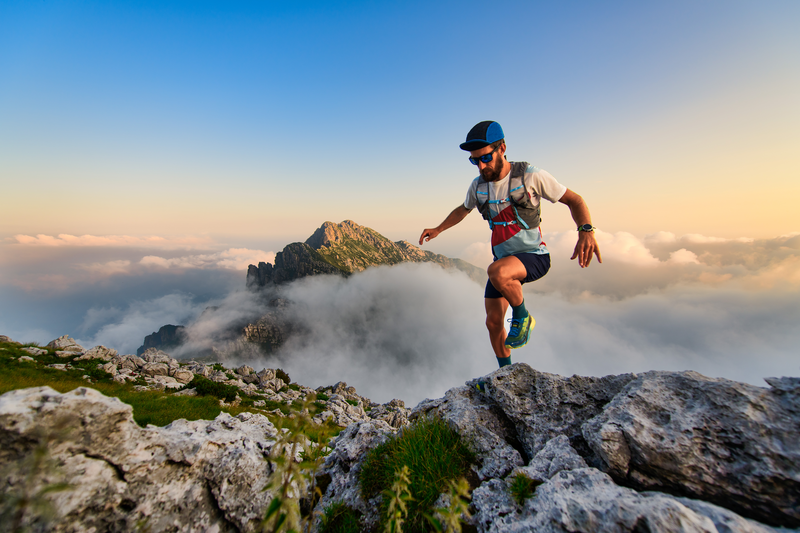
95% of researchers rate our articles as excellent or good
Learn more about the work of our research integrity team to safeguard the quality of each article we publish.
Find out more
REVIEW article
Front. Plant Sci. , 18 June 2021
Sec. Plant Abiotic Stress
Volume 12 - 2021 | https://doi.org/10.3389/fpls.2021.677611
Basic helix-loop-helix proteins (bHLHs) comprise one of the largest families of transcription factors in plants. They have been shown to be involved in responses to various abiotic stresses, such as drought, salinity, chilling, heavy metal toxicity, iron deficiency, and osmotic damages. By specifically binding to cis-elements in the promoter region of stress related genes, bHLHs can regulate their transcriptional expression, thereby regulating the plant’s adaptive responses. This review focuses on the structural characteristics of bHLHs, the regulatory mechanism of how bHLHs are involved transcriptional activation, and the mechanism of how bHLHs regulate the transcription of target genes under various stresses. Finally, as increasing research demonstrates that flavonoids are usually induced under fluctuating environments, the latest research progress and future research prospects are described on the mechanisms of how flavonoid biosynthesis is regulated by bHLHs in the regulation of the plant’s responses to abiotic stresses.
Basic helix-loop-helix proteins (bHLHs), are one of the largest transcription factor (TF) families. They are widely distributed in plants, fungi, and animals (Riechmann and Muyldermans, 1999; Carretero-Paulet et al., 2010). In Ludwig et al. (1989), the first plant bHLH TF was observed in maize. Since then, a great many bHLHs have been proven to regulate plant responses to various abiotic stresses. bHLHs are involved in regulating the synthesis of flavonoids (Ludwig et al., 1989), which play important roles in the ROS homeostasis under these stresses. As an illustration of the size of the bHLHs family in various plant species, 164 bHLH TFs have been found in Arabidopsis (Arabidopsis thaliana L.), while there are 180 in rice (Oryza sativa L.), 190 in tobacco (Nicotiana tabacum L.), 191 in grapes (Vitis vinifera L.), 102 in walnut (Juglans regia L.), 85 in Ginkgo biloba, 268 in Brassica oleracea, 440 in Brassica napus, and 251 Brassica rapa (Xiong et al., 2005; Jaillon et al., 2007; Rushton et al., 2008; Carretero-Paulet et al., 2010; Miao et al., 2020; Zhou et al., 2020; Zhao et al., 2021).
TFs, also known as trans-acting factors, are a category of proteins that specifically bind to cis-acting elements in the promoter region of eukaryotic genes. They regulate specific physiological or biochemical processes in cells at the transcriptional level. The protein structure of TFs generally contains four functional domains: a DNA-binding domain, a transcriptional regulation domain, an oligomerization site, and a nuclear localization domain. The transcriptional regulatory domain may include both an activation domain and an inhibitory domain (Yang et al., 2020).
The bHLH transcription factor conservatively contains two connected sub-regions, the N-terminal basic region directly followed by the HLH (helix-loop-helix) domain (Atchley et al., 1999). More than 50% of bHLHs that have been found in plants possess a highly conserved HER motif (His5-Glu9-Arg13) to achieve DNA binding and regulate the transcription of their target genes (Atchley and Fitch, 1997; Massari and Murre, 2000; Toledo-Ortiz et al., 2003). The HLH region is composed of 40∼50 amino acid residues, which is required for the formation of dimers (Sharker et al., 2020).
The animal bHLHs were first categorized into six subfamilies (A∼F) by Atchley et al. (1999) based on characteristics of the HLH and other conversed domains. The phylogenetic tree of plant bHLH was first constructed in Arabidopsis thaliana. The bHLH family of Arabidopsis was divided into 12 subfamilies (Gao et al., 2017). Later, these subfamilies were expanded into 32 subgroups by phylogenetic analysis based on the 638 bHLH genes extracted from Arabidopsis, Populus trichocarpa, Oryza sativa, Physcomitrella patens, and five algae species (Carretero-Paulet et al., 2010). Evolutionary and functional relationships within subfamilies are supported by intron patterns, predicted DNA-binding motifs, and the architecture of conserved protein motifs. Some subfamilies may modulate biological responses critically for the development of terrestrial plants.
As one of the largest TF families, bHLHs regulate the expression of downstream target genes usually in a binary or ternary complex form (Figure 1). The ternary MBW complex has been analyzed extensively in various biological processes (Dixon et al., 2005; Shoji and Hashimoto, 2011; An et al., 2012). The bHLH proteins of the IIIf subgroup (TT8, GL3, EGL3, and AtMYC1) can interact with R2R3-MYBs from various subgroups (TT2, PAP1, or PAP2), and form ternary complexes with a WD-repeat protein (TTG1) (Baudry et al., 2004). The MYB5-TT8-TTG1 complex is activated in the endothelium to regulate DFR (dihydroflavonol reductase), LDOX (leucoanthocyanidin dioxygenase), and TT12 expression, whereas the TT2-EGL3/GL3-TTG1 complexes regulate the expression of LDOX, BAN (BANYULS; anthocyanidin reductase), AHA10 (autoinhibited H+-ATPase isoform 10), and DFR in the chalaza (Xu et al., 2015). Regulatory complexes composed of MYB10, bHLH3, and WD40 may control the biosynthesis of anthocyanins in peaches (Ravaglia et al., 2013). BoPAP2 (MYB), BoTT8, BoEGL3.1, BoMYC1.2 (bHLH), and BoTTG1 (WD40) have been identified as candidate genes for regulating anthocyanin biosynthetic activity in cabbage (Jin et al., 2018).
There are also many studies that address the activation mechanism of the MYB-bHLH binary complex (Zimmermann et al., 2004). The C-terminal domain of many bHLHs have been found to contain a MYB-binding site. Through binding to this site, MYB could heterodimerize with bHLHs (Heim et al., 2003). For example, VvMYC1 in grapes cannot activate the promoters of CHI, UFGT, and ANR genes in the flavonoid biosynthetic pathway by itself, but the co-transfection of VvMYC1 with a MYB transcription factor can significantly activate the expression of the genes for these three enzymes (Hichri et al., 2010). Rosea1 (ROS1, MYB-type) and Delila (DEL, bHLH-type) from Snapdragon (Antirrhinum majus) can specifically induce anthocyanin accumulation when co-expressed in tomato fruits (Outchkourov et al., 2014). Xiang et al. (2015) found that CmbHLH2 significantly upregulated CmDFR transcriptional expression and triggered anthocyanin accumulation when co-expressed with CmMYB6. Using transient assays in both Nicotiana tabacum leaves or Actinidia arguta fruits and stable transformation in Arabidopsis, Wang et al. (2019) demonstrated that co-expression of AcMYB123 and AcbHLH42 is a prerequisite for anthocyanin production by activating transcription of AcF3GT1 and AcANS or their homologous genes. Li L. et al. (2019) found that AabHLH1 interacted with AaMYB3 to regulate the accumulation of procyanidine. In addition, DhMYB2 interacted with DhbHLH1 to regulate anthocyanin production in Dendrobium hybrid petals (Li et al., 2017). As well, the bHLH transcription factor PPLS1 interacts with SiMYB85 to control the color of leaf sheath and pulvinus by regulating anthocyanin biosynthesis in Setaria italica (Bai et al., 2020).
The transcriptional activities of bHLHs can also be regulated through dimerization, and a series of post-translational modifications including phosphorylation and ubiquitination (Figure 1; Xu et al., 2015). The Arabidopsis bHLH transcription factor FER-LIKE IRON DEFICIENCY-INDUCED TRANSCRIPTION FACTOR (FIT) displays a pivotal role in the Fe-deficiency response, and its activity is regulated by heterodimerizing with bHLH039 (Gratz et al., 2019). Interestingly, this heterodimerization capacity is affected by differential phosphorylation. Specifically, the heterodimerization is activated through Ser and deactivated through Tyr site phosphorylation (Gratz et al., 2020). Another Arabidopsis bHLH transcription factor, SPEECHLESS (SPCH), initiates the stomatal lineage (Simmons and Bergmann, 2016). Recent research revealed that the protein stability of SPCH could be positively regulated by phosphorylation mediated via the MITOGEN-ACTIVATED PROTEIN KINASE (MAPK) cascade or GSK3-like kinase BRASSINOSTEROID INSENSITIVE 2 (BIN2) protein phosphatase 2A phosphatase (Yang et al., 2015; Bian et al., 2020). Chenopodium glaucum CgbHLH001 can positively regulate plant stress tolerance via clearing excessive ROS and accumulating transcripts of stress-related genes (Wang et al., 2017). A recent study indicates that CgbHLH001 activity might be affected by phosphorylation through interaction with CgCDPK (Zhou et al., 2021). Ubiquitination can likewise directly regulate bHLH activity via the 26S proteasome protein degradation pathway (Figure 1). The BTB-BACK-TAZ domain protein MdBT2 in apple can target MdCIbHLH1 for ubiquitination, so as to regulate malate accumulation and vacuolar acidification (Zhang et al., 2020a, b). Pyrus pyrifolia CONSTITUTIVE PHOTOMORPHOGENIC1 (PpCOP1) could ubiquitinate PpbHLH64 and trigger its proteolysis, further reducing the accumulation of anthocyanins (Tao et al., 2020). bHLH activity can also be indirectly regulated by ubiquitination through the degradation of its partners in the MBW complex. The Arabidopsis thaliana MYB Interaction Factor 1 (AtMIF1) is a member of the ubiquitin-protein ligase E3 complex involved in the 26S proteasome protein degradation pathway. AtMIF1 ubiquitinates and degrades AtMYB5, so that transcriptional activation of the MYB/bHLH/WD-repeat (MBW) complex further increases oil content by attenuating GL2 inhibition (Cheng et al., 2021).
The latest reports reveal an emerging regulatory mechanism of MBW transcriptional activation by antagonistic interactions with MBW components (Figure 1). MYB paralogs or ROS-related proteins could disrupt the activated MBW complex by competitively interacting with bHLHs. In Medicago truncatula, two R2R3 MYB paralogs, RED HEART1 (RH1) and RH2, play vital roles in patterned pigmentation. These two antagonistic paralogous proteins competitively bind the MtTT8-MtWD40-1 complex to fine tune the phenotypes of leaf anthocyanin spot marking (Wang et al., 2021). In Zea mays, the ROS-related protein ZmSRO1e (TO RCD-ONEs) interacts with ZmPL1 (MYB)/AtPAP1 (bHLH) to inhibit the formation of an activated MBW complex, thereby repressing the over-accumulation of anthocyanins under abiotic stress (Qin et al., 2021). These two reports provide a multidimensional antagonistic regulatory paradigm for MBW activation. It’s reasonable to speculate that bHLH paralogs or other stress/growth-related proteins might also regulate the MBW activity by competitively interacting with MYB in the complex.
