- 1Instituto de Agrobiotecnología (CSIC/Gobierno de Navarra), Nafarroa, Spain
- 2Centre of the Region Haná for Biotechnological and Agricultural Research, Czech Advanced Technology and Research Institute, Olomouc, Czechia
- 3Department of Chemical Biology, Faculty of Science, Palacký University Olomouc, Olomouc, Czechia
- 4Laboratory of Growth Regulators, Institute of Experimental Botany of the Czech Academy of Sciences, Faculty of Science, Palacký University Olomouc, Olomouc, Czechia
- 5ADM Biopolis, Valencia, Spain
- 6Instituto de Hortofruticultura Subtropical y Mediterránea “La Mayora” (IHSM-UMA-CSIC) Campus de Teatinos, Málaga, Spain
Plants communicate with microorganisms by exchanging chemical signals throughout the phytosphere. Such interactions are important not only for plant productivity and fitness, but also for terrestrial ecosystem functioning. It is known that beneficial microorganisms emit diffusible substances including volatile organic compounds (VOCs) that promote growth. Consistently, soil application of cell-free culture filtrates (CF) of beneficial soil and plant-associated microorganisms enhances plant growth and yield. However, how this treatment acts in plants and whether it alters the resident soil microbiota, are largely unknown. In this work we characterized the responses of pepper (Capsicum annuum L.) plants cultured under both greenhouse and open field conditions and of soil microbiota to soil application of CFs of beneficial and phytopathogenic fungi. To evaluate the contribution of VOCs occurring in the CFs to these responses, we characterized the responses of plants and of soil microbiota to application of distillates (DE) of the fungal CFs. CFs and their respective DEs contained the same potentially biogenic VOCs, and application of these extracts enhanced root growth and fruit yield, and altered the nutritional characteristics of fruits. High-throughput amplicon sequencing of bacterial 16S and fungal ITS rRNA genes of the soil microbiota revealed that the CF and DE treatments altered the microbial community compositions, and led to strong enrichment of the populations of the same beneficial bacterial and fungal taxa. Our findings show that CFs of both beneficial and phytopathogenic fungi can be used as biostimulants, and provide evidence that VOCs occurring in the fungal CFs act as mediators of the plants’ responses to soil application of fungal CFs through stimulation of the beneficial soil microbiota.
Introduction
Plants are metaorganisms that host a complex and dynamic consortium of bacteria, fungi, archaea and protists that communicate with plants by exchanging chemical signals throughout the rhizosphere and endosphere. Such interactions are important not only for plant productivity and fitness, but also for terrestrial ecosystem functioning (Philippot et al., 2013; De-la-Peña and Loyola-Vargas, 2014; Delgado-Baquerizo et al., 2016; Huang et al., 2019; Yu et al., 2019; Prudent et al., 2020). Therefore, understanding the relationships between plant growth and soil microbial populations is necessary when seeking new and efficient ecological intensification and agricultural strategies based on manipulation of the soil microbiota. A safe and environmentally friendly approach to increase crop yield and/or protect plants from abiotic stress and pests while reducing the use of agrochemicals is based on the inoculation of the soil with plant growth promoting microorganisms that act as biostimulants (López-Bucio et al., 2015; Ahmad et al., 2018). These microorganisms emit diffusible substances including phytohormones and amino acids that promote root branching and nutrient uptake, enhance photosynthesis, alter metabolism, confer resistance to abiotic stresses and pathogens (Badri et al., 2013a; De-la-Peña and Loyola-Vargas, 2014; López-Bucio et al., 2015), and stimulate resident beneficial microbial communities in the phytosphere (Kröber et al., 2014; Fiorentino et al., 2018), thereby boosting plant growth and yield. Consistently, agronomic studies have shown that the application to soil of cell-free filtrates of cultures of plant-associated beneficial microbes enhances seed germination, seedling growth and crop yield (Aldesuquy et al., 1998; Varma et al., 1999; Bagde et al., 2011; Sung et al., 2011; Rahman et al., 2012; Yandigeri et al., 2012; Kaur et al., 2019).
Microorganisms also emit a plethora of volatile compounds (VCs) with molecular masses of less than 300 Da that act as efficient semiochemicals in interkingdom communication, participating in countless interactions among plants and microorganisms, and exerting a strong effect on microbial communities (Schmidt et al., 2016; Werner et al., 2016; Schulz-Bohm et al., 2017). VCs emitted by beneficial microorganisms promote growth and developmental changes, enhance photosynthesis, improve nutrient acquisition, elicit plant defenses and inhibit the growth of plant pathogens when applied via the air (Ryu et al., 2003; Chaurasia et al., 2005; Kai et al., 2007; Zhang et al., 2008, 2009; Gutiérrez-Luna et al., 2010; Kottb et al., 2015; Garnica-Vergara et al., 2016; Sánchez-López et al., 2016; Guo et al., 2020). Recent studies have shown that this capacity also extends to VCs emitted by phytopathogens and microorganisms that do not normally interact mutualistically with plants (Ezquer et al., 2010; Bitas et al., 2015; Sánchez-López et al., 2016; Cordovez et al., 2017; Li et al., 2018; García-Gómez et al., 2019, 2020; Moisan et al., 2019), although several lines of evidence indicate that the mechanisms involved in some plants’ responses to VCs emitted by beneficial and pathogenic microorganisms are different (Hernández-Calderón et al., 2018; García-Gómez et al., 2020). In Arabidopsis, enhanced growth and photosynthesis promoted by air application of fungal VCs is associated with increases in levels of active forms of cytokinins (CKs), photosynthetic pigments and transitory starch in leaves, together with reductions in abscisic acid (ABA) contents, and changes in the transcriptome and proteome through mechanisms involving signaling of redox-activated photosynthesis and long-distance communication between roots and the aerial part of the plant (Zhang et al., 2008; Sánchez-López et al., 2016; Ameztoy et al., 2019, 2021; García-Gómez et al., 2020).
To date, studies on the response of plants to soil application of cell-free filtrates from cultures of beneficial microorganisms have been centered on the effect of these compounds on plant growth and yield, but have not explored their modes/mechanisms of action in plants and/or their effects on resident soil microbial communities (Aldesuquy et al., 1998; Bagde et al., 2011; Sung et al., 2011; Rahman et al., 2012; Yandigeri et al., 2012; Kaur et al., 2019). Using the beneficial fungus Trichoderma harzianum and two fungal phytopathogens (i.e., Alternaria alternata and Penicillium aurantiogriseum) here we conducted studies to address the question of whether soil application of cell-free filtrates of beneficial and pathogenic fungal cultures can improve growth and yield of a plant species of agronomic interest (i.e., pepper [Capsicum annuum L.]) cultured under greenhouse and open field conditions, and whether this response is mediated by mechanisms comparable to those triggered by air application of fungal VCs. In addition, using amplicon sequencing targeting of the fungal ITS and bacterial 16S rRNA genes, we investigated whether filtrates of fungal cultures are capable of altering the indigenous soil microbial communities. Furthermore, using distillates (DEs) obtained from the fungal cultures, we evaluated the contribution made by fungal organic VCs (VOCs) in these responses. Our findings show that soil application of cell-free filtrates of beneficial and pathogenic fungi promotes root growth and enhances yield of pepper plants at least partially through mechanisms wherein activation of beneficial soil microbiota promoted by VOCs occurring in the fungal culture filtrates (CFs) could play important roles.
Materials and Methods
Preparation of Filtrates and Distillates From Fungal Cultures
Trichoderma harzianum (CECT 2413), A. alternata (CECT 20912) and P. aurantiogriseum (CECT 20226) from glycerol stocks were inoculated into 500 mL Erlenmeyer flasks containing 200 mL liquid Murashige and Skoog (MS) medium supplemented with 90 mM sucrose. Cultures were incubated at 28°C with constant shaking at 180 rpm on a rotatory shaker for 7 days. Next, 100 mL of the cultures were poured into 2 L Erlenmeyer flasks containing 1 L MS-sucrose medium and incubated at 28°C with constant shaking at 180 rpm for 3 days, after which the fungal mycelium was removed using Whatman #1 filter paper. The resulting filtrate was sterilized using a 0.22 μm Millipore membrane filter and stored at 4°C whilst awaiting further use. DEs were obtained by distilling the CFs at 50°C using a R3000 (BUCHI) rotavapor following manufacturer’s instructions1.
Plants, Growth Conditions, Soil Application of Fungal Culture Filtrates and Distillates and Sampling
This work was carried out using Sweet Italian and Piquillo pepper plants cultured under greenhouse and field conditions, respectively. Seeds were sown and cultured in the dark at 24°C on filter paper moistened with distilled water. Ten days after sowing, the pepper seedlings were transplanted into small pots (250 mL) filled with peat:sand:vermiculite mixture (1:1:1) and cultured for 4 weeks in growth chambers providing 16 h light (90 μmol photons sec–1 m–2), 25°C /8 h dark, 15°C cycles. For greenhouse trials, plants were transplanted to 6 L pots filled with peat:sand:vermiculite mixture (1:1:1) and cultured for 7 weeks with natural light under 16 h 25°C /8 h 15°C conditions. Treatments with fungal CFs and DEs started when the plants developed their first two true leaves. Increasing volumes (1.5, 3, 6, 10, 15, 20, and 25 mL per plant) of CFs and DEs were sequentially applied on soil once a week over 7 weeks. Each treatment was applied to twelve plants. Controls for DE-treated plants were plants irrigated with distilled water. Controls for CF-treated plants were plants irrigated with diluted (1:3) Murashige and Skoog (MS) medium supplemented with 1.5 mM each of glucose and fructose, which is the approximate concentration of both sugars in the three CFs. At the selected sampling times, leaves and commercial fruits were harvested and immediately ground to a fine powder in liquid nitrogen, then stored at −80°C prior to further biochemical characterization. “Commercial” Sweet Italian pepper fruits are defined as those having a minimum length of 14 cm.
Field trials were conducted at the INTIA’s experimental station located near Sartaguda (Navarre, Spain) between May and September of 2016 and 2018. Plants were transplanted from 250 mL pots (see above) to 22.40 m2 plots. Each plot contained 70 plants. Each treatment was applied to plants in three randomly distributed plots. The distance between plants in the same row was 35 cm and the distance between rows was 160 cm. Using a water meter (Contazara, CZ3000RI), plants were drip-irrigated with a 1:25 dilution of the original CF extract (10,4 L/plot) twice: one month after transplanting and at flowering. Using the same method, control plants were irrigated with a 1:25 dilution of the MS medium supplemented with 1.5 mM each of glucose and fructose stock (10.4 L/plot). Commercial fruits were harvested on three occasions: first, third and fourth week of September. Those destined for compositional analyses were immediately ground to a fine powder in liquid nitrogen and stored at −80°C. “Commercial” Piquillo pepper fruits are defined as those having 9–10 cm length, 40–45 cm width, 40–45 g weight and 1.5–3 mm pericarp thickness.
Analytical Procedures
Soluble sugars in CFs and frozen powders of leaves and fruits were determined by HPLC with pulsed amperometric detection on a IC3000 Dionex system, as described in Bahaji et al. (2015a). Contents of amino acids in CFs, leaves and fruits were measured by HPLC according to Loiret et al. (2009). Levels of CKs, ABA and indole acetic acid (IAA) in CFs and leaves were determined following Novák et al. (2008), Pěnčík et al. (2009), and Floková et al. (2014), respectively. Starch content in leaves was measured using an amyloglucosidase–based test kit (Boehringer Mannheim, Germany). Recovery experiments were carried out by adding known amounts of metabolite standards to the samples immediately after the addition of extraction solutions. The difference between the measurements from samples with and without added standards was used as an estimate of the percentage recovery. All presented concentrations of metabolites were corrected for losses during extraction.
Volatile organic compounds in CFs and DEs were determined by gas chromatography-mass spectrometry (GC-MS) using the solid-phase microextraction (SPME) technique as described by García-Gómez et al. (2019). Briefly, 5 mL of CFs and DEs were transferred into a 10 mL headspace vial (Agilent ref. 5183–4475) containing a magnetic stir bar. After 30 min of equilibration at 50°C with shaking, VOCs were adsorbed for 30 min using solid phase microextraction fiber coated with DVB/CAR/PDMS. The fiber was injected into an Agilent 7890A gas chromatograph containing a 30 m × 0.25 mm fused silica HP-5MS column. Mass spectral analyses were carried out with an Agilent 5975C instrument. Mass spectra of VOCs were compared to those obtained from the NIST library and identifications were confirmed using commercially available standard compounds.
Fruit Texture Analyses
Fruit hardness was determined using a penetrometer (FT-327 Penetrometer, PAMPOLS), by measuring the depth or rate of penetration of a rod driven into the fruit with a known force.
Determinations of Photosynthetic Parameters
Gas exchange rates were determined at 25°C with a photosynthetic photon flux density of 350 μmol m–2 s–1, as described by Bahaji et al. (2015b) using a portable LI-COR 6400 gas exchange photosynthesis system (LI-COR, Lincoln, NE, United States). Net rates of CO2 assimilation (An) and stomatal conductance (gs) were calculated as described by von Caemmerer and Farquhar (1981). Intrinsic water use efficiency (WUEi) was calculated as the ratio of An to gs as described by Flexas et al. (2016). Chlorophyll contents in leaves were determined using a portable chlorophyll meter (SPAD-502 Minolta, Japan).
Soil Sample Preparation, DNA Extraction and High-Throughput Sequencing
Soil microbiome studies were conducted at ADM-Biopolis (Paterna, Valencia, Spain) using soil from the 250 mL pots with plants cultured for 4 weeks in growth chambers (see above). Three days after the third treatment with fungal CFs or DEs, three replicate samples each consisting of all the soil from three pots were collected, mixed, sieved to a < 2 mm particle size, lyophilized and stored at −80°C. Soil DNA was extracted from 10 grams of soil of each replicate using a DNeasy Mericon kit from QIAGEN. The quantity and purity of DNA were examined with a NanoDrop ND-1000 spectrophotometer (NanoDrop Technologies, Delaware, United States). The bacterial 16S rRNA and fungal ITS libraries were constructed following the “16S metagenomics sequencing library preparation” protocol given for the Illumina MiSeq system, and using optimized primers targeting the hypervariable V3 and V4 regions of the 16S rRNA gene for the bacterial profile (Klindworth et al., 2013) and primers that cover ITS1-ITS2 for the fungal profile (Op De Beeck et al., 2014). Libraries were sequenced on an Illumina MiSeq instrument and uploaded to SRA archive with reference PRJNA748689. PCR primers present in the raw sequences were trimmed using cutadapt v2.6 (Martin, 2011), and merged with BBMap v38.26. Sequences were also filtered with BBMap in order to remove those with low quality or shorter than 200 nucleotides. Chimeras were filtered with the UCHIME algorithm in the USEARCH package (Edgar et al., 2011) and high-quality sequences were clustered into operational taxonomic units (OTUs), using cd-hit v4.8.1 (Fu et al., 2012) with a similarity threshold of 99% (Abarenkov et al., 2010). A total of 5,282,314 bacterial sequence reads were obtained from 24 samples. The numbers of high-quality sequences per sample varied from 98,030 to 140,027 and were grouped into 6,241 OTUs. In addition, a total of 3,283,782 fungal sequence reads were obtained. The number of high-quality sequences per sample varied from 50,007 to 90,123, resulting in 1,567 OTUs. Rarefaction curves obtained using R vegan package2 suggested that sequencing depth was sufficient to cover most of the detectable bacterial and fungal OTUs as the curves began to plateau or reached their asymptote for all sample types by treatment (Supplementary Figure 1). OTUs were annotated against NCBI nt database using blast tool and keeping up to the 10 best hits, in order to differentiate species whose 16S region is unique from species that share the sequenced 16S region with closes organisms. This way, species mentioned in this report were those that could be fully identified. Control soils for DE- and CF-treated soils were those irrigated with water and diluted MS medium supplemented with 1.5 mM each of glucose and fructose, respectively.
