- 1Plant EvoDevo Laboratory, Agrotechnology Division, CSIR-Institute of Himalayan Bioresource Technology, Palampur, India
- 2Stress Physiology and Molecular Biology Laboratory, School of Life Sciences, Jawaharlal Nehru University, New Delhi, India
- 3National Agri-Food Biotechnology Institute, Mohali, India
- 4Academy of Scientific and Innovative Research, Ghaziabad, India
Plant carnivory is often manifested as dramatic changes in the structure and morphology of the leaf. These changes appear to begin early in leaf development. For example, the development of the Sarracenia purpurea leaf primordium is associated with the formation of an adaxial ridge, whose growth along with that of the leaf margin resulted in a hollow structure that later developed into a pitcher. In Nepenthes khasiana, pitcher formation occurs during the initial stages of leaf development, although this has not been shown at the primordial stage. The formation of the Utricularia gibba trap resulted from the growth of the dome-shaped primordium in both the longitudinal and transverse directions. Recent research has begun to unfold the genetic basis of the development of the carnivorous leaf. We review these findings and discuss them in relation to the flat-shaped leaves of the model plant Arabidopsis.
Introduction
Carnivorous plants develop a set of morphological features termed “carnivorous syndrome”, which facilitate the capture and digestion of attracted prey (Pavlovič et al., 2007). This syndrome is manifested mostly on the leaves – as innovative morphological structures (Figure 1) – and has been the focus of research ever since the publication of Charles Darwin’s book Insectivorous plants (Darwin, 1875; Ellison and Gotelli, 2009). Studies have now shed light on why plant carnivory evolved, leading to a deeper understanding of the underlying mechanisms governing prey entrapment and digestion. It took a 140 years later, through an excellent study involving the purple pitcher plant S. purpurea (Fukushima et al., 2015), that the developmental basis of