- 1Key Laboratory of Forest Ecology and Environment, National Forestry and Grassland Administration, Ecology and Nature Conservation Institute, Chinese Academy of Forestry, Beijing, China
- 2Co-Innovation Center for Sustainable Forestry in Southern China, Nanjing Forestry University, Nanjing, China
Increasing atmospheric nitrogen (N) deposition has a profound impact on the ecosystem functions and processes. Fine root decomposition is an important pathway for the reentry of nutrients into the soil. However, the effect of N addition on root decomposition and its potential mechanism is not well understood with respect to root branch orders. In this study, we conducted a 30-month decomposition experiment of fine roots under different concentrations of N addition treatments (0, 30, 60, and 90 kg N ha-1 year-1, respectively) in a typical Pinus massoniana plantation in the Three Gorges Reservoir Area of China. In the early stage of decomposition (0−18 months), N addition at all concentrations promoted the decomposition of fine roots, and the average decomposition rates of order 1–2, order 3–4, order 5–6 fine roots were increased by 13.54%, 6.15% and 7.96% respectively. In the late stage of decomposition (18−30 months), high N addition inhibited the decomposition of fine root, and the average decomposition rates of order 1–2, order 3–4, order 5–6 fine roots were decreased by 58.35%, 35.43% and 47.56% respectively. At the same time, N addition promoted the release of lignin, carbon (C), N, and phosphorus (P) in the early-stage, whereas high N addition inhibited the release of lignin, C, N, and the activities of lignin-degrading enzyme (peroxidase and polyphenol oxidase) in the late-stage. The decomposition constant (k) was significantly correlated with the initial chemical quality of the fine roots and lignin-degrading enzyme activities. The higher-order (order 3–4 and order 5–6) fine roots decomposed faster than lower-order (order 1–2) fine roots due to higher initial cellulose, starch, sugar, C concentrations and higher C/N, C/P, lignin/N ratios and lower N, P concentrations. In addition, low N (30 kg N ha-1 year-1) treatments decreased soil organic matter content, whereas high N (90 kg N ha-1 year-1) treatment had the opposite effect. All the N treatments reduced soil pH and total P content, indicating that increased N deposition may led to soil acidification. Our findings indicated that the effect of N addition on decomposition varied with the decomposition stages. The decomposition difference between the lower-order and higher-order fine roots were controlled strongly by the initial chemical quality of the fine roots. This study provides new insights into understanding and predicting possible changes in plant root decomposition and soil properties in the future atmospheric N deposition increase scenarios.
Introduction
Over the past century, food and energy production and other human activities have increased the release of reactive nitrogen (N) into the atmosphere by 3–5-fold (IPCC, 2007). China has become the third-largest N depositor globally, with an average annual total deposition of approximately 21.1 kg N ha-1 year-1, which is expected to increase further (Liu et al., 2013). Increasing atmospheric N deposition has a profound impact on the functions and nutrient cycling processes of ecosystem (Van Groenigen et al., 2017; Liu et al., 2019; Yu et al., 2021; Wu et al., 2022).
Fine root decomposition plays an irreplaceable role in subterranean ecosystems (Dong et al., 2020). Nitrogen, phosphorus (P), and other nutrients released during fine root decomposition affect plant growth and soil biological activity. The organic matter entering the soil through fine root decomposition accounts for 14% ~ 86.8% of the total input (Usman et al., 2000; Parton et al., 2007). The carbon (C) returned to soil from fine roots is 4–5 fold that of from the aboveground litter. Therefore, fine root decomposition is an important pathway for the reentry of nutrients into the soil (Fahey and Arthur, 1994; Steinaker and Wilson, 2005; Xu et al., 2022). It is important to clarify the effects of increased N deposition on fine root decomposition and the ecosystem C balance (Reay et al., 2008; Kou et al., 2015; Sun et al., 2016; Song et al., 2016).
Numerous previous studies on the effect of elevated N deposition on fine root decomposition have made some progress, but the results are controversial (Berg and Matzner, 1997; Kou et al., 2015; Tu et al., 2015; Song et al., 2016; Dong et al., 2020). For example, some studies found that adding N significantly decreased the decomposition rate due to the increase of lignin concentration and the combination of inorganic N ions with acid nonhydrolyzable residues or the change of soil environment (Tu et al., 2014; Kou et al., 2015; Xia et al., 2017). On the contrary, a study in temperate grasslands found that N addition, in either organic or inorganic forms, stimulated the decomposition rate of fine roots (Dong et al., 2020). In addition, Song et al. (2015); Song et al. (2016) suggested that low-level (30 kg N ha-1 year-1) N addition promoted fine root decomposition rate and nutrient elements release, whereas high N (≥60 kg N ha-1 year-1) addition had the opposite effects. Other studies found that N addition promoted the early stage of decomposition due to the higher C concentrations and lower N concentrations, while inhibited the later stage of decomposition due to the increase of lignin concentration and the decrease of lignin degrading enzyme activity (Berg and Matzner, 1997; Hobbie et al., 2012). A meta-analysis found that the effect of N addition on litter decomposition depends on the initial quality of substrate, nitrogen deposition rate in environment and the concentration and rate of nitrogen application (Knorr et al., 2005). Overall, the above studies have proposed some hypotheses to explore the potential mechanisms of N addition on fine roots decomposition, but there appears to be mixed and sometimes even conflicting findings.
Nearly half a century ago, most studies considered fine roots a homogeneous system, which holds that the root of all less than 2 mm are basically the same in structure and physiology. However, recent studies have shown that a single diameter class definition ignores the heterogeneity of internal structure and function of the fine root systems (Pregitzer et al., 2002; Guo et al., 2004; Yang et al., 2019). The root system is composed of different branching levels (Guo et al., 2004), and there are significant differences in the physiological processes of different orders fine roots. These differences in root function affect the histochemical composition, including the C/N ratio and concentrations of non-structural carbohydrates, calcium, phenolic compounds, and lignin (Guo et al., 2004; Goebel et al., 2011). Therefore, the differences in chemical composition between different root branch orders may affect their decomposition rate. A few studies on root decomposition have found that the decomposition rate of the lower-order fine roots was slower than that of the higher-order fine roots (Kou et al., 2015; Yang et al., 2019). These studies suggested that the lower-order fine roots are more easily colonized by ectomycorrhizal (EM) mycorrhizal fungi and the chitin rich mycorrhizal sheath may reduce the decomposition of the fine roots (Langley et al., 2003a; Langley and Hungate, 2003b; Guo et al., 2008). Other studies suggested that the slow decomposition of lower-order fine roots is mainly driven by their high content of acid-insoluble substances (Parton et al., 2007; Xiong et al., 2013; Liu et al., 2019), which is unrelated to the initial N concentration (Sun et al., 2013; Xiong et al., 2013). Yang et al. (2019) indicated that the slow decomposition of the lower-order roots is due to the combined effects of higher initial N content and lower C content. However, Shen et al. (2017) has the opposite conclusion that the lower-order fine roots decomposed faster due to the higher C/N ratio and the lower N concentration during the decomposition process and the higher sensitivity to soil temperature, moisture, Ca concentration. The findings regarding fine root decomposition are controversial, and the potential mechanism is unclear; therefore, more experiments are required for better understanding.
As China’s key sensitive ecological area and the ecological barrier between the middle and lower reaches of the Yangtze River, the Three Gorges Reservoir area has an atmospheric N deposition flux of approximately 30 kg N ha-1 year-1 which is quite high (Zheng et al., 2014). Pinus massoniana plantations are widely distributed and contribute substantially to both the economic and ecological value in the Three Gorges Reservoir area of China (Zeng et al., 2018). The effect of increased nitrogen deposition on root decomposition of Pinus massoniana is bound to change the soil carbon pools of local forest ecosystems. Therefore, in this study, we conducted the decomposition experiments of three orders fine roots under the condition of different concentration N treatments for 30 months in a typical Pinus massoniana plantation. Our aim was to clarify the effect of nitrogen addition on different order fine root decomposition and the reasons for the differences. We proposed the following hypotheses: (1) N addition promotes fine root decomposition in the early decomposition stages but inhibits decomposition in the late stages due to the inhibition of lignin degradation. (2) The higher-order fine roots decompose faster than the lower-order fine roots due to the difference in initial chemical quality.
Materials and methods
Site description
The study was conducted in Shuangshan (30° 46′ N, 110° 55′ E) of Zigui County, Hubei Province, China, at an elevation of approximately 825 m. The site has a subtropical continental monsoon climate with an annual average temperature of 14 to 22°C. The average yearly rainfall is 1400 mm, concentrated from April to September. The Pinus massoniana plantation was established by air sowing in the 1980s. The average height, diameter at breast height and density of the tree were 16.96 m, 18.3cm, 675 trees ha-1, respectively. Yellow-brown and purple are the main soil types, with a depth of approximately 40 cm. The initial soil organic matter content (g/kg) and pH was 22.30 ± 5.9, 4.93 ± 0.06 (mean values ± standard error) at 0−20 cm depth. The nutrient concentration (mg/kg) was 544.03 ± 0.93, 138.68 ± 29.36, 3728.39 ± 89.80, 39.93 ± 0.91, and 68.40 ± 12.78 for total N, P, potassium (K), zinc (Zn), and calcium (Ca), respectively. The main associated shrubs are Camellia sinensis, Eurya nitida, Viburnum erosum, and herbs are Dryopteris fuscipes, Houttuynia cordata, Senecio scandens.
