- 1Beijing Key Laboratory of Seed Disease Testing and Control, College of Plant Protection, China Agricultural University, Beijing, China
- 2Department of Biology, Plant Conservation and Population Biology, KU Leuven, Leuven, Belgium
- 3Biology Department, National Museum of Natural Science, Taichung, Taiwan
- 4Department of Life Science, National Taiwan University, Taipei, Taiwan
Mycorrhizal associations are essential for orchid germination and seedling establishment, and thus may constrain the distribution and abundance of orchids under natural conditions. Previous studies have shown that germination and seedling establishment in several orchids often decline with increasing distance from adult plants, resulting in non-random spatial patterns of seedling establishment. In contrast, individuals of the fully mycoheterotrophic orchid Gastrodia confusoides often tend to have random aboveground spatial patterns of distribution within bamboo forests. Since G. confusoides is parasitic on litter-decaying fungi, its random spatial patterns of distribution may be due to highly scattered patterns of litter-decaying fungi within bamboo forests. To test this hypothesis, we first identified the main mycorrhizal fungi associating with developing seeds and adult plants at a bamboo forest site in Taiwan using Miseq high-throughput DNA sequencing. Next, we combined seed germination experiments with quantitative PCR (qPCR) analyses to investigate to what extent the abundance of mycorrhizal fungi affected spatial patterns of seed germination. Our results show that seed germination and subsequent growth to an adult stage in G. confusoides required a distinct switch in mycorrhizal partners, in which protocorms associated with a single Mycena OTU, while adults mainly associated with an OTU from the genus Gymnopus. A strong, positive relationship was observed between germination and Mycena abundance in the litter, but not between germination and Gymnopus abundance. Fungal abundance was not significantly related to the distance from the adult plants, and consequently germination was also not significantly related to the distance from adult plants. Our results provide the first evidence that the abundance of litter-decaying fungi varies randomly within the bamboo forest and independently from G. confusoides adults.
Introduction
Both dispersal and recruitment limitation represent major factors that affect the distribution and abundance of plant populations (Eriksson and Ehrlén, 1992; Clark et al., 2007). Dispersal of seeds into favorable microsites is a first critical step shaping recruitment and distribution patterns of plants (Condit et al., 1992; Nathan and Muller-Landau, 2000; Wang and Smith, 2002; Getzin et al., 2014). When seeds fail to reach suitable habitats, no recruitment will occur at these sites and plants are considered dispersal or seed limited (Clark et al., 2007; Poulsen et al., 2007). If, on the other hand, seeds are widely dispersed, but fail to recruit because habitats are unsuitable, plants are considered habitat- or microsite-limited (Münzbergová and Herben, 2005; Johansson and Ove Eriksson, 2013). Most seed introduction and addition experiments have shown that supplemental addition of seeds to populations almost always results in increased seedling recruitment, indicating that the majority of plant populations are to some extent seed limited (Clark et al., 2007; Poulsen et al., 2007).
For plant species that critically depend on mycorrhizal symbiosis for seed germination and seedling establishment, the availability of appropriate mycorrhizal fungi may represent an influential portion of favorable microsite, and this is particularly true for orchids (Diez, 2007; Jacquemyn et al., 2007; McCormick and Jacquemyn, 2014; Waud et al., 2016; McCormick et al., 2018). Orchids have dust-like seeds that consist of rudimentary embryos without endosperm at the time of seed dispersal (Arditti and Ghani, 2000). Under natural conditions, the germination of orchid seeds and the early development of protocorms rely on mycorrhizal associations for nutrient supplies (Smith and Read, 2008; Rasmussen and Rasmussen, 2009; Rasmussen et al., 2015). Therefore, the availability of suitable mycorrhizal fungi is considered to be a limiting factor in seedling recruitment that significantly affects the abundance and spatial distribution of orchids (Rasmussen and Whigham, 1998; McKendrick et al., 2002; McCormick and Jacquemyn, 2014; McCormick et al., 2018).
Because orchid mycorrhizal (OrM) fungi can grow well without the association with orchids, it is generally assumed that OrM fungi are distributed independently from orchids and are therefore more widespread than the distribution of orchids suggests (McCormick et al., 2018). Previous studies using seed introduction experiments have indeed shown that the presence of orchids was rarely limited by the availability of suitable OrM fungi at a landscape scale (McCormick and Jacquemyn, 2014). At the local scale, however, seed germination is often unpredictable, suggesting that OrM fungi are not regularly distributed within sites. Results from previous studies have, for example, shown that germination and seedling establishment in photosynthetic orchids often decline with increasing distance from adult orchids (Batty et al., 2001; Diez, 2007; Jacquemyn et al., 2007, 2012a,b; McCormick et al., 2012). Because most green adult orchids maintain associations with mycorrhizal fungi, this may create favorable microsites for seed germination near the rhizosphere of adult orchids (Cameron et al., 2008; Smith and Read, 2008; McCormick et al., 2016; Waud et al., 2016).
Mixed results have been obtained for fully mycoheterotrophic orchids, which are species that are non-photosynthetic throughout their entire life and solely depend on fungal carbon. In Neottia nidus-avis, for example, significantly higher seed germination and seedling establishment were observed in plots containing adult N. nidus-avis plants than in plots situated at least 5 m from the nearest adult, but there was no significant difference in seed germination between seed packets buried within 15 cm of the adults and packets buried at 15–25 cm from adult plants (McKendrick et al., 2002). In Corallorhiza trifida, on the other hand, there was no significant relationship between the extent of germination or seedling establishment and the presence of naturally distributed plants at a spatial scale of 1 m (McKendrick et al., 2000), suggesting that growth of C. trifida seedlings depends more on the ability of the fungal symbionts to transfer carbon from their ectomycorrhizal co-associates than on the distribution of conspecific adult plants.
In this study, we investigated the abundance and distribution of mycorrhizal fungi in bamboo litter and how they relate to spatial patterns of seed germination of the fully mycoheterotrophic orchid Gastrodia confusoides. A number of species of the genus Gastrodia exclusively inhabit bamboo forests and are parasitic on litter-decaying fungi to gain access to nutrients through the degradation of bamboo leaf and twig litter, while the litter-decaying fungi are most likely not dependent on this association for survival (Kinoshita et al., 2016). Given that the ground surface of bamboo forests is densely covered by bamboo leaf and twig litter, and litter-decaying fungi often show highly scattered patterns within the bamboo forest (Y-I Lee, pers. observation), we hypothesized that germination is likely more dependent on fungal abundance in the litter than on the distance to the nearest adult plant. To test this hypothesis, we used high-throughput DNA sequencing to compare the fungal communities associating with protocorms and adults to identify the mycorrhizal fungi promoting seed germination in G. confusoides. We further combined seed germination experiments with quantitative PCR (qPCR) analyses to investigate spatial variation in fungal abundance within the surrounding bamboo litter at different distances from adult plants and to assess how spatial variation in fungal abundance affected seed germination.
Materials and Methods
Study Species
The genus Gastrodia consists of about 66 species that are widely distributed across the Paleotropics, Oceania, and warm-temperate regions in Eastern Asia (Pridgeon et al., 2005; WCSP, 2014). All of them are all fully mycoheterotrophic plants that occur in moist and sheltered forests. Gastrodia species have been reported to associate with litter-decaying fungi and/or wood-decaying fungi (Kusano, 1911; Martos et al., 2009; Ogura-Tsujita et al., 2009; Dearnaley and Bougoure, 2010; Lee et al., 2015). Interestingly, several Gastrodia species, such as Gastrodia appendiculata, Gastrodia confusoides, and Gastrodia nantoensis occur predominantly in bamboo forests in Taiwan (Hsu, 2008), and the litter-decaying fungus, Mycena has been identified as their major mycorrhizal partner (Ogura-Tsujita et al., 2009; Lee et al., 2015).
Gastrodia confusoides is a fully mycoheterotrophic perennial orchid that occurs mainly in bamboo forests in central Taiwan. The species generally flowers in September, producing short flowering stalks that vary in height between 2 and 5 cm (Figure 1A). After successful pollination, the flowering stalks elongate rapidly and reach a height between 20 and 50 cm (Figure 1B). Capsules ripen quickly 1 month after pollination, followed by dehiscence and seed dispersal in October (Figure 1C). Only flowers or elongated stalks with capsules of this fully mycoheterotrophic orchid can be found above-ground from September to October. After the seeds have been dispersed, successful development of underground tubers and roots depends on fungal associations with litter-decaying fungi (Figures 1D,E).
