- 1School of Studies in Botany, Jiwaji University, Gwalior, India
- 2Department of Botany, Government Chhatrasal College, Shivpuri, India
Experiments were conducted to evaluate the effectivity of Tagetes erecta L. leachates on various growth, physiological, and biochemical parameters of wheat at different stages of growth. Results suggested that Triticum aestivum L. seedlings/plants when exposed to higher concentrations of marigold leachates (10%, 20%, and 30% w/v of fresh parts and 5% and 10% w/v of dry parts) exhibited enhanced lipid peroxidation along with an increase in the activity of protease and phenylalanine ammonia lyase. Treatment with higher concentrations of leachates of fresh (30% w/v) and dry (10% w/v) T. erecta upregulated the activity of superoxide dismutase, catalase, ascorbate peroxidase, guaiacol peroxidase, glutathione S-transferase, and glutathione reductase and also increased the non-enzymatic components of antioxidant defense such as glutathione, ascorbic acid, and total phenols along with osmotic constituents comprising free proline, free sugars, and free amino acids in wheat. The growth and yield attributes of wheat exhibited a slight increase at treatments with lower concentrations (1% w/v) of dry leachates, whereas a decrease was recorded at higher concentrations (10% w/v). In general, treatments with flower leachates (higher concentrations) showed greater influence as compared with those with leaf leachates. Identification and understanding the mechanism of function of allelochemicals in these leachates may pave a way for further experimentation on Tagetes erecta L crop while it is cultivated and decomposed in the field.
Introduction
In nature, plants synthesize secondary metabolites/allelochemicals which may be present in all types of plants and tissues and may not be involved in their primary metabolism. These compounds vary with different species, seasons, and age of plants (Tomar et al., 2015; Mir et al., 2018; Mir et al., 2021) and are released into the environment by a variety of mechanisms including decomposition of fallen parts, volatilization, root exudation, and other mechanisms/sources. The action/effect of allelochemicals on their release into the environment have been observed by different workers (Mishra and Nautiyal, 2012; Gallego et al., 2020). The action of these allelocompounds varies with the target plants, affecting various biochemical processes, which results in modification of diverse physiological functions (Gniazdowska and Bogatek, 2005). Survival of plants under such circumstances depends on the plant defense to allelochemical detoxification (Gniazdowska and Bogatek, 2005; Mir et al., 2021).
Environmental stresses biotically/abiotically affect physiological and biochemical processes in plants like photosynthesis, plant water relations, and membrane integrity due to the excess production of reactive oxygen species (ROS) which results in metabolic dysfunction and leakage of cellular components (Gill and Tuteja, 2010; Ahanger et al., 2017b). The immediate production of different osmoprotectants and activation or modification of antioxidant defense system play an important role under such conditions to keep cells away from immediate cellular damage (Jamil et al., 2005; Mir et al., 2020). Accumulation of compatible osmolytes such as proline, amino acids, and sugars (Ahanger et al., 2017a); antioxidant components like superoxide dismutase (SOD), catalase (CAT), ascorbate peroxidase (APX), glutathione reductase (GR), glutathione-S-transferase (GST), ascorbic acid (AsA), and glutathione (GSH); and phenolic compounds protect plants from oxidative damage by scavenging the excess ROS and maintains the cellular homeostasis (Gill and Tuteja, 2010; Ahanger et al., 2017b).
Allelochemicals after getting released into the environment become stressful only when they affect the growth and development of receptor plants in the surrounding, i.e., phytotoxicity (Cruz-Ortega et al., 2007). The different cultivars of crops with allelopathic activity can be grown through intercropping with other crops/as cover crop/crop rotation, by use of mulches, etc., in order to suppress weeds under field conditions (Jabran et al., 2015). Various crops reportedly show allelopathic effects on associated weeds of wheat, e.g., Sorghum and sunflower (Kandhro et al., 2015) and Brassica, Sorghum, and sunflower (Awan et al., 2012; Arif et al., 2015). In the northern plains of India including the states of Punjab, Haryana, Uttar Pradesh, Rajasthan, Madhya Pradesh, and Bihar, wheat is a dominant crop, and on the world scenario India is the fourth major wheat-producing country (Kulshrestha, 1985). It plays an important role in fulfilling food and energy requirements and in the livelihood of farmers. Wheat is the major source of starch and energy providing significant quantity of components which are useful for health like proteins, vitamins, dietary fiber, and important phytochemicals which help to reduce the risk of cardiovascular diseases, type 2 diabetes, etc. (Shewry and Hey, 2015).
Tagetes erecta L. is a commonly grown species mostly for its bloom and natural dye extraction (Raghava, 1998) exhibiting nematicidal, fungicidal, and insecticidal activities (Xu et al., 2011) and has been used as a cover crop to protect plant-parasite nematodes, inhibition of growth of microorganisms, and pesticides (Silveira et al., 2009; Hooks et al., 2010). The important secondary metabolites which Tagetes erecta L. contains include terpenes, essential oils, flavonoids, carotenoids, and polyphenols (Xu et al., 2011). Among different flowering crops, Tagetes is an important traditional flower crop raised in India having adaptability to diverse climatic conditions (Raghava et al., 2013), receiving great attention in scientific research because of its tremendous commercial usage (Mir et al., 2019). Aqueous leaf and stem extracts of Tagetes erecta inhibited its own seed germination and seedling growth, i.e., showing autotoxicity (Kaul, 2000). Inhibition of radish was more compared with lettuce when exposed to aqueous extracts of Tagetes species (Kaul and Bedi, 1995). In India, flowers of marigold (Tagetes erecta L.) are largely used by people on various social and religious occasions such as decoration of Mandaps/Pooja places, temples, marriage ceremonies, and houses and cars during festivals, and after use these flowers may be disposed off in agricultural fields, which may affect the crops growing therein. It is with this background that the present work has been undertaken to evaluate the effect of marigold leachates on wheat.
Material and methods
Plant material and experimental site
Certified seeds of marigold (Tagetes erecta L.) cultivar Pusa Basanti Gainda (PBG) developed by the Indian Agriculture Research Institute, New Delhi, were obtained from the Horticulture and Landscaping Division, Indian Agriculture Research Institute, Pusa, New Delhi, India, and the cultivation was done in the Botanical Garden of School of Studies in Botany, Jiwaji University, Gwalior. The collection of samples for the preparation of leachates was done using these cultivated plants. Similarly, the grains of test crop wheat Triticum aestivum L. variety LOK-1 which is a commonly cultivated variety in the state of Madhya Pradesh were procured from Krishi Vigyan Kendra, Rajmata Vijayaraje Scindia Krishi Vishwa Vidyalaya, Gwalior, and were raised under both laboratory and field conditions in the School of Studies in Botany, Jiwaji University, Gwalior, for evaluating the effects of marigold leachates.
Preparation of leachates
Leachates of fresh parts
Preparation of fresh leachates was done in accordance with Tomar and Agarwal (2013) in which clean fresh parts (leaves and flowers) of marigold cv. PBG were cut into small pieces, weighed, and soaked in distilled water at room temperature for 48 h. The leachates were squeezed after 48 h with the help of double-layered cheese cloth followed by centrifugation. The 30% leachates of fresh leaves (FLL) and fresh flowers (FFL) were made from 30 g of initially weighed plant sample, and the lower concentrations (5%, 10%, and 20%) were prepared by further diluting these solutions with distilled water which were then finally stored at 4°C for bioassay.
Leachates of dry parts
Dry leachates were also prepared in a similar way (Tomar and Agarwal, 2013). Briefly, an oven-dried 10-g (powdered) plant sample was soaked in 100 ml distilled water at room temperature for 48 h, filtered, and centrifuged after 48 h of soaking. A supernatant was made up to 100 ml with distilled water which is considered as 10% leachates of dry leaves (DLL) and dry flowers (DFL), and the lower concentrations (1% and 5%) were prepared by diluting these solutions and were subsequently stored at 4°C for bioassay experiments. Laboratory and pot experiments (using soil and sand) were conducted to evaluate the impact of leachates of fresh/dry leaves and flowers of marigold on wheat.
Experimental setup/design
Three types of experiments conducted are as follows.
Laboratory experiments
For laboratory experiments, 10 surface-sterilized wheat grains using mercuric chloride (0.01%) solution followed by thorough washing with distilled water were put in each petri plate, lined with Whatman no. 1 filter paper. Leachates of dry plant material (leaves/flowers) were applied to petri plates which served as different treatments, and the replicates without leachates supplied with the same quantity of distilled water served as control. The analysis of different antioxidant and osmotic components in seedlings grown under different treatments was carried out on the 7th day of seed wetting.
Pot experiments using sand substrate
Filtered sand using a sieve (2.5–5.0 mm) was washed thoroughly four to five times by tap water and was kept for 24 h in 18% HCl (Hewitt, 1966) followed by repeated washings with distilled water till the whole acid is washed away. Sterilized (LOK-1) wheat grains were sown in sand-filled plastic pots having a diameter of 18 cm. After germination, 200 ml of full-strength Hoagland’s solution was supplied to each pot, and after 10 days of germination, wheat seedlings grown in pots were subjected to different leachate treatments:
● Different concentrations (5%, 10%, 20%, 30%) of leachates (made in full-strength Hoagland’s solution) of fresh leaves and flowers of Tagetes erecta L. cv. PBG in one set
● Different concentrations (1%, 5%, 10%) of leachates (made in full-strength Hoagland’s solution) of dry leaves and flowers of Tagetes erecta L. cv. PBG in another set
Wheat seedlings raised in pots were fed with 200 ml of fresh/dry leachates of different concentrations on every alternate day, and the same quantity of Hoagland’s solution without leachates was supplied to control. The analysis of different growth and antioxidant attributes/components of plants grown under different treatments was carried out after 25 days of seed wetting. Screening experiments on 25 DAS-old plants in sand cultures using both fresh and dry leachates of leaves and flowers of Tagetes erecta L. cv. PBG made it obvious that dry leachates have greater impact as compared with fresh leachates. Therefore, for further experimentation both in laboratory and in field, only dry leachates were used.
Pot experiments using soil substrate
Pot experiments were conducted in polythene bags having an area of 962 cm2. Each time/season, polythene bags were refilled with fresh soil. Wheat plants raised in pots/polythene bags treated with dry leachates of marigold parts were arranged in randomized block design. The plants so raised fall in the following categories, and at least four independent pots/polythene bags were maintained for each treatment:
● Wheat plants without leachates, irrigated with normal water, were considered as control.
● Wheat plants treated with (1%, 5%, and 10%) leachates of dry leaves and flowers of marigold cultivar PBG.
After 15 days of seed sowing, 200 ml leachates of dry parts was supplied to each pot after. The plants so raised were put to analysis as outlined below at the vegetative (pre-flowering) and flowering stages. Morphological parameters like plant height, spike length, and number of spikelets per spike were recorded at the flowering stage, whereas yield parameters like seed weight were recorded after the crop harvest.
Measurement of lipid peroxidation and protease activity
Lipid peroxidation was measured according to the method of Heath and Packer (1998). Briefly, 300 mg of fresh plant tissue was extracted in 0.1% TCA followed by centrifugation at 10,000 g. Thereafter, 1 ml supernatant was reacted with 4 ml (20%) TCA containing 0.5% thiobarbituric acid (TBA) in a boiling water bath. Samples were again centrifuged for 15 min at 10,000 g, and the supernatant was read at 532 and 600 nm. MDA content was calculated using an extinction coefficient (ϵ) of 155 mM-1 cm-1.
Protease (EC 3.4.21.40) activity was determined according to Green and Neurath (1954). To the 1-ml supernatant was added 1 ml casein followed by incubation at 40°C for 1 h. Samples were removed and brought to room temperature followed by the addition of chilled TCA (20%) and kept in a freezer for 2–3 h. Samples were again centrifuged at 5000g for 15 min, and the supernatant was made alkaline by adding 1 mL NaOH (1.5 N) and the volume made to 5 ml using buffer. A 1.0-ml alkaline supernatant was mixed with 5 ml reagent C (prepared as in estimation of protein) and left for 5 min followed by addition of 1.0 ml Folin–Ciocalteu’s reagent and allowed to stand for 30 min, and samples were read at 660 nm. Activity was expressed as µg tyrosine released mg-1 protein.
Assay of antioxidant enzymes
For assay of superoxide dismutase (SOD), catalase (CAT), ascorbate peroxidase (APX), guaiacol peroxidase (GPX), glutathione S-transferase (GST), and glutathione reductase (GR), 100 mg of fresh plant tissue was homogenized using mortar and pestle in a chilled 2.0-ml extraction mixture (100 mM potassium phosphate buffer, pH 7.8) containing PVP (0.1%) and EDTA (0.5 mM) followed by centrifugation at 12,000 g. The supernatant so obtained was utilized for enzyme assay, and the whole process was carried out under cold conditions (4°C). Extraction buffer was supplemented with 2 mM ascorbate for the extraction of APX. SOD (EC 1.15.1.1) activity was estimated as the ability of enzyme extract to inhibit the photochemical reduction of NBT (Dhindsa et al., 1981). CAT (EC 1.11.1.6) activity was measured according to Aebi (1984), and the change in optical density was recorded at 240 nm for 2 min and expressed as EU mg-1 protein. For APX (EC 1.11.1.1) assay, H2O2-dependent oxidation of ascorbate was followed by change in the absorbance at 290 nm (Nakano and Asada, 1981) and expressed as EU mg-1 protein. GPX (EC 1.11.11.11) activity was determined following Chance and Maehly (1955), and an increase in absorbance was observed at 470 nm for 2 min and expressed as EU mg-1 protein. GST activity was determined spectrophotometrically following Habig et al. (1974) The reaction was initiated by the addition of 1-chloro-2,4-dinitrobenzene (CDNB), and the change in absorbance at 340 nm was monitored for 2 min. GR (EC 1.6.4.2) activity was determined following Foyer and Halliwell (1976), and glutathione dependent oxidation of NADPH was measured at 340 nm for 3 min and was expressed as EU mg-1 protein.
