- Crop Stress Molecular Biology Laboratory, College of Agriculture, Heilongjiang Bayi Agricultural University, Daqing, China
Soybean is an important grain and oil crop. In China, there is a great contradiction between soybean supply and demand. China has around 100 million ha of salt-alkaline soil, and at least 10 million could be potentially developed for cultivated land. Therefore, it is an effective way to improve soybean production by breeding salt-alkaline-tolerant soybean cultivars. Compared with wild soybean, cultivated soybean has lost a large number of important genes related to environmental adaptation during the long-term domestication and improvement process. Therefore, it is greatly important to identify the salt-alkaline tolerant genes in wild soybean, and investigate the molecular basis of wild soybean tolerance to salt-alkaline stress. In this review, we summarized the current research regarding the salt-alkaline stress response in wild soybean. The genes involved in the ion balance and ROS scavenging in wild soybean were summarized. Meanwhile, we also introduce key protein kinases and transcription factors that were reported to mediate the salt-alkaline stress response in wild soybean. The findings summarized here will facilitate the molecular breeding of salt-alkaline tolerant soybean cultivars.
Introduction
Soybean is a world-widely grown crop, providing vegetable oils and proteins. Although soybean yield per acre has increased by approximately 40% during the past 20 years, current soybean production in China is still in severe shortage along with the population increase (USDA, 2021). On the other hand, roughly over 6% of the world’s soil resources are affected by saline and alkaline (data from GSASmap- https://www.fao.org/global-soil-partnership/gsasmap/en/). It is an effective way to expand the total soybean production by planting salt-alkaline tolerant soybean cultivars in the salt-alkaline soils. Therefore, it is an urgent demand to breed soybean cultivars with salt-alkaline tolerance.
Cultivated soybean (Glycine max L. Merr.) is domesticated from wild soybean (Glycine soja Siebold & Zucc.). Only approximately 50% of genes in G. soja are selected during long-term domestication and improvement, and many important genes related to environmental adaptation are lost (Kofsky et al., 2018). The narrow genetic variation in cultivated soybean seriously restricts the improvement of salt-alkaline stress tolerance. G. soja retains a higher level of genetic diversity and better adaptation to harsh environments (Kofsky et al., 2018). An effective way to breed stress-tolerant cultivars is to retrieve the stress tolerant genes from wild soybean and reintroduce them into cultivated soybean.
During the past decades, an increasing number of studies have demonstrated that plant cells were severely damaged by the primary ionic toxicity and secondary oxidative damage under salt-alkaline stress (Das and Roychoudhury, 2014). Therefore, researchers have made many efforts to identify genes involved in ion balancing and ROS scavenging (Zhao et al., 2021). Furthermore, current studies in the model plant Arabidopsis have strongly suggested that protein kinases and transcription factors are of paramount importance in governing the signaling transduction of plant adaptation to salt-alkaline stress (Chen et al., 2021a; Pan et al., 2022).
This review summarized the current research progress regarding the salt-alkaline stress response in G. soja. In this review, we summarized the functionally characterized genes in G. soja that regulated the capacity to the ion toxicity and ROS damage caused by salt-alkaline stress. We also introduced the protein kinases and transcription factors in G. soja that modulated the salt-alkaline tolerance. The findings summarized here will give soybean breeders an overall impression concerning the functional characterization of crucial salt-alkaline tolerant genes in G. soja, and will facilitate to illustrate the regulatory mechanism and signaling transduction pathways, as well as the molecular breeding of salt-alkaline tolerant soybean cultivars.
Regulation of ion toxicity under salt-alkaline stress in wild soybean
Under salt stress, ionic toxicity caused by excess Na+ and Cl- is the direct and primary damage to plants. Excess Na+ accumulation in plants further affects the uptake of other ions (such as K+) and causes osmotic stress, leading to water deficit inside plant cells (Assaha et al., 2017). Hence, balancing cytoplasmic ion status is very important for plants to survive under salt-alkaline stress. In this review, we summarized the genes (Table 1) and regulatory mechanism (Figure 1) of ion balance in G. soja response to salt-alkaline stress.
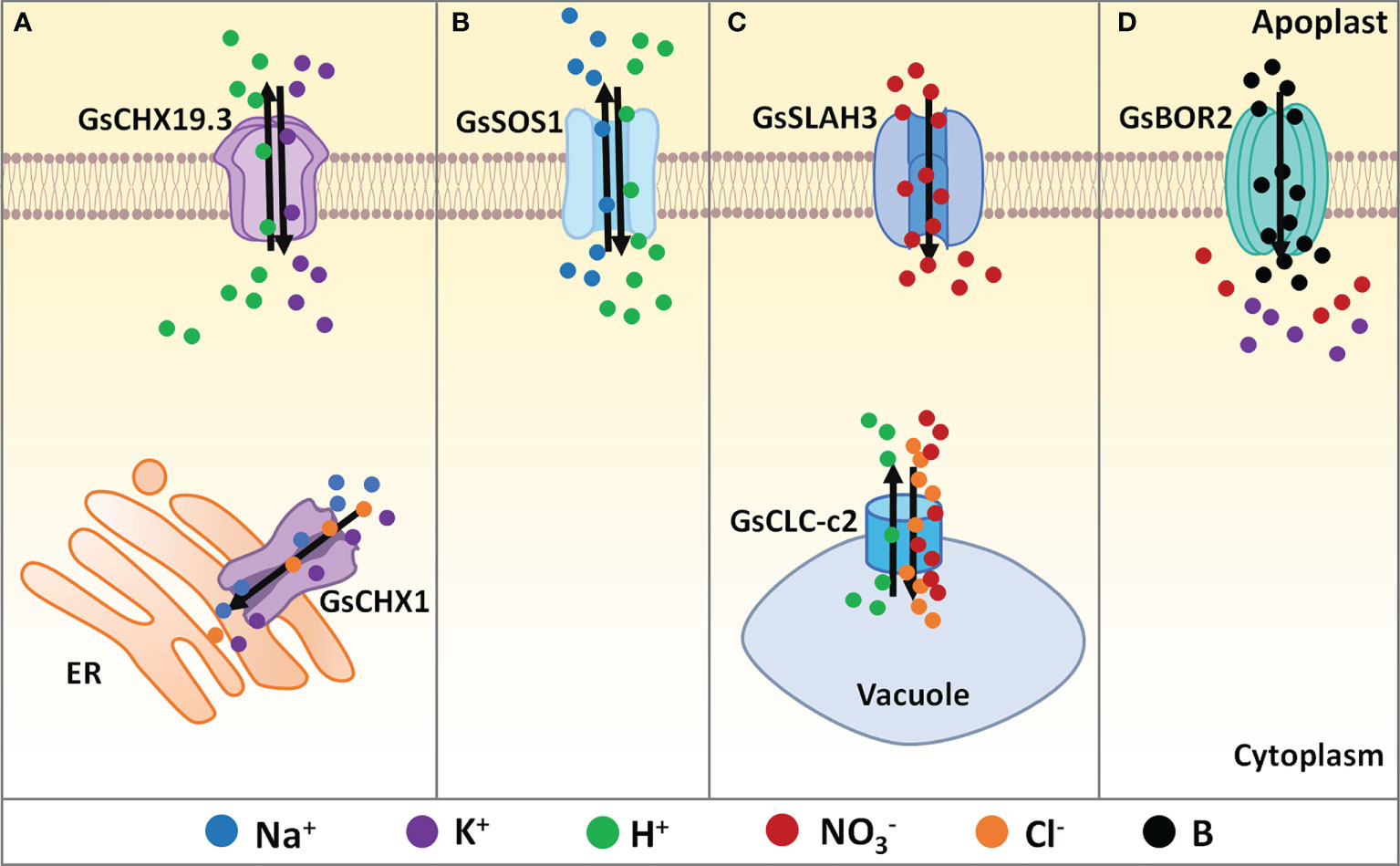
Figure 1 Regulation of ion balance under salt-alkaline stress in wild soybean. (A) Cation/H+ exchangers, (B) Na+/H+ antiporters, (C) Anion channels, (D) Boron transporters identified in G. soja involving in ion balancing under salt-alkaline stress. ER, endoplasmic reticulum. Solid dots with different colors represent different types of ions. Black arrows mean the direction of ion flow across the membrane.
Cation/H+ exchangers
Cation/H+ exchangers (CHXs), belonging to the cation/proton antiporter 2 (CPA2) family, are suggested to mediate K+, Na+, and H+ flow (Isayenkov et al., 2020). In 2014, a salt-tolerant gene Gs/GmCHX1, the homolog of AtCHX20, was identified by whole-genome sequencing of a G. soja accession W05, and genotyping-by-sequencing and phenotypic analyses of a recombinant inbred line (RIL) population (Qi et al., 2014). In G. soja W05, GsCHX1 (Glysoja01g005509) encodes a CHX protein with 811 amino acids in length. In G. max Williams 82 and C08 (one parent of the RIL population), a Ty1/Copia retrotransposon was inserted into the third exon of GmCHX1(Glyma03g32900), resulting in a truncated transcript encoding only 376 residues. In the same year, Guan et al. identified GmSALT3 (salt tolerance-associated gene on chromosome 3) using the RIL population derived from the salt-tolerant variety Tiefeng 8 and the salt-sensitive variety 85-140 (Guan et al., 2014). In 2016, Do et al. isolated a salt tolerance-related QTL from a Brazilian soybean cultivar FT-Abyara, and named the causal gene as GmNcl (Do et al., 2016). Actually, both GmSALT3 and GmNcl are the same gene as GmCHX1. Therefore, GmCHX1/SALT3/Ncl is a critical determinant of salt tolerance in soybean. GmCHX1/SALT3/Ncl contributed to salt tolerance at the seedling stage and conferred improved soybean yield in the field by maintaining a higher seed weight (Do et al., 2016; Liu et al., 2016). However, GmCHX1/SALT3/Ncl does not affect the seedling emergence rate or early vigor under salt stress (Liu et al., 2016). Therefore, identifying the key genes improving the seed germination vigor in saline soil is of great importance for salt-tolerant soybean breeding with GmCHX1/SALT3/Ncl.
In Guan et al. study, 9 haplotypes of GmCHX1/SALT3/Ncl, including 2 salt-tolerant haplotypes and 7 salt-sensitive haplotypes were identified by sequencing 31 soybean landraces and 22 wild soybeans (Guan et al., 2014). In another work, SNPs within the coding region and 2-kb promoter region of Gs/GmCHX1 were highly conserved in the 12 salt-tolerant accessions but varied among the 11 salt-sensitive accessions (Qi et al., 2014). Recently, 40 different haplotypes were identified in 216 G. max and G. soja accessions from Korea, China, and Japan (Lee et al., 2018). In summary, the consequence of these SNPs in salt-sensitive accessions is either the dysfunction of Gs/GmCHX1 protein or the deficient levels of Gs/GmCHX1 transcripts. However, it is still unknown whether there is a regulation of Gs/GmCHX1 at the post-transcriptional level. Besides, the regulatory mechanism of Gs/GmCHX1 transporting activity also remains obscure.
As a CHX family protein, GmCHX1/SALT3/Ncl was found to affect Na+, K+, and Cl– accumulation under salt stress (Figure 1A). Upon salt stress, Na+ accumulation in stems and leaves in the salt-tolerant soybean accessions was significantly less than that in the salt-sensitive accessions (Guan et al., 2014; Do et al., 2016; Liu et al., 2016). The salt-tolerant soybean accumulated less K+ in leaves and stems than the salt-sensitive line (Do et al., 2016). Interestingly, the salt-tolerant NIL line accumulated less Cl− in the leaves and more Cl− in the roots prior to any difference in Na+ (Do et al., 2016; Liu et al., 2016). A recent study showed that GmCHX1/SALT3/Ncl expression contributed to net influx and accumulation of Na+, K+, and Cl– in Xenopus laevis oocytes (Qu et al., 2021). In soybean shoots, GmCHX1/SALT3/Ncl mediated Na+ exclusion by restricting the net xylem loading of Na+, and contributed to Cl- exclusion by re-translocating Cl- back into roots via the phloem. GmCHX1/SALT3/Ncl protein is localized in the endoplasmic reticulum (ER) in plant cells (Guan et al., 2014; Qi et al., 2014). It is still unknown how the ER-localized GmCHX1/SALT3/Ncl affects the Na+ and Cl- exclusion across PM. One possible explanation is that the ER-localized GmCHX1/SALT3/Ncl influences ion gradients across the PM through currently unknown downstream effects. GmCHX1/SALT3/Ncl is also possible to mediate Na+ and Cl- exclusion via an ER-derived vesicle trafficking system.
Similarly, GsCHX19.3 is a plasma membrane localized CHX isolated from bicarbonate stress tolerant G. soja G07256. Overexpression of GsCHX19.3 in Arabidopsis resulted in higher K+ content under normal growth conditions, and lower Na+ accumulation under NaHCO3 treatment (Jia et al., 2017), indicating the role of GsCHX19.3 in both K+ uptake and Na+ excretion (Figure 1A). In Jia et al. study, 34 GsCHXs were identified and phylogenetically clustered into five groups (Group I-V). Only five Group IVa members (GsCHX18.1, GsCHX18.2, GsCHX19.2, GsCHX19.3, and GsCHX20) exhibited enhanced expression at the transcript level under carbonate alkaline stress, indicating the involvement of GsCHX18/19/20s in salt-alkaline stress response. Therefore, further studies are needed to identify the key cis-elements and transcription factors that trigger the expression of GsCHX18/19/20s under salt-alkaline stress.
Na+/H+ antiporters
Na+/H+ antiporters (NHXs) belong to the cation/proton antiporter 1 (CPA1) family. They play critical roles in the exclusion of cytoplasmic Na+ mediated by plasma membrane NHXs and compartmentalization of vacuole Na+ driven by vacuole membrane NHXs (Jia et al., 2018). In Arabidopsis, the plasma membrane SOS1 (Salt Overlay Sensitive 1), also called AtNHX7, is the major transporter removing intracellular Na+ in exchange for extracellular H+. Upon salt stress, SOS3 senses the calcium signal induced by salt stress and interacts with the protein kinase SOS2, thereby activating its kinase activity and recruiting it to the plasma membrane. SOS2 then activates SOS1 Na+/H+ reverse transport activity to exclude cytoplasmic Na+ (Zhu, 2002).
Until now, only one NHX gene GmsSOS1 from G. soja has been functionally reported to modulate salt tolerance (Nie et al., 2015). The GmsSOS1 gene was cloned from G. max and G. soja and encoded a plasma membrane NHX protein. Ectopic expression of GmsSOS1 in the wild type Arabidopsis, as well as the atsos1-1 mutant deleting 14bp (1330-1343bp) (Shi et al., 2000), improved the salt stress tolerance by decreasing Na+ absorption in roots and transportation in shoots (Figure 1A). Considering the importance of NHXs in the regulation of cytoplasmic Na+ concentration, more attention should be paid to identifying G. soja NHX genes and illustrating the main SOS signaling pathway that contributes to salt tolerance.
