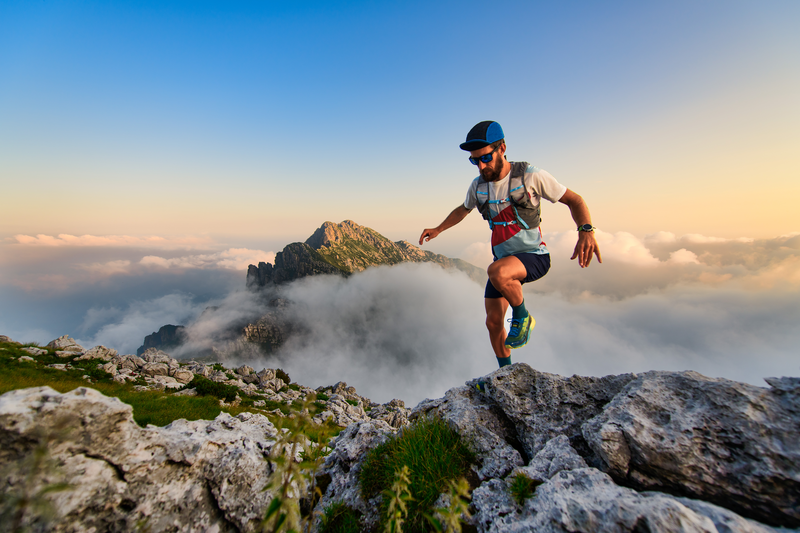
94% of researchers rate our articles as excellent or good
Learn more about the work of our research integrity team to safeguard the quality of each article we publish.
Find out more
ORIGINAL RESEARCH article
Front. Plant Sci. , 02 December 2022
Sec. Plant Symbiotic Interactions
Volume 13 - 2022 | https://doi.org/10.3389/fpls.2022.1017890
Wheat is one of the most important crops in the world. Its production can be influenced by a diversity of beneficial and pathogenic rhizospheric microbes, including fungi. Amongst them, beneficial Trichoderma spp. can be used as alternatives to chemical fertilizers, as they are cheap and harmless to the environment. Our study aimed to isolate, identify, and characterize Trichoderma spp. from Lebanon associated with wheat. Two Trichoderma strains belonging to T. afroharzianum, and T. guizhouense species, were isolated and found to be endophytes, enhancing root growth and producing Indole-3-acetic acid. Inoculation also improved seedling development, and increased plant growth and yield. Furthermore, the two strains inhibit Fusarium growth in vitro. These Trichoderma spp. have thus the capacity to be used as organic fertilizers for wheat.
Wheat (Triticum sp. L.) is one of the most important crops in the world, ranking third behind corn and rice, while providing the basic nutrients (carbohydrates, proteins, vitamins, minerals, and fibers) to humans (Asseng et al., 2011). The world population is projected to exceed 8 billion by the end of 2022, and the demand for wheat is expected to exceed 880 million metric tons by 2050 (https://www.fao.org/worldfoodsituation/csdb/en/). Wheat culture, therefore, needs to be widened to overcome the world’s increasing demand. In addition, recent challenging crises, like the Covid-19 (coronavirus disease 2019) pandemic, and world conflicts negatively impact wheat production and will probably threaten wheat markets in the near future (Glauben et al., 2022; ASCAME, 2022). On the other hand, communities are requesting sustainable, cleaner, and harmless production approaches to avoid more climate change development. In this context, alternative strategies have to be followed, and finding new safer solutions is necessary in order, for example, to reduce the widespread use of chemical fertilizers, which negatively affect earth’s natural resources (Kannojia et al., 2017). As a result, recent studies have aimed to improve biofertilizers and plant bio stimulants using beneficial bacteria, yeasts, or fungi (Berruti et al., 2016; Kashyap et al., 2021). Among fungi, Trichoderma spp. are ubiquitous free-living fungi (Esposito and da Silva, 1998; Almi and Dehimat, 2016) of the Hypocreaceae family, occurring widely in all soils (Ghazanfar et al., 2018) and representing major components of the soil mycoflora (Widden and Abitbol, 1980; Kubicek et al., 2003). These ascomycete fungi, which inhabit root ecosystems, can grow and proliferate using carbohydrates secreted by plant roots. They can also behave as endophytes, penetrating the epidermis of the root tissue (rhizodermis), and few cell layers below (Topolovec-Pintarić, 2019). They proliferate better at mesophilic temperatures (15–35° C) (Carro-Huerga et al., 2021) and tolerate a wide pH range (Topolovec-Pintarić, 2019). These fungi improve plant photosynthetic capacity, and induce defenses (Vargas et al., 2011; Manzar et al., 2021) and many stress-sensitive genes (Brotman et al., 2012; Kashyap et al., 2022). Trichoderma are among the best studied biological control microbes, and are marketed as active ingredients in biofertilizers (plant growth promoters), biopesticides, bioremediates, and natural resistance stimulants. Consequently, their application reduces production costs, and negative environmental impacts (Dennis and Webster, 1971a; Dennis and Webster, 1971b; Harman and Kubicek, 2002; Ghazanfar et al., 2018). Trichoderma fungi improve plant growth through the solubilization of nutrients, and therefore have better effects under nutritional and abiotic stress conditions (Bononi et al., 2020; Chen et al., 2021). The use of Trichoderma in seed coating also improves physiological stresses such as aging, and seed dormancy (Delgado-Sánchez et al., 2010; Mastouri et al., 2010; Delgado-Sánchez et al., 2011). Trichoderma is also able to produce auxin, a plant hormone that controls many aspects of development including plant tissue growth, cell division, cell differentiation, and protein synthesis.
In this study we identified and characterized two Trichoderma strains from wheat plants and rhizosphere sampled in Lebanon. We then tested their capacity to behave as endophyte as well as their ability to promote wheat growth and yield.
The wheat cultivar ‘Apogee’ was used for fungal characterization (Li et al., 2017; Gatti et al., 2018). Apogee has a relatively brief life cycle under long days, and without vernalization, it flowers 25 days after planting.
Samples in this study were collected on May 2020 (late spring and early summer) from three different sites in Lebanon, namely: 1) The international center for agricultural research in the dry areas (ICARDA) -Terbol; 33.81263953421374, 35.99166470541601, 2) Lebanese agricultural research institute (LARI) – Tal Amara; 33.86500666714277, 35.984715713142634, and 3) Doueir; 33.39043029381532, 35.417987723093724. For every location, five samples from soil, together with five plant samples were screened for Trichoderma spp.
Soil samples were taken from the soil section called “horizon”, using a 0 to 15 cm top layer shovel, representing the surface area of the profile, where nutrients and most of the roots are present, and where nutrient absorption takes place. This also corresponds to the area where Trichoderma spp. are generally found. Sampling was carried out within a radius of 10 to 30 cm from the base of the plant depending on the case. Each sample was transferred in an appropriate plastic bag with its reference (place, date, sample number, etc.). Five samples were collected, and homogenized before the final packaging of approximately 1 kg of the sample, placed in plastic bags (to avoid contamination) was kept frozen (-20°C).
For plant sampling, 5-6 independent plant samples (root and stem) spaced at least 10 m apart were placed in plastic bags and kept frozen (-20°C).
