- 1Division of Plant and Soil Sciences, West Virginia University, Morgantown, WV, United States
- 2Department of Biochemistry, Purdue University, West Lafayette, IN, United States
- 3Purdue Center for Plant Biology, Purdue University, West Lafayette, IN, United States
- 4Department of Molecular, Cellular and Developmental Biology, University of Michigan, Ann Arbor, MI, United States
- 5Department of Horticulture and Landscape Architecture, Purdue University, West Lafayette, IN, United States
Terpenoids are a large and diverse class of plant metabolites that also includes volatile mono- and sesquiterpenes which are involved in biotic interactions of plants. Due to the limited natural availability of these terpenes and the tight regulation of their biosynthesis, there is strong interest to introduce or enhance their production in crop plants by metabolic engineering for agricultural, pharmaceutical and industrial applications. While engineering of monoterpenes has been quite successful, expression of sesquiterpene synthases in engineered plants frequently resulted in production of only minor amounts of sesquiterpenes. To identify bottlenecks for sesquiterpene engineering in plants, we have used two nearly identical terpene synthases, snapdragon (Antirrhinum majus) nerolidol/linalool synthase-1 and -2 (AmNES/LIS-1/-2), that are localized in the cytosol and plastids, respectively. Since these two bifunctional terpene synthases have very similar catalytic properties with geranyl diphosphate (GPP) and farnesyl diphosphate (FPP), their expression in target tissues allows indirect determination of the availability of these substrates in both subcellular compartments. Both terpene synthases were expressed under control of the ripening specific PG promoter in tomato fruits, which are characterized by a highly active terpenoid metabolism providing precursors for carotenoid biosynthesis. As AmNES/LIS-2 fruits produced the monoterpene linalool, AmNES/LIS-1 fruits were found to exclusively produce the sesquiterpene nerolidol. While nerolidol emission in AmNES/LIS-1 fruits was 60- to 584-fold lower compared to linalool emission in AmNES/LIS-2 fruits, accumulation of nerolidol-glucosides in AmNES/LIS-1 fruits was 4- to 14-fold lower than that of linalool-glucosides in AmNES/LIS-2 fruits. These results suggest that only a relatively small pool of FPP is available for sesquiterpene formation in the cytosol. To potentially overcome limitations in sesquiterpene production, we transiently co-expressed the key pathway-enzymes hydroxymethylglutaryl-CoA reductase (HMGR) and 1-deoxy-D-xylulose 5-phosphate synthase (DXS), as well as the regulator isopentenyl phosphate kinase (IPK). While HMGR and IPK expression increased metabolic flux toward nerolidol formation 5.7- and 2.9-fold, respectively, DXS expression only resulted in a 2.5-fold increase.
Introduction
Plants produce volatile organic compounds (VOCs) in leaves, flowers and fruits as well as roots and release them into the atmosphere and soil. Emitted VOCs play key roles in attracting pollinators and seed dispersers, directly or indirectly protecting plants against herbivores and pathogens, and mediating plant-plant communication (Dudareva et al., 2013). Based on their biosynthetic origin, plant VOCs are divided into several classes including phenylpropanoids/benzenoids, fatty acid derivatives, amino acid derivatives, and terpenoids. Terpenoids represent one of the largest and most diverse classes of plant metabolites that are involved in various basic physiological processes including photosynthesis, respiration, growth, and development (Vranová et al., 2013; Pichersky and Raguso, 2016). In addition to essential compounds such as sterols, carotenoids, chlorophylls, quinones, and the hormones gibberellins, strigolactones, abscisic acid and brassinosteroids, this metabolite class also includes the volatile mono- and sesquiterpenes that play important roles in biotic interactions of plants.
All terpenoids originate from the C5 building block isomers isopentenyl diphosphate (IPP) and dimethylallyl diphosphate (DMAPP), which in plants are synthesized by two alternative pathways that are localized in different subcellular compartments (Ashour et al., 2010; Hemmerlin et al., 2012). The mevalonic acid (MVA) pathway is localized in the cytosol and partially in peroxisomes (Figure 1), while the methylerythritol phosphate (MEP) pathway operates in plastids. Although these pathways act independently, there is substantial evidence for metabolic crosstalk between them (Hemmerlin et al., 2012; Vranová et al., 2013) with IPP and DMAPP exchange potentially occurring in both directions via yet unidentified transporter(s) in the plastid envelope membranes (Soler et al., 1993; Bick and Lange, 2003; Flügge and Gao, 2005). IPP and DMAPP generated by both pathways are subsequently utilized by prenyltransferases to form larger prenyl diphosphate intermediates that ultimately serve as precursors for the downstream biosynthesis of terpenoid compounds. Farnesyl diphosphate (FPP) is formed in the cytosol by FPP synthase (FPPS), while geranyl diphosphate (GPP) and geranylgeranyl diphosphate (GGPP) are produced in plastids by GPP synthase (GPPS) and GGPP synthase (GGPPS), respectively (Figure 1). While cytosolic FPP serves as a precursor for sterols and brassinosteroids, plastidic GGPP is utilized for chlorophyll, carotenoid, strigolactone, abscisic acid and gibberellin biosynthesis. In addition, FPP is used by cytosolic sesquiterpene synthases, whereas plastidic monoterpene synthases use GPP as substrate. Recently a new regulatory machinery was identified in plants (Figure 1) that is composed of isopentenyl phosphate kinase (IPK) and a subset of Nudix superfamily hydrolases (Henry et al., 2015, 2018). These two enzymes appear to modulate the equilibrium between isopentenyl phosphate (IP)/dimethylallyl phosphate (DMAP) and IPP/DMAPP, and in consequence the metabolic flux toward downstream terpenoid products.
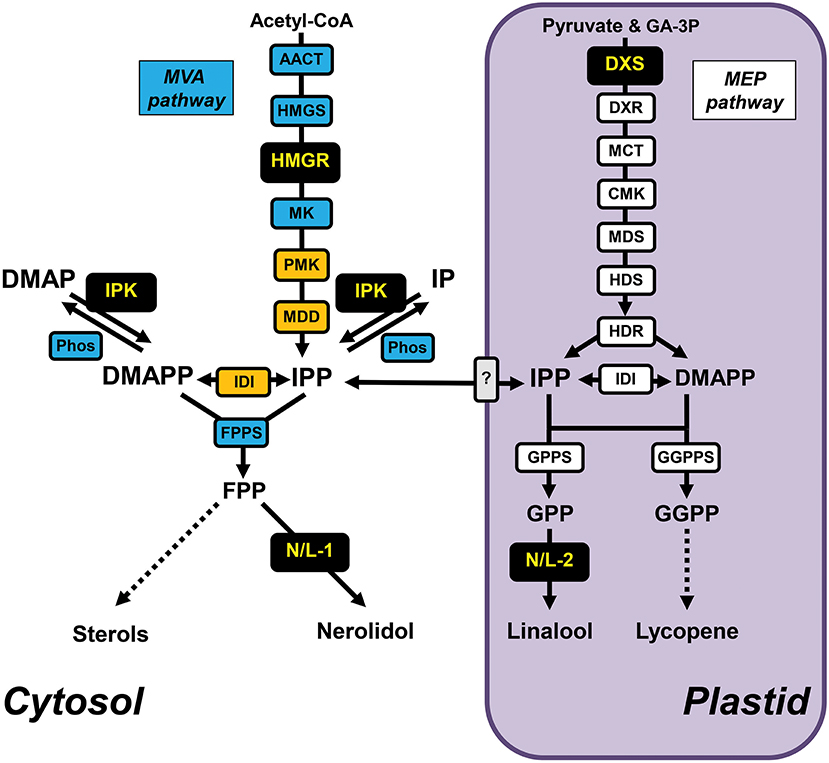
Figure 1. Terpenoid metabolic network in tomato fruits and position of engineered enzymes. Cytosolic and plastidic (highlighted in purple) terpenoid metabolic pathways involved in the biosynthesis of sterols and lycopene in tomato fruits with individual enzymes depicted as boxes. The cytosolic MVA pathway enzymes, prenyl transferase, and Nudix hydrolase are highlighted in blue, peroxisomal MVA pathway enzymes are highlighted in orange, the plastidic MEP pathway enzymes and prenyl transferases are highlighted in white. The unknown transporter(s) involved in IPP exchange across the plastid envelope membranes are shown in gray. The enzymes that have been overexpressed in tomato fruits for metabolic engineering are highlighted in black, including the Antirrhinum majus terpene synthases AmNES/LIS-1 (N/L-1) and AmNES/LIS-2 (N/L-2) catalyzing the formation of nerolidol and linalool, respectively. Dashed lines indicate multiple enzymatic steps. AACT, acetoacetyl-CoA thiolase; CMK, 4-(cytidine 5'-diphospho)-2-C-methyl-D-erythritol kinase; DMAP, dimethylallyl phosphate; DMAPP, dimethylallyl diphosphate; DXR, 1-deoxy-D-xylulose 5-phosphate reductoisomerase; DXS, 1-deoxy-D-xylulose 5-phosphate synthase; FPP, farnesyl diphosphate; FPPS, farnesyl diphosphate synthase; GA-3P, D-glyceraldehyde 3-phosphate; GPP, geranyl diphosphate; GPPS, geranyl diphosphate synthase; GGPP, geranylgeranyl diphosphate; GGPPS, geranylgeranyl diphosphate synthase; HDR, (E)-4-hydroxy-3-methylbut-2-enyl diphosphate reductase; HDS, (E)-4-hydroxy-3-methylbut-2-enyl diphosphate synthase; HMGR, 3-hydroxy-3-methylglutaryl-CoA reductase; HMGS, 3-hydroxy-3-methylglutaryl-CoA synthase; IDI, isopentenyl diphosphate isomerase; IPK, isopentenyl phosphate kinase; IP, isopentenyl phosphate; IPP, isopentenyl diphosphate; MCT, 2-C-methyl-D-erythritol 4-phosphate cytidylyltransferase; MDD, mevalonate diphosphate decarboxylase; MDS, 2-C-methyl-D-erythritol 2,4-cyclodiphosphate synthase; MK, mevalonate kinase; Phos, Nudix hydrolase(s); PMK, phosphomevalonate kinase.