Anion channels
In addition to cation toxicity caused by Na+, Cl- is the major factor resulting in the anionic toxicity under salt stress. Excessive Cl- in cells disturbs the uptake (Rosales et al., 2020). Therefore, under salt stress, plants must simultaneously cope with cation and anionic toxicity.
The chloride channels (CLCs) could simultaneously transport Cl- and (Jentsch and Pusch, 2018). There are eight CLC genes in soybean, among which the tonoplast-localized CLC-c2 showed different expression patterns between the salt-sensitive G. max N23674 and salt-tolerant G. soja BB52 (Wei et al., 2019). Under salt stress, GsCLC-c2 overexpression in soybean composite plants increased Cl- and Na+ content in roots and reduced Cl- and Na+ accumulation in stems and leaves. Furthermore, GsCLC-c2 overexpression in soybean composite plants also resulted in higher content of in shoots, without significant changes in K+ accumulation (Figure 1B). Consequently, GsCLC-c2 contributes to salt stress tolerance by increasing and K+/Na+ ratios, especially in shoots (Wei et al., 2019; Liu et al., 2021b). In the GsCLC-c2 RNAi composite plants, Cl- content was significantly decreased in roots but increased in stems and leaves, while the content was decreased in roots, stems, and leaves, resulting in the increased ratios (Liu et al., 2021b). In addition, GsCLC-c2 overexpression in the wild type and atclc-c mutant Arabidopsis also led to increased and K+/Na+ ratios in roots under salt stress. The K+/Na+ ratio in shoots of GsCLC-c2 transgenic Arabidopsis was increased under salt stress, however, the ratio was not altered (Liu et al., 2021b). Researchers also suggested that GsCLC-c2 could transport both Cl- and by using a two-electrode voltage clamp on Xenopus laevis oocytes (Wei et al., 2019). Therefore, GsCLC-c2 plays a key positive role in regulating G. soja salt-alkaline tolerance by simultaneously transporting Cl- and . More importantly, CLC-c2 is the only gene with non-synonymous changes between G. max N23674 and G. soja BB52. It is important to investigate the sequence variations of the CLC-c2 gene in wild and cultivated soybean populations and identify the salt-alkaline tolerant haplotypes.
Besides CLCs, slow anion channels (SLACs) and SLAC homologs (SLAHs) also play critical roles in anion transport (Barbier-Brygoo et al., 2011). Most characterized SLAC/SLAHs are conductive. An alkaline stress-induced SLAH gene GsSLAH3, the homologous gene to AtSLAH3, was identified in the bicarbonate (NaHCO3) stress tolerant G. soja G07256 based on RNA-seq data. Transgenic Arabidopsis over-expressing GsSLAH3 displayed higher tolerance to stress (NaHCO3 and KHCO3) rather than high pH stress, by increasing accumulation in shoots (Duan et al., 2017). Consistently, under NaHCO3 treatment, the concentrations in the T-DNA insertion Arabidopsis mutants atslah3-1 and atslah3-2 were relatively lower than WT (Zheng et al., 2015) (Figure 1B). It is possible that GsSLAH3 specifically controls the response to carbonate salt-alkaline stress, not high pH stress, by mediating transport. However, direct evidence supporting the conductivity of GsSLAH3 to is still missing. Further studies should also analyze the sequence and expression differences of SLAH genes between G. soja and G. max.
Boron transporters
Boron (B) deficiency decreases uptake and increases K+ leakage across the plasma membrane. Plant B transporters (BORs) belong to the solute carrier (SLC4) family, which is homologous to the human bicarbonate transporter-related protein HsBTR1, and each BOR protein harbors a sodium-coupled bicarbonate transporter domain (Saouros et al., 2021). A recent study reported that a G. soja BOR gene GsBOR2 could improve the tolerance to bicarbonate stress (NaHCO3 and KHCO3) rather than high pH stress (Duan et al., 2018). Functional complementation showed that GsBOR2 restored the sensitivity of Scbor1 mutant yeasts to H3BO3 treatment, suggesting the involvement of boron and BORs in salt-alkaline stress response (Duan et al., 2018) (Figure 1C). However, direct evidence is required to characterize whether BORs affect uptake and K+ leakage under bicarbonate stress.
Till now, only these six G. soja genes encoding ion transporters have been functionally characterized to maintain ion balance and alleviate ion toxicity under salt-alkaline stress. Among them, GmCHX1 has been suggested to transport Na+, K+, and Cl– (Qu et al., 2021), and GsCLC-c2 transported Cl- and (Wei et al., 2019). Even though the regulation of GsCHX19.3, GmsSOS1, GsSLAH3, and GsBOR2 on the cytoplasmic ion accumulation has been studied through functional complementation in yeast mutants and quantification analyses of ion contents in transgenic plants, direct biochemical evidence is still lacking to verify their transporting properties. Therefore, techniques monitoring ion fluctuation in real-time, for example, non-invasive micro-test technology and two photon-total internal refraction fluorescence microscopy, should be applied to directly verify the transporting capability of ion transporters in G. soja. Besides, there are still a few points that need further detailed investigation. Firstly, it is imperative to investigate whether these ion transporter genes could improve soybean yields in the salt-alkaline fields. Secondly, further studies are needed to reveal the regulatory mechanism of the activity of these ion transporters, for example, identifying the protein kinases that trigger their transporting activity and the transcription factors that promote their transcription.
ROS signaling and scavenging under salt-alkaline stress in wild soybean
Under salt-alkaline stress, ion imbalance promotes the generation and accumulation of reactive oxygen species (ROS) in plant cells. ROS play dual roles in plant response to salt-alkaline stress. At low concentrations, it can serve as a signaling molecule, which activates downstream signal transduction under salt-alkaline stress. However, ROS accumulation at high concentrations leads to oxidative damage or apoptotic death and damages plant cells (Liu et al., 2021c). Plants have developed enzymatic and nonenzymatic antioxidant defense systems to protect against oxidative damage. For now, a serial of genes involved in ROS signaling and scavenging have been transcriptionally and functionally characterized to regulate salt-alkaline stress tolerance in G. soja (Table 2; Figure 2).
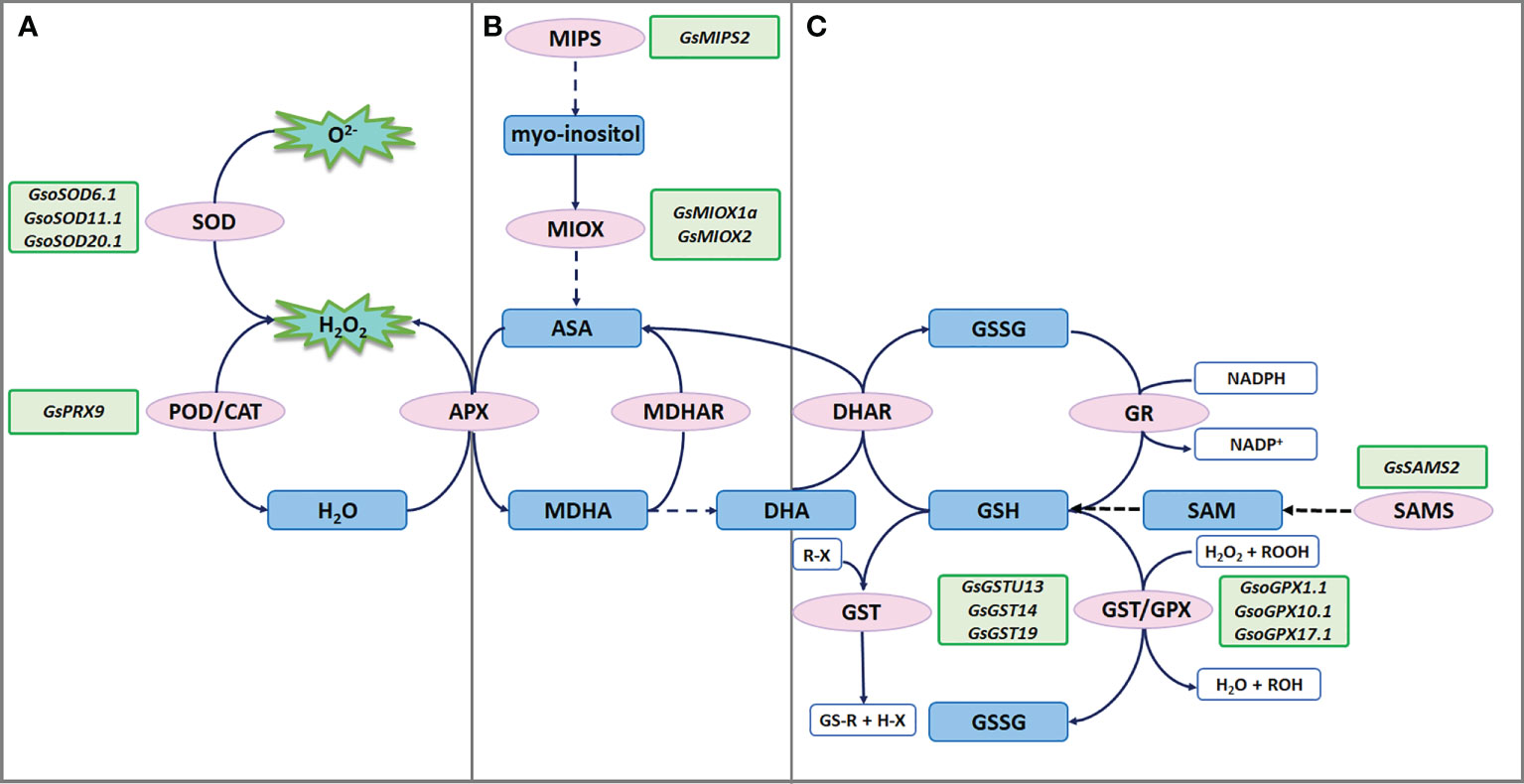
Figure 2 ROS scavenging under salt-alkaline stress in wild soybean. (A) SODs and PRXs in enzymatic antioxidant defense system. (B) MIPSs and MIOXs responsible for AsA biosynthesis. (C) GSTs, GPXs and SAMSs responsible for glutathione mediated nonenzymatic antioxidant defense system.
ROS-activated signaling under salt-alkaline stress in wild soybean
Previous studies have demonstrated the positive role of ROS in salt-alkaline stress response by activating the downstream signaling. Exogenous H2O2 application could improve salt tolerance in rice and maize (Uchida et al., 2002; Azevedo Neto et al., 2005). ROS-production deficiency Arabidopsis mutants atrbohd and atrbohf are sensitive to salt stress (Ben Rejeb et al., 2015). Recently, it has been reported that H2O2 could sulfenylate TSB1 (tryptophan synthase β subunit 1) to increase ABA accumulation, thus increasing salt stress tolerance in Arabidopsis (Liu Y. L. et al., 2022). A recent study in G. max reported that a ROS-involving positive feed-forward loop acted as a signal amplifier in salt stress response (Li et al., 2019). Overexpression of a NAC transcription factor GmSIN1 (SALT INDUCED NAC1) in soybean promoted root growth and salt tolerance and increased yield under salt stress. GmSIN1 upregulated expression of GmRbohB (Respiratory burst oxidase homolog B) genes to generate ROS, and ROS further induced GmSIN1 expression under salt stress (Li et al., 2019). Even though researchers have unraveled the ROS-mediated regulatory mechanism in plant response to salt stress, its role is rarely reported in G. soja. A previous study reported that a methionine sulfoxide reductase B (MSRB) gene in G. soja GsMSRB5a could regulate alkaline stress tolerance by modifying the expression of ROS signaling genes (Sun et al., 2016). Therefore, more studies are needed to clarify the positive regulatory mechanism mediated by ROS on salt-alkaline tolerance in G. soja.
Antioxidant enzymes
Enzymes that detoxify ROS include superoxide dismutases (SODs), catalases (CATs), peroxidases (PODs), glutathione peroxidases (GPXs), ascorbate peroxidases (APXs), monodehydroascorbate reductases (MDHARs), dehydroascorbate reductases (DHARs), and glutathione reductases (GRs). Among them, SODs serve as the front line of antioxidant defense by catalyzing radicals into H2O2 and molecular oxygen (O2). A recent study showed that G. soja W05 had a total of 13 SOD genes (GsoSODs), and the expression of three GsoSODs (GsoSOD6.1, GsoSOD11.1, and GsoSOD20.1) has been found to respond to salt stress via qRT-PCR analyses (Aleem et al., 2022). Under 250mM NaCl treatment, GsoSOD11.1 showed continuously up-regulated expression (Figure 2A). The increase in GsoSODs expression will help to remove radicals.
H2O2 is subsequently removed by CATs and PODs. In plants, PODs are divided into three classes: class I (Ascorbate peroxidase, APXs), class II (lignin peroxidases), and class III (secretory peroxidases, PRXs). Class III PRXs are bifunctional enzymes that could scavenge ROS and also produce ROS. A recent study suggested that overexpression of salt stress-induced G. soja PRX gene GsPRX9 in soybean composite plants improved salt tolerance by increasing both POD and SOD activity, and glutathione levels (Jin et al., 2019) (Figure 2A). The coding sequence of GsPRX9 is identical to that of GmPRX9. Interestingly, in G. max, GmPRX9 expression showed a significantly higher increase in the salt-tolerant varieties than the salt-sensitive varieties after salt treatment. These findings imply that the expression level of PRX9 is related to the salt tolerance of soybean. Therefore, it will be interesting to check the sequences of the PRX9 promoter in the soybean natural population to identify the haplotype that confers higher expression levels of PRX9 and higher salt tolerance.
Even though the expression response and genetic function of CAT and APX genes under salt-alkaline stress have not been reported in G. soja, and CATs and APXs indeed play crucial roles in ROS scavenging in wild soybean response to salt-alkaline stress. For example, compared with a cultivated soybean ZH13, the wild soybean BB52 grown in coastal saline land in the Yellow River Delta displayed higher CAT and APX activities under salt stress (Chen et al., 2013). Therefore, it is essential to identify CATs and APXs genes in G. soja that could improve the tolerance to salt-alkaline stress.
Ascorbic acid
Ascorbic acid (AsA) contributes to abiotic stress tolerance by scavenging ROS through the AsA-GSH cycle. Myo-inositol is one of the main precursors of AsA biosynthesis, while L-myo-inositol-1-phosphate synthases (MIPSs) are rate-limiting enzymes in myo-inositol biosynthesis (Donahue et al., 2010). There are three MIPS genes in soybean. According to the public RNA-seq data of the salt-alkaline tolerant G. soja 07256, GsMIPS2 expression was dramatically increased by about 20 folds at 3 h treated with 50 mM NaHCO3, which was further verified via qRT-PCR analyses. Transcript levels of GsMIPS3 was decreased, while GsMIPS1 expression was not changed by NaHCO3 treatment. Consistently, GsMIPS2 overexpression in Arabidopsis conferred increased tolerance to NaHCO3 and NaCl stresses, and the T-DNA insertion Arabidopsis mutant atmips2 displayed reduced tolerance (Chen et al., 2015b; Nisa, 2016) (Figure 2B).
