- 1Collaborative Innovation Center for Efficient and Green Production of Agriculture in Mountainous Areas of Zhejiang Province, College of Horticultural Science, Zhejiang Agriculture and Forest University, Lin'an, Hangzhou, China
- 2Bashan Management Area of the Management Committee for Taishan Historic and Scenic Area in Tai’an City, Tai’an, China
Leaf senescence, the final stage of leaf development, is one of the adaptive mechanisms formed by plants over a long period of evolution. Leaf senescence is accompanied by various changes in cell structure, physiological metabolism, and gene expressions. This process is controlled by a variety of internal and external factors. Meanwhile, the genes and plant hormones involved in leaf aging affect the quality, yield and stress resistance in horticultural plants. Leaf senescence mediated by plant hormones affected plant quality at both pre-harvest and post-harvest stages. Exogenous plant growth regulators or plant hormone inhibitors has been applied to delay leaf senescence. Modification of related gene expression by over-expression or antisense inhibition could delay or accelerate leaf senescence, and thus influence quality. Environmental factors such as light, temperature and water status also trigger or delay leaf senescence. Delaying leaf senescence could increase chloroplast lifespan and photosynthesis and thus improve source strength, leading to enhanced yield. Accelerating leaf senescence promotes nutrient redistribution from old leaves into young leaves, and may raise yield under certain circumstances. Many genes and transcriptional factors involved in leaf senescence are associated with responses to abiotic and biotic stresses. WRKY transcriptional factors play a vital role in this process and they could interact with JA signalling. This review summarized how genes, plant hormones and environmental factors affect the quality, yield. Besides, the regulation of leaf senescence holds great promise to improving the resistance to plant biotic and abiotic stresses.
1 Introduction
Leaf senescence is the final stage of leaf development. It is the adaptive mechanism formed by plants in their long-term evolutionary process. The most evident morphological indicator of leaf senescence is the change in leaf color from green to yellow. Accompanied by the decrease of chlorophyll content, the protein content and net photosynthesis rate decline during leaf senescence. In general, leaf senescence is mainly controlled by the developmental age (Song et al., 2014). However, leaf senescence may be triggered by environmental clues. It has been suggested that ‘programmed cell death’ (PCD) in plants occurs at a specific stage of intrinsic senescence and becomes irreversible after leaf yellowing reaches a “point of no return” (Sobieszczuk et al., 2018). As the last step of leaf development, leaf senescence affects plant productivity and quality, either positively or negatively.
With the improvement of living standard and yield required for the increasing population, more attention is drawn to pre-harvest management and post-harvest management of plant cultivation. Once horticultural plants are harvested, the leaves have certain similarities with the aging caused by development. The plant leaves are like unique windows (Jan et al., 2018), revealing various changes of cells, tissues, and organs during aging. For instance, Reactive oxygen species (ROS) are accumulated during leaf senescence, contributing to the degradation of chlorophyll. On the contrast, increase Carbohydrate-related metabolism Plasma-Activated Water (PAW) enhanced the resistance to "chilling injury" and delayed leaf senescence in spinach, PAW treatment may contribute to the preservation of valuable nutrients (Uhlig et al., 2021). Plants usually redistribute nutrients from senescent leaves to other organs (Woo et al., 2019), and this strategy could be beneficial for the survive of plants under abiotic stresses, indicating low and high temperatures, drought stress. In addition, plant hormones may delay or advance leaf aging, Zhang et al. elaborated the role of salicylate and ROS in leaf senescence and plant immunity (Zhang et al., 2020). Furthermore, some genes are involved in leaf senescence. These genes are related to the degradation of proteins, nucleic acid and chlorophyll degradation and they are identified mainly by using mutants related to the aging process.
In this review, we summarized the relationship between leaf senescence and the quality, productivity, and stress resistance in horticultural plants (Figure 1). We focused on the molecular mechanism of leaf senescence. This review aimed to gain insights into the regulation of leaf senescence in horticultural industry. This provides a basis for maximizing yield and quality and enhancing stress resistance.
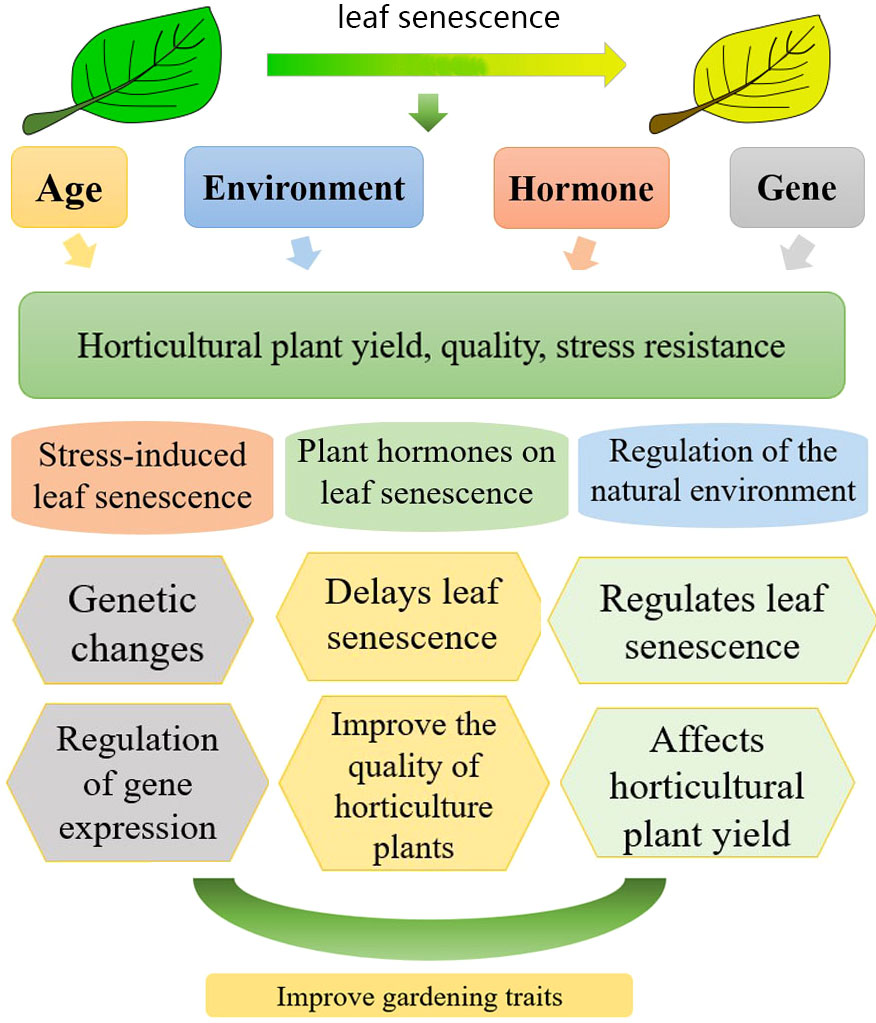
Figure 1 Relationship between leaf aging and the yield, quality and stress resistance in horticultural plants (leaf senescence of horticultural plants is subject to the influence of hormones, genes, exogenous hormones, and growth age control; aging has a certain effect on the yield, quality, and stress resistance of horticultural plants.).
2 Leaf senescence and horticultural plant qualities
2.1 Plant hormones regulate leaf senescence and affect the quality of horticultural plants
Plant hormones affect most stages of the plant life cycle, including leaf senescence. Meanwhile, plant hormones are key players in the quality formation of horticultural plants. Thus modification of phytohormones could affect both leaf senescence and product quality (Figure 2).
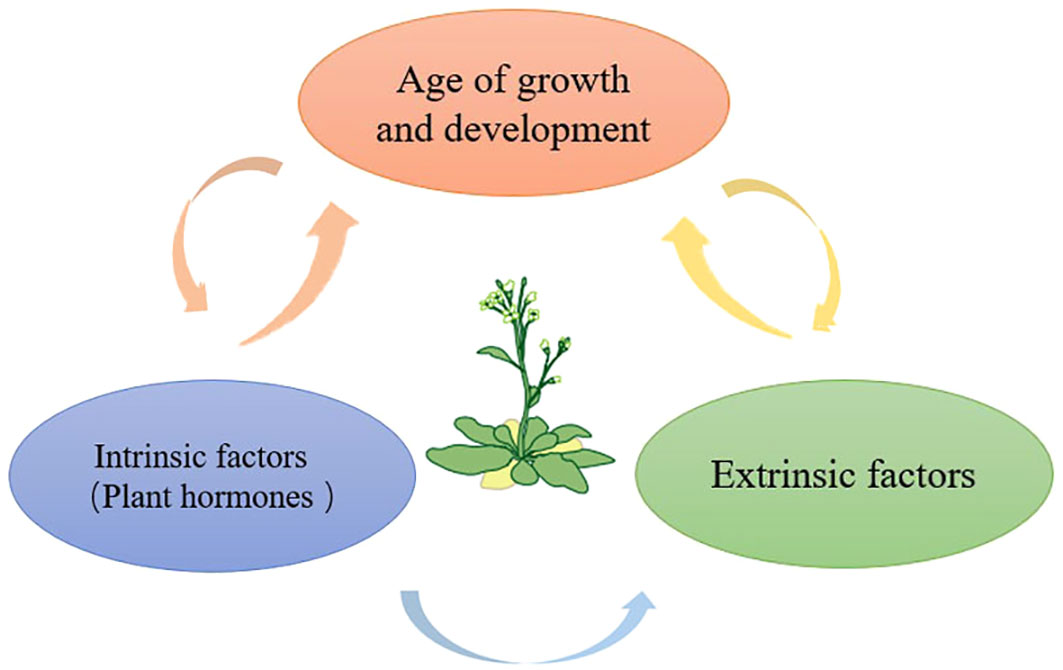
Figure 2 Leaf senescence is the final stage of leaf development, and leaf aging is regulated by a variety of factors, such as strict developmental age, exogenous factors and endogenous factors, thereby regulating the whole process of plant growth and development.
