- 1Division of Biotechnology and Plant Health, Norwegian Institute of Bioeconomy Research, Ås, Norway
- 2Department of Plant Sciences, Norwegian University of Life Sciences (NMBU), Ås, Norway
- 3Department of Agricultural Sciences, University of Helsinki, Helsinki, Finland
- 4Organismal and Evolutionary Biology Research Programme, Faculty of Biological and Environmental Sciences, University of Helsinki, Helsinki, Finland
- 5Department of Genetics, Genomics and Breeding, National Institute of Agricultural Botany- East Malling Research Station, East Malling, United Kingdom
Grey mold caused by the necrotrophic fungal pathogen Botrytis cinerea can affect leaves, flowers, and berries of strawberry, causing severe pre- and postharvest damage. The defense elicitor β-aminobutyric acid (BABA) is reported to induce resistance against B. cinerea and many other pathogens in several crop plants. Surprisingly, BABA soil drench of woodland strawberry (Fragaria vesca) plants two days before B. cinerea inoculation caused increased infection in leaf tissues, suggesting that BABA induce systemic susceptibility in F. vesca. To understand the molecular mechanisms involved in B. cinerea susceptibility in leaves of F. vesca plants soil drenched with BABA, we used RNA sequencing to characterize the transcriptional reprogramming 24 h post-inoculation. The number of differentially expressed genes (DEGs) in infected vs. uninfected leaf tissue in BABA-treated plants was 5205 (2237 upregulated and 2968 downregulated). Upregulated genes were involved in pathogen recognition, defense response signaling, and biosynthesis of secondary metabolites (terpenoid and phenylpropanoid pathways), while downregulated genes were involved in photosynthesis and response to auxin. In control plants not treated with BABA, we found a total of 5300 DEGs (2461 upregulated and 2839 downregulated) after infection. Most of these corresponded to those in infected leaves of BABA-treated plants but a small subset of DEGs, including genes involved in ‘response to biologic stimulus‘, ‘photosynthesis‘ and ‘chlorophyll biosynthesis and metabolism’, differed significantly between treatments and could play a role in the induced susceptibility of BABA-treated plants.
Introduction
Cultivated strawberry (Fragaria × ananassa) is a commercially important soft fruit crop producing berries rich in beneficial vitamins, antioxidants, and nutrients. However, this crop is exposed to malignant viruses, nematodes, fungi and bacteria that can cause severe yield losses (Garrido et al., 2011). The ascomycete fungus Botrytis cinerea is a broad-spectrum necrotrophic pathogen causing severe damage in more than 1000 plant species, including >200 crop species (Williamson et al., 2007; Fillinger and Elad, 2016). Botrytis cinerea can cause grey mold by infecting the berries, flowers and leaves of strawberry and is one of the most economically important pathogens in this crop. Strawberry susceptibility towards B. cinerea varies with tissue type, ripening stage of berries, and leaf age (Meng et al., 2019; Petrasch et al., 2019).
Plants have a standing defense consisting of constitutive physical and chemical barriers such as cell walls, a waxy cuticle, trichomes, and phytoanticipins (Piasecka et al., 2015). Plants also possess inducible defenses that are activated in response to attack or damage. In the first line of inducible defense, pattern-triggered immunity (PTI) is activated when pattern recognition receptors (PRRs) on the plant cell membrane recognize and bind to specific microbe-, pathogen- or damage-associated molecular patterns (MAMPs, PAMPs, and DAMPs, respectively). Pathogens that successfully evade PTI by secreting pathogen effectors can in turn be recognized by intracellular nucleotide-binding leucine-rich-repeat (NB-LRR) proteins, encoded by R-genes. R-proteins/NB-LRR-proteins activate the second line of inducible defense, known as effector-triggered immunity (ETI).
Activation of PTI and ETI may result in local defense response at the site of pathogen attack, as well as systemic defense responses in more distal tissues. Local defense responses are associated with mitogen-activated protein kinase (MPK) signaling, release of reactive oxygen species, expression of PR-proteins, cell-wall modification, phytohormone synthesis and signaling, and accumulation of secondary metabolites with antimicrobial activity such as phytoalexins (Zeilinger et al., 2016; AbuQamar et al., 2017; Wan et al., 2021). Localized infection or perception of MAMPs/PAMPs/DAMPs may also sensitize both local and more distant tissues for enhanced defense following a subsequent challenge in a process known as defense priming (Amil-Ruiz et al., 2011; Conrath et al., 2015; Reimer-Michalski and Conrath, 2016).
Defense priming is established through a signaling cascade where elicitors, consisting of natural or synthetic substances, are recognized by PRRs that in turn activate the innate immune system. Synthetic elicitors may be analogues of defense or signaling molecules, like acibenzolar-S-methyl (BTH/Bion), β-aminobutyric acid (BABA), and methyl jasmonate (MeJA), or natural compounds of biological origin, such as chitosan, laminarin, and substances produced by beneficial bacteria (Wiesel et al., 2014; Bektas and Eulgem, 2015; Tripathi et al., 2019). Chitosan and MeJA have been shown to increase post-harvest resistance to B. cinerea infection in berries when strawberry plants were sprayed before harvesting (Saavedra et al., 2017).
The non-proteinogenic amino acid BABA is an extensively studied defense elicitor. It has been reported to protect some 40 plant species against >80 pests and pathogens (Cohen, 2002; Cohen et al., 2016; Wilkinson et al., 2018) by activating both salicylic acid (SA)-dependent and SA-independent defenses (Ton and Jakab, 2005). BABA can be synthesized chemically for use in crop protection, but is also produced in low concentrations by stressed plants (Thevenet et al., 2017). Studies have shown that BABA induces local and systemic resistance against B. cinerea infection in several plant species, including grape, cucumber, Arabidopsis, grapefruit, tomato and strawberry (Cohen et al., 2016; Martínez-Aguilar et al., 2016; Wilkinson et al., 2018). In strawberry, most studies so far have focused on local effects of BABA in berries. Immersing detached berries in a BABA solution provided dose-dependent protection against B. cinerea infection. High BABA concentrations induced direct defense responses whereas low concentrations induced defense priming, with full activation of plant defenses only occurring upon subsequent B. cinerea infection (Wang et al., 2016). Large-scale transcriptional profiling of strawberry berries infected with B. cinerea has previously identified differential expression of genes involved in pathogen recognition, synthesis of secondary metabolites, signaling transduction, defense responses, and cell transport (Xiong et al., 2018; Haile et al., 2019).
With the aim to identify local and systemic resistance effects of BABA we compared responses to B. cinerea infection in F. vesca leaves on plants sprayed directly with BABA, plants subjected to soil drench with BABA, and untreated control plants. We did a global transcriptomic analysis of leaf tissue using RNA-seq to understand (1) the molecular underpinning of responses to B. cinerea infection in untreated plants and (2) systemic effects of BABA soil drench on B. cinerea-mediated transcription. Our results reveal strawberry-specific and application method-specific responses to BABA that may be important for the practical use of defense elicitors in future crop protection schemes.
Results
Botrytis cinerea infection of leaves and effect of BABA treatment on susceptibility
To establish a working quantification method for B. cinerea infection and verify pathogenicity, F. vesca leaves were drop-inoculated with a spore suspension of B. cinerea isolate Bc101 and incubated under high humidity to promote fungal infection. We detected clear symptoms of infection, in the form of necrotic lesions on leaves, 4-5 days after infection (Supplementary Figure S1). As expected, qPCR quantification of B. cinerea relative to F. vesca using genome-specific primers for B. cinerea (Bc3F and Bc3R) and F. vesca (EF1αF and EF1αR) detected high levels of B. cinerea in infected leaves and negligible levels in mock-infected control leaves, indicating fungal disease progression in the necrotic lesions (Supplementary Figure S1B).
We then sprayed leaves of F. vesca plants with a B. cinerea spore suspension (106 spores mL-1) until run-off. As for the drop inoculation, necrotic lesions began to appear 4-5 days after spraying. Surprisingly, in F. vesca Hawaii-4 plants that had been treated with BABA as a soil drench two days before infection qPCR-quantification showed that B. cinerea levels in the leaves were about twice as high as in untreated control plants (p = 0.061; Figure 1A). The increased susceptibility to B. cinerea after soil drench with BABA corresponded with increased browning and development of larger necrotic lesions (which increased over time) compared to non-treated controls (Figure 1C). BABA soil-drench also induced susceptibility to B. cinerea infection in F. vesca ‘Alexandria’ plants in a dose-dependent manner, with >10-fold increase relative to untreated control in plants treated with 0.6 mM BABA (p = 0.039; Figure 1B). The negative effect of BABA on resistance to B. cinerea was further confirmed in F. ananassa, where plants treated with BABA soil drench suffered larger lesions than untreated controls following B. cinerea infection (p = 0.042; Supplementary Figure S2). Taken together our observations suggest a systemic negative effect on resistance in leaves when BABA was applied to roots as soil drench.
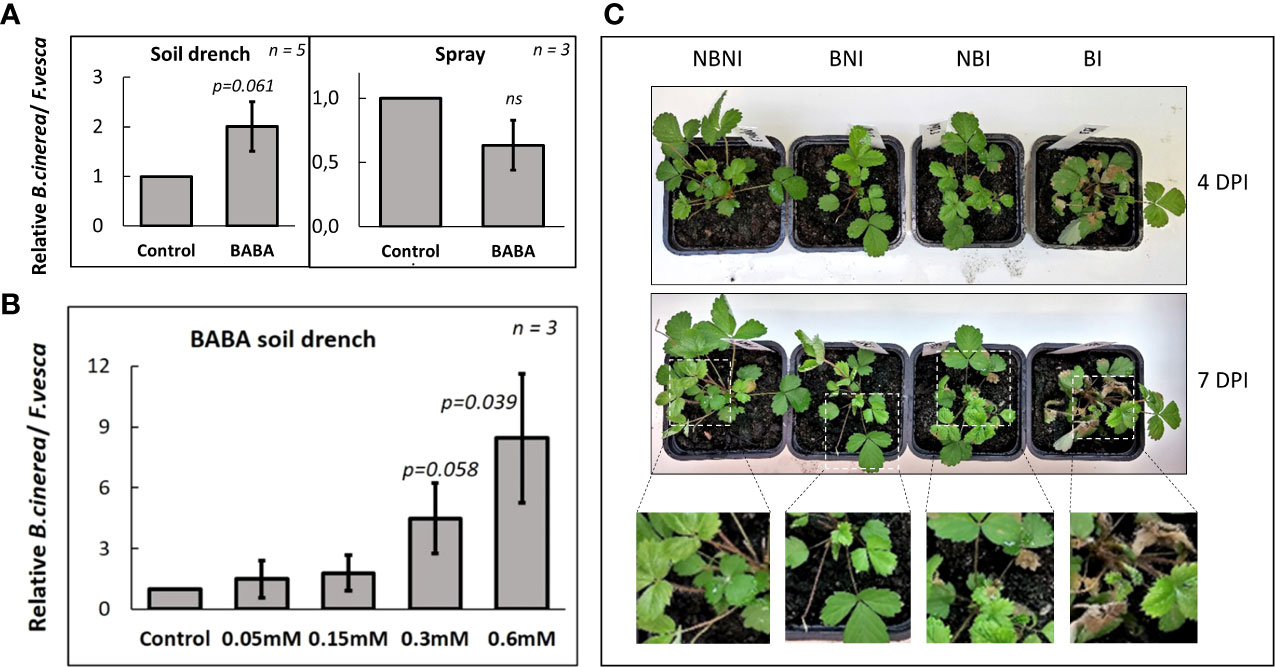
Figure 1 Effects of BABA on Fragaria vesca resistance to Botrytis cinerea infection of leaves. (A) B. cinerea infection 5 days post-inoculation in leaves of F. vesca plants after soil-drenching (left) with 0.2 mM β-aminobutyric acid (BABA) or foliar spraying with 0.3 mM BABA (right), or (B) soil-drenching with 0 mM, 0.05 mM, 0.15 mM, 0.3 mM or 0.6 mM BABA two days before inoculation. Levels of B. cinerea infection was measured as the ratio between B. cinerea and F. vesca DNA using qPCR with genome-specific primers for B. cinerea (Bc3F and Bc3R) and F. vesca (EF1αF and EF1αR). Levels of B. cinerea in untreated control plants are set to 1. ‘n’ = number of biological replicates (i.e. individual plants), and error bars show standard error ( ± 1 SE). P-values report comparisons between control plants and plants subjected BABA using Student’s t-test. ‘ns’ = not statistically significant. (C) Representative pictures of disease progression in F. vesca plants 4 days and 7 days after inoculation, n = 5. Treatment combinations: B. cinerea-infected (NBI; No-BABA and Infected), mock-infected (NBNI; No-BABA and Non-Infected), BABA-treated and B. cinerea-infected (BI; BABA and Infected), and BABA-treated and mock-infected (BNI; BABA and Non-Infected).
