- 1College of Agriculture, Heilongjiang Bayi Agricultural University, Daqing, China
- 2Key Laboratory of Mollisols Agroecology, Northeast Institute of Geography and Agroecology, Chinese Academy of Sciences, Harbin, China
- 3Centre for AgriBioscience, La Trobe University, Bundoora, VIC, Australia
Crop photosynthetic capacity in response to climate change likely constrains crop productivity and adaptability to changing environments, which requests the investigation on the dynamics of photosynthetic parameters over growth season among varieties, especially in cold-temperate regions. Three Japonica rice cultivars i.e., Shoubaimao (SH), Hejiang 19 (HJ); Longjing 31, (LJ). were planted under the control, e[CO2] (700 μmol mol-1), warming (2°C above the air temperature) and the co-elevation of [CO2] and temperature in open-top chambers (OTC). The objective of this study is to examine the rice photosynthetic parameters, water use efficiency (WUE) and yield formation in responses to the co-elevation of [CO2] and temperature which is the main predicted features of future climate. e[CO2] significantly increased An of SH, HJ and LJ by 37%, 39% and 23% in comparison to 34%, 34% and 27% under elevated [CO2] plus warming, respectively. However, An had a weak response to warming for three cultivars. [CO2] and temperature co-elevation significantly decreased the stomatal conductance, resulting in a significant increase of the WUE. e[CO2] significantly increased Vc, max, Jmax and Jmax/Vc, max. e[CO2] significantly increased grain yield and grain number of all cultivars. The positive effect of co-elevation of [CO2] and temperature on grain yield was less than e[CO2]. Warming is likely to partially offset the increased photosynthetic rate caused by e[CO2]. The [CO2] and temperature co-elevation may be favorable to rice crop with increasing the photosynthetic ability of rice crop and improving water use efficiency. The present study provided evidence that the rice genotypic difference in photosynthetic potential under [CO2] and temperature co-elevation. Therefore, it is crucial to explore a broader range of phenotypes and cultivars to be applied to climate change response research, advancing the knowledge that climate change impacts rice crop under the cold-temperate climate region.
Introduction
The Earth System Model (ESM) in the IPCC’s Sixth Assessment Report (AR6) correctly predicted that the atmospheric CO2 concentration ([CO2]) would increase from 414 parts per million (ppm) in 2020 to over 1100 ppm by 2100 if no measures are implemented greenhouse gas emissions. Global warming is mostly attributed to the increase in atmospheric [CO2], which is expected to increase by 1.5 to 2°C in the end of the 21st century (IPCC, 2021). In the face of resource restrictions and the challenges of climate change, it is necessary to provide more food for the world’s rapidly growing population, whereas crop production has been increasing at a decreasing rate, which has largely motivated contemporary photosynthesis research.
Increased [CO2] levels and warming are experiencing a rapid detrimental effect on ecosystems around the world, particularly in agricultural ecosystems, causing changes in plant growth and nutrient acquisition (Carvalho et al., 2020; Guo et al., 2022). As the fundamental substrate in the process of photosynthesis, substantial pieces of evidence have indicated that e[CO2] will stimulate photosynthesis and benefit biomass production and yield, particularly in C3 crops (Cheng et al., 2009; Zhu et al., 2016; Lenka et al., 2019; Brito et al., 2020; Lenka et al., 2021b; Zhu et al., 2022). Considering the warming effect, there are inconsistent conclusions on crop biomass and yield. Previous works have indicated that warming suppressed photosynthesis and shortened the plant growth period, resulting in a reduction of crop biomass (Bhattacharyya et al., 2014; Wang et al., 2016; Cai et al., 2016). However, contrary results have also been reported that warming increased plant growth due to the stimulation of metabolic activities (Arcus et al., 2016; Dusenge et al., 2019). In other words, the optimal temperature for the growth of rice, as a typical thermophilic C3 crop, can reach 35°C, and plants even tolerate 40°C in the vegetative growth stages (Bahuguna et al., 2015; Wang et al., 2015b). Varietal variation and climate region should also be considered to reveal the crop response to climate change.
The productivity of crops depends on physiological and biochemical capacity of photosynthesis. The Farquhar - von Caemmerer - Berry model (the FvCB model, Farquhar et al., 1980) is a method applied to studies on climate-plant, that of two key parameters: the maximum rates of electron transport (Jmax) and carboxylation by Rubisco (Vc, max) are obtained through the measurement and modeling of photosynthetic response to CO2 concentration (An-Ci curves) (Rogers, 2014; Coursolle et al., 2019; Miao et al., 2021). As far as we know, only one report by Cai et al. (2018) was carried out in the subtropical region, and showed that the effect of e[CO2] and warming on An-Ci curves were variable among different growth stages of rice. The results demonstrated that Vc, max and Jmax significantly decreased under the simultaneous increase of CO2 concentration and temperature at the heading and filling stages. Ecosystem modeling may contribute to the understanding of biochemical responses to the interaction between the plant and the future environment (Le et al., 2011; Drewry et al., 2014).
Rice (Oryza sativa L.) is one of the most important sources of daily food for nearly half of the world’s population (Zhu et al., 2018). It is imperative to examine and predict the responsive changes in the physiology and growth of rice to CO2 enrichment and warming. Rice is grown in paddy soils, which maintain a relatively consistent water depth throughout the growing season (Thanawong et al., 2014; Bocchiola, 2015). Water use is continuously concerned under climate change because it reflects the coupled relationship between water loss and carbon gain in the process of plant photosynthetic carbon assimilation (Ullah et al., 2019). Clear pieces of evidence indicated that e[CO2] directly decreased leaf stomatal conductance by approximately 50%, reducing water losses and increasing water use efficiency (Yoshimoto et al., 2005; Ainsworth and Rogers, 2007). Qiao et al. (2010) found that the water use efficiency of C3 crops was promoted by e[CO2] but not by warming. However, the reduction in stomatal conductance in response to e[CO2] can vary depending on plant status, such as growth stage, as well as environmental factors, such as light and temperature (Bunce, 2004). Some recent investigations have concluded that stomatal conductance responds to e[CO2] and warming without a consistent trend (Del Pozo et al., 2005; Mott and Peak, 2013; Urban et al., 2017). Therefore, to date, very limited information is available on the impact of [CO2] and warming co-elevation on stomatal conductance of rice, which would provide new insights into potential agricultural manipulations to reduce water use under future climatic conditions.
The local climate of Northeast China is characterized as a cold temperate and humid and sub-humid climate, where the rice area is expanding rapidly with an increasing rate of 0.16 ×106 ha per year (Xin et al., 2020). Crop productivity is being impacted by climate change. However, as the result of climate change variables, most research focused on photosynthesis responses in the grain yield processes to e[CO2] or elevated temperature instead of their interactions. Previous studies have been carried out in tropical regions, demonstrating that warming negatively affected rice yield (Wang et al., 2016; Wang et al., 2020a; Wang et al., 2020b). We hypothesize that (1) in cold-temperate climate regions, elevated air temperatures within a 2°C increment may not negatively impact rice growth and physiological traits because the temperature is below the critical level during rice growing season; (2) [CO2] and temperature co-elevation would improve photosynthesis with an increase in water use efficiency, thereby contributing to rice production. In this study, the photosynthesis curve response to [CO2] and its parameters were measured in three rice cultivars grown in the open-top chambers. Seasonal variations of transpiration rate and water use efficiency were examined at different growing stages under e[CO2] and increased temperature. The primary objectives of this study were to (1) investigate seasonal and varietal variations of rice leaf photosynthetic performance under [CO2] and temperature co-elevation and (2) examine whether elevated temperature could benefit yield formation and increase rice productivity when combined with e[CO2] in a cold-temperate region. The present study’s findings will be conducive to further understanding the response of yield to e[CO2] and temperature and their interaction in a cold-temperate region of China, and can support decision making for adaptation strategies in rice production under climate change.
Materials and methods
Experimental design
This experiment was conducted in open-top chambers (OTCs) at the Northeast Institute of Geography and Agroecology, Chinese Academy of Sciences, Harbin, China (45°73′N, 126°61′E). A pot experiment was carried out with three rice cultivars and four different environmental treatments. The treatments comprised ambient [CO2] (400 μmol mol-1) and temperature (Control), elevated [CO2] (700 μmol mol-1, e[CO2]), warming (+2°C), and e[CO2] plus warming (e[CO2]+warming). The ambient temperature during the growth season was in a range of 14.2–27.9°C. The structure of OTC was 3.5 m in diameter and 2.0 m in height, with a 0.5 m high canopy that made a 45° angle to the horizontal plane. Covering in OTCs was done with a clear polyethylene film. Zhang et al. (2014) and Li et al. (2018); Li et al. (2019b) illustrated the OTC design in further details. The CO2 concentration inside OTCs was monitored and automatically controlled by supply of 99.9% CO2 gas using a computerized CO2-regulating system (Beijing VK2010, China). The inner temperature in each OTC was effectively regulated via an air conditioner with a pair of thermal probes installed inside and outside of the OTC in order to maintain the temperature inside 2°C higher than or as same as outside. In addition, an inner air flow system was installed to homogenize the CO2 concentration and temperature in the OTCs.
Plant material and growth conditions
Three rice cultivars cv. Shishoubaimao (SH), Hejiang 19 (HJ), and Longjing 31 (LJ) were bred over the historical period, i.e. approximately 7 decades and have been grown on a large scale in the cold-temperate climate region (Table 1). As the first generation, SH was introduced from Japan to northeast China in the 1940s, and then the cultivars HJ and LJ were subsequently bred (Table 1). Rice plants were grown in pots and randomly allocated in OTCs, with three pots for each cultivar under each treatment. The paddy soil is classified as a typical Mollisol (USDA). The soil was collected from field sites in Hailun City, Heilongjiang, China (47°23′N, 126°51′E). The air-dried soil was sieved through a 4-mm sieve and mixed well. Nine kg of sieved soils were placed into each pot (height 23 cm, diameter 30 cm). Basal nutrients (mg nutrient per kilogram soil) of 90 mg kg-1 of N, 113 mg kg-1 of P2O5, and 74 mg kg-1 of K2O were applied before transplanting. Uniform rice seeds were germinated and grown in a tray filled with 5 cm of soil in depth. On the 20th day after emergence, three seedlings of rice with similar size were transplanted into each pot. The paddy soil was always submerged under 3-4 cm of water. The elevated CO2 concentration and temperature treatments were sustained until the end of the experiment.

Table 1 Cultivar name, year of release or introduction, sub-species and days from transplanting to maturity for the chosen three rice cultivars.
Leaf photosynthetic parameter measurements
As reported in the previous studies, the tiller stage is a critical stage when the biomass rapidly accumulated and panicles form. The anthesis stage is more sensitive to environmental factors, such as temperature, light and soil water content. Similarly, carbohydrates are transferred from the source (shoot) to the pool (seed) during the grain filling stage (Jagadish et al, 2007; Sasaki et al, 2007; Wassmann et al., 2009). The varietal response to the climate change would be maximal over those growth stages. Therefore, measurements and samples were taken at the three stages of those cultivars. The three youngest fully expanded leaves (flag leaves at the anthesis and grain-filling stage) were tagged in each treatment for measurement. Photosynthetic measurements were performed during the period from 8:30 to 11:30. We used the LI-Cor 6800 Portable Photosynthesis System (Li-Cor BioScience, Lincoln, NE, USA) with Dynamic Assimilation™, and 6800-01A leaf chamber fluorometers to measure the net photosynthetic rate (An) in response to intercellular carbon dioxide concentration (Ci) by controlling the CO2 concentration in the leaf chamber (An-Ci curves). Detailed information about instructions for equipment and programs was described by Saathoff and Welles (2021). Measurement temperature was set as same as the ambient temperature for the control and e[CO2] treatments, and +2°C above ambient temperature for warming and e[CO2] plus warming (e[CO2]+warming) treatments. Other leaf chamber conditions were as follows: saturating irradiance of 1200 μmol m-2 s-1, the fan speed of 10 000 rpm, the flow rate of 500 μmol mol-1, and relative humidity at leaf of 60%. Steady-state values of An (μmol CO2 m-2 s-1), Gs (mol H2O m-2 s-1), intercellular [CO2] (Ci, μmol mol-1), ambient [CO2] (Ca, μmol mol-1) were recorded during each measurement.
The An-Ci curves. The LI-6800’s Auto Program was used to process: a “down” ramp from 400 to 50 μmol mol-1 at a rate of 400 μmol mol-1 min-1 CO2 and was immediately followed, approximately 10 to 15 s later, by an “up” ramp from 50 to 1 600 μmol mol-1at a rate of 400 μmol mol-1min-1. The data was recorded on a computer every 2 s in all measurements. In the measurement of An-Ci curves, stomatal conductance (Gs), transpiration rate (Tr), and air -to- leaf temperature difference (ΔT) were also automatically measured. The instantaneous water use efficiency (iWUE) is the ratio of An and Tr.
Leaf chlorophyll content measurements
In order to extract chlorophyll, 0.3 g of fresh leaf material was stored in 15 ml of 80% (v/v) ethanol for more than 2 days in the dark at 4°C. The absorbance of the solution was measured using a multi-functional enzyme labeler (CLARIOstar Plus, Germany) at 645 and 663 nm. The Chl a and Chl b content were calculated according to the equations of Arnon (1949) using the absorbance values.
Plant harvest and measurements
The plants were destructively sampled at the tillering, anthesis and grain filling stages. Leaves, stems, and panicles (when present) were separated from samples. After that, the samples were dried at 105°C for 30 min and then dried at 75°C for 72 h, after which the biomass components were recorded. At maturity, grain was harvested to measure grain yield and its components.
