- 1Department of Plant Science and Landscape Architecture, University of Connecticut, Storrs, CT, United States
- 2Institute for System Genomics, University of Connecticut, Storrs, CT, United States
Plant small peptides, including CLAVATA3/EMBRYO SURROUNDING REGION-RELATED (CLE) and Epidermal Patterning Factor-Like (EPFL) peptides, play pivotal roles in coordinating developmental processes through cell-cell communication. Recent studies have revealed that the phloem-derived CLE peptides, CLE41/44 and CLE42, promote (pro-)cambial cell proliferation and inhibit xylem cell differentiation. The endodermis-derived EPFL peptides, EPFL4 and EPFL6, modulate vascular development in the stem. Further, several other peptide ligands CLE9, CLE10, and CLE45 play crucial roles in regulating vascular development in the root. The peptide signaling pathways interact with each other and crosstalk with plant hormone signals. In this mini-review, we summtarize the recent advances on peptides function in vascular development and discuss future perspectives for the research of the CLE and EPFL peptides.
Introduction
Intercellular communication and interaction are fundamentally important for plants to fine-tune growth and development in response to ever-changing environmental conditions (Kucukoglu and Nilsson, 2015). Secreted signaling peptides play crucial roles in regulating vascular development by short-range intercellular communication (Fukuda et al., 2007). Peptides are often referred to as proteins smaller than 100 amino acids and derived from precursor proteins or directly translated from short open reading frames embedded in longer transcripts (Tavormina et al., 2015). The CLAVATA3/EMBRYO SURROUNDING REGION-RELATED (CLE) peptides are essential for plant development and stress responses (Wang and Fiers, 2010; Tavormina et al., 2015). The Arabidopsis genome contains 32 CLE genes encoding 27 unique peptides (Yamaguchi et al., 2016; Fukuda and Hardtke, 2020). These CLE peptides are recognized at the cell surface by transmembrane receptors and activate intracellular signal transduction, therefore regulating plant growth and development (Fletcher, 2020). In this mini-review, we summarize the function and interaction of peptide signaling pathways in vascular development (Table 1) and discuss the gaps in current knowledge and provide perspective for future research.
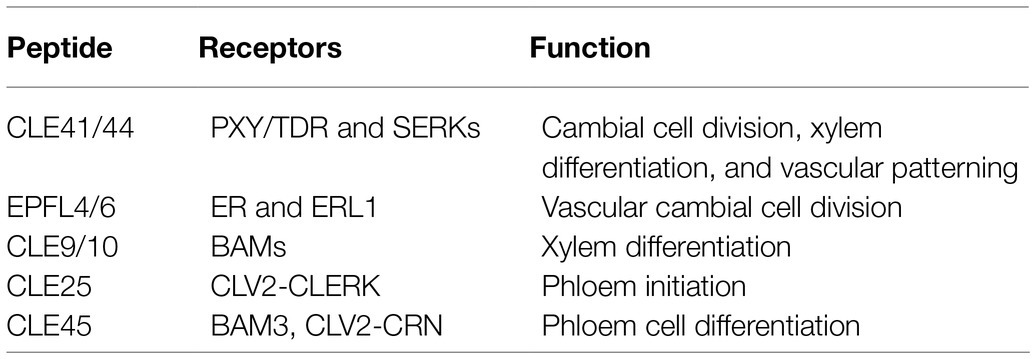
Table 1. CLAVATA3/EMBRYO SURROUNDING REGION-RELATED (CLE) peptides and their receptors in regulating vascular development.
Vascular Development
Plant vasculatures transport water and nutrients to all developing organs. In the Arabidopsis stem, the vascular tissues are organized as bundles, in which the procambium or cambium resides in the middle, xylem inside, and phloem outside of the bundle (Figure 1A). At the shoot tip, vascular bundles are well separated along the stem periphery (Figure 1A). In the more mature stem, cambium starts developing in positions between bundles, connects vascular cambium, and forms a ring of cambial cells (Figure 1A). At the bottom of the stem, cambium cells divide and form xylem precursor cells inward and phloem precursor cells outward of the stem. Eventually, these precursors differentiate into secondary xylem and secondary phloem, respectively (Figure 1A). Other plant organs, such as the root and hypocotyls, also go through secondary growth depending on the activity of cambium cells (Wang, 2020). Regulation of vascular development is vital for plant secondary growth and crop production and therefore remains a critical research area in plant biology.
