- 1State Key Laboratory for Conservation and Utilization of Bio-Resources in Yunnan, Research Center for Perennial Rice Engineering and Technology in Yunnan, School of Agriculture, Yunnan University, Kunming, China
- 2College of Agronomy and Biotechnology, Southwest University, Chongqing, China
- 3State Key Laboratory of Rice Biology, China National Rice Research Institute, Hangzhou, China
- 4Biotechnology Research Center, Chongqing Academy of Agricultural Sciences, Chongqing, China
Gametophytic male sterility (GMS) plays an important role in the study of pollen development and seed propagation of recessive nuclear male sterile lines insensitive to the environmental conditions in hybrid rice breeding. Since the inherent phenotypic and genetic characteristics of GMS, it is very difficult to find and identify the GMS mutants. However, due to the abundance of gene transcription data, a large number of pollen-specific genes have been found, and most of them may be associated with GMS. To promote the study of these genes in pollen development and heterosis utilization, in this study, an easy and efficient method of creating and identifying GMS was established using RNAi and OsMYB76R as a reporter. First, the OsC1/OsMYB76 gene involved in anthocyanin synthesis was modified, and we have validated that the modified OsMYB76R is workable as the same as the pre-modified OsMYB76 gene. Then, the ascorbic acid oxidase gene OsPTD1 was downregulated using RNAi, driven by its own promoter that resulted in abnormal pollen tube growth. Finally, the RNAi elements were linked with OsMYB76R and transformed into an osmyb76 mutant, and the distortion of purple color segregation was found in T1 and F1 generations. This indicates that the OsPTD1 GMS was prepared successfully. Compared to current methods, there are several advantages to this method. First, time is saved in material preparation, as one generation less needs to be compared than in the conventional method, and mutation screening can be avoided. In addition, for identification, the cost is lower; PCR, electrophoresis, and other processes are not needed; and no expensive chemicals or instruments are required. Finally, the results are more accurate, with much lower background effects, and no damage to the plant. The result is an easy, efficient, low-cost, and accurate method of preparing and identifying GMS genes.
Introduction
Plant male sterility plays an important role in the study of the development of the pollen mechanism and heterosis utilization. This includes sporophytic male sterility (SMS) and gametophytic male sterility (GMS). These two types are quite different in their heredity and phenotypes. SMS is caused by the sterility of pollen grains, as determined by sporophyte genotypes. In addition, the SMS gene can be transmitted between generations by either female gametes or male gametes in heterozygotes. All pollens in an SMS anther are sterile. In the field, the SMS anthers are usually abnormal in shape, color, and size, and the sterile plant has few seed settings. It is easy to find and identify homozygous SMS mutants. Due to the accessibility of SMS mutants, many studies have been carried out on the mechanism of pollen development and SMS using abundant SMS mutants (Ma, 2005; Wilson and Zhang, 2009; Ariizumi and Toriyama, 2011; Shi et al., 2015). By contrast, GMS is caused by the sterility of the pollen grains, as determined by gametophyte genotypes. In addition, the GMS gene can only be transmitted through the female gamete in heterozygotes, which leads to a very low probability of a homozygous mutant. Half of the pollen grains in a heterozygous anther are fertile, and the other half are sterile. There is no significant difference in appearance between the anthers of mutants and wild types in field observations, and the seed setting radio for the GMS mutant is normal. It is very difficult to locate and identify GMS mutants directly (Han et al., 2006, 2011; Boavida et al., 2009; Chen et al., 2012; Yang et al., 2012). For these reasons, compared with SMS, the location and identification of GMS are more difficult than for SMS. In officially published reports, GMS mutants have usually been created through spontaneous mutation, induced mutation, T-DNA insertion mutation, and so on. After mutation, the GMS candidates were verified in their crossing or self-crossing generations using PCR or resistance to screening agents, or their traits for detecting the segregation distortion of mutated genes or selectable markers or reporters (SariGorla et al., 1996; Feldmann et al., 1997; Howden et al., 1998; Boavida et al., 2009; Yang et al., 2009; Moon et al., 2013; Liu et al., 2016). These strategies, however, require special equipment, expensive chemicals, or invasive treatment, which entails time-consuming, low-efficiency, low-accuracy, high-cost, sample-damaging practices. Further, fewer GMS mutants are produced by these means, and the study of GMS has fallen behind the SMS research.
Pollen development is regulated not only through sporophytic gene expression but also through a large number of pollen genes (Ma, 2005). The genes associated with GMS are usually expressed in microspores and pollen at a late stage of anther development. Due to the development of the transcriptome and other detection methods for gene expression, an increasing number of pollen-specific genes have been identified (Ma, 2005; Suwabe et al., 2008; Wang et al., 2020). It is speculated that most of these genes are related to gametophyte development, which provides us with an opportunity to understand the mechanism of pollen development and GMS. To identify GMS-related genes from all the pollen-specific genes, it is necessary to develop a simple, rapid, economical, and accurate method for the preparation and identification of GMS.
Anthocyanin pigmentation is visible to the naked eye. The rice coleoptile purple line is a clear purple line that appears on each side of the coleoptile after the seed germination. Due to its early performance stage (budding stage) and stability, it is suitable to use as reporter traits (Xi, 1997; Zhang et al., 2004). OsC1/OsMYB76 is a key gene encoding MYB transcription factor for anthocyanin biosynthesis in almost all organs, such as coleoptile in rice (Reddy, 1998; Zhang et al., 2004; Zhao et al., 2016; Sun et al., 2018; Zheng et al., 2019; Hu et al., 2020). Hence, OsC1/OsMYB76 is an ideal reporter for the indication of transgenic events.
In view of the low efficiency and high cost of the preparation and identification of GMS in commonly used methods, this study aimed to design and validate a new method of creating and verifying GMS using RNAi technology and OsMYB76R (the revised OsMYB76) as a reporter gene. The GMS-related gene OsPTD1 was identified in rice using our new method. We provide an easy, efficient, and low-cost method of creating and identifying the gene for GMS.
