- 1College of Food Science and Technology, Shanghai Ocean University, Shanghai, China
- 2College of Biological and Environmental Sciences, Zhejiang Wanli University, Ningbo, China
Peach fruit was treated with 5 mM γ-aminobutyric acid (GABA) to further investigate the mechanism by which GABA induced chilling tolerance. Here, we found that GABA not only inhibited the occurrence of chilling injury in peach fruit during cold storage but also maintained fruit quality. Most of the ascorbic acid (AsA) and glutathione (GSH) biosynthetic genes were up-regulated by GABA treatment, and their levels were increased accordingly, thus reducing chilling damage in treated peaches. Meanwhile, the increased transcript of genes in the AsA-GSH cycle by GABA treatment was also related to the induced tolerance against chilling. GABA treatment also increased the expression levels of several candidate ERF transcription factors involved in AsA and GSH biosynthesis. In conclusion, our study found that GABA reduced chilling injury in peach fruit during cold storage due to the higher AsA and GSH contents by positively regulating their modifying genes and candidate transcription factors.
Introduction
Peach (Prunus persica (L.) Batsch) is a typical climacteric fruit. Low-temperature storage can inhibit its respiratory and endogenous ethylene peak during postharvest storage, which slows the process of postharvest ripening and senescence (Mahmud et al., 2017). However, when exposed to unsuitable cold temperatures, peach fruit is highly susceptible to chilling damage. Chilling symptoms such as fruit flocculation, flesh browning, and juice reduction can occur under low-temperature storage, resulting in loss of fruit flavor and texture, seriously affecting its edible quality and market economic value.
γ-Aminobutyric acid (GABA) is a naturally occurring amino acid in plants and animals (Nicolas and Hillel, 2004). In plants, GABA plays a role under stress conditions by regulating the activities of related enzymes or the synthesis of metabolites (Bao et al., 2015; Ramesh et al., 2017; Li et al., 2021). For instance, exogenous GABA inhibited chilling injury in tomatoes by scavenging reactive oxygen species (ROS) and increasing antioxidant enzyme activity (Malekzadeh, 2015). Wang et al. (2014a) found that exogenous GABA treatment induced resistance against chilling in melon by promoting polyamine synthesis Additionally, exogenous GABA treatment induced the synthesis of endogenous GABA in bananas (Wang et al., 2014c), persimmon (Niazi et al., 2021) and cucumber (Malekzadeh et al., 2017), thereby increasing the cold resistance and prolonging their low-temperature storage time. There are also many studies on GABA in peach fruit. For example, Aghdam et al. (2016) found that GABA can effectively maintain the content of ascorbic acid, total phenol, flavonoids, and other substances in peach fruit and the ability to eliminate free radicals. Recently, a study found that GABA can improve the cold resistance of peach fruits by promoting the methionine sulfoxide reductase thioredoxin reductase system (Jiao, 2022).
When plants are subjected to stress, ROS are produced rapidly, resulting in tissue damage (Baxte et al., 2014). A complete antioxidant system in plants can rapidly reduce the level of ROS to the average level. AsA and GSH are important antioxidants that can scavenge excess ROS and reduce damage caused by different stresses (Alscher et al., 1997; Song et al., 2016; Hasanuzzaman et al., 2019; Ma et al., 2019). Therefore, investigating the formation and regulation of AsA and GSH is of great significance in improving fruit quality and enhancing stress resistance in postharvest fruit.
Significant progress has been made in investigating the AsA biosynthetic pathway in plants. Four possible pathways of AsA biosynthesis in plants have been proposed. Among these pathways, the L-galactose pathway plays a leading role in plant AsA synthesis (Wheeler et al., 1998), while other pathways such as D-galacturonate, L- gluose and Myo-inositol supplement it (Agius et al., 2003; Lorence et al., 2004; Wolucka et al., 2005). All the genes encoding the enzymes involved in this pathway have been identified, including phosphomannomutase (PMM), GDP-D-mannose pyrophorylase (GMP), GDP-D-mannose-3’,5’-heteroisomerase (GME), GDP-L-galactose phophorylase (GGP), L-galactose-1-phosphate phophoratase (GPP) and L-galactose dehydrogenase (GalDH). PMM and GMP catalyze the synthesis of GDP-D-mannose, providing a substrate for AsA synthesis. GME catalyzes the conversion of GDP-D-mannose to GDP-L- galactose, which is the first step of AsA biosynthesis at the glycoside level. GGP catalyzes GDP-L-galactose to produce GDP-L- galactose -1- phosphate (Wheeler et al., 1998), which is one of the vital catalytic enzymes in the L-galactose pathway. GalDH catalyzes L- galactose to produce L- galactose -1,4-lactone, one of the rate-limiting enzymes in the L-galactose pathway. The specific catalytic steps of the other three AsA synthetic pathways in plants are still at the stage of speculation because the enzyme genes in these pathways have not been fully identified except for inositol oxygenase (MXIO) and D-galactonic acid dehydrogenase (GalUR) (Wolucka and Van Montagu, 2003; Lorence et al., 2004).
GSH biosynthesis is mainly catalyzed by γ-glutamylcysteine ligase (GCS) and glutathione synthase (GS). First, L-glutamic acid and cysteine are catalyzed by GCS to synthesize γ-glutathione cysteine. Then, under the catalysis of glutathione synthase (GS), glycine is added to the C-terminus of γ-glutathione cysteine to produce glutathione.
Our previous study showed that GABA could inhibit chilling injury of peach fruit during cold storage (Shang et al., 2011; Yang et al., 2011). However, the transcriptional activity of genes involved in the metabolism of AsA and GSH remained unstudied. Therefore, this study further investigated the mechanism by which GABA induced cold tolerance in chilled peach fruit by influencing AsA and GSH levels at the molecular level. In addition, we also analyzed the expression of three ERF transcription factors that may be related to ASA-GSH metabolism.
Materials and methods
GABA treatment
Peach fruit (Prunus. Persica Batsch cv. Hujing) were harvested at commercial maturity (120 days after flowering) from experimental farm of Fenghua Peach Fruit Research Institute (Ningbo, China). The peaches were picked and transported to laboratory quickly and then selected in uniform size and randomly divided into two groups with sixty-six fruit in each group. The conforming and undamaged fruit were selected and randomly divided into two groups According to our previous study (Yang et al., 2011), the fruit in first group were immersed in a solution of 5 mM GABA for 20 min, while the other was immersed in water for 20 min as control. Afterwards, both groups of fruit was air-dried for approximately two hours and transfer to 4 °C stored for thirty-five days. Eight peaches from each sampling site in each group were frozen in liquid nitrogen and stored in an ultra-low temperature refrigerator after fruit firmness, total soluble solid (TSS) and relative electrical conductivity were determined. From three weeks of cold storage, another six peaches were taken at each sampling point and transferred from 4 °C to 20 °C for three days to simulate the shelf environment and evaluated the chilling injury index. The whole experiment was repeated three times.
Determination of chilling injury index, fruit firmness, total soluble solid and relative electrical conductivity
The degree of chilling was visually assessed on the mesocarp surface, following a double cut parallel to the axial diameter. The extent of flesh browning was divided into four classes: 0, no browning; 1, browning covering <25% of the cut surface; 2, browning covering ≥25% but <50% of cut surface; 3, browning covering ≥50%. CI index was calculated using the following formula: Chilling injury index = Σ [(browning level) × (number of fruit at the browning level)]/(total number of fruit in the treatment).
A texture analyzer (TMS-Touch, US) with a 7.5 mm diameter probe at a rate of 10 mm s−1 was used to measure the firmness of both sides of peeled peaches. TSS was measured using a handheld refractometer (G-won, GMK-701AC, Korea).