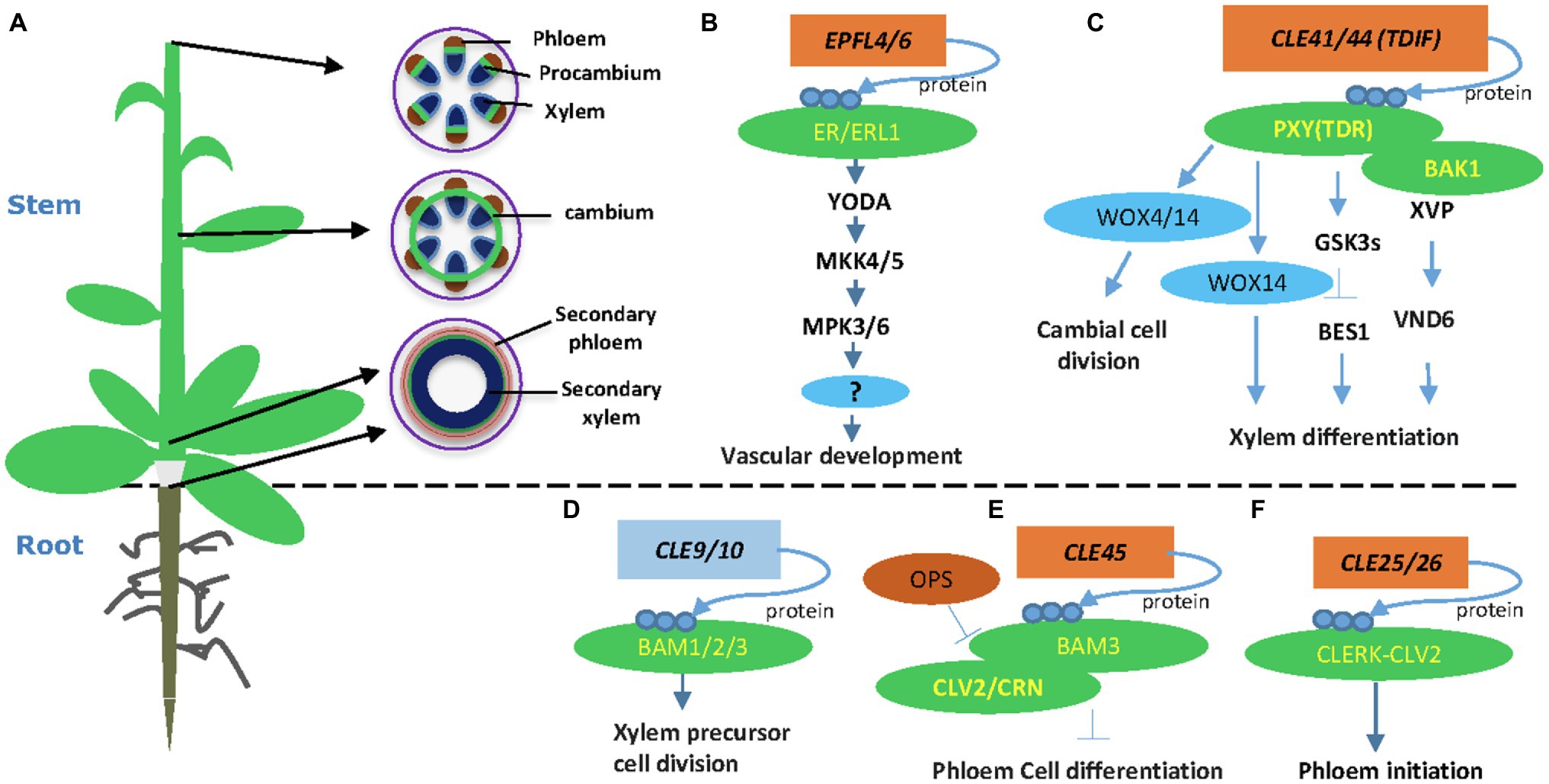
Figure 1. Plant-specific CLE and Epidermal Patterning Factor-Like (EPFL) peptide signaling pathways in vascular development. (A) An Arabidopsis plant and different stages of vascular developmental in the stem. A young stem develops discrete vascular bundles that are comprised of phloem, xylem, and intervening procambium. In developing stems, cambial cells from vascular and interfascicular regions form a closed ring structure. Secondary growth produces secondary phloem and secondary xylem in both mature stem and hypocotyls. (B) The EPFL4 and EPFL6 peptides regulate stem vascular development in Arabidopsis. (C) CLE41/44 regulates vascular cambial cell division and xylem differentiation in the stem. (D) The CLE9/10 peptide regulates xylem formation. (E) The CLE45-BAM3 peptide inhibits root protophloem cell differentiation. (F) The CLE25 peptide promotes root protophloem cell differentiation. Arrows depict positive regulatory relationships and bars depict negative regulatory relationships.
TDIF-PXY Signaling Regulates Vascular Development
The proliferation of cambial stem cells is essential for vascular development and the mechanical strength of the plant stem. Peptide signals play crucial roles in regulating vascular development through cell-to-cell communication (Fukuda and Hardtke, 2020). The tracheary element differentiation inhibitory factor (TDIF) peptide is so far the best-understood peptide in this process (Fletcher, 2020; Wang, 2020). Tracheary element differentiation inhibitory factor is synthesized from the phloem and travels to the cambium, where TDIF binds to its receptor PHLOEM INTERCALATED WITH XYLEM/TDIF RECEPTOR (PXY/TDR) on the plasma membrane (Hirakawa et al., 2008; Etchells et al., 2016). Overexpression of TDIF coding genes CLE41 and CLE44 or exogenous application of synthetic TDIF peptide promote procambial cells proliferation and inhibit xylem cell differentiation (Hirakawa et al., 2008; Whitford et al., 2008; Etchells and Turner, 2010). These experiments established the function of the TDIF-PXY module in cambial cell proliferation, xylem differentiation, and vascular patterning (Hirakawa et al., 2008; Etchells and Turner, 2010). Downstream of the TDIF-PXY module is two WUSCHEL-related HOMEOBOX (WOX) transcription factors, WOX4 and WOX14 (Hirakawa et al., 2010; Etchells et al., 2013; Wang et al., 2013). WOX4 is expressed mainly in the procambium and cambium, and its expression level is upregulated by applying CLE ligand (Hirakawa et al., 2010). WOX14 acts redundantly with WOX4 in regulating vascular cell division (Etchells et al., 2013). The TDIF-PXY-WOX signaling plays a crucial role in the maintenance of the vascular meristem during secondary growth (Hirakawa et al., 2010; Yamaguchi et al., 2016).
The crystal structure of the extracellular domain of PXY/TDR in complex with TDIF peptide has been determined (Morita et al., 2016; Zhang et al., 2016a). The extracellular domain of PXY/TDR adopts a super-helical structure comprising 22 leucine-rich repeats (LRRs) and specifically recognizes TDIF by its inner concave surface (Morita et al., 2016). The TDIF peptide mainly adopts a “Ω”-like conformation binding the inner surface of the LRRs (Zhang et al., 2016a). The highly conserved amino acids of TDIF, including Hyp4, G6, Hyp7, and P9, are critical for the interaction with PXY/TDR (Zhang et al., 2016a). However, there are some discrepancies regarding which amino acids of PXY/TDR are essential to the interaction with TDIF (Morita et al., 2016; Zhang et al., 2016a). Structure-based sequence alignment showed that the TDIF-interacting motifs are conserved among plant species, suggesting a conserved TDIF-PXY recognition mechanism (Morita et al., 2016).
