- 1Botanic Garden, Faculty of Biology, University of Warsaw, Warsaw, Poland
- 2Petersham Lodge, Richmond, United Kingdom
- 3Feature Forest, Gdańk, Poland
Pollinators are often perceived as a primary selective agent influencing flower traits such as colour, size, and nectar properties. The genus Fritillaria L. (Liliaceae), comprising approximately 150 species, is described as generally insect pollinated. However, there are at least three exceptions: two hummingbird-pollinated North American species and one passerine-pollinated Asian species. Despite this variation in pollination, little is known about flower traits that may accompany this shift in fritillaries. In this study, we aimed to assess the attractiveness of the floral traits for (new) pollinators and track the evolution of flowers traits in the context of a shift in the principal pollinator. Therefore, we studied 14 flower traits related to the pollination in 60 Fritillaria species and traced the evolutionary trajectory of these traits. We used a phylogenetic tree of the genus, based on five DNA markers (matK, rpl16, and rbcL, 18S, and ITS) to reconstruct the ancestral state of studied flower traits. The results show that in bird-pollinated species several new traits evolved. For example, flower colouration, nectar sugar, and amino acid concentration and composition fulfil the criteria of ornithophilous flowers, although flower traits do not exclude insect pollinators in bird-pollinated fritillaries. Interestingly, we recorded potential reversals from bird to insect pollination. Our analysis, showing a broad study of flower traits among closely related species in the context of pollinator shift, serves as a starting point for future work exploring the genetic and physiological mechanisms controlling flower traits in the genus Fritillaria.
Introduction
Angiosperms present enormous variation in flower traits, such as colour, shape, size, content and quality of the floral reward. This variation is also encountered among closely related species (e.g., Johnson et al., 1998; Wilson et al., 2004; Smith et al., 2007; Thomson and Wilson, 2008; Guzman et al., 2017; Roguz et al., 2018). Since Darwin (1862), flower divergence has largely been attributed to the selection of pollinators. The phenomenon of adaptation to the preferences of the most common and efficient pollinator has been formalised as pollination syndromes—recurring suites of flower traits associated with different groups of pollinators (Faegri and van der Pijl, 1966). Despite the ongoing debate about the assumptions of this concept (Ollerton, 1996; Waser et al., 1996; Rosas-Guerrero et al., 2014; Dellinger, 2020) it serves as a framework for studies of floral diversity (Kay and Schemske, 2003; Smith et al., 2007).
Several empirical studies have supported the relation between flower traits and pollinators groups (Dellinger, 2020). This relation is even more visible, when pollinators shifts are considered. These new pollinators may be more efficient (Ashworth et al., 2015), or the only present, if e.g., the occurrence of pollinator shift is enforced by the loss of ancestral pollinators (Cox and Elmqvist, 2000). Indeed, significant changes in floral traits, such as corolla symmetry, petal colour, and type of reward, are generally associated with pollinator shifts, strongly supporting the idea that flower traits reflect new pollinators preferences (Bruneau, 1997; Ramírez-Aguirre et al., 2019; Barrionuevo et al., 2021).
Among visual flower traits, colour is one of the key features involved in signalling to pollinators (Willmer, 2007). Colour constitutes to be one of the main traits used in floral syndromes (Faegri and van der Pijl, 1966), and although its attractiveness for specific pollinators groups remains a source of contention, the assumption that e.g., bird pollinated flowers are red remains common (Smith et al., 2007). In Penstemon Schmidel, for example, red petal colour was one of the characters that best predicted hummingbird visitation (Wilson et al., 2004). Flower colour change was also an important step involved in the transition from bee to nocturnal hawk moth pollination in the genus Petunia Juss (Hoballah et al., 2007). Flower colour, however, does not always relate well to pollinator shifts (Smith et al., 2007; Opedal, 2019; Roguz et al., 2020a). For example, red colouration may be a good predictor of bird-pollination in Iris L. species, but not vice-versa (Roguz et al., 2020a). The explanation for this lack of correlation is that ultimate flower choice is based on a combination of stimuli. Moreover, most flower species are pollinated by generalists (Reverté et al., 2016).
Other signal-transmitting flower traits, such as flower size and the arrangement of its reproductive parts, can also be subjected to strong selection pressure (Lavi and Sapir, 2015; Souto-Vilarós et al., 2018). For more efficient pollen transfer, flower size and arrangement of reproductive parts should fit the body and behaviour of the pollinator (Fenster et al., 2006). Studies conducted on Ipomopsis Michx. have shown that the display size was positively correlated with hummingbird importance and negatively correlated with dipteran importance (Smith et al., 2007). However, like in the case of colour, shift to the new pollinator does not always result in a consistent flower size or the arrangement of flower reproductive parts (Dupont et al., 2004).
While visual flower traits are an important signal for potential pollinators, floral rewards are also crucial in shaping the interaction. In most cases plants provide a food reward of either pollen or nectar (Parachnowitsch et al., 2018). In the latter case, animals visiting flowers may also exert selection on floral reward properties, such as volume, sugar, or amino acid (AA) concentration (Dupont et al., 2004). Flower reward chemistry was proposed as a key factor to transition from moth to bee pollination in Satyrium Sw. orchid (Castañeda-Zárate et al., 2021). Also in the case of Ipomopsis reward was positively correlated with hummingbirds importance. However, in Clivia Lindl. the shift from bird- to butterfly pollination was accompanied by the change of flower shape, smaller nectar volume, and emission of scent, while flower colour and nectar chemistry were not substantially modified (Kiepiel and Johnson, 2014).
The set of new flower traits depends on the identity of the new pollinator. The most often recorded pollinator shifts include the transition from insect (bee)-pollinated to bird-pollinated plants (Wilson et al., 2006; Thomson and Wilson, 2008), which is usually caused by the higher efficiency of birds in transferring pollen as well as lower pollen discounting (Thomson and Wilson, 2008). For example, in Penstemon and related genera, there have been at least 10 separate transitions toward hummingbird pollination (Wilson et al., 2007). In Mimulus sect. Erythranthe hummingbird pollination has arisen from melittophily twice (Beardsley et al., 2003). The following genera also include hummingbird-pollinated plants and species that are pollinated by other bird lineages: Agave, which is predominately bat-pollinated (Agavaceae, Good-Avila et al., 2006); Erythrina, which is entirely ornithogamous (Fabaceae; Bruneau, 1997; Etcheverry et al., 2012), Mucuna (Fabaceae; Kalin Arroyo and Penaloza, 1990), and Puya (Bromeliaceae, Hornung-Leoni and Sosa, 2005).
The above examples and other pollinator shift studies show that floral evolution has been highly labile and also directional. Moreover, even specialised pollination systems are not a dead-end (Tripp and Manos, 2008). There have been few reverse transitions from bird to insect pollination (Mast et al., 2012); for example, in the tribe Sinningieae (Gesneriaceae; two transitions from hummingbird to bee pollination, Perret et al., 2003) and in the genus Aquilegia (Ranunculaceae; five transitions from hummingbird to hawk moth pollination, Whittall and Hodges, 2007).
Such shifts to new pollinators, or reverse transitions to an ancestral pollinator, usually result in a different suite of traits, attracting new, more efficient pollinators and repelling less effective pollinators or flower robbers (Castellanos et al., 2004; Salas-Arcos et al., 2017). While flower colour-related traits, which often occur with pollinator shift, are well understood, little is known about the other flower traits, like nectar properties, that are the potential outcome of this shift. To understand the influence of the new pollinators on flower traits, it is necessary to explore plant genera exhibiting pollinator shift.
In our study, we focussed on Fritillaria L. (Liliaceae), which is a genus that includes approximately 150 species of bulbous plants, predominantly found in temperate Holarctic regions of both the Old and New World (Tamura, 1998; Day et al., 2014; Zych et al., 2014). The highest diversity of Fritillaria is observed in the Mediterranean region (Beetle, 1944; Rix, 1984; Zaharof, 1986; Rønsted et al., 2005; Tekñen and Aytaç, 2011; Hill, 2016; Kiani et al., 2017). Fritillaria flowers are generally actinomorphic and have a nodding tulip-like trimerous perianth. Despite the similarity, flowers of fritillaries exist in various sizes and colours, and can be white, pink, greenish, yellow, or purplish/reddish. We still do not fully understand the diversity of fritillaries, but it is possibly related to pollination systems, at least to some extent. Most fritillaries are described as or presumed to be pollinated by insects; however, there have been at least two pollination shifts from insect to bird pollination in the genus. This shift involves distinct bird groups, namely passerines and hummingbirds (Búrquez, 1989; Peters et al., 1995; Pendergrass and Robinson, 2005) and results in new flower traits in fritillaries. For example the only red and orange fritillaries are pollinated by birds. Moreover, properties of nectar reward reflect preferences of their bird pollinators. Hummingbird-pollinated fritillaries produce nectar of medium concentration and with medium AA concentration, while passerine bird pollinated species produce huge amounts of AA rich nectar, dominated by sucrose preferred by passerines. Still, most of the members of this genus, which are presumably insect pollinated, produce smaller amounts of balanced nectar (Roguz et al., 2018, 2019). Also within insect pollinated fritillaries, there may have occurred shifts of the main pollinators. Fritillaria camtschatcensis is a species with distinct flower traits and reward properties (Roguz et al., 2018, 2019), pollinated by flies (Zox and Gold, 2008). Nectaries of this species are covered with protrusions. Traces of hardly accessible, viscous and almost solid nectar are available as a thin film overlying the protrusions (Roguz et al., 2018, 2019). This way of nectar presentation likely excluded insects other than flies with a cushion-like labium.
Fritillaria is also a genus in which reversals from bird to insect pollination may have occurred. Fritillaria raddeana is a species closely related to passerine bird pollinated F. imperialis (Wietsma et al., 2015). However, flowers of F. raddeana are greenish, smaller and this species produces small amounts of highly concentrated, balanced nectar (Roguz et al., 2018, 2019). The question remains, what was the characteristic of the common ancestor of this species.
Given the unique pollination system (at least two transitions from insect to bird pollination, possible reversals), our research goals were to build a phylogenetic framework in which flower traits and reward properties within Fritillaria can be evaluated. We asked the following questions: to what extent do flower traits fulfil criteria of ornithophilous/enthomogamus flowers? Can quantitative traits, for example flower size, influence the diversification dynamic? Do shifts in phenotypic optima of flower traits overlap with the pollinator shift? We tested the assumption that nectar (as an important flower reward) and colour (as an important visual sign) play crucial roles in pollinator shits. We also anticipated reversals from hummingbird-bird to insect-pollination because of the low degree of specialisation of bird-pollinated fritillaries.
Materials and Methods
Phylogenetic Tree
The first step in the analysis of flower trait evolution in fritillaries was to create a phylogenetic tree of the genus. In this study, we prepared a database of five DNA markers: plastid genome (matK, rpl16, and rbcL), nuclear (18S), and internal transcribed spacer (ITS) sequences. These genetic markers have been used successfully to infer phylogenetic relationships in Fritillaria (Rønsted et al., 2005; Day et al., 2014; Khourang et al., 2014; Kim and Kim, 2018). We used Lilium L. as an outgroup based on the established relationship between Fritillaria and this genus (Rønsted et al., 2005; Day et al., 2014). We selected 11 Lilium species with the highest gene coverage (accession numbers in Supplementary Material 1).
The matK region of two Fritillaria species, F. biflora and F. olgae, was sequenced by extracting DNA from the collection of living plants at the University of Warsaw Botanic Garden (BG). We tried to obtain sequences of different regions, but due to methodological difficulties, only those for the matK regions were achieved (methods description Supplementary Material 2). All but two sequences used in the present study were acquired from GenBank. The sequences were downloaded using the MatPhylobi program (Kranas et al., 2018), which is a command-line tool for constructing taxonomic data sets for phylogenetic inference based on NCBI data. To create the sequence database in MatPhylobi, we selected F. michailovski and L. regale as representatives of the studied genera and used them as species to construct the data set. Overall, taxon sampling for Fritillaria totalled 461 accessions (Supplementary Material 1). We prepared two databases with the downloaded sequences. One database was prepared to analyse 60 Fritillaria species, for which we were able to obtain information about flower traits, flower reward properties, and pollinators. The second database was prepared for the flower colour analysis, in which we surveyed 119 species. This analysis was conducted for all species where we were able to obtain both the information about colour and the sequences.