Myo-inositol oxygenases (MIOXs) catalyze the conversion of myo-inositol into D-glucuronic acid (D-GlcUA), which is finally oxidized into AsA (Endres and Tenhaken, 2009). A previous study reported that there were 5 MIOX genes in wild soybean and GsMIOX1a expression displayed a noticeable increase at 6 h treated with 50 mM NaHCO3 (Chen et al., 2015a). Interestingly, expression of GsMIOX1a was dramatically induced by NaHCO3 treatment in G. soja accessions (G07256 and G50109), but not in G. max accessions (Suinong 28 and Hefeng 55). This difference indicates that GsMIOX1a might be a key factor for G. soja response to NaHCO3 stress. Genetic evidence suggests that GsMIOX1a overexpression in Arabidopsis improved POD activity and increased the bicarbonate alkaline stress tolerance (Chen et al., 2015a) (Figure 2B). Taken together, it is absolute that AsA biosynthesis might contribute to soybean tolerance to salt-alkaline stress, possibly via the AsA-GSH cycle.
Glutathione
Glutathione is one important component of the nonenzymatic antioxidant system, having two forms reduced glutathione (GSH) and oxidized glutathione (GSSG). The glutathione peroxidases (GPXs) catalyze the oxidation of GSH to produce GSSG, reducing H2O2 or organic hydroperoxide to H2O and alcohol, which will protect cells against ROS-mediated oxidative damage. A recent study showed that G. soja W05 has 13 GPX (GsoGPXs) genes, three of which were responsive to salt stress (Aleem et al., 2022). Under 250mM NaCl treatment, GsoGPX10.1 showed continuously up-regulated expression (Figure 2C). Even though no genetic evidence supporting the function of GsoGPXs in regulating salt tolerance has been given, it is easy to speculate that the increase in GsoGPXs transcript levels will facilitate the removal of H2O2 or organic hydroperoxide.
S-Adenosyl-L-Methionine Synthetases (SAMSs) catalyze the synthesis of S-Adenosyl-L-Methionine (SAM), which generates glutathione (GSH) through sulfur transfer. Hence, the activity of SAMSs is closely related to the GSH content in plant cells (Hasanuzzaman et al., 2017). It has been reported that ectopic expression of a G. soja SAMS gene GsSAMS2 driven by a stress-inducible promoter RD29A conferred salt tolerance in Medicago sativa (Hua et al., 2012) (Figure 2C). It will be an easy way to improve the GSH content by overexpressing SAMS genes, which can help plants to cope with ROS damage.
The glutathione S-transferases (GSTs) catalyze the conjugation of GSH to the electrophilic groups of a large variety of hydrophobic molecules to detoxify cells. Under abiotic stress, GSTs detoxify the lipid hydroperoxides generated in membrane peroxidation. GSTs could be grouped into six classes, and most GSTs in plants belong to the phi (GSTF) and tau (GSTU) classes. Several studies have revealed the regulatory function of GSTUs in G. soja response to salt-alkaline stress (Figure 2C). A previous study isolated a GSTU gene GsGST from the cDNA library of salt-treated G. soja 50109 seedlings, and overexpression of GsGST in Nicotiana tabacum significantly improved the salt and drought tolerance (Ji et al., 2010). Furthermore, three G. soja GSTUs GsGST13 (also named GsGSTU13), GsGST14 and GsGST19 were found to contribute to higher tolerance under both salt (NaCl), and bicarbonate (NaHCO3) stresses (Wang et al., 2012a; Wang et al., 2012b; Jia et al., 2015). In another study, overexpression of GsGSTU24 and GsGSTU42 in soybean hairy root composite plants and transgenic Arabidopsis seedlings significantly enhanced antioxidant ability to detoxify ROS damage under submergence stress (Li et al., 2020). Experimental data are needed to determine whether GsGSTU24 and GsGSTU42 regulate salt-alkaline tolerance. In addition, a lambda class GST gene GSTL1 was suggested to be induced by salt stress by over 7 folds in G. soja W05, compared to less than 4 folds in G. max C08 (Chan, 2014). GSTL1 expression in tobacco BY-2 cells and Arabidopsis increased survival rates under salt stress by reducing ROS accumulation. In summary, GST genes are important candidates for improving salt-alkaline tolerance via transgene technology.
Flavonoids
Flavonoids are a group of secondary metabolites derived from the phenylpropanoid pathway, including flavonol, flavanol, flavone, isoflavone, flavanone, proanthocyanidins, and anthocyanin (Liu et al., 2021d). Current studies have demonstrated that flavonoids, as antioxidants, are closely associated with salt-alkaline stress tolerance in soybean. For example, the knockout of a flavone synthase (FNS) gene GmFNSII in soybean hairy roots reduced the salt tolerance. GmMYB183 activated the expression of GmCYP81E11 (Cytochrome P450 monooxygenase) and increased the accumulation of monohydroxy B-ring flavonoids, which negatively regulates soybean tolerance to salinity (Pi et al., 2019). Another MYB transcription factor GmMYB173 directly activated the transcription of GmCHS5 and contributed to salt tolerance by enhancing the accumulation of dihydroxy B-ring flavonoids (Pi et al., 2018). Recently, researchers have found that soybean HSFB2b, a class B heat shock factor, improved salt tolerance by promoting flavonoid biosynthesis (Bian et al., 2020). Four haplotypes of HSFB2b in its promoter region were identified among the population consisting of 38 wild soybean and 113 cultivated soybean accessions, and haplotypes II and III from salt-tolerant wild soybean display higher promoter activity under salt stress. The distribution frequency of haplotype III, which showed the highest promoter activity, in cultivated soybeans was very low. Therefore, it will be an effective way to introduce the haplotype III promoter of GsHSFB2b into cultivated soybean to breed salt-tolerant cultivars.
ROS balance is regulated by generation, removal, and transportation. Currently, most studies in G. soja focused on the regulation of ROS scavenging under salt-alkaline stress. Genes responsible for ROS generation in G. soja have not been reported until now. Furthermore, little is known about the positive role of ROS-activated signaling pathways in G. soja. Therefore, future studies regarding ROS-mediated regulation on salt-alkaline response should focus on two points. Firstly, respiratory burst oxidase homologues (Rboh) genes in G. soja should be systematically investigated to uncover the mechanism driving the reduction of ROS generation under salt-alkaline stress. Secondly, identification of target genes that are directly activated by ROS at low concentrations is urgently needed to understand the positive role of ROS by serving as signaling molecules under salt-alkaline stress.
Protein kinases involved in salt-alkaline stress response in wild soybean
Protein kinases (PKs) transfer phosphate groups from adenosine triphosphate (ATP) to serine, threonine, or tyrosine residues of substrate proteins, thereby directly affect substrates’ function by modifying their activity, 3D structure, subcellular localization, or protein stability. The PK superfamily is one of the largest and most highly conserved families in plants. There are 2166 putative PK genes in soybean genome, representing 4.67% of all protein-coding genes (Liu et al., 2015). PKs could be classified into different families based on sequence similarity and domain structure within and outside the catalytic domains. In G. soja, several kinase genes from the RLKs (receptor like kinases), MAPKs (mitogen-activated protein kinases), and SnRKs (sucrose non-fermenting1-related protein kinases) families have been reported to play important roles in signal transduction under salt-alkaline stress (Table 3; Figure 3).
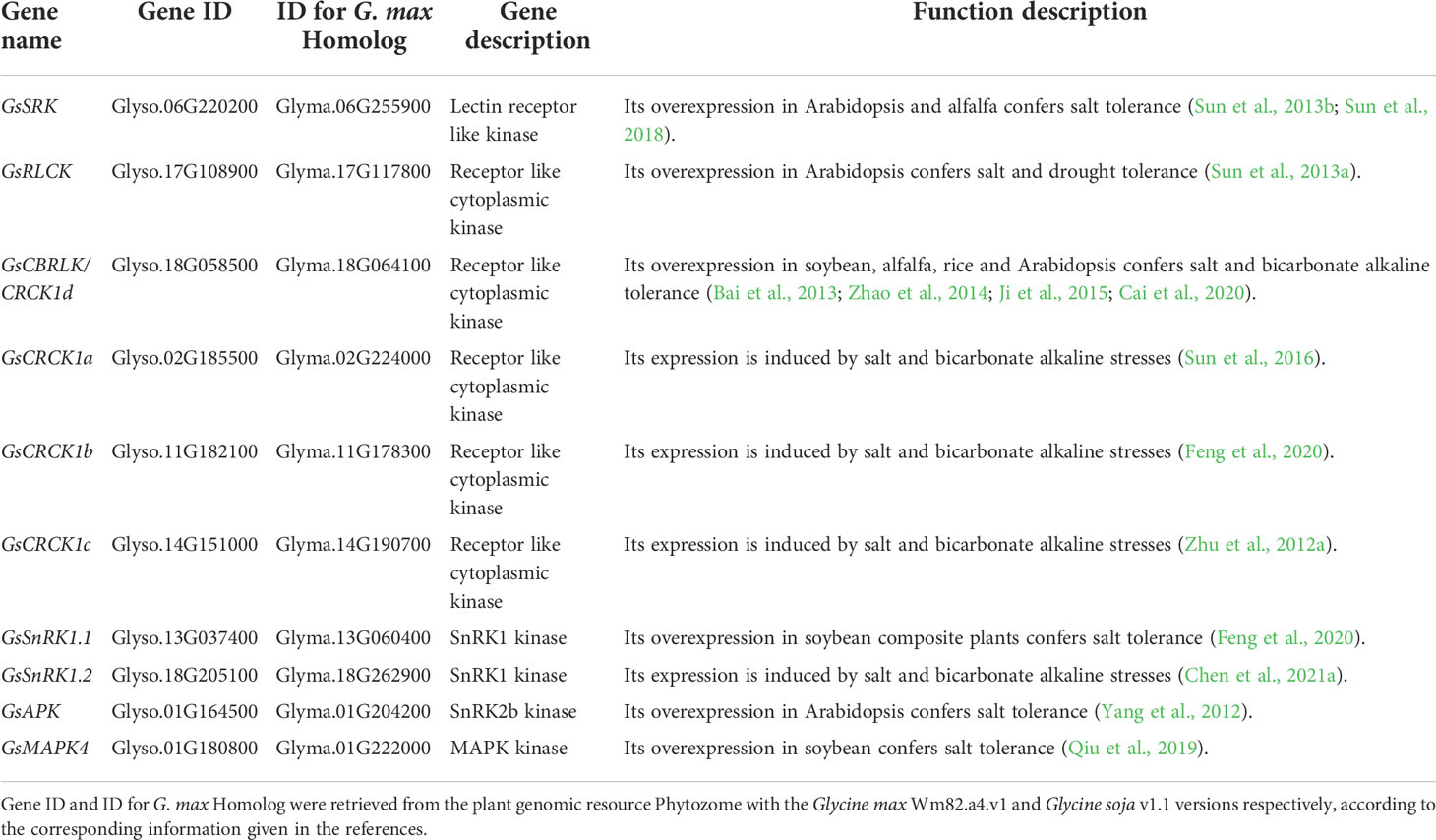
Table 3 The genes encoding protein kinases involved in regulating salt-alkaline stress response in wild soybean.
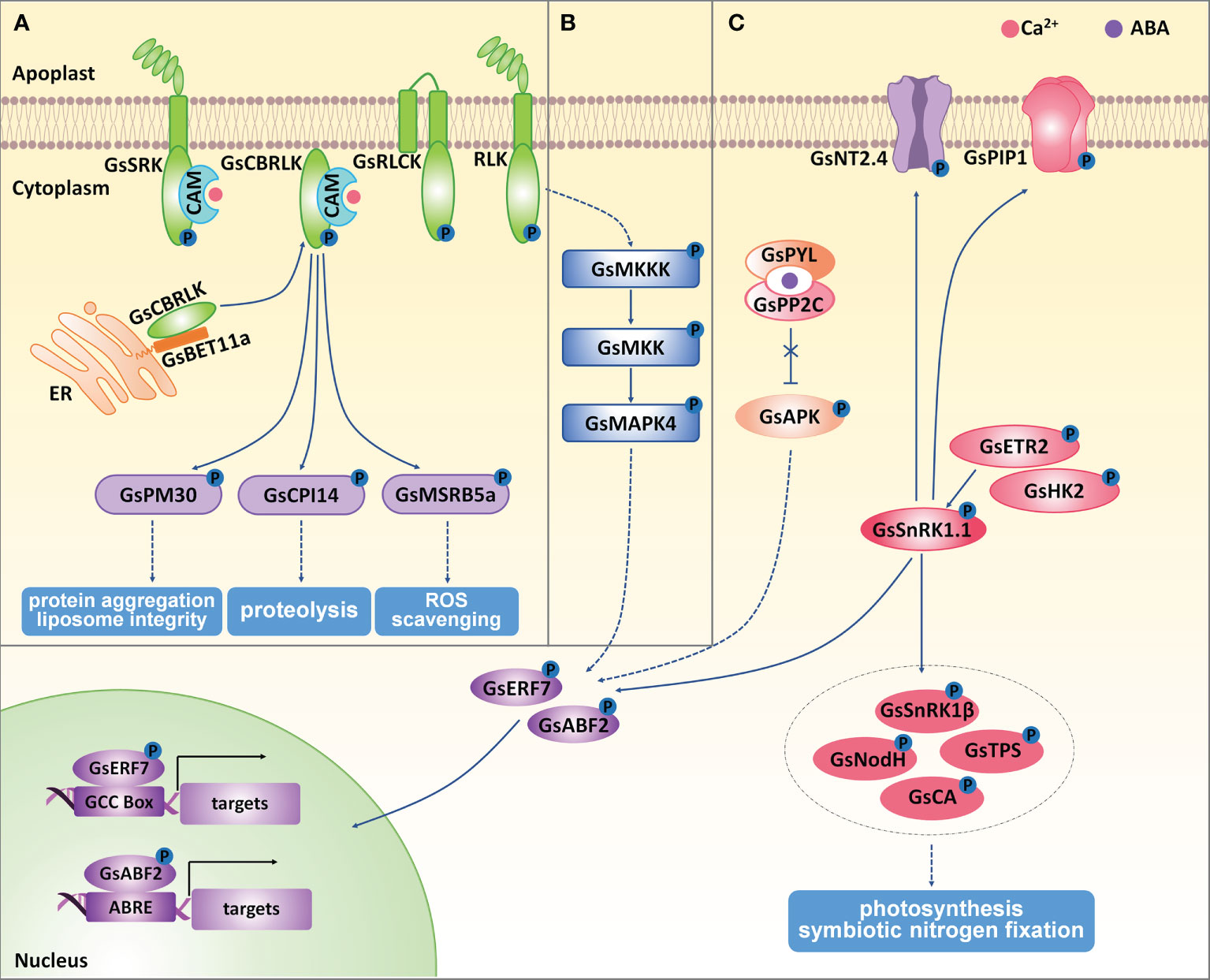
Figure 3 The regulation of protein kinases on wild soybean response to salt-alkaline stress. (A) Receptor like kinases, (B) MAPK kinases, (C) SnRK kinases mediated stress response in G. soja under salt-alkaline stress. ER, endoplasmic reticulum. Solid lines represent direct physical interaction, and dotted lines represent indirect interaction or results. Arrows represent positive effects, and lines ending with a short bar indicate negative effects.
