- 1Guangdong Key Laboratory for New Technology Research of Vegetables/Vegetable Research Institute, Guangdong Academy of Agricultural Sciences, Guangzhou, China
- 2Department of Environmental Science, The Islamia University of Bahawalpur, Bahawalpur, Pakistan
- 3Department of Botany, University of Narowal, Narowal, Pakistan
- 4RO-II Office, University of the Punjab, Lahore, Pakistan
Current research was conducted to explore the effects of liquiritoside on the growth and physiochemical features of Chinese flowering cabbage (Brassica rapa subsp. parachinensis) under lead (Pb) stress. Lead stressed B. rapa plants exhibited decreased growth parameters, chlorophyll, and carotenoid contents. Moreover, Pb toxicity escalated the synthesis of malondialdehyde (MDA), hydrogen peroxide (H2O2), flavonoids, phenolics, and proline in treated plants. Nevertheless, foliar application of liquiritoside mitigated Pb toxicity by decreasing oxidative stress by reducing cysteine, H2O2, and MDA contents in applied plants. Liquiritoside significantly increased plant height, shoot fresh weight and dry weight, number of leaves, and marketable value of Chinese flowering cabbage plants exposed to Pb toxicity. This biotic elicitor also enhanced the proline, glutathione, total phenolics, and flavonoid contents in Chinese flowering cabbage plants exposed to Pb stress compared with the control. Additionally, total glucosinolate content, phytochelatins (PCs), and non-protein thiols were effectively increased in plants grown under Pb regimes compared with the control plants. Overall, foliar application of liquiritoside can markedly alleviate Pb stress by restricting Pb translocation in Chinese flowering cabbage.
Introduction
Vegetables belonging to the Brassicaceae family show cherished health remunerations effects owing to the existence of biologically active and robust antioxidative ingredients. Chinese flowering cabbage (Brassica rapa subsp. parachinensis) is an annual cole crop belonging to the Brassicaceae family. The germination to harvest period of this vegetable is less than 60 days (Peng et al., 2015). Chinese flowering cabbage has valuable biological and nutritional properties (Aixia et al., 2015). Its above-ground parts, including leaves, stem, and inflorescence, can be cooked or consumed raw as salads. Its leaves contain adequate amounts of glucosinolates and polyphenolic compounds(Chen et al., 2008). This rich chemical composition and scientifically proven biological activity has made Chinese flowering cabbage a famous culinary plant (Chung et al., 2016).
Glucosinolates (GLs) are primarily found in plants of the genus Brassica, which include crops of economical and nutritional importance. GLs are rich in sulfur and anionic secondary metabolites (Johnson, 2002). GLs have been extensively studied for their protective effect against herbivory in plants and chemotherapeutic activity in humans (Barba et al., 2016). The consumption of vegetables containing glucosinolates may confer protection against cancer in humans (Johnson, 2002). The hydrolytic breakdown products of glucosinolates have beneficial effects on human health, including cytotoxic and apoptotic effects in damaged cells, and reducing risks of degenerative diseases (Barba et al., 2021).
Lead contamination in soil has rapidly increased during the last decades (Sidhu et al., 2016). Metal pollution severely affects the growth and development of metal-affected plants (Borges et al., 2019). Pesticides, fertilizers, and automobile fuel are the major sources of Pb contamination. This non-essential toxic metal impedes appropriate plant nutrition (Lamhamdi et al., 2013). The edible parts of plants may uptake higher levels of metal contaminant from polluted soils and hence become a health risk for human beings and livestock consumers (Baghaie and Fereydoni, 2019). Metal toxicity enhances oxidative injury by intensifying the synthesis of reactive oxygen species (ROS) (Malar et al., 2014). Metal stressed plants increase the antioxidative system to detoxify ROS and maintain their ionic homeostasis (Fayez et al., 2014). Lead stress also affects photosynthesis, transpiration, and ionic homeostasis in stressed plants (Devi et al., 2013). As well, the plants subjected to Pb toxicity demonstrate a higher level of lipid peroxidation and ROS (Tauqeer et al., 2016). Phenolics are secondary metabolites deposited in plants facing stress and play a defensive role against a higher level of ROS synthesized in these plants (Fayez et al., 2017).
Lethal effects of synthetics pharmaceuticals have augmented the discovery and large-scale production of natural bioactive molecules. But, the resources of natural bioactive compounds are inadequate for various reasons. Conversely, the consumer entreaty for these compounds is growing gradually. Hence, the application of novel approaches to fulfill the current growing demand for natural bioactive compounds is of immense relevance. The use of conventional approaches to accelerate natural biosynthetic pathways in plants is shown to produce high levels of bio-active compounds, without the need of genetic engineering applications (Trejo-Téllez et al., 2019). Further, advances in technology have augmented the discovery of new biotic elicitors capable of increasing the production of secondary metabolites in plants.
Glycyrrhiza uralensis Fisch (Fabaceae), commonly known as licorice, is a traditional plant recognized through the ages for its multiple health benefits and medicinal uses (Kondo et al., 2007; Tanemoto et al., 2015). The roots of this plant are used to treat influenza, coughs, and liver damage in traditional medicinal formulations (Kondo et al., 2007). Liquiritoside also known as liquiritin, is the biologically active component of licorice. This study was designed to investigate the influence of liquiritoside as a foliar spray to increase plant growth and concentration of specific biologically active substances such as proline, glucosinolates, phenolics, and flavonoids in Chinese flowering cabbage plants. In addition, Pb affects the growth of crop plants (Figlioli et al., 2019). However, there is a dearth of research work demonstrating the effect of Pb stress on B. rapa subsp. parachinensis. According to our information, the effect of Pb toxicity and the ameliorative role of liquiritoside in the mitigation of subsequent stress has never been studied before.
In the course of our study, foliar application of liquiritoside showed positive effects on the growth of Chinese flowering cabbage plants (data yet not published). Additionally, we observed that liquiritoside mitigated abiotic stress, improved biosynthesis of, glucosinolates besides, flavonoids and phenolics in treated plants (Akram et al., 2020). Yet, the application of exogenously applied liquiritoside in the mitigation of plant stress has never been inspected. Therefore, it was hypothesized that liquiritoside might also modulate the antioxidative system of plants to alleviate Pb toxicity. Henceforth, the fundamental purpose of the present study was to elucidate the impact of liquiritoside spray on the physiology and growth of the plants under Pb stress. The results of current research will help to identify the differences in physiochemical process in the liquiritoside applied Pb stressed plants, which will perhaps the valuable crop producers planning to use liquiritoside plants growing in Pb contaminated soils.
Materials and Methods
Plant Material
A preliminary study was performed to optimize the dose of liquiritoside. Plants of Chinese flowering cabbage (Brassica rapa subsp. parachinensis) were raised in plastic pots (12-inch) containing sterilized commercial potting mix. The greenhouse experiment conducted in the present study entailed growing plants in pots containing sterilized Tref Jiffy (United States) media, which were placed in greenhouse at 20/25 ± 3°C (night/day) under a 16-h photoperiod. Commercial-grade liquiritoside of 99% purity was obtained from Riotto Botanicals, Shaanxi, China. Foliar formulations of the elicitor were prepared at different concentrations including 0, 0.15, 0.25, 0.50, 0.75 and 1 g/L. Control plants were sprayed with distilled water. The application was performed at the trifoliate stage. The relative growth rate (RGR) was calculated over 5 d time spans, after 1 week of elicitor application using the formula:
Where W1 = initial shoot dry weight, W2 = final shoot dry weight, t2-t1 = growth period.
Greenhouse Experiment
Based on RGR, an experimental set up was designed (Figure 1) under the same greenhouse conditions. Details of treatments are provided in Table 1. Seeds of Chinese flowering cabbage were sown in sterilized pots. An equal amount of water was supplied along with the recommended dose of fertilizers during the trial. The trial was carried out in a RCBD design containing 5 technical replicates of each treatment. Two independent greenhouse trials were conducted. No less than 10 plants were included in each treatment. Pb was added in the potting mix (75 mg/kg of potting mix) for artificial establishment of heavy metal stress according to the experiment design.