1g of soil was homogenized in 10 ml sterile distilled water by thorough vortexing, then filtrated onto sterile Miracloth®. The filtrate was then plated onto 9 cm diameter petri dishes (100 ul filtrate plated per dish), containing potato dextrose agar (PDA) amended with rose Bengal (0.15 g/L), pentachloronitro-benzene (0.15 g/L), chloramphenicol (34 µg/ml), and ampicillin (100 µg/ml). One petri dish was plated per soil mixture. Plates were incubated at 26°c in the dark for 4 days
The internal colonization of roots or stems by potential Trichoderma spp. was detected following surface sterilization of approximately 2 cm root fragments for 10 sec in 95% ethanol, rinsed in sterile water for 10 sec, submerged for 20 sec in 2.5% sodium hypochlorite, then washed three times (2 times for 20 sec, and a third time for 60 sec) in sterile water. Fragments were then left to dry under laminar flow on sterile filter. 0.5 cm fragments were placed on PDA amended with rose Bengal, pentachloronitro- Benzene (0.15 g/L), chloramphenicol (34 µg/ml) and ampicillin (100 µg/ml). Plates were incubated at 24°C in the dark for 4-6 days, until fungal development. After 4 days, a large number of fungal strains appeared and were transferred (incubated at 26°C). Later, a 10-day-old, pre-incubated Petri dish (26°C) was selected, in order to make the 2nd transfer on PDA complemented with Rose Bengal (for Soil and Plant samples). A week after, purification was done by scraping half a colony, depending on the growth rate. Purification was done by plating 1/10, 1/100 or 1/1000 dilutions on PDA complemented with Rose Bengal. After 7 days of fungal development at 26°C, pieces of the grown fungus were transferred each into new Petri dish containing PDA media and again incubated at 26°C for further development.
DNA isolation: Liquid culture were done in potato dextrose broth (PDB) medium (50ml), with 5 plugs (agar + fungi) for 3 days (under agitation) according to (Atoui et al., 2012), with modifications as follows: for approximately 200 mg of powder (ball or mortar grinding). 1 ml of extraction buffer (200 mM Tris – HCl, pH 7.5; 288 mM NaCl; 25 mM EDTA, pH8.0; 0.5% SDS) was added and vortexed vigorously until homogenized. Tubes were centrifuged at 12,000 rpm for 5 minutes (4°C). 750 µL were then transferred to a new tube, and 215 µL of 3M potassium acetate were added, mixed and incubated on ice for 30 min, centrifuged at 12,000 rpm for 15 min at 4°C. 700 µL of the supernatant were collected, transferred to a new tube and 500 µL of cold isopropanol (-20°C) were added. Tubes were centrifuged at 12,000 rpm for 15 min at 4° C. The supernatant was removed, and the pellet washed with 500 µL of cold 70% ethanol (-20°C) for 5 min (4°C). The tubes were vortexed, and centrifuged at 12,000 rpm for 5 min at 4° C. The supernatant was removed, the pellet was briefly dried, and resuspended in 200 μL of sterile milliQ H2O + 1 mg/ml RNase A (1/10 stock solution at 10 mg/ml). The sample was incubated for 1h at 37° C, and DNA concentration was done using NanoDrop. As control, 2 to 5 µL of each sample was run on 0.8% agarose gel.
DNA sequencing: Further Trichoderma identification was done by sending purified PCR product for sanger sequencing to the Eurofins platform (Germany, https://eurofinsgenomics.eu/en/custom-dna-sequencing/). This was done through partial sequencing of the transcribed spacer sites (ITS) (550 bp), the Calmodulin gene (CAL) (458 bp) and the Elongation Factor (EF) gene (350 bp). The oligonucleotides used for amplification and sequencing are: for the calmodulin (CAL-228F: GAGTTCAAGGAGGCCTTCTCCC/CAL-737R: CATCTTTCTGGCCATCATGG), for the elongation factor gene (EF1-728F: CATCGAGAAGTTCGAGAAGG/EF1-986R: TACTTGAAGGAACCCTTACC), and for the internal transcribed space (ITS1: TCCGTAGGTGAACCTGCGG/ITS4: TCCTCCGCTTATTGATATTGC). The amplification conditions were made as follows: 2 min at 95°C; 30 s at 95°C, 30 s at 60°C and 1 min at 72°C for 35 cycles. Each PCR reaction contained a total 50 µl consisting of 10 µl of 5x greenTaq buffer, 2 µl of 10 µM dNTP, 1,25 U of GoTaq®DNA Polymerase, 2 µl of each primer at 10 µM. The nucleotide sequences were compared with those deposited in GenBank using the BLAST program on the NCBI website as well as the similarity index. The sequences were aligned and constructed using the CLUSTALW software (https://npsa-prabi.ibcp.fr/cgi-bin/npsa_automat.pl?page=/NPSA/npsa_clustalwan.html).
The phylogenetic tree was constructed using the MEGA-X program (Kumar et al., 2018). The sequences used correspond to a concatemer of the CAL, EF and ITS sequences. (Supplementary Table 1) shows detailed supplementary information for the strains used in the phylogenetic analyses, with their corresponding geographic origin, substrate, voucher number, and GenBank accession numbers. Briefly, the evolutionary history was inferred by using the Maximum Likelihood method, and Tamura-Nei model (Tamura and Nei, 1993). The bootstrap consensus tree, inferred from 1000 pseudoreplicates (Felsenstein, 1985), is taken to represent the evolutionary history of the taxa analyzed (Felsenstein, 1985). Branches showing bootstrap values lower that 50% were collapsed. The percentage of replicate trees, in which the associated taxa clustered together in the bootstrap test (1000 replicates) were shown next to the branches (Felsenstein, 1985). Initial tree (s) for the heuristic search were obtained automatically by applying Neighbor-Join, and BioNJ algorithms, to a matrix of pairwise distances, and were estimated using the Tamura-Nei model, and then the topology, with superior log likelihood value was selected. This analysis involved 41 nucleotide sequences.
The fungal strains were maintained on PDA with glucose at 26°C for 7 days. Microscopic visualization of Trichoderma conidiophores was done using fungi grown for 3 days on a PDA plate complemented with glucose (Glc). Briefly, the adhesive tape method was used in order to visualize the fungus. The fungus was grown for 3 days and a piece of adhesive tape was added over the fungus in order to stick them for visualization under the microscope.
The endophytic capacity was tested using the Apogee spring wheat variety. The endophytism behavior of the 2 Trichoderma strains was examined using confocal microscopy. Two methods of inoculation were used and described below as the PDA inoculation method and the spore inoculation method. This experiment was repeated twice, and 5 samples were taken for each condition (with and without Trichoderma) for analysis.
For the PDA inoculation method, the seed sterilization protocol was modified from Jaber (2018). Seeds were soaked for 5 min with bleach (0.6% sodium hypochlorite) with stirring (150-200 rpm), then rinsed three times for 10 min with sterile milliQ water. They were then stratified for 3 to 5 days in the dark at 4°C, in distilled sterilized water. The inoculation was done on a PDA with Ampicillin (100 μg/ml) and Chloramphenicol (34 μg/ml) dish. Five wheat seeds were spread over a circle of 3 cm diameter and cultured at 26°C and in the dark. 24 hours later, a Trichoderma plug was added (7 days of culture on PDA) in the center of the petri dish and incubated at 26°C. Finally, roots were harvested after 5 days of culture.
For the spore inoculation method, the seed sterilization was carried out as mentioned above. The coating of the seeds with Trichoderma spores was then done as follows: the seeds and a spore solution coming from a 7-day-old Trichoderma colony on plate. Briefly, the spores were rubbed off using sterile distilled water and then were filtered in order to remove the germinated hyphae. This was followed by measuring the concentration using Thoma’s-cell slide to get a 107 spores/ml final concentration. The solution was placed under shaking for 20-30 min, and then dried for a few minutes under the hood, before sowing. Seeds were then sown in glass tubes containing ½ MS (2.2g/L) supplemented with phytagel (2g/L).
Root samples treatment for imaging: After 5 days of incubation, roots were collected, gently cleaned from the excess of external fungal colonization, cut in 1.5/2.0 cm length, and washed with water or PBS 1X: 20 min. Root fragments were fixed overnight in a mixture of 95% Ethanol - 100% acetic acid (3: 1) (v: v). Samples were then stabilized for 1h in 1X PBS stabilizing solution.
Roots were included in 1X PBS with 3% agarose for 5 min (“Seaken LE Agarose for electrophoresis”) (0.6 g of agarose in 20 ml). For staining, cross-sections were immersed in the staining solution (20 µg/ml propidium iodide; 10µg/ml WGA-Alexa Fluor 488, 0.02% Tween 20 made up in 1X PBS) for 2 min, immersed in water for few seconds to remove the staining solution excess. Cross sections of wheat roots were visualized to check endophytism using Confocal microscope (Zeiss LSM880).
