- 1School of Food Science and Engineering, Hainan University, Haikou, China
- 2Suzhou Key Laboratory of Medical Biotechnology, Suzhou Vocational Health College, Suzhou, China
The role of Sly-miR171d on tomato fruit chilling injury (CI) was investigated. The results showed that silencing the endogenous Sly-miR171d effectively delayed the increase of CI and electrolyte leakage (EL) in tomato fruit, and maintained fruit firmness and quality. After low temperature storage, the expression of target gene GRAS24 increased in STTM-miR171d tomato fruit, the level of GA3 anabolism and the expression of CBF1, an important regulator of cold resistance, both increased in STTM-miR171d tomato fruit, indicated that silencing the Sly-miR171d can improve the resistance ability of postharvest tomato fruit to chilling tolerance.
Introduction
Low temperature storage technology can reduce respiratory metabolism and is widely used in prolonging fruit storage period (Duan et al., 2022). However, fruit from subtropical and tropical regions stored below the temperature of 12°C are susceptible to the risk of chilling injury (CI), resulting in lower product quality and lower sales (Ding et al., 2021). Tomato fruit is the major contributors of minerals, dietary fiber, sugars, essential amino acids, and vitamins in the daily diet (Bai et al., 2020). It widely distributes in tropical and subtropical regions. However, tomato fruit stored at low temperature below 10°C (green ripening stage) and 5°C (red ripening stage) for a long time are susceptible to CI. During storage at low temperatures, incipient CI in tomato is not generally apparent. After returning the fruit to room temperature, CI is very obvious, such as failure to ripen properly, indentation of the surface or damage, discoloration, decay and increased water loss (Tian et al., 2022). CI sharply led to a decline in tomato quality and consumer acceptance, which ultimately led to huge economic losses (Luengwilai et al., 2012). Hence, controlling the postharvest CI of fruit is essential to ensure good quality and minimize losses.
MicroRNAs (miRNAs) is a class of endogenous non-coding small RNAs with a length of about 18–25 nt (Bartel, 2004). The miRNAs binds to mRNA molecules through base pairing, resulting in translation inhibition or cleavage of its target mRNA (Barciszewska-Pacak et al., 2015). Negative regulation of target genes by miRNAs is critical for coordinating the plant responses to cold stress (Zhao et al., 2022b). In recent years, miRNAs has attracted more and more attention in their important role in the post-transcriptional or translational regulation of gene expression and plant stress tolerance (Sunkar et al., 2007). In rice (Oryza sativa), overexpression of miR156 reduced the expression of OsWRKY71, resulting in enhanced expression of MYB2 and MYB3R-2, thereby enhanced cold tolerance (Zhou and Tang, 2019). In Switchgrass (Panicum virgatum), overexpression of miR393 enhanced low temperature tolerance by regulating the auxin signaling pathway in switchgrass (Liu et al., 2017a). In tomato, Sha-miR319d enhances the cold tolerance by reducing the expression of GAMYB-like1 and further changing ROS, heat and cold signal transduction (Shi et al., 2019). In citrus (Citrus reticulata), miR171 plays a key role in the adaptation to long-term B toxicity by regulating the expression of SCARECROW (Huang et al., 2019). In apples (Malus pumila), miR171i and its target gene SCARECROW-LIKE PROTEINS26.1 improves drought stress tolerance by regulating antioxidant gene expression and ascorbic acid metabolism (Wang et al., 2020a). The miR171 regulates members of the GRAS gene family (Huang et al., 2017). GRAS proteins are widely taken part in signal transduction, growth and development, and stress in plants (Peng et al., 1997). In rice, OsGRAS23 increases the transcription of genes related to stress response, especially the protein protection and antioxidant genes, and positively regulates the drought tolerance (Xu et al., 2015). In tomato, overexpression of SlGRAS40 enhances drought resistance and salt resistance by regulating gibberellin (GAs) and auxin homeostasis (Liu et al., 2017b). The down-regulation of SlGRAS10 improves the tolerance of abiotic stresses by regulating complex stress-induced gene expression in physiological modifications (Habib et al., 2021) and overexpression of SlGRAS4 induces the expression of SlCBF and enhances the low temperature tolerance of tomato (Liu et al., 2020). SlGRAS24 is the target gene of miR171d and involved in GAs signal transduction (Huang et al., 2017). Plant-specific GRAS protein family contains important regulatory parts of GA signaling that coordinately participate in plant growth and development (Shan et al., 2012). GRAS proteins have a subgroup that is a GA signaling repressor whose amino acid sequence in the N-terminal region is identical and is therefore called DELLA proteins (Chen et al., 2019). GAs induces the degradation of DELLA repressor protein and controls responses to cold stress (Lantzouni et al., 2020).
To further understand the function of miR171d in tomato, we obtained the up-regulated and down-regulated transgenic lines of Sly-miR171d by constructing the overexpression vector and the short tandem target mimic (STTM) vector. The results revealed the mechanism of Sly-miR171d in promoting cold resistance in tomato through GAs signal and provided help for cultivating stress tolerant germplasm.
Materials and methods
Plant expression vector construction and transformation
Silencing of mature Sly-miR171d was performed with STTM according to the method developed by Yan et al. (2012). The overexpression vector of Sly-miR171d was constructed according to the methods of Zhao et al. (2022a). After sequencing confirmation, all the correct constructs were transformed to Micro-Tom via Agrobacterium-mediated method. All primers and sequences are listed in Supplementary 1, 2.
Sample treatment
Tomato (Solanum lycopersicum cv. Micro-Tom) plant materials used in this study were all homozygous T3 lines of Micro-Tom (MT). The plant growth chamber conditions were as follows: the ambient temperature was maintained at 23 ± 2°C; 16 h light/8 h dark alternate lighting; 60% humidity. Wild-type (WT) Micro-Tom tomato seeds were purchased with Nanjing Fengshuo Horticultural Co., Ltd. All fruit were selected with the same shape and size, without defect and disease. The fruit surface was soaked in 2% (V/V) sodium hypochlorite for 60 s to disinfect, washer with steam water, and dried. Green and red ripening tomato fruit (STTM-miR171d, WT, and miR171d-OE lines) with the same size, maturity and no mechanical damage were selected. The green ripening fruit were stored at 9°C for 30 days and randomly selected every 5 days. The shelf life was simulated at room temperature (25°C storage). Red ripening fruit were stored at 4°C for 25 days and randomly detected every 5 days. Each experiment was repeated two times.
Chilling injury index
Chilling injury index was divided into five grades: 1 = no CI; 2 = slight damage (pitting covered <5% of fruit surface); 3 = moderate damage (pitting covers less than 25%, but >5% of the surface); 4 = severe damage (pitting coverage less than 50% but >25% of the surface); 5 = very severe damage (depression covers 50% of the surface). The CI was expressed as
Each group selected 12 tomato fruit to evaluate the degree of CI.
Electrolyte leakage, firmness, and total soluble solids of tomato fruit
Electrolyte leakage (EL) was detected according to the method of Li et al. (2016). FE30 conductivity meter (Mettler Toledo Instrument Co., Ltd., China) was used to measure the EL of fruit. Select 10 circular pulp tissues (10 mm straight × 4 mm thick in diameter) from the equatorial region of five tomato fruit and measure P1 by shaking in 30 ml of distilled water for 20 min. P2 is then measured after the sample has been boiled for 15 min. where P1 is the initial conductivity value and P2 is the final conductivity value.
Fruit firmness was detected according to the method of El-Mogy et al. (2018). Measuring fruit firmness with a texture analyzer (TA; XT Plus, Stable Micro Systems, United Kingdom) by a 2 mm flat probe at 3 points in the equatorial region, with hardness values of three fruit repeated at a time.
Total soluble solids (TSS) was detected according to the method of Shan et al. (2022). TSS in tomato pulp was determined at room temperature using a hand-held refractometer (WY032R; Chengdu Optical Instrument Factory, China). The results are expressed as %.
Determination of GA3 content
The tomato samples were ground to homogenate and determined according to plant GA3 ELISA kit (Jiangsu Meibiao Biotechnology Co., Ltd., China).
RNA isolation and quantitative real-time PCR analysis
The kits (Tiangen, Beijing, China) were used for total RNA extraction, first-strand cDNA synthesis and real-time quantitative PCR. The expression of Sly-miR171d, SlGRAS24, COR, CBF1, GA20ox1, GA3ox1, GA2ox1, and GAI were calculated by the 2–ΔΔCt method. The internal reference gene is Actin. The qRT-PCR primers are supplemented in Supplementary Table 1. Two grams of fruit pulp tissue were taken from three tomato fruit, ground in liquid nitrogen, and RNA was extracted according to the kit.