Experimental design
In February 2019, based on the local atmospheric N deposition background value (30 kg N ha-1 year-1), 12 plots (2 m × 2 m) were established at the experimental site with four N treatments: control (CK, 0 kg N ha-1 year-1), low N (LN, 30 kg N ha-1 year-1), medium N (MN, 60 kg N ha-1 year-1), and high N (HN, 90 kg N ha-1 year-1). Each treatment was replicated three times. The plots were separated by a 10 m wide buffer zone and isolated with polyvinyl chloride plates inserted into 20 cm depth soil. The forms of nitrogen deposition include dry deposition and wet deposition. The dry deposition is mainly composed of gaseous nitrogen containing pollutants, while the wet deposition is mainly composed of granular ammonium salt and nitrate. In China, ammonium deposition accounts for 68% of the total nitrogen deposition and nitrate deposition accounts for 32% (Zheng et al., 2014; Sun et al., 2016). Therefore, ammonium nitrate (NH4NO3) was selected as the N source in this study. The annual application amount of NH4NO3 was evenly divided into 12 equal parts. An appropriate amount of NH4NO3 was dissolved in 2 L of water and sprayed uniformly in the quadrat at the beginning of each month, starting from February 2019. Precisely the same amount of plain water was sprayed in the control group.
Decomposition and sampling
In early January 2019, taproots were found at the base of the P. massoniana trunk (outside the N addition site). We looked for root order branches approximately 2 m outward along the lateral roots on the taproots. Then, the root system was carefully separated from the soil particles, ensuring that the smallest root tip at the root end was intact. According to the method described by Pregitzer et al. (2002), the roots were divided into different branching orders: order 1-2 (the lower-order fine roots), order 3–4 and order 5–6 (were grouped as the higher-order roots).
All the fine roots of the same order class were mixed, and a 1.0 g sample of each root order class was placed in a nylon 150-mesh litterbag (10 cm ×10 cm). In February 2019, litterbags were randomly placed in the soil of each plot with a depth of 10 cm. Five samplings were conducted during the 30-month decomposition in May, August, November (2019), and August (2020 and 2021). A total of 1260 litterbags were collected in this fine root’s decomposition experiment (4 treatments × 3 replicates × 3 root orders × 5 samplings ×7 replicates). On each harvest date, soil samples (at 0−20 cm depth) from the 12 plots and 7 replicate litterbags for each root order were collected and quickly transferred to the laboratory. The roots were carefully separated from the soil particles, dried in an oven (65 °C for 48 h), and weighed to calculate the dry mass. Some soil samples were screened with a 2 mm mesh and placed in a 4 °C refrigerator to measure soil enzyme activity, whereas others were air-dried indoors and passed 2 mm and 0.149 mm mesh for measuring soil pH and the content of soil organic matter, total nitrogen, and total phosphorus (P).
Chemical analysis
Subsamples of fine roots and soil were analyzed as follows. The concentrations of cellulose, starch and soluble sugar in fine roots were determined by anthrone colorimetry (Ververis et al., 2004; Guo et al., 2022). Lignin concentrations were determined using the acid detergent fiber (ADF) method (Rowland and Roberts, 1994). Total C and organic matter were determined by the potassium dichromate oxidation method (Nelson and Sommers, 1982). Total N, total P and pH-value were determined by the Kjeldahl method, molybdenum antimony colorimetric method and electrode method, respectively (Zhu et al., 2014; Wang et al., 2021). L-3,4-dihydroxyphenylalanine (25000 μmol L-1) was used as the substrate to mark the activities of polyphenol oxidase and peroxidase according to Saiya-Cork et al. (2002), and a multifunctional microplate fluorometer (SpectraMax i3x, Molecular Devices, Beckman Coulter, CA, USA) was used to measure the absorbance.
Statistical analysis
The residual mass rate (MR) of the fine roots at each stage was calculated using equation (1). The percent chemical remaining mass (E) was expressed using equation (2) (Fu et al., 2021). An exponential decay model is used to fit the change process of the remaining mass with time (Olson, 1963) (3).
Here, X0(g) is the initial litter mass, Xt(g) is the mass remaining after t years of decomposition, C0 is the initial nutrient concentration, and Ct is the nutrient concentration at time t, k is decomposition coefficient (Song et al., 2015). All statistical analyses of the data were performed using SPSS 24.0 software (SPSS Inc., Chicago, IL, USA). One-way ANOVA and Duncan’s method were used to analyze variance and multiple comparisons (P < 0.05 used as threshold), and the interactions among N addition, root order classes, and decomposition time were studied by three-way ANOVA. Pearson correlation analysis was used to explore the correlation between fine root decomposition coefficient, nutrient concentration and soil characteristics. Excel 2016 was used for data statistics and plotting.
Results
Initial substrate chemistry
The initial nutrient and C fraction concentrations were significantly (P < 0.05) different among the three root orders before their placement in the litterbags (Table 1). The initial N and P concentrations of the lower-order fine roots were significantly higher than those of higher-order fine roots (P < 0.05). The concentrations of cellulose, soluble sugar, and starch were significantly lower than those of the higher-order fine roots (P < 0.05, Table 1). No significant differences were found in the C and lignin concentrations. The contrasting concentrations of N and P among the three root orders also led to an opposing gradient in C/N, C/P, and lignin/N ratio, with significant (P < 0.05) differences among the root orders, with order 1–2 < order 3–4 < order 5–6 (Table 1).
Fine root mass loss and decomposition rate
The mass remaining of fine roots decreased rapidly in the first 6 months of decomposition and decreased slowly in the 6−30 months of decomposition (Figure 1). The root data of the initial mass remaining in each treatment fitted well with the single exponential model (R2 range 0.846–0.946; Table 2). The ANOVA showed that N addition, root order, decomposition time, and the interactions with each other have significant effects on mass remaining (mostly P < 0.001; Table 3). In the 0−18 months of decomposition, all N addition treatments significantly promoted the decomposition of fine roots (P < 0.05), and the average decomposition rates of order 1–2, order 3–4, order 5–6 fine roots were increased by 13.54%, 6.15% and 7.96% respectively. After 30 months of decomposition, low N treatment promoted the decomposition of fine roots in all orders, whereas high N addition inhibited the decomposition of fine roots, and the average decomposition rates of order 1–2, order 3–4, order 5–6 fine roots were decreased by 58.35%, 35.43% and 47.56% respectively. (Figure 1, Table 2). The lower-order fine roots (order 1–2) decomposed slower than the higher-order fine roots (order 3–4 and order 5–6). The average decomposition constant (k) of the order 1–2 fine roots (0.272) was less than that of the order 3–4 (0.300) and order 5–6 fine roots (0.307) (Table 2, P < 0.05).
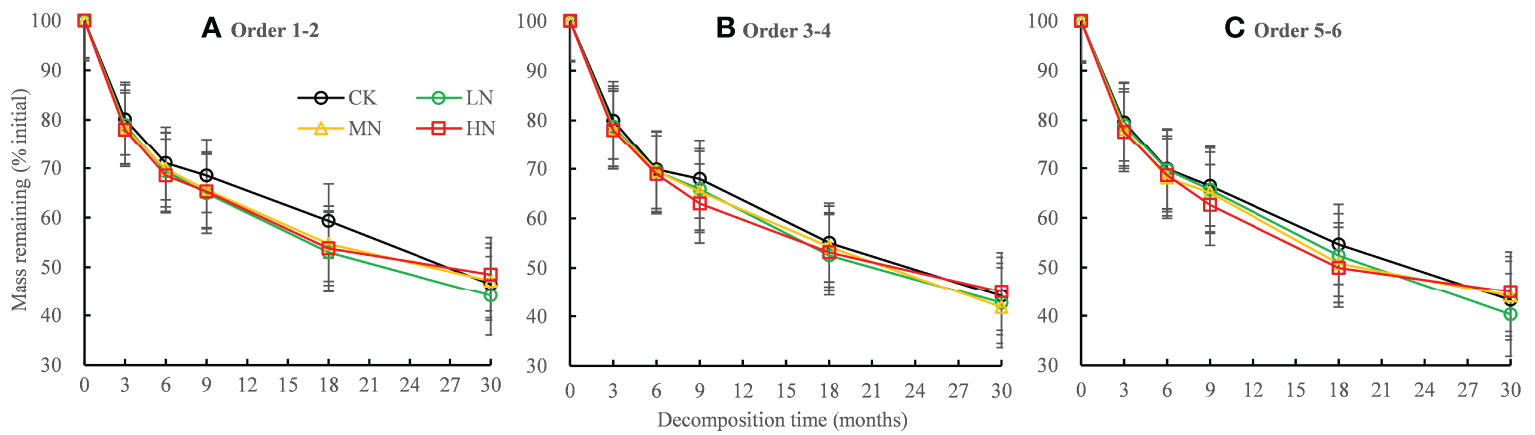
Figure 1 Residual percentages of the initial mass during the 30-month fine root decomposition of Pinus massoniana in different N addition treatments. Values are means ± SE. The different colors denote CK (0 kg ha-1 year-1), LN (30 kg ha-1 year-1), MN (60 kg ha-1 year-1), and HN (90 kg ha-1 year-1). (A–C) represent the mass remaining of order 1-2, order 3-4 and order 5-6 fine roots, respectively.
