- 1Fundo de Defesa da Citricultura, Araraquara, Brazil
- 2Faculdade de Ciências Agrárias e Veterinárias (FCAV), Universidade Estadual Paulista (UNESP), Jaboticabal, Brazil
- 3Empresa Brasileira de Pesquisa Agropecuária, Cruz das Almas, Brazil
- 4CIRAD, UMR AGAP Institut, Montpellier, France
- 5AGAP Institut, Univ. Montpellier, CIRAD, INRAE, Institut Agro, Montpellier, France
- 6Instituto de Biologia Molecular y Celular de Plantas – Consejo Superior de Investigaciones Científicas, Universidad Politécnica de Valencia, Valencia, Spain
Huanglongbing (HLB), the most destructive citrus disease, is associated with unculturable, phloem-limited Candidatus Liberibacter species, mainly Ca. L. asiaticus (Las). Las is transmitted naturally by the insect Diaphorina citri. In a previous study, we determined that the Oceanian citrus relatives Eremocitrus glauca, Microcitrus warburgiana, Microcitrus papuana, and Microcitrus australis and three hybrids among them and Citrus were full-resistant to Las. After 2 years of evaluations, leaves of those seven genotypes remained Las-free even with their susceptible rootstock being infected. However, Las was detected in their stem bark above the scion-rootstock graft union. Aiming to gain an understanding of the full-resistance phenotype, new experiments were carried out with the challenge-inoculated Oceanian citrus genotypes through which we evaluated: (1) Las acquisition by D. citri fed onto them; (2) Las infection in sweet orange plants grafted with bark or budwood from them; (3) Las infection in sweet orange plants top-grafted onto them; (4) Las infection in new shoots from rooted plants of them; and (5) Las infection in new shoots of them after drastic back-pruning. Overall, results showed that insects that fed on plants from the Oceanian citrus genotypes, their canopies, new flushes, and leaves from rooted cuttings evaluated remained quantitative real-time polymerase chain reaction (qPCR)-negative. Moreover, their budwood pieces were unable to infect sweet orange through grafting. Furthermore, sweet orange control leaves resulted infected when insects fed onto them and graft-receptor susceptible plants. Genomic and morphological analysis of the Oceanian genotypes corroborated that E. glauca and M. warburgiana are pure species while our M. australis accession is an M. australis × M. inodora hybrid and M. papuana is probably a M. papuana × M. warburgiana hybrid. E. glauca × C. sinensis hybrid was found coming from a cross between E. glauca and mandarin or tangor. Eremocitrus × Microcitrus hybrid is a complex admixture of M. australasica, M. australis, and E. glauca while the last hybrid is an M. australasica × M. australis admixture. Confirmation of consistent full resistance in these genotypes with proper validation of their genomic parentages is essential to map properly genomic regions for breeding programs aimed to generate new Citrus-like cultivars yielding immunity to HLB.
Introduction
Huanglongbing (HLB), also known as citrus greening, is a ravaging citrus disease that has been concerning citrus growers and researchers across the world, especially during the last two decades, when it spread from South East Asia to West Asia, North Oceania, America, and several African countries (Alquézar et al., 2022). HLB is associated with the presence of the unculturable, Gram-negative, and phloem-limited α-proteobacteria ‘Candidatus Liberibacter africanus’ (Laf), ‘Ca. L. asiaticus’ (Las) and ‘Ca. L. americanus’ (CLam) (Bové, 2006), being Las the most prevalent and aggressive species (Bassanezi et al., 2020). In the field, Las is transmitted by the insect vector Diaphorina citri (Kuwayama) (Hemiptera: Liviidae), commonly known as the Asian citrus psyllid (ACP; Capoor et al., 1967). In Brazil, HLB management relies on the implementation on an area-wide basis of the three-pronged system (TPS) which consists of the elimination of symptomatic trees, use of certified healthy nursery trees for new plantings and resets, and intensive insecticide control of D. citri (Bassanezi et al., 2020). This strategy has been executed since the first description of HLB in Brazil and mainly due to these practices, HLB has only reached 22% of the trees in the citriculture belt of São Paulo State and Triângulo Mineiro (Fundecitrus, 2021), a scenario that would be worst without the implementation of the TPS. However, this strategy has not been adopted and maintained by all growers, ACP resistance to insecticides is arising and thus HLB incidence has gradually increased, demanding new approaches to cope with the disease.
Leaves from HLB-affected trees usually show asymmetrical chlorosis or blotchy mottle, and, at later stages of infection, thicker and enlarged veins (Bové, 2006). Fruits are smaller and misshapen while the juice is highly acidic and poor in soluble solids (Bassanezi et al., 2009). Symptomatic leaves and fruits drop prematurely (Moreira et al., 2022). Leaf symptoms can be confusing, especially at the early stages of infection, and, thus, not always can be used for diagnosis purposes (Gottwald et al., 2007). The quantitative real-time polymerase chain reaction (qPCR) was then developed for Las, becoming the most important and widely used tool for HLB diagnosis and research (Li et al., 2006). However, qPCR does not distinguish the DNA of dead from live bacteria while DNA from dead bacteria is only slowly degraded in plant tissues (Josephson et al., 1993). This can pose problems making the interpretation of results of some works difficult, especially for bacterial titers close to detection limits.
Evaluation by qPCR of citrus genotypes challenge-inoculated with Las in controlled and field conditions has shown that all commercial cultivars are susceptible (Folimonova et al., 2009; Miles et al., 2017). However, some citrus relatives from other genera within the Aurantioideae subfamily have been recently reported as completely or partially resistant to HLB (Ramadugu et al., 2016; Alves et al., 2021a,b). This includes the Australian desert lime, Eremocitrus glauca (Lindl.) Swingle, and several Oceanian Microcitrus species. Both genera belong to the true-citrus fruit trees group, are monoembryonic, and cross-compatible with Citrus (Swingle and Reece, 1967). Furthermore, E. glauca, some Microcitrus species, and their hybrids with Citrus have shown to be suitable citrus rootstocks (Bitters et al., 1964, 1977) and presented good graft compatibility with the rootstock ‘Rangpur’ lime (Citrus × limonia Osbeck) when used as scions (Alves et al., 2021b).
In field experiments, seedlings of E. glauca and some Microcitrus genotypes were identified as potential sources of resistance or tolerance to HLB (Ramadugu et al., 2016). In controlled greenhouse experiments, by using clonal plants grafted onto the Las-susceptible ‘Rangpur’ lime rootstock and upon challenging by graft-inoculation of Las-infected budwood on both the scion and rootstock, Alves et al. (2021b) discerned seven full-resistant citrus genotypes, namely, E. glauca, M. australis, M. warburgiana, and M. papuana and three hybrids among them and with sweet orange within a collection of Citreae species. Although the susceptible rootstock became infected with Las, the bacterium was never detected in the scion leaves of these genotypes thus becoming the most promising germplasm among Oceanian citrus to be used as parents in breeding efforts for generating citrus-like cultivars resistant to Las (Alves et al., 2021b). Because of this, the introgression of HLB resistance into cultivated citrus has become tangible in citrus breeding programs (Alquézar et al., 2021). However, Las was found in the stem bark sampled at 5 cm above the scion-rootstock union of all the seven full-resistant genotypes and unevenly detected at 30 cm height in two of them (Alves et al., 2021b). Las amplifications at these different distances from the infected rootstock led to queries on whether the detected bacterial DNA was viable. Aiming to better characterize the resistance of those genotypes and further understand Las movement within the stem bark of the grafted plant materials, a set of five experiments was carried out. In addition, to support interpretation of the Las resistance phenotype, the genetic background of the seven Oceanian citrus genotypes was assessed by genotyping by sequencing (GBS) analysis.
Materials and methods
Phenotyping of Oceanian citrus genotypes
Morphological analysis of the Oceanian citrus putatively true species was performed with 4–14 healthy plants per genotype propagated onto the ‘Rangpur’ lime rootstock. The aspect of leaves, spines, flowers, and fruits was compared with their description made by Swingle and Reece (1967), also considering the results obtained from their genome analysis, to ascertain the presumed pure or hybrid nature of each genotype.
High-density SNP genotyping of Oceanian citrus genotypes
Aiming to genetically characterize the Fundecitrus collection used for HLB resistance studies (Alves et al., 2021b), a GBS analysis was performed with the seven genotypes described as full-resistant by Alves et al. (2021b) [M. warburgiana (F.M. Bailey) Tanaka, M. papuana Winters (revealed to be actually an M. papuana hybrid in the present work), M. australis (A. Cunn. ex Mudie) Swingle (actually an M. australis hybrid), E. glauca (Lindl.) Swingle, a Microcitrus sp. hybrid (formerly called Microcitrus sp. × E. glauca), an E. glauca × Microcitrus sp. complex hybrid, and a supposed E. glauca × Citrus × sinensis hybrid (eremorange)], and the susceptible sweet orange cultivar ‘Tobias’ [C. × sinensis (L.) Osbeck]. Two finger lime (M. australasica) accessions of the Fundecitrus germplasm (‘True Sanguinea’ and a ‘Green’ one) considered as representative of M. australasica as well as one accession of each Clymenia polyandra, M. inodora, and M. garrawayae were included to check their status of pure species representatives and the potential contribution of the different species to the selected resistant germplasm. Five varieties from the CRB-Citrus collection, San Giuliano (Corsica) whose phylogenomic constitution was previously analyzed by GBS or WGS (Wu et al., 2014, 2018; Ahmed et al., 2019), were also added to the GBS analysis as a reference to check the quality of the results: Nules clementine ICVN 0100389, Eureka lemon ICVN 0100289, Star Ruby grapefruit ICVN 0100293, eremorange SRA871, and Australian finger lime SRA1002. In addition, published WGS data available in NCBI (Wang et al., 2017; Wu et al., 2018) were used to mine diagnostic SNPs (DSNPs) of ancestral taxa from pure representative accessions: C. maxima (SRR5128240 and SRR3824065), C. medica (SRR3938056 and SRR3944139), C. reticulata (SRR3747399 and SRR3747527), M. australis (SRR5128218 and SRR6188449), and M. australasica (SRR5128220).
For GBS analysis, the genomic DNA was isolated using the Plant DNAeasy kit (Qiagen) according to the manufacturer’s instructions. The genomic DNA concentration of each sample was adjusted to 20 ng/μL, and ApekI GBS libraries were prepared following the protocol described by Elshire et al. (2011) with 96 DNA samples multiplexed per GBS library (the accessions used for this paper were included in another larger experiment). Ten μL of each DNA sample (200 ng) was digested with the ApekI enzyme (New England Biolabs, Hitchin, United Kingdom). Digestion took place at 75°C for 2 h and then at 65°C for 20 min for enzyme inactivation. The ligation reaction was completed in the same plate as the digestion, using the T4 DNA ligase enzyme (New England Biolabs, Hitchin, United Kingdom) at 22°C for 1 h. Then, the ligase was inactivated prior to pooling the samples by holding it at 65°C for 20 min. For each library, ligated samples were pooled and PCR-amplified in a single tube. Genome complexity was reduced using PCR primers with one selective base (A) as described by Sonah et al. (2013). Pair-end sequencing was performed on one lane of an Illumina HiSeq4000 platform at Genewiz facilities. RAW sequencing data were cleaned with cutadapt (Martin, 2011) and demultiplexed with GBSX (Herten et al., 2015). Clean demultiplexed sequencing data from GBS are available in the NCBI SRA (Sequence Read Archive), under accession number (Submission ID: PRJNA862483). SNP genotype calling was performed with the GATK4 pipeline (Van der Auwera and O’Connor, 2020). The reference genome was constituted by the nuclear scaffolds of the Clementine v1.0 genome assembly1, the mitochondrial (Yu et al., 2018), and chloroplast (Bausher et al., 2006) reference genomes from C. × sinensis (available in NCBI with reference NC_037463 and NC_008334, respectively). Positions with less than 10 reads were considered as missing data. Polymorphic positions were filtered for diallelic SNPs and minor allele frequency greater than 0.01.
Genetic data analysis
Neighbor-joining analyses for nuclear and mitochondrial data as well as a factorial analysis for nuclear data were performed to describe the genetic diversity organization of the analyzed germplasm. Both analyses are based on a dissimilarity matrix established from SNP genotyping data using the Manhattan dissimilarity index:
where i and j are the two individuals, k is the locus, K is the total number of loci, and xik is the frequency of the alternative allele at locus k for the individual i. All analyses were performed with DARwin software version 6.02.
Ancestral genome contribution along the genome of the admixed genotype was analyzed based on the distribution of homozygosity and heterozygosity of ancestral diagnostic SNPs (DSNPs). Fourteen accessions were used to select diagnostic SNPs for 10 ancestral species: two accessions for C. maxima, C. reticulata, C. medica, and M. australis and one for M. australasica, M. inodora, M. warburgiana, M. garrawayae, E. glauca, and Clymenia polyandra. For each ancestor, DSNPs that fully differentiated the considered ancestor from all other species (i.e., all accessions of the considered ancestor were homozygous for the same allele and all other accessions homozygous for the second allele) were filtered in Excel. For each ancestor, homozygosity and heterozygosity curves along the genome were established with sliding windows of 20 consecutive DSNPs for the considered ancestor (i.e., the proportion of the 20 markers in homozygosity or heterozygosity for the alleles of the considered ancestor). The medium position of the 20 markers of each sliding window was used to draw the curve in Circos representation (Krzywinski et al., 2009) of the true Galaxy interface (Rasche and Hiltemann, 2020).
Plant materials and growth conditions for Las resistance assessment
In this work, the plants of the Oceanian citrus genotypes were the same used in the previous work by Alves et al. (2021b) and included M. warburgiana (F.M. Bailey) Tanaka, M. papuana Winters (revealed to be actually a M. papuana hybrid in the present work), M. australis (A. Cunn. ex Mudie) Swingle (actually a M. australis hybrid), E. glauca (Lindl.) Swingle, a Microcitrus sp. hybrid (formerly called Microcitrus sp. × E. glauca), an E. glauca × Microcitrus sp. complex hybrid, and a supposed E. glauca × Citrus × sinensis hybrid (eremorange), being the susceptible sweet orange cultivar ‘Tobias’ [C. × sinensis (L.) Osbeck] used as control. All these accessions were propagated onto Las-susceptible ‘Rangpur’ lime nucellar rootstocks. Budwood used to propagate each accession came from a single donor mother tree. Plants were kept in a greenhouse with air temperature varying from 20.0 to 30.0°C and natural sunlight conditions. They were grown in 4 L polyethylene bags filled with coir, fertigated twice a week, and sprayed with insecticides and miticides monthly. Other information on the accessions, challenge-inoculation procedures, and plant maintenance practices are described in detail in Alves et al. (2021b). Oceanian citrus and ‘Tobias’ sweet orange control plants used for experiments 1 to 5 reported here (Figure 1) were ∼ 3 to 4-year-old. Las had been detected in all rootstock bark samples by qPCR with an average cycle threshold (Ct) of 30.0 ± 0.4, and in the ‘Tobias’ sweet orange control scion leaves (Ct 23.8 ± 1.4), at 30 months after graft inoculation. Some composite plants from all the seven Oceanian citrus and ‘Tobias’ sweet orange challenge-inoculated genotypes died along the course of the experiments due to the damage caused by Las infection to their ‘Rangpur’ lime root system.
