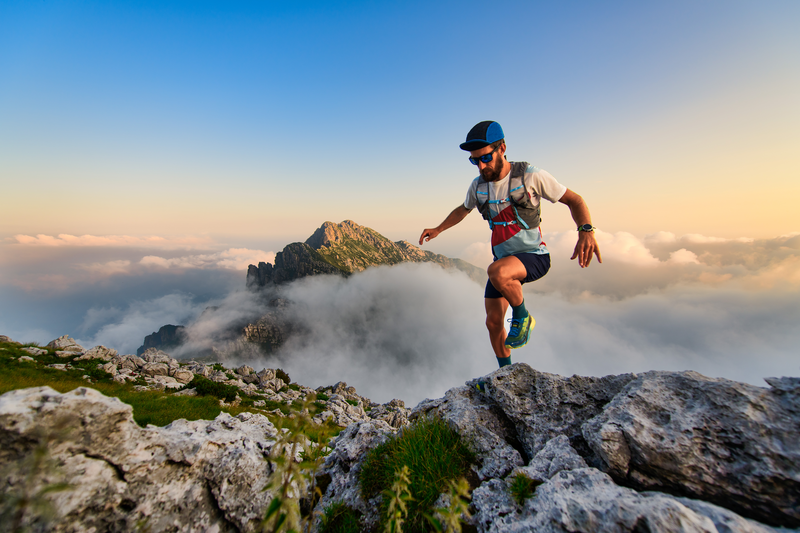
94% of researchers rate our articles as excellent or good
Learn more about the work of our research integrity team to safeguard the quality of each article we publish.
Find out more
ORIGINAL RESEARCH article
Front. Plant Sci. , 03 December 2021
Sec. Crop and Product Physiology
Volume 12 - 2021 | https://doi.org/10.3389/fpls.2021.764978
This article is part of the Research Topic Genetic Improvement of Triticeae using Omics-based Approaches View all 8 articles
Wheat powdery mildew, caused by the fungal pathogen Blumeria graminis f. sp. tritici (Bgt), is a destructive disease leading to huge yield losses in production. Host resistance can greatly contribute to the control of the disease. To explore potential genes related to the powdery mildew (Pm) resistance, in this study, we used a resistant genotype YD588 to investigate the potential resistance components and profiled its expression in response to powdery mildew infection. Genetic analysis showed that a single dominant gene, tentatively designated PmYD588, conferred resistance to powdery mildew in YD588. Using bulked segregant RNA-Seq (BSR-Seq) and single nucleotide polymorphism (SNP) association analysis, two high-confidence candidate regions were detected in the chromosome arm 2B, spanning 453,752,054-506,356,791 and 584,117,809-664,221,850 bp, respectively. To confirm the candidate region, molecular markers were developed using the BSR-Seq data and mapped PmYD588 to an interval of 4.2 cM by using the markers YTU588-004 and YTU588-008. The physical position was subsequently locked into the interval of 647.1–656.0 Mb, which was different from those of Pm6, Pm33, Pm51, Pm52, Pm63, Pm64, PmQ, PmKN0816, MlZec1, and MlAB10 on the same chromosome arm in its position, suggesting that it is most likely a new Pm gene. To explore the potential regulatory genes of the R gene, 2,973 differentially expressed genes (DEGs) between the parents and bulks were analyzed using gene ontology (GO), clusters of orthologous group (COG), and Kyoto Encyclopedia of Genes and Genomes (KEGG) pathway enrichment analysis. Based on the data, we selected 23 potential regulated genes in the enriched pathway of plant-pathogen interaction and detected their temporal expression patterns using an additional set of wheat samples and time-course analysis postinoculation with Bgt. As a result, six disease-related genes showed distinctive expression profiles after Bgt invasion and can serve as key candidates for the dissection of resistance mechanisms and improvement of durable resistance to wheat powdery mildew.
Common wheat (Triticum aestivum L.) is one of the most important cereal crops worldwide (Hoisington et al., 1999). However, powdery mildew, caused by Blumeria graminis f. sp. tritici, (Bgt), can lead to huge yield losses (Everts et al., 2001; Singh et al., 2016; Tsai et al., 2020). To control this disease, farmers traditionally relied on fungicide. But the pathogenic bacteria were easily evolved variation and produced drug resistance due to the overuse of pesticides (Twamley et al., 2019). In addition, environmental pollution along with the use of pesticides become increasingly prominent (Felsenstein et al., 2010). Comparatively speaking, host resistance is generally considered to be the most economical, sustainable, and environmentally friendly approach to control wheat powdery mildew (Johansson et al., 2020; Li Y. et al., 2021).
To improve host resistance, the R genes were commonly identified and used in breeding. To date, more than 100 formally designated powdery mildew resistance (Pm) genes at 63 loci are reported in common wheat and its relatives (Li G. et al., 2019; Maccaferri et al., 2019; Tan et al., 2019; Li Y. et al., 2020; Zhu et al., 2020; Wu et al., 2021). These resistance genes could be classified into two categories: qualitative resistance and quantitative resistance. Most reported Pm genes belonged to qualitative resistance and follow Mendel’s law of segregation (Ma et al., 2014; Zhu et al., 2020). Owing to their high resistance to different virulent isolates, qualitative resistance genes have been widely utilized in wheat cultivars, such as Pm8 and Pm21 (Hurni et al., 2014; Xing et al., 2018; Li H. et al., 2020; Wu et al., 2021). Compared with quantitative resistance, qualitative resistance is often defeated due to the shifts of Bgt virulence in wheat production (Xiao et al., 2013). Thus, it is urgent to discover new Pm genes/alleles and dissect the molecular mechanism of resistance, which is valuable for durable resistance in production.
Due to the large genome (exceed 17 Gb) and allohexaploid property, exploring and dissecting genetic traits of the allohexaploid wheat is difficult (Gupta et al., 2008; Wu et al., 2018). Efficient methods are needed to detect single nucleotide polymorphism (SNP), map the R gene, and assess the Pm gene expression profiles and describe the differential gene expression profiling. In recent years, whole-genome sequencing of common wheat and its relatives has made great progress, and corresponding high-throughput sequencing techniques have been greatly improved and used to construct linkage maps and dissect the molecular expression pattern of functional genes. Especially, bulked segregant analysis-RNA-Seq (BSR-Seq), combined RNA-Seq, and bulked segregant analysis (BSA) are advanced strategies for both holistic studies of the expression profile of complex genome and gene/quantitative trait locus (QTL) mapping (Michelmore et al., 1991; Pearce et al., 2015; Pankievicz et al., 2016). According to this method, mutant candidate genes and a large amount of SNP markers can be identified, and subsequently, comparative genomics analyses and high-density genetic linkage maps can also be carried out (Liu S. et al., 2012; Trick et al., 2012; Li L. et al., 2014; Ramirez-Gonzalez et al., 2014; Wang et al., 2017; Wu et al., 2018; Zhu et al., 2020).
Meanwhile, plants’ resistance to biotic stresses is an extraordinarily complex process. In the long-term interactions between plant and pathogen, plants evolved two layers of innate immunity: the PAMP-triggered immunity (PTI) pathway and the effector-triggered immunity (ETI) pathway (Jones and Dangl, 2006). The PTI is the first layer of the plant immunity system, where cells receive and transduce extracellular stress signals into the intracellular environment. Many receptor-like protein kinases (RLKs) especially leucine-rich repeat (LRR)-RLKs act as sensors and receptors in PTI (Chinchilla et al., 2007; Miya et al., 2007; Postel et al., 2010; Liu T. et al., 2012; Schwessinger and Ronald, 2012). The ETI is triggered by effectors injected into plant cells from pathogens and is often associated with an hypersensitive response (HR) (Jones and Dangl, 2006; Liu C. et al., 2019). In the whole process of plant resistance to pathogenic microorganisms, many factors play direct or indirect roles, including mitogen-activated protein (MAP) kinase signaling cascade system (Asai et al., 2002; He et al., 2006; Gao et al., 2008; Galletti et al., 2011), plant hormone signaling associated proteins (Navarro et al., 2008; Millet et al., 2010; Kim et al., 2014; Naseem et al., 2015), calcium influx (Kwaaitaal et al., 2011), nucleotide-binding LRR (NB-LRR) protein (Zhang et al., 2012), other kinases (Yamada et al., 2016), and transcription factors (Sarris et al., 2015) and so on. However, the immune mechanism in host resistance mainly focuses on the model plant Arabidopsis. Few studies reported the pathogen resistance mechanism in common wheat with a complex genome.
