- Department of Life Sciences, The Biotechnology Centre, The University of the West Indies, Kingston, Jamaica
Aerobic respiration and oxygen consumption are indicators of routine metabolic rate, and dissolved oxygen in plant tissues is one of the most important environmental factors affecting their survival. The reduction of available O2 leads to hypoxia which causes a limitation of the oxidative phosphorylation; when O2 is absent, tissues generate ATP by activating the fermentative glycolysis to sustain glycolysis in the absence of mitochondrial respiration, which results in the production of lactate. Overall, hypoxia was reported to often decrease the respiration rate (O2 uptake) and delay the climacteric rise of ethylene in climacteric fruits by inhibiting action, thus delaying their ripening. Much research has been done on the application of postharvest hypoxia and anoxia treatment to temperate fresh crops (controlled or modified atmosphere), however, very few reported on tropical commodities. Indeed, the physiological mode of action of low or absence of oxygen in fresh crops is not well understood; and the physiological and biochemical bases of the effects low or absence of O2 are also yet to be clarified. Recent investigations using omics technologies, however, have provided useful information on the response of fresh fruits and vegetables to this abiotic stress. The aims of this review are to (i) report on the oxygen exchange in the crops tissue, (ii) discuss the metabolic responses to hypoxia and anoxia, and (iii) report the physiological and biochemical responses of crops tissues to these abiotic stresses and the potential benefits of these environmental conditions.
Introduction
From the botanical point of view, tropical fruits are a diverse group of commodities native to tropical regions which are geographically defined as regions between the latitudes 23° North and South of the equator, with temperatures averaging around 27°C and little variation in photoperiod (Samson, 1986). Tropical fruits present a large biodiversity varying in structure, characteristics, and physiology (Wongs-Areea and Noichinda, 2014). Although the variations of tropical fruits are not well established, banana, pineapples, papaya, and avocado fall within the category of major tropical fruits, while others such as lychee, durian, rambutan, guava, passionfruit, mangosteen, tamarind, and some others are considered minor tropical fruits (FAO, 2003; Paull and Duarte, 2011, 2012).
Atmosphere composition consists of 78% nitrogen (N2), 21% oxygen (O2), 0.04% carbon dioxide (CO2), 0.93% argon, small amounts of other gases, and variable amount of water vapor at 20°C and absolute pressure of 1 atm. Oxygen depletion or hypoxia occurs when the partial pressure of oxygen is low enough to limit the production of ATP by mitochondria, whereas anoxia (absence of O2) is attained when ATP mitochondrial production is insignificant compared to that generated by glycolysis and fermentation. Under normal condition of oxygen level (normoxia) cells run aerobic respiration and energy in the form of ATP is produced by oxidative phosphorylation. However, a reduction in oxygen (hypoxia) reduces the oxidative phosphorylation, while the absence of oxygen (anoxia) stops the phosphorylation process (Wongs-Aree and Noichinda, 2018; Salvatierra et al., 2020). This diverts the production of energy to the fermentation pathway producing fermentative by-products that accumulate in the cells (Boersig et al., 1988; Pfister-Sieber and Brändle, 1994; Cho et al., 2021).
In plant cells, oxygen partial pressure between organs of plant, for example in shoots O2 concentration is much higher in comparison with roots (Kotula et al., 2015; van Dongen and Licausi, 2015). On the other hand, oxygen availability also varies significantly in time and space, and the distribution of active O2 depends on its diffusion and convection, and the conductivity of gas transport in specific tissues (Armstrong et al., 2006; Ho et al., 2011; Licausi, 2011; Wang et al., 2017).
Intrinsically, the oxygen status of cells is variable and depends to a great extent on the concentration or partial pressure of atmospheric oxygen supply. This status differentiates between hypoxia and anoxia. Tissues are under hypoxic conditions when the oxygen partial pressure is the limiting factor of ATP production; anoxic conditions are characterized by a limited production of ATP by oxidative phosphorylation, which is mainly produced by fermentation (Drew, 1997; Atwell et al., 2015).
Under unfavorable conditions of oxygen deprivation, plants develop different structural and metabolic adaptations that are genetically controlled, however, the form of adaptation and shift in metabolism depend on the specific response of each species and its tolerance (Figure 1; Beaudry, 2000; Huang et al., 2008; Ioannidi et al., 2009; Toro and Pinto, 2015; Boecx, 2018). This response causes the molecular mechanisms to react to the low or absence of oxygen (Davies et al., 1974; Jackson et al., 1991; Kennedy et al., 1992; Perata and Alpi, 1993; Ricard et al., 1994; Ratcliffe, 1995; Schmidt et al., 2018), as well as an acclimatization where ethylene was found to play a role (Figure 1; Hartman et al., 2021).
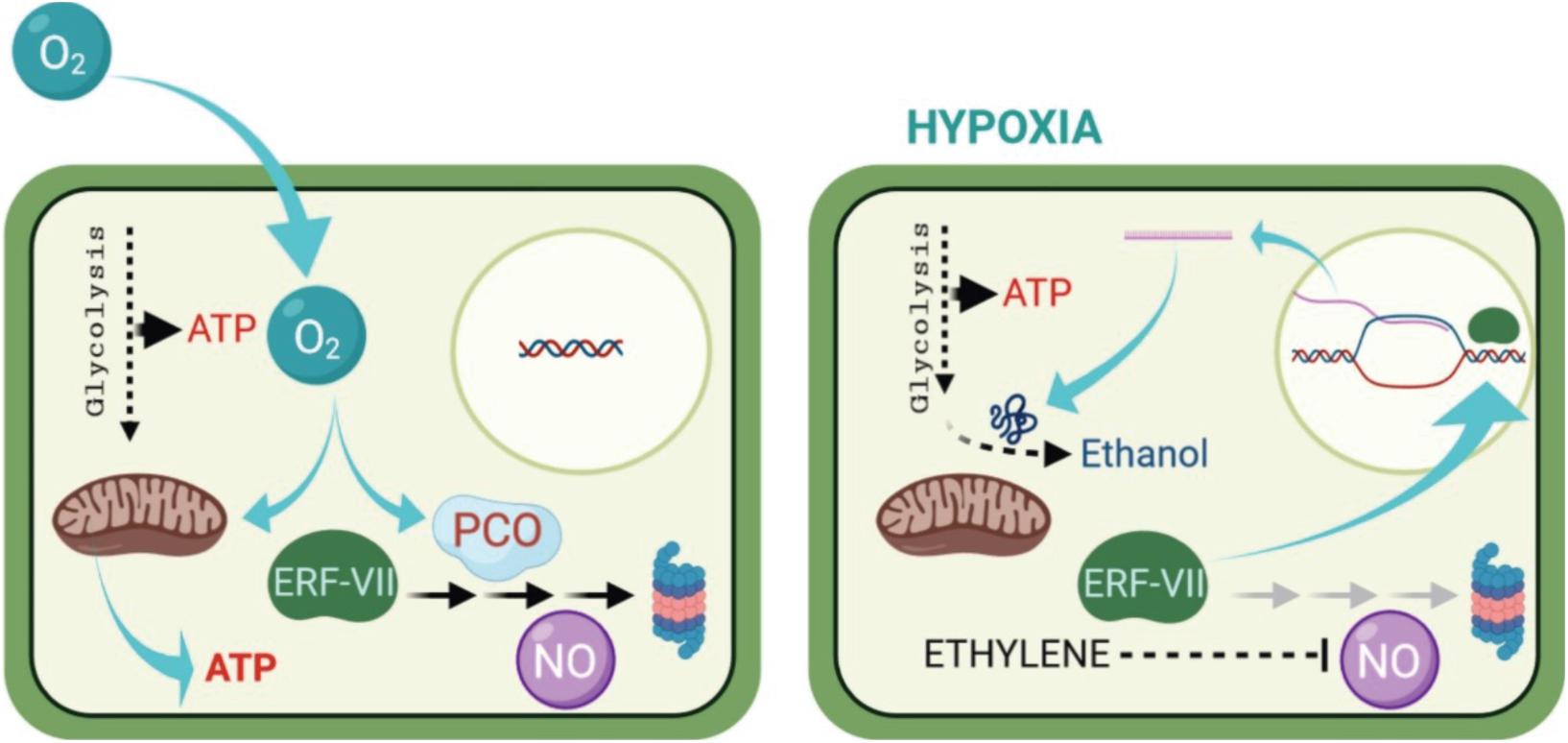
Figure 1. How plants sense oxygen. Under aerobic conditions (left), aerobic respiration in the mitochondria provides most off the energy (ATP) required for the cell metabolism. The ERF—VIII transcription factor genes are constitutively expressed, but their stability is compromised by the activity pf PCOs. Which is a process requiring oxygen, oxidize the N-terminal Cys residue, channeling the ERF-VII proteins to the proteasome, in a process also requiring nitric oxide (NO). Under hypoxia (right), the respiration in the mitochondria is drastically reduced, and AT production can only occur because of enhanced glycolytic activity. The ERF-VII proteins are stabilized because of the absence of oxygen and also thank to ethylene production, which dampers the presence of NO in the cell. The stable ERF-VII proteins migrate to the nucleus where they activate the transcription of Hypoxia-Responsive Genes (HRGs), including genes encoding proteins required for the alcoholic fermentation. (From Loreti and Perata, 2020, published under an open access Creative Common CC BY license).
