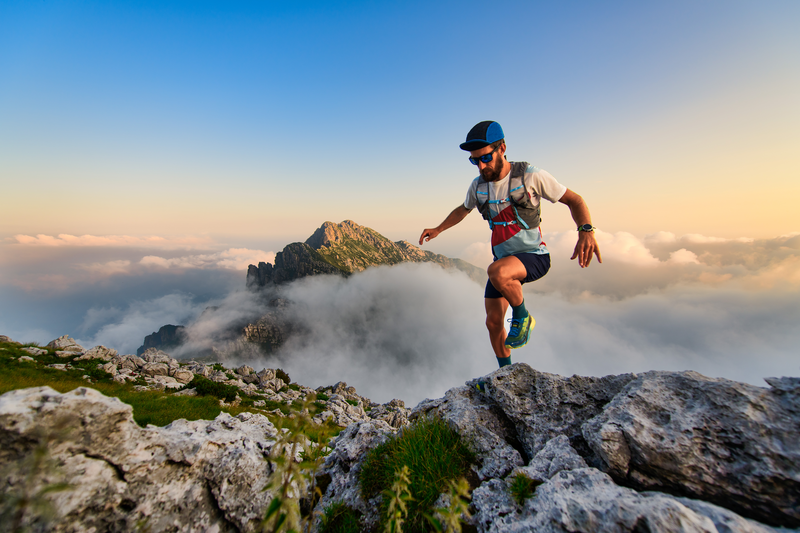
95% of researchers rate our articles as excellent or good
Learn more about the work of our research integrity team to safeguard the quality of each article we publish.
Find out more
ORIGINAL RESEARCH article
Front. Plant Sci. , 22 September 2021
Sec. Plant Breeding
Volume 12 - 2021 | https://doi.org/10.3389/fpls.2021.688937
This article is part of the Research Topic Sorghum and Pearl Millet as Climate Resilient Crops for Food and Nutrition Security View all 28 articles
Pearl millet is a predominant food and fodder crop in West Africa. This study was carried out to test the newly developed open-pollinated varieties (OPVs) for field performance and stability for grain yield, grain iron (Fe), and grain zinc (Zn) contents across 10 locations in West Africa (i.e., Niger, Nigeria, Mali, Burkina Faso, Senegal, and Ghana). The test material consisted of 30 OPVs, of which 8 are Fe/Zn biofortified. The experiment was conducted in a randomized complete block design in three replications. ANOVA revealed highly significant variability for grain yield and micronutrient traits. The presence of genotype × environment (G × E) indicated that the expressions of traits are significantly influenced by both genetic and G × E factors, for grain Fe and Zn contents. Days to 50% flowering and plant height showed less G × E, suggesting these traits are largely under genetic control. The genotypes CHAKTI (46 days), ICTP 8203 (46 days), ICMV 177002 (50 days), ICMV 177003 (48 days), and Moro (53 days) had exhibited early flowering across locations leading to early physiological maturity. CHAKTI (1.42 t/ha yield; 62.24 mg/kg of grain Fe, 47.29 mg/kg of grain Zn) and ICMP 177002 (1.19 t/ha yield, 62.62 mg/kg of grain Fe, 46.62 mg/kg of grain Zn) have performed well for grain yield and also for micronutrients, across locations, compared with the check. Additive Main Effect and Multiplicative Interaction (AMMI) ANOVA revealed the highly significant genotypic differences, the mean sum of squares of environment, and its interaction with the genotypes. Based on the AMMI stability value (ASV), the most stable genotype is SOSAT-C88 (ASV = 0.04) for grain yield and resistance to downy mildew; mean grain yield and stability rankings (YSI) revealed that the genotypes CHAKTI, SOSAT-C88, and ICMV IS 99001 were high yielding and expressed stability across regions. The strong correlation (r = 0.98∗∗) of grain Fe and Zn contents that merits Fe-based selection is highly rewarding. CHAKTI outperformed over other genotypes for grain yield (71% higher), especially with early maturing varieties in West Africa, such as GB 8735, LCIC 9702, and Jirani, and for grain Fe (16.11% higher) and Zn (7% higher) contents across locations, and made a candidate of high-iron variety to be promoted for combating the micronutrient malnutrition in West and Central Africa (WCA).