these highly specialized leaves became known. A few years later, the developing leaf transcriptome of N. khasiana was reported, hinting at the possible link between leaf polarity genes and pitcher formation (Dkhar and Pareek, 2019). Another outstanding study from the Coen lab provided insights on the formation of the U. gibba trap (Whitewoods et al., 2020). These studies have now begun to reveal the molecular mechanisms underpinning the development of the carnivorous leaf, shaped either as a cup (in U. gibba) or a pitcher (in S. purpurea). These innovative leaf morphologies appear to have evolved independently through changes in the existing genetic mechanisms governing flat-shaped leaf development (e.g., Arabidopsis, Figure 2A) (Lee et al., 2019).
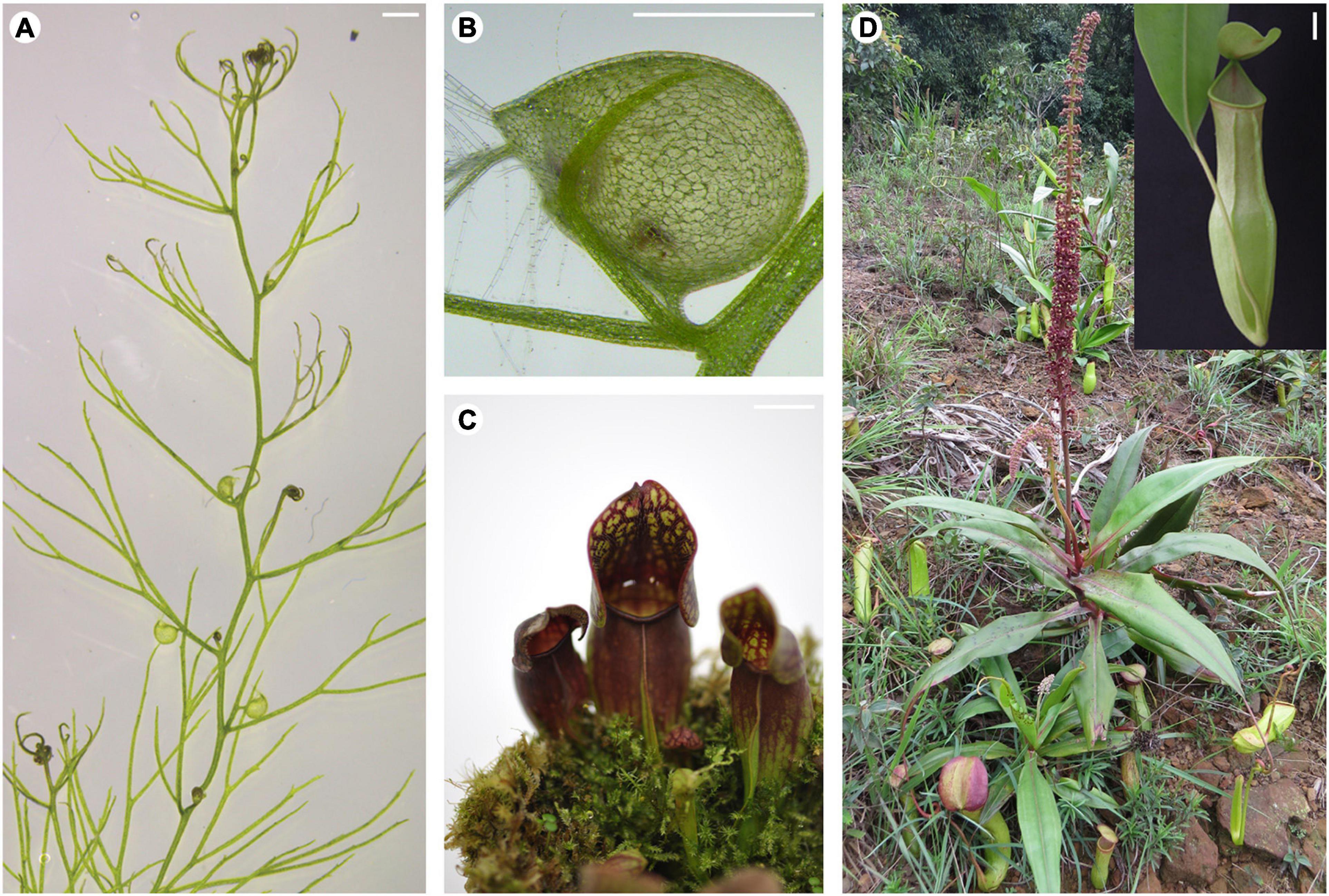
Figure 1. Innovative leaf morphologies of selected carnivorous plants. (A) Stolon of a U. gibba plant showing leaves with or without traps. (B) Close-up view of a U. gibba trap. (C) Pitcher-shaped leaves of S. purpurea. (D) A N. khasiana plant in the wild bearing pitcher-shaped leaves (inset shows close-up view of the N. khasiana pitcher). (A,B) Bar = 1 mm; (C,D) bar = 10 mm.
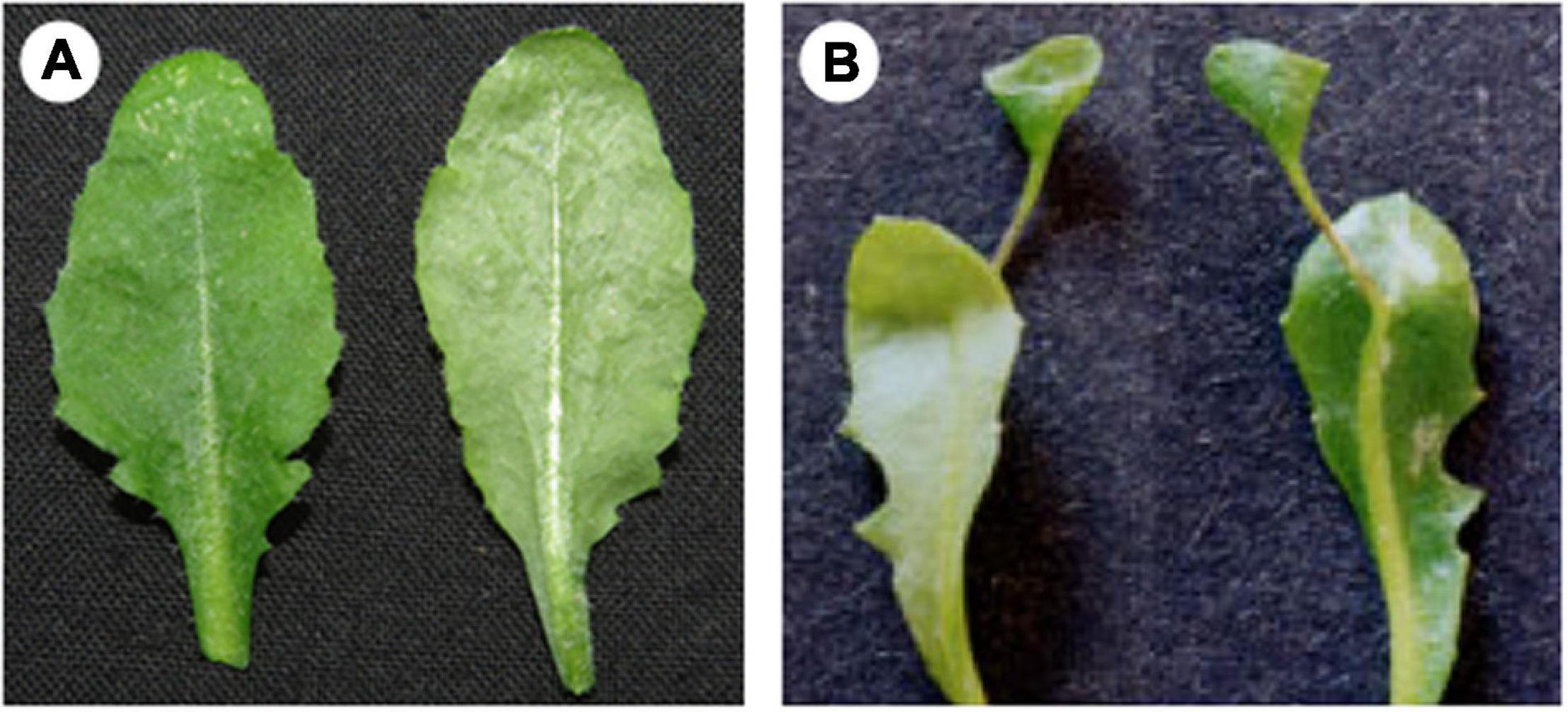
Figure 2. Leaf phenotypes of wild-type and mutant Arabidopsis plants. (A) Flat-shaped leaves of wild-type Arabidopsis plant. (B) Trumpet-shaped leaves of rev gain-of-function mutant Arabidopsis plant. Note the resemblance of the mutant leaf with those of Nepenthes (inset in Figure 1D). Images on the left and right of (A,B) correspond to the adaxial and abaxial sides, respectively. (B) Is reproduced from Zhong and Ye (2004) with permission from Oxford University Press, United Kingdom.
Leaf Organogenesis at the Shoot Apical Meristem of Carnivorous Plants