Data processing and statistical analysis
The An-Ci curves were averaged across three repetitions for the given experiment treatment before further analyses. Using the ‘plantecophys’ package (Duursma, 2015) in R version 3.6.1 (R Core Team, 2019), An-Ci curves were fit to the FvCB model to estimate the photosynthetic parameters including CO2 compensation point (Γ, µmol mol-1), maximum ribulose -1, 5-bisphosphate carboxylase/oxygenase (Rubisco) carboxylation rate (Vc, max, µmol m-2 s-1), and potential light saturated electron transport rate (Jmax, µmol m-2 s-1), respectively. All settings in the ‘fitaci’ function were left at their respective default values, and the default nonlinear fitting method was used, which allows for the calculation of standard errors.
A one-way ANOVA (analysis of variance) was conducted to determine the effects of e[CO2], warming and their interactions on Γ, Vc,max, and Jmax. Treatment means were compared using the Duncan’s multiple range test (p < 0.05) in SPSS 22.
Results
The phenotypes and gas exchange under e[CO2] and warming
Warming increased plant height. The treatments had no significant effect on plant height at the anthesis and grain filling stages, except for HJ at the grain filling stage that warming alone and e[CO2] plus warming decreased plant height, compare to control (Figure 1).
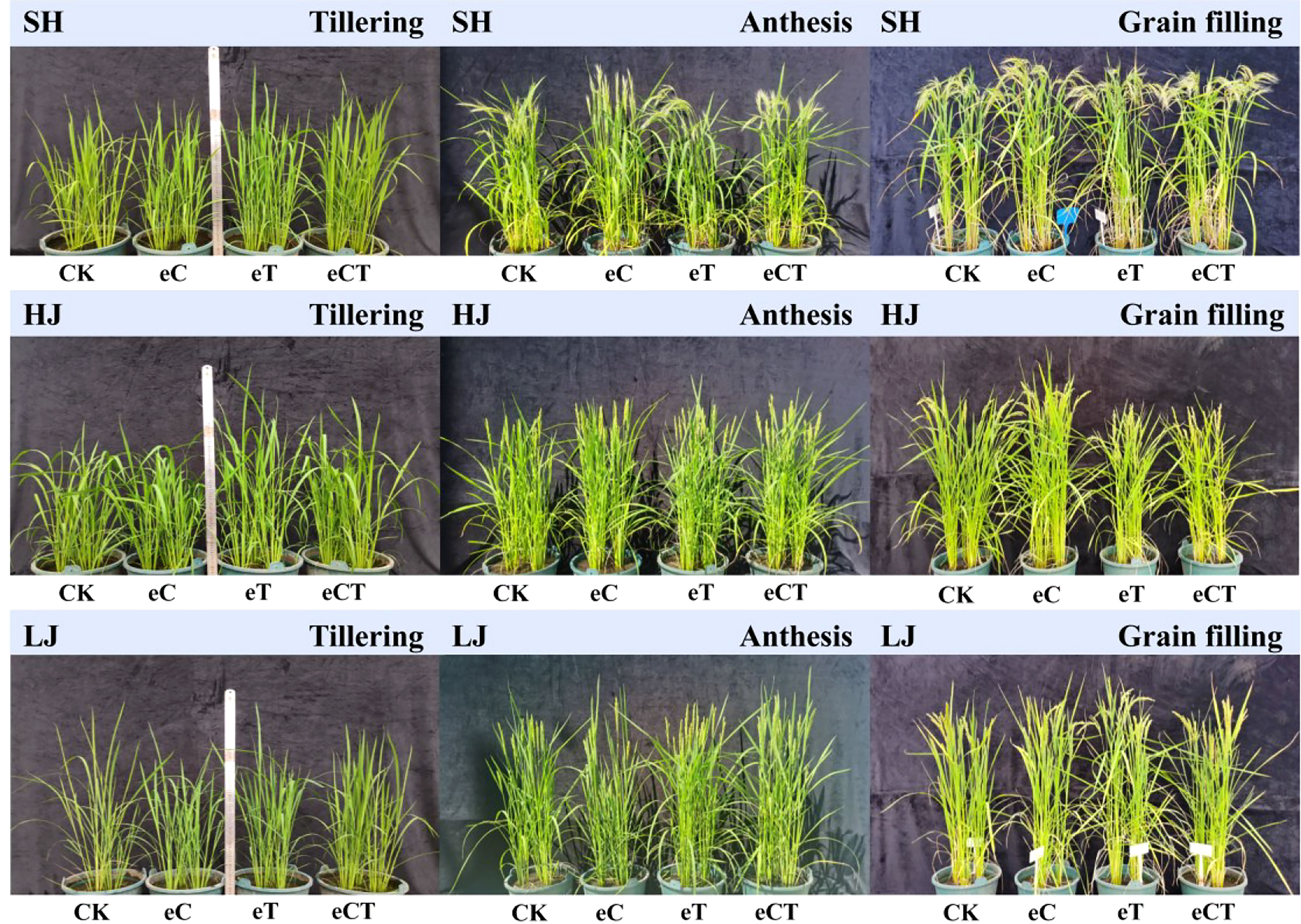
Figure 1 The rice growing conditions under different treatments during the stages of tillering, anthesis and grain filling (control, ambient conditions; eC, eCO2 concentration; eT, elevated air temperature; eCT, the combination of elevated air temperature and [CO2]) at three growth stages (tillering, anthesis and grain filling). SH, HJ and LJ refer to three rice cultivars Shishoubaimao, Hejiang 19, and Longjing 31, respectively.
Compared to the control, e[CO2] resulted in significant increases in leaf An at three sampling stages, in SH, HJ and LJ, ranging from18%-67%, 26%-60% and 11%-37%, respectively (Figure 2). Compared to the control, HJ and LJ leaf An at the anthesis significantly decreased under warming by 20.6% and 15.6% respectively. Compared to the control, e[CO2] plus warming increased An of SH, HJ and LJ by 15%-53%, 18%-47% and 11%-37% throughout all three stages. Compared to control, warming resulted in an increase in leaf Gs among all cultivars. It is interesting to note that e[CO2] alleviated this phenomenon. e[CO2] and e[CO2] plus warming decreased leaf Gs (Figure 2).
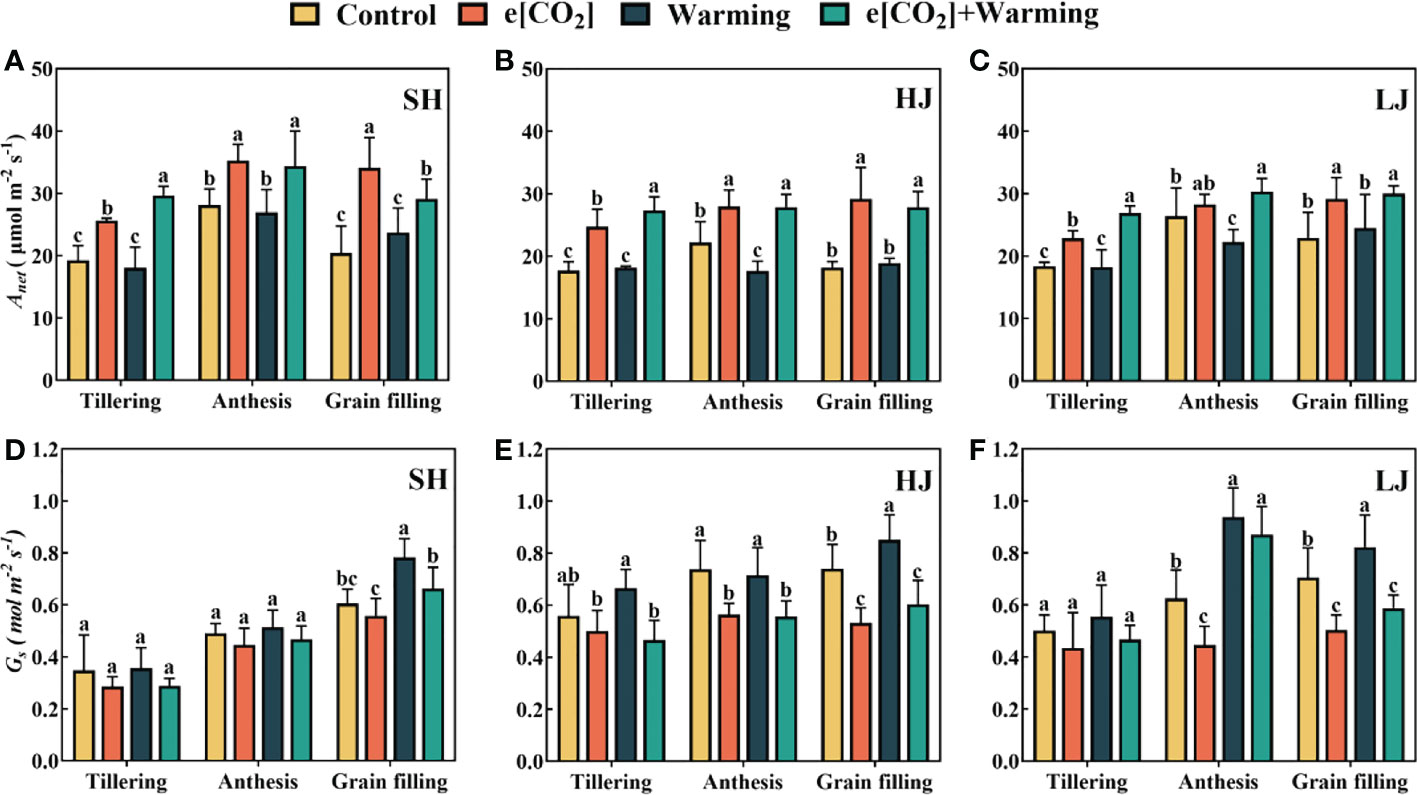
Figure 2 The responses of An (A–C) and Gs (D–F) measured under control, ambient condition; e[CO2], eCO2 concentration; warming, elevated air temperature; e[CO2] plus warming, the combination of elevated air temperature and [CO2] during the stages of tillering, anthesis and grain filling. SH, HJ and LJ refer to three rice cultivars Shishoubaimao, Hejiang 19, and Longjing 31, respectively. Values are the means ± SEs (n = 6). Different lower-case letters indicate significant differences between treatments at P < 0.05 level. An, net photosynthetic rate; Gs, stomatal conductance.
Compared to the control, warming had no significant effect on Ci, but it increased significantly under e[CO2] and e[CO2] plus warming among all cultivars (Figure 3). e[CO2] and e[CO2] plus warming significantly decreased Ci/Ca at the anthesis stage among all cultivars. e[CO2] plus warming had a positive effect on the WUE, and warming weakened the stimulation of WUE caused by e[CO2] was observed at the anthesis stage of SH and grain filling stage of HJ.
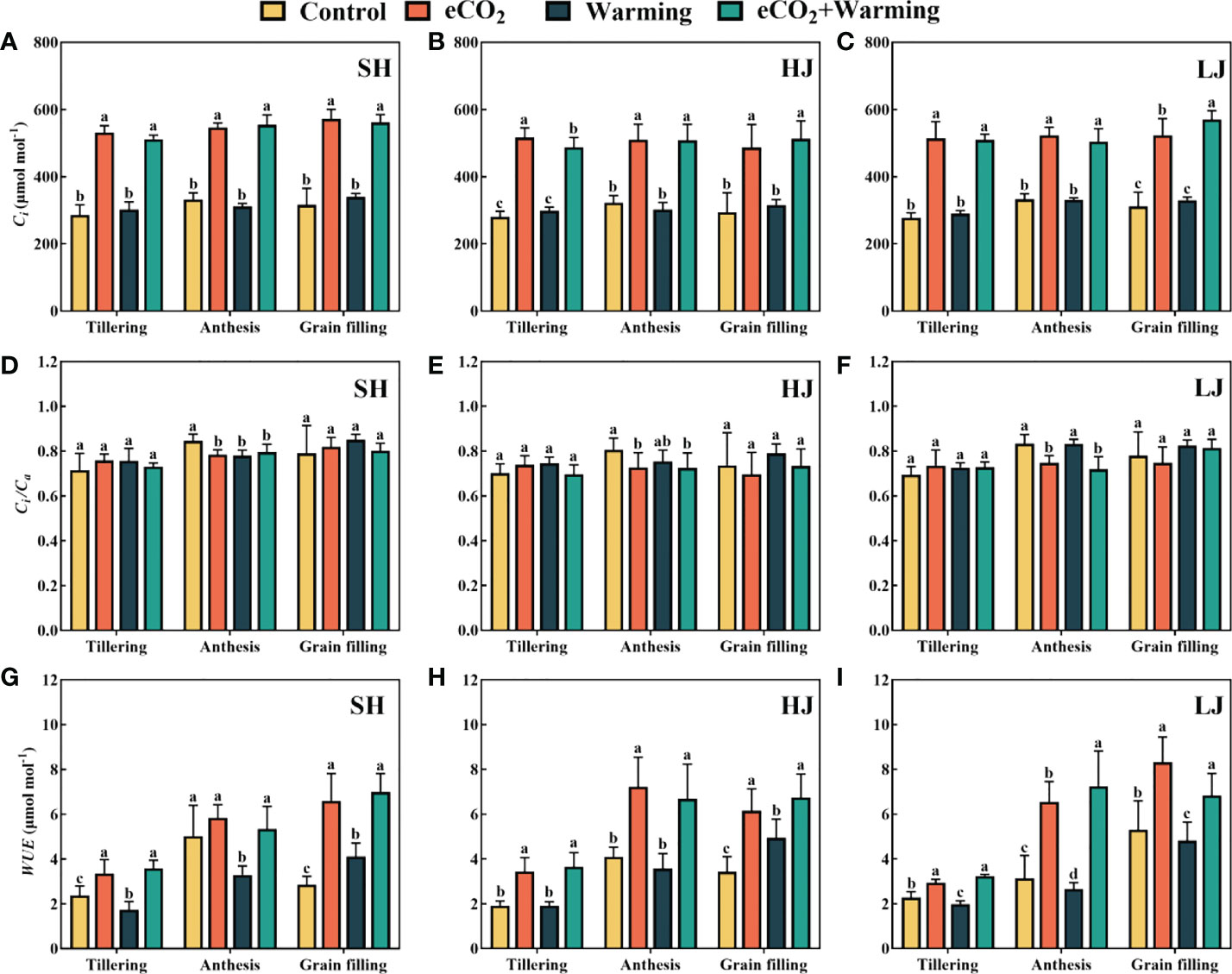
Figure 3 The value of Ci (A-C), Ci/Ca (D-F) and WUE (G-I) of three rice cultivars grown under control, ambient conditions; e[CO2], elevated CO2 concentration; warming, elevated air temperature; e[CO2] plus warming, the combination of elevated air temperature and [CO2]). SH, HJ and LJ represent rice cultivars Shishoubaimao, Hejiang 19, and Longjing 31, respectively. Values are the means ± SEs (n = 6). Different letters indicate significant differences between treatments at P < 0.05 level. Ci, intercellular [CO2]; Ci/Ca, the ratio of intercellular [CO2] to ambient air [CO2]; WUE, water use efficiency.
