- 1Fujian Provincial Key Laboratory for Plant Eco-Physiology, Fujian Normal University, Fuzhou, China
- 2College of Geographical Sciences, Fujian Normal University, Fuzhou, China
- 3State Key Laboratory for Subtropical Mountain Ecology of the Ministry of Science and Technology and Fujian Province, Fujian Normal University, Fuzhou, China
- 4College of Environmental Science and Engineering, Fujian Normal University, Fuzhou, China
- 5Department of Geography and Environmental Science, University of Reading, Reading, United Kingdom
- 6Department of Forestry, Fujian Forestry Vocational Technical College, Nanping, China
- 7College of Ecological and Resources Engineering, Wuyi University, Wuyishan, China
Sapindus mukorossi Gaertn., an important oleaginous woody plant, has garnered increasing research attention owing to its potential as a source of renewable energy (biodiesel). Leaf structural traits are closely related to plant size, and they affect the fruit yield and oil quality. However, plant size factors that predominantly contribute to leaf structural traits remain unknown. Therefore, the purpose of this study was to understand the associations between leaf structural traits and plant size factors in even-aged stands of S. mukorossi. Results showed that leaf length (LL) and leaf area (LA) markedly increased with the increasing diameter at breast height (DBH) and tree height (TH), although other leaf structural traits did not show noticeable changes. Difference in slopes also indicated that the degree of effect of plant size factors on leaf structural traits was in the order of TH > DBH. Leaf structural traits showed no systematic variation with crown width (CW). LA was significantly positively correlated with LL, leaf width (LW), LL/LW, and leaf thickness (LT) and was significantly but negatively correlated with leaf tissue density (LTD) and leaf dry mass content (LDMC). Specific leaf area showed a significantly negative correlation with LT, LDMC, and LTD. LTD showed a significantly positive correlation with LDMC, but a negative correlation with LT. The results were critical to understand the variability of leaf structural traits with plant size, can provide a theoretical foundation for further study in the relationship between leaf structural traits and fruit yield, and regulate leaf traits through artificial management measures to promote plant growth and fruit yield.
Introduction
Leaves are a vital component of the photosynthetic apparatus of a plant and play key roles in long-term adaptations to environmental changes (Liu et al., 2017; Guo et al., 2018; Song et al., 2018; Cai et al., 2020). Leaves are also critical for tree productivity due to their fundamental roles in carbon assimilation through photosynthesis and transpiration (Wright et al., 2004; Lai et al., 2016; Li et al., 2018). Leaf traits are currently gaining high research priority because they are closely linked to many pivotal aspects of growth, reproduction, and ecosystem functions (Garnier et al., 2001; Xue et al., 2012; Qin et al., 2019). Plant growth is driven by factors that affect resource (light, nutrients, and water) gain and utilization (Richards et al., 2010; Li et al., 2017). Leaf traits reflect both resource uptake strategies and resource use efficiency and are thus expected to affect plant growth (Fichtner et al., 2013; Li et al., 2017). Leaf traits as measurable characteristics represent ecological strategies and functional adaptations to environmental conditions (Pérez-harguindeguy et al., 2013; Petter et al., 2016; Qin et al., 2019; Bergholz et al., 2021). Key leaf structural traits include leaf length (LL), leaf width (LW), leaf thickness (LT), leaf area (LA), specific leaf area (SLA), and leaf dry mass content (LDMC), and others (Liu et al., 2006). Leaf structural traits reflect the resource acquisition under a long-term influence of external environment (Xue et al., 2012). LL, LW, and LL/LW are associated with leaf size and shape. LT responds in some way to the light capture and changes in the water status of leaf tissue (Seelig et al., 2015; Griffith et al., 2016). LA is a key variable for physiological studies involving plant growth, light interception, and photosynthetic efficiency (Kandiannan et al., 2009; Rouphael et al., 2010). SLA, as a measure of resource allocation, reflects the potential light capture per unit LA per unit organic matter content invested into leaves (Wright and Westoby, 1999). LDMC is a measure of leaf tissue density (LTD) and reflects the position of a plant on a resource use axis (Wilson et al., 1999). The associations among various leaf traits reflect the plant adaptation under a given constraint or other environmental constraints (Qin et al., 2019). Increasing evidence indicates that leaf traits are highly plastic at various growth stages (Thomas and Ickes, 1995). For example, in Populus euphratica, LT, LA, and LDMC showed a gradually increasing trend, and the SLA showed a decreasing trend with plant growth (Huang et al., 2010). In the same species, LL and LL/LW showed a decreasing trend and the LW showed an increasing trend with increasing tree age (Wang et al., 2019). In some evergreen trees, older leaves showed a significantly greater LA and LDMC but lower SLA than younger leaves (Huang et al., 2013). In Betula platyphylla, LT, SLA, and LDMC showed significant differences between adult trees and saplings at different growth stages (Jin et al., 2018).
Diameter at breast height (DBH) and tree height (TH) are the commonly used measures of tree growth (Sumida et al., 2013), and these are important variables used in forest inventories and management as well as in the forest carbon stock estimation (Li et al., 2015). Crown width (CW) is commonly used to estimate the aboveground biomass of forests and is often regarded as an important indicator of tree growth (Fichtner et al., 2013). These variables directly reflect the plant size and are used as plant size factors. Variation in leaf traits with plant size reflects plant life history and biomass allocation patterns (Huang et al., 2013). Liu et al. (2010) conducted a basic study of differences in leaf traits between small (woody plants shorter than 2 m) and large (woody plants taller than 10 m) trees based on TH. Their study indicated that SLA was higher in small trees than that in large trees (Liu et al., 2010). Along with the DBH growth, the LT, LA, and LDMC of P. euphratica showed a gradually increasing trend, while the SLA showed a decreasing trend (Huang et al., 2010). However, some studies showed that the size and functional traits of leaves are invariant with plant size (West et al., 1999). Most leaf functional traits are substantially less variable than plant size, show little to no systematic variation. However, individual LA itself increases modestly with plant size (Price et al., 2014). DBH is closely related to CW and TH (Dai et al., 2009; Li et al., 2015) because they both serve as general proxies for plant size. However, less is known regarding the specific associations between different plant size factors and leaf structural traits in even-aged forests, although this information may be critical to understand plant life history patterns (Liu et al., 2010).