Electrolyte leakage was evaluated according to Zhao et al. (2019). Cylinders of peach tissue were excised with a 5 mm diameter stainless steel cork borer from the equatorial region. After rinsing three times (2 min to 3 min) with deionized water, 12 small discs (0.1 cm thick, 0.5. cm diameter) were incubated in 25 mL of deionized water at 20°C, followed by shaking for 30 min. Electrolyte leakage of the solution was measured using a conductivity meter (DDS-11A; Shanghai, China). The solution was then heated to 100°C for 15 min and quickly cooled. The total electrolytes of the solution were then measured again. Relative leakage was expressed as a percentage of the total electrolyte leakage.
Determination of levels of AsA, GSH and GSSG
A method of Hu et al. (2016) was used to measure AsA content. Approximately 0.1 g of frozen sample were ground in liquid nitrogen. The homogenates were then extracted with 5 mL of 5% (w/v) trichloroacetic acid (TCA) and centrifuged at 12,000 g at 4° for 15 min to obtain supernatant for AsA determination. Took 1 ml of supernatant after centrifugation at 4°C and added 0.5 ml of 0.4% phosphoric acid ethanol solution, 1.0 ml of 0.5% phenanthroline ethanol solution, 0.5 ml of 0.03% FeCl3 ethanol solution. After mixing, put the solution at 30°C and reacted for 60 min, then measured the absorbance at 534 nm. Two grams of peach fruit was grounded in liquid nitrogen with 2 mL of extraction solution and then centrifuged at 12000 g for 10 min at 4°C. The supernatant was collected for GSH and GSSG determination with a glutathione kit (Beijing Solabao Technology Co., Ltd., Ningbo, China).
Gene expression analysis
Extraction and reverse transcription of total RNA were performed according to the method of Zhang et al. (2021). Gene expression analysis was performed on a StepOnePlus™ real-time PCR instrument (BIO-RAD, Hercules, California, USA) using SYBR Green I Master Mix (Vazyme, Nanjing, Jiangsu, China) and specific primers (Supplementary Table 1). The real-time fluorescence quantitative PCR reaction system is 6.25 μL GreenMaster Mix, 0.5 μL upstream primer, 0.5 μL downstream primer, 5.25 μL template DNA. Reaction range Sequence: 95 °C pre denaturation for 5 min; deformation at 95 °C for 10 s, annealing at 60 °C for the 30 s, and cycling for 40 times. Gene expression was calculated using the 2-ΔCt method.
Statistical analysis
Experimental data were analyzed using GraphPad Prism 9 software. Differences between control and treatment were tested using multiple t tests (* P < 0.05, ** P < 0.01, and *** P < 0.001).
Results
Effect of GABA treatment on CI index in peaches
CI of the non-treated peach fruit increased sharply after three weeks of cold storage. GABA treatment reduced the occurrence of Cl, and the index was considerably lower than that of untreated peach after 28 d of storage (Figures 1A, B). In the 35 d, CI of peach treated with GABA was 44.2% lower than the untreated group.
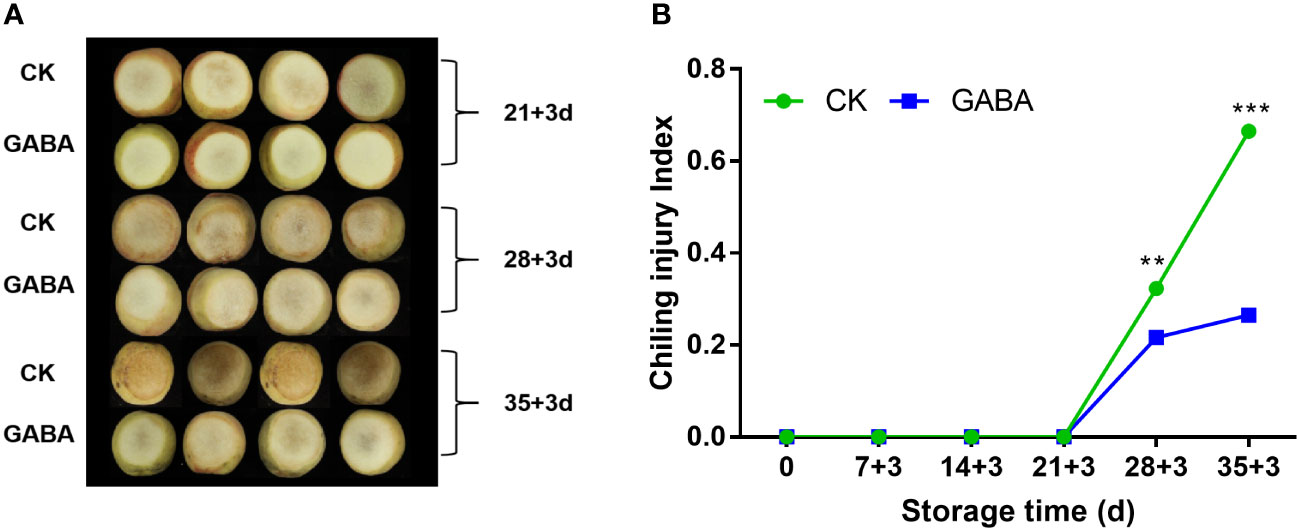
Figure 1 Effect of GABA treatment on the appearance (A) and chilling injury index (B) of peach stored at 4°C. Asterisks indicate significant differences between CK and GABA treatment (*p < 0.05, **p < 0.01, and ***p < 0.001).
Effect of GABA treatment on fruit firmness, TSS and relative electrical conductivity in peaches
Both GABA-treated and non-treated peaches exhibited a decline in fruit firmness with storage time. Compared to the control group, fruit treated with GABA maintained a greater level of firmness over the whole storage (Figure 2A). TSS contents of peach fruit gradually decreased along with storage time, GABA-treated fruit experienced higher TSS levels than the controls (Figure 2B). Relative electrical conductivity increased rapidly in control peaches during the cold storage, which was inhibited with GABA treatment (Figure 2C).
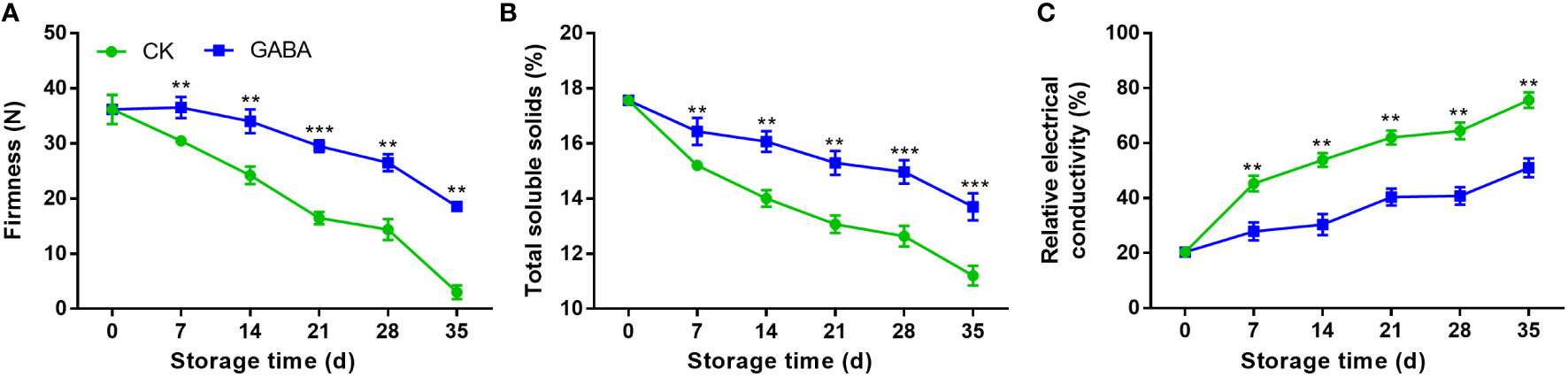
Figure 2 Effect of GABA treatment on fruit firmness (A), total soluble solids (B) and relative electrical conductivity (C) of peach stored at 4°C. Asterisks indicate significant differences between CK and GABA treatment (*p < 0.05, **p < 0.01, and ***p < 0.001).