Pearl millet (Pennisetum glaucum L.) is an important food and fodder crop for people living in the semi-arid regions of Asia and Africa. The people living in the semi-arid regions mostly consume cereal-based foods and do not have access to diverse food, hence, leading to serious malnutrition in children and anemia in women (Underwood, 2000). Micronutrient malnutrition is a major concern in the developing world, where more than 2 billion people are malnourished (World Health Organization, 2006; Tulchinsky, 2010; Kramer and Allen, 2015; Webb et al., 2018). Malnutrition in Africa is a rising concern where children are affected by stunted growth and low IQ. Young children and pregnant women are most vulnerable to micronutrient deficiencies due to their rapid growth and development (Black et al., 2013; Saltzman et al., 2017). At least half of the children worldwide younger than 5 years of age suffer from vitamin and mineral deficiencies, while almost 2 in 3 children between 6 months and 2 years of age are not fed on food that supports their healthy development (UNICEF, 2019). Although only required in small amounts, micronutrients are not produced in the body and must be derived from the diet. It is severe in regions where people depend on staple crops, which are low in micronutrients, to meet their energy requirements. Hence, improving the micronutrient concentration of locally adapted staple food crops will be a sustainable solution to address this malnutrition. Efforts are being made to provide fortified foods to these vulnerable groups, which is a cost-effective strategy, but it is difficult to ensure the availability of these fortified foods to people living in remote areas. Food diversification is another tool to combat micronutrient malnutrition, but the high cost involved and limited food availability make it unsustainable.
Biofortification, the process of increasing the concentration of micronutrients in the staple crops to have a measurable impact on human nutrition after consumption, is therefore important for combating micronutrient malnutrition. Biofortification is becoming more of the breeding process by which the nutrient density is increased through conventional plant breeding and modern biotechnology without altering the preferred traits of the farmers. (1) Iron biofortification of beans, cowpea, and pearl millet, (2) zinc biofortification of maize, rice, and wheat, and provitamin A, and (3) carotenoid biofortification of cassava, maize, rice, and sweet potato are currently underway and at different stages of development (Saltzman et al., 2013). Biofortification has been regarded as a novel and sustainable vehicle in the reduction of micronutrient malnutrition (Bouis et al., 2011).
The HarvestPlus program of the CGIAR is supporting global biofortification efforts, through which the International Crops Research Institute for the Semi-Arid Tropics (ICRISAT) released the ever-first high grain Fe pearl millet variety “Dhanashakti” in 2014 by exploiting the intrapopulation variability (recurrent selection) within ICTP 8203 variety, an early open-pollinated variety (OPV) popularly grown in India (Rai et al., 1990; Govindaraj et al., 2019). Since then, pearl millet hybrids rich in kernel iron and zinc concentrations have been bred and released in India through conventional (i.e., heterosis) breeding methods (Govindaraj et al., 2018, 2019).
Many studies have shown the positive impact of the consumption of biofortified crops, and the results of these studies were also similar to iron fortification and supplementation strategies to fight against hidden hunger (Kodkany et al., 2013; Saltzman et al., 2013; Haas, 2014; Finkelstein et al., 2015). The use of biofortified pearl millet varieties could have a positive effect on Fe and Zn intakes in the long term. The studies conducted by Kodkany et al. (2013) revealed that the quantities of both iron and zinc were absorbed when iron- and zinc-biofortified pearl millet was fed to children aged 2 years as the major staple food and was more than adequate to meet the physiological requirements for these micronutrients.
The regions in West and Central Africa (WCA), where pearl millet is significantly grown and consumed, have no biofortified variety at present. The ICRISAT center in Niamey, Niger with the help of the HarvestPlus program employs in developing high-yielding biofortified pearl millet varieties and hybrids. WCA regions significantly hold the undernutrition populations, and it is very appropriate to breed, test, and identify the Fe/Zn-rich pearl millet varieties besides better yield and resistance to biotic stress across the region of WCA. Most of the pearl millet varieties existing with the farming community have lower levels of grain Fe (<30.0 mg/kg) and Zn (<30.0 mg/kg) contents. Hence, this study was conducted to identify the pearl millet varieties with high grain Fe and Zn contents, their stable performance across the region, and the influence of environmental factors on the inheritance of these traits.
The experimental material consisted of 30 pearl millet varieties, of which 8 varieties had high grain Fe and Zn contents, 20 with moderate levels of grain Fe and Zn, and 2 local checks GB 8735 and Jirani. GB 8735 is the highest acreage cultivated in Niger, Togo, and adjoining countries with its early maturity and stability. This variety was also noticed with higher Fe/Zn in the regions. Using this variety for both early maturity and micronutrient is highly appropriate in this study. The trials were conducted in 10 locations of WCA representing the Sahelian agroecology with an annual rainfall of 400 mm. The test locations were Niger (Sadore, Konni, Maradi, and Magaria), Nigeria (Kano), Mali (Cinzana), Burkina Faso (Gampella), Ghana (Bawku), and Senegal (Bambey and Nioro) in WCA. The material was sown in randomized complete block design in three replications in the rainy season of 2018. A basal dose of fertilizer was applied to the field at the rate of 100 kg/ha. The genotypes were sown in two-row plots with a row length of 3.0 m long and a row-to-row distance of 0.80 and 0.20 m in between the plants. Thinning of the test entries to one plant per hill was carried out at 15–21 days after seedling emergence (DAE). Hand weeding of the test plots was carried out when necessary. Microdosing with urea was carried out at 30 DAE.