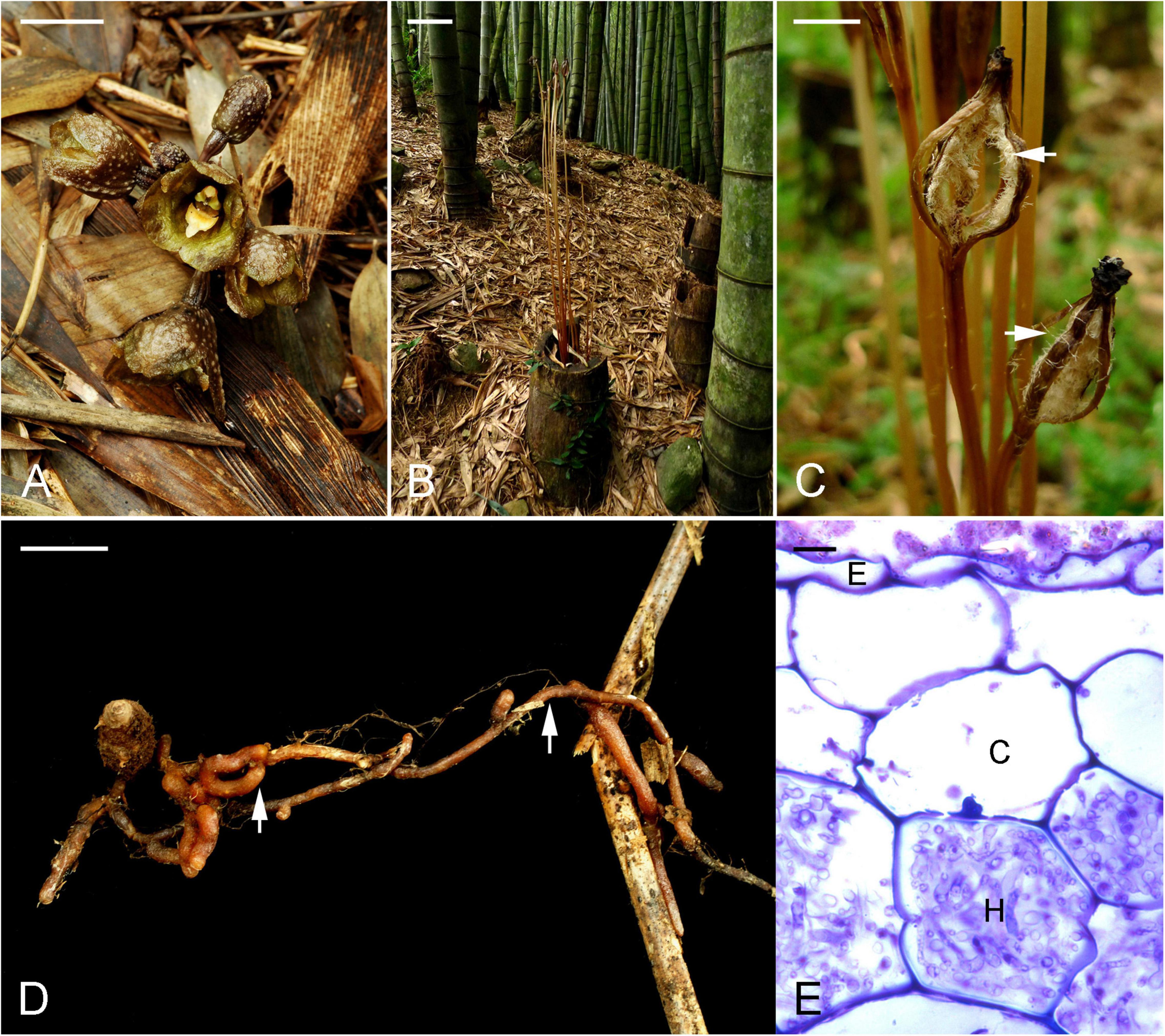
Figure 1. Gastrodia confusoides in its natural habitat. (A) Adult plants with blooming flowers growing between bamboo litter. Scale bar = 1 cm. (B) Stem elongation after flowers have been successfully pollinated. Scale bar = 10 cm. (C) After 2 weeks of pollination, mature fruits have opened, and released seeds (arrows). Scale bar = 1 cm. (D) The tube with mycorrhizal roots (arrows) in decaying bamboo litter. Scale bar = 1 cm. (E) Light microscopy section showing the presence of mycorrhizal fungi in cortical cells of roots. E, epidermal cells; C, cortical cells; H, fungal hyphae. Scale bar = 10 μm.
Seed Germination Experiment
To investigate whether seed germination varied along the distance to the adult plant, a seed germination experiment was established in four plots using the seed packet method described by Rasmussen and Whigham (1993). Experiments were conducted in a bamboo forest located in Heping District, Taichung City, Taiwan at 1,000 m above sea level (24 23′92″N, 120 90′71″E). The bamboo forest was dominated by mature mōsō bamboo (Phyllostachys edulis) with only few understory plants, including Polygonum japonicum and Deparia petersenii. The bamboo forest has been planted and tilled by local farmers for harvesting bamboo shoots for over 50 years. The soils can be classified as Inceptisols, with mostly sandy loam with sand being the dominant particle. Soil pH of the four study plots ranged from 4.0 to 4.5.
When seeds were mature at the beginning of October 2015, capsules were harvested from the studied populations. In order to exclude potential population effects that may create differences in seed quality, all seeds were pooled after harvesting and directly put into seed packets. Per seed packet, approximately 500 seeds were placed within a square of 53-μm mesh phytoplankton netting. Seed packets were buried into the litter layer at a depth of 5 cm in each cardinal direction (north, south, east, west) at five different distances (5, 50, 100, 200, 500 cm) around each sampled orchid adult (McCormick et al., 2016) in the same 10-m2 plot where roots and litter samples were taken (Supplementary Figure 1). Four adult orchids were selected without visible neighboring adult orchids within a radius of 5 m. In total, 5 (distances) × 4 (directions) × 4 (plots) = 80 seed packets were left in the ground for 1 year. In October 2016, seed packets were retrieved, gently washed and maintained moist in paper towel for 1 day until examination. In the laboratory, seed packets were gently rinsed with tap water, then opened and carefully checked under a stereo microscope to count the number of germinated seeds. As orchid seed germination stages can be variable, germination was considered to have occurred when clear signs of mycorrhiza formation were observed and rupture of testa by enlarging embryos was present (Figure 2).
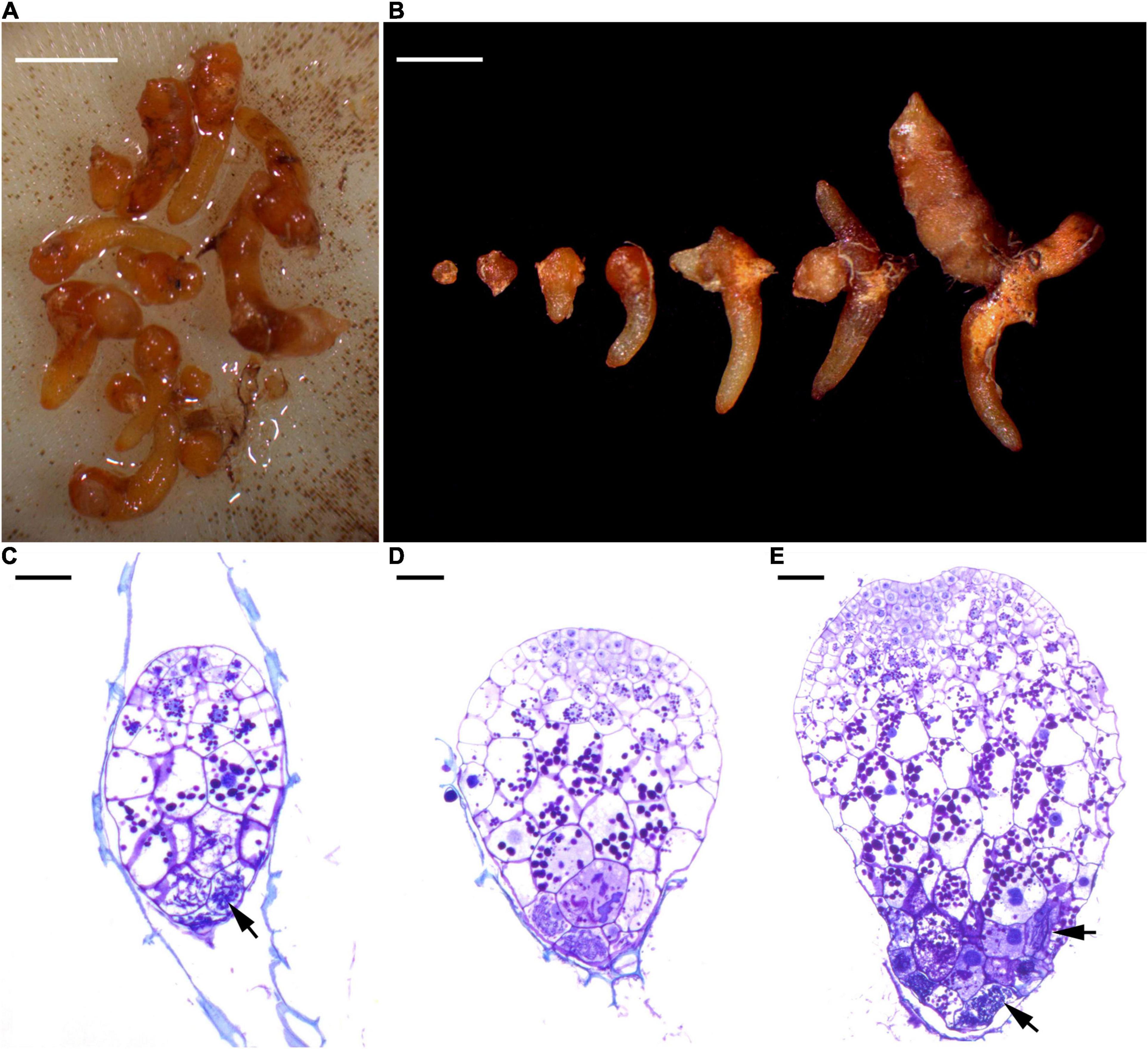
Figure 2. Different stages in the germination process of Gastrodia confusoides seeds and indications of the presence of mycorrhizal fungi. (A) Developing protocorms from a single packet at 12 months after sowing. Scale bar = 2 mm. (B) A selection of different stages of protocorms and young root tubers from the packet. Scale bar = 2 mm. (C) At the early stage of germination, fungal hyphae (arrow) have colonized the basal part of embryo. Scale bar = 50 μm (D) The enlarged embryo has ruptured the seed coat. Scale bar = 50 μm (E) The developing protocorm has elongated with the meristematic cells at the up region and the fungal colonization at the basal region. Fungal hyphae (arrows). Scale bar = 50 μm.