Both databases were prepared following the same procedure. After downloading with MatPhylobi, all five markers were independently aligned using the multiple alignment program MAFFT (version 7; Katoh et al., 2017; method = “localpair” incorporating local pairwise alignment information, maxiterate = 1,000). Then, all alignments were imported into Mesquite for visual inspection (version 3.6; Maddison and Maddison, 2018). Poorly aligned positions and regions with high gap density in each alignment were eliminated using the Gblocks program (Version 0.91b, Castresana, 2000; Talavera and Castresana, 2007). The trimmed alignments were then concatenated with catfasta2phyml into a single combined alignment1. For each locus, we selected the appropriate evolutionary model recommended by the ModelTest-NG (version 0.1.3; Darriba et al., 2020). Selected models were as follows: matK:GTR + I + G4, rpl16:GTR + I + G4, rbcL:TVM + I + G4, 18S:TrN + G4, ITS:GTR + G4.
The obtained alignment was used in RAxML to generate phylogenetic trees. RAxML was used for a maximum-likelihood (ML) analysis (version 8.0; Stamatakis, 2014). Bootstrap (hereafter bsp) analysis with 1000 replicates was conducted on each partition.
Studied Characters: Flower Traits, Reward Properties, and Pollination System
The next step was to prepare a database describing the diversity of Fritillaria flower traits, the divergence of floral reward offered for pollinators, and the pollination system. Since most Fritillaria species are rare and grow in poorly accessible places, collecting data in natural habitats, although crucial, is not possible for most fritillaries. Nonetheless, to gain insight into the evolution of members of this genus, we used flowers from plants cultivated in University of Warsaw Botanic Garden and in the private collections of Colin Everett (Somerton, Somerset, United Kingdom; hereafter CE), one of the co-authors Laurence Hill (Richmond, Surrey, United Kingdom; hereafter LH), and Paweł Kalinowski (Szczeglacin, Korczew, Poland; hereafter PK). Most Fritillaria species are also rare in cultivation; hence, the number of specimens used for each type of analysis varied due to the availability of fresh plant material (the accession numbers and sources of plant material are listed in Table 1).
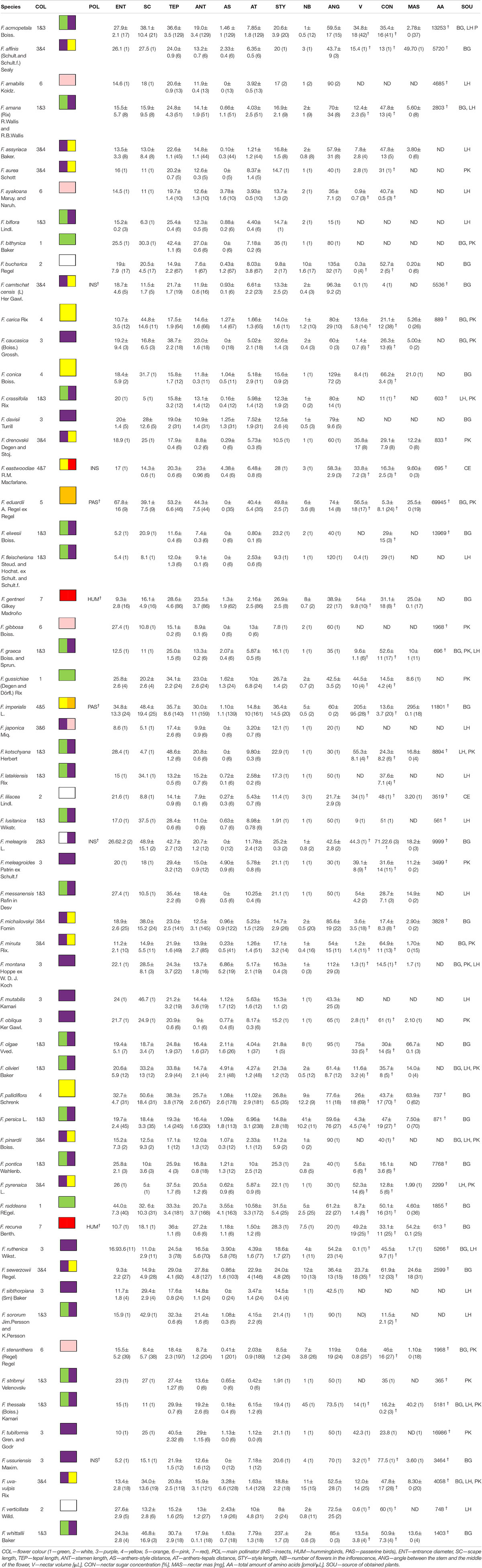
Table 1. Investigated Fritillaria species, all flower measurements in millimetres ± standard deviation, values in () indicate number of measurements, † indicates values obtained from literature.
In the case of flower colour, which is one of the most important traits shaping plant–pollinator interaction, we were not restricted by the availability of plant material. Flower colour was assessed for all 119 species, as we were able to score the colour without plant material (source of information2,3,4). Flower colour was scored by one of the authors, and the most dominant colouration of inner and outer side of the tepals was noted (e.g., we did not consider greenish colouration of the base of the tepals). Fritillary flowers exist in various colours (Figure 1). For the present study, we used simple colour-categories based on flower colour as perceived by humans. Although UV reflecting flower parts may be an important part of petals colouration, therefore shaping plant-pollinator interaction, we were not able to do a genus-level analysis due to a lack of relevant data. The availability of petal reflectance data is limited among fritillaries. In the present study flowers were categorised as green, orange (intermediate tint between yellow and red), pink (intermediate tint between red and white), purple (intermediate tint between blue and violet), red, yellow, or white. In the case of Fritillaria flowers representing similar colour groups e.g., pink and purple the visible differences are unambiguous. Species exhibiting colour polymorphism (e.g., F. persica and F. imperialis), or with bi-coloured flowers (e.g., F. michailovskyi) were coded into multiple categories.
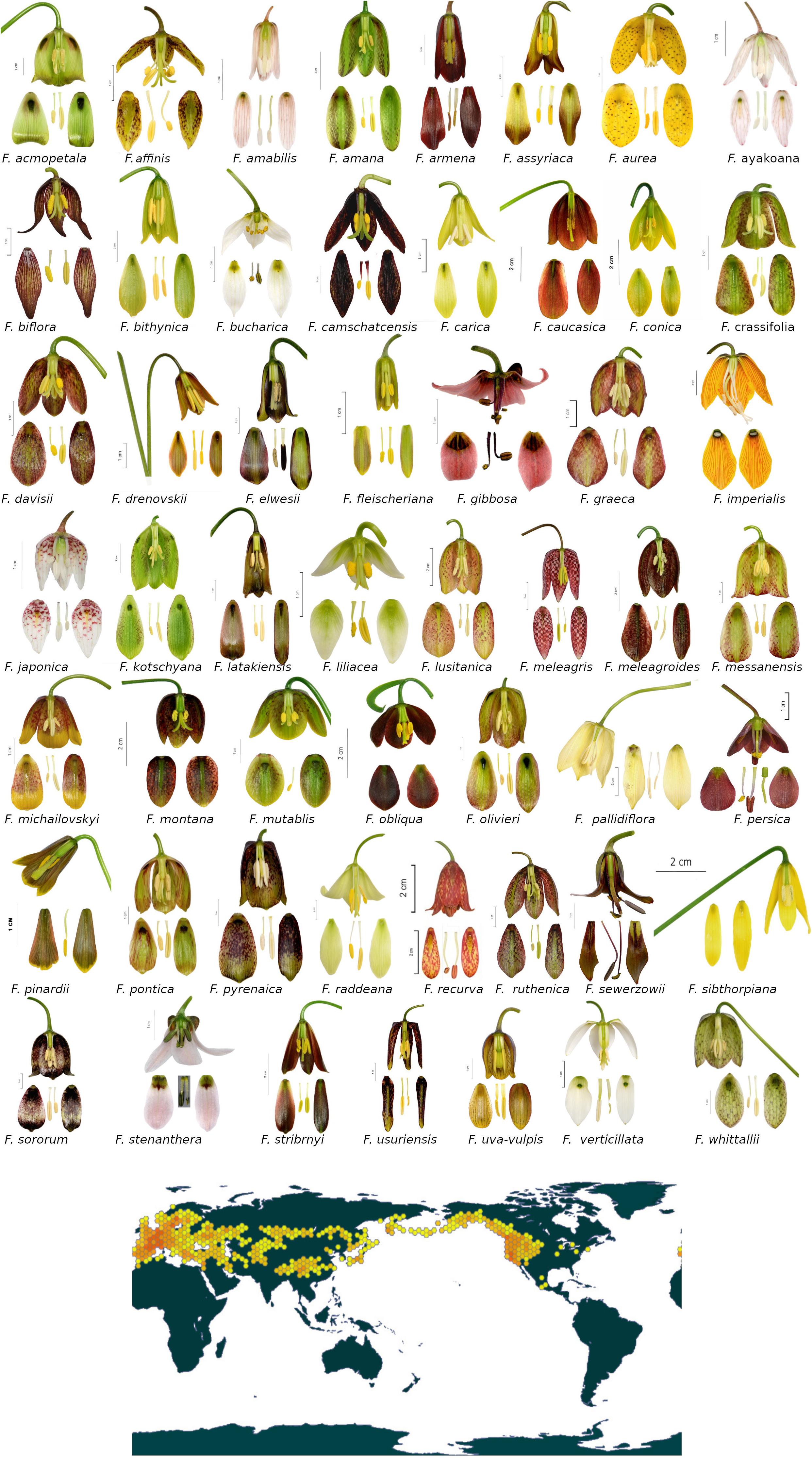
Figure 1. Flowers of some Fritillaria species presented in the study (pictures LH); map showing distribution of Fritillaria species (source: Fritillaria L. in GBIF Secretariat (2019). GBIF Backbone Taxonomy. Checklist dataset https://doi.org/10.15468/39omei accessed via GBIF.org on 2021-02-24).
Fourteen traits for 60 Fritillaria species were included in the floral trait analysis, which were broadly divided into two categories: flower and reward traits (Table 1). We hypothesised that these traits are subject to natural selection by pollinators. To estimate the potential attractiveness of the flower, we assessed the display size (number of flowers per plant). We also assessed tepal length as a measure of flower size (at its longest point; scheme showing the described measurements Supplementary Figure 1). To assess the fit between the pollinators and the flower reproductive parts, we measured the stamen and style length (from the base of the flower to the apex of the studied element), the distance between the anthers and the tepals and the anthers and the style, respectively (measured between the anthers tips and the tepals/style). Flower accessibility may play a crucial role in shaping plant–pollinator interactions; therefore, we recorded the orientation of flowers on the stem (the angle between the stem and the middle of the flower), the length of the scape, and the diameter of the corolla entrance (measured along the stem axis). All measurements were conducted using a digital calliper, Borletti DIN 862 (Borletti, Italy), which was connected to a computer to automatically record the values.
Because of the importance of floral reward for pollinators, we also included nectar properties; that is, volume, mass, sugar and AA concentration. Data on nectar AA concentration for all species, and nectar sugar concentration and volume for 45 species were derived from the literature (Roguz et al., 2018, 2019). In this study we acquired nectar data for F. assyriaca, F. aurea, F. conica, F. drenovskii, F. japonica, F. fleischeriana, F. messanensis, and F. verticillata (Table 1). Nectar was sampled, and the volume and sugar concentration were analysed as described by Roguz et al. (2018).
