwards, G. E. (1999). Feature of photosynthesis in haloxylon species of chenopodiaceae that are dominant plants in central Asian deserts. Plant Cell Physiol. 40, 125–134. doi: 10.1093/oxfordjournals.pcp.a029519
Qian, Y. B., Wu, Z. N. (2010). Gurbantunggut desert environmental research (Beijing: Science Press), P4
Reich, P. B., Cornelissen, H. (2014). The world-wide “ fast-slow “ plant economics spectrum: A traits manifesto. J. Ecol. 102, 275–301. doi: 10.1111/1365-2745.12211
Ren, S. F. (2022). Allometric growth and ecological adaptability of the leaf anatomical structure of nitraria spp. Acta Agrestia Sin. 30, 1150–1158. doi: 10.11733/j.issn.1007-0435.2022.05.015
Shuang, X., Wu, N., Sun, S. (2009). Within-twig biomass allocation in subtropical evergreen broad-leaved species along an altitudinal gradient: Allometric scaling analysis. Trees 23, 637–647. doi: 10.1007/s00468-008-0308-6
Smith, R. J. (2009). Use and misuse of the reduced major axis for line-fitting. Am. J. Phys. Anthropol. 140, 476–486. doi: 10.1002/ajpa.21090
Smith, W. K., Geller, G. N. (1980). Leaf and environmental parameters influencing transpiration: theory and field measurements. Oecologia 46, 308–313. doi: 10.1007/bf00346257
Stubbs, C. J., McMahan, C. S., Tabaracci, K., Kunduru, B., Sekhon, R. S., Robertson, D. J. (2022). Cross-sectional geometry predicts failure location in maize stalks. Plant Methods 18, 56. doi: 10.1186/s13007-022-00887-x
Su, P. X., An, L. Z., Ma, R. J., Liu, X. M. (2005). Kranz anatomy and C4 photosynthetic characteristics of two desert plants, haloxylon ammodendron and calligonum mongolicum. Acta Phytoecol. Sin. 29, 1–7.
Tan, Y. Q., Bai, X. F., Zhu, J. J., Wang, Z. L., Liu, L. D. (2011). An analysis on the water status in twigs and its relations to the drought resistance in five woody plants living in arid zone. Acta Ecol. Sin. 31, 6815–6823.
Tao, Y., Zhang, Y. M., Downing, A. (2013). Similarity and difference in vegetation structure of three desert shrub communities under the same temperate climate but with different microhabitats. Bot. Stud. 54, 59. doi: 10.1186/1999-3110-54-59
Vogel, S. (2009). Leaves in the lowest and highest winds: Temperature, force and shape. New Phytol. 183, 13–26. doi: 10.1111/j.1469-8137.2009.02854.x
Wang, F., Guo, S. J., Zhang, W. X., Wang, F. L., Han, F. G., Zhang, Y. N. (2021). Anatomic structure characteristics of assimilating shoots of haloxylon ammodendron in different ages and their response to soil conditions. Acta Botanica Boreali-Occidental. Sin. 41, 473–479. doi: 10.7606/j.issn.1000-4025.2021.03.0473
Wang, X. Q., Jiang, J., Lei, J. Q. (2003). Distribution of ephemeral plants and their significance in dune stabilization in gurbantunggut desert. J. Geograph. Sci. 58, 598–605.
Wang, L. J., Sun, D. Y., Zhao, C. Y., Li, J. Y., Sheng, Y. (2011). Plant architecture characteristics of haloxylon ammodendron and haloxylon persicum in zhungar basin. Acta Ecol. Sin. 31, 4952–4960.