Estimation of non-enzymatic antioxidants
Reduced glutathione GSH was determined by the method of Moron et al. (1979). A fresh plant sample (0.5 g) was homogenized in 5% TCA (2.5 ml) and centrifuged at 10,000g for 10 min. A volume of 100 µl was made up to 1.0 ml by adding 0.2 M sodium phosphate buffer (pH 8.0) followed by the addition of 2.0 ml freshly prepared 5,5-dithiobis nitrobenzoic acid, and absorbance was measured spectrophotometrically at 412 nm. Computation was done using a standard curve of GSH.
For estimation of ascorbic acid, the method of Omayl et al. (1979) was followed. The 100-mg plant tissue was extracted in 10% TCA and centrifuged at 3,500g for 20 min. A 0.5-ml supernatant mixed with 1 ml DTC reagent [prepared by dissolving 3 g of DNPH, 0.4 g of thiourea, and 0.05 g CuSO4 in 100 ml 9N H2SO4] was added. After incubating for 3 h at 37°C, 0.750 ml of ice-cold H2SO4 (65%) was added. After 30 min, absorbance was read at 520 nm. A standard curve of ascorbic acid was used for calculation and expressed as mg g-1 weight.
Total phenols
Total phenols were estimated following the method of Malick and Singh (1980). Phenols react with phosphomolybdic acid in Folin–Ciocalteau’s reagent in alkaline medium and produce a blue-colored complex. The concentration of phenols was expressed in mg g-1 fresh weight, equivalent to catechol.
Estimation of tannins was done according to the method of Swain and Hills (1959). To a 0.1-ml extract, 1 ml Folin–Denis reagent was added followed by addition of 2 ml sodium bicarbonate (35%) and the resultant was incubated for 45 min in the dark at room temperature. Optical density was recorded at 700 nm, and the computation was done by using a tannic acid standard curve.
Assay of phenylalanine ammonia-lyase
PAL was extracted from fresh tissue in 0.1 M borate buffer followed by centrifugation at 12,000g. An assay mixture containing borate buffer (pH 8.8), 0.2 ml enzyme, and 1 ml phenylalanine (20 mM) was incubated at 30°C followed by addition of TCA (1 M). Optical density was recorded at 290 nm, and the activity was expressed as nmol trans-cinnamic acid min-1 mg-1 protein Zucker (1965).
Total antioxidant activity
The total antioxidant activity was estimated in accordance with Shimada et al. (1992). Tissue was extracted in methanol containing 0.1% HCl and centrifuged at 10,000g. The 0.1-ml supernatant was mixed with 2.0 ml freshly prepared 0.1 mM DPPH (1,1-diphenyl-2-picrylhydrazyl). After 30 min, absorbance was recorded at 517 nm and percent DPPH scavenging activity was calculated using the following equation:
where
Ai = absorbance of extract + DPPH,
Aj = absorbance of extract + methanol, and
Ac = absorbance of DPPH + methanol.
Estimation of osmolytes
Total free sugars were estimated using the anthrone method (Fong et al., 1953; Jain and Guruprasad, 1989), and glucose was used as standard. Free amino acids were estimated according to the method outlined in Sadasivam and Manickam (2004) using glycine as standard. Free proline estimation was done following Bates et al. (1973). Extraction was carried out in 3% sulfosalicylic acid, and the supernatant was reacted with acid ninhydrin reagent at 100°C for 1 h. Reaction was terminated in ice followed by the separation of free proline using toluene, and the optical density was recorded at 520 nm. Computation was done using proline standards.
Relative water content Weatherley’s (1950) method was used to measure the relative water content (RWC) in fully expanded wheat leaves.
Statistical analysis
The appropriate standard error ( ± SE) was calculated using at least four independent replicates. Histograms were prepared using SigmaPlot 11.0 software. Data were statistically analyzed using analysis of variance (ANOVA) with the help of Statistix 8.1 software, and the treatment means were analyzed by using the LSD test at p< 0.05.
Results
Lipid peroxidation and protease activity in wheat seedlings/plants
Triticum aestivum L. seedlings/plants exposed to higher concentrations of leachates 10%, 20%, and 30% w/v of fresh parts and 5% and 10% w/v of dry parts of marigold showed an increase in lipid peroxidation and protease activity (Figures 1–4). Wheat plants raised using sand substrates under field conditions receiving 30% w/v leachates of fresh parts showed maximum enhancement in lipid peroxidation and protease activity; moreover, an increase of 64.35% and 69.27% in lipid peroxidation and an increase of 13.07% and 39.68% in protease activity were observed in plants treated with 30% FLL and 30% FFL, respectively. However, treatments with lower concentrations of 5% FLL and 5% FFL exhibited a decline in lipid peroxidation by 2.09% and 2.17% and protease activity by 12.60% and 18.89%, respectively (Figure 2). On the other hand, a 30.99% and 76.93% increase in lipid peroxidation and a 62.62% and 50.89% increase in protease activity were reported in wheat plants fed with 10% DLL and 10% DFL leachates of dry parts of marigold (Figure 3). More or less similar results were reported in wheat seedlings raised under the laboratory conditions (Figure 1) and the older plants raised under field conditions using soil substrates (Figure 4) receiving treatments with different concentrations of dry leachates of leaves and flowers of marigold.
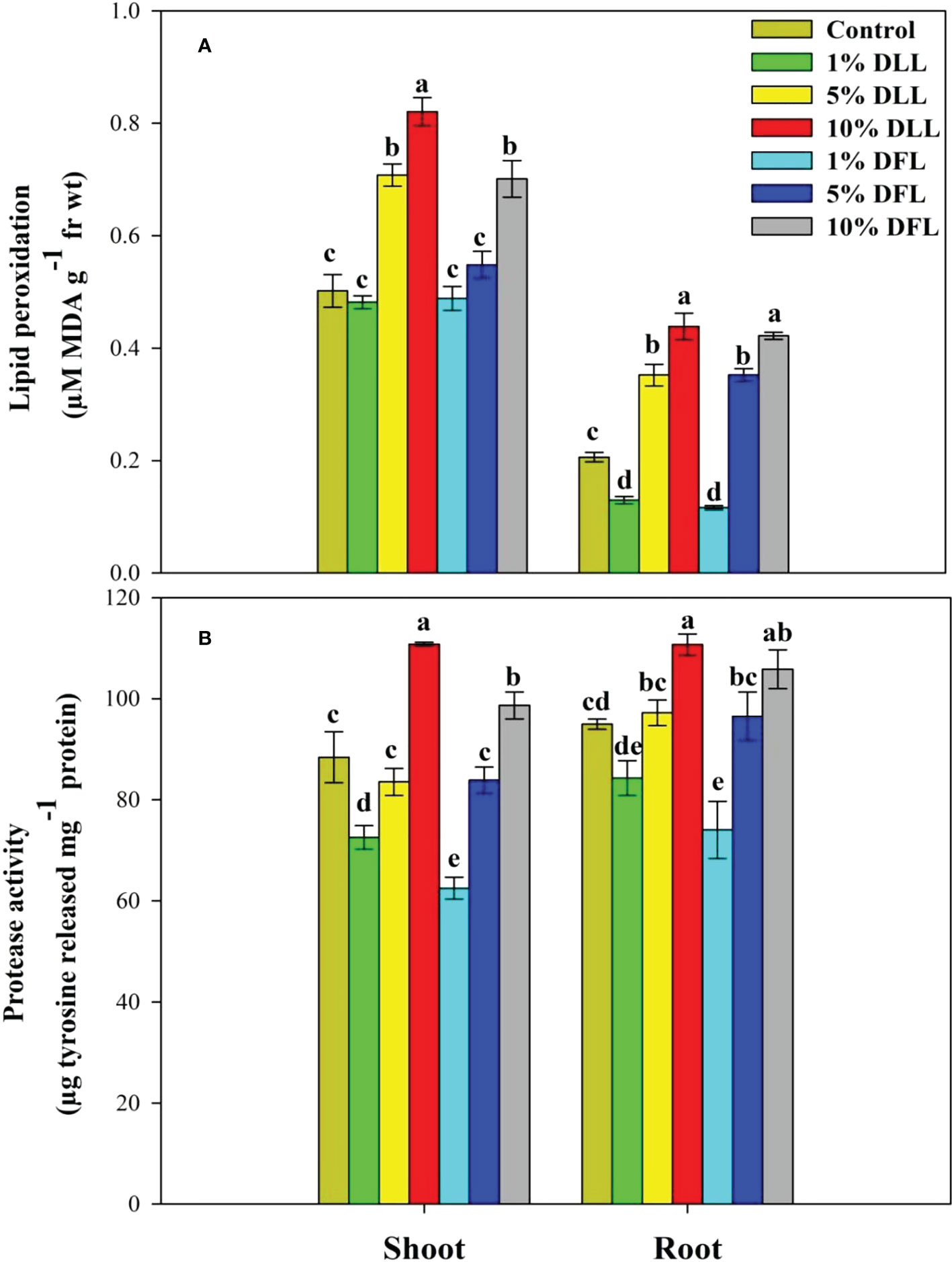
Figure 1 (A) Lipid peroxidation (µM MDA g-1 fr wt) and (B) protease (µg tyrosine released mg-1 protein) activity in Triticum aestivum L. seedlings (7 DAS) treated with leachates of dry leaves and flowers of Tagetes erecta L. Data followed by the same letters are not significantly different at p< 0.05.
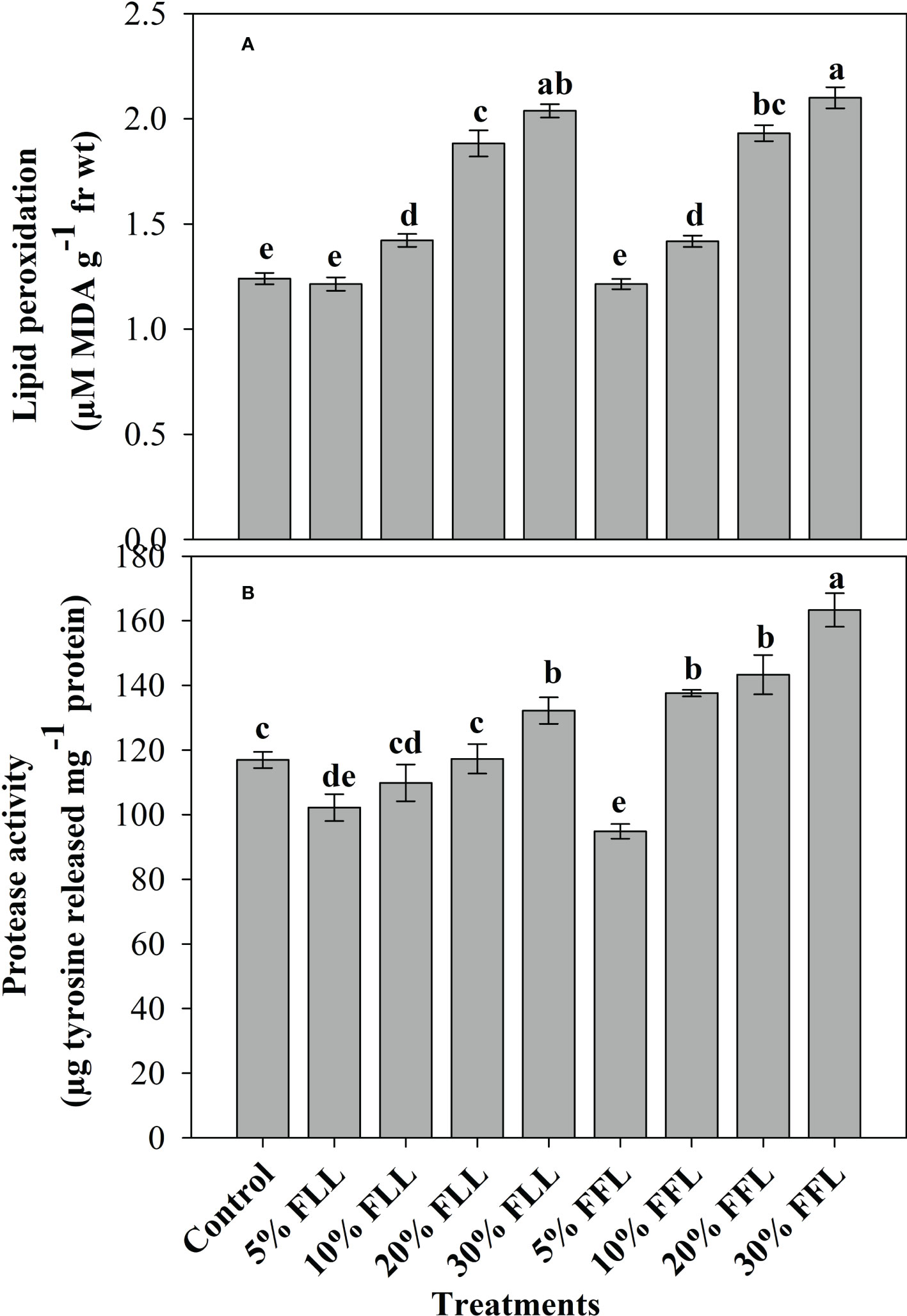
Figure 2 (A) Lipid peroxidation (µM MDA g-1 fr wt) and (B) protease (µg tyrosine released mg-1 protein) activity in leaves of Triticum aestivum L. plants raised using sand substrate (25 DAS) treated with leachates of fresh leaves and flowers of Tagetes erecta L. Data followed by the same letters are not significantly different at p< 0.05.
