- 1College of Horticulture, Gansu Agricultural University, Lanzhou, China
- 2Institute of Vegetables, Gansu Academy of Agricultural Sciences, Lanzhou, China
Cadmium (Cd) contamination is a serious global concern that warrants constant attention. Therefore, a hydroponic study was conducted to evaluate the effect of different concentrations (0, 1, 2.5, 5, 10, 15 mg/l) of zinc oxide nanoparticles (ZnONPs) on the Cd content in lettuce (Lactuca sativa L.) under Cd stress conditions. The results showed that Cd stress triggered a decrease in plant biomass, an increase in relative electrolyte conductivity (REC), a decrease in root activity, accumulation of reactive oxygen species (ROS) accumulation, and nutrient imbalance. The application of ZnONPs reduced the toxicity symptoms of lettuce seedlings under Cd stress, with the most pronounced effect being observed 2.5 mg/l. ZnONPs promoted the growth of lettuce under Cd stress, mainly in terms of increase in biomass, chlorophyll content, antioxidant enzyme activity, and proline content, as well as reduction in Cd content, malondialdehyde, and reactive oxygen species (ROS) in plant tissues. ZnONPs also enhanced the uptake of ions associated with photosynthesis, such as iron, manganese, magnesium, and zinc. In addition, ZnONPs increase the amount of lignin in the roots, which blocks or reduces the entry of Cd into plant tissues.
Introduction
In recent years, Cd contamination in agricultural fields has been escalated by anthropological activities such as mining, irrigation with industrial wastewater, and the application of phosphate fertilizers, herbicides, and pesticides (Seleiman et al., 2020b; Schlutow et al., 2021). Cd is a toxic, non-essential metal that is highly water-soluble and mobile. This eases the uptake of Cd by the roots to take up and send Cd to the leaves through the xylem (Saidi et al., 2014). Trace concentrations of Cd can induce metal toxicity symptoms in plants (Sary Hassan and Ibrahim Ali, 2019). Cadmium complicates the absorption of essential nutrients by plants and accumulates considerably disease in plants (Usman et al., 2019). Eventually, the uptake and transfer of Cd by plants can lead to food chain contamination and health problems, such as anemia, nephrotoxicity and itai-itai Disease (Salem et al., 2018). Therefore, Cd is considered to be one of the most toxic and prevalent heavy metals (Ismael et al., 2019). Cadmium poisoning induces some morphological, physiological, biochemical, and ultrastructural changes in plants (Khan et al., 2021). It causes the causing retarded growth of plant shoots and roots, chlorosis of leaves, and browning of root ends (Zhang et al., 2015). Cd induces the breakdown of proteins and lipids, damages mitochondria, chloroplasts, and cell membrane ultrastructure (Ali et al., 2013b; Howladar et al., 2018), leading to a reduction in chlorophyll content and triggers higher levels of reactive oxygen species (ROS). In addition, it causes an imbalance of nutrient elements (Erdal and Turk, 2016; Santos et al., 2018).Plants mitigate Cd toxicity by modulating their antioxidant mechanisms, such as biomolecules and antioxidant enzymes (Laxa et al., 2019). These stress-related biomolecules and antioxidant enzymes can increase the tolerance of plants to Cd stress. For example, thiols and their metabolites can fix Cd in vesicles to limit uptake (Yan et al., 2020); lignin protects protoplasts by immobilizing Cd into the root cell wall (Parrotta et al., 2015). Peroxidase (POD), involved in lignin synthesis, can help plants to scavenge excess H2O2 and reduce damage caused by oxidative stress (Jalloh et al., 2009). Several studies have shown that plant growth regulators, elemental nutrients, and nanomaterials can reduce Cd toxicity and accumulation in plants (Ali et al., 2013a; Ahmad et al., 2016; Wang et al., 2017). The application of these compounds may be an effective way of reducing Cd uptake by plants.
Nanomaterials are a new class of materials that are being increasingly used in agriculture. NPs are available in sizes ranging from 1–100 nm, and they possess some unique properties owing to their small size (Cele, 2020; Seleiman et al., 2021). Recent research on numerous plant species has highlighted the role of nanotechnology methods in agriculture, including tolerance to abiotic pressures such as heavy metals (Konate et al., 2017; Paramo et al., 2020). Particularly, the application of ZnONPs has attracted considerable interest, which can provide up to 80% of the zinc (Zn) in green leafy vegetables. Certain studies suggest that ZnONPs may have positive effects on plants by promoting plant growth and increasing nutrient accumulation (Rawashdeh et al., 2020; Seleiman et al., 2020a; Faizan et al., 2021a). In addition, ZnONPs reduce oxidative damage induced by salt stress (Ali et al., 2022a). Owing to their large surface area and high adsorption capacity, they can trap free ions of heavy metals like Cd (Khan et al., 2017; Rossi et al., 2018). ZnONPs reduce the negative effects of Cd exposure on plants by enhancing growth and antioxidant capacity, increasing chlorophyll content, reducing ROS compounds, and reducing leaf electrolyte leakage (Bashir et al., 2021; Ali et al., 2022b; Chen et al., 2022). They also reduce the Cd content in plants (Ali et al., 2019; Adrees et al., 2021). However, high concentrations of ZnONPs have been found to enhance the bioavailability of Cd, thus leading to elevated concentrations of Cd in rice, which was deleterious to plant growth (Zhang et al., 2019). However, past studies have focused on food crop foliar sprays to address micronutrient deficiencies in crops (Hussain et al., 2018; Rizwan et al., 2019b). Little is known about the potential of ZnONPs for growth, metal accumulation and resistance to abiotic stresses in green leafy vegetables, particularly low concentrations of Cd stress. Therefore, it is important to investigate the effect of ZnONPs on green leafy vegetables under low concentration of Cd stress. Hydroponics is the current method of choice for growing green vegetables. In addition to the possibility of contamination from hydroponic irrigation systems, leafy greens are particularly vulnerable to urban atmospheric deposition due to their huge leaf area (Sharma et al., 2008). This is concerning because leafy green vegetables are often consumed directly without further processing. Lettuce (Lactuca sativa L.) is a common vegetable that is widely cultivated worldwide (Meng et al., 2009). It is rich in various vitamins, dietary fiber, and various nutritional minerals, with high nutritional value and benefits to human health (Meng et al., 2009). It also has a high ability to absorb Cd from the soil and does not show signs of Cd toxicity, which adversely affect human health (Cobb et al., 2000). This necessitates the identification of methods for reducing Cd uptake and accumulation in lettuce.
In this study, we investigated the effects of ZnONPs on Cd-stressed lettuce using hydroponics and attempted to understand the putative mitigation mechanisms. This study aimed to determine (a) whether the addition of ZnONPs to the nutrient solution could reduce Cd toxicity in lettuce, (b) the optimal concentration of ZnONPs for reducing Cd toxicity, and (c) how ZnONPs might protect lettuce from Cd toxicity.
Materials and methods
Plant material and growing conditions
The experiment was conducted at the College of Horticulture, Gansu Agricultural University, Lanzhou, Gansu Province, PR China (36°03′N, 103°40′E). The ‘Lvsu’ lettuce variety, a Cd-sensitive genotype according to previous studies, was used for this experiment (Dawuda et al., 2019). In an artificial climate chamber at 75%–85% humidity, 24°C day and night, and 250 umol m-2·s-1 light intensity. Germination was carried out using 50-hole cavity trays filled with vermiculite, and a total of 400 seeds were sown: two seeds were sown in each hole. When lettuce seedlings reached the fifth day of growth, four plants of similar size and growth shape were selected and transplanted into hydroponic containers (length 15 cm; width 10 cm; height 10 cm) containing 1.2 l of 1/4 Hoagland nutrient solution. For the first 4 days, a 1/4 strength Hoagland nutrient solution (pH = 6.0) was administered. Afterwards, a half-strength Hoagland nutrient solution was used. Every four days, the nutrient solution was changed until the plants were ready to be picked and data was collected.
Experimental design and treatments
In the first experiment, a 4 × 2 factorial experiment in a completely randomized design was conducted using 7-day-old seedlings in a climate-controlled chamber and replicated three times. A total of seven treatments were set up, there were 30 lettuce seedlings in each treatment, which we describe as (1) control (CK), (2) Cd (with the addition of 1 mg/l of Cd), (3) Cd + 1 NPs (with the addition of 1 mg/l of Cd and 1 mg/l of ZnONPs), (4) Cd + 2.5 NPs (with the addition of 1 mg/l of Cd and 2.5 mg/l of ZnONPs), (5) Cd + 5 NPs (1 mg/l of Cd and 5 mg/l of ZnONPs were added), (6) Cd + 10 NPs (1 mg/l of Cd and 10 mg/l of ZnONPs were added), and (7) Cd + 15 NPs (1 mg/l of Cd and 15 mg/l of ZnONPs were added). ZnONPs were purchased from Bio Yuanye (Shanghai, China) with a purity of 99% and a diameter of 30 ± 10 nm. The Cd concentration was 1 mg/l (CdCl2-2.5 H2O) andwas selected based on the average Cd content recorded in the farmland (Bi et al., 2018). Subsequently, the optimal dose of ZnONPs for the reduction of Cd toxicity was determined based on lettuce growth, chlorophyll content, root activity, reduced Cd accumulation, and relative electrical conductivity (REC) of leaves.
In the second part of the experiment, we selected the optimal concentration of ZnONPs (2.5 mg/l) and observed its effects on growth, oxidative stress, antioxidant enzymes, phytochelatins, and lignin. According to preliminary experiments, the four treatments were described as follows: T0 represented no addition of Cd and ZnONPs; T1 represented 1 mg/l Cd; T2 represented 1 mg/l Cd + 2.5mg/l ZnONPs, and T3 represented 2.5mg/l ZnONPs. The solutions were refreshed daily. Each treatment consisted of three duplicates, each of which had four plants. After 14 days of treatment, the seedlings were harvested for further examination.
Determination of cadmium content, cadmium transfer factor, and trace element content in lettuce
The solution of concentrated HNO3 and perchloric acid (3:1, v: v) was used to digest the dried leaf and root on a hot plate. Prior to heating, the samples were pre-digested for 24 hours in their natural habitat. A Varian Spectra AA 220/Fs atomic absorption spectrophotometer (Mulgrave, Australia) was used to determine the amount of Cd and trace elements in the digests.
The rate of translocation factor (TF) was calculated according to Melo et al. (Melo et al., 2009). TF = Cd concentrationin in leaf/Cd concentrationin in root
Measurement of lettuce biomass, relative leaf conductivity, chlorophyll content and root activity
After treatment for 15 days, lettuce seedlings were collected and photographed. After separating the samples into leaves and roots, their fresh weight was measured immediately using an electronic scale. The samples were dried in an oven at -80°C until they reached a constant weight, and then their dry weight was determined. Leaf REC was measured using the method reported by Zhou (Zhou et al., 2013). Chlorophyll content was measured by 80% acetone extraction after 14 days of lettuce growth (Tang et al., 2021); root activity was analyzed using the triphenyltetrazolium chloride (TTC) method (Zhang et al., 2013).
Determination of malondialdehyde, hydrogen peroxide and superoxide radicals
The levels of lipid peroxidation in leaves and roots were estimated using the MDA content. MDA concentrations in lettuce tissue were determined using the method reported by Li et al. (Li et al., 2013) with modifications. The content of H2O2 and O2- in lettuce tissue was determined using a modified method reported by He et al. (He et al., 2011).
Histochemical detection of hydrogen peroxide and superoxide radicals
The method reported by Tang et al. (Tang et al., 2021) was slightly modified then used for the visualization of H2O2 and O2- levels in the leaves. The H2O2 accumulation in leaves was detected viay the 3-diaminobenzidine (DAB) staining method by incubating leaves with a DAB solution containing 1 mg/mL (pH = 3.8) and then filtering for 60 minutes using a vacuum extractor. The leaves were then left in the dark for 24 hours. The accumulation of O2- in the leaves was observed by staining with nitro blue tetrazolium (NBT). Leaves were removed from the plants and immediately immersed in a solution containing 1 mg/l of NBT (pH = 7.8), vacuum filtered for 20 min, and subsequently incubated for 2 hours at 25°C in the dark.