Warton, D. I., Wright, I. J., Falster, D. S., Westoby, M. (2006). Bivariate line-fitting methods for allometry. Biol. Rev. 81, 259–291. doi: 10.1017/s1464793106007007
Wei, Y. H., Wang, Z. X., Liang, W. Z., Ma, F. L., Han, L. (2020). Response and adaptation of twig-leaf functional traits of populus euphratica to groundwater gradients. Acta Botanica Boreali-Occidental. Sin. 40, 1043–1051. doi: 10.7606/j.issn.1000-4025.2020.06.1043
West, G. B., Brown, J. H., Enquist, B. J. (1997). A general model for the origin of allometric scaling laws in biology. Science 276, 122–126. doi: 10.1126/science.276.5309.122
Westoby, M., Wright, I. J. (2003). The leaf size-twig size spectrum and its relationship to other important spectra of variation among species. Oecologia 135, 621–628. doi: 10.1007/s00442-003-1231-6
Wright, I. J., Reich, P. B., Westoby, M., Ackerly, D. D., Baruch, Z., Bongers, F. (2004). The worldwide leaf economics spectrum. Nature 428, 821–827. doi: 10.1038/nature02403
Wu, X., Zheng, X. J., Mu, X. H., Li, Y. (2021). Differences in allometric relationship of two dominant woody species among various terrains in a desert region of central Asia. Front. Plant Sci. 12, 754887. doi: 10.3389/fpls.2021.754887
Xie, J. B., Tang, L. S., Wang, Z. Y., Xu, G. Q., Li, Y. (2012). Distinguishing the biomass allocation variance resulting from ontogenetic drift or acclimation to soil texture. PloS One 7, e41502. doi: 10.1371/journal.pone.0041502
Xue, L., Cao, H. (2010). Changes of leaf traits of plants under stress resistance. Ecol. Environ. Sci. 19, 2004–2009. doi: 10.16258/j.cnki.1674-5906.2010.08.035
Xu, Y., Yang, X. D., Xie, Y. M., Xu, Y. L., Chang, S. X., Yan, E. R. (2012). Twig size-number trade-off among woody plants in tiantong region, zhejiang province of China. Chin. J. Plant Ecol. 36, 1268–1276. doi: 10.3724/SP.J.1258.2012.01268
Xu, G. Q., Yu, D. D., Xie, J. B., Tang, L. S., Li, Y. (2014). What makes haloxylon persicum grow on sand dunes while haloxylon ammodendron grow on interdune lowlands: a proof from reciprocal transplant experiments. J. Arid Land 6, 581–591. doi: 10.1007/s40333-014-0029-1
Yang, S. D., Chen, G. C., Zhang, C. L., Chen, J., Wang, X. C. (2004). Some difference of capacity of osmotic regulation between lanceolate and broad-ovate leaves in populus euphratica. Acta Botanica Boreali-Occidental. Sin. 24, 1583–1586. doi: 10.3321/j.issn:1000-4025.2004.09.004
Yang, J. Z., Liang, S. M., Li, N. N., Liu, Y. H., Hao, J. P. (2016). Functional traits of maize stems as supporting organs and their plasticity. Scientia Agricultura Sin. 49, 69–79. doi: 10.3864/j.issn.0578-1752.2016.01.006
Yan, Q. D., Su, P. X., Chen, H. B., Zhang, L. M. (2008). Comparative studies on crystal idioblasts of five desert C4 plants. Chin. J. Plant Ecol. 32, 873–882. doi: 10.3773/j.issn.1005-264x.2008.04.016
Yao, G. Q., Nie, Z. F., Turner, N. C., Li, F. M., Gao, T. P., Fang, X. W., et al. (2021). Combined high leaf hydraulic safety and efficiency provides drought tolerance in caragana species adapted to low mean annual precipitation. New Phytol. 229, 230–244. doi: 10.1111/nph.16845
Yuan, S. F., Tang, H. (2010). Research advances in the ecophysiological characteristics of ephemerals adaptation to habitats. Acta Pratacult Sin. 19, 240–247.