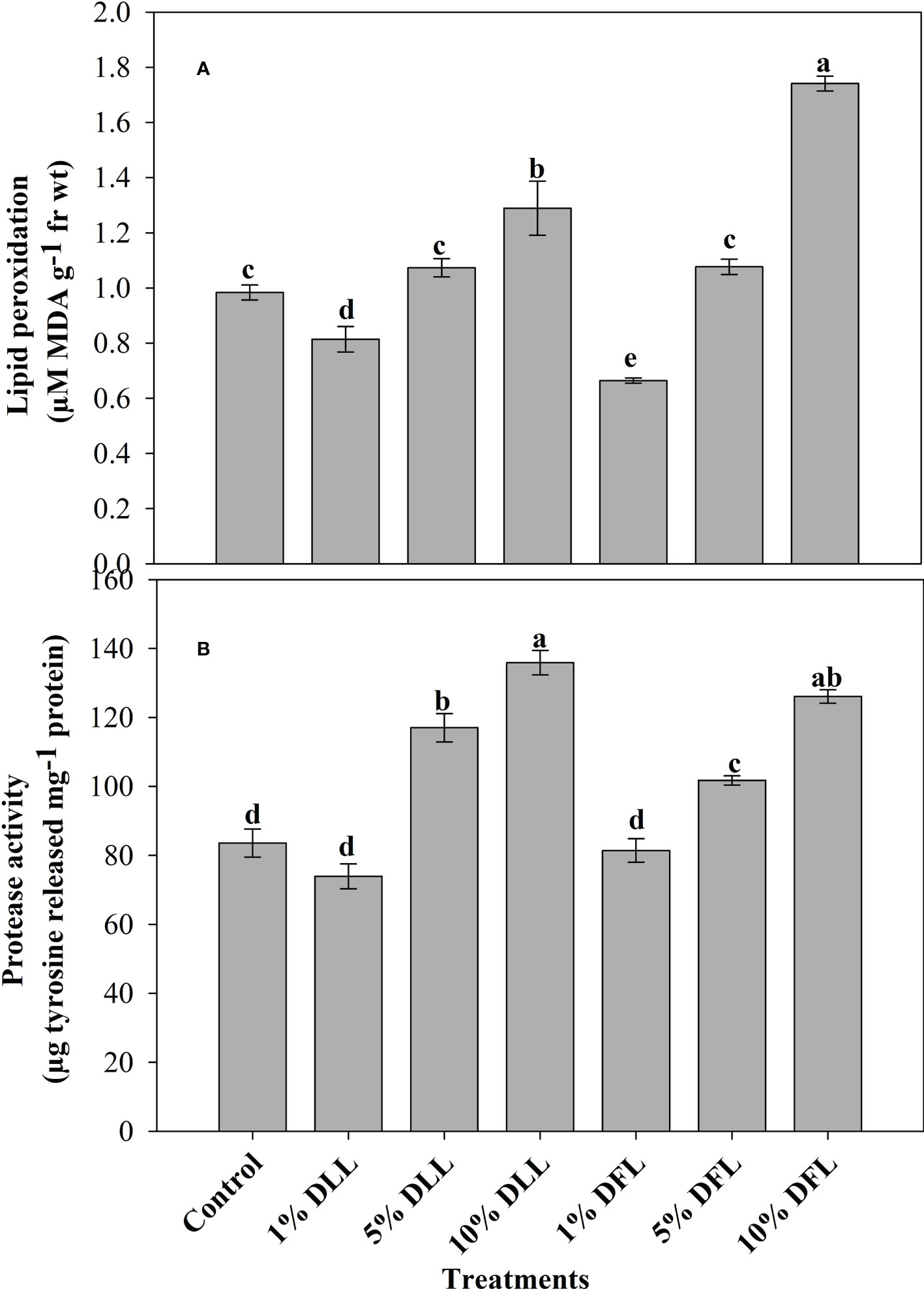
Figure 3 (A) Lipid peroxidation (µM MDA g-1 fr wt) and (B) protease (µg tyrosine released mg-1 protein) activity in leaves of Triticum aestivum L. plants raised using sand substrate (25 DAS) treated with leachates of dry leaves and flowers of Tagetes erecta L. Data followed by the same letters are not significantly different at p< 0.05.
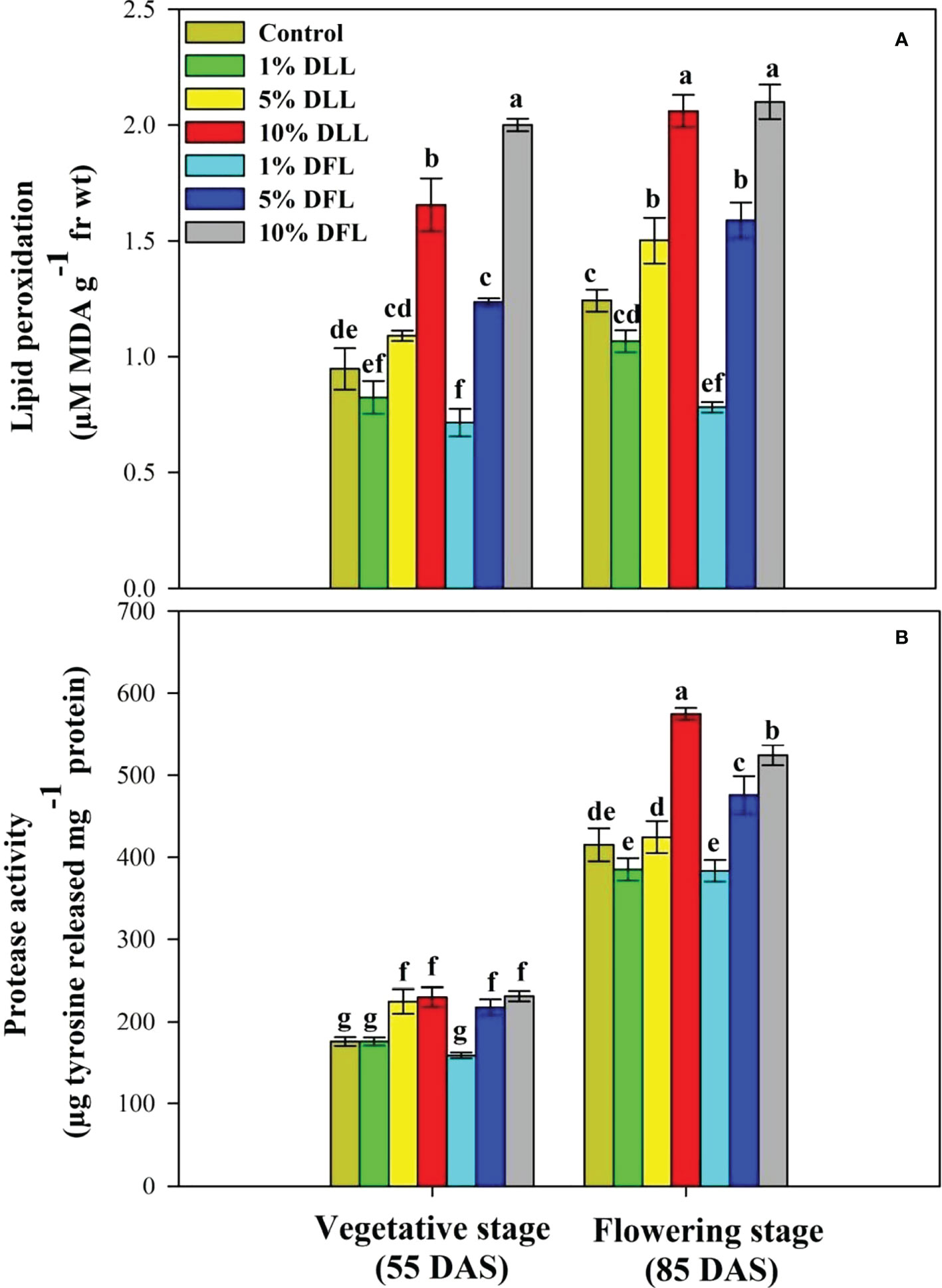
Figure 4 (A) Lipid peroxidation (µM MDA g-1 fr wt) and (B) protease (µg tyrosine released mg-1 protein) activity in flag leaves of Triticum aestivum L. raised using soil substrate at different developmental stages treated with leachates of dry leaves and flowers of Tagetes erecta L. Data followed by the same letters are not significantly different at p< 0.05.
Alterations in antioxidant components of wheat seedlings/plants
Triticum aestivum L. seedlings/plants subjected to treatments with fresh/dry leaf and flower leachates of marigold showed upregulation of the enzymatic antioxidant defense system. Activities of SOD, CAT, APX, GPX, GR, and GST showed a concentration-dependent increase in wheat seedlings/plants fed with different treatments with leachates, and the maximum increase was noticed when subjected to higher concentrations of fresh (30% w/v) and dry (10% w/v) leachate treatments (Figures 5–8). In sand culture experiments, SOD, CAT, APX, GPX, GR, and GST contrary to the control counterparts showed an increase of 85.85%, 20.65%, 82.28%, 180.3%, 39.69%, and 41.71% due to 30% FLL treatments and an increase of 92.34%, 31.01%, 72.18%, 225.4%, 104.9%, and 58.28% due to application of 30% FFL leachates of fresh parts, respectively (Figure 6). On the other hand, increases of 75.32% and 89.74% in SOD, 37.88% and 40.35% in CAT, 87.79% and 131.1% in APX, 36.47% and 60.0% in GPX, 81.92% and 81.52% in GR, and 29.91% and 41.66% in GST were observed upon application of 10% DLL and 10% DFL leachates of marigold, respectively (Figure 7). Treatments with higher concentrations of flower leachates showed more pronounced effects as compared with those of leaf leachates in most of the cases (Figures 5–8). An almost similar trend was observed in both seedlings raised in laboratory conditions (Figure 5) and flag leaves of wheat plants raised in pots (using soil substrate) under field conditions (Figure 8) supplemented with different concentrations of leachates of dry leaves and flowers of marigold. Different developmental stages/age of the plant showed significant variation in the activities of SOD, CAT, APX, GPX, GR, and GST, and in most of the cases, maximum activity was recorded at the flowering stage (Figure 8).