Sampling of Mycorrhizal Communities
Litter samples were collected in each cardinal direction (north, south, east, west) at five different distances (5, 50, 100, 200, 500 cm) around each sampled orchid adult (Supplementary Figure 1). At each sampling point, litter samples (ca. 10 g fresh weight of decaying bamboo leaves) were taken at a depth of 5 cm using a sterile sampling bag, and the litter samples were immediately stored at −80°C for subsequent analysis. Genomic DNA from litter samples was extracted using the PowerSoil® DNA Isolation Kit (MO BIO Laboratories Inc., Carlsbad, CA). Additionally, at least three roots from each sampled orchid adult plant were surface sterilized with a 1% sodium hypochlorite solution for 30 s, followed by three 30-s rinse steps in sterile distilled water and microscopically checked for mycorrhizal colonization. Subsequently, the distal 5-cm portions of colonized roots were sectioned into 3–5 mm fragments, which were then pooled to create a homogeneous mycorrhizal root sample for each individual plant. The protocorms from seed packets in each plot were pooled because of the small amount of tissue. The protocorms were rinsed with water to remove excess soil and litter fragments and were surface sterilized as root samples described above. In each protocorm sample, 20 protocorms were pooled for DNA extraction. Afterward, DNA was extracted from 0.1 g mycorrhizal root fragments or protocorms using the Plant Genomic DNA Purification Kit as described by the manufacturer (GMbiolab Co., Ltd., Taichung, Taiwan). Altogether, there were four plots containing 88 unique samples (4 adult root samples, 4 protocorm samples, 80 litter samples) for DNA extraction and subsequent molecular analysis.
Molecular Methods
The ITS1 region of the nuclear ribosomal RNA genes was amplified using the primer pair ITS1F and ITS2R (Supplementary Table 1; Adams et al., 2013). ITS1F/ITS2R has high amplification efficiency of a broad range of basidiomycetes and ascomycetes, and it produces a wide variety of OTUs corresponding to different orchid-associating mycorrhizal families (Waud et al., 2014). PCR was carried out in 20 μL reactions containing 10 ng of genomic DNA, 0.8 μL of each primer (5 μM), 2 μL of 2.5 mM dNTPs, 4 μL of 5x TransStart® FastPfuBuffer (TransGen Biotech Co., Ltd., Beijing, China), and 0.4 μL of TransStart® FastPfu Polymerase. The parameters of reactions consisted of an initial denaturation at 95°C for 3 min, then 35 cycles of 95°C for 30 s, and 55°C for 30 s and a final extension of 72°C for 45 s. The purified PCR products of the fungal internal transcribed spacer (ITS) plus the 5′ part of the 28S rDNA of Mycena or Gymnopus from our previous study on Gastrodia species (Lee et al., 2015) were included as positive control reactions, while negative control reactions (no template DNA) were included to verify target-specific amplification and the absence of contaminants. PCR products were separated by gel electrophoresis and amplicons within the appropriate size range were cut and purified using the AxyPrep DNA Gel Extraction Kit (Axygen Biosciences, Union City, CA) and quantified using QuantiFluor™ Fluorometer (Promega Corporation, Madison, WI). Samples were then pooled in equimolar concentrations and paired-end sequenced (2 × 250 bp) on an Illumina Miseq.
Bioinformatics
Raw fastq files were demultiplexed and quality-filtered using QIIME (version 1.17) with the following criteria. First, 300 bp reads were truncated at any site receiving an average quality score < 20 over a 50 bp sliding window, discarding the truncated reads that were shorter than 50 bp. Second, reads with one or two nucleotide mismatches in primer matching measurements and reads containing ambiguous characters were removed. Third, only sequences that had an overlap longer than 10 bp were assembled according to their overlap sequence. Reads that could not be assembled were discarded.
Sequences obtained from the Illumina Miseq run were clustered into operational taxonomic units (OTUs) using the UPARSE algorithm implemented in USEARCH version 7 (Edgar, 2013). OTUs were clustered with 97% similarity cutoff using UPARSE (version 7.1)1 and chimeras were identified and removed using the UNITE UCHIME reference data set. The taxonomy of each ITS1 region was analyzed by RDP Classifier2 against the UNITE fungal ITS database using confidence threshold of 70%. Remaining OTUs were assigned taxonomic identities based on the top 10 BLAST (megablast algorithm) (Altschul et al., 1990) results of the OTU representative sequences (selected by UPARSE) using the GenBank nucleotide (nt) database (Benson et al., 2009), including uncultured/environmental entries. OTUs identified by BLAST were then manually screened for potential orchid-associating mycorrhizal families following a step-wise process. OTUs represented by short sequences (<150 bp) or having a low sequence similarity (<90%) with fungal species across their sequence length were removed. The results were put in an OTU table (sample × OTU table with each cell containing read numbers), which was used in the further analyses.
Assessment of Fungal Abundance
Illumina Miseq sequencing revealed that the most abundant (in terms of read counts) OrM fungi associating with Gastrodia confusoides corresponded to two distinct saprotrophic non-rhizoctonia fungal taxa, i.e., Mycena (OTU3) and Gymnopus (OTU5). For both OTUs, a real-time quantitative PCR (qPCR) assay was developed and validated based on the internal transcribed spacer (ITS) 2 region (Supplementary Table 1). The qPCR assays were utilized to quantify Mycena (OTU3) and Gymnopus (OTU5) DNA from each protocorm, root or litter DNA sample on an ABI QuantStudio 6 Flex real-time PCR instrument (Life Technologies, Carlsbad, CA, United States). Reactions were performed in a total volume of 25 μL consisting of 12.5 μL of iQ SYBR Green PCR Super Mix (Bio-Rad Laboratories, Hercules, CA, United States), 1.0 μL DNA template, 9.0 μL H2O and 1.25 μL (10 mM) of each forward and reverse primer, depending on the target OTU (Supplementary Table 1). Amplifications were run as follows: initial denaturation for 5 min at 95°C, followed by 40 cycles of 15 s of denaturation at 94°C, 30 s of annealing at 59°C (OTU3 and OTU5), and 30 s of elongation at 72°C. Each sample extract was amplified in duplicate and quantified using a standard curve amplified in triplicate. Standard curves (range, 1.0E + 02 to 1.0E + 06 molecules μ1–1) were generated using five 10-fold dilutions of target DNA amplified from protocorms (OTU3) or roots (OTU5). A melting curve analysis was performed after each analysis to confirm product specificity (Supplementary Figures 2A,C). In addition, amplification accuracy was verified by Sanger sequencing of a number of generated amplicons. In this way, product identity was confirmed for all samples considered to be positive using the qPCR assays (Ct value < 29 and a single melting peak at the expected melting temperature; Supplementary Figure 2). Additional positive (DNA extract from protocorms colonized with OTU3 or roots colonized with OTU5) and negative control reactions (no template DNA) were included in triplicate with all analyses to verify target-specific amplification and the absence of contaminants, respectively. The positive control showed that the amplification of target DNA was accurate and sensitive, while the negative control showed that no contaminants were present.
Data Analysis
To investigate whether fungal richness (the number of OTUs) differed between protocorms, adults, and soil samples collected at different distances from adult plants, we used a Linear Mixed Model, with the number of OTUs as dependent variable and sample type as fixed factor. Plot was included as a random factor in the analyses. To see how fungal communities differed between protocorms, adult plants, and soil samples, non-metric multidimensional scaling (NMDS) was performed using read abundance data as explanatory variables and the Bray–Curtis coefficient as distance measure. Additionally, permutational multivariate analysis of variance (PERMANOVA; Anderson, 2001) was performed to test whether the fungal communities differed significantly between protocorm, adult, and soil samples. All analyses were performed in the vegan package (Oksanen et al., 2013) in R (R Core Team, 2020). The same analyses were used to test whether fungal communities found in the soil differed between the four sampling plots and between cardinal directions and distances within plots.
To investigate spatial variation in mycorrhizal abundance and seed germination, we used Generalized Linear Mixed Models and Linear Mixed Models. First, a Linear Mixed Model was used to test the hypothesis that fungal abundance was related to the distance of the nearest adult plant. Fungal concentration (expressed in terms of molecules per μL) was used as dependent variable, while distance to adult plants was used a fixed factor. Plot was included in the model as a random factor. Analyses were performed separately for the main fungus associating with protocorms and the fungus associating with adult plants. A Generalized Linear Mixed Model was used to see whether seed germination (at least one seed developing into protocorm) was related to the distance of the nearest adult plant or to the abundance of key fungi (Mycena and Gymnopus) in the litter. Presence/absence of at least one protocorm in seed packets was used as dependent variable, while plot was included as a random effect in the model. In a second analysis, we related germination success (i.e., the percentage of seeds developing into a protocorm) to the distance of the nearest adult plant and the abundance of fungi in the soil.
