- 1College of Horticulture Science and Engineering, Shandong Agricultural University, Taian, China
- 2State Key Laboratory of Crop Biology, Shandong Agricultural University, Taian, China
- 3Shandong Province Collaborative Innovation Center for High-Quality and High-Efficiency Vegetable Production, Taian, China
- 4College of Life Sciences, Shandong Agricultural University, Taian, China
Drought stress is a serious abiotic stress source that affects the growth and fruit quality of peach trees. However, the molecular mechanism of the NUDIX hydrolase family in peaches in response to drought stress is still unclear. Here, we isolated and identified the PpNUDX8 (Prupe.5G062300.1) gene from the peach NUDIX hydrolase family, and found that PpNUDX8 has a typical NUDIX hydrolase domain. In this study, we performed 15% PEG6000 drought treatment on peach seedlings, and qRT–PCR analysis showed that 15% PEG6000 induced the transcription level of PpNUDX8. Overexpression of PpNUDX8 reduced the tolerance of calli to 4% PEG6000 treatment. Compared with wild-type apple calli, PpNUDX8 transgenic apple calli had a lower fresh weight and higher MDA content. After 15% PEG6000 drought treatment, PpNUDX8 transgenic tobacco had a greater degree of wilting and shorter primary roots than Under control conditions. The chlorophyll, soluble protein, and proline contents in the transgenic tobacco decreased, and the MDA content and relative conductivity increased. At the same time, PpNUDX8 negatively regulated ABA signal transduction and reduced the transcriptional expression of stress response genes. In addition, PpNUDX8 was not sensitive to ABA, overexpression of PpNUDX8 reduced the expression of the ABA synthesis-related gene NCED6 and increases the expression of the ABA decomposition-related gene CYP1 in tobacco, which in turn leads to a decrease in the ABA content in tobacco. In addition, Under control conditions, overexpression of PpNUDX8 destroyed the homeostasis of NAD and reduced nicotinamide adenine dinucleotide (NADH) in tobacco. After 15% PEG6000 drought treatment, the changes in NAD and NADH in PpNUDX8 transgenic tobacco were more severe than those in WT tobacco. In addition, PpNUDX8 also interacted with PpSnRk1γ (Prupe.6G323700.1).
Introduction
Plants live in a constantly changing environment, which leads to abiotic stresses during plant growth and development, and drought and water shortages are abiotic stressors that have a great impact on plants (Guo et al., 2016; Zhao et al., 2019). With the development of global warming, the environment in which plants live is gradually deteriorating, and climate change may increase the frequency of drought stress (Fedoroff et al., 2010). When plants face drought or water shortages, they form corresponding defense mechanisms at the morphological, physiological and molecular levels to protect themselves from drought stress. For example, closing the stomata reduces water loss (Ramachandra Reddy et al., 2004; Hu and Xiong, 2014), by increasing the root to cap ratio and forming more capillary roots to better absorb water (Xiong et al., 2006; Schachtman and Goodger, 2008) and to maintain the osmotic pressure of cells by accumulating proline, soluble sugar, soluble protein, and other osmotic substances (Seki et al., 2007). Improving the activity of a variety of antioxidant enzymes [such as superoxide dismutase (SOD), catalase (CAT), and peroxidase (POD)], to remove the reactive oxygen species (ROS) generated by drought stress (Ramachandra Reddy et al., 2004; Goswami et al., 2013), regulating the synthesis and decomposition pathways of ABA and increasing the content of plant endogenous ABA enhances the drought resistance of plant (Qin and Zeevaart, 2002).
The NUDIX hydrolase family is widely present in various organisms. The members of NUDIX hydrolase usually contain the conserved NUDIX motif GX5EX7REUXEEXGU (where U is a hydrophobic group and X represents any residue)(Bessman et al., 1996; McLennan, 2006). The NUDIX motif is an essential catalytic site for enzymes, and the glutamine residue in its core sequence REUXEE needs to bind to the necessary divalent cations to function. NUDIX hydrolase (NUDX) is a pyrophosphate hydrolase that can hydrolyze nucleoside sugars, dinucleoside polyphosphates, nucleoside triphosphates, and other organic pyrophosphates (Mildvan et al., 2005). These NUDX proteins can be adjusted according to changes in substrate content, by degrading substances that are harmful to plants or accumulating excessive metabolic intermediates, and playing important biological functions in the process of body protection, regulation, and signal transmission (Xu et al., 2004; Ogawa et al., 2005; Yoshimura and Shigeoka, 2015; Ogawa and Yoshimura, 2019).
In recent years, increasing evidence has shown that the NUDX protein plays an important regulatory role in a variety of physiological and biochemical processes, such as cell homeostasis, immune regulation, and abiotic stress response (Xu et al., 2004; Yoshimura and Shigeoka, 2015; Dong and Wang, 2016). Overexpressed AtNUDX2 maintains NAD + and ATP levels by recovering nucleotides from free ADP-ribose molecules under oxidative stress conditions, thereby increasing tolerance to oxidative stress (Ogawa et al., 2009). Both the AtNUDX6 and AtNUDX7 genes are involved in the regulation of the stress response and plant defense. AtNUDX6, as a positive regulator of the SA signaling pathway dependent on NPR1 (non-boosting factor of pathogenicity related gene 1), directly participates in plant immune response by regulating the level of reduced nicotinamide adenine dinucleotide (NADH). Knockout (KO) of AtNUDX6 and the overexpression of AtNUDX6 showed that the expression of several genes involved in SA-induced NPR1 activation-dependent and TRX-h5 decreased and increased respectively (Ishikawa et al., 2010). The expression of AtNUDX7 is induced by various types of abiotic stresses, such as drought, salinity, injury and strong light, as well as various oxidation treatments (such as PQ, ozone, O2–, and H2O2). Overexpression of AtNUDX7 gene and KO (knockout) lead to increased and decreased tolerance to oxidative stress, respectively (Davletova et al., 2005; Jambunathan and Mahalingam, 2006; Ge et al., 2007; Ishikawa et al., 2009, 2010; Jambunathan et al., 2010). According to reports, KO-NUDX7 plants accumulate high levels of ABA, resulting in a decrease in seed germination rate (Zeng et al., 2014). AtNUDX7 also plays an indispensable role in seed germination, growth, and development by regulating NAD: NADH homeostasis (Klaus et al., 2005). In grapes, VvNUDXs participate in the detoxification process of abiotic and biotic stress, and regulate disease immunity and resistance pathways (Wang et al., 2020).
Although the NUDIX hydrolase family has more been extensively researched on in terms of the abiotic stress response in Arabidopsis, knowledge of the NUDIX hydrolase family involved in the regulation of abiotic stress in peach is still very limited. As one of the most globally important fruits, peach has high nutritional and economic value. Due to the increase in the frequency of extreme weather caused by global warming, abiotic stresses often occur during the growth and development of peaches, resulting in a decline in the yield and quality of peaches. Therefore, understanding the molecular mechanism of the NUDIX hydrolase family in peaches in respond to abiotic stress is of great significance for the further cultivation of new peach varieties with strong adaptability.
Here, we isolated and identified the PpNUDX8 gene from the peach NUDIX hydrolase family. This study found that PpNUDX8 played a negative regulatory role in drought stress response. Overexpression of PpNUDX8 changed the steady state of NAD and NAD/NADH in tobacco and reduced the content of endogenous ABA in tobacco. In addition, we also found that PpNUDX8 interacts with PpSnRk1γ. These findings can provide references for genetic engineering breeding.
Results
Bioinformatic Analysis of PpNUDX8
PpNUDX8 was amplified with the cDNA library of peach as a template. The coding sequence (CDS) of PpNUDX8 is 1122 bp in length, encodes a protein of 373 amino acids, and has a predicted molecular weight of 41.67 kD. The results of protein multiple sequence alignment showed that the PpNUDX8 protein has a typical NUDIX hydrolase domain (Figure 1A). Phylogenetic tree analysis showed that PpNUDX8 is similar to apricot and plum (Figure 1C). Analysis of the cis-elements of the PpNUDX8 promoter showed that PpNUDX8 has ABA-responsive originals, methyl -jasmonate-responsive originals, gibberellin-responsive originals, drought-induced response originals, and light-responsive originals, etc (Figure 1B). Since PpNUDX8 contains ABA response elements and drought-induced response elements, we speculated that PpNUDX8 may be involved in the response to drought stress. Therefore, we performed 15% PEG 6000 and ABA treatments on 4-week-old peach seedlings. Through qRT–PCR analysis, we found that PpNUDX8 responds to drought and ABA signals (Figure 1D).
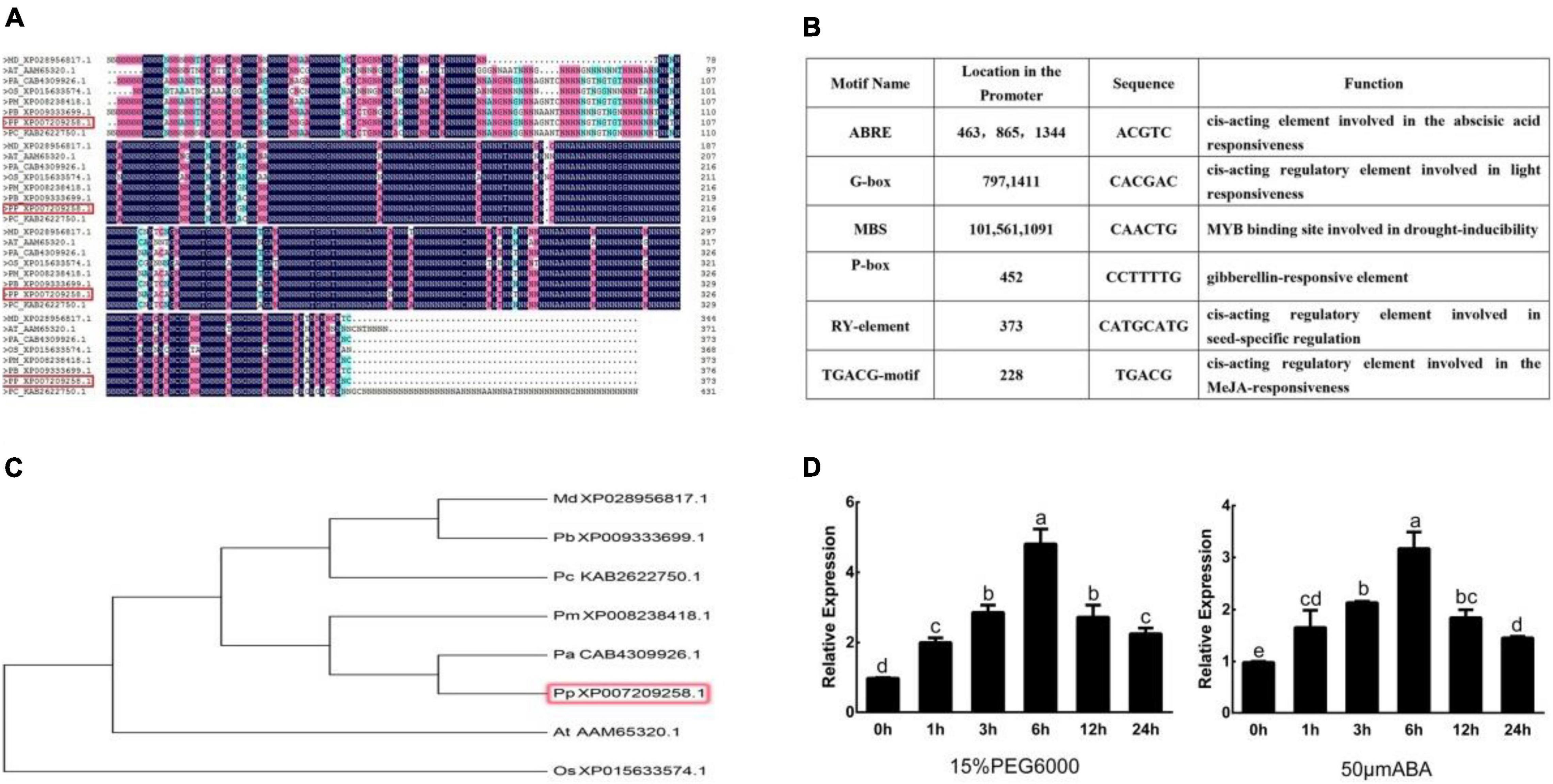
Figure 1. Bioinformatics analysis of PpNUDX8. (A) Protein multiple sequence alignment of PpNUDX8. (B) Analysis of cis-elements in the promoter of PpNUDX8. (C) Phylogenetic tree analysis of PpNUDX8. (D) Expression profile of PpNUDX8 in peach seedlings under 15% PEG 6000 and ABA treatment. These error bars represent the mean ± SD of independent biological triplicates. According to the analysis of variance and Duncan’s test, different letters represent significant differences (P < 0.05).