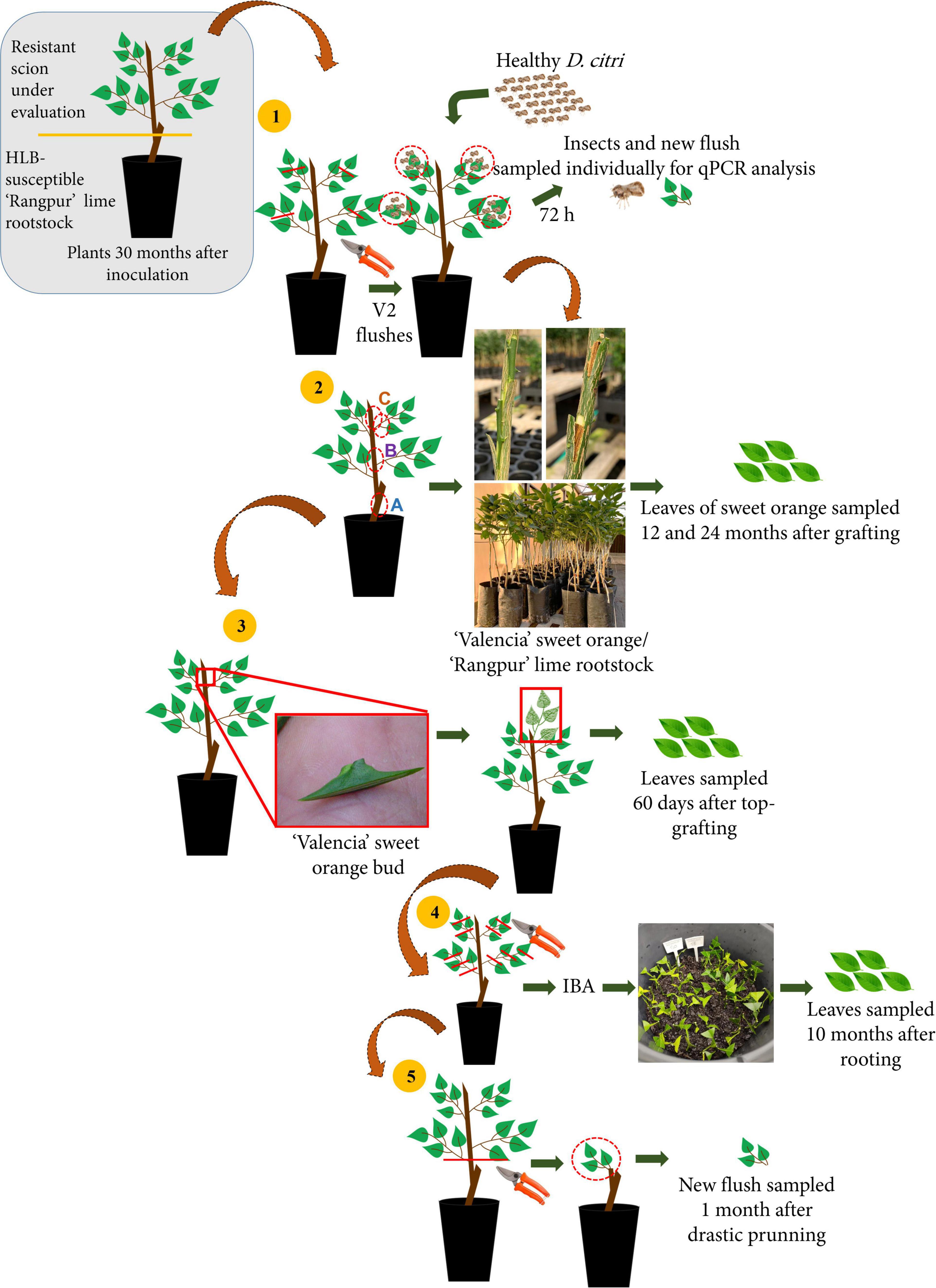
Figure 1. Schematic design of the five experiments carried out (1) Las acquisition by Diaphorina citri reared on Oceanian citrus genotypes; (2) Las indexing in ‘Valencia’ sweet orange plants grafted with (A) bark tissue from 5 cm below the graft union of Las-infected ‘Rangpur’ lime used as rootstock, (B) bark tissue from 8 cm above the graft union, and (C) budwood from the top of the Oceanian citrus genotypes; (3) Top-grafting of ‘Valencia’ sweet orange plants on scions from the Oceanian citrus genotypes; (4) Las multiplication through stem cuttings of Oceanian citrus genotypes; and (5) Forcing Las colonization from the ‘Rangpur’ lime rootstock to new sprouted shoots of Oceanian citrus genotypes after severe back-pruning.
Experiment 1: Las acquisition by Diaphorina citri reared on Oceanian citrus genotypes
Thirty months after Las graft challenge-inoculation and the categorization of the seven Oceanian citrus accessions as resistant, the tip of the branches from the canopy of those plants that remained alive was pruned to promote flushing. Three to four flushes per plant in the V2 phenological stage of development (Cifuentes-Arenas et al., 2018) were selected and used to confine five 20-day-old Las-free D. citri adults per flush for 72 h of the acquisition access period (Figure 1.1). The insects were obtained from a colony that had been reared on Las-free Murraya paniculata (L.) Jack seedlings. After the confinement, insects and 5–10 leaves of each flush were sampled individually, DNA was extracted and Las detection was assessed by qPCR. As a control, Las-infected ‘Tobias’ sweet orange plants were used.
Experiment 2: Las infection in ‘Valencia’ sweet orange plants grafted with bark and budwood from the Oceanian citrus genotypes
About 174 2-year-old plants of ‘Valencia’ sweet orange on ‘Rangpur’ lime rootstock were grafted with bark patches and budwood from the seven Oceanian citrus genotypes and ‘Tobias’ sweet orange as a control to assess the eventual transmission of Las from bark and budwood pieces of challenge-inoculated plants to healthy ‘Valencia’ sweet orange plants (Figures 1.2, 2A). All ‘Valencia’ sweet orange plants were grown in 4 L containers. Two patches of bark tissue (Figure 2B) or budwood (Figure 2C) with ca. 3–5 cm long by 0.8 cm wide size from 4 to 11 individual plants of each Oceanian citrus genotype were grafted at 15 cm above the scion-rootstock union of each ‘Valencia’ sweet orange tree. The bark and budwood tissues were collected from three different regions of each plant from the Oceanian citrus genotypes evaluated: at 5 cm below the scion-rootstock union (‘Rangpur’ lime trunk) and 8 cm above it (Oceanian citrus genotype trunk) and at the highest shoot of the scion canopy of all available plants (budwood). As a control, the same approach was used with bark and budwood tissue from Las-infected ‘Tobias’ sweet orange plants. A sample of 0.2 g from each bark and budwood piece to be grafted in ‘Valencia’ sweet orange plants was used to assess Las by qPCR analysis. Three months after grafting, the ‘Valencia’ sweet orange plants were top pruned at about 1.5 cm distant from the apex to stimulate the development of new shoots and, consequently, to force Las movement from the bark and budwood tissue used as the eventual inoculum source to the new tissues of ‘Valencia’ sweet orange (Raiol-Junior et al., 2021a). The union of the bark and budwood tissue with the ‘Valencia’ sweet orange stems was visually observed to evaluate the graft success (Figure 2D). After 12 and 24 months of grafting, four to six leaves were randomly sampled in the ‘Valencia’ sweet orange scion of all tested plants and processed for DNA extraction and qPCR analysis for Las detection.
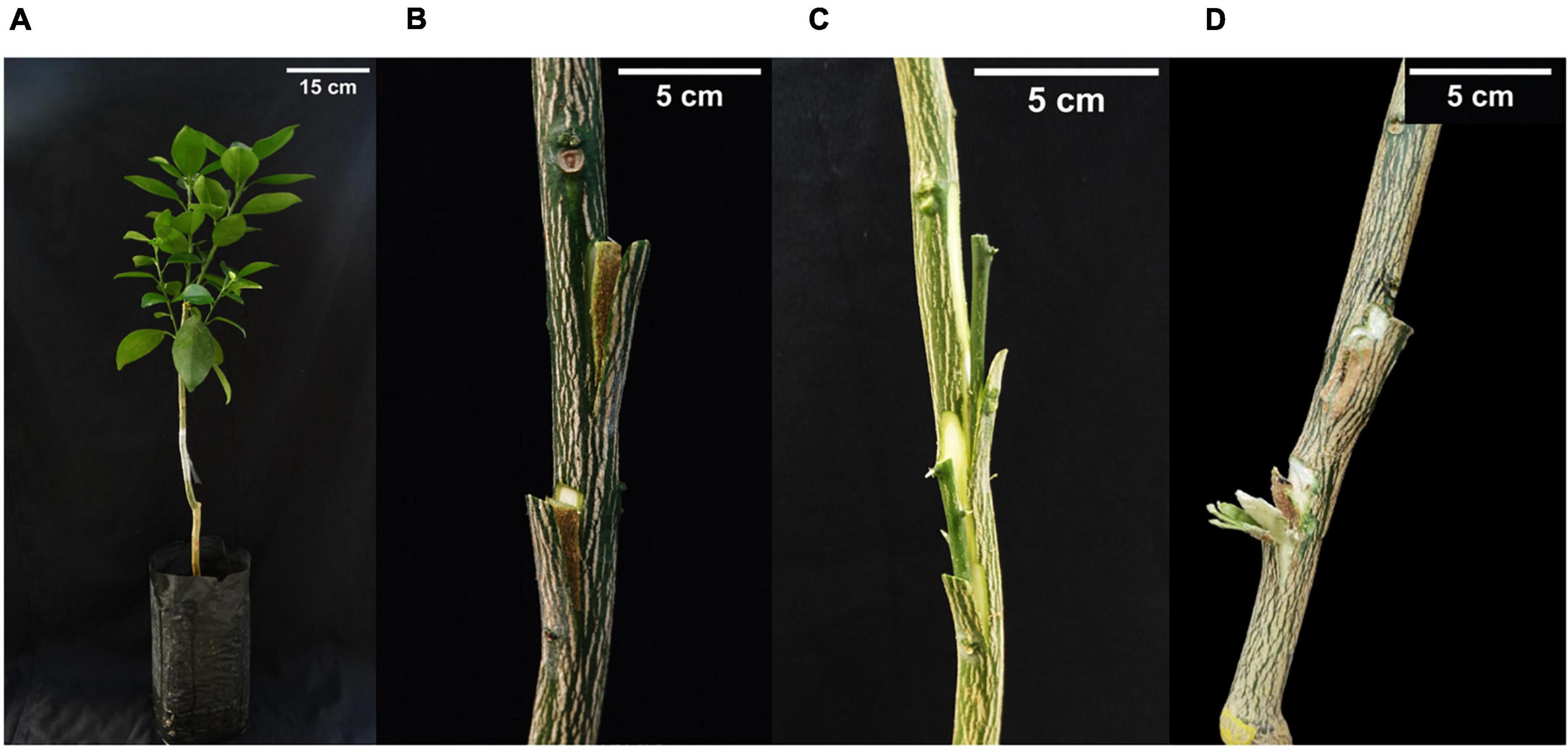
Figure 2. Representative aspect (A) of a ‘Valencia’ sweet orange plant grafted with bark or budwood from the Oceanian citrus genotypes in Experiment 2; (B) of bark tissue taken from 5 cm below the graft union of Las-infected ‘Rangpur’ lime used as rootstock or from 8 cm above the graft union; (C) of budwood removed from the top of the Oceanian citrus genotypes; (D) and from a failed bark graft in experiment 2.
Experiment 3: Top-grafting of ‘Valencia’ sweet orange plants on scions from the Oceanian citrus genotypes
Buds from a Las-free greenhouse-grown ‘Valencia’ sweet orange plant were collected and grafted onto the highest point of the scion top of 4–11 plants from the seven Oceanian citrus genotypes and ‘Tobias’ sweet orange, used as a control (Figure 1.3). This experiment aimed to determine whether Las would be able to translocate from the infected ‘Rangpur’ lime rootstock through the Oceanian citrus genotypes, now used as interstock and colonize the top-grafted ‘Valencia’ sweet orange scions. Sixty days after the ‘Valencia’ buds had grown and developed as scions, their leaves were randomly sampled for DNA extraction and qPCR analysis.
Experiment 4: Las multiplication in cuttings of Oceanian citrus genotypes
To further assess whether the scion tissues of the Oceanian citrus genotypes were free of Las, stem cuttings were propagated for rooting (Figure 1.4). Twenty cuttings with 5-8 cm were collected from partially lignified shoots of the canopy from the Oceanian citrus genotypes M. australis hybrid, E. glauca, eremorange, Microcitrus sp. hybrid, and E. glauca × Microcitrus sp. hybrid and ‘Tobias’ sweet orange as a control. Two leaves were kept in each cutting top and they were cut in half to reduce transpiration. Cutting basal ends were immersed for 0.5 s in a rooting aqueous solution of synthetic auxin indole-3-butyric acid (IBA) (5,000 ppm), then they were disposed inside 12 L polyethylene plastic containers filled with decomposed pine bark substrate, (Terra do Paraíso, Holambra, São Paulo, Brazil) and were manually irrigated whenever necessary. The containers were maintained inside a wet chamber for 90 days. Ten months after cutting propagation, four to six leaves of each cutting-derived plant remaining alive were sampled for Las detection through qPCR.
Experiment 5: Forcing Las colonization in shoots of Oceanian citrus genotypes
All original plants remaining alive from M. australis hybrid, E. glauca, eremorange, Microcitrus sp. hybrid, and E. glauca × Microcitrus sp. hybrid and ‘Tobias’ sweet orange as control were drastically back-pruned at 5 cm above the scion-rootstock union to force new flush growth and Las movement from the Las-infected ‘Rangpur’ lime rootstock to new leaves on the forced grown scion flush. Thirty days after pruning, 5–10 leaves of the newly emerged flushes per plant were randomly sampled for DNA extraction and qPCR analysis (Figure 1.5).
Evaluation by quantitative real-time polymerase chain reaction
Samples were processed as described by Teixeira et al. (2005) using a TissueLyser II system (Qiagen, Valencia, CA, United States) at 45 Hz for 30 s in a 2.0-ml microtube containing 5-mm steel beads. For insect processing, one insect and a 3-mm bead were used in a 1.5 mL microtube. Total DNA were obtained from 0.5 g of leaf midribs, or 0.3 g of root and bark tissue, using a CTAB (cetyltrimethylammonium bromide) buffer (2% CTAB; 1.4 M NaCl; 2% PVP 10,000; 0.5 M EDTA pH 8; 1 M Tris–HCl pH 8; 0.2% b-mercaptoethanol) as mentioned in Murray and Thompson (1980). DNA was resuspended in Milli-Q® water. DNA quality and concentration were measured using a nanodrop (Thermo Fisher Scientific, Massachusetts, EUA) (Desjardins and Conklin, 2010). For qPCR and detection of the 16S rDNA from Las, 1 μL of total DNA (100 ηg/μL), TaqMan® PCR Master Mix (1×) (Invitrogen, Carlsbad, CA, United States), and Las-specific primers/probe (0.5 μM/0.2 μM) were used in a StepOnePlus thermocycler (Applied Biosystems, CA, United States), as described by Li et al. (2006). As an internal control for the quality of plants and psyllids DNA, the mitochondrial gene cytochrome oxidase (COX) (Li et al., 2006) and an insect wingless (Wg) (Manjunath et al., 2008) gene region were used, respectively. Furthermore, leaf and insect samples, negative and positive for Las, were used during DNA extractions and qPCR assays as negative and positive controls, respectively. qPCR cycle threshold (Ct) values higher than 34.0 produce variable results (Lopes et al., 2013); therefore, samples were classified as ‘positive’ with Ct ≤ 34.0, suspected to be positive with 34.0 < Ct values < 36.0, and negative with Ct > 36 and/or when non-detected. Average Ct and the standard deviation values of treatments were calculated using positive Ct values.
