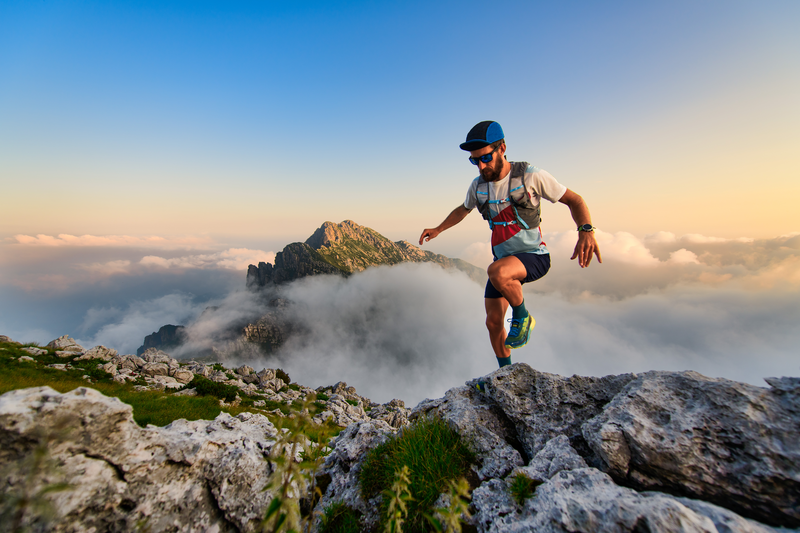
94% of researchers rate our articles as excellent or good
Learn more about the work of our research integrity team to safeguard the quality of each article we publish.
Find out more
ORIGINAL RESEARCH article
Front. Plant Sci. , 13 October 2022
Sec. Plant Systems and Synthetic Biology
Volume 13 - 2022 | https://doi.org/10.3389/fpls.2022.1021088
This article is part of the Research Topic Systems biology of maturation and senescence in horticultural plants View all 10 articles
The medicinal plant Cannabis sativa L. (C. sativa) accumulates plant cytotoxic but medicinally important cannabinoids in glandular trichomes and flowers of female plants. Although the major biosynthetic pathway of cannabinoids has been revealed, their transportation mechanism is still unknown. Multidrug and toxic compound extrusion proteins (MATEs) can transport plant metabolites, ions and phytohormones intra and inter-cellularly. MATEs could have the potential to translocate cannabinoids or their synthetic intermediates to cellular compartment, thus protecting them from unwanted modifications and cytotoxicity. In this study, we performed a genome-wide identification and expression analysis of Cannabis sativa MATEs (CsMATEs) and revealed 42 CsMATEs that were classified phylogenetically into four conserved subfamilies. Forty-two CsMATEs were unevenly distributed on 10 chromosomes, with 50% CsMATEs were physically adjacent to at least one another CsMATEs and 83% CsMATEs localized on plasma membrane. Tandem duplication is the major evolutionary driving force for CsMATEs expansion. Real-time quantitative PCR revealed CsMATE23, CsMATE28 and CsMATE34 mainly expressed in flower, whereas CsMATE17 and CsMATE27 showed strong transcription in root. Light responsive cis-acting element was most abundant in promoters of CsMATE23, CsMATE28 and CsMATE34. Finally, the contents of cannabinoids and corresponding biosynthetic intermediates as well as expressions of CsMATE28 and CsMATE34 were determined under UV-B treatment, among which strong correlation was found. Our results indicates that CsMATEs might involve in biosynthesis of cannabinoids and has the potential to be used in heterologous production of cannabinoids.
The annual dioecious herb Cannabis sativa L. (C. sativa) from Cannabis family accumulates terpenophenolic cannabinoids in glandular trichomes and flowers of female plants (Andre et al., 2016). Till now, over 113 cannabinoids have been isolated from C. sativa and many are derived from non-enzymatic decarboxylaion of their acidic forms by heat or UV irradiation (Güllck and Moller, 2020). The well-studied cannabinoids cannabigerol (CBG), Δ9-tetrahydrocannabinol (THC), cannabidiol (CBD) and cannabichromene (CBC) share the same biosynthetic precursors of olivetolic acid (OA) and geranyl diphosphate (GPP) (Güllck and Moller, 2020). Phenolic OA is condensed from one molecule of hexanoyl-CoA and three molecules of malonyl-CoA by coordinated work of olivetol synthase (OLS) (Taura et al., 2009) and olivetolic acid synthase (OAC) (Gagne et al., 2012) in cytosol. The acyl-activating enzyme 1 (AAE1) provides hexanoyl-CoA by converting C6-hexanoic acid which is derived from fatty acid pathway (Stout et al., 2012), while C10-isoprenoid GPP is synthesized by 2-C-methyl-D-erythritol 4-phosphate (MEP) pathway in plastid (Fellermeier et al., 2001). Aromatic prenyltransferase 4 (aPT4) from UbiA protein superfamily subsequently prenylates OA by GPP to produce cannabichromenic acid (CBGA) (Luo et al., 2019; Güllck et al., 2020), which is further oxidized to CBG. CBGA could be converted to Δ9-tetrahydrocannabinolic acid (THCA) or cannabidiolic acid (CBDA) by flavoproteins Δ9-tetrahydrocannabinolic acid synthase (THCAS) (Sirikantaramas et al., 2005) or cannabidiolic acid synthase (CBDAS) (Taura et al., 2007), respectively, and subsequently decarboxylated to THC or CBD by light or heat.