Overexpression of PpNUDX8 Reduces the Tolerance of Transgenic Apple Calli to Drought
In the 15% PEG6000 drought treatment of peach seedlings, we found that PpNUDX8 responds to drought. Therefore, to further study the role of PpNUDX8 in drought stress, we constructed the overexpression plasmid PpNUDX8-OE, which was transferred into Wanglin apple calli through Agrobacterium tumefaciens (LBA4404). Subsequently, through qRT–PCR analysis, we found that the expression of PpNUDX8 in apple calli was significantly higher than that in wild-type (WT) calli (Figure 2B), indicating that the plasmid PpNUDX8-OE was successfully transferred into Wanglin apple calli. In addition, we used 4% PEG6000 to simulate drought treatment of PpNUDX8 transgenic calli. After 10 days of 4% PEG6000 treatment, we found that the callus growth of PpNUDX8 transgenic apples was significantly worse than that of WT apples (Figure 2A), and the fresh weight was lower (Figure 2C). Subsequently, we measured the MDA content in the PpNUDX8 transgenic callus and WT calli and found that the MDA content in the transgenic calli was higher than that in the WT callus (Figure 2D). These date indicate that overexpression of PpNUDX8 reduces the tolerance of transgenic apple calli to drought.
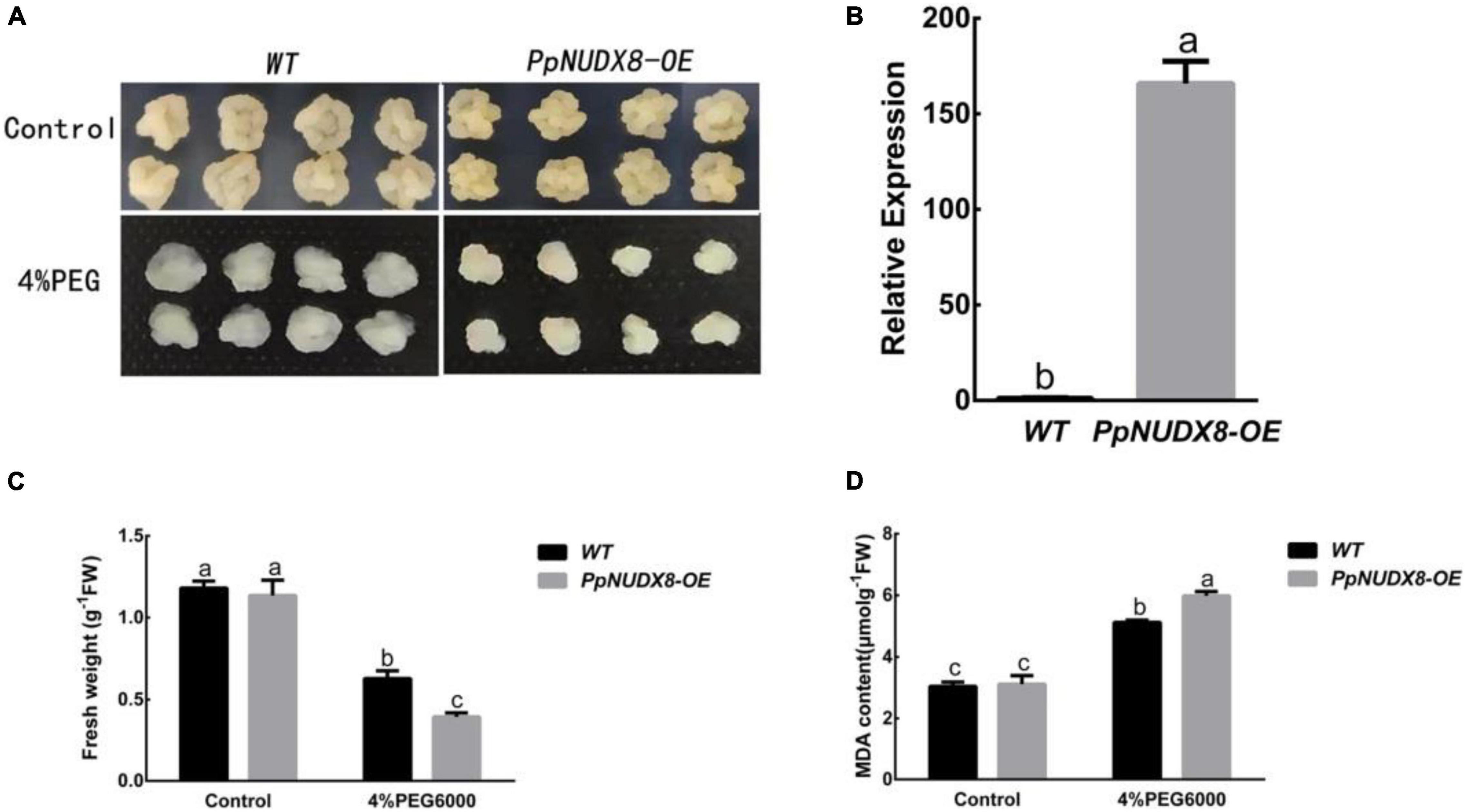
Figure 2. Determination of resistance of PpNUDX8 transgenic apple calli to 4% PEG6000. (A) The growth phenotype of WT and transgenic apple calli treated on MS and MS + 4% PEG 6000 medium for 15 days. (B) Analysis of PpNUDX8 expression in WT and PpNUDX8-OE transgenic calli by qRT–PCR. (C) The fresh weight of apple callus in panel (A). (D) The MDA content of apple callus in panel (A). The error bars represent the mean ± SD extracted from independent biological triplicates. According to the analysis of variance and Duncan’s test, different letters represent significant differences (P < 0.05).
Overexpression of PpNUDX8 Reduces the Drought Tolerance of Transgenic Tobacco
To further study the physiological functions of PpNUDX8, we obtained three PpNUDX8 overexpression transgenic tobacco lines (OE-1, OE-2, and OE-3) through Agrobacterium (LBA3101) transformation. qRT–PCR analysis showed that in these tobacco overexpression lines, the expression level of PpNUDX8 was significantly higher than that of the WT seedlings (Figure 3C). To study the role of PpNUDX8 in drought stress, we transferred 4-day-old WT and PpNUDX8 transgenic tobacco seedlings to MS medium containing 4% PEG6000 for 10 days. As shown in the figure, the growth of WT and transgenic seedlings under the control conditions was basically the same (Figure 3A). After treatment with 4% PEG6000, the growth of PpNUDX8 transgenic tobacco was significantly lower than that of WT seedlings (Figure 3A), and the primary root length of PpNUDX8 transgenic tobacco was significantly shorter than that of WT tobacco (Figure 3D). At the same time, the total chlorophyll content of WT leaves was 2.214266 mg/g, while the chlorophyll content of PpNUDX8 transgenic tobacco was between 1.53 and 1.64 mg/g, which was significantly lower than that of WT seedlings under 4% PEG6000 conditions (Figure 3E). In addition, we used 15% PEG6000 solution to treat 4-week-old WT and PpNUDX8 transgenic tobacco seedlings that grew consistently. After 4 h of treatment, both WT and transgenic seedlings showed symptoms of wilting and leaf curling, but the degree of wilting of transgenic seedlings was significantly greater than that of WT seedlings (Figure 3B). In addition, we compared the changes in soluble protein, proline, malondialdehyde (MDA) content and relative conductivity of WT and PpNUDX8 transgenic seedlings under control and 15% PEG6000 conditions. The results showed that Under control conditions, the soluble protein, proline, MDA contents and relative conductivity of WT and PpNUDX8 transgenic tobacco seedlings were similar. After 15% PEG6000 treatment, the soluble protein, proline, MDA contents and relative conductivity of all plants increased, but proline and the soluble protein contents of WT seedlings were higher than that those of transgenic seedlings (Figures 3F,G). Moreover, the relative conductivity contents and MDA of the WT seedlings were lower than those of the transgenic lines (Figures 3H,I). These date indicate that PpNUDX8 transgenic tobacco is less drought tolerant than WT tobacco.