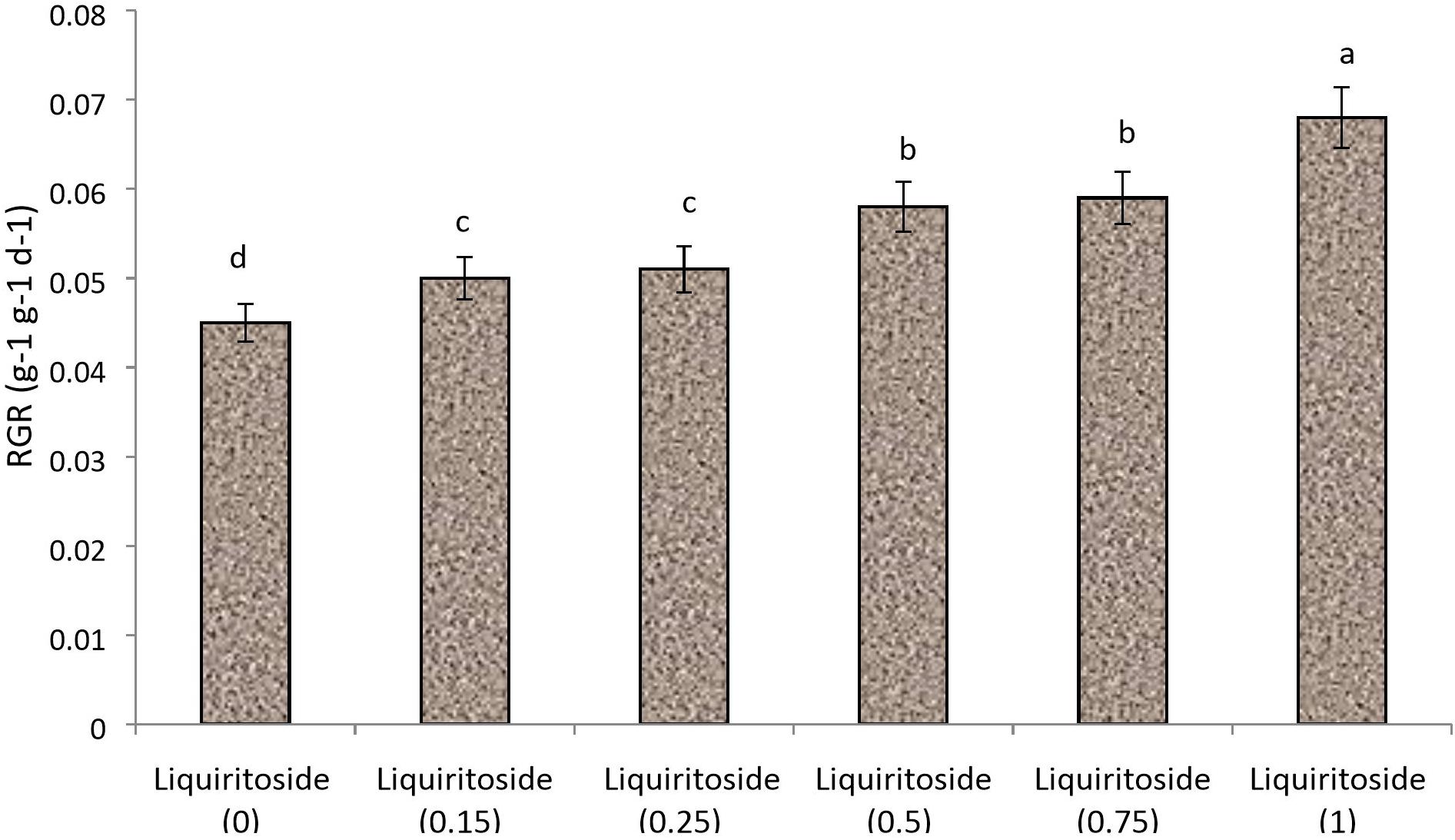
Figure 1. Effect of various doses of liquiritoside (g/L) on the relative growth rate (RGR) of Chinese flowering cabbage. Mean values of two independent experiments are presented. Vertical bars show standard error between different replicates of the same treatment. Data marked by different letters are significantly different at p < 0.05.
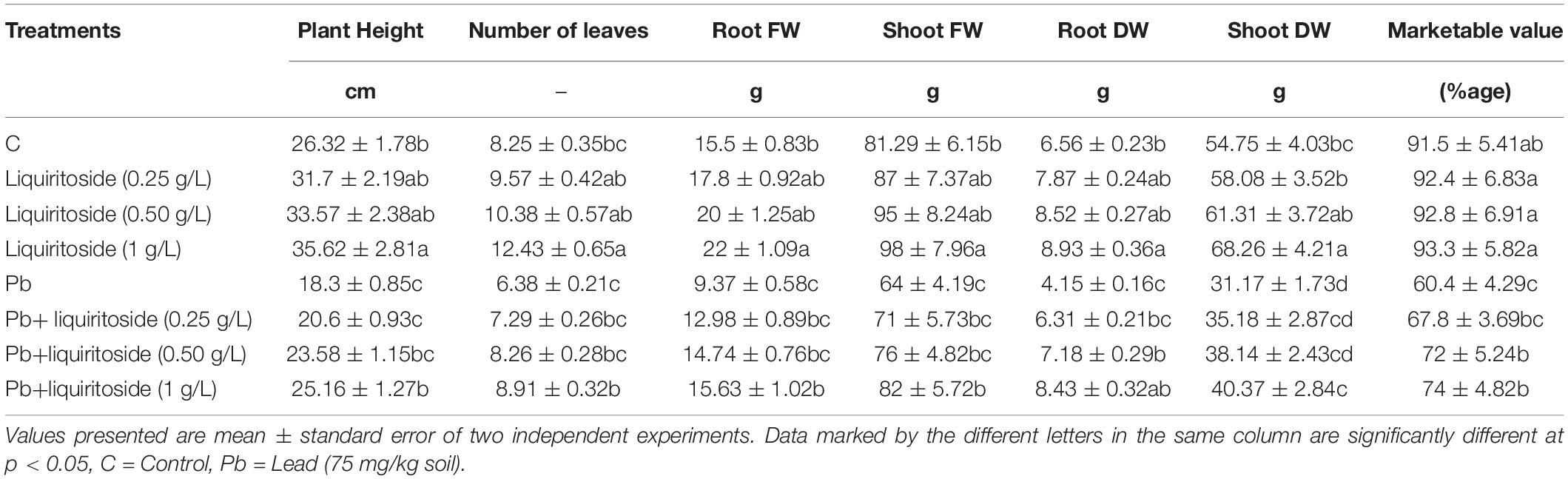
Table 1. Effect of liquiritoside on growth attributes of Chinese flowering cabbage plants under lead (Pb) stress.
The elicitor was applied as a foliar application starting at the four-leaf stage and repeated on a fortnight basis. Plant growth parameters such as root/shoot length, root/shoot biomass, and the number of leaves were evaluated after 2 months of first foliar spray. The marketable value of plants was calculated using the following formula:
A minimum of 20 plants was harvested from each treatment and was used for morphological and metabolomic analysis. Plant samples intended for metabolomics analysis were frozen in liquid nitrogen and kept at –80°C till examined.
Assessment of Total Phenolic, and Flavonoids and Photosynthetic Pigments
Chlorophyll and carotenoids content was determined by using a spectrophotometer according to Metzner et al. (1965). The total phenolic contents were analyzed by employing the standard Folin-Ciocalteau method (Pavel et al., 2006) and expressed as milligrams gallic acid equivalent per gram of dry weight tissue. The total flavonoid content was estimated using the aluminum chloride colorimetric method of Chang et al. (2002).
Analysis of the Nutritional Values of Leaves
Leaf samples for nutritional analysis were prepared as described by Mahmoud et al. (2019). Leaf samples were washed, stretched on paper towels, and air-dried for 60 min at room temperature. Thereafter, leaf samples were oven-dried at 70°C to ensure persistent weight. These dried samples were ground in a stainless-steel grinder and the following nutritional analyses were performed.
Estimation of H2O2 and MDA
The amount of H2O2 content was estimated with the help of a spectrophotometer as described by Jana and Choudhuri (1981). The MDA level, a product of lipid peroxidation was quantified according to Heath and Packer (1968), with slight alterations as suggested by Zhang and Kirkham (1994).
Assessment of Antioxidative Enzymes
The enzymatic activities of POD, SOD, and CAT were analyzed as described by Gao et al. (2005).
Estimation of Phytochelatins (PCs), Non-protein Thiols (NPT), Cysteine, and Glutathione (GSH)
The cysteine content in the plant sample was measured according to the methodology of Gaitonde (1967). The amount of non-protein thiols content was estimated by employing the technique of Ellmann (1959). The quantity of sulfur-assimilating compounds was analyzed by adopting the technique of Nagalakshmi and Prasad (2001).
The amount of phytochelatins in treated plants was assessed according to Bhargava et al. (2005) by eliminating the quantity of GSH from the amount of NPTs as follows:
Quantification of Proline Content
The technique of Bates et al. (1973) was used for the estimation of proline content.
Estimation of Lead
The quantity of Pb from digested plant samples was evaluated according to Khan et al. (2017) by using Atomic Absorption Spectrophotometer.
Quantification of Glucosinolate Content
We used our recently devised method (Akram et al., 2020) for the identification and quantification of different types of glucosinolates from the leaves of Chinese flowering cabbage plants. Leaves from 10 plants were taken from each treatment and pooled together. Analysis was performed on an API 4000 QTrap mass spectrometer equipped with a TurboIonSpray probe (AB Sciex; Foster City, CA, United States) connected to a Shimadzu UFLC (Shimadzu, Kyoto, Japan). The mass spectrometer worked with triple quadrupole analyzer in the Multiple Reaction Monitoring (MRM) mode. Sinigrin does not exist in B. rapa and B. napus (Rangkadilok et al., 2002). In this study, it was used as an internal standard for quantitative analysis of glucosinolates (Jacobo-Velázquez et al., 2011).
Statistical Analysis
The data obtained were analyzed by taking variance with the help of DSAASTAT software. The Duncan’s new multiple range test was employed for evaluation of the significant difference between means of all treatments. The study trials having three replicates were repeated twice, and values of means acquired are exhibited.
Results
Influence of Liquiritoside on Plant Height, Number of Leaves, Root Biomass, Shoot Biomass, and Marketable Value of Chinese Flowering Cabbage Subjected to Pb Stress
In the absence of Pb, the liquiritoside (1 g/L) treatment significantly increased the plant height, the number of leaves, marketable value, dry weight of the roots and shoots by 26, 34, 3, 27, and 20%, respectively, as compared to untreated control (Table 1). Liquiritoside (0.5 g/L) treatment had no significant effect on plant height, the number of leaves, marketable value, shoot, and root biomass after and before Pb exposure. In the presence of Pb, liquiritoside (1 g/L) application remarkably increased plant length, number of leaves, marketable value, the dry weight of the shoots and roots of Chinese flowering cabbage in comparison with Pb control (Table 1).