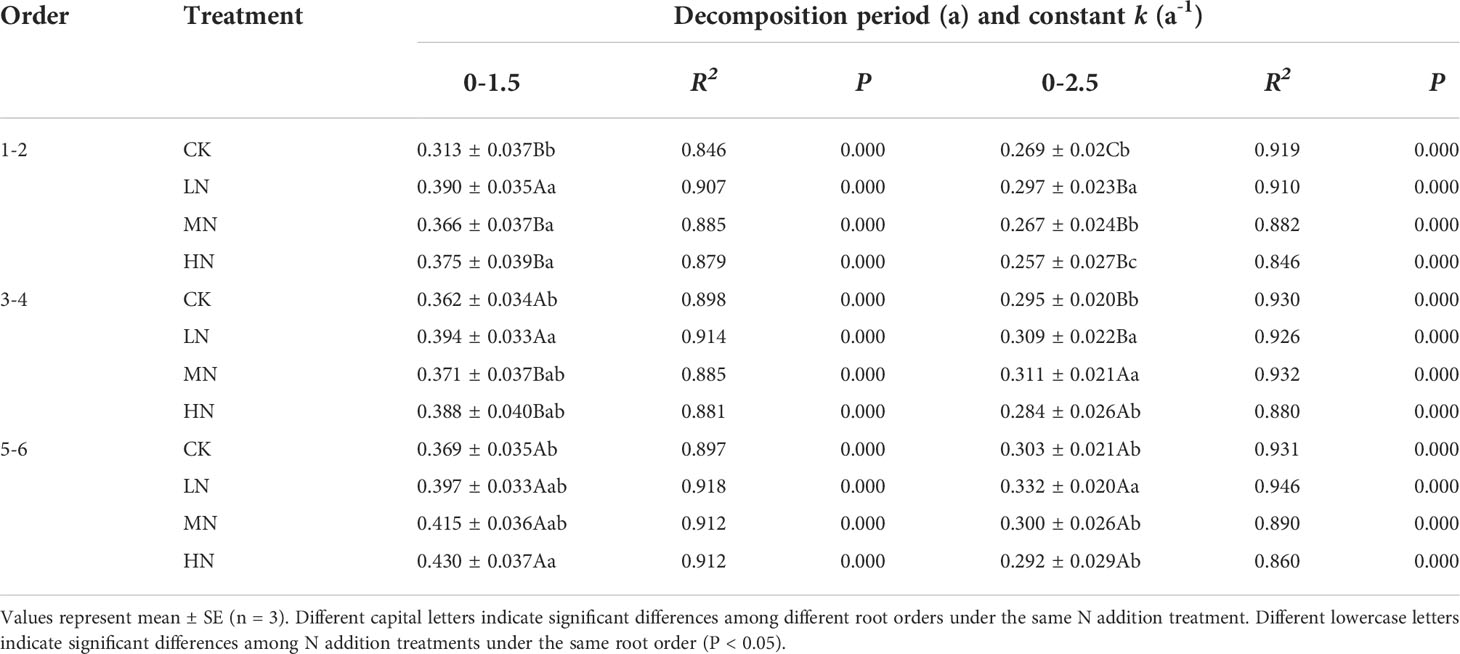
Table 2 Mean decomposition rates (k) of the three orders of roots in different N addition treatments.
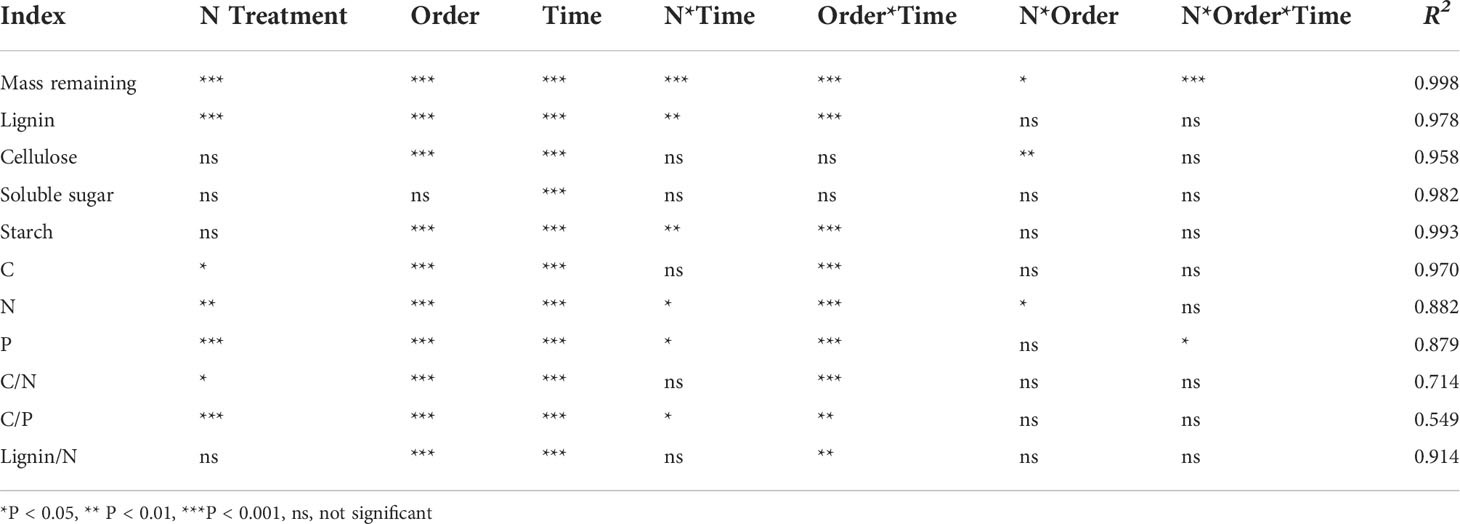
Table 3 Effects of N treatment, root order, decomposition time and their interaction on the residual rate of fine root decomposition.
Root nutrient dynamics during root decomposition
Residual C and P in all orders fine roots decreased rapidly in the first 6 months but increased slowly in the 6−9 months period and then decreased in the 9−30 months (Figures 2A–C, G–I). The variation trends of residual N in the different root orders were dissimilar. Residual N in the lower-order fine roots decreased with time (Figure 2D), while N fixation occurred in the higher-order fine roots in the first 9 months of decomposition, and then decreased in the late decomposition stage (Figures 2E, F). The ANOVA showed that N addition, root order, and decomposition time, and the interaction between root orders and time had significant effects on C, N, and P residue rates (mostly P < 0.001, Table 3). After 30 months of decomposition, LN treatment promoted the release of C, N, while HN treatment has opposite effects. All the N addition treatments promoted P release.
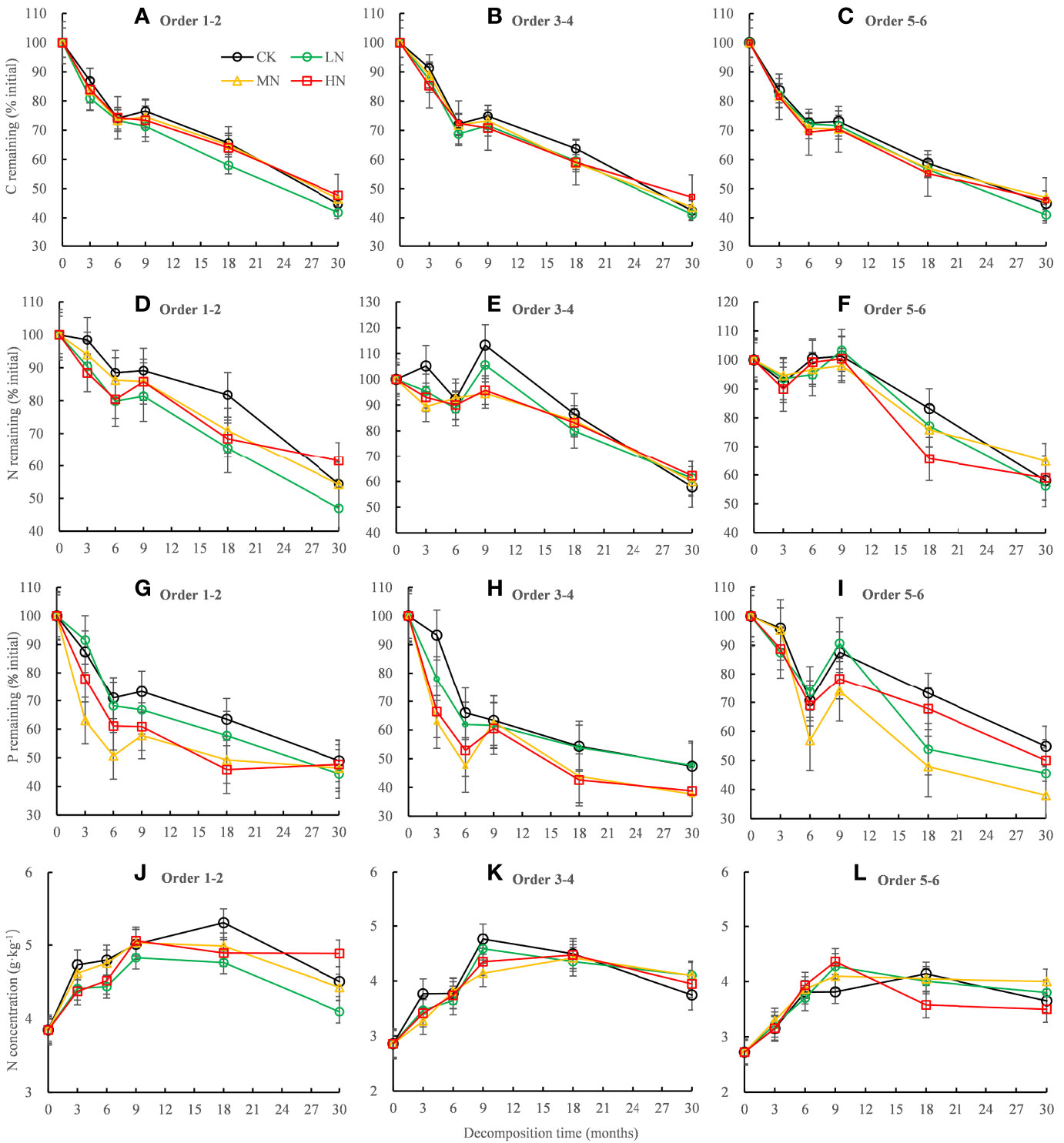
Figure 2 Residual percentages of C, N, and P and absolute concentrations of N during the 30-month fine root decomposition of Pinus massoniana in different N addition treatments. Values are means ± SE. The different colors denote CK (0 kg ha-1 year-1), LN (30 kg ha-1 year-1), MN (60 kg ha-1 year-1), and HN (90 kg ha-1 year-1). (A–I) represent the residual percentages of C, N, and P in order 1-2, order 3-4 and order 5-6 fine roots, respectively. (J–L) represent the absolute concentrations of N in order 1-2, order 3-4 and order 5-6 fine roots, respectively.