The TDIF-PXY-WOX4 signaling is conserved in plants. The TDIF peptide was first discovered from an analysis in zinnia (Ito et al., 2006). Later, TDIF peptide was found in Arabidopsis (Hirakawa et al., 2008) and the genomes of Euphyllophytes (Hirakawa and Bowman, 2015). The function of TDIF-PXY-WOX4 signaling has been investigated in poplar. Transgenic studies showed that the tissue-specific expression of PttPXY and PttCLE41 genes resulted in enhanced secondary growth in hybrid aspen, indicating that TDIF-PXY signaling is conserved in poplar (Etchells et al., 2015). Consistent with this study, PttWOX4 gene is specifically expressed in the cambial region and controls cell division activity in the vascular cambium (Kucukoglu et al., 2017).
In a recent study, a regulatory network downstream of TDIF-PXY was identified using an enhanced yeast one-hybrid system (Smit et al., 2020). Among the transcription factor-promoter interactions, WOX14 positively regulated the expression of TARGET OF MONOPTEROS 6 (TMO6), which encodes a Dof-type transcription factor (Schlereth et al., 2010). In addition, WOX4 and TMO6 both positively regulate the expression of a third transcription factor gene, LATERAL ORGAN BOUNDARIES DOMAIN4 (Smit et al., 2020). These data support a mechanism that this feed-forward loop controls vascular stem cell proliferation and helps to define the function of PXY signaling in determining the shape of the vascular bundle (Fletcher, 2020; Smit et al., 2020).
The TDIF-PXY signaling module regulates cell division in the (pro-)cambium and controls vascular organization via interacting with other factors (Etchells et al., 2016). The SOMATIC EMBRYOGENESIS RECEPTOR KINASE (SERK) family regulates plant growth and development, as well as many other biological processes (Albrecht et al., 2008). The SERK genes are expressed in procambial cells, suggesting that the SERK family may have a function in regulating vascular development (Kwaaitaal and De Vries, 2007). Indeed, the TDIF receptor PXY forms heterodimers with SERKs, including SERK1, SERK2, and SERK3 (BAK1), in regulating procambial cell proliferation (Zhang et al., 2016b). A NAC domain transcription factor XVP is localized on the plasma membrane and forms a complex with TDIF co-receptor PXY-BAK1 (Yang et al., 2020a). The XVP is expressed in the cambium and regulates xylem differentiation and vascular patterning (Yang et al., 2020a). Further study showed that XVP regulates xylem differentiation through a crucial factor VASCULAR-RELATED NAC-DOMAIN6. The XVP promotes the expression of TDIF-encoding gene CLE44, whereas TDIF negatively regulates the expression of XVP, suggesting a feedback regulating loop (Figure 1). However, it is still not clear whether and how XVP proteins are translocated to the nucleus.
EPFL4/6-ER Signaling in the Regulation of Vascular Cambium
The EPIDERMAL PATTERNING FACTOR-LIKE 4 (EPFL4) and EPFL6/CHALLAH (CHAL) secretory peptides were first identified as regulators of inflorescence development through activating the ER signaling (Uchida et al., 2012). Two additional ER paralogs, ER-LIKE 1 (ERL1) and ERL2, together with ER, play crucial roles in regulating inflorescence architecture, organ shape, and epidermal stomatal patterning in Arabidopsis (Shpak et al., 2004; Uchida et al., 2012). In vascular development, the endodermis-produced EPFL4 and EPFL6 peptides function as ligands for phloem-located ER in regulating procambial cell division and stem elongation (Fischer and Teichmann, 2017; Ikematsu et al., 2017). Therefore, the cell-to-cell communication between the endodermis and the phloem plays a crucial role in procambial development (Ikematsu et al., 2017). Both ER and ERL1 are expressed in inflorescence stem and function redundantly in regulating procambial development and xylem differentiation (Ikematsu et al., 2017; Tameshige et al., 2017). The mpk3/mpk6 and mkk4/mkk5 mutants showed short pedicels and a corymb-like inflorescence similar to the phenotypes of the er mutant (Meng et al., 2013). Further evidence showed that the MAPK cascade functions downstream of the ER receptor in regulating pedicel length and inflorescence architecture (Meng et al., 2013). Therefore, the MAPK cascade, involving YDA-MKK4/MKK5-MPK3/MPK6, functions downstream of ER in regulating cell proliferation and plant organ development (Figure 1). It would be interesting to investigate if this MAPK pathway also regulates procambium development in the stem and hypocotyl.
Other CLE Peptide Signaling Pathways in Vascular Development
Besides CLE41/CLE44, EPFL4, and EPFL6, other peptides, including CLE9/10, CLE25, and CLE45, also play critical roles in vascular development (Fukuda and Hardtke, 2020). The CLE9 and CLE10 genes are preferentially expressed in xylem precursor cells of the root vasculature (Kondo et al., 2011). Interestingly, the peptide CLE9/10 forms a complex with the receptor kinase HAESA-LIKE 1 (HSL1) to regulate stomatal lineage cell division. In the root xylem development, CLE9/10 is perceived by BARELY ANY MERISTEM (BAM) class receptor kinases in regulating periclinal cell division (Figure 1; Qian et al., 2018). BARELY ANY MERISTEM 1 and its homologs, BAM2 and BAM3, are class XI LRR receptor-like kinases similar to CLV1 and PXY (DeYoung et al., 2006). The bam1bam2 and bam1bam3 mutants exhibited increased xylem files similarly to the cle9 mutant (Qian et al., 2018). Indeed, physical interaction between CLE9/10 and BAM1 was demonstrated by three independent approaches (Shinohara et al., 2012; Anne et al., 2018; Qian et al., 2018), confirming the BAMs as CLE9 receptors in root xylem development.