Leaves arise from the peripheral zone of the SAM (Bowman and Floyd, 2008). In Arabidopsis and other model plant organisms, the process begins with the recruitment of leaf founder cells (Dkhar and Pareek, 2014). In carnivorous plants, initiation of the leaf primordium might also involve founder cells recruitment. This recruitment is critical to determine the region at the flanks of SAM where primordia will initiate. Initiation is then followed by the establishment of the adaxial/abaxial polarity along with the bulging of the leaf primordia (Tsukaya, 2013). The developmental processes leading to the protrusion of the incipient leaf primordia appear to be common among land plants. As development progresses, structural changes begin to appear on the leaf primordia of carnivorous plants. In S. purpurea, the adaxial side of the developing leaf primordium becomes elevated, forming a ridge (Fukushima et al., 2015). Further growth and development of the adaxial ridge resulted in the formation of a hollow structure at the distal part of the primordium, which eventually develops into a pitcher (Fukushima et al., 2015). In Arabidopsis, the developing leaf primordium remains flat until maturity (Matsumoto and Okada, 2001) (Figure 2A). In U. gibba, trap develops laterally on a leaf. But not all U. gibba leaf bears a trap; rather, filiform-shaped leaflets develop. What specifies a U. gibba leaf to develop a trap instead of a leaflet is inherently genetic (discussed below). Interestingly, both leaflet and trap primordia look alike during the initial stages of development (Whitewoods et al., 2020). Changes appear at later stages: leaflet primordia turn into cylinders with tapered ends whereas trap primordia become spherical in shape, due to growth in both the longitudinal and transverse directions (Whitewoods et al., 2020). In Nepenthes, pitchers are attached to a flattened leaf base lamina via a tendril. It was previously thought that pitcher initiation occurs at the tip of a tendril (Owen and Lennon, 1999). However, recent evidence suggests that pitcher formation in N. khasiana occurs early in leaf development and shares anatomical features with the young in-rolled leaf base lamina (Dkhar and Pareek, 2019). It remains to be seen though, how pitcher initiation occurs on the leaf primordium of Nepenthes.