Materials and Methods
Plant Materials and Growth Conditions
The Japonica rice line Zhonghua 11 was kindly provided by Dr. Li Ping at Shanghai University. The Indica line R25, with multiple organs displaying purple color, is a self-breeding restorer, and YR25, without purple color, is an osmyb76 mutant from R25. Zhongjiu B, another osmyb76 mutant without purple color in all organs, is a breeding material that features a shorter growth period than Zhonghua 11, and it was kindly provided by the China National Rice Research Institute. Zhongjiu B-osabcg15 is nuclear male sterility material, an osabcg15 near-isogenic line of Zhongjiu B, derived from crosses and backcrosses between Zhongjiu B (the recurrent parent) and osabcg15 (Wu et al., 2014). All rice plants used in this study were grown in the fields in Jinghong, Yunnan, China.
Vector Construction
OsMYB76R was synthesized by Shanghai Generay Biotech Co., Ltd. (Shanghai, China), after eliminating the restriction sites of OsMYB76, and its code was optimized according to the rice preference. The target fragment was amplified using the synthesized OsMYB76R as a template. The GUS gene in the pCAMBIA1301 vector was substituted with the PCR product of OsMYB76R using a homologous recombination strategy described in the user manual of the In-Fusion HD Cloning Kit (Takara, Otsu, Japan), which generated the pP35S-OsMYB76R-OE vector. This vector was transformed into the Zhongjiu B calli using an agrobacterium-mediated method. The sequences of OsMYB76R and primers 76F/R are shown in the Supplementary Material 3.
For the RNAi of the OsPTD1 gene, all of the RNAi elements, such as the promoter of POsPTD1 or P35S, target sequence (left arm), stem-loop structure (first intron of OsMYB76), complementary sequence of target (right arm), and Tnos, were synthesized as one fusion sequence by Shanghai Generay Biotech Co., Ltd. The whole RNAi sequence was loaded between the restriction sites SalI and SbfI of pCAMBIA1301, which generated the vectors pPOsPTD1-OsPTD1-RNAi and pP35S-OsPTD1-RNAi_P35S-OsC1. These two vectors were separately transformed into Zhonghua 11.
For the preparation and identification of OsPTD1 GMS, the synthesized RNAi sequences were inserted into pP35S-OsMYB76R-OE using SalI and SbfI, which generated pPOsPTD1-OsPTD1_P35S-OsMYB76R and pP35S-OsPTD1_P35S-OsMYB76R. These were transformed into Zhongjiu B.
Pollen Germination in vitro
The germination medium was composed of 18% sucrose, 5% potato starch, and 0.005% H3BO3. The solid medium was prepared in advance following these steps: evenly mixing the ingredients, heating and melting them in a microwave oven, cooling, and evenly spreading them on the slide. Immediately after the floret opened, pollen grains were shed onto the solid germination medium. After 30 min incubation at 28–30°C, the slides were observed with a Nikon (Tokyo, Japan) SMZ1500 stereoscope and photographed with a Nikon DS-5Mc digital camera.
Coleoptile Purple Color Observation and Inheritance Analyses
The transgenic positive plants with anthocyanin color were self-crossed to obtain the T1 generations. The same positive plants were used as male parents to pollinate the male sterile of Zhongjiu B-osabcg15 to obtain F1 generations. After being soaked and pregerminated, the seeds were evenly planted in a mud tray, covered with plastic cling to keep them wet, and placed under natural light. The purple color of the coleoptile was investigated after 2–3 days of growth. The members of the segregated groups purple and no-purple were counted and calculated, and a chi-square test was conducted to establish whether the observed values were consistent with the theoretical values.
Results
OsMYB76R, Revised From OsC1/OsMYB76, Is Workable When Replacing the GUS Gene as a Reporter in Rice
The OsC1/OsMYB76 gene is a functional gene for anthocyanin biosynthesis in rice. In the previous studies, the CDS (coding sequence) of the OsC1/OsMYB76 gene was used to replace the GUS gene in the vector pCAMBIA1301. The function of the OsC1/OsMYB76 gene was verified with a complement test (data not shown). Transgenic positive plants showed a purple color in many organs, such as the stem base of the seedling, the leaf sheath, the auricle, the stigma apiculus, and the coleoptile, which shows that it can be used as a reporter gene to indicate positive transgenic events. The CDS of OsMYB76 contains common restriction sites, such as PstI, SacI, and SalI, but if OsMYB76 is directly used as a reporter, it must influence the freedom of restriction site selection when the target gene is loaded. To remove these restriction sites, we optimized and modified the gene with artificial synthesis and obtained OsMYB76R, after that we replaced the GUS gene in pCAMBIA1301 with homologous recombination, which resulted in the functional verification vector pP35S-OsMYB76R-OE (Figure 1A). The plasmid was transformed into Zhongjiu B without any purple in any organs, and the results showed that the stem base of the seedling, the leaf sheath, the auricle, the stigma and apiculus, and the coleoptile of the transgenic positive plants were all purple in color (Figures 1B–E). The distribution of purple coloration of the coleoptile of the transgenic-positive plants was wider and easier to observe than that of most wild-type lines, such as R25 (an Indica restorer line) (Figures 1E,F). The results showed that the revised OsMYB76R gene has the equivalent of a genetic function for OsMYB76 in rice anthocyanin biosynthesis, and OsMYB76R is suitable for reporting transformants.