Determination of assays for antioxidant enzymes
The extraction solution was 100 mM potassium phosphate buffer (pH 7.0) with 1 mM EDTA-2Na and 1% polyvinylpyrrolidone (PVP). The homogenate was centrifuged at 12,000 g for 20 minutes, and the supernatant was used for the enzyme assay that followed. All operations were carried out at 4°C.
SOD activity was determined using the modified nitro-blue tetrazolium (NBT) method (Wang et al., 2021). First, 0.1 mL of the extract was added to a 50 mM potassium phosphate buffer (pH 7.8) test combination containing 750 mM NBT, 130 mM methionine, 20 mM riboflavin, and 100 mM EDTA. For 20 minutes, the reaction system was illuminated at a light intensity of 4000 lux. Using a spectrophotometer, the amount of enzyme needed to stop 50% of the photoreduction of NBT at 560 nm was set as one unit of SOD.
The POD activity was determined using the modified guaiacol method reported by Iannone et al. (Iannone et al., 2021). The enzyme extract was added to a reaction mixture containing 50 mM potassium phosphate buffer (pH 7.8), 25 mmol/L guaiacol, and 200 mmol/L H2O2. A spectrophotometer was used to look at the change in absorbance at 470 nm caused by the oxidation of guaiacol.
The determination of CAT activity was performed in the manner described by Tang et al., with modifications. First 0.2 ml of 200 mM H2O2 was added to a tube containing 50 mM Tris-HCl buffer (pH =7.0) and 0.1 ml of enzyme extract. Then watch the reaction mixture’s absorbance change at 240 nm was observed for 1 minute using a spectrophotometer.
Determination of phenylalanine, proline, and phenylalanine ammonia lyase activity in lettuce
Lettuce sample preparation and LC-MS analysis of phenylalanine (Phe) and proline (Pro) components were carried out according to the method described by Jin et al. (Jin et al., 2021). The activity of the PAL was determined using the method described by Patel et al. (Patel et al., 2020) with modifications.
Determination of lettuce total thiol, glutathione, and phytochelatin
The total thiol of the plants was determined using the method described by Krippner and Schubert et al. (Krippner and Schubert, 2021), with minor changes. The contents of GSH were determined using the method described by Jinadasa et al. (Jinadasa et al., 2016). The PCs were measured using the following formula: PCs = total thiol – GSH (Bhargava et al., 2005).
Histochemical detection of lignin and determination of peroxidase activity levels in roots and determination of lignin contents
A phloroglucinol-HCl solution was used to determine the lignin content of roots (Fukushima and Hatfield, 2004). Roots were incubated in a solution of 1% (w/v) phloroglucinol generated in 6N HCl for 5 minutes before being washed with distilled water and placed on glass slides. Pyrogallol was utilized to determine the peroxidase activity of the cell wall (cwPODs) (Rogers et al., 2005). For 15 minutes, the lettuce root tips were immersed in this solution, cleaned thoroughly, and mounted on glass slides.
As for lignin, lettuce roots were extracted to obtain protein-free cell wall fractions (Finger-Teixeira et al., 2010). Then, the bromoacetylation method (Eleftheriou et al., 2015) was used to measure the amount of lignin in the roots (Eleftheriou et al., 2015).
Statistical analysis
The experiment was repeated three times in a completely randomized fashion. The dataset was normalized using IBM SPSS Statistic 20 software before being analyzed using the analysis of variance (ANOVA) test. Differences were considered to be statistically significant according to Duncan’s test at P < 0.05.
Results
Effects of zinc oxide nanoparticles on biomass, chlorophyll content, leaf relative electrical conductivity and root activity of lettuce under cadmium stress
As shown in Figure 1, Cd stress decreased biomass, chlorophyll content, and root activity of lettuce seedlings and increased electrolyte leakage from leaves compared with the control. In experimental concentrations, the effect of ZnONPs at 2.5 mg/l was the most significant. It increased the growth of leaves (34%) and roots (37%), chlorophyll a content (26%), chlorophyll b content (30%), chlorophyll ab content (26%), and root activity (19%); but reduced electrolyte leakage (24%). In addition, the fresh weight of lettuce seedlings, decreased root activity, and increased REC were determined following the addition of 10 mg/l and 15 mg/l of ZnONPs.
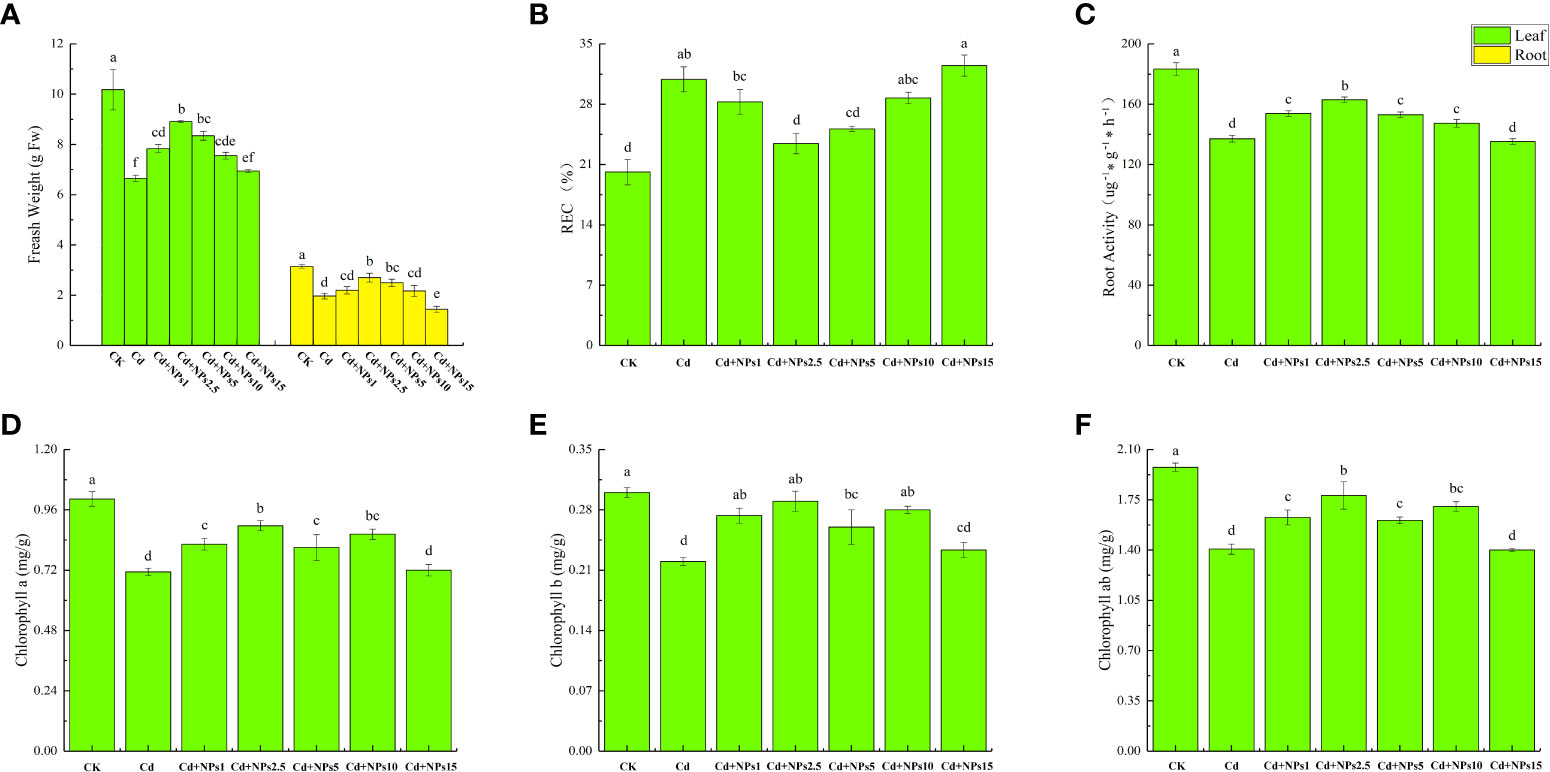
Figure 1 Effects of different concentrations of zinc oxide nanoparticles (ZnONPs) on biomass accumulation (A), leaf relative electrical conductivity (REC) (B), root activity (C) and chlorophyll content (D–F) in lettuce under cadmium (Cd) stress. CK, no treatment; Cd, exposed to 1 mg/l of Cd; Cd + 1 Nps, exposed to 1 mg/l of Cd and 1 mg/l of ZnONPs; Cd + 2.5 Nps, exposed to 1 mg/l of Cd and 2.5 mg/l of ZnONPs; Cd + 5 Nps, exposed to 1 mg/l of Cd and 5 mg/l of ZnONPs; Cd + 10 Nps, exposed to 1 mg/l of Cd and 10 mg/l of ZnONPs; Cd + 15 Nps, exposed to 1 mg/l of Cd and 15 mg/l of ZnONPs. Data are shown as means ± SE of three replicates. Bars marked by different letters indicate significant differences according to Duncan’s test (p < 0.05).
Effect of zinc oxide nanoparticles on trace elemental uptake of lettuce under cadmium stress
The effects of ZnONPs on Cd content and translocation factor in lettuce seedlings are shown in Figure 2. The addition of 1 mg/l and 2.5 mg/l of ZnONPs significantly reduced the Cd content and translocation factor in lettuce seedlings, and decreased the Cd content in leaves and roots by 27%/12% and 33%/17%, respectively. The addition of 5 mg/l ZnONPs reduced the Cd content in leaves (8%), but had no significant effect on the Cd content in roots and the translocation factor. However, the addition of 10 mg/l and 15 mg/l of ZnONPs increased the Cd content in leaves and roots. Application of 1 mg/l, 2.5 mg/l, and 5 mg/l ZnONPs reduced the Cd translocation factor, with 1 mg/l and 2.5 mg/l ZnONPs, thus reducing the Cd translocation factor considerably.
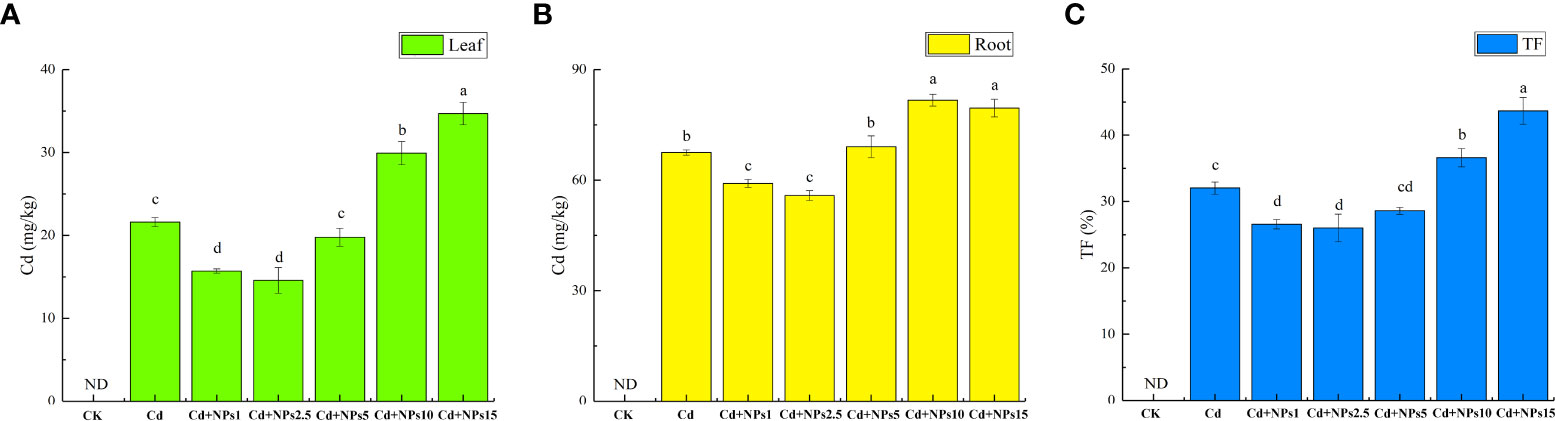
Figure 2 Effect of ZnONPs on Cd content in lettuce leaves (A), Cd content in lettuce roots (B), and Cd translocation factor (TF) under Cd stress (C). T0, no treatment; T1, exposure to 1 mg/l Cd; T2, exposure to 1 mg/l Cd and 2.5 mg/l ZnONPs; T3, exposure to 2.5 mg/l ZnONPs. Bars marked by different letters indicate significant differences according to Duncan’s test (p < 0.05).