Data Analyses
The significance of differences in growth, yield, number of leaves and fruits per plant, photosynthesis and metabolite contents between CF- and DE-treated and non-treated plants was statistically evaluated with Student’s t-test using SPSS software. Differences were considered significant if P < 0.05. Both microbiome α- and β-diversity statistical analyses were performed using the R package “Phyloseq” v1.23.0 (De Mendiburu and Simon, 2015) on log-normalized data in order to avoid an increase in error rates due to rarefaction. In particular, α-diversity was measured using Shannon, Simpson and Richness indexes, and β-diversity was studied using Bray-curtis distance and PCoA. ANOVA test was used to check the differences in α-diversity, and PERMANOVA test was used with β-diversity contrasts. All differential abundance contrasts were done with DESeq2 package (Love et al., 2014) and validated using Wald text.
Results
Effects of Soil Application of Fungal Cell-Free Culture Filtrates on Plant Growth and Yield
We characterized growth and fruit yield responses of pepper plants (cv. Sweet Italian) grown under greenhouse conditions to soil application of CFs from T. harzianum, A. alternata and P. aurantiogriseum. In two independent experiments, the three CFs stimulated root growth (Figure 1A; Supplementary Figure 2), induced fruit development (Figures 1B,C) and enhanced the number of commercial fruits per plant and yield (Table 1). Furthermore, these treatments induced the accumulation of free amino acids in fruits. The most prominent ones were Ser, the long-distance nitrogen transport amino acids Asp, Glu, Asn and Gln and the stress-responsive GABA (Table 2). Soil application of the three CFs did not alter plant height, number of leaves per plant, stem thickness (Supplementary Figure 3) or fruit size and weight (Table 1). Soil application of A. alternata CFs, but not P. aurantiogriseum and T. harzianum CFs, enhanced ABA content in leaves (Supplementary Table 1). In addition, P. aurantiogriseum and T. harzianum CFs, but not A. alternata CFs, enhanced active CK contents in leaves (Supplementary Table 1). Moreover, soil application of the three fungal CFs did not affect leaf chlorophyll content, An and gs in leaves (Supplementary Figure 4). Consistently, WUEi and levels of starch and soluble sugars, regarded here as primary photosynthates, were not affected by the CF treatment (Supplementary Figure 4).
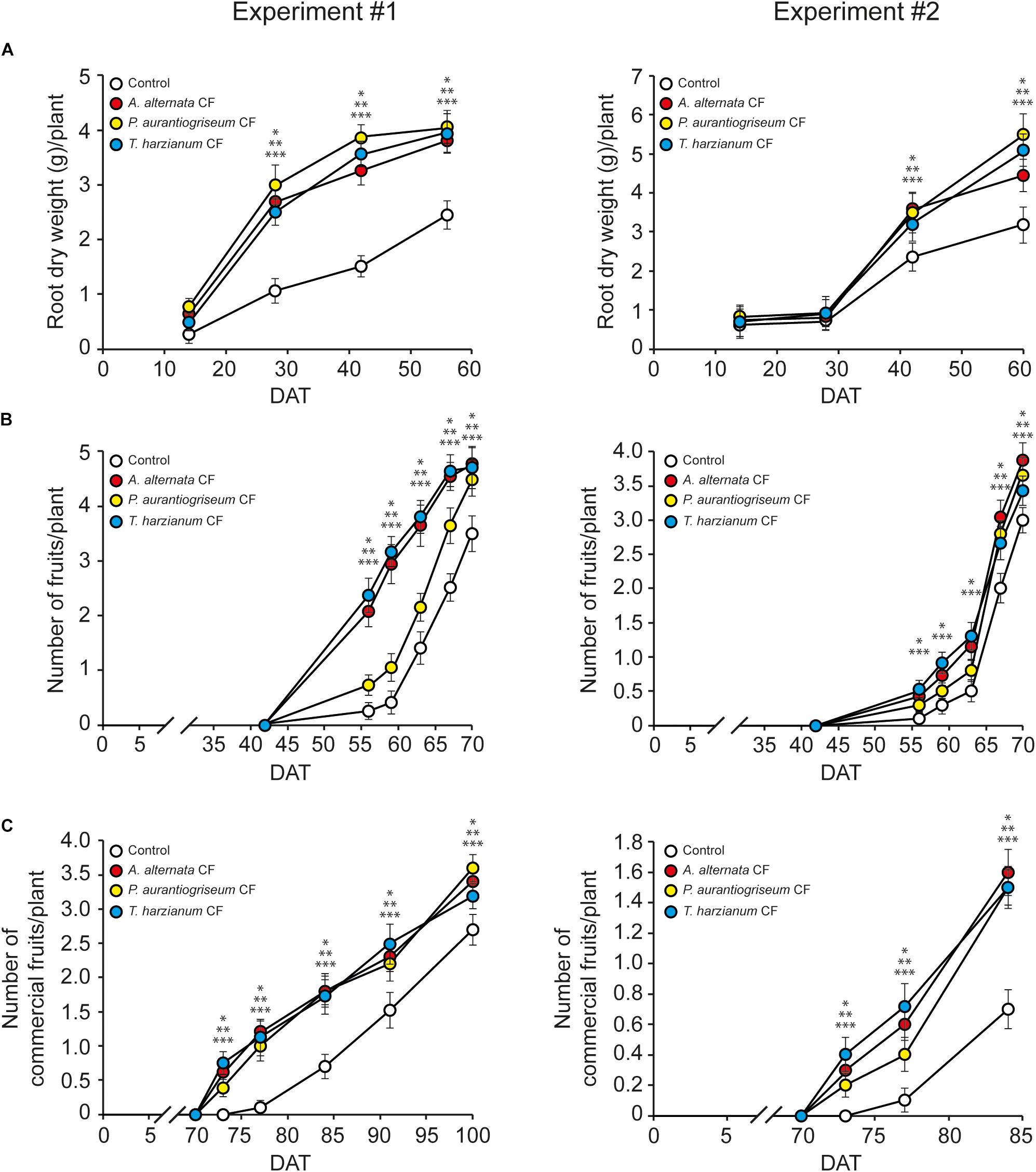
Figure 1. Soil application of fungal CFs stimulates root growth and induces early fruit development in pepper plants. Data over the time for root dry weight (A) and number of total fruits (B) and commercial fruits (C) per pepper plant (cv. Sweet Italian) after the first soil application of A. alternata, P. aurantiogriseum, and T. harzianum CFs. Plants were grown under greenhouse conditions. Values were obtained from two independent experiments and represent the mean ± SD of 12 different plants. Asterisks indicate significant differences between CF-treated plants and plants irrigated with CF control according to a Student’s t-test (*P < 0.05, + A. alternata CF; **P < 0.05, + P. aurantiogriseum CF; ***P < 0.05, + T. harzianum CF). DAT, Days after treatment.
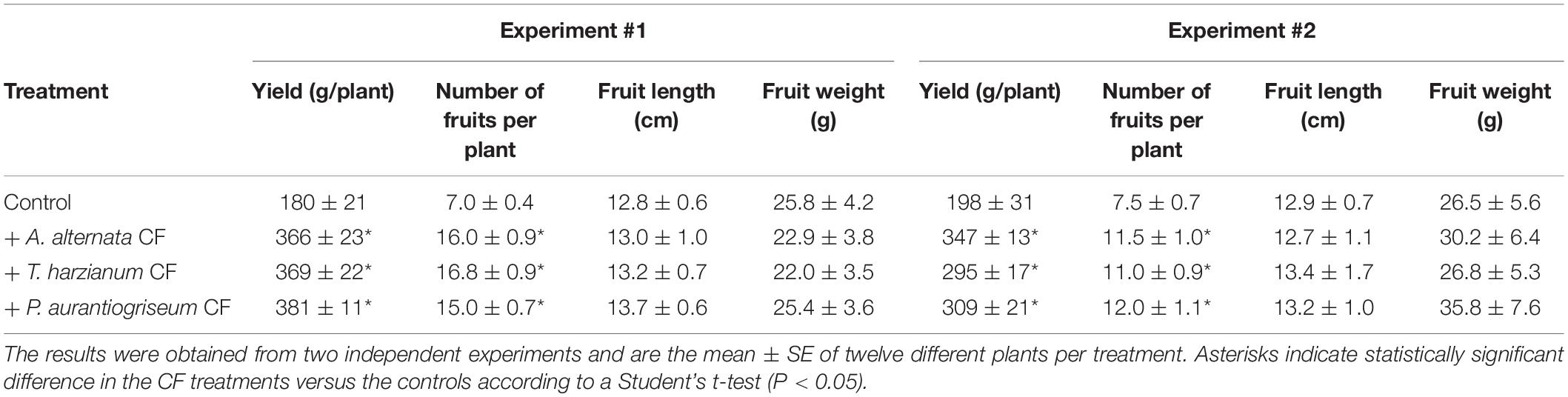
Table 1. Soil application of A. alternata, P. aurantiogriseum, and T. harzianum CFs enhances commercial fruit number and yield of pepper plants (cv. Sweet Italian) cultured under greenhouse conditions.
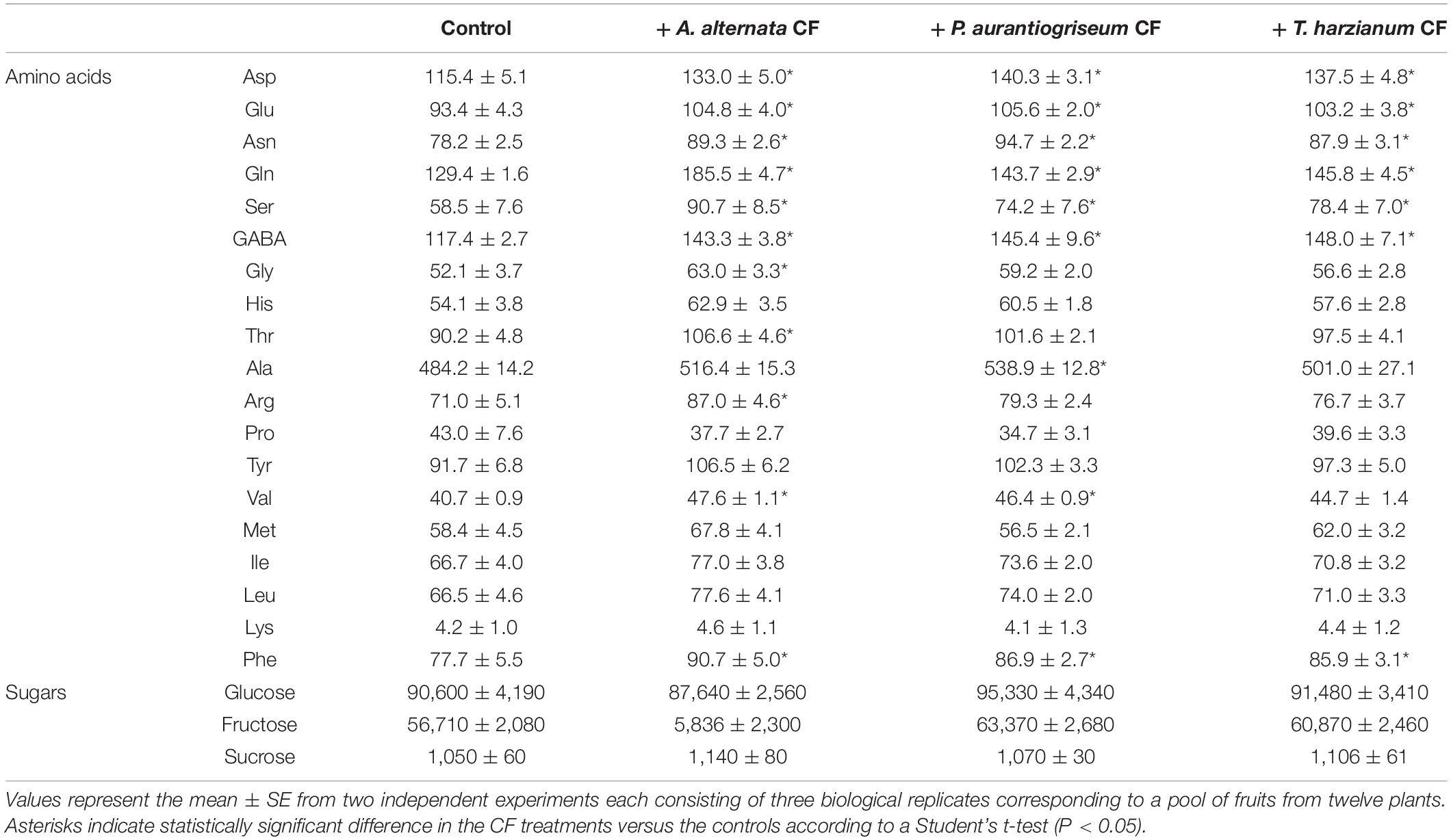
Table 2. Free amino acids and sugar contents (expressed as nmol g–1 DW) in commercial fruits of pepper plants (cv. Sweet Italian) cultured under greenhouse conditions with or without drench application of fungal CFs.
We also characterized the responses of pepper plants (cv. Piquillo) grown in the field to soil application of the three fungal CFs. In two independent experiments, these treatments enhanced the number of commercial fruits per plant and yield (Table 3) and altered fruit composition. As shown in Table 4, the CFs induced the accumulation of sucrose and long-distance nitrogen transport amino acids in fruits. CF treatments did not exert any significant effect on agronomic traits such as fruit size and weight (Table 3) and pericarp texture and thickness (Supplementary Figure 5).
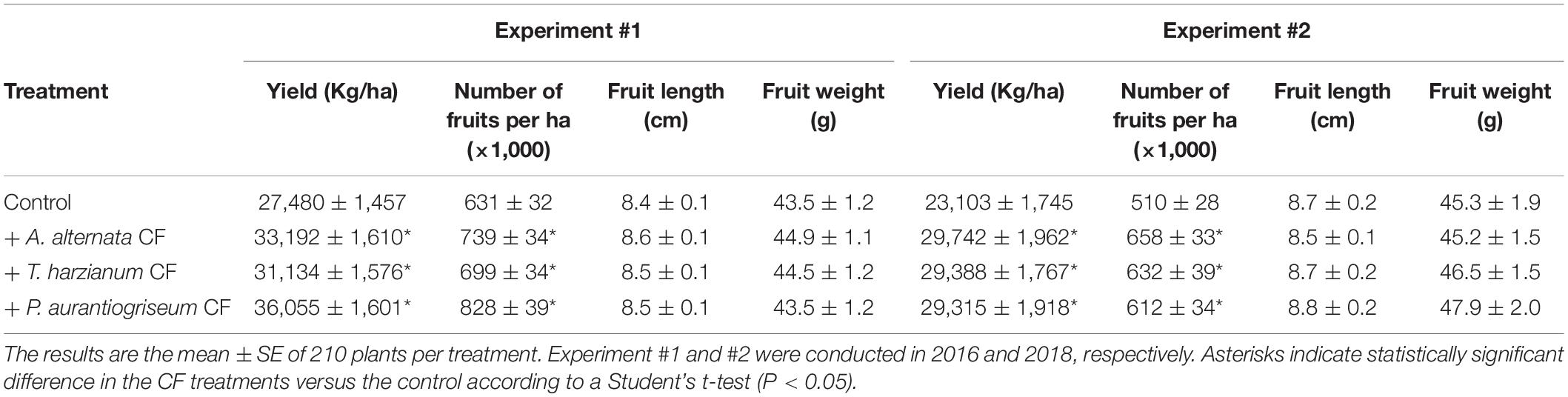
Table 3. Soil application of A. alternata, P. aurantiogriseum, and T. harzianum CFs enhances commercial fruit number and yield of pepper plants (cv. Piquillo) cultured under open field conditions.
