- 1State Key Laboratory of Severe Weather, Chinese Academy of Meteorological Sciences, China Meteorological Administration, Beijing, China
- 2Institute of Agricultural Resources and Regional Planning, Chinese Academy of Agricultural Sciences, Beijing, China
- 3College of Teacher Education, Capital Normal University, Beijing, China
- 4State Key Laboratory of Vegetation and Environmental Change, Institute of Botany, Chinese Academy of Sciences, Beijing, China
Grasslands are structurally and functionally controlled by water availability. Ongoing global change is threatening the sustainability of grassland ecosystems through chronic alterations in climate patterns and resource availability, as well as by the increasing frequency and intensity of anthropogenic perturbations. Compared with many studies on how grassland ecosystems respond during drought, there are far fewer studies focused on grassland dynamics after drought. Compensatory growth, as the ability of plants to offset the adverse effects of environmental or anthropogenic perturbations, is a common phenomenon in grassland. However, compensatory growth induced by drought and its underlying mechanism across grasslands remains not clear. In this review, we provide examples of analogous compensatory growth from different grassland types across drought characteristics (intensity, timing, and duration) and explain the effect of resource availability on compensatory growth and their underlying mechanisms. Based on our review of the literature, a hypothetic framework for integrating plant, root, and microbial responses is also proposed to increase our understanding of compensatory growth after drought. This research will advance our understanding of the mechanisms of grassland ecosystem functioning in response to climate change.
Introduction
Grasslands, as one of the world’s most widespread vegetation types, cover approximately 30% of the Earth’s land surface (Parton et al., 2012) and 69% of agricultural land area (Dixon et al., 2014), respectively. Grasslands not only serve as an important global reservoir of food production (Schirpke et al., 2019), but also play a critical role in the global carbon and water cycle, as well as plant-soil feedback to climate change (Bowman et al., 2015; Putten et al., 2016; Pugnaire et al., 2019). Grassland growth and productivity are largely regulated by temperature and soil water content, particularly the amount and timing of precipitation events (Knapp and Smith, 2001; Knapp et al., 2002; Huxman et al., 2004; Hufkens et al., 2016). In recent decades, ongoing global changes in temperature and precipitation have significantly increased the frequency, severity, and duration of drought events (Dai, 2012; IPCC, 2013; Trenberth et al., 2014; Cook et al., 2015), which also projected to continue to increase in the near future (Vicente-Serrano et al., 2020). The alterations in water availability before or during the growing season are weakening the stability and functionality of grassland ecosystems around the world, particularly in arid and semi-arid regions (Macdougall et al., 2013; Song and Yu, 2015). Because drought events could directly or indirectly affect plant community structure (Knapp et al., 2008; Cherwin and Knapp, 2012; Carlyle et al., 2014; Tielborger et al., 2014), threaten grassland productivity (Knapp and Smith, 2001; Volaire et al., 2014; Frank et al., 2015) and even cause grassland degradation (Breshears et al., 2005), and then alter carbon and nitrogen dynamics (Mackie et al., 2019). Naturally, to deal with the negative impacts of drought on grasslands functions and services, it is urgent to understand how grasslands respond to drought.
Many studies on grassland responses during drought have been well synthesized in both reviews (Grman et al., 2010; Niu et al., 2014; Hoover et al., 2018) and meta-analyses (Matos et al., 2019; Deng et al., 2021), which have considerably improved our understandings of the impacts of drought on grassland biotic and abiotic processes. For example, the mean effect of drought on aboveground net primary production (ANPP) is demonstrated to be negative (Hoover et al., 2014; Niboyet et al., 2017; Li et al., 2022). Droughts have legacy effects on bacterial and fungal community composition, which could, in turn, influence plant growth and ecosystem through plant-soil feedback (De Vries et al., 2012b; Kaisermann et al., 2017; Griffin-Nolan et al., 2018). Except for the ability of grasslands to resist drought (e.g., resistance), the recovery ability of grasslands after drought (e.g., resilience) is another important entry point for clarifying the responses of grasslands to drought (Vogel et al., 2012; Mori et al., 2013; Hoover et al., 2014; Oliver et al., 2015; Xu et al., 2021). However, the recovery ability of grasslands to different drought characteristics (e.g., timing, intensity, and duration) and climate contexts were rarely studied (Vilonen et al., 2022). Besides, our knowledge of grassland drought response is incomplete without understanding the responses after drought and to what extent grasslands can recover. Therefore, understanding the change patterns in the structure and function of grassland ecosystems during the period of after drought and exploring their underlying mechanisms, are crucial for forecasting grassland ecosystem function and dynamics under climate change.
Compensatory growth (CG), defined as the accelerated growth response of plants to damage (Belsky, 1986), which is implied the ability of plants to offset the adverse effects of tissue damage, restore organic functionality, and maintain their original growth state after perturbations (Mcnaughton, 1983). CG has received wide acceptance as a survival strategy of organisms under stressful conditions and a fundamental mechanism for ecosystem stability (Mangel and Munch, 2005; Gonzalez and Loreau, 2009). In fact, it sometimes takes different names, like resilience, recovery, and compensatory dynamic; they all share the essential meaning that accelerated growth organism when recovering from a period of unfavorable conditions (Li et al., 2021). According to the relative strength of growth rate after disturbance compared to the undisturbed group, CG can be classified into three types: under-compensation, exact-compensation, and over-compensation (Figure 1) (Belsky, 1986; Li et al., 2021). Although the existence of CG is widely acknowledged in ecological systems but has received little attention in stress-ecological studies (Metcalfe and Monaghan, 2001; Gessler et al., 2020). In general, it is conventionally to constrain the period of CG assessment through a pre-defined post-drought period or to the status where growth returns to a historic norm (Ovenden et al., 2021). The capacities of grassland CG are different among species (Knapp et al., 2015), community components (Carlsson et al., 2017; Stampfli et al., 2018; Wilcox et al., 2020), life forms (Volaire, 2003; Nippert and Knapp, 2007), nutrient stress tolerances (Macgillivray et al., 1995; Bharath et al., 2020), interactions among soil microbes (De Vries et al., 2012a; Fry et al., 2018) and disturbance’s properties (Chen et al., 2020; Saeidnia et al., 2020; Li et al., 2022). Besides, CG could be evaluated by a variety of quantitative indicators, such as productivity, biomass, species number, coverage, and so on (Yuan et al., 2020). Recent research on the prevalence and detection of CG leaves a large gap in the knowledge of the mechanisms that affect the temporal and scale of CG (Kahl et al., 2019; Li et al., 2020; Saeidnia et al., 2020; Hossain and Li, 2021; Jiao et al., 2021; Li et al., 2021; Ovenden et al., 2021; Vilonen et al., 2022). Thus, a deeper understanding of the pattern of grassland CG and its variation is a major challenge for the emerging extreme climate events and human disturbance.
