- 1Key Laboratory for Subtropical Mountain Ecology (Ministry of Science and Technology and Fujian Province Funded), College of Geographical Sciences, Fujian Normal University, Fuzhou, China
- 2Key Laboratory for Humid Subtropical Eco-Geographical Processes of the Ministry of Education, Fujian Normal University, Fuzhou, China
- 3Fujian Provincial Key Laboratory for Plant Eco-physiology, Fuzhou, China
Carbon isotope composition (δ13C) has been widely used to estimate the intrinsic water-use efficiency (iWUE) of plants in ecosystems around the world, providing an ultimate record of the functional response of plants to climate change. This approach relies on established relationships between leaf gas exchange and isotopic discrimination, which are reflected in different formulations of 13C-based iWUE models. In the current literature, most studies have utilized the simple, linear equation of photosynthetic discrimination to estimate iWUE. However, recent studies demonstrated that using this linear model for quantitative studies of iWUE could be problematic. Despite these advances, there is a scarcity of review papers that have comprehensively reviewed the theoretical basis, assumptions, and uncertainty of 13C-based iWUE models. Here, we 1) present the theoretical basis of 13C-based iWUE models: the classical model (iWUEsim), the comprehensive model (iWUEcom), and the model incorporating mesophyll conductance (iWUEmes); 2) discuss the limitations of the widely used iWUEsim model; 3) and make suggestions on the application of the iWUEmes model. Finally, we suggest that a mechanistic understanding of mesophyll conductance associated effects and post-photosynthetic fractionation are the bottlenecks for improving the 13C-based estimation of iWUE.
Introduction
During photosynthesis, plant stomata act as a control valve for the diffusion of CO2 and water vapor, regulating the rates of water and carbon exchange between the biosphere and the atmosphere (de Boer et al., 2011; Adams et al., 2020; Walker et al., 2021). Intrinsic water-use efficiency (iWUE), defined as the ratio of net photosynthetic rate (An) to stomatal conductance for water vapor (gsw), plays a key role in quantifying carbon uptake and water loss at leaf to continental scales (Seibt et al., 2008; Keenan et al., 2013). The response of iWUE is fundamental to climate change research since small changes in iWUE can have profound impacts on global carbon and water cycles. Furthermore, iWUE can provide insights into the mechanisms of plant physiological responses to climate change and support the screening and breeding of climate-resilient crops (Farquhar and Richards, 1984; von Caemmerer et al., 2014; Gresset et al., 2014). Central to these research domains is the quantification of iWUE.
Stable carbon isotope discrimination (Δ) can be used as an integrated measure of iWUE in C3 plants (Farquhar et al., 1989). Plants discriminate against 13C in favour of 12C during photosynthetic CO2 assimilation in C3 leaves, and the variation in carbon isotope composition (δ13C) from source CO2 to photosynthetic products (e.g., bulk leaf organic carbon or sugars) is termed as Δ, following Farquhar et al. (1982b); Farquhar et al. (1989):
where atmospheric δ13Ca is approximately -7~-8‰ during the 20th century. Δ can also be estimated from δ13C of CO2 entering (δin and Cin) and leaving (δout and Cout) the cuvette during gas exchange, termed as online 12C/13C discrimination (Evans et al., 1986):
where ξ= Cin/(Cin-Cout). In this way, Δ can be measured nondestructively to probe real-time responses of photosynthesis at high temporal resolution. Changes in photosynthetic parameters (An and gs) are captured in Δonline and the isotopic signatures are further imprinted on plant tissues during biosynthesis. As such, biomass-based Δ reflects physiological status of plants throughout the growth period of plant tissues (Cernusak et al., 2013; Soh et al., 2019). Different from classical approaches such as gas exchange or growth analysis, biomass-based Δ can be applied retrospectively, providing a useful record of iWUE at large spatial and temporal scales (Frank et al., 2015; Adams et al., 2020; Gong et al., 2022).
Inferring iWUE from isotopic records relies on theoretical models. In the current literature, most studies have utilized the simple, linear equation of photosynthetic discrimination to estimate iWUE. However, it can be problematic to interpret iWUE using this linear model which ignores effects other than diffusion through stomata and carboxylation. For instance, Seibt et al. (2008) suggested that the uncertainty in iWUE-13C models was related to the simplification of mesophyll conductance (gm). gm represents the conductance to CO2 diffusion from the intercellular space to the carboxylation site in chloroplasts, a key limiting factor of photosynthesis in addition to stomatal conductance and biochemical capacity (Tholen et al., 2012; Stangl et al., 2019). However, recent advances in δ13C-based iWUE estimation have not been systematically reviewed. The main objective of this mini review is to concisely summarize the theoretical basis and uncertainties of δ13C-based iWUE models. We (i) present different formulations of Δ and the associated assumptions, (ii) present Δ-based iWUE models derived from those formulations: the classical model (iWUEsim), the comprehensive model (iWUEcom), the model incorporating gm (iWUEmes), (iii) discuss the limitations of the widely used iWUEsim model; and make suggestions on the application of the iWUEmes model.