Results
Phenotyping analysis of Oceanian citrus genotypes
The M. warburgiana accession used in this study showed axillary solitary spines and elongate-elliptical leaves as well as small and globular fruit with six segments (Figure 3A), coincident with its description by Swingle and Reece (1967). The M. papuana accession used by us showed small and slightly elongated leaves and yielded semi-globose fruit (Figure 3B), which resembled a hybrid rather than a pure species. According to Winters (1976), M. papuana produces narrower, elongated leaves as well as long, cylindrical fruit.
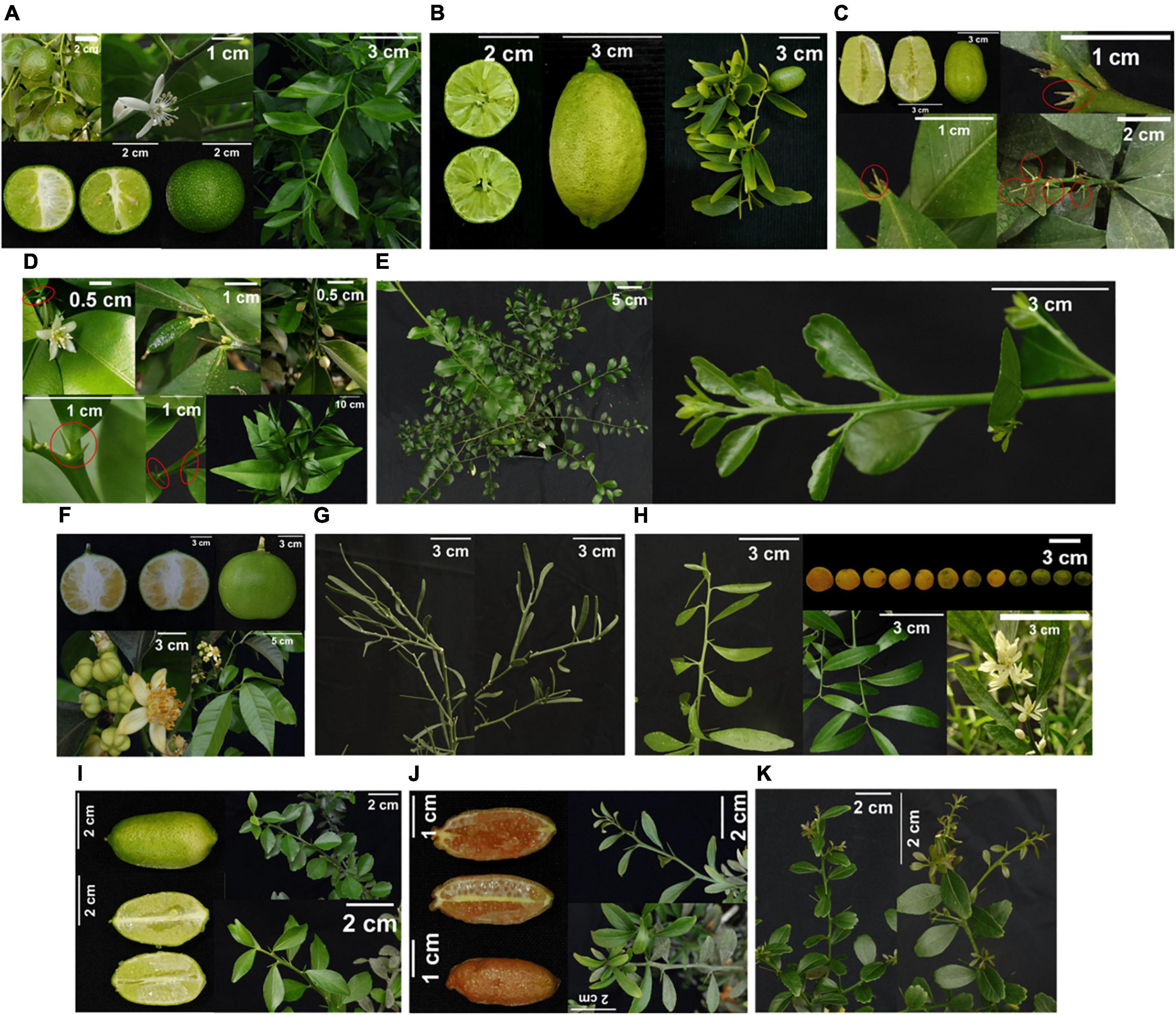
Figure 3. Morphological characterization of (A) M. warburgiana; (B) M. papuana × M. warburgiana hybrid; (C) M. australis × M. inodora hybrid; (D) M. inodora; (E) M. garrawayae; (F) Clymenia polyandra; (G) E. glauca; (H) E. glauca × Citrus sp. hybrid; (I) M. australasica × (M. australis × M. australasica) hybrid; (J) [E. glauca × (M. australis × M. australasica)] × M. australasica hybrid; and (K) M. australasica.
The Australian round lime M. australis is easily distinguished from all the other Microcitrus species by its globose or slightly pear-shaped, rough-skinned fruit. Our M. australis genotype showed slightly pear-shaped fruit (Figure 3C), and instead solitary, paired spines were predominant in its leaf axils, which are characteristic of M. inodora (Figure 3D), suggesting that the M. australis hybrid used in this study may be an M. australis × M. inodora hybrid.
The phenotypic traits of M. garrawayae (Figure 3E) and Clymenia polyandra (Figure 3F) accessions from Fundecitrus also fit with the description of the species by Swingle and Reece (1967). The M. garrawayae accession presents small narrow, thick and leathery, lozenge-shaped to broadly lanceolate leaves, and the C. polyandra one has spineless twigs with leaves being very peculiar, with elliptical shape, acuminating at both ends, and with a wingless petiole. In addition, C. polyandra produces single flowers in the axils of the leaves.
Eremocitrus genus is one of the most distinctive among near Citrus relatives. Morphological traits were similar in our E. glauca accession (Figure 3G) and the one described by Swingle and Reece (1967). In general, the hybrids described between Eremocitrus and Citrus (Swingle and Reece, 1967) showed unifoliolate leaves of intermediate size between those of the two parental species, but thinner and bifacial, thus more similar to Citrus leaves, which coincided with the characteristics presented by the E. glauca × C. × sinensis accession used in this study (Figure 3H).
Regarding the other Microcitrus hybrids, Microcitrus sp. (Figure 3I) and E. glauca × Microcitrus sp. (Figure 3J) hybrids showed leaf, spines and fruit traits resembling the Australian finger lime M. australasica (Figure 3K). However, Microcitrus sp. fruit is subglobose, not fusiform as that of typical finger limes, suggesting that it may be a hybrid with another Microcitrus species yielding globose fruit as the Australian round lime. In the case of E. glauca × Microcitrus sp. hybrid, its leaves showed a narrow, thicker, coriaceous aspect resembling those of the eremorange but the fruits were long, cylindric, and slender, with red color, indicating that this genotype may be a hybrid between E. glauca and M. australasica (Figure 3I). Accumulation of anthocyanins providing red color to fruit is common in some M. australasica accessions.
Genotyping analysis
After filtering the diallelic SNPs for less than 20% of missing data with the VCF tool, we obtained a genotyping matrix of 49187 diallelic SNPs for 27 accessions for the nuclear genome and a matrix with 40 SNPs for the mitochondrial genome. Only five SNPs were obtained for the chloroplast genome and were not found useful for maternal inheritance analysis.
The analysis of heterozygosity performed for the Fundecitrus and Corsican accessions in comparison with already published accessions (Figure 4A) allowed to identify pure species representative with most of the heterozygosity distribution curve under 10% (Figure 4B) from admixed and interspecific hybrid accessions (Figure 4C).
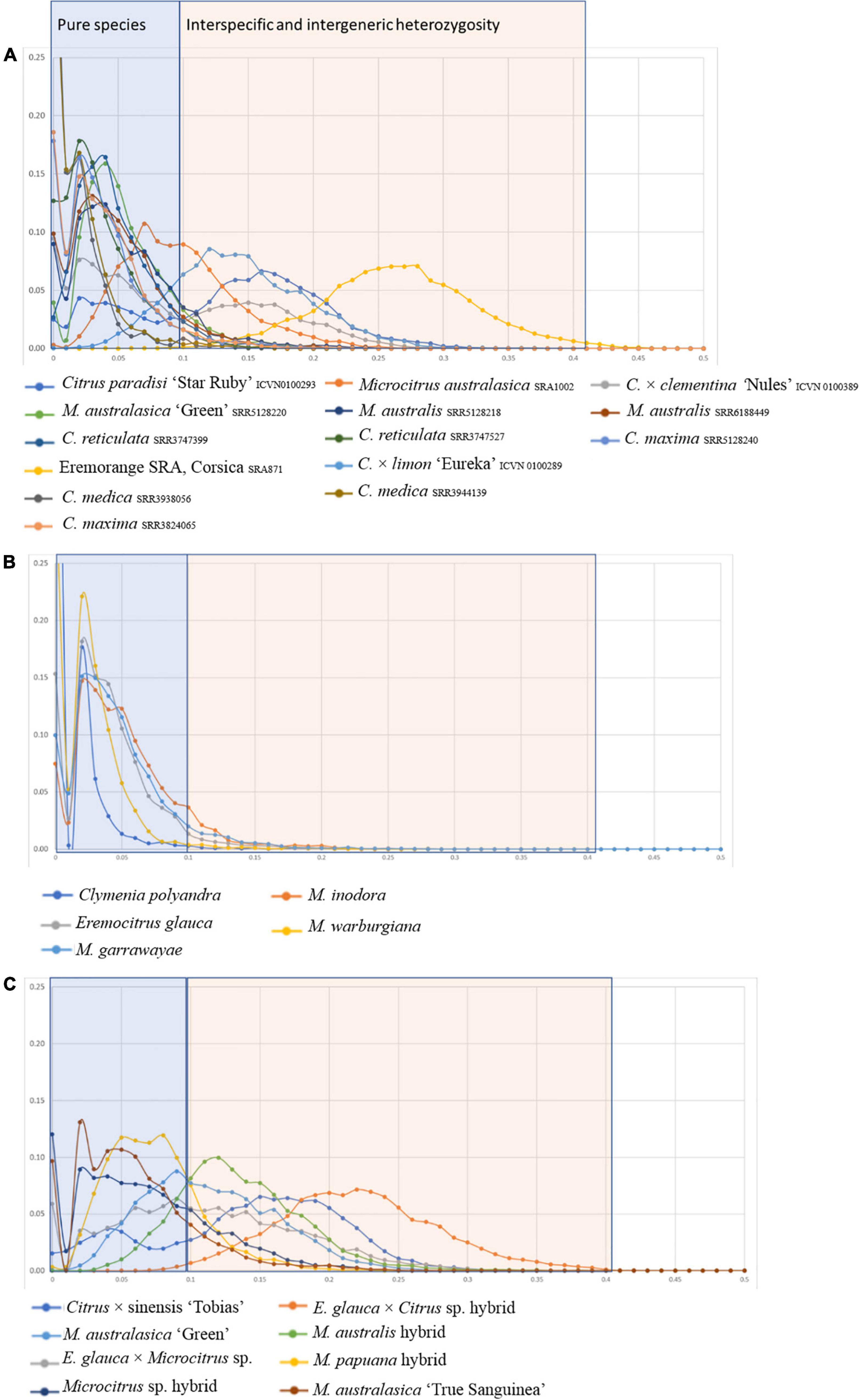
Figure 4. Distribution of heterozygosity values for the 27 accessions, established from sliding windows of 100 successive SNPs. (A) Already published genome with known phylogenomic status; (B) pure specific accessions from Fundecitrus Oceanian germplasm; (C) interspecific and admixed accessions of Fundecitrus Oceanian germplasm.
Among the Fundecitrus germplasm M. warburgiana, M. garrawayae, M. inodora, E. glauca, and C. polyandra appear as pure representatives of their species while M. australis, Eremocitrus × Microcitrus, Microcitrus sp., M. papuana, and the three finger limes (the ‘True Sanguinea’ and the ‘Green’ accessions from Fundecitrus and the accession from Corsica) appeared to result from interspecific admixture. E. glauca × C. × sinensis from Fundecitrus displayed a very similar curve than one of the eremorange accessions of Corsica with a high value of heterozygosity resulting from the strong differentiation between E. glauca and the Asian Citrus species.
Factorial analyses of a subset of the data provided the first elements on the origin of the admixed accessions. A first factorial analysis focused in the Oceanian accessions (Figure 5A). The first axis (28.9%) opposes the finger limes accessions that are close to the true M. australasica representative and the species from Papua-New Guinea (M. warburgiana and M. papuana). The second axis (26.0%) discriminate the E. glauca accessions from the Microcitrus ones. The Eremocitrus × Microcitrus hybrid has an intermediate position in this axis confirming the intergeneric admixture and its position on the first axis suggests a major contribution of M. australasica. The position of Microcitrus sp. accession suggests no, or very low, E. glauca contribution and a major M. australasica one. M. australis, M. inodora, and M. garrawayae were still poorly resolved in this analysis and were subjected to an independent factorial analysis (Figure 5B). The first axis (48%) opposes M. garrawayae and M. australis pure representative accessions while the second axis discriminates M. inodora from all other pure species representatives. The intermediate position of the M. australis accession of Fundecitrus between the two true M. australis accessions and M. inodora, in addition to its important heterozygosity, suggests that it may be an interspecific F1 hybrid of M. australis × M. inodora. The last analysis (Figure 5C) concerned the supposed intergeneric hybrids between Eremocitrus and C. × sinensis, the representative accessions of the corresponding ancestors (E. glauca, C. maxima, and C. reticulata) as well as some admixed genotypes between these two citrus species. The first axis (61.1% of the total diversity) differentiates E. glauca from the Citrus species while axis 2 (26.9%) separates C. maxima from C. reticulata with clementine, sweet orange, and grapefruit in intermediate positions fully in agreement with their known phylogenomic constitution (Wu et al., 2014, 2018; Ahmed et al., 2019). The supposed intergeneric E. glauca × C. × sinensis accession from Fundecitrus is close to the eremorange from Corsica. On axis 1, these two accessions have an intermediate position between Eremocitrus and Citrus species while their relative positions on axis 2 suggest that the eremorange from Corsica has a greater C. maxima contribution than the E. glauca × C. × sinensis from Fundecitrus. Taking into account the probable admixture or hybrid status of accessions revealed by the heterozygosity and factorial analysis, the diagnostic SNP mining for the different ancestors was performed using only pure representatives for 10 considered ancestral species. A total of 20,708 diagnostic SNPs were identified and the number of DSNPs for each ancestor is given in the NJ tree of the 14 used accessions (Figure 6). The distribution over the nine chromosomes is available as Supplementary Table 1.