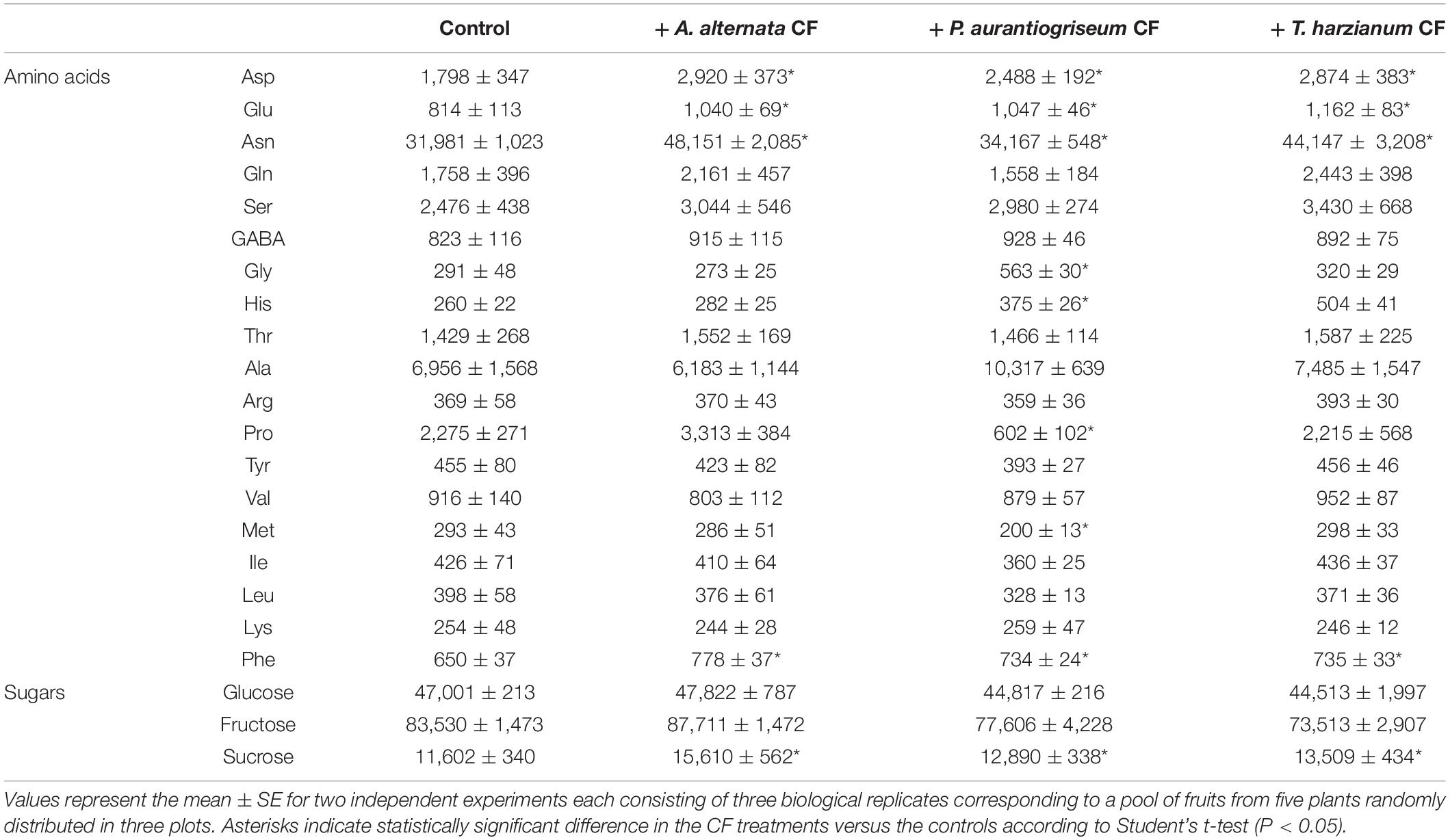
Table 4. Free amino acids and sugar contents (expressed as nmol g–1 DW) in commercial fruits of pepper plants (cv. Piquillo) cultured under open field conditions with or without drench application of fungal CFs.
Compositional Analysis of the Fungal Cell-Free Culture Filtrates
Culture filtrates of the three fungal species contained complex mixtures of amino acids (Supplementary Table 2). Some of these amino acids (e.g., Glu, GABA, Pro, and Ala) are known to be involved in signaling mechanisms for environmental changes that confer stress resistance to plants and/or affect their growth and development (Forde, 2014; Podlešáková et al., 2019). The hormones of the three CFs showed contrasting differences. P. aurantiogriseum CFs contained very much higher levels of CKs than those of A. alternata and T. harzianum (Supplementary Table 3). In addition, P. aurantiogriseum CFs contained higher levels of IAA (16,188 ± 239 pmol/L) than those of A. alternata and T. harzianum (1,049 ± 23 and 1,475 ± 530 pmol/L, respectively). Moreover, ABA content in T. harzianum CF (20.1 ± 5.5 pmol/L) was higher than in A. alternata and P. aurantiogriseum CFs (7.4 ± 1.6 and 7.2 ± 1.6, respectively). Analyses of the organic volatilomes of the three CFs identified a total of 80 VOCs (Table 5). Although the volatilomes were highly different in each of the CFs, 13 compounds (i.e., ethanol, 1-propanol, 1-butanol-3-methyl, benzaldehyde-2-methyl, 2,4-dimethyl-1-heptene, 2-phenylethyl alcohol, benzene-1,3-bis(1,1-dimethylethyl), acetic acid, propanoic acid ethyl ester, butyrolactone, 2-heptanone-4-methyl, 2-heptanone-4,6-dimethyl and 2-nonanone) could be identified in all three (Table 5). No sucrose could be detected in the three CFs, but all these extracts contained 0.5–1.5 mM each of glucose and fructose, likely due to strong extracellular fungal sucrolytic activity.
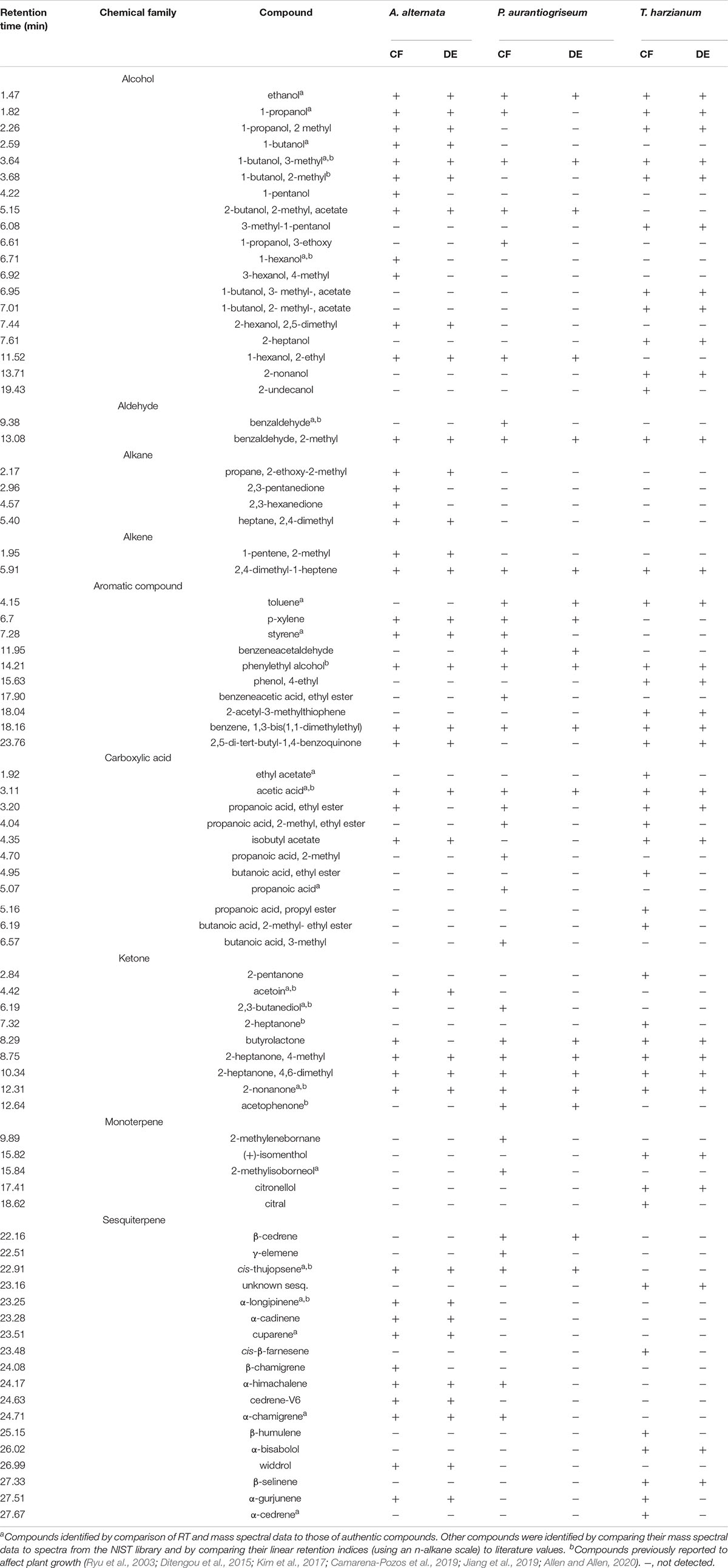
Table 5. Headspace analysis (SPME/GC-MS) of VOCs collected from filtrates and DEs obtained from A. alternata, P. aurantiogriseum, and T. harzianum liquid cultures.
Soil Application of Fungal Distillates Stimulates Root Growth and Enhances Fruit Yield of Pepper Plants
We next investigated the contribution of VOCs to the responses of pepper plants to CFs. To this end, we characterized growth and yield responses of Sweet Italian pepper plants grown under greenhouse conditions to soil application of DEs obtained from cultures of the three fungal species. We reasoned that if the plant’s responses to CFs were solely due to non-volatile bioactive compounds such as hormones and amino acids, DEs should trigger at most a weak response. Conversely, if VOCs have high action potentials, DEs should still trigger strong responses in plants. As a first step in these studies, we conducted compositional analyses of the VOCs in the DEs. These analyses revealed that ca. 75% of the VOCs identified in the CFs were also identified in the DEs (Table 5). The responses of pepper plants to DEs were comparable to those of CF-treated plants as the three DEs stimulated root growth (Figure 2A; Supplementary Figure 2), induced fruit production (Figures 2B,C), and increased the number of commercial fruits per plant and yield (Table 6). DEs did not significantly affect fruit size (Table 6). The overall data indicate that VOCs are important determinants of pepper plants’ responses to soil application of fungal CFs.
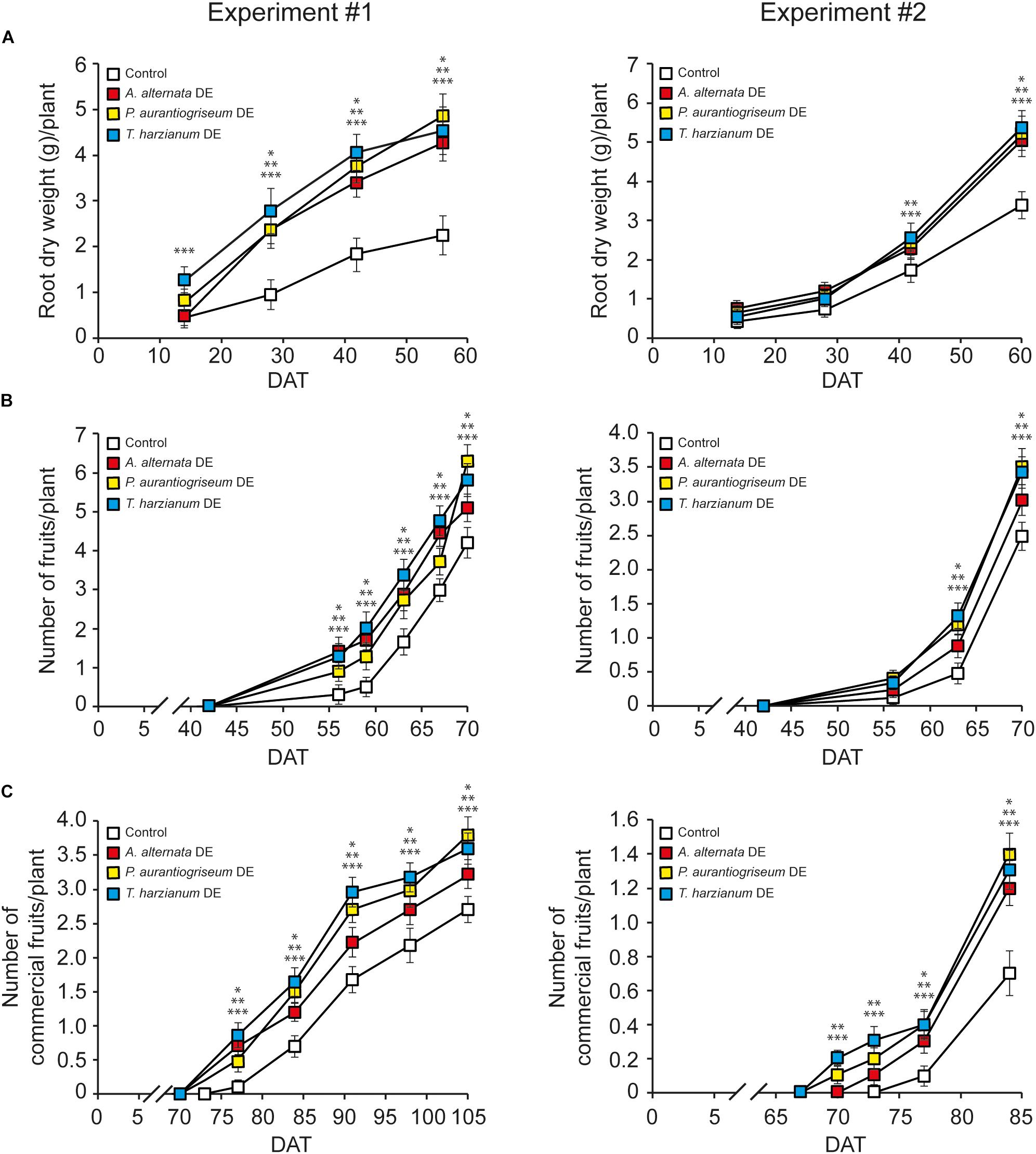
Figure 2. Soil application of fungal DEs stimulates root growth and induces early fruit development in pepper plants. Data over the time for root dry weight (A) and number of total fruits (B) and commercial fruits (C) per pepper plant (cv. Sweet Italian) after the first application of A. alternata, P. aurantiogriseum, and T. harzianum DEs on soil. Plants were grown under greenhouse conditions. Values were obtained from two independent experiments and represent the mean ± SD of 12 different plants. Asterisks indicate significant differences between DE-treated plants and plants irrigated with DE control according to a Student’s t-test (*P < 0.05, + A. alternata DE; **P < 0.05, + P. aurantiogriseum DE; ***P < 0.05, + T. harzianum DE). DAT, Days after treatment.
