- 1School of Life Sciences, Chongqing Normal University, Chongqing, China
- 2Key Laboratory of Plant Hormones and Development Regulation of Chongqing, School of Life Sciences, Chongqing University, Chongqing, China
- 3College of Bioengineering, Chongqing University, Chongqing, China
Botrytis cinerea is one of the most destructive fungal pathogens causing tremendous losses in fresh fruit or vegetables. 3-Methylthio-1-propanol (3-MP) is a naturally occurring food-borne sulfide, which is mainly used to increase the flavor in food. However, the potential application of 3-MP in the postharvest phase to manage fruit fungal diseases has not been explored. In this study, the antifungal activity of 3-MP against B. cinerea was evaluated, and the possible mechanism involved was explored. In vitro 3-MP treatment could effectively inhibit the mycelial growth, spore germination, and germ tube elongation of B. cinerea. 3-MP also impaired the spore viability and membrane integrity of B. cinerea as well as increased the leakage of nucleic acids, proteins, and malondialdehyde (MDA) in B. cinerea. In vivo 3-MP fumigation treatment inhibited the infection of B. cinerea on tomato fruits. Also, the fruits with 3-MP fumigation treatment exhibited higher antioxidant enzyme activity, lower MDA content, and a significant delay of induction of the expression of most of the stress-related genes when compared to the control group. Moreover, a cytotoxicity evaluation revealed that 3-MP had no toxicity to normal cells in a certain concentration range. Collectively, our research results will provide evidence for the development of food-borne sulfide 3-MP as a fungicide in food and agriculture and will provide an important reference for the formulation of B. cinerea biocontrol strategies.
Highlights
- 3-MP inhibited the infection and growth of Botrytis cinerea in vitro and in vivo.
- 3-MP fumigation treatment destroyed mycelial structure of Botrytis cinerea.
- 3-MP impaired the cell viability and membrane integrity of Botrytis cinerea.
- 3-MP affected the defense systems of tomato fruit.
- 3-MP had no toxicity to normal cells.
Introduction
Botrytis cinerea is one of the most serious and widespread postharvest pathogens of much fresh fruit, vegetables, and ornamentals (Chaouachi et al., 2021). Botrytis cinerea is recognized as a “high-risk” pathogen for its characteristics of the short life cycle, high reproduction, and genetic variation, which are not beneficial for maintaining the resistance of fungicides (Haidar et al., 2016; Saito et al., 2019). At present, several control strategies have been applied to control B. cinerea. Among them, chemical fungicides were considered to be the most widely and economical measure. However, many hazards or risks have been caused by the excessive application of chemical fungicides, mainly such as pollution of the environment, food safety threats, and the production of fungal-resistant strains (Tian et al., 2016; Morita et al., 2019; Liu X. et al., 2020). Hence, there is an urgent need for safe bioderived antimicrobial agents to control B. cinerea and reduce the loss of postharvest fruit.
Recently, a large number of bioderived antimicrobial agents that were generally regarded as safe were demonstrated to be effective in controlling postharvest pathogens, such as carvacrol (Pei et al., 2020), methyl thujate (Ma et al., 2020), and others. Among them, a kind of sulfur-containing spice compound was noticeable, which was mainly obtained from plants or microorganisms (Schöller et al., 2002; Hongman et al., 2018; Xie et al., 2020). The study in pear showed that the infection and development of black spots caused by Alternaria alternata were effectively inhibited by benzyl isothiocyanate (BITC) fumigation (Wang, 2020). The in vivo study showed that both dimethyl disulfide (DMDS) and dimethyl trisulfide (DMTS) exhibit excellent antifungal activity, which can completely inhibit the infection and growth of blue mold on citrus fruits (Wang et al., 2021). In addition, the growth of Colletotrichum gloeosporioides, Aspergillus ochraceus, Aspergillus flavus, and Sclerotinia minor could be effectively inhibited by DMTS, and further study indicated that the mechanism involved in this process was mainly due to the suppression of mycelial growth and spore germination with the destruction of the cell membrane (Zuo et al., 2017; Tyagi et al., 2020). Consistently, a recent study revealed the wide use of sulfur-containing spice compounds in controlling fungal diseases in various fruit or vegetables: its excellent role is attributed to the antioxidant and antimicrobial activity (Shang et al., 2019). Therefore, given the uniqueness and effectiveness of food-borne volatile sulfur compounds, more food-borne sulfides should be discovered as microbial inhibitors.
The existed studies indicated that 3-methylthio-1-propanol (3-MP) was first reported in the volatile flavor compounds in wine (0.86–4.91 mg L–1) (Schreier et al., 1974; Fedrizzi et al., 2007; González-Álvarez et al., 2012). 3-MP was found widely in some fruit, beer, and malt whisky as a natural sulfur-containing spice compound (Etschmann et al., 2008; Moreira et al., 2010). A previous report showed that 3-MP can significantly contribute to the flavor quality of wine, bread, and other foods (Moreira et al., 2011) and can enhance the saltiness of weak salt content and the umami taste of monosodium glutamate solution (Zhou et al., 2021). To our knowledge, whether 3-MP has an antimicrobial activity is still unknown so far. Given this, the objective of this study was to investigate the inhibitory effect of 3-MP against B. cinerea by conducting in vitro and in vivo assays, and the possible mechanisms involved in this process were also explored through microscopic observation, physiological detection, and gene expression analysis. The results obtained would lay a foundation for the development of 3-MP as a new fungicide and also provide an important reference for developing an eco-friendly strategy to control B. cinerea.
Materials and Methods
Materials and Chemicals
The fungal pathogen B. cinerea B05.10 used in this study was obtained from the School of Life Sciences, Chongqing University, China. Cherry tomato (Solanum lycopersicum) fruits that were free from wounds with comparable size and stage of maturity were harvested from Shiyi Bai experimental orchard for infection experiments.
For the chemicals, 3-MP can be easily dissolved into the water and purchased from Sigma (St. Louis, MO, United States). The fluorescein diacetate (FDA, Yeasen Biotechnology Co., Ltd., Shanghai, China) and propidium iodide (PI, Yeasen Biotechnology Co., Ltd., Shanghai, China) were purchased from Sangon Biotechnology Co., Ltd. (Shanghai, China).
Evaluation in vitro and in vivo of the Antifungal Activity of 3-Methylthio-1-Propanol Fumigation Treatment
For in vitro assay, B. cinerea mycelial plugs were placed on the side of the two-part plates containing potato dextrose agar (PDA) medium, and 0, 10, 25, 50, 100, and 200 μl of 3-MP was added to the other side of the two-part plates. After inoculation, the Petri dishes were quickly sealed and placed in 25°C constant temperature incubators for 5 days, and then, the colony diameters were measured and recorded. According to the modified method of Elsherbiny et al. (2020), the effects of 3-MP on mycelium morphology were analyzed. The mycelium exposed to 3-MP treatment was fixed with 2.5% glutaraldehyde for 2 h and washed three times for 10 min in 0.1 M phosphate buffer (pH 7.0). After fixation, the samples were dehydrated in graded ethanol series (30, 50, 70, 80, 90, and 100%), for 15 min in each process. The samples were then washed in 3:1, 1:1, and 1:3 mixtures of ethanol and tert-butyl alcohol and finally dipped into pure tert-butyl alcohol, for 10 min in each process. The samples were dried by sublimation in a freeze dryer (ES-2030; HITACHI, Japan) and coated with gold by a sputter coating (E1010, HITACHI, Japan) for 30 s. The samples of the same treatment were sampled three times independently and observed after preparation. A scanning electron microscope (TM4000Plus II, HITACHI, Japan) was used to observe the morphological characteristics of B. cinerea mycelium.
For in vivo assay, tomato fruits that were uniform in size and disease-free were washed and dried, and 10 fruits were placed in each large glass Petri dish (15 cm in diameter and 5.5 cm in height). After the tomato (equatorial position) was scratched by a sterile toothpick, a gray-mold mycelial plug (3.0 mm) was inoculated on the tomato wound. Then, a small Petri dish (i.e., 6.0 cm in diameter, with a piece of filter paper) was placed in the center of the large glass Petri dish (Figure 1A). The Petri dishes were immediately sealed after adding 50 μl 3-MP or sterile water to the filter paper and placed in a 25°C constant temperature incubator for cultivation. Each treatment consisted of 12 large Petri dishes. Tomato lesions were monitored at 0, 24, 48, and 96 h following treatment. For sampling collection, the fruit tissue with a diameter of 1.5 cm around the inoculation point was collected after removing the mycelial plugs on the surface of the tomato, and the tissues of every 10 fruits were gathered and mixed, put into liquid nitrogen immediately, and finally stored at –80°C for further use. This experiment was repeated three times independently from fumigation treatment to sampling.
