- 1College of Agronomy, Sichuan Agricultural University, Chengdu, China
- 2Key Laboratory of Crop Eco-Physiology and Farming System, Sichuan Engineering Research Center for Crop Strip Intercropping System, Chengdu, China
- 3Crop Research Institute, Chengdu Academy of Agricultural and Forestry Sciences, Chengdu, China
- 4National Center of Industrial Biotechnology (NCIB), PMAS Arid Agriculture University, Rawalpindi, Pakistan
- 5Plant and Food Research, Blenheim, New Zealand
- 6College of Life Science, Sichuan Agricultural University, Chengdu, China
- 7College of Grassland Science and Technology, Sichuan Agricultural University, Chengdu, China
Soybean (Glycine max) is a legume species that is widely used in intercropping. Quantitative analyses of plasticity and genetic differences in soybean would improve the selection and breeding of soybean in intercropping. Here, we used data of 20 varieties from one year artificial shading experiment and one year intercropping experiment to characterize the morphological and physiological traits of soybean seedlings grown under shade and full sun light conditions. Our results showed that shade significantly decreased biomass, leaf area, stem diameter, fraction of dry mass in petiole, leaf mass per unit area, chlorophyll a/b ratio, net photosynthetic rate per unit area at PAR of 500 μmol m–2 s–1 and 1,200 μmol m–2 s–1 of soybean seedling, but significantly increased plant height, fraction of dry mass in stem and chlorophyll content. Light × variety interaction was significant for all measured traits, light effect contributed more than variety effect. The biomass of soybean seedlings was positively correlated with leaf area and stem diameter under both shade and full sunlight conditions, but not correlated with plant height and net photosynthetic rate. The top five (62.75% variation explained) most important explanatory variables of plasticity of biomass were that the plasticity of leaf area, leaf area ratio, leaflet area, plant height and chlorophyll content, whose total weight were 1, 0.9, 0.3, 0.2, 0.19, respectively. The plasticity of biomass was positively correlated with plasticity of leaf area and leaflet area but significant negative correlated with plasticity of plant height. The principal component one account for 42.45% variation explain. A cluster analysis further indicated that soybean cultivars were classified into three groups and cultivars; Jiandebaimaodou, Gongdou 2, and Guixia 3 with the maximum plasticity of biomass. These results suggest that for soybean seedlings grown under shade increasing the capacity for light interception by larger leaf area is more vital than light searching (plant height) and light conversion (photosynthetic rate).
Introduction
Intercropping, defined as the cultivation of two or more crop species simultaneously in the same field (Willey, 1979; Francis, 1989), is widely practiced by smallholder farmers across the world, and is attracting attention in the context of ecological intensification of agriculture (Vandermeer, 2011; Brooker et al., 2015; Tanveer et al., 2017). Compared with sole cropping, intercropping often leads to higher yield because of the full use of time, space, and resources (Willey, 1990; Keating and Carberry, 1993; Szumigalski and Van Acker, 2006; Malézieux et al., 2009; Raza et al., 2021). Adaptive plant morphological and physiological responses to intercropping environments are likely to contribute to the yield advantage (Li et al., 2021; Wang et al., 2021). It has been shown that plasticity of the shoot traits of wheat contribute significantly to the enhanced light capture in wheat-maize intercropping systems (Zhu et al., 2015, 2016). This suggests that phenotypic plasticity, which is defined as the ability of a genotype to alter its expressed trait values in response to environmental conditions (Bradshaw, 1965; Valladares et al., 2007; Sultan, 2010), may improve crop performance in intercropping systems. However, detailed information about the genetic differences in phenotypic plasticity in the context of intercropping is still lacking. Such information can be used as a guide in variety selection and breeding to optimize the benefits of intercropping systems (Zhu et al., 2016).
The cereal-legume system is one of the most common types of intercropping systems (Yu et al., 2015), and soybean is one of the most widely used legumes in these systems, which include maize–soybean (Wu et al., 2021) and sorghum–soybean (Ghosh et al., 2009). Due to the shorter plant height and late sowing time than maize and sorghum, soybean plants are often grown under shade conditions in these intercropping systems (Wu et al., 2022). Plants adapt to shade through either shade tolerance (Yang et al., 2018) or shade avoidance mechanisms (Valladares et al., 2007; Valladares and Niinemets, 2008; Niinemets, 2010). Shade tolerance mechanisms, which can help plants survive under low light conditions, increase light harvesting or light use efficiency. These mechanisms include increasing chlorophyll (Chl) content, increasing specific leaf area, and reducing the Chl a/b ratio (Givnish, 1988; Valladares and Niinemets, 2008; Niinemets, 2010). Shade avoidance mechanisms, which can help plants escape from shade and likely increase light capture, include responses such as enhanced stem and petiole elongation, higher dry mass allocation to the stem than to the leaf and root, and develop a small leaf angles (Ballaré, 1999; Smith, 2000; Franklin and Whitelam, 2005; Vandenbussche et al., 2005; Casal, 2012; de Wit et al., 2012).
Phenotypic plasticity plays a remarkable role in the ecological distribution and evolutionary diversification of plants (Enbody et al., 2021). The degrees of plasticity differ among species or populations from contrasting habitats. It has been reported that shade-grown Impatiens capensis possesses longer stems and internodes than its counterparts grown under full sunshine and that I. capensis populations originating from open habitats are more sensitive to shade than those from shade habitats (Dudley and Schmitt, 1995; Anten et al., 2009). Other studies comparing the plasticity of morphological and physiological variables in different species found that shade-intolerant species from light habitats are more sensitive to light and exhibit a greater degree of plasticity than shade-tolerant species from shade habitats (Valladares et al., 2000a,b; Portsmuth and Niinemets, 2007). Comparisons of the plasticity of different functional traits revealed that shade-intolerant species have relatively higher plasticity for physiological traits, but lower plasticity for morphological traits than shade-tolerant species (Valladares et al., 2000b; Li et al., 2012; Naseer et al., 2022). It has been pointed out that light-favoring plants have enhanced physiological plasticity for variables related to photosynthesis, while shade tolerant species rely on enhanced plasticity in light-harvesting traits (Valladares et al., 2002; Poorter et al., 2019).
It has been shown that soybean plants display a suite of shade-avoidance responses when co-grown with maize; these responses result in a lower photosynthetic capacity, an elongated stem, reduced branching, a higher lodging rate and lower yield (Yan et al., 2010; Su et al., 2014; Yang et al., 2014; Wu et al., 2022). Previously, we showed that there are genetic differences in the shade responses of two soybean varieties (Gong et al., 2015). However, it remains unclear how these responses reflect the inherent strategies for coping with shade, and which traits should be displayed in the ideotype for breeding soybean varieties with better performance in intercropping. The aim of this study was: (1) to characterize the phenotypic variation in morphological and physiological traits of soybean grown under both shade and full-light conditions; (2) to quantify the relationship between the biomass of soybean and morphological and physiological traits under the different light conditions; and (3) to develop approaches to establish an ideotype contributing to higher biomass accumulation under shade based on plasticity in morphological and physiological traits.
Materials and methods
Experimental design and field management
Experiment 1: Experiment 1 was conducted in 2014 under field conditions at the Teaching and Experimental Farm of Sichuan Agricultural University in Ya’an (29°59’N, 103°00’E), which is located at the western border of the Sichuan Basin. The soil of the experimental field is a purple clay loam (pH 7.5). On June 19th, the seeds of 20 soybean varieties (names refer to Supplementary Table 1) were sown, each varieties were sown in one plot with a row spacing of 0.5 m and a row length of 2.5 m, and three rows of each variety were planted for each condition. The shade and full sun light treatments were started immediately after sowing. The shade treatment was achieved by installing green shading nets above the experimental field at a height of 2 m, ∼40% transmittance, PAR was around 500 μmol m–2 s–1, average daily temperature was 30.7 °C, average daily humidity was 71.9%. The average daily temperature and humidity of full sun light treatment was 32.9 °C and 64.5% (Yang et al., 2014; Wu et al., 2017b; Figures 1A,B). After the first trifoliolate leaves expanded, soybean seedlings were thinned to 0.1 m between plants in each row.
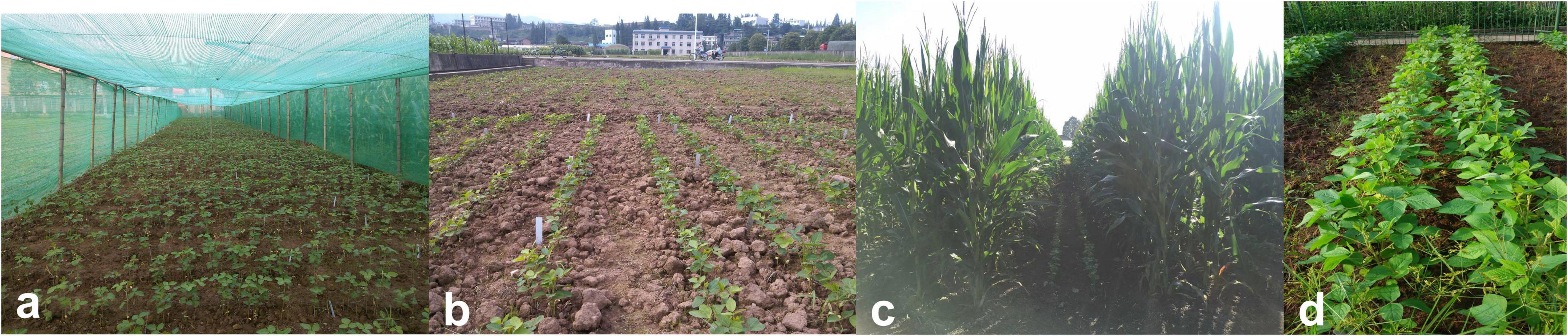
Figure 1. Photographes of the experiment. (a) Shade treatment in 2014; (b) full sun light treatment in 2014; (c) maize-soybean relay strip intercropping in 2015; (d) soybean monoculture in 2015.