Low abundance in C. sativa and important pharmaceutical use evokes tremendous interests for heterologous biosynthesis of cannabinoids. A notable example is the de novo production of THCA and CBDA in yeast Saccharomyces cerevisiae system (Luo et al., 2019). Co-expression of OA synthetic pathway (CsAAE1, CsOAC and CsOLS) along with plastid-localization-signal-free CsaPT4, secretory-signal-free CsTHCAS or CsCBDAS in a GPP-overproducing yeast strain yielded THCA or CBDA, respectively. When those genes were transiently expressed in tobacco (Nicotiana benthamiana) cells, however, either OA, CBGA or THCA was severely glucosylated (Güllck et al., 2020). In addition to been easily glucosylated, CBGA and THCA are cytotoxic (Morimoto et al., 2007). Therefore, mechanisms might exist in C. sativa cells that protect cannabinoids from glucosylation and inhibit their cytotoxicity.
Multidrug and toxic compound extrusion proteins (MATEs) are known to translocate second metabolites, ions, and phytohormones intra and intercellularly, and are associated with exnobiotic efflux, aluminum detoxification and disease resistance (Upadhyay et al., 2019). Moreover, MATEs are widely distributed in prokaryotes and eukaryotes, including plants. YdhE was the first isolated MATE from E.coli as a multidrug efflux protein that confers bacteria drug resistance (Morita et al., 1998). Soon after, ALF, the first plant MATE from Arabidopsis thaliana (A. thaliana) (Diener et al., 2001) and many other plant MATEs were sequentially discovered (Wang et al., 2016; Santos et al., 2017; Wang et al., 2017; Huang et al., 2021). Most MATEs consist of about 400-700 amino acids with 12 transmembrane helices and a common MatE domain (pfam 01554), but no consensus sequence thus far has been found in all MATEs (Kusakizako et al., 2020). MATEs transport second metabolites into and out of the membranes through the employment of either Na+ or H+ electrochemical gradient (Hvorup et al., 2003). For instance, NtMATE1 and NtMATE2 that localize on the vacuolar membrane of tobacco root cells are believed to sequestrate alkaloid nicotine within the vacuole of the roots, then nicotine is transported to the above-ground organs of the plant in response to plant biotic stresses (Shoji et al., 2009). Similar function in alkaloid transportation is also found for tobacco MATEs Nt-JAT1 and Nt-JAT2, which localize on the vacuolar membrane of leaf cells (Morita et al., 2009). GhTT2, GhMATE12, GhMATE16 and GhMATE38 from Gossypium hirsutum L. localized on the tonoplast are proposed to translocate phenolic pro-anthocyanidins (Gao et al., 2016; Xu et al., 2019). VvAM1 and VvAM2 expressed in berry skins of Vitis vinifera and subcellularly localized on tonoplast are responsible of translocating flavonoid anthocyanins (Perez-Diaz et al., 2014). Thus, we hypothesis that MATEs from C. sativa might have the ability to bind and translocate terpenophenolic cannabinoids or corresponding biosynthetic intermediates, protecting them from glucosylation and inhibiting their cytotoxicity in C. sativa cells.
In this study, we identified 42 MATEs in C. sativa genome and characterized their physical-chemical properties, gene structure, motif composition and gene expression patterns. Furthermore, qRT-PCR verification and cis-acting elements analysis of CsMATEs that predominantly expressed in cannabinoids accumulation tissues were conducted. In addition, effects of UV-B on accumulation of cannabinoids and corresponding biosynthetic substrates as well as the expression of CsMATE23, CsMATE28 and CsMATE34 were studied. Together, our result indicates that CsMATE28 and CsMATE34 might be involved in biosynthesis of cannabinoids or its biosynthetic intermediates.
In this study, we used the C. sativa variety Dinamed Kush (DK) for transcriptome sequencing (Yang et al., 2021). DK were grown in the experimental field of the Institute of Chinese Materia Medica of the Chinese Academy of Chinese Medical Sciences, China. Three-weeks-old C. sativa grown in green house at 65% humidity and light wavelength of 380-780 nm (520-860 uM m-2 s-1) was used for UV-B light treatment.
Genome of female C. sativa CRBRx (GCA_900626175.1) and transcriptional data of other nine C. sativa varieties (Zager et al., 2019) were obtained from the NCBI database (https://www.ncbi.nlm.nih.gov). Arabidopsis thaliana MATE gene family members were obtained from the Uniprot database (www.uniprot.org).
CDS sequences and protein sequences of CRBRx were extracted using TBtools (Chen et al., 2020). Protein sequences of A. thaliana MATEs were used as queries to perform homology search by BLASTp method (score value of ≥100, e-value ≤ e−10). Duplicated proteins were manually removed. Recognizable domains were initially retrieved using BLAST-based NCBI conserved domain searches (https://www.ncbi.nlm.nih.gov/Structure/cdd/wrpsb.cgi). Molecular weights and isoelectric points were predicted using ExPASy (Duvaud et al., 2021). Subcellular localizations were predicted by WOLF PSORT (Horton et al., 2007).
Visualization of CsMATEs structures and chromosome locations were conducted using TBtools (Chen et al., 2020). The amino acid sequences were aligned using MEGA 7.0 software (Kumar et al., 2016). Phylogenetic trees of C. sativa and A. thaliana were constructed using the neighborjoining (NJ) method with 1000 bootstrap replicates. Conserved motif information of CsMATEs was analyzed using MEME (Bailey et al., 2006). Cis-acting elements in 2000 bp upstream of the start codon of each CsMATEs were analyzed using PlantCARE (Lescot et al., 2002).
mRNA from five different tissues or organs of female C. sativa Dinamed Kush were sequenced. TBtools (Chen et al., 2020) was used to analyze and visualize the differential expression data of CsMATEs. RNA was extracted using a kit (Tiangen Biotech, Beijing, China) according to manufacturer’s instructions. Three biological replicates of each sample were performed. Extracted RNA was examined by agarose gel electrophoresis and concentrations were determined using Nanodrop (Thermo fisher scientific, Beijing, China). cDNA synthesis was performed using the Reverse Transcription Kit (TransGen, Beijing, China) as described in instruction. qRT-PCR was designed using NCBI-Primer blast (Supplementary Table 1) with EF1-α as the reference gene. qRT-PCR reaction included StarLighter SYBR green qPCR mix (Qi Heng Xing, Beijing, China) 10 μL, cDNA template 1 μL, 0.4 μL of each primer and ddH2O 8.2 μL. The CFX96™ real-time system (Roter-Gene Q MDx, QIAGENBio-Rad, Germany) was used for qRT-PCR. The reaction conditions were: 95°C for 5 min, 35 cycles of 95°C for 30 s, 55°C for 30 s, and 72°C for 90 s. Data were processed using 2-ΔΔCT (Livak and Schmittgen, 2001).