To test the effect of the Trichoderma strains on wheat root development, Trichoderma spores (107 spores/10 ml) were incubated with wheat seeds in a tube containing 0.5X MS medium (Kapusi and Stoger, 2022) with phytagel without sugar. 10 days after germination, we measured the primary root growth. To evaluate wheat yield under controlled conditions, thirty-six 10-day-old plantlets, from seeds, pre-cultured on both, PDA (PDA approach), and phytagel ½ MS media (tube approach), were used. 32 plantlets from each treatment (with, and without Trichoderma) were transplanted to sterilized peatmoss soil (www.jiffygroup.com- Substrates for the professional horticulture) with perlite (3:1). All plants were kept in a growth chamber, under 16h: 8h light to dark period, with 265 μE m−2 s−1, at 22 °C, and 20 °C, day to night temperatures, and 60% relative humidity (RH). Regular irrigation was practiced, in parallel with fertilization practices at 14:12:32 N:P:K, plus 41 g Hortrilon micronutrients (B 0.5%, Cu 2.5%, Fe 5.0%, Mn 2.5%, Mo 0.5%, and Zn 0.6%) (https://www.fertil.fr/).
Wheat yield and yield components, including number of spikes/plants, number of grains/plants, grain weight/plant (g), whole plant dry weight (g), hundred grain weight (g), and harvesting index (HI%) were then measured.
Auxin production was measured using the Gusmiaty method (Gusmiaty et al., 2019) with some modifications in presence or absence of tryptophan.
Briefly, five plugs of each Trichoderma strain with, and without tryptophan were incubated in 0.5X MS liquid medium (2151,45 mg/L), 2-(N-morpholino) ethanesulfonic acid (MES) (0.25 g/L), glucose 2%, pH = 5.78 adjusted with 1M KOH (3 biological repeats).
To determine the auxin concentration (Indole-3-acetic acid or IAA in our case), Salkowski reagent, and IAA standard curve were done, according to (Gusmiaty et al., 2019). A standard IAA solution curve showing the relationship between the standard IAA solution (x), and its absorbance (y), was made, and allowed the quantification of IAA production by Trichoderma using the following equation: Y= a+ bX (a = Intersep, b = Slope (Regression Coefficient), Y = Absorbance, X = Concentration).
Total protein extracts were prepared from wheat grains grounded in liquid nitrogen using a mortar and pestle. From 50 mg of flour, total soluble proteins were extracted at room temperature in 960 μl thiourea/urea lysis buffer (Harder et al., 1999) containing 7 M urea, 2 M thiourea, 6 mM Tris-HCl, 4.2 mM Trizma® base (Sigma-Aldrich, Lyon, France), 4% (w/v) CHAPS) supplemented with 162 μl of the protease inhibitor cocktail Complete Mini (Roche Diagnostics France, Meylan, France). Then, 22,5 μl of dithiothreitol (DTT, 1 M, Sigma-Aldrich), 4 μl of DNase I (Roche Diagnostics) and 10 μl of RNase A (Sigma-Aldrich) were added to the sample. For each sample, the protein extract was stirred for 30 min at 4°C and then centrifuged (35,000g, 15 min) at 4°C. The supernatant was submitted to a second clarifying centrifugation as above. The final supernatant corresponded to the total protein extract. Protein concentration in each extract was measured according to Bradford (1976). Bovine serum albumin was used as a standard. Separation of proteins was performed by sodium dodecyl sulphate polyacrylamide gel electrophoresis (SDS-PAGE) with a 4% (w/v) polyacrylamide stacking gel and a 12% (w/v) polyacrylamide resolving gel as described by Laemmli (1970). Electrophoresis was carried out at a constant current of 110 volts (10 mA) for 2 h using a Tris-glycine running buffer with of 0.2%. After electrophoresis, proteins were stained with GelCode® Blue Stain Reagent (Thermo Scientific, Rockford, IL).
The capacity of the two Trichoderma strains to inhibit growth of another fungus was assessed against the plant pathogen Fusarium graminearum. Antagonism was tested by growth inhibition using 12 cm square plates. A plug of each fungus was placed in opposite corners on the plate and the growth inhibition was calculated by comparing the fungal growth (colony radius) from a plate inoculated with only one fungus. The growth medium was PDA.
Statistical analysis was conducted for the treatments, on a completely randomized design (CRD), and a factorial completely randomized design, with 32 replicates. The R software (R 3.3.4; R development core team, 2017) was used to analyze experimental data, calculate means, standard deviations, bar charts, and interaction, using the R package “ggplot2” (Wickham, 2009). Analysis of variance (ANOVA) was performed using the “lmerTest” R package (Kuznetsova et al., 2017).
In order to isolate Trichoderma fungi associated to wheat in Lebanon, 40 fungal isolates were isolated in total from three sampling sites. 21 were isolated from soil and 19 were isolated from plants. DNA was prepared from them and the ITS sequences amplified and sequenced. Based on the ITS sequences from these 40 colonies, 2 fungal isolates of the genus Trichoderma were identified (Supplementary Table 2). The two isolates were derived from site 3 (Doueir) and were named S3PA (isolated from stem base), and S3SB (isolated from soil). The other isolates included fungi from the genus: Alternaria, Aspergillus, Boeremia, Cladorrhinum, Fusarium, Macrophomina, Microdochium, Mucor, Penicillium, Stromatinia, and Talaromyces (Supplementary Table 2).
A more precise molecular description of these two Trichoderma fungi was done by characterizing the partial sequences of their Elongation factor (EF-1) and Calmodulin (Cal) gene sequences. The three partial sequences (ITS, EF and Cal) were then concatemerized (550, 350, and 489 bp, respectively) and compared to the same concatemerized sequences of 39 strains representing 14 Trichoderma species (Supplementary Table 1) from the public database and representing the Trichoderma diversity. The result of this analysis (Figure 1) showed that the two isolates probably belong to the two species T. afroharzianum and T. guizhouense belonging to the Harzianum species complex.
Figure 1 Phylogenetic tree of CAL-EF-ITS branch includes only species in the T. harzianum complex. Two species are identified from Lebanon and are placed in this tree: T. afroharzianum (T. S3PA) and T. guizhouense (T. S3SB). Strains used in this phylogenetic analysis are described in Supplementary Table 1 with their corresponding geographic origin, substrate, voucher number and GenBank accession numbers. T. agressivum is used as an outgroup in this analysis.
After 48h at 26°C on PDA, the T. guizhouense formed an abundant aerial mycelium, cottony and radiating a white color. At 72 h, the formation of a greenish ring was observed, which increased by time of incubation, and diffused in the media, until covering almost the whole petri dish at 168 h. The conidia were visualized from 48h in broad concentric bands (green), in the aerial hyphae (Figure 2A). After 72h of growth on PDA (26°C), T. afroharzianum produced an abundant aerial mycelium, cottony, radiating a white color (Figure 2B). A dense hyphae growth in the petri dish center was observed after transplantation of Trichoderma, while 96 h later, the formation of a greenish ring was observed, which increased with the time of incubation, and diffused in the media, until covering almost the whole petri dish at 168 h.
Figure 2 Growth of T. guizhouense (A) and T. afroharzianum (B) for one week on PDA supplemented with glucose. The age of the culture is indicated below the picture in hours. Microscopic visualization of conidiophores of T. guizhouense (C) T. afroharzianum (D) (x1000). (I) hyphae, (II) conidiophores, (III) Phialides and (IV) conidia (the scale bar corresponds to 10μm).