2.1.1 Plant hormones regulate leaf senescence to affect pre-harvest quality
Exogenous hormones can delay the aging of pre-harvest leaves, increase photosynthesis, promote the accumulation of assimilates, and thus improve quality. For example, 10~80 mg/L 1-Naphthaleneacetic acid (NAA) and Gibberellin A3 (GA3) have significant effects on preventing A4 seedless lychee from falling fruit before harvest and improving the quality of fruit. Among them, treatment at 40 mg/L had the best effect on reducing the pre-harvest fruit drop rate. Treatment with GA3 at a concentration of 20 mg/L increased the single fruit weight of lychee (Yang et al., 2015). Cut flower roses sprayed with 1-Methylcyclopropene (1-MCP) before harvest have a storage period of up to 26 days. The 1-MCP treatment significantly extends the life of the bottle insert for 0 or 6 days for refrigerated flowers, extending them by 27.1% and 42.6%, respectively (Huang et al., 2017). During the growth process of thick-skinned melon, the leaves usually appear after fruit setting, and leaf aging causes their yellowing. Thus, photosynthesis rate cannot maintained, resulting in decreased yield and fruit quality. Exogenous 6-BA (Zhu, 2015) and exogenous calcium nitrate (Zhang et al., 2014) affected the aging of thick-skinned melon leaves and thus influenced their quality. Zhu et al. observed the soluble solid content and vitamin C content of T2 treated fruits were 12.3% and 11.3% higher than those of the control. (Zhu, 2015). Comprehensive previous studies have revealed that exogenous hormones slowed down the aging degree of leaves to a certain extent, prolonged photosynthesis, promoted the accumulation of assimilates, and improved the quality of horticultural plants.
2.1.2 Plant hormones regulate leaf senescence to affect post-harvest quality
For foliage plants and leafy vegetables, the status of leaves is an important attribute of product quality. For instance, the leafy vegetables should not be wilt, and have green color (Hassan and Mahfouz, 2012; Miret et al., 2018). Ethylene(ET) are considered to be involved in post-harvest process and leaf senescence (Tomotsugu, 2018). The release of ET during post-harvest promoted the over-accumulation of reactive oxygen species (ROS), leading to the yellowing of cabbage leaves (Yu and Xi, 1997). Low temperatures can inhibit ET production, thereby slowing down the yellowing of leaves and inhibiting chlorophyll degradation (Yu and Xi, 1997). Exogenous ET promoted the leaf yellowing and senescence and degradation of chlorophyll in fresh-cut chrysanthemums (Liu et al., 2022b). Treatment with 1-MCP at a concentration of 0.5 μl/L reduced respiration in the Glebionis coronaria, alleviated the decline in protein content, and extended shelf life. However, low concentration of 1-MCP (< 0.5 μl/L) did not affect shelf life of the Glebionis coronaria. (Ma, 2006).Gibberellic acid (GA) interacts with ET via DELLA proteins (Achard et al., 2006), and thus affect leaf senescence. During the storage process of Chinese cabbage, exogenous GA inhibited the expression of aging-related genes, delayed leaf aging and maintained a high chlorophyll content (Xiao et al., 2019). Pre-harvest spray of 20 mg·L-1GA3 significantly reduced the rot rate of fruits during storage period, increased the accumulation of malondialdehyde (MDA), and delayed the degradation of vitamin C, thereby inhibiting the aging of Gongci fruit (Ma et al., 2009). Moreover, GA3-treated cabbage maintained a high maximum quantum yield (Fv/Fm) and total chlorophyll content (Fan et al., 2018).
Abscisic acid (ABA) is a key player in stomatal closure, and thus influence the water loss during post-harvest. Manipulation of ABA levels has an important effect on postharvest quality of leafy vegetables. Miret observed ABA can inhibit leaf senescence during storage, thereby prolonging the shelf life of vegetables (Miret et al., 2017). ABA reactive protein MdBT2 interacted with MdbHLH93 to induce ubiquitination and degradation of MdbHLH93 protein, thereby delaying leaf senescence in apple (An et al., 2019). The ABA pretreatment significantly reduced the brown detergentilence of the ‘Yellow Crown’ pear, improved the ability of POD, APX and peel free radical to varying degrees, and reduced the MDA content (Liu et al., 2017). ABA treatment is used to inhibit the aging of leafy vegetables, improve the postharvest quality of storage at room temperature, and delay the loss of sensory metabolites.
Cytokinins bear upon leaf senescence in horticultural plants. An increase in cytokinin level is thought to block the aging process of leaves associated with age and stress, thereby preserving the photosynthetic properties of the leaves (Lira et al., 2017). Chen et al. discovered that after Forchlorfenuron (CPPU) treatment, the degradation rate of soluble proteins after 3 d of storage at room temperature was significantly slower than that of the control cabbage. CPPU can inhibit the activity of cabbage heart protein hydrolases and slow down yellowing, thereby improving the quality of postharvest gardening plants (Chen et al., 2001). 6-BA can delay the aging of yellow flowers of cauliflower (Gao, 1998).
Based on the analysis of previous studies, exogenous hormones can delay leaf aging, thereby improving the photosynthesis of plant leaves, which has an important effect on postharvest storage. This property extends the shelf life and promotes the postharvest quality of horticultural plants, which helps to increase in their market value.
2.2 Leaf senescence under genetic regulation affects the quality of horticultural plants
In modern horticultural production, genetic modification could become a key strategy for improving biomass and nutritional quality. Genes that change in expression levels during leaf senescence are called leaf senescence- associated genes (SGAs). In previous studies, a large number of SAGs have been isolated in rice (Table 1). These senescent genes are involved in macromolecular degradation, nutrient redistribution, transcriptional regulation, stress defense, etc. (Leng et al., 2017; Woo et al., 2019). In tomatoes, promoters SGA12/SGA13 come into play, flowering earlier and increasing chlorophyll content, thereby increasing yield (Swartzberg et al., 2006). Antisense inhibition of GIGANTEA(BoGI) in kale (Brassica oleracea var. sabellica) delayed both leaf senscence and flowering (Thiruvengadam et al., 2015).
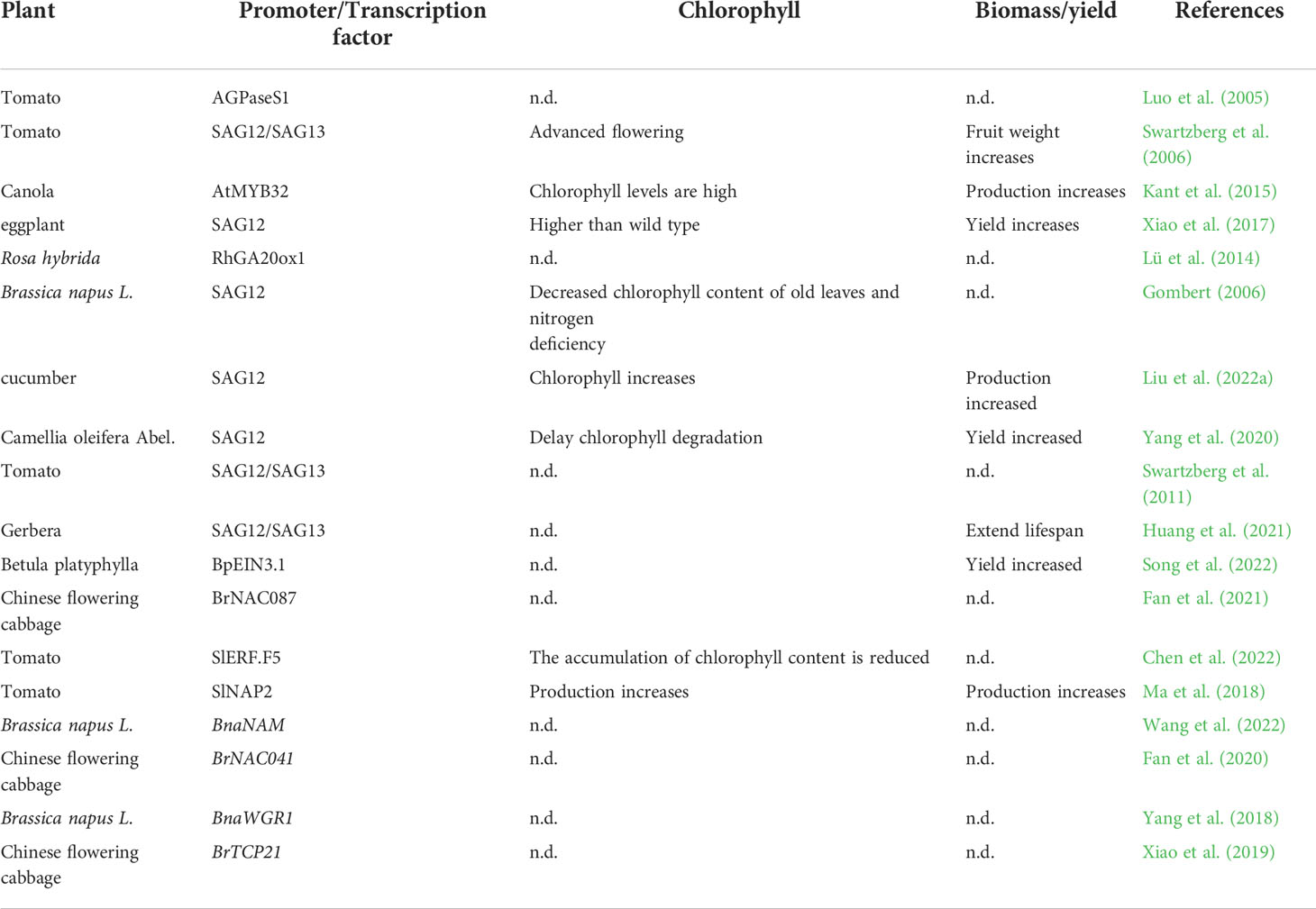
Table 1 Promoter/Transcription factor regulates the effects of leaf senescence on horticultural plants (n.d. = not determined).