In contrast, foliar application of BABA two days before infection had a positive, but non-significant, effect on resistance to B. cinerea infection relative to control plants that had not been treated with BABA (Figure 1A, right), suggesting that locally BABA had a neutral or weakly positive effect on resistance. Botrytis cinerea grew normally on potato dextrose agar amended with high concentrations of BABA, suggesting that the local effect observed in planta was not due to direct negative effects of BABA on B. cinerea growth and survival, but rather was due to induced defense responses (Supplementary Figure S3). As defense elicitors may repress plant growth, we investigated potential growth effects on uninfected F. vesca plants treated with BABA. Soil drench with BABA significantly inhibited plant growth (Supplementary Figure S4A), whereas foliar application did not have any significant effect (Supplementary Figure S4B).
Transcriptional analysis of Fragaria vesca leaves infected with Botrytis cinerea
RNA-seq was performed to contrast the molecular mechanisms involved in defense against B. cinerea in untreated plants and plants treated with 0.2 mM BABA soil-drench two days before infection. We analyzed young leaf samples (n = 5) from plants that had been subjected to four different treatments: B. cinerea-infected only (No BABA and Infected; NBI), mock-infected only (No BABA and Non-Infected; NBNI), BABA-treated and B. cinerea-infected (BABA and Infected; BI), and BABA-treated and mock-infected (BABA and Non-Infected; BNI). Leaf samples were collected 24 hours after infection (i.e., before any visual symptoms appeared for the infected plants). Illumina sequencing generated an average of 26,176,008 reads per plant (median: 25,291,981) with mapping efficiencies ranging from 75 to 97% (median: 83%). The data were submitted to NCBI’s Sequence Read Archive (SRA) as BioProject PRJNA818508. Reads could be mapped to a total of 28,588 annotated genes in the F. vesca genome, and DEGs were identified by pairwise comparisons of gene expression for the different treatments using the R package EdgeR with false discovery rate < 0.05 and absolute log2-fold change values > 1.
Among the differentially expressed genes (DEGs) in non-BABA treated plants infected with B. cinerea, 2461 were upregulated and 2839 were downregulated after infection (comparison of NBI vs. NBNI). In plants treated with BABA before infection, we identified a total of 5518 DEGs after infection (2483 upregulated and 3035 downregulated in BI vs. NBNI and 2237 upregulated and 2968 downregulated in BI vs. BNI). A small but significant subset of DEGs were identified for the direct BI vs. NBI comparison (9 downregulated and 3 upregulated) and for the BNI vs. NBNI comparison (7 downregulated and 4 upregulated) (Supplementary Table S1, S2).
qRT-PCR quantification of gene expression of selected DEGs between NBI and NBNI plants showed similar regulation as the RNA-seq data (Figure 2 and Table 1).
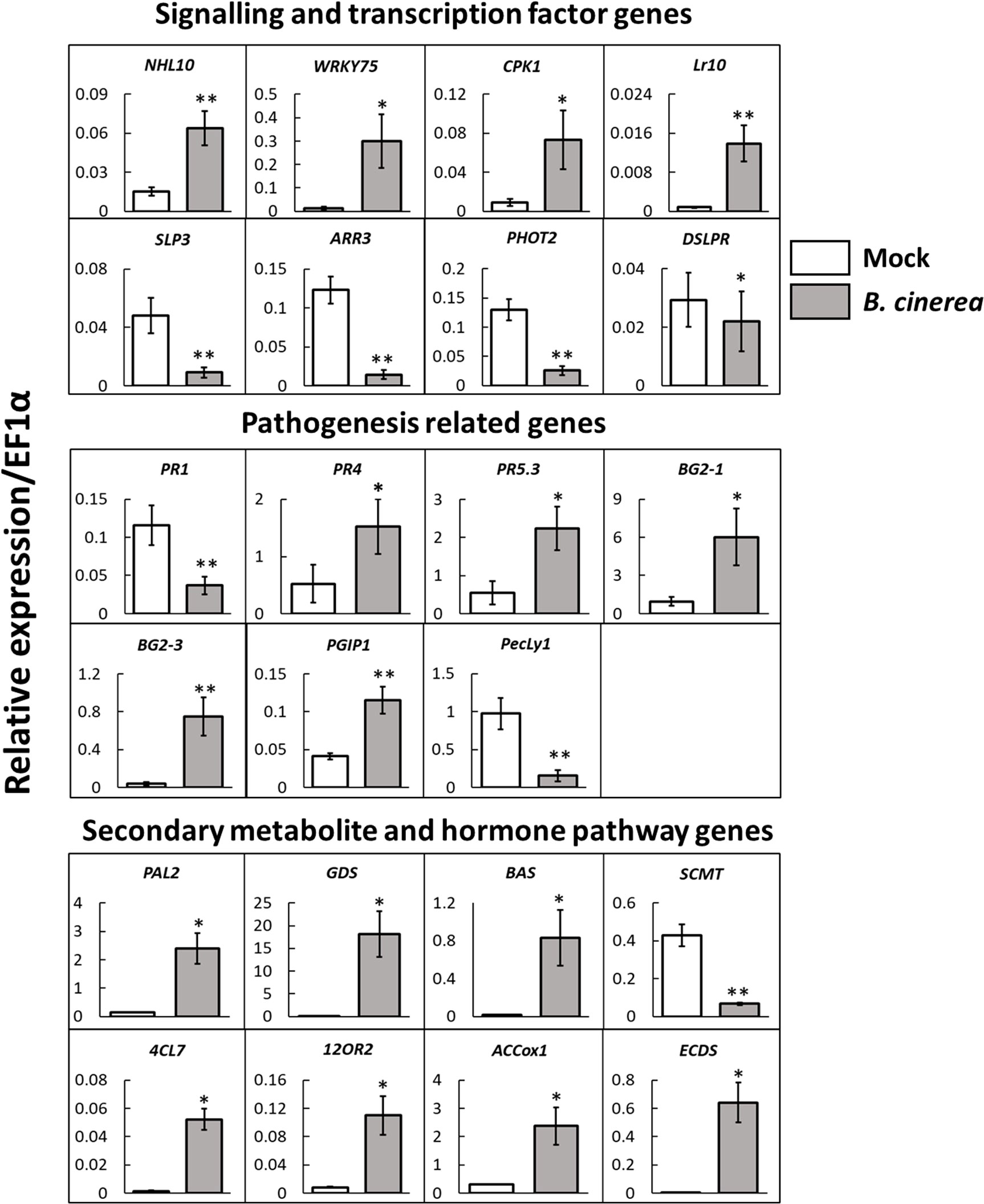
Figure 2 Validation of expression levels by qRT-PCR of selected genes in leaves of Fragaria vesca infected with Botrytis cinerea. White bars; mock-infected plants, grey bars; plants infected with B cinerea. The housekeeping gene EF1α was used as an endogenous control gene and expression values were calculated using the ΔCt method. n = 5 plants per treatment. Error bars show standard error ( ± 1 SE). Student’s t-test: *p < 0.05, **p < 0.01.
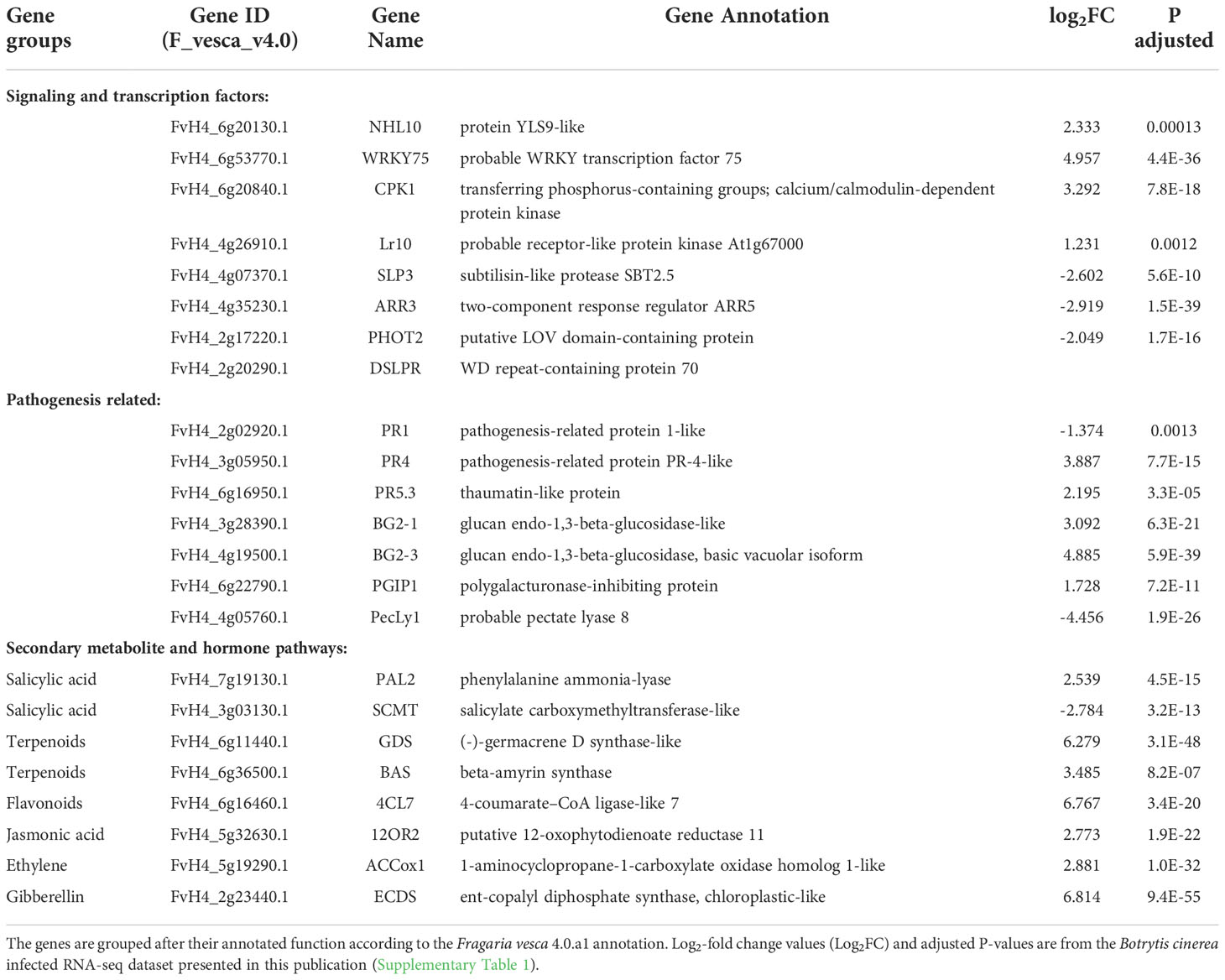
Table 1 Differentially expressed genes selected for qRT-PCR expression analysis from Fragaria vesca leaves infected with Botrytis cinerea.