Results
Mycorrhizal Associations
The quality-filtered Miseq data set contained samples from protocorms, adult roots, and surrounding bamboo litter. The numbers of OTUs were close to saturation after 15,000 sequence counts (Supplementary Figure 3). The four composite protocorm samples comprised a total of 129 OTUs (172,552 sequences), while the 4 adult root samples comprised 427 OTUs (168,843 sequences) (Supplementary Table 2). The surrounding 80 bamboo litter samples comprised 3,925,341 sequences with a total of 1,640 OTUs (Supplementary Table 4, excluding OTUs with < 1,000 total sequences). The number of OTUs per sample differed significantly (χ2 = 100.9, p < 0.0001) between protocorms, adults, and soil samples. While soil samples contained on average > 210 OTUs, the number of OTUs was much lower in protocorms (20.8 ± 4.5) and adult plants (47.0 ± 24.01). Soil samples taken at a distance of 200 and 500 cm contained significantly (p < 0.01) more OTUs than samples taken at 5 and 100 cm (Supplementary Figure 4).
At the class level, the predominant fungi belonged to Agaricomycetes, Sordariomycetes, Leotiomycetes, Eurotiomycetes, Archaeorhizomycetes, accounting for 31.7, 25.9, 11.97, 8.34, and 8.22%, respectively (Supplementary Figure 5A). At the order level, the five most abundant orders were Agaricales, Trechisporales, Helotiales, Hypocreales, and Chaetothyriales, accounting for 15.93, 15.8, 15.04, 11.02, and 10.6%, respectively (Supplementary Figure 5B). At the family level, the predominant fungi belonged to Hydnodontaceae, Mortierellaceae, Myxotrichaceae, Herpotrichiellaceae, and Archaeorhizomycetaceae, accounting for 18.94, 12.38, 12, 11.86, and 4.72%, respectively (Supplementary Figure 5C). Fungal communities differed significantly [F(2, 85) = 7.30, p < 0.001] between protocorms, adult plants, and soil samples (Figure 3). Besides, fungal communities in the soil also differed significantly [F(3, 76) = 6.95, p < 0.0001] between the four plots that were sampled (Supplementary Figure 6), but no spatial differences in fungal communities were found within plots.
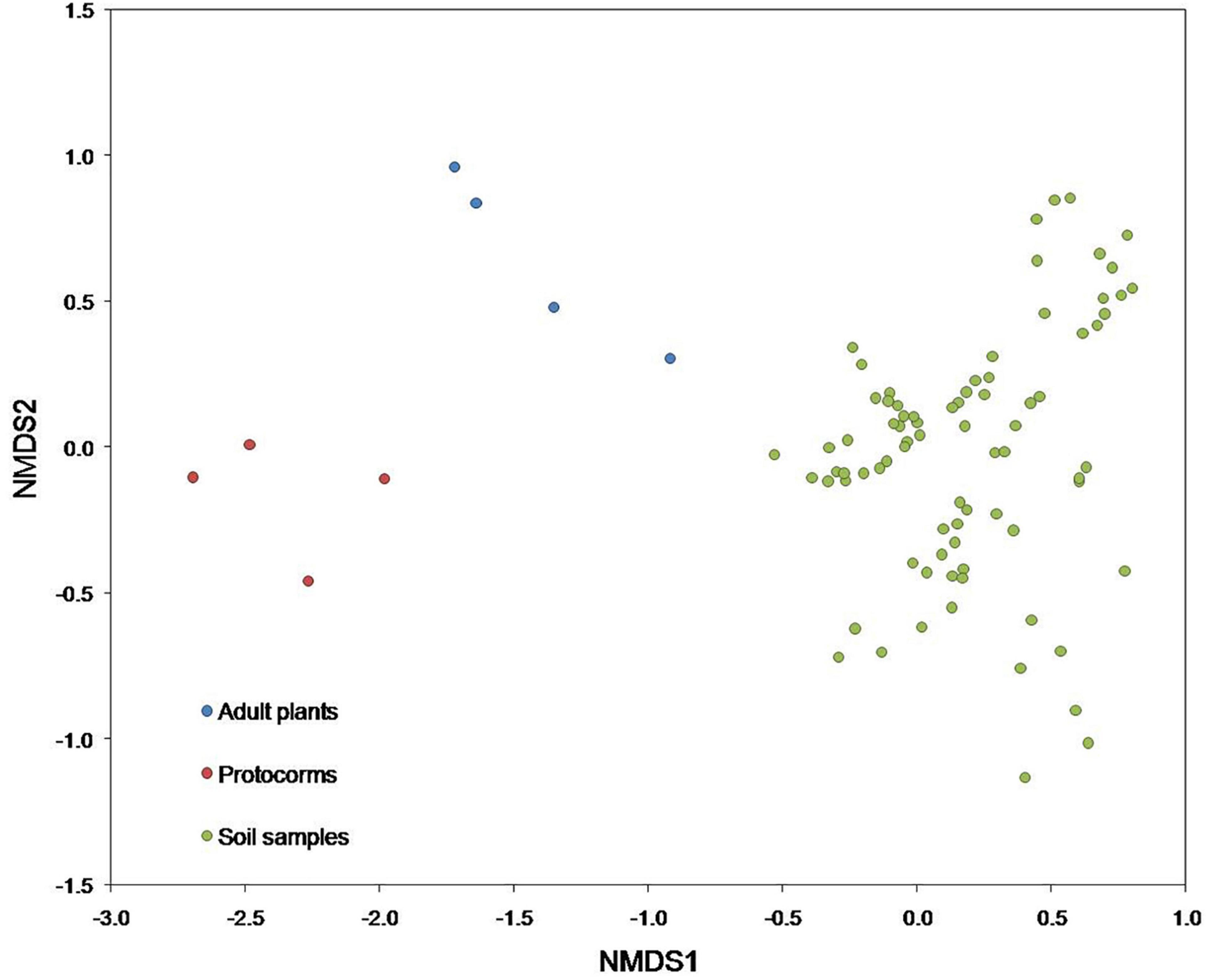
Figure 3. Non-metric multidimensional scaling (NMDS) plot illustrating differences in fungal communities detected in protocorms, adult plants and soil samples. Each data point represents an individual protocorm (red), adult (blue), or soil (green) sample.
Most orchid mycorrhizal sequences were related to members of Mycena (one OTU—164,772 sequences—95.5%) in the protocorm samples, and Gymnopus (one OTU -90,028 sequences—53.3%) in the adult root samples. It is noteworthy that fungal partners differed between protocorm and adult stages in G. confusoides, in which a single Mycena (OTU3) was dominant and occurred exclusively in protocorms, and one prevailing Gymnopus (OTU5) that occurred solely in roots (Supplementary Table 2 and Supplementary Figure 7). Raw sequences reads were deposited in NCBI Sequence Read Archive database (accession numbers PRJNA753675 and PRJNA778455).
qPCR standard curves based on known concentrations of target DNA showed a linear correlation (OTU3, r2 = 0.998; OTU5, r2 = 0.997) between log values of input DNA and qPCR threshold cycles over at least five orders of magnitude (Supplementary Figures 2B,D), enabling accurate quantification of our target fungi in terms of target DNA molecules (mol) per μL DNA extract (Supplementary Table 3). The highest concentrations of target OrM DNA were obtained from G. confusoides protocorm and root samples, with the average OTU3 concentration being 640,129.5 mol μ1–1 DNA extract (SE = 43787.6 mol μ1–1) and the average OTU5 concentration being 484626.5 mol μ1–1 DNA extract (SE = 62,923 mol μ1–1), respectively. For these two target OTUs, no significant relationship between the distance from the orchid plant and fungal concentration was observed in bamboo litter surrounding the orchid plant (χ2 = 4.40 and 6.39, p > 0.05 for OTU3 and OTU5, respectively) (Supplementary Figure 8). In both cases, plot explained a minor part (<10%) of the total variation, indicating that the results were consistent among plots.
Germination
Seed germination was regularly encountered at the study site and distinct stages of seedling development (protocorms and young root tubers) were observed (Figures 2A,B). The percentage of packets containing germinating seeds varied between 70% in plot 1, 90% in plot 2, 85% in plot 3, and 65% in plot 4. On average, 3.2 protocorms (0.64% seed germination) were found within individual seed packets. Analysis of data for germination of seed packets showed no significant effect (χ2 = 4.58; p = 0.33) of distance to adult plants within the 10-m2 plots (Figure 4), nor was there any relationship between the four directions in which seed packets were buried in the litter (data not shown). A strong, significant relationship (χ2 = 51.91; p < 0.001) was observed between seed germination and abundance of Mycena in the litter, but not between seed germination and abundance of Gymnopus (χ2 = 0.04, p = 0.84) (Figure 5).