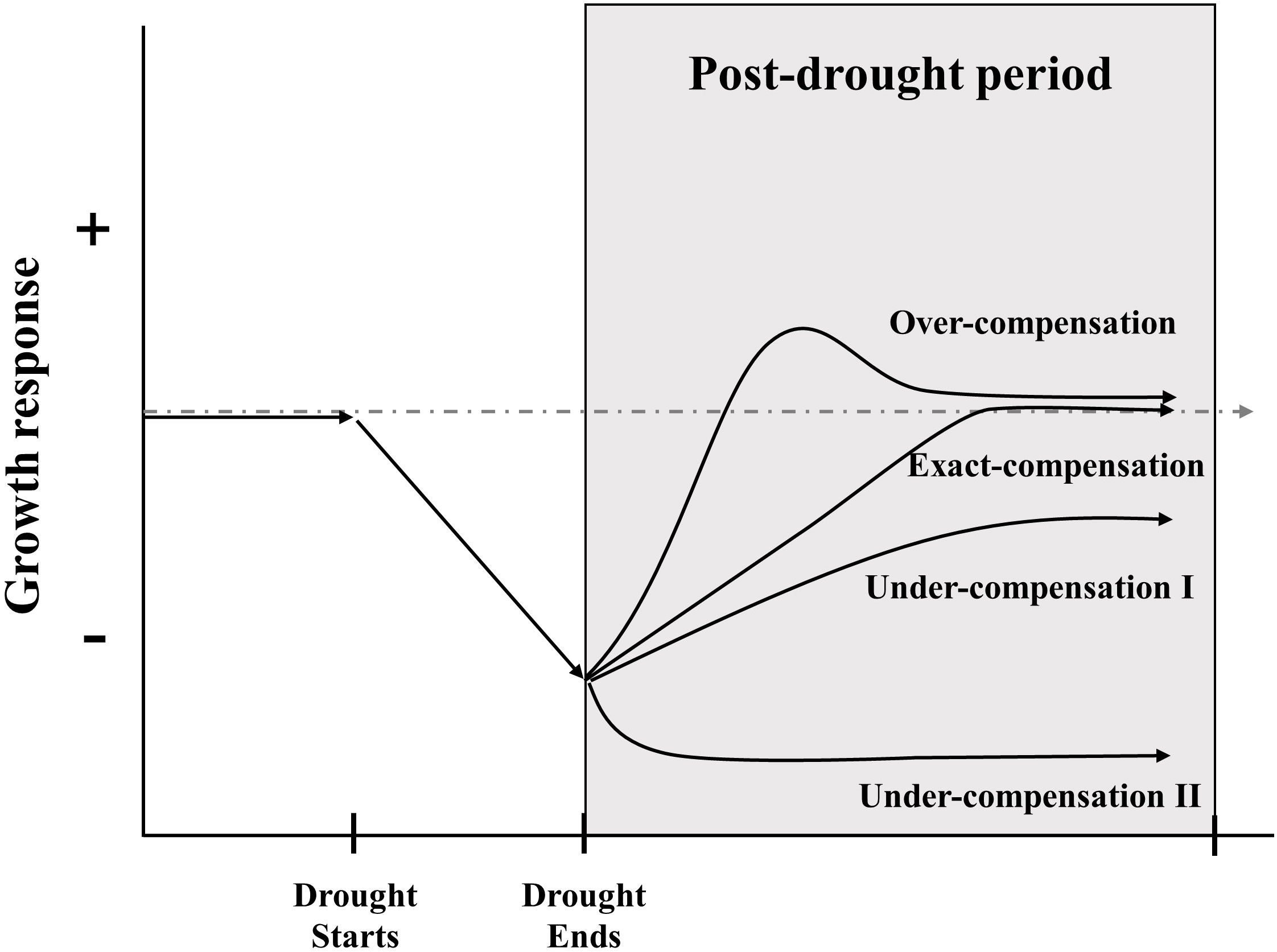
Figure 1 Framework for describing the growth response of grassland ecosystem after drought ends. The grey and dashed line represents growth response in condition without drought, the solid line indicated possible compensatory patterns under drought. Over-compensation means the growth rate increase rapidly after the end of a drought, then exceed the response level of control treatment to some extent, and finally reach the same response level of control treatment; exact-compensation represents the growth rate return to be comparable with control treatment after drought; under-compensation I indicates that the growth rate cannot reach the same level of control treatment with slow growth rate; under-compensation II denotes the collapse of grassland ecosystem with much slower growth rate (Frank et al., 2015; Li et al., 2021; Vilonen et al., 2022).
Here we reviewed current knowledge on the CG of grasslands to drought stress. We firstly discuss differences in CG response to drought imposed by manipulated experiments or natural precipitation variations. Then, CG patterns under different drought timing, intensity, and duration were compared and discussed. Meanwhile, as plant-soil feedback plays a key role in the CG response to drought, resource availability was also addressed in the text. In the following section, we concluded the underlying mechanisms of CG in response to drought across biotic and abiotic reasons. Finally, suggestions for future research were also given to deepen our understanding of the responses during the period after drought and benefit for forecasting grassland ecosystem function and dynamics under climate change.
Compensatory growth among different grassland ecosystems
Numerous rainfall manipulation experiments have been conducted to investigate the growth responses of different grassland ecosystems after drought (Hoover et al., 2018; Matos et al., 2019). In the mesic grasslands of North America and Switzerland, ANPP can fully recover (the same as exact-compensation) within a single year after a short-term extreme drought with grass species compensating for the decreased forb productivity (Hoover et al., 2014; Stampfli et al., 2018; Mackie et al., 2019; Wilcox et al., 2020). Besides, a study focused on belowground net primary productivity (BNPP) also suggested that drought-induced reductions in root production can recover rapidly in a coming wet year even though the drought legacy effects may persist for years after drought (Slette et al., 2022). By contrast, the CG of burned sites was mostly contributed to annual forb ANPP compensating for reduced grass ANPP, while the CG of unburned sites was promoted by subdominant annual and perennial grass species in a savanna grassland in South Africa (Wilcox et al., 2020). The opposite roles played by forbs and grass species mentioned above might be mainly due to the difference in community composition in these sites. As forbs are often less resistant to drought than grasses, if the plant community was dominated by forbs, then the CG of the total ANPP may decrease (Xu et al., 2021). Additionally, annual forbs are more resilient than perennial forbs, which are characterized by limited seedling recruitment and slow regrowth from surviving belowground organs after drought (Wilcox et al., 2020; Xu et al., 2021).
Meanwhile, semi-arid grassland seems to require more time for the ANPP to achieve exact-compensation from drought. Xu et al. (2021) explored the recovery potential of ANPP by inducing two years of extreme drought (66% reduction in ambient growing season precipitation) followed by two years of recovery (ambient precipitation) in a semi-arid grassland ecosystem in Inner Mongolia, China. The results show that ANPP decreased by approximately 33% during the two years of extreme drought. However, one year after the extreme droughts, the ANPP of the drought plots returned to 83% of the ambient plots and fully recovered to ambient ANPP by the second year. The authors attributed these differences to three points: (a) the lower precipitation efficiently limits CG in the semi-arid regions; (b) the reduction of ANPP in the semiarid grassland is much higher than that in the mesic grassland (Ma et al., 2020), which increase the recovery time; (c) a large proportion of high resistance and low resilience of perennial forb species may delay the recovery time of the semiarid grassland (Tello-García et al., 2020).
As for arid grasslands, the CG rate may more slowly due to greater resource limitations and more severe impacts (Stuart-Haentjens et al., 2018). A study in Inner Mongolia suggested that the net primary productivity was less affected by light to moderate drought than moderate to severe drought (Liu et al., 2021). At the same time, another study conducted in the Chihuahuan Desert found that drought consistently and strongly decreased the cover of a dominant C4 grass (Bouteloua eriopoda), whereas water addition slightly increased the cover, even with little variation between years (Báez et al., 2013). The limited CG of Bouteloua eriopoda responding to increased water availability may reflect morphological constraints on this rhizomatous grass (Báez et al., 2013).
In general, grasslands are composed of two dominant herbaceous functional groups: grass and forb, which show great differences in their vulnerability to extreme drought (Taylor et al., 2011; Wilcox et al., 2020). Grass species are generally better able to tolerate drought, especially C4 grasses, whereas forb species may avoid drought via deeper rooting profiles (Nippert and Knapp, 2007). Besides, the growth responses of annual and perennial species may be different during and after drought (Volaire, 2003). Therefore, CG in grasslands may depend on function diversity in predrought communities (Stampfli et al., 2018; Wilcox et al., 2020). However, a study conducted in 13 extreme natural-drought experiments spreading over two biogeographic regions (five sites in annual-dominated grasslands in California and eight sites in perennial-dominated grasslands in the Great Plains) suggested there was no correlation between pre-drought plant diversity and post-drought resilience (Bharath et al., 2020). More importantly, the productivity of grassland ecosystems is simultaneously co-limited by nutrients and water across a wide range of precipitation (Bharath et al., 2020). For example, species-rich semi-natural grasslands exhibited a lower CG compared with intensively managed agricultural grasslands (De Keersmaecker et al., 2016).