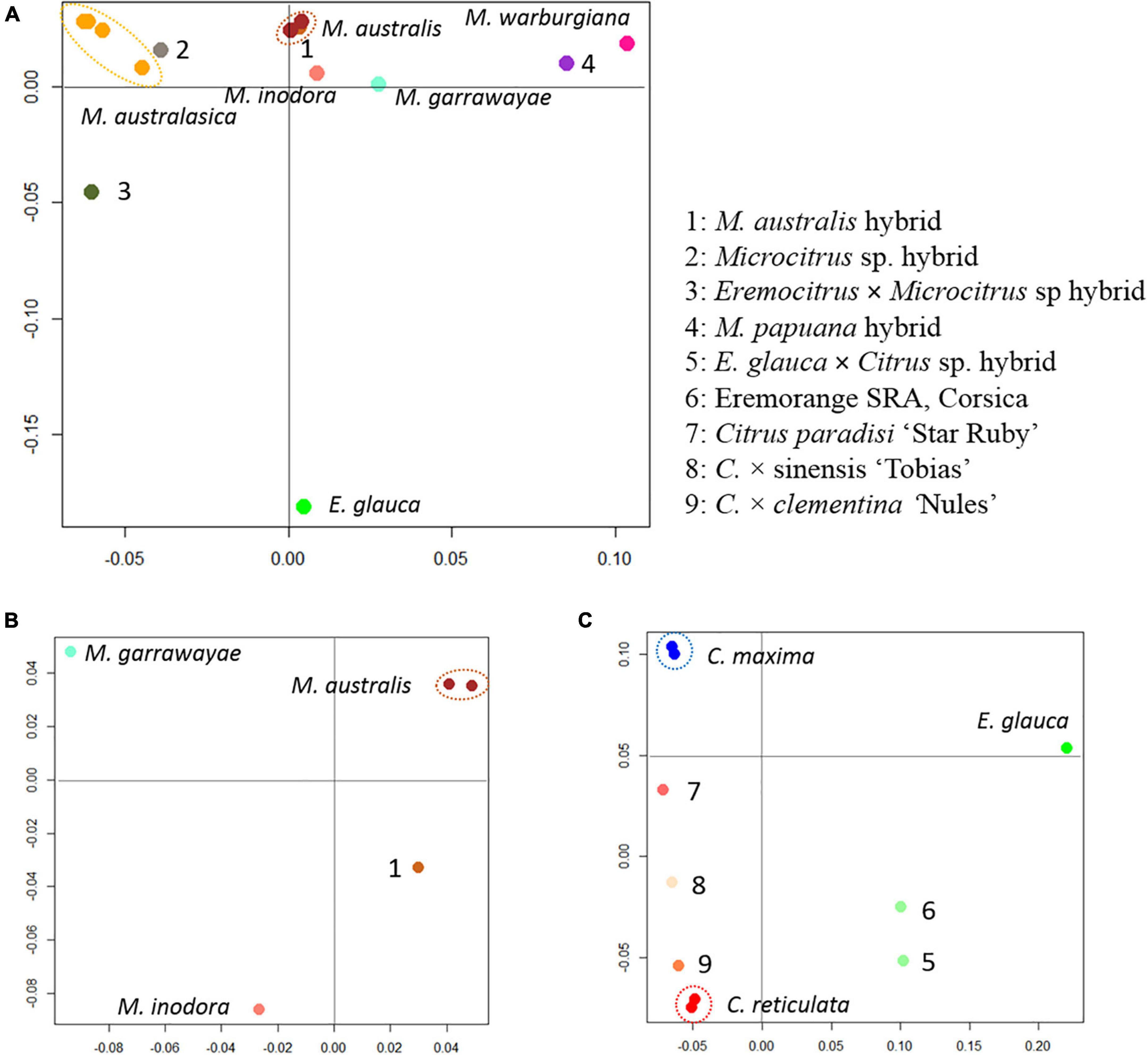
Figure 5. Factorial analysis from the genotyping of 49,187 diallelic SNPs. (A) Organization of the genetic diversity of the Oceanian germplasm. (B) Focus on the relationship of M. australis hybrid accession with M. australis, M. garrawayae, and M. inodora. (C) Focus on the relationship of the two supposed eremoranges with the C. reticulata/C. maxima gene pools.
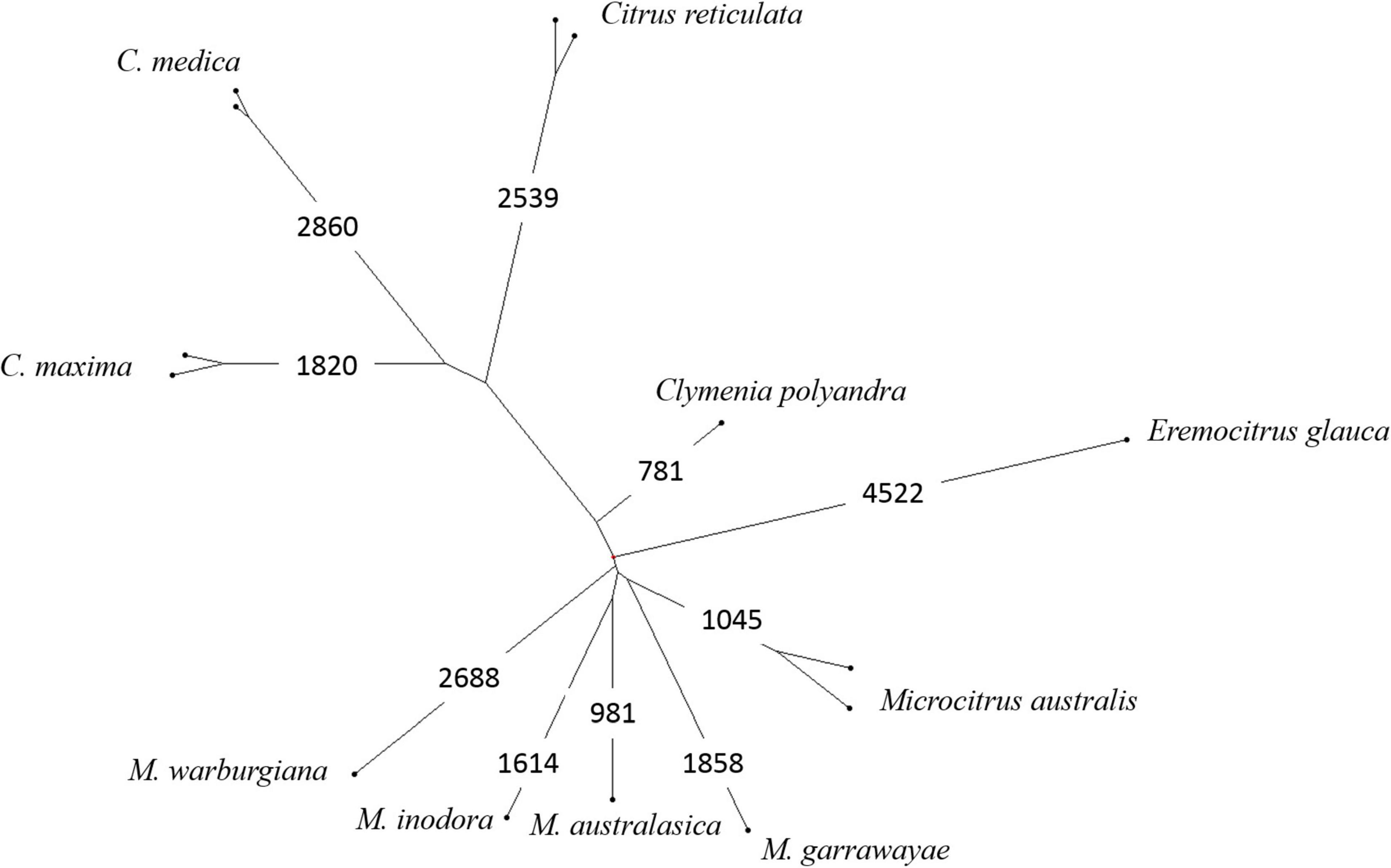
Figure 6. Organization of the nuclear diversity of 10 Asian and Oceanian species; NJ tree analysis from the genotyping of 49,187 diallelic SNPs and number of DSNPs for each species.
The global contribution of the 10 ancestors to the interspecific hybrids or admixed accessions was estimated from the proportion of specific alleles over the set of DSNPs of each ancestor (Table 1). Contributions below 1.5% were considered as artifactual. The contributions of C. maxima and C. reticulata to sweet orange, grapefruit, and clementine are in full agreement with previous works (Wu et al., 2014, 2018; Oueslati et al., 2017) as well as the contributions of C. medica, C. maxima, and C. reticulata to lemon (Wu et al., 2018; Ahmed et al., 2019). The two supposed E. glauca × C. × sinensis hybrids displayed a contribution of 50% from E. glauca. Both displayed also C. reticulata and C. maxima contributions with a greater proportion of C. reticulata (47%) in the Fundecitrus accession than in the Corsican one (40%). All the finger limes accessions displayed introgressions of M. australis in an M. australasica background. The estimated contributions of M. australis and M. inodora to the M. australis accession from Fundecitrus are, respectively, 47 and 40%. The Eremocitrus × Microcitrus accession displayed a major contribution of M. australasica (69%) followed by E. glauca (23%) and M. australis (5.9%). This analysis confirms the absence of E. glauca contribution for the supposed Microcitrus × Eremocitrus hybrid. The M. papuana accession displayed a major but incomplete contribution of M. warburgiana (65%) without any signal for the other ancestors considered in our study.
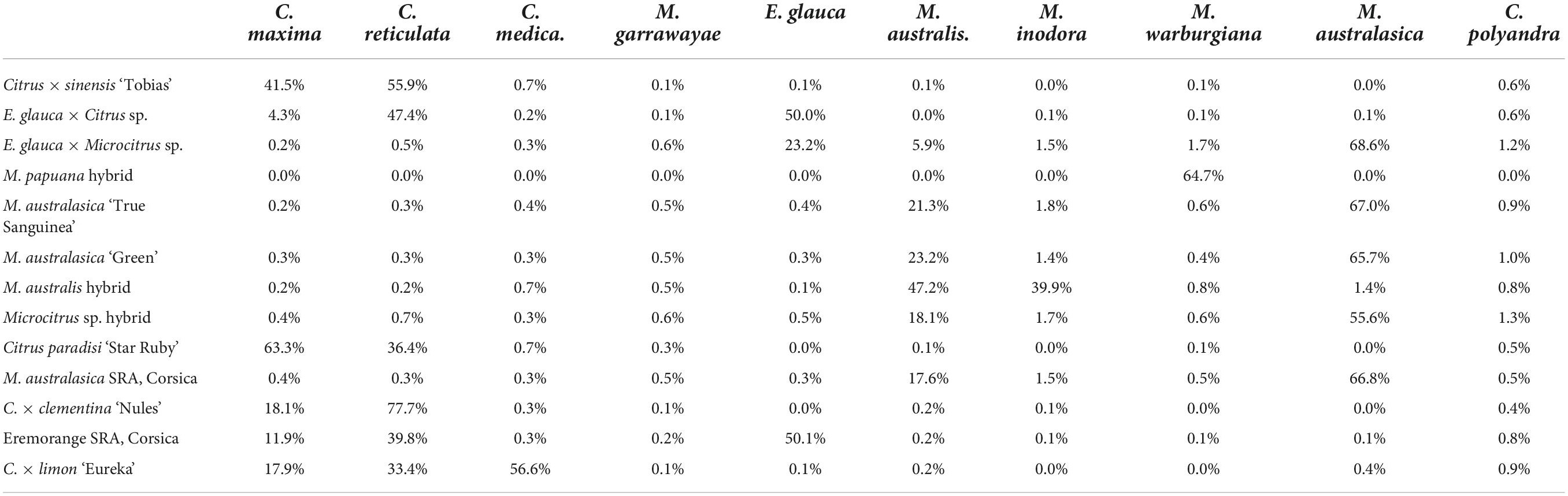
Table 1. Contribution of 10 ancestral species to the admixed and interspecific hybrids of Fundecitrus and Corsican Asian and Oceanian citrus germplasm.
Phylogenomic karyotypes are proposed for the admixed accessions analyzed for HLB resistance. They were inferred from the curve, all along the genome, for homozygosity and heterozygosity for the specific alleles of each ancestor involved in the admixture. Figure 7 provides an example of the supposed E. glauca × C. × sinensis accession. One haplotype is fully constituted by E. glauca genome while the other is a mixture of C. reticulata and C. maxima with a major contribution of C. reticulata. This accession is clearly a direct hybrid between E. glauca and an Asian variety with C. reticulata/C. maxima admixture. The presence of C. reticulata heterozygosity signal at the end of chromosome 2, while sweet orange is homozygous for C. maxima in the same region, discards sweet orange as the second parent. It is more probable that a cultivated mandarin or a tangor (mandarin × sweet orange hybrid) may be the second parent. The distribution of C. maxima genome in the clementine one (Wu et al., 2014) is compatible with the hypothesis that the second parent is a clementine. The Circos representation for M. australis hybrid, Microcitrus × Eremocitrus, Eremocitrus × Microcitrus, M. papuana hybrid, ‘Tobias’ sweet orange and the eremorange from Corsica are given in Supplementary Figure 1. The heterozygosity all along the genome for M. australis and M. inodora confirms the hypothesis that the M. australis accession from Fundecitrus results from a direct hybridization between M. australis and M. inodora. Among the two accessions supposed to result from admixture between E. glauca and Microcitrus species, this background is confirmed for the Eremocitrus × Microcitrus accessions. It displays homozygous regions for M. australasica as well as heterozygous areas of M. australasica/M. australis and M. australasica/E. glauca. It may be a hybrid between M. australasica and a second parent resulting from a complex admixture between E. glauca, M. australis, and M. australasica. The genome of the supposed Microcitrus × Eremocitrus hybrid is constituted in large part of M. australasica in homozygosity, some regions of M. australasica/M. australis heterozygosity, and a small portion of M. australis homozygosity at the start of the chromosome 9. M. papuana displays a significant signal for M. warburgiana heterozygosity in most of the genome but also a signal for M. warburgiana homozygosity. If it is a hybrid of M. papuana, as suggested by the morphology analysis, this concomitant signal for M. warburgiana DSNPs homozygosity and heterozygosity may be explained by the fact that M. papuana and M. warburgiana share numerous specific mutations differentiating the Papua-New Guinea species from the Australian ones. Indeed, in the absence of a pure representative of M. papuana for the DSNPs mining, these Papua-New Guinea-specific SNPs should have been assimilated as M. warburgiana DSNPs resulting in a false homozygosity signal for M. warburgiana in a hybrid between two Papua-New Guinea species.
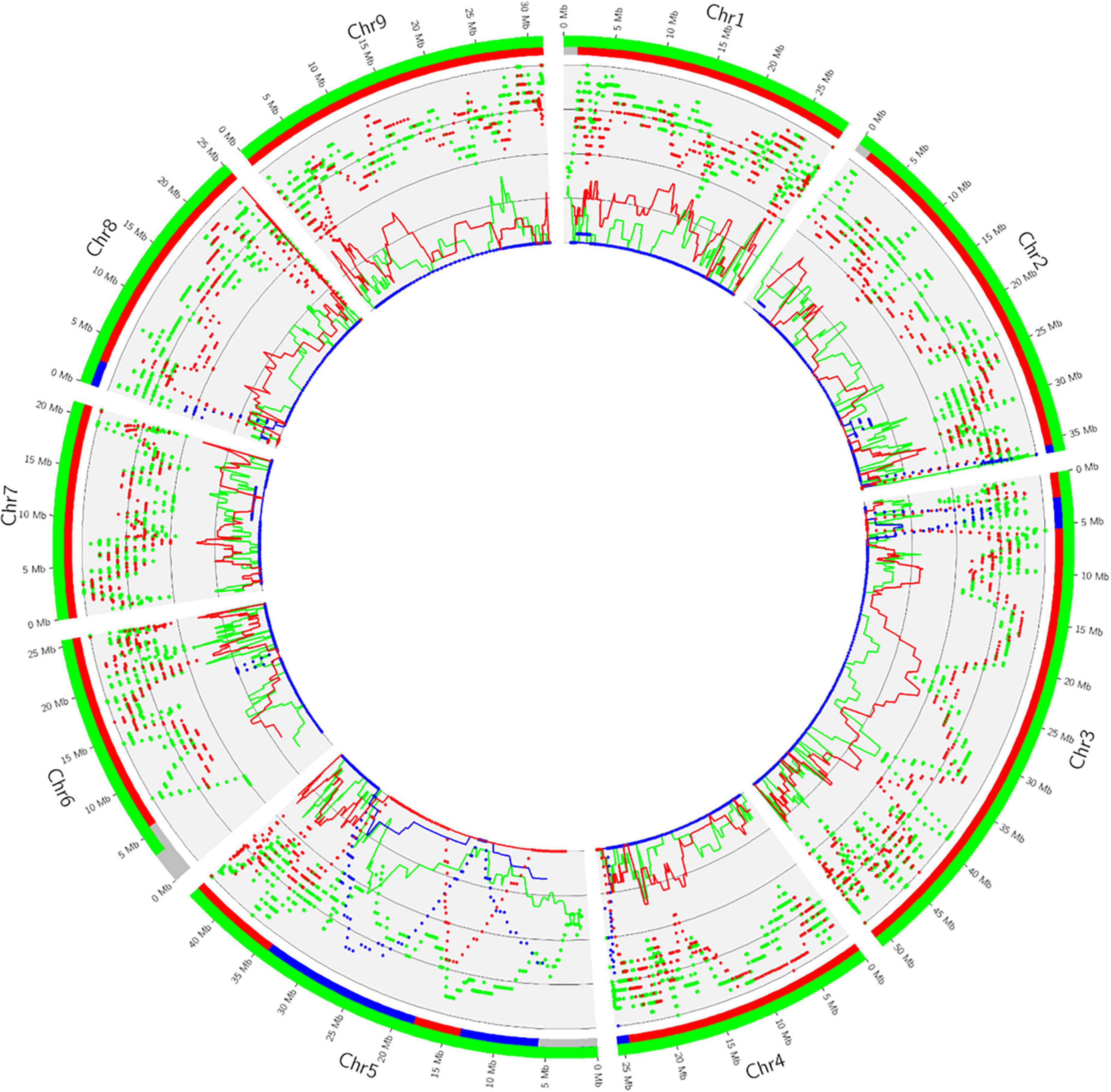
Figure 7. Distribution of heterozygosity (dashes) and homozygosity (line) of the ancestral alleles of E. glauca (green), C. reticulata (red), and C. maxima (blue) along the genome of the supposed E. glauca × C. × sinensis hybrid and phylogenomic karyotype inference (external ribbons with the same color code). Gray corresponds to undetermined regions.