Statistical analysis
Values were expressed as means ± standard deviation (SD). Data analysis using SPSS 20.0 (SPSS, Chicago, IL, United States). A one-way analysis of variance (ANOVA) was performed to test significant differences among the means (P < 0.05).
Results
Characterization of STTM-miR171d and miR171d-OE fruit
Stable transformation of tomato plant STTM-miR171d and miR171d-OE lines were produced by Agrobacterium-mediated method. A total of 25 independent tomato transgenic lines (16 STTM-miR171d and 9 miR171d-OE plants) were detected in the T1 generation. After PCR amplification with hygromycin (STTM-miR171d lines) and kanamycin (miR171d-OE lines), cell lines with target bands were detected by agarose gel electrophoresis (Supplementary 1). This indicated that the vector had been successfully integrated into the tomato chromosome. Expression analysis of miR171d-OE and STTM-miR171d in T3 transgenic plants and identify them as transgenic fruit, and selected as experimental materials. Green tomato fruit was harvested at 35 dpa and red tomato fruit was harvested at 45 dpa.
Chilling injury index
Silencing of Sly-miR171d with STTM delayed CI symptoms in fruit compared to WT (Figures 1A, 2A). The red miR171d-OE tomato showed obvious epidermal atrophy on the 20 days under the condition of 4°C. With the storage time Prolonged, the phenomenon of skin atrophy deepened. WT tomato fruit showed CI symptoms after 20 days, and the symptoms worsened at 25 days, while STTM-miR171d fruit did not show obvious abnormalities on the surface of fruit after 25 days of storage. During the green ripening stage, the miR171d-OE tomato fruit were stored at 9°C for 30 days, and the skin wrinkles appeared after the 12 days of the shelf life. WT tomato fruit and STTM-miR171d fruit did not show obvious symptoms of CI.
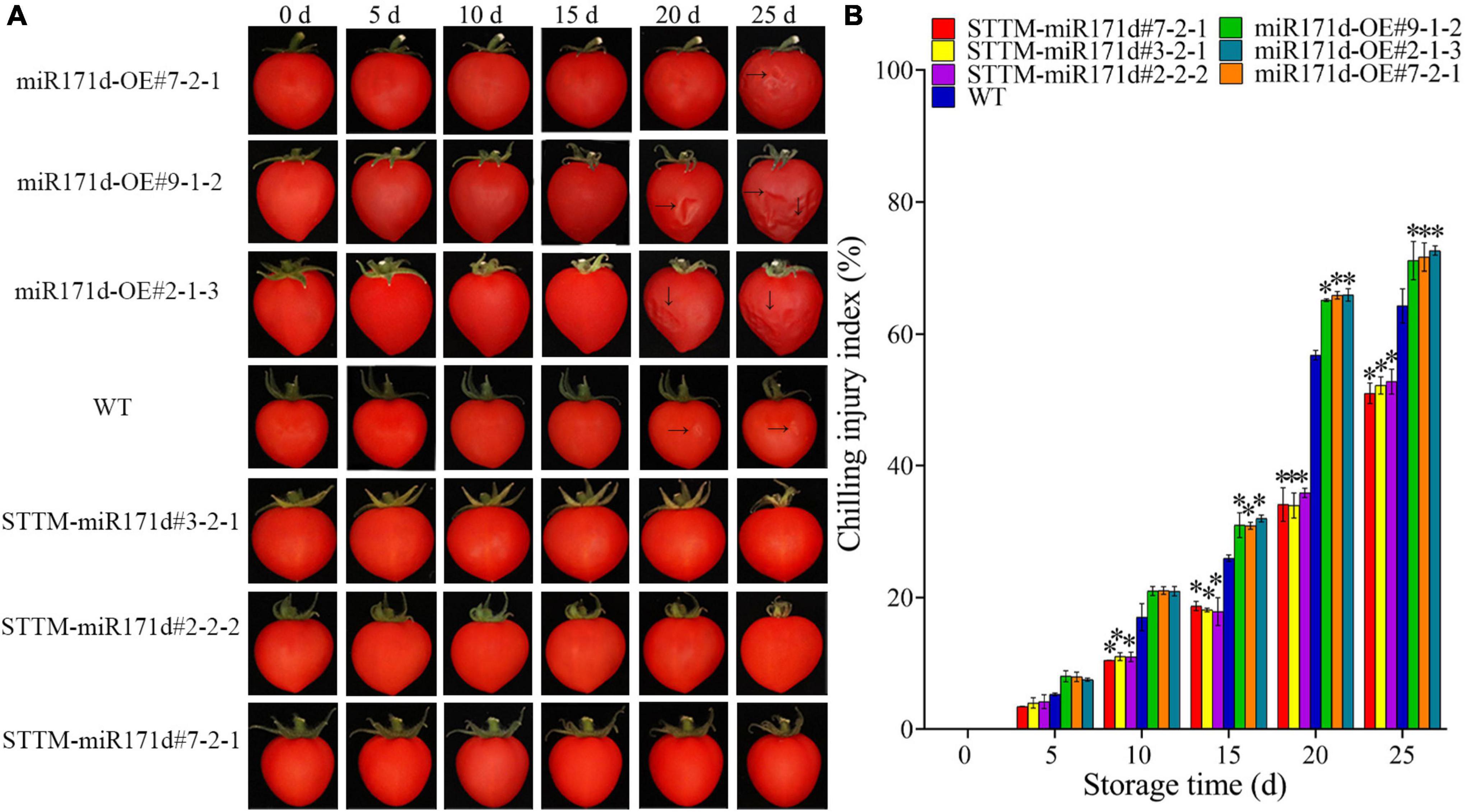
Figure 1. Surface CI symptoms and CI index of red ripe tomato fruit. (A) Symptoms of CI on the surface of tomato fruit. (B) CI index. The error bar represents the independent repeat SDs (n = 3). The asterisk indicates that the data difference with P < 0.05 is statistically significant.
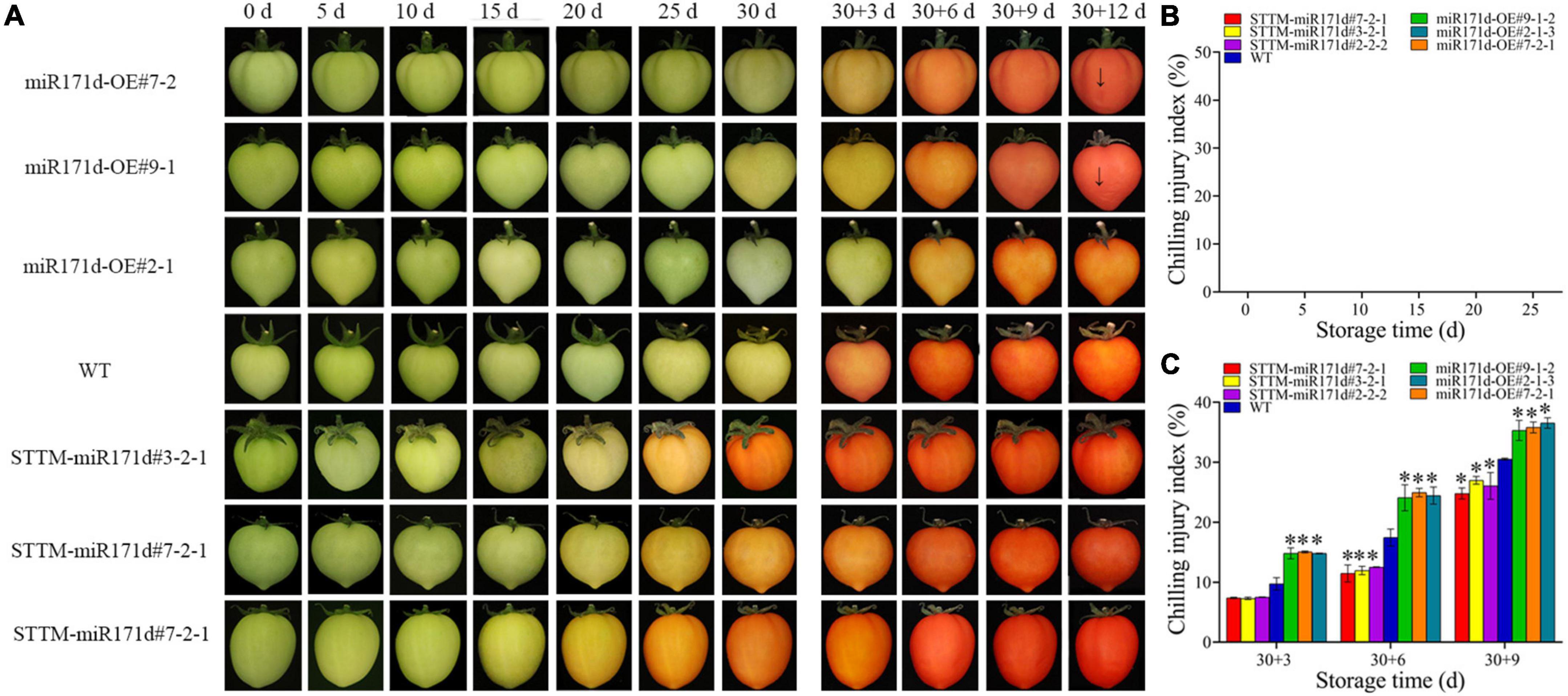
Figure 2. Surface CI symptoms and CI index of green ripe tomato fruit. (A) Symptoms of CI on the surface of tomato fruit. (B,C) CI index. The error bar represents the independent repeat SDs (n = 3). The asterisk indicates that the data difference with P < 0.05 is statistically significant.