Table 1 shows the effect of ZnONP application on the uptake of elemental nutrients by lettuce exposed to Cd. Cd stress resulted in a significant decrease in the uptake of copper (Cu), iron (Fe), manganese (Mn), and zinc (Zn) by lettuce leaves (10%, 42%, 30% and 34%, respectively), and an increase in the uptake of calcium (Ca) (3%), however and no significant effect on the uptake of magnesium (Mg). The accumulation levels of Fe, Mn, Cu and Zn in the roots decreased by 10%, 28%, 44%, and 11%, respectively, while the accumulation levels of Mg and Ca in the roots did not change significantly.The effects of ZnONPs application on different elemental nutrients in lettuce leaves varied, with the uptake of Cu, Fe, and Mn by leaves initially increasing and then decreasing. The uptake of Zn, Mg, and Ca was positively correlated with the concentration of ZnONPs. The application of ZnONPs also promoted nutrient uptake in the roots, where the accumulation of Zn and Ca was positively correlated with the concentration of ZnONPs. The accumulation of Cu, Fe, and Mn increased under the treatment of low and moderate concentrations of ZnONPs but decreased under the treatment of high concentrations of ZnONPs.
Effect of zinc oxide nanoparticles on oxidative stress of lettuce under cadmium stress
The overproduction of reactive oxygen species is one of the main adverse effects of Cd stress on plants. The accumulation of ROS in lettuce seedlings with different treatments is shown in Figure 3. The levels of H2O2 in the shoots and roots of T1 treatment lettuce seedlings increased by 50% and 72%, respectively. Furthermore, the levels of O2 in shoots and roots increased by 45% and 59%, respectively. In T2 treatment, H2O2 and O2 content of lettuce seedlings decreased by 16% and 14%, respectively, in lettuce seedling leaves and by 14% and 14% in root, respectively.
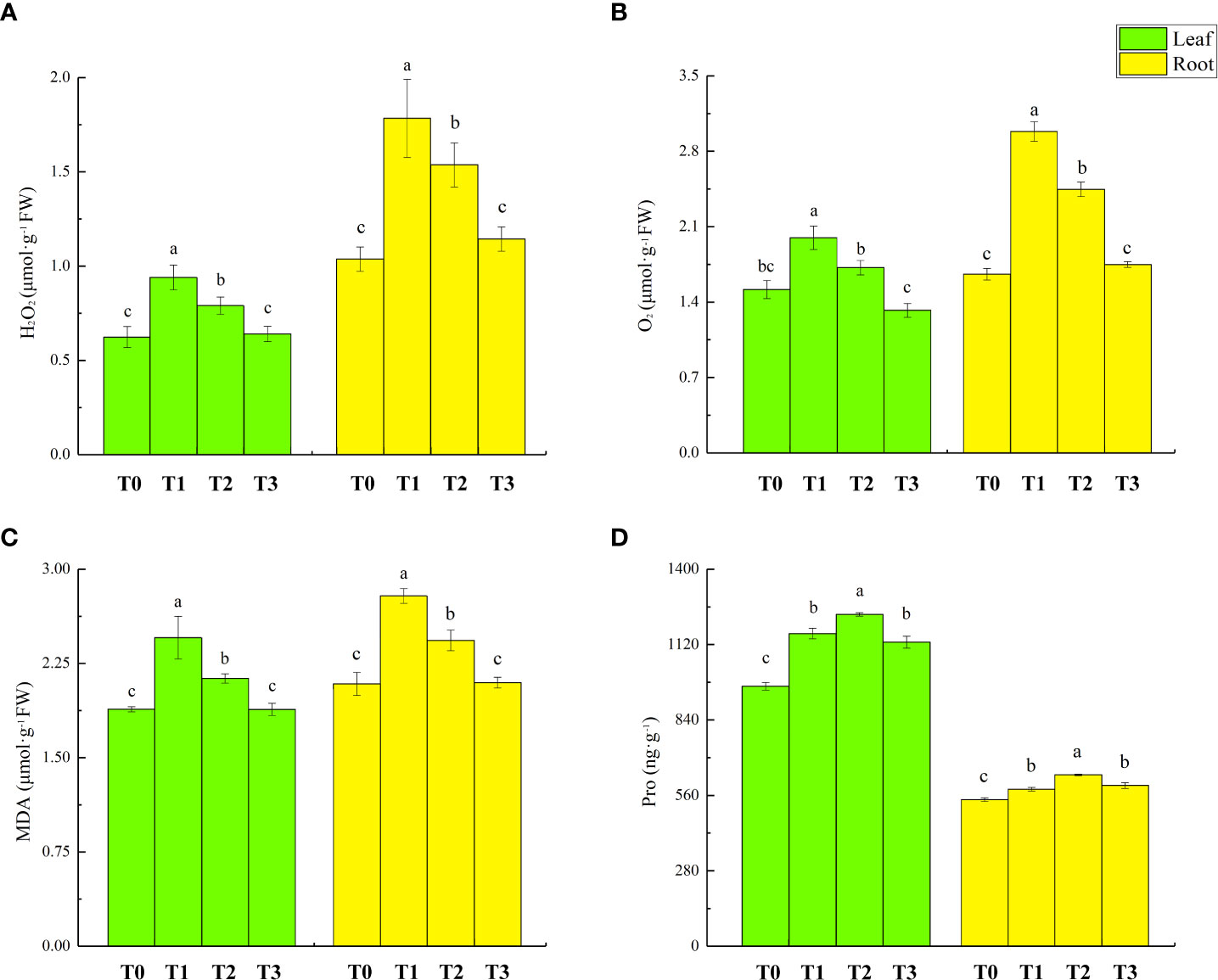
Figure 3 Effect of ZnONPs on hydrogen peroxide (H2O2) content (A), superoxide radicals (O2-) content (B), malondialdehyde (MDA) content (C) and proline (Pro) content (D), in leaves and roots of lettuce. T0, no treatment; T1, exposure to 1 mg/l Cd; T2, exposure to 1 mg/l Cd and 2.5 mg/l ZnONPs; T3, exposure to 2.5 mg/l ZnONPs. Data are shown as means ± SE of three replicates. Bars marked by different letters indicate significant differences according to Duncan’s test (p < 0.05).
The accumulation of malondialdehyde (MDA) content in lettuce seedlings with different treatments is shown in Figure 3C. Compared to T0 treatment, the MDA levels in lettuce seedling’s leaves and roots of T1 treatment increased by 30% and 34%, respectively. There was no significant change in the Pro of lettuce seedling tissues in T3 treatment compared to T1 treatment. The accumulation of malondialdehyde (MDA) content in lettuce seedlings following different treatments is shown in Figure 3D. Compared with T0 treatment, the Pro levels in the leaves and roots under T1 treatment’s lettuce seedlings increased by 20% and 7%, respectively; T2 treatment further increased the Pro content in leaves and roots by 6% and 9%, respectively, from the levels in T1 treatment. Compared to T0 treatment, T3 treatment increased the leaf and root Pro by 17% and 10%, respectively.
The leaf H2O2 and O2- detection of DAB and NBT staining are shown in Figure 4. In DAB staining, there was less brown color in the leaves in T2 treatment than those in T1 treatment. In contrast, T3 treatment showed no significant color change compared to T0 treatment. In NBT staining however, T2 treatment had less blue color compared to T1 treatment. There was no significant color change in T3 treatment compared to T0 treatment.
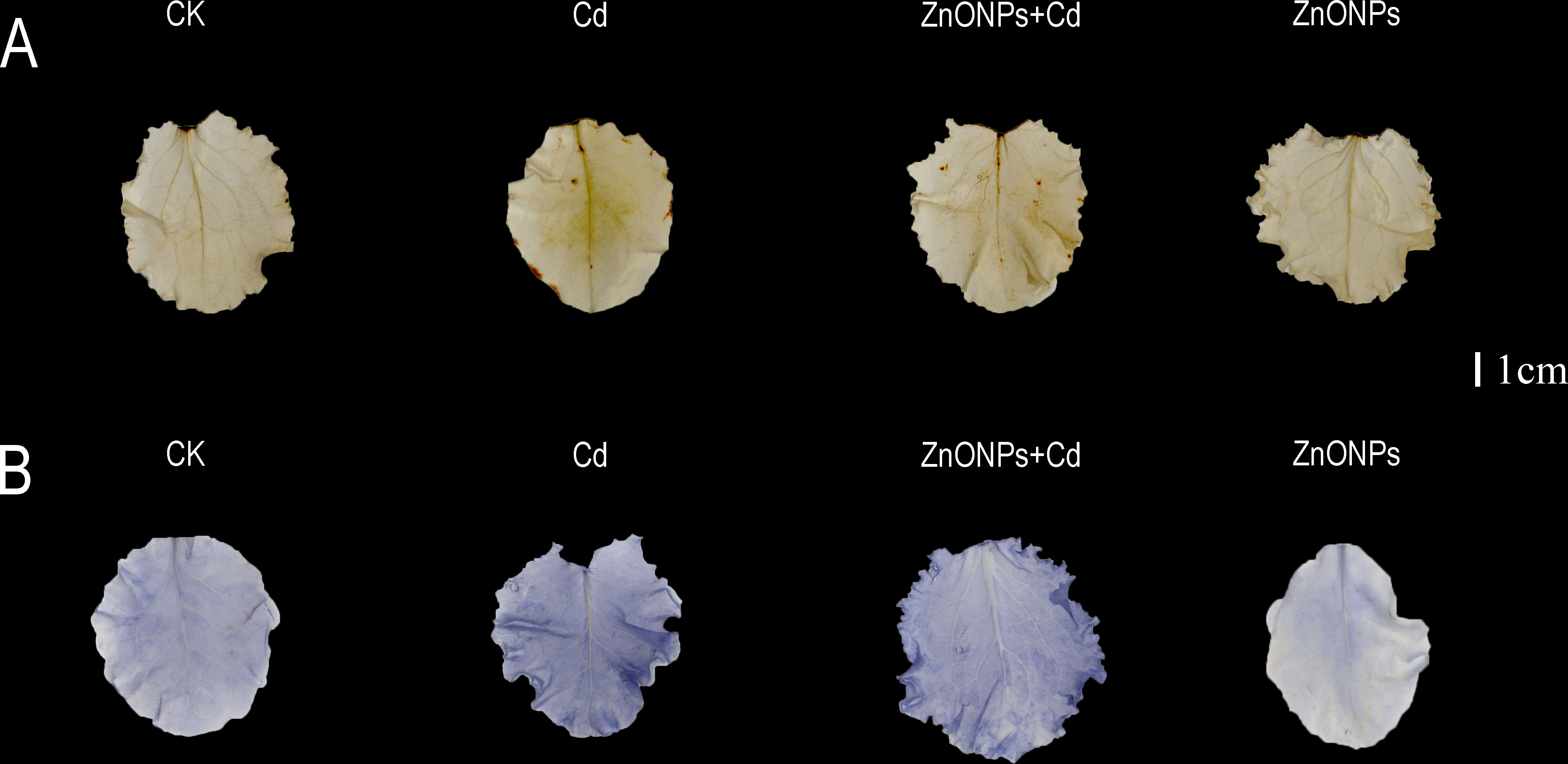
Figure 4 H2O2 (A) and O2- (B) detection of leaf diaminobenzidine and nitro blue tetrazolium staining. Bar= 1 cm. Brown and blue colors indicate the amount of H2O2 and O2- in lettuce leaves, and small amounts of brown and blue colors indicate less accumulation of H2O2 and O2- in the leaves.
Effect of zinc oxide nanoparticles on antioxidant enzymes of lettuce under cadmium stress
The activities of antioxidant enzyme in lettuce seedlings following different treatments are shown in Figure 5. Compared to T0 treatment, the activities of catalase (CAT), superoxide dismutase (SOD) and peroxidase (POD) in leaves and roots of plants that received T1 treatment increased by 32%/33%, 12%/12% and 29%/15%, respectively. Compared to T1 treatment, T2 treatment decreased CAT activity in lettuce leaves and roots by 13% and 10%, and increased SOD and POD activity in leaves and roots by 10%/8% and 13% in root. However, there was no significant change in leaf POD activity. T3 treatment had no significant effect on the antioxidant enzymes’ activity compared to T0 treatment. The histochemical staining of root cwPOD activity is shown in Figure 5D, with more brown indicating relatively strong cwPOD activity. T1 and T3 treatment resulted in more brown color than T0 treatment. The brown fraction was relatively more in T2 treatment, indicating the highest cwPOD activity.