Data on agronomic and morphological traits were recorded in the test plots. Data on days to 50% flowering, plant height (cm), number of downy mildew plants, panicle length (cm), circumference (cm), and grain yield (t/ha) were recorded. Downy mildew incidence were calculated based on the percentage incidence using the number of plants affected to the total number of plants in the plot multiplied by 100. Grain samples for Fe and Zn analysis were collected before harvesting on the standing crop eliminating the plants grounded and contaminated with soil. Proper care was taken in cleaning the grain samples for analysis to avoid contamination from metals and dust.
Pearl millet grain micronutrient estimation was carried out using the energy dispersive X-ray fluorescence (EDXRF) (Paltridge et al., 2012a,b; Guild and Stangoulis, 2016) method. The EDXRF analysis was carried out using Oxford X-Supreme 8000 (Oxford Instruments plc, United Kingdom) with a 10-sample carousel. Analyses were conducted in supplied sample cups prepared as reported previously (Paltridge et al., 2012a,b; Guild and Stangoulis, 2016), with a 4-μm Poly-4 XRF sample film used to seal one end of the cup. Sample cups were cleaned and prepared before each analysis to minimize cross-contamination between samples. Samples of >5 g were used for all analyses to ensure that samples were “infinitely thick” in terms of EDXRF analysis (Paltridge et al., 2012b). Calibrations for Fe and Zn in pearl millet were achieved using 20 standards based on the data of inductively coupled plasma optical emission spectrophotometry (ICP-OES).
The replicated data from each location are subjected to perform ANOVA and Additive Main Effects and Multiplicative Interaction (AMMI) analysis using GenStat software version 18.0 (GenStat, 2015). The significant differences between the genotypes were tested by using the F-test, and the genotypic means were compared by the least significant difference at p ≤ 0.05. AMMI analysis permits the estimation of the interaction effect of a genotype in each environment, and it helps to identify genotypes best suited for specific environmental conditions. The trait phenotypic correlation coefficient was analyzed using Excel. Heritability estimates were calculated using H2 = Vg/Vp, where H2 is the heritability estimate; Vg, the variation in genotype; and Vp, the variation in phenotype.
The ANOVA has revealed a highly significant (P ≤ 0.01) mean sum of squares of entries, locations (hereafter referred to as environment), and genotypes × location [hereafter referred to as genotype × environment (G × E)] for all the studied traits (Table 1). The mean sum of squares of genotypes was higher than the mean sum of squares of environments. The G × E influenced the trait determination in these 10-environment testing; however, it was very much lower in variance magnitude for all the traits. For instance, flowering, grain yield, and grain Fe and Zn contents were largely controlled by genotypic variance. Days to 50% flowering showed 29 times higher mean square magnitude than the G × E source of variation. The heritability for agronomic and morphological traits revealed presence of high heritability for all the studied traits. The estimation of genetic advance percentage mean showed high genetic advance for downy mildew resistance (106.15%), panicle length (66.50%), grain yield (49.32%), and grain Fe content (39.01%).
The mean performance of the pearl millet genotypes indicated significant F probabilities (P ≤ 0.01) for all the studied traits (Table 2). The genotypes CHAKTI, Faringuero, IBMV 8402, ICMV 167005, ICMV 167006, ICMV 177001, ICMV 177002, ICMV 177003, ICMV 177004, ICMV IS 85327, ICMV IS 99001, ICTP 8203, Moro, SARIA-1, SOSAT-C88, Souna 3, and WAAP-NAARA had shown lower (<10%) downy mildew damage percentage when compared with the susceptible check, Sadore local (17.69 %).
Table 2. Mean performance of the pearl millet (Pennisetum glaucum L.) genotypes across locations in West Africa.
The genotypes CHAKTI, ICMV 221, ICTP 8203, and Jirani had attained days to 50% flowering within 45 days, which is very early (Table 2). Other 11 genotypes also exhibited lower days to 50% flowering when compared with the overall mean (55.00 days) and with the local check (66.60 days). The mean plant height of 202.00 cm is recorded across locations. Fourteen genotypes exhibited greater plant height when compared with the mean plant height. Most of the genotypes with the lower days to 50% flowering exhibited moderate plant height.
The test genotypes exhibited a mean panicle length of 32.00 cm across locations (Table 2). The genotypes Gamoji (41.10 cm, 9.91 cm; panicle length and panicle circumference, respectively) and ICMV 177004 (32.97, 9.12) have shown greater panicle length and circumference across locations. The genotype ICMV IS 94222 had exhibited the highest panicle length of 60.01 cm, and AKAD-KOM has the least panicle length of 17.56 cm.
The mean performance of genotypes for grain yield across locations is given in Table 2. The overall mean for grain yield is 0.96 t/ha. The genotype CHAKTI (1.42 t/ha) has performed well for grain yield when compared with the other genotypes. The genotypes Gamoji, IBMV 8402, ICMP 177002, ICMV 167005, ICMV 167006, ICMV 167001, ICMV IS 85327, ICMV IS 94222, ICMV IS 99001, SOSAT-C88, and Souna 3 yielded high across locations when compared with the check Sadore local (0.73 t/ha).
