Corrigendum: Defeating Huanglongbing Pathogen Candidatus Liberibacter asiaticus With Indigenous Citrus Endophyte Bacillus subtilis L1-21
- 1State Key Laboratory for Conservation and Utilization of Bio-Resources in Yunnan, Yunnan Agricultural University, Kunming, China
- 2Binchuan Institute for Food and Medicine Inspection and Testing, Binchuan, China
- 3College of Life Sciences, Gannan Normal University, Ganzhou, China
- 4Institute of Upland Crops, Wenshan Academy of Agricultural Sciences, Wenshan, China
- 5Institute of Crop Fertilization, Yuxi Academy of Agricultural Sciences, Yuxi, China
- 6Key Laboratory of Economic Plants and Biotechnology, Kunming Institute of Botany, Chinese Academy of Sciences (CAS), Kunming, China
- 7Institute of Tropical and Subtropical Cash Crops, Yunnan Academy of Agricultural Sciences, Baoshan, China
- 8Center for Mountain Futures (CMF), Kunming Institute of Botany, Chinese Academy of Sciences (CAS), Kunming, China
Huanglongbing (HLB) has turned into a devastating botanical pandemic of citrus crops, caused by Candidatus Liberibacter asiaticus (CLas). However, until now the disease has remained incurable with very limited control strategies available. Restoration of the affected microbiomes in the diseased host through the introduction of an indigenous endophyte Bacillus subtilis L1-21 isolated from healthy citrus may provide an innovative approach for disease management. A novel half-leaf method was developed in vitro to test the efficacy of the endophyte L1-21 against CLas. Application of B. subtilis L1-21 at 104 colony forming unit (cfu ml−1) resulted in a 1,000-fold reduction in the CLas copies per gram of leaf midrib (107 to 104) in 4 days. In HLB-affected citrus orchards over a period of 2 years, the CLas incidence was reduced to < 3%, and CLas copies declined from 109 to 104 g−1 of diseased leaf midribs in the endophyte L1-21 treated trees. Reduction in disease incidence may corroborate a direct or an indirect biocontrol effect of the endophytes as red fluorescent protein-labeled B. subtilis L1-21 colonized and shared niche (phloem) with CLas. This is the first large-scale study for establishing a sustainable HLB control strategy through citrus endophytic microbiome restructuring using an indigenous endophyte.
Introduction
Huanglongbing (HLB) disease, a major uncontrollable disease of citrus trees resulting in significant yield losses, is caused by one or a combination of the phloem-inhabiting α-proteobacteria Candidatus Liberibacter asiaticus (CLas), Ca. Liberibacter africanus (CLaf), and Ca. Liberibacter americanus (CLam) (Bové, 2006). The bacterium CLas may cause imbalances in host metabolism due to consumption, competition, and depletion of host nutrients (Duan et al., 2009). The main vector of CLas is Asian citrus psyllid (ACP), Diaphorina citri (Narouei-Khandan et al., 2016), and understanding the mechanisms involved in plant-pathogen interaction is essential for the development of novel HLB management strategies (Albrecht and Bowman, 2008; Fan et al., 2011).
The characteristics of HLB disease symptoms include blotchy mottled and pale yellow leaves, followed by distinct yellow shoots, corky veins, stunting, lopsided fruits with color inversion, and twig dieback (Ajene et al., 2020). Roots are also observed with a dramatic reduction in the fibrous root mass of infected plants, which leads to poorly developed root systems (Johnson et al., 2014; Li et al., 2019). Citrus-infected plants with HLB are not always but confused easily with symptoms of nutrient deficiency and without professional training (Tian et al., 2014) because the pathogen distribution is always highly patchy, as leaves and stems contain most of the bacterial titers (Li et al., 2006). In the beginning, the titer may be the highest in the roots (Johnson et al., 2014; da Rocha et al., 2019). The symptoms are clearer and more apparent in the cooler season compared to warmer months. Shimwela et al. (2019) estimated that the incubation period can be several months to years. It is still unknown that how long the trees have been infected before the appearance of symptoms, but symptomatic citrus trees eventually show disease symptoms (Dala-Paula et al., 2019).
So far, there are no curative methods to control this pathogen; although, scientists have used a few control measures to eliminate or reduce the pathogen growth. Approaches to control HLB include frequent use of antibiotics such as penicillin (Shin et al., 2016; Ascunce et al., 2019; McVay et al., 2019) and oxytetracycline (Blaustein et al., 2018), screening for small molecule inhibitors (Pagliai et al., 2015), combinations of stock and scion (Albrecht et al., 2016), graft-based chemotherapy (Zhang et al., 2012; Yang et al., 2016) and transgenic technology (Hao et al., 2016; Zou et al., 2017), control of psyllid vector (Tomaseto et al., 2019), and, finally, the most extreme measure is chopping off and burning down the diseased trees, which results in polluting environment even more. However, this method may slow down the disease, but with huge losses.
However, biocontrol of plant diseases can be the most promising alternative to continuously failing treatments and endophyte-mediated biocontrol offers consistent results due to intimate association with its host and shared niche with the pathogen where endophyte could fully manifest their antagonistic potential, whereas the non-endophytic biocontrol agents often fail under field conditions due to fierce competition in the rhizosphere and harsh environmental conditions (Ahmed et al., 2020; Blacutt et al., 2020; Trivedi et al., 2020). In biocontrol programs, native endophytes could be used to maintain long-term colonization inside the citrus host and eliminate CLas through niche and nutrient competition (Munir et al., 2018b). Biocontrol of plant pathogens has yielded effective results using bacteria under laboratory conditions (Herschkovitz et al., 2005; Andreote et al., 2010; Dematheis et al., 2013) and moderate effects under field conditions (Mattos-Jr et al., 2020), but the potential of indigenous endophytic bacteria as economically useful biocontrol agents against citrus pathogens has not been investigated, especially for HLB control (Munir et al., 2021). This approach may bring a revolution in managing citrus disease caused by phloem-limited α-proteobacteria (Bové, 2006).
Plant diseases and various plant protection measures lead to perturbations of the host microbiome (Irigoyen et al., 2020); it is also evident that CLas is associated with changes in microbial population dynamics during the HLB disease progression (Zhang et al., 2013a, 2016; Munir et al., 2019); therefore, it is of immense importance to understand the impact of key endophytic bacteria in the citrus health and defense against HLB. It is suggested that the key inhibitors of CLas play an important role in pathogen suppression (Barnett et al., 2019).
In an effort to gain in-depth insight, the aim of this study was to quantify the effects of regular applications of an indigenous endophyte, Bacillus subtilis L1-21 isolated from healthy citrus trees (selected based on potential biocontrol activities against several bacterial and fungal pathogens) to diseased trees for CLas reduction. This study highlights the promising research findings of using a novel citrus half-leaf method to reduce the CLas in the presence of B. subtilis L1-21. Most importantly, the evidence was provided from the two field experiments in the presence of endophyte L1-21, where citrus was diseased for more than 5 and 2 years, respectively. Finally, we point out that the red fluorescent protein (RFP)-tagged endophyte L1-21 shared the same niche with CLas inside citrus phloem. To the best of our knowledge, this is the first study undertaking a citrus endophyte-mediated management against HLB disease on a large field scale with consistent control.
Materials and Methods
Bacterial Strain and Culture Conditions
The endophytic strain B. subtilis L1-21, an indigenous endophyte isolated from a healthy citrus host (Munir et al., 2020a) and deposited in the Chinese Culture Collection Bank, Beijing (Accession number: CGMCC15726), was selected for the greenhouse and citrus field experiments (Binchuan, Yunnan Province, China). The endophyte B. subtilis L1-21 and its RFP-tagged strain were stored in 40% glycerol (v/v) at −80°C; stock culture was renewed every 4 months on Luria Bertani (LB) agar and cultured for 24–48 h to check the stability of the potential endophyte. A pure culture of endophyte L1-21 was grown until the late log phase in LB broth for 24–48 h at 37°C in a shaking incubator (150 rpm). The colony forming units (cfu ml−1) of the endophyte were checked using contrasting dilutions in sterile distilled water with 0.1% Tween 20 and plated on LB agar before foliar spraying on the citrus trees.