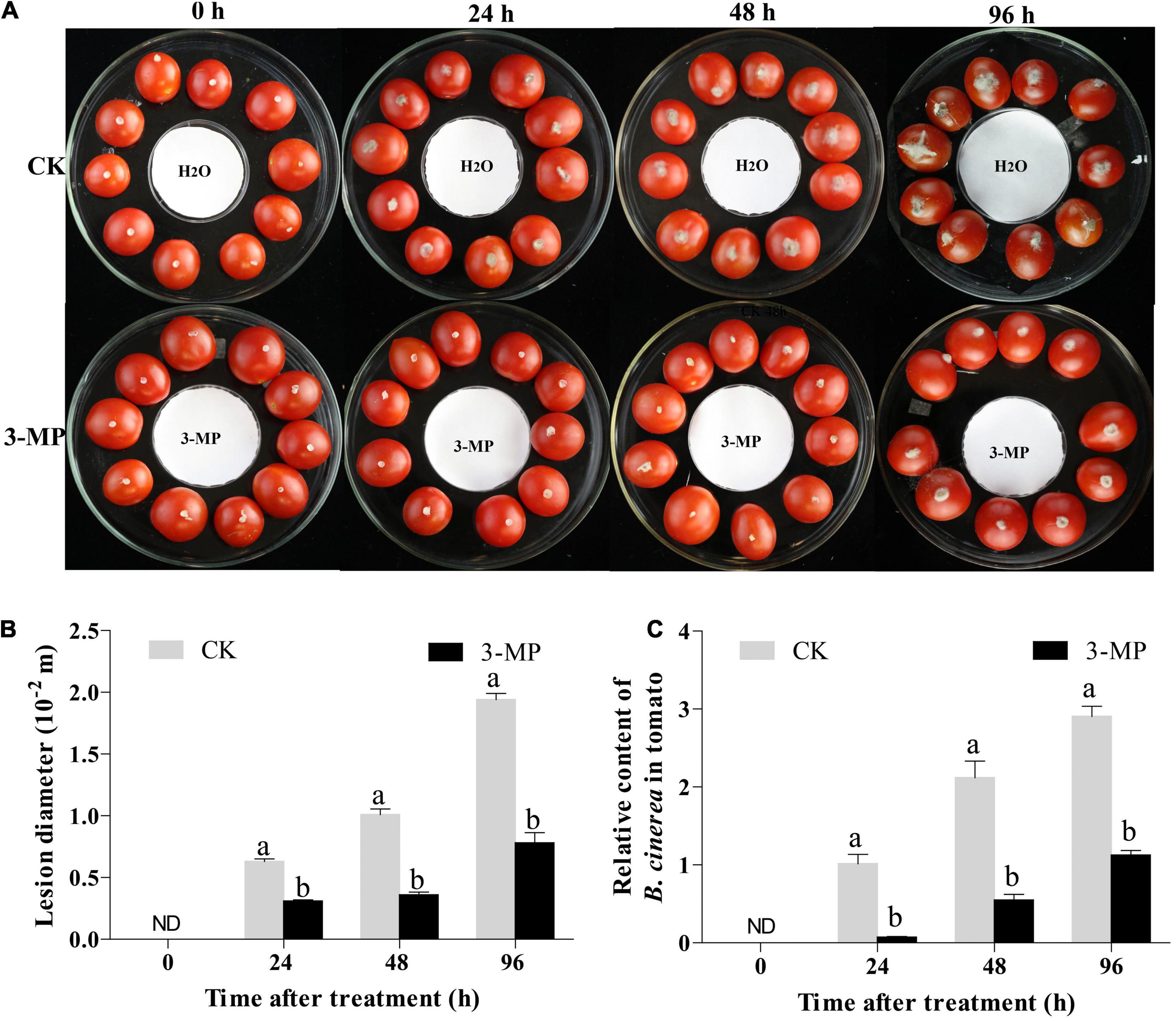
Figure 1. 3-Methylthio-1-propanol (3-MP) decreased the symptoms of gray mold in tomato fruit. (A) Disease symptoms on tomato treated with sterile water or 3-MP for 0, 24, 48, and 96 h. (B) Effect of 3-MP on lesion diameter of gray mold. (C) Accumulation of Botrytis cinerea in fruit after inoculation for 0, 24, 48, and 96 h. ND means not detection. B. cinerea content was calculated by the comparison of the ratio of B. cinerea DNA to tomato DNA. The experiment was performed in triplicates, and values represent the means ± SD of 10 individual fruit. The different lowercase letter means statistically significant (Student’s t-test).
Extraction of Total RNA, DNA, and Quantitative Real-Time PCR
Total RNA was extracted from tomato fruits with different treatments using an RNeasy Plant Mini Kit (Tiangen, China) according to the protocol of the manufacturer.
The DNA of tomato fruits with different treatments was extracted using a Plant Genomic DNA Kit (CWBio, China) to quantify the amount of gray mold.
To measure the expression level of fungal pathogenicity genes, B. cinerea was cultured as the method described earlier (Wang et al., 2020). In brief, mycelium was collected after culturing in potato dextrose broth (PDB) medium containing 0, 25, and 100 μl L–1 3-MP for 12 h.
For the quantitative real-time PCR (qRT-PCR) assay, NanoDrop Lite (Thermo Scientific, California, United States) was used to detect the centration and quality of the total RNA or DNA. The first-strand cDNA was synthesized by using PrimeScript™ RT Reagent Kit with gDNA Eraser (Perfect Real Time) (TAKARA, Japan). The qRT-PCR mixture was performed using an SYBR® Premix Ex Taq™ (Tli RNaseH Plus) (TAKARA, Japan), and the reaction was conducted with a Bio-Rad CFX system (Bio-Rad, California, United States). Each sample was performed in triplicate, and the relative fold differences were calculated using a comparative Ct method. SlUBI and BcActin were used as the internal reference in tomato and B. cinerea background, respectively (Hua et al., 2019; Jian et al., 2019). All the primers used in the whole experiment were listed in Supplementary Table 1.
Measurement of Antioxidant Enzymes Activity
The tissue sample (3.0 g) was weighed and then placed together with less silica sand (0.3 g) in a prefrozen mortar, and 10 ml precooled phosphate-buffered saline (PBS; 50 mM, pH 7.8) with 1% polyvinylpyrrolidone was added to grind gently (Zhao et al., 2020). The ground tissue sample that was obtained above was fully mixed with 9 ml of PBS and then transferred to a centrifuge tube for centrifugation at 12,000 × g for 8 min at 4°C. Then, the supernatant was collected to evaluate the activity of antioxidant enzyme Superoxide Dismutase (SOD), Peroxidase (POD), and Catalase (CAT) according to the operating instruction provided by the Nanjing Construction Bioengineering Research1 kit (Liu Y. et al., 2020). The experiment was repeated two times, and each treatment was conducted in triplicate. All the tomato tissue weights that were mentioned in the whole manuscript represent the fresh weight. Enzyme activity is defined as U kg–1 of fresh weight.
Cytoplasmic Leakage Assay
The spore suspension of B. cinerea (107 spores per ml) was inoculated in a PDB medium placed in a shaker incubator with the condition of 180 rpm, 25 ± 1°C (Wang et al., 2020). After 3 days, 2.0 g mycelium was collected with filter paper and resuspended in sterile distilled water containing 3-MP (0, 25, 100 μl L–1). After incubation at 25 ± 1°C for different times (i.e., 0, 3, 6, 9, and 12 h), the supernatant was collected for the detection of cytoplasmic leakage. For the measurement of leakage of nucleic acids, the optical density at 260 nm (OD260) of the supernatant was measured. The leakage of proteins was measured with a Bradford Protein Assay Kit (Nanjing Construction Bioengineering Research). The malondialdehyde (MDA) content was tested by the method of thiobarbituric acid (TBA) (Pereira et al., 2003). The experiment was repeated two times, and each treatment was conducted in triplicate.
Fluorescence Microscopy
Both FDA and PI were used to examine the spore viability and membrane integrity by counting stained spores (Wang et al., 2020). After being in a PDB medium with 3-MP (0, 25, 100 μl L–1) for 3 h at 25°C, the spores were treated with 50 mg L–1 FDA and 20 mg L–1 PI for 10 min at 25°C, respectively, and then observed by using a fluorescence microscope (Leica, Germany). Spores were counted using a hemocytometer. Five fields of view were randomly observed per treatment, and the experiment was repeated two times.
Effects of 3-Methylthio-1-Propanol on Cell Viability and the Measurement of Half-Maximal Inhibitory Concentration
To evaluate the security and application potential of 3-MP, a cytotoxicity assay was conducted. 3-MP was diluted to 0, 2, 4, 8, 16, 32, 64, 128, 256, and 512 mg L–1, respectively, with cell culture medium (Gibco, California, United States). Mouse fibroblast cells (L929), human non-small cell lung cancer cells (A549), and mouse breast cancer cells (4T1) were chosen in this experimental section. Notably, 100 μl cell suspension (about 10,000 cells) was added to 96-well plates, and three repeated experiments were set at each concentration. Cells were cultured in the incubator for 24 h (37°C and 5% CO2), and then, 10 μl each of different concentrations of 3-MP was added. The effects of 3-MP on cell viability were measured according to the Cell Counting Kit-8 (CCK8) method (Bioground, China). Later, cells were cultured in the incubator for 12 h. Finally, the viability of three different cells was analyzed to acquire the IC50 value of 3-MP.
Statistical Analysis
The statistical tests were performed using SPSS software (version 17.0) (IBM, New York, United States) with a one-way ANOVA. Duncan’s multiple range test and Student’s t-tests were used to analyze the data, and a difference was considered to be statistically significant when P < 0.05.
Results
3-Methylthio-1-Propanol Inhibited the Growth of Botrytis cinerea in vitro
The mycelial growth of B. cinerea was gradually increased in the control plates throughout the incubation period. However, the mycelial growth in the 3-MP fumigation treatment was significantly inhibited and exhibited a dose-dependent fashion (Figure 2A). The 3-MP fumigation treatment also destroyed the ultrastructure of B. cinerea mycelium. The degree of mycelial damage is positively correlated with the concentration of 3-MP (Figure 2B). Especially at 5 days following treatment, in comparison with the control group, the mycelial growth was significantly inhibited to 13.7, 22.2, and 50.8% by 50, 100, and 200 μl 3-MP, respectively (Figures 2C,D).
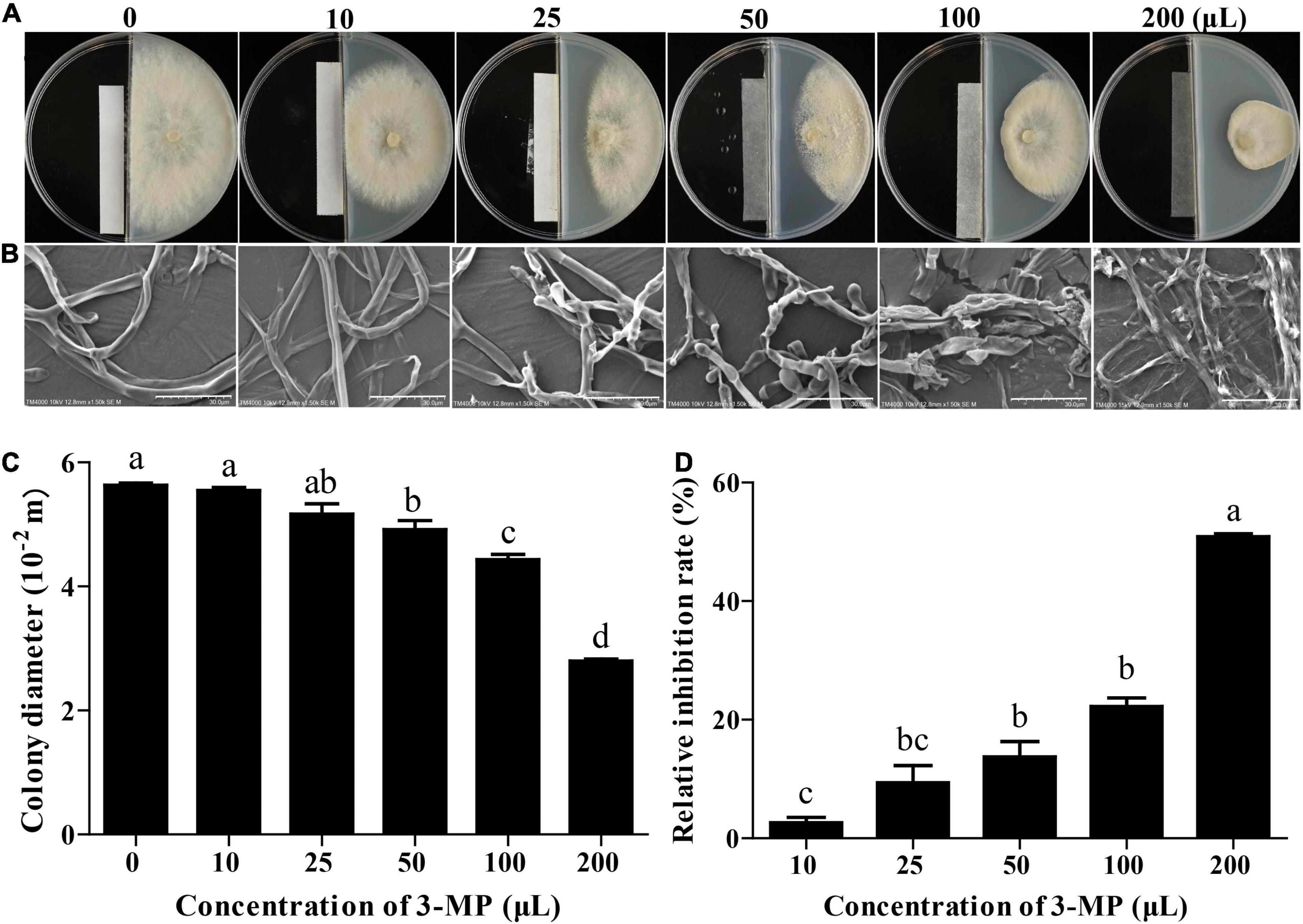
Figure 2. The antifungal ability of 3-methylthio-1-propanol (3-MP) against B. cinerea on PDA medium. Phenotypic (A) and scanning electron microscopic (B) characterization of colony expansion of B. cinerea treated with or without 3-MP fumigation treatment. Bar = 30 μm. Detection of colony diameter (C) and relative inhibition rate (D) in control and 3-MP fumigation treatment group. Values are the means ± SD of three biological replicates analyzed by Duncan’s multiple range test. The different lowercase letter means statistically significant.