Comprehensive model of photosynthetic 13C discrimination and simplifications
A comprehensive description of 13C discrimination (Δcom) during C3 photosynthesis was given by Farquhar et al. (1982b) and extended to include ternary effects of transpiration on CO2 assimilation by Farquhar and Cernusak (2012):
and
where ab (2.9‰) and as (4.4‰) are fractionations associated with the diffusion of CO2 through leaf boundary layer and in the air, respectively. am (1.8‰) is the fractionation associated with the dissolution and diffusion of CO2 in mesophyll (see Table S1 for the list of parameters). Ca, Cs, Ci and Cc represent the mole fraction of CO2 in air, at leaf surface, in the intercellular spaces and chloroplast, respectively (Figure 1). Δcom can be separated into a series of fractionation components of leaf boundary layer conductance (Δgbc), stomatal conductance (Δgsc), mesophyll conductance (Δgm), Rubisco (ribulose-1,5-bisphosphate carboxylase/oxygenase) carboxylation (Δb), day respiration (Δe), and photorespiration (Δf). Note that t is included to account for the ternary effects of transpiration rate (E) on photosynthetic discrimination (Farquhar and Cernusak, 2012). Usually, the effect of t is small and can be omitted under low or moderate vapor pressure deficit (VPD) (Farquhar and Cernusak, 2012; Evans and Caemmerer, 2013). If Δgbc is also omitted (Ca=Cs and gac=gsc), the Δcom model is simplified as:
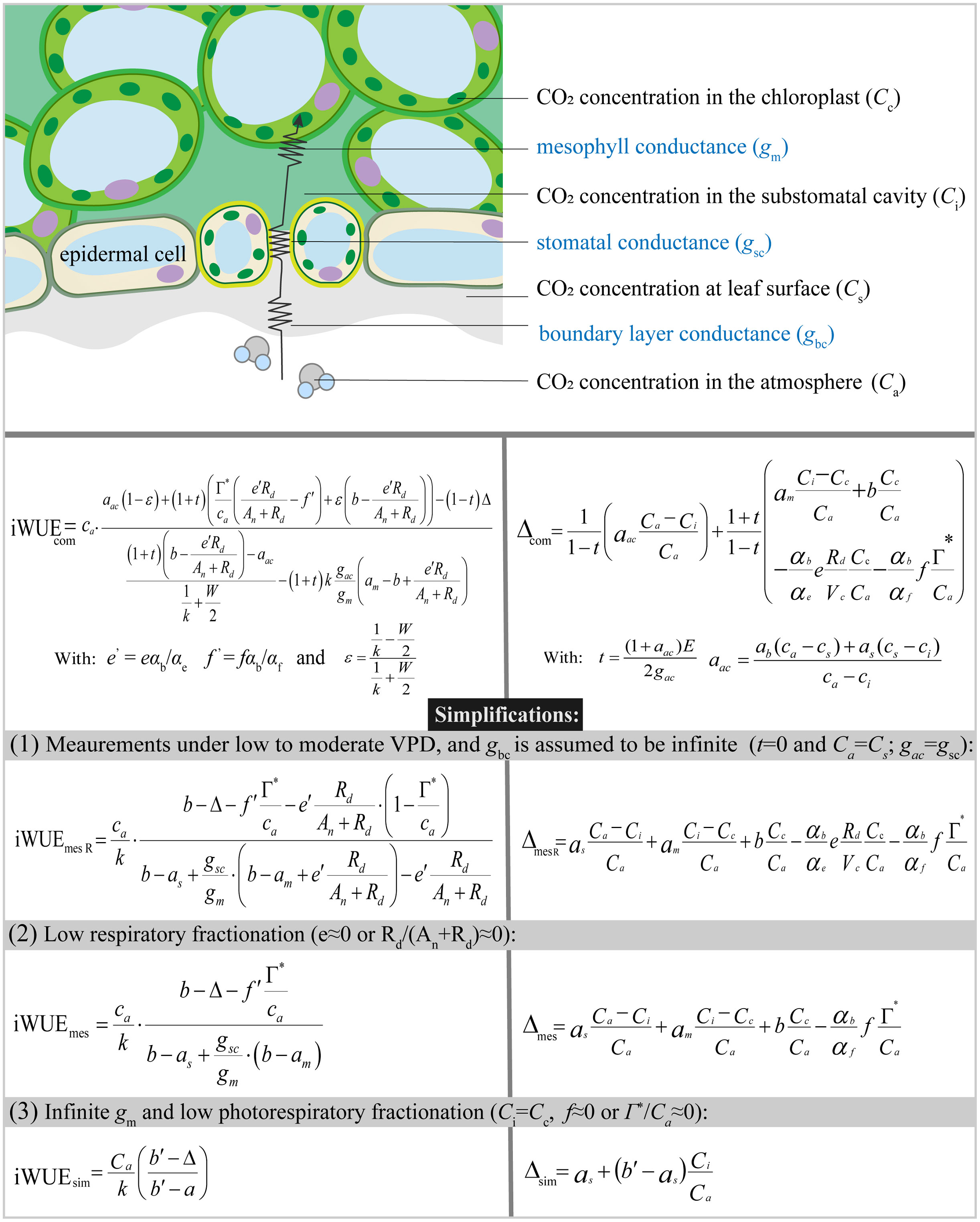
Figure 1 Diagram of the CO2 diffusion pathway in C3 leaves and different formulations of iWUE (iWUEcom, iWUEmes R, iWUEmes, and iWUEsim) derived from the Farquhar et al. model for photosynthetic 13C discrimination.
where the subscript “mes R” indicates that the expression takes mesophyll conductance, day respiration and photorespiration into account.