The N concentration in the fine roots increased continuously in the rapid stage of decomposition and then decreased gradually in the slow stage but was always higher than the initial N concentration (Figures 2J–L). After 30 months of decomposition, the absolute concentrations of N and P in the lower-order roots were significantly (P < 0.05) higher than those in the higher-order roots (Figures 3B, C), but C concentration was lower than that in the higher-order roots (Figure 3A). The two-way ANOVA showed that root order affected C, N, and P concentrations, while N addition only affected the final P concentration, resulting in a significant decrease (P < 0.01) (Figure 3).
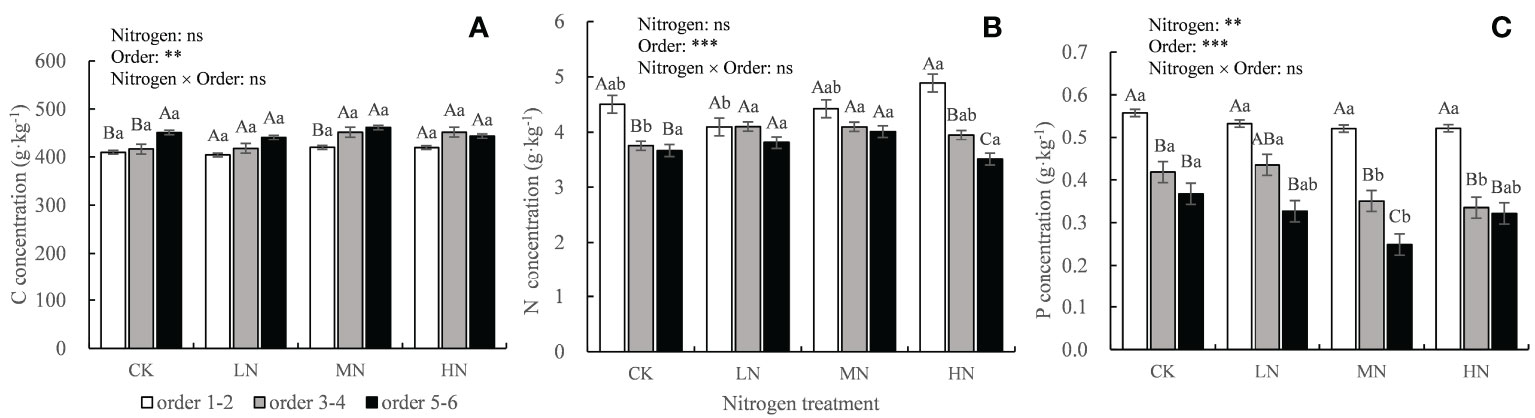
Figure 3 Absolute concentrations of C, N, and P in the decomposing roots in each treatment after 30 months of decomposition. Different capital letters indicate significant differences among different root orders under the same N addition treatment, and different lowercase letters indicate significant differences among the N addition treatments under the same root order (P < 0.05). **P < 0.01, ***P < 0.001, ns, no significant. CK: 0 kg ha-1 year-1; LN: 30 kg ha-1 year-1; MN: 60 kg ha-1 year-1; HN: 90 kg ha-1 year-1. (A–C) represent the absolute concentrations of C, N, and P in fine roots, respectively.
Root C fraction dynamics during root decomposition
Residual lignin decreased rapidly in the first 6 months of decomposition, increased in the following 6−9 months, and then decreased in 9−30 months (Figures 4A–C). Residual cellulose decreased rapidly by 50.61% ~ 67.33% in the first 18 months of decomposition, while it decreased very slowly in 18−30 months of decomposition (Figures 4D–F). The residue rates of soluble sugar and starch decreased rapidly in the first 3 months of decomposition, fluctuated in the following 3−18 months, and remained stable in the 18−30 months period (Figures 4G–L). The ANOVA showed that N treatment had a significant (P < 0.001) effect on lignin residue rate, but no significant effect on the cellulose, starch, and sucrose residue (Table 3). After 30 months of decomposition, LN treatment promoted lignin release, whereas HN treatment inhibited lignin release (Figures 4A–C).
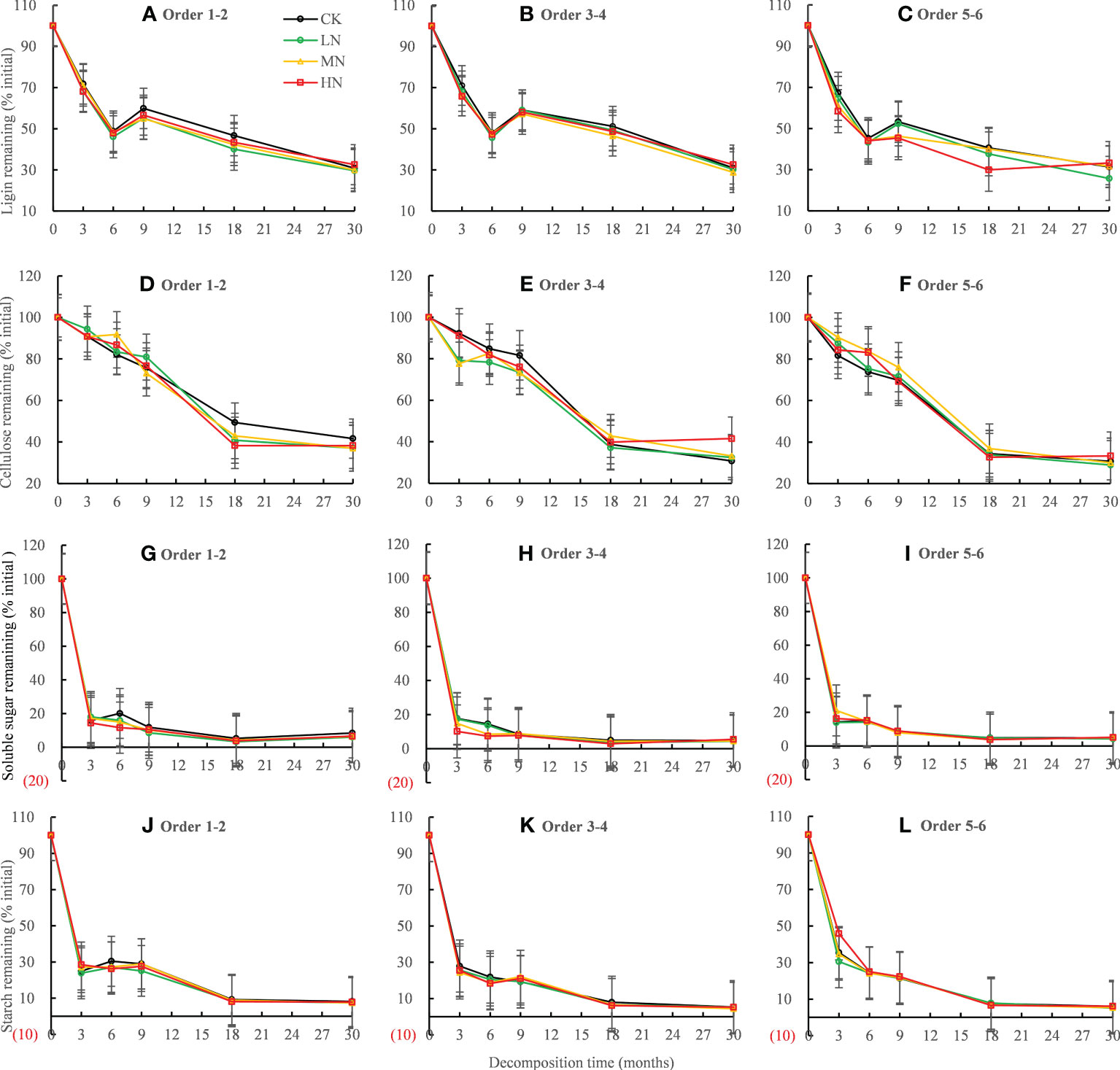
Figure 4 Residual percentages of lignin, cellulose, soluble sugar, and starch during the 30-month fine root decomposition of Pinus massoniana in different N addition treatments. Values are means ± SE. The different colors denote CK (0 kg ha-1 year-1), LN (30 kg ha-1 year-1), MN (60 kg ha-1 year-1), and HN (90 kg ha-1 year-1). (A–L) represent the lignin, cellulose, soluble sugar, and starch remaining in order 1-2, order 3-4 and order 5-6 fine roots, respectively.
Soil properties and enzyme activities under N addition treatments
After 30 months of decomposition, LN treatment decreased the contents of soil organic matter and total N, whereas HN treatment had the opposite effect. All N treatments decreased soil pH, total P content, and polyphenol oxidase and peroxidase activities (Figure 5).