To further analyze the physiological changes, the influence of 3-MP on spore germination and germ tube elongation of B. cinerea was observed. The results indicated that the spore germination and germ tube elongation of B. cinerea was suppressed by treatment of 3-MP (Figure 3). At 12 h following treatment, more than 90.2% of the spores germinated on the control media, while the germination rate was decreased to 61.6% on the media treated by 25 μl L–1 3-MP. Also, it is worth specifically mentioning that the spore germination and germ tube elongation of B. cinerea was completely suppressed by 100 μl L–1 3-MP.
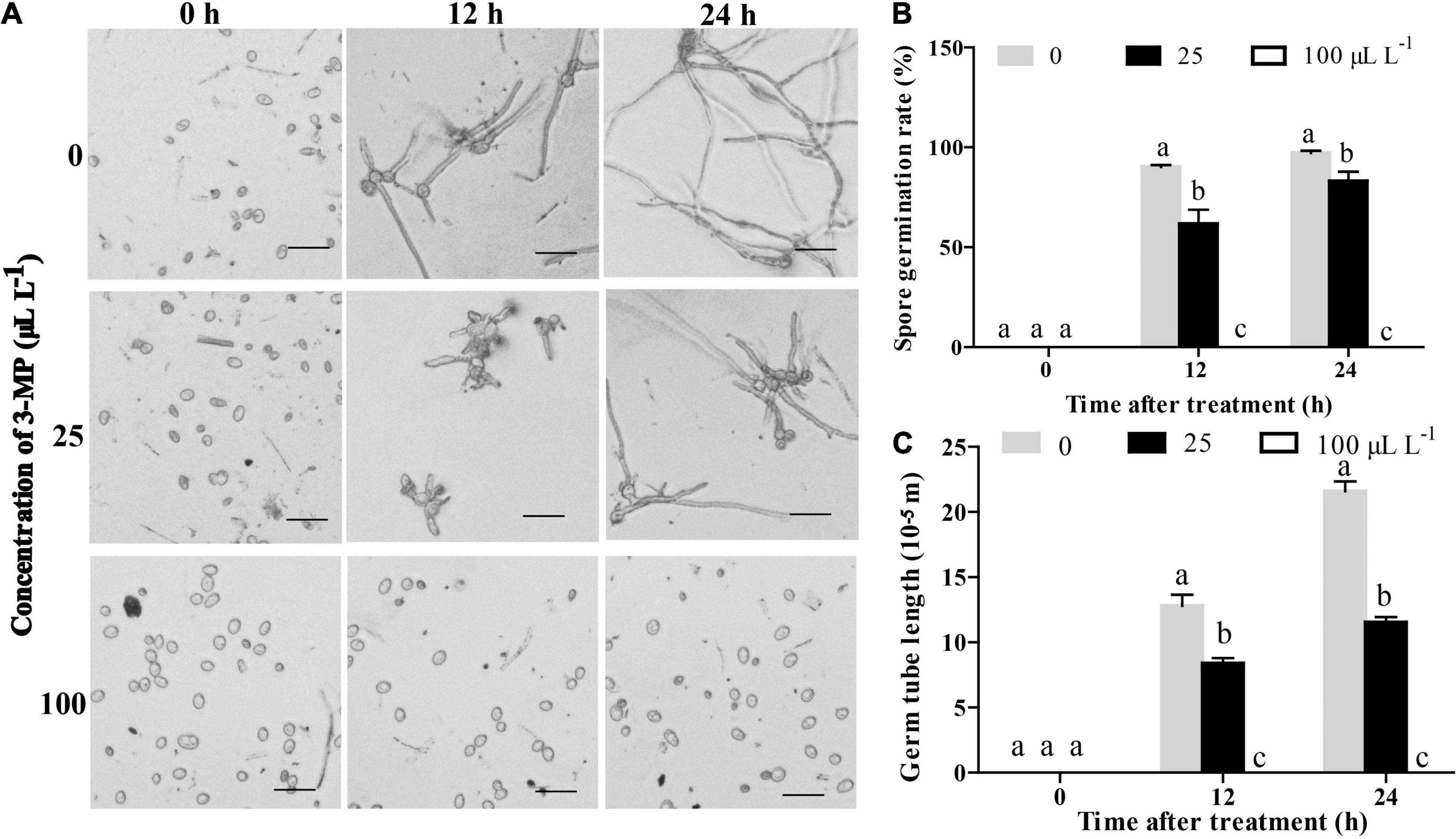
Figure 3. Analysis of the antifungal role of 3-MP on B. cinerea germination and growth. (A) Phenotypic characterization of spore germination of B. cinerea treated with sterile water or different concentration of 3-MP for 0, 12, and 24 h. Bar = 40 μm. (B) The spore germination rate and (C) the germ tube elongation of B. cinerea treated with sterile water or different concentration of 3-MP for 0, 12, and 24 h. Approximately 100 spores were randomly observed in each treatment. The experiment was performed in triplicates, and the values represent means ± SD. The different lowercase letter means statistically significant.
3-Methylthio-1-Propanol Fumigation Treatment Decreased the Disease Phenotype of Gray Mold in Tomato Fruit
To evaluate whether 3-MP fumigation treatment has an antifungal activity on B. cinerea in vivo, the tomato fruit was chosen in this study. As shown in Figure 1A, the lesions in tomato fruits developed after inoculation for 24 h and the lesions in the control fruits were significantly larger than that 50 μl 3-MP treatment. Especially at 96 h following incubation, the diameter of the lesion in 3-MP-treated fruit was 7.8 mm, while the diameter of the lesion in the control fruit was 1.94 mm, which was 2.49-fold higher than the former (Figure 1B). Consistent with this, the quantitative results of qPCR showed that the accumulation of B. cinerea in control fruits was significantly higher than that in 3-MP-treated fruits at all the monitoring times (Figure 1C). In addition, we also detected the content of 3-MP in the fumigated tomato fruit. The results showed that the content of 3-MP in the fumigated tomato fruit was 1.6–2.24 mg L–1 (Supplementary Figure 1). These results indicated that the fumigation treatment of 3-MP can greatly inhibit the development of rot disease caused by B. cinerea.
3-Methylthio-1-Propanol Impaired Spore Viability and Plasma Membrane Integrity of Botrytis cinerea
The percentage of B. cinerea spores stained by FDA in the control was 78.16% (Figures 4A,B), suggesting that most of the fungal spores were survival. However, the percentage of staining spores in 3-MP treatment was 15.27 and 2.47%, suggesting that most of the fungal spores were dead. It has been suggested that PI can penetrate the plasma membrane of dead spores that have lost membrane integrity and can be stained with red fluorescence. As shown in Figures 4C,D, in comparison with the control, more spores were stained by PI after treatment of 25 or 100 μl L–1 3-MP (64.57 and 92.56%, respectively), implying that most spores lost membrane integrity. These results indicated that both the spore survival and membrane integrity of B. cinerea were greatly inhibited by treatment of 3-MP.
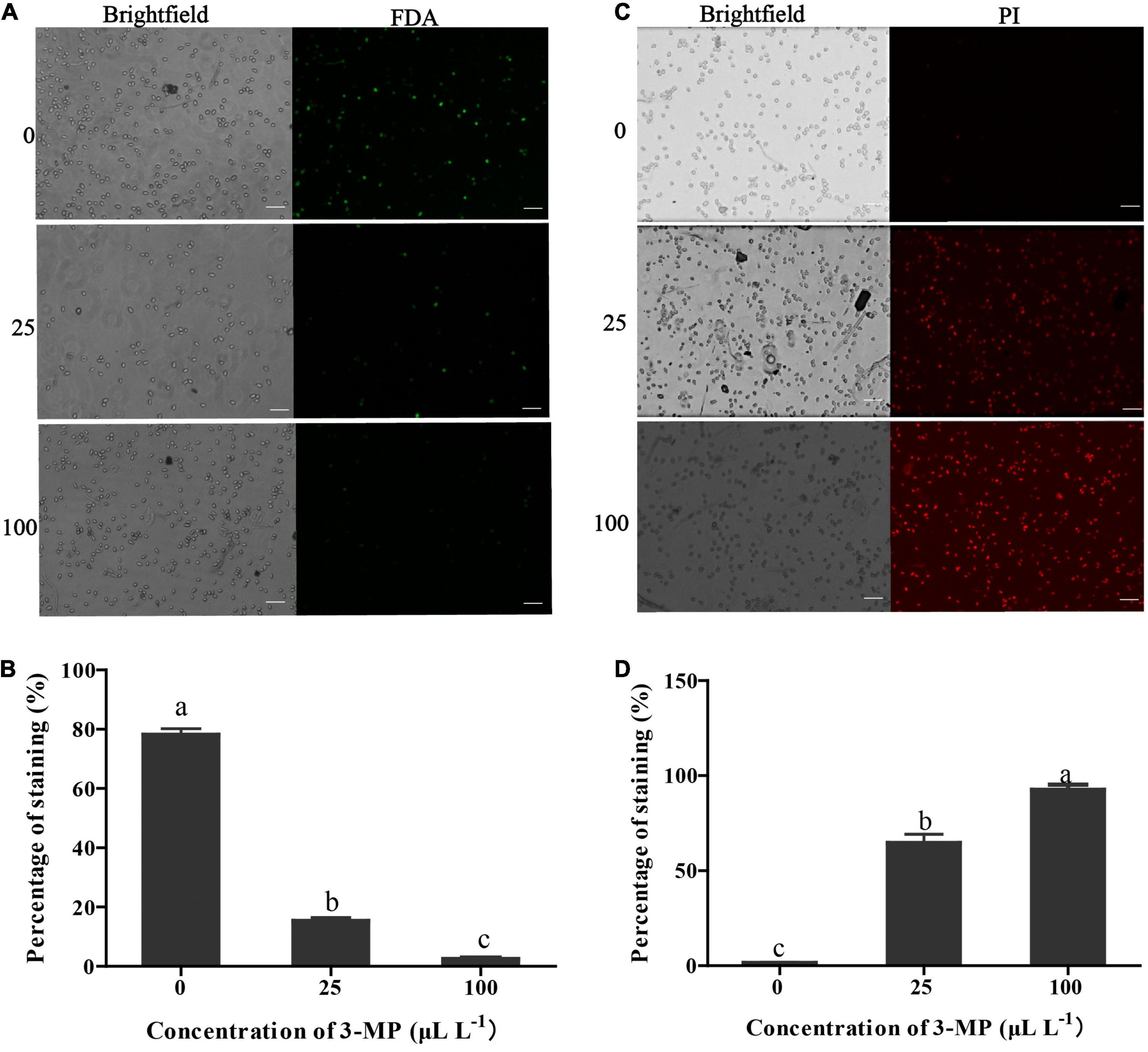
Figure 4. Evaluation of the spore viability and membrane integrity of B. cinerea. (A) Micro-observation of the spores stained with FDA. Bar = 50 μm. (B) The percentage of stained spores with fluorescein diacetate (FDA). (C) Micro-observation of the spores stained with propidium iodide (PI). Bar = 50 μm. (D) The percentage of stained spores with PI. Values are the means ± SD of three biological replicates. The different lowercase letter means statistically significant (Duncan’s multiple range test).