In plants, it has been established that anaerobic metabolic pathways other than ethanol production exist (Perata and Alpi, 1993; Ricard et al., 1994; Kolb and Joly, 2010; Ventura et al., 2020). First, plants respond to hypoxia/anoxia by producing lactate and the reaction consists of reducing pyruvate by the lactate dehydrogenase (LDH) (Mithran et al., 2010). Under prolonged exposure to hypoxia/anoxia, pyruvate is converted to acetaldehyde by pyruvate decarboxylase (PDC), and then the acetaldehyde is converted to ethanol by the acetaldehyde dehydrogenase (LDH). The lactate-ethanol transition pathway depends on the initial pH of the cytoplasmic compartment (Felle, 2005, 2010; Hossain and Uddin, 2011), and the lower the pH, the faster this transition occurs because LDH has an optimal alkaline pH of 8.0 while PDC has an optimal acidic pH of 5.8 (Davies et al., 1974; Davies, 1980; Morrell et al., 1990; Fox et al., 1994; Kato-Noguchi and Morokum, 2007; Cukrov et al., 2016).
Since tolerance of fresh crops to hypoxia/anoxia is of great economic importance in postharvest science and modified atmosphere packaging (MAP) technology, numerous investigations have been carried out to elucidate the mechanisms underlying the effect of oxygen deprivation on the physiological, biochemical, and organoleptic parameters of crops and their shelf-life. This review aims to describe the effects of low oxygen availability -hypoxia/anoxia- on the physiology, the biochemistry and the quality attributes of tropical fruits in order to determine the optimal condition of MAP application in postharvest handling and storage of these commodities.
Anoxia/Hypoxia and Cell Metabolic Changes
Under hypoxic/anoxic conditions, the electron transport chain in the mitochondria leads to the progressive suppression and the inhibition of ATP synthesis. To compensate this lack of aerobic energy, the cell switches to produce ATP by anaerobic glycolysis. Basically, hypoxia/anoxia causes a decrease in ATP production, but this decrease is more significant in the anoxia-intolerant plants; this suggests that the ability of the anoxia-tolerant species to sustain their energy supply might be the key factor for survival under anoxia/hypoxia (Figure 2; Crawford, 1992; Hanhijärvi and Fagerstedt, 1994, 1995; Nakamura and Noguchi, 2020; Zahra et al., 2021). Nevertheless, response to oxygen deprivation is more complex than it seems and requires further investigation at different plants levels. Tolerance to hypoxia/anoxia, however, appears to depend on a dual morphological and metabolic adaptations which are specific to species and tissue types (Kennedy et al., 1992; Perata et al., 1993; Ratcliffe, 1995; Mariani and Ferrante, 2017; Nakamura and Noguchi, 2020; Zahra et al., 2021).
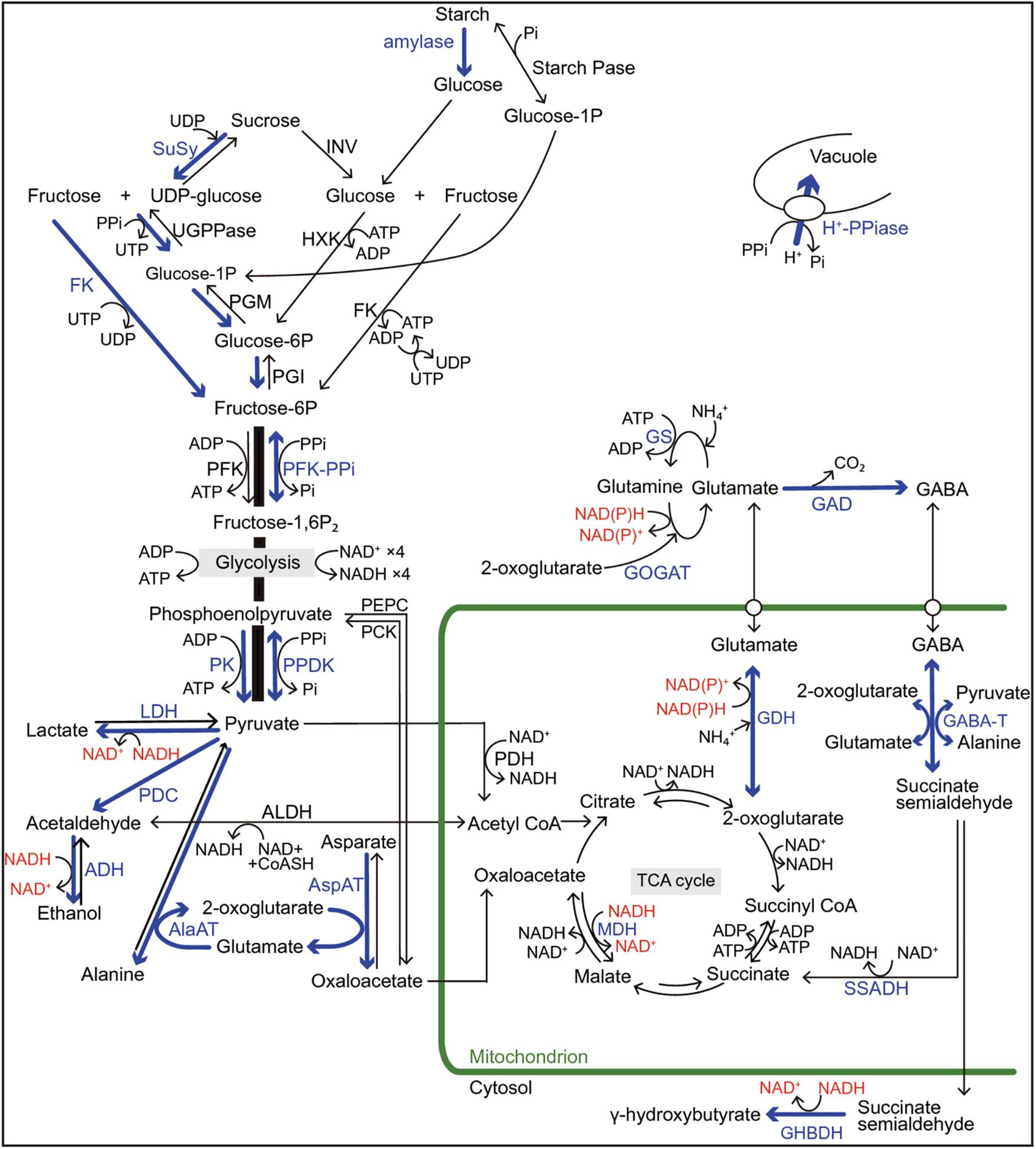
Figure 2. Regulations of sugar catabolism, fermentation, glycolysis, and major amino acid metabolism associated with NAD(P) + regeneration and ATP production in terrestrial and wetland plants under O2-deficient conditions. Blue arrows and letters indicate the reactions and enzymes in the up-regulated pathways when the mitochondrial electron transport and the TCA-cycle flux decrease under O2-deficient conditions. Red letters indicate the regeneration of NAD(P)+ from NAD(P)H. In rice plants, the blue pathways contribute to their tolerance to long-term O2 deficiency compared with the terrestrial plants. Some wetland plants such as rice also have a high ability to optimally regulate the pyruvate level by activation of pyrophosphate (PPi)-dependent phosphofructokinase (PFK-PPi) and pyruvate phosphate dikinase (PPDK) that consume PPi instead of ATP for energy conservation. Besides glycolysis, PPi is consumed to regulate the cytosolic pH by the tonoplast H+-pumping pyrophosphatase (H+-PPiase) instead of H+-ATPase in wetland plants. Although two independent pathways for sucrose degradation contribute to the regulation of glycolytic flux in both terrestrial and wetland plants, the UDP-dependent sucrose synthase (SuSy) pathway is regarded as energetically more advantageous for survival under O2-deficient conditions than the invertase (INV) pathway because here, PPi is utilized instead of ATP. Sugar supply to glycolysis through starch mobilization is observed in species with developed storage organs such as tuber, rhizome, and endosperm. In NAD(P)H regeneration during the metabolisms of 2-oxoglutarate and glutamate associated with γ-aminobutyric acid (GABA) production, the glutamate dehydrogenase (GDH) pathway without ATP consumption is more efficient in energy consumption than the NAD(P)H-dependent glutamine: 2-oxoglutarate aminotransferase (GOGAT) pathway with ATP consumption. The accumulation of some amino acids such as GABA, alanine, and glutamate play an important role in avoiding carbohydrate loss not only during O2-deficient conditions but also during the recovery phase of re-oxygenation after hypoxia/anoxia. Alanine accumulation by alanine aminotransferase (AlaAT) can operate non-circular TCA-cycle and gluconeogenesis under O2 deficiency and re-oxygenation. ADH, alcohol dehydrogenase; AlaAT, alanine aminotransferase; ALDH, acetaldehyde dehydrogenase; AspAT, aspartate aminotransferase; CoASH, coenzyme A; FK, fructokinase; GABA-T, GABA transaminase; GAD, glutamate decarboxylase; GHBDH, γ-aminobutyrate dehydrogenase; Glucose-1-P, glucose-1-phosphate; GS, glutamine synthetase; HXK, hexokinase; LDH lactate dehydrogenase; MDH, malate dehydrogenase; PCK, phosphoenolpyruvate carboxykinase; PDC, pyruvate decarboxylase; PDH; pyruvate dehydrogenase; PEPC, phosphoenolpyruvate carboxylase; PFK, ATP-dependent phosphofructokinase; PFK-PPi, PPi-dependent phosphofructokinase; PGI, phosphoglucoisomerase; PGM, phosphoglucomutase; Pi, phosphate; PK, pyruvate kinase; PPDK, pyruvate Pi dikinase; SSADH, succinate semialdehyde dehydrogenase; Starch Pase, starch phosphorylase; TCA, tricarboxylic acid; UDP, uridine diphosphate; UGPPase, UDP-glucose pyrophosphorylase; UTP, uridine triphosphate. (From Nakamura and Noguchi, 2020; an open access article distributed under the terms of the Creative Commons CC BY license).