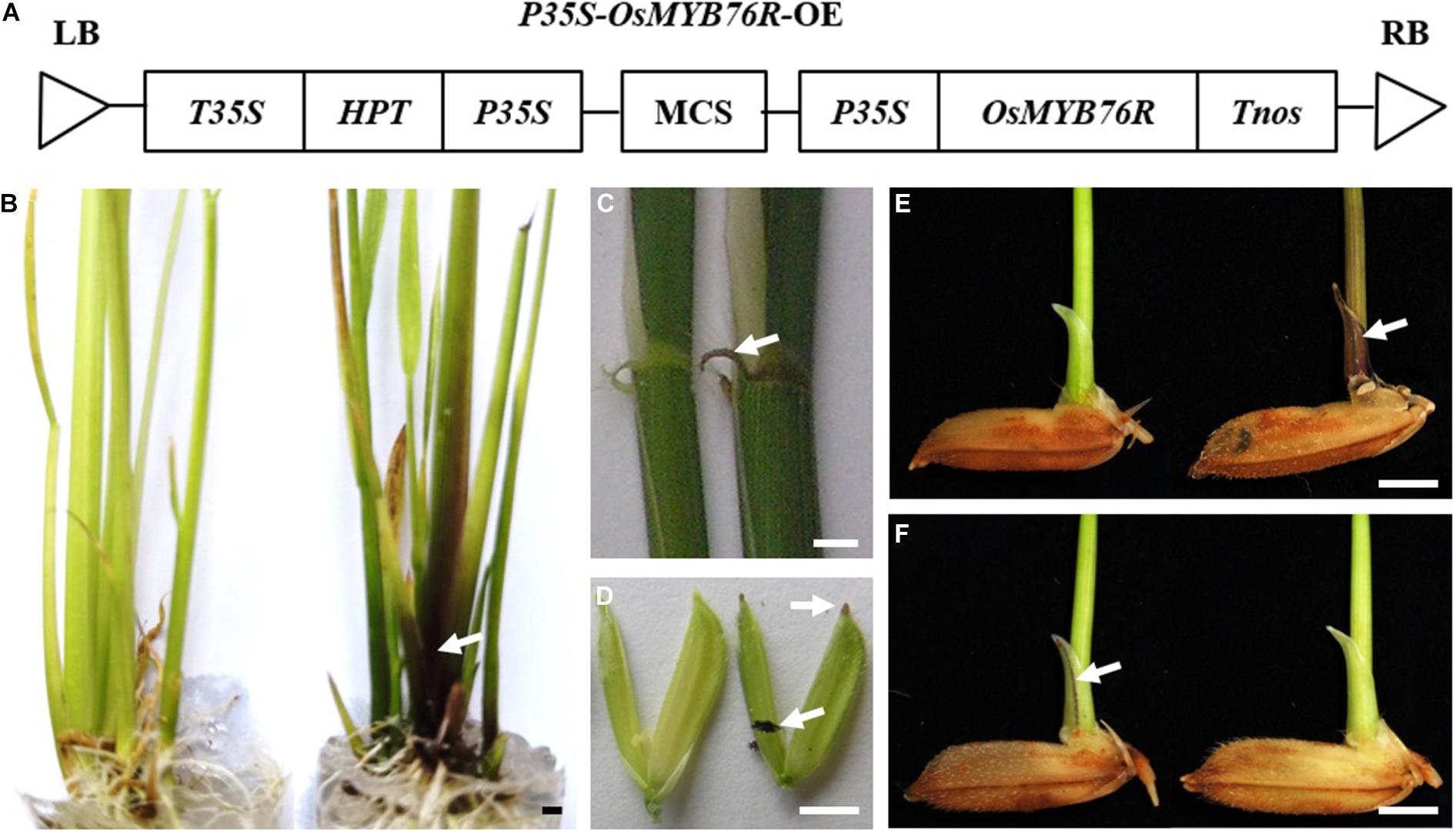
Figure 1. Phenotype of the OsMYB76R gene. (A) The structure of the pP35S-OsMYB76R-OE vector. The left sides of panels (B–E) refer to the color of different organs in Zhongjiu B, and the right sides refer to the color of different organs in a plant that is positive for OsMYB76R overexpression. The traits in panels (B–D) are from T0 plants. The trait in panel (E) is from the T1 plant. The left side of the panel (F) is purple wild type R25, and the right side of the panel (F) shows osmyb76 mutant YR25. The arrows point to the purple tissue. Scale bars, 0.2 cm.
RNA Interference of OsPTD1 Damages Pollen Tube Development
The ascorbate acid oxidase (AAO) gene NPT303 plays an important role in the growth of the tobacco pollen tube, and the silencing of NPT303 results in the abnormal growth of the pollen tube (de Groot et al., 2004). There are more than 10 AAO genes in the rice genome,1 and most of them have an unclear function. Among these AAO genes, Os05g0485800 encodes a protein that is highly homologous to NPT303, may be related to pollen tube growth, and is named pollen tube development gene 1 (OsPTD1). To determine whether it plays a role in pollen germination and growth, two vectors for OsPTD1 RNA interference were constructed, promoted by the constitutive 35S CaMV promoter (P35S) and the specific promoter of POsPTD1 (Figures 2A,B), and were transferred into Zhonghua 11. The T0 pollen germination assay showed that all the pollen from the RNAi promoted by P35S and the wild type could be germinated and grown normally, but only about half of the pollen from the RNAi promoted by POsPTD1 germinated and grew normally. The germination of other pollen grains was abnormal, with most of the abnormally germinated pollen tubes rupturing without growing (Figures 2C–E). These results show that the RNAi under the OsPTD1 promoter broke the growth of the pollen tube, and this may result in GMS.