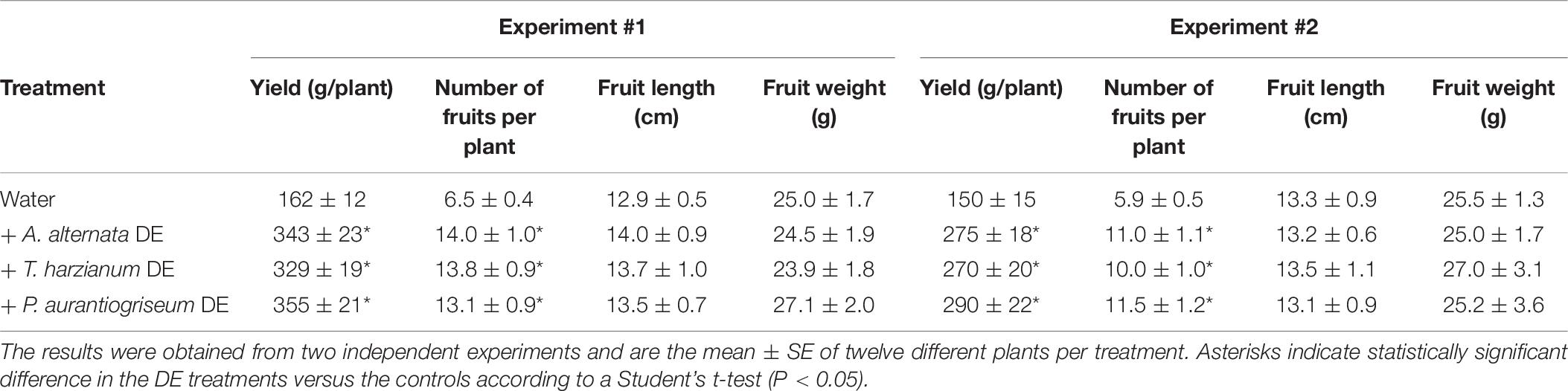
Table 6. Soil application of DEs obtained from A. alternata, P. aurantiogriseum, and T. harzianum cultures enhances commercial fruit number and yield of pepper plants (cv. Sweet Italian) cultured under greenhouse conditions.
Impact of Soil Application of Fungal Culture Filtrates and Distillates on the Indigenous Soil Microbial Abundance, Diversity and Composition
We next investigated the impact of soil application of the filtrates and DEs obtained from cultures of the three fungal species on the composition of the indigenous soil bacterial and fungal communities.
The bacterial microbiome profile based on amplicon sequencing of the bacterial 16S rRNA gene is shown in Supplementary Tables 4, 5. Bacterial abundances in CF- and DE-treated soils were similar to those of control soils (Supplementary Table 6). At class level, most OTUs were assigned to Acidobacteria, Actinobacteria, Chitinophagia, Alphaproteobacteria, Betaproteobacteria, and Gammaproteobacteria, representing ca. 90% of all assigned sequences (Supplementary Table 4; Figure 3). Results of the DESeq2 differential abundance test suggest that the application of the three CFs and DEs significantly altered the soil bacterial community composition (Supplementary Table 5). The most obvious change caused by the treatments was the increase in the relative abundance of Betaproteobacteria (Supplementary Table 4; Figure 3). At genus level, the three CF and DE treatments enriched populations of Pseudomonas (P. brassicacearum and P. mediterranea), Paraburkholderia (P. paradisi and P. silvatlantica), Burkholderia (B. arboris), Rhodoferax, Rhodanobacter (R. glycinis), and Massilia (M. varians), and reduced populations of Cellulosimicrobium (C. funkei) (Figures 3, 4A). Soil application of the fungal CFs and DEs did not alter the number of species, as measured by the Richness index (Supplementary Figure 6A). At species level, the T. harzianum CFs and DEs reduced α-bacterial diversity, as measured by the Shannon and Simpson indexes (Supplementary Figure 6A). At the same level, the 3 CFs and the DE from T. harzianum altered the bacterial β-diversity, as shown in the Bray-curtis PCoA plot (Figure 5A), indicating that these extracts have strong capacity to alter the bacterial community composition in soil. In general, the different CF and DE treatments strongly enriched the populations of Caballeronia udeis and Duganella ginsengisoli (Supplementary Table 4; Figures 3, 4A, 6), and impoverished those of Streptomyces yanglinensis, Frankia inefficax, and Nitrosospira multiformis (Supplementary Table 4; Figures 3, 4A).
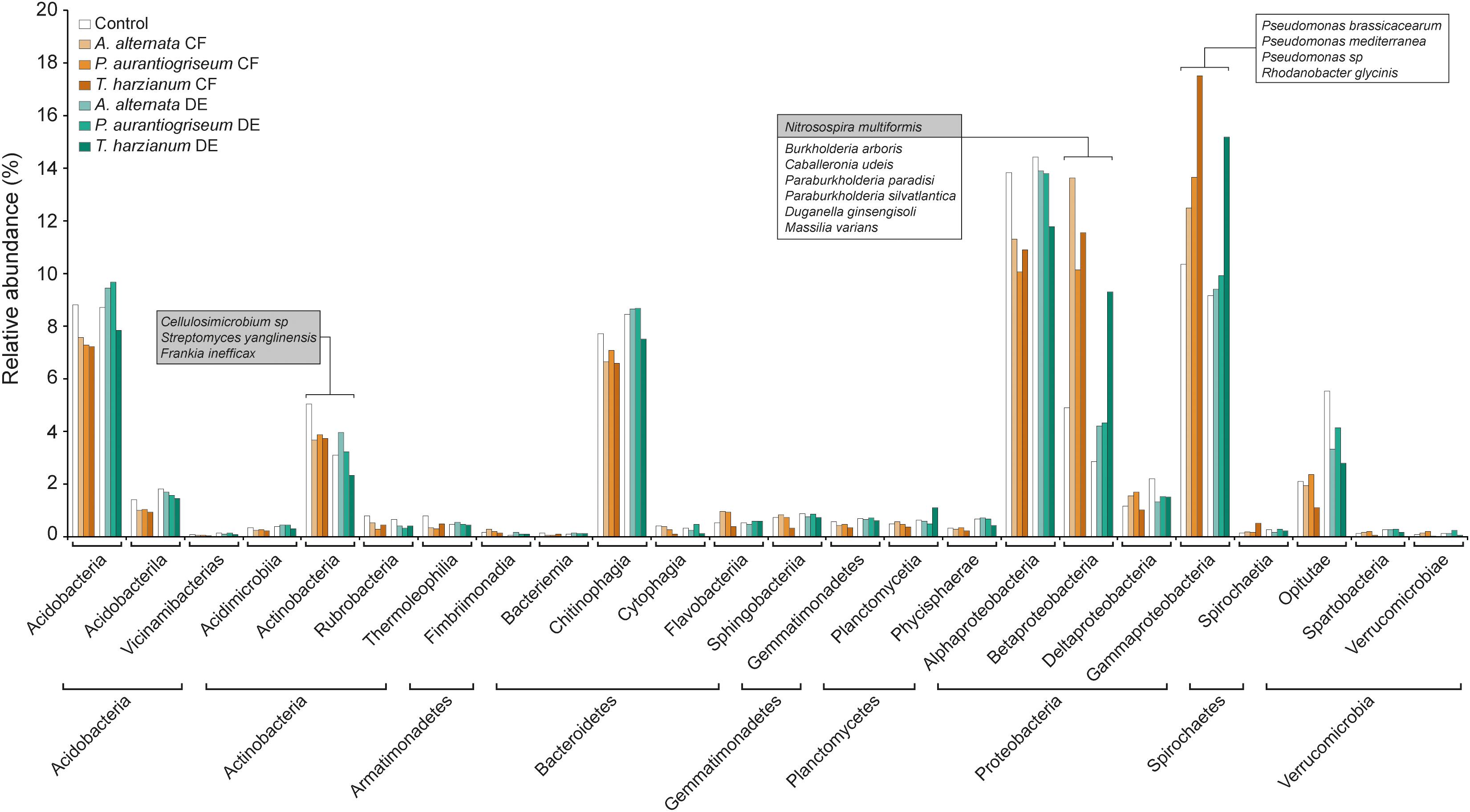
Figure 3. Soil application of fungal CFs and DEs alters the composition of the soil bacterial microbiota. The figure shows the relative abundances of bacteria in soils irrigated with fungal CFs (highlighted in brown) and DEs (highlighted in green). Relative abundances of bacteria in control soils are shown in white bars. Bacteria were arranged according to their phylum and class. Species discussed here whose relative abundances are significantly altered by the CF and DE treatments are boxed. Names of species enriched and impoverished by soil application of CFs and DEs are included in white and gray boxes, respectively. Data obtained from Supplementary Table 4.
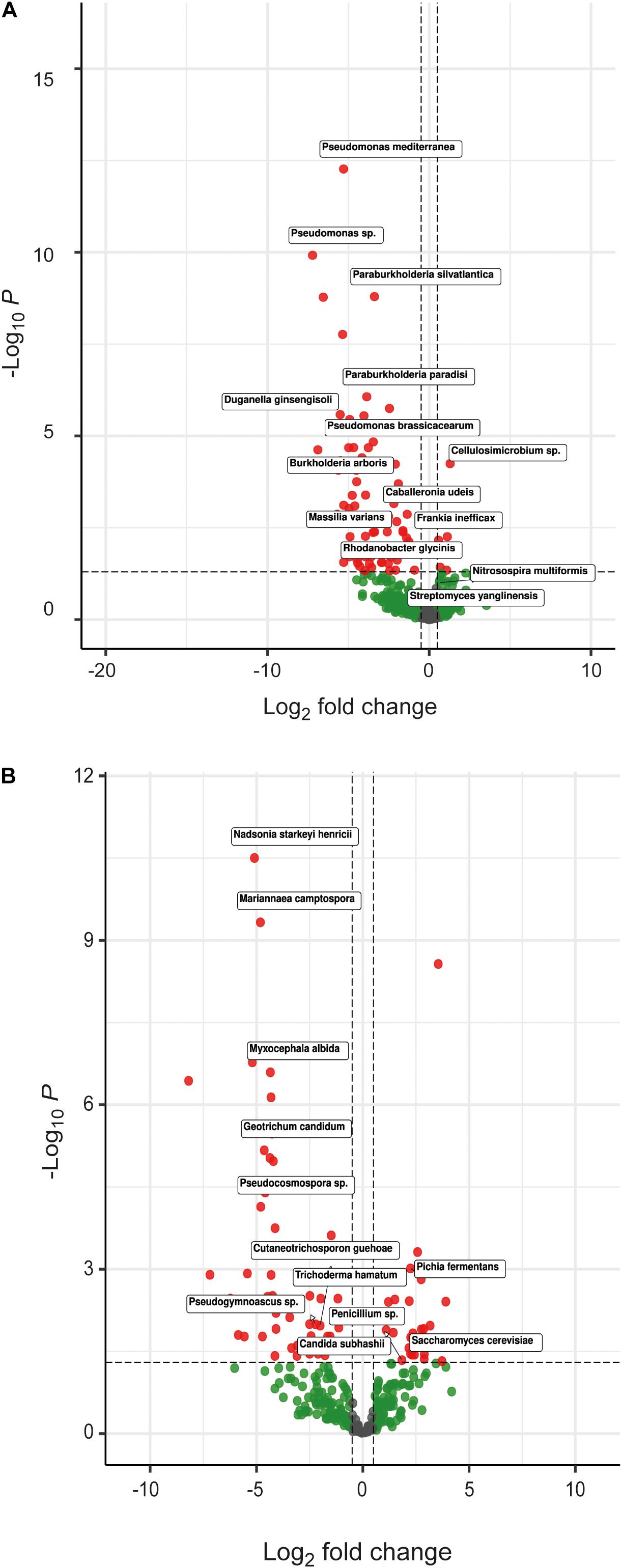
Figure 4. Volcano plot of the differential abundances of microbial profiles at species level, based on DESeq2 results. This plot shows the (A) bacterial and (B) fungal species that were significantly up- and down-regulated by soil application of fungal CFs and DEs (negative and positive log2FC, respectively). Species discussed here are boxed. Thresholds of the volcano plot were established at 0.5 log2Foldchange and 0.05 adjusted p-value (red dots).
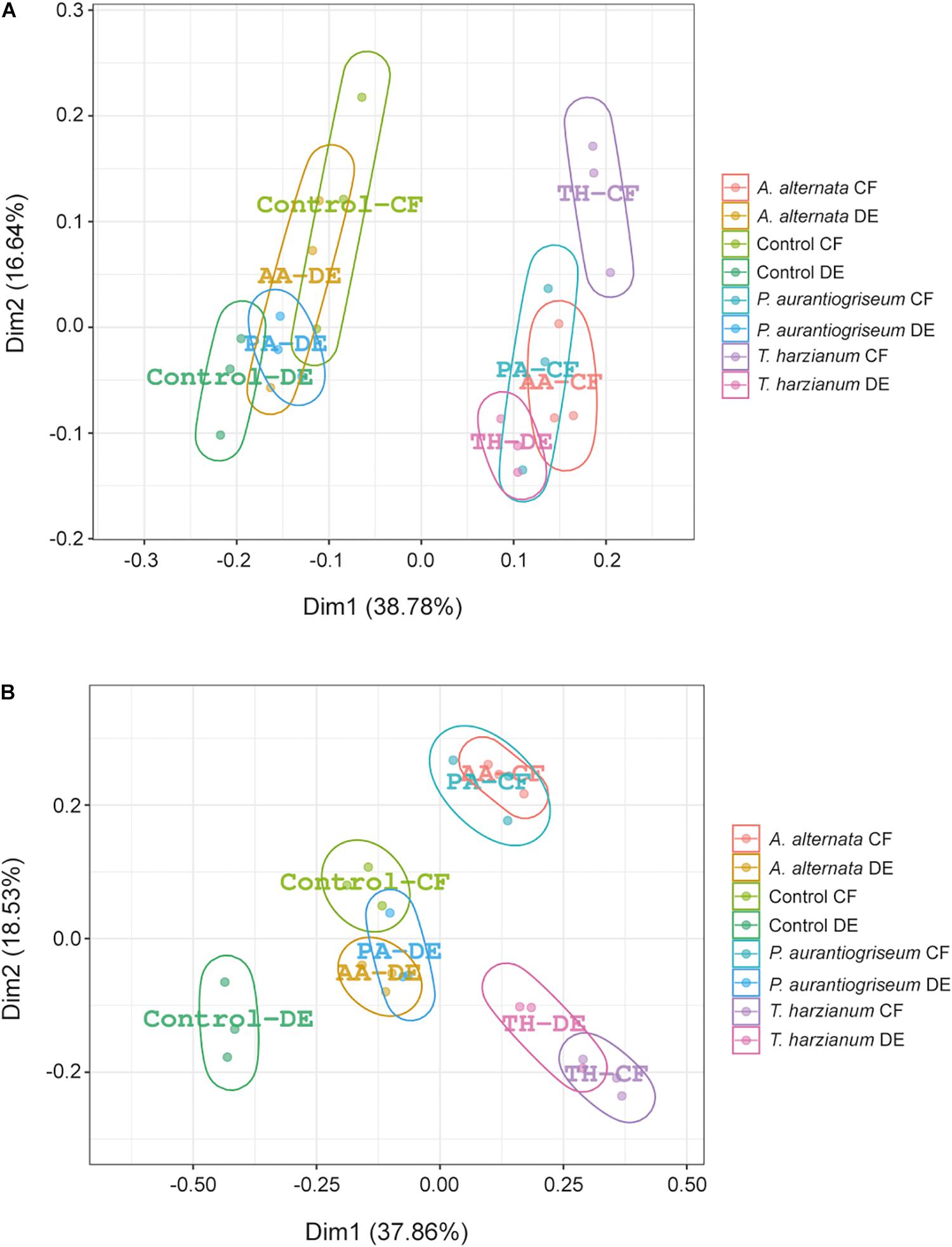
Figure 5. Effect of soil application of fungal CFs and DEs on microbial diversity in soil. The graphics represent the results of principal coordinates analyses (PCoA) showing the β-diversities of the (A) bacterial and (B) fungal communities in soils treated with CFs and DEs of A. alternata (AA-CF and AA-DE, respectively), P. aurantiogriseum (PA-CF and PA-DE, respectively) and T. harzianum (TH-CF and TH-DE, respectively) and control soils.