Effects of Liquiritoside on Chlorophyll and Carotenoid Contents, Flavonoids, and Total Phenolics in Chinese Flowering Cabbage Under Pb Stress
As shown in Table 2, the contents of chlorophyll a, chlorophyll b, and carotenoids in plant tissues of the control treatment were 1.17, 0.47, and 2.78 mg g–1, respectively. The Pb treatment decreased the chlorophyll a and b and carotenoids contents in Chinese flowering cabbage by 27, 28, and 24%, respectively, than that of the untreated control. On the other hand, liquiritoside (1 g/L) supplementation significantly improved the chlorophyll a and b and carotenoids levels of plants exposed to Pb stress compared with relevant control.
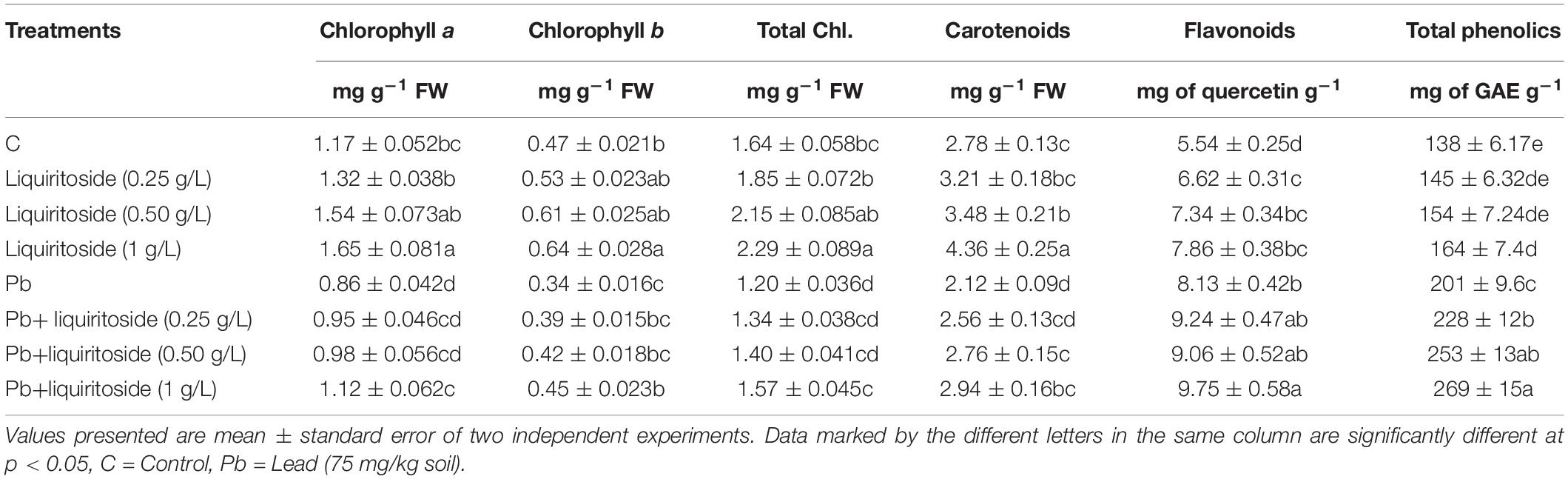
Table 2. Effect of liquiritoside on chlorophyll, carotenoids, flavonoids, and phenolics levels of Chinese flowering cabbage plants under lead (Pb) stress.
The results showed that Pb toxicity markedly augmented the level of both flavonoids, and phenolics by 32 and 38%, respectively, in Chinese flowering cabbage plants as compared to untreated controls. However, liquiritoside supplementation further boosted the magnitude of both flavonoids, and phenolics in the subject plants under Pb toxic and nontoxic conditions (Table 2). The Pb+liquiritoside (1 g/L) treatment accelerated the quantities of both flavonoids, and phenolics by 17 and 31%, respectively, in Chinese flowering cabbage with respect to only Pb treated plants.
Impact of Added Liquiritoside on Antioxidant Enzyme Activities in Chinese Flowering Cabbage Under Pb Treatment
Lead stress attenuated the activities of antioxidant enzymes like SOD, CAT, and POD in Chinese flowering cabbage plants when compared with untreated control. While supplementation of liquiritoside at different concentrations further modulated the level of these antioxidant enzymes (SOD, CAT, and POD) in plants subjected to Pb stressed and non-stressed regimes. The Pb+liquiritoside (0.5 g/L) exhibited remarkable increment in the level of SOD, CAT, and POD by 24, 16, and 37, respectively, in Chinese flowering cabbage plants in contrast with only Pb treated ones (Figure 2).
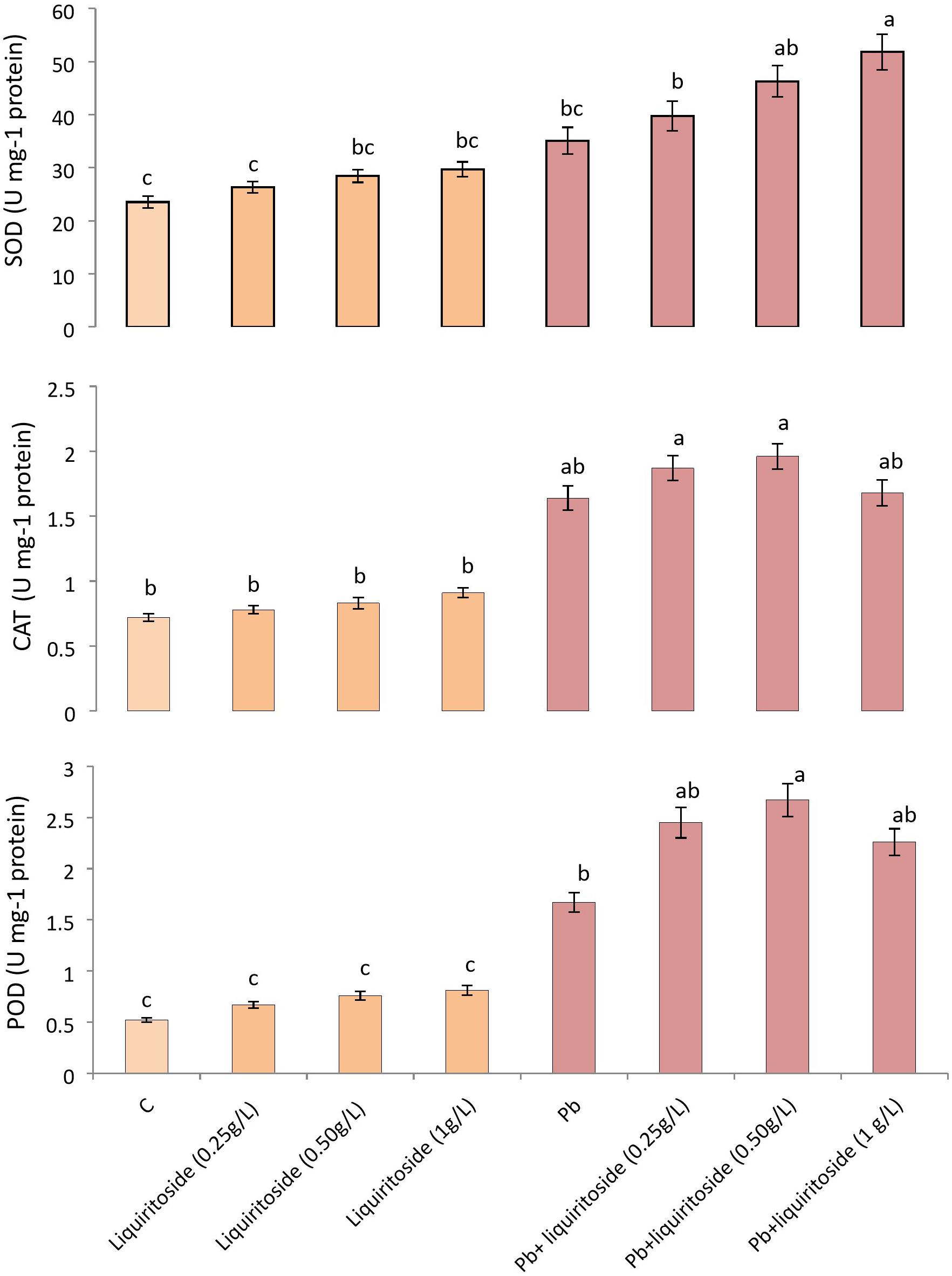
Figure 2. Effect of liquiritoside on the magnitudes of antioxidant enzymes such as SOD, POD, and CAT in Chinese flowering cabbage plants under lead stress. Mean values of two independent experiments are presented. Vertical bars show standard error between different replicates of the same treatment. Data marked by different letters are significantly different at p < 0.05. Pb, lead; C, Control.
Implications of Liquiritoside in the Modulation of GSH, PCs, Cysteine, and NPT Contents in Chinese Flowering Cabbage Exposed to Pb Toxicity
In the absence of Pb treatments, no obvious changes were observed in the GSH contents of plants with increased liquiritoside application, but the GSH contents were meaningfully different in plants raised under various liquiritoside treatments during Pb stressed conditions (Table 3). The pre-incubation of liquiritoside at the dose of 0.25, 0.50, and 1 g/L increased glutathione contents by 11, 14, and 18%, respectively, in Chinese cabbage plants raised under Pb regimes with respect to relevant controls. In the presence of Pb toxicity, sufficient and excessive liquiritoside treatment obviously increased the PCs and NPT contents in Chinese flowering cabbage plants compared with those plants in the liquiritoside deficiency treatment group. The, Pb+liquiritoside (1 g/L) treatment significantly enhanced the level of PCs and NPT in subjected plants by 14 and 17%, respectively, with respect to only Pb treated ones (Table 3). These findings signposted that the biosynthesis of thiol metabolic compounds (NPT, GSH, and PCs) enhanced with increased liquiritoside application in Chinese flowering cabbage to alleviate Pb stress. During the current study, liquiritoside (1 g/L) treatment noticeably attenuated the cysteine contents of Chinese flowering cabbage under nontoxic and toxic regimes (Table 3).