Experiment 2: In order to further verify the shade response of soybean and variety performance of 2014 in a real intercropping shade environment, we conducted Experiment 2 in 2015 at the same field of 2014, so the soil of the experimental field is a purple clay loam (pH 7.5). It was conducted by a split-plot experiment design with three replications. The planting pattern was set as main plot with two levels: maize-soybean relay strip intercropping (shade) and soybean monoculture (full sun light) (Figures 1C,D). 14 soybean varieties (in order to reduce the field work, we only selected 14 varieties with large difference in growth in shade) were used in sub plot based on the results of 2014 (Supplementary Table 1). The field arrangement of relay intercropping was carried out as follows. Briefly, For the full sun light treatment in sole cropping, soybeans were planted as solid rows with a 0.5-m row spacing. For the shade treatment in intercropping, soybean and maize were planted as alternating strips, and every soybean strip was relay intercropped between the maize strips. Each plot contained two maize and two soybean strips, and each soybean strip and maize strip consisted of two soybean and two maize rows. The strip spacing (distance between maize and soybean rows), soybean row spacing and maize row spacing were all 0.5 m (Wu et al., 2017b). Each plot was 6 m long. The maize cultivar, Zhenghong 505, was sown on 9 April and harvested on 9 August, the soybeans were sown on 20 June and harvested on 23 October. When soybean was planted, maize was 2.5 meters high and reduced the light interception rate of soybean by 66%, the average daily temperature and humidity of intercropping treatment was 30.1 °C and 68.6%. The weather condition (rainfall and temperature) of two years are shown in Supplementary Figure 1.
Measurements
In Experiment 1, three individual plants grown in the middle section of the middle row were tagged on August 5th, 2014 (47 days after sowing); these plants were used for all measurements and each individual plant was a biological replicate. Photosynthetic indexes were mearsured on on the 47 days after sowing, and the middle leaflets of the latest fully expanded trifoliolate leaves were used for measurements. Photosynthesis was measured using a portable photosynthesis system (LI-6400XT, Li-Cor Inc., USA) equipped with an LED Light Source (6400-02B). Net photosynthetic rate per unit area was measured under light intensities of 500 (PN500) and 1,200 (PN1200) μmol m–2 s–1, a CO2 concentration of 380 μmol mol–1 sample, an air flow rate of 500 ml min–1, 60–75% relative humidity and a temperature of 30°C. The net photosynthetic rate value was taken when the range is between 0.1 and 0.2 after 1–2 min. After the measurement of photosynthesis, the same trifoliolate leaves were collected in white ziplock bag, and put in a ice box and brought to the laboratory no more than 30 min. First, leaves were scanned using a flatbed scanner (CanoScan LiDE 200, Canon Inc., Japan), and the captured images were used for later analysis of the leaflet area in ImageJ 1.45s. Second, two leaf discs (diameter = 1 cm) from the middle leaflet were punched out, and extracted in a centrifuge tube with 80% aqueous acetone solvent for 24 h in a dark environment with 20 °C indoor temperature, and then used spectrophotometer to determine the total Chl concentration and the Chl a/b ratio (Lichtenthaler, 1987). The remaining leaves were oven-dried and weighed to calculate the leaf mass per unit area and the Chl concentration per unit dry mass. Dried leaves were finally ground into a fine powder for the measurement of nitrogen (N) and carbon (C) concentration using an elemental analyzer (CE-440 Elemental Analyzer, Exeter Analytical Inc., USA). N concentrations were expressed based on unit content per unit area.
On August 6th, 2014 (48 days after sowing), the aboveground parts of the tagged plants were sampled and brought back to the laboratory to measure biomass and morphological traits. Plants were divided into three parts: stem, lamina and petiole. Laminas were scanned, and then the total leaf area per plant was determined using ImageJ. Plant height and stem diameter (measured at the middle point of the first internode) were also measured. The separate parts of the leaf were oven-dried at 70°C to a constant weight (∼72 h). Biomass, fraction of dry mass in the stem, fraction of dry mass in the lamina, and fraction of dry mass in the petiole were then calculated.
In Experiment 2, all sampling and measurement were conducted on July 27th, 2015 (37 days after sowing). First, PN1200 was measured on five newly expanded leaves in five individual plants grown in the middle section of the middle row per plot, other parameters were maintained as described in experiment 1. Second, the measured plant for photosynthetic rates were sampled and brought to laboratory, leaf area, plant height, stem diameter, biomass were measured as described in experiment 1. The mean value per plot were calculated and used for statistics.
Data processing and analysis
The plasticity of plants in response to shade was calculated as the dimensionless slope of norm of reaction as previously reported (Sadras et al., 2009). Briefly, Plasticity = (Ti_shade-Ti_fulllight)/(shade-fulllight), where Ti is the value of one trait (e.g., biomass) of the ith variety, and is the mean value of the trait across all varieties. Thus, slope = 1 indicates average plasticity over the two light environments, slope > 1 indicates above-average plasticity, and slope < 1 indicates below-average plasticity (Sadras et al., 2009).
Two-way ANOVA was used to test for the effects of light and variety on the measured traits. Light was set as a fixed factor, and variety was set as a random factor. Before analysis, trait values were transformed by taking the natural logarithm. Correlation and regression analysis were used to explore associations between traits and plasticity (Supplementary Tables 2, 3 and Supplementary Figures 3, 4). Analyses were performed using SPSS 19.0 software (SPSS, Chicago, USA).
R version 4.0.5 was used to reveal the quantitative relationships between the plasticity of biomass and the plasticity of morphological and physiological traits. Features selection was performed with the train function in R package caret v6.0-91. Then, features importance was obtained with varImp function in R package caret v6.0-91 (Figure 2). The critical features (p < 0.05) were selected, and correlation analysis was performed with ggpairs function in R package GGally v2.1.2 (Figure 3). Similarly, the critical features were performed with principle component analysis with the PCA function in R package FactoMineR package v1.34. The results of PCA were visualized with the fviz_pca_biplot function in R factoextra package v1.0.7 (Figure 6A). Meanwhile, hierarchical cluster analysis (Figure 6B) was performed with the hclust function and visualized with the fviz_dend function in R package factoextra.
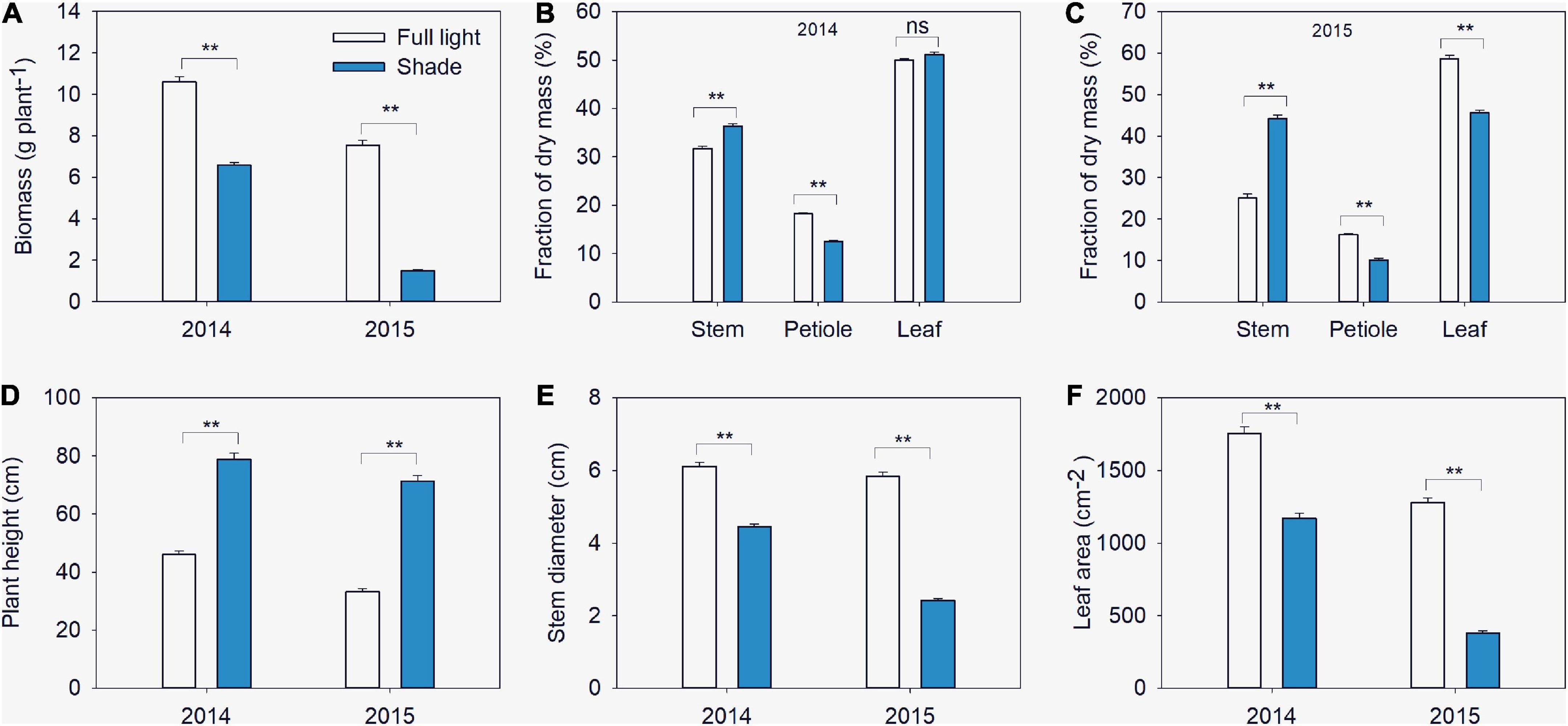
Figure 2. Biomass (A), fractions of dry mass in stem, petiole and leaf (B,C), plant height (D), stem diameter (E) and leaf area (F) of different soybean varieties grown under shade and the control conditions in 2014–2015. Traits were expressed as mean ± standard errors from 20 cultivars in 2014 and 14 cultivars in 2015. ** represent significant difference at 0.01 level.