From the NJ tree based on mitochondrial data (Figure 8), it appears that: the E. glauca × C. × sinensis and Eremocitrus × Microcitrus accessions inherited E. glauca mitochondria as well as the eremorange from Corsica. All analyzed finger limes and the Microcitrus hybrid accessions have M. australasica mitochondria while the M. australis from Fundecitrus has M. australis mitochondria. The mitochondria of the M. papuana accession are differentiated from the M. warburgiana one and may be representative of the M. papuana species. To summarize, the genotypes used in this study, their common names and phylogenomic constitution after genotyping by sequencing (GBS) analysis are listed in Table 2.
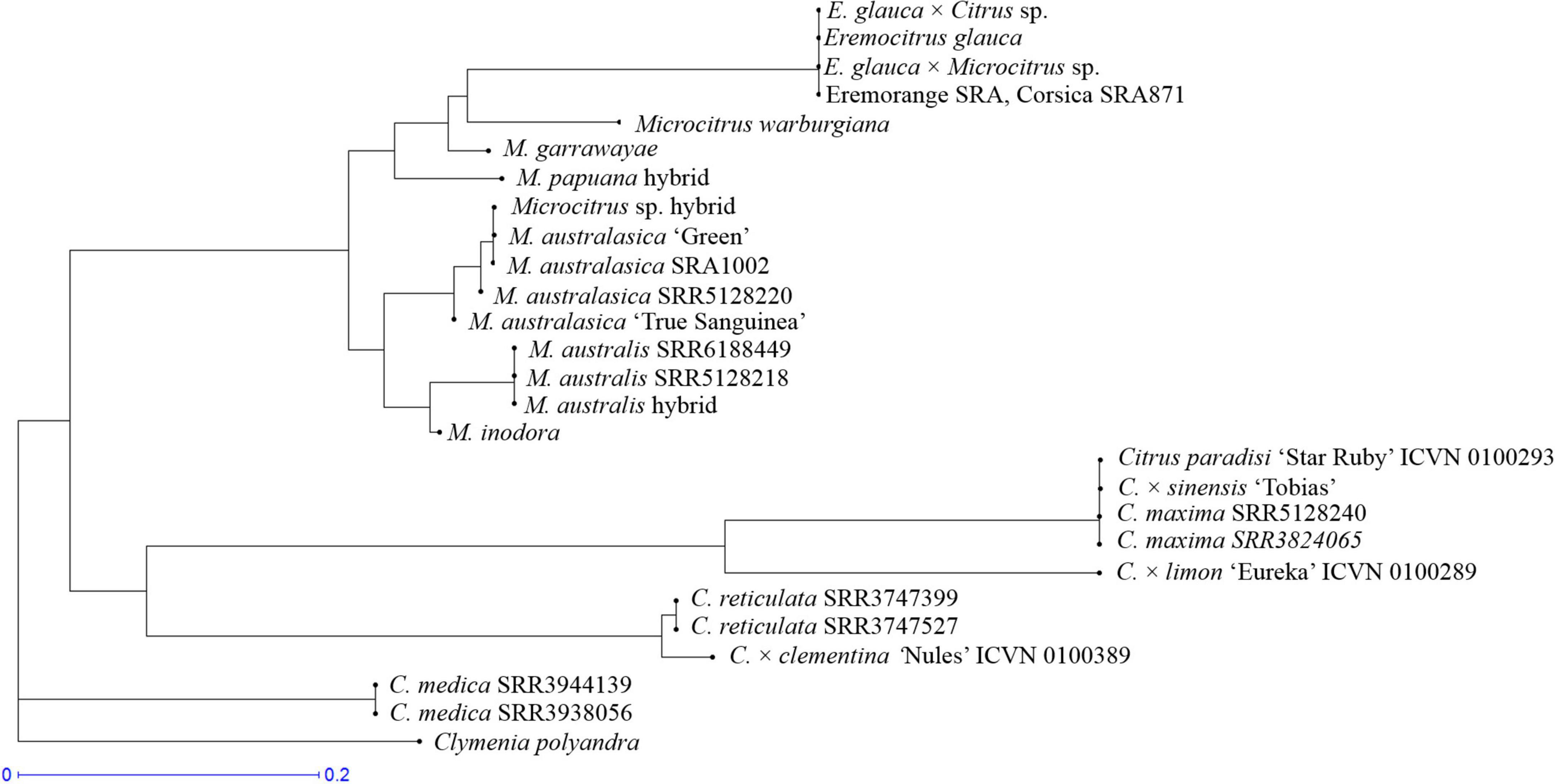
Figure 8. Assignation of the mitochondrial genome of hybrids and admixed Oceanian accessions in relation to ancestral mitochondrial ones; NJ tree based on the genotyping for 40 mitochondrial diallelic DSNPs.
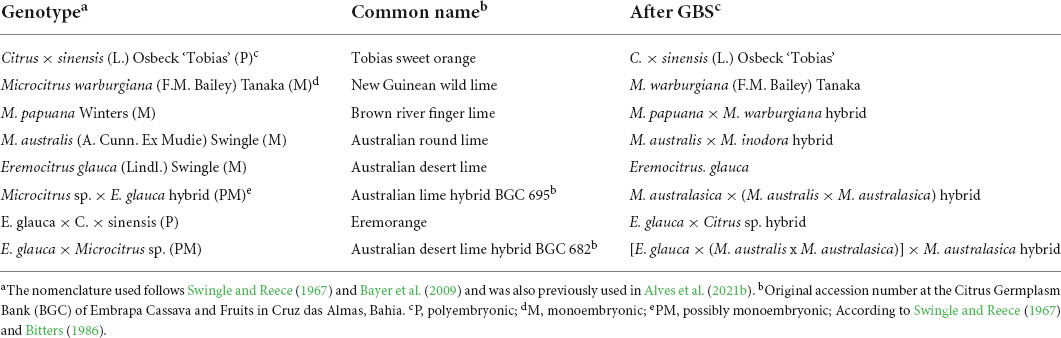
Table 2. Genotypes used in this study, their common names, and phylogenomic constitution after genotyping by sequencing (GBS) analysis.
Experiment 1: Las acquisition by Diaphorina citri reared on Oceanian citrus genotypes
After 72 h of D. citri confinement, none of the new shoot flushes evaluated from plants of all Oceanian citrus genotypes resulted positive by qPCR, while in the case of ‘Tobias’ sweet orange, 100% of the flushes were Las-positive (Table 3). Insects reared on plant flushes followed the same tendency, with none of those fed on Oceanian citrus showing Ct values for Las < 34, but 97.14% (133 of 140) of those fed on ‘Tobias’ sweet orange being clearly Las-positive (Ct ≤ 34). However, a small number of insects were considered suspicious to be positive as they showed Ct values between 34 and 36, specifically two of 160 fed on M. australis hybrid, seven of 140 on the Microcitrus sp. hybrid, six of 220 on the E. glauca × Citrus sp. hybrid and two of 140 on ‘Tobias’ sweet orange. All insects fed on M. warburgiana, M. papuana hybrid, E. glauca, and E. glauca × Microcitrus sp. hybrid were Las-negative (Supplementary Table 2).
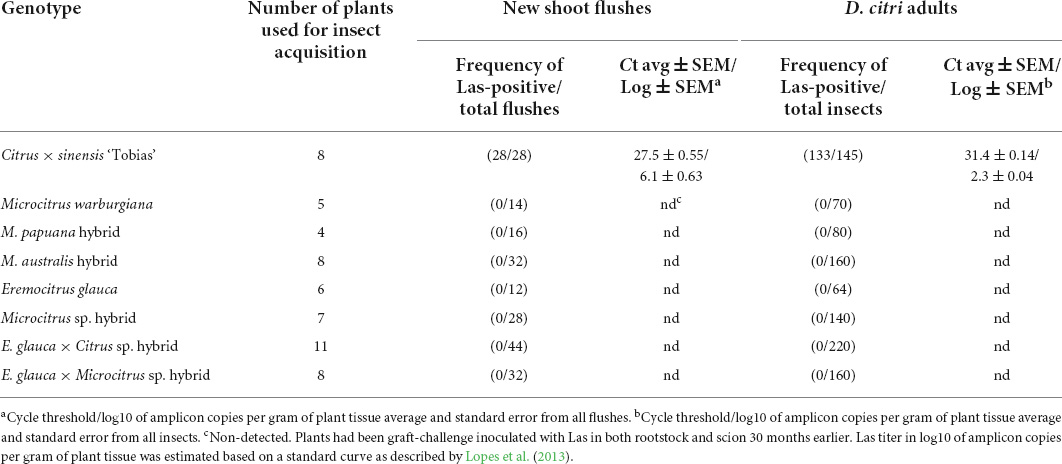
Table 3. Acquisition of ‘Candidatus Liberibacter asiaticus’ (Las) by Diaphorina citri reared for 72 h on new shoot flushes from plants of seven Oceanian citrus genotypes and ‘Tobias’ sweet orange as control, all grafted onto Las-infected ‘Rangpur’ lime rootstocks.
Experiment 2: Las infection in ‘Valencia’ sweet orange plants grafted with bark and budwood from the Oceanian citrus genotypes
Las infection was confirmed in the ‘Rangpur’ lime rootstock bark patches from all composite plants remaining alive with scions of the seven Oceanian citrus genotypes and ‘Tobias’ sweet orange control, with Ct average values ranging from 28.5 ± 0.91 to 32.6 ± 0.25 (Table 4). Las was also detected in the bark patches of the scions taken at 8 cm above the scion-rootstock union, with Ct average values ranging from 26.4 ± 0.64 to 32.8 ± 0.50, with the exception of all those coming from E. glauca which showed Ct values > 34. Moreover, bark pieces at 8 cm from most M. warburgiana and M. australis hybrid scions were also Las-negative (Supplementary Table 3). In budwood collected from the apex, Las was detected in all but one (7 of 8) ‘Tobias’ sweet orange, and only two of 11 E. glauca × Citrus sp. hybrid samples with Ct average values ranging from 22.5 ± 1.09 to 24.0 ± 0.05, while Las was hardly found in few samples of M. australis hybrid (2 of 8), Microcitrus sp. hybrid (3 of 7) and E. glauca × Microcitrus sp. hybrid (1 of 8), with Ct averages ranging from 30.7 ± 2.74 to 33.6 ± 0.00 in Las-positive samples. Las was not detected in budwood of M. warburgiana, M. papuana hybrid, and E. glauca scions.
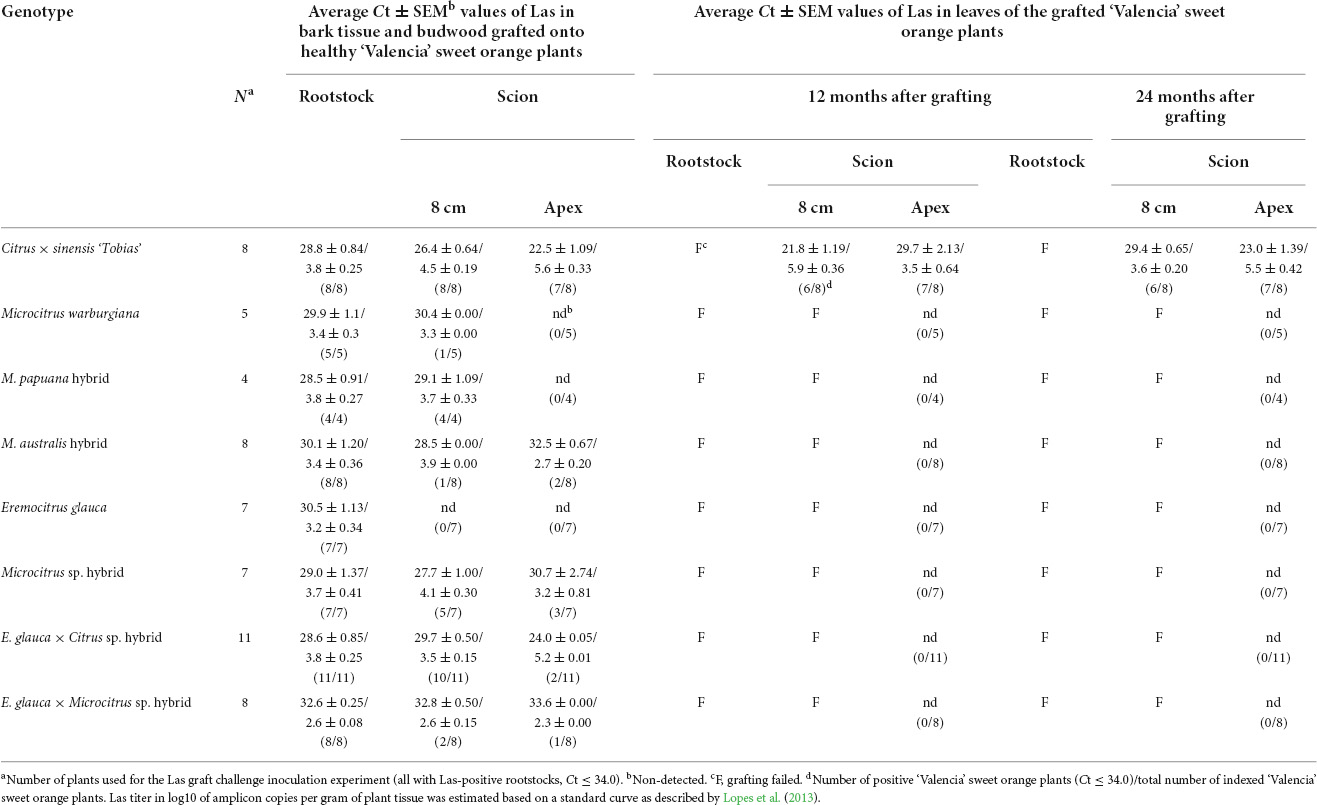
Table 4. ‘Candidatus Liberibacter asiaticus’ (Las) infection, determined by quantitative polymerase chain reaction, in bark patches and budwood collected from different regions (8 cm above the graft union and at the top, respectively) of Oceanian citrus and ‘Tobias’ sweet orange plants, as well as from the stem (5 cm below the graft union) of Las-infected ‘Rangpur’ lime used as rootstock, graft success and Las transmission from those bark patches and budwood to ‘Valencia’ sweet orange plants, 12 and 24 months after grafting.
