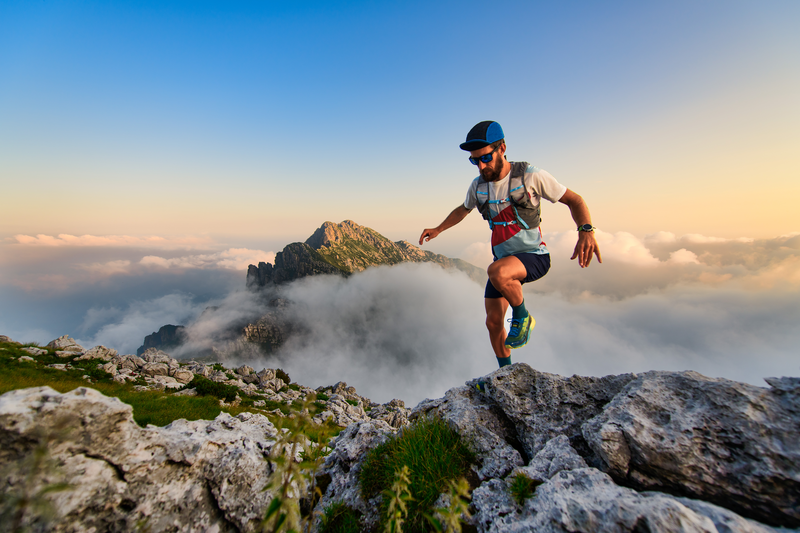
94% of researchers rate our articles as excellent or good
Learn more about the work of our research integrity team to safeguard the quality of each article we publish.
Find out more
REVIEW article
Front. Plant Sci. , 30 September 2021
Sec. Plant Biotechnology
Volume 12 - 2021 | https://doi.org/10.3389/fpls.2021.734776
This article is part of the Research Topic Methods in Phytohormone Detection and Quantification: 2022 View all 6 articles
Phytohormones affect plant growth and development. Many phytohormones are involved in the initiation of trichome development, which can help prevent damage from UV radiation and insect bites and produce fragrance, flavors, and compounds used as pharmaceuticals. Phytohormones promote the participation of transcription factors in the initiation of trichome development; for example, the transcription factors HDZIP, bHLH and MYB interact and form transcriptional complexes to regulate trichome development. Jasmonic acid (JA) mediates the progression of the endoreduplication cycle to increase the number of multicellular trichomes or trichome size. Moreover, there is crosstalk between phytohormones, and some phytohormones interact with each other to affect trichome development. Several new techniques, such as the CRISPR-Cas9 system and single-cell transcriptomics, are available for investigating gene function, determining the trajectory of individual trichome cells and elucidating the regulatory network underlying trichome cell lineages. This review discusses recent advances in the modulation of trichome development by phytohormones, emphasizes the differences and similarities between phytohormones initially present in trichomes and provides suggestions for future research.
- Reviewed the phytohormones regulation the development of trichome.
- New technologies may accelerate the study of trichome development.
Trichomes are a type of tissue often localized on plant leaves, buds, and stems and are classified into two types: glandular trichomes and non-glandular trichomes. Glandular trichomes can synthesize and deposit many kinds of secondary metabolites, such as terpenoids, polyketides, phenylpropanoids and alkaloids, which are very useful to humans for the production of commercial products, such as medicines, fragrances, and pigments. Glandular trichomes are multicellular organs and are considered bioengineering reactors, while non-glandular trichomes are usually single cells and are treated as physical barriers to insects or diffusers of ultraviolet light.
Many researchers have focused on trichome development in recent years. Arabidopsis is a model plant genus, and much progress in the understanding of trichome development has been achieved in this genus. The GLABRA 1 (GL1)-GLABRA 3 (GL3)/ENHANCER OF GLABRA 3 (EGL3)-TRANSPARENT TESTA GLABRA 1 (TTG1) trichome development complex has been well studied (Zhao et al., 2008); however, knowledge of the interaction between this complex and phytohormones is limited. Sweet wormwood (Artemisia annua), tomato (Solanum lycopersicum), and cucumber (Cucumis sativus) are the main plant species used as models for studying glandular trichome development (Lv et al., 2017; Du et al., 2020; Xiong et al., 2020), and many studies have indicated that trichome development involves phytohormones (Yan et al., 2017; Chen et al., 2020; Yuan et al., 2021).
Phytohormones are important signaling molecules that play key roles in plant growth and development and determine plant resistance against insects. Both non-glandular trichomes (providing physical defense) and glandular trichomes (providing chemical defense) serve as defenses against herbivorous insects. Many phytohormones are involved in trichome development, such as salicylic acid (SA), jasmonic acid (JA), gibberellins (GAs), abscisic acid (ABA), brassinosteroids (BRs), auxin and cytokinin (CK) (Zhou et al., 2013; Yan et al., 2018; Liu S. et al., 2020; Tan et al., 2020).
Some trichome tissue can produce useful secondary metabolites with commercial value, and trichomes constitute an ideal model system for studying plant cell development and differentiation. Phytohormones are key regulators of trichome development and differentiation, but the understanding of their roles in trichome initiation and development is limited. Nevertheless, recent progress has been made highlighting trichome development, which is summarized in this review.
Since model plants have a clear genetic background, ethyl methanesulfonate-induced mutation is a useful method for screening trichome development-related genes in model plants (Payne et al., 2000). However, research on most plant species is lacking; thus, the use of mutagenesis in most plants is limited. The transcriptome is an effective means for screening trichome development-related genes, but choosing the right stage of trichome development is very important. The genes involved in trichome development are dominantly expressed at the primary stage of trichome development; when trichome development is complete, the expression of trichome development-related genes is low or has stopped altogether. Cotton fibers are single-celled trichomes that have differentiated from the ovule epidermis and are considered a model system for studying cell elongation and cell wall biogenesis. The development of fibers can be classified into four stages: the initiation stage [2–5 days post anthesis (DPA)], elongation stage (3–20 DPA), secondary cell wall-deposition stage (16–40 DPA) and maturation stage (40–50 DPA) (Basra and Malik, 1984). Many genes involved in fiber development are expressed predominantly in fibers at 2–20 DPA, such as GhFP1, which is highly expressed in fibers at 9–10 DPA (Liu Z. H. et al., 2020); PAG1, which regulates fiber elongation and is highly expressed in fibers at 15 DPA (Yang et al., 2014); and Gh14-3-3L/e/h, which are expressed mainly in fibers at 3–10 DPA and are positively correlated with the rapid elongation of fibers (Yang et al., 2014). We classified the development of trichome initiation in A. annua into three stages: stage I, stage II and stage III (Figures 1A–C). Many genes involved in trichome development in A. annua are not expressed or exhibit low expression at the callus stage (stage I); however, at the primary stage of trichome development (stage II), trichome-related genes are highly expressed. At stage III, the expression of genes related to trichome development may be downregulated. Some of these genes have been screened from transcriptome data (Figure 1D), such as AA335470 (AaMYB1), which is a positive regulator of the development of trichomes in A. annua (Matias-Hernandez et al., 2017). In addition, new genes with potential roles in the development of trichome development may be found in the phylogenetic tree (Figure 1E), such as AA629630 (closely related to AaMYB1), AA503070 and AA615110 (closely related to AaMIXTA1) (Shi et al., 2018). The raw RNA-seq data are available from the NCBI Sequence Read Archive (accession number PRJNA754709). Therefore, choosing the right stage of trichome development is a key factor for studying trichome development.
Figure 1. Different developmental stages of A. annua calli. (A) After 2 weeks of culture on media, calli appeared on the leafstalk (explants). (B) Trichomes present on calli after 3 weeks of culture on media. (C) Additional trichomes present on calli after 4 weeks of culture on media. (D) Nine MYB transcription factors obtained from RNA-seq data across three stages, p < 0.05. One of these transcription factors is AaMYB61, which is a positive regulator of trichome development. (E) Phylogenetic tree showing that AaMIXTA1 and AA335470 (AaMYB1) are closely related, suggesting that AA503070, AA615110, and AA629630 may play roles in trichome development.
A recent study indicated that auxin is involved in trichome development during tomato and fiber elongation in cotton (Zhang X. et al., 2015; Zhang et al., 2017; Tian and Zhang, 2021). Cotton is not only an important natural-fiber economic crop in the textile industry but also an ideal system for studying genome evolution, polyploidization and cell elongation. Many studies have indicated that auxin plays a positive role in fiber development. A previous study suggested that auxin accumulates in the initial cotton fibers but not in other epidermal cells, indicating that auxin modulates trichome initiation (Zhang et al., 2011). Targeted overexpression of the indole-3-acetic acid (IAA) biosynthesis pathway gene iaaM may increase the IAA content in the epidermis of cotton ovules at the fiber initiation stage. The compound 1-N-naphthylphthalamic acid (NPA) can specifically inhibit auxin transport and leads to impaired IAA accumulation and further repression of fiber initiation (Zhang et al., 2011). Repression of the auxin transporter gene GhPIN in transgenic cotton has been shown to decrease the expression of the fiber elongation-related genes GhMYB109, GhMYB25, GhMYB25-like and GhHD1 and to decrease fiber initiation and elongation (Zhang et al., 2017). NUMEROUS SPINES, which encodes an auxin transporter protein, negatively modulates cucumber fruit spine density (Xie et al., 2018) and may be a target gene that can be used as a marker to improve the quality of cucumber. Taken together, these observations indicate that auxin transporter proteins play important roles in trichome development.