Figure 3. Net photosynthetic rates at 500 μmol m– 2 s– 1 (PN500) and 1,200 μmol m– 2 s– 1 (PN1200) (A), chlorophyll concentration (Chl) and Chl a/b ratio (Chl a/b) (B), nitrogen (N) concentration and leaf mass per unit area (LMA) (C) of 20 soybean varieties grown under shade and the control conditions in 2014. Traits were expressed as mean ± standard errors from 20 cultivars in 2014 and 14 cultivars in 2015. ** represent significant difference at 0.01 level.
Results
Plant biomass and biomass allocation
In 2014, shade significantly reduced biomass, the mean biomass of soybean seedlings grown under shade across all varieties was 6.6 g per plant, which was 37.8% less than that of seedlings grown under full light (10.6 g per plant) (Figure 2A). Similar variations trend were observed in 2015 when soybean plants grown under shade in intercropping and under full light in sole cropping. The mean biomass of soybean seedling was 1.5 g per plant, the corresponding grown under full light was 7.6 g per plant (Figure 2A). Significant interactions between light and variety were found for biomass. Partitioning of total sum of squares (SS) indicated that the light effect was the major contributor (Table 1).
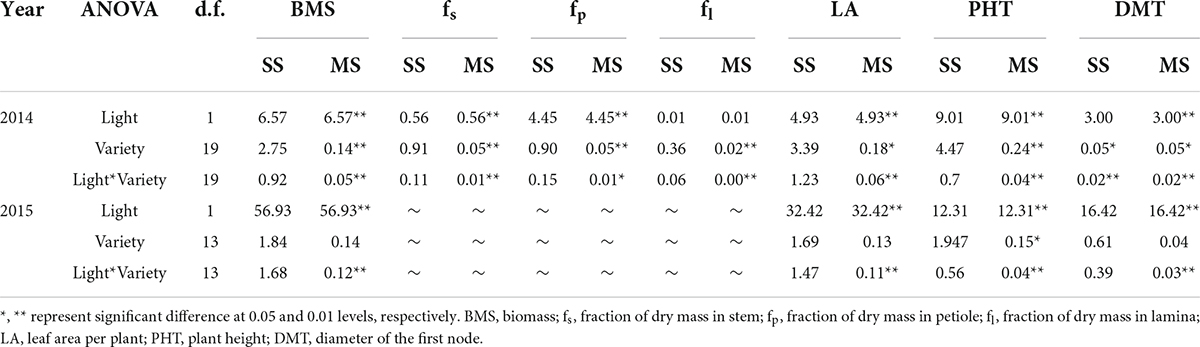
Table 1. The sum of squares (SS) and mean squares (MS) of two-way ANOVAs of the effects of light and variety on morphological traits.
In 2014, the mean fraction of dry mass in stem increased from 31.7% under full light to 36.4% under shade. Meanwhile, the mean fraction of dry mass in petiole decreased from 18.3% under full light to 12.5% under shade (Figure 2B). There was no difference in fraction of dry mass in leaf between plants grown under the two treatments. Significant varietal differences and interactions between light and variety were found in fraction of dry mass. The total SS indicated that the variety effect was the major contributor for stem and leaf, while petiole variation was caused by light (Table 1). In 2015, Similar variations trend of fraction of dry mass in stem and petiole were observed. While the mean fraction of dry mass in leaf decreased from 58% under full light to 45% under shade.
Morphological traits
In 2014, plant height greatly increased under shade conditions, while stem diameter decreased. The mean plant height of soybean seedlings grown under shade across all varieties was 79 cm, which was 71.5% higher than that for seedlings grown under full light (46 cm), while the mean stem diameter reduced from 6.1 mm in full light to 4.5 mm in shade (Figures 2D,E). leaf area was significantly reduced in shade. The mean value of leaf area across all varieties grown under shade was 1,171.1 cm2, which was 33.3% less than that under full light (1,755.4 cm2) (Figure 2F). In 2015, when grown under shade in intercropping, morphological traits significantly varied. Mean plant height of soybean seedling was 71.3 cm, but the value of seedlings grown under full light was 33.3 cm (Figure 2D). Meanwhile, the leaf area of soybean seedlings reduced from 1,278.4 cm2 in full light to 379.9 cm2 in shade, and the stem diameter reduced from 5.84 mm in full light to 2.42 mm in shade (Figures 2E,F). Significant interaction between light and variety were found for those traits in both two years, the partition of the total SS revealed that the major percentage was attributable to light (Table 1). The yields of the 14 soybean varieties in two treatments are also shown in Supplementary Table 4.
Physiological traits
Leaf physiological traits related to light use efficiency were affected by shade. Leaf mass per unit area, PN500, PN1200 and the Chl a/b ratio decreased under the shade condition, while Chl content increased (Figure 3). Leaf mass per unit area and Chl content differed between varieties, while PN500, PN1200 and leaf nitrogen content did not. The interactions between light and variety for leaf mass per unit area, PN500, PN1200, Chl content, Chl a/b ratio and N content were significant. ANOVA results revealed that light effect was the major contributor for Leaf mass per unit area, PN500, PN1200, Chl a/b ratio and nitrogen content, while Chl content variation was caused by variety (Table 2).
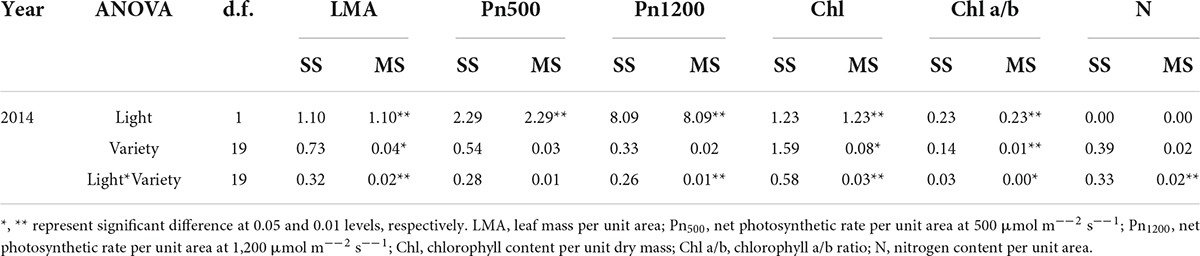
Table 2. The sum of squares (SS) and mean squares (MS) of two-way ANOVAs of the effects of light and variety on physiological traits.
Relationships between the biomass and the morphological and physiological traits
In 2014, the biomass of soybean seedlings was positively correlated with leaf area (Supplementary Figure 3A), as well as with the stem diameter under both shade and full light conditions (Supplementary Figure 3B). But biomass was not correlated with plant height (Supplementary Figure 3C), PN500 (Supplementary Figure 3E) and PN1200 (Supplementary Figure 3F). Plant height was positively correlated with fS under both shade and full light conditions (Supplementary Table 2). Interestingly, leaf mass per unit area was negatively correlated with biomass only under the shade condition (Supplementary Figure 3D).
In 2015, for the variation between shade in intercropping and full light in sole cropping, biomass of soybean seedlings positively correlated with leaf area (Supplementary Figure 4A). For the relationship between biomass and plant height, negative correlations were found only in shade conditions (Supplementary Figure 4B).