The bHLH TFs can form homologous or heterologous dimers and bind to target genes on specific cis-acting elements. Among them, the most frequently studied cis-acting elements are the E-box (5′-CANNTG-3′) and the N-box [5′-CACG(A/C)G-3′] (Li et al., 2006). bHLHs DNA binding domains containing at least five basic amino acids in the N-terminal basic region are expected to bind these DNA motifs (Massari and Murre, 2000).
The E-box is one of the most common targets of the bHLH subfamilies 1 and 27. According to three-dimensional structural analysis of bHLHs, Glu-13 and Arg-16 are essential for E-box-binding recognition (Shimizu et al., 1997; Wang et al., 2003; Carretero-Paulet et al., 2010). This cis-acting element is usually located in the promoter of genes involved in multiple physiological pathways. Nims et al. (2009) found that the yew bHLH TcJAMYC can bind to the E-box in the promotor of paclitaxel-related genes to activate their transcriptional expression. Huang et al. (2013) demonstrated that the Poncirus trifoliata PtrbHLH can bind to an E-box in the promoter region of the POD gene. Wu et al. (2015) showed that the rice OsbHLH062 can bind to an E-box in the promoter of ion transport genes such as OsHAK21. Zhang et al. (2017) found that PnbHLH1 can interact with the E-box core sequence in Panax notoginseng, a traditional Chinese medicine, to induce the triterpenoid saponins. Geng and Liu (2018) experimentally showed that CsbHLH18 bound to the promoter of the sweet orange (Citrus sinensis) CsPOD gene through the E-box. Recent studies have found that GhbHLH18 strongly binds to the E-box in the promoter region of the GhPER8 gene coding for a peroxidase from cotton (Gao et al., 2019). Finally, Chakraborty et al. (2019) demonstrated through DNA-protein interactions both in vitro and in vivo that the bHLH transcription factor MYC2 can bind to the cis-acting E-box element in the HY5 promoter to negatively regulate HY5 expression.
A specific member of the E-box family, the G-box (5′-CACGTG-3′) could be recognized by about 81% of bHLHs (Toledo-Ortiz et al., 2003; Li et al., 2006; Pires and Dolan, 2010) mainly from subfamilies 4, 5, 10, 11, 13, 14, 24, 25, and 26 (Bovy et al., 2002; Yi et al., 2005; Carretero-Paulet et al., 2010; Fu et al., 2014; Chen et al., 2018; Gao et al., 2018; Jiang et al., 2018). Qian et al. (2007) confirmed the specific interaction between the pea bHLH transcription factor PsGBF and the G-box of PsCHS1 by transient expression experiments in tobacco. In Arabidopsis, the bHLH transcription factor PIL5 has a high affinity with the G-box as well (Kang et al., 2010). Arabidopsis bHLH106 was characterized through its association with salt tolerance. Transcriptional analysis of an AtbHLH106 overexpression line showed 198 genes positively were regulated and 36 genes were negatively regulated; these genes possessed one or more G-box sequences in their promoter regions, and many of them are associated with the abiotic stress response (Ahmad et al., 2015). Zhang et al. (2011) isolated CrMYC2 from a Catharanthus roseus cDNA library and found that its corresponding protein could specifically bind to G-box of the ORCA3 gene. In addition, tobacco NtMYC2 and NtbHLH123 bind directly to the G-box region of the PMT2 and CBF, respectively, and activated their transcription (Shoji and Hashimoto, 2011; Zhao et al., 2018).Besides, Tamarix hispida ThbHLH1 specifically binds to the G-box of P5CS and the ALDH gene (Ji et al., 2016). The apple MdMYC2 homodimer also binds to the G-box motif of the AtJAZ3 gene (An et al., 2016). Moreover, the Hevea brasiliensis bHLHs HbMYC2, HbMYC3, and HbMYC4 of interacted with the G-box of the HbPIP2 promotor (Zhai et al., 2018).
The N-box is associated with bHLHs possessing an enhancer activity. bHLHs that bind to the N-box usually have a proline in the N-terminal basic domain and a “WRPW” sequence at its C-terminus (Cordeiro et al., 2016). OsPIF14 binds to the OsDREB1B promoter on two N-boxes [CACG(A/C)G] (Cordeiro et al., 2016). Diterpenoid phytoalexin factor (DPF) is a rice bHLH transcription factor which positively regulates CPSCPS2 transcription through N-box binding. DPF can also regulate CYP99A2 through the N-box, thereby affecting the biosynthesis of diterpenoid phytoalexins (DP) (Yamamura et al., 2015). In addition, some bHLHs can bind to other cis-acting elements, for example, AtbHLH112 can not only bind the E-box, but also bind the GCG-box [5′-GG(G/T)CC(G/T)(GA)(TA)C-3′] (Liu et al., 2015).
Heterodimers can be formed between different bHLHs. Fairchild et al. (2000) discovered that two bHLH TFs, PIF3/AtbHLH008 and HFR1, could heterodimerize. This interaction effectively prevented monomer PIF3/AtbHLH008 from binding to the E-box of phytochrome A (phyA), thereby modulating phyA signaling. In addition, bHLHs can heterodimerize with other TFs, such as R2R3-MYB/BZR1-BES1 as well as other signal transduction proteins (Yin et al., 2005; Dubos et al., 2008). The formation of heterodimers makes it possible for different TFs to interact with each other to co-regulate the expression of target genes.
In summary, bHLHs bind directly to cis-elements in the promoter of target genes or form heterodimers to co-regulate their expression. To date, E-box cis-acting elements, in particular, the G-box of this family, are the widest binding targets of bHLHs (Table 1). We found that most bHLHs in subfamilies 1, 2, 12, 13, 15, 19, 24, 25, 26, 27 can bind to the G-box; and members in subfamilies 1, 2, 13, 15, 22, 26, 27 can recognize other cis-elements in the E-box family, except for the G-box; members of subgroup 15 could also bind to the GCG-box (Abe et al., 1997; Wang et al., 2013; Gao et al., 2016; Yao et al., 2018; Xi et al., 2021).
Previous studies found that bHLHs were involved in a variety of pathways regulating the adaptation to stress in plants, including resistance to mechanical damage, drought, high salt, oxidative stress, low temperature stresses, heavy metal stress, iron deficiency, and osmotic stress (Babitha et al., 2015; Zhao et al., 2016). One of the most common responses of plants to stress is to enhance the biosynthesis of different types of compatible organic solutes. In general, this mechanism protects plants from stresses in multiple different ways through regulating cell osmotic homeostasis, eliminating excessive ROS, maintaining the integrity of the plasma membrane, and stabilizing enzymes and structural proteins (Esmaeilpour et al., 2015).
To uncover the potential roles of bHLHs from different subfamilies in various stress responses, most of the functionally annotated bHLH TFs were categorized into the abovementioned 32 subfamilies (Figure 2 and Table 2). The response function of different bHLH subfamilies to abiotic stress is different. We found that nearly half of the bHLHs subfamilies participated in abiotic stress responses. Among them, there are nine subfamilies of bHLHs involved in drought response, including subfamilies 1, 2, 4, 5, 13, 15, 24, 26, and 30, in which most members are from subfamilies 1, 2, and 15. As well, there are nine subfamilies of bHLHs participating in salinity resistance, including subfamilies 1, 2, 4, 5, 7, 13, 15, 26, and 27. The majority of salinity responsive bHLHs are from subfamilies 1 and 4. In addition, five subfamilies were found to regulate the cold tolerance of plants, including 1, 2, 13, 15, and 26. Interestingly, members in subfamily 1 were found to be able to take over the regulation of chilling responses. Furthermore, bHLHs of subfamilies 1, 3, 4, 12, 28, and 31 are involved in the response to iron deficiency. In short, bHLHs of subfamily 1 seem to participate in most kinds of abiotic stresses, such as drought, salinity, chilling and iron deficiency. Moreover, members of subfamily 2 mainly participate in drought, salt, and cold stress, while that of subfamily 4 mainly regulates responses to drought, salt, and iron deficiency stress.
Figure 2. Phylogenetic relationships of bHLH family members. The phylogenetic tree was constructed using MEGA7.0 with the neighbor-joining method and 1000 bootstrap replicates (Huang and Dai, 2015; Wang et al., 2016b). Then the trees were visualized using iTOL (https://itol.embl.de/). Group names were marked outside the circle. The bHLH protein sequences were downloaded from the JGI (http://www.jgi.doe.gov/) and NCBI (https://www.ncbi.nlm.nih.gov) databases. The Gene and Protein IDs of all these bHLHs are list in Supplementary Tables 1, 2. And the bHLH members that have been functional annotated are labeled with a star marker.
The effects of drought stress on plants are mainly manifested in decreased photosynthesis efficiency, disordered hormone metabolism, and reduced enzyme activities, causing irreversible damage to plant growth and yield (Sun et al., 2018). The bHLH TFs regulate the plant drought-tolerant responses mainly through modulation of the sensitivity to Abscisic acid (ABA) or by regulating the development of stomata, leaf trichome, and root hair (Castilhos et al., 2014). Li et al. (2007) found that the expression of the bHLH-type transcription factor AtAIB was temporarily induced by ABA, and that plants overexpressing AtAIB showed a stronger drought tolerance. Le Hir et al. (2017) found that AtbHLH68 may play a role in responding to drought stress, likely through an ABA-dependent pathway by either directly or indirectly regulating components of ABA signaling and/or metabolism. Yao et al. (2017) demonstrated that heterologous expression of tartary buckwheat FtbHLH3 in Arabidopsis could positively regulate drought/oxidative stress tolerance in an ABA dependent manner. Rao et al. (2020) reported that a large number of bHLHs are involved in ABA signaling and positively regulated stress resistance in Lycium ruthenicum.
In Arabidopsis, AtbHLH006/rd22BP1/AtMYC2 can regulate the expression of RD22. Interestingly, AtbHLH006 can bind to different cis-acting elements under different conditions. AtbHLH006 binds to the E-box (but not G-box) on the PLETHORA gene promoter in JA mediated regulation of the Arabidopsis root stem cell niche (Chen et al., 2011); it could also bind to the G-box motif of the SAG29 promoter to activate its expression during JA mediated leaf senescence (Qi et al., 2015). Another bHLH transcription factor, ZmPTF1 in maize, regulates drought tolerance by promoting root development and the synthesis of ABA (Li Z.X. et al., 2019). Dong et al. (2014) found that plants overexpressing Populus euphratica PebHLH35 had significantly lower stomatal density and decreased stomatal opening than wild-type plants, so their transpiration was significantly reduced. Zhao et al. (2020) illustrated that Apple MdbHLH130 acts as a positive regulator of water stress response by regulating stomatal closure and ROS-scavenging in tobacco.
High salt stress caused by ionic and osmotic stressors eventually results in the suppression of plant growth and a reduction in crop productivity. Some bHLH TFs have a regulatory effect under saline conditions. Under salt stress, plant cells successively face the challenges of osmotic stress, ion toxicity, and oxidative stress (Rozema and Flowers, 2008). As discussed previously, bHLH TFs usually enhance a plant’s resistances to these secondary stresses. AtNIG1 in Arabidopsis was the first bHLH transcription factor shown to be involved salt stress signaling pathways from plants. The survival rate and dry weight of the atnig1 mutant decreased significantly under salt stress (Kim and Kim, 2006). Fu et al. (2014) showed that the expression level of the wheat (Triticum aestivum L.) TabHLH13 gene was significantly up-regulated with the increase of salt ion concentration in the environment. Wu et al. (2015) showed that OsbHLH062 could regulate ion transport genes, such as OsHAK21, and modulate the Jasmonic acid (JA) signaling pathway, so as to endow plants with some resistance to salt stress. One of the most important mechanisms by which bHLH TFs participate in salt tolerance is to regulate ROS balance by directly regulating the expression of a group of peroxidase genes. Jiang et al. (2009) found that AtbHLH92 elevated Arabidopsis tolerance to salt and osmotic stresses through a partial dependence on ABA and SOS2. The beet homolog of AtbHLH92, BvbHLH92, was found to be expressed in both the beet root and leaf in response to salt stress (Jiang et al., 2009). In Tamarix hispida, ThbHLH1 was highly expressed under high salt induction, significantly increasing peroxidase (POD) and superoxide dismutase (SOD) activities (Ji et al., 2016). AtbHLH112 increased the expression of the POD and SOD genes, while concomitantly reduced the expression of the P5CDH and ProDH genes, so as to enhance the resistance of Arabidopsis to high salt (Liu et al., 2014). In addition, bHLHs could also regulate the accumulations of resistance-related secondary metabolites in order to improve plant salinity tolerance. Verma et al. (2020) found that salt stress activated AtMYC2 through a mitogen-activated protein kinase (MAPK) cascade. Then, the AtMYC2 could bind to the promotor of rate-limiting enzyme P5CS1 in the biosynthesis of proline, thereby regulating the biosynthesis of proline, and thus regulating salt tolerance. AtbHLH122 inhibited the expression of CYP707A3 gene under NaCl stress (Liu et al., 2014). Furthermore, Krishnamurthy et al. (2019) identified AtMYC2 and AtbHLH122 as upstream regulators of the ABA-mediated AtNHX1 and AtNHX6, which are both Na+/H+ exchangers, by chromatin immunoprecipitation. Moreover, overexpression of EcbHLH57 can enhance the resistance of tobacco to salt stress by elevating the expression of stress responsive genes such as LEA14, rd29A, rd29B, SOD, APX, ADH1, HSP70, and also PP2C (Babitha et al., 2015). OsbHLH068 has a similar effect as AtbHLH112 in regulating the salt stress response. In Arabidopsis, heterogenic overexpression of OsbHLH068 can reduce salt-induced accumulation of H2O2 (Chen et al., 2017).