The auxin-based regulation of gene expression depends on AUXIN RESPONSE FACTOR (ARF) and AUXIN/INDOLE-3-ACETIC ACID (Aux/IAA) proteins, both of which bind to promoter regions via auxin response elements (AuxREs) (Yamauchi et al., 2019). Aux/IAA proteins have four highly conserved domains, I, II, III and IV, and each determines the functional properties of the protein. Aux/IAA proteins usually act as transcriptional repressors by interacting with ARF proteins (Deng W. et al., 2012). Inhibition of IAA15 in tomato increases the density of glandular trichome types I and VI and non-glandular trichome type V, indicating that auxin-dependent transcriptional regulation is required for trichome development. Some genes involved in trichome differentiation, such as GAMYB-like1 and GAI, may regulate trichome initiation by interacting with GA signaling (Deng W. et al., 2012). In addition, ARFs can regulate trichome density. In tomato, ARF3 is expressed mainly in the trichomes. When its expression was inhibited by RNA interference (RNAi), the density of type I, V, and VI trichomes on the leaves decreased, indicating that ARF3 plays a positive role in trichome development (Zhang X. et al., 2015).
Auxin increases trichome density by modulating transcription factors. GhTCP14 may bind to the promoters of the auxin efflux carriers PIN-FORMED 2 (PIN2) and IAA3 and the auxin uptake carrier AUXIN 1 to increase auxin concentrations, thereby increasing trichome density (Wang et al., 2013; Figure 2).
Figure 2. Regulatory networks of cotton fiber and trichome development induced by auxin. NPA negatively regulates the expression of GhPIN2. GhPIN2 is a negative regulator of GhTCP14, and GhTCP14 directly modulates AUX1- or IAA3-promoted fiber initiation in cotton. SlIAA15 is an inhibitor of IAA signaling in tomato and is degraded when the auxin level increases, thus releasing ARFs and activating the transcriptional response.
Previous studies have indicated that abiotic stress-related genes may affect plant growth by decreasing endogenous plant hormone production, thereby increasing trichome density (Johnson et al., 2002; Plett et al., 2010). Overexpression of Bna.TTG2, a WRKY transcription factor from Brassica napus, increases sensitivity to salt stress and decreases endogenous IAA contents by directly binding to the promoter of the auxin biosynthesis-related genes TRYPTOPHAN BIOSYNTHESIS 5 and YUCCA2 (Li et al., 2015). It is suggested that some phytohormones may interact with IAA during trichome development. PtaMYB186, an insect resistance gene, inhibits auxin flow and increases ethylene-responsive gene expression, thereby leading to increased foliar trichome density in Populus (Plett et al., 2010). This finding indicates that ethylene may induce trichome development. These genes associated with the abiotic stress response may share the common feature of redirecting the metabolites of auxin to other abiotic stress-related plant hormones, while the production of trichomes may be the result of plant adaptation to the environment.
Jasmonic acid is a universal phytohormone that regulates trichome development; for example, JA induces trichome initiation in Arabidopsis, A. annua, and tomato and trichome elongation in cotton (Traw and Bergelson, 2003; Hu et al., 2016; Yan et al., 2017, 2018; Hua et al., 2021a, b). JA-deficient plants may have reduced numbers of trichomes (Yan et al., 2013); the JA-isoleucine (JA-Ile) receptor may be involved in trichome development (Li et al., 2004). Regulation of the expression of AOS, a JA biosynthesis pathway gene, may increase trichome number (Wu et al., 2008). In the aos mutant, mechanical wounding does not lead to an increased number of trichomes. Adding exogenous JA to the gl3 mutant can cause trichomes to regenerate on Arabidopsis leaves, indicating that JA plays an essential role in trichome development (Yoshida et al., 2009).
In JA signal transduction, JASMONATE-ZIM DOMAIN (JAZ) family proteins serve as JA coreceptors and act as repressors of transcription factors (Kazan and Manners, 2012). Overexpression of JAZ proteins can repress the initiation and elongation of trichomes (Hu et al., 2016); therefore, JAZ proteins may be negative regulators of trichome development. To inhibit trichome initiation, JAZ proteins usually interact together with modulators of trichome development, such as GhMYB25-like in cotton (Hu et al., 2016) and GL3/EGL3, GL1 and TTG1 in Arabidopsis (Qi et al., 2011).
Most trichome regulators reported are transcription factors, such as HDZIP transcription factors, basic helix-loop-helix (bHLH) transcription factors or MYB transcription factors; these include AtGL2, AaHD1, AaHD8, GhMYB25-like, and AtGL3/AtEGL3. HDZIP transcription factors play key roles in trichome development. AtGL2 in Arabidopsis (Szymanski et al., 1998), SIWo in tomato (Yang et al., 2011), AaHD1 and AaHD8 in A. annua (Yan et al., 2017, 2018), and CsTril in cucumber (Du et al., 2020) are HDZIP transcription factors that modulate trichome development.
The development of trichomes may involve an endoreduplication cycle. Usually, the cell cycle in eukaryotes involves four phases: G1, S, G2, and M. D-type cyclin (CYCD) and cyclin-dependent kinase (CDKA) complexes play important roles in regulating G1→S and G2→M transitions (Ishida et al., 2008; Wang et al., 2020). Trichome initiation occurs at the G1→S and G2→M stages, and regulation of the endoreduplication cycle may be a promising way to modulate multicellular trichomes (Schnittger et al., 2002a, b). Methyl jasmonate (MeJA) may specifically delay the switch from G1→S and prolong the G1 phase (Noir et al., 2013; Wang et al., 2020; Figure 3). Therefore, MeJA may regulate trichome development by modulating endoreduplication.
Figure 3. Regulatory networks of cotton fiber and trichome development induced by JA. JAZ proteins are degraded when JA levels increase, releasing ARFs and activating transcription factors such as AaHD1 in A. annua, SlWoolly in tomato, and GhMML3 or GhMYB109/GhMYB2 in cotton, thus regulating trichome development or fiber initiation. JA regulates trichome development by affecting the BMW complex to promote the expression of AtGL2 in Arabidopsis. AtGL1 and AtGl3 are involved in cell endoreduplication and induce trichome development. In the development of tomato trichomes, the cyclin protein SlCycB2 interacts with SlWoolly to modulate trichome initiation. SlMYB31 regulates trichome development by modulating lipid loading in trichomes.
The BMW complex is the core element that regulates trichome development in Arabidopsis (Ishida et al., 2008). Trichome initiation is inhibited in GA-deficient mutants, whereas exogenous GA can increase trichome density by upregulating the expression of GL1, TTG1 and GL3 (Chien and Sussex, 1996; Traw and Bergelson, 2003; Yu et al., 2015). In addition, the mutant spy-5 (the GA signaling pathway of which is repressed) displays more trichomes than Columbia wild-type Arabidopsis (Col). GL1 may act downstream of the GA signal because the gl1 mutant is epistatic to the spy mutation (An et al., 2012); results consistent with this observation have been obtained in previous studies. Exogenous application of GA or an increase in the endogenous GA content by overexpression of GhGA20ox1 may increase endogenous GA levels (especially GA4) in fibers and ovules and promote cotton fiber initiation and elongation (Xiao et al., 2010). These observations show that GA is the main phytohormone involved in trichome development.
GA regulates trichome development mainly by activating the expression of transcription factors that are involved in trichome development. ZFP5, a recently discovered C2H2 zinc finger protein involved in GA signaling, plays an important role in trichome development. The number of trichomes on sepals, caulines, and paraclades has been found to be reduced in zfp5 mutants compared with wild-type plants, while in ZFP5-overexpression plants, the trichome number is increased. zfp5 acts upstream of GL1, GL3, GIS, GIS2, and ZFP8 and directly targets ZFP8 to modulate epidermal cell differentiation (Zhou et al., 2011). In addition, ZFP8 and GIS2 are targets of GIS3 (Sun et al., 2015). The mRNA level of ZFP5 is regulated by ZFP6, which modulates trichome development by integrating GA and CK signaling (Zhou et al., 2013). However, the relationship between GIS3 and ZFP6 is poorly understood. Genetic information has suggested that the R3 MYB transcription factor TCL1 may act downstream of the BMW complex, while the mRNA level of TCL1 is not affected by the BMW complex (Zhang et al., 2018). Thus, posttranscriptional regulation of TCL1 may occur.
DELLA proteins are inhibitors of GA signal transduction. DELLAs bind to GhHOX3 and inhibit its binding activity to target genes or its transcriptional activation activity. GA triggers the degradation of DELLAs, which causes the release of GhHOX3; GhHOX3 in turn interacts with other proteins (such as GhHD1) and activates downstream genes to promote cotton fiber elongation (Shan et al., 2014; Figure 4).
Figure 4. Regulatory networks of cotton fiber and trichome development induced by GA. GA20ox2 is the key enzyme that affects GA content and promotes trichome development. Modulation of GA20ox2 by SlbHLH95 in tomato or by AaMYB1 in A. annua affects trichome initiation.
Ethylene is involved in trichome development (Zhang Y. et al., 2021). Ethylene stimulates endoreduplication of epidermal cells and increases the rate of cell division, resulting in an abundance of trichomes. Ethylene also alters cell division polarity, leading to the production of branched trichomes (Kazama et al., 2004). Lotus japonicus lot1 mutant seedlings are insensitive to ethylene treatment and exhibit a phenotype that has fewer trichomes than wild-type seedlings (Ooki et al., 2005). Thus, there may be a direct relationship between ethylene and trichome development.