Principal component analysis (PCA) was performed to visualize the variability between and within experimental treatments (Figure 3A). The first principal component separated infected (BI and NBI) and non-infected (BNI and NBNI) samples, irrespective of BABA-treatment. The fact that the two infected and non-infected treatments grouped together suggests that BABA had little global effect on gene expression. A similar pattern was shown in the cluster dendrogram (Figure 3B), where transcriptomes from infected and uninfected samples clustered together, while BABA-treated samples did not cluster.
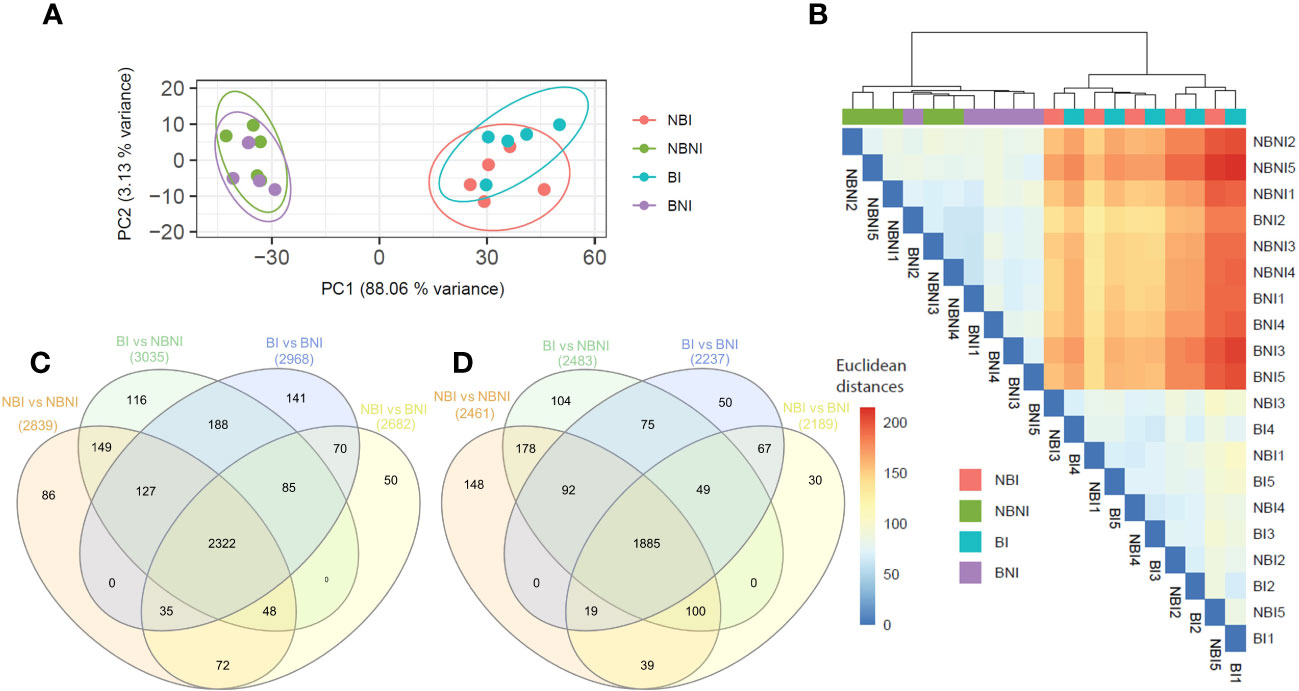
Figure 3 Co-expressed and unique Fragaria vesca transcripts after β-aminobutyric acid (BABA) treatment and Botrytis cinerea infection. (A) Principal component analysis (PCA) analyses of samples based on RNA-Seq data (confidence ellipses with 95% confidence interval). (B) Euclidean distances between samples. Analyses were performed using the R package pcaExplorer (version 2.22.0). (C, D) Venn diagrams of co-expressed and uniquely expressed genes (Log2 > 1) after pairwise comparison of different treatments: (C) downregulated and (D) upregulated differentially expressed genes. Treatment combinations: B. cinerea-infected (NBI; No-BABA and Infected), mock-infected (NBNI; No-BABA and Non-Infected), BABA-treated and B. cinerea-infected (BI; BABA and Infected), and BABA-treated and mock-infected (BNI; BABA and Non-Infected).
For a more detailed analysis at the single gene level, we made a Venn diagram to display DEGs from the pairwise treatment comparisons (Supplementary Table S2) that were significantly up- or downregulated after BABA treatment and infection (BI) relative to non-infected controls (BNI and/or NBNI). A total of 229 (104 + 75 + 50) genes were upregulated (Figure 3C), and 445 (116 + 188 + 141) were downregulated (Figure 3D and Supplementary Table S3).
GO enrichment and MAPMAN analysis of DEGs after Botrytis cinerea infection and BABA treatment
We identified the biological processes, cellular components, and molecular functions that were most strongly affected by B. cinerea infection in leaf tissue of plants that had not been treated with BABA. We did this by computing the enrichment of GO (Gene Ontology) terms of DEGs in infected and mock-infected plants (NBI vs. NBNI). Among upregulated genes (absolute log2-fold change values > 1) in the ‘biological process’ category we identified 124 significantly enriched GO categories (P-value < 0.05). The most enriched GO terms with the lowest P-values were ‘response to biological stimulus’, ‘ribosome biogenesis’ and ‘defense response’. (Supplementary Table 4 and Figure 4A). Among the most enriched GO terms for downregulated genes (Figure 4B) in the ‘biological process’ category were ‘photosynthesis’, ‘photosynthesis, light harvesting’ and ‘response to auxin’. For molecular function, the most enriched GO terms for upregulated DEGs included ‘oxidoreductase activity’ and ‘terpene synthase activity’, while ‘hydrolase’ and ‘glycogen (starch) synthase activity’ were significantly downregulated DEGs. For cellular components, ‘extracellular matrix’ and ‘ribosome’ were among the most enriched upregulated categories, while ‘thylakoid’, ‘extracellular region’ and ‘photosystem’ were among the most enriched downregulated DEGs.
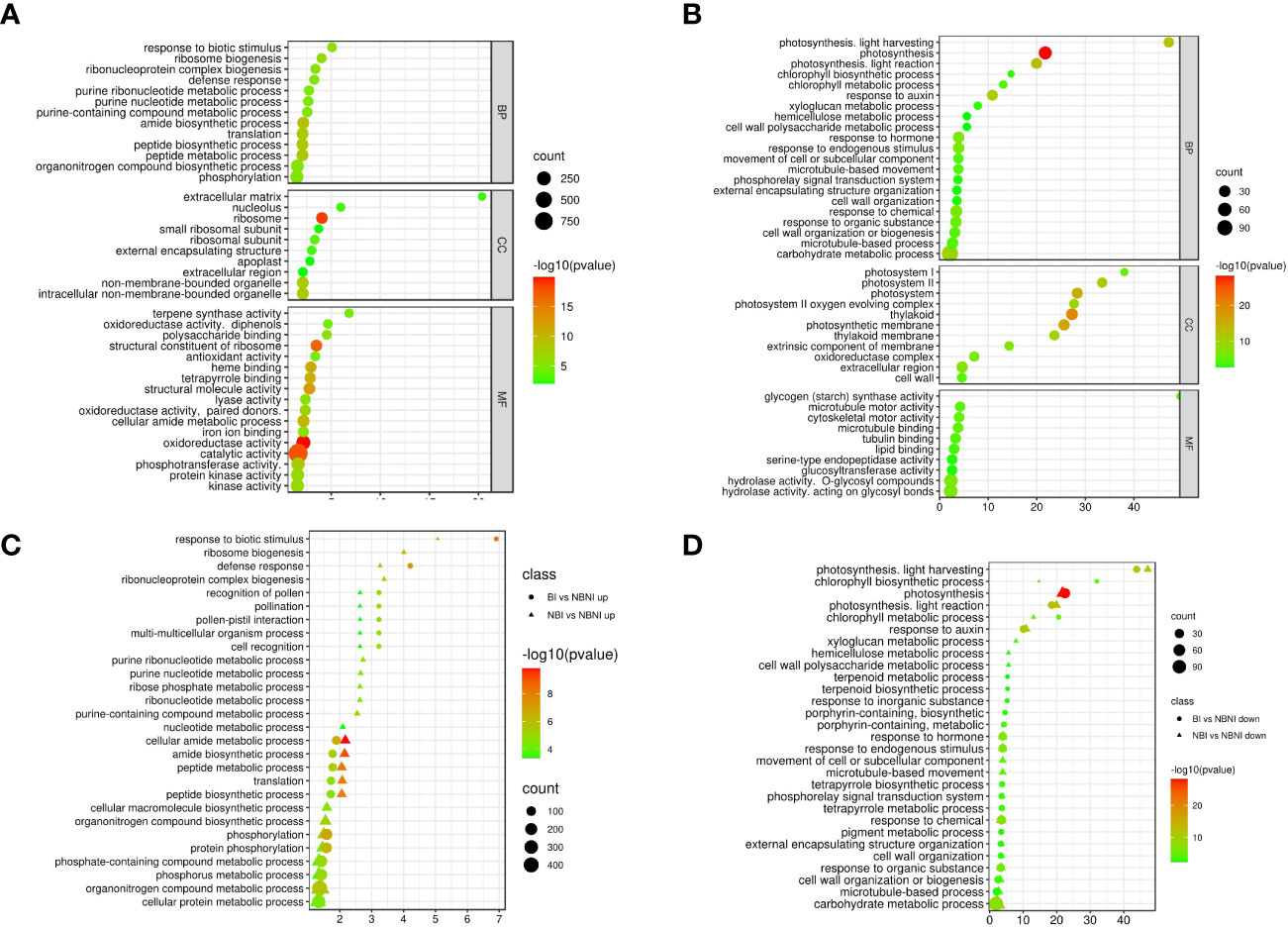
Figure 4 GO enrichment analysis for functional annotation of differentially expressed genes (DEGs). Pathway-enrichment bubble plots of upregulated (A) and downregulated (B) DEGs in Fragaria vesca Hawaii-4 leaves that were not treated with β-aminobutyric acid (BABA) and then infected with Botrytis cinerea. BP; biological process, MF; molecular function, CC; cellular compartment. Comparison of enriched GO-terms in the biological process category for NBI vs. NBNI (triangles) and BI vs. NBNI (circles) for upregulated (C) and downregulated (D) DEGs. Treatment combinations: B. cinerea-infected (NBI; No-BABA and Infected), mock-infected (NBNI; No-BABA and Non-Infected), BABA-treated and B. cinerea infected (BI; BABA and Infected), and BABA-treated and mock-infected (BNI; BABA and Non-Infected). X-axis shows log2-fold enrichment values. Plotted terms are selected based on log2 > 1 enrichment and P < 0.005. A comprehensive list of enriched terms can be found in Supplementary Table S4).