Compensatory growth response to drought intensity, timing, and duration
Drought intensity, timing, and duration are fundamental characteristics of experimental or natural drought events. Drought stress can cause a series of reductions in morphological and physiological functional traits (e.g., plant height, specific leaf area, length of roots, leaf water potential, and photosynthetic capacity), which may finally lead to a reduction in productivity (Cenzano et al., 2013; Wellstein et al., 2017). Response diversity, describing the variation of responses to environmental change among species in a particular community, maybe a key determinant of ecosystem stability and functionality (Elmqvist et al., 2003; Mori et al., 2013). For instance, perennial caespitose grasses and rhizomatous grasses showed different growth response strategies to drought, as the CG of rhizomatous grasses declined with increasing water stress intensity while caespitose grasses displayed little CG with strong drought resistance (Zhang et al., 2018). Besides, defoliation could stimulate the CG of rhizomatous grasses under wet conditions, but the positive effects of defoliation can be weakened by drought intensity (Zhang et al., 2018). Even though, the CG of grassland dominated by perennial species almost remains constant with increasing drought intensity (Ruppert et al., 2015). The main reason may be contributed to the degree of drought intensity being below the upper limit that could cause ecosystem collapse (Dechant and Moradkhani, 2015).
The responses of grassland ecosystems to drought may vary with different seasonal drought timing. When droughts occur in the early season, the reductions in current-year biomass appear to be large enough due to the limitation length of a peak growth period for biomass accumulation (Meng et al., 2019). On contrary, when droughts happen in the late season, the decreased biomass will be reflected in the following year because of large negative legacy effects (Jiao et al., 2021). For example, the timing of drought significantly decreased ANPP (18%~26% reduction compared to the control treatments) during the growing season in a mesic grassland, with later droughts (early summer drought and late summer drought, respectively) having a larger effect than earlier drought (late spring drought), while BNPP was not significantly affected by any manipulated drought timing (Denton et al., 2016). Similar findings were also confirmed in a meadow steppe where spring and summer droughts decreased ANPP but did not affect BNPP (Meng et al., 2019). Furthermore, grasslands subjected to mid-summer drought tend to be primed for greater CG in the following year than grasslands experiencing earlier drought in the season (De Vries et al., 2012b; Denton et al., 2016).
The duration of drought is important to explain the variability of CG, with longer droughts resulting in slower grassland CG, through causing the depletion of seedbank and stored resources needed for re-establishment and resprouting of the drought-sensitive species (Ruppert et al., 2015; Estiarte et al., 2016; Matos et al., 2019). In the North American semi-arid grassland biome, reductions in ANPP appeared to be greater when the rainfall patterns of the growing season were dominated by many small events (that is, chronic drought), while it turned out to be not when rainfall patterns were characterized by large rain events (Cherwin and Knapp, 2012). Compared with wood biomes, grasslands exhibited a stronger CG when exposed to chronic drought, by contrast, displayed a weaker CG when exposed to intense drought (Jiao et al., 2021). Furthermore, the adverse effects of intense drought on ANPP were found to be more significant than chronic drought, additionally, drought duration appeared to hardly alter this pattern (Carroll et al., 2021). Therefore, the compensation of grassland ANPP in response to future droughts may be reduced when the rainfall regimes of the growing season being more extreme.
Compensatory growth under different resource availability
The amount of CG can be also affected by resource availability through the plant-soil feedback (Van Staalduinen et al., 2009). Due to changes in the soil water availability during and after drought, the turnover of C and N in soils is also altered. Increased duration and intensity of drought are usually associated with decreasing C and N mineralization and inorganic N fluxes (Borken and Matzner, 2009; Deng et al., 2021). Even though, a pulse in net C and N mineralization following the wetting of dry soil is generally observed (Wu and Brookes, 2005). Previous grassland studies indicate that drought stresses alleviate N limitation and have a positive effect on forage quality (Dumont et al., 2015). Additionally, increasing N deposition resulting from anthropogenic N emissions can improve grassland CG after a drought even in arid environments (Kinugasa et al., 2012). Besides, the wetting pulses have a greater impact on C and N mineralization or flux rates in arid and semiarid grasslands than that in humid and subhumid grassland (Borken and Matzner, 2009). Whereas, it is worth noting that the cumulative C and N mineralization are most likely less compared with soil under optimum moisture even with wetting pulses, which implies that wetting pulse cannot compensate for small mineralization rates during periods (Borken and Matzner, 2009). In another word, the grassland CG pulsed by the short-term C and N mineralization may be not sustainable if the droughts become frequent.
The dynamics of soil C and N are affected not only by plant organic matter input but also by microbial activities (Deng et al., 2021). Soil microorganisms participate in all aspects of C and N dynamics, regulate the formation of soil organic matter and release extracellular enzymes through C and N turnover (Ren et al., 2017). Meanwhile, microbial decomposition of soil organic matter can cause CO2 efflux and gaseous N emissions by producing C and N-degrading extracellular enzymes (Ren et al., 2017). Interestingly, both microbial and extracellular enzyme activities appear to be more sensitive to soil water content and temperature than to their nutritional resources (Nielsen and Ball, 2015). Therefore, drought can alter soil microbial composition and enzyme activities, then affect the soil C and N balance (Ren et al., 2017), and finally influence both the belowground and aboveground performances of plants in response to drought.
However, previous studies have no consistent results on the relationship between CG and resource availability. Some studies suggested that plants tend to overcompensate more frequently under unfavorable growth conditions (Coughenour et al., 1990; Hawkes and Sullivan, 2001). In contrast, some researcher insisted that CG only occur under abundant even optimal conditions (Belsky et al., 1993), known as the compensatory continuum hypothesis (CCH). Furthermore, even for the same functional type, CG varies among different resource levels (Hawkes and Sullivan, 2001). For instance, Leymus chinensis had less CG under dry conditions compared with wet conditions, while it is opposite for Stipa krylovii (Van Staalduinen and Anten, 2005). Besides, the chronic nutrient addition in the Great Plains reduced grassland drought resistance and increased drought resilience regardless of annual-dominated or perennial-dominated grassland (Bharath et al., 2020). Based on CCH, a limited resource model (LRM) was once introduced to explain the range of observed effects of resource levels on and prediction for compensation for herbivory (Wise and Abrahamson, 2005), which suggested that CG depends on the type of resource and disturbance intensity (e.g., drought, heat stress, herbivore) under consideration (Metcalfe and Monaghan, 2001; Michael and Warren, 2007). The LRM model introduced the roles of limiting and non-limiting resources, and analyzed which resource was affected by the disturbance. If the disturbance mainly affects the first limiting resource, then higher CG is expected to occur at high resource availability. The application of the LRM model needs to take certain experimental requirements into account, including full factorial experimental design, consistent levels of disturbance across environments, determining whether a focal resource is liming plant fitness, and identifying the resource affected by disturbance (Wise and Abrahamson, 2005). Even though, the application of LRM to predict CG in response to drought still remain with many uncertainties and need to be tested in future study, with the complex backgrounds of climate region, grassland type, and species diversity (Monaghan, 2008).
Underlying mechanisms of compensatory growth in response to drought
The CG response of grassland to drought is a synthesis result of plant, root, and soil feedback (Figure 2). With respect to aboveground plant responses, CG under drought conditions may be triggered by the recovery of existing individuals within a grassland ecosystem, or by compensatory dynamics where particular individuals or species increase in abundance to counterbalance reductions in other individuals or species (Tilman and Downing, 1994). Compensatory effects, arising from various responses of different plants or functional groups to perturbations, are an important mechanism for sustaining ecosystem stability (Gonzalez and Loreau, 2009; Song and Yu, 2015). Besides, compensatory changes in species population in response to environmental fluctuations can maintain an appreciated steady state between the rate of resource supply and its consumption (Morgan Ernest and Brown, 2001). The difference in performance between functional traits is a good indicator of plant growth strategy in response to post-drought. For example, Leymus chinensis exhibited a greater capacity for CG than Stipa krylovii, because it has a stronger ability in storing carbohydrates and reallocating them after leaf losses, and a more positive effect of defoliation on light penetration through the canopy (Van Staalduinen and Anten, 2005). The CG of the different grassland ecosystems responding to drought may occur through two fundamentally different biotic mechanisms (Wilcox et al., 2020): (1) drought-tolerant plants increase in abundance and functionally compensate for declines in drought-intolerant species, which is called compensatory dynamics (Gonzalez and Loreau, 2009; Hoover et al., 2014); (2) all individuals within the community recover fully after drought, which is defined as physiological compensatory (Connell and Ghedini, 2015). Physiological compensation often happens with short-term and (or) moderate drought, while compensatory dynamics are more likely to occur under long-term and (or) extreme drought by rearranging species abundances (Smith, 2011).