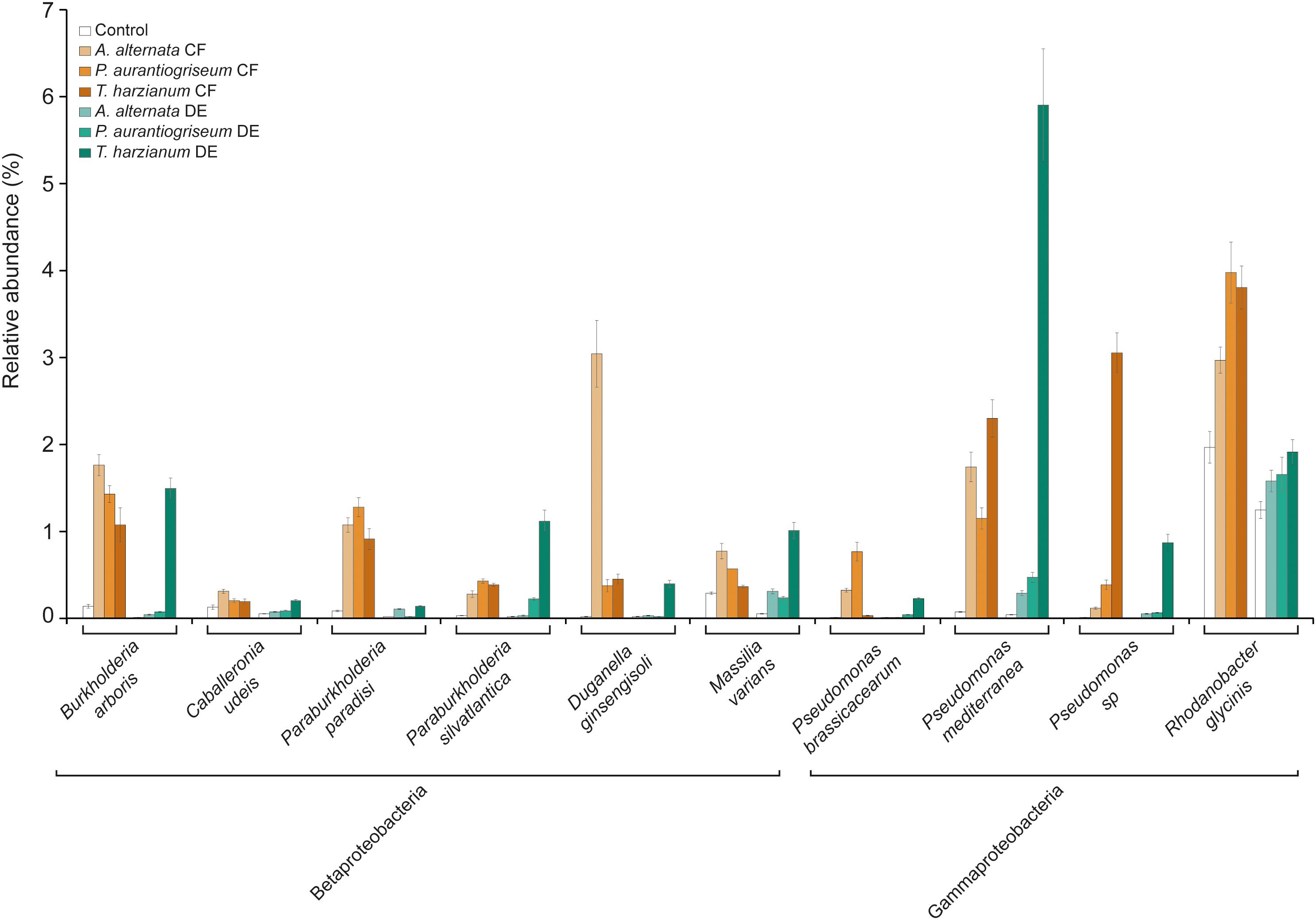
Figure 6. Soil application of fungal CFs and DEs strongly enriches the same beneficial soil bacterial species. The figure shows the relative abundances of bacterial species in soil discussed here that were significantly up-regulated by soil application of fungal CFs (highlighted in brown) and DEs (highlighted in green) according to a Student’s t-test (P < 0.05). Values represent the mean ± SD. Relative abundances of bacteria in control soils are shown in white bars. Data obtained from Supplementary Table 4.
The fungal microbiome profile based on amplicon sequencing of the fungal ITS1-ITS2 gene is shown in Supplementary Tables 4, 7. Fungal abundances in CF- and DE-treated soils were similar to those of control soils (Supplementary Table 6). At class level, the dominant taxa were Saccharomycetes, Sordariomycetes and Tremellomycetes, representing 30–90% of all assigned sequences (Supplementary Table 4; Figure 7). Results of the DESeq2 differential abundance test suggest that the application of the three CFs and DEs significantly altered the soil fungal community composition (Supplementary Table 7). The largest shifts caused by the CFs and DEs treatments in the fungal microbiota were the increases of the relative abundances of Mucoromycetes, Saccharomycetes and Sordariomycetes (Supplementary Table 4; Figure 7). At genus level, CF and DE treatments enriched populations of Geotrichum (G. candidum), Candida (C. subhashii), Trichoderma (T. hamatum) and Myxocephala (M. albida), and reduced those of Saccharomyces (S. cerevisiae) (Figure 4B). At species level, the CF, but not DE treatments altered the fungal α-diversity of the samples (Supplementary Figure 6B). However, the three CF and DE treatments significantly altered β-diversity, as shown in the Bray-curtis PCoA plot (Figure 5B). The CF and the DE treatments strongly enriched the populations of Pseudogymnoascus sp., Nadsonia starkeyi-henricii, Mariannaea camptospora, Pseudocosmospora rogersonii and Myxocephala albida, (Supplementary Table 4; Figures 7, 8), and reduced those of Penicillium sp. and Pichia fermentans (Supplementary Table 4; Figures 4B, 7).
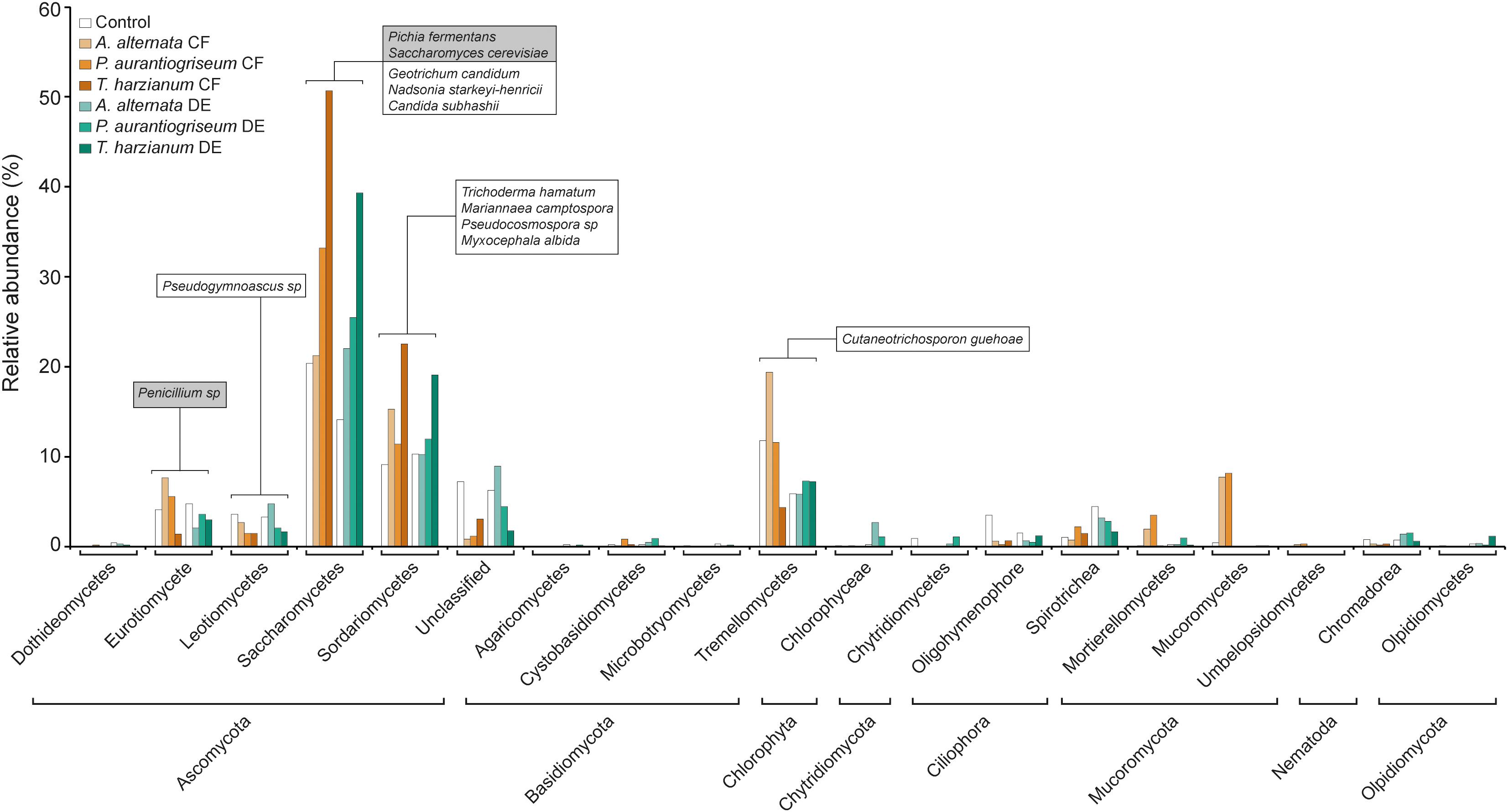
Figure 7. Soil application of fungal CFs and DEs alters the composition of the soil fungal microbiota. The figure shows the relative abundances of fungi in soils irrigated with fungal CFs (highlighted in brown) and DEs (highlighted in green). Relative abundances of fungi in control soils are shown in white bars. Fungi were arranged according to their phylum and class. Species discussed here whose relative abundances are significantly altered by the CF and DE treatments are boxed. Names of species enriched and impoverished by soil application of CFs and DEs are included in white and gray boxes, respectively. Data obtained from Supplementary Table 4.
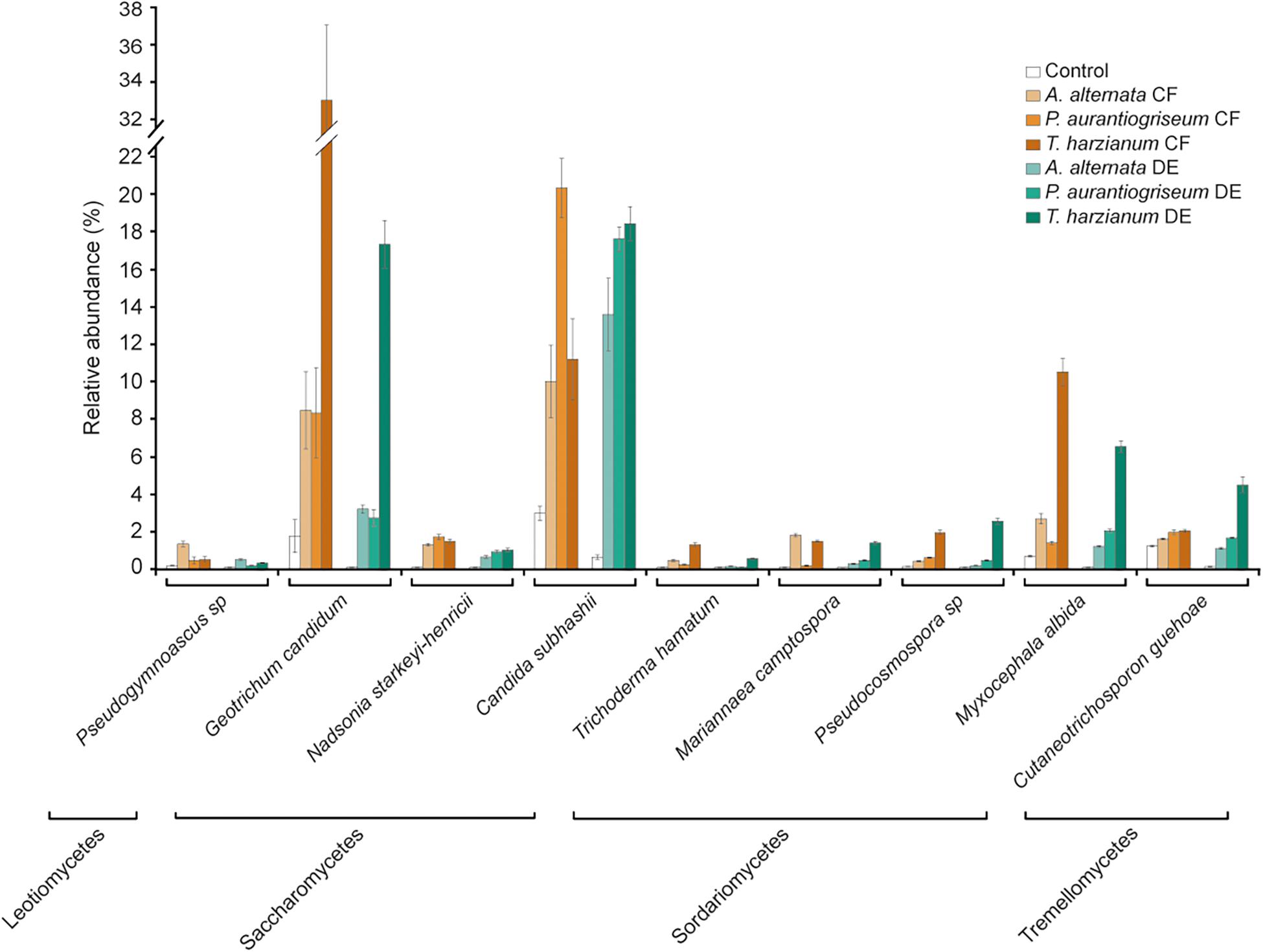
Figure 8. Soil application of fungal CFs and DEs strongly enriches the same beneficial soil fungal species. The figure shows the relative abundances of fungal species in soil discussed here that were significantly up-regulated by soil application of fungal CFs (highlighted in brown) and DEs (highlighted in green) according to a Student’s t-test (P < 0.05). Values represent the mean ± SD. Relative abundances of fungi in control soils are shown in white bars. Data obtained from Supplementary Table 4.
Discussion
Culture Filtrates of Both Beneficial and Phytopathogenic Fungi Can Be Used as Biostimulants to Improve Crop Yield
Here, we showed that soil application of CFs of beneficial and pathogenic fungi promotes root growth, accelerates fruit development, and increases the yield of commercial fruits of pepper plants cultured under greenhouse and field conditions. The differences observed between non-induced plants of different independent experiments could be ascribed to non-controlled environmental factors, such as differences in temperature and light intensity and quality. Therefore, CFs of both beneficial and phytopathogenic fungi can be used as biostimulants to improve crop yield. Because CFs alter fruit composition, they can potentially be used to improve fruit quality.