Although no fundamental metabolic differences have been observed between anoxia tolerant and intolerant plant species (Pfister-Sieber and Brändle, 1994), sugar availability is important since some tissues (e.g., roots) suffer more from sugar starvation under anoxia (Saglio, 1985). Indeed, when O2 becomes less available starch is rapidly hydrolyzed and channeled to the fermentative pathway for ATP production in order to compensate the lack of oxidative phosphorylation (Perata and Alpi, 1993). Although it was noted that starch reserve mobilization is affected by anoxia, anoxia-tolerant plants have been shown to break down starch much easier than anoxia-intolerant species (Perata et al., 1992).
From the metabolic point of view, oxygen unavailability and the production of ATP by fermentative glycolysis cause the acidification of the cytoplasm (Summers et al., 2000; Gout et al., 2001; Greenway and Gibbs, 2003) and, depending on the tolerance to low oxygen partial pressure, the cellular pH remains stable but drops when energy becomes short (Felle, 2005, 2010). This drop in the pH leads to the accumulation of lactate which in turn inhibits LDH and activates PDC producing acetaldehyde, which is converted to ethanol by alcohol dehydrogenase (ADH). However, cytoplasm acidification is not caused solely by lactate accumulation, but also by the possible passive H+ and potassium (K+) leakage from the vacuole and the protoplasm under limited ATP availability and inhibition of vacuolar H+-ATPase (Ratcliffe, 1995; Gout et al., 2001; Kulichikhin et al., 2007; Yemelyanov et al., 2020). Indeed, ethanol is the primary end product of fermentation in tissues of higher plants under to low oxygen, even though its catalysis and regulation involve components that will be identified in the future (Bui et al., 2019). However, other studies suggest that other fermentation pathways exist, such as the alanine pathway which is quantitatively minor (Davies et al., 1974; Nover, 1989; Jackson et al., 1991; Ricard et al., 1994). Under anaerobic fermentation, pyruvate can therefore be converted into products other than ethanol such as alanine, malate, and succinate which have been detected under early anoxic conditions. Although these diversified glycolytic pathways are recommended for enhancing the tolerance of plants to anoxia using molecular engineering (Lee et al., 2014; Diab and Limami, 2016), this physiological mechanism is not fully clear (Pfister-Sieber and Brändle, 1994).
Indeed, the effects of hypoxia/anoxia do not solely depend on the quantity of oxygen availability for ATP production, but also on the exposure time. Under prolonged anoxia, ethanol was found to be the most abundant end product of fermentation, but interestingly some plants showed their ability to release it into their surrounding environment, thereby increasing their tolerance to anoxia (Crawford and Zochowski, 1984; Kato-Noguchi and Morokum, 2007). Another metabolic consequence under anoxia is the alteration of the cellular redox state of the cell, and the ability of the plant to survive under hypoxia/anoxia consists of their capacity to maintain the cell redox (i.e., NADH/NAD+-ratio), since a decrease in NADH/NAD+ was noted in anoxia-intolerant plants (Chirkova et al., 1992; Nakamura and Noguchi, 2020). However, under oxygen deprivation anoxia-tolerant plants have higher ability to oxidize NADPH to NADP+ via glycolysis and fermentation, and this oxidation leads to less accumulation of reducing equivalents (Guglielminetti et al., 1995, 1999). Furthermore, prolonged hypoxia/anoxia, ATP needs also triggers the fermentation pathway and LDH and ADH generate NAD+.
From the molecular point of view, the mechanisms signaling the response to anoxia still remain unclear and not well elucidated, and the sensors of hypoxia/anoxia in plants are not clearly understood (Geigenberger, 2003; Gibbs and Greenway, 2003). Molecular responses to oxygen deprivation have focused on the regulation of genes expression and activation of enzymes involved in acclimation of metabolism such amylases (Bailey-Serres and Chang, 2005). So far, a direct oxygen sensor has not been established in plants, but some studies have demonstrated that increased ADH gene expression and fermentative metabolism are triggered under hypoxia/anoxia (Koch et al., 2000). Other mechanisms which use different sets of transcription factors (TFs) and ethylene responsive factor family (ERF) to perceive low-oxygen derived signals have been reported to play a primordial role in the determination of survival with reduced oxygen availability (Licausi et al., 2010b; Licausi, 2011).
Cytosolic calcium patterns (Ca2+)cyt have also been recognized as important elements in signaling, and there has been increased interest in identifying the calcium fate involved in (Ca2+)cyt changes in specific signaling pathways (Bush, 1995; Subbaiah et al., 1998; Yemelyanov et al., 2011; Lindberg et al., 2012; Hironari and Takashi, 2014). In this regard, one of the suggested hypotheses is the role of Ca2+ as a messenger. This hypothesis is supported by the work of Subbaiah et al. (1998) who observed an increase of Ca2+ in the cytosol suggesting its possible participation in anoxic signaling (Sedbrook et al., 1996; Bose et al., 2011). In a recent study, Igamberdiev and Hill (2018) suggested the possible release of Ca2+ from cell compartments to the cytosol under the decrease of ATP concentration resulting in the suppression of ATPases and activation of calcium ion channels.
Effects of Hypoxia/Anoxia on the Respiration Rate and Ethylene Production
Unlike animal products, crops are living organisms and they continue to respire and are metabolically active during, either, before or after harvest. This respiration is the chemical process of energy production by converting glucose to carbon dioxide, water and heat. The respiration rate (RR) of a fresh crop is therefore determined by the speed at which this chemical process occurs, and a high RR leads to a faster depletion of glucose and loss of freshness. Indeed, under aerobic condition oxygen exchange in fruit depends greatly on its physiology (type of fruit and vegetable) (Platenius, 1942; Brady et al., 1970; Varoquaux et al., 1992; Kader, 1994) and it relies on O2 diffusion from the atmosphere to the fruit following Fick’s law (Palmes and Lindenboom, 1979; Kader and Saltveit, 2003).
However, as a dynamic process resulting from a chemical reaction, following van’t Hoff’s law (van’t Hoff, 1884; Kemp, 1987), RR is influenced by different factors mainly temperature (Waghmare et al., 2013). Atmospheric oxygen concentration also significantly affects RR depending on CO2 accumulation during respiration, regulation of the respiratory metabolism in addition to O2 diffusion limitation and availability which reduces significantly RR (Gupta et al., 2009; Ho et al., 2018). However, at low temperatures it was observed that RR was likely reduced by a specific response to a signal generated by a plant oxygen sensor (Ho et al., 2018).
From the metabolic point of view, more information is readily available on the effects of O2 than on CO2 (Watkins, 2000). Atmospheric carbon dioxide also influences the RR of crop commodities, however, the mechanisms on how CO2 affect RR are still not well understood, and CO2 might increase or decrease RR depending on the type of fruit (Mathooko, 1996). Overall, the RR of commodities decreases with the decrease in oxygen partial pressure below 21 kPa, and the effect of hypoxia becomes more significant below 10 kPa oxygen partial pressure (Chervin et al., 1996).
Practically, extensive literature has reported on the effects of hypoxia/anoxia on numerous temperate fruits (Ho et al., 2014; Boeckx et al., 2019), while limited references are readily available on tropical fruits in comparison. When exposed to low oxygen concentration, hypoxia/anoxia significantly reduced the RR of avocado (El-Mir et al., 2001), banana (Wade, 1974; Yi et al., 2006), Japanese persimmon (Imahori et al., 1998), mango (Yahia and Vazquez-Moreno, 1993), kiwi (Botondi et al., 2012; Huang et al., 2014), pear (Ho et al., 2018), and dragon fruit (Ho et al., 2021), while the absence of oxygen increased the RR of lychee (Liu et al., 2015).
On the other hand, oxygen is closely linked to the rate of respiration of fresh crops and is required for the biosynthesis of ethylene, and ERFs have been shown to play a role under hypoxia/anoxia (Licausi et al., 2010a,b; Licausi, 2011). In an interesting study, Sanders and de Wild (2003) modelized in vivo ethylene production rate in relation to O2 partial pressure using tomato, and their findings are in agreement with the molecular findings since they noted that ethylene biosynthesis and perception was positively related to O2 partial pressure (Mustroph et al., 2010). Thus, similar to the effects on RR, hypoxia/anoxia reduced levels of oxygen uptake and slowed down ethylene production in avocado (Zhang et al., 2011), saskatoon fruit (Rogiers and Knowles, 1998), banana (Imahori et al., 2013), and kiwi fruit (Botondi et al., 2012).
Nevertheless, a decrease in the RR does not change the respiratory quotient (RQ = CO2/O2) –which is a good indicator of the trigger of the fermentation pathway– until O2 partial pressure reaches the compensation point c.a. 2 kPa (Figures 3A,B; Beaudry et al., 1992; Banks et al., 1993; Peppenlenbos et al., 1996). Therefore, this suggests that commodities are not significantly stressed by oxygen depletion since carbohydrates are still completely oxidized to CO2 and the end products of anoxia, namely lactate and acetaldehyde, do not accumulate until the oxygen partial pressure falls below 2 kPa (Beaudry et al., 1992).