The trichomes of the Arabidopsis ethylene receptor mutant etr2-3 are abnormally shaped and unbranched. ETR2 affects the microtubule cytoskeleton, stabilizing or depolymerizing it (Plett et al., 2009). Microtubule cytoskeleton proteins are involved in the regulation of trichome cell expansion, trichome branch number and the elongation of trichome branches (Tian et al., 2015; Chen et al., 2016; Chang et al., 2019). Therefore, the ethylene signaling pathway may affect trichome development via microtubule cytoskeleton proteins. Inhibition of GbPDF1, a fiber initiation- and early elongation-related gene, decreases the mRNA levels of several genes related to ethylene, revealing a direct relationship between ethylene and trichome development (Deng F. et al., 2012).
Further investigation of the relationship between ethylene and trichome development has revealed that the TINY BRANCHED HAIR (TBH) gene is preferentially expressed in multicellular trichomes of cucumber. TBH can restore the trichome phenotype of tbh mutants. Moreover, exogenous ethylene can rescue the trichome defects of tbh mutants. TBH may directly bind to the promoter of the 1-aminocyclopropane-1-carboxylate synthase (ACS) gene to modulate the accumulation of ethylene, thereby affecting trichome development (Zhang Y. et al., 2021; Figure 5A).
Figure 5. Regulatory networks of trichome development induced by ethylene and for cotton fiber regulated by BRs. (A) Ethylene regulates the expression of AaTRY, which is a negative regulator of trichome development in Arabidopsis. It also affects trichome initiation via its involvement in cell endoreduplication. (B) BRs activate GhBZR1, which is an important transcription factor that regulates BR-responsive gene expression. GhBZR1 promotes the expression of Ghxth1 and GhEXP to modulate fiber initiation in cotton.
Cotton fibers are single-celled trichomes derived from the epidermal cells of seeds, and BRs are related to the elongation of these fibers, with genes of the BR biosynthesis pathway being upregulated during the initial fiber and elongation stages (Yang et al., 2014). Exogenous BRs promote fiber elongation from cotton ovules, while treatment with brassinazole, a BR biosynthesis inhibitor, can inhibit fiber elongation (Sun et al., 2005). The BR biosynthesis pathway gene PAG1 is a homolog of Arabidopsis CYP734A1/BAS1 and plays an important role in the inactivation of BRs through C-26 hydroxylation. PAG1 affects the endogenous biological activation of BRs, thereby affecting ethylene signal transduction by mediating very long-chain fatty acid (VLCFA) levels and regulating fiber elongation (Yang et al., 2014). Another BR biosynthesis pathway-related gene, DET2, which encodes a steroid 5d-reductase, may participate in a rate-limiting step in BR biosynthesis. Altering the metabolic flux of the BR biosynthesis pathway by overexpression of GhDET2 affects fiber initiation and fiber elongation. Inhibition of GhDET2 by an antisense method has been shown to reduce fiber initiation and fiber elongation, while the addition of finasteride, a steroid 5a-reductase inhibitor, represses fiber elongation (Luo et al., 2007).
The BR biosynthesis pathway has been thoroughly characterized (Huaxun, 2011). Therefore, the regulation of BR biosynthesis is a promising way to modulate the development of cotton fibers. Liu (Liu Z. H. et al., 2020) overexpressed the bHLH transcription factor GhFP1 in Arabidopsis to increase trichome length. Moreover, the overexpression of GhFP1 in cotton can promote fiber elongation. GhFP1 can directly bind to the promoters of GhCPD and GhDWF4, both of which are BR biosynthesis pathway genes; in turn, this binding positively regulates BR biosynthesis and increases fiber elongation (Figure 5B).
14-3-3 acidic regulatory proteins are highly conserved among eukaryotes. These proteins usually form dimers and alter the relocation, activity, phosphorylation state and stability of their target proteins (Zhang et al., 2010). Gh14-3-3a, Gh14-3-3e, and Gh14-3-3L in cotton may function synergistically in BR signal transduction by altering the nuclear export of BZR1 and promoting the atypical longitudinal growth of cells, thus promoting cotton fiber elongation (Zhang et al., 2010). Overexpression of Gh14-3-3L in cotton can promote fiber elongation and increase mature fiber length, whereas inhibition of the expression of Gh14-3-3L, Gh14-3-3e, and Gh14-3-3 leads to short cotton fibers. 2,4-Epibrassinolide treatment can rescue the short-fiber phenotype in cotton plants in which 14-3-3 proteins are silenced via RNAi. Gh14-3-3 interacts with GhBZR1 via protein–protein interactions to modulate BR signaling, ultimately affecting fiber initiation and elongation (Zhou et al., 2015).
Previous studies have indicated that CKs may induce trichome development (Greenboim-Wainberg et al., 2005). Overexpression of the CK biosynthesis-related gene isopentenyl transferase driven by the carpel-specific CRABS CLAW promoter of Arabidopsis produces numerous ectopic trichomes in hairless carpels (Greenboim-Wainberg et al., 2005). ARR is a type-B response regulator that acts as a transcription factor in CK signaling (Kim and Hwang, 2012). Specifically, ARR1, ARR2, ARR10 and ARR12 can interact with SPLs (SPL2, SPL9 and SPL10) to modulate shoot regenerative capacity (Zhang T. et al., 2015). SPL2, SPL9, and SPL10 participate in trichome development by interacting with ARR2 (Shikata et al., 2009; Zhang T. et al., 2015). SPL9 is the direct target of miR156 and regulates trichome initiation by binding to the promoters of TRY and TCL1 (Yu et al., 2010). Mutation of the ARR2 gene in rice leads to a substantial decrease in trichome initiation and elongation in grain hulls and decreased expression of GL3A (a homologous gene of Arabidopsis GL3) (Worthen et al., 2019). 6-Benzylaminopurine (6-BA) is a CK that can strongly increase the transcript levels of ARR5, leading to significant increases in the expression of GIS2, GL1, and GIS3 (Gan et al., 2007; Sun et al., 2015).
In a study, the promoter of GIS3 was fused to β-glucuronidase (GUS), after which pGIS3:GUS transgenic plants were generated and treated with 6-BA. Histochemical staining of GUS activity indicated that the CK signal could induce GIS3 expression at the protein level (Sun et al., 2015). GIS3 directly targets GIS2, one of the C2H2 transcription factors that respond to CKs, and regulates trichome development. However, the factors downstream of GIS2 remain unclear. In addition to the C2H2 zinc finger proteins ZFP5, ZFP6, ZFP8, GIS, GIS2 and GIS3, which regulate trichome development (Gan et al., 2007; Zhou et al., 2011, 2013; Sun et al., 2015), another member of the C2H2 zinc finger protein in tomato can regulate trichome development and interact with CKs via an unknown mechanism (Chang et al., 2018). In addition, the 26S proteasome can regulate trichome development by modulating endoreduplication (Sako et al., 2010). RPN1a, a subunit of the 26S proteasome, has been shown to be repressed by CK and to inhibit trichome development by downregulating the expression of ZFP6, ZFP5, GIS, GL1, GL2, GL3, TTG1 and MYB23 (Yu et al., 2015). GL3 and RHL1 may be involved in the positive modulation of endocycle progression in trichomes (Sako et al., 2010), and RPN1a may promote the interaction between CK and endoreduplication to regulate trichome development.
The interaction between GA and CK affects the expression of Pd1 (a homolog of soybean GL2), thereby activating the expression of lipid transfer protein P1 (Figure 6), which is localized to the cytoplasm and cell membrane; the transferred lipids affect trichome development (Liu Z. H. et al., 2020).
Figure 6. Regulatory networks of cotton fiber and trichome development induced by CKs. The CK receptor AtARR2 interacts with AaSPL9, which is involved in trichome development in Arabidopsis. In soybean, the expression of GmPd1 is induced by CKs, and GmPd1 binds directly to the promoter of GmP1 (which encodes a lipid transporter) and promotes trichome development. ARR, nuclear-localized B-type ARABIDOPSIS RESPONSE REGULATOR.
Salicylic acid plays an important role in resistance to pathogens; it also regulates trichome density. SA at a concentration of 0.1 or 1 mM can reduce trichome density, while different concentrations of SA have no differential effects on trichome density; different varieties of Arabidopsis yield similar results (Traw and Bergelson, 2003). SA can interact with JA to exert various functions (Liu et al., 2016). JA is a positive regulator of trichome size and trichome density (Maes et al., 2011; Hua et al., 2021a, b), and SA can inhibit JA signaling by degrading ORA59 in Arabidopsis. In trichome development, SA negatively regulates trichome density and constitutively weakens the effect of JA, indicating that crosstalk occurs between the SA- and JA-dependent trichome development pathways (Traw and Bergelson, 2003).
Constitutive pathogen response 5 (cpr5) may be involved in trichome development (Bowling et al., 1997; Kirik et al., 2001; Aki et al., 2007). Compared with the wild type, the cpr5 mutant has fewer trichome branches, and the number and size of the trichomes are much smaller.
The DNA content in the trichomes highly significantly differs between the wild type (32C) and cpr5 mutant (8C), indicating that endoreduplication cycles stop prematurely in the mutant (Kirik et al., 2001). In cpr5 mutants, the content of SA is 20-fold higher than that of Col-0 (Aki et al., 2007). The high concentrations of SA inhibit trichome development (Traw and Bergelson, 2003), leading to fewer trichomes in the cpr5 mutant. Interactions also occur between SA and GAs. GAs have been shown to increase the number of trichomes by 72.0%; however, this increase was reduced to 29.6% when SA was also added (Traw and Bergelson, 2003). The mechanism underlying these interaction effects is still unknown.