Quantitative relationships between the plasticity of biomass and the plasticity of morphological and physiological traits
A multi-variable analysis and features selection were conducted to evaluate the relative importance of plasticity variables of biomass. Results indicated that the plasticity of leaf area, leaf area ratio, leaflet area, plant height, and Chl content were the top five explanatory variables, which significantly affected the plasticity of biomass (Figure 4). A further pearson correlations between the plasticity of biomass and the plasticity of the top five explanatory variables indicated that only leaf area, leaflet area, and plant height were significantly positively correlated with biomass (Figure 5). However, leaf area and leaflet area were significant positive correlated with biomass, plant height was significant negative correlated with the biomass (Figure 5). Results of principal component analysis (PCA) showed that the relationships between soybean cultivars’ biomass and explanatory variables, i.e., leaf area, leaflet area, Chl content, leaf area ratio, and plant height. A total of 62.7% variation explained by the explanatory variables and the principal component one account for 42.5% variation explain (Figure 6A), this was constant with the results of features selection (Figure 4) and correlation analysis (Figure 5). On the basis of the top five explanatory variables, cluster analysis indicated that soybean cultivars were classified into three groups (Figure 6B). Cluster one, including cultivars 3 (Jiandebaimaodou), 13 (Gongdou 2), and 20 (Guixia), with the maximum plasticity of biomass.
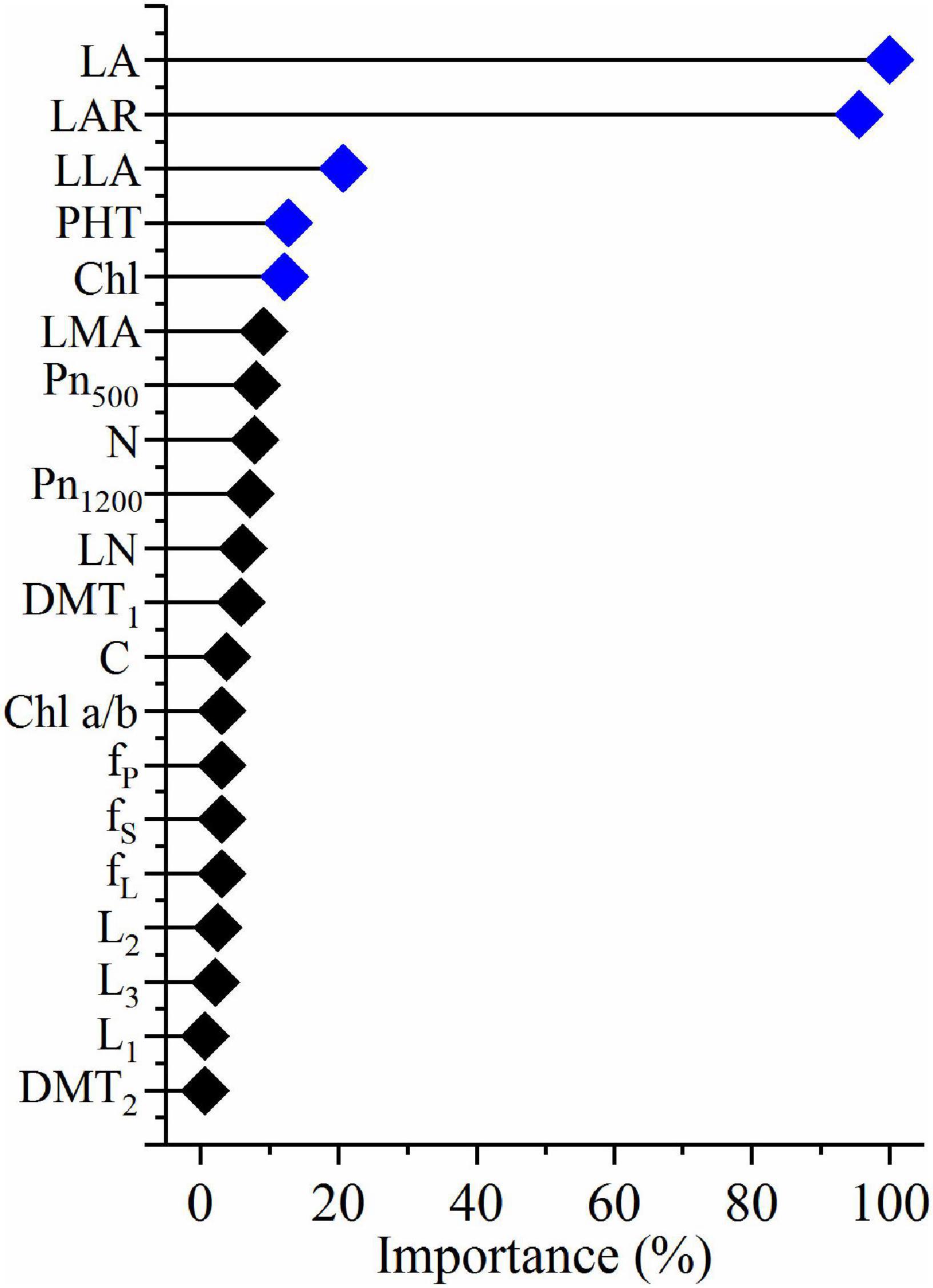
Figure 4. Relative importance of the plasticity of morphological and physiological variables that affect the plasticity of biomass in shade. LA, leaf area per plant; LAR, leaf area ratio; LLA, leaflet area; LN, leaflet number; PHT, plant height; Chl, chlorophyll content per unit dry mass; Chl a/b, chlorophyll a/b ratio; LMA, leaf mass per unit area; Pn500, net photosynthetic rate per unit area at 500 μmol m– 2 s– 1; Pn1200, net photosynthetic rate per unit area at 1,200 μmol m– 2 s– 1; N, nitrogen content per unit area; LN, leaflet number; C, carbon content per unit area; DMT1, diameter of the first node; DMT2, diameter of the second node; fS, fraction of dry mass in stem; fP, fraction of dry mass in petiole; fLr, fraction of dry mass in lamina; L1, first internode length; L2, second internode length; L3, third internode length.
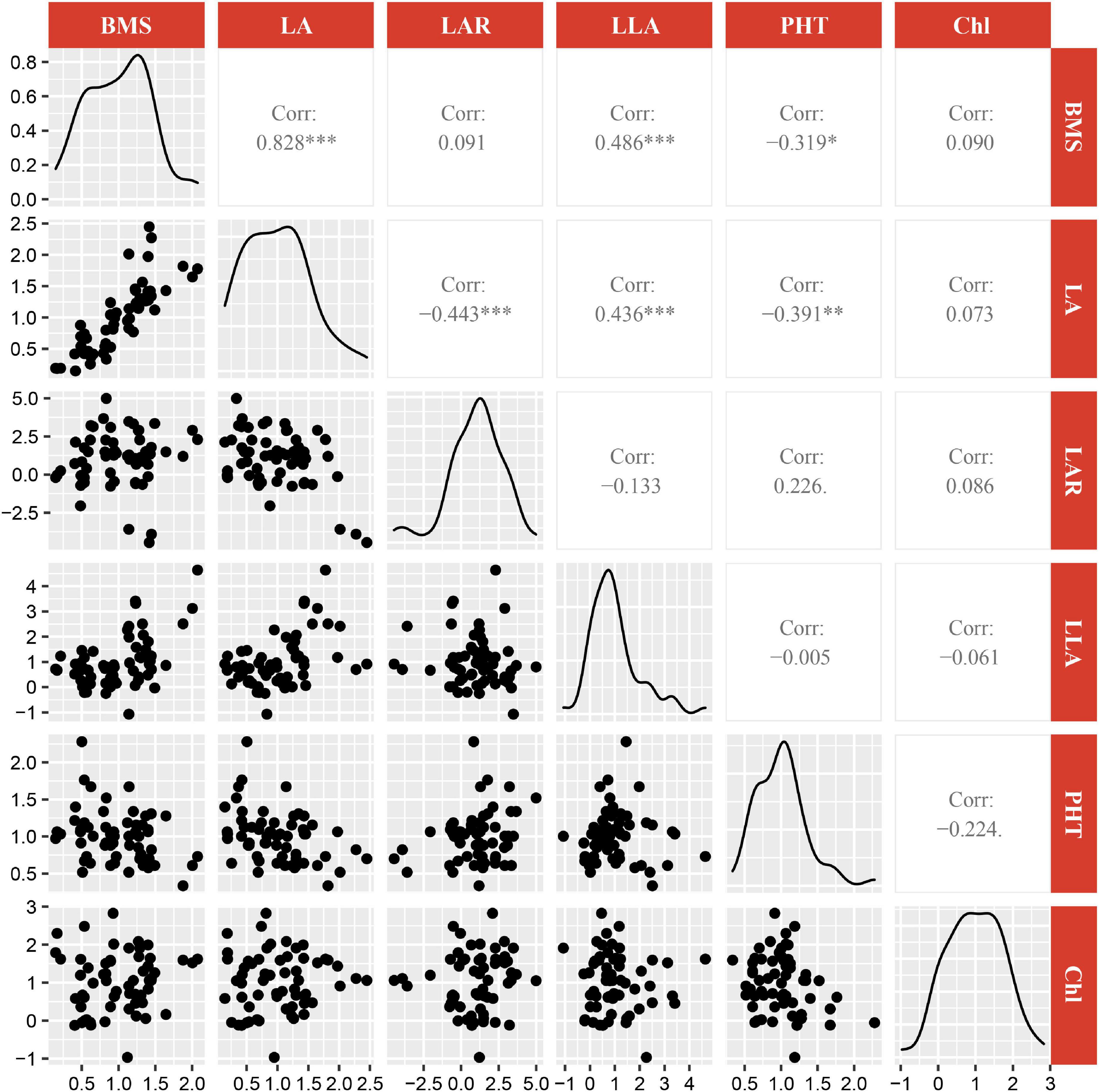
Figure 5. Correlations between the plasticity of biomass and the plasticity of top five most important explanatory variables. BMS, biomass;LA, leaf area; LAR, leaf area ratio; LLA, leaflet area; PHT, plant height; Chl, Chl content. *, **, *** represent significant difference at 0.05, 0.01, and 0.001 levels, respectively.