Due to their roles in attracting beneficial insects, defending against pests and pathogens, and contributing to the aromas of fruits and other edible parts of plants, volatile mono- and sesquiterpenes are increasingly considered as valuable agronomic traits. In addition, these plant-derived terpenes are widely used by humans as flavors, fragrances, preservatives, biofuels and pharmaceuticals (Ajikumar et al., 2008; Immethun et al., 2013; Tippmann et al., 2013). However, because of the involvement of volatile monoterpenes and sesquiterpenes in specialized biological processes, their biosynthesis in plants is often restricted to specific tissues and developmental stages or induced by environmental stimuli (summarized in Gutensohn and Dudareva, 2016). Moreover, the lack of attention to terpene volatiles as traits in conventional crop breeding programs has led to the loss of such traits and as a result current varieties of many crops produce little to no terpene compounds (Köllner et al., 2008).
Metabolic engineering represents an efficient approach to either increase or modify terpene formation in naturally producing plant species, or to introduce a terpene biosynthetic pathway into a plant species naturally devoid of a compound of interest. Over the last decade numerous attempts have been made to engineer the formation of mono- and sequiterpene compounds in various plant species and tissues (summarized in Lange and Ahkami, 2013; Vickers et al., 2014). While engineering of monoterpenes in plants has been successful, the overexpression of sesquiterpene synthases in transgenic plants frequently resulted in the production of only small amounts of sesquiterpenes. Indeed, when a number of diverse sesquiterpene synthases, including a fungal trichodiene synthase (Hohn and Ohlrogge, 1991), germacrene A synthase from chicory (Aharoni et al., 2003), amorpha-4,11-diene synthase from Artemisia annua (Wallaart et al., 2001; Wu et al., 2006) and patchoulol synthase from Pogostemon cablin (Wu et al., 2006), were expressed in Arabidopsis or tobacco plants only trace to low levels of the target sesquiterpene products were observed.
Likewise, the overexpression of the cytosolic maize terpene synthase 10 (TPS10) (Schnee et al., 2006) or mint (E)-β-farnesene synthase (Beale et al., 2006) in Arabidopsis and oregano (E)-β-caryophyllene synthase in maize (Degenhardt et al., 2009) resulted in the formation of only moderate levels of the respective sesquiterpenes, although these levels were sufficient to elicit potent effects on the behavior of herbivores, predators, and parasitoids. In contrast, the expression of α-zingiberene synthase (ZIS), a multiproduct sesquiterpene synthase from Ocimum basilicum, in tomato fruits led to the accumulation of a significant amount of sesquiterpene products (Davidovich-Rikanati et al., 2008). However, the total amount of sesquiterpenes produced in these ZIS tomato fruits was ~3.5-fold lower than the total amount of monoterpenes that accumulated in tomato fruits expressing Ocimum basilicum geraniol synthase (GES) (Davidovich-Rikanati et al., 2007) even though both terpene synthases were expressed under the control of the same fruit ripening specific promoter. Together, these results suggest that often FPP, the immediate precursor for all sesquiterpenes, is not readily available for catalysis by the introduced sesquiterpene synthases, although FPP is expected to be produced efficiently in the cytosol to support the demand for sterol biosynthesis in many plant tissues.
The internal concentrations of FPP and GPP in the cytosolic and plastid compartments of plant tissues and organs involved in terpene biosynthesis have been proven extremely difficult to measure. Previous attempts (McCaskill and Croteau, 1993; Nogues et al., 2006) have only succeeded in ascertaining that the concentrations of these compounds are relatively low, which could be underestimated due to their high instability, and sensitivity to enzymatic and non-enzymatic hydrolysis. Alternative approaches via genetic engineering in which a heterologous terpene synthase (TPS) gene was introduced under a strong promoter into a host plant also provided some indirect information on the availability of these substrates. However, due to the different affinity of any single terpene synthase toward FPP and GPP, results from such experiments are difficult to interpret, even when the enzyme is directed to both the cytosol and the plastid in separate experiments. A better comparison could be made by using two enzymes that have similar kinetic properties for the prenyl diphosphate substrates, one directed to the cytosol and the other to plastids.
While for many TPSs their sesquiterpene-forming activities significantly exceed their monoterpene-forming activities or vice versa, in this study we took advantage of two closely related snapdragon terpene synthases, AmNES/LIS-1 and AmNES/LIS-2 (nerolidol/linalool synthase-1 and -2), which exhibit very similar kinetic properties, to indirectly determine the pool sizes of GPP and FPP precursors in the cytosolic and plastidic compartments. These two TPSs share 95% amino acid sequence identity and were shown to accept both GPP and FPP substrates with similar catalytic efficiencies (kcat/Km ratio) of ~2.8 mM−1s−1 and ~4.1 mM−1s−1, respectively (Nagegowda et al., 2008). The difference between these two enzymes is that AmNES/LIS-1 has no N-terminal transit peptide and is localized in the cytosol, while AmNES/LIS-2 has a transit peptide directing it to plastids.
Here, we expressed the cytosolic AmNES/LIS-1 and plastidic AmNES/LIS-2 in ripening tomato fruits to better understand the availability of each substrate, FPP and GPP, in both compartments. Tomato fruit is a good model system for these experiments since the terpenoid metabolic network, in particular the plastidic MEP pathway, is active providing precursors for the accumulation of lycopene and other carotenoids (Lawrence et al., 1997; Lois et al., 2000; Botella-Pavia et al., 2004; Paetzold et al., 2010), while only very little mono- and sesquiterpenes are formed in ripening tomato fruit (Zhou and Pichersky, 2020). There is ample evidence that the precursors of terpenoids, namely IPP and DMAPP, can be shuttled between plastids and cytosol. Thus, an analysis of FPP and GPP availability for terpene biosynthesis has to consider that the substrate depletion by a TPS introduced in one compartment, could potentially be ameliorated by transport from the other compartment. To evaluate the effect of increased flux through the cytosolic MVA and plastidic MEP pathways on the availability of FPP and GPP substrates in the two cellular compartments, we also co-expressed the key pathway-enzymes 3-hydroxy-3-methyl-glutaryl-CoA reductase (HMGR) and 1-deoxy-D-xylulose 5-phosphate synthase (DXS), respectively, in AmNES/LIS-1 and -2 tomato fruits. In addition, we tested whether the recently identified key regulator isopentenyl phosphate kinase can further enhance the metabolic flux toward cytosolic sesquiterpene formation in AmNES/LIS-1 tomato fruits.
Materials and Methods
Plant Material and Growth Conditions
Tomato (Solanum lycopersicum) line MP1 (Barg et al., 1997) was used for generation of transgenic plants and as control for all analyses. Plants were grown under a 14 h photoperiod in standard greenhouse conditions. MP1 plants were grown from seeds, while all transgenic lines (AmNES/LIS-1, AmNES/LIS-2) were propagated by re-rooting of cuttings.
Vector Construction and Stable Plant Transformation
The coding regions of Antirrhinum majus AmNES/LIS-1 and AmNES/LIS-2 (Nagegowda et al., 2008) were cloned into the binary vector pBIN19 carrying the tomato PG promoter and terminator, and the kanamycin-resistance marker NPTII driven by the CaMV 35S promoter (Nicholass et al., 1995). Transgenic tomato plants were generated via Agrobacterium tumefaciens (strain c58C1/pMP90) (Koncz and Schell, 1986) mediated transformation as described (McCormick et al., 1986). Transgenic plants rooted on kanamycin (100 mg L−1) selection medium were transferred to soil and adapted to greenhouse conditions.
Initial Screening of Transgenic Plants
Genomic DNA was extracted from tomato leaves as described previously (Ausubel et al., 1994). Tomato lines were screened for the presence of transgenes by PCR on genomic DNA using gene-specific primers for AmNES/LIS-1 and -2 (Supplementary Table 1). Confirmed transgenic AmNES/LIS-1 and -2 lines were further analyzed for nerolidol and linalool formation, respectively, in ripe tomato fruits using a solid-phase microextraction (SPME) system and gas chromatography-mass spectrometry (GC-MS).
RNA Isolation and qRT-PCR Analysis
Total RNA was isolated from tomato fruits as described (Eggermont et al., 1996). For quantitative real-time PCR (qRT-PCR) analysis, RNA was pretreated with RNase-free DNase (Promega) and cDNA was synthesized using Reverse Transcriptase (Superscript II, Invitrogene). Gene specific primers were designed using the PrimerExpress software (Applied Biosystems) and showed more than 90% efficiency at final concentrations of 500 nM (Supplementary Table 1). qRT-PCR reactions were performed and transcript levels were determined as previously described (Orlova et al., 2009; Gutensohn et al., 2013) using the StepOne Real-Time PCR system (Applied Biosystems). For the absolute quantification of AmNES/LIS-1 and -2 transcript levels by qRT-PCR, respective cDNA fragments were purified, diluted from 2 ng/ml to 3.2 pg/ml, and used as templates to obtain standard curves in qRT-PCR with gene specific primers (Supplementary Table 1). Based on standard curves, absolute quantities of individual transcripts were calculated and expressed as percentage of total mRNA. Each data point represents an average of at least three independent biological samples with three technical replicates for each sample.