Stress is an external condition that can affect plant growth and development. Stress can activate the SA, ABA and JA biosynthesis pathways, generating phytohormone responses to external conditions. JA increases the size of trichomes in both A. annua and Arabidopsis, GA3 increases the size of trichomes only in A. annua, and 6-BA increases the size of trichomes in Arabidopsis (Maes et al., 2008, 2011). Enhancing BR biosynthesis or increasing endogenous GA content can positively regulate fiber elongation in cotton (Xiao et al., 2010; Liu Z. H. et al., 2020). Drought can increase ABA signal transduction, which participates in the initial formation of trichomes by inducing the expression of MYB41, which modulates cuticle loading on the trichome surface (Cominelli et al., 2010). However, ABA can antagonize JA, which regulates trichome size (Maes et al., 2011); thus, trichomes decrease in size in response to ABA (Yadav et al., 2014).
Endoreduplication has an important role in modulating plant organ or tissue size (Peng et al., 2015; Li et al., 2020). Most studies on this topic have indicated that cyclin proteins are downstream target genes involved in organ size (Breuer et al., 2009, 2012). The trihelix transcription factor GT2-like 1 (GTL1) can directly inhibit the expression of CDH1/FZR/CCS52, which is an activator of the APC/C complex/loop body, preventing the development of the endothelial cycle and ploidy-dependent cell growth (Breuer et al., 2012). Furthermore, GTL1 participates in SA metabolism and signaling by directly binding to the promoter region or genomic region (Völz et al., 2018). SA can antagonize JA during trichome development (Traw and Bergelson, 2003), so SA can enhance the negative effect of GTL1 on trichome size in Arabidopsis and lead to a decrease in trichome size. However, SA increases the size of glandular trichomes in A. annua (Kumari et al., 2018). Thus, models of trichome development can differ between glandular trichomes and non-glandular trichomes.
Phytohormones can affect the expression of the MEP pathway genes 1-deoxy-D-xylulose 5-phosphate synthase and 1-deoxy-D-xylulose-5-phosphate reductoisomerase (Paetzold et al., 2010; Xing et al., 2010), which regulate the contents of terpenoids such as monoterpenes and sesquiterpenes, leading to feedback regulation of trichome development. The monoterpene β-ocimene acts as a phytohormone and can induce trichome development and increase trichome size (Xiao et al., 2020).
In this review, we discuss the plant hormones that regulate the development of trichomes (Table 1), describe the potential mechanisms affecting trichome density, and emphasize that transcription factors play an important role in the initial development of trichomes. In addition, the mechanisms of development differ between unicellular and multicellular trichomes. Elucidating this difference may help efforts to convert unicellular trichomes to multicellular trichomes and thereby increase the production of secondary metabolites.
Fragrances, pigments, and medicines have economic value, and many of these are produced in the glandular trichomes of plants. In A. annua, there are two kinds of trichomes: 10-celled glandular trichomes and non-glandular trichomes (Duke and Paul, 1993). Artemisinin is synthesized mainly in glandular trichome cells, although it has been found that non-glandular trichomes produce some artemisinin (Duke and Paul, 1993; Judd et al., 2019). Increasing the number of multicellular trichomes may be a promising method to increase artemisinin content. Cyclin proteins involved in the process of the endoreduplication cycle have the potential to reshape single-celled trichomes into multicellular trichomes, and promising methods to leverage this capacity have the potential to achieve ideal production capacity, offering economic value (Ishida et al., 2008).
CYCD proteins can induce cellular growth and trigger the reprogramming of mitosis or endoreduplication, leading to the production of multicellular trichomes. The cells of plants with a mutation in CYCD3;1 cannot enter S phase (Perazza et al., 1999). Overexpression of CYCD3;1 can shorten the G1 phase and promote the entry of S phase and induce mitosis, resulting in multicellular trichomes (Schnittger et al., 2002a; Menges et al., 2006). The function of CYCD3 (CYCD3;1-3) is dependent on CKs, as evidenced by the impairment of the cycd3;1-3 mutant in the absence of CKs (Dewitte et al., 2007). These observations indicate that CKs can affect multicellular trichomes through endoreduplication.
CYCB also modulates multicellular trichomes. Ectopic expression of CYCLIN B1;2 in Arabidopsis can trigger mitotic divisions and lead to multicellular trichomes (Schnittger et al., 2002b). Dominant-negative cyclin-dependent kinase (CDK) or CDK inhibitor proteins (ICK/KRPs) can affect the endoreduplication of trichomes. Misexpression of ICK1/KRP1 inhibits cell differentiation and triggers cell death (Schnittger et al., 2003). Overexpression of ICK1/KRP1 has a positive role in the regulation of multicellular trichomes. SIAMESE (SIM) is a CDK inhibitor involved in endoreplication in Arabidopsis. sim mutants exhibit promoted transition from S phase to G2 phase and have an increased number of multicellular trichomes (Churchman et al., 2006). Moreover, overexpression of SIM can inhibit CDK complexes and repress the transition from the S phase to G2 phase (Wang et al., 2020).
The bacterial clustered, regularly interspaced, short palindromic repeat (CRISPR)/CRISPR-associated 9 (Cas9) system stems from Streptococcus pyogenes and involves specific binding to a DNA sequence (20 bp) guided by an engineered single-guide RNA through RNA–DNA interactions (Li et al., 2013; Nekrasov et al., 2013). CRISPR-Cas9 is a tool for base editing involving nucleotide substitution. It is widely used in genome editing and is characterized by low cost and ease of targeting genetic manipulations. This method can be used to improve the economically important traits of crop species; examples of such use include editing the MILDEW-RESISTANCE LOCUS gene to obtain powdery mildew-resistant wheat (Wang et al., 2014); knocking out GW2, GW5, and TGW6 to increase the grain weight of rice (Xu et al., 2016); and targeting the waxy allele to accelerate the breeding process of corn (Gao et al., 2020).
There are many negative regulators during trichome development, such as CPC, ETC1, ETC1, ETC3, MYBL2, myb82, TCL1, TCL2, and TRY (Wada et al., 1997; Szymanski and Marks, 1998; Sawa, 2002; Kirik et al., 2004a, b; Wang et al., 2007; Tominaga et al., 2008; Gan et al., 2011; Liang et al., 2014). Simultaneous mutations in try/cpc/etc1 lead to a dozenfold increase in the number of trichomes (Wang et al., 2008). Therefore, simultaneous editing of negative regulators of trichomes may be a promising method for obtaining trichome-rich plants (Figure 7A).
Figure 7. CRISPR-Cas9 and single-cell sequencing may help reveal the development of trichomes. (A) CRISPR-Cas9 may be a promising technique for producing multiple trichomes by editing the redundant cyclin genes involved in trichome development. G1, gap 1; G2, gap 2; M, mitosis; S, synthesis. (B) Single-cell sequencing can be used to elucidate the mechanism underlying trichome development. Autofluorescence of glandular trichomes on the buds of A. annua, as revealed via a fluorescent isothiocyanate (FITC) filter (λex = 480 nm; λem = 535 nm). Each trichome of A. annua is composed of ten cells: one pair of apical cells, two pairs of subapical cells, one pair of stalk cells and one pair of base cells. The base cells do not autofluorescence. Advanced single-cell mRNA sequencing (mRNA-seq) can be used to restore the developmental process of each cell of glandular trichomes, and the main genes involved in trichome development encode cyclins. St, stalk cell; B, base cell.
In multicellular organisms, different types of cells with the same genome have unique developmental programs and different functions. It is therefore very important to reveal how genes reach the right state at the right place and at the right time. Although many RNA-seq techniques have been used to study the development of glandular trichomes (Li et al., 2020; Liao et al., 2020), progress in understanding glandular trichome development has been very slow. Since many glandular trichomes are multicellular, the function of each cell is different, resulting in different levels of mRNA in each cell. Detection of mRNA of single-cell or glandular trichomes may be a promising method to study trichome development. Single-cell transcriptomics is a promising method applicable for cells with high or low expression to study new cell types and improve the understanding of the relationship between time and space in real time (Figure 7B).
Glandular trichomes are multicellular trichomes, and each cell can have different functions in the biosynthesis or deposition of secondary metabolites (Olsson et al., 2009). The glandular trichomes of A. annua comprise 10 cells: one pair of apical cells, two pairs of subapical cells, one pair of stalk cells and one pair of base cells. The four pairs of cells have different cellular contents according to slicing data (Duke et al., 1994). Therefore, the five classes of cells can exhibit heterogeneity and coregulation of artemisinin biosynthesis. What are the relationships among the five types of cells in regulating the synthesis of artemisinin? What role does each cell play in artemisinin biosynthesis? Single-cell transcriptomics can be a good way to reveal the developmental trajectory of each type of trichome cell and study dynamically expressed genes. The single-cell sequencing is based on protoplast sequencing or transposase-accessible chromatin sequencing (ATAC-seq). The protoplast method was derived from cell wall digestion and dissociation by cellulase and pectinase (Shen et al., 2014). Trichome cells are resistant to cell wall digestion due to the wax and cutin on their surface. Enzymatic digestion procedures can affect gene expression and therefore may not reveal the true level of gene expression (Denyer et al., 2019). Single-cell ATAC-seq can measure the dynamic accessibility of chromatin to gene expression (Farmer et al., 2021; Zhang T. Q. et al., 2021); thus, it can be used to avoid obtaining protoplasts and for single-cell sequencing of glandular trichomes.
Therefore, single-cell sequencing can accurately reveal the function of each cell of glandular trichomes in real time, paving the way for regulating the number of glandular trichomes or for the artificial targeted synthesis of glandular trichomes, thereby leading to increased numbers of economically valuable products.
ZL and WC conceived and designed the entire research plans. JL, XW, and SF performed most of the work. QL provided technical assistance. JL and XW wrote the manuscript. WC, RJ, and ZL helped with the organization and editing. All authors contributed to the article and approved the submitted version.