Δe, the respiratory contribution to discrimination is mainly determined by respiratory fractionation (e), and Rd/(An+ Rd). Δe has rarely been accurately quantified largely due to the difficulty of estimating Rd (Tcherkez et al., 2017; Gong et al., 2018). Moreover, fractionation of day respiration has rarely been reported, and e estimated from respiration in the dark varies between 0 and -6‰ (Ghashghaie et al., 2003; Tcherkez et al., 2010). Under natural conditions, Δe is usually small and negligible (Seibt et al., 2008; Ubierna and Farquhar, 2014). Notably, a significant apparent respiratory fractionation may occur when the CO2 source used for combined gas exchange and isotopic measurements has a δ13C differed from that of the ambient air (Gillon and Griffiths, 1997; Gong et al., 2015). Under such conditions, e should be corrected to account for the isotopic disequilibria between photosynthetic and respiratory fluxes (Wingate et al., 2007; Gong et al., 2015). Assuming Δe=0, Equation 6 can be simplified as:
Cc is usually unknown since its calculation requires gm which cannot be directly measured. gm is assumed to be infinite in early studies (for a review see Flexas et al., 2012); that is, CO2 mole fraction in the chloroplast is equal to that in the intercellular space. Assuming Ci=Cc and Δf=0, Equation 7 is simplified as:
Comprehensive model of iWUE and simplifications
The comprehensive model of iWUE which includes all fractionation components of Equation 3 was first derived by Ma et al. (2021):
where e’=eab/ae, f’=fab/af and є=(1/k-W/2)/(1/k+W/2). This formulation is particularly useful for assessing the contribution of each fractionation component to iWUE estimates. Ma et al. (2021) performed sensitivity tests using theoretical data of the standard photosynthetic scenarios. Their results indicated that ternary correction and Δgbc had little influence on iWUEcom estimates (error< 2 μmol mol-1), which is in agreement with Seibt et al. (2008). Neglecting the contribution of t and Δgbc, Equation 9 can be simplified as:
Δe in the iWUEmes R model could be ignored as it caused an error of less than 2 μmol mol-1 in typical photosynthetic scenarios (Ma et al., 2021). Excluding the contribution of day respiratory, Equation 10 can be simplified as:
The iWUEmes model provided iWUE estimates that are numerically very similar with iWUEcom (error< 3 μmol mol-1) (Ma et al., 2021). Neglecting the contribution of gm and photorespiration, the simplified equation for iWUE is given as:
This linear relationship between iWUE and photosynthetic 13C discrimination is the most used to estimate iWUE, however, the limitations of this formulation have been raised (Seibt et al., 2008; Ubierna and Farquhar, 2014; Ma et al., 2021).
Uncertainty in iWUEsim estimation associated with mesophyll conductance
Experimental evidence shows that gm exerts a significant limitation on CO2 diffusion and leads to a significant drawdown from Ci to Cc (Loreto et al., 1992; Flexas et al., 2008; Cano et al., 2014). It is apparent from Equation 11 that, assuming an infinite gm will lead to overestimation of iWUE, this is supported by experimental observations (Barbour et al., 2010; Stangl et al., 2019; Adams et al., 2020). Experimental results showed that the relationship between Δ and water-use efficiency is at least partly a function of gm (Warren and Adams, 2006). Therefore, it is important to incorporate gm in the parameterization of the iWUE model. Ma et al. (2021) showed that iWUEsim overestimated iWUE by c. 65%. Importantly, the magnitude of overestimation is dependent on Δ, making correction using empirical relations difficult. These results raise concerns regarding the accuracy of iWUEsim estimations.
The overestimation of the iWUEsim model has also been observed in recent studies using 13C series of environmental archives (Baca Cabrera et al., 2021; Bing et al., 2022). More importantly, iWUEsim model could provide biased estimations of historical iWUE trend. Gong et al. (2022) analyzed tree ring 13C series across the globe using the iWUEmes model, and reported that iWUEsim model significantly overestimated iWUE (by c. 100%) and the rate of iWUE gain with time or Ca (by c. 70%) during the 20th century. This finding has been confirmed by studies carried out in distinct ecosystems (Bing et al., 2022; Mathias and Hudiburg, 2022). Failure to consider gm must lead to an overestimated historical trend as implied by the partial derivative of iWUEmes (Equation 11) (Gong et al., 2022):
Given that 13C series of environmental archives (e.g. tree rings) provide a unique proxy for benchmarking the output of land surface models (Frank et al., 2015; Wang et al., 2017; Lavergne et al., 2022), cautions should be paid when iWUEsim model is used to predict historical trend of iWUE.
Uncertainty in iWUEsim estimation associated with b’
The value and physiological meaning of b’ in the equation of Δsim or iWUEsim remain subjects of debate. Initially, Farquhar et al. (1982a) proposed that b’ (27‰) could be derived from early in vitro estimations of Rubisco carboxylation. As it agreed well with the relationship between measured biomass-based Δ and Ci/Ca, it was interpreted as a fitted value. However, when measured Δ from online instantaneous measurements was used to fit Equation 8, the fitted b’ appears to be lower than 27‰ (Caemmerer and Evans, 1991; Ma et al., 2021).
b’ was also explained as the net fractionation caused by Rubisco and PEPC (phosphoenolpyruvate carboxylase). Farquhar and Richards (1984) described b’ as a function of relative contribution of Rubisco and PEPC carboxylation:
where b (29-30‰) and b4 (usually taken as -5.7‰ at 25°C) are fractionation factors of Rubisco and PEPC carboxylation, respectively. β is the proportion of carbon fixation through PEPC carboxylation. PEPC uses produced by CO2 hydration as the substrate for the synthesis of aspartate or malate, which is important for the control of cellular pH (Davies, 1979). Generally, carboxylation by Rubisco contributes a greater fraction of carbon in plants and respiratory substrates. But it is also suggested that the PEPC carboxylation could be important under the conditions of low stomatal conductance or carboxylation in darkness (Ikeda and Yamada, 1981; Gupta et al., 1994; Hibberd and Quick, 2002). Furthermore, several studies have revealed that N source and concentration were potential factors affecting carbon fixation by PEPC, which indicates that b’ could vary with nitrogen metabolism (Raven and Farquhar, 1990; Douthe et al., 2012; Lian et al., 2021). That is, Equation 14 is not particularly useful for iWUE estimation because β is variable and difficult to quantify.
b’ has also been described by Ubierna and Farquhar (2014) as a parameter that included carboxylation, mesophyll conductance, and photorespiration:
According to Equation 15, b’ is largely dependent on Cc/Ci which is modulated by gm. It should be noted that the most used b’=27‰ is consistent with the Cc/Ci value of 0.9, higher than the common values of 0.7-0.8 (Caemmerer and Evans, 1991; Warren et al., 2003). So far, Equations 14 and 15 have only been used to discuss the potential origin of variation in b’, but have not been incorporated in the model of iWUE estimation. In short, there is still no consensus concerning the interpretation of b’, and current discussion on b’ (Equations 14, 15) illustrated that it should not be treated as a constant value of 27‰.