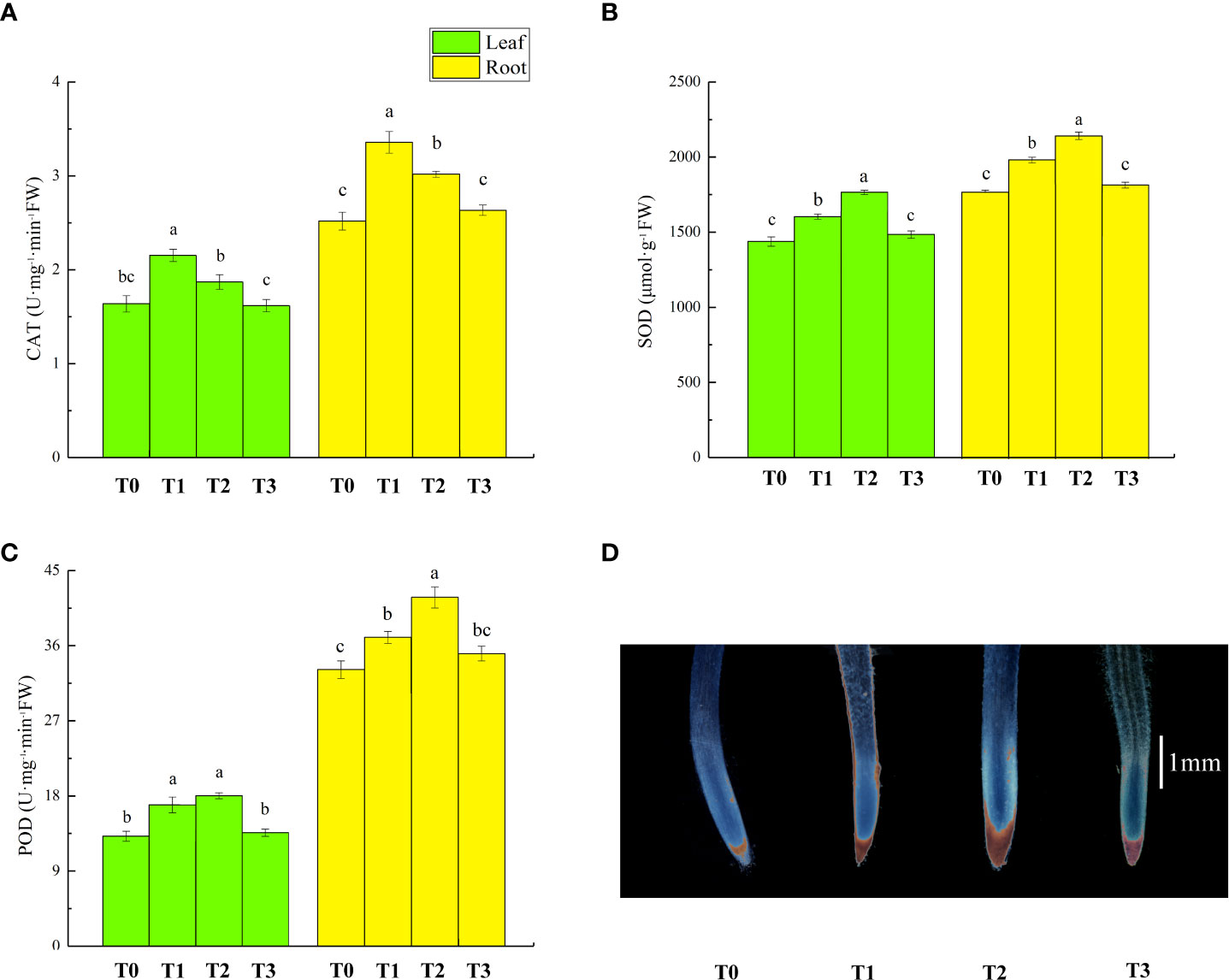
Figure 5 Effects of ZnONPs on the activities of catalase (CAT, A), superoxide dismutase (SOD, B), and peroxidase (POD, C) in lettuce seedlings and pyrogallol staining of lettuce root tips (D). T0, no treatment; T1, exposure to 1 mg/l Cd; T2, exposure to 1 mg/l Cd and 2.5 mg/l ZnONPs; T3, exposure to 2.5 mg/l ZnONPs. Brown color indicates the activity of cwPOD in lettuce roots and a large amount of brown color indicates higher cwPOD activity in roots, bar= 1 mm. Data are shown as means ± SE of three replicates. Bars marked by different letters indicate significant differences according to Duncan’s test (p < 0.05).
Effect of zinc oxide nanoparticles on phenylalanine content, phenylalanine ammonia lyase activity, and lignin content of lettuce under Cd cadmium
The Phe content, PAL activity in lettuce sedding’s leaves and roots, and Lignin content in roots are shown in Figure 6. The Phe content in leaves and roots decreased by 5% and 21% in T1 treatment compared with T0 treatment; PAL activity increased by 10% in roots and did not change significantly in leaves; and lignin content in roots increased by 18%. T2 treatment further reduced Phe content in lettuce leaves and roots (8% and 10%) compared to T1 treatment, increased PAL activity (10% and 11%), and increased root lignin content (14%). Compared to T0 treatment, T3 treatment decreased the Phe content in leaves and roots (5% and 17%) and increased PAL activity (11% and 14%) and lignin content (6%).
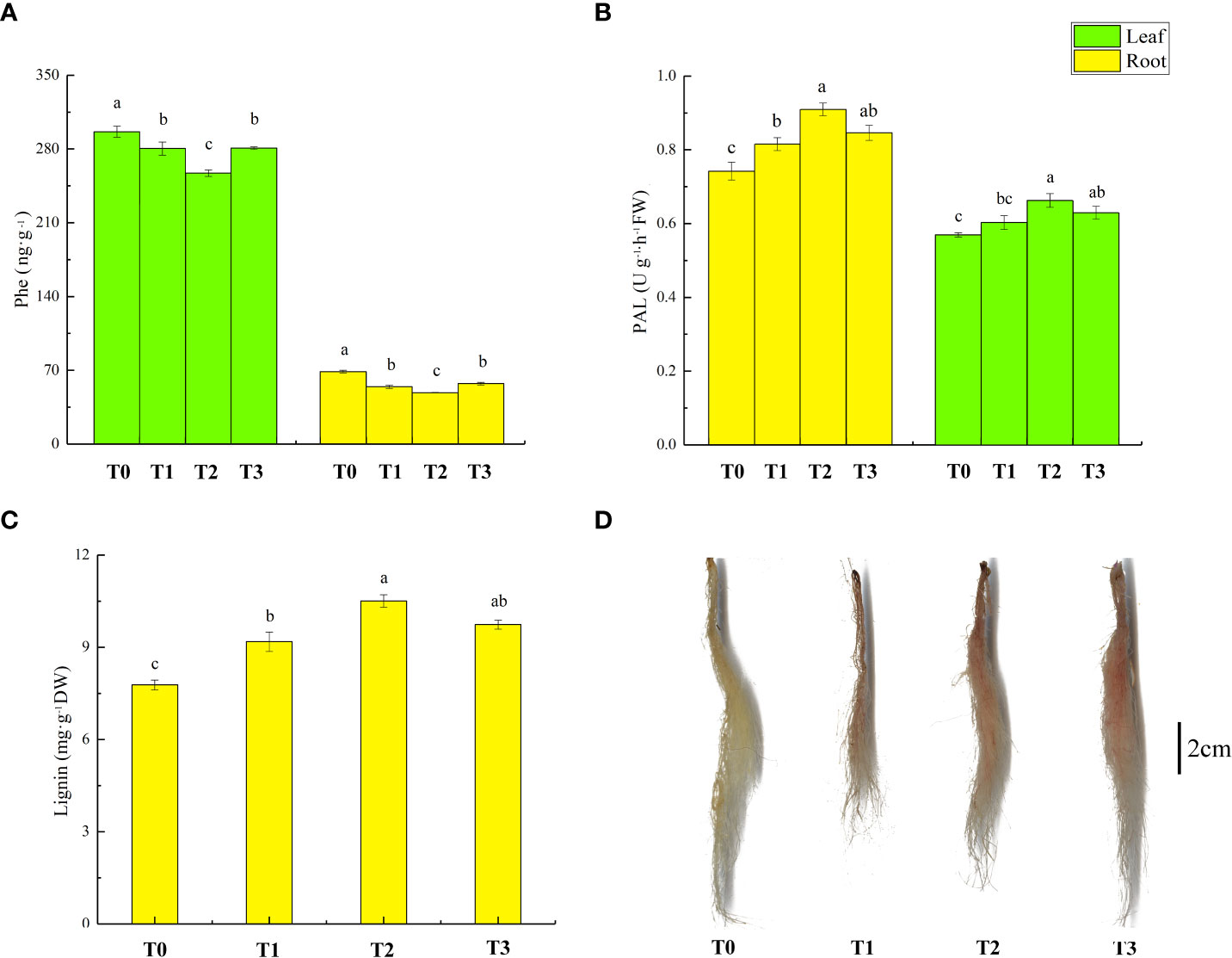
Figure 6 Effect of ZnONPs on Phe content (A) and PAL activity (B) in leaves and roots of lettuce, and lignin content (C) roots of lettuce and visualization of lignin staining in lettuce roots (D). T0, no treatment; T1, exposure to 1 mg/l Cd; T2, exposure to 1 mg/l Cd and 2.5 mg/l ZnONPs; T3, exposure to 2.5 mg/l ZnONPs. The small amount of reddish-brown color indicates that the lignin accumulation in the roots is low, bar = 2cm. Data are shown as means ± SE of three replicates. Bars marked by different letters indicate significant differences according to Duncan’s test (p < 0.05).
The staining of the root lignin is shown in Figure 6D, with more red color representing higher lignin content. T1 and T3 treatment increased the red color of the roots. The red color was more in T2 treatment than in T1 treatment.
Effect of zinc oxide nanoparticles on the content of total thiol, glutathione and phytochelatins in different parts of lettuce under cadmium stress
Figure 7 shows the contents of total thiol, GSH, and PCs in the leaves and roots of lettuce seedlings under different treatments. Compared to T0 treatment, the content of total thiol, GSH and PCs in the leaves under T1 treatment increased by 22%, 11%, and 61%, respectively. The amount of total thiol, GSH, and PCs in the roots increased by 28%, 23%, and 44%, respectively. The content of total thiol and PCs in the leaves further increased by 11% and 20.5% following T2 treatment compared to T1 treatment. In the root, the amount of total thiol and PCs increased by 17% and 36%, respectively. However, the GSH content did not increase significantly. Compared to T0 treatment, T3 treatment increased the total thiol content of lettuce leaves and roots (9% and 20%) and the PC content of roots (38%). There was no significant effect on the GSH content of leaves and roots.