In the red ripening stage, at 4°C for 25 days, the CI of miR171d-OE, STTM-miR171d, and WT tomato fruit were 71.80, 52.02, and 64.30%. The CI of WT tomato fruit was 12.28% higher than that of STTM-miR171d (Figure 1B). The CI of miR171d-OE, STTM-miR171d, and WT tomato fruit were 35.89, 25.96, and 30.53%, respectively, when green tomato fruit were refrigerated and stored at 25°C for 9 days. The CI of WT tomato fruit was 4.57% higher than that of STTM-miR171d (Figure 2C).
Electrolyte leakage, firmness, total soluble solids, and GA3 content of tomato fruit
The accumulation of EL in red and green fruit increased under low temperature stress. At 4°C for 25 days, The EL of miR171d-OE tomato red fruit was 4.18 higher than WT, and that of STTM-miR171d tomato was 7.09 lower than WT. After the green tomato fruit was refrigerated and stored at 9°C, the EL content of STTM-miR171d fruit was 4.83 lower than WT. The EL content of miR171d-OE fruit was 5.23 higher than WT (Figure 3).
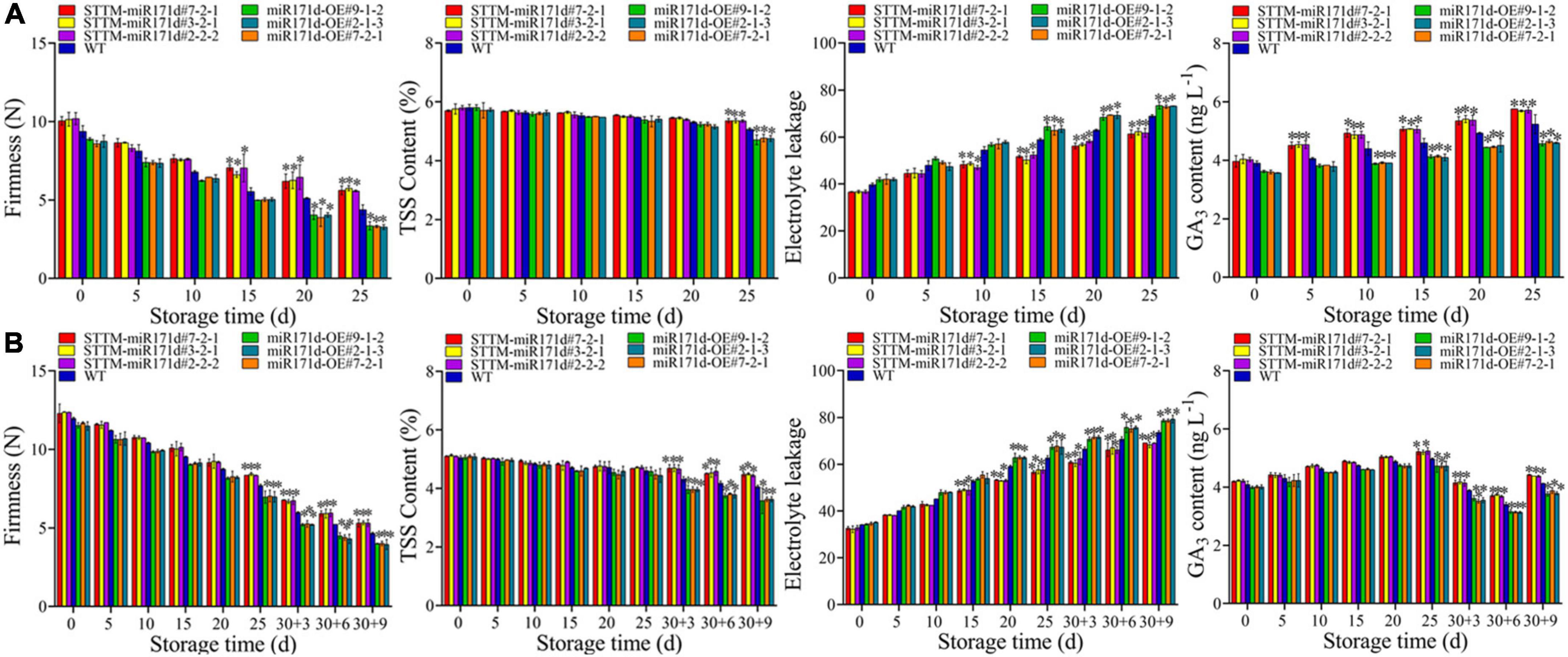
Figure 3. Electrolyte leakage, Firmness, TSS, and GA3 content of tomato fruit in red ripening stage (A) and green ripening stage (B). The error bar represents the independent repeat SDs (n = 3). The asterisk indicates that the data difference with P < 0.05 is statistically significant.
In tomato fruit, firmness decreased gradually with storage time. After 25 days of low temperature stress, the firmness of red STTM-miR171d tomato fruit decreased to 5.65 N. The hardness of miR171d-OE decreased to 3.32 N and that of WT was 4.38 N. The firmness of STTM-miR171d fruit was 1.29 times that of WT. After storage at 25°C for 9 days at green ripening stage, fruit hardness of STTM-miR171d, miR171d-OE, and WT were 5.32, 3.99, and 4.66 N (Figure 3).
The TSS in tomato fruit gradually decreased. The red fruit is stored for 25 days, miR171d-OE fruit decreased from 5.75 to 4.74%, and WT fruit decreased from 5.81 to 5.07%, while STTM-miR171d fruit still maintained 5.36% at 25 days. The TSS content of green tomato fruit showed significant differences after the shelf life, miR171d-OE fruit decreased from 5.08 to 3.61%, WT fruit decreased from 5.05 to 4.06%, while STTM-miR171d fruit remained at 4.48% at the end (Figure 3).
During low temperatures, the GA3 content of red tomato fruit showed an overall upward trend, but the content of miR171d-OE was lower than that of STTM-miR171d. After 25 days of storage, the contents of STTM-miR171d, WT, and miR171d-OE increased to 5.73, 5.24, and 4.61 ng L–1. The GA3 content of green tomato fruit showed an increasing trend throughout the storage period and gradually decreased during the shelf life. During the shelf life, the fruit content of STTM-miR171d decreased from 5.23 to 4.40 ng L–1, and the fruit content of WT decreased from 4.97 to 4.13 ng L–1. The fruit content of miR171d-OE decreased from 4.73 to 3.81 ng L–1, and the content of STTM-miR171d was 6.54% higher than WT (Figure 3).
The expressions of CBF1 and COR in tomato
In red tomato fruit, the expression of COR genes and CBF1 genes showed an upward trend from 0 to 15 days and then sharply decreased, as shown in Figure 4A. At 25 days, the CBF1 gene expression levels of STTM-miR171d#7-2-1, STTM-miR171d#3-2-1, and STTM-miR171d#2-2-2 were 2.55, 2.65, and 2.62 times than WT. The COR gene expression of STTM-miR171d#7-2-1, STTM-miR171d#3-2-1, and STTM-miR171d#2-2-2 were 1.75, 1.90, and 1.83 times than WT, respectively. CBF1 and COR genes in green tomato fruit showed an increasing trend during storage, but sharply decreased during shelf life, as shown in Figure 4B. At the end of 9 days shelf life, the CBF1 gene expression levels of STTM-miR171d#7-2-1, STTM-miR171d#3-2-1, and STTM-miR171d#2-2-2 were 1.61, 1.59, and 1.63 times than WT. The COR gene expression of STTM-miR171d#7-2-1, STTM-miR171d#3-2-1, and STTM-miR171d#2-2-2 were 1.56, 1.38, and 1.48 times than WT (Figure 4).