Finally, we collected data on the pollination system of the fritillaries. Pollinators were determined based on the literature (White, 1789; Búrquez, 1989; Peters et al., 1995; Pendergrass and Robinson, 2005; Zox and Gold, 2008; Zhang et al., 2010; Zych et al., 2014; Guo et al., 2017), in which flower visitors were assessed through direct observations.
Phylogenetic Studies
The obtained Fritillaria tree and trait database were used to reconstruct the ancestral state of studied flower traits and floral reward properties. The ancestral states were inferred on an ultrametric tree, generated using the chronos function in the “ape” package (with the age of the tree set to one, value of smoothing parameter lambda = 0 based on log-likelihoods Paradis et al., 2004). For each trait, we first determined the appropriate transition probability model. Transitions among all possible states may occur at the same rate, but it is supposed that it is easier to lose a complex character than to gain one. To include possibility for such asymmetries in rates we determined the transition probabilities using a log-likelihood ratio analysis, choosing from: ER (equal rate), SYM (symmetrical rate), and ARD (all-rates different). The ARD transition ratio model was chosen in all cases because it had the highest likelihood value (make.simmap function in the “phytools” package, Revell, 2012).
To infer the ancestral states of one polymorphic flower trait in our database, that is, flower colour, we used maximum likelihood estimation of the rates to determine the state probabilities (rayDISC function—this function specifically accommodates characters with polymorphic states; “corHMM” package; Beaulieu et al., 2013). To reconstruct the ancestral states of the continuous traits (e.g., tepals length or nectar volume), we used maximum likelihood estimation for the continuous traits (FastAnc function; “phytools” package; Revell, 2012).
Since pollinator shifts are often accompanied by changes in flower traits, we estimated the shifts in phenotypic trait optima of the studied traits. To do so, we used a least absolute shrinkage and selection operator (LASSO) procedure as proposed by Khabbazian et al. (2016) (estimate_shift_configuration function in the “l1ou” package) and phylogenetic-aware information criterion (pBIC) to perform model selection. We chose to use the phylogenetic pBIC for model selection as it minimises the inference of false shifts (Khabbazian et al., 2016). This method detects past changes in the expected means of the trait values using the Ornstein-Uhlenbeck process. The number of shifts in phenotypic trait optima was selected with the use of the Akaike information criterion (AIC, Ingram et al., 2013). Due to missing data in the reward properties, shifts in phenotypic trait optima were calculated for two groups. In the first variant, shifts were calculated on the basis of flower traits (except for anther-style distance where too many 0 values hampered the analysis) and in the second, shifts were calculated only on the basis of reward properties.
Flowers play an important role in shaping plant–pollinator interactions, and consequently, in plant reproduction. Therefore, floral traits have been hypothesised to influence the diversification dynamics of plant lineages. To test this, we performed a trait-dependent diversification analysis. Some studies have shown that for quantitative traits, state-dependent speciation-extinction models (e.g., Quantitative State Speciation and Extinction) may result in elevated false discovery rates. Therefore, in our study, for the quantitative traits we used an alternative trait-dependent diversification method, which does not model the relationship between traits, but tests for correlations between summary statistics of phylogenetic branching patterns and trait variation at the tips of a phylogenetic tree (ES-sim function, Harvey et al., 2017). The test assumptions were the same as for fitting a Brownian motion model to phylogenetic comparative data.
Finally, we assessed if there is a tendency for closely related Fritillaria species to resemble one another more than distantly related ones, that is, if there is a phylogenetic signal. To assess the strength of the phylogenetic signal on continuous data, we applied Blomberg’s K (Blomberg et al., 2003) (phylosig function in the “phytools” package, Revell, 2012). Blomberg’s K is a scaled ratio of the variance among species over the contrast variance. To incorporate estimation error, we used within-species variance. Species with a single observation and missing values were excluded from the analysis.
It is important to note that our study had inherent bias. Several studies have shown that Fritillaria diversity is centred on East Asia (Day et al., 2014; Huang et al., 2018; Kim and Kim, 2018; Li et al., 2018), the area from which the Liliaceae family originated (Huang et al., 2018; Kim and Kim, 2018). Unfortunately, this study included little material from SW China; therefore, any hypothesis of the ancestral form could change if material from this region was included. We also did not include hybridisation, which probably played an important role in the evolution of the studied genus (Zaharof, 1986), causing some incongruence in the phylogenetic analysis (Rønsted et al., 2005). This is because hybridisation has a small impact on the ancestral state reconstruction and on the parameter estimation (Bastide et al., 2018).
Results
Phylogenetic Tree
The phylogeny analysis included in our study covered approximately 76% of the species currently recognised in the Fritillaria genus (we discuss a phylogenetic tree obtained for all species sequences available in GenBank; Royal Botanic Gardens, Kew, 2020), with matK having the highest coverage (97%), and ITS and 18S having the lowest coverage (both 59%). The analysis resulted in a tree based on five sequences resolving the Fritillaria genus as monophyletic (the final tree presented on Figure 2 and contributing sub-trees are presented in Supplementary Figure 2). However, subtrees inferred from plastid sequence data do not resolve Fritillaria as monophyletic, with the Lilium species nested within Fritillaria. Our final phylogenetic tree showed one large, polyphyletic Fritillaria subgenus (containing approximately 100 species, bsp values 59 and 71, respectively), and several smaller subgenera: Liliorhiza (66 bsp), Rhinopetilium (52 bsp), Japonica (99 bsp), and Petilium (62 bsp). There were also three subgenera consisting of one species: Korolkovia (63 bsp), Theresia (79 bsp), and Davidii (54 bsp). The polyphyletic Fritillaria subgenus consists of two clades: one containing mainly European, Middle Eastern, and North African species (including some species with ranges extending to China), and the second containing mostly Asian species, forming a separate clade (Figure 2).
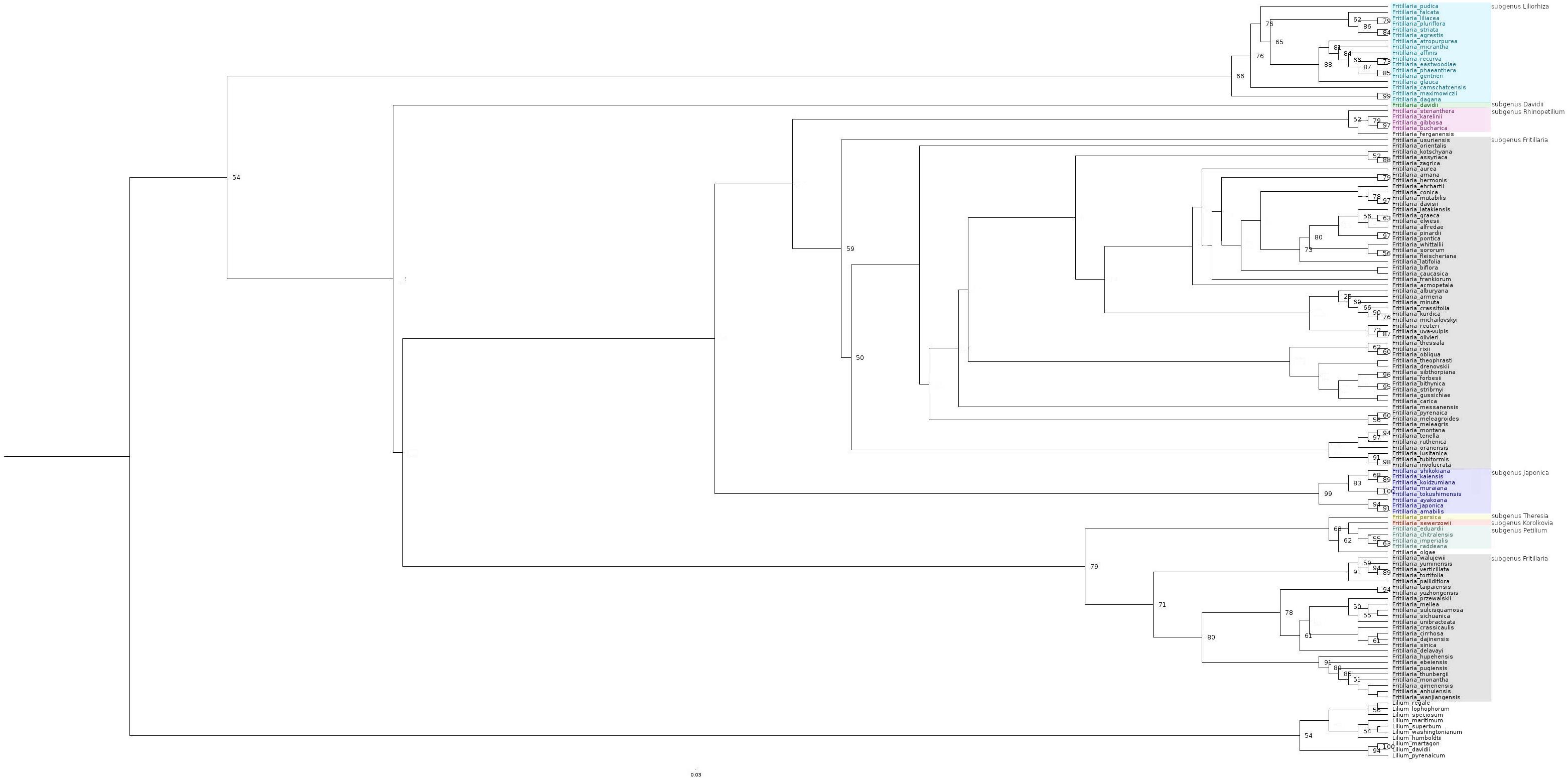
Figure 2. Maximum likelihood tree inferred from analysis combined of five DNA markers: plastid genome (matK, rpl16, and rbcL), nuclear (18S), and internal transcribed spacer (ITS) sequences. The bootstrap values are given along the branches (only values > 50 presented). Species representing different subgenera marked with different colours. Species not classified to any subgenus are left uncoloured.
Studied Characters: Flower Traits, Reward Properties, and Pollination System
Of the 119 Fritillaria species included in the colour analysis, 47.9% had flowers in shades of purple and 18.5% had flowers in shades of green. The rest was distributed among yellow, pink, orange, red, and white, with several species representing two categories. Seven species were categorised as having bi-coloured or polymorphic-coloured flowers (Supplementary Material 3). The only red and orange flowers were found among bird pollinated species.
Most of the Fritillaria species had only one flower (4.98 ± 8.25 [(hereafter mean ± SD], range 1–52); however, there were several species with a large flower display, including, F. persica with more than 50 flowers in the inflorescence. Species described as bird pollinated always had more than one flower. In most cases, the tepal length in Fritillaria ranged from 10 to 50 mm (26.8 ± 9.23 mm), except for the Petilium subgenus, which had comparatively large flowers (40.4. ± 8.27 mm). Tepals were usually longer than anthers (18.2 ± 35.2 mm, range 5–63 mm), and anthers were shorter than stigmas (18.5 ± 10.5, range 1.72–67 mm). The arrangement of the reproductive element was variable. The distance between the anthers and tepals was usually larger (8.02 ± 7.68 mm, range 0–57 mm) than that between the anthers and stigmas (3.19 ± 6.00 mm). In several dozen fritillaries, the anthers touched the stigma, ranging between 0 and 32.2 mm. Fritillaries often presented nodding flowers on a long stem (27.1 ± 17.3 mm, range 3.88–87.6 mm). The angle between flower diameter and stem was 70 ± 35° (range 15°–180°). In some species, nodding flowers were also accompanied by a narrow entrance (22.6 ± 13 mm), however, some Fritillaria flowers have a wide entrance (range 5.4–83 mm).
The properties of the reward offered for flower visitors varied. We found differences in sugar concentration (38.5 ± 20.2%), with a large difference between the lowest (3.25%) and the highest (78%) values. Similarly, in the case of AA concentration (8137 ± 16,229 pmol/μL), with the lowest and highest values being 220 and 79,840.62 pmol/μL, respectively. Differences in the amount of nectar produced were also prominent, with the lowest value being less than 1 μL and the highest being 390 μL (32.5 ± 52.7 μL). Fritillaria flowers produced an average of 33.7 ± 7.9 mg of nectar (range 0.1–480 mg, Table 1).