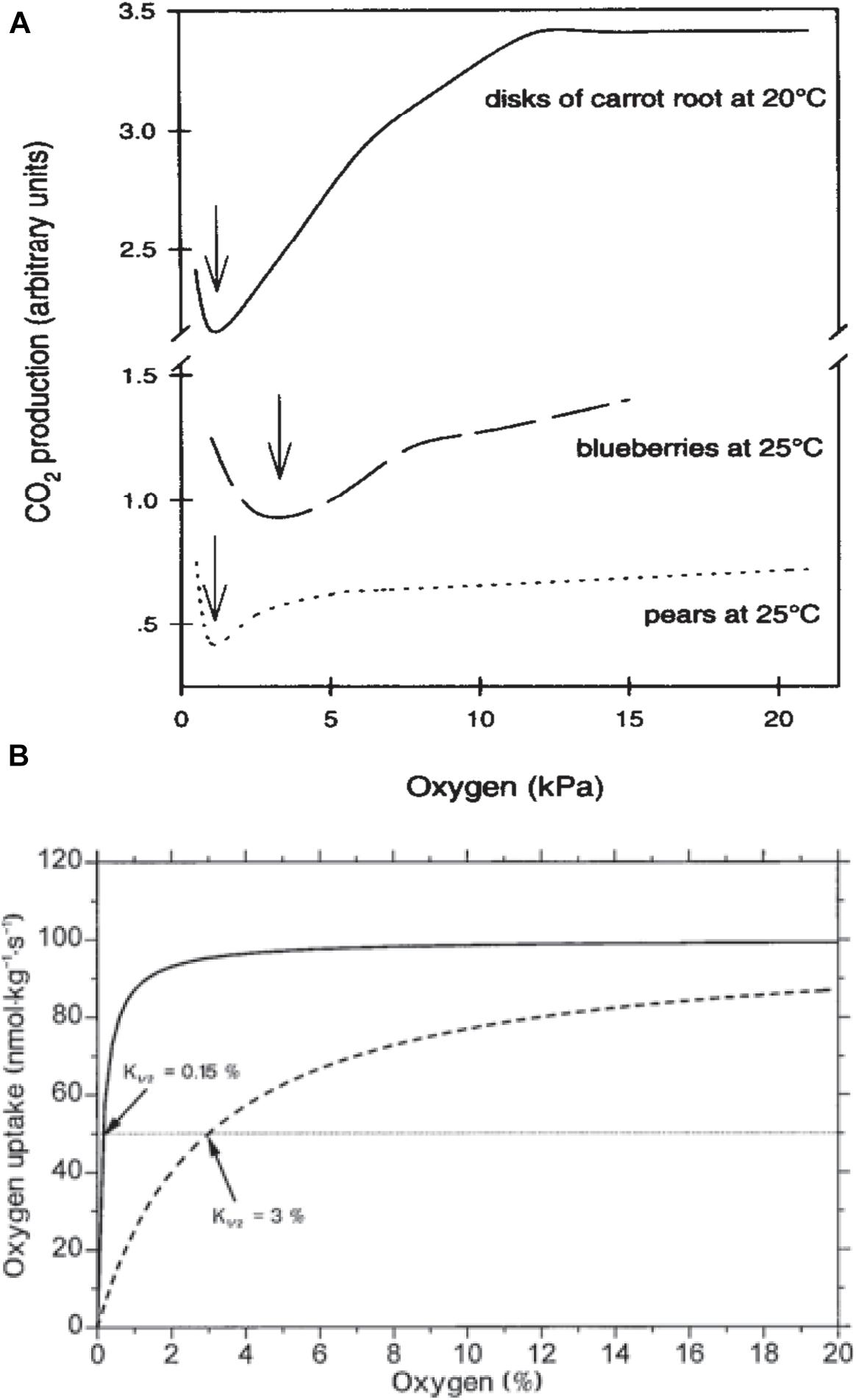
Figure 3. (A) Plant CO2 production in response to oxygen depletion. Data on carrot are from Leshuk and Saltveit (1990), on blueberries from Beaudry et al. (1992) and on pears from Boersig et al. (1988). Arrows indicate ACPs (Chervin et al., 1996, with permission of Elsevier). (B) Hypothetical respiratory responses to O2 for a respiratory system of low diffusive resistance exhibiting a K1/2 of about 0.15% O2, representative of single cells or tissues, and a tissue with significant diffusive resistance to gas exchange with an K1/2 of 3% O2 (with open permission).
Hypoxia/Anoxia and Fresh Crops Ripening, Quality, and Storability
As described above, hypoxia/anoxia may have beneficial effects, such as slowing down the RR, ethylene production, and even reducing the incidence of some physiological disorders (Mojević and Tešanović, 2011; Lurie and Tonutti, 2014; Kongpatjirak et al., 2016) such as delaying ripening (Pesis et al., 1994; Pegoraro et al., 2012), softening (Wu et al., 2020), deastringency (Zhu et al., 2018), browning (Phonyiam et al., 2016), internal breakdown (Lizada and Rumbaoa, 2017), and also diseases (Fallik et al., 2003). However, it is important to note that following exposure of the fruit to anoxic conditions, the accumulation of the ethanol fermentation metabolites -acetaldehyde and ethanol- may be enhanced to extreme levels. The occurrence of anaerobic conditions in the internal atmospheres of fruit and vegetables, and their tendency to develop off-flavors also depend on their anatomical structure and morphology (Porat and Falik, 2008).
The modification of atmosphere was first developed by Kidd and West (1927a, b), and a general consensus was reached on hypoxia/anoxia and the effects in reducing rate of respiration, ethylene synthesis and sensitivity, and lipid catabolism and other oxidative reactions (Burton, 1974; Knee, 1991). Hypoxic/anoxic conditions have shown to delay ripening and senescence of many fruits and these effects were behind the development of MAP and controlled atmosphere (CA) postharvest storage technologies. Extensive literature is readily available on the effects of hypoxia/anoxia on crops, but little on tropical fruits. Green banana fruit stored under low (2%) and absence (0%) of oxygen for 7 days showed high ADH activity and accumulated more ethanol under total anoxia (0% O2), while low oxygen showed a delayed onset of the climacteric peak and extended the shelf-life of banana but reduced remarkably the production of ester volatiles, i.e., ethyl acetate, isoamyl acetate, and isobutyl acetate (Imahori et al., 2013). Similar results on banana were obtained and the application of pure nitrous oxide (100% N2O) or combined to low O2 levels delayed ripening and extended the shelf-life of the fruit (Palomer et al., 2005; Yi et al., 2006).
Short anoxic treatment of pineapple maintained flesh and pulp color; delayed the increase in total sugar content and enhanced total ascorbic acid content during storage; and maintained overall postharvest quality of the fruit stored at ambient temperature (Techavuthiporn et al., 2017). When hypoxically pre-treated (3% O2 for 24 h) or exposed to low oxygen (1 and 0.25% O2 for 1–3 days), avocado fruit increased their tolerance to hypoxia (El-Mir et al., 2001). Litchi is known to have a very short shelf-life, and its short exposure to anoxia (0% O2) markedly delayed skin browning and reduced rotting while maintaining the physical quality of the fruit during storage (Jiang et al., 2004; Liu et al., 2007). Mango is also one of the most widely consumed but perishable tropical fruit. Interestingly, anoxic treatment for 24 h prior to storage showed to be effective in retarding ripening, maintaining firmness, and delaying color change (Kongpatjirak et al., 2016). In a study on cherimoya, Palma et al. (1993) noted that hypoxia (5% O2) delayed ripening and the edible condition differed with oxygen treatment and was inversely proportional to O2 concentration. In a recent study, Ho et al. (2021) investigated the effect of different hypoxic treatments on dragon fruit (Hylocereus undatus) and their results showed that 2 kPa O2 + 5 kPa CO2 was the optimal hypoxic treatment in maintaining the shelf-life of the fruit during storage.
Another benefit of hypoxia/anoxia is the improvement of the quality attributes of some fruits. Hypoxia was shown to be a beneficial treatment for the removal of astringency from persimmon to improve the fruit quality after harvest. Although the longer the fruit is stored, the less effective is the treatment (Salvador et al., 2008), the application of hypoxic treatment with high CO2 (95%) effectively reduced high soluble tannins (SCTs) content which is one of the most important causes of persimmon fruit astringency (Min et al., 2014). Table 1 summarizes the main findings of hypoxia/anoxia treatments effects on tropical fruits.
Conclusion and Future Directions
In postharvest science, hypoxia/anoxia treatments combined with packaging technology have been used as one of the most inexpensive non-chemical technology to prevent physiological disorders, reduce diseases incidence, delay ripening, extending shelf-life, and even improving some quality attributes of a large number of temperate fresh commodities during transportation and storage. Nevertheless, few studies have been focusing on how oxygen deprivation affects tropical fruits. These atmospheric conditions and treatments with low or total absence of oxygen have shown different efficacy levels which depend on composition of the atmosphere, the treatment and storage duration, and the type of commodity treated and their tolerance and response to total or partial absence of oxygen. Overall, on several tropical fruits low or absence of oxygen has shown its involvement in reducing the RR and ethylene biosynthesis, prolonging therefore the shelf-life of these commodities and maintaining some quality attributes and freshness. However, data showed that changes in oxygen availability and ethylene emission rates in reaction to the surrounding hypoxic or anoxic atmosphere varies with different intrinsic and extrinsic factors particularly oxygen levels and temperatures. On the other hand and from the fundamental point of view, the timing of the converging pathways to acetaldehyde and ethanol production and the distinct energy-dependent signaling pathways operating during hypoxia/anoxia are still unclear and not fully understood since most tropical fruits are known to have higher RRs and many of them are climacteric fruits. Besides the progress and advances in plant physiology and hypoxia/anoxia effects on plant tissues, fruits admittedly react differently to extremely low or total absence of oxygen conditions during exposure and storage in terms of tolerance, RR, ethylene production, volatiles biosynthesis, and acetaldehyde, alanine, and ethanol production and accumulation. In order to decipher the effects of hypoxia/anoxia mechanisms on tissues in general, it is first crucial to elucidate the different oxygen sensing mechanisms involved, the specific molecular and metabolic changes occurring at the earliest stages of the hypoxic/anoxic conditions, and establish whether these changes are a sort of adaptation response to oxygen deprivation. Another direction in understanding hypoxia/anoxia effects on plants is to elucidate whether the different oxygen-sensing mechanisms have different activation thresholds, and how these sophisticated sensing and signaling networks likely enable plants to tailor their adaptive responses to face the severity and duration of hypoxic/anoxic conditions. Elucidating these mechanisms will be of great importance and applications in enhancing our understanding of crop physiology in these extreme conditions. It will also a key step in determining which low oxygen atmospheric conditions may be worth testing on tropical fruits to determine the optimal postharvest handling and storage conditions of these sensitive commodities and maintaining longer their freshness and quality attributes. Indeed, anoxia/hypoxia should be further investigated for its potential application to extend the shelf-life and preserve the quality attributes of numerous less known tropical fruits by setting optimal O2 concentration either as a pre-treatment for a very short time span prior to storage or in MAP or CA storage technologies.