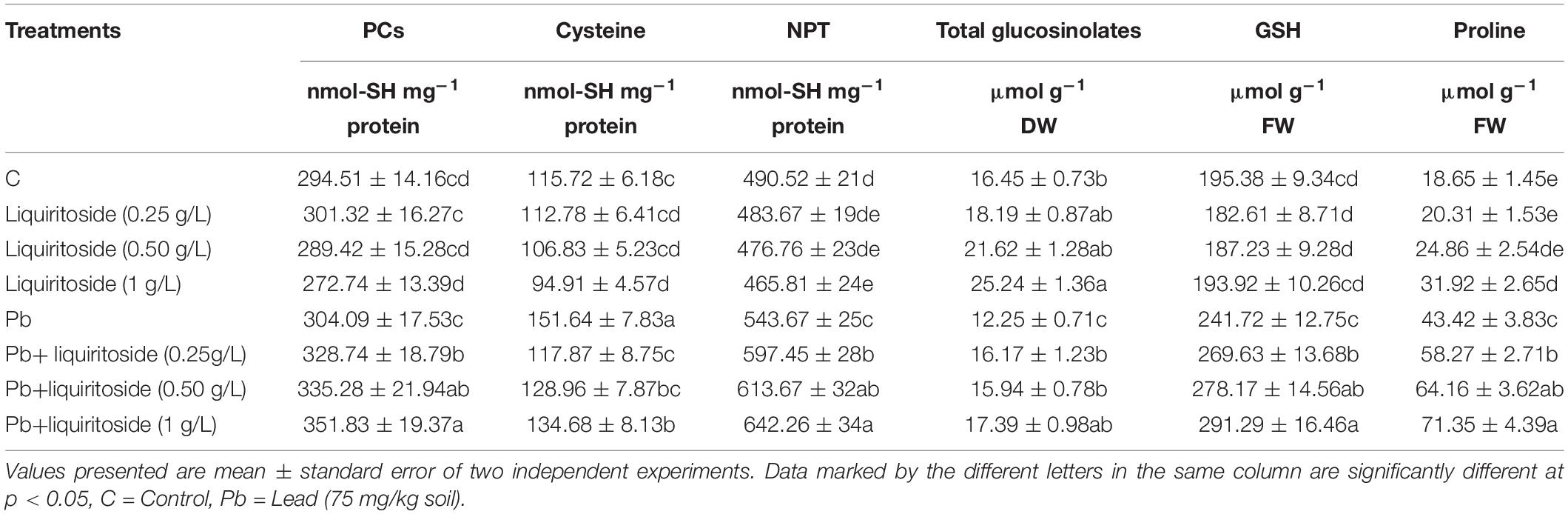
Table 3. Effect of liquiritoside on the levels of phytochelatins (PCs), cysteine, non-protein thiols (NPT), glucosinolates, glutathione (GSH) and proline in Chinese flowering cabbage plants under lead (Pb) stress.
Role of Liquiritoside on Total Glucosinolates and Proline Contents of Chinese Flowering Cabbage
Lead toxicity reduced the total glucosinolates by 26% in Chinese flowering cabbage plants than that of the untreated control. However, liquiritoside supplementation augmented the level of total glucosinolates during stressed and non-stressed conditions. The application of liquiritoside at the concentration of 0.5 and 1 g/L increased the value of total glucosinolates by 23 and 29%, respectively, in plants grown under metal regimes with respect to Pb control (Table 3). Present results depicted that lead stress remarkably declined the level of glucosinolate contents including Progoitrin, Glucoalyssin, Gluconapin, Glucobrassicin, Neoglucobrassin, 4- Hydroxyglucobrassicin and, 4-Methoxyglucobrassicin in Chinese flowering cabbage plants with respect to analogous untreated controls, respectively. Nevertheless, liquiritoside application enhanced the values of these glucosinolate contents in Chinese flowering cabbage plants under stressed and non-stressed regimes (Table 4).
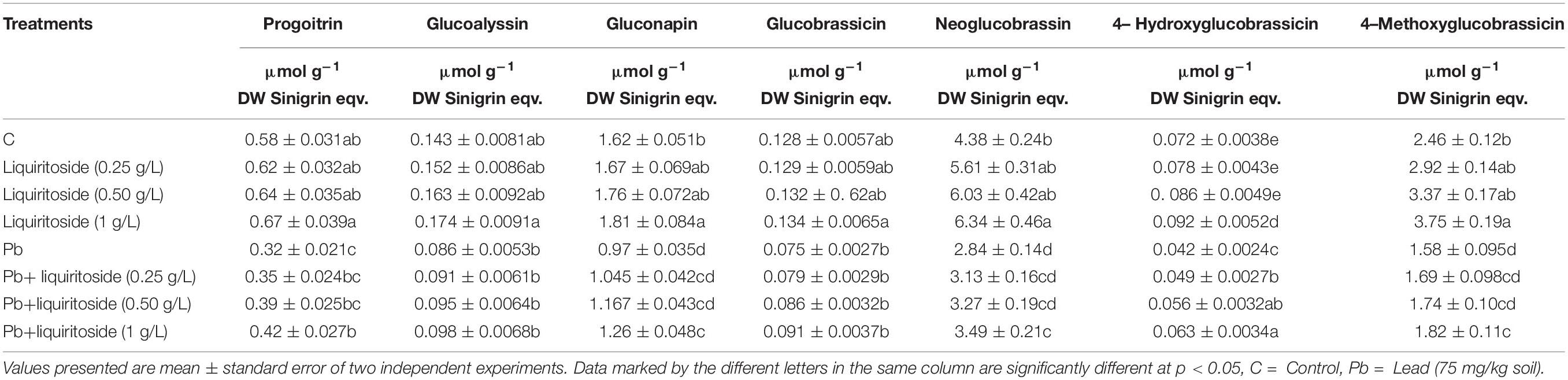
Table 4. Effect of liquiritoside on glucosinolate contents in Chinese flowering cabbage plants under lead (Pb) stress.
During the present research, proline content was enhanced by 57% in Chinese flowering cabbage exposed to Pb stress when compared with untreated control. While, differential liquiritoside (0.25, 0.5, and 1 g/L) supplementation further increased the level of proline by 26, 32, and 39%, respectively, in the subject plants under contaminated regimes than that of Pb control treatment (Table 3).
Effect of Liquiritoside on MDA and H2O2 Concentrations in Chinese Flowering Cabbage Under Pb Stress
During the present investigation, Pb toxicity considerably augmented the contents of MDA and H2O2 in Chinese flowering cabbage with respect to untreated control. Nevertheless, excessive liquiritoside supplementation meaningfully declined the level of both MDA and H2O2 in plants during toxic and nontoxic circumstances. The Pb+ liquiritoside (1 g/L) treatment diminished the quantities of both H2O2 and MDA in Chinese flowering cabbage by 38 and 29%, respectively, as compared to concerned Pb treated groups (Figure 3).
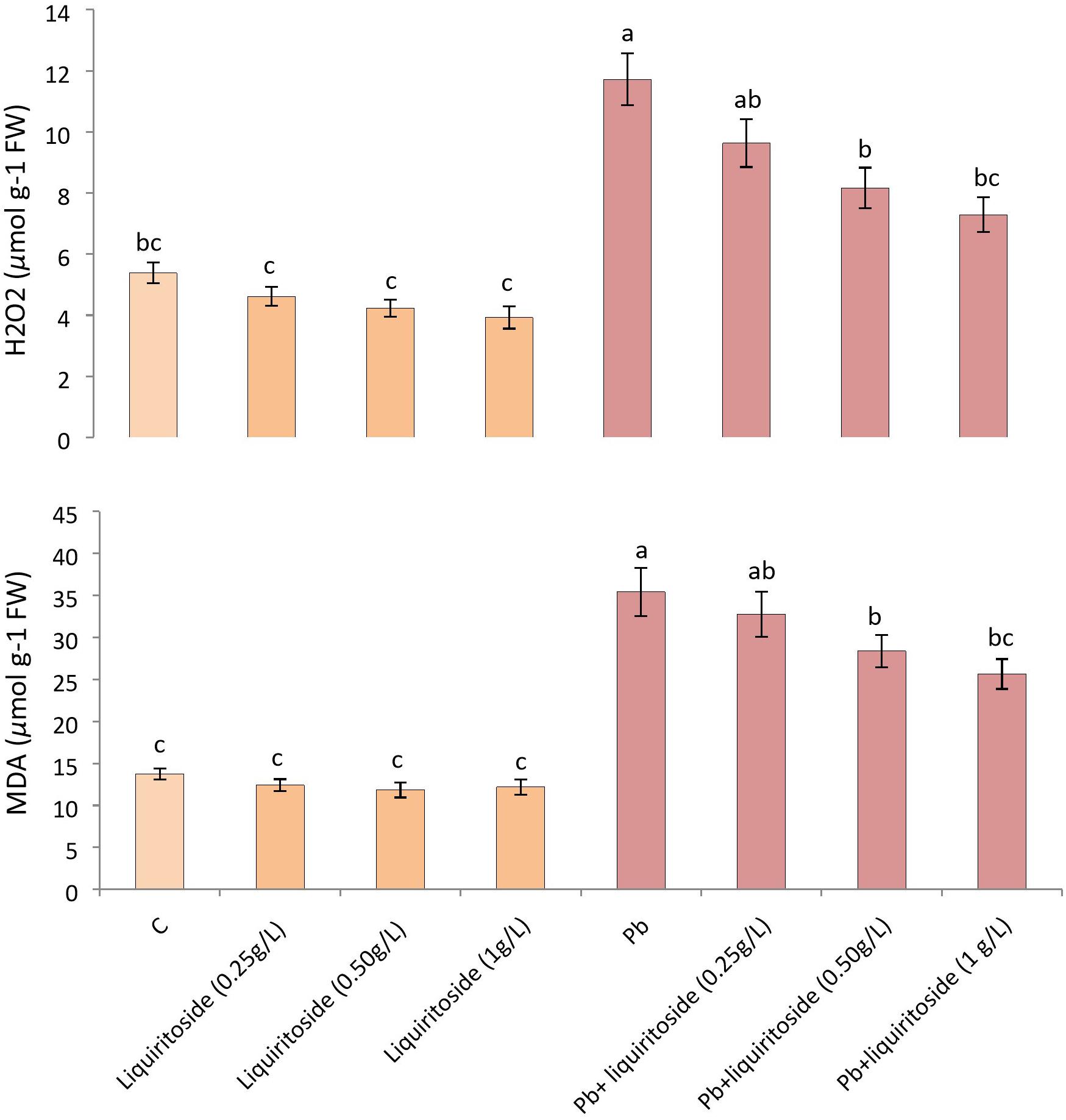
Figure 3. Effect of liquiritoside on the amounts of malondialdehyde (MDA) and hydrogen peroxide (H2O2) in Chinese flowering cabbage plants under lead stress. Mean values of two independent experiments are presented. Vertical bars show standard error between different replicates of the same treatment. Data marked by the different letters in the same column are significantly different at p < 0.05. Pb, lead; C, Control.