Effect of GABA treatment on levels of AsA, GSH and GSSG in peaches
At the beginning of cold storage, AsA levels increased, but then decreased until the end (Figure 3A). On day 7, the contents of AsA content in both control and GABA treated peaches reached the maximum values. After that, the content in the untreated group decreased rapidly, while the GABA-treated group maintained a higher level. Overall, the AsA content in the GABA-treated peach were higher than those of the controls throughout the whole storage. The levels of GSH and GSSG in the non-treated peach decreased gradually within the storage. The results showed that GABA treatment up-regulated peach fruit’s GSH and GSSG contents throughout storage (Figures 3B, C).
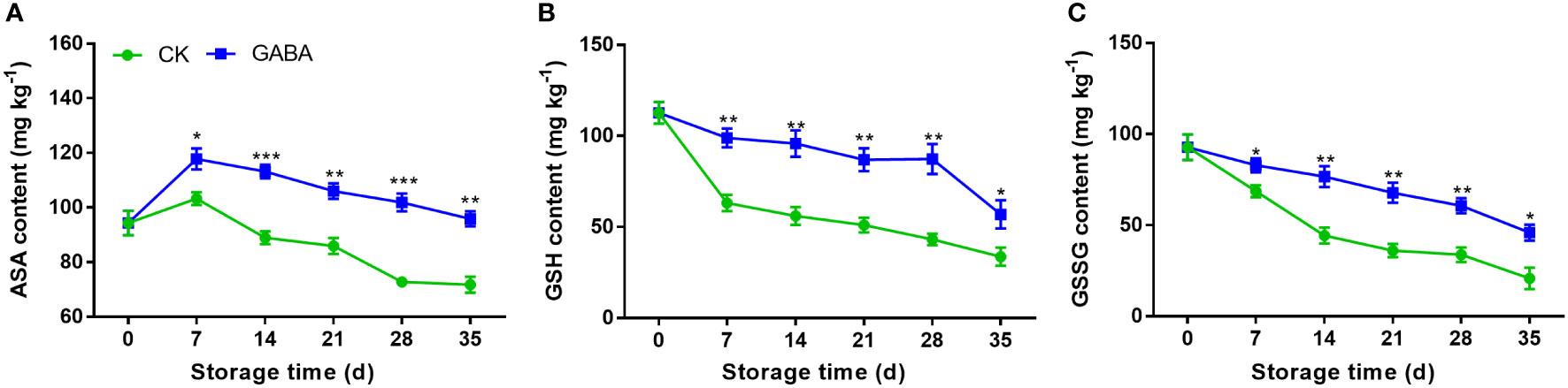
Figure 3 Effect of GABA treatment on AsA (A), GSH (B) and GSSG (C) contents of peach stored at 4°. Asterisks indicate significant differences between CK and GABA treatment (*p < 0.05, **p < 0.01, and ***p < 0.001).
Effect of GABA treatment on expression of genes involved in AsA biosynthesis in peaches
During storage, the expression of PpPMM gradually decreased, GABA treatment induced its expression on day 7, 21 and 35 (Figure 4A). The transcripts of PpGMP and PpGME1 increased firstly and then declined thereafter. GABA upregulated PpGMP after fourteen days of storage but PpGME1 during the whole storage (Figures 4B, C). The expression of PpGME2 declined dramatically during the first seven days of storage and then remained unchanged. A higher level of its expression was observed on day 7 in GABA-treated peaches (Figure 4D). The transcript abundance of PpGGP and PpGPP increased during first 21 d of storage followed by a decline during the remaining storage time. Higher transcripts of PpGGP were observed on day 14 and 28 of storage after GABA treatment (Figure 4E). The treatment also upregulated PpGPP expression on day 14 and 21 as compared to the controls (Figure 4F). A decrease of PpGALDH expression was observed in non-treated peaches, GABA treatment induced it after 28 d of storage (Figure 4G). GABA treatment also upregulated the expression of PpMXIO4 during the first 14 d of storage but inhibited it after 28 d (Figure 4H). Regarding to the gene PpGALUR in D-galacturonate pathway and gene PpAO2 in AsA degradation, both of their expression increased within the storage in peaches, GABA induced PpGALUR after 28 d of storage, however, no difference was observed in PpAO2 expression between the control and treated peaches (Figures 4I, J).
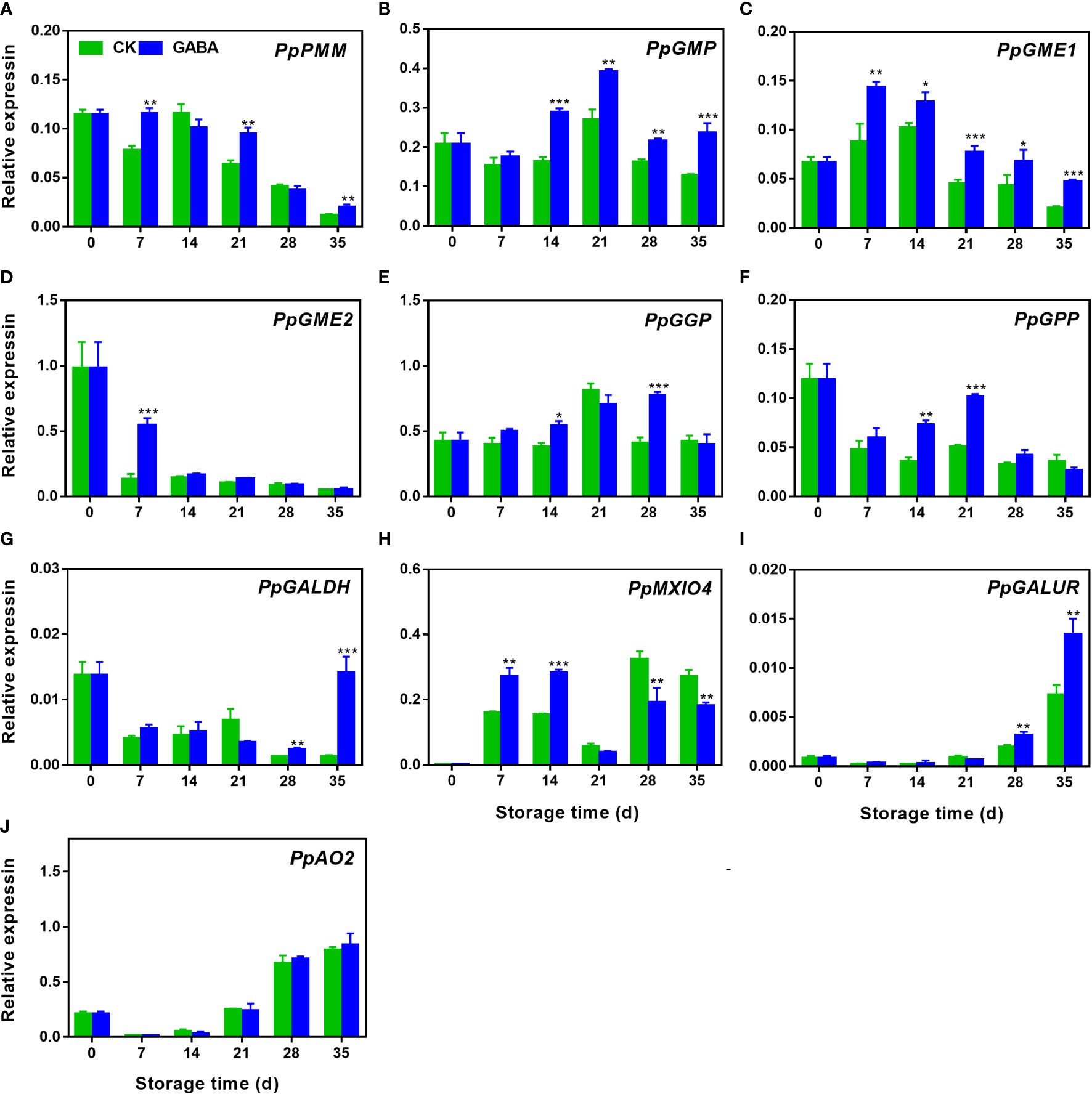
Figure 4 Effect of GABA treatment on expression of genes involved in AsA biosynthesis of peach stored at 4°C. Expression levels of PpPMM(A),PpGMP(B), PpGME1 (C), PpGME2 (D), PpGGP (E), PpGPP (F), PpGALDR (G), PpGALUR (H), PpMXIO4 (I) and PpAO2 (J) were analysed. Asterisks indicate significant differences between CK and GABA treatment (*p < 0.05, **p < 0.01, and ***p < 0.001).