Cold stress is a severe threat for plant productivity and crop production, particularly so when it occurs during the growth phase (Albertos et al., 2019). The DREB1/CBF (dehydration-responsive element binding/C-repeat binding factors) gene is considered as the main regulator of plant’s cold stress response. Under low temperature stress, OsDREBL and OsTPP1 genes were significantly up-regulated at the transcriptional level after overexpression of RsICE1 (inducer of CBF expression) in rice, indicating that RsICE1 is involved in the DREB1/CBF cold regulation signaling network (Man et al., 2017). In Arabidopsis the AtICE1/AtbHLH116 protein binds to the CBF promoter region at low temperatures to affect transcription initiation, and AtICE1/AtbHLH116 overexpressing plants showed higher tolerance to cold (Chinnusamy et al., 2003). The bHLH transcription factor DlICE1 from Dimocarpus longan Lour has a positive regulatory effect on cold tolerance. Overexpression of DlICE1 in Arabidopsis conferred enhanced cold tolerance via increased proline content, decreased ion leakage, and reduced malondialdehyde (MDA) and reactive oxygen species (ROS) accumulation. The expressions of the ICE1-CBF cold signaling pathway genes, including AtCBF1/2/3 and cold-responsive genes (AtRD29A, AtCOR15A, AtCOR47, and AtKIN1), were also significantly higher in DlICE1-overexpressing lines than in wild-type (WT) plants under cold stress (Yang et al., 2019). ICE1 proteins in other plants have similar functions. PuICE1 of can elevate the transcriptional expression of PuDREBa by interacting with PuHHP1, thus improving the cold resistance of Pyrus ussuriensis (Huang et al., 2015). The overexpression of BcICE1 in tobacco can positively regulate the expression of stress-related genes such as CBFs (C-repeat binding factor) and enhance the antioxidant activity and osmotic ability of plants (Zhang et al., 2018). Zuo et al. (2019) showed that the transgenic Arabidopsis with overexpressed Zoysia japonica ZjICE1 showed an enhanced tolerance to cold stress with an increase in SOD, POD, as well as higher free proline content and decreased MDA content. They also upregulated the transcript abundance of cold-responsive genes (CBF1, CBF2, CBF3, COR47A, KIN1, and RD29A). ZjICE2 from Zoysia japonica enhanced the tolerance of transgenic plants to cold stress by activating DREB/CBF regulators and enhancing reactive oxygen species scavenging (Zuo et al., 2019). In addition to ICE proteins, some bHLH proteins are also responsible for plant resistance to low temperatures. As a homolog of ICE1, the rice OrbHLH001 could enhance the tolerance of transgenic Arabidopsis to freezing stress. However, the function of OrbHLH001 was different from that of ICE1 and is independent of a CBF/DREB1 cold-response pathway (Li et al., 2010). The grape VvbHLH1 and VabHLH1 are positive regulators of the cold stress response, and the overexpression of these two genes could enhance the expression level of the COR gene (Xu et al., 2014). The apple (Malus domestica Borkh.) MdCIbHLH1 gene was recently identified as a hub in the transcriptional regulation of bark freezing tolerance Liang et al. (2020). Moreover, Zhao et al. (2018) demonstrated that NtbHLH123 is a transcriptional activator that plays a positive regulatory role in cold tolerance by activating the reactive oxygen species scavenging-related gene NtCBF. Geng and Liu (2018) found that CsbHLH18 can regulate ROS homeostasis at least partially by directly regulating the antioxidant gene CsPOD, thus playing an active role in cold tolerance.
Excessive heavy metals in agricultural lands cause declines in crop productivity (Thao et al., 2015). Song et al. (2014) found that excessive expression of GmbHLH30 in tobacco can enhance its resistance to aluminum toxicity through the maintenance of osmotic pressure. Manganese (Mn) toxicity is also an important factor for limiting crop production in acidic soils. Sun et al. (2019) found that ZmbHLH105 may improve maize tolerance to Mn stress by regulating antioxidant mechanism-mediated ROS clearance and the expression of Mn/Fe-related transporters in plants.
The key bHLH transcription factor in iron (Fe) uptake, FER-LIKE IRON DEFICIENCY-INDUCED TRANSCRIPTION FACTOR (FIT), is critical for adjusting Fe acquisition to plant growth and environmental constraints (Gratz et al., 2020). Kool et al. (1994) found that the bHLH133 transcription factor in rice can regulate the transport of Fe from roots to young leaves, revealing the important role of bHLH proteins in maintaining iron homeostasis in plant cells. Ling et al. (2002) found that the bHLH transcription factor FER from tomato played a role in the regulation of plant root iron nutrition. Yuan et al. (2008) found that the FIT/bHLH29 (FER-like iron deficiency-induced transcription factor) gene played an important role in maintaining intracellular Fe balance through regulating the expression of downstream iron absorption genes. The plant hormone ethylene is one of the signals that trigger iron deficiency responses at both the transcriptional level and the post-transcriptional level. Through ethylene signal transduction, the FIT/bHLH29 protein is protected from degradation by proteasomes (Lingam et al., 2011). Increased FIT levels subsequently leads to the high level of expression of genes required for Fe acquisition. Cui et al. (2018) found that the bHLH18, bHLH19, bHLH20, and bHLH25 in the Iva subgroup are FIT/bHLH29 interactors, which can promote JA induced FIT/bHLH29 protein degradation. Previously, Wang et al. (2017, 2019) demonstrated that four Ib bHLH genes (AtbHLH38, AtbHLH39, AtbHLH100, and AtbHLH101) played important roles in the iron-deficiency responses, though they are not induced by FIT/bHLH29 under iron deficiency conditions. Actually, these four Iva bHLHs mainly antagonized Ib bHLHs, so as to regulate the stability of the FIT/bHLH29 protein under iron deficiency conditions. Furthermore, Lei et al. (2020) found that bHLH121 interplayed with another bHLH transcription factor in the Ivc subgroup to positively regulate FIT/bHLH29 expression, thus playing a key role in maintaining Fe homeostasis in Arabidopsis. Zhang et al. (2015) identified the Arabidopsis AtbHLH104 as a member in subfamily Ivc and demonstrated that bHLH104 acted as a key component positively regulating Fe deficiency responses via targeting Ib subgroup bHLH genes and PYE/bHLH47 expression. Li X. et al. (2016) supplemented this study and proposed that bHLH34, bHLH104, and bHLH105 (IAA-LEUCINE RESISTANT3) can be used as homologous dimers or heterodimers to regulate the stable state of Fe without redundancy. ILR3/bHLH105, alone was shown to stimulate Fe uptake by inhibiting ferritin expression (Kroh and Pilon, 2019; Tissot et al., 2019). Long et al. (2010) showed through chromatin immunoprecipitation-on-chip analysis and transcriptional profiling that PYE/bHLH47 helped maintain iron homeostasis by regulating the expression of known iron homeostasis genes. In addition, AtbHLH115 is also a positive regulator of iron deficiency responses, being negatively regulated by the E3 ligase BTS (Liang et al., 2017). In poplar, transgenic line (TL2) overexpressing PtFIT showed a higher chlorophyll content and Chl a/b ratio than the control plant under the conditions of iron deficiency, indicating that PtFIT was involved in the iron deficiency reaction (Zhai et al., 2018). Ogo et al. (2006) found that overexpression of OsIRO2/OsbHLH056 could promote the absorption of iron in rice. Zhao et al. (2014) demonstrated that Chrysanthemum CmbHLH1 promoted iron absorption through H+-ATPase mediated rhizosphere acidification. Recent research demonstrated that the overexpression of GmbHLH57 and GmbHLH300 up-regulated the iron absorption genes and increased the iron content of transgenic soybean plants (Li L. et al., 2018). Moreover, overexpression of NtbHLH1 results in longer roots, altered rhizosphere pH, and increased ferric-chelate reductase activity under iron deficient conditions (Li et al., 2020). Wang et al. (2020) characterized the role of a novel rice bHLH type transcription factor OsbHLH156 in Fe homeostasis and found that OsbHLH156 is mainly expressed in roots and transcription is greatly increased by iron deficiency. Loss of function of OsbHLH156 resulted in Fe-deficiency-induced chlorosis and reduced Fe concentration in the shoots under upland or Fe(III) supplied conditions.
Plants must maintain the homeostasis of Fe and Cu. When plants are under Fe-deficiency conditions, bHLH IVC not only directly activates bHLH Ib expression but also promotes bHLH Ib and FIT gene expression through interaction with bHLH121. FIT and bHLH Ib members initiate transcription of Fe-uptake genes (IRT1 and FRO2) and Cu-uptake genes (COPT2, FRO4, and FRO5). An increase in Cu concentration alleviates Fe-deficiency stress. Under Cu-deficiency, Cu-uptake genes (COPT2, FRO4, and FRO5) are activated in response to SPL7. CITF1 also regulates the expression of COPT2, FRO4, and FRO5. SPL7 not only regulated the Cu homeostasis signaling pathway, but also suppressed (IPON MANs) IMAS and bHLH Ib of the Fe homeostasis signaling pathway (Cai et al., 2021).
Proper osmoregulation is important for plant response to environmental changes (Lin et al., 2020). Drought, high salinity, low temperature, and other conditions will affect the water content of plants, thus leading to osmotic stress. Therefore, osmotic stress is often accompanied by other types of environmental stresses. Under osmotic stress, RD29A can be regulated through both ABA-independent and ABA-dependent pathways, thus improving plant stress resistance (Yang et al., 2016). Zhai et al. (2016) found that the expression level of RD29A was upregulated in transgenic plants overexpressing TabHLH39. The overexpression of the wild rice gene OrbHLH2 (a homolog protein of ICE1) in Arabidopsis up-regulates the expression of stress response genes DREB1A/CBF3, RD29A, COR15A, and KIN1, thus enhancing tolerance to osmotic stress (Zhou et al., 2009). A MYC-type ICE1-like transcription factor SlICE1a in tomatoes was induced in response to osmotic stress (Feng et al., 2013).
In vegetative tissues, the flavonoid pathway is usually induced in response to physiological and environmental fluctuations as a protective mechanism against oxidative stresses induced by pathogen infections, high-light, UV, extreme temperature, drought, salt, and deficiency of N, P, or C nutrition (Xu et al., 2015). The bHLH transcription factor family is important for regulating the biosynthetic pathway of flavonoids (Hichri et al., 2011). Flavonoids are generally divided into six categories: flavone, flavonol, isoflavone, flavanone, flavanol, and anthocyanidin (Hichri et al., 2011). In Ludwig et al. (1989) the Lc protein was initially isolated from maize and showed transcriptional activity on genes involved in anthocyanin synthesis. Heterologous overexpression of the Lc gene significantly enhanced the accumulation of flavonoids in mature fruits of cherry tomato (Bovy et al., 2002). Since then, the vital roles of bHLH in flavonoid synthesis have attracted growing attention. Flavonoids have strong biological activity and significant antioxidant capacity (Mitchell et al., 1998; Xu et al., 2015), and they play protective roles when plants are subjected to single or multiple stresses such as ultraviolet radiation, salt, temperature, and drought. The level of protection is determined by the position of free hydroxyl groups in the structure of flavonoids and the carbon-carbon double bond in the C ring.