This work was funded by the National Natural Science Foundation of China (32070332 and 31770329), Shanghai Natural Science Foundation in China (20ZR1453800), and National Key R&D Program of China (2019YFC1711000).
The authors declare that the research was conducted in the absence of any commercial or financial relationships that could be construed as a potential conflict of interest.
All claims expressed in this article are solely those of the authors and do not necessarily represent those of their affiliated organizations, or those of the publisher, the editors and the reviewers. Any product that may be evaluated in this article, or claim that may be made by its manufacturer, is not guaranteed or endorsed by the publisher.
Aki, T., Konishi, M., Kikuchi, T., Fujimori, T., Yoneyama, T., and Yanagisawa, S. (2007). Distinct modulations of the hexokinase1-mediated glucose response and hexokinase1-independent processes by HYS1/CPR5 in Arabidopsis. J. Exp. Bot. 58, 3239–3248. doi: 10.1093/jxb/erm169
An, L., Zhou, Z., Su, S., Yan, A., and Gan, Y. (2012). GLABROUS INFLORESCENCE STEMS (GIS) is required for trichome branching through gibberellic acid signaling in Arabidopsis. Plant Cell Physiol. 53, 457–469. doi: 10.1093/pcp/pcr192
An, L., Zhou, Z., Yan, A., and Gan, Y. (2011). Progress on trichome development regulated by phytohormone signaling. Plant Signal. Behav. 6, 1959–1962. doi: 10.4161/psb.6.12.18120
Basra, A. S., and Malik, C. P. (1984). Development of the cotton fiber. Int. Rev. Cytol. 89, 65–113.
Bowling, S. A., Clarke, J. D., Liu, Y., Klessig, D. F., and Dong, X. (1997). The cpr5 mutant of Arabidopsis expresses both NPR1-dependent and NPR1-independent resistance. Plant Cell 9, 1573–1584. doi: 10.1105/tpc.9.9.1573
Breuer, C., Kawamura, A., Ichikawa, T., Tominaga-Wada, R., Wada, T., Kondou, Y., et al. (2009). The trihelix transcription factor GTL1 regulates ploidy-dependent cell growth in the Arabidopsis trichome. Plant Cell 21, 2307–2322. doi: 10.1105/tpc.109.068387
Breuer, C., Morohashi, K., Kawamura, A., Takahashi, N., Ishida, T., Umeda, M., et al. (2012). Transcriptional repression of the APC/C activator CCS52A1 promotes active termination of cell growth. EMBO J. 31, 4488–4501. doi: 10.1038/emboj.2012.294
Chang, J., Xu, Z., Li, M., Yang, M., Qin, H., Yang, J., et al. (2019). Spatiotemporal cytoskeleton organizations determine morphogenesis of multicellular trichomes in tomato. PLoS Genet. 15:e1008438. doi: 10.1371/journal.pgen.1008438
Chang, J., Yu, T., Yang, Q., Li, C., Xiong, C., Gao, S., et al. (2018). Hair, encoding a single C2H2 zinc-finger protein, regulates multicellular trichome formation in tomato. Plant J. 96, 90–102. doi: 10.1111/tpj.14018
Chen, L., Peng, Y., Tian, J., Wang, X., Kong, Z., Mao, T., et al. (2016). TCS1, a Microtubule-Binding protein, interacts with KCBP/ZWICHEL to regulate trichome cell shape in Arabidopsis thaliana. PLoS Genet. 12:e1006266. doi: 10.1371/journal.pgen.1006266
Chen, Y., Su, D., Li, J., Ying, S., Deng, H., He, X., et al. (2020). Overexpression of bHLH95, a basic helix-loop-helix transcription factor family member, impacts trichome formation via regulating gibberellin biosynthesis in tomato. J. Exp. Bot. 71, 3450–3462. doi: 10.1093/jxb/eraa114
Chien, J. C., and Sussex, I. M. (1996). Differential regulation of trichome formation on the adaxial and abaxial leaf surfaces by gibberellins and photoperiod in Arabidopsis thaliana (L.) Heynh. Plant Physiol. 111, 1321–1328. doi: 10.1104/pp.111.4.1321
Churchman, M. L., Brown, M. L., Kato, N., Kirik, V., Hulskamp, M., Inzé, D., et al. (2006). SIAMESE, a plant-specific cell cycle regulator, controls endoreplication onset in Arabidopsis thaliana. Plant Cell 18, 3145–3157. doi: 10.1105/tpc.106.044834
Cominelli, E., Sala, T., Calvi, D. G., and Tonelli, C. (2010). Over-expression of the Arabidopsis AtMYB41 gene alters cell expansion and leaf surface permeability. Plant J. 53, 53–64.
Deng, F., Tu, L., Tan, J., Li, Y., Nie, Y., and Zhang, X. (2012). GbPDF1 is involved in cotton fiber initiation via the core cis-Element HDZIP2ATATHB2. Plant Physiol. 158, 890–904. doi: 10.1104/pp.111.186742
Deng, W., Yang, Y., Ren, Z., Audran Delalande, C., Mila, I., Wang, X., et al. (2012). The tomato SlIAA15 is involved in trichome formation and axillary shoot development. New Phytol. 2, 379–390.
Denyer, T., Ma, X., Klesen, S., Scacchi, E., Nieselt, K., and Timmermans, M. C. P. (2019). Spatiotemporal developmental trajectories in the Arabidopsis root revealed using High-throughput single-Cell RNA sequencing. Dev. Cell 48, 840–852. doi: 10.1016/j.devcel.2019.02.022
Dewitte, W., Scofield, S., Alcasabas, A. A., Maughan, S. C., Menges, M., Braun, N., et al. (2007). Arabidopsis CYCD3 D-type cyclins link cell proliferation and endocycles and are rate-limiting for cytokinin responses. Proc. Natl. Acad. Sci. U.S.A. 104, 14537–14542. doi: 10.1073/pnas.0704166104
Du, H., Wang, G., Pan, J., Chen, Y., Xiao, T., Zhang, L., et al. (2020). The HD-ZIP IV transcription factor Tril regulates fruit spine density through gene dosage effects in cucumber. J. Exp. Bot. 71, 6297–6310. doi: 10.1093/jxb/eraa344
Duke, M. V., Paul, R. N., Elsohly, H. N., Sturtz, G., and Duke, S. O. (1994). Localization of artemisinin and artemisitene in foliar tissues of glanded and glandless biotypes of Artemisia annua L. Int. J. Plant Sci. 5, 365–372.
Duke, S. O., and Paul, R. N. (1993). Development and fine structure of the glandular trichomes of Artemisia annua L. Int. J. Plant Sci. 154, 107–118.
Farmer, A., Thibivilliers, S., Ryu, K. H., Schiefelbein, J., and Libault, M. (2021). Single-nucleus RNA and ATAC sequencing reveals the impact of chromatin accessibility on gene expression in Arabidopsis roots at the single-cell level. Mol. Plant 14, 372–383. doi: 10.1016/j.molp.2021.01.001
Gan, L., Xia, K., Chen, J., and Wang, S. (2011). Functional characterization of TRICHOMELESS2, a new single-repeat R3 MYB transcription factor in the regulation of trichome patterning in Arabidopsis. BMC Plant Biol. 11:176. doi: 10.1186/1471-2229-11-176
Gan, Y., Liu, C., Yu, H., and Broun, P. (2007). Integration of cytokinin and gibberellin signalling by Arabidopsis transcription factors GIS, ZFP8 and GIS2 in the regulation of epidermal cell fate. Development 134, 2073–2081. doi: 10.1242/dev.005017
Gao, H., Gadlage, M. J., Lafitte, H. R., Lenderts, B., Yang, M., Schroder, M., et al. (2020). Superior field performance of waxy corn engineered using CRISPR-Cas9. Nat. Biotechnol. 38, 579–581. doi: 10.1038/s41587-020-0444-0
Greenboim-Wainberg, Y., Maymon, I., Borochov, R., Alvarez, J., Olszewski, N., Ori, N., et al. (2005). Cross talk between gibberellin and cytokinin: the Arabidopsis GA response inhibitor SPINDLY plays a positive role in cytokinin signaling. Plant Cell 17, 92–102. doi: 10.1105/tpc.104.028472
Hu, H., He, X., Tu, L., Zhu, L., Zhu, S., Ge, Z., et al. (2016). GhJAZ2 negatively regulates cotton fiber initiation by interacting with the R2R3-MYB transcription factor GhMYB25-like. Plant J. 88, 921–935. doi: 10.1111/tpj.13273
Hua, B., Chang, J., Wu, M., Xu, Z., Zhang, F., Yang, M., et al. (2021a). Mediation of JA signalling in glandular trichomes by the woolly/SlMYC1 regulatory module improves pest resistance in tomato. Plant Biotechnol. J. 19, 375–393. doi: 10.1111/pbi.13473
Hua, B., Chang, J., Xu, Z., Han, X., Xu, M., Yang, M., et al. (2021b). HOMEODOMAIN PROTEIN 8 mediates jasmonate-triggered trichome elongation in tomato. New Phytol. 230, 1063–1077. doi: 10.1111/nph.17216
Huaxun, Y. L. L. Y. (2011). Recent advances in the regulation of brassinosteroid signaling and biosynthesis pathways. J. Integr. Plant Biol. 53, 455–468. doi: 10.1111/j.1744-7909.2011.01046.x
Ishida, T., Kurata, T., Okada, K., and Wada, T. (2008). A genetic regulatory network in the development of trichomes and root hairs. Annu. Rev. Plant Biol. 59, 365–386. doi: 10.1146/annurev.arplant.59.032607.092949