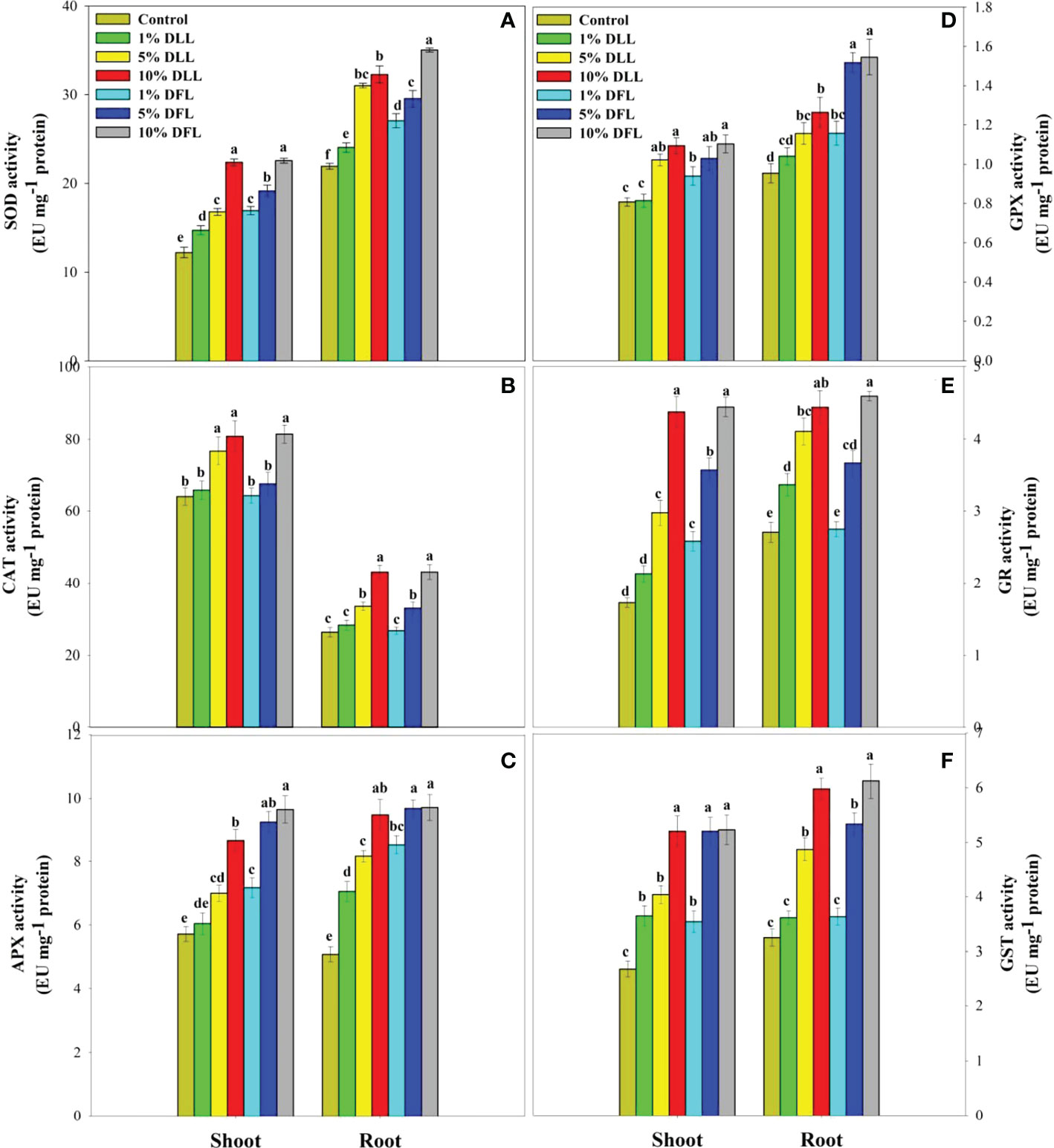
Figure 5 Superoxide dismutase, catalase, ascorbate peroxidase, guaiacol peroxidase, glutathione reductase, and glutathione S-transferase (EU mg-1 protein) activity (A–F) in Triticum aestivum L. seedlings (7 DAS) treated with leachates of dry leaves and flowers of Tagetes erecta L. Data followed by the same letters are not significantly different at p< 0.05.
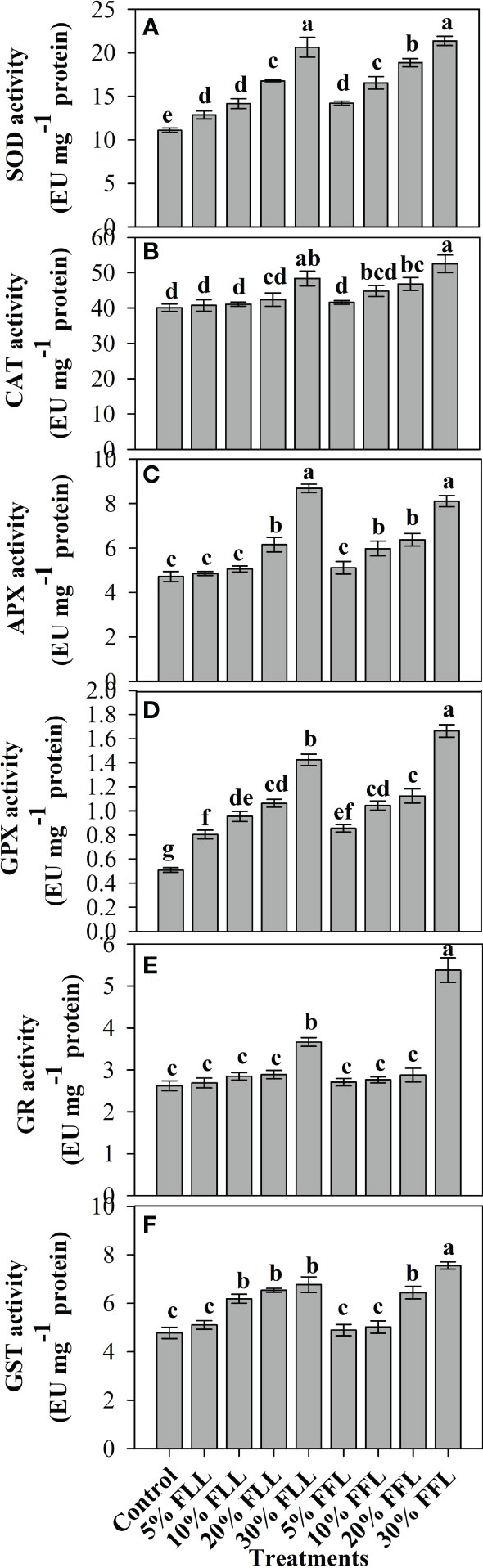
Figure 6 Superoxide dismutase, catalase, ascorbate peroxidase, guaiacol peroxidase, glutathione reductase, and glutathione S-transferase (EU mg-1 protein) activity (A–F) in leaves of Triticum aestivum L. plants raised using sand substrate (25 DAS) treated with leachates of fresh leaves and flowers of Tagetes erecta L. Data followed by the same letters are not significantly different at p< 0.05.
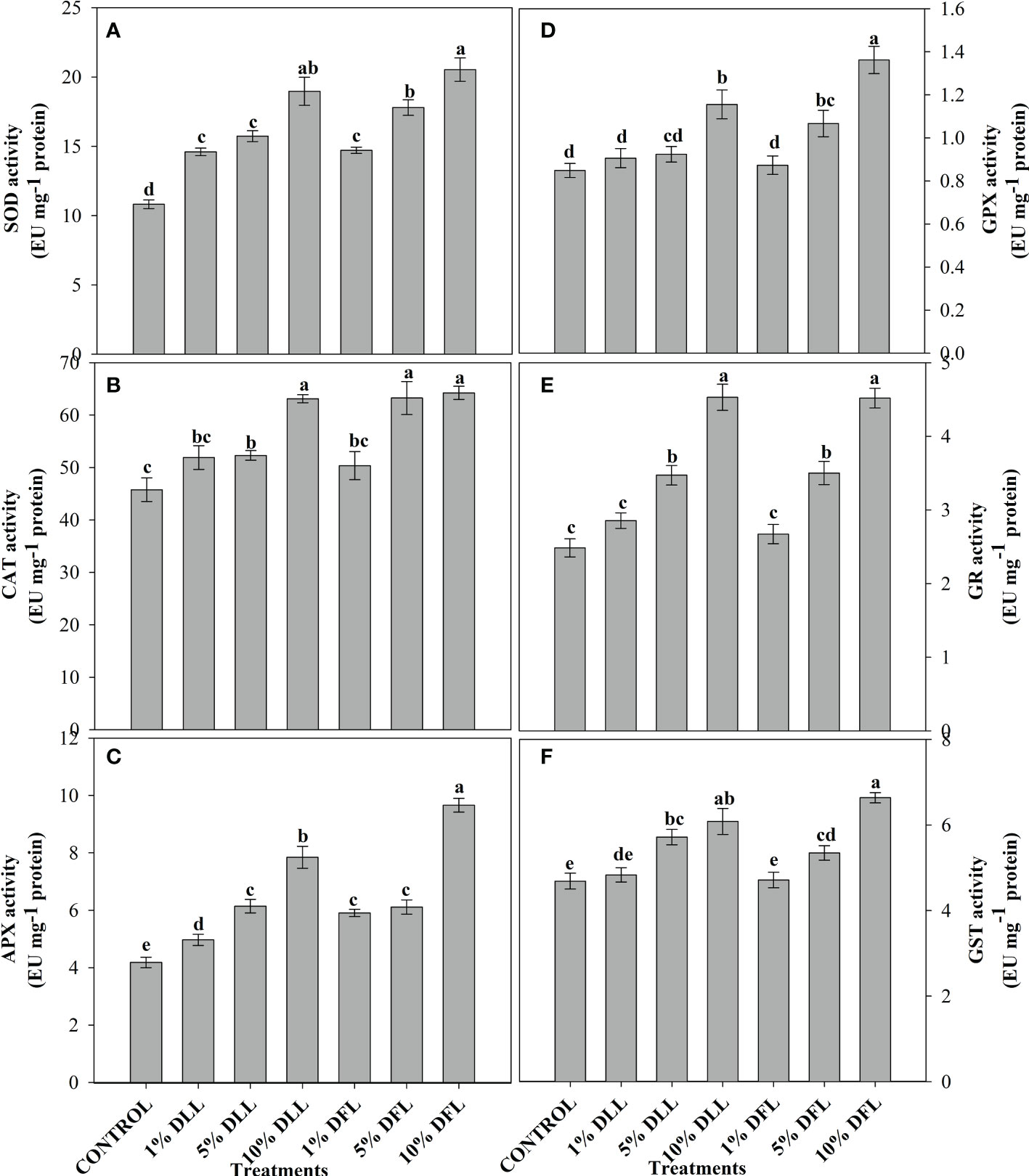
Figure 7 Superoxide dismutase, catalase, ascorbate peroxidase, guaiacol peroxidase, glutathione reductase, and glutathione S-transferase (EU mg-1 protein) activity (A–F) in leaves of Triticum aestivum L. plants raised using sand substrate (25 DAS) treated with leachates of dry leaves and flowers of Tagetes erecta L. Data followed by the same letters are not significantly different at p< 0.05.
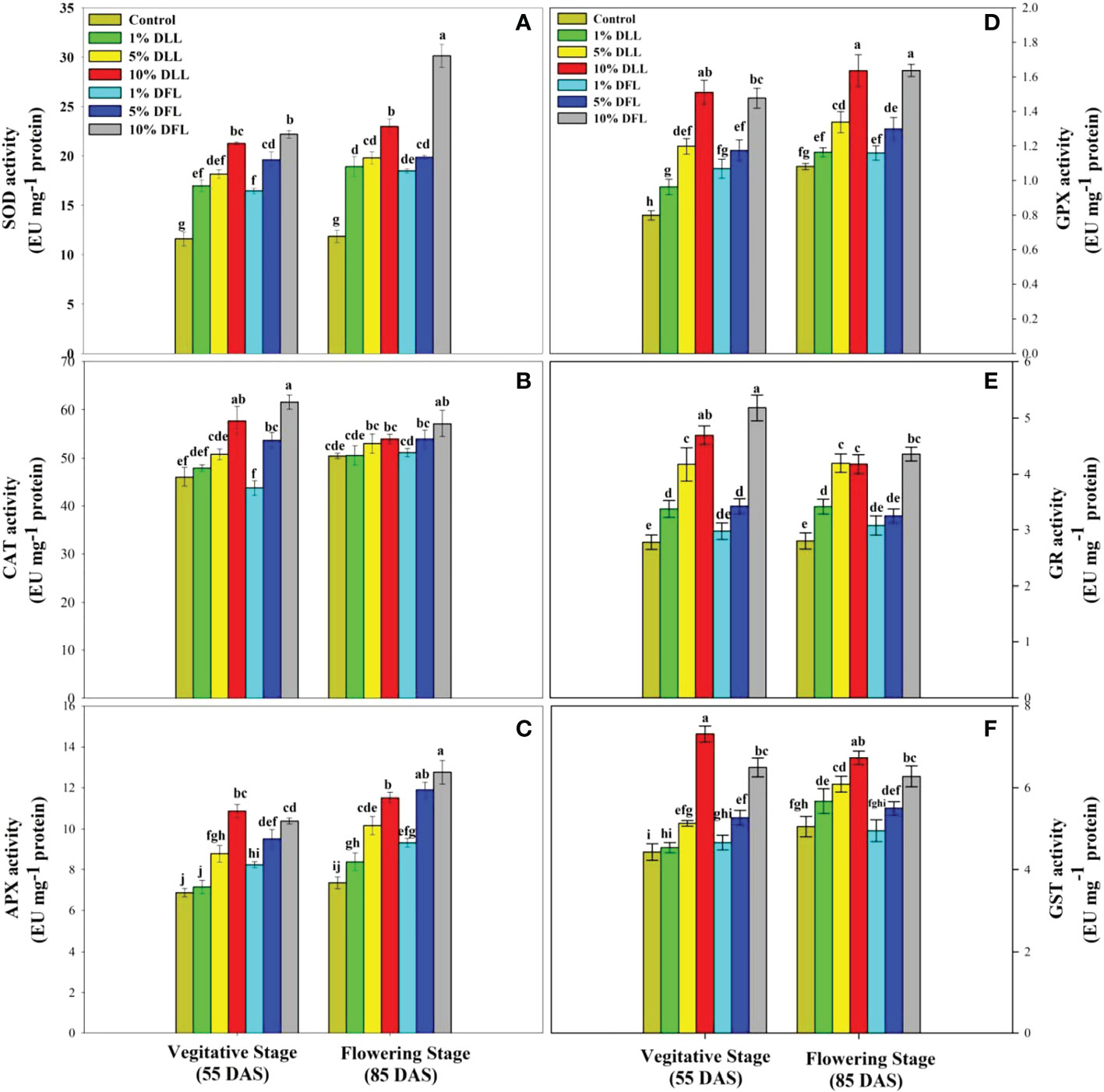
Figure 8 Superoxide dismutase, catalase, ascorbate peroxidase, guaiacol peroxidase, glutathione reductase, and glutathione S-transferase (EU mg-1 protein) activity (A–F) in flag leaves of Triticum aestivum L. raised using soil substrate at different developmental stages treated with leachates of dry leaves and flowers of Tagetes erecta L. Data followed by the same letters are not significantly different at p< 0.05.