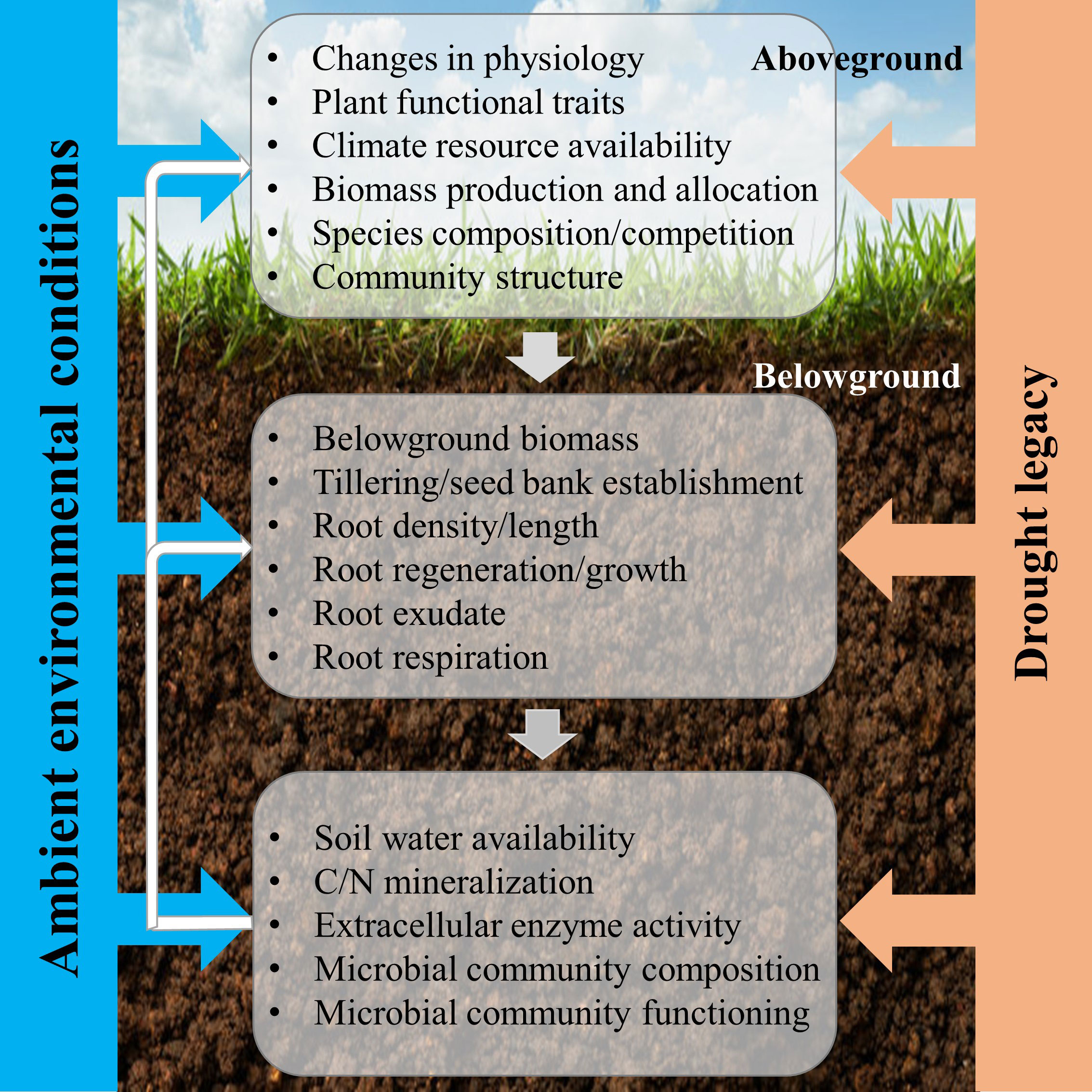
Figure 2 Conceptual framework of the responses of aboveground and belowground processes to post-drought for compensatory growth. The three main three components include aboveground plant, root, and soil microorganism, which are determined by plant community trait, soil type and climate (ambient environmental conditions). The effects of drought on the three components are achieved individually, but we hypothesize that the responses of the aboveground plant determine the responses of root after drought, thereby, the microbial community responses, which react on aboveground plant growth, community composition, ultimately, ecosystem stability and function (Williams and De Vries, 2020).
Nevertheless, plant roots and microbial community also play a key role in mediating the post-drought responses (Figure 2). Root traits, like specific root length, root dry matter content, and toot tissue density, are important for shaping post-drought responses and vary across a range of grassland species with different growth strategies (De Vries et al., 2016; Williams and De Vries, 2020). In addition, root exudates form a pathway for plant-microbial communication and have the potential to influence plant tolerance and recovery during and/or after drought (Williams and De Vries, 2020). The drought-induced changes in the quality of root exudates might have implications for the recovery of plants and microbes (De Vries et al., 2019). Besides, the structure of the microbial community can determine the functional responses of the grassland ecosystems, through the expression of functional genes. Furthermore, CG was almost certainly promoted by a drought-induced increase in soil N availability as a higher mineral N supply rate appeared in the month after rewetting, and then increased plant nitrogen content two months after rewetting (Mackie et al., 2019). Due to an increase in plant-available N, the plant photosynthetic activities are upregulated during post-drought, and then drive a short-term increase in forage quality (Bloor and Bardgett, 2012; Niboyet et al., 2017). Therefore, this field of research will need to be driven forward by studying general mechanisms, focusing on mechanisms that link below- and aboveground processes and responses (Figure 2).
Limitations and suggestions for future research
With growing concerns about grassland vulerability, a comprehensive understanding of grassland response to drought is becoming increasingly important. Previous studies have mainly focused on the plant or ecosystem responses during drought, however, there is still limited understanding of the period after drought. Here, we reviewed compensatory growth across grassland types, drought characteristics, and resource availabilities. Besides, the underlying mechanisms of CG were also summed up. However, there is still a lot of work to achieve the ultimate target on how to accurately quantify CG and predict its direction and strength under changing ambient environmental conditions. The followings are some suggestions for further research on CG.
Firstly, the assessment method of CG mentioned above implicitly assumes that the reference growth level (the pre-defined post-drought period or the historic norm status) is where the drought legacy ends. However, the legacy of drought might be extending far beyond a return to reference growth level under some conditions (Ingrisch and Bahn, 2018; Ovenden et al., 2021). Thus, when CG is activated and then how long it will take are still debatable, which need to be finely defined in future study. Besides, as plant functional traits play an important role in determining net carbon assimilation and allocation, therefore, a better understanding of the post-drought recovery performance of plant functional traits could improve our ability to predict grassland ecosystem production in a rapidly changing climate (Yin and Bauerle, 2017). In order to improve the evaluation accuracy of CG, filtering out suitable plant-soil functional traits may be a good pathway (Figure 2).
Secondly, the adverse impacts of a single drought might be reflected in plant water and nutrient acquisition than in ecosystem carbon cycling, while both sides could be emphasized by a second drought or repeated droughts (Slette et al., 2022). The potential consequences of repeated drought on CG may range from increased adaptation to increased sensitivity, which remains unclear (Slette et al., 2022). Some studies have suggested that the adaptation of soil microbial communities to a previous drought can increase the drought tolerance of plants in facing a subsequent drought event (Lau and Lennon, 2012; Meisner et al., 2013). Besides, drought-exposure history could increase complementarity between plant species in response to future droughts (Chen et al., 2022). Due to more frequent droughts are expected in many parts of the world in the future, studies on the CG response to repeated droughts are needed to improve our knowledge of grassland stability.
Thirdly, even though extensive studies have been focusing on the effects of drought on grasslands, there is limited understanding of the period after drought due to a lack of studies on belowground responses and an undue emphasis on aboveground ecosystem responses (Vilonen et al., 2022). ANPP is the most common indicator or function for evaluating the aboveground ecosystem responses to drought (Hoover et al., 2014; Knapp et al., 2015), because aboveground biomass is easier to obtain than belowground biomass. In fact, grasslands allocate a substantial portion of total net primary production to roots and then store most of their carbon belowground (Hui and Jackson, 2006; Silver et al., 2010). Belowground responses, such as BNPP and soil CO2 flux, are of particular importance in determining the size of the soil carbon pool (Post et al., 1982; Slette et al., 2021). Previous studies have demonstrated that the CG of ANPP and BNPP is different over time, like average precipitation amounts are sufficient for CG in ANPP after extreme drought, while CG in BNPP might be more resource-demanding (Slette et al., 2022). Due to the different patterns of ANPP and BNPP response to changes in water availability, belowground processes and their underlying mechanisms should be addressed in future work modeling ecosystem responses to climate change (Denton et al., 2016).