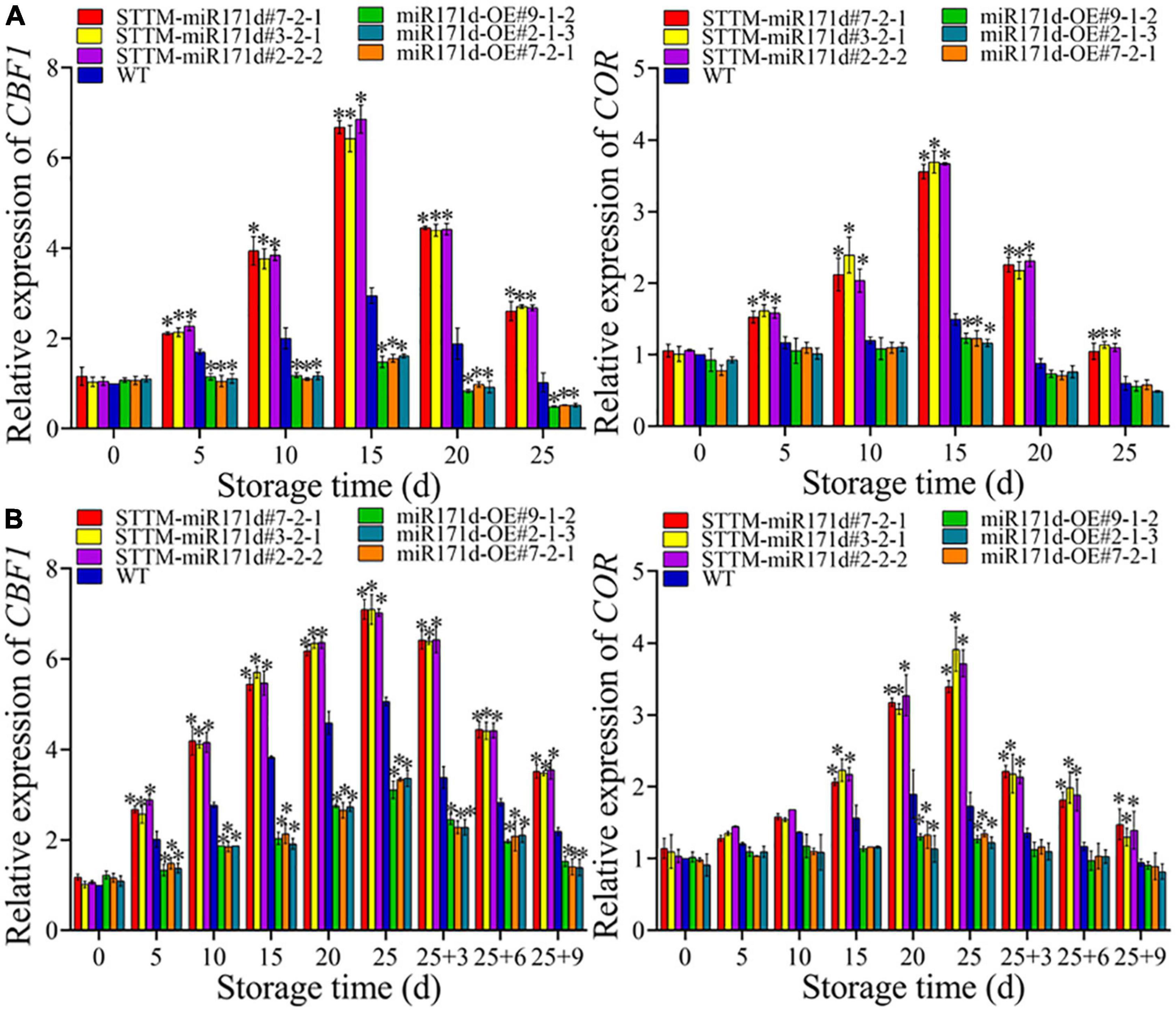
Figure 4. The expression levels of CBF1 and COR genes in red ripe tomato fruit (A) and green ripe tomato fruit (B). The error bar represents the independent repeat SDs (n = 3). The asterisk indicates that the data difference with P < 0.05 is statistically significant.
The expressions of miR171d and SlGRAS24 in tomato
During the low temperature, the expression level of miR171d in STTM-miR171d fruit was not significant and lower than that in WT fruit. Red tomato fruit was refrigerated at 4°C, STTM-miR171d#7-2-1, STTM-miR171d#3-2-1, and STTM-miR171d#2-2-2 the expression of miR171d were 0.34, 0.30, and 0.34. After green tomato fruit was refrigerated at 9°C and stored at 25°C for 9 days, the expression levels of miR171d in STTM-miR171d#7-2-1, STTM-miR171d#3-2-1, and STTM-miR171d#2-2-2 were 0.35, 0.36, and 0.31. The miR171d gene was significantly expressed in tomato fruit miR171d-OE, and the gene expression decreased with an extended storage period. At 25 days in red tomato fruit, the miR171d of miR171d-OE#9-1-2, miR171d-OE#7-2-1, and miR171d-OE#2-1-3 were 9.76, 9.74, and 9.50 times that of WT. After the shelf life of green tomato fruit, the miR171d in miR171d-OE#9-1-2, miR171d-OE#7-2-1, and miR171d-OE#2-1-3 were 9.52, 9.34, and 9.59 times than WT (Figure 5).
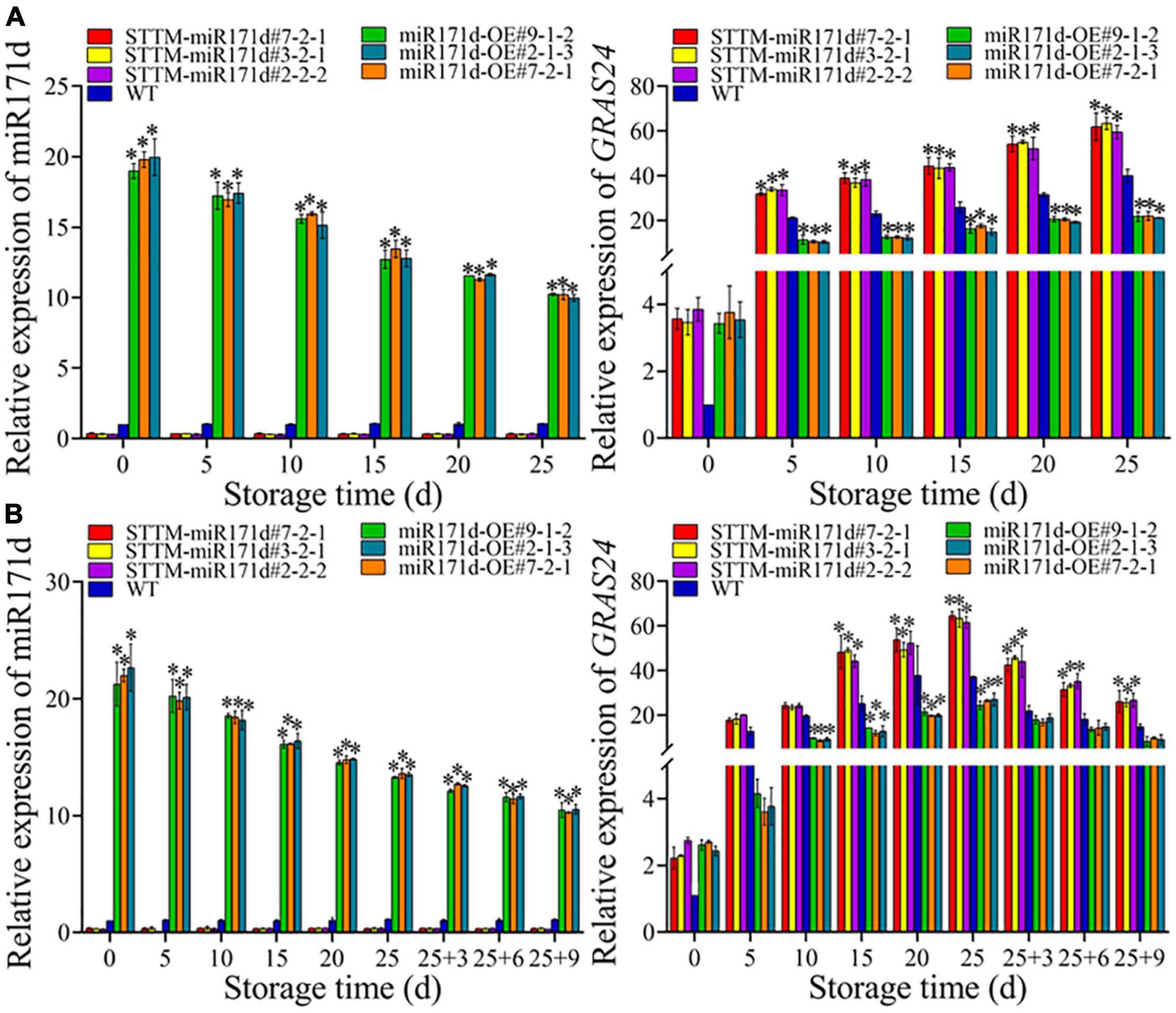
Figure 5. The expression levels of Sly-miR171d and SlGRAS24 genes in red ripe tomato fruit (A) and green ripe tomato fruit (B). The error bar represents the independent repeat SDs (n = 3). The asterisk indicates that the data difference with P < 0.05 is statistically significant.