Role of Liquiritoside on Pb Uptake in Roots and Shoots of Chinese Flowering Cabbage
The findings of the current study depicted that root tissues showed more Pb uptake compared with shoot tissues of Chinese flowering cabbage (Table 5). The higher Pb accumulation was noticed in roots and shoots of plants grown under only Pb treatment. The liquiritoside supplementation exhibited a reduction of Pb uptake in the root and the shoot tissues of Chinese flowering cabbage as compared to only the Pb treatment group (Table 5). With the increase in liquiritoside doses (0.25, 0.5, and 1 g/L), a Pb uptake reduction was recorded in analyzed root and shoot tissues. The excessive dose liquiritoside (1 g/L) caused 28 and 43% decline of Pb uptake in root and shoot organs of Chinese flowering cabbage in contrast with non -supplemented stressed plants. The application of liquiritoside (1 g/L) significantly reduced the translocation factor and bio-concentration factor of Pb in subjected plants than that of non-supplemented ones. The plants grown under Pb regimes exhibited decreased value flowering cabbage plants in a dose dependent manner.
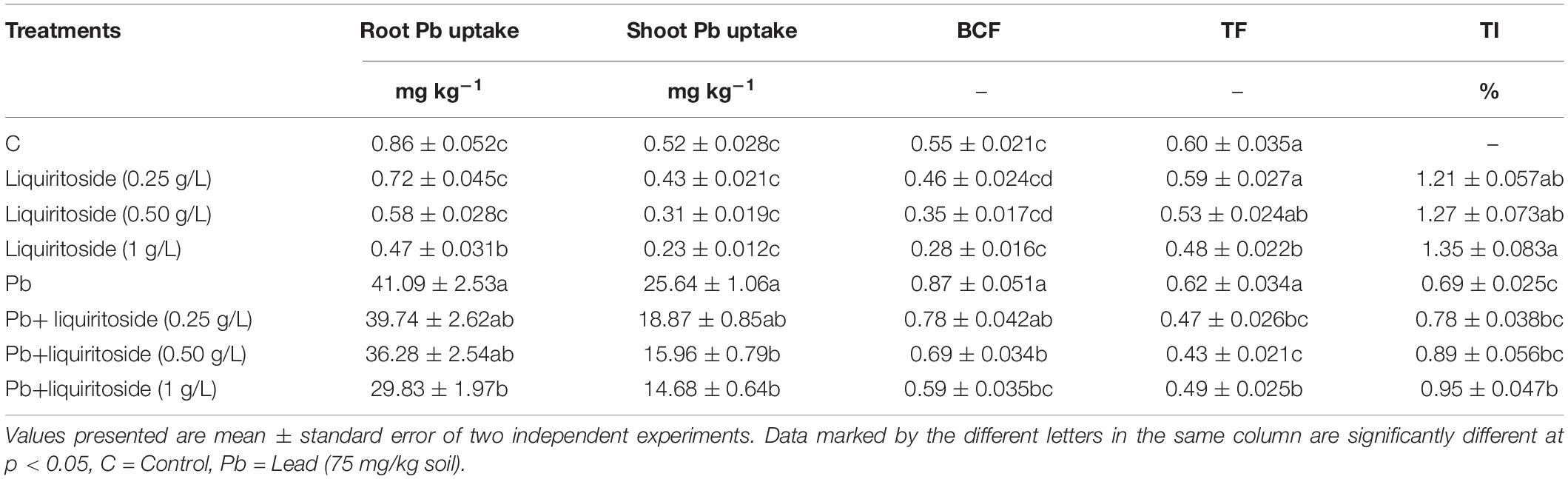
Table 5. Role of liquiritoside on Pb uptake in root and shoot tissues, bio-concentration factor (BCF), translocation factor (TF) and tolerance index (TI) of Chinese flowering cabbage plants under lead (Pb) stress.
Discussion
In the past few years, several studies have shown that exogenous elicitors can mediate plant growth and productivity (Bibi et al., 2016; Brockman and Brennan, 2017; Colla et al., 2017). To the best of our knowledge, the present study is the first to report the positive effects of liquiritoside on the growth and health-promoting elements of Chinese flowering cabbage grown under Pb stress. The application of genistein, a flavonoid improved growth and production of salted soya bean plants (Miransari and Smith, 2007). Likewise, coumarin, a phenolic compound enhanced the growth of wheat plants exposed to salt stress. Similarly, apigenin also enhanced biomass production and the growth of paddy plants under salt stress (Mekawy et al., 2018). Similarly, it was revealed that liquiritoside enhanced growth-related attributes and marketable value of Chinese flowering cabbage exposed to Pb regimes.
The increased growth rate of Chinese flowering cabbage plants observed under the influence of the foliar elicitor could be attributed to the capability of liquiritoside to modulate phytohormones, soluble sugars, amino acids, and mineral elements in applied plants. The liquiritoside may improve the yield and quality of Chinese flowering cabbage by affecting cellular metabolism. For example, it is known that sugars act as signaling molecules and improve plant growth and development (Smeekens et al., 2010). Amino acids provide improved stress tolerance in plants (Karabudak et al., 2014). Some organic acids present in plant extracts can chelate metal ions to stimulates root growth (Battacharyya et al., 2015). All these together could supply nutrition for cell growth, with a resulting increase in growth and vigor.
Chinese flowering cabbage plants under Pb stress exhibited a reduced level of photosynthetic pigments. Several other studies have revealed that Pb deteriorated chlorophyll structure and decreased chlorophyll synthesis by replacing Fe, Mg, and Cu (Akinci et al., 2010; Ashraf et al., 2016). Foliar application of liquiritoside positively affected total chlorophyll and carotenoids content in a dose-dependent manner (Table 2). The positive effect of liquiritoside on leaf pigment content could be attributed to the delay in leaf senescence or enhancement in leaf pigment biosynthesis (Fan et al., 2013; Jannin et al., 2013). These beneficial effects are possibly due to the effect of liquiritoside on phytohormones. The physiological parameter of leaf pigment content also acts as indicators of improved quality of Chinese flowering cabbage that can be obtained by the application of exogenous elicitors. Analogous to our results, it was observed that apigenin-treated plants showed increased biosynthesis of photosynthetic pigments which improved the growth of paddy plants subjected to salinity stress (Mekawy et al., 2018). Moreover, the cinnamic acid applied plants also exhibited an increased amount of photosynthetic pigments besides increased growth of maize plants exposed to salt toxicity (Araniti et al., 2018).
The enzymatic and non-enzymatic antioxidants enable plants to thrive under abiotic stress conditions (Usman et al., 2020). The antioxidative compounds perform the role of sacrificial agents through their activity on ROS, thus defending plant biomolecules. Rutin as an antioxidative flavonoid, scavenge ROS, and enhance the growth of leguminous plants (Ismail et al., 2016). Quercetin synthesized by rutin has ROS scavenging capability because it acts as a substrate of guaiacol peroxidase (Amako et al., 1994). The detoxification of ROS in quercitin applied plants mitigated salt-induced stress and improved the growth of plants. The antioxidant enzymes such as POD, CAT, and SOD consume phenolics, including cinnamate, ellagate and ferulate to alleviate plant stress (Abu Taleb et al., 2013; Singh et al., 2013). Similarly, our finding also exhibited obvious modulations in the activities of POD, CAT, and SOD for dilution of Pb toxicity in liquiritoside supplemented Chinese flowering cabbage plants.
Fayez et al. (2014) demonstrated that abiotic stress modulates physiochemical attributes of plants. Plants synthesize an elevated level of MDA and H2O2 under stress (Noctor et al., 2015). Lead stress enhanced the biosynthesis of ROS, leading to increased oxidative injury in plants (Hattab et al., 2016). Other studies also showed that Pb enhanced lipid peroxidation in plants causing oxidative injuries (Li et al., 2013). Plants engage the antioxidant system to mitigate metal-triggered oxidative stress (Shahid et al., 2014). Phenolics and flavonoids may reduce the biosynthesis of ROS, EL H2O2, and MDA to alleviate plant stress (Mekawy et al., 2018). The reduced level of ROS helps in the mitigation of oxidative injury (Hossain et al., 2019). The improved synthesis of phenolics and flavonoids as well as antioxidant enzymes detoxify ROS and mitigate stress in gallic acid, and rutin treated plants under stress. Pheomphun et al. (2019) observed that reduced H2O2, MDA, and enhanced activity of antioxidant enzymes in catechin supplemented plants for the alleviation of stress.