Collection, Extraction, and Analysis of Terpenes From Tomato Fruits
Volatile terpenes emitted from ripe tomato fruits (Breaker +10 days) were collected for 24 h using a closed-loop stripping method as described previously (Dudareva et al., 2005; Gutensohn et al., 2013; Wang et al., 2020). For analysis of internal terpene pools pericarp of ripe tomato fruits was extracted with methyl tert-butyl ether (MTBE) as described previously (Davidovich-Rikanati et al., 2007; Gutensohn et al., 2013). Terpene glucosides were extracted with methanol and enzymatically hydrolyzed to release the terpene aglycone as described previously (Boachon et al., 2019). Emitted and extracted volatiles were analyzed by GC-MS as described (Dudareva et al., 2005) using naphthalene as internal standard. Nerolidol and linalool standards were used to determine response factors, which were applied for the quantification.
Transient Transformation of Tomato Fruits
Open reading frames of AtHMGR1 (At1g76490), AtDXS (At4g15560), and AtIPK (At1g26640) in the pENTR vector were obtained from the Arabidopsis Biological Resource Center (G12571, Gc104921, GC104863, respectively) and transferred into the binary vector pB2GW7 under control of the CaMV 35S promoter using the Gateway LR Clonase II (Invitrogen). The resulting binary vectors were introduced into A. tumefaciens (strain LBA 4404) and those subsequently used for transient transformation of tomato fruits as described (Orzaez et al., 2006). Agrobacterium suspensions were injected into ripening fruits (Breaker +3 days) of transgenic AmNES/LIS-1 and AmNES/LIS-2 lines using a syringe with hypodermic needle. After 7 days ripe fruits (Breaker +10 days) were harvested, and used for collection and analysis of emitted terpenes as described above. As control AmNES/LIS-1 and -2 fruits were injected with Agrobacterium carrying the empty pB2GW7 vector and subsequently analyzed in the same way.
Results
Overexpression of AmNES/LIS-1 and -2 in Tomato Fruits Results in Different Levels of Nerolidol and Linalool Formation
To determine the level of available FPP and GPP precursors for sesquiterpene and monoterpene production, respectively, in tomato fruits, we overexpressed the cytosolic AmNES/LIS-1 and plastidic AmNES/LIS-2, the two nearly identical bifunctional terpene synthases from snapdragon (Nagegowda et al., 2008), in tomato plants under control of the fruit ripening specific PG promoter (Nicholass et al., 1995). This promoter limits transgene expression to the pericarp of ripening fruit, thus avoiding negative effects on vegetative tissues and general plant development (Orlova et al., 2009). After an initial screening of 29 tomato lines for the presence of the AmNES/LIS-1 transgene as well as nerolidol formation, four independent lines (B5, B7, C6, and C13) with various AmNES/LIS-1 expression levels, as determined by quantitative real-time PCR (Figure 2A), were chosen for further characterization. Likewise, four independent AmNES/LIS-2 overexpressing lines (B12, C14, D13, and U8) with different expression levels (Figure 3A) were selected for detailed analysis from 56 transgenic tomato lines after an initial screening for the presence of the AmNES/LIS-2 transgene and linalool formation.
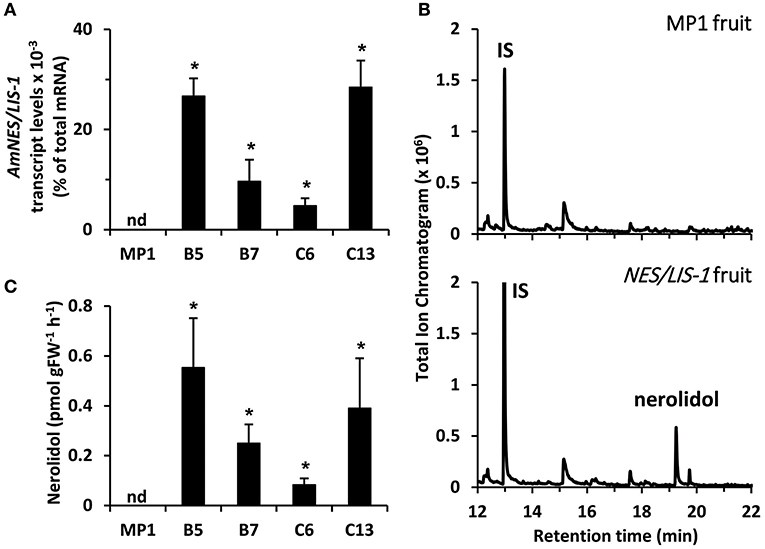
Figure 2. Profiling of transgenic tomato lines overexpressing AmNES/LIS-1 under control of the PG promoter. (A) Transcript levels of AmNES/LIS-1 in fruits (Br + 3 stage) of MP1 control and AmNES/LIS-1 lines B5, B7, C6, and C13 determined by qRT-PCR (means ± SEM, n = 3 biological replicates). (B) Profiling of terpenes collected as emitted volatiles from ripe tomato fruits (MP1 control and AmNES/LIS-1 line B5) and analyzed by GC-MS. IS, internal standard. (C) Quantitative changes in amounts of nerolidol collected as emitted volatile from fruits of MP1 and AmNES/LIS-1 lines. Data are means ± SEM (n ≥ 3). *P < 0.05 AmNES/LIS-1 lines relative to MP1 by Student's t-test. FW, fresh weight; nd, not detected.
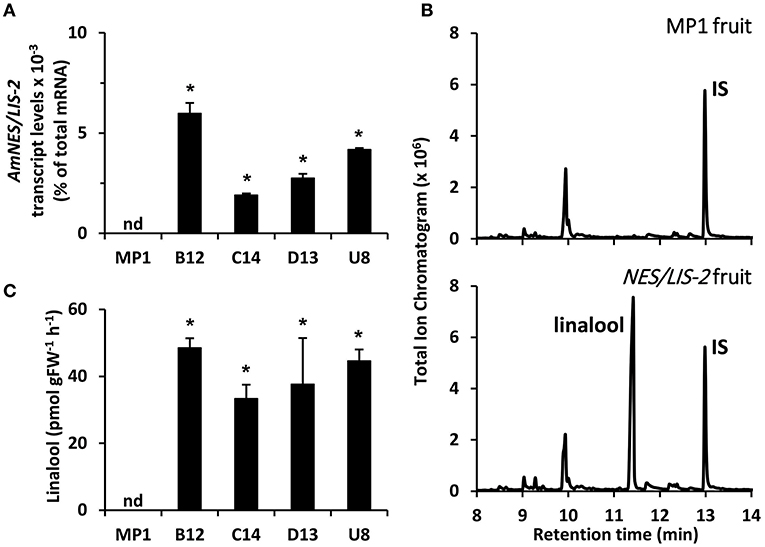
Figure 3. Profiling of transgenic tomato lines overexpressing AmNES/LIS-2 under control of the PG promoter. (A) Transcript levels of AmNES/LIS-2 in fruits (Br + 3 stage) of MP1 control and AmNES/LIS-2 lines B12, C14, D13, and U8 determined by qRT-PCR (means ± SEM, n = 3 biological replicates). (B) Profiling of terpenes collected as emitted volatiles from ripe tomato fruits (MP1 control and AmNES/LIS-2 line U8) and analyzed by GC-MS. IS, internal standard. (C) Quantitative changes in amounts of linalool collected as emitted volatile from fruits of MP1 and AmNES/LIS-2 lines. Data are means ± SEM (n ≥ 3). *P < 0.05 AmNES/LIS-2 lines relative to MP1 by Student's t-test. FW, fresh weight; nd, not detected.
To examine sesquiterpene and monoterpene formation upon AmNES/LIS-1 and AmNES/LIS-2 overexpression, respectively, emitted volatiles were collected from ripe tomato fruits (Br +10 stage) and analyzed by gas chromatography-mass spectrometry (GC-MS). The only difference in the volatile profile between transgenic lines and control was the formation of the sesquiterpene nerolidol and the monoterpene linalool in AmNES/LIS-1 (Figure 2B) and AmNES/LIS-2 (Figure 3B) transgenic fruits, respectively, which were absent in the MP1 control fruits (Figures 2B, 3B). The amounts of nerolidol emitted from AmNES/LIS-1 fruits positively correlated with the AmNES/LIS-1 expression level in the different transgenic lines (Figures 2A,C). A similar positive correlation between the amounts of linalool emitted from fruits and the respective AmNES/LIS-2 transcript levels was observed in the different transgenic AmNES/LIS-2 lines (Figures 3A,C). No formation of linalool was observed in AmNES/LIS-1 fruits and likewise AmNES/LIS-2 fruits did not produce nerolidol (Supplementary Figure 1). The most remarkable outcome of the characterization of these transgenic AmNES/LIS-1 and AmNES/LIS-2 fruits was that despite the almost identical kinetic properties of the two expressed terpene synthases (Nagegowda et al., 2008), and similar or higher expression levels of AmNES/LIS-1 (4.78 ± 1.50 to 28.43 ± 5.31 × 10−3% of total mRNA) relative to that of AmNES/LIS-2 (1.90 ± 0.08 to 5.97 ± 0.52 × 10−3% of total mRNA) (Figures 2A, 3A), nerolidol emission in AmNES/LIS-1 fruits was 60- to 584-fold lower than linalool emission in AmNES/LIS-2 fruits (Figures 2C, 3C).