We then morphologically characterized the two newly isolated strains. After 3 days, pyramidal conidiophores were repeatedly branched, irregularly arranged in whorls, and showed clusters of divergent, usually unevenly folded, barrel shaped to nearly sub-globose phialides. The ellipsoidal to globose conidia tend to be greenish to light turquoise, sometimes hyaline to cluster in aggregates at the terminal of the phialides (Zhu and Zhuang, 2015). Conidiophores of T. guizhouense, showed opposite branches. The main axis and each branch terminated at a cruciate whorl of 3 ampulliform phialides (Figure 2C). Conidia, appearing within 48–72 h, were typically abundant, and disposed in one, or three concentric rings, around the point of inoculation. Green pigment sometimes diffused in the medium (Figure 2B). After 3 days of incubation on PDA, the pyramidal conidiophores of T. afroharzianum showed opposite branches. The main axis, and each branch terminated at a cruciate whorl of 3 ampulliform phialides green to dark green when aging (Figure 2D).
The two isolated strains thus grow rapidly on PDA medium with phenotypic characteristics of other Trichoderma stains (Chaverri et al., 2015).
Trichoderma fungi were previously described as endophytes (Tseng et al., 2020). In order to know if the two Lebanese strains isolated from wheat behave also as endophytes, two modes of inoculation were tested, either by inoculating the seeds by co-cultivation on PDA medium (PDA inoculation method), or by spore coating of the seeds and germination of the seeds in vitro (spore inoculation method, see Material and Method section). The difference between the two methods is that in the PDA method the seeds were inoculated with undetermined high concentrations of spores and fungi whereas with the spore coating method the spore number was controlled. In both cases the root sections of the plants were stained with propidium iodide and WGA-Alexa Fluor 488, staining the fungus in green. These sections were observed using confocal microscopy. Using both inoculation methods, fungal hyphae were observed inside the root for the two Trichoderma strains (Figure 3). The infection level observed using the PDA inoculation method seemed to be higher than the spore inoculation method that had a lower concentration of the spore solution. In addition, T. guizhouense was isolated from soil infected rhizodermis and cortex cells using the first methods (Figures 3A, B) and mostly rhizodermis using spore coating (Figures 3E, F). The T. afroharzianum strain appears less efficient in root colonization, invading mostly the rhizodermis cell layer using the PDA inoculation (Figures 3C, D) and colonizing only the root surface using spore coating (Figures 3G, H). Using leaves of 4-weeks-old plants, we were unable to reisolate the inoculated fungi, suggesting that these Trichoderma strains are not able to colonize the aerial part of the plant.
Figure 3 (A–D) cross section of wheat root seedlings inoculated with T. guizhouense (A, B) or T. afroharzianum (C, D) using the PDA inoculation method. E-H: cross section of wheat root seedlings inoculated with T. guizhouense (E, F), with T. afroharzianum (G, H) using the spore inoculation method. (Ep) epidermis, (C) cortex (Cc) central cylinder, (Hy) fungal hyphae. The fungal hyphae are stained green using WGA-Alexa Fluor 488, and the root cell walls are stained red using propidium iodide, (BDFH correspond to the boxes of ACEG respectively).
All together this experiment shows that the two strains can behave as fungal wheat root endophytes but that T. guizhouense can invade root tissue more efficiently than T. afroharzianum and that the inoculation method can influence the level of colonization.
Trichoderma strains have been often used as plant growth promoting microorganisms (Tseng et al., 2020). In order to know if the two strains studied here can also behave as plant growth promoting fungi (PGPF), we tested their growth promotion effect on 36 young seedlings in vitro, using the spore coating method and ½ MS medium without sucrose.
This experiment shows that the presence of the two Trichoderma strain resulted in a significant increase of the primary, and secondary root growth, as well as the aerial part of the plant as early as 10 days post germination (Figure 4A and Supplementary Figure 1). Total root, and aerial length (cm) of inoculated wheat plants, with and without T. guizhouense and T. afroharzianum respectively, is shown in Figure 4B. The results indicated that plants incubated with either T. guizhouense or T. afroharzianum had increased root growth and aerial development as compared to non-inoculated plants. Root length was increased by 3.5x and 3.5x for plants inoculated by T. afroharzianum T. guizhouense respectively. For the aerial part we observed a 1.5x increase in length with both fungi. In this experiment, the inoculation by the Trichoderma strains enhanced principal root length (2.5x), total root length (3.4x), and aerial part lengths (1.5x) as well as total aerial plant weight (Figure 4B and Supplementary Figures 1A, B).
Figure 4 Growth of roots and aerial parts after 10 days of culture, with and without Trichoderma (A); I) not inoculated, II) a representative seedling inoculated with T. guizhouense, III) a representative seedling inoculated with T. afroharzianum, (B) Total root, and aerial length (cm) of non-inoculated (N.I.) or inoculated wheat plants with T. afroharzianum (T.A) and T. guizhouense (T.G), respectively, (C) IAA production capability of the two Trichoderma isolates (T.G and T.A) with and without tryptophan. Values in the same graph having different letters are significantly different, at P ≤ 0.01 (ANOVA test).
Total root, and aerial mass of wheat plants, with and without T. guizhouense and T. afroharzianum respectively are shown in Supplementary Figure 1B. The inoculation with T. afroharzianum resulted in an increase of 2.6x for the total root mass and 1.9x, for total aerial mass, while the treatment with T. guizhouense resulted in an increase of 2.7x for total root mass and 2x, for the aerial mass.
We further measured the principal root length of inoculated wheat plants over a 10 days period (kinetics), with and without T. guizhouense or T. afroharzianum. The root growth promoting effect of the two strains was already detectable 2 days after sawing and continued to increase over the 10 days period (Supplementary Figure 1B).
The increased root growth observed in presence of the two strains might result from fungal auxin production. We thus measured auxin production by the two Trichoderma strains in culture, in presence and absence of tryptophan, an auxin precursor. This experiment (Figure 4C) shows that the two strains can produce auxin in culture and that addition of tryptophan enhances IAA production by 1.2x, and 1.4x, for T. guizhouense and T. afroharzianum respectively.
These experiments show that wheat inoculation by the Trichoderma Lebanese strains enhances root growth and this might result from auxin production by the fungi.
As shown above, Trichoderma inoculation by the two Trichoderma strains enhanced wheat seedling growth. We thus tested their effect on the complete development of the wheat plants grown in soil. The two inoculation methods described above were used before plant transplantation in pots but no significant difference could be observed as a result of the inoculation method. Plants were grown to maturity and the different wheat yield components were measured (Figure 5; Supplementary Figure 2). This analysis showed that the plant weight (Figure 5A), the number of spikes per plant (Figure 5B), the total grain weight per plant (Figure 5C) and the grain number per plant (Figure 5D) increased following inoculation by the two fungi. In contrast the 100 grains weight remained unchanged (Supplementary Figure 2A). Altogether, yield harvest index (HI%) increased around 1.1x (PDA inoculation method) and 1.2x (spore inoculation method) by the fungal inoculations (Supplementary Figure 2B).
Figure 5 Yield parameters: (A) Total dry plant weight (g)/plant; (B) Number of spikes/plant; (C) Total grains weight (g)/plant; (D) Number of grains/plant, as influenced by PDA and spore inoculation methods. N.I.: T.A: wheat plants inoculated with T. afroharzianum; T.G: wheat plants inoculated with T. guizhouense Values in the same graph having different letters are significantly different, at P ≤ 0.01 (ANOVA test). 32 plants were analyzed per sample.
In order to know whether fungal inoculation could change seed protein quantity as well as the seed protein pattern of the grains we analyzed them in plants inoculated by the two strains and following the two inoculation methods. As shown in Supplementary Figure 2C neither the protein contents nor the protein profiles were modified in the wheat grains by the Trichoderma inoculation.
Thus, inoculation of plants, independently of the method used increased wheat yield including number of spikes/plants, number of grains/plants, grain weight/plant, whole plant dry weight, and harvesting index (HI%), but did not modify the seed protein content.