Cannabinoid content was determined using an Agilent UPLC 1290II-G6400 triple quadrupole mass spectrometer (QQQ; Agilent Technologies, Santa Clara, CA). The autosampler was set to 4°C and a 3-μl sample volume was injected. The chromatographic column was a (2.1 * 100 mm, 1.8 μm) C18 column. Mobile phase A contained water with 0.1% formic acid; phase B was 100% methanol. Elution was performed at 0.3 mL/min.
All the data were analyzed using Prism 8 Statistics programs. One-way analysis of variance (ANOVA) followed by Tukey’s multiple range test was used both for metabolic data and gene expression data.
We performed the homology search against the Arabidopsis MATE sequences and finally 42 CsMATE candidates were identified and named for their position on the chromosomes (Table 1). Forty-two CsMATEs are unevenly scattered on ten chromosomes, with chromosome four contains the highest number of CsMATEs and chromosome six contains the least (Table 1 and Figure 1). It’s worth noting that twenty-one CsMATEs genes are physically adjacent to at least one another CsMATEs, such as CsMATE6–CsMATE8, CsMATE11–CsMATE12, CsMATE17–CsMATE22, CsMATE23–CsMATE25, CsMATE29–CsMATE30, CsMATE33–CsMATE35, and CsMATE40–CsMATE41 (Figure 1). Those CsMATEs account for ~50% of the total CsMATE genes. Moreover, near half of the CsMATEs are located in the proximal region of chromosome telomere (Figure 1).
Figure 1 Distribution of CsMATEs on chromosomes of C. sativa. The y-axis indicates the length of chromosome in megabase (Mb).
We also predicted the physical-chemical properties and subcellular localization of the CsMATEs and found that the longest MATE is CsMATE10, consisting of 621 amino acids, while the shortest CsMATE16 is comprised of 319 amino acids (Table 1). The isoelectric points (pI) and molecular weights (MW) of CsMATEs range from 5.12–9.56 and 35.38–68 kilodalton (kDa), respectively (Table 1). Thirty-five CsMATEs were localized in the plasma membrane, six in the vacuole, and only CsMATE39 localized in the chloroplast (Table 1).
To investigate of the evolutionary relationship of the CsMATEs, we constructed an interspecifc phylogenic tree using C. sativa and A. thaliana MATEs sequences with phylogenetic inference of neighbor-joining (Figure 2). The topology of the phylogenic tree divides CsMATEs into four major subfamilies. Subfamily I contains 14 CsMATEs, subfamily II has 15 CsMATEs, subfamily III possesses five CsMATES, and subfamily IV owns eight CsMATEs (Figure 2). Importantly, physically adjacent CsMATEs were all clustered in the same subfamily (Figure 1 and Figure 2), which indicates tandem duplication is the major evolutionary driving force for CsMATEs expansion (Wang et al., 2019).
Figure 2 Phylogenic tree of MATE proteins in Cannabis sativa (CsMATEs) and Arabidopsis thaliana (AtDTXs). Phylogenic tree was built using MEGA 7.0 software neighbor-joining method (Kumar et al., 2016) with a bootstrap analysis of 1000 replicates.
Conserved protein motifs are associated with gene function and protein subcellular localizations. We isolated 10 predicted conserved motifs (Supplementary Figure S1) using MEME (Bailey et al., 2006) and studied their distributions within CsMATEs. Phylogenetic analysis grouped CsAMTEs into four subfamilies, which is consistent with the interspecific phylogenetic tree in Figure 2 (Figure 3A). We found that 32 CsMATEs (76% of total identified) from subfamily I, II and IV included all 10 motifs and those motifs shared the same order. CsMATE6, 7 and 9 were lack of motif 8; CsMATE8 is without of moitf6 and motif8. CsMATEs from subfamily III had motifs less than six, with CsMATE15 and CsMATE17 only containing motifs 7, 9 and 10 (Figure 3A).
Figure 3 Conserved motifs and gene structure of CsMATEs. (A) CsMATEs Phylogenic tree and motifs distributions. Motifs are indicated as top right. (B) Gene structure and domain distribution. UTR, untranslated region; CDS, coding region; yellow boxes, exons; gray lines, introns; pink boxes, MATE eukaryotic domains; dark green boxes, DinF like domain. Purple dots indicates CsMATE from subfamily I; rose red dots, Subfamily II; pink dots, subfamily III; yellow dots, subfamily IV.
To further examine the evolutionary lineages of CsMATEs, we compared the gene structure of CsMATEs. The results showed that phylogenetically close CsAMTEs shared the same exon number, length and composition (Figure 3B). For instance, CsMATEs from subfamily IV had no intron except for CsMATE2 and CsMATE38 that contained one and two introns, respectively. CsMATEs from subfamily III owned most exons of at least 10. A longest intron that over 20 kb was found in CsMATE35 from subfamily I (Figure 3B). In addition, as tandem duplication is the main force of CsMATEs expansion, physically adjacent CsMATEs also showed same exon/intron pattern, such as CsMATE6–CsMATE8 and CsMATE23–CsMATE25 (Figure 1 and Figure 3B). Although gene structures between different subfamilies were divergent, we found two crucial domains that closed to 5’ end of the gene bodies (Figure 3B).