YD588, a Chinese wheat breeding line, exhibits high-level resistance to powdery mildew at both seedling and adult stages. In order to rapidly confirm the genetic model, candidate interval of the R gene(s), and profile the expression of resistance-related regulatory genes, using BSR-Seq, we (1) conformed the candidate interval of the R gene by analyzing the distribution of SNPs and molecular mapping and (2) profiled the key regulatory genes that may mediate powdery mildew resistance following Bgt inoculation.
The wheat breeding line YD588 was used as the resistant parent against powdery mildew and the susceptible cultivar Yannong 21 (YN21) was used as susceptible to be crossed with YD588 in order to produce F1. For the F2 and F2:3 families, 210 F2 plants and 205 generated F2:3 families were harvested for analyzing the inheritance of the resistance gene and BSR-Seq. Wheat cultivar Huixianhong was used as the susceptible control in evaluating the powdery mildew resistance. Twenty-six wheat genotypes with documented Pm genes were used to evaluate the powdery mildew resistance in YD588.
To evaluate the host resistance, YD588 was tested against 16 Bgt isolates with different virulence using 26 wheat genotypes with documented Pm genes as controls. To confirm the inheritance of the host resistance in YD588, YD588, YN21, and their derived F1, F2, and F2:3 progenies were inoculated with the Bgt isolate Y03 for phenotypic assessment at the one-leaf stage. The test seedlings were infected using the dusting method based on the previous studies (Ma et al., 2014). Seedlings to be evaluated were grown in a high humidity environment at 18°C/12°C (day/night) with a 12/14 h photoperiod. The test seedlings were inoculated with fresh conidiospores previously amplified on Huixianhong seedlings, and immediately incubated in a dark and 100% humidity space at 18oC for 24 h, and then the growth chamber was set to 20oC with a daily photoperiod of 14 h. For the next two days, the above inoculation process was repeated two times in the dark. When the spores were fully developed on the first leaf of the susceptible control Huixianhong (about 10–14 days after inoculation), infection types (ITs) were scored according to a 0–4 scale based on the previous studies (Si et al., 1992; An et al., 2013). The IT scores of 0–2 were considered as resistant type, while the scores 3 and 4 were considered as susceptible type. Under the same procedure, three parallel experiments were conducted to confirm the phenotype.
For YD588, YN21, and their derived F1 hybrids, 10 seeds of each genotype were sown. For the F2 population and F2:3 families, 210 F2 plants and 205 F2:3 families (20 seeds for each family) were sown for genetic analysis and preparation of the samples for BSR-Seq. As susceptible control, Huixianhong was planted randomly in each tray. When the spores were fully developed on the first leaf of Huixianhong, the first leaves of two patents YD588 and YN21, 40 resistant plants from 40 homozygous-resistant F2:3 families, and 40 susceptible plants from 40 homozygous-susceptible F2:3 families were sampled. Using the Spectrum Plant Total RNA kit (Sigma-Aldrich, Shanghai), RNA from the above materials was extracted following the protocol of the manufacturer. Resistant and susceptible RNA bulks were pooled by separately mixing equal amounts of mRNA from the 40 homozygous resistant and susceptible F2;3 plants, respectively. The mRNA of YD588, YN21, resistant bulks, and susceptible bulks were used for subsequent RNA-Seq.
Using RNA samples with Integrity Number (RIN) ≥ 7, cDNA libraries of YD588, YN21, and the resistant and susceptible bulks were constructed. RNA integrity assessment and cDNA libraries construction were performed based on the previous studies (Zhu et al., 2020). Then, using the Agilent 2100 Bioanalyzer (Agilent Technologies, Santa Clara, CA, United States), the quality of the cDNA libraries was assessed. The cDNA libraries sequencing was carried out on the Illumina HiSeq sequencing platform (Illumina HiSeq4000) by Beijing Biomics Technology Company Limited (Beijing, China). High-quality clean data were obtained after raw data filtering, sequences joint, and poor-quality reads elimination. The 10 and 20 Gb clean data were set for parents and bulks, respectively, in the sequencing indicator. Following mapping, the clean data to the reference genome of Chinese Spring (v1.1),1 SNP calling, DEG analysis, and gene ontology (GO) and Kyoto Encyclopedia of Genes and Genomes (KEGG) pathway analyses were performed in Cloud Platform developed by Beijing Biomics Technology Company Limited.
After aligning the clean reads of YD588, YN21, and resistance and susceptible bulks with the Chinese Spring reference genome (IWGSC RefSeq, version 1.1; The International Wheat Genome Sequencing Consortium [IWGSC], 2018) using the STAR (version 2.3.0e; International Rice Genome Sequencing Project, and Sasaki, 2005), SNP calling was carried out by using the GATK software (version 3.1-1; McKenna et al., 2010) following the reference flowchart aimed at RNA-Seq. SNP index values were calculated using the SNPs in YN21 as a reference using the MutMap method (Abe et al., 2012). Subsequently, the ΔSNP index between resistant and susceptible parents and bulks for each SNP was calculated (Takagi et al., 2013) using the following formula:
ΔSNP_index = (SNP_index of resistance parent/bulk) - (SNP_index of susceptible parent/bulk).
Using a 5-Mb size as a step, the average value of ΔSNP index in each window was calculated by sliding the window. The threshold for SNP screening was set as a test of 100,000 permutations coupling with 99.0% confidence (Takagi et al., 2013). Candidate regions with higher confidence (exceeding 99.0%) and SNPs with larger than threshold ΔSNP index value (set as 0.75) in candidate regions were considered to be candidate loci related to powdery mildew resistance in YD588.
To further verify the result of the ΔSNP index, the Euclidean distance (ED) algorithm was also used to calculate the candidate region based on the method of Trapnell et al. (2010).
To further locate and validate the interval of the Pm gene(s) in YD588, simple sequence repeat (SSR) in the candidate intervals obtained from the BSR-Seq were used to develop molecular markers, which were then tested for polymorphisms between YD588, YN21, and their derived resistant and susceptible bulks. The resulting markers were then genotyped on the segregation population of YD588 × YN21. PCR amplification, separation, and visualization of the PCR products were done using the method by Ma et al. (2014). After genotyping, the χ2 test was carried out to evaluate the deviation of the observed phenotypic data from theoretically expected segregation ratios for the goodness-of-fit analysis. The linkage map of the Pm gene(s) in YD588 was finally constructed using the MAPMAKER 3.0 and the Kosambi function based on the studies by Lincoln et al. (1993) and Kosambi (1943).
After SNP calling, fragments per kilo bases of exon per million fragments mapped (FPKM) was used to calculate the expression level of functional genes mapped to the reference genome of Chinese Spring (version 1.1) (Garber et al., 2011). DEGs were defined as fold change ≥ 2 and false discovery rate (FDR) < 0.01 using the EBSeq software (Leng et al., 2013). Statistical significance of DEGs was determined using a combination of multiple tests and FDR (Reiner et al., 2003). The statistic and cluster analysis of DEGs between resistance and susceptible parents and bulks were performed, and then differential expression patterns in the whole genome including the candidate interval was presented.