Uncertainty in iWUE associated with post-photosynthetic fractionation
Post-photosynthetic fractionation (Δpost) includes the discrimination processes that follow photosynthetic carbon fixation, altering δ13C signals in plant organs and leaves at different development stages (Badeck et al., 2005; Vogado et al., 2020). In general, heterotrophic organs (branches, stems and roots) are 13C-enriched compared with autotrophic organs (leaves) (Badeck et al., 2005; Bowling et al., 2008; Cernusak et al., 2009; Lamade et al., 2016), and the immature leaves (heterotrophic phase) are 13C-enriched (by c. 2‰) compared to mature leaves (autotrophic phase) in both deciduous and evergreen species (Lamade et al., 2009; Vogado et al., 2020). However, the contribution of Δpost to δ13C of plant tissues and its influence on iWUE estimation are poorly understood.
Several studies has accounted for Δpost to estimate iWUE (Table S2). Gimeno et al. (2021) found an improvement in correlation between iWUE estimated from gas exchange and that from Δ when gm and Δpost were accounted for. In that study, Δpost was taken as -2.5‰ estimated from the δ13C difference between phloem contents and whole-tree photosynthesis. Similarly, δ13C of wood and cellulose have been corrected by -3.2‰ and -1.3‰, respectively, to account for the offset from leaf δ13C (Thomas et al., 2013; Brownlee et al., 2016). In other studies, iWUE was calculated from tree-ring with a δ13C offset of -2‰ to account for Δpost (Michelot et al., 2011; Frank et al., 2015). Without correcting a Δpost of about 2.5‰, iWUE estimated from the δ13C of tree-ring could be overestimated by 20% (Gessler et al., 2009).
The likely mechanisms underlying Δpost include fractionation associated with respiration, transport and mixing of assimilates (Tcherkez et al., 2003; Brüggemann et al., 2011; Bögelein et al., 2019). Respiratory fractionation ranges from -6 to 0‰ in various species (Duranceau et al., 1999; Ghashghaie et al., 2001; Tcherkez et al., 2003). Also, there are some variations in the apparent fractionation during transport processes (e.g., day-night differences in δ13C of leaf-export organic matter and different leaf-to-phloem δ13C signatures along vertical canopy gradients) and very few direct measurements of isotopic differences between components at molecule/atom scale (Gessler et al., 2008; Mauve et al., 2009; Gilbert et al., 2011; Gilbert et al., 2012; Bögelein et al., 2019). In addition, post-photosynthetic fractionation is complicated by ontogenic effects (e.g., size, height, and age of individuals) that can confound the relationship between iWUE and environmental factors (Vadeboncoeur et al., 2020). That is, the influence of Δpost could accumulate over time and lead to age-dependent patterns (Cernusak et al., 2009). Therefore, using a constant, empirical value of Δpost could be unreliable. A mechanistic description of Δpost should be very useful to improve the iWUE estimates, which requires further study on the fractionations associated with respiration, transport and allocation of assimilates.
iWUEmes, a useful, simplified model for estimating iWUE
iWUEmes takes the influence of mesophyll conductance and photorespiratory fractionation into account. We propose to use the iWUEmes model (Equation 11) since it considers the components that have a significant influence on the iWUE estimation. In particular, it includes gm effect which is known to affect iWUE prediction.
Parameterizing the iWUEmes model requires gsc/gm rather than gm (Ma et al., 2021). Some studies use a constant gm in the equation to estimate iWUE (Keeling et al., 2017), which makes more sense than disregarding gm. However, the assumption of constant gm is not supported by experimental evidence. The positive relationships between gsc and gm have been reported in many studies (Flexas et al., 2013; Gong et al., 2018), and this relationship is rather conserved across levels of CO2, irradiance, and drought stress and functionally distinct species (Flexas et al., 2008; Ma et al., 2021; Gong et al., 2022). Incorporating the gsc/gm ratio improves the predictive accuracy of the iWUE model, as demonstrated in gas exchange experiments (Ma et al., 2021). Without knowing gm, it is preferable to use the average gsc/gm of 0.79 ( ± 0.07) derived from a global synthesis to parameterize the iWUEmes model rather than using the iWUEsim model.
We acknowledge that using a constant gsc/gm is not always adequate. As more gm data become available, interspecific differences in gsc/gm can be identified and should be accounted for in the estimated iWUE. Theoretically, species-specific gsc/gm is more appropriate to be used in the iWUEmes estimation. It is also noteworthy that short-term responses of gm are still not well defined, implying that neglecting short-term variation in gsc/gm might lead to errors in estimating iWUE at nonsteady-states, thus should be addressed in further work. Moreover, the iWUEmes model does not account for the post-photosynthetic fractionation, due to a lack of knowledge on post-photosynthetic fractionation. Therefore, we recommend for biomass-based analyses to distinguish the age of organs to minimize the influence of post-photosynthetic fractionation.
Conclusion remarks
The comprehensive model of photosynthetic 13C discrimination of Farquhar and Cernusak (2012) is a synthesis of current understanding, and provides the theoretical basis for estimating iWUE from the 13C composition of plant materials. The classical iWUEsim model has been shown to strongly overestimate iWUE and its historical trends due to the neglect of gm associated effect, limiting its use in quantitative studies. iWUEmes is suggested as a useful, simplified model for quantitative estimation of iWUE, which has been included in a standardized, open-source tool (R package) for calculation of iWUE from stable isotope signatures (Mathias and Hudiburg, 2022). Nonetheless, the formulation of iWUEmes could still be further improved. For example, a fixed, empirical gsc/gm value could be replaced by species-specific values or mechanistic relationships derived from experimental results. 13C discrimination of plant material, combining with appropriate iWUE models, is also an ultimate tool for screening genetic resources to enhance the iWUE of crops under climate change scenarios. One of the primary questions is how gsc and gm of plants will respond to changes in temperature, water availability, and carbon dioxide concentration. Furthermore, the response of post-photosynthetic fractionation to climate change factors remains unknown. We conclude that mechanistic descriptions of gm associated effect and post-photosynthetic fractionation are the bottlenecks for improving the 13C-based estimation of iWUE.
