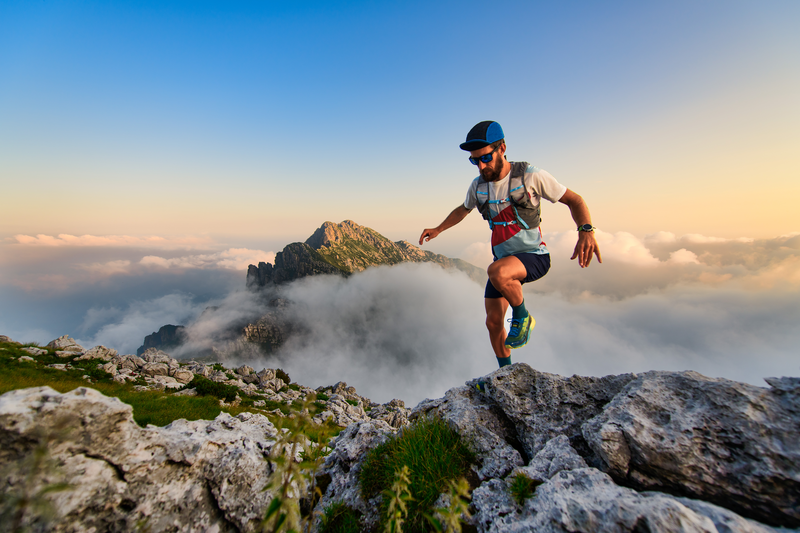
94% of researchers rate our articles as excellent or good
Learn more about the work of our research integrity team to safeguard the quality of each article we publish.
Find out more
ORIGINAL RESEARCH article
Front. Plant Sci. , 28 December 2022
Sec. Plant Pathogen Interactions
Volume 13 - 2022 | https://doi.org/10.3389/fpls.2022.1061520
This article is part of the Research Topic Biointeractions among host plant, wood borers and pathogens/their associated microbes View all 7 articles
Entomopathogen-based biocontrol is crucial for blocking the transmission of vector-borne diseases; however, few cross-latitudinal investigations of entomopathogens have been reported for vectors transmitting woody plant diseases in forest ecosystems. The pine sawyer beetle Monochamus alternatus is an important wood borer and a major vector transmitting pine wilt disease, facilitating invasion of the pinewood nematode Bursaphelenchus xylophilus (PWN) in China. Due to the limited geographical breadth of sampling regions, species diversity of fungal associates (especially entomopathogenic fungi) on M. alternatus adults and their potential ecological functions have been markedly underestimated. In this study, through traditional fungal isolation with morphological and molecular identification, 640 fungal strains (affiliated with 15 genera and 39 species) were isolated from 81 beetle cadavers covered by mycelia or those symptomatically alive across five regional populations of this pest in southern China. Multivariate analyses revealed significant differences in the fungal community composition among geographical populations of M. alternatus, presenting regionalized characteristics, whereas no significant differences were found in fungal composition between beetle genders or among body positions. Four region-representative fungi, namely, Lecanicillium attenuatum (Zhejiang), Aspergillus austwickii (Sichuan), Scopulariopsis alboflavescens (Fujian), and A. ruber (Guangxi), as well as the three fungal species Beauveria bassiana, Penicillium citrinum, and Trichoderma dorotheae, showed significantly stronger entomopathogenic activities than other fungi. Additionally, insect-parasitic entomopathogenic fungi (A. austwickii, B. bassiana, L. attenuatum, and S. alboflavescens) exhibited less to no obvious phytopathogenic activities on the host pine Pinus massoniana, whereas P. citrinum, Purpureocillium lilacinum, and certain species of Fusarium spp.—isolated from M. alternatus body surfaces—exhibited remarkably higher phytopathogenicity. Our results provide a broader view of the entomopathogenic fungal community on the vector beetle M. alternatus, some of which are reported for the first time on Monochamus spp. in China. Moreover, this beetle might be more highly-risk in pine forests than previously considered, as a potential multi-pathogen vector of both PWN and phytopathogenic fungi.
Vector-borne diseases occur in humans and agro-ecosystems, causing severe global health problems and economic losses. In recent decades, a variety of human and animal diseases transmitted by mosquitoes and ticks (Zellner and Huntley, 2019; Gao et al., 2020), together with notorious crop pathogen outbreaks vectored by destructive agricultural pests such as psyllids, thrips, and whiteflies (Navas-Castillo et al., 2011; He et al., 2020; Moreno et al., 2021), have increased the demand for advanced vector control technologies and strategies. Due to the environmental risks of excessive use of chemical pesticides and vector insecticidal resistance, sympatric natural enemies of vectors, including parasitoids, predatory arthropods, and entomopathogenic microbes, were explored in their distribution areas at cross-latitudinal scales (Garcia et al., 2020; Nechols, 2021), some of which have been newly discovered and developed as effective, safe, and economically acceptable alternatives to chemical control (Wang et al., 2019; Islam et al., 2021). Compared to other biocontrol agents, features, such as easy multiplication, host specificity, and high survival in varied environments, of natural and gene-engineered entomopathogenic fungi are more favorably adopted to supplement the arsenal of biological control to manage medical and agricultural insect vectors (Leger and Wang, 2010; Fang et al., 2012; Javed et al., 2019; Lovett et al., 2019).
Forest insect pests, especially wood borers, carry the assembly of phytopathogens or have tight relationships with specific associates that amplify the negative effects of their insect hosts or even dominate significant forest collapses (Linnakoski and Forbes, 2019). For example, bark beetle Scolytus multistriatus, a vector of the pathogen Ophiostoma ulmi, causes Dutch Elm Disease (Jürisoo et al., 2021), and the wood wasp Sirex noctilio transmits several phytopathogenic fungi (Corley et al., 2019). Moreover, a large number of forest insect pests have intimate connections with tree pathogens as potential vectors (Humble and Allen, 2006; Hulcr and Dunn, 2011). However, few cross-latitudinal investigations have been conducted on the distribution and diversity of entomopathogenic fungal species of insect vectors transmitting important woody plant diseases in natural forest ecosystems, substantially restricting the resource exploration of promising entomopathogenic fungi. At present, a strikingly limited number of entomopathogenic fungal species (e.g., Beauveria bassiana and Metarhizium anisopliae) constitutes the main microbial biocontrol agents for application in natural fields, especially in forest ecosystems (Dara et al., 2019; Rajula et al., 2020; Shehzad et al., 2021; Shehzad et al., 2022), which would be insufficient to encounter the increasing challenges from both indigenous species outbreaks and exotic species invasions.
The stem-borer of pine trees, Monochamus alternatus, not only bores into branches and trunks of host pine to hinder the transportation of nutrients and water, but also facilitates the invasion of plant-parasitic pinewood nematode (PWN, Bursaphelenchus xylophilus) as the main vector beetle in pine forest systems in China and adjacent countries (Kobayashi et al., 1984; Futai, 2013; Zhao and Sun, 2017). Investigations of natural entomopathogenic fungi for Monochamus spp. in Spain, Japan, and Anhui/Zhejiang Province of China showed very similar results, demonstrating that Beauveria species were the most abundant isolates, followed by Metarhizium or Lecanicillium (Verticillium) (Shimazu, 2004; Han et al., 2007; Ma et al., 2009; Álvarez-Baz et al., 2015). In contrast to the middle-high latitudinal regions covered by previous surveys, low latitudinal regions, especially southern China, are habitats where pine wilt disease (PWD, caused by PWN) initially emerged and lasts for long time (Tang et al., 2021). Considering that higher fungal diversity occurs in regions with high temperature and humidity and that several kinds of natural enemies evolve relationships with target insects over a longer period, the cross-latitudinal regions in southern China are considered an ideal and rich resource reservoir for exploring novel entomopathogenic fungi with previously unknown functions in the biocontrol of PWD transmission. However, few studies have been conducted in this area.
To explore underlying natural resource of entomopathogenic fungi on M. alternatus from low latitudinal regions of southern China, in the present study, we firstly investigated the diversity and community composition of fungal species associated with naturally infected M. alternatus across five geographical populations in southern China and assessed their relationships with the latitudinal regions. Then, we evaluated entomopathogenic activities and infection phenotypes of representative fungal isolates, followed by measurements of compatibility of these fungi with the host pine P. massoniana. Results showed that variation of fungal community composition significantly couples with the geographical origin of naturally infected M. alternatus and the main fungal species from each population are region-specific. Enzymatic and in vivo interactive bioassays combined with morphological and molecular identifications revealed strongly entomopathogenic fungi functioning in parasitic or non-parasitic mode, which are well compatible with host pine. These findings provide new insights into the distribution of M. alternatus entomopathogenic fungi across geographical regions as well as their promising application in the field to break down transmission of the pine wilt disease and potentially vectoring phytopathogenic fungi by the beetle.
The pine sawyer beetle M. alternatus was collected using commercial traps (FEILUOMENG Co., China) baited with attractants in naturally infested pine forests from five geographical regions of southern China (Figure S1). The specimens were labeled and transported to the laboratory in individual sterilized tubes for each beetle with fresh twigs. During rearing of the collected beetles (approximately 500 beetles in total), 81 beetle cadavers or symptomatically living ones with fungal infections were observed and stored at 4°C for use in this study.
Fungal isolation was performed under aseptic conditions following previously described procedures (Parker et al., 2003). The beetle body was divided into seven positions using sterilized scissors: antennae, head, thorax, abdomen, eggs (for females), wings, and legs. Tissues were inoculated onto potato dextrose agar (PDA) plates containing 0.05 g/L streptomycin, penicillin G and tetracycline and then cultured in an incubator at 25°C until the tissues were covered and surrounded by mycelia. Fungal purification was repeated twice on PDA with antibiotics, and pure isolates were cultured on conventional PDA and deposited in the Key Laboratory of Vector Biology and Pathogen Control of Zhejiang Province at Huzhou University.
The fungal isolate was cultured in 50 ml sterile potato dextrose broth (PDB) at 25°C with shaking at 180 rpm in a 250ml flask for 5–7 days. Mycelia harvested from the PDB were separated by filtration and homogenized in liquid nitrogen using a pre-cooled mortar and pestle. Genomic DNA was extracted using the Rapid Fungi Genomic DNA Isolation Kit (Sangon Biotech Co., China), and the rDNA-ITS region was amplified using primer pairs ITS1 and ITS4 (White et al., 1990) via the following procedure: initial denaturing at 95°C for 4 min, followed by 35 cycles of denaturing at 94°C for 60 s, annealing at 58°C for 60 s, elongation at 72°C for 2 min, and a final elongation at 72°C for 10 min. Amplified PCR products were sequenced and compared with the ITS sequence database in GenBank using BLAST software on the National Center for Biotechnology Information (NCBI) website. The gene sequences were deposited in NCBI under GenBank accession numbers OP321299–OP321543.
