- 1Key Laboratory of Mountain Surface Processes and Ecological Regulation, Institute of Mountain Hazards and Environment, Chinese Academy of Sciences, Chengdu, China
- 2University of the Chinese Academy of Sciences, Chinese Academy of Sciences, Beijing, China
A change in precipitation can profoundly change the structure of soil microbial communities, especially in arid and semi-arid areas which are limited by moisture conditions. Therefore, it is crucial to explore how soil bacterial community composition and diversity will respond to variation in precipitation. Here we conducted a precipitation control experiment to simulate precipitation change by reducing and increasing rainfall by 25%, 50%, and 75% in the alpine grasslands of northern Tibet. The composition, diversity, and species interaction network of soil microbial community were studied by high-throughput sequencing, and the relationship between microbial community species and soil environmental factors was analyzed. Our results showed that Proteobacteria (45%–52%) and Actinobacteria (37%–45%) were the dominant bacteria in the soil. The alpha diversity index based on Shannon, Chao1, and Simpson indices revealed that precipitation change had no significant effect on richness and evenness of soil microbial communities. Non-metric multidimensional scaling (NMDS) and analysis of similarities (ANOSIM) showed that a clear separation of soil microbial communities between D2(-50%),D3(-75%) and W2(+50%), W3(+75%) treatments. The microbial interaction network indicated that the water-increasing treatment group had closer connections, and Proteobacteria and Actinomycetes were the core species. Furthermore, there was a stronger positive correlation between species in the water-reducing treatment group, the contribution of Proteobacteria decreased significantly, the role of connecting hub decreased, and Actinomycetes became the most important core microbial species. In addition, soil water content (SWC) and available phosphorus (AP) were closely related to the variations in soil microbial compositions. The findings of this study provide a theoretical basis for the driving mechanism of global climate change on soil microbial community and grassland ecosystem in alpine grassland.
Introduction
Ongoing global climate change is expected to alter soil microbial communities through direct and indirect means (Brockett et al., 2012). The direct means will change microbial metabolism and substrate availability, while the indirect means will affect the interaction between soil microorganisms and plants (Brockett et al., 2012; Classen et al., 2015). In the second case, the soil microbial community changes, which may contribute to changes in terrestrial ecosystem processes, include the decomposition of soil organic matter, carbon sequestration, and nutrient cycling (Allison and Treseder, 2008; Schindlbacher et al., 2011). These processes reflect climate change-induced changes in plant growth, phenology, and species composition. Precipitation change is the main content and research focus of global climate change (Nyström et al., 2019; Ault, 2020). Variations in precipitation and soil moisture are of great importance to microorganisms, and even have a greater impact than other consequences of global climate change, such as rising temperature and CO2 concentration (Schimel and Schaeffer, 2012). Therefore, understanding how soil microbial communities change with the increase or decrease of precipitation is the key to further explore the ecological physiological and biogeochemical trajectories (Felsmann et al., 2015).
At present, the characteristics of soil microbial community responses to precipitation change have been largely reported (Clark et al., 2009; Landesman and Dighton, 2010; Nielsen and Ball, 2015; Gao et al., 2016; Zhou et al., 2018), but the experimental results are very different. A study of drought simulation experiments in two continents, North America and Australia, showed that bacteria within the phyla Acidobacteria, Chloroflexi, and Nitrospirae were more abundant in conditions of decreased precipitation. These groups are oligotrophic organisms that grow slowly and tend to form small colonies. This allows them to maintain high abundance even in dry environments and have a strong tolerance to water stress (Evans and Wallenstein, 2014; Ochoa-Hueso et al., 2018). In addition, meta-analysis on the effects of global precipitation changes on soil microbial communities showed that reduced precipitation shifts bacterial communities to Gram-positive bacteria (with strong, thick, interconnected peptidoglycan cell walls), so that they can resist dry conditions in some ways (Uhlírová et al., 2005; Zhou et al., 2018). With the increase of precipitation, the relative abundance of Proteobacteria and Bacteroidetes gradually increased. This may be due to the fact that Proteobacteria and Bacteroidetes are generally considered to be symbiotic trophic organisms, which follow trends consistent with changes in ambient precipitation (Fierer et al., 2009; Li et al., 2022a). At the same time, soil microbial communities may respond differently in different ecosystems. In alpine steppe, there was no change found in species composition in bacterial communities after reduced precipitation (Williams, 2007). Studies in temperate steppe confirmed that precipitation can have a significant effect on the relative abundance of soil microorganisms, although it did not have a significant effect on their diversity (Zhao et al., 2016; Wang et al., 2017). Studies in desert grassland emphasized that the response of soil bacterial community diversity to precipitation gradient was not apparent or delayed (Banu et al., 2004; Cruz-Martínez et al., 2009; Bell et al., 2014; Yang et al., 2021). In general, soil microbial communities show different characteristics in response to precipitation increase/decrease and ecosystem differences. At present, there is no general consensus and understanding of regularity on the response of soil microbial communities to precipitation conditions and specific habitats, which needs to be further supplemented. Those studies will also help us better understand the water-related life strategies of soil bacterial communities.
Generally speaking, soil microorganisms form complex interspecific networks, which greatly regulate ecological community structure and ecosystem function, rather than living in isolation (He et al., 2017). Microbial co-occurrence networks have been widely used to study microbial interactions in ecosystems and to identify microbial keystone groups (Barberán et al., 2012; Williams et al., 2014; De Menezes et al., 2017; Bingjian et al., 2020), which play an important role in shaping community structure and driving ecosystem function (Banerjee et al., 2018; Mikhailov et al., 2019). The more compact and closely connected the network is, the more deterministic processes determine the composition of the microbial community; conversely, the looser and more isolated the network is, the more random processes determine the composition of the microbial community (Jiang et al., 2018). In summary, co-occurrence network analysis is an important means to understand microbial community structure and interactions between microorganisms (Barberán et al., 2012). For example, network analysis can predict under-characterized phyla between members to find novel interactions, thus providing valuable information for co-culture of different species (Faust and Raes, 2012). Actinobacteria was found to be the keystone phylum for connecting other bacterial members (Zhou et al., 2011). Sordariales and Hypocreales played similar “connecting” roles in one study of fungal co-occurrence networks (Lu et al., 2013). However, studies examining and comparing changes in network structure in response to altered precipitation regimes are still lacking, and information on the effects of climate change and microbial feedbacks in soil is limited.
Although we pay a lot of attention to microbial communities, abiotic factors such as soil temperature (Feng and Simpson, 2009), soil moisture (Brockett et al., 2012), and soil pH often play a significant role in driving changes in microbial community structure (Fierer and Jackson, 2006). In addition, affected by differences in soil water conditions, the dissolution of soil nutrients would be accelerated or slowed down, resulting in changes in soil nutrients, thus changing the structure of soil microorganisms (Parton et al., 1995; Zhang et al., 2005). Niu et al. (2021) found that nitrogen content was significantly correlated with Fibrobacteres, Bacteroidetes, Patescibacteria, Gemmatimonadetes, and Actinobacteria, while soil phosphorus content was also significantly correlated with Fibrobacteres, Bacteroidetes, and Actinobacteria. Soil available potassium was significantly related to the Entomophthoromycota and Chytridiomycota. Furthermore, soil available nitrogen was significantly associated with the phylum, Entomophthoromycota, while soil available phosphorus had a negative correlation with Cryptomycota (Niu et al., 2021)
The Qinghai-Tibet Plateau has become the region with the greatest uncertainty of environmental change due to global climate change (Tandong, 2019). The basic feature of climate change is warming and humidification. Recently, precipitation has shown an overall increase trend, with an increase of 2.2% every 10 years. Near- (present–2050) and long-term (2051–2100) precipitation is projected to increase by 10.4%–11% and 14.2%–21.4%, respectively, over the current base period (Chen et al., 2015). In the context of continuous global warming, precipitation has rapidly increased along with the accelerated warming of the Qinghai-Tibet Plateau, accompanied by the increase of extreme rainfall events (drought and rewetting), which will directly affect the plant species diversity and community composition of the plateau ecosystem (Zhang et al., 2020). However, due to the complexity of grassland ecosystems, how precipitation changes microbial communities and co-occurrence network patterns in alpine grassland is still controversial, which needs further exploration to deepen our understanding. In this study, we conducted a precipitation control experiment in the alpine steppe of northern Tibet to answer the following two questions:(1) how do the increase and decrease of precipitation change soil microbial community structure and co-occurrence network? (2) What factors drive the change of microbial community under precipitation change?
Material and methods
Study site
The study was conducted in Xainza Alpine Grassland and the Wetland Ecosystem Observation and Experimental Station (30°57’N, 88°42’E, 4675 m above sea level) in Xainza County, Nagqu City, Tibet Autonomous Region (Figure 1A). The research area belongs to the semi-arid monsoon climate of the sub-frigid plateau with cold and dry climate. The annual average temperature is 0.4 °C, and the highest monthly average temperature is not higher than 14 °C (July) and the lowest is below 10 °C (January) (Xiong et al., 2016). The annual precipitation of 298.6 mm is concentrated in June to August. In addition, the annual evaporation is as high as 2181.1 mm, and the annual evaporation of rain is much greater than the annual precipitation (Ping et al., 2021). The vegetation type of the study area belongs to alpine steppe, and Stipa Purpurea community is the dominant community in this area. Associated plants include Carex moocroftii, Artemisia Capillaris, Leontopodium nanum, Oxytropis microphylla, Kentucky bulegrass, and Stellera chamaejasme (Ma et al., 2016b). Plants turn green in the middle and late may of each year, the growing period is from June to August, and gradually stops growing in September. In October, plants basically stop growing and enter the yellow stage, which lasts until the beginning of May of the following year. The main soil type is alpine steppe soil, with thin soil layer and easy erosion, and the soil has obvious coarse-grained characteristics (Cai et al., 2014).
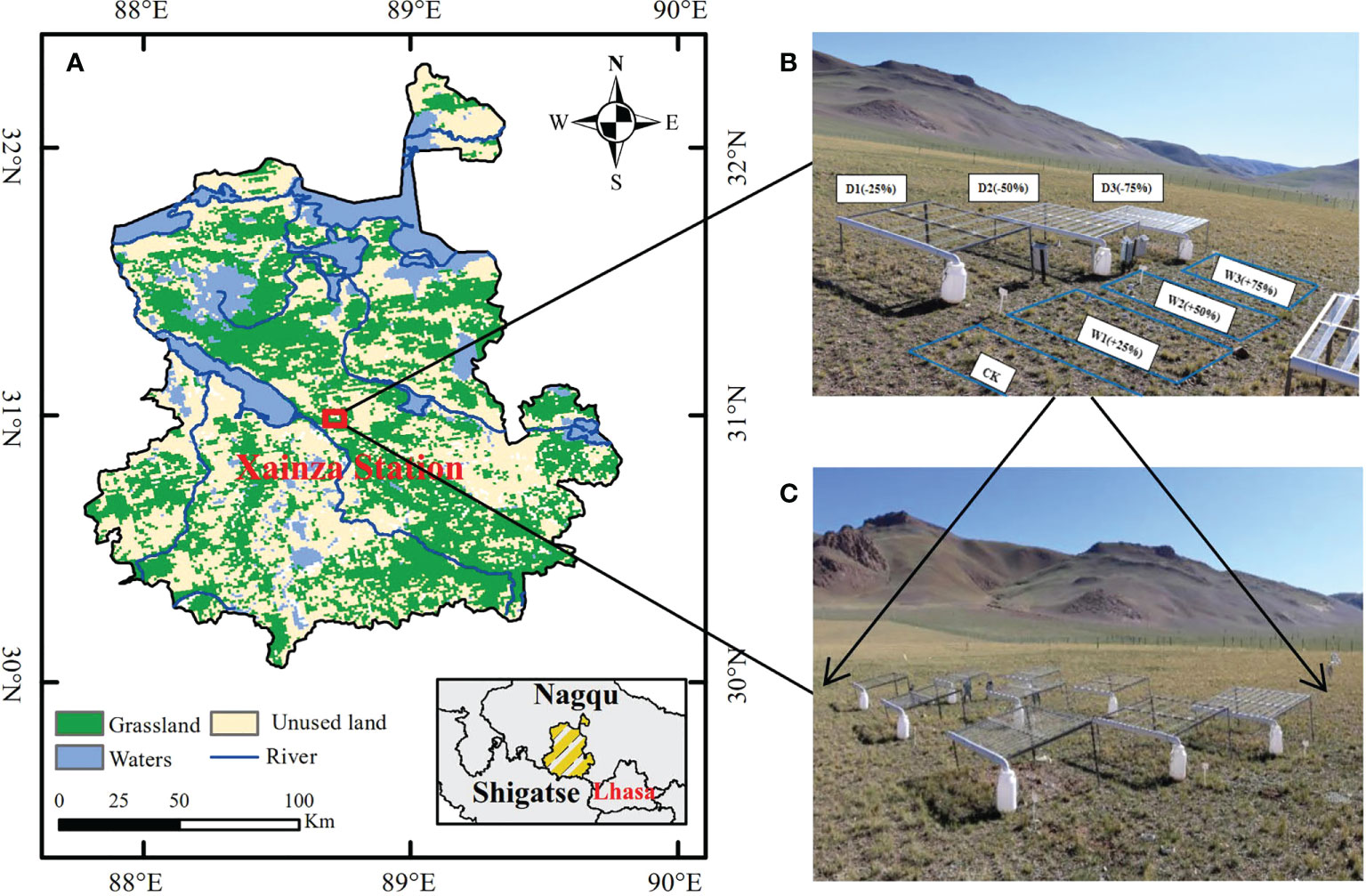
Figure 1 Location of the study area and design of the experiment. (A) Location of Xainza Station; (B) Diagram of the seven precipitation treatments; (C) Complete experimental site with three blocks and 21 plots.