AmNES/LIS-1 Tomato Fruits Accumulate Internal Pools of Nerolidol Derivatives
Earlier metabolic engineering attempts to produce volatile terpenes in different plant tissues, including tomato fruits, resulted in formation not only of the desired product but also of other terpenes, which are derived from the primary product by the action of endogenous plant enzymes (Lewinsohn et al., 2001; Lücker et al., 2001; Davidovich-Rikanati et al., 2007). These product modifications often affect the volatility of the derived compounds and result in their accumulation inside the tissue. Thus, as a first step to further evaluate the underlying mechanisms causing the low levels of nerolidol emission in AmNES/LIS-1 fruits relative to the linalool emission in AmNES/LIS-2 fruits, we analyzed internal terpene pools in AmNES/LIS-1 fruits (Figure 4). GC-MS analysis of terpenes extracted from ripe tomato fruits with methyl tert-butyl ether revealed four different terpene compounds in AmNES/LIS-1 fruits that were absent in the MP1 control fruits (Figure 4A). Besides nerolidol, the transgenic fruits accumulated nerolidyl acetate as well as two unknown terpene compounds (mass spectra shown in Supplementary Figure 2). Comparative analysis of the internal pools of nerolidol and its three derivatives in the lines B5 and C6 with high and low AmNES/LIS-1 expression levels, respectively (Figure 2A), revealed a trend toward accumulating larger amounts of these terpene compounds in line B5 vs. line C6 (Figure 4B). However, only the internal nerolidol pool was statistically higher in line B5. While the metabolic profiling suggests that some of the nerolidol produced in transgenic AmNES/LIS-1 fruits is indeed converted to other compounds by endogenous enzymes, such as acetyl transferases, the accumulated compounds (in the pmol/g FW range) cannot explain the roughly 100-fold difference in nerolidol vs. linalool emission observed in AmNES/LIS-1 and AmNES/LIS-2 fruits, respectively (Figures 2C, 3C).
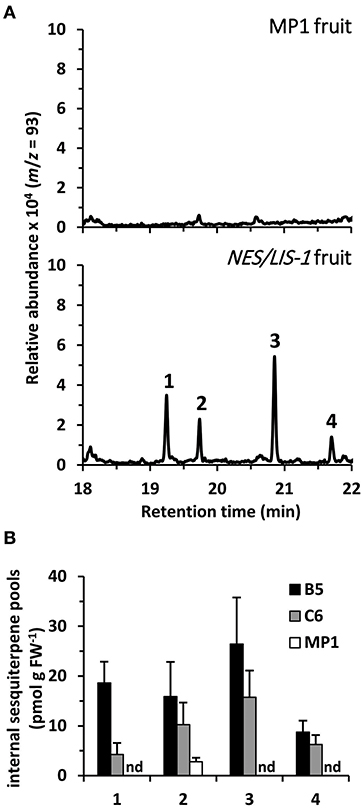
Figure 4. Metabolic profiling of internal sesquiterpene pools in AmNES/LIS-1 fruits. (A) Terpenes were directly extracted from ripe fruits of MP1 control and AmNES/LIS-1 transgenic tomato (line B5). Extracts were analyzed by GC-MS and traces obtained for m/z = 93 (characteristic for terpenes) are shown. Based on their mass spectra and retention time the following compounds were identified: 1, nerolidol; 2, unknown 1; 3, unknown 2; 4, nerolidyl acetate. (B) Quantitative analysis of nerolidol and its derivatives extracted from fruits of the AmNES/LIS-1 lines B5 and C6, and the MP1 control. Data are means ± SEM (n ≥ 3). FW, fresh weight; nd, not detected.
AmNES/LIS-1 and -2 Tomato Fruits Accumulate Glycosylated Terpenes
Terpenes with hydroxyl functional groups, such as nerolidol and linalool, can be glycosylated by non-specific glucotransferases to form non-volatile, inert end products. To test whether the apparent discrepancy between production of nerolidol and linalool in AmNES/LIS-1 and -2 fruits, respectively, may be the consequence of an unequal flux toward respective glucoside derivatives, we analyzed terpene glucoside accumulation in ripe tomato fruits (Br + 10 stage) (Figure 5) from the MP1 control, and representative AmNES/LIS-1 and -2 lines showing high transgene expression. Nerolidol-glucosides were detected only in fruits of the AmNES/LIS-1 lines, and linalool-glucosides were only present in fruits of the AmNES/LIS-2 lines (Figures 5A,B). Remarkably, a substantial part of both, the nerolidol and linalool formed in AmNES/LIS-1 and -2 lines, respectively, appears to be converted to glucosides and accumulated as internal pools (in the nmol/g FW range) in these fruits. However, consistent with the difference in emission of the volatile free nerolidol and linalool (Figures 2C, 3C), the amount of nerolidol-glucosides in the AmNES/LIS-1 lines was 4- to 14-fold lower than the amount of linalool-glucosides in the AmNES/LIS-2 lines (Figure 5). Therefore, these results further confirm a significant difference in the potential to produce sesquiterpenes and monoterpenes in tomato fruits.
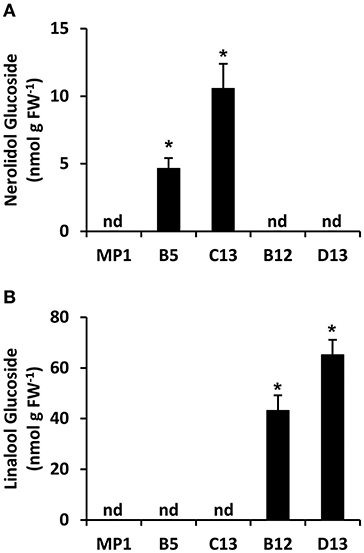
Figure 5. Metabolic profiling of terpene glucosides in AmNES/LIS-1 (lines B5 and C13) and AmNES/LIS-2 (lines B12 and D13) fruits. Glucosides were extracted from ripe fruits of MP1 control and representative transgenic lines, then enzymatically hydrolyzed. The released nerolidol (A) and linalool (B) aglycones were analyzed by GC-MS. Data are means ± SEM (n = 3). *P < 0.05 AmNES/LIS-1/-2 lines relative to the MP1 control by Student's t-test. FW, fresh weight; nd, not detected.
Co-expression of HMGR and DXS in AmNES/LIS-1 and -2 Fruits Has Different Effects on Nerolidol and Linalool Formation
Next, we analyzed whether an increase in the flux through the upstream metabolic pathways providing precursors for the introduced AmNES/LIS-1 can enhance nerolidol production in transgenic tomato fruits. It is generally accepted that HMGR catalyzes the rate limiting step in the MVA pathway (Hemmerlin et al., 2012) (Figure 1). Thus, to increase the level of precursors available for the cytosolic AmNES/LIS-1, we transiently overexpressed Arabidopsis thaliana AtHMGR1 (Enjuto et al., 1994) in ripening AmNES/LIS-1 fruits using Agrobacterium injection (Gutensohn and Dudareva, 2016). Upon co-expression of AtHMGR1, emission of nerolidol was found to be 5.7-fold higher in AmNES/LIS-1 fruits when compared to fruits injected with Agrobacterium carrying the empty vector control (Figure 6A), thus indicating that the metabolic flux through the MVA pathway is indeed limiting in ripening tomato fruits.
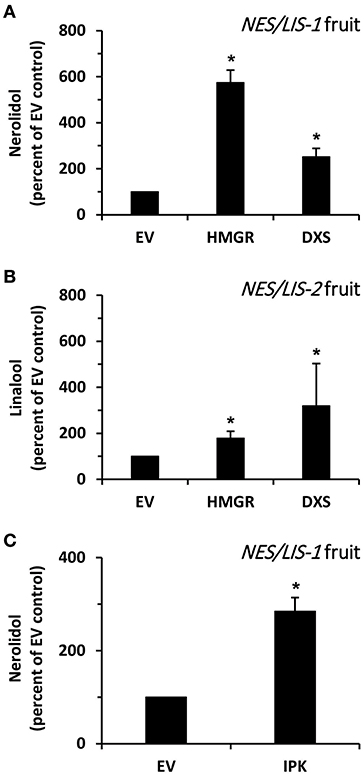
Figure 6. The effect of HMGR, DXS, and IPK overexpression on nerolidol formation in AmNES/LIS-1 fruits. (A) Quantitative changes in amounts of nerolidol emitted from AmNES/LIS-1 fruits (line C13) upon transient overexpression of AtHMGR (HMGR) and AtDXS (DXS). Nerolidol contents are presented as percentage of the nerolidol content in AmNES/LIS-1 fruits injected with Agrobacterium carrying the empty pB2GW7 vector (EV), set as 100%. (B) Quantitative changes in amounts of linalool emitted from AmNES/LIS-2 fruits (line U8) upon transient overexpression of AtHMGR (HMGR) and AtDXS (DXS). Linalool contents are presented as percentage of the linalool content in AmNES/LIS-2 fruits injected with Agrobacterium carrying the empty pB2GW7 vector (EV), set as 100%. (C) Quantitative changes in amounts of nerolidol emitted from AmNES/LIS-1 fruits upon transient overexpression of AtIPK (IPK). The nerolidol content is presented as percentage of the nerolidol content in AmNES/LIS-1 fruits injected with Agrobacterium carrying the empty pB2GW7 vector (EV), set as 100%. Data are means ± SEM (n ≥ 3). *P < 0.05 AmNES/LIS-1/-2 lines relative to the EV controls by Student's t-test.
To analyze if an increased flux through the plastidic MEP pathway could also provide precursors for the cytosolic AmNES/LIS-1 via metabolic crosstalk between plastids and cytosol, we transiently overexpressed A. thaliana AtDXS (Estévez et al., 2000), a key enzyme of the MEP pathway (Figure 1), in ripening AmNES/LIS-1 fruits by Agrobacterium injection. Upon co-expression of AtDXS in AmNES/LIS-1 fruits nerolidol emission was increased 2.5-fold compared to fruits injected with Agrobacterium carrying the empty vector control (Figure 6A), suggesting that an enhanced metabolic flux through the MEP pathway also supports cytosolic sesquiterpene formation although not to the same extent as an increased flux through the MVA pathway.