Effect of GABA treatment on expression of genes involved in GSH biosynthesis in peaches
As shown in Figure 5, the transcripts of all three genes such as PpGCS1, PpGCS2 and PpGS increased firstly and declined gradually thereafter. GABA treatment induced PpGCS1 expression in peach during the whole storage (Figure 5A). Higher transcripts of PpGCS2 were observed on day 14 and 28 of storage after GABA treatment (Figure 5B). The treatment also upregulated PpGS expression on day 7 and 21 as compared with the control (Figure 5C).
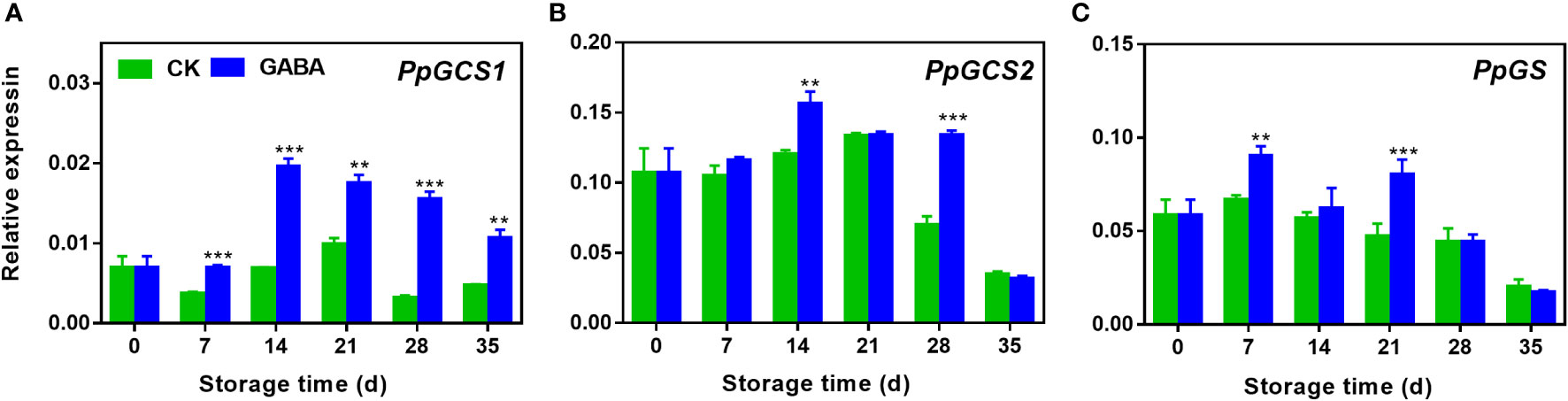
Figure 5 Effect of GABA treatment on expression of genes involved in GSH biosynthesis of peach stored at 4°C. Expression levels of PpGCS1 (A)> , PpGCS2 (B) and PpGS (C) were analysed. Asterisks indicate significant differences between CK and GABA treatment (*p < 0.05, **p < 0.01, and ***p < 0.001).
Effect of GABA treatment on expression of genes involved in AsA-GSH recycling in peaches
Expression of PpGR2 increased initially and then began to decrease while the expression of PpDHAR2 decreased gradually during storage. GABA promoted the transcription level of both genes over the storage (Figure 6A, B). During the first 21 d of storage, PpMDHAR and PpGPX2 transcript abundance increased but then decreased quickly. Up-regulations of PpMDHAR was observed on day 7 and 28 in GABA-treated peaches (Figure 6C) and GABA also induced PpGPX2 expression on day 21 and 28 (Figure 6D). Compared with the control peach, there was no difference in the levels of PpAPX expression between the treated and control fruit in the early storage stage. However, GABA treatment increased the abundance of PpAPX transcripts after 28 d of cold storage (Figure 6E).
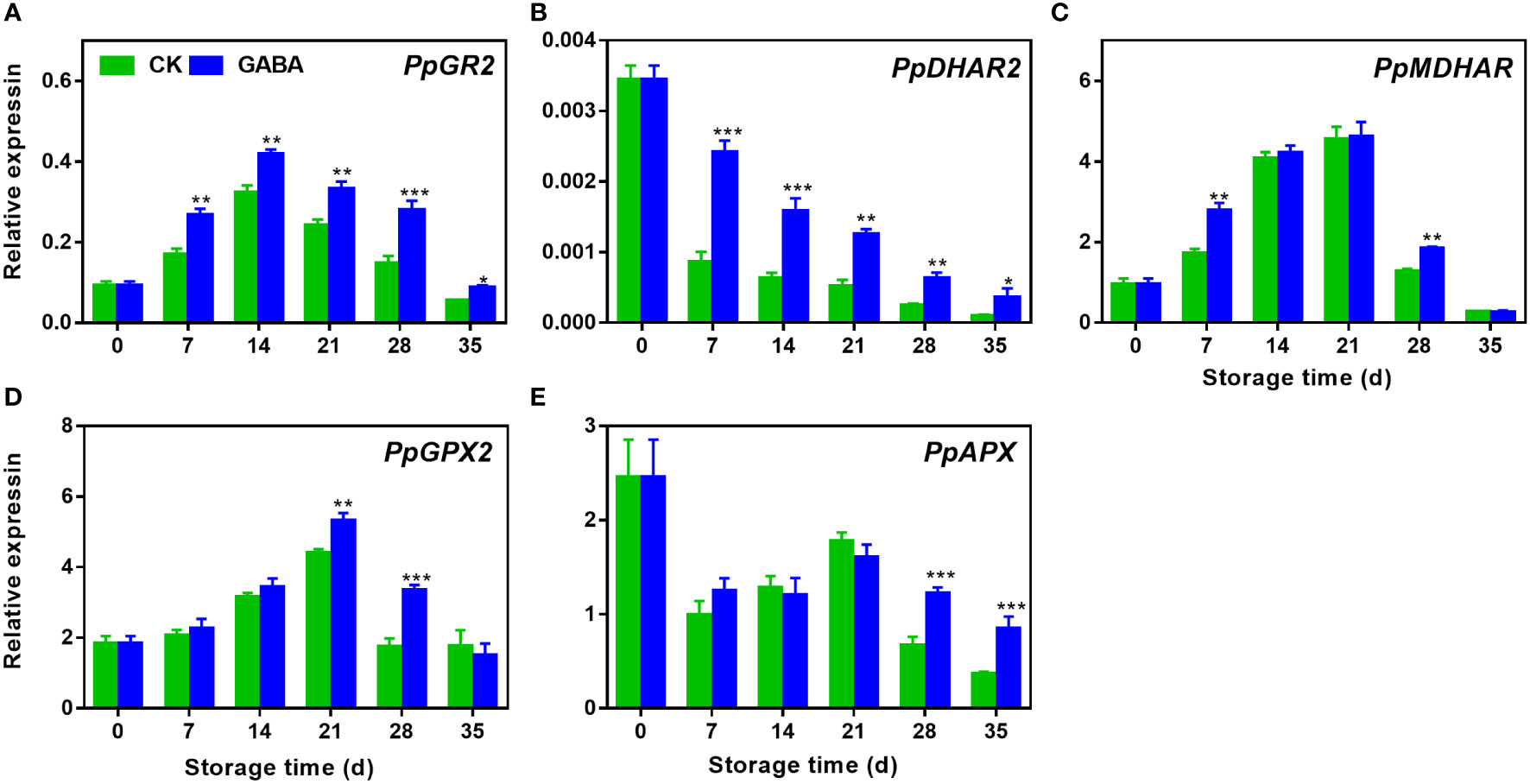
Figure 6 Effect of GABA treatment on expression of genes involved in ASA-GSH cycle of peach stored at 4°C. Expression levels of PpGR2 (A), PpDHAR2 (B), PpMDHAR (C), PpGPX2 (D) and PpAPX (E) were analysed. Asterisks indicate significant differences between CK and GABA treatment (*p < 0.05, **p < 0.01, and ***p < 0.001).