Root growth stimulation and enhanced fruit yield promoted by soil application of A. alternata and P. aurantiogriseum CFs was surprising as it is well known that many fungal phytopathogens release secondary metabolites and proteins in their culture media that display phytotoxicity in tests on tissue culture germinating seeds, plantlets, calli and cellular suspensions (McLean, 1996; Lou et al., 2013; Rao et al., 2014; Ogórek, 2016; Parveen et al., 2019). Phytotoxin formation by microbes is sensitive to a number of diverse environmental factors, including the microbial culture medium composition and the culturing duration and conditions (e.g., temperature, light and aeration) (Berestetskiy, 2008). Here, we report data obtained using filtrates extracted from A. alternata and P. aurantiogriseum grown for 3 days on MS-sucrose, a minimal medium normally used for in vitro culture of plants, but not for microbiology studies. Filtrates of 7 day-old cultures exert a negative effect on plant growth (not shown). Therefore, the contrasting differences between our results and those of previous studies can be ascribed to the fact that, under the experimental conditions used in this study, A. alternata and P. aurantiogriseum are not active with respect to phytotoxin production.
Volatile Organic Compounds Are Strong Determinants of Pepper Plants’ Responses to Soil Application of Fungal Culture Filtrates
Here, we showed that the vast majority of VOCs released by fungi to aqueous culture media can be “rescued” by distillation, and analyzed the effect of soil application of these compounds in plants. This is, to our knowledge, the first study of the response of plants to soil application of VOCs distilled from microbial cultures. Under greenhouse conditions, the responses of pepper plants to soil application of the three DEs were comparable to those of CF-treated plants. Thus, in our experimental system, VOCs appear to be strong determinants of the plants’ responses to fungal CFs. Unlike air VC application, soil DE application did not promote shoot growth and photosynthesis, did not enhance WUEi and the contents of photosynthetic pigments, starch and soluble sugars in leaves, and did not reduce leaf ABA content, indicating that the mechanisms involved in the responses of plants to soil and air applications of volatiles are different.
Some potentially relevant bioactive VOCs present in at least one of the CFs are 1-butanol-3-methyl, 1-butanol-2-methyl, 1-hexanol, benzaldehyde, 2-phenylethyl alcohol, acetic acid, acetoin, 2,3-butanediol, 2-heptanone, 2-nonanone, acetophenone, cis-thujopsene and α-longipinene, as they have been shown to promote shoot growth and root developmental changes when exogenously supplied to plants (Ryu et al., 2003; Ditengou et al., 2015; Kanchiswamy et al., 2015; Fincheira et al., 2017; Kim et al., 2017; Camarena-Pozos et al., 2019; Jiang et al., 2019; Allen and Allen, 2020). Some of these compounds (i.e., 1-butanol-3-methyl, 2-phenylethyl alcohol, acetic acid, and 2-nonanone) were present in the three DEs used in this study. It is likely that these compounds play important roles in the response of pepper plants to soil application of fungal DEs.
Distillates from fungal cultures used in this study are free from bioactive compounds such as phytohormones and amino acids. We must emphasize that the data obtained in this study do not imply that components of the CFs other than VOCs are not involved in the response of pepper plants to soil application of CFs. Soil application of amino acids and hormones affects growth and yield of plants and they are the main constituents of some biostimulants (Sudadi, 2012; Calvo et al., 2014; du Jardin, 2015; Koprna et al., 2016; Khan et al., 2019). On the other hand, microbially produced amino acids and phytohormones such as CKs, ABA and auxins are determinants of plant growth and yield (Arkhipova et al., 2005; Spaepen et al., 2007; Contreras-Cornejo et al., 2009; Ortíz-Castro et al., 2009; Zahir et al., 2010; Radhakrishnan et al., 2014; Chanclud and Morel, 2016). Because CFs of the three fungal species contain CK, ABA, IAA and amino acids, it is conceivable that the response of pepper plants to soil application of fungal CFs is due not only to VOCs, but also to the actions of fungal hormones and/or amino acids. The specific contributions of either amino acids and hormones of the CFs on the plant response to the CFs remains to be tested.
Soil Application of Fungal Culture Filtrates Increases the Relative Abundance of Resident Beneficial Soil Microbiota at Least Partially Through Mechanisms Involving Volatile Organic Compound Action
To our knowledge, this is the first report showing that soil application of fungal CFs and VOCs leads to restructuring of the soil microbiota. These treatments altered the bacterial and fungal β-diversities in soil, and enhanced the relative abundances of several taxa of the dominant soil bacterial classes Betaproteobacteria and Gammaproteobacteria and those of the fungal classes Saccharomycetes and Sordariomycetes. In addition, soil applications of CFs and DEs altered the abundance of the same bacterial and fungal species. Therefore, in our experimental system, VOCs appear to be important mediators in the response of the soil microbiota to fungal CFs. It is well known that soil and foliar application of non-volatile phytohormones and amino acid-enriched extracts promotes changes in the composition of soil and plant microbiota (Lebeis et al., 2015; Colla et al., 2017; Wang et al., 2019). Because CFs of the three fungal species used in this study contain amino acids and hormones, it is conceivable that the response of the soil microbiota to soil application of fungal CFs is also due to the action of these compounds. This is consistent with the fact that CFs promote stronger changes in bacterial β-diversity than DEs.
It has recently been proposed that benefits derived from some biostimulants might be due to shifts in the composition of the plant-associated and soil microbial communities (Mahnert et al., 2018; Luziatelli et al., 2019; Renaut et al., 2019; Wang et al., 2019). Here, we found that soil application of the three fungal CFs and DEs enriches the populations of the same bacterial species. Some of them (e.g., P. silvatlantica, C. udeis, P. mediterranea, P. brassicacearum, and R. glycinis) have been shown to act as plant growth promoters that solubilize mineral phosphate, fix nitrogen, produce siderophores, synthesize hormones (e.g., IAA and CKs) and/or antagonize microbial phytopathogens (Perin et al., 2006; Belimov et al., 2007; Kalita and Małek, 2017; Rabhi et al., 2018; Deng et al., 2019; Lee et al., 2019; Mannaa et al., 2019; Gu et al., 2020). In addition, all CF and DE treatments strongly enriched the populations of C. subhashii, a yeast antagonist of filamentous fungal phytopathogens (Hilber-Bodmer et al., 2017), and that of G. candidum. Although the latter fungus is known to be the causal agent of decays of numerous fruits (Palou et al., 2009; Talibi et al., 2012), it has been shown to promote growth when inoculated in plants (Waqas et al., 2017) probably due to its capacity to synthesize IAA, solubilize mineral phosphate and produce VOCs that inhibit microbial phytopathogens (Wu et al., 2012; Fu et al., 2016; George et al., 2019; Chen et al., 2020). It is worth noting that the relative abundances of most of the above microbial species were very low in the microbial populations of non-treated soils and became very high after the CF and DE treatments. In the case of G. candidum, for instance, relative abundances increased from 0.06 to 1.78% in non-treated soils to up to ca. 20 and 35% in DE- and CF-treated soils, respectively. C. subhashii relative abundances increased from 0.6 to 18.4% after fungal DE treatments whereas those of P. mediterranea increased from 0.04% to 0.07% in non-treated soils to up to 5.9 and 2.3% in DE- and CF-treated soils, respectively. Therefore, we propose that enhanced root growth and yield of pepper plants and changes in the fruit composition promoted by fungal CFs and DEs are at least partly due to strong activation of the beneficial soil microbiota. It remains to be determined if any of the bacterial or fungal species that are enriched in the rhizosphere upon application of the biostimulants may have a dominant effect over the microbial population, modulating root growth, architecture and biomass partitioning for fruit yield.
Ten compounds (i.e., ethanol, 1-butanol-3-methyl, benzaldehyde-2-methyl, 2,4-dimethyl-1-heptene, 2-phenylethyl alcohol, benzene-1,3-bis(1,1-dimethylethyl), acetic acid, 2-heptanone-4-methyl, 2-heptanone-4,6-dimethyl and 2-nonanone) are present in the three fungal DEs. It is likely that, individually or collectively, these compounds play important roles in the VOC-mediated activation of the beneficial microbiota in CF-treated soils. It is noteworthy that the response of the soil microbial community to T. harzianum DEs was stronger than those of soils treated with A. alternata and P. aurantiogriseum DEs, indicating that T. harzianum DEs contain higher levels of bioactive VOCs than those of A. alternata and P. aurantiogriseum and/or different proportions of the same VOCs. Additionally, and/or alternatively, it is possible that T. harzianum DEs contain VOCs with strong action potential that are not present in A. alternata and P. aurantiogriseum DEs. Such compounds could include 3-methyl-1-pentanol; 1-butanol, 3- methyl-, acetate; 1-butanol, 2- methyl-, acetate; 2-heptanol; 2-nonanol; phenol, 4 ethyl; 2-acetyl-3-methylthiophene; (+)-isomenthol; citronellol; α-bisabolol and β-selinene. It is evident that further efforts will be necessary to characterize the action potentials of these compounds on beneficial microbial populations.
Concluding and Additional Remarks
Results presented in this work show that CFs of both beneficial and phytopathogenic fungi can be used as biostimulants, and provide evidence that VOCs occurring in the fungal CFs act as mediators of the plants’ responses to soil application of fungal CFs through stimulation of the beneficial soil microbiota. Data provided here should motivate the applications of translational biology needed to reinforce agricultural practices with more natural, cheaper and sustainable approaches.
The discovery that soil application of VOCs from both beneficial and pathogenic microorganisms can enhance root growth and yield and promote similar changes in the soil microbial communities extends knowledge of the diversity and complexity of the interactions involved in modulation of the physiology of the plant and its interaction with surrounding microbes, raising questions regarding the evolution of the processes, their ecological significance and potential applications. Because microbes respond to microbial VOCs (Schmidt et al., 2016; Werner et al., 2016; Schulz-Bohm et al., 2017), we propose that enrichment of the soil beneficial microbiota promoted by application of fungal VOCs is due, at least partly, to direct action of these compounds on the microorganisms. It is well known that roots have the ability to influence the rhizosphere microbiota by releasing metabolites including amino acids, flavonoids, terpenes, organic acids, phenolic compounds, VOCs, etc. (Badri et al., 2013b; Schulz-Bohm et al., 2017; Sasse et al., 2018; Huang et al., 2019). Air application of microbial VCs enhances root growth (Gutiérrez-Luna et al., 2010; Ditengou et al., 2015; García-Gómez et al., 2019) and ethylene production (García-Gómez et al., 2020), and promotes changes in root exudate composition (Hernández-Calderón et al., 2018). Recent studies have shown that ethylene emitted by roots can control the rhizosphere microbial community assembly, indicating that this volatile hormone can be used by plants as a cue to interact with soil microorganisms (Chen et al., 2020). Therefore, it is likely that VOC-promoted changes of the soil microbiota are also due to enhanced root growth, altered composition of exudates and enhanced ethylene emissions by roots. Clearly, further experiments are necessary to test these hypotheses.
Microbes and their volatiles contribute in the various aspects of biotic stress management. The release of volatiles by beneficial microbes directly protects plants by inhibiting growth of pathogenic organisms, and indirectly elicit plant defense response by enhancing immune response against pathogen attack (De-la-Peña and Loyola-Vargas, 2014; Kanchiswamy et al., 2015). It has been suggested that phytopathogens or their constituents may provide opportunities for plant production or be useful for specific biotechnological applications (Tarkowski and Vereecke, 2014). It remains to be determined if soil application of CFs and DEs from fungal phytopathogens cross-protect plants from other pathogens.
One Sentence Summary
We show that soil application of cell-free filtrates of beneficial and pathogenic fungi promotes root growth and enhances yield of pepper plants through mechanisms wherein activation of beneficial soil microbiota by VOCs occurring in the fungal culture filtrates could play important roles.
Data Availability Statement
The original contributions presented in the study are publicly available. This data can be found here: National Center for Biotechnology Information (NCBI) BioProject database under accession number PRJNA748689.
Author Contributions
EB-F and JP-R designed the experiments, analyzed the data, and wrote the article with contributions from all authors. EB-F, GA, ÁS-L, AB, SG-A, ND, KD, and FJM performed most of the experiments. ECS performed the microbiome statistical analyses. JP-R supervised the experiments and conceived the project and research plans. All authors contributed to the article and approved the submitted version.
Funding
This work was supported by the Agencia Estatal de Investigación (AEI) and Fondo Europeo de Desarrollo Regional (Spain) (grants BIO2013-49125-C2-1-P, BIO2016-78747-P, and PID2019-104685GB-100), the Government of Navarra (refs. P1044 AGROESTI, P1004 PROMEBIO, and P1046 MICROBIOME), and the project “Plants as a tool for sustainable global development” (registration number: CZ.02.1.01/0.0/0.0/16_019/0000827) within the program Research, Development and Education (OP RDE).
Conflict of Interest
The authors declare that the research was conducted in the absence of any commercial or financial relationships that could be construed as a potential conflict of interest.
Publisher’s Note
All claims expressed in this article are solely those of the authors and do not necessarily represent those of their affiliated organizations, or those of the publisher, the editors and the reviewers. Any product that may be evaluated in this article, or claim that may be made by its manufacturer, is not guaranteed or endorsed by the publisher.
Supplementary Material
The Supplementary Material for this article can be found online at: https://www.frontiersin.org/articles/10.3389/fpls.2021.752653/full#supplementary-material
Abbreviations
An, Net rates of CO2 assimilation; CFs, culture filtrates; CKs, cytokinins; DEs, distillates; MS, Murashige and Skoog; OTUs, operational taxonomic units; SPME, solid-phase microextraction; gs, stomatal conductance; VCs, volatile compounds; VOCs, organic VCs; WUEi, intrinsic water use efficiency.