In parallel, we also evaluated the effect of higher metabolic fluxes through the MVA and MEP pathways on the linalool formation by AmNES/LIS-2 introduced into transgenic tomato fruits. Therefore, AtHMGR1 and AtDXS were transiently overexpressed in ripening AmNES/LIS-2 fruits by Agrobacterium injection. Subsequent analysis of emitted volatiles showed that linalool formation was 1.8- and 3.2-fold higher upon co-expression of AtHMGR1 and AtDXS, respectively, in AmNES/LIS-2 fruits compared to fruits injected with Agrobacterium carrying the empty vector control (Figure 6B). These results indicate that increased metabolic fluxes through the MVA and MEP pathway both support monoterpene formation in plastids, although the cytosolic MVA pathway contributes to a lesser extent.
Co-expression of IPK in AmNES/LIS-1 Fruits Significantly Enhances Nerolidol Formation
Isopentenyl phosphate kinase (IPK) was recently identified as a new member of the plant terpenoid metabolic network (Figure 1) and shown to play an important role in modulating the formation of FPP-derived terpenoid compounds, including sesquiterpenes (Henry et al., 2015). In order to find if IPK could also positively influence the FPP levels for sesquiterpene formation in tomato fruits, we transiently overexpressed A. thaliana AtIPK (Henry et al., 2015) in ripening AmNES/LIS-1 fruits by Agrobacterium injection. The analysis of emitted volatiles showed that nerolidol formation was 2.9-fold higher upon AtIPK co-expression in AmNES/LIS-1 fruits compared to control fruits injected with Agrobacterium carrying the empty vector control (Figure 6C). This result indicates that overexpression of IPK indeed supports nerolidol formation in engineered AmNES/LIS-1 fruits by shifting the ratio of IP/DMAP to IPP/DMAPP and thus increasing the pool of precursors available for sesquiterpene formation.
Discussion
To date, there is an increasing demand to introduce or enhance mono- and sesquiterpene traits in crop plants either to provide more sustainable ways of protecting against pests and pathogens, or to achieve improved and lasting aromas of fruits and other edible plant produce. In addition, crop systems can be used as biotechnological platforms for the large-scale production of terpene compounds desired by industry as flavors, fragrances, pharmaceuticals, and biofuels (Ajikumar et al., 2008; Immethun et al., 2013; Tippmann et al., 2013). Naturally the biosynthesis of monoterpenes and sesquiterpenes in plants is often restricted to specific tissues such as glandular trichomes, internal ducts, and flower organs, and is induced by environmental stimuli like herbivore and pathogen attacks (summarized in Gutensohn and Dudareva, 2016). Thus, efforts to improve endogenous terpene traits are limited to such specialized tissues. In contrast, introduction of new terpene traits in crop plants by metabolic engineering often targets tissues in abundant plant organs such as leaves, fruits and seeds. In either case, it is not sufficient to just introduce or increase the expression of a terpene synthase enzyme catalyzing the formation of the terpene product of interest, but it requires a detailed understanding of the availability of the prenyl diphosphate substrates GPP and FPP in the targeted plant tissue and subcellular compartment.
In this study, we utilized two closely related snapdragon bifunctional terpene synthases AmNES/LIS-1 and AmNES/LIS-2 (Nagegowda et al., 2008) that are localized in the cytosol and plastids, respectively. Since these two TPSs, aside from their different subcellular localization, have similar catalytic efficiencies and both accept GPP as well as FPP, their expression in a target tissue allows to indirectly determine the availability of the prenyl diphosphate substrates in the cytosolic and plastidic compartments. Expression of AmNES/LIS-1 and AmNES/LIS-2 in tomato plants under control of the fruit ripening specific PG promoter (Figures 2A, 3A), resulted in formation of the sesquiterpene nerolidol (Figure 2B) and the monoterpene linalool (Figure 3B) in transgenic fruits, respectively. Despite the fact that these snapdragon TPSs are capable to use both prenyl diphosphate substrates (Nagegowda et al., 2008), no linalool and nerolidol production was found in AmNES/LIS-1 and AmNES/LIS-2 fruits, respectively (Supplementary Figure 1). In particular, the absence of linalool formation in AmNES/LIS-1 fruits was surprising. Earlier studies (Davidovich-Rikanati et al., 2008; Gutensohn et al., 2013) had demonstrated that expression of the cytosolic bifunctional TPS, Ocimum basilicum α-zingiberene synthase (ZIS), in ripening tomato fruits resulted not only in the expected formation of sesquiterpenes driven by the cytosolic FPP, but also some monoterpene formation, suggesting the presence of a small GPP pool that appears to be derived from plastids. However, the contrasting results obtained with AmNES/LIS-1 and ZIS fruits could be explained by the kinetic properties of the introduced TPSs. Remarkably, both AmNES/LIS enzymes were found to have relatively high Km values for GPP and FPP compared to many other TPSs, as well as slightly higher catalytic efficiency with FPP (Nagegowda et al., 2008) suggesting that the small cytosolic GPP pool in tomato fruits is likely not sufficient to result in any detectable linalool formation in the AmNES/LIS-1 fruits. In line with this notion, feeding of cut snapdragon flowers with [2H2] mevalolactone labeled nerolidol, however, did not show incorporation into linalool (Dudareva et al., 2005), suggesting that in snapdragon flower tissue a potentially existing cytosolic GPP pool is also too small to drive monoterpene formation.
The nerolidol emission in AmNES/LIS-1 fruits was substantially lower (between 60-fold and almost 600-fold) than the linalool emission in AmNES/LIS-2 fruits (Figures 2C, 3C). Remarkably, a large fraction of both terpene products, nerolidol and linalool, accumulated as glucoside derivatives in the transgenic tomato fruits (Figure 5). Since hyperaccumulation of VOCs in plant tissues was recently shown to have a detrimental effect on the integrity of cellular membranes (Adebesin et al., 2017), the observed glycosylation of the engineered terpenes might represent a mechanism to prevent autotoxicity from these compounds accumulating in the ripening transgenic fruits. In addition, glycosylated derivatives of terpenes, such as linalool, have been found in many fruits (Fan et al., 2009; Bönisch et al., 2014; Yauk et al., 2014; Wu et al., 2017) and are known to play a role in retronasal flavor perception upon fruit consumption (Parker et al., 2017). However, similar to the difference in emission of the volatile free nerolidol and linalool (Figures 2C, 3C), our analysis of these internal pools of terpene derivatives also demonstrated that the accumulation of nerolidol-glucosides in AmNES/LIS-1 fruits was significantly lower (4- to 14-fold) than that of linalool-glucosides in AmNES/LIS-2 fruits (Figure 5). In summary the observed differences in emission and accumulation of nerolidol and linalool in AmNES/LIS-1 and AmNES/LIS-2 fruits, respectively, suggest that only relatively small amounts of FPP are available for sesquiterpene formation in the cytosol, while comparatively larger amounts of GPP are available for monoterpene formation in plastids in pericarp cells of ripening tomato fruits. These results are consistent with the high activity of the plastidic MEP pathway during the ripening process of tomato fruits (Lois et al., 2000; Botella-Pavia et al., 2004), which provides precursors for the massive accumulation of carotenoids, the tetraterpene pigments (Fraser et al., 1994), and can be utilized for monoterpene formation by the introduced TPS. In contrast, the MVA pathway is highly active only during early stages of tomato fruit development (Narita and Gruissem, 1989; Jelesko et al., 1999) providing precursors for the production of sterols, which are required for the rapid cell division and expansion (Gillaspy et al., 1993). However, during the later fruit ripening stage the MVA pathway is not highly active and thus apparently provides only a limited amount of FPP that can be used for sesquiterpene formation by the introduced TPS. Similar results were obtained when two unrelated cytosolic and plastidic TPS from Ocimum basilicum, α-zingiberene synthase (ZIS) and geraniol synthase (GES), respectively, were expressed in tomato fruits under the control of the same ripening specific PG promoter (Davidovich-Rikanati et al., 2007, 2008). Although the data could not be directly compared due to the different kinetic properties of these two TPSs, the higher production of monoterpenes in GES fruits relative to a lower production of sesquiterpenes in ZIS fruits suggested a difference in the pool sizes of FPP and GPP available in the cytosol and plastids, respectively.
Moreover, a similar trend was found earlier when a number of mono- and sesquiterpene synthases were overexpressed in tobacco and Arabidopsis, and the formation of respective terpenes was analyzed in leaves. Expression of a cytosolic germacrene A synthase in Arabidopsis leaves resulted in a sesquiterpene formation that was at least 1000-fold lower compared to the monoterpene formation observed upon the expression of a plastid-targeted dual linalool/nerolidol synthase (Aharoni et al., 2003). Likewise, only trace amounts of sesquiterpenes were found upon expression of amorpha-4,11-diene synthase in tobacco leaves, while the expression of a plastid localized limonene synthase resulted in the production of significantly higher amounts of the corresponding monoterpene (Wu et al., 2006). Upon expression of a second sesquiterpene synthase, patchoulol synthase, in tobacco leaves significantly higher product levels were measured than in the amorpha-4,11-diene synthase expressing tobacco lines (Wu et al., 2006) indicating that the different kinetic properties of the introduced terpene synthases also affect the outcome. The differences in sesquiterpene and monoterpene formation that were observed when respective terpene synthases were expressed in leaves suggested the availability of only a limited pool of FPP in the cytosol and a larger pool of GPP in plastids, similar to the situation found in ripening tomato fruits. In line with these results MEP pathway genes in Arabidopsis are predominantly expressed in photosynthetic tissues, such as leaves, while MVA pathway genes are highly expressed in roots and reproductive organs containing meristematic tissue (Vranová et al., 2013).