Experimental design
Precipitation gradient experiment
The precipitation control experiment was conducted with canopy (Kundel et al., 2018). Seven precipitation gradients were set according to the precipitation variability in the study area in the past 30 years. On the basis of natural precipitation (CK) increase 25%, 50% and 75% water (W1, W2, W3, respectively), decrease 25%, 50%, and 75% water (D1, D2, D3, respectively). We set up a total of seven precipitation gradients above to simulate different water conditions, and laid out three replicates for each gradient, a total of 21 quadrats, the size of each quadrat was 3 m×2 m. In order to avoid the marginal effect between plants and water infiltration between quadrats, the interval between two adjacent quadrats was set as 2 m. In addition, random block design was used for the layout of quadrats, and precipitation gradient experiment was carried out during the 2020–2021 growing season (From May to September) (Figures 1B, C).
Water-reducing treatment: Square steel welded at 8 cm × 8 cm was used as a rain shield. The two ends of the canopy were 0.7 m and 1.05 m from the ground respectively, so that the canopy was inclined. The transparent PVC drainage pipe (diameter of 12 cm) was cut along the diameter direction, so that it could become a rainwater collecting trough, and the top of each canopy was evenly fixed with 5 (D1), 10 (D2), 15 (D3) V-type acrylic plates to be interception of precipitation by 25%, 50%, and 75% of occlude sample area (Zhang et al., 2018b; Wang et al., 2020).
Water-increasing treatment: The rainwater collecting trough led the trapped rainwater through the water pipe to the rainwater collecting barrel. Then, 24 hours after the rainfall stopped, the 25%, 50%, and 75% of the collected precipitation in the water reduction area were uniformly introduced to the corresponding plots through drip irrigation pipes, so that the plots increased precipitation by 25% (W1), 50% (W2), and 75% (W3) to ensure the synchronicity of rainfall under each rainfall treatment.
Sample collection
In August 2021, we carefully excavated the rhizosphere soil of the dominant species (Stipa purpurea) in the community under seven precipitation gradients, with four soil samples from each quadrat, and a total of 28 soil samples were collected. We labeled the soil samples and placed them in a freezer to keep them cold until they were processed for metagenomic sequencing. At the same time, we also collected dry soil samples under seven precipitation gradients to determine soil physical and chemical properties. The roots and other debris in the soil were picked out, dried, ground, and screened through 200 mesh. The screened soil samples were taken to determine the physical and chemical properties of the soil, including pH, soil organic matter (SOM), total nitrogen (TN), total potassium (K), total phosphorus (P), available potassium (AK), available phosphorus (AP), and available nitrogen (AN). Table 1 shows the method used to gather this data.
DNA extraction and DNBSEQ sequencing of soil samples
The metagenomic sequencing of our soil samples was commissioned by BGI Shenzhen Co., Ltd. The experimental process was mainly divided into DNA extraction and DNBSEQ sequencing library construction. A 96-well deep-well plate was prepared and swabs were loaded from the soil sample. DNA was extracted from each soil sample using the magnetic bead method, and then purified automatically using the Kingfisher purification system. At the end of the above procedure, the DNA solution in the deep well plate was transferred to a 1.5-mL centrifuge tube for storage, in order to prepare the DNA solution for library construction and sequencing on DNBSEQ sequencing platform. Library construction of DNBSEQ sequencing platform mainly consists of nine steps, including sample testing, sample interruption, fragment size selection, end repair, preparation reaction system, PCR reaction and product recovery, product cyclization, library detection, and generation of sequencing data. The detailed process of each step has been previously described (Fu et al., 2022).
Processing of sequencing data
To obtain clean data, the raw sequencing data were processed using SOAPnuke software as follows: (1) exclude reads containing 10% uncertain bases (N bases); (2) exclude reads containing adapter sequences (15 bases or longer sequence aligned to the adapter sequence); (3) exclude reads containing low-quality bases of 20% (bases of Q<20); (4) for the sample with the host or environment sources, a filtering step was added here to remove the host genome sequence to reduce interference of the host sequence on the subsequent analysis (Soap2, more than 90% similarity, a host reference sequence is required) (Li et al., 2009). High-quality short reads of each DNA sample were assembled by the MEGAHIT. The parameters of MEGAHIT was ‘megahit_opts = –min-count 2 –k-min 33 –k-max 53 –k-step 10 –no-mercy –memory 0.9 –min-contig-len 200 –num-cpu-threads 5’ (Li et al., 2015). MetaGeneMark was used for metagenomic genes prediction (Zhu et al., 2010). The assembled reads were clustered by CD-HIT to eliminate redundancy (Fu et al., 2012), and finally unigenes were obtained with a similarity threshold of 95%. The parameter of CD-HIT was ‘CD_HitOpts = -aS 0.9 -c 0.95 -M 0 -d 0 -g 1 -T 12’. Salmon software (version 1.4.0) was used to standardize sequences to determine gene abundance by the transcripts per million (TPM) method. Species annotations and species abundances were calculated using Kraken2(DB2020), and the filter options were ‘-l 20 -q 0.5 -n 0.1 -d -Q 2 -5 0’ (Wood and Salzberg, 2014).
Our raw data has been successfully uploaded to NCBI database, the accession number is PRJNA881773, and click on the link below to see “https://www.ncbi.nlm.nih.gov/sra/PRJNA881773”.
Statistical analysis
One-way ANOVA was used to compare the relative abundance of phylum level and genus level species in different precipitation gradients. Three commonly used α-diversity indices of the bacterial community were calculated by QIIME (Version 1.80) (BGI Shenzhen Co., Ltd) (Caporaso et al., 2010). Chao1 is the species richness index, and its value largely reflects the variation of rare species (Chao and Bunge, 2002). Shannon index reflects species richness and evenness, and the individual weight of rare species is higher than that of common species (Hill et al., 2003). The Simpson index reflects the degree of consistency in a community (Zhong et al., 2010). The formula for calculating the α- diversity indices are as follows:
Where Sobs is the number of species observed in the sample; F1 and F2 represent the number of singletons and doubletons, respectively; N is the total number of individuals in all species; ni represents the total number of individuals of species i; Ai is the relative abundance of species.
β-diversity was represented by Bray–Curtis dissimilarity between different water conditions (Chen et al., 2019), and then pairwise differences between different water conditions in low-dimensional space were explained using non-metric multidimensional (NMDS). In addition, the analysis of similarity (ANOSIM) was used to determine whether the water gradients were significantly separated (Ren et al., 2018b). The graphs were generated using the “ggplot” package (Version 3.3.5). The microbial co-occurrence network was constructed based on data of species for the top 200 absolute abundance microbial genera after removing rare species (the number of detected 0 values more than 3) in the soil samples of seven precipitation gradients. A strong and significant correlation at the threshold of Spearman’s |r|>0.7 and p<0.01 were commonly used and recognized to be valid and robust (Steinhauser et al., 2008, Barberán et al., 2012, Ma et al., 2017). The nodes and edges file calculated using package “WGCNA” in R platform (http://www.r-project.org), and visualized in Gephi software (Version 0.9.3) (Bastian et al., 2009). The topological features of nodes-level (including clustering coefficient), edge-level (including path percentage of positive and negative edges, Average path length), and network-level (including network diameter, average degree, modularity) were calculated in Gephi (Bastian et al., 2009).
Redundancy analysis (RDA) was used to analyze the relationship between soil bacterial composition and environmental factors. The selected environmental factors included SWC, ST, pH, SOM, TN, AN, K, AK, P and AP. Monte Carlo permutation test was then used to test the significance of RDA results. The analysis process was performed in Canoco5.0. The map displaying geographical locations of the study area was constructed based on the remote sensing monitoring data of land use in Tibet in 2020 downloaded by the Resource and Environmental Science and Data Center and the software ArcGIS (version 10.5, Environmental Systems Research Institute, Inc., CA, USA).
Results
Soil bacterial community structures and diversity
Relative abundence
A total of 17,739,185,700–18,816,624,900 clean base with high-quality metagenome sequences were identified from the 28 samples from Northern Tibet. After chimera detection and removal, 503,015–1,040,699 contig numbers with an average size of 507–652 bp were obtained. Sequencing data of all soil samples showed that bacteria accounted for more than 98%, while archaea, eukaryotes, and viruses accounted for less than 2%. Therefore, the main research object of this paper was soil bacterial community.
According to the relative abundance ranking of top15 taxa at phylum level (Figure 2), the bacteria with relative abundance ranking lower than 15 were classified as others, and the results showed that the bacterial proportion characteristics among the seven precipitation gradients were relatively consistent. Proteobacteria and Actinobacteria are the two most abundant phyla, accounting for 45%–52% and 37%–45% of all phylum, respectively. Firmicutes (2.1–2.3%), Cyanobacteria (0.64%-0.81%), and Deinococcus-Thermus (0.43%–0.47%) account for a very small proportion (Figure 2A). One-way ANOVA showed that Proteobacteria, Actinobacteria, and Candidatus_Cloacimonetes had significant differences among the seven precipitation gradients (Table 2). The relative abundance of Proteobacteria in water-increasing treatment was higher than that in water-reducing treatment, while the relative abundance of Actinobacteria was lower (P <0.05).
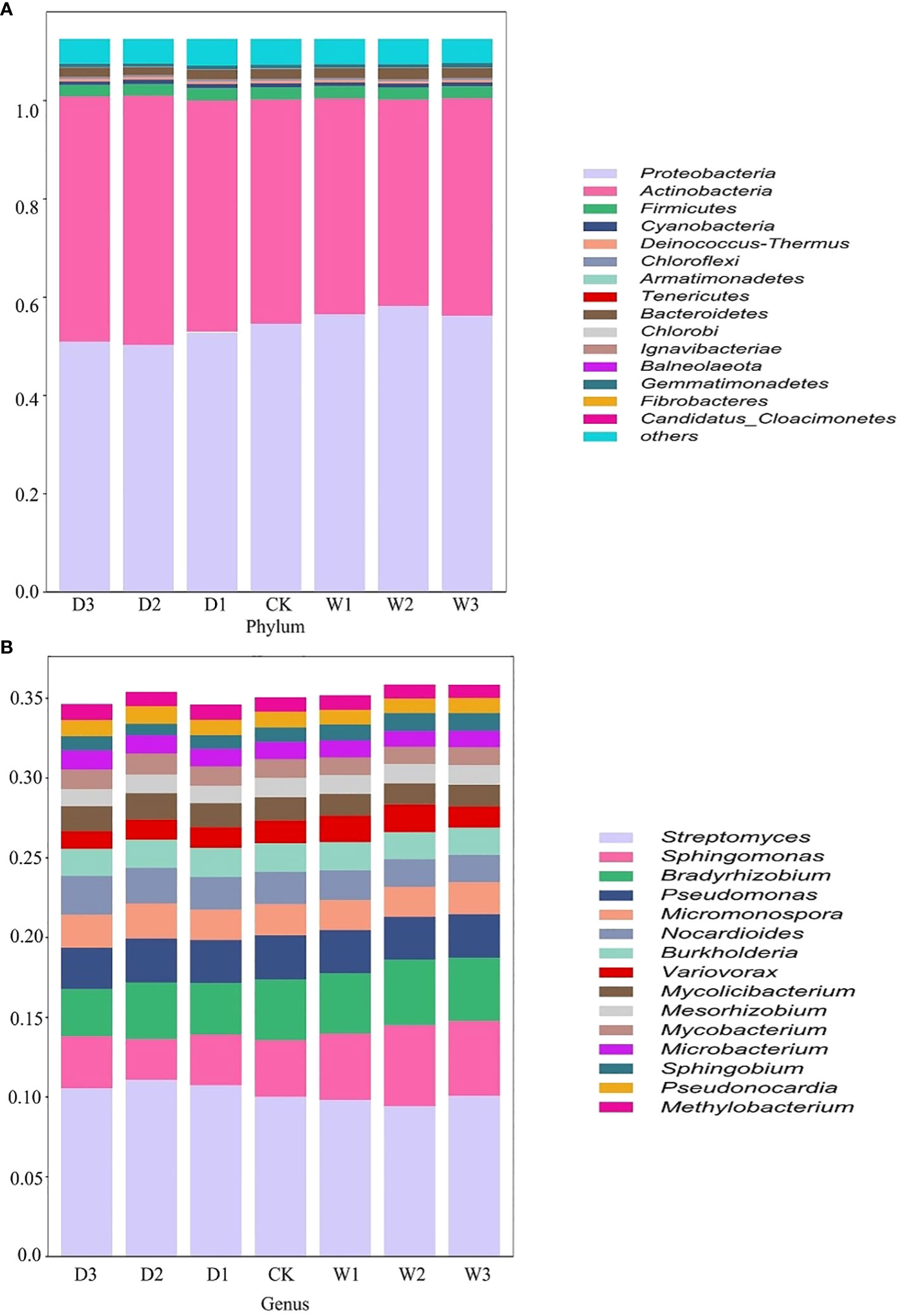
Figure 2 Relative abundance of bacteria in different precipitation gradients at the phylum and genus levels on the Northern Tibet. The bacteria whose relative abundance are in the top 15 at phylum (A) and genus (B) levels. D3, water reducing 75%; D3, water reducing 50%; D1, water reducing 25%; CK, control treatment; W1, water increasing 25%; W2, water increasing 50%; W3, water increasing 75%.