CO2 response curves and model parameters
Compared with the control, e[CO2] increased An across three varieties at three stages, after the carboxylation rate reaches its maximum (Figure 4). However, warming decreased An across three varieties, but not for SH and HJ at the grain-filling stage. The stimulating effect of e[CO2] on An was counteracted by warming.
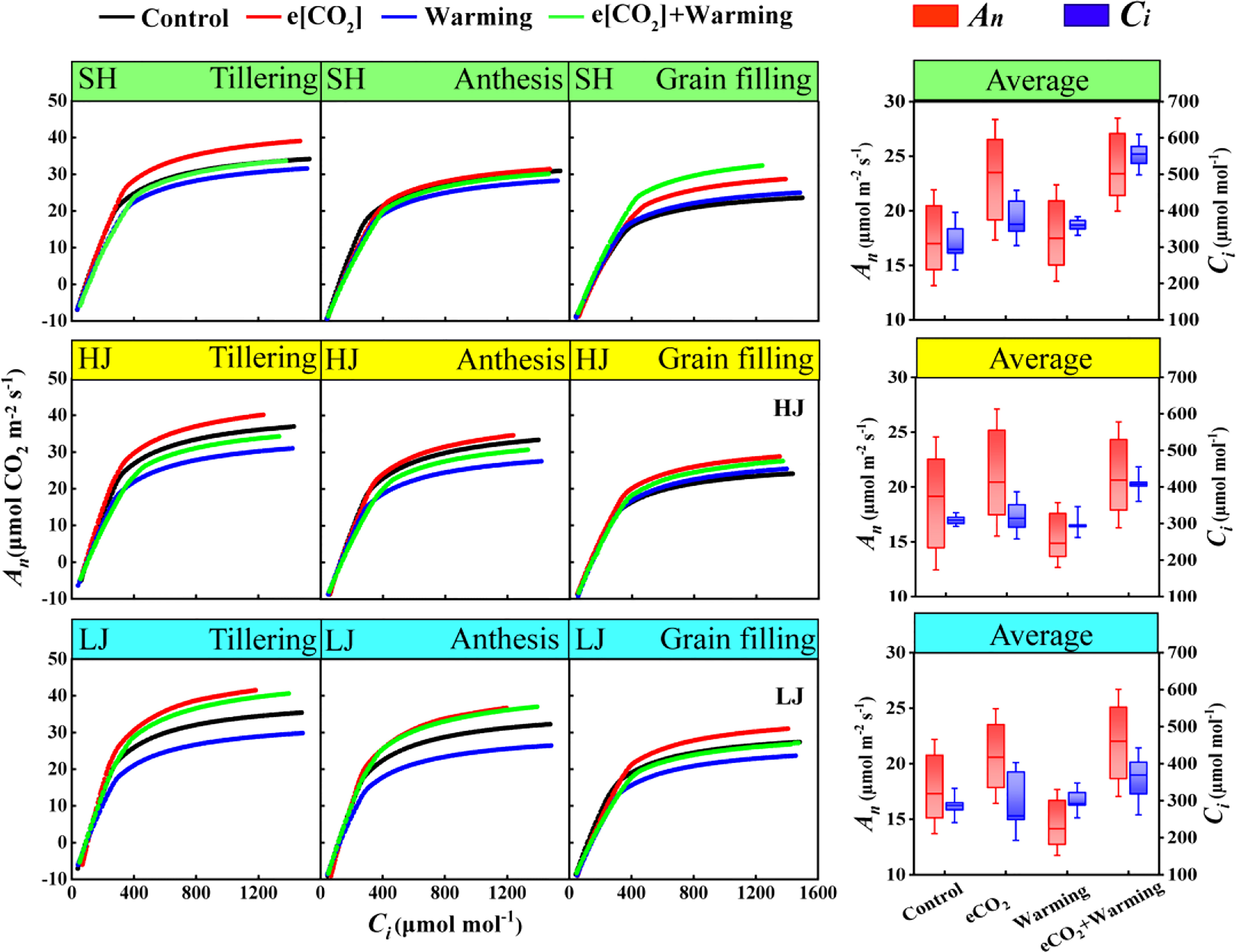
Figure 4 The line graph represents the net photosynthetic rate (An)- intercellular carbon dioxide concentration (Ci) curves under ambient [CO2] and temperature (black dots, Control), e[CO2] (red dots, e[CO2]), warming (blue dots, warming), and e[CO2] plus warming (green dot, e[CO2] +warming) at the tillering, anthesis, and grain-filling stages (from left to right) in SH (Shishoubaimao), HJ (Hejiang 19), and LJ (Longjing 31) (from top to bottom). The box graph represents the average net photosynthetic rate (An, red boxes) and intercellular carbon dioxide concentration (Ci, blue boxes) when the carboxylation rate is maximum.
e[CO2] plus warming increased Ci and An by 43% and 38% at the maximum of the carboxylation rate throughout three stages of SH, respectively, compared with the control (Figure 4). A similar trend was found with a 31% and 14% of increase for Ci of HJ and maximal An, and 18% and 22% of LJ, respectively (Figure 4).
e[CO2] plus warming significantly (P < 0.05) increased Γ by 24% and 30% at the tillering and anthesis stages of SH (Figure 5), by 21% and 17% at the grain-filling stage of HJ and LJ compared with the control, respectively. The effect of e[CO2[ plus warming on Γ varied among the three varieties and stages.
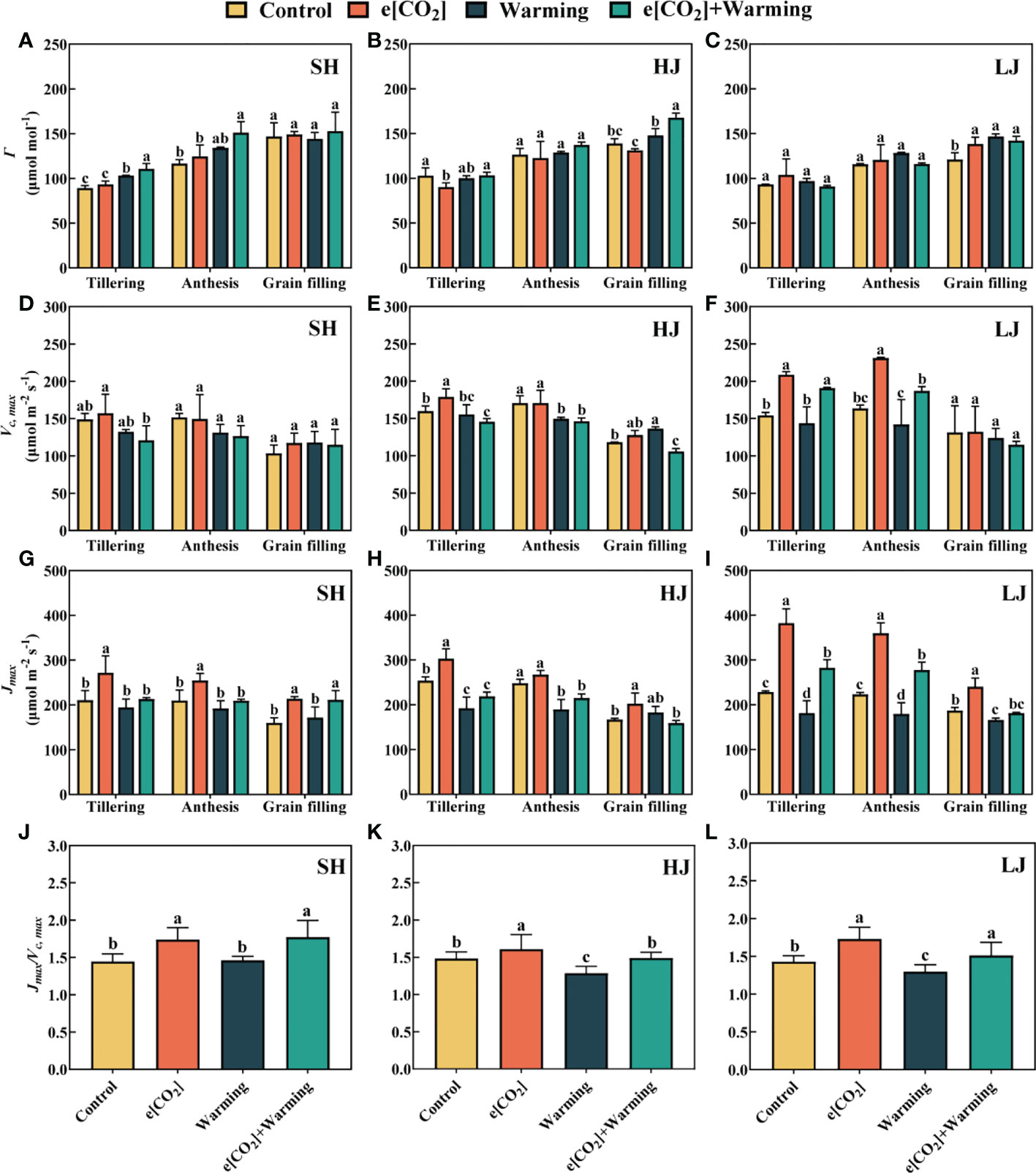
Figure 5 Model parameters were determined from An-Ci data. Carbon dioxide compensation point (Γ, A–C), maximum ribulose-1, 5-bisphosphate carboxylase/oxygenase (Rubisco) carboxylation rate (Vc, max, D–F), and potential light saturated electron transport rate (Jmax, G–I) under e[CO2] and warming at the tillering, anthesis, and grain-filling stages of SH (Shishoubaimao), HJ (Hejiang 19), and LJ (Longjing 31). Data are means ± standard errors (n = 3). The data of (Jmax/Vc, max, J–L) radio is the average means ± standard errors (n = 9) at three stages in each cultivar. Different letters indicate significant differences between mean values at P < 0.05 level.
The response of maximum ribulose -1, 5-bisphosphate carboxylase/oxygenase (Rubisco) carboxylation rate (Vc, max) to climate change depended on varieties and growth stages (Figure 5). e[CO2] plus warming significantly decreased Vc, max by 8.9% and 14% at the tillering and anthesis stages of HJ, while significantly increased Vc, max by 24% at the grain-filling stage of LJ. e[CO2] also increased the Vc, max at the tillering and anthesis stages of LJ.
Similarly, Jmax increased by 32% in response to e[CO2] plus warming at the grain-filling stage of SH (Figure 5), and increased by 24% and 24% of LJ but reduced by 13% and 14% at the tillering and anthesis stages of HJ, respectively, compared with control. Compared with the control, e[CO2] significantly increased the average Jmax: Vc, max ratio across three varieties at three stages.
Leaf chlorophyll content
Compared to the control, the leaf chlorophyll a (Chl a) content under e[CO2] and warming in three cultivars had an average increase of 10% and 15%, respectively (Figure 6). Whereas the effect of e[CO2] plus warming on the leaf Chl a content varied among the varieties. For example, e[CO2] plus warming significantly decreased the leaf Chl a content by 18%-25% at the grain filling across the three varieties. The effect of climatic treatments on chlorophyll b (Chl b) and chlorophyll a+b (Chl a+b) is similar to that of Chl a.