To identify any differences in GO-enrichment for BABA-treated plants compared to non-treated plants, we ranked enriched GO terms for biological process for the NBI vs. NBNI and BI vs. NBNI comparisons of up- and downregulated genes (Supplementary Table 5) according to log2-values (odds ratio) in the same enrichment bubble plot (Figures 4C, D). Most GO terms showed similar enrichment in infected plants (both BI and NBI) relative to non-infected plants not treated with BABA (NBNI). This was true for both up- and downregulated DEGs. However, a few terms showed different enrichment: for BI relative to NBI, terms like ‘defense response’, and ‘response to biologic stimulus’ were enriched in upregulated DEGs, while for NBI ‘purine ribonucleotide biosynthetic process’ and ‘ribosome biogenesis’ were enriched. For downregulated DEGs ‘chlorophyll biosynthetic and metabolic process’ was most enriched for BI, while ‘xyloglucan metabolic process’ was most enriched for NBI (Figures 4C, D). For the direct BI vs. NBI comparison, there was a significant enrichment of genes involved in photosynthesis, suggesting that BABA strenghtened the B. cinerea -mediated downregulation of this biological process.
We then did a Mapman analysis for a more detailed functional categorization of DEGs and visualization of associated metabolic pathways. This analysis confirmed the downregulation of photosynthesis-related genes (Supplementary Figure S7), carbohydrate metabolism, and cell wall-related genes in infected vs. uninfected plants not treated with BABA (NBI vs. NBNI), while genes involved in secondary metabolism, like phenolics and phenylpropanoid pathway, flavonoids and terpenoids, were upregulated (Figure 5A and Supplementary Figures S5, S6). Visualization of DEGs involved in the biotic stress pathway showed that many bins involved in defense signaling were differently regulated, and that genes involved in auxin signaling were downregulated while JA-signaling were upregulated (Figure 5C). Genes involved in defense, like R-proteins and PR-proteins and WRKY transcription factors, and secondary metabolites were generally upregulated, while genes involved in proteolysis and cell wall organization were downregulated. Like the GO enrichment analysis, the Mapman and KEGG analysis (Supplementary Figure S9) showed that the same pathways were induced in all infected plants, irrespective of BABA treatment, although individual bins within each pathway were differently expressed (Figures 5B, D and Supplementary Figures S5–S7).
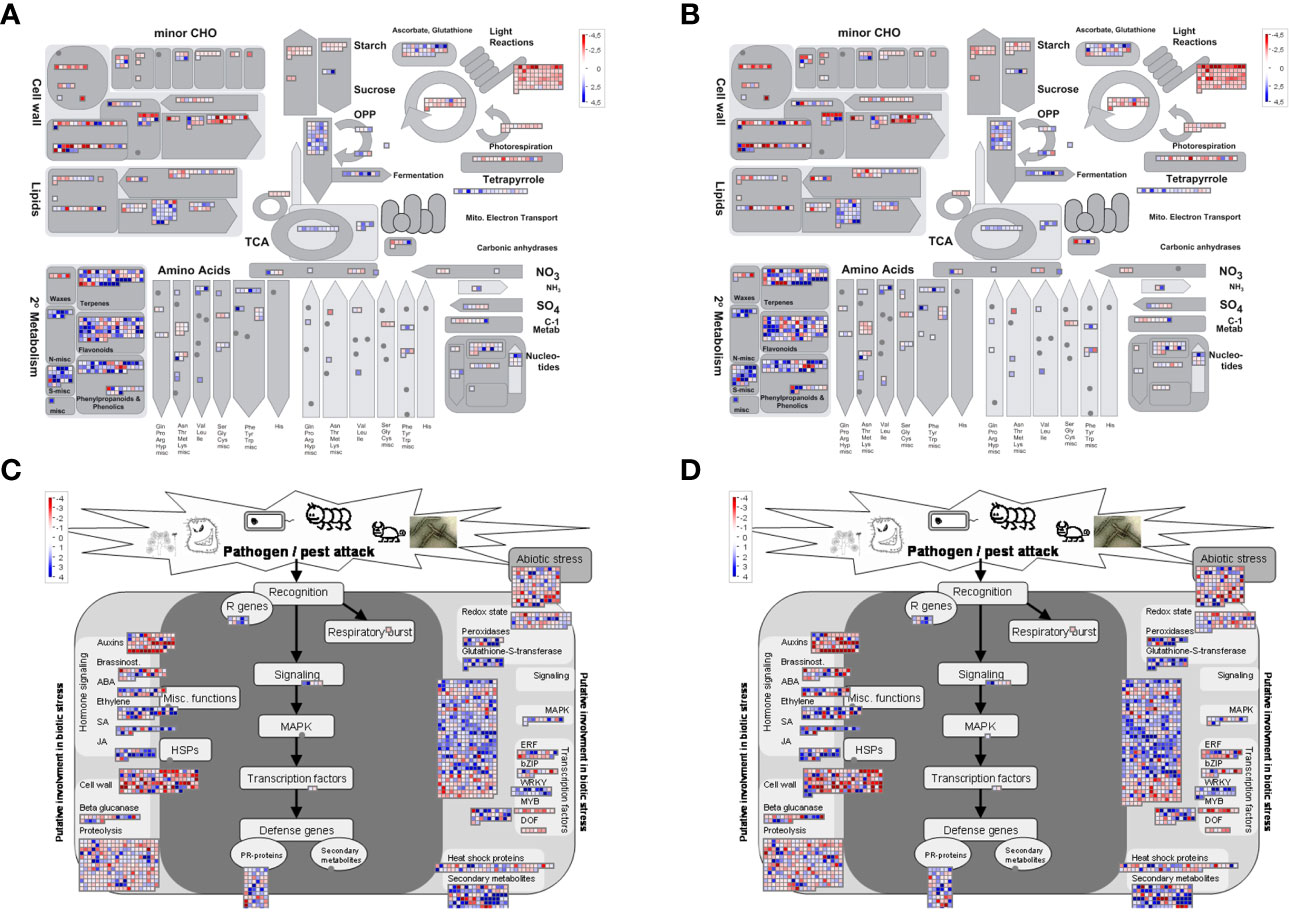
Figure 5 Visualization of enriched metabolic pathways in Fragaria vesca leaves after β-aminobutyric acid (BABA) treatment and Botrytis cinerea infection. Differentially expressed genes (DEGs) were displayed onto metabolic pathways using the MAPMAN software (A, B: central metabolism pathways; C, D: biotic stress pathways). (A, C) DEGs in Botrytis cinerea-infected vs. mock-infected plants [No-BABA and infected (NBI) vs. No-BABA and non-infected (NBNI)]. (B, D) DEGs in BABA-treated and Botrytis cinerea-infected plants vs. mock-infected plants (BABA and infected (BI) vs. NBNI). Blue cells: upregulation compared to NBNI; red cells: downregulation compared to NBNI.
Discussion
Susceptibility of F. vesca to B. cinerea infection depends on genotype, ontogenic stage, tissue type, fruit ripening stage, and leaf age. Analogous to white berries and open flowers (Haile et al., 2019), leaves have a relatively slow disease progression with symptoms appearing 4-5 days after B. cinerea infection. In this study we characterized systemic effects of the well-characterized defense elicitor BABA applied as soil drench, by analyzing B. cinerea infection and molecular response in leaves of F. vesca plants. Surprisingly, we found that BABA induced increased susceptibility to this pathogen.
BABA induces systemic susceptibility in strawberry
When applied as a soil drench BABA has previously been reported to induce systemic resistance against B. cinerea in distal organs like leaves and fruits in tomato (Luna et al., 2016; Wilkinson et al., 2018), and against bacteria in leaves of Arabidopsis and common bean (Po-Wen et al., 2013; Martínez-Aguilar et al., 2016). However, one previous report has shown that BABA seed treatment increased susceptibility to B. cinerea in leaves of tomato (Worrall et al., 2012). Still, it was a surprise to us that BABA soil drench induced systemic susceptibility in F. vesca leaves. As reported for several other crops, we observed that F. vesca plants soil drenched with BABA showed significantly reduced plant growth compared to control plants. When applied as a foliar spray BABA did not induce susceptibility but rather moderate (but non-significant) local resistance in F. vesca leaves, and plant growth was not affected. Because the BABA concentrations used in our foliar applications had no direct antifungal effect (Supplementary Figure S3), the reduced colonization by B. cinerea in these experiments was probably due to locally induced defense responses. Although most previous studies have shown a positive effect of BABA on plant resistance, the systemic susceptibility versus local resistance effect we observed is in line with some previous studies showing that the effect of BABA may depend on the application method, the developmental stage of the plant, and on plant genotype (Sharma et al., 2010; Alexandersson et al., 2016; Wilkinson et al., 2018; Yassin et al., 2021).
No large transcriptional differences between untreated and BABA-treated plants after infection
RNA-Seq showed that B. cinerea induced similar transcriptional reprogramming in leaves of untreated F. vesca plants (NBI vs. BNI) and BABA-treated plants (BI vs. NBNI). The GO enrichment analysis of all DEGs showed that all the major pathways were similarly enriched between BABA-treated and untreated plants (Figure 4) after infection, although a low number of genes involved in ‘response to biologic stimulus’ were significantly upregulated in BABA-treated plants, while genes involved in ‘cell wall polysaccharide metabolic process’, ‘hemicellulose metabolic process’ and ‘xyloglucan metabolic process’ were significantly downregulated in untreated plants. Genes involved in photosynthesis and primary carbohydrate metabolism were downregulated in F. vesca leaves upon B. cinerea infection. Similar responses to B. cinerea infection are seen in Arabidopsis, grape berries, lettuce, and cucumber (Windram et al., 2012; De Cremer et al., 2013; Agudelo- Romero et al., 2015; Kong et al., 2015). Thus, it appears that plants infected by B. cinerea shut down carbohydrate metabolism, possibly to reduce the availability of easily metabolized carbohydrates to the pathogen (Göhre et al., 2012). For downregulated genes, BABA-treated plants showed enrichment for GO-terms like ‘chlorophyll biosynthetic’ and ‘chlorophyll metabolic’ process compared to non-treated plants, and for the direct comparison of DEGs between BI and NBI the term ‘photosynthesis’ was highly enriched, suggesting photosynthesis is more downregulated in BABA-treated plants. Photosynthesis play an important role in resistance to disease and can be another explanation for the increased susceptibility we observed. For example, genes related to photosynthesis were maintained at a relatively stable level in leaves of a resistant rice cultivar compared to leaves of a susceptible cultivar in the early stage of Rhizoctonia solani infection (Zhang et al., 2017). Interestingly, a recent study concluded that in addition to leaf age and low hydrogen peroxide levels, high chlorophyll and carotenoids levels were the best indicators for leaf resistance to B. cinerea in strawberry leaves (Meng et al., 2019). The Mapman analysis confirmed the GO enrichment data, showing that major metabolic pathways were similarly induced following infection in both BABA-treated and untreated plants, although some bins within each pathway were differently expressed between treatments. BABA has been shown to induce expression of genes involved in phenylpropanoid and salicylic acid (SA) biosynthesis associated with reduced decay incidence when applied locally (Li et al., 2021). However, except for the reduced expression of genes involved in photosynthesis the induced susceptibility observed in our study does not seem to be explained by induction or repression of major metabolic pathways, but rather by differential expression of individual genes that might induce susceptibility. For example, our Venn-analysis identified 229 upregulated and 445 downregulated genes that were unique for BABA-treated and infected plants (BI) vs. mock-infected plants with or without BABA treatment (NBNI and BNI). Some of the downregulated genes encoded proteins involved in isoprenoid metabolic and catabolic processes that could partly explain the increased susceptibility compared to untreated plants, as isoprenoids are secondary metabolites with a role in defense.