Leaf aging affects the quality of apple fruit, Wang et al. cloned the MhYTP1 and MhYTP2 genes, and found that their expressions were positively correlated with plant leaf senescence, suggesting that these genes can be indicator of plant senescence (Wang et al., 2017).Tomato transcription factor SlWRKY37 can regulate dark leaf senescence, which can reduce tomato sensitivity to external aging signals, thus providing target genes for delayed leaf yellowing (Wang et al., 2022). Tomato MYC2, as a key factor in leaf senescence, transduces jasmonic acid (JA) signaling and induces JA-mediated senescence in tomato leaves by accelerating chlorophyll degradation and inhibiting carbon fixation (Ding et al., 2022). BnaNTL1 is a membrane-bound NAC (NAM, ATAF and CUC) transcription factor (TF) in rape (Yan et al., 2021) and it is expressed primarily in senescent leaves and can actively regulate ROS production. By regulating key genes, BnaNTL1 can promote the fruit ripening period and postharvest storage, regulate the ripening time, and extend shelf life. Through the regulation of leaf aging genes, the quality of horticultural plants is improved to a certain extent and the shelf life is extended.
2.3 Leaf senescence and horticultural plant quality under environmental regulation
Environmental stresses such as drought, waterlogging, high-temperature, low-temperature, soil salinity stresses, are important factors restricting plant productivity. Environmental stress regulates leaf aging, affecting both quality and yield in horticultural plants.
2.3.1 Temperature
In general, low temperature during post-harvest inhibits the leaf respiration and delays leaf senescence. Low temperature provides a way for spinach post-harvest preservation storage by delaying the decline of antioxidant enzyme activity and leaf aging (Tian et al., 2014). Under low-temperature storage, especially at 0° conditions, it can maintain the high nutrient content of slow down leaf aging, prolong shelf life, and solve the problem of rapid yellowing of leaves (An et al., 2020). In Arabidopsis thaliana, low temperature inhibited the phyB nucleus output, thereby inhibiting the transcriptional activity of ET signaling media and delaying leaf senescence (Lee et al., 2022). On the contrast, high temperature acceleated leaf aging, decreasedthe soluble protein content of strawberries, which directly affected the photosynthetic ability of strawberries (Yang et al., 2012).
2.3.2 Light
Both light intensity and light quality affect the leaf senescence and plant quality in horticultural plants. Extremely low light intensity such as darkness accelerates leaf aging and affects grain and oil yield in sunflower (Dosio et al., 2020). During low light intensity, carbon fixation efficiency determines leaf senescence; however, plant photoreceptor pigment A has a regulatory effect on carbon fixation efficiency (Brower et al., 2012). High light intensity could induce light inhibition and damage. Shading net is a routine measure in horticulture production. Under the color shading net, the quality of the grapes is better than those under black shading nets because the absence of high light intensity causes premature leaf failure, which improves the quality of grapes; the soluble solids are also affected differently under various shade nets, whereas unshashed grapes show thermal damage (Zha et al., 2022). Studies have shown that Supplemental lighting(SL) sources affect the physiology and productivity of plants. Supplemental lighting (SL) within the canopy increases the overall tomato yield and maintains the nutrient content of plants (Gómez and Mitchell, 2016). Besides, light quality has a certain influence on the growth of rhododendrons. Exposure to short period of ultraviolet radiation per day improved water utilization inside plants, thereby delaying the aging of tomato leaves, favoring plants to cope with drought conditions, and playing a key role in water-saving irrigation in agriculture (Mannucci et al., 2022). RBL( red and blue lights) can increase the synthesis of photosynthetic pigments and improve antioxidant enzyme activity. This process delays leaf aging and ensures plant growth. (Wang,Y. et al.,2017)
2.3.3 Moisture
Maximizing leaf lifespan is the key to tea quality; drought and cold have a combined effect on leaf senescence and tea quality (Zheng et al., 2016). Zhao et al. (2016) reported that PYL9 and leaf senescence play an important role in coping with drought stress. PYL9 inhibits drought by limiting water loss during respiration, and the combination of ABA receptor PYL9 and other transcription factors accelerates leaf senescence, thereby improving drought tolerance and survival in plants. The upregulation of SAG12 gene and downregulation of Cab gene reflects the transition of sinks/sources in cabbage-type rape leaves, which are accurate physiological indicators of leaf aging and can effectively identify the degree of nitrogen deficiency in leaves (Gombert, 2006).
The environment is essential for the adaptability and productivity of plants. Leaf aging is an important stage of development for productivity and quality. Natural environmental factors such as light, temperature, and moisture all regulate leaf aging, which affects the quality of horticultural plants.
3 Leaf senescence and horticultural plant yield
3.1 Increasing horticultural plant yield by increasing chloroplast lifespan
Leaf senescence is caused by the gradual loss of green pigment and the degradation of chlorophyll (Gan and Hörtensteiner, 2013). Thomas hypothesized that extending the maximum photosynthetic period delays the aging process, thereby increasing yield (Thomas and Stoddart, 1980). Photosynthesis is the basis of plant growth and material accumulation and important for nutrient accumulation, quality formation and sustainable development of production capacity. Manipulating leaf aging-related genes is an effective strategy to improve pulp yield (Lira et al., 2017), that is, by delaying leaf aging to prolong the photosynthetic characteristics of leaves, thereby enhancing source activity. Zhao et al. (2022) revealed the changes in photosynthetic ability of grape leaves during development and aging and analyzed the nutrient absorption of leaves during the growing season; their results showed significance for improving the yield of horticultural plants. ROS has been identified as a dependent signal to mediate leaf aging, analyzed the differences between dark-induced and natural aging, and proposed to increase plant yield by increasing the chloroplast lifespan (Bartoli et al., 2013; Weaver et al., 1998; Buchanan-Wollaston et al., 2005).
The shortage of assimilates in leaves eventually accelerates the maturation process of plants (Gan, 2003), strongly affecting plant productivity. The most evident manifestation of leaf senescence is the breakdown of chlorophyll into non-green derivatives, which causes the yellowing of leaves (Mayta et al., 2019). As the keys to nutrient cycling, chloroplast degradation and Rubisco play important roles, with protein degradation starting in chloroplasts (Feller et al., 2008). Given that chloroplasts contain most of the nutrients, they are the main source during plant carbon and nitrogen cycles. The disintegration of photosynthetic organs is a developmental stage in the aging process. ROS production is likely to be caused by changes in the electronic pathway and photosynthetic organs. Chloroplasts are the most important site of ROS production (Laloi and Havaux, 2015). The subcellular localization of OsPLS4, which is found in chloroplasts, chloroplast development and biosynthesis of rice cuticle waxes are affected by OsPLS4 mutations, decreased photosynthetic rate, and premature leaf aging (Zhou et al., 2020). Cytokinin slows down leaf senescence and prevents chlorophyll degradation and destruction (Talla et al., 2016). Delaying leaf aging and maintaining the green color of leaves has various meanings, including provision of more carbon and promoted root growth; thus, the onset of aging promotes the increase in plant yield.
3.2 Raising yield via delaying of leaf senescence
Improving the photosynthetic efficiency is the basis for increasing yields. Delaying the aging of plant leaves, which increases the leaf area to a certain extent, is conducive to plant photosynthesis, thus increasing the accumulation of assimilates (Figure 3).
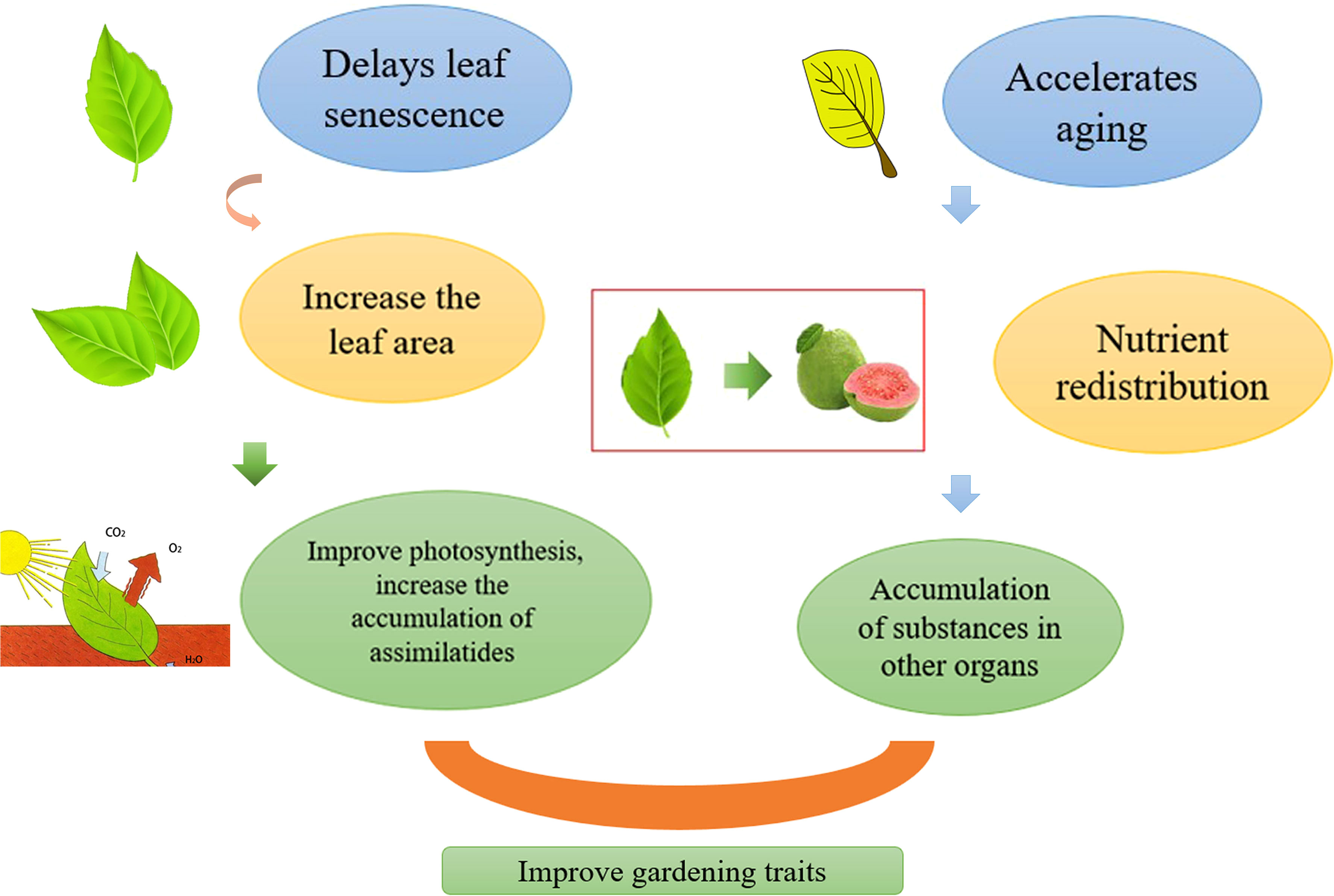
Figure 3 Regulation of leaf aging affects horticultural plant yield, delays leaf aging, increases leaf area, thereby improving photosynthesis and increasing the accumulation of assimilates. Accelerates leaf aging, redistributes nutrients, and promotes the accumulation of substances in other organs. Both pathways can increase yields.