Functional annotation of DEGs was performed based on the information from IWGSC RefSeq (version 1.1; The International Wheat Genome Sequencing Consortium [IWGSC], 2018). Then, GO, clusters of orthologous group (COG), and KEGG pathway enrichment analysis of the DEGs were implemented using the R package based on the previous studies (Yu et al., 2012). Tools and databases used in the GO, COG, and KEGG pathway analysis, respectively, were as follows: GO Term Finder (Boyle et al., 2004), UniGene sequences,2 and KEGG database.3
Differentially expressed genes used in the key signal pathway about disease resistance or stress tolerance were selected to profile their expression patterns via quantitative reverse transcription (qRT)-PCR, using an additional set of wheat samples and time-course analysis postinoculation with Bgt. The first leaves of YD588 and YN21 seedlings were sampled at 3, 6, 12, 24, 36, 48, and 72 h after inoculation with the Bgt isolate Y03. The collected leaves were immediately frozen in liquid nitrogen and ground to a fine powder in a pestle and mortar. Total RNA was extracted using RNAiso Plus, Beijing (TaKaRa Cat#9109) following the instruction manual of the manufacturer and quantified by measuring absorbance using a NanoDrop 1000 spectrophotometer (Thermo Scientific, Beijing). cDNA was synthesized from total RNA using the Fastking kit (Tiangen, KR118-02, Beijing).
The qRT-PCR analysis was performed using SYBR Green Master Mix (Tiangen) based on the previous studies (He et al., 2016, 2018). Amplification was followed by melt curve analysis. The 2–ΔΔCt method was used for relative quantification (He et al., 2018). Primers for specific genes were designed based on the coding sequences of the selected genes. The housekeeping gene Tubulin was used for normalization. Three parallel experiments were set up.
YD588 was resistant to 14 of the 16 Bgt isolates, accounting for a proportion of 87.5% (Figure 1 and Supplementary Table 1). Compared with the documented Pm genes, especially the Pm2 and Pm4 that were mainly used in the production in China, YD588 showed a higher potential in resistance breeding. Then, the Bgt isolate Y03 was selected to analyze the inheritance of the powdery mildew resistance in YD588. When tested against Y03, YD588 had no visible symptoms and displayed high resistance to Bgt when the IT score is 0. In contrast, over 80% of the leaf area was covered with aerial hyphae in YN21 and was considered to be highly susceptible when the IT score is 4. Subsequently, F1, F2, and F2:3 seedlings crossed by YD588 and YN21 were also inoculated with the same isolate. All the 10 tested F1 plants showed the same resistance pattern as YD588, suggesting a dominant inheritance pattern. The 210 tested F2 plants segregated into 155 resistant and 55 susceptible ones, following the theoretical ratio for monogenic segregation (χ2 = 0.16; P = 0.69). Subsequently, 205 generated F2:3 families harvested from the 210 tested F2 plants (five F2 plants died and cannot harvest F3 seeds) further confirmed the ratio for monogenic segregation, with a segregation ratio of 53 homozygous resistant (RR): 98 segregating (Rr): 54 homozygous susceptible (rr) families (χ2 = 0.40; P = 0.82). In conclusion, a single dominant gene, tentatively designated PmYD588, controls the powdery mildew resistance in YD588.
Figure 1. Seedling reaction patterns of YD588 and Yannong 21 (YN21) to the selected four Blumeria graminis f. sp. tritici (Bgt) isolates collected from different regions of China.
After filtering low-quality data, adapter reads, and rRNA, a total of 13.35, 10.43, 28.59, and 24.87 Gb clean data for YD588, YN21, resistant, and susceptible bulks, respectively, were obtained, containing 44,657,220, 34,873,857, 95,638,544, and 83,147,454 clean reads, respectively. All the clean reads from the four samples account for more than 98.99% of total raw data, with Q30 >94.84%, and the GC content ranges from 52.49 to 56.12%. Following alignment of the four sets of clean reads to IWGSC RefSeq (version 1.1; The International Wheat Genome Sequencing Consortium [IWGSC], 2018) individually, 61.88–86.43% reads were mapped on the reference sequence (Supplementary Table 2). In summary, we obtained high-quality sequence data suitable for the subsequent analysis.
A total of 39,995 SNPs between parents and 50,644 SNPs between the bulks were detected, and 12,004 SNPs with consistent differences between resistant and susceptible parents and bulks were further obtained for the subsequent ΔSNP index analysis (Supplementary Table 3). Using the SNP index, six adjacent candidate regions on chromosome arm 2B were identified (Figure 2 and Table 1). To confirm the result, the ED algorithm was also performed, and similar results containing four adjacent candidate regions were obtained (Figure 3 and Table 2). Combining the two results, two high-confidence candidate regions were confirmed, spanning 453,752,054-506,356,791 and 584,117,809-664,221,850 bp, respectively.
Figure 2. Distribution of the single nucleotide polymorphisms (SNPs) with consistent differences between the resistant parent YD588 and susceptible parent Yannong 21 (YN21) and their derived bulked pools on 21 chromosomes (A) and on chromosome arm 2B (B) based on the ΔSNP index value.
Table 1. The candidate intervals of the R gene PmYD588 and gene numbers in these intervals using SNP index.
Figure 3. Distribution of the SNPs with consistent differences between the resistant parent YD588 and susceptible parent YN21 and their derived bulked pools on 21 chromosomes (A) and on the chromosome arm 2B (B) based on the Euclidean distance (ED) algorithm.
Table 2. The candidate intervals of the R gene PmYD588 and gene numbers in these intervals using the Euclidean distance (ED) algorithm.
To map PmYD588, 18 SSR markers were developed using the BSR-Seq data. As a result, five markers (YTU588-003, YTU588-004, YTU588-008, YTU588-012, and YTU588-016) showed consistent polymorphism between the parents and bulks (Table 3). After genotyping the segregation population of YD588 and YN21, these markers were also linked with PmYD588 (Figure 4). A linkage map was then constructed, and PmYD588 was flanked by YTU588-004 and YTU588-008 with genetic distances of 2.8 and 1.4 cM, respectively. The corresponding physical interval was 647.7–656.0 Mb in the chromosome arm 2BL (Figure 4).
Table 3. Molecular markers that were newly developed using BSR-Seq for the powdery mildew resistance gene PmYD588.
Figure 4. Linkage map of PmYD588 using the F2:3 families of YD588 × YN21. Genetic distances in cM are showed to the left. The black arrows point to the centromere.
After SNP calling, DEGs were annotated between the two parents and two bulks, respectively. A total of 12,118 DEGs were identified from the parents and bulks (Figure 5). Among them, 10,816 DEGs were identified between parents YD588 and YN21, where 6,305 ones were downregulated and 4,511 ones were upregulated using the expression index of YN21 as a standard (Supplementary Table 4). Furthermore, 3,360 DEGs were detected between the resistance and susceptible bulks, where 2,642 and 718 were downregulated and upregulated, respectively (Supplementary Table 5). Finally, 2,973 DEGs showed a consistent difference between parents and bulks, which can be used for subsequent GO, COG, and KEGG analysis (Supplementary Table 6).
Figure 5. Heat map of the differentially expressed genes (DEGs) between the resistance and susceptible parents YD588 and YN21 and their derived resistance and susceptible bulks from their derived F2:3 families. RB, resistance bulk; SB, susceptible bulk.
Gene ontology database is a standard biological annotation system. DEGs showed a consistent difference between parents, and bulks were classified using the GO analysis. The results indicated that these DEGs were mainly involved in cell components, including cell, membrane, organelle, and cell part; in molecular functions, including catalytic activity, binding, and transporter activity; in biological processes, including metabolic and cellular processes, single-organism processes, and response to stimulus and biological regulation (Figure 6). It is worth noting that the functional classification in response to stimulus in biological progress was significantly enriched, and these genes may directly participate in the disease defense process.
Figure 6. Gene ontology (GO) analysis of the DEGs with consistent differences between the resistance and susceptible parents YD588 and YN21 and their derived resistance and susceptible bulks from their derived F2:3 families.