Gene expression pattern is to some extent indicative for its potential function, especially for transporter proteins that mostly interact with substrates in tissues where metabolites are synthesized (Nogia and Pati, 2021). We visualized the CsMATEs expressions from different tissues of hemp variety Dinamed Kush, a species with high cannabinoids content, using FPKM (Fragments Per Kilobase of exon model per Million mapped fragments) and cluster analysis, and indeed found the divergent expression patterns (Figure 4A). Specifically, only CsMATE1 showed higher expression in seed compared with other tissues, CsMATE7, CsMATE13, CsMATE21, CsMATE24, CsMATE25, CsMATE29 and CsMATE30 exhibit relatively strong expression in root, CsMATE22 and CsMATE36 have the moderate stronger expression in stem, transcripts of CsMATE4, CsMATE9, CsMATE11, CsMATE14, CsMATE37, CsMATE39 and CsMATE42 are abundantly detected in leaf, and CsMATE03, CsMATE05 CsMATE17, CsMATE23, CsMATE28, CsMATE31, CsMATE34 and CsMATE40 are the genes that expressed mainly in flowers (Figure 4A).
Figure 4 Expression of CsAMTEs and cannabinoid biosynthetic genes. (A) Expression profile of CsMATEs in seed, root, stem, leaf and flower. PFKM values were used to configure the heatmap. Red dots, genes selected for expression verification. CsOAC, olivetolic acid synthase; CsPT1 and CsPT4, prenyltransferase 1 and 4 gene, repectively. (B) quantitative reverse transcription PCR verification. y-axis, relative gene expression normalized to CsEF1-alpha. x-axis, different tissues. Data, mean ± SD.
As we are interested in CsMATEs that involved in transportation of cannabinoids, and to further verify the accuracy of the transcriptomic data, we analyzed the expressions of representative MATE genes by qRT-PCR (real-time quantitative reverse transcription PCR) including CsMATE17, CsMATE23, CsMATE28 and CsMATE34 which had a highest expression in flower but also expressed moderately in stem and leaf according to transcriptome data, as well as CsMATE21 that showed strong root specificity in transcriptomic heatmap (Figure 4A). CsMATE17, unexpectedly, was predominantly expressed in root, inconsistent with the RNA-seq data, while the other four gene showed the same expression pattern as in RNA-seq (Figure 4B). Additionally, we also examined the expression patterns of cannabinoid biosynthetic pathway genes including CsOAC, CsPT1 and CsPT4 (Figure 4A). The result showed that CsMATE23, CsMATE28 and CsMATE34 exhibited similar expression patterns with cannabinoids synthetic genes, suggesting those genes might involve in cannabinoids synthesis (Figure 4A).
Alternative splicing is widespread in plants as a transcriptional regulatory mechanism that allows a single gene to encode a variety of different transcripts and protein products (Roy et al., 2013). To further understand the transcriptional mechanisms of CsMATEs, we performed alternative splicing analysis. We observed 15 CsMATEs have alternative splicing events, accounting for about 1/3 of CsMATEs, with a total of 43 alternative splicing isoforms. Numbers of alternative splicing isoforms of a single CsMATE range from one to seven, and most of the alternative splicing isoforms form the same pre-mRNA were differentially expressed in the same tissues. Of note, although CsMATE23 and CsMATE34 both have an alternative splicing isoform, the alternative splicing isoforms barely express in the tissues we examined (Figure 5A).
Figure 5 Expression of alternative splicing isoforms (A) and cis-acting elements in CsMATEs (B). Red dots, alternative splicing isoform used for analysis in this study. Heatmap was configured using PFKM value. Length of gene promoters in bp.
Light is known to affect the content of cannabinoids (Eichhorn et al., 2019). The cis-acting elements in the promoter region (2 kb upstream of ATG codon) of CsMATE23, CsMATE28 and CsMATE34 were analyzed using plantCARE software (Lescot et al., 2002). We found that the most abundant cis-acting element is the light responsive element, and at least six light responsive cis-acting elements are found in each of the CsMATE23, CsMATE28 and CsMATE34 promoter regions (Figure 5B). Phytohormones responsive cis-acting elements are the second abundant found in their promoter regions (Figure 5B). We also revealed one and two defense and stress responsive cis-acting elements in CsMATE17 and CsMATE28 promoters, respectively, and one low temperature-responsive cis-element in CsMATE23 promoter (Figure 5B).
As we found numerous light responsive cis-acting elements in the promoters of CsMATE23, CsMATE28 and CsMATE34 (Figure 5B) and lights are known to affect accumulation of cannabinoids (Eichhorn et al., 2019), we then studied the content of cannabinoids, cannabinoids biosynthetic intermediates and the expressions of CsMATE23, CsMATE28 and CsMATE34 under UV-B light treatment.
Three-weeks-old cannabis seedlings were subjected to UV-B light treatment, and quantitative analysis of cannabinoid contents from leaves was performed by QQQ-MS/MS. In summary, content of OA decreased significantly after two hours or six hours UV-B treatment and reached highest at 12 hours, whereas we only observed a decrease of GPP at 12 hours (Figure 6A). Except for CBD that showed a decrease at two hours treatment and an increase after 12 hours UV-B treatment, the contents of CBGA, CBG, THCA, THC and CBDA increased after two hours, then decreased at six hours, reached the highest at 12 hours (Figure 6A).