Novel Citrus Half-Leaf Method
We designed a novel citrus half-leaf method to quantify CLas copies in leaves prior to and after treatment based on conventional and quantitative PCR (qPCR). Diseased citrus leaves [Citrus reticulata (C. reticulata)] were collected randomly from 16 years old citrus grove and brought to the laboratory in ice and immediately processed for experiment or stored in 4°C till further use. Firstly, DNA was extracted from one-half of a diseased leaf using the cetyltrimethylammonium bromide (CTAB) method (Araújo et al., 2002; Munir et al., 2019) and conventional PCR analysis, using the primer set Cal-R/Cal-F (Jagoueix et al., 1996), was followed by nested PCR analysis, using the primer set CG03F/CG05R (Zhou et al., 2007), for confirmation of “Ca. Liberibacter asiaticus.” Primers used in this study are given in Supplementary Table S1. Once confirmed as positive for CLas, the other half of the citrus leaf (4–5 cm) was suspended with 104-106 cfu ml−1 of endophytes in a 5-ml Eppendorf tube and the different antibiotics, i.e., penicillin (100 μg μl−1) dissolved in distilled water, spinosad (100 μg μl−1) dissolved in hexane solution, and shenqimycin (100 μg μl−1) dissolved in distilled water as chemical control (positive). LB broth and sterilized distilled water were used as uninfected control (negative). The Eppendorf tubes were kept for 96 h at room temperature, followed by extraction of DNA from the leaf midribs. The CLas 16S ribosomal RNA (16S rRNA) gene was amplified as discussed previously (Munir et al., 2019). The weight of each half leaf was recorded prior to the experiment. Each treatment comprised three replicate Eppendorf tubes that each contained six diseased citrus leaves midribs. The experiment was repeated five times. Treatment effects on CLas were tested by loading the amplified product on 1% agarose gel. In another experiment testing for CLas and endophytes abundance, diseased leaves were placed in Petri dishes (180 mm) and different treatments were performed. DNA was extracted from one-half of a diseased leaf using the CTAB method (Araújo et al., 2002) and amplified with the same primers as described previously. Once the leaf was confirmed as infected with CLas, the other half of the leaf was kept in a 180-mm Petri dish with 104 and 106 cfu ml−1 or penicillin (100 μg μl−1) dissolved in distilled water. LB and distilled water were used as controls. The Petri dishes were kept at room temperature for an interval of 1 day for collected leaves to be checked for pathogen concentration and endophytes for 4–5 days; then, DNA was extracted from the midribs and present endophytes were isolated (Araújo et al., 2002; Munir et al., 2018b). The weight of each half leaf was recorded prior to the experiment. Each treatment comprised three replicates of 12 diseased citrus leaves (six random top and bottom half leaves, each) and the experiment was repeated five times. The presence of the pathogen was confirmed using standard qPCR analysis and density (copies g−1) was calculated based on a standard curve generated by cloning 382 bp of a specific DNA fragment located in the ribosomal protein (rplJ) (Munir et al., 2020a). The PCR reaction was performed in a 25-μl reaction mixture containing 1 × PCR buffer (SYBR Green Master Mix; Bio-Rad, United States), 0.8 μl of each primer (CQULA04R/CQULA04F), and the appropriate amount of DNA template in an RT-PCR system (StepOne Real-Time PCR System, Applied Biosystems, United States) as reported previously (Wang et al., 2006). Changes in CLas copies were tested from 0 to 4 days, based on CT values of pathogen copies g−1 of the citrus leaf that were calculated using the standard curve generated previously; changes in endophyte density were recorded daily as log cfu g−1 of citrus leaf midrib.
Phloem Colonization of Bacillus subtilis L1-21 RFP
For confirmation of phloem colonization of the endophyte, the RFP-tagged strain of B. subtilis L1-21 was generated using genomic DNA by amplifying the mKate2 coding sequence with ribosome-binding site. Bam H1 and Hind III enzymes were used for product digestion and ligated with the plasmid pYC127 as discussed previously (Chai et al., 2008). The resulting plasmid designated as PY69 was used to make overexpression RFP strain of B. subtilis L1-21. A strain expressing red fluorescent reporter gene (mKate2) was grown until late log phase in LB medium with 10 μg μl−1 chloramphenicol in shaking incubator at 37°C. Citrus plants (2 years old) in the greenhouse were foliar sprayed with RFP-tagged endophytic strain L1-21 and its colonization was visualized by confocal laser scanning microscope (CLSM) after 2 days. Citrus leaves were detached and gently washed for surface sterilization as performed previously to remove unattached cells from the citrus leaves (Munir et al., 2021). Leaves midribs were separated and put on the specimen holder by adding optimal cutting temperature (OCT) compound (Sakura, Europe). Specimen holder containing citrus leaves midribs were sliced briefly on the microtome-cryostat (Leica Biosystems, United States). Slice midribs were examined using CLSM (Olympus, FV10-ASW). The emission of RFP was measured at 405 to 635 nm after excitation at 559 nm, while citrus plant autofluorescence was measured after excitation at 405 and 635 nm for blue and green fluorescence, respectively. Images were captured using an automatic picture program FV1000 Viewer. The experiment was repeated three times, each time with three replicates.
Study Site and Sample Processing From HLB-Affected Citrus Groves
The experimental field site was located at Binchuan, Dali City, Yunnan Province, China (100.5754° E, 25.8272° N), with an average annual temperature of 33–35°C and total annual rainfall of about 1,000 mm. Foliar symptoms in one citrus grove (C. reticulata), 16 years old, indicated > 90% CLas disease incidence, and the second citrus grove (C. reticulata), 4 years old, which was located 2 km away from the first citrus grove, exhibited 100% CLas infection as confirmed through conventional PCR and qPCR before the start of experiments. Two field trials were conducted from 2017 to 2019: in one citrus grove, 162 trees (Supplementary Figure S1) were treated with contrasting treatments and in the second citrus grove, 93 of 525 trees were selected (three replicates of 31 trees) for CLas titer test. Prior to the start of each trial, a field survey was carried out to visually determine individual tree HLB severity and detected the CLas pathogen using CLas-specific primers in PCR and qPCR analyses. The soil treatments at the citrus groves comprised fertilizer 1 (rapeseed cape with Bacillus amyloliquefaciens Y2, as a root growth promoting agent); fertilizer 2 (rapeseed cape without Bacillus amyloliquefaciens Y2); and no rapeseed cape as a control (F0). Antibiotic penicillin (100 μg μl−1) dissolved in distilled water was applied one time through trunk injection at the start of the experiment as shown in Supplementary Table S2. In addition, we applied 5 kg of fertilizer to each tree around a water drip of about 20 cm deep. The antibiotics and endophytes used in this study are given in Supplementary Table S2.
Field Endophyte Treatment Application
The endophyte application was carried in the field experiments. Two injection ports per tree were made by drilling 30 mm into the xylem tissue using a 7.14-cm drill bit, located at the opposite sides of the trunk at ~20 cm above the union bud. Antibiotic and endophyte (B. subtilis L1-21) treatments were injected from a pressurized bottle into each port using a micro drip infusioner at the recommended pressure (<50 psi). The first injection was done with penicillin (0.1%) for 4 days on 54 trees, with 27 trees as a control. The second injection was done 2 weeks after the antibiotic treatment with the endophyte (106 cfu ml−1) on 54 trees. We also applied 106 cfu ml−1 of the endophyte or penicillin as a monthly foliar spray on 27 trees for each treatment, until all leaves were wet, in the early morning or late evening. All the experiments were arranged as three replicates of three trees in a randomized complete block design.
Isolation of Endophytes From Citrus Trees
Candidatus Liberibacter asiaticus causing HLB disease negatively affected the indigenous endophytic microbes. Therefore, we estimated the density of endophytic bacteria from three replicates of six citrus tree leaves that were sampled from the trees and transported to the laboratory in a cooler with ice. Endophytes were isolated from three replicates of treatment samples collected on the first week of each month in 2017–2018 as isolated previously (Araújo et al., 2002).
Candidatus Liberibacter asiaticus DNA Extraction and PCR Amplification
Leaf samples from all the treatments were collected monthly from the citrus groves to quantify CLas titer. Leaves were treated as above before DNA extraction. Methods for DNA extraction, PCR, and qPCR were those described previously.
Statistical Analysis
Endophytic bacteria populations were calculated, based on average logarithm (base 10) cfu values, and analyzed using the GraphPad Prism version 8 (San Diego, California, USA). Pathogen copies g−1 of diseased citrus leaf material were calculated using a standard curve value (Munir et al., 2020a). Treatment effects were tested using the ANOVA in the SPSS Statistics version 22.0 (IBM Corporation, Armonk, New York, USA) and treatment means were compared using Duncan's multiple range test at p < 0.05.
Results
Bacillus subtilis L1-21 Suppression of CLas in the Laboratory
We used the indigenous endophyte B. subtilis L1-21 against CLas in the leaf midrib by means of citrus half-leaf method (Figures 1A,B) maintained under room temperature in Eppendorf tube. CLas copies in leaf material were reduced by 5 days after treatment with the endophyte at 104 and 106 cfu ml−1 (Figure 1C); similarly, the antibiotics used in this experiment also reduced CLas copies (Figure 1D). Subsequent dilutions of the endophyte validated the results, confirming that CLas copies inside the citrus leaf midrib were reduced by a single application of the endophyte. Since the leaves midribs were cut and put on shaking in water, we suggested that pathogen ooze out of the midribs and no positive band was observed.