Higher proline has been observed in plants facing stress (Ahmad et al., 2018). However, exogenously applied quercetin and coumarin improve proline content, and LRWC in plants to mitigate stress (Saleh and Madany, 2015). The increased proline biosynthesis was attributed to reduced activity of proline dehydrogenase and increased activity of pyrroline-5-carboxylate synthase in coumarin supplemented plants (Pérez-Arellano et al., 2010; Szabados and Savoure, 2010). Hence, it is assumed that liquiritoside may mitigate metal stress in applied Chinese flowering cabbage plants in the same manner.
Phenolics scavenge ROS to reduce oxidative injury in plants (Soares et al., 2019). Our results showed that liquiritoside enhanced phenolic contents and triggered the activity of antioxidant enzymes. Phenolics detoxify ROS and metal toxicity by making metal complex in plants (Tolrà et al., 2009). The results of this study are in agreement with the findings of Ashraf et al. (2016), who observed that the foliar application of plant extracts increased the total phenolics and flavonoid content of Raphanus sativus plants. Baenas et al. (2014) showed that the nutritional quality of sprouts of brassica vegetables was improved by foliar application of biotic elicitors. Agati and Tattini (2010) reported that flavonoids decline in ROS levels in plants affected by abiotic stress. Similarly, other researchers have described the importance of GSH, flavonoids, and ascorbic acid in mitigation of plant stress through reducing ROS synthesis (Liang et al., 2018). The augmented synthesis of flavonoid alleviates drought stress in plants (Varela et al., 2016).
Current results showed that the levels of total GLS in Chinese flowering cabbage plants exposed to Pb toxicity were significantly increased under the influence of the foliar elicitor (1 g/L) (Table 3). Same types of increments of GLSs have been reported in Chinese flowering cabbage in previous studies (Bhandari et al., 2015; Liang et al., 2018). Metal stressed plants modulate the synthesis of thiol ligands, including phytochelatins (PCs), non-protein thiols, cysteine, and GSH for detoxification and chelation of metal (Kumar et al., 2016; Ahmad et al., 2020). Higher synthesis of thiols in root tissues compared to foliage of plants declines uptake and translocation of injurious metals from roots to shoots (Hasan et al., 2015). The thiol-containing groups of plants such as cysteine, NPT and PCs, have a higher affinity for metals and hence assist in homeostasis and detoxification of metals (Rabêlo et al., 2018). Similarly, cysteine, NPT, GSH, and PCs may have played their part reducing Pb translocation and subsequent detoxification in Chinese cabbage flowering plants.
Plant roots immediately come in contact with metals and hence exhibit relatively more metal content as compared to above-ground parts of plants. The increased demethylation and pectin level help in reduced translocation besides the fixation of metal within the root cell walls (Liu et al., 2019). Perhaps, this strategy reduced Pb translocation from root to shoot of the plants and should be explored in further studies (Bharwana et al., 2013).
Conclusion
This study demonstrates that liquiritoside could be used as an effective plant growth bio-stimulant. Our findings indicate that the nutritional and medicinal contents in leaves of Chinese flowering cabbage plants can be augmented by foliar application of liquiritoside at a rate of 0.5 g/L. The supplementation of liquiritoside alleviated Pb stress of plants by improving growth/photosynthetic pigments, glucosinolates, antioxidants, and reducing MDA, H2O2, cysteine, and Pb uptake. Further studies are required to understand the mechanism underlying the crop’s growth effect, promoting biotic elicitor’s use in organic agriculture.
Data Availability Statement
The original contributions presented in the study are included in the article/supplementary material, further inquiries can be directed to the corresponding author/s.
Author Contributions
WA: suggest the idea and perform the experiments. WUK: carry out statistical analysis. NAY: writing manuscript. AS: data analysis and manuscript drafting. GL: research designing and supervision. All authors contributed to the article and approved the submitted version.
Funding
This study was supported by the Science and Technology Foundation of Guangdong Province (Project No. 2020B0202090002) and Guangdong Agriculture Department of China (Project No. 2021KJ122).
Conflict of Interest
The authors declare that the research was conducted in the absence of any commercial or financial relationships that could be construed as a potential conflict of interest.
Publisher’s Note
All claims expressed in this article are solely those of the authors and do not necessarily represent those of their affiliated organizations, or those of the publisher, the editors and the reviewers. Any product that may be evaluated in this article, or claim that may be made by its manufacturer, is not guaranteed or endorsed by the publisher.
References
Abu Taleb, A., Tharwat, N., and El-Mohamedy, R. (2013). Induction of resistance in tomato plants against fusarium crown and root rot disease by Trichoderma harzianum and chitosan. Egypt. J. Phytopathol. 41, 13–26. doi: 10.21608/ejp.2013.101965
Agati, G., and Tattini, M. (2010). Multiple functional roles of flavonoids in photoprotection. New Phytol. 186, 786–793. doi: 10.1111/j.1469-8137.2010.03269.x
Ahmad, A., Khan, W. U., Shah, A. A., Yasin, N. A., Ali, A., Rizwan, M., et al. (2020). Dopamine alleviates hydrocarbon stress in Brassica oleracea through modulation of physio-biochemical attributes and antioxidant defense systems. Chemosphere 270:128633. doi: 10.1016/j.chemosphere.2020.128633
Ahmad, P., Abass Ahanger, M., Nasser Alyemeni, M., Wijaya, L., Alam, P., and Ashraf, M. (2018). Mitigation of sodium chloride toxicity in Solanum lycopersicum L. by supplementation of jasmonic acid and nitric oxide. J. Plant Interact. 13, 64–72. doi: 10.1080/17429145.2017.1420830
Aixia, G., Jianjun, Z., Yanhua, W., Xiaofeng, L., Shuxin, X., and Shuxing, S. (2015). Glucosinolates in self-crossed progenies of monosomic cabbage alien addition lines in Chinese cabbage. Hortic. Plant J. 1, 86–92.
Akinci, I. E., Akinci, S., and Yilmaz, K. (2010). Response of tomato (Solanum lycopersicum L.) to lead toxicity: growth, element uptake, chlorophyll and water content. Afr. J. Agric. Res. 5, 416–423.
Akram, W., Saeed, T., Ahmad, A., Yasin, N. A., Akbar, M., Khan, W. U., et al. (2020). Liquiritin elicitation can increase the content of medicinally important glucosinolates and phenolic compounds in Chinese kale plants. J. Sci. Food Agric. 100, 1616–1624. doi: 10.1002/jsfa.10170
Amako, K., Chen, G.-X., and Asada, K. (1994). Separate assays specific for ascorbate peroxidase and guaiacol peroxidase and for the chloroplastic and cytosolic isozymes of ascorbate peroxidase in plants. Plant Cell Physiol. 35, 497–504.
Araniti, F., Lupini, A., Mauceri, A., Zumbo, A., Sunseri, F., and Abenavoli, M. R. (2018). The allelochemical trans-cinnamic acid stimulates salicylic acid production and galactose pathway in maize leaves: a potential mechanism of stress tolerance. Plant Physiol. Biochem. 128, 32–40. doi: 10.1016/j.plaphy.2018.05.006
Ashraf, R., Sultana, B., Iqbal, M., and Mushtaq, M. (2016). Variation in biochemical and antioxidant attributes of Raphanus sativus in response to foliar application of plant leaf extracts as plant growth regulator. J. Genetic Eng. Biotechnol. 14, 1–8. doi: 10.1016/j.jgeb.2016.08.003
Baenas, N., García-Viguera, C., and Moreno, D. A. (2014). Biotic elicitors effectively increase the glucosinolates content in Brassicaceae sprouts. J. Agric. Food Chem. 62, 1881–1889. doi: 10.1021/jf404876z
Baghaie, A. H., and Fereydoni, M. (2019). The potential risk of heavy metals on human health due to the daily consumption of vegetables. Environ. Health Eng. Manag. 6, 11–16. doi: 10.15171/ehem.2019.02
Barba, F. J., Nikmaram, N., Roohinejad, S., Khelfa, A., Zhu, Z., and Koubaa, M. (2016). Bioavailability of glucosinolates and their breakdown products: impact of processing. Front. Nutr. 3:24. doi: 10.3389/fnut.2016.00024
Barba, F., Cravotto, G., and Munekata, P. E. S. (2021). Design and Optimization of Innovative Food Processing Techniques Assisted by Ultrasound. New York, NY: Elsevier Science.
Bates, L. S., Waldren, R. P., and Teare, I. D. (1973). Rapid determination of free proline for water-stress studies. Plant Soil 39, 205–207. doi: 10.1007/bf00018060
Battacharyya, D., Babgohari, M. Z., Rathor, P., and Prithiviraj, B. (2015). Seaweed extracts as biostimulants in horticulture. Sci. Hortic. 196, 39–48. doi: 10.1016/j.scienta.2015.09.012
Bhandari, S. R., Jo, J. S., and Lee, J. G. (2015). Comparison of glucosinolate profiles in different tissues of nine brassica crops. Molecules 20, 15827–15841. doi: 10.3390/molecules200915827
Bhargava, P., Srivastava, A. K., Urmil, S., and Rai, L. C. (2005). Phytochelatin plays a role in UV-B tolerance in N2-fixing cyanobacterium Anabaena doliolum. J. Plant Physiol. 162, 1220–1225. doi: 10.1016/j.jplph.2004.12.006
Bharwana, S. A., Ali, S., Farooq, M. A., Iqbal, N., Abbas, F., and Ahmad, M. S. A. (2013). Alleviation of lead toxicity by silicon is related to elevated photosynthesis, antioxidant enzymes suppressed lead uptake and oxidative stress in cotton. J. Bioremed. Biodeg. 4:187.