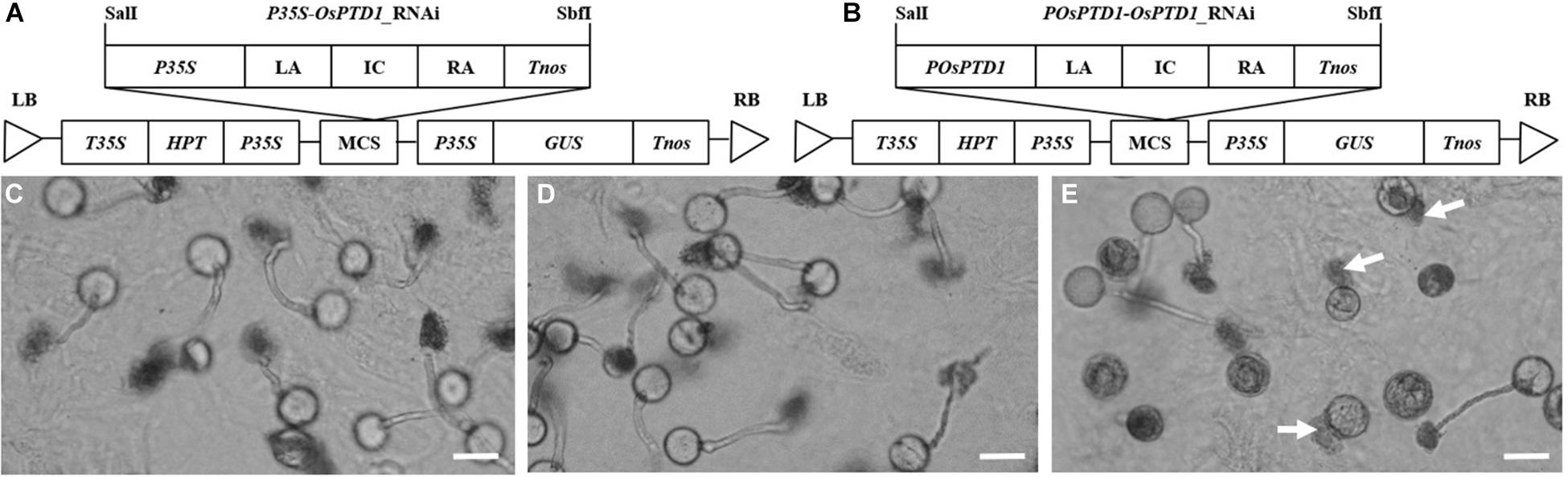
Figure 2. RNAi pollen germination in vitro. (A) The structure of pP35S-OsPTD1-RNAi. (B) The structure of pPOsPTD1-OsPTD1-RNAi. (C) The germination of wild pollen. (D) The germination of the P35S-promoted RNAi pollen. (E) The germination of POsPTD1-promoted RNAi pollen; arrows indicate the rupture of RNAi pollen. All images were created 30 min after germination. Scale bars, 20 μm.
Construction and Verification of a System for GMS Preparation and Identification
To confirm OsPTD1 as a gene related to GMS, two vectors for the preparation and identification of GMS were designed and constructed, based on the OsPTD1 gene. The P35S- and POsPTD1-promoted OsPTD1 RNAi elements were linked with OsMYB76R that generated the RNAi vectors of P35S-OsPTD1_P35S-OsMYB76R and POsPTD1-OsPTD1_P35S-OsMYB76R, respectively (Figures 3A,E). In addition, they were separately transferred into Zhongjiu B (Figures 3B,F). Two types of RNAi-positive plants (purple) were crossed as male parents with a male-sterile Zhongjiu B-osabcg15 mutant with no purple color. The male parents were also allowed to self-cross. Purple segregations in the coleoptile of F1 and T1 were investigated 3 days after the seed germination in wet soil in a disk (Figures 3C,D,G,H). The results showed that the T1 and F1 of P35S promoted RNAi exhibiting 3:1 and 1:1 segregation (purple: no purple), respectively, which did not match with the genetic characteristics of GMS. However, the color segregation ratio of the T1 of the POsPTD1 promoted RNAi that did not show a 3:1 ratio but a 1:1 ratio (Table 1). Only a few seeds, namely, F1 produced by POsPTD1-promoted RNAi male plants, displayed a purple coleoptile, which indicated a distorted segregation ratio, compared with the expected 1:1 (Table 1). The distorted segregation ratio of the POsPTD1-promoted RNAi generation is just the genetic characteristics of GMS, which indicates that POsPTD1-driven RNAi produced a partial gametophytic defect. The above results indicate that the preparation of OsPTD1-related GMS materials using the newly designed method was successful. Hence, this method can identify GMS easily, rapidly, and economically, 3 days after the seed germination.
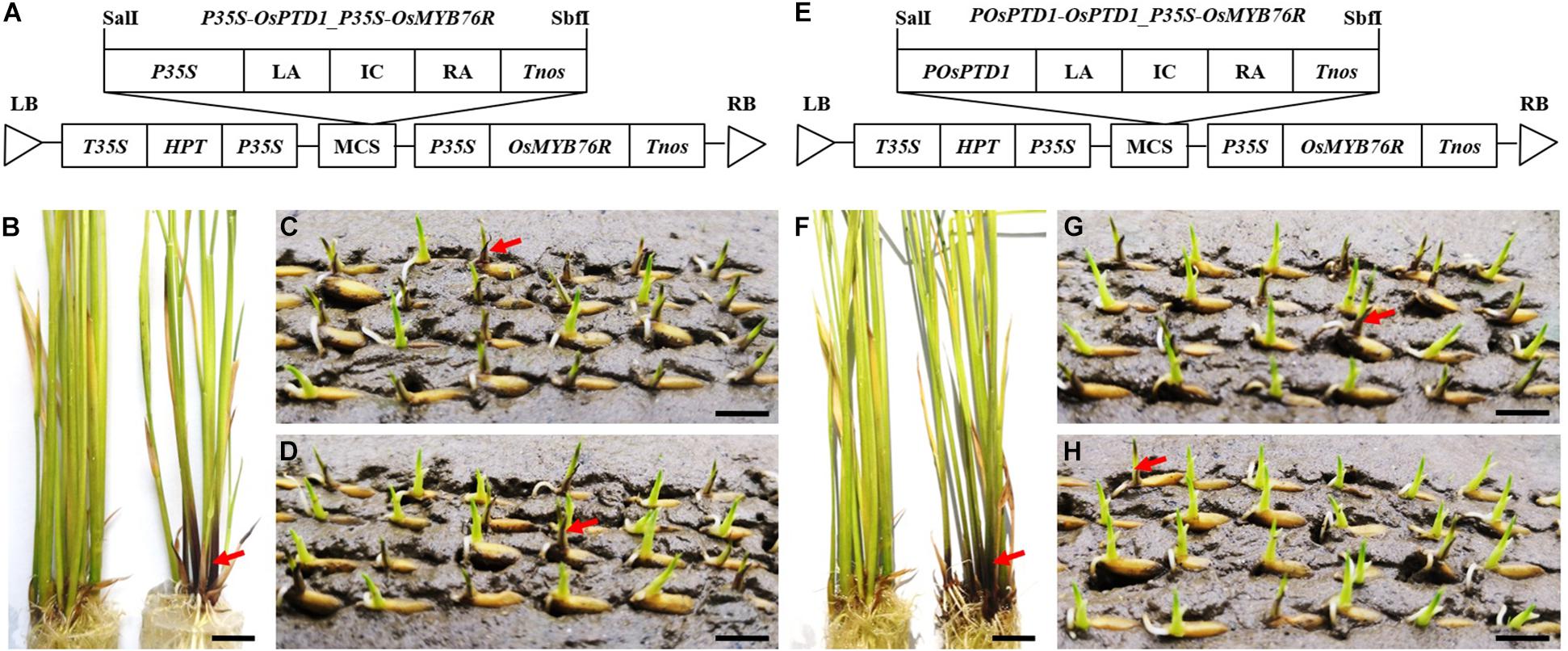
Figure 3. Inheritance characteristics of OsPTD1 RNAi. Panels (A,E) depict the structure of the RNAi vectors pP35S-OsPTD1_P35S-OsMYB76R and pPOsPTD1-OsPTD1_P35S-OsMYB76R, respectively. Panels (B–D) show a T0-positive plant, and a T1 and F1 (positive plants as the male parents) of P35S-OsPTD1_P35S-OsMYB76R. Panels (F–H) show the T0-positive plants and T1 and F1 (positive plants as the male parent) of POsPTD1-OsPTD1_P35S-OsMYB76R. Scale bars, 0.8 cm.