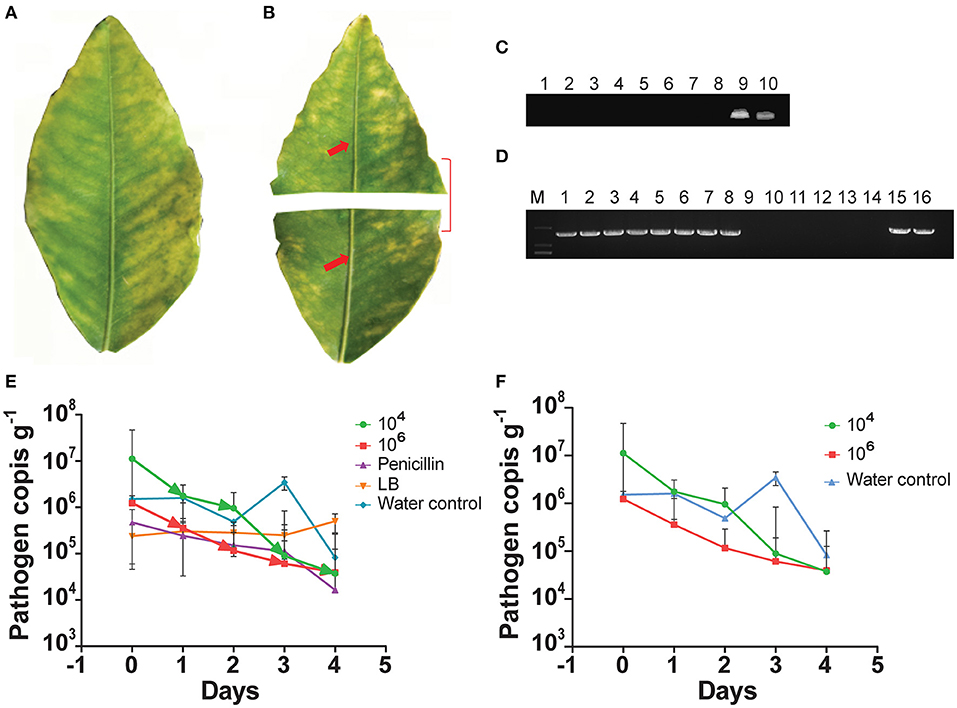
Figure 1. Citrus half-leaf method for reduction of Candidatus Liberibacter asiaticus (CLas) in the diseased citrus leaves from 0 to 4 days. Sketch of citrus leaf used in novel citrus half-leaf experiment. (A) Whole citrus leaves showing Huanglongbing (HLB) symptoms; (B) Leaf was parted into two parts; the first part was used to detect the CLas pathogen using conventional and quantitative PCR (qPCR); after confirmation, the second part was used in the experiment for Bacillus subtilis (B. subtilis) L1-21 treatment. Red arrows indicate leaf midribs used for extraction of DNA for conventional and qPCR; (C) Conventional PCR and diseased citrus leaves were treated with endophytes (103-106 cfu ml−1) and antibiotics penicillin (100 μg μl−1 and 500 μg μl−1), shenqinmycin (100 μg μl−1), and spinosad (100 μg μl−1); lane 9, water control; lane 10, positive control; (D) Conventional PCR, before application; Lane 1, citrus leaf sample treated with penicillin; Lane 2, leaf samples treated with spinosad; Lane 3, citrus leaves treated with shenqinmycin; Lane 4, sample in Luria Broth (LB) medium treated with endophytes without shaking; Lanes 5 and 6, samples in LB medium and water, respectively, on shaker; Lanes 7 and 15, diseased citrus leaf in water only; Lanes 8 and 16, positive control; after application: Lane 9, indicated reduction of CLas pathogen with different treatments including penicillin; Lane 10, spinosad; Lane 11, shenqinmycin; Lane 12, endophyte treatment in LB medium without shaking; Lane 13, endophyte treatment in LB medium with shaking; Lane 14, samples in water with shaking; (E) qPCR, pathogen copies per gram of leaves midrib using 104 and 106 colony forming unit (cfu) ml−1 of endophyte treatment one time. Pathogen copies g−1 was calculated based on the standard curve of recombinant plasmid pUC18-382-HLB generated through qPCR. Penicillin was used as a positive control. LB medium and water were used as negative control treatments. Three biological replicates were used for each treatment and each replicate consisted of 12 diseased leaves (including 6 top and bottom half leaves each). The experiment was repeated five times; and (F) Pathogen copies g−1 of leaves using 104 and 106 cfu ml−1 of endophyte and water treatment one time. The values are means ± SD of five replicated experiments.
To further confirm the efficacy of the endophyte against CLas, we used the half-leaf method to test pathogen copies in one half of the leaf present in Petri dish treated with a single application of the B. subtilis L1-21 (104 and 106 cfu ml−1) or penicillin antibiotic (as a positive/chemical control) and LB broth and water (as negative/uninfected controls). The presence of the pathogen was confirmed using standard qPCR analysis and density (copies g−1) was calculated based on a standard curve (Munir et al., 2020a). CLas pathogen copies in the leaf midribs treated with the endophyte L1-21 (104 and 106 cfu ml−1), penicillin, LB, and water were 1.12 × 107, 1.23 × 106, 4.71 × 105, 2.38 × 105, and 1.51 × 106, respectively (Figure 1E; Supplementary Table S3). Application of the endophyte reduced CLas copies in the leaf midribs 1,000-fold (1.12 × 107 to 3.72 × 104) and 100-fold (1.23 × 106 to 3.93 × 104) by 4 days after a single treatment of 106cfu ml−1 and 104 cfu ml−1 (Figure 1F; Supplementary Table S3) of the endophyte, respectively. Application of penicillin reduced CLas in diseased citrus leaves 10-fold (4.71 × 105 to 1.64 × 104) and there was no effect of LB or water controls by 4 days after treatment. These results clearly indicated that application of the endophytes reduced CLas pathogen in diseased citrus leaves. The half-leaf method was also employed to check the pathogen reduction in individual leaves using top and bottom parts to confirm validation of results and we showed that CLas could be reduced to more than 90% after 3–4 days (Supplementary Table S4).
Bacillus subtilis L1-21-Derived Indigenous Endophytes Inside Citrus Leaves
Prior to application of the endophyte, indigenous citrus endophyte density in the citrus leaves was 105 cfu g−1; following a single treatment with endophytic strain L1-21, the density of other endophytes present inside leaves increased to 109 cfu g−1 (Figure 2A). Treated endophyte L1-21, which displayed pinkish color on LB media, was recovered from the diseased citrus leaves during different time intervals with different treatments mentioned (Figure 2B). The application of penicillin initially decreased leaf midrib microbe density, but this effect subsided with time as the endophyte density returned to pretreatment levels. The endophyte density was unaffected by the LB and water treatments.
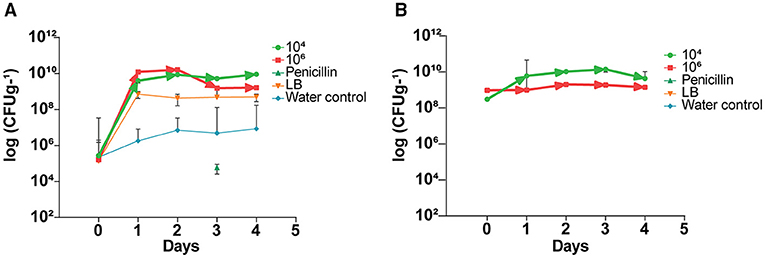
Figure 2. Elevation of indigenous endophytes derived after application of B. subtilis L1-21 in citrus half-leaf method. (A) Native endophytes density in the diseased citrus leaves after application of endophytes and penicillin and negative control. (B) Recovery of treated endophytes from the diseased citrus leaves during different time intervals with different treatments. The populations of endophytic bacteria were calculated based on the average logarithm (base 10) of bacteria recovered from the plant leaves. The detection limit (1 × 102 cfu leaves−1) was treated as log 0 for mean calculation. The log cfu values were analyzed with the GraphPad Prism version 8 (San Diego, California, USA). The values are means ± SD of five replicated experiments.
Bacillus subtilis L1-21 Colonization in Citrus Phloem
To prove the hypothesis that the indigenous endophyte B. subtilis L1-21 colonization inside citrus midribs phloem helps in excluding the CLas, which resides in citrus phloem, an RFP-tagged L1-21 strain was constructed that can express mKate2 genes. After observation with CLSM, we found that RFP-tagged L1-21 successfully colonizes the citrus midribs (Figure 3). Two days after inoculation, we observed that a lot of cells are present inside the phloem. These results indicated that the endophyte not only colonizes the citrus phloem completely but also reduces the pathogen load present in the citrus phloem as proved above.
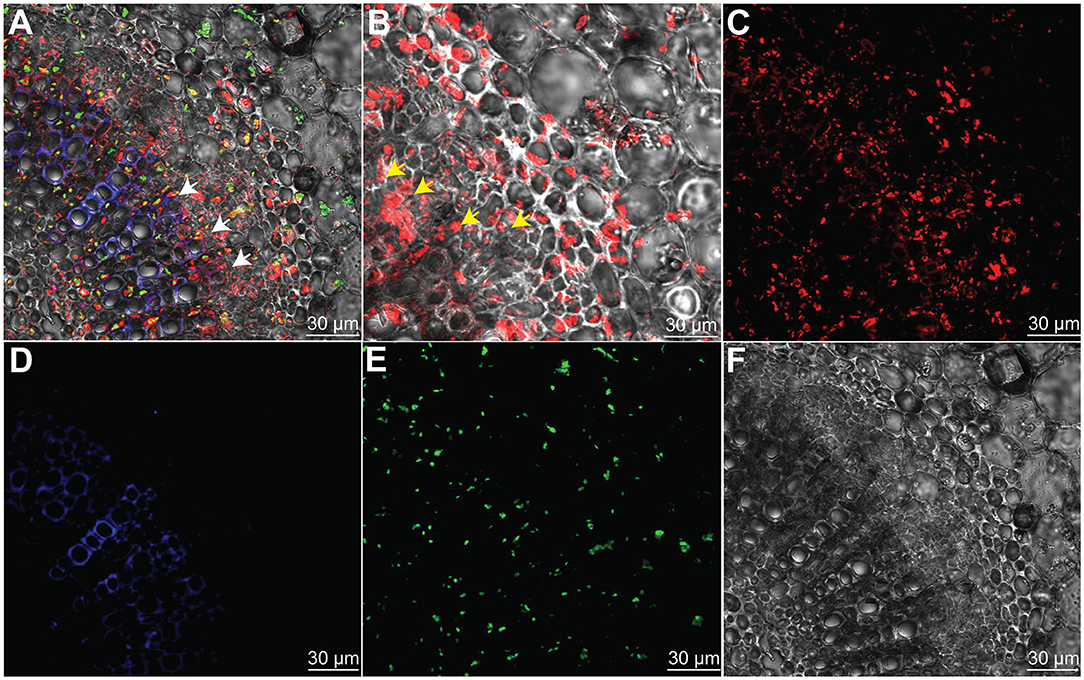
Figure 3. Bacillus subtilis L1-21 colonization in the phloem of citrus leaves midribs. (A–E) Indigenous citrus endophyte expressing mKate2 was grown until the late log phase and foliar sprayed on citrus plants in the greenhouse. Confocal laser scanning microscope was carried for visualization of B. subtilis L1-21 red fluorescent protein (RFP) in phloem; (A) Transverse section of merged citrus leaves midrib displaying red fluorescence (phloem); green autofluorescence (chlorophyll); blue fluorescence depicted the xylem; (B) Enlarge part from panel A observed for details colonization of endophyte inside citrus leaves phloem; (C) Individual RFP of B. subtilis L1-21; (D) Xylem with blue autofluorescence; (E) Green autofluorescence generated by chlorophyll; and (F) Dark field showing citrus phloem. The scale represents the 30 μM. The white and yellow arrows represent the localization of RFP-tagged B. subtilis L1-21 inside phloem.