Receptor like kinases
RLKs consist of an extracellular domain to receive signal molecules, a transmembrane domain and a cytoplasmic kinase catalytic domain. RLKs could be further classified into different groups, for example, LRR-RLK (leucine-rich repeat RLK), LecRLK (Lectin RLK), RLCK (cytoplasmic RLK). In soybean, 1418 genes were identified as RLKs (Liu et al., 2015), including 467 LRR-RLKs (Zhou et al., 2016) and 185 LecRLKs (Liu et al., 2018). Until now, only a few G. soja RLKs were reported to involve in salt-alkaline stress (Figure 3A).
GsSRK encodes a LecRLK protein from G. soja, whose extracellular domain consists of an N-terminal signal peptide, a G-type lectin domain, an S-locus-glycop domain, and a PAN-AP domain (Sun et al., 2013b). GsSRK expression was greatly and rapidly induced by ABA, salt, and drought stresses. GsSRK overexpression in Arabidopsis enhanced salt stress tolerance and promoted seed yields under salt stress (Sun et al., 2013b). Furthermore, ectopic expression of the full-length (GsSRK-f) and truncated GsSRK deleting the G-type lectin domain (GsSRK-t) in alfalfa both increased salt tolerance by improving the capacity in ion homeostasis, ROS scavenging, and osmotic regulation. Interestingly, GsSRK-t transgenic lines were more tolerant to salt stress than GsSRK-f lines, showing more branches and higher fresh weight under salt stress. However, no difference in the physiological indices under salt stress was detected between GsSRK-f and GsSRK-t transgenic lines (Sun et al., 2018). Interestingly, overexpression of GsSRK-t, but not GsSRK-f, caused more branches and shorter shoots, indicating a potential role of the extracellular G-type lectin domain in regulating plant architecture. Therefore, GsSRK is an ideal target to improve yield under salt stress.
Current studies also demonstrated the important roles of G. soja RLCKs in regulating salt-alkaline stress tolerance. GsRLCK encodes a plasma membrane localized RLCK, containing a conserved catalytic domain and two transmembrane domains at the N-terminus (Sun et al., 2013a). The expression of GsRLCK was induced by ABA, salt, alkaline, and drought stresses. GsRLCK overexpression in Arabidopsis increased salt and drought tolerance by promoting the expression of salt responsive marker genes. Furthermore, a group of calcium/calmodulin-binding receptor-like cytoplasmic kinases (CRCKs) in G. soja (GsCRCK1a-d) were reported to function in salt-alkaline stress response. GsCRCK1s, without any extracellular or transmembrane domain, contained only intracellular catalytic domains whose amino acid sequences are highly conserved. The N-terminal sequences of GsCRCK1s were quite variable, named the variable domain. This domain has been demonstrated to fulfill a crucial role in mediating the protein-protein interaction and subcellular localization (Sun et al., 2016; Sun et al., 2019; Sun et al., 2021). It has been suggested that GsCRCK1d, also named GsCBRLK (G. soja calcium/calmodulin-binding receptor-like kinase), localized on plasma membrane in plant cells. The N-terminal variable domain was responsible for its plasma membrane localization (Yang et al., 2010; Sun et al., 2021). GsCBRLK was initially characterized to confer salt and carbonate alkaline tolerance by overexpressing in Arabidopsis (Yang et al., 2010; Sun et al., 2016). Further studies verified its positive regulation on soybean (Ji et al., 2015), alfalfa (Bai et al., 2013; Zhao et al., 2014), and rice (Cai et al., 2020) tolerance to both salt and carbonate alkaline stresses.
Current studies have identified the interacting partners of GsCBRLK and illustrated the functional mechanism and signal transduction mediated by GsCBRLK in salt-alkaline stress response (Yang et al., 2010; Sun et al., 2014; Sun et al., 2016; Sun et al., 2019; Sun et al., 2021). Upon salt-alkaline stress, cytoplasmic free Ca2+ ([Ca2+]cyt) was rapidly accumulated in a short time, and this [Ca2+]cyt oscillation was sensed by calmodulins (CaMs). The Ca2+/CaM complex activated GsCBRLK kinase activity by directly interacting with CaM and the CaM binding domain within GsCBRLK (Yang et al., 2010). Recently, a group of BET1-like soluble NSF attachment protein receptor (SNARE) proteins (GsBET11a/b/c and GsBET12a/b) were suggested to mediate the plasma membrane localization of GsCBRLK by directly interacting with the N-terminal variable domain of GsCBRLK, and contributed to salt stress tolerance (Sun et al., 2021). For now, three types of GsCBRLK interacting partners, including a cysteine proteinase inhibitor GsCPI14 (Sun et al., 2014), several group 3A late embryogenesis abundant (LEA) proteins (Sun et al., 2019), methionine sulfoxide reductase B (MSRB) subfamily proteins (Sun et al., 2016), have been identified as potential downstream substrates of GsCBRLK. Among them, GsCPI14 was suggested to regulate the proteolysis process and confer the tolerance to carbonate alkaline stress. The group 3A LEA gene GsPM30 was found to improve salt tolerance, possibly by preventing protein aggregation and maintaining liposome integrity. GsMSRB5a overexpression in Arabidopsis could increase the tolerance to carbonate alkaline stress by enhancing the ROS scavenging capacity. Even though no biochemical evidence has been given to show the direct phosphorylation of GsCBRLK on these proteins, there are two pieces of evidence supporting that GsCBRLK might work upstream of GsMSRBs. Firstly, GsCBRLK overexpression in Arabidopsis, soybean and alfalfa conferred tolerance to both salt and carbonate alkaline stresses, while GsMSRB5a only activated carbonate alkaline stress responses when overexpressed in Arabidopsis. Secondly, the total MSR enzyme activity was obviously increased in both GsCBRLK and GsMSRB5a transgenic lines. Therefore, future studies are needed to determine the phosphorylation of GsCBRLK on its substrates. Moreover, it will be helpful to identify the upstream regulators that directly interact with and activate GsCBRLK.
MAPK kinases
The MAPK kinase cascade has been well documented to play important roles in abiotic stress response in plants (Lin et al., 2021). A MAPK module generally comprises three kinases: a MAPKKK (MAPK kinase), a MKK (MAPK kinase), and a MAPK. In this module, MAPKKKs phosphorylate and activate MKKs, and MKKs then phosphorylate and activate MAPKs. In soybean, there are 38 MAPKs, 11 MKKs, and 150 MKKKs. According to the amino acid sequences, these 38 MAPKs could be clustered into four groups (Group A-D) (Neupane et al., 2013). Even though a number of researches have strongly demonstrated the important roles of MAPK pathway in abiotic stress response in Arabidopsis and rice, only two MAPK kinases in G. max, GMK1/GmMPK6 (Group D) (Im et al., 2012; Im et al., 2021) and GmMMK1 (Group B) (Liao et al., 2021), have been functionally characterized to regulate salt tolerance. By association analysis of GmMKK1 sequence variations and salt tolerance in the natural soybean population, researchers showed that the sequence variation in GmMKK1 promoter was the main reason for the functional differences of GmMKK1 in the natural population in terms of the germination rates under salt treatment. In G. soja, GsMAPK4 (Group B) was the only MAPK kinase reported to participate in salt stress response. GsMAPK4 expression was up-regulated under salt stress, and overexpression of GsMAPK4 in G. max significantly improved the tolerance to salt stress (Qiu et al., 2019) (Figure 3B). However, the upstream MKKs and MKKKs of GsMAPK4 are still unknown. Therefore, it is vital to identify the core MAPK components in soybean response to salt-alkaline stress.
SnRK kinases
In plants, SnRK family kinases are classified into SnRK1, SnRK2, and SnRK3 subfamilies (Wang et al., 2019b). In G. soja, there are four SnRK1 genes named GsSnRK1.1-1.4 (Chen et al., 2021b). Among them, the expression of GsSnRK1.1 and GsSnRK1.2 were induced by both salt (NaCl) and bicarbonate alkaline (NaHCO3) stresses, while GsSnRK1.3 and GsSnRK1.4 were not affected by either salt or bicarbonate alkaline stress. By using the Agrobacterium rhizogenes-mediated transformation of soybean hairy roots, GsSnRK1 (identical to GsSnRK1.1) overexpression contributed to higher salt tolerance (Feng et al., 2020). Researchers have identified the putative substrates of GsSnRK1 by using the yeast two-hybrid assays (Song et al., 2019) and the quantitative phosphoproteomics technology (Li et al., 2022) (Figure 3C). Phosphorylation assays found that GsSnRK1 phosphorylated plasma membrane-localized GsNT2.4 (Nitrate transporter 2.4) and GsPIP1 (Plasma membrane intrinsic protein 1), which might be involved in the nitrate and water uptake under salt-stress. GsSnRK1β (SnRK1 beta subunit), GsNodH (Sulfotransferase NodH), GsTPS (Alpha, alpha-trehalose‐phosphate synthase) and GsCA (Carbonic anhydrase) were also phosphorylated by GsSnRK1, suggesting the possible role of GsSnRK1 in the regulation of photosynthesis and symbiotic nitrogen fixation. Furthermore, two transcription factors GsERF7 (ethylene-responsive factor 7) and GsABF2 (ABRE‐binding bZIP factor 2) were also demonstrated to be phosphorylation substrates of GsSnRK1 (Li et al., 2022). The phosphorylation of GsERF7 by GsSnRK1 could facilitate its translocation from the cytoplasm to the nucleus and enhance its transactivation activity. GsERF7 could bind the GCC cis-acting element and up-regulate the expression levels of abiotic stress-responsive and hormone synthetic genes. GsERF7 overexpression, especially co-overexpression with GsSnRK1, could significantly improve soybean tolerance to salt and bicarbonate alkaline stresses (Feng et al., 2020). In addition, two phytohormone‐related histidine kinases GsHK2 (Histidine Kinase 2-like) and GsETR2 (Ethylene receptor 2-like), whose C‐terminal kinase domain interacted with GsSnRK1, were suggested to phosphorylate GsSnRK1.1 in plant cells (Song et al., 2019). In the future, more genetic and physiological experiments are needed to clearly show the regulatory function of these GsSnRK1 interacting partners in salt-alkaline stress response.
SnRK2 kinases have been well-demonstrated as core components of ABA-mediated stress signaling. An SnRK2b-type kinase from G. soja, GsAPK, was found to contribute to salt stress tolerance when ectopically expressed in Arabidopsis (Yang et al., 2012). GsAPK exhibited ABA-activated and Ca2+-independent kinase activity (Figure 3C). The first abscisic acid-activated and Ca2+-independent protein kinase (AAPK) was identified in the fava bean (Li and Assmann, 1996). AtSnRK2.6/OST1 (Open stomatal 1) is the homologous protein to fava bean AAPK, and also displayed ABA-activated and Ca2+-independent kinase activity (Maszkowska et al., 2021). It is possible that the negative regulator PP2Cs was inhibited upon ABA treatment, thereby resulting in the successful activation of GsAPK. As expected, GsAPK overexpression Arabidopsis displayed higher ABA insensitivity, suggesting that GsAPK regulated salt tolerance via the ABA dependent signaling pathway. In Arabidopsis, SnRK2.2, SnRK2.3, and SnRK2.6 acted as redundant ABA-activated SnRK2s and functioned together in ABA-mediated stress response (Nakashima et al., 2009). There are 22 SnRK2 kinases in soybean (Zhao et al., 2017). Therefore, more work is needed for the functional characterization of soybean SnRK2 genes in salt-alkaline stress.
In summary, current research has characterized the function of several protein kinase genes, including GsSRK, GsRLCK, GsCRCK1d, GsSnRK1, GsAPK2, and GsMPK4, in regulating salt-alkaline tolerance. Several studies have identified interacting partners of GsCRCK1d and GsSnRK1 to illustrate their regulatory role in salt-alkaline stress signal transduction. However, more work is needed to depict the regulatory network in which PKs occupy a core connecting role. Based on the current reports in G. soja, some interesting hypotheses are needed to verify. For example, it is an interesting work to determine whether these functionally characterized PKs could phosphorylate the ion transporters or antioxidant enzymes reported in G. soja to mediate the salt-alkaline stress responses. Besides, a multi-year filed trial is needed to evaluate the potential application of these G. soja PK genes in improving soybean yields on salt-alkaline fields.
Transcription factors regulating salt-alkaline stress response in wild soybean
Transcription factors (TFs) are key regulators of transcriptional changes and core components of signal transduction in plant response to abiotic stress. Researchers have identified a great deal of TFs in soybean genome, which belong to different families, such as APETALA2/ethylene-responsive factors (AP2/ERF), TIFY, WRKY, homeodomain leucine zipper (HD-Zip), basic helix-loop-helix (bHLH), Cys2/His2-type zinc finger (C2H2), NAC (no apical meristem (NAM), Arabidopsis thaliana transcription activation factor (ATAF1/2) and cup-shaped cotyledon (CUC2)). Up to now, genetic evidence has been reported to show the function of AP2/ERFs, TIFYs, WRKYs, and NACs in G. soja response to salt-alkaline stress (Table 4; Figure 4).
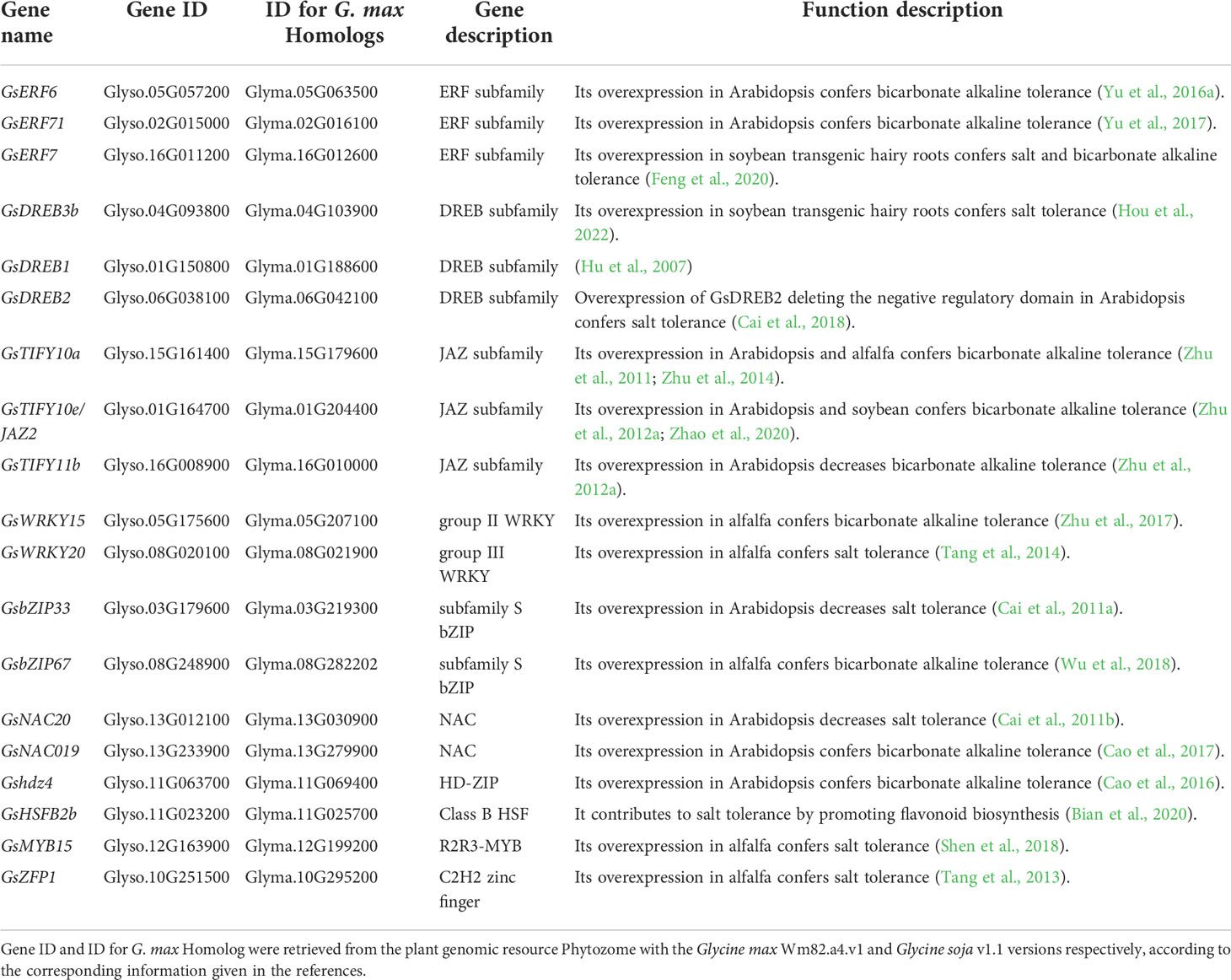
Table 4 The genes encoding transcription factors involved in regulating salt-alkaline stress response in wild soybean.