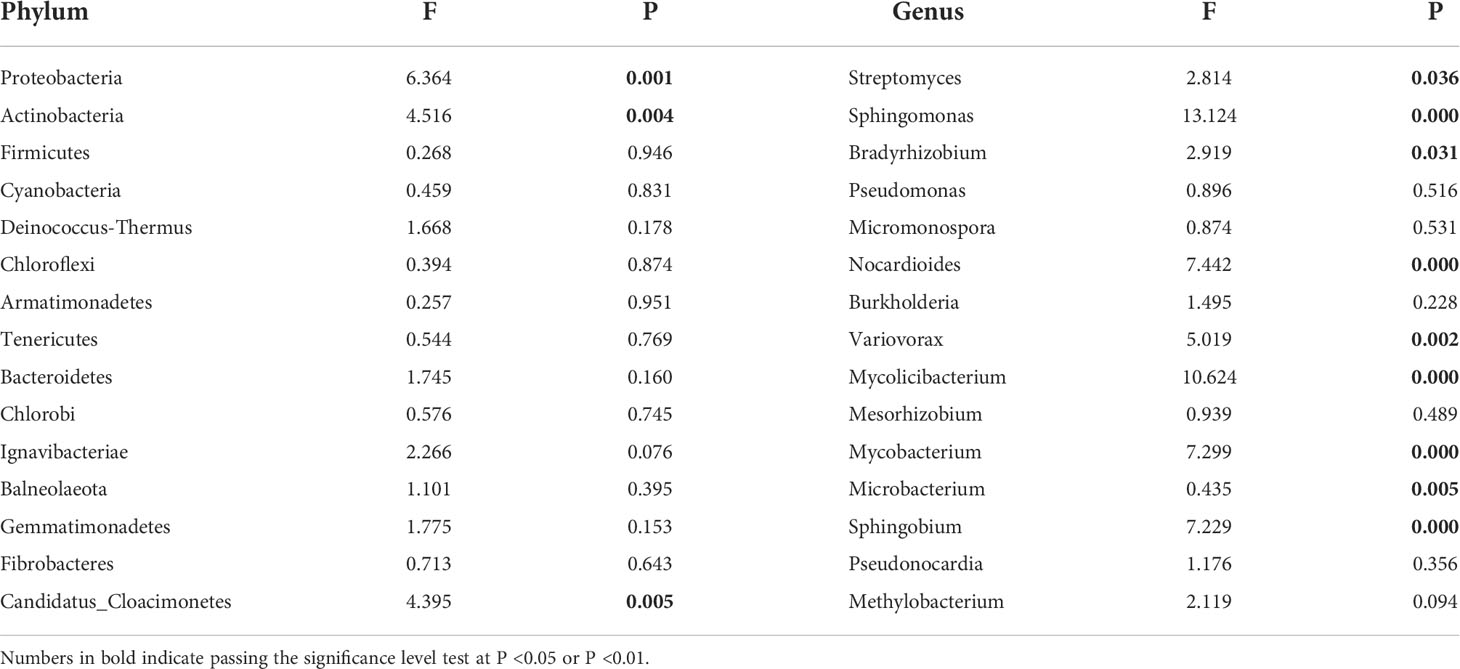
Table 2 Differences in community composition of phylum and genus of soil microorganisms under different water conditions.
At the genus level, overall dominant genera account for 34% to 36% of the relative abundance of all taxonomies, the dominant bacteria was Streptomyces (9.4%–11%), the secondary dominant bacterial genus were Sphingomonas (2.56%–5.08%), Bradyrhizobium (2.96%–4.09%), and Pseudomonas (2.59%–2.77%). Other genera occupy a smaller proportion (Figure 2B). One-way ANOVA showed that Streptomyces, Sphingomonas, Bradyrhizobium, Nocardioides, Variovorax, Mesorhizobium, Mycobacterium, Microbacterium, and Sphingobium showed significant differences among the seven precipitation gradients (Table 2). The distribution of Streptomyces from large to small was D2 (11.06%), D1 (10.72%), D3 (10.52), W3 (10.05%), CK (9.99%), W1 (9.79%), and W2 (9.4%), which decreased with the increase of precipitation. Further, Sphingomonas regularity of distribution from large to small was W2 (5.08%), W3 (4.69%), W1 (4.17%), CK (3.55%), D3 (3.26%), D1 (3.17%), and D2 (2.55%). The distribution pattern of Bradyrhizobium from large to small was W2 (4.09%), W3 (3.96%), CK (3.8%), W1 (3.78%), D2 (3.54%), D1 (3.23%), and D3 (2.96%), Sphingomonas and Bradyrhizobium both increased roughly with the increase in precipitation (Figure 2B).
Alpha diversity
We calculated the Chao1 index, Shannon index, and Simpson index of soil microbial community under seven precipitation gradients, and the results showed that there were no significant differences among the three indexes under different water conditions, which also indicated the similarity of alpha diversity under seven precipitation gradients (Figure 3). The Chao1 index of all samples in the current study was more than 50, and the Shannon and Simpson indices of all samples were more than 1.16 and 0.59, respectively.This indicated that the number of species in the seven precipitation gradient communities was more than 50, but the community diversity was low (a larger Shannon index indicated a higher community diversity, while a larger Simpson index indicated a lower community diversity).
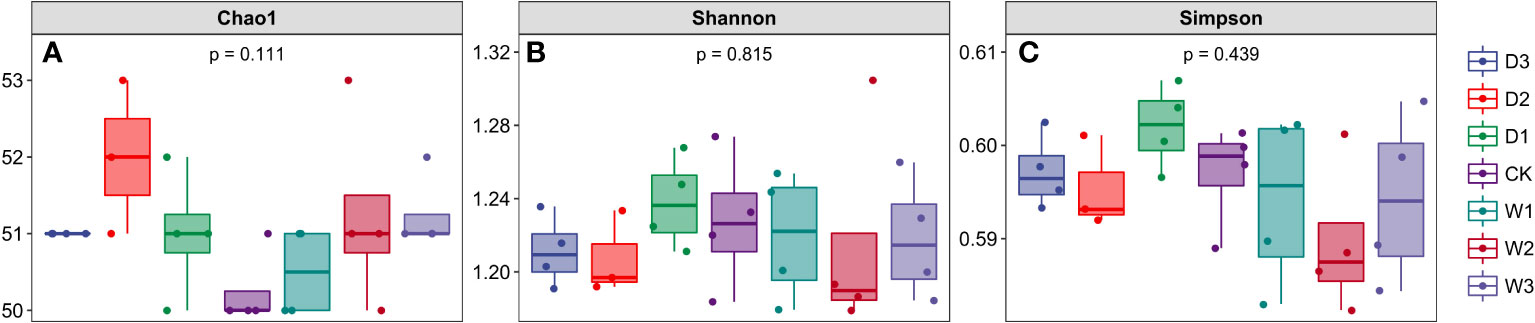
Figure 3 Alpha diversity index of soil bacterial community under seven precipitation gradients in the alpine steppe of Northern Tibet. (A) Chao1 index; (B) Shannon index; (C) Simpson index.
Beta diversity
NMDS analysis was conducted based on Bray–Curtis dissimilarities to reflect the soil bacteria beta diversity of alpine steppe in the Northern Tibet. The results showed that the taxa of soil bacterial communities were significantly separated among seven precipitation gradients(Stress=0.0524, Figure 4A). For instance, the Bray–Curtis dissimilarity from the W2, W3 to the D2, D3 was relatively far, highlighting the significant difference of soil bacterial communities between them. In addition, the Bray–Curtis dissimilarity of W1, CK, and D1 was close, implying the similar soil bacteria community structures, especially the relative abundance of genus. Furthermore, the ANOSIM highlighted the differences in soil bacterial communities in alpine steppe (Figure 4B and Table 3). The soil bacteria community of D3 was substantially different from those of W1, W2, and W3 (P<0.05). Moreover, the soil bacterial community at D2 was different from that at W1, W3 (P<0.05). The results showed large differences in precipitation did change the structure of soil bacterial community, which was significantly different between the increase and decrease of 50% and 75% moisture.
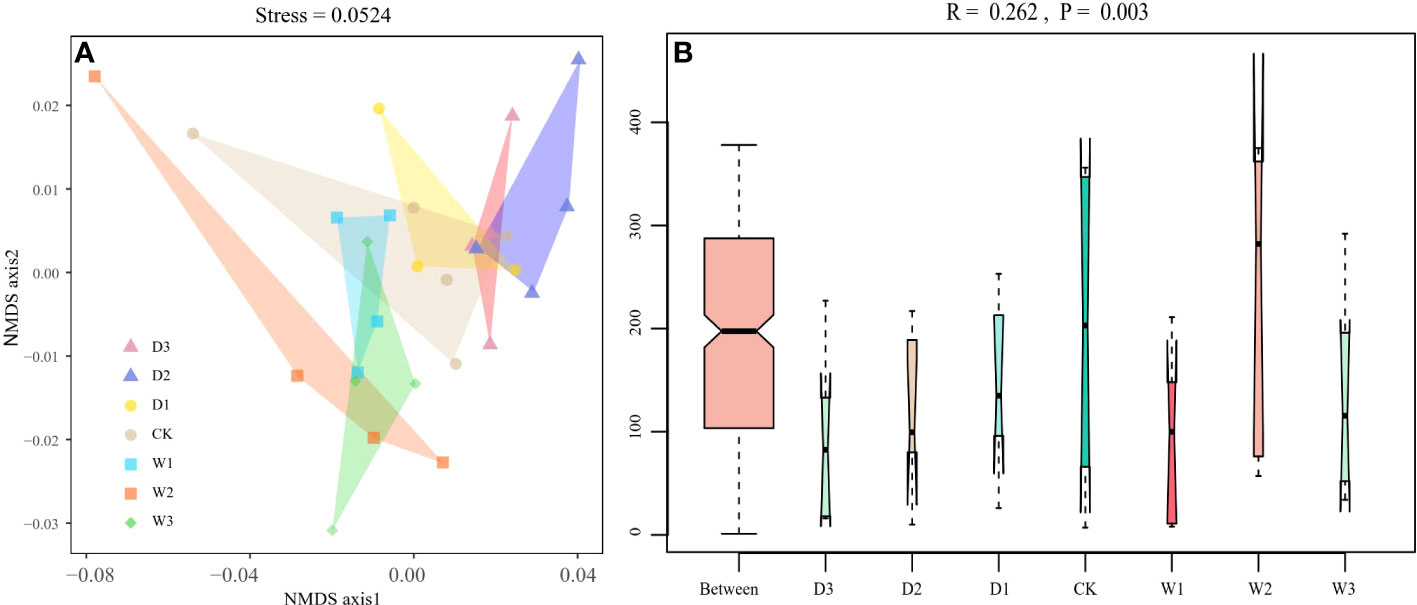
Figure 4 Beta diversity of soil bacterial community under seven precipitation gradients in the alpine steppe of Northern Tibet. (A) Non-metric multidimensional scaling (NMDS) analysis. Different shaped dots represent the samples from different alpine grassland types, and the bumps of the samples bound the faces of different colors. (B) Analysis of similarity (ANOSIM). Between reflects the differences between groups, and groups D3, D2, D1, CK, W1, W2 and W3 represent the differences within groups.
Co-occurrence network analysis of soil bacterial community
In this study, network analysis was applied to illustrate the differences in soil bacterial community of water-increasing and water-reducing treatments in the growing seasons (Figure 5), and the network properties of soil bacterial communities under different water conditions were summarized in the Table 4. Water-increasing and water-reducing treatments had formed unique co-occurrence network of soil bacterial community, respectively. In the water-increasing treatment, the nodes and edges of the microbial network were 197 and 6654, respectively, with an average degree (AD) of 33.77 (Figure 5A and Table 4). In the water-reducing treatment, the microbial network had 198 nodes, but had fewer edges (4822) and a smaller average degree (24.354) (Figure 5B). This may indicate that the network structure of the water-increasing treatment was more closely connected, but the complexity of the network structure of the two water treatments was similar. In the co-occurrence network, the water-increasing treatment presented a shorter average path length (the shortest among all possible pairs of nodes) and a lower network diameter (the longest of the shortest paths among all pairs of nodes) (2.437 and 7.00) than those in water-reducing treatment (3.128 and 8.00), which reflected higher sensitivity and more rapid response to water addition. Furthermore, the higher clustering coefficient (0.708) of the water-reducing treatment was higher than water-increasing treatment (0.674). The modularity indices of two sample types were all greater than 0.40, which suggested that the co-occurrence networks of bacterial communities had a strong modular structure and complex species interaction across the two sample types in alpine steppe on the Northern Tibet.