The genotypes AFRIBEH-NAARA, AKAD-KOM, GB 8735, ICMP 177001, ICMV 177003, ICMV 221, ICTP 8203, and Jirani exhibited high grain Fe and Zn contents across locations when compared with the check Sadore local (33.71 mg/kg Fe and 29.84 mg/kg Zn), expressing lower than the overall mean (Table 2). The genotypes CHAKTI and ICMP 177002 have the highest grain Fe content of >62.0 mg/kg and grain Zn content of >45.0 mg/kg.
The AMMI ANOVA carried out to partition the total variances into its components revealed existence of highly significant (P ≤ 0.01) genotypic differences among all the traits (Table 3). The mean sum of squares of the environment and its interaction with the genotypes were also significant at P ≤ 0.01. The G × E interaction was further partitioned into IPCA 1 and IPCA 2, which were significant at P ≤ 0.01 for all the studied traits. The mean sum of squares of G × E interactions was lower than the mean sum of squares of the genotypes.
Table 3. Analysis of variance for AMMI model of pearl millet genotypes across locations in West Africa.
In the AMMI biplot, the IPCA 1 (PC1) and IPCA 2 (PC2) scores of genotype and environments were plotted against each other for grain yield (Figure 1) and grain Fe content (Figure 2). The IPCA 1 component accounted for 40.26 and 27.54% of G × E interaction, while IPCA 2 accounted for 12.75 and 22.40%, respectively, for grain yield and grain Fe content. In the biplot for grain yield, the genotypes ICMP 177002, IBMV 8402, Souna 3, ICMV IS 85327, and Moro are scattered away from the origin and away from the environmental spikes. Most of the genotypes are close to the origin in the biplot for grain Fe content. The genotypes AFRIBEH-NAARA, ICMV 177001, ICMV 177002, and SOSAT-C88 are scattered away from the origin and also away from the environment spikes.
Figure 1. Additive Main Effects and Multiplicative Interaction (AMMI) biplot for grain yield across environments in West and Central Africa. 1. AFRIBEH-NAARA, 2. AKAD-KOM, 3. CHAKTI, 4. Faringuero, 5. Gamoji, 6. GB 8735, 7. IBMV 8402, 8. ICMP 177001, 9. ICMP 177002, 10. ICMV 167005, 11. ICMV 167006, 12. ICMV 177001, 13. ICMV 177002, 14. ICMV 177003, 15. ICMV 177004, 16. ICMV 221, 17. ICMV 167001, 18. ICMV IS 85327, 19. ICMV IS 94222, 20. ICMV IS 99001, 21. ICTP 8203, 22. Jirani, 23. KAANATI, 24. LCIC 9702, 25. Moro, 26. Sadore Local, 27. SARIA-1, 28. SOSAT-C88, 29. Souna 3, 30. WAAPP-NAARA.
Figure 2. Additive Main Effects and Multiplicative Interaction (AMMI) biplot for grain Fe content across environments in West and Central Africa. 1. AFRIBEH-NAARA, 2. AKAD-KOM, 3. CHAKTI, 4. Faringuero, 5. Gamoji, 6. GB 8735, 7. IBMV 8402, 8. ICMP 177001, 9. ICMP 177002, 10. ICMV 167005, 11. ICMV 167006, 12. ICMV 177001, 13. ICMV 177002, 14. ICMV 177003, 15. ICMV 177004, 16. ICMV 221, 17. ICMV 167001, 18. ICMV IS 85327, 19. ICMV IS 94222, 20. ICMV IS 99001, 21. ICTP 8203, 22. Jirani, 23. KAANATI, 24. LCIC 9702, 25. Moro, 26. Sadore Local, 27. SARIA-1, 28. SOSAT-C88, 29. Souna 3, 30. WAAPP-NAARA.
The AMMI stability value (ASV) ranked the genotypes based on the least score (Table 4). Low scores represent the most stable genotypes across locations. Based on the ASV, the most stable genotype is SOSAT-C88 (ASV = 0.04) followed by LCIC 9702 (ASV = 0.13) for grain yield, ICMV 221 (ASV = 0.05) followed by ICMV IS 99001 (ASV = 0.23) for grain Fe content, and WAAP-NAARA (ASV = 0.28) followed by GB 8735 (ASV = 0.50) for grain Zn content. The sum of mean grain yield and stability rankings (YSI) revealed that the genotypes SOSAT-C88, ICMV IS 99001, and CHAKTI were high yielding and expressed stability across regions. The genotypes ICMV 167001 and ICMV 167005 were high yielding but were unstable, which has a high YSI rank.
Table 4. Interaction principal component axis scores of agronomic and morphological traits of pearl millet genotypes across locations in West Africa.
Additive main effects and multiplicative interaction analysis identified four high-yielding genotypes in each of the environments (Table 5). CHAKTI performed well and yielded high at Bambey (Senegal), Nioro (Senegal), Kano (Nigeria), Konni (Niger), Bawku (Ghana), and Gampella (Burkina Faso). The genotype ICMV 167005 performed well at Magaria, Sadore in Niger, and Cinzana location of Mali.