Jacobsen, S. E., and Olszewski, N. E. (1993). Mutations at the SPINDLY locus of Arabidopsis alter gibberellin signal transduction. Plant Cell 5, 887–896. doi: 10.1105/tpc.5.8.887
Johnson, C. S., Kolevski, B., and Smyth, D. R. (2002). TRANSPARENT TESTA GLABRA2, a trichome and seed coat development gene of Arabidopsis, encodes a WRKY transcription factor. Plant Cell 14, 1359–1375. doi: 10.1105/tpc.001404
Judd, R., Bagley, M. C., Li, M., Zhu, Y., Lei, C., Yuzuak, S., et al. (2019). Artemisinin biosynthesis in non-glandular trichome cells of Artemisia annua. Mol. Plant 12, 704–714. doi: 10.1016/j.molp.2019.02.011
Kazama, H., Dan, H., Imaseki, H., and Wasteneys, G. O. (2004). Transient exposure to ethylene stimulates cell division and alters the fate and polarity of hypocotyl epidermal cells. Plant Physiol. 134, 1614–1623. doi: 10.1104/pp.103.031088
Kazan, K., and Manners, J. M. (2012). JAZ repressors and the orchestration of phytohormone crosstalk. Trends Plant Sci. 17, 22–31. doi: 10.1016/j.tplants.2011.10.006
Kim, K., and Hwang, I. (2012). Attenuation of cytokinin signaling via proteolysis of a type-B response regulator. Plant Signal. Behav. 7, 756–759. doi: 10.4161/psb.20469
Kirik, V., Bouyer, D., Schöbinger, U., Bechtold, N., Herzog, M., Bonneville, J. M., et al. (2001). CPR5 is involved in cell proliferation and cell death control and encodes a novel transmembrane protein. Curr. Biol. 11, 1891–1895. doi: 10.1016/s0960-9822(01)00590-5
Kirik, V., Simon, M., Huelskamp, M., and Schiefelbein, J. (2004a). The ENHANCER of TRY and CPC1 gene acts redundantly with TRIPTYCHON and CAPRICE in trichome and root hair cell patterning in Arabidopsis. Dev. Biol. 268, 506–513. doi: 10.1016/j.ydbio.2003.12.037
Kirik, V., Simon, M., Wester, K., Schiefelbein, J., and Hulskamp, M. (2004b). ENHANCER of TRY and CPC 2 (ETC2) reveals redundancy in the region-specific control of trichome development of Arabidopsis. Plant Mol. Biol. 55, 389–398. doi: 10.1007/s11103-004-0893-8
Kumari, A., Pandey, N., and Pandey-Rai, S. (2018). Exogenous salicylic acid-mediated modulation of arsenic stress tolerance with enhanced accumulation of secondary metabolites and improved size of glandular trichomes in Artemisia annua L. Protoplasma 255, 139–152. doi: 10.1007/s00709-017-1136-6
Li, J., Norville, J. E., Aach, J., McCormack, M., Zhang, D., Bush, J., et al. (2013). Multiplex and homologous recombination-mediated genome editing in Arabidopsis and Nicotiana benthamiana using guide RNA and Cas9. Nat. Biotechnol. 31, 688–691.
Li, L., Zhao, Y., McCaig, B. C., Wingerd, B. A., Wang, J., Whalon, M. E., et al. (2004). The tomato homolog of CORONATINE-INSENSITIVE1 is required for the maternal control of seed maturation, Jasmonate-Signaled defense responses, and glandular trichome development. Plant Cell 16, 126–143. doi: 10.1105/tpc.017954
Li, Q., Yin, M., Li, Y., Fan, C., Yang, Q., Wu, J., et al. (2015). Expression of Brassica napus TTG2, a regulator of trichome development, increases plant sensitivity to salt stress by suppressing the expression of auxin biosynthesis genes. J. Exp. Bot. 66, 5821–5836. doi: 10.1093/jxb/erv287
Li, S., Zhang, J., Liu, L., Wang, Z., Li, Y., Guo, L., et al. (2020). SlTLFP8 reduces water loss to improve water-use efficiency by modulating cell size and stomatal density via endoreduplication. Plant Cell Environ. 43, 2666–2679. doi: 10.1111/pce.13867
Liang, G., He, H., Li, Y., Ai, Q., and Yu, D. (2014). MYB82 functions in regulation of trichome development in Arabidopsis. J. Exp. Bot. 65, 3215–3223. doi: 10.1093/jxb/eru179
Liao, X., Wang, J., Zhu, S., Xie, Q., Wang, L., Yu, H., et al. (2020). Transcriptomic and functional analyses uncover the regulatory role of lncRNA000170 in tomato multicellular trichome formation. Plant J. 104, 18–29. doi: 10.1111/tpj.14902
Liu, L., Sonbol, F. M., Huot, B., Gu, Y., Withers, J., Mwimba, M., et al. (2016). Salicylic acid receptors activate jasmonic acid signalling through a non-canonical pathway to promote effector-triggered immunity. Nat. Commun. 7:13099. doi: 10.1038/ncomms13099
Liu, S., Fan, L., Liu, Z., Yang, X., Zhang, Z., Duan, Z., et al. (2020). A Pd1-Ps-P1 feedback loop controls pubescence density in soybean. Mol. Plant 13, 1768–1783. doi: 10.1016/j.molp.2020.10.004
Liu, Z. H., Chen, Y., Wang, N. N., Chen, Y. H., Wei, N., Lu, R., et al. (2020). A basic helix-loop-helix protein (GhFP1) promotes fibre elongation of cotton (Gossypium hirsutum) by modulating brassinosteroid biosynthesis and signalling. New Phytol. 225, 2439–2452. doi: 10.1111/nph.16301
Luo, M., Xiao, Y., Li, X., Lu, X., Deng, W., Li, D., et al. (2007). GhDET2, a steroid 5α-reductase, plays an important role in cotton fiber cell initiation and elongation. Plant J. 51, 419–430. doi: 10.1111/j.1365-313X.2007.03144.x
Lv, Z., Zhang, L., and Tang, K. (2017). New insights into artemisinin regulation. Plant Signal. Behav. 7:e1366398. doi: 10.1080/15592324.2017.1366398
Maes, L., Inzé, D., and Goossens, A. (2008). Functional specialization of the TRANSPARENT TESTA GLABRA1 network allows differential hormonal control of laminal and marginal trichome initiation in Arabidopsis rosette leaves. Plant Physiol. 148, 1453–1464. doi: 10.1104/pp.108.125385
Maes, L., Van Nieuwerburgh, F. C. W., Zhang, Y., Reed, D. W., Pollier, J., Vande Casteele, S. R., et al. (2011). Dissection of the phytohormonal regulation of trichome formation and biosynthesis of the antimalarial compound artemisinin in Artemisia annua plants. New Phytol. 12, 176–189. doi: 10.1111/j.1469-8137.2010.03466.x
Matias-Hernandez, L., Jiang, W., Yang, K., Tang, K., Brodelius, P. E., and Pelaz, S. (2017). AaMYB1 and its orthologue AtMYB61 affect terpene metabolism and trichome development in Artemisia annua and Arabidopsis thaliana. Plant J. 90, 520–534. doi: 10.1111/tpj.13509
Menges, M., Samland, A. K., Planchais, S., and Murray, J. A. (2006). The D-type cyclin CYCD3;1 is limiting for the G1-to-S-phase transition in Arabidopsis. Plant Cell 18, 893–906. doi: 10.1105/tpc.105.039636
Nekrasov, V., Staskawicz, B., Weigel, D., Jones, J. D., and Kamoun, S. (2013). Targeted mutagenesis in the model plant Nicotiana benthamiana using Cas9 RNA-guided endonuclease. Nat. Biotechnol. 31, 691–693. doi: 10.1038/nbt.2655
Noir, S., Bömer, M., Takahashi, N., Ishida, T., Tsui, T., Balbi, V., et al. (2013). Jasmonate controls leaf growth by repressing cell proliferation and the onset of endoreduplication while maintaining a potential Stand-By mode. Plant Physiol. 161, 1930–1951. doi: 10.1104/pp.113.214908
Olsson, M. E., Olofsson, L. M., Lindahl, A. L., Lundgren, A., Brodelius, M., and Brodelius, P. E. (2009). Localization of enzymes of artemisinin biosynthesis to the apical cells of glandular secretory trichomes of Artemisia annua L. Phytochemistry 70, 1123–1128.
Ooki, Y., Banba, M., Yano, K., Maruya, J., Sato, S., Tabata, S., et al. (2005). Characterization of the Lotus japonicus symbiotic mutantlot1 that shows a reduced nodule number and distorted trichomes. Plant Physiol. 137, 1261–1271. doi: 10.1104/pp.104.056630
Paetzold, H., Garms, S., Bartram, S., Wieczorek, J., Urós-Gracia, E., Rodríguez-Concepción, M., et al. (2010). The isogene 1-Deoxy-D-Xylulose 5-Phosphate synthase 2 controls isoprenoid profiles, precursor pathway allocation, and density of tomato trichomes. Mol. Plant 3, 904–916. doi: 10.1093/mp/ssq032
Payne, C. T., Zhang, F., and Lloyd, A. M. (2000). GL3 encodes a bHLH protein that regulates trichome development in Arabidopsis through interaction with GL1 and TTG1. Genetics 156, 1349–1362.
Peng, Y., Chen, L., Lu, Y., Wu, Y., Dumenil, J., Zhu, Z., et al. (2015). The ubiquitin receptors DA1, DAR1, and DAR2 redundantly regulate endoreduplication by modulating the stability of TCP14/15 in Arabidopsis. Plant Cell 27, 649–662. doi: 10.1105/tpc.114.132274
Perazza, D., Herzog, M., Hülskamp, M., Brown, S., Dorne, A. M., and Bonneville, J. M. (1999). Trichome cell growth in Arabidopsis thaliana can be derepressed by mutations in at least five genes. Genetics 152, 461–476.