Conclusion
Overall, in light of the persistence and intensification of climate change, the responses of ecosystems to drought need to be paid more attention. In past decades, a series of analogous compensatory growth of net primary productivity and community stability to drought disturbance in different grassland ecosystems were discussed based on effective indicators, like recovery and resilience. In this review, we discussed compensatory growth across different grassland ecosystems and drought characteristics, explained the effect of resource availability on compensatory growth, and summed up the mechanism by which compensatory growth may occur. The review suggests that the CG is likely to be primarily due to the different responses of plant functional groups and their interactions with soil microbes to water availability. We propose identifying the starting time and duration of compensatory growth; better describing the symbol of CG with plant-soil functional traits; conducting more research on the plant-soil feedback and the decoupling of above- and belowground processes. These proposed researches would expand our understanding of compensatory growth, and increase our ability to evaluate the stability and sustainability of grassland ecosystems in the face of climate change.
Author contributions
HZ, LH, XL, GY, YW and XW jointly conceived and wrote the paper. All authors contributed to the article and approved the submitted version.
Funding
This work was financially supported by the Second Tibetan Plateau Scientific Expedition and Research (STEP) program (2019QZKK0106) and the National Natural Science Foundation of China (42205126, 41975145, 41775108).
Acknowledgments
The authors thank the reviewers for the constructive comments on an earlier version of this manuscript.
Conflict of interest
The authors declare that the research was conducted in the absence of any commercial or financial relationships that could be construed as a potential conflict of interest.
Publisher’s note
All claims expressed in this article are solely those of the authors and do not necessarily represent those of their affiliated organizations, or those of the publisher, the editors and the reviewers. Any product that may be evaluated in this article, or claim that may be made by its manufacturer, is not guaranteed or endorsed by the publisher.
References
Báez, S., Collins, S. L., Pockman, W. T., Johnson, J. E., Small, E. E. (2013). Effects of experimental rainfall manipulations on chihuahuan desert grassland and shrubland plant communities. Oecologia 172, 1117–1127. doi: 10.1007/s00442-012-2552-0
Belsky, A. J. (1986). Does herbivory benefit plants? a review of the evidence. Am. Nat. 127, 870–892. doi: 10.1086/284531
Belsky, A. J., Carson, W. P., Jensen, C. L., Fox, G. A. (1993). Overcompensation by plants: Herbivore optimization or red herring? Evol. Ecol. 7, 109–121. doi: 10.1007/BF01237737
Bharath, S., Borer, E. T., Biederman, L. A., Blumenthal, D. M., Fay, P. A., Gherardi, L. A., et al. (2020). Nutrient addition increases grassland sensitivity to droughts. Ecology 101, e02981. doi: 10.1002/ecy.2981
Bloor, J. M. G., Bardgett, R. D. (2012). Stability of above-ground and below-ground processes to extreme drought in model grassland ecosystems: Interactions with plant species diversity and soil nitrogen availability. Perspect. Plant Ecol. Evol. Syst. 14, 193–204. doi: 10.1016/j.ppees.2011.12.001
Borken, W., Matzner, E. (2009). Reappraisal of drying and wetting effects on c and n mineralization and fluxes in soils. Glob Chang Biol. 15, 808–824. doi: 10.1111/j.1365-2486.2008.01681.x
Bowman, D. M., Perry, G. L., Marston, J. B. (2015). Feedbacks and landscape-level vegetation dynamics. Trends Ecol. Evol. 30, 255–260. doi: 10.1016/j.tree.2015.03.005
Breshears, D. D., Cobb, N. S., Rich, P. M., Price, K. P., Allen, C. D., Balice, R. G., et al. (2005). Regional vegetation die-off in response to global-change-type drought. Proc. Natl. Acad. Sci. U.S.A. 102, 15144–15148. doi: 10.1073/pnas.0505734102
Carlsson, M., Merten, M., Kayser, M., Isselstein, J., Wrage-Mönnig, N. (2017). Drought stress resistance and resilience of permanent grasslands are shaped by functional group composition and n fertilization. Agric. Ecosyst. Environ. 236, 52–60. doi: 10.1016/j.agee.2016.11.009
Carlyle, C. N., Fraser, L. H., Turkington, R. (2014). Response of grassland biomass production to simulated climate change and clipping along an elevation gradient. Oecologia 174, 1065–1073. doi: 10.1007/s00442-013-2833-2
Carroll, C. J. W., Slette, I. J., Griffin-Nolan, R. J., Baur, L. E., Hoffman, A. M., Denton, E. M., et al. (2021). Is a drought a drought in grasslands? productivity responses to different types of drought. Oecologia 197, 1017–1026. doi: 10.1007/s00442-020-04793-8
Cenzano, A. M., Varela, M. C., Bertiller, M. B., Luna, M. V. (2013). Effect of drought on morphological and functional traits of poa ligularis and pappostipa speciosa, native perennial grasses with wide distribution in Patagonian rangelands, Argentina. Aust. J. Bot. 61, 383. doi: 10.1071/bt12298
Chen, Y., Vogel, A., Wagg, C., Xu, T., Iturrate-Garcia, M., Scherer-Lorenzen, M., et al. (2022). Drought-exposure history increases complementarity between plant species in response to a subsequent drought. Nat. Commun. 13, 3217. doi: 10.1038/s41467-022-30954-9
Chen, N., Zhang, Y., Zu, J., Zhu, J., Zhang, T., Huang, K., et al. (2020). The compensation effects of post-drought regrowth on earlier drought loss across the tibetan plateau grasslands. Agric. For. Meteorol. 281, 107822. doi: 10.1016/j.agrformet.2019.107822
Cherwin, K., Knapp, A. (2012). Unexpected patterns of sensitivity to drought in three semi-arid grasslands. Oecologia 169, 845–852. doi: 10.1007/s00442-011-2235-2
Connell, S. D., Ghedini, G. (2015). Resisting regime-shifts: the stabilising effect of compensatory processes. Trends Ecol. Evol. 30, 513–515. doi: 10.1016/j.tree.2015.06.014
Cook, B. I., Ault, T. R., Smerdon, J. E. (2015). Unprecedented 21st century drought risk in the American southwest and central plains. Sci. Adv. 1, e1400082. doi: 10.1126/sciadv.1400082
Coughenour, M. B., Detling, J. K., Bamberg, I. E., Mugambi, M. M. (1990). Production and nitrogen responses of the African dwarf shrub indigofera spinosa to defoliation and water limitation. Oecologia 83, 546–552. doi: 10.1007/BF00317208
