- 1Key Laboratory of Bio-Resources and Eco-Environment of Ministry of Education, College of Life Sciences, Sichuan University, Chengdu, China
- 2Sichuan Key Laboratory of Conservation Biology on Endangered Wildlife, College of Life Sciences, Sichuan University, Chengdu, China
- 3CAS Key Laboratory of Mountain Ecological Restoration and Bioresource Utilization & Ecological Restoration and Biodiversity Conservation Key Laboratory of Sichuan Province, Chengdu Institute of Biology, Chinese Academy of Sciences, Chengdu, China
Tribe Lilieae, encompassing Lilium, Notholirion, Cardiocrinum, and Fritillaria, includes economically important crops with a horticultural and medicinal value. It is considered to be a core lineage of Liliaceae, but phylogenetic relationships within it, and the timing of the origin of individual clades, remain incompletely resolved. To address these issues, we reconstructed the evolutionary history of the tribe. We sequenced 45 Liliaceae plastomes and combined them with publicly available data (for a total of 139 plastomes) to explore the systematics, origin, divergence, and evolution of Lilieae. Our taxon sampling covers all ten sections of Lilium, all Cardiocrinum species, three Notholirion species, and major phylogenetic clades of Fritillaria. Our phylogenetic analysis confirms the monophyly of major sections/subgenera of Lilium and Fritillaria with strong support. We dated the origin of Lilieae to the Eocene, with genera and species radiations inferred to have occurred in the Miocene. The reconstruction of the ancestral area implies that Lilieae may have originated from the Qinghai-Tibet Plateau (QTP): the Himalayas and Hengduan Mountains and uplifting of the QTP likely promoted divergence within the tribe. Ancestral-state reconstructions of the bulb component number (including bulblets and scales) show a strong correlation with the genus-level phylogenetic diversity in Lilieae. They also predict that the most recent common ancestor of Lilieae had bulbs with numerous bulblets. Based on these observations, we predicted that climatic oscillations associated with the QTP uplift played an important role in the evolution of the Lilieae bulb. Our findings provide a well-supported picture of evolutionary relationships and a useful framework for understanding the pathway of bulb evolution within Lilieae, contributing to a better understanding of the evolutionary history of lilies.
Introduction
Liliaceae (Liliales) have a substantial importance in the systematic history of the monocotyledons, and the family itself has a complicated taxonomic history (Cronquist, 1988; Chase et al., 1995; APG, 1998, 2003, 2009, 2016; Patterson and Givnish, 2002; Givnish et al., 2016, 2018, 2020; Peruzzi, 2016; Kim and Kim, 2018). The classification of the family has changed substantially in the last three decades (Patterson and Givnish, 2002; Peruzzi et al., 2009; Givnish et al., 2016; Peruzzi, 2016; Kim and Kim, 2018). Tribe Lilieae is considered to be a core member of Liliaceae and encompasses four closely related genera, namely, Lilium (including Nomocharis, which was accommodated in Lilium; Gao and Gao, 2016), Notholirion, Cardiocrinum, and Fritillaria (Gao et al., 2013; APG, 2016; Peruzzi, 2016). Lilieae has 260 species distributed in northern hemisphere temperate regions (Boufford, 1993; Chen et al., 2000), including many well-known horticultural and medicinal species. For example, longiflorum lily (L. longiflorum), orange lily (L. bulbiferum), and Crown Imperial (F. imperialis) are widely cultivated (Pelkonen et al., 2010; Wietsma et al., 2015; Tuyl et al., 2018), and the bulbs of F. cirrhosa and F. thunbergii, known as “beimu,” are used in traditional Chinese medicine (Chen et al., 2000; Wang et al., 2009). Despite the importance of lilies, the origin, divergence, and evolution of Lilieae are still quite poorly understood.
The species of Lilieae are herbaceous geophytes with rounded bulbs. They have showy flowers with six free tepals and stamens, dorsifixed anthers, and a trilocular superior ovary (Chen et al., 2000; Patterson and Givnish, 2002). Within Lilieae, Cardiocrinum species have cordate leaves (Ohara et al., 2006), and Notholirion species have numerous bulblets and a raceme inflorescence (Li et al., 2020). Fritillaria generally differs from Lilium in that their bulbs comprise two or three farinaceous scales and nodding solitary flowers (Mustafa and Abdul-Razaq, 2015). Within Lilium, the bulbs have many fleshy scales, and the flowers are quite diverse in shape and ornamentation (Comber, 1949; Hayashi and Kawano, 2000). Thus, members of Lilieae vary in leaves, flowers, and bulbs, all features of considerable taxonomic and evolutionary significance. However, the evolution of these morphological characteristics is still poorly understood (Peruzzi, 2016; Huang et al., 2018).
Previous studies have confirmed the monophyly of Lilieae (Patterson and Givnish, 2002; Fay et al., 2006; Kim et al., 2013; Petersen et al., 2013; Givnish et al., 2016; Kim and Kim, 2018; Do et al., 2020). However, these studies only involved a small number of Lilieae species. Phylogenetic relationships between the two large genera in Lilieae, namely, Fritillaria and Lilium, have been controversial with observed phylogenetic incongruences and a lack of strong support when taxon sample sizes were increased (Hayashi and Kawano, 2000; Ronsted et al., 2005; Lkinci et al., 2006; Huang et al., 2018). In previous phylogenetic examination by Huang et al. (2018) that involved more than half of all Lilieae species, Fritillaria was divided into two clades, and Lilium was nested within Fritillaria. Similarly, the infrageneric classifications of Fritillaria and Lilium have been much studied (Hayashi and Kawano, 2000; Wang et al., 2009; Muratovic et al., 2010; Lee et al., 2011; Gao et al., 2013; Day et al., 2014; Mustafa and Abdul-Razaq, 2015; Givnish et al., 2020). Fritillaria is currently divided into eight subgenera that are generally supported by phylogenetic analyses (Rix, 2001; Ronsted et al., 2005; Day et al., 2014). For Lilium, phylogenetic studies are often built on the seven morphological sections of Comber (1949). Nonetheless, previous studies focused on partial clades and lack strong support, and so details of the infrageneric relationships for Fritillaria and Lilium remain controversial (Gao et al., 2013; Du et al., 2014; Ghanbari et al., 2018; Tuyl et al., 2018; Givnish et al., 2020). Thus, with an increasing phylogenetic conflict being published, the resulting uncertainty in overall Lilieae phylogeny (Huang et al., 2018; Kim and Kim, 2018) has limited further evolutionary study of the tribe.
A key difficulty in Lilieae phylogenetic reconstruction relates to the development or application of suitable molecular markers for addressing these issues. Until recently, the nuclear ribosomal internal transcribed spacer (ITS) sequence was widely used to reconstruct phylogenetic relationships in Liliaceae (Lkinci et al., 2006; Muratovic et al., 2010; Lee et al., 2011; Nursel, 2011; Gao et al., 2013; Du et al., 2014; Ghanbari et al., 2018). However, most ITS-based clades are weakly supported and so may not adequately reconstruct the phylogeny and evolution of Lilieae. Similarly, individual plastid regions or genes (i.e., one or a few) have been widely applied in molecular phylogenetic studies of Liliales families and genera (Patterson and Givnish, 2002; Ronsted et al., 2005; Petersen et al., 2013; Mustafa and Abdul-Razaq, 2015; Kiani et al., 2017; Givnish et al., 2020), and they also did not provide fully clear relationships in Lilieae, especially in Fritillaria and Lilium (Ronsted et al., 2005; Day et al., 2014; Huang et al., 2018).
Using the complete plastid genome (i.e., plastome) much more detailed molecular information data than traditional nuclear or plastid markers (e.g., Goremykin et al., 2003; Leebens-Mack et al., 2005; Raman and Park, 2016; Xie et al., 2018; Guo et al., 2020) are provided. The data from plastomes have been widely used to infer the phylogenetic relationships and phylogeographic histories of plant taxa considering their relatively small size, conservation of gene content and order, and effectively high copy number (Givnish et al., 2010, 2016, 2018; Raman and Park, 2016; Sancho et al., 2017; Li et al., 2019; Xie et al., 2019, 2020b; Guo et al., 2020). Given technological advances in sequencing, recent studies have used plastomes to resolve phylogenetic uncertainties in monocotyledons (Givnish et al., 2010, 2016, 2018, 2020; Barrett et al., 2013, 2014; Mennes et al., 2015; Ross et al., 2016; Lam et al., 2018; Liu et al., 2018; Xie et al., 2018; Soto Gomez et al., 2020; Su et al., 2021). Here, we sequenced 45 Liliaceae plastomes and combined 94 publicly available plastomes to explore the origin, divergence, and evolution of Lilieae. Our study determines (1) whether Lilium and Fritillaria are monophyletic, (2) when and how Lilieae originated and diverged, and (3) the history of morphological trait evolution in Lilieae.
Materials and Methods
Taxon Sampling
We included 139 plastid genomes of monocots representing nine families of Liliales and 11 monocot outgroups (GenBank accessions: Supplementary Table 1). We studied 79 species of Lilieae, which encompassed three of four Notholirion species (excluding N. koeiei), all three Cardiocrinum species, 26 of 152 Fritillaria species, and 47 of 118 Lilium species (Supplementary Table 1). Samples covered all ten sections of Lilium and major phylogenetic clades (six of eight subgenera) of Fritillaria (Comber, 1949; Ronsted et al., 2005; Gao et al., 2013; Day et al., 2014). Among all 139 plastomes, we assembled 45 plastomes, with this study including the first publication of molecular data from F. fusca. Fresh material from adult plants was collected in the field between 2009 and 2020 and dried in the silica gel for further DNA extraction. Voucher specimens are deposited in the Sichuan University Herbarium [SZ, the Herbarium code refers to Thiers (2016)]. Detailed information of materials included in this study is listed in Supplementary Table 1. In addition, we downloaded 82 ITS sequences of Lilieae species from GenBank.
DNA Sequencing and Genome Assembly
We extracted total genomic DNAs from 100 mg desiccated leaves using a modified cetyltrimethylammonium bromide method (Doyle, 1987). Total genomic DNAs were sent to Novogene Technologies, Inc. (Beijing, China) for genome library construction and sequencing. The sequencing library was generated using NEB Next® Ultra™ DNA Library Prep Kit for Illumina (NEB, United States) following the recommendations of the manufacturer, and index codes were added to each sample. Sequencing was performed using an Illumina Novaseq2500 sequencer (Illumina, San Diego, CA, United States).
The plastomes were de novo assembled using the NOVOPlasty 2.7.2 program (Dierckxsens et al., 2017) with raw data. To minimize the impact of distant starting seed sequences on the plastomes, we used a consistent seed sequence within each genus, specifically L. henryi (NC035570), F. cirrhosa (MH244906), N. macrophyllum (MH011354), Tulipa sylvestris (MT261172, which was also used as a seed of Lloydia tibetica), and Smilax china (HM536959). Genome annotation and IR region searches were processed using PGA software (Qu et al., 2019). Manual modifications for the uncertain start and stop codons were conducted based on comparison with homologous genes from other plastomes of other species using GENEIOUS R11 (Kearse et al., 2012).
Phylogenetic Analyses
We reconstructed phylogenetic relationships based on the data of Lilieae species (including a 79-taxon plastome data set and a separate data set comprising 77 nuclear ITS sequences) to analyze the phylogeny of Lilieae, using five species from the tribe Tulipeae as an outgroup in each case. For plastomes, all sequences including protein-coding genes (CDS), ribosomal RNA genes (rDNA), transfer RNA genes (tRNA), and non-coding regions (NCR) were extracted using the PhyloSuite platform (Zhang et al., 2020) and then aligned using the multiple alignments using Fast Fourier transform (MAFFT) program (Katoh et al., 2005). Because infA, ycf1, ycf15, and ycf68 have a high-sequence variability, we excluded these genes from the analysis (Givnish et al., 2018; Li et al., 2019; Lu et al., 2021). We adjusted all alignments manually using the GENEIOUS R11 software (Kearse et al., 2012) and then concatenated them into supermatrices using the PhyloSuite platform (Zhang et al., 2020). We created two matrices, namely, CDS matrix (i.e., CDSs only) and a whole plastid supermatrix (WP: CDS + tRNA + rDNA + NCR).