The study revealed that pSARK-IPT transgenic plants can increase CO2 fixation under drought conditions and delay leaf aging, thereby increasing tolerance to extreme drought. Moreover, the yield of genetically modified plants has increased significantly (Blumwald et al., 2009). For the peach tree, the aging of roots and leaves is delayed, and the nutrient storage level is increased, thereby increasing the fruit yield (Zhang et al., 2021). Shading delays the aging of peony leaves, thereby increasing photosynthetic capacity. The development of seeds and accumulation of nutrients are prolonged, thereby increasing the yield (Han et al., 2020). Improving nitrogen utilization can delay the aging of rapeseed leaves. Varieties with high nitrogen use can adapt to low light conditions during flowering. Studies have also shown a positive correlation between nitrogen use and delayed leaf senescence (Schulte auf’m Erley et al., 2007). Precise regulation of leaf aging enables plants to respond quickly to environmental signals. In apples, MdAB15 inhibits the transcriptional activity of downstream target genes MdNYE1 and MdNYC1. This condition slows down the aging of ABA-induced leaves (An et al., 2021). DCPTA is sprayed on the foliar surface of sugar beet to enhance leaf development and photosynthetic productivity. Rubisco activity is increased to a certain extent, demonstrating an increase in plant net photosynthesis. Delayed leaf aging increases net carbon assimilation of leaves (Keithly et al., 1990). In the late stage of growth and development, especially in the period of yield formation, the delayed leaf aging and maintenance of the leaf area are keys to improving the accumulation of dry matter. In summary, delaying the onset of leaf aging can improve photosynthesis, which in turn increases horticultural plant yields.
3.3 Improving yield through nutrient redistribution mediated by accelerated leaf senescence
As leaves begin to age, the materials they have accumulated during the growth phase are converted into nutrients that can be exported and supplied to other tissues and organs (Woo et al., 2019). Aging is accompanied by the reuse of nutrients, which can increase plant yield to a certain extent (Figure 3). When a plant is subjected to adversity stress, the leaves age early, allowing the plant to adapt better to the environment. For several annual plants, nutrients are transferred to the fruit and grain as the leaves age. A study showed that C2H2-type zinc finger MdZAT10 can accelerate the aging of apple leaves (Yang et al., 2021). In Arabidopsis, knocking out HDA15 can accelerate leaf aging and promote plant flowering (Huang et al., 2022). In safflower, CtCP1 promotes the hydrolysis of the CtbHLH41 protein, accelerating the transcriptional activity of related genes and leaf senescence (Hong et al., 2022). A study revealed that MdHHO3 promotes early leaf aging under nitrate deficiency, which proves that the lack of nitrates can cause aging of apple leaves (Wen et al., 2022). The sink-source relationship affects leaf aging, and the large source of the sink or the large source of the sink will lead to abnormal leaf maturity. Both the sink and the source are important factors that determine yield. If plant vegetative growth is too vigorous, reproductive growth is restricted, resulting in reduced yield and quality. Therefore, to ensure the coordination of the relationship between the sink and the source, we can obtain high-yield and high-quality horticultural crops. Reproductive growth also promotes leaf senescence to a certain extent. A competition for nutrition exists between vegetative and reproductive organs. In summary, accelerating leaf senescence can increase yield to a certain extent.
3.4 Genes regulate leaf senescence and horticultural plant yield
Genetic modification can delay leaf aging, maintain photosynthesis for a long time, and sustain leaf activity, thereby increasing yield. According to Kant et al., the IPT gene encodes cytokinin synthesis to delay the aging of rapeseed leaves under flooding conditions. During long periods, transgenic rapeseed maintains the high chlorophyll levels, which improves crop yield under water stress conditions (Kant et al., 2015). At the genetic level, plant responses to stresses differ significantly from aging procedures and are associated with accelerated aging process (Guo and Gan, 2011). Leaf senescence caused by environmental stress or intrinsic inheritance can reduce photosynthesis, lead to premature cell death, and cause the supply of anabolites to decrease before flowering, thus negatively regulating the final grain weight (Gregersen et al., 2008). In numerous annual plants, such as monocotyledonous plants, aging is usually controlled by reproductive structures. Mature leaves are regulated by environmental and other organ physiological signals that trigger reversible events, which eventually lead leaves at to a “point of no return”, which is a sign of the onset of irreversible aging (Lim et al., 2007). Genes, such as the NAC transcription factor S1NAP2 that controls the aging of tomato leaves. This prolongs the photosynthesis time of the leaves, which increases the fruit yield and sugar content of tomatoes (Ma et al., 2018). In cucumbers, transient expression of the MT and MT synthetic gene CsASMT inhibits leaf senescence under dark conditions. MT delays leaf aging, reduces the rate of decline in chlorophyll, and promotes photosynthesis. This condition speeds up the growth of cucumbers and increases yield (Liu et al., 2022a). In Chinese cabbage leaf senescence, ET reactive factor (ERF) works in conjunction with JA. The transcription factor BrERF72 is a nucleus-localizing protein that activates the expression of JA biosynthetic genes by directly targeting its promoter (Tan et al., 2018). In summary, leaf senescence is not controlled by a single gene, but by a polygenic regulatory system. Predecessors revealed that the aging gene, which regulates leaf aging at the molecular level, provides a basis for anti-aging breeding of horticultural plants and increasing yields.
4 Leaf senescence and plant stress resistance
4.1 Leaf senescence and disease resistance of horticultural plants
For plants that are frequently exposed to adverse conditions, biological and abiotic stresses are inevitable during the growing season (Gregersen et al., 2013). Leaf senescence is an important component of plant development and an adaptation strategy for plants to cope with stress. It can also reduce pathogen transmission. Zhang et al. observed that powdery mildew and propabenzole (PBZ)-activated ethylene (ET) signal transduction, upregulates the expressions of CSEIN3 and CsCCGs and CsRBOHs, leads to leaf senescence, and improves the plant’s resistance to powdery. An invading pathogen causes a “suicide” hypersensitivity reaction in leaves, which triggers a cascade of immune responses and a slow green loss phenomenon, which is indicative of the rapid aging process of cells (Zhang et al., 2022). Wang et al. have also shown that cucumber anthrax and downy mildew resistance is directly regulated by NYE1/SGR1 (Wang et al., 2019).
The improvement of leaf disease resistance is an important factor for the increase in plant yield. Plant leaf aging reduces the invasion of pathogens to a certain extent. Numerous genes that control leaf aging are also involved in plant stress resistance. In eggplant, P-SAG12-IPT overexpression plays a role in delayed leaf aging while enhancing tolerance to drought and cold; the activity of ROS-scavenging enzymes is higher than that of wild-type eggplant, and growth rate and yield are relatively improved (Xiao et al., 2018).
WRKY18 works with WRKY40 and WRKY60 and can modulate disease resistance. WRKY18, WRKY40, WRKY60 three mutants and double mutants WRKY18, WRKY40 and WRKY18, WRKY60 are significantly more resistant to lilac. In addition, in terms of resistance to powdery mildew fungi, two mutants, WRKY18, are more resistant to WRKY40 (Xu et al., 2006). WRKY70 expression has a diametrically opposite effect on the resistance of Arabidopsis necrotrophic bacteria and biotrophic bacteria (Li et al., 2006). Mutant nore1-1was found in EMS mutagenesis of Arabidopsis thaliana; it is associated with leaf senescence, where the mutant activates the defense system, accelerates leaf senescence, and improves the plant’s disease resistance (Lee et al., 2016). Salicylic acid (SA) and reactive oxygen species (ROS) can regulate leaf senescence and defend against pathogen invasion. The WRKY55 transcription factor accelerates leaf senescence and improves resistance to Pseudomonas syringum by modulating these two factors (Wang et al., 2020). In rice, the overexpressed OsNBL1 interacts with the localized protein OsClpP6, delays leaf senescence, contains a higher chlorophyll content than wild-type plant leaves, and is critical for salt and disease resistance (Zhao et al., 2021). Leaf aging plays a key role in disease resistance. Regulating leaf aging is conducive to horticultural plants to cope with different diseases, and to a certain extent, the resistance of plants is improved.