Based on the above results, eleven representative fungal species, namely, Aspergillus austwickii, A. ruber, Beauveria bassiana, Clonostachys rosea, Lecanicillium attenuatum, L. aphanocladii, Penicillium citrinum, Pestalotiopsis disseminata, Purpureocillium lilacinum, Scopulariopsis alboflavescens, and Trichoderma dorotheae, were used to test their entomopathogenic activities. Considering that it is difficult to collect an adequate quantity of adult M. alternatus with long-lasting alive status from the field to match this bioassay, the population of the model insect beetle Tribolium castaneum, successfully reared in the laboratory with a common genetic background, was used to determine the entomopathogenicity of the eleven fungal isolates. Their infection phenotypes on M. alternatus were also further confirmed in the subsequent experiments. Conidia obtained from 14-day-old fungi were suspended in a sterile aqueous solution of 0.01% Tween-80 (Orduño-Cruz et al., 2015). This suspension was shaken with glass beads and filtered through two layers of gauze to remove the mycelia. The conidia were counted with a hemocytometer and adjusted to 1×108 conidial/ml with sterile 0.01% Tween-80.
Adult T. castaneum was reared on wheat bran at 25°C in a climate-controlled incubator. Beetles were surface-sterilized with bleach, ethanol, and distilled water [10:10:80 (vol:vol)] and starved for 24 h before use. Aliquots of wheat bran were UV-sterilized, placed in sterile Petri dishes, and then treated with conidial suspension. T. castaneum beetles were also immersed in the conidial suspension for 10 s and transferred to a Petri dish (n = 20 in each Petri dish). The wheat bran was replaced with a new conidial treatment every 4 days. Beetles and wheat bran in the control group were treated with sterile 0.01% Tween-80 solution. Each fungal and control group was replicated three times. In a pilot assay, beetles were checked for mortality 9 days after fungal treatments and in the subsequent survival monitoring assay, beetles were recorded daily for 15 days. Dead beetles from these assays were transferred to sterile moist centrifugal tubes and inspected for fungal growth on the cadavers. The aerial hyphae of each fungal species on cadavers were confirmed using Koch’s postulate.
Fungal entomopathogenic activity correlated well with the activities of three enzymes including protease, chitinase, and lipase, in M. alternatus and many other insect pests (Cortez-Madrigal et al., 2014; Dhawan and Joshi, 2017; Moharram et al., 2021). The enzyme activities of the fungal species in this study were measured following previously reported procedures (Sharma et al., 2016; Li et al., 2018; Alabdalall et al., 2020) with minor modifications. To test protease activity, conidia (1×108 conidia/ml) of each isolated fungus were cultured in 50 ml of protease-inducing medium with shaking at 180 rpm at 25°C for 10 days. One milliliter of fermentation supernatant was obtained from each culture solution every 2 days and incubated with 1 ml of 1% casein solution at 37°C for 30 min. The quantities of the reaction products were determined by measuring the optical density at 600 nm (OD600) using the Folin-phenol reagent method. The negative control of each sample was prepared by adding trichloroacetic acid to the reaction solution before incubation to inhibit putative enzymatic activities. Five concentrations of tyrosine were used to create a standard curve, and one protease unit was defined as the amount of fermentation supernatant required to produce 1 µg of tyrosine from casein per minute. To test chitinase activity, conidia (1×108 conidia/ml) of fungi were cultured in liquid chitin medium for 10 days and at every 2 d-interval, 1 ml of fermentation supernatant was incubated with 1 ml of 1% colloidal chitin at 50°C for 1 h. The negative control for each sample was prepared by heating the reaction solution in a boiling water bath before incubation. Reaction product quantities were determined by measuring the OD540 using the 3,5-dinitrosalicylic acid (DNS) method. Five concentrations of N-acetyl-D-glucosamine were used to create a standard curve, and one chitinase unit was defined as the amount of fermentation supernatant required to produce 1 µg of N-acetyl-D-glucosamine per minute. To test lipase activity, conidia (1×108 conidia/ml) of fungi were cultured in Sabouraud’s dextrose agar (SDA) medium for 10 days and 0.2 ml of fermentation supernatant was pipetted every 2 days and incubated with 0.2 ml matrix solution containing 4-nitrophenyl palmitate (Gopinath et al., 2005) at 37°C for 30 min. The negative control for each sample was prepared by adding trichloroacetic acid to the reaction solution before incubation. The quantity of the reaction product p-nitrophenol was calculated by comparing the OD410 value with the linear standard curve, and one lipase unit was defined as the amount of fermentation supernatant required to produce 1 µg of p-nitrophenol per minute. Three biological replicates were used for enzymatic bioassays.
The fungal species with remarkable entomopathogenic activities were cultured on PDA plates for 7–11 days at 25°C. Circular agar blocks (5 mm in diameter) from colonies were transferred to new PDA plates to observe colony morphology. For asexual morphological descriptions, conidia, conidiophores and nutritional hyphae were sampled from colonies on glass slides, and their traits were observed and measured using a Leica DM2000 microscope (Leica Co., Germany).
Fungal infection phenotypes in live M. alternatus adults (sampled from Zhejiang Province) were confirmed following the procedure described above. Beetles were surface-sterilized, dipped in conidia suspension, transferred to 50-ml sterile tubes (one beetle per tube), and provided with fresh pine twigs (also immersed in conidia suspension for 10 s). Beetle activity was assessed based on the quantity of the frass produced. When feeding ceased, the beetle was transferred to a new 50-ml tube (with sterile moist cotton) for fungal growth for 7 days. Each fungal species was applied to five living beetles. The infected beetles were observed as described above and fulfilled by Koch’s postulates.
For scanning electron microscopy (SEM) observation, colony plugs and infected beetles were fixed in pre-chilled 2.5% glutaraldehyde at 4°C for 2 days. Samples were washed thrice in 0.1% phosphate buffer (pH 7.2–7.4) for 5 min and dehydrated in 30%, 50%, 70%, 80%, 90%, 95%, 100% ethanol for 10 min. The samples were dried in a vacuum freeze dryer (Yamato Scientific Co., Japan), coated with platinum using a sputter coater, and observed using an S-3400N scanning electron microscopy (Hitachi, Japan).
The primer pairs NS1/NS4 (White et al., 1990), LR7/LROR (Vilgalys and Hester, 1990; Rehner and Samuels, 1994), EF-983F/EF-2218R (Aphidech and Kusavadee, 2013), RPB2-5’F/RPB2-5’R (Wang et al., 2015), and TUB1/TUB22 (Qi et al., 2021), were used to amplify a region spanning of the nuclear ribosomal SSU gene, a segment of the large subunit rRNA gene (LSU), part of the elongation factor 1-alpha (EF-1α) gene, the second largest subunit sequences of RNA polymerase ІІ (rpb2), and part of the β-tubulin gene, respectively. The PCR products were examined by 1.5% agarose gel electrophoresis and then subjected to sequencing with the GenBank accession numbers listed in Table S1. Sequences of ITS and the five genes were then aligned using Clustal X2.0 and MEGA 6 (Larkin et al., 2007; Tamura et al., 2013). Ambiguously aligned sites were excluded, and gaps were treated as missing data during sequence alignment. The aligned sequences of the six genes were concatenated to construct Maximum Likelihood (ML) phylogenetic trees using MEGA 6.
Two- to three-year-old P. massoniana seedlings were used to determine the phytopathogenic activities of the entomopathogenic fungi. Fungal inoculation was conducted by making a wound on the main stem of each P. massoniana seedling using a sterile cork borer with a 5-mm diameter at 15 cm above the soil line (one seedling with one inoculation point). A plug with a 5-mm diameter was taken from the margin of one actively growing fungal species cultured on PDA and transferred to the cambium layer of seedling inoculation point. The inoculation points were wrapped in a laboratory film (Parafilm M, USA) to prevent contamination and desiccation. Mock inoculation, mimicked using a plug of PDA alone (without fungi), was applied to the seedlings in the same manner as a control. The Fusarium species, which are broad-spectral plant pathogens, together with other non-entomopathogenic fungi were also included in the experiment. Each fungal isolate and the control were replicated thrice. After 3 weeks, lesion length was measured both downward and upward from the inoculation point. The fungi were re-isolated from the infected areas to complete Koch’s postulates.
To measure pectinase and cellulase activities, conidia (1×108 conidia/ml) of each fungus were cultured in 50 ml of pectinase- or cellulase-inducing medium (0.2% KNO3, 0.05% KCl, 0.001% FeSO4, 0.1% K2HPO4, 0.05% MgSO4·7H2O, 1% pectin, or 1% carboxymethyl cellulose sodium; pH 5.0) for 10 days. For every 2 days, 1 ml of fermentation supernatant was pipetted and incubated with 1 ml of 0.1% pectin solution or 0.5% sodium carboxymethyl cellulose solution at 50°C for 1 h. The negative control for each sample was prepared by heating the reaction solution in a boiling water bath for 5 min before incubation. After incubation, the reaction solutions were bathed in boiling water, incubated with 2 ml of DNS, boiled again at 100°C for 5 min, and finally refrigerated on ice. Reaction product quantities were determined by measuring the OD540 using the 3,5-dinitrosalicylic acid (DNS) method (Pereira et al., 2002). Five concentrations of D-galacturonic acid and D-glucose were used to generate the standard curves. One pectinase unit and one cellulase unit were defined as the amounts of fermentation supernatant to produce 1 µg of D-galacturonic acid and 1 µg of D-glucose per minute, respectively. The enzymatic bioassays were performed in triplicate.
Variations in α-diversity indices of fungal communities among geographical regions, mortality of beetles caused by fungi at 9 d, enzymatic activity levels, and phytopathogenicity among fungi were assessed using one-way ANOVA followed by the Bonferroni approach for pair-wise comparisons. Where normality and/or equal variance were not assumed, nonparametric Kruskal-Wallis one-way ANOVAs were performed, followed by pair-wise comparisons using the Mann-Whitney U test. Absolute abundance was estimated as the number of isolates per fungal taxa, whereas the ratio of isolates from each fungal taxa to total fungal isolates was considered the relative abundance of certain taxa. Venn diagrams and upset plots were used to display the intersection of fungal species in beetle geographical populations (or body positions or genders) using R software with the package ggplots2. Principal component analysis (PCA) was used to investigate the variation patterns of the fungal community structures among geographical locations. Sörensen’s similarity index (Cs) was calculated using the equation: () (Sörensen, 1948), where a and b are the numbers of species unique to each geographical location and c is the number of shared species between the two locations. Principal coordinate analysis (PCoA) was applied to visualize the relationship between the variation in fungal community composition and beetle geographical populations (or body positions or genders), followed by significance tests using one-way PERMANOVA. The survival of beetles was calculated using Kaplan-Meier survival analysis. Comparisons between survival curves were further tested using the Log Rank (Mantel-Cox) method. GraphPad Prism 6 and PAST software were used for statistical analyses.