Determination of Cytoplasmic Leakages and Membrane Lipid Peroxidation
The results showed that, at 3 h following treatment, an increase in the content of nucleic acids and proteins was observed in B. cinerea treated by 3-MP when compared to the control group. Noteworthy, at 6 h following treatment, the content of nucleic acids and proteins in the culture medium reached the highest value, suggesting that most of the mycelium had been disrupted by treatment of 3-MP (Figures 5A,B). Consistently, although the MDA content in the control group was relatively stable at all the monitoring times, the MDA content in B. cinerea treated by 3-MP was higher than that in the control group at all the corresponding monitoring times (Figure 5C). These results indicated that 3-MP can impair the membrane integrity of B. cinerea mycelium, which resulted in cytoplasmic leakages and membrane lipid peroxidation of B. cinerea.
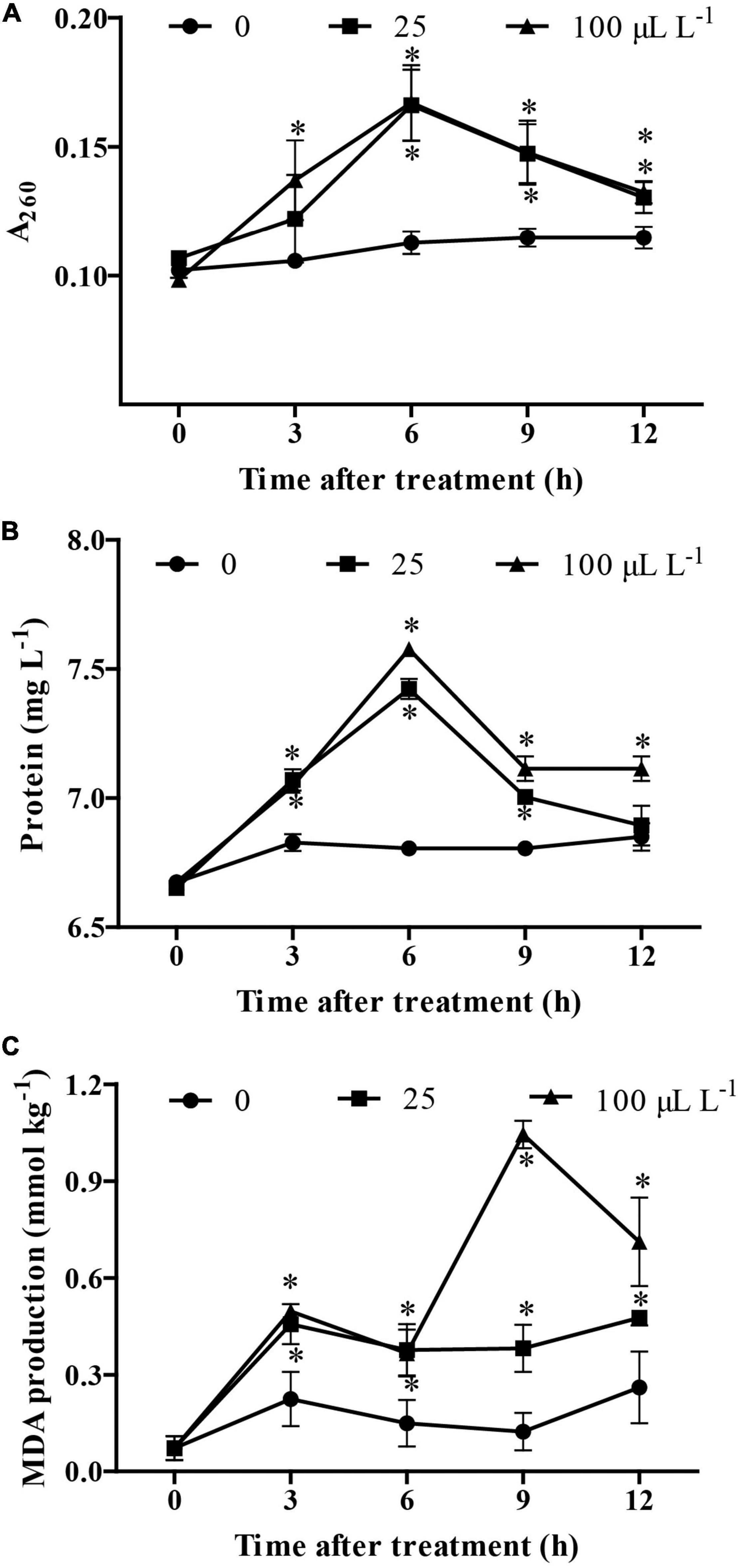
Figure 5. Measurement of cytoplasmic leakages and lipid peroxidation of B. cinerea. (A) Nucleic acids, (B) soluble proteins, and (C) malondialdehyde (MDA) content were determined. Values are the means ± SD of three biological replicates. *P < 0.05.
3-Methylthio-1-Propanol Downregulated the Expression Level of Genes Related to Pathogenicity
To evaluate whether the virulence or ability of infection of B. cinerea was affected by 3-MP, the expression level of BcPG1, BcBMP1, BcPLC1, BcPLS1, and BcPME1, which are key genes in determining the infection and growth of B. cinerea, was analyzed (Gourgues et al., 2004; Schumacher et al., 2008; Cong et al., 2019). As shown in Figure 6, the qRT-PCR results indicated that the expression level of all these genes was differentially downregulated in the 3-MP-treated group. The expression level of BcPG1, BcBMP1, and BcPLS1 was markedly downregulated by treatment of 25 μl L–1 3-MP but exhibited a slight increase when the concentration of 3-MP reached 100 μl L–1. Interestingly, the expression level of BcPLC1 was greatly downregulated when treated by 25 μl L–1 3-MP but not further changed when the concentration of 3-MP reached 100 μl L–1. In addition, 3-MP greatly downregulated the expression level of BcPME1 and exhibited a negative correlation with the concentration of 3-MP.
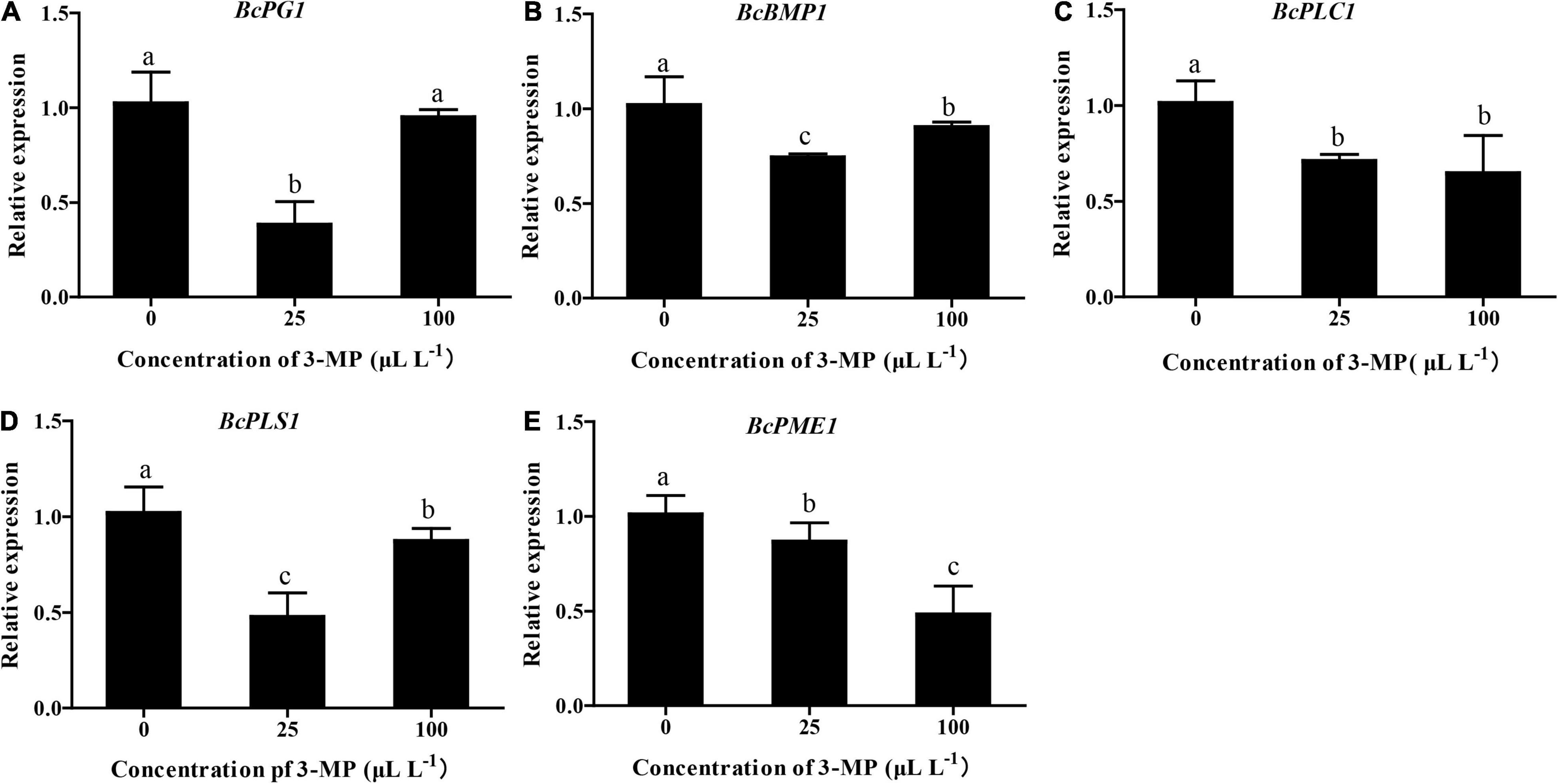
Figure 6. Evaluation of the expression level of genes related to pathogenesis. (A) BcPG1, (B) BcBMP1, (C) BcPLC1, (D) BcPLS1, and (E) BcPME1 genes involved in the virulence and infection process of B. cinerea were selected. The experiment was performed in triplicates, and values represent means ± SD. The different lowercase letter means statistically significant (Duncan’s multiple range test).