Figure 6 Cannabinoids contents (A) and expression of CsMATE23, CsMATE38 and CsMATE34 under UV-B light (B). Leaves from UV-B treated three-weeks-old C. sativa seedlings were collected at 0, 2, 6 and 12 hours. Cannabinoids contents were measured using QQQ MS/MS. gene expressions were detected by qRT-PCR with CsEF1-alpha as internal reference. Three biological repeats were performed. Different letters above the bars indicate significantly different values (p < 0.05) calculated using one-way analysis of variance (ANOVA) followed by Tukey’s multiple range test.
Correspondingly, expressions of CsMATE23, CsMATE28 and CsMATE34 also exhibited significant changes under UV-B treatment (Figure 6B). CsMATE23 was greatly reduced after two hours UV-B treatment and restored its expression at 12 hours. The expression of CsMATE28 and CsMATE34 displayed no significant change during the first six hours treatment, but significantly increased at 12 hours. (Figure 6B).
MATEs have been identified in a variety of plant species, including 56 MATEs in Arabidopsis, 55 in rice (Wang et al., 2016). 67 in tomato (Santos et al., 2017), 64 in potato (Huang et al., 2021), 70 in Medicago truncatula (Wang et al., 2017), 72 in cotton (Xu et al., 2019) and 117 in soybean (Liu et al., 2016). In the present study, we identified 42 CsMATEs and investigated their physical-chemical properties, gene distribution, evolutionary relationships, conserved motifs, gene structures and gene expressions. CsMATEs contains only 42 members (Table 1) and is the fewest when compared with other plant species, which suggests CsMATEs may have undergone contraction during evolution. Those 42 CsMATEs were divided into four subfamilies and distributed on 10 chromosomes, with ~50% CsMATEs adjacent to at least one another CsMATEs (Figure 1), thus the expansion of CsMATEs might be largely due to tandem duplication (Wang et al., 2019).
Although cannabinoids synthetic pathways have already been illustrated, the translocation of the cannabinoids and biosynthetic intermediates are not known (Güllck and Moller, 2020). This inevitably hinders cannabinoids heterologous biosynthesis (Güllck et al., 2020). Cannabinoids are synthesized in glandular trichomes and flowers of female C. sative and their biosynthetic processes undergo cellular compartmentalization (Güllck and Moller, 2020). For instance, plastid localized CsaPT4 prenylates cytosolic synthesized OA in chloroplast to yield CBGA (Taura et al., 2009; Gagne et al., 2012; Luo et al., 2019; Güllck et al., 2020). Metabolite transporter proteins that could shuttle from cytosol to chloroplast should exist in C sativa cells. However, subcellular localization prediction indicates that vast majority of CsMATEs locate in vacuole or plasma membrane (Table 1). Although CsMATE39 contains putative chloroplast localization signal (Table 1), it expresses mainly in leaf (Figure 4A), the tissue that is not the most abundant cannabinoids accumulate. We assume that transporters from other families might be involved in translocating phenolic OA such as ATP binding cassette transporters which could translocate plant phenolic metabolites (Hwang et al., 2016). THCAS is secreted to apoplastic space of the glandular trichome to carry out oxidative cyclization of CBGA (Sirikantaramas et al., 2005). The plastid produced CBGA thus should be translocated across the plasm membrane. Thirty-five CsMATEs are predicted to localized on plasm membrane (Table 1). Of them, CsMATE03, CsMATE05, CsMATE23, CsMATE28, CsMATE31, CsMATE34 and CsMATE40 had a highest expression in flower but also expressed moderately in stem and leaf (the tissues that have glandular trichomes) according to transcriptome data (Figure 4A) and qRT-PCR (Figure 4B). Although we don’t have the trichome-specific transcriptional data of those seven genes in variety DK, they uniformly expressed in the trichomes of the other nine C. sativa varieties (Zager et al., 2019, Supplementary Figure S2). Moreover, the seven genes exhibited similar expression pattern in different tissues as that of cannabinoid synthetic genes (Figure 4A), which further indicates the involvement CsMATEs in biosynthesis of cannabinoids.
Within the seven genes, except for CsMATE23, CsMATE28, CsMATE34 and CsMATE40 all have an unexpressed alternative spliceform in tissues we studied (Figure 5A). Light responsive cis-acting elements were abundantly detected in promoters of the representative CsMATEs (CsMATE23, CsMATE28 and CsMATE34), reflects those genes could be regulated by lights. UV-B is known to affect accumulation of cannabinoids (Lydon et al., 1987). In accordance with this, the contents of cannabinoids and corresponding intermediates were changed under UV-B treatment (Figure 6A). As expected, the variation trend of CBGA was analogous to its direct downstream THCA, CBDA and CBG (Figure 6A), reflects the precursor nature of the CBGA (Güllck et al., 2020). Notably, although overall variation of OA is similar to CBGA, accumulation of OA is opposed to CBGA at two hours treatment where OA content was decreased but CBGA contents was increased compared to zero hours treatment (Figure 6A). Meanwhile, GPP did not show any change at two hours treatment (Figure 6A). Therefore, increase of CBGA after two hours UV-B treatment could be largely caused by over-usage of OA for synthesizing CBGA either due to enhanced CBGAS activity or more efficient translocation of OA form cytosol to plastid. Unfortunately, none of the CsMATEs we tested was up-regulated after two hours treatment (Figure 6B). We reasoned that plastid located ATP binding cassette transporters that translocate phenolic metabolites might responsible for OA transportation (Hwang et al., 2016). Moreover, Although the transcriptional variation of CsMATE23 is analogous to the content of OA (Figure 6B), OA is synthesized in cytosol and CsMATE23 is localized in plasma membrane (Table 1). Meanwhile, CsMATE23 is barely transcribed in C sativa root, however its Arabidopsis ortholog AtRHS2 (AtDTX31) located in plasma membrane of root is required for hairy root elongation (Won et al., 2009). Hence, we may also exclude participation of CsMATE23 in transporting OA precursors.