The CLE45 peptide forms a complex with BARELY ANY MERISTEM 3 (BAM3) to regulate Arabidopsis protophloem differentiation (Depuydt et al., 2013). Protophloem consists of narrow thin-walled cells and is developed from procambium. Studies revealed that CLE45 is expressed from protophloem sieve elements in root meristems and perceived fully by CLAVATA 2 (CLV2) and the CORYNE (CRN) receptor proteins (Hazak et al., 2017). CRN is required for BAM3-mediated CLE45 signaling via stabilizing BAM3 expression. In addition, a protophloem-specific CRN expression rescues the resistance of the crn mutant to root-active CLE peptides (Hazak et al., 2017). Collectively, these data support a vital function of the CLE45 and CLV/BAM receptors in vascular development (Hazak et al., 2017). Downstream of BAM3, a MEMBRANE-ASSOCIATED KINASE REGULATOR protein, positively regulates CLE45 signaling (Kang and Hardtke, 2016). Further, OCTOPUS (OPS), a polarly localized membrane-associated protein, promotes root protophloem differentiation by antagonizing CLE45 signaling through direct interference with BAM3 and CLV2-CRN (Figure 1; Truernit et al., 2012; Rodriguez-Villalon et al., 2014; Breda et al., 2019).
The CLE25 and CLE26 play crucial roles in regulating root protophloem development (Czyzewicz et al., 2015; Anne et al., 2018; Ren et al., 2019). CLE25 expression is tightly associated with phloem initiation, and the loss-of-function mutant cle25 shows a delayed protophloem differentiation phenotype (Ren et al., 2019). Genetic screening for suppressors identified CLV2 as a potential receptor for CLE25 (Ren et al., 2019). Further peptide assay and protein interaction experiment found that CLE-RESISTANT RECEPTOR KINASE (CLERK) interacts with CLV2 and possibly forms a receptor complex for CLE25 (Ren et al., 2019). However, direct binding between CLE25 and the CLERK-CLV2 receptor complex remains to be established experimentally.
The CLE45 and CLE25/26 peptides are all expressed in root and repress phloem development as shown by expression analyses and synthetic peptides treatment experiments (Kinoshita et al., 2007; Jun et al., 2010). CLE25 may be involved in the formative cell division during protophloem differentiation. It is still unclear which division step is regulated by CLE25 in the two successive formative cell division steps during protophloem development (Rodriguez-Villalon et al., 2014; Ren et al., 2019). In addition, there are some differences in terms of perception of these peptides. The CLE25 and CLE26 peptides could be perceived by CLERK (Anne et al., 2018; Ren et al., 2019), while CLE45 is perceived by BAM3 (Kang and Hardtke, 2016; Hazak et al., 2017). Further, CLE25 may be involved in response to detrimental environmental signals (Takahashi et al., 2018). Further investigation is needed to clarify the functional difference of these peptides in protophloem development.
The Crosstalk Between TDIF-TDR Signaling and Other Signaling Pathways
The development of vascular tissue involves interactions between TDIF-PXY and other peptide signaling pathways. The receptor kinases PXY and ER genetically interact to coordinate cell division and organization (Uchida and Tasaka, 2013; Etchells et al., 2016; Wang et al., 2019). Gene expression analysis suggested that PXL1 and PXL2 expression is upregulated in er mutant stems (Wang et al., 2019). The expression of EPFL4 and EPFL6 is altered in pxf (mutation of the PXY family, including pxy, pxl1, and pxl2) and er pxy mutants (Wang et al., 2019). Further study showed that the mutant of er pxf had considerably fewer cells per vascular bundle than either pxf, er, or pxy er, suggesting that PXL1 and PXL2 are functionally redundant with ER to regulate vascular proliferation in the stem (Wang et al., 2019). These results demonstrate that PXY and ER coordinately regulate vascular proliferation and organization via inter-tissue signaling (Wang et al., 2019).
TDFI-PXY signaling also interacts with plant hormonal signals, such as brassinosteroids (BR), auxin, and ethylene signaling. The GLYCOGEN SYNTHASE KINASE 3 (GSK3) family members, including BRASSINOSTEROID-INSENSITIVE 2 (BIN2), are crucial downstream components of the TDIF signaling pathway in suppressing xylem differentiation (Kondo et al., 2014). The PXY/TDR receptor interacts with GSK3s on the plasma membrane and activates GSK3s in a TDIF-dependent fashion (Kondo et al., 2014). The interaction between PXY/TDR and BIN2 inhibits the transcription factor BES1 to prevent procambial cell differentiation into the xylem (Kondo et al., 2014). Recently, an additional study showed that the GSK3 protein BIN2-LIKE 1 (BIL1) integrates the PXY/TDR signaling through phosphorylating the auxin response factor MP/ARF5, which limits the effect of MP/ARF5 on ARR7 and ARR15 expression, thus enhancing vascular cambial activity (Han et al., 2018). Together, these results suggest that BIL1 mediated the crosstalk between peptide signaling and hormone signaling to maintain the cambial activity (Figure 1; Han et al., 2018). Ethylene promotes cambium activity (Love et al., 2009; Yang et al., 2020b) and interacts with TDIF-PXY signaling (Etchells et al., 2012). The interaction between TDIF-PXY and plant hormone may help plants better perceive and respond to long-distance developmental and environmental signals.