Bibi, A., Ullah, F., Mehmood, S., Bibi, K., Khan, S. U., Khattak, A., et al. (2016). Moringa oleifera Lam. leaf extract as bioregulator for improving growth of maize under mercuric chloride stress. Acta Agric. Scand. Sec. B Soil Plant Sci. 66, 469–475. doi: 10.1080/09064710.2016.1173225
Borges, K. L. R., Hippler, F. W. R., Carvalho, M. E. A., Nalin, R. S., Matias, F. I., and Azevedo, R. A. (2019). Nutritional status and root morphology of tomato under Cd-induced stress: comparing contrasting genotypes for metal-tolerance. Sci. Hortic. 246, 518–527. doi: 10.1016/j.scienta.2018.11.023
Brockman, H. G., and Brennan, R. F. (2017). The effect of foliar application of Moringa leaf extract on biomass, grain yield of wheat and applied nutrient efficiency. J. Plant Nutr. 40, 2728–2736. doi: 10.1080/01904167.2017.1381723
Chang, C. C., Yang, M. H., Wen, H. M., and Chern, J. C. (2002). Estimation of total flavonoid content in propolis by two complementary colorimetric methods. J. Food Drug Anal. 10, 178–182.
Chen, X., Zhu, J., Gerendás, J., and Zimmermann, N. (2008). Glucosinolates in Chinese Brassica campestris vegetables: Chinese cabbage, purple cai-tai, choysum, pakchoi, and turnip. HortScience 43, 571–574. doi: 10.21273/hortsci.43.2.571
Chung, I. M., Rekha, K., Rajakumar, G., and Thiruvengadam, M. (2016). Production of glucosinolates, phenolic compounds and associated gene expression profiles of hairy root cultures in turnip (Brassica rapa ssp. rapa). 3 Biotech 6:175.
Colla, G., Cardarelli, M., Bonini, P., and Rouphael, Y. (2017). Foliar applications of protein hydrolysate, plant and seaweed extracts increase yield but differentially modulate fruit quality of greenhouse tomato. HortScience 52, 1214–1220. doi: 10.21273/hortsci12200-17
Devi, R., Munjral, N., Gupta, A. K., and Kaur, N. (2013). Effect of exogenous lead on growth and carbon metabolism of pea (Pisum sativum L) seedlings. Physiol. Mol. Biol. Plants. 19, 81–89. doi: 10.1007/s12298-012-0143-5
Ellmann, G. L. (1959). Tissue sulfhydryl groups. Arch Biochem. Biophys. 82, 70–77. doi: 10.1016/0003-9861(59)90090-6
Fan, D., Hodges, D., Critchley, A., and Prithiviraj, B. (2013). A commercial extract of brown macroalga (Ascophyllum nodosum) affects yield and the nutritional quality of spinach in vitro. Commun. Soil Sci. Plant Anal. 44, 1873–1884. doi: 10.1080/00103624.2013.790404
Fayez, K. A., El-Deeb, B. A., and Mostafa, N. Y. (2017). Toxicity of biosynthetic silver nanoparticles on the growth, cell ultrastructure and physiological activities of barley plant. Acta Physiol. Plant. 39:155.
Fayez, K. A., Radwan, D. E. M., Mohamed, A. K., and Abdelrahman, A. M. (2014). Fusilade herbicide causes alterations in chloroplast ultrastructure, pigment content and physiological activities of peanut leaves. Photosynthetica 52, 548–554. doi: 10.1007/s11099-014-0062-5
Figlioli, F., Sorrentino, M. C., Memoli, V., Arena, C., Maisto, G., Giordano, S., et al. (2019). Overall plant responses to Cd and Pb metal stress in maize: growth pattern, ultrastructure, and photosynthetic activity. Environ. Sci. Pollut. Res. 26, 1781–1790. doi: 10.1007/s11356-018-3743-y
Gaitonde, M. K. (1967). A spectrophotometric method for the direct determination of cysteine in the presence of other naturally occurring amino acids. Biochem. J. 104, 627–633. doi: 10.1042/bj1040627
Gao, H., Chen, G., Han, L., and Lin, H. (2005). Calcium influence on chilling resistance of grafting eggplant seedlings. J. Plant Nutr. 27, 1327–1339. doi: 10.1081/pln-200025833
Hasan, M., Ahammed, G. J., Yin, L., Shi, K., Xia, X., Zhou, Y., et al. (2015). Melatonin mitigates cadmium phytotoxicity through modulation of phytochelatins biosynthesis, vacuolar sequestration, and antioxidant potential in Solanum lycopersicum L. Front. Plant Sci. 6:601. doi: 10.3389/fpls.2015.00601
Hattab, S., Hattab, S., Flores-Casseres, M. L., Boussetta, H., Doumas, P., Hernandez, L. E., et al. (2016). Characterisation of lead-induced stress molecular biomarkers in Medicago sativa plants. Environ. Exp. Bot. 123, 1–12. doi: 10.1016/j.envexpbot.2015.10.005
Heath, R. L., and Packer, L. (1968). Photoperoxidation in isolated chloroplasts: I. Kinetics and stoichiometry of fatty acid peroxidation. Arch. Biochem. Biophys. 125, 189–198. doi: 10.1016/0003-9861(68)90654-1
Hossain, M. S., Hasanuzzaman, M., Sohag, M. M. H., Bhuyan, M. B., and Fujita, M. (2019). Acetate-induced modulation of ascorbate: glutathione cycle and restriction of sodium accumulation in shoot confer salt tolerance in Lens culinaris Medik. Physiol. Mol. Biol. Plants 25, 443–455. doi: 10.1007/s12298-018-00640-6
Ismail, H., Maksimoviý, J. D., Maksimoviý, V., Shabala, L., Živanoviý, B. D., Tian, Y., et al. (2016). Rutin, a flavonoid with antioxidant activity, improves plant salinity tolerance by regulating K+ retention and Na+ exclusion from leaf mesophyll in quinoa and broad beans. Funct. Plant Biol. 43, 75–86. doi: 10.1071/fp15312
Jacobo-Velázquez, D. A., Martínez-Hernández, G. B., del, C., Rodríguez, S., Cao, C.-M., and Cisneros-Zevallos, L. (2011). Plants as biofactories: physiological role of reactive oxygen species on the accumulation of phenolic antioxidants in carrot tissue under wounding and hyperoxia stress. J. Agric. Food Chem. 59, 6583–6593. doi: 10.1021/jf2006529
Jana, S., and Choudhuri, M. A. (1981). Glycolate metabolism of three submersed aquatic angiosperms: effect of heavy metals. Aquat. Bot. 11, 67–77. doi: 10.1016/0304-3770(81)90047-4
Jannin, L., Arkoun, M., Etienne, P., Laîné, P., Goux, D., Garnica, M., et al. (2013). Brassica napus growth is promoted by Ascophyllum nodosum (L.) Le Jol. seaweed extract: microarray analysis and physiological characterization of N, C, and S metabolisms. J. Plant Growth Regul. 32, 31–52. doi: 10.1007/s00344-012-9273-9
Johnson, I. T. (2002). Glucosinolates: bioavailability and importance to health. Int. J. Vit. Nutr. Res. 72, 26–31. doi: 10.1024/0300-9831.72.1.26
Karabudak, T., Bor, M., Ozdemir, F., and Turkan, I. (2014). Glycine betaine protects tomato (Solanum lycopersicum) plants at low temperature by inducing fatty acid desaturase7 and lipoxygenase gene expression. Mol. Biol. Rep. 41, 1401–1410. doi: 10.1007/s11033-013-2984-6
Khan, W. U., Ahmad, S. R., Yasin, N. A., Ali, A., and Ahmad, A. (2017). Effect of Pseudomonas fluorescens RB4 and Bacillus subtilis 189 on the phytoremediation potential of Catharanthus roseus (L.) in Cu and Pb-contaminated soils. Int. J. Phytoremed. 19, 514–521. doi: 10.1080/15226514.2016.1254154
Kondo, K., Shiba, M., Nakamura, R., Morota, T., and Shoyama, Y. (2007). Constituent properties of licorices derived from Glycyrrhiza uralensis, G. glabra, or G. inflata identified by genetic information. Biol. Pharm. Bull. 30, 1271–1277. doi: 10.1248/bpb.30.1271
Kumar, A., Dixit, G., Singh, A. P., Dwivedi, S., Srivastava, S., Mishra, K., et al. (2016). Selenate mitigates arsenite toxicity in rice (Oryza sativa L.) by reducing arsenic uptake and ameliorates amino acid content and thiol metabolism. Ecotoxicol. Environ. Safety 133, 350–359. doi: 10.1016/j.ecoenv.2016.06.037
Lamhamdi, M., El Galiou, O., Bakrim, A., Nóvoa-Muñoz, J. C., Arias-Estévez, M., Aarab, A., et al. (2013). Effect of lead stress on mineral content and growth of wheat (Triticum aestivum) and spinach (Spinacia oleracea) seedlings. Saudi J. Biol. Sci. 20, 29–36. doi: 10.1016/j.sjbs.2012.09.001
Li, D. M., Nie, Y. X., Zhang, J., Yin, J. S., Li, Q., Wang, X. J., et al. (2013). Ferulic acid pretreatment enhances dehydration-stress tolerance of cucumber seedlings. Biol. Plant. 57, 711–717. doi: 10.1007/s10535-013-0326-0
Liang, X., Lee, H. W., Li, Z., Lu, Y., Zou, L., and Ong, C. N. (2018). Simultaneous quantification of 22 glucosinolates in 12 brassicaceae vegetables by hydrophilic interaction chromatography-tandem mass spectrometry. ACS Omega 3, 15546–15553. doi: 10.1021/acsomega.8b01668