Plett, J. M., Mathur, J., and Regan, S. (2009). Ethylene receptor ETR2 controls trichome branching by regulating microtubule assembly in Arabidopsis thaliana. J. Exp. Bot. 60, 3923–3933. doi: 10.1093/jxb/erp228
Plett, J. M., Wilkins, O., Campbell, M. M., Ralph, S. G., and Regan, S. (2010). Endogenous overexpression of Populus MYB186 increases trichome density, improves insect pest resistance, and impacts plant growth. Plant J. 64, 419–432. doi: 10.1111/j.1365-313X.2010.04343.x
Qi, T., Song, S., Ren, Q., Wu, D., Huang, H., Chen, Y., et al. (2011). The Jasmonate-ZIM-Domain proteins interact with the WD-Repeat/bHLH/MYB complexes to regulate Jasmonate-Mediated anthocyanin accumulation and trichome initiation in Arabidopsis thaliana. Plant Cell 23, 1795–1814. doi: 10.1105/tpc.111.083261
Sako, K., Maki, Y., Aoyama, T., Goto, D. B., and Yamaguchi, J. (2010). Control of endoreduplication of trichome by RPT2a, a subunit of the 19S proteasome in Arabidopsis. J. Plant Res. 123, 701–706. doi: 10.1007/s10265-010-0321-x
Sawa, S. (2002). Overexpression of the AtmybL2 gene represses trichome development in Arabidopsis. DNA Res. 9:31. doi: 10.1093/dnares/9.2.31
Schnittger, A., Schöbinger, U., Bouyer, D., Weinl, C., Stierhof, Y., and Hülskamp, M. (2002a). Ectopic D-type cyclin expression induces not only DNA replication but also cell division in Arabidopsis trichomes. Proc. Natl. Acad. Sci. U.S.A. 99, 6410–6415. doi: 10.1073/pnas.092657299
Schnittger, A., Schöbinger, U., Stierhof, Y., and Hülskamp, M. (2002b). Ectopic B-Type cyclin expression induces mitotic cycles in endoreduplicating a Abidopsis trichomes. Curr. Biol. 12, 415–420. doi: 10.1016/S0960-9822(02)00693-0
Schnittger, A., Weinl, C., Bouyer, D., Schöbinger, U., and Hülskamp, M. (2003). Misexpression of the cyclin-dependent kinase inhibitorICK1/KRP1 in single-celled Arabidopsis trichomes reduces endoreduplication and cell size and induces cell death. Plant Cell 15, 303–315. doi: 10.1105/tpc.008342
Shan, C., Shangguan, X., Zhao, B., Zhang, X., Chao, L., Yang, C. Q., et al. (2014). Control of cotton fibre elongation by a homeodomain transcription factor GhHOX3. Nat. Commun. 5, 5519. doi: 10.1038/ncomms6519
Shen, J., Fu, J., Ma, J., Wang, X., Gao, C., Zhuang, C., et al. (2014). Isolation, culture, and transient transformation of plant protoplasts. Curr. Protoc. Cell Biol. 63, 2–8. doi: 10.1002/0471143030.cb0208s63
Shi, P., Fu, X., Shen, Q., Liu, M., Pan, Q., Tang, Y., et al. (2018). The roles of AaMIXTA1 in regulating the initiation of glandular trichomes and cuticle biosynthesis in Artemisia annua. New Phytol. 217, 261–276. doi: 10.1111/nph.14789
Shikata, M., Koyama, T., Mitsuda, N., and Ohme-Takagi, M. (2009). Arabidopsis SBP-box genes SPL10, SPL11 and SPL2 control morphological change in association with shoot maturation in the reproductive phase. Plant Cell Physiol. 50, 2133–2145. doi: 10.1093/pcp/pcp148
Sun, L., Zhang, A., Zhou, Z., Zhao, Y., Yan, A., Bao, S., et al. (2015). Glabrous inflorescence stems3 (GIS3) regulates trichome initiation and development in Arabidopsis. New Phytol. 206, 220–230. doi: 10.1111/nph.13218
Sun, Y. T. T. U., Veerabomma, S., Abdel-Mageed, H. A., Fokar, M., Asami, T., Yoshida, S., et al. (2005). Brassinosteroid regulates fiber development on cultured cotton ovules. Plant Cell Physiol. 46, 1384–1391. doi: 10.1093/pcp/pci150
Szymanski, D. B., Jilk, R. A., Pollock, S. M., and Marks, M. D. (1998). Control of GL2 expression in Arabidopsis leaves and trichomes. Development 125, 1161–1171.
Szymanski, D. B., and Marks, M. D. (1998). GLABROUS1 overexpression and TRIPTYCHON alter the cell cycle and trichome cell fate in Arabidopsis. Plant Cell 10, 2047–2062. doi: 10.1105/tpc.10.12.2047
Tan, Y., Barnbrook, M., Wilson, Y., Molnár, A., Bukys, A., and Hudson, A. (2020). Shared mutations in a novel glutaredoxin repressor of multicellular trichome fate underlie parallel evolution of antirrhinum species. Curr. Biol. 30, 1357–1366. doi: 10.1016/j.cub.2020.01.060
Tian, J., Han, L., Feng, Z., Wang, G., Liu, W., Ma, Y., et al. (2015). Orchestration of microtubules and the actin cytoskeleton in trichome cell shape determination by a plant-unique kinesin. eLife 4:e09351. doi: 10.7554/eLife.09351
Tian, Y., and Zhang, T. (2021). MIXTAs and phytohormones orchestrate cotton fiber development. Curr. Opin. Plant Biol. 59, 101975. doi: 10.1016/j.pbi.2020.10.007
Tominaga, R., Iwata, M., Sano, R., Inoue, K., Okada, K., and Wada, T. (2008). Arabidopsis CAPRICE-LIKE MYB 3 (CPL3) controls endoreduplication and flowering development in addition to trichome and root hair formation. Development 135, 1335–1345. doi: 10.1242/dev.017947
Traw, M. B., and Bergelson, J. (2003). Interactive effects of jasmonic acid, salicylic acid, and gibberellin on induction of trichomes in Arabidopsis. Plant Physiol. 133, 1367–1375. doi: 10.1104/pp.103.027086
Völz, R., Kim, S. K., Mi, J., Mariappan, K. G., Guo, X., Bigeard, J., et al. (2018). The Trihelix transcription factor GT2-like 1 (GTL1) promotes salicylic acid metabolism, and regulates bacterial-triggered immunity. PLoS Genet. 14:e1007708. doi: 10.1371/journal.pgen.1007708
Wada, T., Tachibana, T., Shimura, Y., and Okada, K. (1997). Epidermal cell differentiation in Arabidopsis determined by a Myb homolog. CPC Sci. 277, 1113–1116.