Efficacy of Short-Term Field Applications of Bacillus subtilis L1-21 Against CLas
In 2017–2018, the efficacy of potential endophyte B. subtilis L1-21 was tested against CLas in two diseased citrus groves (>90% and 100% HLB prevalence, respectively) containing 16- and 4-year-old citrus trees, respectively. We treated the CLas-infected mandarin groves with contrasting applications of the endophyte, antibiotic, and biofertilizers to determine the most effective management strategy (Supplementary Figure S1). HLB-affected citrus grove was completely recovered after 6–7 months of successful treatment with the indigenous endophyte (Supplementary Figures S1B,C). Antibiotic and biofertilizers were applied once at the start of the experiment and endophytes were applied monthly, following leaf sampling to check densities of CLas and the endophyte. Prior to the start of the experiment, all the citrus trees were tested for the total number of endophytes present inside each tree (Figure 4A). Bacillus subtilis L1-21 applied to the citrus trees spreads to neighboring, untreated trees (Supplementary Figure S2); therefore, the endophytic density of all the citrus trees were presented on monthly basis. The density of CLas and endophytes was measured monthly in leaf samples collected from each of the 162 citrus trees using conventional and nested PCR techniques (Figures 4B–D). The health of leaves was improved following treatment with the endophyte for 1 year, where they changed from yellow to green, and we found negative effects of CLas on the endophytic microbiomes of the citrus tree. Citrus trees were monitored monthly to assess visual disease symptoms and following 1 year of monthly applications of B. subtilis L1-21, CLas density in the trees reduced significantly. In the first quarter of the experiment, the number of diseased trees reduced to <100, where trees that had been characterized by yellow and mottled leaves began to develop more robust shoots and leaves. The leaf density of citrus endophytes was initially reduced by the application of antibiotic but later recovered to more than 107 cfu g−1. By the second quarter of the experiment, the number of diseased trees reduced to 69, in which the endophyte density was similarly increased; by the final quarter, the number of diseased citrus trees reduced to three, and trees in the grove finally yielded fruit suitable for the commercial market. Thus, we confirmed that the endophyte B. subtilis L1-21 was the most effective control agent for the management of HLB and a reliable yet cheap option for citrus growers worldwide. Strengthening citrus microbe density using an endophyte with diverse antagonistic activities through trunk injection and foliar spray application may elevate microbe density in diseased citrus trees to levels where CLas may more easily be controlled (Figure 4E). Previously, we found the number of the endophyte types was lower in diseased citrus trees, indicating that greater density of indigenous citrus endophytes may contribute to the control of CLas in diseased trees (Munir et al., 2019).
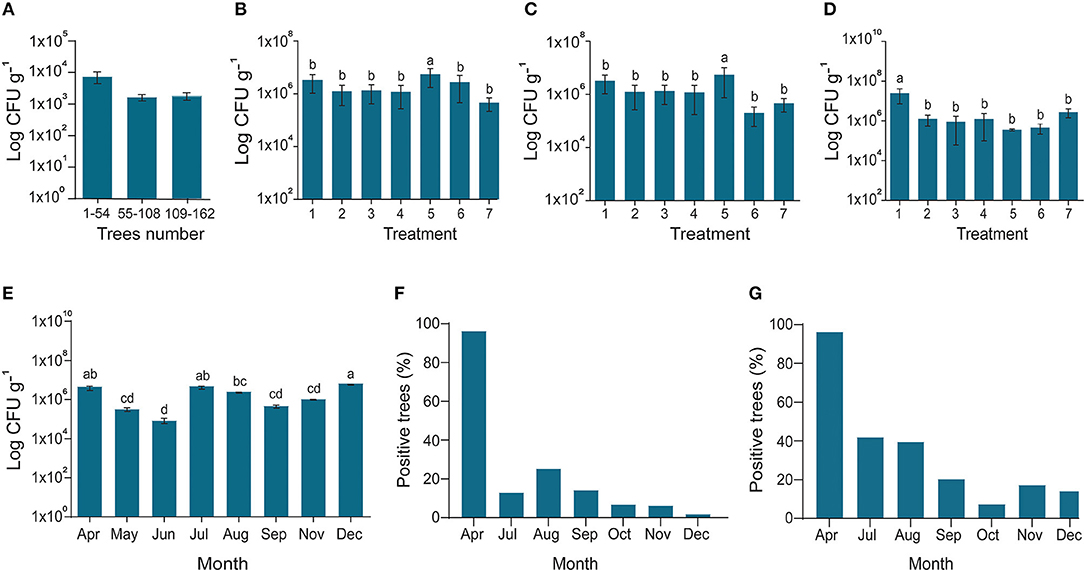
Figure 4. Endophytes density and reduction of CLas pathogen. (A) In March 2017, the diseased citrus trees were checked for possible assessment endophyte before starting experiment; (B) In April 2017, all the trees treated with fertilizer 1 with Bacillus amyloliquefaciens Y2; the X-axis represents endophytes and penicillin injection through the trunk (1); endophyte injection (2); Penicillin spray (3); Endophyte spray (4); Penicillin injection (5); Control CK1 and CK2 (6,7); (C) Fertilizer 2 with B. amyloliquefaciens Y2; CK2 and CK3 (6,7); (D) No organic fertilizer (F0) was used as control; (6,7) indigenous endophytes showing increase in the number of endophytes due to dispersal of endophytes in all the trees even control samples; (E) Endophytes population during different sampling times along with; and (F) Successive reduction of CLas pathogen inside disease trees using conventional PCR and (G) Nested PCR. The populations of endophytic bacteria were calculated based on the average logarithm (base 10) of bacteria recovered from the plant leaves. The log cfu values were analyzed with the GraphPad Prism version 8 (San Diego, California, USA). The values are means ± SD with statistically significant difference among different treatments with different letters (p ≤ 0.05).
The copies of CLas in leaf samples were reduced following 6 months of monthly treatments with B. subtilis L1-21 (Figures 4F,G), confirming our hypothesis that indigenous citrus endophytes may reduce disease incidence in the field by >95%. After a year of monthly applications of the endophyte, population of B. subtilis L1-21 in citrus leaf midribs increased from 103 to around 109 cfu g−1 in most trees, showing that indigenous endophytes may represent a novel management strategy for the control of CLas in citrus plants (Figure 5). We have found similar effects of this endophyte on CLas in 2 other citrus groves in the study region and one each in the Genma county of Yunnan Province (Figure 6).
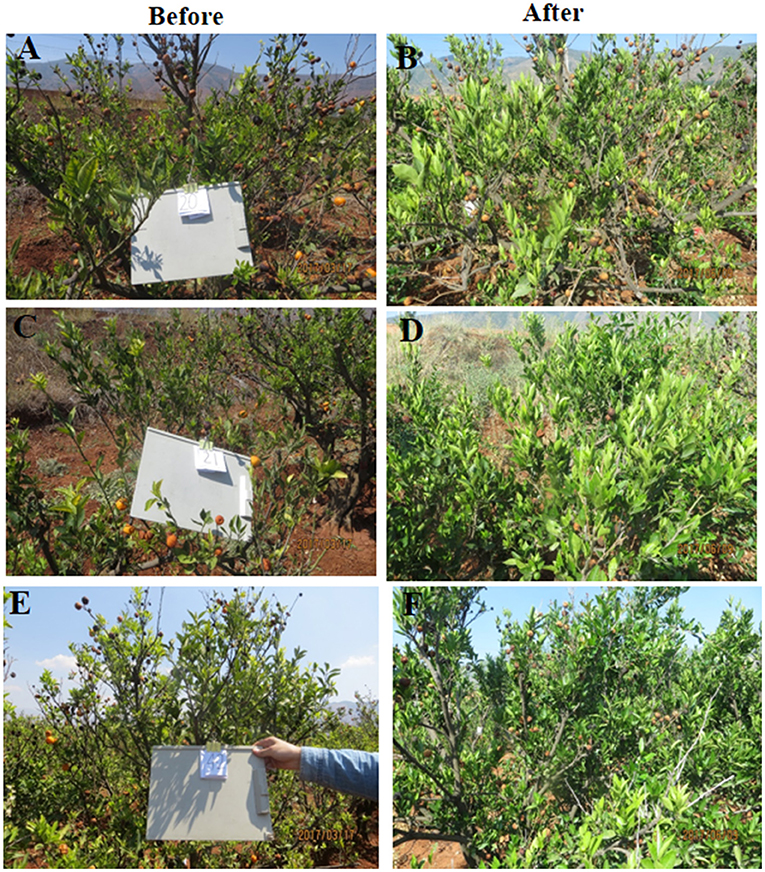
Figure 5. Huanglongbing affected diseased citrus groves before and after application of endophyte L1-21 in Binchuan county, Yunnan Province, China. The citrus groves were checked for CLas pathogen detection before the start of the experiment to make sure that all the citrus trees are affected with HLB. (A,C,E) In March 2017, the citrus grove was affected with HLB and (B,D,F) In June 2017, trees displayed more robust growth with green leaves after Bacillus subtilis L1-21 application.