Dai, A. (2012). Increasing drought under global warming in observations and models. Nat. Clim. Change 3, 52–58. doi: 10.1038/nclimate1633
Dechant, C. M., Moradkhani, H. (2015). Analyzing the sensitivity of drought recovery forecasts to land surface initial conditions. J. Hydrol. 526, 89–100. doi: 10.1016/j.jhydrol.2014.10.021
De Keersmaecker, W., Van Rooijen, N., Lhermitte, S., Tits, L., Schaminée, J., Coppin, P., et al. (2016). Species-rich semi-natural grasslands have a higher resistance but a lower resilience than intensively managed agricultural grasslands in response to climate anomalies. J. Appl. Ecol. 53, 430–439. doi: 10.1111/1365-2664.12595
Deng, L., Peng, C., Kim, D.-G., Li, J., Liu, Y., Hai, X., et al. (2021). Drought effects on soil carbon and nitrogen dynamics in global natural ecosystems. Earth Sci. Rev. 214, 103501. doi: 10.1016/j.earscirev.2020.103501
Denton, E. M., Dietrich, J. D., Smith, M. D., Knapp, A. K. (2016). Drought timing differentially affects above- and belowground productivity in a mesic grassland. Plant Ecol. 218, 317–328. doi: 10.1007/s11258-016-0690-x
De Vries, F. T., Brown, C., Stevens, C. J. (2016). Grassland species root response to drought: consequences for soil carbon and nitrogen availability. Plant Soil 409, 297–312. doi: 10.1007/s11104-016-2964-4
De Vries, F. T., Liiri, M. E., Bjørnlund, L., Bowker, M. A., Christensen, S., Setälä, H. M., et al. (2012a). Land use alters the resistance and resilience of soil food webs to drought. Nat. Clim. Change 2, 276–280. doi: 10.1038/nclimate1368
De Vries, F. T., Liiri, M. E., Bjørnlund, L., Setälä, H. M., Christensen, S., Bardgett, R. D. (2012b). Legacy effects of drought on plant growth and the soil food web. Oecologia 170, 821–833. doi: 10.1007/s00442-012-2331-y
De Vries, F. T., Williams, A., Stringer, F., Willcocks, R., Mcewing, R., Langridge, H., et al. (2019). Changes in root-exudate-induced respiration reveal a novel mechanism through which drought affects ecosystem carbon cycling. New Phytol. 224, 132–145. doi: 10.1111/nph.16001
Dixon, A. P., Faber-Langendoen, D., Josse, C., Morrison, J., Loucks, C. J., Ebach, M. (2014). Distribution mapping of world grassland types. J. Biogeogr. 41, 2003–2019. doi: 10.1111/jbi.12381
Dumont, B., Andueza, D., Niderkorn, V., Lüscher, A., Porqueddu, C., Picon-Cochard, C. (2015). A meta-analysis of climate change effects on forage quality in grasslands: specificities of mountain and Mediterranean areas. Grass Forage Sci. 70, 239–254. doi: 10.1111/gfs.12169
Elmqvist, T., Folke, C., Nyström, M., Peterson, G., Bengtsson, J., Walker, B., et al. (2003). Response diversity, ecosystem change, and resilience. Front. Ecol. Environ. 1, 488–494. doi: 10.1890/1540-9295(2003)001[0488:RDECAR]2.0.CO;2
Estiarte, M., Vicca, S., Peñuelas, J., Bahn, M., Beier, C., Emmett, B. A., et al. (2016). Few multiyear precipitation–reduction experiments find a shift in the productivity–precipitation relationship. Glob Chang Biol. 22, 2570–2581. doi: 10.1111/gcb.13269
Frank, D., Reichstein, M., Bahn, M., Thonicke, K., Frank, D., Mahecha, M. D., et al. (2015). Effects of climate extremes on the terrestrial carbon cycle: concepts, processes and potential future impacts. Glob Chang Biol. 21, 2861–2880. doi: 10.1111/gcb.12916
Fry, E. L., Johnson, G. N., Hall, A. L., Pritchard, W. J., Bullock, J. M., Bardgett, R. D. (2018). Drought neutralises plant-soil feedback of two mesic grassland forbs. Oecologia 186, 1113–1125. doi: 10.1007/s00442-018-4082-x
Gessler, A., Bottero, A., Marshall, J., Arend, M. (2020). The way back: recovery of trees from drought and its implication for acclimation. New Phytol. 228, 1704–1709. doi: 10.1111/nph.16703
Gonzalez, A., Loreau, M. (2009). The causes and consequences of compensatory dynamics in ecological communities. Annu. Rev. Ecol. Evol. Syst. 40, 393–414. doi: 10.1146/annurev.ecolsys.39.110707.173349
Griffin-Nolan, R. J., Carroll, C. J. W., Denton, E. M., Johnston, M. K., Collins, S. L., Smith, M. D., et al. (2018). Legacy effects of a regional drought on aboveground net primary production in six central US grasslands. Plant Ecol. 219, 505–515. doi: 10.1007/s11258-018-0813-7
Grman, E., Lau, J. A., Schoolmaster, D. R., Jr., Gross, K. L. (2010). Mechanisms contributing to stability in ecosystem function depend on the environmental context. Ecol. Lett. 13, 1400–1410. doi: 10.1111/j.1461-0248.2010.01533.x
Hawkes, C. V., Sullivan, J. J. (2001). The impact of herbivory on plants in different resource conditions: A meta-analysis. Ecology 82, 2045–2058. doi: 10.1890/0012-9658(2001)082[2045:TIOHOP]2.0.CO;2
Hoover, D. L., Knapp, A. K., Smith, M. D. (2014). Resistance and resilience of a grassland ecosystem to climate extremes. Ecology 95, 2646–2656. doi: 10.1890/13-2186.1
Hoover, D. L., Wilcox, K. R., Young, K. E. (2018). Experimental droughts with rainout shelters: a methodological review. Ecosphere 9, e02088. doi: 10.1002/ecs2.2088
Hossain, M. L., Li, J. (2021). NDVI-based vegetation dynamics and its resistance and resilience to different intensities of climatic events. Glob. Ecol. Conserv. 30, e01768. doi: 10.1016/j.gecco.2021.e01768
Hufkens, K., Keenan, T. F., Flanagan, L. B., Scott, R. L., Bernacchi, C. J., Joo, E., et al. (2016). Productivity of north American grasslands is increased under future climate scenarios despite rising aridity. Nat. Clim. Change 6, 710–714. doi: 10.1038/nclimate2942
Hui, D., Jackson, R. B. (2006). Geographical and interannual variability in biomass partitioning in grassland ecosystems: a synthesis of field data. New Phytol. 169, 85–93. doi: 10.1111/j.1469-8137.2005.01569.x
Huxman, T. E., Snyder, K. A., Tissue, D., Leffler, A. J., Ogle, K., Pockman, W. T., et al. (2004). Precipitation pulses and carbon fluxes in semiarid and arid ecosystems. Oecologia 141, 254–268. doi: 10.1007/s00442-004-1682-4
Ingrisch, J., Bahn, M. (2018). Towards a comparable quantification of resilience. Trends Ecol. Evol. 33, 251–259. doi: 10.1016/j.tree.2018.01.013
IPCC (2013). “Climate change 2013,” in The physical science basis: Working group I contribution to the fifth assessment report of the intergovernmental panel on climate change (Cambridge: Cambridge University Press).