We used the PartitionFinder 2 program (Lanfear et al., 2017) to determine optimal partitions for plastomes by the Akaike information criterion method, considering each gene or individual non-coding sequence as an initial partition. A total of 57 partitions of the WP were designated for the across-Lilieae analysis; 41 had a generalized time-reversible (GTR) + I model, 12 had a GTR + G model, and the remaining four had a GTR model (Supplementary Table 2). We performed partitioned maximum likelihood (ML) analyses using the IQ-TREE v1.6.11 program (Nguyen et al., 2015). The ML analyses of ITS were performed using the RAxML v.8 software (Stamatakis, 2014) under the GTR + G model selected by jModelTest (Posada, 2008). All the ML analyses used 1000 rapid-search bootstrap replicates. Bayesian inference (BI) was performed using the MrBayes v3.2 software (Ronquist et al., 2012). The models of partitions and jModelTest (the same set used in the likelihood analyses) for BI analyses were parameterized independently. Two independent runs of 10 million generations were performed using the BI analyses. Trees were sampled once every 1,000 generations with the first 25% trees of each run discarded as the burn-in. The stationarity was reached when the average standard deviation (SD) of split frequencies remained below 0.001 and effective sample size (ESS) >200.
Estimation of Divergence Time
There are currently no well-documented fossils in Liliaceae, and thus fossil constraints were limited to Liliales. We used 128 Liliales plastomes (more taxa than for the main phylogenetic analyses, which focused on Lilieae) and 11 additional outgroup plastomes (Supplementary Table 1) to estimate the origin times of Lilieae and other allied taxa. Referring to previous studies (Li et al., 2019; Xie et al., 2020a) and to minimize the effects of missing data, we only used combined single-copy CDS genes data set derived from 139 plastomes for the estimation of divergence time. The data set included nine of ten families of Liliales (excluding mycoheterotrophic Corsiaceae) and all tribes (including 12 genera) of Liliaceae. Estimations of divergence time were performed using an uncorrelated lognormal relaxed molecular clock method implemented in the BEAST 1.10.4 program (Suchard et al., 2018); a Yule process (Gernhard, 2008) was specified as the tree prior. An optimal partitioning scheme was determined using the PartitionFinder 2 program (Lanfear et al., 2017). The information for model settings is presented in Supplementary Table 3. Six calibration points (i.e., four from fossils) were used to calibrate time as follows (Supplementary Table 4; see Iles et al., 2015; Givnish et al., 2016; Huang et al., 2018). (1) Based on the fossilized leaves of Luzuriaga peterbannisteri (Conran et al., 2014), the crown node of Luzuriaga (Drymophila/Luzuriaga clade: Alstroemeriaceae) was constrained to a minimum age of 23.2 Ma (Lindqvist and Lee, 2009; Conran et al., 2014). (2) The stem node of Ripogonaceae was constrained to a minimum age of 51.5 Ma (Carpenter et al., 2007; Conran et al., 2009) based on the fossilized leaves of Ripogonum tasmanicum (Conran et al., 2009). (3) Based on previous studies about fossilized seeds of Spirematospermum chandlerae (Friis, 1988; Iles et al., 2015; Givnish et al., 2018), the crown node of Zingiberales was set as 83 Ma. (4) Based on the fossilized leaves of S. trinervis (Huzioka, 1963), which is close to S. china (Morita, 1932), the stem node of S. china clade was set as 7.2–5.33 Ma age (Denk et al., 2015). These four fossil prior calibrations were set as the mean in lognormal distributions with an SD of 0.5 million years. More details about parameters related to calibration points are shown in Supplementary Table 4. (5) Referring to the review of Chacón et al. (2012), the crown node of Smilax was set to 46 Ma [95% highest probability density (HPD) 54.8–37.2 Ma, a lognormal prior distribution], which represents a conservative minimal age. This considered that Smilax-like fossils are known from the Early/Lower Eocene (55.8–48.6 Ma; Edelman, 1975; Wilf, 2000) and the Middle Eocene (48.6–37.2 Ma; MacGinitie, 1941; Wilde and Frankenhauser, 1998). (6) According to previous research of the evolutionary timescale of monocots (Givnish et al., 2016), 123.8 Ma was implemented as a minimum age of Liliales and as the zero offset of lognormal distribution with a log mean of 123 (95% HPD: 131.1–115.6) and SD of 4.3 in the uncorrelated lognormal analysis. This time is equal to the stem group of the Liliales and is congruent with several studies about Liliales/monocots (Eguchi and Tamura, 2016; Barba-Montoya et al., 2018; Givnish et al., 2018; Xie et al., 2020a). We ran an empty analysis without the data to evaluate the interactions of the priors first. The result of the empty run produced reasonable ages of the calibrated nodes according to the fossils. For each BEAST analysis, the Markov chain Monte Carlo (MCMC) algorithm was run for 100 million generations with sampling every 10,000 generations, followed with a burn-in of the initial 10% cycles. MCMC samples were inspected in TRACER to confirm sampling adequacy and convergence of the chains to a stationary distribution.
Reconstruction of Ancestral Area
The following five regions were defined for biogeographic analyses based on the paleogeographic and climatic evidence (Lkinci et al., 2006; Tu et al., 2010; Buerki et al., 2012) and also according to the distribution of Lilieae: (A) the Qinghai-Tibet Plateau-Himalayas-Hengduan Mountains (QTP-HHM), (B) East Asia and Siberia, (C) Northern America, (D) Irano-Turanian region (central and western Asia, north-east Africa, and north-west China), and (E) Europe and the Mediterranean Basin. All these regions are separated by physical barriers or climatological differences. Reconstructions of the ancestral area of Lilieae were conducted using the Statistical Dispersal-Vicariance (S-DIVA) analysis as implemented in the RASP v4 software (Yu et al., 2020). We used the BI tree based on the WP data (obtained by phylogenetic analyses) for the S-DIVA analyses. We tested the implemented biogeographical models DEC, DIVALIKE, and BAYAREALIKE with and without the J-parameter modeling jump dispersal (Matzke, 2013). The BAYAREALIKE + J model for biogeographical reconstruction yielded the best model fit. Because uncertainty in the root areas of an outgroup can lead to biased inferences for the crown node of the ingroups (Yu et al., 2015), we removed outgroups before ancestral-state reconstruction. To explore the effects of area constraints, the maximum number of areas at each node was set to three.
Ancestral Character-State Reconstructions
Lilieae species vary in traits associated with bulbs, flowers, and leaves that have considerable ecological and evolutionary significance (Stevenson and Loconte, 1995; Patterson and Givnish, 2002; Peruzzi et al., 2012). We conducted reconstructions of seven vegetative features, namely, (i) bulb component (including bulblets and scales) number, (ii) stem height, (iii) leaf length, (iv) leaf width, (v) flower number per inflorescence, (vi) tepal length, and (vii) tepal width, all based on field observations, specimen study, and information in the literature (Shoe, 1958; Boufford, 1993; Chen et al., 2000; Ohara et al., 2006; Li et al., 2020). The details of the seven characters are provided in Supplementary Table 5. All trait measurements of specimens were carried out using MATO (Altınordu et al., 2016). We used the mean values of seven traits as qualitative character states. The Comparison Trees and States method in the RASP v4 software (Yu et al., 2020) was used to assess the phylogenetic signal in the seven traits. The phylogenetic signal is the tendency of related species to resemble each other in a specific character more than species drawn at random from the same tree (Munkemuller et al., 2012; Yu et al., 2020). After phylogenetic signal analyses, we found that the bulb component number was the only character to show a strong phylogenetic signal (see below). We conducted the reconstruction of an ancestral trait of the bulb type using MultiState Reconstruction with the Bayes Traits method implemented in RASP. Lilieae bulbs were divided into three types, namely, (i) few scales (scale numbers < 5), (ii) many scales (scale numbers ≥ 5), and (iii) numerous bulblets, coded as A, B, and C, respectively, in Supplementary Table 5 (letter line). The MCMC iterations were set as 100 million, sampled every 10,000, and the first 50,000 iterations were set as burn-in.
Results
Plastome Features and Sequence Divergence of Lilieae
Plastome features are conserved (Supplementary Table 6) among the 80 included plastomes (79 species) of Lilieae. Their total length ranges from 151,009 bp (F. ussuriensis) to 153,235 bp (L. fargesii); their GC content is very similar (36.9–37.1%). There are 115 unique genes, comprising 81 CDS genes, 4 rDNA genes, and 30 tRNA genes (of these, nine, four, and eight genes, are located in the inverted repeats). We obtained 53 non-coding regions excluding lengths of less than 200 bp. The length of single sequences ranged from 71 bp (trnG-UCC) to 6,660 bp (ycf2).
Phylogenetic Analysis
For plastomes, the topologies from ML (Supplementary Figures 1, 2) and BI (Figure 1A and Supplementary Figure 3) trees are congruent, and from here on, we referred to the BI tree (Figure 1A). The trees reconstructed from CDSs (Supplementary Figures 2, 3) and WP matrices (Figure 1A and Supplementary Figure 1) were topologically consistent with each other with little difference in well-supported branches in terms of posterior probabilities (PP) or bootstrap support values (BS). The ITS tree (Figure 1B and Supplementary Figure 4) is roughly comparable to the WP tree regarding intergeneric relationships but is weakly supported regarding subgeneric clades (cf. Figures 1A,B). In all analyses, Lilieae is monophyletic. In the WP tree (Figure 1A), four genera are monophyletic with robust support (PP = 1.00, BS = 100), and Fritillaria is monophyletic with moderate support (PP = 0.82, BS = 84). Fritillaria comprises seven subgenera, which all have robust support (Figure 1A). Lilium is divided into two main lineages (marked as “L1” and “L2” in Figure 1A) with strong support. Lilium species are divided into ten sections based on morphology and molecular results following previous studies on Lilium (Comber, 1949; Nursel, 2011; Gao et al., 2013; Du et al., 2014; Huang et al., 2018; Tuyl et al., 2018), but only four sections (i.e., Leucolirion I, Sinomartagon I and III, Archelirion) are inferred to be monophyletic here.