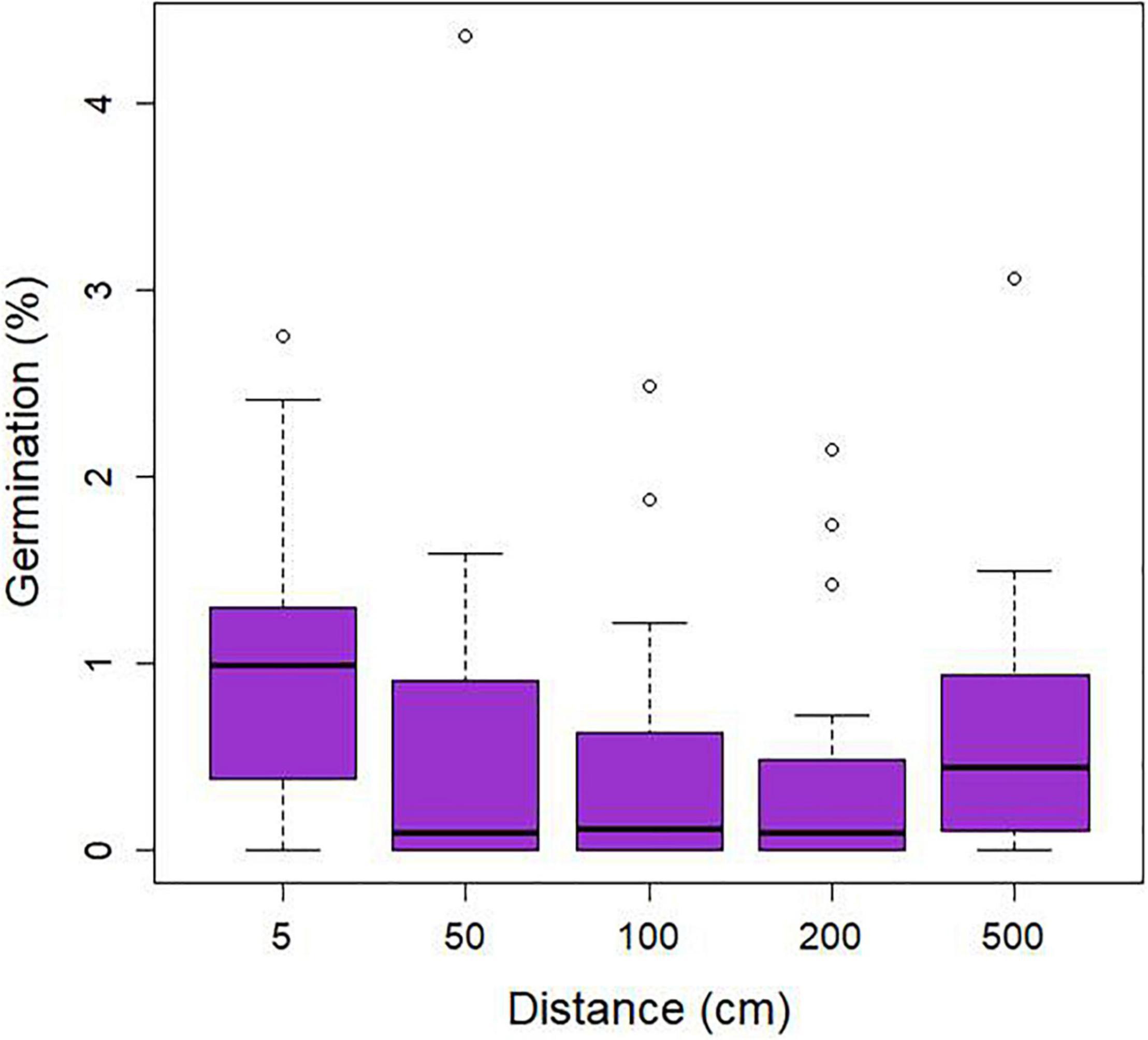
Figure 4. Relationship between the percentage seed germination of Gastrodia confusoides and distance from adult plants (cm) measured in four plots in bamboo forest in Taiwan.
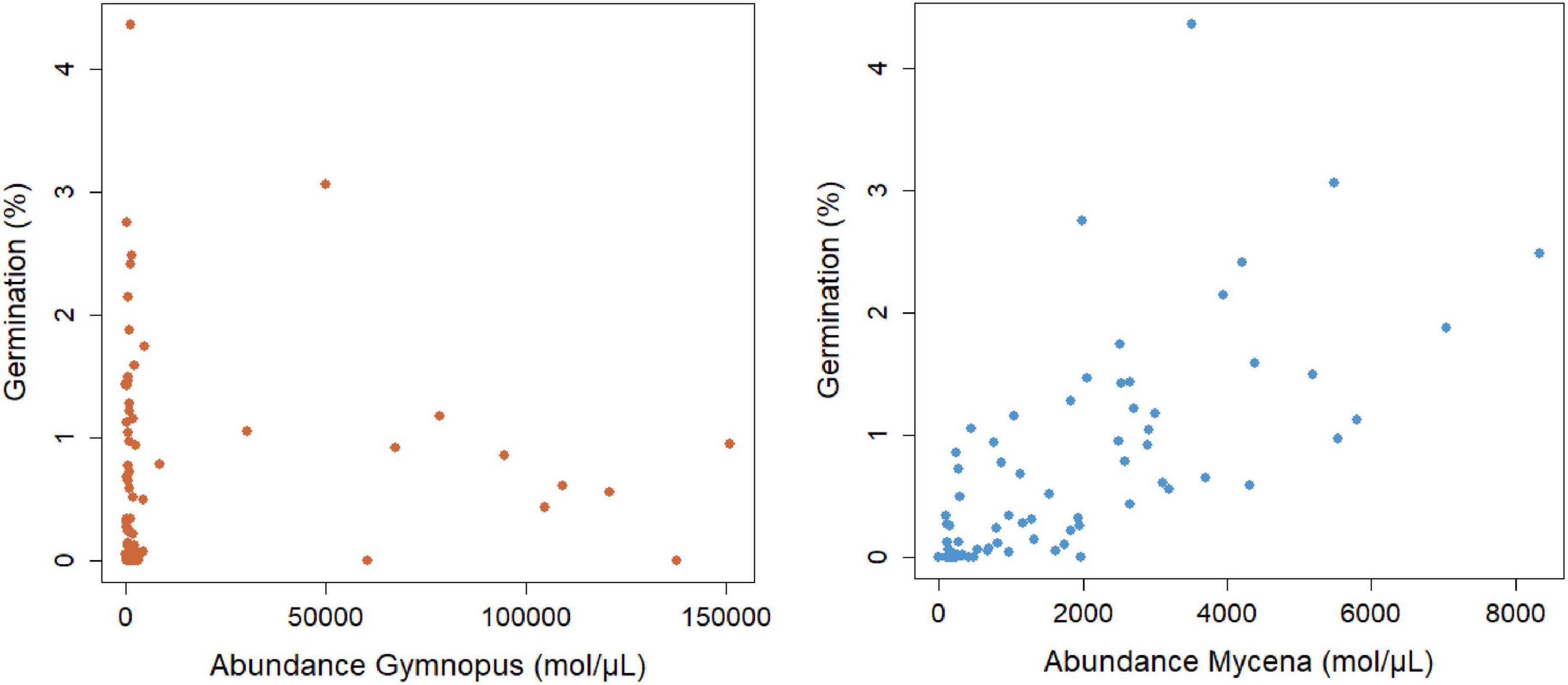
Figure 5. Relationship between the abundance of Mycena (OTU3) and Gymnopus (OTU5) DNA molecules μL–1 DNA extract and percentage seed germination of Gastrodia confusoides. Seed germination was expressed as the percentage of seeds developing into a protocorm.
Discussion
Mycorrhizal Communities Associating With Gastrodia confusoides
Autotrophic and mycoheterotrophic orchids tend to associate with different sets of mycorrhizal fungi (Jacquemyn and Merckx, 2019; Wang et al., 2021). Whereas most autotrophic orchids typically associate with a wide variety of fungi including so-called rhizoctonia fungi (i.e., members of Tulasnellaceae, Ceratobasidiaceae, and Serendipitaceae), partially and fully mycoheterotrophic orchids associate predominantly with ectomycorrhizal fungi (Tesitelova et al., 2015; Yagame et al., 2016; Ogura-Tsujita et al., 2021) and saprotrophic fungi (Lee et al., 2015; Ogura-Tsujita et al., 2018). Our results are in line with these observations and showed that the dominant fungi associating with G. confusoides were saprotrophic fungi of the Mycenaceae and Marasmiaceae (Supplementary Table 2).
These results further confirm previous reports that have shown that fungi from Mycenaceae and Marasmiaceae are the two major fungal families associating with Gastrodia species (Ogura-Tsujita et al., 2009; Dearnaley and Bougoure, 2010; Lee et al., 2015; Kinoshita et al., 2016). Individuals of Gastrodia nipponica inhabiting broad leaved forests were shown to simultaneously associate with ectomycorrhizal fungi from Russulaceae and Sebacinaceae, suggesting that orchid mycorrhizal communities in Gastrodia species can vary depending on the vegetation type where the species grow. A similar result was found in Gastrodia elata, which is mainly found in broadleaved forests. Chen et al. (2019) showed that besides Mycenaceae, a broader spectrum of fungal associations, such as Resinicium, Hyphodontia, Sistotrema, Tricholoma, and Russula was detected in the juvenile stage of G.elata. A higher fungal diversity in the juvenile stage may enable G. elata to access a range of carbon pools for germination and/or protocorm development from various mycobiont communities in different vegetation types.