Author contributions
XG conceptualized the topic of this review, WM and YY wrote the first draft, and all authors contributed to the writing and revision of the manuscript. All authors contributed to the article and approved the submitted version.
Funding
This work was supported by the National Natural Science Foundation of China (NSFC 31870377, 32120103005, 32201277).
Conflict of interest
The authors declare that the research was conducted in the absence of any commercial or financial relationships that could be construed as a potential conflict of interest.
Publisher’s note
All claims expressed in this article are solely those of the authors and do not necessarily represent those of their affiliated organizations, or those of the publisher, the editors and the reviewers. Any product that may be evaluated in this article, or claim that may be made by its manufacturer, is not guaranteed or endorsed by the publisher.
Supplementary material
The Supplementary Material for this article can be found online at: https://www.frontiersin.org/articles/10.3389/fpls.2022.1037972/full#supplementary-material
References
Adams, M. A., Buckley, T. N., Turnbull, T. L. (2020). Diminishing CO2-driven gains in water-use efficiency of global forests. Nat. Clim. Change 10 (5), 466–471. doi: 10.1038/s41558-020-0747-7
Baca Cabrera, J. C., Hirl, R. T., Schäufele, R., Macdonald, A., Schnyder, H. (2021). Stomatal conductance limited the CO2 response of grassland in the last century. BMC Biol. 19 (1), 50. doi: 10.1186/s12915-021-00988-4
Badeck, F. W., Tcherkez, G., Nogués, S., Piel, C., Ghashghaie, J. (2005). Post-photosynthetic fractionation of stable carbon isotopes between plant organs–a widespread phenomenon. Rapid Commun. Mass Spectrom 19 (11), 1381–1391. doi: 10.1002/rcm.1912
Barbour, M. M., Warren, C. R., Farquhar, G. D., Forrester, G., Brown, H. (2010). Variability in mesophyll conductance between barley genotypes, and effects on transpiration efficiency and carbon isotope discrimination. Plant Cell Environ. 33 (7), 1176–1185. doi: 10.1111/j.1365-3040.2010.02138.x
Bing, X., Fang, K., Gong, X., Wang, W., Xu, C., Li, M., et al. (2022). The intra-annual intrinsic water use efficiency dynamics based on an improved model. Clim Change 172 (1), 16. doi: 10.1007/s10584-022-03368-1
Bögelein, R., Lehmann, M. M., Thomas, F. M. (2019). Differences in carbon isotope leaf-to-phloem fractionation and mixing patterns along a vertical gradient in mature European beech and Douglas fir. New Phytol. 222 (4), 1803–1815. doi: 10.1111/nph.15735
Bowling, D. R., Pataki, D. E., Randerson, J. T. (2008). Carbon isotopes in terrestrial ecosystem pools and CO2 fluxes. New Phytol. 178 (1), 24–40. doi: 10.1111/j.1469-8137.2007.02342.x
Brownlee, A. H., Sullivan, P. F., Csank, A. Z., Sveinbjörnsson, B., Ellison, S. B. Z. (2016). Drought-induced stomatal closure probably cannot explain divergent white spruce growth in the brooks range, Alaska, USA. Ecology 97 (1), 145–159. doi: 10.1890/15-0338.1
Brüggemann, N., Gessler, A., Kayler, Z., Keel, S. G., Badeck, F., Barthel, M., et al. (2011). Carbon allocation and carbon isotope fluxes in the plant-soil-atmosphere continuum: A review. Biogeosciences 8 (11), 3457–3489. doi: 10.5194/bg-8-3457-2011
Caemmerer, S.v., Evans, J. R. (1991). Determination of the average partial pressure of CO2 in chloroplasts from leaves of several C3 plants. Aust. J. Plant Physiol. 18 (3), 287–305. doi: 10.1071/PP9910287
von Caemmerer, S.v., Ghannoum, O., Pengelly, J. J. L., Cousins, A. B. (2014). Carbon isotope discrimination as a tool to explore C4 photosynthesis. J. Exp. Bot. 65 (13), 3459–3470. doi: 10.1093/jxb/eru127
Cano, F. J., Lopez, R., Warren, C. R. (2014). Implications of the mesophyll conductance to CO2 for photosynthesis and water-use efficiency during long-term water stress and recovery in two contrasting eucalyptus species. Plant Cell Environ. 37 (11), 2470–2490. doi: 10.1111/pce.12325
Cernusak, L. A., Tcherkez, G., Keitel, C., Cornwell, W. K., Santiago, L. S., Knohl, A., et al. (2009). Why are non-photosynthetic tissues generally 13C enriched compared with leaves in C3 plants? Review and synthesis of current hypotheses. Funct. Plant Biol. 36 (3), 199–213. doi: 10.1071/FP08216
Cernusak, L. A., Ubierna, N., Winter, K., Holtum, J. A. M., Marshall, J. D., Farquhar, G. D. (2013). Environmental and physiological determinants of carbon isotope discrimination in terrestrial plants. New Phytol. 200 (4), 950–965. doi: 10.1111/nph.12423
Davies, D. D. (1979). The central role of phosphoenolpyruvate in plant metabolism. Ann. Rev. Plant Physiol. 30 (1), 131–158. doi: 10.1146/annurev.pp.30.060179.001023
de Boer, H. J., Lammertsma, E. I., Wagner-Cremer, F., Dilcher, D. L., Wassen, M. J., Dekker, S. C. (2011). Climate forcing due to optimization of maximal leaf conductance in subtropical vegetation under rising CO2. Proc. Natl. Acad. Sci. U.S.A. 108 (10), 4041–4046. doi: 10.1073/pnas.1100555108