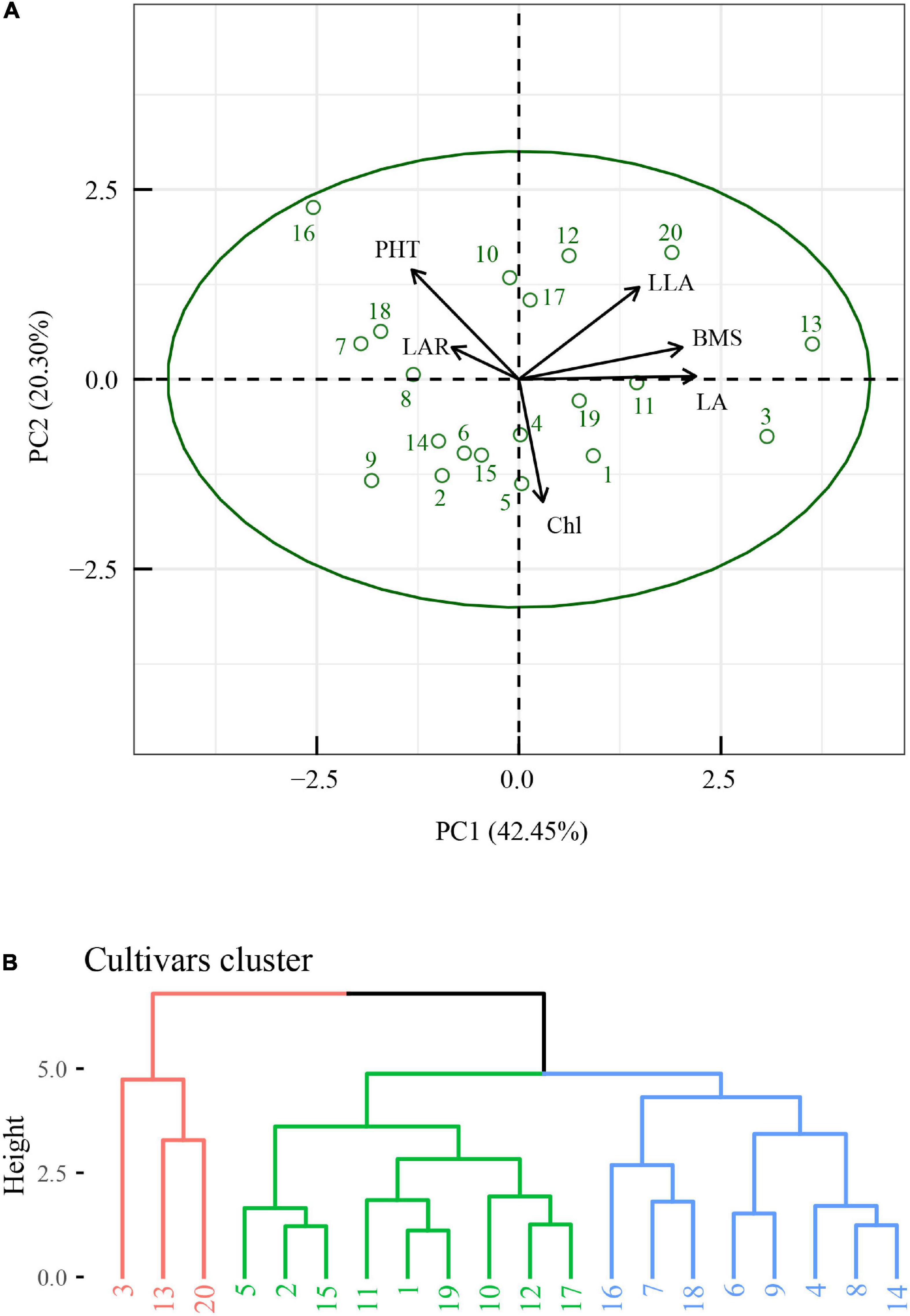
Figure 6. Principal component analysis (PCA) of the plasticity of biomass and the plasticity of top five most important explanatory variables (A), and clustering analysis of the soybean cultivars (B). BMS, biomass; LA, leaf area; LAR, leaf area ratio; LLA, leaflet area; PHT, plant height; Chl, Chl content. The numbers in panel (B) represent cultivars.
Discussion
Soybean seedling responses to shade
Compared with seedlings grown in full light, shade-grown soybean seedlings showed increased plant height, internode length and fraction of dry mass in stem and reduced leaf area, leaflet area and leaflet number (Figure 2). These phenotypes are typical shade avoidance symptoms that allow plants to search for light and escape from shade (Franklin, 2008). The presence of these symptoms indicates that soybean seedlings escape from shade by increasing stem elongation at the cost of reduced leaf expansion (Valladares et al., 2011). We also found that plant height was positively correlated with fraction of dry mass in stem under both light treatments and that fraction of dry mass in stem was inversely related to fraction of dry mass in lamina (Supplementary Table 2). These results indicate a trade-off between increased plant height which allows plants to search for light, and leaf expansion which increases light capture and utilization. Under shading this trade-off was balanced toward light searching.
Other findings in this study were that biomass was positively correlated with stem diameter under both light conditions (Supplementary Figures 3B and Supplementary Table 2) and that the plasticity of biomass was correlated with the plasticity of stem diameter under shading net (Supplementary Table 3). In addition, the stem diameter was positively correlated with leaf area under both light conditions, but it was negatively correlated with the length of the first internode under shade. Because the measurement of stem diameter was taken at the middle point of the first internode, these results indicate that the growth in the horizontal direction led to an increase in internode length in seedling development, which was consistent with Zhang et al. (2020). As the trifoliolate leaves of soybean develop at the apex of stem, a wider stem might lead to bigger leaf primordia due to the presence of more cells produced by cell division (Reinhardt et al., 2005; Wu et al., 2017a). Whether the thicker stem could produce larger leaf and the stem diameter of seedling grown under shade could be used to predict the leaf area still need to be testified in future studies.
Besides avoiding shade via stem elongation, plants often cope with shade by increasing light use efficiency via a shade tolerance strategy (Gong et al., 2015). We found that a reduced Chl a/b, a lower leaf mass per unit area and a higher Chl concentration were beneficial for photosynthesis under low light conditions, consistent with previous studies (Hussain et al., 2020; Tan et al., 2022). A higher Chl concentration indicates that there are more pigment-binding proteins for photons captured by photosystem II per unit N content. As the light-harvesting complex of photosystem II contains mostly Chl b, increased accumulation of the light-harvesting complex under shade causes a decline in the Chl a/b ratio (Boardman, 1977; Anderson, 1986; Evans, 1989).
Leaf mass per unit area reflects the trade-off between the functions of the leaf lamina in light radiation interception and conversion. A decline in leaf mass per unit area is beneficial for receiving more light per unit of leaf mass; however, low leaf mass per unit area negatively affects carboxylation because the reduced thickness of the palisade mesophyll resulting in less area for CO2 exchange (Terashima et al., 2001, 2006, 2011). Leaf mass per unit area is also strongly correlated with leaf thickness (especially palisade thickness) and the chemical composition of the leaf (i.e., N content and Chl concentration) (Poorter et al., 2009). In this study, leaf mass per unit area was positively correlated with PN1200 (r = 0.572**) under full light, indicating that a thicker leaf mesophyll layer leads to increased CO2 exchange as discussed above. But, we did not find a relationship between leaf mass per unit area and PN500 when soybean was grown under the shade treatment, which suggesting that when leaf mass per unit area was reduced, photosynthetic rate of all soybean genotypes declined to the similar levels. It is notable that the biomass of shade-grown soybean was negatively correlated with leaf mass per unit area (Supplementary Figure 3D), suggesting that soybean seedlings respond to shade by increasing leaf mass per unit area, which increases light interception at the cost of light conversion.
Although the plasticity of plant morphological and leaf physiological traits were helpful for acclimating to shade, the biomass of soybean seedlings was still severely reduced under the shade treatment (Figure 2A). The plasticity of biomass was positively correlated with the plasticity in leaf area and stem diameter under both light treatments (Supplementary Table 3), although leaf area and stem diameter were significantly reduced under the shade condition (Figures 2E,F). However, the plasticity of biomass was not correlated with plant height, PN500, PN1200, or Chl concentration in the present study. Thus, the increase in plant height and Chl concentration, as well as the decrease in Chl a/b ratio could not offset the light deficit. Based on these findings, we speculate that the formation of a canopy that increases light interception is more important than increasing light conversion for soybean seedlings that cannot escape from shade.