To further explore the regulatory genes that may relate to powdery mildew, COG analysis and KEGG pathway enrichment analyses were performed on DEGs that showed consistent differences between parents and corresponding bulks (Figure 7). From the data of COG, 0.8% of candidate genes were found to be directly involved in the plant defense mechanism, while most of the candidate genes account for amino acid and carbohydrate transport and metabolism, and DNA duplication, recombination, and repair. This probably indicated that more diverse or alternative metabolism and synthesis processes are being activated during plant immune response, which is consistent with previous studies (Rudd et al., 2015; Verbancic et al., 2018).
Figure 7. Clusters of orthologous groups (COG) analysis of the DEGs with consistent differences between the resistance and susceptible parents YD588 and YN21 and their derived resistance and susceptible bulks from their derived F2:3 families.
Using the KEGG analysis, 115 significantly enriched pathways accounting for 20 categories in cellular processes, environmental processing, genetic information processing, metabolism, and organismal system were found (Supplementary Figure 1 and Supplementary Table 7). In particular, one plant-pathogen interaction pathway was enriched, which can be used to select key regulatory genes for further time-course analysis postinoculation with Bgt (Figure 8).
Figure 8. The plant-pathogen interaction pathway enriched from DEGs with consistent differences between the resistance and susceptible parents YD588 and YN21 and their derived resistance and susceptible bulks from their derived F2:3 families.
In the enriched plant-pathogen interaction pathway, 23 genes were involved, and the sequence information of one new gene was not clear (Supplementary Table 8). Therefore, we monitored the expression level of the remaining 22 genes in this pathway following Bgt inoculation. As a result, six of them showed significant differences between YD588 and YN21 in the time-course analysis following Bgt inoculation (Figure 9).
Figure 9. Expression profiles of TraesCS2A02G461900 (A), TraesCS2B02G401300 (B), TraesCS2B02G367500 (C), TraesCS4B02G321800 (D), TraesCS6A02G417900 (E), and TraesCS5B02G442600 (F) in each corresponding stage of YD588 and YN21 after Bgt infection.
The transcriptional levels of two LRR genes (TraesCS2A02G461900 and TraesCS2B02G401300) were upregulated in YD588 but not in YN21 despite different patterns in the time scale. TraesCS2B02G401300 was induced at an early stage within 3 h and lasted until 48 and 72 h, while TraesCS2A02G461900 was significantly upregulated only after 48 h (Figures 9A,B). Two calcium-associated genes TraesCS4B02G321800 and TraesCS2B02G367500 were obviously induced at 24 h after inoculation, while the expression of TraesCS4B02G321800 was elevated from 3 h, and TraesCS2B02G367500 was induced from 24 h (Figures 9C,D). The transcription of TraesCS6A02G417900 showed an obvious periodicity, which was stimulated at 3 h, downregulated at 6 h, and peaked two times at 24 and 72 h (Figure 9E). In contrast to the expression trend of the above genes, the transcript of TraesCS5B02G442600, one of the pathogenesis-related 1 proteins (CAP) superfamily proteins, was not induced any time after Bgt inoculation in YD588 (Figure 9F).
In this study, the wheat breeding line YD588 was proved to be resistant to powdery mildew. Using BSR-Seq and molecular markers, a dominant gene, tentatively designated PmYD588, confers the broad-spectrum seedling resistance to different Bgt isolates. To preliminarily dissect the resistance mechanism that participated in the resistance process, we also profiled the gene expression pattern after Bgt invasion in a wheat breeding line YD588.
Using BSR-Seq, the R gene PmYD588 was first proved to be associated with two adjacent candidate intervals 453,752,054-506,356,791 and 584,117,809-664,221,850 bp in the chromosome arm 2B. Then, using molecular markers derived from the BSR-Seq, it was further located at an interval of 4.2 cM corresponding with the 647.1-656.0 Mb physical interval based on the reference genome of Chinese Spring. Prior to PmYD588, ten Pm genes, namely, Pm6 (Wan et al., 2020), Pm33 (Zhu et al., 2005), Pm51 (Zhan et al., 2014), Pm52 (Wu et al., 2019), Pm63 (Tan et al., 2019), Pm64 (Zhang et al., 2019), PmQ (Li Y. et al., 2020), PmKN0816 (Wang et al., 2021), MlZec1 (Mohler et al., 2005), and MlAB10 (Maxwell et al., 2010) have previously been reported on the chromosome arm 2BL. However, PmYD588 can be distinguished from Pm6 (698.3–699.2 Mb), Pm33 (779.1–784.3 Mb), Pm51 (709.8–739.4 Mb), Pm52 (581.0–585.0 Mb), Pm63 (710.3–723.4 Mb), Pm64 (699.2–705.5 Mb), PmQ (710.7–715.0 Mb), PmKN0816 (700.4–710.3 Mb), MlZec1 (796.7–780.0 Mb), and MlAB10 (796.7–780.0 Mb) in its physical interval, suggesting PmYD588 is most likely a new Pm gene. In spite of this, more evidence is still needed in the future to clarify the relationship of these Pm genes on the chromosome arm 2BL, such as mutual allelism tests and even cloning of these genes.
In the process of plant disease resistance, the functional exhibition not only depends on the R gene but also regulates a mass of genes in the innate immune system of the plants. To further describe the potential resistance mechanism in the YD588, we enriched a plant-pathogen interaction pathway that may be related to the functional exhibition of the R gene and selected 22 genes for time-course analysis postinoculation with Bgt.
Among the 22 candidate genes in the plant-pathogen interaction pathway, five genes were described as LRR-RLK family protein and two genes labeled as NBS-LRR disease resistance protein homolog. These genes with the LRR domain accounted for ∼32% of all candidate genes. It has been reported that pattern-recognition receptor-like kinases (RLKs) or proteins (RLPs) possessing LRR domain activate effector-triggered immunity (ETI) in several plant species (Saur et al., 2000; Cui et al., 2015; Pruitt et al., 2015; Couto and Zipfel, 2016; Mott et al., 2016; Yan et al., 2018; Yuan et al., 2021). In our study, the transcriptional levels of two LRR genes (TraesCS2A02G461900 and TraesCS2B02G401300) were upregulated in YD588 but not in YN21 because of different patterns. At the beginning of Bgt inoculation, there was no significant expression difference of TraesCS2A02G461900 in YD588 and YN21, but after 48 h of treatment, the expression was significantly upregulated only in YD588. In contrast, TraesCS2B02G401300 was induced at an early stage within 3 h and lasted for 48 and 72 h. The difference in response time of these two genes may be due to the direct or indirect regulation of the two genes in the process of powdery mildew resistance, and considering TraesCS2A02G461900 was a kinase, it may be functioned by phosphorylating other ligands while TraesCS2B02G401300 was not (Figures 9A,B).
Calcium-binding protein, calcium-dependent protein kinase (CDPKs), or calmodulin protein account for about 27% of the 22 selected genes in the in the plant-pathogen interaction pathway (6/22). Calcium-binding protein and CDPKs are known to play pivotal roles during abiotic and biotic stress responses (Coca and Segundo, 2010; Feng et al., 2011; Asano et al., 2012). It has been reported that CDPKs regulated host cell entry in barley powdery mildew resistance (Freymark et al., 2007), and calcium-binding protein TaCab1 was induced during stripe rust infection (Feng et al., 2011). In our result, the transcriptional levels of TraesCS4B02G321800 described as CDPK and TraesCS2B02G367500 encoding a calcium-binding protein were detected. These two genes were both obviously induced 24 h after inoculation, while the expression of TraesCS4B02G321800 was elevated from 3 h and TraesCS2B02G367500 was induced from 24 h (Figures 9C,D).
In our remaining candidate genes, TraesCS6A02G417900 encoded a Lysin Motif (LysM) receptor-like kinase protein. LysMs have been reported to bind or percept N-acetylglucosamine (GlcNAc)-containing molecules produced by microorganisms and play a vital role in the plant-pathogen interaction (Radutoiu et al., 2003; Buendia et al., 2018). The transcription of TraesCS6A02G417900 was stimulated at an early time after Bgt inoculation (3 h) and then downregulated at 6 h. Subsequently, two peaks emerged in the expression trend, one at 24 h and another at 72 h (Figure 9E). The fluctuant expression of TraesCS6A02G417900 implied the periodical function during resistance response.