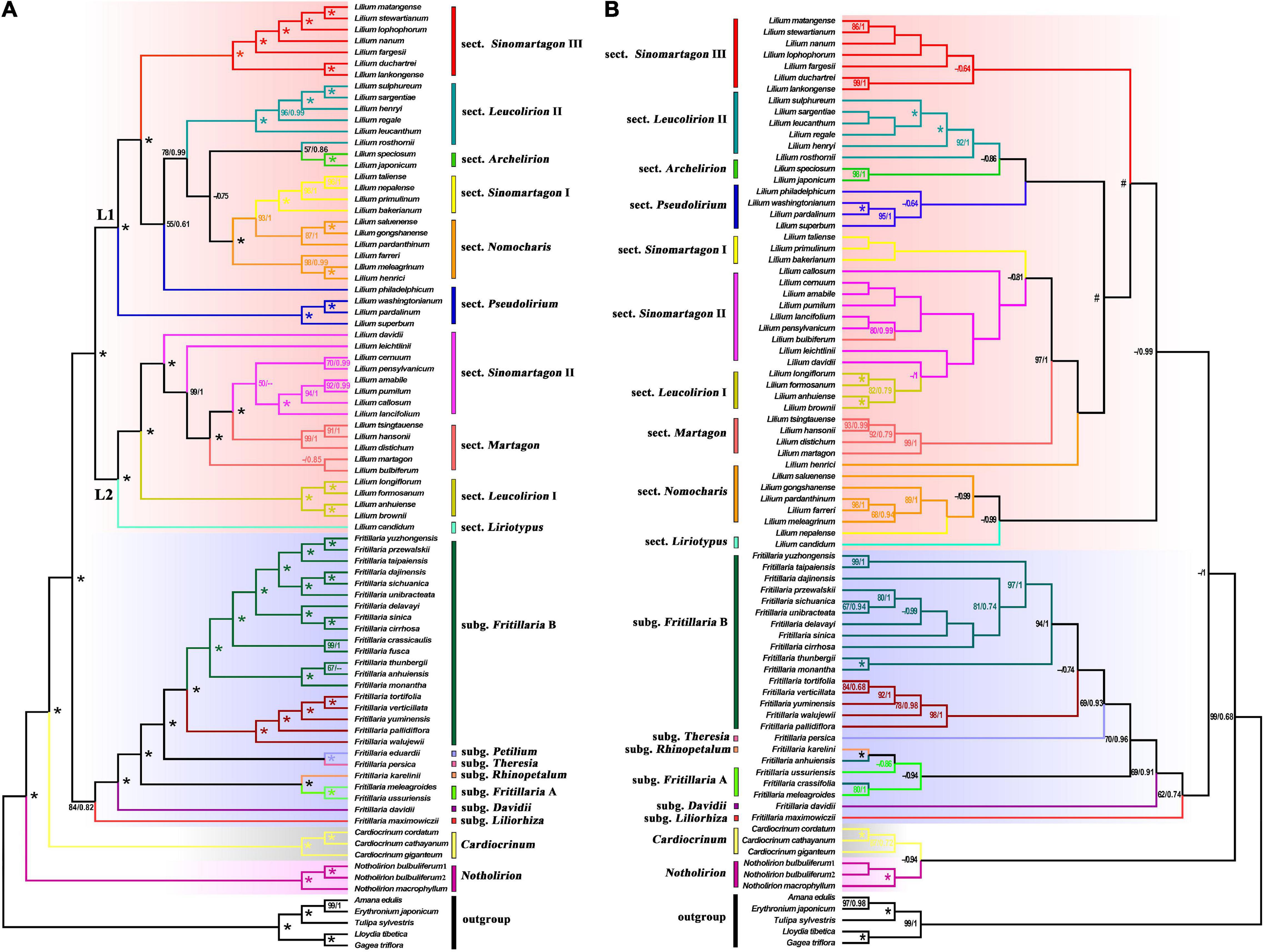
Figure 1. Phylogenetic relationships of Lilieae species inferred using whole plastomes (WP) and nuclear ribosomal internal transcribed spacer (ITS) sequence data. Maximum-likelihood bootstrap support/Bayesian posterior probability values are shown near corresponding nodes (“-” indicates support values less than 50%; “*” represents 100%/1 support). The genus, subgenus, and sectional classifications of Lilieae are indicated to the middle of trees and colored within trees. (A) Phylogenetic relationships inferred from WP data and (B) Phylogenetic relationships inferred from ITS data.
Estimation of Divergence Time and Reconstruction of Ancestral Area
Divergence time analyses based on 139 plastomes representing 127 Liliales species and 11 outgroups, with four fossil calibration points and two other calibration points, resulted in an inferred crown-group age of Liliaceae of ∼64.67 Ma (95% HPD: 78.61–51.87 Ma), and the stem age of Liliaceae was estimated as ∼79.87 Ma (95% HPD: 95.66–66.89 Ma, Figure 2 and Table 1). The stem age of Lilieae was estimated as ∼43.04 Ma (95% HPD: 54.07–32.50 Ma), and the age of the crown group was ∼25.16 Ma (95% HPD: 32.60–18.43 Ma, Figure 2 and Table 1). Within Lilieae, the stem age of genus Notholirion is predicted to have originated at ∼25.16 Ma (95% HPD: 32.60–18.43 Ma), and the genus Cardiocrinum originated at ∼22.89 Ma (95% HPD: 29.73–16.77 Ma). The timing of the divergence between Lilium and Fritillaria was ∼18.60 Ma (95% HPD: 23.99–13.79 Ma), and then Lilium diverged into two lineages (i.e., L1 and L2; Figure 2) at ∼15.79 Ma (95% HPD: 20.87–11.41 Ma). In Fritillaria, F. maximowiczii stem age was∼18.41 Ma (95% HPD: 23.74–13.68 Ma, Figure 2). We predicted that the tribe Lilieae originated around the Eocene (∼53–36 Ma), with genus and species diversity in the tribe significantly increasing since the Miocene (∼23–5 Ma).
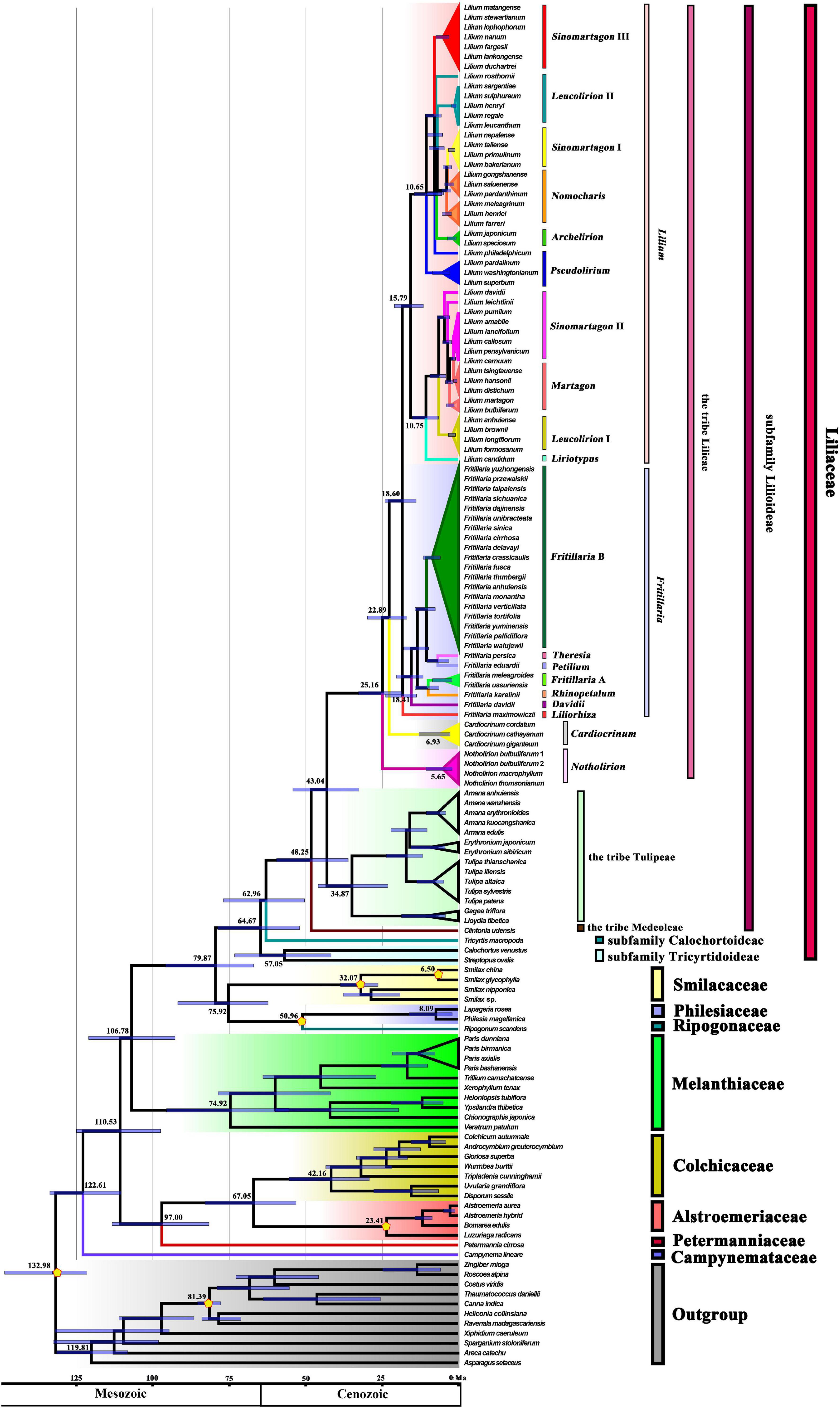
Figure 2. The estimation of divergence time based on 139 plastomes of Liliales and outgroups. The 95% highest posterior density (HPD) estimates for each well-supported clade are represented by bars. The APG (2016) classification is indicated to the right of the tree and colored within the tree. Yellow pentagons indicate six calibration points (see text).
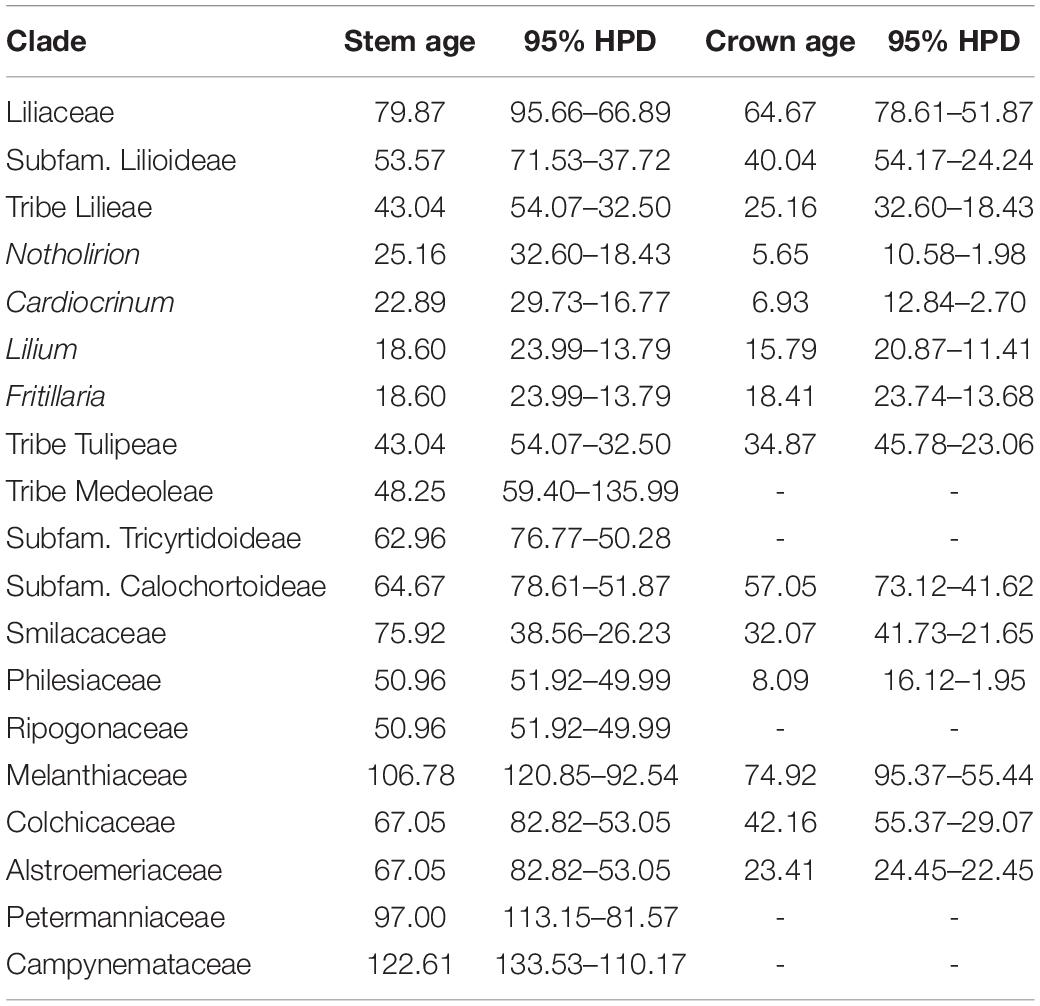
Table 1. Inferred stem and crown ages (Ma) of Lilieae and other Liliales taxa, with upper and lower bounds of the 95% higher posterior density (HPD) for mean ages based on the analysis with 139 plastomes and six fossils calibration points (four based on fossils) (see Figure 2).