Phenotypic plasticity in response to light is the remarkable ability of plants to adjust morphology and physiology under different light conditions (Delagrange et al., 2004; Valladares and Niinemets, 2008). Previous studies have found that mean plasticity in morphological traits in response to light (e.g., elongation of the stem and internodes) is lower for shade-tolerant plants than for shade-intolerant plants (Dudley and Schmitt, 1995; Valladares et al., 2000b; Sánchez-Gómez et al., 2006; Portsmuth and Niinemets, 2007). Most plants from open habitats show shade avoidance responses, such as searching for light and escaping shade, when grown under shade (Gommers et al., 2013). Therefore, plasticity in plant height and internode length could be explained as the capacity for light searching. In this study, all soybean varieties showed the capacity for light searching via stem elongation. However, the plasticity of biomass was negative correlated to the plasticity of plant height (Supplementary Table 3). This is probably because soybean seedlings could not escape from shade in our shading net treatment or intercropping. Thus, the elongation of the stem could not enhance light interception. It can be inferred that under intercropping conditions where there is a large companion crop, e.g., maize, a strategy that enhances leaf area and allows more light to be captured, would be more beneficial than escaping shade through stem elongation.
Leaves are involved in both shade avoidance and tolerance strategies, as the leaf lamina directly captures light and converts the light into carbohydrates. Leaf area as the most important factor that affect plasticity of biomass and has strong positive relationship between plasticity of biomass, suggesting that light capture by the leaf determines light utilization, rather than the light search by elongating the stem. The abundance of climbing plants in deep shade was found to be directly related to their ability to intercept light efficiently but not to their ability to increase carbon fixation by increasing leaf area (Valladares et al., 2011). Hence, these findings suggest that capacity for light capture and absorption by the leaf is different from the ability to search for light by elongating the stem under deep shade, although both strategies might help increase light capture.
Phenotypic plasticity of light searching, capturing, and conversion and their relationships to agronomic practices
Soybean is a light-favoring crop and is usually grown in a sole cropping system without shade from other plants. Soybean seedlings display a suite of architectural and physiological changes in response to shade, and there are genetic differences in these responses as shown in this study. In the maize-soybean relay strip intercropping system, soybean grew under shade environment during the seedling stages and the morphological traits were more plastic. Our previous study pointed out that most of the genetic differences in morphological traits are only expressed during the seedling stages (Hussain et al., 2020). So, we infer that plant seedlings have higher plasticity in architectural and morphological traits than in leaf physiological traits. It has been reported that plasticity in architectural traits leads to increased light interception and a yield advantage in intercropping systems (Zhu et al., 2015, 2016; Li et al., 2021),but it depends on the planting configurations (Li et al., 2020). This previous finding, combined with our results, indicates that the performance of component crops in intercropping systems might be based on light capture rather than photosynthesis and that increasing light capture might be a more feasible approach for increasing the total light intercepted in field production.
Plasticity in leaf physiological traits, such as photosynthetic rates and Chl concentration, is associated with changes in the capacity for photosynthesis in the leaf mesophyll. However, the plasticity of leaf physiological traits were not correlated with plasticity of biomass. Among the 20 varieties that we investigated in experiment 1, there was less variation in photosynthetic rate, Chl content and Chl a/b ratio compared with morphological traits, consistent with the findings of our previous study on two soybean varieties (Gong et al., 2015; Wu et al., 2022). These findings suggest that the capacity to adjust photosynthetic rate seems to be a conserved resource use strategy (Valladares et al., 2000a); thus, the soybean genotypes with contrasting capacities for light conversion in the mesophyll did not differ in their ability to accumulate biomass. Therefore, the selection of soybean varieties possessing higher photosynthesis rates might have little effect on improving shade tolerance.
The evolution of crops is not only driven by natural forces but also by selection by humans to meet the demand for food; crops became less shade tolerant over the past few thousands of years when grown in sole cropping systems, and fewer studies have focused on the genotypic differences in the shade tolerance of crops. It had been reported that shade reduced tillering in maize (Rotili et al., 2021), leaf size and morphology in tomato (Chitwood et al., 2015) and also negatively affected biomass in grasses (Warnasooriya and Brutnell, 2014). Many shade avoidance on crops focused on discovery and validation of molecular function. Cultivated soybean was domesticated from its wild progenitor Glycine soja, which is a typical climbing species that usually grows in shade environments. It is obvious that cultivated soybean was domesticated by humans to grow in high-light conditions. However, the capacities for light searching, light capture and light conversion were maintained in soybean. When modern soybean is grown under shade conditions such as those found in intercropping systems, elongation of the main stem seems to be an atavism.
The responses of the investigated traits in this study can be classified into three functional strategies: light searching, light capture and light conversion. Among these strategies, increasing light searching via elongation of the stem easily led to lodging (Liu et al., 2016), and increasing light conversion by increasing Chl content and photosynthesis in the mesophyll could not offset the light limitation and seems to be a conserved response among genotypes (Gong et al., 2015). The strategy of searching for light via stem elongation might increase the opportunity to escape from shade or to intercept more light; however, this trait is probably not acceptable in agriculture for several reasons. Firstly, it is hard for soybean plants to escape from shading in intercropping systems due to the presence of a tall companion crop, e.g., maize (Fan et al., 2018). Thus, enhanced stem elongation will not contribute to biomass accumulation. Secondly, over-elongated plants have increased rates of lodging (Liu et al., 2015, 2016). Soybean varieties with less stem elongation have a lower capacity to search for light. As we found negative correlation between the plasticity of biomass and plasticity of plant height, soybean varieties with low stem elongation plasticity might perform better in intercropping systems. A previous study of climbing plants grown under deep shade found that those plants with a higher capacity to intercept light were more abundant (Valladares et al., 2011), consistent with our finding that light capture contributed more to growth when soybeans could not escape from shade. In wheat/maize and wheat/cotton intercropping, although the leaf area of intercropped wheat and maize was lower compared with that of wheat and maize grown as single crops, the productivity of the intercrops was still increased by increased light interception (Zhang et al., 2008; Gou et al., 2017). In short, when selecting soybean varieties for intercropping, the focus should be on those traits related to light capture, such as leaf area per plant and leaflet area.
Conclusion
A suite of shade responses for soybean varieties was documented. The biomass of soybean seedlings was positively correlated with leaf area per plant and stem diameter under both shade and full-light conditions. Although plant height increased significantly under shade, it was unrelated to the changes of biomass in this study. The top three most important explanatory variables of plasticity of biomass were leaf area, leaf area ratio and leaflet area. Plasticity of biomass was positively correlated with plasticity of leaf area and leaflet area and negatively correlated with plasticity of plant height, but it was not associated with plasticity of photosynthetic rate. These results suggest that increasing the capacity to capture light by increasing leaf area, rather than increasing the capacity to search for light by elongating the stem or by increasing light conversion in the leaf mesophyll, was more vital for light utilization by soybean seedlings grown under shade. Increasing light capture via the production of larger leaves gave rise to higher biomass accumulation in seedlings under shade. Therefore, selection and breeding of soybean varieties for future intercropping systems should focus on traits contributing to light capture, such as the production of more and larger leaves.
Data availability statement
The original contributions presented in this study are included in the article/Supplementary material, further inquiries can be directed to the corresponding author.
Author contributions
YW, WG, and WY designed the experiments. YW performed the experiments and data collection and wrote the manuscript. All authors contributed to the article and approved the submitted version.
Funding
This study was supported by Sichuan Science and Technology Program (No. 2021YJ0496) and National Natural Science Foundation of China (No. 32001416).
Conflict of interest
The authors declare that the research was conducted in the absence of any commercial or financial relationships that could be construed as a potential conflict of interest.
Publisher’s note
All claims expressed in this article are solely those of the authors and do not necessarily represent those of their affiliated organizations, or those of the publisher, the editors and the reviewers. Any product that may be evaluated in this article, or claim that may be made by its manufacturer, is not guaranteed or endorsed by the publisher.