Trap Morphogenesis in Utricularia gibba is a Result of Restricted Gene Expression
How does a U. gibba plant direct its leaf to develop a trap, instead of a leaflet? The answer lies in the expression pattern of the PHAVOLUTA (PHV) gene, a member of the class III HD-ZIP gene family known to specify the adaxial identity of lateral organs (McConnell et al., 2001). The Arabidopsis genome contains five HD-ZIPIII genes namely PHABOLUSA (PHB), REVOLUTA (REV), ATHB8, ATHB15, and PHV (Emery et al., 2003). In U. gibba, PHV showed extended as well as restricted expression patterns on the adaxial side of the developing leaf primordia (Whitewoods et al., 2020). This differential gene expression pattern carries biological significance: primordia showing restricted PHV expression developed into traps. When this restricted expression pattern is perturbed, through heat-shock-induced ectopic expression of PHV that is altered to prevent recognition by miRNA (preferably miR165), allowing PHV expression throughout the leaf tissue, trap development is significantly reduced (Whitewoods et al., 2020). Thus, trap initiation in U. gibba is a result of restricted PHV expression. In S. purpurea, however, no distinct PHB or FIL (FILAMENTOUS FLOWER, a gene specifying abaxial identity) expression patterns were seen between the hollow and the ridge regions, indicating that neither of the two genes play a role in pitcher initiation. Instead, changes in the orientation of cell division in the developing leaf primordium led to the development of the pitcher-shaped leaf in S. purpurea (Fukushima et al., 2015).
Arabidopsis REVOLUTA Mutants Display Nepenthes Leaf Phenotype
Arabidopsis mutants of the HD-ZIPIII genes viz. PHB, PHV and REV display leaf phenotypes similar to those seen in pitcher plants, particularly Nepenthes. These gain-of-function mutations transform the flat-shaped leaves of Arabidopsis into trumpet-shaped (McConnell and Barton, 1998; McConnell et al., 2001; Emery et al., 2003; Zhong and Ye, 2004). In severe cases, these trumpet-shaped leaves grew out from the abaxial midvein of the slightly narrower leaf lamina (Figure 2B), resembling the pitcher-shaped leaves of Nepenthes. Unlike phb and phv gain-of-function mutants, the inside surface of the trumpet-shaped leaf of gain-of-function rev mutants is adaxial, as is the case with Nepenthes pitchers. The rev phenotype is due to a single nucleotide change in the putative lipid/sterol-binding START domain of the REV gene, which prevented recognition and thereby negative regulation by miR165 resulting in the expanded expression of REV (Emery et al., 2003). In N. khasiana, REV showed increased expression in the tip of the young N. khasiana leaf that later developed into a pitcher (Dkhar and Pareek, 2019). We now know that increased and restricted expression pattern of PHV resulted in trap formation in U. gibba. It is likely then that the increased and expanded expression of REV probably led to the development of the Nepenthes pitcher.