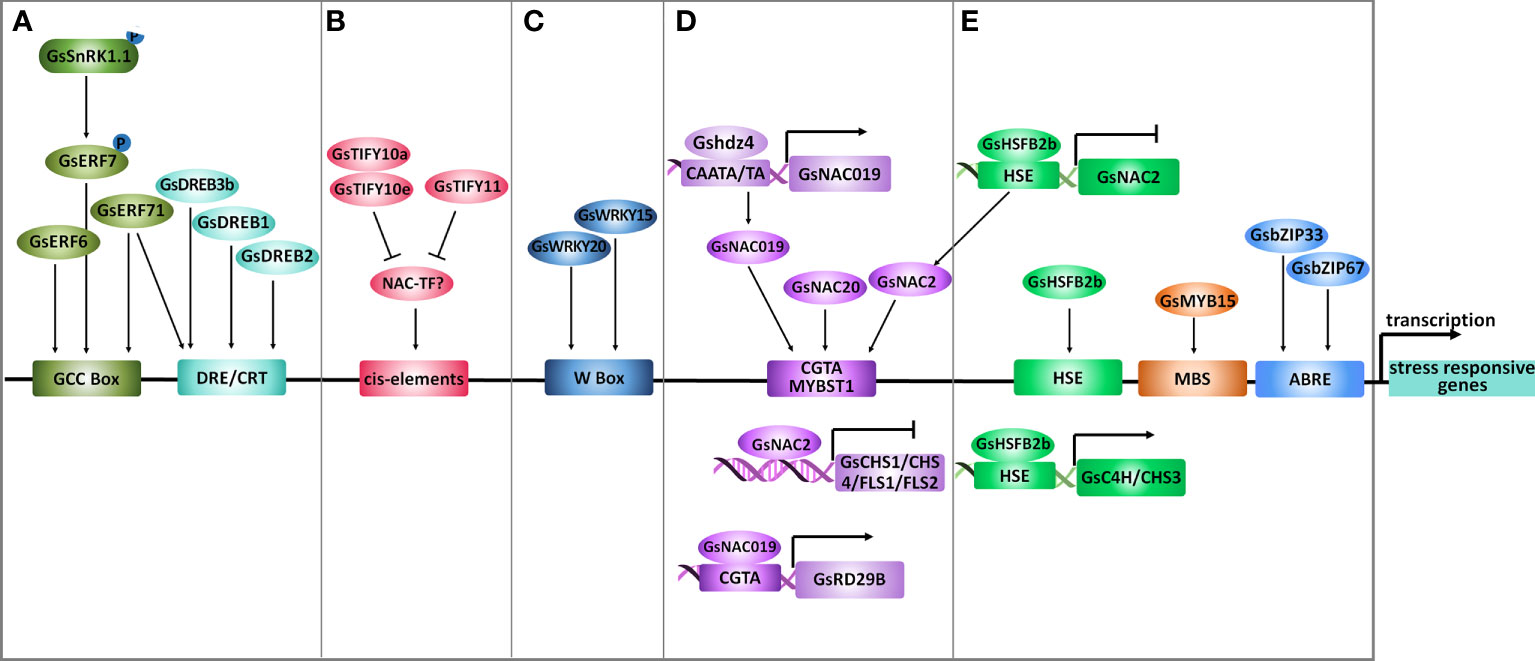
Figure 4 Transcriptional regulation under salt-alkaline stress in wild soybean. (A) AP2/ERFs, (B) TIFYs, (C) WRKYs, (D) NACs and (E) other transcription factors mediating the transcriptional response of G. soja to salt-alkaline stress. Arrows represent positive effects, and lines ending with a short bar indicate negative effects.
AP2/ERF transcription factors
The AP2/ERF family TFs are characterized by an AP2 DNA binding domain, consisting of 60 highly conserved amino acids. The AP2/ERF family in plants could be divided into five major subfamilies, AP2, ERF, RAV (Related to Abscisic acid insensitive 3/Viviparous 1), DREB (Dehydration-Responsive Element Binding), and Soloist (Xie et al., 2019). According to a recent study, the soybean genome contains 301 AP2/ERF genes, among which 153 were classified into the ERF subfamily (Wang H. et al., 2022a). In G. soja, only three ERF subfamily TFs (GsERF7, GsERF71 and, GsERF6) have been suggested to participate in salt-alkaline stress response (Figure 4A). GsERF7, belonging to the ERF subfamily, was identified as a phosphorylation substrate of GsSnRK1 kinase, and the phosphorylation of GsERF7 on S36 was necessary for its nucleus localization and transactivation activity (Feng et al., 2020). Transformation of GsERF7 in soybean hairy roots improved the dry weight of composite soybean plants under salt stress. At the same time co-transformation of GsERF7 and GsSnRK1 further promoted the tolerance of composite soybean to salt and bicarbonate alkaline stress. GsERF71 and GsERF6 also encode ERF subfamily TFs (Yu et al., 2016a; Yu et al., 2017). By overexpressing in Arabidopsis, GsERF71 and GsERF6 were demonstrated to specifically confer plant tolerance to bicarbonate (NaHCO3 and KHCO3) alkaline stress, but not to high pH stress caused by KOH. Both GsERF71 and GsERF6 showed nucleus localization and transactivation activity, and GsERF71 was suggested to bind the GCC box (core sequence AGCCGCC). Interestingly, under bicarbonate stress, GsERF71 overexpression in Arabidopsis could up-regulate AHA2 (Arabidopsis H+-ATPase 2) expression to facilitate the neutralization of rhizosphere pH and increase the expression of auxin biosynthesis and transporting genes to promote auxin accumulation in roots (Yu et al., 2016a; Yu et al., 2017).
The dehydration-responsive element-binding (DREB) TFs are classified as a subfamily of the AP2/ERF family and have been well-documented to modulate multiple abiotic stress responses. There are 103 DREB genes in the soybean genome. Recently, a DREB transcription factor DREB3b was identified to contribute to salt stress tolerance of G. soja by combining RNA-sequencing and population genetics analysis (Hou et al., 2022) (Figure 4A). Among the 103 soybean DREB genes, 24 showed differential expression under salt stress, including 14 up-regulated and 10 down-regulated genes. Out of the 14 up-regulated DREBs, 9 displayed natural sequence variation detected by a panel of 424 accessions comprised of 85 wild soybeans, 153 landraces, and 186 cultivars. The natural variation in DREB3a and DREB3b is statistically related to differences in salt tolerance, and DREB3b, undergoes artificial selection during soybean domestication. Soybean plants carrying the G. soja DREB3b allele (DREB3b39Del) are more salt tolerant than those containing the reference genome allele (DREB3bRef). Consistently, DREB3b39Del improved salt tolerance more than DREB3bRef in transgenic soybean hairy roots. An InDel marker was developed to identify the DREB3b39Del allele, and could facilitate the molecular breeding of salt-tolerant soybean varieties in the future. In 2007, Hu et al. also found a Gypsy-like retrotransposon in the 5’ upstream region of GsDREB1 (GenBank Accession EF051460.1) of individuals among the saline population of wild soybean (Hu et al., 2007). Therefore, these two DREB genes are likely targets for molecular breeding to improve soybean salt-alkaline tolerance.
Cai et al. reported that GsDREB2 deleting the negative regulatory domain (NRD, amino acid residues 140-204) confers salt tolerance in transgenic Arabidopsis, while the full-length GsDREB2 could not (Cai et al., 2018). The NRD negatively controlled the transactivation ability and DRE element (core sequence A/GCCGAC) binding affinity of GsDREB2, thereby inhibited the function of GsDREB2 in stress response (Figure 4A). Similarly, in Arabidopsis, deletion of the NRD (residues 136 and 165) within AtDREB2A could transform it to a constitutively active form (Sakuma et al., 2006), and the NRD is important for AtDREB2A protein degradation via the BPM-CUL3 E3 ligase (Morimoto et al., 2017). In this context, it will be a good choice for soybean breeding through gene manipulation of DREB2s by deleting the NRD domain to improve its regulatory effect on salt-alkaline stress tolerance.
TIFY transcription factors
The TIFY family TFs are characterized by a conserved TIFY motif (TIFF/YXG) and have been demonstrated to widely participate in plant response to various abiotic stresses (Singh and Mukhopadhyay, 2021; Liu W. C. et al., 2022). The TIFY family was divided into TIFY, Jasmonate ZIM (JAZ), ZIM-like (ZML), and PEAPOD (PPD) subfamilies. A previous genome-wide analysis identified 34 TIFY genes in G. soja (Zhu et al., 2013), and a recent study reported 38 TIFY genes in G. max (Liu X. et al., 2022). In G. soja, GsTIFY10s (10a, 10b, 10c, 10d, 10e, and 10f) and GsTIFY11s (11a and 11b) shared the highest sequence identity and were clustered together in the JAZ subfamily. Notably, all GsTIFY10/11s were dramatically up-regulated at the early stage of NaHCO3 treatment (Zhu et al., 2013) (Figure 4B). Among them, overexpression of GsTIFY10 (identical to GsTIFY10a) and GsJAZ2 (identical to GsTIFY10e) in Arabidopsis improved the tolerance to both salt (NaCl) and bicarbonate (NaHCO3) alkaline stress (Zhu et al., 2011; Zhu et al., 2012b). The positive regulation of GsTIFY10a and GsTIFY10e on bicarbonate alkaline tolerance was further verified by overexpressing in alfalfa (Zhu et al., 2014) and soybean (Zhao et al., 2020), respectively. Recently, a study in G. max showed that GmTIFY10s expression displayed an apparent response to abiotic stresses, and overexpression of GmTIFY10e and GmTIFY10g in Arabidopsis and soybean improved salt tolerance (Liu Y. L. et al., 2022). In summary, the GsTIFY10 genes are key positive regulators of soybean tolerance to salt-alkaline stress. Intriguingly, even though GsTIFY11b was highly homologous to GsTIFY10s, its overexpression in Arabidopsis resulted in an obvious decrease in salt tolerance (Zhu et al., 2012a). GsTIFY10s and GsTIFY11s may regulate different signaling pathways under salt-alkaline stress.
Further research is needed to illustrate the regulatory mechanism mediated by GsTIFY10/11s. The TIFY proteins generally localize in the nucleus and are considered to be transcriptional repressors even though without a DNA binding domain. Both GsTIFY10a and GsTIFY10e were localized to the nucleus, and neither showed transcriptional activity in yeasts. All G. soja TIFY10/11 proteins contain a TIFY domain and a Jas domain. The TIFY domain has been found to mediate homo- and hetero-dimerization of TIFYs and protein interaction with other TFs, such as MYCs. GsTIFY10a could form homodimers or heterodimers with GsTIFY10e. Therefore, screening the interacting TFs of GsTIFY10s is extremely important to understand the GsTIFY10s-mediated responsive mechanism under salt-alkaline stress. Besides, more experiments are needed to investigate the background molecular basis to reveal the opposite function between GsTIFY10s and GsTIFY11s in salt-alkaline stress.
WRKY transcription factors
The WRKY family TFs are characterized by a conserved DNA-binding domain that harbors the WRKYGQK heptapeptide followed by a C2H2 or C2HC zinc finger. WRKY TFs specifically recognize and bind to the W-Box (core sequence TTGAC) to regulate gene transcription. Several studies have focused on the genome-wide analysis of soybean WRKY genes and their response to abiotic stress (Song et al., 2016; Yu et al., 2016b). The soybean genome contains around 180 genes encoding WRKY transcription factors, which were classified into three groups (Group I-III). Until now, only one WRKY gene in G. soja, GsWRKY20 (group III) has been reported to be involved in salt-alkaline stress response (Figure 4C). GsWRKY20 conferred drought tolerance when overexpressing in Arabidopsis via an ABA-dependent signaling pathway and by increasing the thickness of the cuticle (Luo et al., 2013a; Tang et al., 2014). Overexpression of GsWRKY20 and GsWRKY15 in alfalfa also increased tolerance to salt stress and bicarbonate alkaline stress, respectively (Tang et al., 2014; Zhu et al., 2017). Notably, expression of GsWRKY20 also resulted in earlier flowering (Luo et al., 2013b), which is an effective strategy for avoiding adverse environmental stress. These studies strongly suggested the multiple regulatory roles of GsWRKY20 in stress response, which makes GsWRKY20 a perfect target for soybean molecular breeding to improve the tolerance to diverse abiotic stress simultaneously. In addition, a G. soja group III WRKY transcription factor GsWRKY57 was also suggested to contribute to drought tolerance (Wang et al., 2019a). Considering the high conservation between GsWRKY20 and GsWRKY57, it is possible that GsWRKY57 also acts as a positive regulator of salt tolerance.
NAC transcription factors
The plant-specific NAC family is one of the largest TF families, with 226 members in soybean (Hussain et al., 2017). A number of studies have shown that NACs play important roles in the salt-alkaline stress response in plants. Two NAC genes in G. soja have been suggested to be involved in salt-alkaline stress response. A recent study reported that a NAC family gene NAC2 functioned downstream of a class B heat shock factor HSFB2b to negatively regulate salt tolerance (Bian et al., 2020). Overexpression of the NAC2 gene in soybean hairy roots decreased salt tolerance. Under salt stress, GsHSFB2b could promote flavonoid biosynthesis by directly inhibiting GsNAC2, which repressed the expression of GsCHS1/CHS4/FLS1/FLS2 (Figure 4D). In addition, GsHSFB2b can also directly bind to the promoter of GsC4H and GsCHS3 and activate their expression to trigger the flavonoid biosynthesis pathway.