Also, individual genes that might induce susceptibility were differently expressed: our BABA-treated plants showed induction of WRKY40-like transcription factors that are similar to the Arabidopsis WRKY40 gene which results in increased susceptibility to B. cinerea when overexpressed with WRKY18 and WRKY60 (Xu et al., 2006).
Major defense pathways are induced by Botrytis cinerea infection in leaves
In contrast to the unexpected systemic susceptibility induced by BABA soil drench, B. cinerea induced well-known transcriptional defense responses in leaves of both BABA-treated and untreated plants. These responses included changes in genes involved in pathogen recognition, cell-wall modification, defense signaling, biosynthesis and metabolism of jasmonic acid (JA), ethylene (ET) and auxin, and secondary metabolite pathways (Figures 4, 5 and Supplementary Table 4). The JA- and ET-pathway genes are known to be involved in positive regulation of defense against B. cinerea and were also induced in infected leaves in our study. In contrast, auxin-responsive genes were downregulated, as was also seen in resistant white berries (Haile et al., 2019).
Pattern recognition receptors (PRR) are receptor-like kinases (RLKs) that are localized in the plasma membrane and involved in the first line of defense against pathogens. We found that several of the RLK-subclasses previously reported to be involved in pathogen perception also were induced in F. vesca leaves upon B. cinerea infection. Wall-associated receptor kinases (WAKs) are involved in pathogen recognition, cell wall integrity, and defense against plant diseases (Kohorn, 2015; Bacete et al., 2017). For example, silencing of WAK4 in Rosa chinensis reduces pathogen resistance to B. cinerea (Liu et al., 2021). In F. vesca leaves, we found that 11 out of 40 wall-associated kinase/wall-associated kinase-like (WAK/WAKL) transcripts were upregulated upon B. cinerea infection, suggesting they have a role in defense. WAKs have also been shown to be upregulated by B. cinerea in berries (Haile et al., 2019), but these are not identical to the WAKs we detected in leaves, suggesting tissue-specific regulation of WAKs in F. vesca. Leucine-rich repeats (LRR)-RLKs have been shown to perceive DAMPs that are released after cell wall damage and are thus thought to be involved in cell wall-mediated immune responses. We found induction of 18 probable LRR receptor-like serine/threonine-protein kinases and 11 L-type lectin-domain-containing receptor kinases (LecRKs) in F. vesca leaves. Like in berries, several of the MAPK-kinases (20 out of 61) involved in intracellular immune signaling following pathogen infection were induced.
Transcription factors induced by Botrytis cinerea in leaves
Transcription factors (TFs) play an important role in defense against pathogens by regulating expression of genes involved in stress responses, such as cell wall modulation, pathogen perception and response, hormone pathways, and production of secondary metabolites. The most highly upregulated TF-classes in F. vesca leaves were the ethylene-responsive (ERFs), WRKY-class, bHLH, and MYB TFs (Figures 5C, D and Supplementary Table 2). In Arabidopsis, negative regulation of ABA signaling by WRKY33 and positive regulation of the jasmonate-mediated signaling pathway are critical for defense against B. cinerea (Liu et al., 2015; Chen et al., 2021). In our study, WRKY75 was significantly upregulated in leaves infected with B. cinerea and may thus play a similar role in F. vesca as in Arabidopsis. However, the WRKYs we identified in F. vesca leaves were not identical to those previously identified in white berries (Haile et al., 2019), again suggesting tissue-specific responses to infection in F. vesca.
Cell wall modification by Botrytis cinerea
Regulation of cell wall assembly and disassembly is important for resistance against B. cinerea in tomato and strawberry berries (Cantu et al., 2007; Haile et al., 2019). Many genes involved in cell wall metabolism were differently expressed in F. vesca leaves upon B. cinerea infection, suggesting that infection induces structural rearrangements of the cell wall. For example, five probable xyloglucan endotransglucosylases (XTHs), which are involved in cell wall modulation and repair, were induced in response to infection, while seven were downregulated. Several studies have demonstrated fungus-mediated transcriptional shut-down of these enzymes, probably to facilitate fungal colonization (Miedes et al., 2014), while in other studies, the GO term ‘xyloglucan metabolic process’ was enriched for genes induced by fungal infection of susceptible plants (Ksiażkiewicz et al., 2021). Interestingly, plants not treated with BABA were also more enriched for ‘xyloglucan metabolic process’ in downregulated genes than BABA-treated plants and this might partly explain the difference in susceptibility to B. cinerea between BABA-treated and untreated plants.
Microbial pathogens, including B. cinerea, secrete polygalacturonases (PGs) which depolymerize pectine polymers in the plant cell wall. In response, plants produce polygalacuronase-inhibiting proteins (PGIPs) that counteract the activity of polygalacturonases and confer resistance against B. cinerea infection in a number of species, including grape and tomato (Kalunke et al., 2015). In Fragaria chilesensis, upregulation of PGIP and the β-1,3-glucanase BG correlated with reduced incidence of postharvest grey mold after preharvest application of chitosan and MeJA (Saavedra et al., 2017). The only two PGIPs we detected in the leaf transcriptome were upregulated after infection, suggesting a similar response in F. vesca leaves. The level of methylesterification of pectins regulated by pectin methylesterases (PMEs) and pectin methylesterase-inhibiting proteins (PMEIs) affects susceptibility to pathogen attack (Lionetti et al., 2012; Bellincampi et al., 2014). PMEIs have been shown to reduce susceptibility to pathogens such as B. cinerea by inhibiting PMEs to increase pectin methylesterification (Lionetti et al., 2017). However, PMEs can also induce defense against pathogens by generating demethylesterified oligogalacturonides (OGAs) (Brutus et al., 2010). In F. vesca, ectopic expression of the F. ananassa pectin methylesterase PE1 partly demethylates OGAs that are perceived as DAMPs by WAKs and confer partial resistance against B. cinerea (Osorio et al., 2008; Osorio et al., 2011).
In our study both PMEs and PMEIs were highly differently regulated, suggesting strong regulation of pectin methylesterification upon B. cinerea infection in F. vesca leaves. Thus, transcripts controlling pectin methylesterification in F. vesca seem to be partly activated and partly deactivated following leaf infection by B. cinerea.
We found several expansins to be downregulated in F. vesca leaves after B. cinerea infection, although two expansin-like B1 transcripts were strongly induced. Most expansins are cell wall remodeling agents which enables cell expansion through non-enzymatically relaxation of the cell wall (S. AbuQamar, 2014; Marowa et al., 2016). The role of cell wall disassembly in ripening-associated susceptibility to B. cinerea infection was demonstrated in tomato, where suppression of the polygalacturonase LePG and the expansin LeExp1 in ripening fruits increased resistance to B. cinerea (Cantu et al., 2007).
Fragaria vesca leaves infected with B. cinerea also showed upregulation of most lignin-associated cinnamoyl-CoA reductase (CCR) or reductase like genes, which belong to the large group of oxidoreductases - one of the most enriched GO-terms in upregulated DEGs. Upregulation of these genes, that encode an enzyme in the monolignol pathway, suggests that cell-wall strengthening processes were activated in the leaf in response to infection. In a previous study, upregulation of CCR correlated with cell wall strengthening and production of the antimicrobial phytoalexin resveratrol and inhibition of B cinerea growth in unripe grapevine berries (Kelloniemi et al., 2015). The GO term ‘oxidoreductase’ includes ‘peroxidases’ which was also enriched upon B. cinerea infection in F. vesca leaves. Peroxidases fortify the cell wall by polymerizing monolignols into lignin.
PR proteins are important for leaf defense
Pathogenesis-related (PR) proteins are primarily induced by pathogens and can be divided into 17 families based on their primary structure, function, and biological properties. Several main classes of PR proteins cause damage to fungal cell walls (Sels et al., 2008; AbuQamar et al., 2016; Zeilinger et al., 2016). Our Mapman-analysis showed a strong enrichment for PR-proteins among the upregulated genes, including most major allergen Pru ar 1-like genes, a type of PR-10 protein involved in response to biotic stimulus. These genes are more highly expressed in resistant, white F. vesca berries than in the more susceptible red berries (Haile et al., 2019). Furthermore, we found three Fra a 1 allergen of the PR-10 class to be highly upregulated in F. vesca leaves. Knock-down of these genes in F. ananassa reduces expression of FvPAL and FvCHS as well as metabolic intermediates of the flavonoid pathway, suggesting that these genes activate the flavonoid biosynthesis pathway which is involved in defense (Muñoz et al., 2010).
The GO term oxidoreductase was enriched after B. cinerea infection in leaves. Germin-like proteins (GLPs) are classified as PR proteins in the PR-16 family. GLPs can be induced by fungal pathogens and have oxidoreductase activity resulting in H2O2 production, induction of JA-mediated signaling, and activation of defense against pathogens (Pei et al., 2019). In contrast to what has previously been demonstrated in white berries (Haile et al., 2019), expression of five out of 14 GLPs were strongly induced in infected F. vesca leaves, suggesting that leaves have a stronger H2O2 defense response upon infection than berries.
Genes involved in secondary metabolite production are induced by Botrytis cinerea in leaves
As in white berries, important steps in the phenylpropanoid pathways were upregulated in F. vesca leaves upon infection (Supplementary Figure S5). Plants activate the phenylpropanoid pathway when pathogens breach the cell wall, leading to the synthesis of precursors for many secondary metabolites important for defense. This includes signaling molecules, antimicrobial phenolic compounds like flavonoids and ellagitannins, and monolignol precursors for lignin biosynthesis (Yadav et al., 2020).
Phenolic compounds play a major role in resistance in immature berries of strawberry, and the levels of flavonoids like proanthocyanidins and flavan-3-ols diminish as the berries ripen, increasing their susceptibility to B. cinerea (Mikulic-Petkovsek et al., 2013; Nagpala et al., 2016). Two shikimate dehydrogenase genes were also induced by B. cinerea infection in F. vesca leaves in our study. These genes are involved in the production of ellagitannin, which is enriched in unmature berries and leaves after pathogen infection (Mamaní et al., 2012). In addition, genes involved in the biosynthesis of defense metabolites such as terpenoids were upregulated, reflected by the enrichment of the GO-term ‘terpene synthase’ in upregulated genes of non-BABA treated plants, suggesting that several defense systems were activated in F. vesca leaves upon B. cinerea infection. Terpenes are a diverse group of compounds including molecules with antimicrobial activity. The importance of terpene synthases in defense was recently demonstrated in berries of F. ananassa, where overexpression of the terpenoid synthase FaTPS1 increased production of the sesquiterpene germacrene D, and improved resistance to B. cinera in strawberry fruits (Zhang et al., 2022).