Most of the Fritillaria species are described as pollinated by insects, with various bee species being the common observed visitors. However, there are several pollinator shifts noted. According to literature data, there is one shift to pollination by flies in the case of F. camtschatcensis. There are also at least two shifts toward ornithophily. Asian F. imperialis is pollinated by passerine birds, while North American F. gentneri and F. recurva are pollinated by hummingbirds (White, 1789; Búrquez, 1989; Peters et al., 1995; Pendergrass and Robinson, 2005; Zox and Gold, 2008; Zhang et al., 2010; Zych et al., 2014; Guo et al., 2017; Figure 3).
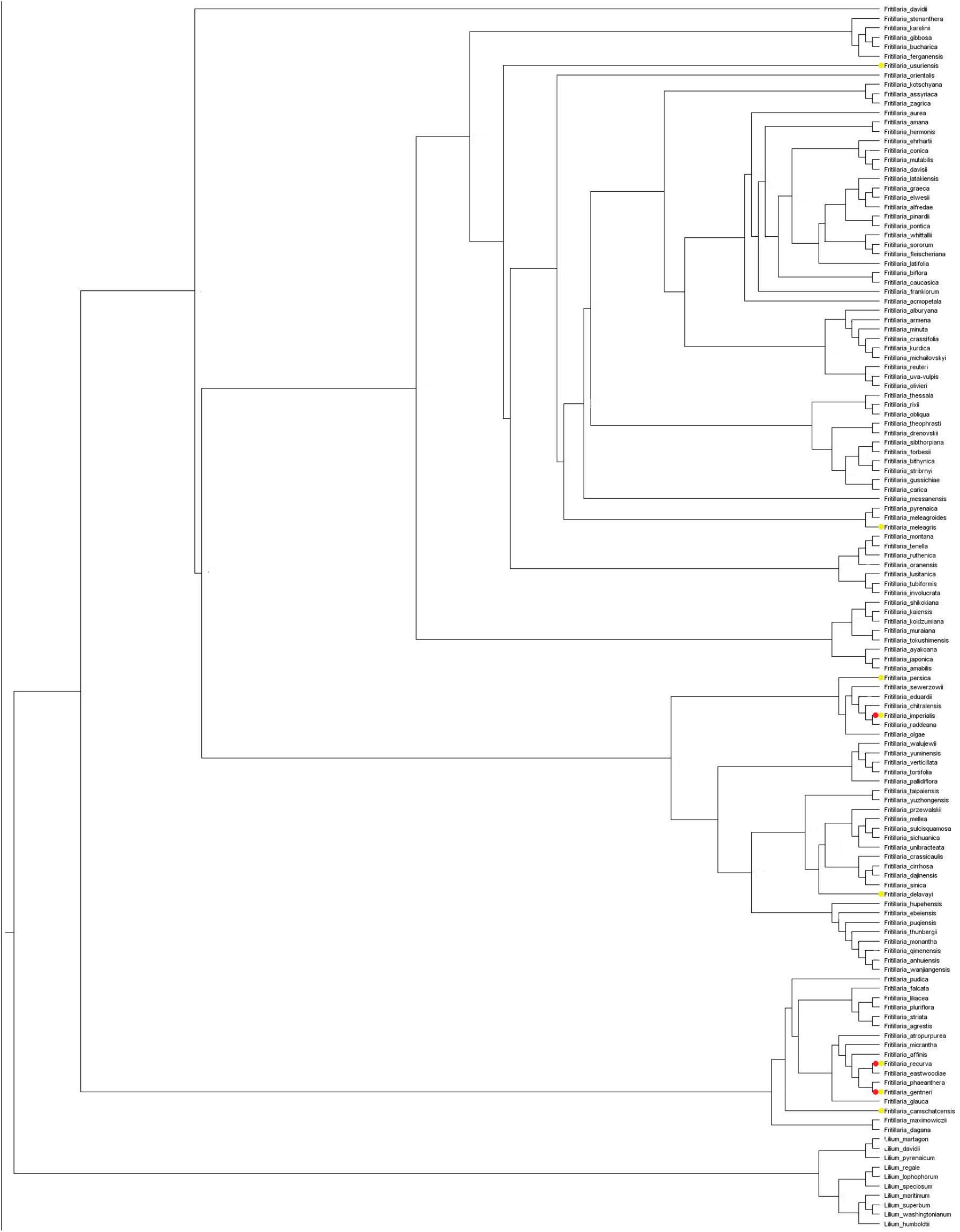
Figure 3. Maximum likelihood tree showing available information about pollinators of Fritillaria flowers (based on the literature data). Species marked with yellow dot are described as pollinated by insects, with red dot are described as pollinated by insects and birds. For species with no colour sign information about pollinators are not available.
Phylogenetic Studies
The ancestor of the fritillaries probably had purple or pink flowers. The internal nodes exhibit flowers with variable pigments, with purple colouration being the most common among both internal nodes and modern species. The results of our analysis indicate that several transitions were indeed reversals, for example, from yellow or green back to purple flowers (Figure 4).
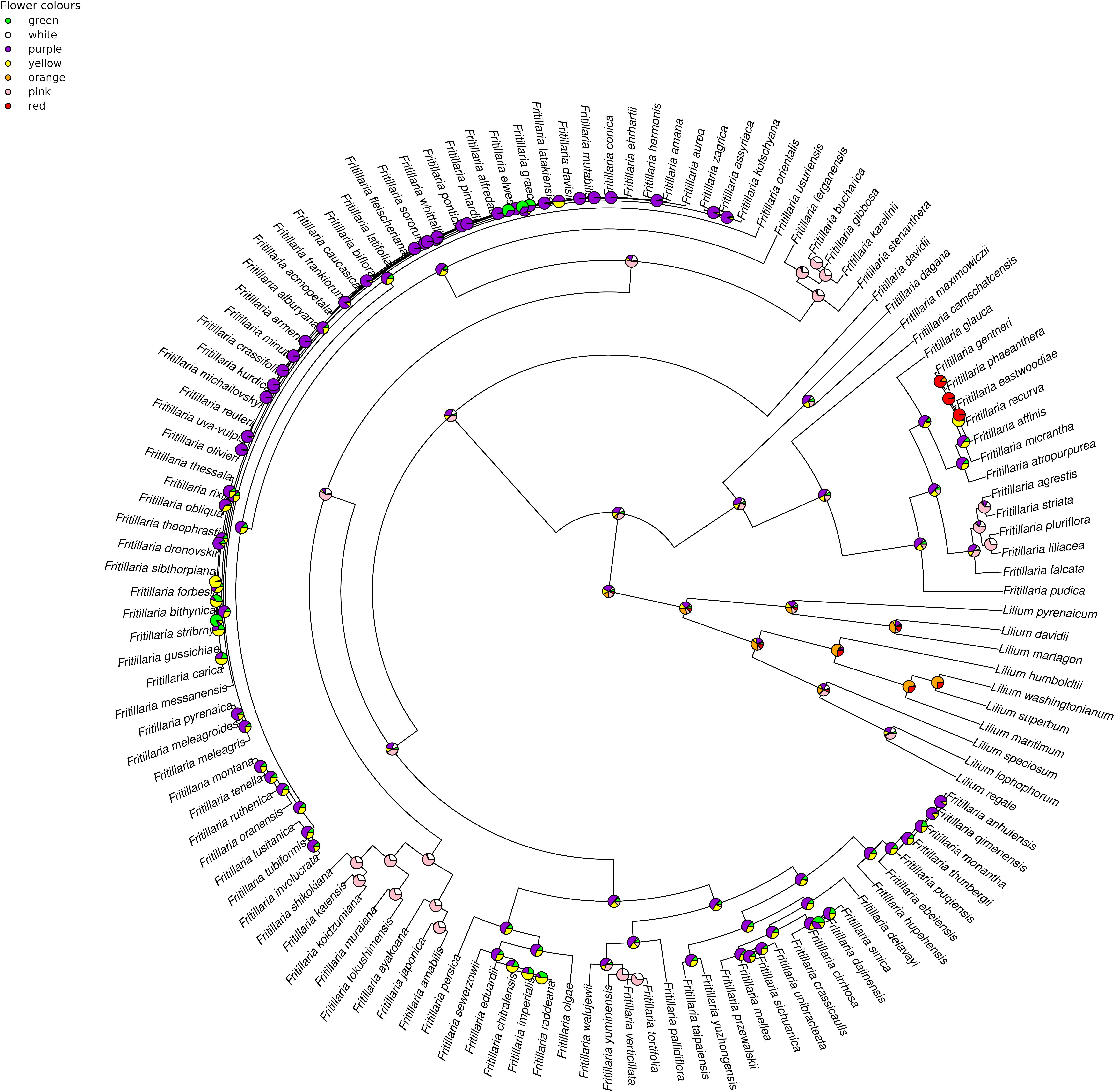
Figure 4. Estimation of ancestral states of flower colours among studied Fritillaria species calculated using maximum likelihood. Pie charts represent the proportion of the likeliest state at each internal node.
The most recent common ancestor of fritillaries probably had more than one flower in the inflorescence, with the tepals, stamen, and styles of medium length (Supplementary Figure 3). In the case of subgenus Japonica, we observed a tendency for the size of the flower elements to decrease, while for the subgenus Petilium we noted the opposite tendency, that is, flowers and flower parts elongated in most species. Both the tepals and the stigmas were probably close to the anthers with variable tendencies in modern Fritillaria species (Supplementary Figure 4). The small distance between the reproductive elements in the most recent common ancestor may have also been related to the relatively small diameter of the nodding flowers, growing on a few centimetres-long scape (Supplementary Figure 5).
Ancestral state reconstruction of the characters related to the floral reward revealed that the ancestor probably produced a relatively small amount of nectar, which was rich in sugars but low in AAs (Figure 5). The most notable adaptations in flower reward properties were found among species representing the subgenus Petilium, e.g., large amounts of nectar with a low sugar concentration, but a high AA concentration.