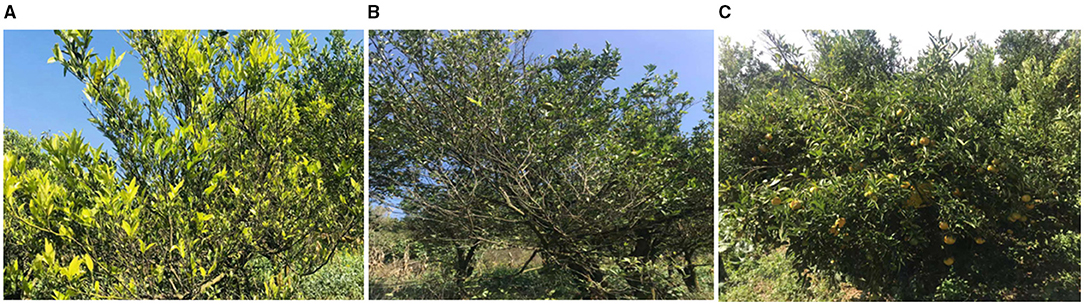
Figure 6. Management of HLB in diseased citrus fields using endophyte Bacillus subtilis L1-21: In 2018, an experiment was performed to check the reduction of diseased severity in a citrus grove in Genma county, Yunnan Province, China. Trees showed diseased symptoms to a healthy state after 7–8 months regular treatment with indigenous citrus endophyte L1-21. (A) April 2018; (B) June 2018; and (C) November 2018 (Genma county, Yunnan Province, China).
Effects of Long-Term Field Applications of Bacillus subtilis L1-21 on CLas
To confirm the results from the 1-year field experiments, we regularly treated diseased citrus groves, comprising 525 trees, with B. subtilis L1-21 in 2018–2019; these regular applications reduced copies of CLas in the citrus trees so that the number of diseased trees was reduced. Finally, we selected 93 among the 525 trees, which represented three replicates of 31 trees and found that after 1 year, regular applications of the endophyte had reduced the CLas from 109 to 104 g−1 of leaf midrib (Figure 7A) (99% control; Table 1). CLas copies were very high in April 2018 but decreased within 3 months (Figure 7B). In April 2019, we found 16 of the 93 diseased trees contained 100 copies of the pathogen g−1 of leaf material, while 39 diseased trees contained < 100 copies, representing a 91.39% reduction in CLas (Figure 7C) and there was a decrease in the number of yellow leaves and shoots and an increase in the growth of new shoots (Figure 7D). Our findings indicated that CLas density remained constant in around 25% of the citrus trees during the endophyte treatment and was undetected in 16 of the diseased citrus trees in April 2019. Thus, we suggested that CLas was successfully eliminated from diseased citrus trees by B. subtilis L1-21.
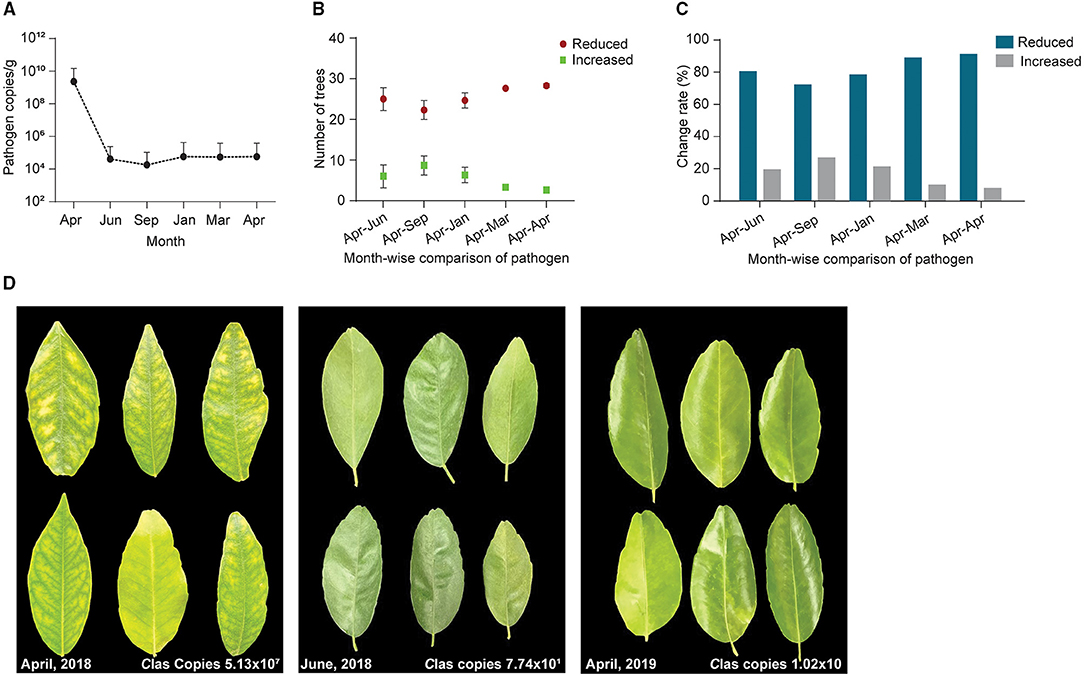
Figure 7. A total of 93 citrus diseased sampled trees were treated with endophytes and CLas pathogen was monitored throughout the study period from April 2018 to April 2019. (A) CT values obtained through qPCR were changed to pathogen copies per gram of citrus leaf using a standard curve generated from pUC18-382 recombinant plasmid; (B) Number of citrus trees displayed a reduction of CLas pathogen during different time intervals, April to September 2018 and January, March, and April 2019. Each repeat contains 31 citrus trees; (C) Percentage reduction of pathogen across month-wise indicating reduction of the pathogen from time to time; and (D) Citrus leaves showing yellow symptoms before application of endophytes (April 2018) to more greenish leaves with the different time intervals decreasing pathogen copies 107 to 10 CLas/gram citrus leaf midribs.
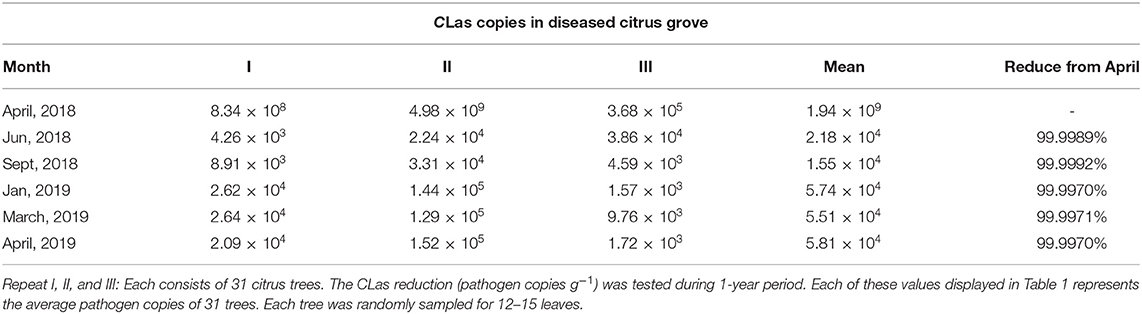
Table 1. Reduction of Candidatus Liberibacter asiaticus (CLas) pathogen copies during different time intervals 2018–2019.
Discussion
In order to demonstrate the specific role of endophytes to manage the alarmingly devastating disease of citrus, we isolated and identified indigenous endophytes from symptomatic, asymptomatic, and healthy leaves from different locations of citrus growing regions in China (Munir et al., 2020a). We tested the efficacy of B. subtilis L1-21, which showed marked pink colonies differed among all the isolated endophytes, used as a potential endophyte isolated from citrus trees in the experimental fields. The novel half-leaf method advanced the understanding of pathogen exclusion within the midrib. The endophyte application positively affected other microbes in citrus leaves, indicating native bacterial density was unaffected. It is likely that healthy and asymptomatic citrus trees harbor disease suppressive endophytic bacteria that may secrete antimicrobial compounds in the host phloem and, perhaps, contribute to protection against CLas. We found that there was no effect on CLas copies in diseased citrus leaves of treatment with LB and water as controls, indicating that reduction of the pathogen within the leaf was due to application of B. subtilis L1-21. Previously, citrus endophytes, such as Methylobacterium and Sphingomonas, were also found to be involved in the protection of plants against various devastating pathogens (Innerebner et al., 2011; Ardanov et al., 2012).
The pathogen reduction is associated with competition for the nutrients among pathogen and endophytes and substances released by endophytes that could have possible interference with the pathogen quorum sensing signaling (Miller and Bassler, 2001; Piewngam et al., 2018). Endophytic strain B. subtilis L1-21 was labeled with RFP that can express mKate2 gene. In light of our finding, CLSM visualization clearly showed successful colonization in the phloem of citrus leaves, which may open more doors for future research to study the mechanisms involved in the interaction of the endophyte with non-culturable CLas. The endophyte colonization in a similar niche as CLas might have employed direct inhibition of pathogen by producing antibiotics or lipopeptides, bacteriocin proteins (Kamada et al., 2013). On the other hand, core microbiomes present in the citrus host may be regulated by these endophytes, creating the possibility of pathogen elimination, most probably through induced systemic resistance (ISR) (Pertot et al., 2013; Zhao et al., 2018).