Sapindus mukorossi Gaertn. is a deciduous oleaginous woody tree, which is widely distributed in the tropical and subtropical regions of Asia (Zhang et al., 2019). Its fruit pericarp contains saponin, which is an efficient natural surfactants used for developing high-quality shampoos and detergents (Bahar and Singh, 2007; Attri et al., 2015; Bhatta et al., 2021). The oil content of its seeds and kernels is high, and the seed oil meets the standards for biodiesel production, making it as a source of bioenergy (Zhao et al., 2014). Therefore, S. mukorossi is considered a valuable and promising industrial crop owing to the high content and quality of its seed oil (Zhang et al., 2019). Previous studies have mainly focused on S. mukorossi breeding, genetic diversity, biochemical analyses for identifying varieties that produce high seed oil and saponin yield (Shao et al., 2013; Diao et al., 2014; Sun et al., 2017), extraction techniques of saponins and its application in industry (Singh and Sharma, 2019a) and medicine (Huang et al., 2008; Li et al., 2013; Singh and Kumari, 2015; Singh and Sharma, 2019b; Zhao et al., 2021), and extraction techniques for seed kernel oil (Liu et al., 2019; Hu et al., 2021). Leaf size and shape can predict single fruit weight and other qualitative traits and can therefore be used as indicators for evaluating fruit quality (Jin et al., 2016; Long et al., 2017; Rowland et al., 2020). The saponins yield and oil content are important fruit traits. Studies have shown that the effect of plant size on the seed oil content of S. mukorossi was in the order of TH>DBH>CW, and the effect of plant size factor on the saponin content of S. mukorossi decreased in the order of DBH>TH>CW (Fan et al., 2013, 2014). The size of an oleaginous tree affects its fruit yield and oil quality to a certain extent (Wu et al., 2012; Guo et al., 2014). The inherent association between leaf traits and fruit characteristics has been explored in some previous studies (Wang et al., 2014; Zhang et al., 2020a). Therefore, leaf structural traits may also affect the fruit yield and oil quality of oleaginous trees. Although previous studies on leaf traits have laid a foundation for the selection of excellent fruit quality traits, no study has assessed the leaf structural traits of plants of different sizes in an even-aged forest in this species. In homogeneous even-aged bioenergy plantations, the plant size factors directly reflect the plant size and possibly indicate their effects on fruit yield and oil quality. In this study, we measured various leaf structural traits and assessed their associations with three plant size factors in S. mukorossi. The main objectives are to determine (1) whether there are notable correlations between leaf structural traits with plant size; (2) whether DBH, TH, and CW had consistent effects on leaf structural traits; and (3) which plant size factors directly reflect more leaf structural traits. By addressing these questions, we were able to understand the potentiality and sensibility of S. mukorossi leaf structural traits responding to plant size. Our findings are of great significance to provide a basis for studying the associations of leaf traits with fruit yield and quality, be helpful for predicting the yield to select superior individuals, and provide a theoretical basis for regulating plant growth and improving fruit yield with artificial management measures regulating the leaf traits of oleaginous trees.
Materials and Methods
Study Area
The field experiment was conducted in Nanping city, Yanping District (26°29′N, 118°13′E), northwest Fujian Province, southeast China. This region is characterized by a subtropical humid monsoon climate, with a mean annual temperature of 19.3°C, mean annual precipitation of 1,669 mm, and relative humidity of 82%. Summers are long, hot, and rainy, while winters are short and moderate. The soil in this zone is classified as red soil as per the Chinese Soil Taxonomic Classification (Fan et al., 2015). The initial total soil C, N, and P contents were 17.49, 1.12, and 0.45 g/kg, respectively. The S. mukorossi forest was established at an altitude of 120 m. The planting density was approximately 1,100 plants per hectare in 2013. Mean TW, DBH, and CW were 5.05 ± 1.26 m, 6.79 ± 2.53 cm, and 3.80 ± 1.04 m, respectively.
Experimental Design
This study was performed during the reproductive growth period during October 2019. Three 25.82 × 25.82 m sample plots were established, with a 10-m-wide buffer zone separating the plots. DBH (≥2 cm), CW, and TH were measured and recorded for all the trees. Ten trees were selected in each plot, and the distribution of plant characteristics was determined in 30 trees. The selected trees were categorized into individual size factor classes (DBH, CW, and TH) based on the upper limit exclusion method (Zhang et al., 2015a). The DBH classes were formed based on the second (1–2.9 cm), fourth (3–4.9 cm), sixth (5–6.9 cm), eighth (7–8.9 cm), tenth (9–10.9 cm), and twelfth (11–12.9 cm)-order diameters. The TH classes were formed based on the third (2.5–3.4 m), fourth (3.5–4.4 m), fifth (4.5–5.4 m), sixth (5.5–6.4 m), and seventh (6.5–7.4 m)-grade heights. The CW classes were formed based on the values at the 2.75th (<3 m), 3.25th (3–3.49 m), 3.75th (3.5–3.99 m), 4.25th (4–4.49 m), 4.75th (4.5–4.99 m), and 5.25th (>5 m) CW stages (Zhang et al., 2015a). The distribution of plant size values of the S. mukorossi forest was normal (Figure 1).
Sampling and Data Collection
Pinnately compound leaves were collected from the east-, west-, north-, and south-facing sides of the middle-outer crown of each selected plant using a lopping machine. Eight fully expanded, mature, and healthy leaves were selected per plant and were taken to the laboratory. A single relatively complete, moderate-sized, and well-grown representative leaflet was selected for each pinnately compound leaf.
For each sample, leaf fresh weight (LFW, g) was measured immediately using an electronic balance. The LL (cm, the maximum value along the midrib), LW (cm, the maximum value perpendicular to the midrib), and LA (cm2) were measured by scanning the leaves (Epson Perfection V30) and analyzing the scans using the ImageJ software (National Institutes of Health, Bethesda, MD, United States). The LT was measured by using the Vernier calipers (precision of 0.01 mm) at three points on the upper, middle, and lower sides at an intermediate position between the leaf margin and midrib (avoiding the main vein), and the average LT value of each leaf was calculated. The leaves were then soaked in deionized water for over 12 h in a ziplock bag, and the leaf saturated fresh weight (LSFW, g) was measured after absorbing water from the surface. All leaves were oven-dried at 70°C for 48 h to a constant mass, and the leaf dry mass (LDM, g) was measured.
Leaf shape index, SLA, LDMC, LTD, and leaf relative water content (LRWC) were calculated using the following formulas (Zhou et al., 2017; Yu et al., 2018):
where LL is the leaf length (cm), LW is the leaf width (cm), LA is the leaf area (cm2), LDM is the leaf dry mass (g), LSFW is the leaf saturated fresh weight (g), LFW is the leaf fresh weight (g), and LT is the leaf thickness (cm).
Statistical Analysis
The relationships between leaf structural traits and plant size factors (e.g., DBH, TH, and CW) were analyzed by using Model II Standardized Major Axis (SMA) regression as follows:
To linearize the relationship, this formula was log-transformed to obtain the following equation:
where y is the leaf structural traits, x refers to the plant size factor, a is the normalization constant (the intercept), and b is the scaling exponent (the slope). When b = 1, the equation describes an isometric relationship; when b ≠ 1, the equation describes an allometric relationship. Model Type II regression was used to determine the numerical values of a and b using the package “smatr” in R.3.6.1 (R Core Team, 2019). When the data among different groups showed no significant numerical differences for the scaling exponents (the slope, b), a common scaling exponent was calculated (Warton et al., 2006, 2012). The common slope was calculated from a pooled variance/covariance matrix. The significance level for testing slope heterogeneity was p < 0.05. In addition, the correlations among key leaf structural traits were examined by using Pearson's correlation analysis. Pearson's correlation coefficients for all leaf structural traits were calculated using the “corrplot” package in R.3.6.1.
Results
Leaf Structural Traits and Plant Size
LL and LA were significantly and positively correlated with DBH (p < 0.05), while there were no significant correlation between the LW, LL/LW, LT, SLA, LDMC, LTD, and LRWC with DBH (p > 0.05). LL, LL/LW, and LA showed a modest increase with TH (p < 0.05), and there were no significant correlation between LW, LT, SLA, LDMC, LTD, and LRWC with TH (p > 0.05); these relationships showed low R2 values. The steeper slopes of the relationships between LL, LL/LW, and LA with TH and with DBH indicate that LL, LL/LW, and LA increase more rapidly with TH than those with DBH. All leaf structural traits approach invariance with CW, indicating that it is not associated noticeably with leaf structural traits (Table 1 and Figure 2).