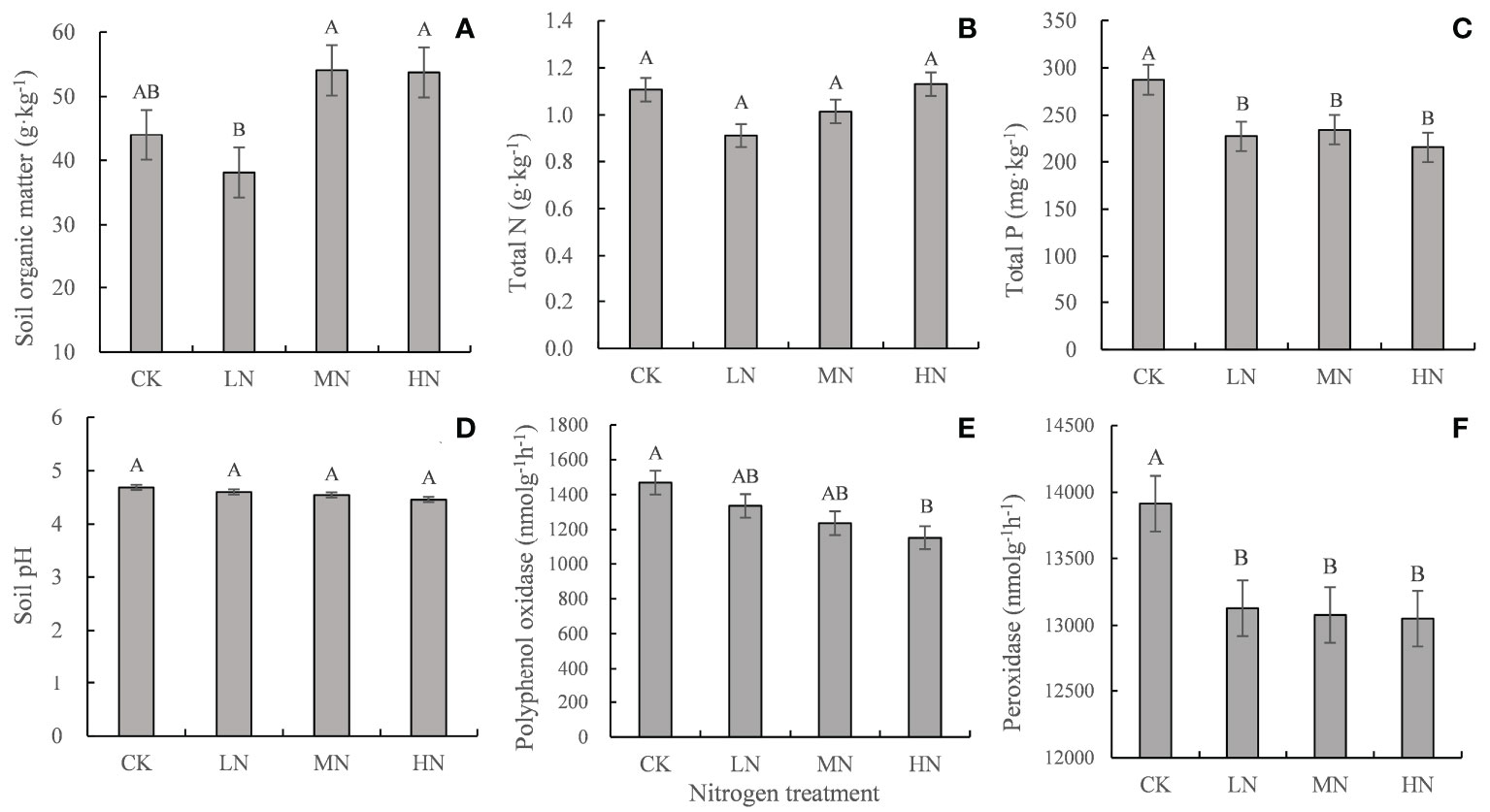
Figure 5 Soil properties and enzyme activities in the different treatments after 30 months of decomposition. Values represent the means ± SE (n = 3). Different capital letters indicate significant differences among different N addition treatments (P < 0.05). CK (0 kg ha-1 year-1), LN (30 kg ha-1 year-1), MN (60 kg ha-1 year-1), and HN (90 kg ha-1 year-1). (A–C) represent the content of soil organic matter, total N, total P, respectively. (D–F) represent the soil pH and the activity of polyphenol oxidase and peroxidase, respectively.
Relationships between fine root decomposition and nutrient elements and soil characteristics
After 30 months of decomposition, the decomposition constant (k) is closely related to the concentration of nutrient elements (Table 4). For example, the k-value was significantly positively correlated with the initial concentrations of cellulose, starch, soluble sugar, C and the ratio of C/N, C/P, and lignin/N (P < 0.01), and significantly negatively correlated with the initial and final concentration of N, P (P < 0.01). In addition, the k-value was also significantly positively correlated with soil peroxidase and polyphenol oxidase activities, and significantly negatively correlated with soil organic matter content (Table 5, P < 0.05). There was a significant positive linear correlation between residual mass and residual lignin and nitrogen during the 30-month decomposition process (Figure 6, P < 0.001).

Table 4 Correlation between the decomposition constant (k) and nutrient concentration of fine roots.
Discussion
Effect of N addition on fine root decomposition
The main factors affecting the decomposition rate of the fine roots varied depending on the decomposition phase. The early decomposition stage was dominated by the degradation of soluble and relatively unstable compounds in the litter, including the initial total non-structural carbohydrates. The degradation of cellulose followed this, and finally, the acid-insoluble substances and lignin degradation became dominant (Berg and McClaugherty, 2008; Tu et al., 2014). In our study, two stages of decomposition with different mass-loss rates were observed. In the first 6 months of decomposition, the mass remaining of fine roots decreased rapidly with an average loss of 30.60% of the initial mass, whereas slower decomposition rates were observed in the following 6−30 months, with an average loss of 25.01% (Figure 1). Consistent with many previous studies (Yang et al., 2004; Berg and McClaugherty, 2008; Tu et al., 2015), we observed the degradation of starch and soluble sugar first, followed by a rapid decline in cellulose (Figures 4D−L). During 18−30 months of decomposition, the above nutrient residue rate remained unchanged, and the lignin residue rate continued to decline (Figures 4A−C), indicating that lignin decomposition was dominant in the late stage of decomposition.
Our first hypothesis was confirmed as we found that all concentrations of N addition promoted the decomposition rate of the fine roots in the early stage of decomposition (0−18 months), whereas HN treatment inhibited the degradation of the fine roots in the late stage of decomposition (18−30 months) (Table 2). The stimulating effect of N addition on the decomposition rates in the early stages may be partly explained by the altered soil enzyme activities that were involved in polysaccharide breakdown due to increased N inputs (Sun et al., 2016). Our previous research showed that N addition increased the activities of hydrolases (β-1-4 glucosidase, N-acetylglucosaminosidase, and acid phosphatase) and oxidase (polyphenol oxidase and peroxidase) after 9 months of decomposition (Wang et al., 2021). The increase in hydrolase and oxidase activities promoted soluble sugar, cellulose, and hemicellulose degradation in the early decomposition stage (Li et al., 2011; Sun et al., 2016; Dong et al., 2019). As our study found that nitrogen addition promoted the release of C, N, P, and lignin in the early decomposition stage (Figures 2, 4). These results were largely consistent with those of studies showing that exogenous nitrogen stimulated the initial decomposition rate in the N-fertilizer experiment (Hobbie et al., 2012; Sun et al., 2016; Gill et al., 2021). With the progress of fine root decomposition, lignin decomposition became dominant in the late stage of decomposition (Berg and McClaugherty, 2008; Tu et al., 2015). There was a significant positive linear correlation between residual lignin and residual mass, which explained 76.13% of the variation in the residual mass (Figure 6). We observed that HN treatment inhibited the degradation of lignin (Figures 4A−C), which may be the main reason for the slow decomposition of fine roots caused by nitrogen addition. The inhibition of nitrogen addition on lignin decomposition may be related to the following mechanisms.
Firstly, a high-N environment may inhibit the synthesis of lignin-decomposing enzymes. The range of soil pH was 4.45−4.69 after 30 months of N addition (Figure 5D). However, the optimum pH ranges for the growth of Basidiomycetes and Ascomycetes related to lignin decomposition are 4.0−5.0 and 6.0−7.5, respectively (Sinsabaugh, 2010). Therefore, Basidiomycetes might be the leading lignin-degrading fungal community in this ecosystem. A previous study reported that Basidiomycetes do not synthesize lignin-degrading enzymes in the presence of ammonium or other low molecular weight N-rich compounds such as amino acids (Keyser et al., 1978). In addition, we found that N addition inhibited the activities of peroxidase and polyphenol oxidase related to the lignin degradation in the soil after 30 months of nitrogen addition experiment (Figure 5). As a matter of fact, many previous studies have found that N additions inhibited the activities of lignin-degrading enzymes in the later stages of fine root decomposition (Manzoni et al., 2010; Tu et al., 2014; Xia et al., 2018; Dong et al., 2022). Secondly, the added N reacts with the secondary compounds (mostly polyphenols) produced during lignin decomposition and is fixed into the fine roots, resulting in the continuous accumulation of anti-decomposition substances and N in fine roots, which further slows down the decomposition rate of the fine roots (Hansen et al., 2009; Hobbie et al., 2012). In the present study, the higher residual N (46.90% ~ 65.90%) in the fine roots observed after 30 months of decomposition may be due to the interaction between exogenous inorganic N addition and residual lignin (Figures 2D−F). Some studies have found that exogenous inorganic N ions will be adsorbed by acidic non hydrolyzable residues in the substrates to form new insoluble substances, and directly polymerize with lignin to produce more refractory humus, thus inhibiting fine root decomposition (Preston et al., 2009; Berg et al., 2013; Kou et al., 2018). By contrast, other studies showed that nitrogen addition had no significant effect on the activity of lignin decomposing enzymes in litter layer or soil (Hobbie, 2008; Keeler et al., 2009). In addition, some studies have also found that the effect of nitrogen addition on oxidase activity depends on the availability of nitrogen in the environment, and even get opposite results in different forest types. For example, a study found that the activity of polyphenol oxidase decreased in the black oak-white oak mixed forest but increased in the mixed forest of sugar maple-red oak and sugar maple-basswood mixed forest after one year of nitrogen application (Waldrop et al., 2004). Therefore, the mechanism of inhibition of N addition on late-stage decomposition may vary depending on specific litter types and/or forest sites (Keeler et al., 2009).