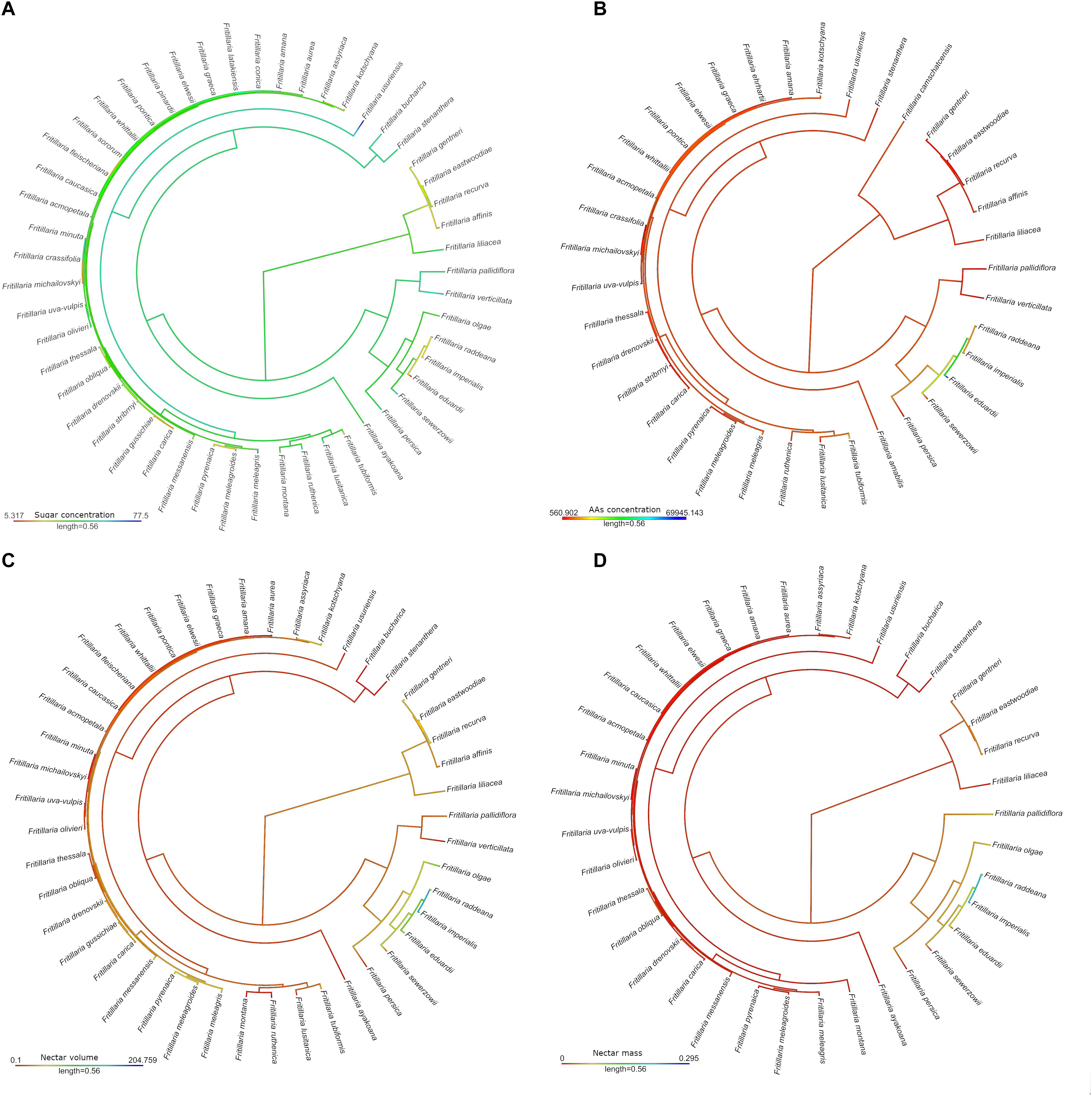
Figure 5. Maximum-likelihood ancestral state reconstruction for continuous traits among studied Fritillaria species: nectar sugar (%) (A) and amino acids concertation (pmol/μL) (B), nectar volume (μL) (C) and mass (mg) (D). Colour intensity shows the level of presented trait.
Using a LASSO procedure, we found support for 13 independent shifts in flower phenotypic trait optima and four shifts in the reward properties. For pollinator shift toward pollination by passerine birds both shift in phenotypic trait optima of flower traits and nectar properties coincide. Adaptation to pollination by new pollinators does not always coincide with the shift in the phenotypic optima of studied traits. For the species pollinated by hummingbirds we found shifts in phenotypic trait optima only for flower traits of F. recurva, while there were no shifts in the reward properties. We also did not notice such a shift in phenotypic trait optima in the case of e.g., F. camtschatcensis, a species pollinated by flies (Figure 6).
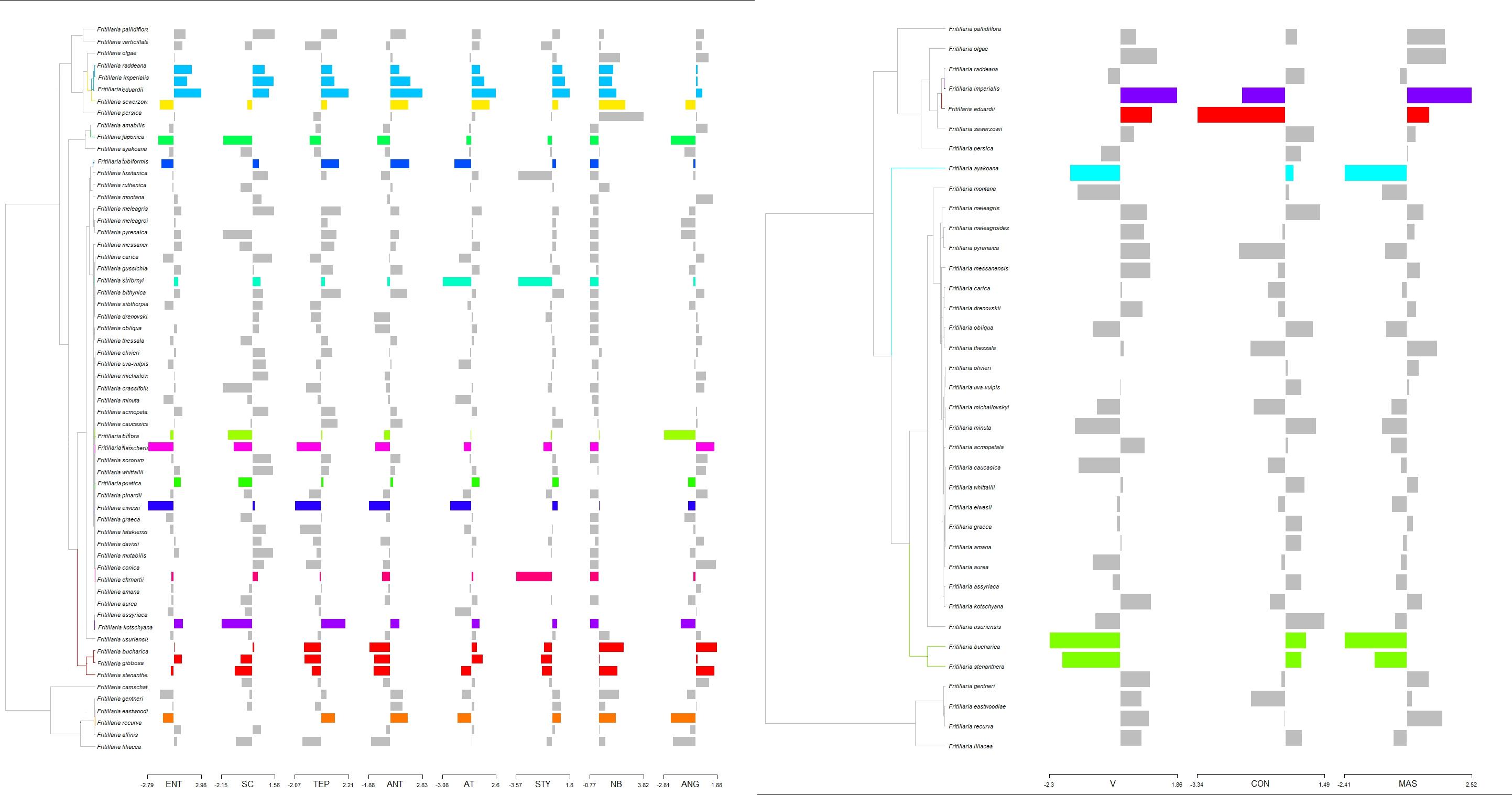
Figure 6. Estimated shifts in phenotypic optima of flower traits assessed for studied Fritillaria traits. Shifts in adaptive peaks are indicated with asterisks and convergent shifts in adaptive peaks have the same branch colour. Left: shift configuration. Right: bar graphs showing the traits combined for analysis. (A) Shifts calculated on the base of flower traits (with the exception of anthers-stigmas distance where too many 0 values hampered the analysis) and (B) reward properties. Difference in number of species in (A,B) results from missing data in the case of reward properties.
The Es-sim tests, which search for the relationship between change in continuous traits and diversification, showed no correlation between the summary statistics of phylogenetic branching patterns and studied traits. We found a statistically significant, although very weak, phylogenetic signal for Blomberg’s K (Blomberg et al., 2003) for the number of flowers (0.12). This parameter was not calculated for AA concentration, since we only had one sample in most cases (Supplementary Material 4).
Discussion
Most of the previously described large-scale phylogenetic relationships in Fritillaria were also found in our study (Rønsted et al., 2005; Day et al., 2014). The phylogenetic tree of fritillaries, based on five sequences, resolved the genus as monophyletic, which was also obtained in previous studies. However, subtrees inferred from plastid sequence data, also as in previous studies (Rønsted et al., 2005; Day et al., 2014), do not resolve Fritillaria as monophyletic, with the Lilium species nested within the studied genus.
The diversity of flower traits and pollination systems observed in fritillaries is likely due to several changes in the ecology and evolution of the studied genus. The most recent common ancestors of Fritillaria probably had purple or pink flowers of medium size, with the reproductive elements arranged in a similar manner to those of modern species. The ancestor likely had flowers with medium amounts of nectar rich in sugars, but with lower AA amount compared to modern species. We did not find phylogenetic signal for most of the studied traits, which indicate lack of tendency for closely related Fritillaria species to resemble one another. This result may suggest that several important shifts in floral characteristics e.g., flower colour in Fritillaria may be related to plant–pollinator interactions or other habitat-related factors. We were especially curious to study the new flower traits, e.g., reward properties in the context of new pollinators preferences. Our study revealed, for example, that flower colour is particularly good at separating bird- from insect-pollinated flowers. Colour shift may have been one of the triggers for bird pollination in Fritillaria. Red colour appeared among the modern, hummingbird-pollinated members of the subgenus Rhinopetalum. Similarly, in Asian species pollinated by passerine birds, orange flowers appeared. Red and orange flowers in Fritillaria are only found among bird-pollinated species, and in contrast to studies on Iochroma (Smith et al., 2007), Iris (Roguz et al., 2020a), and Polemonium (Landis et al., 2018), there seems to be a clear correlation between pollinator type and flower colour in the case of ornithophilous Fritillaria.
The two most common colour shifts were purple/green and purple/yellow, in both directions. We assume that these transitions types may have had little or no association with the influence of interacting animals. Green-coloured floral parts, common among Fritillaria species and most probably not visually attractive for pollinators (Roguz et al., 2020b), may be related to plant physiology. The photosynthetic activity of green flowers may be sufficient to maintain their own structures (Bazzaz and Carlson, 1979). Additionally, transition to a purple, anthocyanin-based colour, can result from environmental stress. Increased anthocyanin production may correlate with the level of sun damage or protection against herbivory (Chalker-Scott, 1999; Winkel-Shirley, 2002; Arista et al., 2013; Irwin et al., 2013). However, purple colouration may reduce visual attractiveness for insect pollinators, since several bee species have preference toward yellow flowers (Lunau and Maier, 1995; Spaethe et al., 2001). In some Fritillaria species, such as F. persica, both colour morphs (green and purple) are maintained, which is not typical. In most cases, one of the colour morphs will eventually be lost and the species will again be monomorphic (Ellis and Field, 2016). While gene flow usually leads to the fixation of one colour morph over the long term (Gray and McKinnon, 2007), some factors, including environmental heterogeneity (Tucić et al., 1998) and vegetative reproduction by rhizomes, may also maintain colour polymorphism in Fritillaria.
Tracking flower colour shifts in fritillaries suggests that colour loss in this genus may be reversible, in contrast to studies of other plant families (Smith and Goldberg, 2015; Landis et al., 2018). For example, the common ancestor of F. eduardii and F. sewerzowii most likely had orange flowers, while F. sewerzowii regains the purple colour present in the deeper nodes. The exception to this rule seems to be white flower colouration, which, similarly to Iris, is probably irreversible (Roguz et al., 2020a).
Other flower traits may also be related to plant–pollinator interactions in Fritillaria. Strong corolla constriction found in American hummingbird-pollinated fritillaries, and anthers and stigmas exerted beyond the corolla may promote contact between the birds’ bodies and the flower reproductive parts (Temeles et al., 2006; Martén-Rodríguez et al., 2009, 2010). A shift to bird pollination may also account for the increased size of the corolla entrance in the subgenus Petilium. These passerine bird-pollinated species have large flowers, growing close to the stem on a relatively short scape, with long stigma and anthers, distant from the tepals. These new traits may enable both to exploit the reward and make contact with anthers and stigmas. Nevertheless, similar to Dupont et al. (2004), we found no unique set of differences in the arrangement of flower reproductive parts between ornithophilous species and their entomophilous relatives. For example, long, exerted styles and stamens often associated with bird pollination (Beardsley et al., 2003), were found in both insect- and bird-pollinated Fritillaria species. These results may also suggest that some of the studied flower traits are just by change present in the bird-pollinated Fritillaria and do not result from pollinator driven trait evolution.