Supplementary material
The Supplementary Material for this article can be found online at: https://www.frontiersin.org/articles/10.3389/fpls.2022.1015414/full#supplementary-material
References
Anderson, J. M. (1986). Photoregulation of the composition, function, and structure of thylakoid membranes. Annu. Rev. Plant Physiol. 37, 93–136. doi: 10.1146/annurev.pp.37.060186.000521
Anten, N. P., von Wettberg, E. J., Pawlowski, M., and Huber, H. (2009). Interactive effects of spectral shading and mechanical stress on the expression and costs of shade avoidance. Am. Nat. 173, 241–255. doi: 10.1086/595761
Ballaré, C. L. (1999). Keeping up with the neighbours: Phytochrome sensing and other signalling mechanisms. Trends Plant Sci. 4, 97–102. doi: 10.1016/S1360-1385(99)01383-7
Boardman, N. K. (1977). Comparative photosynthesis of sun and shade plants. Annu. Rev. Plant Physiol. 28, 355–377. doi: 10.1146/annurev.pp.28.060177.002035
Bradshaw, A. D. (1965). Evolutionary significance of phenotypic plasticity in plants. Adv. Genet. 13, 115–155. doi: 10.1016/S0065-2660(08)60048-6
Brooker, R. W., Bennett, A. E., Cong, W.-F., Daniell, T. J., George, T. S., Hallett, P. D., et al. (2015). Improving intercropping: A synthesis of research in agronomy, plant physiology and ecology. N. Phytol. 206, 107–117. doi: 10.1111/nph.13132
Chitwood, D. H., Kumar, R., Ranjan, A., Pelletier, J. M., Townsley, B., Ichihashi, Y., et al. (2015). Light-induced indeterminacy alters shade avoiding tomato leaf morphology. Plant Physiol. 169, 2030–2047. doi: 10.1104/pp.15.01229
de Wit, M., Kegge, W., Evers, J. B., Vergeer-van Eijk, M. H., Gankema, P., Voesenek, L. A. C. J., et al. (2012). Plant neighbor detection through touching leaf tips precedes phytochrome signals. Proc. Natl. Acad. Sci. U.S.A. 109, 14705–14710. doi: 10.1073/pnas.1205437109
Delagrange, S., Messier, C., Lechowicz, M. J., and Dizengremel, P. (2004). Physiological, morphological and allocational plasticity in understory deciduous trees: Importance of plant size and light availability. Tree Physiol. 24, 775–784. doi: 10.1093/treephys/24.7.775
Dudley, S. A., and Schmitt, J. (1995). Genetic differentiation in morphological responses to simulated foliage shade between populations of impatiens capensis from open and woodland sites. Funct. Ecol. 9, 655–666. doi: 10.2307/2390158
Enbody, E. D., Pettersson, M. E., Sprehn, C. G., Palm, S., Wickström, H., and Andersson, L. (2021). Ecological adaptation in European eels is based on phenotypic plasticity. Proc. Natl. Acad. Sci. U.S.A. 118:e2022620118. doi: 10.1073/pnas.2022620118
Evans, J. R. (1989). Photosynthesis and nitrogen relationships in leaves of C3 plants. Oecologia 78, 9–19. doi: 10.1007/BF00377192
Fan, Y., Chen, J., Cheng, Y., Raza, M. A., Wu, X., Wang, Z., et al. (2018). Effect of shading and light recovery on the growth, leaf structure, and photosynthetic performance of soybean in a maize-soybean relay-strip intercropping system. PLoS One 13:e0198159. doi: 10.1371/journal.pone.0198159
Francis, C. A. (1989). Biological efficiencies in multiple-cropping systems. Adv. Agron. 42, 1–42. doi: 10.1016/S0065-2113(08)60522-2
Franklin, K. A. (2008). Shade avoidance. N. Phytol. 179, 930–944. doi: 10.1111/j.1469-8137.2008.02507.x
Franklin, K. A., and Whitelam, G. C. (2005). Phytochromes and shade-avoidance responses in plants. Ann. Bot. 96, 169–175. doi: 10.1093/aob/mci165
Ghosh, P. K., Tripathi, A. K., Bandyopadhyay, K. K., and Manna, M. C. (2009). Assessment of nutrient competition and nutrient requirement in soybean/sorghum intercropping system. Eur. J. Agron. 31, 43–50. doi: 10.1016/j.eja.2009.03.002
Givnish, T. J. (1988). Adaptation to sun and shade: A whole-plant perspective. Funct. Plant Biol. 15, 63–92. doi: 10.1071/PP9880063
Gommers, C. M. M., Visser, E. J. W., Onge, K. R. S., Voesenek, L. A. C. J., and Pierik, R. (2013). Shade tolerance: When growing tall is not an option. Trends Plant Sci. 18, 65–71. doi: 10.1016/j.tplants.2012.09.008
Gong, W. Z., Jiang, C. D., Wu, Y. S., Chen, H. H., Liu, W. Y., and Yang, W. Y. (2015). Tolerance vs. avoidance: Two strategies of soybean (Glycine max) seedlings in response to shade in intercropping. Photosynthetica 53, 259–268. doi: 10.1007/s11099-015-0103-8
Gou, F., van Ittersum, M. K., Simon, E., Leffelaar, P. A., van der Putten, P. E. L., Zhang, L., et al. (2017). Intercropping wheat and maize increases total radiation interception and wheat RUE but lowers maize RUE. Eur. J. Agron. 84, 125–139. doi: 10.1016/j.eja.2016.10.014
Hussain, S., Pang, T., Iqbal, N., Shafiq, I., Skalicky, M., Brestic, M., et al. (2020). Acclimation strategy and plasticity of different soybean genotypes in intercropping. Funct. Plant Biol. 47, 592–610. doi: 10.1071/FP19161
Keating, B. A., and Carberry, P. S. (1993). Resource capture and use in intercropping: Solar radiation. Field Crops Res. 34, 273–301. doi: 10.3389/fpls.2022.848893
Li, S., Evers, J. B., van der Werf, W., Wang, R., Xu, Z., Guo, Y., et al. (2020). Plant architectural responses in simultaneous maize/soybean strip intercropping do not lead to a yield advantage. Ann. Appl. Biol. 177, 195–210. doi: 10.1111/aab.12610
Li, S., van der Werf, W., Zhu, J., Guo, Y., Li, B., Ma, Y., et al. (2021). Estimating the contribution of plant traits to light partitioning in simultaneous maize/soybean intercropping. J. Exp. Bot. 72, 3630–3646. doi: 10.1093/jxb/erab077
Li, X., Cai, J., Li, H., Bo, Y., Liu, F., Jiang, D., et al. (2012). Effect of shading from jointing to maturity on high molecular weight glutenin subunit accumulation and glutenin macropolymer concentration in grain of winter wheat. J. Agron. Crop Sci. 198, 68–79. doi: 10.1111/j.1439-037X.2011.00484.x
Lichtenthaler, H. K. (1987). Chlorophylls and carotenoids: Pigments of photosynthetic biomembranes. Methods Enzymol. 148, 350–382. doi: 10.1515/znc-2001-11-1225
Liu, W., Deng, Y., Hussain, S., Zou, J., Yuan, J., Luo, L., et al. (2016). Relationship between cellulose accumulation and lodging resistance in the stem of relay intercropped soybean [Glycine max (L.) Merr.]. Field Crops Res. 196, 261–267. doi: 10.1016/j.fcr.2016.07.008
Liu, W., Zou, J., Zhang, J., Yang, F., Wan, Y., and Yang, W. (2015). Evaluation of soybean (Glycine max) stem vining in maize-soybean relay strip intercropping system. Plant Prod. Sci. 18, 69–75. doi: 10.1626/pps.18.69
Malézieux, E., Crozat, Y., Dupraz, C., Laurans, M., Makowski, D., Ozier-Lafontaine, H., et al. (2009). Mixing plant species in cropping systems: Concepts, tools and models. A review. Agron. Sustain. Dev. 29, 43–62. doi: 10.1051/agro:2007057
Naseer, M. A., Hussain, S., Nengyan, Z., Ejaz, I., Ahmad, S., Farooq, M., et al. (2022). Shading under drought stress during grain filling attenuates photosynthesis, grain yield and quality of winter wheat in the Loess Plateau of China. J. Agron. Crop Sci. 208, 255–263. doi: 10.1111/jac.12563
Niinemets, Ü (2010). A review of light interception in plant stands from leaf to canopy in different plant functional types and in species with varying shade tolerance. Ecol. Res. 25, 693–714. doi: 10.1007/s11284-010-0712-4
Poorter, H., Niinemets, Ü, Ntagkas, N., Siebenkäs, A., Mäenpää, M., Matsubara, S., et al. (2019). A meta-analysis of plant responses to light intensity for 70 traits ranging from molecules to whole plant performance. N. Phytol. 223, 1073–1105. doi: 10.1111/nph.15754
Poorter, H., Niinemets, Ü, Poorter, L., Wright, I. J., and Villar, R. (2009). Causes and consequences of variation in leaf mass per area (LMA): A meta-analysis. N. Phytol. 182, 565–588. doi: 10.1111/j.1469-8137.2009.02830.x
Portsmuth, A., and Niinemets, Ü (2007). Structural and physiological plasticity in response to light and nutrients in five temperate deciduous woody species of contrasting shade tolerance. Funct. Ecol. 21, 61–77. doi: 10.1111/j.1365-2435.2006.01208.x
Raza, M. A., Gul, H., Wang, J., Yasin, H. S., Qin, R., Bin Khalid, M. H., et al. (2021). Land productivity and water use efficiency of maize-soybean strip intercropping systems in semi-arid areas: A case study in Punjab Province, Pakistan. J. Clean Prod. 308:127282. doi: 10.1016/j.jclepro.2021.127282
Reinhardt, D., Frenz, M., Mandel, T., and Kuhlemeier, C. (2005). Microsurgical and laser ablation analysis of leaf positioning and dorsoventral patterning in tomato. Development 132, 15–26. doi: 10.1242/dev.01544
Rotili, D. H., Sadras, V. O., Abeledo, L. G., Ferreyra, J. M., Micheloud, J. R., Duarte, G., et al. (2021). Impacts of vegetative and reproductive plasticity associated with tillering in maize crops in low-yielding environments: A physiological framework. Field Crops Res. 265:108107. doi: 10.1016/j.fcr.2021.108107