In red STTM-miR171d tomato fruit the expression of GRAS24 gene increased with storage time under low temperature stress. Red tomato fruit after 25 days, the expression of GRAS24 in STTM-miR171d#7-2-1, STTM-miR171d#3-2-1, STTM-miR171d#2-2-2, and WT were 61.89, 63.38, 59.49, and 40.06. The expression levels of GRAS24 in red fruit of miR171d-OE#9-1-2, miR171d-OE#7-2-1, and miR171d-OE#2-1-3 were 21.78, 22.07, and 21.15. Under low temperature stress in green STTM-miR171d tomato, the expression of GRAS24 gene increased with the storage time during the storage period, and the shelf life decreased gradually. At the end of the shelf life at 25°C, the expression of GRAS24 in STTM-miR171d#7-2-1, STTM-miR171d#3-2-1, STTM-miR171d#2-2-2, and WT were 26.06, 25.50, 26.65, and 14.78. The expression levels of GRAS24 in green fruit of miR171d-OE#9-1-2, miR171d-OE#7-2-1, and miR171d-OE#2-1-3 were 8.20, 9.76, and 9.07 (Figure 5).
The expressions of GA20ox1, GA3ox1, GA2ox1, and GAI in tomato
GA20ox1, GA3ox1, and GA2ox1 are key genes that affect bioactive GA levels in plants. GA20ox1 and GA3ox1 are synthetic genes, and GA2ox1 is the catabolic gene. At 25 days in red tomato fruit, the expression of GA20ox1 and GA3ox1 genes in STTM-miR171d were 1.24 and 1.12 times that of WT. After 9 days of shelf life, the expression of GA20ox1 and GA3ox1 genes in STTM-miR171d were 1.23 and 1.38 times that of WT. The expression of the GA2ox1 gene increased rapidly and then decreased during the storage period. At 25 days of red tomato fruit, the GA2ox1 gene expression level in STTM-miR171d fruit was 1.50 times that of WT. Green tomato after 9 days of shelf life, the expression level of GA2ox1 gene in STTM-miR171d fruit was 1.25 times that of WT (Figure 6).
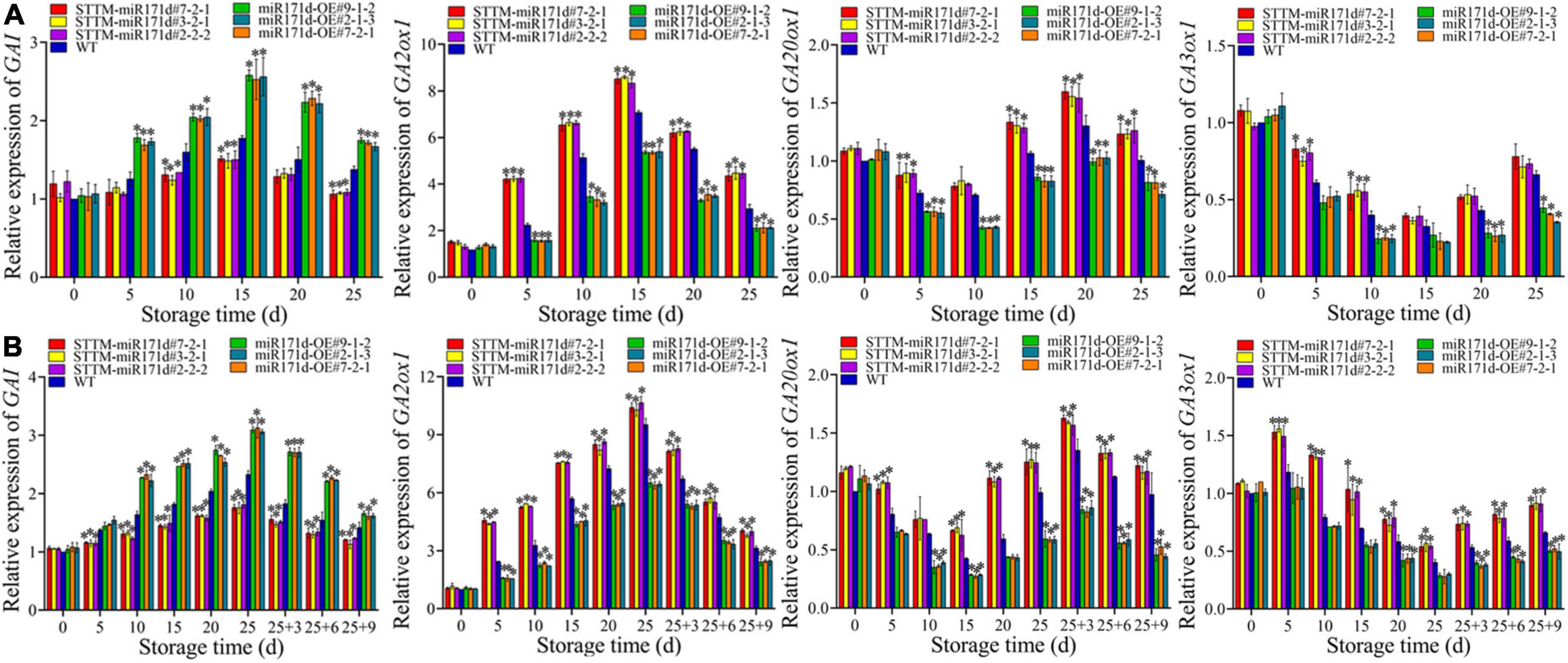
Figure 6. The expression levels of GA20ox1, GA3ox1, GA2ox1, and GAI genes in red ripe tomato fruit (A) and green ripe tomato fruit (B). The error bar represents the independent repeat SDs (n = 3). The asterisk indicates that the data difference with P < 0.05 is statistically significant.
In tomato fruit the expression of the GAI gene first increased and then decreased. The expression of GAI in red tomato fruit reached the peak at 15 days. On 25 days, miR171d-OE #9-1-2, miR171d-OE #7-2-1, and miR171d-OE #2-1-3 were 1.26, 1.25, and 1.21 times than those in WT fruit, respectively. The expression of GAI in green tomato fruit reached its peak at the end of storage period. After 9 days of shelf life, miR171d-OE #9-1-2, miR171d-OE #7-2-1, and miR171d-OE #2-1-3 were 1.18, 1.14, and 1.14 times than WT, respectively (Figure 6).
Discussion
Refrigeration is currently the most commonly used technique for extending the shelf life of postharvest fruit. However, most tropical and subtropical fruit are susceptible to CI during refrigeration (Wang et al., 2021). As important regulatory factors of various physiological processes in plants, miRNA also plays a significant role in plants resistance to cold tolerance (Zhou and Tang, 2019). Low temperature stress can increase membrane permeability and damage the membrane system, leading to cell metabolism and dysfunction in plants (Mekontso et al., 2021; Wang et al., 2022). EL is one of the main outcomes caused by CI, and elevated EL is considered an important marker of cell membrane damage, with lower EL indicating higher membrane integrity (Duan et al., 2022). CI index reflects the degree of CI symptoms and judges cold tolerance, and the development of CI symptoms increases with long-term storage (Zhang et al., 2022). The contents of TSS reflects the intracellular mass and solute concentration, and is a protective substance in the cells under the conditions of CI, and its content is positively correlated with the cold resistance of most plants (Jia et al., 2018). The present results showed that STTM-miR171d tomato fruit surface did not change significantly, WT fruit showed epidermis shrank, while miR171d-OE tomato fruit showed epidermis atrophy and surface depression. The accumulation of EL in tomato fruit increased with storage time, and the accumulation of EL in STTM-miR171d fruit did not increase significantly. TSS content in STTM-miR171d fruit was higher than that of miR171d-OE and WT fruit, and remained at a higher level. Inhibition of Sly-miR171d can slow down the development of CI symptoms in tomato fruit, reduce the accumulation of EL, maintain high cell membrane integrity and high TSS content to delay the decline of fruit tissue firmness. Therefore, silencing Sly-miR171d improved the cold resistance of tomato fruit.