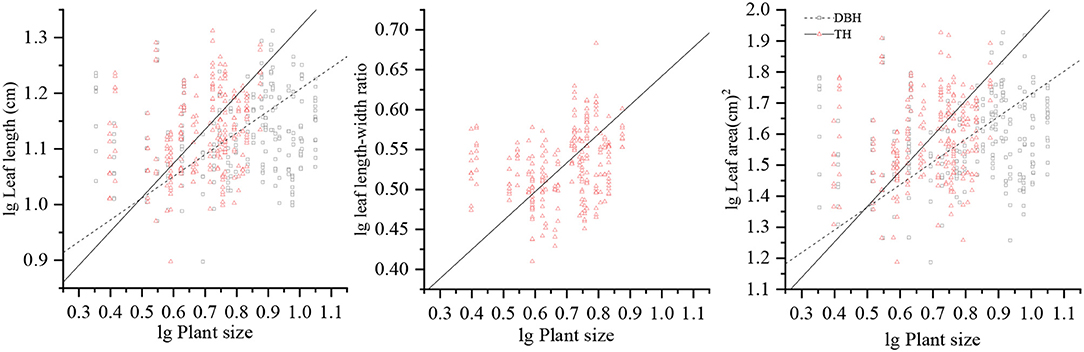
Figure 2. Allometric relationships of LL, LL/LW, and LA with respect to DBH and TH. LL, leaf length; LW, leaf width; LL/LW, leaf length–leaf width ratio; LA, leaf area; DBH, diameter at breast height; TH, tree height.
Associations Among Leaf Structural Traits
Leaf traits are not independent of each other; therefore, correlations among leaf structural traits have been observed. LL was significantly and positively correlated with LW, LL/LW, LT, and LA and significantly but negatively correlated with LDMC and LTD. LW was significantly and positively correlated with LA and LT and significantly but negatively correlated with LL/LW, LDMC, and LTD. LL/LW was significantly and positively correlated with LA and SLA. LA was positively correlated with LT but negatively correlated with LDMC and LTD. SLA was significantly but negatively correlated with LT, LDMC, and LTD. LT was negatively correlated with LTD. LDMC was positively correlated with LTD (Figure 3).
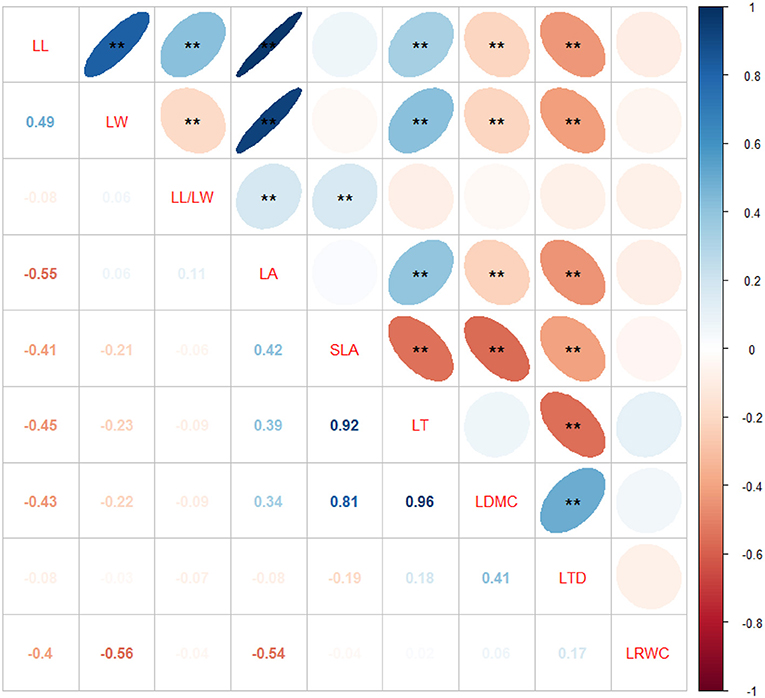
Figure 3. Correlations among leaf structural traits of Sapindus mukorossi. Correlation coefficients of the blue and red ellipses are positive and negative, respectively. Darker the color and smaller the area of the ellipse, greater the degree of correlation, and larger the absolute value of correlation coefficient. Variable names are on the diagonal. **p < 0.01.
Discussion
Effects of TH, DBH, and CW on Leaf Structural Traits
Leaf traits may reflect adaptations to environmental variations and can thus elucidate the associations between environmental drivers and ecosystem functions (Guo et al., 2020). Leaf traits are significantly correlated with plant growth and development. Previous studies indicated that the pine nut yield had a linear correlation with plant crown size (Zhang et al., 2015b), and the inherent association between leaf traits and fruit characteristics has also been found. Assessing the leaf structure characteristics of oleaginous trees of different sizes can help to better regulate leaf traits and improve fruit yield. In general, LL, LW, LL/LW, and LA can accurately reflect the leaf shape and size, and changes, which may represent adaptive strategies of plants to the changing environments. Collectively, our results suggest that LL, LL/LW, and LA vary as a function of TH or DBH, and this is consistent with previous evaluations (Fonseca et al., 2000; Wright et al., 2007; Price et al., 2014). The variability in LL in short trees reflects the adaptability of leaves to extrinsic micro- and macroenvironmental factors and stresses (Jensen and Zwieniecki, 2013). Jensen and Zwieniecki (2013) focused only on trees taller than 20 m and proposed that the factors limiting leaf size can be understood by subjecting the plants to physical constraints imposed by intrinsic (biological and geometrical) properties of the carbohydrate transport network. The trees included in our study were not large enough (shorter than 10 m), so without the restriction of water potential transport, longer sieve tubes of leaves enable somewhat greater transport efficiency and better nutrient uptake. Meanwhile, LA can change the photosynthetic capacity by affecting the optical light interception efficiency, and plants can increase the total photosynthetic area by increasing LA to promote plant growth. However, the positive correlation between leaf size and plant size was reversed in other studies (Price et al., 2014; Wang et al., 2019). A possible explanation for the reversed trend may be the greater support and hydraulic costs of larger leaves (Price et al., 2014). In P. euphratica, LL and LL/LW decreased with plant growth (Wang et al., 2019), and changes in leaf shape may be an adaptive strategy to the desert environments. Another reason for the different result may be the variation in tree species or habitats. We observed a decrease in the amount of variance for each functional trait with DBH than that with TH. Difference in slopes indicated that the effects of plant size factors on leaf structural traits were in the order of TH > DBH. As TH increases, the volume and depth of soil explored by the root system also increase; therefore, taller trees can take up more nutrients to supply to their leaves (Liu et al., 2010). Some studies have shown that the root system of taller plants is more competitive and can acquire more resources (Thomas and Winner, 2002). They also showed greater LA to enhance optical radiation absorption, improve photosynthesis, and increase biomass for survival and growth. However, shorter trees usually have shallower roots and lower trunk runoff; therefore, they cannot acquire sufficient light and water (Ou and Liu, 2017). The leaf structural traits that we evaluated showed no systematic variation with CW. The major reason may be that leaf structural traits were linked to canopy thickness, permeability of the canopy, and leaf area index (Lü et al., 2007). Moreover, CW was in close relation with planting density, indicating that planting density has no significant influences on leaf structural traits (Gong et al., 2010).