To reveal the relationship between bHLH subfamilies and flavonoid biosynthesis, all the functional annotated bHLHs were categorized into the 32 known subgroups in this research (Figure 2 and Table 3). It seems that only bHLHs in subfamilies 1, 2, 5, 13, 14, 15 are involved in the regulation of flavonoid metabolism (Table 3). Among them, more than half of the annotated bHLHs involved in flavonoid metabolism regulation come from subfamilies 2 and 5. As flavonoids are important metabolites involved in plant responses to abiotic stresses, bHLHs of subfamilies 2 and 5 may regulate a series of physiological stress responses. The typical bHLH transcription factor in the IIIf subfamily [equivalent to the subfamily 5] AtTT8/AtbHLH042 can regulate the expression of DFR and BAN, two flavonoid late biosynthetic genes, thus affecting the synthesis of anthocyanins and procyanidins (Nesi et al., 2000). AtTT8/AtbHLH042 constitutes a major regulatory step in the specific activation of the expression of flavonoid structural genes (Baudry et al., 2006). Similarly, MtTT8 in Medicago truncatula is considered to be the central component of a ternary complex (MYB-bHLH-WD40) that controls the biosynthesis of anthocyanins and procyanidins (Li P. et al., 2016). In addition to AtTT8, three other bHLH genes are also categorized into the IIIf clade: AtGL3, AtEGL3, and AtMYC1. They are also known to be involved in biosynthesis of flavonoids (Maes et al., 2008; Symonds et al., 2011). Together with MYB factors, especially PAP2 (AtMYB90), GL3 seems to be a partner of bHLHs in anthocyanin accumulation under nitrogen-deficient conditions (Lea et al., 2007). In contrast, nightshade GLABRA3 (SlGL3), the homolog to AtGL3, inhibited anthocyanin accumulation in Arabidopsis (Tominaga-Wada et al., 2018).
Homologous or heterologous overexpression of bHLHs can exhibit a significant regulatory effect on the synthesis of various flavonoids. Actually, most bHLH have been found to possess a positive regulatory function on plant flavonoid synthesis. In Arabidopsis, transgenic plants overexpressing AtMYC3 and AtMYC4 showed higher levels of anthocyanin than that of wild-type plants (Niu et al., 2011). Heterologous overexpression of the grape VvbHLH1 gene increases the activity of key enzymes in the flavonoid synthesis pathway such as Phenylalanine Ammonia-Lyase (PAL), Chalcone Synthase (CHS), Chalcone Isomerase (CHI), and Flavanone-3-Hydroxylase (F3H) as well as enhancing the salt and drought tolerance of transgenic Arabidopsis seedlings (2016a). VvbHLH003 and VvbHLH007 were also found to be related to anthocyanin or flavonoids synthesis (Wang et al., 2018). Likewise, the heterologous expression of eggplant (Solanum melongena) SmbHLH13 can promote anthocyanin biosynthesis by positively regulating the expression of the structural genes SmCHS and SmF3H. The heterologous expression of Arabidopsis PAP2 induces anthocyanin accumulation in tomato (Li N. et al., 2018), while the heterogeneous overexpression of liverwort (Plagiochasma appendiculatum) PabHLH1 in Arabidopsis can activate the synthesis of both flavonoids and anthocyanins, through the up-regulation of early and late structural genes in the synthesis pathway of flavonoids (Zhao et al., 2019). The ectopic overexpression of apple MdMYC2 also significantly up-regulated the transcriptional expression of these structural genes in transgenic Arabidopsis lines (An et al., 2016). Recent studies have shown that both triticale (Triticum × Secale) TsMYC2 and wheat (Triticum aestivum) TaMYC1 can regulate anthocyanin biosynthesis and control the grain properties (Zong et al., 2019). On the other hand, several bHLHs could negatively regulate flavonoid synthesis. For example, the wintersweet (Chimonanthus praecox L.) CpbHLH13 was found to reduce the anthocyanin content when ectopically overexpressed in the tobacco inflorescence (Aslam et al., 2020).
It is generally believed that the mechanism of action of flavonoids in the stress-resistance process is through their antioxidant properties. Under abiotic stresses, such as low temperature, drought, high salt, or heavy metal exposure, a series of physiological and biochemical changes will occur in plant cells, which are mainly manifested as the reduction of photosynthesis efficiency and the generation of a large number of reactive oxygen radicals, as well as serious damage to cell structure (Winkel-Shirley, 2001). The oxidative stress generated by these reactive oxygen species can also induce a large amount of flavonoids synthesis, thereby quenching the reactive oxygen species and protecting cells from oxidative damage (Treutter, 2006). Qin et al. (2021) found that abiotic stress promotes the synthesis of anthocyanins by inhibiting SPL9 through miR156, thereby facilitating the neutralization of reactive oxygen species (ROS) and simultaneously inducing ZmSRO1e. ZmSRO1e interacts with ZmPL1/AtPAP1 to inhibit the formation of an activated MBW complex, thus repressing the over-accumulation of anthocyanins under abiotic stress. These two pathways balance the relationship between development and abiotic stress tolerance via their control of ROS accumulation. Overexpression of the Antirrhinum majus bHLH gene AmDEL led to the up-regulation of genes related to the biosynthesis of flavonoids, proline biosynthesis, and reactive oxygen scavenging under both salt and drought stress (Wang et al., 2016b). Moreover, flavonoids can also bind with copper irons to reduce the toxic damage of these ions to cytoplasmic structures and organelles such as chloroplasts (Rice-Evans et al., 1996). The role of flavonoids in plant stress tolerance is not only limited to the removal of reactive oxygen species, but also can act as signaling molecules (Ribeiro et al., 2015).
As one of the most numerous TFs in eukaryotes, the bHLH family has many members and diverse functions. Many studies have shown that bHLHs can regulate plant resistances to various abiotic stresses (Babitha et al., 2015; Huang and Dai, 2015; An et al., 2016; Jin et al., 2016). As well, there are many reports that show that flavonoids in plants are synthesized in large quantities to effectively eliminate ROS, to enhance the plant’s tolerances to survive in an adverse environment. This naturally leads to an interesting question: Can bHLHs regulate plant tolerance by regulating the synthesis of flavonoids? A large number of studies have confirmed that bHLHs are involved in the synthesis of flavonoids. Specially, bHLHs in subfamily 1, 2, 13, and 15 could bind to G-box or E-box in the promoter of cold, drought, and salinity responsive genes (Tables 1, 2); members of this subfamily also could modulate the synthesis of some flavonoids (Table 3). Since members of this group share similarly conversed protein motifs (Supplementary Figure 1), it is reasonable to hypothesize that plant bHLHs in subfamily 1, 2, 13, and 15 could bind to G-box or E-box of cold, drought and salt responsive genes to further regulate the synthesis of flavonoids. Similarly, it also makes sense that bHLHs in subfamily 5 could regulate the synthesis of flavonoids to resist salinity and drought stresses (Tables 1-3). However, these hypotheses still need to be verified.
YQ, TZ, YY, JY, JX, and LG analyzed the phylogenetic relationships of bHLH family members. EP conceived the original idea for the review. All authors wrote the manuscript.
This work was supported by the grants 31970286 and 31301053 from the National Science Foundation of China, LY17C020004 from the Natural Science Foundation of Zhejiang Province, and 20170432B01 from the Hangzhou Science and Technology Bureau.
The authors declare that the research was conducted in the absence of any commercial or financial relationships that could be construed as a potential conflict of interest.
The Supplementary Material for this article can be found online at: https://www.frontiersin.org/articles/10.3389/fpls.2021.677611/full#supplementary-material
Abe, H., Yamaguchi-Shinozaki, K., Urao, T., Iwasaki, T., Hosokawa, D., and Shinozaki, K. (1997). Role of arabidopsis MYC and MYB homologs in drought- and abscisic acid-regulated gene expression. Plant Cell 9, 1859–1868. doi: 10.1105/tpc.9.10.18591997
Ahmad, A., Niwa, Y., Goto, S., Ogawa, T., Shimizu, M., Suzuki, A., et al. (2015). bHLH106 Integrates Functions of Multiple Genes through Their G-Box to Confer Salt Tolerance on Arabidopsis. PLoS One 10:e0126872. doi: 10.1371/journal.pone.0126872
Albertos, P., Wagner, K., and Poppenberger, B. (2019). Cold stress signalling in female reproductive tissues. Plant Cell Environ. 42, 846–853. doi: 10.1111/pce.13408
An, J. P., Li, H. H., Song, L. Q., Su, L., Liu, X., You, C. X., et al. (2016). The molecular cloning and functional characterization of MdMYC2, a bHLH transcription factor in apple. Plant Physiol. Biochem. 108, 24–31. doi: 10.1016/j.plaphy.2016.06.032
An, X. H., Tian, Y., Chen, K. Q., Wang, X. F., and Hao, Y. J. (2012). The apple WD40 protein MdTTG1 interacts with bHLH but not MYB proteins to regulate anthocyanin accumulation. J. Plant Physiol. 169, 710–717. doi: 10.1016/j.jplph.2012.01.015
Aslam, M. Z., Lin, X., Li, X., Yang, N., and Chen, L. (2020). Molecular Cloning and Functional Characterization of CpMYC2 and CpBHLH13 Transcription Factors from Wintersweet (Chimonanthus praecox L.). Plants 9:785. doi: 10.3390/plants9060785
Atchley, W. R., and Fitch, W. M. (1997). A natural classification of the basic helix-loop-helix class of transcription factors. Proc. Natl. Acad. Sci. U. S. A. 94, 5172–5176. doi: 10.1073/pnas.94.10.5172
Atchley, W. R., Terhalle, W., and Dress, A. (1999). Positional dependence, cliques, and predictive motifs in the bHLH protein domain. J. Mol. Evol. 48, 501–516. doi: 10.1007/PL00006494
Babitha, K. C., Vemanna, R. S., Nataraja, K. N., and Udayakumar, M. (2015). Overexpression of EcbHLH57 Transcription Factor from Eleusine coracana L. in Tobacco Confers Tolerance to Salt, Oxidative and Drought Stress. PLoS One 10:e0137098. doi: 10.1371/journal.pone.0137098
Bai, H., Song, Z. J., Zhang, Y., Li, Z. Y., Wang, Y. F., and Liu, X. (2020). The bHLH transcription factor PPLS1 regulates the color of pulvinus and leaf sheath in foxtail millet (Setaria italica). Theor. Appl. Gene. 133, 1911–1926. doi: 10.1007/s00122-020-03566-4
Baudry, A., Caboche, M., and Lepiniec, L. (2006). TT8 controls its own expression in a feedback regulation involving TTG1 and homologous MYB and bHLH factors, allowing a strong and cell-specific accumulation of flavonoids in Arabidopsis thaliana. Plant J. 46, 768–779. doi: 10.1111/j.1365-313X.2006.02733.x
Baudry, A., Heim, M. A., Dubreucq, B., Caboche, M., Weisshaar, B., and Lepiniec, L. (2004). TT2, TT8, and TTG1 synergistically specify the expression of BANYULS and proanthocyanidin biosynthesis in Arabidopsis thaliana. Plant J. 39, 366–380. doi: 10.1111/j.1365-313X.2004.02138.x