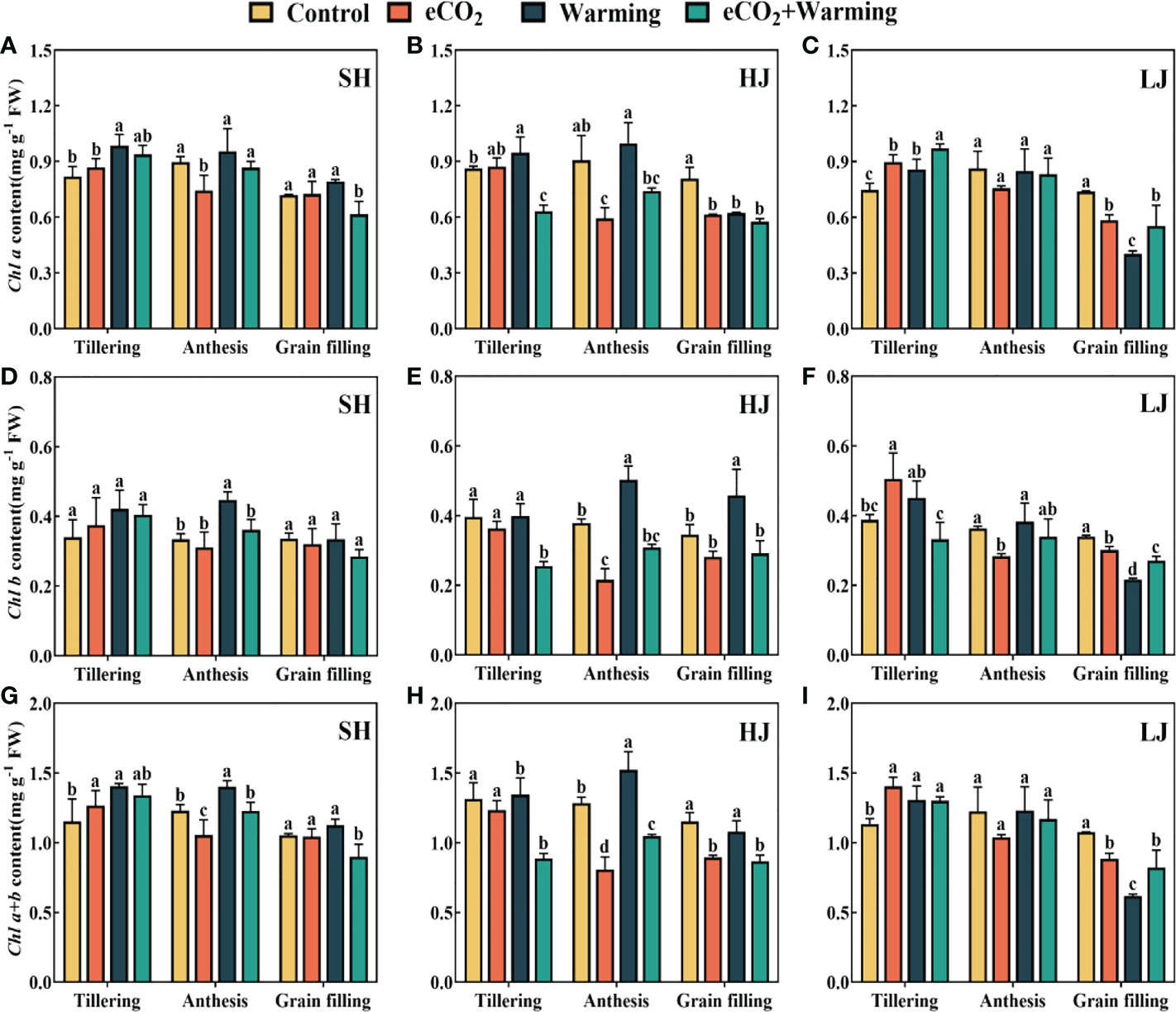
Figure 6 The leaf chlorophyll a (Chl a, A-C), chlorophyll b (Chl b, D-F) and chlorophyll a+b (Chl a+b, G-I) under control, ambient conditions; e[CO2], elevated [CO2] concentration; warming, elevated air temperature; e[CO2] plus warming, the combination of elevated air temperature and [CO2]) at the growth stage of tillering, anthesis and grain filling. SH, HJ and LJ refer to three rice cultivars Shishoubaimao, Hejiang 19, and Longjing 31, respectively. Values are the means ± SEs (n =6 ).Different lower-case letters indicate significant differences between treatments at P < 0.05 level.
Dynamic response of stomatal conductance to Ci
The Gs tended to decrease with the increase of Ci. Compared with the control, e[CO2] plus warming notably decreased Gs irrespective of rice variety and growth stage (Figure 7). Interestingly, Gs gradually decreased over the growing season, meanwhile, the effect of e[CO2] plus warming on Gs reduced over time.
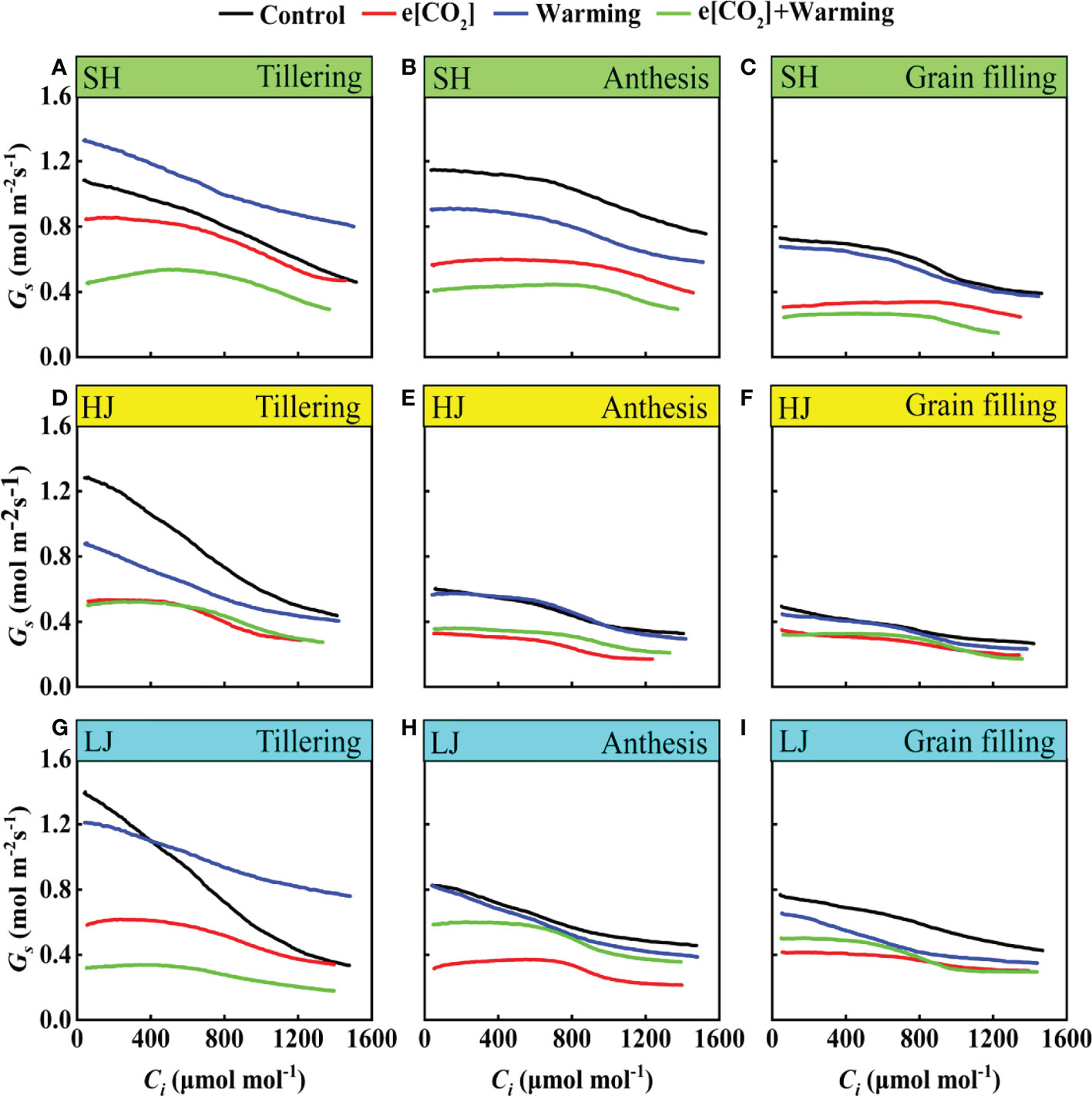
Figure 7 The Gs-Ci Curves under ambient CO2 and temperature (black dots, Control), elevated CO2 concentration (red dots, e[CO2]), elevated temperature (blue dots, warming), and elevated CO2 concentration plus warming (green dot, e[CO2] + warming) for cv. SH (A-C), HJ (D-F), and LJ (G, H, I) during the tillering stage, anthesis stage, and grain-filling stage. Gs represents stomatal conductance, Ci represents intercellular carbon dioxide concentration.
Transpiration rate changes in response to e[CO2] and warming
With the advancement of Ci, the values of transpiration rate (Tr) gradually decreased across three varieties. Except for the anthesis stage, e[CO2] plus warming significantly decreased Tr (Figure 8) across three growth stages, compared with the control. Interestingly, with the growth period, the response of Tr to climate change gradually weakened.
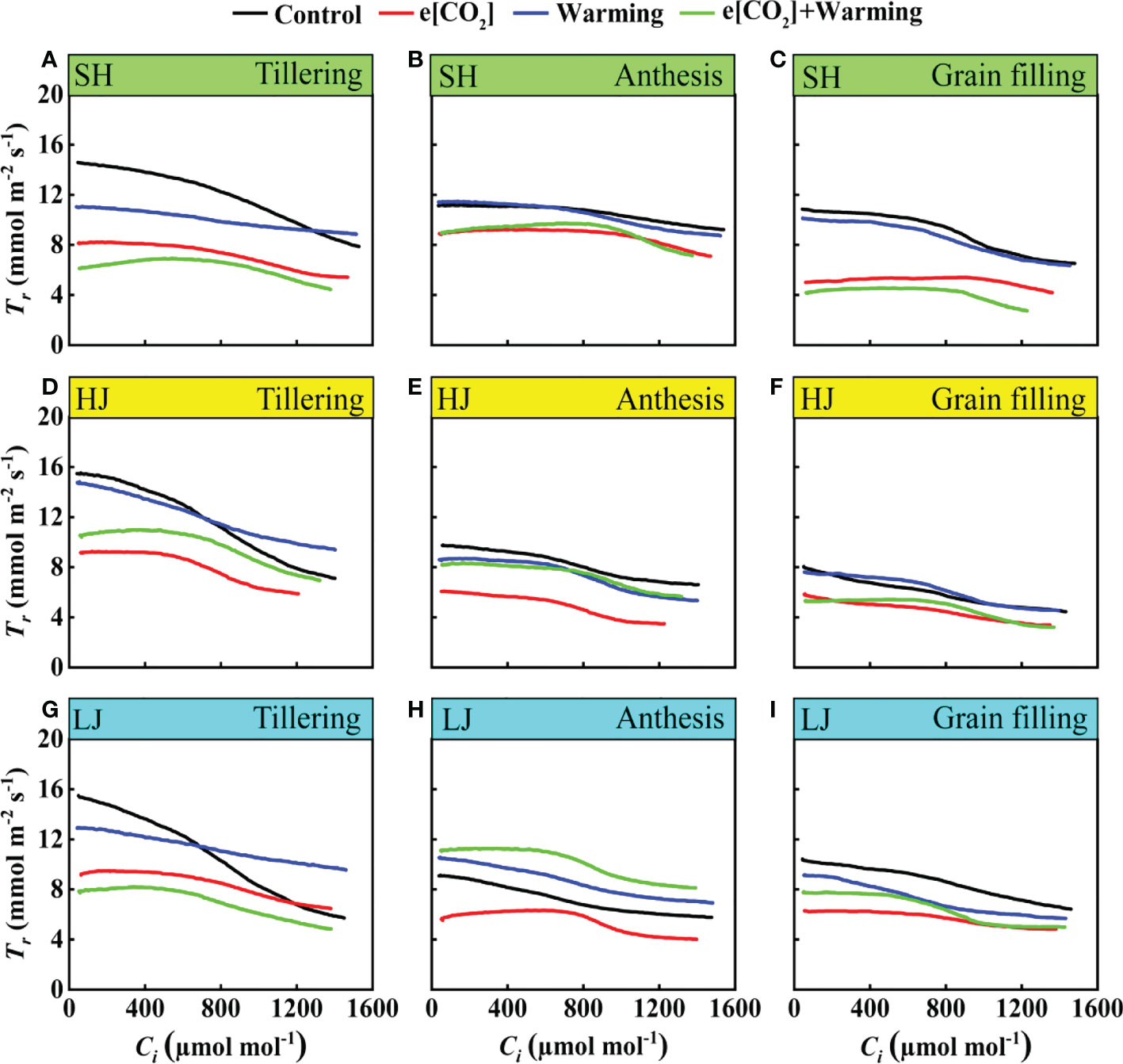
Figure 8 The Tr-Ci curves under ambient CO2 and temperature (black dots, Control), elevated CO2 (red dots, e[CO2]), elevated temperature (blue dots, warming), and eCO2 plus warming (green dot, e[CO2]+warming) for cv. SH (A-C), HJ (D-F), and LJ (G-I) during the tillering stage, anthesis stage, and grain-filling stage. Tr represents transpiration rate, Ci represents intercellular carbon dioxide concentration.
Response of instantaneous water use efficiency under e[CO2] and warming
In the present study, instantaneous water use efficiency (iWUE) in response to climate change varied among three varieties (Figure 9). With an increase in the intercellular carbon dioxide (Ci), instantaneous water use efficiency of rice leaves showed an increasing trend with a greater increase under e[CO2] plus warming than the control, except for the anthesis stage of LJ. Taken together, these results indicated that climate change could pose a positive effect on the iWUE.