A total of 640 fungal strains were isolated from 81 beetle cadavers or those symptomatically alive in five geographical regions, belonging to 15 fungal genera and 39 species (Table 1). Genus Aspergillus was highest in relative abundance (35.47%), followed by Penicillium (25.31%), Scopulariopsis (9.69%), Lecanicillium (8.75%), Fusarium (7.66%), and Trichoderma (6.42%). These genera accounted for 93.30% of the total identified strains. The most dominant species was A. ruber (21.72%), followed by P. citrinum (9.84%), A. sydowii (9.84%), S. alboflavescens (9.69%), L. attenuatum (8.44%), and F. annulatum (5.16%). These collectively represented 64.69% of the total identified strains.
Table 1 GenBank accession numbers of fungal isolates from M. alternatus in this study and similarity scores to closest (type) strains in NCBI according to the rDNA-ITS region.
Significant differences in fungal community composition were found among geographical regions/provinces in which M. alternatus adults were sampled. In Zhejiang, a total of 197 fungal strains were isolated from the beetles, belonging to 11 genera and 22 species, in which fungal species L. attenuatum and P. citrinum were dominant, with relative abundances of 25.38% and 14.72%, respectively (Table S2; Figure S2). In Sichuan, a total of 36 fungal strains were isolated, belonging to 6 genera and 12 species, in which fungal species A. austwickii and T. dorotheae were dominant, with relative abundances of 41.67% and 19.44%, respectively (Table S2; Figure S2). In Fujian, 172 fungal strains belonging to 12 species and 6 genera were isolated, in which fungal species S. alboflavescens and A. sydowii were higher in relative abundances than others, at 31.40% and 29.07%, respectively (Table S2; Figure S2). In Guangdong, 74 fungal strains belonging to 14 species and 9 genera were isolated, and P. citrinum with a relative abundance of 22.97%, was higher than other species (Table S2; Figure S2). In Guangxi, 161 fungal strains belonging to 6 species and 5 genera were isolated, of which A. ruber with a relative abundance of 85.09% was the dominant species (Table S2; Figure S2). The fungal species, namely, L. attenuatum, A. austwickii, S. alboflavescens, and A. ruber, appeared to be region-representative in Zhejiang, Sichuan, Fujian, and Guangxi, respectively, since their relative abundances were highest in one population while strikingly low in the others. Since P. citrinum was also abundant in Zhejiang, no region-representative species were found in Guangdong.
Additionally, except for the fungi isolated from M. alternatus in Fujian population, fungal communities from the rest of geographical populations contained distinct species (exclusively isolated species) in their respective populations (Figure 1A). Furthermore, the number of shared fungal species in the Fujian and Guangdong population was higher than that in the other populations (Figure 1A).
Figure 1 Community composition variations of fungal associates of M. alternatus populations from five geographical regions. (A) Venn diagram and upset plot. (B) PCA of Bray-Curtis distance differentiating patterns of samples from different populations according to fungal community composition. (C) PCoA of Bray-Curtis distance showing variation in fungal community composition among geographical populations.
The α-diversity index comparisons of fungal communities among populations and multivariate analyses further supported the geographical variation in community composition. An obvious decrease in fungal species diversity was found in high- to low-latitudinal populations (Table S3). Species richness and diversity indices were significantly higher in the population of Zhejiang than those of Guangxi, whereas dominance and evenness indices were higher in the Guangxi (Table S3). Principal component analysis (PCA) showed that fungal community compositions of M. alternatus in Zhejiang, Fujian, and Guangxi populations diverged remarkably from each other (Figure 1B), and the pair-wise similarity coefficient calculation further indicated that fungal communities from the three regions were dissimilar to each other, while the fungal composition of the Fujian population was more similar to the Guangdong than other populations (Table S4). Principal coordinate analysis (PCoA) elucidated the distances of fungal community composition between geographical population groups and inter-sample variations within groups (Figure 1C; one-way PERMANOVA; F = 9.180, P = 0.0001). Pair-wise comparisons also demonstrated strikingly significant mutual dissimilarities in fungal composition between different M. alternatus geographical populations, except for those isolated from Fujian and Sichuan populations, which did not show notable differences with that from Guangdong population (Table S5).
Fungal communities from the five geographical populations were integrated and re-categorized according to body position (Table S6; Figures S3A, B) and gender (Table S7; Figures S4A, B). However, no significant differences were found in the fungal community composition among M. alternatus body positions (one-way PERMANOVA; F = 1.135, P = 0.217) and among genders (one-way PERMANOVA; F = 1.167, P = 0.277), as visualized by PCoA analyses (Figures S3C, S4C).
Fungal isolate Beauveria bassiana, a well-known entomopathogenic fungus, as well as four more fungal isolates with activities reported previously in insects including Clonostachys rosea (Mohammed et al., 2021), L. aphanocladii (Nedveckytė et al., 2021), Pestalotiopsis disseminata (Lv et al., 2011) and Purpureocillium lilacinum (Panyasiri et al., 2022), together with A. austwickii, A. ruber, L. attenuatum, P. citrinum, S. alboflavescens and T. dorotheae—which were the main species associated with their corresponding M. alternatus populations—were used to evaluate their entomopathogenic activities. In a pilot assay, after 9 days of infection, four fungal species, namely, A. austwickii, B. bassiana, L. attenuatum, and S. alboflavescens showed significantly higher mortality on the model insect beetle T. castaneum than the control (Figure S5; Kruskal-Wallis test; χ10 2 = 25.86, P = 0.0039). In the subsequent 15-day-time course assay, there was a significant difference in the survival curves among treatments (Figure 2A; Kaplan-Meier test; χ11 2 = 288.9, P = 0.0001). No significant differences were found between the survival of T. castaneum adults inoculated with Tween 80 (control group) and those inoculated with conidial suspension of P. lilacinum, P. disseminata, C. rosea, and L. aphanocladii (Figure 2A; Log-rank tests; Pl, χ1 2 = 0.22, P = 0.6398; Pd, χ1 2 = 0.44, P = 0.5080; Cr, χ1 2 = 0.57, P = 0.4511; Lap, χ1 2 = 1.35, P = 0.2456), indicating that the four fungal species did not influence beetle fitness. However, the survival of T. castaneum adults inoculated with B. bassiana, A. austwickii, S. alboflavescens, T. dorotheae, A. ruber, and L. attenuatum was significantly lower than that of the beetles in the control group (Figure 2A; Log-rank tests; Bb, χ1 2 = 96.23, P< 0.0001; Aa, χ1 2 = 101.6, P< 0.0001; Sa, χ1 2 = 41.75, P< 0.0001; Td, χ1 2 = 29.89, P< 0.0001; Ar, χ1 2 = 26.11, P< 0.0001; Lat, χ1 2 = 20.78, P< 0.0001), demonstrating the potent insecticidal activities of the six fungal species. Another fungus, P. citrinum, showed a relatively moderate efficacy in killing T. castaneum adults (Figure 2A; Log-rank test; χ1 2 = 7.70, P = 0.0055).
Figure 2 Entomopathogenic activities of representative fungal species isolated from M. alternatus populations with naturally fungal infection. (A) Kaplan-Meier survival curves of T. castaneum beetles inoculated with conidia suspension (1×108 conidia/ml). Log-rank tests were performed and the levels of differences were denoted: ns, not significant, P > 0.05; **P< 0.01; ****P< 0.0001. (B) Protease activities of fermentation supernatants from fungal species. (C) Chitinase activities of fermentation supernatants from fungal species. (D) Lipase activities of fermentation supernatants from fungal species. In B-D, different letters mean significant differences among fungi at each time point (P< 0.05) and ns means not significant. Data were represented as Mean ± SD.
The entomopathogenic activities of these fungal species were determined using enzymatic activity assays. A. austwickii and B. bassiana had consistently higher protease activities with incubation time than the other fungal species (Figure 2B). B. bassiana performed better in chitinase activity than A. austwickii, reaching a peak level higher than that of other fungi on day 8 (Figure 2C). Except for B. bassiana, the other fungi showed decreased chitinase activity from day 4 until the end, and S. alboflavescens exhibited a relatively higher chitinase activity during this time (Figure 2C). As for lipase activity, A. austwickii reached a peak level higher than other fungi on day 2 and then exhibited a decrease in activity to a stable level (Figure 2D). Both B. bassiana and L. attenuatum showed the highest lipase activity levels on day 6, compared to other fungi (Figure 2D). P. citrinum did not exhibit an increase in lipase activity until day 8–10 of the incubation (Figure 2D).
Among the seven entomopathogenic fungi that caused significant fitness loss in T. castaneum adults, three fungi (A. ruber, P. citrinum, and T. dorotheae; grown on PDA medium; Figures S6–S8) did not display visible infection phenotypes on the beetle, neither did they on M. alternatus. However, the other four (A. austwickii, B. bassiana, L. attenuatum, and S. alboflavescens) showed conspicuous infection symptoms on T. castaneum body surface (Figure S9), indicating strong parasitic capacities of these fungi. Their infection phenotypes were also observed in M. alternatus adult bodies, with mycelia of the four fungal species penetrating the body surface from the inside of the beetle, carrying asexual conidiophores (Figures 3–6).
Figure 3 Morphology of A. austwickii and M. alternatus cadaver infected by A. austwickii under optical microscope and SEM. (A) Colonial morphology cultured on PDA. (B) Reverse of colony on PDA. (C) Hyphae and conidiophores (OM). (D) Conidia (OM). (E) Conidiophores (SEM). (F) Conidia (SEM). (G) M. alternatus cadaver surrounded by mycelia. (H) Conidiophores grown from M. alternatus cuticle (SEM). (I) Conidia on M. alternatus cuticle surface (SEM).