Footnotes
- ^ www.manualslib.com/manual/1589626/Buchi-Rotavapor-R-3000.html
- ^ https://cran.r-project.org/web/packages/vegan
References
Abarenkov, K., Nilsson, R. H., Larsson, K. H., Alexander, I. J., Eberhardt, U., Erland, S., et al. (2010). The UNITE database for molecular identification of fungi - recent updates and future perspectives. New Phytol. 186, 281–285. doi: 10.1111/j.1469-8137.2009.03160.x
Ahmad, M., Pataczek, L., Hilger, T. H., Zahir, Z. A., Hussain, A., Rasche, F., et al. (2018). Perspectives of microbial inoculation for sustainable development and environmental management. Front. Microbiol. 9:2992. doi: 10.3389/fmicb.2018.02992
Aldesuquy, H. S., Mansour, F. A., and Abo-Hamed, S. A. (1998). Effect of the culture filtrates of Streptomyces on growth and productivity of wheat plants. Folia Microbiol. 43, 465–470. doi: 10.1007/BF02820792
Allen, M. M., and Allen, D. J. (2020). Biostimulant potential of acetic acid under drought stress is confounded by pH-dependent root growth inhibition. Front. Plant Sci. 11:213. doi: 10.3389/fpls.2020
Ameztoy, K., Baslam, M., Sánchez-López, ÁM., Muñoz, F. J., Bahaji, A., Almagro, G., et al. (2019). Plant responses to fungal volatiles involve global post-translational thiol redox proteome changes that affect photosynthesis. Plant. Cell Environ. 42, 2627–2644. doi: 10.1111/pce.13601
Ameztoy, K., Sánchez-López, ÁM., Muñoz, F. J., Bahaji, A., Almagro, G., Baroja-Fernández, E., et al. (2021). Proteostatic regulation of MEP and shikimate pathways by redox-activated photosynthesis signaling in plants exposed to small fungal volatiles. Front. Plant Sci. 12:637976. doi: 10.3389/fpls.2021.637976
Arkhipova, T. N., Veselov, S. U., Melentiev, A. I., Martynenko, E. V., and Kudoyarova, G. R. (2005). Ability of bacterium Bacillus subtilis to produce cytokinins and to influence the growth and endogenous hormone content of lettuce plants. Plant Soil 272, 201–209. doi: 10.1007/s11104-004-5047-x
Badri, D. V., Chaparro, J. M., Zhang, R., Shen, Q., and Vivanco, J. M. (2013a). Application of natural blends of phytochemicals derived from the root exudates of Arabidopsis to the soil reveal that phenolic-related compounds predominantly modulate the soil microbiome. J. Biol. Chem. 288, 4502–4512. doi: 10.1074/jbc.M112.433300
Badri, D. V., Zolla, G., Bakker, M. G., Manter, D. K., and Vivanco, J. M. (2013b). Potential impact of soil microbiomes on the leaf metabolome and on herbivore feeding behavior. New Phytol. 198, 264–273. doi: 10.1111/nph.12124
Bagde, U. S., Prasad, R., and Varma, A. (2011). Influence of culture filtrate of Piriformospora indica on growth and yield of seed oil in Helianthus annus. Symbiosis 53:83. doi: 10.1007/s13199-011-0114-6
Bahaji, A., Baroja-Fernández, E., Ricarte-Bermejo, A., Sánchez-López, ÁM., Muñoz, F. J., Romero, J. M., et al. (2015a). Characterization of multiple SPS knockout mutants reveals redundant functions of the four Arabidopsis sucrose phosphate synthase isoforms in plant viability, and strongly indicates that enhanced respiration and accelerated starch turnover can alleviate the. Blockage of sucrose biosynthesis. Plant Sci. 238, 135–147. doi: 10.1016/j.plantsci.2015.06.009
Bahaji, A., Sánchez-López, ÁM., De Diego, N., Muñoz, F. J., Baroja-Fernández, E., Li, J., et al. (2015b). Plastidic phosphoglucose isomerase is an important determinant of starch accumulation in mesophyll cells, growth, photosynthetic capacity, and biosynthesis of plastidic cytokinins in Arabidopsis. PLoS One 10:e0119641. doi: 10.1371/journal.pone.0126531
Belimov, A. A., Dodd, I. C., Safronova, V. I., Hontzeas, N., and Davies, W. J. (2007). Pseudomonas brassicacearum strain Am3 containing 1-aminocyclopropane-1- carboxylate deaminase can show both pathogenic and growth-promoting properties in its interaction with tomato. J. Exp. Bot. 58, 1485–1495. doi: 10.1093/jxb/erm010
Berestetskiy, A. O. (2008). A review of fungal phytotoxins: from basic studies to practical use. Appl. Biochem. Microbiol. 44, 453–465. doi: 10.1134/S0003683808050013
Bitas, V., McCartney, N., Li, N., Demers, J., Kim, J. E., Kim, H. S., et al. (2015). Fusarium oxysporum volatiles enhance plant growth via affecting auxin transport and signaling. Front. Microbiol. 10:1248. doi: 10.3389/fmicb.2015.01248
Calvo, P., Nelson, L., and Kloepper, J. W. (2014). Agricultural uses of plant biostimulants. Plant Soil 383, 3–41. doi: 10.1007/s11104-014-2131-8
Camarena-Pozos, D. A., Flores-Núñ;ez, V. M., López, M. G., López-Bucio, J., and Partida-Martínez, L. P. (2019). Smells from the desert: microbial volatiles that affect plant growth and development of native and non-native plant species. Plant Cell Environ. 42, 1368–1380. doi: 10.1111/pce.13476
Chanclud, E., and Morel, J. B. (2016). Plant hormones: a fungal point of view. Mol. Plant Pathol. 17, 1289–1297. doi: 10.1111/mpp.12393
Chaurasia, B., Pandey, A., Palni, L. M. S., Trivedi, P., Kumar, B., and Colvin, N. (2005). Diffusible and volatile compounds produced by an antagonistic Bacillus subtilis strain cause structural deformations in pathogenic fungi in vitro. Microbiol. Res. 160, 75–81. doi: 10.1016/j.micres.2004.09.013
Chen, Y., Bonkowski, M., Shen, Y., Griffiths, B. S., Jiang, Y., Wang, X., et al. (2020). Root ethylene mediates rhizosphere microbial community reconstruction when chemically detecting cyanide produced by neighbouring plants. Microbiome 8, 4. doi: 10.1186/s40168-019-0775-6
Colla, G., Hoagland, L., Ruzzi, M., Cardarelli, M., Bonini, P., Canaguier, R., et al. (2017). Biostimulant action of protein hydrolysates: unraveling their effects on plant physiology and microbiome. Front. Plant Sci. 8:2202. doi: 10.3389/fpls.2017.02202
Contreras-Cornejo, H. A., Macías-Rodríguez, L., Cortés-Penagos, C., and López-Bucio, J. (2009). Trichoderma virens, a plant beneficial fungus, enhances biomass production and promotes lateral root growth through an auxin-dependent mechanism in Arabidopsis. Plant Physiol. 149, 1579–1592. doi: 10.1104/pp.108.130369
Cordovez, V., Mommer, L., Moisan, K., Lucas-Barbosa, D., Pierik, R., Mumm, R., et al. (2017). Plant phenotypic and transcriptional changes induced by volatiles from the fungal root pathogen Rhizoctonia solani. Front. Plant Sci. 8:1262. doi: 10.3389/fpls.2017.01262
De Mendiburu, F., and Simon, R. (2015). Agricolae – Ten years of an open source statistical tool for experiments in breeding, agriculture and biology. PeerJ Prepr. 3, 1–17. doi: 10.7287/peerj.preprints.1404v1
De-la-Peña, C., and Loyola-Vargas, V. M. (2014). Biotic interactions in the rhizosphere: a diverse cooperative enterprise for plant productivity. Plant Physiol. 166, 701–709. doi: 10.1104/pp.114.241810
Delgado-Baquerizo, M., Maestre, F. T., Reich, P. B., Jeffries, T. C., Gaitan, J. J., Encinar, D., et al. (2016). Microbial diversity drives multifunctionality in terrestrial ecosystems. Nat. Commun. 7:10541. doi: 10.1038/ncomms10541
Deng, S., Wipf, H. M.-L., Pierroz, G., Raab, T. K., Khanna, R., and Coleman-Derr, D. (2019). A plant growth-promoting microbial soil amendment dynamically alters the strawberry root bacterial microbiome. Sci. Rep. 9, 17677. doi: 10.1038/s41598-019-53623-2
Ditengou, F. A., Müller, A., Rosenkranz, M., Felten, J., Lasok, H., Van Doorn, M. M., et al. (2015). Volatile signalling by sesquiterpenes from ectomycorrhizal fungi reprogrammes root architecture. Nat. Commun. 6, 6279. doi: 10.1038/ncomms7279
du Jardin, P. (2015). Plant biostimulants: definition, concept, main categories and regulation. Sci. Hortic. 196, 3–14. doi: 10.1016/j.scienta.2015.09.021
Edgar, R. C., Haas, B. J., Clemente, J. C., Quince, C., and Knight, R. (2011). UCHIME improves sensitivity and speed of chimera detection. Bioinformatics 27, 2194–2200. doi: 10.1093/bioinformatics/btr381
Ezquer, I., Li, J., Ovecka, M., Baroja-Fernández, E., Muñoz, F. J., Montero, M., et al. (2010). Microbial volatile emissions promote accumulation of exceptionally high levels of starch in leaves in mono- and dicotyledonous plants. Plant Cell Physiol. 51, 1674–1693. doi: 10.1093/pcp/pcq126
Fincheira, P., Parra, L., Mutis, A., Parada, M., and Quiroz, A. (2017). Volatiles emitted by Bacillus sp. BCT9 act as growth modulating agents on Lactuca sativa seedlings. Microbiol. Res. 203, 47–56. doi: 10.1016/j.micres.2017.06.007
Fiorentino, N., Ventorino, V., Woo, S. L., Pepe, O., De Rosa, A., Gioia, L., et al. (2018). Trichoderma-based biostimulants modulate rhizosphere microbial populations and improve N uptake efficiency, yield, and nutritional quality of leafy vegetables. Front. Plant Sci. 9:743. doi: 10.3389/fpls.2018.00743
Flexas, J., Díaz-Espejo, A., Conesa, M. A., Coopman, R. E., Douthe, C., Gago, J., et al. (2016). Mesophyll conductance to CO2 and Rubisco as targets for improving intrinsic water use efficiency in C3 plants. Plant Cell Environ. 39, 965–982. doi: 10.1111/pce.12622
Floková, K., Tarkowská, D., Miersch, O., Strnad, M., Wasternack, C., and Novák, O. (2014). UHPLC-MS/MS based target profiling of stress-induced phytohormones. Phytochemistry 105, 147–157. doi: 10.1016/j.phytochem.2014.05.015
Forde, B. G. (2014). Glutamate signalling in roots. J. Exp. Bot. 65, 779–787. doi: 10.1093/jxb/ert335
Fu, L., Niu, B., Zhu, Z., Wu, S., and Li, W. (2012). CD-HIT: accelerated for clustering the next-generation sequencing data. Bioinformatics 28, 3150–3152. doi: 10.1093/bioinformatics/bts565
Fu, S.-F., Sun, P.-F., Lu, H.-Y., Wei, J.-Y., Xiao, H.-S., Fang, W.-T., et al. (2016). Plant growth-promoting traits of yeasts isolated from the phyllosphere and rhizosphere of Drosera spatulata Lab. Fungal Biol. 120, 433–448. doi: 10.1016/j.funbio.2015.12.006
García-Gómez, P., Almagro, G., Sánchez-López, ÁM., Bahaji, A., Ameztoy, K., Ricarte-Bermejo, A., et al. (2019). Volatile compounds other than CO2 emitted by different microorganisms promote distinct posttranscriptionally regulated responses in plants. Plant Cell Environ. 42, 1729–1746. doi: 10.1111/pce.13490
García-Gómez, P., Bahaji, A., Gámez-Arcas, S., Muñoz, F. J., Sánchez-lópez, ÁM., Almagro, G., et al. (2020). Volatiles from the fungal phytopathogen Penicillium aurantiogriseum modulate root metabolism and architecture through proteome resetting. Plant Cell Environ. 43, 2551–2570.
Garnica-Vergara, A., Barrera-Ortiz, S., Muñoz-Parra, E., Raya-González, J., Méndez-Bravo, A., Macías-Rodríguez, L., et al. (2016). The volatile 6-pentyl-2H-pyran-2-one from Trichoderma atroviride regulates Arabidopsis thaliana root morphogenesis via auxin signaling and ETHYLENE INSENSITIVE 2 functioning. New Phytol. 209, 1496–1512. doi: 10.1111/nph.13725
George, T. K., SubaidaBeevi, S., Asok, A. K., and Shaikmoideen, J. M. (2019). Plant growthpromoting endophytic yeast Geotrichum candidum (Jx 477426) from roots of Bruguiera cylindrica. J. Microbiol. Biotechnol. Food Sci. 9, 267–272. doi: 10.15414/jmbfs.2019.9.2.267-272
Gu, Y., Wang, J., Xia, Z., and Wei, H. L. (2020). Characterization of a versatile plant growth-promoting rhizobacterium Pseudomonas mediterranea strain s58. Microorganisms 8:334. doi: 10.3390/microorganisms8030334
Guo, Y., Jud, W., Ghirardo, A., Antritter, F., Benz, J. P., Schnitzler, J.-P., et al. (2020). Sniffing fungi – phenotyping of volatile chemical diversity in Trichoderma species. New Phytol. 227, 244–259. doi: 10.1111/nph.16530
Gutiérrez-Luna, F. M., López-Bucio, J., Altamirano-Hernández, J., Valencia-Cantero, E., Reyes de La Cruz, H., and Macías-Rodríguez, L. (2010). Plant growth-promoting rhizobacteria modulate root-system architecture in Arabidopsis thaliana through volatile organic compound emission. Symbiosis 51, 75–83. doi: 10.1007/s13199-010-0066-2
Hernández-Calderón, E., Aviles-Garcia, M. E., Castulo-Rubio, D. Y., Macías-Rodríguez, L., Ramírez, V. M., Santoyo, G., et al. (2018). Volatile compounds from beneficial or pathogenic bacteria differentially regulate root exudation, transcription of iron transporters, and defense signaling pathways in Sorghum bicolor. Plant Mol. Biol. 96, 291–304. doi: 10.1007/s11103-017-0694-5
Hilber-Bodmer, M., Schmid, M., Ahrens, C. H., and Freimoser, F. M. (2017). Competition assays and physiological experiments of soil and phyllosphere yeasts identify Candida subhashii as a novel antagonist of filamentous fungi. BMC Microbiol. 17:4. doi: 10.1186/s12866-016-0908-z
Huang, A. C., Jiang, T., Liu, Y. X., Bai, Y. C., Reed, J., Qu, B., et al. (2019). A specialized metabolic network selectively modulates Arabidopsis root microbiota. Science 36, eaau6389. doi: 10.1126/science.aau6389
Jiang, C.-H., Xie, Y.-S., Zhu, K., Wang, N., Li, Z.-J., Yu, G.-J., et al. (2019). Volatile organic compounds emitted by Bacillus sp. JC03 promote plant growth through the action of auxin and strigolactone. Plant Growth Regul. 87, 317–328. doi: 10.1007/s10725-018-00473-z
Kai, M., Effmert, U., Berg, G., and Piechulla, B. (2007). Volatiles of bacterial antagonists inhibit mycelial growth of the plant pathogen Rhizoctonia solani. Arch. Microbiol. 187, 351–360. doi: 10.1007/s00203-006-0199-0
Kalita, M., and Małek, W. (2017). Molecular phylogeny of Bradyrhizobium bacteria isolated from root nodules of tribe Genisteae plants growing in southeast Poland. Syst. Appl. Microbiol. 40, 482–491. doi: 10.1016/j.syapm.2017.09.001
Kanchiswamy, C. N., Malnoy, M., and Maffei, M. E. (2015). Chemical diversity of microbial volatiles and their potential for plant growth and productivity. Front. Plant Sci. 6:151. doi: 10.3389/fpls.2015.00151
Kaur, T., Rani, R., and Manhas, R. K. (2019). Biocontrol and plant growth promoting potential of phylogenetically new Streptomyces sp. MR14 of rhizospheric origin. AMB Express 9, 125. doi: 10.1186/s13568-019-0849-7
Khan, S., Yu, H., Li, Q., Gao, Y., Sallam, B. N., Wang, H., et al. (2019). Exogenous Application of amino acids improves the growth and yield of lettuce by enhancing photosynthetic assimilation and nutrient availability. Agron 9:266. doi: 10.3390/agronomy9050266
Kim, J.-M., To, T. K., Matsui, A., Tanoi, K., Kobayashi, N. I., Matsuda, F., et al. (2017). Acetate-mediated novel survival strategy against drought in plants. Nat. Plants 3, 17097. doi: 10.1038/nplants.2017.97