Douthe, C., Dreyer, E., Brendel, O., Warren, C. R. (2012). Is mesophyll conductance to CO2 in leaves of three eucalyptus species sensitive to short-term changes of irradiance under ambient as well as low O2? Funct. Plant Biol. 39 (5), 435–448. doi: 10.1071/FP11190
Duranceau, M., Ghashghaie, J., Badeck, F., Deleens, E., Cornic, G. (1999). δ13C of CO2 respired in the dark in relation to δ13C of leaf carbohydrates in phaseolus vulgaris L. under progressive drought. Plant Cell Environ. 22 (5), 515–523. doi: 10.1046/j.1365-3040.1999.00420.x
Evans, J. R., Caemmerer, S. V. (2013). Temperature response of carbon isotope discrimination and mesophyll conductance in tobacco. Plant Cell Environ. 36 (4), 745–756. doi: 10.1111/j.1365-3040.2012.02591.x
Evans, J. R., Sharkey, T. D., Berry, J. A., Farquhar, G. D. (1986). Carbon isotope discrimination measured concurrently with gas exchange to investigate CO2 diffusion in leaves of higher plants. Aust. J. Plant Physiol. 13 (2), 281–292. doi: 10.1071/PP9860281
Farquhar, G. D., Ball, M. C., von Caemmerer, S., Roksandic, Z. (1982a). Effect of salinity and humidity on δ13C value of halophytes–evidence for diffusional isotope fractionation determined by the ratio of intercellular/atmospheric partial pressure of CO2 under different environmental conditions. Oecologia 52 (1), 121–124. doi: 10.1007/BF00349020
Farquhar, G. D., Cernusak, L. A. (2012). Ternary effects on the gas exchange of isotopologues of carbon dioxide. Plant Cell Environ. 35 (7), 1221–1231. doi: 10.1111/j.1365-3040.2012.02484.x
Farquhar, G. D., Ehleringer, J. R., Hubick, K. T. (1989). Carbon isotope discrimination and photosynthesis. Annu. Rev. Plant Physiol. Plant Mol. Biol. 40 (1), 503–537. doi: 10.1146/annurev.pp.40.060189.002443
Farquhar, G. D., O'Leary, M. H., Berry, J. A. (1982b). On the relationship between carbon isotope discrimination and the intercellular carbon dioxide concentration in leaves. Aust. J. Plant Physiol. 9 (2), 121–137. doi: 10.1071/PP9820121
Farquhar, G. D., Richards, R. A. (1984). Isotopic composition of plant carbon correlates with water-use efficiency of wheat genotypes. Aust. J. Plant Physiol. 11 (6), 539–552. doi: 10.1071/PP9840539
Flexas, J., Barbour, M. M., Brendel, O., Cabrera, H. M., Carriquí, M., Díaz-Espejo, A., et al. (2012). Mesophyll diffusion conductance to CO2: an unappreciated central player in photosynthesis. Plant Sci. 193-194, 70–84. doi: 10.1016/j.plantsci.2012.05.009
Flexas, J., Niinemets, Ü., Gallé, A., Barbour, M. M., Centritto, M., Diaz-Espejo, A., et al. (2013). Diffusional conductances to CO2 as a target for increasing photosynthesis and photosynthetic water-use efficiency. Photosynth. Res. 117 (1-3), 45–59. doi: 10.1007/s11120-013-9844-z
Flexas, J., Ribas-Carbo, M., Diaz-Espejo, A., Galmes, J., Medrano, H. (2008). Mesophyll conductance to CO2: Current knowledge and future prospects. Plant Cell Environ. 31 (5), 602–621. doi: 10.1111/j.1365-3040.2007.01757.x
Frank, D. C., Poulter, B., Saurer, M., Esper, J., Huntingford, C., Helle, G., et al. (2015). Water-use efficiency and transpiration across European forests during the anthropocene. Nat. Clim. Change 5 (6), 579–583. doi: 10.1038/nclimate2614
Gessler, A., Brandes, E., Buchmann, N., Helle, G., Rennenberg, H., Barnard, R. L. (2009). Tracing carbon and oxygen isotope signals from newly assimilated sugars in the leaves to the tree-ring archive. Plant Cell Environ. 32, 780–795. doi: 10.1111/j.1365-3040.2009.01957.x
Gessler, A., Tcherkez, G., Peuke, A. D., Ghashghaie, J., Farquhar, G. D. (2008). Experimental evidence for diel variations of the carbon isotope composition in leaf, stem and phloem sap organic matter in ricinus communis. Plant Cell Environ. 31 (7), 941–953. doi: 10.1111/j.1365-3040.2008.01806.x
Ghashghaie, J., Badeck, F.-W., Lanigan, G., Nogués, S., Tcherkez, G., Deléens, E., et al. (2003). Carbon isotope fractionation during dark respiration and photorespiration in C3 plants. Phytochem. Rev. 2 (1), 145–161. doi: 10.1023/B:PHYT.0000004326.00711.ca
Ghashghaie, J., Duranceau, M., Badeck, F.-W., Cornic, G., Adeline, M.-T., Deleens, E. (2001). δ13C of CO2 respired in the dark in relation to δ13C of leaf metabolites: comparison between nicotiana sylvestris and helianthus annuus under drought. Plant Cell Environ. 24 (5), 505–515. doi: 10.1046/j.1365-3040.2001.00699.x
Gilbert, A., Robins, R. J., Remaud, G. S., Tcherkez, G. G. (2012). Intramolecular 13C pattern in hexoses from autotrophic and heterotrophic C3 plant tissues. Proc. Natl. Acad. Sci. U.S.A. 109, 18204–18209. doi: 10.1073/pnas.1211149109
Gilbert, A., Silvestre, V., Robins, R. J., Tcherkez, G., Remaud, G. S. (2011). A 13C NMR spectrometric method for the determination of intramolecular δ13C values in fructose from plant sucrose samples. New Phytol. 191, 579–588. doi: 10.1111/j.1469-8137.2011.03690.x
Gillon, J. S., Griffiths, H. (1997). The influence of (photo)respiration on carbon isotope discrimination in plants. Plant Cell Environ. 20 (10), 1217–1230. doi: 10.1046/j.1365-3040.1997.d01-24.x