The asexual morphological features of parasitic entomopathogenic fungi grown on PDA medium and the bodies of the two beetle species were observed and as follows: A. austwickii colony was initially white, and then became dark green due to the production of spores (Figure 3A). The reverse color was brown and green (Figure 3B). The conidiophores were smooth-walled and contained pyriform-shaped vesicles (Figure 3C, by optical microscope, OM). The conidia were globose, 3.5–4.4 μm×3.1–3.6 μm in diameter (Figure 3D, by OM) and grown from ampulliform phialides on the conidiophore (Figure 3E, by SEM). The conidia were shown with rough walls (Figure 3F, by SEM). M. alternatus cadaver was covered with yellowish green mycelia of A. austwickii (Figure 3G; mainly on its abdomen). Conidiophores grown from M. alternatus (Figure 3H, by SEM) and T. castaneum beetles (Figure S9A) had radial conidial heads typical of the Genus Aspergillus, producing spherical conidia with rough and echinulate walls (Figure 3I, by SEM; Figure S9B). B. bassiana formed a downy white colony with a powdery texture (Figure 4A), and the reverse was milky white (Figure 4B). Hyphae were septate and branched (Figure 4C, by OM) and the subglobose conidia were 2.9–3.4 μm×1.7–2.1 μm in diameter (Figure 4D, by OM; Figure 4F, by SEM). Conidiophores consisted of dense and spherical lateral clusters of globose to flask-shaped conidiogenous cells on top of an elongating and geniculate rachis (Figure 4C, by OM; Figure 4E, by SEM). M. alternatus cadaver was wholly covered by B. bassiana mycelia (Figure 4G), and the characteristics of conidiophores (Figure 4H, by SEM) and conidia (Figure 4I, by SEM) on the beetle were the same as those on PDA medium and T. castaneum (Figures S9C, D). L. attenuatum colony was white and cottony (Figure 5A), and the reverse side was light yellow in the center with a white margin (Figure 5B). Hyphae were smooth-walled, carrying conidiophores in solitary, opposite, or verticillate, with long and sharp-tipped phialides that sometimes branched (Figure 5C, by OM; Figure 5E; by SEM). The conidia were cylindrical with slightly narrowed ends or elliptical sharp, and they were 3.2–4.4 μm×1.6–1.9 μm in diameter (Figure 5D, by OM; Figure 5F, by SEM). M. alternatus cadaver was covered with a thin layer of mycelia, especially on its head and antennae (Figure 5G). The conidiophores with thorn-like phialides were grown from infecting mycelia on M. alternatus (Figure 5H, by SEM) and T. castaneum (Figure S9E), and cylindrical to oval conidia were found attached to the cuticles of the two beetle species (Figure 5I, by SEM; Figure S9F). S. alboflavescens initially formed a powdery and translucent colony, which then turned yellowish brown with an irregular margin (Figure 6A). The reverse of the colony had a pale yellowish margin around the tawny center (Figure 6B). Cylindrical to slightly flask-shaped phialides were clustered or occasionally grown solitarily on the main stem or branches of the conidiophores (Figure 6C, by OM). The conidia were globose to subglobose, 6.0–7.9 μm×5.6–6.9 μm in diameter (Figure 6D, by OM), and arranged in chain (Figure 6E, by SEM) with truncate base (Figure 6F, by SEM). The mouthparts and leg base nodes of M. alternatus cadaver were covered with pale yellowish mycelia (Figure 6G). Clustered conidiophores with long and slim phialides were grown from M. alternatus (Figure 6H, by SEM) and T. castaneum (Figure S9G) bodies. Conidia on beetle cuticles were rough-walled, having a truncate base (Figure 6I, by SEM; Figure S9H), similar to those on PDA medium.
Figure 4 Morphology of B. bassiana and M. alternatus cadaver infected by B. bassiana under optical microscope and SEM. (A) Colonial morphology cultured on PDA. (B) Reverse of colony on PDA. (C) Hyphae and conidiophores (OM). (D) Conidia (OM). (E) Conidiophores (SEM). (F) Conidia (SEM). (G) M. alternatus cadaver surrounded by mycelia. (H) Conidiophores grown from M. alternatus cuticle (SEM). (I) Conidia on M. alternatus cuticle surface (SEM).
Figure 5 Morphology of L. attenuatum and M. alternatus cadaver infected by L. attenuatum under optical microscope and SEM. (A) Colonial morphology cultured on PDA. (B) Reverse of colony on PDA. (C) Hyphae and conidiophores (OM). (D) Conidia (OM). (E) Conidiophores (SEM). (F) Conidia (SEM). (G) M. alternatus cadaver surrounded by mycelia. (H) Conidiophores grown from M. alternatus cuticle (SEM). (I) Conidia on M. alternatus cuticle surface (SEM).
Figure 6 Morphology of S. alboflavescens and M. alternatus cadaver infected by S. alboflavescens under optical microscope and SEM. (A) Colonial morphology cultured on PDA. (B) Reverse of colony on PDA. (C) Hyphae and conidiophores (OM). (D) Conidia (OM). (E) Conidiophores (SEM). (F) Conidia (SEM). (G) M. alternatus cadaver surrounded by mycelia. (H) Conidiophores grown from M. alternatus cuticle (SEM). (I) Conidia on M. alternatus cuticle surface (SEM).
In the ML phylogenetic analysis, the six-gene sequences of 65 species (including the seven entomopathogenic fungal species isolated in this study) were used to reconstruct the phylogenetic framework (Table S1; Figure S10). A. austwickii strain HUZU6 and A. ruber strain HUZU28 were clustered with their respective reference strains and were separated from other Aspergillus species. P. citrinum strain HUZU144 clustered into the clade of P. citrinum CBS 139.45, was distinct from the other Penicillium species (Figure S10). S. alboflavescens strain HUZU190 clustered well into a clade with S. alboflavescens CBS 399.34, which was distinct from the other Scopulariopsis species (Figure S10). B. bassiana strain HUZU62, L. attenuatum strain HUZU100, and T. dorotheae strain HUZU218 matched to their corresponding reference strains and were less distinct from their phylogenetically related species (Figure S10). The results of the multi-gene phylogenetic analysis conformed to the morphological features of the entomopathogenic fungal species.
Fusarium is an important Genus of pine pathogenic fungi with a relatively high isolation frequency in M. alternatus. Therefore, Fusarium species were included as positive controls for entomopathogenic fungi, to evaluate their potential ability to damage the host pine P. massoniana. Five fungal species, namely, F. annulatum, F. circinatum, P. citrinum, P. lilacinum, and T. dorotheae, showed dramatic increases in cellulase activity levels with incubation time. In contrast, cellulase activity levels of the insect-parasitic entomopathogens A. austwickii, B. bassiana, L. attenuatum and S. alboflavescens did not increase over time and were lower than those of the above five fungal species (Figure 7A). Regarding pectinase activity, all fungal species elevated their activity levels and remained stable at the end of the incubation, although these species showed variable time points to reach their peak activity (Figure 7B). F. annulatum, F. circinatum, and P. citrinum exhibited significantly higher pectinase activity than B. bassiana and S. alboflavescens, on days 8 and 10. With the exception of A. austwickii, which expressed the highest level of pectinase, the other three insect-parasitic entomopathogens showed almost the lowest pectinase activity among all fungi (Figure 7B).
Figure 7 Phytopathogenic activities of the entomopathogenic fungi to the host pine P. massoniana. (A) Cellulase activities of fermentation supernatants from fungal species. (B) Pectinase activity of fermentation supernatants from fungal species. (C) Lesion lengths caused by fungi after 3 weeks. In A and B, different letters mean significant differences among fungi at each time point (P< 0.05); In C, asterisks on bars mean significant difference between the control group and fungal species (* P< 0.05; ** P< 0.01). Data were represented as Mean ± SD.
Lesion lengths caused by fungal associates on P. massoniana seedlings further demonstrated lower pine pathogenicity of insect-parasitic entomopathogenic fungi than the other fungal species (Figure 7C; Kruskal-Wallis test; χ20 2 = 59.80, P< 0.0001). One insect-parasitic entomopathogenic fungus, S. alboflavescens, slightly induced necrosis in the cambial zone of host pine, while exhibiting the lowest cellulase and pectinase activities among all fungi. A. austwickii had high pectinase activity but did not cause obvious lesions on the pine. Some fungal associates isolated from M. alternatus, such as F. polyphialidicum in Genus Fusarium, P. lilacinum, and P. citrinum, induced significantly greater lesions than the mock inoculation control (Figure 7C).
In this study, the pine sawyer beetle M. alternatus of naturally fungal infection is associated with a strikingly high diversity of fungal communities, with 640 fungal strains in total affiliated to 7 orders, 13 families, 15 genera, and 39 species (Table 1). This is distinct from previously considered, which held that entomopathogenic fungal species of M. alternatus are restricted to genera mainly in Beauveria and Metarhizium (Kim et al., 2020; Kim et al., 2022). B. bassiana was reported as the dominant entomopathogenic fungus of M. alternatus in Japan, Korea, and the Anhui/Zhejiang Province of China (Shimazu, 2004; Han et al., 2007; Ma et al., 2009; Shin et al., 2009), and B. pseudobassiana, which is closely related to it, was the most frequently isolated species from infected adults of M. galloprovincialis in Spain (Álvarez-Baz et al., 2015), implying Beauveria spp. to be the main infecting fungi of Monochamus vector beetles in natural fields. However, in this survey, only two strains of B. bassiana were isolated from the body surface of M. alternatus adults in the Zhejiang population, and no strains were found in the other populations. Lecanicillium species are pathogenic parasites to various insect species (Goettel et al., 2008). In Monochamus insect pests, L. lecanii (formerly named Verticillium lecanii) was reported to associate with naturally infected M. alternatus in Anhui/Zhejiang regions of China (Han et al., 2007; Ma et al., 2009). Its closely related species, L. attenuatum, was found to accompany B. pseudobassiana in M. galloprovincialis populations in Spain (Álvarez-Baz et al., 2015), and to the best of our knowledge, our study is the first report to elucidate such an association of L. attenuatum with Monochamus spp. in China. It is highly possible that the identity of L. lecanii determined by morphological traits is that of L. attenuatum in the Zhejiang population of M. alternatus, which needs to be further confirmed. Scopulariopsis is commonly found in various habitats, and some species, such as S. asperula and S. brevicaulis, have been isolated from mites and insects with entomopathogenic activities (Perrucci et al., 2008; Woudenberg et al., 2017). The species S. alboflavescens isolated from M. alternatus in this survey was reported to be hosted only by mammals as a pathogenic fungus (Woudenberg et al., 2017; Pérez-Cantero and Guarro, 2020), with no record on insects. The two dominant fungal genera Aspergillus and Penicillium of M. alternatus found in this study are eminent producers of secondary metabolites with diverse structures, many possessing excellent insecticidal properties that target insect metabolic systems (Frisvad et al., 2018; Toghueo and Boyom, 2020). Representative species such as A. ruber, A. austwickii, and P. citrinum among the collected isolates formed the first report of their associations with Monochamus spp., as per published record.