The Mycena (OTU3) sequences isolated from G. confusoides protocorms shared high sequence similarity with sequences isolated from G. nantoensis and G. appendiculata growing in other bamboo forests (Lee et al., 2015). These results suggest that this Mycena strain is particularly adapted to grow in bamboo forest, where it decomposes bamboo litter, and therefore could be shared among Gastrodia species. It has been reported that only a limited group of saprotrophic fungi can occur in bamboo forests in Japan, and the fungal community in bamboo forests is different from that in other forest types (Shidei, 1974; Yashima and Nose, 2010). These results suggest the adaptation of “the bamboo forest Gastrodia species” to particular fungal groups specific to bamboo thickets.
As compared to the protocorm (OTU3, 95.5%), the adult root of G. confusoides possessed a higher diversity of fungi (OTU5, 53.3% of all sequences with the remaining 46.7% of various fungal communities) (Supplementary Table 2). Most of the remaining fungal OTUs belonged to Ascomycota that were not typical OrM fungi, except for Fusarium oxysporum. Although a F. oxysporum strain has been confirmed to serve as an OrM fungus in a terrestrial orchid, Bletilla striata (Jiang et al., 2019), the role of F. oxysporum in Gastrodia mycorrhizal symbiosis is not clear.
Shift in Fungal Partners Between Different Developmental Stages
Although it is clear that mycorrhizal interactions play a crucial role in the life cycle of orchids (Rasmussen, 1995), there is remarkable variation in the number of fungi orchids associate with, ranging from highly specific interactions with one or a few OrM fungi (McCormick et al., 2009) to very broad interactions with orchids associating with multiple fungi simultaneously (e.g., Jacquemyn et al., 2012c; De Long et al., 2013; Waud et al., 2017; Shefferson et al., 2019). Furthermore, there is growing evidence that orchid mycorrhizal interactions are not stable, but can vary substantially both in space (Lin et al., 2020; Xing et al., 2020) and time (Bidartondo and Read, 2008; Ventre-Lespiaucq et al., 2021), with some species continuously associating with the same partner while others show almost complete switching of partners through space or time (Ventre-Lespiaucq et al., 2021). Spatial and temporal turnover may reflect variation in ecological conditions or in fungal availability. Temporal turnover can occur throughout the growing season, between subsequent years (e.g., McCormick et al., 2006; Ercole et al., 2015; Ojá et al., 2015), or between ontogenetic stages (e.g., McCormick et al., 2004; Bidartondo and Read, 2008; Jacquemyn et al., 2011). For example, Tipularia discolor adults associate with a variety of fungi, while fungal diversity detected in protocorms is much lower (McCormick et al., 2004). A recent meta-analysis has shown that temporal turnover of mycorrhizal partners occurs frequently in orchids and that in most cases partial replacement, whereby an individual retains a subset of its fungal partners and replaces others, was the most frequent scenario (Ventre-Lespiaucq et al., 2021).
Our high-throughput sequencing data demonstrated that G. confusoides switched fungal partners from the protocorm stage (Mycenaceae) to the adult stage (Marasmiaceae). A similar shift in fungal partner across ontogenetic stages has been observed in G. elata using an in vitro culture system, in which Mycena promoted seed germination and the further development of the tuber required the association with Armillaria (Xu and Guo, 2000). However, high-throughput sequencing of both juvenile and adult stages of G. elata showed that the juvenile stage associated with more diverse fungal groups than Mycena alone (Chen et al., 2019). A similar pattern of total replacement has been found in Cyrtosia septentrionalis, another mycoheterotrophic orchid associated with wood-decaying fungi. In this case, Physisporinus induces seed germination, while the adult stage associates with Armillaria (Umata et al., 2013).
Complete partner turnover seems to occur primarily in subtropical Asian mycoheterotrophic orchids associated with litter- or wood-decaying fungi, but not in temperate mycoheterotrophic orchids. In temperate forests, mycoheterotrophic orchids, such as Cephalanthera austiniae, Corallorhiza species, and Neottia nidus-avis usually associate with narrow clades of ectomycorrhizal fungi during their entire life cycle (Taylor and Bruns, 1997; McKendrick et al., 2000, 2002). Although the precise reasons for mycorrhizal switching are not well understood, previous research has shown that shifts in fungal partners are risky and may come with a cost, constraining the population dynamics of orchids (McCormick et al., 2006; Ogura-Tsujita et al., 2021; Ventre-Lespiaucq et al., 2021). In the case of mycoheterotrophic orchids, switching partners may occur when it provides benefits in terms of resource acquisition (Ogura-Tsujita et al., 2021). For example, in the fully mycoheterotrophic G. elata, changing fungal partners from Mycena to Armillaria may enable the utilization of a larger carbon pool, i.e., the wood in the forest (Ogura-Tsujita et al., 2021). Similar differences in resource acquisition abilities may explain the observed switch in mycorrhizal partners in protocorms and adult plants of G. confusoides.
Abundance and Spatial Distribution of Mycorrhizal Fungi and Seed Germination
The distribution and abundance of suitable OrM fungi in the soil are critical factors determining seed germination and hence the spatial distribution of orchids within sites (McCormick and Jacquemyn, 2014). Whereas a number of studies have shown strong relationships between the presence/absence of adult plants and seed germination (McKendrick et al., 2000, 2002; Batty et al., 2001; Diez, 2007; Jacquemyn et al., 2007, 2012a,b), we found no significant relationship between the distance from the mother plant and germination (Figure 4), indicating that locations closer to adult plants are not necessarily locations supporting higher abundances of mycorrhizal fungi required for seed germination. This can be explained by the fact that adults plants almost exclusively associate with Gymnopus, which was completely absent in germinating seeds and protocorms. We also found no correlation between seed germination and the abundance of Gymnopus (OTU5) (Figure 5), suggesting that this fungus is not involved in seed germination, but only starts to play a role in the subsequent growth of seedlings into adult plants. In contrast, protocorms associated mainly with Mycena (OTU3) and in this case there was a highly significant, positive relationship between seed germination and the abundance of Mycena (Figure 5), indicating that the local distribution of this fungus is the determining factor driving spatial patterns of seed germination.
These differences in seed germination patterns between autotrophic and mycoheterotrophic orchids may be explained by the nutritional needs of different groups of OrM fungi they associate with. In orchids associating with rhizoctonia fungi, the accumulation of fungi around adults plants and the associated strong relationship between germination and the location of adult plants (Waud et al., 2016) may be explained by the fact that the orchids contribute carbon to and hence to some extent support the fungi, although clear evidence showing that orchids contribute carbon to the fungi is still very limited (Cameron et al., 2008; Liebel et al., 2015). In orchids associating with ectomycorrhizal fungi, the fungi are supported by trees, and hence the distribution and abundance of the orchids is for a large part related to the distribution and abundance of the fungi and their ectomycorrhizal trees (McCormick et al., 2009). In bamboo forests, the ground is usually covered with a thick layer of bamboo litter. Since Mycena and Gymnopus are efficient decomposers of bamboo leaves, sticks and trunks, they are expected to be widely distributed within the bamboo forest.
Conclusion
Seed germination and subsequent growth to an adult plant in the mycoheterotrophic orchid G. confusoides represents a two-stage process that is accompanied by a switch in mycorrhizal partners. Our results further show that the main mycorrhizal fungi associating with protocorms and adult plants are randomly distributed within the bamboo forest and independently from G. confusoides adults. Future research should investigate in more detail the functional and physiological basis of the interaction with both fungi and assess the cost of switching. In particular, more details about resource transport patterns between symbionts are needed to understand why adult plants switch to a different partner.
Data Availability Statement
The datasets presented in this study can be found in online repositories. The names of the repository/repositories and accession number(s) can be found below: SRA, PRJNA753675, PRJNA778455.
Author Contributions
Y-IL designed the research. Y-YL and Y-HC performed the molecular analyses. Y-IL, Y-YL, and MB performed bioinformatic analyses. HJ conducted statistical analyses. Y-IL and HJ wrote the manuscript. All authors contributed to the article and approved the submitted version.
Conflict of Interest
The authors declare that the research was conducted in the absence of any commercial or financial relationships that could be construed as a potential conflict of interest.
Publisher’s Note
All claims expressed in this article are solely those of the authors and do not necessarily represent those of their affiliated organizations, or those of the publisher, the editors and the reviewers. Any product that may be evaluated in this article, or claim that may be made by its manufacturer, is not guaranteed or endorsed by the publisher.
Acknowledgments
We thank C. K. Yang for providing the photograph of flowering Gastrodia confusoides plants. Two reviewers provided useful comments that significantly improved the quality of the manuscript.
Supplementary Material
The Supplementary Material for this article can be found online at: https://www.frontiersin.org/articles/10.3389/fpls.2021.775290/full#supplementary-material
Footnotes
References
Adams, R. I., Miletto, M., Taylor, J. W., and Bruns, T. D (2013). Dispersal in microbes: fungi in indoor air are dominated by outdoor air and show dispersal limitation at short distances. ISME J. 7, 1262–1273. doi: 10.1038/ismej.2013.28
Anderson, M. J. (2001). A new method for non-parametric multivariate analysis of variance. Austral. Ecol. 26, 32–46.
Altschul, S. F., Gish, W., Miller, W., Myers, E. W., and Lipman, D. J. (1990). Basic local alignment search tool. J. Mol. Biol. 215, 403–410.