The leaf thickness reflects plant resource acquisition and water conservation. SLA can be used as an indicator of the carbon acquisition strategy as well as of the ability of the plant to acquire resources, such as light, and protect itself under strong light (Zhang et al., 2020b). LDMC can be used to characterize the ability of the plant to conserve nutrients and resist physical damage (Zhang et al., 2016). LTD is related to plant drought tolerance and damage resistance (Guo et al., 2020). LRWC is closely related to photosynthesis and water use efficiency, and the photosynthetic rate could be improved by reducing LRWC (Duan et al., 2017). These traits did not change significantly with plant size. These trends are contrary to those reported in other studies (Huang et al., 2010; Zhang et al., 2020b). For P. euphratica, the LT and LDMC showed a gradually increasing trend and the SLA showed a decreasing trend with plant growth (Huang et al., 2010; Wang et al., 2019). Increases in LT, developed palisade tissue, cuticle thickness, and a number of mucus cells reduce water loss in plants to a certain extent by decreasing the transpiration through leaves and improving the water retention capacity (Huang et al., 2010). In a previous study in a tropical montane rainforest, the SLA of 127 small individuals (woody plants shorter than 2 m) and 47 large trees (woody plants taller than 10 m) was measured; SLA was higher in small individuals than that in large trees (Liu et al., 2010). A small SLA in large individuals can be attributed to their responses to the changing light competition and water conditions. Our results may also be because of the difference in survival environment; S. mukorossi is distributed in the tropical and subtropical regions, indicating the absence of water scarcity. Another possible explanation is the inclusion of different tree species in various studies.
Overall, there were no definite trends of changes in leaf structural traits with different plant size factors, except for the positive correlations of TH and DBH with LL, LL/LW, and LA. CW also has little explanatory power with respect to leaf structural traits. Looking at the variance also suggests that the leaf traits we examined displayed consistently low variance, with shallow slopes and low R2 values. This may be explained by several reasons. First, S. mukorossi is a deciduous tree, and the collected leaves were current-year leaves of even-aged stands. Studies have found that the leaf shape index and water content did not differ significantly between the small and large current-year fruiting twigs in Morus alba L. (Wang et al., 2014). Moreover, since the leaves were selected during the same reproductive growth period, the photosynthetic products were gradually shifted to the reproductive organs to ensure their growth for normal flowering and fruiting, and the leaf growth became stagnant (Zhang et al., 2016). In our study, DBH was significantly and positively correlated with CW and TH (Figure 4), and an increase in the DBH and TH increased the total biomass. Some biomass estimation models based on CW, DBH, and TH have also been established (Xu et al., 2013; Dong et al., 2015). Therefore, individual biomass likely increased with plant growth, although leaf traits did not change significantly with increasing DBH, TH, and CW. As the leaf biomass increases, LA becomes larger and more light can be received, and as the light energy utilization becomes more efficient, more photosynthetic products can be accumulated.
Correlations Among Leaf Structural Traits
Leaf structural traits do not function independently and are correlated with one another. The relationships among leaf traits are closely linked to plant life history patterns. LL, LW, and LL/LW can accurately reflect the leaf shape. LA represents the contact area between leaves and the external environment, and this trait is affected by the balance of gas and energy exchange between the plants and the atmosphere. In this study, LA was positively correlated with LL, LW, LL/LW, and LT and negatively correlated with LTD and LDMC. In general, plants with a larger LA have thicker leaves, which prevents the heat exchange between leaves and the surrounding air and slows the diffusion rates of water vapor and CO2 through the leaves. Many studies have reported a positive correlation between LA and LT, but trends of association of LA with LDMC, LTD, and LRWC vary across studies (Zhang et al., 2016; Duan et al., 2017; Yu et al., 2018). These results indicate that correlations among leaf traits vary across research scales, plant species, and habitats.
SLA and LDMC are important leaf traits, which can reflect the survival strategies of plants for adapting to changing environments. Our study showed that SLA was significantly but negatively correlated with LT, LDMC, and LTD. These trends are consistent with previous reports (Huang et al., 2010; Guo et al., 2020) and indicate that S. mukorossi balances different leaf functional traits to adapt to the environment. Plants with a smaller SLA usually possess leaves with a greater LT (Reich et al., 1998). LT reflects the strategies of the plant for acquiring and utilizing resources. The thinner the leaves, the faster the plant growth and the stronger the light interception ability. For plants with a smaller SLA, a larger part of the materials in leaves is used to construct a protective structure or increase the density of mesophyll cells; thus, these plants often possess thicker leaves (Huang et al., 2010). From the perspectives of survival strategies and energy required to develop organs, if the plants use more energy to build the defense structures of the leaves, there is no additional energy to increase LA (Huang et al., 2010). Therefore, there was no significant correlation observed between LA and SLA in this study. However, many studies have reported a significant positive or negative correlation between LA and SLA (Zhang et al., 2016; Huang et al., 2020). LDMC reflects LTD as well as ecological functions and resource acquisition of plants (Dao et al., 2016). Our data demonstrated that LDMC was positively correlated with LTD (Cornelissen et al., 2003). Increased LDMC decreases the leaf water content, which in turn increases LTD. Increased LTD reduces the light transmission and decreases the photosynthetic capacity, which further reduces SLA. These results are consistent with the reports of Wang et al. (2015). LTD was significantly but negatively correlated with LT and LA, indicating that increases in LTD decreased LT and LA, thereby increasing LDMC. LRWC is closely linked to photosynthesis, and reduced LRWC can improve the water use efficiency and photosynthesis (Duan et al., 2017). In this study, the LRWC was not significantly correlated with any leaf traits. This may be because S. mukorossi grows in subtropical humid regions, where water is not a limiting factor.
The study has some potential limitations that should be addressed in future research. Leaf traits may exhibit considerable plasticity in response to environmental changes. Waigwa et al. (2020) found that LT had a positive correlation with soil phosphorous, and SLA had a strong negative relationship with soil total nitrogen. Morphological traits of tropical evergreen oaks were also correlated with the mean annual temperature, mean annual precipitation sum, and soil pH (Lin et al., 2021). However, environmental parameters were unavailable in this study. Meanwhile, leaf nutrient traits are also key functional traits, and focusing only on leaf structural traits might provide limited information. Therefore, the relationships between leaf traits (leaf structural traits and leaf nutrient traits) and environmental parameters (especially rhizosphere soil properties, temperature, and precipitation) should receive more attention in future research. In addition, leaf traits can predict fruit traits, and therefore, they can be used as indicators for evaluating fruit quality (Rowland et al., 2020). In hybrid pear, LA was significantly correlated with fruit vertical diameter and fruit type (Zhang et al., 2009). In Chinese bayberry, LL, LW, and LT played important roles in establishing the acid–sugar (AS) ratio (Liang et al., 2010). Further research is also warranted into the inherent association between leaf traits and fruit characteristics of S. mukorossi.