The most interesting finding we observed is the correlation between transcriptional alterations of CsMATEs with the accumulation of CBGA, THCA and CBDA (Figure 6). As the precursor of THCA and CBDA, CBGA content did not show much alteration at 12 hours treatment compared to the control zero hour (Figure 6A). However, the contents of THCA, THC, CBDA and CBD were all increased at least more than four times at 12 hours compared to zero hours and transcriptions of CsMATE28 and CsMATE34 were also significantly enhanced at 12 hours (Figure 6B). This could be explained by that increased CsMATE28 or CsMATE34 more inefficiently transport CBGA to the apoplastic space to enable its subsequent conversions (Sirikantaramas et al., 2005).
We identified 42 CsMATEs and analyzed their structural features, evolutionary relationships and expression patterns. We found number of CsMATEs was subjected to contraction and its expansion within the family was mainly due to tandem duplication. Though RNA-seq and qRT-PCR analysis, we found two root-specifically transcribed CsMATEs (CsMATE17 and CsMATE27) and three CsMATEs (CsMATE23, CsMATE28 and CsMATE34) whose transcription pattern were correlated with transcriptions of cannabinoids biosynthetic genes. In addition, although CBGA content was not much affected under UV-B treatment at 12 hours, accumulations of THCA, CBDA and CBG were increased. This could be due to the increased expression of CsMATE28 or CsMATE34. Although multiple evidences suggest that CsMATEs may play an important role in the synthesis and transport of cannabinoids, the transport of cannabinoids is a complex process. Therefore. The functions of candidate genes (CsMATE28 and CsMATE34) in cannabinoid transportations should be investigated in depth in the future.
The original contributions presented in the study are included in the article/Supplementary Material. Further inquiries can be directed to the corresponding authors.
WC and HW designed the study. WC, HW, SW, XC and XM wrote the manuscript. SW, XC, XM, YW, AW, MA and QD conducted the bioinformatic analysis. XC and XM performed the qRT-PCR and UV-B treatment. All authors contributed to the article and approved the submitted version.
This work is supported by Scientific and technological innovation project of China Academy of Chinese Medical Sciences (CI2021A04113).
The authors declare that the research was conducted in the absence of any commercial or financial relationships that could be construed as a potential conflict of interest.
All claims expressed in this article are solely those of the authors and do not necessarily represent those of their affiliated organizations, or those of the publisher, the editors and the reviewers. Any product that may be evaluated in this article, or claim that may be made by its manufacturer, is not guaranteed or endorsed by the publisher.
The Supplementary Material for this article can be found online at: https://www.frontiersin.org/articles/10.3389/fpls.2022.1021088/full#supplementary-material
Andre, C. M., Hausman, J.-F., Guerriero, G. (2016). Cannabis sativa: The plant of the thousand and one molecules. Front. Plant Sci. 7. doi: 10.3389/fpls.2016.00019
Bailey, T. L., Williams, N., Misleh C.and Li, W. W. (2006). MEME: discovering and analyzing DNA and protein sequence motifs. Nucleic Acids Res. 34, W369–W373. doi: 10.1093/nar/gkl198
Chen, C., Chen, H., Zhang, Y., Thomas, H. R., Frank, M. H., He, Y., et al. (2020). TBtools: An integrative toolkit developed for interactive analyses of big biological data. Mol. Plant 13 (8), 1194–1202. doi: 10.1016/j.molp.2020.06.009
Diener, A. C., Gaxiola, R. A., Fink, G. R. (2001). Arabidopsis ALF5, a multidrug efflux transporter gene family member, confers resistance to toxins. Plant Cell. 13 (7), 1625–1638. doi: 10.1105/tpc.010035
Duvaud, S., Gabella, C., Lisacek, F., Stockinger, H., Ioannidis V.and Durinx, C. (2021). Expasy, the Swiss bioinformatics resource portal, as designed by its users. Nucleic Acids Res. 49 (W1), W216–W227. doi: 10.1093/nar/gkab225
Eichhorn, B. S., Wu, B. S., Rufyikiri, A. S., MacPherson, S., Lefsrud, M. (2019). An update on plant photobiology and implications for cannabis production. Front. Plant Sci. 10. doi: 10.3389/fpls.2019.00296
Fellermeier, M., Eisenreich W., A., Bacher A., Zenk, M. H. (2001). Biosynthesis of cannabinoids. incorporation experiments with (13)C-labeled glucoses. Eur. J. Biochem. 268 (6), 1596–1604. doi: 10.1046/j.1432-1033.2001.02030
Gagne, S. J., Stout, J. M., Liu, E., Boubakir, Z., Clark, S. M., Page, et al. (2012). Identification of olivetolic acid cyclase from cannabis sativa reveals a unique catalytic route to plant polyketides. Proc. Natl. Acad. Sci. U. S. A. 109 (31), 12811–12816. doi: 10.1073/pnas.1200330109
Gao, J. S., Wu, N., Shen, Z. L., Lv, K., Qian, S. H., Guo, N., et al. (2016). Molecular cloning, expression analysis and subcellular localization of a transparent testa 12 ortholog in brown cotton (Gossypium hirsutum l.). Gene 576 (2 Pt 2), 763–769. doi: 10.1016/j.gene.2015.11.002