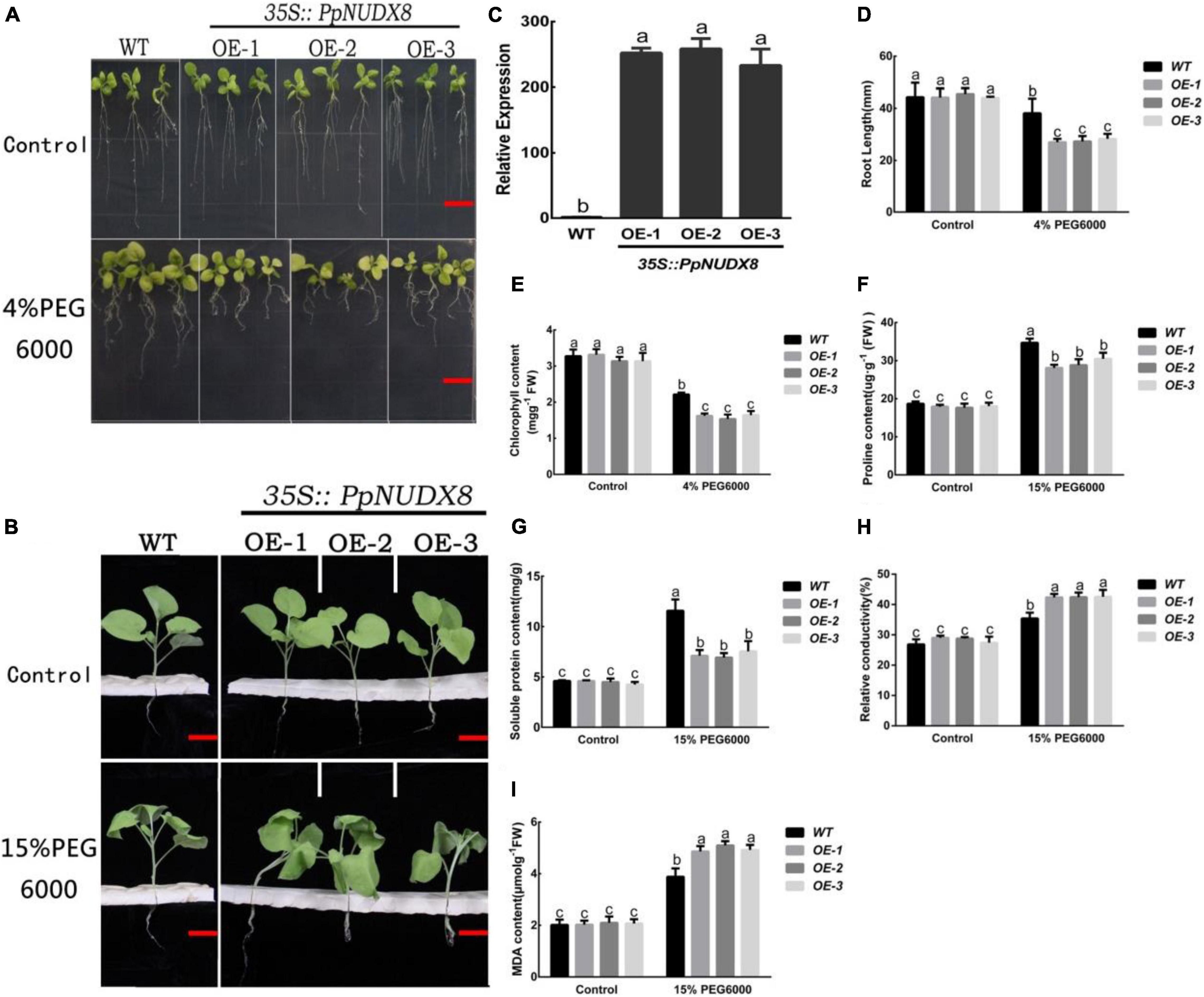
Figure 3. Measurement of drought tolerance of PpNUDX8 transgenic tobacco and WT. (A) The growth phenotype of WT and PpNUDX8 transgenic tobacco treated on MS and MS + 4% PEG 6000 medium for 10 days. Scale bar = 15 mm. (B) The growth phenotype of WT and PpNUDX8 transgenic tobacco treated with 15% PEG6000 for 4 h. Scale bar = 25 mm. (C) Expression analysis of WT and PpNUDX8-OX transgenic tobacco lines by RT-qPCR. (D) Analysis of the primary root length in panel (A). (E) Chlorophyll content in panel (A). (F–I) The proline content (F), soluble protein content (G), relative conductivity (H), and MDA content (I) in panel (B). The error bars represent the mean ± SD extracted from independent biological triplicates. According to the analysis of variance and Duncan’s test, different letters represent significant differences (P < 0.05).
Overexpression of PpNUDX8 Changed the Steady State of Tobacco Nicotinamide Adenine Dinucleotide and Reduced Nicotinamide Adenine Dinucleotide
Nicotinamide adenine dinucleotide (NAD), as a coenzyme for transferring electrons, participates in many redox reactions in cells. Some studies have shown that NAD plays an important role in the response of plants to environmental signals. The dynamic balance of NAD and NADH is essential for plants to cope with abiotic stress (Hashida et al., 2010; Wu et al., 2018).
As a member of the peach NUDIX hydrolase family, PpNUDX8 may be involved in the homeostasis of NAD in cells. Therefore, we determined the changes in NAD and NADH contents of WT and PpNUDX8 transgenic tobacco lines before and after 15% PEG6000 treatment. Under control conditions, the NAD content and NAD/NADH ratio of the PpNUDX8 transgenic line were higher than those of the WT plants, and the NADH content was lower than that of the WT (Figures 4A–C). After 15% PEG6000 treatment, the NAD content, NADH content, and NAD/NADH ratio of the PpNUDX8 transgenic and WT seedlings decreased, but the NAD content, NADH content, and NAD/NADH ratio of the PpNUDX8 transgenic lines decreased significantly (Figures 4A–C). At the same time, we determined the expression level of the enzyme QS, which plays a key role in NAD biosynthesis. The results showed that under normal circumstances, the expression of QS2 in the PpNUDX8 transgenic line was higher than that in the WT line (Figure 4D). After 15% PEG6000 treatment, the QS2 expression of WT and PpNUDX8 transgenic lines decreased, but the QS2 of PpNUDX8 transgenic lines decreased more than the WT plants (Figure 4D). These date indicate that the overexpression of PpNUDX8 may change the steady state of NAD and NADH in transgenic tobacco by changing the expression of QS2, which plays a key role in NAD biosynthesis. Drastic changes in their steady state may lead to a reduction in the drought tolerance of PpNUDX8 transgenic tobacco.
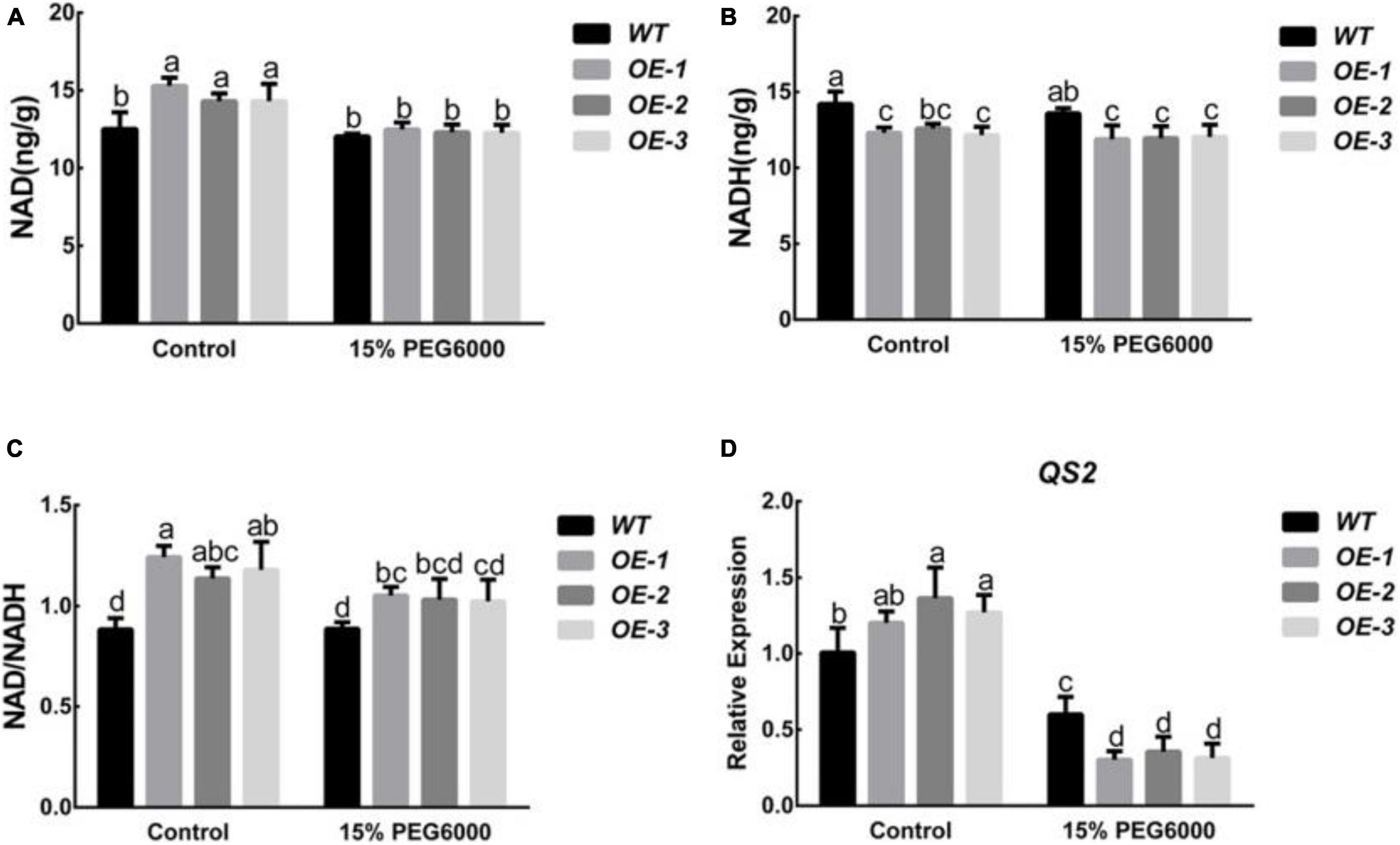
Figure 4. Measurement of NAD and NADH content in PpNUDX8 transgenic tobacco and WT before and after 15% PEG6000 treatment for 4 h, and analysis of QS2 expression. (A) NAD content. (B) NADH content. (C) The ratio of NAD and NADH was calculated based on the values shown in panels (A,B). (D) Expression analysis of QS2. The error bars represent the mean ± SD extracted from independent biological triplicates. According to the analysis of variance and Duncan’s test, different letters represent significant differences (P < 0.05).
PpNUDX8 Negatively Regulates Abscisic Acid Signal Transduction and Expression of Stress-Related Genes
Research on ABA signal transduction and the mechanism of action has always been an important subject in plant stress biology research. In order to further study the role of PpNUDX8 in drought stress, we detected the transcription levels of several ABA signaling pathway-related genes (SRK2.2, PP2C6, and PP2C53) and stress-related genes (DREBA1, RAB18, RD29A, RD29B, and LEA5) in tobacco by RT-qPCR. Under control conditions, the expression levels of tobacco genes SRK2.2, PP2C6, and PP2C53 in WT and PpNUDX8 transgenic lines were slightly different, but after 15% PEG6000 treatment, the transcription level of SRK2.2 in WT was significantly higher In the PpNUDX8 transgenic lines (Figure 5A). The transcription levels of WT and PpNUDX8 transgenic lines PP2C6 and PP2C53 were reduced, but the transcription levels of PpNUDX8 transgenic lines PP2C6 and PP2C53 were still higher than that of WT (Figures 5B,C). In addition, under control conditions, there was only a slight difference in the transcription levels of stress-related genes (DREBA1, RAB18, RD29A, RD29B, and LEA5) between the PpNUDX8 transgenic lines and WT. After 15% PEG6000 treatment, the transcription level of stress-related genes (DREBA1, RAB18, RD29A, RD29B, and LEA5) in WT was significantly higher than that of PpNUDX8 transgenic lines (Figures 5D–H). In conclusion, these data indicate that overexpression of PpNUDX8 may reduce the resistance of tobacco to drought stress by negatively regulating ABA signal transduction and expression of stress-related genes.