The reconstructions of the ancestral area based on the BEAST plastome analyses (Figure 3) supported the most likely ancestral distribution of Lilieae as being in QTP-HHM. Notholirion and Cardiocrinum are predicted to have diversified in situ there, and the most recent common ancestor (MRCA) of Lilium was probably distributed in the QTP-HHM or East Asia. The MRCA of sect. Pseudolirium of Lilium was inferred to have dispersed from Asia to North America, while the MRCA of other Lilium lineages diversified in the QTP-HHM and East Asia. For the largest genus of Lilieae, Fritillaria, the QTP-HHM and North Asia are reconstructed as the most likely ancestral ranges. More recent dispersal events were inferred from QTP-HHM to the lrano-Turanian region for Fritillaria. The lrano-Turanian region is inferred to be the area of origin and diversification for subg. Rhinoperalum, Theresia, Petilium, and Fritillaria clade B in Fritillaria.
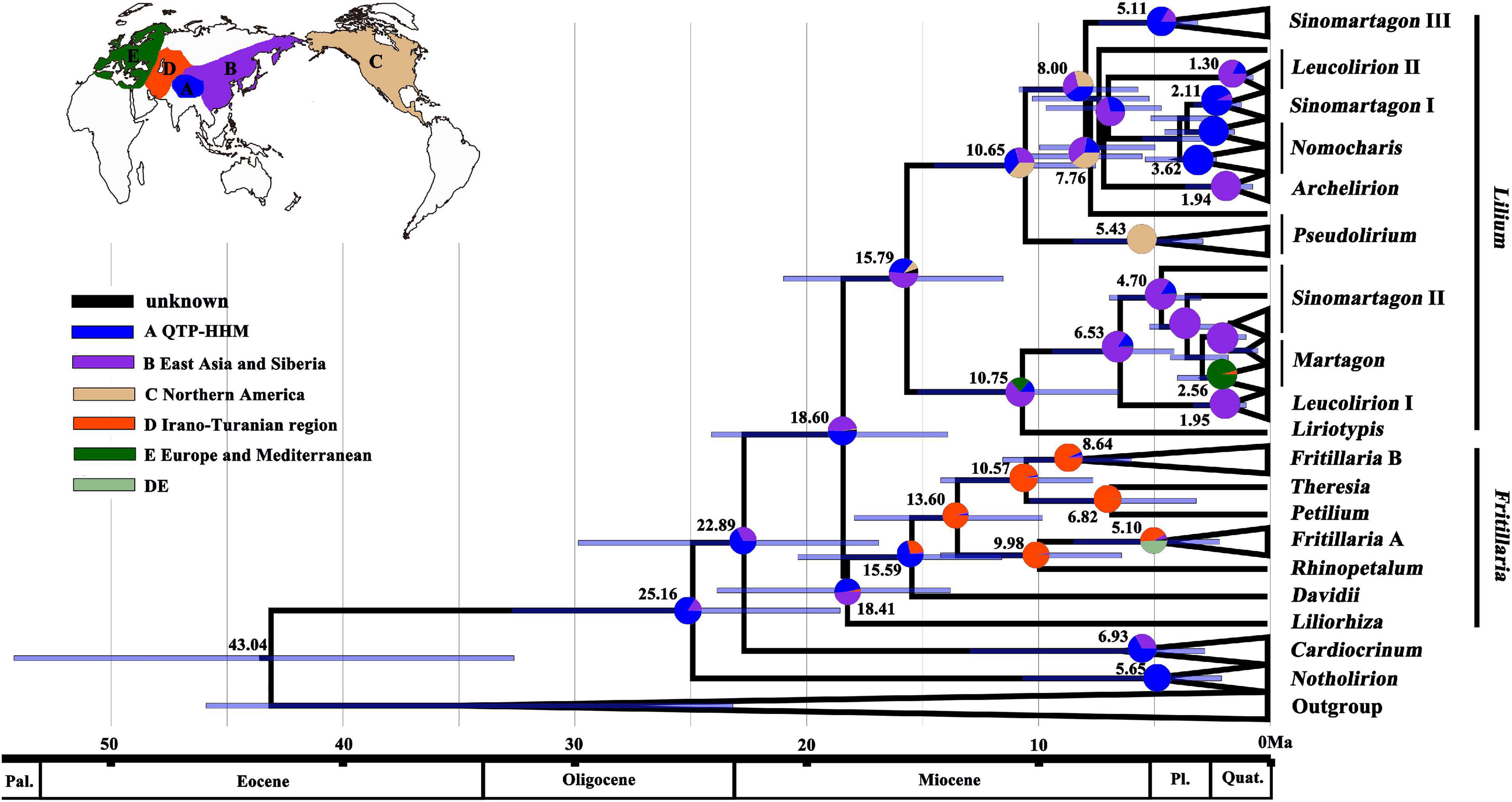
Figure 3. Reconstructions of the ancestral area based on plastome-based phylogeny of Lilieae (see Figure 1A). Pie charts show proportions of inferred ancestral ranges, pie slice colors to regions defined in the caption and inset world map. Mean divergence ages are given on nodes (see Figure 2). Bars on nodes indicate the 95% HPD of divergence ages.
Evolution of Lilieae Traits
Indices of the phylogenetic signal are shown in Supplementary Table 7 for seven traits in Lilieae. RASP calculated Pagel’s λ (λ; Pagel, 1999), and Blomberg’s K value (K; Blomberg et al., 2003), which can be used to gage the amount of phylogenetic signal relative to the amount expected for a character undergoing Brownian motion evolution along with the specified topology and branch lengths. The K of bulb component number was 1.08 (λ, 1.04), indicating a good correlation between phylogenetic and bulb component numbers. We inferred a little phylogenetic signal in flower and leaf traits during the diversification of Lilieae (Supplementary Table 7, both K and λ < 1). The reconstruction of an ancestral trait for the bulb type is presented in Figure 4 and Table 2. The results from RASP proposed one possible evolutionary route for Lilieae bulbs. First, the MRCA of Lilieae possibly had numerous bulblets (node 1); second, a phenotype with many scales may have appeared in the MRCA of Cardiocrinum, Fritillaria, and Lilium (node 2). Third, the MRCA of Fritillaria and Lilium differentiated into bulbs with few scales (node 3). The information for pivotal nodes 1–5 (Figure 4) that represent important ancestors are recorded in Table 2.
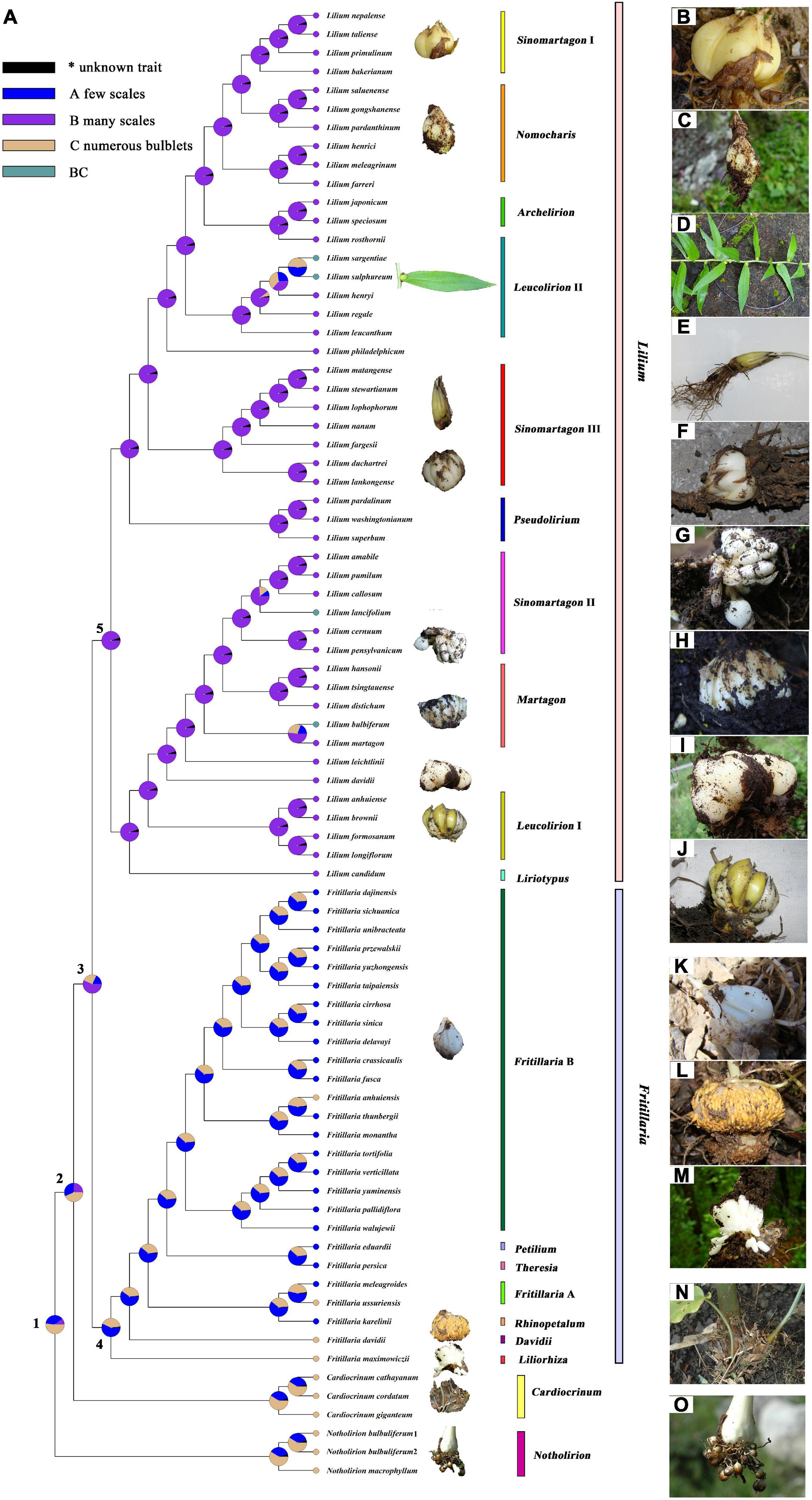
Figure 4. Reconstruction of the ancestral trait of Liliaea bulbs. (A) Reconstruction of the ancestral trait; (B–O) Bulb types of selected Lilieae species: (B) L. taliense, (C) L. pardanthinum, (D) L. sulphureum, (E) L. lophophorum, (F) L. duchartrei, (G) L. pensylvanicum, (H) L. distichum, (I) L. davidii, (J) L. brownii, (K) F. delavayi, (L) F. davidii, (M) F. maximowiczii, (N) C. giganteum, and (O) N. bulbuliferum.
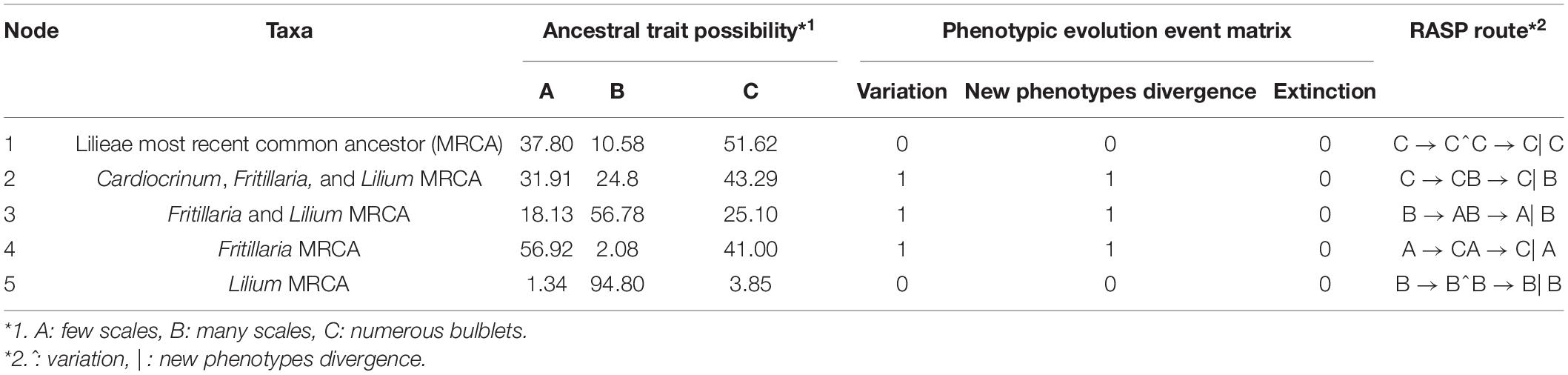
Table 2. Bulb ancestral trait transitions across Lilieae (see Figure 4).