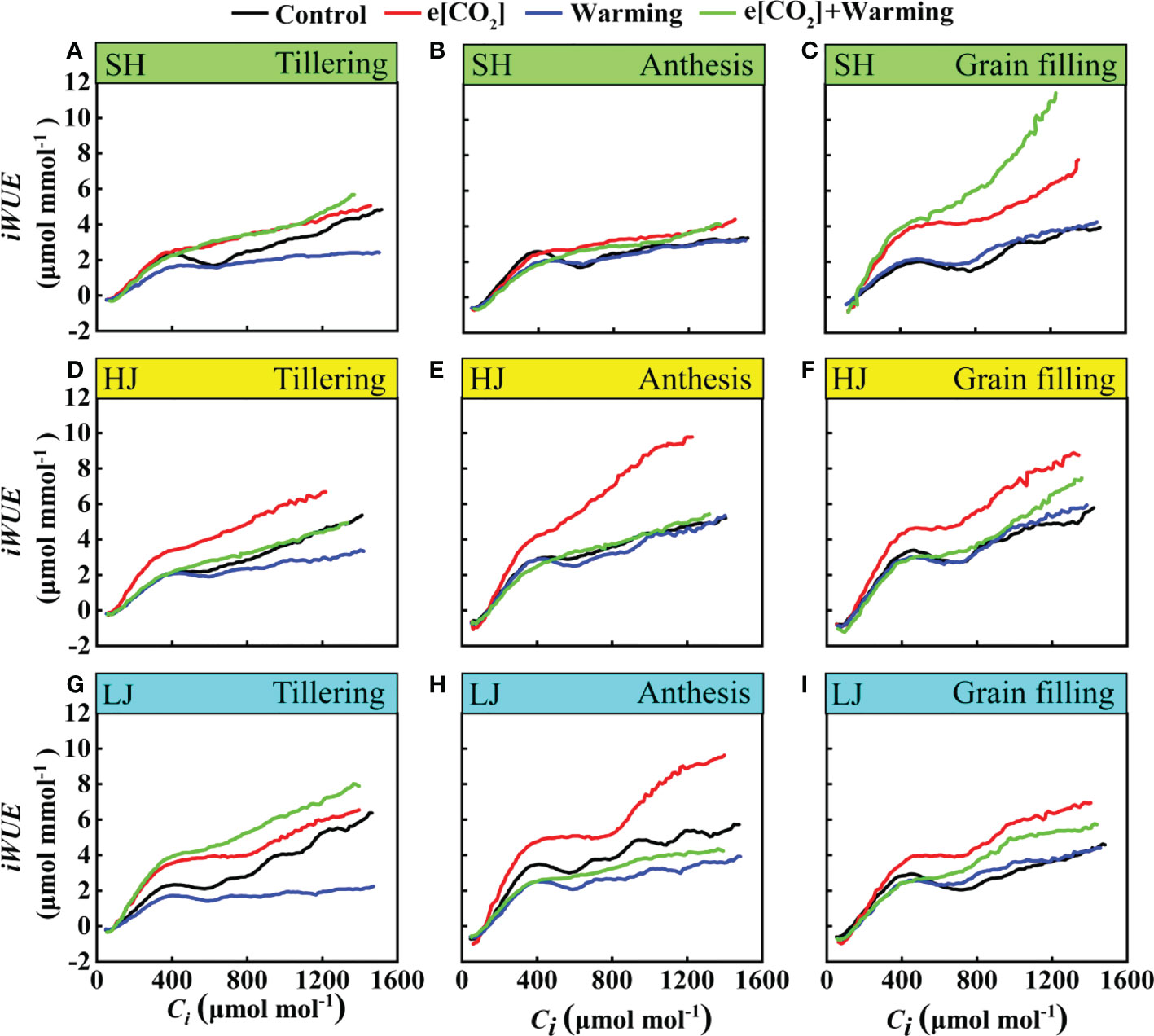
Figure 9 The iWUE-Ci Curves under ambient [CO2] and temperature (black dots, Control), elevated [CO2] (red dots, e[CO2]), elevated temperature (blue dots, warming), and e[CO2] plus warming (green dot, e[CO2]+warming) for cv. SH (A-C), HJ (D-F), and LJ (G-I) during the stage of tillering anthesis, and grain-filling. iWUE represents instantaneous water use efficiency, Ci represents intercellular carbon dioxide concentration.
Temperature differences between air and leaves in response to e[CO2] and warming
The air-to-leaf temperature difference of all treatments showed a gradual decrease with the increase of Ci (Figure 10). Here, a clear reduction of ΔT was observed at the tillering stage across three varieties under e[CO2] plus warming, but there is little difference at the anthesis stage. However, compared to the control, e[CO2] plus warming notably increased ΔT across three varieties at the grain-filling stage. e[CO2] plus warming altered the trend that air-to-leaf temperature difference decreased gradually with the reproductive process.
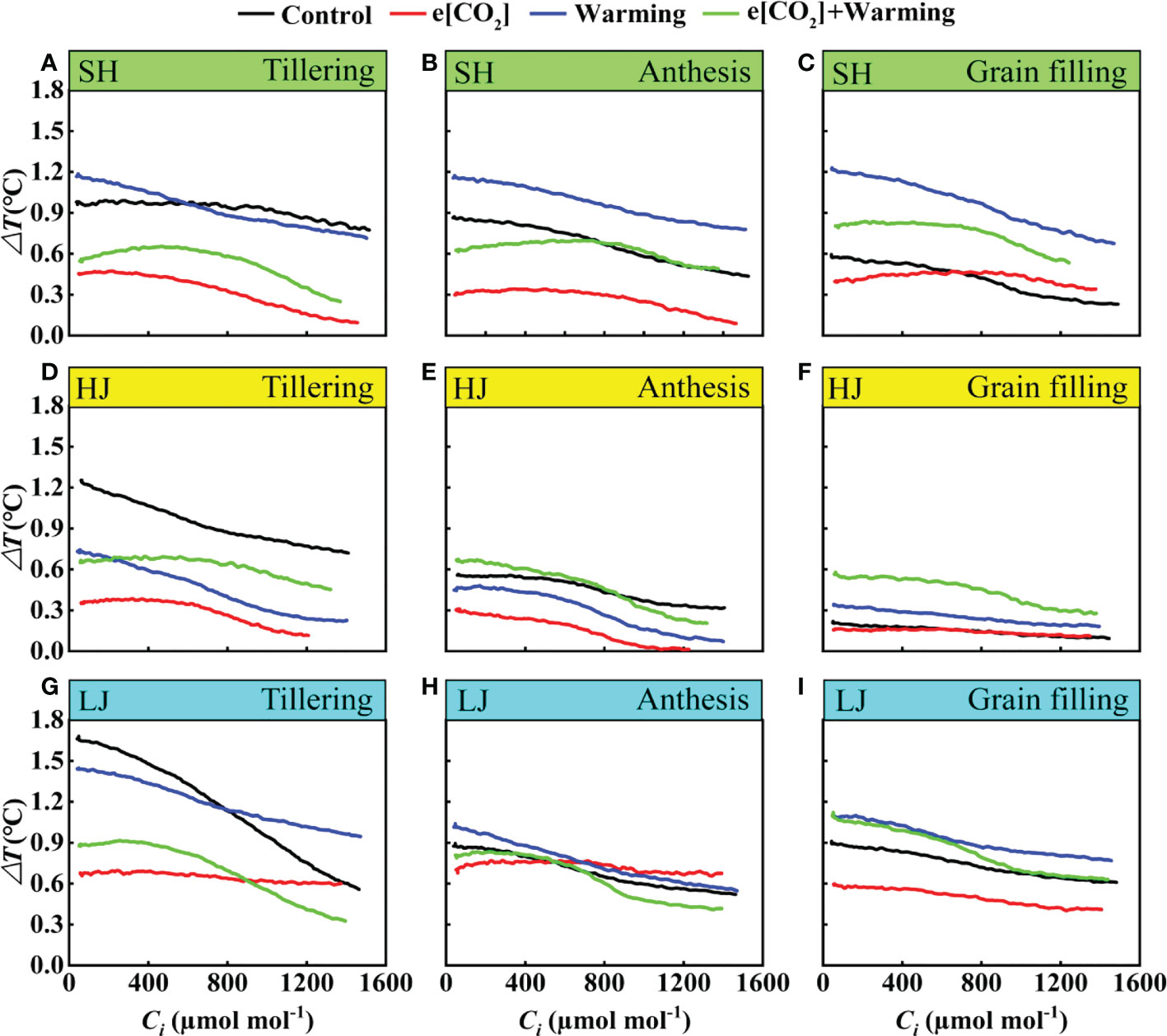
Figure 10 The ΔT-Ci Curves under ambient CO2 and temperature (black dots, Control), elevated [CO2] (red dots, e[CO2]), elevated temperature (blue dots, warming), and e[CO2] plus warming (green dot, e[CO2] +warming) for cv. SH (A-C), HJ (D-F), and LJ (G-I) during the tillering, anthesis, and grain-filling stages. ΔT represents the air-to-leaf temperature difference, Ci represents intercellular carbon dioxide concentration.
Aboveground biomass, grain yield and its components
In comparison to the control, the aboveground biomass at the mature stage tended to increase under e[CO2], warming, and e[CO2] plus warming. (Figure 11). In general, the aboveground biomass of SH, HJ, and LJ increased by 28%, 28%, and 36% under e[CO2] plus warming. At maturity, the dry matter weight of leaves, stems, and panicles under e[CO2] plus warming increased by 13%-50%, 21%-73%, and 4%-34%, respectively, compared to the control.
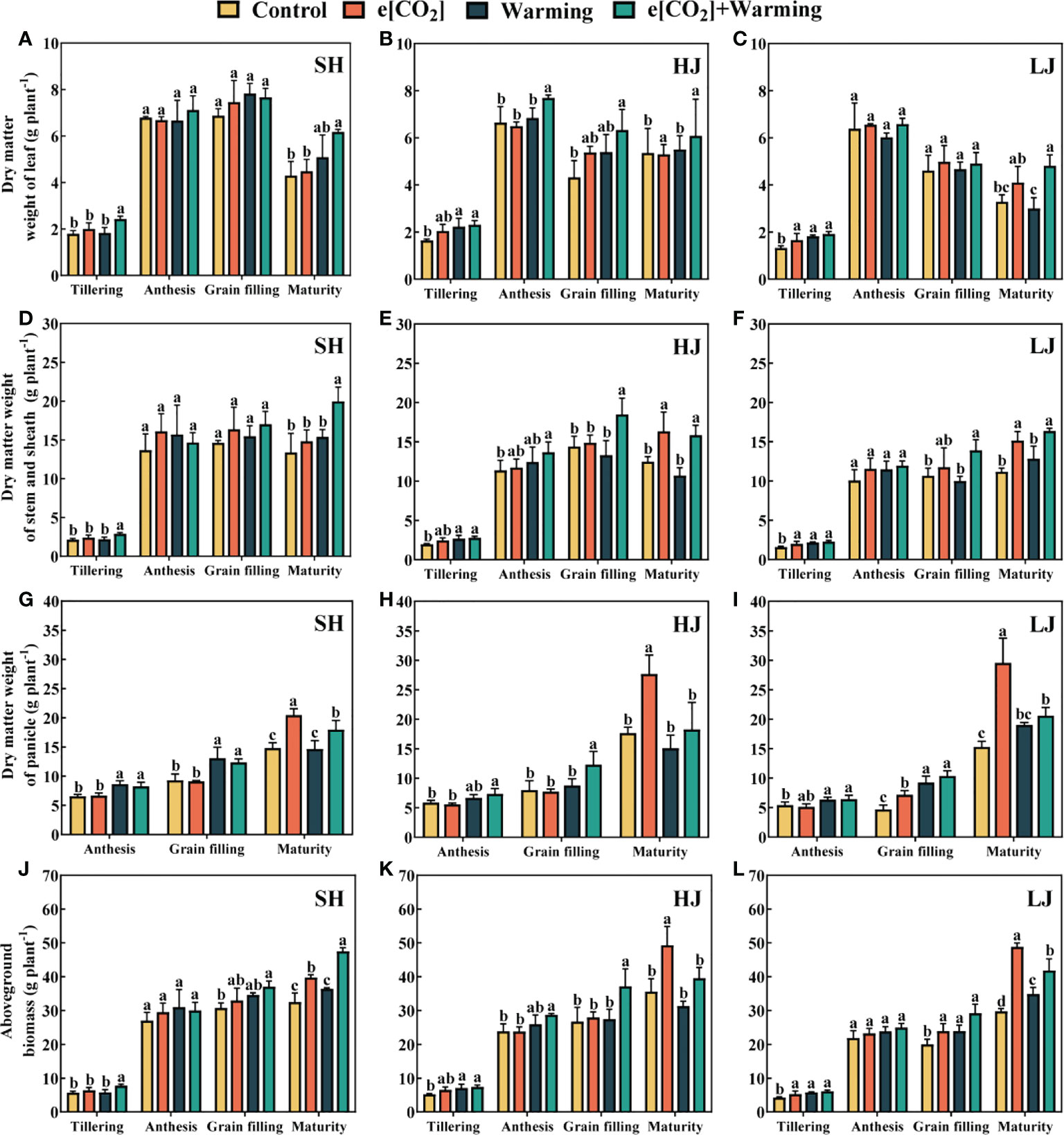
Figure 11 The dry matter weight of leaf (A–C), stem (D–F), panicle (G–I) and aboveground plant (J–L) at the stage of tillering, anthesis, grain filling and maturity under control, ambient conditions; e[CO2], elevated [CO2]; warming, elevated air temperature; e[CO2] plus warming, the combination of elevated air temperature and [CO2]. Values are the means ± SEs (n=3). Lower-case letters indicate significant differences between treatments at P < 0.05 level.
In most cases, leaf dry weight decreased slightly with warming, which could offset the positive effect of e[CO2] on rice dry matter accumulation. For example, compared to e[CO2], a 12%-34% reduction of the panicle dry matter weight was observed under e[CO2] plus warming.
In comparison to the control, e[CO2] significantly increased grain yields of SH, HJ and LJ by 39%, 46% and 59%, respectively (Figure 12). Rice yield did not change significantly in SH and LJ under warming, but increased by 11% in HJ, compared to the control. The increase in rice yield under e[CO2] plus warming was less than under e[CO2] but higher than that under warming. Furthermore, e[CO2] resulted in increases of 44%, 47%, and 88% in grain number in SH, HJ, and LJ. In contrast to the control, 1000-grain weight did not significantly differ among treatments except for a decrease under e[CO2] plus warming.