Glutathione and ascorbic acid contents also exhibited a similar trend (Tables 1–4) and showed increases of 190.6% and 33.72% by 30% FLL treatments and 211.6% and 45.55% due to 30% FFL leachates of fresh parts (Table 2). Increases of 134% and 71.33% were recorded due to 10% DLL treatments and 160% and 82.25% due to 10% DFL leachates of dry parts (Table 3). A more or less similar trend was observed in flag leaves of wheat plants at the pre-flowering and flowering stages exposed to 10% DLL and 10% DFL leachates of dry parts of marigold (Table 4).
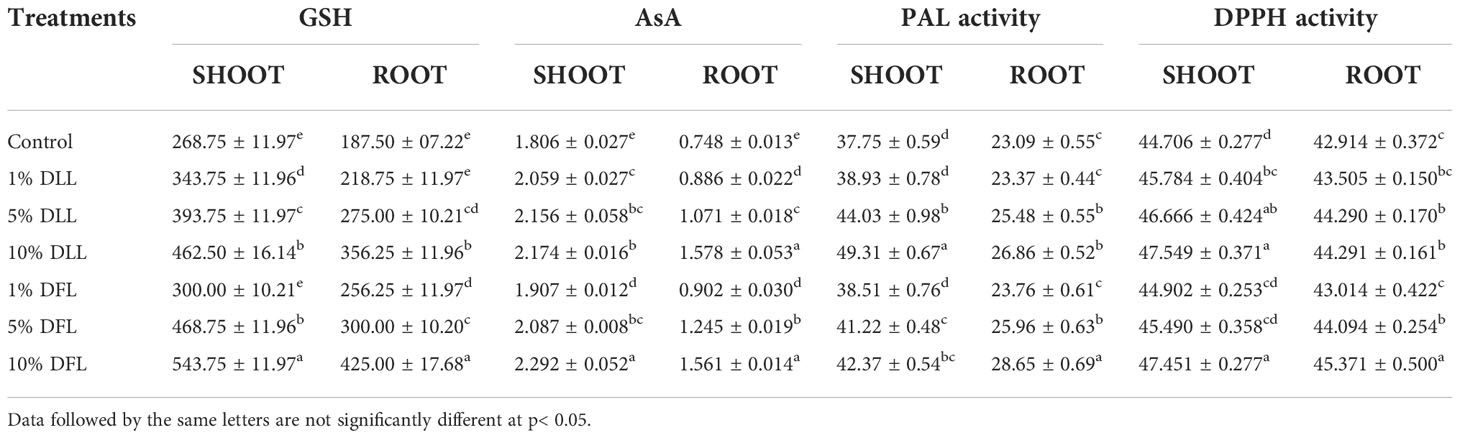
Table 1 Reduced glutathione (nM g-1 fr wt), ascorbic acid (mg g-1 fr wt), phenyl alanine ammonia lyase (nM trans-cinnamic acid min-1 mg-1 protein), and total antioxidant (percent) activity in Triticum aestivum L. seedlings (7 DAS) treated with leachates of dry leaves and flowers of Tagetes erecta L.
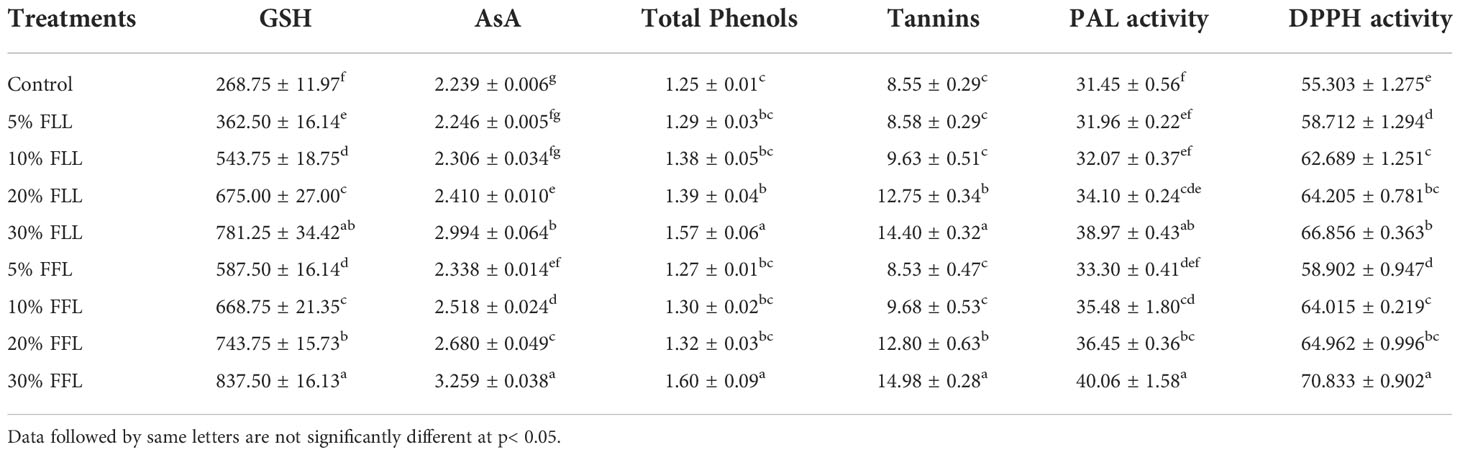
Table 2 Reduced glutathione (nM g-1 fr wt), ascorbic acid, total phenols, tannins (mg g-1 fr wt), phenyl alanine ammonia lyase (nM trans-cinnamic acid min-1 mg-1 protein), and total antioxidant (percent) activity in leaves of Triticum aestivum L. plants raised using sand substrate (25 DAS) treated with leachates of fresh leaves and flowers of Tagetes erecta L.
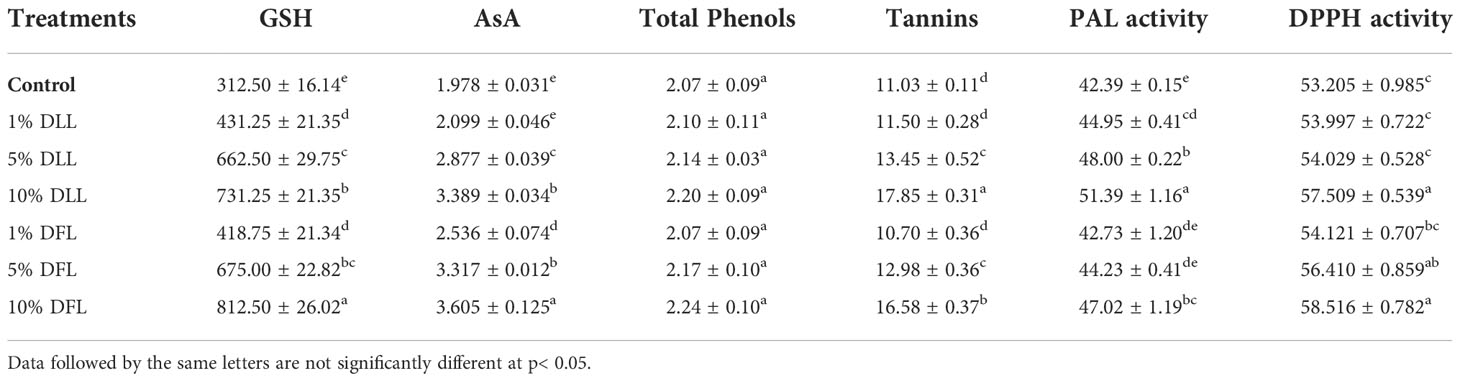
Table 3 Reduced glutathione (nM g-1 fr wt), ascorbic acid, total phenols, tannins (mg g-1 fr wt), phenyl alanine ammonia lyase (nM trans-cinnamic acid min-1 mg-1 protein), and total antioxidant (percent) activity in leaves of Triticum aestivum L. plants raised using sand substrate (25 DAS) treated with leachates of dry leaves and flowers of Tagetes erecta L.
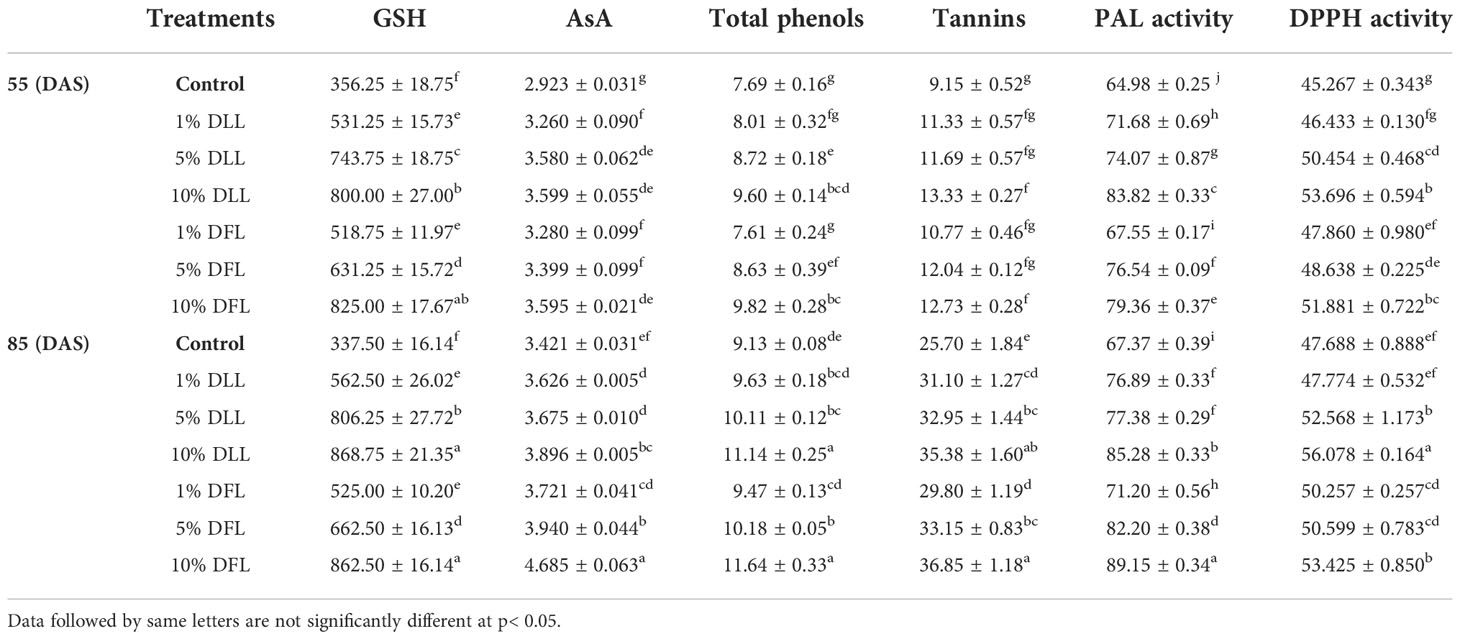
Table 4 Reduced glutathione (nM g-1 fr wt), ascorbic acid content (mg g-1 fr wt), total phenols, tannins (mg g-1 dr wt), phenyl alanine ammonia lyase (nM trans-cinnamic acid min-1 mg-1 protein), and total antioxidant (percent) activity in flag leaves of Triticum aestivum L. raised using soil substrate at different developmental stages treated with leachates of dry leaves and flowers of Tagetes erecta L.
Effect on the total phenols and tannins
Total phenols and tannins increased in Triticum aestivum L. plants exposed to higher concentrations of treatments, i.e., 10%, 20%, and 30% w/v of fresh parts and 5% and 10% w/v of dry parts of marigold (Tables 2–4); however, the maximum increase was noticed in wheat plants treated with 30% w/v of fresh parts and 10% w/v of dry parts of marigold. An increase in total phenols and tannins by 25.6% and 68.42% due to 30% FLL treatments and by 28% and 75.20% due to 30% FFL leachates of fresh leaves and flowers of marigold (Table 2) and by 6.28% and 61.83% due to 10% DLL treatments and 8.21% and 50.31% due to 10% DFL leachates of dry leaves and flowers of marigold was found (Table 3). Contents of total phenols and tannins were more at the flowering stage (85 DAS) as compared with the pre-flowering stage (55 DAS). An increase of 22.01% and 27.49% in total phenols and 37.66% and 43.38% in tannins was observed in wheat plants at the flowering stage exposed to 10% DLL and DFL leachates of dry marigold parts (Table 4).
Increased contents of phenols in wheat plants treated with leachates of marigold plant parts may impart greater total antioxidant activity (Tables 1–4). Leachates of fresh parts of 30% FLL and 30% FFL caused an increase in the activity of total antioxidants (DPPH) by 20.89% and 28.08% relative to the control (Table 2) and at the same time by 8.08% and 9.98% when supplied with 10% DLL and 10% DFL treatments (Table 3) in the sand culture setup. This improvement of metabolites in wheat plants treated with leaf and flower leachates of marigold was accompanied by an increase in activity of (PAL) phenylalanine ammonia lyase (Tables 1–4). PAL activity increased by 23.91% and 27.37% in wheat plants due to application of 30% FLL and 30% FFL treatments (Table 2) and by 21.23% and 10.92% when exposed to 10% DLL and 10% DFL treatments (Table 3). Total antioxidant activity and the activity of phenylalanine ammonia lyase showed a more or less similar trend in wheat seedlings/plants raised in laboratory conditions and pots (using soil substrate) under field conditions (Tables 1, 4).