The Crosstalk Between EPFL4/6-ER Signaling and Other Signaling Pathways
The LRR receptor-like kinase ER is a stem growth regulator and involves in diverse developmental processes, including epidermal and mesophyll development, stomatal density, and vascular development (Tameshige et al., 2017). For example, hydrogen peroxide induces cortex proliferation, while SPINDLY, an O-linked glucosamine acetyltransferase, functions downstream of ER to fine-tune cortex proliferation, indicating a connection between ER signaling and cellular redox homeostasis (Cui et al., 2014). Another study showed that an LRR receptor-like kinase SUPPRESSOR OF BIR1-1, previously known to control plant immune responses and abscission, appears downstream of BREVIPEDICELLUS (BP) and ER to regulate the precocious differentiation of xylem fiber (Milhinhos et al., 2019). In addition, ER signaling pathway and the SWR1 chromatin remodeling complex jointly regulate inflorescence architecture by promoting the expression of the PACLOBUTRAZOL RESISTANCE (PRE) gene family (Cai et al., 2017). Further study showed that a growth-related transcription factor HOMOLOG OF BEE2 INTERACTING WITH IBH1 (HBI1) functions downstream of PRE1 to regulate inflorescence architecture by promoting pedicel elongation (Cai et al., 2021). These studies indicate potential crosstalks between ERFL4/6 and other pathways in regulating vascular development.
Conclusion
Significant progress has been made in our understanding of peptide signaling, including the TDIF-PXY/TDR module and the EPFL4/6–ER/ERL1 module, in regulating vascular development (Fukuda and Hardtke, 2020; Wang, 2020; Wang and Wang, 2020). While the TDIF-PXY signaling in vascular development has been the subject of much research since the discovery of TDIF, many questions remain. For instance, compared to the wild type, pxy mutants showed more fiber cells indicating that PXY has a potential function in regulating xylem development (Fisher and Turner, 2007). Moreover, environmental factors, including light, carbon dioxide concentration, and drought, all affect the expression pattern of several EPFL family genes, indicating that ER signaling could respond to external environmental stimuli (Richardson and Torii, 2013). Therefore, studying the crosstalk between environmental factors and peptide signaling in vascular development is an exciting field in the future.
Further, the EPFL4/6–ER/ERL1 module regulates procambial maintenance in inflorescence stems (Uchida et al., 2012), and a MAPK signaling module YDA-MKK4/5-MPK3/6 functions downstream of ER (Meng et al., 2013). Identification of the substrates of MPK3/6 would be crucial to understand the molecular mechanism of the EPFL-ER signaling. The crosstalk between TDIF-PXY and EPFL-ER signaling has been proposed, but the mechanistic interaction has not been established. It is possible that a common downstream signaling hub may connect both pathways in regulating cambial activity.
In addition, the BAM1 receptor can potentially bind both CLV3 and CLE25 peptides, indicating that the same receptors can perceive different CLE peptides in various biological contexts (Takahashi et al., 2018; Fletcher, 2020). In contrast, the CLE9/10 peptide ligand is recognized by two different receptor kinases HSL1 and BAM3 in regulating stomatal lineage cell division and periclinal cell division of xylem precursor cells, respectively (Qian et al., 2018). Therefore, the same receptor can perceive different CLE peptides, while the same peptide can bind to different receptors, indicating extremely complex peptide signal transduction networks in plants. The complexity may be necessary for peptide signaling to have developmental and organ-specific functions. Further dissection of the peptide signaling pathways will provide new insight into our understanding of vascular development.
Author Contributions
BY and HW wrote the review. All authors contributed to the article and approved the submitted version.
Funding
This work is supported by the National Science Foundation (IOS-1453048) and in part, by USDA NIFA Hatch project #CONS00990 to HW.
Conflict of Interest
The authors declare that the research was conducted in the absence of any commercial or financial relationships that could be construed as a potential conflict of interest.
Publisher’s Note
All claims expressed in this article are solely those of the authors and do not necessarily represent those of their affiliated organizations, or those of the publisher, the editors and the reviewers. Any product that may be evaluated in this article, or claim that may be made by its manufacturer, is not guaranteed or endorsed by the publisher.