Conclusions
A key result of our study is that LL, LL/LW, and LA of the oleaginous deciduous broad-leaved woody tree S. mukorossi increased with increasing DBH and TH. Furthermore, TH has more explanatory with respect to leaf traits than DBH. TH was the key plant size factor affecting the leaf structural traits of S. mukorossi. All leaf structural traits did not vary significantly with increasing CW. LA was positively correlated with LL, LW, LL/LW, and LT and negatively correlated with LDMC and LTD. SLA showed a significantly negative correlation with LT, LDMC, and LTD. LDMC was positively correlated with LTD. The covariation indicated that S. mukorossi adjusted and balanced the combination of leaf structural traits in response to environmental changes. Considering that climate, soil, and topographic variables can influence leaf traits, future progress requires more research on the effects of environmental factors on leaf traits. The influence of leaf traits on fruit characteristics also differs among various species. Further studies are needed to investigate the inherent association between leaf traits and fruit characteristics of S. mukorossi to predict and increase fruit yield.
Data Availability Statement
The original contributions presented in the study are included in the article/supplementary material, further inquiries can be directed to the corresponding authors.
Author Contributions
YC: Data curation, investigation, methodology, software, visualization, and writing (original draft). CX: Conceptualization, investigation, methodology, visualization, and writing (review and editing). HY: Conceptualization and writing (review and editing). JZ: Conceptualization, data curation, and investigation. WH: Software and visualization. SZ: Data curation and investigation. QZ: Conceptualization, funding acquisition, investigation, and writing (review and editing). BL: Conceptualization, methodology, supervision, validation, and writing (review and editing). All authors contributed to the article and approved the submitted version.
Funding
This study was funded by the National Natural Science Foundation of China (31971643), the Industry-University Cooperation Project of Fujian Science and Technology Department (2020N5008, 2019N5009), Special Funding Project of Fujian Provincial Department of Finance (SC-299), the General Program of Natural Science Foundation of Fujian Province of China (2018J01737), and the Minjiang Scholar Program.
Conflict of Interest
The authors declare that the research was conducted in the absence of any commercial or financial relationships that could be construed as a potential conflict of interest.
Acknowledgments
We thank the Fujian Forestry Vocational Technical College for their help during the implementation of our study.
References
Attri, V., Pant, K. S., Dhiman, R., Lal, C., and Sarvade, S. (2015). Effect of seed size and pre-sowing treatments on growth parameters and biomass of Sapindus mukorossi (Gaertn) seedlings under nursery condition. Environ. Ecol. 33, 46–49.
Bahar, N., and Singh, V. R. R. (2007). Seed source selection of Sapindus mukorossi in Himachal Pradesh. Indian For. 133, 731–736.
Bergholz, K., Kober, K., Jeltsch, F., Schmidt, K., and Weiss, L. (2021). Trait means or variance—What determines plant species' local and regional occurrence in fragmented dry grasslands? Ecol. Evol. 11, 3357–3365. doi: 10.1002/ece3.7287
Bhatta, S., Joshi, L. R., Khakurel, D., and Bussmann, R. W. (2021). “Sapindus mukorossi Gaertn. Sapindaceae,” in Ethnobotany of the Himalayas, eds R. M. Kunwar, H. Sher, R. W. Bussmann (Cham: Springer), 1–9.
Cai, L. L., Sun, X. Y., Hao, D., Li, S. Y., Gong, X. Q., Ding, H., et al. (2020). Sugarcane bagasse amendment improves the quality of green waste vermicompost and the growth of Eisenia fetida. Front. Environ. Sci. Eng. 14, 63–73. doi: 10.1007/s11783-020-1240-2
Cornelissen, J. H. C., Lavorel, S., Garnier, E., Díaz, S., Buchmann, N., Gurvich, D. E., et al. (2003). A handbook of protocols for standardised and easy measurement of plant functional traits worldwide. Aust. J. Bot. 51, 335–380. doi: 10.1071/BT02124
Dai, F., Li, F. R., Jia, W. W., and Chen, Y. (2009). Research crown diameter and diameter at breast height relations of ten kinds of major natural secondary broad-leaved tree in Maoershan. Bull. Bot. Res. 29, 597–602. doi: 10.7525/j.issn.1673-5102.2009.05.016
Dao, R. N., Song, Y. T., Wu, Y. N., Huo, G. W., Wang, X. M., and Xu, Z. C. (2016). Response of plant leaf traits to grazing intensity in Stipa krylovii steppe. Chin. J. Appl. Ecol. 27, 2231–2238. doi: 10.13287/j.1001-9332.201607.034
Diao, S. F., Shao, W. H., Jiang, J. M., Dong, R. X., and Li, Y. J. (2014). Phenotypic traits of Sapindus mukorossi seedling population for association analysis. Bull. Bot. Res. 34, 458–464. doi: 10.7525/j.issn.1673-5102.2014.04.006
Dong, L. H., Li, F. R., and Song, Y. W. (2015). Error structure and additivity of individual tree biomass model for four natural conifer species in Northeast China. Chin. J. Appl. Ecol. 26, 704–714. doi: 10.13287/j.1001-9332.20150106.011
Duan, Y. Y., Song, L. J., Niu, S. Q., Huang, T., Yang, G. H., and Hao, W. F. (2017). Variation in leaf functional traits of different-aged Robinia pseudoacacia communities and relationships with soil nutrients. Chin. J. Appl. Ecol. 28, 28–36. doi: 10.13287/j.1001-9332.201701.036
Fan, H. B., Wu, J. P., Liu, W. F., Yuan, Y. H., Hu, L., and Cai, Q. K. (2015). Linkages of plant and soil C:N:P stoichiometry and their relationships to forest growth in subtropical plantations. Plant Soil. 392, 127–138. doi: 10.1007/s11104-015-2444-2
Fan, H. H., Yao, X. M., Zhang, T. Y., Lin, X. Q., Tang, X. H., and Ma, L. Z. (2013). The selection of high-saponi-yield plus tree of Sapindus mukorossi. J. Fujian For. Sci. Tech. 40, 69–73. doi: 10.3969/j.issn.1002-7351.2013.04.14
Fan, H. H., Zhang, T. Y., Yao, X. M., Lin, X. Q., Tang, X. H., and Ma, L. Z. (2014). Selection of superior individuals of Sapindus mukorossi for high yield of oil. J. Central South Univ. For. Technol. 34, 4–8. doi: 10.14067/j.cnki.1673-923x.2014.05.021
Fichtner, A., Sturm, K., Rickert, C., Von Oheimb, G., and Hardtle, W. (2013). Crown size-growth relationships of European beech (Fagus sylvatica L.) are driven by the interplay of disturbance intensity and inter-specific competition. For. Ecol. Manage. 302:178–184. doi: 10.1016/j.foreco.2013.03.027
Fonseca, C. R., Overton, J. M., Collins, B., and Westoby, M. (2000). Shifts in trait-combinations along rainfall and phosphorus gradients. J. Ecol. 88, 964–977. doi: 10.1046/j.1365-2745.2000.00506.x