Auxin and Its Role in Pitcher Development
Trumpet-shaped leaves were also seen in Arabidopsis plants with defective PIN1 and REV genes, but not on single pin1 or rev Arabidopsis mutants (Qi et al., 2014). PIN1 encodes for an auxin efflux carrier protein that mediates local auxin accumulation during leaf initiation (Reinhardt et al., 2003). Based on their observations, Qi et al. (2014) suggested that auxin and REV act independently to promote leaf polarity. Fukushima et al. (2015) evaluated the effects of auxin on pitcher development in S. purpurea. Neither the addition of 1-Naphthaleneacetic acid (NAA) nor 1-N-Naphthylphthalamic acid (NPA), an auxin transport inhibitor, prevented the formation of the ridge and the hollow regions in the leaf primordia of S. purpurea. In N. khasiana, addition of NPA at 5, 10, 20, and 40 μM concentrations to the growing 1/4 strength MS medium increases shoot branching, as a result of axillary bud growth (Figures 3A,B). At higher NPA concentration, pitchers of N. khasiana failed to develop, although a tiny structure at the tip of the leaf can still be seen (Figure 3A). Altogether, these results suggest that auxin might have an indirect role in the formation (or the expansion) of the N. khasiana pitcher.
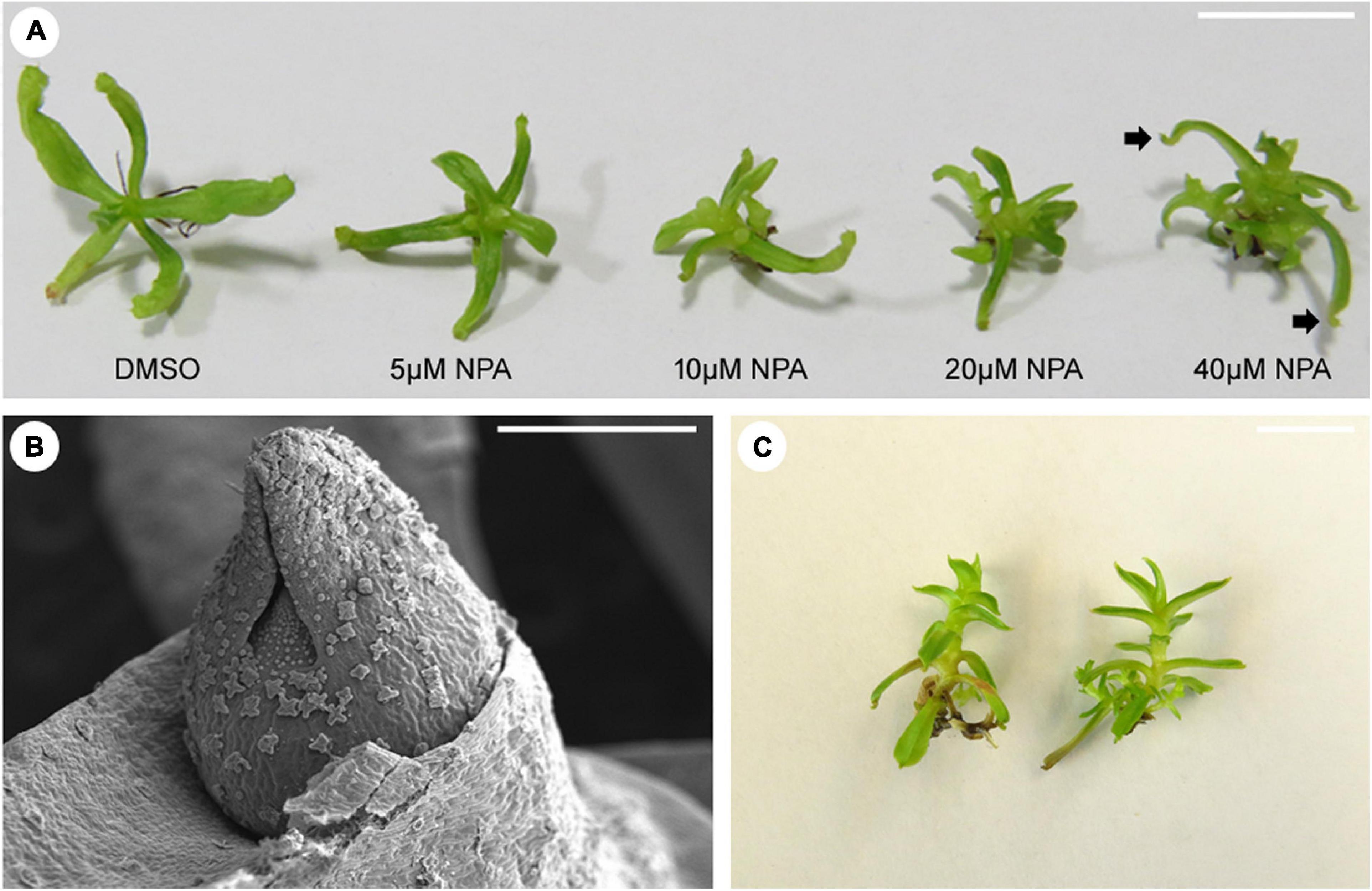
Figure 3. Effects of auxin transport inhibition and nutrient availability on pitcher formation in N. khasiana. (A) Morphological phenotypes of N. khasiana plantlets after 1 month of treatment with varying concentrations of NPA (arrow points to tiny structures at the tip of the leaf). (B) A scanning electron micrograph of an axillary bud of N. khasiana, arising as a result of NPA treatment. (C) N. khasiana plantlets grown in 2 MS (twice the strength of full MS i.e., 1 MS). Note the dramatic changes in leaf morphology. (A,C) Bar = 10 mm; (B) bar = 0.5 mm.
Temperature and Nutrient Availability Affect Pitcher Formation
Leaf shape can vary to change in environmental conditions such as temperature and light (Dkhar and Pareek, 2014). Interestingly, carnivorous plants also respond to change in temperature and nutrient availability. When the Australian pitcher plant Cephalotus follicularis was grown at 15°C under continuous light conditions, more flat leaves developed than pitcher-shaped leaves (Fukushima et al., 2017). At 25°C, however, the reverse was observed. Could this be a result of the plant’s response to higher temperatures? We know