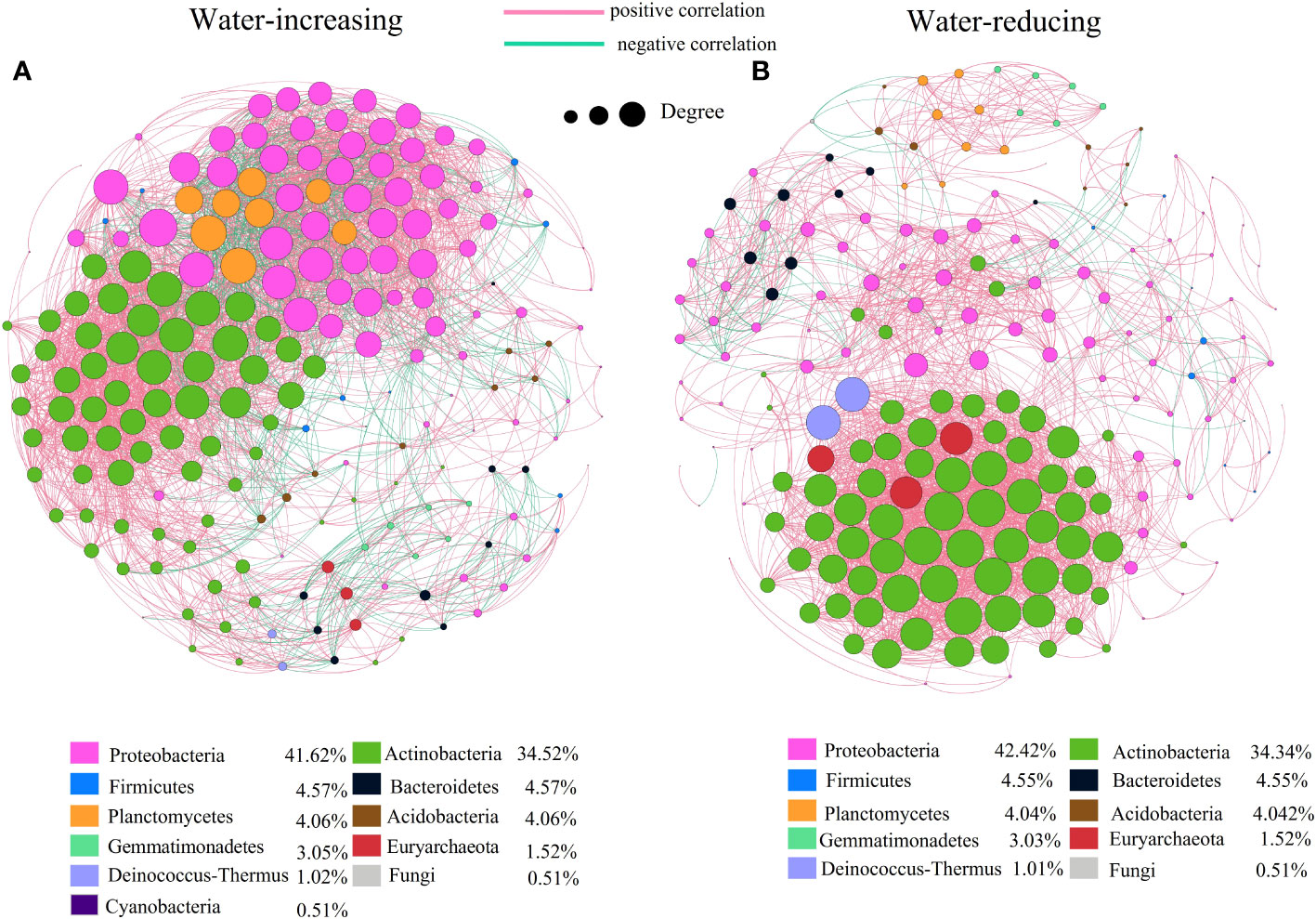
Figure 5 Network of co-occurring genera based on correlations analysis for water-increasing and water-reducing. A connection stands for a strong (Spearman’s p>0.7) and significant (p<0.01) correlation. The size of each node is proportional to the degree. Nodes colored by taxonomy, lines colored by positive and negative correlation, (A) water-increasing treatment, (B) water-reducing treatment.
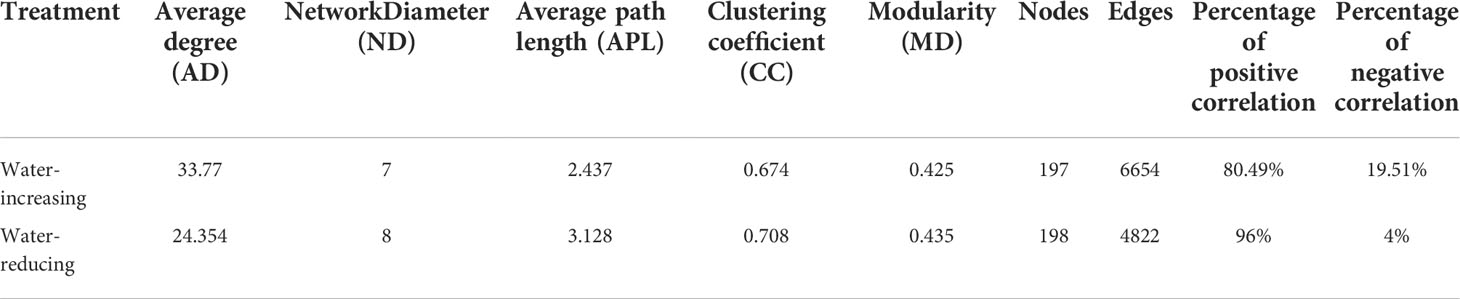
Table 4 Network properties of soil bacterial communities for water-increasing and water-reducing treatments.
In the network diagram of water-reducing treatment, positive correlation edges accounted for 96% (greater than water-increasing treatment, 80.49%) and negative correlation edges accounted for 4% (less than water-increasing treatment, 19.51%). The mutual exclusion effect of water-reducing treatment was small among species. The bacteria corresponding to the key species were predominantly Proteobacteria (41.62%,42.42%) and Actinobacteria (34.52%, 34.34%) in two types of treatment (Figure 5). However, the numbers of Proteobacteria in the network of water-reducing treatment was significantly reduced, indicating that the connectivity of Proteobacteria was gradually weakened (Figure 5B).
Relationships between soil bacterial community and the environmental factors
The RDA showed that the variations in the soil bacterial community were determined by environmental factors (Figure 6). The permutation test indicated significance in the ordination diagram (P=0.004). Environmental variables had an explanation rate of 58.96% for the soil bacterial community, with the first and second axes accounting for 58.75% and 0.21% of the variation, respectively. The results also revealed that correlations between each phylum and environmental variations. Environmental factors, especially SWC and AP were significantly related to the soil bacterial community (P < 0.05, Table 5). The difference in environmental factors in alpine steppes can explain the differences in soil bacterial communities among different water conditions. Further, the dominant factors for different soil bacteria were also different. For example, soil water content (SWC) was positively correlated to Bacteroidetes, Candidatus_Cloacimonetes, Ignavibacteriae, Proteobacteria, Fibrobacteres, Chlorobi, and Firmicutes, but negatively correlated to Actinobacteria, Deinococcus-Thermus, Chloroflexi and Tenericutes. Available phosphorus (AP) was positively correlated to Deinococcus-Thermus, Actinobacteria, and Chloroflexi, but negatively correlated to Proteobacteria, Gemmatimonatetes and Chlorobi. Total nitrogen (TN) was positively correlated to Deinococcus-Thermus, Cyanobacteria and Actinobacteria, but negatively correlated to Gemmatimonatetes, Proteobacteria, and Chlorobi. Soil temperature (ST) was positively correlated to Actinobacteria and Chloroflexi, but negatively correlated to Proteobacteria, Candidatus_Cloacimonetes, Bacteroidetes, and Ignavibacteriae. In addition, the soil bacterial abundance at different points also showed variability of water conditions. For instance, the projection point of the corresponding sample points in W2 on the corresponding vector of Proteobacteria was ahead of other points, suggesting that Proteobacteria has greater abundance potential in the W2 than in other points.
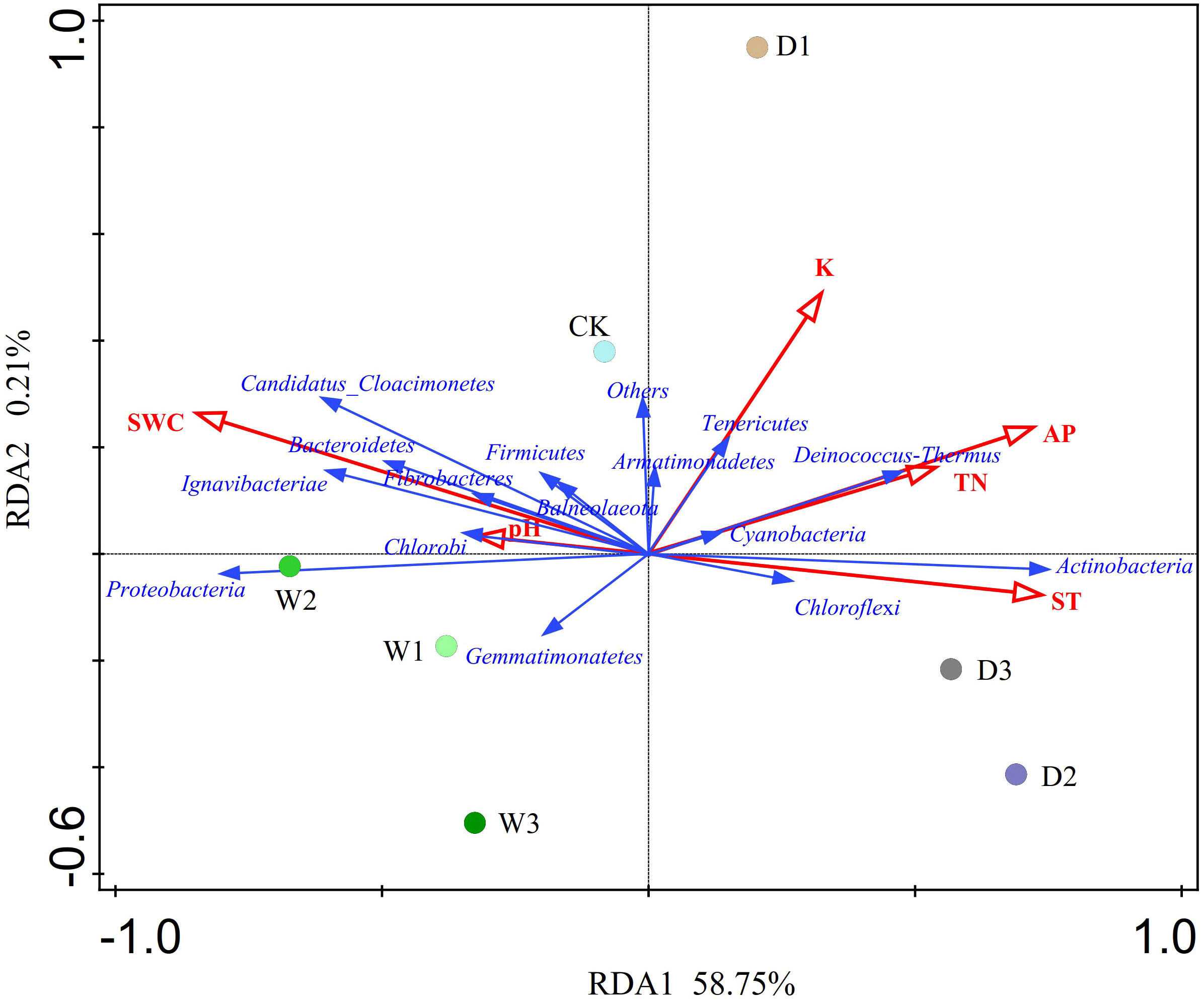
Figure 6 Redundancy analysis (RDA) of soil bacterial communities and environmental factors of different water conditions in alpine steppe on the Northern Tibet. Points denote sampling sites of seven precipitation gradients. The blue arrows represent soil bacteria (phylum), the red arrows represent environmental factors, and the cosine of the angle within two vector arrows represents the correlation between vectors. SWC, soil water content; AP, available phosphorus; K, kalium; ST, soil temperature; TN, total nitroge; P, phosphorus.