The Antioxidant Enzymes Activity Was Promoted by 3-Methylthio-1-Propanol in Tomato Fruit
As shown in Figure 7A, the POD activity in control and 3-MP-treated fruits showed a similar trend, both of their activity increased as time went on, and the activity of POD in fruits treated by 3-MP was significantly higher than that in the control fruits at all the monitoring times. Consistently, the changing pattern of the activity of SOD and CAT was similar, both of their activity exhibited a slight increase at first and then a decrease. Also, both of their activities increase to a maximum peak at 24 and 48 h subjected to treatment of 3-MP, respectively. Notably, the activity of SOD and CAT in 3-MP-treated fruits was always higher than that in control fruits during the whole time (Figures 7B,C). Furthermore, the MDA content in 3-MP-treated fruits increased all the time, and it was significantly lower when compared to that in the control fruits at all the monitoring times (Figure 7D). These results indicated that the antioxidant systems in tomatoes were activated by treatment of 3-MP.
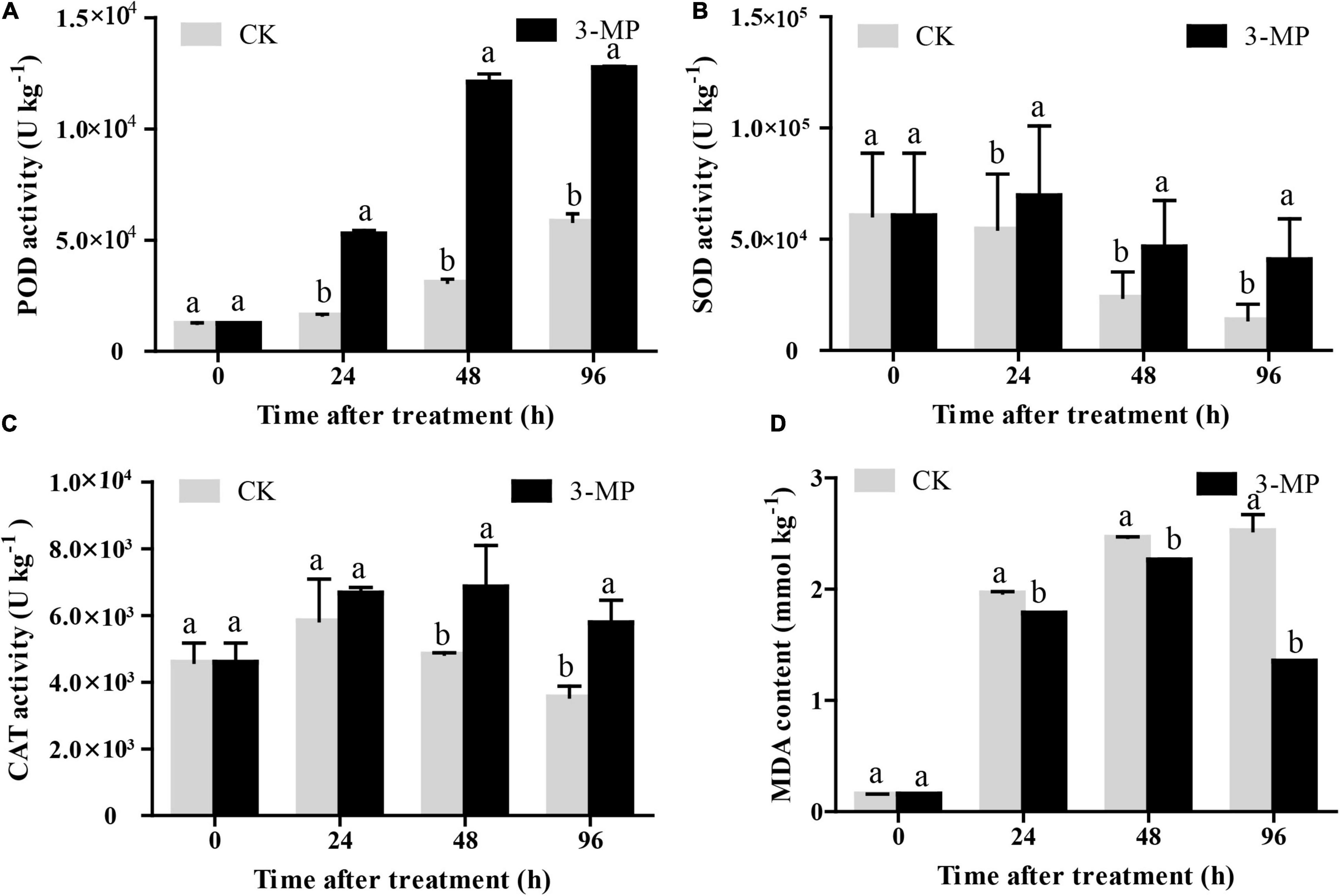
Figure 7. Analysis of the activity of (A) POD, (B) SOD, (C) CAT, and (D) MDA content in tomato fruit. Values are the means ± SD of three biological replicates. The different lowercase letter means statistically significant (Student’s t-test).
Measurement of the Expression Level of Defense-Related Genes in Tomato Fruit
To analyze the molecular processes underlying the positive effect of 3-MP on the resistance of tomato fruit to B. Cinerea, the transcripts of previously reported stress-related genes involved in jasmonic acid (JA), salicylic acid (SA), ethylene signaling pathway, and non-antioxidant pathway, which are usually chosen as biomarkers for plant stress response, were evaluated in tomato fruit treated with or without 3-MP. As shown in Figure 8, the expression level of SlNPR1, SlPR5, SlJAZ1, SlCOI1, SlPI II, and SlLapA1 gene at 24 h in control fruits was higher than that in the fruits treated with 3-MP, while the expression level of remaining genes exhibited no significant difference between control and treatment groups. It should specifically be mentioned that this phenomenon was reversed at 48 h to some extent, as the expression level of SlNPR1, SlJAZ1, SlPI II, SlGST, and SlAPX gene in control fruits was significantly lower than that in the tomato fruits treated with 3-MP. However, the transcripts of other genes, mainly such as SlEDS1, SlPAD4, SlAOC, SlLapA1, and SlERF1, were higher in the control fruits when compared to that in the 3-MP-treated fruits. Most importantly, the expression level of most of the genes in the fruits treated with 3-MP for 96 h was significantly higher than that in control fruits, especially SlEDS1 and SlPR5 gene, whose transcripts in the 3-MP-treated fruits were 3.85 and 12.6 times higher than that in the control fruits, respectively. These results indicated that various defense pathways were activated in response to B. cinerea infection, and the damage caused by B. cinerea was greatly alleviated by treatment of 3-MP.
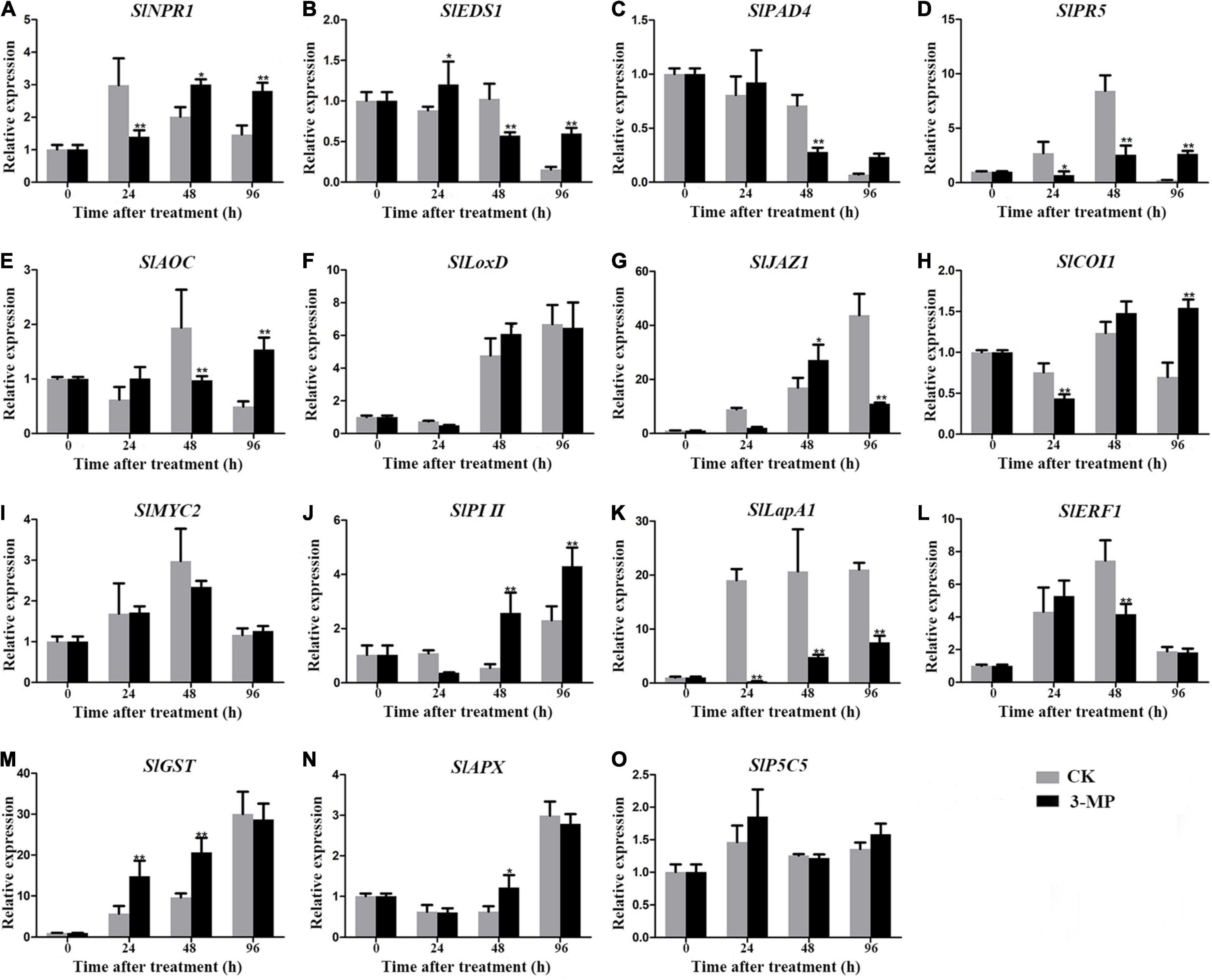
Figure 8. Detection of the expression level of stress-related genes in tomato fruit treated with or without 3-MP. Four salicylic acid signaling-related (SlNPR1, SlEDS1, SlPAD4, and SlPR5), seven jasmonic acid signaling-related (SlAOC, SlLoxD, SlJAZ1, SlCOI1, SlMYC2, SlPI II, and SlLapA1), one ethylene signaling-related (SlERF1) and three non-antioxidant pathway (SlGST, SlAPX, and SlP5CS) genes were selected. (A) SlNPR1, (B) SlEDS1, (C) SlPAD4, (D) SlPR5, (E) SlAOC, (F) SlLoxD, (G) SlJAZ1, (H) SlCOI1, (I) SlMYC2, (J) SlPI II, (K) SlLapA1, (L) SlEF1, (M) SlGST, (N) SlAPX, (O) SlP5C5. Values represent the means ± SD of three independent samples (Student’s t-test, *P < 0.05 and **P < 0.01).