Gimeno, T. E., Campany, C. E., Drake, J. E., Barton, C. V. M., Tjoelker, M. G., Ubierna, N., et al. (2021). Whole-tree mesophyll conductance reconciles isotopic and gas-exchange estimates of water-use efficiency. New Phytol. 229 (5), 2535–2547. doi: 10.1111/nph.17088
Gong, X. Y., Ma, W. T., Yu, Y. Z., Fang, K., Yang, Y., Tcherkez, G., et al. (2022). Overestimated gains in water-use efficiency by global forests. Global Change Biol. 28 (16), 4923–4934. doi: 10.1111/gcb.16221
Gong, X. Y., Schäufele, R., Feneis, W., Schnyder, H. (2015). 13CO2/12CO2 exchange fluxes in a clamp-on leaf cuvette: disentangling artefacts and flux components. Plant Cell Environ. 38 (11), 2417–2432. doi: 10.1111/pce.12564
Gong, X. Y., Tcherkez, G., Wenig, J., Schäufele, R., Schnyder, H. (2018). Determination of leaf respiration in the light: Comparison between an isotopic disequilibrium method and the laisk method. New Phytol. 218 (4), 1371–1382. doi: 10.1111/nph.15126
Gresset, S., Westermeier, P., Rademacher, S., Ouzunova, M., Presterl, T., Westhoff, P., et al. (2014). Stable carbon isotope discrimination is under genetic control in the C4 species maize with several genomic regions influencing trait expression. Plant Physiol. 164 (1), 131–143. doi: 10.1104/pp.113.224816
Gupta, S., Ku, M., Lin, J.-H., Zhang, D., Edwards, G. (1994). Light/dark modulation of phosphoenolpyruvate carboxylase in C3 and C4 species. Photosynth. Res. 42, 133–143. doi: 10.1007/BF02187124
Hibberd, J. M., Quick, W. P. (2002). Characteristics of C4 photosynthesis in stems and petioles of C3 flowering plants. Nature 415 (6870), 451–454. doi: 10.1038/415451a
Ikeda, M., Yamada, Y. (1981). Dark CO2 fixation in leaves of tomato plants grown with ammonium and nitrate as nitrogen sources. Plant Soil 60 (2), 213–222. doi: 10.1007/BF02374106
Keeling, R. F., Graven, H. D., Welp, L. R., Resplandy, L., Bi, J., Piper, S. C., et al. (2017). Atmospheric evidence for a global secular increase in carbon isotopic discrimination of land photosynthesis. Proc. Natl. Acad. Sci. U.S.A. 114 (39), 10361–10366. doi: 10.1073/pnas.1619240114
Keenan, T. F., Hollinger, D. Y., Bohrer, G., Dragoni, D., Munger, J. W., Schmid, H. P., et al. (2013). Increase in forest water-use efficiency as atmospheric carbon dioxide concentrations rise. Nature 499 (7458), 324–327. doi: 10.1038/nature12291
Lamade, E., Setiyo, I. E., Girard, S., Ghashghaie, J. (2009). Changes in 13C/12C of oil palm leaves to understand carbon use during their passage from heterotrophy to autotrophy. Rapid Commun. Mass Spectrom 23 (16), 2586–2596. doi: 10.1002/rcm.4169
Lamade, E., Tcherkez, G., Darlan, N. H., Rodrigues, R. L., Fresneau, C., Mauve, C., et al. (2016). Natural 13C distribution in oil palm (Elaeis guineensis jacq.) and consequences for allocation pattern. Plant Cell Environ. 39 (1), 199–212. doi: 10.1111/pce.12606
Lavergne, A., Hemming, D., Prentice, I. C., Guerrieri, R., Oliver, R. J., Graven, H. (2022). Global decadal variability of plant carbon isotope discrimination and its link to gross primary production. Global Change Biol. 28 (2), 524–541. doi: 10.1111/gcb.15924
Lian, L., Lin, Y., Wei, Y., He, W., Cai, Q., Huang, W., et al. (2021). PEPC of sugarcane regulated glutathione s-transferase and altered carbon–nitrogen metabolism under different n source concentrations in oryza sativa. BMC Plant Biol. 21, 287. doi: 10.1186/s12870-021-03071-w
Loreto, F., Harley, P. C., Di Marco, G., Sharkey, T. D. (1992). Estimation of mesophyll conductance to CO2 flux by three different methods. Plant Physiol. 98 (4), 1437–1443. doi: 10.1104/pp.98.4.1437
Ma, W. T., Tcherkez, G., Wang, X. M., Schäufele, R., Schnyder, H., Yang, Y., et al. (2021). Accounting for mesophyll conductance substantially improves 13C-based estimates of intrinsic water-use efficiency. New Phytol. 229 (3), 1326–1338. doi: 10.1111/nph.16958
Mathias, J. M., Hudiburg, T. W. (2022). isocalcR: An r package to streamline and standardize stable isotope calculations in ecological research. Global Change Biol. 28 (24), 7428–7436. doi: 10.1111/gcb.16407
Mauve, C., Bleton, J., Bathellier, C., Lelarge-Trouverie, C., Guérard, F., Ghashghaie, J., et al. (2009). Kinetic 12C/13C isotope fractionation by invertase: evidence for a small in vitro isotope effect and comparison of two techniques for the isotopic analysis of carbohydrates. Rapid Commun. Mass Spectrom 23, 2499–2506. doi: 10.1002/rcm.4068
Michelot, A., Eglin, T., Dufrêne, E., Lelarge-Trouverie, C., Damesin, C. (2011). Comparison of seasonal variations in water-use efficiency calculated from the carbon isotope composition of tree rings and flux data in a temperate forest. Plant Cell Environ. 34 (2), 230–244. doi: 10.1111/j.1365-3040.2010.02238.x
Raven, J. A., Farquhar, G. D. (1990). The influence of n metabolism and organic acid synthesis on the natural abundance of isotopes of carbon in plants. New Phytol. 116 (3), 505–529. doi: 10.1111/j.1469-8137.1990.tb00536.x