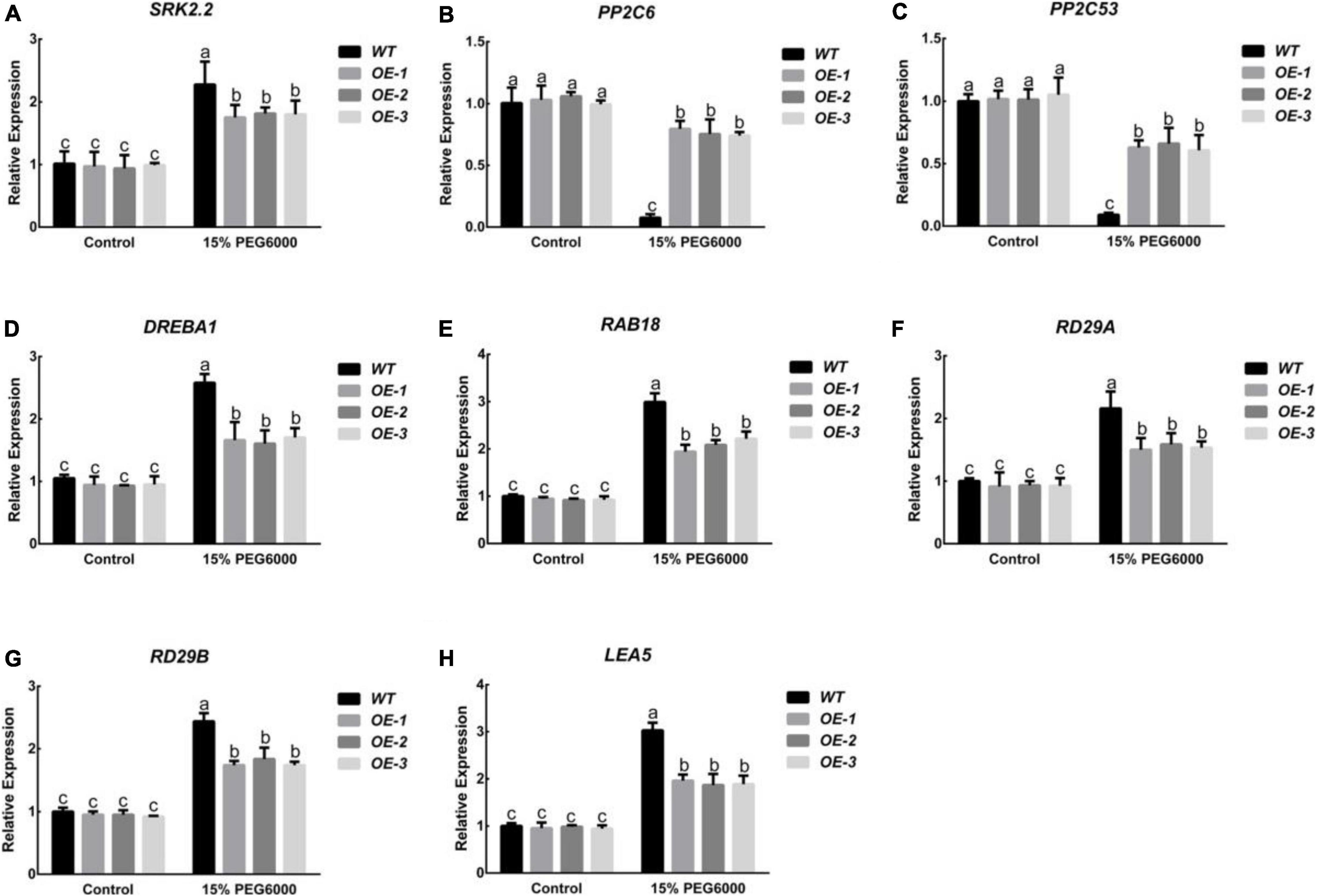
Figure 5. Analysis of the expression levels of SnRK2.2, PP2C6, PP2C53, DREBA1, RAB18, RD29A, RD29B, and LEA5 in PpNUDX8 transgenic tobacco and WT before and after treatment with 15% PEG6000 for 4 h. (A) SnRK2.2. (B) PP2C6. (C) PP2C53. (D) DREBA1. (E) RAB18. (F) RD29A. (G) RD29B. (H) LEA5. The error bars represent the mean ± SD extracted from independent biological triplicates. According to the analysis of variance and Duncan’s test, different letters represent significant differences (P < 0.05).
Overexpression of PpNUDX8 Reduces the Sensitivity of Tobacco to Abscisic Acid
To determine the effect of PpNUDX8 under ABA treatment, we tested the sensitivity of PpNUDX8 transgenic tobacco lines under abscisic acid (ABA) treatment. To determine the effect of PpNUDX8 under ABA treatment, we tested the sensitivity of PpNUDX8 transgenic tobacco lines under ABA treatment. In the MS plate seed germination test, the germination rates of WT and PpNUDX8 transgenic tobacco seeds on normal MS medium were similar (Figure 6B). After 1 μm/L ABA treatment, the germination rate of PpNUDX8 transgenic tobacco seeds was higher than that of WT seeds (Figure 6B). In addition, ABA also significantly inhibited the early growth of WT and PpNUDX8 transgenic seedlings, but the inhibition of WT seedlings was more serious. We found that the percentage of green cotyledons of PpNUDX8 transgenic tobacco seedlings was much higher than that of WT seedlings (Figure 6A). In addition, we further tested the sensitivity of WT and PpNUDX8 transgenic tobacco lines to ABA at the seedling growth stage after germination. After transferring 4-day-old tobacco seedlings to MS medium containing 10 μm/L ABA for 15 days, the PpNUDX8 transgenic line had longer primary roots than the WT (Figures 6C,D). These date indicate that overexpression of PpNUDX8 may reduce the sensitivity of tobacco to ABA.
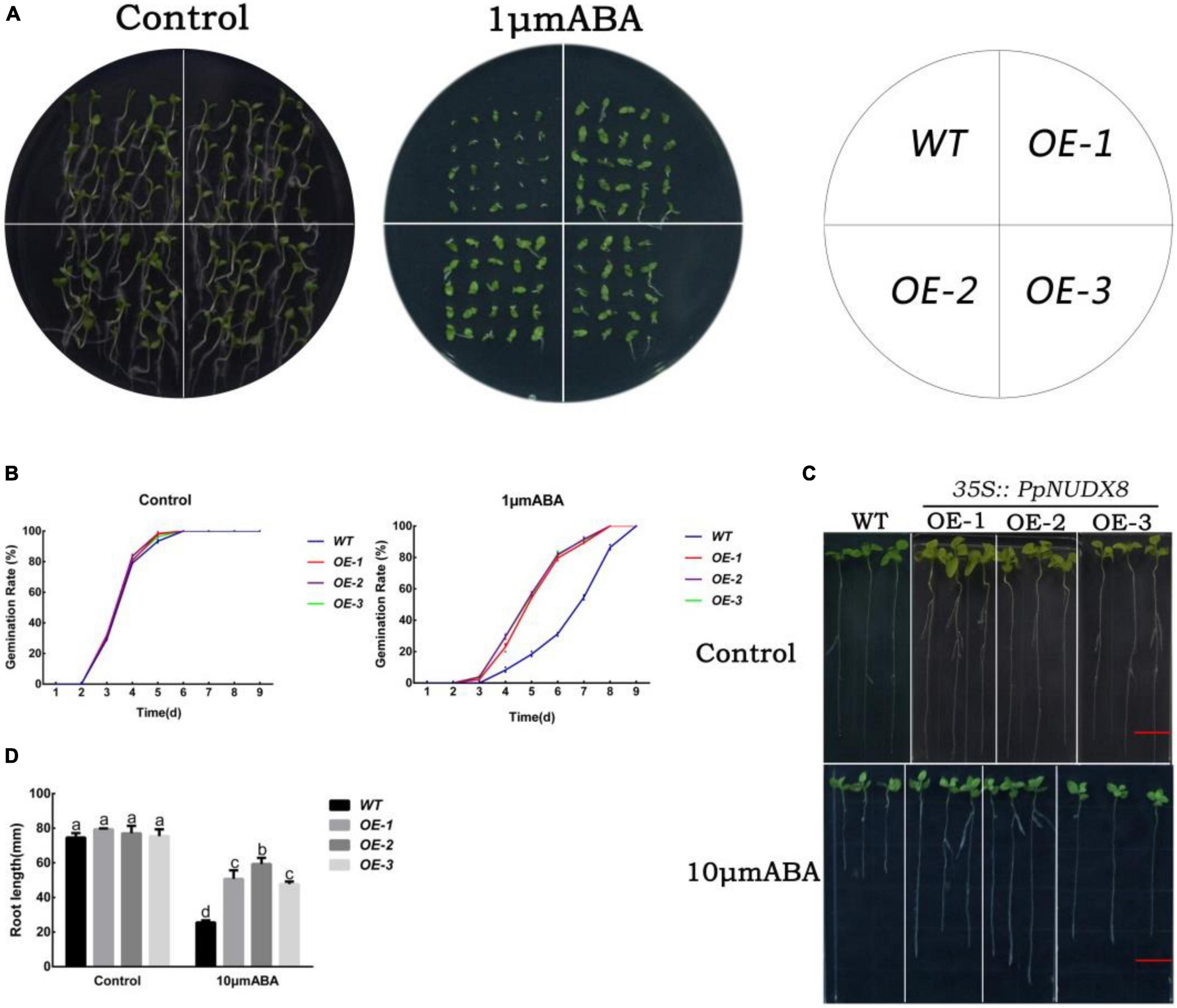
Figure 6. Analysis of abscisic acid (ABA) sensitivity of PpNUDX8 transgenic tobacco. (A) Phenotypes of WT and PpNUDX8 transgenic lines treated with 0 or 1 μm/L ABA during seed germination. (B) Seed germination rate in panel (A). (C) Root growth phenotypes of WT and PpNUDX8 transgenic lines treated with 0 or 10 μm/L ABA in the seedling growth stage. Scale bar = 15 mm. (D) The primary root growth in panel (C). The error bars represent the mean ± SD extracted from independent biological triplicates. According to the analysis of variance and Duncan’s test, different letters represent significant differences (P < 0.05).
Overexpression of PpNUDX8 Reduces the Abscisic Acid Content of Tobacco
Studies have shown that ABA can enhance the drought resistance of plants. After the plants are subjected to drought stress, their endogenous ABA content will increase significantly. Under control conditions, the ABA content of the PpNUDX8 transgenic line was lower than that of the WT. After 15% PEG6000 for 4 h, the ABA content in all plants increased, but the increase in the PpNUDX8 transgenic lines was lower than that in the WT seedlings (Figure 7A). In addition, since NCED and CYP are enzymes that play key roles in the pathways of ABA synthesis and decomposition, respectively, we measured their transcription levels before and after 15% PEG6000 treatment. The results showed that Under control conditions, the expression level of the CYP1 gene of the PpNUDX8 transgenic line was much higher than that of WT tobacco, and the expression level of the NCED6 gene was lower than that of WT tobacco. After 15% PEG6000 treatment, the expression of CYP1 in both WT and PpNUDX8 transgenic lines decreased, but the gene expression in PpNUDX8 transgenic lines was still higher than that of WT (Figure 7B). NCED6 gene expression increased, but the NCED6 gene expression of the PpNUDX8 transgenic line was still much lower than that of WT tobacco (Figure 7C). These date indicate that overexpression of PpNUDX8 may reduce the ABA content in tobacco by changing the expression of NCED6 and CYP1.