Güllck, T., Booth, J. K., Carvalho, A., Khakimov, B., Crocoll, C., Motawia, M. S., et al. (2020). Synthetic biology of cannabinoids and cannabinoid glucosides in nicotiana benthamiana and saccharomyces cerevisiae. J. Nat. Prod. 83 (10), 2877–2893. doi: 10.1021/acs.jnatprod.0c00241
Güllck, T., Moller, B. L. (2020). Phytocannabinoids: Origins and biosynthesis. Trends Plant Sci. 25 (10), 985–1004. doi: 10.1016/j.tplants.2020.05.005
Horton, P., Park, K. J., Obayashi, T., Fujita, N., Harada, H., Adams-Collier, C. J., et al. (2007). WoLF PSORT: protein localization predictor. Nucleic Acids Res. 35, W585–W587. doi: 10.1093/nar/gkm259
Huang, Y., He, G., Tian, W., Li, D., Meng, L., Wu, D., et al. (2021). Genome-wide identification of MATE gene family in potato (Solanum tuberosum l.) and expression analysis in heavy metal stress. Front. Genet. 12. doi: 10.3389/fgene.2021.650500
Hvorup, R. N., Winnen, B., Chang, A. B., Jiang, Y., Zhou, X. F., Saier, M. H., Jr. (2003). The multidrug/oligosaccharidyl-lipid/polysaccharide (MOP) exporter superfamily. Eur. J. Biochem. 270 (5), 799–813. doi: 10.1046/j.1432-1033.2003.03418.x
Hwang, J.-U., Song, W.-Y., Hong, D., Ko, D., Yamaoka, Y., Jang, S., et al. (2016). Plant ABC transporters enable many unique aspects of a terrestrial plant’s lifestyle. Mol. Plant 9, 338–355. doi: 10.1016/j.molp.2016.02.003
Kumar, S., Stecher, G., Tamura, K. (2016). MEGA7: Molecular evolutionary genetics analysis version 7.0 for bigger datasets. Mol. Biol. Evol. 33 (7), 1870–1874. doi: 10.1093/molbev/msw054
Kusakizako, T., Miyauchi, H., Ishitani R.and Nureki, O. (2020). Structural biology of the multidrug and toxic compound extrusion superfamily transporters. Biochim. Biophys. Acta Biomembr. 1862 (12), 183154. doi: 10.1016/j.bbamem.2019.183154
Lescot, M., Dehais, P., Thijs, G., Marchal, K., Moreau, Y., Van de Peer, Y., et al. (2002). PlantCARE, a database of plant cis-acting regulatory elements and a portal to tools for in silico analysis of promoter sequences. Nucleic Acids Res. 30 (1), 325–327. doi: 10.1093/nar/30.1.325
Liu, J., Li, Y., Wang, W., Gai, J., Li, Y. (2016). Genome-wide analysis of MATE transporters and expression patterns of a subgroup of MATE genes in response to aluminum toxicity in soybean. BMC Genomics 17, 223. doi: 10.1186/s12864-016-2559-8
Livak, K. J., Schmittgen, T. D. (2001). Analysis of relative gene expression data using real-time quantitative PCR and the 2(-delta delta C(T)) method. Methods 25 (4), 402–408. doi: 10.1006/meth.2001.1262
Luo, X., Reiter, M. A., d'Espaux, L., Wong, J., Denby, C. M., Lechner, A., et al. (2019). Complete biosynthesis of cannabinoids and their unnatural analogues in yeast. Nature 567 (7746), 123–126. doi: 10.1038/s41586-019-0978-9
Lydon, J., Teramura, A. H., Coffman, C. B. (1987). UV-B radiation effects on photosynthesis, growth and cannabinoid production of two cannabis sativa chemotypes. Photochem. Photobiol. 46 (2), 201–206. doi: 10.1111/j.1751-1097.1987.tb04757.x
Morimoto, S., Tanaka, Y., Sasaki, K., Tanaka, H., Fukamizu, T., Shoyama, Y., et al. (2007). Identification and characterization of cannabinoids that induce cell death through mitochondrial permeability transition in cannabis leaf cells. J. Biol. Chem. 282 (28), 20739–20751. doi: 10.1074/jbc.M700133200
Morita, Y., Kodama, K., Shiota, S., Mine, T., Kataoka, A., Mizushima, T., et al. (1998). NorM, a putative multidrug efflux protein, of vibrio parahaemolyticus and its homolog in escherichia coli. Antimicrob. Agents Chemother. 42 (7), 1778–1782. doi: 10.1128/AAC.42.7.1778
Morita, M., Shitan, N., Sawada, K., Van Montagu, M. C., Inze, D., Rischer, H., et al. (2009). Vacuolar transport of nicotine is mediated by a multidrug and toxic compound extrusion (MATE) transporter in nicotiana tabacum. Proc. Natl. Acad. Sci. U. S. A. 106 (7), 2447–2452. doi: 10.1073/pnas.0812512106
Nogia, P., Pati, P. K. (2021). Plant secondary metabolite transporters: Diversity, functionality, and their modulation. Front. Plant Sci. 12. doi: 10.3389/fpls.2021.758202
Perez-Diaz, R., Ryngajllo, M., Perez-Diaz, J., Pena-Cortes, H., Casaretto, J. A., Gonzalez-Villanueva, E., et al. (2014). VvMATE1 and VvMATE2 encode putative proanthocyanidin transporters expressed during berry development in vitis vinifera l. Plant Cell Rep. 33 (7), 1147–1159. doi: 10.1007/s00299-014-1604-9
Roy, B., Haupt, L. M., Griffiths, L. R. (2013). Review: Alternative splicing (AS) of genes as an approach for generating protein complexity. Curr. Genomics 14 (3), 182–194. doi: 10.2174/1389202911314030004
Santos, A. L. D., Chaves-Silva, S., Yang, L., Maia, L. G. S., Chalfun-Junior, A., Sinharoy, S., et al. (2017). Global analysis of the MATE gene family of metabolite transporters in tomato. BMC Plant Biol. 17 (1), 185. doi: 10.1186/s12870-017-1115-2