In general, the distribution of C from plant to soil is mainly completed through plant fine roots in forest ecosystems (Van Groenigen et al., 2006; Song et al., 2016). C input primarily exists in the form of active rhizosphere sediments (Boddy et al., 2007). Recent findings show that the transformation of root-derived matters into stable soil organic matter components is greater than that of leaf litter (Bird et al., 2008; Mambelli et al., 2011). Therefore, the variation of root decomposition may affect the accumulation of soil organic matter in forest ecosystem. Tu et al. (2015) found that the later stage of root decomposition (the stage when mass loss tends to be stable) is mainly the stage of humus formation. N addition may promote the accumulation of stable C components in the soil by inhibiting the later stages of fine root decomposition. After 30 months of N addition, we observed that low N treatment promoted fine root decomposition and decreased the soil organic matter content, whereas high N inhibited fine root decomposition and increased soil organic matter content (Figure 5A). This is consistent with the previous conclusion that low nitrogen (30 kg N ha−1 year−1) reduces soil C storage and high nitrogen (≥90 kg N ha−1 year−1) has the opposite effect (Song et al., 2016). In fact, many previous studies have shown that the increase of soil organic matter is generally related to the slower decomposition of plant residues rather than the more significant litter input (Zak et al., 2008; Janssens et al., 2010; Xia et al., 2018). N addition can increase soil carbon storage by inhibiting heterotrophic respiration, reducing microbial biomass and altering microbial community composition and carbon degrading enzyme activity (Xia et al., 2018; Dong et al., 2022). Correlation analysis showed that soil organic matter content was significantly negatively correlated with fine roots decomposition coefficient (k) (Table 4). Therefore, we suggested that the inhibition of N addition on the late decomposition stage of fine roots may partly explain the increase of soil organic matter under high N deposition. In addition, a previous study showed that long-term simulated N deposition accelerated soil acidification (Yu et al., 2021). Our previous studies found that the soil pH and total P content decreased after 9 months of N addition (Wang et al., 2021). This phenomenon recurred after 30 months of N addition, indicating that increasing N deposition will lead to soil acidification. Due to the low solubility of P compounds at low pH, N deposition-induced acidification may limit the release of P radicals (Chapin et al., 2011). Furthermore, N inputs can enhance phosphatase production and consequently cause some ecosystems to shift from relative N limitation to P limitation (Marklein and Houlton, 2012). Our study also found that N addition enhanced acid phosphatase activity (Figure S1), indicating that the soil needs more enzyme hydrolysis to obtain the required phosphorus.
Differences in fine root decomposition in the different order classes
Studies have shown significant differences in the physiological functions of different root orders (Guo et al., 2004; Kou et al., 2015). For example, the higher-order roots mainly perform the functions of transportation, storage, and structural support, while the lower-order roots are used primarily to obtain nutrients and absorb water. These differences in root function are reflected in the histochemical composition and decomposition rate (Fan and Guo, 2010; Wang et al., 2014; McCormack et al., 2015).
Our second hypothesis was confirmed as we found that the decomposition rate of the higher-order roots was faster than that of the lower-order roots during the 30−month decomposition (Table 1, Figure 1). This is consistent with many previous conclusions (Sun et al., 2013; Yang et al., 2019). The mycorrhizal hypothesis suggests that EM mycorrhizal fungi are more likely to infect lower-order fine roots during decomposition, and mycorrhizal sheaths are rich in chitin. The high chitin content may reduce the decomposition of fine roots, resulting in slower decomposition of the lower-order fine roots than the higher-order fine roots (Langley et al., 2003a; Langley and Hungate 2003b; Guo et al., 2008). In addition, a previous study has shown that microbial decomposition will slow down due to the limitation of carbon quality (Moorhead and Sinsabaugh, 2006). The low decomposition rate of the lower-order fine roots may be due to the combined effect of high nitrogen concentration and low carbon concentration (Yang et al., 2019). In our study, we observed that the higher-order roots contained higher concentrations of initial cellulose and soluble sugars, starch, C and higher C/N, C/P, and lignin/N ratios (Table 1). Correlation analysis showed that the decomposition constant (k) was significantly positively correlated with the above indicators, and significantly negatively correlated with the initial and final N and P concentrations (Table 4). After 30 months of decomposition, the absolute concentrations of N and P in the higher-order roots were significantly slower than those in the lower-order roots (Figures 3B, C). Therefore, the higher-order fine roots decomposed faster than the lower-order fine roots due to higher initial concentrations of cellulose, starch, soluble sugars, C and higher C/N, C/P, and lignin/N ratios and lower N and P concentrations. Same as our conclusion, a 23-month decomposition experiment also found that the litter decomposition rate was controlled strongly by the initial nutrient content and C/N, C/P ratios (Zhang et al., 2021).
Previous research showed that the global reserves of litter estimated by the short-term decomposition coefficient is underestimated by at least one-third (Harmon et al., 2009; Moore et al., 2017). Our study observed that the fine root system retained a considerable part of the initial mass (40.29%~ 48.46%) after 30 months of decomposition. In the first 18 months of this study, the root decomposition coefficient was significantly greater than that in the entire decomposition stage (Table 2), indicating that the short-term root decomposition coefficient would overestimate the root decomposition rate. These studies show that root decomposition is a long-term process and long-term work is needed to fully understand the effects of N deposition on decomposition.
Conclusion
The effect of nitrogen addition on decomposition varied with decomposition stages. N addition promotes early-stage and inhibits late-stage decomposition of fine roots. The inhibition of lignin-degrading enzyme activity by N addition may be the mechanism of the observed negative effect on the late decomposition stage. The decomposition difference between the lower-order and higher-order fine roots were controlled strongly by the initial chemical quality of the fine roots. High N addition increased soil organic matter content and decreased soil pH and P content, indicating that increasing N deposition would increase soil C sequestration, but also lead to soil acidification in Pinus massoniana plantations. This study provides new insights into understanding and predicting possible changes in plant root decomposition and soil properties in the future atmospheric N deposition increase scenarios. However, due to the fine root system retained a considerable part of the initial mass (40.29%~ 48.46%) after 30 months of decomposition, short-term root decomposition coefficient would overestimate the root decomposition rate. Thus, long-term work is needed to fully understand the effects of N deposition on decomposition.
Data availability statement
The original contributions presented in the study are included in the article/Supplementary Material. Further inquiries can be directed to the corresponding author.
Author contributions
LW and RC designed the experiments and wrote the original draft manuscript. YS, PS, TC, and MZ conducted the field trial and the laboratory analysis. RC, LZ, and WX critically reviewed and edited the preliminary draft. All authors contributed to the article and approved the submitted version.
Funding
This study was supported by the Fundamental Research Fund of the Chinese Academy of Forestry (No. CAFYBB2021ZE003) and the National Key Research and Development Program of Ministry of Science and Technology of China (No. 2016YFD0600204).
Acknowledgments
We thank the National Forest Ecosystem Station of Three Gorges Reservoir in Zigui County for their support in our field work. We also thank Editage (www.editage.cn) for English language editing.
Conflict of interest
The authors declare that the research was conducted in the absence of any commercial or financial relationships that could be construed as a potential conflict of interest.
Publisher’s note
All claims expressed in this article are solely those of the authors and do not necessarily represent those of their affiliated organizations, or those of the publisher, the editors and the reviewers. Any product that may be evaluated in this article, or claim that may be made by its manufacturer, is not guaranteed or endorsed by the publisher.
Supplementary material
The Supplementary Material for this article can be found online at: https://www.frontiersin.org/articles/10.3389/fpls.2022.1048153/full#supplementary-material
References
Berg, B., Liu, C. J., Laskowski, R., Davey, M. (2013). Relationships between nitrogen, acid-unhydrolyzable residue, and climate among tree foliar litters. Can. J. For. Res. 43 (1), 103–107. doi: 10.1139/cjfr-2012-0385
Berg, B., Matzner, E. (1997). Effect of n deposition on decomposition of plant litter and soil organic matter in forest systems. Environ. Rev. 5 (1), 1–25. doi: 10.1139/a96-017
Berg, B., McClaugherty, C. (2008). Plant litter: Decomposition, humus formation, carbon sequestration. 2nd edn (Heidelberg: Springer).