The phenotypic correlation patterns of micronutrient traits of pearl millet indicated the presence of a highly significant and positive correlation between grain Fe and Zn contents (r = 0.98; P < 0.01) (Table 6). The grain Fe and Zn contents exhibited significant and negative correlations with days to 50% flowering, plant height, and panicle length and a significant positive correlation with panicle circumference. It is observed that both these micronutrients are not correlated with grain yield (Table 6).
Table 6. Association of agronomic and morphological traits of pearl millet (Pennisetum glaucum L.) across locations in West Africa.
Micronutrient malnutrition is a major concern in West and Central Africa. Breeding OPVs with grain Fe and Zn micronutrients will be helpful in combating the dietary-based malnutrition among the poor households in this region. Significant differences for grain Fe and Zn densities and grain yield indicate the presence of variability for these traits in the material tested. Environment is one of the major factors that influences the performance of the genotypes. As G × E interaction is important for any trait breeding, ANOVA revealed a higher magnitude of mean squares for genotypes than the mean squares of G × E interaction, marking the lower impact of the environment on the inheritance of these agronomic and micronutrient traits studied across locations.
In the Sahelian zone of West Africa, the farmers prefer to have the early maturing genotypes with long panicles and dual-purpose pearl millet where the rainfall is 300–400 mm (Blümmel et al., 2003; Omanya et al., 2007; Anna Ida Pucher, 2018). In this study, most of the genotypes exhibited early flowering, which is very important for drought-prone areas of Sub-Saharan Africa, especially in the Sahelian zone of West Africa where millet is grown in around 8 million ha out of 16 million ha of total millet cultivation in Africa (Zakari et al., 2019).
CHAKTI and ICMP 177002 have performed well with high grain yield and grain Fe and Zn contents. CHAKTI has iniadi background, which was developed using the intrapopulation method after four cycles of recurrent selections for high Fe. CHAKTI is proposed for the large-scale farmer trials and release in WCA. Previous studies reported that iniadi germplasm has been found to be the best source for grain Fe and Zn contents (Velu et al., 2011; Rai et al., 2014; Govindaraj et al., 2020). The identification of new sources of grain Fe and Zn is equally important as depending on one type of germplasm may be counterproductive in achieving micronutrient genetic gains in pearl millet.
The AMMI ANOVA also indicated the broad range of diversity that existed among genotypes, and the significance of environments and their interactions indicated that there was a differential response of genotypes to environments and will help breeder in choosing genotypes to a specific environment. The significance of IPCA1 and IPCA2 indicated the presence of a complex, multidimensional variation in the G × E data. The AMMI biplot analysis is a predominant method to find the G × E interaction for different studied traits. Low G × E interaction indicates the stability of the genotypes over the range of environments. A genotype showing high positive interaction in an environment can exploit the agroecological or agromanagement conditions of the specific environment and is, therefore, best suited to that environment. Heritability across the locations is very significant indicating a relatively lesser G × E influence; thus, simple selection for micronutrients is highly feasible. These magnitudes of heritability are affected by the type of genetic material, traits variability, and environmental conditions to which the trials are conducted (Dabholkar, 1992; Falconer and Mackay, 1996). Therefore, the heritability estimate is relatively high for grain yield due to conducive rainfall and other environmental conditions such as the adaptability of the new variety.
The most powerful interpretive tool in the analysis of G × E interaction in the AMMI model is the biplot analysis. The biplots permit easy visualization of differences in interaction effects. In the AMMI biplot, the genotypes with high mean performance and large value of IPCA scores are conceived as specific adaptability to the environment. Purchase et al. (2000) proposed an ASV measure to quantify and classify genotypes according to their per se potential. The ASV has identified the genotype CHAKTI as the most prominent genotype that outperformed across the majority of the locations, indicating this as a stable performing genotype across environments. The ASV parameter has been successfully used in several studies to find stable performers (Mallikarjuna et al., 2015; Govindaraj et al., 2018). CHAKTI is the top-ranking OPV that had a lower ASV of 0.5–2.0 for grain yield and micronutrients, and still, it ranked first in 6 out of 10 locations and second in only 1 location. Based on the multilocational testing and superior performance, recently in 2018, the first biofortified pearl millet variety “CHAKTI” in Africa was released in Niger (Jayashree and Meyer, 2018) and the ECOWAS regional catalog in 20191. This variety is gaining importance in the farming community in Niger, Mali, Burkina Faso, and Senegal due to its extra early maturity, high yield, and high grain Fe and Zn contents (Mavindidze, 2020).