Effect of GABA treatment on expression of ERF transcription factors in peaches
The transcript levels of all the three candidate ERF transcription factors exhibited a sharp increase and subsequent decrease during the cold storage in both treated and non-treated peach. GABA treatment increased PpERF4 expression during the whole storage (Figure 7A). Higher transcripts of PpERF106 were observed on day 7 and 14 of storage after GABA treatment (Figure 7B). The treatment also upregulated PpERF115 expression on day 21 and 28 as compared to the controls (Figure 7C).
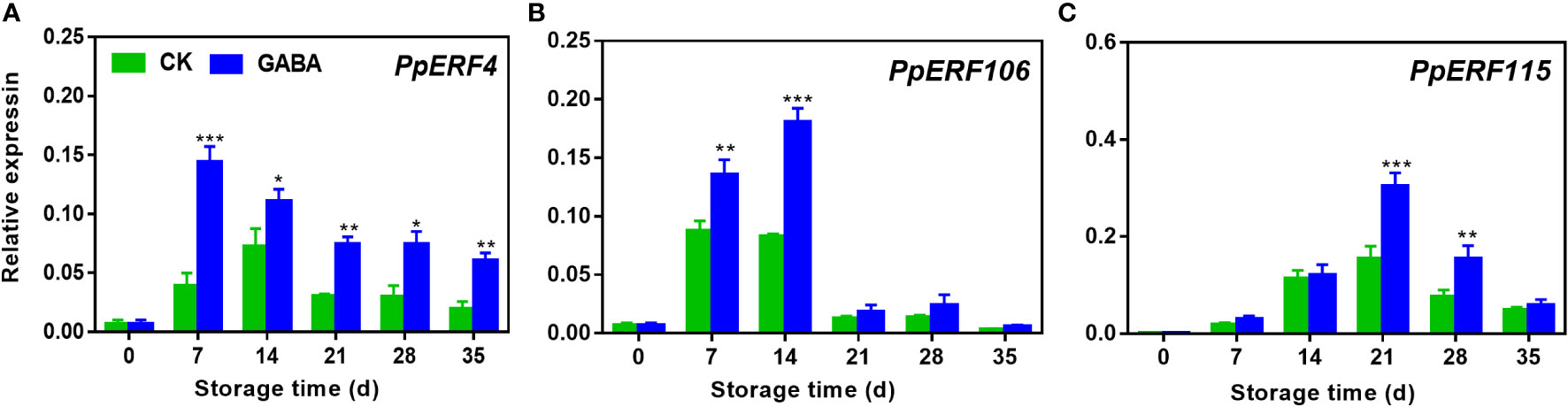
Figure 7 Effect of GABA treatment on expression of ERF transcription factors of peach stored at 4°C. Expression levels of PpERF4 (A), PpERF106 (B) and PpERF115 (C) were analysed. Asterisks indicate significant differences between CK and GABA treatment (*p < 0.05, **p < 0.01, and ***p < 0.001).
Discussion
GABA is a non-protein amino acid that accumulates rapidly in plants when stressed (Ramos-Ruiz et al., 2019). It plays a crucial role in regulating pH in the cytosol, controlling carbon and nitrogen metabolism, and protecting cells from biotic and abiotic stresses (Hijaz et al., 2018). In recent years, there have also been many related researches on GABA-inducing chilling tolerance in post-harvest in fruit and vegetables (Ali et al., 2022; Fan et al., 2022; Jiao, 2022). Same as our previous studies (Yang et al., 2011), here we also found GABA could effectively reduce chilling incidence and maintain fruit quality during postharvest storage.
AsA plays a critical role in oxidative stress defense and membrane degradation in plants. Increased plant resistance against cold stress due to enhanced hydrogen peroxide scavenging system by AsA, is widely reported in postharvest bananas, kiwifruit and peach (Wang et al., 2014b; Yang et al., 2016; Lo’Ay and El-Khateeb, 2018). Here, our research found the AsA levels in GABA-treated peach increased during storage which was crucial to relieve oxidative stress and reduce the chilling injury in peach fruit.
The biosynthesis of AsA in plant is mainly divided into four pathways, namely L-galactose (Wheeler et al., 1998), D-galacturonate (Agius et al., 2003), L-glucose (Wolucka et al., 2005) and myo-inositol (Lorence et al., 2004). Among them, the L-galactose pathway has been officially recognized as the leading synthetic pathway of AsA biosynthesis (Zhang et al., 2015). Gómez-García and Ochoa-Alejo (2016) found that it was the primary mechanism for AsA biosynthesis in fruit and leaves of peppers. Li et al. (2011) revealed that the expression of four L-galactose genes such as MdGME, MdGPP and MdGaLDH) in young apple fruit was correlated well with the AsA content. In our current study, most genes of the L-galactose pathway were up-regulated by GABA treatment, consequently responsible for the corresponding increase in AsA levels to some extent.
Besides the L-galactose pathway, the D-galacturonate pathway is also reported to be the major pathway for AsA biosynthesis in plants, in which GALUR is a crucial enzyme (Agius et al., 2003), converting D-galacturonate into L-galactose acid (Badejo et al., 2012). In Arabidopsis overexpressed with strawberry FaGALUR gene ectopically, AsA content was increased (Wolucka and Van Montagu, 2003). However, there was no apparent relationship between AsA content and AdGALUR expression in kiwifruit (Bulley et al., 2009). Our experiment here showed that GABA treatment did not enhance the transcription level of PpGALUR in the early stage of storage but considerably increased expression was observed at the end of storage. Therefore, we speculated that PpGALUR in GABA-treated peach might play a role in inducing AsA accumulation during the late period of storage.
In addition to the two AsA biosynthetic pathways described above, we also studied the transcriptional abundance of some genes in the myo-inositol and degradation pathways. As a key enzyme in the myo-inositol pathway, the role of MIOX in the plant AsA biosynthesis pathway is still unclear (Munir et al., 2020). Previous studies have suggested that overexpression of AtMIOX in Arabidopsis increased the AsA level by 2-to-3 fold (Lorence et al., 2004). A study has shown, however, that AtMIOX regulated the Myo-inositol level without increasing AsA content (Endres and Tenhaken, 2009). In our present study, the different responses of PpMIOX4 transcripts in response to GABA treatment were observed indicating the precise mechanism of PpMIOX4 in AsA biosynthesis in peach fruit needs to be further explored. In respect to the AsA degradation, no differential expression of PpAO2 was found in peaches treated with GABA. Therefore, we believed that the GABA signal was not involved in the regulation of AsA degradation.
GSH is another well-known antioxidant in plants that can reduce the accumulation of ROS produced in various adversity stresses (Chen and Yang, 2020). It was found that increasing GSH content could reduce chilling injury in postharvest fruit such as iwifruit, pears and bell pepper (Wei et al., 2019; Yao et al., 2021; Jiao et al., 2022). Similarly, in GABA-treated peach treatment, higher GSH content was observed, which was also related to the induced chilling tolerance in peach treated with GABA.