Entomopathogenic fungi present a geographical distribution preference for specific M. alternatus populations. Results of this field investigation revealed that the fungal species with insecticidal activities, namely, L. attenuatum (50.8%), A. austwickii (41.7%), S. alboflavescens (31.4%), and A. ruber (85.1%), were mainly found or dominant in Zhejiang, Sichuan, Fujian, and Guangxi, respectively, in M. alternatus populations (Table S2). This implied that variation in optimal growth ranges of abiotic factors, such as temperature and humidity, might directly determine their distributions. Multivariate analysis confirmed a significant geographical distribution pattern in the community composition of fungal associates in naturally infected M. alternatus (Figure 1). More significant differences in fungal community composition were found in geographically distant M. alternatus populations. Latitudinal geographies with distinct environmental factors, such as climate and vegetation, could shape the diversity and composition of many types of fungal communities (Větrovský et al., 2019; Mukhtar et al., 2021), including insect-associated fungi (such as ophiostomatoid fungal symbionts with bark beetles) (Roe et al., 2011). Referring to the potential distribution preferences of entomopathogenic fungi, certain species or their phylogenetically close species matched well with the high frequencies at which they were isolated and the environmental conditions for their optimal growth. For example, L. attenuatum was isolated from soil in Korea (Woo et al., 2020) and from M. galloprovincialis in Spain, which are located at high latitudes. L. flavidum, its congeneric species, was demonstrated to have a narrow growth temperature range from 18°C to 21°C, with no growth at 27°C (Zare and Gams, 2008). In contrast, the growth temperatures of Aspergillus and Scopulariopsis appear to be much higher than those of Lecanicillium species. Although Aspergillus can grow across a broad temperature range, many species of this Genus occur more frequently at tropical latitudes, with growth at temperatures from 30°C to 37°C (Klich, 2002; Belli et al., 2004). Another study pointed out that the optimal growth temperature of S. brevicaulis is 30°C, which is also optimal for enzyme production (Anbu et al., 2007). The vector beetle M. alternatus has a wide range of potential distribution areas in China, providing diverse reservoirs of natural resources for exploring original entomopathogenic fungal strains with huge economic value. As shown in this study, more entomopathogenic fungi will be found in M. alternatus through deeper field investigation and developed as novel biopesticides to control pine wilt disease in the future.
The behavioral phenotypes of the seven fungi with strong insecticidal activities against T. castaneum adults were consistent with those against M. alternatus. The four parasitic entomopathogenic fungi, namely, A. austwickii, B. bassiana, L. attenuatum, and S. alboflavescens, could be re-isolated from T. castaneum with artificial infection in the laboratory, and the SEM results confirmed that the asexual characteristics of conidia and conidiophores grown on T. castaneum were the same as those grown on M. alternatus (Figure S9; Figures 3–6). Mycelia of these four fungal species grew intensively on the abdomen, whole body, head and antenna, and mouthpart and leg base nodes of adult M. alternatus, respectively (Figures 3G, 4G, 5G, 6G), suggesting that these fungal species have spatial preference and localized niches on host insect bodies. However, multivariate analysis showed that the fungal community composition did not diverge significantly among different body positions, although there was a slight variation among the groups (Figure S3). The other three insecticidal fungal species with no visible parasitism on M. alternatus, namely, A. ruber, P. citrinum, and T. dorotheae, failed re-isolation from artificially infected T. castaneum and also were not observed by SEM. T. castaneum is a Coleopteran model insect widely used in genetic editing, toxicological and immunological research, and active natural product screening (Wang et al., 2007; Klingler and Bucher, 2022), owing to its advantages of short generation-time and simple rearing techniques (Rösner et al., 2020). The consistent results found here further indicate that the laboratory population of T. castaneum could be a good substitute or a tool to evaluate insecticidal activities and infection phenotypes of entomopathogenic fungi for M. alternatus and other Coleopterans.
Entomopathogenic fungi harbor diverse biological “weapons” and apply several strategies to kill target insect hosts, including enzymatic degradation, physical penetration of integuments, propagation within host body cavity (hemocoel), and/or mycotoxin excretion (Mannino et al., 2019; Litwin et al., 2020). For parasitic entomopathogenic fungi, the first step to colonize host body surface relies on the efficient release of protease, chitinase, and lipase to degrade cuticles (Sevim et al., 2012; Umaru and Simarani, 2022), and these three extracellular enzymes are direct indicators of their insecticidal activity (Mustafa and Kaur, 2009; Grewal et al., 2021). In this study, A. austwickii isolated from M. alternatus showed moderate chitinase activity while higher protease and lipase activities, which correlated with its obvious infection phenotype and higher mortality rate, compared to other species (Figures 2, 3). Similar result was found in B. bassiana, which expressed particularly high level of protease, causing high mortality of the test beetle T. castaneum (Figures 2, 4). This conformed with a previous study, which found that mortality rates of M. alternatus positively correlated with protease activity levels of B. bassiana (Lin et al., 2008). L. attenuatum presented significant parasitism on M. alternatus (Figure 5), analogous to A. austwickii and B. bassiana, while the performance of L. attenuatum—in terms of the three enzymatic activities—was lower than that of the other two species, which could explain the weaker virulence of L. attenuatum on the test beetle (Figure 2) as well as on M. galloprovincialis adults and Oryctes agamemnon larvae (Álvarez-Baz et al., 2015; Saleem and Ibrahim, 2019). S. alboflavescens expressed relatively high level of chitinase activity, but its low protease and lipase productivities were not equivalent to its marked infection phenotype and strong pathogenicity to beetles (Figures 2, 6). Determination of the exact roles of enzymes involved in S. alboflavescens pathogenesis requires further investigation. Fungal secretion of toxic secondary metabolites could accelerate the death of insect hosts alongside enzyme-initiating infection. The chemical action might even be the principal mode for non-parasitic entomopathogenic fungi, such as Trichoderma species, which were reported to capable of producing insecticidal secondary metabolites, antifeedant compounds, and repellent chemicals (Poveda, 2021). The structural nature of these secondary metabolites is worthy of exploring for their efficacies against M. alternatus in the future.
Interactions between insect hosts and entomopathogenic fungi exhibit host specificity and strain-level variation in certain fungal species. For example, in this study, the P. lilacinum strain isolated from M. alternatus expressed low-to-moderate level of protease (Figure 2B), but no obvious parasitism or mortality was found when interacting with the test beetles (Figure 2A). However, P. lilacinum has been reported to be a strong parasitic entomopathogen of aphid and moth insect pests that secretes proteases and chitinases (Liu et al., 2022). Similarly, P. disseminata and C. rosea did not show any insecticidal activity to the test beetles (Figure 2A), whereas they were natural parasites of the scale insect Hemiberlesia pitysophila and psyllid Diaphorina citri via enzymatic degradation of cuticles (Huang et al., 2014; Yang et al., 2021). Their low mortality in beetles and weak enzymatic behavior may serve the purpose of adhesion to the body surface without killing the host. The components and structure of cuticle of Coleopteran beetles are distinct from those of Hemipteran and other insect cuticles (Fraenkel and Rudall, 1947; Balabanidou et al., 2018), which could explain the variation of specific fungal species on different host insects. Strain-level genetic variation of entomopathogenic fungal species originating from different insect orders could also shape their specificity in host parasitism (Brodeur, 2012; Wang and Wang, 2017; Rohrlich et al., 2018), implying that the parasitic fungi on M. alternatus might fail to function on other distantly related hosts, and vice versa.
Many entomopathogenic fungal species have an endophytic lifestyle to provide chemical defense and growth promotion for host plants, and in return, gain benefits, such as space and nutrition from the plants (Jaber and Ownley, 2018; Bamisile et al., 2018; Bamisile et al., 2021). In this study, upon measuring levels of digestive enzymes that target plant cell wall components, A. austwickii consistently exhibited the highest level of pectinase activity, whereas the other three parasitic entomopathogenic fungi showed almost the lowest activity, which increased gradually with incubation time; however, all the four fungal species secreted tiny amounts of cellulase throughout the time (Figures 7A, B). In contrast, T. dorotheae and P. citrinum balanced well between the cellulase and pectinase activities (Figures 7A, B) and have been reported as endophytic fungi of other woody plants (Huh et al., 2011; Sadeghi et al., 2019). The phytopathogenicity assay showed that these entomopathogenic fungi are capable of growing in the host pine in a relatively mild manner (Figure 7C). This suggested that moderate expression of the two enzymes—under the threshold of pathogenesis—could facilitate the colonization of entomopathogenic fungi on the host pine P. massoniana, conferring them high compatibility with the host pine and meanwhile expanding their contact area with M. alternatus in the field. P. citrinum was an exception found in the assay, inducing much longer wounds in the host pine phloem (Figure 7C), which might be partially attributed to specific virulent factors of this fungus interactions with the pine immune system. Further study is needed to evaluate the non-host (especially natural enemy insects of M. alternatus) infection potential of the entomopathogenic fungi, which will provide more valuable views for their application prospect in biocontrol of the pine wilt disease.
In addition to transmitting the pine wood nematode, the adult beetle M. alternatus appears to be a multi-pathogen vector potentially carrying destructive pine-pathogenic fungi. Fusarium fungal species isolated from M. alternatus in this study showed extraordinarily high cellulase and pectinase production, and inoculation with F. polyphialidicum triggered a significant lesion on the host pine (Figure 7). Fusarium pathogenesis in pine forests, which leads to substantial economic losses worldwide, has attracted increased attention in recent years (Herron et al., 2015; Elvira-Recuenco et al., 2019). The survey in this study revealed that Fusarium spp. accounted for approximately 8% of all isolates from M. alternatus adults (Table 1), and the frequencies of association between Fusarium and M. alternatus or other Monochamus beetles have been recorded to be more than 30% (Han et al., 2007). Another fungal associate, P. lilacinum, caused much heavier damage to the P. massoniana phloem than the other fungi (Figure 7C), which is generally regarded as an effective protector of plants against various pest infestations (Lopez et al., 2014; Lopez and Sword, 2015). Its pathogenicity in the plantation system was observed only as a mushroom parasite (Masaphy, 2022). Nevertheless, considering the obvious symptom of P. lilacinum infection initially found in P. massoniana, its potential adverse effects on pine trees should be evaluated in future studies. These findings suggested a new underlying threat from fungal phytopathogens harbored by the vector M. alternatus, further highlighting the importance of developing novel fungal entomopathogenic bioagents for vector beetle management.