Jiao, T., Williams, C. A., De Kauwe, M. G., Schwalm, C. R., Medlyn, B. E. (2021). Patterns of post-drought recovery are strongly influenced by drought duration, frequency, post-drought wetness, and bioclimatic setting. Glob Chang Biol. 27, 4630–4643. doi: 10.1111/gcb.15788
Kahl, S. M., Lenhard, M., Joshi, J., Teller, B. (2019). Compensatory mechanisms to climate change in the widely distributed species silene vulgaris. J. Ecol. 107, 1918–1930. doi: 10.1111/1365-2745.13133
Kaisermann, A., De Vries, F. T., Griffiths, R. I., Bardgett, R. D. (2017). Legacy effects of drought on plant-soil feedbacks and plant-plant interactions. New Phytol. 215, 1413–1424. doi: 10.1111/nph.14661
Kinugasa, T., Tsunekawa, A., Shinoda, M. (2012). Increasing nitrogen deposition enhances post-drought recovery of grassland productivity in the Mongolian steppe. Oecologia 170, 857–865. doi: 10.1007/s00442-012-2354-4
Knapp, A. K., Beier, C., Briske, D. D., Classen, A. T., Luo, Y., Reichstein, M., et al. (2008). Consequences of more extreme precipitation regimes for terrestrial ecosystems. Bioscience 58, 811–821. doi: 10.1641/b580908
Knapp, A. K., Carroll, C. J. W., Denton, E. M., La Pierre, K. J., Collins, S. L., Smith, M. D. (2015). Differential sensitivity to regional-scale drought in six central US grasslands. Oecologia 177, 949–957. doi: 10.1007/s00442-015-3233-6
Knapp, A. K., Fay, P. A., Blair, J. M., Collins, S. L., Smith, M. D., Carlisle, J. D., et al. (2002). Rainfall variability, carbon cycling, and plant species diversity in a mesic grassland. Science 298, 2202–2205. doi: 10.1126/science.1076347
Knapp, A. K., Smith, M. D. (2001). Variation among biomes in temporal dynamics of aboveground primary production. Science 291, 481–484. doi: 10.1126/science.291.5503.481
Lau, J. A., Lennon, J. T. (2012). Rapid responses of soil microorganisms improve plant fitness in novel environments. Proc. Natl. Acad. Sci. U. S. A. 109, 14058–14062. doi: 10.1073/pnas.1202319109
Li, C., Barclay, H., Roitberg, B., Lalonde, R. (2020). Forest productivity enhancement and compensatory growth: A review and synthesis. Front. Plant Sci. 11. doi: 10.3389/fpls.2020.575211
Li, C., Barclay, H., Roitberg, B., Lalonde, R. (2021). Ecology and prediction of compensatory growth: From theory to application in forestry. Front. Plant Sci. 12. doi: 10.3389/fpls.2021.655417
Li, L., Qian, R., Liu, W., Wang, W., Biederman, J. A., Zhang, B., et al. (2022). Drought timing influences the sensitivity of a semiarid grassland to drought. Geoderma 412, 115714. doi: 10.1016/j.geoderma.2022.115714
Liu, X., Zhu, Z., Yu, M., Liu, X. (2021). Drought-induced productivity and economic losses in grasslands from inner Mongolia vary across vegetation types. Reg. Environ. Change 21, 1–12. doi: 10.1007/s10113-021-01789-9
Macdougall, A. S., Mccann, K. S., Gellner, G., Turkington, R. (2013). Diversity loss with persistent human disturbance increases vulnerability to ecosystem collapse. Nature 494, 86–89. doi: 10.1038/nature11869
Macgillivray, C. W., Grime, J. P., The Integrated Screening Programme, T. (1995). Testing predictions of the resistance and resilience of vegetation subjected to extreme events. Funct. Ecol. 9, 640–649. doi: 10.2307/2390156
Mackie, K. A., Zeiter, M., Bloor, J. M. G., Stampfli, A., De Vries, F. (2019). Plant functional groups mediate drought resistance and recovery in a multisite grassland experiment. J. Ecol. 107, 937–949. doi: 10.1111/1365-2745.13102
Ma, W., Liang, X., Wang, Z., Luo, W., Yu, Q., Han, X. (2020). Resistance of steppe communities to extreme drought in northeast China. Plant Soil, 473, 181–194. doi: 10.1007/s11104-020-04767-y
Mangel, M., Munch, S. B. (2005). A life-history perspective on short- and long-term consequences of compensatory growth. Am. Nat. 166, E155–E176. doi: 10.1086/444439
Matos, I. S., Menor, I. O., Rifai, S. W., Rosado, B. H. P., Grytnes, J. A. (2019). Deciphering the stability of grassland productivity in response to rainfall manipulation experiments. Glob. Ecol. Biogeogr. 29, 558–572. doi: 10.1111/geb.13039
Mcnaughton, S. J. (1983). Compensatory plant growth as a response to herbivory. Oikos 40, 329–336. doi: 10.2307/3544305
Meisner, A., De Deyn, G. B., De Boer, W., van der Putten, W. H. (2013). Soil biotic legacy effects of extreme weather events influence plant invasiveness. Proc. Natl. Acad. Sci. U. S. A. 110, 9835–9838. doi: 10.1073/pnas.1300922110
Meng, B., Shi, B., Zhong, S., Chai, H., Li, S., Wang, Y., et al. (2019). Drought sensitivity of aboveground productivity in leymus chinensis meadow steppe depends on drought timing. Oecologia 191, 685–696. doi: 10.1007/s00442-019-04506-w
Metcalfe, N. B., Monaghan, P. (2001). Compensation for a bad start: grow now, pay later? Trends Ecol. Evol. 16, 254–260. doi: 10.1016/S0169-5347(01)02124-3
Michael, J. W., Warren, G. A. (2007). Effects of resource availability on tolerance of herbivory: A review and assessment of three opposing models. Am. Nat. 169, 443–454. doi: 10.1086/512044
Monaghan, P. (2008). Early growth conditions, phenotypic development and environmental change. Philos. Trans. R. Soc Lond. B Biol. Sci. 363, 1635–1645. doi: 10.1098/rstb.2007.0011
Morgan Ernest, S. K., Brown, J. H. (2001). Homeostasis and compensation: The role of species and resources in ecosystem stability. Ecology 82, 2118–2132. doi: 10.1890/0012-9658(2001)082[2118:HACTRO]2.0.CO;2
Mori, A. S., Furukawa, T., Sasaki, T. (2013). Response diversity determines the resilience of ecosystems to environmental change. Biol. Rev. Camb. Philos. Soc 88, 349–364. doi: 10.1111/brv.12004
Niboyet, A., Bardoux, G., Barot, S., Bloor, J. M. G. (2017). Elevated CO2 mediates the short-term drought recovery of ecosystem function in low-diversity grassland systems. Plant Soil 420, 289–302. doi: 10.1007/s11104-017-3377-8
Nielsen, U. N., Ball, B. A. (2015). Impacts of altered precipitation regimes on soil communities and biogeochemistry in arid and semi-arid ecosystems. Glob Chang Biol. 21, 1407–1421. doi: 10.1111/gcb.12789
Nippert, J. B., Knapp, A. K. (2007). Soil water partitioning contributes to species coexistence in tallgrass prairie. Oikos 116, 1017–1029. doi: 10.1111/j.0030-1299.2007.15630.x
Niu, S., Luo, Y., Li, D., Cao, S., Xia, J., Li, J., et al. (2014). Plant growth and mortality under climatic extremes: An overview. Environ. Exp. Bot. 98, 13–19. doi: 10.1016/j.envexpbot.2013.10.004
Oliver, T. H., Heard, M. S., Isaac, N. J. B., Roy, D. B., Procter, D., Eigenbrod, F., et al. (2015). Biodiversity and resilience of ecosystem functions. Trends Ecol. Evol. 30, 673–684. doi: 10.1016/j.tree.2015.08.009
Ovenden, T. S., Perks, M. P., Clarke, T. K., Mencuccini, M., Jump, A. S. (2021). Life after recovery: Increased resolution of forest resilience assessment sheds new light on post-drought compensatory growth and recovery dynamics. J. Ecol. 109, 3157–3170. doi: 10.1111/1365-2745.13576
Parton, W., Morgan, J., Smith, D., Del Grosso, S., Prihodko, L., Lecain, D., et al. (2012). Impact of precipitation dynamics on net ecosystem productivity. Glob Chang Biol. 18, 915–927. doi: 10.1111/j.1365-2486.2011.02611.x
Post, W. M., Emanuel, W. R., Zinke, P. J., Stangenberger, A. G. (1982). Soil carbon pools and world life zones. Nature 298, 156–159. doi: 10.1038/298156a0
Pugnaire, F. I., Morillo, J. A., Peñuelas, J., Reich, P. B., Bardgett, R. D., Gaxiola, A., et al. (2019). Climate change effects on plant-soil feedbacks and consequences for biodiversity and functioning of terrestrial ecosystems. Sci. Adv. 5, eaaz1834. doi: 10.1126/sciadv.aaz1834
Putten, W. H., Bradford, M. A., Pernilla Brinkman, E., Voorde, T. F. J., Veen, G. F., Bailey, J. K. (2016). Where, when and how plant–soil feedback matters in a changing world. Funct. Ecol. 30, 1109–1121. doi: 10.1111/1365-2435.12657
Ren, C., Zhao, F., Shi, Z., Chen, J., Han, X., Yang, G., et al. (2017). Differential responses of soil microbial biomass and carbon-degrading enzyme activities to altered precipitation. Soil Biol. Biochem. 115, 1–10. doi: 10.1016/j.soilbio.2017.08.002