It is well documented that GSH biosynthesis is mainly a free amino acid-dependent enzymatic synthesis of ATP and Mg2+. γ-Glutamylcysteine ligase (GCS) is a key rate-limiting enzyme in GSH biosynthesis catalyzing γ-COOH and cysteine to glutamate -NH-binding acid while consuming ATP to generate glutamylcysteine (γ-EC) (Noctor et al., 2012). Then, glutathione synthase (GS) catalyzes γ-EC to generate GSH (Jobbagy et al., 2019). In our experiments, GABA treatment increased the expression of all of the three GSH biosynthetic genes in peach, thus inducing GSH accumulation.
The AsA-GSH cycle is an essential H2O2 scavenging system in plants (Hasanuzzaman et al., 2019). AsA and GSH are important non-enzymatic antioxidants in this cycle, and their redox states can represent the redox states in the cellular environment (Wang et al., 2013). GPX, MDHAR, DHAR, GR and APX are critical enzymes in the metabolism of the AsA-GSH cycle. GPX has a defensive role against oxidative damage in plant cells (Lushchak, 2012). MDHAR and DHAR can reduce MDHA and DHA respectively to form AsA, ensuring the efficient regeneration of AsA, while GSH is oxidized to GSSG (Aravind and Prasad, 2005; Ma et al., 2019). GR can use NAD (P) H as an electron donor to regenerate GSSG into GSH and maintain the reduced state of GSH. APX can use AsA as an electron donor to reduce H2O2 to H2O, and remove excess H2O2 in cells (Yin et al., 2010). Studies have shown that Glycine betaine treatment reduced oxidative stress damage and improved cold resistance by regulating the metabolism of the AsA-GSH cycle in plants (Einset and Connolly, 2009). In peach, we found that GABA treatment up-regulated the expression of PpGR2, PpDHAR2, PpMDHAR, PpGPX2 and PpAPX genes in the AsA-GSH cycle during cold storage, which was similar to the results obtained by Wang et al. (Wang et al., 2016), who reported that Glycine betaine treatment also induced associated genes in the AsA-GSH cycle, thus improving cold resistance in pepper fruit. Our results showed that GABA treatment up-regulated the expression of related genes in the AsA-GSH cycle, thereby maintaining higher AsA and GSH levels during cold storage and inhibiting the occurrence of chilling injury in peach fruit.
Many studies have found transcription factors such as ERFs have been identified to be involved in AsA metabolic pathway in Arabidopsis, tomato and citrus fruit (Zhang et al., 2009; Hu et al., 2016; Ye et al., 2019). AtERF98 triggers the expression of genes involved in the D-Man/L-Gal synthesis pathway and the MIOX4 gene in the myo-inositol pathway (Yuan et al., 2020). The function of AtERF98-regulated AsA synthesis was severely impaired in vtc1-1 mutants, suggesting that AtERF98 is involved in the D-Man/L-Gal synthesis pathway and plays a vital role in regulating the synthesis of AsA (Zhang et al., 2012). In this study, we found that the expression of three PpERF genes was up-regulated in the peach GABA-treated group when compared with the untreated group, showing that ERFs might also participate in the metabolic regulation of AsA and GSH contents.
Conclusion
In conclusion, after GABA treatment, the chilling injury of peach fruit was decreased. GABA increased AsA and GSH levels by regulating the expression of AsA and GSH-related genes in cold-stored peach. Therefore, we believed that GABA treatment could enhance chilling tolerance due to the increased levels of AsA and GSH. Furthermore, our results also suggested three candidate ERF transcription factors might be involved in AsA and GSH biosynthesis. However, more evidence is needed to determine the relationship of ERF transcription factors and their networks to hormone signalling and hormone-mediated stress responses, which may help provide insight into the transcriptional regulation of AsA-GSH metabolic processes in postharvest peach.
Data availability statement
The original contributions presented in the study are included in the article/Supplementary Material. Further inquiries can be directed to the corresponding author.
Author contributions
CZ, ZY and SC contributed to conception and design of the study. SJ organized the database. QL performed the statistical analysis. CZ wrote the first draft of the manuscript. LS, SL, WC and WD wrote sections of the manuscript. All authors contributed to manuscript revision, read, and approved the submitted version.
Funding
The work was supported by the National Natural Science Foundation of China (32172646), the Key Projects of Natural Science Foundation of Zhejiang Province (LZ21C200002) and the Natural Science Foundation of Zhejiang Province (LQ20C200006).
Conflict of interest
The authors declare that the research was conducted in the absence of any commercial or financial relationships that could be construed as a potential conflict of interest.
Publisher’s note
All claims expressed in this article are solely those of the authors and do not necessarily represent those of their affiliated organizations, or those of the publisher, the editors and the reviewers. Any product that may be evaluated in this article, or claim that may be made by its manufacturer, is not guaranteed or endorsed by the publisher.
Supplementary material
The Supplementary Material for this article can be found online at: https://www.frontiersin.org/articles/10.3389/fpls.2022.1059979/full#supplementary-material
References
Aghdam, M. S., Razavi, F., Karamneghad, F. (2016). Maintaining the postharvest nutritional quality of peach fruits by γ-aminobutyric acid. Plant Physiol. 5 (4), 1457–1463. doi: 10.30495/IJPP.2015.539673
Agius, F., Gonzalez-Lamothe, R., Caballero, J. L., Munoz-Blanco, J., Botella, M. A., Valpuesta, V. (2003). Engineering increased vitamin c levels in plants by overexpression of a d-galacturonic acid reductase. Nat. Biotechnol. 21 (2), 177–181. doi: 10.1038/nbt777
Ali, S., Anjum, M. A., Nawaz, A., Ejaz, S., Anwar, R., Khaliq, G., et al. (2022). Postharvest gamma-aminobutyric acid application mitigates chilling injury of aonla (Emblica officinalis gaertn.) fruit during low temperature storage. Postharvest Biol. Technol. 185, 111803. doi: 10.1016/j.postharvbio.2021.111803
Alscher, R. G., Donahue, J. L., Cramer, C. L. (1997). Reactive oxygen species and antioxidants: Relationships in green cells. Physiol. Plant 100, 224–233. doi: 10.1111/j.1399-3054.1997.tb04778.x
Aravind, P., Prasad, M. N. V. (2005). Modulation of cadmium-induced oxidative stress in Ceratophyllum demersum by zinc involves ascorbate–glutathione cycle and glutathione metabolism. Postharvest Biol. Technol. 43 (2), 107–116. doi: 10.1016/j.plaphy.2005.01.002
Badejo, A. A., Wada, K., Gao, Y., Maruta, T., Sawa, Y., Shigeoka, S., et al. (2012). Translocation and the alternative d-galacturonate pathway contribute to increasing the ascorbate level in ripening tomato fruits together with the d-mannose/L-galactose pathway. J. Exp. Bot. 63 (1), 229–239. doi: 10.1093/jxb/err275