Discussion
Plastome-Based Inference of Well-Supported Phylogenetic Trees for Lilieae
Our plastome analyses inferred well-supported relationships among genera and species of Lilieae. Among the four Lilieae genera, the monophyly of Notholirion and Cardiocrinum was reconfirmed, and the uncertain phylogenetic relationships of Fritillaria and Lilium were resolved (Figure 1). In previous studies (Ronsted et al., 2005; Huang et al., 2018), Fritillaria was partitioned into two clades, namely, subgenus Liliorhiza (including F. maximowiczii) and other subgenera, with Lilium nested in two Fritillaria clades based on a few plastid sequences. However, Fritillaria was monophyletic according to previous ITS-based studies (Hayashi and Kawano, 2000; Ronsted et al., 2005; Lee et al., 2011; Nursel, 2011; Gao et al., 2013; Ghanbari et al., 2018; Huang et al., 2018), which we confirmed here for our ITS-based inferences (Figure 1B). Also, Fritillaria is confirmed to be monophyletic (PP = 0.82, BS = 84) based on the plastome-based tree (Figure 1A). In addition, our plastome analysis also revealed generally well-supported subgeneric relationships in Fritillaria and Lilium. Relationships among the six subgenera of Fritillaria are generally well supported, in line with previous Fritillaria studies (Day et al., 2014; Mustafa and Abdul-Razaq, 2015; Mucciarelli et al., 2016; Kiani et al., 2017; Huang et al., 2018). Within Lilium, the plastome analyses split the ten sections of this genus into two clades (i.e., L1 and L2) with strong support. Our tree provided a better-supported picture of Lilium evolution than many previous analyses (Hayashi and Kawano, 2000; Gao et al., 2013; Du et al., 2014; Ghanbari et al., 2018) and supports a previous plastome study (Givnish et al., 2020). However, the subgeneric clades reconstructed by ITS were weakly supported, especially within Lilium (Figure 1B). Nonetheless, our plastome analysis reconstructed a well-supported tree for Lilieae, contributing to a better understanding of Lilieae phylogeny.
Uplift of the Qinghai-Tibet Plateau Promoted the Divergence Within Lilieae
The Qinghai-Tibet Plateau is one of the important biodiversity hotspots in the world, and climate changes caused its uplifting are thought to have exerted important influences on species evolution in Eurasia (Favre et al., 2015; Renner, 2016; Xing and Ree, 2017; Peterson et al., 2019; Xie et al., 2020b). Even though many details of geological history for the QTP remain controversial, a general consensus has emerged (Wang et al., 2014; Deng and Ding, 2015; Favre et al., 2015; Renner, 2016; Xing and Ree, 2017). For example, the QTP initially uplifted at 45–35 Ma to form a “proto-QTP,” with subsequent extension at 23–15 Ma; uplifts initiated the monsoon system (Wang et al., 2014; Favre et al., 2015; Renner, 2016). By the Middle Miocene, high mountain ranges formed and were accompanied by the aridification of Central Asia (Deng and Ding, 2015; Favre et al., 2015; Renner, 2016). The final extensions of the surrounding mountains, such as the Hengduan Mountains, to current elevations occurred from approximately 10 Ma to the present (Xing and Ree, 2017).
The QTP is an important distribution area and a center of origination and diversity for members of the tribe Lilieae (Gao et al., 2013; Huang et al., 2018; Givnish et al., 2020). Species of Lilieae dispersed in the temperate climatic regions of the northern hemisphere (Boufford, 1993; Chen et al., 2000; Kiani et al., 2017): Cardiocrinum species spread over east Asia (Ohara et al., 2006; Yang et al., 2016; Lu et al., 2020) and Notholirion species evolved as endemics in the Himalaya mountains (Li et al., 2018, 2020). Lilium and Fritillaria species are widely distributed in the northern hemisphere, and many Lilium species are densely distributed in east Asia (Gao et al., 2013; Gao and Gao, 2016, Gao et al., 2020; Givnish et al., 2016; Liu et al., 2018; Su et al., 2021), whereas species of Fritillaria are mainly distributed in central Asia and the Mediterranean region (Wang et al., 2009; Day et al., 2014; Mustafa and Abdul-Razaq, 2015; Mucciarelli et al., 2016). Many species of Lilieae are distributed mainly in the southern and south-eastern regions of the QTP (Wang et al., 2009; Gao et al., 2013; Li et al., 2020), consistent with the QTP being an intensive distribution center of Lilieae species (Chen et al., 2000; Gao and Gao, 2016; Huang et al., 2018; Li et al., 2020). Furthermore, several studies (Gao et al., 2013; Givnish et al., 2020) have concluded that the QTP is a center of origin and diversification for Lilium. Other studies have shown that the divergence of Cardiocrinum and Fritillaria species was promoted by the orogeny of the Hengduan Mountains and QTP, respectively (Yang et al., 2016; Huang et al., 2018). Huang et al. (2018) revealed that the uplift of the QTP and associated climatic changes probably drove early diversification of Lilieae in the QTP region, but this requires verification using age estimates analysis and reconstructions of the ancestral area.
Here, we estimated the timing of the origin of Liliaceae to be around 79.87 Ma (95% HPD: 95.66–66.89 Ma, Figure 2 and Table 1), approximately congruent with several studies, such as 70.428 (44.204–89.435) Ma (Xie et al., 2020a), and 79.8 (59.3–103.0) Ma (Givnish et al., 2016). Divergence time estimates of angiosperms have been heavily influenced by differences in the gene sampling and number and the fossil calibrations used (Foster et al., 2017; Barba-Montoya et al., 2018; Li et al., 2019). Although the divergence time of Lilieae has been estimated previously (Gao et al., 2013; Givnish et al., 2016, 2020; Huang et al., 2018), no study has been conducted on the origin times based on the genome data at the level of the order Liliales. Here, we used 139 plastomes and six calibration points (including four based on fossils) in our age estimates and estimated that Lilieae originated during the Eocene [∼43.04 (54.07–32.50) Ma, Figure 2 and Table 1]. Considering our molecular dating and reconstructions of the ancestral area, this points to several origin and divergence events in Lilieae during the uplifting of the QTP. First, Lilieae may have originated from the QTP-HHM region in the Eocene. Second, a subsequent extension of the QTP and the monsoon system possibly promoted the differentiation between Cardiocrinum [∼22.89 (29.73–16.77) Ma] and Fritillaria and Lilium [∼18.60 (23.99–13.79) Ma]. Third, the pivotal clades and subgenera of Lilium and Fritillaria diverged from each other in the Middle Miocene. Finally, the diversification of Lilium and Fritillaria expanded nearly 10 million years ago in the regions surrounding the QTP uplift, which occurred mainly between the Late Miocene and Late Pliocene. Thus, our findings add support to the idea that the uplifting of the QTP promoted the divergence within Lilieae.
The Most Recent Common Ancestor of Lilieae May Have Had Numerous Bulblets
The bulb is an important taxonomic identifier of Lilieae (Comber, 1949; Boufford, 1993; Chen et al., 2000; Tuyl et al., 2018; Li et al., 2020) and for species reproduction by vegetative propagation (Shoe, 1958; Takayama and Misawa, 1980; Miller and Langhans, 1990; Patterson and Givnish, 2002; Shin et al., 2002; Yan et al., 2019; Givnish et al., 2020). Bulbs are a reliable trait for classification within Lilieae at any time of year, as flowers and leaves are only present for part of the year. Within Lilieae, Notholirion species have a bulb with numerous bulblets (Figure 4O), small bulblike organs of vegetative reproduction (Chen et al., 2000; Li et al., 2018, 2020). Cardiocrinum species also have bulblets but fewer than Notholirion species (Ohara et al., 2006; Yang et al., 2016; Figure 4N). Bulbs of Lilium species are composed of many fleshy scales (Chen et al., 2000; Tuyl et al., 2018; Figures 4B–J), and those in Fritillaria only possess 1–3 farinaceous scales (Chen et al., 2000; Mucciarelli et al., 2016; Figure 4K). Generally, bulbs of Lilium and Fritillaria have no bulblets. However, subgenus Liliorhiza (including F. maximowiczii, Figure 4M) and subgenus Davidii (F. davidii, Figure 4L) of Fritillaria both have bulblets. Previous research revealed that the number of scales per bulb was different in Lilieae and Tulipeae (Patterson and Givnish, 2002; Givnish et al., 2016, 2018). Therefore, to focus on different numbers of storage components of bulbs, we reconstructed the evolution of bulb traits in Lilieae. Figure 4 documents a conceivable evolutionary pathway for the Lilieae bulb. The Lilieae MRCA may have had numerous bulblets, and then bulb type diverged twice in four genera. Furthermore, some species of Lilium (e.g., L. lancifolium, L. sulphureum, L. sargentiae, and L. bulbiferum) have bulbils (Figure 4D), a type of bulblet growing in leaf axils. This might indicate that bulbs evolved independently at least three times during the Lilium diversification. Bulbs with many scales and few scales likely originated after bulbs with bulblets, but this requires verification by increasing taxon sampling and more information on bulb types across taxa.
Bulb evolution in Lilieae may relate to adaptation to the photosynthetic environment during climate oscillation (Patterson and Givnish, 2002; Givnish et al., 2018, 2020). In particular, adaptations related to sexual/asexual reproductive adaptation are important ways for coping with changing environmental conditions in plants (Bengtsson, 2003; Otto and Gerstein, 2006; Silvertown, 2008). As is well known, Lilieae species have both types of reproduction, but each aspect may be emphasized differently across the species (Comber, 1949; Shoe, 1958; Takayama and Misawa, 1980; Tuyl et al., 2018). For example, taxa, such as N. bulbuliferum, L. lancifolium, and F. davidii frequently undergo asexual reproduction using bulblets (Chen et al., 2000; Ohara et al., 2006; Luo et al., 2012; Li et al., 2020). However, bulbs with scales generally provide more nutrients for the organism to generate seeds, and so this form appears to be more focused on sexual reproduction. Because clones are less genetically diverse, they are more vulnerable to habitat disturbance, and so asexual reproduction may be less advantageous during prolonged environmental upheaval (Silvertown, 2008). Sexual reproduction and its potential for recombination should be advantageous when the environment is undergoing perturbation (Baker, 1959; Bender et al., 1984; Bengtsson, 2003; Otto and Gerstein, 2006). Our analysis provides new insights into the evolutionary history of lilies and patterns of QTP species evolution. Significant uplift of the QTP is thought to have greatly intensified the seasonality of monsoonal Asia and initiated a general global decrease in temperature and increase in thermal and moisture seasonality at higher latitudes (Raymo and Ruddiman, 1992; Graham, 1999; Patterson and Givnish, 2002). Combined with the above age estimates, bulb scales might have arisen to enhance sexual reproduction in the MRCA of Fritillaria and Lilium during the Miocene, allowing adaptation to climatic oscillations due to the QTP uplift.
Data Availability Statement
The original contributions presented in the study are publicly available. This data can be found at NCBI under accession PRJNA784653.
Author Contributions
JL, S-DZ, and X-JH conceived the study. JL composed the article. JC and H-HQ performed experiments. YY and ZZ performed the data analysis. D-FX, MP, and X-FG revised the manuscript. All authors read and approved the final manuscript.
Funding
This work was supported by the National Natural Science Foundation of China (Grant Nos. 31872647, 32070221, and 32170209), National Plant Specimen Resource Center, Digitization and Sharing for the Herbarium of the Sichuan University (Grant No. 2020GJZWBB-SC), the Fourth National Survey of Traditional Chinese Medicine Resources (Grant No. 2019PC002), the China Post-doctoral Science Foundation (Grant No. 2020M683303), and the Fundamental Research Funds for the Central Universities (Grant No. 20826041E4158).
Conflict of Interest
The authors declare that the research was conducted in the absence of any commercial or financial relationships that could be construed as a potential conflict of interest.