that Nepenthes, including N. khasiana, grow in nutrient-deficient soil. So, upon adding sufficient nutrients to the substratum, prey-deprived N. talangensis plants respond to this change in condition by developing leaves that lack pitchers (Pavlovič et al., 2010). We replicated this experiment in vitro by growing dissected shoot apices of 3-months old N. khasiana seedlings in MS medium of varying strengths (Dkhar et al., unpublished data). Most shoot apices of N. khasiana grown in 2 MS (twice the strength of full MS i.e., 1 MS) failed to grow, but if they survive, the plantlets develop narrower leaves completely devoid of pitchers (Figure 3C). By modifying nutrient content in the growing medium, we are now presented with N. khasiana plants lacking pitchers. It would be interesting to know how nutrient availability signals the plant to develop or not to develop pitchers.
Conclusion
Carnivorous plants evolved pitcher- or cup-shaped leaves independently in four angiosperm lineages viz. Cephalotaceae (Cephalotus), Lentibulariaceae (Utricularia), Nepenthaceae (Nepenthes) and Sarraceniaceae (Sarracenia) (Whitewoods and Coen, 2017). Although these leaves may look complex in shape and form, research suggests that their formation may be a result of simple modification – through changes in gene expression patterns and growth modulation – of existing leaf developmental programs at work in simpler leaf forms. Studies on the model plant Arabidopsis have led to a deeper understanding of the underlying mechanisms controlling leaf development, which now form the basis of ongoing research in the study of the evolution and development of innovative leaf morphologies seen across angiosperms, including carnivorous plants. The tremendous progress seen in Arabidopsis is, in part, attributed to the ease at which this plant can be genetically transformed, allowing various genes for leaf development to be functionally validated. Recent progress in this direction, such as the establishment of Agrobacterium-mediated transformation protocols in N. mirabilis (Miguel et al., 2020) and U. gibba (Oropeza-Aburto et al., 2020), offers much-needed hope for a complete genetic dissection of these complex leaf shapes.
Author Contributions
JD conceived the review. AA wrote the initial manuscript, which was then corrected and finalized by JD. AP did the final editing. All authors read and agreed with the final content of the manuscript.