Flower size or arrangement of the reproductive elements often enable legitimate pollinators to access the reward. It’s properties seem to play an important role in the evolution of new pollination systems in fritillaries (Roguz et al., 2018, 2019). Our study revealed that the ability to produce AA-rich nectar with low sugar concentration evolved only once, in the case of the subgenus Petilium. These tendencies fulfil many of the criteria that are characteristic of passerine bird-pollinated flowers. On the other hand, the ability to produce both highly concentrated and/or copious nectar evolved several times, for example, in F. raddeana and F. recurva. The ability to produce highly concentrated nectar has never been lost, which may be related to the huge proportion of bee-pollinated fritillaries. The scarlet flowers of hummingbird pollinated species also produce reward attractive for its bird pollinators—copious amounts of relatively diluted nectar, with low concentrations of AA.
It is important to note that flower size and entrance, the arrangement of reproductive elements, or reward properties do not exclude insect pollinators in bird-pollinated fritillaries. Moreover, balanced proportions of different sugar types found in hummingbird-pollinated flowers may attract both insects and birds (Roguz et al., 2018). This scenario is also supported by the fact that F. eastwoodiae, which is a cross between F. micrantha (insect-pollinated) and F. recurva (bird-pollinated), must have arisen as a hybrid between two insect-visited species (Dewoody et al., 2013), since the flowers of F. micrantha are too small for other pollen vectors.
We also assume a pollinator shift within insect-pollinated species. In the case of fly-pollinated, typically purple-flowered F. camtschatcensis the reward properties (i.e., small amounts of highly concentrated nectar) and the petal structure (uneven surface, covered with numerous protrusions) may act as a trigger for pollinator shift. Flowers of this species, looking like and emitting the smell of rotting flesh, may attract new pollinators. On the other hand, the way of nectar presentation (thin film, almost solid) excludes most of the insect groups. Observed pollinator shifts in Fritillaria follow the predominant directionality in angiosperms, from insect to bird pollination (Wilson et al., 2007; Rausher, 2008; Van der Niet and Johnson, 2012). This transition can be explained by the more efficient transfer of pollen in bird-pollinated plants (Thomson and Wilson, 2008). However, we recorded potential for several reversals, from hummingbird- to insect-pollination, and from passerine bird- to insect-pollination. The most striking example is F. raddeana, a member of the subgenus Petilium. Flowers of this species resemble those of F. eduardii and F. imperialis but are half the size and pale-yellow-green in colour. The reward is also typical for insect-pollinated species, with a small volume of highly concentrated nectar, rich in sucrose and with low AA content (Roguz et al., 2018, 2019).
In addition to F. raddeana, it is likely that most of the floral diversity in Fritillaria is the result of adaptation to insect pollinators. Several species, even distantly related, for example F. pudica and F. carica, have similar flower traits, including colour, flower size, nectary shape (Rix and Strange, 2014), and reward properties. These similarities may reflect adaptation to pollinator preferences, especially taking into consideration species similarity and lack of phylogenetic signal. Insect-pollinated Fritillaria flowers are also of medium size, with anthers and stigmas that are often shorter than tepals, enabling small insects to touch the reproductive parts while foraging. Insect-pollinators often seek flowers with hexose-dominated nectar of medium volume and AA concentration, which are common in Fritillaria flowers (Roguz et al., 2018, 2019).
Finally, it is important to note the potential influence of other environmental factors on the evolution of Fritillaria flowers. Our study suggests that changes in Fritillaria flowers occurred rather recently, with more than 10 changes in the phenotypic optima of studied flower traits. Many flower traits were similar at the level of the deep internal nodes, whereas most changes in floral display and nectar properties appeared in shallow internal nodes. This evolutionary pattern and lack of phylogenetic signal for most traits suggest forces that act on an ecological time scale, rather than changes associated with deep phylogenetic relationships (Gómez et al., 2016). Fritillaries are found in a variety of climatic regions and in different habitats, including coasts, riparian zones, meadows, woodland, steppe, deserts, mountain screes, and alpine zones (Tomović et al., 2007; Tekñen and Aytaç, 2008; Zox and Gold, 2008; Hill, 2011, 2016; Tekñen and Aytaç, 2011; Rix and Strange, 2014; Zych et al., 2014; Gao et al., 2019). Consequently, some aspects of the floral display may have arisen by selection pressure exerted by abiotic factors related to the habitat type, such as temperature, altitudinal gradients, or water stress (Zhao and Wang, 2015; Landis et al., 2018; Gao et al., 2019). In addition, some of the observed variability should be attributed to the potential for natural hybridisation (Naruhashi et al., 2006; Kawano et al., 2008; Hill, 2011).
Further studies of Fritillaria flower traits and pollination systems in natural habitats, as well as molecular analyses of flower traits, would be of great importance. The results obtained in such studies may be crucial for understanding the influence of pollinators on the flower traits evolution.
Data Availability Statement
The datasets presented in this study can be found in online repositories. The names of the repository/repositories and accession number(s) can be found below: https://www.ncbi.nlm.nih.gov/genbank/, MW081399 and https://www.ncbi.nlm.nih.gov/genbank/, MW081400.
Author Contributions
KR and MZ conceived the study and wrote the draft version of the manuscript. KR and LH assembled field data. KR performed the nectar analysis. KR performed phylogenetic analyses. KR, MZ, AR, and LH analysed the data. All authors contributed to the final version.
Funding
This work was supported by the Polish National Science Centre, grant no. 4786 2013/11/N/NZ8/00611 (to KR).
Conflict of Interest
The authors declare that the research was conducted in the absence of any commercial or financial relationships that could be construed as a potential conflict of interest.
Acknowledgments
We thank Paweł Pstrokoński, Piotr Woźniak, Justyna Ryniewicz, Mateusz Skłodowski, and Anna Szaciłło for their help in the collection of field data and laboratory analyses; Paweł Kalinowski, Colin Everett, Paul Cumbleton, and U.S. Fish and Wildlife Service for plant material; Dorota Szubierajska for the help in the maintenance of experimental plants.
Supplementary Material
The Supplementary Material for this article can be found online at: https://www.frontiersin.org/articles/10.3389/fpls.2021.656783/full#supplementary-material
Supplementary Figure 1 | Schema of flower traits measurements (TEP, tepal length; ANT, stamen length; STY, stigma length; AT, anthers-tepals distance; AS, anthers-stigma distance; ANG, orientation of the flowers on the stem expressed as the angle between stem and the middle of the flower; SC, scape length; ENT, flower diameter (measured along the stem axis).
Supplementary Figure 2 | Maximum likelihood trees inferred from analysis of (A) nuclear genome 18S subtree, (B) internal transcribed spacer ITS subtree, and plastid genomes (C) matK subtree, (D) rbcL subtree, (E) rpl16 subtree. The incongruences found in trees based on plastid markers are marked with an ∗.
Supplementary Figure 3 | Maximum-likelihood ancestral state reconstruction for number of flowers (A), and the length of tepals (in mm) (B), stamens (in mm) (C) and stigmas (in mm) (D).
Supplementary Figure 4 | Maximum-likelihood ancestral state reconstruction for the distance between anthers and tepals (in mm) (A), and anthers and stigmas (in mm) (B).
Supplementary Figure 5 | Maximum-likelihood ancestral state reconstruction the angle between the stem and middle of the flower (A), and length of the scape (in mm) (B).
Supplementary Material 1 | Table with accession numbers of the used DNA regions: (matK, rpl16, and rbcL), nuclear (18S) and internal transcribed spacer (ITS).
Supplementary Material 2 | The description of the methods used for DNA extraction, amplification, and sequencing of matK regions for F. biflora and F. olgae.
Supplementary Material 3 | List of used Fritillaria species with defined flower colours used in the colour-related analyses.
Supplementary Material 4 | Supplementary Table 1 Results of trait-dependent diversification analysis Es-sim test for continuous traits for investigated Fritillaria species; Supplementary Table 2 The strength of the phylogenetic signal on continuous data (Blomberg’s K) for investigated Fritillaria species.
Footnotes
- ^ https://github.com/nylander/catfasta2phyml
- ^ www.fritillariaicones.com
- ^ http://www.efloras.org
- ^ http://www.fritillaria.org.uk
References
Arista, M., Talavera, M., Berjano, R., and Ortiz, P. L. (2013). Abiotic factors may explain the geographical distribution of flower colour morphs and the maintenance of colour polymorphism in the scarlet pimpernel. J. Ecol. 101, 1613–1622. doi: 10.1111/1365-2745.12151
Ashworth, L., Aguilar, R., Martén-Rodríguez, S., et al. (2015). “Pollination syndromes: a global pattern of con- vergent evolution driven by the most effective pollinator,” in Evolutionary Biology: Biodiversification from Genotype to Phenotype, ed. P. Pontarotti (Marseille: Springer), 203–224. doi: 10.1007/978-3-319-19932-0_11
Barrionuevo, C. N., Benitez-Vieyra, S., and Sazatornil, F. (2021). Floral biology of Salvia stachydifolia, a species visited by bees and birds: connecting sexual phases, nectar dynamics and breeding system to visitors’ behaviour. J. Plant Ecol. rtab012. doi: 10.1093/jpe/rtab012
Bastide, P., Solís-Lemus, C., Kriebel, R., William Sparks, K., and Ané, C. (2018). Phylogenetic comparative methods on phylogenetic networks with reticulations. Syst. Biol. 67, 800–820. doi: 10.1093/sysbio/syy033
Bazzaz, F. A., and Carlson, R. W. (1979). Photosynthetic contribution of flowers and seeds to reproductive effort of an annual colonizer. New Phytol. 82, 223–232. doi: 10.1111/j.1469-8137.1979.tb07577.x
Beardsley, P. M., Yen, A., and Olmstead, R. G. (2003). AFLP phylogeny of Mimulus section Erythranthe and the evolution of hummingbird pollination. Evolution 57, 1397–1410. doi: 10.1111/j.0014-3820.2003.tb00347.x
Beaulieu, J. M., O’Meara, B. C., and Donoghue, M. J. (2013). Identifying hidden rate changes in the evolution of a binary morphological character: the evolution of plant habit in campanulid angiosperms. Syst. Biol. 62, 725–737. doi: 10.1093/sysbio/syt034
Blomberg, S. P., Garland, T., and Ives, A. R. (2003). Testing for phylogenetic signal in comparative data: behavioral traits are more labile. Evolution 57, 717–745. doi: 10.1111/j.0014-3820.2003.tb00285.x
Bruneau, A. (1997). Evolution and homology of bird pollination syndromes in Erythrina (Leguminosae). Am. J. Bot. 84, 54–71. doi: 10.2307/2445883
Búrquez, A. (1989). Blue tits, Parus caeruleus, as pollinators of the crown imperial, Fritillaria imperialis, in Britain. Oikos 55, 335–340. doi: 10.2307/3565592
Castañeda-Zárate, M., Johnson, S., and van der Nier, T. (2021). Food reward chemistry explains a novel pollinator shift and vestigialization of long floral spurs in an orchid. Curr. Biol. 31, 238–246. doi: 10.1016/j.cub.2020.10.024
Castellanos, M. C., Wilson, P., and Thomson, J. D. (2004). ‘Anti-bee’ and ‘pro-bird’ changes during the evolution of hummingbird pollination in Penstemon flowers. J. Evol. Biol. 17, 876–885. doi: 10.1111/j.1420-9101.2004.00729.x
Castresana, J. (2000). Selection of conserved blocks from multiple alignments for their use in phylogenetic analysis. Mol. Biol. Evol. 17, 540–552. doi: 10.1093/oxfordjournals.molbev.a026334
Chalker-Scott, L. (1999). Environmental significance of anthocyanins in plant stress responses. Photochem. Photobiol. 70, 1–9. doi: 10.1111/j.1751-1097.1999.tb01944.x
Cox, P. A., and Elmqvist, T. (2000). Pollinator extinction in the Pacific Islands. Conserv. Biol. 14, 1237–1239. doi: 10.1046/j.1523-1739.2000.00017.x
Darriba, D., Posada, D., Kozlov, A. M., Stamatakis, A., Morel, B., and Flouri, T. (2020). ModelTest-NG: a new and scalable tool for the selection of DNA and protein evolutionary models. Mol. Biol. Evol. 37, 291–294. doi: 10.1093/molbev/msz189
Darwin, C. (1862). On the Various Contrivances by Which British and Foreign Orchids are Fertilised by Insects, and on the Good Effects of Intercrossing. London: John Murray.