Effects of 3-Methylthio-1-Propanol on L929, A549, and 4T1 Cell Viability
To our knowledge, the existed reports indicated that 3-MP mainly comes from fruit and beer and is mainly used as a food flavoring additive (Moreira et al., 2011). However, there is no report to evaluate whether 3-MP is harmful to humans. As shown in Figure 9, 3-MP exhibited the inhibitory effects on two different tumor cells (i.e., A549 and 4T1) compared to the normal cell (i.e., L929), and this inhibitory effect was more significant with its increasing concentration. According to the result of cell viability, the IC50 value of 3-MP on L929, A549, and 4T1 was 499.19, 91.12, 239.94 μg ml–1, respectively. Compared to 4T1 cells, 3-MP showed higher effectiveness in inhibiting A549 proliferation from the perspective of cell level, and this phenomenon deserved further study.
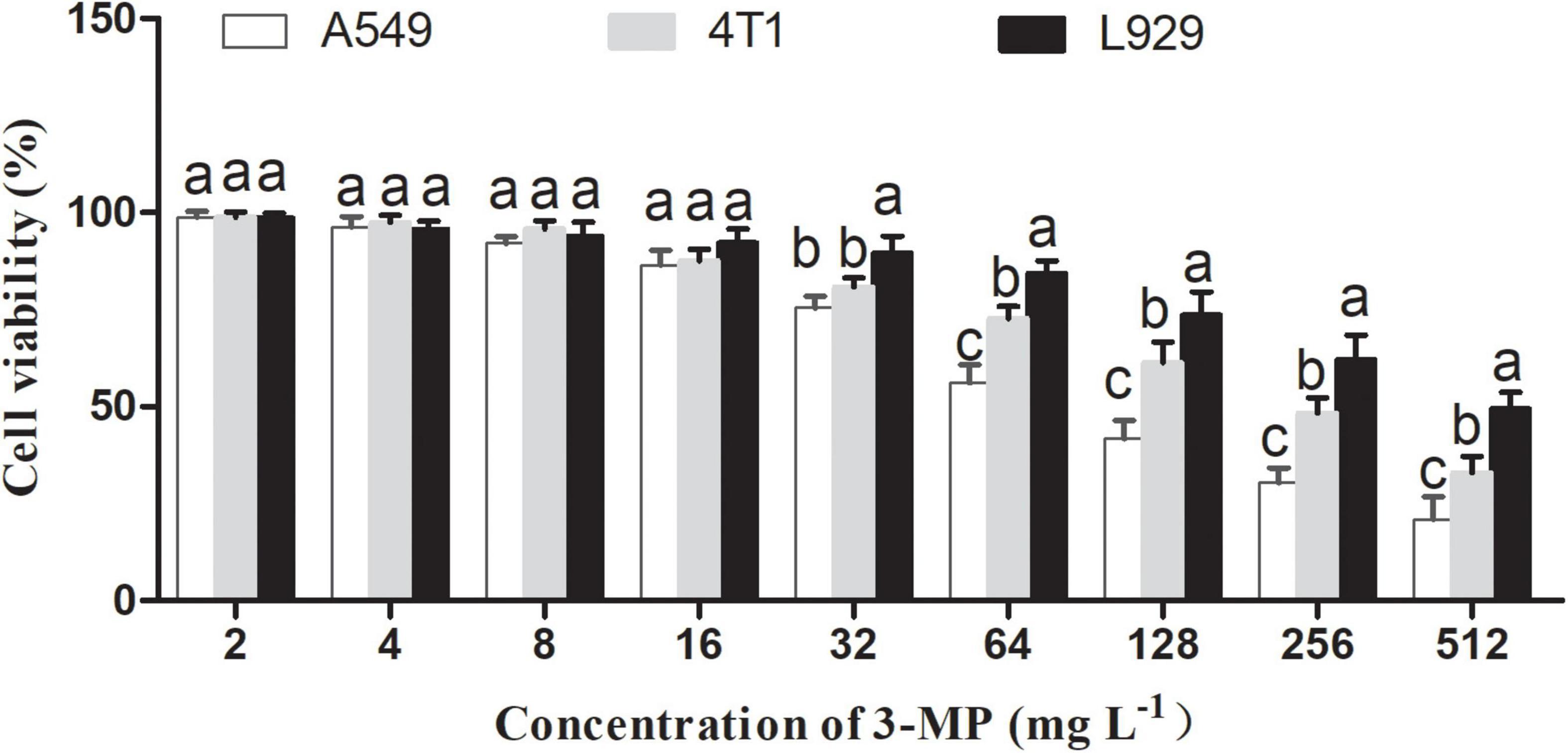
Figure 9. Effects of 3-MP on L929, A549, and 4T1 cell viability. Values are the means ± SD of three biological replicates. The different lowercase letter means statistically significant (Duncan’s multiple range test).
Discussion
The food-borne sulfur-containing spice compounds are the ideal substitutes for chemical fungicides due to their excellent characteristics of antioxidant, anticancer, and antimicrobial activity (Schöller et al., 2002; Hongman et al., 2018; Xie et al., 2020). However, the existed studies on sulfur-containing spice compounds are not matched their important roles, it is necessary to explore some new sulfur-containing spice compounds to be chosen. 3-MP was found widely in many foods as a natural sulfur-containing spice compound (Etschmann et al., 2008; Moreira et al., 2010) and also used as an important food flavoring additive (Zhou et al., 2021). However, its biological control effect and mechanism on fungal diseases have not been explored yet. In this study, we demonstrated that 3-MP has excellent antifungal activity against B. cinerea by using in vitro and in vivo experiments, and further study showed 3-MP can affect the infection and growth of B. cinerea, as well as the defense systems of tomato fruit.
Mycelium is the main form of B. cinerea infecting plant hosts (Bika et al., 2021), and the germination of spores is necessary for the formation of the next round of infection (Castillo et al., 2017). The inhibition of mycelial growth and spore germination is an important antifungal mechanism of fungicides or compounds (Pande et al., 2006; Romanazzi et al., 2016). In our current research, 3-MP effectively inhibited the mycelial growth and spore germination of B. cinerea. This inhibitory effect of 3-MP was positively correlated with the concentration of 3-MP. This dose dependence is consistent with the previous studies of other compounds such as hinokitiol and epsilon-poly-L-lysine (Dou et al., 2021).
Importantly, spores can contribute not only to a new round of infection but also to the long-distance transmission of pathogens (Etxebeste et al., 2019). It is a very important way to inhibit the spread and infection of the pathogen by destroying the cell membrane structure and reducing the spore viability. Many studies indicated that various antifungal agents can destroy the integrity of the cell membrane, which would lead to the leakages of cytoplasmic contents and finally result in a decrease in vitality and even death of the spores (Guo et al., 2020; Wang et al., 2020). Similarly, our results showed that 3-MP treatment has greatly impaired the spore viability and membrane integrity of B. cinerea as well as increased the leakage of cellular components such as the content of nucleic acids, protein, and the MDA content in B. cinerea. This damage results in cell dysfunction and the suppression of mycelial growth and spore germination, all of which reduce pathogenicity.
Further studies have shown that 3-MP can differentially downregulate the expression level of key genes related to pathogenicity and growth of the pathogen. BcPG1, BcBMP1, BcPLC1, BcPLS1, and BcPME1 are key genes in determining the infection and growth of B. cinerea, and many studies demonstrated that the knockout of these genes can greatly affect the virulence and infection process of B. cinerea (Zheng et al., 2000; Valette-Collet et al., 2003; Gourgues et al., 2004; Brito et al., 2006; Schumacher et al., 2008; Wang et al., 2020). Given this, the transcript level of these genes was evaluated, and our data indicated that 3-MP can differentially downregulate the expression level of the five genes, suggesting that the downregulation of these genes might partly contribute to the antifungal effects of 3-MP.