Sánchez-Gómez, D., Valladares, F., and Zavala, M. A. (2006). Functional traits and plasticity in response to light in seedlings of four Iberian forest tree species. Tree Physiol. 26, 1425–1433. doi: 10.1093/treephys/26.11.1425
Sadras, V. O., Reynolds, M. P., de la Vega, A. J., Petrie, P. R., and Robinson, R. (2009). Phenotypic plasticity of yield and phenology in wheat, sunflower and grapevine. Field Crops Res. 110, 242–250. doi: 10.1016/j.fcr.2008.09.004
Smith, H. (2000). Phytochromes and light signal perception by plants—an emerging synthesis. Nature 407, 585–591. doi: 10.1038/35036500
Su, B. Y., Song, Y. X., Song, C., Cui, L., Yong, T. W., and Yang, W. Y. (2014). Growth and photosynthetic responses of soybean seedlings to maize shading in relay intercropping system in Southwest China. Photosynthetica 52, 332–340. doi: 10.1007/s11099-014-0036-7
Sultan, S. E. (2010). Plant developmental responses to the environment: Eco-devo insights. Curr. Opin. Plant Biol. 13, 96–101. doi: 10.1016/j.pbi.2009.09.021
Szumigalski, A. R., and Van Acker, R. C. (2006). Nitrogen yield and land use efficiency in annual sole crops and intercrops. Agron. J. 98, 1030–1040. doi: 10.2134/agronj2005.0277
Tan, T., Li, S., Fan, Y., Wang, Z., Ali Raza, M., Shafiq, I., et al. (2022). Far-red light: A regulator of plant morphology and photosynthetic capacity. Crop J. 10, 300–309. doi: 10.1016/j.cj.2021.06.007
Tanveer, M., Anjum, S. A., Hussain, S., Cerdà, A., and Ashraf, U. (2017). Relay cropping as a sustainable approach: Problems and opportunities for sustainable crop production. Environ. Sci. Pollut. Res. 24, 6973–6988. doi: 10.1007/s11356-017-8371-4
Terashima, I., Hanba, Y. T., Tazoe, Y., Vyas, P., and Yano, S. (2006). Irradiance and phenotype: Comparative eco-development of sun and shade leaves in relation to photosynthetic CO2 diffusion. J. Exp. Bot. 57, 343–354. doi: 10.1093/jxb/erj014
Terashima, I., Hanba, Y. T., Tholen, D., and Niinemets, U. (2011). Leaf functional anatomy in relation to photosynthesis. Plant Physiol. 155, 108–116. doi: 10.1104/pp.110.165472
Terashima, I., Miyazawa, S.-I., and Hanba, Y. T. (2001). Why are sun leaves thicker than shade leaves? — Consideration based on analyses of CO2 diffusion in the leaf. J. Plant Res. 114, 93–105. doi: 10.1007/PL00013972
Valladares, F., Chico, J., Aranda, I., Balaguer, L., Dizengremel, P., Manrique, E., et al. (2002). The greater seedling high-light tolerance of Quercus robur over Fagus sylvatica is linked to a greater physiological plasticity. Trees 16, 395–403. doi: 10.1007/s00468-002-0184-4
Valladares, F., Gianoli, E., and Gómez, J. M. (2007). Ecological limits to plant phenotypic plasticity. N. Phytol. 176, 749–763. doi: 10.1111/j.1469-8137.2007.02275.x
Valladares, F., Gianoli, E., and Saldaña, A. (2011). Climbing plants in a temperate rainforest understorey: Searching for high light or coping with deep shade? Ann. Bot. 108, 231–239. doi: 10.1093/aob/mcr132
Valladares, F., Martinez-Ferri, E., Balaguer, L., Perez-Corona, E., and Manrique, E. (2000a). Low leaf-level response to light and nutrients in Mediterranean evergreen oaks: A conservative resource-use strategy? N. Phytol. 148, 79–91.
Valladares, F., and Niinemets, Ü (2008). Shade tolerance, a key plant feature of complex nature and consequences. Annu. Rev. Ecol. Evol. Syst. 39, 237–257.
Valladares, F., Wright, S. J., Lasso, E., Kitajima, K., and Pearcy, R. W. (2000b). Plastic phenotypic response to light of 16 congeneric shrubs from a panamanian rainforest. Ecology 81, 1925–1936. doi: 10.1890/0012-9658(2000)081[1925:PPRTLO]2.0.CO;2
Vandenbussche, F., Pierik, R., Millenaar, F. F., Voesenek, L. A. C. J., and Van Der Straeten, D. (2005). Reaching out of the shade. Curr. Opin. Plant Biol. 8, 462–468. doi: 10.1016/j.pbi.2005.07.007
Wang, R., Sun, Z., Bai, W., Wang, E., Wang, Q., Zhang, D., et al. (2021). Canopy heterogeneity with border-row proportion affects light interception and use efficiency in maize/peanut strip intercropping. Field Crops Res. 271:108239. doi: 10.1016/j.fcr.2021.108239
Warnasooriya, S. N., and Brutnell, T. P. (2014). Enhancing the productivity of grasses under high-density planting by engineering light responses: From model systems to feedstocks. J. Exp. Bot. 65, 2825–2834. doi: 10.1093/jxb/eru221
Willey, R. W. (1979). Intercropping: Its importance and research needs. I. Competition and yield advantages. Field Crop Abstr. 32, 1–10. doi: 10.2307/1941795
Willey, R. W. (1990). Resource use in intercropping systems. Agric. Water Manag. 17, 215–231. doi: 10.1016/0378-3774(90)90069-B
Wu, Y., Gong, W., Yang, F., Wang, X., Yong, T., Liu, J., et al. (2022). Dynamic of recovery growth of intercropped soybean after maize harvest in maize–soybean relay strip intercropping system. Food Energ. Secur. 11:e350. doi: 10.1002/fes3.350
Wu, Y., Gong, W., and Yang, W. (2017a). Shade inhibits leaf size by controlling cell proliferation and enlargement in soybean. Sci. Rep. 7:9259. doi: 10.1038/s41598-017-10026-5
Wu, Y., He, D., Wang, E., Liu, X., Huth, N. I., Zhao, Z., et al. (2021). Modelling soybean and maize growth and grain yield in strip intercropping systems with different row configurations. Field Crops Res. 265:108122. doi: 10.1016/j.fcr.2021.108122
Wu, Y., Yang, F., Gong, W., Ahmed, S., Fan, Y., Wu, X., et al. (2017b). Shade adaptive response and yield analysis of different soybean genotypes in relay intercropping systems. J. Integr. Agricult. 16, 1331–1340. doi: 10.1016/S2095-3119(16)61525-3
Yan, Y., Gong, W., Yang, W., Wan, Y., Chen, X., Chen, Z., et al. (2010). Seed treatment with uniconazole powder improves soybean seedling growth under shading by corn in relay strip intercropping system. Plant Prod. Sci. 13, 367–374. doi: 10.1626/pps.13.367
Yang, F., Feng, L., Liu, Q., Wu, X., Fan, Y., Raza, M. A., et al. (2018). Effect of interactions between light intensity and red-to-far-red ratio on the photosynthesis of soybean leaves under shade condition. Environ. Exp. Bot. 150, 79–87. doi: 10.1016/j.envexpbot.2018.03.008
Yang, F., Huang, S., Gao, R., Liu, W., Yong, T., Wang, X., et al. (2014). Growth of soybean seedlings in relay strip intercropping systems in relation to light quantity and red:far-red ratio. Field Crops Res. 155, 245–253. doi: 10.1016/j.fcr.2013.08.011
Yu, Y., Stomph, T.-J., Makowski, D., and van der Werf, W. (2015). Temporal niche differentiation increases the land equivalent ratio of annual intercrops: A meta-analysis. Field Crops Res. 184, 133–144. doi: 10.1016/j.fcr.2015.09.010
Zhang, L., van der Werf, W., Bastiaans, L., Zhang, S., Li, B., and Spiertz, J. H. J. (2008). Light interception and utilization in relay intercrops of wheat and cotton. Field Crops Res. 107, 29–42. doi: 10.1016/j.fcr.2007.12.014
Zhang, R., Shan, F., Wang, C., Yan, C., Dong, S., Xu, Y., et al. (2020). Internode elongation pattern, internode diameter and hormone changes in soybean (Glycine max) under different shading conditions. Crop Pasture Sci. 71, 679–688. doi: 10.1071/CP20071
Zhu, J., van der Werf, W., Anten, N. P. R., Vos, J., and Evers, J. B. (2015). The contribution of phenotypic plasticity to complementary light capture in plant mixtures. N. Phytol. 207, 1213–1222. doi: 10.1111/nph.13416
Keywords: shade, soybean, plasticity, morphological, physiological
Citation: Wu Y, Chen P, Gong W, Gul H, Zhu J, Yang F, Wang X, Yong T, Liu J, Pu T, Yan Y and Yang W (2022) Morphological and physiological variation of soybean seedlings in response to shade. Front. Plant Sci. 13:1015414. doi: 10.3389/fpls.2022.1015414
Received: 09 August 2022; Accepted: 26 August 2022;
Published: 06 October 2022.
Edited by:
Muhammad Ali Raza, Islamia University of Bahawalpur, PakistanReviewed by:
Sabin Saurav Pokharel, Nanjing Agricultural University, ChinaTing Peng, Henan Agricultural University, China
Copyright © 2022 Wu, Chen, Gong, Gul, Zhu, Yang, Wang, Yong, Liu, Pu, Yan and Yang. This is an open-access article distributed under the terms of the Creative Commons Attribution License (CC BY). The use, distribution or reproduction in other forums is permitted, provided the original author(s) and the copyright owner(s) are credited and that the original publication in this journal is cited, in accordance with accepted academic practice. No use, distribution or reproduction is permitted which does not comply with these terms.
*Correspondence: Wenyu Yang, bXNzaXlhbmd3eUBzaWNhdS5lZHUuY24=
†These authors have contributed equally to this work and share first authorship