Bird, J. A., Kleber, M., Torn, M. S. (2008). 13C and15N stabilization dynamics among soil organic matter fractions during needle and fine root decomposition. Organic Geochem 39 (4), 465–477. doi: 10.1016/j.orggeochem.2007.12.003
Boddy, E., Hill, P. W., Farrar, J., Jones, D. L. (2007). Fast turnover of low molecular weight components of the dissolved organic carbon pool of temperate grassland field soils. Soil Biol. Biochem. 39, 827–835. doi: 10.1016/j.soilbio.2006.09.030
Chapin, F. S., III, Matson, P. A., Vitousek, P. M. (2011). Principles of terrestrial ecosystem ecology. Springer Sci. Business Media London, 215–219. doi: 10.1007/978-1-4419-9504-9
Dong, L. L., Berg, B., Gu, W. P., Wang, Z. P., Sun, T. (2022). Effects of different forms of nitrogen addition on microbial extracellular enzyme activity in temperate grassland soil. Ecol. Processes 11, 36. doi: 10.1186/s13717-022-00380-2
Dong, L. L., Berg, B., Sun, T., Zheng, W. W., Han, X. G. (2020). Response of fine root decomposition to different forms of n deposition in a temperate grassland. Soil Biol. Biochem. 147, 107845. doi: 10.1016/j.soilbio.2020.107845
Dong, L. L., Sun, T., Berg, B., Zhang, L., Zhang, Q., Wang, Z. W. (2019). Effects of different forms of n deposition on leaf litter decomposition and extracellular enzyme activities in a temperate grassland. Soil Biol. Biochem. 134, 78–80. doi: 10.1016/j.soilbio.2019.03.016
Fahey, T. J., Arthur, M. A. (1994). Further studies of root decomposition following harvest of a northern hardwoods forest. For. Sci. 4, 618–629. doi: 10.1093/forestscience/40.4.618
Fan, P. P., Guo, D. L. (2010). Slow decomposition of lower order roots: a key mechanism of root carbon and nutrient retention in the soil. Oecologia 163 (2), 509–515. doi: 10.1007/s00442-009-1541-4
Fu, Y. M., Feng, F. J., Zhang, X. Y., Qi, D. D. (2021). Changes in fine root decomposition of primary pinus koraiensis forest after clear cutting and restoration succession into secondary broad-leaved forest. Appl. Soil Ecol. 158, 103785. doi: 10.1016/j.apsoil.2020.103785
Gill, A., Schilling, J., Hobbie, S. E. (2021). Experimental nitrogen fertilisation globally accelerates, then slows decomposition of leaf litter. Ecol. Lett. 24, 802–811. doi: 10.1111/ele.13700
Goebel, M., Hobbie, S. E., Bulaj, B., Zadworny, M., Archibald, D. D., Oleksyn, J., et al. (2011). Decomposition of the finest root branching orders: linking belowground dynamics to fine-root function and structure. Ecol. Monogr. 81 (1), 89–102. doi: 10.1890/09-2390.1
Guo, X. W., Liu, S. R., Wang, H., Chen, Z. C., Zhang, J. L., Chen, L., et al. (2022). Divergent allocations of nonstructural carbohydrates shape growth response to rainfall reduction in two subtropical plantations. For. Ecosyst. 9, 100021. doi: 10.1016/j.fecs.2022.100021
Guo, D. L., Mitchell, R. J., Hendricks, J. J. (2004). Fine root branch orders respond differentially to carbon source-sink manipulations in a longleaf pine forest. Oecologia 140 (3), 450–457. doi: 10.1007/s00442-004-1596-1
Guo, D., Xia, M., Wei, X., Chang, W., Liu, Y., Wang, Z. (2008). Anatomical traits associated with absorption and mycorrhizal colonization are linked to root branch order in twenty-three chinese temperate tree species. New Phytol. 180 (3), 673–683. doi: 10.1111/j.1469-8137.2008.02573.x
Hansen, K., Vesterdal, L., Schmidt, I. K., Gundersen, P., Sevel, L., Bastrup-Birk, A., et al. (2009). Litterfall and nutrient return in five tree species in a common garden experiment. For. Ecol. Manage. 257 (10), 2133–2144. doi: 10.1016/j.foreco.2009.02.021
Harmon, M. E., Silver, W. L., Fasth, B., Chen, H., Burke, I. C., Partons, W. J., et al. (2009). Long-term patterns of mass loss during the decomposition of leaf and fine root litter: An intersite comparison. Global Change Biol. 15 (5), 1320–1338. doi: 10.1111/j.1365-2486.2008.01837.x
Hobbie, S. E. (2008). Nitrogen effects on decomposition: A five-year experiment in eight temperate sites. Ecology 89 (9), 2633–2644. doi: 10.1890/07-1119.1
Hobbie, S. E., Eddy, W. C., Buyarski, C. R., Adair, E. C., Ogdahl, M. L., Weisenhorn, P. (2012). Response of decomposing litter and its microbial community to multiple forms of nitrogen enrichment. Ecol. Monogr. 82 (3), 389–405. doi: 10.1890/11-1600.1
IPCC (2007). Climate change 2007: The physical science basis (Cambridge, UK: Cambridge University Press). New York, NY, USA.
Janssens, I. A., Dieleman, W., Luyssaert, S., Subke, J. A., Reichstein, M., Ceulemans, R., et al. (2010). Reduction of forest soil respiration in response to nitrogen deposition. Nat. Geosci. 3, 315–322. doi: 10.1038/ngeo844
Keeler, B. L., Hobbie, S. E., Kellogg, L. E. (2009). Effects of long-term nitrogen addition on microbial enzyme activity in eight forested and grassland sites: Implications for litter and soil organic matter decomposition. Ecosystems 12, 1–15. doi: 10.1007/s10021-008-9199-z
Keyser, P., Kirk, T. K., Zeikus, I. G. (1978). Ligninolytic enzyme system of phanerochaete chrysosporium: Synthesized in the absence of lignin in response to nitrogen starvation. J. Bacteriol 135, 790–797. doi: 10.1128/jb.135.3.790-797.1978
Knorr, M., Frey, S. D., Curtis, P. S. (2005). Nitrogen additions and litter decomposition: a meta-analysis. Ecology 86, 3252–3257. doi: 10.1890/05-0150
Kou, L., Chen, W., Zhang, X. (2015). Differential responses of needle and branch order-based root decay to nitrogen addition: Dominant effects of acid-unhydrolyzable residue and microbial enzymes. Plant Soil 394, 315–327. doi: 10.1007/s11104-015-2517-2
Kou, L., Lei, J., Fu, X. L., Dai, X. Q., Wang, H. M., Li, S. G. (2018). Nitrogen deposition increases root production and turnover but slows root decomposition in pinus elliottii plantations. New Phytol. 218, 1450–1461. doi: 10.1111/nph.15066
Langley, J. A., Dijkstra, P., Drake, B. G., Hungate, B. A. (2003a). Ectomycorrhizal colonization, biomass, and production in a regenerating scrub oak forest in response to elevated CO2. Ecosystems 6 (5), 424–430. doi: 10.1007/PL00021509
Langley, J. A., Hungate, B. A. (2003b). Mycorrhizal controls on belowground litter quality. Ecology 84 (9), 2302–2312. doi: 10.1890/02-0282
Liu, X., Zhang, Y., Han, W., Tang, A., Shen, J., Cui, Z., et al. (2013). Enhanced nitrogen deposition over China. Nature 494, 459–462. doi: 10.1038/nature11917
Liu, H. M., Zhou, G. Y., Bai, S. H., Song, J. J., Shang, Y. J., He, M., et al. (2019). Differential response of soil respiration to nitrogen and phosphorus addition in a highly phosphorus-limited subtropical forest, China. For. Ecol. Manage. 448 (C), 499–508. doi: 10.1016/j.foreco.2019.06.020
Liu, Q., Zhuang, L., Yin, R., Ni, X., You, C., Yue, K., et al. (2019). Root diameter controls the accumulation of humic substances in decomposing root litter. Geoderma 348, 68–75. doi: 10.1016/j.geoderma.2019.04.016
Li, L. J., Zeng, D. H., Yu, Z. Y., Fan, Z. P., Yang, D., Liu, Y. X. (2011). Impact of litter quality and soil nutrient availability on leaf decomposition rate in a semi-arid grassland of northeast China. J. Arid Environ. 75 (9), 787–792. doi: 10.1016/j.jaridenv.2011.04.009
Mambelli, S., Bird, J. A., Gleixner, G., Dawson, T. E., Torn, M. S. (2011). Relative contribution of foliar and fine root pine litter to the molecular composition of soil organic matter after in situ degradation. Organic Geochem 42, 1099–1108. doi: 10.1016/j.orggeochem.2011.06.008
Manzoni, S., Trofymow, J. A., Jackson, R. B., Porporato, A. (2010). Stoichiometric controls on carbon, nitrogen, and phosphorus dynamics in decomposing litter. Ecol. Monogr. 80, 89–106. doi: 10.1890/09-0179.1
Marklein, A. R., Houlton, B. Z. (2012). Nitrogen inputs accelerate phosphorus cycling rates across a wide variety of terrestrial ecosystems. New Phytol. 193 (3), 696–704. doi: 10.1111/j.1469-8137.2011.03967.x
McCormack, M. L., Dickie, I. A., Eissenstat, D. M., Fahey, T. J., Fernandez, C. W., Guo, D., et al. (2015). Redefining fine roots improves understanding of belowground contributions to terrestrial biosphere processes. New Phytol. 207, 505–518. doi: 10.1111/nph.13363
Moore, T. R., Trofymow, J. A., Prescott, C. E., Titus, B. D. (20172017). Can short-term litter-bag measurements predict long-term decomposition in northern forests? Plant Soil 416, 419–426. doi: 10.1007/s11104-017-3228-7
Moorhead, D. L., Sinsabaugh, R. L. (2006). A theoretical model of litter decay and microbial interaction. Ecol. Monogr. 76, 151–174. doi: 10.1890/0012-9615(2006)076[0151:ATMOLD]2.0.CO;2
Nelson, D. W., Sommers, L. E. (1982). “Total carbon, organic carbon, and organic matter,” in Methods of soil analysis (Madison, WI, USA: ASA-SSSA), 539–579.