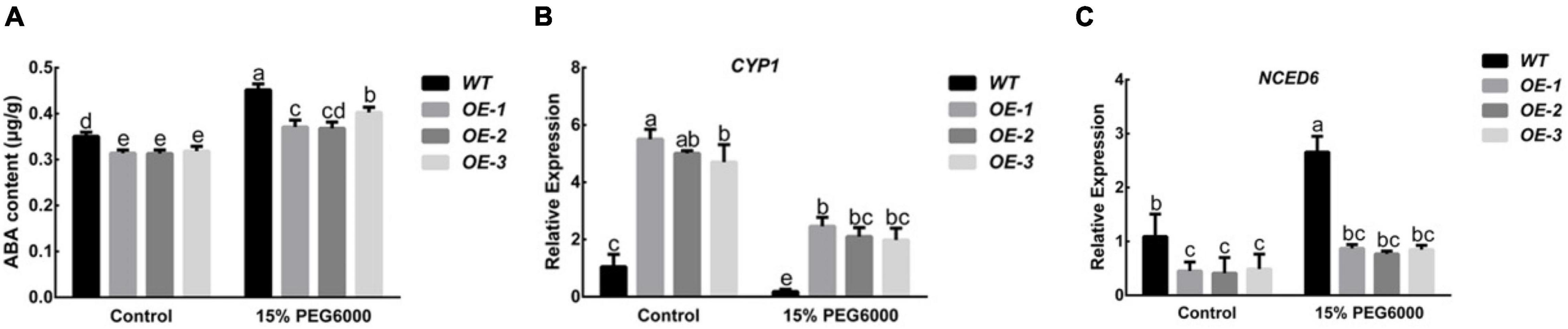
Figure 7. Determination of ABA content in PpNUDX8 transgenic tobacco before and after 15% PEG6000 drought treatment and expression analysis of NCED6 and CYP1. (A) The content of ABA. (B) CYP1. (C) NCED6. The error bars represent the mean ± SD extracted from independent biological triplicates. According to the analysis of variance and Duncan’s test, different letters represent significant differences (P < 0.05).
PpNUDX8 Interacts With PpSnRk1γ
To further explore the biochemical functions of PpNUDX8 in peaches, we used PpNUDX8-pGBKT7 as the “bait” to screen the interacting proteins in the peach cDNA library. After multiple transformations of PpNUDX8-pGBKT7 and library plasmids, we screened the candidate gene PpSnRk1γ. To confirm the interaction between PpNUDX8 and PpSnRk1γ, yeast two-hybrid (Y2H) assays were performed. The full-length cDNA of PpNUDX8 was inserted into the yeast vector pGBKT7 and the full-length cDNA of PpSnRk1γ was inserted into the yeast vector pGADT7. The results showed that PpNUDX8 interacted with PpSnRk1γin yeast (Figure 8A).
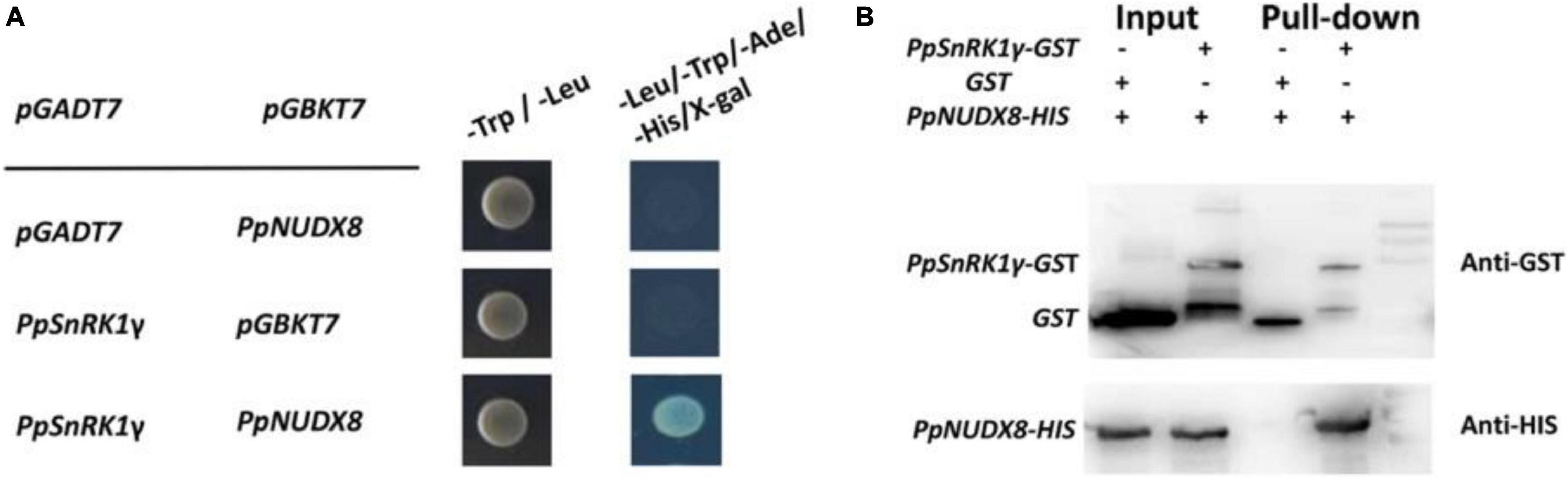
Figure 8. Interaction between PpNUDX8 and PpSnRk1γ. (A) Y2H test shows that PpNUDX8 and PpSnRk1γ interact in yeast. (B) The interaction between PpNUDX8 and PpSnRk1γ protein in a pull-down assay.
To further verify the accuracy of the interaction between PpNUDX8 and PpSnRk1γ, we performed a pull-down experiment. PpNUDX8-His and PpSnrk1γ-GST fusion proteins were prepared. As shown in Figure 8B, PpSnrk1γ was found to be pulled down by PpNUDX8, indicating that PpSnrk1γ interacts with PpNUDX8.
Materials and Methods
Plant Materials and Treatments
In the drought and ABA treatment of peach seedlings, 4 weeks old peach seedlings were soaked in a 15% PEG6000 solution and sprayed with 50 μmol/L ABA on peach seedlings, and at 0, 1, 3, 6, and 12 h, the leaves of peach seedlings were collected. WT and transgenic tobacco seeds were sterilized with 4% sodium hypochloride for 10 min, and sown on MS medium containing 3% sucrose and 0.7% agar. After vernalization at 4°C for 4 days, the MS medium plate was transferred to a 24°C light incubator with a 16/8 h light/dark schedule, and a relative humidity of 70%. In the drought treatment apple callus experiment, 15-day-old apple calli were crushed and transferred to MS medium containing 4% PEG6000, and the phenotype was observed and recorded after 10 days of treatment. The relative electrical conductivity and MDA contents of apple calli before and after treatment were measured. For the seed germination test, tobacco seeds were germinated on MS medium supplemented with 0 or 1 μmol/L ABA, and the germination rate was calculated for 10 consecutive days after sowing. One hundred seeds per plant were used to calculate the germination rate and green cotyledons. For the determination of tobacco root length, 4-day-old tobacco seedlings of similar size were vertically cultured in MS medium containing 10 μmol/LABA for another 10 days, and then the primary root length was measured. For MS plate drought treatment, 4-day-old tobacco seedlings were transferred to MS medium containing 4% PEG6000 and cultured for 15 days. In the 15% PEG6000 simulated drought treatment, 3-week-old tobacco seedlings were immersed in a 15% PEG6000 solution, and samples were taken at 0 and 4 h to measure relative conductivity and the contents of MDA, proline, and soluble protein.
Vector Construction and Genetic Transformation
The full-length CDS of the peach gene PpNUDX8 was obtained on the Phytozome website1. Using the cDNA of peach as a template, the full length PpNUDX8 gene was cloned, inserted it into the required vector, and DNAMAN software was used for sequence alignment. The primers used for vector construction are listed in Supplementary Table 1. Subsequently, the obtained vector 35S:PpNUDX8 was transformed into Agrobacterium tumefaciens strains GV4404 and GV3101, and Agrobacterium infection was used to transform Wanglin calli and Nicotiana tabacum (Xie et al., 2012). T0 seeds were sown on MS medium containing kanamycin (50 mg/L) for initial selection, and 4 weeks after the seedlings were moved into the plugs, DNA was extracted for transgenic selection. Three homozygous T3 transgenic lines (OE-1, OE-2, and OE-3) were selected for the subsequent experiment.
RNA Extraction and qRT–PCR
According to the manufacturer’s instructions, a RNA plant extraction kit (Chinese Tiangen) was used to extract the total RNA from apple calli and tobacco and peach seedlings. Reverse transcription was performed with HiScript QRT SuperMix (Vazyme, Nanjing, China). MdACTIN (apple), NtACTIN (tobacco), and PpACTIN (peach) was used as internal controls to complete the qRT–PCR analysis. Each sample was subjected to independent biological triplicate analysis. The primers used for qRT–PCR are listed in Supplementary Table 1.
Y2H and Pull-Down Experiments
The full-length CDS fragments of PpNUDX8, and PpSnrk1γ were inserted into pGBKT7 or pGADT7 to generate the PpNUDX8-PpGBKT7, and PpSnrk1γ-pGADT7 plasmids. Then, transfer the different combinations of pGBD and PGAD were transferred into yeast strains (Y2H) and spread on to SD/-Trp/-Leu and SD/-Trp/-Leu/-Ade-/-His/X-gal selection media at 28°C for 3 days.
The PpNUDX8 clone was connected to the PET32a vector with a HIS tag, PpSnRk1γwas connected to the PGEX4T-1 vector with GST tag, and the recombinant vectors PpNUDX8-HIS, PpSnRk1γ-GST, and empty PGEX4T-1 were transferred into the BL21 protein expression strain. Then, prokaryotic expression was used to induce the fusion protein and the corresponding kit was used to purify the protein. The purified proteins PpNUDX8-HIS and PpSnRk1γ-GST were mixed and incubated, and a control (PpNUDX8-HIS and empty PGEX4T-1 mixed incubation) was set at the same time. After incubation, part of the mixed solution Input was collected and applied it to the GST column, and the effluent was collected subjected to pull-down experiments after elution.
Abscisic Acid Content Determination
High performance liquid chromatography-tandem mass spectrometry (HPLC–MS/MS) was used to determine the ABA content of tobacco leaves. Extraction method: Approximately, 0.1 g of tobacco leaf sample was weighed, 1 mL of pre-cooled reagent was added, and the sample was extracted overnight at 4°C prior to centrifugation at 8000 g for 10 min, and the residue was extracted with 0.5 mL of reagent for 2 h. The supernatant was removed after centrifugation and the process was repeated. The two supernatants were combined and evaporated under nitrogen at 40°C until the organic phase was removed. Then, 0.5 mL of reagent was added for the second extraction and decolorization three times, and the upper ether phase was discarded. Reagent three was added to adjust the pH to 2.8. Reagent four was used for three extraction cycles, and the organic phases were combined and evaporated to dryness under nitrogen. A total of 0.5 mL of reagent five was added to the residue and vortexed and shaken to achieve dissolution. After passing the solution through a syringe filter, the sample was analyzed (reagent 1: 80:20:1 methanol/water/acetic acid; reagent 2: petroleum ether; reagent 3: saturated citric acid solution; reagent 4; ethyl acetate; and reagent 5: methanol).