Discussion
OsMYB76R Is a Favorable Reporter Gene
It is best to identify the positive transformants as soon as possible after transformation. The reporter gene is a visible indicator of whether the target gene is transferred or not, and it can also be widely used for identifying positive transgenic plants. An ideal reporting gene should have the following characteristics: stable phenotype, easy detection, less background affect, low cost, and no damage to plants, the environment, or health (Ziemienowicz, 2001; Rosellini, 2012; He et al., 2020).
The endogenous genes of some plants have been screened and used as reporter genes. For example, anthocyanin-related genes are among the most widely used endogenous reporters in plants, such as maize, wheat, Arabidopsis, tobacco, and tomato (Rosellini, 2012; He et al., 2020). The anthocyanin biosynthesis pathway involves many genes, and at least 16 are involved in the anthocyanin biosynthesis in rice (Sharma and Dixon, 2005; Oshima et al., 2019). Due to the limited carrying capacity of the vector, it is impossible to load all genes controlling the anthocyanin synthesis into the same vector as the reporter genes. Scientists often use a compromise strategy to solve this problem: selecting the appropriate genetic background material, one in which most anthocyanin synthesis genes are functional as the transgenic host, and using one to several anthocyanin genes as the reporter (Lloyd et al., 1992; Goldsbrough et al., 1996; Rosellini, 2012; Oshima et al., 2019).
OsC1/OsMYB76 is a homologous gene of the anthocyanin biosynthesis gene C1 in maize, and it is a functional gene for anthocyanin biosynthesis in rice. The purple color phenotype controlled by OsC1/OsMYB76 is visible and stable, which indicates that it is suitable for use as a reporter gene (Xi, 1997; Reddy, 1998; Zhang et al., 2004; Zheng et al., 2019; Hu et al., 2020). To make it easier to use this gene, OsMYB76R was optimized from OsMYB76 and the usual restriction sites in OsMYB76 CDS were removed. The revised OsMYB76R had normal functions, such as those of OsMYB76, and could indicate positive transformants quickly, directly, accurately, and harmlessly in the early T0 generation. Additionally, it was easy to separate the positive plants from the next generations of transgenic plants. The OsMYB76R sequence was only 819 bp that saved space for the loading of more and longer target genes. Moreover, the usual restriction sites in OsMYB76R were removed, which did not affect the freedom of restriction-site selection, when the target gene is loaded. For this reason, OsMYB76R is a desirable reporter gene.
In addition, Zhongjiu B is an Indica-type rice with a shorter growth period than Zhonghua 11 (a commonly used Japonica variety in rice transgene). The transgenic efficiency of Zhongjiu B is higher than the two popular Indica rice types, Minghui 63 and 9311. Zhongjiu B, transformed by only one reporter gene OsMYB76R, displays purple color in multiple organs, which indicates that other anthocyanin pigment genes in Zhongjiu B meet genetic conditions sufficient to anthocyanin expression in multiple organs. Therefore, Zhongjiu B is a good Indica rice host for the transformation of the OsMYB76R gene as a reporter.
Combining Candidate Gene RNAi and OsMYB76R to Enable High-Efficiency, Accurate, and Low-Cost Preparation and Identification of GMS
Gametophytic male sterility mutants have traditionally been obtained through natural mutation, induced mutation, T-DNA insertion mutation, and gene editing. After mutagenesis, it is necessary to determine whether the plants are GMSs according to the genetic characteristics of their offspring. This can be done with genotype analyses through PCR or chemical resistance analyses using screening-marker genes in self-crossing generation and the F1 generation (mutant as the male parent). Thereafter, segregation distortion is used to determine whether the mutant is GMS. In other words, if the segregation ratio of the self-bred offspring is 1:1 instead of 3:1, and the mutated alleles do not transfer through pollen learned from F1, it is GMS (Feldmann et al., 1997; Howden et al., 1998; Boavida et al., 2009). For example, TMS1 (Yang et al., 2009), OsGT1 (Moon et al., 2013), and gaMS-1 (SariGorla et al., 1996) were prepared and determined as GMS using the above methods. These methods are cumbersome and involve the use of multiple reagents and equipment, which inevitably leads to the problems of low efficiency, high cost, low accuracy, and sample damage. For the gene-editing strategy, scientists must spend another generation to remove the editing component to overcome continuous editing and screen out mutants with no transgenic elements that undoubtedly lengthen the identification time by at least one generation.