Publisher’s Note
All claims expressed in this article are solely those of the authors and do not necessarily represent those of their affiliated organizations, or those of the publisher, the editors and the reviewers. Any product that may be evaluated in this article, or claim that may be made by its manufacturer, is not guaranteed or endorsed by the publisher.
Acknowledgments
We thank Hai-Ying Liu, Dan-Mei Su, Jiao Huang, Jin-Bo Tan, Xian-Lin Guo, Xin Yang, Qiu-Pin Jiang, and Jun-Pei Chen for their help in materials collection. We acknowledge Chang-Bao Wang and Jin-Bo Tan, who provided bulb pictures of F. maximowiczii and F. davidii, respectively, in Figure 4. We also thank two reviewers for their comments during the review process.
Supplementary Material
The Supplementary Material for this article can be found online at: https://www.frontiersin.org/articles/10.3389/fpls.2021.699226/full#supplementary-material
Supplementary Figure 1 | Phylogenetic tree resulting from a maximum likelihood (ML) analysis based on the Lilieae whole plastome (WP) matrix.
Supplementary Figure 2 | Phylogenetic tree resulting from an ML analysis based on the Lilieae plastid coding sequences (CDSs) matrix.
Supplementary Figure 3 | Phylogenetic tree resulting from a Bayesian inference (BI) analysis based on the Lilieae plastid CDSs matrix.
Supplementary Figure 4 | Phylogenetic tree resulting from an ML analysis based on Lilieae nuclear ITS data.
References
Altınordu, F., Peruzzi, L., Yu, Y., and He, X. J. (2016). A tool for the analysis of chromosomes: karyotype. Taxon 65, 586–592. doi: 10.12705/653.9
APG (1998). An ordinal classification for the families of flowering plants. Ann. Mo. Bot. Gard. 85, 531–553.
APG (2003). An update of the Angiosperm Phylogeny Group classification for the orders and families of flowering plants: APG II. Bot. J. Linn. Soc. 141, 399–436. doi: 10.1046/j.1095-8339.2003.t01-1-00158.x
APG (2009). An update of the Angiosperm Phylogeny Group classification for the orders and families of flowering plants: APG III. Bot. J. Linn. Soc. 161, 105–121. doi: 10.1111/j.1095-8339.2009.00996.x
APG (2016). An update of the Angiosperm Phylogeny Group classification for the orders and families of flowering plants: APG IV. Bot. J. Linn. Soc. 181, 1–20. doi: 10.1111/boj.12385
Baker, H. G. (1959). Reproductive methods as factors in speciation in flowering plants. cold spring harb. Symp. Quant. Biol. 24, 177–191. doi: 10.1101/sqb.1959.024.01.019
Barba-Montoya, J., Reis, M. D., Schneider, H., Donoghue, P. C. J., and Yang, Z. (2018). Constraining uncertainty in the timescale of angiosperm evolution and the veracity of a Cretaceous Terrestrial Revolution. New Phytol. 218, 819–834. doi: 10.1111/nph.15011
Barrett, C. F., Davis, J. I., Leebens-Mack, J., Conran, J. G., and Stevenson, D. W. (2013). Plastid genomes and deep relationships among the commelinid monocot angiosperms. Cladistics 29, 65–87. doi: 10.1111/j.1096-0031.2012.00418.x
Barrett, C. F., Specht, C. D., Leebens-Mack, J., Stevenson, D. W., Zomlefer, W. B., and Davis, J. I. (2014). Resolving ancient radiations: can complete plastid gene sets elucidate deep relationships among the tropical gingers (Zingiberales)? Ann. Bot. 113, 119–133. doi: 10.1093/aob/mct264
Bender, E. A., Case, T. J., and Gilpin, M. E. (1984). Perturbation experiments in community ecology: theory and practice. Ecology 65, 1–13. doi: 10.2307/1939452
Bengtsson, B. O. (2003). Genetic variation in organisms with sexual and asexual reproduction. J. Evolution Biol. 16, 189–199. doi: 10.1046/j.1420-9101.2003.00523.x
Blomberg, S. P., Garland, T., and Ives, A. R. (2003). Testing for phylogenetic signal in comparative data: behavioral traits are more labile. Evolution 57, 717–745. doi: 10.1111/j.0014-3820.2003.tb00285.x
Buerki, S., Jose, S., Yadav, S. R., Goldblatt, P., Manning, J. C., and Forest, F. (2012). Contrasting biogeographic and diversification patterns in two mediterranean-type ecosystems. PLoS One 7:e39377. doi: 10.1371/journal.pone.0039377
Carpenter, R. J., Jordan, G. J., and Hill, R. S. (2007). A toothed Lauraceae leaf from the early Eocene of Tasmania, Australia. Int. J. Plant Sci. 168, 1191–1198. doi: 10.1086/520721
Chacón, J. de Assis, M. C., Meerow, A. W., and Renner, S. S. (2012). From east Gondwana to central America: historical biogeography of the Alstroemeriaceae. J. Biogeogr. 39, 1806–1818. doi: 10.1111/j.1365-2699.2012.02749.x
Chase, M. W., Duvall, M. R., Hills, H. G., Conran, J. G., and Hoot, S. (1995). “Molecular phylogenetics of Lilianae,” in Monocotyledons: Systematics and Evolution, eds P. J. Rudall, P. J. Cribb, D. F. Cutler, and C. J. Humphries (London: Kew: The Royal Botanic Gardens), 109–137.
Chen, S. C., Liang, S. J., Xu, J. M., and Minoru, N. T. (2000). “Liliaceae A. L. Jussieu,” in Flora of China, eds Z. Y. Wu, D. Y. Hong, and P. H. Raven (Beijing, Saint Louis: Science Press, Beijing & Missouri Botanical garden Press), 73–263.
Comber, H. F. (1949). A New Classification of the Genus Lilium. London: The Royal Horticultural Society, 86–105.
Conran, J. G., Bannister, J. M., Mildenhall, D. C., Lee, D. E., Chacon, J., and Renner, S. S. (2014). Leaf fossils of Luzuriaga and a monocot flower with in situ pollen of Liliacidites contortus Mildenh. & Bannister sp. nov. (Alstroemeriaceae) from the Early Miocene. Am. J. Bot. 101, 141–155. doi: 10.3732/ajb.1300351
Conran, J. G., Carpenter, R. J., and Jordan, G. J. (2009). Early Eocene Ripogonum (Liliales: Ripogonaceae) leaf macrofossils from southern Australia. Aust. Syst. Bot. 22, 219–228. doi: 10.1071/SB08050
Cronquist, A. (1988). The Evolution and Classification of Flowering Plants. New York, NY: The New York Botanical Garden: Columbia University Press.
Day, P. D., Berger, M., Hill, L., Fay, M. F., and Kelly, L. J. (2014). Evolutionary relationships in the medicinally important genus Fritillaria L. (Liliaceae). Mol. Phylogenet. Evol. 80, 11–19. doi: 10.1016/j.ympev.2014.07.024
Deng, T., and Ding, L. (2015). Paleoaltimetry reconstructions of the Tibetan Plateau: progress and contradictions. Natl. Sci. Rev. 2, 417–437. doi: 10.1093/nsr/nwv062
Denk, T., Velitzelos, D., Güner, H. T., and Ferrufino Acosta, L. (2015). Smilax (smilacaceae) from the miocene of western eurasia with caribbean biogeographic affinities. Am. J. Bot. 102, 423–438. doi: 10.3732/ajb.1400495
Dierckxsens, N., Mardulyn, P., and Smits, G. (2017). NOVO plasty: de novo assembly of organelle genomes from whole genome data. Nucleic Acids Res. 45:e18. doi: 10.1093/nar/gkw955
Do, H. D. K., Kim, C., Chase, M., and Kim, J. H. (2020). Implications of plastome evolution in the true lilies (monocot order Liliales). Mol. Phylogenet. Evol. 148:106818. doi: 10.1016/j.ympev.2020.106818
Doyle, J. J. (1987). A rapid DNA isolation procedure for small quantities of fresh leaf tissue. Phytochem. Bull. 19, 11–15.
Du, Y. P., He, H. B., Wang, Z. X., Li, S., and Wei, C. (2014). Molecular phylogeny and genetic variation in the genus Lilium native to China based on the internal transcribed spacer sequences of nuclear ribosomal DNA. J. Plant Res. 127, 249–263. doi: 10.1007/s10265-013-0600-4
Edelman, D. W. (1975). The Eocene Germer Basin Flora of Southcentral Idaho. Ph. D, Thesis. University of Idaho.
Eguchi, S., and Tamura, M. N. (2016). Evolutionary timescale of monocots determined by the fossilized birth-death model using a large number of fossil records. Evolution 70, 1136–1144. doi: 10.1111/evo.12911
Favre, A., Packert, M., Pauls, S. U., Jahnig, S. C., Uhl, D., Michalak, I., et al. (2015). The role of the uplift of the Qinghai-Tibetan Plateau for the evolution of Tibetan biotas. Biol. Rev. 90, 236–253. doi: 10.1111/brv.12107
Fay, M. F., Chase, M. W., Ronsted, N., Devey, D. S., and Davis, J. I. (2006). Phylogenetics of Liliales: summarized evidence from combined analyses of five plastid and one mitochondrial loci. Aliso 22, 559–565.
Foster, C. S. P., Sauquet, H., Merwe, M. M. V. D., and Mcpherson, H. (2017). Evaluating the impact of genomic data and priors on Bayesian estimates of the angiosperm evolutionary timescale. Syst. Biol. 66:syw086. doi: 10.1093/sysbio/syw086
Friis, E. M. (1988). Spirematospermum chandlerae sp. nov., an extinct species of Zingiberaceae from the North American Cretaceous. Tertiary Res. 9, 7–12.
Gao, Y. D., and Gao, X. F. (2016). Accommodating Nomocharis in Lilium (Liliaceae). Phytotaxa 277, 205–210. doi: 10.11646/phytotaxa.277.2.8
Gao, Y. D., Harris, A. J., Zhou, S. D., and He, X. J. (2013). Evolutionary events in Lilium (including Nomocharis, Liliaceae) are temporally correlated with orogenies of the Q–T plateau and the Hengduan Mountains. Mol. Phylogenet. Evol. 68, 443–460. doi: 10.1016/j.ympev.2013.04.026
Gao, Y. D., Harris, A., Li, H. C., and Gao, X. F. (2020). Hybrid speciation and introgression both underlie the genetic structures and evolutionary relationships of three morphologically distinct species of Lilium (Liliaceae) forming a hybrid zone along an elevational gradient. Front. Plant Sci. 11:576407. doi: 10.3389/fpls.2020.576407
Gernhard, T. (2008). The conditioned reconstructed process. J. Theor. Biol. 253, 769–778. doi: 10.1016/j.jtbi.2008.04.005
Ghanbari, S., Fakheri, B. A., Naghavi, M. R., and Mahdinezhad, N. (2018). Evaluating phylogenetic relationships in the Lilium family using the ITS marker. J. Plant Biotechnol. 45, 236–241. doi: 10.5010/JPB.2018.45.3.236
Givnish, T. J., Ames, M., McNeal, J. R., McKain, M. R., Steele, P. R., dePamphilis, C. W., et al. (2010). Assembling the tree of the monocotyledons: plastome sequence phylogeny and evolution of Poales. Ann. Mo. Bot. Gard. 97, 584–616. doi: 10.3417/2010023
Givnish, T. J., Skin-Ner, M., Reetnik, I., IKinci, N., Kriebel, R., Lemmon, A. R., et al. (2020). Evolution, geographic spread and floral diversification of the genus Lilium with special reference to the Lilies of North America. North Am. Lily Soc. Year Book 74, 26–44.