Author Contributions
The author confirms being the sole contributor of this work and has approved it for publication.
Conflict of Interest
The author declares that the research was conducted in the absence of any commercial or financial relationships that could be construed as a potential conflict of interest.
Acknowledgments
I thank Suchetta Stephenson for her critical reading and editing of the manuscript.
References
Armstrong, J., Jones, R. E., and Armstrong, W. (2006). Rhizome phyllosphere oxygenation in Phragmites and other species in relation to redox potential, convective gas flow, submergence and aeration pathways. New Phytol. 172, 719–731. doi: 10.1111/j.1469-8137.2006.01878.x
Atwell, B. J., Greenway, H., and Colmer, T. D. (2015). Efficient use of energy in anoxia-tolerant plants with focus on germinating rice seedlings. New Phytol. 206, 35–56. doi: 10.1111/nph.13173
Bailey-Serres, J., and Chang, R. (2005). Sensing and signalling in response to oxygen deprivation in plants and other organisms. Ann. Bot. 96, 507–518. doi: 10.1093/aob/mci206
Banks, N. H., Dadzie, B. K., and Cleland, D. J. (1993). Reducing gas exchange of fruits with surface coatings. Postharvest Biol. Technol. 3, 269–284. doi: 10.1016/0925-5214(93)90062-8
Beaudry, R. M. (2000). Responses of horticultural commodities to low oxygen: Limits to the expanded use of modified atmosphere packaging. HortTechnology 10, 491–500. doi: 10.21273/HORTTECH.10.3.491
Beaudry, R. M., Cameron, A. C., Shirazi, A., and Dostal-Lange, D. L. (1992). Modified-atmosphere packaging of blueberry fruit: effect of temperature on package O2 and CO2. J. Am. Soc. Hortic. Sci. 117, 436–441. doi: 10.21273/JASHS.117.3.436
Boecx, J. (2018). Regulation of the Respiratory Metabolism of Apple During (dynamic) Controlled Atmosphere Storage. Ph.D. thesis. Belgium: Katholieke Universiteit Leuven.
Boeckx, J., Pols, S., Hertog, M. L. A. T. M., and Nicolaï, B. M. (2019). Regulation of the central carbon metabolism in apple fruit exposed to postharvest low-oxygen stress. Front. Plant Sci. 10:1384. doi: 10.3389/fpls.2019.01384
Boersig, M. R., Kader, A. A., and Romani, R. J. (1988). Aerobic-anaerobic respiratory transition in pear fruit and cultured pear fruit cells. J. Am. Soc. Hortic. Sci. 113, 869–873.
Bose, B., Pottosin, I. I., Shabala, S. S., Palmgren, M. G., and Shabala, S. (2011). Calcium efflux systems in stress signaling and adaptation in plants. Front. Plant Sci. 02:85. doi: 10.3389/fpls.2011.00085
Botondi, R., Russo, V., and Mencarelli, F. (2012). Anaerobic metabolism during short and long term storage of kiwifruit. Postharvest Biol. Technol. 64, 83–90. doi: 10.1016/j.postharvbio.2011.09.017
Brady, C. J., O’connell, P. B. H., Smydzuk, J., and Wade, N. L. (1970). Permeability, sugar accumulation, and respiration rate in ripening banana fruits. Aust. J. Biol. Sci. 23, 1143–1152. doi: 10.1071/BI9701143
Broughton, W. J., and Guat, T. (1979). Storage conditions and ripening of the custard apple Annona squamosa L. Sci. Hortic. 10, 73–82. doi: 10.1016/0304-4238(79)90071-2
Bui, L. T., Novi, G., Lombardi, L., Iannuzzi, C., Rossi, J., Santaniello, A., et al. (2019). Conservation of ethanol fermentation and its regulation in land plants. J. Exp. Bot. 70, 1815–1827. doi: 10.1093/jxb/erz052
Burton, W. G. (1974). Some biophysical principles underlying the controlled atmosphere storage of plant material. Ann. Appl. Biol. 78, 149–168. doi: 10.1111/j.1744-7348.1974.tb01494.x
Bush, D. S. (1995). Calcium regulation in plant cells and its role in signaling. Annu. Rev. Plant Physiol. Plant Mol. Biol. 46, 95–122. doi: 10.1146/annurev.pp.46.060195.000523
Chervin, C., Brady, C. J., Patterson, B. D., and Faragher, J. D. (1996). Could studies on cell responses to low oxygen levels provide improved options for fruit storage and disinfestation? Postharvest Biol. Technol. 7, 289–299. doi: 10.1016/0925-5214(95)00048-8
Chirkova, T. V., Zhukova, T. M., and Bugrova, M. P. (1992). Redox reactions of plant cells in response to short-term anaerobiosis. Vestnik SPBGU 3, 82–86.
Cho, H. Y., Loreti, E., Shih, M. C., and Perata, P. (2021). Energy and sugar signaling during hypoxia. New Phytol. 229, 57–63. doi: 10.1111/nph.16326
Cukrov, D., Zermiani, M., Brizzolara, S., Cestaro, A., Licausi, F., Luchinat, C., et al. (2016). Extreme hypoxic conditions induce selective molecular responses and metabolic reset in detached apple fruit. Front. Plant Sci. 7:146. doi: 10.3389/fpls.2016.00146
Crawford, R. M. M. (1992). Oxygen availability as an ecological limit to plant distribution. Adv. Ecol. Res. 23, 93–185. doi: 10.1016/S0065-2504(08)60147-6
Crawford, R. M. M., and Zochowski, Z. M. (1984). Tolerance of anoxia and ethanol toxicity in chickpea seedlings (Cicer arietinum L.). J. Exp. Bot. 35, 1472–1480. doi: 10.1093/jxb/35.10.1472
Davies, D. D. (1980). Biochemistry of Plants. A Comprehensive Treatise. Vol. 2: Metabolism and Respiration. New York, NY: Academic Press.
Davies, D. D., Grego, S., and Kenworthy, P. (1974). The control of the production of lactate and ethanol by higher plants. Planta 118, 297–310. doi: 10.1007/BF00385580
Diab, H., and Limami, A. M. (2016). Reconfiguration of N metabolism upon hypoxia stress and recovery: Roles of alaninea aminotransferase (AlaAT) and glutamate dehydrogenase (GDH). Plants 5:25. doi: 10.3390/plants5020025
Drew, M. C. (1997). Oxygen deficiency and root metabolism: Injury and acclimation under hypoxia and anoxia. Annu. Rev. Plant Physiol. Plant Mol. Biol. 48, 223–250. doi: 10.1146/annurev.arplant.48.1.223
El-Mir, M., Gerasopoulos, D., Metzidakis, I., and Kanellis, A. K. (2001). Hypoxic acclimation prevents avocado mesocarp injury caused by subsequent exposure to extreme low oxygen atmospheres. Postharvest Biol. Technol. 23, 215–226. doi: 10.1016/S0925-5214(01)00124-7
Escribano, M. I., Del Cura, B., Munõz, T., and Merodio, C. (1997). The effect of high carbon dioxide at low temperature on ribulose 1,5-biphosphate carboxylase and polygalacturonase protein levels in cherimoya fruit. J. Amer. Soc. Hort. Sci. 122, 258–262. doi: 10.21273/JASHS.122.2.258
Fallik, E., Polevaya, Y., Tuvia-Alkalai, S., Shalom, Y., and Zuckermann, H. (2003). A 24-h anoxia treatment reduces decay development while maintaining tomato fruit quality. Postharvest Biol. Technol. 29, 233–236. doi: 10.1016/S0925-5214(03)00109-1
FAO (2003). Medium-Term Prospects for Agricultural Commodities. Projections for the year 2010. Rome: Food and Agriculture Organization.