Due to the relatively small nerolidol production in AmNES/LIS-1 fruits and the known low activity of the MVA pathway in ripening tomato fruits (Narita and Gruissem, 1989; Jelesko et al., 1999), we tested if an increase in the metabolic flux through this pathway would result in higher sesquiterpene levels. Since HMGR is generally considered to catalyze the rate-limiting step in the MVA pathway (Hemmerlin et al., 2012), AtHMGR1 was transiently overexpressed in ripening AmNES/LIS-1 fruits. AtHMGR1 expression increased nerolidol emission 5.7-fold (Figure 6A) relative to fruits injected with Agrobacterium carrying the empty vector control, suggesting that the metabolic flux through the MVA pathway is indeed limiting in ripening tomato fruits and provides insufficient amounts of the FPP precursor to support high levels of sesquiterpene formation. Although the plastidic MEP pathway is already quite active in ripening tomato fruits (Lois et al., 2000; Botella-Pavia et al., 2004), overexpression of the key MEP pathway enzyme DXS in tomato fruits was shown to lead to higher carotenoid levels (Enfissi et al., 2005), indicating that the metabolic flux through this pathway can be even further increased. Earlier studies have shown that cytosolic synthesis of terpenoids, including sesquiterpenes, can also be supported by precursors derived from the plastidic MEP pathway (Hemmerlin et al., 2003; Laule et al., 2003; Dudareva et al., 2005). Therefore, we tested if increased flux through the MEP pathway in ripening tomato fruits would affect cytosolic production of nerolidol and increase its level. The transient expression of AtDXS in AmNES/LIS-1 fruits indeed resulted in an increased nerolidol formation relative to the empty vector control (Figure 6A), thus confirming that an enhanced metabolic flux through the MEP pathway can support cytosolic sesquiterpene formation as well. However, the 2.5-fold increase in nerolidol formation achieved by AtDXS co-expression in AmNES/LIS-1 fruits was significantly lower than the 5.7-fold increase observed upon AtHMGR1 co-expression (Figure 6A).
We utilized the same co-expression approach in ripening tomato fruits to verify if increased metabolic fluxes through the MVA and MEP pathways likewise support monoterpene formation in plastids. Transient expression of AtHMGR1 and AtDXS in ripening AmNES/LIS-2 fruits resulted in 1.8- and 3.2-fold higher linalool formation, respectively, compared to the empty vector control (Figure 6B). These results provide further evidence that the MVA and MEP pathways both in principle can provide precursors for terpene biosynthesis in the cytosol and plastids. This is consistent with previous studies demonstrating the exchange of MVA and MEP pathway derived precursors in both directions supporting production of various terpenoids in both compartments (Kasahara et al., 2002; Nagata et al., 2002; Hemmerlin et al., 2003; Laule et al., 2003; Schuhr et al., 2003; Dudareva et al., 2005). However, by comparing the extent to which increased fluxes through the MVA and MEP pathway supported nerolidol and linalool formation in AmNES/LIS-1 and AmNES/LIS-2 fruits, respectively (Figures 6A,B), it became evident that the pathway localized in the same compartment as the introduced terpene synthase had a more profound effect on product outcome than the pathway providing precursors via metabolic crosstalk across the plastid envelope membranes. This suggested that the yet unknown plastid envelope transporter(s) involved in this metabolite exchange potentially also represent a limiting factor for the metabolic engineering of sesquiterpenes in tomato fruits and plants in general.
Isopentenyl phosphate kinase (IPK) is a newly discovered member of the terpenoid metabolic network in plants, which is co-expressed with MVA pathway and FPPS genes (Henry et al., 2015). IPK catalyzes the conversion of IP to IPP and thus together with Nudix hydrolases regulates the ratio of these two metabolites and subsequently the metabolic flux toward prenyl diphosphate intermediates and downstream terpenoid products (Henry et al., 2015, 2018). Indeed, AtIPK overexpression in tobacco leaves led to up to 3.4-fold higher β-caryophyllene and 5-epi-aristolochene formation (Henry et al., 2015). When AtIPK was transiently co-expressed in AmNES/LIS-1 tomato fruits the level of nerolidol increased by 2.9-fold relative to the empty vector control (Figure 6C), suggesting that overexpression of IPK enhances the available cytosolic FPP pool. This is likely the outcome of two processes, an increase in cytosolic IPP and DMAPP as well as an increase in FPPS activity which was shown to be inhibited by IP (Henry et al., 2015), thus further confirming the essential role of IPK in regulating and potentially enhancing the metabolic flux toward sesquiterpenes.
Conclusion
In this study, we have taken a novel approach to evaluate the availability of prenyl diphosphate substrates for the metabolic engineering of terpene production in plants by utilizing two nearly identical bifunctional terpene synthases, AmNES/LIS-1 and -2, with similar catalytic properties toward GPP and FPP, that are localized in the cytosol and plastids, respectively. The variable outcome in mono- and sesquiterpene engineering observed in our and previous studies seems to be the result of a combination of two factors: the kinetic properties of the introduced terpene synthases and the availability of the required prenyl diphosphate substrates in the respective subcellular compartment. The limited production of the sesquiterpene nerolidol observed in our experiments appears to be due to the low metabolic activity of the MVA pathway in the targeted tissue, which cannot be fully compensated by plastidic MEP pathway despite it being highly active. This conclusion was supported by the increased nerolidol formation upon co-expression of HMGR and IPK resulting in an enhanced metabolic flux through the MVA pathway toward cytosolic FPP. In contrast, increasing the metabolic flux through the MEP pathway via expression of the key pathway enzyme DXS had only a minor effect on nerolidol formation suggesting a potential limitation in the metabolic crosstalk between plastids and cytosol by the metabolite transport across the envelope membranes. Thus, future approaches toward metabolic engineering of sesquiterpene production in plants should consider the co-expression of respective TPSs with multiple key MVA pathway enzymes including HMGR and PMK (Henry et al., 2018), the central regulator IPK, as well as FPP synthase. In addition, targeted efforts toward the identification of plastid envelope transporters are required before the full potential of metabolites provided by the MEP pathway can be utilized to support engineered sesquiterpene production in the cytosol.
Data Availability Statement
The original data presented in the study are included in the article/Supplementary Material, further inquiries can be directed to the corresponding author.
Author Contributions
MG, EP, and ND planned and designed the research. MG, LH, SG, JL, and TN performed the experiments. MG and ND analyzed the data and wrote the manuscript with contributions from JL and EP. All authors contributed to the article and approved the submitted version.
Funding
This work was supported by the U.S. Agriculture and Food Research Initiative competitive Grant Number 2008-35318-04541 to ND and EP. This study was also supported by the West Virginia Agricultural and Forestry Experiment Station Hatch Project WVA00730 to MG, and by the USDA National Institute of Food and Agriculture Hatch Project number 177845 to ND. In addition, the work of MG was supported by the Ray Marsh and Arthur Pingree Dye Professorship.
Conflict of Interest
The authors declare that the research was conducted in the absence of any commercial or financial relationships that could be construed as a potential conflict of interest.
Acknowledgments
The authors would like to acknowledge the greenhouse staff at Purdue University and West Virginia University for their support in cultivating and maintaining the tomato lines used in this study and Pan Liao (Purdue University) for help with the manuscript revision.
Supplementary Material
The Supplementary Material for this article can be found online at: https://www.frontiersin.org/articles/10.3389/fpls.2021.691754/full#supplementary-material
Supplementary Figure 1. Metabolic profiling of transgenic tomato lines overexpressing AmNES/LIS-1 and AmNES/LIS-2 under control of the PG promoter. Emission of the sesquiterpene nerolidol (A) and the monoterpene linalool (B) from ripe tomato fruits (AmNES/LIS-1 line B5 and AmNES/LIS-2 line U8) were analyzed by GC-MS. IS, internal standard.
Supplementary Figure 2. Mass spectra of two unknown terpene compounds observed in the internal pools extracted from AmNES/LIS-1 fruits (see Figure 4A). Extracts were analyzed by GC-MS and mass spectra are shown for: (A) unknown terpene 1; (B) unknown terpene 2.
Supplementary Table 1. Primers for genotyping and qRT-PCR analyses of transgenic tomato lines.
References
Adebesin, F., Widhalm, J. R., Boachon, B., Lefèvre, F., Pierman, B., Lynch, J. H., et al. (2017). Emission of volatile organic compounds from petunia flowers is facilitated by an ABC transporter. Science 356, 1386–1388. doi: 10.1126/science.aan0826
Aharoni, A., Giri, A. P., Deuerlein, S., Griepink, F., de Kogel, W. J., Verstappen, F. W., et al. (2003). Terpenoid metabolism in wild-type and transgenic Arabidopsis plants. Plant Cell 15, 2866–2884. doi: 10.1105/tpc.016253
Ajikumar, P. K., Tyo, K., Carlsen, S., Mucha, O., Phon, T. H., and Stephanopoulos, G. (2008). Terpenoids: opportunities for biosynthesis of natural product drugs using engineered microorganisms. Mol. Pharm. 5, 167–190. doi: 10.1021/mp700151b
Ashour, M., Wink, M., and Gershenzon, J. (2010). Biochemistry of terpenoids: monoterpenes, sesquiterpenes, and diterpenes. Annu. Plant Rev. 40, 258–303. doi: 10.1002/9781444320503.ch5
Ausubel, F. M., Brent, R., Kingston, R. E., Moore, D. D., Seidmann, J. G., Smith, J. A., et al. (1994). Current Protocols in Molecular Biology. New York, NY: John Wiley and Sons.