Accumulation of osmotic constituents in wheat plants
Free sugars in wheat leaves treated with fresh leachates of leaves and flowers of T. erecta L. showed little variation (Table 5); however, wheat plants fed with higher concentrations, i.e., 10% DLL and 10% DFL of dry parts T. erecta L., showed an increase in free sugars by 22.65% and 17.67% (Table 6). Nevertheless, an increase in free sugars by 26.82% and 34.13% was also found in flag leaves of 85 DAS-old wheat plants fed with 10% DLL and 10% DFL of dry parts (Table 7). Free amino acids and free proline contents in wheat plants raised in sand cultures registered a maximum increase by 17% and 113.4% up on application of 30% FLL treatments and by 34.01% and 92.32% due to application of 30% FFL leachates of fresh parts (Table 5). On the other hand, the enhancement in free amino acids and free proline contents in wheat plants was 243.5% and 46.77% when exposed to 10% DLL treatments and 297% and 44.41% when exposed to 10% DFL leachates of dry parts of Tagetes erecta L. (Table 6). Wheat plants raised in pots using soil substrates under a field setup also showed a similar trend (Table 7).
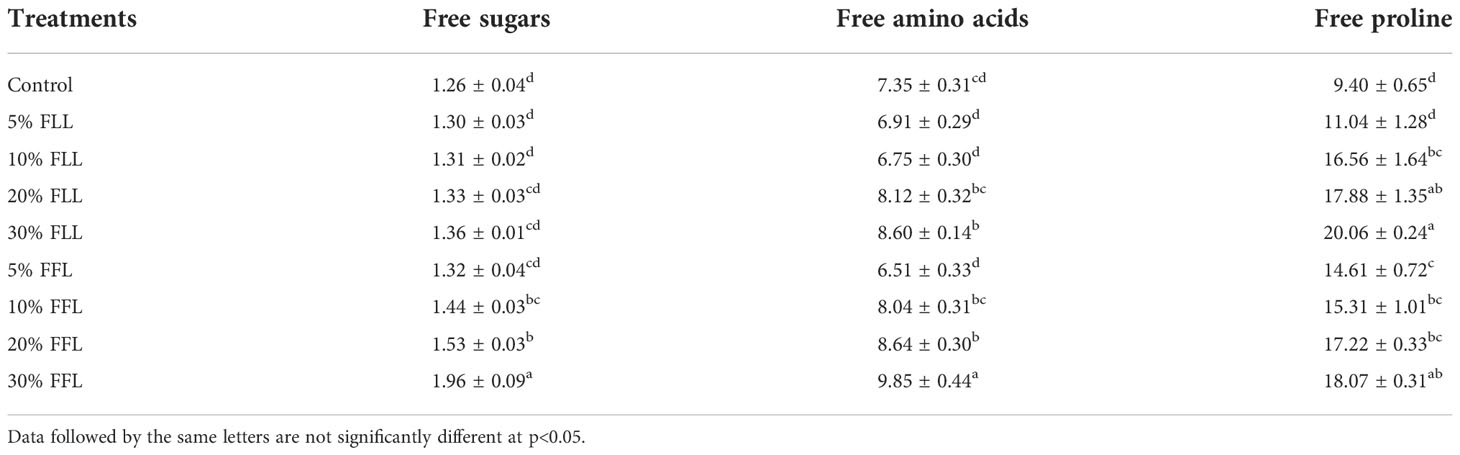
Table 5 Free sugars, free amino acids (mg g-1 fr wt), and free proline (μ mole g-1 fr wt) contents in leaves of Triticum aestivum L. plants raised using sand substrate (25 DAS) treated with leachates of fresh leaves and flowers of Tagetes erecta L.
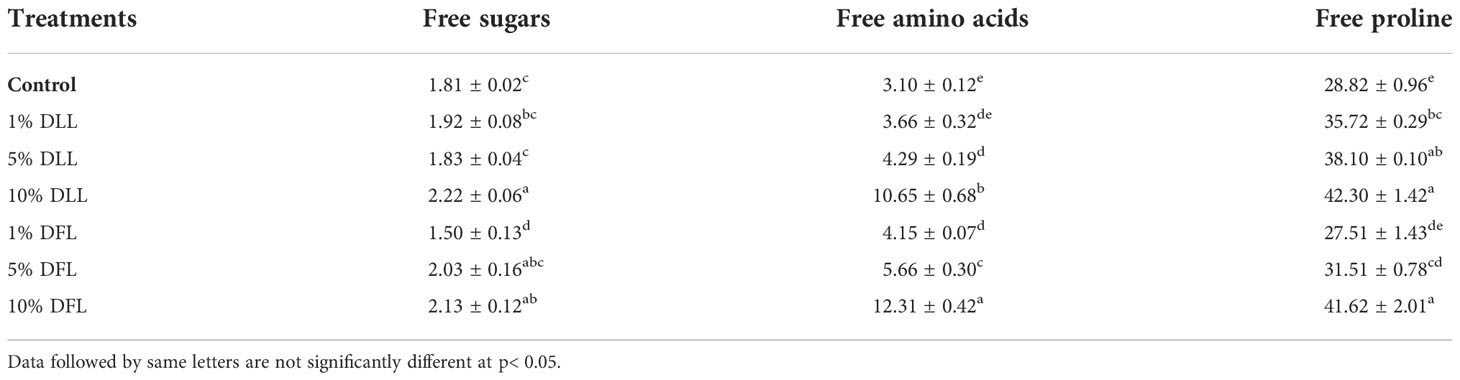
Table 6 Free sugars, free amino acids (mg g-1 fr wt) and free proline (μ mol g-1 fr wt) contents in leaves of Triticum aestivum L. plants raised using sand substrate (25 DAS) treated with leachates of dry leaves and flowers of Tagetes erecta L.
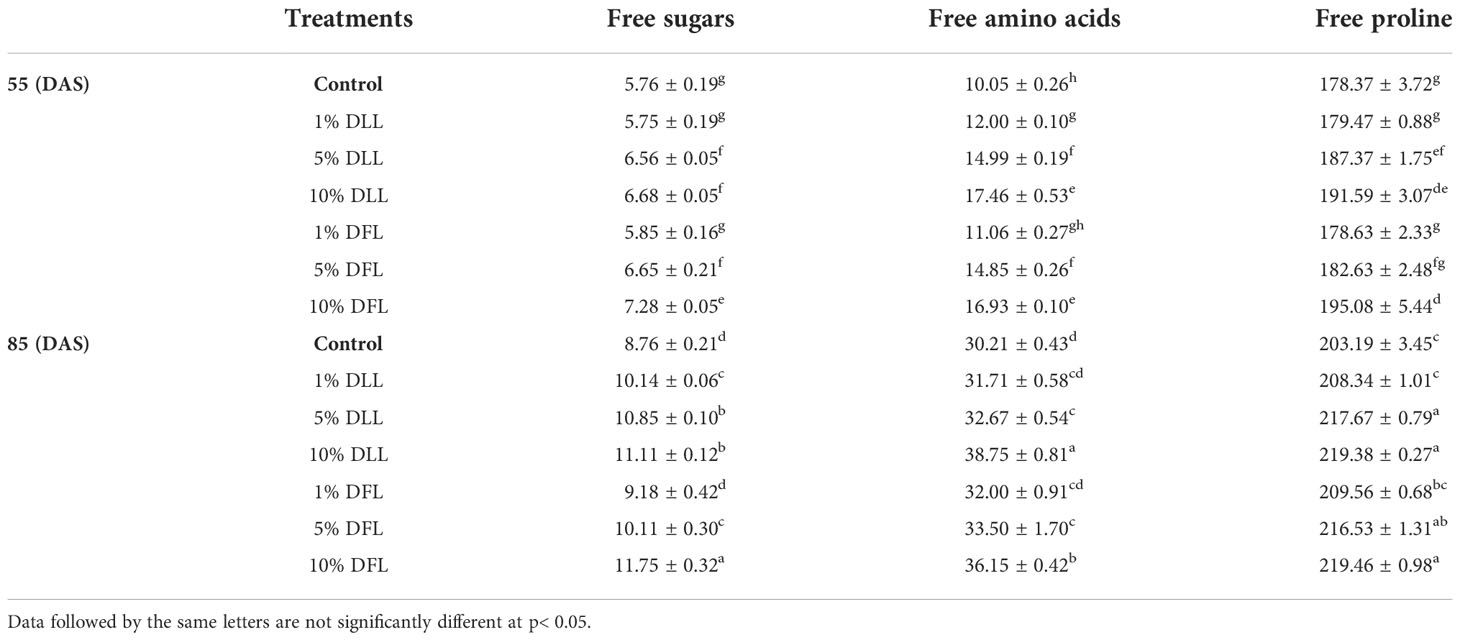
Table 7 Free sugars, free amino acids (mg g-1 dr wt), and free proline (μ mole g-1 dr wt) contents in flag leaves of Triticum aestivum L. raised using soil substrate at different developmental stages treated with leachates of dry leaves and flowers of Tagetes erecta L.
Variation in relative water content, length, biomass, and yield attributes in wheat plants
RWC (relative water content) decreases in wheat plants with the increase in the concentration of leachates, and a maximum decline was observed in wheat plants treated with 10% DLL and 10% DFL leachates of dry parts. Plant height, spike length, number of spikelets per spike, and 100 grain weight of the wheat plants registered an increase of 5.94%, 14.25%, 30.30%, and 3.49% due to application of 1% DLL and of 6.42%, 20.21%, 33.33%, and 4.19% due to 1% DFL leachate treatments, whereas a reduction in plant height by 7.39% and 5.83%, spike length by 5.74% and 4.89%, number of spikelets per spike by 27.27% and 33.33%, and 100 grain weight by 3.84% and 3.14% was recorded due to application of 10% DLL and 10% DFL leachates of dry leaves and flowers of Tagetes erecta L. (Table S1).
Discussion
Allelopathic effects on plant species are directly reflected in altered physiology and biochemistry. Both inhibitory and stimulatory modulations do have a direct relationship with the cellular structural and functional stability. In the present study, it was observed that Triticum aestivum L. seedlings/plants showed significant enhancement in lipid peroxidation when exposed to higher concentrations of fresh/dry leachates of marigold leaves and flowers. Stress imposition exhibited increased generation of H2O2 imparting greater peroxidation of lipids, which results in leakage of important cellular components (Sofo et al., 2004). Zhang et al. (2007), while working on mangrove plants, noticed that the lipid peroxidation level in leaves may serve as a biomarker in Bruguiera gymnorrhiza whereas POD activity may be used as heavy metal biomarker for Kandelia candel. The enhancement in lipid peroxidation has also been reported in different plants facing extreme environmental conditions like salt stress (Borzouei et al., 2012; Ahanger et al., 2020), stress imposed by heavy metals (Singh et al., 2017), and allelopathic stress as well (Al-Hawas and Azooz, 2018; da Silva and Vieira, 2019). In addition, the oxidative effects of leachates were also evident from the altered protease activity reflecting in greater damage to protein structures and hence to their functioning. It was evident that exposure to higher concentrations of leachates resulted in increased protease activity in both laboratory and pot experiments (Figures 1B, 2B, 3B, 4B). Plant proteases play an important role in many different biological processes including stomatal development and distribution, as well as systemic stress response. They are also related to the increase in ROS production detected during plant exposure and response to environmental stress (Mangena, 2022). Proteases mitigate this process by degradation of damaged, denatured proteins, remobilizing amino acids and generating molecules involved in signal transduction (D’Ippólito et al., 2021). The upregulation of protease activity in plants facing extreme environments has been reported earlier as well (Ahanger et al., 2020; Tittal et al., 2020).
An increase of total phenols and tannins was observed in Triticum aestivum L. at different developmental stages up on application of leachates of leaves and flowers of Tagetes erecta L., which may be due to the presence of allelochemicals in these leachates. The metabolite accumulation increases with the increase in the concentration of leachates. Increased contents of phenols in treated wheat plants may impart greater total antioxidant activity, and this improvement of metabolites in wheat plants was accompanied by an increase in activity of phenylalanine ammonia lyase (PAL). This increase in the antioxidant components in wheat plants in response to treatments of leaf and flower leachates of marigold indicated stress imposition by allelochemicals present in these leachates of plant parts. Plants exposed to a variety of environmental stresses results in oxidative stress; the uncontrolled production and accumulation of ROS is one of the effects of allelochemicals on target plants which affect biological processes triggering changes in cellular metabolism accompanied by activation of the antioxidant defense system. The inappropriate balance between ROS production and the counterbalance of free radicals by the antioxidant defense system causes damage to the cellular components which may lead to cell death. Antioxidants’ components are important for the maintenance of ROS at the physiological level (Xie et al., 2019) maintaining cellular homeostasis (Gill and Tuteja, 2010; Das and Roychoudhury, 2014) by scavenging ROS and also preventing the formation of toxic radicals.