Association studies among traits revealed the presence of a significant positive correlation between the grain Fe and Zn contents, indicating that they are inherited together and both traits can be selected in every generation. Similar results were earlier reported in pearl millet (Govindaraj et al., 2013; Anand et al., 2014; Govindaraj et al., 2020). This high correlation may be due to the overlapping and co-segregating quantitative trait loci reported in pearl millet (Kumar, 2011), implying that selecting high-Fe lines would likely increase Zn as an associated trait. Grain Fe and Zn contents have exhibited no significant correlation with grain yield, which implies that there is no yield limitation in increasing micronutrients in pearl millet. Earlier studies in pearl millet reported a significant negative correlation with grain yield (Rai et al., 2012; Anand et al., 2014) and no correlations (Govindaraj et al., 2019; Pujar et al., 2020), indicating that conscious selection for both traits is required in segregating population to avoid any negative linkages. Also, it is important to develop the experimental population involving diverse high-yield and high-Fe/Zn parents to study the linkage between yield and nutrient traits in the segregating population or by using genomic tools.
The biofortification in pearl millet intends to contribute towards the prevention of micronutrient deficiencies by increasing the daily adequacy of micronutrient intakes among the poor farming population in the semi-arid regions. A stable performing high-Fe variety “CHAKTI” was identified across 10 locations, with high grain yield (71% higher) and grain Fe (16% higher) and Zn (7.4% higher) contents than the currently growing OPVs (35 ppm mean Fe and 28 ppm mean Zn). The correlation between Fe and Zn and no correlation with yield indicate that both micronutrients can be improved simultaneously in high-yielding backgrounds. Further study is needed to explore the progeny test-based selections from the identified high-Fe/Zn OPVs for breeding hybrid parents prospecting biofortification in WCA with competitive yield in near future.
The original contributions presented in the study are included in the article/supplementary material, further inquiries can be directed to the corresponding authors.
PG and MG conceived the concept and provided the genetic materials. MR and PG helped in designing the trial, data curation, conducted the research, statistical data analyses, and drafting the manuscript. DI conducted trials in Burkina Faso and contributed to the data analysis and data curation. OS conducted trials in Senegal and contributed to the data curation and data analysis from Senegal. KAI helped in conducting multisite data from Niger. PAA contributed to conducting the trial and data curation from the Ghana trial. MDS helped in conducting the trial and data curation from Mali locations. AII conducted trials in Nigeria and contributed to the data curation from Nigeria. All authors read and agreed to the content of the manuscript.
The authors declare that the research was conducted in the absence of any commercial or financial relationships that could be construed as a potential conflict of interest.
All claims expressed in this article are solely those of the authors and do not necessarily represent those of their affiliated organizations, or those of the publisher, the editors and the reviewers. Any product that may be evaluated in this article, or claim that may be made by its manufacturer, is not guaranteed or endorsed by the publisher.
We are thankful to the ICRISAT pearl millet breeding team Hamadou Adamou, Issa Karimou, Tahirou Boye, and Ada Abarachi based in Niamey, Niger for their help in setting the trial, and carrying out the field experiments at Sadore, Konni, and Magaria stations. We are also thankful to ICRISAT, Kano, Nigeria for conducting trials in Nigeria. NARS partners from INRAN, Niger; INERA, Burkina Faso; ISRA, Senegal; CSIR-SARI, Ghana; and IER, Mali who have helped us in multilocation trials and the release committee, Government of Niger for their efforts in the release of “CHAKTI.” Special thanks to millet team members. We also thank the CGIAR HarvestPlus program, Bill and Melinda Gates Foundation, and CRP Grain Legumes and Dryland Cereals for providing the financial support.
Anand, K., Rai, K. N., Radhika, K., Govindaraj, M., Sahrawat, K. L., and Rao, A. S. (2014). Grain iron and zinc density in pearl millet: combining ability, heterosis and association with grain yield and grain size. Springerplus 3:763. doi: 10.1186/2193-1801-3-763
Anna Ida Pucher (2018). Pearl Millet Breeding in West Africa – Steps Towards Higher Productivity and Nutritional Value. Dissertation Submitted in Fulfilment of the Requirements for the Degree. Germany: Universität Hohenheim.
Black, R. E., Victora, C. G., Walker, S. P., Bhutta, Z. A., Christian, P., de Onis, M., et al. (2013). Maternal and child undernutrition and overweight in low-income and middle-income countries. Lancet 382, 427–451. doi: 10.1016/S0140-6736(13)60937-X
Blümmel, M., Zerbini, E., Reddy, B. V. S., Charles Thomas, H. Jr., Bidinger, F., Khan, A. A., et al. (2003). Improving the production and utilization of sorghum and pearl millet as livestock feed: progress towards dual-purpose genotypes. F. Crop Res. 84, 143–158. doi: 10.1016/S0378-4290(03)00146-1
Bouis, H. E., Hotz, C., McClafferty, B., Meenakshi, J. V., and Pfeiffer, W. H. (2011). Biofortification: a new tool to reduce micronutrient malnutrition. Food Nutr. Bull. 32, S31–S40.
Dabholkar, A. R. (1992). Elements of Biometrical Genetics. New Delhi: Concept Publishing Company, 431.
Falconer, D. S., and Mackay, T. F. C. (1996). Quantitative Genetics, 4th Edn. London: Longman Group Limited, 464.