Barg, R., Pilowsky, M., Shabtai, S., Carmi, N., Szechtman, A. D., Dedicova, B., et al. (1997). The TYLCV-tolerant tomato line MP1 is characterized by superior transformation competence. J. Exp. Bot. 48, 1919–1923. doi: 10.1093/jxb/48.11.1919
Beale, M. H., Birkett, M. A., Bruce, T. J., Chamberlain, K., Field, L. M., Huttly, A. K., et al. (2006). Aphid alarm pheromone produced by transgenic plants affects aphid and parasitoid behavior. Proc. Natl. Acad. Sci. U.S.A. 103, 10509–10513. doi: 10.1073/pnas.0603998103
Bick, J. A., and Lange, B. M. (2003). Metabolic cross talk between cytosolic and plastidial pathways of isoprenoid biosynthesis: unidirectional transport of intermediates across the chloroplast envelope membrane. Arch. Biochem. Biophys. 415, 146–154. doi: 10.1016/S0003-9861(03)00233-9
Boachon, B., Lynch, J. H., Ray, S., Yuan, J., Caldo, K. P. M., Junker, R. R., Sharon, A., et al. (2019). Natural fumigation as a mechanism for volatile transport between flower organs. Nat. Chem. Biol. 15, 583–588. doi: 10.1038/s41589-019-0287-5
Bönisch, F., Frotscher, J., Stanitzek, S., Rühl, E., Wüst, M., Bitz, O., et al. (2014). Activity-based profiling of a physiologic aglycone library reveals sugar acceptor promiscuity of family 1 UDP-glucosyltransferases from grape. Plant Physiol. 166, 23–39. doi: 10.1104/pp.114.242578
Botella-Pavia, P., Besumbes, O., Phillips, M. A., Carretero-Paulet, L., Boronat, A., and Rodríguez-Concepción, M. (2004). Regulation of carotenoid biosynthesis in plants: evidence for a key role of hydroxymethylbutenyl diphosphate reductase in controlling the supply of plastidial isoprenoid precursors. Plant J. 40, 188–199. doi: 10.1111/j.1365-313X.2004.02198.x
Davidovich-Rikanati, R., Lewinsohn, E., Bar, E., Iijima, Y., Pichersky, E., and Sitrit, Y. (2008). Overexpression of the lemon basil alpha-zingiberene synthase gene increases both mono- and sesquiterpene contents in tomato fruit. Plant J. 56, 228–238. doi: 10.1111/j.1365-313X.2008.03599.x
Davidovich-Rikanati, R., Sitrit, Y., Tadmor, Y., Iijima, Y., Bilenko, N., Bar, E., et al. (2007). Enrichment of tomato flavor by diversion of the early plastidial terpenoid pathway. Nat. Biotechnol. 25, 899–901. doi: 10.1038/nbt1312
Degenhardt, J., Hiltpold, I., Köllner, T. G., Frey, M., Gierl, A., Gershenzon, J., et al. (2009). Restoring a maize root signal that attracts insect-killing nematodes to control a major pest. Proc. Natl. Acad. Sci. U.S.A. 106, 13213–13218. doi: 10.1073/pnas.0906365106
Dudareva, N., Andersson, S., Orlova, I., Gatto, N., Reichelt, M., Rhodes, D., et al. (2005). The nonmevalonate pathway supports both monoterpene and sesquiterpene formation in snapdragon flowers. Proc. Natl. Acad. Sci. U.S.A. 102, 933–938. doi: 10.1073/pnas.0407360102
Dudareva, N., Klempien, A., Muhlemann, J. K., and Kaplan, I. (2013). Biosynthesis, function, and metabolic engineering of plant volatile organic compounds. New Phytol. 198, 16–32. doi: 10.1111/nph.12145
Eggermont, K., Goderis, I. J., and Broekaert, W. F. (1996). High-throughput RNA extraction from plant samples based on homogenisation by reciprocal shaking in the presence of a mixture of sand and glass beads. Plant Mol. Biol. Rep. 14, 273–279. doi: 10.1007/BF02671663
Enfissi, E. M., Fraser, P. D., Lois, L. M., Boronat, A., Schuch, W., and Bramley, P. M. (2005). Metabolic engineering of the mevalonate and non-mevalonate isopentenyl diphosphate-forming pathways for the production of health-promoting isoprenoids in tomato. Plant Biotechnol. J. 3, 17–27. doi: 10.1111/j.1467-7652.2004.00091.x
Enjuto, M., Balcells, L., Campos, N., Caelles, C., Arró, M., and Boronat, A. (1994). Arabidopsis thaliana contains two differentially expressed 3-hydroxy-3-methylglutaryl-CoA reductase genes, which encode microsomal forms of the enzyme. Proc. Natl. Acad. Sci. U.S.A. 91, 927–931. doi: 10.1073/pnas.91.3.927
Estévez, J. M., Cantero, A., Romero, C., Kawaide, H., Jiménez, L. F., Kuzuyama, T., et al. (2000). Analysis of the expression of CLA1, a gene that encodes the 1-deoxyxylulose 5-phosphate synthase of the 2-C-methyl-D-erythritol-4-phosphate pathway in Arabidopsis. Plant Physiol. 124, 95–104. doi: 10.1104/pp.124.1.95
Fan, G., Qiao, Y., Yao, X., Mo, D., Wang, K., and Pan, S. (2009). Free and bound volatile compounds in juice and peel of Jincheng oranges. Eur. Food Res. Technol. 229, 571–578. doi: 10.1007/s00217-009-1080-6
Flügge, U. I., and Gao, W. (2005). Transport of isoprenoid intermediates across chloroplast envelope membranes. Plant Biol. 7, 91–97. doi: 10.1055/s-2004-830446
Fraser, P. D., Truesdale, M. R., Bird, C. R., Schuch, W., and Bramley, P. M. (1994). Carotenoid biosynthesis during tomato fruit development. Plant Physiol. 105, 405–413. doi: 10.1104/pp.105.1.405
Gillaspy, G., Ben-David, H., and Gruissem, W. (1993). Fruits: a developmental perspective. Plant Cell 5, 1439–1451. doi: 10.2307/3869794
Gutensohn, M., and Dudareva, N. (2016). Tomato fruits–a platform for metabolic engineering of terpenes. Methods Enzymol. 576, 333–359. doi: 10.1016/bs.mie.2016.03.012
Gutensohn, M., Orlova, I., Nguyen, T. T., Davidovich-Rikanati, R., Ferruzzi, M. G., Sitrit, Y., et al. (2013). Cytosolic monoterpene biosynthesis is supported by plastid-generated geranyl diphosphate substrate in transgenic tomato fruits. Plant J. 75, 351–363. doi: 10.1111/tpj.12212
Hemmerlin, A., Harwood, J. L., and Bach, T. J. (2012). A raison d'être for two distinct pathways in the early steps of plant isoprenoid biosynthesis? Prog. Lipid Res. 51, 95–148. doi: 10.1016/j.plipres.2011.12.001
Hemmerlin, A., Hoeffler, J. F., Meyer, O., Tritsch, D., Kagan, I. A., Grosdemange-Billiard, C., et al. (2003). Cross-talk between the cytosolic mevalonate and the plastidial methylerythritol phosphate pathways in tobacco Bright Yellow-2 cells. J. Biol. Chem. 278, 26666–26676. doi: 10.1074/jbc.M302526200
Henry, L. K., Gutensohn, M., Thomas, S. T., Noel, J. P., and Dudareva, N. (2015). Orthologs of the archaeal isopentenyl phosphate kinase regulate terpenoid production in plants. Proc. Natl. Acad. Sci. U.S.A. 112, 10050–10055. doi: 10.1073/pnas.1504798112
Henry, L. K., Thomas, S. T., Widhalm, J. R., Lynch, J. H., Davis, T. C., Kessler, S. A., et al. (2018). Contribution of isopentenyl phosphate to plant terpenoid metabolism. Nat. Plants 4, 721–729. doi: 10.1038/s41477-018-0220-z
Hohn, T. M., and Ohlrogge, J. B. (1991). Expression of a fungal sesquiterpene cyclase gene in transgenic tobacco. Plant Physiol. 97, 460–462. doi: 10.1104/pp.97.1.460
Immethun, C. M., Hoynes-O'Connor, A. G., Balassy, A., and Moon, T. S. (2013). Microbial production of isoprenoids enabled by synthetic biology. Front. Microbiol. 4:75. doi: 10.3389/fmicb.2013.00075
Jelesko, J. G., Jenkins, S. M., Rodríguez-Concepción, M., and Gruissem, W. (1999). Regulation of tomato HMG1 during cell proliferation and growth. Planta 208, 310–318. doi: 10.1007/s004250050564
Kasahara, H., Hanada, A., Kuzuyama, T., Takagi, M., Kamiya, Y., and Yamaguchi, S. (2002). Contribution of the mevalonate and methylerythritol phosphate pathways to the biosynthesis of gibberellins in Arabidopsis. J. Biol. Chem. 277, 45188–45194. doi: 10.1074/jbc.M208659200
Köllner, T. G., Held, M., Lenk, C., Hiltpold, I., Turlings, T. C., Gershenzon, J., et al. (2008). A maize (E)-β-caryophyllene synthase implicated in indirect defense responses against herbivores is not expressed in most American maize varieties. Plant Cell 20, 482–494. doi: 10.1105/tpc.107.051672
Koncz, C., and Schell, J. (1986). The promoter of TL-DNA gene 5 controls the tissue-specific expression of chimaeric genes carried by a novel type of Agrobacterium binary vector. Mol. Gen. Genet. 204, 383–396. doi: 10.1007/BF00331014
Lange, B. M., and Ahkami, A. (2013). Metabolic engineering of plant monoterpenes, sesquiterpenes and diterpenes–current status and future opportunities. Plant Biotechnol. J. 11, 169–196. doi: 10.1111/pbi.12022
Laule, O., Fürholz, A., Chang, H. S., Zhu, T., Wang, X., Heifetz, P. B., et al. (2003). Crosstalk between cytosolic and plastidial pathways of isoprenoid biosynthesis in Arabidopsis thaliana. Proc. Natl. Acad. Sci. U.S.A. 100, 6866–6871. doi: 10.1073/pnas.1031755100