4.2 Leaf senescence and stress resistance of horticultural plants
Regulation of the aging process in leaves is a part of the adaptation of plants to different environmental conditions, especially drought stress. Usually, numerous transgenic plants exhibit matching drought resistance and green leaf traits. Darkness can induce leaf senescence, and shading is an important way to improve plant performance (Brower et al., 2012). Field crops will benefit from shady and dark conditions, resulting in nutrient remobilization after leaf aging, which is conducive to the photosynthesis of plants. Under the induction of dark environment and JA, the aging rate of plant leaves significantly improves. Specifically, SlWRKY37 plays a positive role in regulating leaf aging in tomatoes. Wang et al. revealed the function of SlWRKY37 in leaf aging, and delayed leaf yellowing by reducing the sensitivity to external aging signals through a target gene (Wang, Z. et al. 2022). Sucrose phosphatase prevents plant leaf senescence under drought stress conditions (Sami et al., 2016). Cytokinin levels in roots are suppressed due to nutrient deficiencies, causing leaf senescence and allowing nutrient transport (Maillard et al., 2015; Havé et al., 2016). Delays the onset of leaf senescence, improves photosynthesis, and as nutrients are transferred from old leaves to young leaves, the antioxidant capacity of plants increases to resist stress and allow normal growth to return (Jan et al., 2018). WRKY transcription factors have an important influence on leaf senescence and environmental stress. Gu et al. isolated the gene GhWRKY91 from WRKY, and its transgenic plants showed a strong drought tolerance, delayed degree of leaf aging in an arid environment, enhanced gene expression associated with drought stress, and relatively weakened aging gene (Gu et al., 2019). Tea plants generally grow under cold and drought stress conditions, which affect their quality and leaf aging. Zheng et al. discovered that drought induction delays leaf aging, thus enhancing antioxidant capacity, prolonging photosynthesis, and revealing the relationship between quality, stress resistance, and leaf life (Zheng et al., 2016). Lee et al. identified the drought-responsive NAC transcription factor NTL4, which promotes the production of ROS in senescent leaves under drought stress and accelerates leaf aging; however, mutants lacking NTL4 show delayed leaf senescence and improved drought resistance (Lee et al., 2012). Accelerate leaf senescence and improve nutrient reuse, pepper leaves, after inoculation with CMV, accelerate senescence and improve resistance, and the difference between CMV-inoculated resistant peppers and sensitive peppers and changes in their photosynthesis was studied (Stefanov et al., 2011). Hu et al. reviewed the relationship between JA-mediated leaf senescence and tolerance to cold stress and observed that JA treatment can increase the expression of senescent genes (Hu et al., 2017). The drought tolerance of sorghum after flowering is tightly bound to the greening of leaves, thereby increasing biomass (Harris et al., 2019). Overexpressed SlERF5 in transgenic tomatoes can maintain leaf moisture status and chlorophyll concentrations, giving plants strong salt stress and drought tolerance; however, studies have shown the minimal influence of these findings on production (Pan et al., 2012). The short period of ultraviolet radiation per day has a certain effect on improving water utilization inside the plant, thereby delaying the aging of Tom tomato leaves, favoring plants to cope with drought conditions, and playing a key role in water-saving irrigation in agriculture (Mannucci et al., 2022). The use of ABA has a slight effect on the yield composition and vegetative growth of grapes, but it can lead to aging, shedding, and dormancy of grape leaves, which increases freezing tolerance (Li and Dami, 2015). Delaying the aging of plant leaves can improve the stress resistance of plants to a certain extent. After the aging of the leaves is slowed down, photosynthesis is enhanced, which is conducive to plants coping with various stressful environments.
5 Conclusions and perspectives
This paper reviews the relationship between leaf aging and horticultural plant quality, yield, and stress resistance. Through the quality analysis of horticultural plants before and after harvest, most of the reports revealed a certain relationship with leaf aging. Delayed leaf aging plays an important role in prolonging the shelf life of vegetable fruits and is conducive to occupying a huge market potential. We summarized the different roles of various plant hormones which are an important part of regulating plant quality, in the storage of horticultural plants. Through the analysis of gene regulation, that of the WRKY family, NAC family, etc., the potential mechanism of regulating leaf aging, which is the key to maintaining optimal productivity and improving the survival of offspring, can be studied to optimize the productivity of horticultural plants. In addition, we analyzed the possible effects of various environmental conditions on the quality of horticultural plants, and concluded that light, temperature, and moisture all played a pivotal role. Through the summary analysis of leaf senescence and plant yield, delaying the onset of leaf senescence and improves photosynthesis, thereby promoting the accumulation of assimilates to increase yield. Meanwhile, accelerating the aging rate of leaves, improves the reuse of nutrients and promotes the accumulation and maturation of fruit nutrients. Although photosynthesis and photoconjugates play important roles in leaf aging, the molecular mechanisms of aging induction need to be further studied. Controlling leaf aging has important implications for agricultural and horticultural production. Screening mutants with missing functions during leaf aging is beneficial to unravel the mystery of the molecular mechanism of leaf senescence. We believe that by delaying leaf aging, coupled with improved photosynthesis and tolerance to stress, it is possible for horticultural plants to achieve stable yields and yield potential. Leaf senescence is an important part of plant development, but also an adaptation strategy for plants to cope with stress, and the regulation of the aging process is an important method for plants to adapt to different environmental conditions. In summary, the relationship between aging and productivity, quality, and stress tolerance is complex. To achieve high yield and quality of horticultural crops, experts in different fields must work together.
Author contributions
YH, WZ and HW conceived and designed the review. WZ wrote the manuscript, and YH proposed revisions to the manuscript. All authors contributed to the article and approved the submitted version.
Funding
This study was supported by Lingyan Project of Science and Technology Department of Zhejiang Province (Grant No.2022C02051), the National Natural Science Foundation of China (Grant Nos.31872105, 31972221, 32002048), Ministry of Agriculture, and the National Key Research and Development Program of China (Nos.2018YFD1000800 and 2019YFD1000300)
Conflict of interest
The authors declare that the research was conducted in the absence of any commercial or financial relationships that could be construed as a potential conflict of interest.
Publisher’s note
All claims expressed in this article are solely those of the authors and do not necessarily represent those of their affiliated organizations, or those of the publisher, the editors and the reviewers. Any product that may be evaluated in this article, or claim that may be made by its manufacturer, is not guaranteed or endorsed by the publisher.
References
Achard, P., Cheng, H., De Grauwe, L., Decat, J., Schoutteten, H., Moritz, T., et al. (2006). Integration of plant responses to environmentally activated phytohormonal signals. Science 311, 91–94. doi: 10.1126/science.1118642
An, R., Chen, W., Hu, H., Li, P. (2020). Effects of storage temperature on senescence and active ingredients of postharvest shanghai green leaves. Packaging Eng. 41 (09), 7–16. doi: 10.19554/j.cnki.1001-3563.2020.09.002
An, J. P., Zhang, X. W., Bi, S. Q., You, C. X., Wang, X. F., Hao, Y. J. (2019). Mdb HLH 93, an apple activator regulating leaf senescence, is regulated by ABA and md BT 2 in antagonistic ways. New Phytol. 222 (2), 735–751. doi: 10.1111/nph.15628
An, J. P., Zhang, X. W., Liu, Y. J., Zhang, J. C., Wang, X. F., You, C. X., et al. (2021). MdABI5 works with its interaction partners to regulate abscisic acid-mediated leaf senescence in apple. Plant J. 105 (6), 1566–1581. doi: 10.1111/tpj.15132
Bartoli, C. G., Casalongué, C. A., Simontacchi, M., Marquez-Garcia, B., Foyer, C. H. (2013). Interactions between hormone and redox signalling pathways in the control of growth and cross tolerance to stress. Environ. Exp. Bot. 94, 73–88. doi: 10.1016/j.cbpa.2009.04.414
Blumwald, E., Rivero, R. M., Wadia, H., Peleg, Z., Szczerba, M., Jauregui, R.N., Li, L., et al. (2009). Delayed leaf senescence induces extreme drought tolerance incrop plants. Comparative Biochemistry and Physiology Part A: Molecular & Integrative Physiology, 153 (2), S188. doi: 10.1016/j.cbpa.2009.04.414
Brower, B., Ziolkowska, A., Bagard, M., Keech, O., Gardestrom, P. (2012). The impact of light intensity on shade-induced leaf senescence. Plant Cell Environ. 35 (6), 1084–1098. doi: 10.1111/j.1365-3040.2011.02474.x
Buchanan-Wollaston, V., Page, T., Harrison, E., Breeze, E., Lim, P. O., Nam, H. G., et al. (2005). Comparative transcriptome analysis reveals significant differences in gene expression and signalling pathways between developmental and dark/starvation-induced senescence in arabidopsis. Plant J. 42 (4), 567–585. doi: 10.1111/j.1365-313x.2005.02399.x
Chen, Y., Feng, P., Tang, B., Hu, Z., Xie, Q., Zhou, S., et al. (2022). The AP2/ERF transcription factor SlERF. F5 functions in leaf senescence in tomato. Plant Cell Rep. 41 (5), 1181–1195. doi: 10.1007/s00299-022-02846-1
Chen, Q., Ye, H., Liu, W., LI, Y., Chen, J. (2001). The role of CPPU in delaying the aging of cabbage leaves. Northwest Bot. Sin. 21 (06), 197–200. doi: 10.3321/j.issn:1000-4025.2001.06.032
Ding, F., Wang, C., Xu, N., Zhang, S., Wang, M. (2022). SlMYC2 mediates jasmonate-induced tomato leaf senescence by promoting chlorophyll degradation and repressing carbon fixation. Plant Physiol. Biochem. 180, 27–34. doi: 10.1016/j.jplph.2008.11.002
Dosio, G. A. A., Izquierdo, N. G., Núñez Bordoy, E. I., Aguirrezábal, L. A. N. (2020). Leaf senescence did not account for variations in grain and oil yield observed in sunflower under radiation limiting conditions. Agric. For. Meteorology 291, 108032. doi: 10.1016/j.agrformet.2020.108032
Fan, Z. Q., Tan, X. L., Shan, W., Kuang, J. F., Lu, W. J., Chen, J. Y. (2018). Characterization of a transcriptional regulator, BrWRKY6, associated with gibberellin-suppressed leaf senescence of Chinese flowering cabbage. J. Agric. Food Chem. 66 (8), 1791–1799. doi: 10.1021/acs.jafc.7b06085
Fan, Z. Q., Tan, X. L., Shan, W., Kuang, J. F., Lu, W. J., Lin, H. T., et al. (2020). Involvement of BrNAC041 in ABA-GA antagonism in the leaf senescence of Chinese flowering cabbage. Postharvest Biol. Technol. 168, 111254. doi: 10.1016/j.postharvbio.2020.111254
Fan, Z., Wei, W., Tan, X., Shan, W., Kuang, J., Lu, W., et al. (2021). A NAC transcription factor BrNAC087 is involved in gibberellin-delayed leaf senescence in Chinese flowering cabbage. Postharvest Biol. Technol. 181, 111673. doi: 10.1016/j.postharvbio.2021.11167
Feller, U., Anders, I., Mae, T. (2008). Rubiscolytics: Fate of rubisco after its enzymatic function in a cell is terminated. J. Exp. Bot. 59, 1615–1624. doi: 10.1093/jxb/erm242
Gan, S. (2003). Mitotic and postmitotic senescence in plants. Sci. Aging Knowledge Environ. 2003 (38), re7–re7. doi: 10.1126/sageke.2003.38.re7
Gan, S.-S., Hörtensteiner, S. (2013). Frontiers in plant senescence research: from bench to bank. Plant Mol. Biol. 82 (6), 503–504. doi: 10.1007/s11103-013-0098-0
Gao, X. (1998). Research on broccoli storage technology. Yangtze River Vegetables 11, 36–37. Available at: https://kns-cnki-net-443.webvpn.zafu.edu.cn/kcms/detail/detail.aspx?FileName=CJSC199811025&DbName=CJFQ1998.