Cysteine-rich secretory proteins, antigen 5, and pathogenesis-related 1 protein (CAP) superfamily protein was another relatively large family protein in our candidate pool, accounting for ∼23% (5/22). CAP superfamily is involved in cancer and immune defense in animals (Gibbs et al., 2008). Small peptides derived from the CAP superfamily play important roles in salt stress and immune signaling in Arabidopsis and tomato, respectively (Chien et al., 2015). The transcript of TraesCS5B02G442600, one of the CAP superfamily proteins, was monitored in the time course following Bgt inoculation. However, induced expression was not observed any time after Bgt inoculation in YD588. In contrast, high-level expression was detected in YN21 at 72 h. Considering the previous studies in other plant species, we concluded that CAP superfamily protein might not function directly and might be activated after splicing or other modification processing.
In conclusion, using a resistant wheat breeding line YD588, we identified a powdery mildew resistance gene PmYD588, investigated the resistance expression profile to powdery mildew, and further selected several key candidate genes for time-course analysis postinoculation with Bgt. Our study can be valuable for enhancing the genetic diversity, understanding resistance pathways, and preliminarily dissecting the expression profiles after Bgt invasion.
The datasets presented in this study can be found in online repositories. The names of the repository/repositories and accession number(s) can be found below: NCBI Sequence Read Archive (SRA), PRJNA761248.
PM, CL, and BW conceived the research. PM, BW, LW, YX, XZ, and WW performed the experiments. PM and BW analyzed the data. PM and HX performed phenotypic assessments. BW and PM wrote the manuscript. All authors read and approved the final manuscript.
This research was financially supported by the National Natural Science Foundation of China (32072053, 31900265), Key Research and Development Program of Shandong Province (2020CXGC010805), and Open Project Funding of the State Key Laboratory of Crop Stress Adaptation and Improvement (CX1130A0920014).
The authors declare that the research was conducted in the absence of any commercial or financial relationships that could be construed as a potential conflict of interest.
All claims expressed in this article are solely those of the authors and do not necessarily represent those of their affiliated organizations, or those of the publisher, the editors and the reviewers. Any product that may be evaluated in this article, or claim that may be made by its manufacturer, is not guaranteed or endorsed by the publisher.
We are grateful to Paula Jafmeson, the University of Canterbury, financially supported by the “Double Hundred” Plan for Foreign Experts in Shandong Province, China, for constructive comments and English language editing of this manuscript.
The Supplementary Material for this article can be found online at: https://www.frontiersin.org/articles/10.3389/fpls.2021.764978/full#supplementary-material
Abe, A., Kosugi, S., Yoshida, K., Natsume, S., Takagi, H., Kanzaki, H., et al. (2012). Genome sequencing reveals agronomically important loci in rice using MutMap. Nat. Biotechnol. 30, 174–178. doi: 10.1038/nbt.2095
An, D., Zheng, Q., Zhou, Y., Ma, P., Lv, Z., Li, L., et al. (2013). Molecular cytogenetic characterization of a new wheat-rye 4R chromosome translocation line resistant to powdery mildew. Chromosome Res. 21, 419–432. doi: 10.1007/s10577-013-9366-8
Asai, T., Tena, G., Plotnikova, J., Willmann, M. R., Chiu, W. L., Gomez-Gomez, L., et al. (2002). MAP kinase signalling cascade in Arabidopsis innate immunity. Nature 415, 977–983. doi: 10.1038/415977a
Asano, T., Hayashi, N., Kikuchi, S., and Ohsugi, R. (2012). CDPK-mediated abiotic stress signaling. Plant Signal. Behav. 7, 817–821. doi: 10.4161/psb.20351
Boyle, E. I., Weng, S., Gollub, J., Jin, H., Botstein, D., Cherry, J. M., et al. (2004). GO::TermFinder—open source software for accessing gene ontology information and finding significantly enriched gene ontology terms associated with a list of genes. Bioinformatics 20, 3710–3715. doi: 10.1093/bioinformatics/bth456
Buendia, L., Girardin, A., Wang, T., Cottret, L., and Lefebvre, B. (2018). LysM receptor-like kinase and LysM receptor-like protein families: an update on phylogeny and functional characterization. Front Plant Sci. 9:1531. doi: 10.3389/fpls.2018.01531
Chien, P. S., Nam, H. G., and Chen, Y. R. (2015). A salt-regulated peptide derived from the CAP superfamily protein negatively regulates salt-stress tolerance in Arabidopsis. J. Exp. Bot. 66, 5301–5313. doi: 10.1093/jxb/erv263
Chinchilla, D., Zipfel, C., Robatzek, S., Kemmerling, B., Nurnberger, T., Jones, J. D., et al. (2007). A flagellin-induced complex of the receptor FLS2 and BAK1 initiates plant defence. Nature 448, 497–500. doi: 10.1038/nature05999
Coca, M., and Segundo, B. S. (2010). AtCPK1 calcium-dependent protein kinase mediates pathogen resistance in Arabidopsis. Plant J. 63, 526–540. doi: 10.1111/j.1365-313X.2010.04255.x
Couto, D., and Zipfel, C. (2016). Regulation of pattern recognition receptor signalling in plants. Nat. Rev. Immunol. 16, 537–552. doi: 10.1038/nri.2016.77
Cui, H., Tsuda, K., and Parker, J. E. (2015). Effector-triggered immunity: from pathogen perception to robust defense. Annu. Rev. Plant Biol. 66, 487–511. doi: 10.1146/annurev-arplant-050213-040012
Everts, K. L., Leath, S., and Finney, P. L. (2001). Impact of powdery mildew and leaf rust on milling and baking quality of soft red winter wheat. Plant Dis. 85, 423–429. doi: 10.1094/PDIS.2001.85.4.423
Felsenstein, F., Semar, M., and Stammler, G. (2010). Sensitivity of wheat powdery mildew (Blumeria graminis f. sp. tritici) towards metrafenone. Gesunde Pflanzen 62, 29–33. doi: 10.1007/s10343-010-0214-x