Seibt, U., Rajabi, A., Griffiths, H., Berry, J. A. (2008). Carbon isotopes and water use efficiency: sense and sensitivity. Oecologia 155 (3), 441–454. doi: 10.1007/s00442-007-0932-7
Soh, W. K., Yiotis, C., Murray, M., Parnell, A., Wright, I. J., Spicer, R. A., et al. (2019). Rising CO2 drives divergence in water use efficiency of evergreen and deciduous plants. Sci. Adv. 5 (12), eaax7906. doi: 10.1126/sciadv.aax7906
Stangl, Z. R., Tarvainen, L., Wallin, G., Ubierna, N., Räntfors, M., Marshall, J. D. (2019). Diurnal variation in mesophyll conductance and its influence on modelled water-use efficiency in a mature boreal pinus sylvestris stand. Photosynth. Res. 141 (no. 1), 53–63. doi: 10.1007/s11120-019-00645-6
Tcherkez, G., Gauthier, P., Buckley, T. N., Busch, F. A., Barbour, M. M., Bruhn, D., et al. (2017). Leaf day respiration: low CO2 flux but high significance for metabolism and carbon balance. New Phytol. 216 (4), 986–1001. doi: 10.1111/nph.14816
Tcherkez, G., Nogués, S., Bleton, J., Cornic, G., Badeck, F., Ghashghaie, J. (2003). Metabolic origin of carbon isotope composition of leaf dark-respired CO2 in french bean. Plant Physiol. 131 (1), 237–244. doi: 10.1104/pp.013078
Tcherkez, G., Schäufele, R., Nogués, S., Piel, C., Boom, A., Lanigan, G., et al. (2010). On the 13C/12C isotopic signal of day and night respiration at the mesocosm level. Plant Cell Environ. 33 (6), 900–913. doi: 10.1111/j.1365-3040.2010.02115.x
Tholen, D., Ethier, G., Genty, B., Pepin, S., Zhu, X. G. (2012). Variable mesophyll conductance revisited: Theoretical background and experimental implications. Plant Cell Environ. 35 (12), 2087–2103. doi: 10.1111/j.1365-3040.2012.02538.x
Thomas, R. B., Spal, S. E., Smith, K. R., Nippert, J. B. (2013). Evidence of recovery of juniperus virginiana trees from sulfur pollution after the clean air act. Proc. Natl. Acad. Sci. U.S.A. 110, 15319–15324. doi: 10.1073/pnas.1308115110
Ubierna, N., Farquhar, G. (2014). Advances in measurements and models of photosynthetic carbon isotope discrimination in C3 plants. Plant Cell Environ. 37. (7), 1494–1498. doi: 10.1111/pce.12346
Vadeboncoeur, M. A., Jennings, K. A., Ouimette, A. P., Asbjornsen, H. (2020). Correcting tree-ring δ13C time series for tree-size effects in eight temperate tree species. Tree Physiol. 40 (3), 333–349. doi: 10.1093/treephys/tpz138
Vogado, N. O., Winter, K., Ubierna, N., Farquhar, G. D., Cernusak, L. A. (2020). Directional change in leaf dry matter δ 13C during leaf development is widespread in C3 plants. Ann. Bot. 126 (6), 981–990. doi: 10.1093/aob/mcaa114
Walker, A. P., De Kauwe, M. G., Bastos, A., Belmecheri, S., Georgiou, K., Keeling, R. F., et al. (2021). Integrating the evidence for a terrestrial carbon sink caused by increasing atmospheric CO2. New Phytol. 229 (5), 2413–2445. doi: 10.1111/nph.16866
Wang, H., Prentice, I. C., Keenan, T. F., Davis, T. W., Wright, I. J., Cornwell, W. K., et al. (2017). Towards a universal model for carbon dioxide uptake by plants. Nat. Plants 3 (9), 734–741. doi: 10.1038/s41477-017-0006-8
Warren, C. R., Adams, M. A. (2006). Internal conductance does not scale with photosynthetic capacity: implications for carbon isotope discrimination and the economics of water and nitrogen use in photosynthesis. Plant Cell Environ. 29 (2), 192–201. doi: 10.1111/j.1365-3040.2005.01412.x
Warren, C. R., Ethier, G. J., Livingston, N. J., Grant, N. J., Turpin, D. H., Harrison, D. L., et al. (2003). Transfer conductance in second growth Douglas-fir (Pseudotsuga menziesii (Mirb.)Franco) canopies. Plant Cell Environ. 26 (8), 1215–1227. doi: 10.1046/j.1365-3040.2003.01044.x
Keywords: water-use efficiency, carbon isotope discrimination, mesophyll conductance, post-photosynthetic fractionation, climate change, photosynthesis
Citation: Ma WT, Yu YZ, Wang X and Gong XY (2023) Estimation of intrinsic water-use efficiency from δ13C signature of C3 leaves: Assumptions and uncertainty. Front. Plant Sci. 13:1037972. doi: 10.3389/fpls.2022.1037972
Received: 06 September 2022; Accepted: 19 December 2022;
Published: 12 January 2023.
Edited by:
Boris Rewald, University of Natural Resources and Life Sciences Vienna, AustriaReviewed by:
Junpeng Mu, Mianyang Normal University, ChinaCopyright © 2023 Ma, Yu, Wang and Gong. This is an open-access article distributed under the terms of the Creative Commons Attribution License (CC BY). The use, distribution or reproduction in other forums is permitted, provided the original author(s) and the copyright owner(s) are credited and that the original publication in this journal is cited, in accordance with accepted academic practice. No use, distribution or reproduction is permitted which does not comply with these terms.
*Correspondence: Xiao Ying Gong, eGdvbmdAZmpudS5lZHUuY24=
†ORCID: Xuming Wang, orcid.org/0000-0001-5291-9332
Xiao Ying Gong, orcid.org/0000-0002-4983-5645