Gombert, J. (2006). The expression patterns of SAG12/Cab genes reveal the spatial and temporal progression of leaf senescence in brassica napus l. with sensitivity to the environment. J. Exp. Bot. 57 (9), 1949–1956. doi: 10.1093/jxb/erj142
Gómez, C., Mitchell, C. A. (2016). Physiological and productivity responses of high-wire tomato as affected by supplemental light source and distribution within the canopy. J. Am. Soc. Hortic. Sci. 141 (2), 196–208. doi: 10.21273/JASHS.141.2.196
Gregersen, P. L., Culetic, A., Boschian, L., Krupinska, K. (2013). Plant senescence and crop productivity. Plant Mol. Biol. 82 (6), 603–622. doi: 10.1007/s11103-013-0013-8
Gregersen, P. L., Holm, P. B., Krupinska, K. (2008). Leaf senescence and nutrient remobilisation in barley and wheat. Plant Biol. 10, 37–49. doi: 10.1111/j.1438-8677.2008.00114.x
Gu, L., Ma, Q., Zhang, C., Wang, C., Wei, H., Wang, H., et al. (2019). The cotton GhWRKY91 transcription factor mediates leaf senescence and responses to drought stress in transgenic arabidopsis thaliana. Front. Plant Sci. 10. doi: 10.3389/fpls.2019.01352
Guo, Y., Gan, S.-S. (2011). Convergence and divergence in gene expression profiles induced by leaf senescence and 27 senescence-promoting hormonal, pathological and environmental stress treatments. Plant Cell Environ. 35 (3), 644–655. doi: 10.1111/j.1365-3040.2011.02442.x
Han, C., Yu, M., Wang, Q., Wang, L., Yang, H., Zhao, Y., et al. (2020). Leaf structure and seed histochemistry analyses provided structural insights into the improved yield and quality of tree peony seed under light shading conditions. Sci. Rep. 10 (1), 1–9. doi: 10.1038/s41598-020-61366-8
Harris-Shultz, K. R., Hayes, C. M., Knoll, J. E. (2019). Mapping QTLs and identification of genes associated with drought resistance in sorghum. Sorghum 1931, 11–40. doi: 10.1007/978-1-4939-9039-9_2
Hassan, F. A. S., Mahfouz, S. A. (2012). Effect of 1-methylcyclopropene (1-MCP) on the postharvest senescence of coriander leaves during storage and its relation to antioxidant enzyme activity. Scientia Hortic. 141, 69–75. doi: 10.1016/j.scienta.2012.04.021
Havé, M., Marmagne, A., Chardon, F., Masclaux-Daubresse, C. (2016). Nitrogen remobilisation during leaf senescence: lessons from arabidopsis to crops. J. Exp. Bot. 68 (10), erw365. doi: 10.1093/jxb/erw365
Hong, Y., Zhang, J., Lv, Y., Yao, N., Liu, X. (2022). The safflower bHLH transcription factor CtbHLH41 negatively regulates SA-induced leaf senescence through interaction with CtCP1. Environ. Exp. Bot. 199, 104883. doi: 10.1016/j.envexpbot.2022.104883
Huang, S., Gong, B., Wei, F., Ma, H. (2017). Pre-harvest 1-methylcyclopropene application affects post-harvest physiology and storage life of the cut rose cv. Carola. Horticulture Environment Biotechnol. 58 (2), 144–151. doi: 10.1007/s13580-017-0081-9
Huang, D., Lan, W., Ma, W., Huang, R., Lin, W., Li, M., et al. (2022). WHIRLY1 recruits the histone deacetylase HDA15 repressing leaf senescence and flowering in arabidopsis. J. Integr. Plant Biol 64 (7), 1411–1429. doi: 10.1111/jipb.13272
Huang, S., Zhu, K., Chen, Y., Wang, X., Wang, Y. (2021). Gerbera POE1 (GhPOE1) is involved in leaf senescence in arabidopsis. South Afr. J. Bot. 143, 33–41. doi: 10.1016/j.sajb.2021.06.039
Hu, Y., Jiang, Y., Han, X., Wang, H., Pan, J., Yu, D. (2017). Jasmonate regulates leaf senescence and tolerance to cold stress: crosstalk with other phytohormones. J. Exp. Bot. 68 (6), 1361–1369. doi: 10.1093/jxb/erx004
Jan, S., Abbas, N., Ashraf, M., Ahmad, P. (2018). Roles of potential plant hormones and transcription factors in controlling leaf senescence and drought tolerance. Protoplasma 256 (2), 313–329. doi: 10.1007/s00709-018-1310-5
Kant, S., Burch, D., Badenhorst, P., Palanisamy, R., Mason, J., Spangenberg, G. (2015). Regulated expression of a cytokinin biosynthesis gene IPT delays leaf senescence and improves yield under rainfed and irrigated conditions in canola (Brassica napus l.). PloS One 10 (1), e0116349. doi: 10.1371/journal.pone.0116349
Keithly, J. H., Yokoyama, H., Gausman, H. W. (1990). Effect of 2-(3,4-dichlorophenoxy) triethylamine (DCPTA) on the growth and development of sugarbeet. Plant Science, 68 (1), 57–64. doi: 10.1016/0168-9452(90)90152-e
Laloi, C., Havaux, M. (2015). Key players of singlet oxygen-induced cell death in plants. Front. Plant Sci. 6. doi: 10.3389/fpls.2015.00039
Lee, I. H., Lee, I. C., Kim, J., Kim, J. H., Chung, E.-H., Kim, H. J., et al. (2016). NORE1/SAUL1 integrates temperature-dependent defense programs involving SGT1b and PAD4 pathways and leaf senescence in arabidopsis. Physiologia Plantarum 158 (2), 180–199. doi: 10.1111/ppl.12434
Lee, J. H., Park, Y. J., Kim, J. Y., Park, C. M. (2022). Phytochrome b conveys low ambient temperature cues to the ethylene-mediated leaf senescence in arabidopsis. Plant Cell Physiol. 63 (3), 326–339. doi: 10.1093/pcp/pcab178
Lee, S., Seo, P. J., Lee, H.-J., Park, C.-M. (2012). A NAC transcription factor NTL4 promotes reactive oxygen species production during drought-induced leaf senescence in arabidopsis. Plant J. 70 (5), 831–844. doi: 10.1111/j.1365-313x.2012.04932.X
Leng, Y., Ye, G., Zeng, D. (2017). Genetic dissection of leaf senescence in rice. Int. J. Mol. Sci. 18 (12), 2686. doi: 10.3390/ijms18122686
Li, J., Brader, G., Kariola, T., Tapio Palva, E. (2006). WRKY70 modulates the selection of signaling pathways in plant defense. Plant J. 46 (3), 477–491. doi: 10.1111/j.1365-313x.2006
Li, S., Dami, I. E. (2015). Responses of vitis vinifera “Pinot gris” grapevines to exogenous abscisic acid (ABA): I. yield, fruit quality, dormancy, and freezing tolerance. J. Plant Growth Regul. 35 (1), 245–255. doi: 10.1007/s00344-015-9529-2
Lim, P. O., Kim, H. J., Gil Nam, H. (2007). Leaf senescence. Annu. Rev. Plant Biol. 58, 115–136. doi: 10.1146/annurev.arplant.57.032905.105316
Lira, B. S., Gramegna, G., Trench, B. A., Alves, F. R. R., Silva, E. M., Silva, G. F. F., et al. (2017). Manipulation of a senescence-associated gene improves fleshy fruit yield. Plant Physiol. 175 (1), 77–91. doi: 10.1104/pp.17.00452
Liu, K., Jing, T., Wang, Y., Ai, X., Bi, H. (2022a). Melatonin delays leaf senescence and improves cucumber yield by modulating chlorophyll degradation and photoinhibition of PSII and PSI. Environ. Exp. Bot. 200, 104915. doi: 10.1016/j.envexpbot.2022.104915
Liu, R., Zuo, X., Chen, Y., Qian, Z., Xu, C., Wang, L., et al. (2022b). Transcriptional regulation in leaves of cut chrysanthemum (Chrysanthemum morifolium) ‘Fen dante’ in response to post-harvest ethylene treatment. Horticulturae 8 (7), 573. doi: 10.3390/horticulturae8070573
Liu, P., Wang, X., Wang, Q., Liu Xu, T. X. (2017). Effects of abscisic acid treatment on the quality and browning of the peel of 'yellow crown' pear after harvesting. Journal of Agriculture (02), 84–90. Available at: https://kns-cnki-net-443.webvpn.zafu.edu.cn/kcms/detail/detail.aspx?FileName=XKKJ201702018&DbName=CJFQ2017.