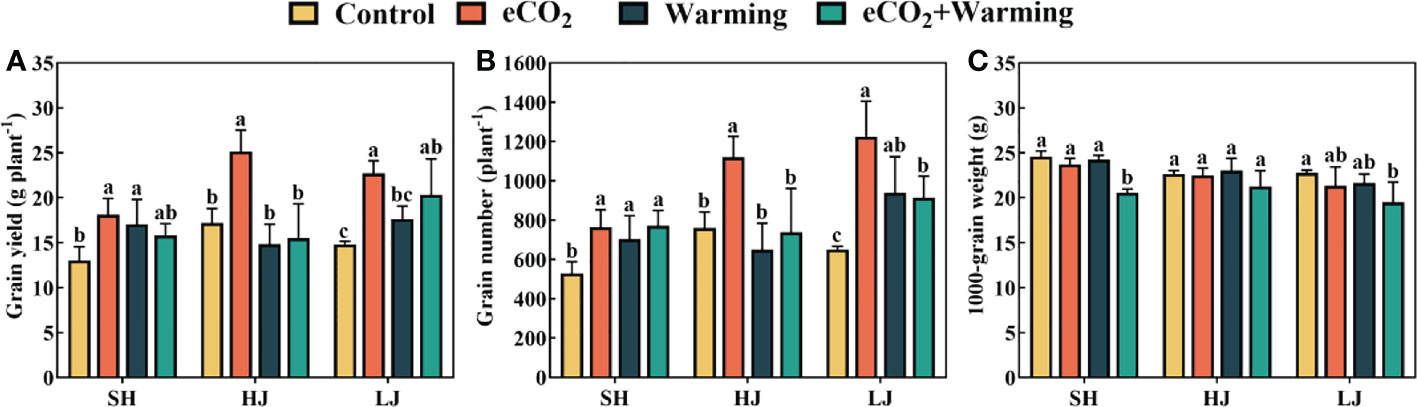
Figure 12 The rice yield (A), grain number (B) and 1000-grain weight (C) of three rice cultivars grown under control, ambient conditions; e[CO2], elevated CO2 concentration; warming, elevated air temperature; e[CO2] plus warming, the combination of elevated air temperature and [CO2]. SH, HJ and LJ refer to three rice cultivars Shishoubaimao, Hejiang 19, and Longjing 31, respectively. Values are the means ± SEs (n = 3). Lower-case letters indicate significant differences between treatments at P < 0.05 level.
Discussion
Vc, max and Jmax in response to e[CO2] and temperature
Previous studies demonstrated that e[CO2] led to a decrease in Vc, max (Medlyn et al., 1999; Ainsworth and Long, 2005; Cai et al., 2018). For example, a FACE study by Cai et al. (2018) indicated a significant reduction in Vc, max at several growth stages due to the interaction effect of e[CO2] (104-199 μmol/mol) and increased canopy temperature (1.0-1.7°C). Moreover, abiotic stress may constrain the effect of e[CO2] on Vc, max and Jmax, as Liu et al. (2018) found that 7 days of e[CO2] increased cucumber Vc, max and Jmax by 12.0% and 14.7%, respectively, but this effect disappeared under mild and severe drought stresses (Liu et al., 2018). However, in this study the Vc, max and Jmax increased in response to e[CO2] and/or warming treatments, indicating that e[CO2] and warming favor rice photosynthetic potential in cold temperate regions. It is probably because the increased temperature in the warming treatment is still in the range of temperature for optimal growth of rice (Zhang et al., 2022).
The Vc, max and Jmax in FvCB in response to [CO2] and temperature co-elevation varied among rice varieties. In this study, [CO2] and temperature co-elevation significantly increased Vc, max and Jmax in LJ but decreased in HJ (Figure 5). The increase in Vc, max and Jmax was greater in LJ compared to SH and HJ, especially for intercellular CO2 concentrations above 400 ppm, consistent with the performance of photosynthetic rates, as can be observed from An-Ci curve (Figure 4). Similar results were also found in a study by Miao et al. (2021) who found that Yangdao6 (a more CO2-responsive indica cultivar) had a stronger Vc, max and Jmax from heading to maturity under e[CO2] (+200) compared to other cultivars. Previous studies found that the response of Vc, max and Jmax to climate change differed between species (Wullschleger, 1993). The various responses of Vc, max and Jmax in C3 plants grown at e[CO2] were either positive such as Liquidambar styraciflflua (DeLucia and Thomas, 2000), or negative such as Oryza sativa L. and Lolium perenne L. (Ainsworth et al., 2003; Borjigidai et al., 2006). Interestingly, warming (1-2°C increase) and deficient soil nitrogen resulted in the decrease in Vc, max and Jmax of rice and cotton species (Pettersson and McDonald, 1994; Cai et al., 2018). However, in the present study, the increased Vc, max and Jmax in specific cultivars in response to e[CO2] and warming indicates its strong adaptability to climate change.
The Jmax: Vc, max ratio is critical for understanding plant photosynthetic processes. (Miao et al., 2009). Compared to the Vc, max, the response of Jmax to e[CO2] was more pronounced in this study. Some studies found that the enrichment of CO2 led to an increase of Jmax: Vc, max ratio (Ainsworth and Long, 2005), but warming reduced this ratio (Kattge and Knorr, 2007; Lin et al., 2013). This is consistent with our findings that the Jmax: Vc, max ratio was significantly increased under e[CO2] for three cultivars (Figure 5). Despite that [CO2] and temperature co-elevation only significantly increased the Jmax: Vc, max ratio in SH, but had no remarkable effect on Jmax: Vc, max ratio for HJ and LJ. It might explain the greater improvement in net photosynthetic rate for SH than HJ and LJ. Thus, the Jmax: Vc, max ratio may be imperative to the instinct photosynthetic and yield performance under the changing environments.
Leaf photosynthesis and water use efficiency under different treatments
The impact of e[CO2] on photosynthesis has been well documented in rice (Roy et al., 2012; Chen et al., 2014; Zhu et al., 2014; Cai et al., 2018). e[CO2] notably increased the net photosynthetic rate (An) in three varieties, especially at the grain filling stage. These results were consistent with previous studies (Chen et al., 2014; Yuan et al., 2021; Wang et al., 2015a). While the average temperature at the test site did not exceed the suitable temperature for rice under the warming scenario in this study, but high temperatures and heat waves frequently occurred in July and August. This may increase photorespiration and respiration, which cause a reduction in the net photosynthesis rate (An). Furthermore, Previous studies have shown that the anthesis stage of rice is more sensitive to environmental factors, such as temperature, etc. (Jagadish et al, 2007; Wassmann et al., 2009). Therefore, in the present study, a significant decrease in An was found under warming at the anthesis stage, which may result in a decrease in rice yield formation or offset the positive effect of e[CO2] on rice yield.
In this study, the stomatal conductance was decreased with the continuous increase of intercellular CO2 concentration under [CO2] and temperature co-elevation (Figure 2). It is well recognized that increasing CO2 concentration led to a reduction in stomatal conductance (Bunce, 2004; Ainsworth and Rogers, 2007; Lenka et al., 2021a). However, previous studies indicated that the warming effect on stomatal conductance was either positive or negative (Mott and Peak, 2013; Lahr et al., 2015; Urban et al., 2017). This is likely attributed to the increase extent of the environmental temperature. Previous studies demonstrated that stomatal conductance was 4.3 times higher at 35°C than that at 15°C for the warm climate species such as soybean and tomato (Bunce, 2000a). However, several studies have also demonstrated that warming led to the reduction of stomatal conductance in rice and many other species (Lewis et al., 2002; Lahr et al., 2015; Göbel et al., 2019; Huang et al., 2021). These findings are in line with the present study. The differences in stomatal performance in response to warming may be related to the plant species or original habitats. Furthermore, the response of stomatal conductance to warming also varied with the phenological process, for instance, warming increased stomatal conductance for SH at the tillering stage except at anthesis and grain filling stages, indicating that stomatal conductance was not consistently characteristic in response to climate change throughout the growth phase (Wang et al., 2020b). Additionally, there was an offsetting effect on stomatal conductance under e[CO2] and warming at anthesis and grain filling stages (Del Pozo et al., 2005; Wang et al., 2020b).
The treatment of [CO2] and temperature co-elevation resulted in a higher water use efficiency (Figures 3 and 9). Numerous studies have shown that higher water use efficiency under e[CO2] (Bunce, 2000b; Allen et al., 2003; Ruiz-Vera et al., 2013; Li et al., 2019a). The water use efficiency can be mediated by both photosynthetic rate and transpiration rate, as previously observed in rice (Gauthami et al., 2014; Mishra et al., 2018). In the current study, under [CO2] and temperature co-elevation, the increase in water use efficiency is a result of a combination of increased photosynthetic rate and decreased transpiration rate, such as SH and LJ at the grain filling stage. Meanwhile, the water use efficiency of SH and LJ was more responsive to climate change than that of HJ, implying that improvement of water use efficiency under climate change could be improved by breeding new rice varieties in the future.
Stomatal conductance response to climate change may pose an essential role on the water use efficiency. A study in free air CO2 enrichment system (FACE) by Bernacchi et al. (2007) reported that e[CO2] decreased the evapotranspiration by 9-16% and as a result of the decrease in Gs. Similarly, in a study on soybean in environment-controlled chambers, doubling [CO2] caused a 9% decline in evapotranspiration at a day/night temperature combination of 28/18°C, while there was the limited effect at 40/30°C and 44/34°C temperature treatments (Allen et al., 2003). In the present study, the transpiration rate of all cultivars was significantly decreased under e[CO2]. It may mainly be related to the significantly decreased stomatal conductance (Bernacchi et al., 2007; Kimball, 2016; Lenka et al., 2020). The reduction of stomatal conductance mainly occurred under e[CO2] rather than the warming condition (Figures 2 and 7), highlighting that enhanced water use efficiency under e[CO2] plus warming is the e[CO2]-induced reduction of stomatal conductance.
In the present study, the air -to- leaf temperature difference (ΔT) showed gradual decrease with the intercellular CO2 concentration increased under e[CO2], warming and e[CO2] plus warming (Figure 10). In general, a deceased stomatal conductance reduces leaf water loss and increases leaf temperature, resulting in a decrease in air-to-leaf temperature differences. (Allen et al., 2003; Kimball, 2016; Urban et al., 2017). The air -to- leaf temperature difference reduced under e[CO2] across three growth stages for all species. However, Lenka et al. (2020) reported that temperature increase above ambient 1.5°C along with e[CO2] was unlikely to lead to a difference in leaf temperature in soybean cultivar. In the present study, the air -to- leaf temperature difference in SH cultivar under e[CO2] plus warming is greater than that in HJ and LJ. The relatively stable response of the air -to- leaf temperature difference in HJ and LJ indicated a potential for physiological adaptation of rice cultivars to climate change.
Warming alters biomass and yield under e[CO2]
Elevated temperature often reduces rice dry matter accumulation due to enhanced plant respiration, shortens growth duration and increases floral sterility (Ziska et al., 1996; Dong et al., 2011; Cai et al., 2016). However, warming has also been reported to benefit rice growth in regions with low ambient temperature (Keiichi et al., 2009; Adachi et al., 2014; Chen et al., 2017; Wang et al., 2020a). In the present study, warming promoted leaf photosynthesis, and panicle dry matter accumulation in SH and LJ at the stage of anthesis and grain filling. Additionally, there is substantial evidence that e[CO2] positively impacts rice dry matter accumulation which is consistent with the present study. (Sakai et al., 2001; Roy et al., 2012; Cai et al., 2018; Wang et al., 2020a). For example, the rice yield increased by 15% under e[CO2] (550 ppm) in a free-air [CO2] enrichment study (Wang et al., 2018). The results showed that while warming did not reduce or slightly reduced rice yield, the increase in yield under e[CO2] plus warming was less than that under e[CO2]. Nevertheless, considering the possible increase in rice biomass caused by the co-elevation of e[CO2] and temperature in this region, the rice varieties adapting to e[CO2] plus warming should receive more attention to improve rice production in the future.
Conclusion
Warming by 2°C is likely to partially offset increases in photosynthetic rate attributed to e[CO2]. The [CO2] and temperature co-elevation may be favorable increasing photosynthetic ability of rice crop and improving water use efficiency. Although increased temperature in this study had a minor effect on grain yield, the effect may increase under future climate change. This study provided evidence that the rice has genotypic differences in photosynthetic potential under [CO2] and temperature co-elevation. Therefore, it is crucial to explore a broader range of phenotypes and cultivars in climate change adaption research, in order to advance the knowledge that climate change impacts rice crop resulting in a prospective increase of grain yield induced by [CO2] and temperature co-elevation in the cold-temperate climate regions.
Data availability statement
The original contributions presented in the study are included in the article/supplementary material. Further inquiries can be directed to the corresponding authors.
Author contributions
JJ and YL designed the experiments and managed the projects. YL, CZ, ZY, and JDL performed the experiments. CZ, KY, GW, XL, JLL and XZ performed the data analysis. JJ and CZ wrote the first draft of the manuscript, and YL and CZ edited and revised the manuscript. All authors contributed to the article and approved the submitted version.
Funding
The project was funded by the Strategic Priority Research Program of the Chinese Academy of Sciences (XDA28100200, XDA28020201), the National Natural Science Foundation of China (32172123), Key Program of Natural Science Foundation of Heilongjiang Province of China (ZD2021D001), the International Partnership Program of Chinese Academy of Sciences (131323KYSB20210004), and Youth Innovation Promotion Association of Chinese Academy of Sciences (2019233), the International Partnership Program of Chinese Academy of Sciences (131323KYSB20210004), and Youth Innovation Promotion Association of Chinese Academy of Sciences (2019233).