Day, P. D., Berger, M., Hill, L., Fay, M. F., Leitch, A. R., Leitch, I. J., et al. (2014). Evolutionary relationships in the medicinally important genus Fritillaria L. (Liliaceae). Mol. Phylogenet. Evol. 80, 11–19. doi: 10.1016/j.ympev.2014.07.024
Dellinger, A. S. (2020). Pollination syndromes in the 21st century: where do we stand and where may we go? New Phytol. 228, 1193–1213. doi: 10.1111/nph.16793
Dewoody, J., Janeway, L., O’brien, J., Puentes, S., Hipkins, V., and Nelson, J. (2013). “Genetic differentiation among species of Fritillaria in Northern California: is F. eastwoodiae an endangered species or a hybrid swarm?,” in Botany 2013: Conference (New Orleans), 27–31. doi: 10.14492/hokmj/1381758488
Dupont, Y. L., Hansen, D. M., Rasmussen, J. T., and Olesen, J. M. (2004). Evolutionary changes in nectar sugar composition associated with switches between bird and insect pollination: the Canarian bird-flower element revisited. Funct. Ecol. 18, 670–676. doi: 10.1111/j.0269-8463.2004.00891.x
Ellis, T. J., and Field, D. L. (2016). Repeated gains in yellow and anthocyanin pigmentation in flower colour transitions in the Antirrhineae. Ann. Bot. 117, 1133–1140. doi: 10.1093/aob/mcw043
Etcheverry, ÁV., Figueroa-Castro, D., Figueroa-Fleming, T., Alemán, M. M., Juárez, V. D., López-Spahr, D., et al. (2012). Generalised pollination system of Erythrina dominguezii (Fabaceae: Papilionoideae) involving hummingbirds, passerines and bees. Aust. J. Bot. 60, 484–494. doi: 10.1071/bt11325
Faegri, K., and van der Pijl, L. (1966). The Principles of Pollination Ecology. Oxford: Pergamon Press.
Fenster, C. B., Cheely, G., Dudash, M. R., and Reynolds, R. J. (2006). Nectar reward and advertisement in hummingbird. Am. J. Bot. 93, 1800–1800. doi: 10.3732/ajb.93.12.1800
Gao, Y., Wang, C., Song, B., and Du, F. (2019). Corolla retention after pollination facilitates the development of fertilized ovules in Fritillaria delavayi (Liliaceae). Sci. Rep. 9:729.
Good-Avila, S., Souza, V., Gaut, B., and Eguiarte, L. (2006). Timing and rate of speciation in Agave (Agavaceae). Proc. Natl. Acad. Sci.U.S.A. 103, 9124–9129. doi: 10.1073/pnas.0603312103
Gómez, J. M., Torices, R., Lorite, J., Klingenberg, C. P., and Perfectti, F. (2016). The role of pollinators in the evolution of corolla shape variation, disparity and integration in a highly diversified plant family with a conserved floral bauplan. Ann. Bot. 117, 889–904. doi: 10.1093/aob/mcv194
Gray, S. M., and McKinnon, J. S. (2007). Linking color polymorphism maintenance and speciation. Trends Ecol. Evol. 22, 71–79. doi: 10.1016/j.tree.2006.10.005
Guo, F. X., Chen, Y., Zhang, J. F., and Bai, G. (2017). A bloom phenology and pollination mode of endangered plant Fritillaria unibracteata. Acta Pratacult. Sin. 25, 1324–1332. doi: 10.11733/j.issn.1007-0435.2017.06.024
Guzman, B., Gomez, J. M., and Vargas, P. (2017). Is floral morphology a good predictor of floral visitors to Antirrhineae (snapdragons and relatives)? Plant Biol. (Stuttgart, Germany) 19, 515–524. doi: 10.1111/plb.12567
Harvey, M. G., Rabosky, D. L., and Cooper, N. (2017). Continuous traits and speciation rates: alternatives to state-dependent diversification models. Methods Ecol. Evol. 9, 984–993. doi: 10.1111/2041-210x.12949
Hill, L. (2011). A taxonomic history of Japanese endemic Fritillaria (Liliaceae). Kew Bulletin. 66, 227–240. doi: 10.1007/s12225-011-9285-9
Hill, L. (2016). 847. Fritillaria Kiusiana: Liliaceae. Curtiss Bot. Mag. 33, 275–285. doi: 10.1111/curt.12159
Hoballah, M. E., Gübitz, T., Stuurman, J., Broger, L., Barone, M., Mandel, T., et al. (2007). Single gene-mediated shift in pollinator attraction in Petunia. Plant Cell 19, 779–790. doi: 10.1105/tpc.106.048694
Hornung-Leoni, C., and Sosa, V. (2005). Morphological variation in Puya (Bromeliaceae): an allometric study. Plant Syst. Evol. 256, 35–53. doi: 10.1007/s00606-005-0302-z
Huang, J., Yang, L. Q., Yu, Y., Liu, Y. M., Xie, D. F., Li, J., et al. (2018). Molecular phylogenetics and historical biogeography of the tribe Lilieae (Liliaceae): bi-directional dispersal between biodiversity hotspots in Eurasia. Ann. Bot. 122, 1245–1262.
Ingram, T., Mahler, D. L., and Hansen, T. (2013). SURFACE: detecting convergent evolution from comparative data by fitting Ornstein-Uhlenbeck models with stepwise Akaike information criterion. Methods Ecol. Evol. 4, 416–425. doi: 10.1111/2041-210x.12034
Irwin, R. E., Strauss, S. Y., Storz, S., Emerson, A., and Guilbert, G. (2013). The Role of herbivores in the maintenance of a flower color polymorphism in wild radish. Ecology 84, 1733–1743. doi: 10.1890/0012-9658(2003)084[1733:trohit]2.0.co;2
Johnson, S. D., Linder, H. P., and Steiner, K. E. (1998). Phylogeny and radiation of pollination systems in Disa (Orchidaceae). Am. J. Bot. 85, 402–411. doi: 10.2307/2446333
Kalin Arroyo, M. T., and Penaloza, A. (1990). Genetic self-compatibility in a South American species of Ourisia (Scrophulariaceae). N. Z. J. Bot. 28, 467–470. doi: 10.1080/0028825x.1990.10412330
Katoh, K., Rozewicki, J., and Yamada, K. (2017). MAFFT online service: multiple sequence alignment, interactive sequence choice and visualization. Brief. Bioinform. 20, 1160–1166. doi: 10.1093/bib/bbx108
Kawano, S., Masuda, J., and Hayashi, K. (2008). Life-history monographs of Japanese plants. 10: Fritillaria koidzumiana Ohwi (Liliaceae). Plant Species Biol. 23, 51–57. doi: 10.1111/j.1442-1984.2008.00208.x
Kay, K. M., and Schemske, D. W. (2003). Pollinator assemblages and visitation rates for 11 species of Neotropical Costus. Biotropica 35, 198–207. doi: 10.1646/02159
Khabbazian, M., Kriebel, R., Rohe, K., Ané, C., and Hansen, T. (2016). Fast and accurate detection of evolutionary shifts in Ornstein-Uhlenbeck models. Methods Ecol. Evol. 7, 811–824. doi: 10.1111/2041-210x.12534
Khourang, M., Babaei, A., Sefidkon, F., Naghavi, M. R., Asgari, D., and Potter, D. (2014). Phylogenetic relationship in Fritillaria spp. of Iran inferred from ribosomal ITS and chloroplast trnL-trnF sequence data. Biochem. Syst. Ecol. 57, 451–457. doi: 10.1016/j.bse.2014.10.001
Kiani, M., Mohammadi, S., Babaei, A., Sefidkon, F., Naghavi, M. R., Ranjbar, M., et al. (2017). Iran supports a great share of biodiversity and floristic endemism for Fritillaria spp. (Liliaceae): a review. Plant Div. 39, 245–262. doi: 10.1016/j.pld.2017.09.002
Kiepiel, I., and Johnson, S. D. (2014). Shift from bird to butterfly pollination in Clivia (Amaryllidaceae). Am. J. Bot. 101, 190–200. doi: 10.3732/ajb.1300363
Kim, J. S., and Kim, J. H. (2018). Updated molecular phylogenetic analysis, dating and biogeographical history of the lily family (Liliaceae: Liliales). Bot. J. Linn. Soc. 187, 579–593. doi: 10.1093/botlinnean/boy031
Landis, J. B., Bell, C. D., Hernandez, M., Zenil-Ferguson, R., McCarthy, E. W., Soltis, D. E., et al. (2018). Evolution of floral traits and impact of reproductive mode on diversification in the phlox family (Polemoniaceae). Mol. Phylogenet. Evol. 127, 878–890. doi: 10.1016/j.ympev.2018.06.035
Lavi, R., and Sapir, Y. (2015). Are pollinators the agents of selection for the extreme large size and dark color in Oncocyclus irises? New Phytol. 205, 369–377. doi: 10.1111/nph.12982
Li, Y., Zhang, Z., Yang, J., and Lv, G. (2018). Complete chloroplast genome of seven Fritillaria species, variable DNA markers identification and phylogenetic relationships within the genus. PLoS One 13:e0194613. doi: 10.1371/journal.pone.0194613
Lunau, K., and Maier, E. J. (1995). Innate colour preferences of flower visitors. J. Comp. Physiol. A 177, 1–19. doi: 10.1007/BF00243394
Maddison, W. P., and Maddison, D. R. (2019). Mesquite: A Modular System for Evolutionary Analysis. Version 3.61.
Martén-Rodríguez, S., Almarales-Castro, A., and Fenster, C. B. (2009). Evaluation of pollination syndromes in Antillean Gesneriaceae: evidence for bat, hummingbird and generalized flowers. J. Ecol. 97, 348–359. doi: 10.1111/j.1365-2745.2008.01465.x
Martén-Rodríguez, S., Fenster, C. B., Agnarsson, I., Skog, L. E., and Zimmer, E. A. (2010). Evolutionary breakdown of pollination specialization in a Caribbean plant radiation. New Phytol. 188, 403–417. doi: 10.1111/j.1469-8137.2010.03330.x
Mast, A. R., Milton, E. F., Jones, E. H., Barker, R. M., Barker, W. R., and Weston, P. H. (2012). Time-calibrated phylogeny of the woody Australian genus Hakea (Proteaceae) supports multiple origins of insect-pollination among bird-pollinated ancestors. Am. J. Bot. 99, 472–487. doi: 10.3732/ajb.1100420
Naruhashi, N., Takata, Y., and Negoro, H. (2006). Pollinators and dispersing insects of seeds in Fritillaria koidzumiana (Liliaceae). J. Phytogeogr. And Taxon. 54, 57–63.
Ollerton, J. (1996). Reconciling ecological processes with phylogenetic patterns: the apparent paradox of plant-pollinator systems. J. Ecol. 84, 767–769. doi: 10.2307/2261338
Opedal, Ø. H. (2019). The evolvability of animal-pollinated flowers: towards predicting adaptation to novel pollinator communities. New Phytol. 221, 1128–1135. doi: 10.1111/nph.15403
Parachnowitsch, A. L., Manson, J. S., and Sletvold, N. (2018). Evolutionary ecology of nectar. Ann. Bot. 123, 247–261. doi: 10.1093/aob/mcy132
Paradis, E., Claude, J., and Strimmer, K. (2004). APE: analyses of phylogenetics and evolution in R language. Bioinformatics 20, 289–290. doi: 10.1093/bioinformatics/btg412
Pendergrass, K., and Robinson, A. (2005). Recovery Plan for Fritillaria Gentneri (Gentner’s fritillary). Washington, D.C: U.S. Fish and Wildlife Service.