References
Albrecht, C., Russinova, E., Kemmerling, B., Kwaaitaal, M., and De Vries, S. C. (2008). Arabidopsis Somatic Embryogenesis receptor kinase proteins serve brassinosteroid-dependent and -independent signaling pathways. Plant Physiol. 148, 611–619. doi: 10.1104/pp.108.123216
Anne, P., Amiguet-Vercher, A., Brandt, B., Kalmbach, L., Geldner, N., Hothorn, M., et al. (2018). CLERK is a novel receptor kinase required for sensing of root-active CLE peptides in arabidopsis. Development 145:dev162354. doi: 10.1242/dev.162354
Breda, A. S., Hazak, O., Schultz, P., Anne, P., Graeff, M., Simon, R., et al. (2019). A cellular insulator against CLE45 peptide signaling. Curr. Biol. 29, 2501–2508.e3. doi: 10.1016/j.cub.2019.06.037
Cai, H., Chai, M., Chen, F., Huang, Y., Zhang, M., He, Q., et al. (2021). HBI1 acts downstream of ERECTA and SWR1 in regulating inflorescence architecture through the activation of the brassinosteroid and auxin signaling pathways. New Phytol. 229, 414–428. doi: 10.1111/nph.16840
Cai, H., Zhao, L., Wang, L., Zhang, M., Su, Z., Cheng, Y., et al. (2017). ERECTA signaling controls Arabidopsis inflorescence architecture through chromatin-mediated activation of PRE1 expression. New Phytol. 214, 1579–1596. doi: 10.1111/nph.14521
Cui, H., Kong, D., Wei, P., Hao, Y., Torii, K. U., Lee, J. S., et al. (2014). SPINDLY, ERECTA, and its ligand STOMAGEN have a role in redox-mediated cortex proliferation in the arabidopsis root. Mol. Plant 7, 1727–1739. doi: 10.1093/mp/ssu106
Czyzewicz, N., Shi, C.-L., Vu, L. D., Van De Cotte, B., Hodgman, C., Butenko, M. A., et al. (2015). Modulation of Arabidopsis and monocot root architecture by CLAVATA3/EMBRYO SURROUNDING REGION 26 peptide. J. Exp. Bot. 66, 5229–5243. doi: 10.1093/jxb/erv360
Depuydt, S., Rodriguez-Villalon, A., Santuari, L., Wyser-Rmili, C., Ragni, L., and Hardtke, C. S. (2013). Suppression of Arabidopsis protophloem differentiation and root meristem growth by CLE45 requires the receptor-like kinase BAM3. Proc. Natl. Acad. Sci. U. S. A. 110, 7074–7079. doi: 10.1073/pnas.1222314110
DeYoung, B. J., Bickle, K. L., Schrage, K. J., Muskett, P., Patel, K., and Clark, S. E. (2006). The CLAVATA1-related BAM1, BAM2 and BAM3 receptor kinase-like proteins are required for meristem function in Arabidopsis. Plant J. 45, 1–16. doi: 10.1111/j.1365-313X.2005.02592.x
Etchells, J. P., Mishra, L. S., Kumar, M., Campbell, L., and Turner, S. R. (2015). Wood formation in trees is increased by manipulating PXY-regulated cell division. Curr. Biol. 25, 1050–1055. doi: 10.1016/j.cub.2015.02.023
Etchells, J. P., Provost, C. M., Mishra, L., and Turner, S. R. (2013). WOX4 and WOX14 act downstream of the PXY receptor kinase to regulate plant vascular proliferation independently of any role in vascular organisation. Development 140, 2224–2234. doi: 10.1242/dev.091314
Etchells, J. P., Provost, C. M., and Turner, S. R. (2012). Plant vascular cell division is maintained by an interaction between PXY and ethylene signalling. PLoS Genet. 8:e1002997. doi: 10.1371/journal.pgen.1002997
Etchells, J. P., Smit, M. E., Gaudinier, A., Williams, C. J., and Brady, S. M. (2016). A brief history of the TDIF-PXY signalling module: balancing meristem identity and differentiation during vascular development. New Phytol. 209, 474–484. doi: 10.1111/nph.13642
Etchells, J. P., and Turner, S. R. (2010). The PXY-CLE41 receptor ligand pair defines a multifunctional pathway that controls the rate and orientation of vascular cell division. Development 137, 767–774. doi: 10.1242/dev.044941
Fischer, U., and Teichmann, T. (2017). The ERECTA and ERECTA-like genes control a developmental shift during xylem formation in Arabidopsis. New Phytol. 213, 1562–1563. doi: 10.1111/nph.14440
Fisher, K., and Turner, S. (2007). PXY, a receptor-like kinase essential for maintaining polarity during plant vascular-tissue development. Curr. Biol. 17, 1061–1066. doi: 10.1016/j.cub.2007.05.049
Fletcher, J. C. (2020). Recent advances in Arabidopsis CLE peptide signaling. Trends Plant Sci. 25, 1005–1016. doi: 10.1016/j.tplants.2020.04.014
Fukuda, H., and Hardtke, C. S. (2020). Peptide signaling pathways in vascular differentiation. Plant Physiol. 182, 1636–1644. doi: 10.1104/pp.19.01259
Fukuda, H., Hirakawa, Y., and Sawa, S. (2007). Peptide signaling in vascular development. Curr. Opin. Plant Biol. 10, 477–482. doi: 10.1016/j.pbi.2007.08.013
Han, S., Cho, H., Noh, J., Qi, J., Jung, H. J., Nam, H., et al. (2018). BIL1-mediated MP phosphorylation integrates PXY and cytokinin signalling in secondary growth. Nat. Plants 4, 605–614. doi: 10.1038/s41477-018-0180-3
Hazak, O., Brandt, B., Cattaneo, P., Santiago, J., Rodriguez-Villalon, A., Hothorn, M., et al. (2017). Perception of root-active CLE peptides requires CORYNE function in the phloem vasculature. EMBO rep. 18, 1367–1381. doi: 10.15252/embr.201643535
Hirakawa, Y., and Bowman, J. L. (2015). A role of TDIF peptide signaling in vascular cell differentiation is conserved among euphyllophytes. Front. Plant Sci. 6:1048. doi: 10.3389/fpls.2015.01048