Klindworth, A., Pruesse, E., Schweer, T., Peplies, J., Quast, C., Horn, M., et al. (2013). Evaluation of general 16S ribosomal RNA gene PCR primers for classical and next-generation sequencing-based diversity studies. Nucleic Acids Res. 41:e1. doi: 10.1093/nar/gks808
Koprna, R., De Diego, N., Dundálková, L., and Spíchal, L. (2016). Use of cytokinins as agrochemicals. Bioorg. Med. Chem. 24, 484–492. doi: 10.1016/j.bmc.2015.12.022
Kottb, M., Gigolashvili, T., Großkinsky, D. K., and Piechulla, B. (2015). Trichoderma volatiles effecting Arabidopsis: from inhibition to protection against phytopathogenic fungi. Front. Microbiol. 6:995. doi: 10.3389/fmicb.2015.00995
Kröber, M., Wibberg, D., Grosch, R., Eikmeyer, F., Verwaaijen, B., Chowdhury, S. P., et al. (2014). Effect of the strain Bacillus amyloliquefaciens FZB42 on the microbial community in the rhizosphere of lettuce under field conditions analyzed by whole metagenome sequencing. Front. Microbiol. 5:252. doi: 10.3389/fmicb.2014.00252
Lebeis, S. L., Paredes, S. H., Lundberg, D. S., Breakfield, N., Gehring, J., McDonald, M., et al. (2015). Salicylic acid modulates colonization of the root microbiome by specific bacterial taxa. Science 349, 860–864. doi: 10.1126/science.aaa8764
Lee, S. A., Kanth, B. K., Kim, H. S., Kim, T.-W., Sang, M. K., Song, J., et al. (2019). Complete genome sequence of the plant growth-promoting endophytic bacterium Rhodanobacter glycinis T01E-68 isolated from tomato Solanum lycopersicum L. plant roots. Korean J. Microbiol. 55, 422–424. doi: 10.7845/kjm.2019.9115
Li, N., Wang, W., Bitas, V., Subbarao, K., Liu, X., and Kang, S. (2018). Volatile compounds emitted by diverse Verticillium species enhance plant growth by manipulating auxin signaling. Mol. Plant Microbe Interact. 31, 1021–1031. doi: 10.1094/MPMI-11-17-0263-R
Loiret, F. G., Grimm, B., Hajirezaei, M. R., Kleiner, D., and Ortega, E. (2009). Inoculation of sugarcane with Pantoea sp. increases amino acid contents in shoot tissues; serine, alanine, glutamine and asparagine permit concomitantly ammonium excretion and nitrogenase activity of the bacterium. J. Plant Physiol. 166, 1152–1161. doi: 10.1016/j.jplph.2009.01.002
López-Bucio, J., Pelagio-Flores, R., and Herrera-Estrella, A. (2015). Trichoderma as biostimulant: exploiting the multilevel properties of a plant beneficial fungus. Sci. Hortic. 196, 109–123. doi: 10.1016/j.scienta.2015.08.043
Lou, J., Fu, L., Peng, Y., and Zhou, L. (2013). Metabolites from Alternaria fungi and their bioactivities. Molecules 18, 5891–5935. doi: 10.3390/molecules18055891
Love, M. I., Huber, W., and Anders, S. (2014). Moderated estimation of fold change and dispersion for RNA-seq data with DESeq2. Genome Biol. 15:550. doi: 10.1186/s13059-014-0550-8
Luziatelli, F., Ficca, A. G., Colla, G., Baldassarre Švecová, E., and Ruzzi, M. (2019). Foliar application of vegetal-derived bioactive compounds stimulates the growth of beneficial bacteria and enhances microbiome biodiversity in lettuce. Front. Plant Sci. 10:60. doi: 10.3389/fpls.2019.00060
Mahnert, A., Haratani, M., Schmuck, M., and Berg, G. (2018). Enriching beneficial microbial diversity of indoor plants and their surrounding built environment with biostimulants. Front. Microbiol. 9:2985. doi: 10.3389/fmicb.2018.02985
Mannaa, M., Park, I., and Seo, Y. S. (2019). Genomic features and insights into the taxonomy, virulence, and benevolence of plant-associated Burkholderia species. Int. J. Mol. Sci. 20:121. doi: 10.3390/ijms20010121
Martin, M. (2011). Cutadapt removes adapter sequences from high-throughput sequencing reads. EMBnet J. 17, 10–12. doi: 10.14806/ej.17.1.200
McLean, M. (1996). The phytotoxicity of Fusarium metabolites: an update since 1989. Mycopathologia 133, 163–179. doi: 10.1007/BF02373024
Moisan, K., Cordovez, V., van de Zande, E. M., Raaijmakers, J. M., Dicke, M., and Lucas-Barbosa, D. (2019). Volatiles of pathogenic and non-pathogenic soil-borne fungi affect plant development and resistance to insects. Oecologia 190, 589–604. doi: 10.1007/s00442-019-04433-w
Novák, O., Hauserová, E., Amakorová, P., Doležal, K., and Strnad, M. (2008). Cytokinin profiling in plant tissues using ultra-performance liquid chromatography-electrospray tandem mass spectrometry. Phytochemistry 69, 2214–2224. doi: 10.1016/j.phytochem.2008.04.022
Ogórek, R. (2016). Enzymatic activity of potential fungal plant pathogens and the effect of their culture filtrates on seed germination and seedling growth of garden cress (Lepidium sativum L.). Eur. J. Plant Pathol. 145, 469–481. doi: 10.1007/s10658-016-0860-7
Op De Beeck, M., Lievens, B., Busschaert, P., Declerck, S., Vangronsveld, J., and Colpaert, J. V. (2014). Comparison and validation of some ITS primer pairs useful for fungal metabarcoding studies. PLoS One 9:e97629. doi: 10.1371/journal.pone.0097629
Ortíz-Castro, R., Contreras-Cornejo, H. A., Macías-Rodríguez, L., and López-Bucio, J. (2009). The role of microbial signals in plant growth and development. Plant Signal. Behav. 4, 701–712. doi: 10.4161/psb.4.8.9047
Palou, L., Smilanick, J., and Crisosto, C. (2009). Evaluation of food additives as alternative or complementary chemicals to conventional fungicides for the control of major postharvest diseases of stone fruit. J. Food Prot. 72, 1037–1046. doi: 10.4315/0362-028X-72.5.1037
Parveen, S., Wani, A. H., and Bhat, M. Y. (2019). Effect of culture filtrates of pathogenic and antagonistic fungi on seed germination of some economically important vegetables. Braz. J. Biol. Sci. 6, 133–139. doi: 10.21472/bjbs.061212
Pěnčík, A., Rolčík, J., Novák, O., Magnus, V., Barták, P., Buchtík, R., et al. (2009). Isolation of novel indole-3-acetic acid conjugates by immunoaffinity extraction. Talanta 80, 651–655. doi: 10.1016/j.talanta.2009.07.043
Perin, L., Martínez-Aguilar, L., Paredes-Valdez, G., Baldani, J. I., Estrada-de los Santos, P., Reis, V. M., et al. (2006). Burkholderia silvatlantica sp. nov., a diazotrophic bacterium associated with sugar cane and maize. Int. J. Syst. Evol. Microbiol. 56, 1931–1937. doi: 10.1099/ijs.0.64362-0
Philippot, L., Raaijmakers, J. M., Lemanceau, P., and Van Der Putten, W. H. (2013). Going back to the roots: the microbial ecology of the rhizosphere. Nat. Rev. Microbiol. 11, 789–799. doi: 10.1038/nrmicro3109
Podlešáková, K., Ugena, L., Spíchal, L., Doležal, K., and De Diego, N. (2019). Phytohormones and polyamines regulate plant stress responses by altering GABA pathway. N. Biotechnol. 48, 53–65. doi: 10.1016/j.nbt.2018.07.003
Prudent, M., Dequiedt, S., Sorin, C., Girodet, S., Nowak, V., Duc, G., et al. (2020). The diversity of soil microbial communities matters when legumes face drought. Plant Cell Environ. 43, 1023–1035. doi: 10.1111/pce.13712
Rabhi, N. E. H., Silini, A., Cherif-Silini, H., Yahiaoui, B., Lekired, A., Robineau, M., et al. (2018). Pseudomonas knackmussii MLR6, a rhizospheric strain isolated from halophyte, enhances salt tolerance in Arabidopsis thaliana. J. Appl. Microbiol. 125, 1836–1851. doi: 10.1111/jam.14082
Radhakrishnan, R., Kang, S.-M., Baek, I.-Y., and Lee, I.-J. (2014). Characterization of plant growth-promoting traits of Penicillium species against the effects of high soil salinity and root disease. J. Plant Interact. 9, 754–762. doi: 10.1080/17429145.2014.930524
Rahman, A., Sultana, R., Ferdousi Begum, M., and Firoz Alam, M. (2012). Effect of culture filtrates of Trichoderma on seed germination and seedling growth in chili. Int. J. Biosci. 2, 46–55.
Rao, V. K., Girisham, S., and Reddy, S. M. (2014). Influence of different species of Penicillium and their culture filtrates on seed germination and seedling growth of sorghum. J. Biochem. Technol. 5, 832–837.
Renaut, S., Masse, J., Norrie, J. P., Blal, B., and Hijri, M. (2019). A commercial seaweed extract structured microbial communities associated with tomato and pepper roots and significantly increased crop yield. Microb. Biotechnol. 12, 1346–1358. doi: 10.1111/1751-7915.13473
Ryu, C.-M., Farag, M. A., Hu, C.-H., Reddy, M. S., Wei, H.-X., Paré, P. W., et al. (2003). Bacterial volatiles promote growth in Arabidopsis. Proc. Natl. Acad. Sci. U.S.A. 100, 4927–4932. doi: 10.1073/pnas.0730845100
Sánchez-López, ÁM., Baslam, M., De Diego, N., Muñoz, F. J., Bahaji, A., Almagro, G., et al. (2016). Volatile compounds emitted by diverse phytopathogenic microorganisms promote plant growth and flowering through cytokinin action. Plant Cell Environ. 39, 2592–2608. doi: 10.1111/pce.12759
Sasse, J., Martinoia, E., and Northen, T. (2018). Feed your friends: do plant exudates shape the root microbiome? Trends Plant Sci. 23, 25–41. doi: 10.1016/j.tplants.2017.09.003
Schmidt, R., Etalo, D. W., de Jager, V., Gerards, S., Zweers, H., de Boer, W., et al. (2016). Microbial small talk: volatiles in fungal–bacterial interactions. Front. Microbiol. 6:1495. doi: 10.3389/fmicb.2015.01495
Schulz-Bohm, K., Martín-Sánchez, L., and Garbeva, P. (2017). Microbial volatiles: small molecules with an important role in intra- and inter-kingdom interactions. Front. Microbiol. 8:2484. doi: 10.3389/fmicb.2017.02484
Spaepen, S., Vanderleyden, J., and Remans, R. (2007). Indole-3-acetic acid in microbial and microorganism-plant signaling. FEMS Microbiol. Rev. 31, 425–448. doi: 10.1111/j.1574-6976.2007.00072.x
Sudadi, S. (2012). Exogenous application of tryptophan and indole acetic acid (IAA) to induce root nodule formation and increase yield of soybean. Agric. Sci. Res. J. 2, 134–139.
Sung, G. H., Shrestha, B., Park, K. B., Han, S. K., and Sung, J. M. (2011). Enhancing effect of Shimizuomyces paradoxus on seed germination and seedling growth of canola, plant growth of cucumber, and harvest of tomato. Mycobiology 39, 7–11. doi: 10.4489/MYCO.2011.39.1.007
Talibi, I., Askarne, L., Boubaker, H., Boudyach, E. H., Msanda, F., Saadi, B., et al. (2012). Antifungal activity of some Moroccan plants against Geotrichum candidum, the causal agent of postharvest citrus sour rot. Crop Prot. 35, 41–46. doi: 10.1016/j.cropro.2011.12.016
Tarkowski, P., and Vereecke, D. (2014). Threats and opportunities of plant pathogenic bacteria. Biotechnol. Adv. 32, 215–229. doi: 10.1016/j.biotechadv.2013.11.001
Varma, A., Savita, V., Sudha, Sahay, N., Butehorn, B., and Franken, P. (1999). Piriformospora indica, a cultivable plant-growth-promoting root endophyte. Appl. Environ. Microbiol. 65, 2741–2744. doi: 10.1128/AEM.65.6.2741-2744.1999
von Caemmerer, S., and Farquhar, G. D. (1981). Some relationships between the biochemistry of photosynthesis and the gas exchange of leaves. Planta 153, 376–387. doi: 10.1007/BF00384257
Wang, D., Deng, X., Wang, B., Zhang, N., Zhu, C., Jiao, Z., et al. (2019). Effects of foliar application of amino acid liquid fertilizers, with or without Bacillus amyloliquefaciens SQR9, on cowpea yield and leaf microbiota. PLoS One 14:e0222048. doi: 10.1371/journal.pone.0222048
Waqas, M., Kim, Y.-H., Khan, A. L., Shahzad, R., Asaf, S., Hamayun, M., et al. (2017). Additive effects due to biochar and endophyte application enable soybean to enhance nutrient uptake and modulate nutritional parameters. J. Zhejiang Univ. B 18, 109–124. doi: 10.1631/jzus.B1500262
Werner, S., Polle, A., and Brinkmann, N. (2016). Belowground communication: impacts of volatile organic compounds (VOCs) from soil fungi on other soil-inhabiting organisms. Appl. Microbiol. Biotechnol. 100, 8651–8665. doi: 10.1007/s00253-016-7792-1
Wu, Y., He, Y., Yin, H., Chen, W., Wang, Z., Xu, L., et al. (2012). Isolation of phosphate-solubilizing fungus and its application in solubilization of rock phosphates. Pakistan J. Biol. Sci. PJBS 15, 1144–1151. doi: 10.3923/pjbs.2012.1144.1151
Yandigeri, M. S., Meena, K. K., Singh, D., Malviya, N., Singh, D. P., Solanki, M. K., et al. (2012). Drought-tolerant endophytic actinobacteria promote growth of wheat (Triticum aestivum) under water stress conditions. Plant Growth Regul. 68, 411–420. doi: 10.1007/s10725-012-9730-2
Yu, K., Pieterse, C. M. J., Bakker, P. A. H. M., and Berendsen, R. L. (2019). Beneficial microbes going underground of root immunity. Plant. Cell Environ. 42, 2860–2870. doi: 10.1111/pce.13632
Zahir, Z. A., Shah, M. K., Naveed, M., and Akhter, M. J. (2010). Substrate-dependent auxin production by Rhizobium phaseoli improves the growth and yield of Vigna radiata L. under salt stress conditions. J. Microbiol. Biotechnol. 20, 1288–1294. doi: 10.4014/jmb.1002.02010
Zhang, H., Sun, Y., Xie, X., Kim, M. S., Dowd, S. E., and Paré, P. W. (2009). A soil bacterium regulates plant acquisition of iron via deficiency-inducible mechanisms. Plant J. 58, 568–577. doi: 10.1111/j.1365-313X.2009.03803.x
Keywords: biostimulant, fruit yield, fungal phytopathogen, plant growth promoting microorganism, plant-microbe interaction, soil microbiota, volatile organic compounds
Citation: Baroja-Fernández E, Almagro G, Sánchez-López ÁM, Bahaji A, Gámez-Arcas S, De Diego N, Dolezal K, Muñoz FJ, Climent Sanz E and Pozueta-Romero J (2021) Enhanced Yield of Pepper Plants Promoted by Soil Application of Volatiles From Cell-Free Fungal Culture Filtrates Is Associated With Activation of the Beneficial Soil Microbiota. Front. Plant Sci. 12:752653. doi: 10.3389/fpls.2021.752653
Received: 03 August 2021; Accepted: 30 September 2021;
Published: 21 October 2021.
Edited by:
Maria Jose Soto, Consejo Superior de Investigaciones Científicas (CSIC), SpainReviewed by:
José López-Bucio, Universidad Michoacana de San Nicolás de Hidalgo, MexicoDayakar Venkata Badri, Hill’s Pet Nutrition, Inc., United States
Copyright © 2021 Baroja-Fernández, Almagro, Sánchez-López, Bahaji, Gámez-Arcas, De Diego, Dolezal, Muñoz, Climent Sanz and Pozueta-Romero. This is an open-access article distributed under the terms of the Creative Commons Attribution License (CC BY). The use, distribution or reproduction in other forums is permitted, provided the original author(s) and the copyright owner(s) are credited and that the original publication in this journal is cited, in accordance with accepted academic practice. No use, distribution or reproduction is permitted which does not comply with these terms.
*Correspondence: Edurne Baroja-Fernández, e.baroja@csic.es; Javier Pozueta-Romero, javier.pozueta@csic.es