In order to know if the two Lebanese strains also possess antifungal activity, we tested their growth inhibition activity against F. graminearum in vitro. To do this, a plug of a fungal colony was place in one corner of a square petri dish plate (12cm) containing PDA. To test the inhibition, the two fungi were inoculated on opposite corners (Figure 6A). Doing this, the contact between the two fungi was observed at day 7 (contact day). Beyond this period and after 10 days, T. guizhouense and T. afroharzianum invades the colonies of F. graminearum and even sporulates on them (6A). The percentage of growth inhibition was calculated using plates only inoculated by F. graminearum and measuring the radius of the colony each day. This experiment shows that T. guizhouense and T. afroharzianum inhibit F. graminearum growth even before the contact between the fungal colonies, and that the % of inhibition of mycelial growth reached 50% and 58% respectively, after 7 days (contact day; Figure 6B).
Figure 6 Growth antagonism: (A) Antagonistic effect of T. guizhouense (TG) and T. afroharzianum (TA) against the plant pathogenic fungi, Fusarium graminearum (FG), 10 days post inoculation. (B) Kinetics of mycelial growth inhibition of F. graminearum in the presence of T. guizhouense and T. afroharzianum. The inhibition is expressed as the percentage (%) of growth inhibition compared to plates inoculated with only one fungus. Fungi were inoculated in opposite corners of the square petri dishes.
Plants interact with many microorganisms in nature and it is believed that a better understanding of these interactions can help develop sustainable agriculture less dependent on pesticides and polluting fertilizers. Among interacting microorganisms, some are deleterious (pathogens) but others are beneficial (Singh et al., 2018; Kakabouki et al., 2021). The fungus Trichoderma is widely studied as a beneficial microorganism, promoting plant growth but also protecting plants against pathogens through a variety of mechanisms (Stewart and Hill, 2014; Mukhopadhyay and Kumar, 2020; Sood et al., 2020; Shahriar et al., 2022). Among crops, wheat is one of the major staple foods around the world. Previous studies have shown that it can benefit from interaction with Trichoderma fungi for growth promotion or pathogen protection (TariqJaveed et al., 2021).
In this study we have isolated two Trichoderma strains from Lebanese soils, characterized them, and evaluated their growth promotion effect on wheat. These two strains were isolated amongst 40 fungal isolates from soil or plant tissues. The storage and/or isolation methods can explain the low frequency (5%) of Trichoderma strains isolated in this experiment. Molecular and taxonomic characterization showed that the two strains are T. guizhouense, and T. afroharzianum isolates belonging to the Harzianum complex (Chaverri et al., 2015; Stummer et al., 2020). The morphology of the two strains is in agreement with Trichoderma morphology (Zhu and Zhuang, 2015). In addition, these two isolates were also able to colonize the root rhizoderm, and external cortex, while markedly enhancing seedling root growth. In contrast, our study suggested that the two Lebanese isolates lack the ability to colonize wheat aerial tissues under the conditions used. Growth conditions might influence colonization as the T. afroharzanium strain was isolated from the base of the aerial part of a field grown plant.
The influence of the two Trichoderma strains inoculation on wheat root development suggested that they may produce auxin (Nieto-Jacobo et al., 2017). IAA is the main auxin promoting root system development, and able to modify root architecture in the Trichoderma plant interaction (Illescas et al., 2021). Additional Trichoderma metabolites, and proteins (effectors) are also known to significantly modulate IAA production in plants, resulting in root hair growth and enhanced root mass development (Illescas et al., 2021). IAA biosynthesis in fungi is tryptophan-dependent, and supplying this amino acid to the medium, increases IAA production (Nieto-Jacobo et al., 2017). We indeed could enhance IAA production in culture of T. afroharzianum, and T. guizhouense by adding tryptophan to the medium. These results are in agreement with previous studies showing that auxin-generating Trichoderma strains interact with wheat and other plants (Pelagio-Flores et al., 2017; Illescas et al., 2021).
Approaches of inoculating Trichoderma to promote plant growth varied between seed/seedling treatments, soil processing, and foliar spray (Bhandari et al., 2021). Recent studies caried out on model plants like Arabidopsis thaliana inoculated by T. atroviride, and T. guizhouense strains showed that Trichoderma volatiles increased endogenous sugar contents in shoots, roots and root exudates, and could enhance Arabidopsis root development, root architecture, and promote the symbiosis efficiency. These effects were suggested to be related to auxin transport and signaling (Esparza-Reynoso et al., 2021; Li et al., 2022). Trichoderma fungi can also stimulate root development and modify plant metabolism, by releasing numerous secondary compounds (Kakabouki et al., 2021). For example, harzianic acid is one of T. harzianum metabolites that is responsible for promoting plant growth (Vinale et al., 2014; Xie et al., 2021) and acting as biocontrol agent. Further studies will indicate which mechanisms are responsible for the wheat growth promotion by our two strains.
In this work we tested the ability of T. afroharzianum and T. guizhouense to enhance wheat growth (plant growth promotion) using two seed inoculation methods: 1) PDA mediated inoculation, and 2) spore inoculation. Our results showed a notable increased in seedling development during the first 10 days following seed inoculation by the T. afroharzianum, and T. guizhouense fungi (Figures 4A, B). This result is in agreement with previous studies (Kucuk, 2014), which showed that root and shoot growth of wheat could be improved by T. harzianum, isolated from Turkey.
Remarkably, the wheat seedling growth was greatly increased when plants were inoculated by the two Lebanese strains, indicating that the interaction can be established very early during wheat development. Similar effects were observed when inoculating seedlings with other Trichoderma spp. (Mahato, et al., 2018; Oliveira et al., 2018; Anjum et al., 2020; Kthiri et al., 2020). These studies indicated that many growth or yield parameters like the percentage of germination, root length, shoot length, total length, fresh root mass, fresh shoot mass, total fresh mass, dry root mass, dry shoot mass, total biomass, root mass ratio, shoot mass ratio and aerial part/root system ratio, were augmented. Similarly, T. yunnanense, and T. afroharzianum isolates produced IAA under salt stress (200 mM), and could stimulate photosynthesis, water utilization, and growth of wheat in saline conditions (Zhang et al., 2019; Oljira et al., 2020).
Our study is also in agreement with previous studies (Colla et al., 2015; Silletti et al., 2021), where seed coating encouraged seed germination, and the quality of yield and grain, including protein and mineral composition in durum wheat. Xue et al. (2017) inoculated six different Trichoderma strains on wheat seedlings and found that the six strains had the ability of increasing plant dry weight, and total yield, over a three-year experiment. This is in line with our data showing that treatment with the two Lebanese Trichoderma strains could significantly affect plant yield. The HI% indicated an increased yield, as a response to treatments with both T. guizhouense and T. afroharzianum strains, but T. afroharzianum showed higher impact on yield and its components. The fungal growth promotion effects are not completely described but it is known that Trichoderma can stimulate plant food scanning by enhancing root elongation in order to invade more, either shallow surface, or even deeper underneath areas, seeking for nutrients uptake. This capacity probably essentially relies on auxin production (Zin and Badaluddin, 2020). In agreement with this, in our study T. afroharzianum and T. guizhouense isolated strains strongly promoted primary and secondary root elongation.
Protein content of grains is an important agronomic character for wheat (Illescas et al., 2022). Protein analysis of the grains revealed that in our experiments the inoculation by Trichoderma spp. did not modify their protein nature and content. This shows that Trichoderma can promote wheat growth and enhance grain production without changing the grain protein composition. This observation might be correlated with the other part of our study, indicating that the weight of a hundred grains is not changed despite yield increase.
The growth inhibition against F. graminearum in vitro indicates that like other Trichoderma strains, the two Lebanese strains can probably be used as biocontrol agents. Further experiments will be needed to show that these biological control activities are relevant in field conditions.
In conclusion, in this study, two Trichoderma strains were isolated from wheat plants and wheat rhizospheric soil in Lebanon. These fungi were identified as T. guizhouense and T. afroharzianum and behaves as root endophytes. In addition, they promote wheat seedling growth and yield.
The datasets presented in this study can be found in online repositories. The names of the repository/repositories and accession number(s) can be found in the article/Supplementary Material.