Luo, Y. Y., Gianfagna, T. J., Janes, H. W., Huang, B., Wang, Z., Xing, J. (2005). Expression of the ipt gene with the AGPase S1 promoter in tomato results in unbranched roots and delayed leaf senescence. Plant Growth Regul. 47 (1), 47–57. doi: 10.1007/s10725-005-8647-4
Lü, P., Zhang, C., Liu, J., Liu, X., Jiang, G., Jiang, X., et al. (2014). Rh HB 1 mediates the antagonism of gibberellins to ABA and ethylene during rose (Rosa hybrida) petal senescence. Plant J. 78 (4), 578–590. doi: 10.1111/tpj.12494
Ma, S. (2006). Study on the effect of 1-MCP treatment on postharvest aging and storage of leafy vegetables (Nanjing Agricultural University: MA thesis).
Maillard, A., Diquélou, S., Billard, V., Laîné, P., Garnica, M., Prudent, M., et al. (2015). Leaf mineral nutrient remobilization during leaf senescence and modulation by nutrient deficiency. Front. Plant Sci. 6. doi: 10.3389/fpls.2015.00317
Mannucci, A., Scartazza, A., Santaniello, A., Castagna, A., Santin, M., Quartacci, M. F., et al. (2022). Short daily ultraviolet exposure enhances intrinsic water-use efficiency and delays senescence in micro-tom tomato plants. Funct. Plant Biol. 49 (9), 810–821. doi: 10.1071/fp22013
Ma, P., Wu, W., Tang, X., Huang, Y., Wei, Y. (2009). Effects of pre-harvest spraying salicylic acid (SA) and gibberellin (GA3) on the physiology and storage effect of gongci fruit after harvesting. J. Fruit Trees 6, 891–894.
Mayta, M. L., Hajirezaei, M.-R., Carrillo, N., Lodeyro, A. F. (2019). Leaf senescence: The chloroplast connection comes of age. Plants 8 (11), 495. doi: 10.3390/plants8110495
Ma, X., Zhang, Y., Turečková, V., Xue, G.-P., Fernie, A. R., Mueller-Roeber, B., et al. (2018). The NAC transcription factor SlNAP2 regulates leaf senescence and fruit yield in tomato. Plant Physiol. 177 (3), 1286–1302. doi: 10.1104/pp.18.00292
Miret, J. A., Munné-Bosch, S., Dijkwel, P. P. (2017). ABA signalling manipulation suppresses senescence of a leafy vegetable stored at room temperature. Plant Biotechnol. J. 16 (2), 530–544. doi: 10.1007/s00299-011-1170-3
Miret, J. A., Munné-Bosch, S., Dijkwel, P. P. (2018). ABA signalling manipulation suppresses senescence of a leafy vegetable stored at room temperature. Plant Biotechnol. J. 16 (2), 530–544. doi: 10.1111/pbi.12793
Pan, Y., Seymour, G. B., Lu, C., Hu, Z., Chen, X., Chen, G. (2012). An ethylene response factor (ERF5) promoting adaptation to drought and salt tolerance in tomato. Plant cell rep. 31 (2), 349–360. doi: 10.1007/s00299-011-1170-3
Sami, F., Yusuf, M., Faizan, M., Faraz, A., Hayat, S. (2016). Role of sugars under abiotic stress. Plant Physiol. Biochem. 109, 54–61. doi: 10.1016/j
Schulte auf’m Erley, G., Wijaya, K.-A., Ulas, A., Becker, H., Wiesler, F., Horst, W. J. (2007). Leaf senescence and n uptake parameters as selection traits for nitrogen efficiency of oilseed rape cultivars. Physiologia Plantarum 130 (4), 519–531. doi: 10.1111/j.1399-3054.2007.00921.x
Sobieszczuk-Nowicka, E., Wrzesiński, T., Bagniewska-Zadworna, A., Kubala, S., Rucińska-Sobkowiak, S. R., Polcyn, W., et al. (2018). Physio-Genetic Dissection of Dark-Induced Leaf Senescence and Timing Its Reversal in Barley. Plant Physiol. 178 (2), 654–671. doi: 10.1104/pp.18.00516
Song, S., Ge, M., Wang, W., Gu, C., Chen, K., Zhang, Q., et al. (2022). BpEIN3. 1 represses leaf senescence by inhibiting synthesis of ethylene and abscisic acid in betula platyphylla. Plant Sci. 321, 111330. doi: 10.1016/j.plantsci.2022.111330
Song, Y., Yang, C., Gao, S., Zhang, W., Li, L., Kuai, B. (2014). Age-triggered and dark-induced leaf senescence require the bHLH transcription factors PIF3, 4, and 5. Mol. Plant 7 (12), 1776–1787. doi: 10.1093/mp/ssu109
Stefanov, D., Stoimenova, E., Marinova, G., Ivanova, B., Edreva, A. (2011). Accelerated leaf senescence takes part in enhanced resistance in cucumber mosaic virus inoculated pepper leaves. Acta Physiologiae Plantarum 34 (1), 181–190. doi: 10.1007/s11738-011-0816-7
Swartzberg, D., Dai, N., Gan, S., Amasino, R., Granot, D. (2006). Effects of cytokinin production under two SAG promoters on senescence and development of tomato plants. Plant Biol. 8 (5), 579–586. doi: 10.1055/s-2006-924240
Swartzberg, D., Hanael, R., Granot, D. (2011). Relationship between hexokinase and cytokinin in the regulation of leaf senescence and seed germination. Plant Biol. 13 (3), 439–444. doi:10.1111/j.1438-8677.2010.00376.x
Talla, S. K., Panigrahy, M., Kappara, S., Nirosha, P., Neelamraju, S., Ramanan, R. (2016). Cytokinin delays dark-induced senescence in rice by maintaining the chlorophyll cycle and photosynthetic complexes. J. Exp. Bot. 67 (6), 1839–1851. doi: 10.1093/jxb/erv575
Tan, X., Fan, Z., Shan, W., Yin, X., Kuang, J., Lu, W., et al. (2018). Association of BrERF72 with methyl jasmonate-induced leaf senescence of Chinese flowering cabbage through activating JA biosynthesis-related genes. Hortic. Res. 5 (1), 22. doi: 10.1038/s41438-018-0028-z
Thomas, H., Stoddart, J.L. (2018). Leaf senescence. Annu. Rev. Plant Physiol. 31 (1), 83–111, doi: 10.1146/annurev.pp.31.060180.000503
Tian, Y., Hou, J., Li, D. (2014). Effects of water stress on thephysiological physiology of spinach leaves during refrigeration. Preservation and processing 14 (4), 10–15. doi: 10.3969/j.issn.1009-6221.2014.04.003
Tomotsugu, K. (2018). A hidden link between leaf development and senescence. Plant Sci. 276, 105–110. doi: 10.1016/j.plantsci.2018.08.006
Thiruvengadam, M., Shih, C. F., Yang, C.H. (2015). Expression of an antisense Brassica oleracea GIGANTEA (BoGI) gene in transgenic broccoli causes delayed flowering, leaf senescence, and post-harvest yellowing retardation. Plant Mol Biol Rep. 33 (5), 1499–1509. doi: 10.1007/s11105-015-0852-3
Tian, Y., Hou, J., Li, D. (2014). “Effects of water stress on spinach leaf senescence during refrigeration,” in Proceedings of the 9th national food refrigeration chain conference and the 6th national annual conference on innovation and development of refrigerated and refrigerated industry. 64–70.