Discussion
Responses of soil microbial community to precipitation change
Soil microbial communities have different response strategies to different precipitation gradients in semi-arid ecosystems (Placella et al., 2012). This is because precipitation directly affects soil water availability and indirectly affects plant productivity, carbon allocation and above-ground and below-ground litter input, which ultimately changes soil microbial communities, especially in semi-arid regions with water shortage (Sierra et al., 2017; Sihi et al., 2018; Zhou et al., 2018). In this study, we found that Proteobacteria and Actinobacteria, which accounted for 45–52% and 37–45% of the seven precipitation gradients at the phylum level, respectively, were the dominant bacteria, while Firmicutes were the secondary dominant bacteria, and their relative abundance was only about 2% (Figure 2A). Actinobacteria constitute one of the largest bacterial phyla in many types of soil ecosystems (Barka et al., 2016). They often live as plant commensals and nitrogen-fixing symbionts in soil (Fitzpatrick et al., 2018), participating in the formation of soil organic matter and biogeochemical cycles (Yu et al., 2020). Proteobacteria were one of the major phyla of ammoniated microorganisms and are also abundant in grassland ecosystems (Chen et al., 2020). Proteobacteria and Actinobacteria are the dominant bacteria in grassland, which is consistent with previous studies (Zeng et al., 2017; Nan et al., 2020). However, their relative abundance ratio of Proteobacteria and Actinobacteria were different due to environmental differences caused by different study areas and ecosystem types. For example, a study of the high-altitude Gangotri soil ecosystem confirmed that Proteobacteria and Actinobacteria accounted for 38.49% and 14.48%, respectively (Kumar et al., 2019). Proteobacteria and Actinobacteria accounted for 47.19% and 42.2% of the soil microbial communities in the alpine grassland of northern Tibet, respectively (Fu et al., 2022). Ding et al. (2013) found that Actinomycetes were more dominant in arid and semi-arid habitats than in agricultural ones (Ding et al., 2013).
The relative abundance of Proteobacteria in water-increasing treatment was significantly higher than that in water-reducing treatment, while Actinobacteria was significantly lower than that in water-reducing treatment (Figure 2A and Table 1). This may be due to the different evolutionary and physiological adaptation mechanisms that made the phylum of soil bacteria respond differently to precipitation changes (Cregger et al., 2012). Proteobacteria belong to a group of water sensitive bacteria and keep strong consistent change with precipitation. These groups are generally copiotrophic and fast-growing bacteria, and respond to unstable carbon availability due to changes in precipitation (Fierer et al., 2009). Actinomycetes are Gram-positive bacteria, which have a strong tolerance to drought and can grow in dry conditions. Therefore, the relatively high abundance of Actinomycetes in the water-reducing treatment may be related to their stronger cell walls, ability to produce spores and grow in filamentous form, which helps to effectively reduce the damage caused by drought and high temperature (Koyama et al., 2018; Li et al., 2018).
At the genus level, Streptomyces was confirmed to be the dominant genus in seven precipitation gradients (9.4%–11%), but it decreased with increasing precipitation. The proportion of Sphingomonas and Bradyrhizobium was very small (2.56%–5.08% and 2.96%–4.09%, respectively), but both increased along with the increase in precipitation (Figure 2B). Streptomyces is the largest bacterial group and is widely distributed in nature, especially in soil (Barka et al., 2016). Streptomyces was previously reported to be the dominant bacterium in the alpine grassland environment of northern Tibet (Yin et al., 2016), which is consistent our data. However, Streptomyces did not always increase with the amount of water available, which may be driven by competitive interactions between soil fungi and soil bacteria or predator-prey dynamics between soil microbes and soil protozoa (Cregger et al., 2012). Sphingomonas was the main antibacterial agent in the soil community, which had an inhibitory effect on pathogenic plant fungi (Kyselková et al., 2009; Wachowska et al., 2013). Bradyrhizobium is a common soil microorganism that establishes a symbiotic relationship with plant roots and fix nitrogen (Chen, 2016). Sphingomonas and Bradyrhizobium were higher in water-increasing treatment, indicating that they also maintained a higher consistency with soil moisture.
The α-diversity mainly measures the diversity of microbiota within individuals, while β-diversity is an index to characterize the similarity of microbial composition between individuals (Yang et al., 2022).We analyzed soil microbial community diversity under seven precipitation gradients and found that there were no significant differences in the three α-diversity indices (Figure 3), indicating that the α-diversity of soil bacterial communities was not affected by short-term precipitation changes. Bachar et al. (2010) and Wang et al. (2014c) showed that changes in precipitation did not alter bacterial community diversity in Mediterranean, semiarid, and arid regions, and they found that bacterial richness fluctuated little between seasons with different water availability, which was consistent with our results (Bachar et al., 2010; Wang et al., 2014c). Zhang et al. (2019b) found that the frequency of Acidobacteria decreased with the addition of water (Zhang et al., 2018a), which may be because the number and abundance of bacterial communities in arid and semi-arid areas were low, and the composition of bacterial communities was relatively similar (Wu et al., 2020). Compared to other soils, Yang and Li, (2020) pointed out that grassland soils had a greater ability of microorganisms to maintain their functions in response to repeated water stress (Yang and Li, 2020).In addition, some studies have shown that changes in precipitation have no significant effect on bacterial community composition in surface soil, but does affect bacterial abundance, which is consistent with our results (Wang et al., 2014c; Shi et al., 2018).The variation of precipitation had no significant effect on the composition of soil bacterial community at phylum level, but only affected a few soil water-sensitive species (Jia et al., 2021). Bray–Curtis dissimilarities represent β-diversity, and the results revealed the differentiation of soil microbial communities among the seven precipitation gradients of alpine grasslands. The significant difference in soil bacterial communities between D2, D3, and W2, W3 was demonstrated because the Bray–Curtis distance from D2, D3 to W2, W3 was relatively far (Figure 4). These results indicated that β-diversity had a significant effect on the treatments with large precipitation variations ( ± 50% and ±75%). This indicated that the composition of microbial communities among them was quite inconsistent. Sudden changes in soil moisture stress microorganisms because they must expend energy to regulate the osmotic pressure of their microenvironment (Evans and Wallenstein, 2012) and affect REDOX regulation (Bubier and Moore, 1994). In addition, climatic factors can alter soil geochemistry and thus affect microbial community structure. In general, the inconsistent responses of microorganisms to precipitation changes may be caused by ecosystem specific responses, historical precipitation mechanisms, or indirect water-induced environmental factors (She et al., 2018).
Differences of co-occurrence network between water-increasing and water-reducing treatment
Soil microorganisms exist in complex ecological networks, and also show different types of network relationships, including symbiosis, competition, predation, partially beneficial, or partially harmful relationships (Faust and Raes, 2012). Understanding their co-occurrence patterns can help to understand microbial interactions from a new perspective (Ma et al., 2016a), and explain microbial spatial niches (Freilich et al., 2018), which is of great significance for understanding biodiversity and community stability (Zhang et al., 2019b). Our results showed that there was little difference in the nodes between the two soil sample types, while the edges in the water-reducing treatment was much smaller than that in the water-increasing treatment (Figure 5 and Table 4). This indicated that the stability (complexity) of bacterial communities in two soil sample types was similar, but the communities in the water-increasing treatment were more closely connected and the connections in the water-reducing treatment were more distant (Hu et al., 2022). This suggested that the interaction, or at least the potential of mutual influence, between species in water-increasing treatment may be more intense or effective, indicating that synergies or competition between different bacterial species may be enhanced by up-regulating wetting disturbance in this study (He et al., 2017). In addition, the average path length and network diameter of water-increasing treatment were lower, which may indicate higher sensitivity and faster response of water addition among species under wet conditions (Jia et al., 2021). The longer average path distance indicated a lower sensitivity and slower response to water reduction (Table 4). This might be related to the adaptation of bacterial communities to the drought conditions of alpine steppe (Jia et al., 2021). Both water-increasing and water-reducing treatments had stable modular structures, which confirmed that short-term drought or wetting disturbance did not damage the stability of microbial community in the region. The weak interaction was stronger with increasing water, which indicated that the ecological network among species treated with increasing water was more stable (Guan et al., 2021). A precipitation gradient experiment in Inner Mongolia desert steppe also confirmed that the robustness of microbial networks increased with increasing precipitation (Li et al., 2022a). However, we found that decreased precipitation enhanced positive interactions between microbial species (Figure 5 and Table 4), possibly because limited soil water availability may have improved interactions and cooperation between functional groups of soil biota (Holtkamp et al., 2011); Another explanation is that the ecological niches of microbial groups may overlap under the soil moisture condition of water shortage and reduced precipitation (Hernandez et al., 2021).The degree of Proteobacteria decreased in water reduction treatment, and the role of module center or connector was weakened, which may be due to characteristics of Proteobacteria. Importantly, Proteobacteria belong to a group of water sensitive bacteria, which maintain a strong and consistent change with precipitation. These groups are generally copiotrophic, fast-growing bacteria, and respond to unstable carbon availability due to changes in precipitation (Fierer et al., 2009; Li et al., 2022a). In all network interactions, most of the core nodes were corresponding to Proteobacteria and Actinomycetes, and their relative abundance in soil microbial pathways was also the highest among all detected phyla (Fu et al., 2022). Therefore, Proteobacteria and Actinomycetes are the most important key bacterial species in the alpine grassland of northern Tibet, which are crucial for maintaining the stability of microbial communities in the study area.
The key factor driving the change of soil microbial community
Changes in precipitation lead to changes in soil water content (Zhang et al., 2019a), which leads to changes in other soil physical and chemical indexes. Subsequently, this leads to a change in soil redox conditions, which affects the structure and diversity of soil microbial community (Vanegas et al., 2013). Because changes in precipitation affect the soil available water and material migration, this results in changes the substrate supply of soil microorganisms (Apostel et al., 2015; Ren et al., 2018a). We found that soil temperature promote growth of Actinomycetes (Yang et al., 2022) and that soil moisture was negatively correlated with Actinomycetes. Because Actinomycetes are aerobic bacteria, soil moisture content was reduced in water-reducing treatment, evaporation was increased, and there was more oxygen content in the soil air, all of which are conditions that are conducive to Actinomycetes growth (Li et al., 2020). Other studies have shown that Actinomycetes can adapt to any extreme environment (such as drought, infertility and malnutrition), mainly determined by their diversity (Grover et al., 2016; Takahashi and Nakashima, 2018; Das et al., 2021), which is consistent with our results (Mickan et al., 2018). Another study of three genomes with similar 16S rRNA sequences of Acidobacteria revealed that the presence of fiber synthesis genes and a large number of novel high molecular excretion proteins proved that Acidobacteria had certain drought tolerance (Ward et al., 2009). Nitrogen elements is widely distributed limiting environmental factors in terrestrial ecosystems, and the dynamic change of soil nitrogen content has an important impact on the ecosystem (Wang et al., 2014b). In the current study, we found that total nitrogen was positively correlated to Deinococcus-Thermus, Cyanobacteria and Actinobacteria, which was consistent with a previous study on the newly reclaimed mudflat paddy soils (Li et al., 2022b). The reason was that nitrogen could promote the increase of the relative abundance of some bacterial communities and induced a shift in bacterial community composition towards a more copiotrophic type (Wu et al., 2021; Yao et al., 2021; You et al., 2021). In addition, this study found that there was a certain degree of correlation between available phosphorus (AP) and Actinomycetes, Deinococcus-Thermus, Chloroflexi, Proteobacteria, Gemmatimonatetes and Chlorobi. AP was an important nutrient in the soil of the alpine steppe in Northern Tibet, and its distribution in the soil was affected by precipitation change, which also had some effects on microbial species.
Changes in soil temperature might directly or indirectly kill microorganisms and reduce microbial abundance by limiting soil water and nutrient availability; But the tolerant/adaptive species that survived increased their advantage (Walker et al., 2006; Wilson and Walker, 2010). Secondly, the amount of substrate at higher soil temperatures may be very conducive to the rapid proliferation of microorganisms (Sharma et al., 2006). Zhang et al. (2017) pointed out that the high altitude area was only suitable for the survival of cold-tolerant bacteria, which reflected the restrictive effect of temperature on microbial flora (Zhang et al., 2017). In the current study, soil temperature was significantly different under seven water gradients due to the precipitation control experiment (supplement), which also affected the abundance of bacterial communities. At present, different research groups have drawn different conclusions on the influence of soil environmental factors on microbial community (Sun et al., 2012; Liu et al., 2016). Therefore, the response of soil microbial community to precipitation change in the alpine steppe of northern Tibet needs to be further studied.
Conclusion
In general, we can draw the following four conclusions from current studies. (1) Actinomycetes and Actinomycetes are the most important key bacterial taxa in the alpine steppe of northern Tibet and they play a crucial role in maintaining the stability of microbial community. (2) The α-diversity of soil microbial community is not significantly affected by precipitation change, while the β-diversity is significantly affected by drastic changes in water supply. (3) In the co-occurrence network, the communities in the water- increasing treatment were more closely connected than those in the water-reducing treatment. The network patterns of water-increasing/reducing treatment both showed strong positive correlation, but the water-reducing treatment was greater. (4) SWC and AP were key environmental factors affecting soil microbial community composition. Furthermore, the results also highlighted the tolerance of Actinobacteria to arid environments and the positively sensitive response of Proteobacteria to soil moisture. The results of this study are key to aiding our understanding of the response of the soil microbial community structure to precipitation changes in northern Tibet. Moreover, these data provide a more in-depth reference for studying the driving mechanism of global climate change on soil microbial community and grassland ecosystem in alpine grassland.