Yue, L. J., Ma, Q., Zhou, X. R., Wang, P., Wang, S. M. (2013). Sodium compound fertilizer in improving the growth and drought resistance of desert plants halaxylon ammodendron, nitraria tangutorum and reaumuria soongorica. J. Lanzhou Univ. (Natural Sciences) 49, 666–674. doi: 10.3969/j.issn.0455-2059.2013.05.013
Yu, T., Ren, C., Zhang, J. P., He, X. L., Ma, L., Chen, Q. J., et al. (2012). Effect of high desert surface layer temperature stress on haloxylon aammodendron (C. a. mey.). Bunge. Flora 207, 572–580. doi: 10.1016/j.flora.2012.06.008
Zan, D. D., Zhuang, L. (2017). Drought resistance analysis based on anatomical structure of assimilating shoots of haloxylon ammodendron from xinjiang. J. Arid Land Resour. Environ. 31, 146–152. doi: 10.13448/j.cnki.jalre.2017.159
Zhang, L. Y. (2002). Haloxylon ammodendron and haloxylon persicum in xinjiang desert (Part 1). Plants 4, 4–6.
Zhang, L. Y., Chen, C. D. (2002). On the general characteristics of plant diversity of gurbantunggut desert. Acta Ecol. Sin. 22, 1923–1932. doi: 10.3321/j.issn:1000-0933.2002.11.018
Zhang, Y. M., Wang, X. Q. (2008). Study on the biological soil crust of junggar desert (Beijing: Science Press).
Zhang, H., Wu, R., Kang, Y. R. (2018). Photosynthetic, physiological, and morphological characteristics of haloxylon ammodendron assimilation twigs in minqin oasis. Pratacultural Sci. 35, 371–379. doi: 10.11829/j.issn.1001-0629.2017-0133
Zhang, X. L., Wu, M. D., Wu, Q. T., Wang, L. D., Zhang, S. Y., Li, L., et al. (2022). Reviewing the adaptation strategies of clonal plants to heterogeneous habitats. Acta Ecol. Sin. 42, 4255–4266. doi: 10.5846/stxb202009212451
Zhou, Z. J., Su, P. X., Wu, X. K., Zhang, H. N., Shi, R., Yang, J. P. (2022). Leaf and canopy photosynthesis of four desert plants: Considering different photosynthetic organs. Photosynthesis Res. 151, 265–277. doi: 10.1007/s11120-021-00884-6
Keywords: allometry, desert shrubs, ecological adaptability, convergent adaptation, assimilative branches
Citation: Meng H-H, Yin B-F, Li Y-G, Zhou X-B, Zhang Y-M, Tao Y and Zhou D-Q (2022) Differences and allometric relationships among assimilative branch traits of four shrubs in Central Asia. Front. Plant Sci. 13:1064504. doi: 10.3389/fpls.2022.1064504
Received: 08 October 2022; Accepted: 29 November 2022;
Published: 13 December 2022.
Edited by:
Runguo Zang, Chinese Academy of Forestry, ChinaReviewed by:
Xuemin He, Xinjiang University, ChinaCopyright © 2022 Meng, Yin, Li, Zhou, Zhang, Tao and Zhou. This is an open-access article distributed under the terms of the Creative Commons Attribution License (CC BY). The use, distribution or reproduction in other forums is permitted, provided the original author(s) and the copyright owner(s) are credited and that the original publication in this journal is cited, in accordance with accepted academic practice. No use, distribution or reproduction is permitted which does not comply with these terms.
*Correspondence: Ye Tao, dGFveWVAbXMueGpiLmFjLmNu; Duo-Qi Zhou, ZHVvcWl6aG91QDE2My5jb20=
Disclaimer: All claims expressed in this article are solely those of the authors and do not necessarily represent those of their affiliated organizations, or those of the publisher, the editors and the reviewers. Any product that may be evaluated in this article or claim that may be made by its manufacturer is not guaranteed or endorsed by the publisher.
Research integrity at Frontiers
Learn more about the work of our research integrity team to safeguard the quality of each article we publish.