Physiological Measurement
Total chlorophyll (a + b) measurement (Qin and Zeevaart, 2002). Tobacco leaves and soak were soaked in 95% ethanol under dark conditions for 24 h (during this period, the solution was shaken once every 8 h). Finally, a spectrophotometer was used to determine the total chlorophyll concentration at wavelengths of 665, 646, and 470 nm. Relative conductivity measurement (Lurie and Ben-Arie, 1983). First, the tobacco leaves were rinsed with distilled water then cut into small pieces, and 0.5 g of leaves was put in distilled water. The air was pumped three times, placed at room temperature for 3∼4 h, and the conductivity S1 was measured with a conductivity meter. The sample was boiled for 20 min and cooled to room temperature, and the electrolyte conductivities S2 and S0 were measured. Relative conductivity (%) = 100 × (S1-S0)/(S2-S0). MDA content determination (Ma et al., 2017). The thiobarbituric acid method was used to detect the MDA content. The soluble protein content was determined by the Coomassie Brilliant Blue method.
Determination of Nicotinamide Adenine Dinucleotide and Reduced Nicotinamide Adenine Dinucleotide Enzyme Activity
According to the instructions of the NAD/NADH detection kit (Shanghai Fanyin Biological), the double antibody sandwich method enzyme-linked immunosorbent assay (ELISA) method was used for NAD and NADH enzyme activity determination.
Statistical Analysis
SPSS software version 19.0 (SPSS Corp, Chicago, IL, United States) was used for statistical analysis. Duncan’s multiple range test was performed for significant difference analysis with p < 0.05 designated as a significant difference.
Discussion
Drought stress causes great damage to the growth and development of plants and may lead to premature death of plants in severe cases (Tiwari et al., 2010). Therefore, studying the molecular mechanism of drought stress and the identification of stress response genes should be the focus of plant genetic engineering breeding (Liu et al., 2016; Tan et al., 2016; He et al., 2017). NUDIX hydrolase is a kind of pyrophosphate hydrolase whose hydrolysis substrate mainly includes some coenzymes [NAD(P)H, FAD and coenzyme A] and nucleotide sugars. These compounds are not only metabolic intermediates and coenzymes but also contain some toxic organic compounds, so they must be properly regulated. In addition to participating in the removal of harmful substances and metabolic regulation, NUDIX hydrolase is also closely related to the response of plants to biotic and abiotic stress (Ogawa et al., 2005, 2008; Munoz et al., 2006; Xu et al., 2006; Yoshimura and Shigeoka, 2015). For example, in Arabidopsis, knocking out AtNUDX6 and overexpressing AtNUDX6 showed that the expression of several genes involved in SA-induced NPR1 activation-dependent and TRX-h5 decreased and increased, respectively (Ishikawa et al., 2010). The expression of AtNUDX7 is induced by various types of abiotic stresses, such as drought, salinity, injury and strong light, as well as various oxidation treatments (such as PQ, ozone, O2–, and H2O2). Overexpression of the AtNUDX7 gene and KO lead to increased and decreased tolerance to oxidative stress, respectively (Davletova et al., 2005; Jambunathan and Mahalingam, 2006; Ge et al., 2007; Ishikawa et al., 2009, 2010; Jambunathan et al., 2010).
In this study, the NUDIX hydrolase PpNUDX8 was isolated and identified from peaches. After 4% PEG6000 drought treatment of PpNUDX8 transgenic apple calli and tobacco seedlings, it was found that overexpression of PpNUDX8 reduced the drought tolerance of transgenic apple calli and tobacco (Figures 2A, 3A,B). This result indicates that PpNUDX8, as a negative regulator, participates in the tolerance of plants in response to drought stress.
Plants have evolved a variety of mechanisms to resist drought stress (Miller et al., 2010), such as the root system of plants. Some plants have longer root systems under drought stress, which can absorb deep soil water (Hu and Xiong, 2014). The root system is an important organ for plants to absorb water (Wang et al., 2003). Good development of the root system is conducive to the absorption and utilization of water in the soil, thereby enhancing the resistance of plants to drought stress (Wang et al., 2017). In this study, the primary roots of PpNUDX8 transgenic tobacco after 4% PEG6000 treatment were significantly shorter than those of WT tobacco (Figure 3D), which would not be conducive to improving the water absorption capacity of transgenic tobacco. In addition, under drought stress conditions, the membrane structure related to photosynthesis will be destroyed, which may lead to a decrease in chlorophyll content. According to reports, as the soil moisture content decreases, the chlorophyll content will decrease significantly (Kocheva et al., 2004; Li et al., 2006; Guo et al., 2007). Therefore, maintaining a high chlorophyll content can make more efficient use of light energy, thereby improving the drought resistance of plants. In this study, the chlorophyll content of PpNUDX8 transgenic tobacco after 4% PEG6000 treatment was lower than that of WT (Figure 3E), which would reduce the photosynthetic capacity of the transgenic tobacco and reduce the drought tolerance of the plant. Plants undergo osmotic adjustment after being subjected to drought stress. Plants accumulate a variety of organic and inorganic substances to increase their concentration in cells, reduce osmotic potential, and improve the water retention capacity of cells under drought stress (Bartels and Sunkar, 2005). Proline and soluble protein act as osmotic regulators to maintain the osmotic balance of cells (Hong-Bo et al., 2005; Ashraf and Foolad, 2007; Maurel et al., 2008). In this study, the proline and soluble protein contents of the PpNUDX8 transgenic line under 15% PEG6000 treatment were lower than those of WT tobacco (Figures 3F,G), which indicated that the osmotic adjustment ability of PpNUDX8 transgenic tobacco under 15% PEG6000 treatment was not as good as that of WT tobacco.
Therefore, PpNUDX8 may be involved in osmotic adjustment. Under stress conditions, the transcription levels of many stress-related genes will be induced (Yamaguchi-Shinozaki and Shinozaki, 2006). DREBA1 participates in dehydration stress response through ABA-independent signaling pathway (Umezawa et al., 2006). In this experiment, after 15% PEG6000 treatment, the transcription level of DREBA1 in the PpNUDX8 transgenic line was significantly lower than that of WT (Figure 5D). This means that PpNUDX8 transgenic tobacco reduced drought tolerance through ABA-dependent and ABA-independent signaling pathways. Advanced Embryogenesis Abundant Protein (LEA) can protect biological macromolecules, redirect the distribution of water in cells, combine with inorganic ions, and avoid damage caused by the accumulation of high concentrations of ions under drought stress conditions (Close, 2010). After 15% PEG6000 treatment, the transcription level of LEA5 in the PpNUDX8 transgenic line was significantly lower than that of WT (Figure 5H). In addition, the RAB18, RD29A and RD29B genes are very sensitive to various abiotic stressors and are generally involved in drought stress responses (Msanne et al., 2011; Li et al., 2020). After 15% PEG6000 treatment, the expression levels of PpNUDX8 transgenic lines RAB18, RD29A, and RD29B were significantly lower than WT (Figures 5E–G). These data indicate that PpNUDX8 reduces drought resistance by regulating the expression level of stress-related genes.
In addition, plants produce a large amount of ROS after being subjected to adverse stress, which aggravates the degree of membrane lipid peroxidation, produces MDA, destroys the integrity of cell membranes, and leads to an increase in electrical conductivity. MDA content and relative conductivity can reflect the degree of damage to the integrity of cell membranes (Hodges et al., 1999; Ozgur and Gevrek, 2008). Therefore, to explore the degree of damage to the cell membrane of PpNUDX8 transgenic tobacco under 15% PEG6000 treatment, we measured the MDA content and relative conductivity of the transgenic tobacco. The MDA content and relative conductivity of PpNUDX8 transgenic tobacco were significantly higher than those of WT tobacco (Figures 3H,I). This result indicates that the cell membrane of transgenic tobacco is more severely damaged than WT tobacco under 15% PEG6000 treatment, and the resistance of PpNUDX8 transgenic tobacco to oxidative stress is weaker than that of WT tobacco (Figure 3B).
Abscisic acid, as a plant hormone that has been extensively studied, can not only regulate seed dormancy and germination, but also respond to abiotic stresses such as drought and water shortage (Kermode, 2005; Zhu, 2016; Nonogaki, 2017). In addition, drought stress can trigger ABA-dependent signaling pathways (Zhu, 2002). According to reports, some NUDIX hydrolases may be involved in the ABA signaling pathway. For example, KO-AtNUDX7 accumulates high levels of ABA, resulting in a decrease in the seed germination rate (Zeng et al., 2014). In this experiment, after ABA plate treatment on PpNUDX8 transgenic tobacco seeds, we found that PpNUDX8 transgenic tobacco was very insensitive to ABA, and the percentage of green cotyledons of PpNUDX8 transgenic tobacco seedlings was much higher than that of WT seedlings (Figure 6A), and PpNUDX8 Compared with WT seedlings, the transgenic seedlings had longer primary roots (Figures 6C,D). This observation indicates that PpNUDX8 may be involved in the ABA signaling pathway. Therefore, we tested the expression levels of PP2Cs and SnRK2s, the core components of ABA signal transduction. In the ABA signal transduction pathway, PP2Cs play a negative regulatory role in ABA signal transduction (Ma et al., 2009; Park et al., 2009). SnRK2s positively regulate the ABA signal transduction pathway, and SnRK2s can improve plant tolerance to abiotic stress (Huai et al., 2008; Mao et al., 2010; Zhang et al., 2011). After 15% PEG6000 treatment, the expression level of SnRK2.2 in the PpNUDX8 transgenic seedlings was lower than that in the WT seedlings (Figure 5A), while the transcription levels of PP2C6 and PP2C53 were higher than those in the WT seedlings (Figures 5B,C). This result indicates that PpNUDX8 inhibited ABA signal transduction. According to reports, under drought stress, ABA content in plants will quickly accumulate to resist drought stress (Fujita et al., 2011). In this experiment, we measured the ABA content of the PpNUDX8 transgenic and WT seedlings and found that the ABA content of the PpNUDX8 transgenic seedlings was lower than that of the WT seedlings before and after drought treatment (Figure 7A). NCED and CYP are enzymes that play key roles in ABA synthesis and decomposition pathways, respectively. Therefore, we analyzed the transcription levels of NCED6 and CYP1 by qRT–PCR. The results showed that the expression of CYP1 in PpNUDX8 transgenic tobacco was much higher than that of WT, while the expression of PpNUDX8 transgenic tobacco NCED6 was lower than that of WT tobacco (Figures 7B,C). These results indicate that overexpression of PpNUDX8 may affect the transcription levels of NCED6 and CYP1 in transgenic tobacco, leading to a lower ABA content in PpNUDX8 transgenic lines than in WT plants, which in turn leads to drought tolerance in transgenic plants.
Nicotinamide adenine dinucleotide and NADH are key metabolic intermediates between redox reactions and energy metabolism. The destruction of the dynamic balance of NAD and NADH is likely to affect the accumulation of reactive oxygen species, plant growth, and adaptation to unfavorable environmental conditions (Hashida et al., 2009; Chini et al., 2017; Bockwoldt et al., 2019). For example, deletion of the AtNUDX7 gene disrupts NADH homeostasis, and KO-AtNUDX7 accumulates high levels of ABA, resulting in a decrease in seed germination (Zeng et al., 2014). In addition, the NADH content decreased and increased in Arabidopsis overexpressing AtNUDX6 and knocking out AtNUDX6, respectively (Ishikawa et al., 2010). In this experiment, overexpression of PpNUDX8 affected changes in tobacco NAD, NADH content and NAD biosynthetic enzyme QS2 expression, both of which were higher than in WT tobacco (Figures 4A,B,D). After 15% PEG 6000 treatment, the NAD and NADH ratios of WT and PpNUDX8 transgenic lines decreased, but that in PpNUDX8 transgenic tobacco changed to a greater degree (Figures 4A,B).