In this study, using a strategy that combines RNAi technology and OsMYB76R reporting technology, a method was designed that allows GMS preparation and identification at the same time by transferring the linked OsPTD1-RNAi element and OsMYB76R into Zhongjiu B with no purple color in any organ, the GMS genetic resources of OsPTD1 were prepared successfully. The genetic identification of GMS was completed only 3 days after the seed germination, using purple segregation distortion in the T1 and F1 progenies, derived from T0 positive plants of RNAi.
There are three strategies used to create GMS: random mutations (such as spontaneous mutation, induced mutation, and T-DNA insertion mutation), gene editing (ZFNs, TALENs, CRISPR-Cas, and so forth), and downregulation of gene expression (with RNAi and anti-RNA). The third strategy was used in this study. RNAi is a much more efficient, easier, and cheaper means of GMS preparation than the random mutation strategy, because the burdensome mutant screening work can be avoided in RNAi. This step is necessary for random mutation because there is no definite target gene. Furthermore, the RNAi strategy is quicker than gene editing, where editing elements must be removed in generation T1 to avoid any influence on the flowing study by persistent editing. So, if a gene-editing strategy is used to produce GMS, the genetic population for segregation distortion analysis could only be constructed in T1, not in T0, while it could be finished in T0 when the RNAi strategy is adopted. This means that the time required for one generation will be saved. In identification, the first two strategies require PCR, resistance detection, or fluorescence testing to analyze the inheritance behavior, which entails the use of specialized equipment and expensive chemicals and invasive sampling procedures. However, in the RNAi-linked OsMYB76R strategy, we use anthocyanin-related genes as reporter genes to analyze their genetic characteristics, in a more direct, simple, timeless, harmless, low-cost, and high-accuracy way than commonly used methods based on PCR detection, resistance testing, or widely used reporting genes, such as GUS, FPs, and Luc.
In conclusion, our strategy for the preparation and identification of GMS has the advantages of being harmless, quick, efficient, cheap, accurate, and reliable. In addition, pollen lethal factor and fluorescent protein are the two key components in the present technology of nuclear sterility seed production. If GMS produced by this method is entirely aborted (no transgenic element transfer through pollen), the RNAi elements and linked OsMYB76R can be used to replace the lethal pollen factor and the fluorescent protein gene in the current system that is useful for developing a new nuclear-sterility seed-preparation system. Obviously, this strategy can also be used to prepare and identify GMS in other crops. A simple sketch map for understanding this method is shown in Figure 4.
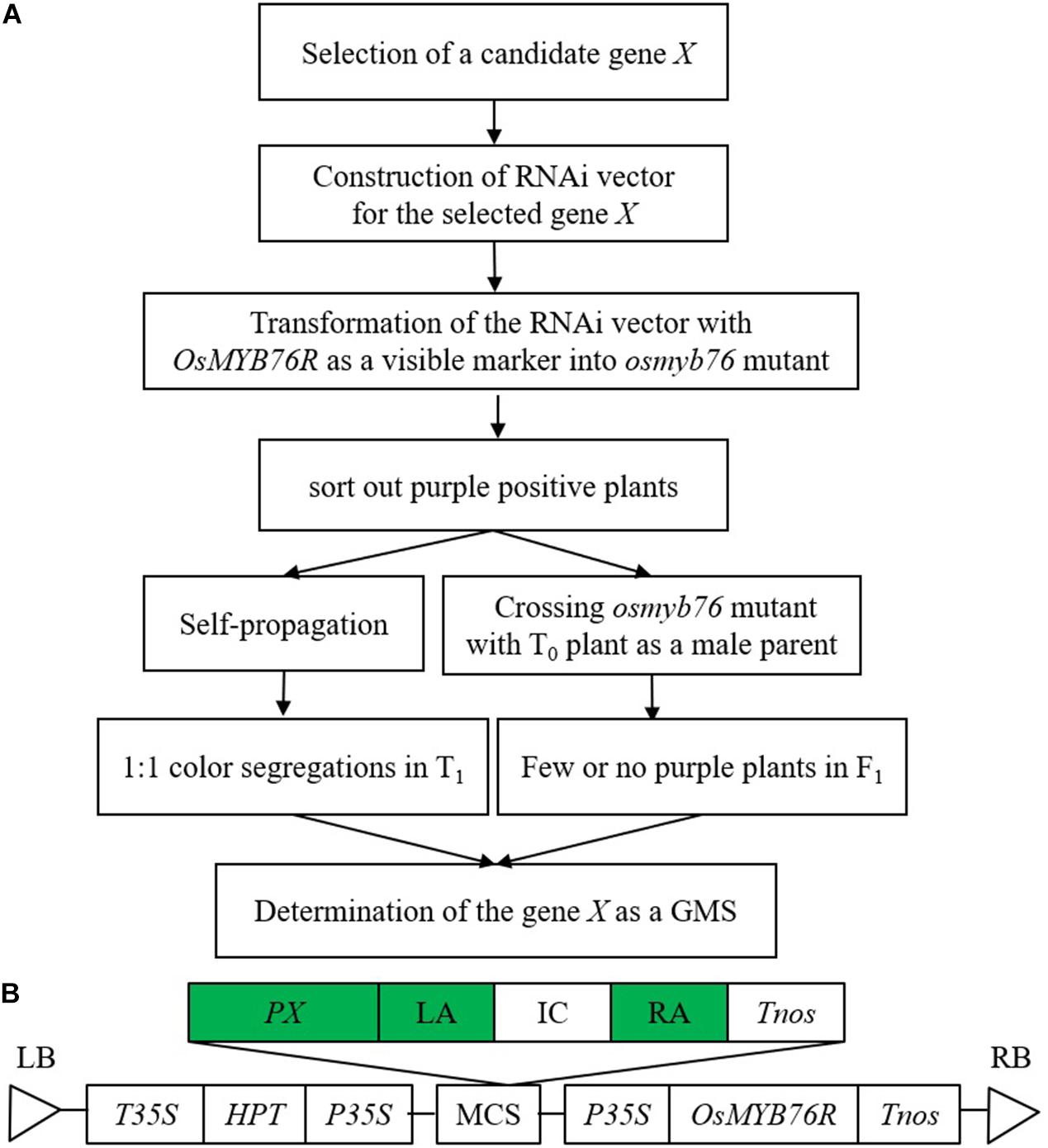
Figure 4. Strategy for gametophytic male sterility preparation and identification. (A) Schematic diagram of gametophytic male sterility (GMS) preparation and identification. (B) Vector structure of this strategy. When this strategy is used, the green highlighting should be replaced according to the candidate GMS-related gene. X, GMS candidate; PX, promoter of X; LA, left arm of RNAi-X; IC, the first intron of OsC1; RA, right arm of RNAi-X.