Funding
Research in JD lab is supported by the Council of Scientific and Industrial Research (CSIR), Government of India (MLP 202) and Science and Engineering Research Board (SERB), Government of India [(SRG/2020/001139) (GAP 273)].
Conflict of Interest
The authors declare that the research was conducted in the absence of any commercial or financial relationships that could be construed as a potential conflict of interest.
Publisher’s Note
All claims expressed in this article are solely those of the authors and do not necessarily represent those of their affiliated organizations, or those of the publisher, the editors and the reviewers. Any product that may be evaluated in this article, or claim that may be made by its manufacturer, is not guaranteed or endorsed by the publisher.
Acknowledgments
The authors acknowledge the Editor and a Reviewer for critical reading and suggestions to improve the manuscript. JD acknowledges Sanjay Kumar, Director, CSIR-IHBT, Palampur, for providing the necessary facilities needed during the preparation of this review article, Kamlesh Kant Nutan (Pareek Lab) for the Arabidopsis image in Figure 2A, and the permission granted by Oxford University Press, United Kingdom to use the image in Figure 2B, which is reproduced from Zhong and Ye (2004). This review would not have been complete without the help of Enrico Coen, Department of Cell and Developmental Biology, John Innes Centre, United Kingdom and Kenji Fukushima, Department for Molecular Plant-Physiologie and Biophysics, University of Wuerzburg, Germany. Special thanks to Chris Whitewoods and Karen Lee (Coen Lab) for the U. gibba images used in Figures 1A,B and Matthias Freund (Fukushima Lab) for the S. purpurea image used in Figure 1C. This mini review represents CSIR-IHBT communication number 4969.
References
Bowman, J. L., and Floyd, S. K. (2008). Patterning and polarity in seed plant shoots. Ann. Rev. Plant Biol. 59, 67–88. doi: 10.1146/annurev.arplant.57.032905.105356
Darwin, C. (1875). Insectivorous Plants. New York: D. Appleton and Company. doi: 10.5962/bhl.title.99933
Dkhar, J., and Pareek, A. (2014). What determines a leaf’s shape? EvoDevo 5:47. doi: 10.1186/2041-9139-5-47
Dkhar, J., and Pareek, A. (2019). ASYMMETRIC LEAVES1 and REVOLUTA are the key regulatory genes associated with pitcher development in Nepenthes khasiana. Sci. Rep. 9:6318. doi: 10.1038/s41598-019-42779-6
Ellison, A. M., and Gotelli, N. J. (2009). Energetics and the evolution of carnivorous plants—Darwin’s ‘most wonderful plants in the world’. J. Exp. Bot. 60, 19–42. doi: 10.1093/jxb/ern179
Emery, J. F., Floyd, S. K., Alvarez, J., Eshed, Y., Hawker, N. P., Izhaki, A., et al. (2003). Radial patterning of Arabidopsis shoots by class III HD-ZIP and KANADI genes. Curr. Biol.? 13, 1768–1774. doi: 10.1016/j.cub.2003.09.035
Fukushima, K., Fang, X., Alvarez-Ponce, D., Cai, H., Carretero-Paulet, L., Chen, C., et al. (2017). Genome of the pitcher plant Cephalotus reveals genetic changes associated with carnivory. Nat. Ecol. Evol. 1:59. doi: 10.1038/s41559-016-0059
Fukushima, K., Fujita, H., Yamaguchi, T., Kawaguchi, M., Tsukaya, H., and Hasebe, M. (2015). Oriented cell division shapes carnivorous pitcher leaves of Sarracenia purpurea. Nat. Commun. 6:6450. doi: 10.1038/ncomms7450
Lee, K. J. I., Bushell, C., Koide, Y., Fozard, J. A., Piao, C., Yu, M., et al. (2019). Shaping of a three-dimensional carnivorous trap through modulation of a planar growth mechanism. PLoS Biol. 17:e3000427. doi: 10.1371/journal.pbio.3000427