Finkelstein, J. L., Mehta, S., Udipi, S. A., Ghugre, P. S., Luna, S. V., Wenger, M. J., et al. (2015). A randomized trial of iron-biofortified pearl millet in school children in India. J. Nutr. 145, 1576–1581. doi: 10.3945/jn.114.208009
Govindaraj, M., Rai, K. N., Kanatti, A., Rao, A. S., and Shivade, H. (2019). Nutritional security in drylands: fast-track intra-population genetic improvement for grain iron and zinc densities in pearl millet. Front. Nutr. 6:74. doi: 10.3389/fnut.2019.00074
Govindaraj, M., Rai, K. N., Kanatti, A., and Shivade, H. (2018). Terminal drought and a d 2 dwarfing gene affecting grain iron and zinc concentration in pearl millet. J. Cereal Sci. (TSI) 79, 247–252. doi: 10.1016/j.jcs.2017.11.005
Govindaraj, M., Rai, K. N., Kanatti, A., Upadhyaya, H. D., Shivade, H., and Rao, A. S. (2020). Exploring the genetic variability and diversity of pearl millet core collection germplasm for grain nutritional traits improvement. Sci. Rep. 10:21177.
Govindaraj, M., Rai, K. N., Shanmugasundaram, P., Dwivedi, S. L., Sahrawat, K. L., Muthaiah, A. R., et al. (2013). Combining ability and heterosis for grain iron and zinc densities in pearl millet. Crop Sci. 53, 507–517. doi: 10.2135/cropsci2012.08.0477
Guild, G. E., and Stangoulis, J. C. R. (2016). Non-matrix matched glass disk calibration standards improve XRF micronutrient analysis of wheat grain across five Laboratories in India. Front. Plant Sci. 7:784. doi: 10.3389/fpls.2016.00784
Haas, J. E. (2014). Efficacy and other nutrition evidence for iron crops. Biofortification Prog. Briefs, 20, Washington, D.C.: International Food Policy Research Institute (IFPRI). 39–40.
ICRISAT archives Available online at: http://www.icrisat.org/what-we-do/crops/PigeonPea/Archives/Earlymillet.htm
Jayashree, B., and Meyer, C. (2018). Africa’s First Biofortified Pearl Millet Variety Aims to Combat Anaemia. Available online at: https://www.icrisat.org/africas-first-biofortified-pearl-millet-variety-aims-to-combat-anaemia/ (accessed July 19, 2020).
Kodkany, B. S., Bellad, R. M., Mahantshetti, N. S., Westcott, J. E., Krebs, N. F., Kemp, J. F., et al. (2013). Biofortification of pearl millet with iron and zinc in a randomized controlled trial increases absorption of these minerals above physiologic requirements in young children. J. Nutr. 143, 1489–1493. doi: 10.3945/jn.113.176677
Kramer, C. V., and Allen, S. (2015). Malnutrition in developing countries. Paediatr. Child Health 25, 422–427.
Kumar, S. (2011). Development of New Mapping Population and Marker-assisted Improvement of Iron and Zinc Grain Density in Pearl Millet [Pennisetum glaucum (L.) R. Br.]. Dissertation, Bikaner: Swami Keshwanand Rajasthan Agricultural University.
Mallikarjuna, M. G., Thirunavukkarasu, N., Hossain, F., Bhat, J. S., Jha, S. K., Rathore, A., et al. (2015). Stability performance of inductively coupled plasma mass spectrometry-phenotyped kernel minerals concentration and grain yield in maize in different agro-climatic zones. PLos One 10:e0139067. doi: 10.1371/journal.pone.0139067
Mavindidze, D. (2020). Iron Pearl Millet: Crop of the Future for Improving Nutrition in Sahel. Available online at: https://www.harvestplus.org/knowledge-market/in-the-news/iron-pearl-millet-crop-future-improving-nutrition-sahel (accessed July 27, 2020).
Omanya, G. O., Weltzien-Rattunde, E., Sogodogo, D., Hanssens, N., Guero, Y., Zangre, R. G., et al. (2007). Participatory varietal selection with improved pearl millet in West Africa. Exp. Agric. 43, 5–19. doi: 10.1017/S0014479706004248
Paltridge, N. G., Milham, P. J., Ortiz-Monasterio, J. I., Velu, G., Yasmin, Z., et al. (2012a). Energy-dispersive X-ray fluorescence spectrometry as a toolfor zinc, iron and selenium analysis in whole grain wheat. Plant Soil 361, 261–269. doi: 10.1007/s11104-012-1423-0
Paltridge, N. G., Palmer, L. J., Milham, P. J., Guild, G. E., and Stangoulis, J. C. R. (2012b). Energy-dispersive X-ray fluorescence analysis of zinc and iron concentration in rice and pearl millet grain. Plant Soil 361, 251–260. doi: 10.1007/s11104-011-1104-4
Pujar, M., Govindaraj, M., Gangaprasad, S., Kanatti, A., and Shivade, H. (2020). Genetic variation and diversity for grain iron, zinc, protein and agronomic traits in advanced breeding lines of pearl millet [Pennisetum glaucum (L.) R. Br.] for biofortification breeding. Genet. Resour. Crop. Evol. 67, 2009–2022. doi: 10.1007/s10722-020-00956-x
Purchase, J. L., Hatting, H., and van Deventer, C. S. (2000). Genotype x environment interaction of winter wheat (Triticum aestivum L.) in South Africa: II. Stability analysis of yield performance. South Afr. J. Plant Soil 17, 101–107.