Bao, H., Chen, X., Lv, S., Jiang, P., Feng, J., Fan, P., et al. (2015). Virus-induced gene silencing reveals control of reactive oxygen species accumulation and salt tolerance in tomato by gamma-aminobutyric acid metabolic pathway. Plant Cell Environ. 38 (3), 600–613. doi: 10.1111/pce.12419
Baxte, A., Mittler, R., Suzuki, N. (2014). ROS as key players in plant stress signaling. J. Exp. Bot. 65, 1229–1240. doi: 10.1093/jxb/ert375
Bulley, S. M., Rassam, M., Hoser, D., Otto, W., Schünemann, N., Wright, M., et al. (2009). Gene expression studies in kiwifruit and gene over-expression in Arabidopsis indicates that GDP-l-galactose guanyltransferase is a major control point of vitamin c biosynthesis. J. Exp. Bot. 60 (3), 765–778. doi: 10.1093/jxb/ern327
Chen, Q., Yang, G. (2020). Signal function studies of ROS, especially RBOH-dependent ROS, in plant growth, development and environmental stress. J. Plant Growth Regul. 39 (1), 157–171. doi: 10.1007/s00344-019-09971-4
Einset, J., Connolly, E. L. (2009). Glycine betaine enhances extracellular processes blocking ROS signaling during stress. Plant Signal. Behav. 4 (3), 197–199. doi: 10.4161/psb.4.3.7725
Endres, S., Tenhaken, R. (2009). Myoinositol oxygenase controls the level of myoinositol in arabidopsis, but does not increase ascorbic acid. Plant Physiol. 149 (2), 1042–1049. doi: 10.1104/pp.108.130948
Fan, Z., Lin, B., Lin, H., Lin, M., Chen, J., Lin, Y. (2022). γ-aminobutyric acid treatment reduces chilling injury and improves quality maintenance of cold-stored Chinese olive fruit. Food Chem. X 13, 100208. doi: 10.1016/j.fochx.2022.100208
Gómez-García, M., Ochoa-Alejo, N. (2016). Predominant role of the l-galactose pathway in l-ascorbic acid biosynthesis in fruits and leaves of the Capsicum annuum l. chili pepper. Braz. J. Bot. 39 (1), 157–168. doi: 10.1007/s40415-015-0232-0
Hasanuzzaman, M., Bhuyan, M. B., Anee, T. I., Parvin, K., Nahar, K., Mahmud, J. A., et al. (2019). Regulation of ascorbate-glutathione pathway in mitigating oxidative damage in plants under abiotic stress. Antioxidants 8 (9), 384. doi: 10.3390/antiox8090384
Hijaz, F., Nehela, Y., Killiny, N. (2018). Application of gamma-aminobutyric acid increased the level of phytohormones in citrus sinensis. Planta 248 (4), 909–918. doi: 10.1007/s00425-018-2947-1
Hu, T., Ye, J., Tao, P., Li, H., Zhang, J., Zhang, Y., et al. (2016). The tomato HD-zip I transcription factor SlHZ24 modulates ascorbate accumulation through positive regulation of the d-mannose/L-galactose pathway. Plant J. 85 (1), 16–29. doi: 10.1111/tpj.13085
Jiao, C. (2022). γ-aminobutyric acid boosts chilling tolerance by promoting the methionine sulfoxide reductase-thioredoxin reductase system in peach fruit. Hortic. Environ. Biotechnol. 63, 353–361. doi: 10.1007/s13580-021-00408-0
Jiao, J., Jin, M., Liu, H., Suo, J., Yin, X., Zhu, Q., et al. (2022). Application of melatonin in kiwifruit (Actinidia chinensis) alleviated chilling injury during cold storage. Sci. Hortic. 296 (5), 110876. doi: 10.1016/j.scienta.2022.110876
Jobbagy, S., Vitturi, D. A., Salvatore, S. R., Turell, L., Pires, M. F., Kansanen, E., et al. (2019). Electrophiles modulate glutathione reductase activity via alkylation and upregulation of glutathione biosynthesis. Redox Biol. 21, 101050. doi: 10.1016/j.redox.2018.11.008
Li, M. J., Chen, X. S., Wang, P. P., Ma, F. W. (2011). Ascorbic acid accumulation and expression of genes involved in its biosynthesis and recycling in developing apple fruit. J. Am. Soc Hortic. Sci. 136 (4), 231–238. doi: 10.21273/JASHS.136.4.231
Li, L., Dou, N., Zhang, H., Wu, C. (2021). The versatile GABA in plants. Plant Signal. Behav. 16 (3), 1862565. doi: 10.1080/15592324.2020.1862565
Lo’Ay, A., El-Khateeb, A. (2018). Antioxidant enzyme activities and exogenous ascorbic acid treatment of ‘Williams’ banana during long-term cold storage stress. Sci. Hortic. 234 (14), 210–219. doi: 10.1016/j.scienta.2018.02.038
Lorence, A., Chevone, B. I., Mendes, P., Nessler, C. L. (2004). Myo-inositol oxygenase offers a possible entry point into plant ascorbate biosynthesis. Plant Physiol. 134 (3), 1200–1205. doi: 10.1104/pp.103.033936
Lushchak, V. I. (2012). Glutathione homeostasis and functions: potential targets for medical interventions. Amino Acids 2012, 1–26. doi: 10.1155/2012/736837
Mahmud, J. A., Hasanuzzaman, M., Nahar, K., Rahman, A., Hossain, M., Fujita, M. (2017). γ-aminobutyric acid (GABA) confers chromium stress tolerance in Brassica juncea l. by modulating the antioxidant defense and glyoxalase systems. Ecotoxicology 26 (5), 675–690. doi: 10.1007/s10646-017-1800-9
Ma, Y., Huang, D., Chen, C., Zhu, S., Gao, J. (2019). Regulation of ascorbate-glutathione cycle in peaches via nitric oxide treatment during cold storage. Sci. Hortic. 247 (15), 400–406. doi: 10.1016/j.scienta.2018.12.039
Malekzadeh, P. (2015). Influence of exogenous application of glycinebetaine on antioxidative system and growth of salt-stressed soybean seedlings (Glycine max l.). Physiol. Mol. Biol. Plants 21 (2), 225–232. doi: 10.1007/s12298-015-0292-4
Malekzadeh, P., Khosravi-Nejad, F., Hatamnia, A. A., Sheikhakbari Mehr, R. (2017). Impact of postharvest exogenous γ-aminobutyric acid treatment on cucumber fruit in response to chilling tolerance. Physiol. Mol. Biol. Plants 23 (4), 827–836. doi: 10.1007/s12298-017-0475-2
Munir, S., Mumtaz, M. A., Ahiakpa, J. K., Liu, G., Chen, W., Zhou, G., et al. (2020). Genome-wide analysis of myo-inositol oxygenase gene family in tomato reveals their involvement in ascorbic acid accumulation. BMC Genom. 21 (1), 1–15. doi: 10.1186/s12864-020-6708-8
Niazi, Z., Razavi, F., Khademi, O., Aghdam, M. S. (2021). Exogenous application of hydrogen sulfide and γ-aminobutyric acid alleviates chilling injury and preserves quality of persimmon fruit (Diospyros kaki, cv. karaj) during cold storage. Sci. Hortic. 285 (27), 110198. doi: 10.1016/j.scienta.2021.110198
Nicolas, B., Hillel, F. (2004). GABA in plants: just a metabolite. Trends Plant Sci. 9 (3), 110–115. doi: 10.1016/j.tplants.2004.01.006
Noctor, G., Mhamdi, A., Chaouch, S., Han, Y., Neukermans, J., Marquez-Garcia, B., et al. (2012). Glutathione in plants: an integrated overview. Plant Cell Environ. 35 (2), 454–484. doi: 10.1111/j.1365-3040.2011.02400.x
Ramesh, S. A., Tyerman, S. D., Gilliham, M., Xu, B. (2017). γ-aminobutyric acid(GABA) signalling in plants. Cell. Mol. Life Sci. 74 (9), 1577–1603. doi: 10.1007/s00018-016-2415-7
Ramos-Ruiz, R., Martinez, F., Knauf-Beiter, G. (2019). The effects of GABA in plants. Cogent. Food Agr. 5 (1), 1670553. doi: 10.1080/23311932.2019.1670553