NM, PR, MD, FA-S and NB conceived and designed the experiments. NM, CM, PR, MD, GA, AP, BC and LR conducted the experiments and analyses. PR, NM, GA, and FA drafted the manuscript. All authors have read and approved the final version of the manuscript.
This work was funded by the ‘AUF-CNRSL-UL’, Lebanon Francophonie University Agency (AUF), the National Council for Scientific Research in Lebanon (CNRS-L) and the Lebanese University (UL). This work was also supported by the CNRS and has benefited from the support of the LabEx Saclay Plant Sciences (ANR-10-LABX-0040-SPS, LabEx SPS and ANR-17-EUR-0007, EUR SPS-GSR) which is managed by the French National Research Agency under the program ‘Investissements d’avenir’ (ANR-11-IDEX-0003-02) and the Plant2Pro, Carnot Institute project TrichoKissCool.
The authors would like to thank ICARDA and LARI for supporting this research by providing samples and materials. Also thanks to Dr Muhsin Parson for careful reading and editing of the manuscript.
The authors declare that the research was conducted in the absence of any commercial or financial relationships that could be construed as a potential conflict of interest.
All claims expressed in this article are solely those of the authors and do not necessarily represent those of their affiliated organizations, or those of the publisher, the editors and the reviewers. Any product that may be evaluated in this article, or claim that may be made by its manufacturer, is not guaranteed or endorsed by the publisher.
The Supplementary Material for this article can be found online at: https://www.frontiersin.org/articles/10.3389/fpls.2022.1017890/full#supplementary-material
Almi, H., Dehimat, L. (2016) Etude des myco-pathogènes de Lens culinaris et évaluation de l’effet de deux souches de Trichoderma harzianum. Available at: http://depot.umc.edu.dz/handle/123456789/5076.
Anjum, A., Yazid, M. D. I., Fauzi Daud, M., Idris, J., Ng, A. M. H., Naicker, S., et al. (2020). Spinal cord injury: Pathophysiology, multimolecular interactions, and underlying recovery mechanisms. Int. J. Mol. Sci. 21 (20), 7533. doi: 10.3390/ijms21207533
ASCAME. (2022). “Association des Chambres de Commerce et d'Industrie de la Méditerranée,” in COVID-19 and the Russian invasion of Ukraine. Available at: https://www.ascame.org/wp-content/uploads/2022/04/COVID-19-and-the-Russianinvasion-of-Ukraine-2.pdf.
Asseng, S., Foster, I., Turner, N. C. (2011). The impact of temperature variability on wheat yields. Global Change Biol. 17 (2), 997−1012. doi: 10.1111/j.1365-2486.2010.02262.x
Atoui, A., El Khoury, A., Kallassy, M., Lebrihi, A. (2012). Quantification of Fusarium graminearum and Fusarium culmorum by real-time PCR system and zearalenone assessment in maize. Int. J. Food Microbiol. 154 (1−2), 59−65. doi: 10.1016/j.ijfoodmicro.2011.12.022
Berruti, A., Lumini, E., Balestrini, R., Bianciotto, V. (2016). Arbuscular Mycorrhizal Fungi as Natural Biofertilizers: Let’s Benefit from Past Successes. Front. Microbiol. 6. doi: 10.3389/fmicb.2015.01559
Bhandari, S., Pandey, K. R., Joshi, Y., Lamichhane, S. (2021). An overview of multifaceted role of Trichoderma spp. For sustainable agriculture. Arch. Agric. Environ. Sci. 6 (1), 72–79. doi: 10.26832/24566632.2021.0601010
Bononi, L., Chiaramonte, J. B., Pansa, C. C., Moitinho, M. A., Melo, I. S. (2020). Phosphorus-solubilizing Trichoderma spp. from Amazon soils improve soybean plant growth. Sci. Rep. 10 2858 (1). doi: 10.1038/s41598-020-59793-8
Bradford, M. M. (1976). A rapid and sensitive method for the quantitation of microgram quantities of protein utilizing the principle of protein-dye binding. Anal. Biochem. 72, 248–254. doi: 10.1006/abio.1976.9999
Brotman, Y., Lisec, J., Méret, M., Chet, I., Willmitzer, L., Viterbo, A. (2012). Transcript and metabolite analysis of the Trichoderma-induced systemic resistance response to Pseudomonas syringae in Arabidopsis thaliana. Microbiol. (Reading England) 158 (Pt 1), 139−146. doi: 10.1099/mic.0.052621-0
Carro-Huerga, G., Mayo-Prieto, S., Rodríguez-González, Á., Álvarez-García, S., Gutiérrez, S., Casquero, P. A. (2021). The Influence of Temperature on the Growth, Sporulation, Colonization, and Survival of Trichoderma spp. In Grapevine Pruning Wounds. Agronomy. doi: 10.3390/agronomy11091771
Chaverri, P., Branco-Rocha, F., Jaklitsch, W., Gazis, R., Degenkolb, T., Samuels, G. J. (2015). Systematics of the Trichoderma harzianum species complex and the re-identification of commercial biocontrol strains. Mycologia 107 (3), 558−590. doi: 10.3852/14-147
Chen, D., Hou, O., Jia, L., Sun, K. (2021). Combined Use of Two Trichoderma Strains to Promote Growth of Pakchoi (Brassica chinensis L.). Agronomy 11, 726. doi: 10.3390/agronomy11040726
Colla, G., Rouphael, J., Bonini, P., Cardarelli, M. (2015). Coating seeds with endophytic fungi enhances growth, nutrient uptake, yield and grain quality of winter wheat. Int. J. Plant Production 9, 171−189. doi: 10.22069/IJPP.2015.2042
Delgado-Sánchez, P., Ortega-Amaro, M. A., Jiménez-Bremont, J. F., Flores, J. (2011). Are fungi important for breaking seed dormancy in desert species? Experimental evidence in Opuntia streptacantha (Cactaceae). Plant Biol. (Stuttgart Germany) 13 (1), 154−159. doi: 10.1111/j.1438-8677.2010.00333.x
Delgado-Sánchez, P., Ortega, A., Rodríguez-Hernánde, A. A., Jimenez, J. F., Flores, J. (2010). Further evidence from the effect of fungi on breaking Opuntia seed dormancy. Plant Signaling Behav. 5 (10), 1229−1230. doi: 10.4161/psb.5.10.12835
Dennis, C., Webster, J. (1971a). Antagonistic properties of species-groups of Trichoderma : I. production of non-volatile antibiotics. Trans. Br. Mycological Soc. 57 (1), 25–IN3. doi: 10.1016/S0007-1536(71)80077-3
Dennis, C., Webster, J. (1971b). Antagonistic properties of species-groups of Trichoderma : II. production of volatile antibiotics. Trans. Br. Mycological Soc. 57 (1), 41–IN4. doi: 10.1016/S0007-1536(71)80078-5
Esparza-Reynoso, S., Ruíz-Herrera, L. F., Pelagio-Flores, R., Macías-Rodríguez, L. I., Martínez-Trujillo, M., López-Coria, M., et al. (2021). Trichoderma atroviride-emitted volatiles improve growth of Arabidopsis seedlings through modulation of sucrose transport and metabolism. Plant Cell Environ. 44 (6), 1961−1976. doi: 10.1111/pce.14014
Esposito, E., da Silva, M. (1998). Systematics and Environmental Application of the Genus Trichoderma. Crit. Rev. Microbiol. 24 (2), 89–98. doi: 10.1080/10408419891294190
Felsenstein, J. (1985). Confidence Limits on Phylogenies: An Approach Using the Bootstrap. Evolution 39 (4), 783−791. doi: 10.2307/2408678
Gatti, M., Choulet, F., Macadré, C., Guérard, F., Seng, J.-M., Langin, T., et al. (2018). Identification, molecular cloning and functional characterization of a wheat UDP-glucosyltransferase involved in deoxynivalenol glucosylation and resistance to Fusarium Head Blight. Front. Plant Sci. 9. doi: 10.3389/fpls.2018.0185
Ghazanfar, M., Raza, M., Raza, W., Qamar, M. (2018). Trichoderma as potential biocontrol agent, its exploitation in agriculture: A review. Plant Prot. 2 (3), 109–135.