Rai, K. N., Govindaraj, M., and Rao, A. S. (2012). Genetic enhancement of grain iron and zinc content in pearl millet. Qual. Assur. Saf. Crop. 4, 119–125. doi: 10.1111/j.1757-837X.2012.00135.x
Rai, K. N., Gupta, S. K., Sharma, R., Govindaraj, M., Rao, A. S., Shivade, H., et al. (2014). Pearl millet breeding lines developed at ICRISAT: a reservoir of variability and useful source of non-target traits. SAT e-J. 1, 1–13.
Rai, K. N., Kumar, K. A., Andrews, D. J., Rao, A. S., Raj, A. G. B., and Witcombe, J. R. (1990). Registration of ‘ICTP 8203’ pearl millet. Crop Sci. 30:959. doi: 10.2135/cropsci1990.0011183x003000040048x
Saltzman, A., Birol, B., Bouis, H. E., Boy, E., De Moura, F. F., Islam, Y., et al. (2013). Biofortification: progress toward a more nourishing future. Glob Food Secur. 2, 9–17. doi: 10.1016/j.gfs.2012.12.003
Saltzman, A., Birol, E., Oparinde, A., Andersson, M. S., Asare-Marfo, D., Diressie, M. T., et al. (2017). Availability, production, and consumption of crops biofortified by plant breeding: current evidence and future potential. Ann. N. Y. Acad. Sci. 1390, 104–114. doi: 10.1111/nyas.13314
Tulchinsky, T. H. (2010). Micronutrient deficiency conditions: global health issues. Public Health Rev. 32, 243–255. doi: 10.1007/BF03391600
Underwood, B. A. (2000). Overcoming micronutrient deficiencies in developing countries: is there a role for agriculture? Food Nutr. Bull. 21, 356–360.
UNICEF (2019). The State of the World’s Children 2019. Children, Food and Nutrition: Growing Well in a Changing World. New York, NY: UNICEF.
Velu, G., Ortiz-Monasterio, I., Singh, R. P., and Payne, T. (2011). Variation for grain micronutrients in wheat core collections accession of diverse origin. Asian J. Crop Sci. 3, 43–48. doi: 10.3923/ajcs.2011.43.48
Webb, P., Stordalen, G. A., Singh, S., Wijesinha-Bettoni, R., Shetty, P., and Lartey, A. (2018). Hunger and malnutrition in the 21st century. BMJ 361:k2238. doi: 10.1136/bmj.k2238
World Health Organization (2006). Workshop to Review the Results of Studies Evaluating the Impact of Zinc Supplementation on Childhood Mortality and Severe Morbidity: Conclusions and Next Steps. Geneva: World Health Organization.
Keywords: pearl millet, micronutrient malnutrition (MNM), CHAKTI, AMMI analysis, grain Fe and Zn
Citation: Gangashetty PI, Riyazaddin M, Sanogo MD, Inousa D, Issoufou KA, Asungre PA, Sy O, Govindaraj M and Ignatius AI (2021) Identification of High-Yielding Iron-Biofortified Open-Pollinated Varieties of Pearl Millet in West Africa. Front. Plant Sci. 12:688937. doi: 10.3389/fpls.2021.688937
Received: 31 March 2021; Accepted: 26 July 2021;
Published: 22 September 2021.
Edited by:
Palak Chaturvedi, University of Vienna, AustriaReviewed by:
Karthikeyan Adhimoolam, Jeju National University, South KoreaCopyright © 2021 Gangashetty, Riyazaddin, Sanogo, Inousa, Issoufou, Asungre, Sy, Govindaraj and Ignatius. This is an open-access article distributed under the terms of the Creative Commons Attribution License (CC BY). The use, distribution or reproduction in other forums is permitted, provided the original author(s) and the copyright owner(s) are credited and that the original publication in this journal is cited, in accordance with accepted academic practice. No use, distribution or reproduction is permitted which does not comply with these terms.
*Correspondence: Prakash I. Gangashetty, cC5nYW5nYXNoZXR0eUBjZ2lhci5vcmc=; Mahalingam Govindaraj, bS5nb3ZpbmRhcmFqQGNnaWFyLm9yZw==
Disclaimer: All claims expressed in this article are solely those of the authors and do not necessarily represent those of their affiliated organizations, or those of the publisher, the editors and the reviewers. Any product that may be evaluated in this article or claim that may be made by its manufacturer is not guaranteed or endorsed by the publisher.
Research integrity at Frontiers
Learn more about the work of our research integrity team to safeguard the quality of each article we publish.