Tomotsugu, K. (2018). A hidden link between leaf development and senescence. Plant Science. doi: 10.1016/j.plantsci.2018.08.006
Uhlig, S., Fæste, C. K., Ivanova, L., Noriega Fernández, E., Sone, I., Sivertsvik, M. (2021). Impact of plasma-activated water treatment on quality and shelf-life of fresh spinach leaves evaluated by comprehensive metabolomic analysis. Foods 10 (12), 3067 doi: 10.3390/foods10123067
Wang, Y., Cui, X., Yang, B., Xu, S., Wei, X., Zhao, P., et al. (2020). WRKY55 transcription factor positively regulates leaf senescence and defense response through modulating the transcription of genes implicated in ROS and SA biosynthesis in arabidopsis. Development, 147 (16), dev.189647. doi: 10.1242/dev.189647
Wang, Z., Gao, M., Li, Y., Zhang, J., Su, H., Cao, M., et al. (2022). The transcription factor SlWRKY37 positively regulates jasmonic acid-and dark-induced leaf senescence in tomato. J. Exp. Botany. erac258. doi: 10.1093/jxb/erac258
Wang, X., Rehmani, M. S., Chen, Q., Yan, J., Zhao, P., Li, C., et al. (2022). Rapeseed NAM transcription factor positively regulates leaf senescence via controlling senescence-associated gene expression. Plant Sci. 323, 111373. doi: 10.1016/j.plantsci.2022.111373
Wang, Y., Tan, J., Wu, Z., Vanden Langenberg, K., Wehner, T. C., Wen, C., et al. (2019). STAYGREEN, STAY HEALTHY: a loss-of-susceptibility mutation in the STAYGREEN gene provides durable, broad-spectrum disease resistances for over 50 years of US cucumber production. New Phytol. 221 (1), 415–430. doi: 10.1111/nph.15353
Wang, N., Guo, T., Wang, P., Sun, X., Shao, Y., Liang, B., et al (2017). Functional analysis of apple MhYTP1 and MhYTP2 genes in leaf senescence and fruit ripening. Scientia Horticulturae. 221, 23–32. doi: 10.1016/j.scienta.2017.04.018
Weaver, L. M., Gan, S., Quirino, B., Amasino, R. M. (1998). A comparison of the expression patterns of several senescence-associated genes in response to stress and hormone treatment. Plant Mol. Biol. 37 (3), 455–469. doi: 10.1023/a:1005934428906
Wen, B., Gong, X., Deng, W., Chen, X., Li, D., Fu, X., et al. (2022). The apple GARP family gene MdHHO3 regulates the nitrate response and leaf senescence. Front. Plant Sci. 13. doi: 10.3389/fpls.2022.932767
Woo, H. R., Kim, H. J., Lim, P. O., Nam, H. G. (2019). Leaf senescence: Systems and dynamics aspects. Annu. Rev. Plant Biol. 70 (1), 347–376. doi: 10.1146/annurev-arplant-050718-095859
Xiao, X. M., Xu, Y. M., Zeng, Z. X., Tan, X. L., Liu, Z. L., Chen, J. W., et al. (2019). Activation of the transcription of BrGA20ox3 by a BrTCP21 transcription factor is associated with gibberellin-delayed leaf senescence in chinese flowering cabbage during storage. Int. J. Mol. Sci. 20 (16), 3860. doi: 10.3390/ijms20163860
Xiao, F., Yang, Z., Han, W., Li, Y., Qiu, Y., Sun, Q., et al. (2018). Effects of day and night temperature on photosynthesis, antioxidant enzyme activities, and endogenous hormones in tomato leaves during the flowering stage. J. Hortic. Sci. Biotechnol. 93 (3), 306–315. doi: 10.1080/14620316.2017.1287529
Xiao, X. O., Zeng, Y. M., Cao, B. H., Lei, J. J., Chen, Q. H., Meng, C. M., et al. (2017). PSAG12-IPT overexpression in eggplant delays leaf senescence and induces abiotic stress tolerance. J. Hortic. Sci. Biotechnol. 92 (4), 349–357. doi: 10.1080/14620316.2017.1287529
Xu, X., Chen, C., Fan, B., Chen, Z. (2006). Physical and functional interactions between pathogen-induced arabidopsis WRKY18, WRKY40, and WRKY60 transcription factors. Plant Cell 18 (5), 1310–1326. doi: 10.1105/tpc.105.037523
Yang, K., An, J. P., Li, C. Y., Shen, X. N., Liu, Y. J., Wang, D. R., et al. (2021). The apple C2H2-type zinc finger transcription factor MdZAT10 positively regulates JA-induced leaf senescence by interacting with MdBT2. Hortic. Res. 8, 159. doi: 10.1038/s41438-021-00593-0
Yang, M., Hu, F., Lin, Y., Wang, X. (2015). Effects of NAA and GA3 on fruit drop and quality of A4 seedless lychee before harvesting. Guangdong Agric. Sci. 04), 19–22. doi: 10.16768/j
Yang, S., Liang, K., Wang, A., Zhang, M., Qiu, J., Zhang, L. (2020). Physiological characterization and transcriptome analysis of camellia oleifera abel. during leaf senescence. Forests 11 (8), 812. doi: 10.3390/f11080812
Yang, L., Ye, C., Zhao, Y., Cheng, X., Wang, Y., Jiang, Y. Q., et al. (2018). An oilseed rape WRKY-type transcription factor regulates ROS accumulation and leaf senescence in nicotiana benthamiana and arabidopsis through modulating transcription of RbohD and RbohF. Planta 247 (6), 1323–1338. doi: 10.1007/s00425-018-2868-z
Yang, Z., Zhu, J., Zhang, B., Gu, L., Zhang, J. (2012). Effect of high temperature treatment on aging characteristics of strawberry leaves at the fruiting stage. Chin. Agric. Meteorology 04), 512–518.
Yan, J., Li, Y., Zhao, P., Mu, B., Chen, Q., Li, X., et al. (2021). Membrane-bound transcriptional activator NTL1 from rapeseed positively modulates leaf senescence through targeting genes involved in reactive oxygen species production and programmed cell death. J. Agric. Food Chem. 69 (17), 4968–4980. doi: 10.1021/acs.jafc.1c00182
Yu, T., Xi, Y. (1997). Effect of temperature on ethylene release and reactive oxygen species metabolism in Chinese cabbage. Zhejiang J. Agric. 06), 11–15. Available at: https://kns-cnki-net-443.webvpn.zafu.edu.cn/kcms/detail/detail.aspx?FileName=ZJNB706.002&DbName=CJFQ1997.
Zhang, Li Y., Liu, H. H, Zhang, Y. P., Zhu, Li B. (2014). Effects of calcium nitrate on leaf senescence and fruit yield and quality of pachyderm melon fruit setting nodes. Plant Nutr. Fertilizer Sci. 02), 490–495. doi: 10.13320/j.cnki.jauh.2014.0008
Zhang, Y., Luo, J., Peng, F., Xiao, Y., Du, A. (2021). Application of bag-controlled release fertilizer facilitated new root formation, delayed leaf, and root senescence in peach trees and improved nitrogen utilization efficiency. Front. Plant Sci. 12. doi: 10.3389/fpls.2021.627313
Zhang, Y., Wang, H. L., Li, Z., Guo, H. (2020). Genetic network between leaf senescence and plant immunity: crucial regulatory nodes and new insights. Plants 9 (4), 495. doi: 10.3390/plants9040495
Zhang, D., Wu, S., Li, N., Gao, J., Liu, S., Zhu, S., et al. (2022). Chemical induction of leaf senescence and powdery mildew resistance involves ethylene-mediated chlorophyll degradation and ROS metabolism in cucumber. Hortic. Res. 9, uhac101. doi: 10.1093/hr/uhac101
Zhao, Y., Chan, Z., Gao, J., Xing, L., Cao, M., Yu, C., et al. (2016). ABA receptor PYL9 promotes drought resistance and leaf senescence. Proc. Natl. Acad. Sci. 113 (7), 1949–1954. doi: 10.1073/pnas.1522840113
Zhao, S., Deng, C., Zeng, Z., Wu, M., Shi, X., Duan, X., et al. (2022). Changes in mineral nutrition and photosynthetic capacity during leaf development and aging of sunshine rose' grapes and related properties. J. Pomology. 1–14 doi: 10.13925/j.cnki.gsxb.20220001
Zhao, X., Zhang, T., Feng, H., Qiu, T., Li, Z., Yang, J., et al. (2021). OsNBL1, a multi-organelle localized protein, plays essential roles in rice senescence, disease resistance, and salt tolerance. Rice 14 (1), 1–17. doi: 10.1186/s12284-020-00450-z
Zha, Q., Yin, X., Xi, X., Jiang, A. (2022). Colored shade nets can relieve abnormal fruit softening and premature leaf senescence of “Jumeigui” grapes during ripening under greenhouse conditions. Plants 11 (9), 1227. doi: 10.3390/plants11091227
Zheng, C., Wang, Y., Ding, Z., Zhao, L. (2016). Global transcriptional analysis reveals the complex relationship between tea quality, leaf senescence and the responses to cold-drought combined stress in camellia sinensis. Front. Plant Sci. 7. doi: 10.3389/fpls.2016.01858
Zhou, D., Li, T., Yang, Y., Qu, Z., Ouyang, L., Jiang, Z., et al. (2020). OsPLS4 is involved in cuticular wax biosynthesis and affects leaf senescence in rice. Front. Plant Sci. 11. doi: 10.3389/fpls.2020.00782
Keywords: leaf senescence, yield, quality, stress resistance, post-harvest storage
Citation: Zhao W, Zhao H, Wang H and He Y (2022) Research progress on the relationship between leaf senescence and quality, yield and stress resistance in horticultural plants. Front. Plant Sci. 13:1044500. doi: 10.3389/fpls.2022.1044500
Received: 14 September 2022; Accepted: 12 October 2022;
Published: 24 October 2022.
Edited by:
Peitao Lü, Fujian Agriculture and Forestry University, ChinaReviewed by:
Zheng Li, Northwest A&F University, ChinaQiang Li, Agricultural University of Hebei, China
Copyright © 2022 Zhao, Zhao, Wang and He. This is an open-access article distributed under the terms of the Creative Commons Attribution License (CC BY). The use, distribution or reproduction in other forums is permitted, provided the original author(s) and the copyright owner(s) are credited and that the original publication in this journal is cited, in accordance with accepted academic practice. No use, distribution or reproduction is permitted which does not comply with these terms.
*Correspondence: Huasen Wang, whsych66@163.com; Yong He, heyong@zafu.edu.cn