Shoji, T., Inai, K., Yazaki, Y., Sato, Y., Takase, H., Shitan, N., et al. (2009). Multidrug and toxic compound extrusion-type transporters implicated in vacuolar sequestration of nicotine in tobacco roots. Plant Physiol. 149 (2), 708–718. doi: 10.1104/pp.108.132811
Sirikantaramas, S., Taura, F., Tanaka, Y., Ishikawa, Y., Morimoto, S., Shoyama, Y. (2005). Tetrahydrocannabinolic acid synthase, the enzyme controlling marijuana psychoactivity, is secreted into the storage cavity of the glandular trichomes. Plant Cell Physiol. 46 (9), 1578–1582. doi: 10.1093/pcp/pci166
Stout, J. M., Boubakir, Z., Ambrose, S. J., Purves, R. W., Page, J. E. (2012). The hexanoyl-CoA precursor for cannabinoid biosynthesis is formed by an acyl-activating enzyme in cannabis sativa trichomes. Plant J. 71 (3), 353–365. doi: 10.1111/j.1365-313X.2012.04949.x
Taura, F., Sirikantaramas, S., Shoyama, Y., Yoshikai, K., Shoyama, Y., Morimoto, S. (2007). Cannabidiolic-acid synthase, the chemotype-determining enzyme in the fiber-type cannabis sativa. FEBS Lett. 581 (16), 2929–2934. doi: 10.1016/j.febslet.2007.05.043
Taura, F., Tanaka, S., Taguchi, C., Fukamizu, T., Tanaka, H., Shoyama, Y., et al. (2009). Characterization of olivetol synthase, a polyketide synthase putatively involved in cannabinoid biosynthetic pathway. FEBS Lett. 583 (12), 2061–2066. doi: 10.1016/j.febslet.2009.05.024
Upadhyay, N., Kar, D., Deepak Mahajan, B., Nanda, S., Rahiman, R., Panchakshari, N., et al. (2019). The multitasking abilities of MATE transporters in plants. J. Exp. Bot. 70 (18), 4643–4656. doi: 10.1093/jxb/erz246
Wang, L., Bei, X., Gao, J., Li, Y., Yan, Y., Hu, Y. (2016). The similar and different evolutionary trends of MATE family occurred between rice and arabidopsis thaliana. BMC Plant Biol. 16 (1), 207. doi: 10.1186/s12870-016-0895-0
Wang, J., Hou, Q., Li, P., Yang, L., Sun, X., Benedito, V. A., et al. (2017). Diverse functions of multidrug and toxin extrusion (MATE) transporters in citric acid efflux and metal homeostasis in medicago truncatula. Plant J. 90 (1), 79–95. doi: 10.1111/tpj.13471
Wang, X., Lin, S., Liu, D., Wang, Q., McAvoy, R., Ding, J., et al. (2019). Characterization and expression analysis of ERF genes in fragaria vesca suggest different divergences of tandem ERF duplicates. Front. Genet. 10. doi: 10.3389/fgene.2019.00805
Won, S. K., Lee, Y. J., Lee, H. Y., Heo, Y. K., Cho, M., Cho, H. T. (2009). Cis-element- and transcriptome-based screening of root hair-specific genes and their functional characterization in arabidopsis. Plant Physiol. 150 (3), 1459–1473. doi: 10.1104/pp.109.140905
Xu, L., Shen, Z. L., Chen, W., Si, G. Y., Meng, Y., Guo, N., et al. (2019). Phylogenetic analysis of upland cotton MATE gene family reveals a conserved subfamily involved in transport of proanthocyanidins. Mol. Biol. Rep. 46 (1), 161–175. doi: 10.1007/s11033-018-4457-4
Yang, L., Meng, X., Chen, S., Li, J., Sun, W., Chen, W., et al. (2021). Identification of the histone deacetylases gene family in hemp reveals genes regulating cannabinoids synthesis. Front. Plant Sci. 12. doi: 10.3389/fpls.2021.755494
Keywords: Cannabis sativa, cannabinoids, transporter, MATEs, genome-wide, heterologous biosynthesis
Citation: Wang S, Cao X, Meng X, Aili M, Dou Q, Wang Y, Wahab AT, Chen S, Sun W, Wan H and Chen W (2022) Characterization and expression analysis of MATEs in Cannabis sativa L. reveals genes involving in cannabinoid synthesis. Front. Plant Sci. 13:1021088. doi: 10.3389/fpls.2022.1021088
Received: 17 August 2022; Accepted: 29 September 2022;
Published: 13 October 2022.
Edited by:
Peitao Lü, Fujian Agriculture and Forestry University, ChinaReviewed by:
Yuanyuan Liu, Fujian Agriculture and Forestry University, ChinaCopyright © 2022 Wang, Cao, Meng, Aili, Dou, Wang, Wahab, Chen, Sun, Wan and Chen. This is an open-access article distributed under the terms of the Creative Commons Attribution License (CC BY). The use, distribution or reproduction in other forums is permitted, provided the original author(s) and the copyright owner(s) are credited and that the original publication in this journal is cited, in accordance with accepted academic practice. No use, distribution or reproduction is permitted which does not comply with these terms.
*Correspondence: Weiqiang Chen, d3FjaGVuQGljbW0uYWMuY24=; Huihua Wan, aGh3YW5AaWNtbS5hYy5jbg==
†These authors have contributed equally to this work
Disclaimer: All claims expressed in this article are solely those of the authors and do not necessarily represent those of their affiliated organizations, or those of the publisher, the editors and the reviewers. Any product that may be evaluated in this article or claim that may be made by its manufacturer is not guaranteed or endorsed by the publisher.
Research integrity at Frontiers
Learn more about the work of our research integrity team to safeguard the quality of each article we publish.