Data availability statement
The original contributions presented in the study are publicly available. This data can be found here: NCBI, PRJNA881773.
Author contributions
YY designed the experiment. XQL, LF, and YL carried out the experiment and collected the soil samples. XQL analyzed the data, and completed the writing and modification of the first draft. XYL and YY participated in the discussion of the content of the first draft and put forward valuable suggestions. All authors contributed to manuscript revision, read, and approved the final submitted version of the manuscript.
Funding
This research was supported by the National Natural Science Foundation of China (41871049 and 41877338).
Acknowledgments
We would like to thank the Xainza Alpine Grassland and the Wetland Ecosystem Observation and Experimental Station for providing us with the experimental site and the station staff, as well as Yiduo for his help in the sampling work.
Conflict of interest
The authors declare that the research was conducted in the absence of any commercial or financial relationships that could be construed as a potential conflict of interest.
Publisher’s note
All claims expressed in this article are solely those of the authors and do not necessarily represent those of their affiliated organizations, or those of the publisher, the editors and the reviewers. Any product that may be evaluated in this article, or claim that may be made by its manufacturer, is not guaranteed or endorsed by the publisher.
References
Allison, S. D., Treseder, K. K. (2008). Warming and drying suppress microbial activity and carbon cycling in boreal forest soils. Glob. Chang Biol. 14, 2898–2909. doi: 10.1111/j.1365-2486.2008.01716.x
Apostel, C., Dippold, M., Kuzyakov, Y. (2015). Biochemistry of hexose and pentose transformations in soil analyzed by position-specific labeling and 13C-PLFA. Soil Biol. Biochem. 80, 199–208. doi: 10.1016/j.soilbio.2014.09.005
Ault, T. R., Al-Ashhab, A, Soares, MIM, Sklarz, MY, Angel, R, Ungar, ED (2020). On the essentials of drought in a changing climate. Science 368, 256–260. doi: 10.1126/science.aaz5492
Bachar, A., Al-Ashhab, A., Soares, M. I. M., Sklarz, MY, Angel, R, Ungar, ED, et al. (2010). Soil microbial abundance and diversity along a low precipitation gradient. Microb. Ecol. 60, 453–461. doi: 10.1007/s00248-010-9727-1
Banerjee, S., Schlaeppi, K., van der Heijden, M. G. A. (2018). Keystone taxa as drivers of microbiome structure and functioning. Nat. Rev. Microbiol. 16, 567–576. doi: 10.1038/s41579-018-0024-1
Banu, N., Singh, B., Copeland, L. (2004). Microbial biomass and microbial biodiversity in some soils from new south Wales, Australia. Aust. J. Soil Res. - Aust. J. Soil Res. 42, 777–82. doi: 10.1071/SR03132
Barberán, A., Bates, S. T., Casamayor, E. O., Fierer, N. (2012). Using network analysis to explore co-occurrence patterns in soil microbial communities. ISME J. 6, 343–351. doi: 10.1038/ismej.2011.119
Barka, E. A., Vatsa, P., Sanchez, L., Gaveau-Vaillant, N, Jacquard, C, Meier-Kolthoff, JP, et al. (2016). Taxonomy, physiology, and natural products of Actinobacteria. Microbiol. Mol. Biol. Rev. 80, 1–43. doi: 10.1128/MMBR.00019-15
Bastian, M, Heymann, S, Jacomy, M. Gephi: An Open Source Software for Exploring and 680 Manipulating Networks Proceedings of the Third International Conference on 681 Weblogs and Social Media. (San Jose, California, USA) (2009) doi: 10.13140/2.1.1341.1520.
Bell, C. W., Tissue, D. T., Loik, M. E., Wallenstein, MD, Acosta Martinez, - V, Erickson, RA, et al. (2014). Soil microbial and nutrient responses to 7 years of seasonally altered precipitation in a chihuahuan desert grassland. Glob. Chang Biol. 20, 1657–1673. doi: 10.1111/gcb.12418
Bingjian, Z., Li, C., Wang, J., Li, J, Li, X. (2020). Elevation rather than season determines the assembly and co-occurrence patterns of soil bacterial communities in forest ecosystems of mount Gongga. Appl. Microbiol. Biotechnol. 104, 7589–602. doi: 10.1007/s00253-020-10783-w
Bu, Y., Magdoff, F. R. (2003). A comparison of ten methods for determination of available phosphorus in soils. Acta Pedologica Sin., 140–146. doi.org/CNKI:SUN:TRXB.0.2003-01-019
Bubier, J. L., Moore, T. R. (1994). An ecological perspective on methane emissions from northern wetlands. Trends Ecol. Evol. 9, 460–464. doi: 10.1016/0169-5347(94)90309-3
Brockett, B. F. T., Prescott, C. E., Grayston, S. J. (2012). Soil moisture is the major factor influencing microbial community structure and enzyme activities across seven biogeoclimatic zones in western Canada. Soil Biol. Biochem. 44, 9–20. doi: 10.1016/j.soilbio.2011.09.003
Cai, Y., Du, Z., Wang, X., Zhao, H, Yan, Y, Lu, X. (2014). Effects of excretal returns on CH4 emissions from an alpine steppe soil in northern Tibet,China. Mountain Res. 32, 393–400. doi: 10.16089/j.cnki.1008-2786.2014.04.006
Caporaso, J. K., Kuczynski, J., Stombaugh, J., Bittinger, K., Stombaugh, J, Stombaugh, J, Fau Bittinger, - K, Stombaugh, J, Stombaugh, J, Fau Bittinger, - K, et al. (2010). QIIME allows analysis of high-throughput community sequencing data. Nat. Methods 5, 1548–7105. doi: 10.1038/nmeth.f.303
Chao, A., Bunge, J. (2002). Estimating the number of species in a stochastic abundance model. Biometrics 58, 531–539. doi: 10.1111/j.0006-341X.2002.00531.x
Chen, D., Xu, B., Yao, T., Guo, Z, Cui, P, Chen, F, et al. (2015). Assessment of past,present and future environmental changes on the Tibetan Plateau. Chin. Sci. Bull. 60, 3025–3035+3021-3022. doi: 10.1360/n972014-01370
Chen, L., Xiang, W., Wu, H., Ouyang, S, Zhou, B, Zeng, Y, et al. (2019). Tree species identity surpasses richness in affecting soil microbial richness and community composition in subtropical forests. Soil Biol. Biochem. 130, 113–121. doi: 10.1016/j.soilbio.2018.12.008
Chen, Q., Niu, B., Hu, Y., Luo, T, Zhang, G. (2020). Warming and increased precipitation indirectly affect the composition and turnover of labile-fraction soil organic matter by directly affecting vegetation and microorganisms The Science of the Total Environment 714, 136787. doi: 10.1016/j.scitotenv.2020.136787
Chen, W. (2016). Progress and perspective of systematics of rhizobia;Rhizobia: from saprophytes to endosymbionts. Microbiol. China 43, 1095–1100. doi: 10.13344/j.microbiol.china.150994
Chen, Y., Hou, M., Ma, D., Han, X, Zhang, R, Zhang, X. (2018). Determination of tatal phosphorus in soil by alkali fusion-Mo-Sb anti spectrophotometric method. China Standardization, 193–196. doi: doi.org/CNKI:SUN:ZGBZ.0.2018-S1-041
Clark, J. S., Campbell, J. H., Grizzle, H., Acosta-Martìnez, V, Zak, JC. (2009). Soil microbial community response to drought and precipitation variability in the chihuahuan Desert. Microb. Ecol. 57, 248–260. doi: 10.1007/s00248-008-9475-7
Classen, A. T., Sundqvist, M. K., Henning, J. A., Newman, GS, Moore, JAM, Cregger, MA, et al. (2015). Direct and indirect effects of climate change on soil microbial and soil microbial-plant interactions: What lies ahead? Ecosphere 6, art130. doi: 10.1890/ES15-00217.1
Cregger, M. A., Schadt, C. W., McDowell, N. G., Pockman, WT, Classen, AT. (2012). Response of the soil microbial community to changes in precipitation in a semiarid ecosystem. Appl. Environ. Microbiol. 78, 8587–8594. doi: 10.1128/AEM.02050-12
Cruz-Martínez, K., Suttle, K. B., Brodie, E. L., Power, ME, Andersen, GL, Banfield, JF. (2009). Despite strong seasonal responses, soil microbial consortia are more resilient to long-term changes in rainfall than overlying grassland. ISME J. 3, 738–744. doi: 10.1038/ismej.2009.16
Das, P., Singh, S. K., Singh, P., Zeyad, MT, Aamir, M, Upadhyay, RS. (2021). “Chapter 15 - actinomycetes as biostimulants and their application in agricultural practices[C],” in Microbiome stimulants for crops. Eds. WHITE, J., KUMAR, A., DROBY, S. (Woodhead Publishing), 267–282. doi: 10.1016/B978-0-12-822122-8.00021-2
De Menezes, A. B., Richardson, A. E., Thrall, P. H. (2017). Linking fungal–bacterial co-occurrences to soil ecosystem function. Curr. Opin. Microbiol. 37, 135–141. doi: 10.1016/j.mib.2017.06.006
Ding, G. C., Piceno, Y. M., Heuer, H., Weinert, N, Dohrmann, AB, Carrillo, A, et al. (2013). Changes of soil bacterial diversity as a consequence of agricultural land use in a semi-arid ecosystem. PLos One 8, e59497. doi: 10.1371/journal.pone.0059497
Evans, S., Wallenstein, M. (2012). Soil microbial community response to drying and rewetting stress: Does historical precipitation regime matter? Biogeochemistry 109, 1–16. doi: 10.1007/s10533-011-9638-3
Faust, K., Raes, J. (2012). Microbial interactions: from networks to models. Nat. Rev. Microbiol. 10, 538–550. doi: 10.1038/nrmicro2832
Felsmann, K., Baudis, M., Gimbel, K., Kayler, ZE, Ellerbrock, R, Bruelheide, H, et al. (2015). Soil bacterial community structure responses to precipitation reduction and forest management in forest ecosystems across Germany. PLos One 10, e0122539. doi: 10.1371/journal.pone.0122539
Feng, X., Simpson, M. J. (2009). Temperature and substrate controls on microbial phospholipid fatty acid composition during incubation of grassland soils contrasting in organic matter quality. Soil Biol. Biochem. 41, 804–812. doi: 10.1016/j.soilbio.2009.01.020
Fierer, N., Jackson, R. B. (2006). The diversity and biogeography of soil bacterial communities. Proc. Natl. Acad. Sci. 103, 626–631. doi: 10.1073/pnas.0507535103
Fierer, N., Strickland, M. S., Liptzin, D., Bradford, MA, Cleveland, CC.. (2009). Global patterns in belowground communities. Ecol. Lett. 12, 1238–1249. doi: 10.1111/j.1461-0248.2009.01360.x
Fitzpatrick, C. R., Copeland, J., Wang, P. W., Guttman, DS, Kotanen, PM, Johnson, MTJ. (2018). Assembly and ecological function of the root microbiome across angiosperm plant species. Proc. Natl. Acad. Sci. 115, E1157–E1165. doi: 10.1073/pnas.1717617115
Freilich, M. A., Wieters, E., Broitman, B. R., Marquet, PA, Navarrete, SA. (2018). Species co-occurrence networks: Can they reveal trophic and non-trophic interactions in ecological communities? Ecology 99, 690–699. doi: 10.1002/ecy.2142
Fu, L., Niu, B., Zhu, Z., Wu, S, Li, W. (2012). CD-HIT: accelerated for clustering the next-generation sequencing data. Bioinformatics 28, 3150–3152. doi: 10.1093/bioinformatics/bts565
Fu, L., Yan, Y., Li, X., Liu, Y, Lu, X. (2022). Rhizosphere soil microbial community and its response to different utilization patterns in the semi-arid alpine grassland of northern Tibet. Front. Microbiol. doi: 10.3389/fmicb.2022.931795
Gao, C., Kim, Y.-C., Zheng, Y., Yang, W., Chen, L., Ji, N-N., et al. (2016). Increased precipitation, rather than warming, exerts a strong influence on arbuscular mycorrhizal fungal community in a semiarid steppe ecosystem1. Botany v.94 no.6, 459–469. doi: 10.1139/cjb-2015-0210
Grover, M., Bodhankar, S., Maheswari, M., Srinivasarao, C. (2016). “Actinomycetes as mitigators of climate change and abiotic Stress[C],” in Plant growth promoting actinobacteria: A new avenue for enhancing the productivity and soil fertility of grain legumes. Eds. SUBRAMANIAM, G., ARUMUGAM, S., RAJENDRAN, V. (Singapore: Springer Singapore), 203–212. doi: 10.1007/978-981-10-0707-1_13
Guan, Y., Jiang, N., Wu, Y., Yang, Z, Bello, A, Yang, W. (2021). Disentangling the role of salinity-sodicity in shaping soil microbiome along a natural saline-sodic gradient. Sci. Total Environ. 765, 142738. doi: 10.1016/j.scitotenv.2020.142738
Guo, X., Wang, J., Luan, G., Kang, Z. (2012). Comparison of two kinds of instruments for the determination of soil available potassium. Modern Agric. Sci. Technol., 221+223.