In addition, the Gshdz4-GsNAC019-GsRD29B module was reported to confer tolerance to bicarbonate (NaHCO3 and KHCO3) alkaline stress but not to high pH stress caused by KOH (Cao et al., 2016; Cao et al., 2017). Gshdz4 binds to the CAATA/TA motif in the GsNAC019 promoter region and activates the expression of GsNAC019; thereby, GsNAC019 could possibly bind to the CGTA core sequence in the GsRD29B promoter (Figure 4D). However, further evidence is needed to confirm some details in this module. There is still no genetic data supporting the GsRD29B regulation on bicarbonate stress tolerance. Further experiments, for example, EMSA or ChiP-qPCR, are required to verify the binding affinity of Gshdz4 to the GsNAC019 promoter, and the binding and regulation of GsNAC019 to GsRD29B.
It is widely accepted that transcription factors function downstream of protein kinases in the signal transduction network in response to salt-alkaline stress. Phosphorylation is critical for the subcellular localization, DNA binding, and transcriptional activity of transcription factors. Till now, fourteen G. soja TF genes have been functionally characterized to participate in salt-alkaline stress response. However, only GsERF7 has been suggested to be directedly targeted and phosphorylated by GsSnRK1. Therefore, identifying protein kinases that interact with and phosphorylate these reported transcription factors in G. soja will greatly facilitate to unravel the transcriptional regulation under salt-alkaline stress. Moreover, downstream genes that are directly targeted and transcriptionally regulated by these G. soja transcription factors also need to be identified to establish the transcriptionally regulatory network under salt-alkaline stress.
Conclusions and future prospects
Soybean is one of the most vital beans in the world, providing high-quality vegetable protein, edible oil, and industrial raw materials. Under the background of repeated COVID-19 and the increasing worldwide population, the demand for soybean is increasing day by day. Therefore, it is urgently demanded to improve the soybean production, especially in China. However, its yield is greatly restricted by adverse environmental stresses. Compared with cultivated soybean, G. soja retains much higher genetic diversity and displays much higher salt-alkaline stress tolerance. Therefore, G. soja is considered as genetic reservoir for breeding new salt-alkaline tolerant soybean cultivars with profitable agronomic traits (Kofsky et al., 2018).
Over the years, researchers have demonstrated that the regulation of ion balance and ROS scavenging is critical for wild soybean to survive on saline-alkaline soil. Genes involved in ion uptake, exclusion, and transport, as well as ROS signaling, and scavenging, have been functionally characterized in G. soja. Furthermore, regulatory components of signal transduction pathways, including protein kinases and transcription factors were also revealed. However, these G. soja genes were functionally characterized mainly by ectopically expressed in the model plant Arabidopsis or transiently expressed in soybean hairy roots. Considering the potential dysfunction of ectopic expression and functional redundancy of homologues genes, functional characterization of G. soja genes in future studies should directly be conducted in transgenic soybean plants.
Although current studies have depicted the roles of some protein kinases, transcription factors and functional genes (such as antioxidant enzymes and ion channels) in salt-alkaline stress, few studies focus on the genetic interplay and protein interaction of these regulators. Therefore, the molecular mechanism and signal pathways of soybean response to salt-alkaline stress are still vague. To facilitate the soybean breeding by multigene pyramiding breeding technology, further studies should be concentrated on characterizing the genetic interplay and protein interaction of these genes to construct the signal transduction pathways of soybean salt-alkaline stress response.
Phytohormones, especially ABA, play key roles in regulating plant tolerance to salt-alkaline stress. Current research has well-illustrated the core components of the hormone signal transduction pathway in plants and has demonstrated the regulation of hormone signaling genes on the tolerance to salt-alkaline stress (Fang et al., 2021; Wang N. et al., 2022b). In G. soja, several genes were characterized to modulate salt-alkaline tolerance via hormone-dependent signaling pathways. For instance, the expression of ABA-induced genes was up-regulated in GsWRKY20 and GsCBRLK transgenic plants under salt-alkaline treatment (Yang et al., 2010; Tang et al., 2014). GsTIFY10a and GsTIFY10e mediated the sensitivity to MeJA treatment by suppressing the expression of JA responsive and biosynthesis genes (Zhu et al., 2011; Zhu et al., 2012a). Moreover, GsERF71 enhanced alkaline stress tolerance by modifying auxin accumulation (Yu et al., 2017). The expression of auxin biosynthesis genes and IAA contents were downregulated by GsERF71 under alkaline stress. However, how hormone signaling activates salt-alkaline stress response in G. soja is still lacking. Therefore, more genetic and biochemical experiments should be conducted to unravel the regulatory mechanism of hormone signaling in G. soja response to salt-alkaline stress.
In addition, taking advantage of high-throughput genomic sequencing of hundreds of soybean varieties, more and more attention has been paid to the identification of sequence variations in key genes that regulate salt-alkaline tolerance, for example, GsCHX1 (Qi et al., 2014), GsERD15B (Jin et al., 2021), GsDREB3b (Hou et al., 2022) and GsSALT18 (Guo et al., 2021). Currently, molecular markers of GsCHX1 have been developed for breeding salt-tolerant soybean cultivars (Lee et al., 2018; Guan et al., 2021). Genes harboring sequence variations between G. soja and G. max are potential targets for molecular breeding of salt-alkaline tolerant soybean. Therefore, future work should concentrate on identifying salt-alkaline tolerant haplotypes in G. soja and re-introduce them into G. max to improve the salt-alkaline tolerance.
Author contributions
Conceptualization, XS and MS. Original draft preparation, XC and BJ. Manuscript review and editing, XS and MS. Funding acquisition, XS and MS. All authors contributed to the article and approved the submitted version.
Funding
This research was funded by Natural Science Foundation of Heilongjiang Province (grant number JQ2021C002), National Natural Science Foundation of China (grant number 32101672), Special Funds from the Central Finance to Support the Development of Local Universities (To XS, no grant number), and Postdoctoral Science Foundation of Heilongjiang Province (grant number LBH-Q21161).
Conflict of interest
The authors declare that the research was conducted in the absence of any commercial or financial relationships that could be construed as a potential conflict of interest.
Publisher’s note
All claims expressed in this article are solely those of the authors and do not necessarily represent those of their affiliated organizations, or those of the publisher, the editors and the reviewers. Any product that may be evaluated in this article, or claim that may be made by its manufacturer, is not guaranteed or endorsed by the publisher.
References
Aleem, M., Aleem, S., Sharif, I., Wu, Z., Aleem, M., Tahir, A., et al. (2022). Characterization of SOD and GPX gene families in the soybeans in response to drought and salinity stresses. Antioxid. (Basel) 11 (3), 460. doi: 10.3390/antiox11030460
Assaha, D. V. M., Ueda, A., Saneoka, H., Al-Yahyai, R., Yaish, M. W. (2017). The role of Na+ and K+ transporters in salt stress adaptation in glycophytes. Front. Physiol. 8, 509. doi: 10.3389/fphys.2017.00509
Azevedo Neto, A. D., Prisco, J. T., Enéas-Filho, J., Rolim Medeiros, J.-V., Gomes-Filho, E. (2005). Hydrogen peroxide pre-treatment induces salt-stress acclimation in maize plants. J. Plant Physiol. 162 (10), 1114–1122. doi: 10.1016/j.jplph.2005.01.007
Bai, X., Liu, J., Tang, L. L., Cai, H., Chen, M., Ji, W., et al. (2013). Overexpression of GsCBRLK from Glycine soja enhances tolerance to salt stress in transgenic alfalfa (Medicago sativa). Funct. Plant Biol. 40 (10), 1048–1056. doi: 10.1071/fp12377
Barbier-Brygoo, H., De Angeli, A., Filleur, S., Frachisse, J.M., Gambale, F., Thomine, S., et al. (2011). Anion channels/transporters in plants: from molecular bases to regulatory networks. Annu. Rev. Plant Biol. 62 (1), 25–51. doi: 10.1146/annurev-arplant-042110-103741
Ben Rejeb, K., Lefebvre-De Vos, D., Le Disquet, I., Leprince, A.-S., Bordenave, M., Maldiney, R., et al. (2015). Hydrogen peroxide produced by NADPH oxidases increases proline accumulation during salt or mannitol stress in Arabidopsis thaliana New Phytol. 208 (4), 1138–1148. doi: 10.1111/nph.13550
Bian, X., Li, W., Niu, C., Wei, W., Hu, Y., Han, J., et al. (2020). A class b heat shock factor selected for during soybean domestication contributes to salt tolerance by promoting flavonoid biosynthesis. New Phytol. 225 (1), 268–283. doi: 10.1111/nph.16104
Cai, X., Shen, Y., Hu, B., Wang, Y., Chen, Y., Sun, M., et al. (2020). Overexpression of a Glycine soja receptor-like protein kinase gene GsCBRLK in rice increases salt-alkaline tolerance. Plant Physiol. J. 56, 2683–2694. doi: 10.13592/j.cnki.ppj.2020.0266
Cai, H., Sun, N., Song, T. (2018). Modification of GsDREB2 from Glycine soja increases plant tolerance to salt and osmotic stress. Acta Pratac Sin. 27, 168–176. doi: CYXB20180601&DbName=CJFQ2018
Cai, H., Zhu, Y., Bai, X., Ji, W., Li, Y., Wang, D., et al. (2011a). Isolation and tolerance analysis of GsbZIP33 gene linked to response on stress in Glycine soja. Mol. Plant Breed. 9, 397–401. doi: FZZW201104002&DbName=CJFQ2011
Cai, H., Zhu, Y., Li, Y., Bai., X., Ji, W., Wang, D., et al. (2011b). Isolation and tolerance analysis of GsNAC20 gene linked to response to stress in Glycine soja. Acta Agron. Sin. 37, 1351–1359. doi: 10.3724/SP.J.1006.2011.01351
Cao, L., Yu, Y., Ding, X., Zhu, D., Yang, F., Liu, B., et al. (2017). The Glycine soja NAC transcription factor GsNAC019 mediates the regulation of plant alkaline tolerance and ABA sensitivity. Plant Mol. Biol. 95 (3), 253–268. doi: 10.1007/s11103-017-0643-3
Cao, L., Yu, Y., DuanMu, H., Chen, C., Duan, X., Zhu, P., et al. (2016). A novel Glycine soja homeodomain-leucine zipper (HD-zip) I gene, Gshdz4, positively regulates bicarbonate tolerance and responds to osmotic stress in Arabidopsis. BMC Plant Biol. 16 (1), 184. doi: 10.1186/s12870-016-0872-7
Chan, C. (2014). Biochemical and functional study of a putative lambda class glutathione-s-transferase gene in the wild soybean (The Chinese University of Hong Kong: Doctor of Philosophy).