Felle, H. H. (2010). “pH signaling during anoxia,” in Waterlogging Signalling and Tolerance in Plants, eds S. Mancuso and S. Shabala (Berlin: Springer), 79–96. doi: 10.1007/978-3-642-10305-6_5
Fox, G. G., McCallan, N. R., Ratcliffe, R. G. (1994). Manipulating cytoplasmic pH under anoxia: a critical test of the role of pH in the switch from aerobic to anaerobic metabolism. Planta 190, 210–217. doi: 10.1007/BF00202588
Geigenberger, P. (2003). Response of plant metabolism to too little oxygen. Curr. Opin. Plant Biol. 6, 247–256. doi: 10.1016/s1369-5266(03)00038-4
Gibbs, J., and Greenway, H. (2003). Mechanisms of anoxia tolerance in plants. I. Growth, survival and anaerobic catabolism. Funct. Plant Biol. 30, 1–37. doi: 10.1071/PP98095_ER
González-Aguilar, G. A., Buta, J. G., and Wang, C. Y. (2003). Methyl jasmonate and modified atmosphere packaging (MAP) reduce decay and maintain postharvest quality of papaya “Sunrise. Postharvest Biol. Technol. 28, 361–370. doi: 10.1016/S0925-5214(02)00200-4nb
Gout, E., Boisson, A. M., Aubert, S., Douce, R., and Bligny, R. (2001). Origin of the cytoplasmic pH changes during anaerobic stress in higher plant cells. Carbon-13 and phosphorous-31 nuclear magnetic resonance studies. Plant Physiol. 125, 912–925. doi: 10.1104/pp.125.2.912
Greenway, H., and Gibbs, J. (2003). Mechanisms of anoxia tolerance in plants. II. Energy requirements for maintenance and energy distribution to essential processes. Funct. Plant Biol. 30, 999–1036. doi: 10.1071/PP98096
Guglielminetti, L., Yamaguchi, J., Perata, P., and Alpi, A. (1995). Amylolytic activities in cereal seeds under aerobic and anaerobic conditions. Plant Physiol. 109, 1069–1076. doi: 10.1104/pp.109.3.1069
Guglielminetti, L., Loreti, E., Perata, P., and Alpi, A. (1999). Sucrose synthesis in cereal grains under oxygen deprivation. J. Plant Res. 112, 353–359. doi: 10.1007/PL00013889
Gupta, K. J., Zabalza, A., and Van Dongen, J. T. (2009). Regulation of respiration when the oxygen availability changes. Physiol. Plant. 137, 383–391. doi: 10.1111/j.1399-3054.2009.01253.x
Hanhijärvi, A. M., and Fagerstedt, K. V. (1994). Comparison of the effect of natural and experimental anoxia on carbohydrate and energy metabolism in Iris pseudacorus rhizomes. Physiol. Plant. 90, 437–444. doi: 10.1111/j.1399-3054.1994.tb08799.x
Hanhijärvi, A. M., and Fagerstedt, K. V. (1995). Comparison of carbohydrate utilization and energy charge in the yellow flag iris (Iris pseudacorus) and garden iris (Iris germanica) under anoxia. Physiol. Plant. 93, 493–497. doi: 10.1111/j.1399-3054.1995.tb06848.x
Hartman, S., Sasidharan, R., and Voesenek, L. A. C. J. (2021). The role of ethylene in metabolic acclimations to low oxygen. New Phytol. 229, 64–70. doi: 10.1111/nph.16378
Hironari, H., and Takashi, S. (2014). Calcium signaling in plant endosymbiotic organelles: Mechanism and role in physiology. Mol. Plant 7, 1094–1104. doi: 10.1093/mp/ssu020
Ho, P. L., Tran, D. T., Hertog, M. L. A. T. M., and Nicolaï, B. M. (2021). Effect of controlled atmosphere storage on the quality attributes and volatile organic compounds profile of dragon fruit (Hylocereus undatus). Postharvest Biol. Technol. 173:111406. doi: 10.1016/j.postharvbio.2020.111406
Ho, Q. T., Hertog, M. L. A. T. M., Verboven, P., Ambaw, A., Rogge, S., Verlinden, B. E., et al. (2018). Down-regulation of respiration in pear fruit depends on temperature. J. Exp. Bot. 69, 2049–2060. doi: 10.1093/jxb/ery031
Ho, Q. T., Buts, K., Herremans, E., Hertog, M. L. A. T. M., Verboven, P., and Nicolai, B. M. (2014). “Hypoxic storage of fruit”,” in Low-Oxygen Stress in Plants, eds J. T. van Dongen and F. Licausi (Wien: Springer-Verlag), 353–369. doi: 10.1007/978-3-7091-1254-0_18
Ho, Q. T., Verboven, P., Verlinden, B. E., Herremans, E., Wevers, M., Carmeliet, J., et al. (2011). A three-dimensional multiscale model for gas exchange in fruit. Plant Physiol. 155, 1158–1168. doi: 10.1104/pp.110.169391
Hossain, M. A., and Uddin, S. N. (2011). Mechanisms of waterlogging tolerance in wheat: Morphological and metabolic adaptations under hypoxia or anoxia. Aust. J. Crop Sci. 5, 1094–1101. doi: 10.3316/informit.044652841339657
Huang, Z., Guo, L., Wang, H., Qu, H., Ma, S., Liu, Y., et al. (2014). Energy status of kiwifruit stored under different temperatures or exposed to long-term anaerobic conditions or pure oxygen. Postharvest Biol. Technol. 98, 56–64. doi: 10.1016/j.postharvbio.2014.07.008
Huang, S., Colmer, T. D., and Millar, A. H. (2008). Does anoxia tolerance involve altering the energy currency towards PPi? Trends Plant Sci. 13, 221–227. doi: 10.1016/j.tplants.2008.02.007
Igamberdiev, A. U., and Hill, R. D. (2018). Elevation of cytosolic Ca2+ in response to energy deficiency in plants: the general mechanism of adaptation to low oxygen stress. Biochem. J. 475, 1411–1425. doi: 10.1042/BCJ20180169
Imahori, Y., Kohei Yamamoto, K., Tanaka, H., and Bai, J. (2013). Residual effects of low oxygen storage of mature green fruit on ripening processes and ester biosynthesis during ripening in bananas. Postharvest Biol. Technol. 77, 19–27. doi: 10.1016/j.postharvbio.2012.11.004
Imahori, Y., Kota, M., Ueda, Y., Yoshioka, H., and Chachin, K. (1998). Relationship between low-oxygen induced injury and respiration in several fruits under hypoxia. J. JPN. Food Stor. Sci. Soc. 5, 303–308. doi: 10.5891/jafps.24.303
Ioannidi, E., Kalamaki, M. S., Engineer, C., Pateraki, I., Alexandrou, D., Mellidou, I., et al. (2009). Expression profiling of ascorbic acid-related genes during tomato fruit development and ripening and in response to stress conditions. J. Exp. Bot. 60, 663–678. doi: 10.1093/jxb/ern322
Jackson, M. B., Davis, D. D., and Lambers, H. (1991). Plant Life Under Oxygen Deprivation: Ecology, Physiology and Biochemistry. The Hague: SAB Academic Publishing.
Jiang, Y., Su, X., Duan, X., Lin, W., and Li, W. (2004). Anoxia treatment for delaying skin browning, inhibiting disease development and maintaining the quality of litchi fruit. Food Technol. Biotechnol. 42, 131–134.
Kader, A. A. (1994). “Modified and controlled atmosphere storage of tropical fruits,” in Postharvest Handling of Tropical Fruits, eds B. R. Champ, E. Highley, and J. I. Johnson (Australia: ACIAR Proceedings), 239–249.
Kader, A. A., and Saltveit, M. E. (2003). “Respiration and gas exchange,” in Postharvest Physiology and Pathology of Vegetables, eds J. A. Bartz and J. K. Brecht (New York: Marcel Dekker), 7–29.
Kato-Noguchi, H., and Morokum, M. (2007). Ethanolic fermentation and anoxia tolerance in four rice cultivars. J. Plant Physiol. 164, 168–173. doi: 10.1016/j.jplph.2005.09.017
Kemp, H. R. (1987). The effect of temperature and pressure on equilibria: A derivation of the van’t Hoff rules. J. Chem. Educ. 64, 482–484. doi: 10.1021/ed064p482
Kennedy, R. A., Rumpho, M. E., and Fox, T. C. (1992). Anaerobic metabolism in plants. Plant Physiol. 100, 1–6. doi: 10.1104/pp.100.1.1
Kidd, F., and West, C. (1927a). Relation Between the Respiratory Activity and the Keeping Quality of Apples. Report of the Food Investigation Board for 1925. London, UK: DSIR.
Kidd, F., and West, C. (1927b). A Relation between the Concentration of Oxygen and Carbon Dioxide in the Atmosphere, Rate of Respiration, and Length of Storage Life of Apples. Report of the Food Investigation Board for 1925, 1926. London, UK: DSIR.
Koch, K. E., Ying, Z., Wu, Y., and Avigne, W. (2000). Multiple paths of sugar-sensing and a sugar/oxygen overlap for genes of sucrose and ethanol metabolism. J. Exp. Bot. 51, 417–427. doi: 10.1093/jexbot/51.suppl_1.417
Knee, M. (1991). “Fruit metabolism and practical problems of fruit storage under hypoxia and anoxia,” in Plant Life under Oxygen Deprivation: Ecology, Physiology and Biochemistry, eds M. B. Jackson, D. D. Davies, and H. Lamberts (The Hague: SPB Academic Publishing), 229–243.
Kolb, R. M., and Joly, C. A. (2010). Germination and anaerobic metabolism of seeds of Tabebuia cassinoides (Lam.) DC subjected to flooding and anoxia. Flora Morphol. Dist. Func. Ecol. Plants 205, 112–117. doi: 10.1016/j.flora.2009.01.001
Kongpatjirak, P., Safitri, A. A., and Setha, S. (2016). Pre-storage anoxia treatment affects fruit quality, antioxidant properties, and shelf life of mango. J. Food Sci. Agric. Technol. 2, 1–5.