Feng, H., Wang, X., Sun, Y., Wang, X., Chen, X., Guo, J., et al. (2011). Cloning and characterization of a calcium binding EF-hand protein gene TaCab1 from wheat and its expression in response to Puccinia striiformis f. sp. tritici and abiotic stresses. Mol. Biol. Rep. 38, 3857–3866. doi: 10.1007/s11033-010-0501-8
Freymark, G., Diehl, T., Miklis, M., Romeis, T., and Panstruga, R. (2007). Antagonistic control of powdery mildew host cell entry by barley calcium-dependent protein kinases (CDPKs). Mol. Plant Microbe Interact. 20, 1213–1221. doi: 10.1094/MPMI-20-10-1213
Galletti, R., Ferrari, S., and De Lorenzo, G. (2011). Arabidopsis MPK3 and MPK6 play different roles in basal and oligogalacturonide- or flagellin-induced resistance against Botrytis cinerea. Plant Physiol. 157, 804–814. doi: 10.1104/pp.111.174003
Gao, M., Liu, J., Bi, D., Zhang, Z., Cheng, F., Chen, S., et al. (2008). MEKK1, MKK1/MKK2 and MPK4 function together in a mitogen-activated protein kinase cascade to regulate innate immunity in plants. Cell Res. 18, 1190–1198. doi: 10.1038/cr.2008.300
Garber, M., Grabherr, M. G., Guttman, M., and Trapnell, C. (2011). Computational methods for transcriptome annotation and quantification using RNA-seq. Nat. Methods 8, 469–477. doi: 10.1038/nmeth.1613
Gibbs, G. M., Roelants, K., and O’Bryan, M. K. (2008). The CAP superfamily: cysteine-rich secretory proteins, antigen 5, and pathogenesis-related 1 proteins–roles in reproduction, cancer, and immune defense. Endocr. Rev. 29, 865–897. doi: 10.1210/er.2008-0032
Gupta, P. K., Mir, R. R., Mohan, A., and Kumar, J. (2008). Wheat genomics: present status and future prospects. Int. J. Plant Genomics 2008:896451. doi: 10.1155/2008/896451
He, H., Zhu, S., Jiang, Z., Ji, Y., Wang, F., Zhao, R., et al. (2016). Comparative mapping of powdery mildew resistance gene Pm21 and functional characterization of resistance-related genes in wheat. Theor. Appl. Genet. 129, 819–829. doi: 10.1007/s00122-016-2668-4
He, H., Zhu, S., Zhao, R., Jiang, Z., Ji, Y., Ji, J., et al. (2018). Pm21, encoding a typical CC-NBS-LRR protein, confers broad-spectrum resistance to wheat powdery mildew disease. Mol. Plant. 11, 879–882. doi: 10.1016/j.molp.2018.03.004
He, P., Shan, L., Lin, N. C., Martin, G. B., Kemmerling, B., Nurnberger, T., et al. (2006). Specific bacterial suppressors of MAMP signaling upstream of MAPKKK in Arabidopsis innate immunity. Cell 125, 563–575. doi: 10.1016/j.cell.2006.02.047
Hoisington, D., Khairallah, M., Reeves, T., Ribaut, J. M., Skovmand, B., Taba, S., et al. (1999). Plant genetic resources: what can they contribute toward increased crop productivity? Proc. Natl. Acad. Sci. U.S.A. 96, 5937–5943. doi: 10.1073/pnas.96.11.5937
Hurni, S., Brunner, S., Stirnweis, D., Herren, G., Peditto, D., McIntosh, R. A., et al. (2014). The powdery mildew resistance gene Pm8 derived from rye is suppressed by its wheat ortholog Pm3. Plant J. 79, 904–913. doi: 10.1111/tpj.12593
International Rice Genome Sequencing Project, and Sasaki, T. (2005). The map-based sequence of the rice genome. Nature 436, 793–800. doi: 10.1038/nature03895
Johansson, E., Henriksson, T., Prieto-Linde, M. L., Andersson, S., Ashraf, R., and Rahmatov, M. (2020). Diverse wheat-alien introgression lines as a basis for durable resistance and quality characteristics in bread wheat. Front Plant Sci. 11:1067. doi: 10.3389/fpls.2020.01067
Jones, J., and Dangl, J. (2006). The plant immune system. Nature 444, 323–329. doi: 10.1038/nature05286
Kim, H. G., Kwon, S. J., Jang, Y. J., Chung, J. H., Nam, M. H., and Park, O. K. (2014). GDSL lipase 1 regulates ethylene signaling and ethylene-associated systemic immunity in Arabidopsis. FEBS Lett. 588, 1652–1658. doi: 10.1016/j.febslet.2014.02.062
Kosambi, D. D. (1943). The estimation of map distance from recombination values. Ann. Eugen. 12, 172–175. doi: 10.1111/j.1469-1809.1943.tb02321.x
Kwaaitaal, M., Huisman, R., Maintz, J., Reinstadler, A., and Panstruga, R. (2011). Ionotropic glutamate receptor (iGluR)-like channels mediate MAMP-induced calcium influx in Arabidopsis thaliana. Biochem. J. 440, 355–365. doi: 10.1042/BJ20111112
Leng, N., Dawson, J. A., Thomson, J. A., Ruotti, V., Rissman, A. I., Smits, B. M. G., et al. (2013). EBSeq: an empirical Bayes hierarchical model for inference in RNA-seq experiments. Bioinformatics 29:2073. doi: 10.1093/bioinformatics/btt337
Li, G., Cowger, C., Wang, X., Carver, B. F., and Xu, X. (2019). Characterization of Pm65, a new powdery mildew resistance gene on chromosome 2AL of a facultative wheat cultivar. Theor. Appl. Genet. 132, 2625–2632. doi: 10.1007/s00122-019-03377-2
Li, H., Dong, Z., Ma, C., Xia, Q., Tian, X., Sehgal, S., et al. (2020). A spontaneous wheat-Aegilops longissima translocation carrying Pm66 confers resistance to powdery mildew. Theor. Appl. Genet. 133, 1149–1159. doi: 10.1007/s00122-020-03538-8
Li, L., Li, D., Liu, S., Ma, X., Dietrich, C. R., Hu, H. C., et al. (2014). The maize glossy13 gene, cloned via BSR-Seq and Seq-Walking encodes a putative ABC transporter required for the normal accumulation of epicuticular waxes. PLoS One 12:e82333. doi: 10.1371/journal.pone.0082333
Li, Y., Shi, X., Hu, J., Wu, P., Qiu, D., Qu, Y., et al. (2020). Identification of a recessive gene PmQ conferring resistance to powdery mildew in wheat landrace Qingxinmai using BSR-seq analysis. Plant Dis. 104, 743–751. doi: 10.1094/PDIS-08-19-1745-RE
Li, Y., Wei, Z. Z., Fatiukha, A., Jaiwar, S., Wang, H., Hasan, S., et al. (2021). TdPm60 identified in wild emmer wheat is an ortholog of Pm60 and constitutes a strong candidate for PmG16 powdery mildew resistance. Theor. Appl. Genet. 9, 2777–2793. doi: 10.1007/s00122-021-03858-3
Lincoln, S., Daly, M., and Lander, E. (1993). Constructing Genetic Maps with Mapmaker/EXP3.0 Whitehead Institute Techn Rep, 3rd Edn. Cambridge, MA: Whitehead Institute.