To tolerate the environmental stimulus, a series of alterations occur at molecular or physiological levels in host tissues, mainly such as the activation of ROS regulatory systems and various hormone signaling pathways (Suzuki, 2016). The excessive ROS accumulation can disrupt the redox homeostasis and then lead to oxidative damage to the host cell, and many reports indicated that necrotrophic pathogens would stimulate to generate the masses of ROS into host tissues to induce cell death (Glazebrook, 2005; van Kan, 2006; Sun et al., 2021). POD, SOD, and CAT are the pivotal antioxidant enzymes that demonstrated to play important roles in redundant ROS-scavenging, and many previous studies showed that antifungal agents or biocontrol strains can greatly improve the activity of these enzymes to cope with the invasion of pathogens (Yan et al., 2018; Liu et al., 2019; Sun et al., 2021). Similarly, our results demonstrated that the activity of POD, SOD, and CAT in the fruits of the 3-MP-treated group was still higher than that in the control group at all the monitoring times, which were well reflected by the MDA data, as the MDA content in the fruits treated by 3-MP was significantly lower than that in the control fruit (Figure 7). These results indicate that the relatively high activity of POD, SOD, and CAT in the 3-MP-treated group might partly contribute to tomato fruit that suffers less oxidative damage. SA and JA are important hormones in plant defense responses, and SA and JA signaling pathways would be activated in response to pathogen infections (Zhang et al., 2016; Arnao and Hernández-Ruiz, 2017; Sun et al., 2021). In this study, the expression of most of the stress-related genes was first induced in the control fruit, while their expression in 3-MP fumigation treatment exhibited a significant delay. However, this phenomenon was broken at 96 h subjected to B. cinerea infection, as the transcript of most of the genes in the fruits by 3-MP fumigation treatment was significantly higher than that in the control fruits (Figure 8). We speculated that these phenomena might attribute to the faster colonization and higher accumulation of B. cinerea in the control fruits than that in the 3-MP-treated group, which would activate the various host defense routes to resist the invasion of B. cinerea. Also, with the accumulation of B. cinerea, it reached maximized at 96 h in the control fruits, which would lead to the collapse of the defense routes in the control tomato. Overall, these results indicated that both the antioxidant enzyme system and the defense signaling pathways in host tissues were activated in response to B. cinerea infection, and the antioxidant enzyme system might partly contribute to alleviate the damage caused by B. cinerea in the fruits of the 3-MP-treated group.
In this study, we also evaluated the safety of 3-MP by the cell survival assay. It was considered that the material had no obvious toxicity to cells when the cell survival rate is more than 80% (Atkinson et al., 2019). The results of cytotoxicity demonstrated that 3-MP can specifically inhibit the proliferation of tumor cells and had no toxicity to normal cells in a certain range of concentration (0–89.93 mg L–1). Similarly, apoptin specifically killed cancer cells but had no toxicity to normal cells (Cui et al., 2020). This indicated that 3-MP had the potential to become a targeted drug for inhibiting cancer cells. Furthermore, the content of 3-MP in the fumigated tomato fruit (1.6–2.24 mg L–1) (Supplementary Figure 1) was far below the threshold of cytotoxicity (89.93 mg L–1) (Figure 9), and it was also lower than that in most wines (4.91 mg L–1) (Fedrizzi et al., 2007; González-Álvarez et al., 2012). These results indicated that 3-MP may not damage our bodies as a kind of food additive and also indirectly proved that 3-MP was a safe compound that could be used during postharvest storage.
Conclusion
Our study revealed the excellent antifungal roles of sulfur-containing spice compound 3-MP in both in vitro and in vivo conditions. The mechanism involved in the antifungal effect of 3-MP against B. cinerea may be attributed to its capability to inhibit mycelial growth and spore germination, impair the spore viability and membrane integrity, and downregulate the disease-related genes. In addition, the antioxidant enzyme system and the defense signaling pathways in host tissues might also partly contribute to the antifungal activity of 3-MP. Most importantly, the cytotoxicity assay revealed that 3-MP had no toxicity to normal cells in a certain concentration range. To our knowledge, this is the first report showing the potential of 3-MP as an antifungal agent against gray mold on tomato fruit, and further research on formulations and application methods for developing 3-MP as a new eco-friendly fungicide is necessary.
Data Availability Statement
The original contributions presented in the study are included in the article/Supplementary Material, further inquiries can be directed to the corresponding authors.
Author Contributions
SF, WL, and YJ contributed to the experimental design and operation, data analysis, and writing. YC, RM, JD, and QL contributed to the experiment operation and data analysis. TY, LJ, and XY contributed to the suggestion, editing, and adjustment of the format. ZL and WJ contributed to the experimental design, supervision, writing and editing, and funding acquisition. All authors contributed to the article and approved the submitted version.
Funding
This study was supported by the National Natural Science Foundation of China (32001845), the Committee of Science and Technology of Chongqing (cstc2020jcyj-msxmX0774), the Chongqing Education Commission Science and Technology Research Project (KJQN202000520), and Fundamental Research Funds for the Central Universities (2021CDJZYJH-002).
Conflict of Interest
The authors declare that the research was conducted in the absence of any commercial or financial relationships that could be construed as a potential conflict of interest.
Publisher’s Note
All claims expressed in this article are solely those of the authors and do not necessarily represent those of their affiliated organizations, or those of the publisher, the editors and the reviewers. Any product that may be evaluated in this article, or claim that may be made by its manufacturer, is not guaranteed or endorsed by the publisher.
Supplementary Material
The Supplementary Material for this article can be found online at: https://www.frontiersin.org/articles/10.3389/fpls.2021.763755/full#supplementary-material
Footnotes
References
Arnao, M. B., and Hernández-Ruiz, J. (2017). Melatonin and its relationship to plant hormones. Ann. Bot. 121, 195–207. doi: 10.1093/aob/mcx114
Atkinson, I., Anghel, E. M., Petrescu, S., Seciu, A. M., Stefan, L. M., Mocioiu, O. C., et al. (2019). Cerium-containing mesoporous bioactive glasses: material characterization, in vitro bioactivity, biocompatibility and cytotoxicity evaluation. Micropor. Mesopor. Mat. 276, 76–88. doi: 10.1016/j.micromeso.2018.09.029
Bika, R., Baysal-Gurel, F., and Jennings, C. (2021). Botrytis cinerea management in ornamental production: a continuous battle. Can. J. Plant Pathol. 43, 345–365. doi: 10.1080/07060661.2020.1807409
Brito, N., Espino, J. J., and González, C. (2006). The endo-β-1,4-xylanase Xyn11A is required for virulence in Botrytis cinerea. Mol. Plant Microbe Interact. 19, 25–32. doi: 10.1094/mpmi-19-0025
Castillo, L., Plaza, V., Larrondo, L. F., and Canessa, P. (2017). Recent advances in the study of the plant pathogenic fungus botrytis cinerea and its interaction with the environment. Curr. Protein Pept. Sci. 18, 976–989. doi: 10.2174/1389203717666160809160915
Chaouachi, M., Marzouk, T., Jallouli, S., Elkahoui, S., Gentzbittel, L., Ben, C., et al. (2021). Activity assessment of tomato endophytic bacteria bioactive compounds for the postharvest biocontrol of Botrytis cinerea. Postharvest Biol. Tech. 172:111389. doi: 10.1016/j.postharvbio.2020.111389
Cong, M., Zhang, B., Zhang, K., Li, G., and Zhu, F. (2019). Stimulatory effects of sublethal doses of carbendazim on the virulence and sclerotial production of Botrytis cinerea. Plant Dis. 103, 2385–2391. doi: 10.1094/pdis-01-19-0153-re
Cui, Y., Li, Y., Li, S., Li, W., Zhu, Y., Wang, J., et al. (2020). Anti-tumor effect of a dual cancer-specific recombinant adenovirus on ovarian cancer cells. Exp. Cell Res. 396:112185. doi: 10.1016/J.Yexcr.2020.112185
Dou, Y., Routledge, M. N., Gong, Y. Y., Godana, E. A., Dhanasekaran, S., Yang, Q. Y., et al. (2021). Efficacy of epsilon-poly-L-lysine inhibition of postharvest blue mold in apples and potential mechanisms. Postharvest Biol. Tech. 171:111346. doi: 10.1016/j.postharvbio.2020.111346
Elsherbiny, E. A., Amin, B. H., Aleem, B., Kingsley, K. L., and Bennett, J. W. (2020). Trichoderma volatile organic compounds as a biofumigation tool against late blight pathogen phytophthora infestans in postharvest potato tubers. J. Agr. Food Chem. 68, 8163–8171. doi: 10.1021/acs.jafc.0c03150
Etschmann, M., Kötter, P., Hauf, J., Bluemke, W., Entian, K. D., and Schrader, J. (2008). Production of the aroma chemicals 3-(methylthio)-1-propanol and 3-(methylthio)-propylacetate with yeasts. Appl. Microbiol. Biot. 80, 579–587. doi: 10.1007/s00253-008-1573-4
Etxebeste, O., Otamendi, A., Garzia, A., Espeso, E. A., and Cortese, M. S. (2019). Rewiring of transcriptional networks as a major event leading to the diversity of asexual multicellularity in fungi. Crit. Rev. Microbiol. 45, 548–563. doi: 10.1080/1040841X.2019.1630359
Fedrizzi, B., Magno, F., Badocco, D., Nicolini, G., and Versini, G. (2007). Aging effects and grape variety dependence on the content of sulfur volatiles in wine. J. Agri. Food Chem. 55, 10880–10887. doi: 10.1021/jf072145w
Glazebrook, J. (2005). Contrasting mechanisms of defense against biotrophic and necrotrophic pathogens. Annu. Rev. Phytopathol. 43, 205–227. doi: 10.1146/annurev.phyto.43.040204.135923
González-Álvarez, M., González-Barreiro, C., Cancho-Grande, B., and Simal-Gándara, J. (2012). Impact of phytosanitary treatments with fungicides (cyazofamid, famoxadone, mandipropamid and valifenalate) on aroma compounds of Godello white wines. Food Chem. 131, 826–836. doi: 10.1016/j.foodchem.2011.09.053