Kotula, L., Clode, P. L., Striker, G. G., Pedersen, O., Lauchli, A., Shabala, S., et al. (2015). Oxygen deficiency and salinity affect cell-specific ion concentrations in adventitious roots of barley (Hordeum vulgare). New Phytol. 208, 1114–1125. doi: 10.1111/nph.13535
Kulichikhin, K. Y., Aitio, O., Chirkova, T. V., and Fagerstedt, K. V. (2007). Effect of oxygen concentration on intracellular pH, glucose-6-phosphate and NTP content in rice (Oryza sativa) and wheat (Triticum aestivum) root tips: In vivo 31P-NMR study. Physiol. Plant. 129, 507–518. doi: 10.1111/j.1399-3054.2006.00819.x
Lee, D. J., Chi, Y. T., Kim, D. M., Choi, S. H., Lee, J. Y., and Choi, G. W. (2014). Ectopic expression of CaRLK1 enhances hypoxia tolerance with increasing alanine production in Nicotiana spp. Plant Mol. Biol. 86, 255–270. doi: 10.1007/s11103-014-0227-4
Leshuk, J. A., and Saltveit, M. E. Jr. (1990). A simple system for the rapid determination of the anaerobic compensation point of plant tissue. HortScience 25, 480–482. doi: 10.21273/HORTSCI.25.4.480
Licausi, F. (2011). Regulation of the molecular response to oxygen limitations in plants. New Phytol. 190, 550–555. doi: 10.1111/j.1469-8137.2010.03562.x
Licausi, F., Weits, D. A., Pant, B. D., Scheible, W. R., Geigenberger, P., and van Dongen, J. T. (2010b). Hypoxia responsive gene expression is mediated by various subsets of transcription factors and miRNAs that are determined by the actual oxygen availability. New Phytol. 190, 442–456. doi: 10.1111/j.1469-8137.2010.03451.x
Licausi, F., Van Dongen, J. T., Giuntoli, B., Novi, G., Santaniello, A., Geigenberger, P., et al. (2010a). HRE1 and HRE2, two hypoxia−inducible ethylene response factors, affect anaerobic responses in Arabidopsis thaliana. Plant J. 62, 302–305. doi: 10.1111/j.1365-313X.2010.04149.x
Lindberg, S., Kader, M. A., and Yemelyanov, V. (2012). “Calcium signalling in plant cells under environmental stress,” in Environmental Adaptations and Stress Tolerance of Plants in the Era of Climate Change, eds P. Ahmad and M. Prasad (New York, NY: Springer), 325–360. doi: 10.1007/978-1-4614-0815-4_15
Liu, T., Wang, H., Kuang, J., Sun, C., Shi, J., Duan, X., et al. (2015). Short-term anaerobic, pure oxygen and refrigerated storage conditions affect the energy status and selective gene expression in litchi fruit. LWT Food Sci. Technol. 60, 1254–1261. doi: 10.1016/j.lwt.2014.09.003
Liu, H., Song, L. L., Jiang, Y. M., Joyce, D. C., Zhao, M. M., You, Y., et al. (2007). Short-term anoxia treatment maintains tissue energy levels and membrane integrity and inhibits browning of harvested litchi fruit. J. Sci. Food Agric. 87, 1767–1771. doi: 10.1002/jsfa.2920
Lizada, M. C. C., and Rumbaoa, R. A. (2017). Ethylene and the adaptive response of mango to hypoxia. Acta Hortic. 1178, 143–146. doi: 10.17660/ActaHortic.2017.1178.25
Loreti, E., and Perata, P. (2020). The many facets of hypoxia in plants. Plants 9:745. doi: 10.3390/plants9060745
Lurie, S., and Tonutti, P. (2014). Heat and hypoxia stress and their effects on stored fruits. Stewart Postharvest Rev. 3, 1–7.
Mariani, L., and Ferrante, A. (2017). Agronomic management for enhancing plant tolerance to abiotic stresses–drought, salinity, hypoxia, and lodging. Horticulturae 3:52. doi: 10.3390/horticulturae3040052
Mathooko, F. M. (1996). Regulation of ethylene biosynthesis in higher plants by carbon dioxide. Postharvest Biol. Technol. 7, 1–26. doi: 10.1016/0925-5214(95)00026-7
Min, T., Fang, F., Ge, H., Shi, Y. N., Luo, Z. R., Yao, Y. C., et al. (2014). Two novel anoxia-induced ethylene response factors that interact with promoters of deastringency-related genes from persimmon. PLoS One 9:e97043. doi: 10.1371/journal.pone.0097043
Minh, N. T. (2017). Investigation of MAP for durian preservation. Int. J. Appl. Eng. Res. 24, 15298–15303.
Mithran, M., Paparelli, E., Novi, G., Perata, P., and Loreti, E. (2010). Analysis of the role of the pyruvate decarboxylase gene family in Arabidopsis thaliana under low-oxygen conditions. Plant Biol. 16, 28–34. doi: 10.1111/plb.12005
Mojeviæ, M. V., and Tešanoviæ, D. B. (2011). Influence of short anoxia treatment and maturation on quality and storage of tomatoes. J. Agric. Sci. 56, 121–131. doi: 10.2298/JAS1102121M
Morrell, S., Greenway, H., and Davis, D. D. (1990). Regulation of pyruvate decarboxylase in vitro and in vivo. J. Exp. Bot. 41, 131–139. doi: 10.1093/jxb/41.2.131
Mustroph, A., Lee, S. C., Oosumi, T., Zanetti, M. E., Yang, H., Ma, K., et al. (2010). Cross-kingdom comparison of transcriptomic adjustments to low-oxygen stress highlights conserved and plant-speci?c responses. Plant Physiol. 152, 1484–1500. doi: 10.1104/pp.109.151845
Nakamura, M., and Noguchi, K. (2020). Tolerant mechanisms to O2 deficiency under submergence conditions in plants. J. Plant Res. 133, 343–371. doi: 10.1007/s10265-020-01176-1
Nover, L. (1989). “Anaerobic stress,” in Heat Shock and Other Stress Responses Systems of Plants, eds L. Nover, D. Neumann, and D. D. Scharf (New York: Springer Verlag), 69–90.
O’Hare, T. J., Prasad, A., and Cooke, A. W. (1994). Low temperature and controlled atmosphere storage of rambutan. Postharvest Biol. Technol. 4, 147–157. doi: 10.1016/0925-5214(94)90016-7
Palma, T., Stanley, D. W., Aguillera, J. M., and Zoffoli, J. P. (1993). Respiratory behavior of cherimoya (Annona cherimola Mill.) under controlled atmospheres. HortScience 8, 647–549. doi: 10.21273/HORTSCI.28.6.647
Palmes, E. D., and Lindenboom, R. H. (1979). Ohm’s law, Fick’s law, and diffusion samplers for gases. Anal. Chem. 51, 2400–2401. doi: 10.1021/ac50050a026
Palomer, X., Roig-Villanova, I., Grima-Calvo, D., and Vendrell, M. (2005). Effects of nitrous oxide (N2O) treatment on the postharvest ripening of banana fruit. Postharvest Biol. Technol. 36, 167–175. doi: 10.1016/j.postharvbio.2004.12.008
Pegoraro, P., Schreinert dos Santos, R., Krüger, M. M., Tieche, A., Carlos da Maia, L., Rombaldi, C. V., et al. (2012). Effects of hypoxia storage on gene transcript accumulation during tomato fruit ripening. Braz. J. Plant Physiol. 24, 141–148. doi: 10.1590/S1677-04202012000200007
Peppenlenbos, H. W., Tijskens, L. M. M., Vant’Leven, J., and Wilkinson, E. C. (1996). Modelling oxidative and fermentation carbon dioxide production of fruits and vegetables. Postharvest Biol. Technol. 9, 283–295. doi: 10.1016/S0925-5214(96)00029-4
Perata, P., and Alpi, A. (1993). Plant responses to anaerobiosis. Plant Sci. 93, 1–17. doi: 10.1016/0168-9452(93)90029-Y
Perata, P., Geshi, N., Akazawa, T., and Yamaguchi, J. (1993). Effect of anoxia on the induction of α-amylase in cereal seeds. Planta 191, 402–408. doi: 10.1007/BF00195699
Perata, P., Pozueta-Romero, J., Akazawa, T., and Yamaguchi, J. (1992). Effect of anoxia on starch breakdown in rice and wheat seeds. Planta 188, 611–618. doi: 10.1007/BF00197056
Pesis, E., Marinansky, R., Zauberman, G., and Fuchs, Y. (1994). Prestorage low-oxygen atmosphere treatment reduces chilling injury symptoms in ‘Fuerte’ avocado fruit. HortScience 29, 1042–1046. doi: 10.21273/HORTSCI.29.9.1042
Pfister-Sieber, M., and Brändle, R. (1994). Aspects of plant behaviour under anoxia and post-anoxia. P. Roy. Soc. Edinb. B 102B, 313–324. doi: 10.1017/S0269727000014305
Phonyiam, O., Kongsuwan, A., and Setha, S. (2016). Effect of short-term anoxic treatment on internal browning and antioxidant ability in pineapple cv. Phulae. Int. Food Res. J. 23, 521–527.
Platenius, H. (1942). The effects of respiration rate and respiratory quotient of some vegetables. Plant Physiol. 17, 179–197. doi: 10.1104/pp.17.2.179
Porat, R., and Falik, E. (2008). “Production of off-flavours in fruit and vegetables under fermentative conditions,” in Fruit and Vegetable Flavour. Recent Advances and Future Prospects, eds B. Brückner and S. G. Wyllie (Cambridge: Woodhead Publishing), 150–164. doi: 10.1533/9781845694296.2.150
Ratcliffe, R. G. (1995). “Metabolic aspects of the anoxic response in plant tissue,” in Environment and Plant Metabolism, ed. N. Smirnoff (London: Bios Scientific Publishers), 111–127.