Currently, CLas is being treated with antibiotics (oxytetracycline hydrochloride, penicillin G potassium, ampicillin, and others) in the greenhouse and field experiments, but these are phytotoxic and persist as residues inside fruits (Zhang et al., 2014; Munir et al., 2018a). Proper use of antibiotics at the right time, adequate dosage, and application techniques can avoid problems related to the residue and phytotoxicity (McVay et al., 2019). Dissemination of antibiotics in the agriculture system is a potential threat to human health, agricultural productivity, natural ecosystem functioning, development of resistance in the pathogen, and negative effects on native microbiota (Williams-Nguyen et al., 2016), which urge the implementation of environmental-friendly alternatives for disease control. The use of antibiotics in citrus groves is common practice, which has been shown to drastically reduce the bacterial community richness and diversity (Zhang et al., 2013b); however, studies focusing on antibiotic effect solely on an endophytic community are scarce. Other common management practices such as glyphosate applications between the trees likely affect the endophytic microbial community over time. Our field experiment demonstrated successful management of CLas in HLB-diseased citrus with regular applications of B. subtilis L1-21. Pathogen copies in the diseased trees were reduced to <10 g−1 of leaf midrib and growth of green leaves and shoots was increased, including in previously moribund trees; similarly, marketable fruit yields also improved. However, the underlying mechanisms of endophytes on reductions in CLas copies and disease development remain unclear. These results indicated disease suppressive activities, such as quorum sensing attenuation by pathogen diffusible antibacterial compounds, niche and nutrient competition, synthesis of plant growth promoting hormones, siderophores or other bioactive compounds, and induction of plant defense, may have occurred with field applications of the endophyte, which limited in planta transmission of the pathogen, but warrants detailed study (Munir et al., 2021). Further, we assume that reduction of the pathogen may be related to increasing within plant endophyte density because the previous study has documented that CLas present inside citrus leaves regulated HLB symptoms positively and the leaf microbiome negatively (Blaustein et al., 2017; Munir et al., 2019). Pathogen exclusion effects were related to the increased density of B. subtilis L1-21 within the diseased trees. To determine in planta endophyte interactions with CLas, we first quantified their pretreatment densities in the diseased trees. Following treatment with B. subtilis L1-21, the endophyte densities in the citrus trees increased and the density of pathogen reduced. Notably, high copies of CLas remained present in untreated diseased trees. Thus, this study confirmed that the population dynamics of native citrus bacteria affect CLas titers (Sagaram et al., 2009; Zhang et al., 2016). It has been suggested that the endophyte application may also inhibit pathogen indirectly through activation of plant defense or recruitment of other endophytes in the citrus that causes the CLas reduction. We found significant genes and pathways associated with the elimination of CLas. Some pathways were effectively regulated in the diseased host, but not in healthy trees after endophytes treatment confirming different roles of endophytes in the host (data not shown).
Timing and effective dosage of application are much important to use biocontrol in field conditions (Brilli et al., 2019). Desired results can be achieved using this endophyte in the field during any time of the year in citrus fields. Later, we found that this endophyte can colonize for a longer duration inside citrus plants and maximum abundance was present even after 3 months, which makes it economically feasible for farmers. Using antibiotics to manage HLB disease could raise major concerns with respect to the uptake and distribution of antibiotics in citrus plants (Hijaz et al., 2020). Antibiotics such as penicillin and oxytetracycline showed uneven distribution in different parts of citrus plants and activity against the disease was also not stable (Al-Rimawi et al., 2019). In addition, endophyte-based biocontrol products will cost 100 dollars/hectares during 3 months of application and citrus growers and overall industry could minimize the use of high costing antibiotic applications (Sundin and Wang, 2018).
Candidatus Liberibacter asiaticus density in citrus trees is driven by an inversely proportional density of endophytes. Potential endophyte B. subtilis L1-21 for the management of citrus HLB using novel half-leaf method in vitro demonstrated that treatment of diseased citrus leaves reduced pathogen density in leaf midribs by 1,000-fold. In addition, phloem localization of endophyte L1-21 has also been confirmed in citrus revealed the possible role of pathogen exclusion. In light of our finding, this endophyte can efficiently colonize the phloem of citrus leaves, which may open more doors for future research to study the mechanisms involved in the interaction of endophytes with vascular pathogens. Based on the results, how the potential endophyte leads to a restructuring of the bacterial endophytic microbiome in diseased citrus groves is given in Figure 8. We suggested that indigenous endophyte application reduces the pathogen inside diseased citrus canopy from >90 to <3% within a year to a level that will bring benefit to the farmer in the future. Although the suppressive mechanism of endophyte against pathogen requires further exploration, however, we gained valuable insights about multiple metabolites variations in HLB-affected citrus trees, which mounted a defense against pathogen posttreatment with endophyte (Munir et al., 2020b). This study is the first initiative to highlight the restructuring of the citrus bacterial endophytic microbiomes by indigenous endophytes to mitigate the devastating disease HLB in economically important citrus crops.
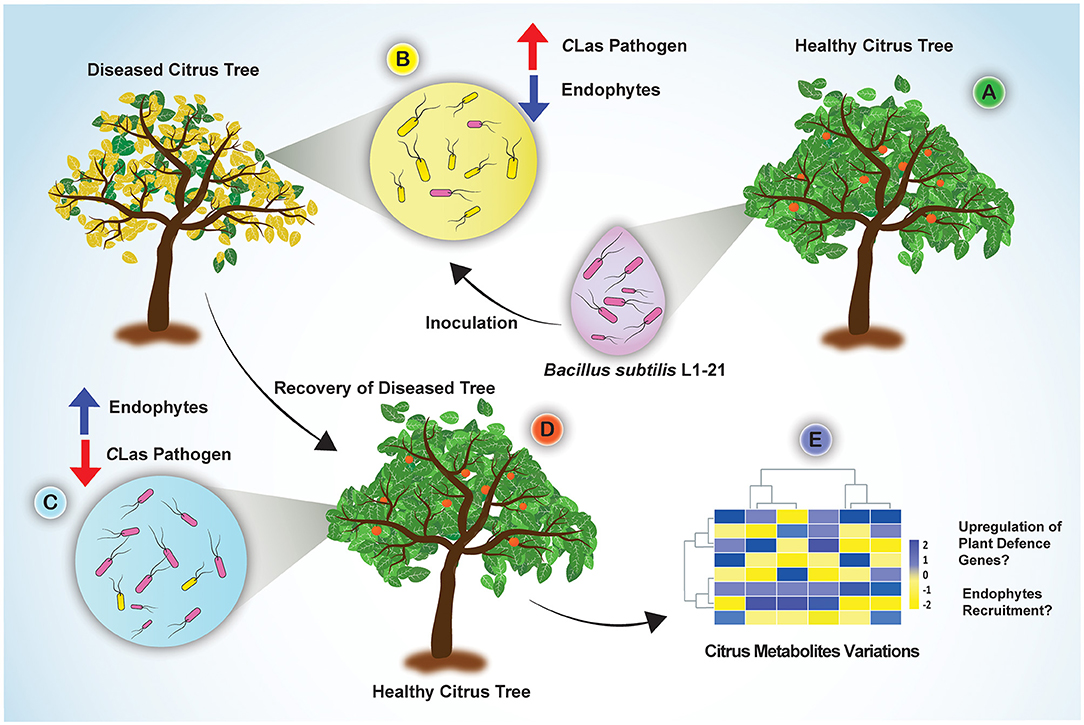
Figure 8. Concluding sketch illustrating the different events taking place in the diseased citrus fields through restructuring bacterial endophytes for management of HLB disease. (A) Indigenous endophyte B. subtilis L1-21, displaying marked pinkish colonies, was isolated from healthy citrus plants; (B) Diseased citrus trees with more pathogen and less endophytes were inoculated with the endophyte B. subtilis L1-21; (C) Endophyte L1-21 shifted the bacterial endophytic community and excluded/restricted the pathogen colonization in diseased citrus trees from two different regions by regular application for 1 year; (D) The recovered tree showing more robust leaves and fruits; (E) Significant citrus metabolites were upregulated in the diseased citrus host after application of potential endophyte B. subtilis L1-21 (Munir et al., 2020b).
Data Availability Statement
The original contributions presented in the study are included in the article/Supplementary Material, further inquiries can be directed to the corresponding author.
Author Contributions
SM, YL, PengfH, PengbH, PengjH, WC, YiW, YuW, JG, SK, and XL performed the laboratory experiments and data analyses. SM, SZ, ZL, YX, WW, KZ, ZL, YY, QL, SY, CM, HW, and YH performed field experiments and collected samples, and supervised the study. SM and YH drafted the manuscript. All authors contributed to the article and approved the submitted version.
Funding
This study was financially supported by the National Natural Science Foundation of China (32050410307), the Central Government Fund for Local Science and Technology Development (202107AA110007), the China Postdoctoral Science Foundation (No. 2020M683664XB), and the Yunnan First Level Research Fund for Post-Doctorate Researchers (202103).
Conflict of Interest
The authors declare that the research was conducted in the absence of any commercial or financial relationships that could be construed as a potential conflict of interest.
Publisher's Note
All claims expressed in this article are solely those of the authors and do not necessarily represent those of their affiliated organizations, or those of the publisher, the editors and the reviewers. Any product that may be evaluated in this article, or claim that may be made by its manufacturer, is not guaranteed or endorsed by the publisher.
Acknowledgments
We thank all the members of the Binchuan Institute of Food and Medicines Inspection and Testing, Yunnan Province, China for their valuable time during all the field experiments. In addition, we are also thankful to Dr Yiyang Yu from Nanjing Agricultural University, China for providing PY69 plasmid.