GA3 is a natural plant hormone that plays a crucial role in various abiotic stresses (Alonso-Ramírez et al., 2009). GA inactivation and positive and negative feedback regulation of GA biosynthesis to maintain GA homeostasis (Hedden and Thomas, 2012). The down-regulation of GA biosynthesis genes GA20ox1 and GA3ox1 in tomato fruit stored at low temperature is related to the decrease of GA content (Zhu et al., 2016). Exogenous SA treatment of tomato fruit induced GA3ox1 expression, increased GA3 content, stimulated the degradation of DELLA protein, and alleviated CI symptoms in tomato fruit (Ding et al., 2015). GRAS transcription factors are essential in plant responses to stress. DELLA belongs to the GRAS protein family, and GA can stimulate DELLA accumulation, inhibit protein degradation, and control key developmental processes to enhance stress tolerance (Achard et al., 2008). Exogenous GA3 may resist phytoplasma infection of potato purple top disease by down-regulating the DELLA gene (Ding et al., 2013). Under cold stress, SlPIF4 activates the SlDELLA gene and inhibits GA biosynthesis to partially enhance the cold tolerance of tomato plants (Wang et al., 2020b). Overexpression of tomato transcription factor SlGRAS40 affects auxin and GA pathways in the process of tomato nutrition and reproduction, promotes the accumulation of DELLA protein, and enhances plants stress resistance (Liu et al., 2017b). In the study, the expression levels of key GA metabolizing genes and signal transduction genes in red and green ripe tomato fruit were analyzed. After low temperature storage, the expression level of target gene GRAS24 was positively correlated with GA biosynthesis key genes GA20ox1, GA3ox1, and GA2ox1. It was negatively correlated with the DELLA protein gene GAI. These results suggested that Sly-miR171d negatively regulated target gene GRAS24, increased GA content in tomato fruit and down-regulated DELLA protein gene GAI. Conversely, the expression of key genes GA20ox1 and GA3ox1 for GA biosynthesis and the GA decomposition gene GA2ox1 were up-regulated. The target gene GRAS24 may inhibit growth by downregulating the relative expression of GAI or degrading DELLA protein, thereby activating the cold tolerance of tomato fruit (Figure 7).
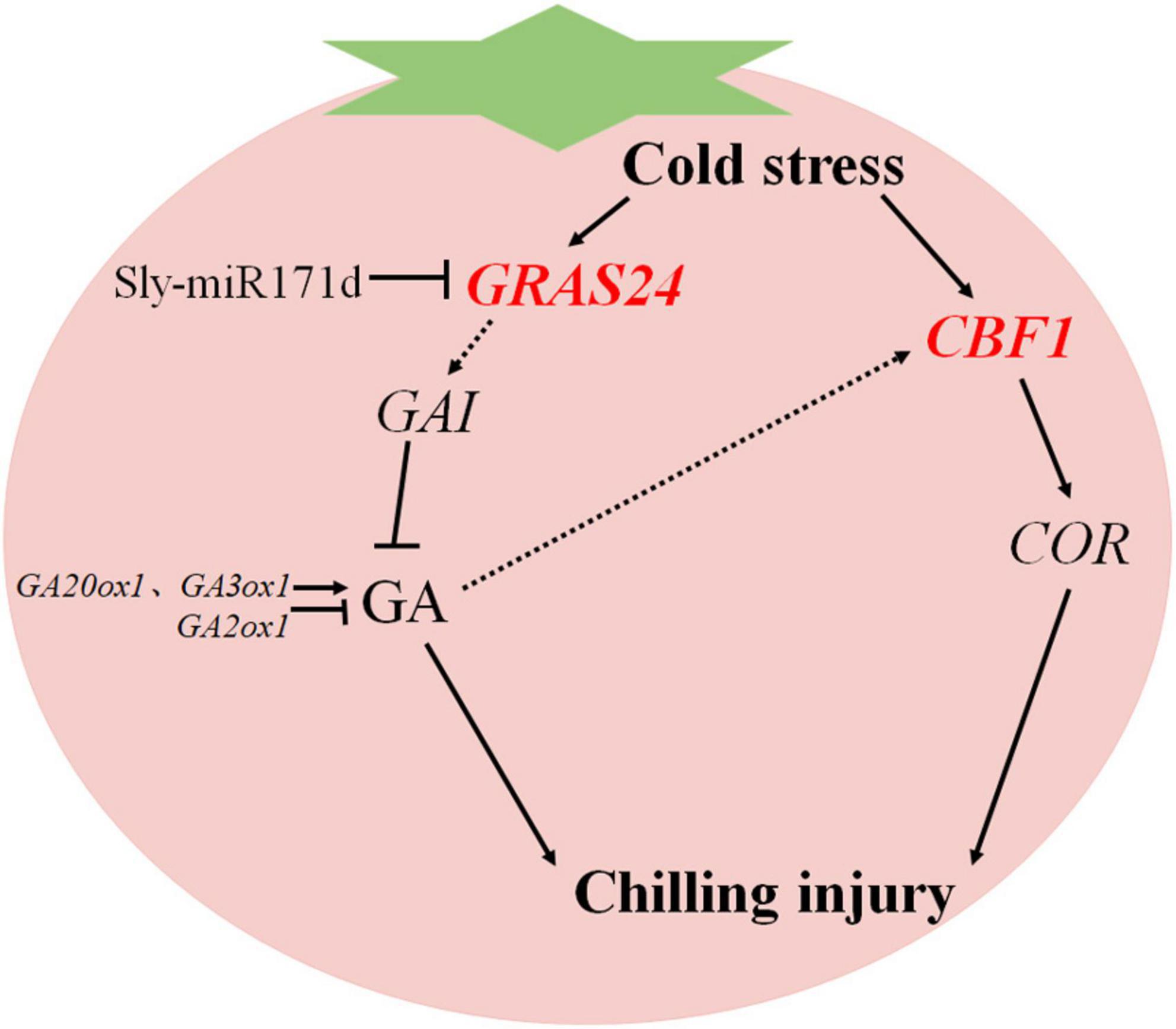
Figure 7. A simplified model of the regulatory mechanism of Sly-miR171d on cold tolerance of tomato fruit stored at low temperature.
The CBF pathway is essential in plant resistance to cold stress. The transcript level of CBF1 is positively correlated with tomato chilling resistance and negatively correlated with tomato CI index, which is for assessing the chilling resistance of tomato (Zhao et al., 2011). The expression of CBF-related genes in Arabidopsis thaliana can actively regulate low temperature stress resistance (Jiang et al., 2020). Overexpression of CmICE2 in Arabidopsis induced up-regulation of CBF and COR genes, and enhanced the response to freezing stress (Zhang et al., 2020). Overexpression of AtCBF1 in potato induces physiological modifications associated with cold adaptation to enhance freezing resistance (Pino et al., 2008). CBF has been shown to fully activate COR gene expression and induce resistance to low temperature stress (Stockinger et al., 1997). Application of exogenous GA3 increased the expression of the CBF1 gene and improved low temperature tolerance of tomato fruit by regulating GA catabolism through the CBF pathway (Zhu et al., 2016). Exogenous SA induced the expression of CBF1, which in turn induced the expression of the GA2ox1 gene, thereby activated the biosynthesis of GA and alleviated the CI of tomato fruit during low temperature storage (Ding et al., 2016). In this study, CBF1 and COR were higher expressed in STTM-miR171d than that in miR171-OE and WT fruit, which was consistent with their phenotype. The expression patterns of CBF1 and GA2ox1 are similar, and the CBF pathway may enhance the low temperature tolerance of tomato fruit by regulating GA catabolism (Figure 7).
Conclusion
In conclusion, silencing of Sly-miR171d and enhancing the expression of target gene GRAS24 effectively maintained the stability of cell membrane and increased the GA3 level in tomato fruit under low temperature, thereby improved postharvest chilling tolerance of tomato fruit.