Gourgues, M., Brunet-Simon, A., Lebrun, M. H., and Levis, C. (2004). The tetraspanin BcPls1 is required for appressorium-mediated penetration of Botrytis cinerea into host plant leaves. Mol. Microbiol. 51, 619–629. doi: 10.1046/j.1365-2958.2003.03866.x
Guo, H., Qiao, B., Ji, X., Wang, X., and Zhu, E. (2020). Antifungal activity and possible mechanisms of submicron chitosan dispersions against Alteraria alternata. Postharvest Biol. Tech. 161:110883. doi: 10.1016/j.postharvbio.2019.04.009
Haidar, R., Fermaud, M., Calvo-Garrido, C., Roudet, J., and Deschamps, A. (2016). Modes of action for biological control of Botrytis cinerea by antagonistic bacteria. Phytopathol. Mediterr. 55, 301–322. doi: 10.14601/Phytopathol_Mediterr-18079
Hongman, H., Yifang, W., Gongliang, Z., Yaolei, Z., Longquan, X., Hongshun, H., et al. (2018). Effects of sulfide flavors on AHL-mediated quorum sensing and biofilm formation of hafnia alvei. J. Food Sci. 83, 2550–2559. doi: 10.1111/1750-3841.14345
Hua, C., Kai, K., Wang, X., Shi, W., Zhang, D., and Liu, Y. (2019). Curcumin inhibits gray mold development in kiwifruit by targeting mitogen-activated protein kinase (MAPK) cascades in Botrytis cinerea. Postharvest Biol. Tech. 151, 152–159. doi: 10.1016/j.postharvbio.2019.02.006
Jian, W., Cao, H., Yuan, S., Liu, Y., Lu, J., Lu, W., et al. (2019). SlMYB75, an MYB-type transcription factor, promotes anthocyanin accumulation and enhances volatile aroma production in tomato fruits. Hortic. Res. 6:22. doi: 10.1038/s41438-018-0098-y
Liu, C., Chen, L., Zhao, R., Li, R., Zhang, S., Yu, W., et al. (2019). Melatonin induces disease resistance to Botrytis cinerea in tomato fruit by activating jasmonic aacid signaling pathway. J. Agri. Food Chem. 67, 6116–6124. doi: 10.1021/acs.jafc.9b00058
Liu, X., Ji, D., Cui, X., Zhang, Z., Li, B., Xu, Y., et al. (2020). P-coumaric acid induces antioxidant capacity and defense responses of sweet cherry fruit to fungal pathogens. Postharvest Biol. Tech. 169:111297. doi: 10.1016/j.postharvbio.2020.111297
Liu, Y., Shi, Y., Zhu, N., Zhong, S., Bouzayen, M., and Li, Z. (2020). SlGRAS4 mediates a novel regulatory pathway promoting chilling tolerance in tomato. Plant Biotechnol. J. 18, 1620–1633. doi: 10.1111/pbi.13328
Ma, D., Ji, D., Liu, J., Xu, Y., Chen, T., and Tian, S. (2020). Efficacy of methyl thujate in inhibiting Penicillium expansum growth and possible mechanism involved. Postharvest Biol. Tech. 161:111070. doi: 10.1016/j.postharvbio.2019.111070
Moreira, N., de Pinho, P. G., Santos, C., and Vasconcelos, I. (2011). Relationship between nitrogen content in grapes and volatiles, namely heavy sulphur compounds, in wines. Food Chem. 126, 1599–1607. doi: 10.1016/j.foodchem.2010.12.030
Moreira, N., Guedes de Pinho, P., Santos, C., and Vasconcelos, I. (2010). Volatile sulphur compounds composition of monovarietal white wines. Food Chem. 123, 1198–1203. doi: 10.1016/j.foodchem.2010.05.086
Morita, T., Tanaka, I., Ryuda, N., Ikari, M., Ueno, D., and Someya, T. (2019). Antifungal spectrum characterization and identification of strong volatile organic compounds produced by Bacillus pumilus TM-R. Heliyon 5:e01817. doi: 10.1016/j.heliyon.2019.e01817
Pande, S., Galloway, J., Gaur, P. M., Siddique, K. H. M., Tripathi, H. S., Taylor, P., et al. (2006). Botrytis grey mould of chickpea: a review of biology, epidemiology, and disease management. Aust. J. Agric. Res. 57, 1137–1150. doi: 10.1071/AR06120
Pei, S., Liu, R., Gao, H., Chen, H., Wu, W., Fang, X., et al. (2020). Inhibitory effect and possible mechanism of carvacrol against Colletotrichum fructicola. Postharvest Bio. Tech. 163:111126. doi: 10.1016/j.postharvbio.2020.111126
Pereira, E. D. J., Panek, A. D., and Eleutherio, E. C. A. (2003). Protection against oxidation during dehydration of yeast. Cell Stress Chaperon. 8, 120–124. doi: 10.1379/1466-12682003008<0120:paoddo<2.0.co;2
Romanazzi, G., Smilanick, J. L., Feliziani, E., and Droby, S. (2016). Integrated management of postharvest gray mold on fruit crops. Postharvest Biol. Tech. 113, 69–76. doi: 10.1016/j.postharvbio.2015.11.003
Saito, S., Michailides, T. J., and Xiao, C. L. (2019). Fungicide-resistant phenotypes in Botrytis cinerea populations and their impact on control of gray mold on stored table grapes in California. Eur. J. Plant Pathol. 154, 203–213. doi: 10.1007/s10658-018-01649-z
Schöller, C. E. G., Gürtler, H., Pedersen, R., Molin, S., and Wilkins, K. (2002). Volatile metabolites from actinomycetes. J. Agric. Food Chem. 50, 2615–2621. doi: 10.1021/jf0116754
Schreier, P., Drawert, F., and Junker, A. (1974). Glc-mass-spectrometrical investigation of volatile constituents of wine. 2. thioether-compounds of wines. Z. Lebensm. Unters. Forsch. 154, 279–284. doi: 10.1007/Bf01083423
Schumacher, J., Viaud, M., Simon, A., and Tudzynski, B. (2008). The Gα subunit BCG1, the phospholipase C (BcPLC1) and the calcineurin phosphatase co-ordinately regulate gene expression in the grey mould fungus Botrytis cinerea. Mol. Microbiol. 67, 1027–1050. doi: 10.1111/j.1365-2958.2008.06105.x
Shang, A., Cao, S., Xu, X., Gan, R., Tang, G., Corke, H., et al. (2019). Bioactive compounds and biological functions of garlic (Allium sativum L.). Foods 8:246. doi: 10.3390/foods8070246
Sun, C., Huang, Y., Lian, S., Saleem, M., Li, B., and Wang, C. (2021). Improving the biocontrol efficacy of Meyerozyma guilliermondii Y-1 with melatonin against postharvest gray mold in apple fruit. Postharvest Biol. Tech. 171:111351. doi: 10.1016/j.postharvbio.2020.111351
Suzuki, N. (2016). Hormone signaling pathways under stress combinations. Plant Signal. Behavi. 11:e1247139. doi: 10.1080/15592324.2016.1247139
Tian, S., Torres, R., Ballester, A. R., Li, B., Vilanova, L., and González-Candelas, L. (2016). Molecular aspects in pathogen-fruit interactions: virulence and resistance. Postharvest Bio. Tech. 122, 11–21. doi: 10.1016/j.postharvbio.2016.04.018
Tyagi, S., Lee, K. J., Shukla, P., and Chae, J. C. (2020). Dimethyl disulfide exerts antifungal activity against Sclerotinia minor by damaging its membrane and induces systemic resistance in host plants. Sci. Rep. 10:6547. doi: 10.1038/s41598-020-63382-0
Valette-Collet, O., Cimerman, A., Reignault, P., Levis, C., and Boccara, M. (2003). Disruption of Botrytis cinerea pectin methylesterase gene bcpme1 reduces virulence on several host plants. Mol. Plant Microbe Interact. 16, 360–367. doi: 10.1094/mpmi.2003.16.4.360
van Kan, J. A. L. (2006). Licensed to kill: the lifestyle of a necrotrophic plant pathogen. Trends Plant Sci. 11, 247–253. doi: 10.1016/j.tplants.2006.03.005
Wang, T. (2020). Benzyl isothiocyanate fumigation inhibits growth, membrane integrity and mycotoxin production in Alternaria alternata. RSC Adv. 10, 1829–1837. doi: 10.1039/c9ra09225k
Wang, Y., Liu, X., Chen, T., Xu, Y., and Tian, S. (2020). Antifungal effects of hinokitiol on development of Botrytis cinerea in vitro and in vivo. Postharvest Biol. Tech. 159:111038. doi: 10.1016/j.postharvbio.2019.111038
Wang, Z., Zhong, T., Chen, K., Du, M., Chen, G., Chen, X., et al. (2021). Antifungal activity of volatile organic compounds produced by Pseudomonas fluorescens ZX and potential biocontrol of blue mold decay on postharvest citrus. Food Control 120:107499. doi: 10.1016/j.foodcont.2020.107499
Xie, J., Liao, B., and Tang, R. (2020). Functional application of sulfur-containing spice compounds. J. Agric. Food Chem. 68, 12505–12526. doi: 10.1021/acs.jafc.0c05002
Yan, Y., Zhang, X., Zheng, X., Apaliya, M. T., Yang, Q., Zhao, L., et al. (2018). Control of postharvest blue mold decay in pears by Meyerozyma guilliermondii and it’s effects on the protein expression profile of pears. Postharvest Biol. Tech. 136, 124–131. doi: 10.1016/j.postharvbio.2017.10.016
Zhang, Y., Shi, X., Li, B., Zhang, Q., Liang, W., and Wang, C. (2016). Salicylic acid confers enhanced resistance to glomerella leaf spot in apple. Plant Physiol. Bioch. 106, 64–72. doi: 10.1016/j.plaphy.2016.04.047
Zhao, L., Wang, Y., Wang, Y., Li, B., Gu, X., Zhang, X., et al. (2020). Effect of β-glucan on the biocontrol efficacy of cryptococcus podzolicus against postharvest decay of pears and the possible mechanisms involved. Postharvest Biol. Tech. 160:111057. doi: 10.1016/j.postharvbio.2019.111057
Zheng, L., Campbell, M., Murphy, J., Lam, S., and Xu, J. R. (2000). The BMP1 gene is essential for pathogenicity in the gray mold fungus Botrytis cinerea. Mol. Plant Microbe Interact. 13, 724–732. doi: 10.1094/mpmi.2000.13.7.724
Zhou, T., Feng, Y., Thomas-Danguin, T., and Zhao, M. (2021). Enhancement of saltiness perception by odorants selected from Chinese soy sauce: a gas chromatography/olfactometry-associated taste study. Food Chem. 335:127664. doi: 10.1016/j.foodchem.2020.127664
Zuo, C., Zhang, W., Chen, Z., Chen, B., and Huang, Y. (2017). RNA sequencing reveals that endoplasmic reticulum stress and disruption of membrane integrity underlie dimethyl trisulfide toxicity against Fusarium oxysporum f. sp. cubense tropical race 4. Front. Microbiol. 8:1365. doi: 10.3389/fmicb.2017.01365
Keywords: Botrytis cinerea, 3-methylthio-1-propanol, antifungal activity, fumigation, sulfide
Citation: Feng S, Lu W, Jian Y, Chen Y, Meng R, Deng J, Liu Q, Yu T, Jin L, Yang X, Li Z and Jian W (2021) Biocontrol Effect and Possible Mechanism of Food-Borne Sulfide 3-Methylthio-1-Propanol Against Botrytis cinerea in Postharvest Tomato. Front. Plant Sci. 12:763755. doi: 10.3389/fpls.2021.763755
Received: 01 September 2021; Accepted: 25 October 2021;
Published: 14 December 2021.
Edited by:
Giuseppe Colla, University of Tuscia, ItalyReviewed by:
Alessandra Di Francesco, University of Bologna, ItalyQingguo Wang, Shandong Agricultural University, China
Copyright © 2021 Feng, Lu, Jian, Chen, Meng, Deng, Liu, Yu, Jin, Yang, Li and Jian. This is an open-access article distributed under the terms of the Creative Commons Attribution License (CC BY). The use, distribution or reproduction in other forums is permitted, provided the original author(s) and the copyright owner(s) are credited and that the original publication in this journal is cited, in accordance with accepted academic practice. No use, distribution or reproduction is permitted which does not comply with these terms.
*Correspondence: Wei Jian, amlhbndlaTc5NTZAMTYzLmNvbQ==; Zhengguo Li, emhlbmdndW9saUBjcXUuZWR1LmNu
†These authors have contributed equally to this work