He, D., Shen, W., Eberwein, J., Zhao, Q, Ren, L, Wu, QL., et al. (2017). Diversity and co-occurrence network of soil fungi are more responsive than those of bacteria to shifts in precipitation seasonality in a subtropical forest. Soil Biol. Biochem. 115, 499–510. doi: 10.1016/j.soilbio.2017.09.023
Hernandez, D. J., David, A. S., Menges, E. S., Searcy, CA, Afkhami, ME.. (2021). Environmental stress destabilizes microbial networks The ISME Journal. 15, 1722–34 doi: 10.1038/s41396-020-00882-x
Hill, T. C. J., Walsh, K. A., Harris, J. A., Moffett, BF. (2003). Using ecological diversity measures with bacterial communities. FEMS Microbiol. Ecol. 43, 1–11. doi: 10.1111/j.1574-6941.2003.tb01040.x
Holtkamp, R., van der Wal, A., Kardol, P., van der Putten, WH, de Ruiter, PC, Dekker, SC. (2011). Modelling c and n mineralisation in soil food webs during secondary succession on ex-arable land. Soil Biol. Biochem. 43, 251–260. doi: 10.1016/j.soilbio.2010.10.004
Hu, Z., Xiao, M., Ding, J., Ji, J, Chen, J, Ge, T, et al. (2022). Response characteristics of soil microbial community under long-term film mulching. Environ. Sci., 1–17. doi: 10.13227/j.hjkx.202201237
Huang, X., Du, L., Hong, J., Chen, G, Wang, S, Lian, Z, et al. (2020). Study on the correlation between potassium dichromate external heating method and ASI for soil organic matter determination. Hubei Agric. Sci. 59, 122–125. doi: 10.14088/j.cnki.issn0439-8114.2020.15.024
Jia, M., Gao, Z., Gu, H., Zhao, C, Liu, M, Liu, F, et al. (2021). Effects of precipitation change and nitrogen addition on the composition, diversity, and molecular ecological network of soil bacterial communities in a desert steppe. PLos One 16, e0248194. doi: 10.1371/journal.pone.0248194
Jiang, Y., Lei, Y., Yang, Y., Korpelainen, H, Niinemets, Ü, Li, C.. (2018). Divergent assemblage patterns and driving forces for bacterial and fungal communities along a glacier forefield chronosequence. Soil Biol. Biochem. 118, 207–216. doi: 10.1016/j.soilbio.2017.12.019
Koyama, A., Steinweg, J. M., Haddix, M. L., Dukes, JS, Wallenstein, MD. (2018). Soil bacterial community responses to altered precipitation and temperature regimes in an old field grassland are mediated by plants. FEMS Microbiol Ecol 94, 1 .doi: 10.1093/femsec/fix156
Kumar, S., Suyal, D. C., Yadav, A., Shouche, Y, Goel, R. (2019). Microbial diversity and soil physiochemical characteristic of higher altitude. PLos One 14, e0213844. doi: 10.1371/journal.pone.0213844
Kundel, D, Meyer, S, Birkhofer, H, Fliessbach, A, Mäder, P, Scheu, S, et al. Design and Manual to 834 Construct Rainout-Shelters for Climate Change Experiments in Agroecosystems. Frontiers in Environmental Science 2018, 6. doi: 10.3389/fenvs.2018.00014
Kyselková, M., Kopecky, J., Frapolli, M., Defago, G, Mareckova, M, Grundmann, G, et al. (2009). Comparison of rhizobacterial community composition in soil suppressive or conducive to tobacco black root rot disease. ISME J. 3, 1127–38. doi: 10.1038/ismej.2009.61
Landesman, W. J., Dighton, J. (2010). Response of soil microbial communities and the production of plant-available nitrogen to a two-year rainfall manipulation in the new Jersey Pinelands. Soil Biol. Biochem. 42, 1751–1758. doi: 10.1016/j.soilbio.2010.06.012
Li, D., Liu, C. M., Luo, R., Sadakane, K, Lam, TW. (2015). MEGAHIT: an ultra-fast single-node solution for large and complex metagenomics assembly via succinct de bruijn graph. Bioinformatics 31, 1674–1676. doi: 10.1093/bioinformatics/btv033
Li, J., Benti, G., Wang, D., Yang, Z, Xiao, R. (2022a). Effect of alteration in precipitation amount on soil microbial community in a semi-arid Grassland. Front. Microbiol. 13, 842446. doi: 10.3389/fmicb.2022.842446
Li, N., Wang, B., An, S., Jiao, F, Huang, Q. (2020). Response of soil bacterial community structure to precipitation change in grassland of loess Plateau. Environ. Sci. 41, 4284–4293.
Li, Q., Zhang, Y., Hu, J., Dai, Q. (2022b). Response of bacterial communities and nitrogen-cycling genes in newly reclaimed mudflat paddy soils to nitrogen fertilizer gradients. Environ. Sci. pollut. Res. 29, 71113–23. doi: 10.1007/s11356-022-20770-5
Li, R., Yu, C., Li, Y., Lam, T-W, Yiu, S-M, Kristiansen, K, et al. (2009). SOAP2: an improved ultrafast tool for short read alignment. Bioinformatics 25, 1966–1967. doi: 10.1093/bioinformatics/btp336
Li, T., Wei, Z., Liu, G., Chen, T.. (2018). Advances in the study of microbial ecology in desert Soil. J Journal of Desert Research 38, 329–38. doi: 10.7522/j.issn.1000-694X.2017.00113
Liu, Y. (2020). Improvement of kjeldahl method for determination of total nitrogen in soil quality. Chem. Manage., 45–46. doi: doi.org/CNKI:SUN:FGGL.0.2020-14-029
Liu, Y., Zeng, Q., Huang, Y. (2016). Soil microbial communities by 454 prosequencing under different arbor forests on the loess Plateau. China Environ. Sci. 36, 3487–3494. doi: 10.3969/j.issn.1000-6923.2016.11.035
Lu, L., S-x, Y., Liu, X., Zhang, W-M, Gu, T, Shen, Q, et al. (2013). Fungal networks in yield-invigorating and -debilitating soils induced by prolonged potato monoculture Soil Biology Biochemistry 65, 186–194. doi: 10.1016/j.soilbio.2013.05.025
Ma, B., Wang, H., Dsouza, M., Lou, J, He, Y, Dai, Z, et al. (2016a). Geographic patterns of co-occurrence network topological features for soil microbiota at continental scale in eastern China. ISME J. 10, 1891–1901. doi: 10.1038/ismej.2015.261
Ma, L, Li, B, Jiang, XT, Wang, YL, Xia, Y, Li, AD, et al. Catalogue of antibiotic resistome and host-876 tracking in drinking water deciphered by a large scale survey. Microbiome (2017) 5, 154. doi: 10.1186/s40168-017-0369-0
Ma, X., Yan, Y., Lu, X., Wang, X.. (2016b). Dynamics of belowground biomass and its relationship with soil moisture in alpine grassland on the north Tibetan Plateau. Ecol. Environ. Sci. 25, 189–195. doi: 10.16258/j.cnki.1674-5906.2016.02.002
Mickan, B. S., Abbott, L. K., Fan, J., Hart, MM, Siddique, KHM, Solaiman, ZM, et al. (2018). Application of compost and clay under water-stressed conditions influences functional diversity of rhizosphere bacteria. Biol. Fertil. Soils 54, 55–70. doi: 10.1007/s00374-017-1238-5
Mikhailov, I. S., Zakharova, Y. R., Bukin, Y. S., Galachyants, YP, Petrova, DP, Sakirko, MV, et al. (2019). Co-Occurrence networks among bacteria and microbial eukaryotes of lake baikal during a spring phytoplankton Bloom. Microb. Ecol. 77, 96–109. doi: 10.1007/s00248-018-1212-2
Nan, J., Chao, L., Ma, X., Xu, D, Mo, L, Zhang, X, et al. (2020). Microbial diversity in the rhizosphere soils of three stipa species from the eastern inner Mongolian grasslands. Global Ecol. Conserv. 22, e00992. doi: 10.1016/j.gecco.2020.e00992
Nielsen, U. N., Ball, B. A. (2015). Impacts of altered precipitation regimes on soil communities and biogeochemistry in arid and semi-arid ecosystems. Glob. Chang Biol. 21, 1407–1421. doi: 10.1111/gcb.12789
Niu, H., Pang, Z., Fallah, N., Zhou, Y., Zhang, C., Hu, C., et al. (2021). Diversity of microbial communities and soil nutrients in sugarcane rhizosphere soil under water soluble fertilizer. PLos One 16, e0245626. doi: 10.1371/journal.pone.0245626
Nyström, M., Jouffray, J. B., Norström, A. V., Crona, B, Søgaard Jørgensen, P, Carpenter, SR, et al. (2019). Anatomy and resilience of the global production ecosystem. Nature 575, 98–108. doi: 10.1038/s41586-019-1712-3
Ochoa-Hueso, R., Collins, S. L., Delgado-Baquerizo, M., Hamonts, K, Pockman, WT, Sinsabaugh, RL, et al. (2018). Drought consistently alters the composition of soil fungal and bacterial communities in grasslands from two continents. Glob. Chang Biol. 24, 2818–2827. doi: 10.1111/gcb.14113
Parton, W. J., Scurlock, J. M. O., Ojima, D. S., Schimel, DS, Hall, DO, Scopegram Group, M. (1995). Impact of climate change on grassland production and soil carbon worldwide. Glob. Chang Biol. 1, 13–22. doi: 10.1111/j.1365-2486.1995.tb00002.x
Ping, C., Sun, G., Ci, D., Li, J, Chi, L, Hua, K, et al. (2021). Study on growth performance of short legged yak in shenzha Couty. Tibet J. Agric. Sci. 43, 55–56. doi: 10.3969/j.issn.1005-2925.2021.01.014
Placella, S. A., Brodie, E. L., Firestone, M. K. (2012). Rainfall-induced carbon dioxide pulses result from sequential resuscitation of phylogenetically clustered microbial groups. Proc. Natl. Acad. Sci. 109, 10931–10936. doi: 10.1073/pnas.1204306109
Ren, C., Chen, J., Lu, X., Doughty, R, Zhao, F, Zhong, Z, et al. (2018a). Responses of soil total microbial biomass and community compositions to rainfall reductions. Soil Biol. Biochem. 116, 4–10. doi: 10.1016/j.soilbio.2017.09.028
Ren, C., Zhang, W., Zhong, Z., Han, X, Yang, G, Feng, Y, et al. (2018b). Differential responses of soil microbial biomass, diversity, and compositions to altitudinal gradients depend on plant and soil characteristics. Sci. Total Environ. 610-611, 750–758. doi: 10.1016/j.scitotenv.2017.08.110
Schimel, J. P., Schaeffer, S. M. (2012). Microbial control over carbon cycling in soil. Front. Microbiol. 3, 348. doi: 10.3389/fmicb.2012.00348
Schindlbacher, A., Rodler, A., Kuffner, M., Kitzler, B, Sessitsch, A, Zechmeister-Boltenstern, S., et al. (2011). Experimental warming effects on the microbial community of a temperate mountain forest soil. Soil Biol. Biochem. 43, 1417–1425. doi: 10.1016/j.soilbio.2011.03.005
Sharma, S., Szele, Z., Schilling, R., Munch, JC, Schloter, M. (2006). Influence of freeze-thaw stress on the structure and function of microbial communities and denitrifying populations in soil. Appl. Environ. Microbiol. 72, 2148–2154. doi: 10.1128/AEM.72.3.2148-2154.2006
She, W., Bai, Y., Zhang, Y., Qin, S, Feng, W, Sun, Y, et al. (2018). Resource availability drives responses of soil microbial communities to short-term precipitation and nitrogen addition in a desert Shrubland. Front. Microbiol. 9, 186. doi: 10.3389/fmicb.2018.00186
Shi, L., Zhang, H., Liu, T., Mao, P, Zhang, W, Shao, Y, et al. (2018). An increase in precipitation exacerbates negative effects of nitrogen deposition on soil cations and soil microbial communities in a temperate forest. Environ. pollut. 235, 293–301. doi: 10.1016/j.envpol.2017.12.083
Sierra, C., Malghani, S., Loescher, H. (2017). Interactions among temperature, moisture, and oxygen concentrations in controlling decomposition rates in a boreal forest soil. Boigeosciences 14, 703–710. doi: 10.5194/bg-14-703-2017
Sihi, D., Davidson, E. A., Chen, M., Savage, KE, Richardson, AD, Keenan, TF, et al. (2018). Merging a mechanistic enzymatic model of soil heterotrophic respiration into an ecosystem model in two AmeriFlux sites of northeastern USA. Agric. For. Meteorology 252, 155–166. doi: 10.1016/j.agrformet.2018.01.026
Steinhauser, D, Krall, L, Müssig, C, Büssis, D, Usadel, B. Correlation Networks Analysis of 947 Biological Networks (2008) 305–33. doi: 10.1002/9780470253489.ch13.