Acknowledgments
The authors are grateful to Drs. Ying Xu, Zhuxiu Liu and Haidong Gu for assisting with the data analysis, as well as Drs. Qingyun Bu and Xiufeng Li for providing the rice cultivar materials. We also appreciate the valuable work and comments of the reviewers and the editor.
Conflict of interest
The authors declare that the research was conducted in the absence of any commercial or financial relationships that could be construed as a potential conflict of interest.
Publisher’s note
All claims expressed in this article are solely those of the authors and do not necessarily represent those of their affiliated organizations, or those of the publisher, the editors and the reviewers. Any product that may be evaluated in this article, or claim that may be made by its manufacturer, is not guaranteed or endorsed by the publisher.
References
Adachi, M., Hasegawa, T., Fukayama, H., Tokida, T., Sakai, H., Okada, M. (2014). Soil and water warming accelerates phenology and down-regulation of leaf photosynthesis of rice plants grown under free-air CO2 enrichment (FACE). Plant Cell Physiol. 55, 370–380. doi: 10.1093/pcp/pcu005
Ainsworth, E. A., Davey, P. A., Hymus, G. J., Osborne, C. P., Rogers, A., Blum, H., et al. (2003). Is stimulation of leaf photosynthesis by elevated carbon dioxide concentration maintained in the long term? a test with Lolium perenne grown for 10 years at two nitrogen fertilization levels under free air CO2 enrichment (FACE). Plant Cell Environ. 26, 705–714. doi: 10.1046/j.1365-3040.2003.01007.x
Ainsworth, E. A., Long, S. P. (2005). What have we learned from 15 years of free-air CO2 enrichment (FACE)? a meta-analytic review of the responses of photosynthesis, canopy properties and plant production to rising CO2. New Phytol. 165, 351–372. doi: 10.1111/j.1469-8137.2004.01224.x
Ainsworth, E. A., Rogers, A. (2007). The response of photosynthesis and stomatal conductance to rising [CO2]: mechanisms and environmental interactions. Plant Cell Environ. 30, 258–270. doi: 10.1111/j.1365-3040.2007.01641.x
Allen, L. H., Pan, D., Boote, K. J., Pickering, N. B., Jones, J. W. (2003). Carbon dioxide and temperature effects on evapotranspiration and water use efficiency of soybean. Agron. J. 95, 1071–1081. doi: 10.2134/agronj2003.1071
Arcus, V. L., Prentice, E. J., Hobbs, J. K., Mulholland, A. J., van der Kamp, M. W., Pudney, C. R., et al. (2016). On the temperature dependence of enzyme-catalyzed rates. Biochemistry 55, 1681–1688. doi: 10.1021/acs.biochem.5b01094
Arnon, D. I. (1949). Copper enzymes in isolated chloroplasts. polyphenoloxidase in beta vulgaris. Plant Physiol. 24, 1. doi: 10.1104/pp.24.1.1
Bahuguna, R. N., Jha, J., Pal, M., Shah, D., Lawas, L. M., Khetarpal, S. (2015). Physiological and biochemical characterization of NERICA-L-44: a novel source of heat tolerance at the vegetative and reproductive stages in rice. Physiol. Plantarum 154, 543–559. doi: 10.1111/ppl.12299
Bernacchi, C. J., Kimball, B. A., Quarles, D. R., Long, S. P., Ort, D. R. (2007). Decreases in stomatal conductance of soybean under open-air elevation of [CO2] are closely coupled with decreases in ecosystem evapotranspiration. Plant Physiol. 143, 134–144. doi: 10.1104/pp.106.089557
Bhattacharyya, P., Roy, K. S., Dash, P. K., Neogi, S., Shahid, M. D., Nayak, A. K., et al. (2014). Effect of elevated carbon dioxide and temperature on phosphorus uptake in tropical flooded rice (Oryza sativa l.). Eur. J. Agron. 53, 28–37. doi: 10.1016/j.eja.2013.10.008
Bocchiola, D. (2015). Impact of potential climate change on crop yield and water footprint of rice in the po valley of Italy. Agr Syst. 139, 223–237. doi: 10.1016/j.agsy.2015.07.009
Borjigidai, A., Hikosaka, K., Hirose, T., Hasegawa, T., Okada, M., Kobayashi, K. (2006). Seasonal changes in temperature dependence of photosynthetic rate in rice under a free-air CO2 enrichment. Ann. Bot-London 97, 549–557. doi: 10.1093/aob/mcl001
Brito, F. A., Pimenta, T. M., Henschel, J. M., Martins, S. C., Zsögön, A., Ribeiro, D. M. (2020). Elevated CO2 improves assimilation rate and growth of tomato plants under progressively higher soil salinity by decreasing abscisic acid and ethylene levels. Environ. Exp. Bot. 176, 104050. doi: 10.1016/j.envexpbot.2020.104050
Bunce, J. A. (2000a). Acclimation of photosynthesis to temperature in eight cool and warm climate herbaceous C3 species: temperature dependence of parameters of a biochemical photosynthesis model. Photosynth Res. 63, 59–67. doi: 10.1023/A:1006325724086
Bunce, J. A. (2000b). Responses of stomatal conductance to light, humidity and temperature in winter wheat and barley grown at three concentrations of carbon dioxide in the field. Global Change Biol. 6, 371–382. doi: 10.1046/j.1365-2486.2000.00314.x
Bunce, J. A. (2004). Carbon dioxide effects on stomatal responses to the environment and water use by crops under field conditions. Oecologia 140, 1–10. doi: 10.1007/s00442-003-1401-6
Cai, C., Li, G., Yang, H., Yang, J., Liu, H., Struik, P. C., et al. (2018). Do all leaf photosynthesis parameters of rice acclimate to elevated CO2, elevated temperature, and their combination, in FACE environments? Global Change Biol. 24, 1685–1707. doi: 10.1111/gcb.13961
Cai, C., Yin, X., He, S., Jiang, W., Si, C., Struik, P. C., et al. (2016). Responses of wheat and rice to factorial combinations of ambient and elevated CO2 and temperature in FACE experiments. Global Change Biol. 22, 856–874. doi: 10.1111/gcb.13065
Carvalho, J. M., Barreto, R. F., Prado, R. D. M., Habermann, E., Martinez, C. A., Branco, R. B. F. (2020). Elevated [CO2] and warming increase the macronutrient use efficiency and biomass of Stylosanthes capitata vogel under field conditions. J. Agron. Crop Sci. 206, 597–606. doi: 10.1111/jac.12398
Chen, J., Chen, C., Tian, Y., Zhang, X., Dong, W., Zhang, W. (2017). Differences in the impacts of nighttime warming on crop growth of rice-based cropping systems under field conditions. Eur. J. Agron. 82, 80–92. doi: 10.1016/j.eja.2016.10.006
Cheng, W., Sakai, H., Yagi, K., Hasegawa, T. (2009). Interactions of elevated [CO2] and night temperature on rice growth and yield. Agr For. Meteorol 149, 51–58. doi: 10.1016/j.agrformet.2008.07.006
Chen, C. P., Sakai, H., Tokida, T., Usui, Y., Nakamura, H., Hasegawa, T. (2014). Do the rich always become richer? characterizing the leaf physiological response of the high-yielding rice cultivar takanari to free-air CO2 enrichment. Plant Cell Physiol. 55, 381–391. doi: 10.1093/pcp/pcu009
Coursolle, C., Otis Prud’homme, G., Lamothe, M., Isabel, N. (2019). Measuring rapid a–ci curves in Boreal conifers: Black spruce and balsam fir. Front. Plant Sci. 10. doi: 10.3389/fpls.2019.01276
Del Pozo, A., Pérez, P., Morcuende, R., Alonso, A., Martínez-Carrasco, R. (2005). Acclimatory responses of stomatal conductance and photosynthesis to elevated CO2 and temperature in wheat crops grown at varying levels of n supply in a Mediterranean environment. Plant Sci. 169, 908–916. doi: 10.1016/j.plantsci.2005.06.009
DeLucia, E. H., Thomas, R. B. (2000). Photosynthetic responses to CO2 enrichment of four hardwood species in a forest understory. Oecologia 122, 11–19. doi: 10.1007/PL00008827
Dong, W., Chen, J., Zhang, B., Tian, Y., Zhang, W. (2011). Responses of biomass growth and grain yield of midseason rice to the anticipated warming with FATI facility in East China. Field Crop Res. 123, 259–265. doi: 10.1016/j.fcr.2011.05.024
Drewry, D. T., Kumar, P., Long, S. P. (2014). Simultaneous improvement in productivity, water use, and albedo through crop structural modification. Global Change Biol. 20, 1955–1967. doi: 10.1111/gcb.12567
Dusenge, M. E., Duarte, A. G., Way, D. A. (2019). Plant carbon metabolism and climate change: elevated CO2 and temperature impacts on photosynthesis, photorespiration and respiration. New Phytol. 221, 32–49. doi: 10.1111/nph.15283
Duursma, R. A. (2015). Plantecophys-an r package for analysing and modelling leaf gas exchange data. PloS One 10, e0143346. doi: 10.1371/journal.pone.0143346
Farage, P. K., McKee, I. F., Long, S. P. (1998). Does a low nitrogen supply necessarily lead to acclimation of photosynthesis to elevated CO2? Plant Physiol. 118, 573–580. doi: 10.1104/pp.118.2.573
Farquhar, G. D., von Caemmerer, S., Berry, J. A. (1980). A biochemical model of photosynthetic CO2 assimilation in leaves of C3 species. Planta 149, 78–90. doi: 10.1007/BF00386231
Gauthami, P., Subrahmanyam, D., Padma, V., Kiran, T. V., Rao, Y. V., Rao, P. R., et al. (2014). Variation in leaf photosynthetic response of rice genotypes to post-anthesis water deficit. Indian J. Plant Physiol. 19, 127–137. doi: 10.1007/s40502-014-0086-7
Göbel, L., Coners, H., Hertel, D., Willinghöfer, S., Leuschner, C. (2019). The role of low soil temperature for photosynthesis and stomatal conductance of three graminoids from different elevations. Front. Plant Sci. 10. doi: 10.3389/fpls.2019.00330
Guo, L., Yu, Z., Li, Y., Xie, Z., Wang, G., Liu, X., et al. (2022). Plant phosphorus acquisition links to phosphorus transformation in the rhizospheres of soybean and rice grown under CO2 and temperature co-elevation. Sci. Total Environ. 823, 153558. doi: 10.1016/j.scitotenv.2022.153558
Huang, G., Yang, Y., Zhu, L., Peng, S., Li, Y. (2021). Temperature responses of photosynthesis and stomatal conductance in rice and wheat plants. Agr For. Meteorol 300, 108322. doi: 10.1016/j.agrformet.2021.108322
IPCC (2021). “Climate Change 2021: The Physical Science Basis,” in Contribution of Working Group I to the Sixth Assessment Report of the Intergovernmental Panel on Climate Change. Eds. Masson-Delmotte, V., Zhai, P., Pirani, A., Connors, S. L., Péan, C., Berger, S., et al (Cambridge, United Kingdom and New York, NY, USA: Cambridge University Press) 2391 pp. doi: 10.1017/9781009157896
Jagadish, S. K., Craufurd, P. Q., Wheeler, T. (2007). High temperature stress and spikelet fertility in rice (Oryza sativa l.). J. Exp. Bot. 58, 1627–1635. doi: 10.1093/jxb/erm003
Kanno, K., Mae, T., Makino, A. (2009). High night temperature stimulates photosynthesis, biomass production and growth during the vegetative stage of rice plants. Soil Sci. Plant Nutr. 55, 124–131. doi: 10.1111/j.1747-0765.2008.00343.x
Kattge, J., Knorr, W. (2007). Temperature acclimation in a biochemical model of photosynthesis: a reanalysis of data from 36 species. Plant Cell Environ. 30, 1176–1190. doi: 10.1111/j.1365-3040.2007.01690.x
Kimball, B. A. (2016). Crop responses to elevated CO2 and interactions with H2O, n, and temperature. Curr. Opin. Plant Biol. 31, 36–43. doi: 10.1016/j.pbi.2016.03.006
Lahr, E. C., Schade, G. W., Crossett, C. C., Watson, M. R. (2015). Photosynthesis and isoprene emission from trees along an urban–rural gradient in Texas. Global Change Biol. 21, 4221–4236. doi: 10.1111/gcb.13010
Le, P. V., Kumar, P., Drewry, D. T. (2011). Implications for the hydrologic cycle under climate change due to the expansion of bioenergy crops in the Midwestern united states. P Natl. Acad. Sci. U.S.A. 108, 15085–15090. doi: 10.1073/pnas.110717710
Lenka, N. K., Lenka, S., Mahapatra, P., Sharma, N., Kumar, S., Aher, S. B., et al. (2019). The fate of 15N labeled urea in a soybean-wheat cropping sequence under elevated CO2 and/or temperature. Agr Ecosyst. Environ. 282, 23–29. doi: 10.1016/j.agee.2019.04.033
Lenka, N. K., Lenka, S., Thakur, J. K., Yashona, D. S., Shukla, A. K., Elanchezhian, R., et al. (2020). Carbon dioxide and temperature elevation effects on crop evapotranspiration and water use efficiency in soybean as affected by different nitrogen levels. Agr Water Manage 230, 105936. doi: 10.1016/j.agwat.2019.105936