Conclusion
Fragaria vesca leaves showed similar transcriptional reprogramming following B. cinerea infection as previously seen in flowers and unmature berries of F. vesca, with induction of major metabolic pathways important for defense. However, we also found leaf-specific responses to B. cinerea infection that might reflect the higher resistance of leaves compared to mature berries. Furthermore, we show that, similar to berries in strawberry and grapes, BABA increased disease resistance when applied locally to leaves. However, in contrast to the Arabidopsis-B. cinerea pathosystem, BABA reduced plant growth and induced susceptibility to B. cinerea infection when applied systemically as a soil drench. This effect was associated with reduced expression of genes involved in photosynthesis compared to non-treated plants, suggesting that BABA enhanced the B. cinerea induced downregulation of this biological process. Thus, our study adds to the evidence that the effect of defense elicitors such as BABA on plant resistance varies among tissue types, development stages, application methods, and genotypes.
Experimental procedures
Plant growth
Fragaria vesca ‘Hawaii-4’ and F. vesca ‘Alexandria’ accessions were cultivated in a growth room with 14 h light (~100 μmol m-2 s-1 photosynthetically active radiation (PAR) at 24 °C) and 10 h darkness (19°C) at 40-45% relative humidity. We also used Fragaria × ananassa in one experiment to test if BABA soil drench affected resistance in commercial strawberry. Plants were grown in topsoil in 400 mL pots and were not subjected to any kind of chemical treatments (pesticides, fungicides, or fertilizers) that could interact with the plants’ phenotypic responses to BABA. For the infection experiments we used 8- to 12-week-old plants grown from seeds to ensure that the plants in each experimental batch were in the same developmental stage. Plants were about 10-12 cm tall (measured from the soil surface) at the start of the experiments, bearing 6-10 trifoliate leaves.
Treatment with BABA
β-aminobutyric acid (BABA, Sigma-Aldrich, #A44207-5G) was dissolved in distilled H2O. For soil-drench treatments, 50 ml of BABA solution was added to each pot to a final concentration of 0 mM (control), 0.05 mM, 0.15 mM, 0.2 mM, 0.3 mM or 0.6 mM. Each pot contained one plant and received a single soil-drench treatment. For above-ground treatment, 0.3 mM BABA solution was sprayed onto leaves until run-off. BABA treatment was always performed two days before infection with B. cinerea.
Botrytis cinerea inoculum preparation and infection
Botrytis cinerea isolate Bc101 was grown on potato dextrose agar plates in darkness. Spores on 4- to 5-week-old plates were harvested by pouring liquid potato dextrose broth (PDB) media onto the plates and collecting the liquid with a pipette. The spore suspension was passed through a sterile 70 µM nylon mesh and centrifuged at 3000g for 1 min, before the spore pellet was re-suspended in fresh PDB media. Spores were counted using a hemocytometer and the suspension was adjusted to a concentration of 106 spores mL-1. The spore suspension was supplemented with 0.02% Tween-20 and sprayed on plants using a hand sprayer until run-off. PDB media supplemented with 0.02% Tween-20 was used for mock infections. Sprayed plants were placed in a plastic tray, covered with transparent polypropylene bags to maintain high humidity that promotes infection, and incubated in a plant growth room (14 h light and 10 h darkness at room temperature, ~100 μmol m-2 s-1 PAR) until symptoms appeared. Necrotic lesions on leaves usually began to appear 4-5 days after spore spraying. Student’s t-tests were used for statistical comparison of different treatment combinations for all phenotypic data.
Isolation of RNA and DNA
About 100 mg of leaf tissue harvested 5 days post infection was ground in liquid N2 and used for DNA extraction using the DNeasy Plant Kit (#69106) in a QIAcube automated sample prep system according to the manufacturer’s instructions. Total RNA was isolated from 100 mg leaf tissue harvested 24 hours post infection using Spectrum™ Plant Total RNA Kit with minor modifications (Badmi et al., 2018). Briefly, 100 mg of tissue powder was mixed with preheated lysis buffer containing CTAB (2%), PVPP (2%), Tris-Cl (pH 8.0, 100 mM), EDTA (pH 8.0, 25 mM), NaCl (1 M) and β-mercaptoethanol (1%). The mixture was incubated at 65 °C for 8 min, with vortexing for the first 60 seconds. The lysate was then centrifuged for 10 min at 13,000 rpm, the supernatant was mixed with an equal volume of chloroform:isoamyl alcohol (24:1) and centrifuged again for 10 min at 4 °C. The supernatant was transferred to the kit’s filtration column (blue retainer ring), and from this step we followed the manufacturer’s instructions. On-column DNase I treatment was done to ensure DNA-free total RNA. Total RNA (500 ng) was used to prepare cDNA using iScript cDNA Synthesis Kit (#170-8891). SsoAdvanced Universal SYBR Green Supermix (#1725271) was mixed with genomic DNA or cDNA to perform RT-qPCR on the Applied Biosystems ViiA 7 system and determine the Ct values for each primer pair.
RNA-seq analysis
Fragaria vesca Hawaii-4 plants were either drenched with 50 ml water or soil-drenched with 50 ml 0.2 mM BABA. After 48 hours, five plants of each treatment (water or BABA) were either mock-infected or infected with B. cinerea spores as described above, and leaves were harvested for RNA isolation 24 h later. Each plant served as a single biological replicate (n = 5). Isolated total RNA (0.3 µg) was used to prepare samples using TruSeq Stranded mRNA (Illumina, San Diego, CA, USA) according to the manufacturer’s instructions. Library preparation and cDNA sequencing was performed at the Norwegian Sequencing Centre (Oslo, Norway) on the Illumina NextSeq 500 system with 75 bp single end reads. Demultiplexed reads were trimmed and filtered using trimmomatic (version 0.38) with recommended settings (Bolger et al., 2014). Trimmed and filtered reads were aligned to the chromosome-continuity version 4.0.a1 of the F. vesca transcriptome (rosaceae.org) using hisat2 (version 2.1.0) (Putri et al., 2022). Gene count data were extracted using the htseq-count function from the HTSeq package (version 0.11.2) (Anders et al., 2015). Differentially expressed genes were identified using the R package EdgeR (version 3.32.1) and filtered using false discovery rate (alpha) < 0.05 and absolute log2-fold change values > 1 (Varet et al., 2016). Normalization was performed using the ‘trimmed mean of M values’ (TMM) method and p-values were adjusted using the Benjamini-Hochberg method (Benjamini, 2014). Differential expressed genes are visualized as volcano plot (adjusted p-values versus log2 fold-change based on edgeR data) (Supplementary Figure S8).
QPCR DNA-based Botrytis cinerea quantification
Quantification of B. cinerea was performed using genomic DNA isolated from infected F. vesca leaves 5 days after infection, when symptoms started to appear. Ct values from B. cinerea-specific genomic DNA primers (Bc3F and Bc3R, Supplementary Table S6) were normalized against F. vesca-specific EF1α primers to obtain the relative B. cinerea levels in the tissue. For RNA-seq validation using qPCR, EF1α was used as the housekeeping control gene and relative expression levels of genes were determined using the ΔCt method (Pfaffl, 2001) using primers in Supplementary Table S6.
Functional categorization of DEGs using GO and Mapman enrichment analysis
Differential expressed genes with False discovery rate (FDR) FDR < 0.05 were analyzed for enriched GO-terms based on Fragaria vesca Whole Genome v4.0.a1 Assembly & Annotation (www.rosaceae.org) and Blast2GO. GO pathway enrichment plot was plotted with SRplot (www.bioinformatics.com.cn/en). The Mercator4 online tool (Schwacke et al., 2019) was used to functionally annotate and classify all the F. vesca transcripts into hierarchically structured bins, and combined with DEG analysis and displayed onto metabolic pathways with the MAPMAN software (Thimm et al., 2004). DEGs with FDR < 0.05 were plotted in Venn diagrams using InteractiVenn (Heberle et al., 2015).
Data availability statement
The datasets presented in this study can be found in online repositories. The names of the repository/repositories and accession number(s) can be found below: https://www.ncbi.nlm.nih.gov/, PRJNA818508.
Author contributions
RB, MB, and TTh designed the experiments. CF contributed with design of some experiments. RB cultivated the plants and performed the experiments. LH did infection assays on F. ananassa and helped with experiments. AE isolated DNA from leaf tissues and cultivated plants, YZ helped with performing the experiments, TTe did most of the bioinformatical work and TTe, TTh, and RB analyzed the RNA-seq data. TH provided plants and greenhouse space. RB wrote the first draft of the manuscript. PK critically reviewed and contributed to the writing of the manuscript. TTh wrote the final manuscript. All authors contributed to the article and approved the submitted version.
Funding
This work was funded by a Toppforsk grant (249958/F20) from the Research Council of Norway.
Acknowledgments
Botrytis cinerea isolate Bc101 (Gordon et al., 2011) was provided by Gunn Mari Strømeng. We thank Birgit Schaller, Monica Skogen, Magne Nordang Skårn, Arti Rai, and Andrew Dobson for technical help with experiments.
Conflict of interest
The authors declare that the research was conducted in the absence of any commercial or financial relationships that could be construed as a potential conflict of interest.
Publisher’s note
All claims expressed in this article are solely those of the authors and do not necessarily represent those of their affiliated organizations, or those of the publisher, the editors and the reviewers. Any product that may be evaluated in this article, or claim that may be made by its manufacturer, is not guaranteed or endorsed by the publisher.