Chen, X., Ding, Y., Yang, Y., Song, C., Wang, B., Yang, S., et al. (2021a). Protein kinases in plant responses to drought, salt, and cold stress. J. Integr. Plant Biol. 63 (1), 53–78. doi: 10.1111/jipb.13061
Chen, J., Li, Q., Zhang, P., Lu, H., Bian, Y., Jian, Y., et al. (2021b). Cloning and functional characterization of two GsSnRK1 gene promoters from wild soybean. Plant Biotechnol. Rep. 15 (5), 627–639. doi: 10.1007/s11816-021-00700-6
Chen, C., Sun, X., Duanmu, H., Yu, Y., Liu, A., Xiao, J., et al. (2015a). Ectopic expression of a Glycine soja myo-inositol oxygenase gene (GsMIOX1a) in Arabidopsis enhances tolerance to alkaline stress. PloS One 10 (6), e0129998. doi: 10.1371/journal.pone.0129998
Chen, C., Sun, X., Liu, A., Duan Mu, H., Yu, Y., Xiao, J., et al. (2015b). Cloning and functional analysis of Glycine soja bicarbonate stress responsive gene GsMIPS2. Acta Agron. Sin. 41, 1343–1352. doi: 10.3724/SP.J.1006.2015.01343
Chen, P., Yan, K., Shao, H., Zhao, S. (2013). Physiological mechanisms for high salt tolerance in wild soybean (Glycine soja) from yellow river delta, China: photosynthesis, osmotic regulation, ion flux and antioxidant capacity. PloS One 8 (12), e83227. doi: 10.1371/journal.pone.0083227
Das, K., Roychoudhury, A. (2014). Reactive oxygen species (ROS) and response of antioxidants as ROS-scavengers during environmental stress in plants. Front. Environ. Sci. 2, 53. doi: 10.3389/fenvs.2014.00053
Do, T., Chen, H., Hien, V. T., Hamwieh, A., Yamada, T., Sato, T., et al. (2016). Ncl synchronously regulates NA+, K+, and Cl- in soybean and greatly increases the grain yield in saline field conditions. Sci. Rep. 6, 19147. doi: 10.1038/srep19147
Donahue, J. L., Alford, S. R., Torabinejad, J., Kerwin, R. E., Nourbakhsh, A., Ray, W. K., et al. (2010). The Arabidopsis thaliana myo-inositol 1-phosphate synthase1 gene is required for myo-inositol synthesis and suppression of cell death. Plant Cell 22 (3), 888–903. doi: 10.1105/tpc.109.071779
Duan, X., Yu, Y., Duanmu, H., Chen, C., Sun, X., Cao, L., et al. (2017). GsSLAH3, a Glycine soja slow type anion channel homologue, positively modulates plant bicarbonate stress tolerance. Physiol. Plant 164 (2), 145–162. doi: 10.1111/ppl.12683
Duan, X., Yu, Y., Zhang, Y., Chen, C., Duanmu, H., Cao, L., et al. (2018). A potential efflux boron transporter gene GsBOR2, positively regulates Aarabidopsis bicarbonate tolerance. Plant Sci. 274, 284–292. doi: 10.1016/j.plantsci.2018.05.032
Endres, S., Tenhaken, R. (2009). Myoinositol oxygenase controls the level of myoinositol in Arabidopsis, but does not increase ascorbic acid. Plant Physiol. 149 (2), 1042–1049. doi: 10.1104/pp.108.130948
Fang, S., Hou, X., Liang, X. (2021). Response mechanisms of plants under saline-alkali stress. Front. Plant Sci. 12, 667458. doi: 10.3389/fpls.2021.667458
Feng, X., Feng, P., Yu, H., Yu, X., Sun, Q., Liu, S., et al. (2020). GsSnRK1 interplays with transcription factor GsERF7 from wild soybean to regulate soybean stress resistance. Plant Cell Environ. 43 (5), 1192–1211. doi: 10.1111/pce.13726
Guan, R., Qu, Y., Guo, Y., Yu, L., Liu, Y., Jiang, J., et al. (2014). Salinity tolerance in soybean is modulated by natural variation in GmSALT3. Plant J. 80 (6), 937–950. doi: 10.1111/tpj.12695
Guan, R., Yu, L., Liu, X., Li, M., Chang, R., Gilliham, M., et al. (2021). Selection of the salt tolerance gene GmSALT3 during six decades of soybean breeding in China. Front. Plant Sci. 12, 794241. doi: 10.3389/fpls.2021.794241
Guo, X., Jiang, J., Liu, Y., Yu, L., Chang, R., Guan, R., et al. (2021). Identification of a novel salt tolerance-related locus in wild soybean (Glycine soja sieb. & zucc.). Front. Plant Sci. 12, 791175. doi: 10.3389/fpls.2021.791175
Hasanuzzaman, M., Nahar, K., Anee, T. I., Fujita, M. (2017). Glutathione in plants: biosynthesis and physiological role in environmental stress tolerance. Physiol. Mol. Biol. Pla. 23 (2), 249–268. doi: 10.1007/s12298-017-0422-2
Hou, Z., Li, Y., Cheng, Y., Li, W., Li, T., Du, H., et al. (2022). Genome-wide analysis of DREB genes identifies a novel salt tolerance gene in wild soybean (Glycine soja). Front. Plant Sci. 13, 821647. doi: 10.3389/fpls.2022.821647
Hua, Y., Zhang, B., Cai, H., Li, Y., Bai, X., Ji, W., et al. (2012). Stress-inducible expression of GsSAMS2 enhances salt tolerance in transgenic Medicago sativa. Afr. J. Biotechnol. 11 (17), 4030–4038. doi: 10.5897/AJB11.2583
Hu, Z., Jiang, G., Deng, X., Wang, H. (2007). Moleculer adaptation through diversity of retrotransposons and transcriptional factors in populations of wild soybean (Glycine soja). Chin. J. Plant Ecol. 31, 952–959. doi: 10.17521/cjpe.2007.0121
Hussain, R. M., Ali, M., Feng, X., Li, X. (2017). The essence of NAC gene family to the cultivation of drought-resistant soybean (Glycine max l. merr.) cultivars. BMC Plant Biol. 17 (1), 55. doi: 10.1186/s12870-017-1001-y
Im, J. H., Lee, H., Kim, J., Kim, H. B., Seyoung, K., Kim, B. M., et al. (2012). A salt stress-activated mitogen-activated protein kinase in soybean is regulated by phosphatidic acid in early stages of the stress response. J. Plant Biol. 55 (4), 303–309. doi: 10.1007/s12374-011-0036-8
Im, J. H., Son, S., Ko, J. H., Kim, K. H., An, C. S., Han, K. H. (2021). Nuclear translocation of soybean MPK6, GmMPK6, is mediated by hydrogen peroxide in salt stress. Plants (Basel) 10 (12), 2611. doi: 10.3390/plants10122611
Isayenkov, S. V., Dabravolski, S. A., Pan, T., Shabala, S. (2020). Phylogenetic diversity and physiological roles of plant monovalent Cation/H+ antiporters. Front. Plant Sci. 11, 573564. doi: 10.3389/fpls.2020.573564
Jentsch, T. J., Pusch, M. (2018). CLC chloride channels and transporters: structure, function, physiology, and disease. Physiol. Rev. 98 (3), 1493–1590. doi: 10.1152/physrev.00047.2017
Jia, B., Sun, M., DuanMu, H., Ding, X., Liu, B., Zhu, Y., et al. (2017). GsCHX19.3, a member of cation/H+ exchanger superfamily from wild soybean contributes to high salinity and carbonate alkaline tolerance. Sci. Rep. 7 (1), 9423. doi: 10.1038/s41598-017-09772-3
Jia, B., Sun, M., Sun, X., Li, R., Wang, Z., Wu, J., et al. (2015). Overexpression of GsGSTU13 and SCMRP in Medicago sativa confers increased salt-alkaline tolerance and methionine content. Physiol. Plant 156, 176–189. doi: 10.1111/ppl.12350
Jia, Q., Zheng, C., Sun, S., Amjad, H., Liang, K., Lin, W. (2018). The role of plant cation/proton antiporter gene family in salt tolerance. Biol. Plantarum 62 (4), 617–629. doi: 10.1007/s10535-018-0801-8
Ji, W., Koh, J., Li, S., Zhu, N., Dufresne, C. P., Zhao, X., et al. (2015). Quantitative proteomics reveals an important role of GsCBRLK in salt stress response of soybean. Plant Soil 402 (1-2), 159–178. doi: 10.1007/s11104-015-2782-0
Jin, T., Sun, Y., Shan, Z., He, J., Wang, N., Gai, J., et al. (2021). Natural variation in the promoter of GsERD15B affects salt tolerance in soybean. Plant Biotechnol. J. 19 (6), 1155–1169. doi: 10.1111/pbi.13536
Jin, T., Sun, Y., Zhao, R., Shan, Z., Gai, J., Li, Y. (2019). Overexpression of peroxidase gene GsPRX9 confers salt tolerance in soybean. Int. J. Mol. Sci. 20, 3745. doi: 10.3390/ijms20153745
Ji, W., Zhu, Y., Li, Y., Yang, L., Zhao, X., Cai, H., et al. (2010). Over-expression of a glutathione s-transferase gene, GsGST, from wild soybean (Glycine soja) enhances drought and salt tolerance in transgenic tobacco. Biotechnol. Lett. 32 (8), 1173–1179. doi: 10.1007/s10529-010-0269-x
Kofsky, J., Zhang, H., Song, B. H. (2018). The untapped genetic reservoir: the past, current, and future applications of the wild soybean (Glycine soja). Front. Plant Sci. 9, 949. doi: 10.3389/fpls.2018.00949
Lee, S., Kim, J. H., Sundaramoorthy, J., Park, G. T., Lee, J. D., Kim, J. H., et al. (2018). Identification of GmSALT3 haplotypes and development of molecular markers based on their diversity associated with salt tolerance in soybean. Mol. Breed. 38 (7), 86. doi: 10.1007/s11032-018-0845-7
Liao, X., Shi, M., Zhang, W., Ye, Q., Li, Y., Feng, X., et al. (2021). Association analysis of GmMAPKs and functional characterization of GmMMK1 to salt stress response in soybean. Physiol. Plantarum 173 (4), 2026–2040. doi: 10.1111/ppl.13549
Li, J., Assmann, S.M. (1996). An abscisic acid-activated and calcium-independent protein kinase from guard cells of fava bean. Plant Cell 8 (12), 2359–2368. doi: 10.1105/tpc.8.12.2359
Lin, F., Bai, X., Fan, C., Zhao, C., Cai, H., Ji, W., et al. (2013). Investigation and analysis of the main agronomic traits of different transgenic soybean lines with GsGST14 gene. Soybean Sci. 32, 56–58. doi: DDKX201301016&DbName=CJFQ2013
Lin, L., Wu, J., Jiang, M., Wang, Y. (2021). Plant mitogen-activated protein kinase cascades in environmental stresses. Int. J. Mol. Sci. 22 (4), 1543. doi: 10.3390/ijms22041543
Li, Q., Sun, Q., Wang, D., Liu, Y., Zhang, P., Lu, H., et al. (2022). Quantitative phosphoproteomics reveals the role of wild soybean GsSnRK1 as a metabolic regulator under drought and alkali stresses. J. Proteomics 258, 104528. doi: 10.1016/j.jprot.2022.104528
Liu, J., Chen, N., Grant, J. N., Cheng, Z., Stewart, C. N., Hewezi, T. (2015). Soybean kinome: functional classification and gene expression patterns. J. Exp. Bot. 66 (7), 1919–1934. doi: 10.1093/jxb/eru537
Liu, W., Feng, Y., Yu, S., Fan, Z., Li, X., Li, J., et al. (2021d). The flavonoid biosynthesis network in plants. Int. J. Mol. Sci. 22 (23), 12824. doi: 10.3390/ijms222312824
Liu, J., Fu, C., Li, G., Khan, M., Wu, H. (2021c). ROS homeostasis and plant salt tolerance: plant nanobiotechnology updates. Int. J. Mol. Sci. 13 (6), 3552. doi: 10.3390/su13063552
Liu, P., Huang, Y., Shi, P., Yu, M., Xie, J., Xie, L. (2018). Duplication and diversification of lectin receptor-like kinases (LecRLK) genes in soybean. Sci. Rep. 8 (1), 5861. doi: 10.1038/s41598-018-24266-6
Liu, X., Liu, F., Zhang, L., Cheng, C., Wei, P., Yu, B. (2021b). GsCLC-c2 from wild soybean confers chloride/salt tolerance to transgenic Arabidopsis and soybean composite plants by regulating anion homeostasis. Physiol. Plant 172 (4), 1867–1879. doi: 10.1111/ppl.13396
Liu, W., Song, R., Zheng, S., Li, T., Zhang, B., Gao, X., et al. (2022). Coordination of plant growth and abiotic stress responses by tryptophan synthase β; subunit 1 through modulation of tryptophan and ABA homeostasis in Arabidopsis. Mol. Plant 15 (6), 973–990. doi: 10.1016/j.molp.2022.04.009
Liu, Y., Yu, L., Qu, Y., Chen, J., Liu, X., Hong, H., et al. (2016). GmSALT3, which confers improved soybean salt tolerance in the field, increases leaf Cl- exclusion prior to Na+ exclusion but does not improve early vigor under salinity. Front. Plant Sci. 7, 1485. doi: 10.3389/fpls.2016.01485
Liu, X., Yu, F., Yang, G., Liu, X., Peng, S. (2022). Identification of TIFY gene family in walnut and analysis of its expression under abiotic stresses. BMC Genomics 23 (1), 190. doi: 10.1186/s12864-022-08416-9
Liu, Y., Zheng, L., Jin, L., Liu, Y., Kong, Y., Wang, Y., et al. (2022). Genome-wide analysis of the soybean TIFY family and identification of GmTIFY10e and GmTIFY10g response to salt stress. Front. Plant Sci. 13, 845314. doi: 10.3389/fpls.2022.845314
Li, S., Wang, N., Ji, D., Zhang, W., Wang, Y., Yu, Y., et al. (2019). A GmSIN1/GmNCED3s/GmRbohBs feed-forward loop acts as a signal amplifier that regulates root growth in soybean exposed to salt stress. Plant Cell 31 (9), 2107–2130. doi: 10.1105/tpc.18.00662
Li, X., Wang, Y., Liu, F., Pi, B., Zhao, T., Yu, B. (2020). Transcriptomic analysis of Glycine soja and G. max seedlings and functional characterization of GsGSTU24 and GsGSTU42 genes under submergence stress. Environ. Exp. Bot. 171, 103963. doi: 10.1016/j.envexpbot.2019.103963
Luo, X., Bai, X., Sun, X., Zhu, D., Liu, B., Ji, W., et al. (2013a). Expression of wild soybean WRKY20 in Arabidopsis enhances drought tolerance and regulates ABA signalling. J. Exp. Bot. 64 (8), 2155–2169. doi: 10.1093/jxb/ert073
Luo, X., Sun, X., Liu, B., Zhu, D., Bai, X., Cai, H., et al. (2013b). Ectopic expression of a WRKY homolog from Glycine soja alters flowering time in Arabidopsis. PloS One 8 (8), e73295. doi: 10.1371/journal.pone.0073295
Maszkowska, J., Szymanska, K.P., Kasztelan, A., Krzywinska, E., Sztatelman, O., Dobrowolska, G. (2021). The multifaceted regulation of SnRK2 kinases. Cells 10 (9), 2180. doi: 10.3390/cells10092180
Morimoto, K., Ohama, N., Kidokoro, S., Mizoi, J., Takahashi, F., Todaka, D., et al. (2017). BPM-CUL3 E3 ligase modulates thermotolerance by facilitating negative regulatory domain-mediated degradation of DREB2A in Arabidopsis. Proc. Natl. Acad. Sci. U.S.A. 114 (40), E8528–E8536. doi: 10.1073/pnas.1704189114
Nakashima, K., Fujita, Y., Kanamori, N., Katagiri, T., Umezawa, T., Kidokoro, S., et al. (2009). Three Arabidopsis SnRK2 protein kinases, SRK2D/SnRK2.2, SRK2E/SnRK2.6/OST1 and SRK2I/SnRK2.3, involved in ABA signaling are essential for the control of seed development and dormancy. Plant Cell Physiol. 50 (7), 1345–1363. doi: 10.1093/pcp/pcp083
Neupane, A., Nepal, M.P., Piya, S., Subramanian, S., Rohila, J.S., Reese, R.N., et al. (2013). Identification, nomenclature, and evolutionary relationships of mitogen-activated protein kinase (MAPK) genes in soybean. Evol. Bioinform. Online 9, 363–386. doi: 10.4137/EBO.S12526
Nie, W., Xu, L., Yu, B. (2015). A putative soybean GmsSOS1 confers enhanced salt tolerance to transgenic Arabidopsis sos1-1 mutant. Protoplasma 252 (1), 127–134. doi: 10.1007/s00709-014-0663-7
Nisa (2016). Functional analysis of GsMIPS2 and GsSNAP33 genes under salt and alkali stress conditions (Northeast Agricultural University: Doctor of Philosophy).