Liu, C., Cui, D., Zhao, J., Liu, N., Wang, B., Liu, J., et al. (2019). Two Arabidopsis receptor-like cytoplasmic kinases SZE1 and SZE2 associate with the ZAR1-ZED1 complex and are required for effector-triggered immunity. Mol. Plant 12, 967–983. doi: 10.1016/j.molp.2019.03.012
Liu, S., Yeh, C. T., Tang, H. M., Dan, N., and Schnable, P. S. (2012). Gene mapping via bulked segregant RNA-Seq (BSR-Seq). PLoS One 7:e36406. doi: 10.1371/journal.pone.0036406
Liu, T., Liu, Z., Song, C., Hu, Y., Han, Z., She, J., et al. (2012). Chitin-induced dimerization activates a plant immune receptor. Science 336, 1160–1164. doi: 10.1126/science.1218867
Ma, P., Xu, H., Luo, Q., Qie, Y., Zhou, Y., Xu, Y., et al. (2014). Inheritance and genetic mapping of a gene for seedling resistance to powdery mildew in wheat line X3986-2. Euphytica 200, 149–157. doi: 10.1007/s10681-014-1178-1
Maccaferri, M., Harris, N. S., Twardziok, S. O., Pasam, R. K., Gundlach, H., Spannagl, M., et al. (2019). Durum wheat genome highlights past domestication signatures and future improvement targets. Nat. Genet. 51, 885–895. doi: 10.1038/s41588-019-0381-3
Maxwell, J. J., Lyerly, J. H., Srnic, G., Parks, R., Cowger, C., Marshall, D., et al. (2010). MlAB10: a Triticum turgidum subsp. Dicoccoides derived powdery mildew resistance gene identified in common wheat. Crop Sci. 50, 2261–2267. doi: 10.2135/cropsci2010.04.0195
McKenna, A., Hanna, M., Banks, E., Sivachenko, A., Cibulskis, K., Kernytsky, A., et al. (2010). The Genome Analysis Toolkit: a MapReduce framework for analyzing next-generation DNA sequencing data. Genome Res. 20, 1297–1303. doi: 10.1101/gr.107524.110
Michelmore, R. W., Paran, I., and Kesseli, R. V. (1991). Identification of markers linked to disease-resistance genes by bulked segregant analysis: a rapid method to detect markers in specific genomic regions by using segregating populations. Proc. Natl. Acad. Sci. U.S.A. 88, 9828–9832. doi: 10.1073/pnas.88.21.9828
Millet, Y. A., Danna, C. H., Clay, N. K., Songnuan, W., Simon, M. D., Werck-Reichhart, D., et al. (2010). Innate immune responses activated in Arabidopsis roots by microbe-associated molecular patterns. Plant Cell 22, 973–990. doi: 10.1105/tpc.109.069658
Miya, A., Albert, P., Shinya, T., Desaki, Y., Ichimura, K., Shirasu, K., et al. (2007). CERK1, a LysM receptor kinase, is essential for chitin elicitor signaling in Arabidopsis. Proc. Natl. Acad. Sci. U.S.A. 104, 19613–19618. doi: 10.1073/pnas.0705147104
Mohler, V., Zeller, F. J., Wenzel, G., and Hsam, S. L. K. (2005). Chromosomal location of genes for resistance to powdery mildew in common wheat (Triticum aestivum L. em Thell.). 9. Gene MlZec1 from the Triticum dicoccoides-derived wheat line Zecoi-1. Euphytica 142, 161–167. doi: 10.1007/s10681-005-1251-x
Mott, G. A., Thakur, S., Smakowska, E., Wang, P. W., Belkhadir, Y., Desveaux, D., et al. (2016). Genomic screens identify a new phytobacterial microbe-associated molecular pattern and the cognate Arabidopsis receptor-like kinase that mediates its immune elicitation. Genome Biol. 17:98. doi: 10.1186/s13059-016-0955-7
Naseem, M., Srivastava, M., Tehseen, M., and Ahmed, N. (2015). Auxin crosstalk to plant immune networks: a plant-pathogen interaction perspective. Curr. Protein Pept. Sci. 16, 389–394. doi: 10.2174/1389203716666150330124911
Navarro, L., Bari, R., Achard, P., Lisón, P., Nemri, A., Harberd, N. P., et al. (2008). DELLAs control plant immune responses by modulating the balance of jasmonic acid and salicylic acid signaling. Curr. Biol. 18, 650–655. doi: 10.1016/j.cub.2008.03.060
Pankievicz, V. C. S., Camilios-Neto, D., Bonato, P., Balsanelli, E., Tadra-Sfeir, M. Z., Faoro, H., et al. (2016). RNA-seq transcriptional profiling of Herbaspirillum seropedicae colonizing wheat (Triticum aestivum) roots. Plant Mol. Biol. 90, 589–603. doi: 10.1007/s11103-016-0430-6
Pearce, S., Vazquez-Gross, H., Herin, S. Y., Hane, D., Wang, Y., Gu, Y. Q., et al. (2015). WheatExp: an RNA-seq expression database for polyploid wheat. BMC Plant Biol. 15:299. doi: 10.1186/s12870-015-0692-1
Postel, S., Küfner, I., Beuter, C., Mazzotta, S., Schwedt, A., Borlotti, A., et al. (2010). The multifunctional leucine-rich repeat receptor kinase BAK1 is implicated in Arabidopsis development and immunity. Eur. J. Cell Biol. 89, 169–174. doi: 10.1016/j.ejcb.2009.11.001
Pruitt, R. N., Schwessinger, B., Joe, A., Thomas, N., Liu, F., Albert, M., et al. (2015). The rice immune receptor XA21 recognizes a tyrosine-sulfated protein from a Gram-negative bacterium. Sci. Adv. 1:e1500245. doi: 10.1126/sciadv.1500245
Radutoiu, S., Madsen, L. H., Madsen, E. B., Felle, H. H., Umehara, Y., Gronlund, M. S. S., et al. (2003). Plant recognition of symbiotic bacteria requires two LysM receptor-like kinases. Nature 425, 585–592. doi: 10.1038/nature02039
Ramirez-Gonzalez, R., Segovia, V., Bird, N., Fenwick, P., and Uauy, C. (2014). RNA-Seq bulked segregant analysis enables the identification of high-resolution genetic markers for breeding in hexaploid wheat. Plant Biotechnol. J. 13, 613–624. doi: 10.1111/pbi.12281
Reiner, A., Yekutieli, D., and Benjamini, Y. (2003). Identifying differentially expressed genes using false discovery rate controlling procedures. Bioinformatics 19, 368–375. doi: 10.1093/bioinformatics/btf877
Rudd, J. J., Kanyuka, K., Hassani-Pak, K., Derbyshire, M., Andongabo, A., Devonshire, J., et al. (2015). Transcriptome and metabolite profiling of the infection cycle of Zymoseptoria tritici on wheat reveals a biphasic interaction with plant immunity involving differential pathogen chromosomal contributions and a variation on the hemibiotrophic lifestyle definition. Plant Physiol. 167, 1158–1185. doi: 10.1104/pp.114.255927
Sarris, P., Duxbury, Z., Huh, S., Ma, Y., Segonzac, C., Sklenar, J., et al. (2015). A plant immune receptor detects pathogen effectors that target WRKY transcription factors. Cell 161, 1089–1100. doi: 10.1016/j.cell.2015.04.024
Saur, M. L. I., Kadota, Y., Sklenar, J., Nicholas, N. J., and Smakowska, E. (2000). NbCSPR underlies age-dependent immune responses to bacterial cold shock protein in Nicotiana benthamiana. Proc. Natl. Acad. Sci. U.S.A. 113, 3389–3394. doi: 10.1073/pnas.1511847113
Schwessinger, B., and Ronald, P. C. (2012). Plant innate immunity: perception of conserved microbial signatures. Annu. Rev. Plant Biol. 63, 451–482. doi: 10.1146/annurev-arplant-042811-105518
Si, Q. M., Zhang, X. X., Duan, X. Y., Sheng, B. Q., and Zhou, Y. L. (1992). On gene analysis and classification of powdery mildew (Erysiphe graminis f. sp. tritici) resistant wheat varieties. Acta Phytopathol. Sin. 22, 349–355. doi: 10.13926/j.cnki.apps.1992.04.021
Singh, R. P., Singh, P. K., Rutkoski, J., Hodson, D. P., He, X., Jorgensen, L. N., et al. (2016). Disease impact on wheat yield potential and prospects of genetic control. Annu. Rev. Phytopathol. 54, 303–322. doi: 10.1146/annurev-phyto-080615-095835
Takagi, H., Abe, A., Yoshida, K., Kosugi, S., Natsume, S., Mitsuoka, C., et al. (2013). QTL-seq: rapid mapping of quantitative trait loci in rice by whole genome resequencing of DNA from two bulked populations. Plant J. 74, 174–183. doi: 10.1111/tpj.12105
Tan, C., Li, G., Cowger, C., Carver, B. F., and Xu, X. (2019). Characterization of Pm63, a powdery mildew resistance gene in Iranian landrace PI 628024. Theor. Appl. Genet. 132, 1137–1144. doi: 10.1007/s00122-018-3265-5