Bian, C., Guo, X. Y., Zhang, Y., Wang, L., Xu, T. D., Delong, A., et al. (2020). Protein phosphatase 2A promotes stomatal development by stabilizing SPEECHLESS in Arabidopsis. Proc. Natl. Acad. Sci. U. S. A. 117, 13127–13137. doi: 10.1073/pnas.1912075117
Bovy, A., De Vos, R., Kemper, M., Schijlen, E., Almenar Pertejo, M., and Muir, S. (2002). High-flavonol tomatoes resulting from the heterologous expression of the maize transcription factor genes LC and C1. Plant Cell 14, 2509–2526. doi: 10.1105/tpc.004218
Cai, Y., Li, Y., and Liang, G. (2021). FIT and bHLH Ib transcription factors modulate iron and copper crosstalk in Arabidopsis. Plant Cell Environ. 44, 1679–1691. doi: 10.1111/pce.14000
Carretero-Paulet, L., Galstyan, A., Roig-Villanova, I., Martinez-Garcia, J. F., Bilbao-Castro, J. R., and Robertson, D. L. (2010). Genome-wide classification and evolutionary analysis of the bHLH family of transcription factors in Arabidopsis, poplar, rice, moss, and algae. Plant Physiol. 153, 1398–1412. doi: 10.1104/pp.110.153593
Castilhos, G., Lazzarotto, F., Spagnolo-Fonini, L., Bodanese-Zanettini, M. H., and Margis-Pinheiro, M. (2014). Possible roles of basic helix-loop-helix transcription factors in adaptation to drought. Plant Sci. 223, 1–7. doi: 10.1016/j.plantsci.2014.02.010
Chakraborty, M., Gangappa, S. N., Maurya, J. P., Sethi, V., Srivastava, A. K., and Singh, A. (2019). Functional interrelation of MYC2 and HY5 plays an important role in Arabidopsis seedling development. Plant J. 99, 1080–1097. doi: 10.1111/tpj.14381
Chen, H. C., Cheng, W. H., Hong, C. Y., Chang, Y. S., and Chang, M. C. (2018). The transcription factor OsbHLH035 mediates seed germination and enables seedling recovery from salt stress through ABA-dependent and ABA-independent pathways, respectively. Rice 11:50. doi: 10.1186/s12284-018-0244-z
Chen, H. C., Hsieh-Feng, V., Liao, P. C., Cheng, W. H., Liu, L. Y., and Yang, Y. W. (2017). The function of OsbHLH068 is partially redundant with its homolog, AtbHLH112, in the regulation of the salt stress response but has opposite functions to control flowering in Arabidopsis. Plant Mol. Biol. 94, 531–548. doi: 10.1007/s11103-017-0624-6
Chen, Q., Sun, J., Zhai, Q., Zhou, W., Qi, L., and Xu, L. (2011). The basic helix-loop-helix transcription factor MYC2 directly represses PLETHORA expression during jasmonate-mediated modulation of the root stem cell niche in Arabidopsis. Plant Cell 23, 3335–3352. doi: 10.1105/tpc.111.089870
Cheng, T., Zhao, P., Ren, Y., Zou, J., and Sun, M. X. (2021). AtMIF1 increases seed oil content by attenuating GL2 inhibition. New Phytol. 229, 2152–2162. doi: 10.1111/nph.17016
Chinnusamy, V., Ohta, M., Kanrar, S., Lee, B. H., Hong, X., and Agarwal, M. (2003). ICE1: a regulator of cold-induced transcriptome and freezing tolerance in Arabidopsis. Genes. Dev. 17, 1043–1054. doi: 10.1101/gad.1077503
Cordeiro, A. M., Figueiredo, D. D., Tepperman, J., Borba, A. R., Lourenco, T., and Abreu, I. A. (2016). Rice phytochrome-interacting factor protein OsPIF14 represses OsDREB1B gene expression through an extended N-box and interacts preferentially with the active form of phytochrome B. Biochim. Biophys. Acta Gene Regul. Mech. 1859, 393–404. doi: 10.1016/j.bbagrm.2015.12.008
Cui, Y., Chen, C. L., Cui, M., Zhou, W. J., Wu, H. L., and Ling, H. Q. (2018). Four IVa bHLH Transcription Factors Are Novel Interactors of FIT and Mediate JA Inhibition of Iron Uptake in Arabidopsis. Mol. Plant 11, 1166–1183. doi: 10.1016/j.molp.2018.06.005
Dixon, R. A., Xie, D. Y., and Sharma, S. B. (2005). Proanthocyanidins–a final frontier in flavonoid research? New Phytol. 165, 9–28. doi: 10.1111/j.1469-8137.2004.01217.x
Dong, Y., Wang, C. P., Han, X., Tang, S., Liu, S., and Xia, X. L. (2014). A novel bHLH transcription factor PebHLH35 from Populus euphratica confers drought tolerance through regulating stomatal development, photosynthesis and growth in Arabidopsis. Biochem. Biophys. Res. Commun. 450, 453–458. doi: 10.1016/j.bbrc.2014.05.139
Dubos, C., Le Gourrierec, J., Baudry, A., Huep, G., Lanet, E., and Debeaujon, I. (2008). MYBL2 is a new regulator of flavonoid biosynthesis in Arabidopsis thaliana. Plant J. 55, 940–953. doi: 10.1111/j.1365-313X.2008.03564.x
Esmaeilpour, A., Van Labeke, M. C., Samson, R., and Van Damme, P. (2015). Osmotic stress affects physiological responses and growth characteristics of three pistachio cultivars. Acta Physiol. Plant. 37:123. doi: 10.1007/s11738-015-1876-x
Fairchild, C. D., Schumaker, M. A., and Quail, P. H. (2000). HFR1 encodes an atypical bHLH protein that acts in phytochrome A signal transduction. Genes Dev. 14, 2377–2391. doi: 10.1101/gad.828000
Feng, H. L., Ma, N. N., Meng, X., Zhang, S., Wang, J. R., and Chai, S. (2013). A novel tomato MYC-type ICE1-like transcription factor, SlICE1a, confers cold, osmotic and salt tolerance in transgenic tobacco. Plant Physiol. Biochem. 73, 309–320. doi: 10.1016/j.plaphy.2013.09.014
Fu, S. L., Liu, G. X., Zhang, L. C., Xia, C., Zhao, G. Y., Jia, J. Z., et al. (2014). Cloning and Analysis of a Salt Stress Related Gene TabHLH13 in Wheat. J. Plant Genet. Resour. 15, 1006–1011. doi: 10.13430/j.cnki.jpgr.2014.05.013
Gao, C., Sun, J., Wang, C., Dong, Y., Xiao, S., Wang, X., et al. (2017). Genome-wide analysis of basic/helix-loop-helix gene family in peanut and assessment of its roles in pod development. PLoS One 12:e0181843. doi: 10.1371/journal.pone.0181843
Gao, L. H., Liu, B. X., Li, J. B., Wu, Y. M., and Tang, Y. X. (2016). Cloning and function analysis of bHLH transcription factor gene GhMYC4 from Gossypium hirsutism L. Chin. Acad. Agricult. Sci. 18, 33–41. doi: 10.13304/j.nykjdb.2015.729
Gao, Y., Wu, M., Zhang, M., Jiang, W., Liang, E., and Zhang, D. (2018). Roles of a maize phytochrome-interacting factors protein ZmPIF3 in regulation of drought stress responses by controlling stomatal closure in transgenic rice without yield penalty. Plant Mol. Biol. 97, 311–323. doi: 10.1007/s11103-018-0739-4
Gao, Z. Y., Sun, W. J., Wang, J., Zhao, C. Y., and Zuo, K. J. (2019). GhbHLH18 negatively regulates fiber strength and length by enhancing lignin biosynthesis in cotton fibers. Plant Sci. 286, 7–16. doi: 10.1016/j.plantsci.2019.05.020
Geng, J., and Liu, J. H. (2018). The transcription factor CsbHLH18 of sweet orange functions in modulation of cold tolerance and homeostasis of reactive oxygen species by regulating the antioxidant gene. J. Exp. Bot. 69, 2677–2692. doi: 10.1093/jxb/ery065
Gratz, R., Brumbarova, T., Ivanov, R., Trofimov, K., Tunnermann, L., and Ochoa-Fernandez, R. (2020). Phospho-mutant activity assays provide evidence for alternative phospho-regulation pathways of the transcription factor FER-LIKE IRON DEFICIENCY-INDUCED TRANSCRIPTION FACTOR. New Phytol. 225, 250–267. doi: 10.1111/nph.16168
Gratz, R., Manishankar, P., Ivanov, R., Koster, P., Mohr, I., and Trofimov, K. (2019). CIPK11-Dependent Phosphorylation Modulates FIT Activity to Promote Arabidopsis Iron Acquisition in Response to Calcium Signaling. Dev. Cell 48, 726–740. doi: 10.1016/j.devcel.2019.01.006
Heim, M. A., Jakoby, M., Werber, M., Martin, C., Weisshaar, B., and Bailey, P. C. (2003). The basic helix-loop-helix transcription factor family in plants: a genome-wide study of protein structure and functional diversity. Mol. Biol. Evol. 20, 735–747. doi: 10.1093/molbev/msg088
Hichri, I., Barrieu, F., Bogs, J., Kappel, C., Delrot, S., and Lauvergeat, V. (2011). Recent advances in the transcriptional regulation of the flavonoid biosynthetic pathway. J. Exp. Bot. 62, 2465–2483. doi: 10.1093/jxb/erq442
Hichri, I., Heppel, S. C., Pillet, J., Leon, C., Czemmel, S., and Delrot, S. (2010). The Basic Helix-Loop-Helix Transcription Factor MYC1 Is Involved in the Regulation of the Flavonoid Biosynthesis Pathway in Grapevine. Mol. Plant 3, 509–523. doi: 10.1093/mp/ssp118
Huang, D. Q., and Dai, W. H. (2015). Molecular characterization of the basic helix-loop-helix (bHLH) genes that are differentially expressed and induced by iron deficiency in Populus. Plant Cell Rep. 34, 1211–1224. doi: 10.1007/s00299-015-1779-8
Huang, X., Li, K., Jin, C., and Zhang, S. (2015). ICE1 of Pyrus ussuriensis functions in cold tolerance by enhancing PuDREBa transcriptional levels through interacting with PuHHP1. Sci. Rep. 5:17620. doi: 10.1038/srep17620
Huang, X. S., Wang, W., Zhang, Q., and Liu, J. H. (2013). A basic helix-loop-helix transcription factor, PtrbHLH, of Poncirus trifoliata confers cold tolerance and modulates peroxidase-mediated scavenging of hydrogen peroxide. Plant Physiol. 162, 1178–1194. doi: 10.1104/pp.112.210740
Jaillon, O., Aury, J. M., Noel, B., Policriti, A., Clepet, C., and Casagrande, A. (2007). The grapevine genome sequence suggests ancestral hexaploidization in major angiosperm phyla. Nature 449, 463–467. doi: 10.1038/nature06148
Ji, X., Nie, X., Liu, Y., Zheng, L., Zhao, H., and Zhang, B. (2016). A bHLH gene from Tamarix hispida improves abiotic stress tolerance by enhancing osmotic potential and decreasing reactive oxygen species accumulation. Tree Physiol. 36, 193–207. doi: 10.1093/treephys/tpv139
Jiang, W., Liu, T., Nan, W., Jeewani, D. C., Niu, Y., Li, C., et al. (2018). Two transcription factors TaPpm1 and TaPpb1 co-regulate anthocyanin biosynthesis in purple pericarps of wheat. J. Exp. Bot. 69, 2555–2567. doi: 10.1093/jxb/ery101
Jiang, Y. Q., Yang, B., and Deyholos, M. K. (2009). Functional characterization of the Arabidopsis bHLH92 transcription factor in abiotic stress. Mol. Genet. Genom. 282, 503–516. doi: 10.1007/s00438-009-0481-3
Jin, C., Huang, X. S., Li, K. Q., Yin, H., Li, L. T., Yao, Z. H., et al. (2016). Overexpression of a bHLH1 Transcription Factor of Pyrus ussuriensis Confers Enhanced Cold Tolerance and Increases Expression of Stress-Responsive Genes. Front. Plant Sci. 7:441. doi: 10.3389/fpls.2016.00441
Jin, S. W., Rahim, M. A., Kim, H. T., Park, J. I., Kang, J. G., and Nou, I. S. (2018). Molecular analysis of anthocyanin-related genes in ornamental cabbage. Genome 61, 111–120. doi: 10.1139/gen-2017-0098
Kang, H., Oh, E., Choi, G., and Lee, D. (2010). Genome-wide DNA-binding specificity of PIL5, an Arabidopsis basic Helix-Loop-Helix (bHLH) transcription factor. Int. J. Data Min. Bioinform. 4, 588–599. doi: 10.1504/IJDMB.2010.035902
Kim, J., and Kim, H. Y. (2006). Functional analysis of a calcium-binding transcription factor involved in plant salt stress signaling. FEBS Lett. 580, 5251–5256. doi: 10.1016/j.febslet.2006.08.050
Kool, M., Ahrens, C. H., Goldbach, R. W., Rohrmann, G. F., and Vlak, J. M. (1994). Identification of genes involved in DNA replication of the Autographa californica baculovirus. Proc. Natl. Acad. Sci. U. S. A. 91, 11212–11216. doi: 10.1073/pnas.91.23.11212
Krishnamurthy, P., Vishal, B., Khoo, K., Rajappa, S., Loh, C. S., and Kumar, P. P. (2019). Expression of AoNHX1 increases salt tolerance of rice and Arabidopsis, and bHLH transcription factors regulate AtNHX1 and AtNHX6 in Arabidopsis. Plant Cell Rep. 38, 1299–1315. doi: 10.1007/s00299-019-02450-w