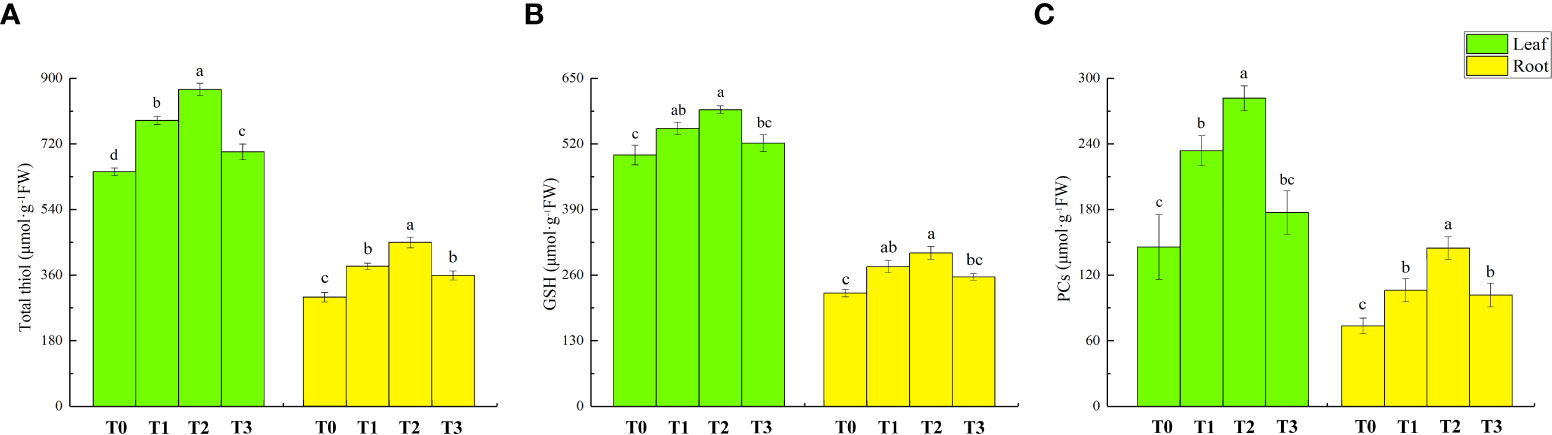
Figure 7 Effect of ZnONPs on total thiol content (A), GSH content (B) and PCs content (C) and proline (Pro) content in leaves and roots of lettuce. T0, no treatment; T1, exposure to 1 mg/l Cd; T2, exposure to 1 mg/l Cd and 2.5 mg/l ZnONPs; T3, exposure to 2.5 mg/l ZnONPs. Data are shown as means ± SE of three replicates. Bars marked by different letters indicate significant differences according to Duncan’s test (p < 0.05).
Discussion
Recent research has confirmed the role of nanomaterials in enhancing plant tolerance to abiotic stresses like metals (Faizan et al., 2021b; Ali et al., 2022a). ZnONPs are widely used nanomaterials. They release Zn, an essential micronutrient for regulating plant development and exhibits lowtoxicity compared to other forms of Zn (Kouhi et al., 2014; Khoshgoftarmanesh and Markarian, 2022). Many studies have reported the growth-promoting effects of ZnONPs in a variety of plants. Song and kimfound that it increased the biomass of lettuce and carrots (Song and Kim, 2020).Venkatachalam et al. (Venkatachalam et al., 2017) found that it increased the photosynthetic pigment content of the leaves of silver acacia seedlings. The negative effects of Cd on plants have been described in the introduction section. Owing to its resemblance to phosphorus, Cd is easily transported by plants and accumulates in edible parts, whichnegatively impacts plant growth (Huang et al., 2020). In addition, Cd stress causes imbalances of trace elements (Wang et al., 2019). As a result, this study aims to elucidate potential mechanisms of ZnONPs regulation of Cd tolerance in lettuce. The study results show that Cd stress significantly reduced the biomass, chlorophyll, and root activity of lettuce seedlings, and significantly increased REC (Figure 1). Cd causes ultrastructural changes and induces programmed cell death, which is one of the main factors contributing to decreased chlorophyll content, root activity and increased leaf REC (Ali et al., 2015; Rizwan et al., 2016). Moreover, Cd competes with other trace elements for binding sites, thus reducing the uptake of trace elements (Rizwan et al., 2017; Ronzan et al., 2018). In this study, the supply of ZnONPs alleviated the toxic effects of Cd stress on lettuce seedlings, reduced Cd uptake and translocation, and increased the trace elements in lettuce. Similar findings have been recorded in different studies onplants (Rizwan et al., 2019b; Faizan et al., 2020). Zn released from ZnONPs maintains the stability of biological cell membranes, reduces electrolyte leakage and loss of cell viability caused by Cd stress, and positively regulates the expression of metal transport proteins in plants (Mustafa and Komatsu, 2016; Rajapakse et al., 2017; Hussain et al., 2018). Furthermore, appropriate concentrations of Zn can reduce the transfer of Cd from roots to shoots by altering the expression pattern of ZIP genes (Palusinska et al., 2020). This may be the reason underlying the alleviation of Cd stress in lettuce seedlings by ZnONPs. However, in this experiment, high concentrations of ZnONPs were found to be toxic to lettuce seedling growth and to increase Cd content and transport. This could be the result of toxic effects caused by a large accumulation of Zn, with potential phytotoxicity occurring when the Zn concentration in plant leaf tissue exceeds 200 mg/kg dry matter. High concentrations of ZnONPs have been observed in previous studies to lead to a significant accumulation of Zn in plants, leading to toxicity (Bonnet et al., 2000; Srivastav et al., 2021; Hong et al., 2022). High concentrations of Zn can also have indirect effects on the uptake and mutual elemental interactions of other elements, thus increasing the accumulation of Cd (Zhang et al., 2019; Li et al., 2021; Xiao et al., 2022). Therefore, attention should be paid to the concentration of ZnONPs to avoid their toxic effects on plants. Notably, low and moderate concentrations of ZnONPs have a significant effect on Fe uptake, and improve Fe accumulation in leaves more than in roots, which is consistent with the findings of Sharifan et al. (Sharifan et al., 2019). Because ZnONPs at a concentration of 2.5 mg/l alleviated the toxicity of Cd to lettuce seedlings and minimized the accumulation of Cd, were selected for subsequent experiments to investigate the possible mechanism of Cd stress alleviation by ZnONPs.
The most common effect of Cd toxicity is the induction of ROS production and accumulation, which can cause oxidative damage to plants, leading to biofilm damage and promoting increased MDA content owing to lipid peroxidation (Noman et al., 2018a). Generally, higher plants scavenge excess ROS by increasing antioxidant enzyme activity to overcome ROS accumulation and membrane lipid peroxidation caused by Cd toxicity. When plants experience an oxidative burst, SOD first converts O2 to the less toxic H2O2, after which CAT and POD are responsible for scavenging H2O2 (Noman et al., 2018b). The H2O2, O2 and MDA content of lettuce seedlings were significantly increased under Cd stress, and significant enhancement of SOD, POD, and CAT activities were observed. Previous studies have obtained similar results for other plants (Xu et al., 2015; Zouari et al., 2016).The use of ZnONPs reduced H2O2 content, O2 and MDA content and enhanced SOD and POD activities in lettuce seedlings. Numerous studies have documented ZnONP-mediated induction of antioxidant enzyme activities in different plants. Rizwan et al. found that ZnONPs increased the activity of antioxidant enzymes and reduced the accumulation of reactive oxygen species in cowpea seedlings (Rizwan et al., 2019a). Pavani et al. found that ZnONPs increased SOD and POD activities in response to different types of abiotic stress (Pavani et al., 2020). However, CAT activity decreased after the application of ZnONPs, which could be due to the decrease in H2O2 content. Mitigation of Cd stress toward ZnONPs was mainly achieved by enhancing SOD and POD activities and decreasing ROS content. The sole application of ZnONPs had no significant effect on ROS content and antioxidant enzyme activity in lettuce seedlings, indicating that the concentrations used were appropriate and did not cause Zn toxicity.
To withstand metal stress, plants accumulate a variety of metabolites. Pro is an important amino acid metabolite that plays a crucial role in plant tolerance to stress conditions and can act as a plant osmoregulatory substance, metal chelator, and ROS quencher (Ghasemi-Omran et al., 2021). It can stabilize and protect the functions of various enzymes, regulate plant growth, and play an important role in mitigating heavy metal toxicity (Torabian et al., 2016; Hussain et al., 2021). In this study, both Cd stress and ZnONPs increased the amount of proline in lettuce. The highest levels of proline were found in lettuce seedlings with ZnONPs applied under Cd stress, and similar results were found in a previous study (Faizan et al., 2021b). In addition, ZnONPs could potentially increase proline content, and previous studies have found that the supply of ZnONPs in previous reports increases Pro content in plants (Faizan et al., 2020), which may be related to the increased expression of proline biosynthesis genes (Faizan et al., 2021a). Generally, there are two pathways of Cd transport to xylem: the symplastic pathway and the apoplast pathway (Dong et al., 2017; Qi et al., 2020). The transport of Cd through the apoplast pathway mainly involves the transmembrane transport of thiol compounds and ions (Silveira Rabelo et al., 2017; Song et al., 2017). Plants minimize the toxicity of Cd using thiols, glutathione (GSH), and cysteine-rich metal-binding peptides (PCs) in root hairs to bind to Cd and immobilize it in vesicles thus limiting its transportation (Yan et al., 2020). This study documented a significant increase in thiol, GSH, and PCs content in lettuce seedlings under Cd stress and ZnONPs alone. In addition, the supply of ZnONPs further increased thiol, GSH, and PCs content in the tissues of lettuce seedlings under Cd stress. This suggests that ZnONPs could promote the synthesis of thiol compounds as a way of reducing the toxic effects of Cd. This may be the effect of Zn released from ZnONPs. Zn induces the synthesis of sulfur-containing compounds including GSH and PCs, thereby increasing the levels of plant PCs and GSH (Sharifan et al., 2020). Similar results have been reported in previous studies. Wei et al. found that Zn promotes the accumulation of sulfate in plants and increases the content of cysteine, which in turn increases the content of thiols and PCs (Wei et al., 2022); Howladar et al. found that ZnONPs promote the ASA-GSH cycle and increase GSH content in tissues (Howladar, 2022; Prakash et al., 2022). Therefore, ZnONPs increased the accumulation of thiols in lettuce seedlings, which is significant to improving their tolerance to Cd toxicity.
In the symplastic pathway Cd was absorbed by the root system; it was first transported to the xylem and then to the stem and leaves (Tao and Lu, 2022). PAL plays an important role because it catalyzes the conversion of phenylalanine to lignin, thereby increasing the level of lignification. The increased level of lignification enhances the plastid barrier, reducing cell wall penetration and preventing Cd entry into the cytoplasm. In addition, lignin in the root cell wall provides Cd binding sites that can protect protoplasts from Cd stress by anchoring Cd in the cell wall, which is an effective way of preventing Cd transport into the leaves (Kidwai et al., 2019). This study found that both Cd stress and ZnONPsincreased the lignin content in the roots of lettuce seedlings. Moreover, the highest lignin content was found in the roots of lettuce seedlings with ZnONPs applied under Cd stress, which is consistent with the results of previous experiments. Similar findings have been found in previous experiments. Zhao et al. found that plants could reduce the toxicity of Cd by increasing lignin to fix Cd (Zhao et al., 2021). Benakova et al. found that low concentrations of ZnONPs were also able to increase the lignin content of roots (Benakova et al., 2017). In contrast, Molnar et al. (Molnar et al., 2020) found maximum lignin content in co-treatments of Zn and Cd in Brassica napus. This could be related to the increased activity of PAL and cwPOD, both key enzymes in the synthesis of plant lignin. Cd stress and ZnONPs also increased PAL activity in the present study. A decrease in Phe content was observed owing to Cd stress and the use of ZnONPs, which could be attributed to the increase in PAL activity. PAL is the rate-limiting enzyme of lignin anabolism and can to catalyze the conversion of Phe to cinnamic acid (Hose et al., 2001; Garza-Alonso et al., 2021). This could explain the decrease in Phe content in lettuce seedlings, as a large amount of Phe is involved in lignin synthesis. However, additional studies at the molecular and biochemical levels are required to lucidate the role of ZnONPs in thiol metabolism.
Conclusion
Cd stress adversely affects lettuce through the inhibition of growth, ROS production, and mineral element uptake. The ZnONPs in the nutrient solutions played a significant role in boosting growth and lowering Cd toxicity. ZnONPs reduce oxidative stress caused by Cd by enhancing the antioxidant system to scavenge ROS production in Cd-exposed plants. ZnONPs also reduce the toxicity of Cd by speeding up thiol metabolism and creating more lignin, which inhibits the movement of Cd from roots to leaves. Additionally, ZnONPs can regulate the uptake and transport of trace elements. This effect is most pronounced in the photosynthetic-related elements, such as Fe, Mg, and Mn. The ability of ZnONPs to attenuate Cd toxicity in lettuce may be achieved by removing excess ROS, inhibiting Cd uptake, and immobilizing it in the roots to reduce its translocation to the leaves. However, more studies at the molecular level are required to fully explain the involvement of ZnONPs in the Cd stress response of lettuce. In conclusion, the application of lower concentrations of ZnONPs to lettuce under Cd stress is an effective detoxification method that can be investigated in phytoremediation.