In short, these findings can give us a deeper understanding of the regulatory mechanism of PpNUDX8 under drought stress. This research is helpful to further understand the role of NUDIX hydrolase family in drought stress response, and is expected to be applied to drought improvement in horticultural crop breeding.
Data Availability Statement
The original contributions presented in the study are included in the article/Supplementary Material, further inquiries can be directed to the corresponding authors.
Author Contributions
HH, XC, and LL conceived and designed the research. All authors contributed to the article and approved the submitted version.
Funding
This work was partially supported by National Natural Science Foundation of China (31872041), National Key Research and Development Plan (2018YFD1000104), Shandong Province Agricultural Good Seed Project grant nos. 2020LZGC007 and 2020LZGC00702, and Shandong Provincial Fruit Industry Technology System – Cultivation and Soil Fertilization Post (SDAIT-06-04).
Conflict of Interest
The authors declare that the research was conducted in the absence of any commercial or financial relationships that could be construed as a potential conflict of interest.
Publisher’s Note
All claims expressed in this article are solely those of the authors and do not necessarily represent those of their affiliated organizations, or those of the publisher, the editors and the reviewers. Any product that may be evaluated in this article, or claim that may be made by its manufacturer, is not guaranteed or endorsed by the publisher.
Supplementary Material
The Supplementary Material for this article can be found online at: https://www.frontiersin.org/articles/10.3389/fpls.2021.831883/full#supplementary-material
Footnotes
References
Ashraf, M., and Foolad, M. R. (2007). Roles of glycine betaine and proline in improving plant abiotic stress resistance. Environ. Exp. Bot. 59, 206–216. doi: 10.1016/j.envexpbot.2005.12.006
Bartels, D., and Sunkar, R. (2005). Drought and salt tolerance in plants. Crit. Rev. Plant Sci. 24, 23–58. doi: 10.1080/07352680590910410
Bessman, M. J., Frick, D. N., and O’Handley, S. F. (1996). The MutT proteins or “Nudix” hydrolases, a family of versatile, widely distributed, “housecleaning” enzymes. J. Biol. Chem. 271, 25059–25062. doi: 10.1074/jbc.271.41.25059
Bockwoldt, M., Houry, D., Niere, M., Gossmann, T. I., Reinartz, I., Schug, A., et al. (2019). Identification of evolutionary and kinetic drivers of NAD-dependent signaling. Proc. Natl. Acad. Sci. U.S.A. 116, 15957–15966. doi: 10.1073/pnas.1902346116
Chini, C. C. S., Tarrago, M. G., and Chini, E. N. (2017). NAD and the aging process: role in life, death and everything in between. Mol. Cell. Endocrinol. 455, 62–74. doi: 10.1016/j.mce.2016.11.003
Close, T. J. (2010). Dehydrins: emergence of a biochemical role of a family of plant dehydration proteins. Physiol. Plant. 97, 795–803.
Davletova, S., Rizhsky, L., Liang, H., Shengqiang, Z., Oliver, D. J., Coutu, J., et al. (2005). Cytosolic ascorbate peroxidase 1 is a central component of the reactive oxygen gene network of Arabidopsis. Plant Cell 17, 268–281. doi: 10.1105/tpc.104.026971
Dong, S., and Wang, Y. (2016). nudix effectors: a common weapon in the arsenal of plant pathogens. PLoS Pathog. 12:e1005704. doi: 10.1371/journal.ppat.1005704
Fedoroff, N. V., Battisti, D. S., Beachy, R. N., Cooper, P. J., Fischhoff, D. A., Hodges, C. N., et al. (2010). Radically rethinking agriculture for the 21st century. Science 327, 833–834. doi: 10.1126/science.1186834
Fujita, Y., Fujita, M., Shinozaki, K., and Yamaguchi-Shinozaki, K. (2011). ABA-mediated transcriptional regulation in response to osmotic stress in plants. J. Plant Res. 124, 509–525. doi: 10.1007/s10265-011-0412-3
Ge, X., Li, G. J., Wang, S. B., Zhu, H., Zhu, T., Wang, X., et al. (2007). AtNUDT7, a negative regulator of basal immunity in Arabidopsis, modulates two distinct defense response pathways and is involved in maintaining redox homeostasis. Plant Physiol. 145, 204–215. doi: 10.1104/pp.107.103374
Goswami, A., Banerjee, R., and Raha, S. (2013). Drought resistance in rice seedlings conferred by seed priming : role of the anti-oxidant defense mechanisms. Protoplasma 250, 1115–1129. doi: 10.1007/s00709-013-0487-x
Guo, P., Baum, M., Varshney, R. K., Graner, A., Grando, S., and Ceccarelli, S. (2007). QTLs for chlorophyll and chlorophyll fluorescence parameters in barley under post-flowering drought. Euphytica 163, 203–214. doi: 10.1007/s10681-007-9629-6
Guo, Y. Y., Yu, H. Y., Kong, D. S., Yan, F., and Zhang, Y. J. (2016). Effects of drought stress on growth and chlorophyll fluorescence of Lycium ruthenicum Murr. seedlings. Photosynthetica 54, 524–531. doi: 10.1007/s11099-016-0206-x
Hashida, S. N., Itami, T., Takahashi, H., Takahara, K., Nagano, M., Kawai-Yamada, M., et al. (2010). Nicotinate/nicotinamide mononucleotide adenyltransferase-mediated regulation of NAD biosynthesis protects guard cells from reactive oxygen species in ABA-mediated stomatal movement in Arabidopsis. J. Exp. Bot. 61, 3813–3825. doi: 10.1093/jxb/erq190
Hashida, S. N., Takahashi, H., and Uchimiya, H. (2009). The role of NAD biosynthesis in plant development and stress responses. Ann. Bot. 103, 819–824. doi: 10.1093/aob/mcp019
He, Y., Zhang, Y., Chen, L., Wu, C., Luo, Q., Zhang, F., et al. (2017). A Member of the 14-3-3 gene family in Brachypodium distachyon, BdGF14d, confers salt tolerance in transgenic tobacco plants. Front. Plant Sci. 8:340. doi: 10.3389/fpls.2017.00340
Hodges, D. M., Delong, J. M., and Prange, F. (1999). Improving the thiobarbituric acid-reactive-substances assay for estimating lipid peroxidation in plant tissues containing anthocyanin and other interfering compounds. Planta 207, 604–611. doi: 10.1007/s004250050524
Hong-Bo, S., Zong-Suo, L., and Ming-An, S. (2005). LEA proteins in higher plants: structure, function, gene expression and regulation. Colloids Surf. B Biointerfaces 45, 131–135. doi: 10.1016/j.colsurfb.2005.07.017
Hu, H., and Xiong, L. (2014). Genetic engineering and breeding of drought-resistant crops. Annu. Rev. Plant Biol. 65, 715–741. doi: 10.1146/annurev-arplant-050213-040000
Huai, J., Wang, M., He, J., Zheng, J., Dong, Z., Lv, H., et al. (2008). Cloning and characterization of the SnRK2 gene family from Zea mays. Plant Cell Rep. 27, 1861–1868. doi: 10.1007/s00299-008-0608-8
Ishikawa, K., Ogawa, T., Hirosue, E., Nakayama, Y., Harada, K., Fukusaki, E., et al. (2009). Modulation of the Poly(ADP-ribosyl)ation reaction via the Arabidopsis ADP-Ribose/NADH pyrophosphohydrolase, AtNUDX7, Is involved in the response to oxidative stress. Plant Physiol. 151, 741–754. doi: 10.1104/pp.109.140442
Ishikawa, K., Yoshimura, K., Harada, K., Fukusaki, E., Ogawa, T., Tamoi, M., et al. (2010). AtNUDX6, an ADP-ribose/NADH pyrophosphohydrolase in Arabidopsis, positively regulates NPR1-dependent salicylic acid signaling. Plant Physiol. 152, 2000–2012. doi: 10.1104/pp.110.153569
Jambunathan, N., and Mahalingam, R. (2006). Analysis of Arabidopsis growth factor gene 1 (GFG1) encoding a nudix hydrolase during oxidative signaling. Planta 224, 1–11. doi: 10.1007/s00425-005-0183-y
Jambunathan, N., Penaganti, A., Tang, Y., and Mahalingam, R. (2010). Modulation of redox homeostasis under suboptimal conditions by Arabidopsis nudix hydrolase 7. BMC Plant Biol. 10:173. doi: 10.1186/1471-2229-10-173
Kermode, A. R. (2005). Role of abscisic acid in seed dormancy. J. Plant Growth Regul. 24, 319–344. doi: 10.1007/s00344-005-0110-2
Klaus, S. M., Wegkamp, A., Sybesma, W., Hugenholtz, J., Gregory, J. F. III, and Hanson, A. D. (2005). A nudix enzyme removes pyrophosphate from dihydroneopterin triphosphate in the folate synthesis pathway of bacteria and plants. J. Biol. Chem. 280, 5274–5280. doi: 10.1074/jbc.M413759200
Kocheva, K., Lambrev, P., Georgiev, G., Goltsev, V., and Karabaliev, M. (2004). Evaluation of chlorophyll fluorescence and membrane injury in the leaves of barley cultivars under osmotic stress. Bioelectrochemistry 63, 121–124. doi: 10.1016/j.bioelechem.2003.09.020
Li, R.-H., Guo, P.-G., Michael, B., Stefania, G., and Salvatore, C. (2006). Evaluation of chlorophyll content and fluorescence parameters as indicators of drought tolerance in barley. Agric. Sci. China 5, 751–757. doi: 10.1016/s1671-2927(06)60120-x
Li, X., Tang, Y., Zhou, C., Zhang, L., and Lv, J. (2020). A Wheat WRKY transcription Factor TaWRKY46 enhances tolerance to osmotic stress in transgenic Arabidopsis Plants. Int. J. Mol. Sci. 21:1321. doi: 10.3390/ijms21041321
Liu, Q., Zhang, S., and Liu, B. (2016). 14-3-3 proteins: macro-regulators with great potential for improving abiotic stress tolerance in plants. Biochem. Biophys. Res. Commun. 477, 9–13. doi: 10.1016/j.bbrc.2016.05.120
Lurie, S., and Ben-Arie, R. (1983). Microsomal membrane changes during the ripening of apple fruit. Plant Physiol. 73, 636–638. doi: 10.1104/pp.73.3.636
Ma, Q. J., Sun, M. H., Lu, J., Liu, Y. J., You, C. X., and Hao, Y. J. (2017). An apple CIPK protein kinase targets a novel residue of AREB transcription factor for ABA-dependent phosphorylation. Plant Cell Environ. 40, 2207–2219. doi: 10.1111/pce.13013