Shang, H. T., Cao, S. F., Yang, Z. F., Cai, Y. T., Zheng, Y. H. (2011). Effect of exogenous gamma-aminobutyric acid treatment on proline accumulation and chilling injury in peach fruit after long-term cold storage. J. Agric. Food Chem. 59 (4), 1264–1268. doi: 10.1021/jf104424z
Song, L., Wang, J., Shafi, M., Liu, Y., Wang, J., Wu, J., et al. (2016). Hypobaric treatment effects on chilling injury, mitochondrial dysfunction, and the ascorbate-glutathione (AsA-GSH) cycle in postharvest peach fruit. J. Agric. Food Chem. 64 (22), 4665–4674. doi: 10.1021/acs.jafc.6b00623
Wang, Q., Ding, T., Zuo, J., Gao, L., Fan, L. (2016). Amelioration of postharvest chilling injury in sweet pepper by glycine betaine. Postharvest Biol. Technol. 112, 114–120. doi: 10.1016/j.postharvbio.2015.07.008
Wang, C., Fan, L., Gao, H., Wu, X., Li, J., Lv, G., et al. (2014a). Polyamine biosynthesis and degradation are modulated by exogenous gamma-aminobutyric acid in root-zone hypoxia-stressed melon roots. Plant Physiol. Biochem. 82, 17–26. doi: 10.1016/j.plaphy.2014.04.018
Wang, Y., Luo, Z., Huang, X., Yang, K., Gao, S., Du, R. (2014c). Effect of exogenous γ-aminobutyric acid (GABA) treatment on chilling injury and antioxidant capacity in banana peel. Sci. Hortic. 168 (26), 132–137. doi: 10.1016/j.scienta.2014.01.022
Wang, K., Shao, X., Gong, Y., Xu, F., Wang, H. (2014b). Effects of postharvest hot air treatment on gene expression associated with ascorbic acid metabolism in peach fruit. Plant Mol. Biol. Rep. 32 (4), 881–887. doi: 10.1007/s11105-014-0699-z
Wang, J., Zeng, Q., Zhu, J., Liu, G., Tang, H. (2013). Dissimilarity of ascorbate-glutathione (AsA–GSH) cycle mechanism in two rice (Oryza sativa l.) cultivars under experimental free-air ozone exposure. Agric. Ecosyst. Environ. 165 (15), 39–49. doi: 10.1016/j.agee.2012.12.006
Wei, C., Ma, L., Cheng, Y., Guan, Y., Guan, J. (2019). Exogenous ethylene alleviates chilling injury of ‘Huangguan’ pear by enhancing the proline content and antioxidant activity. Sci. Hortic. 257 (17), 108671. doi: 10.1016/j.scienta.2019.108671
Wheeler, G. L., Jones, M. A., Smirnoff, N. (1998). The biosynthetic pathway of vitamin c in higher plants. Nature 393, 365–369. doi: 10.1038/30728
Wolucka, B. A., Goossens, A., Inzé, D. (2005). Methyl jasmonate stimulates the de novo biosynthesis of vitamin c in plant cell suspensions. J. Exp. Bot. 56, 2527–2538. doi: 10.1093/jxb/eri246
Wolucka, B. A., Van Montagu, M. (2003). GDP-Mannose 3’, 5’-epimerase forms GDP-l-gulose, a putative intermediate for the de novo biosynthesis of vitamin c in plants. J. Biol. Chem. 278 (48), 47483–47490. doi: 10.1074/jbc.M309135200
Yang, A., Cao, S., Yang, Z., Cai, Y., Zheng, Y. (2011). γ-aminobutyric acid treatment reduces chilling injury and activates the defence response of peach fruit. Food Chem. 129 (4), 1619–1622. doi: 10.1016/j.foodchem.2011.06.018
Yang, Q., Wang, F., Rao, J. (2016). Effect of putrescine treatment on chilling injury, fatty acid composition and antioxidant system in kiwifruit. PloS One 11 (9), e0162159. doi: 10.1371/journal.pone.0162159
Yao, M., Ge, W., Zhou, Q., Zhou, X., Luo, M., Zhao, Y., et al. (2021). Exogenous glutathione alleviates chilling injury in postharvest bell pepper by modulating the ascorbate-glutathione (AsA-GSH) cycle. Food Chem. 352 (1), 129458. doi: 10.1016/j.foodchem.2021.129458
Ye, J., Li, W., Ai, G., Li, C., Liu, G., Chen, W., et al. (2019). Genome-wide association analysis identifies a natural variation in basic helix-loop-helix transcription factor regulating ascorbate biosynthesis via d-mannose/L-galactose pathway in tomato. PloS Genet. 15 (5), e1008149. doi: 10.1371/journal.pgen.1008149
Yin, L., Wang, S., Eltayeb, A. E., Uddin, M., Yamamoto, Y., Tsuji, W., et al. (2010). Overexpression of dehydroascorbate reductase, but not monodehydroascorbate reductase, confers tolerance to aluminum stress in transgenic tobacco. Planta 231 (3), 609–621. doi: 10.1007/s00425-009-1075-3
Yuan, J., Yu, Z., Lin, T., Wang, L., Chen, X., Liu, T., et al. (2020). BcERF070, a novel ERF (ethylene-response factor) transcription factor from non-heading Chinese cabbage, affects the accumulation of ascorbic acid by regulating ascorbic acid-related genes. Mol. Breed. 40 (1), 1–18. doi: 10.1007/s11032-019-1065-5
Zhang, G.-Y., Liu, R.-R., Zhang, C.-Q., Tang, K.-X., Sun, M.-F., Yan, G.-H., et al. (2015). Manipulation of the rice l-galactose pathway: evaluation of the effects of transgene overexpression on ascorbate accumulation and abiotic stress tolerance. PloS One 10 (5), e0125870. doi: 10.1371/journal.pone.0125870
Zhang, W. Y., Lorence, A., Gruszewski, H. A., Chevone, B. I., Nessler, C. L. (2009). AMR1, an arabidopsis gene that coordinately and negatively regulates the mannose/L-galactose ascorbic acid biosynthetic pathway. Plant Physiol. 150 (1), 942–950. doi: 10.1104/pp.108.134767
Zhang, Y., Wang, K., Xiao, X., Cao, S., Chen, W., Yang, Z., et al. (2021). Effect of 1-MCP on the regulation processes involved in ascorbate metabolism in kiwifruit. Postharvest Biol. Technol. 179, 111563. doi: 10.1016/j.postharvbio.2021.111563
Zhang, Z. J., Wang, J., Zhang, R. X., Huang, R. F. (2012). The ethylene response factor AtERF98 enhances tolerance to salt through the transcriptional activation of ascorbic acid synthesis in arabidopsis. Plant J. 71 (2), 273–287. doi: 10.1111/j.1365-313X.2012.04996.x
Zhao, Y., Zhu, X., Hou, Y., Wang, X., Li, X. (2019). Effects of nitric oxide fumigation treatment on retarding cell wall degradation and delaying softening of winter jujube (Ziziphus jujuba mill. cv. dongzao) fruit during storage. Postharvest Biol. Technol. 156, 110954. doi: 10.1016/j.postharvbio.2019.110954
Keywords: γ-aminobutyric acid, ascorbic acid, glutathione, chilling injury, peach
Citation: Zhou C, Dong W, Jin S, Liu Q, Shi L, Cao S, Li S, Chen W and Yang Z (2022) γ-Aminobutyric acid treatment induced chilling tolerance in postharvest peach fruit by upregulating ascorbic acid and glutathione contents at the molecular level. Front. Plant Sci. 13:1059979. doi: 10.3389/fpls.2022.1059979
Received: 02 October 2022; Accepted: 21 November 2022;
Published: 07 December 2022.
Edited by:
María Serrano, Miguel Hernández University of Elche, SpainReviewed by:
Gholamreza Gohari, University of Maragheh, IranMesbah Babalar, University of Tehran, Iran
Copyright © 2022 Zhou, Dong, Jin, Liu, Shi, Cao, Li, Chen and Yang. This is an open-access article distributed under the terms of the Creative Commons Attribution License (CC BY). The use, distribution or reproduction in other forums is permitted, provided the original author(s) and the copyright owner(s) are credited and that the original publication in this journal is cited, in accordance with accepted academic practice. No use, distribution or reproduction is permitted which does not comply with these terms.
*Correspondence: Zhenfeng Yang, eWFuZ3pmQHp3dS5lZHUuY24=