Wang, K., Ndathe, R. W., Kumar, N., Zeringue, E. A., Kato, N., and Larkin, J. C. (2020). The CDK inhibitor SIAMESE targets both CDKA;1 and CDKB1 complexes to establish endoreplication in trichomes. Plant Physiol. 184, 165–175. doi: 10.1104/pp.20.00271
Wang, M., Zhao, P., Cheng, H., Han, L., Wu, X., Gao, P., et al. (2013). The cotton transcription factor TCP14 functions in Auxin-Mediated epidermal cell differentiation and elongation. Plant Physiol. 162, 1669–1680. doi: 10.1104/pp.113.215673
Wang, S., Hubbard, L., Chang, Y., Guo, J., Schiefelbein, J., and Chen, J. G. (2008). Comprehensive analysis of single-repeat R3 MYB proteins in epidermal cell patterning and their transcriptional regulation in Arabidopsis. BMC Plant Biol. 8:81. doi: 10.1186/1471-2229-8-81
Wang, S., Kwak, S., Zeng, Q., Ellis, B. E., Chen, X., Schiefelbein, J., et al. (2007). TRICHOMELESS1 regulates trichome patterning by suppressing GLABRA1 in Arabidopsis. Development 134, 3873–3882. doi: 10.1242/dev.009597
Wang, Y., Cheng, X., Shan, Q., Zhang, Y., Liu, J., Gao, C., et al. (2014). Simultaneous editing of three homoeoalleles in hexaploid bread wheat confers heritable resistance to powdery mildew. Nat. Biotechnol. 32, 947–951. doi: 10.1038/nbt.2969
Worthen, J. M., Yamburenko, M. V., Lim, J., Nimchuk, Z. L., Kieber, J. J., and Schaller, G. E. (2019). Type-B response regulators of rice play key roles in growth, development and cytokinin signaling. Development 146:dev174870. doi: 10.1242/dev.174870
Wu, J., Wu, Q., Wu, Q., Gai, J., and Yu, D. (2008). Constitutive overexpression of AOS-like gene from soybean enhanced tolerance to insect attack in transgenic tobacco. Biotechnol. Lett. 30, 1693–1698. doi: 10.1007/s10529-008-9742-1
Xiao, M., Liu, R., Long, C., Ruan, Y., and Liu, C. (2020). Using β-ocimene to increase the artemisinin content in juvenile plants of Artemisia annua L. Biotechnol. Lett. 42, 1161–1167. doi: 10.1007/s10529-020-02849-6
Xiao, Y. H., Li, D. M., Yin, M. H., Li, X. B., Zhang, M., Wang, Y. J., et al. (2010). Gibberellin 20-oxidase promotes initiation and elongation of cotton fibers by regulating gibberellin synthesis. J. Plant Physiol. 167, 829–837. doi: 10.1016/j.jplph.2010.01.003
Xie, Q., Liu, P., Shi, L., Miao, H., Bo, K., Wang, Y., et al. (2018). Combined fine mapping, genetic diversity, and transcriptome profiling reveals that the auxin transporter gene ns plays an important role in cucumber fruit spine development. Theor. Appl. Genet. 131, 1239–1252. doi: 10.1007/s00122-018-3074-x
Xing, S., Miao, J., Li, S., Qin, G., Tang, S., Li, H., et al. (2010). Disruption of the 1-deoxy-D-xylulose-5-phosphate reductoisomerase (DXR) gene results in albino, dwarf and defects in trichome initiation and stomata closure in Arabidopsis. Cell Res. 20, 688–700. doi: 10.1038/cr.2010.54
Xiong, C., Xie, Q., Yang, Q., Sun, P., Gao, S., Li, H., et al. (2020). WOOLLY, interacting with MYB transcription factor MYB31, regulates cuticular wax biosynthesis by modulating CER6 expression in tomato. Plant J. 103, 323–337. doi: 10.1111/tpj.14733
Xu, R., Yang, Y., Qin, R., Li, H., Qiu, C., Li, L., et al. (2016). Rapid improvement of grain weight via highly efficient CRISPR/Cas9-mediated multiplex genome editing in rice. J. Genet. Genom. 43, 529–532. doi: 10.1016/j.jgg.2016.07.003
Yadav, R. K., Sangwan, R. S., Sabir, F., Srivastava, A. K., and Sangwan, N. S. (2014). Effect of prolonged water stress on specialized secondary metabolites, peltate glandular trichomes, and pathway gene expression in Artemisia annua L. Plant Physiol. Biochem. 74, 70–83. doi: 10.1016/j.plaphy.2013.10.023
Yamauchi, T., Tanaka, A., Inahashi, H., Nishizawa, N. K., Tsutsumi, N., Inukai, Y., et al. (2019). Fine control of aerenchyma and lateral root development through AUX/IAA- and ARF-dependent auxin signaling. Proc. Natl. Acad. Sci. U.S.A. 116, 20770–20775. doi: 10.1073/pnas.1907181116
Yan, L., Zhai, Q., Wei, J., Li, S., Wang, B., Huang, T., et al. (2013). Role of tomato lipoxygenase D in wound-induced jasmonate biosynthesis and plant immunity to insect herbivores. PLoS Genet. 9:e1003964. doi: 10.1371/journal.pgen.1003964
Yan, T., Chen, M., Shen, Q., Li, L., Fu, X., Pan, Q., et al. (2017). HOMEODOMAIN PROTEIN 1 is required for jasmonate-mediated glandular trichome initiation in Artemisia annua. New Phytol. 213, 1145–1155. doi: 10.1111/nph.14205
Yan, T., Li, L., Xie, L., Chen, M., Shen, Q., Pan, Q., et al. (2018). A novel HD-ZIP IV/MIXTA complex promotes glandular trichome initiation and cuticle development in Artemisia annua. New Phytol. 218, 567–578. doi: 10.1111/nph.15005
Yang, C., Li, H., Zhang, J., Luo, Z., Gong, P., Zhang, C., et al. (2011). A regulatory gene induces trichome formation and embryo lethality in tomato. Proc. Natl. Acad. Sci. U.S.A. 108, 11836–11841. doi: 10.1073/pnas.1100532108
Yang, Z., Zhang, C., Yang, X., Liu, K., Wu, Z., Zhang, X., et al. (2014). PAG1, a cotton brassinosteroid catabolism gene, modulates fiber elongation. New Phytol. 203, 437–448. doi: 10.1111/nph.12824
Yoshida, Y., Sano, R., Wada, T., Takabayashi, J., and Okada, K. (2009). Jasmonic acid control of GLABRA3 links inducible defense and trichome patterning in Arabidopsis. Development 136, 1039–1048. doi: 10.1242/dev.030585
Yu, D., Yu, F., Du, C., Li, X., Zhao, X., and Liu, X. (2015). RPN1a, a subunit of the 26S proteasome, controls trichome development in Arabidopsis. Plant Physiol. Biochem. 88, 82–88. doi: 10.1016/j.plaphy.2015.01.012
Yu, N., Cai, W. J., Wang, S., Shan, C. M., Wang, L. J., and Chen, X. Y. (2010). Temporal control of trichome distribution by microRNA156-targeted SPL genes in Arabidopsis thaliana. Plant Cell 22, 2322–2335.
Yuan, Y., Xu, X., Luo, Y., Gong, Z., Hu, X., Wu, M., et al. (2021). R2R3 MYB-dependent auxin signalling regulates trichome formation, and increased trichome density confers spider mite tolerance on tomato. Plant Biotechnol. J. 19, 138–152. doi: 10.1111/pbi.13448
Zhang, J., Zeng, Q., Luo, X., Li, X., Xiang, C., Li, D., et al. (2011). Spatiotemporal manipulation of auxin biosynthesis in cotton ovule epidermal cells enhances fiber yield and quality. Nat. Biotechnol. 29, 453–458. doi: 10.1038/nbt.1843
Zhang, M., Zeng, J., Long, H., Xiao, Y., Yan, X., and Pei, Y. (2017). Auxin regulates cotton fiber initiation via GhPIN-mediated auxin transport. Plant Cell Physiol. 58, 385–397. doi: 10.1093/pcp/pcw203
Zhang, N., Yang, L., Luo, S., Wang, X., Wang, W., Cheng, Y., et al. (2018). Genetic evidence suggests that GIS functions downstream of TCL1 to regulate trichome formation in Arabidopsis. BMC Plant Biol. 18:63. doi: 10.1186/s12870-018-1271-z
Zhang, T., Lian, H., Tang, H., Dolezal, K., Zhou, C., Yu, S., et al. (2015). An intrinsic MicroRNA timer regulates progressive decline in shoot regenerative capacity in plants. Plant Cell 27, 349–360. doi: 10.1105/tpc.114.135186
Zhang, T. Q., Chen, Y., and Wang, J. W. (2021). A single-cell analysis of the Arabidopsis vegetative shoot apex. Dev. Cell 56, 1056–1074. doi: 10.1016/j.devcel.2021.02.021
Zhang, X., Yan, F., Tang, Y., Yuan, Y., Deng, W., and Li, Z. (2015). Auxin response gene SlARF3 plays multiple roles in tomato development and is involved in the formation of epidermal cells and trichomes. Plant Cell Physiol. 56, 2110–2124. doi: 10.1093/pcp/pcv136
Zhang, Y., Shen, J., Bartholomew, E. S., Dong, M., Chen, S., Yin, S., et al. (2021). TINY BRANCHED HAIR functions in multicellular trichome development through an ethylene pathway in Cucumis sativus L. Plant J. 106, 753–765. doi: 10.1111/tpj.15198
Zhang, Z., Zhou, Y., Li, Y., Shao, S., Li, B., Shi, H. Y., et al. (2010). Interactome analysis of the six cotton 14-3-3s that are preferentially expressed in fibres and involved in cell elongation. J. Exp. Bot. 61, 3331–3344. doi: 10.1093/jxb/erq155
Zhao, M., Morohashi, K., Hatlestad, G., Grotewold, E., and Lloyd, A. (2008). The TTG1-bHLH-MYB complex controls trichome cell fate and patterning through direct targeting of regulatory loci. Development 135, 1991–1999. doi: 10.1242/dev.016873
Zhou, Y., Zhang, Z. T., Li, M., Wei, X. Z., Li, X. J., Li, B. Y., et al. (2015). Cotton (Gossypium hirsutum) 14-3-3 proteins participate in regulation of fibre initiation and elongation by modulating brassinosteroid signalling. Plant Biotechnol. J. 13, 269–280. doi: 10.1111/pbi.12275
Zhou, Z., An, L., Sun, L., Zhu, S., Xi, W., Broun, P., et al. (2011). Zinc finger protein 5 is required for the control of trichome initiation by acting upstream of zinc finger protein 8 in Arabidopsis. Plant Physiol. 157, 673–682. doi: 10.1104/pp.111.180281
Keywords: phytohormones, trichome, gibberellins, endoreduplication, CRISPR/Cas9, sing cell transcriptome
Citation: Li J, Wang X, Jiang R, Dong B, Fang S, Li Q, Lv Z and Chen W (2021) Phytohormone-Based Regulation of Trichome Development. Front. Plant Sci. 12:734776. doi: 10.3389/fpls.2021.734776
Received: 01 July 2021; Accepted: 27 August 2021;
Published: 30 September 2021.
Edited by:
Ahmad M. Alqudah, Aarhus University, DenmarkReviewed by:
Anshu Alok, University of Minnesota Twin Cities, United StatesCopyright © 2021 Li, Wang, Jiang, Dong, Fang, Li, Lv and Chen. This is an open-access article distributed under the terms of the Creative Commons Attribution License (CC BY). The use, distribution or reproduction in other forums is permitted, provided the original author(s) and the copyright owner(s) are credited and that the original publication in this journal is cited, in accordance with accepted academic practice. No use, distribution or reproduction is permitted which does not comply with these terms.
*Correspondence: Zongyou Lv, em9uZ3lvdWx2QDE2My5jb20=; Wansheng Chen, Y2hlbndzMTI2QDEyNi5jb20=
Disclaimer: All claims expressed in this article are solely those of the authors and do not necessarily represent those of their affiliated organizations, or those of the publisher, the editors and the reviewers. Any product that may be evaluated in this article or claim that may be made by its manufacturer is not guaranteed or endorsed by the publisher.
Research integrity at Frontiers
Learn more about the work of our research integrity team to safeguard the quality of each article we publish.