Glauben, T., Svanidze, M., Götz, L., Prehn, S., Jamali Jaghdani, T., Djuric, I., et al. (2022). The War in Ukraine, Agricultural Trade and Risks to Global Food Security. Intereconomics 57, 157−163. doi: 10.1007/s10272-022-1052-7
Gusmiaty, Restu, A. M., Payangan, R. Y. (2019). Production of IAA (Indole Acetic Acid) of the rhizosphere fungus in the Suren community forest stand. IOP Conf. Series: Earth Environ. Sci. 343, 12058. doi: 10.1088/1755-1315/343/1/012058
Harder, A., Wildgruber, R., Nawrocki, A., Fey, S. J., Larsen, P. M., Görg, A. (1999). Comparison of yeast cell protein solubilization procedures for two dimensional electrophoresis. Electrophoresis 20, 826–829.
Harman, G. E., Kubicek, C. P. (2002). “Trichoderma And Gliocladium,” in Basic Biology, Taxonomy and Genetics, vol. 1. (London: CRC Press). doi: 10.1201/9781482295320
Illescas, M., Morán-Diez, M. E., Martínez de Alba, Á. E., Hermosa, R., Monte, E. (2022). Effect of Trichoderma asperellum on Wheat Plants’ Biochemical and Molecular Responses, and Yield under Different Water Stress Conditions. Int. J. Mol. Sci. 23 (12), 6782. doi: 10.3390/ijms23126782
Illescas, M., Pedrero-Méndez, A., Pitorini-Bovolini, M., Hermosa, R., Monte, E. (2021). Phytohormone Production Profiles in Trichoderma Species and Their Relationship to Wheat Plant Responses to Water Stress. Pathogens 10 (8), 991. doi: 10.3390/pathogens10080991
Jaber, L. R. (2018). Seed inoculation with endophytic fungal entomopathogens promotes plant growth and reduces crown and root rot (CRR) caused by Fusarium culmorum in wheat. Planta 248 (6), 1525−1535. doi: 10.1007/s00425-018-2991-x
Kakabouki, I., Tataridas, A., Mavroeidis, A., Kousta, A., Karydogianni, S., Zisi, C., et al. (2021). Effect of Colonization of Trichoderma harzianum on Growth Development and CBD Content of Hemp (Cannabis sativa L.) 9, 518, 518. doi: 10.3390/microorganisms9030518
Kannojia, P., Sharma, P. K., Kashyap, A. K., Manzar, N., Singh, U. B., Chaudhary, K., et al. (2017). “Microbe-Mediated Biotic Stress Management in Plants,” in book: Plant-Microbe Interactions in Agro-Ecological Perspectives (Singapore: Springer), 627–648. doi: 10.1007/978-981-10-6593-4_26
Kapusi, E., Stoger, E. (2022). (Éds.). Recombinant Proteins Plants: Methods Protoc. (New York, NY, US: Springer), 49−60. doi: 10.1007/978-1-0716-2241-4_3
Kashyap, A. S., Manzar, N., Nebapure, S. M., Rajawat, M. V. S., Deo, M. M., Singh, J. P., et al. (2022). Unraveling microbial volatile elicitors using a transparent methodology for induction of systemic resistance and regulation of antioxidant genes at expression levels in Chili against bacterial wilt disease. Antioxidants (Basel) 11 (2), 404. doi: 10.3390/antiox11020404
Kashyap, A. S., Manzar, N., Rajawat, M. V. S., Kesharwani, A. K., Singh, R. P., Dubey, S. C., et al. (2021). Screening and biocontrol Potential of Rhizobacteria native to gangetic plains and hilly regions to induce systemic resistance and promote plant Growth in Chilli against bacterial wilt disease. Plants (Basel) 10 (10), 2125. doi: 10.3390/plants10102125
Kthiri, Z., Jabeur, M. B., Machraoui, M., Gargouri, S., Hiba, K., Hamada, W. (2020). Coating seeds with Trichoderma strains promotes plant growth and enhance the systemic resistance against Fusarium crown rot in durum wheat. Egyptian J. Biol. Pest Control 30, 139. doi: 10.1186/s41938-020-00338-6
Kubicek, C. P., Bissett, J., Druzhinina, I., Kullnig-Gradinger, C., Szakacs, G. (2003). Genetic and metabolic diversity of Trichoderma : A case study on South-East Asian isolates. Fungal Genet. Biology: FG B 38 (3), 310−319. doi: 10.1016/s1087-1845(02)00583-2
Kucuk, C. (2014). Enhanced root and shoot growth of wheat (Triticum aestivum L.) by Trichoderma harzianum from Turkey. Pakistan J. Biol. sciences PJBS 17, 122–125. doi: 10.3923/pjbs.2014.122.125
Kumar, S., Stecher, G., Li, M., Knyaz, C., Tamura, K. (2018). MEGA X : Molecular Evolutionary Genetics Analysis across Computing Platforms. Mol. Biol. Evol. 35 (6), 1547−1549. doi: 10.1093/molbev/msy096
Kuznetsova, A., Brockhoff, P., Christensen, R. (2017). lmerTest Package : Tests in Linear Mixed Effects Models. J. Stat. Softw. 82, 1–26. doi: 10.18637/JSS.V082.I13
Laemmli, U. K. (1970). Cleavage of structural proteins during the assembly of the head of bacteriophage T4. Nature 227 (5259), 680–685.
Li, G., Boontung, R., Powers, C., Belamkar, V., Huang, T., Miao, F., et al. (2017). Genetic basis of the very short life cycle of « Apogee » wheat. BMC Genomics 18 (1), 838. doi: 10.1186/s12864-017-4239-8
Li, Y., Shao, J., Fu, Y., Chen, Y., Wang, H., Xu, Z., et al. (2022). The volatile cedrene from Trichoderma guizhouense modulates Arabidopsis root development through auxin transport and signalling. Available at: https://pubag.nal.usda.gov/catalog/7687078.
Mahato, S., Bhuju, S., Shrestha, J. (2018). Effect of Trichoderma viride as biofertilizer on growth and yield of wheat. Malays. J. Sustain. Agric. 2 (2), 1–5. doi: 10.26480/mjsa.02.2018.01.05
Manzar, N., Singh, Y., Kashyap, A. S., Sahu, P. K., Rajawat, M. V. S., Bhowmik, A., et al. (2021). Biocontrol potential of native Trichoderma spp. against anthracnose of great millet (Sorghum bicolour L.) from Tarai and hill regions of India. Biol. Control 152, 104474. doi: 10.1016/j.biocontrol.2020.104474
Mastouri, F., Björkman, T., Harman, G. (2010). Seed Treatment with Trichoderma harzianum Alleviates Biotic, Abiotic, and Physiological Stresses in Germinating Seeds and Seedlings. Phytopathology 100, 1213−1221. doi: 10.1094/PHYTO-03-10-0091
Mukhopadhyay, R., Kumar, D. (2020). Trichoderma : A beneficial antifungal agent and insights into its mechanism of biocontrol potential. Egyptian J. Biol. Pest Control 30 (1), 133. doi: 10.1186/s41938-020-00333-x
Nieto-Jacobo, M. F., Steyaert, J. M., Salazar-Badillo, F. B., Nguyen, D. V., Rostás, M., Braithwaite, M., et al. (2017). Environmental Growth Conditions of Trichoderma spp. Affects Indole Acetic Acid Derivatives, Volatile Organic Compounds, and Plant Growth Promotion. Front. Plant Sci. 8. doi: 10.3389/fpls.2017.00102
Oliveira, J. B. D., Muniz, P. H. P. C., Peixoto, G. H. S., Oliveira, T. A. S. D., Duarte, E. A. A., Rodrigues, F., et al. (2018). Promotion of Seedling Growth and Production of Wheat by Using Trichoderma spp. J. Agric. Sci. 10 (8), 267–276. doi: 10.5539/jas.v10n8p267
Oljira, A. M., Hussain, T., Waghmode, T., Zhao, H., Sun, H., Liu, X., et al. (2020). Trichoderma Enhances Net Photosynthesis, Water Use Efficiency, and Growth of Wheat (Triticum aestivum L.) under Salt Stress. Microorganisms 8, 1565. doi: 10.3390/microorganisms8101565
Pelagio-Flores, R., Esparza-Reynoso, S., Garnica-Vergara, A., López-Bucio, J., Herrera-Estrella, A. (2017). Trichoderma-Induced Acidification Is an Early Trigger for Changes in Arabidopsis Root Growth and Determines Fungal Phytostimulation. Front. Plant Sci. 8. doi: 10.3389/fpls.2017.00822
Shahriar, S. A., Islam, M. N., Chun, C. N. W., Kaur, P., Rahim, M. A., Islam, M. M., et al. (2022). Microbial Metabolomics Interaction and Ecological Challenges of Trichoderma Species as Biocontrol Inoculant in Crop Rhizosphere. Agron. (Basel) 12 (4), 900. doi: 10.3390/agronomy12040900
Silletti, S., Di Stasio, E., Van Oosten, M. J., Ventorino, V., Pepe, O., Napolitano, M., et al. (2021). Biostimulant Activity of Azotobacter chroococcum and Trichoderma harzianum in Durum Wheat under Water and Nitrogen Deficiency. Agronomy 11 (2), 380. doi: 10.3390/agronomy11020380
Singh, A., Kashyap, A. S., Kesharwani, A. K., Tomar, S. (2018). “Biointensive management for soil borne diseases in cucurbitaceous crops,” in Edit on: First Edition Chapter: Biointensive management for soil borne diseases in cucurbitaceous crops (Today and Tomorrow's (Darya Ganj, Delhi) Printers and Publisher), 630.