Supplementary material
The Supplementary Material for this article can be found online at: https://www.frontiersin.org/articles/10.3389/fpls.2022.1025422/full#supplementary-material
References
AbuQamar, S. (2014). Expansins: Cell wall remodeling proteins with a potential function in plant defense. J. Plant Biochem. Physiol. 02 (01), 1–2. doi: 10.4172/2329-9029.1000e118
AbuQamar, S. F., Moustafa, K., Tran, L.-S. P. (2016). ‘Omics’ and plant responses to Botrytis cinerea. Front. Plant Sci. 7 (November). doi: 10.3389/fpls.2016.01658
AbuQamar, S., Moustafa, K., Tran, L.-S. P. (2017). Mechanisms and strategies of plant defense against. Botrytis cinerea Crit. Rev. Biotechnol. 37 (2), 262–274. doi: 10.1080/07388551.2016.1271767
Agudelo-Romero, P., Erban, A., Rego, C., Carbonell-Bejerano, P., Nascimento, T., Sousa, L., et al. (2015). Transcriptome and metabolome reprogramming in Vitis vinifera cv. trincadeira berries upon infection with botrytis cinerea. J. Exp. Bot. 66 (7), 1769–1785. doi: 10.1093/jxb/eru517
Alexandersson, E., Mulugeta, T., Lankinen, Å., Liljeroth, E., Andreasson, E. (2016). Plant resistance inducers against pathogens in Solanaceae species-from molecular mechanisms to field application. Int. J. Mol. Sci. 17 (10), 1–25. doi: 10.3390/ijms17101673
Amil-Ruiz, F., Blanco-Portales, R., Muñoz-Blanco, J., Caballero, J. L. (2011). The strawberry plant defense mechanism: A molecular review. Plant Cell Physiol. 52 (11), 1873–1903. doi: 10.1093/pcp/pcr136
Anders, S., Pyl, P. T., Huber, W. (2015). Genome analysis HTSeq — a Python framework to work with high-throughput sequencing data. Bioinformatics 31 (2), 166–169. doi: 10.1093/bioinformatics/btu638
Bacete, L., Mélida, H., Miedes, E., Molina, A. (2017). Plant cell wall-mediated immunity: Cell wall changes trigger disease resistance responses. Plant J. 12 (10), 3218–3221. doi: 10.1111/tpj.13807
Badmi, R., Payyavula, R. S., Bali, G., Guo, H. B., Jawdy, S. S., Gunter, L. E., et al. (2018). A new calmodulin-binding protein expresses in the context of secondary cell wall biosynthesis and impacts biomass properties in Populus. Front. Plant Sci. 871 (December). doi: 10.3389/fpls.2018.01669
Bektas, Y., Eulgem, T. (2015). Synthetic plant defense elicitors. Front. Plant Sci. 5,1–17. doi: 10.3389/fpls.2014.00804
Bellincampi, D., Cervone, F., Lionetti, V. (2014). Plant cell wall dynamics and wall-related susceptibility in plant-pathogen interactions. Front. Plant Sci. 5 (MAY). doi: 10.3389/fpls.2014.00228
Benjamini, Y. (2014). Controlling the false discovery rate - a practical and powerful approach to multiple testing. Journal of the Royal Statistical Society. Series B (Methodological). 57 (1), 289–300. doi: 10.2307/2346101
Bolger, A. M., Lohse, M., Usadel, B. (2014). Genome analysis Trimmomatic : A flexible trimmer for Illumina sequence data 30 (15), 2114–2120. doi: 10.1093/bioinformatics/btu170
Brutus, A., Sicilia, F., Macone, A., Cervone, F., De Lorenzo, G. (2010). A domain swap approach reveals a role of the plant wall-associated kinase 1 (WAK1) as a receptor of oligogalacturonides. Proc. Natl. Acad. Sci. United States America 107 (20), 9452–9457. doi: 10.1073/pnas.1000675107
Cantu, D., Vicente, A. R., Greve, L. C., Dewey, F. M., Bennett, A. B., Labavitch, J. M., et al. (2007). The intersection between cell wall disassembly, ripening, and fruit susceptibility to Botrytis cinerea. PNAS. 105 (3), 859–864. doi: 10.1073/pnas.0709813105
Chen, L., Zhang, L., Xiang, S., Chen, Y., Zhang, H., Yu, D. (2021). The transcription factor WRKY75 positively regulates jasmonate-mediated plant defense to necrotrophic fungal pathogens. J. Exp. Bot. 72 (4), 1473–1489. doi: 10.1093/jxb/eraa529
Cohen, Y. R. (2002). β-aminobutyric acid-induced resistance against plant pathogens. Plant Dis. 86 (5), 448–457. doi: 10.1094/PDIS.2002.86.5.448
Cohen, Y., Vaknin, M., Mauch-Mani, B. (2016). BABA-induced resistance: milestones along a 55-year journey. Phytoparasitica 44 (4), 513–538. doi: 10.1007/s12600-016-0546-x
Conrath, U., Beckers, G. J. M., Langenbach, C. J. G., Jaskiewicz, M. R. (2015). Priming for enhanced defense. Annu. Rev. Phytopathol. 53 (1), 150612172936006. doi: 10.1146/annurev-phyto-080614-120132
De Cremer, K., Mathys, J., Vos, C., Froenicke, L., Michelmore, R. W., Cammue, B. P. A., et al. (2013). RNAseq-based transcriptome analysis of Lactuca sativa infected by the fungal necrotroph Botrytis cinerea. Plant Cell Environ. 36 (11), 1992–2007. doi: 10.1111/pce.12106
Fillinger, S., Elad, Y. B. (2016). Botrytis - The Fungus, the Pathogen and its Management in Agricultural Systems. Switzerland: Springer Inter- national Publishing.
Garrido, C., Carbú, M., Fernández-Acero, F. J., González-Rodríguez, V. E., Cantoral, J. M. (2011). “New insights in the study of strawberry fungal pathogens,” in Genes, Genomes and Genomics ,24–39. Global Science Books. Available at: http://www.globalsciencebooks.info/Online/GSBOnline/OnlineGGG_5_SI1.html.
Göhre, V., Jones, A. M. E., Sklenář, J., Robatzek, S., Weber, A. P. M. (2012). Molecular crosstalk between PAMP-triggered immunity and photosynthesis. Mol. Plant-Microbe Interact. 25 (8), 1083–1092. doi: 10.1094/MPMI-11-11-0301
Gordon, L., Gunn, H., Strømeng, M., Tronsmo, A., Sønsteby, A., Stensvand, A. (2011). Attempts to reduce strawberry grey mould (Botrytis cinerea) in Norway using fungal antagonists. Eur. J. Plant Sci. Biotechnol. 5 (Special(Engstedt 2007), 78–85.
Haile, Z. M., Nagpala-De Guzman, E. G., Moretto, M., Sonego, P., Engelen, K., Zoli, L., et al. (2019). Transcriptome profiles of strawberry (Fragaria vesca) fruit interacting with Botrytis cinerea at different ripening stages. Front. Plant Sci. 10 (September). doi: 10.3389/fpls.2019.01131
Heberle, H., Meirelles, V. G., da Silva, F. R., Telles, G. P., Minghim, R. (2015). InteractiVenn: A web-based tool for the analysis of sets through Venn diagrams. BMC Bioinf. 16 (1), 1–7. doi: 10.1186/s12859-015-0611-3
Kalunke, R. M., Tundo, S., Benedetti, M., Cervone, F., De Lorenzo, G., D’Ovidio, R. (2015). An update on polygalacturonase-inhibiting protein (PGIP), a leucine-rich repeat protein that protects crop plants against pathogens. Front. Plant Sci. 6 (March). doi: 10.3389/fpls.2015.00146
Kelloniemi, J., Trouvelot, S., Héloir, M.-C., Simon, A., Dalmais, B., Frettinger, P., et al. (2015). Analysis of the Molecular Dialogue Between Gray Mold (Botrytis cinerea) and Grapevine (Vitis vinifera) Reveals a Clear Shift in Defense Mechanisms During Berry Ripening. Molecular Plant-Microbe Interactions 28 (ja), MPMI-02-15-0039. doi: 10.1094/MPMI-02-15-0039-R
Kohorn, B. D. (2015). The state of cell wall pectin monitored by wall associated kinases: A model. Plant Signaling Behav. 10 (7), e1035854-1–e1035854-3. doi: 10.1080/15592324.2015.1035854
Kong, W., Chen, N., Liu, T., Zhu, J., Wang, J., He, X., et al. (2015). Large-Scale transcriptome analysis of cucumber and Botrytis cinerea during infection. PloS One 10 (11), 1–16. doi: 10.1371/journal.pone.0142221
Ksiażkiewicz, M., Rychel-Bielska, S., Plewiński, P., Nuc, M., Irzykowski, W., Jędryczka, M., et al. (2021). The resistance of narrow-leafed lupin to Diaporthe toxica is based on the rapid activation of defense response genes. Int. J. Mol. Sci. 22 (2), 1–30. doi: 10.3390/ijms22020574
Lionetti, V., Cervone, F., Bellincampi, D. (2012). Methyl esterification of pectin plays a role during plant-pathogen interactions and affects plant resistance to diseases. J. Plant Physiol. 169 (16), 1623–1630. doi: 10.1016/j.jplph.2012.05.006
Lionetti, V., Fabri, E., De Caroli, M., Hansen, A. R., Willats, W. G. T., Piro, G., et al. (2017). Three pectin methylesterase inhibitors protect cell wall integrity for Arabidopsis immunity to Botrytis. Plant Physiol. 173 (3), 1844–1863. doi: 10.1104/pp.16.01185
Liu, S., Kracher, B., Ziegler, J., Birkenbihl, R. P., Somssich, I. E. (2015). Negative regulation of ABA signaling by WRKY33 is critical for Arabidopsis immunity towards Botrytis cinerea 2100. ELife 4, 1–27. doi: 10.7554/eLife.07295
Liu, X., Wang, Z., Tian, Y., Zhang, S., Li, D., Dong, W., et al. (2021). Characterization of wall-associated kinase/wall-associated kinase-like (WAK/WAKL) family in rose (Rosa chinensis) reveals the role of RcWAK4 in Botrytis resistance. BMC Plant Biol. 21 (1), 1–12. doi: 10.1186/s12870-021-03307-9
Li, C., Wang, K., Lei, C., Cao, S., Huang, Y., Ji, N., et al. (2021). Alterations in sucrose and phenylpropanoid metabolism affected by BABA-primed defense in postharvest grapes and the associated transcriptional mechanism. Mol. Plant-Microbe Interact. 34 (11), 1250–1266. doi: 10.1094/MPMI-06-21-0142-R
Luna, E., Beardon, E. G., Ravnskov, S., Scholes, J. D., Ton, J. (2016). Optimizing chemically induced resistance in tomato against Botrytis cinerea. Plant Dis. 100 (4), 704–710. doi: 10.1094/PDIS-03-15-0347-RE
Mamaní, A., Filippone, M. P., Grellet, C., Welin, B., Castagnaro, A. P., Ricci, J. C. D. (2012). Pathogen-induced accumulation of an ellagitannin elicits plant defense response. Mol. Plant-Microbe Interact. 25 (11), 1430–1439. doi: 10.1094/MPMI-12-11-0306
Marowa, P., Ding, A., Kong, Y. (2016). Expansins: roles in plant growth and potential applications in crop improvement. Plant Cell Rep. 35 (5), 949–965. doi: 10.1007/s00299-016-1948-4
Martínez-Aguilar, K., Ramírez-Carrasco, G., Hernández-Chávez, J. L., Barraza, A., Alvarez-Venegas, R. (2016). Use of BABA and INA as activators of a primed state in the common bean (Phaseolus vulgaris L.). Front. Plant Sci. 7 (May). doi: 10.3389/fpls.2016.00653
Meng, L., Höfte, M., Van Labeke, M. C. (2019). Leaf age and light quality influence the basal resistance against Botrytis cinerea in strawberry leaves. Environ. Exp. Bot. 157 (June 2018), 35–45. doi: 10.1016/j.envexpbot.2018.09.025
Miedes, E., Vanholme, R., Boerjan, W., Molina, A. (2014). The role of the secondary cell wall in plant resistance to pathogens. Front. Plant Sci. 5 (AUG). doi: 10.3389/fpls.2014.00358