Givnish, T. J., Zuluaga, A., Marques, I., Lam, V. K. Y., Gomez, M. S., Iles, W. J. D., et al. (2016). Phylogenomics and historical biogeography of the monocot order Liliales: out of Australia and through Antarctica. Cladistics 32, 581–605. doi: 10.1111/cla.12153
Givnish, T. J., Zuluaga, A., Spalink, D., Gomez, M. S., Lam, V. K. Y., Saarela, J. M., et al. (2018). Monocot plastid phylogenomics, timeline, net rates of species diversification, the power of multi-gene analyses, and a functional model for the origin of monocots. Am. J. Bot. 105, 1888–1910. doi: 10.1002/ajb2.1178
Goremykin, V. V., Hirsch-Ernst, K. I., Wölfl, S., and Hellwig, F. H. (2003). Analysis of the Amborella Trichopoda chloroplast genome sequence suggests that amborella is not a basal angiosperm. Mol. Biol. Evol. 20, 1499–1505. doi: 10.1093/molbev/msg159
Graham, A. (1999). Late Cretaceous and Cenozoic History of North American Vegetation. London: Oxford Univ. Press.
Guo, X. L., Zheng, H. Y., Price, M., Zhou, S. D., and He, X. J. (2020). Phylogeny and comparative analysis of Chinese Chamaesium species revealed by the complete plastid genome. Plants 9:965. doi: 10.3390/plants9080965
Hayashi, K., and Kawano, S. (2000). Molecular systematics of Lilium and allied genera (Liliaceae): phylogenetic relationships among Lilium and related genera based on the rbcL and matK gene sequence data. Plant Spec. Biol. 15, 73–93. doi: 10.1046/j.1442-1984.2000.00025.x
Huang, J., Yang, L. Q., Yu, Y., Liu, Y. M., Xie, D. F., Li, J., et al. (2018). Molecular phylogenetics and historical biogeography of the tribe Lilieae (Liliaceae): bi-directional dispersal between biodiversity hotspots in Eurasia. Ann. Bot. 122, 1245–1262. doi: 10.1093/aob/mcy138
Huzioka, K. (1963). “The Utto flora of northern Honshu,” in Tertiary Floras of Japan, Miocene floras, The Collaborating Association to Commemorate the 80 th Anniversary of the Geological Survey of Japan, ed. R. W. Chaney (Tokyo, Japan: Geological Survey of Japan), 151–216.
Iles, W. J. D., Smith, S. Y., Gandolfo, M. A., and Graham, S. W. (2015). Monocot fossils suitable for molecular dating analyses. Bot. J. Linn. Soc. 178, 346–374. doi: 10.1111/boj.12233
Katoh, K., Kuma, K.-I., Toh, H., and Miyata, T. (2005). MAFFT version 5: improvement in accuracy of multiple sequence alignment. Nucleic Acids Res. 33, 511–518. doi: 10.1093/nar/gki198
Kearse, M., Moir, R., Wilson, A., Stones-Havas, S., Cheung, M., Sturrock, S., et al. (2012). Geneious basic: an integrated and extendable desktop software platform for the organization and analysis of sequence data. Bioinformatics 28, 1647–1649. doi: 10.1093/bioinformatics/bts199
Kiani, M., Mohammadi, S., Babaei, A., and Sefidkon, F. (2017). Iran supports a great share of biodiversity and floristic endemism for Fritillaria spp. (Liliaceae): a review. Plant Div. 39, 245–262. doi: 10.1016/j.pld.2017.09.002
Kim, J. S., Hong, J. K., Chase, M. W., Fay, M. F., and Kim, J. H. (2013). Familial relationships of the monocot order Liliales based on a molecular phylogenetic analysis using four plastid loci: matK, rbcL, atpB and atpF-H. Bot. J. Linn. Soc. 172, 5–21. doi: 10.1111/boj.12039
Kim, J. S., and Kim, J. H. (2018). Updated molecular phylogenetic analysis, dating and biogeographical history of the lily family (Liliaceae: Liliales). Bot. J. Linn. Soc. 187, 579–593. doi: 10.1093/botlinnean/boy031
Lam, V. K. Y., Darby, H., Merckx, V. S. F. T., Lim, G., Yukawa, T., Neubig, K. M., et al. (2018). Phylogenomic inference in extremis: a case study with mycoheterotroph plastomes. Am. J. Bot. 105, 480–494. doi: 10.1002/ajb2.1070
Lanfear, R., Frandsen, P. B., Wright, A. M., Senfeld, T., and Calcott, B. (2017). Partition Finder 2: new methods for selecting partitioned models of evolution for molecular and morphological phylogenetic analyses. Mol. Biol. Evol. 34, 772–773. doi: 10.1093/molbev/msw260
Lee, C. S., Kim, S. C., Yeau, S. H., and Lee, N. S. (2011). Major lineages of the genus Lilium (Liliaceae) based on nrDNA ITS sequences, with special emphasis on the korean species. J. Plant Biol. 54, 159–171. doi: 10.1007/s12374-011-9152-0
Leebens-Mack, J., Raubeson, L. A., Cui, L., Kuehl, J. V., Fourcade, M. H., Chumley, T. W., et al. (2005). Identifying the basal angiosperm node in chloroplast genome phylogenies: sampling one’s way out of the felsenstein zone. Mol. Biol. Evol. 22, 1948–1963. doi: 10.1093/molbev/msi191
Li, H. T., Yi, T. S., Gao, L. M., Ma, P. F., Zhang, T., Yang, J. B., et al. (2019). Origin of angiosperms and the puzzle of the Jurassic gap. Nat. Plants 5, 461–470. doi: 10.1038/s41477-019-0421-0
Li, J., Liu, H. Y., Xie, D. F., Zhou, S. D., and He, X. J. (2018). The complete chloroplast genome of Notholition macrophyllum. Mitochondrial DNA B 3, 1102–1103. doi: 10.1080/23802359.2018.1463825
Li, J., Zhou, S. D., Yang, M., Xie, D. F., and He, X. J. (2020). Notholirion campanulatum is co-specific with N. bulbuliferum (Liliaceae) based on morphology and molecular data. Phytotaxa 471, 234–246. doi: 10.11646/phytotaxa.471.3.5
Lindqvist, J. K., and Lee, D. E. (2009). High-frequency paleoclimate signals from Foulden Maar, Waipiata Volcanic Field, Southern new Zealand: an Early Miocene varved lacustrine diatomite deposit. Sediment. Geol. 222, 98–110. doi: 10.1016/j.sedgeo.2009.07.009
Liu, H. Y., Yu, Y., Deng, Y. Q., Li, J., Huang, Z. X., and Zhou, S. D. (2018). The chloroplast genome of Lilium henrici: genome structure and comparative analysis. Molecules 23:1276. doi: 10.3390/molecules23061276
Lkinci, N., Oberprieler, C., and Guner, A. (2006). On the origin of European lilies: phylogenetic analysis of Lilium section Liriotypus (Liliaceae) using sequences of the nuclear ribosomal transcribed spacers. Willdenowia 36, 647–656. doi: 10.3372/wi.36.36201
Lu, R. S., Chen, Y., Tamaki, I., Sakaguchi, S., Ding, Y. Q., Takahashi, D., et al. (2020). Pre-quaternary diversification and glacial demographic expansions of Cardiocrinum (Liliaceae) in temperate forest biomes of Sino-Japanese Floristic Region. Mol. Phylogenet. Evol. 143:106693. doi: 10.1016/j.ympev.2019.106693
Lu, R. S., Yang, T., Chen, Y., Wang, S. Y., Cai, M., Cameron, K. M., et al. (2021). Comparative plastome genomics and phylogenetic analyses of Liliaceae. Bot. J. Linn. Soc. 196, 279–293. doi: 10.1093/botlinnean/boaa109
Luo, J., Li, L., and Kong, L. (2012). Preparative separation of phenylpropenoid glycerides from the bulbs of Lilium lancifolium by high-speed counter-current chromatography and evaluation of their antioxidant activities. Food Chem. 131, 1056–1062. doi: 10.1016/j.foodchem.2011.09.112
MacGinitie, H. D. (1941). A Middle Eocene Flora from the Central Sierra Nevada. Washington: Carnegie Institution of Washington Publication.
Matzke, N. (2013). Probabilistic historical biogeography: new models for founder-event speciation, imperfect detection, and fossils allow improved accuracy and model-testing. Front. Biogeogr. 5:242–248. doi: 10.21425/F55419694
Mennes, C. B., Lam, V. K. Y., Rudall, P. J., Lyon, S. P., Graham, S. W., Smets, E. F., et al. (2015). Ancient Gondwana break-up explains the distribution of the mycoheterotrophic family Corsiaceae (Liliales). J. Biogeogr. 42, 1123–1136. doi: 10.1111/jbi.12486
Miller, W. B., and Langhans, R. W. (1990). Low temperature alters carbohydrate metabolism in easter lily bulbs. Hortscience 25, 463–465. doi: 10.21273/HORTSCI.25.4.463
Morita, H. (1932). On new species of the genera Cinnamomum and Smilax from the miocene deposits of Oguni-Machi, Uzen province, Japan. Japanese J. Geol. Geogr. 9, 1–8.
Mucciarelli, M., Rosso, P., Noble, V., Bartolucci, F., and Peruzzi, L. (2016). A morphometric study and taxonomic revision of Fritillaria tubaeformis complex (Liliaceae). Plant Syst. Evol. 302, 1329–1343. doi: 10.1007/s00606-016-1334-2
Munkemuller, T., Lavergne, S., Bzeznik, B., Dray, S., Jombart, T., Schiffers, K., et al. (2012). How to measure and test phylogenetic signal. Methods Ecol. Evol. 3, 743–756. doi: 10.1111/j.2041-210X.2012.00196.x
Muratovic, E., Hidalgo, O., Garnatje, T., and Siljak-Yakovlev, S. (2010). Molecular phylogeny and genome size in European lilies (genus Lilium, Liliaceae). J. Comput. Theor. Nanos. 3, 180–189. doi: 10.1166/asl.2010.1116
Mustafa, L. H., and Abdul-Razaq, R. T. (2015). A comparative systematic study of the genus Fritillaria l. (Liliaceae) in Iraqi Kurdistan Region. Adv. Bio. 6, 14–24. doi: 10.15515/abr.0976-4585.6.4.14-24
Nguyen, L.-T., Schmidt, H. A., Haeseler, A. V., and Minh, B. Q. (2015). IQ-TREE: a fast and effective stochastic algorithm for estimating maximum-likelihood phylogenies. Mol. Biol. Evol. 32, 268–274. doi: 10.1093/molbev/msu300
Nursel, I. (2011). Molecular phylogeny and divergence times estimates of Lilium L. section Liriotypus Asch. et Graebn. (Liliaceae) based on plastid and nuclear ribosomal ITS DNA sequence data. Turk. J. Bot. 35, 319–330. doi: 10.3906/bot-1003-29
Ohara, M., Narumi, T., Yoshizane, T., Okayasu, T., Masuda, J., and Kawano, S. (2006). Life-history monographs of Japanese plants. 7: Cardiocrinum cordatum (Thunb.) Makino (Liliaceae). Plant Spec. Biol. 21, 201–207. doi: 10.1111/j.1442-1984.2006.00166.x
Otto, S. P., and Gerstein, A. C. (2006). Why have sex? The population genetics of sex and recombination. Biochem. Soc. T. 34, 519–522. doi: 10.1042/BST0340519
Patterson, T. B., and Givnish, T. J. (2002). Phylogeny, concerted convergence, and phylogenetic niche conservatism in the core Liliales: insights from rbcL and ndhF sequence data. Evolution 56, 233–252.