Sood, M., Kapoor, D., Kumar, V., Sheteiwy, M. S., Ramakrishnan, M., Landi, M., et al. (2020). Trichoderma: The “Secrets” of a Multitalented Biocontrol Agent. Plants 9 (6), 762. doi: 10.3390/plants9060762
Stewart, A., Hill, R. (2014). Applications of Trichoderma in Plant Growth Promotion. Biotechnol. Biol. Trichoderma 31, 415−428. doi: 10.1016/B978-0-444-59576-8.00031-X
Stummer, B., Zhang, Q., Zhang, X., Warren, R., Harvey, P. (2020). Quantification of Trichoderma afroharzianum, Trichoderma harzianum and Trichoderma gamsii inoculants in soil, the wheat rhizosphere and in planta suppression of the crown rot pathogen Fusarium pseudograminearum. J. Appl. Microbiol. 129 (4), 971–990. doi: 10.1111/jam.14670
Tamura, K., Nei, M. (1993). Estimation of the number of nucleotide substitutions in the control region of mitochondrial DNA in humans and chimpanzees. Mol. Biol. Evol. 10 (3), 512−526. doi: 10.1093/oxfordjournals.molbev.a040023
TariqJaveed, M., Farooq, T., Al-Hazmi, A. S., Hussain, M. D., Rehman, ,. A. U. (2021). Role of Trichoderma as a biocontrol agent (BCA) of phytoparasitic nematodes and plant growth inducer. J. Invertebrate Pathol. 183, 107626. doi: 10.1016/j.jip.2021.107626
Topolovec-Pintarić, S. (2019). “Trichoderma : Invisible Partner for Visible Impact on Agriculture,” in Trichoderma - The Most Widely Used Fungicide (London, UK: IntechOpen). doi: 10.5772/intechopen.83363
Tseng, Y.-H., Rouina, H., Groten, K., Rajani, P., Furch, A. C. U., Reichelt, M., et al. (2020). An Endophytic Trichoderma Strain Promotes Growth of Its Hosts and Defends Against Pathogen Attack. Front. Plant Sci. 11. doi: 10.3389/fpls.2020.573670
Vargas, W. A., Crutcher, F. K., Kenerley, C. M. (2011). Functional characterization of a plant-like sucrose transporter from the beneficial fungus Trichoderma virens. Regulation of the symbiotic association with plants by sucrose metabolism inside the fungal cells. New Phytol. 189 (3), 777−789. doi: 10.1111/j.1469-8137.2010.03517.x
Vinale, F., Manganiello, G., Nigro, M., Mazzei, P., Piccolo, A., Pascale, A., et al. (2014). A Novel Fungal Metabolite with Beneficial Properties for Agricultural Applications. Molecules 19 (7), 9760–9772. doi: 10.3390/molecules19079760
Wickham, H. (2009). “Getting started with qplot,” in Ggplot2: Elegant Graphics for Data Analysis, 2nd Edition. Ed. Wickham, H. (New York: Springer), 9−26. doi: 10.1007/978-0-387-98141-3_2
Widden, P., Abitbol, J.-J. (1980). Seasonality of Trichoderma Species in a Spruce-Forest Soil. Mycologia 72 (4), 775−784. doi: 10.1080/00275514.1980.12021246
Xie, L., Zang, X., Cheng, W., Zhang, Z., Zhou, J., Chen, M., et al. (2021). Harzianic Acid from Trichoderma afroharzianum Is a Natural Product Inhibitor of Acetohydroxyacid Synthase. J. Am. Chem. Soc. 143 (25), 9575–9584. doi: 10.1021/jacs.1c03988
Xue, A., Guo, W., Chen, Y., Siddiqui, I., Marchand, G., Liu, J., et al. (2017). Effect of seed treatment with novel strains of Trichoderma spp. On establishment and yield of spring wheat. Crop Prot. 96, 97–102. doi: 10.1016/J.CROPRO.2017.02.003
Zhang, S., Gan, Y., Xu, B. (2019). Mechanisms of the IAA and ACC-deaminase producing strain of Trichoderma longibrachiatum T6 in enhancing wheat seedling tolerance to NaCl stress. BMC Plant Biol. 19 (1), 22. doi: 10.1186/s12870-018-1618-5
Zhu, Z., Zhuang, W. (2015). Trichoderma (Hypocrea) species with green ascospores from China. Persoonia 34, 113–129. doi: 10.3767/003158515X686732
Keywords: auxins, 3-indole-acetic acid, plant root growth promotion, wheat yield, antifungal activity
Citation: Matar N, Macadré C, Ammar GAG, Peres A, Collet B, Boustany NE, Rajjou L, As-Sadi F, Dufresne M and Ratet P (2022) Identification of beneficial Lebanese Trichoderma spp. wheat endophytes. Front. Plant Sci. 13:1017890. doi: 10.3389/fpls.2022.1017890
Received: 12 August 2022; Accepted: 08 November 2022;
Published: 02 December 2022.
Edited by:
Sofia I. A. Pereira, Universidade Católica Portuguesa, PortugalReviewed by:
Abhijeet Shankar Kashyap, National Bureau of Agriculturally Important Microorganisms (ICAR), IndiaCopyright © 2022 Matar, Macadré, Ammar, Peres, Collet, Boustany, Rajjou, As-Sadi, Dufresne and Ratet. This is an open-access article distributed under the terms of the Creative Commons Attribution License (CC BY). The use, distribution or reproduction in other forums is permitted, provided the original author(s) and the copyright owner(s) are credited and that the original publication in this journal is cited, in accordance with accepted academic practice. No use, distribution or reproduction is permitted which does not comply with these terms.
*Correspondence: Pascal Ratet, cGFzY2FsLnJhdGV0QHVuaXZlcnNpdGUtcGFyaXMtc2FjbGF5LmZy
Disclaimer: All claims expressed in this article are solely those of the authors and do not necessarily represent those of their affiliated organizations, or those of the publisher, the editors and the reviewers. Any product that may be evaluated in this article or claim that may be made by its manufacturer is not guaranteed or endorsed by the publisher.
Research integrity at Frontiers
Learn more about the work of our research integrity team to safeguard the quality of each article we publish.