Olson, J. S. (1963). Energy storage and the balance of producers and decomposers in ecological systems. Ecology 44, 322–331. doi: 10.2307/1932179
Parton, W., Silver, W. L., Burke, I. C., Grassens, L., Harmon, M. E., Currie, W. S., et al. (2007). Global-scale similarities in nitrogen release patterns during long-term decomposition. Science 315 (5810), 361–364. doi: 10.1126/science.1134853
Pregitzer, K. S., Deforest, J. L., Burton, A. J., Allen, M. F., Ruess, R. W., Hendrick, R. L. (2002). Fine root architecture of nine north American trees. Ecol. Monogr. 72 (2), 293–309. doi: 10.1890/0012-9615(2002)072[0293:FRAONN]2.0.CO;2
Preston, C. M., Nault, J. R., Trofymow, J. A. (2009). Chemical changes during 6 years of decomposition of 11 litters in some Canadian forest sites. part 2. C13abundance, solid-state C13NMR spectroscopy and the meaning of "lignin. Ecosystems 12, 1078–1102. doi: 10.1007/s10021-009-9266-0
Reay, D. S., Dentener, F., Smith, P., Grace, J., Feely, R. (2008). Global nitrogen deposition and carbon sinks. Nat. Geosci. 1 (7), 430–437. doi: 10.1038/ngeo230
Rowland, A. P., Roberts, J. D. (1994). Lignin and cellulose fractions in decomposition studies using acid detergent fibre methods. Commun. Soil Sci. Plant Anal. 25 (3), 269–277. doi: 10.1080/00103629409369035
Saiya-Cork, K. R., Sinsabaugh, R. L., Zak, D. R. (2002). The effects of long-term nitrogen deposition on extracellular enzyme activity in an acer saccharum forest soil. Soil Biol. Biochem. 34 (9), 1309–1315. doi: 10.1016/S0038-0717(02)00074-3
Shen, Y. F., Wang, N., Cheng, R. M., Xiao, W. F., Yang, S., Guo, Y., et al. (2017). Characteristics of fine roots of pinus massoniana in the three gorges reservoir area, China. Forests 8, 183. doi: 10.3390/f8060183
Sinsabaugh, R. L. (2010). Phenol oxidase, peroxidase and organic matter dynamics of soil. Soil Biol. Biochem. 42, 391–404. doi: 10.1016/j.soilbio.2009.10.014
Song, X. Z., Gu, H., Wang, M., Zhou, G., Li, Q. (2016). Management practices regulate the response of moso bamboo foliar stoichiometry to nitrogen deposition. Sci. Rep. 6, 24107. doi: 10.1038/srep24107
Song, X. Z., Zhou, G. M., Gu, H. H., Qi, L. H. (2015). Management practices amplify the effects of n deposition on leaf litter decomposition of the moso bamboo forest. Plant Soil 395, 391–400. doi: 10.1007/s11104-015-2578-2
Steinaker, D. F., Wilson, S. D. (2005). Belowground litter contributions to nitrogen cycling at a northern grassland-forest boundary. Ecology 86, 2825–2833. doi: 10.1890/04-0893
Sun, T., Dong, L. L., Wang, Z. W., Lü, X. T., Mao, Z. J. (2016). Effects of long-term nitrogen deposition on fine root decomposition and its extracellular enzyme activities in temperate forests. Soil Biol. Biochem. 93, 50–59. doi: 10.1016/j.soilbio.2015.10.023
Sun, T., Mao, Z., Han, Y. (2013). Slow decomposition of very fine roots and some factors controlling the process: A 4-year experiment in four temperate tree species. Plant Soil 372 (1-2), 445–458. doi: 10.1007/s11104-013-1755-4
Tu, L. H., Hu, H. L., Chen, G., Peng, Y., Xiao, Y. L., Hu, T. X., et al. (2014). Nitrogen addition significantly affects forest litter decomposition under high levels of ambient nitrogen deposition. PLoS One 9 (2), e88752. doi: 10.1371/journal.pone.0088752
Tu, L. H., Peng, Y., Chen, G., Hu, H. L., Xiao, Y. L., Hu, T. X., et al. (2015). Direct and indirect effects of nitrogen additions on fine root decomposition in a subtropical bamboo forest. Plant Soil 389 (1-2), 273–288. doi: 10.1007/s11104-014-2353-9
Usman, S., Singh, S. P., Rawat, Y. S., Bargali, S. S. (2000). Fine root decomposition and nitrogen mineralisation patterns in quercus leucotrichophora and pinus roxburghii forests in central himalaya. For. Ecol. Manage. 131 (1-3), 191–199. doi: 10.1016/S0378-1127(99)00213-3
Van Groenigen, J. W., Kessel, C. V., Hungate, B. A., Oenema, O., Powlson, D. S., Van Groenigen, K. J. (2017). Sequestering soil organic carbon: a nitrogen dilemma. Environ. Sci. Technol. 51 (9), 4738–4739. doi: 10.1021/acs.est.7b01427
Van Groenigen, K. J., Six, J., Hungate, B. A., de Graaff, M. A., van Breemen, N., van Kessel, C. (2006). Element interactions limit soil carbon storage. Proc. Natl. Acad. Sci. 103 (17), 6571–6574. doi: 10.1073/pnas.0509038103
Ververis, C., Georghiou, K., Christodoulakis, N., Santas, P., Santas, R. (2004). Fiber dimensions, lignin and cellulose content of various plant materials and their suitability for paper production. Ind. Crops Products 19 (3), 245–254. doi: 10.1016/j.indcrop.2003.10.006
Waldrop, M. P., Zak, D. R., Sinsabaugh, R. L. (2004). Microbial community response to nitrogen deposition in northern forest ecosystems. Soil Biol. Biochem. 36 (9), 1443–1451. doi: 10.1016/j.soilbio.2004.04.023
Wang, L. J., Cheng, R. M., Xiao, W. F., Shen, Y. F., Zeng, L. X., Yang, S., et al. (2021). Seasonal responses of soil enzyme activities and microbial biomass to nitrogen addition at different levels in pinus massoniana plantation in the three gorges reservoir area. Acta Ecologica Sin. 41 (24), 9857–9868. doi: 10.5846/stxb202010272751
Wang, W., Zhang, X., Na, T., Ao, D., Zeng, H. (2014). Effects of litter types, microsite and root diameters on litter decomposition in pinus sylvestris plantations of northern China. Plant Soil 374 (1-2), 677–688. doi: 10.1007/s11104-013-1902-y
Wu, F. T., Peng, C. H., Wang, C. Y., Chen, H., Liu, W. G., Liu, Z. H., et al. (2022). Responses of soil CH4 fluxes to nitrogen addition in two tropical montane rainforests in southern China. For. Ecosyst. 9, 100031. doi: 10.1016/j.fecs.2022.100031
Xia, M. X., Talhelm, A. F., Pregitzer, K. S. (2017). Chronic nitrogen deposition influences the chemical dynamics of leaf litter and fine roots during decomposition. Soil Biol. Biochem. 112, 24–34. doi: 10.1016/j.soilbio.2017.04.011
Xia, M. X., Talhelm, A. F., Pregitzer, K. S. (2018). Long-term simulated atmospheric nitrogen deposition alters leaf and fine root decomposition. Ecosystems 21, 1–14. doi: 10.1007/s10021-017-0130-3
Xiong, Y., Fan, P., Fu, S., Zeng, H., Guo, D. (2013). Slow decomposition and limited nitrogen release by lower order roots in eight chinese temperate and subtropical trees. Plant Soil 363 (1-2), 19–31. doi: 10.1007/s11104-012-1290-8
Xu, Y. W., Huang, R. X., Zhou, B. Z., Ge, X. G. (2022). Fine-root decomposition and nutrient return in moso bamboo (Phyllostachys pubescens J.Houz.) plantations in southeast China. Front. Plant Sci. 13. doi: 10.3389/fpls.2022.735359
Yang, Y. S., Chen, G. S., Guo, J. F., Lin, P. (2004). Decomposition dynamic of fine roots in a mixed forest of cunninghamia lanceolata and tsoongiodendron odonum in mid-subtropics. Ann. For. Sci. 61 (1), 65–72. doi: 10.1051/forest:2003085
Yang, S., Cheng, R. M., Xiao, W. F., Shen, Y. F., Wang, L. J., Guo, Y., et al. (2019). Heterogeneity in decomposition rates and nutrient release in fine-root architecture of pinus massoniana in the three gorges reservoir area. Forests 11 (1), 14. doi: 10.3390/f11010014
Yu, X. Y., Zhu, Y. J., Wang, B., Liu, D., Bai, H., Jin, L., et al. (2021). Effects of nitrogen addition on rhizospheric soil microbial communities of poplar plantations at different ages. For. Ecol. Manage. 494 (1), 119328. doi: 10.1016/j.foreco.2021.119328
Zak, D. R., Holmes, W. E., Burton, A. J., Pregitzer, K. S., Talhelm, A. F. (2008). Simulated atmospheric NO- 3deposition increases soil organic matter by slowing decomposition. Ecol. Appl. 18, 2016–2027. doi: 10.1890/07-1743.1
Zeng, L. X., He, W., Teng, M. J., Luo, X., Yan, Z. H., Huang, Z. L., et al. (2018). Effects of mixed leaf litter from predominant afforestation tree species on decomposition rates in the three gorges reservoir, China. Sci. Total Environ. 639, 679–686. doi: 10.1016/j.scitotenv.2018.05.208
Zhang, J. H., Li, H., Zhang, H. F., Zhang, H., Tang, Z. Y. (2021). Responses of litter decomposition and nutrient dynamics to nitrogen addition in temperate shrublands of north China. Front. Plant Sci. 11. doi: 10.3389/fpls.2020.618675
Zheng, D. N., Wang, X. S., Xie, S. D., Duan, L., Chen, D. S. (2014). Simulation of atmospheric nitrogen deposition in China in 2010. China Environ. Sci. 34 (5), 1089–1097. doi: 10.3969/j.issn.1000-6923.2014.05.001
Keywords: nitrogen addition, root orders, decomposition, initial chemical quality, lignin degradation
Citation: Wang L, Shen Y, Cheng R, Xiao W, Zeng L, Sun P, Chen T and Zhang M (2022) Nitrogen addition promotes early-stage and inhibits late-stage decomposition of fine roots in Pinus massoniana plantation. Front. Plant Sci. 13:1048153. doi: 10.3389/fpls.2022.1048153
Received: 19 September 2022; Accepted: 24 October 2022;
Published: 14 November 2022.
Edited by:
Yuanrun Zheng, Institute of Botany (CAS), ChinaReviewed by:
Xiaoqin Cheng, Beijing Forestry University, ChinaQuan Li, Zhejiang Agriculture and Forestry University, China
Copyright © 2022 Wang, Shen, Cheng, Xiao, Zeng, Sun, Chen and Zhang. This is an open-access article distributed under the terms of the Creative Commons Attribution License (CC BY). The use, distribution or reproduction in other forums is permitted, provided the original author(s) and the copyright owner(s) are credited and that the original publication in this journal is cited, in accordance with accepted academic practice. No use, distribution or reproduction is permitted which does not comply with these terms.
*Correspondence: Ruimei Cheng, Y2FmY2hlbmdAc2luYS5jb20=