Hirakawa, Y., Kondo, Y., and Fukuda, H. (2010). TDIF peptide signaling regulates vascular stem cell proliferation via the WOX4 homeobox gene in Arabidopsis. Plant Cell 22, 2618–2629. doi: 10.1105/tpc.110.076083
Hirakawa, Y., Shinohara, H., Kondo, Y., Inoue, A., Nakanomyo, I., Ogawa, M., et al. (2008). Non-cell-autonomous control of vascular stem cell fate by a CLE peptide/receptor system. Proc. Natl. Acad. Sci. U. S. A. 105, 15208–15213. doi: 10.1073/pnas.0808444105
Ikematsu, S., Tasaka, M., Torii, K. U., and Uchida, N. (2017). ERECTA-family receptor kinase genes redundantly prevent premature progression of secondary growth in the Arabidopsis hypocotyl. New Phytol. 213, 1697–1709. doi: 10.1111/nph.14335
Ito, Y., Nakanomyo, I., Motose, H., Iwamoto, K., Sawa, S., Dohmae, N., et al. (2006). Dodeca-CLE as peptides as suppressors of plant stem cell differentiation. Science 313, 842–845. doi: 10.1126/science.1128436
Jun, J., Fiume, E., Roeder, A. H. K., Meng, L., Sharma, V. K., Osmont, K. S., et al. (2010). Comprehensive analysis of CLE polypeptide signaling gene expression and overexpression activity in arabidopsis. Plant Physiol. 154, 1721–1736. doi: 10.1104/pp.110.163683
Kang, Y. H., and Hardtke, C. S. (2016). Arabidopsis MAKR 5 is a positive effector of BAM 3-dependent CLE 45 signaling. EMBO Rep. 17, 1145–1154. doi: 10.15252/embr.201642450
Kinoshita, A., Nakamura, Y., Sasaki, E., Kyozuka, J., Fukuda, H., and Sawa, S. (2007). Gain-of-function phenotypes of chemically synthetic CLAVATA3/ESR-related (CLE) peptides in Arabidopsis thaliana and Oryza sativa. Plant Cell Physiol. 48, 1821–1825. doi: 10.1093/pcp/pcm154
Kondo, Y., Hirakawa, Y., Kieber, J. J., and Fukuda, H. (2011). CLE peptides can negatively regulate protoxylem vessel formation via cytokinin signaling. Plant Cell Physiol. 52, 37–48. doi: 10.1093/pcp/pcq129
Kondo, Y., Ito, T., Nakagami, H., Hirakawa, Y., Saito, M., Tamaki, T., et al. (2014). Plant GSK3 proteins regulate xylem cell differentiation downstream of TDIF-TDR signalling. Nat. Commun. 5:3504. doi: 10.1038/ncomms4504
Kucukoglu, M., Nilsson, J., Zheng, B., Chaabouni, S., and Nilsson, O. (2017). WUSCHEL-RELATED HOMEOBOX4 (WOX4)-like genes regulate cambial cell division activity and secondary growth in Populus trees. New Phytol. 215, 642–657. doi: 10.1111/nph.14631
Kucukoglu, M., and Nilsson, O. (2015). CLE peptide signaling in plants - the power of moving around. Physiol. Plant. 155, 74–87. doi: 10.1111/ppl.12358
Kwaaitaal, M. A. C. J., and De Vries, S. C. (2007). The SERK1 gene is expressed in procambium and immature vascular cells. J. Exp. Bot. 58, 2887–2896. doi: 10.1093/jxb/erm103
Love, J., Björklund, S., Vahala, J., Hertzberg, M., Kangasjärvi, J., and Sundberg, B. (2009). Ethylene is an endogenous stimulator of cell division in the cambial meristem of Populus. Proc. Natl. Acad. Sci. 106, 5984–5989. doi: 10.1073/PNAS.0811660106
Meng, X., Wang, H., He, Y., Liu, Y., Walker, J. C., Torii, K. U., et al. (2013). A MAPK cascade downstream of ERECTA receptor-like protein kinase regulates Arabidopsis inflorescence architecture by promoting localized cell proliferation. Plant Cell 24, 4948–4960. doi: 10.1105/tpc.112.104695
Milhinhos, A., Vera-Sirera, F., Blanco-Touriñán, N., Mari-Carmona, C., Carrió-Seguí, À., Forment, J., et al. (2019). SOBIR1/EVR prevents precocious initiation of fiber differentiation during wood development through a mechanism involving BP and ERECTA. Proc. Natl. Acad. Sci. U. S. A. 116, 18710–18716. doi: 10.1073/pnas.1807863116
Morita, J., Kato, K., Nakane, T., Kondo, Y., Fukuda, H., Nishimasu, H., et al. (2016). Crystal structure of the plant receptor-like kinase TDR in complex with the TDIF peptide. Nat. Commun. 7:12383. doi: 10.1038/ncomms12383
Qian, P., Song, W., Yokoo, T., Minobe, A., Wang, G., Ishida, T., et al. (2018). The CLE9/10 secretory peptide regulates stomatal and vascular development through distinct receptors. Nat. Plants 4, 1071–1081. doi: 10.1038/s41477-018-0317-4
Ren, S. C., Song, X. F., Chen, W. Q., Lu, R., Lucas, W. J., and Liu, C. M. (2019). CLE25 peptide regulates phloem initiation in Arabidopsis through a CLERK-CLV2 receptor complex. J. Integr. Plant Biol. 61, 1043–1061. doi: 10.1111/jipb.12846
Richardson, L. G. L., and Torii, K. U. (2013). Take a deep breath: peptide signalling in stomatal patterning and differentiation. J. Exp. Bot. 64, 5243–5251. doi: 10.1093/jxb/ert246
Rodriguez-Villalon, A., Gujas, B., Kang, Y. H., Breda, A. S., Cattaneo, P., Depuydt, S., et al. (2014). Molecular genetic framework for protophloem formation. Proc. Natl. Acad. Sci. U. S. A. 111, 11551–11556. doi: 10.1073/pnas.1407337111
Schlereth, A., Möller, B., Liu, W., Kientz, M., Flipse, J., Rademacher, E. H., et al. (2010). MONOPTEROS controls embryonic root initiation by regulating a mobile transcription factor. Nature 464, 913–916. doi: 10.1038/nature08836
Shinohara, H., Moriyama, Y., Ohyama, K., and Matsubayashi, Y. (2012). Biochemical mapping of a ligand-binding domain within Arabidopsis BAM1 reveals diversified ligand recognition mechanisms of plant LRR-RKs. Plant J. 70, 845–854. doi: 10.1111/j.1365-313X.2012.04934.x