Garnier, E., Laurent, G., Bellmann, A., Debain, S., Berthelier, P., Ducout, B., et al. (2001). Consistency of species ranking based on functional leaf traits. New Phytol. 152, 69–83. doi: 10.1046/j.0028-646x.2001.00239.x
Gong, N. N., Ma, L. Y., Jia, L. M., and Duan, J. (2010). Effects of different stand densities and site conditions on crown of Pinus tabulaeformis plantations in Beijing Mountain Area. J. Northeast For. Univ. 38, 9–12. doi: 10.13759/j.cnki.dlxb.2010.05.018
Griffith, D. M., Quigley, K. M., and Anderson, T. M. (2016). Leaf thickness controls variation in leaf mass per area (LMA) among grazing-adapted grasses in Serengeti. Oecologia. 181, 1035–1040. doi: 10.1007/s00442-016-3632-3
Guo, H. L., Li, Z. W., and Xiao, Z. S. (2014). Factors influencing the fruit crops and seed fates of Pistacia chinensis: the results of structural equation modeling. Biodiversity Sci. 22, 174–181. doi: 10.3724/SP.J.1003.2014.08139
Guo, W., Zhang, J., Qi, L. H., Shang, Z. A., Wang, R., and Yang, C. (2020). Leaf functional traits and influencing factors of Phyllostachys edulis and its varieties. Journal of Forest and Environment. 40, 260–268. doi: 10.13324/j.cnki.jfcf.2020.03.005
Guo, Z. W., Lin, H., and Chen, S. L. (2018). Altitudinal Patterns of Leaf Traits and Leaf Allometry in Bamboo Pleioblastus amarus. Front. Plant Sci. 9:1110. doi: 10.3389/fpls.2018.01110
Hu, B., Xi, X. H., Li, H. C., Qin, Y. X., Li, C., Zhang, Z. Q., et al. (2021). A comparison of extraction yield, quality and thermal properties from Sapindus mukorossi seed oil between microwave assisted extraction and Soxhlet extraction. Ind. Crop Prod. 161:113185. doi: 10.1016/j.indcrop.2020.113185
Huang, H. C., Wu, M. D., Tsai, W. J., Liao, S. C., Liaw, C. C., Hsu, L. C., et al. (2008). Triterpenoid saponins from the fruits and galls of Sapindus mukorossi. Phytochemistry. 69, 1609–1616. doi: 10.1016/j.phytochem.2007.10.033
Huang, H. X., Yang, X. D., Sun, B. W., Zhang, Z. H., and Yan, E. R. (2013). Variability and association of leaf traits between current-year and former-year leaves in evergreen trees in Tiantong, Zhejiang, China. Chin. J. Plant Ecol. 10, 912–921. doi: 10.3724/SP.J.1258.2013.00094
Huang, W. J., Li, Z. J., Yang, Z. P., Liang, J. Y., and Bai, G. Z. (2010). Heteromorphic leaf structural characteristics and their correlations with diameter at breast height of Populus euphratica. Chin. J. Ecol. 29, 2347–2352. doi: 10.13292/j.1000-4890.2010.0385
Huang, Y. R., Ma, Y. B., Hao, Y. G., Zhang, J. Q., Zhang, J. P., Li, S., et al. (2020). Study on leaf trait of poplar in protection forest at Ulan Buh Desert Oasis. J. Agric. Sci. Technol. 22, 42–50. doi: 10.13304/j.nykjdb.2019.0156
Jensen, K. H., and Zwieniecki, M. A. (2013). Physical limits to leaf size in tall trees. Phys. Rev. Lett. 110:018104. doi: 10.1103/PhysRevLett.110.018104
Jin, F. L., Zhang, F. W., Yue, X., Li, M., and Ao, X. X. (2016). Correlation between leaf size and fruit quality of Kiwi. Agric. Sci. Technol. 17, 2469-2472+2488. doi: 10.16175/j.cnki.1009-4229.2016.11.008
Jin, M. Y., Jiang, F., Jin, G. Z., and Liu, Z. L. (2018). Variations of specific leaf area in different growth periods and canopy positions of Betula platyphylla at different ages. Sci. Silvae Sin. 54, 18–26. doi: 10.11707/j.1001-7488.20180903
Kandiannan, K., Parthasarathy, U., Krishnamurthy, K. S., Thankamani, C. K., and Srinivasan, V. (2009). Modeling individual leaf area of ginger (Zingiber officinale Roscoe) using leaf length and width. Sci. Hortic. 120, 532–537. doi: 10.1016/j.scienta.2008.11.037
Lai, L., Huang, X., Yang, H., Chuai, X., Zhang, M., Zhong, T., et al. (2016). Carbon emissions from land-use change and management in China between 1990 and 2010. Sci. Adv. 2:e1601063. doi: 10.1126/sciadv.1601063
Li, Q., Ma, M., Wu, X., and Yang, H. (2018). Snow cover and vegetation-induced decrease in global albedo from 2002 to 2016. J. Geophys. Res. Atmos. 123, 124–138. doi: 10.1002/2017JD027010
Li, R., Wu, Z. L., Wang, Y. J., and Li, L. L. (2013). Separation of total saponins from the pericarp of Sapindus mukorossi Gaerten. by foam fractionation. Ind. Crops Prod. 51, 163–170. doi: 10.1016/j.indcrop.2013.08.079
Li, Y., Krober, W., Bruelheide, H., Hardtle, W., and Von Oheimb, G. (2017). Crown and leaf traits as predictors of subtropical tree sapling growth rates. J. Plant Ecol. 10, 136–145. doi: 10.1093/jpe/rtw041
Li, Y. Q., Deng, X. W., Huang, Z. H., Xiang, W. H., Yan, W. D., Lei, P. F., et al. (2015). Development and evaluation of models for the relationship between tree height and diameter at breast height for Chinese-fir plantations in Subtropical China. PLoS ONE. 10, 1–21. doi: 10.1371/journal.pone.0125118
Liang, S. M., Zhang, S. W., Zheng, X. L., Ren, H. Y., Zhu, T. T., and Qi, X. J. (2010). Correlation between growth indexes and fruit quality traits of Chinese bayberry (Myrica rubra sieb.et zucc.). J. Nucl. Agric. Sci. 33, 751–758. doi: 10.11869/j.issn.100-8551.2019.04.0751
Lin, Y. T., Kuang, L. H., Tang, S. B., Mou, Z. J., Phillips, O. L., Lambers, H., et al. (2021). Leaf traits from stomata to morphology are associated with climatic and edaphic variables for dominant tropical forest evergreen oaks. J. Plant Ecol. doi: 10.1093/jpe/rtab060
Liu, F. D., Yang, W. J., Wang, Z. S., Xu, Z., Liu, H., Zhang, M., et al. (2010). Plant size effects on the relationships among specific leaf area, leaf nutrient content, and photosynthetic capacity in tropical woody species. Acta Oecol. 36, 149–159. doi: 10.1016/j.actao.2009.11.004
Liu, J. H., Zeng, D. H., and Lee, D. K. (2006). Leaf traits and their interrelationships of main plant species in southeast Horqin sandy land. Chin. J. Ecol. 25, 921–925. doi: 10.13292/j.1000-4890.2006.0175
Liu, Z. L., Zhu, Y., Li, F. R., and Jin, G. Z. (2017). Non-destructively predicting leaf area, leaf mass and specific leaf area based on a linear mixed-effect model for broadleaf species. Ecol. Indic.78, 340–350. doi: 10.1016/j.ecolind.2017.03.025
Liu, Z. Z., Gui, M. L., Xu, T. T., Zhang, L., Kong, L. T., Qin, L., et al. (2019). Efficient aqueous enzymatic-ultrasonication extraction of oil from Sapindus mukorossi seed kernels. Ind. Crops Prod. 134, 124–133. doi: 10.1016/j.indcrop.2019.03.065
Long, J. C., Nuermaimaiti, A., Zhou, X. W., Jiao, H. M., Chen, Q., and Wang, X. J. (2017). The correlation relationship analysis about leaf and fruit traits of thin-skinned walnut in Xinjiang. J. Tarim Univ. 29, 46-54. doi: 10.3969/j.issn.1009-0568.2017.04.008
Lü, C. G., Zou, J. S., Hu, N., and Yao, K. M. (2007). Effects of leaf morphological factors on canopy structure and transmission of photosynthetically active radiation in rice. JiangSu J. Agric. Sci. 23, 501–508.