Ma, Y., Szostkiewicz, I., Korte, A., Moes, D., Yang, Y., Christmann, A., et al. (2009). Regulators of PP2C phosphatase activity function as abscisic acid sensors. Science 324, 1064–1068. doi: 10.1126/science.1172408
Mao, X., Zhang, H., Tian, S., Chang, X., and Jing, R. (2010). TaSnRK2.4, an SNF1-type serine/threonine protein kinase of wheat (Triticum aestivum L.), confers enhanced multistress tolerance in Arabidopsis. J. Exp. Bot. 61, 683–696. doi: 10.1093/jxb/erp331
Maurel, C., Verdoucq, L., Luu, D. T., and Santoni, V. (2008). Plant aquaporins: membrane channels with multiple integrated functions. Annu. Rev. Plant Biol. 59, 595–624. doi: 10.1146/annurev.arplant.59.032607.092734
McLennan, A. G. (2006). The Nudix hydrolase superfamily. Cell. Mol. Life Sci. 63, 123–143. doi: 10.1007/s00018-005-5386-7
Mildvan, A. S., Xia, Z., Azurmendi, H. F., Saraswat, V., Legler, P. M., Massiah, M. A., et al. (2005). Structures and mechanisms of Nudix hydrolases. Arch. Biochem. Biophys. 433, 129–143. doi: 10.1016/j.abb.2004.08.017
Miller, G., Suzuki, N., Ciftci-Yilmaz, S., and Mittler, R. (2010). Reactive oxygen species homeostasis and signalling during drought and salinity stresses. Plant Cell Environ. 33, 453–467. doi: 10.1111/j.1365-3040.2009.02041.x
Msanne, J., Lin, J., Stone, J. M., and Awada, T. (2011). Characterization of abiotic stress-responsive Arabidopsis thaliana RD29A and RD29B genes and evaluation of transgenes. Planta 234, 97–107. doi: 10.1007/s00425-011-1387-y
Munoz, F. J., Baroja-Fernandez, E., Moran-Zorzano, M. T., Alonso-Casajus, N., and Pozueta-Romero, J. (2006). Cloning, expression and characterization of a Nudix hydrolase that catalyzes the hydrolytic breakdown of ADP-glucose linked to starch biosynthesis in Arabidopsis thaliana. Plant Cell Physiol. 47, 926–934. doi: 10.1093/pcp/pcj065
Nonogaki, H. (2017). Seed biology updates - highlights and new discoveries in seed dormancy and germination research. Front. Plant Sci. 8:524. doi: 10.3389/fpls.2017.00524
Ogawa, T., and Yoshimura, K. (2019). Modulation of the subcellular levels of redox cofactors by Nudix hydrolases in chloroplasts. Environ. Exp. Bot. 161, 57–66. doi: 10.1016/j.envexpbot.2018.11.002
Ogawa, T., Ishikawa, K., Harada, K., Fukusaki, E., Yoshimura, K., and Shigeoka, S. (2009). Overexpression of an ADP-ribose pyrophosphatase, AtNUDX2, confers enhanced tolerance to oxidative stress in Arabidopsis plants. Plant J. 57, 289–301. doi: 10.1111/j.1365-313X.2008.03686.x
Ogawa, T., Ueda, Y., Yoshimura, K., and Shigeoka, S. (2005). Comprehensive analysis of cytosolic Nudix hydrolases in Arabidopsis thaliana. J. Biol. Chem. 280, 25277–25283. doi: 10.1074/jbc.M503536200
Ogawa, T., Yoshimura, K., Miyake, H., Ishikawa, K., Ito, D., Tanabe, N., et al. (2008). Molecular characterization of organelle-type Nudix hydrolases in Arabidopsis. Plant Physiol. 148, 1412–1424. doi: 10.1104/pp.108.128413
Ozgur, T., and Gevrek, M. N. (2008). Influence of water stress on proline accumulation, lipid peroxidation and water content of wheat. Asian J. Plant Sci. 7, 409–412. doi: 10.3923/ajps.2008.409.412
Park, S. Y., Fung, P., Nishimura, N., Jensen, D. R., Fujii, H., Zhao, Y., et al. (2009). Abscisic acid inhibits type 2C protein phosphatases via the PYR/PYL family of START proteins. Science 324, 1068–1071. doi: 10.1126/science.1173041
Qin, X., and Zeevaart, J. A. (2002). Overexpression of a 9-cis-epoxycarotenoid dioxygenase gene in Nicotiana plumbaginifolia increases abscisic acid and phaseic acid levels and enhances drought tolerance. Plant Physiol. 128, 544–551. doi: 10.1104/pp.010663
Ramachandra Reddy, A., Chaitanya, K. V., and Vivekanandan, M. (2004). Drought-induced responses of photosynthesis and antioxidant metabolism in higher plants. J. Plant Physiol. 161, 1189–1202. doi: 10.1016/j.jplph.2004.01.013
Schachtman, D. P., and Goodger, J. Q. (2008). Chemical root to shoot signaling under drought. Trends Plant Sci. 13, 281–287. doi: 10.1016/j.tplants.2008.04.003
Seki, M., Umezawa, T., Urano, K., and Shinozaki, K. (2007). Regulatory metabolic networks in drought stress responses. Curr. Opin. Plant Biol. 10, 296–302. doi: 10.1016/j.pbi.2007.04.014
Tan, T., Cai, J., Zhan, E., Yang, Y., Zhao, J., Guo, Y., et al. (2016). Stability and localization of 14-3-3 proteins are involved in salt tolerance in Arabidopsis. Plant Mol. Biol. 92, 391–400. doi: 10.1007/s11103-016-0520-5
Tiwari, J. K., Munshi, A. D., Kumar, R., Pandey, R. N., Arora, A., Bhat, J. S., et al. (2010). Effect of salt stress on cucumber: Na+–K+ ratio, osmolyte concentration, phenols and chlorophyll content. Acta Physiol. Plant. 32, 103–114. doi: 10.1007/s11738-009-0385-1
Umezawa, T., Fujita, M., Fujita, Y., Yamaguchi-Shinozaki, K., and Shinozaki, K. (2006). Engineering drought tolerance in plants: discovering and tailoring genes to unlock the future. Curr. Opin. Biotechnol. 17, 113–122. doi: 10.1016/j.copbio.2006.02.002
Wang, C., Lu, G., Hao, Y., Guo, H., Guo, Y., Zhao, J., et al. (2017). ABP9, a maize bZIP transcription factor, enhances tolerance to salt and drought in transgenic cotton. Planta 246, 453–469. doi: 10.1007/s00425-017-2704-x
Wang, W., Vinocur, B., and Altman, A. (2003). Plant responses to drought, salinity and extreme temperatures: towards genetic engineering for stress tolerance. Planta 218, 1–14. doi: 10.1007/s00425-003-1105-5
Wang, Z., Wang, P., Guan, L., Haider, M. S., Nasim, M., Fang, J., et al. (2020). Versatile physiological functions of the Nudix Hydrolase family in berry development and stress response in grapevine. Res. Squ. [Preprint]. doi: 10.21203/rs.2.22110/v1
Wu, R., Zhang, F., Liu, L., Li, W., Pichersky, E., and Wang, G. (2018). MeNA, controlled by reversible methylation of Nicotinate, is an NAD precursor that undergoes long-distance transport in Arabidopsis. Mol. Plant 11, 1264–1277. doi: 10.1016/j.molp.2018.07.003
Xie, X. B., Li, S., Zhang, R. F., Zhao, J., Chen, Y. C., Zhao, Q., et al. (2012). The bHLH transcription factor MdbHLH3 promotes anthocyanin accumulation and fruit colouration in response to low temperature in apples. Plant Cell Environ. 35, 1884–1897. doi: 10.1111/j.1365-3040.2012.02523.x
Xiong, L., Wang, R. G., Mao, G., and Koczan, J. M. (2006). Identification of drought tolerance determinants by genetic analysis of root response to drought stress and abscisic Acid. Plant Physiol. 142, 1065–1074. doi: 10.1104/pp.106.084632
Xu, J., Yang, J.-Y., Niu, Q.-W., and Chua, N.-H. (2006). Arabidopsis DCP2, DCP1, and VARICOSE form a decapping complex required for postembryonic development. Plant Cell 18, 3386–3398. doi: 10.1105/tpc.106.047605
Xu, W., Dunn, C. A., Jones, C. R., D’Souza, G., and Bessman, M. J. (2004). The 26 Nudix hydrolases of Bacillus cereus, a close relative of Bacillus anthracis. J. Biol. Chem. 279, 24861–24865. doi: 10.1074/jbc.M403272200
Yamaguchi-Shinozaki, K., and Shinozaki, K. (2006). Transcriptional regulatory networks in cellular responses and tolerance to dehydration and cold stresses. Annu. Rev. Plant Biol. 57, 781–803. doi: 10.1146/annurev.arplant.57.032905.105444
Yoshimura, K., and Shigeoka, S. (2015). Versatile physiological functions of the Nudix hydrolase family in Arabidopsis. Biosci. Biotechnol. Biochem. 79, 354–366. doi: 10.1080/09168451.2014.987207
Zeng, X., Li, Y. F., and Mahalingam, R. (2014). Arabidopsis nudix hydrolase 7 plays a role in seed germination. Planta 239, 1015–1025. doi: 10.1007/s00425-014-2035-0
Zhang, H., Mao, X., Jing, R., Chang, X., and Xie, H. (2011). Characterization of a common wheat (Triticum aestivum L.) TaSnRK2.7 gene involved in abiotic stress responses. J. Exp. Bot. 62, 975–988. doi: 10.1093/jxb/erq328
Zhao, P., Hou, S., Guo, X., Jia, J., Yang, W., Liu, Z., et al. (2019). A MYB-related transcription factor from sheepgrass, LcMYB2, promotes seed germination and root growth under drought stress. BMC Plant Biol. 19:564. doi: 10.1186/s12870-019-2159-2
Zhu, J. K. (2002). Salt and drought stress signal transduction in plants. Annu. Rev. Plant Biol. 53, 247–273. doi: 10.1146/annurev.arplant.53.091401.143329
Keywords: peach, NUDIX hydrolase, drought stress, ABA, NAD/NADH
Citation: He H, Zhang Y, Wen B, Meng X, Wang N, Sun M, Zhang R, Zhao X, Tan Q, Xiao W, Li D, Fu X, Chen X and Li L (2022) PpNUDX8, a Peach NUDIX Hydrolase, Plays a Negative Regulator in Response to Drought Stress. Front. Plant Sci. 12:831883. doi: 10.3389/fpls.2021.831883
Received: 09 December 2021; Accepted: 29 December 2021;
Published: 16 February 2022.
Edited by:
Zhaojun Ding, Shandong University, ChinaReviewed by:
Liangyu Liu, Capital Normal University, ChinaBingsheng Lv, Qingdao Agricultural University, China
Copyright © 2022 He, Zhang, Wen, Meng, Wang, Sun, Zhang, Zhao, Tan, Xiao, Li, Fu, Chen and Li. This is an open-access article distributed under the terms of the Creative Commons Attribution License (CC BY). The use, distribution or reproduction in other forums is permitted, provided the original author(s) and the copyright owner(s) are credited and that the original publication in this journal is cited, in accordance with accepted academic practice. No use, distribution or reproduction is permitted which does not comply with these terms.
*Correspondence: HuaJie He, MjQ0NzI3ODczN0BxcS5jb20=; XiuDe Chen, Y2hlbnhpdWRlQDE2My5jb20=; Ling Li, bGlsaW5nc2RhdUAxNjMuY29t