The activities of antioxidant enzymes such as SOD, CAT, APX, GR, and GPX along with the non-enzymatic antioxidant components such as AsA and GSH were considerably affected in wheat seedlings when exposed to shoot extracts of Achillea santolina (Hatata and El-Darier, 2009). In the present study also, it was observed that activity of enzymatic antioxidants and the contents of non-enzymatic ones showed an apparent increase reflecting the potential to induce the counteractive mechanisms for lessening the allelopathic effects. Increased peroxidation of lipids and alterations in antioxidant enzymes in different plants upon exposure to allelochemical stress have been reported earlier as well (Cruz-Ortega et al., 2007; Tomar et al., 2015). The concentration-dependent enhancement in the activity of enzymatic antioxidants like superoxide dismutase, catalase, and peroxidase has been reported in Brassica oleracea seedlings when subjected to the treatment of Calotropis procera extracts (Gulzar and Siddiqui, 2017) and in pea seedlings also when exposed to Artemisia monosperma and Thymus vulgaris extracts (Al-Hawas and Azooz, 2018). Sesamum indicum L. has also shown a significant increase in the activity of antioxidant enzymes up on exposure to sorghum aqueous leaf and stem extract (Murimwa et al., 2019).
In the present study, it was also noticed that osmotic components/osmolytes including sugars, proline, and free amino acids increased in wheat seedlings grown with leachate treatments. Osmolytes are low-molecular-weight organic solutes, the biosynthesis of which is influenced by the environment. Osmolytes protect subcellular structures from ROS and contribute toward turgor maintenance and osmotic adjustments (Slama et al., 2015). The accumulation of free proline has also been reported in lettuce seedlings subjected to different stages of shoot aqueous extracts of diploid and mixoploid fenugreek (Omezzine et al., 2014). Singh et al. (2006) have reported the accumulation of free proline in Cassia occidentalis as a result of treatment of α-pinene. These osmoregulators like sugars and amino acids play a significant part in stabilization of cells and tissues of plants particularly under harsh conditions by adjusting the osmotic pressure (Hasegawa et al., 2000). Accumulation of soluble sugars increased in Zea mays by the allelopathic potential of aqueous extract of air-dried olive processing waste in a concentration-dependent way (Saleh, 2013). Aqueous extract of roots and leaves of Heliotropium bacciferum Forssk gradually increased the proline and soluble sugars in Oryza sativa and Teucrium polium plants (Al-Taisan, 2014), and in this respect leaves of Heliotropium bacciferum were more effective than roots. An increased amount of proline and soluble sugars served as a defense mechanism in the growth of Raphanus sativus L. against the allelochemical stress induced by water extracts of aerial parts of peppermint having phenolic compounds like trans-ferulic acid (10.8 mg/g), hesperidin (9.3 mg/g), ellagic acid (6.8 mg/g), and sinapic acid (4.2 mg/g) detected using HPLC (Mahdavikia et al., 2017). Unal et al. (2017) have reported an increase in proline content in wild mustard plants against the allelopathic effects of Palustriella falcata. An enhancement of proline has also been reported in Phaseolus vulgaris seedlings against allelopathic effects of an alkaloid fraction of Crotalaria retusa Linn (Ogunsusi et al., 2018).
RWC (relative water content), height, spike length, number of spikelets per spike, and 100 grain weight of the wheat plants raised with the leachates of dry leaves and flowers of Tagetes erecta L. exhibited a slight increase at lower concentrations of 1% w/v, whereas a decrease was recorded at higher concentrations of 10% w/v. A decrease in relative water content has been reported earlier in Brassica campestris L. (mustard) treated with aqueous extracts of the roots, leaves, and seeds of Cassia tora L. by Sarkar et al. (2012). Similarly, a decrease in RWC was also reported in Chenopodium album L., Melilotus alba, and Nicotiana plumbaginifolia plants subjected to allelopathic stress imposed by Cassia sophera L. extracts (Gulzar et al., 2014) and in Brassica oleracea plants exposed to Calotropis procera extracts (Gulzar and Siddiqui, 2017).
A decline in length, biomass, and yield attributes was also observed in Oryza sativa L. and Triticum aestivum L. plants in response to incorporation of sunflower residues (Bashir et al., 2012). A reduction in growth of lettuce was registered when exposed to shoot aqueous extracts of diploid and mixoploid fenugreek (Omezzine et al., 2014). A decrease in germination, length, and biomass accumulation has been observed in Chenopodium album L., Melilotus alba, and Nicotiana plumbaginifolia plants in response to Cassia sophera L. extracts (Gulzar et al., 2014), in wheat plants in response to Jatropha curcas leachates/extracts (Tomar et al., 2015), in Brassica oleracea plants in response to Calotropis procera extracts (Gulzar and Siddiqui, 2017), and in Eleusine coracana in response to higher concentrations of leaf leachate extracts of (mangrove) Excoecaria agallocha L. The inhibition can be related to the allelochemicals like flavonoids and phenolic acids (Desai et al., 2017).
Conclusion
The above results indicate that the leachates of fresh/dry parts of Tagetes erecta L. at higher concentrations enhance the antioxidant and osmotic components of wheat (Triticum aestivum L.) seedlings/plants, which probably reflects on their growth and yield parameters. Treatments of dry leachates have greater impact than fresh leachates, and the effect of flower leachates in general was more than that of leaf leachates. The leachate treatments show inhibitory effects on the growth and yield of wheat at higher concentrations only, probably indicating that continuous/mismanaged decomposition of Tagetes erecta L. in the agriculture field may need monitoring. Moreover, understanding the mechanisms of possible allelochemical action may impart a basis for the development of new natural biodegradable herbicides to control weeds maintaining an ecofriendly environment for crops in order to boost production in sustainable agriculture; however, further experiments are needed in this context.
Data availability statement
The raw data supporting the conclusions of this article will be made available by the authors, without undue reservation.
Author contributions
This manuscript contains part of the PhD work of RM. This study was conceived and designed by Prof. RA. RM performed the experiments and wrote the first draft of the manuscript, and RA cross-checked the results and manuscript. SA and MA helped in the experiments, and KJ helped in the literature survey. All authors contributed to the article and approved the submitted version.
Acknowledgments
The authors are thankful to Head, School of Studies in Botany, Jiwaji University, Gwalior, for providing necessary facilities.
Conflict of interest
The authors declare that the research was conducted in the absence of any commercial or financial relationships that could be construed as a potential conflict of interest.
Publisher’s note
All claims expressed in this article are solely those of the authors and do not necessarily represent those of their affiliated organizations, or those of the publisher, the editors and the reviewers. Any product that may be evaluated in this article, or claim that may be made by its manufacturer, is not guaranteed or endorsed by the publisher.
Supplementary material
The Supplementary Material for this article can be found online at: https://www.frontiersin.org/articles/10.3389/fpls.2022.1001394/full#supplementary-material
References
Ahanger, M. A., Mir, R. A., Alyemeni, M. N., Ahmad, P. (2020). Combined effects of brassinosteroid and kinetin mitigates salinity stress in tomato through the modulation of antioxidant and osmolyte metabolism. Plant Physiol. Biochem. 147, 31–42. doi: 10.1016/j.plaphy.2019.12.007
Ahanger, M. A., Tittal, M., Mir, R. A., Agarwal, R. M. (2017a). Alleviation of water and osmotic stress –induced changes in nitrogen metabolizing enzymes in Triticum aestivum l. cultivars by potassium. Protoplasma 254, 1953–1963. doi: 10.1007/s00709-017-1086-z
Ahanger, M. A., Tomar, N. S., Tittal, M., Argal, S., Agarwal, R. M. (2017b). Plant growth under water/salt stress: ROS production; antioxidants and significance of added potassium under such conditions. Physiol. Mol. Biol. Plants 23, 731–744. doi: 10.1007/s12298-017-0462-7
Al-Hawas, G. H. S., Azooz, M. M. (2018). Allelopathic potentials of Artrmisia monosperma and Thymus vulgaris on growth and physio-biochemical characteristics of pea seedlings. Pak. J. Biol. Sci. 21, 187–198. doi: 10.3923/pjbs.2018.187.198
Al-Taisan, W. A. (2014). Allelopathic effects of Heliotropium bacciferum leaf and roots on Oryza sativa and Teucrium polium. Life. Sci. J. 11, 41–50.
Arif, M., Cheema, Z. A., Khaliq, A., Hassan, A. (2015). Organic weed management in wheat through allelopathy. Int. J. Agric. Biol. 17, 127–134.
Awan, F. K., Rasheed, M., Ashraf, M., Khurshid, M. Y. (2012). Efficacy of brassica, sorghum and sunflower aqueous extracts to control wheat weeds under rain fed conditions of pothwar, Pakistan. J. Anim. Plant Sci. 22, 715–721.
Bashir, U., Javai, A., Bajwa, R. (2012). Allelopathic effects of sunflower residue on growth of rice and subsequent wheat crop. Chil. J. Agr. Res. 72, 326–331. doi: 10.4067/S0718-58392012000300004
Bates, L. S., Waldren, R. P., Teare, I. D. (1973). Rapid determination of free proline for water stress studied. Plant Soil. 39, 205–207. doi: 10.1007/BF00018060
Borzouei, A., Kafi, M., Akbari-Ghogdi, E., Mousavi-Shalmani, M. (2012). Long term salinity stress in relation to lipid peroxidation, super oxide dismutase activity and proline content of salt-sensitive and salt-tolerant wheat cultivars. Chil. J. Agr. Res. 72, 476–482. doi: 10.4067/S0718-58392012000400003
Chance, B., Maehly, C. (1955). Assay of catalase and peroxidases. Meth. Enzymol. 11, 764–775. doi: 10.1016/S0076-6879(55)02300-8
Cruz-Ortega, R., Lara-Nunez, A., Anaya, A. L. (2007). Allelochemical stress can trigger oxidative damage in receptor plants: mode of action of phytotoxicity. Plant Signal. Behav. 2, 269–270. doi: 10.4161/psb.2.4.3895
da Silva, I. F., Vieira, E. A. (2019) Phytotoxic potential of Senna occidentalis (L.) link extracts on seed germination and oxidative stress of ipe seedlings. Plant Biol. 21, 770–779. doi: 10.1111/plb.12969
Das, K., Roychoudhury, A. (2014). Reactive oxygen species (ROS) and response of antioxidants as ROS-scavengers during environmental stress in plants. Front. Environ. Sci. 2. doi: 10.3389/fenvs.2014.00053
Desai, N., Dethe, U., Gaikwad, D. (2017). Allelopathic effect of Excoecaria agallocha l. mangrove leaf leachate on germination and growth behavior of Eleusine coracana (Finger millet). Am. J. Plant Physiol. 12, 38–44. doi: 10.3923/ajpp.2017.38.44
Dhindsa, R. H., Plumb-Dhindsa, R., Thorpe, T. A. (1981). Leaf senescence correlated with increased level of membrane permeability, lipid peroxidation and decreased level of SOD and CAT. J. Exp. Bot. 32, 93–101. doi: 10.1093/jxb/32.1.93
D’Ippólito, S., Rey-Burusco, M. F., Feingold, S. E., Guevara, M. G. (2021). Role of proteases in the response of plants to drought. Plant Physiol. Biochem. 168, 1–9. doi: 10.1016/j.plaphy.2021.09.038
Fong, J., Schaffer, F. L., Kirk, P. L. (1953). The ultramicrodetermination of glycogen in liver. A comparison of the anthrone and reducing-sugar methods. Arch. Biochem. Biophy. 45, 319–326. doi: 10.1016/S0003-9861(53)80009-3
Foyer, C. H., Halliwell, B. (1976). The presence of glutathione and glutathione reductase in chloroplasts: A proposed role in ascorbic acid metabolism. Planta 133, 21–25. doi: 10.1007/BF00386001
Gallego, J. C. A., Caro, J. G., Campos, V. H., Lobon, N. C. (2020). Effect of leaf litter from Cistus ladanifer l. on the germination and growth of accompanying shrubland species. Plants 9, 593. doi: 10.3390/plants9050593
Gill, S. S., Tuteja, N. (2010). Reactive oxygen species and antioxidant machinery in abiotic stress tolerance in crop plants. Plant Physiol. Biochem. 48, 909–930. doi: 10.1016/j.plaphy.210.08.016
Gniazdowska, A., Bogatek, R. (2005). Allelopathic interactions between plants. multisite action of allelochemicals. Acta Physiol. Plant 27, 395–407. doi: 10.1007/s11738-005-0017-3
Green, N. M., Neurath, H. (1954). “Proteolytic enzymes,” in The proteins. Eds. Neurath, H., Vailey, K. (New York: Academic Press), 1057–1198.
Gulzar, A., Siddiqui, M. B. (2017). Allelopathic effect of Calotropis procera (Ait.) r. br. on growth and antioxidant activity of Brassica oleracea var. botrytis. J. Saudi. Society. Agric. Sci. 16, 375–382. doi: 10.1016/j.jssas.2015.12.003
Gulzar, A., Siddiqui, M. B., Shazia, B. (2014). Assessment of allelopathic potential of Cassia sophera l. @ on seedling growth and physiological basis of weed plants. Afr. J. Biotechnol. 13, 1037–1046. doi: 10.5897/AJB2013.13512
Habig, W. H., Pabst, M. J., Jakoby, W. B. (1974). Glutathione s-transferases: The first enzymatic step in mercapturic acid formation. J. Biol. Chem. 249, 7130–7139. doi: 10.1016/S0021-9258(19)42083-8
Hasegawa, P. M., Bressan, R. A., Zhu, J. K., Bohnert, H. J. (2000). Plant cellular and molecular responses to high salinity. Ann. Rev. Plant Physiol. Plant Mol. Biol. 51, 463–499. doi: 10.1146/annurev.arplant.51.1.463
Hatata, M. M., El-Darier, S. M. (2009). Allelopathic effect and oxidative stress induced by aqueous extract of Achillea santolina l. shoot on Triticum aestivum l. plant. Egypt. J. Exp. Biol. Bot. 5, 131–141.