Matsumoto, N., and Okada, K. (2001). A homeobox gene, PRESSED FLOWER, regulates lateral axis-dependent development of Arabidopsis flowers. Genes Dev. 15, 3355–3364. doi: 10.1101/gad.931001
McConnell, J. R., and Barton, M. K. (1998). Leaf polarity and meristem formation in Arabidopsis. Development 125, 2935–2942. doi: 10.1242/dev.125.15.2935
McConnell, J. R., Emery, J., Eshed, Y., Bao, N., Bowman, J., and Barton, M. K. (2001). Role of PHABULOSA and PHAVOLUTA in determining radial patterning in shoots. Nature? 411, 709–713. doi: 10.1038/35079635
Miguel, S., Michel, C., Biteau, F., Hehn, A., and Bourgaud, F. (2020). In vitro plant regeneration and Agrobacterium-mediated genetic transformation of a carnivorous plant, Nepenthes mirabilis. Sci. Rep. 10:17482. doi: 10.1038/s41598-020-74108-7
Oropeza-Aburto, A., Cervantes-Pérez, S. A., Albert, V. A., and Herrera-Estrella, L. (2020). Agrobacterium tumefaciens mediated transformation of the aquatic carnivorous plant Utricularia gibba. Plant Methods 16:50. doi: 10.1186/s13007-020-00592-7
Owen, T. P., and Lennon, K. A. (1999). Structure and development of the pitchers from the carnivorous plant Nepenthes alata (Nepenthaceae). Am. J. Bot. 86, 1382–1390. doi: 10.2307/2656921
Pavlovič, A., Masarovičová, E., and Hudák, J. (2007). Carnivorous syndrome in Asian pitcher plants of the genus Nepenthes. Ann. Bot. 100, 527–536. doi: 10.1093/aob/mcm145
Pavlovič, A., Singerová, L., Demko, V., Šantrůček, J., and Hudák, J. (2010). Root nutrient uptake enhances photosynthetic assimilation in prey-deprived carnivorous pitcher plant Nepenthes talangensis. Photosynthetica 48, 227–233. doi: 10.1007/s11099-010-0028-1
Qi, J., Wang, Y., Yu, T., Cunha, A., Wu, B., Vernoux, T., et al. (2014). Auxin depletion from leaf primordia contributes to organ patterning. Proc. Natl. Acad. Sci. U. S. A. 111, 18769–18774. doi: 10.1073/pnas.1421878112
Reinhardt, D., Pesce, E. R., Stieger, P., Mandel, T., Baltensperger, K., Bennett, M., et al. (2003). Regulation of phyllotaxis by polar auxin transport. Nature 426, 255–260. doi: 10.1038/nature02081
Whitewoods, C. D., and Coen, E. (2017). Growth and development of three-dimensional plant form. Curr. Biol. 27, R910–R918. doi: 10.1016/j.cub.2017.05.079
Whitewoods, C. D., Gonçalves, B., Cheng, J., Cui, M., Kennaway, R., Lee, K., et al. (2020). Evolution of carnivorous traps from planar leaves through simple shifts in gene expression. Science 367, 91–96. doi: 10.1126/science.aay5433
Keywords: carnivorous plants, leaf development, Nepenthes, Utricularia, Sarracenia
Citation: Agrawal A, Pareek A and Dkhar J (2022) Genetic Basis of Carnivorous Leaf Development. Front. Plant Sci. 12:825289. doi: 10.3389/fpls.2021.825289
Received: 30 November 2021; Accepted: 23 December 2021;
Published: 13 January 2022.
Edited by:
Jeremy D. Rentsch, Francis Marion University, United StatesReviewed by:
Andrej Pavlovič, Palacký University Olomouc, CzechiaCopyright © 2022 Agrawal, Pareek and Dkhar. This is an open-access article distributed under the terms of the Creative Commons Attribution License (CC BY). The use, distribution or reproduction in other forums is permitted, provided the original author(s) and the copyright owner(s) are credited and that the original publication in this journal is cited, in accordance with accepted academic practice. No use, distribution or reproduction is permitted which does not comply with these terms.
*Correspondence: Jeremy Dkhar, amVyZW15ZGtoYXJAaWhidC5yZXMuaW4=