Supplementary Material
The Supplementary Material for this article can be found online at: https://www.frontiersin.org/articles/10.3389/fpls.2021.789065/full#supplementary-material
References
Ahmed, A., Munir, S., He, P., Li, Y., He, P., Yixin, W., et al. (2020). Biocontrol arsenals of bacterial endophyte: an imminent triumph against clubroot disease. Microbiol. Res. 241:126565. doi: 10.1016/j.micres.2020.126565
Ajene, I. J., Khamis, F., Van Asch, B., Pietersen, G., Rasowo, B. A., Ekesi, S., et al. (2020). Habitat suitability and distribution potential of Liberibacter species (“Candidatus Liberibacter asiaticus” and “Candidatus Liberibacter africanus”) associated with citrus greening disease. Divers. Distrib. 26, 575–588. doi: 10.1111/ddi.13051
Albrecht, U., and Bowman, K. D. (2008). Gene expression in Citrus sinensis (L.) Osbeck following infection with the bacterial pathogen Candidatus Liberibacter asiaticus causing Huanglongbing in Florida. Plant Sci. 175, 291–306. doi: 10.1016/j.plantsci.2008.05.001
Albrecht, U., Fiehn, O., and Bowman, K. D. (2016). Metabolic variations in different citrus rootstock cultivars associated with different responses to Huanglongbing. Plant Physiol. Biochem. 107, 33–44. doi: 10.1016/j.plaphy.2016.05.030
Al-Rimawi, F., Hijaz, F., Nehela, Y., Batuman, O., and Killiny, N. (2019). Uptake, translocation, and stability of oxytetracycline and streptomycin in citrus plants. Antibiotics 8:196. doi: 10.3390/antibiotics8040196
Andreote, F. D., Da Rocha, U. N., Araújo, W. L., Azevedo, J. L., and Van Overbeek, L. S. (2010). Effect of bacterial inoculation, plant genotype and developmental stage on root-associated and endophytic bacterial communities in potato (Solanum tuberosum). Antonie Van Leeuwenhoek 97, 389–399. doi: 10.1007/s10482-010-9421-9
Araújo, W. L., Marcon, J., Maccheroni, W., Van Elsas, J. D., Van Vuurde, J. W., and Azevedo, J. L. (2002). Diversity of endophytic bacterial populations and their interaction with Xylella fastidiosa in citrus plants. Appl. Environ. Microbiol. 68, 4906–4914. doi: 10.1128/AEM.68.10.4906-4914.2002
Ardanov, P., Sessitsch, A., Häggman, H., Kozyrovska, N., and Pirttil,ä, A. M. (2012). Methylobacterium-induced endophyte community changes correspond with protection of plants against pathogen attack. PLoS ONE 7:e46802. doi: 10.1371/journal.pone.0046802
Ascunce, M. S., Shin, K., Huguet-Tapia, J. C., Poudel, R., Garrett, K. A., Van Bruggen, A. H., et al. (2019). Penicillin trunk injection affects bacterial community structure in citrus trees. Microbial Ecol. 78, 457–469. doi: 10.1007/s00248-018-1302-1
Barnett, M. J., Solow-Cordero, D. E., and Long, S. R. (2019). A high-throughput system to identify inhibitors of Candidatus Liberibacter asiaticus transcription regulators. Proc. Natl. Acad. Sci. 116, 18009–18014. doi: 10.1073/pnas.1905149116
Blacutt, A., Ginnan, N., Dang, T., Bodaghi, S., Vidalakis, G., Ruegger, P., et al. (2020). An in vitro pipeline for screening and selection of citrus-associated microbiota with potential anti-“Candidatus Liberibacter asiaticus” properties. Appl. Environ. Microbiol. 86, e02883–e02819. doi: 10.1128/AEM.02883-19
Blaustein, R. A., Lorca, G. L., Meyer, J. L., Gonzalez, C. F., and Teplitski, M. (2017). Defining the core citrus leaf-and root-associated microbiota: factors associated with community structure and implications for managing huanglongbing (citrus greening) disease. Appl. Environ. Microbiol. 83, e00210–e00217. doi: 10.1128/AEM.00210-17
Blaustein, R. A., Lorca, G. L., and Teplitski, M. (2018). Challenges for managing Candidatus Liberibacter spp. (huanglongbing disease pathogen): current control measures and future directions. Phytopathology 108, 424–435. doi: 10.1094/PHYTO-07-17-0260-RVW
Bové, J. M. (2006). Huanglongbing: a destructive, newly-emerging, century-old disease of citrus. J. Plant Pathol. 88, 7−37. doi: 10.4454/jpp.v88i1.828
Brilli, F., Loreto, F., and Baccelli, I. (2019). Exploiting plant volatile organic compounds (VOCs) in agriculture to improve sustainable defense strategies and productivity of crops. Front. Plant Sci. 10:264. doi: 10.3389/fpls.2019.00264
Chai, Y., Chu, F., Kolter, R., and Losick, R. (2008). Bistability and biofilm formation in Bacillus subtilis. Mol. Microbiol. 67, 254–263. doi: 10.1111/j.1365-2958.2007.06040.x
da Rocha, U. N., Shin, K., Timilsina, S., Jones, J. B., Singer, B. H., and Van Bruggen, A. H. (2019). Potential soil transmission of a novel Candidatus Liberibacter strain detected in citrus seedlings grown in soil from a huanglongbing infested citrus grove. bioRxiv. doi: 10.1101/821553
Dala-Paula, B. M., Plotto, A., Bai, J., Manthey, J. A., Baldwin, E. A., Ferrarezi, R. S., et al. (2019). Effect of huanglongbing or greening disease on orange juice quality, a review. Fron. Plant Sci. 9:1976. doi: 10.3389/fpls.2018.01976
Dematheis, F., Kurtz, B., Vidal, S., and Smalla, K. (2013). Multitrophic interactions among Western Corn Rootworm, Glomus intraradices and microbial communities in the rhizosphere and endorhiza of maize. Front. Microbiol. 4:357. doi: 10.3389/fmicb.2013.00357
Duan, Y., Zhou, L., Hall, D. G., Li, W., Doddapaneni, H., Lin, H., et al. (2009). Complete genome sequence of citrus huanglongbing bacterium,‘Candidatus Liberibacter asiaticus’ obtained through metagenomics. Mol. Plant Microbe Interact. 22, 1011–1020. doi: 10.1094/MPMI-22-8-1011
Fan, J., Chen, C., Yu, Q., Brlansky, R. H., Li, Z. G., and Gmitter, F. G. Jr. (2011). Comparative iTRAQ proteome and transcriptome analyses of sweet orange infected by “Candidatus Liberibacter asiaticus.” Physiol. Plant. 143, 235–245. doi: 10.1111/j.1399-3054.2011.01502.x
Hao, G., Stover, E., and Gupta, G. (2016). Overexpression of a modified plant thionin enhances disease resistance to citrus canker and huanglongbing (HLB). Front. Plant Sci. 7:1078. doi: 10.3389/fpls.2016.01078
Herschkovitz, Y., Lerner, A., Davidov, Y., Rothballer, M., Hartmann, A., Okon, Y., et al. (2005). Inoculation with the plant-growth-promoting rhizobacterium Azospirillum brasilense causes little disturbance in the rhizosphere and rhizoplane of maize (Zea mays). Microb. Ecol. 50, 277–288. doi: 10.1007/s00248-004-0148-x
Hijaz, F., Nehela, Y., Al-Rimawi, F., Vincent, C. I., and Killiny, N. (2020). the role of the xylem in oxytetracycline translocation within citrus trees. Antibiotics 9:691. doi: 10.3390/antibiotics9100691
Innerebner, G., Knief, C., and Vorholt, J. A. (2011). Protection of Arabidopsis thaliana against leaf-pathogenic Pseudomonas syringae by Sphingomonas strains in a controlled model system. Appl. Environ. Microbiol. 77, 3202–3210. doi: 10.1128/AEM.00133-11
Irigoyen, S., Ramasamy, M., Pant, S., Niraula, P., Bedre, R., Gurung, M., et al. (2020). Plant hairy roots enable high throughput identification of antimicrobials against Candidatus Liberibacter spp. Nat. Commun. 11, 1–14. doi: 10.1038/s41467-020-19631-x
Jagoueix, S., Bov,é, J. M., and Garnier, M. (1996). PCR detection of the two Candidatus liberobacter species associated with greening disease of citrus. Mol. Cell. Probes 10, 43–50. doi: 10.1006/mcpr.1996.0006
Johnson, E., Wu, J., Bright, D., and Graham, J. (2014). Association of Candidatus Liberibacter asiaticus root infection, but not phloem plugging with root loss on huanglongbing-affected trees prior to appearance of foliar symptoms. Plant Pathol. 63, 290–298. doi: 10.1111/ppa.12109
Kamada, N., Chen, G. Y., Inohara, N., and Núñez, G. (2013). Control of pathogens and pathobionts by the gut microbiota. Nat. Immunol. 14:685. doi: 10.1038/ni.2608
Li, J., Li, L., Pang, Z., Kolbasov, V. G., Ehsani, R., Carter, E. W., et al. (2019). Developing citrus huanglongbing (HLB) management strategies based on the severity of symptoms in HLB-endemic citrus-producing regions. Phytopathology 109, 582–592. doi: 10.1094/PHYTO-08-18-0287-R
Li, W., Hartung, J. S., and Levy, L. (2006). Quantitative real-time PCR for detection and identification of Candidatus Liberibacter species associated with citrus huanglongbing. J. Microbiol. Methods 66, 104–115. doi: 10.1016/j.mimet.2005.10.018
Mattos-Jr, D., Kadyampakeni, D. M., Da Silva, J. R., Vashisth, T., and Boaretto, R. M. (2020). Reciprocal effects of huanglongbing infection and nutritional status of citrus trees: a review. Trop. Plant Pathol. 45, 586-596. doi: 10.1007/s40858-020-00389-y
McVay, J., Sun, X., Jones, D., Urbina, H., Aldeek, F., Cook, J. M., et al. (2019). Limited persistence of residues and metabolites in fruit and juice following penicillin trunk infusion in citrus affected by Huanglongbing. Crop Prot. 125:104753. doi: 10.1016/j.cropro.2019.03.001