Data availability statement
The datasets presented in this study can be found in online repositories. The names of the repository/repositories and accession number(s) can be found in the article/Supplementary material.
Author contributions
ZX, XX, and SL conceived and designed the experiments. ZX, TH, and KZ conducted the experiments. ZX, TH, KZ, LM, HS, ZZ, XX, and SL wrote and edited the manuscript. All authors approved the publication of final version of the manuscript.
Funding
This study was supported by the National Natural Science Foundation of China (31872160), the Hainan Provincial Natural Science Foundation of China (2019RC127, 321RC1025, and 322RC565), Jiangsu Higher Education Institution Innovative Research Team for Science and technology (2021), and Qing-Lan Project of Jiangsu Province in China (2021 and 2022).
Conflict of interest
The authors declare that the research was conducted in the absence of any commercial or financial relationships that could be construed as a potential conflict of interest.
Publisher’s note
All claims expressed in this article are solely those of the authors and do not necessarily represent those of their affiliated organizations, or those of the publisher, the editors and the reviewers. Any product that may be evaluated in this article, or claim that may be made by its manufacturer, is not guaranteed or endorsed by the publisher.
Supplementary material
The Supplementary Material for this article can be found online at: https://www.frontiersin.org/articles/10.3389/fpls.2022.1006940/full#supplementary-material
References
Achard, P., Renou, J. P., Berthomé, R., Harberd, N. P., and Genschik, P. (2008). Plant DELLAs restrain growth and promote survival of adversity by reducing the levels of reactive oxygen species. Curr. Biol. 18, 656–660. doi: 10.1016/j.cub.2008.04.034
Alonso-Ramírez, A., Rodríguez, D., Reyes, D., Jiménez, J. A., Nicolás, G., López-Climent, M., et al. (2009). Evidence for a role of gibberellins in salicylic acid-modulated early plant responses to abiotic stress in Arabidopsis seeds. Plant Physiol. 150, 1335–1344. doi: 10.1104/pp.109.139352
Bai, C., Zheng, Y., Watkins, C. B., Fu, A., Ma, L., Gao, H., et al. (2020). Revealing the specific regulations of brassinolide on tomato fruit chilling injury by integrated Multi-Omics. Front. Nutr. 8:769715. doi: 10.3389/fnut.2021.769715
Barciszewska-Pacak, M., Milanowska, K., Knop, K., Bielewicz, D., Nuc, P., Plewka, P., et al. (2015). Arabidopsis microRNA expression regulation in a wide range of abiotic stress responses. Front Plant Sci. 6:410. doi: 10.3389/fpls.2015.00410
Bartel, D. P. (2004). MicroRNAs: Genomics, biogenesis, mechanism, and function. Cell 116, 281–297. doi: 10.1016/S0092-8674(04)00045-5
Chen, H., Li, H. H., Lu, X. Q., Chen, L. Z., Liu, J., and Wu, H. (2019). Identification and expression analysis of GRAS transcription factors to elucidate candidate genes related to stolons, fruit ripening and abiotic stresses in woodland strawberry (Fragaria vesca). Int. J. Mol. Sci. 20:4593. doi: 10.3390/ijms20184593
Ding, F., Ren, L., Xie, F., Wang, M., and Zhang, S. (2021). Jasmonate and melatonin act synergistically to potentiate cold tolerance in tomato plants. Front. Plant Sci. 12:763284. doi: 10.3389/fpls.2021.763284
Ding, Y., Sheng, J. P., Li, S. Y., Nie, Y., Zhao, J. H., Zhu, Z., et al. (2015). The role of gibberellins in the mitigation of chilling injury in cherry tomato (Solanum lycopersicum L.) fruit. Postharvest Biol. Technol. 101, 88–95. doi: 10.1016/j.postharvbio.2014.12.001
Ding, Y., Wei, W., Wu, W., Davis, R. E., Jiang, Y., Lee, I. M., et al. (2013). Role of gibberellic acid in tomato defence against potato purple top phytoplasma infection. Ann. Appl. Biol. 162, 191–199. doi: 10.1111/aab.12011
Ding, Y., Zhao, J. H., Nie, Y., Fan, B., Wu, S. J., Zhang, Y., et al. (2016). Salicylic-acid-induced chilling-and oxidative-stress tolerance in relation to gibberellin homeostasis, C-repeat/dehydration-responsive element binding factor pathway, and antioxidant enzyme systems in cold-stored tomato fruit. J. Agric. Food Chem. 64, 8200–8206. doi: 10.1021/acs.jafc.6b02902
Duan, W. H., Mekontso, F. N., Li, W., Tian, J. X., Li, J. K., Wang, Q., et al. (2022). Alleviation of postharvest rib-edge darkening and chilling injury of carambola fruit by brassinolide under low temperature storage. Sci. Hortic. 299:111015. doi: 10.1016/j.scienta.2022.111015
El-Mogy, M. M., Garchery, C., and Stevens, R. (2018). Irrigation with salt water affects growth, yield, fruit quality, storability and marker-gene expression in cherry tomato. Acta Agric. Scand. B Soil Plant Sci. 68, 727–737. doi: 10.1080/09064710.2018.1473482
Habib, S., Lwin, Y. Y., and Li, N. (2021). Down-Regulation of SlGRAS10 in tomato confers abiotic stress tolerance. Gene 12:623. doi: 10.3390/genes12050623
Hedden, P., and Thomas, S. G. (2012). Gibberellin biosynthesis and its regulation. Biochem. J. 444, 11–25. doi: 10.1042/BJ20120245
Huang, J. H., Lin, X. J., Zhang, L. Y., Wang, X. D., Fan, G. C., and Chen, L. S. (2019). MicroRNA sequencing revealed Citrus adaptation to long-term boron toxicity through modulation of root development by miR319 and miR171. Int. J. Mol. Sci. 20:1422. doi: 10.3390/ijms20061422
Huang, W., Peng, S. Y., Xian, Z. Q., Lin, D. B., Hu, G. J., Yang, L., et al. (2017). Overexpression of a tomato miR171 target gene SlGRAS24 impacts multiple agronomical traits via regulating gibberellin and auxin homeostasis. Plant Biotechnol. J. 15, 472–488. doi: 10.1111/pbi.12646
Jia, B., Zheng, Q. L., Zuo, J. H., Gao, L. P., Wang, Q., Guan, W. Q., et al. (2018). Application of postharvest putrescine treatment to maintain the quality and increase the activity of antioxidative enzyme of cucumber. Sci. Hortic. 239, 210–215. doi: 10.1016/j.scienta.2018.05.043
Jiang, B. C., Shi, Y. T., Peng, Y., Jia, Y. X., Yan, Y., Dong, X. J., et al. (2020). Cold-induced CBF–PIF3 interaction enhances freezing tolerance by stabilizing the phyB thermosensor in Arabidopsis. Mol. Plant 13, 894–906. doi: 10.1016/j.molp.2020.04.006
Lantzouni, O., Alkofer, A., Falter-Braun, P., and Schwechheimer, C. (2020). GROWTH-REGULATING FACTORS interact with DELLAs and regulate growth in cold stress. Plant Cell 32, 1018–1034. doi: 10.1105/tpc.19.00784
Li, P. Y., Yin, F., Song, L. J., and Zheng, X. L. (2016). Alleviation of chilling injury in tomato fruit by exogenous application of oxalic acid. Food Chem. 202, 125–132. doi: 10.1016/j.foodchem.2016.01.142
Liu, Y. D., Huang, W., Xian, Z. Q., Hu, N., Lin, D. B., Ren, H., et al. (2017b). Overexpression of SlGRAS40 in tomato enhances tolerance to abiotic stresses and influences auxin and gibberellin signaling. Front. Plant Sci. 8:1659. doi: 10.3389/fpls.2017.01659
Liu, Y. D., Shi, Y., Zhu, N., Zhong, S. L., Bouzayen, M., and Li, Z. G. (2020). SlGRAS4 mediates a novel regulatory pathway promoting chilling tolerance in tomato. Plant Biotechnol. J. 18, 1620–1633. doi: 10.1111/pbi.13328
Liu, Y. R., Wang, K., Li, D. Y., Yan, J. P., and Zhang, W. J. (2017a). Enhanced cold tolerance and tillering in switchgrass (Panicum virgatum L.) by heterologous expression of Osa-miR393a. Plant Cell Physiol. 58, 2226–2240. doi: 10.1093/pcp/pcx157