Lawrence, S. D., Cline, K., and Moore, G. A. (1997). Chromoplast development in ripening tomato fruit: identification of cDNAs for chromoplast-targeted proteins and characterization of a cDNA encoding a plastid-localized low-molecular-weight heat shock protein. Plant Mol. Biol. 33, 483–492. doi: 10.1023/A:1005785321165
Lewinsohn, E., Schalechet, F., Wilkinson, J., Matsui, K., Tadmor, Y., Nam, K. H., et al. (2001). Enhanced levels of the aroma and flavor compound S-linalool by metabolic engineering of the terpenoid pathway in tomato fruits. Plant Physiol. 127, 1256–1265. doi: 10.1104/pp.010293
Lois, L. M., Rodríguez-Concepción,., M, Gallego, F., Campos, N., and Boronat, A. (2000). Carotenoid biosynthesis during tomato fruit development: regulatory role of 1-deoxy-D-xylulose 5-phosphate synthase. Plant J. 22, 503–513. doi: 10.1046/j.1365-313x.2000.00764.x
Lücker, J., Bouwmeester, H. J., Schwab, W., Blaas, J., van der Plas, L. H., and Verhoeven, H. A. (2001). Expression of Clarkia S-linalool synthase in transgenic petunia plants results in the accumulation of S-linalyl-β-D-glucopyranoside. Plant J. 27, 315–324. doi: 10.1046/j.1365-313x.2001.01097.x
McCaskill, D., and Croteau, R. (1993). Procedures for the isolation and quantification of the intermediates of the mevalonic acid pathway. Anal. Biochem. 215, 142–149. doi: 10.1006/abio.1993.1566
McCormick, S., Niedermeyer, J., Fry, J., Barnason, A., Horsch, R., and Fraley, R. (1986). Leaf disc transformation of cultivated tomato (L. esculentum) using Agrobacterium tumefaciens. Plant Cell Rep. 5, 81–84. doi: 10.1007/BF00269239
Nagata, N., Suzuki, M., Yoshida, S., and Muranaka, T. (2002). Mevalonic acid partially restores chloroplast and etioplast development in Arabidopsis lacking the non-mevalonate pathway. Planta 216, 345–350. doi: 10.1007/s00425-002-0871-9
Nagegowda, D. A., Gutensohn, M., Wilkerson, C. G., and Dudareva, N. (2008). Two nearly identical terpene synthases catalyze the formation of nerolidol and linalool in snapdragon flowers. Plant J. 55, 224–239. doi: 10.1111/j.1365-313X.2008.03496.x
Narita, J. O., and Gruissem, W. (1989). Tomato hydroxymethylglutaryl-CoA reductase is required early in fruit development but not during ripening. Plant Cell 1, 181–190. doi: 10.1105/tpc.1.2.181
Nicholass, F. J., Smith, C. J., Schuch, W., Bird, C. R., and Grierson, D. (1995). High levels of ripening-specific reporter gene expression directed by tomato fruit polygalacturonase gene-flanking regions. Plant Mol. Biol. 28, 423–435. doi: 10.1007/BF00020391
Nogues, I., Brilli, F., and Loreto, F. (2006). Dimethylallyl diphosphate and geranyl diphosphate pools of plant species characterized by different isoprenoid emissions. Plant Physiol. 141, 721–730. doi: 10.1104/pp.105.073213
Orlova, I., Nagegowda, D. A., Kish, C. M., Gutensohn, M., Maeda, H., Varbanova, M., et al. (2009). The small subunit snapdragon geranyl diphosphate synthase modifies the chain length specificity of tobacco geranylgeranyl diphosphate synthase in planta. Plant Cell 21, 4002–4017. doi: 10.1105/tpc.109.071282
Orzaez, D., Mirabel, S., Wieland, W. H., and Granell, A. (2006). Agroinjection of tomato fruits. A tool for rapid functional analysis of transgenes directly in fruit. Plant Physiol. 140, 3–11. doi: 10.1104/pp.105.068221
Paetzold, H., Garms, S., Bartram, S., Wieczorek, J., Urós-Gracia, E. M., Rodríguez-Concepción, M., et al. (2010). The isogene 1-deoxy-D-xylulose 5-phosphate synthase 2 controls isoprenoid profiles, precursor pathway allocation, and density of tomato trichomes. Mol. Plant 3, 904–916. doi: 10.1093/mp/ssq032
Parker, M., Black, C. A., Barker, A., Pearson, W., Hayasaka, Y., and Francis, I. L. (2017). The contribution of wine-derived monoterpene glycosides to retronasal odour during tasting. Food Chem. 232, 413–424. doi: 10.1016/j.foodchem.2017.03.163
Pichersky, E., and Raguso, R. A. (2016). Why do plants make so many terpenoid compounds? New Phytol. 220, 692–702. doi: 10.1111/nph.14178
Schnee, C., Köllner, T. G., Held, M., Turlings, T. C., Gershenzon, J., and Degenhardt, J. (2006). The products of a single maize sesquiterpene synthase form a volatile defense signal that attracts natural enemies of maize herbivores. Proc. Natl. Acad. Sci. U.S.A. 103, 1129–1134. doi: 10.1073/pnas.0508027103
Schuhr, C. A., Radykewicz, T., Sagner, S., Latzel, C., Zenk, M. H., Arigoni, D., et al. (2003). Quantitative assessment of crosstalk between the two isoprenoid biosynthesis pathways in plants by NMR spectroscopy. Phytochem. Rev. 2, 3–16. doi: 10.1023/B:PHYT.0000004180.25066.62
Soler, E., Clastre, M., Bantignies, B., Marigo, G., and Ambid, C. (1993). Uptake of isopentenyl diphosphate by plastids isolated from Vitis vinifera L. cell suspensions. Planta 191, 324–329. doi: 10.1007/BF00195689
Tippmann, S., Chen, Y., Siewers, V., and Nielsen, J. (2013). From flavors and pharmaceuticals to advanced biofuels: production of isoprenoids in Saccharomyces cerevisiae. Biotechnol. J. 8, 1435–1444. doi: 10.1002/biot.201300028
Vickers, C. E., Bongers, M., Liu, Q., Delatte, T., and Bouwmeester, H. (2014). Metabolic engineering of volatile isoprenoids in plants and microbes. Plant Cell Environ. 37, 1753–1775. doi: 10.1111/pce.12316
Vranová, E., Coman, D., and Gruissem, W. (2013). Network analysis of the MVA and MEP pathways for isoprenoid synthesis. Annu. Rev. Plant Biol. 64, 665–700. doi: 10.1146/annurev-arplant-050312-120116
Wallaart, T. E., Bouwmeester, H. J., Hille, J., Poppinga, L., and Maijers, N. C. (2001). Amorpha-4,11-diene synthase: cloning and functional expression of a key enzyme in the biosynthetic pathway of the novel antimalarial drug artemisinin. Planta 212, 460–465. doi: 10.1007/s004250000428
Wang, F., Park, Y. L., and Gutensohn, M. (2020). Glandular trichome-derived sesquiterpenes of wild tomato accessions (Solanum habrochaites) affect aphid performance and feeding behavior. Phytochemistry 180:112532. doi: 10.1016/j.phytochem.2020.112532
Wu, B. P., Gao, L. X., Gao, J., Xu, Y. Y., Liu, H. R., Cao, X. M., et al. (2017). Genome-wide identification, expression patterns, and functional analysis of UDP glycosyltransferase family in peach (Prunus persica L. Batsch). Front. Plant Sci. 8:389. doi: 10.3389/fpls.2017.00389
Wu, S., Schalk, M., Clark, A., Miles, R. B., Coates, R., and Chappell, J. (2006). Redirection of cytosolic or plastidic isoprenoid precursors elevates terpene production in plants. Nat. Biotechnol. 24, 1441–1447. doi: 10.1038/nbt1251
Yauk, Y. K., Ged, C., Wang, M. Y., Matich, A. J., Tessarotto, L., Cooney, J. M., et al. (2014). Manipulation of flavour and aroma compound sequestration and release using a glycosyltransferase with specificity for terpene alcohols. Plant J. 80, 317–330. doi: 10.1111/tpj.12634
Keywords: sesquiterpenes, monoterpenes, metabolic engineering, tomato fruit, nerolidol/linalool synthase, MEP pathway, MVA pathway, isopentenyl phosphate kinase
Citation: Gutensohn M, Henry LK, Gentry SA, Lynch JH, Nguyen TTH, Pichersky E and Dudareva N (2021) Overcoming Bottlenecks for Metabolic Engineering of Sesquiterpene Production in Tomato Fruits. Front. Plant Sci. 12:691754. doi: 10.3389/fpls.2021.691754
Received: 07 April 2021; Accepted: 17 May 2021;
Published: 17 June 2021.
Edited by:
Reuben J. Peters, Iowa State University, United StatesReviewed by:
Raimund Nagel, University of Leipzig, GermanyQiang Wang, Sichuan Agricultural University, China
Matthias Wüst, University of Bonn, Germany
Axel Schmidt, Max Planck Institute for Chemical Ecology, Germany
Copyright © 2021 Gutensohn, Henry, Gentry, Lynch, Nguyen, Pichersky and Dudareva. This is an open-access article distributed under the terms of the Creative Commons Attribution License (CC BY). The use, distribution or reproduction in other forums is permitted, provided the original author(s) and the copyright owner(s) are credited and that the original publication in this journal is cited, in accordance with accepted academic practice. No use, distribution or reproduction is permitted which does not comply with these terms.
*Correspondence: Michael Gutensohn, bWljaGFlbC5ndXRlbnNvaG4mI3gwMDA0MDttYWlsLnd2dS5lZHU=
†Present address: Joseph H. Lynch, Division of Plant and Soil Sciences, West Virginia University, Morgantown, WV, United States
Thuong T. H. Nguyen, Department of Biochemistry, Faculty of Biology and Biotechnology, University of Science, Vietnam National University, Ho Chi Minh City, Vietnam