Data availability statement
The original contributions presented in the study are included in the article/supplementary material. Further inquiries can be directed to the corresponding author.
Author contributions
FG and conceived and designed the experiments. analyzed the data. wrote the manuscript. JX conceived, and designed the experiments. JZ contributed reagents/materials/analysis tools. JL contributed towards, execution of different experiments, analyzed the data. XZ experimental design, data processing, related discussions and manuscripts writing improvement. CW contributed towards, execution of different experiments. TN contributed towards execution of different experiments. CT contributed towards, execution of different experiments. All authors contributed to the article and approved the submitted version.
Funding
This research was supported by the National Key Research and Development Program (2016YFD0201005), the Gansu Province Special Fund Project for Guiding Science and Technology Development (2018ZX-02), and the Research and Industrialization Demonstration of Greenhouse Structure and Construction Technology for Horticultural Crop Cultivation in Northwest Non-Arable Land (201203002).
Acknowledgments
I thank my supervisor and colleagues for their help in my experiments. I also thank the laboratory for providing me with the experimental conditions.
Conflict of interest
The authors declare that the research was conducted in the absence of any commercial or financial relationships that could be construed as a potential conflict of interest.
Publisher’s note
All claims expressed in this article are solely those of the authors and do not necessarily represent those of their affiliated organizations, or those of the publisher, the editors and the reviewers. Any product that may be evaluated in this article, or claim that may be made by its manufacturer, is not guaranteed or endorsed by the publisher.
References
Adrees, M., Khan, Z. S., Hafeez, M., Rizwan, M., Hussain, K., Asrar, M., et al. (2021). Foliar exposure of zinc oxide nanoparticles improved the growth of wheat (Triticum aestivum l.) and decreased cadmium concentration in grains under simultaneous cd and water deficient stress. Ecotoxicology Environ. Saf. 208. doi: 10.1016/j.ecoenv.2020.111627
Ahmad, P., Abdel Latef, A. A., Abd_Allah, E. F., Hashem, A., Sarwat, M., Anjum, N. A., et al. (2016). Calcium and potassium supplementation enhanced growth, osmolyte secondary metabolite production, and enzymatic antioxidant machinery in cadmium-exposed chickpea (Cicer arietinum l.). Front. Plant Sci. 7, 513. doi: 10.3389/fpls.2016.00513
Ali, B., Gill, R. A., Yang, S., Gill, M. B., Farooq, M. A., Liu, D., et al. (2015). Regulation of cadmium-induced proteomic and metabolic changes by 5-aminolevulinic acid in leaves of brassica napus l. PloS One 10 (4), e0123328. doi: 10.1371/journal.pone.0123328
Ali, B., Huang, C. R., Qi, Z. Y., Ali, S., Daud, M. K., Geng, X. X., et al. (2013a). 5-aminolevulinic acid ameliorates cadmium-induced morphological, biochemical, and ultrastructural changes in seedlings of oilseed rape. Environ. Sci. pollut. Res. 20 (10), 7256–7267. doi: 10.1007/s11356-013-1735-5
Ali, S., Mfarrej, M. F. B., Hussain, A., Akram, N. A., Rizwan, M., Wang, X., et al. (2022b). Zinc fortification and alleviation of cadmium stress by application of lysine chelated zinc on different varieties of wheat and rice in cadmium stressed soil. Chemosphere 295. doi: 10.1016/j.chemosphere.2022.133829
Ali, S., Rizwan, M., Noureen, S., Anwar, S., Ali, B., Naveed, M., et al. (2019). Combined use of biochar and zinc oxide nanoparticle foliar spray improved the plant growth and decreased the cadmium accumulation in rice (Oryza sativa l.) plant. Environ. Sci. pollut. Res. 26 (11), 11288–11299. doi: 10.1007/s11356-019-04554-y
Ali, B., Saleem, M. H., Ali, S., Shahid, M., Sagir, M., Tahir, M. B., et al. (2022a). Mitigation of salinity stress in barley genotypes with variable salt tolerance by application of zinc oxide nanoparticles. Front. Plant Sci. 13. doi: 10.3389/fpls.2022.973782
Ali, B., Wang, B., Ali, S., Ghani, M., Hayat, M., Yang, C., et al. (2013b). 5-aminolevulinic acid ameliorates the growth, photosynthetic gas exchange capacity, and ultrastructural changes under cadmium stress in brassica napus l. J. Plant Growth Regul. 32 (3), 604–614. doi: 10.1007/s00344-013-9328-6
Bashir, A., Rehman, M. Z. U., Hussaini, K. M., Adrees, M., Qayyum, M. F., Sayal, A. U., et al. (2021). Combined use of zinc nanoparticles and co-composted biochar enhanced wheat growth and decreased cd concentration in grains under cd and drought stress: A field study. Environ. Technol. Innovation 23. doi: 10.1016/j.eti.2021.101518
Benakova, M., Ahmadi, H., Ducaiova, Z., Tylova, E., Clemens, S., Tuma, J. (2017). Effects of cd and zn on physiological and anatomical properties of hydroponically grown brassica napus plants. Environ. Sci. pollut. Res. 24 (25), 20705–20716. doi: 10.1007/s11356-017-9697-7
Bhargava, P., Srivastava, A. K., Urmil, S., Rai, L. C. (2005). Phytochelatin plays a role in UV-b tolerance in N2-fixing cyanobacterium anabaena doliolum. J. Plant Physiol. 162 (11), 1220–1225. doi: 10.1016/j.jplph.2004.12.006
Bi, C., Zhou, Y., Chen, Z., Jia, J., Bao, X. (2018). Heavy metals and lead isotopes in soils, road dust and leafy vegetables and health risks via vegetable consumption in the industrial areas of shanghai, China. Sci. Total Environ. 619, 1349–1357. doi: 10.1016/j.scitotenv.2017.11.177
Bonnet, M., Camares, O., Veisseire, P. (2000). Effects of zinc and influence of acremonium lolii on growth parameters, chlorophyll a fluorescence and antioxidant enzyme activities of ryegrass (Lolium perenne l. cv Apollo). J. Exp. Bot. 51 (346), 945–953. doi: 10.1093/jexbot/51.346.945
Cele, T. (2020). “Preparation of nanoparticles,” in Silver nanoparticles-health and safety IntechOpen, vol. 15. doi: 10.5772/intechopen.90771
Chen, F., Bashir, A., Zia-Ur-Rehman, M., Adrees, M., Qayyum, M. F., Ma, J., et al. (2022). Combined effects of green manure and zinc oxide nanoparticles on cadmium uptake by wheat (Triticum aestivum l.). Chemosphere 298. doi: 10.1016/j.chemosphere.2022.134348
Cobb, G. P., Sands, K., Waters, M., Wixson, B. G., Dorward-King, E. (2000). Accumulation of heavy metals by vegetables grown in mine wastes. Environ. Toxicol. Chemistry: Int. J. 19 (3), 600–607. doi: 10.1002/etc.5620190311
Dawuda, M. M., Liao, W., Hu, L., Yu, J., Xie, J., Calderon-Urrea, A., et al. (2019). Root tolerance and biochemical response of Chinese lettuce (Lactuca sativa l.) genotypes to cadmium stress. Peerj 7. doi: 10.7717/peerj.7530
Dong, Q., Xu, P., Wang, Z. (2017). Differential cadmium distribution and translocation in roots and shoots related to hyper-tolerance between tall fescue and Kentucky bluegrass. Front. Plant Sci. 8, 113. doi: 10.3389/fpls.2017.00113
Eleftheriou, E. P., Adamakis, I.-D. S., Panteris, E., Fatsiou, M. (2015). Chromium-induced ultrastructural changes and oxidative stress in roots of arabidopsis thaliana. Int. J. Mol. Sci. 16 (7), 15852–15871. doi: 10.3390/ijms160715852
Erdal, S., Turk, H. (2016). Cysteine-induced upregulation of nitrogen metabolism-related genes and enzyme activities enhance tolerance of maize seedlings to cadmium stress. Environ. Exp. Bot. 132, 92–99. doi: 10.1016/j.envexpbot.2016.08.014
Faizan, M., Bhat, J. A., Chen, C., Alyemeni, M. N., Wijaya, L., Ahmad, P., et al. (2021a). Zinc oxide nanoparticles (ZnO-NPs) induce salt tolerance by improving the antioxidant system and photosynthetic machinery in tomato. Plant Physiol. Biochem. 161, 132–140. doi: 10.1016/j.plaphy.2021.02.002
Faizan, M., Faraz, A., Mir, A. R., Hayat, S. (2021b). Role of zinc oxide nanoparticles in countering negative effects generated by cadmium in lycopersicon esculentum. J. Plant Growth Regul. 40 (1), 101–115. doi: 10.1007/s00344-019-10059-2
Faizan, M., Hayat, S., Pichtel, J. (2020). “Effects of zinc oxide nanoparticles on crop plants: A perspective analysis,” in Sustainable agriculture reviews Hayat, S., Pichtel, J., Faizan, M., Fariduddin., Q. eds., vol. 41. (Cham: Springer International Publishing), 83–99.