Ruppert, J. C., Harmoney, K., Henkin, Z., Snyman, H. A., Sternberg, M., Willms, W., et al. (2015). Quantifying drylands' drought resistance and recovery: the importance of drought intensity, dominant life history and grazing regime. Glob Chang Biol. 21, 1258–1270. doi: 10.1111/gcb.12777
Saeidnia, F., Majidi, M. M., Mirlohi, A., Spanani, S., Karami, Z., Abdollahi Bakhtiari, M. (2020). A genetic view on the role of prolonged drought stress and mating systems on post-drought recovery, persistence and drought memory of orchardgrass (Dactylis glomerata l.). Euphytica 216. doi: 10.1007/s10681-020-02624-8
Schirpke, U., Kohler, M., Leitinger, G., Fontana, V., Tasser, E., Tappeiner, U. (2019). Future impacts of changing land-use and climate on ecosystem services of mountain grassland and their resilience. Ecosyst. Serv. 26, 79–94. doi: 10.1016/j.ecoser.2017.06.008
Silver, W. L., Ryals, R., Eviner, V. (2010). Soil carbon pools in california’s annual grassland ecosystems. Rangel Ecol. Manag 63, 128–136. doi: 10.2111/REM-D-09-00106.1
Slette, I. J., Blair, J. M., Fay, P. A., Smith, M. D., Knapp, A. K. (2021). Effects of compounded precipitation pattern intensification and drought occur belowground in a mesic grassland. Ecosystems 25, 1265–1278. doi: 10.1007/s10021-021-00714-9
Slette, I. J., Hoover, D. L., Smith, M. D., Knapp, A. K. (2022). Repeated extreme droughts decrease root production, but not the potential for post-drought recovery of root production, in a mesic grassland. Oikos. doi: 10.1111/oik.08899
Smith, M. D. (2011). An ecological perspective on extreme climatic events: a synthetic definition and framework to guide future research. J. Ecol. 99, 656–663. doi: 10.1111/j.1365-2745.2011.01798.x
Song, M. H., Yu, F. H. (2015). Reduced compensatory effects explain the nitrogen-mediated reduction in stability of an alpine meadow on the Tibetan plateau. New Phytol. 207, 70–77. doi: 10.1111/nph.13329
Stampfli, A., Bloor, J. M. G., Fischer, M., Zeiter, M. (2018). High land-use intensity exacerbates shifts in grassland vegetation composition after severe experimental drought. Glob Chang Biol. 24, 2021–2034. doi: 10.1111/gcb.14046
Stuart-Haentjens, E., De Boeck, H. J., Lemoine, N. P., Mand, P., Kroel-Dulay, G., Schmidt, I. K., et al. (2018). Mean annual precipitation predicts primary production resistance and resilience to extreme drought. Sci. Total Environ. 636, 360–366. doi: 10.1016/j.scitotenv.2018.04.290
Taylor, S. H., Ripley, B. S., Woodward, F. I., Osborne, C. P. (2011). Drought limitation of photosynthesis differs between C(3) and C(4) grass species in a comparative experiment. Plant Cell Environ. 34, 65–75. doi: 10.1111/j.1365-3040.2010.02226.x
Tello-García, E., Huber, L., Leitinger, G., Peters, A., Newesely, C., Ringler, M.-E., et al. (2020). Drought- and heat-induced shifts in vegetation composition impact biomass production and water use of alpine grasslands. Environ. Exp. Bot. 169, 103921. doi: 10.1016/j.envexpbot.2019.103921
Tielborger, K., Bilton, M. C., Metz, J., Kigel, J., Holzapfel, C., Lebrija-Trejos, E., et al. (2014). Middle-Eastern plant communities tolerate 9 years of drought in a multi-site climate manipulation experiment. Nat. Commun. 5, 5102. doi: 10.1038/ncomms6102
Tilman, D., Downing, J. A. (1994). Biodiversity and stability in grasslands. Nature 367, 363–365. doi: 10.1038/367363a0
Trenberth, K. E., Dai, A., van der Schrier, G., Jones, P. D., Barichivich, J., Briffa, K. R., et al. (2014). Global warming and changes in drought. Nat. Clim. Change 4, 17–22. doi: 10.1038/nclimate2067
Van Staalduinen, M. A., Anten, N. P. (2005). Differences in the compensatory growth of two co-occurring grass species in relation to water availability. Oecologia 146, 190–199. doi: 10.1007/s00442-005-0225-y
Van Staalduinen, M. A., Dobarro, I., Peco, B. (2009). Interactive effects of clipping and nutrient availability on the compensatory growth of a grass species. Plant Ecol. 208, 55–64. doi: 10.1007/s11258-009-9686-0
Vicente-Serrano, S. M., Quiring, S. M., Peña-Gallardo, M., Yuan, S., Domínguez-Castro, F. (2020). A review of environmental droughts: Increased risk under global warming? Earth Sci. Rev. 201, 102953. doi: 10.1016/j.earscirev.2019.102953
Vilonen, L., Ross, M., Smith, M. D. (2022). What happens after drought ends: synthesizing terms and definitions. New Phytol. 235, 420–431. doi: 10.1111/nph.18137
Vogel, A., Scherer-Lorenzen, M., Weigelt, A. (2012). Grassland resistance and resilience after drought depends on management intensity and species richness. PLoS One 7, e36992. doi: 10.1371/journal.pone.0036992
Volaire, F. (2003). Seedling survival under drought differs between an annual (Hordeum vulgare) and a perennial grass (Dactylis glomerata). New Phytol. 160, 501–510. doi: 10.1046/j.1469-8137.2003.00906.x
Volaire, F., Barkaoui, K., Norton, M. (2014). Designing resilient and sustainable grasslands for a drier future: Adaptive strategies, functional traits and biotic interactions. Eur. J. Agron. 52, 81–89. doi: 10.1016/j.eja.2013.10.002
Wellstein, C., Poschlod, P., Gohlke, A., Chelli, S., Campetella, G., Rosbakh, S., et al. (2017). Effects of extreme drought on specific leaf area of grassland species: A meta-analysis of experimental studies in temperate and sub-Mediterranean systems. Glob Chang Biol. 23, 2473–2481. doi: 10.1111/gcb.13662
Wilcox, K. R., Koerner, S. E., Hoover, D. L., Borkenhagen, A. K., Burkepile, D. E., Collins, S. L., et al. (2020). Rapid recovery of ecosystem function following extreme drought in a south African savanna grassland. Ecology 101, e02983. doi: 10.1002/ecy.2983
Williams, A., De Vries, F. T. (2020). Plant root exudation under drought: implications for ecosystem functioning. New Phytol. 225, 1899–1905. doi: 10.1111/nph.16223
Wise, M. J., Abrahamson, W. G. (2005). Beyond the compensatory continuum: environmental resource levels and plant tolerance of herbivory. Oikos 109, 417–428. doi: 10.1111/j.0030-1299.2005.13878.x
Wu, J., Brookes, P. C. (2005). The proportional mineralisation of microbial biomass and organic matter caused by air-drying and rewetting of a grassland soil. Soil Biol. Biochem. 37, 507–515. doi: 10.1016/j.soilbio.2004.07.043
Xu, C., Ke, Y., Zhou, W., Luo, W., Ma, W., Song, L., et al. (2021). Resistance and resilience of a semi-arid grassland to multi-year extreme drought. Ecol. Indic. 131, 108139. doi: 10.1016/j.ecolind.2021.108139
Yin, J., Bauerle, T. L. (2017). A global analysis of plant recovery performance from water stress. Oikos 126, 1377–1388. doi: 10.1111/oik.04534
Yuan, J., Li, H., Yang, Y. (2020). The compensatory tillering in the forage grass hordeum brevisubulatum after simulated grazing of different severity. Front. Plant Sci. 11. doi: 10.3389/fpls.2020.00792
Keywords: compensatory growth, grassland ecosystem, drought, resilience, recovery, mechanism
Citation: Zhou H, Hou L, Lv X, Yang G, Wang Y and Wang X (2022) Compensatory growth as a response to post-drought in grassland. Front. Plant Sci. 13:1004553. doi: 10.3389/fpls.2022.1004553
Received: 27 July 2022; Accepted: 21 November 2022;
Published: 01 December 2022.
Edited by:
Bernard Roitberg, Simon Fraser University, CanadaReviewed by:
E. Tobias Krause, Friedrich-Loeffler-Institute, GermanyYong Zhang, Southwest Forestry University, China
Wenping Yuan, Sun Yat-sen University, China
Copyright © 2022 Zhou, Hou, Lv, Yang, Wang and Wang. This is an open-access article distributed under the terms of the Creative Commons Attribution License (CC BY). The use, distribution or reproduction in other forums is permitted, provided the original author(s) and the copyright owner(s) are credited and that the original publication in this journal is cited, in accordance with accepted academic practice. No use, distribution or reproduction is permitted which does not comply with these terms.
*Correspondence: Yuhui Wang, eWh3YW5nQGliY2FzLmFjLmNu; Guang Yang, NTQ3NUBjbnUuZWR1LmNu; Xu Wang, d2FuZ3h1MDFAY2Fhcy5jbg==