Experiment 3: Top-grafting of ‘Valencia’ sweet orange plants on scions from the Oceanian citrus genotypes
Budwood from healthy ‘Valencia’ sweet orange plants was grafted on the top of the original plants remaining alive from the Oceanian citrus genotypes and ‘Tobias’ sweet orange growing on Las-infected ‘Rangpur’ lime rootstock. Unfortunately, at the time this experiment was conducted, no plants of M. warburgiana were alive, and thus, this genotype was not evaluated. In addition, no ‘Valencia’ sweet orange budwood sprouted on Microcitrus sp. hybrid plants (Table 5), likely due to their highly debilitated root system. Rates of budwood sprouting varied from 1/8 for ‘Tobias’ sweet orange, 1/4 for M. papuana hybrid, 2/8 for M. australis hybrid, 2/6 for E. glauca, 4/11 for E. glauca × Citrus sp. hybrid, and 3/8 for E. glauca × Microcitrus sp. hybrid. Las was detected only in ‘Valencia’ sweet orange samples top-grafted on the ‘Tobias’ sweet orange with a Ct of 26.9 (Table 5 and Supplementary Table 4).
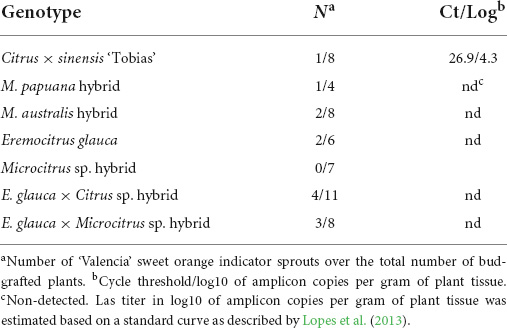
Table 5. Graft success and titer of ‘Candidatus Liberibacter asiaticus’ (Las) in new shoots grown from budwood of ‘Valencia’ sweet orange top-grafted on Oceanian citrus genotypes and ‘Tobias’ sweet orange, all growing onto Las-infected ‘Rangpur’ lime rootstocks, as determined through detection of Las16S DNA by qPCR 60 days after bud grafting.
Experiment 4: Las multiplication in cuttings of Oceanian citrus genotypes
No plants of M. warburgiana or M. papuana hybrid were alive to attempt establishing stem cuttings for rooting and all cuttings performed from M. australis hybrid, E. glauca, and E. glauca × Citrus sp. hybrid stems did not survive as the root system of the original plants was almost dead by aggressive Las infection of the ‘Rangpur’ lime rootstock. However, 32 and five cutting-derived rooted plants were obtained from Microcitrus sp. and E. glauca × Microcitrus sp. hybrids, respectively, which were all Las-free 10 months after propagation (Table 6 and Supplementary Table 5). As controls, 33 of 39 cutting-derived plants of ‘Tobias’ sweet orange, had Ct average values between 21.6 ± 0.28 and 29.9 ± 1.13.
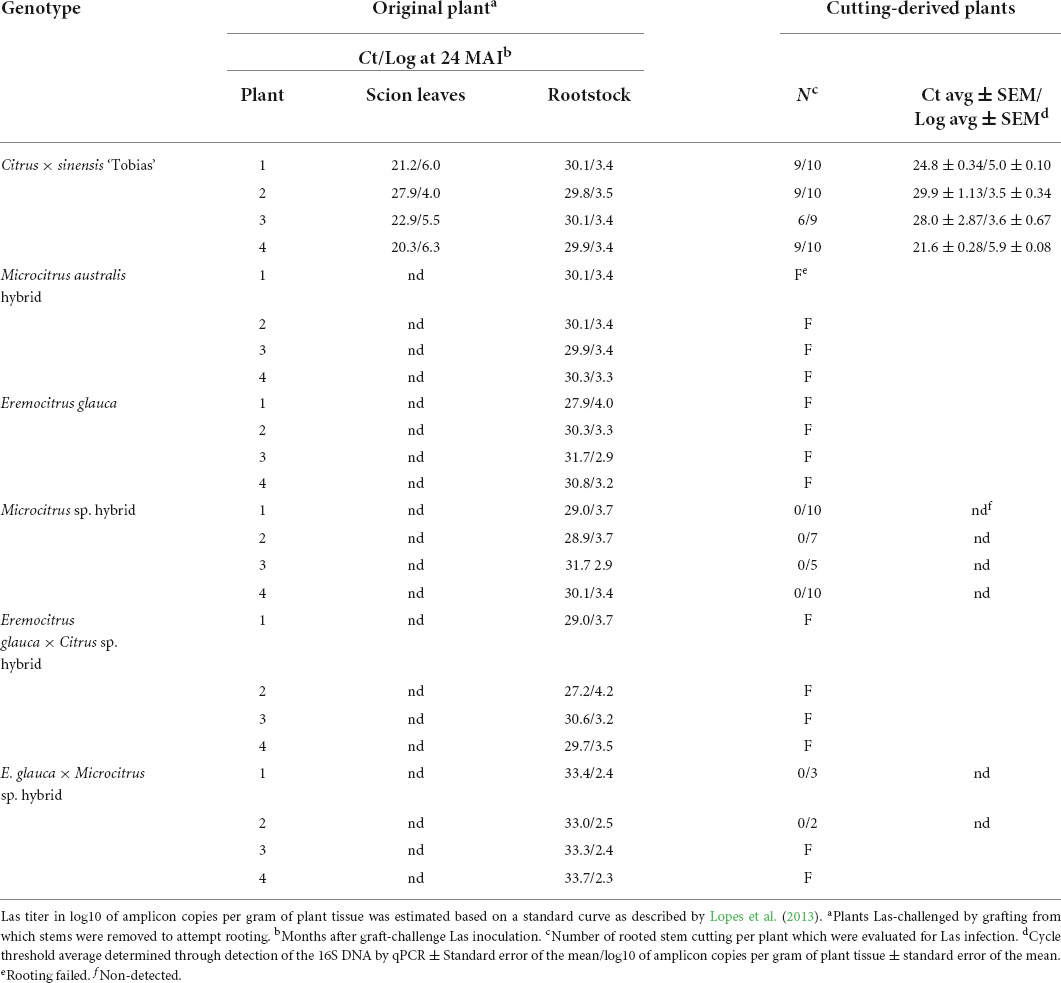
Table 6. ‘Candidatus Liberibacter asiaticus’ (Las) infection in leaves of cutting-derived plantlets obtained from Oceanian citrus genotypes and ‘Tobias’ sweet orange control, all grafted onto Las-infected ‘Rangpur’ lime rootstocks, 2 years before and 10 months after cutting propagation.
Experiment 5: Forcing Las colonization in new shoots of Oceanian citrus genotypes
The stems of all surviving Oceanian citrus plants growing onto Las-infected ‘Rangpur’ lime rootstock were pruned exhaustively in attempts to force Las movement from the rootstock to the new growing tissues in the scions. New shoots grew on stems of most plants, except E. glauca × Microcitrus sp. hybrid for which only one out of eight plants sprouted. Las was detected in six of the eight ‘Tobias’ plants with an average Ct value of 26.8 ± 1.48 (Table 7 and Supplementary Table 6), but new shoots from M. australis hybrid, E. glauca, Microcitrus sp. × E. glauca hybrid, and E. glauca × Citrus sp. hybrid plants were all Las-negative. All original plants of M. warburgiana and M. papuana hybrid were dead before performing this experiment, as mentioned above.
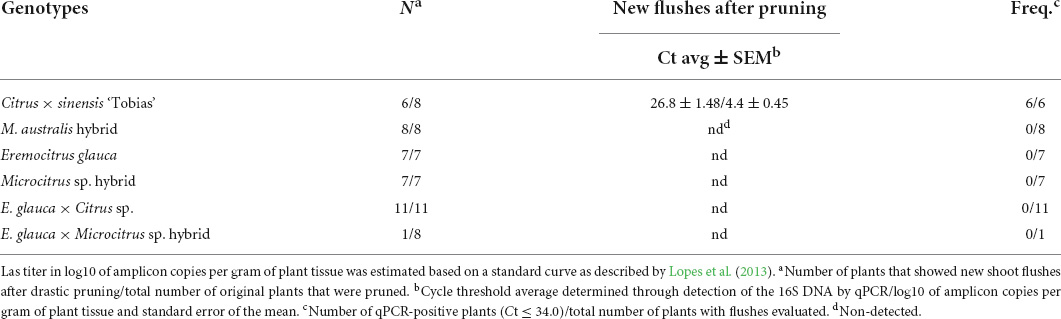
Table 7. ‘Candidatus Liberibacter asiaticus’ detection in new flushes from Oceanian citrus genotypes and ‘Tobias’ sweet orange as scions onto Las-infected ‘Rangpur’ lime rootstocks, one month after drastic pruning of the canopy at 5 cm above the scion-rootstock union.
Discussion
In view of the huge losses in citrus production and quality caused by HLB, as well as the high costs of the existing management strategies, finding a durable and sustainable solution for this disease has been the objective of different research programs worldwide. Genetic resistance to HLB has been found in several citrus relatives of the Rutaceae family, Aurantioideae subfamily, when seedling trees in the field were subjected for 6 years to natural disease challenge conditions in Florida, an HLB endemic region (Ramadugu et al., 2016). However, the experimental system did not reveal whether immune germplasm was actually resistance to the bacterium or to the insect vector. For example, the most HLB field-resistant species belonging to the Clauseneae tribe, such as Clausena lansium and Glycosmis pentaphylla, are resistant to Diaphorina citri under controlled greenhouse conditions (Felisberto et al., 2019). Conversely, other Clauseneae species such as Bergera koenigii and Murraya paniculata are excellent D. citri hosts (Tomaseto et al., 2019; Eduardo et al., 20223) but non- or transient Las hosts, respectively (Cifuentes-Arenas et al., 2019; Alves et al., 2021a). Among genera from the true citrus species, partial resistance to D. citri has been found in Poncirus (Westbrook et al., 2011; Richardson and Hall, 2013; Hall et al., 2015; Felisberto et al., 2019) and in Oceanian Microcitrus and Eremocitrus species (Eduardo et al., 2022). Therefore, to assess resistance within Citrus and Citrus relatives to either the bacterium, the insect vector or both, experiments performed under controlled environmental conditions and using challenging procedures rendering unequivocal results are advisable.
In a previous study, after propagation of over 20 citrus relatives within the Citrinae subtribe, Citreae tribe, onto the Las-susceptible ‘Rangpur’ lime rootstock and graft-inoculation with Las-infected budwood on both the scion and rootstock in controlled greenhouse conditions, we selected seven Oceanian citrus genotypes as full-resistant to Las, that is, Las was never detected in their scion leaves over a period of 24 months after inoculation but it did in all rootstocks tested (Alves et al., 2021b). However, as Las was identified through qPCR in the stem tissue at 5 cm above the scion-rootstock union in most plants of the resistant genotypes, and also in stem bark tissue at 30 cm height in a few plants of two of the genotypes under evaluation, we wondered whether these genotypes were actually partial-resistant to Las or transient hosts. Moreover, we performed a phenotypic description and a fine phylogenomic characterization of the seven Oceanian citrus accessions.
The phenotypic analysis of the Fundecitrus accessions considered as representative of M. papuana and M. australis revealed that they should be rather ascribed as interspecific hybrids. Moreover, the characteristics of the supposed interspecific or intergeneric hybrids did not allow concluding on their origin accurately. Therefore, we performed, by GBS, a fine genetic characterization of their nuclear and mitochondrial genomes in comparison with those of pure representatives of 10 ancestral species of Asian and Oceanian citrus. The identification of 20708 DSNPs allowed us to efficiently infer ancestral karyotypes of the Fundecitrus germplasm, including full-resistant accessions. It extended to Oceanian species the TraceAncestor approach on GBS data proposed by Ahmed et al. (2019) for Asian citrus. The DSNP set should be improved by analyzing several true representatives of the different species and particularly representatives of M. papuana that we missed in the present study. In agreement with the phenotypic observations, this analysis confirmed that the M. inodora, M. garrawayae, M. warburgiana, E. glauca, and C. polyandra accessions of Fundecitrus germplasm were pure representative of these species. According to nuclear and cytoplasmic genome data, the M. australis accession should be an M. australis × M. inodora hybrid while the M. papuana accession is a probable admixture between M. papuana and M. warburgiana with M. papuana mitochondria. It would be necessary to include a true M. papuana representative in the phylogenomic analysis to conclude this unquestionably. The supposed E. glauca × C. × sinensis appeared to result from an intergeneric cross between E. glauca as a female parent and an admixed C. reticulata/C. maxima Asian variety as a male parent. However, the phylogenomic profiles discarded sweet orange as a male parent and suggested that the second parent was a clementine. This accession is different from the eremorange from Corsica whose phylogenomic profile is fully compatible with an E. glauca × C. × sinensis origin (Wu et al., 2018). The Eremocitrus × Microcitrus accession is a complex hybrid involving, in order of importance, M. australasica, E. glauca, and M. australis. According to the nuclear and mitochondrial data, it should have resulted from [E. glauca × (M. australis × M. australasica)] × M. australasica hybridizations. The Microcitrus hybrid may have resulted from a kind of backcross M. australasica × (M. australis × M. australasica) with, however, a small M. australis introgression in the M. australasica recurrent parent. It is interesting to notice that the three Australian finger limes from Fundecitrus and Corsica are not pure M. australasica representatives but all of them display M. australis introgressions. As a whole, these results highlight the important inclination of the Oceanian species to produce interspecific and even intergeneric hybrids, likely favored by the absence of facultative apomixis in these species. Consequently, this results sometimes in confusing classifications of these accessions in germplasm collections, particularly if the accessions were introduced by seeds. It also stresses the importance of clonal propagation of this germplasm for any analysis of genetic resistance to Las using Oceanian citrus species.
Next, to better characterize the resistance phenotype of the seven full-resistant Oceanian citrus species and hybrids, new experiments with the same plants were designed. We first considered whether D. citri adults were able to acquire Las when fed on Las challenge-inoculated Oceanian citrus scions still surviving on Las-infected ‘Rangpur’ lime rootstocks. Adult D. citri can acquire Las within 5–7 h feeding on infected plants (Xu et al., 1988). In our study, after 72 h of confinement on new flushes of the Las-susceptible ‘Tobias’ sweet orange in an environment proper for Las acquisition (Alves et al., 2021a), 97% of the insects acquired the bacteria. Conversely, Las was not detected in any insect fed on the shoot flushes of the seven Oceanian citrus genotypes, which, as expected, were also negative. These results suggested that Las detected in the Oceanian citrus stems did not reach the scions canopy or did at titers insufficient for insect acquisition and then transmission. However, Las was detected in budwood of the canopy of at least some plants from the M. australis hybrid and the other three hybrids with M. australasica or sweet orange background in concentrations theoretically sufficient to be acquired by D. citri and transmitted to other host plants (Lopes and Cifuentes-Arenas, 2021).