Pine wilt disease, caused by the pinewood nematode (PWN), is a devastating vector-borne disease that has been infesting pine forests in several continents. Nevertheless, little attention has been paid to the diversity of entomopathogenic fungi naturally associated with vector beetles Monochamus spp. Our study initiated a preliminary investigation of entomopathogenic fungal associations in five geographical populations of the disease vector M. alternatus in southern China. This cross-latitudinal survey demonstrated significant variation in fungal community composition, coupled with the geographical origin of naturally infected M. alternatus. Furthermore, the main fungal species from the geographical populations were region-representative and strongly entomopathogenic, functioning in parasitic and non-parasitic modes. Among them, the natural distributions of insect-parasitic fungal species were discovered for the first time on Monochamus spp. in China, some of which showed potent insecticidal activities comparable to B. bassiana. These parasitic entomopathogenic fungi could hardly cause visible lesions on the host pine, which further supported their promising application in the field to break down vector transmission of pine wilt disease. Our findings reflect that cross-latitudinal resource exploration of entomopathogenic fungi is crucial for the development of novel biocontrol strategies for insect vector-borne diseases.
The original contributions presented in the study are included in the article and/or Supplementary Material. Further inquiries can be directed to the corresponding author.
SW, LZ, and CC designed the research. CC supervised the experiments. SW, JW, YW, YQ, YH, JYW, and JC performed the experiments. SW and CC performed the bioinformatic and statistical analyses. SW and CC wrote the manuscript with all authors contributing to the discussion of the data. LZ and CC provided funds for the research. All authors contributed to the article and approved the submitted version.
This research was supported by the National Key Research and Development Program of China (2021YFC2600100), Natural Science Foundation of Zhejiang Province (LY21C040001), and the National Natural Science Foundation of China (31702018).
The authors thank Jianting FAN (School of Forestry and Biotechnology, Zhejiang A&F University) for his great support in field sampling, Minhong XU (School of Engineering, Huzhou University) for her excellent technical assistance in SEM and Xudong JIANG (Chongqing Municipal Academy of Forestry) for his advice in experimental methods.
The authors declare that the research was conducted in the absence of any commercial or financial relationships that could be construed as a potential conflict of interest.
All claims expressed in this article are solely those of the authors and do not necessarily represent those of their affiliated organizations, or those of the publisher, the editors and the reviewers. Any product that may be evaluated in this article, or claim that may be made by its manufacturer, is not guaranteed or endorsed by the publisher.
The Supplementary Material for this article can be found online at: https://www.frontiersin.org/articles/10.3389/fpls.2022.1061520/full#supplementary-material
Alabdalall, A. H., ALanazi, N. A., Aldakeel, S. A., AbdulAzeez, S., Borgio, J. F. (2020). Molecular, physiological, and biochemical characterization of extracellular lipase production by Aspergillus niger using submerged fermentation. Peer J. 8, e9425. doi: 10.7717/peerj.9425
Álvarez-Baz, G., Fernández-Bravo, M., Pajares, J., Quesada-Moraga, E. (2015). Potential of native Beauveria pseudobassiana strain for biological control of pine wood nematode vector Monochamus galloprovincialis. J. Invertebr. Pathol. 132, 48–56. doi: 10.1016/j.jip.2015.08.006
Anbu, P., Gopinath, S. C. B., Hilda, A., Lakshmipriya, T., Annadurai, G. (2007). Optimization of extracellular keratinase production by poultry farm isolate Scopulariopsis brevicaulis. Bioresour. Technol. 98 (6), 1298–1303. doi: 10.1016/j.biortech.2006.05.047
Aphidech, S., Kusavadee, S. (2013). Isolation, identification, culture and production of adenosine and cordycepin from cicada larva infected with entomopathogenic fungi in Thailand. Afr. J. Microbiol. Res. 7 (2), 137–146. doi: 10.5897/AJMR12.1038
Balabanidou, V., Grigoraki, L., Vontas, J. (2018). Insect cuticle: a critical determinant of insecticide resistance. Curr. Opin. Insect Sci. 27, 68–74. doi: 10.1016/j.cois.2018.03.001
Bamisile, B. S., Akutse, K. S., Siddiqui, J. A., Xu, Y. (2021). Model application of entomopathogenic fungi as alternatives to chemical pesticides: Prospects, challenges, and insights for next-generation sustainable agriculture. Front. Plant Sci. 12. doi: 10.3389/fpls.2021.741804
Bamisile, B. S., Dash, C. K., Akutse, K. S., Keppanan, R., Wang, L. (2018). Fungal endophytes: beyond herbivore management. Front. Microbiol. 9. doi: 10.3389/fmicb.2018.00544
Belli, N., Marin, S., Sanchis, V., Ramos, A. J. (2004). Influence of water activity and temperature on growth of isolates of Aspergillus section Nigri obtained from grapes. Int. J. Food Microbiol. 96 (1), 19–27. doi: 10.1016/j.ijfoodmicro.2004.03.004
Brodeur, J. (2012). Host specificity in biological control: insights from opportunistic pathogens. Evol. Appl. 5 (5), 470–480. doi: 10.1111/j.1752-4571.2012.00273.x
Corley, J. C., Lantschner, M. V., Martínez, A. S., Fischbein, D., Villacide, J. M. (2019). Management of Sirex noctilio populations in exotic pine plantations: critical issues explaining invasion success and damage levels in south America. J. Pest Sci. 92 (1), 131–142. doi: 10.1007/s10340-018-1060-3
Cortez-Madrigal, H., Sánchez-Saavedra, J. M., Díaz-Godínez, G., Mora-Aguilera, G. (2014). Enzymatic activity and pathogenicity of entomopathogenic fungi from central and southeastern Mexico to Diaphorina citri (Hemiptera: Psyllidae). Southwestern Entomol. 39 (3), 491–502. doi: 10.3958/059.039.0310
Dara, S. K., Montalva, C., Barta, M. (2019). Microbial control of invasive forest pests with entomopathogenic fungi: A review of the current situation. Insects 10 (10), 341. doi: 10.3390/insects10100341
Dhawan, M., Joshi, N. (2017). Enzymatic comparison and mortality of Beauveria bassiana against cabbage caterpillar Pieris brassicae Linn. Braz. J. Microbiol. 48 (3), 522–529. doi: 10.1016/j.bjm.2016.08.004
Elvira-Recuenco, M., Cacciola, S. O., Sanz-Ros, A. V., Garbelotto, M., Aguayo, J., Solla, A., et al. (2019). Potential interactions between invasive Fusarium circinatum and other pine pathogens in Europe. Forests 11 (1), 7. doi: 10.3390/f11010007
Fang, W., Azimzadeh, P., Leger, R. J. S. (2012). Strain improvement of fungal insecticides for controlling insect pests and vector-borne diseases. Curr. Opin. Microbiol. 15 (3), 232–238. doi: 10.1016/j.mib.2011.12.012
Fraenkel, G., Rudall, K. M. (1947). The structure of insect cuticles. Proc. R. Soc. B: Biol. Sci. 134 (874), 111–143. doi: 10.1098/rspb.1947.0006
Frisvad, J. C., Hubka, V., Ezekiel, C. N., Hong, S. B., Nováková, A., Chen, A. J. (2018). Taxonomy of Aspergillus section flavi and their production of aflatoxins, ochratoxins and other mycotoxins. Stud. Mycol. 91 (1), 37–59. doi: 10.1016/j.simyco.2018.06.001
Futai, K. (2013). Pine wood nematode, Bursaphelenchus xylophilus. Annu. Rev. Phytopathol. 51, 61–83. doi: 10.1146/annurev-phyto-081211-172910
Gao, H., Cui, C., Wang, L., Jacobs-Lorena, M., Wang, S. (2020). Mosquito microbiota and implications for disease control. Trends Parasitol. 36 (2), 98–111. doi: 10.1016/j.pt.2019.12.001
Garcia, F. R., Ovruski, S. M., Suárez, L., Cancino, J., Liburd, O. E. (2020). Biological control of tephritid fruit flies in the americas and Hawaii: A review of the use of parasitoids and predators. Insects 11 (10), 662. doi: 10.3390/insects11100662
Goettel, M. S., Koike, M., Kim, J. J., Aiuchi, D., Shinya, R., Brodeur, J. (2008). Potential of Lecanicillium spp. for management of insects, nematodes and plant diseases. J. Invertebr. Pathol. 98 (3), 256–261. doi: 10.1016/j.jip.2008.01.009
Gopinath, S. C., Hilda, A., Anbu, P. (2005). Extracellular enzymatic activity profiles in fungi isolated from oil-rich environments. Mycoscience 46 (2), 119–126. doi: 10.1007/S10267-004-0221-9
Grewal, G. K., Joshi, N., Suneja, Y. (2021). Pathogenicity of Metarhizium rileyi (Farlow) Kepler, S.A. rehner and Humber isolates against Spodoptera litura (Fabricius) and their extracellular enzymatic activities. Egyptian J. Biol. Pest Control 31 (1), 1–7. doi: 10.1186/S41938-021-00407-4
Han, B., Piao, C. G., Wang, L. F., Li, Y., Zheng, R. Z. (2007). Survey, identification and virulence test of pathogens of the pine sawyer beetle, Monochamus alternatus, at forest farm of maanshan, anhui province. For. Res. 20 (2), 204–208. doi: 10.3321/j.issn:1001-1498.2007.02.010
He, Z., Guo, J. F., Reitz, S. R., Lei, Z. R., Wu, S. Y. (2020). A global invasion by the thrip, Frankliniella occidentalis: Current virus vector status and its management. Insect Sci. 27 (4), 626–645. doi: 10.1111/1744-7917.12721
Herron, D. A., Wingfield, M. J., Wingfield, B. D., Rodas, C. A., Marincowitz, S., Steenkamp, E. T. (2015). Novel taxa in the Fusarium fujikuroi species complex from Pinus spp. Stud. Mycol. 80, 131–150. doi: 10.1016/j.simyco.2014.12.001