Pelkonen, V. P., Niittyvuopio, A., Pirttila, A. M., Laine, K., and Hohtola, A. (2010). Phylogenetic background of orange lily (Lilium bulbiferum s.l.) cultivars from a genetically isolated environment. Plant Biol. 9, 534–540. doi: 10.1055/s-2007-965042
Peruzzi, L. (2016). A new infrafamilial taxonomic setting for Liliaceae, with a key to genera and tribes. Plant Biosyst. 150, 1341–1347. doi: 10.1080/11263504.2015.1115435
Peruzzi, L., Leitch, I. J., and Caparelli, K. F. (2009). Chromosome diversity and evolution in Liliaceae. Ann. Bot. 103, 459–475. doi: 10.1093/aob/mcn230
Peruzzi, L., Peterson, A., Tison, J. M., and Harpke, D. (2012). New light on phylogeny and taxonomy of the Eurasian Gagea villosa-G. fragifera complex (Liliaceae). Nord. J. Bot. 29, 722–733. doi: 10.1111/j.1756-1051.2011.01187.x
Petersen, G., Seberg, O., and Davis, J. I. (2013). Phylogeny of the Liliales (Monocotyledons) with special emphasis on data partition congruence and RNA editing. Cladistics 29, 274–295. doi: 10.1111/j.1096-0031.2012.00427.x
Peterson, A., Harpke, R., Peterson, J., Harpke, A., and Peruzzi, L. (2019). A pre-Miocene Irano-Turanian cradle: origin and diversification of the species-rich monocot genus Gagea (Liliaceae). Ecol. Evol. 9, 5870–5890. doi: 10.5061/dryad.97np7bt
Posada, D. (2008). jModel test: phylogenetic model averaging. Mol. Biol. Evol. 25, 1253–1256. doi: 10.1093/molbev/msn083
Qu, X. J., Moore, M. J., Li, D. Z., and Yi, T. S. (2019). PGA: a software package for rapid, accurate, and flexible batch annotation of plastomes. Plant Methods 15, 1–12. doi: 10.1186/s13007-019-0435-7
Raman, G., and Park, S. (2016). The complete chloroplast genome sequence of Ampelopsis: gene organization, comparative analysis, and phylogenetic relationships to other angiosperms. Front. Plant Sci. 7:341. doi: 10.3389/fpls.2016.00341
Raymo, M. E., and Ruddiman, W. F. (1992). Tectonic forcing of late Cenozoic climate. Nature 359, 117–122.
Renner, S. S. (2016). Available data point to a 4-km-high Tibetan Plateau by 40 ma, but 100 molecular-clock papers have linked supposed recent uplift to young node ages. J. Biogeogr. 43, 1479–1487. doi: 10.1111/jbi.12755
Rix, E. M. (2001). Fritillaria: A Revised Classification Together With an Updated List of Species. London: The Fritillaria Group of the Alpine Garden Society Press.
Ronquist, F., Teslenko, M., Mark, P. V. D., Ayres, D. L., Darling, A., Höhna, S., et al. (2012). MrBayes 3.2: Efficient Bayesian phylogenetic inference and model choice across a large model space. Syst. Biol. 61, 539–542. doi: 10.1093/sysbio/sys029
Ronsted, N., Law, S., Thornton, H., Fay, M. F., and Chase, M. W. (2005). Molecular phylogenetic evidence for the monophyly of Fritillaria and Lilium (Liliaceae; Liliales) and the infrageneric classification of Fritillaria. Mol. Phylogenet. Evol. 35, 509–527. doi: 10.1016/j.ympev.2004.12.023
Ross, T. G., Barrett, C. F., Gomez, M. S., Lam, V. K. Y., Henriquez, C. L., Les, D. H., et al. (2016). Plastid phylogenomics and molecular evolution of Alismatales. Cladistics 32, 160–178. doi: 10.1111/cla.12133
Sancho, R., Cantalapiedra, C. P., Lopez-Alvarez, D., Gordon, S. P., Vogel, J. P., Catalan, P., et al. (2017). Comparative plastome genomics and phylogenomics of Brachypodium: flowering time signatures, introgression and recombination in recently diverged ecotypes. New Phytol. 218, 1631–1644. doi: 10.1111/nph.14926
Shin, K. S., Chakrabarty, D., and Paek, K. Y. (2002). Sprouting rate, change of carbohydrate contents and related enzymes during cold treatment of lily bulblets regenerated in vitro. Sci. Hortic. 96, 195–204. doi: 10.1016/S0304-4238(02)00087-0
Shoe, R. (1958). Phyllotaxis of kniphofia and lilium candidum. New Phytol. 57, 160–167. doi: 10.1111/j.1469-8137.1958.tb05302.x
Silvertown, J. (2008). The evolutionary maintenance of sexual reproduction: evidence from the ecological distribution of asexual reproduction in clonal plants. Int. J. Plant Sci. 169, 157–168. doi: 10.1086/523357
Soto Gomez, M., Lin, Q., Leal, E. D. S., Gallaher, T. J., Scherberich, D., Mennes, C. B., et al. (2020). A bi-organellar phylogenomic study of Pandanales: inference of higher-order relationships and unusual rate-variation patterns. Cladistics 36, 481–504. doi: 10.1111/cla.12417
Stamatakis, A. (2014). RAxML version 8: a tool for phylogenetic analysis and post-analysis of large phylogenies. Bioinformatics 30, 1312–1313. doi: 10.1093/bioinformatics/btu033
Stevenson, D. W., and Loconte, H. (1995). “Cladistic analysis of monocot families,” in Monocoteledons: Sysytematics and Evolution, eds P. J. Rudall, P. J. Cribb, D. F. Cutler, and C. J. Humphries (London: Royal Botanic Gardens), 543–578.
Su, D. M., Xie, F. M., Liu, H. Y., Xie, D. F., Li, J., He, X. J., et al. (2021). Comparative analysis of complete plastid genomes from Lilium lankongense Franchet and its closely related species and screening of Lilium-specific primers. Peer J 9:e10964. doi: 10.7717/peerj.10964
Suchard, M. A., Lemey, P., Baele, G., Ayres, D. L., Drummond, A. J., and Rambaut, A. (2018). Bayesian phylogenetic and phylodynamic data integration using BEAST 1.10. Virus Evol. 4:vey016. doi: 10.1093/ve/vey016
Takayama, S., and Misawa, M. (1980). Differentiation in Lilium bulbscales grown in vitro. effects of activated charcoal, physiological age of bulbs and sucrose concentration on differentiation and scale leaf formation in vitro. Physiol. Plantarum 48, 121–125. doi: 10.1111/j.1399-3054.1980.tb03229.x
Thiers, B. (2016). Index Herbariorum: A Global Directory of Public Herbaria and Associated Staff. New York, NY: Botanical Garden’s Virtual Herbarium.
Tu, T., Volis, S., Dillon, M. O., Hang, S., and Wen, J. (2010). Dispersals of Hyoscyameae and Mandragoreae (Solanaceae) from the New World to Eurasia in the early Miocene and their biogeographic diversification within Eurasia. Mol. Phylogenet. Evol. 57, 1226–1237. doi: 10.1016/j.ympev.2010.09.007
Tuyl, J. M. V., Arens, P., Shahin, A., Marasek-Ciołakowska, A., Barba-Gonzalez, R., Kim, H. T., et al. (2018). “Lilium,” in Ornamental Crops, Handbook of Plant Breeding, ed. V. H. Johan (Cham: Springer International Publishing AG), 481–512.
Wang, C. S., Dai, J. G., Zhao, X. X., Li, Y. L., Graham, S. A., He, D. F., et al. (2014). Outward-growth of the Tibetan Plateau during the Cenozoic: a review. Tectonophysics 621, 1–43. doi: 10.1016/j.tecto.2014.01.036
Wang, Q., Zhou, S. D., Deng, X. Y., Zheng, Q., and He, X. J. (2009). Comparative morphology of the leaf epidermis in Fritillaria (Liliaceae) from China. Bot. J. Linn. Soc. 160, 93–109. doi: 10.1111/j.1095-8339.2009.00855.x
Wietsma, W. A., Deinum, D., Teunissen, H. A. S., and Berg, R. G. V. D. (2015). Phylogenetic relationships within Fritillaria section Petilium based on AFLP fingerprints. Plant Syst. Evol. 301, 1043–1054. doi: 10.1007/s00606-014-1135-4
Wilde, V., and Frankenhauser, H. (1998). The Middle Eocene plant taphocoenosis from Eckfeld (Eifel, Germany). Rev. Palaeobot. Palyno. 101, 7–28.
Wilf, P. (2000). Late Paleocene-early eocene climate changes in southwestern Wyoming: Paleobotanical analysis. Geol. Soc. Am. Bull. 112, 292–307. doi: 10.1130/0016-76062000112<0292:LPEECC<2.3.CO;2
Xie, D. F., Yu, Y., Wen, J., Huang, J., Chen, J. P., Li, J., et al. (2020b). Phylogeny and highland adaptation of chinese species in Allium section Daghestanica (Amaryllidaceae) revealed by transcriptome sequencing. Mol. Phylogenet. Evol. 146:106737. doi: 10.1016/j.ympev.2020.106737
Xie, D. F., Tan, J. B., Yu, Y., Gui, L. J., Su, D. M., Zhou, S. D., et al. (2020a). Insights into phylogeny, age and evolution of Allium (Amaryllidaceae) based on the whole plastome sequences. Ann. Bot. 125, 1039–1055. doi: 10.1093/aob/mcaa024
Xie, D. F., Yu, H. X., Price, M., Xie, C., Deng, Y. Q., Chen, J. P., et al. (2019). Phylogeny of chinese Allium species in section Daghestanica and adaptive evolution of Allium (Amaryllidaceae, Allioideae) species revealed by the chloroplast complete genome. Front. Plant Sci. 10:460. doi: 10.3389/fpls.2019.00460
Xie, D. F., Yu, Y., Deng, Y. Q., Li, J., Liu, H. Y., Zhou, S. D., et al. (2018). Comparative analysis of the chloroplast genomes of the chinese endemic genus Urophysa and their contribution to chloroplast phylogeny and adaptive evolution. Int. J. Mol. Sci. 19:1847. doi: 10.3390/ijms19071847
Xing, Y. W., and Ree, R. H. (2017). Uplift-driven diversification in the Hengduan Mountains, a temperate biodiversity hotspot. Proc. Natl. Acad. Sci. U.S.A. 114, E3444–E3451. doi: 10.1073/pnas.1616063114
Yan, Z. Q., He, X. F., Guo, K., Li, X. Z., Yang, X. Y., Jin, H., et al. (2019). Allelochemicals from the rhizosphere of Lanzhou lily: discovery of the autotoxic compounds of a bulb crop. Sci. Hortic. 250, 121–126. doi: 10.1016/j.scienta.2019.02.038
Yang, L. Q., Hu, H. Y., Xie, C., Lai, S. P., Yang, M., He, X. J., et al. (2016). Molecular phylogeny, biogeography and ecological niche modelling of Cardiocrinum (Liliaceae): insights into the evolutionary history of endemic genera distributed across the Sino-Japanese floristic region. Ann. Bot. 119, 59–72. doi: 10.1093/aob/mcw210
Yu, Y., Blair, C., and He, X. J. (2020). RASP 4: ancestral state reconstruction tool for multiple genes and characters. Mol. Biol. Evol. 37, 604–606. doi: 10.1093/molbev/msz257
Yu, Y., Harris, A. J., Blair, C., and He, X. J. (2015). RASP (Reconstruct Ancestral State in Phylogenies): a tool for historical biogeography. Mol. Phylogenet. Evol. 87, 46–49. doi: 10.1016/j.ympev.2015.03.008
Keywords: Lilium, Fritillaria, divergence time estimate, QTP, Liliales, trait evolution
Citation: Li J, Cai J, Qin H-H, Price M, Zhang Z, Yu Y, Xie D-F, He X-J, Zhou S-D and Gao X-F (2022) Phylogeny, Age, and Evolution of Tribe Lilieae (Liliaceae) Based on Whole Plastid Genomes. Front. Plant Sci. 12:699226. doi: 10.3389/fpls.2021.699226
Received: 23 April 2021; Accepted: 08 December 2021;
Published: 01 February 2022.
Edited by:
Sean W. Graham, University of British Columbia, CanadaReviewed by:
Domingos Cardoso, Federal University of Bahia, BrazilTom Givnish, University of Wisconsin-Madison, United States
Copyright © 2022 Li, Cai, Qin, Price, Zhang, Yu, Xie, He, Zhou and Gao. This is an open-access article distributed under the terms of the Creative Commons Attribution License (CC BY). The use, distribution or reproduction in other forums is permitted, provided the original author(s) and the copyright owner(s) are credited and that the original publication in this journal is cited, in accordance with accepted academic practice. No use, distribution or reproduction is permitted which does not comply with these terms.
*Correspondence: Xing-Jin He, eGpoZUBzY3UuZWR1LmNu; Song-Dong Zhou, enNkQHNjdS5lZHUuY24=