Mikulic-Petkovsek, M., Schmitzer, V., Slatnar, A., Weber, N., Veberic, R., Stampar, F., et al. (2013). Alteration of the content of primary and secondary metabolites in strawberry fruit by Colletotrichum nymphaeae infection. J. Agric. Food Chem. 61 (25), 5987–5995. doi: 10.1021/jf402105g
Muñoz, C., Hoffmann, T., Escobar, N. M., Ludemann, F., Botella, M. A., Valpuesta, V., et al. (2010). The strawberry fruit fra a allergen functions in flavonoid biosynthesis. Mol. Plant 3 (1), 113–124. doi: 10.1093/mp/ssp087
Nagpala, E. G., Guidarelli, M., Gasperotti, M., Masuero, D., Bertolini, P., Vrhovsek, U., et al. (2016). Polyphenols variation in fruits of the susceptible strawberry cultivar alba during ripening and upon fungal pathogen interaction and possible involvement in unripe fruit tolerance. J. Agric. Food Chem. 64 (9), 1869–1878. doi: 10.1021/acs.jafc.5b06005
Osorio, S., Bombarely, A., Giavalisco, P., Usadel, B., Stephens, C., Aragüez, I., et al. (2011). Demethylation of oligogalacturonides by FaPE1 in the fruits of the wild strawberry Fragaria vesca triggers metabolic and transcriptional changes associated with defence and development of the fruit. J. Exp. Bot. 62 (8), 2855–2873. doi: 10.1093/jxb/erq465
Osorio, S., Castillejo, C., Quesada, M. A., Medina-Escobar, N., Brownsey, G. J., Suau, R., et al. (2008). Partial demethylation of oligogalacturonides by pectin methyl esterase 1 is required for eliciting defence responses in wild strawberry (Fragaria vesca). Plant J. 54 (1), 43–55. doi: 10.1111/j.1365-313X.2007.03398.x
Pei, Y., Li, X., Zhu, Y., Ge, X., Sun, Y., Liu, N., et al. (2019). Ghabp19, a novel germin-like protein from Gossypium hirsutum, plays an important role in the regulation of resistance to verticillium and fusarium wilt pathogens. Front. Plant Sci. 10 (May). doi: 10.3389/fpls.2019.00583
Petrasch, S., Knapp, S. J., van Kan, J. A. L., Blanco-Ulate, B. (2019). Grey mould of strawberry, a devastating disease caused by the ubiquitous necrotrophic fungal pathogen Botrytis cinerea. Mol. Plant Pathol. 20 (6), 877–892. doi: 10.1111/mpp.12794
Pfaffl, M. W. (2001). A new mathematical model for relative quantification in real-time RT–PCR. Nucleic Acids Res. 29 (9), 2003–2007. doi: 10.1111/j.1365-2966.2012.21196.x
Piasecka, A., Jedrzejczak-Rey, N., Bednarek, P. (2015). Secondary metabolites in plant innate immunity: Conserved function of divergent chemicals. New Phytol. 206 (3), 948–964. doi: 10.1111/nph.13325
Po-Wen, C., Singh, P., Zimmerli, L. (2013). Priming of the Arabidopsis pattern-triggered immunity response upon infection by necrotrophic Pectobacterium carotovorum bacteria. Mol. Plant Pathol. 14 (1), 58–70. doi: 10.1111/j.1364-3703.2012.00827.x
Putri, G. H., Anders, S., Pyl, P. T., Pimanda, J. E. (2022). Analysing high-throughput sequencing data in Python with HTSeq 2.0. Bioinformatics 38 (10), 2943–2945, doi: 10.1093/bioinformatics/btac166
Reimer-Michalski, E. M., Conrath, U. (2016). Innate immune memory in plants. Semin. Immunol. 28 (4), 319–327. doi: 10.1016/j.smim.2016.05.006
Saavedra, G., Sanfuentes, E., Figueroa, P., Figueroa, C. (2017). Independent preharvest applications of methyl jasmonate and chitosan elicit differential upregulation of defense-related genes with reduced incidence of gray mold decay during postharvest storage of Fragaria chiloensis fruit. Int. J. Mol. Sci. 18 (7), 1420. doi: 10.3390/IJMS18071420
Schwacke, R., Ponce-Soto, G. Y., Krause, K., Bolger, A. M., Arsova, B., Hallab, A., et al. (2019). MapMan4: A refined protein classification and annotation framework applicable to multi-omics data analysis. Mol. Plant 12 (6), 879–892. doi: 10.1016/j.molp.2019.01.003
Sels, J., Mathys, J., De Coninck, B. M. A., Cammue, B. P. A., De Bolle, M. F. C. (2008). Plant pathogenesis-related (PR) proteins: A focus on PR peptides. Plant Physiol. Biochem. 46 (11), 941–950. doi: 10.1016/j.plaphy.2008.06.011
Sharma, K., Butz, A. F., Finckh, M. R. (2010). Effects of host and pathogen genotypes on inducibility of resistance in tomato (Solanum lycopersicum) to Phytophthora infestans. Plant Pathol. 59 (6), 1062–1071. doi: 10.1111/j.1365-3059.2010.02341.x
Thevenet, D., Pastor, V., Baccelli, I., Balmer, A., Vallat, A., Neier, R., et al. (2017). The priming molecule β-aminobutyric acid is naturally present in plants and is induced by stress. New Phytol. 213 (2), 552–559. doi: 10.1111/nph.14298
Thimm, O., Bläsing, O., Gibon, Y., Nagel, A., Meyer, S., Krüger, P., et al. (2004). MAPMAN: A user-driven tool to display genomics data sets onto diagrams of metabolic pathways and other biological processes. Plant J. 37 (6), 914–939. doi: 10.1111/j.1365-313X.2004.02016.x
Ton, J., Jakab, G. (2005). Dissecting the β-aminobutyric acid–induced priming phenomenon in Arabidopsis. Plant J. 17 (March 2005), 987–999. doi: 10.1105/tpc.104.029728.2
Tripathi, D., Raikhy, G., Kumar, D. (2019). Chemical elicitors of systemic acquired resistance–salicylic acid and its functional analogs. Curr. Plant Biol. 17 (March), 48–59. doi: 10.1016/j.cpb.2019.03.002
Varet, H., Brillet-guéguen, L., Coppée, J., Dillies, M. (2016). SARTools : A DESeq2- and EdgeR-based r pipeline for comprehensive differential analysis of RNA-seq data. PLoS ONE 11(6), e0157022. doi: 10.1371/journal.pone.0157022
Wan, J., He, M., Hou, Q., Zou, L., Yang, Y., Wei, Y., et al. (2021). Cell wall associated immunity in plants. Stress Biol. 1 (1), 1–15. doi: 10.1007/s44154-021-00003-4
Wang, K., Liao, Y., Xiong, Q., Kan, J., Cao, S., Zheng, Y. (2016). Induction of Direct or Priming Resistance against Botrytis cinerea in Strawberries by β-Aminobutyric Acid and Their Effects on Sucrose Metabolism. Journal of Agricultural and Food Chemistry. 64 (29), 5855–5865. doi: 10.1021/acs.jafc.6b00947
Wiesel, L., Newton, A. C., Elliott, I., Booty, D., Gilroy, E. M., Birch, P. R. J., et al. (2014). Molecular effects of resistance elicitors from biological origin and their potential for crop protection. Front. Plant Sci. 5 (November). doi: 10.3389/fpls.2014.00655
Williamson, B, Tudzynski, B, Tudzynski, P. (2007). Botrytis cinerea:the cause of grey mould disease. Mol Plant Pathol. 8, 561–80.
Wilkinson, S. W., Pastor, V., Paplauskas, S., Pétriacq, P., Luna, E. (2018). Long-lasting β-aminobutyric acid-induced resistance protects tomato fruit against Botrytis cinerea. Plant Pathol. 67 (1), 30–41. doi: 10.1111/ppa.12725
Windram, O., Madhou, P., McHattie, S., Hill, C., Hickman, R., Cooke, E., et al. (2012). Arabidopsis defense against Botrytis cinerea: Chronology and regulation deciphered by high-resolution temporal transcriptomic analysis. Plant Cell 24 (9), 3530–3557. doi: 10.1105/tpc.112.102046
Worrall, D., Holroyd, G. H., Moore, J. P., Glowacz, M., Croft, P., Taylor, J. E., et al. (2012). Treating seeds with activators of plant defence generates long-lasting priming of resistance to pests and pathogens. New Phytol. 193 (3), 770–778. doi: 10.1111/j.1469-8137.2011.03987.x
Xiong, J.-S., Zhu, H.-Y., Bai, Y.-B., Liu, H., Cheng, Z.-M. (2018). RNA Sequencing-based transcriptome analysis of mature strawberry fruit infected by necrotrophic fungal pathogen Botrytis cinerea. Physiol. Mol. Plant Pathol. 104, 77–85. doi: 10.1016/j.pmpp.2018.08.005
Xu, X., Chen, C., Fan, B., Chen, Z. (2006). Physical and functional interactions between and WRKY60 transcription factors. The Plant Cell 18 (5), 1310–1326. doi: 10.1105/tpc.105.037523
Yadav, V., Wang, Z., Wei, C., Amo, A., Ahmed, B., Yang, X., et al. (2020). Phenylpropanoid pathway engineering: An emerging approach towards plant defense. Pathogens 9 (4), 1–25. doi: 10.3390/pathogens9040312
Yassin, M., Ton, J., Rolfe, S. A., Valentine, T. A., Cromey, M., Newton, A. C. (2021). The rise, fall and resurrection of chemical-induced resistance agents. Pest Manag Sci. 77, 3900–3909. doi: 10.1002/ps.6370
Zeilinger, S., Gupta, V. K., Dahms, T. E. S., Silva, R. N., Singh, H. B., Upadhyay, R. S., et al. (2016). Friends or foes? emerging insights from fungal interactions with plants. FEMS Microbiol. Rev. 40 (2), 182–207. doi: 10.1093/femsre/fuv045
Zhang, J., Chen, L., Fu, C., Wang, L., Liu, H., Cheng, Y., et al. (2017). Comparative transcriptome analyses of gene expression changes triggered by Rhizoctonia solani AG1 IA infection in resistant and susceptible rice varieties. Front. Plant Sci. 8 (August). doi: 10.3389/fpls.2017.01422
Keywords: strawberry, β-aminobutyric acid, Botrytis cinerea, crop protection, disease resistance, necrotroph, transcriptomics
Citation: Badmi R, Tengs T, Brurberg MB, Elameen A, Zhang Y, Haugland LK, Fossdal CG, Hytönen T, Krokene P and Thorstensen T (2022) Transcriptional profiling of defense responses to Botrytis cinerea infection in leaves of Fragaria vesca plants soil-drenched with β-aminobutyric acid. Front. Plant Sci. 13:1025422. doi: 10.3389/fpls.2022.1025422
Received: 22 August 2022; Accepted: 21 November 2022;
Published: 08 December 2022.
Edited by:
Pramod Prasad, ICAR-Indian Institute of Wheat and Barley Research, Regional Station, IndiaReviewed by:
Zeraye Mehari Haile, Ethiopian Institute of Agricultural Research, EthiopiaSilvia Proietti, University of Tuscia, Italy
Copyright © 2022 Badmi, Tengs, Brurberg, Elameen, Zhang, Haugland, Fossdal, Hytönen, Krokene and Thorstensen. This is an open-access article distributed under the terms of the Creative Commons Attribution License (CC BY). The use, distribution or reproduction in other forums is permitted, provided the original author(s) and the copyright owner(s) are credited and that the original publication in this journal is cited, in accordance with accepted academic practice. No use, distribution or reproduction is permitted which does not comply with these terms.
*Correspondence: Tage Thorstensen, dGFnZS50aG9yc3RlbnNlbkBuaWJpby5ubw==
†Present address: Raghuram Badmi, University College Cork, School of Biological Earth and Environmental Sciences, Cork, Ireland