Ou, X. L., and Liu, Y. H. (2017). Effect of age, slope aspects and diameter classes on leaf functional traits of Pinus tabulaeformis in Songshan, Beijing. J. Nanjing For. Univ. Nat. Sci. Ed. 41, 80–88. doi: 10.3969/j.issn.1000-2006.201605021
Pérez-harguindeguy, N., Díaz, S., Garnier, E., Lavorel, S., Poorte, H., Jaureguiberry, P., et al. (2013). New handbook for standardised measurement of plant functional traits worldwide. Aust. J. Bot. 61, 167–234. doi: 10.1071/BT12225
Petter, G., Wagner, K., Wanek, W., Sánchez Delgado, E. J., Zotz, G., Cabral, J. S., et al. (2016). Functional leaf traits of vascular epiphytes: vertical trends within the forest, intra- and interspecific trait variability, and taxonomic signals. Funct. Ecol. 30, 188–198. doi: 10.1111/1365-2435.12490
Price, C. A., Wright, I. J., Ackerly, D. D., Niinemets, Ü., Reich, P. B., and Veneklaas, E. J. (2014). Are leaf functional traits 'invariant' with plant size and what is 'invariance' anyway? Funct. Ecol. 28, 1330–1343. doi: 10.1111/1365-2435.12298
Qin, J., Shangguan, Z., and Xi, W. (2019). Seasonal variations of leaf traits and drought adaptation strategies of four common woody species in South Texas, USA. J. For. Res. 30, 1715–1725. doi: 10.1007/s11676-018-0742-2
R Core Team (2019). R: A language and environment for statistical computing. R Foundation for Statistical Computing, Vienna, Austria. Available online at:https://www.R-project.org/ (accessed July 30, 2020).
Reich, P. B., Ellsworth, D. S., and Walters, M. B. (1998). Leaf structure (specific leaf area) modulates photosynthesis–nitrogen relations: evidence from within and across species and functional groups. Funct. Ecol. 12, 948–958. doi: 10.1046/j.1365-2435.1998.00274.x
Richards, A. E., Forrester, D. I., Bauhus, J., and Scherer-Lorenzen, M. (2010). The influence of mixed tree plantations on the nutrient of individual species: a review. Tree Physiol. 30, 1193–1208. doi: 10.1093/treephys/tpq035
Rouphael, Y., Mouneimne, A. H., Ismail, A., Gyves, M. D., Rivera, C. M., and Colla, G. (2010). Modeling individual leaf area of rose (Rosa hybrida L.) based on leaf length and width measurement. Photosynthetica 48, 9–15. doi: 10.1007/s11099-010-0003-x
Rowland, S. D., Zumstein, K., Nakayama, H., Cheng, Z. Z., Flores, A. M., Chitwood, D. H., et al. (2020). Leaf shape is a predictor of fruit quality and cultivar performance in tomato. New Phytol. 226, 851–865. doi: 10.1111/nph.16403
Seelig, H. D., Wolter, A., and Schröder, F. G. (2015). Leaf thickness and turgor pressure in bean during plant desiccation. Sci. Hortic. 184, 55–62. doi: 10.1016/j.scienta.2014.12.025
Shao, W. H., Diao, S. F., Dong, R. X., Jiang, J. M., and Yue, H. F. (2013). Study on geographic variation of morphology and economic character of fruit and seed of Sapindus mukorossi. For. Res. 5, 603–608. doi: 10.13275/j.cnki.lykxyj.2013.05.012
Singh, R., and Kumari, N. (2015). Comparative determination of phytochemicals and antioxidant activity from leaf and fruit of Sapindus mukorrossi Gaertn.—a valuable medicinal tree. Ind. Crops Prod. 73, 1–8. doi: 10.1016/j.indcrop.2015.04.012
Singh, R., and Sharma, B. (2019a). “Phytochemical analysis and pharmaceutical development from Sapindus spp.,” in Biotechnological Advances, Phytochemical Analysis and Ethnomedical Implication of Sapindus Species (Singapore: Springer), 55–88.
Singh, R., and Sharma, B. (2019b). “Pharmacological activities and medicinal implications of Sapindus spp.” in Biotechnological Advances, Phytochemical Analysis and Ethnomedical Implication of Sapindus Species (Singapore: Springer), 89–105.
Song, Z., Li, R., Qiu, R., Liu, S., Tan, C., Li, Q., et al. (2018). Global land surface temperature influenced by vegetation cover and PM2.5 from 2001 to 2016. Remote Sens. 10:2034. doi: 10.3390/rs10122034
Sumida, A., Miyaura, T., and Torii, H. (2013). Relationships of tree height and diameter at breast height revisited: analyses of stem growth using 20-year data of an even-aged Chamaecyparis obtusa stand. Tree Physiol. 33, 106–118. doi: 10.1093/treephys/tps127
Sun, C. W., Wang, J. W., Duan, J., Zhao, G. C., Weng, X. H., and Jia, L. M. (2017). Association of fruit and seed traits of Sapindus mukorossi germplasm with environmental factors in Southern China. Forests 8:491. doi: 10.3390/f8120491
Thomas, S. C., and Ickes, K. (1995). Ontogenetic changes in leaf size in Malaysian rain forest trees. Biotropica 27, 427–434. doi: 10.2307/2388954
Thomas, S. C., and Winner, W. E. (2002). Photosynthetic differences between saplings and adult trees: an integration of field results by meta-analysis. Tree Physiol. 22, 117–127. doi: 10.1093/treephys/22.2-3.117
Waigwa, A. N., Mwangi, B. N., Wahiti, G. R., Omengo, F., Zhou, Y., and Wang, Q. F. (2020). Variation of morphological and leaf stoichiometric traits of two endemic species along the elevation gradient of mount Kenya, East Africa. J. Plant Ecol. 13, 785–792. doi: 10.1093/jpe/rtaa067
Wang, W. J., Lv, H., Zhong, Y. M., Chen, L. J., Li, J. W., and Ma, Q. (2019). Relationship between heteromorphic leaf traits of Populus euphratica and its individual development. J. Beijing For. Univ. 41, 62–69. doi: 10.13332/j.1000-1522.20180364
Wang, X. J., Zhang, K., Xiao, D., and Hou, J. H. (2015). Leaf traits and their interrelationships of main plant species in Liangshui natural broadleaved Korean pine mixed forest. J. Central South Univ. For. Technol. 9, 52–58. doi: 10.14067/j.cnki.1673-923x.2015.09.009
Wang, Y., Xu, X., Liu, G., Qin, F., and Chen, M. H. (2014). Relationship between fruit characters and leaf features of current-year fruiting twigs of Morus alba L. Chin. Agric. Sci. Bull. 30, 46–52. doi: 10.11924/j.issn.1000-6850.2014-1718
Warton, D. I., Duursma, R. A., Falster, D. S., and Taskinen, S. (2012). Smatr 3-an R package for estimation and inference about allometric lines. Methods Ecol. Evol. 3, 257–259. doi: 10.1111/j.2041-210X.2011.00153.x