Kroh, G. E., and Pilon, M. (2019). Connecting the negatives and positives of plant iron homeostasis. New Phytol. 223, 1052–1055. doi: 10.1111/nph.15933
Le Hir, R., Castelain, M., Chakraborti, D., Moritz, T., Dinant, S., and Bellini, C. (2017). AtbHLH68 transcription factor contributes to the regulation of ABA homeostasis and drought stress tolerance in Arabidopsis thaliana. Physiol. Plant 160, 312–327. doi: 10.1111/ppl.12549
Lea, U. S., Slimestad, R., Smedvig, P., and Lillo, C. (2007). Nitrogen deficiency enhances expression of specific MYB and bHLH transcription factors and accumulation of end products in the flavonoid pathway. Planta 225, 1245–1253. doi: 10.1007/s00425-006-0414-x
Lei, R. H., Li, Y., Cai, Y. R., Li, C. Y., Pu, M. N., and Lu, C. K. (2020). bHLH121 Functions as a Direct Link that Facilitates the Activation of FIT by bHLH IVc Transcription Factors for Maintaining Fe Homeostasis in Arabidopsis. Mol. Plant 13, 634–649. doi: 10.1016/j.molp.2020.01.006
Li, C. H., Qiu, F., Ding, L., Huang, M. Z., Huang, S. R., and Yang, G. S. (2017). Anthocyanin biosynthesis regulation of DhMYB2 and DhbHLH1 in Dendrobium hybrids petals. Plant Physiol. Biochem. 112, 335–345. doi: 10.1016/j.plaphy.2017.01.019
Li, F., Guo, S., Zhao, Y., Chen, D., Chong, K., and Xu, Y. (2010). Overexpression of a homopeptide repeat-containing bHLH protein gene (OrbHLH001) from Dongxiang Wild Rice confers freezing and salt tolerance in transgenic Arabidopsis. Plant Cell Rep. 29, 977–986. doi: 10.1007/s00299-010-0883-z
Li, H. M., Sun, J. Q., Xu, Y. X., Jiang, H. L., Wu, X. Y., and Li, C. Y. (2007). The bHLH-type transcription factor AtAIB positively regulates ABA response in Arabidopsis. Plant Mol. Biol. 65, 655–665. doi: 10.1007/s11103-007-9230-3
Li, L., Gao, W., Peng, Q., Zhou, B., Kong, Q., Ying, Y., et al. (2018). Two soybean bHLH factors regulate response to iron deficiency. J. Integr. Plant Biol. 60, 608–622. doi: 10.1111/jipb.12651
Li, N., Wu, H., Ding, Q., Li, H., Li, Z., Ding, J., et al. (2018). The heterologous expression of Arabidopsis PAP2 induces anthocyanin accumulation and inhibits plant growth in tomato. Funct. Integr. Genomics 18, 341–353. doi: 10.1007/s10142-018-0590-3
Li, L., Hao, X. L., Liu, H., Wang, W., Fu, X. Q., and Ma, Y. N. (2019). Jasmonic acid-responsive AabHLH1 positively regulates artemisinin biosynthesis in Artemisia annua. Biotechnol. Appl. Biochem. 66, 369–375. doi: 10.1002/bab.1733
Li, Z. X., Liu, C., Zhang, Y., Wang, B. M., Ran, Q. J., and Zhang, J. R. (2019). The bHLH family member ZmPTF1 regulates drought tolerance in maize by promoting root development and abscisic acid synthesis. J. Exp. Bot. 70, 5471–5486. doi: 10.1093/jxb/erz307
Li, W. F., Ning, G. X., Mao, J., Guo, Z. G., Zhou, Q., and Chen, B. H. (2019). Whole-genome DNA methylation patterns and complex associations with gene expression associated with anthocyanin biosynthesis in apple fruit skin. Planta 250, 1833–1847. doi: 10.1007/s00425-019-03266-4
Li, P., Chen, B., Zhang, G., Chen, L., Dong, Q., and Wen, J. (2016). Regulation of anthocyanin and proanthocyanidin biosynthesis by Medicago truncatula bHLH transcription factor MtTT8. New Phytol. 210, 905–921. doi: 10.1111/nph.13816
Li, X., Zhang, H., Ai, Q., Liang, G., and Yu, D. (2016). Two bHLH Transcription Factors, bHLH34 and bHLH104. Regulate Iron Homeostasis in Arabidopsis thaliana. Plant Physiol. 170, 2478–2493. doi: 10.1104/pp.15.01827
Li, X., Duan, X., Jiang, H., Sun, Y., Tang, Y., and Yuan, Z. (2006). Genome-wide analysis of basic/helix-loop-helix transcription factor family in rice and Arabidopsis. Plant Physiol. 141, 1167–1184. doi: 10.1104/pp.106.080580
Li, Y. Y., Sui, X. Y., Yang, J. S., Xiang, X. H., Li, Z. Q., and Wang, Y. Y. (2020). A novel bHLH transcription factor, NtbHLH1, modulates iron homeostasis in tobacco (Nicotiana tabacum L.). Biochem. Biophys. Res. Commun. 522, 233–239.
Liang, G., Zhang, H., Li, X., Ai, Q., and Yu, D. (2017). bHLH transcription factor bHLH115 regulates iron homeostasis in Arabidopsis thaliana. J. Exp. Bot. 68, 1743–1755. doi: 10.1093/jxb/erx043
Liang, Y., Wang, S., Zhao, C., Ma, X., Zhao, Y., and Shao, J. (2020). Transcriptional regulation of bark freezing tolerance in apple (Malus domestica Borkh.). Hortic. Res. 7:205. doi: 10.1038/s41438-020-00432-8
Lin, Z., Li, Y., Zhang, Z., Liu, X., Hsu, C. C., and Du, Y. (2020). A RAF-SnRK2 kinase cascade mediates early osmotic stress signaling in higher plants. Nat. Commun. 11:613. doi: 10.1038/s41467-020-14477-9
Ling, H. Q., Bauer, P., Bereczky, Z., Keller, B., and Ganal, M. (2002). The tomato fer gene encoding a bHLH protein controls iron-uptake responses in roots. Proc. Natl. Acad. Sci. U. S. A. 99, 13938–13943. doi: 10.1073/pnas.212448699
Lingam, S., Mohrbacher, J., Brumbarova, T., Potuschak, T., Fink-Straube, C., and Blondet, E. (2011). Interaction between the bHLH Transcription Factor FIT and ETHYLENE INSENSITIVE3/ETHYLENE INSENSITIVE3-LIKE1 Reveals Molecular Linkage between the Regulation of Iron Acquisition and Ethylene Signaling in Arabidopsis. Plant Cell 23, 1815–1829. doi: 10.1105/tpc.111.084715
Liu, W. W., Tai, H. H., Li, S. S., Gao, W., Zhao, M., and Xie, C. X. (2014). bHLH122 is important for drought and osmotic stress resistance in Arabidopsis and in the repression of ABA catabolism. New Phytol. 201, 1192–1204. doi: 10.1111/nph.12607
Liu, Y. J., Ji, X. Y., Nie, X. G., Qu, M., Zheng, L., and Tan, Z. L. (2015). Arabidopsis AtbHLH112 regulates the expression of genes involved in abiotic stress tolerance by binding to their E-box and GCG-box motifs. New Phytol. 207, 692–709. doi: 10.1111/nph.13387
Long, T. A., Tsukagoshi, H., Busch, W., Lahner, B., Salt, D. E., and Benfey, P. N. (2010). The bHLH transcription factor POPEYE regulates response to iron deficiency in Arabidopsis roots. Plant Cell 22, 2219–2236. doi: 10.1105/tpc.110.074096
Ludwig, S. R., Habera, L. F., Dellaporta, S. L., and Wessler, S. R. (1989). Lc, a member of the maize R gene family responsible for tissue-specific anthocyanin production, encodes a protein similar to transcriptional activators and contains the myc-homology region. Proc. Natl. Acad. Sci. U. S. A. 86, 7092–7096. doi: 10.1073/pnas.86.18.7092
Maes, L., Inze, D., and Goossens, A. (2008). Functional specialization of the TRANSPARENT TESTA GLABRA1 network allows differential hormonal control of laminal and marginal trichome initiation in Arabidopsis rosette leaves. Plant Physiol. 148, 1453–1464. doi: 10.1104/PP.108.125385
Man, L. L., Xiang, D. J., Wang, L. N., Zhang, W. W., Wang, X. D., and Qi, G. C. (2017). Stress-responsive gene RsICE1 from Raphanus sativus increases cold tolerance in rice. Protoplasma 254, 945–956. doi: 10.1007/s00709-016-1004-9
Massari, M. E., and Murre, C. (2000). Helix-loop-helix proteins: regulators of transcription in eucaryotic organisms. Mol. Cell Biol. 20, 429–440. doi: 10.1128/MCB.20.2.429-440.2000
Miao, L. M., Gao, Y. Y., Zhao, K., Kong, L. J., Yu, S. B., and Li, R. R. (2020). Comparative analysis of basic helix-loop-helix gene family among Brassica oleracea, Brassica rapa, and Brassica napus. BMC Genomics 21:178. doi: 10.1186/s12864-020-6572-6
Mitchell, J. H., Gardner, P. T., Mcphail, D. B., Morrice, P. C., Collins, A. R., and Duthie, G. G. (1998). Antioxidant efficacy of phytoestrogens in chemical and biological model systems. Arch. Biochem. Biophys. 360, 142–148. doi: 10.1006/abbi.1998.0951
Nesi, N., Debeaujon, I., Jond, C., Pelletier, G., Caboche, M., and Lepiniec, L. (2000). The TT8 gene encodes a basic helix-loop-helix domain protein required for expression of DFR and BAN genes in Arabidopsis siliques. Plant Cell 12, 1863–1878. doi: 10.2307/3871198
Nims, E., Vongpaseuth, K., Roberts, S. C., and Walkerbot, E. L. (2009). WITHDRAWN: TCJAMYC: A bHLH transcription factor that activates paclitaxel biosynthetic pathway genes in yew. J. Biol. Chem. 290:20104. doi: 10.1074/jbc.M109.026195
Niu, Y. J., Figueroa, P., and Browse, J. (2011). Characterization of JAZ-interacting bHLH transcription factors that regulate jasmonate responses in Arabidopsis. J. Exp. Bot. 62, 2143–2154. doi: 10.1093/jxb/erq408
Ogo, Y., Itai, R. N., Nakanishi, H., Inoue, H., Kobayashi, T., and Suzuki, M. (2006). Isolation and characterization of IRO2, a novel iron-regulated bHLH transcription factor in graminaceous plants. J. Exp. Bot. 57, 2867–2878. doi: 10.1093/jxb/erl054
Outchkourov, N. S., Carollo, C. A., Gomez-Roldan, V., De Vos, R. C. H., Bosch, D., and Hall, R. D. (2014). Control of anthocyanin and non-flavonoid compounds by anthocyanin-regulating MYB and bHLH transcription factors in Nicotiana benthamiana leaves. Front. Plant Sci. 5:519. doi: 10.3389/fpls.2014.00519
Pires, N., and Dolan, L. (2010). Origin and diversification of basic-helix-loop-helix proteins in plants. Mol. Biol. Evol. 27, 862–874. doi: 10.1093/molbev/msp288
Qi, T. C., Wang, J. J., Huang, H., Liu, B., Gao, H., and Liu, Y. L. (2015). Regulation of Jasmonate-Induced Leaf Senescence by Antagonism between bHLH Subgroup IIIe and IIId Factors in Arabidopsis. Plant Cell 27, 1634–1649. doi: 10.1105/tpc.15.00110
Qian, W., Tan, G., Liu, H., He, S., Gao, Y., and An, C. (2007). Identification of a bHLH-type G-box binding factor and its regulation activity with G-box and Box I elements of the PsCHS1 promoter. Plant Cell Rep. 26, 85–93. doi: 10.1007/s00299-006-0202-x
Qin, L., Sun, L., Wei, L., Yuan, J., Kong, F., and Zhang, Y. (2021). Maize SRO1e represses anthocyanin synthesis through regulating the MBW complex in response to abiotic stress. Plant J. 105, 1010–1025. doi: 10.1111/tpj.15083
Rao, S., Tian, Y., Xia, X., Li, Y., and Chen, J. (2020). Chromosome doubling mediates superior drought tolerance in Lycium ruthenicum via abscisic acid signaling. Hortic. Res. 7:40. doi: 10.1038/s41438-020-0260-1
Ravaglia, D., Espley, R. V., Henry-Kirk, R. A., Andreotti, C., Ziosi, V., and Hellens, R. P. (2013). Transcriptional regulation of flavonoid biosynthesis in nectarine (Prunus persica) by a set of R2R3 MYB transcription factors. BMC Plant Biol. 13:68. doi: 10.1186/1471-2229-13-68
Ren, Y. R., Zhao, Q., Zhao, X. Y., Hao, Y. J., and You, C. X. (2016). Expression analysis of the MdCIbHLH1 gene in apple flower buds and seeds in the process of dormancy. Hortic. Plant J. 2, 61–66. doi: 10.1016/j.hpj.2016.06.007
Ribeiro, D., Freitas, M., Lima, J. L. F. C., and Fernandes, E. (2015). Proinflammatory Pathways: The Modulation by Flavonoids. Med. Res. Rev. 35, 877–936. doi: 10.1002/med.21347
Rice-Evans, C. A., Miller, N. J., and Paganga, G. (1996). Structure-antioxidant activity relationships of flavonoids and phenolic acids. Free Radic. Biol. Med. 20, 933–956. doi: 10.1016/0891-5849(95)02227-9
Riechmann, L., and Muyldermans, S. (1999). Single domain antibodies: comparison of camel VH and camelised human VH domains. J. Immunol. Methods 231, 25–38. doi: 10.1016/S0022-1759(99)00138-6
Rohrmann, G. F. (2019). Baculovirus Molecular Biology [Internet], 4th Edn. Bethesda, MD: National Center for Biotechnology Information.