Finger-Teixeira, A., Ferrarese, M. D. L., Soares, A. R., da Silva, D., Ferrarese, O. (2010). Cadmium-induced lignification restricts soybean root growth. Ecotoxicology Environ. Saf. 73 (8), 1959–1964. doi: 10.1016/j.ecoenv.2010.08.021
Fukushima, R. S., Hatfield, R. D. (2004). Comparison of the acetyl bromide spectrophotometric method with other analytical lignin methods for determining lignin concentration in forage samples. J. Agric. Food Chem. 52 (12), 3713–3720. doi: 10.1021/jf035497l
Garza-Alonso, C. A., Gonzalez-Garcia, Y., Cadenas-Pliego, G., Olivares-Saenz, E., Trejo-Tellez, L. I., Benavides-Mendoza, A. (2021). Seed priming with ZnO nanoparticles promotes early growth and bioactive compounds of moringa oleifera. Notulae Botanicae Horti Agrobotanici Cluj-Napoca 49 (4). doi: 10.15835/nbha49412546
Ghasemi-Omran, V. O., Ghorbani, A., Sajjadi-Otaghsara, S. A. (2021). Melatonin alleviates NaCl-induced damage by regulating ionic homeostasis, antioxidant system, redox homeostasis, and expression of steviol glycosides-related biosynthetic genes in in vitro cultured stevia rebaudiana bertoni. In Vitro Cell. Dev. Biology-Plant 57 (2), 319–331. doi: 10.1007/s11627-021-10161-9
He, J., Qin, J., Long, L., Ma, Y., Li, H., Li, K., et al. (2011). Net cadmium flux and accumulation reveal tissue-specific oxidative stress and detoxification in populus x canescens. Physiologia Plantarum 143 (1), 50–63. doi: 10.1111/j.1399-3054.2011.01487.x
Hong, M., Gong, J. L., Cao, W. C., Fang, R., Cai, Z., Ye, J., et al. (2022). The combined toxicity and mechanism of multi-walled carbon nanotubes and nano zinc oxide toward the cabbage. Environ. Sci. pollut. Res. 29 (3), 3540–3554. doi: 10.1007/s11356-021-15857-4
Hose, E., Clarkson, D. T., Steudle, E., Schreiber, L., Hartung, W. (2001). The exodermis: a variable apoplastic barrier. J. Exp. Bot. 52 (365), 2245–2264. doi: 10.1093/jexbot/52.365.2245
Howladar, S. M. (2022). Nano-zinc oxide effects on eggplant (Solanum melongena l.) transplant quality in comparison with conventional zinc oxide. Pakistan J. Bot. 54 (1), 113–120. doi: 10.30848/pjb2022-1(27
Howladar, S. M., Al-Robai, S. A., Al-Zahrani, F. S., Howladar, M. M., Aldhebiani, A. Y. (2018). Silicon and its application method effects on modulation of cadmium stress responses in triticum aestivum (L.) through improving the antioxidative defense system and polyamine gene expression. Ecotoxicology Environ. Saf. 159, 143–152. doi: 10.1016/j.ecoenv.2018.05.004
Huang, Y., Guo, X., Hu, X.-Y. (2020). Effects of biochar on bioavailability of two elements in phosphorus and cadmium-enriched soil and accumulation of cadmium in crops. Huan jing ke xue= Huanjing kexue 41 (6), 2861–2868. doi: 10.13227/j.hjkx.201911278
Hussain, A., Ali, S., Rizwan, M., Zia Ur Rehman, M., Javed, M. R., Imran, M., et al. (2018). Zinc oxide nanoparticles alter the wheat physiological response and reduce the cadmium uptake by plants. Environ. pollut. 242, 1518–1526. doi: 10.1016/j.envpol.2018.08.036
Hussain, F., Hadi, F., Qiu, R. L. (2021). Effects of zinc oxide nanoparticles on antioxidants, chlorophyll contents, and proline in persicaria hydropiper l. and its potential for Pb phytoremediation. Environ. Sci. pollut. Res. 28 (26), 34697–34713. doi: 10.1007/s11356-021-13132-0
Iannone, M. F., Groppa, M. D., Zawoznik, M. S., Coral, D. F., van Raap, M. B. F., Benavides, M. P. (2021). Magnetite nanoparticles coated with citric acid are not phytotoxic and stimulate soybean and alfalfa growth. Ecotoxicology Environ. Saf. 211. doi: 10.1016/j.ecoenv.2021.111942
Ismael, M. A., Elyamine, A. M., Moussa, M. G., Cai, M., Zhao, X., Hu, C. (2019). Cadmium in plants: uptake, toxicity, and its interactions with selenium fertilizers. Metallomics 11 (2), 255–277. doi: 10.1039/C8MT00247A
Jalloh, M. A., Chen, J., Zhen, F., Zhang, G. (2009). Effect of different n fertilizer forms on antioxidant capacity and grain yield of rice growing under cd stress. J. Hazardous Materials 162 (2-3), 1081–1085. doi: 10.1016/j.jhazmat.2008.05.146
Jinadasa, N., Collins, D., Holford, P., Milham, P. J., Conroy, J. P. (2016). Reactions to cadmium stress in a cadmium-tolerant variety of cabbage (Brassica oleracea l.): is cadmium tolerance necessarily desirable in food crops? Environ. Sci. pollut. Res. 23 (6), 5296–5306. doi: 10.1007/s11356-015-5779-6
Jin, N., Jin, L., Luo, S., Tang, Z., Liu, Z., Wei, S., et al. (2021). Comprehensive evaluation of amino acids and polyphenols in 69 varieties of green cabbage (Brassica oleracea l. var. capitata l.) based on multivariate statistical analysis. Molecules 26 (17). doi: 10.3390/molecules26175355
Khan, M. N., Mobin, M., Abbas, Z. K., AlMutairi, K. A., Siddiqui, Z. H. (2017). Role of nanomaterials in plants under challenging environments. Plant Physiol. Biochem. 110, 194–209. doi: 10.1016/j.plaphy.2016.05.038
Khan, I., Seleiman, M. F., Chattha, M. U., Jalal, R. S., Mahmood, F., Hassan, F. A. S., et al. (2021). Enhancing antioxidant defense system of mung bean with a salicylic acid exogenous application to mitigate cadmium toxicity. Notulae Botanicae Horti Agrobotanici Cluj-Napoca 49 (2). doi: 10.15835/nbha49212303
Khoshgoftarmanesh, A. H., Markarian, S. (2022). Antioxidant response of wheat to tire rubber ash and ZnO nanoparticles and ionic zinc exposure in nutrient solution culture. Acta Physiologiae Plantarum 44 (4). doi: 10.1007/s11738-022-03384-9
Kidwai, M., Dhar, Y. V., Gautam, N., Tiwari, M., Ahmad, I. Z., Asif, M. H., et al. (2019). Oryza sativa class HI peroxidase (OsPRX38) overexpression in arabidopsis thaliana reduces arsenic accumulation due to apoplastic lignification. J. Hazardous Materials 362, 383–393. doi: 10.1016/j.jhazmat.2018.09.029
Konate, A., He, X., Zhang, Z., Ma, Y., Zhang, P., Alugongo, G. M., et al. (2017). Magnetic (Fe3O4) nanoparticles reduce heavy metals uptake and mitigate their toxicity in wheat seedling. Sustainability 9 (5), 790. doi: 10.3390/su9050790
Kouhi, S. M. M., Lahouti, M., Ganjeali, A., Entezari, M. H. (2014). Comparative phytotoxicity of ZnO nanoparticles, ZnO microparticles, and Zn2+ on rapeseed (Brassica napus l.): investigating a wide range of concentrations. Toxicological Environ. Chem. 96 (6), 861–868. doi: 10.1080/02772248.2014.994517
Krippner, J., Schubert, S. (2021). Elevated zinc concentrations did not induce thiols in spinach (Spinacia oleracea) and parsley (Petroselinum crispum). J. Plant Nutr. Soil Sci. 184 (4), 439–447. doi: 10.1002/jpln.202000537
Laxa, M., Liebthal, M., Telman, W., Chibani, K., Dietz, K. J.. (2019). The Role of the PlantAntioxidant System in Drought Tolerance. Antioxidants 8 (4). doi: 10.3390/antiox8040094
Li, Y., Liang, L., Li, W., Ashraf, U., Ma, L., Tang, X., et al. (2021). ZnO nanoparticle-based seed priming modulates early growth and enhances physio-biochemical and metabolic profiles of fragrant rice against cadmium toxicity. J. Nanobiotechnology 19 (1). doi: 10.1186/s12951-021-00820-9
Li, Y., Zhang, S., Jiang, W., Liu, D. (2013). Cadmium accumulation, activities of antioxidant enzymes, and malondialdehyde (MDA) content in pistia stratiotes l. Environ. Sci. pollut. Res. 20 (2), 1117–1123. doi: 10.1007/s11356-012-1054-2
Melo, E., Costa, E., Guilherme, L., Faquin, V., Nascimento, C. (2009). Accumulation of arsenic and nutrients by castor bean plants grown on an as-enriched nutrient solution. J. Hazardous Materials 168 (1), 479–483. doi: 10.1016/j.jhazmat.2009.02.048
Meng, H., Hua, S., Shamsi, I. H., Jilani, G., Li, Y., Jiang, L., et al. (2009). Cadmium-induced stress on the seed germination and seedling growth of Brassica napus L., and its alleviation through exogenous plant growth regulators. Plant Growth Regul. 58 (1), 47–59. doi: 10.1007/s10725-008-9351-y
Molnar, A., Ronavari, A., Belteky, P., Szollosi, R., Valyon, E., Olah, D., et al. (2020). ZnO nanoparticles induce cell wall remodeling and modify ROS/RNS signalling in roots of brassica seedlings. Ecotoxicology Environ. Saf. 206. doi: 10.1016/j.ecoenv.2020.111158
Mustafa, G., Komatsu, S. (2016). Toxicity of heavy metals and metal-containing nanoparticles on plants. Biochim. Biophys. Acta (BBA)-Proteins Proteomics 1864 (8), 932–944. doi: 10.1016/j.bbapap.2016.02.020
Noman, A., Ali, Q., Maqsood, J., Iqbal, N., Javed, M. T., Rasool, N., et al. (2018a). Deciphering physio-biochemical, yield, and nutritional quality attributes of water-stressed radish (Raphanus sativus l.) plants grown from zn-lys primed seeds. Chemosphere 195, 175–189. doi: 10.1016/j.chemosphere.2017.12.059
Noman, A., Ali, Q., Naseem, J., Javed, M. T., Kanwal, H., Islam, W., et al. (2018b). Sugar beet extract acts as a natural bio-stimulant for physio-biochemical attributes in water stressed wheat (Triticum aestivum l.). Acta Physiologiae Plantarum 40 (6), 1–17. doi: 10.1007/s11738-018-2681-0
Palusinska, M., Barabasz, A., Kozak, K., Papierniak, A., Maslinska, K., Antosiewicz, D. M. (2020). Zn/Cd status-dependent accumulation of zn and cd in root parts in tobacco is accompanied by specific expression of ZIP genes. BMC Plant Biol. 20 (1). doi: 10.1186/s12870-020-2255-3
Paramo, L. A., Feregrino-Pérez, A. A., Guevara, R., Mendoza, S., Esquivel, K. (2020). Nanoparticles in agroindustry: Applications, toxicity, challenges, and trends. Nanomaterials 10 (9), 1654. doi: 10.3390/nano10091654
Parrotta, L., Guerriero, G., Sergeant, K., Cai, G., Hausman, J.-F. (2015). Target or barrier? the cell wall of early-and later-diverging plants vs cadmium toxicity: differences in the response mechanisms. Front. Plant Sci. 6, 133. doi: 10.3389/fpls.2015.00133
Patel, K. V., Nath, M., Bhatt, M. D., Dobriyal, A. K., Bhatt, D. (2020). Nanofomulation of zinc oxide and chitosan zinc sustain oxidative stress and alter secondary metabolite profile in tobacco. 3 Biotech. 10 (11). doi: 10.1007/s13205-020-02469-x
Pavani, K. V., Beulah, M., Sai Poojitha, G. U. (2020). The effect of zinc oxide nanoparticles (ZnO NPs) on vigna mungo l. seedling growth and antioxidant activity. Nanoscience Nanotechnology-Asia 10 (2), 117–122. doi: 10.2174/2210681208666180820150647
Prakash, V., Rai, P., Sharma, N. C., Singh, V. P., Tripathi, D. K., Sharma, S., et al. (2022). Application of zinc oxide nanoparticles as fertilizer boosts growth in rice plant and alleviates chromium stress by regulating genes involved in regulating oxidative stress. Chemosphere 134554. doi: 10.1016/j.chemosphere.2022.134554
Qi, X., Tam, N. F.-Y., Li, W. C., Ye, Z. (2020). The role of root apoplastic barriers in cadmium translocation and accumulation in cultivars of rice (Oryza sativa l.) with different cd-accumulating characteristics. Environ. pollut. 264. doi: 10.1016/j.envpol.2020.114736
Rajapakse, D., Curtis, T., Chen, M., Xu, H. (2017). Zinc protects oxidative stress-induced RPE death by reducing mitochondrial damage and preventing lysosome rupture. Oxid. Med. Cell. Longevity 2017. doi: 10.1155/2017/6926485
Rawashdeh, R. Y., Harb, A. M., AlHasan, A. M. (2020). Biological interaction levels of zinc oxide nanoparticles; lettuce seeds as case study. Heliyon 6 (5). doi: 10.1016/j.heliyon.2020.e03983
Rizwan, M., Ali, S., Adrees, M., Ibrahim, M., Tsang, D. C., Zia-ur-Rehman, M., et al. (2017). A critical review on effects, tolerance mechanisms and management of cadmium in vegetables. Chemosphere 182, 90–105. doi: 10.1016/j.chemosphere.2017.05.013
Rizwan, M., Ali, S., Ali, B., Adrees, M., Arshad, M., Hussain, A., et al. (2019a). Zinc and iron oxide nanoparticles improved the plant growth and reduced the oxidative stress and cadmium concentration in wheat. Chemosphere 214, 269–277. doi: 10.1016/j.chemosphere.2018.09.120
Rizwan, M., Ali, S., Zia Ur Rehman, M., Adrees, M., Arshad, M., Qayyum, M. F., et al. (2019b). Alleviation of cadmium accumulation in maize (Zea mays l.) by foliar spray of zinc oxide nanoparticles and biochar to contaminated soil. Environ. pollut. 248, 358–367. doi: 10.1016/j.envpol.2019.02.031
Rizwan, M., Meunier, J.-D., Davidian, J.-C., Pokrovsky, O., Bovet, N., Keller, C. (2016). Silicon alleviates cd stress of wheat seedlings (Triticum turgidum l. cv. Claudio) grown in hydroponics. Environ. Sci. pollut. Res. 23 (2), 1414–1427. doi: 10.1007/s11356-015-5351-4
Rogers, L. A., Dubos, C., Surman, C., Willment, J., Cullis, I. F., Mansfield, S. D., et al. (2005). Comparison of lignin deposition in three ectopic lignification mutants. New Phytol. 168 (1), 123–140. doi: 10.1111/j.1469-8137.2005.01496.x
Ronzan, M., Piacentini, D., Fattorini, L., Della Rovere, F., Eiche, E., Rieman, M., et al. (2018). Cadmium and arsenic affect root development in oryza sativa l. negatively interacting with auxin. Environ. Exp. Bot. 151, 64–75. doi: 10.1016/j.envexpbot.2018.04.008
Rossi, L., Sharifan, H., Zhang, W., Schwab, A. P., Ma, X. (2018). Mutual effects and in planta accumulation of co-existing cerium oxide nanoparticles and cadmium in hydroponically grown soybean (Glycine max (L.) merr.). Environ. Science: Nano 5 (1), 150–157. doi: 10.1039/c7en00931c
Saidi, I., Chtourou, Y., Djebali, W. (2014). Selenium alleviates cadmium toxicity by preventing oxidative stress in sunflower (Helianthus annuus) seedlings. J. Plant Physiol. 171 (5), 85–91. doi: 10.1016/j.jplph.2013.09.024
Salem, H. M., Abdel-Salam, A., Abdel-Salam, M. A., Seleiman, M. F. (2018). “"Phytoremediation of metal and metalloids from contaminated soil,",” in Plants under metal and metalloid stress: Responses, tolerance and remediation. Eds. Hasanuzzaman, M., Nahar, K., Fujita, M. (: Springer Singapore: Singapore), 249–262.