Warton, D. I., Wright, I. J., Falster, D. S., and Westoby, M. (2006). Bivariate line-fitting methods for allometry. Biol. Rev. 81, 259–291. doi: 10.1017/S1464793106007007
West, G. B., Brown, J. H., and Enquist, B. J. (1999). A general model for the structure and allometry of plant vascular systems. Nature 400, 664–667. doi: 10.1038/23251
Wilson, P. J., Thompson, K., and Hodgson, J. G. (1999). Specific leaf area and leaf dry matter content as alternative predictors of plant strategies. New Phytol. 143, 155–162. doi: 10.1046/j.1469-8137.1999.00427.x
Wright, I. J., Ackerly, D. D., Bongers, F., Harms, K. E., Ibarra-Manriquez, G., Martinez-Ramos, M., et al. (2007). Relationships among ecologically important dimensions of plant trait variation in seven Neotropical forests. Ann. Bot. 99, 1003–1015. doi: 10.1093/aob/mcl066
Wright, I. J., Reich, P. B., Westoby, M., Ackerly, D. D., Bamch, Z., Bongers, F., et al. (2004). The worldwide leaf economics spectmm. Nature 428, 821–827. doi: 10.1038/nature02403
Wright, I. J., and Westoby, M. (1999). Differences in seedling growth behavior among species: trait correlations across species, and trait shifts along nutrient compared to rainfall gradients. J Ecol. 87, 85–97. doi: 10.1046/j.1365-2745.1999.00330.x
Wu, Z. Z., Li, Y. Q., Wang, Z. J., Liu, Q. Z., and Zhang, Z. X. (2012). Analysis on influence factors of individual yield in Pistacia chinensis Bunge. Nonwood For. Res. 1, 23–27. doi: 10.14067/j.cnki.1003-8981.2012.01.025
Xu, C. B., Zhong, Q. L., Cheng, D. L., Hu, S. Z., Chang, Y. N., Zhang, Z., et al. (2013). Comparison of the regression models of Machilus pauhoi biomass based on C2H and D2H. J. South China Agric. Univ. 34, 543–547. doi: 10.7671/j.issn.1001-411X.2013.04.017
Xue, L., Zhang, R., Xi, R. C., Guo, S. H., Yang, Z. Y., Liu, B., et al. (2012). Seasonal change of leaf morphological traits of six broadleaf seedlings in South China. Acta Ecol. Sin. 32, 123–134. doi: 10.5846/stxb201011291691
Yu, H., Zhong, Q. L., Huang, Y. B., Cheng, D. L., Pei, P., Zhang, Z. R., et al. (2018). Relationships between leaf functional traits of Machilus pauhoi understory seedlings from different provenances and geographical environmental factors. Chin. J. Appl. Ecol. 29, 449–458. doi: 10.13287/j.1001-9332.201802.008
Zhang, L. L., Zhong, Q. L., Cheng, D. L., Zhang, Z. R., Fei, L., Xu, C. B., et al. (2015a). Characteristics of leaf carbon, nitrogen and phosphorus stoichiometry in relation to plant size of Machilus pauhoi. Chin. J. Appl. Ecol. 26, 1928–1934. doi: 10.13287/j.1001-9332.20150506.015
Zhang, Q., Dong, L. H., and Li, F. R. (2015b). The relationship between crown size and pine nut yield for Korean pine plantation. Bull. Bot. Res. 35, 443–449. doi: 10.7525/j.issn.1673-5102.2015.03.019
Zhang, S. M., Xi, J. W., Hong, J. Y., Xia, G. H., Li, Y., Huang, X. Z., et al. (2020a). A study on phenotypic diversity of fruit and leaf traits in Carya dabieshanensis. For Res. 33, 152−161. doi: 10.13275/j.cnki.lykxyj.2020.01.020
Zhang, X., Wang, Z. N., Lu, J. Y., Yang, M., and Yang, H. M. (2016). Responses of leaf traits to drought at different growth stages of alfalfa. Acta Ecol. Sin. 36, 2669–2676. doi: 10.5846/stxb201406261324
Zhang, X., Zhong, Q. L., Li, B. Y., Yao, X. M., Xu, C. B., Cheng, D. L., et al. (2020b). Relationship between the leaf functional traits and the diameter growth in even-aged stands of Zenia insignis. Sci. Silvae Sin. 56, 168–175. doi: 10.11707/j.1001-7488.20200519
Zhang, X. P., Zhang, Q., Zhang, J. L., Feng, B., and Jiang, X. (2009). Study on correlation between leaf character and fruit character of hybrid pear. Acta Agric. Jiangxi 21, 53–54. doi: 10.19386/j.cnki.jxnyxb.2009.04.017
Zhang, Y. Q., Wen, Y., Bai, Q., Ma, Z., Ye, H. L., and Su, S. C. (2019). Spatio-temporal effects of canopy microclimate on fruit yield and quality of Sapindus mukorossi Gaertn. Sci. Hortic. 251, 136–149. doi: 10.1016/j.scienta.2019.02.074
Zhao, L., Zhang, L., and Zafar, M. N. (2021). Synthesis and performance characterization of an efficient environmental-friendly Sapindus mukorossi saponins based hybrid coal dust suppressant. J. Clean Prod. 306, 127261. doi: 10.1016/j.jclepro.2021.127261
Zhao, X. X., Dong, Z. H., Liu, G. B., Hu, D. N., Liu, Y. Q., Meng, D. Y., et al. (2014). Difference analysis of seed qualities and composition of fatty acid of oil of Sapindus mukorossi Gaertn from different provenances. Acta Agric. Univ. Jiangxiensis 36, 575–581. doi: 10.13836/j.jjau.2014094
Keywords: leaf structural traits, plant size, even-aged stands, Sapindus mukorossi, allometric relationships
Citation: Chang Y, Xu C, Yang H, Zhou J, Hua W, Zhang S, Zhong Q and Li B (2021) Leaf Structural Traits Vary With Plant Size in Even-Aged Stands of Sapindus mukorossi. Front. Plant Sci. 12:692484. doi: 10.3389/fpls.2021.692484
Received: 08 April 2021; Accepted: 21 June 2021;
Published: 22 July 2021.
Edited by:
Mashura Shammi, Jahangirnagar University, BangladeshReviewed by:
Lina Fusaro, National Research Council (CNR), ItalyReetika Singh, Allahabad University, India
Yunfei Xie, Jiangnan University, China
Copyright © 2021 Chang, Xu, Yang, Zhou, Hua, Zhang, Zhong and Li. This is an open-access article distributed under the terms of the Creative Commons Attribution License (CC BY). The use, distribution or reproduction in other forums is permitted, provided the original author(s) and the copyright owner(s) are credited and that the original publication in this journal is cited, in accordance with accepted academic practice. No use, distribution or reproduction is permitted which does not comply with these terms.
*Correspondence: Quanlin Zhong, qlzhong@126.com; Baoyin Li, liby@fjnu.edu.cn
†These authors have contributed equally to this work