Liu, Z., Pi, F., Guo, X., Guo, X., and Yu, S. (2019). Characterization of the structural and emulsifying properties of sugar beet pectins obtained by sequential extraction. Food Hydrocoll. 88, 31–42. doi: 10.1016/j.foodhyd.2018.09.036
Mahmoud, S. H., Salama, D. M., El-Tanahy, A. M. M., and Abd El-Samad, E. H. (2019). Utilization of seaweed (Sargassum vulgare) extract to enhance growth, yield and nutritional quality of red radish plants. Ann. Agricult. Sci. 64, 167–175. doi: 10.1016/j.aoas.2019.11.002
Malar, S., Manikandan, R., Favas, P. J. C., Sahi, S. V., and Venkatachalam, P. (2014). Effect of lead on phytotoxicity, growth, biochemical alterations and its role on genomic template stability in Sesbania grandiflora: a potential plant for phytoremediation. Ecotoxicol. Environ. Safety 108, 249–257. doi: 10.1016/j.ecoenv.2014.05.018
Mekawy, A. M. M., Abdelaziz, M. N., and Ueda, A. (2018). Apigenin pretreatment enhances growth and salinity tolerance of rice seedlings. Plant Physiol. Biochem. 130, 94–104. doi: 10.1016/j.plaphy.2018.06.036
Metzner, H., Rau, H., and Senger, H. (1965). Untersuchungen zur synchronisierbarkeit einzelner pigmentmangel-mutanten von Chlorella. Planta 65, 186–194. doi: 10.1007/bf00384998
Miransari, M., and Smith, D. L. (2007). Overcoming the stressful effects of salinity and acidity on soybean nodulation and yields using signal molecule genistein under field conditions. J. Plant Nutr. 30, 1967–1992. doi: 10.1080/01904160701700384
Nagalakshmi, N., and Prasad, M. N. V. (2001). Responses of glutathione cycle enzymes and glutathione metabolism to copper stress in Scenedesmus bijugatus. Plant Sci. 160, 291–299. doi: 10.1016/s0168-9452(00)00392-7
Noctor, G., Lelarge-Trouverie, C., and Mhamdi, A. (2015). The metabolomics of oxidative stress. Phytochemistry 112, 33–53. doi: 10.1016/j.phytochem.2014.09.002
Pavel, S., Klejdus, B., and Kubáò, V. (2006). Determination of total content of phenolic compounds and their antioxidant activity in vegetablesevaluation of spectrophotometric methods. J. Agric. Food Chem. 54, 607–616. doi: 10.1021/jf052334j
Peng, Y., Shi, D., Zhang, T., Li, X., Fu, T., Xu, Y., et al. (2015). Development and utilization of an efficient cytoplasmic male sterile system for Cai-xin (Brassica rapa L.). Sci. Hortic. 190, 36–42. doi: 10.1016/j.scienta.2015.04.002
Pérez-Arellano, I., Carmona-Álvarez, F., Martínez, A. I., Rodríguez-Díaz, J., and Cervera, J. (2010). Pyrroline-5-carboxylate synthase and proline biosynthesis: from osmotolerance to rare metabolic disease. Protein Sci. 19, 372–382. doi: 10.1002/pro.340
Pheomphun, P., Treesubsuntorn, C., and Thiravetyan, P. (2019). Effect of exogenous catechin on alleviating O3 stress: the role of catechin-quinone in lipid peroxidation, salicylic acid, chlorophyll content, and antioxidant enzymes of Zamioculcas zamiifolia. Ecotoxicol. Environ. Safety 180, 374–383. doi: 10.1016/j.ecoenv.2019.05.002
Rabêlo, F. H. S., Fernie, A. R., Navazas, A., Borgo, L., Keunen, E., da Silva, B. K. D. A., et al. (2018). A glimpse into the effect of sulfur supply on metabolite profiling, glutathione and phytochelatins in Panicum maximum cv. Massai exposed to cadmium. Environ. Exp. Bot. 151, 76–88. doi: 10.1016/j.envexpbot.2018.04.003
Rangkadilok, N., Nicolas, M. E., Bennett, R. N., Premier, R. R., Eagling, D. R., and Taylor, P. W. J. (2002). Determination of sinigrin and glucoraphanin in Brassica species using a simple extraction method combined with ion-pair HPLC analysis. Sci. Hortic. 96, 27–41. doi: 10.1016/s0304-4238(02)00119-x
Saleh, A. M., and Madany, M. M. Y. (2015). Coumarin pretreatment alleviates salinity stress in wheat seedlings. Plant Physiol. Biochem. 88, 27–35. doi: 10.1016/j.plaphy.2015.01.005
Shahid, M., Pourrut, B., Dumat, C., Nadeem, M., Aslam, M., and Pinelli, E. (2014). Heavy-metal-induced reactive oxygen species: phytotoxicity and physicochemical changes in plants. Rev. Environ. Contam. Toxicol. 232, 1–44. doi: 10.1007/978-3-319-06746-9_1
Sidhu, G. P. S., Singh, H. P., Batish, D. R., and Kohli, R. K. (2016). Effect of lead on oxidative status, antioxidative response and metal accumulation in Coronopus didymus. Plant Physiol. Biochem. 105, 290–296. doi: 10.1016/j.plaphy.2016.05.019
Singh, P. K., Singh, R., and Singh, S. (2013). Cinnamic acid induced changes in reactive oxygen species scavenging enzymes and protein profile in maize (Zea mays L.) plants grown under salt stress. Physiol. Mol. Biol. Plants 19, 53–59. doi: 10.1007/s12298-012-0126-6
Smeekens, S., Ma, J., Hanson, J., and Rolland, F. (2010). Sugar signals and molecular networks controlling plant growth. Curr. Opin. Plant Biol. 13, 274–279.
Soares, C., Carvalho, M. E. A., Azevedo, R. A., and Fidalgo, F. (2019). Plants facing oxidative challenges—A little help from the antioxidant networks. Environ. Exp. Bot. 161, 4–25. doi: 10.1016/j.envexpbot.2018.12.009
Szabados, L., and Savoure, A. (2010). Proline: a multifunctional amino acid. Trends Plant Sci. 15, 89–97. doi: 10.1016/j.tplants.2009.11.009
Tanemoto, R., Okuyama, T., Matsuo, H., Okumura, T., Ikeya, Y., and Nishizawa, M. (2015). The constituents of licorice (Glycyrrhiza uralensis) differentially suppress nitric oxide production in interleukin-1β-treated hepatocytes. Biochem. Biophys. Rep. 2, 153–159. doi: 10.1016/j.bbrep.2015.06.004
Tauqeer, H. M., Ali, S., Rizwan, M., Ali, Q., Saeed, R., Iftikhar, U., et al. (2016). Phytoremediation of heavy metals by Alternanthera bettzickiana: growth and physiological response. Ecotoxicol. Environ. Safety. 126, 138–146. doi: 10.1016/j.ecoenv.2015.12.031
Tolrà, R., Barceló, J., and Poschenrieder, C. (2009). Constitutive and aluminium-induced patterns of phenolic compounds in two maize varieties differing in aluminium tolerance. J. Inorgan. Biochem. 103, 1486–1490. doi: 10.1016/j.jinorgbio.2009.06.013
Trejo-Téllez, L. I., Estrada-Ortiz, E., Gómez-Merino, F. C., Becker, C., Krumbein, A., and Schwarz, D. (2019). Flavonoid, nitrate and glucosinolate concentrations in Brassica species are differentially affected by photosynthetically active radiation, phosphate and phosphite. Front. Plant Sci. 10:371. doi: 10.3389/fpls.2019.00371
Usman, K., Abu-Dieyeh, M. H., Zouari, N., and Al-Ghouti, M. A. (2020). Lead (Pb) bioaccumulation and antioxidative responses in Tetraena qataranse. Sci. Rep. 10:17070.
Varela, M. C., Arslan, I., Reginato, M. A., Cenzano, A. M., and Luna, M. V. (2016). Phenolic compounds as indicators of drought resistance in shrubs from Patagonian shrublands (Argentina). Plant Physiol. Biochem. 104, 81–91. doi: 10.1016/j.plaphy.2016.03.014
Keywords: Chinese flowering cabbage, flavonoids, glucosinolates, liquiritoside, lead
Citation: Akram W, Khan WU, Shah AA, Yasin NA and Li G (2021) Liquiritoside Alleviated Pb Induced Stress in Brassica rapa subsp. Parachinensis: Modulations in Glucosinolate Content and Some Physiochemical Attributes. Front. Plant Sci. 12:722498. doi: 10.3389/fpls.2021.722498
Received: 08 June 2021; Accepted: 30 July 2021;
Published: 26 August 2021.
Edited by:
Youssef Rouphael, University of Naples Federico II, ItalyReviewed by:
Arafat Abdel Hamed Abdel Latef, South Valley University, EgyptReyes Rodenas, UMR 5546 Laboratoire de Recherche en Sciences Vegetales (LRSV), France
Copyright © 2021 Akram, Khan, Shah, Yasin and Li. This is an open-access article distributed under the terms of the Creative Commons Attribution License (CC BY). The use, distribution or reproduction in other forums is permitted, provided the original author(s) and the copyright owner(s) are credited and that the original publication in this journal is cited, in accordance with accepted academic practice. No use, distribution or reproduction is permitted which does not comply with these terms.
*Correspondence: Nasim Ahmad Yasin, nasimhort@gmail.com; Guihua Li, liguihua@gdaas.cn