Huang, R., Lyu, C. Q., Huang, B. L., Guo, L. J., Yao, J. M., Li, R. L., et al. (2014). Relationships between virulence and activities of protease, chitinase and lipase produced by entomogenous Pestalotiopsis disseminata. J. South. Agric. 45 (7), 1172–1177. doi: 10.3969/j:issn.2095-1191.2014.7.1172
Huh, N., Jang, Y., Lee, J., Kim, G., Kim, J. (2011). Phylogenetic analysis of major molds inhabiting woods and their discoloration characteristics. part 1. genus Trichoderma. Holzforschung 65 (2), 257–263. doi: 10.1515/hf.2011.018
Hulcr, J., Dunn, R. R. (2011). The sudden emergence of pathogenicity in insect–fungus symbioses threatens naive forest ecosystems. Proc. R. Soc. B: Biol. Sci. 278 (1720), 2866–2873. doi: 10.1098/rspb.2011.1130
Humble, L. M., Allen, E. A. (2006). Forest biosecurity: alien invasive species and vectored organisms. Can. J. Plant Pathol. 28 (S1), S256–S269. doi: 10.1080/07060660609507383
Islam, W., Adnan, M., Shabbir, A., Naveed, H., Abubakar, Y. S., Qasim, M., et al. (2021). Insect-fungal-interactions: A detailed review on entomopathogenic fungi pathogenicity to combat insect pests. Microbial Pathog. 159, 105122. doi: 10.1016/j.micpath.2021.105122
Jaber, L. R., Ownley, B. H. (2018). Can we use entomopathogenic fungi as endophytes for dual biological control of insect pests and plant pathogens? Biol. Control 116, 36–45. doi: 10.1016/j.biocontrol.2017.01.018
Javed, K., Javed, H., Mukhtar, T., Qiu, D. (2019). Efficacy of Beauveria bassiana and Verticillium lecanii for the management of whitefly and aphid. Pakistan J. Agric. Sci. 56 (3), 669–674. doi: 10.21162/PAKJAS/19.8396
Jürisoo, L., Selikhovkin, A. V., Padari, A., Shevchenko, S. V., Shcherbakova, L. N., Popovichev, B. G., et al. (2021). The extensive damage to elms by Dutch elm disease agents and their hybrids in northwestern Russia. Urban For. Urban Greening 63, 127214. doi: 10.1016/j.ufug.2021.127214
Kim, J. C., Lee, S. J., Kim, S., Lee, M. R., Baek, S., Park, S. E., et al. (2020). Management of pine wilt disease vectoring Monochamus alternatus adults using spray and soil application of Metarhizium anisopliae JEF isolates. J. Asia-Pacific Entomol. 23 (1), 224–233. doi: 10.1016/j.aspen.2019.12.012
Kim, J. C., Lee, M. R., Yu, J. S., Park, S. E., Ha, P., Kim, J. S. (2022). Management of overwintering pine sawyer beetle, Monochamus alternatus with colonized Beauveria bassiana ERL836. PloS One 17 (9), e0274086. doi: 10.1371/journal.pone.0274086
Klich, M. A. (2002). Biogeography of Aspergillus species in soil and litter. Mycologia 94 (1), 21–27. doi: 10.1080/15572536.2003.11833245
Klingler, M., Bucher, G. (2022). The red flour beetle T. castaneum: elaborate genetic toolkit and unbiased large scale RNAi screening to study insect biology and evolution. EvoDevo 13 (1), 1–11. doi: 10.1186/s13227-022-00201-9
Kobayashi, F., Yamane, A., Ikeda, T. (1984). The Japanese pine sawyer beetle as the vector of pine wilt disease. Annu. Rev. Entomol. 29 (1), 115–135. doi: 10.1146/annurev.en.29.010184.000555
Larkin, M. A., Blackshields, G., Brown, N. P., Chenna, R., Mcgettigan, P. A., Mcwilliam, H., et al. (2007). Clustal W and clustal X version 2.0. Bioinformatics 23 (21), 2947–2948. doi: 10.1093/bioinformatics/btm404
Leger, R. J. S., Wang, C. (2010). Genetic engineering of fungal biocontrol agents to achieve greater efficacy against insect pests. Appl. Microbiol. Biotechnol. 85 (4), 901–907. doi: 10.1007/s00253-009-2306-z
Li, C., Li, X., Bai, C., Zhang, Y., Wang, Z. (2018). A chitinase with antifungal activity from naked oat (Avena chinensis) seeds. J. Food Biochem. 43 (2), e12713. doi: 10.1111/jfbc.12713
Linnakoski, R., Forbes, K. M. (2019). Pathogens–the hidden face of forest invasions by wood-boring insect pests. Front. Plant Sci. 10. doi: 10.3389/fpls.2019.00090
Lin, H. P., Wei, J. Y., Mao, S. F., Chen, X., Ma, L. J. (2008). Correlation between protease, chitinase and lipase activities and virulence of Beauveria bassiana against Monochamus alternatus. Chin. J. Biol. Control 24 (3), 290. doi: 10.16409/j.cnki.2095-039x.2008.03.018
Litwin, A., Nowak, M., Różalska, S. (2020). Entomopathogenic fungi: unconventional applications. Rev. Environ. Sci. Bio/Technology 19 (1), 23–42. doi: 10.1007/s11157-020-09525-1
Liu, Z., Liu, F. F., Li, H., Zhang, W. T., Wang, Q., Zhang, B. X., et al. (2022). Virulence of the bio-control fungus Purpureocillium lilacinum against Myzus persicae (Hemiptera: Aphididae) and Spodoptera frugiperda (Lepidoptera: Noctuidae). J. Econ. Entomol. 115 (2), 462–473. doi: 10.1093/jee/toab270
Lopez, D. C., Sword, G. A. (2015). The endophytic fungal entomopathogens Beauveria bassiana and Purpureocillium lilacinum enhance the growth of cultivated cotton (Gossypium hirsutum) and negatively affect survival of the cotton bollworm (Helicoverpa zea). Biol. Control 89, 53–60. doi: 10.1016/j.biocontrol.2015.03.010
Lopez, D. C., Zhu-Salzman, K., Ek-Ramos, M. J., Sword, G. A. (2014). The entomopathogenic fungal endophytes Purpureocillium lilacinum (formerly Paecilomyces lilacinus) and Beauveria bassiana negatively affect cotton aphid reproduction under both greenhouse and field conditions. PloS One 9 (8), e103891. doi: 10.1371/journal.pone.0103891
Lovett, B., Bilgo, E., Millogo, S. A., Ouattarra, A. K., Sare, I., Gnambani, E. J., et al. (2019). Transgenic Metarhizium rapidly kills mosquitoes in a malaria-endemic region of Burkina Faso. Science 364 (6443), 894–897. doi: 10.1126/science.aaw8737
Lv, C., Huang, B., Qiao, M., Wei, J., Ding, B. (2011). Entomopathogenic fungi on Hemiberlesia pitysophila. PloS One 6 (8), e23649. doi: 10.1371/journal.pone.0023649
Mannino, M. C., Huarte-Bonnet, C., Davyt-Colo, B., Pedrini, N. (2019). Is the insect cuticle the only entry gate for fungal infection? insights into alternative modes of action of entomopathogenic fungi. J. Fungi 5 (2), 33. doi: 10.3390/jof5020033
Masaphy, S. (2022). First report on Purpureocillium lilacinum infection of indoor-cultivated morel primordia. Agriculture 12 (5), 695. doi: 10.3390/agriculture12050695
Ma, L., Zhang, L., Lin, H., Mao, S. (2009). Investigation of pathogens of Monochamus alternatus in East China and virulence. Chin. J. Biol. Control 25 (3), 220–224. doi: 10.16409/j.cnki.2095-039x.2009.03.018
Mohammed, A. A., Younus, A. S., Ali, A. N. (2021). Efficacy of Clonostachys rosea, as a promising entomopathogenic fungus, against coleopteran stored product insect pests under laboratory conditions. Egyptian J. Biol. Pest Control 31 (1), 1–6. doi: 10.1186/s41938-021-00405-6
Moharram, A. M., Abdel-Galil, F. A., Hafez, W. M. M. (2021). On the enzymes' actions of entomopathogenic fungi against certain indigenous and invasive insect pests. Egyptian J. Biol. Pest Control 31 (1), 51. doi: 10.1186/s41938-021-00397-3
Moreno, A., Miranda, M. P., Fereres, A. (2021). Psyllids as major vectors of plant pathogens. Entomol. Generalis 41 (5), 419–438. doi: 10.1127/entomologia/2021/1289
Mukhtar, H., Lin, C. M., Wunderlich, R. F., Cheng, L. C., Ko, M. C., Lin, Y. P. (2021). Climate and land cover shape the fungal community structure in topsoil. Sci. Total Environ. 751, 141721. doi: 10.1016/j.scitotenv.2020.141721
Mustafa, U., Kaur, G. (2009). Extracellular enzyme production in Metarhizium anisopliae isolates. Folia Microbiol. 54 (6), 499–504. doi: 10.1007/s12223-009-0071-0
Navas-Castillo, J., Fiallo-Olivé, E., Sánchez-Campos, S. (2011). Emerging virus diseases transmitted by whiteflies. Annu. Rev. Phytopathol. 49, 219–248. doi: 10.1146/annurev-phyto-072910-095235
Nechols, J. R. (2021). The potential impact of climate change on non-target risks from imported generalist natural enemies and on biological control. BioControl 66 (1), 37–44. doi: 10.1007/s10526-020-10032-z
Nedveckytė, I., Pečiulytė, D., Būda, V. (2021). Fungi associated with horse-chestnut leaf miner moth Cameraria ohridella mortality. Forests 12 (1), 58. doi: 10.3390/f12010058
Orduño-Cruz, N., Guzmán-Franco, A. W., Rodríguez-Leyva, E., Alatorre-Rosas, R., González-Hernández, H., Mora-Aguilera, G. (2015). In vivo selection of entomopathogenic fungal isolates for control of Diaphorina citri (Hemiptera: Liviidae). Biol. Control 90, 1–5. doi: 10.1016/j.biocontrol.2015.05.011
Panyasiri, C., Supothina, S., Veeranondha, S., Chanthaket, R., Boonruangprapa, T., Vichai, V. (2022). Control efficacy of entomopathogenic fungus Purpureocillium lilacinum against chili thrips (Scirtothrips dorsalis) on chili plant. Insects 13 (8), 684. doi: 10.3390/insects13080684
Parker, B. L., Skinner, M., Costa, S. D., Gouli, S., Reid, W., Bouhssini, M. E. (2003). Entomopathogenic fungi of Eurygaster integriceps puton (Hemiptera: Scutelleridae): collection and characterization for development. Biol. Control 27 (3), 260–272. doi: 10.1016/S1049-9644(03)00017-3
Pereira, J. F., Queiroz, M. V. D., Gomes, E. A., Muro-Abad, J. I., Araújo, E. F. D. (2002). Molecular characterization and evaluation of pectinase and cellulase production of penicillium spp. Biotechnol. Lett. 24 (10), 831–838. doi: 10.1023/A:1015502721909
Pérez-Cantero, A., Guarro, J. (2020). Current knowledge on the etiology and epidemiology of Scopulariopsis infections. Med. Mycol. 58 (2), 145–155. doi: 10.1093/mmy/myz036
Perrucci, S., Zini, A., Donadio, E., Mancianti, F., Fichi, G. (2008). Isolation of Scopulariopsis spp. fungi from Psoroptes cuniculi body surface and evaluation of their entomopathogenic role. Parasitol. Res. 102 (5), 957–962. doi: 10.1007/s00436-007-0860-9