Ricard, B., Couee, I., Raymon, P., Saglio, P. H., Stanet-Ges, V., and Pradet, A. (1994). Plant metabolism under anoxia. Plant Physiol. Biochem. 32, 1–10.
Rogiers, S. Y., and Knowles, N. R. (1998). Effects of storage temperature and atmosphere on saskatoon (Amelanchier alnifolia Nutt.) fruit quality, respiration and ethylene production. Postharvest Biol. Technol. 13, 183–190. doi: 10.1016/S0925-5214(98)00012-X
Rohani, M. Y., Zaipun, M. Z., and Norhayati, M. (1997). Effect of modified atmosphere on the storage life and quality of Eksotika papaya. J. Trop. Agric. Food Sci. 25, 103–113.
Saglio, P. H. (1985). Effect of path or sink anoxia on sugar translocation in roots of maize seedlings. Plant Physiol. 77, 285–290. doi: 10.1104/pp.77.2.285
Salvador, A., Arnal, L., Besada, C., Larrea, V., Hernando, I., and Pérez-Munuera, I. (2008). Reduced effectiveness of the treatment for removing astringency in persimmon fruit when stored at 15°C: Physiological and microstructural study. Postharvest Biol. Technol. 49, 340–347. doi: 10.1016/j.postharvbio.2008.01.015
Salvatierra, A., Toro, G., Mateluna, P., Opazo, I., Ortiz, M., and Pimentel, P. (2020). Keep calm and survive: Adaptation strategies to energy crisis in fruit trees under root hypoxia. Plants 9:1108. doi: 10.3390/plants9091108
Sanders, M. G., and de Wild, H. P. J. (2003). The relation between in vivo ethylene production and oxygen partial pressure. Postharvest Biol. Technol. 30, 143–151. doi: 10.1016/S0925-5214(03)00102-9
Schmidt, R. S., Fulda, M., Paul, M. V., Anders, M., Plum, F., Weits, D. A., et al. (2018). Low-oxygen response is triggered by an ATP-dependent shift in oleoyl-CoA in Arabidopsis. Proc. Natl. Acad. Sci. U. S. A. 115, E12101–E12110. doi: 10.1073/pnas.1809429115
Sedbrook, J. C., Kronebusch, P. J., Borisy, G. G., Trewavas, A. J., and Masson, P. H. (1996). Transgenic AEQUORIN reveals organ-specific cytosolic Ca2+ responses to anoxia in Arabidopsis thaliana seedlings. Plant Physiol. 111, 243–257. doi: 10.1104/pp.111.1.243
Subbaiah, C. C., Bush, D. S., and Sachs, M. M. (1998). Mitochondrial contribution to the anoxic Ca2+ signal in maize suspension-cultured cells. Plant Physiol. 118, 759–771. doi: 10.1104/pp.118.3.759
Summers, J. E., Ratcliffe, R. G., and Jackson, M. B. (2000). Anoxia tolerance in the aquatic monocot Potamogeton pectinatus: absence of oxygen stimulates elongation in association with an unusually large Pasteur effect. J. Exp. Bot. 51, 1413–1422. doi: 10.1093/jexbot/51.349.1413
Techavuthiporn, C., Boonyaritthongchai, P., and Supabvanich, S. (2017). Physicochemical changes of “Phulae” pineapple fruit treated with short-term anoxia during ambient storage. Food Chem. 228, 388–393. doi: 10.1016/j.foodchem.2017.02.028
Toro, G., and Pinto, M. (2015). Plant respiration under low oxygen. Chil. J. Agric. Res. 75, 57–70. doi: 10.4067/S0718-58392015000300007
van Dongen, J. T., and Licausi, F. (2015). Oxygen sensing and signaling. Annu. Rev. Plant Biol. 66, 345–367. doi: 10.1146/annurev-arplant-043014-114813
van’t Hoff, M. J. H. (1884). Etudes de dynamique chimique. Rec. Trav. Chim. Pays Bas 3, 333–336. doi: 10.1002/recl.18840031003
Varoquaux, P., Gouble, B., Baron, C., and Yildiz, F. (1992). Respiratory parameters and sugar catabolism of mushroom (Agaricus bisporus Lange). Postharvest Biol. Technol. 16, 51–61. doi: 10.1016/S0925-5214(99)00004-6
Venkatram, A., Bhagwan, A., Pratap, M., and Reddy, D. V. V. (2016). Effect of modified atmosphere package on physicochemical characteristics of ‘Balanagar” custard apple (Annona squamosa L.) fruits stored at 15 ±1 °C. Int. J. Sci. Nat. 7, 332–338.
Ventura, I., Brunello, L., Iacopino, S., Valeri, M. C., Novi, G., Dornbusch, T., et al. (2020). Arabidopsis phenotyping reveals the importance of alcohol dehydrogenase and pyruvate decarboxylase for aerobic plant growth. Sci. Rep. 10:16669. doi: 10.1038/s41598-020-73704-x
Wade, N. L. (1974). Effects of oxygen concentration and ethephon upon the respiration and ripening of banana fruits. J. Exp. Bot. 25, 955–964. doi: 10.1093/jxb/25.5.955
Waghmare, R. B., Mahajan, P. V., and Annapure, U. S. (2013). Modelling the effect of time and temperature on respiration rate of selected fresh-cut produce. Postharvest Biol. Technol. 80, 25–30. doi: 10.1016/j.postharvbio.2013.01.012
Watkins, C. B. (2000). Responses of horticultural commodities to high carbon dioxide as related to modified atmosphere packaging. HortTechnol 10, 502–506. doi: 10.21273/HORTTECH.10.3.501
Wang, F., Chen, Z. H., and Shabala, S. (2017). Hypoxia sensing in plants: On a quest for ion channels as putative oxygen sensors. Plant Cell Physiol. 58, 1126–1142. doi: 10.1093/pcp/pcx079
Wongs-Aree, C., and Noichinda, S. (2018). “Glycolysis fermentative by-products and secondary metabolites involved in plant adaptation under hypoxia during pre- and postharvest,” in Hypoxia and Anoxia, eds K. Das and M. S. Biradar (London: IntechOpen Publisher), 59–72. doi: 10.5772/intechopen.80226
Wongs-Areea, C., and Noichinda, S. (2014). ““Postharvest physiology and quality maintenance of tropical fruits”,” in Postharvest Handling. A System Approach, eds W. J. Florowski, R. L. Shewfelt, S. E. Prussia, and N. Banks (Amsterdam: Academic Press), 275–312.
Wu, W., Wang, M. M., Gong, H., Liu, X. F., Guo, D. L., Sun, N. J., et al. (2020). High CO2/hypoxia-induced softening of persimmon fruit is modulated by DkERF8/16 and DkNAC9 complexes. J. Exp. Bot. 71, 2690–2700. doi: 10.1093/jxb/eraa009
Yahia, E. M., and Vazquez-Moreno, L. (1993). Responses of mango to insecticidal oxygen and carbon dioxide atmospheres. LWT Food Sci. Technol. 26, 42–48. doi: 10.1006/fstl.1993.1008
Yemelyanov, V. V., Chirkova, T. V., Shishova, M. F., and Lindberg, S. M. (2020). Potassium efflux and cytosol acidification as primary anoxia-induced events in wheat and rice seedlings. Plants 9:1216. doi: 10.3390/plants9091216
Yemelyanov, V. V., Shishova, M. F., Chirkova, T. V., and Lindberg, S. M. (2011). Anoxia-induced elevation of cytosolic Ca2+ concentration depends on different Ca2+ sources in rice and wheat protoplasts. Planta 234, 271–280. doi: 10.1007/s00425-011-1396-x
Yi, C., Jiang, Y. M., Sun, J., Luo, Y. B., Jiang, W. B., and Macnish, A. (2006). Effects of short-term N2 treatments on ripening of banana fruit. J. Hortic. Sci. Biotechnol. 81, 1025–1028. doi: 10.1080/14620316.2006.11512166
Zahra, N., Hafeez, M. B., Shaukat, K., Wahid, A., Hussain, S., Naseer, R., et al. (2021). Hypoxia and Anoxia Stress: Plant responses and tolerance mechanisms. J. Agro. Crop Sci. 207, 249–284. doi: 10.1111/jac.12471
Zhang, Z., Huber, D. J., and Rao, J. (2011). Ripening delay of mid-climacteric avocado fruit in response to elevated doses of 1-methylcyclopropene and hypoxia-mediated reduction in internal ethylene concentration. Postharvest Biol. Technol. 60, 83–91. doi: 10.1016/j.postharvbio.2011.01.001
Keywords: tropical fruits, physiology, biochemistry, hypoxia, anoxia
Citation: Benkeblia N (2021) Physiological and Biochemical Response of Tropical Fruits to Hypoxia/Anoxia. Front. Plant Sci. 12:670803. doi: 10.3389/fpls.2021.670803
Received: 22 February 2021; Accepted: 23 June 2021;
Published: 16 July 2021.
Edited by:
Natalia Marina Villarreal, CONICET Instituto Tecnológico de Chascomús (INTECH), ArgentinaReviewed by:
Yueming Jiang, South China Botanical Garden, Chinese Academy of Sciences, ChinaQing-gang Zhu, Northwest A&F University, China
Copyright © 2021 Benkeblia. This is an open-access article distributed under the terms of the Creative Commons Attribution License (CC BY). The use, distribution or reproduction in other forums is permitted, provided the original author(s) and the copyright owner(s) are credited and that the original publication in this journal is cited, in accordance with accepted academic practice. No use, distribution or reproduction is permitted which does not comply with these terms.
*Correspondence: Noureddine Benkeblia, noureddine.benkeblia@uwimona.edu.jm