Miller, M. B., and Bassler, B. L. (2001). Quorum sensing in bacteria. Annu. Rev. Microbiol. 55, 165–199. doi: 10.1146/annurev.micro.55.1.165
Munir, S., Ahmed, A., Li, Y., He, P., Singh, B. K., He, P., et al. (2021). The hidden treasures of citrus: finding huanglongbing cure where it was lost. Crit. Rev. Biotechnol. doi: 10.1080/07388551.2021.1942780
Munir, S., He, P., Wu, Y., He, P., Khan, S., Huang, M., et al. (2018a). Huanglongbing control: perhaps the end of the beginning. Microb. Ecol. 76, 192–204. doi: 10.1007/s00248-017-1123-7
Munir, S., Li, Y., He, P., He, P., He, P., Cui, W., et al. (2018b). Bacillus subtilis L1-21 possible assessment of inhibitory mechanism against phytopathogens and colonization in different plant hosts. Pak. J. Agric. Sci. 55, 996–1002. doi: 10.21162/pakjas/18.7750
Munir, S., Li, Y., He, P., He, P., He, P., Cui, W., et al. (2019). Seasonal variation and detection frequency of Candidatus Liberibacter asiaticus in Binchuan, Yunnan province China. Physiol. Mol. Plant Pathol. 106, 137–144. doi: 10.1016/j.pmpp.2019.01.004
Munir, S., Li, Y., He, P., Huang, M., He, P., He, P., et al. (2020a). Core endophyte communities of different citrus varieties from citrus growing regions in China. Sci. Rep. 10, 1–12. doi: 10.1038/s41598-020-60350-6
Munir, S., Li, Y., He, P., He, P., Ahmed, A., Wu, Y., et al. (2020b). Unraveling the metabolite signature of citrus showing defense response towards Candidatus Liberibacter asiaticus after application of endophyte Bacillus subtilis L1-21. Microbiol. Res. 234, 126425. doi: 10.1016/j.micres.2020.126425
Narouei-Khandan, H. A., Halbert, S. E., Worner, S. P., and Van Bruggen, A. H. (2016). Global climate suitability of citrus huanglongbing and its vector, the Asian citrus psyllid, using two correlative species distribution modeling approaches, with emphasis on the USA. Eur. J. Plant Pathol. 144, 655–670. doi: 10.1007/s10658-015-0804-7
Pagliai, F. A., Gonzalez, C. F., and Lorca, G. L. (2015). Identification of a ligand binding pocket in LdtR from Liberibacter asiaticus. Front. Microbiol. 6:1314. doi: 10.3389/fmicb.2015.01314
Pertot, I., Puopolo, G., Hosni, T., Pedrotti, L., Jourdan, E., and Ongena, M. (2013). Limited impact of abiotic stress on surfactin production in planta and on disease resistance induced by Bacillus amyloliquefaciens S499 in tomato and bean. FEMS Microbiol. Ecol. 86, 505–519. doi: 10.1111/1574-6941.12177
Piewngam, P., Zheng, Y., Nguyen, T. H., Dickey, S. W., Joo, H.-S., Villaruz, A. E., et al. (2018). Pathogen elimination by probiotic Bacillus via signalling interference. Nature 562, 532-537. doi: 10.1038/s41586-018-0616-y
Sagaram, U. S., Deangelis, K. M., Trivedi, P., Andersen, G. L., Lu, S.-E., and Wang, N. (2009). Bacterial diversity analysis of Huanglongbing pathogen-infected citrus, using PhyloChip arrays and 16S rRNA gene clone library sequencing. Appl. Environ. Microbiol. 75, 1566–1574. doi: 10.1128/AEM.02404-08
Shimwela, M., Halbert, S., Keremane, M., Mears, P., Singer, B., Lee, W., et al. (2019). In-grove spatiotemporal spread of citrus huanglongbing and its psyllid vector in relation to weather. Phytopathology 109, 418–427. doi: 10.1094/PHYTO-03-18-0089-R
Shin, K., Ascunce, M. S., Narouei-Khandan, H. A., Sun, X., Jones, D., Kolawole, O. O., et al. (2016). Effects and side effects of penicillin injection in huanglongbing affected grapefruit trees. Crop Prot. 90, 106–116. doi: 10.1016/j.cropro.2016.08.025
Sundin, G. W., and Wang, N. (2018). Antibiotic resistance in plant-pathogenic bacteria. Annu. Rev. Phytopathol. 56, 161–180. doi: 10.1146/annurev-phyto-080417-045946
Tian, S., Lu, L., Labavitch, J. M., Webb, S. M., Yang, X., Brown, P. H., et al. (2014). Spatial imaging of Zn and other elements in Huanglongbing-affected grapefruit by synchrotron-based micro X-ray fluorescence investigation. J. Exp. Bot. 65, 953–964. doi: 10.1093/jxb/ert450
Tomaseto, A. F., Marques, R. N., Fereres, A., Zanardi, O. Z., Volpe, H. X., Alquézar, B., et al. (2019). Orange jasmine as a trap crop to control Diaphorina citri. Sci. Rep. 9, 1–11. doi: 10.1038/s41598-019-38597-5
Trivedi, P., Leach, J. E., Tringe, S. G., Sa, T., and Singh, B. K. (2020). Plant–microbiome interactions: from community assembly to plant health. Nat. Rev. Microbiol. 18, 607–621. doi: 10.1038/s41579-020-0412-1
Wang, Z., Yin, Y., Hu, H., Yuan, Q., Peng, G., and Xia, Y. (2006). Development and application of molecular-based diagnosis for ‘Candidatus Liberibacter asiaticus’, the causal pathogen of citrus huanglongbing. Plant Pathol. 55, 630–638. doi: 10.1111/j.1365-3059.2006.01438.x
Williams-Nguyen, J., Sallach, J. B., Bartelt-Hunt, S., Boxall, A. B., Durso, L. M., Mclain, J. E., et al. (2016). Antibiotics and antibiotic resistance in agroecosystems: state of the science. J. Environ. Qual. 45, 394–406. doi: 10.2134/jeq2015.07.0336
Yang, C., Powell, C. A., Duan, Y., Shatters, R., Fang, J., and Zhang, M. (2016). Deciphering the bacterial microbiome in huanglongbing-affected citrus treated with thermotherapy and sulfonamide antibiotics. PLoS ONE 11:e0155472. doi: 10.1371/journal.pone.0155472
Zhang, M., Guo, Y., Powell, C., Doud, M., Yang, C., Zhou, H., et al. (2016). Zinc treatment increases the titre of ‘Candidatus Liberibacter asiaticus’ in huanglongbing-affected citrus plants while affecting the bacterial microbiomes. J. Appl. Microbiol. 120, 1616–1628. doi: 10.1111/jam.13102
Zhang, M., Guo, Y., Powell, C. A., Doud, M. S., Yang, C., and Duan, Y. (2014). Effective antibiotics against ‘Candidatus Liberibacter asiaticus’ in HLB-affected citrus plants identified via the graft-based evaluation. PLoS ONE 9:e111032. doi: 10.1371/journal.pone.0111032
Zhang, M., Powell, C. A., Benyon, L. S., Zhou, H., and Duan, Y. (2013a). Deciphering the bacterial microbiome of citrus plants in response to ‘Candidatus Liberibacter asiaticus’-infection and antibiotic treatments. PLoS ONE 8:e76331. doi: 10.1371/journal.pone.0076331
Zhang, M., Powell, C. A., Guo, Y., Benyon, L., and Duan, Y. (2013b). Characterization of the microbial community structure in Candidatus Liberibacter asiaticus-infected citrus plants treated with antibiotics in the field. BMC Microbiol. 13, 1–10. doi: 10.1186/1471-2180-13-112
Zhang, M., Powell, C. A., Guo, Y., Doud, M. S., and Duan, Y. (2012). A graft-based chemotherapy method for screening effective molecules and rescuing huanglongbing-affected citrus plants. Phytopathology 102, 567–574. doi: 10.1094/PHYTO-09-11-0265
Zhao, L., Xu, Y., and Lai, X. (2018). Antagonistic endophytic bacteria associated with nodules of soybean (Glycine max L.) and plant growth-promoting properties. Braz. J. Microbiol. 49, 269–278. doi: 10.1016/j.bjm.2017.06.007
Zhou, L., Gabriel, D., Duan, Y., Halbert, S., and Dixon, W. (2007). First report of dodder transmission of huanglongbing from naturally infected Murraya paniculata to citrus. Plant Dis. 91, 227–227. doi: 10.1094/PDIS-91-2-0227B
Keywords: Citrus, Bacillus subtilis, endophyte, pathogen, restructuring, microbiome
Citation: Munir S, Li Y, He P, He P, He P, Cui W, Wu Y, Li X, Li Q, Zhang S, Xiong Y, Lu Z, Wang W, Zong K, Yang Y, Yang S, Mu C, Wen H, Wang Y, Guo J, Karunarathna SC and He Y (2022) Defeating Huanglongbing Pathogen Candidatus Liberibacter asiaticus With Indigenous Citrus Endophyte Bacillus subtilis L1-21. Front. Plant Sci. 12:789065. doi: 10.3389/fpls.2021.789065
Received: 04 October 2021; Accepted: 26 November 2021;
Published: 21 January 2022.
Edited by:
Mengcen Wang, Zhejiang University, ChinaReviewed by:
Xiaoan Sun, Weifang University of Science and Technology, ChinaMubbashir Hussain, Kohat University of Science and Technology, Pakistan
Copyright © 2022 Munir, Li, He, He, He, Cui, Wu, Li, Li, Zhang, Xiong, Lu, Wang, Zong, Yang, Yang, Mu, Wen, Wang, Guo, Karunarathna and He. This is an open-access article distributed under the terms of the Creative Commons Attribution License (CC BY). The use, distribution or reproduction in other forums is permitted, provided the original author(s) and the copyright owner(s) are credited and that the original publication in this journal is cited, in accordance with accepted academic practice. No use, distribution or reproduction is permitted which does not comply with these terms.
*Correspondence: Yueqiu He, ynfh2007@163.com
†These authors have contributed equally to this work