Santos, L., Batista, B., Lobato, A. (2018). Brassinosteroids mitigate cadmium toxicity in cowpea plants. Photosynthetica 56 (2), 591–605. doi: 10.1007/s11099-017-0700-9
Sary Hassan, M. B., Ibrahim Ali, A. A. (2019). The combined use of beneficial soil microorganisms enhanced the growth and efficiently reduced lead content in leaves of lettuce (Lactuca sativa l.) plant under lead stress. Alexandria J. Agric. Sci. 64 (1), 41–51. doi: 10.21608/ALEXJA.2019.41854
Schlutow, A., Schroeder, W., Scheuschner, T. (2021). Assessing the relevance of atmospheric heavy metal deposition with regard to ecosystem integrity and human health in Germany. Environ. Sci. Europe 33 (1). doi: 10.1186/s12302-020-00391-w
Seleiman, M. F., Almutairi, K. F., Alotaibi, M., Shami, A., Alhammad, B. A., Battaglia, M. L. (2021). Nano-fertilization as an emerging fertilization technique: Why can modern agriculture benefit from its use? Plants-Basel 10 (1). doi: 10.3390/plants10010002
Seleiman, M. F., Alotaibi, M. A., Alhammad, B. A., Alharbi, B. M., Refay, Y., Badawy, S. A. (2020a). Effects of ZnO nanoparticles and biochar of rice straw and cow manure on characteristics of contaminated soil and sunflower productivity, oil quality, and heavy metals uptake. Agronomy-Basel 10 (6). doi: 10.3390/agronomy10060790
Seleiman, M. F., Santanen, A., Makela, P. S. A. (2020b). Recycling sludge on cropland as fertilizer - advantages and risks. Resour. Conserv. Recycling 155. doi: 10.1016/j.resconrec.2019.104647
Sharifan, H., Ma, X., Moore, J. M., Habib, M. R., Eyans, C. (2019). Zinc oxide nanoparticles alleviated the bioavailability of cadmium and lead and changed the uptake of iron in hydroponically grown lettuce (Lactuca sativa l. var. longifolia). ACS Sustain. Chem. Eng. 7 (19), 16401–16409. doi: 10.1021/acssuschemeng.9b03531
Sharifan, H., Moore, J., Ma, X. (2020). Zinc oxide (ZnO) nanoparticles elevated iron and copper contents and mitigated the bioavailability of lead and cadmium in different leafy greens. Ecotoxicology Environ. Saf. 191. doi: 10.1016/j.ecoenv.2020.110177
Sharma, R. K., Agrawal, M., Marshall, F. M. (2008). Heavy metal (Cu, zn, cd and Pb) contamination of vegetables in urban India: A case study in varanasi. Environ. pollut. 154 (2), 254–263. doi: 10.1016/j.envpol.2007.10.010
Silveira Rabelo, F. H., Jordao, L. T., Lavres, J. (2017). A glimpse into the symplastic and apoplastic cd uptake by massai grass modulated by sulfur nutrition: Plants well-nourished with s as a strategy for phytoextraction. Plant Physiol. Biochem. 121, 48–57. doi: 10.1016/j.plaphy.2017.10.018
Song, Y., Jin, L., Wang, X. (2017). Cadmium absorption and transportation pathways in plants. Int. J. Phytoremediation 19 (2), 133–141. doi: 10.1080/15226514.2016.1207598
Song, U., Kim, J. (2020). Zinc oxide nanoparticles: a potential micronutrient fertilizer for horticultural crops with little toxicity. Horticulture Environment Biotechnol. 61 (3), 625–631. doi: 10.1007/s13580-020-00244-8
Srivastav, A., Ganjewala, D., Singhal, R. K., Rajput, V. D., Minkina, T., Voloshina, M., et al. (2021). Effect of ZnO nanoparticles on growth and biochemical responses of wheat and maize. Plants-Basel 10 (12). doi: 10.3390/plants10122556
Tang, C., Xie, J., Lv, J., Li, J., Zhang, J., Wang, C., et al. (2021). Alleviating damage of photosystem and oxidative stress from chilling stress with exogenous zeaxanthin in pepper (Capsicum annuum l.) seedlings. Plant Physiol. Biochem. 162, 395–409. doi: 10.1016/j.plaphy.2021.03.010
Tao, J., Lu, L. (2022). Advances in genes-encoding transporters for cadmium uptake, translocation, and accumulation in plants. Toxics 10 (8). doi: 10.3390/toxics10080411
Torabian, S., Zahedi, M., Khoshgoftarmanesh, A. (2016). Effect of foliar spray of zinc oxide on some antioxidant enzymes activity of sunflower under salt stress. J. Agric. Sci. Technol. 18 (4), 1013–1025. doi: 10.1001.1.16807073.2016.18.4.10.4
Usman, K., Al-Ghouti, M. A., Abu-Dieyeh, M. H. (2019). The assessment of cadmium, chromium, copper, and nickel tolerance and bioaccumulation by shrub plant tetraena qataranse. Sci. Rep. 9 (1), 1–11. doi: 10.1038/s41598-019-42029-9
Venkatachalam, P., Jayaraj, M., Manikandan, R., Geetha, N., Rene, E. R., Sharma, N. C., et al. (2017). Zinc oxide nanoparticles (ZnONPs) alleviate heavy metal-induced toxicity in leucaena leucocephala seedlings: A physiochemical analysis. Plant Physiol. Biochem. 110, 59–69. doi: 10.1016/j.plaphy.2016.08.022
Wang, W., Cang, L., Zhou, D. M., Yu, Y. C. (2017). Exogenous amino acids increase antioxidant enzyme activities and tolerance of rice seedlings to cadmium stress. Environ. Prog. Sustain. Energy 36 (1), 155–161. doi: 10.1002/ep.12474
Wang, Y., Cui, T., Niu, K., Ma, H. (2021). Comparison and characterization of oxidation resistance and carbohydrate content in cd-tolerant and -sensitive Kentucky bluegrass under cd stress. Agronomy-Basel 11 (11). doi: 10.3390/agronomy11112358
Wang, Y. M., Yang, R. X., Zheng, J. Y., Shen, Z. G., Xu, X. M. (2019). Exogenous foliar application of fulvic acid alleviate cadmium toxicity in lettuce (Lactuca sativa l.). Ecotoxicology Environ. Saf. 167, 10–19. doi: 10.1016/j.ecoenv.2018.08.064
Wei, C., Jiao, Q., Agathokleous, E., Liu, H., Li, G., Zhang, J., et al. (2022). Hormetic effects of zinc on growth and antioxidant defense system of wheat plants. Sci. Total Environ. 807. doi: 10.1016/j.scitotenv.2021.150992
Xiao, Y. M., Li, Y., Shi, Y., Li, Z. Q., Zhang, X. Y., Liu, T., et al. (2022). Combined toxicity of zinc oxide nanoparticles and cadmium inducing root damage in phytolacca americana l. Sci. Total Environ. 806. doi: 10.1016/j.scitotenv.2021.151211
Xu, L., Fan, Z., Dong, Y., Kong, J., Liu, S., Hou, J., et al. (2015). Effects of exogenous NO supplied with different approaches on cadmium toxicity in lettuce seedlings. Plant Biosystems-An Int. J. Dealing all Aspects Plant Biol. 149 (2), 270–279. doi: 10.1080/11263504.2013.822030
Yan, A., Wang, Y., Tan, S. N., Yusof, M. L. M., Ghosh, S., Chen, Z. (2020). Phytoremediation: A promising approach for revegetation of heavy metal-polluted land. Front. Plant Sci. 11. doi: 10.3389/fpls.2020.00359
Zhang, X., Huang, G., Bian, X., Zhao, Q. (2013). Effects of root interaction and nitrogen fertilization on the chlorophyll content, root activity, photosynthetic characteristics of intercropped soybean and microbial quantity in the rhizosphere. Plant Soil Environ. 59 (2), 80–88. doi: 10.17221/613/2012-pse
Zhang, W., Long, J. H., Li, J., Zhang, M., Xiao, G. L., Ye, X. Y., et al. (2019). Impact of ZnO nanoparticles on cd toxicity and bioaccumulation in rice (Oryza sativa l.). Environ. Sci. pollut. Res. 26 (22), 23119–23128. doi: 10.1007/s11356-019-05551-x
Zhang, Y., Xu, S., Yang, S., Chen, Y. (2015). Salicylic acid alleviates cadmium-induced inhibition of growth and photosynthesis through upregulating antioxidant defense system in two melon cultivars (Cucumis melo l.). Protoplasma 252 (3), 911–924. doi: 10.1007/s00709-014-0732-y
Zhao, L., Zhu, Y. H., Wang, M., Ma, L. G., Han, Y. G., Zhang, M. J., et al. (2021). Comparative transcriptome analysis of the hyperaccumulator plant phytolacca americana in response to cadmium stress. 3 Biotech. 11 (7). doi: 10.1007/s13205-021-02865-x
Zhou, B., Deng, Y. S., Kong, F. Y., Li, B., Meng, Q. W. (2013). Overexpression of a tomato carotenoid epsilon-hydroxylase gene alleviates sensitivity to chilling stress in transgenic tobacco. Plant Physiol. Biochem. 70, 235–245. doi: 10.1016/j.plaphy.2013.05.035
Zouari, M., Ahmed, C. B., Elloumi, N., Bellassoued, K., Delmail, D., Labrousse, P., et al. (2016). Impact of proline application on cadmium accumulation, mineral nutrition and enzymatic antioxidant defense system of olea europaea l. cv chemlali exposed to cadmium stress. Ecotoxicology Environ. Saf. 128, 195–205. doi: 10.1016/j.ecoenv.2016.02.024
Keywords: cadmium, nanoparticles, lettuce, antioxidants, stress
Citation: Gao F, Zhang X, Zhang J, Li J, Niu T, Tang C, Wang C and Xie J (2022) Zinc oxide nanoparticles improve lettuce (Lactuca sativa L.) plant tolerance to cadmium by stimulating antioxidant defense, enhancing lignin content and reducing the metal accumulation and translocation. Front. Plant Sci. 13:1015745. doi: 10.3389/fpls.2022.1015745
Received: 10 August 2022; Accepted: 04 October 2022;
Published: 27 October 2022.
Edited by:
Mahmoud F. Seleiman, King Saud University, Saudi ArabiaReviewed by:
Juraci Alves Oliveira, Universidade Federal de Viçosa, BrazilImran Khan, Lanzhou University, China
Basharat Ali, Khwaja Fareed University of Engineering and Information Technology (KFUEIT), Pakistan
Copyright © 2022 Gao, Zhang, Zhang, Li, Niu, Tang, Wang and Xie. This is an open-access article distributed under the terms of the Creative Commons Attribution License (CC BY). The use, distribution or reproduction in other forums is permitted, provided the original author(s) and the copyright owner(s) are credited and that the original publication in this journal is cited, in accordance with accepted academic practice. No use, distribution or reproduction is permitted which does not comply with these terms.
*Correspondence: Jianming Xie, eGllamlhbm1pbmdAZ3NhdS5lZHUuY24=
†These authors have contributed equally to this work and share first authorship