Perret, M., Chautems, A., Spichiger, R., Kite, G., and Savolainen, V. (2003). Systematics and evolution of tribe Sinningieae (Gesneriaceae): evidence from phylogenetic analyses of six plastid DNA regions and nuclear ncpGS. Am. J. Bot. 90, 445–460. doi: 10.3732/ajb.90.3.445
Peters, W. S., Pirl, M., Gottsberger, G., and Peters, D. S. (1995). Pollination of the Crown Imperial Fritillaria imperialis by Great Tits Parus major. J. Ornithol. 136, 207–212.
Ramírez-Aguirre, E., Martén-Rodríguez, S., Quesada-Avila, G., Quesada, M., Martínez-Díaz, Y., Oyama, K., et al. (2019). Reproductive isolation among three sympatric Achimenes species: pre- and post-pollination components. Am. J. Bot. 106, 1–11. doi: 10.1002/ajb2.1324
Rausher, M. D. (2008). Evolutionary transitions in floral color. Int. J. Plant Sci. 169, 7–21. doi: 10.1086/523358
Revell, L. J. (2012). phytools: an R package for phylogenetic comparative biology (and other things). Methods Ecol. Evol. 3, 217–223. doi: 10.1111/j.2041-210x.2011.00169.x
Reverté, S., Retana, J., Gómez, J. M., and Bosch, J. (2016). Pollinators show flower colour preferences but flowers with similar colours do not attract similar pollinators. Ann. Bot. 118, 249–257. doi: 10.1093/aob/mcw103
Rix, E. (1984). “Fritillaria L,” in Flora of Turkey and the East Aegean Islands, Vol. 8, ed. P. H. Davis (Edinburgh: Edinburgh University Press), 284–302.
Rix, M., and Strange, K. (2014). 791. Fritillaria sororum. Curtis’s Bot. Mag. 31, 214–222. doi: 10.1111/curt.12075
Roguz, K., Bajguz, A., Chmur, M., Gołȩbiewska, A., Roguz, A., and Zych, M. (2019). Diversity of nectar amino acids in the Fritillaria (Liliaceae) genus: ecological and evolutionary implications. Sci. Rep. 23:15209.
Roguz, K., Bajguz, A., Gołȩbiewska, A., Chmur, M., Hill, L., Kalinowski, P., et al. (2018). Functional diversity of nectary structure and nectar composition in the genus Fritillaria (Liliaceae). Front. Plant Sci. 9:1246. doi: 10.3389/fpls.2018.01246
Roguz, K., Gallagher, M., Senden, E., Bar-Lev, Y., Lebel-Vine, M., Heliczer, R., et al. (2020a). All the colours of the rainbow: diversification of flower colour and intraspecific colour variation in the genus Iris. Front. Plant Sci. 11:569811. doi: 10.3389/fpls.2020.569811
Roguz, K., Koethe, S., Hill, L., Lunau, K., Roguz, A., and Zych, M. (2020b). Evaluating Honest Signalling in Spring Flowering Fritillaria (Liliaceae): Flower Colour, Size and Reward. (in press).
Rønsted, N., Law, S., Thornton, H., Fay, M. F., and Chase, M. W. (2005). Molecular phylogenetic evidence for the monophyly of Fritillaria and Lilium (Liliaceae; Liliales) and the infrageneric classification of Fritillaria. Mol. Phylogenet. Evol. 35, 509–527. doi: 10.1016/j.ympev.2004.12.023
Rosas-Guerrero, V., Aguilar, R., Martén-Rodríguez, S., Ashworth, L., Lopezaraiza-Mikel, M., and Bastida, J. M., et al. (2014). A quantitative review of pollination syndromes: do floral traits predict effective pollinators? Ecol. Lett. 17, 388–400. doi: 10.1111/ele.12224
Royal Botanic Gardens, Kew (2020). World Checklist of Selected Plant Families. Kew: Royal Botanic Gardens.
Salas-Arcos, L., Lara, C., and Ornelas, J. F. (2017). Reproductive biology and nectar secretion dynamics of Penstemon gentianoides (Plantaginaceae): a perennial herb with a mixed pollination system? PeerJ 5:e3636. doi: 10.7717/peerj.3636
Smith, S. D., Ané, C., and Baum, D. (2007). The role of pollinator shifts in the floral diversification of Iochroma (Solanaceae). Evolution 62, 793–806. doi: 10.1111/j.1558-5646.2008.00327.x
Smith, S. D., and Goldberg, E. E. (2015). Tempo and mode of flower color evolution. Am. J. Bot. 102, 1014–1025. doi: 10.3732/ajb.1500163
Souto-Vilarós, D., Vuleta, A., Jovanović, S. M., Budečević, S., Wang, H., Sapir, Y., et al. (2018). Are pollinators the agents of selection on flower colour and size in irises? Oikos 127, 834–846. doi: 10.1111/oik.04501
Spaethe, J., Tautz, J., and Chittka, L. (2001). Visual constraints in foraging bumblebees: flower size and color affect search time and flight behavior. Proc. Natl. Acad. Sci. U.S.A. 98, 3898–3903. doi: 10.1073/pnas.071053098
Stamatakis, A. (2014). RAxML version 8: a tool for phylogenetic analysis and post-analysis of large phylogenies. Bioinformatics 30, 1312–1313. doi: 10.1093/bioinformatics/btu033
Talavera, G., and Castresana, J. (2007). Improvement of phylogenies after removing divergent and ambiguously aligned blocks from protein sequence alignments. Syst. Biol. 56, 564–577. doi: 10.1080/10635150701472164
Tamura, M. (1998). “Liliaceae,” in The Families and Genera of Vascular Plants, ed. K. Kubitzki (Berlin: Springer).
Tekñen, M., and Aytaç, Z. (2008). Fritillaria mughlae (Liliaceae), a new species from Turkey. Ann. Bot. Fennici 45, 141–147. doi: 10.5735/085.045.0209
Tekñen, M., and Aytaç, Z. (2011). The revision of the genus Fritillaria L. (Liliaceae) in the Mediterranean region (Turkey). Turk. J. Bot. 35, 447–478.
Temeles, E. J., Linhart, Y. B., Masonjones, M., and Masonjones, H. D. (2006). The role of flower width in hummingbird bill length-flower length relationships1. Biotropica 34, 68–80. doi: 10.1646/0006-3606(2002)034[0068:trofwi]2.0.co;2
Thomson, J. D., and Wilson, P. (2008). Explaining evolutionary shifts between bee and hummingbird pollination: convergence, divergence, and directionality. Int. J. Plant Sci. 169, 23–38. doi: 10.1086/523361
Tomović, G., Vukojičić, S., Niketić, M., Zlatković, B., and Stevanović, V. (2007). Fritillaria (Liliaceae) in Serbia: distribution, habitats and some taxonomic notes. Phytol. Balc. 13, 359–370.
Tripp, E. A., and Manos, P. S. (2008). Is floral specialization an evolutionary dead-end? Pollination system transitions in Ruellia (Acanthaceae). Evolution 62, 1712–1737. doi: 10.1111/j.1558-5646.2008.00398.x
Tucić, B., Tomić, V., Avramov, S., and Pemac, D. (1998). Testing the adaptive plasticity of Iris pumila leaf traits to natural light conditions using phenotypic selection analysis. Acta Oecol. 19, 473–481. doi: 10.1016/s1146-609x(99)80001-0
Van der Niet, T., and Johnson, S. D. (2012). Phylogenetic evidence for pollinator-driven diversification of angiosperms. Trends Ecol. Evol. 27, 353–361. doi: 10.1016/j.tree.2012.02.002
Waser, N. M., Chittka, L., Price, M. V., Williams, N. M., and Ollerton, J. (1996). Generalization in pollination systems, and why it matters. Ecology 77, 1043–1060. doi: 10.2307/2265575
White, G. (1789). The Natural History & Antiquities of Selborne. New York, NY: J. M. Dent & Sons Ltd.
Whittall, J. B., and Hodges, S. A. (2007). Pollinator shifts drive increasingly long nectar spurs in columbine flowers. Nature 447, 706–709. doi: 10.1038/nature05857
Wietsma, W. A., Deinum, D., Teunissen, H. A. S., and van der Berg, R. (2015). Phylogenetic relationships within Fritillaria section Petilium based on AFLP fingerprints. Plant Syst. Evol. 301, 1043–1054. doi: 10.1007/s00606-014-1135-4
Wilson, P., Castellanos, M., Wolfe, A. D., and Thomson, J. D. (2006). “Shifts between bee and bird pollination in penstemons,” in Plant-Pollinator Interactions: From Specialization to Generalization, eds J. Ollerton and N. M. Waser (Cambridge: Cambridge University Press), 318–335.
Wilson, P., Castellanos, M. C., Hogue, J. N., Thomson, J. D., and Armbruster, W. S. (2004). A multivariate search for pollination syndromes among penstemons. Oikos 104, 345–361. doi: 10.1111/j.0030-1299.2004.12819.x
Wilson, P., Wolfe, A. D., Armbruster, W. S., and Thomson, J. D. (2007). Constrained lability in floral evolution: Counting convergent origins of hummingbird pollination in Penstemon and Keckiella. New Phytol. 176, 883–890. doi: 10.1111/j.1469-8137.2007.02219.x
Winkel-Shirley, B. (2002). Biosynthesis of flavonoids and effects of stress. Curr. Opin. Plant Biol. 5, 218–223. doi: 10.1016/s1369-5266(02)00256-x
Zaharof, E. (1986). Variation and taxonomy of Fritillaria graeca (Liliaceae) in Greece. Plant Syst. Evol. 154, 41–61. doi: 10.1007/bf00984867
Zhang, X. J., Cui, D. L., Zong, X. C., Ren, R. Y., Wei, J. C., Situ, L. L., et al. (2010). Pollination biology and breeding system of Fritillaria ussuriensis Maxim. Acta Bot. Boreali Occidentalia Sin. 30, 1404–1408.
Zhao, Z. G., and Wang, Y. K. (2015). Selection by pollinators on floral traits in generalized Trollius ranunculoides (Ranunculaceae) along altitudinal gradients. PLoS One 10:e0118299. doi: 10.1371/journal.pone.0118299
Zox, H., and Gold, W. (2008). Ecology of black liliy (Fritillaria camschatcensis) a Washington State sensitive species. Douglasia Fall 32, 17–22.
Keywords: pollinator shift, nectar, ancestral state, flower colour, floral syndrome, diversification, ornithophily
Citation: Roguz K, Hill L, Roguz A and Zych M (2021) Evolution of Bird and Insect Flower Traits in Fritillaria L. (Liliaceae). Front. Plant Sci. 12:656783. doi: 10.3389/fpls.2021.656783
Received: 21 January 2021; Accepted: 03 March 2021;
Published: 31 March 2021.
Edited by:
Marcial Escudero, Seville University, SpainReviewed by:
Belinda Kahnt, Martin Luther University of Halle-Wittenberg, GermanyKlaus Lunau, Heinrich Heine University of Düsseldorf, Germany
Antonio Jesús Muñoz Pajares, Universidad de Granada, Spain
Copyright © 2021 Roguz, Hill, Roguz and Zych. This is an open-access article distributed under the terms of the Creative Commons Attribution License (CC BY). The use, distribution or reproduction in other forums is permitted, provided the original author(s) and the copyright owner(s) are credited and that the original publication in this journal is cited, in accordance with accepted academic practice. No use, distribution or reproduction is permitted which does not comply with these terms.
*Correspondence: Katarzyna Roguz, ay5yb2d1ekBiaW9sLnV3LmVkdS5wbA==