Sun, L., Qi, Y., Dong, Y., Peng, Q, He, Y, Liu, X, et al. (2012). Research progress on the effects of global change on microbial community diversity of grassland soils. Prog. Geogr. 31, 1715–1723. doi: 10.11820/dlkxjz.2012.12.018
Takahashi, Y., Nakashima, T. (2018). Actinomycetes, an inexhaustible source of naturally occurring Antibiotics. Antibiotics (Basel) 7, 45. doi: 10.3390/antibiotics7020045
Tandong, Y. (2019). A comprehensive study of water-Ecosystem-Human activities reveals unbalancing Asian water tower and accompanying potential risks. Chin. Sci. Bull. 64, 2761–2762. doi: 10.1360/TB-2019-0532
Uhlírová, E., Elhottová, D., Tríska, J., Santrůcková, H. (2005). Physiology and microbial community structure in soil at extreme water content. Folia Microbiol. (Praha) 50, 161–166. doi: 10.1007/BF02931466
Vanegas, J., Landazabal, G., Melgarejo, L. M., Beltran, M, Uribe-Vélez, D. (2013). Structural and functional characterization of the microbial communities associated with the upland and irrigated rice rhizospheres in a neotropical Colombian savannah. Eur. J. Soil Biol. 55, 1–8. doi: 10.1016/j.ejsobi.2012.10.008
Wachowska, U., Irzykowski, W., Jędryczka, M., Stasiulewicz-Paluch, AD, Głowacka, K.. (2013). Biological control of winter wheat pathogens with the use of antagonistic sphingomonas bacteria under greenhouse conditions. Biocontrol Sci. Technol. 23, 1110–1122. doi: 10.1080/09583157.2013.812185
Walker, V. K., Palmer, G. R., Voordouw, G. (2006). Freeze-thaw tolerance and clues to the winter survival of a soil community. Appl. Environ. Microbiol. 72, 1784–1792. doi: 10.1128/AEM.72.3.1784-1792.2006
Wang, A., Duan, Z., Dong, J., Zhao, Y, Tang, Y, Li, X.. (2014a). Comparative study of different pH determination methods for assessing soil acidification in greenhouse system. J. Agro-Environment Sci. 33, 2164–2170. doi: doi.org/CNKI:SUN:NHBH.0.2014-11-017
Wang, C., Wang, X., Liu, D., Wu, H, Lü, X, Fang, Y, et al. (2014b). Aridity threshold in controlling ecosystem nitrogen cycling in arid and semi-arid grasslands. Nat. Commun. 5, 4799. doi: 10.1038/ncomms5799
Wang, N., Wang, M., Li, S., Sui, X, Han, S, Feng, F. (2014c). Effects of variation in precipitation on the distribution of soil bacterial diversity in the primitive Korean pine and broadleaved forests. World J. Microbiol. Biotechnol. 30, 2975–2984. doi: 10.1007/s11274-014-1725-x
Wang, N, Han, D, Sun, X, Guo, W, Ma, H, Feng, F., et al Effects of precipitation change in soil microbial 982 functional diversity primitive Korean pine and broadleaved forests. Acta Ecologica Sinica (2017) 37, 868–76. doi: 10.5846/stxb201509101873
Wang, J., Zhang, L., Zhao, R., Xie, Z. (2020). Responses of plant growth of different life-forms to precipitation changes in desert steppe. Chin. J. Appl. Ecol. 31, 778–786. doi: 10.13287/j.1001-9332.202003.001
Ward, N. L., Challacombe, J. F., Janssen, P. H., Henrissat, B, Coutinho, PM, Wu, M, et al. (2009). Three genomes from the phylum acidobacteria provide insight into the lifestyles of these microorganisms in soils. Appl. Environ. Microbiol. 75, 2046–2056. doi: 10.1128/AEM.02294-08
Williams, M. A. (2007). Response of microbial communities to water stress in irrigated and drought-prone tallgrass prairie soils. Soil Biol. Biochem. 39, 2750–2757. doi: 10.1016/j.soilbio.2007.05.025
Williams, R. J., Howe, A., Hofmockel, K. S. (2014). Demonstrating microbial co-occurrence pattern analyses within and between ecosystems. Front. Microbiol. 5, 358. doi: 10.3389/fmicb.2014.00358
Wilson, S. L., Walker, V. K. (2010). Selection of low-temperature resistance in bacteria and potential applications. Environ. Technol. 31, 943–956. doi: 10.1080/09593331003782417
Wood, D. E., Salzberg, S. L. (2014). Kraken: ultrafast metagenomic sequence classification using exact alignments. Genome Biol. 15, R46. doi: 10.1186/gb-2014-15-3-r46
Wu, K., Xu, W., Yang, W. (2020). Effects of precipitation changes on soil bacterial community composition and diversity in the junggar desert of xinjiang, China. PeerJ 8, e8433 doi: 10.7717/peerj.8433
Wu, L., Wang, Y., Zhang, S., Wei, W, Kuzyakov, Y, Ding, X.. (2021). Fertilization effects on microbial community composition and aggregate formation in saline-alkaline soil. Plant Soil 463, 523–535. doi: 10.1007/s11104-021-04909-w
Xiong, D., Zhao, G., Wu, J., Shi, P, Zhang, X. (2016). The relationship between species diversity and ecosystem multifunctionality in alpine grasslands on the Tibetan changtang Plateau. Acta Ecologica Sin. 36, 3362–3371. doi: 10.5846/stxb201506301361
Yang, O., Li, X. (2020). Effect of repeated drying-rewetting cycles on soil extracellular enzyme activities and microbial community composition in arid and semi-arid ecosystems. Eur. J. Soil Biol. 98, 103187. doi: 10.1016/j.ejsobi.2020.103187
Yang, Y., Chen, K., Zhang, N., Jiang, L.. (2022). Responses of soil microbial community to different precipitation gradients in the alpine wetlands of qinghai lake Basin. Chin. J. Appl. Environ. Biol. 28, 290–299.
Yang, Y., Ni, Z., Lili, J., Kelong, C.. (2021). Effects of simulated precipitation on soil edaphic physicochemical factors and microbial community characteristics in bird island if qinghai lake on the Tibet Plateau. Acta Agrestia Sin. 29, 1043–1052. doi: 10.11733/j.issn.1007-0435.2021.05.021
Yao, R., Yang, J., Wang, X., Xie, W, Zheng, F, Li, H, et al. (2021). Response of soil characteristics and bacterial communities to nitrogen fertilization gradients in a coastal salt-affected agroecosystem. Land Degradation Dev. 32, 338–353. doi: 10.1002/ldr.3705
Ye, X., Zhao, Z. (2011). Comparison of soil available nitrogen concentration between flow injection method and alkali-diffusion method. J. Anhui Agric. Sci. 39, 12166–12167+12178. doi: 10.13989/j.cnki.0517-6611.2011.20.170
Yin, M., He, J., Zhang, G. (2016). Biological activity and diversity of alkali tolerant actinomycetes in northern Tibet alpine grassland. J. Northwest A F University(Natural Sci. Edition) 44, 142–148. doi: 10.13207/j.cnki.jnwafu.2016.04.020
You, X., Yin, S., Suo, F., Xu, Z, Chu, D, Kong, Q, et al. (2021). Biochar and fertilizer improved the growth and quality of the ice plant (Mesembryanthemum crystallinum l.) shoots in a coastal soil of yellow river delta, China. Sci. Total Environ. 775, 144893. doi: 10.1016/j.scitotenv.2020.144893
Yu, J., Liu, F., Tripathi, B. M., Steinberger, Y. (2020). Changes in the composition of soil bacterial and fungal communities after revegetation with caragana microphylla in a desertified semiarid grassland. J. Arid Environments 182, 104262. doi: 10.1016/j.jaridenv.2020.104262
Zeng, Q., An, S., Liu, Y. (2017). Soil bacterial community response to vegetation succession after fencing in the grassland of China. Sci. Total Environ. 609, 2–10. doi: 10.1016/j.scitotenv.2017.07.102
Zhang, H., Liu, H., Zhao, J., Li, G, Lai, X, Li, J, et al. (2018a). Effects of simulated nitrogen deposition and precipitation change on soil bacterial community structure in a stipa baicalensis steppe. Acta Ecologica Sin. 38, 10. doi: 10.5846/stxb201612092539
Zhang, J., Miao, Y., Zhang, T., Wei, Y, Qiao, X, Miao, R, et al. (2020). Drought timing and primary productivity in a semiarid grassland. Land Degradation Dev. 31, 2185–2195. doi: 10.1002/ldr.3603
Zhang, L., Wang, A., Yang, W., Xu, Z, Wu, F, Tan, B, et al. (2017). Soil microbial abundance and community structure vary with altitude and season in the coniferous forests, China. J. Soils Sediments 17, 2318–2328. doi: 10.1007/s11368-016-1593-0
Zhang, L., Xie, Z., Zhao, R., Zhang, Y. (2018b). Plant, microbial community and soil property responses to an experimental precipitation gradient in a desert grassland. Appl. Soil Ecol. 127, 87–95. doi: 10.1016/j.apsoil.2018.02.005
Zhang, W., Parker, K. M., Luo, Y., Wan, S., Wallace, L. L, Hu, S. (2005). Soil microbial responses to experimental warming and clipping in a tallgrass prairie. Glob. Chang Biol. 11, 266–277. doi: 10.1111/j.1365-2486.2005.00902.x
Zhang, Y., Zhang, S., Kong, W., Yang, L, Wang, M., et al. (2019a). Soil moisture-precipitation coupling in summer in China and its surrounding areas. J. Lanzhou University(Nature Sciences) 55, 443–448+454. doi: 10.13885/j.issn.0455-2059.2019.04.004
Zhang, Z., Deng, Y., Feng, K., Cai, W, li, S, Yin, H, et al. (2019b). Deterministic assembly and diversity gradient altered the biofilm community performances of Bioreactors. Environ. Sci. Technol. 53, 1315–24. doi: 10.1021/acs.est.8b06044
Zhao, C., Miao, Y., Yu, C., Zhu, L, Wang, F, Jiang, L, et al. (2016). Soil microbial community composition and respiration along an experimental precipitation gradient in a semiarid steppe. Sci. Rep. 6, 24317. doi: 10.1038/srep24317
Zhong, W., Gu, T., Wang, W., Zhang, B, Lin, X, Huang, Q, et al. (2010). The effects of mineral fertilizer and organic manure on soil microbial community and diversity. Plant Soil 326, 511–522. doi: 10.1007/s11104-009-9988-y
Zhou, G., Wei, X., Wu, Y., Liu, S, Huang, Y, Yan, J, et al. (2011). Quantifying the hydrological responses to climate change in an intact forested small watershed in southern China. Glob. Chang Biol. 17, 3736–3746. doi: 10.1111/j.1365-2486.2011.02499.x
Zhou, Z., Wang, C., Luo, Y. (2018). Response of soil microbial communities to altered precipitation: A global synthesis. Global Ecol. Biogeography 27, 1121–1136. doi: 10.1111/geb.12761
Zhu, B., Ni, J., Gao, L., Lu, G.. (2018). Study on simultaneous determination of total phosphorus and total potassium in soil. J. Anhui Agric. Sci. 46, 110–111. doi: 10.13989/j.cnki.0517-6611.2018.15.034
Keywords: Precipitation change, Alpine grassland, soil microbial, community structure, co-occurrence network
Citation: Li X, Yan Y, Lu X, Fu L and Liu Y (2022) Responses of soil bacterial communities to precipitation change in the semi-arid alpine grassland of Northern Tibet. Front. Plant Sci. 13:1036369. doi: 10.3389/fpls.2022.1036369
Received: 04 September 2022; Accepted: 26 September 2022;
Published: 17 October 2022.
Edited by:
Jian Sun, Institute of Tibetan Plateau Research, Chinese Academy of Sciences (CAS), ChinaReviewed by:
Dong Wang, Henan University, ChinaZhenchao Zhang, Qingdao Agricultural University, China
Xiangtao Wang, Tibet University, China
Copyright © 2022 Li, Yan, Lu, Fu and Liu. This is an open-access article distributed under the terms of the Creative Commons Attribution License (CC BY). The use, distribution or reproduction in other forums is permitted, provided the original author(s) and the copyright owner(s) are credited and that the original publication in this journal is cited, in accordance with accepted academic practice. No use, distribution or reproduction is permitted which does not comply with these terms.
*Correspondence: Yan Yan, eXlAaW1kZS5hYy5jbg==; Xuyang Lu, eHlsdUBpbWRlLmFjLmNu