Pan, L., Ma, J., LI, J., Fu, C. (2022). Advances of salt stress-responsive transcription factors in plants. Chin. J. Biotech. 38 (1), 50–65. doi: 10.13345/j.cjb.210135
Pi, E., Xu, J., Li, H., Fan, W., Zhu, C., Zhang, T., et al. (2019). Enhanced salt tolerance of rhizobia-inoculated soybean correlates with decreased phosphorylation of the transcription factor GmMYB183 and altered flavonoid biosynthesis. Mol. Cell Proteomics 18 (11), 2225–2243. doi: 10.1074/mcp.RA119.001704
Pi, E., Zhu, C., Fan, W., Huang, Y., Qu, L., Li, Y., et al. (2018). Quantitative phosphoproteomic and metabolomic analyses reveal GmMYB173 optimizes flavonoid metabolism in soybean under salt stress. Mol. Cell Proteomics 17 (6), 1209–1224. doi: 10.1074/mcp.RA117.000417
Qi, X., Li, M. W., Xie, M., Liu, X., Ni, M., Shao, G., et al. (2014). Identification of a novel salt tolerance gene in wild soybean by whole-genome sequencing. Nat. Commun. 5, 4340. doi: 10.1038/ncomms5340
Qiu, Y., Feng, Z., Fu, M., Yuan, X., Luo, C., Yu, Y., et al. (2019). GsMAPK4, a positive regulator of soybean tolerance to salinity stress. J. Integr. Agr. 18 (2), 372–380. doi: 10.1016/s2095-3119(18)61957-4
Qu, Y., Guan, R., Bose, J., Henderson, S. W., Wege, S., Qiu, L., et al. (2021). Soybean CHX-type ion transport protein GmSALT3 confers leaf na+ exclusion via a root derived mechanism, and cl- exclusion via a shoot derived process. Plant Cell Environ. 44 (3), 856–869. doi: 10.1111/pce.13947
Rosales, M. A., Franco-Navarro, J. D., Peinado-Torrubia, P., Díaz-Rueda, P., Álvarez, R., Colmenero-Flores, J. M. (2020). Chloride improves nitrate utilization and NUE in plants. Front. Plant Sci. 11, 442. doi: 10.3389/fpls.2020.00442
Sakuma, Y., Maruyama, K., Osakabe, Y., Qin, F., Seki, M., Shinozaki, K., et al. (2006). Functional analysis of an Arabidopsis transcription factor, DREB2A, involved in drought-responsive gene expression. Plant Cell 18 (5), 1292–1309. doi: 10.1105/tpc.105.035881
Saouros, S., Mohan, T.C., Cecchetti, C., Lehmann, S., Barrit, J.D., Scull, N.J., et al. (2021). Structural and functional insights into the mechanism of action of plant borate transporters. Sci. Rep. 11 (1), 12328. doi: 10.1038/s41598-021-91763-6
Shen, X., Wang, Y., Zhang, Y., Guo, W., Jiao, Y., Zhou, X. (2018). Overexpression of the wild soybean R2R3-MYB transcription factor GsMYB15 enhances resistance to salt stress and Helicoverpa armigera in transgenic Arabidopsis. Int. J. Mol. Sci. 19 (12), 3958. doi: 10.3390/ijms19123958
Shi, H., Ishitani, M., Kim, C., Zhu, J. (2000). The Arabidopsis thaliana salt tolerance gene SOS1 encodes a putative Na+/H+ antiporter. P. Natl. Acad. Sci. U.S.A. 97 (12), 6896–6901. doi: 10.1073/pnas.120170197
Singh, P., Mukhopadhyay, K. (2021). Comprehensive molecular dissection of TIFY transcription factors reveal their dynamic responses to biotic and abiotic stress in wheat (Triticum aestivum l.). Sci. Rep. 11 (1), 9739. doi: 10.1038/s41598-021-87722-w
Song, H., Wang, P., Hou, L., Zhao, S., Zhao, C., Xia, H., et al. (2016). Global analysis of WRKY genes and their response to dehydration and salt stress in soybean. Front. Plant Sci. 7, 9. doi: 10.3389/fpls.2016.00009
Song, Y., Zhang, H., You, H., Liu, Y., Chen, C., Feng, X., et al. (2019). Identification of novel interactors and potential phosphorylation substrates of GsSnRK1 from wild soybean (Glycine soja). Plant Cell Environ. 42 (1), 145–157. doi: 10.1111/pce.13217
Sun, X., Cai, X., Yin, K., Gu, L., Shen, Y., Hu, B., et al. (2021). Wild soybean SNARE proteins BET1s mediate the subcellular localization of the cytoplasmic receptor-like kinases CRCK1s to modulate salt stress responses. Plant J. 105 (3), 771–785. doi: 10.1111/tpj.15072
Sun, M., Qian, X., Chen, C., Cheng, S., Jia, B., Zhu, Y., et al. (2018). Ectopic expression of GsSRK in Medicago sativa reveals its involvement in plant architecture and salt stress responses. Front. Plant Sci. 9, 226. doi: 10.3389/fpls.2018.00226
Sun, M., Shen, Y., Yin, K., Guo, Y., Cai, X., Yang, J., et al. (2019). A late embryogenesis abundant protein GsPM30 interacts with a receptor like cytoplasmic kinase GsCBRLK and regulates environmental stress responses. Plant Sci. 283, 70–82. doi: 10.1016/j.plantsci.2019.02.015
Sun, X., Sun, M., Jia, B., Qin, Z., Yang, K., Chen, C., et al. (2016). A Glycine soja methionine sulfoxide reductase B5a interacts with the Ca2+/CAM-binding kinase GsCBRLK and activates ROS signaling under carbonate alkaline stress. Plant J. 86 (6), 514–529. doi: 10.1111/tpj.13187
Sun, X., Sun, M., Luo, X., Ding, X., Ji, W., Cai, H., et al. (2013a). A Glycine soja ABA-responsive receptor-like cytoplasmic kinase, GsRLCK, positively controls plant tolerance to salt and drought stresses. Planta 237 (6), 1527–1545. doi: 10.1007/s00425-013-1864-6
Sun, X., Yang, S., Sun, M., Wang, S., Ding, X., Zhu, D., et al. (2014). A novel Glycine soja cysteine proteinase inhibitor GsCPI14, interacting with the calcium/calmodulin-binding receptor-like kinase GsCBRLK, regulated plant tolerance to alkali stress. Plant Mol. Biol. 85 (1-2), 33–48. doi: 10.1007/s11103-013-0167-4
Sun, X., Yu, Q., Tang, L., Ji, W., Bai, X., Cai, H., et al. (2013b). GsSRK, a G-type lectin s-receptor-like serine/threonine protein kinase, is a positive regulator of plant tolerance to salt stress. J. Plant Physiol. 170 (5), 505–515. doi: 10.1016/j.jplph.2012.11.017
Tang, L., Cai, H., Ji, W., Luo, X., Wang, Z., Wu, J., et al. (2013). Overexpression of GsZFP1 enhances salt and drought tolerance in transgenic alfalfa (Medicago sativa l.). Plant Physiol. Biochem. 71, 22–30. doi: 10.1016/j.plaphy.2013.06.024
Tang, L., Cai, H., Zhai, H., Luo, X., Wang, Z., Cui, L., et al. (2014). Overexpression of Glycine soja WRKY20 enhances both drought and salt tolerance in transgenic alfalfa (Medicago sativa l.). Plant Cell Tiss Org. Cul. 118 (1), 77–86. doi: 10.1007/s11240-014-0463-y
Uchida, A., Jagendorf, A.T., Hibino, T., Takabe, T., Takabe, T. (2002). Effects of hydrogen peroxide and nitric oxide on both salt and heat stress tolerance in rice. Plant Sci. 163 (3), 515–523. doi: 10.1016/S0168-9452(02)00159-0
USDA (2021). “Soybeans: Acreage planted, harvested, yield, production, value, and loan rate, U.S 1980-2021,” in Oil crops yearbook (Agriculture United States Department of Agriculture: Economic Research Service, USDA). Available at: https://www.ers.usda.gov/data-products/oil-crops-yearbook/.
Wang, Z., Cai, H., Bai, X., Ji, W., Li, Y., Wei, Z., et al. (2012a). Isolation of GsGST19 from Glycine soja and analysis of saline-alkaline tolerance for transgenic Medicago sativa. Acta Agron. Sin. 38, 971–979. doi: 10.3724/SP.J.1006.2012.00971
Wang, H., Ni, D., Shen, J., Deng, S., Xuan, H., Wang, C., et al. (2022a). Genome-wide identification of the AP2/ERF gene family and functional analysis of GmAP2/ERF144 for drought tolerance in soybean. Front. Plant Sci. 13, 848766. doi: 10.3389/fpls.2022.848766
Wang, N., Fan, X., Lin, Y., Li, Z., Wang, Y., Zhou, Y., et al. (2022b). Alkaline stress induces different physiological, hormonal and gene expression responses in diploid and autotetraploid rice. Int. J. Mol. Sci. 23 (10), 5561. doi: 10.3390/ijms23105561
Wang, Z., Song, F., Cai, H., Zhu, Y.M., Bai, X., Ji, W., et al. (2012b). Over-expressing GsGST14 from Glycine soja enhances alkaline tolerance of transgenic Medicago sativa. Biol. Plantarum 56 (3), 516–520. doi: 10.1007/s10535-012-0075-5
Wang, Y., Yan, H., Qiu, Z., Hu, B., Zeng, B., Zhong, C., et al. (2019b). Comprehensive analysis of SnRK gene family and their responses to salt stress in eucalyptus grandis. Int. J. Mol. Sci. 20 (11), 2786. doi: 10.3390/ijms20112786
Wang, Y., Zhang, Y., Guo, W., Dai, W., Zhou, X., Jiao, Y., et al. (2019a). Cloning and function of GsWRKY57 transcription factor gene response to drought stress. Chin. J. Oil Crop Sci. 41, 524–530.
Wei, P., Che, B., Shen, L., Cui, Y., Wu, S., Cheng, C., et al. (2019). Identification and functional characterization of the chloride channel gene, GsCLC-c2 from wild soybean. BMC Plant Biol. 19 (1), 121. doi: 10.1186/s12870-019-1732-z
Wu, J., Cai, H., Bai, X., Ji, W., Wei, Z., Tang, L., et al. (2014). An analysis of salt tolerance of transgenic alfalfa with the GsGST13/SCMRP gene. Acta Pratac Sin. 23, 257–265.
Wu, S., Zhu, P., Jia, B., Yang, J., Shen, Y., Cai, X., et al. (2018). A Glycine soja group S2 bZIP transcription factor GsbZIP67 conferred bicarbonate alkaline tolerance in Medicago sativa. BMC Plant Biol. 18 (1), 234. doi: 10.1186/s12870-018-1466-3
Xie, Z., Nolan, T.M., Jiang, H., Yin, Y. (2019). AP2/ERF transcription factor regulatory networks in hormone and abiotic stress responses in Arabidopsis. Front. Plant Sci. 10, 228. doi: 10.3389/fpls.2019.00228
Yang, L., Ji, W., Gao, P., Li, Y., Cai, H., Bai, X., et al. (2012). GsAPK, an ABA-activated and calcium-independent SnRK2-type kinase from G. soja, mediates the regulation of plant tolerance to salinity and ABA stress. PloS One 7 (3), e33838. doi: 10.1371/journal.pone.0033838
Yang, L., Ji, W., Zhu, Y. M., Gao, P., Li, Y., Cai, H., et al. (2010). GsCBRLK, a calcium/calmodulin-binding receptor-like kinase, is a positive regulator of plant tolerance to salt and ABA stress. J. Exp. Bot. 61 (9), 2519–2533. doi: 10.1093/jxb/erq084
Yu, Y., Duan, X., Ding, X., Chen, C., Zhu, D., Yin, K., et al. (2017). A novel AP2/ERF family transcription factor from Glycine soja, GsERF71, is a DNA binding protein that positively regulates alkaline stress tolerance in Arabidopsis. Plant Mol. Biol. 94 (4-5), 509–530. doi: 10.1007/s11103-017-0623-7
Yu, Y., Liu, A., Duan, X., Wang, S., Sun, X., Duanmu, H., et al. (2016a). GsERF6, an ethylene-responsive factor from Glycine soja, mediates the regulation of plant bicarbonate tolerance in Arabidopsis. Planta 244 (3), 681–698. doi: 10.1007/s00425-016-2532-4
Yu, Y., Wang, N., Hu, R., Xiang, F. (2016b). Genome-wide identification of soybean WRKY transcription factors in response to salt stress. Springerplus 5 (1), 920. doi: 10.1186/s40064-016-2647-x
Zhao, W., Cheng, Y., Zhang, C., Shen, X., You, Q., Guo, W., et al. (2017). Genome-wide identification and characterization of the GmSnRK2 family in soybean. Int. J. Mol. Sci. 18 (9), 1834. doi: 10.3390/ijms18091834
Zhao, C., Pan, X., Yu, Y., Zhu, Y., Kong, F., Sun, X., et al. (2020). Overexpression of a TIFY family gene, GsJAZ2, exhibits enhanced tolerance to alkaline stress in soybean. Mol. Breed. 40 (3), 33. doi: 10.1007/s11032-020-01113-z
Zhao, S., Zhang, Q., Liu, M., Zhou, H., Ma, C., Wang, P. (2021). Regulation of plant responses to salt stress. Int. J. Mol. Sci. 22 (9), 4609. doi: 10.3390/ijms22094609
Zhao, Y., Zhu, Y. M., Xi, B., Wei, J., Hua, C. (2014). Over-expressing GsCBRLK/SCMRP enhances alkaline tolerance and methionine content in transgenic Medicago sativa. Acta Agron. Sin. 40 (3), 431. doi: 10.3724/SP.J.1006.2014.00431
Zheng, X., He, K., Kleist, T., Chen, F., Luan, S. (2015). Anion channel SLAH3 functions in nitrate-dependent alleviation of ammonium toxicity in Arabidopsis. Plant Cell Environ. 38 (3), 474–486. doi: 10.1111/pce.12389
Zhou, F., Guo, Y., Qiu, L. (2016). Genome-wide identification and evolutionary analysis of leucine-rich repeat receptor-like protein kinase genes in soybean. BMC Plant Biol. 16, 58. doi: 10.1186/s12870-016-0744-1
Zhu, J. (2002). Salt and drought stress signal transduction in plants. Annu. Rev. Plant Biol. 53 (1), 247–273. doi: 10.1146/annurev.arplant.53.091401.143329
Zhu, D., Bai, X., Chen, C., Chen, Q., Cai, H., Li, Y., et al. (2011). GsTIFY10, a novel positive regulator of plant tolerance to bicarbonate stress and a repressor of jasmonate signaling. Plant Mol. Biol. 77 (3), 285–297. doi: 10.1007/s11103-011-9810-0
Zhu, D., Bai, X., Luo, X., Chen, Q., Cai, H., Ji, W., et al. (2013). Identification of wild soybean (Glycine soja) TIFY family genes and their expression profiling analysis under bicarbonate stress. Plant Cell Rep. 32 (2), 263–272. doi: 10.1007/s00299-012-1360-7
Zhu, D., Bai, X., Zhu, Y., Cai, H., Li, Y., Ji, W., et al. (2012a). Isolation and functional analysis of GsTIFY11b relevant to salt and alkaline stress from Glycine soja. Hereditas 34, 230–239. doi: 10.3724/SP.J.1005.2012.00230
Zhu, D., Cai, H., Luo, X., Bai, X., Deyholos, M. K., Chen, Q., et al. (2012b). Over-expression of a novel JAZ family gene from Glycine soja, increases salt and alkali stress tolerance. Biochem. Biophys. Res. Commun. 426 (2), 273–279. doi: 10.1016/j.bbrc.2012.08.086
Zhu, P., Chen, R., Yu, Y., Song, X., Li, H., Du, J., et al. (2017). Cloning of gene GsWRKY15 related to alkaline stress and alkaline tolerance of transgenic plants. Acta Agron. Sin. 43, 1319–1327. doi: 10.3724/SP.J.1006.2017.01319
Keywords: wild soybean, salt stress, carbonate stress, ion balance, ROS scavenging, protein kinase, transcription factor
Citation: Cai X, Jia B, Sun M and Sun X (2022) Insights into the regulation of wild soybean tolerance to salt-alkaline stress. Front. Plant Sci. 13:1002302. doi: 10.3389/fpls.2022.1002302
Received: 25 July 2022; Accepted: 29 September 2022;
Published: 19 October 2022.
Edited by:
PingWu Liu, Hainan University, ChinaReviewed by:
Ling Xu, Zhejiang Sci-Tech University, ChinaAgnieszka Kiełbowicz-Matuk, Institute of Plant Genetics (PAN), Poland
Wen-Cheng Liu, Henan University, China
Quanle Xu, Northwest A&F University, China
Copyright © 2022 Cai, Jia, Sun and Sun. This is an open-access article distributed under the terms of the Creative Commons Attribution License (CC BY). The use, distribution or reproduction in other forums is permitted, provided the original author(s) and the copyright owner(s) are credited and that the original publication in this journal is cited, in accordance with accepted academic practice. No use, distribution or reproduction is permitted which does not comply with these terms.
*Correspondence: Mingzhe Sun, c3VubWluZ3poZUBieWF1LmVkdS5jbg==; Xiaoli Sun, Y3NtYmwyMDE2QDEyNi5jb20=
†These authors have contributed equally to this work