Luengwilai, K., Beckles, D. M., and Saltveit, M. E. (2012). Chilling-injury of harvested tomato (Solanum lycopersicum L.) cv. Micro-Tom fruit is reduced by temperature pre-treatments. Postharvest Biol. Technol. 63, 123–128. doi: 10.1016/j.postharvbio.2011.06.017
Mekontso, F. N., Duan, W. H., Cisse, E. H. M., Chen, T. Y., and Xu, X. B. (2021). Alleviation of postharvest chilling injury of carambola fruit by γ-aminobutyric acid: Physiological, biochemical, and structural characterization. Front. Nutr. 752:538. doi: 10.3389/fnut.2021.752583
Peng, J. R., Carol, P., Richards, D. E., King, K. E., Cowling, R. J., Murphy, G. P., et al. (1997). The Arabidopsis GAI gene defines a signaling pathway that negatively regulates gibberellin responses. Genes Dev. 11, 3194–3205. doi: 10.1101/gad.11.23.3194
Pino, M. T., Skinner, J. S., Jeknić, Z., Hayes, P. M., Soeldner, A. H., Thomashow, M. F., et al. (2008). Ectopic AtCBF1 over-expression enhances freezing tolerance and induces cold acclimation-associated physiological modifications in potato. Plant Cell Environ. 31, 393–406. doi: 10.1111/j.1365-3040.2008.01776.x
Shan, S. S., Wang, Z. Q., Pu, H. L., Duan, W. H., Song, H. M., Li, J. K., et al. (2022). DNA methylation mediated by melatonin was involved in ethylene signal transmission and ripening of tomato fruit. Sci. Hortic. 291:110566. doi: 10.1016/j.scienta.2021.110566
Shan, X. Y., Yan, J. B., and Xie, D. X. (2012). Comparison of phytohormone signaling mechanisms. Curr. Opin. Plant Biol. 15, 84–91. doi: 10.1016/j.pbi.2011.09.006
Shi, X. P., Jiang, F. L., Wen, J. Q., and Wu, Z. (2019). Overexpression of Solanum habrochaites microRNA319d (sha-miR319d) confers chilling and heat stress tolerance in tomato (S. lycopersicum). BMC Plant Biol. 19:214. doi: 10.1186/s12870-019-1823-x
Stockinger, E. J., Gilmour, S. J., and Thomashow, M. F. (1997). Arabidopsis thaliana CBF1 encodes an AP2 domain-containing transcriptional activator that binds to the C-repeat/DRE, a cis-acting DNA regulatory element that stimulates transcription in response to low temperature and water deficit. Proc. Natl. Acad. Sci. U.S.A. 94, 1035–1040. doi: 10.1073/pnas.94.3.1035
Sunkar, R., Chinnusamy, V., Zhu, J. H., and Zhu, J. K. (2007). Small RNAs as big players in plant abiotic stress responses and nutrient deprivation. Trends Plant Sci. 12, 301–309. doi: 10.1016/j.tplants.2007.05.001
Tian, J. X., Xie, S. Y., Zhang, P., Wang, Q., Li, J. K., and Xu, X. B. (2022). Attenuation of postharvest peel browning and chilling injury of banana fruit by Astragalus polysaccharides. Postharvest Biol. Technol. 184:111783. doi: 10.1016/j.postharvbio.2021.111783
Wang, F., Chen, X. X., Dong, S. J., Jiang, X. C., Wang, L. Y., Yu, J. Q., et al. (2020b). Crosstalk of PIF4 and DELLA modulates CBF transcript and hormone homeostasis in cold response in tomato. Plant Biotechnol. J. 18, 1041–1055. doi: 10.1111/pbi.13272
Wang, Y. T., Feng, C., Zhai, Z. F., Peng, X., Wang, Y. Y., Sun, Y. T., et al. (2020a). The apple microR171i-SCARECROW-LIKE PROTEINS26. 1 module enhances drought stress tolerance by integrating ascorbic acid metabolism. Plant Physiol. 184, 194–211. doi: 10.1104/pp.20.00476
Wang, Z. Q., Pu, H. L., Shan, S. S., Zhang, P., Li, J. K., Song, H. M., et al. (2021). Melatonin enhanced chilling tolerance and alleviated peel browning of banana fruit under low temperature storage. Postharvest Biol Technol. 179:111571. doi: 10.1016/j.postharvbio.2021.111571
Wang, Z. Q., Zhang, L., Duan, W. H., Li, W., Wang, Q., Li, J. K., et al. (2022). Melatonin maintained higher contents of unsaturated fatty acid and cell membrane structure integrity in banana peel and alleviated postharvest chilling injury. Food Chem. 397:133836. doi: 10.1016/j.foodchem.2022.133836
Xu, K., Chen, S. J., Li, T. F., Ma, X. S., Liang, X. H., Ding, X. F., et al. (2015). OsGRAS23, a rice GRAS transcription factor gene, is involved in drought stress response through regulating expression of stress-responsive genes. BMC Plant Biol. 15:141. doi: 10.1186/s12870-015-0532-3
Yan, J., Gu, Y. Y., Jia, X. Y., Kang, W. J., Pan, S. J., Tang, X. Q., et al. (2012). Effective small RNA destruction by the expression of a short tandem target mimic in Arabidopsis. Plant Cell 24, 415–427. doi: 10.1105/tpc.111.094144
Zhang, L., Cao, X., Wang, Z. Q., Zhang, Z. K., Li, J. K., Wang, Q., et al. (2022). Brassinolide alleviated chilling injury of banana fruit by regulating unsaturated fatty acids and phenolic compounds. Sci Hortic. 297:110922. doi: 10.1016/j.scienta.2022.110922
Zhang, Z. H., Zhu, L., Song, A. P., Wang, H. B., Chen, S. M., Jiang, J. F., et al. (2020). Chrysanthemum (Chrysanthemum morifolium) CmICE2 conferred freezing tolerance in Arabidopsis. Plant Physiol. Biochem. 146, 31–41. doi: 10.1016/j.plaphy.2019.10.041
Zhao, K. Y., Chen, R. L., Duan, W. H., Meng, L. H., Song, H. M., Wang, Q., et al. (2022b). Chilling injury of tomato fruit was alleviated under low-temperature storage by silencing Sly-miR171e with short tandem target mimic technology. Front. Nutr. 9:906227. doi: 10.3389/fnut.2022.906227
Zhao, K. Y., Song, H. M., Wang, Z. Q., Xing, Z. T., Tian, J. X., Wang, Q., et al. (2022a). Knockdown of Sly-miR164a by short tandem target mimic (STTM) enhanced postharvest chilling tolerance of tomato fruit under low temperature storage. Postharvest Biol. Technol. 187:111872. doi: 10.1016/j.postharvbio.2022.111872
Zhao, R. R., Sheng, J. P., Lv, S. N., Zheng, Y., Zhang, J., Yu, M. M., et al. (2011). Nitric oxide participates in the regulation of LeCBF1 gene expression and improves cold tolerance in harvested tomato fruit. Postharvest Biol. Technol. 62, 121–126. doi: 10.1016/j.postharvbio.2011.05.013
Zhou, M. Q., and Tang, W. (2019). MicroRNA156 amplifies transcription factor-associated cold stress tolerance in plant cells. Mol. Genet. Genomics 294, 379–393. doi: 10.1007/s00438-018-1516-4
Keywords: Sly-miR171d, tomato, chilling tolerance, GRAS, GA
Citation: Xing Z, Huang T, Zhao K, Meng L, Song H, Zhang Z, Xu X and Liu S (2022) Silencing of Sly-miR171d increased the expression of GRAS24 and enhanced postharvest chilling tolerance of tomato fruit. Front. Plant Sci. 13:1006940. doi: 10.3389/fpls.2022.1006940
Received: 29 July 2022; Accepted: 23 August 2022;
Published: 09 September 2022.
Edited by:
Zhenfeng Yang, Zhejiang Wanli University, ChinaReviewed by:
Feng Xu, Ningbo University, ChinaYuquan Duan, Chinese Academy of Agricultural Sciences (CAAS), China
Copyright © 2022 Xing, Huang, Zhao, Meng, Song, Zhang, Xu and Liu. This is an open-access article distributed under the terms of the Creative Commons Attribution License (CC BY). The use, distribution or reproduction in other forums is permitted, provided the original author(s) and the copyright owner(s) are credited and that the original publication in this journal is cited, in accordance with accepted academic practice. No use, distribution or reproduction is permitted which does not comply with these terms.
*Correspondence: Xiangbin Xu, xbxuibcas@126.com; Songbai Liu, liusongbai@126.com