Heath, R. L., Packer, L. (1998). Photoperoxidation in isolated chloroplasts. i. kinetics and stoichiometry of fatty acid peroxidation. Arch. Biochem. Biophy. 125, 189–198. doi: 10.1016/0003-9861(68)90654-1
Hewitt, E. J. (1966). Sand and water culture methods used in the study of plant nutrition. 2nd Ed (England: Common w. Agric. Bur. Farnham Royal-Bucks), 547.
Hooks, C. R. R., Wang, K. H., Ploeg, A., Mc Sorley, R. (2010). Using marigold (Tagetes spp.) as a cover crop to protect crops from plant-parasitic nematodes. App. Soil. Ecol. 46, 307–320. doi: 10.1016/j.apsoil.2010.09.005
Jabran, A. K., Mahajan, G., Sardana, V., Chauhan, B. S. (2015). Allelopathy for weed control in agricultural systems. Crop Prot. 72, 57–65. doi: 10.1016/j.cropro.2015.03.004
Jain, V. K., Guruprasad, K. N. (1989). Effect of chlorocholin chloride and gibberellic acid on the anthocyanin synthesis in radish seedlings. Physiol. Plant 75, 233–236. doi: 10.1111/j.1399-3054.1989.tb06174.x
Jamil, A., Anwer, F., Ashraf, M. (2005). “Plants tolerance to biotic and abiotic stresses through modern genetic engineering techniques,” in Crops: Growth, quality and biotechnology (Helsinki, Finland: WFL Publishers), 1276–1299.
Kandhro, M. N., Shah, A. N., Kubar, M. I., Laghari, M. (2015). Weed management and yield improvement in cotton through allelopathic mulches of sorghum and sunflower straw. Pak. J. Weed. Sci. Res. 21, 523–532.
Kaul, K. (2000) . Autotoxicity in Tagetes erecta L. on its own germination and seedling growth. Allelopathy J. 7, 109–113.
Kaul, K., Bedi, Y. S. (1995) . Allelopathic influence of tagetes species on germination and seedling growth of radish (Raphanus sativus) and lettuce (Lectuca sativa). Indian J. Agric. Sci. 65, 599–601.
Kulshrestha, V. P. (1985). History and ethnobotany of wheat in India J. Agric. Trop. Bot. Appl. 32, 61–71. doi: 10.3406/jatba.1985.3929
Mahdavikia, F., Saharkhiz, M. J., Karami, A. (2017). Defensive response of radish seedlings to the oxidative stress arising from phenolic compounds in the extract of peppermint (Mentha× piperita l.). Sci. Hortic. 214, 133–140. doi: 10.1016/j.scienta.2016.11.029
Malick, C. P., Singh, M. B. (1980). Plant enzymology and histo-enzymology (New Delhi: Kalyani Publishers).
Mangena, P. (2022). Pleiotropic effects of recombinant protease inhibitors in plants. Front. Plant Sci. 13. doi: 10.3389/fpls.2022.994710
Mir, R. A., Ahanger, M. A., Agarwal, R. M. (2019). Marigold: From mandap to medicine and from ornamentation to remediation. Am. J. Plant Sci. 10, 309–338. doi: 10.4236/ajps.2019.102024
Mir, R. A., Argal, S., Agarwal, R. M. (2018). Accumulation of secondary metabolites and osmotica in different parts of Tagetes erecta l. and its ecophysiological relevance. Int. J. Sci. Res. Rev. 7, 198–209.
Mir, R. A., Argal, S., Ahanger, M. A. (2020). “Role of osmolytes in regulation of growth and photosynthesis under environmental stresses,” in Improving abiotic stress tolerance in plants. Eds. Khan, M. I. R., Singh, A., Poor, P. (Boca Raton: CRC, Press), 99–118.
Mir, R. A., Argal, S., Ahanger, M. A., Tomar, N. S., Agarwal, R. M. (2021). Variation in phenolic compounds, antioxidant activity and osmotica of different cultivars of Tagetes erecta l. at different growth stages and effect of its leachates on germination and growth of wheat (Triticum aestivum l.). J. Plant Growth Regul. 41, 907–921. doi: 10.1007/s00344-021-10348-9
Mishra, S., Nautiyal, C. S. (2012). Reducing the allelopathic effect of Parthenium hysterophorus l. @ on wheat (Triticum aestivum l.) by Pseudomonas putida. Plant Growth Regul. 66, 155–165. doi: 10.1007/s10725-011-9639-1
Moron, M. S., Dipierre, J. W., Mannervik, B. (1979). Levels of glutathione reductase and glutathione-s-transferase activities in rat lung and liver. Biochem. Biophy. Acta 582, 67–68. doi: 10.1016/0304-4165(79)90289-7
Murimwa, J. C., Js., T., Mabasa, S., Mandumbu, R. (2019). Allelopathic effects of aqueous extracts of sorghum (Sorghum bicolor l. Moench) on the early seedling growth of sesame (Sesamum indicum l.) varieties and selected weeds. Int. J. Agron 2019, 1–12. doi: 10.1155/2019/5494756
Nakano, Y., Asada, K. (1981). Hydrogen peroxide is scavenged by ascorbate specific peroxidase in spinach chloroplast. Plant Cell Physiol. 22, 867–880. doi: 10.1093/oxfordjournals.pcp.a076232
Ogunsusi, M., Akinlalu, A. O., Komolafe, I. J., Oyedapo, O. O. (2018). Allelopathic effects of alkaloid fraction of Crotalaria retusa linn. on growth and some biochemical parameters of bean seedlings (Phaseolus vulgaris). Int. J. Plant Physiol. Biochem. 10, 1–9. doi: 10.5897/IJPPB2017.0261
Omayl, S. T., Turubull, J. D., Saubexilich, H. E. (1979). “Selected methods for determination of ascorbic acid in animal cells, tissues and fluids,” in Methods in enzymology (New York: Academic Press), 3–11.
Omezzine, F., Ladhari, A., Haouala, R. (2014). Physiological and biochemical mechanisms of allelochemicals in aqueous extracts of diploid and mixoploid Trigonella foenum-graecum l. S. Afr. J. Bot. 93, 167–178. doi: 10.1016/j.sajb.2014.04.009
Raghava, S. P. S. (1998). Pusa narangi gainda and pusa basanti gainda: New marigolds. Indian. Hortic. 43, 31.
Raghava, S. P. S., Singh, K. P., Dantuluri, V. S. R. (2013). “Marigold,” in Ornamental plants for gardening. Eds. Chopra, V. L., Singh, M. (New Delhi, India: Scientific publishers), 257–267.
Sadasivam, S., Manickam, A. (2004). Biochemical methods. 2nd ed. (New Delhi: New Age International Limited Publishers).
Saleh, A. M. (2013). In vitro assessment of allelopathic potential of olive processing waste on maize (Zea mays l.). Egypt. J. Exp. Biol. 9, 35–39.
Sarkar, E., Chatterjee, S. N., Chakraborty, P. (2012). Allelopathic effect of Cassia tora on seed germination and growth of mustard. Turk. J. Bot. 36, 488–494. doi: 10.3906/bot-1103-15
Shewry, P. R., Hey, S. J. (2015). The contribution of wheat to human diet and health. Food Energy Secur. 4, 178–202. doi: 10.1002/fes3.64
Shimada, K., Fujikawa, K., Yahara, K., Nakamura, T. (1992). Antioxidative properties of xanthone on the auto oxidation of soybean in cylcodextrin emulsion. J. Agr. Food Chem. 40, 945–948. doi: 10.1021/jf00018a005
Silveira, L. C. P., Filho, E. B., Pierre, L. S. R., Peres, F. S. C., Louzada, J. N. C. (2009). Marigold (Tagetes erecta l.) as an attractive crop to natural enemies in onion fields. Sci. Agric. (Piracicaba Braz) 66, 780–787. doi: 10.1590/S0103-90162009000600009
Singh, H. P., Batish, D. R., Kaur, S., Arora, K., Kohli, R. K. (2006) α-pinene inhibits growth and induces oxidative stress in roots. Ann. Bot. 98, 1261–1269. doi: 10.1093/aob/mcl213
Singh, H., Singh, A., Hussain, I., Yadav, V. (2017). Oxidative stress induced by lead in Vigna radiata l. seedling attenuated by exogenous nitric oxide. Trop. Plant Res. 4, 225–234. doi: 10.22271/tpr.2017.v4.i2.031
Slama, I., Abdelly, C., Bouchereau, A., Flowers, T., Savoure, A. (2015). Diversity, distribution and roles of osmoprotective compounds accumulated in halophytes under abiotic stress. Ann. Bot. 115, 433–447. doi: 10.1093/aob/mcu239
Sofo, A., Dichio, B., Xiloyannis, C., Masia, A. (2004). Lipoxygenase activity and proline accumulation in leaves and roots of olive trees in response to drought stress. Physiol. Plant 121, 58–65. doi: 10.1111/j.0031-9317.2004.00294.x
Swain, T., Hills, W. E. (1959). The phenolic constituents of Purnus domestica: the quantitative analysis of phenolic constituents. J. Sci. Food. Agric. 10, 63–68. doi: 10.1002/jsfa.2740100110
Tittal, M., Mir, R. A., Jatav, K. S., Agarwal, R. M. (2020). Supplementation of potassium alleviates water stress-induced changes in Sorghum bicolor l. Physiol. Plant 172, 1149–1161. doi: 10.1111/ppl.13306
Tomar, N. S., Agarwal, R. M. (2013). Influence of treatment of Jatropha curcas l. leachates and potassium on growth and phytochemical constituents of wheat (Triticum aestivum l). Am. J. Plant Sci. 4, 1134–1150. doi: 10.4236/ajps.2013.45140
Tomar, N. S., Sharma, M., Agarwal, R. M. (2015). Phytochemical analysis of Jatropha curcas l. during different seasons and developmental stages and seedling growth of wheat (Triticum aestivum l) as affected by extracts/leachates of Jatropha curcas l. Physiol. Mol. Biol. Plants. 21, 83–92. doi: 10.1007/s12298-014-0272-0
Unal, B. T., Islek, C., Ezer, T., Duzelten, Z. (2017) Allelopathic effects of Palustriella falcata (Bryophyta) extracts on wild mustard plants. Int. J. Agron. Agric. R. 4, 37–45.
Weatherley, P. E. (1950). Studies in water relations of cotton plant. in: the field measurements of water deficits in leaves. New Phytol. 49, 81–97. doi: 10.1111/j.1469-8137.1950.tb05146.x
Xie, X., He, Z., Chen, N., Tang, Z., Wang, Q., Cai, Y. (2019). The roles of environmental factors in regulation of oxidative stress in plant. Biomed. Res. Int 2019 9732325. doi: 10.1155/2019/9732325
Xu, L. W., Wang, G. Y., Shi, Y. P. (2011). Chemical constituents from Tagetes erecta flowers. Chem. Nat. Compd. 47, 281–283. doi: 10.1007/s10600-011-9905-5
Zhang, F. Q., Wang, Y. S., Lou, Z. P., Dong, J. D. (2007). Effect of heavy metal stress on antioxidative enzymes and lipid peroxidation in leaves and roots of two mangrove plant seedlings (Kandelia candel and Bruguiera gymnorrhiza). Chemosphere 67, 44–50. doi: 10.1016/j.chemosphere.2006.10.007
Keywords: allelopathy, antioxidant system, osmolytes, Tagetes erecta L., wheat
Citation: Mir RA, Argal S, Ahanger MA, Jatav KS and Agarwal RM (2022) Differential activity of wheat antioxidant defense system and alterations in the accumulation of osmolytes at different developmental stages as influenced by marigold (Tagetes erecta L.) leachates. Front. Plant Sci. 13:1001394. doi: 10.3389/fpls.2022.1001394
Received: 23 July 2022; Accepted: 14 November 2022;
Published: 01 December 2022.
Edited by:
Zhiqiang Pan, Agricultural Research Service (USDA), United StatesReviewed by:
Laishram Rajendrakumar Singh, University of Delhi, IndiaMona F. A. Dawood, Assiut University, Egypt
Nafees A. Khan, Aligarh Muslim University, India
Copyright © 2022 Mir, Argal, Ahanger, Jatav and Agarwal. This is an open-access article distributed under the terms of the Creative Commons Attribution License (CC BY). The use, distribution or reproduction in other forums is permitted, provided the original author(s) and the copyright owner(s) are credited and that the original publication in this journal is cited, in accordance with accepted academic practice. No use, distribution or reproduction is permitted which does not comply with these terms.
*Correspondence: Rayees Ahmad Mir, rayeesmir89@gmail.com