Data Availability Statement
The original contributions presented in the study are included in the article/Supplementary Material, further inquiries can be directed to the corresponding authors.
Author Contributions
YC, WZ, and SS designed and performed the experiments. LJ, KY, WZ, and JY performed the experiments and analyzed the data. LW and SD designed the experiments. LG, ZW, and YZ designed the experiments and wrote the manuscript. All authors read and approved the final manuscript.
Funding
This study was supported by the National Natural Science Foundation of China (31701390 and 31370349), Special Project on Performance Incentive Guidance of Chongqing Scientific Research Institution (cstc2018jxjl80021), Fund of Agriculture Development (NKY-2021AC003), and Recruitment Announcement for High-level Talents of Yunnan University (KL180018).
Conflict of Interest
The authors declare that the research was conducted in the absence of any commercial or financial relationships that could be construed as a potential conflict of interest.
Publisher’s Note
All claims expressed in this article are solely those of the authors and do not necessarily represent those of their affiliated organizations, or those of the publisher, the editors and the reviewers. Any product that may be evaluated in this article, or claim that may be made by its manufacturer, is not guaranteed or endorsed by the publisher.
Supplementary Material
The Supplementary Material for this article can be found online at: https://www.frontiersin.org/articles/10.3389/fpls.2021.728193/full#supplementary-material
Supplementary Material 1 | Sequence of synthesized OsMYB76R.
Supplementary Material 2 | Sequence of synthesized OsPTD1 RNAi elements.
Supplementary Material 3 | Primer sequences for OsMYB76R amplification.
Abbreviations
GMS, gametophytic male sterility; SMS, sporophytic male sterility; MYB, myeloblastosis; AAO, ascorbic acid oxidase.
Footnotes
References
Ariizumi, T., and Toriyama, K. (2011). Genetic regulation of sporopollenin synthesis and pollen exine development. Annu. Rev. Plant Biol. 62, 437–460. doi: 10.1146/annurev-arplant-042809-112312
Boavida, L. C., Shuai, B., Yu, H. J., Pagnussat, G. C., Sundaresan, V., and Mccormick, S. (2009). A collection of ds insertional mutants associated with defects in male gametophyte development and function in Arabidopsis thaliana. Genetics 181, 1369–1385. doi: 10.1534/genetics.108.090852
Chen, R., Fa-Ke, Y. U., Liu, H. Q., Yang, S. H., and Wang, F. (2012). Characterization of male gametophytic sterile mutant collections in rice by t-dna insertion. Chin. J. Rice Sci. 26, 173–181.
Feldmann, K. A., Coury, D. A., and Christianson, M. L. (1997). Exceptional segregation of a selectable marker (kanr) in Arabidopsis identifies genes important for gametophytic growth and development. Genetics 147, 1411–1422. doi: 10.1093/genetics/147.3.1411
Goldsbrough, A. P., Tong, Y., and Yoder, J. I. (1996). Lc as a nondestructive visual reporter and transposition excision marker gene for tomato. Plant J. 9, 927–933. doi: 10.1046/j.1365-313x.1996.9060927.x
Groot, P. D., Weterings, K., Been, M. D., Wittink, F., and Wullems, G. (2004). Silencing of the pollen-specific gene ntp303 and its family members in tobacco affects in vivo pollen tube growth and results in male sterile plants. Plant Mol. Biol. 55, 715–726. doi: 10.1007/s11103-004-1964-6
Han, M. J., Jung, K. H., Yi, G., and An, G. (2011). Rice importin β1 gene affects pollen tube elongation. Mol. Cells 31, 523–530. doi: 10.1007/s10059-011-2321-7
Han, M. J., Jung, K. H., Yi, G., Lee, D. Y., and An, A. G. (2006). Rice immature pollen 1 (rip1) is a regulator of late pollen development. Plant Cell Physiol. 47, 1457–1472. doi: 10.1093/pcp/pcl013
He, Y., Zhang, T., Sun, H., Zhan, H., and Zhao, Y. (2020). A reporter for noninvasively monitoring gene expression and plant transformation. Hortic. Res. 7:152.
Howden, R., Park, S. K., Moore, J. M., Orme, J., and Twell, D. (1998). Selection of t-dna-tagged male and female gametophytic mutants by segregation distortion in Arabidopsis. Genetics 149, 621–631. doi: 10.1093/genetics/149.2.621
Hu, W., Zhou, T., Han, Z., Tan, C., and Xing, Y. (2020). Dominant complementary interaction between osc1 and two tightly linked genes, rb1 and rb2, controls the purple leaf sheath in rice. Theor. Appl. Genet. 133, 2555–2566. doi: 10.1007/s00122-020-03617-w
Liu, L., Zheng, C., Kuang, B., Wei, L., and Tai, W. (2016). Receptor-like kinase rupo interacts with potassium transporters to regulate pollen tube growth and integrity in rice. PLoS Genet. 12:e1006085. doi: 10.1371/journal.pgen.1006085
Lloyd, A., Walbot, V., and Davis, R. (1992). Arabidopsis and nicotiana anthocyanin production activated by maize regulators r and c1. Science 258, 1773–1775. doi: 10.1126/science.1465611
Ma, H. (2005). Molecular genetic analyses of microsporogenesis and microgametogenesis in flowering plants. Annu. Rev. Plant Biol. 56, 393–434.
Moon, S., Kim, S. R., Zhao, G., Yi, J., Yoo, Y., Jin, P., et al. (2013). Rice glycosyltransferase1 encodes a glycosyltransferase essential for pollen wall formation. Plant Physiol. 161, 663–675.