Then, we attempted Las transmission by grafting bark pieces and budwood from the Oceanian citrus scions onto ‘Valencia’ sweet orange healthy plants. Although bark grafting failed for all Oceanian species and hybrids, budwood grafting was highly successful in all cases, revealing that Las was efficiently transmitted from Las-positive ‘Tobias’ to ‘Valencia’ sweet orange but, if present, it was not transmitted in any case from the Oceanian citrus genotypes to ‘Valencia’ sweet orange receptor plants. The efficiency of Las transmission by grafting infective budwood is usually high. Notwithstanding, its frequency depends on the bacterial titer in the plant tissue used for grafting, the kind, number, and size of the infected materials used for inoculation, the season of the year, and the environmental conditions (Lopes et al., 2009). The frequency of successful Las transmission in graft-inoculated, greenhouse-grown C. × sinensis plants of ‘Hamlin’, ‘Natal,’ ‘Pera,’ and ‘Valencia’ sweet orange cultivars was reported to span from 54 to 88% (Lopes et al., 2009; Albrecht et al., 2014). The failure to transmit Las from apical budwood of the Oceanian citrus genotypes suggests that Las detected by qPCR on them was at low titer, with few or no cells alive, and that viable cells were below a minimum threshold number insufficient to be transmitted by grafting.
Afterward, we tried to force Las infection by either top-grafting healthy ‘Valencia’ sweet orange onto the Oceanian citrus scions or by establishing Oceanian citrus scion stem cuttings in pots for rooting. Once either ‘Valencia’ sweet orange or Oceanian citrus cuttings started to grow, they were evaluated for Las detection. However, few plants survived after attempting top-grafting or set up cuttings, mainly because the ‘Rangpur’ lime rootstock was so much compromised by Las infection that Oceanian citrus scions were already dying when these experiments were carried out, about 36–48 months after the challenge inoculation. Even with this, ‘Valencia’ sweet orange plants were Las-negative on M. papuana hybrid, M. australis hybrid, E. glauca, Microcitrus sp. hybrid, E. glauca × Citrus sp. hybrid, and E. glauca × Microcitrus sp. hybrid remaining alive while only ‘Tobias’ sweet orange interstock was able to infect the top-grafted ‘Valencia’ sweet orange. The graft compatibility of those Las-resistant Oceanian citrus genotypes with a citrus elite variety such as ‘Valencia’ opens the possibility for their use as putatively HLB-resistant rootstocks/interstocks for other citrus cultivars. In citriculture, there are several examples of successful disease control by using resistant/tolerant rootstocks, such as in the cases of citrus tristeza (Garnsey et al., 1987; Yoshida, 1996; Yokomi et al., 2017), citrus sudden death (Román et al., 2004; Yamamoto et al., 2011) and Phytophthora spp. gummosis (Wutscher, 1979; Matheron et al., 1998), though for all these scion-rootstock combinations, scion cultivars used should be also disease resistant or tolerant as a premise. For HLB, the situation would be different because there are no citrus cultivars resistant to Las, and the most important varieties are quite sensitive to the bacterium. However, the use of Las-resistant rootstocks may alleviate symptoms and perhaps delay tree decline. Specific experiments should be planned to test this.
On the other hand, rooted cuttings were successfully established only for Microcitrus sp. and E. glauca × Microcitrus sp. hybrids as well as for ‘Tobias’ sweet orange, being 84% of the latter Las-positive while none of the Oceanian citrus hybrid cuttings were Las-infected. The lack of infection in 16% of ‘Tobias’ sweet orange cuttings may be explained by the uneven distribution of Las within the infected cuttings and composite trees (Tatineni et al., 2008; Li et al., 2009; Raiol-Junior et al., 2021a). Lack of Las-infection in rooted cuttings of the Oceanian citrus hybrids remaining alive may be indicative of the inability of the bacterium to accumulate in the phloem of these genotypes and suggested indirectly that erratic detection of Las in Oceanian citrus may result from bacterial movement from the infected rootstock favored under specific physiological conditions.
Aiming to press the canopy of Oceanian citrus to become invaded by viable bacteria from the rootstock, we pruned severely the Oceanian citrus genotypes close to the bud union with the rootstock to promote vigorous scion sprouting and sap movement up with the new flush. Most of the pruned plants produced new growth successfully. Whereas new flushes from ‘Tobias’ sweet orange were all Las-positive with high bacterial titers, those from M. australis hybrid, E. glauca, and three other Oceanian citrus hybrids were all Las-negative further indicating that the bacterium was unable to infect consistently Oceanian citrus species and hybrids previously considered by us as full-resistant to Las. Under greenhouse conditions, Las moves 2.9–3.9 cm day–1 in a citrus host (Raiol-Junior et al., 2021b), preferably toward new flushes and newly developed roots, by following the source:sink driven sap flow (Raiol-Junior et al., 2021a). The presence of Las only in ‘Tobias’ sweet orange shoot flushes after 30 days of sprouting confirmed the viability and the fast movement of Las within the phloem of susceptible plants. On the other hand, the absence of Las in new flushes of M. australis hybrid, E. glauca, Microcitrus sp. hybrid, E. glauca × Citrus sp. Hybrid, and E. glauca × Microcitrus sp. hybrid proved the inability of Las to colonize new tissues of these resistant genotypes.
Overall, these experiments together confirmed that the Oceanian true species, M. warburgiana and E. glauca together with the M. papuana × M. warburgiana hybrid were full-resistant to Las, as their canopies resulted Las-negative in all our thorough tests, D. citri was unable to acquire Las from their young leaves and attempts to force Las flow from the ‘Rangpur’ lime rootstocks to them as scions or interstocks were unsuccessful. Based on Swingle and Reece (1967) all known species of Microcitrus and Eremocitrus emerged from a common ancestor likely resembling M. warburgiana, the Papua/New Guinea species, from which different lines of evolution produced the quite unique, pronounced xerophytic E. glauca and other Microcitrus species adapted to different regions of Australia. In the context of resistance to Las, it should be noticed that the two Microcitrus species from Papua/New Guinea (M. warburgiana and M. papuana) as well as E. glauca are close phylogenetically according to their mitochondrial genomes and this correlates with these three Oceanian species or hybrids being the most consistently full-resistant to Las, which suggests that full-resistance to Las within Oceanian citrus may have arisen within these few related species.
The response of the other four Oceanian citrus hybrids to Las-aggressive challenge inoculation conceived to compel infection or at least Las translocation from the infected rootstock to the Oceanian hybrids scions was trickier to interpret. Las-positive samples were detected not only close to the rootstock-scion bud unions but also at their canopies, at titers high enough to indicate bacterial infection. As these four genotypes are hybrids between full-resistant and either partial-resistance or susceptible parents, it could be claimed that they may have inherited partial-resistant actually. However, D. citri hardly acquired the bacterium from their young leaves and their budwood was unable to transmit Las to ‘Valencia’ sweet orange healthy plants used for biological indexing. This together with the unfeasibility to detect Las in rooted cuttings and in new flushes after hard pruning from some of them suggested that most of the bacterium found in Oceanian citrus hybrid tissues was unviable, likely multiplied at the ‘Rangpur’ lime root systems and moved to sink scion leaves through the phloem vessels under proper physiological conditions and after several years of vascular connections between the heavily Las-infected rootstocks and the Oceanian hybrid scions. Being phloem vessels from Oceanian citrus hybrids incompetent as culture media to support Las survival and multiplication, bacterial cells flowing from the rootstock would progressively decline and die, although unviable bacteria could be detectable by qPCR during months (Josephson et al., 1993). Assessing the cell viability of bacterial pathogens in plant cells is important to understand their interactions and disease progress or resistance responses. There are several methods to study bacterial cell viability, including plating assays, serological techniques, in vivo imaging markers, and flow cytometry, among others (Chitarra and van den Bulk, 2003) but they are mostly applicable to culturable bacteria. Although it has been recently proposed a method to discriminate damaged and viable cells from unculturable bacteria in infected plants based on PCR and plant cell dye staining (Sicard et al., 2020), it is not applicable to phloem vessels-restricted Las. Therefore, distinguishing live and dead bacteria is still unresolved for Las. qPCR provides great sensitivity for Las detection but does not allow to get conclusions on bacterial viability. Another characteristic of qPCR detection for Las is that, in our conditions, Ct values higher than 34 produce variable, inconsistent results (Lopes et al., 2013). Moreover, Ct values between 34 and 36 may result from amplification of non-specific genetic material of other microorganisms also living in the phloem (Bao et al., 2019) as high sequence homology between 16S rDNA from Las and citrus-associated bacteria has already been described (Lin et al., 2008). In our study, only Ct values lower than 34 were considered Las-positive to avoid over-interpretation of Las infection in specific samples on plants that resulted Las-negative repeatedly over years.
Consistent and durable full-resistance in the seven Oceanian citrus genotypes together with the proper characterization of their genomic backgrounds and parentages are essential to be able to properly mapping their genomic regions involved in resistance to Las as well as to use them in breeding programs aimed to generate new citrus-like cultivars yielding immunity or high resistance to HLB. The Las-resistance mechanism in Oceanian citrus species and the nature of Las cells detected in budwood from the Oceanian citrus hybrid genotypes are worth further research.
Data availability statement
The datasets presented in this study can be found in online repositories. The names of the repository/repositories and accession number(s) can be found in the article/Supplementary material.
Author contributions
MA, LP, EG, NW, SL, and LR-J conceptualized and designed the work. MA, LR-J, and EC collected the data. MM generated GBS data. PO performed genetic and phylogenomic analysis. MA, LR-J, EC, NW, EG, PO, SL, JF, and LP contributed to data analysis and interpretation, drafting, and critical revision of the article. All authors contributed to the article and approved the submitted version.
Funding
This study was financed in part by Fundecitrus, grant no. 817526 from the European Union H2020 Innovation Action Program and Project PID2019-104569RB-I00 from the AEI-Spain, the Coordenação de Aperfeiçoamento de Pessoal de Nível Superior – Brazil (CAPES) – Finance Code 001. MA was recipient of a Ph.D. fellowship from CAPES. JF (#312089/2019-8) and NW (#313812/2021-7) were recipients of a fellowship grant from Conselho Nacional de Desenvolvimento Científico e Tecnológico – Brazil (CNPq).
Acknowledgments
We thank André Luís Sanches, Miguel Antonio Mendes, and Sidnei Ferreira Alkimim for their excellent help with grafting for propagation, Las challenge inoculations, and plant maintenance; Fernanda Benedito for assistance with DNA extraction; Juan Cifuentes-Arenas for suggestions and critical comments; Tamiris Garcia and Afranio da Silva Aquino for photograph assistance; and Embrapa Cassava and Fruits for providing some genetic resources.
Conflict of interest
The authors declare that the research was conducted in the absence of any commercial or financial relationships that could be construed as a potential conflict of interest.
Publisher’s note
All claims expressed in this article are solely those of the authors and do not necessarily represent those of their affiliated organizations, or those of the publisher, the editors and the reviewers. Any product that may be evaluated in this article, or claim that may be made by its manufacturer, is not guaranteed or endorsed by the publisher.
Supplementary material
The Supplementary Material for this article can be found online at: https://www.frontiersin.org/articles/10.3389/fpls.2022.1009350/full#supplementary-material
Footnotes
- ^ https://phytozome-next.jgi.doe.gov/info/Cclementina_v1_0
- ^ https://darwin.cirad.fr/
- ^ Eduardo, W. I., Silva, A. C., Volpe, H. X. L., Alquézar, B., Peña, L., and Miranda, M. P. (2022). Push-pull and kill strategy for Diaphorina citri control in citrus orchards. Submitted.
References
Ahmed, D., Comte, A., Curk, F., Costantino, G., Luro, F., Dereeper, A., et al. (2019). Genotyping by sequencing can reveal the complex mosaic genomes in gene pools resulting from reticulate evolution: A case study in diploid and polyploid citrus. Ann. Bot. 123, 1231–1251. doi: 10.1093/aob/mcz029
Albrecht, U., Hall, D. G., and Bowman, K. D. (2014). Transmission efficiency of Candidatus Liberibacter asiaticus and progression of huanglongbing disease in graft- and psyllid-inoculated Citrus. Hortscience 49, 367–377. doi: 10.21273/HORTSCI.49.3.367
Alquézar, B., Carmona, L., Bennici, S., Miranda, M. P., Bassanezi, R. B., and Peña, L. (2022). Cultural management of huanglongbing: Current status and ongoing research. Phytopathology 112, 11–25. doi: 10.1094/PHYTO-08-21-0358-IA
Alquézar, B., Carmona, L., Bennici, S., and Peña, L. (2021). Engineering of citrus to obtain huanglongbing resistance. Curr. Opin. Biotechnol. 70, 196–203. doi: 10.1016/j.copbio.2021.06.003
Alves, M. N., Cifuentes-Arenas, J. C., Raiol-Junior, L. L., Ferro, J. A., and Peña, L. (2021a). Early population dynamics of “Candidatus Liberibacter asiaticus” in susceptible and resistant genotypes after inoculation with infected Diaphorina citri feeding on young shoots. Front. Microbiol. 12:683923. doi: 10.3389/fmicb.2021.683923
Alves, M. N., Lopes, S. A., Raiol-Junior, L. L., Wulff, N. A., Girardi, E. A., Ollitrault, P., et al. (2021b). Resistance to ‘Candidatus Liberibacter asiaticus’, the Huanglongbing associated bacterium, in sexually and/or graft-compatible Citrus relatives. Front. Plant Sci. 11:617664. doi: 10.3389/fpls.2020.617664
Bao, M., Zheng, Z., Sun, X., Chen, J., and Deng, X. (2019). Enhancing PCR capacity to detect “Candidatus Liberibacter asiaticus” utilizing whole genome sequence information. Plant Dis. 2020, 527–532. doi: 10.1094/PDIS-05-19-0931-RE
Bassanezi, R. B., Lopes, S. A., Miranda, M. P., Wulff, N. A., Volpe, H. X. L., and Ayres, A. J. (2020). Overview of citrus huanglongbing spread and management strategies in Brazil. Trop. Plant Pathol. 45, 251–264. doi: 10.1007/s40858-020-00343-y
Bassanezi, R. B., Montesino, L. H., and Stuchi, E. S. (2009). Effects of huanglongbing on fruit quality of sweet orange cultivars in Brazil. Eur. J. Plant Pathol. 125, 565–572. doi: 10.1007/s10658-009-9506-3
Bausher, M. G., Singh, N. D., Lee, S.-B., Jansen, R. K., and Daniell, H. (2006). The complete chloroplast genome sequence of Citrus sinensis (L.) Osbeck var “Ridge Pineapple”: Organization and phylogenetic relationships to other angiosperms. BMC Plant Biol. 6:21. doi: 10.1186/1471-2229-6-21
Bayer, R. J., Mabberley, D. J., Morton, C., Miller, C. H., Sharma, I. K., Pfeil, B. E., et al. (2009). A molecular phylogeny of the orange subfamily (Rutaceae: Aurantioideae) using nine cpDNA sequences. Am. J. Bot. 96, 668–685. doi: 10.3732/ajb.0800341
Bitters, W. P. (1986). Citrus Rootstocks: Their Characters and Reactions. Riverside, CA: UC Riverside Science Library. Available online at: https://citrusvariety.ucr.edu/links/documents/Bitters.pdf (accessed on May 16, 2022).
Bitters, W. P., Brusca, J. A., and Cole, D. A. (1964). The search for new citrus rootstocks. Citrograph 49, 443–448.
Bitters, W. P., Cole, D. A., and McCarty, C. D. (1977). Citrus relatives are not irrelevant as dwarfing stocks or interstocks for citrus. Proc. Int. Soc. Citriculture 2, 561–567.
Bové, J. M. (2006). Huanglongbing: A destructive, newly-emerging, century-old disease of citrus. J. Plant Pathol. 88, 7–37. doi: 10.4454/jpp.v88i1.828
Capoor, S., Rao, D., and Viswanath, S. (1967). Diaphorina citri Kuway., a vector of the greening disease of citrus in India. Indian J. Agric. Sci. 37, 572–579.