Rozema, J., and Flowers, T. (2008). Ecology. Crops for a salinized world. Science 322, 1478–1480. doi: 10.1126/science.1168572
Rushton, P. J., Bokowiec, M. T., Han, S. C., Zhang, H. B., Brannock, J. F., and Chen, X. F. (2008). Tobacco transcription factors: Novel insights into transcriptional regulation in the Solanaceae. Plant Physiol. 147, 280–295. doi: 10.1104/pp.107.114041
Sharker, M. R., Sukhan, Z. P., Kim, S. C., Lee, W. K., and Kho, K. H. (2020). Identification, characterization, and expression analysis of a serotonin receptor involved in the reproductive process of the Pacific abalone. Haliotis discus hannai. Mol. Biol. Rep. 47, 555–567. doi: 10.1007/s11033-019-05162-2
Shimizu, T., Toumoto, A., Ihara, K., Shimizu, M., Kyogoku, Y., and Ogawa, N. (1997). Crystal structure of PHO4 bHLH domain-DNA complex: flanking base recognition. EMBO J. 16, 4689–4697. doi: 10.1093/emboj/16.15.4689
Shoeva, O. Y. (2018). Complex regulation of the TaMyc1 gene expression in wheat grain synthesizing anthocyanin pigments. Mol. Biol. Rep. 45, 327–334. doi: 10.1007/s11033-018-4165-0
Shoji, T., and Hashimoto, T. (2011). Tobacco MYC2 Regulates Jasmonate-Inducible Nicotine Biosynthesis Genes Directly and By Way of the NIC2-Locus ERF Genes. Plant Cell Physiol. 52, 1117–1130. doi: 10.1093/pcp/pcr063
Simmons, A. R., and Bergmann, D. C. (2016). Transcriptional control of cell fate in the stomatal lineage. Curr. Opin. Plant Biol. 29, 1–8. doi: 10.1016/j.pbi.2015.09.008
Song, Q., Qian, S. F., Chen, X. Q., Chen, L. M., and Li, K. Z. (2014). Study on the Function of Transcription Factor GmbHLH30 on Aluminum Tolerance Preliminary in Tampa Black Soybean. Life Sci. Res. 18, 332–337. doi: 10.16605/j.cnki.1007-7847.2014.04.007
Sun, K., Wang, H., and Xia, Z. (2019). The maize bHLH transcription factor bHLH105 confers manganese tolerance in transgenic tobacco. Plant Sci. 280, 97–109. doi: 10.1016/j.plantsci.2018.11.006
Sun, X., Wang, Y., and Sui, N. (2018). Transcriptional regulation of bHLH during plant response to stress. Biochem. Biophys. Res. Commun. 503, 397–401. doi: 10.1016/j.bbrc.2018.07.123
Symonds, V. V., Hatlestad, G., and Lloyd, A. M. (2011). Natural allelic variation defines a role for ATMYC1: trichome cell fate determination. PLoS Genet 7:e1002069. doi: 10.1371/journal.pgen.1002069
Tao, R., Yu, W., Gao, Y., Ni, J., Yin, L., Zhang, X., et al. (2020). Light-Induced Basic/Helix-Loop-Helix64 Enhances Anthocyanin Biosynthesis and Undergoes CONSTITUTIVELY PHOTOMORPHOGENIC1-Mediated Degradation in Pear. Plant Physiol. 184, 1684–1701. doi: 10.1104/pp.20.01188
Thao, N. P., Khan, M. I., Thu, N. B., Hoang, X. L., Asgher, M., and Khan, N. A. (2015). Role of Ethylene and Its Cross Talk with Other Signaling Molecules in Plant Responses to Heavy Metal Stress. Plant Physiol. 169, 73–84. doi: 10.1104/pp.15.00663
Tissot, N., Robe, K., Gao, F., Grant-Grant, S., Boucherez, J., and Bellegarde, F. (2019). Transcriptional integration of the responses to iron availability in Arabidopsis by the bHLH factor ILR3. New Phytol. 223, 1433–1446. doi: 10.1111/nph.15753
Toledo-Ortiz, G., Huq, E., and Quail, P. H. (2003). The Arabidopsis basic/helix-loop-helix transcription factor family. Plant Cell 15, 1749–1770. doi: 10.1105/tpc.013839
Tominaga-Wada, R., Masakane, A., and Wada, T. (2018). Effect of phosphate deficiency-induced anthocyanin accumulation on the expression of Solanum lycopersicum GLABRA3 (SlGL3) in tomato. Plant Signal Behav. 13:e1477907. doi: 10.1080/15592324.2018.1477907
Treutter, D. (2006). Significance of flavonoids in plant resistance: a review. Environ. Chem. Lett. 4, 147–157. doi: 10.1007/s10311-006-0068-8
Verma, D., Jalmi, S. K., Bhagat, P. K., Verma, N., and Sinha, A. K. (2020). A bHLH transcription factor, MYC2, imparts salt intolerance by regulating proline biosynthesis in Arabidopsis. Febs J. 287, 2560–2576. doi: 10.1111/febs.15157
Wang, C., Ji, W., Liu, Y., Zhou, P., Meng, Y., and Zhang, P. (2021). The antagonistic MYB paralogs RH1 and RH2 govern anthocyanin leaf markings in Medicago truncatula. New Phytol. 229, 3330–3344. doi: 10.1111/nph.17097
Wang, F. B., Zhu, H., Chen, D. H., Li, Z. J., Peng, R. H., and Yao, Q. H. (2016a). A grape bHLH transcription factor gene, VvbHLH1, increases the accumulation of flavonoids and enhances salt and drought tolerance in transgenic Arabidopsis thaliana. Plant Cell Tissue Organ. Cult. 125, 387–398. doi: 10.1007/s11240-016-0953-1
Wang, F. B., Zhu, H., Kong, W. L., Peng, R. H., Liu, Q. C., and Yao, Q. H. (2016b). The Antirrhinum AmDEL gene enhances flavonoids accumulation and salt and drought tolerance in transgenic Arabidopsis. Planta 244, 59–73. doi: 10.1007/s00425-016-2489-3
Wang, H. Y., Klatte, M., Jakoby, M., Baumlein, H., Weisshaar, B., and Bauer, P. (2007). Iron deficiency-mediated stress regulation of four subgroup Ib BHLH genes in Arabidopsis thaliana. Planta 226, 897–908. doi: 10.1007/s00425-007-0535-x
Wang, J., Cheng, G., Wang, C., He, Z. Z., Lan, X. X., and Zhang, S. Y. (2017). The bHLH transcription factor CgbHLH001 is a potential interaction partner of CDPK in halophyte Chenopodium glaucum. Scient. Rep. 7:8441. doi: 10.1038/s41598-017-06706-x
Wang, L., Tang, W., Hu, Y., Zhang, Y., Sun, J., and Guo, X. (2019). A MYB/bHLH complex regulates tissue-specific anthocyanin biosynthesis in the inner pericarp of red-centered kiwifruit Actinidia chinensis cv. Hongyang. Plant J. 99, 359–378. doi: 10.1111/tpj.14330
Wang, N., Cui, Y., Liu, Y., Fan, H., Du, J., and Huang, Z. (2013). Requirement and functional redundancy of Ib subgroup bHLH proteins for iron deficiency responses and uptake in Arabidopsis thaliana. Mol. Plant 6, 503–513. doi: 10.1093/mp/sss089
Wang, P. F., Su, L., Gao, H. H., Jiang, X. L., Wu, X. Y., and Li, Y. (2018). Genome-Wide Characterization of bHLH Genes in Grape and Analysis of their Potential Relevance to Abiotic Stress Tolerance and Secondary Metabolite Biosynthesis. Front. Plant Sci. 9:64. doi: 10.3389/fpls.2018.00064
Wang, S., Li, L., Ying, Y., Wang, J., Shao, J. F., and Yamaji, N. (2020). A transcription factor OsbHLH156 regulates Strategy II iron acquisition through localising IRO2 to the nucleus in rice. New Phytol. 225, 1247–1260. doi: 10.1111/nph.16232
Wang, Y. J., Zhang, Z. G., He, X. J., Zhou, H. L., Wen, Y. X., and Dai, J. X. (2003). A rice transcription factor OsbHLH1 is involved in cold stress response. Theor. Appl. Genet. 107, 1402–1409. doi: 10.1007/s00122-003-1378-x
Winkel-Shirley, B. (2001). Flavonoid biosynthesis. A colorful model for genetics, biochemistry, cell biology, and biotechnology. Plant Physiol. 126, 485–493. doi: 10.1104/pp.126.2.485
Wu, H., Ye, H. Y., Yao, R. F., Zhang, T., and Xiong, L. Z. (2015). OsJAZ9 acts as a transcriptional regulator in jasmonate signaling and modulates salt stress tolerance in rice. Plant Sci. 232, 1–12. doi: 10.1016/j.plantsci.2014.12.010
Xi, H. C., He, Y. J., and Chen, H. Y. (2021). Functional Characterization of SmbHLH13 in Anthocyanin Biosynthesis and Flowering in Eggplant. Hortic. Plant J. 7, 73–80. doi: 10.1016/j.hpj.2020.08.006
Xiang, L. L., Liu, X. F., Li, X., Yin, X. R., Grierson, D., and Li, F. (2015). A Novel bHLH Transcription Factor Involved in Regulating Anthocyanin Biosynthesis in Chrysanthemums (Chrysanthemum morifolium Ramat.). PLoS One 10:e0143892. doi: 10.1371/journal.pone.0143892
Xiong, Y., Liu, T., Tian, C., Sun, S., Li, J., and Chen, M. (2005). Transcription factors in rice: a genome-wide comparative analysis between monocots and eudicots. Plant Mol. Biol. 59, 191–203. doi: 10.1007/s11103-005-6503-6
Xu, H. F., Wang, N., Liu, J. X., Qu, C. Z., Wang, Y. C., Jiang, S. H., et al. (2017). The molecular mechanism underlying anthocyanin metabolism in apple using the MdMYB16 and MdbHLH33 genes. Plant Mol. Biol. 94, 149–165. doi: 10.1007/s11103-017-0601-0
Xu, W., Dubos, C., and Lepiniec, L. (2015). Transcriptional control of flavonoid biosynthesis by MYB-bHLH-WDR complexes. Trends Plant Sci. 20, 176–185. doi: 10.1016/j.tplants.2014.12.001
Xu, W., Zhang, N., Jiao, Y., Li, R., Xiao, D., and Wang, Z. (2014). The grapevine basic helix-loop-helix (bHLH) transcription factor positively modulates CBF-pathway and confers tolerance to cold-stress in Arabidopsis. Mol. Biol. Rep. 41, 5329–5342. doi: 10.1007/s11033-014-3404-2
Yamamura, C., Mizutani, E., Okada, K., Nakagawa, H., Fukushima, S., and Tanaka, A. (2015). Diterpenoid phytoalexin factor, a bHLH transcription factor, plays a central role in the biosynthesis of diterpenoid phytoalexins in rice. Plant J. 84, 1100–1113. doi: 10.1111/tpj.13065