Arditti, J., and Ghani, A. K. A. (2000). Tansley Review No. 110. Numerical and physical properties of orchid seeds and their biological implications. New Phytol. 145, 367–421. doi: 10.1046/j.1469-8137.2000.00587.x
Batty, A. L., Dixon, K. W., Brundrett, M., and Sivasithamparam, K. (2001). Constraints to symbiotic germination of terrestrial orchid seed in a Mediterranean bush land. New Phytol. 152, 511–520. doi: 10.1046/j.0028-646X.2001.00277.x
Benson, D. A., Karsch-Mizrachi, I., Lipman, D. J., Ostell, J., and Sayers, E. W. (2009). GenBank. Nucleic Acids Res. 37, D26–D31.
Bidartondo, M. I., and Read, D. J. (2008). Fungal specificity bottlenecks during orchid germination and development. Mol. Ecol. 17, 3707–3716. doi: 10.1111/j.1365-294X.2008.03848.x
Cameron, D. D., Johnson, I., Read, D. J., and Leake, J. R. (2008). Giving and receiving: measuring the carbon cost of mycorrhizas in the green orchid, Goodyera repens. New Phytol. 180, 176–184. doi: 10.1111/j.1469-8137.2008.02533.x
Chen, L., Wang, Y. C., Qin, L. Y., He, H. Y., Yu, X. L., Yang, M. Z., et al. (2019). Dynamics of fungal communities during Gastrodia elata growth. BMC Microbiol. 19:158. doi: 10.1186/s12866-019-1501-z
Clark, C. J., Poulsen, J. R., Levey, D. J., and Osenberg, C. W. (2007). Are plant populations seed limited? A critique and meta-analysis of seed addition experiments. Am. Nat. 170, 128–142. doi: 10.1086/518565
Condit, R., Hubbell, S. P., and Foster, R. B. (1992). Recruitment near conspecific adults and the maintenance of tree and shrub diversity in a neotropical forest. Am. Nat. 140, 261–286. doi: 10.1086/285412
Dearnaley, J. D. W., and Bougoure, J. J. (2010). Isotopic and molecular evidence for saprotrophic Marasmiaceae mycobionts in rhizomes of Gastrodia sesamoides. Fungal Ecol. 3, 288–294. doi: 10.1016/j.funeco.2009.11.003
De Long, J. R., Swarts, N. D., Dixon, K. W., and Egerton-Warburton, L. M. (2013). Mycorrhizal preference promotes habitat invasion by a native Australian orchid: Microtis media. Ann. Bot. 111, 409–418. doi: 10.1093/aob/mcs294
Diez, J. M. (2007). Hierarchical patterns of symbiotic orchid germination linked to adult proximity and environmental gradients. J. Ecol. 95, 159–170.
Edgar, R. C. (2013). UPARSE: highly accurate OTU sequences from microbial amplicon reads. Nat. Methods 10:996. doi: 10.1038/nmeth.2604
Ercole, E., Adamo, M., Rodda, M., Gebauer, G., Girlanda, M., and Perotto, S. (2015). Temporal variation in mycorrhizal diversity and carbon and nitrogen stable isotope abundance in the wintergreen meadow orchid Anacamptis morio. New Phytol. 205, 1308–1319. doi: 10.1111/nph.13109
Eriksson, O., and Ehrlén, J. (1992). Seed and microsite limitation of recruitment in plant populations. Oecologia 91, 360–364. doi: 10.1007/BF00317624
Getzin, S., Wiegand, T., and Hubbell, S. P. (2014). Stochastically driven adult–recruit associations of tree species on Barro Colorado Island. Proc. R. Soc. B 281:20140922. doi: 10.1098/rspb.2014.0922
Hsu, T. C. (2008). Taxonomy of Gastrodia (Orchidaceae) in Taiwan. Ph. D. Thesis. Taipei: National Taiwan University.
Jacquemyn, H., Brys, R., Vandepitte, K., Honnay, O., Roldan-Ruiz, I., and Wiegand, T. (2007). A spatially explicit analysis of seedling recruitment in the terrestrial orchid Orchis purpurea. New Phytol. 176, 448–459. doi: 10.1111/j.1469-8137.2007.02179.x
Jacquemyn, H., Merckx, V., Brys, R., Tyteca, D., Cammue, B. P. A., Honnay, O., et al. (2011). Analysis of network architecture reveals phylogenetic constraints on mycorrhizal specificity in the genus Orchis (Orchidaceae). New Phytol. 192, 518–528. doi: 10.1111/j.1469-8137.2011.03796.x
Jacquemyn, H., Brys, R., Honnay, O., Roldan-Ruiz, I., Lievens, B., and Wiegand, T. (2012a). Non-random spatial structuring of orchids in a hybrid zone of three Orchis species. New Phytol. 193, 454–464. doi: 10.1111/j.1469-8137.2011.03913.x
Jacquemyn, H., Brys, R., Lievens, B., and Wiegand, T. (2012b). Spatial variation in belowground seed germination and divergent mycorrhizal associations correlate with spatial segregation of three co-occurring orchid species. J. Ecol. 100, 1328–1337.
Jacquemyn, H., Deja, A., De hert, K., Cachapa, B. B., and Lievens, B. (2012c). Variation in mycorrhizal associations with Tulasnelloid fungi among populations of five Dactylorhiza species. PLoS One 7:e42212. doi: 10.1371/journal.pone.0042212
Jacquemyn, H., and Merckx, V. S. F. T. (2019). Mycorrhizal symbioses and the evolution of trophic modes in plants. J. Ecol. 107, 1567–1581.
Jiang, J., Zhang, K., Cheng, S., Nie, Q., Zhou, S., Chen, Q, et al. (2019). Fusarium oxysporum KB-3 from Bletilla striata: an orchid mycorrhizal fungus. Mycorrhiza 29, 531–540. doi: 10.1007/s00572-019-00904-3
Johansson, V. A., and Ove Eriksson, O. (2013). Recruitment limitation, germination of dust seeds, and early development of underground seedlings in six Pyroleae species. Botany 91, 17–24. doi: 10.1139/cjb-2012-0153
Kinoshita, A., Ogura-Tsujita, Y., Umata, H., Sato, H., Hashimoto, T., and Yukawa, T. (2016). How do fungal partners affect the evolution and habitat preferences of mycoheterotrophic plants? A case study in Gastrodia. Am. J. Bot. 103, 207–220. doi: 10.3732/ajb.1500082
Kusano, S. (1911). Gastrodia elata and its symbiotic association with Armilllaria mellea. J. Col. Agr. Imp. Univ. Tokyo 4, 1–66.
Lee, Y. I., Yang, C. K., and Gebauer, G. (2015). The importance of associations with saprotrophic non-Rhizoctonia fungi among fully mycoheterotrophic orchids is currently under-estimated: novel evidence from sub-tropical Asia. Ann. Bot. 116, 423–435. doi: 10.1093/aob/mcv085
Liebel, H. T., Bidartondo, M. I., and Gebauer, G. (2015). Are carbon and nitrogen exchange between fungi and the orchid Goodyera repens affected by irradiance? Ann. Bot. 115, 251–261. doi: 10.1093/aob/mcu240
Lin, M., Xiong, H., Xiang, X., Zhou, Z., Liang, L., and Mei, Z. (2020). The effect of plant geographical location and developmental stage on root-associated microbiomes of Gymnadenia conopsea. Front. Microbiol. 11:1257. doi: 10.3389/fmicb.2020.01257
Martos, F., Dulormne, M., Pailler, T., Bonfante, P., Faccio, A., Fournel, J., et al. (2009). Independent recruitment of saprotrophic fungi as mycorrhizal partners by tropical achlorophyllous orchids. New Phytol. 184, 668–681. doi: 10.1111/j.1469-8137.2009.02987.x
McCormick, M. K., Whigham, D. F., and O’Neill, J. P. (2004). Mycorrhizal diversity in photosynthetic terrestrial orchids. New Phytol. 163, 425–438. doi: 10.1111/j.1469-8137.2004.01114.x
McCormick, M. K., Whigham, D. F., Sloan, D., O’Malley, K., and Hodkinson, B. (2006). Orchid-fungus fidelity: a marriage meant to last? Ecology 87, 903–911. doi: 10.1890/0012-9658(2006)87[903:ofammt]2.0.co;2
McCormick, M. K., Whigham, D. F., O’Neill, J. P., et al. (2009). Abundance of Corallorhiza odontorhiza (Orchidaceae) within an established population reflects abundance of ectomycorrhizal root tips and fungal community composition. Ecol. Monogr. 79, 619–635.
McCormick, M. K., Taylor, D. L., Juhaszova, K., Burnett, R. K., Whigham, D. F., and O’Neill, J. (2012). Limitations on orchid recruitment: not a simple picture. Mol. Ecol. 21, 1511–1523. doi: 10.1111/j.1365-294X.2012.05468.x
McCormick, M. K., and Jacquemyn, H. (2014). What constrains the distribution of orchid populations? New Phytol. 202, 392–400. doi: 10.1371/journal.pone.0129890
McCormick, M. K., Taylor, D. L., Whigham, D. F., and Burnett, R. K. Jr. (2016). Germination patterns in three terrestrial orchids relate to abundance of mycorrhizal fungi. J. Ecol. 104, 744–754.