Chitarra, L. G., and van den Bulk, R. W. (2003). The application of flow cytometry and fluorescent probe technology for detection and assessment of viability of plant pathogenic bacteria. Eur. J. Plant Pathol. 109, 407–417. doi: 10.1023/A:1024275610233
Cifuentes-Arenas, J. C., Beattie, G. A. C., Peña, L., and Lopes, S. A. (2019). Murraya paniculata and Swinglea glutinosa as short-term transient hosts of ‘Candidatus Liberibacter asiaticus’ and implications for the spread of huanglongbing. Phytopatology 109, 2064–2073. doi: 10.1094/PHYTO-06-19-0216-R
Cifuentes-Arenas, J. C., de Goes, A., de Miranda, M. P., Beattie, G. A. C., and Lopes, S. A. (2018). Citrus flush shoot ontogeny modulates biotic potential of Diaphorina citri. PLoS One 13:e0190563. doi: 10.1371/journal.pone.0190563
Desjardins, P., and Conklin, D. (2010). NanoDrop microvolume quantitation of nucleic Acids. J. Vis. Exp. 45:e2565. doi: 10.3791/2565
Eduardo, W. I., Miranda, M. P., Volpe, H. X. L., Garcia, R. B., Girardi, E. A., Alquezar, B., et al. (2022). Resistance of True Citrus species to Diaphorina citri. Pest Manag. Sci. doi: 10.1002/ps.7098
Elshire, R. J., Glaubitz, J. C., Sun, Q., Poland, J. A., Kawamoto, K., Buckler, E. S., et al. (2011). A Robust Simple Genotyping-by-Sequencing (GBS) Approach for High Diversity Species. PLoS One 6:e19379. doi: 10.1371/journal.pone.0019379
Felisberto, P. A. C., Girardi, E. A., Peña, L., Felisberto, G., Beattie, G. A. C., and Lopes, S. A. (2019). Unsuitability of indigenous South American rutaceae as potential hosts of Diaphorina citri. PestManag. Sci. 75, 1911–1920. doi: 10.1002/ps.5304
Folimonova, S. Y., Robertson, C. J., Gamsey, S. M., and Dawson, W. O. (2009). Examination of the responses of different genotypes of citrus to huanglongbing (citrus greening) under different conditions. Phytopathology 23, 1346–1354. doi: 10.1094/PHYTO-99-12-1346
Fundecitrus. (2021). Doenças: Greening/HLB. Available online at: https://www.fundecitrus.com.br/levantamentos/greening (Acsessed on Mar 20, 2021)
Garnsey, S. M., Barret, H. C., and Hutchison, D. J. (1987). Identification of citrus tristeza virus resistance in citrus relatives and its potential applications. Phylophylaclica 19, 187–191.
Gottwald, T. R., da Graça, J. V., and Bassanezi, R. B. (2007). Citrus huanglongbing: The pathogen and its impact. Plant Health Prog. 8:31. doi: 10.1094/PHP-2007-0906-01-RV
Hall, D. G., George, J., and Lapointe, S. L. (2015). Further investigations on colonization of Poncirus trifoliata by the Asian citrus psyllid. Crop. Prot. 72, 112–118. doi: 10.1016/j.cropro.2015.03.010
Herten, K., Hestand, M. S., Vermeesch, J. R., and Van Houdt, J. K. (2015). GBSX: A toolkit for experimental design and demultiplexing genotyping by sequencing experiments. BMC Bioinform. 16:73. doi: 10.1186/s12859-015-0514-3
Josephson, K. L., Gerba, C. P., and Pepper, I. L. (1993). Polymerase chain reaction detection of nonviable bacterial pathogens. Appl. Environ. Microbiol. 59, 3513–3515.
Krzywinski, M., Schein, J., Birol, I., Connors, J., Gascoyne, R., Horsman, D., et al. (2009). Circos: An Information Aesthetic for Comparative Genomics. Genome Res. 19, 1639–1645. doi: 10.1101/gr.092759.109
Li, W., Levy, L., and Hartung, J. S. (2009). Quantitative distribution of “Candidatus Liberibacter asiaticus” in citrus plants with citrus huanglongbing. Phytopathology 99, 139–144. doi: 10.1094/PHYTO-99-2-0139
Li, W., Li, W., Hartung, J. S., and Levy, L. (2006). Quantitative real-time PCR for detection and identification of Candidatus Liberibacter species associated with citrus huanglongbing. J. Microbiol. Method. 66, 104–115. doi: 10.1016/j.mimet.2005.10.018
Lin, H., Doddapaneni, H., Bai, X., Yao, J., Zhao, X., and Civerolo, E. (2008). Acquisition of uncharacterized sequences from Candidatus Liberibacter, an unculturable bacterium, using an improved genomic walking method. Mol. Cell.Probes 22, 30–37. doi: 10.1016/j.mcp.2007.06.006
Lopes, S. A., and Cifuentes-Arenas, J. C. (2021). A protocol for successful transmission of ‘Candidatus Liberibacter asiaticus’ from citrus to citrus using Diaphorina citri. Phytopathology 111, 2367–2374.
Lopes, S. A., Frare, G. F., Bertolni, E., Cambra, M., Fernandes, N. G., Ayres, A. J., et al. (2009). Liberibacters associated with citrus huanglongbing in Brazil: ‘Candidatus Liberibacter asiaticus’ is heat tolerant, ‘Ca. L. americanus’ is heat sensitive. Plant Dis. 3, 257–262. doi: 10.1094/PDIS-93-3-0257
Lopes, S. A., Luiz, F. Q. B. Q., Martins, E. C., Fassini, C. G., Barbosa, J. C., and Beattie, G. A. C. (2013). Candidatus Liberibacter asiaticus titers in citrus and acquisition rates by Diaphorina citri are decreased by higher temperature. Plant Dis. 97, 1563–1570. doi: 10.1094/PDIS-11-12-1031-RE
Manjunath, K. L., Halbert, S. E., Ramadugu, C., Weeb, S., and Lee, R. F. (2008). Detection of ‘Candidatus Liberibacter asiaticus’ in Diaphorina citri and its importance in the management of Citrus huanglongbing in Florida. Phytopathology 98, 387–396. doi: 10.1094/PHYTO-98-4-0387
Martin, M. (2011). Cutadapt removes adapter sequences from high-throughput sequencing reads. EMBnet. J. 17, 10–12. doi: 10.14806/ej.17.1.200
Matheron, M. E., Wright, G. C., and Porchas, M. (1998). Resistance to Phytophthora citrophthora and P. parasitica and nursery characteristics of several citrus rootstocks. Plant Dis. 82, 1217–1225. doi: 10.1094/pdis.1998.82.11.1217
Miles, G. P., Stover, E., Ramadugu, C., Keremane, M. L., and Lee, R. F. (2017). Apparent tolerance to huanglongbing in citrus and citrus-related germplasm. HortScience 52, 31–39. doi: 10.21273/HORTSCI11374-16
Moreira, R. R., Machado, F. J., Lanza, F. E., Trombin, V. G., Bassanezi, R. B., Miranda, M. P., et al. (2022). Impact of diseases and pests on premature fruit drop in sweet orange orchards in São Paulo state citrus belt, Brazil. Pest. Manag. Sci. 78, 2643-2656. doi: 10.1002/ps.6894
Murray, M. G., and Thompson, W. F. (1980). Rapid isolation of high molecular weight plant DNA. Nucleic Acids Res. 8, 4321–4325. doi: 10.1093/nar/8.19.4321
Oueslati, A., Salhi-Hannachi, A., Luro, F., Vignes, H., Mournet, P., and Ollitrault, P. (2017). Genotyping by sequencing reveals the interspecific C. maxima / C. reticulata admixture along the genomes of modern citrus varieties of mandarins, tangors, tangelos, orangelos and grapefruits. PloS One 12:e0185618. doi: 10.1371/journal.pone.0185618
Raiol-Junior, L. L., Cifuentes-Arenas, J. C., Carvalho, E. V., Girardi, E. A., and Lopes, S. A. (2021a). Evidence that ‘Candidatus Liberibacter asiaticus’ moves predominantly towards new tissue growth in citrus plants. Plant Dis. 105, 34–42. doi: 10.1094/PDIS-01-20-0158-RE
Raiol-Junior, L. L., Cifuentes-Arenas, J. C., Cunniffe, N. J., Turgeon, R., and Lopes, S. A. (2021b). Modelling ‘Candidatus Liberibacter asiaticus’ movement within citrus plants. Phytopathology 111, 1711–1719. doi: 10.1094/PHYTO-12-20-0559-R
Ramadugu, C., Keremane, M. L., Halbert, S. E., Duan, Y. P., Roose, M. L., Stover, E., et al. (2016). Long-term field evaluation reveals huanglongbing resistance in Citrus relatives. Plant Dis 9, 1858–1869. doi: 10.1094/PDIS-03-16-0271-RE
Rasche, H., and Hiltemann, S. (2020). Galactic Circos: User-Friendly Circos Plots within the Galaxy Platform. GigaScience 9:giaa065. doi: 10.1093/gigascience/giaa065
Richardson, M. L., and Hall, D. G. (2013). Resistance of Poncirus and Citrus × Poncirus germplasm to the Asian Citrus Psyllid. Crop Sci. 53, 183–188. doi: 10.2135/cropsci2012.02.0091
Román, M. P., Cambra, M., Juárez, J., Moreno, P., Duran-Vila, N., Tanaka, F. A. O., et al. (2004). Sudden death of citrus in Brazil: A graft-transmissible bud union disease. Plant Dis. 88, 453–467. doi: 10.1094/PDIS.2004.88.5.453
Sicard, A., Castillo, A. I., Voeltz, M., Chen, H., Zeilinger, A. R., De La Fuente, L., et al. (2020). Inference of bacterial pathogen instantaneous population growth dynamics. MPMI 33, 402–411. doi: 10.1094/MPMI-10-19-0274-TA
Sonah, H., Bastien, M., Iquira, E., Tardivel, A., Légaré, G., and Boyle, B. (2013). An Improved Genotyping by Sequencing (GBS) Approach Offering Increased Versatility and Efficiency of SNP Discovery and Genotyping. PLoS One 8:e54603. doi: 10.1371/journal.pone.0054603
Swingle, W. T., and Reece, C. (1967). “The botany of Citrus and its wild relatives,” in The Citrus Industry, eds W. Reuther, H. J. Webber, and L. D. Batchelor (Berkeley and Los Angeles: University of California Press), 191–430.
Tatineni, S., Sagaram, U. S., Gowda, S., Robertson, C. J., Dawson, W. O., Iwanami, T., et al. (2008). In planta distribution of ‘Candidatus Liberibacter asiaticus’ as revealed by polymerase chain reaction (PCR) and real-time PCR. Phytopathology 98, 592–599. doi: 10.1094/PHYTO-98-5-0592
Teixeira, D. C., Danet, J. L., Eveillard, S., Martins, E. C., Junior, W. C. J., Yamamoto, P. T., et al. (2005). Citrus huanglongbing in São Paulo State, Brazil: PCR detection of the ‘Candidatus’ Liberibacter species associated with the disease. Mol. Cell. Probes 19, 173–179. doi: 10.1016/j.mcp.2004.11.002
Tomaseto, A. F., Marques, R. N., Fereres, A., Zanardi, O. A., Volpe, H. X. L., Alquézar, B., et al. (2019). Orange jasmine as a trap crop to control Diaphorina citri. Sci. Rep. 9:2070. doi: 10.1038/s41598-019-38597-5
Van der Auwera, G. A., and O’Connor, B. D. (2020). Genomics in the Cloud: Using Docker, GATK, and WDL in Terra, 1st Edn. Sebastopol, CA: O’Reilly Media.
Wang, X., Xu, Y., Zhang, S., Cao, L., Huang, Y., Cheng, J., et al. (2017). Genomic analyses of primitive, wild and cultivated citrus provide insights into asexual reproduction. Nat. Genet. 49, 765–772. doi: 10.1038/ng.3839
Westbrook, C. J., Hall, D. G., Stover, E., Duan, Y. P., and Lee, R. F. (2011). Colonization of Citrus and Citrus-related germplasm by Diaphorina citri (Hemiptera: Psyllidae). Hortscience 46, 997–1005. doi: 10.21273/HORTSCI.46.7.997
Winters, H. F. (1976). Microcitrus papuana, a new species from Papua New Guinea (Rutaceae). Baileya 20, 19–24. doi: 10.1016/j.ijantimicag.2010.05.008
Wu, G. A., Prochnik, S., Jenkins, J., Salse, J., Hellsten, U., Murat, F., et al. (2014). Sequencing of diverse mandarin, pummelo and orange genomes reveals complex history of admixture during citrus domestication. Nat. Biotechnol. 32, 656–662. doi: 10.1038/nbt.2906
Wu, G. A., Terol, J., Ibanez, V., López-García, A., Pérez-Román, E., Borredá, C., et al. (2018). Genomics of the origin and evolution of Citrus. Nature 554, 311–316. doi: 10.1038/nature25447
Xu, C. F., Xia, Y. H., Li, K. B., and Ke, C. (1988). Further study of the transmission of Citrus huanglungbin by a psyllid, Diaphorina citri Kuwayama. Int. Organ. Citrus Virol. Conf. Proc. 10, 1957–2010.
Yamamoto, P. T., Bassanezi, R. B., Wulff, N. A., Santos, M. A., Sanches, A. L., Toloy, R. S., et al. (2011). Citrus sudden death is transmitted by graft-inoculation and natural transmission is prevented by individual insect-proof cages. Plant Dis. 95, 104–112. doi: 10.1094/PDIS-04-10-0307
Yokomi, R. K., Selvaraj, V., Maheshwary, Y., Sponari, M., Giampetruzzi, A., Chiumenti, M., et al. (2017). Identification and characterization of Citrus tristeza virus isolates breaking resistance in trifoliate orange in California. Phytopathology 107, 901–908. doi: 10.1094/PHYTO-01-17-0007-R
Yoshida, T. (1996). Graft compatibility of Citrus with plants in the Aurantioideae and their susceptibility to citrus tristeza virus. Plant Dis. 80, 414–417. doi: 10.1094/PD-80-0414
Keywords: greening, HLB, Rutaceae, Aurantioideae, Microcitrus, Eremocitrus, citrus breeding
Citation: Alves MN, Raiol-Junior LL, Girardi EA, Miranda M, Wulff NA, Carvalho EV, Lopes SA, Ferro JA, Ollitrault P and Peña L (2022) Insight into resistance to ‘Candidatus Liberibacter asiaticus,’ associated with Huanglongbing, in Oceanian citrus genotypes. Front. Plant Sci. 13:1009350. doi: 10.3389/fpls.2022.1009350
Received: 01 August 2022; Accepted: 22 August 2022;
Published: 09 September 2022.
Edited by:
Agata Gadaleta, University of Bari Aldo Moro, ItalyReviewed by:
Freddy Ibanez-Carrasco, Texas A&M AgriLife Research and Extension Center at Weslaco, United StatesYasir Iftikhar, University of Sargodha, Pakistan
Copyright © 2022 Alves, Raiol-Junior, Girardi, Miranda, Wulff, Carvalho, Lopes, Ferro, Ollitrault and Peña. This is an open-access article distributed under the terms of the Creative Commons Attribution License (CC BY). The use, distribution or reproduction in other forums is permitted, provided the original author(s) and the copyright owner(s) are credited and that the original publication in this journal is cited, in accordance with accepted academic practice. No use, distribution or reproduction is permitted which does not comply with these terms.
*Correspondence: Leandro Peña, bHBlbnlhQGlibWNwLnVwdi5lcw==