Shpak, E. D., Berthiaume, C. T., Hill, E. J., and Torii, K. U. (2004). Synergistic interaction of three ERECTA-family receptor-like kinases controls Arabidopsis organ growth and flower development by promoting cell proliferation. Development 131, 1491–1501. doi: 10.1242/dev.01028
Smit, M. E., McGregor, S. R., Sun, H., Gough, C., Bågman, A. M., Soyars, C. L., et al. (2020). A PXY-mediated transcriptional network integrates signaling mechanisms to control vascular development in Arabidopsis. Plant Cell 32, 319–335. doi: 10.1105/tpc.19.00562
Takahashi, F., Suzuki, T., Osakabe, Y., Betsuyaku, S., Kondo, Y., Dohmae, N., et al. (2018). A small peptide modulates stomatal control via abscisic acid in long-distance signaling. Nature 556, 235–238. doi: 10.1038/s41586-018-0009-2
Tameshige, T., Ikematsu, S., Torii, K. U., Uchida, N., and Etchells, P. (2017). Stem development through vascular tissues: EPFL-ERECTA family signaling that bounces in and out of phloem. J. Exp. Bot. 68, 45–53. doi: 10.1093/jxb/erw447
Tavormina, P., De Coninck, B., Nikonorova, N., De Smet, I., and Cammuea, B. P. A. (2015). The plant peptidome: an expanding repertoire of structural features and biological functions. Plant Cell 27, 2095–2118. doi: 10.1105/tpc.15.00440
Truernit, E., Bauby, H., Belcram, K., Barthélémy, J., and Palauqui, J. C. (2012). OCTOPUS, a polarly localised membrane-associated protein, regulates phloem differentiation entry in Arabidopsis thaliana. Development 139, 1306–1315. doi: 10.1242/dev.072629
Uchida, N., Lee, J. S., Horst, R. J., Lai, H. H., Kajita, R., Kakimoto, T., et al. (2012). Regulation of inflorescence architecture by intertissue layer ligand-receptor communication between endodermis and phloem. Proc. Natl. Acad. Sci. U. S. A. 109, 6337–6342. doi: 10.1073/pnas.1117537109
Uchida, N., and Tasaka, M. (2013). Regulation of plant vascular stem cells by endodermisderived EPFL-family peptide hormones and phloemexpressed ERECTA-family receptor kinases. J. Exp. Bot. 64, 5335–5343. doi: 10.1093/jxb/ert196
Wang, G., and Fiers, M. (2010). CLE peptide signaling during plant development. Protoplasma 240, 33–43. doi: 10.1007/s00709-009-0095-y
Wang, H. (2020). Regulation of vascular cambium activity. Plant Sci. 291:110322. doi: 10.1016/j.plantsci.2019.110322
Wang, J., Kucukoglu, M., Zhang, L., Chen, P., Decker, D., Nilsson, O., et al. (2013). The Arabidopsis LRR-RLK, PXC1, is a regulator of secondary wall formation correlated with the TDIF-PXY/TDR-WOX4 signaling pathway. BMC Plant Biol. 13:94. doi: 10.1186/1471-2229-13-94
Wang, N., Bagdassarian, K. S., Doherty, R. E., Kroon, J. T., Connor, K. A., Wang, X. Y., et al. (2019). Organ-specific genetic interactions between paralogues of the PXY and ER receptor kinases enforce radial patterning in Arabidopsis vascular tissue. Development 146:dev177105. doi: 10.1242/dev.181750
Wang, S., and Wang, H. (2020). Coordination of Multilayered Signalling Pathways on Vascular Cambium Activity. Annu. Plant Rev. Online 3, 457–472. doi: 10.1002/9781119312994.APR0754
Whitford, R., Fernandez, A., De Groodt, R., Ortega, E., and Hilson, P. (2008). Plant CLE peptides from two distinct functional classes synergistically induce division of vascular cells. Proc. Natl. Acad. Sci. U. S. A. 105, 18625–18630. doi: 10.1073/pnas.0809395105
Yamaguchi, Y. L., Ishida, T., and Sawa, S. (2016). CLE peptides and their signaling pathways in plant development. J. Exp. Bot. 67, 4813–4826. doi: 10.1093/jxb/erw208
Yang, J. H., Lee, K. H., Du, Q., Yang, S., Yuan, B., Qi, L., et al. (2020a). A membrane-associated NAC domain transcription factor XVP interacts with TDIF co-receptor and regulates vascular meristem activity. New Phytol. 226, 59–74. doi: 10.1111/nph.16289
Yang, S., Wang, S., Li, S., Du, Q., Qi, L., Wang, W., et al. (2020b). Activation of ACS7 in Arabidopsis affects vascular development and demonstrates a link between ethylene synthesis and cambial activity. J. Exp. Bot. 71, 7160–7170. doi: 10.1093/jxb/eraa423
Zhang, H., Lin, X., Han, Z., Qu, L. J., and Chai, J. (2016a). Crystal structure of PXY-TDIF complex reveals a conserved recognition mechanism among CLE peptide-receptor pairs. Cell Res. 26, 543–555. doi: 10.1038/cr.2016.45
Keywords: vascular development, peptide, cambium, signaling, Arabidopsis
Citation: Yuan B and Wang H (2021) Peptide Signaling Pathways Regulate Plant Vascular Development. Front. Plant Sci. 12:719606. doi: 10.3389/fpls.2021.719606
Edited by:
Qingyu Wu, Chinese Academy of Agricultural Sciences (CAAS), ChinaReviewed by:
Yuki Hirakawa, Gakushuin University, JapanGuodong Wang, Shaanxi Normal University, China
Copyright © 2021 Yuan and Wang. This is an open-access article distributed under the terms of the Creative Commons Attribution License (CC BY). The use, distribution or reproduction in other forums is permitted, provided the original author(s) and the copyright owner(s) are credited and that the original publication in this journal is cited, in accordance with accepted academic practice. No use, distribution or reproduction is permitted which does not comply with these terms.
*Correspondence: Huanzhong Wang, huanzhong.wang@uconn.edu, orcid.org/0000-0002-9004-3420