Oshima, M., Taniguchi, Y., Akasaka, M., Abe, K., Ichikawa, H., Tabei, Y., et al. (2019). Development of a visible marker trait based on leaf sheath-specific anthocyanin pigmentation applicable to various genotypes in rice. Breeding Sci. 69, 244–254.
Reddy, V. (1998). Cloning and characterization of the rice homologue of the maize C1 anthocyanin regulatory gene. Plant Mol. Biol. 36, 497–498.
Rosellini, D. (2012). Selectable markers and reporter genes: a well furnished toolbox for plant science and genetic engineering. Crit. Rev. Plant Sci. 31, 401–453.
SariGorla, M., Ferrario, S., Villa, M., and Pe, M. E. (1996). gaMS-1: a gametophytic male sterile mutant in maize. Sex Plant Reprod. 9, 216–220. doi: 10.1007/BF02173101
Sharma, S. B., and Dixon, R. A. (2005). Metabolic engineering of proanthocyanidins by ectopic expression of transcription factors in Arabidopsis thaliana. Plant J. 44, 62–75. doi: 10.1111/j.1365-313X.2005.02510.x
Shi, J., Cui, M., Yang, L., Kim, Y. J., and Zhang, D. (2015). Genetic and biochemical mechanisms of pollen wall development. Trends Plant Sci. 20, 741–753. doi: 10.1016/j.tplants.2015.07.010
Sun, X., Zhang, Z., Chen, C., Wu, W., Ren, N., Jiang, C., et al. (2018). The C-S-A gene system regulates hull pigmentation and reveals evolution of anthocyanin biosynthesis pathway in rice. J. Exp. Bot. 69, 1485–1498. doi: 10.1093/jxb/ery001
Suwabe, K., Suzuki, G., Takahashi, H., Shiono, K., Endo, M., Yano, K., et al. (2008). Separated transcriptomes of male gametophyte and tapetum in rice: validity of a laser microdissection (LM) microarray. Plant Cell Physiol. 49, 1407–1416. doi: 10.1093/pcp/pcn124
Wang, M. L., Yan, W., Peng, X. Q., Chen, Z. F., Xu, C. J., Wu, J. X., et al. (2020). Identification of late-stage pollen-specific promoters for construction of pollen-inactivation system in rice. J. Integr. Plant Biol. 62, 1246–1263. doi: 10.1111/jipb.12912
Wilson, Z. A., and Zhang, D. B. (2009). From Arabidopsis to rice: pathways in pollen development. J. Exp. Bot. 60, 1479–1492. doi: 10.1093/jxb/erp095
Wu, L. N., Guan, Y. S., Wu, Z. G., Yang, K., Lv, J., Converse, R., et al. (2014). OsABCG15 encodes a membrane protein that plays an important role in anther cuticle and pollen exine formation in rice. Plant Cell Rep. 33, 1881–1899. doi: 10.1007/s00299-014-1666-8
Xi, J. (1997). The discovery and primary research of coleoptile purple lines in rice. Hybrid Rice 12:41.
Yang, K. Z., Xia, C., Liu, X. L., Dou, X. Y., Wang, W., Chen, L. Q., et al. (2009). A mutation in THERMOSENSITIVE MALE STERILE 1, encoding a heat shock protein with DnaJ and PDI domains, leads to thermosensitive gametophytic male sterility in Arabidopsis. Plant J. 57, 870–882. doi: 10.1111/j.1365-313X.2008.03732.x
Yang, S. H., Chen, R., Liu, H. Q., Zhou, S. F., and Wang, F. (2012). Identification and preliminary analysis of a male gametophytic mutant mga36 in rice. Fujian J. Agric. Sci. 27, 1039–1043.
Zhang, Y., Li, Y. F., Liu, X. F., Lin, M. X., Shen, F. C., He, G. H., et al. (2004). Analysis on the inheritance of coleoptile purple line in rice. Sci. Agric. Sin. 37, 1693–1698.
Zhao, S. S., Wang, C. H., Ma, J., Wang, S., Tian, P., Wang, J. L., et al. (2016). Map-based cloning and functional analysis of the chromogen gene C in rice (Oryza sativa L.). J. Plant Biol. 59, 496–505. doi: 10.1007/s12374-016-0227-9
Zheng, J., Wu, H., Zhu, H. B., Huang, C. Y., Liu, C., Chang, Y. S., et al. (2019). Determining factors, regulation system, and domestication of anthocyanin biosynthesis in rice leaves. New Phytol. 223, 705–721. doi: 10.1111/nph.15807
Keywords: Oryza sativa, gametophytic male sterility, OsMYB76R, RNAi, GMS preparation and identification
Citation: Chen Y, Zhu W, Shi S, Wu L, Du S, Jin L, Yang K, Zhao W, Yang J, Guo L, Wang Z and Zhang Y (2021) Use of RNAi With OsMYB76R as a Reporter for Candidate Genes Can Efficiently Create and Verify Gametophytic Male Sterility in Rice. Front. Plant Sci. 12:728193. doi: 10.3389/fpls.2021.728193
Received: 21 June 2021; Accepted: 13 August 2021;
Published: 06 September 2021.
Edited by:
Kazuki Matsubara, Institute of Crop Science (NARO), JapanReviewed by:
Tomohiko Kazama, Kyushu University, JapanJunxing Lu, Chongqing Normal University, China
Copyright © 2021 Chen, Zhu, Shi, Wu, Du, Jin, Yang, Zhao, Yang, Guo, Wang and Zhang. This is an open-access article distributed under the terms of the Creative Commons Attribution License (CC BY). The use, distribution or reproduction in other forums is permitted, provided the original author(s) and the copyright owner(s) are credited and that the original publication in this journal is cited, in accordance with accepted academic practice. No use, distribution or reproduction is permitted which does not comply with these terms.
*Correspondence: Longbiao Guo, Z3VvbG9uZ2JpYW9AY2Fhcy5jbg==; Zhongwei Wang, c2hlbmdyYW40QDEyNi5jb20=; Yi Zhang, emhhbmd5aTYxMTZAeW51LmVkdS5jbg==
†These authors have contributed equally to this work