The International Wheat Genome Sequencing Consortium [IWGSC] (2018). Shifting the limits in wheat research and breeding using a fully annotated reference genome. Science 361:eaar7191. doi: 10.1126/science.aar7191
Trapnell, C., Williams, B. A., Pertea, G., Mortazavi, A., Kwan, G., Baren, M. J., et al. (2010). Transcript assembly and quantification by RNA Seq reveals unannotated transcripts and isoform switching during cell differentiation. Nat. Biotechnol. 28, 511–515. doi: 10.1038/nbt.1621
Trick, M., Adamski, N. M., Mugford, S. G., Jiang, C. C., Febrer, M., and Uauy, C. (2012). Combining SNP discovery from next-generation sequencing data with bulked segregant analysis (BSA) to fine-map genes in polyploid wheat. BMC Plant Biol. 12:14. doi: 10.1186/1471-2229-12-14
Tsai, H. Y., Janss, L. L., Andersen, J. R., Orabi, J., Jensen, J. D., Jahoor, A., et al. (2020). Genomic prediction and GWAS of yield, quality and disease-related traits in spring barley and winter wheat. Sci. Rep. 10:3347. doi: 10.1038/s41598-020-60203-2
Twamley, T., Gaffney, M., and Feechan, A. (2019). A microbial fermentation mixture primes for resistance against powdery mildew in wheat. Front. Plant Sci. 10:1241. doi: 10.3389/fpls.2019.01241
Verbancic, J., Lunn, J. E., Stitt, M., and Persson, S. (2018). carbon supply and the regulation of cell wall synthesis. Mol. Plant. 11, 75–94. doi: 10.1016/j.molp.2017.10.004
Wan, W. T., Xiao, J., Li, M. L., Tang, X., Wen, M. X., Cheruiyot, A. K., et al. (2020). Fine mapping of wheat powdery mildew resistance gene Pm6 using 2B/2G homoeologous recombinants induced by the ph1b mutant. Theor. Appl. Genet. 133, 1265–1275. doi: 10.1007/s00122-020-03546-8
Wang, W. R., He, H. G., Gao, H. G., Xu, H. X., Song, W. Y., Zhang, X., et al. (2021). Characterization of the powdery mildew resistance gene in wheat breeding line KN0816 and its evaluation in marker-assisted selection. Plant Dis. doi: 10.1094/PDIS-05-21-0896-RE Online ahead of print,
Wang, Y., Xie, J., Zhang, H., Guo, B., Ning, S., Chen, Y., et al. (2017). Mapping stripe rust resistance gene YrZH22 in Chinese wheat cultivar Zhoumai 22 by bulked segregant RNA-Seq (BSR-Seq) and comparative genomics analyses. Theor. Appl. Genet. 130, 2191–2201. doi: 10.1007/s00122-017-2950-0
Wu, P., Hu, J., Zou, J., Qiu, D., Qu, Y., Li, Y., et al. (2019). Fine mapping of the wheat powdery mildew resistance gene Pm52 using comparative genomics analysis and the Chinese Spring reference genomic sequence. Theor. Appl. Genet. 132, 1451–1461. doi: 10.1007/s00122-019-03291-7
Wu, P., Xie, J., Hu, J., Qiu, D., Liu, Z., Li, J., et al. (2018). Development of molecular markers linked to powdery mildew resistance gene Pm4b by combining SNP discovery from transcriptome sequencing data with bulked segregant analysis (BSR-Seq) in Wheat. Front. Plant Sci. 9:95. doi: 10.3389/fpls.2018.00095
Wu, X., Bian, Q., Gao, Y., Ni, X., Sun, Y., Xuan, Y., et al. (2021). Evaluation of resistance to powdery mildew and identification of resistance genes in wheat cultivars. PeerJ 9:e10425. doi: 10.7717/peerj.10425
Xiao, M., Song, F., Jiao, J., Wang, X., Xu, H., and Li, H. (2013). Identification of the gene Pm47 on chromosome 7BS conferring resistance to powdery mildew in the Chinese wheat landrace Hongyanglazi. Theor. Appl. Genet. 126, 1397–1403. doi: 10.1007/s00122-013-2060-6
Xing, L., Hu, P., Liu, J., Witek, K., Zhou, S., Xu, J., et al. (2018). Pm21 from Haynaldia villosa encodes a CC-NBS-LRR protein conferring powdery mildew resistance in wheat. Mol. Plant. 11, 874–878. doi: 10.1016/j.molp.2018.02.013
Yamada, K., Yamaguchi, K., Shirakawa, T., Nakagami, H., Mine, A., Ishikawa, K., et al. (2016). The Arabidopsis CERK1-associated kinase PBL27 connects chitin perception to MAPK activation. EMBO J. 35, 2468–2483. doi: 10.15252/embj.201694248
Yan, W., Xu, Y., Sun, Y., Wang, H., and Wang, Y. (2018). Leucine-rich repeat receptor-like gene screen reveals that Nicotiana RXEG1 regulates glycoside hydrolase 12 MAMP detection. Nat. Commun. 9:594. doi: 10.1038/s41467-018-03010-8
Yu, G., Wang, L. G., Han, Y., and He, Q. Y. (2012). clusterProfiler: an R package for comparing biological themes among gene clusters. OMICS 16, 284–287. doi: 10.1089/omi.2011.0118
Yuan, M., Jiang, Z., Bi, G., Nomura, K., Liu, M., Wang, Y., et al. (2021). Pattern-recognition receptors are required for NLR-mediated plant immunity. Nature 592, 105–109. doi: 10.1038/s41586-021-03316-6
Zhan, H. X., Li, G. R., Zhang, X. J., Li, X., Guo, H. J., Gong, W. P., et al. (2014). Chromosomal location and comparative genomics analysis of powdery mildew resistance gene Pm51 in a putative wheat-Thinopyrum ponticum introgression line. PLoS One 9:e113455. doi: 10.1371/journal.pone.0113455
Zhang, D. Y., Zhu, K. Y., Dong, L. L., Liang, Y., Li, G. Q., Fang, T. L., et al. (2019). Wheat powdery mildew resistance gene Pm64 derived from wild emmer (Triticum turgidum var. dicoccoides) is tightly linked in repulsion with stripe rust resistance gene Yr5. Crop J. 7, 761–770. doi: 10.1016/j.cj.2019.03.003
Zhang, Z., Wu, Y., Gao, M., Zhang, J., Kong, Q., Liu, Y., et al. (2012). Disruption of PAMP-induced MAP kinase cascade by a Pseudomonas syringae effector activates plant immunity mediated by the NB-LRR protein SUMM2. Cell Host Microbe 11, 253–263. doi: 10.1016/j.chom.2012.01.015
Zhu, T., Wu, L., He, H., Song, J., Jia, M., Liu, L., et al. (2020). Bulked segregant RNA-Seq reveals distinct expression profiling in Chinese wheat cultivar Jimai 23 responding to powdery mildew. Front. Genet. 11:474. doi: 10.3389/fgene.2020.00474
Keywords: wheat, powdery mildew, BSR-seq, expression profiling, DEG
Citation: Ma P, Wu L, Xu Y, Xu H, Zhang X, Wang W, Liu C and Wang B (2021) Bulked Segregant RNA-Seq Provides Distinctive Expression Profile Against Powdery Mildew in the Wheat Genotype YD588. Front. Plant Sci. 12:764978. doi: 10.3389/fpls.2021.764978
Received: 26 August 2021; Accepted: 03 November 2021;
Published: 03 December 2021.
Edited by:
Maoqun Yu, Chengdu Institute of Biology, Chinese Academy of Sciences (CAS), ChinaReviewed by:
Jian Ma, Sichuan Agricultural University, ChinaCopyright © 2021 Ma, Wu, Xu, Xu, Zhang, Wang, Liu and Wang. This is an open-access article distributed under the terms of the Creative Commons Attribution License (CC BY). The use, distribution or reproduction in other forums is permitted, provided the original author(s) and the copyright owner(s) are credited and that the original publication in this journal is cited, in accordance with accepted academic practice. No use, distribution or reproduction is permitted which does not comply with these terms.
*Correspondence: Pengtao Ma, cHRtYUB5dHUuZWR1LmNu; Cheng Liu, bGNoNjY4ODQwN0AxNjMuY29t; Bo Wang, d2FuZ2JvQHl0dS5lZHUuY24=
†These authors have contributed equally to this work
Disclaimer: All claims expressed in this article are solely those of the authors and do not necessarily represent those of their affiliated organizations, or those of the publisher, the editors and the reviewers. Any product that may be evaluated in this article or claim that may be made by its manufacturer is not guaranteed or endorsed by the publisher.
Research integrity at Frontiers
Learn more about the work of our research integrity team to safeguard the quality of each article we publish.