Lenka, N. K., Lenka, S., Yashona, D. S., Jat, D. (2021a). Elevated temperature and low nitrogen partially offset the yield, evapotranspiration, and water use efficiency of winter wheat under carbon dioxide enrichment. Agr Water Manage 250, 106856. doi: 10.1016/j.agwat.2021.106856
Lenka, N. K., Lenka, S., Yashona, D. S., Shukla, A. K., Elanchezhian, R., Dey, P. (2021b). Carbon dioxide and/or temperature elevation effect on yield response, nutrient partitioning and use efficiency of applied nitrogen in wheat crop in central India. Field Crop Res. 264, 108084. doi: 10.1016/j.fcr.2021.108084
Lewis, J. D., Lucash, M., Olszyk, D. M., Tingey, D. T. (2002). Stomatal responses of Douglas-fir seedlings to elevated carbon dioxide and temperature during the third and fourth years of exposure. Plant Cell Environ. 25, 1411–1421. doi: 10.1046/j.1365-3040.2002.00923.x
Li, X., Kang, S., Niu, J., Huo, Z., Liu, J. (2019a). Improving the representation of stomatal responses to CO2 within the penman–monteith model to better estimate evapotranspiration responses to climate change. J. Hydrol 572, 692–705. doi: 10.1016/j.jhydrol.2019.03.029
Lin, Y. S., Medlyn, B. E., De Kauwe, M. G., Ellsworth, D. S. (2013). Biochemical photosynthetic responses to temperature: how do interspecific differences compare with seasonal shifts? Tree Physiol. 33, 793–806. doi: 10.1093/treephys/tpt047
Liu, B. B., Li, M., Li, Q. M., Cui, Q. Q., Zhang, W. D., Ai, X. Z., et al. (2018). Combined effects of elevated CO2 concentration and drought stress on photosynthetic performance and leaf structure of cucumber (Cucumis sativus l.) seedlings. Photosynthetica 56, 942–952. doi: 10.1007/s11099-017-0753-9
Li, Y., Yu, Z., Jin, J., Zhang, Q., Wang, G., Liu, C., et al. (2018). Impact of elevated CO2 on seed quality of soybean at the fresh edible and mature stages. Front. Plant Sci. 9. doi: 10.3389/fpls.2018.01413
Li, Y., Yu, Z., Yang, S., Wang, G., Liu, X., Wang, C., et al. (2019b). Impact of elevated CO2 on c: N: P ratio among soybean cultivars. Sci. Total Environ. 694, 133784. doi: 10.1016/j.scitotenv.2019.133784
Medlyn, B. E., Badeck, F. W., De Pury, D. G. G., Barton, C. V. M., Broadmeadow, M., Jstbid, P. G. (1999). Effects of elevated [CO2] on photosynthesis in European forest species: a meta-analysis of model parameters. Plant Cell Environ. 22, 1475–1495. doi: 10.1046/j.1365-3040.1999.00523.x
Miao, Y., Cai, Y., Wu, H., Wang, D. (2021). Diurnal and seasonal variations in the photosynthetic characteristics and the gas exchange simulations of two rice cultivars grown at ambient and elevated CO2. Front. Plant Sci. 12. doi: 10.3389/fpls.2021.651606
Miao, Z., Xu, M., Lathrop, R. G., Jr., Wang, Y. (2009). Comparison of the a–cc curve fitting methods in determining maximum ribulose 1·5-bisphosphate carboxylase/oxygenase carboxylation rate, potential light saturated electron transport rate and leaf dark respiration. Plant Cell Environ. 32, 109–122. doi: 10.1111/j.1365-3040.2008.01900.x
Mishra, S. S., Behera, P. K., Kumar, V., Lenka, S. K., Panda, D. (2018). Physiological characterization and allelic diversity of selected drought tolerant traditional rice (Oryza sativa l.) landraces of koraput, India. Physiol. Mol. Biol. Pla 24, 1035–1046. doi: 10.1007/s12298-018-0606-4
Mott, K. A., Peak, D. (2013). Testing a vapour-phase model of stomatal responses to humidity. Plant Cell Environ. 36, 936–944. doi: 10.1111/pce.12026
Pettersson, R., McDonald, A. J. S. (1994). Effects of nitrogen supply on the acclimation of photosynthesis to elevated CO2. Photosynth Res. 39, 389–400. doi: 10.1007/BF00014593
Qiao, Y., Zhang, H., Dong, B., Shi, C., Li, Y., Zhai, H., et al. (2010). Effects of elevated CO2 concentration on growth and water use efficiency of winter wheat under two soil water regimes. Agr Water Manage 97, 1742–1748. doi: 10.1016/j.agwat.2010.06.007
Rogers, A. (2014). The use and misuse of vc, max in earth system models. Photosynth Res. 119, 15–29. doi: 10.1007/s11120-013-9818-1
Roy, K. S., Bhattacharyya, P., Neogi, S., Rao, K. S., Adhya, T. K. (2012). Combined effect of elevated CO2 and temperature on dry matter production, net assimilation rate, c and n allocations in tropical rice (Oryza sativa l.). Field Crop Res. 139, 71–79. doi: 10.1016/j.fcr.2012.10.011
Ruiz-Vera, U. M., Siebers, M., Gray, S. B., Drag, D. W., Rosenthal, D. M., Kimball, B. A., et al. (2013). Global warming can negate the expected CO2 stimulation in photosynthesis and productivity for soybean grown in the Midwestern united states. Plant Physiol. 162, 410–423. doi: 10.1104/pp.112.211938
Saathoff, A. J., Welles, J. (2021). Gas exchange measurements in the unsteady state. Plant Cell Environ. 44, 3509–3523. doi: 10.1111/pce.14178
Sasaki, H., Hara, T., Ito, S., Uehara, N., Kim, H. Y., Kobayashi, K. (2007). Effect of free-air CO2 enrichment on the storage of carbohydrate fixed at different stages in rice (Oryza sativa l.). Field Crop Res. 100, 24–31. doi: 10.1016/j.fcr.2006.05.003
Sakai, H., Yagi, K., Kobayashi, K., Kawashima, S. (2014). Rice carbon balance under elevated CO2. New Phytol. 150, 241–249. doi: 10.1046/j.1469-8137.2001.00105.x
Thanawong, K., Perret, S. R., Basset-Mens, C. (2014). Eco-efficiency of paddy rice production in northeastern Thailand: a comparison of rain-fed and irrigated cropping systems. J. Clean Prod. 73, 204–217. doi: 10.1016/j.jclepro.2013.12.067
Ullah, H., Santiago-Arenas, R., Ferdous, Z., Attia, A., Datta, A. (2019). Improving water use efficiency, nitrogen use efficiency, and radiation use efficiency in field crops under drought stress: A review. Adv. Agron. 156, 109–157. doi: 10.1016/bs.agron.2019.02.002
Urban, J., Ingwers, M. W., McGuire, M. A., Teskey, R. O. (2017). Increase in leaf temperature opens stomata and decouples net photosynthesis from stomatal conductance in Pinus taeda and Populus deltoides x nigra. J. Exp. Bot. 68, 1757–1767. doi: 10.1093/jxb/erx052
Wang, W., Cai, C., He, J., Gu, J., Zhu, G., Zhang, W., et al. (2020b). Yield, dry matter distribution and photosynthetic characteristics of rice under elevated CO2 and increased temperature conditions. Field Crop Res. 248, 107605. doi: 10.1016/j.fcr.2019.107605
Wang, W., Cai, C., Lam, S. K., Liu, G., Zhu, J. (2018). Elevated CO2 cannot compensate for japonica grain yield losses under increasing air temperature because of the decrease in spikelet density. Eur. J. Agron. 99, 21–29. doi: 10.1016/j.eja.2018.06.005
Wang, B., Cai, W., Li, J., Wan, Y., Guo, C., Wilkes, A., et al. (2020a). Leaf photosynthesis and stomatal conductance acclimate to elevated [CO2] and temperature thus increasing dry matter productivity in a double rice cropping system. Field Crop Res. 248, 107735. doi: 10.1016/j.fcr.2020.107735
Wang, Q., Li, Y., Liu, M., Li, G., Liu, Z., Tang, S. (2015b). Effects of high temperature at vegetative stage on rice growth and dry matter. China Rice 21, 33–37. doi: 10.3969/j.issn.1006-8082.2015.04.006
Wang, J., Liu, X., Zhang, X., Smith, P., Li, L., Filley, T. R., et al. (2016). Size and variability of crop productivity both impacted by CO2 enrichment and warming–a case study of 4 year field experiment in a Chinese paddy. Agr Ecosyst. Environ. 221, 40–49. doi: 10.1016/j.agee.2016.01.028
Wang, B., Li, J., Wan, Y., Cai, W., Guo, C., Wilkes, A. (2019). Variable effects of 2°C air warming on yield formation under elevated [CO2] in a Chinese double rice cropping system. Agr For. Meteorol 278, 107662. doi: 10.1016/j.agrformet.2019.107662
Wang, J., Wang, C., Chen, N., Xiong, Z., Wolfe, D., Zou, J. (2015a). Response of rice production to elevated [CO2] and its interaction with rising temperature or nitrogen supply: a meta-analysis. Climatic Change 130, 529–543. doi: 10.1007/s10584-015-1374-6
Wassmann, R., Jagadish, S. V. K., Heuer, S., Ismail, A., Redona, E., Sumfleth, K. (2009). Climate change affecting rice production: the physiological and agronomic basis for possible adaptation strategies. Adv. Agron. 101, 59–122. doi: 10.1016/S0065-2113(08)00802-X
Wullschleger, S. D. (1993). Biochemical limitations to carbon assimilation in C3 plants–a retrospective analysis of the A/Ci curves from 109 species. J. Exp. Bot. 44, 907–920. doi: 10.1093/jxb/44.5.907
Xin, F., Xiao, X., Dong, J., Zhang, G., Zhang, Y., Wu, X., et al. (2020). Large Increases of paddy rice area, gross primary production, and grain production in northeast China during 2000–2017. Sci. Total Environ. 711, 135183. doi: 10.1016/j.scitotenv.2019.135183
Yoshimoto, M., Oue, H., Kobayashi, K. (2005). Energy balance and water use efficiency of rice canopies under free-air CO2 enrichment. Agr For. Meteorol 133, 226–246. doi: 10.1016/j.agrformet.2005.09.010
Yuan, M., Cai, C., Wang, X., Li, G., Wu, G., Wang, J. (2021). Warm air temperatures increase photosynthetic acclimation to elevated CO2 concentrations in rice under field conditions. Field Crops Res. 262, 108036. doi: 10.1016/j.fcr.2020.108036
Zhang, J., Li, Y., Yu, Z., Adams, J., Tang, C., Wang, G., et al. (2022). Elevated atmospheric CO2 and warming enhance the acquisition of soil-derived nitrogen rather than urea fertilizer by rice cultivars. Agr For. Meteorol 324, 109117. doi: 10.1016/j.agrformet.2022.109117
Zhang, W., Wang, G., Liu, X., Feng, Z. (2014). Effects of elevated O3 exposure on seed yield, n concentration and photosynthesis of nine soybean cultivars (Glycine max (L.) merr.) in northeast China. Plant Sci. 226, 172–181. doi: 10.1016/j.plantsci.2014.04.020
Zhu, C., Kobayashi, K., Loladze, I., Zhu, J., Jiang, Q., Xu, X. (2018). Carbon dioxide (CO2) levels this century will alter the protein, micronutrients, and vitamin content of rice grains with potential health consequences for the poorest rice-dependent countries. Sci. Adv. 4, eaaq1012. doi: 10.1126/sciadv.aaq10
Zhu, C., Langley, J. A., Ziska, L. H., Cahoon, D. R. (2022). Accelerated sea-level rise is suppressing CO2 stimulation of tidal marsh productivity: A 33-year study. Sci. Adv. 8, eabn0054. doi: 10.1126/sciadv.abn0054
Zhu, C., Xu, X., Wang, D., Zhu, J., Liu, G., Seneweera, S. (2016). Elevated atmospheric [CO2] stimulates sugar accumulation and cellulose degradation rates of rice straw. Gcb Bioenergy 8, 579–587. doi: 10.1111/gcbb.12277
Zhu, C., Zhu, J., Cao, J., Jiang, Q., Liu, G., Ziska, L. H. (2014). Biochemical and molecular characteristics of leaf photosynthesis and relative seed yield of two contrasting rice cultivars in response to elevated [CO2]. J. Exp. Bot. 65, 6049–6056. doi: 10.1093/jxb/eru344
Keywords: elevated [CO2], warming, oryza sativa L., photosynthesis capacity, water use efficiency
Citation: Zhang C, Li Y, Yu Z, Wang G, Liu X, Liu J, Liu J, Zhang X, Yin K and Jin J (2022) Co-elevation of atmospheric [CO2] and temperature alters photosynthetic capacity and instantaneous water use efficiency in rice cultivars in a cold-temperate region. Front. Plant Sci. 13:1037720. doi: 10.3389/fpls.2022.1037720
Received: 06 September 2022; Accepted: 08 November 2022;
Published: 23 November 2022.
Edited by:
Quan Xu, Shenyang Agricultural University, ChinaReviewed by:
Lianxuan Shi, Northeast Normal University, ChinaChao Wu, Guangxi Institute of Botany, Chinese Academy of Sciences (CAS), China
Copyright © 2022 Zhang, Li, Yu, Wang, Liu, Liu, Liu, Zhang, Yin and Jin. This is an open-access article distributed under the terms of the Creative Commons Attribution License (CC BY). The use, distribution or reproduction in other forums is permitted, provided the original author(s) and the copyright owner(s) are credited and that the original publication in this journal is cited, in accordance with accepted academic practice. No use, distribution or reproduction is permitted which does not comply with these terms.
*Correspondence: Yansheng Li, bGl5YW5zaGVuZ0BpZ2EuYWMuY24=; Kuide Yin, eWlua3VpZGVAMTYzLmNvbQ==