Yang, J. H., Lee, K. H., Du, Q., Yang, S., Yuan, B., Qi, L., et al. (2020). A membrane-associated NAC domain transcription factor XVP interacts with TDIF co-receptor and regulates vascular meristem activity. New Phytol. 226, 59–74. doi: 10.1111/nph.16289
Yang, K. Z., Jiang, M., Wang, M., Xue, S., Zhu, L. L., and Wang, H. Z. (2015). Phosphorylation of Serine 186 of bHLH Transcription Factor SPEECHLESS Promotes Stomatal Development in Arabidopsis. Mol. Plant 8, 783–795. doi: 10.1016/j.molp.2014.12.014
Yang, T. R., Yao, S. F., Hao, L., Zhao, Y. Y., Lu, W. J., and Xiao, K. (2016). Wheat bHLH-type transcription factor gene TabHLH1 is crucial in mediating osmotic stresses tolerance through modulating largely the ABA-associated pathway. Plant Cell Rep. 35, 2309–2323. doi: 10.1007/s00299-016-2036-5
Yang, X., Wang, R., Hu, Q., Li, S., Mao, X., and Jing, H. (2019). DlICE1, a stress-responsive gene from Dimocarpus longan, enhances cold tolerance in transgenic Arabidopsis. Plant Physiol. Biochem. 142, 490–499. doi: 10.1016/j.plaphy.2019.08.007
Yao, P., Sun, Z., Li, C., Zhao, X., Li, M., and Deng, R. (2018). Overexpression of Fagopyrum tataricum FtbHLH2 enhances tolerance to cold stress in transgenic Arabidopsis. Plant Physiol. Biochem. 125, 85–94. doi: 10.1016/j.plaphy.2018.01.028
Yao, P. F., Li, C. L., Zhao, X. R., Li, M. F., Zhao, H. X., and Guo, J. Y. (2017). Overexpression of a Tartary Buckwheat Gene, FtbHLH3, Enhances Drought/Oxidative Stress Tolerance in Transgenic Arabidopsis. Front. Plant Sci. 8:625. doi: 10.3389/fpls.2017.00625
Yi, K., Wu, Z., Zhou, J., Du, L., Guo, L., Wu, Y., et al. (2005). OsPTF1, a novel transcription factor involved in tolerance to phosphate starvation in rice. Plant Physiol. 138, 2087–2096. doi: 10.1104/pp.105.063115
Yin, Y., Vafeados, D., Tao, Y., Yoshida, S., Asami, T., and Chory, J. (2005). A new class of transcription factors mediates brassinosteroid-regulated gene expression in Arabidopsis. Cell 120, 249–259. doi: 10.1016/j.cell.2004.11.044
Yuan, Y., Wu, H., Wang, N., Li, J., Zhao, W., and Du, J. (2008). FIT interacts with AtbHLH38 and AtbHLH39 in regulating iron uptake gene expression for iron homeostasis in Arabidopsis. Cell Res. 18, 385–397. doi: 10.1038/cr.2008.26
Zhai, J., Hao, H., Xiao, H., Cao, Y., Lin, X., and Huang, X. (2018). Identification of JAZ-interacting MYC transcription factors involved in latex drainage in Hevea brasiliensis. Sci. Rep. 8:909. doi: 10.1038/s41598-018-19206-3
Zhai, Y. Q., Zhang, L. C., Xia, C., Fu, S. L., Zhao, G. Y., and Jia, J. Z. (2016). The wheat transcription factor, TabHLH39, improves tolerance to multiple abiotic stressors in transgenic plants. Biochem. Biophys. Res. Commun. 473, 1321–1327. doi: 10.1016/j.bbrc.2016.04.071
Zhang, H., Hedhili, S., Montiel, G., Zhang, Y., Chatel, G., and Pre, M. (2011). The basic helix-loop-helix transcription factor CrMYC2 controls the jasmonate-responsive expression of the ORCA genes that regulate alkaloid biosynthesis in Catharanthus roseus. Plant J. 67, 61–71. doi: 10.1111/j.1365-313X.2011.04575.x
Zhang, J., Liu, B., Li, M., Feng, D., Jin, H., and Wang, P. (2015). The bHLH transcription factor bHLH104 interacts with IAA-LEUCINE RESISTANT3 and modulates iron homeostasis in Arabidopsis. Plant Cell 27, 787–805. doi: 10.1105/tpc.114.132704
Zhang, Q. Y., Gu, K. D., Cheng, L., Wang, J. H., Yu, J. Q., and Wang, X. F. (2020a). BTB-TAZ Domain Protein MdBT2 Modulates Malate Accumulation and Vacuolar Acidification in Response to Nitrate. Plant Physiol. 183, 750–764. doi: 10.1104/pp.20.00208
Zhang, Q. Y., Gu, K. D., Wang, J. H., Yu, J. Q., Wang, X. F., and Zhang, S. (2020b). BTB-BACK-TAZ domain protein MdBT2-mediated MdMYB73 ubiquitination negatively regulates malate accumulation and vacuolar acidification in apple. Hortic. Res. 7:151. doi: 10.1038/s41438-020-00384-z
Zhang, T., Mo, J., Zhou, K., Chang, Y., and Liu, Z. (2018). Overexpression of Brassica campestris BcICE1 gene increases abiotic stress tolerance in tobacco. Plant Physiol. Biochem. 132, 515–523. doi: 10.1016/j.plaphy.2018.09.039
Zhang, X., Ge, F., Deng, B., Shah, T., Huang, Z. J., Liu, D. Q., et al. (2017). Molecular Cloning and Characterization of PnbHLH1 Transcription Factor in Panax notoginseng. Molecules 22:1268. doi: 10.3390/molecules22081268
Zhao, M., Song, A., Li, P., Chen, S., Jiang, J., and Chen, F. (2014). A bHLH transcription factor regulates iron intake under Fe deficiency in chrysanthemum. Sci. Rep. 4:6694. doi: 10.1038/srep06694
Zhao, Q., Fan, Z., Qiu, L., Che, Q., Wang, T., and Li, Y. (2020). MdbHLH130, an Apple bHLH Transcription Factor, Confers Water Stress Resistance by Regulating Stomatal Closure and ROS Homeostasis in Transgenic Tobacco. Front. Plant Sci. 11:543696. doi: 10.3389/fpls.2020.543696
Zhao, Q., Ren, Y. R., Wang, Q. J., Yao, Y. X., You, C. X., and Hao, Y. J. (2016). Overexpression of MdbHLH104 gene enhances the tolerance to iron deficiency in apple. Plant Biotechnol. J. 14, 1633–1645. doi: 10.1111/pbi.12526
Zhao, Q., Xiang, X., Liu, D., Yang, A., and Wang, Y. (2018). Tobacco Transcription Factor NtbHLH123 Confers Tolerance to Cold Stress by Regulating the NtCBF Pathway and Reactive Oxygen Species Homeostasis. Front. Plant Sci. 9:381. doi: 10.3389/fpls.2018.00381
Zhao, W., Liu, Y., Li, L., Meng, H., Yang, Y., and Dong, Z. (2021). Genome-Wide Identification and Characterization of bHLH Transcription Factors Related to Anthocyanin Biosynthesis in Red Walnut (Juglans regia L.). Front. Genet. 12:632509. doi: 10.3389/fgene.2021.632509
Zhao, Y., Zhang, Y. Y., Liu, H., Zhang, X. S., Ni, R., and Wang, P. Y. (2019). Functional characterization of a liverworts bHLH transcription factor involved in the regulation of bisbibenzyls and flavonoids biosynthesis. BMC Plant Biol. 19:497. doi: 10.1186/s12870-019-2109-z
Zhou, J., Li, F., Wang, J. L., Ma, Y., Chong, K., and Xu, Y. Y. (2009). Basic helix-loop-helix transcription factor from wild rice (OrbHLH2) improves tolerance to salt- and osmotic stress in Arabidopsis. J. Plant Physiol. 166, 1296–1306. doi: 10.1016/j.jplph.2009.02.007
Zhou, X., Liao, Y., Kim, S. U., Chen, Z., Nie, G., and Cheng, S. (2020). Genome-wide identification and characterization of bHLH family genes from Ginkgo biloba. Sci. Rep. 10:13723. doi: 10.1038/s41598-020-69305-3
Zhou, Z. X., Wang, J., Zhang, S. Y., Yu, Q. H., and Lan, H. Y. (2021). Investigation of the Nature of CgCDPK and CgbHLH001 Interaction and the Function of bHLH Transcription Factor in Stress Tolerance in Chenopodium glaucum. Front. Plant Sci. 11:603O298. doi: 10.3389/fpls.2020.603298
Zimmermann, I. M., Heim, M. A., Weisshaar, B., and Uhrig, J. F. (2004). Comprehensive identification of Arabidopsis thaliana MYB transcription factors interacting with R/B-like BHLH proteins. Plant J. 40, 22–34. doi: 10.1111/j.1365-313X.2004.02183.x
Zong, Y., Li, G., Xi, X., Sun, X., Li, S., and Cao, D. (2019). A bHLH transcription factor TsMYC2 is associated with the blue grain character in triticale (Triticum x Secale). Plant Cell Rep. 38, 1291–1298. doi: 10.1007/s00299-019-02449-3
Keywords: plant, bHLH transcription factor, flavonoids, abiotic stresses, cis-elements
Citation: Qian Y, Zhang T, Yu Y, Gou L, Yang J, Xu J and Pi E (2021) Regulatory Mechanisms of bHLH Transcription Factors in Plant Adaptive Responses to Various Abiotic Stresses. Front. Plant Sci. 12:677611. doi: 10.3389/fpls.2021.677611
Received: 08 March 2021; Accepted: 19 May 2021;
Published: 18 June 2021.
Edited by:
Cai-Zhong Jiang, USDA-ARS, United StatesReviewed by:
Qibin Ma, South China Agricultural University, ChinaCopyright © 2021 Qian, Zhang, Yu, Gou, Yang, Xu and Pi. This is an open-access article distributed under the terms of the Creative Commons Attribution License (CC BY). The use, distribution or reproduction in other forums is permitted, provided the original author(s) and the copyright owner(s) are credited and that the original publication in this journal is cited, in accordance with accepted academic practice. No use, distribution or reproduction is permitted which does not comply with these terms.
*Correspondence: Erxu Pi, MjAxMzAwMTRAaHpudS5lZHUuY24=
†These authors have contributed equally to this work
Disclaimer: All claims expressed in this article are solely those of the authors and do not necessarily represent those of their affiliated organizations, or those of the publisher, the editors and the reviewers. Any product that may be evaluated in this article or claim that may be made by its manufacturer is not guaranteed or endorsed by the publisher.
Research integrity at Frontiers
Learn more about the work of our research integrity team to safeguard the quality of each article we publish.