McCormick, M. K., Whigham, D. F., and Canchani-Viruet, A. (2018). Mycorrhizal fungi affect orchid distribution and population dynamics. New Phytol. 219, 1207–1215. doi: 10.1111/nph.15223
McKendrick, S. L., Leake, J. R., Taylor, D. L., and Read, D. J. (2000). Symbiotic germination and development of myco-heterotrophic plants in nature: ontogeny of Corallorhiza trifida and characterization of its mycorrhizal fungi. New Phytol. 145, 523–537. doi: 10.1046/j.1469-8137.2000.00603.x
McKendrick, S. L., Leake, J. R., Taylor, D. L., and Read, D. J. (2002). Symbiotic germination and development of the mycoheterotrophic orchid Neottia nidus-avis in nature and its requirement for locally distributed Sebacina spp. New Phytol. 154, 233–247. doi: 10.1046/j.1469-8137.2002.00372.x
Münzbergová, Z., and Herben, T. (2005). Seed, dispersal, microsite, habitat and recruitment limitation: identification of terms and concepts in studies of limitations. Oecologia 145, 1–8. doi: 10.1007/s00442-005-0052-1
Nathan, R., and Muller-Landau, H. C. (2000). Spatial patterns of seed dispersal, their determinants and consequences for recruitment. Trends Ecol. Evol. 15, 278–285. doi: 10.1016/s0169-5347(00)01874-7
Ogura-Tsujita, Y., Gebauer, G., Hashimoto, T., Umata, H., and Yukawa, T. (2009). Evidence for novel and specialized mycorrhizal parasitism: the orchid Gastrodia confusa gains carbon from saprotrophic Mycena. Proc. R. Soc. B 276, 761–767. doi: 10.1098/rspb.2008.1225
Ogura-Tsujita, Y., Gebauer, G., Xu, H., Fukasawa, Y., Umata, H., Tetsuka, K., et al. (2018). The giant mycoheterotrophic orchid Erythrorchis altissima is associated mainly with a divergent set of wood-decaying fungi. Mol. Ecol. 27, 1324–1337. doi: 10.1111/mec.14524
Ogura-Tsujita, Y., Yukawa, T., and Kinoshita, A. (2021). Evolutionary histories and mycorrhizal associations of mycoheterotrophic plants dependent on saprotrophic fungi. J. Plant Res. 134, 19–41. doi: 10.1007/s10265-020-01244-6
Ojá, J., Kohout, P., Tedersoo, L., Kull, T., and Kõljalg, U. (2015). Temporal patterns of orchid mycorrhizal fungi in meadows and forests as revealed by 454 pyrosequencing. New Phytol. 205, 1608–1618. doi: 10.1111/nph.13223
Oksanen, J., Blanchet, F. G., Kindt, R., Legendre, P., Minchin, P. R., O’Hara, R. B., et al. (2013). Vegan: Community Ecology Package. R Package Version 2. Vienna: R Core Team.
Poulsen, J. R., Osenberg, C. W., Clark, C. J., Levey, D. J., and Bolker, B. M. (2007). Plants as reef fish: fitting the functional form of seedling recruitment. Am. Nat. 170, 167–183. doi: 10.1086/518945
Pridgeon, A. M., Cribb, P. J., Chase, M. W., and Rasmussen, F. N. (2005). Genera Orchidacearum, Vol. 4. Oxford, UK: Oxford University Press.
R Core Team (2020). R: A Language and Environment for Statistical Computing. Vienna: R Foundation for Statistical Computing.
Rasmussen, H. N. (1995). Terrestrial orchids: from seed to mycotrophic plant. New York, NY: Cambridge University Press.
Rasmussen, H. N., and Whigham, D. F. (1993). Seed ecology of dust seeds in situ: a new study technique and its application in terrestrial ecology. Am. J. Bot. 80, 1374–1378. doi: 10.2307/2445665
Rasmussen, H. N., and Whigham, D. F. (1998). The underground phase: a special challenge in studies of terrestrial orchid populations. Bot. J. Linn. Soc. 126, 49–64. doi: 10.1111/j.1095-8339.1998.tb02515.x
Rasmussen, H. N., and Rasmussen, F. N. (2009). Orchid mycorrhiza: implications of a mycophagous life style. Oikos 118, 334–345.
Rasmussen, H. N., Dixon, K. W., Jersáková, J., and Těšitelová, T. (2015). Germination and seedling establishment in orchids: a complex of requirements. Ann. Bot. 116, 391–402. doi: 10.1093/aob/mcv087
Shefferson, R. P., Bunch, W., Cowden, C. C., Lee, Y. I., Kartzinel, T. R., Yukawa, T., et al. (2019). Does evolutionary history determine specificity in broad ecological interactions? J. Ecol. 107, 1582–1593.
Taylor, D. L., and Bruns, T. D. (1997). Independent, specialized invasions of ectomycorrhizal mutualism by two nonphotosynthetic orchids. Proc. Natl. Acad. Sci. U S A. 94, 4510–4515. doi: 10.1073/pnas.94.9.4510
Tesitelova, T., Kotilinek, M., Jersakova, J., et al. (2015). Two widespread green Neottia species (Orchidaceae) show mycorrhizal preference for Sebacinales in various habitats and ontogenetic stages. Mol. Ecol. 24, 1122–1134. doi: 10.1111/mec.13088
Umata, H., Ota, Y., Yamada, M., et al. (2013). Germination of the fully myco-heterotrophic orchid Cyrtosia septentrionalisis characterized by low fungal specificity and does not require direct seed-mycobiont contact. Mycoscience 54, 343–352. doi: 10.1016/j.myc.2012.12.003
Ventre-Lespiaucq, A., Jacquemyn, H., Rasmussen, H. N., and Méndez, M. (2021). Temporal turnover in mycorrhizal interactions: a proof of concept with orchids. New Phytol. 230, 1690–1699. doi: 10.1111/nph.17291
Wang, B. C., and Smith, T. B. (2002). Closing the seed dispersal loop. Trends Ecol. Evol. 17, 379–386. doi: 10.1093/jhered/ess102
Wang, D., Jacquemyn, H., Gomes, S. I. F., Vos, R. A., and Merckx, V. S. F. T. (2021). Symbiont switching and trophic mode shifts in Orchidaceae. New Phytol. [Preprint]. doi: 10.1111/nph.17414
Waud, M., Busschaert, P., Ruyters, S., Jacquemyn, H., and Lievens, B. (2014). Impact of primer choice on characterization of orchid mycorrhizal communities using 454 pyrosequencing. Mol. Ecol. Resour. 14, 679–699. doi: 10.1111/1755-0998.12229
Waud, M., Wiegand, T., Brys, R., Lievens, B., and Jacquemyn, H. (2016). Nonrandom seedling establishment corresponds with distance-dependent decline in mycorrhizal abundance in two terrestrial orchids. New Phytol. 211, 255–264. doi: 10.1111/nph.13894
Waud, M., Brys, R., Van Landuyt, W., Lievens, B., and Jacquemyn, H. (2017). Mycorrhizal specificity does not limit the distribution of an endangered orchid species. Mol. Ecol. 26, 1687–1701. doi: 10.1111/mec.14014
WCSP (2014). World checklist of selected plant families. Facilitated by the Royal Botanic Gardens, Kew. Richmond: Royal Botanic Gardens, Kew.
Xing, X., Liu, Q., Gao, Y., Shao, S., Guo, L., Jacquemyn, H., et al. (2020). The architecture of the network of orchid–fungus interactions in nine co-occurring Dendrobium species. Front. Ecol. Evol. 8:130. doi: 10.3389/fevo.2020.00130
Xu, J., and Guo, S. (2000). Retrospect on the research of the cultivation of Gastrodia elata Bl, a rare traditional Chinese medicine. Chin. Med. J. 113, 686–692.
Yagame, T., Ogura-Tsujita, Y., Kinoshita, A., et al. (2016). Fungal partner shifts during the evolution of mycoheterotrophy in Neottia. Am. J. Bot. 103, 1630–1641. doi: 10.3732/ajb.1600063
Keywords: Gastrodia, mycoheterotrophy, mycorrhiza, orchid, saprotrophic fungi, seed germination
Citation: Li Y-Y, Boeraeve M, Cho Y-H, Jacquemyn H and Lee Y-I (2022) Mycorrhizal Switching and the Role of Fungal Abundance in Seed Germination in a Fully Mycoheterotrophic Orchid, Gastrodia confusoides. Front. Plant Sci. 12:775290. doi: 10.3389/fpls.2021.775290
Received: 13 September 2021; Accepted: 17 December 2021;
Published: 13 January 2022.
Edited by:
Raffaella Balestrini, Institute for Sustainable Plant Protection, National Research Council (CNR), ItalyReviewed by:
Franck Richard, UMR 5175 Centre d’Ecologie Fonctionnelle et Evolutive (CEFE), FranceChihiro Miura, Tottori University, Japan
Copyright © 2022 Li, Boeraeve, Cho, Jacquemyn and Lee. This is an open-access article distributed under the terms of the Creative Commons Attribution License (CC BY). The use, distribution or reproduction in other forums is permitted, provided the original author(s) and the copyright owner(s) are credited and that the original publication in this journal is cited, in accordance with accepted academic practice. No use, distribution or reproduction is permitted which does not comply with these terms.
*Correspondence: Hans Jacquemyn, aGFucy5qYWNxdWVteW5Aa3VsZXV2ZW4uYmU=; Yung-I Lee, bGVleWkyNjIzNEBudHUuZWR1LnR3