Poveda, J. (2021). Trichoderma as biocontrol agent against pests: new uses for a mycoparasite. Biol. Control 159, 104634. doi: 10.1016/j.biocontrol.2021.104634
Qi, M., Xie, C. X., Chen, Q. W., Yu, Z. D. (2021). Pestalotiopsis trachicarpicola, a novel pathogen causes twig blight of Pinus bungeana (Pinaceae: Pinoideae) in China. Antonie van Leeuwenhoek 114 (1), 1–9. doi: 10.1007/s10482-020-01500-8
Rajula, J., Rahman, A., Krutmuang, P. (2020). Entomopathogenic fungi in southeast Asia and Africa and their possible adoption in biological control. Biol. Control 151, 104399. doi: 10.1016/j.biocontrol.2020.104399
Rehner, S. A., Samuels, G. J. (1994). Taxonomy and phylogeny of Gliocladium analysed from nuclear large subunit ribosomal DNA sequences. Mycol. Res. 98 (6), 625–634. doi: 10.1016/s0953-7562(09)80409-7
Roe, A. D., James, P., Rice, A. V., Cooke, J. E., Sperling, F. A. (2011). Spatial community structure of mountain pine beetle fungal symbionts across a latitudinal gradient. Microbial Ecol. 62 (2), 347–360. doi: 10.1007/s00248-011-9841-8
Rohrlich, C., Merle, I., Mze Hassani, I., Verger, M., Zuin, M., Besse, S., et al. (2018). Variation in physiological host range in three strains of two species of the entomopathogenic fungus Beauveria. PloS One 13 (7), e0199199. doi: 10.1371/journal.pone.0199199
Rösner, J., Wellmeyer, B., Merzendorfer, H. (2020). Tribolium castaneum: a model for investigating the mode of action of insecticides and mechanisms of resistance. Curr. Pharm. Design 26 (29), 3554–3568. doi: 10.2174/1381612826666200513113140
Sadeghi, F., Samsampour, D., Seyahooei, M. A., Bagheri, A., Soltani, J. (2019). Diversity and spatiotemporal distribution of fungal endophytes associated with Citrus reticulata cv. siyahoo. Curr. Microbiol. 76 (3), 279–289. doi: 10.1007/s00284-019-01632-9
Saleem, A. R., Ibrahim, R. A. (2019). Assessment of the virulence and proteolytic activity of three native entomopathogenic fungi against the larvae of Oryctes agamemnon (Burmeister) (Coleoptera: Scarabaeidae). Egyptian J. Biol. Pest Control 29 (1), 1–8. doi: 10.1186/s41938-019-0120-1
Sevim, A., Donzelli, B. G., Wu, D., Demirbag, Z., Gibson, D. M., Turgeon, B. G. (2012). Hydrophobin genes of the entomopathogenic fungus, Metarhizium brunneum, are differentially expressed and corresponding mutants are decreased in virulence. Curr. Genet. 58 (2), 79–92. doi: 10.1007/s00294-012-0366-6
Sharma, V., Salwan, R., Sharma, P. N. (2016). Differential response of extracellular proteases of Trichoderma harzianum against fungal phytopathogens. Curr. Microbiol. 73 (3), 419–425. doi: 10.1007/s00284-016-1072-2
Shehzad, M., Tariq, M., Ali, Q., Aslam, A., Mukhtar, T., Akhtar, M. F., et al. (2022). Evaluation of insecticidal activity of Beauveria bassiana against different instar larvae of Plutella xylostella by using two different methods of application. Int. J. Trop. Insect Sci. 42, 1471–1476. doi: 10.1007/s42690-021-00665-7
Shehzad, M., Tariq, M., Mukhtar, T., Gulzar, A. (2021). On the virulence of the entomopathogenic fungi, Beauveria bassiana and Metarhizium anisopliae (Ascomycota: Hypocreales), against the diamondback moth, Plutella xylostella (L.) (Lepidoptera: Plutellidae). Egyptian J. Biol. Pest Control 31 (1), 86. doi: 10.1186/s41938-021-00428-z
Shimazu, M. (2004). Effects of temperature on growth of Beauveria bassiana f-263, a strain highly virulent to the Japanese pine sawyer, Monochamus alternatus, especially tolerance to high temperatures. Appl. Entomol. Zoology 39 (3), 469–475. doi: 10.1303/aez.2004.469
Shin, T. Y., Choi, J. B., Bae, S. M., Cha, Y. R., Oh, J. M., Koo, H. N., et al. (2009). Isolation and identification of entomopathogenic fungus from the pine wilt disease vector, Monochamus alternatus hope (Coleoptera: Cerambycidae) in Korea. Int. J. Ind. Entomol. 18 (2), 125–129.
Sörensen, T. A. (1948). A method of establishing groups of equal amplitude in plant sociology based on similarity of species content and its application to analyses of the vegetation on Danish commons. Biologiske Skrifter 5, 1–34.
Tamura, K., Stecher, G., Peterson, D., Filipski, A., Kumar, S. (2013). MEGA6: Molecular evolutionary genetics analysis version 6.0 Mol. Biol. Evol. 30, (12), 2725–2729. doi: 10.1093/molbev/mst197
Tang, X., Yuan, Y., Li, X., Zhang, J. (2021). Maximum entropy modeling to predict the impact of climate change on pine wilt disease in China. Front. Plant Sci. 12. doi: 10.3389/fpls.2021.652500
Toghueo, R. M. K., Boyom, F. F. (2020). Endophytic Penicillium species and their agricultural, biotechnological, and pharmaceutical applications. 3 Biotech. 10 (3), 107. doi: 10.1007/s13205-020-2081-1
Umaru, F. F., Simarani, K. (2022). Efficacy of entomopathogenic fungal formulations against Elasmolomus pallens (Dallas) (Hemiptera: Rhyparochromidae) and their extracellular enzymatic activities. Toxins 14 (9), 584. doi: 10.3390/toxins14090584
Větrovský, T., Kohout, P., Kopecký, M., Machac, A., Man, M., Bahnmann, B. D., et al. (2019). A meta-analysis of global fungal distribution reveals climate-driven patterns. Nat. Commun. 10 (1), 5142. doi: 10.1038/s41467-019-13164-8
Vilgalys, R., Hester, M. (1990). Rapid genetic identification and mapping of enzymatically amplified ribosomal DNA from several Cryptococcus species. J. Bacteriol. 172 (8), 4238–4246. doi: 10.1128/jb.172.8.4238-4246.1990
Wang, Z. Z., Liu, Y. Q., Shi, M., Huang, J. H., Chen, X. X. (2019). Parasitoid wasps as effective biological control agents. J. Integr. Agric. 18 (4), 705–715. doi: 10.1016/S2095-3119(18)62078-7
Wang, C., Wang, S. (2017). Insect pathogenic fungi: genomics, molecular interactions, and genetic improvements. Annu. Rev. Entomol. 62 (1), 73–90. doi: 10.1146/annurev-ento-031616-035509
Wang, L., Wang, S., Li, Y., Paradesi, M. S., Brown, S. J. (2007). BeetleBase: the model organism database for Tribolium castaneum. Nucleic Acids Res. 35 (suppl_1), D476–D479. doi: 10.1093/nar/gkl776
Wang, Y. B., Yu, H., Dai, Y. D., Chen, Z. H., Zeng, W. B., Yuan, F., et al. (2015). Polycephalomyces yunnanensis (Hypocreales), a new species of Polycephalomyces parasitizing Ophiocordyceps nutans and stink bugs (hemipteran adults). Phytotaxa 208, 34–44. doi: 10.11646/phytotaxa.208.1.3
White, T. J., Bruns, T., Lee, S., Taylor, J. (1990). Amplification and direct sequencing of fungal ribosomal RNA genes for phylogenetics. PCR protocols: guide to Methods Appl. 18 (1), 315–322. doi: 10.1016/B978-0-12-372180-8.50042-1
Woo, R. M., Park, M. G., Choi, J. Y., Park, D. H., Kim, J. Y., Wang, M., et al. (2020). Insecticidal and insect growth regulatory activities of secondary metabolites from entomopathogenic fungi, Lecanicillium attenuatum. J. Appl. Entomol. 144 (7), 655–663. doi: 10.1111/jen.12788
Woudenberg, J. H. C., Meijer, M., Houbraken, J., Samson, R. A. (2017). Scopulariopsis and scopulariopsis-like species from indoor environments. Stud. Mycol. 86 (1), 29–51. doi: 10.1016/j.simyco.2017.03.001
Yang, Z., Wu, Q., Fan, J., Huang, J., Wu, Z., Lin, J., et al. (2021). Effects of the entomopathogenic fungus Clonostachys rosea on mortality rates and gene expression profiles in Diaphorina citri adults. J. Invertebr. Pathol. 179, 107539. doi: 10.1016/j.jip.2021.107539
Zare, R., Gams, W. (2008). A revision of the Verticillium fungicola species complex and its affinity with the genus Lecanicillium. Mycol. Res. 112 (7), 811–824. doi: 10.1016/j.mycres.2008.01.019
Zellner, B., Huntley, J. F. (2019). Ticks and tularemia: do we know what we don't know? Front. Cell. Infect. Microbiol. 9. doi: 10.3389/fcimb.2019.00146
Keywords: entomopathogenic fungi, Monochamus alternatus, cross-latitudinal, multi-pathogen vector, Pinus massoniana, biological control
Citation: Wu S, Wu J, Wang Y, Qu Y, He Y, Wang J, Cheng J, Zhang L and Cheng C (2022) Discovery of entomopathogenic fungi across geographical regions in southern China on pine sawyer beetle Monochamus alternatus and implication for multi-pathogen vectoring potential of this beetle. Front. Plant Sci. 13:1061520. doi: 10.3389/fpls.2022.1061520
Received: 04 October 2022; Accepted: 28 November 2022;
Published: 28 December 2022.
Edited by:
Lilin Zhao, Institute of Zoology (CAS), ChinaReviewed by:
Sufang Zhang, Chinese Academy of Forestry, ChinaCopyright © 2022 Wu, Wu, Wang, Qu, He, Wang, Cheng, Zhang and Cheng. This is an open-access article distributed under the terms of the Creative Commons Attribution License (CC BY). The use, distribution or reproduction in other forums is permitted, provided the original author(s) and the copyright owner(s) are credited and that the original publication in this journal is cited, in accordance with accepted academic practice. No use, distribution or reproduction is permitted which does not comply with these terms.
*Correspondence: Chihang Cheng, Y2hlbmdjaGloYW5nQHpqaHUuZWR1LmNu
Disclaimer: All claims expressed in this article are solely those of the authors and do not necessarily represent those of their affiliated organizations, or those of the publisher, the editors and the reviewers. Any product that may be evaluated in this article or claim that may be made by its manufacturer is not guaranteed or endorsed by the publisher.
Research integrity at Frontiers
Learn more about the work of our research integrity team to safeguard the quality of each article we publish.